- Department of Neurosurgery, Shengjing Hospital of China Medical University, Shenyang, China
Background: Stroke has been the focus of medical research due to its serious consequences and sequelae. Among the tens of millions of new stroke patients every year, cerebral ischemia patients account for the vast majority. While cerebral ischemia drug research and development is still ongoing, most drugs are terminated at preclinical stages due to their unacceptable toxic side effects. In recent years, natural herbs have received considerable attention in the pharmaceutical research and development field due to their low toxicity levels. Numerous studies have shown that natural herbs exert actions that cannot be ignored when treating cerebral ischemia.
Methods: We reviewed and summarized the therapeutic effects and mechanisms of different natural herbal extracts on cerebral ischemia to promote their application in this field. We used keywords such as “natural herbal extract,” “herbal medicine,” “Chinese herbal medicine” and “cerebral ischemia” to comprehensively search PubMed, ScienceDirect, ScienceNet, CNKI, and Wanfang databases, after which we conducted a detailed screening and review strategy.
Results: We included 120 high-quality studies up to 10 January 2024. Natural herbal extracts had significant roles in cerebral ischemia treatments via several molecular mechanisms, such as improving regional blood flow disorders, protecting the blood-brain barrier, and inhibiting neuronal apoptosis, oxidative stress and inflammatory responses.
Conclusion: Natural herbal extracts are represented by low toxicity and high curative effects, and will become indispensable therapeutic options in the cerebral ischemia treatment field.
1 Introduction
Cerebral ischemia (CI) is a complex disease in clinical medicine. To put it simply, due to various reasons, blood in brain tissue cannot support normal metabolism and function, with subsequent symptoms collectively referred to as CI. Worldwide, morbidity and mortality rates due to CI are very high. The disease is characterized by several etiologies, changeable conditions, and serious consequences, which exert extremely heavy burdens on patients and their families. The National Institutes of Health Stroke Scale is commonly used to assess neurological damage in patients with clinical ischemia. Even if a patient avoids death, most will experience severe neurological dysfunction. Currently, the early treatment of patients with CI mainly occurs via the rapid restoration of cerebral blood flow perfusion, however, this restoration increases oxidative stress and inflammatory responses in ischemic tissue, leading to reperfusion injury. One factor that determines the severity of a patient’s condition is ischemia duration, which is a very important determinant when selecting treatment options for patients with acute cerebral ischemia (ACI) (Powers, 2020). Another problem that cannot be ignored is that the recombinant tissue plasminogen activator (rt-PA) drug is currently approved by the U.S. Food and Drug Administration for stroke patients, but its treatment window is very narrow and it has very serious side effects (e.g., cerebral hemorrhaging) (Dhamija and Donnan, 2007). Thus, a lack of drugs is an urgent issue for CI treatment. To remedy this, promoting low-toxicity and high-efficiency drug research and development can alleviate CI patient suffering. Our work is based on this purpose and motivation.
Herbal medicines are gifts from nature, and have helped humans solve medical problems that have plagued humankind for centuries. For example, artemisinin extracted from Artemisia annua L. helps alleviate malaria (Talman et al., 2019). Methanol extracts from Allium turcicum Özhatay and Cowley exert significant anticancer, antioxidant, and antimicrobial activities (łpek et al., 2024). These natural herbal extracts (NHEs) have active roles in many different fields, for example, a Chenopodium quinoa Willd. seed extract restores photosystem II damage caused by toxic metal salts (Ganieva et al., 2023). Also, Pistacia atlantica Desf. extracts effectively inhibit Fusarium oxysporum f. sp. albedinis to rescue infected date palms (Fatiha et al., 2023). NHEs have unique structures and properties, and are roughly divided into alkaloids, flavonoids, polysaccharides, glycosides, organic acids, and volatile oils.
1.1 Alkaloids
Alkaloids are nitrogen-containing organic compounds mainly found in plants, which have similar chemical properties to alkalis. One common property is that they all contain nitrogen as part of their chemical structure, but not all organic compounds containing nitrogen are alkaloids. Thanks to natural herb research and exploration, nearly 10,000 alkaloids have been discovered and collected. Alkaloids are subdivided into more than 60 types, for example, Leonurus japonicus Houtt. total alkaloid (LHA) is an organic amine in alkaloids. LHA and kukoamine A (KuA) are common alkaloids. LHA helps protect the blood-brain barrier (BBB) and inhibits inflammatory reactions and apoptosis in ischemia-reperfusion (IR) injury (Zhang Q.-Y. et al., 2017; Li Y. et al., 2021). KuA impacts CI injury by alleviating brain edema and inhibiting oxidative stress and apoptosis (Liu et al., 2017).
1.2 Flavonoids
Flavonoids are one of the most widespread organic compounds in nature; they exist in almost all green plants, especially higher plants. In the selected studies in this review, many have investigated flavonoid NHEs, such as emodin, scutellarin, and icariin (ICA). From our research, emodin enhances cell viability and inhibits oxidative stress radicalization to alleviate IR injury (Wang et al., 2007; Leung et al., 2020); scutellarin improves neurological dysfunction in rats during IR injury by inhibiting apoptosis, focal death, and necrosis (Wang C. et al., 2023); and ICA inhibits apoptosis and protects neuronal dendrites during chronic cerebral ischemia (CCI) to improve cognitive impairment (Li W.-X. et al., 2015).
1.3 Polysaccharides
Polysaccharides are macromolecules composed of at least 10 monosaccharides, and have important roles maintaining normal life activities. Polysaccharides are divided into plant, animal, and fungal polysaccharides according to extraction sources. Plant polysaccharides include ganoderma polysaccharides, lentinan, ginseng polysaccharides, and other polysaccharides beneficial to humans. Previous studies have reported that some polysaccharides may have anti-tumor effects (Zhang et al., 2021).
1.4 Glycosides
Glycosides have high medicinal value, which not only enhance immunity and antiviral effects, but also inhibit oxidative stress and enhance metabolic function in cells. Astragaloside IV (ASIV), ginsenoside, and notoginsenoside are well-known representative glycosides, especially ASIV. As an Astragalus membranaceus (Fisch.) Bunge extract, ASIV inhibits inflammatory reactions, promotes neurogenesis and angiogenesis, promotes neurotrophic factor expression, protects the BBB, and significantly improves neurological dysfunction caused by IR injury (Li et al., 2013; Li L. et al., 2021; Li S. et al., 2021; Shi et al., 2021). Ginsenoside has protective roles in injury caused by ACI, CCI, and IR. It not only inhibits apoptosis, increases angiogenesis, and improves local blood flow disorders, but also protects the BBB and improves cognitive dysfunction caused by CI (Zhou et al., 2014; Yang et al., 2016; Wang S. et al., 2017; Wan et al., 2017; Zhang et al., 2019; Zhang C. et al., 2020). Notoginsenoside resists injury caused by ACI and IR by alleviating brain edema, enhancing cell viability, protecting the BBB, and inhibiting apoptosis (Tu et al., 2018; Liu B. et al., 2021; Gao et al., 2022; Liu et al., 2022).
