- 1Department of Gynaecology and Obstetrics, Zhongda Hospital, Southeast University, Nanjing, China
- 2Deaprtment of Biochemistry and Molecrla Biology, School of Medicine, Southeast University, Nanjing, China
Protocatechuic acid (PCA) and protocatechuic aldehyde (PAL) are important phenolic compounds in plants. We here investigated their possible beneficial effect against fungal infection and the underlying mechanism. The model animal of Caenorhabditis elegans was used as host, and Candida albicans was used as fungal pathogen. The nematodes were first infected with C. albicans, and the PCA and PAL treatment were then performed. Post-treatment with 10–100 μM PCA and PAL suppressed toxicity of C. albicans infection in reducing lifespan. Accompanied with this beneficial effect, treatment with 10–100 μM PCA and PAL inhibited C. albicans accumulation in intestinal lumen. In addition, treatment with 10–100 μM PCA and PAL suppressed the increase in expressions of antimicrobial genes caused by C. albicans infection. The beneficial effect of PCA and PAL against C. albicans infection depended on p38 MAPK and insulin signals. Moreover, although treatment with 10–100 μM PCA and PAL could not exhibit noticeable antifungal activity, PCA and PAL treatment obviously suppressed biofilm formation, inhibited hyphal growth, and reduced expressions of virulence genes (ALS3, CaVps34, Vma7, Vac1, and/or HWP1) related to biofilm formation and hyphal growth in C. albicans. Therefore, our data demonstrated the potential of PCA and PAL post-treatment against fungal infection and fungal virulence.
Introduction
Based on clinical survey, in the United States, Candida spp are considered as the fourth most common cause for systemic infections with high mortality in hospital (Pfaller and Diekema, 2010). Candida albicans, a fungal pathogen, can be widely detected in human microbiome (Mayer et al., 2013). In clinical, C. albicans can result in some forms of infections, including the life-threatening systemic infection (Nobile and Johnson, 2015; Lopes and Lionakis, 2022). Some virulence factors, such as biofilm formation and hyphal growth, contribute to pathogenic potential of C. albicans (Gow et al., 2003; Pereira et al., 2021). Thus, how to reduce and counteract C. albicans infection is an important issue in the clinical.
The model animal of Caenorhabditis elegans relies on innate immunity mechanism to defend pathogen infection (Martineau et al., 2021). It can provide a useful platform for determining interactions between hosts and bacterial or fungal pathogens (Kumar et al., 2020). Antimicrobial proteins secreted by different tissues act as immune effectors in nematodes after pathogen infection (Millet and Ewbank, 2004; Dierking et al., 2016). In nematodes, several signaling pathways (such as p38 MAPK and insulin) have been identified to regulate the innate immunity (Kim and Ewbank, 2018). It has been suggested that C. elegans can be further used for the study of human infectious diseases (Marsh and May, 2012).
The model animal of C. elegans is an important model for pharmacological discovery for some diseases (Griffin et al., 2017; Bulteriis and Braeckman, 2020). Due to short lifespan and life-cycle and exposure to a small amount of compound, it can be used for large-scale or high-throughput pharmacological and toxicological screens (O’Reilly et al., 2014; Carretero et al., 2017; Wang, 2020). Meanwhile, C. elegans has been widely applied for screening and identifying novel agents or compounds with the functions to enhance host immune response and to attenuate microbial virulence (Arvanitis et al., 2013).
After fungal infection, lifespan of nematodes could be reduced (Kim et al., 2020). Infection with C. albicans can induce antifungal immune defenses by activating expression of some antimicrobial genes (Sun et al., 2016b). Mutation of pmk-1 and daf-16 caused susceptibility to fungal infection (Pukkia-Worley et al., 2011; Kitisin et al., 2022), suggesting that PMK-1/p38 MAPK and DAF-16 in insulin signaling pathway mediate the resistance to C. albicans infection. For C. elegans, it can also be used for pharmacological assessment of compound against fungal infection, including C. albicans infection (Anastassopoulou et al., 2011; Madende et al., 2020).
Protocatechuic acid (PCA) and protocatechuic aldehyde (PAL) are two phenolic compounds. PCA and PLA can be found in herbs, fruits, and vegetables (Kakkar and Bais, 2014). Some aspects of beneficial effects of PCA and PAL, including antioxidation and anti-inflammation, have already been suggested (Zhang et al., 2021; Li et al., 2022). However, the possible usefulness of PCA and PLA treatment against fungal infection and underlying molecular basis remain largely unclear. Thus, we aimed to further determine possible beneficial effect of PCA and PAL against fungal infection in hosts and underlying mechanism. In this study, C. albicans was used as the fungal pathogen, and C. elegans was employed as the host.
Materials and methods
Maintenance of nematodes
Nematodes (wild-type N2) were cultured normally on nematode growth medium (NGM) plates seeded by Escherichia coli OP50 (Brenner, 1974). C. elegans strain was purchased from Caenorhabditis Genetics Center (CGC). The NGM plates were prepared as described (Stiernagle, 2006). To prepare synchronized young adults for fungal infection and following pharmacological treatment, the gravid nematodes were lysed with lysis buffer (2% HOCl and 0.45 M NaOH) to obtain the embryos (Zhao et al., 2022a). Collected embryos were transferred onto new NGM plate to allow to develop into young adults.
Fungal preparation
Information for C. albicans strains was shown in Supplementary Table S1. If not specially indicated, the used C. albicans strain is SC5314, which has been shown to be virulent for nematodes (Sun et al., 2015). Fungal strains were cultured in liquid yeast extract-peptone-dextrose broth or on brain heart infusion agar containing kanamycin (45 mg/mL).
Fungal infection
Young adults were transferred on BHI agar plates containing kanamycin (45 mg/mL) and seeded with C. albicans. PBS buffer (200 μL) was added together with 50 μL SC5314 to facilitate fungal dispersion. C. albicans infection was performed from young adults for 48-h at 20°C.
Pharmacological treatment
The PCA and PAL (purity, ≥98%) were purchased from Weikeqi Bio-Technology Co., Ltd. (China). After C. albicans infection, animals were treated with PCA and PAL for 24-h at 20°C. After pharmacological treatments, animals were cultured on NGM plate. Used concentrations for PCA and PAL were 10, 50, and 100 μM as described (Kong et al., 2014; Han et al., 2019).
Assay of lifespan
Lifespan of C. elegans was analyzed as described (Wang et al., 2023). After PCA or PAL treatment, survival of animals was checked every day. Animals were considered as dead if no responses were observed after prodding using a platinum wire. Median lifespans refer to days at which 50% nematodes survive. Fifty nematodes were tested for lifespan assay. Three replicates were performed.
Colony-forming unit (CFU) assay
C. albicans CFU was quantified in nematodes as described (Sun et al., 2016a). After infection and PCA and PAL treatments, animals were washed for five times using M9 buffer to remove fungal lawn on surface. Each group of fifty animals was homogenized and transferred on a YPD agar containing kanamycin (45 μg/mL), ampicillin (100 μg/mL), and streptomycin (100 μg/mL). After incubation for 48-h at 37°C, numbers of fungal colony were counted. Ten replicates were carried out.
SC5314:GFP accumulation in animal’s body was also examined. Data was expressed as relative fluorescence intensity of SC5314:GFP in intestinal lumen, which was normalized to autofluorescence of intestine. Forty animals were tested for each group. Three replicates were performed.
Transcriptional expression analysis
Using RNeasy Mini Kit (Qiagen), total RNAs of C. elegans and C. albicans were extracted for cDNA synthesis. To isolate biofilm cell RNA, the yeast cell suspensions (1 × 106 CFU/mL) were incubated with fresh RPMI 1640 in 96-well plates for 3-h at 37°C. Supernatants were then removed, and wells were washed using PBS buffer to remove unattached cells. PCA or PAL was added into wells and incubated at 37°C for 24-h. The 430–600 μm glass beads were applied to break adherent cells. To isolate hyphae cell RNA, the suspensions (1 × 106 CFU/mL) were collected and incubated with PCA and PAL diluted with RPMI 1640 containing 10% FBS at 37°C for 10-h. C. albicans cells were collected by centrifugation (3000 rpm, 2-min). Quality of RNAs was assessed by the ratio of OD260/280 in Nanodrop One. SYBR Green master mix was used for quantitative real-time polymerase chain reaction (qRT-PCR). Comparative cycle threshold method was employed. Internal reference gene (tba-1) expression was normalized in nematodes (Zhao et al., 2022b). Gene encoding 18S rRNA was used as internal reference gene in C. albicans (Shin and Eom, 2019). Expression of genes in control group was normalized to 100%. Primer information was shown in Supplementary Table S2, S3. Three replicates were performed.
