- 1Institute of Chronic Disease Risks Assessment, Henan University, Kaifeng, China
- 2School of Basic Medical Sciences, Henan University, Kaifeng, Henan, China
- 3School of Clinical Medicine, Henan University, Kaifeng, Henan, China
- 4School of Nursing and Health, Institute of Nursing and Health, Henan University, Kaifeng, Henan, China
- 5Henan International Joint Laboratory for Nuclear Protein Regulation, School of Basic Medical Sciences, Henan University, Kaifeng, Henan, China
- 6School of Life Sciences, Henan University, Kaifeng, China
With an increasing worldwide prevalence, hepatocellular carcinoma (HCC) is the most common primary malignant tumor of the liver in the world. It is also the primary reason for cancer-related death in the world. The pathogenesis of HCC is complex, such as DNA methylation changes, immune regulatory disorders, cell cycle disorders, chromosomal instability, and so on. Although many studies have been conducted on HCC, the molecular mechanisms of HCC are not completely understood. At present, there is no effective treatment for HCC. Hydrogen sulfide (H2S) has long been regarded as a toxic gas with the smell of rotten eggs, but recent studies have shown that it is an important gasotransmitter along with carbon monoxide (CO) and nitric oxide (NO). Increasing evidence indicates that H2S has multiple biological functions, such as anti-inflammation, anti-apoptosis, anti-oxidative stress, and so on. Recently, a lot of evidence has shown that H2S has a “double-edged sword” effect in HCC, but the mechanism is not fully understood. Here, we reviewed the progress on the role and mechanism of H2S in HCC in recent years, hoping to provide a theoretical reference for future related research.
1 Introduction
Hepatocellular carcinoma (HCC) is an important primary liver cancer and a serious medical problem in the world. At present, HCC has been regarded as the leading cause of death of patients with liver cirrhosis, and its incidence rate is expected to increase in the future (Forner et al., 2018; Sim and Knox, 2018; Llovet et al., 2021). The evidence indicates that by 2025, about 1 million people will be affected by HCC every year. More than 90% of HCC cases occur in the environment of chronic liver diseases (Renne et al., 2021). The main risk factors of HCC include diabetes, alcoholism, chronic hepatitis, nonalcoholic fatty liver disease (NAFLD) and exposure to dietary toxins, such as aflatoxin and aristolochic acid (Kulik and El-Serag, 2019; Yang et al., 2019; Gilles et al., 2022). The pathogenesis of HCC is complex, and involves a variety of molecular faults, including DNA methylation change, immune regulation disorder, cell cycle disorder, chromosome instability, epithelial cell to mesenchymal cell transition (EMT), microRNA (miRNA) disorder, and the increased HCC stem cells (Chidambaranathan-Reghupaty et al., 2021). If diagnosed early, HCC may be cured and have a good long-term prognosis. However, the vast majority of HCC patients are found in the late stage. At this time, the surgical treatment is no longer a choice (Dimitrou et al., 2017). Instead, it requires chemotherapy, using chemicals to destroy cancer cells and inhibit the proliferation of new cancer cells (Chang et al., 2020). Therefore, it is particularly important to find suitable chemotherapy drugs for HCC.
Hydrogen sulfide (H2S) has long been considered as a toxic gas with the rotten egg odor. However, it was regarded as the third gaseous signal molecule after carbon oxide (CO) and nitric oxide (NO) recently (Powell et al., 2018; Zaorska et al., 2020). Currently, there are mainly three enzymes that catalyze endogenous H2S production, namely, cystathionine gamma-lyase (CSE), 3-mercaptopyruvate sulfurtransferase (3-MST), and cystathionine-beta-synthase (CBS) (Coavoy-Sánc et al., 2020; Dilek et al., 2020; Shackelford et al., 2021a). During endogenous H2S production, CBS catalyzes the β-substitution reaction of homocysteine with serine to generate cystathionine. Cysteine is produced through α, γ-cysteine elimination of cystathionine catalyzed by CSE. Cysteine can be transformed into H2S via the β-elimination reaction under the catalysis of CBS and CSE. 3-mercaptopyruvate (3-MP) is formed via transferring amines from cystine into α-ketoglutarate catalyzed by cysteine aminotransferase (CAT). 3-MST catalyzes 3-MP sulfur to produce H2S (Figure 1) (Wang et al., 2020a; Casin and Calvert, 2021; Zhao et al., 2021). The mechanisms of the effects of H2S on cell functions mainly include regulation of the activity of transcription factors, histone modification, DNA damage repair, DNA methylation, and post-translational modification of proteins through the sulfur hydration of H2S (Dongó et al., 2018). The evidence indicates that H2S plays a vital role in multiple pathological and physiological processes, such as anti-inflammation (Mohammed et al., 2021), anti-apoptosis (Fouad et al., 2020), anti-oxidative stress (Tocmo and Parkin, 2019), blood pressure reduction (Greaney et al., 2017; Zaorska et al., 2019), and the regulation of cell survival/death, cell proliferation/hypertrophy and cell differentiation (Zhang et al., 2017). Hence, H2S participates in many diseases, such as lung diseases (Pacitti et al., 2021), ischemia/reperfusion injury (Krylatov et al., 2021), and cancers (Shackelford et al., 2021b; Khattak et al., 2022; Faris et al., 2023). In recent years, many studies have revealed that H2S has the dual effects of anticancer and cancer promotion in HCC, but the relevant mechanisms are not completely understood. Hence, we summarized the recent studies on the role and mechanisms of H2S in HCC through PubMed, hoping to provide a theoretical reference for future related research.
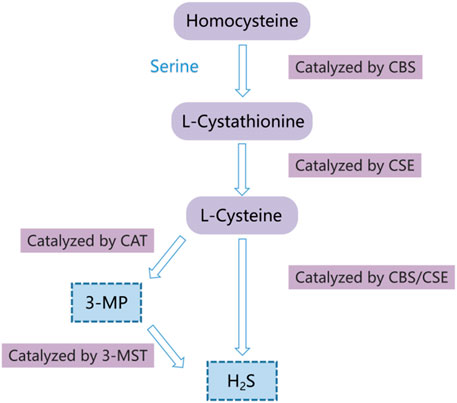
FIGURE 1. Diagram of the endogenous H2S generation process. CSE, cystathionine-gamma-lyase; 3-MST, 3-mercaptopyruvate thiotransferase; CAT, cysteine aminotransferase; CBS, cystathionine-beta-synthase; 3-MP, 3-mercaptopyruvate.
2 H2S inhibits hepatocellular carcinoma
2.1 Exogenous H2S inhibits hepatocellular carcinoma
2.1.1 Exogenous H2S inhibits hepatocellular carcinoma by blocking the STAT3 pathway
The signal transducer and activator of transcription (STAT) protein is a potential cytoplasmic transcription factor, which includes seven members: STAT1, STAT2, STAT3, STAT4, STAT5a, STAT5b, and STAT6 (Loh et al., 2019). STAT3, a main sensor that mediates the signal transmission of interleukin-6 (IL-6) to the nucleus, participates in cell growth, regeneration, survival, differentiation, immune responses, and cell respiration. The STAT3 activation is strictly regulated in normal tissues. However, the abnormal activation of STAT3 is related to the formation, progression, and metastasis of cancers (El-Tanani et al., 2022; Sadrkhanloo et al., 2022). The relevant mechanism remains to be clarified. The results of SEN LU et al. showed that GYY4137(a donor of H2S) suppressed IL-6-induced STAT3 activation through effectively decreasing p-STAT3 levels by reducing JAK2 phosphorylation (an activator of STAT3) in HCC cells. GYY4137 also reduced the expression levels of STAT3 downstream proteins, including cyclin D1, Bcl-2, myeloid cell leukemia sequence 1 (Mcl-1) and survivin. The number of HCC cells in the G0/G1 phase of the cell cycle was increased by GYY4137, which was consistent with GYY4137 inhibition of cyclin D1, suggesting that cell cycle arrest of HCC cells was induced by exogenous H2S. GYY4137 promoted the cleavage of poly (ADP-ribose) polymerase (PARP) and upregulated the levels of cleaved caspase-9 and caspase-3 in HCC cells, suggesting that exogenous H2S induced apoptosis of HCC cells, which was consistent with the inhibition of GYY4137 on Bcl-2, survivin, and Mcl-1. In addition, GYY4137 suppressed HCC cell’s viability time- and dose-dependently and suppressed the angiogenesis by downregulating the levels of vascular endothelial growth factor (VEGF) and hypoxia-inducible factor-1α (HIF-1α) that were STAT3 downstream proteins. Similar to the results in vitro, GYY4137 notably suppressed tumor growth in the model of subcutaneous HCC cells xenotransplantation through suppressing the activation of STAT3 and its downstream target gene expression in vivo. Hence, it could be inferred that exogenous H2S inhibited the proliferation, metastasis and invasion of HCC through inducing cell cycle arrest and apoptosis of HCC cells, and the suppression of the angiogenesis by suppressing the STAT3 pathway (Lu et al., 2014), which is consistent with the fact that the abnormal activation of STAT3 promotes tumor cell proliferation via increasing cyclin D1 level, and inhibits apoptosis via increasing the levels of Bcl-2, survivin, and Mcl-1 (Garcia et al., 2001). In the above study, how H2S inhibits JAK phosphorylation through its sulfur hydration to reduce STAT3 phosphorylation level needs to be further clarified. STAT3 pathway will become a vital target for H2S-related drugs to treat HCC.
