- 1Department of Rheumatology, Shanghai Guanghua Hospital, Shanghai University of Traditional Chinese Medicine, Shanghai, China
- 2Guanghua Clinical Medical College, Shanghai University of Traditional Chinese Medicine, Shanghai, China
- 3Institute of Arthritis Research in Integrative Medicine, Shanghai Academy of Traditional Chinese Medicine, Shanghai, China
- 4Arthritis Institute of Integrated Traditional and Western Medicine, Shanghai Chinese Medicine Research Institute, Shanghai, China
- 5Computation and Informatics in Biology and Medicine, University of Wisconsin-Madison, Madison, WI. United States
- 6Department of Medical Genetics, School of Medicine and Public Health, University of Wisconsin-Madison, Madison, WI, United States
Rheumatic and autoimmune diseases are a group of immune system-related disorders wherein the immune system mistakenly attacks and damages the body’s tissues and organs. This excessive immune response leads to inflammation, tissue damage, and functional impairment. Therapeutic approaches typically involve medications that regulate immune responses, reduce inflammation, alleviate symptoms, and target specific damaged organs. Tripterygium wilfordii Hook. f., a traditional Chinese medicinal plant, has been widely studied in recent years for its application in the treatment of autoimmune diseases, including rheumatoid arthritis, systemic lupus erythematosus, and multiple sclerosis. Numerous studies have shown that preparations of Tripterygium wilfordii have anti-inflammatory, immunomodulatory, and immunosuppressive effects, which effectively improve the symptoms and quality of life of patients with autoimmune diseases, whereas the active metabolites of T. wilfordii have been demonstrated to inhibit immune cell activation, regulate the production of inflammatory factors, and modulate the immune system. However, although these effects contribute to reductions in inflammatory responses and the suppression of autoimmune reactions, as well as minimize tissue and organ damage, the underlying mechanisms of action require further investigation. Moreover, despite the efficacy of T. wilfordii in the treatment of autoimmune diseases, its toxicity and side effects, including its potential hepatotoxicity and nephrotoxicity, warrant a thorough assessment. Furthermore, to maximize the therapeutic benefits of this plant in the treatment of autoimmune diseases and enable more patients to utilize these benefits, efforts should be made to strengthen the regulation and standardized use of T. wilfordii.
1 Introduction
Rheumatic and autoimmune diseases are chronic conditions that affect millions of people worldwide. These diseases are characterized by inflammation, pain, and damage to the affected organs and tissues, and thus significantly affect the quality of life of patients (Schwenzer et al., 2010; Ma et al., 2020). Several treatment modalities are currently available for these diseases, including the administration of non-steroidal anti-inflammatory drugs, disease-modifying antirheumatic drugs, and biological agents (Tsitrouli et al., 2021). However, these therapeutic approaches do not always fully satisfy the clinical needs of patients and may be associated with potential side effects. Additionally, patients may exhibit an inadequate response to these strategies, and the occurrence of adverse events, coupled with their high cost, imposes a substantial burden on clinical healthcare (Shi et al., 2020). Consequently, there is a compelling need to identify effective novel drugs that could be applied in the treatment of rheumatic and autoimmune diseases.
Tripterygium wilfordii Hook. f. is a herb used in traditional Chinese medicine that was first recorded in the Compendium of Materia Medica (Ben Cao Gang Mu Shi Yi), and has been widely used to treat various diseases, including rheumatic and autoimmune disorders, for centuries (Jia et al., 2021). T. wilfordii is used as a herbal medicine in traditional Chinese medicine to treat joint pain, promote blood circulation, relieve rheumatism, and alleviate pain and swelling (Shen et al., 2021). In recent decades, the assessment of the therapeutic potential of T. wilfordii has attracted increased interest, which has led to extensive research on its pharmacological properties and potential clinical applications. Owing to its strong anti-inflammatory and immunomodulatory properties, T. wilfordii is considered a promising candidate for the treatment of rheumatic diseases and autoimmune disorders (Hou et al., 2017).
Preliminary clinical studies have indicated that T. wilfordii and its active metabolites have beneficial effects in the treatment of a range of rheumatic and autoimmune disorders, including rheumatoid arthritis (RA), systemic lupus erythematosus (SLE), and psoriasis (Gonçalves et al., 2020). For example, in a mouse model of collagen-induced arthritis, treatment with triptolide was found to significantly reduce disease severity and inhibit pro-inflammatory cytokine production and immune cell activation (Yang et al., 2016). Similarly, in a mouse model of lupus nephritis, treatment with celastrol was demonstrated to significantly reduce disease severity and reduce the levels of autoantibodies and pro-inflammatory cytokines (Chen et al., 2022). Clinical studies have also reported promising outcomes with the use of T. wilfordii for the treatment of rheumatic diseases and autoimmune disorders (Yu et al., 2020). For example, in a randomized, double-blind, placebo-controlled trial, patients with RA who received T. wilfordii extract showed significant improvements in disease severity and joint function compared with those who received a placebo (Jiang et al., 2017). In a similar trial, patients with psoriasis who received a T. wilfordii extract showed significant improvements in disease severity and skin symptoms compared with those who received a placebo (Lv et al., 2018).
Considering its potent anti-inflammatory and immunomodulatory properties, T. wilfordii has shown significant potential as a therapeutic agent for the treatment of rheumatic diseases and autoimmune disorders (Song CY. et al., 2020). Although further research is needed to gain a more comprehensive understanding of the mechanisms underlying the effects of T. wilfordii and its active metabolites, evidence obtained to date indicates that preparations of this plant may offer a safe and effective alternative to currently available treatment modalities (Lv et al., 2018). However, more studies will be required to determine the optimal dosages and treatment regimens for T. wilfordii, as well as to identify any potential side effects or drug interactions. In this review, we discuss the evidence supporting the use of T. wilfordii in the treatment of rheumatic diseases and autoimmune disorders, with a focus on key findings from preclinical and clinical studies.
2 Historical uses of Tripterygium wilfordii in traditional medicine
T. wilfordii has a long history of use in traditional medicine. Natural populations of this vine are widely distributed in moist regions of the Northern Hemisphere, including China, Japan, North and South Korea, the United States, and Canada, with a total of 1,219 locations confirmed globally (Figure 1) (Cheng et al., 2021). In traditional Chinese medicine, T. wilfordii is considered a potent herbal medicine, which can be used to treat a range of diseases and disorders (Lu et al., 2022). The medicinal use of T. wilfordii can be traced back to the Han Dynasty (220 BC), at which time it was used to treat various ailments such as gout, edemas, and fever. Since then, the plant has also been used to treat rheumatic diseases and promote blood circulation. During the Tang Dynasty (618–907 AD), the use of T. wilfordii became even more widespread and was used to treat a wider range of conditions, including inflammation, swelling, and joint pain (Lu et al., 2022), and the use of this plant in traditional medicine has continued to the present day. Currently, T. wilfordii is commonly administered to treat RA, SLE, and other autoimmune diseases, and its anticancer properties have been recognized (Xue et al., 2012). Furthermore, the traditional use of T. wilfordii has been documented in South Korea and Japan. In South Korea, this plant is referred to as “jeokseong” and has been used in the treatment of RA, while in Japan, T. wilfordii is known as “raiji,” and is recognized not only for its therapeutic properties in RA management (Cheng et al., 2021) but also for its use in the treatment of inflammatory diseases, such as Crohn’s disease and ulcerative colitis (Wang et al., 2016).
3 Chemical composition of Tripterygium wilfordii
Recently, considering its potential use in the treatment of rheumatic and autoimmune diseases, T. wilfordii has attracted the attention of the conventional medical community (Oliner et al., 2016). To date, over 500 metabolites have been isolated and identified from T. wilfordii, including sesquiterpenes, diterpenes, triterpenes, alkaloids, flavonoids, lignans, and glycosides. Among these metabolites, notable compounds, such as triptolide, celastrol, and wilforlide, are believed to contribute to the diverse pharmacological activities of T. wilfordii. The identification and characterization of such compounds can provide valuable insights into the chemical composition and potential therapeutic properties of this plant (Luo et al., 2019; Xu et al., 2019). The following subsections provide an overview of the major metabolites isolated from T. wilfordii in modern medicine and their current status in terms of mechanistic research. (Table 1)
3.1 Triptolide
Triptolide, one of the key active compounds identified in T. wilfordii, is primarily obtained through extraction and isolation from various medicinal plants. First isolated by Kupchan et al., in 1972, triptolide has been established to have significant anti-leukemic activity (Kupchan et al., 1972) in addition to other important biological activities, including prominent anti-inflammatory, immunosuppressive, and anticancer effects (Gao et al., 2021). Triptolide exerts its anticancer effects by modulating different signaling pathways and gene expression at multiple levels, as well as by interacting with miRNAs. Specifically, these therapeutic effects are mediated via the modulation of cell apoptosis, senescence, proliferation, invasion, migration, and angiogenesis (Tong et al., 2021). For example, triptolide has been established to induce apoptosis in prostate cancer cells through the activation of caspases and poly-ADP-ribose polymerase cleavage (Zhao et al., 2013). Moreover, it inhibits nuclear factor kappa-B (NF-κB) activation and downregulates the expression of toll-like receptor 4 (TLR4) (Wen et al., 2012). Reportedly, triptolide can also contribute to the prevention of bone deterioration by reducing the number of osteoclasts in inflamed joints, reducing the expression of receptor activator of NF-κB (RANK) and its ligand RANKL, enhancing the expression of osteoprotegerin (OPG) at the mRNA and protein levels, and lowering the ratio of RANKL to OPG in the serum and inflamed joints of mice with collagen-induced arthritis (Liu C. et al., 2013).
3.2 Celastrol
The pentacyclic triterpenoid celastrol has been identified as a major bioactive metabolite of T. wilfordii that has diverse biological properties, including antitumor, antioxidant, anti-obesity, neuroprotective, and immunosuppressive effects (Hou et al., 2020; Xu et al., 2021). Moreover, celastrol has been identified as a natural proteasome inhibitor, the activity of which accordingly leads to an accumulation of ubiquitinated proteins and proteasome substrates, such as NF-κB (IκBα), Bax, and p27, and induces the ubiquitination of orphan nuclear receptor NR4A1 (Nur77) through its Lys63 linkage (Hernandes et al., 2017). Under inflammatory conditions, ubiquitinated Nur77 accumulates within the mitochondria, thereby rendering these organelles sensitive to autophagy. This event involves an interaction between Nur77 and p62/SQSTM1, thereby contributing to an alleviation of inflammation (Liu et al., 2022). Celastrol has also been shown to target multiple signaling pathways, including those of NF-κB, endoplasmic reticulum Ca2+-ATPase, myeloid differentiation factor 2, TLR4, pro-inflammatory chemokines, DNA damage, cell cycle arrest, and apoptosis (Song X. et al., 2020).
3.3 Wilforlide A
Wilforlide A is a triterpene isolated from T. wilfordii that can serve as a quality control standard for tripterygium glycosides, a class of drugs derived from T. wilfordii. Wilforlide A also exhibits potent anti-inflammatory and immunosuppressive activities (Xue et al., 2010), and has been used alone as a traditional botanical drug for the treatment of autoimmune diseases and is widely used to treat conditions such as RA, SLE, and psoriasis (Wang et al., 2018). Regarding its mechanism of action, it has been established that wilforlide A inhibits the secretion of pro-inflammatory cytokines (MCP1, GM-CSF, and M-CSF) and the M1 biomarker inducible nitric oxide synthase in synovial cells (Cao et al., 2022). In in vitro experiments, wilforlide A was found to inhibit the lipopolysaccharide- and interferon-γ (IFN-γ)-induced upregulation of TLR4, degradation of IκBα, and activation of NF-κB p65 (Cao et al., 2022). Additionally, treatment with the TLR4 inhibitor TAK242 has been demonstrated to have inhibitory effects on M1 polarization similar to those of wilforlide, and the combined administration of TAK242 and wilforlide A has been found to enhance these inhibitory effects (Mao et al., 2021).