RNA interference (RNAi)
RNAi was performed by feeding animals with E. coli HT115 expressing daf-16 or pmk-1. RNAi was carried out after the SC5314 infection. E. coli HT115 expressing L4440 (empty vector) acted as the control (Xu et al., 2022). Efficiency of RNAi was assessed by qRT-PCR (Supplementary Figure S1).
Antifungal activity of PCA and PAL
(1) Time-kill assay. Method was performed as described (Sun et al., 2016a). C. albicans SC5314 cells cultured overnight were suspended in RPMI medium to reach the concentration of 1–5 × 104 cells/mL. PCA and PAL were added to inoculated RPMI medium to obtain anticipated concentrations. Again, the centrifugated SC5314 cells were dispensed into culture tubes containing PCA and PAL in a volume of 5 mL. The C. albicans cells were incubated at 35°C. After PCA or PAL treatment, colony counts of SC5314 were analyzed on YPD agar at 6, 12, 18, and 24-h. Fluconazole was employed as the control. Experiments were carried out in triplicate.
(2) Agar diffusion assay. Method was performed as described (Lafleur et al., 2013). After concentration by centrifugation, 107 cells/mL for C. albicans SC5314 were inoculated in liquid YPD medium. The 10 mL suspension was transferred on YPD agar plates. PCA or PAL (50 μL) was pipetted on filter disks (6-mm diameter) and placed onto agar surfaces. Plates were incubated for 48-h at 35°C. Fluconazole (50 μL) was employed as the control. Experiments were performed in triplicate.
Fungal biofilm formation
After PCA and PAL treatment, C. albicans biofilms in 96-well plate were first washed by PBS buffer. The biofilms were fixed by methanol (200 μL), and stained by crystal violet (0.1%) for 5-min. After staining, each well in the plate was washed with sterile distilled water for three times. The wells were dried for 1-h at 60°C, and the biofilm was dissolved by acetic acid (33%). To further quantify formation of biofilm, absorbances at wavelength of OD600 were measured. Experiments were repeated three times. Biofilm formation was also visualized under the light microscope.
Fungal hyphal growth
The hyphal growth assay was analyzed as described (Manoharan et al., 2017). After incubation of yeast cell suspension (1 × 106 CFU/mL) with PCA or PAL for 10-h with agitation (200 r/min) at 37°C, C. albicans hyphae growth was visualized under a light microscope. RPMI 1640 containing 10% FBS was employed as the control. Experiments were repeated three times.
Data analysis
Statistical analysis was performed by SPSS 12.0 software. Difference between different groups was examined using analysis of variance (ANOVA). Probability level of 0.01 was considered statistically significant. Statistical significances between lifespan curves were analyzed by Kaplan-Meier survival analysis, followed by log-rank test.
Results
Role of PCA and PAL in promoting lifespan in nematodes after fungal infection
After SC5314 infection only, the lifespan was sharply decreased by SC5314 from day-2 (Figure 1). The lifespan reduction observed in SC5314 infected nematodes could be significantly suppressed by treatment with PCA and PAL at concentrations of 10–100 μM (Figure 1). In addition, the effect of PCA and PAL to extend lifespan of SC5314 infected nematodes was concentration dependent (Figure 1). This observation demonstrated the effect of PCA and PAL treatment against toxicity of fungal infection in decreasing lifespan.
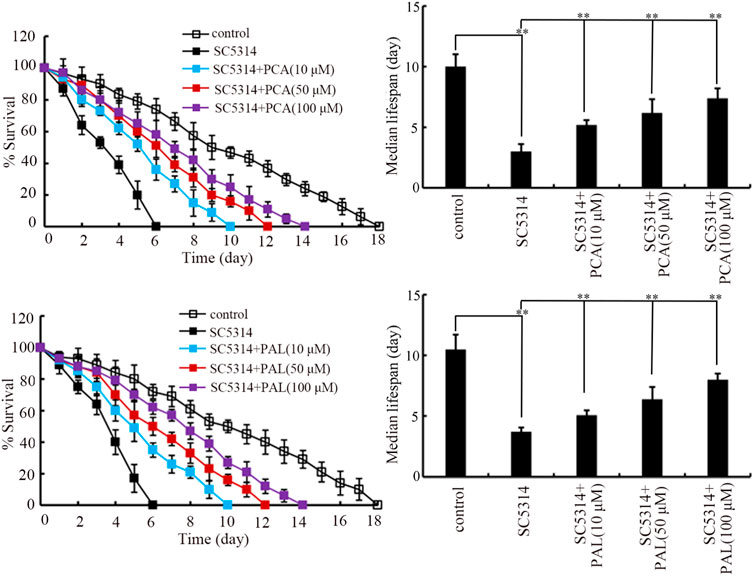
Figure 1. Effect of PCA and PAL treatment on lifespan of nematodes after C. albicans infection. PCA, protocatechuic acid; PAL, protocatechuic aldehyde. **p < 0.01. Lifespan curves of SC4314 showed a significant difference (p < 0.01) compared to control. Lifespan curves of SC5314+PCA (10 μM), SC5314+PCA (50 μM), SC5314+PCA (100 μM), SC5314+PAL (10 μM), SC5314+PAL (50 μM), and SC5314+PAL (100 μM) showed a significant difference (p < 0.01) compared to SC5314.
Role of PCA and PAL against C. albicans colony formation in C. elegans
To determine the underlying mechanism for observed benefits of PCA and PAL against fungal infection, SC5314 accumulation in intestinal lumen was investigated. After the infection, pronounced SC5314:GFP accumulation could be detected in intestinal lumen of nematodes (Figure 2A). The SC5314:GFP accumulation in intestinal lumen could be inhibited by treatment with 10–100 μM PCA and 10–100 μM PAL (Figure 2A). After the infection, a high level of intestinal CFU of SC5314 was further detected (Figure 2B). Moreover, the intestinal CFU of SC5314 after infection could be significantly suppressed by treatment with 10–100 μM PCA and 10–100 μM PAL (Figure 2B). The inhibition in intestinal SC5314:GFP accumulation and intestinal CFU by PCA and PAL was concentration dependent, and the 100 μM PCA and 100 μM PAL showed the most beneficial effect against SC5314 accumulation in intestinal lumen (Figures 2A,B).
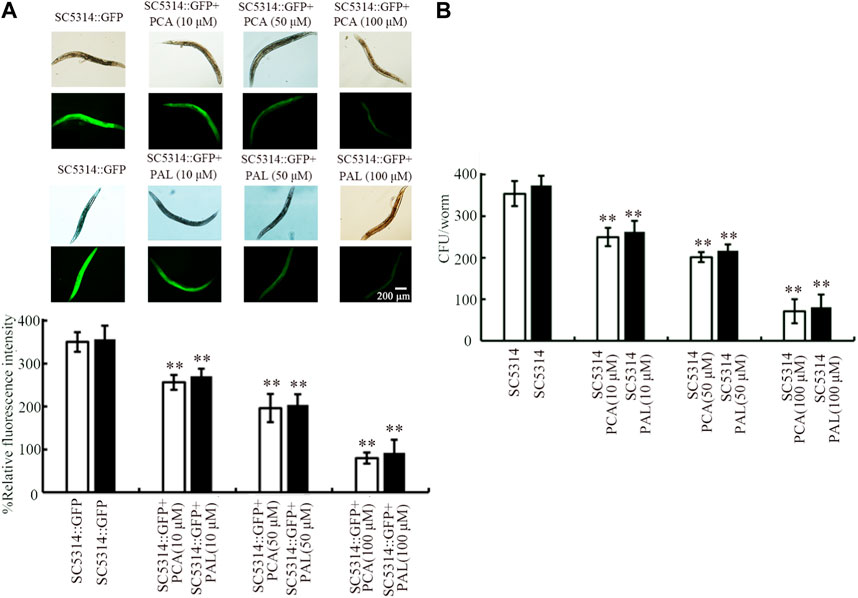
Figure 2. Effect of PCA and PAL treatment on C. albicans accumulation in intestinal lumen of nematodes. (A) Effect of PCA and PAL treatment on relative fluorescence intensity of SC5314:GFP in intestinal lumen of nematodes. (B) Effect of PCA and PAL treatment on CFU of SC5314 in infected nematodes. PCA, protocatechuic acid; PAL, protocatechuic aldehyde. **p < 0.01 vs. SC5314:GFP or SC5314.
