- Department of Ultrasound, Affiliated Hospital of Zunyi Medical University, Zunyi, China
The tumor microenvironment (TME) plays an important role in dynamically regulating the progress of cancer and influencing the therapeutic results. Targeting the tumor microenvironment is a promising cancer treatment method in recent years. The importance of tumor immune microenvironment regulation by ultrasound combined with microbubbles is now widely recognized. Ultrasound and microbubbles work together to induce antigen release of tumor cell through mechanical or thermal effects, promoting antigen presentation and T cells’ recognition and killing of tumor cells, and improve tumor immunosuppression microenvironment, which will be a breakthrough in improving traditional treatment problems such as immune checkpoint blocking (ICB) and himeric antigen receptor (CAR)-T cell therapy. In order to improve the therapeutic effect and immune regulation of TME targeted tumor therapy, it is necessary to develop and optimize the application system of microbubble ultrasound for organs or diseases. Therefore, the combination of ultrasound and microbubbles in the field of TME will continue to focus on developing more effective strategies to regulate the immunosuppression mechanisms, so as to activate anti-tumor immunity and/or improve the efficacy of immune-targeted drugs, At present, the potential value of ultrasound combined with microbubbles in TME targeted therapy tumor microenvironment targeted therapy has great potential, which has been confirmed in the experimental research and application of breast cancer, colon cancer, pancreatic cancer and prostate cancer, which provides a new alternative idea for clinical tumor treatment. This article reviews the research progress of ultrasound combined with microbubbles in the treatment of tumors and their application in the tumor microenvironment.
1 Introduction
The tumour microenvironment (TME) is a complex and rich multicellular environment for tumor growth, which is composed of tumor cells, immune cells, stromal cells, extracellular matrix (ECM), etc (Bilotta et al., 2022). In recent years, the importance of TME in dynamically regulating the progress of cancer and influencing treatment results has been widely recognized, and the rapid development of tumor immunotherapy against TME has established it as an important treatment method for various cancers. Compared with surgery, radiotherapy and chemotherapy, tumor immunotherapy can stimulate the body’s immune system and enhance the body’s immune defense mechanism against tumors, thus indirectly attacking tumor cells and reshaping the immune microenvironment (Xu et al., 2019; Kumar et al., 2021). On the one hand, immune-mediated tumor cell death can be enhanced by promoting immune tumor cells to recognize and eliminate target cells carrying tumor antigens, and on the other hand, immune suppression signals induced by tumor cells can be eliminated or reduced (Zimmermannova et al., 2021).
At present, a variety of immunotherapeutic drugs have been approved for clinical use, and have benefited patients with bladder cancer (Patel et al., 2020), melanoma (Tagliaferri et al., 2022), breast cancer (Keenan and Tolaney, 2020) and lung cancer (Lahiri et al., 2023). However, due to the presence of immunosuppressive TAMs in tumors, which protect cancer cells from treatment-induced cell death, some patients respond poorly to immunotherapy and may even develop highly progressive disease after treatment. However, due to the existence of immunosuppressive TAMs in tumors, which protect cancer cells from treatment-induced cell death, some patients have poor response to immunotherapy, and may even develop into highly advanced diseases after treatment. The positive reaction of immunotherapy usually depends on the dynamic interaction of tumor cells and immunomodulators in the TME (DeBerardinis, 2020). Although immunotherapy targeting the TME is a breakthrough in cancer treatment, the overall effective rate still needs to be improved.
In order to enhance the efficacy of tumor immunotherapy, it is essential to change the tumor microenvironment. The combination of ultrasound and microbubbles regulates the tumor immunosuppressive microenvironment by damaging endothelial cells with generated microfluidics, microfluidics and free radicals, causing microvascular rupture and tumor cell apoptosis, impeding tumor angiogenesis and enhancing the effect of immunotherapy (Alphandéry, 2022; Han et al., 2022; Li et al., 2022). In addition, drugs and exogenous genes can be released targetedly through local ultrasonic irradiation, so as to get hold of better therapeutic curative effect (Ho et al., 2018). Therefore, UTMD shows a excellent application in enhancing the immunotherapy effect. Overview of this article, we summarize the benefits of combined application of ultrasound and microbubbles in immunotherapy of the TME in tumor therapy, and describe the future prospects.
2 Overview of ultrasound
As a simple, safe and noninvasive method, ultrasound has been widely used in the diagnosis and treatment of diseases. UTMD is a new treatment method which has been developed in the field of tumor treatment in recent years. It has the advantages of precision, efficiency and safety, and has good repeatability. It is enhanced in drug release and exogenous genes through ultrasonic cavitation effect (Myers et al., 2018; Awad et al., 2021; Wang et al., 2022). The cavitation is an important physical phenomenon. When the ultrasonic pressure reaches a certain threshold, the surrounding liquid quickly fills the small cavity of gas and steam, forming micro bubbles (MBS), also known as cavitation nuclei. These MBs are excited under the sound field, and the dynamic process of continuous vibration, expansion, contraction and even collapse releases instantaneous energy and causes extreme physical phenomena (Han et al., 2017; Aryal and Porter, 2022; He et al., 2022), such as luminescence, high temperature, high pressure, discharge and micro-jet (Snipstad et al., 2021).
The principle of enhancing ultrasonic cavitation effect is to increase the number of cavitation nuclei by increasing exogenous MBS. With the increase of MBS, The energy required to generate cavitation decreases with the increase in MBS, which results in a decrease in the energy threshold for cavitation effect (Chowdhury et al., 2020) (Figure 1 Ultrasonic cavitation effect). When high-frequency ultrasound is generated, microbubbles will undergo asymmetric oscillation, causing asymmetric expansion and collapse of their volume. The strong compression of gas inside bubbles and the repeated impact of local pressure generated by surrounding fluids have a significant impact on cells or tissues, and will produce thermal effects, which will cause denaturation of various immunoregulatory proteins in tissues (Chen et al., 2021; Entzian and Aigner, 2021) and acting on factors such as VEGF can lead to microvascular rupture in tissues (Mitragotri, 2005; Thomas et al., 2019). In animal models, HIFI was combined with liposomes containing temperature sensitive drugs, and it was found that the permeability of drugs to tumors was significantly enhanced (Sadeghi-Goughari and Kwon, 2019). Due to the low frequency and low intensity of low-frequency ultrasound, under the action of ultrasound, bubbles oscillate uniformly without rupture, resulting in microfluidics characterized by fluid flow (Niu et al., 2020). Microfluids exert shear stress on cells while generating heat, and lead to sound holes which help to open the tissue barrier formed by endothelial cells. Studies have shown that under the action of low-frequency ultrasound, the collapse process of MB caused by cavitation effect produces jets and releases energy, which can instantly rupture adjacent cell membrane, increase their permeability and promote the ingestion of drugs by cells (Dong et al., 2017; Yang et al., 2018). At present, the way of using UTMD to improve the efficiency of drug targeting and delivery in local tissues is widely recognized in research (Feril and Tachibana, 2012; Cai et al., 2022; Yang et al., 2022). Therefore, By combining ultrasound with microbubble therapy, tumor cells can be killed specifically, anti-tumor immune response can be stimulated, and tumor microenvironment can be improved, which is crucial for tumor immunotherapy (Zhang et al., 2017).
3 Tumor microenvironment and immunotherapy
In recent years, researchers have gone deep into the components of the tumor microenvironment and noticed that it is composed of cytotoxic T lymphocytes (CTLs) with natural anti-tumor immunity and cells that inhibit the function of tumor immunotherapy: myeloid derived suppressor cells (MDSCs), tumor associated macrophages (TAMs), and tumor associated fibroblasts (CAFs) (Pitt et al., 2016) (Figure 2 Cell composition within the tumor microenvironment). Therefore, in-depth understanding of the immunosuppressive mechanism of immunosuppressive cells in the TME is of great significance for expanding the idea of immunotherapy and improving the curative effect. Due to the formation of tumor microenvironment, the interstitial pressure of tumor increases, the ability of immune cells to infiltrate tumor tissue is insufficient, and the lack of CTL and other immune cells with antigen stimulation in tumor tissue can cause insufficient effective inhibition of tumor growth and promote tumor immune escape; In addition, The inability to effectively activate antigen-specific T cells that inhibit tumor growth and the poor release of tumor antigens to peripheral lymph nodes leads to the depletion of T cells that are already lacking and insufficient direct or indirect antigen presentation; However, tumor cells and immunosuppressed cells secrete a variety of negative immunoregulatory factors, which lead to immune escape and can not recognize and present tumor antigens. All these factors lead to the formation of an immunosuppressive microenvironment, the clinically observed resistance to immunotherapy, and the tumor promoting effect (Bejarano et al., 2021; Liu et al., 2022). However, at present, in cancer, immunotherapy for effective activation of T cells is mainly a strategy to enhance adaptive immunity by inhibiting immune checkpoints and promoting T cell release (Hope and Salmond, 2019; Ajina et al., 2020; Lim et al., 2020). In recent years, studies have found that t-cell-promoting immune tumors, such as monoclonal antibody trastuzumab (TRA), act on HER2 positive breast cancer and improve antibody cell dependent toxicity. However, as a first-line drug, its drug resistance and targeting are still clinical challenges (Modi et al., 2020; Elamir et al., 2021). Therefore, it is a promising research direction in tumor treatment to seek to improve the anti-tumor efficiency of immune cells such as T cells and reduce the cytotoxicity of drugs.