3.4 Sesquiterpenes
The sesquiterpenes isolated from T. wilfordii are the products of the metabolism of approximately 300 different C15 isoprenoid precursors, which are synthesized from the single substrate farnesyl diphosphate by sesquiterpene synthases (Miller and Allemann, 2012). Two novel sesquiterpenes, namely, 1β,2α,6α,8β,15-pentaacetoxy-9α-benzoyloxy-β-dihydroagarofuran and 1β,2β,6α,15-tetraacetoxy-9β-benzoyloxy-β-dihydroagarofuran, have recently been discovered in T. wilfordii and found to exhibit inhibitory effects against A549 human lung cancer cells, human osteosarcoma cells, and human breast cancer cell lines. Moreover, sesquiterpenes have been reported to have a range of pharmacological properties, including antitumor, anti-inflammatory, antimicrobial, antiparasitic, insecticidal, and antiviral activities (Chen et al., 2020).
3.5 Diterpenes
Diterpenes are among the major active metabolites of T. wilfordii and can be further classified as abietane, tigliane, and ingenane diterpenes (Qu et al., 2022). Among these, triptolide was first isolated from T. wilfordii in 1972 by Kupchan, and exhibits potent pharmacological activities against various immune disorders, cancers, viruses, fibrosis, asthma, depression, and metabolic imbalances (Guo et al., 2016; Yuan et al., 2019).
3.6 Triterpenes
The triterpenes present in T. wilfordii are primarily classified into friedelanes, oleananes, and ursanes (Chang et al., 2016; Hu et al., 2017). Pentacyclic triterpenes are a class of representative plant compounds produced as secondary metabolites, which have been shown to possess diverse biological activities, including anticancer, anti-inflammatory, cardioprotective, and antidiabetic effects (Ghante and Jamkhande, 2019). Bevirimat, a triterpene compound extracted from T. wilfordii, has significant antiviral, antifibrotic, immunosuppressive, metabolic dysfunction-regulating, and spermicidal activities (Song X. et al., 2020). Notably, it is the only triterpene derivative with anti-human immunodeficiency virus properties that has been assessed to date in Phase II clinical trials (Timilsina et al., 2016).
3.7 Alkaloids
The alkaloids present in T. wilfordii can be classified into two main categories, sesquiterpenes and indole alkaloids, both of which are characterized by prominent anti-inflammatory, antiviral, immunosuppressive, and insecticidal properties (Fang et al., 2012). To date, four indole alkaloids have been isolated from T. wilfordii, namely, celabazine, celacinnine, celafurine, and celallocinnine (Horiuch et al., 2006), and the findings of relevant studies have indicated that celacinnine and celallocinnine interact with matrix metalloproteinase-9, thereby contributing to an alleviation of skin inflammation (Tang et al., 2017).
3.8 Glycosides
Glycosides extracted from the roots of T. wilfordii have been demonstrated to exert anti-inflammatory and immunosuppressive effects by inhibiting the release of inflammatory cytokines such as interleukin (IL)-6, IL-1β, and tumor necrosis factor-alpha (TNF-α) (Zhang and Chen, 2017). These glycosides have been used extensively in the treatment of inflammatory diseases (Zhang et al., 2021). Mechanistically, they can reduce the activity of NF-κB, suppress the gene expression of cyclooxygenase-2 and M1 biomarker inducible nitric oxide synthase, and reduce the production of prostaglandin E2 and nitric oxide, thereby exerting anti-inflammatory effects (Yang et al., 2020).
3.9 LLDT-8
5R-Hydroxytriptolide (LLDT-8) is a novel analog of T. wilfordii-derived triptolide characterized by low cytotoxicity and high immunosuppressive activity (Su et al., 2017), and numerous in vitro and in vivo studies have demonstrated the significant anti-inflammatory and immunosuppressive properties of this compound. LLDT-8 has been approved by the China Food and Drug Administration as an immunosuppressive drug in clinical trials for RA treatment (Cui et al., 2019), and is currently being investigated as a low-toxicity immunosuppressive agent in Phase I clinical trials for RA in China. The findings of early studies indicated that the activity of LLDT-8 may be associated with anti-inflammatory, antioxidant, and cytokine effects and that it can prevent bleomycin-induced pulmonary fibrosis in mice (Zhou et al., 2021). Recently, the first long non-coding RNA-transcription factor-mRNA co-expression network was constructed to further elucidate the changes in genome-wide long non-coding RNA and mRNA expression before and after LLDT-8 treatment, which indicated that long non-coding RNAs could serve as biomarkers and targets for LLDT-8 drug development (Guo et al., 2019). Additionally, LLDT-8 has been found to have potent anti-inflammatory effects, including the amelioration of anti-glomerular basement membrane glomerulonephritis by modulating the Fcγ signaling pathway and suppression of renal chemokine expression to inhibit immune cell infiltration, thereby ameliorating lupus nephritis (Ma et al., 2017). The protective effects of LLDT-8 include the elimination of activated T cells by promoting the expression of the pro-apoptotic gene signal transducer and activator of signal transducer and activator of transcription 1 and interferon regulatory factor-1 in the spleen (Ziaei and Halaby, 2016). LLDT-8 has also been established to reduce the production of IFN-γ, IL-2, and TNF-α by peripheral blood mononuclear cells (Ibrahim et al., 2021), and has therapeutic potential for the treatment of neurodegenerative diseases by effectively inhibiting pro-inflammatory cytokines (TNF-α and IL-1β) and suppressing the NF-κB signaling pathway, thereby reducing neuroinflammation (Ziaei and Halaby, 2016). Furthermore, LLDT-8 has been demonstrated to reduce the levels of serum alanine aminotransferase and aspartate aminotransferase, liver balloon cell formation, and macrovesicular steatosis, thereby preventing liver injury (Dong et al., 2019). LLDT-8 also regulates the expression of stearoyl-CoA desaturase-1 and peroxisome proliferator-activated receptor alpha, which contribute to promoting significant degradation of lipids and suppression of lipid synthesis (Gao et al., 2021).
4 Tripterygium wilfordii in the treatment of RA
The earliest studies on T. wilfordii were conducted in the 1970s, during which its efficacy in the treatment of RA was initially discovered. Since then, numerous studies have investigated the therapeutic effects of T. wilfordii in the treatment of other rheumatic diseases and autoimmune disorders, including SLE, psoriasis, and multiple sclerosis (Table 2) (Xu et al., 2008). A meta-analysis of randomized controlled trials conducted to assess the efficacy of T. wilfordii in the treatment of RA found that the use of T. wilfordii glycoside tablets alone could reduce the number of tender and swollen joints in patients with RA, with an efficacy comparable to that of methotrexate (MTX). Furthermore, a combination of T. wilfordii glycoside tablets and MTX was found to be superior to the administration of MTX alone in improving the clinical symptoms of patients with RA (Zhou et al., 2018). Similarly, a further systematic review and network meta-analysis evaluating the efficacy and safety of T. wilfordii in treating RA found that the glycosides of this plant administered in combination with MTX may have been the optimal treatment of choice based on an assessment of the ACR20 response. Moreover, among the different treatments assessed, the use of T. wilfordii glycosides alone ranked second only to the T. wilfordii glycoside + MTX combined treatment (Wang et al., 2022).
4.1 Tripterygium wilfordii and its derivatives inhibit RA-associated inflammation
As discussed in previous sections, triptolide, celastrol, and wilforlide A have been shown to significantly inhibit inflammatory responses, with NF-κB activation in B cells being one of the primary targets (Urits et al., 2019). Targets downstream of NF-κB include pro-inflammatory cytokines such as TNF-β, which mediate the inflammatory response via NF-κB signaling (Lin, 2006). T. wilfordii glycosides, such as triptolide, celastrol, and wilforlide A, can exert anti-inflammatory effects by inhibiting the p56 subunit of the NF-κB signaling pathway, as well as by downregulating the expression of inflammatory factors such as TNF and IFN (Li et al., 2021). Studies have shown that NOD-like receptor protein 3 (NLRP3)-induced inflammation plays a key role in RA pathogenesis, and treatment with T. wilfordii has been demonstrated to inhibit activation of the NLRP3 inflammasome by blocking the NF-κB signaling pathway, thereby contributing reductions in joint swelling, arthritis index score, inflammatory cell infiltration, and synovial hyperplasia induced by complete Freund’s adjuvant in rats (Jing et al., 2023). Additionally, significant reductions in the secretion of IL-1β and IL-18 have been observed in rat serum and T helper 1 cell supernatant exposed to a T. wilfordii extract (Kim et al., 2019). Further research has revealed that celastrol can alleviate RA symptoms and suppress inflammation by inhibiting the reactive oxygen species (ROS)–NF–κB–NLRP3 axis. Moreover T. wilfordii glycosides have been established to upregulate the levels of superoxide dismutase and glutathione peroxidase, which induces the clearance of ROS and reactive nitrogen species, thereby reducing oxidative stress and inflammation (Figure 2) (Kim et al., 2019; Lv et al., 2022).
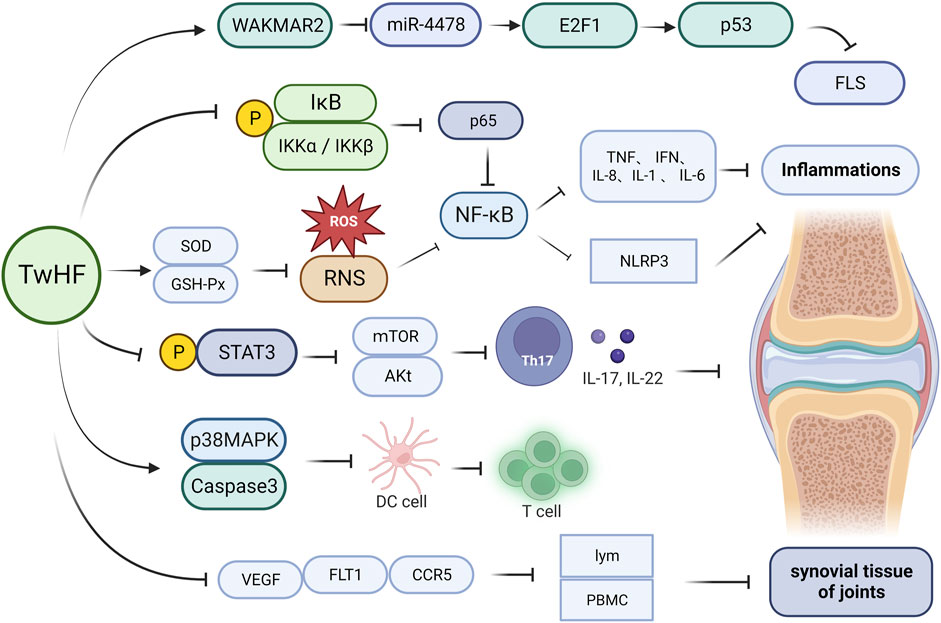
FIGURE 2. Mechanisms underlying the efficacy of Tripterygium wilfordii in the treatment of rheumatoid arthritis. Administration of T. wilfordii preparations can upregulate the levels of SOD and GSH-Px, thereby inducing the clearance of ROS and RNS within organisms. Additionally, T. wilfordii can significantly reduce the phosphorylation of IKKα/IKKβ and IκBα, subsequently inactivating p65. This in turn suppresses the NF-κB signal pathway, leading to a reduction in the release of cytokines, such as IL-1, IL-6, IL-8, TNF, IFN, and NLRP3, and thus achieving an anti-inflammatory effect. Furthermore, T. wilfordii can reduce the phosphorylation of STAT3, thereby reducing the expression of mTOR and Akt. This results in the metabolic disruption of Th17 cells, inhibiting their differentiation and reducing the release of inflammatory cytokines such as IL-17 and IL-22. Moreover, T. wilfordii induces DC apoptosis by activating p38 MAPK and caspase-3, thereby reducing the proliferation and differentiation of T cells, and suppresses the expression of VEGF, FLT1, and CCR5, leading to reduced lymphocyte and peripheral PBMCs of the synovium. This subsequently prevents the damage and deterioration of synovial tissue in the joints. Finally, T. wilfordii upregulates WAKMAR2 to inhibit the expression of miR-4478, subsequently upregulating E2F1 and p53 expression. This cascade leads to the suppression of FLS expression. (SOD, superoxide dismutase; GSH-Px, glutathione peroxidase; ROS, reactive oxygen species; RNS, reactive nitrogen species; NF-κB, nuclear factor kappa-B; TNF, tumor necrosis factor; IFN, interferon; NLRP3, NOD-like receptor protein 3; STAT3, signal transducer and activator of transcription 3; mTOR, mammalian target of rapamycin; AKt, protein kinase B; Th17, T helper cell 17; IL-17, interleukin 17; IL-22, interleukin 22; IL-1, interleukin 1; IL-6, interleukin 6; IL-8, interleukin 8; IκB, inhibitor of NF-κB; IKKα, inhibitor of kappa B kinase alpha; IKKβ, inhibitor of kappa B kinase beta; p65, nuclear factor kappa-B p65; p53, tumor suppressor protein; WAKMAR2, long non-coding RNA; miR-4478, microRNA 4,478; E2F1, E2F transcription factor 1; FLS, fibroblast-like synoviocyte; p38 MAPK, p38 mitogen-activated protein kinase; DC, dendritic cell; CCR5, chemokine receptor 5; PBMC, peripheral blood mononuclear cell; VEGF, vascular endothelial growth factor; FLT1, vascular endothelial growth factor receptor 1; lym, lymphocyte).