2.1.2 Exogenous H2S suppresses hepatocellular carcinoma by promoting autophagy
Besides apoptosis, cell cycle arrest and angiogenesis, autophagy is also involved in anti-tumor effects (Ahmed et al., 2022). Moreover, H2S plays an important role in cancers by regulating autophagy (Iqbal et al., 2021). Therefore, it is natural to speculate that H2S may inhibit HCC by regulating autophagy. Autophagy is a homeostatic process, in which cell components and structures are transferred to lysosomes for degradation and recycling. It can also remove the waste materials from cells, including the damaged organelles and protein aggregation, and help to clear the invading pathogens. From yeast to mammals, the mechanism of autophagy is conservative (Ganzleben et al., 2021; Kumar et al., 2021; Zahedi-Amiri et al., 2021). The disorder of autophagy is often related to the pathogenesis of various cancers, which not only inhibits the cancer but also promotes the cancer (Devis-Jauregui et al., 2021; Rakesh et al., 2022). To study the role and mechanism of exogenous H2S in HCC by regulating autophagy, Shanshan S Wang and colleagues committed a lot of experiments, and the results revealed that NaHS treatment increased the expressions of Atg5 and LC3-II, and decreased p62 expression in HCC cells. The transmission electron microscopy showed that the number of intracellular double-membrane vesicles increased in NaHS-treated HCC cells. This indicated that exogenous H2S upregulated autophagy in HCC cells (Wang et al., 2017). It has been reported that rapamycin, an inhibitor of the mechanistic target of rapamycin (mTOR) and an activator of autophagy, induces autophagy via suppressing protein kinase B (AKT)/phosphatidylinositol-3-kinase (PI3K)/mTOR pathway (Sundarraj et al., 2021; Kamel et al., 2022). Like rapamycin, NaHS also notably downregulated the levels of p-PI3K, p-Akt and mTOR proteins in HCC cells. Moreover, the treatment of NaHS combined with rapamycin further upregulated autophagy, indicating that exogenous H2S promoted autophagy by suppressing the PI3K/AKT/mTOR pathway. In addition, exogenous H2S suppressed the proliferation, cell cycle progression and migration of HCC cells, but induced apoptosis of HCC cells, which was enhanced by rapamycin. Summarily, exogenous H2S suppressed the migration and proliferation of HCC cells via inducing apoptosis and cell cycle arrest through inducing autophagy by suppressing the PI3K/AKT/mTOR pathway (Wang et al., 2017). In addition to the PI3K/AKT/mTOR pathway, our previous study demonstrated that exogenous H2S upregulated autophagy via the AMPK/mTOR pathway. In HCC (Wang et al., 2019), whether exogenous H2S regulates autophagy through other signal pathways, such as the AMPK/mTOR pathway, needs further study. Contrary to some of the above conclusions that exogenous H2S ameliorates HCC by activating autophagy, exogenous H2S improves liver diseases and nervous system diseases by inhibiting autophagy-mediated cell death (Nguyen et al., 2021a; Nguyen et al., 2021b). The reason may be related to the differences in the type and course of diseases, the type of tissue cells and the base level of autophagy of cells, which needs to be further studied.
2.1.3 Exogenous H2S enhances doxorubicin sensitivity to hepatocellular carcinoma cells by inhibiting the outflow of doxorubicin
One of the most vital limitations of cancer chemotherapy is that the anti-cancer response of cancer patients decreases over the extended treatment period. This phenomenon is called multidrug resistance (MDR), which is the primary reason for cancer treatment failure (Nikolaou et al., 2018; Wang et al., 2021a). MDR is associated with drug efflux, particularly through many membrane-binding proteins named ATP binding cassette (ABC) transporters. The overexpression of these proteins decreases the accumulation of chemotherapy drugs in cells, which may contribute to the MDR of some cancers (Gupta et al., 2018; Kopecka et al., 2020). Therefore, it is very important to reduce the drug resistance of cancer cells to improve the anti-cancer efficacy. Eric Stokes and colleagues found that doxorubicin reduced the expression of endogenous H2S-producing enzyme (CSE) in HCC cells. Exogenous H2S promoted doxorubicin inhibition of colony formation and cell survival, while exogenous H2S alone didn’t have this effect. Additionally, exogenous H2S promoted the cellular accumulation of doxorubicin through inhibiting the levels of ABCA1 and ABCG8, which is the underlying mechanism of the synergistic effect of doxorubicin and H2S. Moreover, exogenous H2S notably inhibited the heterodimer formation between retinoid X receptor beta (RXRβ) and liver X receptor alpha (LXRα) induced by doxorubicin and weakened the binding of LXRα/RXRβ with the promoter of ABCG8 and ABCA1 genes. Exogenous H2S S-sulfhydrated RXRβ but not LXRα, and the inhibition of RXRβ S-sulfhydration alleviated H2S inhibition of LXRα/RXRβ heterodimer formation. Collectively, it could be deduced that exogenous H2S reversed doxorubicin resistance of HCC through inhibiting the levels of ABCA1 and ABCG8 by inhibiting the binding of LXRα/RXRβ with ABCG8 and ABCA1 genes promoter via suppression of the heterodimer formation between RXRβ and LXRα, which needed to be further confirmed (Stokes et al., 2018). The above study indicates that doxorubicin downregulates CSE expression in HCC cells, which may be due to its characteristics of DNA embedding (AbuHammad and Zihlif, 2013). The exact mechanism of doxorubicin regulating CSE needs to be further clarified. In addition, the above study shows that H2S S-sulfhydration of RXRβ is the mechanism of reversing MDR of doxorubicin in HCC. It has been reported that the EMT pathway is related to the occurrence of MDR (Erin et al., 2020; Shome and Ghosh, 2021). Therefore, whether H2S can regulate tumor MDR through the EMT pathway is required to be further studied in the future. It has been reported that H2S inhibits cisplatin resistance of cancer through inducing apoptosis, blocking cell cycle, and suppressing cell migration and invasion (Ma et al., 2018). Hence, whether exogenous H2S reversed doxorubicin resistance of HCC through inducing apoptosis, blocking cell cycle, or suppressing cell migration and invasion needs to be studied.