Dendritic cells are a group of highly heterogeneous professional antigen presenting cells (APCs), which have the functions of ingesting, processing and presenting antigens (Verneau et al., 2020; Zimmermannova et al., 2021). Some studies have shown that cDCs can induce a strong anti-tumor CTL response, and then reduce the tumor growth in mice inoculated with cDC2, which is related to the decrease of MDSCs and reprogramming of TME’s natural tam (Gerhard et al., 2021; Marciscano and Anandasabapathy, 2021). In addition, TME can trigger a variety of mechanisms to interfere with DC function, including reducing and inhibiting the recruitment of dendritic cells in tumors through the production of chemoattractants (such as carbon tetrachloride and CCL5), as well as by reducing the survival signals required for DC differentiation and survival growth factors and IL-12 (Xiang et al., 2022). Together, these two factors lead to insufficient activation of T cells, and may induce T cell to tolerate TAA (Garris and Luke, 2020). The increase in the density of immunocytotoxicity-related dendritic cells in the TME makes the prognosis of ovarian cancer, lung cancer and breast cancer better (Batchu et al., 2021; Ding et al., 2021). Dendritic cells can be regarded as a promising target for cancer immunotherapy.
TAMs is an important part of tumor inflammatory infiltration. In the process of tumor formation, the pool of resident macrophages is expanded by in-situ proliferation and supplemented by monocyte-derived macrophages (MDMS) recruited to TME (Mehta et al., 2021; Munir et al., 2021; Koll et al., 2023). However, these TAMs in TME have been further modified, which leads to phenotypic and functional heterogeneity between different tumor types, including bladder cancer, breast cancer and pancreatic cancers (Sun X. et al., 2022; Sun M. et al., 2022; Hezaveh et al., 2022). Several studies have confirmed that TAMs in the TME can regulate cancer progression through various mechanisms, including immune escape, angiogenesis, cancer cell invasion and immunosuppression to promote tumor occurrence and enhance the subsequent inflammatory stimulation of tumor growth and metastasis (Miyake et al., 2016; Bao et al., 2022). In addition, TAMs can affect the poor prognosis, drug resistance and cancer recurrence after routine treatment, for example, due to colony stimulating factor 1 (CSF 1) (Akkari et al., 2020; Garrido-Martin et al., 2020). Therefore, different methods have been developed to target TAMs, including blocking the recruitment and infiltration of TAMs into the TME, inhibiting the induced activation of TAMs on CSF and cytokines such as IL-4 and IL-10, and interfering with the transcription of TAMs function in the TME (Zhang H. et al., 2021; Hezaveh et al., 2022).
In TME, CAFs, as one of the main cell types producing ECM molecules, can support tumor growth through various mechanisms (Ribeiro Franco et al., 2020). CAFs produce matrix remodeling enzymes while depositing into ECM, and play a very important role in tumor invasion, metastasis and drug resistance (Deepak et al., 2020). In addition, CAFs communicates with tumor cells by secreting a variety of cytokines, exosomes and growth factors, and promotes malignant biological behaviors such as proliferation, invasion and metastasis of tumor cells by transmitting signal molecules such as mRNA, and plays an active role in the occurrence and development of tumors (Erdogan and Webb, 2017; Ren et al., 2019; Eckhardt et al., 2020). CAFs also have a greater impact on the vasculature and immune cells in the TME. For example, VEGF secreted and promoted by CAFs can drive angiogenesis (Komi and Redegeld, 2020; Zhao et al., 2022), while secretion of IL 6, CXCL 9 and TGF regulates the T cell responses (Jiang et al., 2017; Yoshida, 2020). At present, in the face of the severe situation of tumor immunotherapy efficacy and drug resistance, CAF can be used as a potential target in tumor diagnosis and treatment, with broad clinical application prospects.
4 Mechanism and application of ultrasound combined with microbubbles in tumor microenvironment immunotherapy
4.1 Promote antigen release from cancer cells
The first step of immune clearance of tumor cells is to activate natural killer cells (NK), macrophages and dendritic cells, which can start the inflammatory and immune reactions by recognizing tumor-associated antigens, thus clearing abnormal expression cells (Mollica Poeta et al., 2019; Blass and Ott, 2021; Lahiri et al., 2023). However, the lack of antigen stimulation in tumor immune microenvironment can the growth of tumor cells cannot be effectively inhibited and immune escape. Therefore, how to induce strong and specific tumor antigen release is a problem faced by tumor treatment. 1In recent years, ultrasound, as a safe and effective method to inhibit tumor growth, can affect tumor hypoxia reaction by inducing mechanical and thermal effects such as oscillation and cavitation, and induce tumor cell apoptosis, thus increasing antigen exposure and stimulating immune response (Chen et al., 2014; Giovagnoli-Vicuña et al., 2022) (Figure 3 Ultrasound combined with microbubbles promotes the release of cancer cell antigens and damages tumor cells). It has been reported that UTMD can lead to apoptosis of tumor cells by acting on ceramide signaling pathway and calcium ion level in tissue environment, while tumor cells that are about to die and undergo stress release immune recognition agents, such as damps, to stimulate the body to produce immune response to tumors (Wang L. et al., 2021; Zhu et al., 2021). However, liposomal microbubbles in animal transplanted tumors after ultrasound irradiation, cellular immunity against cancer cells is triggered. Experimental data suggest that tumor associated antigens released from cancer cells damaged by ultrasound irradiation are increased and recognized by DCs. In addition, the anti-tumorffect depends on the intensity of ultrasound exposure, which suggests that the degree of tumor damage may be related to the antitumor immune response (Oda et al., 2012; Xiong et al., 2020; Zhang et al., 2022). In Wang L’s research, liposome microbubbles were used to deliver paclitaxel to glioblastoma xenografts in nude mice and were treated with low frequency ultrasound irradiation. The results showed that low frequency ultrasound combined with microbubbles could induce apoptosis and inhibit cell proliferation (Wang L. W. X. et al., 2021). In the study of Elamir A encapsulating anti-HER2 monoclonal antibody on liposomes to target the treatment of HER2 positive breast cancer, it was reported that low-frequency ultrasound combined with microbubble therapy was more effective than other groups in inhibiting tumor growth, promoting apoptosis, inhibiting cell proliferation and angiogenesis, and enhancing immune response (Elamir et al., 2021). In recent years, it has been found that high-intensity focused ultrasound is used to focus ultrasound energy from the body to the target area, and has been used as an ablation therapy for cancer. This therapy mainly uses the mechanical effect of boiling cathepsin, that is, high-intensity focused ultrasound, to induce cancer cell damage, thus inducing a strong immune response (Aryal and Porter, 2022; Wu Y. et al., 2023). This approach significantly increases the levels of tumor associated antigens, chemokines (IL-8) and a large number of cell damage related molecules, which in turn further stimulated the uptake and presentation of antigens by DCs (Yang H. et al., 2019; Zhang et al., 2022). Therefore, inducing the death of immunogenic cell by external ultrasonic energy may be a potential immunotherapy for cancer.
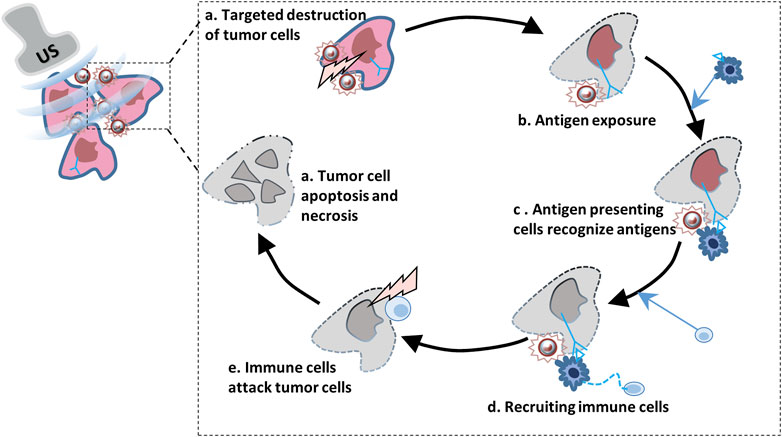
FIGURE 3. Ultrasound combined with microbubbles promotes the release of cancer cell antigens and damages tumor cells.
4.2 Promote the presentation of antigen-presenting cells (APCs)
Tumor immunotherapy refers to the proliferation of T lymphocytes in vivo stimulated by antigens presented by antigen presenting cells, resulting in specific cytotoxicity, thereby killing pathogens. Tumor cells and immunosuppressed cells secrete a variety of negative immunoregulatory factors in tumor tissues, which leads to the inability of immune cells to recognize and present tumor antigens. The general interruption of antigen presentation interrupts tumor antigen recognition, and the defect of antigen presentation provides an immune escape mechanism for tumor cells in the TME (Bishop, 2016; Jhunjhunwala et al., 2021). The presentation of tumor specific antigens by MHC molecules on APCs (such as DCs) is the key to stimulate specific T cell responses (Yang et al., 2023; Zhu and He, 2023). After MHC antigen is presented to APC, the most critical step is to induce CD 8 + T cell responses specific for cancer cells. In the case of TME, CD 8 + T cells can not be effectively triggered (Alrubayyi and Rowland-Jones, 2023; Yang et al., 2023). Therefore, it is necessary to induce dendritic cells to cross present extracellular antigens in DC-based cancer immunotherapy. According to reports, The combination of ultrasound and microbubbles is a new method of tumor specific antigen delivery. By opening the transient pores in the cell membrane of these cells, tumor specific antigens can be directly delivered to dendritic cells, and then the extracellular antigens can be directly recognized in the cytosol. 3The results showed that the antigen delivery system combined with ultrasound and microbubbles could be used for antigen delivery of tumor immunotherapy based on DC (Pelka and Guha, 2023). In the xenotransplantation mouse models of RM 1 (prostate cancer), MC38 (colon cancer) and B16 (melanoma), ultrasound-mediated cavitation induces tumor cell necrosis, which significantly increases the damage related to molecular mode damping release and tumor antigen presentation, thus making the tumor sensitive to ICB treatment (Hu et al., 2022). Sun W’s experiments on 4T1 homologous mouse model proved that ultrasound sensitizer CE 6 and ultrasound irradiation jointly promoted mRNA escape from endosome, thus enhancing antigen presentation, effectively triggering anti-tumor immunity and preventing tumor metastasis (Sun et al., 2023). In addition, a nano-scale cancer vaccine was designed in this study, which was sealed by Ce6 and other materials based on PLGA (lactic co glycolic acid). After subcutaneous injection, the nano-vaccine can be effectively delivered to antigen presenting cells (APCs) of lymph nodes. As is known to all, tumor vaccines is considered as a promising immunotherapy method by inducing specific anti-tumor immune responses. In order to effectively present tumor-associated antigens and improve tumor immunity, a cell-free DCs vaccine has been developed, in which the cell membrane antigen of breast cancer cells and dendritic cells derived from human PBMC cultured in vitro are combined into ultrasound contrast agent microbubbles (MB). The research results show that DC-IMBs can accumulate in lymphoid organs and induce anti-tumormmune responses, and significantly inhibit tumor growth through apoptosis, prolong the survival time of treated animals and exert anti - tumor effect (Jugniot et al., 2022).
