- 1Department of Infection, Guang’an Men Hospital, China Academy of Chinese Medical Sciences, Beijing, China
- 2Graduate school, Beijing University of Chinese Medicine, Beijing, China
Objective: This meta-analysis aimed to determine the efficacy of curcumin in preventing liver fibrosis in animal models.
Methods: A systematic search was conducted on studies published from establishment to November 2023 in PubMed, Web of Science, Embase, Cochrane Library, and other databases. The methodological quality was assessed using Sycle’s RoB tool. An analysis of sensitivity and subgroups were performed when high heterogeneity was observed. A funnel plot was used to assess publication bias.
Results: This meta-analysis included 24 studies involving 440 animals with methodological quality scores ranging from 4 to 6. The results demonstrated that curcumin treatment significantly improved Aspartate aminotransferase (AST) [standard mean difference (SMD) = -3.90, 95% confidence interval (CI) (−4.96, −2.83), p < 0.01, I2 = 85.9%], Alanine aminotransferase (ALT)[SMD = − 4.40, 95% CI (−5.40, −3.40), p < 0.01, I2 = 81.2%]. Sensitivity analysis of AST and ALT confirmed the stability and reliability of the results obtained. However, the funnel plot exhibited asymmetry. Subgroup analysis based on species and animal models revealed statistically significant differences among subgroups. Furthermore, curcumin therapy improved fibrosis degree, oxidative stress level, inflammation level, and liver synthesis function in animal models of liver fibrosis.
Conclusion: Curcumin intervention not only mitigates liver fibrosis but also enhances liver function, while concurrently modulating inflammatory responses and antioxidant capacity in animal models. This result provided a strong basis for further large-scale animal studies as well as clinical trials in humans in the future.Systematic Review Registration: https://www.crd.york.ac.uk/prospero/, identifier CRD42024502671.
1 Introduction
Hepatic fibrosis arises from an imbalanced reparative response to chronic injury, and its progression may result in cirrhosis, liver failure, hepatocellular carcinoma, and other severe conditions (Hernandez-Gea and Friedman, 2011; Ginès et al., 2021). This condition is marked by an abnormal accumulation and irregular distribution of extracellular matrix (ECM) components, including collagen and glycoproteins, disrupting the normal functioning of liver cells. Such disruption triggers myofibroblast activation, leading to structural disorders and the loss of liver function (Asrani et al., 2019). Excessive production of ECM destroys liver structure, impairing organ function, disrupting blood flow, and potentially causing cirrhosis (Y. Wang et al., 2023).
In 2017, the global prevalence of chronic liver disease reached 1.5 billion cases, with non-alcoholic fatty liver disease (NAFLD), hepatitis B virus (HBV) infection, hepatitis C virus (HCV) infection, and alcoholic liver disease (ALD) constituting approximately 60%, 29%, 9%, and 2% of cases, respectively (James et al., 2018; Moon, Singal, and Tapper, 2020). Liver fibrosis represents the inevitable stage in the development of all chronic liver diseases. Anti-fibrotic interventions play a crucial role in delaying the progression of liver disease and enhancing the quality of life for patients. However, progress in the research on anti-fibrosis drugs is sluggish at present. Conventional antiviral, anti-inflammatory therapies and other treatment methods employed in the treatment of liver fibrosis have proven ineffective in ECM deposition or facilitating its degradation (Ma et al., 2020). As a result, they are unable to adequately manage or eliminate liver fibrosis. Over an extended period, both domestically and internationally, significant attention has been directed toward the pharmacological properties and clinical development of curcumin (Sun and Kisseleva, 2015). Curcumin, derived from turmeric, has long captivated the pharmaceutical field globally due to its pharmacological effects and clinical development (Hasanzadeh et al., 2020). Curcumin is a lipophilic polyphenol that is antioxidant, anti-inflammatory, and anti-fibrotic. It has been extensively used as a food seasoning because of its high level of safety (Bavarsad et al., 2019) (Figure 1). Numerous human clinical trials have extensively employed curcumin for interventions in various diseases, such as multiple myeloma, pancreatic cancer, NAFLD, colon cancer, and Alzheimer’s disease, both in vitro and in animal models (Hatcher et al., 2008).
A growing body of evidence suggests that curcumin also holds therapeutic potential for liver fibrosis. It regulates cytokine production by regulating several signaling pathways, including those involved in inflammation. Including the transforming growth factor-beta (TGF-β)/Smad Signaling Pathway (N. Chen et al., 2014), PPAR-γ Signaling Pathway (Jin et al., 2016), NF-κB pathway (Cui et al., 2014), etc. This regulation inhibits hepatic stellate cell (HSC) activation while reducing liver inflammation and tissue oxidative stress levels (Y. Tang, 2015). Therefore, curcumin appears to be a highly effective treatment for liver fibrosis. However, no systematic review on this topic exists. Consequently, we conducted a meta-analysis based on preclinical data to evaluate the efficacy of curcumin, enhancing the credibility of the evidence, fortifying this conclusion, and providing clinical guidance for patients with hepatic fibrosis.
2 Methods
The systematic review and meta-analysis were conducted following the guidelines outlined in the Preferred Reporting Items for Systematic Reviews and Meta-Analysis (PRISMA).
2.1 Search strategy
The systematic search was conducted in four databases—PubMed, Web of Science, Embase, and Cochrane Library—from the establishment to October 2000 to November 2023, confined to English, Primary keywords included ‘liver cirrhosis’, ‘Hepatic Cirrhosis’, ‘Cirrhosis, Hepatic’, as well as ‘Curcumin’, ‘Turmeric Yellow’, ‘Yellow, Turmeric’, and ‘Curcumin Phytosome’. The comprehensive electronic search strategy for all databases is provided in Supplementary S1.
2.2 Inclusion and exclusion criteria
The predetermined inclusion criteria were as follows: 1) papers: describing the number of animals used; 2) language: published in English-language journals; 3) participants: liver fibrosis animal models; 4) intervention: Curcumin as the sole intervention; 5) comparison: a placebo solution or no treatment; 6) primary outcomes: AST and ALT.
Excluded documents criteria were as follows:1) clinical studies, reviews; 2) vitro studies; 3) conference reports and comments; 4) unpublished or duplicate literature; 5) lacking a control group.
2.3 Data extraction
Following predefined inclusion and exclusion criteria, we reviewed both abstracts and full texts of papers to establish the final selection of research literature. Any discrepancies regarding the eligibility of a specific study were resolved through discussions with a third-party reviewer.
Standardized pre-test tables in Excel were employed for data extraction from the included studies to facilitate evidence synthesis. The extracted information encompassed the following aspects:
(a) The first author’s name and the year of publication of the document;
(b) Species, sex, and weight of the animal model, along with the sample size for each group;
(c) Methods employed for modeling liver fibrosis;
(d) Dose (with a focus on the maximum dose when different doses were utilized), time, and duration of administration in the treatment group.
In cases where study data was presented graphically, efforts were made to obtain the original numerical values from the authors. If raw data is not available, use the icon Digital Ruler software to measure the icon data (GetData Graph Digitizer 2.26, https://www.softpedia.com/get/Multimedia/Graphic/Graphic-Others/GetData-Graph-Digitizer.shtml). If the data provided in the text shows SE, convert it to SD, using the formula: SD = SEM*
2.4 Risk of bias assessment
To assess the data quality in the articles included in this review, two researchers (Yan-bo Li and Zi-wen Zhuo) employed SYRGLE’s bias risk tool (Hooijmans et al., 2014). The assessment comprehensively addresses ten bias areas, including those related to selection, detection, performance, attrition, reporting bias, and other biases. In cases of disagreement, a third researcher was consulted. The tool provides three possible responses: "+" signifies a low risk of bias, "-" indicates a high risk of bias, and "?" denotes that the representation concept cannot be definitively attributed.
2.5 Statistical analysis
Summary statistics were assessed using the SMD and 95% CI. The heterogeneity of the studies was evaluated using the I2 statistics. Based on the level of heterogeneity, different effect models were employed. The random effect model was utilized when I2 >50%, while the fixed effect model was employed otherwise. Stata 16.0 was used for all statistical analyses.
The source of heterogeneity was identified through subgroup analysis and sensitivity analysis. When the number of included studies exceeded 10, publication bias was assessed using a funnel plot. A significance level of p < 0.05 indicated a statistically significant difference.
3 Results
3.1 Study selection
A total of 735 articles were initially identified. After removing duplicates and reviewing articles, the remaining studies underwent screening based on titles and abstracts, leading to the exclusion of 687 articles. Subsequently, two independent researchers (Bo-hao Huang and Bo-han Lv) meticulously reviewed the full text of 48 articles, evaluating them against the predetermined eligibility criteria. In total, 24 studies involving 440 animals were included in this meta-analysis. The flow chart of database search and research selection is shown in Figure 2.
3.2 Characteristics of the included studies
Table 1 summarizes key characteristics of the reviewed literature, encompassing: 1) first author; 2) year of publication; 3) species and sex of the animals; 4) weight; 5) number of subjects in each group; 6) modeling method; 7) curcumin dose, route, and duration; 8) histopathology.
In terms of species, the studies involved Sprague-Dawley rats (9 studies), Wistar rats (11 studies), C57 BL/6 mice (1 study), Rattus rattus mice (1 study), ICR mice (1 study), and Mesocricetus auratus mice (1 study). All studies exclusively used male subjects. The methods employed for establishing animal models of liver fibrosis included CCI4 injection (15 studies), BDL surgery (5 studies), NDMA injection (3 studies), and TAA injection (1 study).