Celastrol has been found to significantly inhibit the protein and mRNA expression of the pro-inflammatory cytokines IL-6 and IL-8 and monocyte chemoattractant protein-1 induced by lipopolysaccharide in human retinal pigment epithelial and adult retinal pigment epithelial-19 cells (Tao et al., 2016). Further mechanistic studies have revealed that celastrol significantly reduces the phosphorylation of IKKα/IKKβ and IκBα, leading to the inactivation of p65, and thereby alleviating lipopolysaccharide-induced inflammation by inhibiting the NF-κB signaling pathway (Wang et al., 2017). Administration of T. wilfordii glycosides has also been shown to reduce the expression of the inflammatory factors IL-6, IL-8, NF-κB, and TNF-α (Liu et al., 2014), and promote an upregulation of the levels of IL-10 and CD4+CD25+ regulatory T cells (Tregs), increase the expression of forkhead box protein P3 (FoxP3), downregulate TNF-α and endothelin-1 levels to inhibit paw edema, and reduce the expression of high-mobility group protein 1 and IL-17 in the serum of rats with collagen-induced arthritis (Lei and Jian, 2012; Hao et al., 2022). In other studies, T. wilfordii glycosides have been demonstrated to inhibit the differentiation, maturation, and migration of immature dendritic cells, as well as the secretion of cytokines, thereby suppressing the activation of neutrophils and T cells through the transcriptional signal transducer and activator of STAT pathways. This leads to the downregulation of inducible cyclooxygenase-2, prostaglandins, and metalloproteinases, resulting in an attenuation of the inflammatory responses mediated by these cells (Tian et al., 2020; Kianmehr et al., 2023). Mitochondria play essential roles in cell death, autophagy, immunity, and inflammation, and as mentioned earlier, Nur77 can induce cell apoptosis by targeting mitochondria (Lith and de Vries, 2021). Studies have shown that T. wilfordii glycosides can interact with Nur77, thereby promoting its translocation from the nucleus to mitochondria. Within the mitochondria, Nur77 interacts with toll-like receptor 2, leading to its ubiquitination. The ubiquitinated Nur77 remains within the mitochondria, rendering these organelles sensitive to autophagy and facilitating the clearance of damaged mitochondria, thereby exerting anti-inflammatory effects (Sommer et al., 2021). Furthermore, glycosides of T. wilfordii have been shown to reduce the expression of CD80 and CD86 induced by IFN-γ and inhibit the expression of IL-23 and IL-1 in dendritic cells and T helper 1 cells (Wangchuk et al., 2018), thereby retarding the progression of inflammatory diseases. Furthermore, these glycosides have also been reported to inhibit RA-induced cell autophagy by suppressing activation of the toll-like receptor 2/high-mobility group protein signaling pathway, (Abdin and Hasby, 2014; Elshabrawy et al., 2017).
4.2 Tripterygium wilfordii and its derivatives inhibit the progression of RA via immune cell modulation
Normal functioning of the immune system is essential for maintaining bodily homeostasis; consequently, immune dysregulation can have particularly detrimental effects. Many autoimmune diseases develop as a response to abnormal immune expression, in which the body’s own tissues are mistakenly targeted, thus leading to the development of diseases such as RA, SLE, and psoriasis (Perl, 2017). In this context, T. wilfordii has been demonstrated to inhibit the proliferation of T and B cells, reduce the production of proinflammatory cytokines, and induce the apoptosis of activated lymphocytes (Lu et al., 2017). T. wilfordii glycosides have been shown to downregulate expression of the cell factors TNF-β, IL-6, IL-1, and IL-8 and upregulate the expression of IL-10, thereby inhibiting the activity of Th1 cells and promoting the transformation of Th1 to Th2 cells. These glycosides can also inhibit the synthesis and release of NF-κB, promote apoptosis, and reduce the occurrence and progression of immune reactions (Lin et al., 2001). In addition to these effects, T. wilfordii glycosides can contribute to lowering the levels of immunoglobulins IgE and IgA, enhance the function of CD8+ T cells, and inhibit the function of CD4+ T cells, thereby reducing the CD4+/CD8+ T cell ratio and inhibiting further development of abnormal immune responses (Liu et al., 2019).
The immunosuppressive effects T. wilfordii glycosides can be attributed to an inhibition of STAT3 phosphorylation, thereby suppressing Th17 cell function. Whereas Th17 cells promote inflammation, Tregs have anti-inflammatory functions (Balkrishna et al., 2020); therefore, maintaining an appropriate balance between Th17 cells and Tregs is essential for the maintenance of immune homeostasis. In this regard, it has been found that T. wilfordii can inhibit the expression of mammalian target of rapamycin, hypoxia-inducible factor 1-alpha, c-Myc, and protein kinase B, leading to metabolic blockade of Th17 cells and thus inhibiting their differentiation (Tang et al., 2020). Conversely, by upregulating carnitine palmitoyltransferase 1A and adenosine 5ʹ-monophosphate-activated protein kinase alpha, T. wilfordii promotes lipid fatty acid oxidation, thereby facilitating the generation of inducible Tregs (Marks, 2011). Moreover, it has been demonstrated that the administration of T. wilfordii significantly increases the Bax/Bcl-2 ratio, induces the release of mitochondrial cytochrome C into the cytoplasm, and activates caspase-3 and caspase-9, ultimately inducing T cell apoptosis via the mitochondrial apoptosis pathway (Jin et al., 2022).
Assisting cells, particularly dendritic cells, are professional antigen-presenting cells that have been established to be further targets of T. wilfordii glycosides (Zhang G. et al., 2013). These glycosides inhibit the differentiation, maturation, trafficking, and functioning of immature dendritic cells (Wangchuk et al., 2018). High concentrations (>20 ng/mL) of T. wilfordii glycosides have been observed to induce dendritic cell apoptosis via the sequential phosphorylation of p38 mitogen-activated protein kinase and activation of caspase-3 (Ziaei and Halaby, 2016). The chemotaxis of neutrophils and T cells mediated by dendritic cells has also been shown to be inhibited by a T. wilfordii glycoside-mediated reduction in STAT3 phosphorylation and activation of NF-κB (Zhang and Ma, 2010). Moreover, these glycosides prevent the differentiation of immature monocyte-derived dendritic cells by downregulating the expression of CD1a, CD40, CD80, CD86, and HLA-DR, as well as reduce the capacity of monocyte-derived dendritic cells to stimulate lymphocyte proliferation in allogeneic mixed lymphocyte reactions (Zhao et al., 2021). Recently, it has also been demonstrated that T. wilfordii glycosides inhibit the expression of IL-12/IL-23 in antigen-presenting cells by binding with CCAAT-enhancer-binding protein α, thus providing mechanistic insights into the immunomodulatory properties of these glycosides (Ziaei and Halaby, 2016).
4.3 Tripterygium wilfordii and its derivatives alleviate joint damage in RA
With respect to the treatment of RA, T. wilfordii glycosides have been demonstrated to inhibit the release of chemotactic factors from macrophages, thereby reducing their effects on synovial cells and chondrocytes, and thus inhibiting the abnormal proliferation of synovial cells (Baoqi et al., 2021). Furthermore, treatment with LLDT-8 has been found to suppress matrix metalloproteinase-13 production via the OPG/RANK/RANKL signaling pathway, increase OPG/RANKL expression, and attenuate collagen-induced arthritis (Shen et al., 2015). Moreover, T. wilfordii-derived triptolide can inhibit the expression of vascular endothelial growth factor (VEGF), VEGF receptor 1, and chemokine receptor 5 in the joint tissues of rats with adjuvant-induced arthritis. This activity has the effects of reducing the infiltration of lymphocytes and peripheral blood mononuclear cells into the synovium, thereby suppressing damage and degradation of the synovial tissue. Triptolide also targets neutrophils in mice with RA, promotes neutrophil apoptosis, and inhibits neutrophil autophagy and neutrophil extracellular trap formation, thereby reducing tissue damage and inflammation (Zhang B. et al., 2013). Studies have also shown that T. wilfordii glycosides can significantly alleviate paw swelling in rats (as determined by performing traditional anti-inflammatory tests), downregulate the expression of VEGF and VEGF receptor 2, and reduce vascular permeability and angiogenesis (Ashrafizadeh et al., 2022). Furthermore, by inhibiting the expression of miR-4478, T. wilfordii upregulates WAKMAR2, which in turn promotes an upregulation of E2F transcription factor 1 and p53 expression, with the outcome of this cascade being a suppression of fibroblast-like synoviocyte expression (Zhou et al., 2021).
5 Tripterygium wilfordii treatment of other rheumatic diseases
5.1 Systemic lupus erythematosus
SLE is a chronic autoimmune disease characterized by a wide range of clinical symptoms, notably the abnormal production of self-antibodies, such as antinuclear and anti-double-stranded DNA antibodies (Moulton et al., 2017). SLE predominantly affects women of childbearing age, with a male-to-female patient ratio of approximately 1:9, and is accordingly speculated to be influenced by female hormones (Ameer et al., 2022). Moreover, genetic and environmental factors have been established to contribute to the development of SLE (Li et al., 2010). T. wilfordii has a long history of use in the treatment of SLE and, when administered with appropriate care, patients can experience satisfactory alleviation. In therapeutic strategies for this disease, the use of selected immunosuppressive agents can contribute to reducing the cumulative steroid dose and the prevention of disease relapse. Similarly, in patients with refractory or relapsed SLE, the use of other immunosuppressive agents can contribute to a reduction in steroid dosage, control of disease activity, and improvements in the rates of clinical remission (Li and Yang, 2022). In a study of patients with SLE, treatment with a T. wilfordii extract combined with prednisone was demonstrated to increase the levels of CD4+ and CD25+ T cells, thus enhancing immune tolerance in these patients. Based on the findings of these studies, it can be concluded that the regulatory effects of T. wilfordii extract on T cells further enhance its protective effects in patients with SLE. Studies have shown that neutrophil extracellular traps, web-like structures released by neutrophils, are involved in driving autoimmunity and tissue damage in SLE (Georgakis et al., 2021), and in this regard, it has been found that T. wilfordii can downregulate the activation of spleen tyrosine kinase and phosphorylation of mitogen-activated protein kinase, extracellular signal-regulated kinase, and IκBα, as well as the guanine methylation of histones. By downregulating the spleen tyrosine kinase–mitogen-activated protein kinase kinase–extracellular signal-regulated kinase–NF-κB signaling cascade, T. wilfordii can effectively inhibit neutrophil oxidative bursts and the formation of neutrophil extracellular traps induced by diverse inflammatory stimuli, thereby inhibiting SLE development (Kato et al., 2013).