1.5 Organic acids
Organic acids are widely found in leaves, roots, and especially plant fruits. They have acidic properties, and are widely found in Lonicera japonica Thunb., Schisandra chinensis (Turcz.) Baill., Prunus mume Siebold & Zucc., Rubus idaeus L., and other herbs. Representative organic acid compounds from NHEs include salvianolic acid A (SAA) and betulinic acid (BA). In an ACI model, SAA reduces the incidence of cerebral hemorrhaging, protects the BBB, relieves vascular endothelial dysfunction, promotes neural function recovery, and induces neural progenitor cell proliferation. SAA also alleviates ischemic brain edema, inhibits inflammatory reactions, relieves oxidative stress, inhibits apoptosis, and improves long-term learning and memory defects (Jiang et al., 2011; Chien et al., 2016; Song et al., 2019; Liu C. et al., 2021; Ling et al., 2021; Yang Y. et al., 2022). BA also inhibits neuronal autophagy against IR injury (Zhao et al., 2021).
1.6 Volatile oils
Volatile oils mainly come from aromatic traditional Chinese medicine, with many fragrant plants more or less containing these compounds. The group includes terpenoids and aromatic compounds and also their oxygen-containing derivatives such as alcohols, aldehydes, ketones, phenols, ethers, and lipids. Additionally, the group includes some nitrogen- and sulfur-containing compounds. The most common NHEs are ginkgolide and ligustilide (LIG). Seven ginkgolide species have been found: A, B, C, M, J, K, and L. Ginkgolide B (GB) exerts the greatest effects, so many pharmacological studies have focused on this compound. GB protects the BBB and improves mitochondrial respiratory function against ACI injury (Li et al., 2007). LIG alleviates neurological deficits and inhibits apoptosis, astrocyte activation and proliferation, and oxidative stress responses in a CCI model (Feng et al., 2012; Peng D. et al., 2022).
2 Search strategy
To comprehensively and systematically conduct literature retrieval and data extraction, we preliminarily searched and screened all studies from PubMed, ScienceDirect, Web of Science, CNKI, and WANFANG databases before 10 January 2024 in strict accordance with Preferred Reporting Items for Systematic Review and Meta-analysis (PRISMA) guidelines. The retrieval keywords are as follows: 1) natural herbal extracts, 2) Chinese herbal medicines, 3) herbal medicines, and 4) cerebral ischemia. Constructed retrieval expressions are (natural herbal extracts OR Chinese herbal medicines OR herbal medicines) AND cerebral ischemia. After retrieving pertinent studies (n = 7,018), we preliminarily screened (using titles, keywords, and abstracts) and comprehensively reviewed these studies. Finally, 120 studies were selected for review (Figure 1).
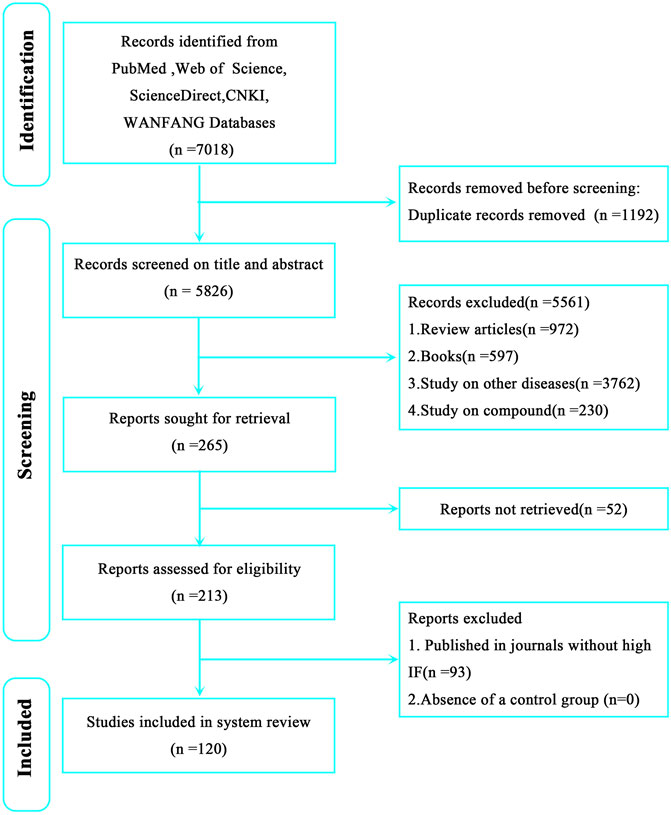
Figure 1. Preferred Reporting Items for Systematic Review and Meta-analysis (PRISMA) flow chart showing literature retrieval and screening in this review.
2.1 Inclusion and exclusion criteria
In view of the therapeutic effects and mechanisms of NHEs toward CI, and the large number of studies, we formulated the following inclusion and exclusion criteria. Inclusion criteria; 1) A cerebral ischemia model and 2) a control group are included; 3) At least one experimental group used NHEs as an intervention; and 4) Research data are published in high impact journals. Exclusion criteria; 1) Reviews or books; and 2) Studies on other diseases and compounds.
2.2 Data extraction and treatment evaluation
The authors independently extracted and summarized information from selected studies, solved any issues via discussion, and finally summarized the information, including; 1) NHEs; 2) The source and voucher numbers of the herbal medicine; 3) Extraction methods 4) Extraction parts and solvents; 5) Toxicity and side effects; 6) Related diseases in the study; 7) Establishing in vitro or in vivo models; 8) Animal or cell models; 9) Dose and time of treatment; 10) Main biological effects; 11) Mechanism of action; 12) Year of publication and first author; and 13) Positive controls.
2.3 Risk of bias
After discussions, the authors referred to the previous literature (Dong et al., 2023) to establish an evaluation scale of bias risk for selected studies. The following questions were posed; 1) Had the study passed peer review; 2) Were randomness principles followed when grouping models; 3) Were blinding methods applied during drug interventions and data collection; 4) Were sample sizes statistically calculated before model establishment; 5) Were animal welfare laws and regulations strictly observed in the research process; and 6) Were potential conflicts of interest between authors declared?
3 Results
From our strategy, 7,018 studies were retrieved, including 2032 Pubmed results, 1,242 Web of Science results, 3,578 ScienceDirect results, 124 CNKI results, and 42 WANFANG results. After preliminary screening and applying inclusion and exclusion, 120 studies were finally selected (Figure 1). Among these, 21 were related to ACI, 28 to CCI, and 71 to IR. In order to avoid disease subtype differences which may have affected our study results, we separately summarized the therapeutic effects of NHEs for ACI, CCI, and IR.
3.1 ACI
In the 21 ACI-related studies were 19 in vivo and nine in vitro studies, from which we summarized the characteristics of each study (Table 1). To ensure reliability, we summarized in vivo and in vitro study characteristics, separately. In the 19 in vivo studies, NHEs mitigated ACI-induced damage via different modes of action (Table 2). In nine in vitro studies, damaged cell models achieved varying degrees of remission after a NHE intervention (Table 3).