To assess the therapeutic impact of curcumin on liver fibrosis, we analyzed data from 17 studies that reported AST levels, 18 studies that reported ALT levels, 4 studies that reported alkaline phosphatase (ALP) levels, 5 studies that reported albumin (ALB) levels, 6 studies that reported total bilirubin (TBil) levels, 3 studies that reported collagen I levels, 5 studies that reported mRNA of alpha-smooth muscle actin (α-SMA) levels, 3 studies that reported malondialdehyde (MDA) levels, 3 studies that reported mRNA of TGF-β mRNA, 4 studies that reported mRNA of nuclear factor κB (NF-κb) levels, 3 studies that reported triglyceride (TG) levels, 3 studies that reported superoxide dismutase (SOD) levels, 8 studies that reported Glutathione (GSH) levels, 2 studies that reported Hydroxyproline (HYP) levels. 3 studies that reported laminin (LN) levels, and 3 studies that reported the degree of liver fibrosis.
3.3 Quality of the included studies
The quality of the literature included in the study underwent evaluation, with two researchers (Yanbo Li and Ziwen Zhuo) conducting a case-by-case assessment. The results revealed that the scores of the included literature varied between 4 and 6. Specifically, 15 studies scored 6 (Gowifel et al., 2020; Wu et al., 2010; Eshaghian et al., 2018; Khodarahmi et al., 2020; Y. Zhao et al., 2014; Qin et al., 2018; Lu et al., 2017; Chen et al., 2014; Wu et al., 2008; Morsy et al., 2012; Zhao et al., 2018; Kabirifar et al., 2018; Barta et al., 2015; Kyung et al., 2018), 8 studies scored 5 (Macías-Pérez et al., 2019; El et al., 2016; Hernández-Aquino et al., 2020; Zhang et al., 2013; Reyes-Gordillo et al., 2008; Fu et al., 2008; George et al., 2006; Abo-Zaid, Shaheen, and Ismail, 2020), and 1 study scored 4 (H.-Y. Lee et al., 2016).
Nineteen studies reported utilizing random assignment of animals, although specific methods of randomization were not mentioned. The remaining five studies did not mention animal randomization. Of the total 19 studies, it was indicated that the animals were maintained under identical conditions, while the remaining five studies did not provide information on the conditions of the animals. All the included literature reported complete data.
In terms of methodology, each study detailed the approach taken to balance inter-group baseline characteristics, and there was no evidence of selective reporting. None of the studies reported hidden assignment, blind intervention, randomization of outcome evaluation, or outcome blindness. Furthermore, no other sources of bias were identified across all studies. Table 2 provides a comprehensive assessment of the methodological quality of the included studies.
A. sequence generation; B. baseline characteristics; C. allocation concealment D. random housing; E. blinding (caregivers/investigators) F. random for outcome assessment; G. blinding (outcome assessor) H. incomplete outcome data; I. selective outcome reporting; J. other biases.
3.4 Outcome measures
3.4.1 Liver function
3.4.1.1 AST
This meta-analysis comprised 17 studies involving 284 animals to assess the impact of curcumin on AST levels (Gowifel et al., 2020; Macías-Pérez et al., 2019; Wu et al., 2010; Eshaghian et al., 2018; Khodarahmi et al., 2020; Wu et al., 2008; Zhao et al., 2014; Qin et al., 2018; Lu et al., 2017; Tu et al., 2012; Morsy et al., 2012; Lee et al., 2016; Fu et al., 2008; Zhao et al., 2018; George et al., 2006; Kyung et al., 2018; Abo-Zaid, Shaheen, and Ismail, 2020). The summary analysis indicated a significant reduction in AST levels with curcumin compared to the CCI4 model group [SMD = −3.90, 95% CI (−4.96, −2.83), p < 0.01, I2 = 85.9%, Figure 3A].
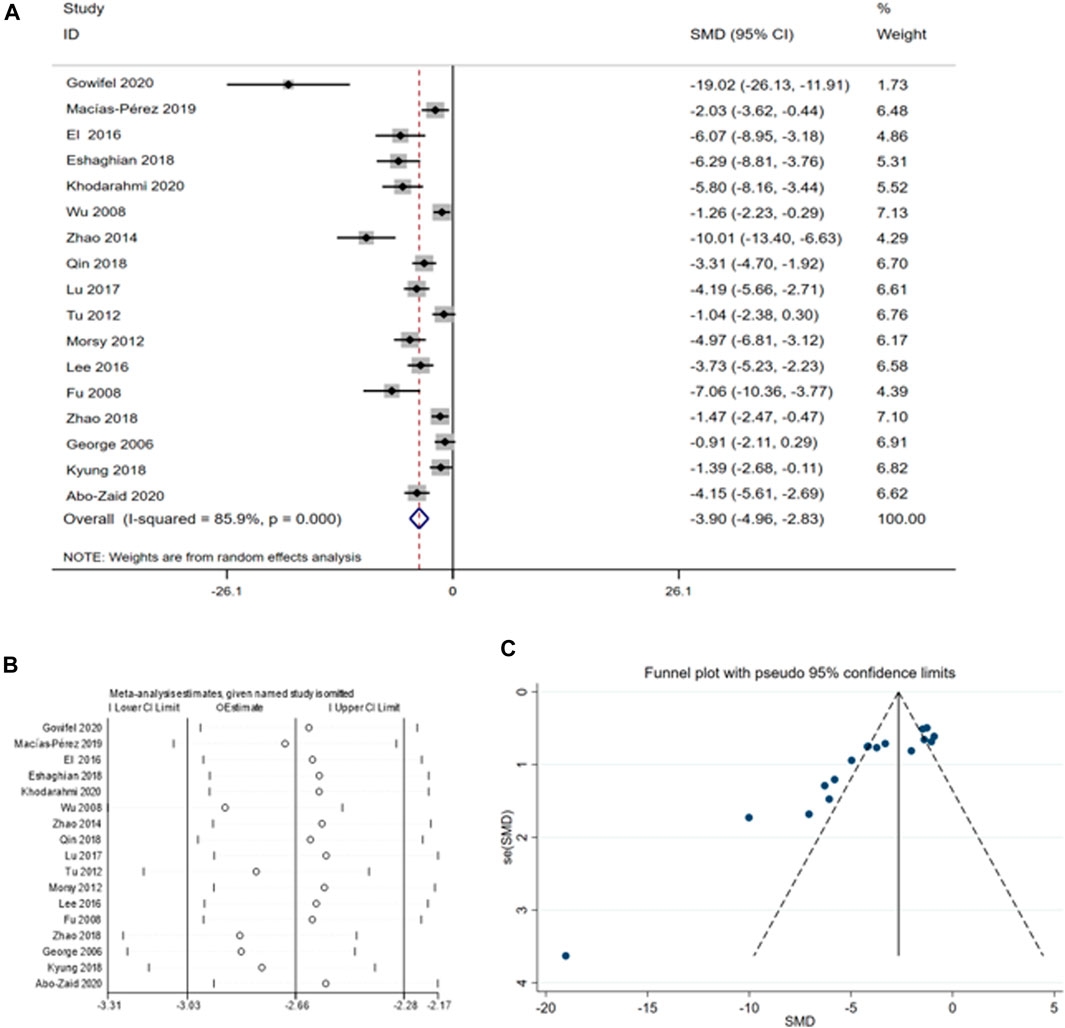
Figure 3. (A) Forest plot: antifibrosis effect of curcumin on AST; (B) sensitive analysis of AST; (C) funnel plot of AST.
A thorough analysis was performed because of the high heterogeneity of the sample, including sensitivity analysis, funnel plot examination, and subgroup analysis. According to the sensitivity analysis, omitting individual studies did not affect the aggregated estimates of AST significantly (Figure 3B). However, the funnel plot for AST displayed asymmetry (Figure 3C), we examined the sources of heterogeneity using subgroup analysis based on species (Supplementary Figure S1) and animal models (Supplementary Figure S2) (Table 3).
3.4.1.2 ALT
To assess the impact of curcumin on ALT in fibrosis-model animals, we conducted a meta-analysis involving 18 studies encompassing a total of 296 animals (Gowifel et al., 2020; Macías-Pérez et al., 2019 El et al., 2016; Eshaghian et al., 2018; (Fu et al., 2008; George et al., 2006; Reyes-Gordillo et al., 2008; Wu et al., 2008; Morsy et al., 2012; Tu et al., 2012; Zhao et al., 2014; Lee et al., 2016; Lu et al., 2017; Kyung et al., 2018; Qin et al., 2018; Abo-Zaid, Shaheen, and Ismail, 2020; Hernández-Aquino et al., 2020; Khodarahmi et al., 2020). The summary analysis revealed a significant reduction in ALT levels [SMD = -4.40, 95% CI (−5.40, −3.40), p < 0.01, I2 = 81.2%, Figure 4A] with curcumin treatment. However, given the asymmetric funnel funnel plot (Figure 4B) and apparent heterogeneity (Figure 4C) among the different studies subgroup analyses were performed according to the species (Supplementary Figure S3) and the method for (Table 4).
