- Key Laboratory of Plant Secondary Metabolism and Regulation of Zhejiang Province, College of Life Sciences, Zhejiang Sci-Tech University, Hangzhou, China
Lung cancer is one of the leading causes of cancer-related deaths worldwide that presents a substantial peril to human health. Non-Small Cell Lung Cancer (NSCLC) is a main subtype of lung cancer with heightened metastasis and invasion ability. The predominant treatment approaches currently comprise surgical interventions, chemotherapy regimens, and radiotherapeutic procedures. However, it poses significant clinical challenges due to its tumor heterogeneity and drug resistance, resulting in diminished patient survival rates. Therefore, the development of novel treatment strategies for NSCLC is necessary. Ferroptosis was characterized by iron-dependent lipid peroxidation and the accumulation of lipid reactive oxygen species (ROS), leading to oxidative damage of cells and eventually cell death. An increasing number of studies have found that exploiting the induction of ferroptosis may be a potential therapeutic approach in NSCLC. Recent investigations have underscored the remarkable potential of natural products in the cancer treatment, owing to their potent activity and high safety profiles. Notably, accumulating evidences have shown that targeting ferroptosis through natural compounds as a novel strategy for combating NSCLC holds considerable promise. Nevertheless, the existing literature on comprehensive reviews elucidating the role of natural products inducing the ferroptosis for NSCLC therapy remains relatively sparse. In order to furnish a valuable reference and support for the identification of natural products inducing ferroptosis in anti-NSCLC therapeutics, this article provided a comprehensive review explaining the mechanisms by which natural products selectively target ferroptosis and modulate the pathogenesis of NSCLC.
1 Introduction
According to the latest data from the Global Cancer Observatory (GCO) (Cancer Today (iarc.fr)), in 2020, lung cancer ranked as the third most common cancer globally, with an incidence rate of approximately 22.4 cases per 100,000 population and a mortality rate of around 18% per 100,000 population (Sung et al., 2021) (Figure 1). Non-Small Cell Lung Cancer (NSCLC) represents the predominant subtype of lung cancer, accounting for approximately 85% of cases, and it is characterized by a poor prognosis, with a 5-year survival rate of only 19% (Rodríguez-Abreu et al., 2021; Mithoowani and Febbraro, 2022). Over the past few decades, various treatment modalities, including surgery, chemotherapy, radiation therapy, targeted therapy, and immunotherapy, have been employed in the clinical management of NSCLC (Hopstaken et al., 2021; Miller and Hanna, 2021; Wang et al., 2021; Alduais et al., 2023). Despite the significant advances achieved for these treatment strategies, the development of therapy resistance in NSCLC remains a considerable challenge (Brown et al., 2019; Patel and Weiss, 2020; Muthusamy et al., 2022), thus necessitating the exploration of novel therapeutic approaches for NSCLC.
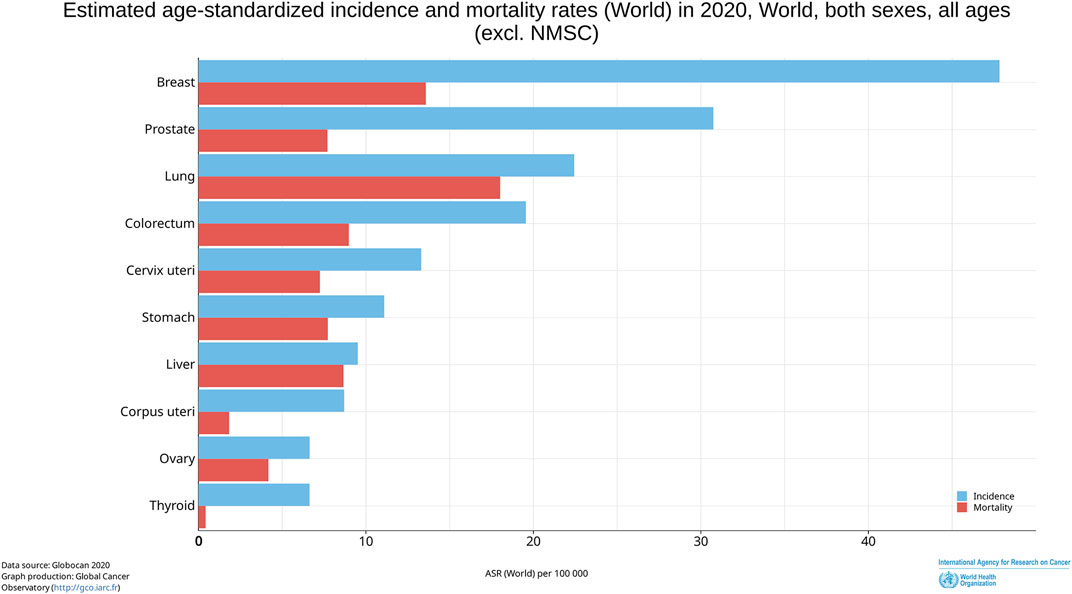
Figure 1. Estimated age-standardized incidence and mortality rates (World) in 2020, World, both sexes, all ages.
Ferroptosis is a novel form of programmed cell death (PCD) that has been recently discovered and differs morphologically, biochemically, and genetically from apoptosis, autophagy, and necrotic (Mou et al., 2019; Chen et al., 2021b; Yuan et al., 2021). The presence of ferroptosis in cells is commonly linked to the accumulation of iron, disturbances in fatty acid metabolism, and lipid peroxidation (Ursini and Maiorino, 2020; Xu et al., 2021; Yao et al., 2021), which play crucial roles in initiating ferroptosis (Li and Li, 2020; Zhang et al., 2022). Previous studies have highlighted the significant role of ferroptosis in the pathogenesis of NSCLC, suggesting ferroptosis maybe a potential and novel approach for NSCLC treatment (Liu et al., 2021; Liu et al., 2022). The mediators or signal pathways regarding to ferroptosis in the pathological progression of NSCLC were presented in Table 1.
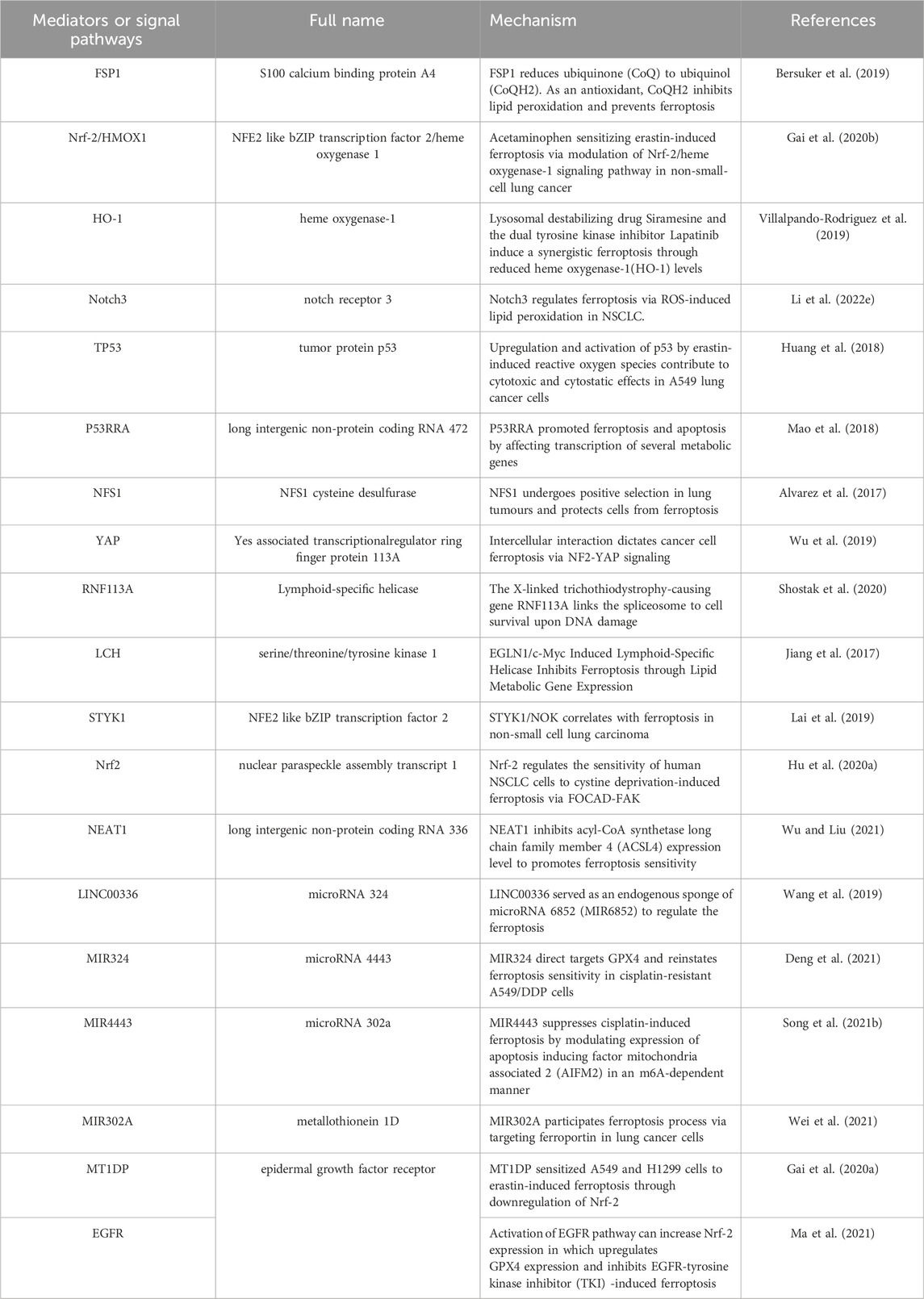
Table 1. The mediators or signal pathways regarding to ferroptosis in the pathological progression of NSCLC.
In recent years, natural products from traditional herbal medicine have emerged as an increasingly important therapy in the prevention and treatment of NSCLC (Zhang et al., 2018; Wan et al., 2019; Li et al., 2021). Furthermore, there is a growing body of research focusing on the modulation of ferroptosis by natural products for the prevention and treatment of NSCLC (Batbold and Liu, 2021). This article discusses the molecular mechanisms underlying ferroptosis and highlights the mechanisms by which different types of natural products induce ferroptosis to exert anti-cancer effects on NSCLC. The aim is to further provide theoretical support for drug development and treatment strategies in NSCLC.
2 Mechanism of ferroptosis
Ferroptosis represents a distinct form of cell death, which was first proposed by Dixon et al., in 2012 (Dixon et al., 2012). Morphologically, ferroptosis is characterized by mitochondrial shrinkage, mitochondrial membrane rupture, increased membrane density, and reduced or vanished mitochondrial cristae (Xie et al., 2016). Biochemically, lipid peroxidation, iron metabolism, redox homeostasis and fatty acid supply are currently thought to be pivotal to the induction of ferroptosis. (Li et al., 2020; Chen et al., 2021c). The following part provides an overview of the extensively studied mechanisms underlying ferroptosis (Figure 2).
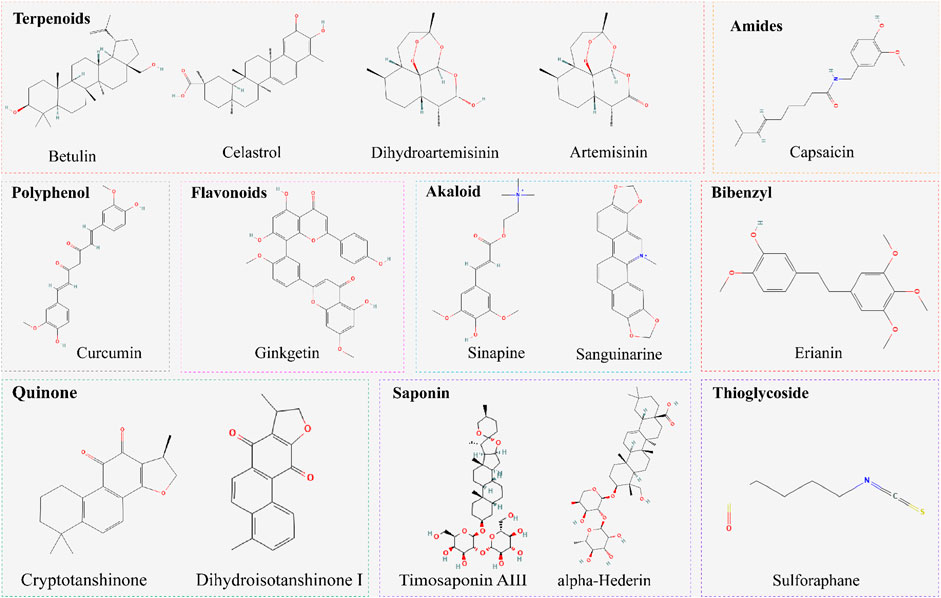
Figure 2. Regulatory mechanism of ferroptosis. General mechanism of ferroptosis associated with System Xc−, GPX4, accumulation of iron, and lipid peroxidation.