4.3 Priming of T cells and infiltration of T cells into tumor tissues
The reasons for the weakening of immune clearance mechanism and tumor growth and metastasis in TME are (Crespo et al., 2013; Kishton et al., 2017; Ma et al., 2019):a) the reduced proliferation of immune cells and the insufficient ability of immune cells to infiltrate tumor tissues; b) Antigen specific T cells are heavily depleted and poorly activated. Therefore, in cancer immunotherapy, it is very essential to stimulate antigen-specific T cell responses to directly attack cancer cells. T cell immunotherapy with chimeric antigen receptor (car T cell) is an adoptive cancer immunotherapy (Boulch et al., 2021), which expresses engineered cells of chimeric antigen receptor (CAR) against specific tumor antigens (TA), that is, it is designed according to the principle of T cell receptor and costimulatory signal transduction, and this therapy can identify and eliminate cancer cells. But at present, its main therapeutic target are hematological tumors, such as leukemia and lymphoma (Agarwal et al., 2020; Yang et al., 2021). The reason is that the amount of cardiac T cells infiltrating into solid tumor tissues is very low. Because it is difficult to achieve a sufficiently effective T cell infiltration in solid tumors, Based on the combination of ultrasound and microbubbles, the permeability and function of endothelial cells can be directly affected by the cavitation produced. Under the mechanical damage of us irradiation, the infiltration of cd8 + t cells in tumor tissues increased significantly, which effectively improved the immunosuppressive TME. It also induces systemic anti-tumor immune responses and enhances the therapeutic effect of anti-PD-L 1 antibody in local and distant tumors. Recently, it was reported that UTMD plays an indispensable role in enhancing the transfection efficiency of tumor suppressor genes and cytokines and promoting the recruitment of T cells at tumor targeted sites. We detected its synergistic efficacy in the treatment of tumors with anti-PD-L1 antibody in a mouse tumor model (Ilovitsh et al., 2020; Tang et al., 2023). By combining ultrasound with tumor targeting microbubbles, Hu J team showed that USNB promoted the infiltration and antitumor activity of CD8+ T cells (Sun et al., 2023). In addition, studies have shown that the new combination therapy of low intensity focused ultrasound targeted microbubble destruction (LIFU-TMD) and PD-L 1 blockade also shows the same effect of reducing tumor and enhancing T cell infiltration (Wu N. et al., 2023). Furthermore, in order to improve the cure rate of solid tumor based on immune adjuvant, some tumor-associated antigens (TAAs) produced by experimental UMC therapy show vaccine activity, especially in the presence of immune adjuvant, which jointly promoted the maturation of dendritic cells and induced the production of cytokines (Zhang N. et al., 2021). Importantly, UMC can downregulate immune checkpoint molecules, such as CD274, Foxp3 and CTLA4, and cooperate with ally to stimulate the activation and proliferation of T cells in vivo, so as to promote tumor treatment (Omata et al., 2001; Suzuki et al., 2010; Hunt et al., 2015). In enhancing HIFU therapy, antigen-sensitized DC prepared by ultrasound and microbubbles can cause a large number of proliferation of T cells and CTL, which has strong anti-tumor effects (Singh et al., 2019; Ye et al., 2022). In summary, the combination of ultrasound and microbubbles can open the tight connection between endothelial cells, induce the secretion of chemokines, promote the infiltration, proliferation, and activation of cytotoxic T cells, and infiltrated immune cells such as T cells and lymphocytes can improve the microenvironment of tumor tissue for further activation of anti-tumor immune responses.
4.4 Enhancing the recognition and killing effect of T cells on cancer cells
When the microenvironment of tumor tissue is in immunosuppression state, it can inhibit the activation of T cells mediated by T cell receptor (TCR), thus inhibiting the activation of effector T lymphocytes, increasing the expression of CD8+T cells through PD-1/PD-L1 signaling pathway, and finally leading to the immune escape of tumor (Jiang X. et al., 2019; Jiang Y. et al., 2019; Yi et al., 2022). In order to improve this environment, it has obtained clinical approval or is being evaluated in trials, including immunotherapy, anti-antigenic drugs, and immune checkpoint blockade (ICB). However, due to the existence of the microenvironment of immunosuppressed tumors, the clinical therapeutic effect of some patients is limited (Bader et al., 2020). As a new tumor treatment method, ultrasound combined with microbubbles has become a hot spot in tumor immunotherapy research because of its advantages of targeting, safety and high efficiency. In a mouse colon cancer model treated with ultrasound combined with microbubble (Tang et al., 2021), tumor perfusion, CD8+T cell infiltration and anti-PD-L1 antibody delivery were also observed to increase. In particular, flow cytometry showed that ultrasound combined with microbubble treatment not only increased the infiltration of CD8+T cells into tumors, but also promoted the expression of apoptosis indexes such as Ki67, which had a stronger killing effect on tumor cells and more obvious inhibitory effect on tumor growth. In addition, NK cells are essential for T cells to mediate tumor immunity. Studies have found that the clearance of NK cells also caused a reduction in the recruitment and activation of tumor specific CD8+T cells (André et al., 2018; Yu et al., 2021). Sta Maria et al. reported (Sta Maria et al., 2015), in the xenograft tumor of human colorectal adenocarcinoma mice, compared with the group that did not receive ldbFUS treatment, the group that injected MBs + NK cells into the tail vein of mice and underwent ultrasound irradiation had significantly more NK cell aggregation. The efficiency of adoptively transferred natural killer cells (NK) in treating TME of solid tumors is hindered. In the experiment of enhancing the adoptive penetration of NK-92MI cells into ovarian tumors by using the combination of focused ultrasound (FUS) and microbubbles, NK cell are targeted to activate and release IL-2 to maintain their viability, so as to increase NK cell-mediated tumor cell killing (Yang C. et al., 2019). In conclusion, the combined treatment of ultrasound and microbubbles promoted the recognition of tumor cells by T cell receptors and led to their death, and enhanced the killing effect of T cells in the tumor immunosuppressive microenvironment. In the near future, we may apply ultrasound and microbubble technology to cancer immunotherapy to achieve ideal results.
4.5 Targeted therapy for tumor vasculature
In the TME, the high proliferation rate of ECs, the decrease of cell tight junctions, the abnormality of peripheral cell coverage and the increase of ECM deposition lead to the abnormality and dysfunction of the tumor vascular system, which provides the conditions for the growth, proliferation, invasion and metastasis of tumors. At present, the use of effective vascular therapy for anti-tumor has been extensively studied. In the past clinical studies, the application of VEGF pathway inhibitors showed more effective anti-tumor effects, such as several inhibitors targeting VEGF pathway (Ranieri et al., 2006; Costache et al., 2015; Kim et al., 2022), including bevacizumab and gemcitabine. In the experiment of pancreatic cancer in mice, blocking BICC1/LCN2 signal pathway can reduce the microvessel density and tumor volume of mouse PAAD cell grafts, enhance the antitumor effect of gemcitabine, and delay tumor growth (Kooiman et al., 2020). In mouse models of breast cancer and neurocytoma, thalidomide, an anti-vascular drug, is related to the reduction of angiogenesis and the inhibition of tumor growth and metastasis (Wan et al., 2021; Al Kawas et al., 2022; Huang et al., 2023). However, blocking VEGF pathway by antibodies to inhibitors such as VEGF and VEGFR, as a tumor inhibition therapy, has poor curative effect, and even tumor resistance is a common event in patients. Therefore, safe and effective improvement of tumor vessel normalization and enhancement of the concentration and efficacy of VEGF targeted therapeutics are expected to become an important part of anti - antigenic therapy (Heath and Bicknell, 2009). Ultrasound combined with microbubbles to enhance the treatment of anti-VEGF and anti-VEGF receptor antibody is helpful for immunosuppression in the tumor microenvironment (Curley et al., 2017). Based on previous studies, ultrasound combined with microbubbles has the ability to improve the composition and integrity of tumor vascular system, improve drug permeability and normalize blood vessels, which is one of the main clinical strategies to improve the drug delivery and treatment effects of tumor TME (Kooiman et al., 2020). For example, in human breast cancer cells, ultrasound mediated disruption of VEGF targeting and paclitaxel (PTX) - loaded lipid microbubbles (VTPLLM + US), VTPLLM + US inhibited MCF-7 cell proliferation and accelerated apoptosis by regulating VEGF expression (Su et al., 2019). In a study of gene therapy for breast cancer, the same results showed that VEGF-CNA could effectively inhibit the expression of VEGF-C protein and mRNA in MCM cf-7l and significantly inhibit the growth of human lymphatic endothelial cells (LECs) and MCF-7 cells in vitro (Xu et al., 2013). In addition, in the study of prostate cancer (PCa), low-frequency ultrasound combined with microbubbles stimulated DCs in the bone marrow of BALB/c mice by adding cytokines (granulocyte macrophage colony stimulating factor (GM-CSF) and interleukin −4 (IL-4)). Cell migration and invasion tests and cell enhancement tests showed that downregulation of VEGF expression inhibited the evolution of PCA cells, and it can be inhibited by VEGF (Zhang et al., 2017). Therefore, ultrasound mediated microbubble destruction (UMMD) plays a key role in the targeted treatment of tumor blood vessels, inhibiting tumor proliferation and enhancing the efficacy of cancer immunotherapy, and may be a powerful and promising technology.