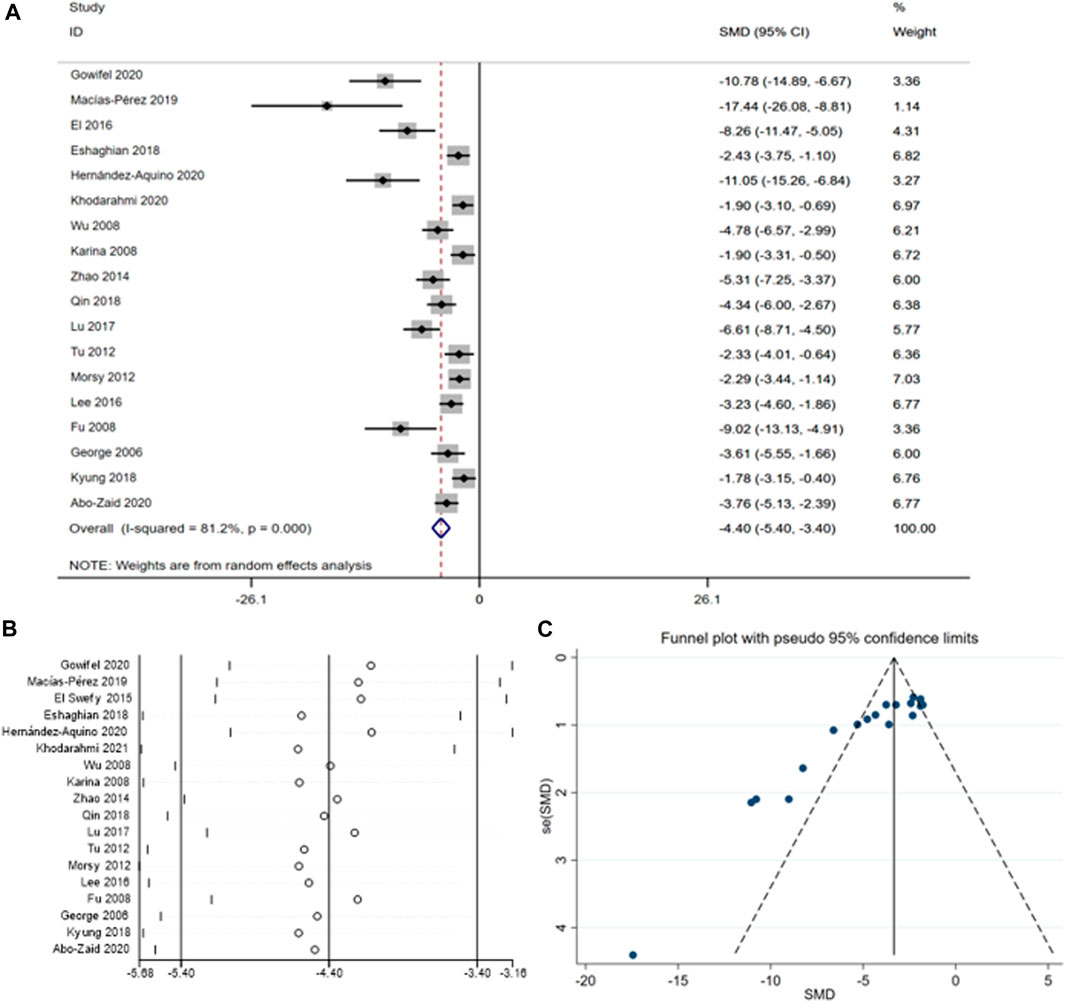
Figure 4. (A) Forest plot: antifibrosis effect of ALT; (B) sensitive analysis of ALT; (C) funnel plot of ALT.
3.4.1.3 ALP
ALP is mainly produced in the liver, liver fibrosis results in liver damage and increased ALP synthesis and secretion (Liu et al., 2021). A total of 4 studies involving 296 animals were included in the analysis of the ALP levels (Eshaghian et al., 2018; Zhao et al., 2014; Fu et al., 2008; Kabirifar et al., 2018). Curcumin significantly reduced liver ALP levels compared with the model groups [SMD = − 6.59, 95% CI (−8.86,4.31), p < 0.05, I2 = 64.0%, Figure 5].
3.4.1.4 ALB
Albumin is synthesized by hepatic parenchymal cells and reflects the function of the liver (Garcia-Martinez et al., 2013). A total of 5 studies involving 84 animals were included in this meta-analysis (Gowifel et al., 2020; Macías-Pérez et al., 2019; Zhao et al., 2014; Qin et al., 2018; Abd-Allah et al., 2016). The result revealed a significant increase in the albumin levels within the curcumin groups [SMD = 3.11, 95%CI (1.91,4.32), p = 0.013, I2 = 68.2%, Figure 6], suggesting that curcumin may contribute to enhancing liver synthetic function in the state of liver fibrosis.
3.4.1.5 TBil
Reduced excretory function of hepatocytes due to hepatic fibrosis, resulting in elevated serum total bilirubin (Tamber et al., 2023). Six studies involving 48 animals were included to assess the influence of curcumin on bilirubin level (Macías-Pérez et al., 2019; Zhao et al., 2014; Abd-Allah et al., 2016; Barta et al., 2015). The results indicate that curcumin can effectively reduce the concentration of TBil [SMD = − 3.15, 95% CI (−4.85,-1.44), p < 0.01, I2 = 86.9%, Figure 7].
3.4.2 Collagen I
Collagen I is an important biomarker for assessing scarring after liver injury (Mu et al., 2018). Three studies with 48 animals for collagen I were included in the meta-analysis (Gowifel et al., 2020; Hernández-Aquino et al., 2020; Eshaghian et al., 2018). The results demonstrated a decrease in the expression of collagen I with the application of curcumin [SMD = - 2.86, 95% CI (−4.63, −1.10), p = 0.015, I2 = 76.2%, Figure 8], indicating its potential anti-fibrotic effect.
3.4.3 mRNA expression levels of α-SMA
α-SMA is a marker protein that indicates activation of HSCs and is indicative of liver fibrosis (Yuan et al., 2022). 5 studies involving 80 animals were included in the meta-analysis to assess the impact of curcumin on mRNA of α-SMA expression (Gowifel et al.; Hernández-Aquino et al., 2020; Zhao et al., 2014; Fu et al., 2008; Kabirifar et al., 2018). The results indicate that curcumin has the potential to enhance apoptosis and reduce mRNA of α-SMA expression [SMD = −3.72 (−5.26,-2.18), p < 0.01, I2 = 75.6%, Figure 9] in the context of hepatic fibrosis.
3.4.4 Oxidative stress levels
Oxidative stress damage is one of factors inducing liver fibrosis. SOD, MDA, GSH and HYP are biomarkers of oxidative stress ((Ruart et al., 2019). To explore the relationship between curcumin treatment and oxidative stress levels, this meta-analysis incorporated three studies involving 52 animals focusing on serum MDA (Gowifel et al., 2020; El et al., 2016; Morsy et al., 2012), three studies involving 54 animals focusing on serum SOD (Gowifel et al., 2020; Wu et al., 2008; Abd-Allah et al., 2016), eight studies involving 134 animals focusing on serum GSH(Gowifel et al., 2020; (Hernández-Aquino et al., 2020; Reyes-Gordillo et al., 2008; James et al., 2018; Morsy et al., 2012; H.-Y. Lee et al., 2016; Fu et al., 2008; El et al., 2016); two studies involving 28 animals focusing on serum HYP((Kabirifar et al., 2018; Soto-Angona et al., 2020).
The result indicated that curcumin treatment reduced serum MDA [SMD = −8.85, 95% CI (−16.77, −0.93), p < 0.01, I2 = 94.0%, Figure 10A], increase serum SOD [SMD = 3.92, 95% CI (0.97,6.87), p < 0.01, I2 = 90.4%, Figure 10B] and serum GSH [SMD = 1.97, 95% CI (1.46, 2.49), p < 0.01, I2 = 90.9%, Figure 10C] in a significant manner, and reduced serum HYP [SMD = −1.29, 95% CI (−2.12, −0.45), p = 0.450, I2 = 0.0%, Figure 10D].
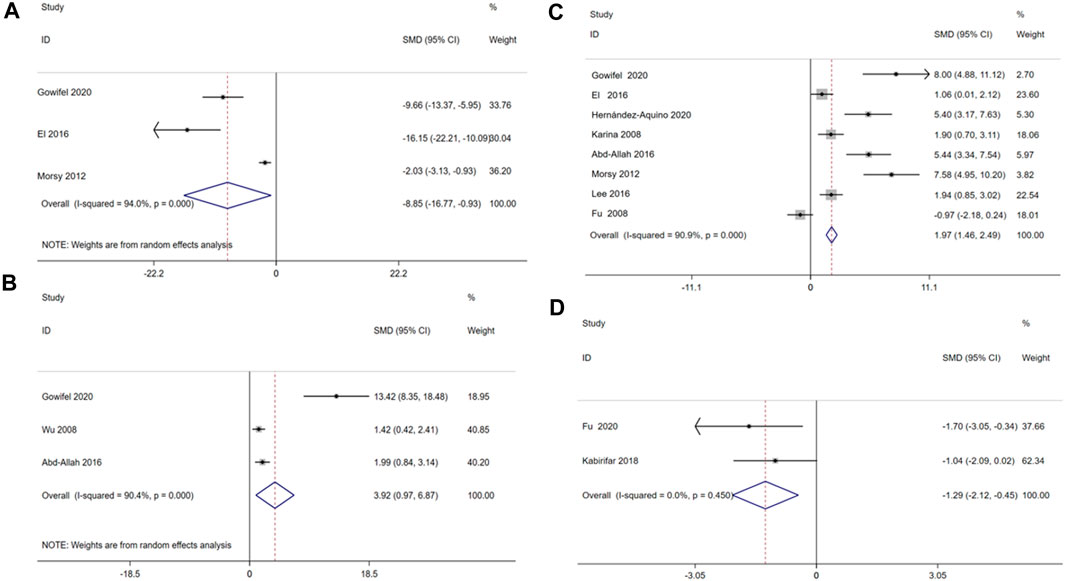
Figure 10. Forest plot: antifibrosis effect of curcumin on the Oxidative stress levels. (A) MDA; (B) SOD; (C) GSH; (D) HYP.