3.2 CCI
We included 28 studies on CCI, including 28 in vivo and four in vitro studies. We summarized CCI-related research characteristics (Table 4). NHEs alleviated damage caused by CCI and improved cognitive dysfunction caused by ischemia (Table 5). Detailed information on four studies outlining in vitro CCI characteristics (extracts, interventions, biological effects, and mechanisms) is shown (Table 6).
3.3 Cerebral IR
Of the 71 cerebral IR-related studies, 68 were in vivo and 31 were in vitro based. A summary of study characteristics is shown (Table 7), and then we describe the studies in vivo and in vitro, separately. Studies showed that NHEs had therapeutic roles in in vivo brain IR models (Supplementary Table S1). NHEs also reduced IR damage in in vitro models (Table 8).
4 Quality evaluation of selected studies
Using our bias risk assessment scale, bias risk assessments were conducted on the 120 studies. All were peer-reviewed publications and they strictly complied with animal welfare regulations, which meant that all studies had at least two points (total score = 6 points). Additionally, 120 studies followed randomization principles (84.17%), 39 adopted blind methods (32.5%), 88 declared conflicts of interest among authors (73.33%), and only one study statistically calculated the sample size (0.83%) (Figure 2). Perhaps some researchers had calculated sample sizes before their studies, but this was not stated. After evaluations, bias risk scores for studies were in the 2–6 range: six studies scored 2 (5%), 29 scored 3 (24.17%), 56 scored 4 (46.67%), 28 scored 5 (23.33%), and one scored 6 (0.83%). Approximately half (46.67%) received four points, which proved that study quality was high. Bias risk evaluations for studies are shown (Table 7).
5 Toxicity
Unfortunately, many studies failed to provide NHE-related toxicity information. While drug toxicity studies are usually conducted at pre-experimental stages, researchers must articulate this. To complement the required NHE toxicity reports for this review, we performed additional NHE safety reviews by summarizing the toxicity reports in selected studies (Supplementary Table S2). However, not all NHEs have accompanying toxicity reports, which undoubtedly confirms a lack of safety studies in the natural herb research field. Reported that the LD50 (median lethal dose, the minimum amount of toxin required to kill half of an animal of a certain weight or age within a specified period of time) of SAA in mice (the dose required to kill half of cells/animal after a specific trial duration) was 1,161.2 mg/kg (Yang M.-Y. et al., 2022). G. biloba extracts may be carcinogenic and caution is recommended for their long-term use (Mei et al., 2017). ASIV is not toxic during maternal and embryonic development, but may inhibit fertility in female rats, suggesting its non-use in perinatal periods (Xuying et al., 2010). Luo et al. reported that emodin reduces and inhibits human sperm motility, suggesting some reproductive toxicity in these cells (Luo et al., 2015). LHA did not generate significant adverse reactions in toxicity tests in multiple experimental animals (Zhu et al., 2018). The main component of an ethanolic extract from Erythrina velutina Willd. (EEEV) is gallic acid, with in vivo studies showing that gallic acid at 210 mg/kg exerted no toxic effects in mice (Li et al., 2019). Ginkgolide A and ginkgolide B reduce mouse blastocyst viability and cause embryonic retardation in mice, leading to embryo death, suggesting caution when using these reagents during pregnancy (Mei et al., 2017). The main component of supercritical CO2 extracts from DanShen (SCED) is tanshinone IIA (TSA); it was found that at high TSA concentrations (25M), zebrafish embryo models exhibited severe growth inhibition, developmental malformations, and cardiotoxicity (Wang T. et al., 2017). Usually there are no obvious side effects when Panax notoginseng is supplemented to patients, but due to its estrogen effects, some patients have reported vaginal bleeding and distending breast pain. Those patients taking high P. notoginseng doses (>2.5 g/day) have central nervous system damaging effects such as insomnia, tachyarrhythmia, hypertension, and tension (Mancuso and Santangelo, 2017). In vivo betulinic acid studies showed no signs of systemic toxicity (Liu C. et al., 2019). Scutellarin has the lowest toxicity in rodents, and can even be said to be non-toxic. Quercetin (Que) toxicity is low; the organ weights and histopathology of rats treated with 400 mg/kg/d Que for 410 consecutive days showed no significant changes. Ginsenoside Rd, ginsenoside Rb1, and notoginsenoside promote cancer cell apoptosis and have significant effects in cancer treatment. Notoginsenoside R1 also inhibits cell proliferation, migration, invasion and angiogenesis, and promotes cell apoptosis at 150 µM. Shikonin is considered safe, but may cause skin allergies at very low doses. Luteolin exerts cytotoxicity at 5 μM and 10 µM doses, and its safety must be further evaluated in animal models and clinical trials. Hydroxysafflor yellow A is sensitive to interactions between herbs and drugs, resulting in no therapeutic effects at certain doses (Guo et al., 2023). Galangin has an IC50 (half-inhibitory concentration, the concentration at which a biological process or activity is inhibited by 50%) value of 275.48 μM in V79 cells, and does not produce genotoxic effects at all concentrations (Bacanlı et al., 2017). Similarly, echinocystic acid (EA) exerts no cytotoxic effects under any conditions in cell viability assays (Joh et al., 2012). Glycyrrhizin is moderately toxic and should be used with caution during pregnancy. It also has selective cytotoxic effects toward cancer cells, and its most important side effects are secondary diseases induced by hypertension and hypokalemia (Nazari et al., 2017). After the maternal application of 1.0 mg/kg ASIV for 28 consecutive days, ASIV delays development in young rats and should be used with caution in perinatal women (Zhang J. et al., 2020). Studies report serious adverse reactions to matrine, the most serious being hepatotoxicity, neurotoxicity, and reproductive toxicity (Wang X. et al., 2023). Acute and subacute toxicity studies report that breviscapine is a safe drug with a potential for widespread use in clinical settings (Wu et al., 2021). Icariin has an IC50 of 20 μM in HeLa cells, and its toxic effects in normal cells are relatively negligible (Huang et al., 2019).