5 Regulation of tumor immune microenvironment by UTMD
Clinical studies have found that the tumor immunosuppression microenvironment plays a key role in the development and treatment response of tumor disease, and is also the main reason for drug resistance and off-target toxicity of immunotherapy. 2Recent studies have found that ultrasound-targeted microbubble sonodynamic therapy (SDT) is a promising strategy to inhibit tumor growth and activate immunotherapy against tumor immune response by inducing microvascular rupture and tumor cell apoptosis to regulate tumor immunosuppression microenvironment, block tumor angiogenesis and enhance the effect of immunotherapy (Kim et al., 2020; Abe et al., 2022; Han et al., 2022). Team Ji C developed ultrasonic microbubble nanoparticles with mitochondrial targeting and sound sensitizer (IR780), which were wrapped in perfluoronalKane (FDC). After mitochondrial targeting and mitochondrial dysfunction in 4T1 tumor-bearing mice, the tumor growth in vivo and in vitro was inhibited, and the immunogenic cell death was enlarged, which led to the effective improvement of the microenvironment of immunosuppressed tumors, the maturation of dendritic cells and the increase of the number of infiltrating immune cells, which further enhanced the immune response to tumor immunotherapy (Ji et al., 2021). In addition, the ultrasonic cavitation effect exhausted tumor blood perfusion through abnormal blood vessel rupture, induced TME to transform and sensitize anti-PD-l immunotherapy, induced some cellular immunogenic cell death (ICD), and significantly inhibits the growth of mouse 4T1 breast cancer. Moreover, it was also found that the levels of dendritic cells (DCs) and CD8+ T cells in tumor tissues increased significantly (Wu N. et al., 2023). More than that, UTMD and small molecule ribonucleic acid have the potential to interfere with the immune system. In the study of hepatocellular carcinoma (HCC) mouse model, UTMD-microRNA combined treatment delivered microRNA-122 and anti-microrna-21 into HCC tumors, which reduced the level of GM-CSF and affected the expression of cytokines in the immune microenvironment, thereby improving the therapeutic effect on liver cancer (Wischhusen et al., 2020). To sum up, the combined application of ultrasound and microbubbles can transform the tumor microenvironment into a state conducive to the infiltration of immune cell, thus promoting the anti-tumor immune response. Therefore, this strategy has the potential to be a tumor immunotherapy based on tumor microenvironment immunomodulation.
6 Expectation
The tumor immunosuppressive microenvironment is the main cause of immune therapy resistance and off target toxicity observed in clinical practice. Although various anticancer drugs and methods have been developed for immunosuppression environment, most cancer treatments involve tumor cells escaping from immune attacks, and drugs can not completely reach the target tumor cells and aggregate to form a killing effect. Adopting a safe, targeted, and effective approach to the treatment of tumor microenvironment has become an important direction for researching and conquering tumors. Ultrasound combined with microbubble therapy is an emerging tumor treatment strategy that has the characteristics of enhancing vascular permeability, non-invasive, and selectivity. In fact, multiple clinical studies have begun to explore the tumor microenvironment of different target organs. At present, a number of clinical studies on solid tumors, such as breast cancer, prostate cancer and pancreatic cancer, are in progress. Focused ultrasound combined with microbubbles can improve tumor vascular permeability, limit the enhancement of anti-tumor immunity in the tumor microenvironment, and is suitable for the treatment of primary tumors. Several articles reported that in order to improve the stability and efficiency of drug delivery and reduce the interference of TME on drugs, targeted microbubbles were used to deliver drugs to tumor cells, thus improving the efficiency. In addition, mechanical fluctuant and thermal effects generated by UTMD are also the research focus of tumor immunotherapy. This combination can release cytokines and enhance the infiltration of immune active cells into tumor tissues, thus achieving effective cancer treatment. In addition, UTMD can induce cancer cells to release antigens and dangerous signals, enhance the induced anti-cancer immune response, and begin boosting cell membrane permeability and causing damage to tumor cells together to initiate effective anti-tumor immunity. Therefore, further study on the effect of ultrasound combined with microbubbles on the tumor microenvironment can provide new ideas for tumor immunotherapy and new development direction for tumor targeted therapy with high efficiency and low side effect.
7 Conclusion
The combined action of ultrasound and microbubbles can induce a variety of biological responses through mechanical fluctuant and thermal effects. TME plays an important role in dynamically regulating the progress of cancer and influencing the therapeutic results. The strategy of taking the TME as a therapeutic target is a promising cancer treatment method that has emerged in recent years. Therefore, the importance of UTMD in the regulation of tumor immune microenvironment has been widely recognized now. Ultrasound and microbubbles work together to induce antigen release of tumor cell through mechanical or thermal effects, promoting antigen presentation and T cells’ recognition and killing effect on tumor cells, and improve tumor immunosuppression microenvironment, which will be a breakthrough to improve the drug resistance, insufficient targeting and toxic and side effects of traditional treatments such as ICB and CAR-T cell therapy. In order to improve the efficacy and immune regulation of TME targeted tumor therapy, it is essential to develop and optimize microbubble ultrasound application systems for organs or diseases. However, improving the targeted curative effect and immune regulation effectiveness of tumor treatment is also one aspect that needs to be improved in ultrasound combined with microbubble therapy. In addition, there is no unified international standard for ultrasound parameters, microbubble types, doses, etc. That need to be applied in tumor experiments, and it needs to be further improved. Therefore, UTMD in the field of TME will continue to focus on developing more effective strategies to regulate the immunosuppression mechanisms, so as to activate anti-tumor immunity and/or improve the efficacy of immune-targeted drugs, which have great potential in targeted treatment of TME and to provide a cutting-edge selection idea and synergistic treatment method for clinical treatment of tumor.
Author contributions
YW: Conceptualization, Data curation, Formal Analysis, Writing–original draft, Writing–review and editing. JL: Formal Analysis, Investigation, Methodology, Supervision, Writing–original draft. LS: Conceptualization, Data curation, Investigation, Writing–review and editing. ZT: Data curation, Formal Analysis, Writing–review and editing. SW: Data curation, Methodology, Writing–review and editing. ZW: Data curation, Formal Analysis, Funding acquisition, Methodology, Resources, Writing–review and editing.
Funding
The author(s) declare that no financial support was received for the research, authorship, and/or publication of this article.
Conflict of interest
The authors declare that the research was conducted in the absence of any commercial or financial relationships that could be construed as a potential conflict of interest.
Publisher’s note
All claims expressed in this article are solely those of the authors and do not necessarily represent those of their affiliated organizations, or those of the publisher, the editors and the reviewers. Any product that may be evaluated in this article, or claim that may be made by its manufacturer, is not guaranteed or endorsed by the publisher.