3.4.5 Regulation of pathway factors
3.4.5.1 Levels of TGF-β mRNA
TGF-β participates in regulating cell growth, proliferation, and differentiation, and is an important regulatory factor in the process of liver fibrosis (Seki et al., 2007). Three studies with 28 animals for mRNA of TGF-β levels were included in the meta-analysis (Khodarahmi et al., 2020; Kabirifar et al., 2018; Yao et al., 2012). The results demonstrated a decrease in the expression of mRNA of TGF-β levels with the treatment of curcumin [SMD = − 1.89, 95% CI (−3.54, −0.24), p < 0.01, I2 = 78.8%, Figure 11].
3.4.5.2 Levels of NF-κb mRNA
NF-κB activation is closely associated with inflammatory responses and can promote the activation of HSCs and proliferation of fibroblasts. (Luedde and Schwabe, 2011). Four studies with 58 animals for mRNA of NF-κb levels were included in the meta-analysis (Macías-Pérez et al., 2019; El et al., 2016; Eshaghian et al., 2018; Kabirifar et al., 2018). The results demonstrated a decrease in the expression of mRNA of NF-κb levels with the treatment of curcumin [SMD = −3.05, 95% CI (−4.80,-1.29), p < 0.01, I2 = 78.7%, Figure 12].
3.4.6 Triglyceride
Three studies with 56 animals for mRNA of TG levels were included in the meta-analysis (Wu et al., 2008; Lee et al., 2016; Kabirifar et al., 2018). The results demonstrated a decrease in the expression of TG levels with the treatment of curcumin [SMD = −2.47, 95% CI (−4.44,-0.51), p < 0.01, I2 = 85.5%, Figure 13].
3.4.7 Laminin
LN is a key component of ECM, which is a marker of liver fibrosis. (Plevris et al., 2018). Two studies with 44 animals for LN levels were included in the meta-analysis (El et al., 2016; Lu et al., 2017; Qin et al., 2018). The results demonstrated a decrease in the expression of LN levels with the treatment of curcumin [SMD = −6.05, 95% CI (−11.92, −0.17), p < 0.01, I2 = 92.9%, Figure 14].
3.4.8 Degree of fibrosis
Three studies with 46 animals for the degree of fibrosis were included in the meta-analysis (Macías-Pérez et al., 2019; Qin et al., 2018). The results demonstrated a decrease in the degree of fibrosis with the treatment of curcumin [SMD = −7.58, 95% CI (−13.60, −1.57), p < 0.01, I2 = 94.5%, Figure 15].
3.4.9 Liver histopathology
Pathological examinations, including hematoxylin and eosin (H&E) staining, Masson trichromatic (MT) staining, and Sirius red staining, were performed in 23 included papers. Specifically, H&E staining was conducted in 21 studies, Sirius red staining in 6 studies, and MT staining in 10 studies. Liver histological examinations using H&E and Compared with the model groups, curcumin groups showed a significant reduction in inflammatory cell infiltration and interstitial collagen fiber deposition. Moreover, it promoted a delay in hepatocyte necrosis and apoptosis, protecting the normal liver tissue structure. MT staining demonstrated a significant reduction in collagen production in the liver, while Sirius red staining indicated that curcumin could reduce collagen fiber generation compared to the model group.
4 Discussion
4.1 Summary of evidence
Curcumin was evaluated in this study for its efficacy in treating experimental liver fibrosis. In our meta-analysis, consisting of 24 articles included 440 animals, and the overall quality of the selected literature was medium to high. A comprehensive analysis revealed that curcumin intervention not only mitigates liver fibrosis but also improves liver function, while concurrently modulating inflammatory responses and antioxidant capacity in animal models. This result provided a strong basis for further large-scale animal studies as well as clinical trials in humans in the future.
4.2 Molecular mechanisms
The pathological process of liver fibrosis encompasses activation of HSCs, inflammation, oxidative stress, etc., (Yang et al., 2023). With its hepatoprotective and anti-fibrotic effects, curcumin exerts its mechanisms through antioxidative and anti-inflammatory actions, inhibition of hepatic stellate cell activation, and the blockade of receptors and signaling pathways (Farzaei et al., 2018). We delved into the molecular mechanisms underlying these processes (Figure 16).
4.2.1 Curcumin improves liver fibrosis by inhibiting HSC activation
HSCs are nonparenchymal cells in the liver, constituting 5%–10% of the total liver cell population. HSCs usually remain in a quiescent state, displaying low proliferative activity and limited collagen synthesis (Higashi, Friedman, and Hoshida, 2017). However, under liver stress, HSCs can undergo activation, transitioning from a ‘static’ to an ‘active’ phenotype. This activation enhances their collagen synthesis capability, leading to the secretion of ECM and pro-inflammatory mediators (Heymann and Tacke, 2016). This process contributes to liver fibrosis, serving as a key driver in liver injury (Kamm and McCommis, 2022).
In the progression of HSC-mediated liver fibrosis, various cytokines and cellular pathways interplay, creating an intricate network of interactions, including TGF-β, PI3K/AKT, MAPK, HIF-1α, Wnt signaling, and downstream pathways ((Xiang et al., 2018) (J. Wang et al., 2018). In addition, HSCs can generate reactive oxygen species and nitrogen compounds (ROS and RNS), which can induce HSC activation, proliferation, and apoptosis. These by-products result from metabolic events associated with HSC activation (Khomich, Ivanov, and Bartosch, 2019).
Numerous studies demonstrated that curcumin effectively targets the inhibition of HSC activation (Shu et al., 2023). intervened with curcumin in the HSC line LX-2 and found that curcumin could inhibit activity and promote apoptosis in LX-2 cells by suppressing autophagy through activation of the PI3K/Akt/mTOR signaling pathway (Shu et al., 2023). Lian et al. discovered that curcumin inhibits glycolysis and regulates metabolism in HSCs by modulating hedgehog signaling (Lian et al., 2015). Qin et al., in their intervention with curcumin on HSC-T6 cells, found that curcumin could protect against activation and migration of hepatic stellate cells by inhibiting the CXCL12/CXCR4 biological axis in liver fibrosis (Qin et al., 2018).
4.2.2 Curcumin prevents liver fibrosis by modulating the inflammatory response
Decades of chronic inflammation can lead to structural damage in the liver, where normal tissue is gradually replaced by scar tissue (Czaja, 2014). This process results in impaired organ function, altered hepatic blood flow, and the development of liver fibrosis. Inflammatory cells found in the liver, including Kupffer cells, macrophages, neutrophils, and hepatic stellate cells, play a crucial role in this progression (Koyama and Brenner, 2017). Under sustained stimulation, these cells release pro-inflammatory mediators such as NF-κB, IL-6, IL-22, or TGF-β. These mediators, in turn, facilitate the recruitment of T cells and neutrophils, while also stimulating the fibrotic activity of HSCs(Hammerich and Tacke, 2023).
In the present study, rat and human fibrotic livers expressed elevated levels of both IL-33 and ST2, compared to healthy livers (Moussion, Ortega, and Girard, 2008). The IL-33 and ST-2 axis contribute to signaling via the ST 2L receptor, which contains a membrane binding domain, an extracellular segment containing three interconnected motifs similar to immunoglobulins, and a cytoplasmic Toll/IL-1 receptor domain.
(Pascual-Figal and Januzzi, 2015; Marvie et al., 2010). In chronic injury, IL-33 serves as a factor contributing to liver fibrosis, and ST2 acts as an important biomarker for liver fibrosis (Mchedlidze et al., 2013; Oztas et al., 2015). Furthermore, their expression demonstrated a significant rise in tandem with the severity of fibrosis (Gao et al., 2016). Additionally, it has been discovered that IL-13 primarily triggers the pro-fibrotic impact of IL-33. By stimulating TGF-β signaling via IL-4 Rα and signal transducers and transcriptional activator 6 (STAT 6) in HSCs, IL-13 can facilitate liver fibrosis (Liang et al., 2019).
The NF-κB signaling plays crucial role in regulating immune and inflammatory responses (Zhang et al., 2019). NF-κB activation triggers the release of inflammatory cytokines and chemokines, such as TGF-β1, TNF-α, IL-1, IL-6, and IFN-γ(Hayden and Ghosh, 2008). Moreover, it participates in the activation of other pathways, such as the NF-κB/IκBα signaling pathway and the downstream NF-κB/NLRP3 signaling pathway (Pariente-Pérez, et al., 2020), thereby exacerbating liver inflammation and fibrosis. NF-κB governs liver fibrosis through three key mechanisms: (A) overseeing liver cell damage, acting as the primary initiator of the fibrotic response; (B) adjusting inflammatory signals initiated in liver macrophages and other inflammatory cells; and (C) impacting the fibrotic response in HSC(Luedde and Schwabe, 2011). Curcumin has been documented to inhibit NF-κB phosphorylation and degradation, decrease p65 expression, hinder the activation of the NF-κB signaling pathway, and alleviate hepatic inflammation (Barta et al., 2015).