Effect of PCA and PAL on innate immune response after fungal infection
Four genes (abf-2, cnc-4, cnc-7, and fipr-22/23) were used as antimicrobial genes in response to SC5314 infection (Sun et al., 2016a). The noticeable increase in expression of these 4 antimicrobial genes was induced by SC5314 infection (Figure 3). After SC5314 infection, the increase in expression of these 4 antimicrobial genes was obviously suppressed by treatment with 10–100 μM PCA (Figure 3). Similarly, the increase in expression of these 4 antimicrobial genes in SC5314 infected nematodes was also significantly inhibited by 10–100 μM PAL (Figure 3). The effect of PCA and PAL to modulate expressions of abf-2, cnc-4, cnc-7, and fipr-22/23 in SC5314 infected nematode was also concentration dependent (Figure 3).
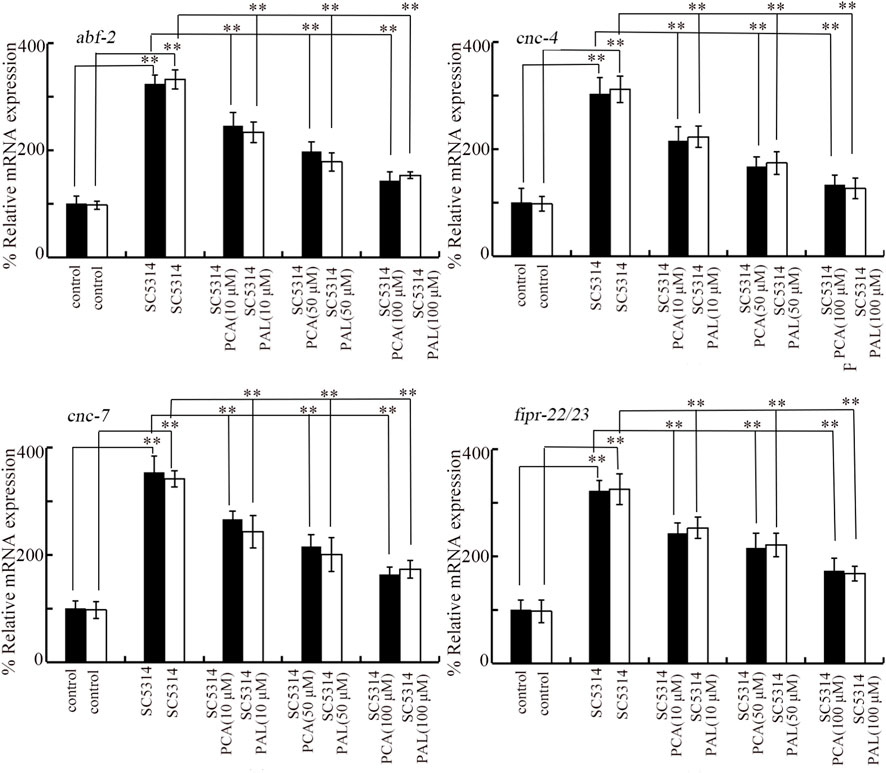
Figure 3. Effect of PCA and PAL treatment on expressions of abf-2, cnc-4, cnc-7, and fipr-22/23 in C. albicans infected nematodes. PCA, protocatechuic acid; PAL, protocatechuic aldehyde. **p < 0.01.
Effect of PCA and PAL against C. albicans infection depended on p38 MAPK signaling and insulin signaling.
Insulin and p38 MAPK are two normally determined signaling pathways required for controlling innate immunity (Millet and Ewbank, 2004). In p38 MAPK signaling pathway, PMK-1 is p38 MAPK. In insulin signaling pathway, DAF-16 is FOXO transcriptional factor. SC5314 infection only could cause the decrease in expressions of daf-16 and pmk-1 (Figure 4A). In SC5314 infected animals, treatment with 100 μM PCA and 100 μM PAL increased expression of daf-16 and pmk-1 (Figure 4A).
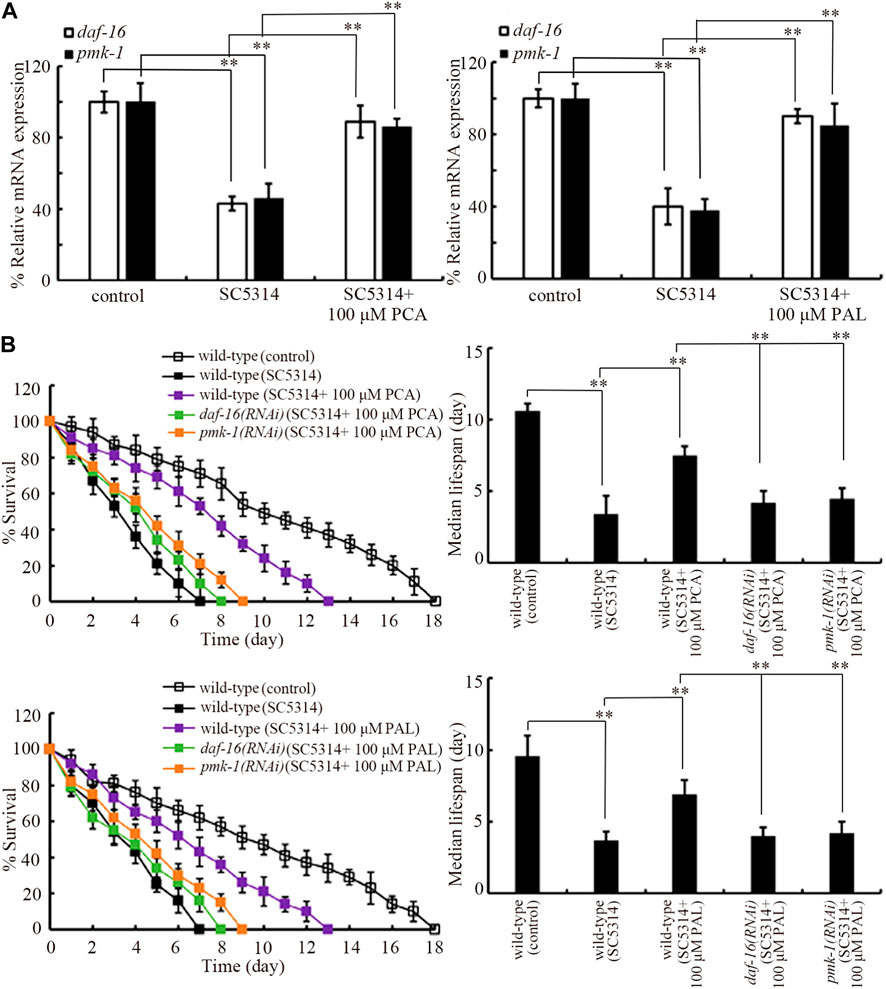
Figure 4. Effect pmk-1 or daf-16 RNAi on function of PCA and PAL against C. albicans infection. (A) Effect of PCA and PAL treatment on expressions of pmk-1 and daf-16 in C. albicans infected nematodes. (B) Effect pmk-1 or daf-16 RNAi on function of PCA and PAL in increasing survival of C. albicans infected nematodes. RNAi of pmk-1 or daf-16 was performed after C. albicans infection. PCA, protocatechuic acid; PAL, protocatechuic aldehyde. **p < 0.01. Lifespan curves of SC4314 showed a significant difference (p < 0.01) compared to control. Lifespan curves of SC5314 + 100 μM PCA and SC5314 + 100 μM PAL showed a significant difference (p < 0.01) compared to SC5314. Lifespan curves of daf-16(RNAi) (SC5314 + 100 μM PCA) and pmk-1(RNAi) (SC5314 + 100 μM PCA) showed a significant difference (p < 0.01) compared to the group of SC5314 + 100 μM PCA. Lifespan curves of daf-16(RNAi) (SC5314 + 100 μM PAL) and pmk-1(RNAi) (SC5314 + 100 μM PAL) showed a significant difference (p < 0.01) compared to the group of SC5314 + 100 μM PAL.
Moreover, the effect of 100 μM PCA and 100 μM PAL in increasing survival of SC5314 infected nematodes was significantly inhibited by RNAi of daf-16 and pmk-1 (Figure 4B). In addition, daf-16 and pmk-1 RNAi also significantly suppressed the function of 100 μM PCA and 100 μM PAL in decreasing both intestinal SC5314:GFP accumulation and intestinal CFU (Supplementary Figures S2A, B). Therefore, both PMK-1 and DAF-16 were required for the effect of PCA and PAL against C. albicans infection.