5.2 Psoriasis
Psoriasis, a chronic skin disease affecting over 100 million people worldwide (Griffiths and Barker, 2007), is characterized by the typical symptoms of erythema, itching, and scaling, leading to physical discomfort and psychological burden (Kamiya et al., 2019). Psoriasis is a disease involving both the innate and adaptive immune systems, in which keratinocytes, dendritic cells, and T cells play key roles in disease development (Li and Yang, 2022). It has been found that an extract of T. wilfordii can inhibit the excessive proliferation of human keratinocytes (HaCaT cells) and significantly reduce the mRNA levels of inflammatory cytokines such as TNF-α and IL-6 in HaCaT cells. By modulating the interactions between keratinocytes and downstream dendritic cells and T cells in the immune system, as well as reducing the expression levels of inflammatory cytokines in the skin and circulation, T. wilfordii has an inhibitory effect on systemic inflammation, thus achieving therapeutic effects in patients with psoriasis (Parveen et al., 2019). Furthermore, T. wilfordii extract has been shown to regulate the activity of dendritic cells and T cells, suppress the generation of CD4 T and Th17 cells, inhibit the development of Th17 cells, and inhibit the proliferation of keratinocytes and epidermal cells, as well as the expression of inflammatory cytokines such as TNF-α and IL-17A (Guo et al., 2020). Similarly, in murine animal models, treatment with T. wilfordii extract has been shown to significantly reduce white scales, erythema, inflammatory cells, excessive keratinization, and incomplete keratinization. Furthermore, this treatment contributes to marked reductions in the release of inflammatory factors, such as IL-17, INF-γ, and IL-22, in the plasma at the mRNA and protein levels (Maqbool et al., 2021).
5.3 Crohn’s disease
Crohn’s disease is a chronic granulomatous inflammatory condition that affects the entire gastrointestinal tract, particularly the ileocecal and perianal areas (Baumgart and Sandborn, 2012). Although the exact etiology of Crohn’s has yet to be sufficiently established, several specific factors are known to be directly associated with the disease, including genetic factors, intestinal immune dysregulation, and alterations in the gut microbiota (Mazal, 2014). Recent findings have emphasized the key role played by the gut microbiota in the development of this disease, which is in turn, closely associated with sustained adverse inflammatory responses (Rodríguez et al., 2020). Currently, the treatment options for patients with CD are rather limited, although the use of natural products, such as extracts of T. wilfordii, which may have fewer side effects than conventional medications, has clear appeal (Zhang et al., 2020). Studies have reported that an extract of T. wilfordii induces apoptosis in T lymphocytes and dendritic cells by inhibiting the NF-κB signaling pathway and reduces the production of pro-inflammatory cytokines such as TNF-α and IL-1, thereby alleviating intestinal inflammation in patients (Ren et al., 2013). It has also been found that FoxP3 Tregs play a vital role in maintaining intestinal immune balance via the actions of IL-10 and transforming growth factor-beta, and that T. wilfordii extract promotes mucosal healing by upregulating FoxP3 Tregs and IL-10, downregulating inflammatory cytokines such as TNF-α, and modulating the local production of other inflammatory cytokines (Li et al., 2014).
5.4 Multiple sclerosis
Multiple sclerosis, also known as demyelinating disease, is a multifocal chronic autoimmune inflammatory disease of the central nervous system (Doshi and Chataway, 2016). Although the etiology of multiple sclerosis has yet to be elucidated, the accumulation and activation of monocytes within the central nervous system have been established to play key roles in its pathogenesis. Chemokines are major players in leukocyte recruitment and activation at the sites of inflammation (Moreira et al., 2006), and studies have shown that neuroinflammation can have both detrimental and beneficial effects on the function of neurons and glial cells, with the NF-κB signaling pathway playing a pivotal role in controlling this process. These findings accordingly indicate that selective anti-NF-κB treatment strategies may contribute to minimizing damage during the acute and chronic inflammatory phases of this disease (Webb et al., 2017; Sanadgol et al., 2018). Experimental autoimmune encephalomyelitis is a T cell-mediated autoimmune disease of the central nervous system that serves as an animal model of human multiple sclerosis (Baecher-Allan et al., 2018), and it has been proposed that T. wilfordii may act as an active suppressor of experimental autoimmune encephalomyelitis, whereas LLDT-8 has been shown to inhibit T cell proliferation and activation (Fu et al., 2006). Specifically, it can attenuate the inflammatory response in the experimental autoimmune encephalomyelitis model by increasing the levels of heat shock protein 70 and stabilizing the NF-κB/IκB complex (Figure 3) (Zheng et al., 2013).
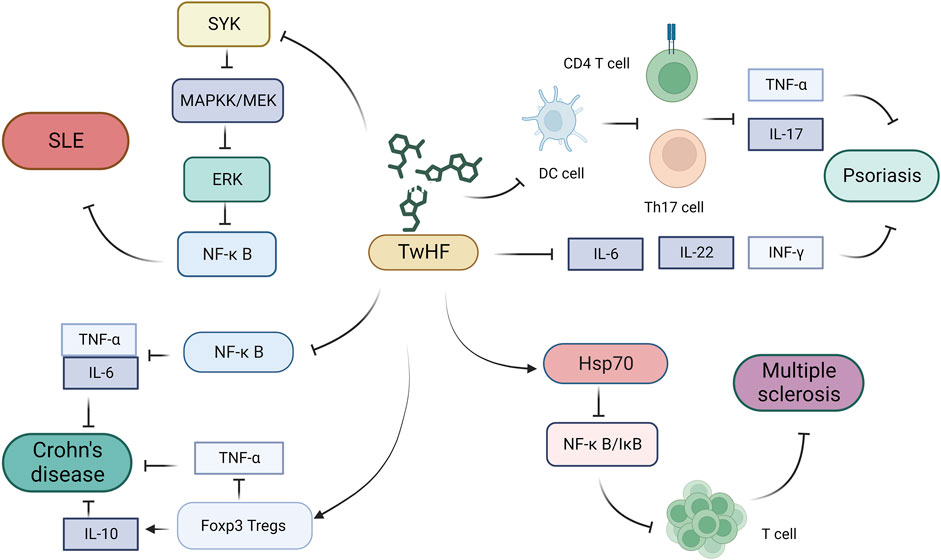
FIGURE 3. Mechanisms underlying the efficacy of Tripterygium wilfordii in the treatment of other rheumatic diseases (Ma et al., 2020). T. wilfordii can downregulate the activation of SYK, exerting an inhibitory effect on the pathogenesis of SLE through downregulation of the SYK–MEK–ERK–NF-κB signaling cascade (Schwenzer et al., 2010). T. wilfordii suppresses the proliferation of CD4+T and Th17 cells, diminishes the expression of inflammatory cytokines, such as TNF-α and IL-17A, and further inhibits the release of inflammatory factors, including IL-6, INF-γ, and IL-22. Consequently, this attenuates the progression of psoriasis (Tsitrouli et al., 2021). T. wilfordii inhibits the NF-κB signaling pathway, leading to reduced production of the pro-inflammatory cytokines TNF-α and ILN-6, thereby alleviating intestinal inflammatory responses in patients with Crohn’s disease. Additionally, T. wilfordii upregulates FoxP3 Tregs and IL-10 expression while downregulating the inflammatory cytokine TNF-α, thereby suppressing the progression of Crohn’s disease (Shi et al., 2020). T. wilfordii elevates Hsp70 levels to inhibit the NF-κB/IκB complex, thereby attenuating T cell proliferation and differentiation, resulting in the suppression of inflammatory responses in multiple sclerosis. (SYK, spleen tyrosine kinase; MAPKK/MEK, mitogen-activated protein kinase; ERK, extracellular signal-regulated kinase; NF-κB, nuclear factor kappa-B; SLE, systemic lupus erythematosus; DC, dendritic cell; Th17, T helper cell 17; IL-17, interleukin 17; IL-6, interleukin 6; IL-10, interleukin 10; IL-22, interleukin 22; IFN-γ, gamma interferon; TNF-α, tumor necrosis factor alpha; FoxP3, forkhead box Protein 3; IκB, inhibitor of NF-κB; Hsp70, heat shock protein 70).
6 Toxic effects of Tripterygium wilfordii
In addition to its therapeutic efficacy, the safety of the medicinal use of T. wilfordii has been extensively studied. Although preparations of this plant are generally well tolerated, they can cause a rage of side effects, including gastrointestinal discomfort, rashes, alopecia, liver and kidney damage, reproductive toxicity, and myelosuppression (Xi et al., 2017). A recent meta-analysis of T. wilfordii in the treatment of renal disease indicated that approximately 11.7% of patients experienced adverse events after taking the T. wilfordii preparations. Briefly, the authors found that 273 studies reported gastrointestinal reactions, in which 4,843 (8.6%) patients had elevated levels of alanine transaminase (twice the normal level), 12.7% of female patients had menstrual disorders, approximately 4.9% of patients experienced cardiovascular events, and approximately 6.5% and 7.8% of patients experienced hematological and mucosal damage, respectively. Moreover, 43 studies reported renal damage in 193 patients and 159 studies reported alopecia, weight loss, and fatigue in 1,007 patients (Zhang et al., 2016). However, despite their potential severity, these adverse effects are generally reversible and can be prevented through careful monitoring and dose adjustment (Baoqi et al., 2021).
6.1 Hepatotoxic side effects
During its hepatic metabolism, metabolites of T. wilfordii undergo lipid peroxidation, which can lead to hepatocytic necrosis. Hepatotoxicity is among the major toxic reactions associated with the use of T. wilfordii, which manifests clinically as liver discomfort, hepatomegaly, and abnormal liver function. This is primarily characterized by elevated activities of alanine aminotransferase and aspartate aminotransferase, increased levels of total bile acids and total bilirubin, lower levels of prealbumin expression, hepatocellular steatosis, necrosis, and inflammatory cell infiltration (Zhong et al., 2013). Mechanistic studies that have examined the hepatotoxicity of T. wilfordii glycosides have revealed that when administered at high doses, these glycosides can inhibit the mRNA expression of key hepatic cytochrome P450 family members, including CYP27A1 and CYP8B1, as well as that of antioxidative enzymes such as SOD-1 and glutathione peroxidase 1. The consequent reduced protein expression of these enzymes results in bile stasis, mitochondrial damage, and a series of oxidative stress responses within the liver (Li et al., 2020).
6.2 Nephrotoxic side effects
The nephrotoxicity associated with T. wilfordii usage is mainly attributable to its diterpenoid, triterpenoid, and alkaloid metabolites, which can damage renal tubular epithelial cells. The clinical manifestations of T. wilfordii-induced nephrotoxicity include oliguria, edema, hematuria, hypotension, and hyperkalemia (Zhang Q. et al., 2018). Administration of a T. wilfordii extract to rats through gavage has be shown to result in elevated levels of blood creatinine, blood urea nitrogen, and uric acid, as well as causing renal glomerular atrophy, liquefaction, and necrosis of the local renal tubules (Shen et al., 2020). Further investigations have revealed that the molecular mechanisms underlying T. wilfordii-induced nephrotoxicity may involve an increase in the levels of the death receptor Fas and its ligand FasL through the mediation of triptolide. Additionally, cytochrome C, an intrinsic pathway mediator, is released into the cytoplasm, leading to alterations in electron transport, loss of mitochondrial membrane potential, and induction of apoptosis through multiple pathways (Feng et al., 2019). It has also been suggested that T. wilfordii-induced renal injury may be attributable to an impairment of angiotensin II receptor synthesis, resulting in elevated levels of ROS in renal tissue and the promotion of cell apoptosis by damaging the renal glomeruli (Zhang C. et al., 2018).
6.3 Reproductive toxicity
Toxic side effects of T. wilfordii have also been reported for the reproductive system, as exemplified by instances of amenorrhea in women and reduced sperm counts in men (Singla and Challana, 2014), and these have been found to be significantly correlated with patient age, drug dosage, and course of treatment. As a cytotoxic medication, T. wilfordii mainly acts on follicular cells, thereby reducing the level of estrogen secreted by these follicles (Deng et al., 2022). Additionally, preparations of this plant can reduce progesterone levels, thereby altering the levels of hormones in the hypothalamus–pituitary–gonadal axis and leading to abnormalities in the menstrual cycle and thus menstrual disorders or amenorrhea. Furthermore, T. wilfordii has been shown to reduce the expression of spermatogenesis-related genes and inhibit the morphological and functional development of spermatozoa, leading to reduced fertility (Ruan et al., 2018), whereas in animal experiments, T. wilfordii has been found to induce mitochondrial apoptosis of ovarian granulosa cells in NIH mice, leading to ovarian damage (Zeng et al., 2017). In the latter case, however, it was established that the perturbation of ovarian function caused by tretinoin was reversible, and that function recovered naturally after discontinuing administration of the drug.