Wu et al. investigated the effect of curcumin on CCI4-induced liver fibrosis in mice and demonstrated that curcumin inhibits NF-κB and IL-6 expression while enhancing the expression of the anti-inflammatory factor IL-10, thereby exerting an anti-fibrotic role (Wu et al., 2008). Similarly, Hernández-Aquino et al. treated CCI4-induced Wistar rats with curcumin and observed a reduction in liver fibrosis. They also noted that curcumin restored protein levels of NF-κB, IL-1, IL-10, TGF-β, CTGF, Col-I, and Smad7 (Hernández-Aquino et al., 2020). Furthermore, Wang et al. found that curcumin treatment increased the ratio of Nrf-2/NF-κB mRNA and its protein expression in liver inflammatory cells, protecting the liver and reversing the process of cirrhosis (Wang et al., 2018).
4.2.3 Curcumin inhibits liver fibrosis by regulating fat metabolism
The development of liver fibrosis is closely linked to disturbances in fat metabolism. According to the “second blow” theory, the accumulation of free fatty acids (FFA), coupled with inflammation or insulin resistance, can give rise to pathological manifestations, including lipid accumulation, hepatocyte ballooning, lobular inflammation, and fibrosis (Soto-Angona et al., 2020). From NAFLD to NASH, oxidative stress, damage-associated molecular patterns (DAMPs), and other byproducts of cellular metabolic disorders, induced by an excessive lipid burden, continuously trigger the process of lipophagy. Lipophagy, a lysosomal-mediated lipid metabolism process, assists the liver in eliminating metabolic waste. The overactivation of HSCs induced by lipophagy promotes ECM deposition, speeding up the advancement of liver fibrosis (Filali-Mouncef et al., 2022).
Research indicates that curcumin reduces serum cholesterol levels by increasing hepatic LDL receptor expression, inhibiting LDL oxidation, and enhancing bile acid secretion and faecal cholesterol excretion (Zhang et al., 2019). Curcumin also suppresses genes involved in cholesterol biosynthesis, proecting against liver injury and fibrogenesis in animal models (Fu et al., 2008; Peschel et al., 2007). Using autophagy inhibitors in NASH treatment prevents in vitro HSC activation and reduces lipid droplet degradation (Thoen et al., 2011), suggesting autophagy as a potential target for NASH and fibrosis treatment. Afrin et al. demonstrated that curcumin mitigates liver damage and inhibits the progression of NASH and fibrosis in model neonatal C57BL/6J male mice induced by streptozotocin by inhibiting HMGB1-NF-κB translocation (Afrin et al., 2017). Furthermore, supplementing curcumin to hyperadipose-induced NASH in experimental rabbits reduced NASH grade and aminotransferase activity, while increasing mitochondrial antioxidant levels (Ramirez-Tortosa et al., 2009).
4.2.4 Curcumin inhibits liver fibrosis by regulating oxidative stress
ROS is produced by oxidative stress in the liver for various reasons, such as chronic viral infection, drug hepatotoxicity and alcohol damage, which induces hepatocyte necrosis and subsequent liver function damage, leading to excessive deposition of ECM and diffuse liver fibrosis (Parola and Pinzani, 2019). Cellular antioxidant mechanisms are triggered in response to oxidative stress injury. ROS overproduction activates the transcription factor nuclear factor E2-related factor 2 (Nrf2). Through antioxidant response elements (ARE), Nrf2 can activate the expression of downstream antioxidant protection genes such as heme oxygenase 1 (HO-1), generating antioxidant enzymes like HO-1, NADH quinone oxidoreductase (NQO1), and glutathione S-transferase (GST), thereby protecting liver cells from oxidative damage (W. Tang, 2014). Prior research has shown that the overexpression of Nrf2, along with downstream antioxidant factors like NQO1 and HO-1, can diminish the expression of α-SMA, collagen I and collagen III in rats with liver fibrosis, exerting an anti-fibrotic effect (Ge et al., 2022).
Excessive levels of ROS can damage cellular lipids and proteins, leading to hepatocyte necrosis and apoptosis. Additionally, ROS promote the production of pro-inflammatory and pro-fibrogenic factors by activating HSCs, Kupffer cells, and other inflammatory cells (Luedde and Schwabe, 2011; Zhang et al., 2013). Studies have demonstrated that ROS signaling also regulates the expression and activity of the transcription factor NF-κB (Luedde and Schwabe, 2011). Inhibition of NF-κB activity has been shown to protect against hepatic fibrosis in vivo (Lee et al., 2020; Rodríguez et al., 2021). Kong et al. showed that curcumin inhibits ROS levels and oxidative stress in hepatocytes by activating PPAR-α and regulating upstream signaling pathways of autophagy, including AMPK and PI3K/AKT/mTOR (Kong et al., 2020). These effects contribute to the anti-liver fibrosis properties of curcumin.
4.3 Limitations
We need to acknowledge several potential limitations in our meta-analysis. Firstly, we limited the inclusion of literature to English, which may introduce selection bias by excluding studies published in other languages. Secondly, some literature overlooked the implementation of randomization and blinding methods, possibly compromising the authenticity of the results. Moreover, the meta-analysis revealed high heterogeneity in AST, ALT, ALP, ALB, and other indicators. Subgroup analysis based on animal species and modeling methods was conducted, but the heterogeneity remained largely unchanged. Through comprehensive literature review identified high heterogeneity as a common issue in experimental meta-analyses. The conduct of experiments may contribute to selection bias, given that positive results are more likely to be reported (Feng et al., 2022). These factors limit the generalizability of our results to clinical settings. Therefore, future studies should enhance methodological rigor, including strict adherence to randomization and blinding methods, provision of accurate experimental data, and appropriate expansion of sample sizes.
Curcumin, a hydrophobic polyphenol extracted from the rhizome of Curcuma longa, is widely utilized in the treatment of cardiovascular diseases, liver diseases, and tumours (Xu et al., 2020; Jabczyk et al., 2021; Pourbagher-Shahri et al., 2021). Despite curcumin exhibits challenges such as low aqueous solubility, poor stability in body fluids, rapid metabolism, and reduced absorption in the gastrointestinal tract, clinical and basic studies have confirmed its remarkable pharmacological efficacy in treating liver diseases like NAFLD, liver fibrosis, and liver cancer (Nelson et al., 2017; Farzaei et al., 2018); (E. S. Lee et al., 2020) The ongoing enhancement of nano-formulations of curcumin holds promise for expanding its clinical applications (Y. Chen et al., 2020).
5 Conclusion
Our meta-analysis showcased curcumin’s effectiveness in preclinical liver fibrosis studies. The potential protective mechanisms observed in animals encompass liver protection, collagen production inhibition, oxidative stress reduction, and inflammatory response regulation. Despite these promising findings, the predominance of animal studies underscores the necessity for clinical trials to validate curcumin’s clinical efficacy and precisely elucidate its mechanisms in liver fibrosis treatment.
Data availability statement
The original contributions presented in the study are included in the article/Supplementary Material, further inquiries can be directed to the corresponding author.
Author contributions
B-HH: Conceptualization, Writing–original draft, Writing–review and editing. Z-WG: Methodology, Writing–original draft, Writing–review and editing. B-HL: Writing–review and editing. XZ: Formal Analysis, Visualization, Writing–review and editing. Y-BL: Conceptualization, Investigation, Writing–original draft. W-LL: Data curation, Funding acquisition, Supervision, Writing–original draft.
Funding
The author(s) declare that financial support was received for the research, authorship, and/or publication of this article. This work was supported by the National Natural Science Foundation of China (No. 82374332).
Conflict of interest
The authors declare that the research was conducted in the absence of any commercial or financial relationships that could be construed as a potential conflict of interest.
Publisher’s note
All claims expressed in this article are solely those of the authors and do not necessarily represent those of their affiliated organizations, or those of the publisher, the editors and the reviewers. Any product that may be evaluated in this article, or claim that may be made by its manufacturer, is not guaranteed or endorsed by the publisher.