PCA and PAL did not exhibit obvious antifungal activity
Firstly, in time-kill assay, compared with strong anti-fungal activity of fluconazole (8 μg/mL), both 10–100 μM PCA and 10–100 μM PAL did not show noticeable anti-fungal activity from 6-h to 24-h (Figure 5A). Moreover, compared with obvious zone of inhibition induced by fluconazole (8 μg/mL), both 10–100 μM PCA and 10–100 μM PAL had no obvious effect on C. albicans SC5314 in the agar diffusion assay (Figure 5B).
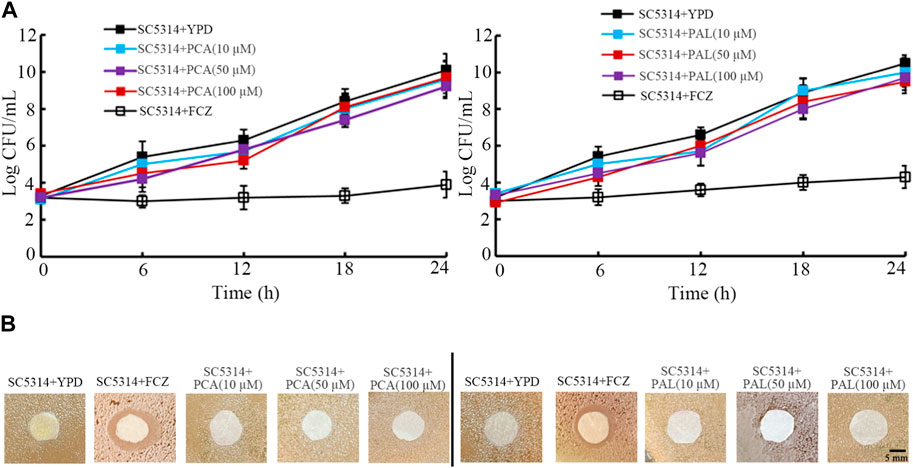
Figure 5. Analysis of antifungal activity of PCA and PAL. (A) Time-killing assay. (B) Disk diffusion assay. PCA, protocatechuic acid; PAL, protocatechuic aldehyde; FCZ, fluconazole. FCZ treatment concentration was 8 μg/mL.
Effect of PCA and PAL on fungal biofilm formation
Biofilm formation contributes to pathogenesis of C. albicans during their infection in hosts (Zeng et al., 2017). Both crystal violet staining and analysis of OD600 absorbance indicated that the biofilm formation of SC5314 was significantly reduced by 10–100 μM PCA and 10–100 μM PAL (Figures 6A,B). The beneficial effect of 10–100 μM PCA and 10–100 μM PAL in reducing biofilm formation of SC5314 was also found by visualization under light microscopy (Figure 6C). In addition, expression of biofilm-related gene ALS3 was significantly decreased by treatment with both 10–100 μM PCA and 10–100 μM PAL (Figure 6D). Therefore, PCA and PAL treatment showed the inhibitory effect on biofilm formation in C. albicans.
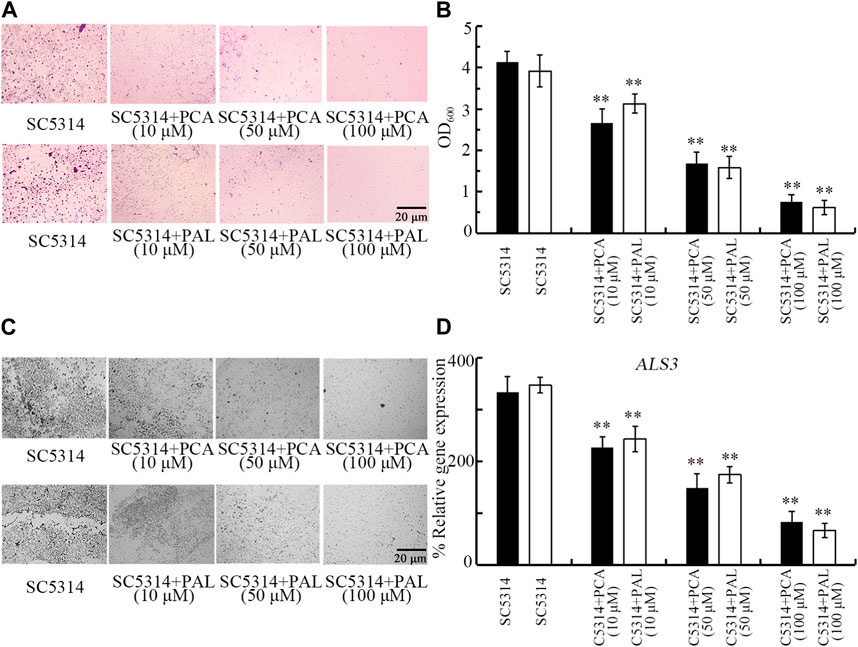
Figure 6. Effect of PCA and PAL on C. albicans biofilm formation. (A) Crystal violate staining images. (B) Effect of PCA and PAL on amount of biofilm formation based on OD600 absorbance analysis. (C) Effect of PCA and PAL on biofilm formation visualized under a light microscope. (D) Effect of PCA and PAL on expression of ALS3. PCA, protocatechuic acid; PAL, protocatechuic aldehyde. **p < 0.01 vs. SC5314.
Effect of PCA and PAL on C. albicans hyphal growth
Transition from yeast to hyphae also contributes to the induction of C. albicans pathogenicity (Gow et al., 2011). Although 10 μM PCA and PAL did not affect hyphal growth, the hyphal growth was obviously inhibited by 50 and 100 μM PCA and PAL (Figure 7A). After 50 and 100 μM PCA and PAL treatment, more yeast cells could be observed than the hyphal cells (Figure 7A).
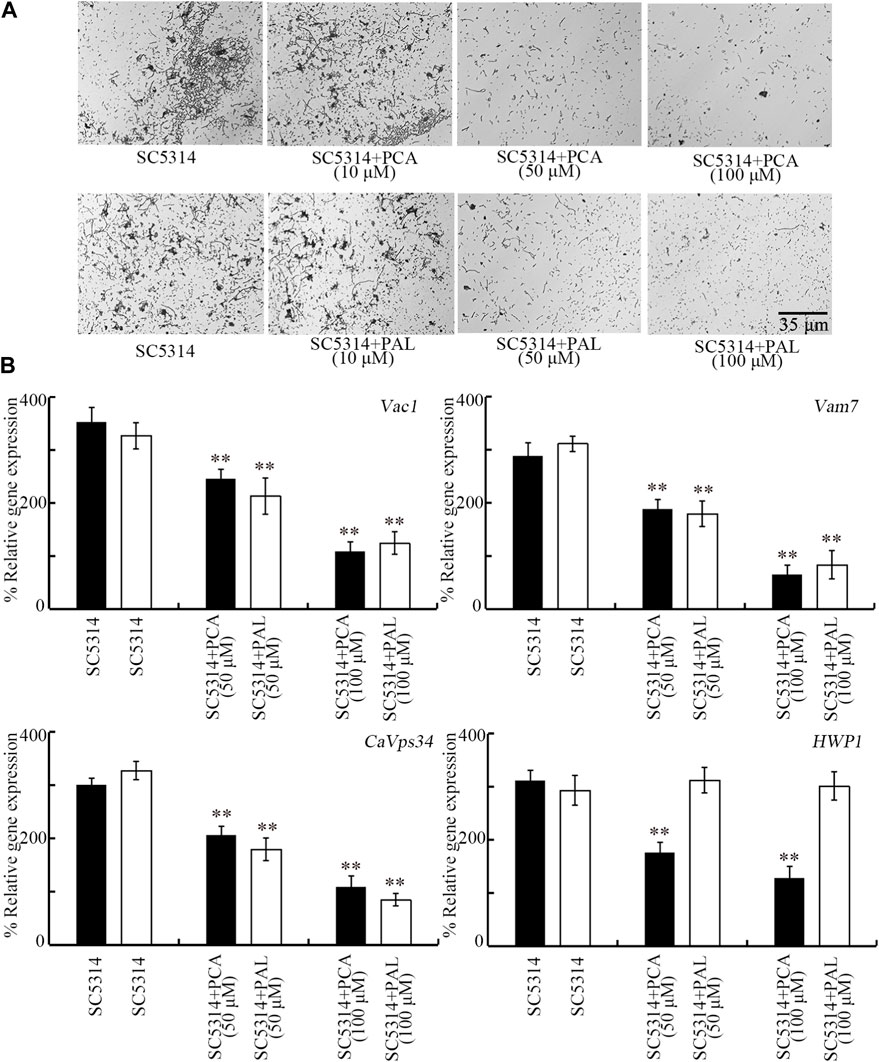
Figure 7. Effect of PCA and PAL on C. albicans hyphal growth. (A) Effect of PCA and PAL on hyphal growth. (B) Effect of PCA and PAL on expressions of Vac1, Vma7, CaVps34, and HWP1. PCA, protocatechuic acid; PAL, protocatechuic aldehyde. **p < 0.01 vs. SC5314.