2.1.4 HA-ADT, a novel donor of hydrogen sulfide, suppresses hepatocellular carcinoma
Given the effective inhibitory properties of exogenous H2S on HCC mentioned above, H2S-related drugs are expected to become new drugs for HCC treatment. At present, the existing H2S release agents cannot completely satisfy the requirements of scientific research and clinical trials. Therefore, developing efficient and safe H2S release agents is crucial for the clinical application of H2S-related drugs. Hyaluronic acid (HA) is a biopolymer that is widely used in many biomedical applications because of its good safety profile, such as drug delivery and tissue engineering (Jung et al., 2014). Methyl derivatives of 5 - (4-hydroxyphenyl) - 3H-1,2-dithio-3-thione (ADT-OH) can be used as H2S-releasing agents to produce H2S through mitochondrial enzyme metabolism (da Costa et al., 2022; Montoya and Pluth, 2016). HA-ADT, a new type of H2S slow-release agent, is a new conjugate formed by connecting HA and ADT-OH through a chemical reaction (Dong et al., 2019). Shao Feng Duan and colleagues studied the effect of HA-ADT on HCC and found that compared to NaHS (a rapidly releasing H2S donor) and GYY4137 (a slowly releasing H2S donor), HA-ADT exhibited stronger suppression of the proliferation, invasion, and cell cycle progress and migration of human HCC cells. In addition, HA-ADT-induced apoptosis was evidenced by the downregulation of the expressions of p-glycogen synthase kinase-3β (GSK-3β), phospho (p)-protein kinase B (PKB/AKT) and p-β-catenin, and suppressed autophagy through decreasing the expressions of transforming growth factor-β (TGF-β) and p-Smad2/p-Smad3 in human HCC cells. In addition, HA-ADT was more effective in inhibiting the proliferation of liver cancer xenograft tumors than GYY4137 and NaHS. Collectively, HA-ADT inhibited HCC via promoting apoptosis through suppressing GSK-3β/AKT/β-catenin and inhibiting autophagy through suppressing TGF-β/Smad2/3 pathways (Duan et al., 2023). The evidence indicates that exogenous H2S suppresses urothelial carcinoma cell proliferation through inducing cell autophagy and apoptosis (Panza et al., 2022), which is inconsistent with the above study that exogenous H2S downregulates autophagy to inhibit HCC. This indicates that autophagy may have different activities in different tumors, and plays a dual role in promoting and inhibiting tumor development, according to the cellular environment.
2.2 Endogenous H2S inhibits hepatocellular carcinoma
Besides exogenous H2S, endogenous H2S also participates in inhibiting HCC. 3-MST is an important enzyme that catalyzes the production of endogenous H2S (Rao et al., 2022). It is located in the vascular endothelium and releases H2S rapidly under different stimuli (Zhang et al., 2020). The evidence indicates that 3-MST may be a tumor suppressor and participates in HCC (Li et al., 2022a). However, the mechanism is not completely understood. Meng Li and colleagues found that compared with matched non-tumor tissues, 3-MST expression was notably downregulated in human HCC tissues. The low 3-MST expression was closely associated with the larger tumor size and the lower survival rate. In HCC patients, the higher expression of 3-MST is associated with better clinical outcomes. 3-MST overexpression in HCC cells suppressed cell proliferation and promoted apoptosis, and also notably restrained the proliferation of tumor xenografts in nude mice. Conversely, the silencing of 3-MST by intratumoral siRNA significantly promoted the growth of HCC. Furthermore, 3-MST gene knockout aggravated HCC in mice. These outcomes indicated that 3-MST inhibited HCC. 3-MST overexpression significantly decreased H2S level, while siRNA-mediated 3-MST downregulation increased H2S level in HCC cells, indicating that endogenous H2S production was involved in 3-MST inhibition of HCC. The in-depth research revealed that 3-MST inhibited the HCC cell cycle through suppressing AKT/forkhead box transcription factor 3a (FOXO3a)/retinoblastoma (Rb, an important transcription inhibitor for G1-S progress) signaling pathway (Li et al., 2022b). As we all know, Rb affects tumor progression by regulating apoptosis (Li et al., 2019; Wang et al., 2020b). In the above study, 3-MST negatively regulates Rb, which may result in the induction of apoptosis of HCC cells. The specific mechanism of 3-MST regulating Rb needs further study. Summarily, 3-MST/H2S inhibits HCC through promoting the apoptosis and cell cycle arrest of HCC cells by inhibiting the AKT/FOXO3a/Rb pathway (Li et al., 2022b). In addition, in the above study, 3-MST negatively regulates the production of H2S in HCC cells. The reason may be the negative feedback between the 3-MST and the CBS/CSE system of H2S, which may also be why CSE/H2S promotes HCC, while 3-MST/H2S has the opposite effect.
3 H2S promotes hepatocellular carcinoma
3.1 Exogenous H2S promotes hepatocellular carcinoma
Contrary to the above study, exogenous H2S can also promote HCC. STAT3 has been found to be activated to promote the occurrence of HCC (Lee and Cheung, 2019). The results of Yulan Zhen et al. showed that the treatment of HCC cells with NaHS significantly increased the expression levels of p-STAT3 and STAT3 mRNA, which led to the increased expression levels of COX-2 and COX-2 mRNA, the increased VEGF level, the reduced cleaved caspase-3 level, the increased viability and migration of HCC cells and the reduction of HCC cells apoptosis. This indicated that exogenous H2S promoted HCC by increasing the migration and proliferation and lessening HCC cell apoptosis. While the treatment of HCC cells with AG490 (a STAT3 inhibitor) or NS-398 (a COX-2 inhibitor) notably abolished the above effects of NaHS. Moreover, the treatment of HCC cells with AG490 significantly weakened the increased COX-2 expression induced by NaHS. Meanwhile, the treatment of HCC cells with NS-398 suppressed the increased p-STAT3 expression induced by NaHS. Collectively, exogenous H2S aggravated HCC by promoting the proliferation and migration of HCC cells through inhibiting apoptosis and increasing angiogenesis via inducing the STAT3-COX-2 pathway (Zhen et al., 2018). These results provide a new insight into the molecular mechanisms underlying H2S promotion of the cell proliferation of HCC cells. Further, the conditions under which exogenous H2S inhibits HCC, including inhibiting the migration and proliferation of HCC cells and facilitating HCC cell apoptosis, and on the contrary, the conditions under which exogenous H2S promotes HCC, need to be clarified. It can be inferred from a previous study that the low concentration of exogenous H2S can promote HCC, while the high concentration of exogenous H2S inhibits HCC. The above speculation was confirmed by the experiments of Dongdong Wu and colleagues. Their results showed that H2S in human HCC cells was increased compared to that in L02 cells (a kind of human normal hepatocyte), indicating that H2S was related to the occurrence and development of HCC. 10–100 μM NaHS promoted the migration and growth of HCC cells, while 600–1,000 μM NaHS had the opposite effect. 25–100 μM NaHS inhibited HCC apoptosis, while 400–1,000 μM NaHS had the opposite effect. These results indicated that the low concentration of H2S promoted HCC, while the high concentration of H2S inhibited HCC. Further research showed that 25–50 μM NaHS upregulated the protein levels of phosphorylated extracellular signal-regulated kinase (p-ERK), phosphorylated epidermal growth factor receptor (p-EGFR), matrix metalloproteinase-2 (MMP-2) and phosphorylated protein kinase B (p-AKT), and downregulated the ratio of Bax/Bcl-2 and the levels of phosphatase and tensin homolog (PTEN). While 800–1,000 μM NaHS had the opposite effect in HCC cells. Similar to that in vitro, the low concentration of H2S promoted the growth and angiogenesis of HCC xenografts in nude mice, while the high concentration of H2S had the opposite effect. These results indicated that the low concentration of H2S activated PTEN/AKT and EGFR/ERK/MMP-2 pathways, while the high concentration of H2S had the opposite effects (Wu et al., 2017). It has been reported that EGFR/ERK/MMP-2 and PTEN/AKT pathways contribute to the development of HCC (Qian et al., 2015; Yang et al., 2020; Wang et al., 2021b). Therefore, it can be deduced that exogenous H2S plays a double-edged sword role in HCC cells through regulating angiogenesis and apoptosis via EGFR/ERK/MMP-2 and PTEN/AKT pathways (Wu et al., 2017).