Supplementary material
The Supplementary Material for this article can be found online at: https://www.frontiersin.org/articles/10.3389/fphar.2024.1396834/full#supplementary-material
References
Abd-Allah, G. A., El-bakry, K., Bahnasawy, M. H., and El-Khodary, E. R. (2016). Protective effects of curcumin and ginger on liver cirrhosis induced by carbon tetrachloride in rats. Int. J. Pharmacol. 12, 361–369. doi:10.3923/ijp.2016.361.369
Abo-Zaid, M. A., Shaheen, E. S., and Ismail, A. H. (2020). Immunomodulatory effect of curcumin on hepatic cirrhosis in experimental rats. J. Food Biochem. 44 (6), e13219. doi:10.1111/jfbc.13219
Afrin, R., Arumugam, S., Rahman, A., Wahed, M. I., Karuppagounder, V., Harima, M., et al. (2017). Curcumin ameliorates liver damage and progression of NASH in NASH-HCC mouse model possibly by modulating HMGB1-NF-κB translocation. Int. Immunopharmacol. 44, 174–182. doi:10.1016/j.intimp.2017.01.016
Asrani, S. K., Devarbhavi, H., Eaton, J., and Kamath, P. S. (2019). Burden of liver diseases in the world. J. Hepatology 70 (1), 151–171. doi:10.1016/j.jhep.2018.09.014
Barta, A., Janega, P., Babál, P., Murár, E., Cebová, M., and Pechánová, O. (2015). The effect of curcumin on liver fibrosis in the rat model of microsurgical cholestasis. Food and Funct. 6 (7), 2187–2193. doi:10.1039/C5FO00176E
Bavarsad, K., Riahi, M. M., Saadat, S., George, B., Atkin, S. L., and Sahebkar, A. (2019). Protective effects of curcumin against ischemia-reperfusion injury in the liver. Pharmacol. Res. 141 (March), 53–62. doi:10.1016/j.phrs.2018.12.014
Chen, N., Geng, Q., Zheng, J., He, S., Huo, X., and Sun, X. (2014). Suppression of the TGF-β/Smad signaling pathway and inhibition of hepatic stellate cell proliferation play a role in the hepatoprotective effects of curcumin against alcohol-induced hepatic fibrosis. Int. J. Mol. Med. 34 (4), 1110–1116. doi:10.3892/ijmm.2014.1867
Chen, Y., Lu, Y., Lee, R. J., and Xiang, G. (2020). Nano encapsulated curcumin: and its potential for biomedical applications. Int. J. Nanomedicine 15 (May), 3099–3120. doi:10.2147/IJN.S210320
Cui, L., Jia, X., Zhou, Q., Zhai, X., Zhou, Y., and Zhu, H. (2014). Curcumin affects β-catenin pathway in hepatic stellate cell in vitro and in vivo. J. Pharm. Pharmacol. 66 (11), 1615–1622. doi:10.1111/jphp.12283
Czaja, A. J. (2014). Hepatic inflammation and progressive liver fibrosis in chronic liver disease. World J. Gastroenterology 20 (10), 2515–2532. doi:10.3748/wjg.v20.i10.2515
El, S., Hasan, R. A., Ibrahim, A., and Mahmoud, M. F. (2016). Curcumin and hemopressin treatment attenuates cholestasis-induced liver fibrosis in rats: role of CB1 receptors. Naunyn-Schmiedeberg’s Archives Pharmacol. 389 (1), 103–116. doi:10.1007/s00210-015-1181-7
Eshaghian, A., Khodarahmi, A., Safari, F., Binesh, F., and Moradi, A. (2018). Curcumin attenuates hepatic fibrosis and insulin resistance induced by bile duct ligation in rats. Brit. J. Nutr. 120 (4), 393–403. doi:10.1017/S0007114518001095
Farzaei, M., Zobeiri, M., Parvizi, F., El-Senduny, F., Marmouzi, I., Coy-Barrera, E., et al. (2018). Curcumin in liver diseases: a systematic review of the cellular mechanisms of oxidative stress and clinical perspective. Nutrients 10 (7), 855. doi:10.3390/nu10070855
Feng, X., Bu, F., Huang, L., Xu, W., Wang, W., and Wu, Q. (2022). Preclinical evidence of the effect of quercetin on diabetic nephropathy: a meta-analysis of animal studies. Eur. J. Pharmacol. 921 (April), 174868. doi:10.1016/j.ejphar.2022.174868
Filali-Mouncef, Y., Hunter, C., Roccio, F., Zagkou, S., Dupont, N., Primard, C., et al. (2022). The Ménage à Trois of Autophagy, Lipid Droplets and Liver Disease. Autophagy 18 (1), 50–72. doi:10.1080/15548627.2021.1895658
Fu, Y., Zheng, S., Lin, J., Ryerse, J., and Chen, A. (2008). Curcumin protects the rat liver from CCl 4 -caused injury and fibrogenesis by attenuating oxidative stress and suppressing inflammation. Mol. Pharmacol. 73 (2), 399–409. doi:10.1124/mol.107.039818
Gao, Y., Liu, Y., Yang, M., Guo, X., Zhang, M., Li, H., et al. (2016). IL-33 treatment attenuated diet-induced hepatic steatosis but aggravated hepatic fibrosis. Oncotarget 7 (23), 33649–33661. doi:10.18632/oncotarget.9259
Garcia-Martinez, R., Caraceni, P., Bernardi, M., Gines, P., Arroyo, V., and Jalan, R. (2013). Albumin: pathophysiologic basis of its role in the treatment of cirrhosis and its complications. Hepatology 58 (5), 1836–1846. doi:10.1002/hep.26338
Ge, C., Tan, J., Lou, D., Zhu, L., Zhong, Z., Dai, X., et al. (2022). Mulberrin confers protection against hepatic fibrosis by trim31/nrf2 signaling. Redox Biol. 51 (May), 102274. doi:10.1016/j.redox.2022.102274
George, J., Lonchin, S., Jayalakshmi, R., and Chandrakasan, G. (2006). Efficacy of silymarin and curcumin on dimethyl nitrosamine induced liver fibrosis in rats. Biomedicine 26, 18–26.
Ginès, P., Krag, A., Abraldes, J. G., Solà, E., Fabrellas, N., and Kamath, P. S. (2021). Liver cirrhosis. Lancet 398 (10308), 1359–1376. doi:10.1016/S0140-6736(21)01374-X
Gowifel, A. M. H., Khalil, M. G., Nada, S. A., Kenawy, S. A., Ahmed, K. A., Salama, M. M., et al. (2020). Combination of pomegranate extract and curcumin ameliorates thioacetamide-induced liver fibrosis in rats: impact on TGF-β/Smad3 and NF-κB signaling pathways. Toxicol. Mech. Methods 30 (8), 620–633. doi:10.1080/15376516.2020.1801926
Hammerich, L., and Tacke, F. (2023). Hepatic inflammatory responses in liver fibrosis. Nat. Rev. Gastroenterology Hepatology 20 (10), 633–646. doi:10.1038/s41575-023-00807-x
Hasanzadeh, S., Read, M. I., Bland, A. R., Majeed, M., Jamialahmadi, T., and Sahebkar, A. (2020). Curcumin: an inflammasome silencer. Pharmacol. Res. 159 (September), 104921. doi:10.1016/j.phrs.2020.104921
Hatcher, H., Planalp, R., Cho, J., Torti, F. M., and Torti, S. V. (2008). Curcumin: from ancient medicine to current clinical trials. Cell. Mol. Life Sci. 65 (11), 1631–1652. doi:10.1007/s00018-008-7452-4
Hayden, M. S., and Ghosh, S. (2008). Shared principles in NF-kappaB signaling. Cell 132 (3), 344–362. doi:10.1016/j.cell.2008.01.020
Hernández-Aquino, E., Quezada-Ramírez, M. A., Silva-Olivares, A., Ramos-Tovar, E., Flores-Beltrán, R. E., Segovia, J., et al. (2020). Curcumin downregulates Smad pathways and reduces hepatic stellate cells activation in experimental fibrosis. Ann. Hepatology 19 (5), 497–506. doi:10.1016/j.aohep.2020.05.006
Hernandez-Gea, V., and Friedman, S. L. (2011). Pathogenesis of liver fibrosis. Annu. Rev. Pathology Mech. Dis. 6 (1), 425–456. doi:10.1146/annurev-pathol-011110-130246
Heymann, F., and Tacke, F. (2016). Immunology in the liver — from homeostasis to disease. Nat. Rev. Gastroenterology Hepatology 13 (2), 88–110. doi:10.1038/nrgastro.2015.200
Higashi, T., Friedman, S. L., and Hoshida, Y. (2017). Hepatic stellate cells as key target in liver fibrosis. Adv. Drug Deliv. Rev. 121 (November), 27–42. doi:10.1016/j.addr.2017.05.007
Hooijmans, C. R., Rovers, M. M., Vries, R.Bm De, Leenaars, M., Ritskes-Hoitinga, M., and Langendam, M. W. (2014). SYRCLE’s risk of bias tool for animal studies. BMC Med. Res. Methodol. 14 (1), 43. doi:10.1186/1471-2288-14-43
Jabczyk, M., Nowak, J., Hudzik, B., and Zubelewicz-Szkodzińska, B. (2021). Curcumin in metabolic Health and disease. Nutrients 13 (12), 4440. doi:10.3390/nu13124440
James, S. L., Abate, D., Abate, K. H., Abay, S. M., Abbafati, C., Abbasi, N., et al. (2018). Global, regional, and national incidence, prevalence, and years lived with disability for 354 diseases and injuries for 195 countries and territories, 1990–2017: a systematic analysis for the global burden of disease study 2017. Lancet 392 (10159), 1789–1858. doi:10.1016/S0140-6736(18)32279-7
Jin, H., Lian, N., Zhang, F., Chen, L., Chen, Q., Lu, C., et al. (2016). Activation of PPARγ/P53 signaling is required for curcumin to induce hepatic stellate cell senescence. Cell Death Dis. 7 (4), e2189. doi:10.1038/cddis.2016.92