References
Abe, S., Nagata, H., Crosby, E. J., Inoue, Y., Kaneko, K., Liu, C. X., et al. (2022). Combination of ultrasound-based mechanical disruption of tumor with immune checkpoint blockade modifies tumor microenvironment and augments systemic antitumor immunity. J. Immunother. cancer 10 (1), e003717. doi:10.1136/jitc-2021-003717
Agarwal, S., Hanauer, J. D. S., Frank, A. M., Riechert, V., Thalheimer, F. B., and Buchholz, C. J. (2020). In vivo generation of CAR T cells selectively in human CD4+ lymphocytes. Mol. Ther. J. Am. Soc. Gene Ther. 28 (8), 1783–1794. doi:10.1016/j.ymthe.2020.05.005
Ajina, R., Zahavi, D. J., Zhang, Y. W., and Weiner, L. M. (2020). Overcoming malignant cell-based mechanisms of resistance to immune checkpoint blockade antibodies. Seminars cancer Biol. 65, 28–37. doi:10.1016/j.semcancer.2019.12.005
Akkari, L., Bowman, R. L., Tessier, J., Klemm, F., Handgraaf, S. M., de Groot, M., et al. (2020). Dynamic changes in glioma macrophage populations after radiotherapy reveal CSF-1R inhibition as a strategy to overcome resistance. Sci. Transl. Med. 12 (552), eaaw7843. doi:10.1126/scitranslmed.aaw7843
Al Kawas, H., Saaid, I., Jank, P., Westhoff, C. C., Denkert, C., Pross, T., et al. (2022). How VEGF-A and its splice variants affect breast cancer development - clinical implications. Cell. Oncol. Dordr. 45 (2), 227–239. doi:10.1007/s13402-022-00665-w
Alphandéry, E. (2022). Ultrasound and nanomaterial: an efficient pair to fight cancer. J. nanobiotechnology 20 (1), 139. doi:10.1186/s12951-022-01243-w
Alrubayyi, A., and Rowland-Jones, S. (2023). Perforin 2 mediates endosomal antigen export for cross-presentation. Nat. Rev. Immunol. 23 (6), 345. doi:10.1038/s41577-023-00883-9
André, P., Denis, C., Soulas, C., Bourbon-Caillet, C., Lopez, J., Arnoux, T., et al. (2018). Anti-NKG2A mAb is a checkpoint inhibitor that promotes anti-tumor immunity by unleashing both T and NK cells. Cell 175 (7), 1731–1743. doi:10.1016/j.cell.2018.10.014
Aryal, M., and Porter, T. (2022). Emerging therapeutic strategies for brain tumors. Neuromolecular Med. 24 (1), 23–34. doi:10.1007/s12017-021-08681-z
Awad, N. S., Paul, V., AlSawaftah, N. M., Ter Haar, G., Allen, T. M., Pitt, W. G., et al. (2021). Ultrasound-Responsive nanocarriers in cancer treatment: a review. ACS Pharmacol. Transl. Sci. 4 (2), 589–612. doi:10.1021/acsptsci.0c00212
Bader, J. E., Voss, K., and Rathmell, J. C. (2020). Targeting metabolism to improve the tumor microenvironment for cancer immunotherapy. Mol. cell 78 (6), 1019–1033. doi:10.1016/j.molcel.2020.05.034
Bao, Z., Zeng, W., Zhang, D., Wang, L., Deng, X., Lai, J., et al. (2022). SNAIL induces EMT and lung metastasis of tumours secreting CXCL2 to promote the invasion of M2-type immunosuppressed macrophages in colorectal cancer. Int. J. Biol. Sci. 18 (7), 2867–2881. doi:10.7150/ijbs.66854
Batchu, R. B., Gruzdyn, O. V., Kolli, B. K., Dachepalli, R., Umar, P. S., Rai, S. K., et al. (2021). IL-10 signaling in the tumor microenvironment of ovarian cancer. Adv. Exp. Med. Biol. 1290, 51–65. doi:10.1007/978-3-030-55617-4_3
Bejarano, L., Jordāo, M. J. C., and Joyce, J. A. (2021). Therapeutic targeting of the tumor microenvironment. Cancer Discov. 11 (4), 933–959. doi:10.1158/2159-8290.CD-20-1808
Bilotta, M. T., Antignani, A., and Fitzgerald, D. J. (2022). Managing the TME to improve the efficacy of cancer therapy. Front. Immunol. 13, 954992. doi:10.3389/fimmu.2022.954992
Bishop, G. A. (2016). B cell-T cell interaction: antigen bridge to antigen presentation. Nat. Rev. Immunol. 16 (8), 467. doi:10.1038/nri.2016.82
Blass, E., and Ott, P. A. (2021). Advances in the development of personalized neoantigen-based therapeutic cancer vaccines. Nat. Rev. Clin. Oncol. 18 (4), 215–229. doi:10.1038/s41571-020-00460-2
Boulch, M., Cazaux, M., Loe-Mie, Y., Thibaut, R., Corre, B., Lemaître, F., et al. (2021). A cross-talk between CAR T cell subsets and the tumor microenvironment is essential for sustained cytotoxic activity. Sci. Immunol. 6 (57). doi:10.1126/sciimmunol.abd4344
Cai, Y., Fan, K., Lin, J., Ma, L., and Li, F. (2022). Advances in BBB on chip and application for studying reversible opening of blood-brain barrier by sonoporation. Micromachines 14 (1), 112. doi:10.3390/mi14010112
Chen, L., Zhang, T., Sun, S., Ren, W., Wu, A., and Xu, H. (2021). Ultrasound-mediated cavitation enhances EGFR-targeting PLGA-PEG nano-micelle delivery for triple-negative breast cancer treatment. Cancers 13 (14), 3383. doi:10.3390/cancers13143383
Chen, Z. Y., Wang, Y. X., Zhao, Y. Z., Yang, F., Liu, J. B., Lin, Y., et al. (2014). Apoptosis induction by ultrasound and microbubble mediated drug delivery and gene therapy. Curr. Mol. Med. 14 (6), 723–736. doi:10.2174/1566524014666140804165245
Chowdhury, S. M., Abou-Elkacem, L., Lee, T., Dahl, J., and Lutz, A. M. (2020). Ultrasound and microbubble mediated therapeutic delivery: underlying mechanisms and future outlook. J. Control. release official J. Control. Release Soc. 326, 75–90. doi:10.1016/j.jconrel.2020.06.008
Costache, M. I., Ioana, M., Iordache, S., Ene, D., Costache, C. A., and Săftoiu, A. (2015). VEGF expression in pancreatic cancer and other malignancies: a review of the literature. Romanian J. Intern. Med. = Revue roumaine de Med. interne 53 (3), 199–208. doi:10.1515/rjim-2015-0027
Crespo, J., Sun, H., Welling, T. H., Tian, Z., and Zou, W. (2013). T cell anergy, exhaustion, senescence, and stemness in the tumor microenvironment. Curr. Opin. Immunol. 25 (2), 214–221. doi:10.1016/j.coi.2012.12.003
Curley, C. T., Sheybani, N. D., Bullock, T. N., and Price, R. J. (2017). Focused ultrasound immunotherapy for central nervous system pathologies: challenges and opportunities. Theranostics 7 (15), 3608–3623. doi:10.7150/thno.21225
DeBerardinis, R. J. (2020). Tumor microenvironment, metabolism, and immunotherapy. N. Engl. J. Med. 382 (9), 869–871. doi:10.1056/NEJMcibr1914890
Deepak, K. G. K., Vempati, R., Nagaraju, G. P., Dasari, V. R., Rao, D. N., et al. (2020). Tumor microenvironment: challenges and opportunities in targeting metastasis of triple negative breast cancer. Pharmacol. Res. 153, 104683. doi:10.1016/j.phrs.2020.104683
Ding, Z., Li, Q., Zhang, R., Xie, L., Shu, Y., Gao, S., et al. (2021). Personalized neoantigen pulsed dendritic cell vaccine for advanced lung cancer. Signal Transduct. Target. Ther. 6 (1), 26. doi:10.1038/s41392-020-00448-5
Dong, Y., Su, H., Jiang, H., Zheng, H., Du, Y., Wu, J., et al. (2017). Experimental study on the influence of low-frequency and low-intensity ultrasound on the permeability of the Mycobacterium smegmatis cytoderm and potentiation with levofloxacin. Ultrason. sonochemistry 37, 1–8. doi:10.1016/j.ultsonch.2016.12.024
Eckhardt, B. L., Cao, Y., Redfern, A. D., Chi, L. H., Burrows, A. D., Roslan, S., et al. (2020). Activation of canonical BMP4-SMAD7 signaling suppresses breast cancer metastasis. Cancer Res. 80 (6), 1304–1315. doi:10.1158/0008-5472.CAN-19-0743
Elamir, A., Ajith, S., Sawaftah, N. A., Abuwatfa, W., Mukhopadhyay, D., Paul, V., et al. (2021). Ultrasound-triggered herceptin liposomes for breast cancer therapy. Sci. Rep. 11 (1), 7545. doi:10.1038/s41598-021-86860-5
Entzian, K., and Aigner, A. (2021). Drug delivery by ultrasound-responsive nanocarriers for cancer treatment. Pharmaceutics 13 (8), 1135. doi:10.3390/pharmaceutics13081135
Erdogan, B., and Webb, D. J. (2017). Cancer-associated fibroblasts modulate growth factor signaling and extracellular matrix remodeling to regulate tumor metastasis. Biochem. Soc. Trans. 45 (1), 229–236. doi:10.1042/BST20160387
Feril, L. B., and Tachibana, K. (2012). Use of ultrasound in drug delivery systems: emphasis on experimental methodology and mechanisms. Int. J. Hyperth. official J. Eur. Soc. Hyperthermic Oncol. North Am. Hyperth. Group 28 (4), 282–289. doi:10.3109/02656736.2012.668640
Garrido-Martin, E. M., Mellows, T. W. P., Clarke, J., Ganesan, A. P., Wood, O., Cazaly, A., et al. (2020). M1(hot) tumor-associated macrophages boost tissue-resident memory T cells infiltration and survival in human lung cancer. J. Immunother. cancer 8 (2), e000778. doi:10.1136/jitc-2020-000778