2.1 Inhibition of the cysteine-glutamate transporter system Xc− induces ferroptosis
The system Xc- is a transport system involved in the regulation of cellular redox balance and the production of GSH (Liu et al., 2021). It is composed of two subunits: the light chain, known as xCT (SLC7A11), and the heavy chain, known as solute carrier family 3 member 2 (SLC3A2) (Tu et al., 2021). xCT is responsible for the transport of cystine (oxidized form of cysteine) into the cell, while SLC3A2 acts as a chaperone and stabilizes the expression of xCT on the cell surface (Wang et al., 2022). The function of system Xc- is to transport cystine into the cell in exchange for glutamate (Glu) export. Cystine is a disulfide form of the cysteine, which is essential for the synthesis of the antioxidant GSH. GSH helps to neutralize reactive oxygen species (ROS) and protects cells from oxidative damage (Albrecht et al., 2010). In the context of ferroptosis, system Xc- plays a central role in maintaining intracellular redox homeostasis. It imports cystine into the cell, which is subsequently reduced to cysteine. By promoting the availability of cystine, system Xc- supports the synthesis of GSH by catalyzing glutathione synthetase (GSS) and glycine (Gly). Throughout this process, GSH undergoes oxidation to form oxidized glutathione (GSSG). However, GSSG is subsequently converted back to its reduced form, GSH, with the assistance of an enzyme called glutathione reductase (GR). Therefore, inhibition or genetic depletion of system Xc- leads to impaired cystine uptake, reduced GSH synthesis, and increased vulnerability to lipid peroxidation, ultimately promoting ferroptosis (Li F. J. et al., 2022).
2.2 Inhibition of GPX4 induce ferroptosis
GPX4, a crucial antioxidant enzyme predominantly localized within cellular organelle membranes, plays a significant role in the elimination of lipid peroxides (Miao et al., 2022). Specifically, it exhibits the capacity to catalyze the reaction between GSH and lipid peroxidation during the process of ferroptosis. This enzyme facilitates the reduction of lipid peroxidation into benign alcohol forms, thereby impeding the buildup of lipid peroxidation (Sui et al., 2018). However, under conditions of inadequate intracellular GSH levels, the functionality of GPX4 becomes hindered, impeding the effective clearance of lipid peroxidation (Xu et al., 2021). During ferroptosis, the inhibition of system Xc- leads to a reduction in GSH synthesis, diminishes the substrate availability for GPX4 and reduces the elimination of lipid peroxidation, ultimately provokes the initiation of ferroptosis (Seibt et al., 2019).
2.3 Accumulation of iron
Iron is an essential bio-element within cells, participating in various physiological processes, including oxygen transport (Lipper et al., 2019), DNA synthesis (Lane et al., 2015), and energy production (Hentze et al., 2004). Under certain conditions, iron can also act as catalysts for cell death, promoting the occurrence of ferroptosis (Ma et al., 2022; Anandhan et al., 2023). Ferric ions (Fe3+) are imported into the cell from the extracellular space through their binding to transferrin (TF), forming the complex “TF–Fe3+–TfR1” with transferrin receptor 1 (TfR1) (Hadzhieva et al., 2014; Basuli et al., 2017). This process involving TF and TfR1 is crucial for the intracellular accumulation of lipid peroxides and the occurrence of ferroptosis. Within the endosome, Fe3+ are converted to ferrous ions (Fe2+) by ferric reductases such as STEAP3 metalloreductase (Ye et al., 2022). Subsequently, Fe2+ are transported from the endosome to the labile iron pool (LIP) via the divalent metal transporter 1 (DMT1) (Aschner et al., 2022). In the cytosol, Fe2+ reacts with hydrogen peroxide (H2O2) through the Fenton reaction, leading to lipid peroxidation and the generation of ROS(Liu et al., 2022). Importantly, various cellular processes that influence iron uptake, storage, utilization, and release can impact cell sensitivity to ferroptosis. For instance, the degradation of ferritin induced by nuclear receptor coactivator 4 (NCOA4) also contributes to ferroptosis promotion (Santana-Codina et al., 2021). Conversely, reduced expression of solute carrier family 40 member 1 (SLC40A1) may result in intracellular Fe2+ accumulation, subsequently increase iron-dependent oxidative stress and facilitate ferroptosis (Hao et al., 2021).
2.4 Lipid peroxidation
Lipid metabolism plays a vital role in the occurrence of ferroptosis, which is characterized by the accumulation of lipid peroxides resulting from the oxidation of polyunsaturated fatty acids (PUFAs), a class of fatty acids characterized by the presence of multiple double bonds, including omega-3 and omega-6 fatty acids (Christie and Harwood, 2020), which plays essential roles in the composition of cell membranes and participate in numerous physiological processes within cells (Wiktorowska-Owczarek et al., 2015). During the process of lipid peroxidation, several enzymes involved in lipid metabolism act as positive regulators of ferroptosis. One such enzyme is Acyl-CoA synthetase long chain family member 4 (ACSL4), which participates in phospholipid metabolism and facilitates the synthesis of PUFA-CoA from PUFAs like arachidonoyl (AA) and adrenal (AdA), thereby activating PUFAs(Doll et al., 2017). Following ACSL4-driven esterification, lysophosphatidic transferase 3 (LPCAT3) incorporates PUFAs into phospholipids, forming phospholipids containing PUFAs (Reed et al., 2022). Subsequently, ALOX15 oxidizes these PUFA-PLs, generating lipid peroxides and ultimately leading to ferroptosis (Ma et al., 2022).
2.5 Others
Ferroptosis can also be regulated by several another protein. Recent publications are summarized in Table 2.
3 Natural products modulating ferroptosis for intervention in NSCLC
Natural products possess multiple pharmacological activities, particular in the treatment of tumors. Recently, researchers have identified certain natural products that can modulate ferroptosis to exert anti-tumor potential (Yang et al., 2022). Figure 3 provides a compilation of natural products with their sources and chemical formulas, which induce ferroptosis to treat NSCLC. The regulatory targets and mechanisms of these natural products are illustrated in Figure 4 and presented in Table 3.
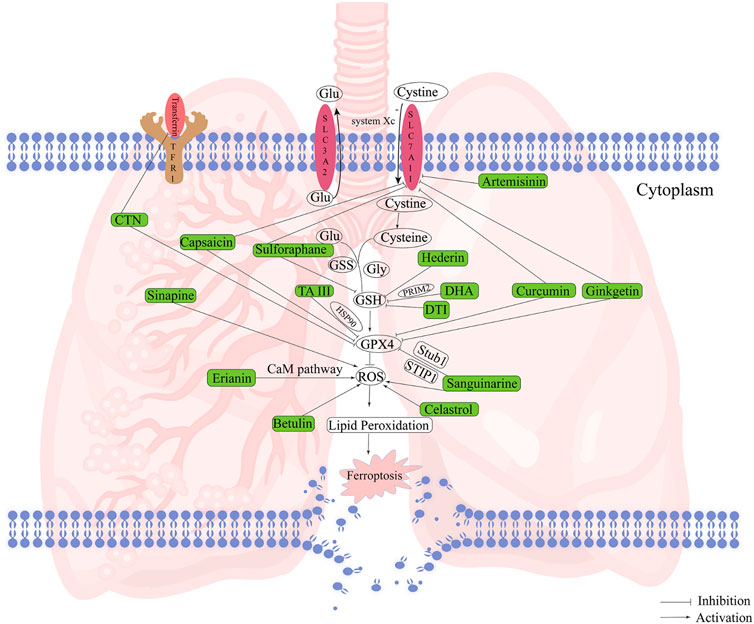
Figure 4. The mechanism of natural products inducing ferroptosis in NSCLC. The activation of ROS or the inhibition of GPX4, GSH, SLC7A11 and transferrin by different natural products can induce ferroptosis to treat NSCLC.
3.1 Timosaponin AIII
Timosaponin AIII(TA III) is a steroidal saponin and major active component derived from the traditional Chinese medicinal herb Schisandra chinensis (Wang et al., 2023). Timosaponin AIII exhibits various pharmacological activities, including anti-inflammatory (Yuan et al., 2016), anti-oxidant (Jiang et al., 2014), and anti-cancer effects (Chien et al., 2022). Zhou (Zhou et al., 2023) discovered that Timosaponin AIII can inhibit the proliferation and migration of NSCLC cells, induce cell cycle arrest at the G2/M phase, and trigger ROS release and iron accumulation. This process is accompanied by the generation of malondialdehyde (MDA) and the depletion of GSH. Furthermore, it was confirmed that heat shock protein 90 (HSP90) is a direct target of Timosaponin AIII. Timosaponin AIII forms a complex with HSP90, leading to the ubiquitination and degradation of GPX4, ultimately inducing ferroptosis. This study confirms that Timosaponin AIII can play a therapeutic role in NSCLC by inducing ferroptosis.
3.2 Curcumin
Curcumin is a naturally occurring compound derived from turmeric (Anand et al., 2007). The curcumin possesses pharmacological properties such as immunomodulation (Gautam et al., 2007), anti-inflammatory (Peng et al., 2021), and anti-cancer activity (Gouda and Bhandary, 2019). In a study conducted by Tang (Tang et al., 2021), characteristic changes associated with ferroptosis were observed in response to curcumin treatment in NSCLC cells. These changes included increased intracellular accumulation of iron and MDA, depletion of GSH, downregulation of SLC7A11 and GPX4 protein levels, negative regulators of ferroptosis, accumulation of lipid peroxidation, curcumin-induced mitochondrial membrane rupture, and reduction of mitochondrial cristae. Furthermore, the curcumin-treated group exhibited increased accumulation of autolysosomes, along with elevated levels of autophagy biomarkers, such as Beclin1 and microtubule-associated protein 1 light chain 3 alpha (LC3). The study also demonstrated that the characteristic changes of ferroptosis were partially reversed when the curcumin-treated NSCLC cells group was treated with CQ (an inhibitor of autophagosome-lysosome fusion) or when the Beclin1 was silenced. In summary, these findings suggest that autophagy contributes to curcumin-induced ferroptosis in NSCLC cells, and inhibiting autophagy can alleviate cellular sensitivity to ferroptosis.
3.3 Sanguinarine
Sanguinarine is a natural small-molecule compound isolated from the bloodroot plant (Lou et al., 2021), which possesses anti-bacterial (Zhang et al., 2020), anti-inflammatory (Niu et al., 2012), and anti-cancer properties (Gaziano et al., 2016). Xu (Xu et al., 2022). conducted a study and found that sanguinarine inhibited the proliferation of NSCLC cells in a dose-dependent and time-dependent manner. In xenograft tumor animal models, sanguinarine effectively suppressed the growth and metastasis of NSCLC cells. Furthermore, it was discovered that sanguinarine induced intracellular accumulation of iron, increased levels of ROS and MDA, and reduced GSH levels. Sanguinarine mediated the ubiquitination and degradation of GPX4 through stress induced phosphoprotein 1 (STIP1) and U-Box containing protein 1 (Stub1), thereby triggering ferroptosis in NSCLC cells. Furthermore, overexpression of GPX4 partially restored the proliferative and invasive inhibitory effects of sanguinarine on NSCLC cells by suppressing ferroptosis. In conclusion, sanguinarine inhibits the growth and metastasis of NSCLC cells by regulating the Stub1/GPX4-dependent iron-dependent cell death pathway.
3.4 Erianin
Erianin, a natural benzylisoquinoline compound extracted from Dendrobium (Li et al., 2023), exhibits anti-cancer activity by inhibiting cell proliferation, inducing apoptosis (Xu et al., 2021), and autophagy (Chen et al., 2020) in various cancers such as cervical cancer (Li et al., 2018), colorectal cancer (Miao et al., 2023), prostate cancer (Trapika et al., 2021), and breast cancer (Xie et al., 2021). Chen (Chen et al., 2020) found that erianin arrested NSCLC cells in the G2/M phase, thereby inhibiting cell proliferation and metastasis. Further investigations revealed that erianin treatment induced accumulation of ROS, consumption of GSH, and occurrence of lipid peroxidation in NSCLC cells. However, the results were reversed by ferroptosis inhibitor. Calmodulin (CaM), a major endogenous calcium-regulating protein, plays a crucial role in regulating L-type voltage-dependent calcium channels, which are important for calcium and iron transport (Zeng et al., 2023). This study confirmed that erianin targeted the CaM signaling pathway, leading to ROS accumulation and upregulation of iron by modulating the calcium-CaM pathway, thereby inducing ferroptosis in NSCLC cells.
3.5 Ginkgetin
Ginkgetin is a natural flavonoid compound derived from the Ginkgo biloba. It exhibits pharmacological activities such as neuroprotection (Singh et al., 2019), cardiovascular protection (Silva and Martins, 2022), anti-oxidant (Li et al., 2022d), and anti-inflammatory effects (Li et al., 2022b). Additionally, research has shown that it exerts anti-cancer effects by inhibiting cell proliferation, angiogenesis, and inducing apoptosis in tumor cells (DeFeudis et al., 2003; Ye et al., 2007; Bai et al., 2015; Han et al., 2016; Kim et al., 2021). Lou (Lou J. S. et al., 2021) conducted a study and found that the combination of Ginkgetin with cisplatin enhanced the cytotoxicity against NSCLC cells. This combination treatment increasesthe accumulation of iron and the occurrence of lipid peroxidation. Further investigations revealed that Ginkgetin reduced the expression of SLC7A11 and GPX4, decreased the GSH/GSSG ratio, increased ROS formation, and reduced the activity of the Nrf-2/HO-1 signaling pathway. These actions collectively induced ferroptosis in NSCLC cells. These results suggest that the combination of Ginkgetin and cisplatin reduces NSCLC development by inducing ferroptosis.