6 Discussion
We comprehensively summarized the molecular mechanisms underlying CI treatment by NHEs (Figure 3). As mentioned, NHE therapeutic effects toward CI are roughly divided in two ways: one reduces damage, mainly by improving local blood flow disturbance, inhibiting oxidative stress, inflammatory responses and apoptosis, relieving cerebral edema, protecting the BBB, and inhibiting excitatory amino acid overexpression. The other way promotes injury recovery, mainly by promoting endothelial cell proliferation and migration, promoting neuron proliferation and differentiation, and promoting neurotrophic factor expression. NHEs may also act on molecules, such as SAA, via several pathways. SAA is a bioactive compound extracted from Salvia miltiorrhiza Bunge. Studies report that SAA has direct or indirect effects on toll-like receptor 2/4 (TLR2/4), phosphoinositide 3-kinase (PI3K), glycogen synthase kinase 3β (GSK3β), vascular endothelial growth factor A (VEGFA), and intercellular adhesion molecule 1 (ICAM1), which means that SAA not only inhibits apoptosis, inflammation, and oxidative stress, and protects the BBB, but also promotes vascular proliferation and recovery (Jiang et al., 2011; Chien et al., 2016; Song et al., 2019; Liu C. et al., 2021; Ling et al., 2021). Additionally, some key factors involved in multiple pathways, such as VEGF, are activated by multiple NHEs. The discovery of VEGF has completely changed our understanding of blood vessel production during development and physiological homeostasis. The biological effects mediated by VEGF are mainly due to its impact on vascular permeability and new blood vessel generation. VEGF has important relationships with tumor growth and metastasis, hypertensive retinopathy, and other pathological conditions (Apte et al., 2019). T-VA is extracted from Ligusticum sinense (Li G. et al., 2015). Several studies report that T-VA, ginsenoside Rb1 (GSRb1), L-borneol, and DL-n-butylphthalide (DL-NBP) can also play a role through VEGF (Li G. et al., 2015; Zhu et al., 2018; Ma et al., 2021; Wang et al., 2020).
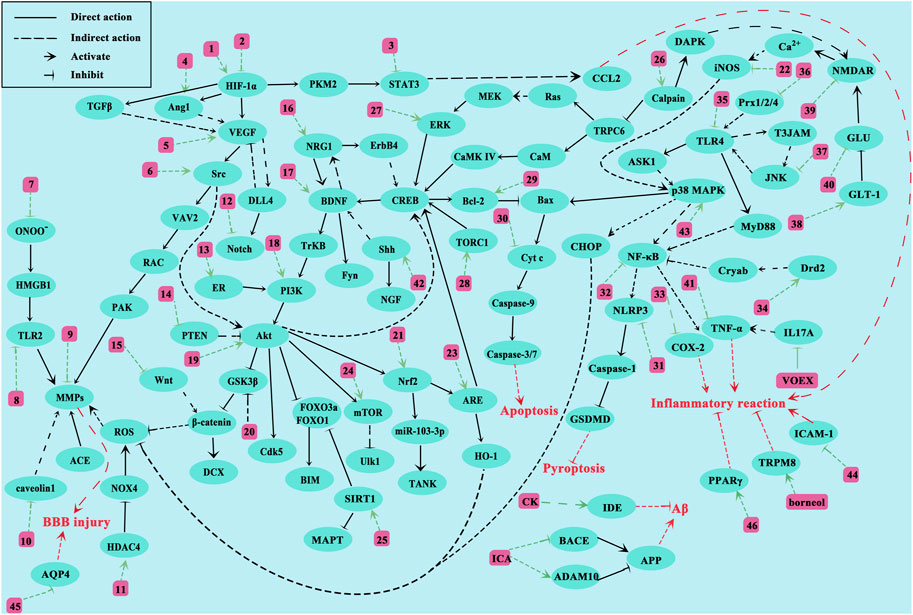
Figure 3. Proposed NHE molecular mechanisms for treating CI. The green line represents the interaction between NHEs and molecules. The red line represents the pathological effects caused by molecules. Numbers represent NHEs (1, DL-NBP; 2, PNS, SAA and SA; 3, ASIV and Formononetin; 4, L-borneol; 5, T-VA and GSRb1; 6, Triptolide; 7, Glycyrrhizin and SAA; 8, SAA; 9, ASIV and Methylophiopogonanone A; 10, NGR1; 11, LHA; 12, L-borneol; 13, NGR1; 14, HAR; 15, L-borneol; 16, EF; 17, GSRd, LHA, PF11, and SAA; 18, EK100, TFCJ, DGMI, and Formononetin; 19, Honokiol, L-NBP, HSYA, and GSF1; 20, CK; 21, Catalpol, KRGP, CZ-7, AA, Rus, PQS, and ASIV; 22, Honokiol; 23, EGB761; 24, EGB761 and Vitexin; 25, LIG, BA and ASIV; 26, Hyperforin and GSRd; 27, emodin; 28, TSA; 29, Catalpol, MO, SHPL-49, Galangin, KuA, Asiaticoside, GSRb1, OLE, EGB761, SA, GB, Sophoricoside and Matrine; 30, PAL extracts and GSRb1; 31, SB extracts; 32, T-VA, Silymarin, Storax, EK100 and Rus; 33, ES; 34, SAA; 35, Fructus extracts, Procyanidins and SAA; 36, Gas-d; 37, YZR extracts and EA; 38, GSRb1 and emodin; 39, GSRb1 and GSRd; 40, EEEV, scutellarin, EDAC and CTRF; 41, MO; 42, SA and PNS; 43, L-borneol, SB extracts and ASD extract; 44, ASIV and Vitexin; 45, GSRg1, ASIV and DSE; 46, Silymarin, SAA and ICA).
CI is a severe nerve injury caused by interrupted cerebral blood flow. The molecular mechanisms underpinning its pathological processes are extremely complex and cannot be fully explained at present. CI involves amino acid excitation, injury oxidative stress, inflammatory responses, BBB injury, mitochondrial dysfunction, cell necrosis, and apoptosis. Many studies report that key molecules are involved in these CI-mediated processes, such as hypoxia-inducible factor alpha (HIF-1α), VEGF, brain-derived neurotrophic factor (BDNF), protein kinase B (Akt), matrix metalloproteinases (MMPs), c-Jun N-terminal kinase (JNK), B-cell lymphoma-2-associated X (Bax), Caspase-3/9, mitogen-activated protein kinases (MAPKs) and nuclear factor kappa-B (NF-κB) (Wang et al., 2020; Li et al., 2022; Liu et al., 2023; Li et al., 2017; Chen H.-S. et al., 2018; Hu et al., 2020; Peng T. et al., 2022; Xu et al., 2021).
Blood flow disturbance is the most fundamental issue in CI, and appears to initiate several pathological conditions, such as BBB damage, mitochondrial dysfunction, cell necrosis, and apoptosis. To reduce the severity and prognosis of stroke onset, doctors must rapidly conduct clinical interventions such as intravenous thrombolysis and surgical thrombectomy to unblock cerebral blood vessels. Anticoagulant and antiplatelet therapies are recommended for patients without contraindications, but their harsh conditions of use and severe side effects have prompted scientists to explore better treatments. Thromboxane A2 (TXA2) is a potent vasoconstrictor and the main cyclooxygenase (COX) product of arachidonic acid (AA). The functional importance of this eicosanoid in acute coronary ischemic syndrome has been demonstrated as it activates platelets (Reilly and Fitzgerald, 1993). Fei et al. extracted natural compounds with TSA as the main component from S. miltiorrhiza Bunge (DanShen); this SCED inhibits platelet aggregation and improves regional blood flow disorders by inhibiting TXA2 activation (Fei et al., 2017). Although the specific molecular mechanisms have not been clarified, and to determine NHE biological effects on blood flow disorders, several studies have used laser speckle imaging to show that P. notoginseng saponins (PNS), GSRb1, L-borneol, and galangin can improve regional blood flow disorders after stroke (Li S. et al., 2012; Wang S. et al., 2017; Gao et al., 2022; Xie et al., 2023).