6.4 Gastrointestinal side effects
Adverse reactions of the human gastrointestinal tract associated with the oral administration of different preparations of T. wilfordii primarily include a loss of appetite, dry mouth, nausea and vomiting, constipation, abdominal pain, and diarrhea (Liu et al., 2018). However, most patients tolerate these symptoms and recover after discontinuing administration of the drug. In laboratory studies, mice administered the LD50 dosage of triptolide, either intraperitoneally and orally, showed significant congestion at the base of the stomach and irregularly scattered intestinal ulcers. In a study on the in vivo activity of triptolide, it was found that although this compound was readily absorbed by the gastrointestinal tract, the absorption was incomplete, which may account for the gastrointestinal tract irritation associated with triptolide usage (Liu Y. et al., 2013).
6.5 Other side effects
Abnormal skin-related symptoms are an additional frequently reported side effect of T. wilfordii usage, among which skin pigmentation, drug eruption, erythema nodosum, oral mucosal herpes, and skin allergic vasculitis are common reactions (Tao et al., 2002). However, most of these responses require no special treatment and resolve after a reduction in dosage or discontinued administration of the drug. Furthermore, celastrol has been shown to inhibit cell viability and migration, block the cell cycle at the G0/G1 phase, promote apoptosis by enhancing the expression of caspase-3 and Bax, and reduce the expression of Bcl-2 in human biliary epithelial cell death, thereby inducing cholangiocytotoxicity (Y-JJCT and Drugs, 2020). Furthermore, triptolide has been found to inhibit the proliferation and viability of inner ear stem cells, and induces apoptosis by enhancing the expression of the DNA damage repair proteins γH2AX and 53BP1. Moreover, it has been speculated that triptolide-induced inner ear stem cell cytotoxicity may be associated with mitochondrial dysfunction caused by optic nerve atrophy and incision (Tang et al., 2019).
7 Discussion
T. wilfordii has a long-standing history and extensive application in the treatment of rheumatic and autoimmune diseases, often with notable clinical efficacy. With continual advances in clinical research and progress in the pharmacology and toxicology of T. wilfordii, a diverse range of bioactive metabolites within this plant have gradually been discovered and demonstrated to have notable anti-inflammatory and immunomodulatory effects. In this review, we have systematically summarized the historical evolution of T. wilfordii in the treatment of rheumatic and autoimmune diseases, along with its global distribution. We also describe the effective metabolites produced by this plant and their derivatives, which have been shown to have notable anti-inflammatory, anticancer, immunosuppressive, and immunomodulatory properties (Table 3). Additionally, we present a comprehensive overview of the mechanistic actions of T. wilfordii in the treatment rheumatoid arthritis, including immune suppressive, anti-inflammatory, anti-angiogenic, and bone and cartilage protective effects, and discuss the mechanisms underlying the efficacy of T. wilfordii treatment for other rheumatic and autoimmune diseases, namely, SLE, psoriasis, Crohn’s disease, and multiple sclerosis. Moreover, we highlight the potential toxic effects of T. wilfordii preparations, primarily in terms of hepatorenal damage. However, although these effects have been observed in clinical settings and are clearly undesirable, many eventually resolve without intervention, or can be partially reversed or alleviated through dose adjustment.
Although significant progress has been made in the treatment of rheumatic and autoimmune diseases using T. wilfordii, particularly in the case of RA, limitations persist in advanced chemical and pharmacological methods, as well as in the accumulation of experience in clinical practice. Despite substantial accomplishments in clinical trials, meta-analyses, experimental studies, and guideline development, gaps remain in our understanding of the pathogenesis and etiology of rheumatic and autoimmune diseases, as well as the precise mechanisms of action of T. wilfordii preparations. Currently, these gaps present a substantial hurdle hampering the more widespread application of T. wilfordii preparations in countries other than China, as well as the realization of personalized treatment for patients. Moreover, T. wilfordii and the extracts thereof contain a diverse range of metabolites that may have synergistic or antagonistic effects, which consequently presents considerable challenges in establishing clear associations between these metabolites and their corresponding biological targets. Accordingly, elucidating the potential molecular mechanisms underlying the effects of T. wilfordii metabolites and delineating their functional and toxicological characteristics are of paramount importance. An integrated approach that combines clinical data with systems biology, network pharmacology, analytical chemistry, and molecular biology approaches is necessary to achieve these goals.
Author contributions
YS and JZ is responsible for the collection, collation, and writing of the original manuscript. KW, PJ, LX, CC, LX, YZ, YS, YZ, LL, YB, SS and for the collection, collation of the original data. SG and DH are responsible for the concept development, revision, and manuscript review. All authors reviewed and accepted the final version.
Funding
The author(s) declare financial support was received for the research, authorship, and/or publication of this article. This work was funded by the National Natural Science Funds of China (82074234, 82004166 and 82071756), Shanghai Chinese Medicine Development Office, National Administration of Traditional Chinese Medicine, Regional Chinese Medicine (Specialist) Diagnosis and Treatment Center Construction Project-Rheumatology, State Administration of Traditional Chinese Medicine, Shanghai Municipal Health Commission, East China Region-based Chinese and Western Medicine Joint Disease Specialist Alliance, and Shanghai He Dongyi Famous Chinese Medicine Studio Construction Project (SHGZS-202220).
Conflict of interest
The authors declare that the research was conducted in the absence of any commercial or financial relationships that could be construed as a potential conflict of interest.
The author(s) declared that they were an editorial board member of Frontiers, at the time of submission. This had no impact on the peer review process and the final decision
Publisher’s note
All claims expressed in this article are solely those of the authors and do not necessarily represent those of their affiliated organizations, or those of the publisher, the editors and the reviewers. Any product that may be evaluated in this article, or claim that may be made by its manufacturer, is not guaranteed or endorsed by the publisher.
References
Abdin, A. A., and Hasby, E. A. (2014). Modulatory effect of celastrol on Th1/Th2 cytokines profile, TLR2 and CD3+ T-lymphocyte expression in a relapsing-remitting model of multiple sclerosis in rats. Eur. J. Pharmacol. 742, 102–112. doi:10.1016/j.ejphar.2014.09.001
Ameer, M. A., Chaudhry, H., Mushtaq, J., Khan, O. S., Babar, M., Hashim, T., et al. (2022). An overview of systemic lupus erythematosus (SLE) pathogenesis, classification, and management. Cureus 14 (10), e30330. doi:10.7759/cureus.30330
Ashrafizadeh, M., Paskeh, M. D. A., Mirzaei, S., Gholami, M. H., Zarrabi, A., Hashemi, F., et al. (2022). Targeting autophagy in prostate cancer: preclinical and clinical evidence for therapeutic response. J. Exp. Clin. cancer Res. CR 41 (1), 105. doi:10.1186/s13046-022-02293-6
Baecher-Allan, C., Kaskow, B. J., and Weiner, H. L. (2018). Multiple sclerosis: mechanisms and immunotherapy. Neuron 97 (4), 742–768. doi:10.1016/j.neuron.2018.01.021
Balkrishna, A., Thakur, P., Singh, S., Chandra Dev, S. N., and Varshney, A. (2020). Mechanistic paradigms of natural plant metabolites as remedial candidates for systemic lupus erythromatosus. Syst. Lupus Erythromatosus. Cells. 9 (4), 1049. doi:10.3390/cells9041049
Baoqi, Y., Dan, M., Xingxing, Z., Xueqing, Z., Yajing, W., Ke, X., et al. (2021). Effect of anti-rheumatic drugs on cardiovascular disease events in rheumatoid arthritis. Front. Cardiovasc. Med. 8, 812631. doi:10.3389/fcvm.2021.812631
Baumgart, D. C., and Sandborn, W. J. (2012). Crohn's disease. Lancet (London, Engl. 380 (9853), 1590–1605. doi:10.1016/s0140-6736(12)60026-9
Cao, Y., Liu, J., Huang, C., Tao, Y., Wang, Y., Chen, X., et al. (2022). Wilforlide A ameliorates the progression of rheumatoid arthritis by inhibiting M1 macrophage polarization. J. Pharmacol. Sci. 148 (1), 116–124. doi:10.1016/j.jphs.2021.10.005
Chang, W., He, W., Li, P. P., Song, S. S., Yuan, P. F., Lu, J. T., et al. (2016). Protective effects of Celastrol on diethylnitrosamine-induced hepatocellular carcinoma in rats and its mechanisms. Eur. J. Pharmacol. 784, 173–180. doi:10.1016/j.ejphar.2016.04.045
Chen, D. L., Li, X., and Zhou, X. J. (2020). Research progress on sesquiterpenes and its pharmacological activities in genus Carpesium. Zhongguo Zhong yao za zhi 45 (1), 37–51. doi:10.19540/j.cnki.cjcmm.20190929.201
Chen, H. Y., Chiang, Y. F., Hong, Y. H., Shieh, T. M., Huang, T. C., Ali, M., et al. (2022). Quercetin ameliorates renal injury and pyroptosis in lupus nephritis through inhibiting IL-33/ST2 pathway in vitro and in vivo. Antioxidants (Basel, Switz. 11 (11), 2238. doi:10.3390/antiox11112238
Cheng, Y., Zhao, Y., and Zheng, Y. (2021). Therapeutic potential of triptolide in autoimmune diseases and strategies to reduce its toxicity. Chin. Med. 16 (1), 114. doi:10.1186/s13020-021-00525-z
Cui, Y. Q., Zheng, Y., Tan, G. L., Zhang, D. M., Wang, J. Y., and Wang, X. M. (2019). (5R)-5-hydroxytriptolide inhibits the inflammatory cascade reaction in astrocytes. Neural Regen. Res. 14 (5), 913–920. doi:10.4103/1673-5374.249240
Deng, D., Yan, J., Li, W., Wu, Y., and Wu, K. (2022). Protective effect of XinJiaCongRongTuSiZiWan on the reproductive toxicity of female rats induced by triptolide. eCAM 2022, 3642349. doi:10.1155/2022/3642349