3.6 Hederin
Hederin belongs to a class of saponin compounds found in the Ginkgo biloba plant, which belongs to the family Ginkgoaceae (Jeong et al., 2019). Numerous studies have indicated that α-Hederin has anti-tumor functions (Belmehdi et al., 2023). For instance, in colorectal cancer cells (Sun et al., 2019), α-Hederin inhibits the epithelial-mesenchymal transition induced by interleukin-6 and the activity of the JAK2/STAT3 signaling pathway, thereby suppressing cell migration and invasion. In gastric cancer cells (Wang et al., 2020), the combination of α-Hederin and cisplatin promotes apoptosis in gastric cancer cells through mitochondria-related apoptotic pathways. Wu (Wu et al., 2022) discovered that α-Hederin inhibits the proliferation and invasion of NSCLC cells in a dose-dependent manner both in vitro and in vivo. Subsequent proteomics, metabolomics, and high-throughput sequencing confirmed that α-Hederin treatment reduces the expression of GSH peroxidase 2 (GPX2) and GSS, inhibits the synthesis of GSH, disrupts the GSH redox system. After the administration of the ferroptosis inhibitor of ferrostatin-1, the study observed a partial restoration of α-Hederin-induced cell death. Meanwhile, ferrostatin-1 treatment recovered α-Hederin-induced disturbance in mitochondrial membrane potential. In summary, α-Hederin could induces ferroptosis in the treatment of NSCLC.
3.7 Dihydroisotanshinone I
Dihydroisotanshinone I (DTI), a diterpenoid compound belonging to the tanshinone class, is extracted from the medicinal herb Salvia miltiorrhiza (Hsu et al., 2021). Salvia miltiorrhiza has been widely used in traditional Chinese medicine and possess diverse pharmacological activities and therapeutic potential (XD et al., 2019; He et al., 2024). Research studies have demonstrated that DTIDTI exhibits anti-oxidant (Ip et al., 2002), and anti-cancer properties (Wu et al., 2017; Lin et al., 2019). Wu (Wu et al., 2021)have shown that DTI inhibits the growth of A549 cells and H460 cells via inducing ferroptosis. The underlying mechanism involves the downregulation of GPX4 protein and GSH levels, accumulation of MDA,ROS, leading toand lipid peroxidation and finally induction of ferroptosis in NSCLC.
3.8 Sulforaphane
Sulforaphane belongs to the class of compounds known as isothiocyanates and is predominantly found in vegetables of the Brassicaceae family, particularly in cruciferous vegetables such as cauliflower, cabbage, and broccoli (Vanduchova et al., 2019). Extensive research has demonstrated that sulforaphane exerts its beneficial effects through multiple mechanisms, including the activation of intracellular antioxidant enzymes (Ishida et al., 2021), modulation of cellular signaling pathways (Zhang et al., 2022c), and anti-cancer activities (Russo et al., 2018). Studies conducted by Yuko Iida (Iida et al., 2021) have shown that sulforaphane significantly inhibits the growth of NSCLC cells, and this growth inhibition can be reversed by ferroptosis inhibitors offerrostatin-1. Furthermore, treatment of NSCLC cells with sulforaphane leads to an increase in iron and ROS levels, as well as collection of lipid peroxidation products, all of which can be attenuated by ferroptosis inhibitors. Subsequent investigations revealed that sulforaphane specifically downregulates the expression of the SLC7A11, resulting in a reduction in GSH accumulation. Collectively, Sulforaphane inhibits the growth of NSCLC cells via inducing ferroptosis.
3.9 Cryptotanshinone
Cryptotanshinone (CTN) is a diterpenoid monomer and a lipophilic component extracted from the dried roots and rhizomes of the traditional Chinese herb S. miltiorrhiza (Li et al., 2021; Zhang et al., 2023). CTN has been proven to possess various biological activities, including antioxidant (Guo et al., 2022), anti-tumor (Dalil et al., 2022), antibacterial (Zhong et al., 2021), and anti-inflammatory effects (Wu et al., 2020). Li (Popa et al., 2021) discovered that CTN effectively suppresses NSCLC cells invasion, proliferation and tumorigenesis. The treatment of CTN resulted in increased iron accumulation within the cells and decreased the expression levelof GPX4 protein. Furthermore, CTN elicited an upregulation of Cytoglobin, a protein known to induce ferroptosis, while downregulats ferroportin expression. Moreover, study demonstrated that CTN induces iron-dependent lipid peroxidation by inhibiting the function of TfR1. These data suggest that the induction of ferroptosis in NSCLC cells, achieved by increasing iron accumulation, Cytoglobin, and iron-dependent lipid peroxidation, or by downregulating the expression levels of ferroportin and GPX4, may be an important mechanism through which CTN attenuates NSCLC.
3.10 Artemisinin
Artemisinin is a sesquiterpene lactone isolated from the Artemisia annual (Ma et al., 2020). It is widely used for the treatment of malaria (Talman et al., 2019). Apart from its well-known role in treating malaria, artemisinin has been reported in numerous studies to possess additional pharmacological activities, including anti-schistosomiasis (Pérez del Villar et al., 2012),anti-cancer (Kiani et al., 2020),anti-inflammation (Yuan et al., 2019),anti-virus (Wani et al., 2021). Zhang (Zhang et al., 2021) discovered that artemisinin downregulates the protein and mRNA levels of xCT. Furthermore, artemisinin upregulates the mRNA level of TfR1, Therefore, Zhang hypothesis that Artemisinin may induces ferroptosis in NSCLC. Consequently, the cell death caused by artemisinin can be partially reversed by N-Acetyl-L-cysteine (NAC), a ROS scavenger, and ferrostatin-1, a ferroptosis inhibitor. The findings demonstrate that the inhibitory effect of artemisinin on NSCLC cells is at least partially attributed to the induction of ferroptosis.
3.11 Sinapine
Sinapine belongs to the class of compounds known as phenethylamines particularly in abundance in sources such as barley, mustard seeds, peas, and rapeseeds (Dang et al., 2023). It exhibits numerous pharmacological activities, such like antioxidant (Yates et al., 2019), anti-inflammatory (Li et al., 2019), and anti-cancer activities (Guo et al., 2014). In the realm of cancer research, Sinapine has shown promise as an anti-cancer agent, displaying inhibitory effects on various cancer cells, including breast cancer (Guo et al., 2016) and colorectal cancer (Yang et al., 2023). Notably, Shao (Shao et al., 2022) found that Sinapine play the anti-tumor effects on NSCLC cells. Induce ferroptosis by increasing intracellular ferrous iron, lipid peroxidation, and ROS in NSCLC cells. Also, treatment with Sinapine upregulates transferrin and transferrin receptor, and inhibits either of them attenuated the ferroptosis induced by Sinapine. Additionally, Sinapine treatment led to a p53-dependent downregulation of SLC7A11. Furthermore, Sinapine also play the inhibition role in the growth of NSCLC in vivo. In conclusion, the findings highlight that Sinapine could be a promising therapeutic approach via triggers ferroptosis in NSCLC.
3.12 Dihydroartemisinin
Dihydroartemisinin (DHA) is a derivative of artemisinin, which is a compound extracted from the Artemisia annua plant (Dai et al., 2021). DHA exhibits its antimalarial activity by rapidly and effectively clearing the malaria parasite from the bloodstream (Hanboonkunupakarn and White, 2022). In addition to its antimalarial properties, DHA has been demonstrated anti-inflammatory (Yang et al., 2022),anti-cancer (Bai et al., 2021) and immunomodulatory (Gao et al., 2020) properties. In DNA replication, the protein encoded by the DNA primase subunit 2 (PRIM2) gene plays a critical role. PRIM2 functions by catalyzing the synthesis of RNA primers, which act as the starting points for DNA synthesis (Wei and Lozano-Durán, 2023). Yuan’s study (Yuan et al., 2020) revealed that DHA reduced the expression of PRIM2, and silencing PRIM2 mimicked the inhibitory effects of DHA on cell proliferation and colony formation, while promoting cell death in NCSLC cells. Additionally, the study found that DHA treatment and the absence of PRIM2 led to a series of ferroptosis characteristic in NSCLC cells. Mechanistically, the combination of DHA treatment and the absence of PRIM2 decrease the level of GSH, increasecellular lipid ROS and MDA levels, as well as downregulats SLC7A11 and β-catenin expressions in NCSLC cells.
3.13 Celastrol
Celastrol is a prominent bioactive compound extracted from the root bark of Tripterygium wilfordii, a plant belonging to the Celastraceae family (Xu et al., 2021). It falls within the class of pentacyclic triterpenoids, possessing a triterpene framework and exhibiting noteworthy biological activity (Li et al., 2022f), such like anti-cancer (Xu et al., 2023), anti-inflammatory effect in liver fibrosis (Wang et al., 2020), and potential pharmacological treatment of obesity (Liu et al., 2015). In a study conducted by Liu(Liu et al., 2021), it was observed that the combination of erastin,a ferroptosis inducer, and celastrol induced cell death in NSCLC cells at concentrations that were not toxic individually. The co-treatment with celastrol and erastin resulted in promotion of ROS generation, disturbance of mitochondrial membrane potential, augmentation of the interaction between dynamin-related protein 1 (DRP1) and mitochondrial fission, mitochondrial 1 (FIS1), and stimulation of mitochondrial fission. Of these, the above results suggest that Celastrol may be a natural compound that effectively induces ferroptosis.
3.14 Betulin
Betulin is a natural triterpene compound that occurs in the bark of specific tree species, including white and silver birch trees (Demets et al., 2022). The emerging evidence has shown that Betulinpossesses various advantageous properties, including anti-inflammatory (Tuli et al., 2021), anti-oxidant (Günther et al., 2021), and anti-cancer (Wang et al., 2017). Yan (Yan et al., 2022) found that betulin in combination with Gefitinib exhibited antagonistic effects on cellular viability on NSCLC cells of A549 and H460. However, the ferroptosis inhibitors of ferrostatin-1, liproxstatin-1 and deferoxamine can completely rescue the viability of A549 and H460 after treatment of betulin in combination with Gefitinib. Moreover, in order to confirm whether ferroptosis contributes to the death under the treatment of betulin in combination with gefetinib, the author performed a series of experiments and found that combination induced ROS accumulation, lipid peroxidation, and GSH depletion. The expression of SLC7A11, GPX4 and ferritin heavy chain 1 (FTH1), negative regulators of ferroptosis, was decreased under the combination treatment of betulin and gefetinib. Whereas, the positive regulatory protein of ferroptosis heme oxygenase 1(HO-1) was increased. , Therefore, Betulin may be a potential therapeutic agent for NSCLC via inducing ferroptosis.
3.15 Capsaicin
Capsaicin, a natural compound present in chili peppers, in fruits of the capsicum genus like cayenne peppers and jalapenos (Chapa-Oliver and Mejía-Teniente, 2016), has attracted attention for its potential health benefits (Sharma et al., 2013). Research suggests that capsaicin may contributes to improved cardiovascular health (Munjuluri et al., 2021) and possess anti-microbial properties (Goci et al., 2021). In a study conducted by Liu(Liu et al., 2022), it was observed that capsaicin exhibited significant inhibitory effects on the proliferation of NSCLC cells. Mechanismlly, capsaicin increased the levels of total iron and ferrous ions, while reducing GSH levels in the treated cells compared to the control group. Additionally, both mRNA and protein levels of SLC7A11 and GPX4 showed significant decreases in NSCLC cells treated with capsaicin compared to the control group. In summary, the treatment potential of capsaicin in NSCLC cells lies in its ability to induce ferroptosis.
3.16 Chinese medicine and preparations
In addition, several studies have indicated that certain Chinese medicine and preparations possess the potential therapeutic ability to stimulate ferroptosis to exhibit potential benefits in the treatment of NSCLC. Hedyotis diffusa (HD), a species of flowering plant in the family Rubiaceae, is a traditional Chinese herbal medicine, which exhibits numerous pharmacological activities, including antioxidant (Lu et al., 2000), anti-inflammatory (Hung et al., 2022), and anti-cancer (Wu et al., 2022). It contains various bioactive compounds, including iridoids, flavonoids, triterpenoids, and phenolic acids, which are believed to contribute to its medicinal effects (Zhang et al., 2021). Huang (Huang et al., 2022)found that HD can inhibit NSCLC cells growth and induce characteristics of ferroptosis, including increase in mitochondrial membrane density, shrunken mitochondria, and decline of cristae. Moreover, HD increase cellular Lipid ROS, the Fe2+ fluorescence intensity and MDA levels. Mechanically, HD-induced ferroptosis in lung adenocarcinoma cells may be related to the voltage dependent anion channel 2/3(VDAC2/3) pathway, (Yang et al., 2020),a group of specific channel proteins, facilitates the exchange of metabolites and ions across the outer mitochondrial membrane and may regulate mitochondrial functions. Furthermore, HD exerts its regulatory effects on the BCL2 apoptosis regulator (Bcl2)/BCL2 associated X, apoptosis regulator (Bax) protein complex, thereby modulating the functional dynamics of VDAC2/3. This modulation results in the activation of VDAC2/3 channels, facilitating the translocation of ions and facilitating the intracellular accumulation of ROS. Above all, HD could induce ferroptosis via Bcl2 inhibition to promote Bax regulation of VDAC2/3 to attentus the NSCLC cells growth. Zhao (Zhao et al., 2022) showed that Fuzheng Kang’ai (FZKA) decoction significantly suppressed the expression of GPX4 and system Xc− and conducted a reduction in the GSH/GSSG ratio to induce ferroptosis in NSCLC treatment. Importantly, the induction of ferroptosis in NSCLC cells by FZKA decoction was significantly reversed when GPX4 was overexpressed. These findings were further confirmed in vivo animal model, validating the observed effects of FZKA on ferroptosis in NSCLC cells.