Kabirifar, R., Ghoreshi, Z.-al-sadat, Rezaifar, A., Binesh, F., Bamdad, K., and Ali, M. (2018). Curcumin, quercetin and atorvastatin protected against the hepatic fibrosis by activating AMP-activated protein kinase. J. Funct. Foods 40 (January), 341–348. doi:10.1016/j.jff.2017.11.020
Kamm, D. R., and McCommis, K. S. (2022). Hepatic stellate cells in physiology and pathology. J. Physiology 600 (8), 1825–1837. doi:10.1113/JP281061
Khodarahmi, A., Javidmehr, D., Eshaghian, A., Ghoreshi, Z. A., Karimollah, A., Yousefi, H., et al. (2020). Curcumin exerts hepatoprotection via overexpression of paraoxonase-1 and its regulatory genes in rats undergone bile duct ligation. J. Basic Clin. Physiology Pharmacol. 32 (5), 969–977. doi:10.1515/jbcpp-2020-0067
Khomich, O., Ivanov, A. V., and Bartosch, B. (2019). Metabolic hallmarks of hepatic stellate cells in liver fibrosis. Cells 9 (1), 24. doi:10.3390/cells9010024
Kong, D, Zhang, Z., Chen, L., Huang, W., Zhang, F., Wang, L>, et al. (2020). Curcumin blunts epithelial-mesenchymal transition of hepatocytes to alleviate hepatic fibrosis through regulating oxidative stress and autophagy. Redox Biol. 36, 101600. doi:10.1016/j.redox.2020.101600
Koyama, Y., and Brenner, D. A. (2017). Liver inflammation and fibrosis. J. Clin. Invest. 127 (1), 55–64. doi:10.1172/JCI88881
Kyung, E. J., Kim, H. B., Hwang, E. S., Lee, S., Choi, B. K., Kim, J. W., et al. (2018). Evaluation of hepatoprotective effect of curcumin on liver cirrhosis using a combination of biochemical analysis and magnetic resonance-based electrical conductivity imaging. Mediat. Inflamm. 2018, 5491797–5491799. doi:10.1155/2018/5491797
Lee, E. S., Kwon, M.-H., Kim, H. M., Woo, Ho B., Ahn, C. M., and Chung, C. H. (2020). Curcumin analog CUR5–8 ameliorates nonalcoholic fatty liver disease in mice with high-fat diet-induced obesity. Metabolism 103 (February), 154015. doi:10.1016/j.metabol.2019.154015
Lee, H.-Y., Kim, S.-W., Lee, G.-H., Choi, M.-K., Jung, H.-W., Kim, Y.-J., et al. (2016). Turmeric extract and its active compound, curcumin, protect against chronic CCl4-induced liver damage by enhancing antioxidation. BMC Complementary Altern. Med. 16 (1), 316. doi:10.1186/s12906-016-1307-6
Lian, N., Jiang, Y., Zhang, F., Jin, H., Lu, C., Wu, X., et al. (2015). Curcumin regulates cell fate and metabolism by inhibiting hedgehog signaling in hepatic stellate cells. Lab. Investig. 95 (7), 790–803. doi:10.1038/labinvest.2015.59
Liang, Y., Yi, P., Yuan, D. M. K., Jie, Z., Kwota, Z., Soong, L., et al. (2019). IL-33 induces immunosuppressive neutrophils via a type 2 innate lymphoid cell/IL-13/STAT6 Axis and protects the liver against injury in LCMV infection-induced viral hepatitis. Cell. Mol. Immunol. 16 (2), 126–137. doi:10.1038/cmi.2017.147
Liu, Y., Cavallaro, P. M., Kim, B.-M., Liu, T., Wang, H., Kühn, F., et al. (2021). A role for intestinal alkaline phosphatase in preventing liver fibrosis. Theranostics 11 (1), 14–26. doi:10.7150/thno.48468
Lu, C., Xu, W., and Zheng, S. (2017). Nrf2 activation is required for curcumin to induce lipocyte phenotype in hepatic stellate cells. Biomed. Pharmacother. 95 (November), 1–10. doi:10.1016/j.biopha.2017.08.037
Luedde, T., and Schwabe, R. F. (2011). NF-κB in the liver—linking injury, fibrosis and hepatocellular carcinoma. Nat. Rev. Gastroenterology Hepatology 8 (2), 108–118. doi:10.1038/nrgastro.2010.213
Ma, X., Jiang, Y., Wen, J., Zhao, Y., Zeng, J., and Guo, Y. (2020). A comprehensive review of natural products to fight liver fibrosis: alkaloids, terpenoids, glycosides, coumarins and other compounds. Eur. J. Pharmacol. 888 (December), 173578. doi:10.1016/j.ejphar.2020.173578
Macías-Pérez, J. R., Vázquez-López, B. J., Muñoz-Ortega, M. H., Aldaba-Muruato, L. R., Martínez-Hernández, S. L., Sánchez-Alemán, E., et al. (2019). Curcumin and α/β-Adrenergic antagonists cotreatment reverse liver cirrhosis in hamsters: participation of Nrf-2 and NF-κB. J. Immunol. Res. (3019794). doi:10.1155/2019/3019794
Marvie, P., Lisbonne, M., L’helgoualc’h, A., Rauch, M., Turlin, B., Preisser, L., et al. (2010). Interleukin-33 overexpression is associated with liver fibrosis in mice and humans. J. Cell Mol. Med. 14 (6B), 1726–1739. doi:10.1111/j.1582-4934.2009.00801.x
Mchedlidze, T., Waldner, M., Zopf, S., Walker, J., Rankin, A. L., Schuchmann, M., et al. (2013). Interleukin-33-Dependent innate lymphoid cells mediate hepatic fibrosis. Immunity 39 (2), 357–371. doi:10.1016/j.immuni.2013.07.018
Moon, A. M., Singal, A. G., and Tapper, E. B. (2020). Contemporary epidemiology of chronic liver disease and cirrhosis. Clin. Gastroenterology Hepatology 18 (12), 2650–2666. doi:10.1016/j.cgh.2019.07.060
Morsy, M. A., Abdalla, A. M., Mahmoud, A. M., Abdelwahab, S. A., and Mahmoud, M. E. (2012). Protective effects of curcumin, α-lipoic acid, and N-acetylcysteine against carbon tetrachloride-induced liver fibrosis in rats. J. Physiology Biochem. 68 (1), 29–35. doi:10.1007/s13105-011-0116-0
Moussion, C., Ortega, N., and Girard, J.-P. (2008). “The IL-1-like cytokine IL-33 is constitutively expressed in the nucleus of endothelial cells and epithelial cells in vivo: a novel ‘alarmin’?” Edited by derya unutmaz. PLoS ONE 3 (10), e3331. doi:10.1371/journal.pone.0003331
Mu, M., Shi, Z., Rong-Min, Wu, Kai-Sheng, D., Shuang, Lu, Juan-Juan, Z., et al. (2018). Ferulic acid attenuates liver fibrosis and hepatic stellate cell activation via inhibition of TGF-β/Smad signaling pathway. Drug Des. Dev. Ther. 12, 4107–4115. doi:10.2147/DDDT.S186726
Nelson, K. M., Dahlin, J. L., Bisson, J., Graham, J., Pauli, G. F., and Walters, M. A. (2017). The essential medicinal chemistry of curcumin: miniperspective. J. Med. Chem. 60 (5), 1620–1637. doi:10.1021/acs.jmedchem.6b00975
Oztas, E., Kuzu, U. B., Zengin, N. I., Kalkan, I. H., Saygili, F., Yildiz, H., et al. (2015). Can serum ST2 levels Be used as a marker of fibrosis in chronic hepatitis B infection? Medicine 94 (47), e1889. doi:10.1097/MD.0000000000001889
Pariente-Pérez, T., Aguilar-Alonso, F., Solano, J. D., Vargas-Olvera, C., Curiel-Muñiz, P., Mendoza-Rodríguez, C. A., et al. (2020). Differential behavior of NF-κB, IκBα and EGFR during the renal carcinogenic process in an experimental model in vivo. Oncol. Lett. 19 (4), 3153–3164. doi:10.3892/ol.2020.11436
Parola, M., and Pinzani, M. (2019). Liver fibrosis: pathophysiology, pathogenetic targets and clinical issues. Mol. Aspects Med. 65, 37–55. doi:10.1016/j.mam.2018.09.002
Pascual-Figal, D. A., and Januzzi, J. L. (2015). The biology of ST2: the international ST2 consensus panel. Am. J. Cardiol. 115 (7), 3B–7B. doi:10.1016/j.amjcard.2015.01.034
Peschel, D., Koerting, R., and Nass, N. (2007). Curcumin induces changes in expression of genes involved in cholesterol homeostasis. J. Nutr. Biochem. 18 (2), 113–119. doi:10.1016/j.jnutbio.2006.03.007
Plevris, N., Sinha, R., Hay, A. W., McDonald, N., Plevris, J. N., and Hayes, P. C. (2018). Index serum hyaluronic acid independently and accurately predicts mortality in patients with liver disease. Alimentary Pharmacol. Ther. 48 (4), 423–430. doi:10.1111/apt.14897
Pourbagher-Shahri, A. M., Farkhondeh, T., Ashrafizadeh, M., Talebi, M., and Samargahndian, S. (2021). Curcumin and cardiovascular diseases: focus on cellular targets and cascades. Biomed. Pharmacother. 136 (April), 111214. doi:10.1016/j.biopha.2020.111214
Qin, L., Qin, J., Xiumei, Z., Yang, Q., and Huang, L. (2018). Curcumin protects against hepatic stellate cells activation and migration by inhibiting the CXCL12/CXCR4 biological Axis in liver fibrosis: a study in vitro and in vivo. Biomed. Pharmacother. 101 (May), 599–607. doi:10.1016/j.biopha.2018.02.091