Dong, Y., Lu, H., Li, Q., Qi, X., Li, Y., Zhang, Z., et al. (2019). (5R)-5-hydroxytriptolide ameliorates liver lipid accumulation by suppressing lipid synthesis and promoting lipid oxidation in mice. Life Sci. 232, 116644. doi:10.1016/j.lfs.2019.116644
Doshi, A., and Chataway, J. (2016). Multiple sclerosis, a treatable disease. Clin. Med. Lond. Engl. 16 (6), s53–s59. doi:10.7861/clinmedicine.16-6-s53
Elshabrawy, H. A., Essani, A. E., Szekanecz, Z., Fox, D. A., and Shahrara, S. (2017). TLRs, future potential therapeutic targets for RA. Autoimmun. Rev. 16 (2), 103–113. doi:10.1016/j.autrev.2016.12.003
Fang, W., Peng, F., Yi, T., Zhang, C., Wan, C., Xu, H., et al. (2012). Biological activity and safety of Tripterygium extract prepared by sodium carbonate extraction. Mol. (Basel, Switz. 17 (9), 11113–11123. doi:10.3390/molecules170911113
Feng, X., Fang, S. N., Liang, N., Liu, J. P., and Chen, W. (2019). Nephrotoxicity of tripterygium wilfordii hook. f preparations: a systematic review and meta-analysis. J. Altern. complementary Med. (New York, NY) 25 (1), 16–31. doi:10.1089/acm.2018.0270
Fu, Y. F., Zhu, Y. N., Ni, J., Zhong, X. G., Tang, W., Zhou, R., et al. (2006). 5R)-5-hydroxytriptolide (LLDT-8), a novel triptolide derivative, prevents experimental autoimmune encephalomyelitis via inhibiting T cell activation. J. Neuroimmunol. 175 (1-2), 142–151. doi:10.1016/j.jneuroim.2006.03.011
Gao, J., Zhang, Y., Liu, X., Wu, X., Huang, L., and Gao, W. (2021). Triptolide: pharmacological spectrum, biosynthesis, chemical synthesis and derivatives. Theranostics 11 (15), 7199–7221. doi:10.7150/thno.57745
Georgakis, S., Gkirtzimanaki, K., Papadaki, G., Gakiopoulou, H., Drakos, E., Eloranta, M. L., et al. (2021). NETs decorated with bioactive IL-33 infiltrate inflamed tissues and induce IFN-α production in patients with SLE. JCI insight 6 (21), e147671. doi:10.1172/jci.insight.147671
Ghante, M. H., and Jamkhande, P. G. (2019). Role of pentacyclic triterpenoids in chemoprevention and anticancer treatment: an overview on targets and underling mechanisms. J. pharmacopuncture 22 (2), 55–67. doi:10.3831/kpi.201.22.007
Gonçalves, E. C. D., Baldasso, G. M., Bicca, M. A., Paes, R. S., Capasso, R., and Dutra, R. C. (2020). Terpenoids, cannabimimetic ligands, beyond the cannabis plant. Mol. (Basel, Switz. 25 (7), 1567. doi:10.3390/molecules25071567
Griffiths, C. E., and Barker, J. N. (2007). Pathogenesis and clinical features of psoriasis. Lancet (London, Engl. 370 (9583), 263–271. doi:10.1016/s0140-6736(07)61128-3
Guo, L., Wang, C., Qiu, X., Pu, X., and Chang, P. (2020). Colorectal cancer immune infiltrates: significance in patient prognosis and immunotherapeutic efficacy. Front. Immunol. 11, 1052. doi:10.3389/fimmu.2020.01052
Guo, S., Liu, J., Jiang, T., Lee, D., Wang, R., Zhou, X., et al. (2019). 5R)-5-Hydroxytriptolide (LLDT-8) induces substantial epigenetic mediated immune response network changes in fibroblast-like synoviocytes from rheumatoid arthritis patients. Sci. Rep. 9 (1), 11155. doi:10.1038/s41598-019-47411-1
Guo, X., Xue, M., Li, C. J., Yang, W., Wang, S. S., Ma, Z. J., et al. (2016). Protective effects of triptolide on TLR4 mediated autoimmune and inflammatory response induced myocardial fibrosis in diabetic cardiomyopathy. J. Ethnopharmacol. 193, 333–344. doi:10.1016/j.jep.2016.08.029
Hao, F., Wang, Q., Liu, L., Wu, L. B., Cai, R. L., Sang, J. J., et al. (2022). Effect of moxibustion on autophagy and the inflammatory response of synovial cells in rheumatoid arthritis model rat. J. traditional Chin. Med. 42 (1), 73–82. doi:10.19852/j.cnki.jtcm.20210324.002
Hernandes, C., Pereira, A. M. S., and Severino, P. (2017). Compounds from celastraceae targeting cancer pathways and their potential application in head and neck squamous cell carcinoma: a review. Curr. genomics 18 (1), 60–74. doi:10.2174/1389202917666160803160934
Horiuch, M., Murakami, C., Fukamiya, N., Yu, D., Chen, T. H., Bastow, K. F., et al. (2006). Tripfordines A-C, sesquiterpene pyridine alkaloids from Tripterygium wilfordii, and structure anti-HIV activity relationships of Tripterygium alkaloids. J. Nat. Prod. 69 (9), 1271–1274. doi:10.1021/np060124a
Hou, H. W., Wang, J. M., Wang, D., Wu, R., and Ji, Z. L. (2017). Triptolide exerts protective effects against fibrosis following ileocolonic anastomosis by mechanisms involving the miR-16-1/HSP70 pathway in IL-10-deficient mice. Int. J. Mol. Med. 40 (2), 337–346. doi:10.3892/ijmm.2017.3016
Hou, W., Liu, B., and Xu, H. (2020). Celastrol: progresses in structure-modifications, structure-activity relationships, pharmacology and toxicology. Eur. J. Med. Chem. 189, 112081. doi:10.1016/j.ejmech.2020.112081
Hu, M., Luo, Q., Alitongbieke, G., Chong, S., Xu, C., Xie, L., et al. (2017). Celastrol-induced Nur77 interaction with TRAF2 alleviates inflammation by promoting mitochondrial ubiquitination and autophagy. Mol. cell 66 (1), 141–153. doi:10.1016/j.molcel.2017.03.008
Ibrahim, S. R. M., Sirwi, A., Eid, B. G., Mohamed, S. G. A., and Mohamed, G. A. (2021). Summary of natural products ameliorate concanavalin A-induced liver injury: structures, sources, pharmacological effects, and mechanisms of action. Plants (Basel, Switz. 10 (2), 228. doi:10.3390/plants10020228
Jia, Y., Lei, L., Luo, X., Zhao, Z., Wang, M., and van Andel, T. (2021). Analysis of historical changes in traditional Chinese medicine based on an Indonesian collection of Chinese materia medica from c. 1870. J. Ethnopharmacol. 269, 113714. doi:10.1016/j.jep.2020.113714
Jiang, Q., Tang, X. P., Chen, X. C., Xiao, H., Liu, P., and Jiao, J. (2017). Will Chinese external therapy with compound Tripterygium wilfordii hook F gel safely control disease activity in patients with rheumatoid arthritis: design of a double-blinded randomized controlled trial. BMC complementary Altern. Med. 17 (1), 444. doi:10.1186/s12906-017-1957-z
Jin, Z. R., Cao, Y. L., Luo, Z. C., Zhao, Q. C., Xi, Y., Weng, J. M., et al. (2022). Therapeutic effects of xianlu oral solution on rats with oligoasthenozoospermia through alleviating apoptosis and oxidative stress. Evidence-based complementary Altern. Med. eCAM 2022, 1269530. doi:10.1155/2022/1269530
Jing, W., Liu, C., Su, C., Liu, L., Chen, P., Li, X., et al. (2023). Role of reactive oxygen species and mitochondrial damage in rheumatoid arthritis and targeted drugs. Front. Immunol. 14, 1107670. doi:10.3389/fimmu.2023.1107670
Kamiya, K., Kishimoto, M., Sugai, J., Komine, M., and Ohtsuki, M. (2019). Risk factors for the development of psoriasis. Int. J. Mol. Sci. 20 (18), 4347. doi:10.3390/ijms20184347
Kato, A., Hulse, K. E., Tan, B. K., and Schleimer, R. P. (2013). B-lymphocyte lineage cells and the respiratory system. J. allergy Clin. Immunol. 131 (4), 933–957. quiz 58. doi:10.1016/j.jaci.2013.02.023
Kianmehr, M., Behdadfard, M., Hedayati-Moghadam, M., and Khazdair, M. R. (2023). Effects of herbs and derived natural products on lipopolysaccharide-induced toxicity: a literature review. Oxidative Med. Cell. Longev. 2023, 7675183. doi:10.1155/2023/7675183
Kim, H. K., Lim, S., Bae, M. J., Lee, W., and Kim, S. (2019). PG102 upregulates IL-37 through p38, ERK, and Smad3 pathways in HaCaT keratinocytes. Mediat. Inflamm. 2019, 6085801. doi:10.1155/2019/6085801
Kupchan, S. M., Court, W. A., Dailey, R. G., Gilmore, C. J., and Bryan, R. F. (1972). Triptolide and tripdiolide, novel antileukemic diterpenoid triepoxides from Tripterygium wilfordii. J. Am. Chem. Soc. 94 (20), 7194–7195. doi:10.1021/ja00775a078
Lei, W., and Jian, L. (2012). Changes of CD4(+) CD25(+) regulatory T cells, FoxP3 in adjuvant arthritis rats with damage of pulmonary function and effects of tripterygium glycosides tablet. Int. J. rheumatology 2012, 348450. doi:10.1155/2012/348450
Li, C., Zhang, H., and Li, X. (2020). The mechanism of traditional Chinese medicine for the treatment of obesity. Diabetes, metabolic syndrome Obes. targets Ther. 13, 3371–3381. doi:10.2147/dmso.S274534
Li, G., Ren, J., Wang, G., Gu, G., Hu, D., Ren, H., et al. (2014). T2 enhances in situ level of Foxp3+ regulatory cells and modulates inflammatory cytokines in Crohn's disease. Int. Immunopharmacol. 18 (2), 244–248. doi:10.1016/j.intimp.2013.12.014
Li, H., and Yang, P. (2022). Identification of biomarkers related to neutrophils and two molecular subtypes of systemic lupus erythematosus. BMC Med. genomics 15 (1), 162. doi:10.1186/s12920-022-01306-9
Li, S., Liu, F., Zhang, K., Tong, Y., and Liu, X. (2021). Research progress on the mechanism of natural product ingredients in the treatment of uveitis. J. Immunol. Res. 2021, 6683411. doi:10.1155/2021/6683411
Li, Y., Lee, P. Y., and Reeves, W. H. (2010). Monocyte and macrophage abnormalities in systemic lupus erythematosus. Archivum Immunol. Ther. Exp. 58 (5), 355–364. doi:10.1007/s00005-010-0093-y
Lin, A. (2006). A five-year itch in TNF-alpha cytotoxicity: the time factor determines JNK action. Dev. cell 10 (3), 277–278. doi:10.1016/j.devcel.2006.02.006
Lin, K. X., Wang, C. Z., and Qian, G. S. (2001). Effect of Tripterygium wilfordii on Th1, Th2 cytokines production in asthma patients. Zhongguo Zhong xi yi jie he za zhi Zhongguo Zhongxiyi jiehe zazhi 21 (1), 42–44. doi:10.1007/bf02935109
Lith, S. C., and de Vries, C. J. M. (2021). Nuclear receptor Nur77: its role in chronic inflammatory diseases. Essays Biochem. 65 (6), 927–939. doi:10.1042/ebc20210004
Liu, C., Zhang, Y., Kong, X., Zhu, L., Pang, J., Xu, Y., et al. (2013a). Triptolide prevents bone destruction in the collagen-induced arthritis model of rheumatoid arthritis by targeting RANKL/RANK/OPG signal pathway. Evidence-based complementary Altern. Med. eCAM 2013, 626038. doi:10.1155/2013/626038
Liu, D., Zhang, Q., Luo, P., Gu, L., Shen, S., Tang, H., et al. (2022). Neuroprotective effects of celastrol in neurodegenerative diseases-unscramble its major mechanisms of action and targets. Aging Dis. 13 (3), 815–836. doi:10.14336/ad.2021.1115
Liu, G., Shen, Y., You, L., Song, W., Lu, K., and Li, Y. (2014). Tripterygium wilfordii polyglycoside suppresses inflammatory cytokine expression in rats with diabetic nephropathy. Xi bao yu fen zi mian yi xue za zhi 30 (7), 721–724.