4 Conclusion and prospects
Ferroptosis is a novel form of cell death that distinguishes itself from apoptosis, necroptosis, and autophagy. Multiple evidences have indicated the significant role of ferroptosis in regulating tumor cell growth and drug resistance, making it a potential new target for anti-tumor interventions (Chen et al., 2021a; Song et al., 2021; Wang et al., 2022). Therefore, ferroptosis inducers hold great promise as highly prospective agents for cancer diagnosis and therapeutic intervention, and they are also of significant importance in the development of anti-cancer drugs (Lei et al., 2022). Here, we have summarized the characteristic ferroptosis inducers and their main anti-cancer mechanisms in Supplementary Table, aiming to provide support for the clinical development of anti-cancer drugs.
The current study summarized the introduction and mechanism of ferroptosis. The mechanism of ferroptosis mainly involves four processes: GPX4, system Xc−, iron metabolism, and lipid peroxidation. In the field of NCSLC research, the ferroptosis as a novel cellular death mechanism has gathered significant attention in recent years (Zhang et al., 2022b; Zhao et al., 2023). We summarized relevant targets or pathways of ferroptosis in NCSLC therapy as shown in Table 1. Additionally, natural products have made certain progress in the prevention and treatment of NCSLC by regulating ferroptosis process. However, the investigation into the modulation of ferroptosis in NSCLC using natural products is currently in its initial exploratory phase, with certain limitations evident in existing research studies. Firstly, most studies have only explored the molecular mechanisms by which natural product from traditional Chinese medicine induces ferroptosis through a single pathway. Nevertheless, traditional Chinese medicine formulas and patent medicines widely used in clinical practice possess the characteristics of “multiple components, multiple targets, and multiple effects” (Yan et al., 2022). In future research, we should explore the molecular mechanisms of traditional Chinese medicine in regulating ferroptosis from multiple pathways and perspectives, construct “components-targets/pathways-disease” pharmacological network, and conduct relevant clinical trials.
Secondly, natural products are typically complex mixtures with diverse chemical structures and compositions, which increases the complexity of studying their pharmacological activities and tissue specificity (Xia et al., 2022). These complexities may make it difficult to accurately assess the absorption, distribution, metabolism, and excretion properties of drugs. Based on this, we need to strengthen the exploration of the potential of nanotechnology (Kaur et al., 2022), encapsulation (Linh et al., 2022), and targeted delivery systems (Gorain et al., 2022) to improve the pharmacokinetics and tissue specificity of natural products.
Thirdly, certain natural products may cause adverse reactions in the digestive system, such as nausea, vomiting, diarrhea, gastrointestinal discomfort, etc. (Menniti-Ippolito et al., 2008; Vasudeva et al., 2012; Zorzela et al., 2021; Andrade et al., 2022). Therefore, the importance of conducting controlled clinical trials should be emphasized to evaluate the safety of treatment methods based on natural products for NSCLC patients.
Finally, it is also necessary to consider combining natural products with other treatment modalities, including chemotherapy drugs such as sulfasalazine (Lay et al., 2007), sorafenib (Groenendijk et al., 2015), zalcitabine (McNeill and Wilson, 2007), and cisplatin (Kryczka et al., 2021), which all can be purposed to induce ferroptosis, to improve the efficiency of natural product-based treatments for NSCLC. We believe that with the progress ferroptosis of research, new effective strategies for the treatment of NSCLC can be provided.
Author contributions
QZ: Writing–original draft, Validation. YX: Data curation, Software, Writing–original draft. FW: Investigation, Writing–original draft. DY: Methodology, Writing–review and editing. ZL: Writing–review and editing, Funding acquisition.
Funding
The author(s) declare that financial support was received for the research, authorship, and/or publication of this article. This work was funded by the R & D project of “Jianbing” and “Lingyan” in Zhejiang Province (2022C02023) and the Key Science and Technology Project of New Agricultural Variety Breeding of Zhejiang Province (2021C02074).
Conflict of interest
The authors declare that the research was conducted in the absence of any commercial or financial relationships that could be construed as a potential conflict of interest.
Publisher’s note
All claims expressed in this article are solely those of the authors and do not necessarily represent those of their affiliated organizations, or those of the publisher, the editors and the reviewers. Any product that may be evaluated in this article, or claim that may be made by its manufacturer, is not guaranteed or endorsed by the publisher.
Supplementary material
The Supplementary Material for this article can be found online at: https://www.frontiersin.org/articles/10.3389/fphar.2024.1385565/full#supplementary-material
References
Albrecht, P., Lewerenz, J., Dittmer, S., Noack, R., Maher, P., and Methner, A. (2010). Mechanisms of oxidative glutamate toxicity: the glutamate/cystine antiporter system xc-as a neuroprotective drug target. CNS Neurol. Disord. Drug Targets 9 (3), 373–382. doi:10.2174/187152710791292567
Alduais, Y., Zhang, H., Fan, F., Chen, J., and Chen, B. (2023). Non-small cell lung cancer (NSCLC): a review of risk factors, diagnosis, and treatment. Med. Baltim. 102 (8), e32899. doi:10.1097/md.0000000000032899
Alvarez, S. W., Sviderskiy, V. O., Terzi, E. M., Papagiannakopoulos, T., Moreira, A. L., Adams, S., et al. (2017). NFS1 undergoes positive selection in lung tumours and protects cells from ferroptosis. Nature 551 (7682), 639–643. doi:10.1038/nature24637
Anand, P., Kunnumakkara, A. B., Newman, R. A., and Aggarwal, B. B. (2007). Bioavailability of curcumin: problems and promises. Mol. Pharm. 4 (6), 807–818. doi:10.1021/mp700113r
Anandhan, A., Dodson, M., Shakya, A., Chen, J., Liu, P., Wei, Y., et al. (2023). NRF2 controls iron homeostasis and ferroptosis through HERC2 and VAMP8. Sci. Adv. 9 (5), eade9585. doi:10.1126/sciadv.ade9585
Andrade, J., Guerrero, B. V., Diaz, C. M. G., Radillo, J. J. V., and Lo Pez, M. A. R. (2022). Natural products and their mechanisms in potential photoprotection of the skin. J. Biosci. 47, 77. doi:10.1007/s12038-022-00314-2
Aschner, M., Skalny, A. V., Martins, A. C., Sinitskii, A. I., Farina, M., Lu, R., et al. (2022). Ferroptosis as a mechanism of non-ferrous metal toxicity. Arch. Toxicol. 96 (9), 2391–2417. doi:10.1007/s00204-022-03317-y
Bai, B., Wu, F., Ying, K., Xu, Y., Shan, L., Lv, Y., et al. (2021). Therapeutic effects of dihydroartemisinin in multiple stages of colitis-associated colorectal cancer. Theranostics 11 (13), 6225–6239. doi:10.7150/thno.55939
Bai, Y., Zhao, F., Li, Y., Wang, L., Fang, X. J., and Wang, C. Y. (2015). Ginkgo biloba extract induce cell apoptosis and G0/G1 cycle arrest in gastric cancer cells. Int. J. Clin. Exp. Med. 8 (11), 20977–20982.
Basuli, D., Tesfay, L., Deng, Z., Paul, B., Yamamoto, Y., Ning, G., et al. (2017). Iron addiction: a novel therapeutic target in ovarian cancer. Oncogene 36 (29), 4089–4099. doi:10.1038/onc.2017.11
Batbold, U., and Liu, J. J. (2021). Artemisia santolinifolia-mediated chemosensitization via activation of distinct cell death modes and suppression of STAT3/survivin-signaling pathways in NSCLC. Molecules 26 (23), 7200. doi:10.3390/molecules26237200
Belmehdi, O., Taha, D., Abrini, J., Ming, L. C., Khalid, A., Abdalla, A. N., et al. (2023). Anticancer properties and mechanism insights of α-hederin. Biomed. Pharmacother. 165, 115205. doi:10.1016/j.biopha.2023.115205
Bersuker, K., Hendricks, J. M., Li, Z., Magtanong, L., Ford, B., Tang, P. H., et al. (2019). The CoQ oxidoreductase FSP1 acts parallel to GPX4 to inhibit ferroptosis. Nature 575 (7784), 688–692. doi:10.1038/s41586-019-1705-2
Brown, S., Banfill, K., Aznar, M. C., Whitehurst, P., and Faivre Finn, C. (2019). The evolving role of radiotherapy in non-small cell lung cancer. Br. J. Radiol. 92 (1104), 20190524. doi:10.1259/bjr.20190524
Chapa-Oliver, A. M., and Mejía-Teniente, L. (2016). Capsaicin: from plants to a cancer-suppressing agent. Molecules 21 (8), 931. doi:10.3390/molecules21080931
Chen, G.-Q., Benthani, F. A., Wu, J., Liang, D., Bian, Z.-X., and Jiang, X. (2019). Artemisinin compounds sensitize cancer cells to ferroptosis by regulating iron homeostasis. Cell Death Differ. 27 (1), 242–254. doi:10.1038/s41418-019-0352-3
Chen, L., Wang, H. J., Xie, W., Yao, Y., Zhang, Y. S., and Wang, H. (2014). Cryptotanshinone inhibits lung tumorigenesis and induces apoptosis in cancer cells in vitro and in vivo. Mol. Med. Rep. 9 (6), 2447–2452. doi:10.3892/mmr.2014.2093
Chen, P., Wu, Q., Feng, J., Yan, L., Sun, Y., Liu, S., et al. (2020a). Erianin, a novel dibenzyl compound in Dendrobium extract, inhibits lung cancer cell growth and migration via calcium/calmodulin-dependent ferroptosis. Signal Transduct. Target Ther. 5 (1), 51. doi:10.1038/s41392-020-0149-3
Chen, X., Kang, R., Kroemer, G., and Tang, D. (2021a). Broadening horizons: the role of ferroptosis in cancer. Nat. Rev. Clin. Oncol. 18 (5), 280–296. doi:10.1038/s41571-020-00462-0
Chen, X., Li, J., Kang, R., Klionsky, D. J., and Tang, D. (2021b). Ferroptosis: machinery and regulation. Autophagy 17 (9), 2054–2081. doi:10.1080/15548627.2020.1810918
Chen, X., Yu, C., Kang, R., Kroemer, G., and Tang, D. (2021c). Cellular degradation systems in ferroptosis. Cell Death Differ. 28 (4), 1135–1148. doi:10.1038/s41418-020-00728-1
Chen, Y. T., Hsieh, M. J., Chen, P. N., Weng, C. J., Yang, S. F., and Lin, C. W. (2020b). Erianin induces apoptosis and autophagy in oral squamous cell carcinoma cells. Am. J. Chin. Med. 48 (1), 183–200. doi:10.1142/s0192415x2050010x
Chien, H. J., Liu, C. J., Ying, T. H., Wu, P. J., Wang, J. W., Ting, Y. H., et al. (2022). Timosaponin AIII inhibits migration and invasion abilities in human cervical cancer cells through inactivation of p38 MAPK-mediated uPA expression in vitro and in vivo. Cancers (Basel) 15 (1), 37. doi:10.3390/cancers15010037
Christie, W. W., and Harwood, J. L. (2020). Oxidation of polyunsaturated fatty acids to produce lipid mediators. Essays Biochem. 64 (3), 401–421. doi:10.1042/ebc20190082
Dai, X., Zhang, X., Chen, W., Chen, Y., Zhang, Q., Mo, S., et al. (2021). Dihydroartemisinin: a potential natural anticancer drug. Int. J. Biol. Sci. 17 (2), 603–622. doi:10.7150/ijbs.50364
Dalil, D., Iranzadeh, S., and Kohansal, S. (2022). Anticancer potential of cryptotanshinone on breast cancer treatment; A narrative review. Front. Pharmacol. 13, 979634. doi:10.3389/fphar.2022.979634
Dang, R., Guan, H., and Wang, C. (2023). Sinapis Semen: a review on phytochemistry, pharmacology, toxicity, analytical methods and pharmacokinetics. Front. Pharmacol. 14, 1113583. doi:10.3389/fphar.2023.1113583