Amino acid excitotoxicity is due to the abnormal accumulation of some excitatory amino acids (such as glutamate) outside neurons after ischemia. Glutamate accumulation leads to sustained Ca2+ channel and N-methyl-D-aspartic acid receptor (NMDAR) activation on neuronal synaptic membranes. NMDAR is an ion channel regulated by glutamate on cell membranes. After glutamate activation, high Ca2+ levels are transported into membranes. Ca2+ accumulates in the cytoplasm and mitochondria, resulting in Ca2+ overload. This alteration affects many biological processes, such as calpain activation, oxidative stress responses, and mitochondrial damage, and also protease, kinase, phosphatase, and other enzyme activities, leading to cell death. As a major transporter of excitatory amino acids, glutamate transporter 1 (GLT-1) is mainly distributed in astrocytes. Usually, GLT-1 mediates glutamate uptake by glial cells to maintain extracellular glutamate concentrations. GLT-1 function is impaired during CI, resulting in high intersynaptic glutamate accumulation (Rao et al., 2001). GSRb1 and emodin reportedly activate GLT-1 receptors on astrocyte membranes, transferring glutamate into astrocytes to reduce its abnormal accumulation outside neurons (Wang S. et al., 2017; Leung et al., 2020). GSRb1 also has the same effects as ginsenoside Rd (GSRd) in inhibiting NMDAR expression (Zhang C. et al., 2020). Glutamine synthetase (GS) catalyzes glutamate conversion to glutamine in vivo and has important roles regulating glutamate levels. KRGP is an active substance extracted from Korean red ginseng(Liu L. et al., 2019). Liu et al. found that GS expression levels are significantly elevated after CI in KRGP-pretreated mice, while GS expression levels are not changed much in nuclear factor erythroid 2-related factor 2 (Nrf2) gene deletion mice, suggesting that the Nrf2 pathway has important roles in glutamate homeostasis after CI, and that KRGP may reduce amino acid excitation damage caused by CI via Nrf2 signaling (Liu L. et al., 2019).
Oxidative stress is a common phenomenon in hypoxic cells. Mitochondria are essential organelles which maintain energy homeostasis in cells. The state and function of mitochondria undergo significant changes during hypoxia, leading to increased intracellular reactive oxygen species (ROS) levels, which severely damage cells and brain tissue. Oxidative stress products directly attack biomacromolecules (amino acids and nucleic acids) to induce apoptosis and increase BBB permeability. Nrf2 has antioxidant and anti-inflammatory effects that activate heme oxygenase-1 (HO-1) after oxidative stress-inducer (e.g., inflammatory chemokines/cytokines) activation or tissue damage (Hassanein et al., 2023). HO-1 is an inducible homolog with antioxidant properties and has important roles regulating oxidative stress, with elevated HO-1 levels detected in almost all oxidatively stressed cells. catalpol, KRGP, CZ-7, AA, and ruscogenin (Rus) increase HO-1 expression by stimulating Nrf2 (Liu L. et al., 2019; Liu D.-D. et al., 2019; Wang et al., 2022; Zhang S. et al., 2023; Kim et al., 2023). Additionally, by detecting mitochondrial energy metabolism-related genes (Atp12a and Atp6v1g3), Liu et al. found that notoginsenoside R1 (NGR1) mitigates mitochondrial energy metabolism abnormalities (Liu et al., 2022). Three Nei-like DNA glycosylases exist in mammalian cells, which protect DNA by removing oxidative bases. GSRd protects neurons by activating Nei-like DNA glycosylase 1/3 (NEIL1/3) to promote DNA hydrolysis of oxidative stress-induced product damage (Yang et al., 2016).
Inflammatory responses are self-defense mechanisms; they are stimulated by endogenous and exogenous inflammatory factors and are closely related to different diseases. Neuroinflammation occurs at almost all stages of ischemic stroke and is caused by damage-associated molecular pattern release by damaged/dead cells. These patterns, including adenosine, heat shock proteins, and interleukin 33 (IL-33) are recognized by corresponding immune cells which trigger multiple downstream signaling pathways (Qin et al., 2022). Additionally, these patterns stimulate inflammation-related cytokine, interferon or chemokine production, leading to increased adhesion molecule expression, helping white blood cells adhere to blood vessel surfaces, and promoting immune cell infiltration. Therefore, for patients with CI, early anti-inflammatory treatment is an important method to reduce ischemic injury and improve prognosis. Pro-inflammatory cytokines induce chemokine secretion immediately after CI. Chemokine-chemokineligand2 (CCL2) and its receptors are involved in regulating inflammation in the ischemic state, and may be recruited to and adhere to cerebral vascular endothelial cells by immune cells. Signal transducer and activator of transcription 3 (STAT3) has positive regulatory effects on chemokines (such as CCL2) and acts as a key transcription factor during inflammation and immunity. Li et al. reports that ASIV inhibits CCL2 functions by inhibiting STAT3 expression and inhibiting NK cell infiltration (Li S. et al., 2021). JNK, TLR4, NF-κB, and MAPKs also have key roles in inflammatory signaling pathways via a vicious cycle between JNK and TLR4 (Cheng et al., 2021a). TLRs are expressed on cell surfaces and in intracellular spaces, and regulate the state and function of many immune cells. Fructus extracts, procyanidins, and SAA inhibit TLR4 expression (Lee et al., 2015; Yang et al., 2020; Ling et al., 2021), while Alpinia oxyphylla Miq. (YZR) extract and EA inhibit JNK activation (Yu et al., 2019; Cheng et al., 2021a). T-VA, silymarin, storax, and EK100 also suppress inflammatory responses by inhibiting NF-κB (Hou et al., 2010; Li G. et al., 2015; Wang et al., 2019; Zhou M. et al., 2021). The MAPK signaling pathway is activated shortly after ischemic injury onset. MAPK is composed of three major effectors, extracellular signal-related kinases (ERK1/2), JNK, and p38 MAPK. Among these, p38 MAPK regulates pro-inflammatory cytokine expression. The activation of MAPK/ERK signaling and the stimulating effects of MMP expression can aggravate BBB injury in ischemic stroke and further enhance pro-inflammatory factor expression. Interestingly, we found that different NHEs have opposite effects on p38 MAPK, but all were protective against CI injury, which we speculate might be due to the activation of different factors downstream of p38 MAPK. L-borneol and Angelica sinensis (Oliv.) Diels (ASD) extracts activate p38 MAPK (Cheng et al., 2021b; Xie et al., 2023), while Honokiol and Scutellaria baicalensis Georgi (SB) extracts inhibit its function (Hwang et al., 2011; Chen et al., 2014).