3.2 Endogenous H2S promotes hepatocellular carcinoma
CSE is a vitamin B6-dependent enzyme that catalyzes the production of endogenous H2S (Chiku et al., 2009). It is generally expressed in the liver, heart, kidney, ileum, pancreatic islet, placenta and vascular system, but not in the central nervous system (Kimura, 2010). The change in CSE expression is related to the change in the level of endogenous H2S, thus participating in the progress of various diseases such as cancer and diabetes (Jia et al., 2022; Omorou et al., 2022). The PI3K/Akt signal pathway is an important signal pathway regulating cell growth, proliferation, metabolism, survival, and movement (Akbarzadeh et al., 2021; Korkmaz et al., 2022). Many studies have demonstrated that the PI3K/AKT pathway regulates HCC (Li et al., 2021; Sun et al., 2021; Zhou et al., 2021). However, the relevant mechanisms are not completely understood. In addition, CSE is upregulated by the PI3K/AKT pathway (Wang et al., 2022a). Therefore, it can be speculated that CSE and PI3K/AKT pathways play a vital role in HCC. Peng Yin and colleagues found that the PI3K/AKT pathway positively regulated the expression of CSE in HCC cells. Akt deletion or PI3K inhibitor could reduce the expression of CSE, while Akt activation could upregulate CSE expression. The PI3K/AKT pathway regulated the expression of CSE at the transcriptional level. The double luciferase transporter analysis showed that the −592/+139 gene fragment was the core promoter of CSE. The specificity protein 1 (SP1) was an important transcription factor and could directly bind to the core promoter of CSE to regulate CSE expression. The mutation of the Sp1 binding core promoter of CSE reversed the PI3K/AKT pathway-induced expression of CSE, indicating that the PI3K/Akt pathway upregulated the expression of CSE through Sp1 binding to the core promoter of CSE. Moreover, the production of endogenous H2S was positively related to the expression of CSE, and CSE/H2S promoted HCC cell proliferation by inducing cell cycle progression. Collectively, the PI3K/Akt pathway upregulated the expression of CSE through Sp1 binding to the core promoter of CSE, thus promoting HCC, indicating that endogenous H2S promoted HCC(86). The Sp1 promoted the transcription of genes encoding cyclinD, p21Cip/WAK-1, and cyclin E, which were involved in cell cycle progression (Sherr and Roberts, 2004; Santiago et al., 2007). This is consistent with the conclusion of the above study that CSE/H2S promotes the growth of HCC cells by promoting cell cycle progression. Another study by Yan Pan et al. further clarified the mechanism of endogenous H2S promotion of HCC. The results revealed that CSE was upregulated in HCC cells. The inhibition of endogenous H2S/CSE pathway by propargylglycine (PPG)/CSE siRNA significantly reduced the proliferation of HCC cells, indicating that the H2S/CSE pathway induced the proliferation of HCC cells. In addition, the inhibition of the H2S/CSE pathway promoted ROS production, DNA damage and mitochondrial disruption, and upregulated the apoptosis of HCC cells. The increased apoptosis was related to the activation of p53 and p21, the decrease of the Bcl-2/Bax ratio, and the increase of caspase-3 and phosphorylated c-Jun N-terminal kinase (p-JNK) activity. Moreover, the suppression of the proliferation of HCC cells by the suppression of the H2S/CSE pathway was related to the inhibition of the epidermal growth factor receptor (EGFR) through suppressing extracellular signal-regulated kinase 1/2 (ERK1/2). Summarily, it could be deduced from the above that CSE/H2S promotes the proliferation of HCC cells by inhibiting mitochondrial ROS-mediated apoptosis through activating the EGFR/ERK1/2 pathway (Pan et al., 2014). ROS has been reported to induce apoptosis (Cui et al., 2021; Fontana et al., 2021). In the above study, H2S/CSE promotes HCC cell proliferation by inhibiting HCC cell apoptosis through suppressing ROS production (Pan et al., 2014).
H2S/CSE can not only promote HCC growth but also promote the metastasis of HCC. With the progress of technology, the accuracy of extracorporeal radiotherapy is getting higher and higher, which allows radiotherapy to be applied to patients with HCC (Chen et al., 2021). Although radiotherapy can significantly improve the survival rate of HCC patients, the metastasis and recurrence of HCC after radiotherapy are more common in clinical practice (Wang et al., 2022b). Therefore, it is particularly important to determine the factors that promote the metastasis of HCC cells after radiotherapy to improve the therapeutic effect of HCC. The study of Hang Zhang et al. showed that in xenograft tumors in vivo, both single-dose and fractionated irradiation promoted the metastasis of HCC cells 20–60 days after irradiation. Radiation upregulated the expressions of epithelial-mesenchymal transition (EMT) marker proteins including N-cadherin and Snail, and downregulated the E-cadherin expression in vivo and in vitro, suggesting that radiation-induced long-term EMT in HCC. The in-depth research revealed that in HCC cells treated with the single-dose irradiation, the expression levels of CSE and CBS, and the phosphorylation of p38 mitogen-activated protein kinases (MAPK) increased significantly, indicating that radiation upregulated endogenous H2S and p38 MAPK pathways. The inhibition of CSE or CBS, two endogenous H2S-producing enzymes, notably abolished the upregulated expressions of EMT marker proteins and p38 MAPK induced by radiation, indicating that H2S/CSE promoted EMT and p38 MAPK signaling pathways in HCC. Furthermore, the inhibition of p38 MAPK also abolished the radiation-induced expressions of EMT marker proteins, indicating that H2S/CSE promoted long-term metastasis of HCC cells after irradiation through promoting EMT by activating the p38 MAPK pathway, therefore inducing the invasion and metastasis of HCC cells and the xenograft tumors (Zhang et al., 2018). Contrary to the conclusion that H2S/CSE promoted EMT, exogenous H2S inhibited transforming growth factor beta (TGF β)-induced EMT of HCC cells (Fang et al., 2010; Guo et al., 2016). The above contradictory imagination may be related to the cell type and H2S concentration. Perhaps the low concentration of endogenous H2S promotes EMT of cells, while the high concentration of endogenous H2S has the opposite effect, which needs to be further studied.
4 Conclusion
H2S plays a vital role in HCC, which has been the research hotspot recently. Here, we summarizes the role and mechanism of H2S in HCC as follows: 1) exogenous H2S improves HCC by inhibiting STAT3 pathway; 2) exogenous H2S aggravates HCC via inducing STAT3-COX-2 pathway; 3) exogenous H2S plays a double-edged sword role in HCC through PTEN/AKT and EGFR/ERK/MMP-2 pathways; 4) exogenous H2S suppresses HCC cells proliferation and migration by inducing autophagy via suppressing PI3K/AKT/mTOR pathway; 5) HA-ADT suppresses HCC cells through inhibiting TGF-β/Smad2/3 and AKT/GSK-3β/β-catenin pathways; 6) PI3K/Akt pathway increases CSE/H2S level through Sp1 binding to CSE core promoter to aggravate HCC through promoting the cell cycle progression; 7) CSE/H2S promotes the proliferation of HCC cells by inhibiting mitochondrial ROS-mediated apoptosis through activating EGFR/ERK1/2 pathway; 8) CSE/H2S promotes long-term metastasis of HCC cells after irradiation through enhancing EMT by activating the p38MAPK pathway; 9) 3-MST/H2S inhibits HCC through promoting the apoptosis and cell cycle arrest of HCC cells by inhibiting AKT/FOXO3a/Rb pathway; 10) exogenous H2S reverses doxorubicin resistance to HCC via inhibiting the expressions of ABCG8 and ABCA1 (Table 1) (Figure 2). It can be seen from the above that several signal pathways, including EGFR/ERK/MMP-2 pathway, STAT3-COX-2 pathway, PI3K/AKT/mTOR pathway, PTEN/AKT signaling pathway, p38 MAPK pathway and AKT/FOXO3a/Rb pathway, participate in the role and mechanism of H2S in HCC. In addition to the signal pathways mentioned in this review, it is worth further studying whether H2S can play a role in HCC through other signal pathways. Moreover, in this review, H2S regulates HCC by regulating the cell cycle, apoptosis, angiogenesis, doxorubicin resistance, metastasis, proliferation, and migration of HCC cells. Can H2S also play a role in HCC through other mechanisms? For example, H2S plays a role in HCC by regulating pyroptosis and ferroptosis. In addition, the cost of the H2S-related drugs is generally cheaper. If they can be used to treat HCC, the economic burden of HCC patients will be greatly reduced. However, the current research shows that H2S plays a dual role in cancers (such as HCC) and inflammation. Therefore, more research is needed in the future to clarify under what conditions H2S promotes HCC and under what conditions H2S has the opposite effect. Furthermore, it is particularly important to avoid the occurrence of side effects such as H2S promoting cancer and inflammation when H2S-related drugs are used to treat HCC patients in the future. At present, the exogenous H2S releaser has many limitations, such as being unable to maintain a high concentration of H2S for a long time. Therefore, it is urgent to find new long-acting H2S-releasing agents so that it is possible to apply H2S-related drugs to the clinical treatment of HCC.