DeFeudis, F. V., Papadopoulos, V., and Drieu, K. (2003). Ginkgo biloba extracts and cancer: a research area in its infancy. Fundam. Clin. Pharmacol. 17 (4), 405–417. doi:10.1046/j.1472-8206.2003.00156.x
Demets, O. V., Takibayeva, A. T., Kassenov, R. Z., and Aliyeva, M. R. (2022). Methods of betulin extraction from birch bark. Molecules 27 (11), 3621. doi:10.3390/molecules27113621
Deng, R., Yu, S., Ruan, X., Liu, H., Zong, G., Cheng, P., et al. (2023). Capsaicin orchestrates metastasis in gastric cancer via modulating expression of TRPV1 channels and driving gut microbiota disorder. Cell Commun. Signal. 21 (1), 364. doi:10.1186/s12964-023-01265-3
Deng, S. H., Wu, D. M., Li, L., Liu, T., Zhang, T., Li, J., et al. (2021). miR-324-3p reverses cisplatin resistance by inducing GPX4-mediated ferroptosis in lung adenocarcinoma cell line A549. Biochem. Biophys. Res. Commun. 549, 54–60. doi:10.1016/j.bbrc.2021.02.077
Dixon, S. J., Lemberg, K. M., Lamprecht, M. R., Skouta, R., Zaitsev, E. M., Gleason, C. E., et al. (2012). Ferroptosis: an iron-dependent form of nonapoptotic cell death. Cell 149 (5), 1060–1072. doi:10.1016/j.cell.2012.03.042
Doll, S., Proneth, B., Tyurina, Y. Y., Panzilius, E., Kobayashi, S., Ingold, I., et al. (2017). ACSL4 dictates ferroptosis sensitivity by shaping cellular lipid composition. Nat. Chem. Biol. 13 (1), 91–98. doi:10.1038/nchembio.2239
Gai, C., Liu, C., Wu, X., Yu, M., Zheng, J., Zhang, W., et al. (2020a). MT1DP loaded by folate-modified liposomes sensitizes erastin-induced ferroptosis via regulating miR-365a-3p/NRF2 axis in non-small cell lung cancer cells. Cell Death Dis. 11 (9), 751. doi:10.1038/s41419-020-02939-3
Gai, C., Yu, M., Li, Z., Wang, Y., Ding, D., Zheng, J., et al. (2020b). Acetaminophen sensitizing erastin-induced ferroptosis via modulation of Nrf2/heme oxygenase-1 signaling pathway in non-small-cell lung cancer. J. Cell Physiol. 235 (4), 3329–3339. doi:10.1002/jcp.29221
Gao, Y., Cui, M., Zhong, S., Feng, C., Nwobodo, A. K., Chen, B., et al. (2020). Dihydroartemisinin ameliorates LPS-induced neuroinflammation by inhibiting the PI3K/AKT pathway. Metab. Brain Dis. 35 (4), 661–672. doi:10.1007/s11011-020-00533-2
Gautam, S. C., Gao, X., and Dulchavsky, S. (2007). Immunomodulation by curcumin. Adv. Exp. Med. Biol. 595, 321–341. doi:10.1007/978-0-387-46401-5_14
Gaziano, R., Moroni, G., Buè, C., Miele, M. T., Sinibaldi-Vallebona, P., and Pica, F. (2016). Antitumor effects of the benzophenanthridine alkaloid sanguinarine: evidence and perspectives. World J. Gastrointest. Oncol. 8 (1), 30–39. doi:10.4251/wjgo.v8.i1.30
Goci, E., Haloci, E., Di Stefano, A., Chiavaroli, A., Angelini, P., Miha, A., et al. (2021). Evaluation of in vitro capsaicin release and antimicrobial properties of topical pharmaceutical formulation. Biomolecules 11 (3), 432. doi:10.3390/biom11030432
Gorain, B., Pandey, M., Leng, N. H., Yan, C. W., Nie, K. W., Kaur, S. J., et al. (2022). Advanced drug delivery systems containing herbal components for wound healing. Int. J. Pharm. 617, 121617. doi:10.1016/j.ijpharm.2022.121617
Gouda, M. M., and Bhandary, Y. P. (2019). Acute lung injury: IL-17A-Mediated inflammatory pathway and its regulation by curcumin. Inflammation 42 (4), 1160–1169. doi:10.1007/s10753-019-01010-4
Groenendijk, F. H., Mellema, W. W., van der Burg, E., Schut, E., Hauptmann, M., Horlings, H. M., et al. (2015). Sorafenib synergizes with metformin in NSCLC through AMPK pathway activation. Int. J. Cancer 136 (6), 1434–1444. doi:10.1002/ijc.29113
Günther, A., Makuch, E., Nowak, A., Duchnik, W., Kucharski, Ł., Pełech, R., et al. (2021). Enhancement of the antioxidant and skin permeation properties of betulin and its derivatives. Molecules 26 (11), 3435. doi:10.3390/molecules26113435
Guo, K., Liu, R., Jing, R., Wang, L., Li, X., Zhang, K., et al. (2022). Cryptotanshinone protects skin cells from ultraviolet radiation-induced photoaging via its antioxidant effect and by reducing mitochondrial dysfunction and inhibiting apoptosis. Front. Pharmacol. 13, 1036013. doi:10.3389/fphar.2022.1036013
Guo, Y., An, H., Feng, L., Liu, Q., Wang, S., and Zhang, T. (2014). Sinapine as an active compound for inhibiting the proliferation of Caco-2 cells via downregulation of P-glycoprotein. Food Chem. Toxicol. 67, 187–192. doi:10.1016/j.fct.2014.02.035
Guo, Y., Ding, Y., Zhang, T., and An, H. (2016). Sinapine reverses multi-drug resistance in MCF-7/dox cancer cells by downregulating FGFR4/FRS2α-ERK1/2 pathway-mediated NF-κB activation. Phytomedicine 23 (3), 267–273. doi:10.1016/j.phymed.2015.12.017
Hadzhieva, M., Kirches, E., and Mawrin, C. (2014). Review: iron metabolism and the role of iron in neurodegenerative disorders. Neuropathol. Appl. Neurobiol. 40 (3), 240–257. doi:10.1111/nan.12096
Han, D., Cao, C., Su, Y., Wang, J., Sun, J., Chen, H., et al. (2016). Ginkgo biloba exocarp extracts inhibits angiogenesis and its effects on Wnt/β-catenin-VEGF signaling pathway in Lewis lung cancer. J. Ethnopharmacol. 192, 406–412. doi:10.1016/j.jep.2016.09.018
Hanboonkunupakarn, B., and White, N. J. (2022). Advances and roadblocks in the treatment of malaria. Br. J. Clin. Pharmacol. 88 (2), 374–382. doi:10.1111/bcp.14474
Hao, L., Mi, J., Song, L., Guo, Y., Li, Y., Yin, Y., et al. (2021). SLC40A1 mediates ferroptosis and cognitive dysfunction in type 1 diabetes. Neuroscience 463, 216–226. doi:10.1016/j.neuroscience.2021.03.009
Hayano, M., Yang, W. S., Corn, C. K., Pagano, N. C., and Stockwell, B. R. (2016). Loss of cysteinyl-tRNA synthetase (CARS) induces the transsulfuration pathway and inhibits ferroptosis induced by cystine deprivation. Cell Death Differ. 23 (2), 270–278. doi:10.1038/cdd.2015.93
He, X., Chen, Y., Xia, Y., Hong, X., You, H., Zhang, R., et al. (2024). DNA methylation regulates biosynthesis of tanshinones and phenolic acids during growth of Salvia miltiorrhiza. Plant Physiol. 194 (4), 2086–2100. doi:10.1093/plphys/kiad573
Hentze, M. W., Muckenthaler, M. U., and Andrews, N. C. (2004). Balancing acts: molecular control of mammalian iron metabolism. Cell 117 (3), 285–297. doi:10.1016/s0092-8674(04)00343-5
Hopstaken, J. S., de Ruiter, J. C., van Diessen, J. N. A., Theelen, W., Monkhorst, K., and Hartemink, K. J. (2021). Treatment of non-small cell lung cancer. Ned. Tijdschr. Geneeskd. 165, D5486.
Hsu, C. M., Yang, M. Y., Tsai, M. S., Chang, G. H., Yang, Y. H., Tsai, Y. T., et al. (2021). Dihydroisotanshinone I as a treatment option for head and neck squamous cell carcinomas. Int. J. Mol. Sci. 22 (16), 8881. doi:10.3390/ijms22168881
Hu, B. Q., Huang, J. F., Niu, K., Zhou, J., Wang, N. N., Liu, Y., et al. (2023). B7-H3 but not PD-L1 is involved in the antitumor effects of Dihydroartemisinin in non-small cell lung cancer. Eur. J. Pharmacol. 950, 175746. doi:10.1016/j.ejphar.2023.175746
Hu, K., Li, K., Lv, J., Feng, J., Chen, J., Wu, H., et al. (2020a). Suppression of the SLC7A11/glutathione axis causes synthetic lethality in KRAS-mutant lung adenocarcinoma. J. Clin. Invest. 130 (4), 1752–1766. doi:10.1172/jci124049
Hu, Z., Zhang, H., Yi, B., Yang, S., Liu, J., Hu, J., et al. (2020b). VDR activation attenuate cisplatin induced AKI by inhibiting ferroptosis. Cell Death Dis. 11 (1), 73. doi:10.1038/s41419-020-2256-z
Huang, C., Yang, M., Deng, J., Li, P., Su, W., and Jiang, R. (2018). Upregulation and activation of p53 by erastin-induced reactive oxygen species contribute to cytotoxic and cytostatic effects in A549 lung cancer cells. Oncol. Rep. 40 (4), 2363–2370. doi:10.3892/or.2018.6585
Huang, F., Pang, J., Xu, L., Niu, W., Zhang, Y., Li, S., et al. (2022). Hedyotis diffusa injection induces ferroptosis via the Bax/Bcl2/VDAC2/3 axis in lung adenocarcinoma. Phytomedicine 104, 154319. doi:10.1016/j.phymed.2022.154319
Hung, H. Y., Cheng, K. C., Kuo, P. C., Chen, I. T., Li, Y. C., Hwang, T. L., et al. (2022). Chemical constituents of hedyotis diffusa and their anti-inflammatory bioactivities. Antioxidants (Basel) 11 (2), 335. doi:10.3390/antiox11020335
Iida, Y., Okamoto-Katsuyama, M., Maruoka, S., Mizumura, K., Shimizu, T., Shikano, S., et al. (2021). Effective ferroptotic small-cell lung cancer cell death from SLC7A11 inhibition by sulforaphane. Oncol. Lett. 21 (1), 71. doi:10.3892/ol.2020.12332
Ip, S. P., Yang, H., Sun, H. D., and Che, C. T. (2002). Dihydroisotanshinone I protects against menadione-induced toxicity in a primary culture of rat hepatocytes. Planta Med. 68 (12), 1077–1081. doi:10.1055/s-2002-36345
Ishida, K., Kaji, K., Sato, S., Ogawa, H., Takagi, H., Takaya, H., et al. (2021). Sulforaphane ameliorates ethanol plus carbon tetrachloride-induced liver fibrosis in mice through the Nrf2-mediated antioxidant response and acetaldehyde metabolization with inhibition of the LPS/TLR4 signaling pathway. J. Nutr. Biochem. 89, 108573. doi:10.1016/j.jnutbio.2020.108573
Jeong, K. B., Luo, K., Lee, H., Lim, M. C., Yu, J., Choi, S. J., et al. (2019). Alpha-Hederin nanopore for single nucleotide discrimination. ACS Nano 13 (2), 1719–1727. doi:10.1021/acsnano.8b07797
Jiang, L. L., Zhou, S. J., Zhang, X. M., Chen, H. Q., and Liu, W. (2016). Sulforaphane suppresses in vitro and in vivo lung tumorigenesis through downregulation of HDAC activity. Biomed. Pharmacother. 78, 74–80. doi:10.1016/j.biopha.2015.11.007
Jiang, S. H., Shang, L., Xue, L. X., Ding, W., Chen, S., Ma, R. F., et al. (2014). The effect and underlying mechanism of Timosaponin B-II on RGC-5 necroptosis induced by hydrogen peroxide. BMC Complement. Altern. Med. 14, 459. doi:10.1186/1472-6882-14-459
Jiang, Y., Mao, C., Yang, R., Yan, B., Shi, Y., Liu, X., et al. (2017). EGLN1/c-Myc induced lymphoid-specific helicase inhibits ferroptosis through lipid metabolic gene expression changes. Theranostics 7 (13), 3293–3305. doi:10.7150/thno.19988
Kaur, A., Kaur, L., Singh, G., Dhawan, R. K., and Mahajan, A. (2022). Nanotechnology-based herbal formulations: a survey of recent patents, advancements, and transformative headways. Recent Pat. Nanotechnol. 16 (4), 295–307. doi:10.2174/1872210515666210428135343
Kiani, B. H., Kayani, W. K., Khayam, A. U., Dilshad, E., Ismail, H., and Mirza, B. (2020). Artemisinin and its derivatives: a promising cancer therapy. Mol. Biol. Rep. 47 (8), 6321–6336. doi:10.1007/s11033-020-05669-z
Kim, J. K., Choi, M. S., Kim, J. Y., Yu, J. S., Seo, J. I., Yoo, H. H., et al. (2021). Ginkgo biloba leaf extract suppresses intestinal human breast cancer resistance protein expression in mice: correlation with gut microbiota. Biomed. Pharmacother. 140, 111712. doi:10.1016/j.biopha.2021.111712
Kryczka, J., Kryczka, J., Czarnecka-Chrebelska, K. H., and Brzeziańska-Lasota, E. (2021). Molecular mechanisms of chemoresistance induced by cisplatin in NSCLC cancer therapy. Int. J. Mol. Sci. 22 (16), 8885. doi:10.3390/ijms22168885
Lai, Y., Zhang, Z., Li, J., Li, W., Huang, Z., Zhang, C., et al. (2019). STYK1/NOK correlates with ferroptosis in non-small cell lung carcinoma. Biochem. Biophys. Res. Commun. 519 (4), 659–666. doi:10.1016/j.bbrc.2019.09.032