MMPs are essential for BBB function and structure, and mainly act on the tight junction component, ZO-1, between adjacent cells. Endothelial cells and their tight junction components are key factors maintaining BBB stability. MMPs disrupt the BBB via enzymatic ZO-1 hydrolysis, so they are potential therapeutic targets for CI (Batra et al., 2010). Glycyrrhizin indirectly inhibits MMPs by reducing peroxynitrite (ONOO−) production (Chen et al., 2020). NGR1 mitigates BBB disruption by MMPs by inhibiting caveolin 1 (Liu B. et al., 2021). LHA promotes histone deacetylase 4 (HDAC4) expression, leading to decreased NADPH oxidase 4 (NOX4) expression, which in turn inhibits MMP expression (Zhang Q.-Y. et al., 2017). Additionally, ginsenoside Rg1 (GSRg1), ASIV, and DSE prevent ischemic cerebral edema and BBB damage by inhibiting AQP4 (Lee K. et al., 2012; Li et al., 2013; Zhou et al., 2014).
Apoptosis is a normal physiological activity, but after CI, the process becomes overactivated and causes neuronal death, which leads to neurological deficits in patients with CI, and seriously affects neurological function recovery in later stages. Bax is a classical apoptosis-promoting gene that promotes cytochrome C (Cyt-C) transfer from the mitochondria to cells, and then activates the caspase cascade to eventually lead to apoptosis. B-cell lymphoma-2 (Bcl-2) is an apoptosis inhibitor protein, which binds to Bax and forms dimers to inhibit apoptosis. Therefore, the balance between Bcl-2 and Bax is key to neuronal survival. Several NHEs protect neurons from apoptosis, such as catalpol, MO, SHPL-49, galangin, KuA, asiaticoside, GSRb1, oleuropein (OLE), EGB761, salvianolic acid (SA), and GB, by increasing Bcl-2 levels (Li S. et al., 2012; Sun et al., 2015; Yu et al., 2016; Liu et al., 2017; Zhao et al., 2017; Yang et al., 2018; Zhang et al., 2018; Zhu et al., 2018; Luan et al., 2020; Wang et al., 2022; Zhang P. et al., 2023). Additionally, SAA also inhibits neuronal apoptosis via the Akt/FOXO3a/BIM pathway (Song et al., 2019).
7 Conclusion and prospects
With deepening research on NHEs, their mechanisms are becoming more complex and multifaceted. Complexity means that a single NHE, such as SAA, can simultaneously act on multiple molecular pathways. Multifaceted means that a NHE acting on the same key factor on a certain pathway may have different regulatory outcomes, such as L-borneol and Honokiol. In such cases, a deeper understanding of study conditions and results is required. Although too many pathways and factors are involved in these studies, key factors such as VEGF, BDNF, Akt, MMPs, JNK, Bax, Caspase-3/9, MAPKs, and NF-κB, may provide reference points for further research. Additionally, although CI pathogenesis is highly complex, the first and most important pathology is disturbed blood flow, which is why early CI treatments should rapidly restore blood supply. Therefore, more attention should be paid to NHEs (TSA or GSRb1) with antiplatelet or antithrombotic effects. For advanced CI treatments, selected studies should mainly focus on inhibiting neuroinflammation, inhibiting neuronal apoptosis, and protecting the BBB. This means that NHE treatment effects for CI are significant, and show how important neuroinflammation and neuronal apoptosis processes are in CI. In addition, the treatment of transient cerebral ischemia and permanent cerebral ischemia is also different. Transient cerebral ischemia is a sudden, transient cerebral vascular insufficiency, usually without brain tissue necrosis. The treatment of transient cerebral ischemia is mainly prevention, such as antiplatelet therapy. Permanent cerebral ischemia often has abnormal pathology such as thrombus, which leads to blood flow interruption and eventually brain cell death. If the vascular recanalization treatment cannot be carried out in time, serious sequelae will often be left.
Although strict review conditions were set, some flaws were identified in selected studies: 1) The elaboration of extraction protocols or NHE sources was not adequately detailed. Due to different NHE extraction methods, resultant NHEs may have different biological activities and effects, which makes the research data unreliable; 2) NHE toxicity was not adequately explored in selected studies. Even if some studies performed toxicity tests, they were in vitro and not in vivo; 3) Although considerable animal data were observed, it is uncertain if these NHEs can be eventually used in clinical practice; therefore, more pharmacological and pharmacological studies are required; and 4) NHE mechanisms were not fully explored. Most studies only explored one or several related factors, but did not examine complete NHE mechanisms.
Clinically observed NHE effects are the result of combined drug actions across a multitude of signaling pathways. Researchers are constantly exploring new treatment options to maximize treatment benefits while minimizing side effects. Traditional Chinese medicine efforts in this area are worthy of recognition, and the synergistic actions of multiple NHEs should be considered in future research. In addition, the reproducibility of drug efficacy is very critical, and it is an important factor affecting whether a drug can be transformed into clinical practice. In order to do this, we need to maintain a rigorous working attitude and record the experimental process in detail. We should strengthen our understanding of the various parts of the experiment and conduct sufficient pre-experiments. The factors affecting the transformation of medical achievements also include the lack of advanced medical equipment, insufficient attention to medical transformation, and lack of communication between the supply and demand sides.
In this review, we retrieved and screened high-quality NHE studies related to CI. We briefly summarized the potential therapeutic effects and mechanisms underpinning NHEs toward CI, which may promote NHE development and their applications in clinical settings. Selecting a clinical medication is a long and complicated process, and any possibilities, to combat CI, must be carefully and comprehensively considered.
Data availability statement
The original contributions presented in the study are included in the article/Supplementary Material, further inquiries can be directed to the corresponding author.
Author contributions
JY: Conceptualization, Data curation, Methodology, Visualization, Writing–original draft, Writing–review and editing. BY: Data curation, Supervision, Writing–review and editing. JZ: Conceptualization, Funding acquisition, Project administration, Supervision, Writing–review and editing.
Funding
The author(s) declare that financial support was received for the research, authorship, and/or publication of this article. This work is supported by the Education Department of Liaoning Provincial Basic Research Projects (JYTMS20230098); and the 30 Project Fund of Shengjing Hospital: M0269.
Conflict of interest
The authors declare that the research was conducted in the absence of any commercial or financial relationships that could be construed as a potential conflict of interest.
Publisher’s note
All claims expressed in this article are solely those of the authors and do not necessarily represent those of their affiliated organizations, or those of the publisher, the editors and the reviewers. Any product that may be evaluated in this article, or claim that may be made by its manufacturer, is not guaranteed or endorsed by the publisher.