We believe that the H2S-related drugs will become a new strategy for HCC treatment.
Author contributions
HZ: Funding acquisition, Writing–original draft. YZ: Writing–original draft. XF: Writing–original draft. CC: Writing–original draft. SK: Writing–original draft. HW: Conceptualization, Funding acquisition, Investigation, Project administration, Supervision, Validation, Writing–original draft, Writing–review and editing.
Funding
The author(s) declare financial support was received for the research, authorship, and/or publication of this article. This work was supported by the grants from the Key Scientific and Technological Projects in Henan Province, China (Grant No. 202102310153).
Conflict of interest
The authors declare that the research was conducted in the absence of any commercial or financial relationships that could be construed as a potential conflict of interest.
Publisher’s note
All claims expressed in this article are solely those of the authors and do not necessarily represent those of their affiliated organizations, or those of the publisher, the editors and the reviewers. Any product that may be evaluated in this article, or claim that may be made by its manufacturer, is not guaranteed or endorsed by the publisher.
References
AbuHammad, S., and Zihlif, M. (2013). Gene expression alterations in doxorubicin resistant MCF7 breast cancer cell line. Genomics 101 (4), 213–220. doi:10.1016/j.ygeno.2012.11.009
Ahmed, S. A., Mendonca, P., Elhag, R., and Soliman, K. F. A. (2022). Anticancer effects of fucoxanthin through cell cycle arrest, apoptosis induction, angiogenesis inhibition, and autophagy modulation. Int. J. Mol. Sci. 23 (24), 16091. doi:10.3390/ijms232416091
Akbarzadeh, M., Mihanfar, A., Akbarzadeh, S., Yousefi, B., and Majidinia, M. (2021). Crosstalk between miRNA and PI3K/AKT/mTOR signaling pathway in cancer. Life Sci. 285, 119984. doi:10.1016/j.lfs.2021.119984
Casin, K. M., and Calvert, J. W. (2021). Harnessing the benefits of endogenous hydrogen sulfide to reduce cardiovascular disease. Antioxidants (Basel). 10 (3), 383. doi:10.3390/antiox10030383
Chang, Y., Jeong, S. W., Young Jang, J., and Jae Kim, Y. (2020). Recent updates of transarterial chemoembolilzation in hepatocellular carcinoma. Int. J. Mol. Sci. 21 (21), 8165. doi:10.3390/ijms21218165
Chen, L., Wang, Z., Song, S., Sun, T., Ren, Y., Zhang, W., et al. (2021). The efficacy of radiotherapy in the treatment of hepatocellular carcinoma with distant organ metastasis. J. Oncol. 2021, 5190611. doi:10.1155/2021/5190611
Chidambaranathan-Reghupaty, S., Fisher, P. B., and Sarkar, D. (2021). Hepatocellular carcinoma (HCC): epidemiology, etiology and molecular classification. Adv. Cancer Res. 149, 1–61. doi:10.1016/bs.acr.2020.10.001
Chiku, T., Padovani, D., Zhu, W., Singh, S., Vitvitsky, V., and Banerjee, R. (2009). H2S biogenesis by human cystathionine gamma-lyase leads to the novel sulfur metabolites lanthionine and homolanthionine and is responsive to the grade of hyperhomocysteinemia. J. Biol. Chem. 284 (17), 11601–11612. doi:10.1074/jbc.M808026200
Coavoy-Sánchez, S. A., Costa, S. K. P., and Muscará, M. N. (2020). Hydrogen sulfide and dermatological diseases. Br. J. Pharmacol. 177 (4), 857–865. doi:10.1111/bph.14699
Cui, Y., Song, M., Xiao, B., Liu, M., Liu, P., Han, Y., et al. (2021). ROS-mediated mitophagy and apoptosis are involved in aluminum-induced femoral impairment in mice. Chem. Biol. Interact. 349, 109663. doi:10.1016/j.cbi.2021.109663
da Costa Marques, L. A., Teixeira, S. A., de Jesus, F. N., Wood, M. E., Torregrossa, R., Whiteman, M., et al. (2022). Vasorelaxant activity of AP39, a mitochondria-targeted H(2)S donor, on mouse mesenteric artery rings in vitro. Biomolecules 12 (2), 280. doi:10.3390/biom12020280
Devis-Jauregui, L., Eritja, N., Davis, M. L., Matias-Guiu, X., and Llobet-Navàs, D. (2021). Autophagy in the physiological endometrium and cancer. Autophagy 17 (5), 1077–1095. doi:10.1080/15548627.2020.1752548
Dilek, N., Papapetropoulos, A., Toliver-Kinsky, T., and Szabo, C. (2020). Hydrogen sulfide: an endogenous regulator of the immune system. Pharmacol. Res. 161, 105119. doi:10.1016/j.phrs.2020.105119
Dimitroulis, D., Damaskos, C., Valsami, S., Davakis, S., Garmpis, N., Spartalis, E., et al. (2017). From diagnosis to treatment of hepatocellular carcinoma: an epidemic problem for both developed and developing world. World J. Gastroenterol. 23 (29), 5282–5294. doi:10.3748/wjg.v23.i29.5282
Dong, Q., Yang, B., Han, J. G., Zhang, M. M., Liu, W., Zhang, X., et al. (2019). A novel hydrogen sulfide-releasing donor, HA-ADT, suppresses the growth of human breast cancer cells through inhibiting the PI3K/AKT/mTOR and Ras/Raf/MEK/ERK signaling pathways. Cancer Lett. 455, 60–72. doi:10.1016/j.canlet.2019.04.031
Dongó, E., Beliczai-Marosi, G., Dybvig, A. S., and Kiss, L. (2018). The mechanism of action and role of hydrogen sulfide in the control of vascular tone. Nitric Oxide 81, 75–87. doi:10.1016/j.niox.2017.10.010
Duan, S. F., Zhang, M. M., Dong, Q., Yang, B., Liu, W., Zhang, X., et al. (2023). A water-soluble hydrogen sulfide donor suppresses the growth of hepatocellular carcinoma via inhibiting the AKT/GSK-3β/β-Catenin and TGF-β/smad2/3 signaling pathways. J. Oncol. 2023, 8456852. doi:10.1155/2023/8456852
El-Tanani, M., Al Khatib, A. O., Aladwan, S. M., Abuelhana, A., McCarron, P. A., and Tambuwala, M. M. (2022). Importance of STAT3 signalling in cancer, metastasis and therapeutic interventions. Cell Signal 92, 110275. doi:10.1016/j.cellsig.2022.110275
Erin, N., Grahovac, J., Brozovic, A., and Efferth, T. (2020). Tumor microenvironment and epithelial mesenchymal transition as targets to overcome tumor multidrug resistance. Drug Resist Updat 53, 100715. doi:10.1016/j.drup.2020.100715
Fang, L. P., Lin, Q., Tang, C. S., and Liu, X. M. (2010). Hydrogen sulfide attenuates epithelial-mesenchymal transition of human alveolar epithelial cells. Pharmacol. Res. 61 (4), 298–305. doi:10.1016/j.phrs.2009.10.008
Faris, P., Negri, S., Faris, D., Scolari, F., Montagna, D., and Moccia, F. (2023). Hydrogen sulfide (H(2)S): as a potent modulator and therapeutic prodrug in cancer. Curr. Med. Chem. 30 (40), 4506–4532. doi:10.2174/0929867330666230126100638