Lane, D. J., Merlot, A. M., Huang, M. L., Bae, D. H., Jansson, P. J., Sahni, S., et al. (2015). Cellular iron uptake, trafficking and metabolism: key molecules and mechanisms and their roles in disease. Biochim. Biophys. Acta 1853 (5), 1130–1144. doi:10.1016/j.bbamcr.2015.01.021
Lay, J. D., Hong, C. C., Huang, J. S., Yang, Y. Y., Pao, C. Y., Liu, C. H., et al. (2007). Sulfasalazine suppresses drug resistance and invasiveness of lung adenocarcinoma cells expressing AXL. Cancer Res. 67 (8), 3878–3887. doi:10.1158/0008-5472.Can-06-3191
Lei, G., Zhuang, L., and Gan, B. (2022). Targeting ferroptosis as a vulnerability in cancer. Nat. Rev. Cancer 22 (7), 381–396. doi:10.1038/s41568-022-00459-0
Li, D., and Li, Y. (2020). The interaction between ferroptosis and lipid metabolism in cancer. Signal Transduct. Target Ther. 5 (1), 108. doi:10.1038/s41392-020-00216-5
Li, F. J., Long, H. Z., Zhou, Z. W., Luo, H. Y., Xu, S. G., and Gao, L. C. (2022a). System X(c) (-)/GSH/GPX4 axis: an important antioxidant system for the ferroptosis in drug-resistant solid tumor therapy. Front. Pharmacol. 13, 910292. doi:10.3389/fphar.2022.910292
Li, G., Zhang, H., Lai, H., Liang, G., Huang, J., Zhao, F., et al. (2023). Erianin: a phytoestrogen with therapeutic potential. Front. Pharmacol. 14, 1197056. doi:10.3389/fphar.2023.1197056
Li, H., Gao, C., Liu, C., Liu, L., Zhuang, J., Yang, J., et al. (2021a). A review of the biological activity and pharmacology of cryptotanshinone, an important active constituent in Danshen. Biomed. Pharmacother. 137, 111332. doi:10.1016/j.biopha.2021.111332
Li, J., Cao, F., Yin, H. L., Huang, Z. J., Lin, Z. T., Mao, N., et al. (2020). Ferroptosis: past, present and future. Cell Death Dis. 11 (2), 88. doi:10.1038/s41419-020-2298-2
Li, M., He, Y., Peng, C., Xie, X., and Hu, G. (2018). Erianin inhibits human cervical cancer cell through regulation of tumor protein p53 via the extracellular signal-regulated kinase signaling pathway. Oncol. Lett. 16 (4), 5006–5012. doi:10.3892/ol.2018.9267
Li, Y., Li, J., Su, Q., and Liu, Y. (2019). Sinapine reduces non-alcoholic fatty liver disease in mice by modulating the composition of the gut microbiota. Food Funct. 10 (6), 3637–3649. doi:10.1039/c9fo00195f
Li, Y., Sheng, Y., Liu, J., Xu, G., Yu, W., Cui, Q., et al. (2022b). Hair-growth promoting effect and anti-inflammatory mechanism of Ginkgo biloba polysaccharides. Carbohydr. Polym. 278, 118811. doi:10.1016/j.carbpol.2021.118811
Li, Y., Wang, Y., Gao, L., Tan, Y., Cai, J., Ye, Z., et al. (2022c). Betulinic acid self-assembled nanoparticles for effective treatment of glioblastoma. J. Nanobiotechnology 20 (1), 39. doi:10.1186/s12951-022-01238-7
Li, Y., Zhu, X., Wang, K., Zhu, L., Murray, M., and Zhou, F. (2022d). The potential of Ginkgo biloba in the treatment of human diseases and the relationship to Nrf2-mediated antioxidant protection. J. Pharm. Pharmacol. 74 (12), 1689–1699. doi:10.1093/jpp/rgac036
Li, Z., Feiyue, Z., and Gaofeng, L. (2021b). Traditional Chinese medicine and lung cancer--From theory to practice. Biomed. Pharmacother. 137, 111381. doi:10.1016/j.biopha.2021.111381
Li, Z., Xiao, J., Liu, M., Cui, J., Lian, B., Sun, Y., et al. (2022e). Notch3 regulates ferroptosis via ROS-induced lipid peroxidation in NSCLC cells. FEBS Open Bio 12 (6), 1197–1205. doi:10.1002/2211-5463.13393
Li, Z., Zhang, J., Duan, X., Zhao, G., and Zhang, M. (2022f). Celastrol: a promising agent fighting against cardiovascular diseases. Antioxidants (Basel) 11 (8), 1597. doi:10.3390/antiox11081597
Lin, Y. S., Shen, Y. C., Wu, C. Y., Tsai, Y. Y., Yang, Y. H., Lin, Y. Y., et al. (2019). Danshen improves survival of patients with breast cancer and dihydroisotanshinone I induces ferroptosis and apoptosis of breast cancer cells. Front. Pharmacol. 10, 1226. doi:10.3389/fphar.2019.01226
Linh, N. T., Qui, N. H., and Triatmojo, A. (2022). The effect of nano-encapsulated herbal essential oils on poultry' s health. Arch. Razi Inst. 77 (6), 2013–2021. doi:10.22092/ari.2022.358842.2318
Lipper, C. H., Stofleth, J. T., Bai, F., Sohn, Y. S., Roy, S., Mittler, R., et al. (2019). Redox-dependent gating of VDAC by mitoNEET. Proc. Natl. Acad. Sci. U. S. A. 116 (40), 19924–19929. doi:10.1073/pnas.1908271116
Liu, J., Kang, R., and Tang, D. (2022a). Signaling pathways and defense mechanisms of ferroptosis. Febs J. 289 (22), 7038–7050. doi:10.1111/febs.16059
Liu, J., Lee, J., Salazar Hernandez, M. A., Mazitschek, R., and Ozcan, U. (2015). Treatment of obesity with celastrol. Cell 161 (5), 999–1011. doi:10.1016/j.cell.2015.05.011
Liu, M., Fan, Y., Li, D., Han, B., Meng, Y., Chen, F., et al. (2021a). Ferroptosis inducer erastin sensitizes NSCLC cells to celastrol through activation of the ROS-mitochondrial fission-mitophagy axis. Mol. Oncol. 15 (8), 2084–2105. doi:10.1002/1878-0261.12936
Liu, M. R., Zhu, W. T., and Pei, D. S. (2021b). System Xc(-): a key regulatory target of ferroptosis in cancer. Invest. New Drugs 39 (4), 1123–1131. doi:10.1007/s10637-021-01070-0
Liu, X. Y., Wei, D. G., and Li, R. S. (2022b). Capsaicin induces ferroptosis of NSCLC by regulating SLC7A11/GPX4 signaling in vitro. Sci. Rep. 12 (1), 11996. doi:10.1038/s41598-022-16372-3
Lou, G., Wang, J., Hu, J., Gan, Q., Peng, C., Xiong, H., et al. (2021a). Sanguinarine: a double-edged sword of anticancer and carcinogenesis and its future application prospect. Anticancer Agents Med. Chem. 21 (16), 2100–2110. doi:10.2174/1871520621666210126091512
Lou, J. S., Zhao, L. P., Huang, Z. H., Chen, X. Y., Xu, J. T., Tai, W. C., et al. (2021b). Ginkgetin derived from Ginkgo biloba leaves enhances the therapeutic effect of cisplatin via ferroptosis-mediated disruption of the Nrf2/HO-1 axis in EGFR wild-type non-small-cell lung cancer. Phytomedicine 80, 153370. doi:10.1016/j.phymed.2020.153370
Lu, C. M., Yang, J. J., Wang, P. Y., and Lin, C. C. (2000). A new acylated flavonol glycoside and antioxidant effects of Hedyotis diffusa. Planta Med. 66 (4), 374–377. doi:10.1055/s-2000-8544
Ma, C. S., Lv, Q. M., Zhang, K. R., Tang, Y. B., Zhang, Y. F., Shen, Y., et al. (2021). NRF2-GPX4/SOD2 axis imparts resistance to EGFR-tyrosine kinase inhibitors in non-small-cell lung cancer cells. Acta Pharmacol. Sin. 42 (4), 613–623. doi:10.1038/s41401-020-0443-1
Ma, J., Zhang, H., Chen, Y., Liu, X., Tian, J., and Shen, W. (2022a). The role of macrophage iron overload and ferroptosis in atherosclerosis. Biomolecules 12 (11), 1702. doi:10.3390/biom12111702
Ma, N., Zhang, Z., Liao, F., Jiang, T., and Tu, Y. (2020). The birth of artemisinin. Pharmacol. Ther. 216, 107658. doi:10.1016/j.pharmthera.2020.107658
Ma, X. H., Liu, J. H., Liu, C. Y., Sun, W. Y., Duan, W. J., Wang, G., et al. (2022b). ALOX15-launched PUFA-phospholipids peroxidation increases the susceptibility of ferroptosis in ischemia-induced myocardial damage. Signal Transduct. Target Ther. 7 (1), 288. doi:10.1038/s41392-022-01090-z
Mao, C., Wang, X., Liu, Y., Wang, M., Yan, B., Jiang, Y., et al. (2018). A G3BP1-interacting lncRNA promotes ferroptosis and apoptosis in cancer via nuclear sequestration of p53. Cancer Res. 78 (13), 3484–3496. doi:10.1158/0008-5472.Can-17-3454
McNeill, D. R., and Wilson, D. M. (2007). A dominant-negative form of the major human abasic endonuclease enhances cellular sensitivity to laboratory and clinical DNA-damaging agents. Mol. Cancer Res. 5 (1), 61–70. doi:10.1158/1541-7786.Mcr-06-0329
Menniti-Ippolito, F., Mazzanti, G., Vitalone, A., Firenzuoli, F., and Santuccio, C. (2008). Surveillance of suspected adverse reactions to natural health products: the case of propolis. Drug Saf. 31 (5), 419–423. doi:10.2165/00002018-200831050-00007
Miao, Q., Deng, W. Q., Lyu, W. Y., Sun, Z. T., Fan, S. R., Qi, M., et al. (2023). Erianin inhibits the growth and metastasis through autophagy-dependent ferroptosis in KRAS(G13D) colorectal cancer. Free Radic. Biol. Med. 204, 301–312. doi:10.1016/j.freeradbiomed.2023.05.008
Miao, Y., Chen, Y., Xue, F., Liu, K., Zhu, B., Gao, J., et al. (2022). Contribution of ferroptosis and GPX4's dual functions to osteoarthritis progression. EBioMedicine 76, 103847. doi:10.1016/j.ebiom.2022.103847
Miller, M., and Hanna, N. (2021). Advances in systemic therapy for non-small cell lung cancer. Bmj 375, n2363. doi:10.1136/bmj.n2363
Mithoowani, H., and Febbraro, M. (2022). Non-small-cell lung cancer in 2022: a review for general practitioners in oncology. Curr. Oncol. 29 (3), 1828–1839. doi:10.3390/curroncol29030150
Mou, Y., Wang, J., Wu, J., He, D., Zhang, C., Duan, C., et al. (2019). Ferroptosis, a new form of cell death: opportunities and challenges in cancer. J. Hematol. Oncol. 12 (1), 34. doi:10.1186/s13045-019-0720-y
Munjuluri, S., Wilkerson, D. A., Sooch, G., Chen, X., White, F. A., and Obukhov, A. G. (2021). Capsaicin and TRPV1 channels in the cardiovascular system: the role of inflammation. Cells 11 (1), 18. doi:10.3390/cells11010018
Muthusamy, B., Patil, P. D., and Pennell, N. A. (2022). Perioperative systemic therapy for resectable non-small cell lung cancer. J. Natl. Compr. Canc Netw. 20 (8), 953–961. doi:10.6004/jnccn.2022.7021
Niu, X., Fan, T., Li, W., Xing, W., and Huang, H. (2012). The anti-inflammatory effects of sanguinarine and its modulation of inflammatory mediators from peritoneal macrophages. Eur. J. Pharmacol. 689 (1-3), 262–269. doi:10.1016/j.ejphar.2012.05.039
Patel, S. A., and Weiss, J. (2020). Advances in the treatment of non-small cell lung cancer: immunotherapy. Clin. Chest Med. 41 (2), 237–247. doi:10.1016/j.ccm.2020.02.010
Peng, Y., Ao, M., Dong, B., Jiang, Y., Yu, L., Chen, Z., et al. (2021). Anti-inflammatory effects of curcumin in the inflammatory diseases: status, limitations and countermeasures. Drug Des. Devel Ther. 15, 4503–4525. doi:10.2147/dddt.S327378
Pérez del Villar, L., Burguillo, F. J., López-Abán, J., and Muro, A. (2012). Systematic review and meta-analysis of artemisinin based therapies for the treatment and prevention of schistosomiasis. PLoS One 7 (9), e45867. doi:10.1371/journal.pone.0045867
Popa, D. E., Caliţa, M., Pîrlog, M. C., Constantin, C., Popp, C. G., and Gheonea, D. I. (2021). The differential diagnosis of ulcerative colitis versus angiodysplasia of the colon with ischemic colitis. Rom. J. Morphol. Embryol. 62 (2), 395–400. doi:10.47162/rjme.62.2.05
Reed, A., Ichu, T. A., Milosevich, N., Melillo, B., Schafroth, M. A., Otsuka, Y., et al. (2022). LPCAT3 inhibitors remodel the polyunsaturated phospholipid content of human cells and protect from ferroptosis. ACS Chem. Biol. 17 (6), 1607–1618. doi:10.1021/acschembio.2c00317
Rodríguez-Abreu, D., Powell, S. F., Hochmair, M. J., Gadgeel, S., Esteban, E., Felip, E., et al. (2021). Pemetrexed plus platinum with or without pembrolizumab in patients with previously untreated metastatic nonsquamous NSCLC: protocol-specified final analysis from KEYNOTE-189. Ann. Oncol. 32 (7), 881–895. doi:10.1016/j.annonc.2021.04.008
Russo, M., Spagnuolo, C., Russo, G. L., Skalicka-Woźniak, K., Daglia, M., Sobarzo-Sánchez, E., et al. (2018). Nrf2 targeting by sulforaphane: a potential therapy for cancer treatment. Crit. Rev. Food Sci. Nutr. 58 (8), 1391–1405. doi:10.1080/10408398.2016.1259983