Supplementary material
The Supplementary Material for this article can be found online at: https://www.frontiersin.org/articles/10.3389/fphar.2024.1424146/full#supplementary-material
References
Ahad, M. A., Chear, N.J.-Y., Keat, L. G., Has, A. T. C., Murugaiyah, V., and Hassan, Z. (2023). Bio-enhanced fraction from Clitoria ternatea root extract ameliorates cognitive functions and in vivo hippocampal neuroplasticity in chronic cerebral hypoperfusion rat model. Ageing Res. Rev. 89, 101990. doi:10.1016/j.arr.2023.101990
Apte, R. S., Chen, D. S., and Ferrara, N. (2019). VEGF in signaling and disease: beyond discovery and development. Cell. 176, 1248–1264. doi:10.1016/j.cell.2019.01.021
Bacanlı, M., Başaran, A. A., and Başaran, N. (2017). The antioxidant, cytotoxic, and antigenotoxic effects of galangin, puerarin, and ursolic acid in mammalian cells. Drug Chem. Toxicol. 40, 256–262. doi:10.1080/01480545.2016.1209680
Bai, X., Qiu, Y., Wang, J., Dong, Y., Zhang, T., and Jin, H. (2024). Panax quinquefolium saponins attenuates microglia activation following acute cerebral ischemia-reperfusion injury via Nrf2/miR-103-3p/TANK pathway. Cell. Biol. Int. 48, 201–215. doi:10.1002/cbin.12100
Batra, A., Latour, L. L., Ruetzler, C. A., Hallenbeck, J. M., Spatz, M., Warach, S., et al. (2010). Increased plasma and tissue MMP levels are associated with BCSFB and BBB disruption evident on post-contrast FLAIR after experimental stroke. J. Cereb. Blood Flow. Metab. 30, 1188–1199. doi:10.1038/jcbfm.2010.1
Cao, Y., Mao, X., Sun, C., Zheng, P., Gao, J., Wang, X., et al. (2011). Baicalin attenuates global cerebral ischemia/reperfusion injury in gerbils via anti-oxidative and anti-apoptotic pathways. Brain Res. Bull. 85, 396–402. doi:10.1016/j.brainresbull.2011.05.002
Chen, C., Zhang, H., Xu, H., Xue, R., Zheng, Y., Wu, T., et al. (2018a). Harpagoside rescues the memory impairments in chronic cerebral hypoperfusion rats by inhibiting PTEN activity. J. Alzheimers Dis. 63, 445–455. doi:10.3233/JAD-171170
Chen, H., Guan, B., Wang, B., Pu, H., Bai, X., Chen, X., et al. (2020). Glycyrrhizin prevents hemorrhagic transformation and improves neurological outcome in ischemic stroke with delayed thrombolysis through targeting peroxynitrite-mediated HMGB1 signaling. Stroke Res. 11, 967–982. doi:10.1007/s12975-019-00772-1
Chen, H.-S., Chen, X., Li, W.-T., and Shen, J.-G. (2018b). Targeting RNS/caveolin-1/MMP signaling cascades to protect against cerebral ischemia-reperfusion injuries: potential application for drug discovery. Acta Pharmacol. Sin. 39, 669–682. doi:10.1038/aps.2018.27
Chen, J.-H., Kuo, H.-C., Lee, K.-F., and Tsai, T.-H. (2014). Magnolol protects neurons against ischemia injury via the downregulation of p38/MAPK, CHOP and nitrotyrosine. Toxicol. Appl. Pharmacol. 279, 294–302. doi:10.1016/j.taap.2014.07.005
Chen, X., Wu, H., Chen, H., Wang, Q., Xie, X.-J., and Shen, J. (2019). Astragaloside VI promotes neural stem cell proliferation and enhances neurological function recovery in transient cerebral ischemic injury via activating EGFR/MAPK signaling cascades. Mol. Neurobiol. 56, 3053–3067. doi:10.1007/s12035-018-1294-3
Cheng, C.-Y., Chiang, S.-Y., Kao, S.-T., and Huang, S.-C. (2021a). Alpinia oxyphylla Miq extract reduces cerebral infarction by downregulating JNK-mediated TLR4/T3JAM- and ASK1-related inflammatory signaling in the acute phase of transient focal cerebral ischemia in rats. Chin. Med. 16, 82. doi:10.1186/s13020-021-00495-2
Cheng, C.-Y., Huang, H.-C., Kao, S.-T., and Lee, Y.-C. (2021b). Angelica sinensis extract promotes neuronal survival by enhancing p38 MAPK-mediated hippocampal neurogenesis and dendritic growth in the chronic phase of transient global cerebral ischemia in rats. J. Ethnopharmacol. 278, 114301. doi:10.1016/j.jep.2021.114301
Chien, M.-Y., Chuang, C.-H., Chern, C.-M., Liou, K.-T., Liu, D.-Z., Hou, Y.-C., et al. (2016). Salvianolic acid A alleviates ischemic brain injury through the inhibition of inflammation and apoptosis and the promotion of neurogenesis in mice. Free Radic. Biol. Med. 99, 508–519. doi:10.1016/j.freeradbiomed.2016.09.006
Choi, M., Lim, C., Lee, B.-K., and Cho, S. (2022). Amelioration of Brain Damage after Treatment with the Methanolic Extract of Glycyrrhizae Radix et Rhizoma in Mice. Pharmaceutics 14, 2776. doi:10.3390/pharmaceutics14122776
Dai, J., Qiu, Y.-M., Ma, Z.-W., Yan, G.-F., Zhou, J., Li, S.-Q., et al. (2018). Neuroprotective effect of baicalin on focal cerebral ischemia in rats. Neural Regen. Res. 13, 2129–2133. doi:10.4103/1673-5374.241464
Damodaran, T., Tan, B. W. L., Liao, P., Ramanathan, S., Lim, G. K., and Hassan, Z. (2018). Clitoria ternatea L. root extract ameliorated the cognitive and hippocampal long-term potentiation deficits induced by chronic cerebral hypoperfusion in the rat. J. Ethnopharmacol. 224, 381–390. doi:10.1016/j.jep.2018.06.020
Dhamija, R. K., and Donnan, G. A. (2007). Time is brain--acute stroke management. Aust. Fam. Physician 36, 892–895.
Dong, X., Zhou, S., and Nao, J. (2023). Kaempferol as a therapeutic agent in Alzheimer’s disease: evidence from preclinical studies. Ageing Res. Rev. 87, 101910. doi:10.1016/j.arr.2023.101910
Fatiha, A., Larbi, B., and Ahmed, M. (2023). Antifungal activity of the Pistacia atlantica tar against Fusarium oxysporum f. Sp. albedinis, the cause of the bayoud of the date palm in Southwest Algeria. Adv. Biol. Earth Sci. 8, 75–82.