During hyphal growth of C. albicans, Vac1, Vam7, CaVps34, and HWP1 play important roles (Sharkey et al., 1999; Bruckmann et al., 2000; Poltermann et al., 2005; Franke et al., 2006). After treatment with 50 and 100 μM PCA, expressions of all these 4 genes were significantly decreased (Figure 7B). In addition, after treatment with 50 and 100 μM PAL, expressions of Vac1, Vam7, and CaVps34 were also significantly decreased (Figure 7B). Therefore, both PCA and PAL treatment suppressed the transition from yeast to hyphae for C. albicans cells.
Discussion
Till now, the reported pharmacological effects of PCA contain inhibition in neurodegenerative diseases, anti-oxidation, anti-inflammation, anti-hyperglycemia, and anti-aging (Khan et al., 2015; Semaming et al., 2015; Krzysztoforska et al., 2019). In addition, PAL treatment has been shown to have the pharmacological effects of neuroprotection, anti-oxidation, and inhibition in pulmonary fibrosis, sepsis, and diabetic nephropathy (Zhang et al., 2015; Chang et al., 2021; Zhang et al., 2021; Guo et al., 2022). We used C. elegans as the host to examine the possible effect of PCA and PAL against fungal infection. Due to high sensitivity to exposure, C. elegans is helpful for detecting pharmacological effects of compounds at different concentrations (Wang, 2020). Besides this, considering the well-described molecular background, C. elegans will provide an important platform to elucidate underlying mechanism for the observed pharmacological effects of certain compound (Bulteriis and Braeckman, 2020).
Previous reports have indicated that treatment with PCA was helpful for nematodes against heat stress, osmotic stress, and oxidative stress (Kim et al., 2014; Schmitt et al., 2021). In addition, treatment with PAL could delay paralysis and inhibit aggregation of Aβ plaques, suggesting its neuroprotective effect (Shi et al., 2023). We found that treatment with both 10–100 μM PCA and 10–100 μM PAL inhibited adverse effect of SC5314 infection in decreasing lifespan (Figure 1). Treatment with 10–100 μM PCA and 10–100 μM PAL could not alter lifespan of nematodes (Supplementary Figure S4), suggesting that this effect of PCA and PAL was not associated with the possible role of PCA and PAL in extending longevity. Our results here demonstrated novel therapeutic potential of PCA and PAL. That is, this suggests that PCA and PAL administration in the clinical may be helpful to reduce fatality rate caused by fungal pathogen infection to a certain degree. Besides this, PCA treatment has also been shown to have the function against virus infection in mice (Wang et al., 2022). Nevertheless, we also noted that treatment with PCA and PAL at the examined concentrations did not recover lifespan of SC5314 infected nematodes to control level (Figure 1).
After fungal infection, the colony formation in intestine normally acts as a crucial contributor to toxicity of pathogen infection (Pukkila-Worley et al., 2011). The identified important cellular contributor to antifungal infection function of PCA and PAL was the inhibition in SC5314 accumulation in intestinal lumen (Figure 2). This suggested that treatment with PCA and PAL may be helpful for the excretion of C. albicans from intestinal lumen in nematodes. The C. albicans accumulation could also be decreased by 64 mg/L thymol (Shu et al., 2016). Similarly, the beneficial effect of treatment with paeoniflorin or Xuebijing (a traditional Chinese medicine) in enhancing excretion of bacterial pathogen from intestine was also observed in nematodes (Zhang et al., 2022; Wang et al., 2023). Different from these, multi-walled carbon nanotubes enhanced toxicity of fungal infection by increasing accumulation of SC5314 in intestinal lumen (Shakoor et al., 2016).
Certain antimicrobial genes of nematodes will be activated by C. albicans infection to be against the adverse effects of fungal pathogen and kill the C. albicans cells (Pukkila-Worley et al., 2011; Sun et al., 2016a). We observed that the increase in expressions of antimicrobial genes (fipr-22/23, cnc-7, cnc-4, and abf-2) in SC5314 infected nematodes was suppressed by the following treatment with 10–100 μM PCA and PAL (Figure 3). The inhibition in colony formation and accumulation of SC5314 in intestine induced by PCA or PAL treatment may lead to this suppression in increase in expression of fipr-22/23, cnc-7, cnc-4, and abf-2 in SC5314 infected animals. This further implies that inhibition in fungal pathogen accumulation in intestinal lumen may be the crucial cellular contributor to PCA and PAL function against fungal infection.
Moreover, RNAi of pmk-1 or daf-16 suppressed the formation of beneficial effect of PCA and PAL against fungal infection (Figure 4B). Therefore, the beneficial effect of PCA and PAL against fungal infection was dependent of p38 MAPK signaling and insulin signaling. p38 MAPK and insulin are conserved signaling pathways involved in controlling both innate immunity and stress responses (Harding and Ewbank, 2010; Zhao et al., 2016; Shao et al., 2019; Wang, 2019). Insulin signaling and p38 MAPK signaling are required for controlling innate immunity to both bacterial and functional infections (Troemel et al., 2006; Evans et al., 2008; Sun et al., 2016a; Kong et al., 2019). Mutation of daf-16 or pmk-1 caused the decrease in lifespan, enhancement in pathogen accumulation in intestinal lumen, and reduction in expression of antimicrobial genes (such as abf-2) in fungal infected nematodes (Sun et al., 2016a). Beneficial effect of thymol against fungal infection also required function of p38 MAPK signaling pathway (Shu et al., 2016). Meanwhile, in SC5314 infected nematodes, expression of pmk-1 and daf-16 could be increased by PCA and PAL treatment (Figure 4A). For the underlying molecular basis, our data suggests that PCA and PAL have the function against C. albicans infection by activating insulin signaling and p38 MAPK signaling in nematodes. It was also reported that PCA treatment could significantly upregulate expression of daf-16 (Dilberger et al., 2019).
Besides the inhibition in fungal pathogen accumulation in intestinal lumen, antifungal activity is another possible mechanism for formation of anti-fungal infection property of bioactive compounds. Nevertheless, time-kill assay indicated that both 10–100 μM PCA and 10–100 μM PAL had no obvious anti-fungal effect (Figure 5A). In addition, we also did not observe obvious zone of inhibition after treatment with 10–100 μM PCA and PAL in the agar diffusion assay (Figure 5B). These observations indicated that anti-fungal infection property of PCA and PAL in nematodes may be not directly associated with possible effect of anti-fungal activity for PCA and PAL.
Biofilm formation potentially protects C. albicans from the defense of host immune system (Chandra and Mukherjee, 2015). More importantly, the formed C. albicans biofilm is very resistant to traditional antifungal agents by strongly attaching to biotic or abiotic surfaces (Oppenheimer-Shaanan et al., 2013). We further observed the obvious beneficial effect of PCA and PAL treatment in reducing SC5314 biofilm formation (Figures 6A–C). Our data suggested that administration with PCA and PAL will be helpful for enhancing antifungal agents during treatment for fungal infections in patients. It has been reported that treatment with PCA showed the inhibitory effects on biofilms formation of E. coli (Bernal-Mercado et al., 2018). In addition, treatment with PAL had the inhibitory effects on biofilms formation of Ralstonia solanacearum, Yersinia enterocolitica and Vibrio parahaemolyticus (Li et al., 2016; Liu and Wang, 2022; Meng et al., 2022).
C. albicans biofilm is a cellular community encased in self-released extracellular polysaccharides (Rodrigues et al., 2018). In C. albicans, ALS3 encode a cell wall glycoprotein, and acts at adherence step of biofilms formation (Roudbarmohammadi et al., 2016). We found that the ALS3 expression was significantly decreased by 10–100 μM PCA and PAL treatment (Figure 6D), which provides an important molecular basis for PCA and PAL treatment in reducing fungal biofilm formation to a certain degree.