Ramirez-Tortosa, M. C., Ramirez-Tortosa, C. L., Mesa, M. D., Granados, S., Gil, A., and Quiles, J. L. (2009). Curcumin ameliorates rabbits’s steatohepatitis via respiratory chain, oxidative stress, and TNF-alpha. Free Radic. Biol. Med. 47 (7), 924–931. doi:10.1016/j.freeradbiomed.2009.06.015
Reyes-Gordillo, K., Shibayama, M., Tsutsumi, V., Vergara, P., Moreno, M. G., Muriel, P., et al. (2008). Curcumin prevents and reverses cirrhosis induced by bile duct obstruction or CCl4 in rats: role of TGF-β modulation and oxidative stress. Fundam. Clin. Pharmacol. 22 (4), 417–427. doi:10.1111/j.1472-8206.2008.00611.x
Rodríguez, M. J., Sabaj, M., Tolosa, G., Herrera Vielma, F., Zúñiga, M. J., González, D. R., et al. (2021). Maresin-1 Prevents Liver Fibrosis by Targeting Nrf2 and NF-κB, Reducing Oxidative Stress and Inflammation. Cells 10 (12), 3406. doi:10.3390/cells10123406
Ruart, M., Chavarria, L., Campreciós, G., Suárez-Herrera, N., Montironi, C., Guixé-Muntet, S., et al. (2019). Impaired endothelial autophagy promotes liver fibrosis by aggravating the oxidative stress response during acute liver injury. J. Hepatology 70 (3), 458–469. doi:10.1016/j.jhep.2018.10.015
Seki, E., De Minicis, S., Österreicher, C. H., Kluwe, J., Osawa, Y., Brenner, D. A., et al. (2007). TLR4 enhances TGF-beta signaling and hepatic fibrosis. Nat. Med. 13 (11), 1324–1332. doi:10.1038/nm1663
Shu, Y., He, Y., Ye, G., Liu, X., Huang, J., Zhang, Q., et al. (2023). Curcumin inhibits the activity and induces apoptosis of activated hepatic stellate cell by suppressing autophagy. J. Cell. Biochem. 124 (11), 1764–1778. doi:10.1002/jcb.30487
Soto-Angona, Ó., Anmella, G., Valdés-Florido, M. J., De Uribe-Viloria, N., Carvalho, A. F., Penninx, B. W. J. H., et al. (2020). Non-alcoholic fatty liver disease (NAFLD) as a neglected metabolic companion of psychiatric disorders: common pathways and future approaches. BMC Med. 18 (1), 261. doi:10.1186/s12916-020-01713-8
Sun, M., and Kisseleva, T. (2015). Reversibility of liver fibrosis. Clin. Res. Hepatology Gastroenterology 39 (September), S60–S63. doi:10.1016/j.clinre.2015.06.015
Tamber, S. S., Bansal, P., Sharma, S., Singh, R. B., and Sharma, R. (2023). Biomarkers of liver diseases. Mol. Biol. Rep. 50 (9), 7815–7823. doi:10.1007/s11033-023-08666-0
Tang, W., Jiang, Y. F., Ponnusamy, M., and Diallo, M. (2014). Role of Nrf2 in chronic liver disease. World J. Gastroenterology 20 (36), 13079–13087. doi:10.3748/wjg.v20.i36.13079
Tang, Y. (2015). Curcumin targets multiple pathways to halt hepatic stellate cell activation: updated mechanisms in vitro and in vivo. Dig. Dis. Sci. 60 (6), 1554–1564. doi:10.1007/s10620-014-3487-6
Thoen, L. F., Guimarães, E. L., Dollé, L., Mannaerts, I., Najimi, M., Sokal, E., et al. (2011). A role for autophagy during hepatic stellate cell activation. J. Hepatol. 55 (6), 1353–1360. doi:10.1016/j.jhep.2011.07.010
Tu, C.-tao, Yao, Q.-yan, Xu, B.-li, Wang, J.-yao, Zhou, C.-hui, and Zhang, S.-cai (2012). Protective effects of curcumin against hepatic fibrosis induced by carbon tetrachloride: modulation of high-mobility group box 1, toll-like receptor 4 and 2 expression. Food Chem. Toxicol. 50 (9), 3343–3351. doi:10.1016/j.fct.2012.05.050
Wang, J.-nian, Li, Li, Li, L.-yun, Qi, Y., Li, J., and Xu, T. (2018). Emerging role and therapeutic implication of Wnt signaling pathways in liver fibrosis. Gene 674 (October), 57–69. doi:10.1016/j.gene.2018.06.053
Wang, Y., Huang, S., Wen, K., Wu, C., Zeng, T., Xie, S., et al. (2023). Corilagin alleviates liver fibrosis in zebrafish and mice by repressing Ido1-mediated M2 macrophage repolarization. Phytomedicine 119 (October), 155016. doi:10.1016/j.phymed.2023.155016
Wu, S.-Ju, Lin, Y.-Ho, Chu, C.-C., Tsai, Y.-H., and Chao, J. C.-J. (2008). Curcumin or saikosaponin a improves hepatic antioxidant capacity and protects against CCl 4 -induced liver injury in rats. J. Med. Food 11 (2), 224–229. doi:10.1089/jmf.2007.555
Wu, S.-Ju, Tam, K.-W., Tsai, Y.-H., Chang, C.-C., and Chao, J. C.-J. (2010). Curcumin and saikosaponin A inhibit chemical-induced liver inflammation and fibrosis in rats. Am. J. Chin. Med. 38 (01), 99–111. doi:10.1142/S0192415X10007695
Xiang, D.-M., Wen, S., Ning, B.-F., Zhou, T.-F., Li, X.-F., Zhong, W., et al. (2018). The HLF/IL-6/STAT3 feedforward circuit drives hepatic stellate cell activation to promote liver fibrosis. Gut 67 (9), 1704–1715. doi:10.1136/gutjnl-2016-313392
Xu, T., Guo, Pu, He, Y., Pi, C., Wang, Y., Feng, X., et al. (2020). Application of curcumin and its derivatives in tumor multidrug resistance. Phytotherapy Res. 34 (10), 2438–2458. doi:10.1002/ptr.6694
Yang, X., Li, Q., Liu, W., Zong, C., Lixin, W., Shi, Y., et al. (2023). Mesenchymal stromal cells in hepatic fibrosis/cirrhosis: from pathogenesis to treatment. Cell. Mol. Immunol. 20 (6), 583–599. doi:10.1038/s41423-023-00983-5
Yao, Q. Y., Xu, B. L., Wang, J. Y., Liu, H. C., Zhang, S. C., and Tu, C. T. (2012). Inhibition by curcumin of multiple sites of the transforming growth factor-beta1 signalling pathway ameliorates the progression of liver fibrosis induced by carbon tetrachloride in rats. BMC complementary and alternative medicine 12, 156. doi:10.1186/1472-6882-12-156
Yuan, S., Wei, C., Liu, G., Zhang, L., Li, J., Li, L., et al. (2022). Sorafenib attenuates liver fibrosis by triggering hepatic stellate cell ferroptosis via HIF-1α/SLC7A11 pathway. Cell Prolif. 55 (1), e13158. doi:10.1111/cpr.13158
Zhang, T., Hu, J., Wang, X., Zhao, X., Li, Z., Niu, J., et al. (2019). MicroRNA-378 promotes hepatic inflammation and fibrosis via modulation of the NF-κB-TNFα pathway. J. Hepatology 70 (1), 87–96. doi:10.1016/j.jhep.2018.08.026
Zhang, Z., Guo, Y., Zhang, S., Zhang, Y., Wang, Y., Ni, W., et al. (2013). Curcumin modulates cannabinoid receptors in liver fibrosis in vivo and inhibits extracellular matrix expression in hepatic stellate cells by suppressing cannabinoid receptor type-1 in vitro. Eur. J. Pharmacol. 721 (1–3), 133–140. doi:10.1016/j.ejphar.2013.09.042
Zhao, X.-An, Chen, G., Liu, Y., Chen, Y., Wu, H., Xiong, Y., et al. (2018). Curcumin reduces Ly6Chi monocyte infiltration to protect against liver fibrosis by inhibiting kupffer cells activation to reduce chemokines secretion. Biomed. Pharmacother. 106 (October), 868–878. doi:10.1016/j.biopha.2018.07.028
Zhao, Y., Ma, X., Wang, J., He, X., Hu, Y., Zhang, P., et al. (2014). Curcumin protects against CCl4-induced liver fibrosis in rats by inhibiting HIF-1α through an ERK-dependent pathway. Molecules 19 (11), 18767–18780. doi:10.3390/molecules191118767
Glossary
Keywords: Chinese medicine, hepatic fibrosis, curcumin against hepatic fibrosis, preclinical meta-study, experiments on animals
Citation: Huang B-H, Guo Z-W, Lv B-H, Zhao X, Li Y-B and Lv W-L (2024) A role for curcumin in preventing liver fibrosis in animals: a systematic review and meta-analysis. Front. Pharmacol. 15:1396834. doi: 10.3389/fphar.2024.1396834
Received: 06 March 2024; Accepted: 30 April 2024;
Published: 24 May 2024.
Edited by:
Chenghai Liu, Shanghai University of Traditional Chinese Medicine, ChinaReviewed by:
Lei Zhang, Qingdao University, ChinaPriyanka Choudhury, Medical College of Wisconsin, United States
Chuantao Tu, Fudan University, China
Copyright © 2024 Huang, Guo, Lv, Zhao, Li and Lv. This is an open-access article distributed under the terms of the Creative Commons Attribution License (CC BY). The use, distribution or reproduction in other forums is permitted, provided the original author(s) and the copyright owner(s) are credited and that the original publication in this journal is cited, in accordance with accepted academic practice. No use, distribution or reproduction is permitted which does not comply with these terms.
*Correspondence: Wen-Liang Lv, bHZ3ZW5saWFuZ0Bzb2h1LmNvbQ==
†These authors have contributed equally to this work