Fontana, F., Marzagalli, M., Raimondi, M., Zuco, V., Zaffaroni, N., and Limonta, P. (2021). δ-Tocotrienol sensitizes and re-sensitizes ovarian cancer cells to cisplatin via induction of G1 phase cell cycle arrest and ROS/MAPK-mediated apoptosis. Cell Prolif. 54 (11), e13111. doi:10.1111/cpr.13111
Forner, A., Reig, M., and Bruix, J. (2018). Hepatocellular carcinoma. Lancet 391 (10127), 1301–1314. doi:10.1016/S0140-6736(18)30010-2
Fouad, A. A., Hafez, H. M., and Hamouda, A. (2020). Hydrogen sulfide modulates IL-6/STAT3 pathway and inhibits oxidative stress, inflammation, and apoptosis in rat model of methotrexate hepatotoxicity. Hum. Exp. Toxicol. 39 (1), 77–85. doi:10.1177/0960327119877437
Ganzleben, I., Neurath, M. F., and Becker, C. (2021). Autophagy in cancer therapy-molecular mechanisms and current clinical advances. Cancers (Basel) 13 (21), 5575. doi:10.3390/cancers13215575
Garcia, R., Bowman, T. L., Niu, G., Yu, H., Minton, S., Muro-Cacho, C. A., et al. (2001). Constitutive activation of Stat3 by the Src and JAK tyrosine kinases participates in growth regulation of human breast carcinoma cells. Oncogene 20 (20), 2499–2513. doi:10.1038/sj.onc.1204349
Gilles, H., Garbutt, T., and Landrum, J. (2022). Hepatocellular carcinoma. Crit. Care Nurs. Clin. North Am. 34 (3), 289–301. doi:10.1016/j.cnc.2022.04.004
Greaney, J. L., Kutz, J. L., Shank, S. W., Jandu, S., Santhanam, L., and Alexander, L. M. (2017). Impaired hydrogen sulfide-mediated vasodilation contributes to microvascular endothelial dysfunction in hypertensive adults. Hypertension 69 (5), 902–909. doi:10.1161/HYPERTENSIONAHA.116.08964
Guo, L., Peng, W., Tao, J., Lan, Z., Hei, H., Tian, L., et al. (2016). Hydrogen sulfide inhibits transforming growth factor-β1-induced EMT via wnt/catenin pathway. PLoS One 11 (1), e0147018. doi:10.1371/journal.pone.0147018
Gupta, P., Zhang, Y. K., Zhang, X. Y., Wang, Y. J., Lu, K. W., Hall, T., et al. (2018). Voruciclib, a potent CDK4/6 inhibitor, antagonizes ABCB1 and ABCG2-mediated multi-drug resistance in cancer cells. Cell Physiol. Biochem. 45 (4), 1515–1528. doi:10.1159/000487578
Iqbal, I. K., Bajeli, S., Sahu, S., Bhat, S. A., and Kumar, A. (2021). Hydrogen sulfide-induced GAPDH sulfhydration disrupts the CCAR2-SIRT1 interaction to initiate autophagy. Autophagy 17 (11), 3511–3529. doi:10.1080/15548627.2021.1876342
Jia, Y., Wang, Y., Zhang, Y., Wang, J., Pei, Y., Wang, Z., et al. (2022). Profiling cystathionine β/γ-Lyase in complex biosamples using novel activatable fluorogens. Anal. Chem. 94 (2), 1203–1210. doi:10.1021/acs.analchem.1c04393
Jung, H. S., Kong, W. H., Sung, D. K., Lee, M. Y., Beack, S. E., Keum, D. H., et al. (2014). Nanographene oxide-hyaluronic acid conjugate for photothermal ablation therapy of skin cancer. ACS Nano 8 (1), 260–268. doi:10.1021/nn405383a
Kamel, R., El Morsy, E. M., Elsherbiny, M. E., and Nour-Eldin, M. (2022). Chrysin promotes angiogenesis in rat hindlimb ischemia: impact on PI3K/Akt/mTOR signaling pathway and autophagy. Drug Dev. Res. 83 (5), 1226–1237. doi:10.1002/ddr.21954
Khattak, S., Rauf, M. A., Khan, N. H., Zhang, Q. Q., Chen, H. J., Muhammad, P., et al. (2022). Hydrogen sulfide biology and its role in cancer. Molecules 27 (11), 3389. doi:10.3390/molecules27113389
Kimura, H. (2010). Hydrogen sulfide: from brain to gut. Antioxid. Redox Signal 12 (9), 1111–1123. doi:10.1089/ars.2009.2919
Kopecka, J., Trouillas, P., Gašparović, A., Gazzano, E., Assaraf, Y. G., and Riganti, C. (2020). Phospholipids and cholesterol: inducers of cancer multidrug resistance and therapeutic targets. Drug Resist Updat 49, 100670. doi:10.1016/j.drup.2019.100670
Korkmaz, R., Yüksek, V., and Dede, S. (2022). The effects of sodium fluoride (NaF) treatment on the PI3K/Akt signal pathway in NRK-52e cells. Biol. Trace Elem. Res. 200 (7), 3294–3302. doi:10.1007/s12011-021-02927-4
Krylatov, A., Maslov, L., Tsibulnikov, S. Y., Voronkov, N., Boshchenko, A., Downey, J., et al. (2021). The role of reactive oxygen species, kinases, hydrogen sulfide, and nitric oxide in the regulation of autophagy and their impact on ischemia and reperfusion injury in the heart. Curr. Cardiol. Rev. 17 (4), e230421186874. doi:10.2174/1573403X16666201014142446
Kulik, L., and El-Serag, H. B. (2019). Epidemiology and management of hepatocellular carcinoma. Gastroenterology 156 (2), 477–491. doi:10.1053/j.gastro.2018.08.065
Kumar, S., Sánchez-Álvarez, M., Lolo, F. N., Trionfetti, F., Strippoli, R., and Cordani, M. (2021). Autophagy and the lysosomal system in cancer. Cells 10 (10), 2752. doi:10.3390/cells10102752
Lee, C., and Cheung, S. T. (2019). STAT3: an emerging therapeutic target for hepatocellular carcinoma. Cancers (Basel) 11 (11), 1646. doi:10.3390/cancers11111646
Li, M., Song, X., Jin, Q., Chen, Y., Zhang, J., Gao, J., et al. (2022b). 3-Mercaptopyruvate sulfurtransferase represses tumour progression and predicts prognosis in hepatocellular carcinoma. Liver Int. 42 (5), 1173–1184. doi:10.1111/liv.15228
Li, M., Song, X., Xu, C., and Yu, C. (2022a). Response to the clinical significance and prognostic value of 3-mercaptopyruvate sulfurtransferase in hepatocellular carcinoma. Liver Int. 42 (7), 1696. doi:10.1111/liv.15280
Li, S., Qi, Y., Huang, Y., Guo, Y., Huang, T., and Jia, L. (2021). Exosome-derived SNHG16 sponging miR-4500 activates HUVEC angiogenesis by targeting GALNT1 via PI3K/Akt/mTOR pathway in hepatocellular carcinoma. J. Physiol. Biochem. 77 (4), 667–682. doi:10.1007/s13105-021-00833-w
Li, T., Xiong, Y., Wang, Q., Chen, F., Zeng, Y., Yu, X., et al. (2019). Ribociclib (LEE011) suppresses cell proliferation and induces apoptosis of MDA-MB-231 by inhibiting CDK4/6-cyclin D-Rb-E2F pathway. Artif. Cells Nanomed Biotechnol. 47 (1), 4001–4011. doi:10.1080/21691401.2019.1670670
Llovet, J. M., Kelley, R. K., Villanueva, A., Singal, A. G., Pikarsky, E., Roayaie, S., et al. (2021). Hepatocellular carcinoma. Nat. Rev. Dis. Prim. 7 (1), 6. doi:10.1038/s41572-020-00240-3
Loh, C. Y., Arya, A., Naema, A. F., Wong, W. F., Sethi, G., and Looi, C. Y. (2019). Signal transducer and activator of transcription (STATs) proteins in cancer and inflammation: functions and therapeutic implication. Front. Oncol. 9, 48. doi:10.3389/fonc.2019.00048
Lu, S., Gao, Y., Huang, X., and Wang, X. (2014). GYY4137, a hydrogen sulfide (H₂S) donor, shows potent anti-hepatocellular carcinoma activity through blocking the STAT3 pathway. Int. J. Oncol. 44 (4), 1259–1267. doi:10.3892/ijo.2014.2305