Moreover, we observed that the C. albicans hyphal growth was significantly inhibited by 50 and 100 μM PCA and PAL treatment (Figure 7A), which suggested the inhibition in transition from yeast to hyphal cells. After the C. albicans biofilm formation, the hyphae will appear together with the extracellular matrix material production (Qian et al., 2020). Hyphal growth is another important virulence factor, since the formed hyphae potentially attach to cells and cause damage on tissues in hosts (Finkel and Mitchell, 2011; Tati et al., 2016). Hyphal growth is closely associated with the biofilm formation, and hyphae are intertwined with biofilms in C. albicans (Finkel and Mitchell, 2011). Our data suggested that PCA and PAL treatment can provide a useful strategy to inhibit formation of both hyphae and biofilms produced by pathogenic C. albicans.
Furthermore, we found that expressions of some genes governing the C. albicans hyphal growth were downregulated by PCA and PAL treatment (Figure 7B), which further provides important molecular basis for PCA and PAL treatment in suppressing fungal hyphal growth. After 50 and 100 μM PCA and PAL treatment, the expressions of hyphae-related genes (Vac1, Vma7, CaVps34, and/or HWP1) were significantly decreased (Figure 7B). In C. albicans, null mutation of Vac1 encoding vesicle transporter caused defective in hyphal growth (Franke et al., 2006). The Vam7, a H+-ATPase subunit, regulates vacuolar ion transport, which is required for normal hyphal growth (Poltermann et al., 2005). Null mutation of CaVps34 encoding a phosphatidylinositol 3-kinase resulted in the significant delay in yeast-to-hyphae transition (Bruckmann et al., 2000). Deletion of HWP1 encoding a hypha-specific cell surface protein caused deficit in hyphal development (Sharkey et al., 1999).
Conclusion
In conclusion, treatment with PCA and PAL effectively inhibited adverse effect of fungal infection in decreasing lifespan of nematodes. This beneficial effect of PCA and PAL treatment was largely due to the suppression in C. albicans accumulation in intestinal lumen. Both p38 MAPK signaling and insulin signaling were required for formation of beneficial effect of PCA and PAL against fungal infection. Moreover, both biofilm formation and hyphal growth of C. albicans were inhibited by PCA and PAL treatment, suggesting their anti-virulence potential. Our data suggested the anti-fungal infection anti-virulence potentials of PCA and PAL treatment. Nevertheless, the underlying mechanism of anti-virulence potential for PCA and PAL needs to be further determined. The further identification of direct pharmacological targets for PCA and PAL against fungal virulence is suggested to be further performed.
Data availability statement
The original contributions presented in the study are included in the article/Supplementary Material, further inquiries can be directed to the corresponding author.
Ethics statement
The manuscript presents research on animals that do not require ethical approval for their study.
Author contributions
CY: Investigation, Writing–original draft. YW: Investigation, Writing–original draft. LZ: Investigation, Writing–original draft. DW: Writing–review and editing.
Funding
The author(s) declare that financial support was received for the research, authorship, and/or publication of this article. This work was supported by the Jiangsu Provincial Key Laboratory of Critical Care Medicine (JSKLCCM-2022-02-007).
Conflict of interest
The authors declare that the research was conducted in the absence of any commercial or financial relationships that could be construed as a potential conflict of interest.
Publisher’s note
All claims expressed in this article are solely those of the authors and do not necessarily represent those of their affiliated organizations, or those of the publisher, the editors and the reviewers. Any product that may be evaluated in this article, or claim that may be made by its manufacturer, is not guaranteed or endorsed by the publisher.
Supplementary material
The Supplementary Material for this article can be found online at: https://www.frontiersin.org/articles/10.3389/fphar.2024.1396733/full#supplementary-material
References
Anastassopoulou, C. G., Fuchs, B. B., and Mylonakis, E. (2011). Caenorhabditis elegans-based model systems for antifungal drug discovery. Curr. Pharm. Des. 17, 1225–1233. doi:10.2174/138161211795703753
Arvanitis, M., Glavis-Bloom, J., and Mylonakis, E. (2013). C. elegans for anti-infective discovery. Curr. Opin. Pharmacol. 13, 769–774. doi:10.1016/j.coph.2013.08.002
Bernal-Mercado, A. T., Vazquez-Armenta, F. J., Tapia-Rodriguez, M. R., Islas-Osuna, M. A., Mata-Haro, V., Gonzalez-Aguilar, G. A., et al. (2018). Comparison of single and combined use of catechin, protocatechuic, and vanillic acids as antioxidant and antibacterial agents against uropathogenic Escherichia coli at planktonic and biofilm levels. Molecules 23, 2813. doi:10.3390/molecules23112813
Brenner, S. (1974). The genetics of Caenorhabditis elegans. Genetics 77, 71–94. doi:10.1093/genetics/77.1.71
Bruckmann, A., Kunkel, W., Hart, A., Wetzker, R., and Eck, R. (2000). A phosphatidylinositol 3-kinase of Candida albicans influences adhesion, filamentous growth and virulence. Microbiology 146, 2755–2764. doi:10.1099/00221287-146-11-2755
Bulterijs, S., and Braeckman, B. P. (2020). Phenotypic screening in C. elegans as a tool for the discovery of new geroprotective drugs. Pharmaceuticals 13, 164. doi:10.3390/ph13080164
Carretero, M., Solis, G. M., and Petrascheck, M. (2017). C. elegans as model for drug discovery. Curr. Top. Med. Chem. 17, 2067–2076. doi:10.2174/1568026617666170131114401
Chandra, J., and Mukherjee, P. K. (2015). Candida biofilms: development, architecture, and resistance. Microbiol. Spectr. 3. doi:10.1128/microbiolspec.MB-0020-2015
Chang, Y., Chung, M., Hsieh, C., Shieh, J., and Wu, M. (2021). Evaluation of the therapeutic effects of protocatechuic aldehyde in diabetic nephropathy. Toxins 13, 560. doi:10.3390/toxins13080560
Dierking, K., Yang, W., and Schulenburg, H. (2016). Antimicrobial effectors in the nematode Caenorhabditis elegans: an outgroup to the Arthropoda. Philos. Trans. R. Soc. Lond. B Biol. Sci. 371, 20150299. doi:10.1098/rstb.2015.0299
Dilberger, B., Passon, M., Asseburg, H., Silaidos, C. V., Schmitt, F., Schmiedl, T., et al. (2019). Polyphenols and metabolites enhance survival in rodents and nematodes - impact of mitochondria. Nutrients 11, 1886. doi:10.3390/nu11081886
Evans, E. A., Kawli, T., and Tan, M. W. (2008). Pseudomonas aeruginosa suppresses host immunity by activating the DAF-2 insulin-like signaling pathway in Caenorhabditis elegans. PLoS Pathog. 4, e1000175. doi:10.1371/journal.ppat.1000175
Finkel, J. S., and Mitchell, A. P. (2011). Genetic control of Candida albicans biofilm development. Nat. Rev. Microbiol. 9, 109–118. doi:10.1038/nrmicro2475
Franke, K., Nguyen, M., Hartl, A., Dahse, H., Vogl, G., Wurzner, R., et al. (2006). The vesicle transport protein Vac1p is required for virulence of Candida albicans. Microbiology 152, 3111–3121. doi:10.1099/mic.0.29115-0
Gow, N. A., van de Veerdonk, F. L., Brown, A. J., and Netea, M. G. (2011). Candida albicans morphogenesis and host defence: discriminating invasion from colonization. Nat. Rev. Microbiol. 10, 112–122. doi:10.1038/nrmicro2711
Gow, N. A. R., Knox, Y., Munro, C. A., and Thompson, W. D. (2003). Infection of chick chorioallantoic membrane (CAM) as a model for invasive hyphal growth and pathogenesis of Candida albicans. Med. Mycol. 41, 331–338. doi:10.1080/13693780310001600859
Griffin, E. F., Caldwell, K. A., and Caldwell, G. A. (2017). Genetic and pharmacological discovery for Alzheimer's disease using Caenorhabditis elegans. ACS Chem. Neurosci. 8, 2596–2606. doi:10.1021/acschemneuro.7b00361
Guo, Y., Yang, J., He, Y., Zhou, H., Wang, Y., Ding, Z., et al. (2022). Protocatechuic aldehyde prevents ischemic injury by attenuating brain microvascular endothelial cell pyroptosis via lncRNA Xist. Phytomedicine 94, 153849. doi:10.1016/j.phymed.2021.153849
Han, L., Yang, Q., Li, J., Cheng, F., Zhang, Y., Li, Y., et al. (2019). Protocatechuic acid-ameliorated endothelial oxidative stress through regulating acetylation level via CD36/AMPK pathway. J. Agri. Food Chem. 67, 7060–7072. doi:10.1021/acs.jafc.9b02647
Harding, B. W., and Ewbank, J. J. (2021). An integrated view of innate immune mechanisms in C. elegans. Biochem. Soc. Trans. 49, 2307–2317. doi:10.1042/BST20210399
Kakkar, S., and Bais, S. (2014). A review on protocatechuic acid and its pharmacological potential. ISRN Pharmacol. 2014, 952943. doi:10.1155/2014/952943
Khan, A. K., Rashid, R., Fatima, N., Mahmood, S., Mir, S., Khan, S., et al. (2015). Pharmacological activies of protocatechuc acid. Acta Pol. Pharm. 72, 643–650.