Santana-Codina, N., Gikandi, A., and Mancias, J. D. (2021). The role of NCOA4-mediated ferritinophagy in ferroptosis. Adv. Exp. Med. Biol. 1301, 41–57. doi:10.1007/978-3-030-62026-4_4
Seibt, T. M., Proneth, B., and Conrad, M. (2019). Role of GPX4 in ferroptosis and its pharmacological implication. Free Radic. Biol. Med. 133, 144–152. doi:10.1016/j.freeradbiomed.2018.09.014
Shao, M., Jiang, Q., Shen, C., Liu, Z., and Qiu, L. (2022). Sinapine induced ferroptosis in non-small cell lung cancer cells by upregulating transferrin/transferrin receptor and downregulating SLC7A11. Gene 827, 146460. doi:10.1016/j.gene.2022.146460
Sharma, S. K., Vij, A. S., and Sharma, M. (2013). Mechanisms and clinical uses of capsaicin. Eur. J. Pharmacol. 720 (1-3), 55–62. doi:10.1016/j.ejphar.2013.10.053
Shostak, K., Jiang, Z., Charloteaux, B., Mayer, A., Habraken, Y., Tharun, L., et al. (2020). The X-linked trichothiodystrophy-causing gene RNF113A links the spliceosome to cell survival upon DNA damage. Nat. Commun. 11 (1), 1270. doi:10.1038/s41467-020-15003-7
Silva, H., and Martins, F. G. (2022). Cardiovascular activity of Ginkgo biloba-an insight from healthy subjects. Biol. (Basel) 12 (1), 15. doi:10.3390/biology12010015
Singh, S. K., Srivastav, S., Castellani, R. J., Plascencia-Villa, G., and Perry, G. (2019). Neuroprotective and antioxidant effect of Ginkgo biloba extract against AD and other neurological disorders. Neurotherapeutics 16 (3), 666–674. doi:10.1007/s13311-019-00767-8
Song, X., Liu, J., Kuang, F., Chen, X., Zeh, H. J., Kang, R., et al. (2021a). PDK4 dictates metabolic resistance to ferroptosis by suppressing pyruvate oxidation and fatty acid synthesis. Cell Rep. 34 (8), 108767. doi:10.1016/j.celrep.2021.108767
Song, Z., Jia, G., Ma, P., and Cang, S. (2021b). Exosomal miR-4443 promotes cisplatin resistance in non-small cell lung carcinoma by regulating FSP1 m6A modification-mediated ferroptosis. Life Sci. 276, 119399. doi:10.1016/j.lfs.2021.119399
Stoyanovsky, D. A., Tyurina, Y. Y., Shrivastava, I., Bahar, I., Tyurin, V. A., Protchenko, O., et al. (2019). Iron catalysis of lipid peroxidation in ferroptosis: regulated enzymatic or random free radical reaction? Free Radic. Biol. Med. 133, 153–161. doi:10.1016/j.freeradbiomed.2018.09.008
Su, L., Jiang, X., Yang, C., Zhang, J., Chen, B., Li, Y., et al. (2019). Pannexin 1 mediates ferroptosis that contributes to renal ischemia/reperfusion injury. J. Biol. Chem. 294 (50), 19395–19404. doi:10.1074/jbc.RA119.010949
Sui, X., Zhang, R., Liu, S., Duan, T., Zhai, L., Zhang, M., et al. (2018). RSL3 drives ferroptosis through GPX4 inactivation and ROS production in colorectal cancer. Front. Pharmacol. 9, 1371. doi:10.3389/fphar.2018.01371
Sun, J., Feng, Y., Wang, Y., Ji, Q., Cai, G., Shi, L., et al. (2019). α-hederin induces autophagic cell death in colorectal cancer cells through reactive oxygen species dependent AMPK/mTOR signaling pathway activation. Int. J. Oncol. 54 (5), 1601–1612. doi:10.3892/ijo.2019.4757
Sun, X., Ou, Z., Xie, M., Kang, R., Fan, Y., Niu, X., et al. (2015). HSPB1 as a novel regulator of ferroptotic cancer cell death. Oncogene 34 (45), 5617–5625. doi:10.1038/onc.2015.32
Sung, H., Ferlay, J., Siegel, R. L., Laversanne, M., Soerjomataram, I., Jemal, A., et al. (2021). Global cancer statistics 2020: GLOBOCAN estimates of incidence and mortality worldwide for 36 cancers in 185 countries. CA Cancer J. Clin. 71 (3), 209–249. doi:10.3322/caac.21660
Talman, A. M., Clain, J., Duval, R., Ménard, R., and Ariey, F. (2019). Artemisinin bioactivity and resistance in malaria parasites. Trends Parasitol. 35 (12), 953–963. doi:10.1016/j.pt.2019.09.005
Tang, X., Ding, H., Liang, M., Chen, X., Yan, Y., Wan, N., et al. (2021). Curcumin induces ferroptosis in non-small-cell lung cancer via activating autophagy. Thorac. Cancer 12 (8), 1219–1230. doi:10.1111/1759-7714.13904
Trapika, I., Liu, X. T., Chung, L. H., Lai, F., Xie, C., Zhao, Y., et al. (2021). Ceramide regulates anti-tumor mechanisms of erianin in androgen-sensitive and castration-resistant prostate cancers. Front. Oncol. 11, 738078. doi:10.3389/fonc.2021.738078
Tu, H., Tang, L. J., Luo, X. J., Ai, K. L., and Peng, J. (2021). Insights into the novel function of system Xc-in regulated cell death. Eur. Rev. Med. Pharmacol. Sci. 25 (3), 1650–1662. doi:10.26355/eurrev_202102_24876
Tuli, H. S., Sak, K., Gupta, D. S., Kaur, G., Aggarwal, D., Chaturvedi Parashar, N., et al. (2021). Anti-inflammatory and anticancer properties of birch bark-derived betulin: recent developments. Plants (Basel) 10 (12), 2663. doi:10.3390/plants10122663
Ursini, F., and Maiorino, M. (2020). Lipid peroxidation and ferroptosis: the role of GSH and GPx4. Free Radic. Biol. Med. 152, 175–185. doi:10.1016/j.freeradbiomed.2020.02.027
Vanduchova, A., Anzenbacher, P., and Anzenbacherova, E. (2019). Isothiocyanate from broccoli, sulforaphane, and its properties. J. Med. Food 22 (2), 121–126. doi:10.1089/jmf.2018.0024
Vasudeva, N., Yadav, N., and Sharma, S. K. (2012). Natural products: a safest approach for obesity. Chin. J. Integr. Med. 18 (6), 473–480. doi:10.1007/s11655-012-1120-0
Villalpando-Rodriguez, G. E., Blankstein, A. R., Konzelman, C., and Gibson, S. B. (2019). Lysosomal destabilizing drug siramesine and the dual tyrosine kinase inhibitor lapatinib induce a synergistic ferroptosis through reduced heme oxygenase-1 (HO-1) levels. Oxid. Med. Cell Longev. 2019, 9561281. doi:10.1155/2019/9561281
Wan, L. Q., Tan, Y., Jiang, M., and Hua, Q. (2019). The prognostic impact of traditional Chinese medicine monomers on tumor-associated macrophages in non-small cell lung cancer. Chin. J. Nat. Med. 17 (10), 729–737. doi:10.1016/s1875-5364(19)30089-5
Wang, F., Oudaert, I., Tu, C., Maes, A., Van der Vreken, A., Vlummens, P., et al. (2022a). System Xc(-) inhibition blocks bone marrow-multiple myeloma exosomal crosstalk, thereby countering bortezomib resistance. Cancer Lett. 535, 215649. doi:10.1016/j.canlet.2022.215649
Wang, J., Deng, H., Zhang, J., Wu, D., Li, J., Ma, J., et al. (2020a). α-Hederin induces the apoptosis of gastric cancer cells accompanied by glutathione decrement and reactive oxygen species generation via activating mitochondrial dependent pathway. Phytother. Res. 34 (3), 601–611. doi:10.1002/ptr.6548
Wang, M., Herbst, R. S., and Boshoff, C. (2021). Toward personalized treatment approaches for non-small-cell lung cancer. Nat. Med. 27 (8), 1345–1356. doi:10.1038/s41591-021-01450-2
Wang, M., Mao, C., Ouyang, L., Liu, Y., Lai, W., Liu, N., et al. (2019). Long noncoding RNA LINC00336 inhibits ferroptosis in lung cancer by functioning as a competing endogenous RNA. Cell Death Differ. 26 (11), 2329–2343. doi:10.1038/s41418-019-0304-y
Wang, X., Lu, X., Zhu, R., Zhang, K., Li, S., Chen, Z., et al. (2017). Betulinic acid induces apoptosis in differentiated PC12 cells via ROS-mediated mitochondrial pathway. Neurochem. Res. 42 (4), 1130–1140. doi:10.1007/s11064-016-2147-y
Wang, X., Yu, Y., Pei, L., and Gao, H. (2023). Comparison of the pharmacokinetics of timosaponin AIII, timosaponin BIII, and mangiferin extracted from crude and salt-processed Anemarrhenae Rhizoma by UPLC-MS/MS. RSC Adv. 13 (18), 11919–11928. doi:10.1039/d2ra07979h
Wang, Y., Li, C., Gu, J., Chen, C., Duanmu, J., Miao, J., et al. (2020b). Celastrol exerts anti-inflammatory effect in liver fibrosis via activation of AMPK-SIRT3 signalling. J. Cell Mol. Med. 24 (1), 941–953. doi:10.1111/jcmm.14805
Wang, Y., Zheng, L., Shang, W., Yang, Z., Li, T., Liu, F., et al. (2022b). Wnt/beta-catenin signaling confers ferroptosis resistance by targeting GPX4 in gastric cancer. Cell Death Differ. 29 (11), 2190–2202. doi:10.1038/s41418-022-01008-w
Wani, K. I., Choudhary, S., Zehra, A., Naeem, M., Weathers, P., and Aftab, T. (2021). Enhancing artemisinin content in and delivery from Artemisia annua: a review of alternative, classical, and transgenic approaches. Planta 254 (2), 29. doi:10.1007/s00425-021-03676-3
Wei, D., Ke, Y. Q., Duan, P., Zhou, L., Wang, C. Y., and Cao, P. (2021). MicroRNA-302a-3p induces ferroptosis of non-small cell lung cancer cells via targeting ferroportin. Free Radic. Res. 55 (7), 821–830. doi:10.1080/10715762.2021.1947503
Wei, H., and Lozano-Durán, R. (2023). The primase subunits of DNA polymerase α, PRIM1 and PRIM2, are required for the replication of the geminivirus tomato yellow leaf curl virus in the host plant. Micropubl. Biol. 2023. doi:10.17912/micropub.biology.000735
Wenzel, S. E., Tyurina, Y. Y., Zhao, J., St Croix, C. M., Dar, H. H., Mao, G., et al. (2017). PEBP1 wardens ferroptosis by enabling lipoxygenase generation of lipid death signals. Cell 171 (3), 628–641. doi:10.1016/j.cell.2017.09.044
Wiktorowska-Owczarek, A., Berezińska, M., and Nowak, J. Z. (2015). PUFAs: structures, metabolism and functions. Adv. Clin. Exp. Med. 24 (6), 931–941. doi:10.17219/acem/31243
Wu, C. Y., Yang, Y. H., Lin, Y. S., Chang, G. H., Tsai, M. S., Hsu, C. M., et al. (2021). Dihydroisotanshinone I induced ferroptosis and apoptosis of lung cancer cells. Biomed. Pharmacother. 139, 111585. doi:10.1016/j.biopha.2021.111585
Wu, C. Y., Yang, Y. H., Lin, Y. Y., Kuan, F. C., Lin, Y. S., Lin, W. Y., et al. (2017). Anti-cancer effect of danshen and dihydroisotanshinone I on prostate cancer: targeting the crosstalk between macrophages and cancer cells via inhibition of the STAT3/CCL2 signaling pathway. Oncotarget 8 (25), 40246–40263. doi:10.18632/oncotarget.14958
Wu, H., and Liu, A. (2021). Long non-coding RNA NEAT1 regulates ferroptosis sensitivity in non-small-cell lung cancer. J. Int. Med. Res. 49 (3), 300060521996183. doi:10.1177/0300060521996183
Wu, J., Minikes, A. M., Gao, M., Bian, H., Li, Y., Stockwell, B. R., et al. (2019). Intercellular interaction dictates cancer cell ferroptosis via NF2-YAP signalling. Nature 572 (7769), 402–406. doi:10.1038/s41586-019-1426-6
Wu, Y., Wang, D., Lou, Y., Liu, X., Huang, P., Jin, M., et al. (2022a). Regulatory mechanism of α-hederin upon cisplatin sensibility in NSCLC at safe dose by destroying GSS/GSH/GPX2 axis-mediated glutathione oxidation-reduction system. Biomed. Pharmacother. 150, 112927. doi:10.1016/j.biopha.2022.112927
Wu, Y. H., Wu, Y. R., Li, B., and Yan, Z. Y. (2020). Cryptotanshinone: a review of its pharmacology activities and molecular mechanisms. Fitoterapia 145, 104633. doi:10.1016/j.fitote.2020.104633
Wu, Z., Yin, B., and You, F. (2022b). Molecular mechanism of anti-colorectal cancer effect of hedyotis diffusa willd and its extracts. Front. Pharmacol. 13, 820474. doi:10.3389/fphar.2022.820474
Xd, M. E., Cao, Y. F., Che, Y. Y., Li, J., Shang, Z. P., Zhao, W. J., et al. (2019). Danshen: a phytochemical and pharmacological overview. Chin. J. Nat. Med. 17 (1), 59–80. doi:10.1016/s1875-5364(19)30010-x
Xia, P., Mao, Y., and Liang, Z. (2022). Two important Ginseng plants in Southeast Asia: a systematic review of their traditional uses, botany, phytochemistry, and pharmacology. Acta Physiologiae Plantarum 44, 105.