Ma, Y., Yan, Z., Deng, X., Guo, J., Hu, J., Yu, Y., et al. (2018). Anticancer effect of exogenous hydrogen sulfide in cisplatin-resistant A549/DDP cells. Oncol. Rep. 39 (6), 2969–2977. doi:10.3892/or.2018.6362
Mohammed, R. A., Shawky, H. M., Rashed, L. A., Elhanbuli, H. M., Abdelhafez, D. N., Said, E. S., et al. (2021). Combined effect of hydrogen sulfide and mesenchymal stem cells on mitigating liver fibrosis induced by bile duct ligation: role of anti-inflammatory, anti-oxidant, anti-apoptotic, and anti-fibrotic biomarkers. Iran. J. Basic Med. Sci. 24 (12), 1753–1762. doi:10.22038/IJBMS.2021.56477.12604
Montoya, L. A., and Pluth, M. D. (2016). Organelle-targeted H2S probes enable visualization of the subcellular distribution of H2S donors. Anal. Chem. 88 (11), 5769–5774. doi:10.1021/acs.analchem.6b00087
Nguyen, T. T. P., Kim, D. Y., Im, S. S., and Jeon, T. I. (2021b). Impairment of ULK1 sulfhydration-mediated lipophagy by SREBF1/SREBP-1c in hepatic steatosis. Autophagy 17 (12), 4489–4490. doi:10.1080/15548627.2021.1968608
Nguyen, T. T. P., Kim, D. Y., Lee, Y. G., Lee, Y. S., Truong, X. T., Lee, J. H., et al. (2021a). SREBP-1c impairs ULK1 sulfhydration-mediated autophagic flux to promote hepatic steatosis in high-fat-diet-fed mice. Mol. Cell 81 (18), 3820–3832.e7. doi:10.1016/j.molcel.2021.06.003
Nikolaou, M., Pavlopoulou, A., Georgakilas, A. G., and Kyrodimos, E. (2018). The challenge of drug resistance in cancer treatment: a current overview. Clin. Exp. Metastasis 35 (4), 309–318. doi:10.1007/s10585-018-9903-0
Omorou, M., Liu, N., Huang, Y., Al-Ward, H., Gao, M., Mu, C., et al. (2022). Cystathionine beta-Synthase in hypoxia and ischemia/reperfusion: a current overview. Arch. Biochem. Biophys. 718, 109149. doi:10.1016/j.abb.2022.109149
Pacitti, D., Scotton, C. J., Kumar, V., Khan, H., Wark, P. A. B., Torregrossa, R., et al. (2021). Gasping for sulfide: a critical appraisal of hydrogen sulfide in lung disease and accelerated aging. Antioxid. Redox Signal 35 (7), 551–579. doi:10.1089/ars.2021.0039
Pan, Y., Ye, S., Yuan, D., Zhang, J., Bai, Y., and Shao, C. (2014). Hydrogen sulfide (H2S)/cystathionine γ-lyase (CSE) pathway contributes to the proliferation of hepatoma cells. Mutat. Res. 763-764, 10–18. doi:10.1016/j.mrfmmm.2014.03.002
Panza, E., Bello, I., Smimmo, M., Brancaleone, V., Mitidieri, E., Bucci, M., et al. (2022). Endogenous and exogenous hydrogen sulfide modulates urothelial bladder carcinoma development in human cell lines. Biomed. Pharmacother. 151, 113137. doi:10.1016/j.biopha.2022.113137
Powell, C. R., Dillon, K. M., and Matson, J. B. (2018). A review of hydrogen sulfide (H(2)S) donors: chemistry and potential therapeutic applications. Biochem. Pharmacol. 149, 110–123. doi:10.1016/j.bcp.2017.11.014
Qian, L., Liu, Y., Xu, Y., Ji, W., Wu, Q., Liu, Y., et al. (2015). Matrine derivative WM130 inhibits hepatocellular carcinoma by suppressing EGFR/ERK/MMP-2 and PTEN/AKT signaling pathways. Cancer Lett. 368 (1), 126–134. doi:10.1016/j.canlet.2015.07.035
Rakesh, R., PriyaDharshini, L. C., Sakthivel, K. M., and Rasmi, R. R. (2022). Role and regulation of autophagy in cancer. Biochim. Biophys. Acta Mol. Basis Dis. 1868 (7), 166400. doi:10.1016/j.bbadis.2022.166400
Rao, S. P., Xie, W., Kwon, Y. I. C., Juckel, N., Xie, J., Dronamraju, V. R., et al. (2022). Sulfanegen stimulates 3-mercaptopyruvate sulfurtransferase activity and ameliorates Alzheimer's disease pathology and oxidative stress in vivo. Redox Biol. 57, 102484. doi:10.1016/j.redox.2022.102484
Renne, S. L., Sarcognato, S., Sacchi, D., Guido, M., Roncalli, M., Terracciano, L., et al. (2021). Hepatocellular carcinoma: a clinical and pathological overview. Pathologica 113 (3), 203–217. doi:10.32074/1591-951X-295
Sadrkhanloo, M., Entezari, M., Orouei, S., Ghollasi, M., Fathi, N., Rezaei, S., et al. (2022). STAT3-EMT axis in tumors: modulation of cancer metastasis, stemness and therapy response. Pharmacol. Res. 182, 106311. doi:10.1016/j.phrs.2022.106311
Santiago, F. S., Ishii, H., Shafi, S., Khurana, R., Kanellakis, P., Bhindi, R., et al. (2007). Yin Yang-1 inhibits vascular smooth muscle cell growth and intimal thickening by repressing p21WAF1/Cip1 transcription and p21WAF1/Cip1-Cdk4-cyclin D1 assembly. Circ. Res. 101 (2), 146–155. doi:10.1161/CIRCRESAHA.106.145235
Shackelford, R., Ozluk, E., Islam, M. Z., Hopper, B., Meram, A., Ghali, G., et al. (2021a). Hydrogen sulfide and DNA repair. Redox Biol. 38, 101675. doi:10.1016/j.redox.2020.101675
Shackelford, R. E., Mohammad, I. Z., Meram, A. T., Kim, D., Alotaibi, F., Patel, S., et al. (2021b). Molecular functions of hydrogen sulfide in cancer. Pathophysiology 28 (3), 437–456. doi:10.3390/pathophysiology28030028
Sherr, C. J., and Roberts, J. M. (2004). Living with or without cyclins and cyclin-dependent kinases. Genes Dev. 18 (22), 2699–2711. doi:10.1101/gad.1256504
Shome, R., and Ghosh, S. S. (2021). Transferrin coated d-penicillamine-Au-Cu nanocluster PLGA nanocomposite reverses hypoxia-induced EMT and MDR of triple-negative breast cancers. ACS Appl. Bio Mater 4 (6), 5033–5048. doi:10.1021/acsabm.1c00296
Sim, H. W., and Knox, J. (2018). Hepatocellular carcinoma in the era of immunotherapy. Curr. Probl. Cancer 42 (1), 40–48. doi:10.1016/j.currproblcancer.2017.10.007
Stokes, E., Shuang, T., Zhang, Y., Pei, Y., Fu, M., Guo, B., et al. (2018). Efflux inhibition by H(2)S confers sensitivity to doxorubicin-induced cell death in liver cancer cells. Life Sci. 213, 116–125. doi:10.1016/j.lfs.2018.10.031
Sun, E. J., Wankell, M., Palamuthusingam, P., McFarlane, C., and Hebbard, L. (2021). Targeting the PI3K/Akt/mTOR pathway in hepatocellular carcinoma. Biomedicines 9 (11), 1639. doi:10.3390/biomedicines9111639
Sundarraj, K., Raghunath, A., Panneerselvam, L., and Perumal, E. (2021). Fisetin inhibits autophagy in HepG2 cells via PI3K/Akt/mTOR and AMPK pathway. Nutr. Cancer 73 (11-12), 2502–2514. doi:10.1080/01635581.2020.1836241
Tocmo, R., and Parkin, K. (2019). S-1-propenylmercaptocysteine protects murine hepatocytes against oxidative stress via persulfidation of Keap1 and activation of Nrf2. Free Radic. Biol. Med. 143, 164–175. doi:10.1016/j.freeradbiomed.2019.07.022