Kim, D. H., and Ewbank, J. J. (2018). Signaling in the innate immune response. WormBook 2018, 1–35. doi:10.1895/wormbook.1.83.2
Kim, G. H., Rosiana, S., Kirienko, N. V., and Shapiro, R. S. (2020). A simple nematode infection model for studying Candida albicans pathogenesis. Curr. Protoc. Microbiol. 59, e114. doi:10.1002/cpmc.114
Kim, Y. S., Seo, H. W., Lee, M., Kim, D., Jeon, H., and Cha, D. S. (2014). Protocatechuic acid extends lifespan and increases stress resistance in Caenorhabditis elegans. Arch. Pharm. Res. 37, 245–252. doi:10.1007/s12272-013-0183-6
Kitisin, T., Muangkaew, W., and Sukphopetch, P. (2022). Regulation of DAF-16-mediated longevity and immune response to Candida albicans infection in Caenorhabditis elegans. New Microbiol. 45, 51–61.
Kong, B. S., Cho, Y. H., and Lee, E. J. (2014). G protein-coupled estrogen receptor-1 is involved in the protective effect of protocatechuic aldehyde against endothelial dysfunction. PLoS One 9, e113242. doi:10.1371/journal.pone.0113242
Kong, Y., Liu, H.-L., Li, W.-J., and Wang, D.-Y. (2019). Intestine-specific activity of insulin signaling pathway in response to microgravity stress in Caenorhabditis elegans. Caenorhabditis Elegans. Biochem. Biophys. Res. Commun. 517, 278–284. doi:10.1016/j.bbrc.2019.07.067
Krzysztoforska, K., Mirowska-Guzel, D., and Widy-Tyszkiewicz, E. (2019). Pharmacological effects of protocatechuic acid and its therapeutic potential in neurodegenerative diseases: review on the basis of in vitro and in vivo studies in rodents and humans. Nutr. Neurosci. 22, 72–82. doi:10.1080/1028415X.2017.1354543
Kumar, A., Baruah, A., Tomioka, M., Iino, Y., Kalita, M. C., and Khan, M. (2020). Caenorhabditis elegans: a model to understand host-microbe interactions. Cell. Mol. Life Sci. 77, 1229–1249. doi:10.1007/s00018-019-03319-7
Lafleur, M. D., Sun, L. M., Lister, I., Keating, J., Nantel, A., Long, L., et al. (2013). Potentiation of azole antifungals by 2-adamantanamine. Antimicrob. Agents Chemother. 57, 3585–3592. doi:10.1128/AAC.00294-13
Li, S., Yu, Y., Chen, J., Guo, B., Yang, L., and Ding, W. (2016). Evaluation of the antibacterial effects and mechanism of action of protocatechualdehyde against Ralstonia solanacearum. Molecules 21, 754. doi:10.3390/molecules21060754
Li, Y., Yang, Y., Kang, X., Li, X., Wu, Y., Xiao, J., et al. (2022). Study on the anti-inflammatory effects of Callicarpa nudiflora based on the spectrum-effect relationship. Front. Pharmacol. 12, 806808. doi:10.3389/fphar.2021.806808
Liu, Y., and Wang, L. (2022). Antibiofilm effect and mechanism of protocatechuic aldehyde against Vibrio parahaemolyticus. Front. Microbiol. 13, 1060506. doi:10.3389/fmicb.2022.1060506
Lopes, J. P., and Lionakis, M. S. (2022). Pathogenesis and virulence of Candida albicans. Virulence 13, 89–121. doi:10.1080/21505594.2021.2019950
Madende, M., Albertyn, J., Sebolai, O., and Pohl, C. H. (2020). Caenorhabditis elegans as a model animal for investigating fungal pathogenesis. Med. Microbiol. Immunol. 209, 1–13. doi:10.1007/s00430-019-00635-4
Manoharan, R. K., Lee, J. H., Kim, Y. G., and Lee, J. (2017). Alizarin and chrysazin inhibit biofilm and hyphal formation by Candida albicans. Front. Cell. Infect. Microbiol. 7, 447. doi:10.3389/fcimb.2017.00447
Marsh, E. K., and May, R. C. (2012). Caenorhabditis elegans, a model organism for investigating immunity. Appl. Environ. Microbiol. 78, 2075–2081. doi:10.1128/AEM.07486-11
Martineau, C. N., Kirienko, N. V., and Pujol, N. (2021). Innate immunity in C. elegans. Curr. Top. Dev. Biol. 144, 309–351. doi:10.1016/bs.ctdb.2020.12.007
Mayer, F. L., Wilson, D., and Hube, B. (2013). Candida albicans pathogenicity mechanisms. Virulence 4, 119–128. doi:10.4161/viru.22913
Meng, X., Han, X., Guo, X., Jiao, K., Jin, T., Li, J., et al. (2022). Inhibitory effect of protocatechualdehyde on Yersinia enterocolitica and its critical virulence factors. Microb. Pathog. 173, 105877. doi:10.1016/j.micpath.2022.105877
Millet, A. C., and Ewbank, J. J. (2004). Immunity in Caenorhabditis elegans. Curr. Opin. Immunol. 16, 4–9. doi:10.1016/j.coi.2003.11.005
Nobile, C. J., and Johnson, A. D. (2015). Candida albicans biofilms and human disease. Annu. Rev. Microbiol. 69, 71–92. doi:10.1146/annurev-micro-091014-104330
Oppenheimer-Shaanan, Y., Steinberg, N., and Kolodkin-Gal, I. (2013). Small molecules are natural triggers for the disassembly of biofilms. Trend. Microbiol. 21, 594–601. doi:10.1016/j.tim.2013.08.005
O'Reilly, L. P., Luke, C. J., Perlmutter, D. H., Silverman, G. A., and Pak, S. C. (2014). C. elegans in high-throughput drug discovery. Adv. Drug Deliv. Rev. 69-70, 247–253. doi:10.1016/j.addr.2013.12.001
Pereira, R., Dos Santos Fontenelle, R. O., de Brito, E. H. S., and de Morais, S. M. (2021). Biofilm of Candida albicans: formation, regulation and resistance. J. Appl. Microbiol. 131, 11–22. doi:10.1111/jam.14949
Pfaller, M. A., and Diekema, D. J. (2010). Epidemiology of invasive mycoses in North America. Crit. Rev. Microbiol. 36, 1–53. doi:10.3109/10408410903241444
Poltermann, S., Nguyen, M., Gunther, J., Wendland, J., Hartl, A., Kunkel, W., et al. (2005). The putative vacuolar ATPase subunit Vma7p of Candida albicans is involved in vacuole acidification, hyphal development and virulence. Microbiology 151, 1645–1655. doi:10.1099/mic.0.27505-0
Pukkila-Worley, R., Ausubel, F. M., and Mylonakis, E. (2011). Candida albicans infection of Caenorhabditis elegans induces antifungal immune defenses. PLoS Pathog. 7, e1002074. doi:10.1371/journal.ppat.1002074
Qian, W., Zhang, J., Wang, W., Wang, T., Liu, M., Yang, M., et al. (2020). Antimicrobial and antibiofilm activities of paeoniflorin against carbapenem-resistant Klebsiella pneumoniae. J. Appl. Microbiol. 128, 401–413. doi:10.1111/jam.14480
Rodrigues, C. F., Rodrigues, M. E., and Henriques, M. (2018). Susceptibility of Candida glabrata biofilms to echinocandins: alterations in the matrix composition. Biofouling 34, 569–578. doi:10.1080/08927014.2018.1472244
Roudbarmohammadi, S., Roudbary, M., Bakhshi, B., Katiraee, F., Mohammadi, R., and Falahati, M. (2016). ALS1 and ALS3 gene expression and biofilm formation in Candida albicans isolated from vulvovaginal candidiasis. Adv. Biomed. Res. 5, 105. doi:10.4103/2277-9175.183666
Schmitt, F., Babylon, L., Dieter, F., and Eckert, G. P. (2021). Effects of pesticides on longevity and bioenergetics in invertebrates - the impact of polyphenolic metabolites. Int. J. Mol. Sci. 22, 13478. doi:10.3390/ijms222413478
Semaming, Y., Pannengpetch, P., Chattipakorn, S. C., and Chattipakorn, N. (2015). Pharmacological properties of protocatechuic Acid and its potential roles as complementary medicine. Evid. Based Complement Alternat. Med 2015, 593902. doi:10.1155/2015/593902