Garris, C. S., and Luke, J. J. (2020). Dendritic cells, the T-cell-inflamed tumor microenvironment, and immunotherapy treatment response. Clin. cancer Res. official J. Am. Assoc. Cancer Res. 26 (15), 3901–3907. doi:10.1158/1078-0432.CCR-19-1321
Gerhard, G. M., Bill, R., Messemaker, M., Klein, A. M., and Pittet, M. J. (2021). Tumor-infiltrating dendritic cell states are conserved across solid human cancers. J. Exp. Med. 218 (1), e20200264. doi:10.1084/jem.20200264
Giovagnoli-Vicuña, C., Briones-Labarca, V., Bernal, G., de la Fuente-Ortega, E., and Macuer, J. (2022). Ultrasound and high hydrostatic pressure extractions on antioxidant capacity, antiproliferative and apoptosis effects in gastric cancer cells by lemon extract treatment. Nat. Prod. Res. 36 (17), 4482–4486. doi:10.1080/14786419.2021.1984910
Han, H., Lee, H., Kim, K., and Kim, H. (2017). Effect of high intensity focused ultrasound (HIFU) in conjunction with a nanomedicines-microbubble complex for enhanced drug delivery. J. Control. release official J. Control. Release Soc. 266, 75–86. doi:10.1016/j.jconrel.2017.09.022
Han, Y., Sun, J., Wei, H., Hao, J., Liu, W., and Wang, X. (2022). Ultrasound-targeted microbubble destruction: modulation in the tumor microenvironment and application in tumor immunotherapy. Front. Immunol. 13, 937344. doi:10.3389/fimmu.2022.937344
He, J., Liu, Z., Zhu, X., Xia, H., Gao, H., and Lu, J. (2022). Ultrasonic microbubble cavitation enhanced tissue permeability and drug diffusion in solid tumor therapy. Pharmaceutics 14 (8), 1642. doi:10.3390/pharmaceutics14081642
Heath, V. L., and Bicknell, R. (2009). Anticancer strategies involving the vasculature. Nat. Rev. Clin. Oncol. 6 (7), 395–404. doi:10.1038/nrclinonc.2009.52
Hezaveh, K., Shinde, R. S., Klötgen, A., Halaby, M. J., Lamorte, S., Ciudad, M. T., et al. (2022). Tryptophan-derived microbial metabolites activate the aryl hydrocarbon receptor in tumor-associated macrophages to suppress anti-tumor immunity. Immunity 55 (2), 324–340.e8. doi:10.1016/j.immuni.2022.01.006
Ho, Y. J., Wang, T. C., Fan, C. H., and Yeh, C. K. (2018). Spatially uniform tumor treatment and drug penetration by regulating ultrasound with microbubbles. ACS Appl. Mater. interfaces 10 (21), 17784–17791. doi:10.1021/acsami.8b05508
Hope, H. C., and Salmond, R. J. (2019). Targeting the tumor microenvironment and T cell metabolism for effective cancer immunotherapy. Eur. J. Immunol. 49 (8), 1147–1152. doi:10.1002/eji.201848058
Hu, J., He, J., Wang, Y., Zhao, Y., Fang, K., Dong, Y., et al. (2022). Ultrasound combined with nanobubbles promotes systemic anticancer immunity and augments anti-PD1 efficacy. J. Immunother. cancer 10 (3), e003408. doi:10.1136/jitc-2021-003408
Huang, C., Li, H., Xu, Y., Xu, C., Sun, H., Li, Z., et al. (2023). BICC1 drives pancreatic cancer progression by inducing VEGF-independent angiogenesis. Signal Transduct. Target. Ther. 8 (1), 271. doi:10.1038/s41392-023-01478-5
Hunt, S. J., Gade, T., Soulen, M. C., Pickup, S., and Sehgal, C. M. (2015). Antivascular ultrasound therapy: magnetic resonance imaging validation and activation of the immune response in murine melanoma. J. ultrasound Med. official J. Am. Inst. Ultrasound Med. 34 (2), 275–287. doi:10.7863/ultra.34.2.275
Ilovitsh, T., Feng, Y., Foiret, J., Kheirolomoom, A., Zhang, H., Ingham, E. S., et al. (2020). Low-frequency ultrasound-mediated cytokine transfection enhances T cell recruitment at local and distant tumor sites. Proc. Natl. Acad. Sci. U. S. A. 117 (23), 12674–12685. doi:10.1073/pnas.1914906117
Jhunjhunwala, S., Hammer, C., and Delamarre, L. (2021). Antigen presentation in cancer: insights into tumour immunogenicity and immune evasion. Nat. Rev. Cancer 21 (5), 298–312. doi:10.1038/s41568-021-00339-z
Ji, C., Si, J., Xu, Y., Zhang, W., Yang, Y., He, X., et al. (2021). Mitochondria-targeted and ultrasound-responsive nanoparticles for oxygen and nitric oxide codelivery to reverse immunosuppression and enhance sonodynamic therapy for immune activation. Theranostics 11 (17), 8587–8604. doi:10.7150/thno.62572
Jiang, J., Ye, F., Yang, X., Zong, C., Gao, L., Yang, Y., et al. (2017). Peri-tumor associated fibroblasts promote intrahepatic metastasis of hepatocellular carcinoma by recruiting cancer stem cells. Cancer Lett. 404, 19–28. doi:10.1016/j.canlet.2017.07.006
Jiang, X., Wang, J., Deng, X., Xiong, F., Ge, J., Xiang, B., et al. (2019). Role of the tumor microenvironment in PD-L1/PD-1-mediated tumor immune escape. Mol. cancer 18 (1), 10. doi:10.1186/s12943-018-0928-4
Jiang, Y., Chen, M., Nie, H., and Yuan, Y. (2019). PD-1 and PD-L1 in cancer immunotherapy: clinical implications and future considerations. Hum. vaccines Immunother. 15 (5), 1111–1122. doi:10.1080/21645515.2019.1571892
Jugniot, N., Dahl, J. J., and Paulmurugan, R. (2022). Immunotheranostic microbubbles (iMBs) - a modular platform for dendritic cell vaccine delivery applied to breast cancer immunotherapy. J. Exp. Clin. cancer Res. CR 41 (1), 299. doi:10.1186/s13046-022-02501-3
Keenan, T. E., and Tolaney, S. M. (2020). Role of immunotherapy in triple-negative breast cancer. J. Natl. Compr. Cancer Netw. JNCCN 18 (4), 479–489. doi:10.6004/jnccn.2020.7554
Kim, D. K., Jeong, J., Lee, D. S., Hyeon, D. Y., Park, G. W., Jeon, S., et al. (2022). PD-L1-directed PlGF/VEGF blockade synergizes with chemotherapy by targeting CD141+ cancer-associated fibroblasts in pancreatic cancer. Nat. Commun. 13 (1), 6292. doi:10.1038/s41467-022-33991-6
Kim, J. H., Wang, X., Zhang, C., Yoon, S. A., Shin, J., et al. (2020). Multifunctional sonosensitizers in sonodynamic cancer therapy. Chem. Soc. Rev. 49 (11), 3244–3261. doi:10.1039/c9cs00648f
Kishton, R. J., Sukumar, M., and Restifo, N. P. (2017). Metabolic regulation of T cell longevity and function in tumor immunotherapy. Cell metab. 26 (1), 94–109. doi:10.1016/j.cmet.2017.06.016
Koll, F. J., Banek, S., Kluth, L., Köllermann, J., Bankov, K., Chun, F. K. H., et al. (2023). Tumor-associated macrophages and Tregs influence and represent immune cell infiltration of muscle-invasive bladder cancer and predict prognosis. J. Transl. Med. 21 (1), 124. doi:10.1186/s12967-023-03949-3
Komi, D. E. A., and Redegeld, F. A. (2020). Role of mast cells in shaping the tumor microenvironment. Clin. Rev. allergy and Immunol. 58 (3), 313–325. doi:10.1007/s12016-019-08753-w
Kooiman, K., Roovers, S., Langeveld, S. A. G., Kleven, R. T., Dewitte, H., O'Reilly, M. A., et al. (2020). Ultrasound-Responsive cavitation nuclei for therapy and drug delivery. Ultrasound Med. Biol. 46 (6), 1296–1325. doi:10.1016/j.ultrasmedbio.2020.01.002
Kumar, A. R., Devan, A. R., Nair, B., Vinod, B. S., and Nath, L. R. (2021). Harnessing the immune system against cancer: current immunotherapy approaches and therapeutic targets. Mol. Biol. Rep. 48 (12), 8075–8095. doi:10.1007/s11033-021-06752-9
Lahiri, A., Maji, A., Potdar, P. D., Singh, N., Parikh, P., Bisht, B., et al. (2023). Lung cancer immunotherapy: progress, pitfalls, and promises. Mol. cancer 22 (1), 40. doi:10.1186/s12943-023-01740-y
Li, X., Gao, Y., Liu, X., Hu, X., Li, Y., Sun, J., et al. (2022). Ultrasound and laser-promoted dual-gas nano-generator for combined photothermal and immune tumor therapy. Front. Bioeng. Biotechnol. 10, 1005520. doi:10.3389/fbioe.2022.1005520
Lim, A. R., Rathmell, W. K., and Rathmell, J. C. (2020). The tumor microenvironment as a metabolic barrier to effector T cells and immunotherapy. eLife 9, e55185. doi:10.7554/eLife.55185
Liu, C., Wang, M., Zhang, H., Li, C., Zhang, T., Liu, H., et al. (2022). Tumor microenvironment and immunotherapy of oral cancer. Eur. J. Med. Res. 27 (1), 198. doi:10.1186/s40001-022-00835-4
Ma, X., Bi, E., Lu, Y., Su, P., Huang, C., Liu, L., et al. (2019). Cholesterol induces CD8(+) T cell exhaustion in the tumor microenvironment. Cell metab. 30 (1), 143–156.e5. doi:10.1016/j.cmet.2019.04.002
Marciscano, A. E., and Anandasabapathy, N. (2021). The role of dendritic cells in cancer and anti-tumor immunity. Seminars Immunol. 52, 101481. doi:10.1016/j.smim.2021.101481