Wang, H., Shi, X., Qiu, M., Lv, S., and Liu, H. (2020a). Hydrogen sulfide plays an important protective role through influencing endoplasmic reticulum stress in diseases. Int. J. Biol. Sci. 16 (2), 264–271. doi:10.7150/ijbs.38143
Wang, H., Zhong, P., and Sun, L. (2019). Exogenous hydrogen sulfide mitigates NLRP3 inflammasome-mediated inflammation through promoting autophagy via the AMPK-mTOR pathway. Biol. Open 8 (7), bio043653. doi:10.1242/bio.043653
Wang, J., Wang, Z., Yuan, J., Wang, Q., and Shen, X. (2021b). Upregulation of miR-137 expression suppresses tumor growth and progression via interacting with DNMT3a through inhibiting the PTEN/Akt signaling in HCC. Onco Targets Ther. 14, 165–176. doi:10.2147/OTT.S268570
Wang, L., Guo, Y., Zhang, H., Li, H., Wang, F., Zhang, J., et al. (2022b). Risk factors for brain metastasis of hepatocellular carcinoma. J. Healthc. Eng. 2022, 7848143. doi:10.1155/2022/7848143
Wang, L., Jiang, W., Wang, J., Xie, Y., and Wang, W. (2022a). Puerarin inhibits FUNDC1-mediated mitochondrial autophagy and CSE-induced apoptosis of human bronchial epithelial cells by activating the PI3K/AKT/mTOR signaling pathway. Aging (Albany NY) 14 (3), 1253–1264. doi:10.18632/aging.203317
Wang, M., Chen, W., Chen, J., Yuan, S., Hu, J., Han, B., et al. (2021a). Abnormal saccharides affecting cancer multi-drug resistance (MDR) and the reversal strategies. Eur. J. Med. Chem. 220, 113487. doi:10.1016/j.ejmech.2021.113487
Wang, S. S., Chen, Y. H., Chen, N., Wang, L. J., Chen, D. X., Weng, H. L., et al. (2017). Hydrogen sulfide promotes autophagy of hepatocellular carcinoma cells through the PI3K/Akt/mTOR signaling pathway. Cell Death Dis. 8 (3), e2688. doi:10.1038/cddis.2017.18
Wang, Y., Chen, S., Sun, S., Liu, G., Chen, L., Xia, Y., et al. (2020b). Wogonin induces apoptosis and reverses sunitinib resistance of renal cell carcinoma cells via inhibiting CDK4-RB pathway. Front. Pharmacol. 11, 1152. doi:10.3389/fphar.2020.01152
Wu, D., Li, M., Tian, W., Wang, S., Cui, L., Li, H., et al. (2017). Hydrogen sulfide acts as a double-edged sword in human hepatocellular carcinoma cells through EGFR/ERK/MMP-2 and PTEN/AKT signaling pathways. Sci. Rep. 7 (1), 5134. doi:10.1038/s41598-017-05457-z
Yang, B., Feng, X., Liu, H., Tong, R., Wu, J., Li, C., et al. (2020). High-metastatic cancer cells derived exosomal miR92a-3p promotes epithelial-mesenchymal transition and metastasis of low-metastatic cancer cells by regulating PTEN/Akt pathway in hepatocellular carcinoma. Oncogene 39 (42), 6529–6543. doi:10.1038/s41388-020-01450-5
Yang, J. D., Hainaut, P., Gores, G. J., Amadou, A., Plymoth, A., and Roberts, L. R. (2019). A global view of hepatocellular carcinoma: trends, risk, prevention and management. Nat. Rev. Gastroenterol. Hepatol. 16 (10), 589–604. doi:10.1038/s41575-019-0186-y
Yin, P., Zhao, C., Li, Z., Mei, C., Yao, W., Liu, Y., et al. (2012). Sp1 is involved in regulation of cystathionine γ-lyase gene expression and biological function by PI3K/Akt pathway in human hepatocellular carcinoma cell lines. Cell Signal 24 (6), 1229–1240. doi:10.1016/j.cellsig.2012.02.003
Zahedi-Amiri, A., Malone, K., Beug, S. T., Alain, T., and Yeganeh, B. (2021). Autophagy in tumor immunity and viral-based immunotherapeutic approaches in cancer. Cells 10 (10), 2672. doi:10.3390/cells10102672
Zaorska, E., Hutsch, T., Gawryś-Kopczyńska, M., Ostaszewski, R., Ufnal, M., and Koszelewski, D. (2019). Evaluation of thioamides, thiolactams and thioureas as hydrogen sulfide (H(2)S) donors for lowering blood pressure. Bioorg Chem. 88, 102941. doi:10.1016/j.bioorg.2019.102941
Zaorska, E., Tomasova, L., Koszelewski, D., Ostaszewski, R., and Ufnal, M. (2020). Hydrogen sulfide in pharmacotherapy, beyond the hydrogen sulfide-donors. Biomolecules 10 (2), 323. doi:10.3390/biom10020323
Zhang, D., Du, J., Tang, C., Huang, Y., and Jin, H. (2017). H2S-Induced sulfhydration: biological function and detection methodology. Front. Pharmacol. 8, 608. doi:10.3389/fphar.2017.00608
Zhang, F., Chen, S., Wen, J. Y., and Chen, Z. W. (2020). 3-Mercaptopyruvate sulfurtransferase/hydrogen sulfide protects cerebral endothelial cells against oxygen-glucose deprivation/reoxygenation-induced injury via mitoprotection and inhibition of the RhoA/ROCK pathway. Am. J. Physiol. Cell Physiol. 319 (4), C720–C733. doi:10.1152/ajpcell.00014.2020
Zhang, H., Song, Y., Zhou, C., Bai, Y., Yuan, D., Pan, Y., et al. (2018). Blocking endogenous H(2)S signaling attenuated radiation-induced long-term metastasis of residual HepG2 cells through inhibition of EMT. Radiat. Res. 190 (4), 374–384. doi:10.1667/RR15074.1
Zhao, S., Li, X., Lu, P., Li, X., Sun, M., and Wang, H. (2021). The role of the signaling pathways involved in the effects of hydrogen sulfide on endoplasmic reticulum stress. Front. Cell Dev. Biol. 9, 646723. doi:10.3389/fcell.2021.646723
Zhen, Y., Wu, Q., Ding, Y., Zhang, W., Zhai, Y., Lin, X., et al. (2018). Exogenous hydrogen sulfide promotes hepatocellular carcinoma cell growth by activating the STAT3-COX-2 signaling pathway. Oncol. Lett. 15 (5), 6562–6570. doi:10.3892/ol.2018.8154
Keywords: hydrogen sulfide, hepatocellular carcinoma, apoptosis, cystathione gamma-lyase, 3-mercaptopyruvate sulfurtransferase
Citation: Zhao H, Zhang Y, Fu X, Chen C, Khattak S and Wang H (2023) The double-edged sword role of hydrogen sulfide in hepatocellular carcinoma. Front. Pharmacol. 14:1280308. doi: 10.3389/fphar.2023.1280308
Received: 21 August 2023; Accepted: 02 October 2023;
Published: 11 October 2023.
Edited by:
Simona Rapposelli, University of Pisa, ItalyReviewed by:
Wuxiyar Otkur, Chinese Academy of Sciences (CAS), ChinaRoberta Ibba, University of Sassari, Italy
Copyright © 2023 Zhao, Zhang, Fu, Chen, Khattak and Wang. This is an open-access article distributed under the terms of the Creative Commons Attribution License (CC BY). The use, distribution or reproduction in other forums is permitted, provided the original author(s) and the copyright owner(s) are credited and that the original publication in this journal is cited, in accordance with accepted academic practice. No use, distribution or reproduction is permitted which does not comply with these terms.
*Correspondence: Honggang Wang, d2hnMTk3MTY3QHZpcC5oZW51LmVkdS5jbg==
†These authors share first authorship