Shakoor, S., Sun, L., and Wang, D. (2016). Multi-walled carbon nanotubes enhanced fungal colonization and suppressed innate immune response to fungal infection in nematodes. Toxicol. Res. 5, 492–499. doi:10.1039/c5tx00373c
Shao, H.-M., Han, Z.-Y., Krasteva, N., and Wang, D.-Y. (2019). Identification of signaling cascade in the insulin signaling pathway in response to nanopolystyrene particles. Nanotoxicology 13, 174–188. doi:10.1080/17435390.2018.1530395
Sharkey, L. L., McNemar, M. D., Saporito-Irwin, S. M., Sypherd, P. S., and Fonzi, W. A. (1999). HWP1 functions in the morphological development of Candida albicans downstream of EFG1, TUP1, and RBF1. J. Bacteriol. 181, 5273–5279. doi:10.1128/JB.181.17.5273-5279.1999
Shi, X., Luo, Y., Yang, L., and Duan, X. (2023). Protective effect of Gastrodia elata Blume in a Caenorhabditis elegans model of Alzheimer's disease based on network pharmacology. Biomed. Rep. 18, 37. doi:10.3892/br.2023.1620
Shin, D., and Eom, Y. (2019). Zerumbone inhibits Candida albicans biofilm formation and hyphal growth. Can. J. Microbiol. 65, 713–721. doi:10.1139/cjm-2019-0155
Shu, C., Sun, L., and Zhang, W. (2016). Thymol has antifungal activity against Candida albicans during infection and maintains the innate immune response required for function of the p38 MAPK signaling pathway in Caenorhabditis elegans. Immunol. Res. 64, 1013–1024. doi:10.1007/s12026-016-8785-y
Sun, L.-M., Liao, K., Li, Y.-P., Zhao, L., Liang, S., Guo, D., et al. (2016b). Synergy between PVP-coated silver nanoparticles and azole antifungal against drug-resistant Candida albicans. J. Nanosci. Nanotechnol. 16, 2325–2335. doi:10.1166/jnn.2016.10934
Sun, L. M., Liao, K., and Wang, D.-Y. (2015). Effects of magnolol and honokiol on adhesion, yeast-hyphal transition, and formation of biofilm by Candida albicans. PLoS One 10, e0117695. doi:10.1371/journal.pone.0117695
Sun, L.-M., Zhi, L.-T., Shakoor, S., Liao, K., and Wang, D.-Y. (2016a). microRNAs involved in the control of innate immunity in Candida infected. Caenorhabditis Elegans. Sci. Rep. 6, 36036. doi:10.1038/srep36036
Tati, S., Davidow, P., McCall, A., Hwang-Wong, E., Rojas, I. G., Cormack, B., et al. (2016). Candida glabrata binding to Candida albicans hyphae enables its development in oropharyngeal candidiasis. PLoS Pathog. 12, e1005522. doi:10.1371/journal.ppat.1005522
Troemel, E. R., Chu, S. W., Reinke, V., Lee, S. S., Ausubel, F. M., and Kim, D. H. (2006). p38 MAPK regulates expression of immune response genes and contributes to longevity in C. elegans. PLoS Genet. 2, e183. doi:10.1371/journal.pgen.0020183
Wang, D.-Y. (2019) Molecular toxicology in Caenorhabditis elegans. Germany: Springer Nature Singapore Pte Ltd.
Wang, D.-Y. (2020) Exposure toxicology in Caenorhabditis elegans. Germany: Springer Nature Singapore Pte Ltd.
Wang, Q., Ren, X., Wu, J., Li, H., Yang, L., Zhang, Y., et al. (2022). Protocatechuic acid protects mice from influenza A virus infection. Eur. J. Clin. Microbiol. Infect. Dis. 41, 589–596. doi:10.1007/s10096-022-04401-y
Wang, Y.-X., Zhang, L., Yuan, X.-A., and Wang, D.-Y. (2023). Treatment with paeoniflorin increases lifespan of Pseudomonas aeruginosa infected Caenorhabditis elegans by inhibiting bacterial accumulation in intestinal lumen and biofilm formation. Front. Pharmacol. 14, 1114219. doi:10.3389/fphar.2023.1114219
Xu, R.-R., Hua, X., Rui, Q., and Wang, D.-Y. (2022). Polystyrene nanoparticles caused dynamic alteration in mitochondrial unfolded protein response from parents to the offspring in C. elegans. Chemosphere 308, 136154. doi:10.1016/j.chemosphere.2022.136154
Xu, Y., Jiang, W., Zhang, S., Zhu, H., and Hou, J. (2012). Protocatechuic aldehyde protects against experimental sepsis in vitro and in vivo. Basic Clin. Pharmacol. Toxicol. 110, 384–389. doi:10.1111/j.1742-7843.2011.00827.x
Zeng, B., Li, J., Wang, Y., Chen, P., Wang, X., Cui, J., et al. (2017). In vitro and in vivo effects of suloctidil on growth and biofilm formation of the opportunistic fungus Candida albicans. Oncotarget 8, 69972–69982. doi:10.18632/oncotarget.19542
Zhang, L., Ji, Y., Kang, Z., Lv, C., and Jiang, W. (2015). Protocatechuic aldehyde ameliorates experimental pulmonary fibrosis by modulating HMGB1/RAGE pathway. Toxicol. Appl. Pharmacol. 283, 50–56. doi:10.1016/j.taap.2015.01.001
Zhang, L., Wang, Y.-X., Cao, C., Zhu, Y.-K., Huang, W., Yang, Y., et al. (2022). Beneficial effect of Xuebijing against Pseudomonas aeruginosa infection in Caenorhabditis elegans. Front. Pharmacol. 13, 949608. doi:10.3389/fphar.2022.949608
Zhang, S., Gai, Z., Gui, T., Chen, J., Chen, Q., and Li, Y. (2021). Antioxidant effects of protocatechuic acid and protocatechuic aldehyde: old wine in a new bottle. Evid. Based Complement Alternat. Med. 2021, 6139308. doi:10.1155/2021/6139308
Zhao, Y., Hua, X., Bian, Q., and Wang, D.-Y. (2022b). Nanoplastic exposure at predicted environmental concentrations induces activation of germline Ephrin signal associated with toxicity formation in the Caenorhabditis elegans offspring. Toxics 10, 699. doi:10.3390/toxics10110699
Zhao, Y.-L., Zhi, L.-T., Wu, Q.-L., Yu, Y.-L., Sun, Q.-Q., and Wang, D.-Y. (2016). p38 MAPK-SKN-1/Nrf signaling cascade is required for intestinal barrier against graphene oxide toxicity in Caenorhabditis elegans. Nanotoxicology. Nanotoxicology 10, 1469–1479. doi:10.1080/17435390.2016.1235738
Keywords: C. elegans, procatechuic acid, protocatechuic aldehyde, C. albicans infection, virulence
Citation: Yuan C, Wang Y, Zhang L and Wang D (2024) Procatechuic acid and protocatechuic aldehyde increase survival of Caenorhabditis elegans after fungal infection and inhibit fungal virulence. Front. Pharmacol. 15:1396733. doi: 10.3389/fphar.2024.1396733
Received: 06 March 2024; Accepted: 30 April 2024;
Published: 22 May 2024.
Edited by:
Judith Maria Rollinger, University of Vienna, AustriaReviewed by:
James Prabhanand Bhaskar, ITC Life Science and Technology Centre, IndiaMahdi Yaghoobi, KU Leuven, Belgium
Copyright © 2024 Yuan, Wang, Zhang and Wang. This is an open-access article distributed under the terms of the Creative Commons Attribution License (CC BY). The use, distribution or reproduction in other forums is permitted, provided the original author(s) and the copyright owner(s) are credited and that the original publication in this journal is cited, in accordance with accepted academic practice. No use, distribution or reproduction is permitted which does not comply with these terms.
*Correspondence: Dayong Wang, ZGF5b25nd0BzZXUuZWR1LmNu