Xie, H., Feng, S., Farag, M. A., Sun, P., and Shao, P. (2021). Synergistic cytotoxicity of erianin, a bisbenzyl in the dietetic Chinese herb Dendrobium against breast cancer cells. Food Chem. Toxicol. 149, 111960. doi:10.1016/j.fct.2020.111960
Xie, Y., Hou, W., Song, X., Yu, Y., Huang, J., Sun, X., et al. (2016). Ferroptosis: process and function. Cell Death Differ. 23 (3), 369–379. doi:10.1038/cdd.2015.158
Xu, C., Sun, S., Johnson, T., Qi, R., Zhang, S., Zhang, J., et al. (2021a). The glutathione peroxidase Gpx4 prevents lipid peroxidation and ferroptosis to sustain Treg cell activation and suppression of antitumor immunity. Cell Rep. 35 (11), 109235. doi:10.1016/j.celrep.2021.109235
Xu, H., Ye, D., Ren, M., Zhang, H., and Bi, F. (2021b). Ferroptosis in the tumor microenvironment: perspectives for immunotherapy. Trends Mol. Med. 27 (9), 856–867. doi:10.1016/j.molmed.2021.06.014
Xu, H., Zhao, H., Ding, C., Jiang, D., Zhao, Z., Li, Y., et al. (2023). Celastrol suppresses colorectal cancer via covalent targeting peroxiredoxin 1. Signal Transduct. Target Ther. 8 (1), 51. doi:10.1038/s41392-022-01231-4
Xu, R., Wu, J., Luo, Y., Wang, Y., Tian, J., Teng, W., et al. (2022). Sanguinarine represses the growth and metastasis of non-small cell lung cancer by facilitating ferroptosis. Curr. Pharm. Des. 28 (9), 760–768. doi:10.2174/1381612828666220217124542
Xu, S., Feng, Y., He, W., Xu, W., Xu, W., Yang, H., et al. (2021c). Celastrol in metabolic diseases: progress and application prospects. Pharmacol. Res. 167, 105572. doi:10.1016/j.phrs.2021.105572
Xu, Y., Fang, R., Shao, J., and Cai, Z. (2021d). Erianin induces triple-negative breast cancer cells apoptosis by activating PI3K/Akt pathway. Biosci. Rep. 41 (6). doi:10.1042/bsr20210093
Yan, W. Y., Cai, J., Wang, J. N., Gong, Y. S., and Ding, X. B. (2022). Co-treatment of betulin and gefitinib is effective against EGFR wild-type/KRAS-mutant non-small cell lung cancer by inducing ferroptosis. Neoplasma 69 (3), 648–656. doi:10.4149/neo_2022_211103N1568
Yang, S., Wang, X., Xiao, W., Xu, Z., Ye, H., Sha, X., et al. (2022). Dihydroartemisinin exerts antifibrotic and anti-inflammatory effects in graves' ophthalmopathy by targeting orbital fibroblasts. Front. Endocrinol. (Lausanne) 13, 891922. doi:10.3389/fendo.2022.891922
Yan, H., Zheng, W., Wang, Y., Wu, Y., Yu, J., and Xia, P. (2022). Integrative Metabolome and Transcriptome Analysis Reveals the Regulatory Network of Flavonoid Biosynthesis in Response to MeJA in Camellia vietnamensis Huang. Int. J. Mol. Sci. 23 (16), 9370. doi:10.3390/ijms23169370
Yang, B., Li, Q., Cheng, K., Fang, J., Mustafa, G., Pan, J., et al. (2022). Proteomics and metabolomics reveal the mechanism underlying differential antioxidant activity among the organs of two base plants of Shiliang tea (Chimonanthus salicifolius and Chimonanthus zhejiangensis). Food Chem. 385, 132698. doi:10.1016/j.foodchem.2022.132698
Yang, Y., Luo, M., Zhang, K., Zhang, J., Gao, T., Connell, D. O., et al. (2020). Nedd4 ubiquitylates VDAC2/3 to suppress erastin-induced ferroptosis in melanoma. Nat. Commun. 11 (1), 433. doi:10.1038/s41467-020-14324-x
Yang, Y., Zeng, Z., Li, L., Lei, S., Wu, Y., Chen, T., et al. (2023). Sinapine thiocyanate exhibited anti-colorectal cancer effects by inhibiting KRT6A/S100A2 axis. Cancer Biol. Ther. 24 (1), 2249170. doi:10.1080/15384047.2023.2249170
Yao, X., Li, W., Fang, D., Xiao, C., Wu, X., Li, M., et al. (2021). Emerging roles of energy metabolism in ferroptosis regulation of tumor cells. Adv. Sci. (Weinh) 8 (22), e2100997. doi:10.1002/advs.202100997
Yates, K., Pohl, F., Busch, M., Mozer, A., Watters, L., Shiryaev, A., et al. (2019). Determination of sinapine in rapeseed pomace extract: its antioxidant and acetylcholinesterase inhibition properties. Food Chem. 276, 768–775. doi:10.1016/j.foodchem.2018.10.045
Ye, B., Aponte, M., Dai, Y., Li, L., Ho, M. C., Vitonis, A., et al. (2007). Ginkgo biloba and ovarian cancer prevention: epidemiological and biological evidence. Cancer Lett. 251 (1), 43–52. doi:10.1016/j.canlet.2006.10.025
Ye, C. L., Du, Y., Yu, X., Chen, Z. Y., Wang, L., Zheng, Y. F., et al. (2022). STEAP3 affects ferroptosis and progression of renal cell carcinoma through the p53/xCT pathway. Technol. Cancer Res. Treat. 21, 15330338221078728. doi:10.1177/15330338221078728
Yuan, B., Liao, F., Shi, Z. Z., Ren, Y., Deng, X. L., Yang, T. T., et al. (2020). Dihydroartemisinin inhibits the proliferation, colony formation and induces ferroptosis of lung cancer cells by inhibiting PRIM2/slc7a11 Axis. Onco Targets Ther. 13, 10829–10840. doi:10.2147/ott.S248492
Yuan, H., Pratte, J., and Giardina, C. (2021). Ferroptosis and its potential as a therapeutic target. Biochem. Pharmacol. 186, 114486. doi:10.1016/j.bcp.2021.114486
Yuan, X., Li, J., Li, Y., Deng, Z., Zhou, L., Long, J., et al. (2019). Artemisinin, a potential option to inhibit inflammation and angiogenesis in rosacea. Biomed. Pharmacother. 117, 109181. doi:10.1016/j.biopha.2019.109181
Yuan, Y. L., Lin, B. Q., Zhang, C. F., Cui, L. L., Ruan, S. X., Yang, Z. L., et al. (2016). Timosaponin B-ii ameliorates palmitate-induced insulin resistance and inflammation via IRS-1/PI3K/akt and IKK/NF-[Formula: see text]B pathways. Am. J. Chin. Med. 44 (4), 755–769. doi:10.1142/s0192415x16500415
Zeng, H., Zhu, Q., Yuan, P., Yan, Y., Yi, K., and Du, L. (2023). Calmodulin and calmodulin-like protein-mediated plant responses to biotic stresses. Plant Cell Environ. 46 (12), 3680–3703. doi:10.1111/pce.14686
Zhang, C., Liu, X., Jin, S., Chen, Y., and Guo, R. (2022a). Ferroptosis in cancer therapy: a novel approach to reversing drug resistance. Mol. Cancer 21 (1), 47. doi:10.1186/s12943-022-01530-y
Zhang, Q., Lyu, Y., Huang, J., Zhang, X., Yu, N., Wen, Z., et al. (2020). Antibacterial activity and mechanism of sanguinarine against Providencia rettgeri in vitro. PeerJ 8, e9543. doi:10.7717/peerj.9543
Zhang, Q., Yi, H., Yao, H., Lu, L., He, G., Wu, M., et al. (2021a). Artemisinin derivatives inhibit non-small cell lung cancer cells through induction of ROS-dependent apoptosis/ferroptosis. J. Cancer 12 (13), 4075–4085. doi:10.7150/jca.57054
Zhang, R., Ma, C., Wei, Y., Wang, X., Jia, J., Li, J., et al. (2021b). Isolation, purification, structural characteristics, pharmacological activities, and combined action of Hedyotis diffusa polysaccharides: a review. Int. J. Biol. Macromol. 183, 119–131. doi:10.1016/j.ijbiomac.2021.04.139
Zhang, S., Lin, Q., Zheng, Y., Wang, W., Zhao, H., and Yang, D. (2023). Comparative transcriptome analysis reveals the regulatory effects of exogenous auxin on lateral root development and tanshinone accumulation in Salvia miltiorrhiza. Planta 258 (2), 33. doi:10.1007/s00425-023-04193-1
Zhang, X. W., Liu, W., Jiang, H. L., and Mao, B. (2018). Chinese herbal medicine for advanced non-small-cell lung cancer: a systematic review and meta-analysis. Am. J. Chin. Med. 46 (5), 923–952. doi:10.1142/s0192415x18500490
Zhang, Y., Guo, R., Li, J., and Zhu, L. (2022b). Research progress on the occurrence and therapeutic mechanism of ferroptosis in NSCLC. Naunyn Schmiedeb. Arch. Pharmacol. 395 (1), 1–12. doi:10.1007/s00210-021-02178-z
Zhang, Y., Wu, Q., Liu, J., Zhang, Z., Ma, X., Zhang, Y., et al. (2022c). Sulforaphane alleviates high fat diet-induced insulin resistance via AMPK/Nrf2/GPx4 axis. Biomed. Pharmacother. 152, 113273. doi:10.1016/j.biopha.2022.113273
Zhao, L. P., Wang, H. J., Hu, D., Hu, J. H., Guan, Z. R., Yu, L. H., et al. (2023). β-Elemene induced ferroptosis via TFEB-mediated GPX4 degradation in EGFR wide-type non-small cell lung cancer. J. Adv. Res. doi:10.1016/j.jare.2023.08.018
Zhao, Y. Y., Yang, Y. Q., Sheng, H. H., Tang, Q., Han, L., Wang, S. M., et al. (2022). GPX4 plays a crucial role in Fuzheng kang'ai decoction-induced non-small cell lung cancer cell ferroptosis. Front. Pharmacol. 13, 851680. doi:10.3389/fphar.2022.851680
Zhong, J., Wang, H., Zhuang, Y., and Shen, Q. (2021). Identification of the antibacterial mechanism of cryptotanshinone on methicillin-resistant Staphylococcus aureus using bioinformatics analysis. Sci. Rep. 11 (1), 21726. doi:10.1038/s41598-021-01121-9
Zhou, C., Yu, T., Zhu, R., Lu, J., Ouyang, X., Zhang, Z., et al. (2023). Timosaponin AIII promotes non-small-cell lung cancer ferroptosis through targeting and facilitating HSP90 mediated GPX4 ubiquitination and degradation. Int. J. Biol. Sci. 19 (5), 1471–1489. doi:10.7150/ijbs.77979
Zorzela, L., Khamba, B., Sparks, E., Necyk, C., Urichuk, L., Katzman, M. A., et al. (2021). Study of natural products adverse reactions (sonar) in adults with mental health conditions: a cross-sectional study. Drug Saf. 44 (9), 999–1006. doi:10.1007/s40264-021-01092-w
Glossary
Keywords: non-small cell lung cancer, ferroptosis, natural products, cancer treatment, drug development
Citation: Zhang Q, Xia Y, Wang F, Yang D and Liang Z (2024) Induction of ferroptosis by natural products in non-small cell lung cancer: a comprehensive systematic review. Front. Pharmacol. 15:1385565. doi: 10.3389/fphar.2024.1385565
Received: 13 February 2024; Accepted: 12 April 2024;
Published: 01 May 2024.
Edited by:
Jianqiang Xu, Dalian University of Technology, ChinaReviewed by:
Xinhui Wang, Zhejiang University, ChinaCharareh Pourzand, University of Bath, United Kingdom
Copyright © 2024 Zhang, Xia, Wang, Yang and Liang. This is an open-access article distributed under the terms of the Creative Commons Attribution License (CC BY). The use, distribution or reproduction in other forums is permitted, provided the original author(s) and the copyright owner(s) are credited and that the original publication in this journal is cited, in accordance with accepted academic practice. No use, distribution or reproduction is permitted which does not comply with these terms.
*Correspondence: Zongsuo Liang, liangzs2022@126.com
†These authors have contributed equally to this work and share first authorship