Mehta, A. K., Kadel, S., Townsend, M. G., Oliwa, M., and Guerriero, J. L. (2021). Macrophage biology and mechanisms of immune suppression in breast cancer. Front. Immunol. 12, 643771. doi:10.3389/fimmu.2021.643771
Mitragotri, S. (2005). Healing sound: the use of ultrasound in drug delivery and other therapeutic applications. Nat. Rev. Drug Discov. 4 (3), 255–260. doi:10.1038/nrd1662
Miyake, M., Hori, S., Morizawa, Y., Tatsumi, Y., Nakai, Y., Anai, S., et al. (2016). CXCL1-Mediated interaction of cancer cells with tumor-associated macrophages and cancer-associated fibroblasts promotes tumor progression in human bladder cancer. Neoplasia (New York, NY) 18 (10), 636–646. doi:10.1016/j.neo.2016.08.002
Modi, S., Saura, C., Yamashita, T., Park, Y. H., Kim, S. B., Tamura, K., et al. (2020). Trastuzumab deruxtecan in previously treated HER2-positive breast cancer. N. Engl. J. Med. 382 (7), 610–621. doi:10.1056/NEJMoa1914510
Mollica Poeta, V., Massara, M., Capucetti, A., and Bonecchi, R. (2019). Chemokines and chemokine receptors: new targets for cancer immunotherapy. Front. Immunol. 10, 379. doi:10.3389/fimmu.2019.00379
Munir, M. T., Kay, M. K., Kang, M. H., Rahman, M. M., Al-Harrasi, A., Choudhury, M., et al. (2021). Tumor-associated macrophages as multifaceted regulators of breast tumor growth. Int. J. Mol. Sci. 22 (12), 6526. doi:10.3390/ijms22126526
Myers, R., Grundy, M., Rowe, C., Coviello, C. M., Bau, L., Erbs, P., et al. (2018). Ultrasound-mediated cavitation does not decrease the activity of small molecule, antibody or viral-based medicines. Int. J. nanomedicine 13, 337–349. doi:10.2147/IJN.S141557
Niu, Z. H., Zhao, C. Y., and Jiang, Y. X. (2020). Synergistic anti-tumor mechanisms of low-frequency ultrasound-targeted microbubble destruction:mechanisms and application. Zhongguo yi xue ke xue yuan xue bao Acta Acad. Med. Sin. 42 (4), 540–545. doi:10.3881/j.issn.1000-503X.11357
Oda, Y., Suzuki, R., Otake, S., Nishiie, N., Hirata, K., Koshima, R., et al. (2012). Prophylactic immunization with Bubble liposomes and ultrasound-treated dendritic cells provided a four-fold decrease in the frequency of melanoma lung metastasis. J. Control. release official J. Control. Release Soc. 160 (2), 362–366. doi:10.1016/j.jconrel.2011.12.003
Omata, D., Munakata, L., Maruyama, K., and Suzuki, R. (2001). Ultrasound and microbubble-mediated drug delivery and immunotherapy. J. Med. ultrasonics, 2022. doi:10.1007/s10396-022-01201-x
Patel, V. G., Oh, W. K., and Galsky, M. D. (2020). Treatment of muscle-invasive and advanced bladder cancer in 2020. CA a cancer J. Clin. 70 (5), 404–423. doi:10.3322/caac.21631
Pelka, S., and Guha, C. (2023). Enhancing immunogenicity in metastatic melanoma: adjuvant therapies to promote the anti-tumor immune response. Biomedicines 11 (8), 2245. doi:10.3390/biomedicines11082245
Pitt, J. M., Marabelle, A., Eggermont, A., Soria, J. C., Kroemer, G., and Zitvogel, L. (2016). Targeting the tumor microenvironment: removing obstruction to anticancer immune responses and immunotherapy. Ann. Oncol. official J. Eur. Soc. Med. Oncol. 27 (8), 1482–1492. doi:10.1093/annonc/mdw168
Ranieri, G., Patruno, R., Ruggieri, E., Montemurro, S., Valerio, P., and Ribatti, D. (2006). Vascular endothelial growth factor (VEGF) as a target of bevacizumab in cancer: from the biology to the clinic. Curr. Med. Chem. 13 (16), 1845–1857. doi:10.2174/092986706777585059
Ren, J., Smid, M., Iaria, J., Salvatori, D. C. F., van Dam, H., Zhu, H. J., et al. (2019). Cancer-associated fibroblast-derived Gremlin 1 promotes breast cancer progression. Breast cancer Res. BCR 21 (1), 109. doi:10.1186/s13058-019-1194-0
Ribeiro Franco, P. I., Rodrigues, A. P., de Menezes, L. B., and Pacheco Miguel, M. (2020). Tumor microenvironment components: allies of cancer progression. . Pathology, Res. Pract. 216 (1), 152729. doi:10.1016/j.prp.2019.152729
Sadeghi-Goughari, M. J. S., and Kwon, H. J. (2019). Enhancing thermal effect of focused ultrasound therapy using gold nanoparticles. IEEE Trans. nanobioscience 18 (4), 661–668. doi:10.1109/TNB.2019.2937327
Singh, M. P., Sethuraman, S. N., Ritchey, J., Fiering, S., Guha, C., Malayer, J., et al. (2019). In-situ vaccination using focused ultrasound heating and anti-CD-40 agonistic antibody enhances T-cell mediated local and abscopal effects in murine melanoma. Int. J. Hyperth. official J. Eur. Soc. Hyperthermic Oncol. North Am. Hyperth. Group 36 (1), 64–73. doi:10.1080/02656736.2019.1663280
Snipstad, S., Hanstad, S., Bjørkøy, A., Mørch, Ý., and de Lange Davies, C. (2021). Sonoporation using nanoparticle-loaded microbubbles increases cellular uptake of nanoparticles compared to Co-incubation of nanoparticles and microbubbles. Pharmaceutics 13 (5), 640. doi:10.3390/pharmaceutics13050640
Sta Maria, N. S., Barnes, S. R., Weist, M. R., Colcher, D., Raubitschek, A. A., and Jacobs, R. E. (2015). Low dose focused ultrasound induces enhanced tumor accumulation of natural killer cells. PloS one 10 (11), e0142767. doi:10.1371/journal.pone.0142767
Su, J. W. J., Luo, J., and Li, H. (2019). Ultrasound-mediated destruction of vascular endothelial growth factor (VEGF) targeted and paclitaxel loaded microbubbles for inhibition of human breast cancer cell MCF-7 proliferation. Mol. Cell. probes 46, 101415. doi:10.1016/j.mcp.2019.06.005
Sun, M., Zeng, H., Jin, K., Liu, Z., Hu, B., Liu, C., et al. (2022). Infiltration and polarization of tumor-associated macrophages predict prognosis and therapeutic benefit in muscle-invasive bladder cancer. Cancer Immunol. Immunother. CII 71 (6), 1497–1506. doi:10.1007/s00262-021-03098-w
Sun, X., He, X., Zhang, Y., Hosaka, K., Andersson, P., Wu, J., et al. (2022). Inflammatory cell-derived CXCL3 promotes pancreatic cancer metastasis through a novel myofibroblast-hijacked cancer escape mechanism. Gut 71 (1), 129–147. doi:10.1136/gutjnl-2020-322744
Sun, W. J. P., Zhou, T., Li, Z., Xing, C., Zhang, L., Wei, M., et al. (2023). Ultrasound responsive nanovaccine armed with engineered cancer cell membrane and RNA to prevent foreseeable metastasis. . Adv. Sci. (Weinheim, Baden-Wurttemberg, Ger. 10 (19), e2301107. doi:10.1002/advs.202301107
Suzuki, R., Namai, E., Oda, Y., Nishiie, N., Otake, S., Koshima, R., et al. (2010). Cancer gene therapy by IL-12 gene delivery using liposomal bubbles and tumoral ultrasound exposure. J. Control. release official J. Control. Release Soc. 142 (2), 245–250. doi:10.1016/j.jconrel.2009.10.027
Tagliaferri, L., Lancellotta, V., Fionda, B., Mangoni, M., Casà, C., Di Stefani, A., et al. (2022). Immunotherapy and radiotherapy in melanoma: a multidisciplinary comprehensive review. Hum. vaccines Immunother. 18 (3), 1903827. doi:10.1080/21645515.2021.1903827
Tang, J., Tang, J., Li, H., Zhou, J., Tang, N., Zhu, Q., et al. (2023). Mechanical destruction using a minimally invasive Ultrasound Needle induces anti-tumor immune responses and synergizes with the anti-PD-L1 blockade. Cancer Lett. 554, 216009, 216009. doi:10.1016/j.canlet.2022.216009
Tang, J., Yang, J., Zhu, B., Wang, X., Luo, Y., et al. (2021). Tumor perfusion enhancement by ultrasound stimulated microbubbles potentiates PD-L1 blockade of MC38 colon cancer in mice. Cancer Lett. 498, 121–129. doi:10.1016/j.canlet.2020.10.046
Thomas, E., Menon, J. U., Owen, J., Skaripa-Koukelli, I., Wallington, S., Gray, M., et al. (2019). Ultrasound-mediated cavitation enhances the delivery of an EGFR-targeting liposomal formulation designed for chemo-radionuclide therapy. Theranostics 9 (19), 5595–5609. doi:10.7150/thno.34669
Verneau, J., Sautés-Fridman, C., and Sun, C. M. (2020). Dendritic cells in the tumor microenvironment: prognostic and theranostic impact. Seminars Immunol. 48, 101410. doi:10.1016/j.smim.2020.101410
Wan, X., Guan, S., Hou, Y., Qin, Y., Zeng, H., Yang, L., et al. (2021). FOSL2 promotes VEGF-independent angiogenesis by transcriptionnally activating Wnt5a in breast cancer-associated fibroblasts. Theranostics 11 (10), 4975–4991. doi:10.7150/thno.55074