Liu, L., Zhao, H., Sun, X., Zheng, Q., Luo, Y., Ru, Y., et al. (2018). Efficacy and safety of Tripterygium wilfordii hook F for chronic urticaria: a systematic review and meta-analysis. BMC complementary Altern. Med. 18 (1), 243. doi:10.1186/s12906-018-2305-7
Liu, L. L., Tian, Y. G., Su, X. H., Fan, Y. F., Li, C., Zhu, X. X., et al. (2019). Comparative study on dose-toxicity-effect of tripterygium glycosides tablets and tripterygium wilfordii tablets on CIA model rats. Zhongguo Zhong yao za zhi 44 (16), 3502–3511. doi:10.19540/j.cnki.cjcmm.20190703.401
Liu, Y., Tu, S., Gao, W., Wang, Y., Liu, P., Hu, Y., et al. (2013b). Extracts of tripterygium wilfordii hook F in the treatment of rheumatoid arthritis: a systemic review and meta-analysis of randomised controlled trials. Evidence-based complementary Altern. Med. eCAM 2013, 410793. doi:10.1155/2013/410793
Lu, Y., Luo, Y., Zhou, J., Hu, T., Tu, L., Tong, Y., et al. (2022). Probing the functions of friedelane-type triterpene cyclases from four celastrol-producing plants. Plant J. cell Mol. Biol. 109 (3), 555–567. doi:10.1111/tpj.15575
Lu, Y., Xie, T., Zhang, Y., Zhou, F., Ruan, J., Zhu, W., et al. (2017). Triptolide Induces hepatotoxicity via inhibition of CYP450s in Rat liver microsomes. BMC complementary Altern. Med. 17 (1), 15. doi:10.1186/s12906-016-1504-3
Luo, D., Zuo, Z., Zhao, H., Tan, Y., and Xiao, C. (2019). Immunoregulatory effects of Tripterygium wilfordii Hook F and its extracts in clinical practice. Front. Med. 13 (5), 556–563. doi:10.1007/s11684-018-0649-5
Lv, M., Deng, J., Tang, N., Zeng, Y., and Lu, C. (2018). Efficacy and safety of tripterygium wilfordii hook F on psoriasis vulgaris: a systematic review and meta-analysis of randomized controlled trials. eCAM 2018, 2623085. doi:10.1155/2018/2623085
Lv, M., Liang, Q., Luo, Z., Han, B., Ni, T., Wang, Y., et al. (2022). UPLC-LTQ-Orbitrap-Based cell metabolomics and network pharmacology analysis to reveal the potential antiarthritic effects of pristimerin: in vitro, in silico and in vivo study. Metabolites 12 (9), 839. doi:10.3390/metabo12090839
Ma, B., Hu, T., Li, P., Yuan, Q., Lin, Z., Tu, Y., et al. (2017). Phylogeographic and phylogenetic analysis for Tripterygium species delimitation. Ecol. Evol. 7 (20), 8612–8623. doi:10.1002/ece3.3344
Ma, L., Xu, C., Paccaly, A., and Kanamaluru, V. (2020). Population pharmacokinetic-pharmacodynamic relationships of sarilumab using disease activity score 28-joint C-reactive protein and absolute neutrophil counts in patients with rheumatoid arthritis. Clin. Pharmacokinet. 59 (11), 1451–1466. doi:10.1007/s40262-020-00899-7
Mao, J. H., Xu, Y., Li, B. W., Yang, Y. L., Peng, Y., and Zhi, F. (2021). Microglia polarization in ischemic stroke: complex mechanisms and therapeutic interventions. Chin. Med. J. 134 (20), 2415–2417. doi:10.1097/cm9.0000000000001711
Maqbool, S., Ihtesham, A., Langove, M. N., Jamal, S., Jamal, T., and Safian, H. A. (2021). Neuro-dermatological association between psoriasis and depression: an immune-mediated inflammatory process validating skin-brain axis theory. AIMS Neurosci. 8 (3), 340–354. doi:10.3934/Neuroscience.2021018
Marks, W. H. (2011). Tripterygium wilfordii Hook F. versus Sulfasalazine in the treatment of rheumatoid arthritis: a well-designed clinical trial of a botanical demonstrating effectiveness. Fitoterapia 82 (1), 85–87. doi:10.1016/j.fitote.2010.11.024
Mazal, J. (2014). Crohn disease: pathophysiology, diagnosis, and treatment. Radiol. Technol. 85 (3), 297–316.
Miller, D. J., and Allemann, R. K. (2012). Sesquiterpene synthases: passive catalysts or active players? Nat. Product. Rep. 29 (1), 60–71. doi:10.1039/c1np00060h
Moreira, M. A., Souza, A. L., Lana-Peixoto, M. A., Teixeira, M. M., and Teixeira, A. L. (2006). Chemokines in the cerebrospinal fluid of patients with active and stable relapsing-remitting multiple sclerosis. Braz. J. Med. Biol. Res. 39 (4), 441–445. doi:10.1590/s0100-879x2006000400003
Moulton, V. R., Suarez-Fueyo, A., Meidan, E., Li, H., Mizui, M., and Tsokos, G. C. (2017). Pathogenesis of human systemic lupus erythematosus: a cellular perspective. Trends Mol. Med. 23 (7), 615–635. doi:10.1016/j.molmed.2017.05.006
Oliner, J. D., Saiki, A. Y., and Caenepeel, S. (2016). The role of MDM2 amplification and overexpression in tumorigenesis. Cold Spring Harb. Perspect. Med. 6 (6), a026336. doi:10.1101/cshperspect.a026336
Parveen, A., Subedi, L., Kim, H. W., Khan, Z., Zahra, Z., Farooqi, M. Q., et al. (2019). Phytochemicals targeting VEGF and VEGF-related multifactors as anticancer therapy. J. Clin. Med. 8 (3), 350. doi:10.3390/jcm8030350
Perl, A. (2017). Review: metabolic control of immune system activation in rheumatic diseases. Arthritis & rheumatology (Hoboken, NJ) 69 (12), 2259–2270. doi:10.1002/art.40223
Qu, B., Liu, Y., Shen, A., Guo, Z., Yu, L., Liu, D., et al. (2022). Combining multidimensional chromatography-mass spectrometry and feature-based molecular networking methods for the systematic characterization of compounds in the supercritical fluid extract of Tripterygium wilfordii Hook F. Analyst. 148 (1), 61–73. doi:10.1039/d2an01471h
Ren, J., Wu, X., Liao, N., Wang, G., Fan, C., Liu, S., et al. (2013). Prevention of postoperative recurrence of Crohn's disease: tripterygium wilfordii polyglycoside versus mesalazine. J. Int. Med. Res. 41 (1), 176–187. doi:10.1177/0300060512474744
Rodríguez, C., Romero, E., Garrido-Sanchez, L., Alcaín-Martínez, G., Andrade, R. J., Taminiau, B., et al. (2020). MICROBIOTA INSIGHTS IN CLOSTRIDIUM DIFFICILE INFECTION AND INFLAMMATORY BOWEL DISEASE. Gut microbes 12 (1), 1725220. doi:10.1080/19490976.2020.1725220
Ruan, Q.-l., Hua, Y.-q., Wang, M., and Duan, J.-aJCTPM (2018). Reproductive toxicity of Triptolide on male Caenorhabditis elegans, a model organism, 1009–1014.
Sanadgol, N., Golab, F., Mostafaie, A., Mehdizadeh, M., Khalseh, R., Mahmoudi, M., et al. (2018). Low, but not high, dose triptolide controls neuroinflammation and improves behavioral deficits in toxic model of multiple sclerosis by dampening of NF-κB activation and acceleration of intrinsic myelin repair. Toxicol. Appl. Pharmacol. 342, 86–98. doi:10.1016/j.taap.2018.01.023
Schwenzer, N. F., Kötter, I., Henes, J. C., Schraml, C., Fritz, J., Claussen, C. D., et al. (2010). The role of dynamic contrast-enhanced MRI in the differential diagnosis of psoriatic and rheumatoid arthritis. AJR Am. J. Roentgenol. 194 (3), 715–720. doi:10.2214/ajr.09.2671
Shen, J., Ma, H., and Wang, C. (2021). Triptolide improves myocardial fibrosis in rats through inhibition of nuclear factor kappa B and NLR family pyrin domain containing 3 inflammasome pathway. Korean J. physiology Pharmacol. 25 (6), 533–543. doi:10.4196/kjpp.2021.25.6.533
Shen, Q. Q., Wang, J. J., Roy, D., Sun, L. X., Jiang, Z. Z., Zhang, L. Y., et al. (2020). Organic anion transporter 1 and 3 contribute to traditional Chinese medicine-induced nephrotoxicity. Chin. J. Nat. Med. 18 (3), 196–205. doi:10.1016/s1875-5364(20)30021-2
Shen, Y., Jiang, T., Wang, R., He, S., Guo, M., Zuo, J., et al. (2015). 5R)-5-Hydroxytriptolide (LLDT-8) inhibits osteoclastogenesis via RANKL/RANK/OPG signaling pathway. BMC complementary Altern. Med. 15, 77. doi:10.1186/s12906-015-0566-y
Shi, Z. C., Fei, H. P., and Wang, Z. L. (2020). Cost-effectiveness analysis of etanercept plus methotrexate vs triple therapy in treating Chinese rheumatoid arthritis patients. Medicine 99 (3), e16635. doi:10.1097/md.0000000000016635
Singla, N., and Challana, S. (2014). Reproductive toxicity of triptolide in male house rat, Rattus rattus. TheScientificWorldJournal 2014, 879405. doi:10.1155/2014/879405
Sommer, N., Pak, O., and Hecker, M. (2021). New avenues for antiinflammatory signaling of Nur77 in acute lung injury. Am. J. Respir. cell Mol. Biol. 65 (3), 236–237. doi:10.1165/rcmb.2021-0210ED
Song, C. Y., Xu, Y. G., and Lu, Y. Q. (2020a). Use of Tripterygium wilfordii Hook F for immune-mediated inflammatory diseases: progress and future prospects. J. Zhejiang Univ. Sci. B 21 (4), 280–290. doi:10.1631/jzus.B1900607
Song, X., Zhang, Y., and Dai, E. (2020b). Therapeutic targets of thunder god vine (Tripterygium wilfordii hook) in rheumatoid arthritis (Review). Mol. Med. Rep. 21 (6), 2303–2310. doi:10.3892/mmr.2020.11052
Su, R., Sun, M., Wang, W., Zhang, J., Zhang, L., Zhen, J., et al. (2017). A novel immunosuppressor, (5r)-5-hydroxytriptolide, alleviates movement disorder and neuroinflammation in a 6-OHDA hemiparkinsonian rat model. Aging Dis. 8 (1), 31–43. doi:10.14336/ad.2016.0929
Tang, C., Livingston, M. J., Liu, Z., and Dong, Z. (2020). Autophagy in kidney homeostasis and disease. Nat. Rev. Nephrol. 16 (9), 489–508. doi:10.1038/s41581-020-0309-2
Tang, H. C., Huang, H. J., Lee, C. C., and Chen, C. Y. C. (2017). Network pharmacology-based approach of novel traditional Chinese medicine formula for treatment of acute skin inflammation in silico. Comput. Biol. Chem. 71, 70–81. doi:10.1016/j.compbiolchem.2017.08.013
Tang, X., Wang, C., Hsieh, Y., Wang, C., Wang, J., Han, Z., et al. (2019). Triptolide induces toxicity in inner ear stem cells via promoting DNA damage. Toxicol. Vitro 61, 104597. doi:10.1016/j.tiv.2019.104597
Tao, L., Qiu, Y., Fu, X., Lin, R., Lei, C., Wang, J., et al. (2016). Angiotensin-converting enzyme 2 activator diminazene aceturate prevents lipopolysaccharide-induced inflammation by inhibiting MAPK and NF-κB pathways in human retinal pigment epithelium. J. neuroinflammation 13, 35. doi:10.1186/s12974-016-0489-7
Tao, X., Younger, J., Fan, F. Z., Wang, B., and Lipsky, P. E. (2002). Benefit of an extract of Tripterygium Wilfordii Hook F in patients with rheumatoid arthritis: a double-blind, placebo-controlled study. Arthritis rheumatism 46 (7), 1735–1743. doi:10.1002/art.10411
Tian, R., Liu, X., Luo, Y., Jiang, S., Liu, H., You, F., et al. (2020). Apoptosis exerts a vital role in the treatment of colitis-associated cancer by herbal medicine. Front. Pharmacol. 11, 438. doi:10.3389/fphar.2020.00438
Timilsina, U., Ghimire, D., Timalsina, B., Nitz, T. J., Wild, C. T., Freed, E. O., et al. (2016). Identification of potent maturation inhibitors against HIV-1 clade C. Sci. Rep. 6, 27403. doi:10.1038/srep27403
Tong, L., Zhao, Q., Datan, E., Lin, G. Q., Minn, I., Pomper, M. G., et al. (2021). Triptolide: reflections on two decades of research and prospects for the future. Nat. Product. Rep. 38 (4), 843–860. doi:10.1039/d0np00054j