Wang, H., Hu, Z., Sukumar, U. K., Bose, R. J., Telichko, A., Dahl, J. J., et al. (2022). Ultrasound-guided microbubble-mediated locoregional delivery of multiple MicroRNAs improves chemotherapy in hepatocellular carcinoma. Nanotheranostics 6 (1), 62–78. doi:10.7150/ntno.63320
Wang, L., Li, G., Cao, L., Dong, Y., Wang, Y., Wang, S., et al. (2021). An ultrasound-driven immune-boosting molecular machine for systemic tumor suppression. Sci. Adv. 7 (43), eabj4796. doi:10.1126/sciadv.abj4796
Wang, L. W. X., Shen, L., Alrobaian, M., Panda, S. K., Almasmoum, H. A., Ghaith, M. M., et al. (2021). Paclitaxel and naringenin-loaded solid lipid nanoparticles surface modified with cyclic peptides with improved tumor targeting ability in glioblastoma multiforme. Biomed. Pharmacother. = Biomedecine Pharmacother. 138, 111461. doi:10.1016/j.biopha.2021.111461
Wischhusen, J. C., Chowdhury, S. M., Lee, T., Wang, H., Bachawal, S., Devulapally, R., et al. (2020). Ultrasound-mediated delivery of miRNA-122 and anti-miRNA-21 therapeutically immunomodulates murine hepatocellular carcinoma in vivo. J. Control. release official J. Control. Release Soc. 321, 272–284. doi:10.1016/j.jconrel.2020.01.051
Wu, N., Cao, Y., Liu, Y., Zhou, Y., He, H., Tang, R., et al. (2023). Low-intensity focused ultrasound targeted microbubble destruction reduces tumor blood supply and sensitizes anti-PD-L1 immunotherapy. Front. Bioeng. Biotechnol. 11, 1173381. doi:10.3389/fbioe.2023.1173381
Wu, Y., Li, X., Wang, Z., Zhang, S., Feng, Y., Sun, L., et al. (2023). Real-time elastography and contrast-enhanced ultrasound for evaluating adventitia in the early diagnosis of vulnerable plaques: an exploratory study based on histopathology. Transl. stroke Res. doi:10.1007/s12975-023-01141-9
Xiang, M., Liu, T., Tian, C., Ma, K., Gou, J., Huang, R., et al. (2022). Kinsenoside attenuates liver fibro-inflammation by suppressing dendritic cells via the PI3K-AKT-FoxO1 pathway. Pharmacol. Res. 2022, 106092, 106092. doi:10.1016/j.phrs.2022.106092
Xiong, J., Jiang, B., Luo, Y., Zou, J., Gao, X., Xu, D., et al. (2020). Multifunctional nanoparticles encapsulating Astragalus polysaccharide and gold nanorods in combination with focused ultrasound for the treatment of breast cancer. Int. J. nanomedicine 15, 4151–4169. doi:10.2147/IJN.S246447
Xu, Q. S. T., Tian, H., Wang, C., and Zhou, H. (2013). Ultrasound-mediated vascular endothelial growth factor C (VEGF-C) gene microbubble transfection inhibits growth of MCF-7 breast cancer cells. Oncol. Res. 20 (7), 297–301. doi:10.3727/096504013x13639794277680
Xu, X., Li, T., Shen, S., Wang, J., Abdou, P., Gu, Z., et al. (2019). Advances in engineering cells for cancer immunotherapy. Theranostics 9 (25), 7889–7905. doi:10.7150/thno.38583
Yang, C., Du, M., Yan, F., and Chen, Z. (2019). Focused ultrasound improves NK-92MI cells infiltration into tumors. Front. Pharmacol. 10, 326. doi:10.3389/fphar.2019.00326
Yang, H., Sun, Y., Wei, J., Xu, L., Tang, Y., Yang, L., et al. (2019). The effects of ultrasound-targeted microbubble destruction (UTMD) carrying IL-8 monoclonal antibody on the inflammatory responses and stability of atherosclerotic plaques. Biomed. Pharmacother. = Biomedecine Pharmacother. 118, 109161. doi:10.1016/j.biopha.2019.109161
Yang, K., Halima, A., and Chan, T. A. (2023). Antigen presentation in cancer - mechanisms and clinical implications for immunotherapy. Nat. Rev. Clin. Oncol. 20 (9), 604–623. doi:10.1038/s41571-023-00789-4
Yang, M., Wang, L., Neuber, B., Wang, S., Gong, W., et al. (2021). Dual effects of cyclooxygenase inhibitors in combination with CD19.CAR-T cell immunotherapy. Front. Immunol. 12, 670088. doi:10.3389/fimmu.2021.670088
Yang, M., Xie, S., Adhikari, V. P., Dong, Y., Du, Y., and Li, D. (2018). The synergistic fungicidal effect of low-frequency and low-intensity ultrasound with amphotericin B-loaded nanoparticles on C. albicans in vitro. Int. J. Pharm. 542 (1-2), 232–241. doi:10.1016/j.ijpharm.2018.03.033
Yang, T., Zhang, Y., Wang, T., Li, M., Zhang, Y., Zhao, D., et al. (2022). Low-frequency ultrasound irradiation increases paclitaxel-induced sarcoma cells apoptosis and facilitates the transmembrane delivery of drugs. Front. Pharmacol. 13, 1065289. doi:10.3389/fphar.2022.1065289
Ye, X., Li, Z., Xue, G., and Wei, Z. (2022). Experimental study on the induction of cytotoxic T lymphocyte killing effects and dendritic-cell-based tumor vaccine prepared by high-intensity focused ultrasound. J. cancer Res. Ther. 18 (5), 1292–1298. doi:10.4103/jcrt.jcrt_2291_21
Yi, M., Zheng, X., Niu, M., Zhu, S., Ge, H., and Wu, K. (2022). Combination strategies with PD-1/PD-L1 blockade: current advances and future directions. Mol. cancer 21 (1), 28. doi:10.1186/s12943-021-01489-2
Yoshida, G. J. (2020). Regulation of heterogeneous cancer-associated fibroblasts: the molecular pathology of activated signaling pathways. J. Exp. Clin. cancer Res. CR 39 (1), 112. doi:10.1186/s13046-020-01611-0
Yu, Z., Liu, W., He, Y., Sun, M., Yu, J., Jiao, X., et al. (2021). HLA-A2.1-restricted ECM1-derived epitope LA through DC cross-activation priming CD8+ T and NK cells: a novel therapeutic tumour vaccine. J. Hematol. Oncol. 14 (1), 71. doi:10.1186/s13045-021-01081-7
Zhang, H., Luo, Y. B., Wu, W., Zhang, L., Wang, Z., Dai, Z., et al. (2021). The molecular feature of macrophages in tumor immune microenvironment of glioma patients. Comput. Struct. Biotechnol. J. 19, 4603–4618. doi:10.1016/j.csbj.2021.08.019
Zhang, N., Wang, J., Foiret, J., Dai, Z., and Ferrara, K. W. (2021). Synergies between therapeutic ultrasound, gene therapy and immunotherapy in cancer treatment. Adv. drug Deliv. Rev. 178, 113906. doi:10.1016/j.addr.2021.113906
Zhang, J., Zhang, H., Zhang, L., Li, D., Qi, M., Zhang, L., et al. (2022). Single-cell transcriptome identifies drug-resistance signature and immunosuppressive microenvironment in metastatic small cell lung cancer. Adv. Genet. Hob. NJ) 3 (2), 2100060. doi:10.1002/ggn2.202100060
Zhang, W., Shou, W. D., Xu, Y. J., Bai, W. K., and Hu, B. (2017). Low-frequency ultrasound-induced VEGF suppression and synergy with dendritic cell-mediated anti-tumor immunity in murine prostate cancer cells in vitro. Sci. Rep. 7 (1), 5778. doi:10.1038/s41598-017-06242-8
Zhao, Y., Guo, S., Deng, J., Shen, J., Du, F., Wu, X., et al. (2022). VEGF/VEGFR-targeted therapy and immunotherapy in non-small cell lung cancer: targeting the tumor microenvironment. Int. J. Biol. Sci. 18 (9), 3845–3858. doi:10.7150/ijbs.70958
Zhu, L., Ren, S., Daniels, M. J., Qiu, W., Song, L., You, T., et al. (2021). Exogenous HMGB1 promotes the proliferation and metastasis of pancreatic cancer cells. Front. Med. 8, 756988. doi:10.3389/fmed.2021.756988
Zhu, Z., and He, L. (2023). Qi Chunjian the regulatory effect of macrophages on NK cells in the tumor microenvironment. Chin. J. Immunol. 39 (4), 849–854. doi:10.3969/j.issn.1000-484X.2023.04.031
Keywords: ultrasound, cavitation effect, tumour microenvironment, immunotherapy, tumor cell
Citation: Wu Y, Li J, Shu L, Tian Z, Wu S and Wu Z (2024) Ultrasound combined with microbubble mediated immunotherapy for tumor microenvironment. Front. Pharmacol. 15:1304502. doi: 10.3389/fphar.2024.1304502
Received: 29 September 2023; Accepted: 11 January 2024;
Published: 29 February 2024.
Edited by:
S. Ilavenil, National Institute of Animal Science, Republic of KoreaReviewed by:
Anis Ahamed, King Saud University, Saudi ArabiaPonmurugan Karuppiah, King Saud University, Saudi Arabia
Copyright © 2024 Wu, Li, Shu, Tian, Wu and Wu. This is an open-access article distributed under the terms of the Creative Commons Attribution License (CC BY). The use, distribution or reproduction in other forums is permitted, provided the original author(s) and the copyright owner(s) are credited and that the original publication in this journal is cited, in accordance with accepted academic practice. No use, distribution or reproduction is permitted which does not comply with these terms.
*Correspondence: Zuohui Wu, Y3N4ZmQxMjM0NUAxNjMuY29t