Tsitrouli, Z., Akritidou, M. A., Genitsaris, S., and Willigen, G. V. (2021). Treatment of rheumatoid arthritis with gene therapy applications: biosafety and bioethical considerations. Biotech. (Basel Switz. 10 (3), 11. doi:10.3390/biotech10030011
Urits, I., Burshtein, A., Sharma, M., Testa, L., Gold, P. A., Orhurhu, V., et al. (2019). Low back pain, a comprehensive review: pathophysiology, diagnosis, and treatment. Curr. pain headache Rep. 23 (3), 23. doi:10.1007/s11916-019-0757-1
Wang, H. L., Zhao, Q., Li, W., Zhu, H. C., Lv, L., Zhu, Z. H., et al. (2022). A systematic review and network meta-analysis about the efficacy and safety of Tripterygium wilfordii hook F in rheumatoid arthritis. eCAM 2022, 3181427. doi:10.1155/2022/3181427
Wang, Q., Meng, J., Dong, A., Yu, J. Z., Zhang, G. X., and Ma, C. G. (2016). The pharmacological effects and mechanism of tripterygium wilfordii hook F in central nervous system autoimmunity. J. Altern. complementary Med. (New York, NY) 22 (7), 496–502. doi:10.1089/acm.2016.0004
Wang, S., Li, R., He, S., He, L., Zhao, H., Deng, X., et al. (2017). Tripterygium wilfordii glycosides upregulate the new anti-inflammatory cytokine IL-37 through ERK1/2 and p38 MAPK signal pathways. Evidence-based complementary Altern. Med. eCAM 2017, 9148523. doi:10.1155/2017/9148523
Wang, Z., Yeung, S., Chen, S., Moatazedi, Y., and Chow, M. S. S. (2018). Bioavailability of wilforlide A in mice and its concentration determination using an HPLC-APCI-MS/MS method. J. Chromatogr. B, Anal. Technol. Biomed. life Sci. 1090, 65–72. doi:10.1016/j.jchromb.2018.05.018
Wangchuk, P., Apte, S. H., Smout, M. J., Groves, P. L., Loukas, A., and Doolan, D. L. (2018). Defined small molecules produced by himalayan medicinal plants display immunomodulatory properties. Int. J. Mol. Sci. 19 (11), 3490. doi:10.3390/ijms19113490
Webb, R., Hughes, M. G., Thomas, A. W., and Morris, K. (2017). The ability of exercise-associated oxidative stress to trigger redox-sensitive signalling responses. Antioxidants (Basel, Switz. 6 (3), 63. doi:10.3390/antiox6030063
Wen, C. C., Chen, H. M., and Yang, N. S. (2012). Developing phytocompounds from medicinal plants as immunomodulators. Adv. botanical Res. 62, 197–272. doi:10.1016/b978-0-12-394591-4.00004-0
Xi, C., Peng, S., Wu, Z., Zhou, Q., and Zhou, J. (2017). Toxicity of triptolide and the molecular mechanisms involved. Biomed. Pharmacother. = Biomedecine Pharmacother. 90, 531–541. doi:10.1016/j.biopha.2017.04.003
Xu, H. Y., Zhang, Y. Q., Liu, Z. M., Chen, T., Lv, C. Y., Tang, S. H., et al. (2019). ETCM: an encyclopaedia of traditional Chinese medicine. Nucleic acids Res. 47 (D1), D976–d82. doi:10.1093/nar/gky987
Xu, L., Pan, J., Chen, Q., Yu, Q., Chen, H., Xu, H., et al. (2008). In vivo evaluation of the safety of triptolide-loaded hydrogel-thickened microemulsion. Food Chem. Toxicol. 46 (12), 3792–3799. doi:10.1016/j.fct.2008.09.065
Xu, S., Feng, Y., He, W., Xu, W., Xu, W., Yang, H., et al. (2021). Celastrol in metabolic diseases: progress and application prospects. Pharmacol. Res. 167, 105572. doi:10.1016/j.phrs.2021.105572
Xue, M., Jiang, Z. Z., Liu, J. P., Zhang, L. Y., Wang, T., Wang, H., et al. (2010). Comparative study on the anti-inflammatory and immune suppressive effect of Wilforlide A. Fitoterapia 81 (8), 1109–1112. doi:10.1016/j.fitote.2010.07.007
Xue, M., Zhao, Y., Li, X. J., Jiang, Z. Z., Zhang, L., Liu, S. H., et al. (2012). Comparison of toxicokinetic and tissue distribution of triptolide-loaded solid lipid nanoparticles vs free triptolide in rats. Eur. J. Pharm. Sci. 47 (4), 713–717. doi:10.1016/j.ejps.2012.05.012
Yang, Y., Ye, Y., Qiu, Q., Xiao, Y., Huang, M., Shi, M., et al. (2016). Triptolide inhibits the migration and invasion of rheumatoid fibroblast-like synoviocytes by blocking the activation of the JNK MAPK pathway. Int. Immunopharmacol. 41, 8–16. doi:10.1016/j.intimp.2016.10.005
Yang, Y. J., Deng, Y., Liao, L. L., Peng, J., Peng, Q. H., and Qin, Y. H. (2020). Tripterygium glycosides combined with leflunomide for rheumatoid arthritis: a systematic review and meta-analysis. Evidence-based complementary Altern. Med. eCAM 2020, 1230320. doi:10.1155/2020/1230320
Y-Jjct, L. I., and Drugs, H. (2020). Toxicity and mechanisms of celastrol on human biliary epithelial cells. Chin. Traditional Herb. Drugs, 3700–3707.
Yu, Y., Yong, B., Xu, C., and Zhang, L. (2020). T-96 attenuates inflammation by inhibiting NF-κB in adjuvant-induced arthritis. Front. Biosci. (Landmark Ed. 25 (3), 498–512. doi:10.2741/4816
Yuan, K., Li, X., Lu, Q., Zhu, Q., Jiang, H., Wang, T., et al. (2019). Application and mechanisms of triptolide in the treatment of inflammatory diseases-A review. Front. Pharmacol. 10, 1469. doi:10.3389/fphar.2019.01469
Zeng, Y., Sun, H., Li, Y., Shao, M., Han, P., Yu, X., et al. (2017). Exposure to triptolide affects follicle development in NIH mice: role of endoplasmic reticulum stress in granulosa cell apoptosis. Hum. Exp. Toxicol. 36 (1), 82–92. doi:10.1177/0960327116638725
Zhang, B., Liu, L., Zhao, S., Wang, X., Liu, L., and Li, S. (2013b). Vitexicarpin acts as a novel angiogenesis inhibitor and its target network. Evidence-based complementary Altern. Med. eCAM 2013, 278405. doi:10.1155/2013/278405
Zhang, C., Sun, P. P., Guo, H. T., Liu, Y., Li, J., He, X. J., et al. (2016). Safety profiles of tripterygium wilfordii hook F: a systematic review and meta-analysis. Front. Pharmacol. 7, 402. doi:10.3389/fphar.2016.00402
Zhang, C., Wang, N., Xu, Y., Tan, H. Y., Li, S., and Feng, Y. (2018b). Molecular mechanisms involved in oxidative stress-associated liver injury induced by Chinese herbal medicine: an experimental evidence-based literature review and network pharmacology study. Int. J. Mol. Sci. 19 (9), 2745. doi:10.3390/ijms19092745
Zhang, G., Chen, J., Liu, Y., Yang, R., Guo, H., and Sun, Z. (2013a). Triptolide-conditioned dendritic cells induce allospecific T-cell regulation and prolong renal graft survival. J. investigative Surg. 26 (4), 191–199. doi:10.3109/08941939.2012.737408
Zhang, H., and Chen, W. (2017). Interleukin 6 inhibition by triptolide prevents inflammation in a mouse model of ulcerative colitis. Exp. Ther. Med. 14 (3), 2271–2276. doi:10.3892/etm.2017.4778
Zhang, J., Huang, Q., Zhao, R., and Ma, Z. (2020). A network pharmacology study on the Tripteryguim wilfordii Hook for treatment of Crohn's disease. BMC complementary Med. Ther. 20 (1), 95. doi:10.1186/s12906-020-02885-9
Zhang, K., Pace, S., Jordan, P. M., Peltner, L. K., Weber, A., Fischer, D., et al. (2021). Beneficial modulation of lipid mediator biosynthesis in innate immune cells by antirheumatic tripterygium wilfordii glycosides. Biomolecules 11 (5), 746. doi:10.3390/biom11050746
Zhang, Q., Li, Y., Liu, M., Duan, J., Zhou, X., and Zhu, H. (2018a). Compatibility with panax notoginseng and rehmannia glutinosa alleviates the hepatotoxicity and nephrotoxicity of tripterygium wilfordii via modulating the pharmacokinetics of triptolide. Int. J. Mol. Sci. 19 (1), 305. doi:10.3390/ijms19010305
Zhang, Y., and Ma, X. (2010). Triptolide inhibits IL-12/IL-23 expression in APCs via CCAAT/enhancer-binding protein alpha. J. Immunol. 184 (7), 3866–3877. doi:10.4049/jimmunol.0903417
Zhao, F., Huang, W., Ousman, T., Zhang, B., Han, Y., Clotaire, D. Z., et al. (2013). Triptolide induces growth inhibition and apoptosis of human laryngocarcinoma cells by enhancing p53 activities and suppressing E6-mediated p53 degradation. PloS one 8 (11), e80784. doi:10.1371/journal.pone.0080784
Zhao, J., Zhang, F., Xiao, X., Wu, Z., Hu, Q., Jiang, Y., et al. (2021). Tripterygium hypoglaucum (lévl.) hutch and its main bioactive components: recent advances in pharmacological activity, pharmacokinetics and potential toxicity. Front. Pharmacol. 12, 715359. doi:10.3389/fphar.2021.715359
Zheng, Y., Zhang, W. J., and Wang, X. M. (2013). Triptolide with potential medicinal value for diseases of the central nervous system. CNS Neurosci. Ther. 19 (2), 76–82. doi:10.1111/cns.12039
Zhong, Y., Deng, Y., Chen, Y., Chuang, P. Y., and Cijiang He, J. (2013). Therapeutic use of traditional Chinese herbal medications for chronic kidney diseases. Kidney Int. 84 (6), 1108–1118. doi:10.1038/ki.2013.276
Zhou, X., Xie, D., Huang, J., Lu, A., Wang, R., Jin, Y., et al. (2021). Therapeutic effects of (5r)-5-hydroxytriptolide on fibroblast-like synoviocytes in rheumatoid arthritis via lncRNA WAKMAR2/miR-4478/E2F1/p53 Axis. Front. Immunol. 12, 605616. doi:10.3389/fimmu.2021.605616
Zhou, Y. Z., Zhao, L. D., Chen, H., Zhang, Y., Wang, D. F., Huang, L. F., et al. (2018). Comparison of the impact of Tripterygium wilfordii Hook F and Methotrexate treatment on radiological progression in active rheumatoid arthritis: 2-year follow up of a randomized, non-blinded, controlled study. Arthritis Res. Ther. 20 (1), 70. doi:10.1186/s13075-018-1563-6
Ziaei, S., and Halaby, R. (2016). Immunosuppressive, anti-inflammatory and anti-cancer properties of triptolide: a mini review. Avicenna J. phytomedicine 6 (2), 149–164.
Glossary
Keywords: rheumatic and autoimmune diseases, Tripterygium wilfordii Hook F, antiinflammatory, immune-modulating, immunosuppressive effects
Citation: Shan Y, Zhao J, Wei K, Jiang P, Xu L, Chang C, Xu L, Shi Y, Zheng Y, Bian Y, Zhou M, Schrodi SJ, Guo S and He D (2023) A comprehensive review of Tripterygium wilfordii hook. f. in the treatment of rheumatic and autoimmune diseases: Bioactive compounds, mechanisms of action, and future directions. Front. Pharmacol. 14:1282610. doi: 10.3389/fphar.2023.1282610
Received: 24 August 2023; Accepted: 19 October 2023;
Published: 01 November 2023.
Edited by:
Nanwen Zhang, Fujian Medical University, ChinaReviewed by:
Rabab Sayed, Cairo University, EgyptZhigang Lu, Nanjing University of Chinese Medicine, China
Copyright © 2023 Shan, Zhao, Wei, Jiang, Xu, Chang, Xu, Shi, Zheng, Bian, Zhou, Schrodi, Guo and He. This is an open-access article distributed under the terms of the Creative Commons Attribution License (CC BY). The use, distribution or reproduction in other forums is permitted, provided the original author(s) and the copyright owner(s) are credited and that the original publication in this journal is cited, in accordance with accepted academic practice. No use, distribution or reproduction is permitted which does not comply with these terms.
*Correspondence: Dongyi He, dongyihe@medmail.com.cn
†These authors have contributed equally to this work