Fei, Y.-X., Wang, S.-Q., Yang, L.-J., Qiu, Y.-Y., Li, Y.-Z., Liu, W.-Y., et al. (2017). Salvia miltiorrhiza Bunge (Danshen) extract attenuates permanent cerebral ischemia through inhibiting platelet activation in rats. J. Ethnopharmacol. 207, 57–66. doi:10.1016/j.jep.2017.06.023
Feng, Z., Lu, Y., Wu, X., Zhao, P., Li, J., Peng, B., et al. (2012). Ligustilide alleviates brain damage and improves cognitive function in rats of chronic cerebral hypoperfusion. J. Ethnopharmacol. 144, 313–321. doi:10.1016/j.jep.2012.09.014
Ferreira, R. S., Ribeiro, P. R., Silva, J. H. C. E., Hoppe, J. B., de Almeida, M. M. A., de Lima Ferreira, B. C., et al. (2023). Amburana cearensis seed extract stimulates astrocyte glutamate homeostatic mechanisms in hippocampal brain slices and protects oligodendrocytes against ischemia. BMC Complement. Med. Ther. 23, 154. doi:10.1186/s12906-023-03959-0
Ganieva, R., Galandarova, T., and Agalarov, R. (2023). Effect of chenopodium quinoa seed extract in protecting the thylakoid membrane under the influence of Cd and Na salts. Adv. Biol. Earth Sci. 8, 288–292.
Gao, J., Yao, M., Zhang, W., Yang, B., Yuan, G., Liu, J.-X., et al. (2022). Panax notoginseng saponins alleviates inflammation induced by microglial activation and protects against ischemic brain injury via inhibiting HIF-1α/PKM2/STAT3 signaling. Biomed. Pharmacother. 155, 113479. doi:10.1016/j.biopha.2022.113479
Guan, T., Liu, Q., Qian, Y., Yang, H., Kong, J., Kou, J., et al. (2013). Ruscogenin reduces cerebral ischemic injury via NF-κB-mediated inflammatory pathway in the mouse model of experimental stroke. Eur. J. Pharmacol. 714, 303–311. doi:10.1016/j.ejphar.2013.07.036
Guang, H.-M., and Du, G.-H. (2006). Protections of pinocembrin on brain mitochondria contribute to cognitive improvement in chronic cerebral hypoperfused rats. Eur. J. Pharmacol. 542, 77–83. doi:10.1016/j.ejphar.2006.04.054
Guo, C., Huang, Q., Wang, Y., Yao, Y., Li, J., Chen, J., et al. (2023). Therapeutic application of natural products: NAD+ metabolism as potential target. Phytomedicine 114, 154768. doi:10.1016/j.phymed.2023.154768
Hassanein, E. H. M., Ibrahim, I. M., Abd-Alhameed, E. K., Sharawi, Z. W., Jaber, F. A., and Althagafy, H. S. (2023). Nrf2/HO-1 as a therapeutic target in renal fibrosis. Life Sci. 334, 122209. doi:10.1016/j.lfs.2023.122209
Hosseinzadeh, H., Sadeghnia, H. R., Ghaeni, F. A., Motamedshariaty, V. S., and Mohajeri, S. A. (2012). Effects of saffron (Crocus sativus L.) and its active constituent, crocin, on recognition and spatial memory after chronic cerebral hypoperfusion in rats. Phytother. Res. 26, 381–386. doi:10.1002/ptr.3566
Hou, Y.-C., Liou, K.-T., Chern, C.-M., Wang, Y.-H., Liao, J.-F., Chang, S., et al. (2010). Preventive effect of silymarin in cerebral ischemia-reperfusion-induced brain injury in rats possibly through impairing NF-κB and STAT-1 activation. Phytomedicine 17, 963–973. doi:10.1016/j.phymed.2010.03.012
Hu, W., Wu, X., Yu, D., Zhao, L., Zhu, X., Li, X., et al. (2020). Regulation of JNK signaling pathway and RIPK3/AIF in necroptosis-mediated global cerebral ischemia/reperfusion injury in rats. Exp. Neurol. 331, 113374. doi:10.1016/j.expneurol.2020.113374
Huai, Y., Dong, Y., Xu, J., Meng, N., Song, C., Li, W., et al. (2013). L-3-n-butylphthalide protects against vascular dementia via activation of the Akt kinase pathway. Neural Regen. Res. 8, 1733–1742. doi:10.3969/j.issn.1673-5374.2013.19.001
Huang, S., Xie, T., and Liu, W. (2019). Icariin inhibits the growth of human cervical cancer cells by inducing apoptosis and autophagy by targeting mTOR/PI3K/AKT signalling pathway. J. BUON 24, 990–996.
Huang, S.-S., Su, H.-H., Chien, S.-Y., Chung, H.-Y., Luo, S.-T., Chu, Y.-T., et al. (2022). Activation of peripheral TRPM8 mitigates ischemic stroke by topically applied menthol. J. Neuroinflammation 19, 192. doi:10.1186/s12974-022-02553-4
Hui, Z., Sha, D.-J., Wang, S.-L., Li, C.-S., Qian, J., Wang, J.-Q., et al. (2017). Panaxatriol saponins promotes angiogenesis and enhances cerebral perfusion after ischemic stroke in rats. BMC Complement. Altern. Med. 17, 70. doi:10.1186/s12906-017-1579-5
Hwang, Y. K., Jinhua, M., Choi, B.-R., Cui, C.-A., Jeon, W. K., Kim, H., et al. (2011). Effects of Scutellaria baicalensis on chronic cerebral hypoperfusion-induced memory impairments and chronic lipopolysaccharide infusion-induced memory impairments. J. Ethnopharmacol. 137, 681–689. doi:10.1016/j.jep.2011.06.025
Ipek, P., Atalar, M. N., Baran, A., Baran, M. F., Ommati, M. M., Karadag, M., et al. (2024). Determination of chemical components of the endemic species Allium turcicum L. plant extract by LC-MS/MS and evaluation of medicinal potentials. Heliyon 10, e27386. doi:10.1016/j.heliyon.2024.e27386
Jiang, J., Dai, J., and Cui, H. (2018b). Vitexin reverses the autophagy dysfunction to attenuate MCAO-induced cerebral ischemic stroke via mTOR/Ulk1 pathway. Biomed. Pharmacother. 99, 583–590. doi:10.1016/j.biopha.2018.01.067
Jiang, M., Liu, X., Zhang, D., Wang, Y., Hu, X., Xu, F., et al. (2018a). Celastrol treatment protects against acute ischemic stroke-induced brain injury by promoting an IL-33/ST2 axis-mediated microglia/macrophage M2 polarization. J. Neuroinflammation 15, 78. doi:10.1186/s12974-018-1124-6
Jiang, M., Wang, X.-Y., Zhou, W.-Y., Li, J., Wang, J., and Guo, L.-P. (2011). Cerebral protection of salvianolic acid A by the inhibition of granulocyte adherence. Am. J. Chin. Med. 39, 111–120. doi:10.1142/S0192415X11008683
Joh, E.-H., Gu, W., and Kim, D.-H. (2012). Echinocystic acid ameliorates lung inflammation in mice and alveolar macrophages by inhibiting the binding of LPS to TLR4 in NF-κB and MAPK pathways. Biochem. Pharmacol. 84, 331–340. doi:10.1016/j.bcp.2012.04.020
Kim, M.-S., Bang, J. H., Lee, J., Han, J.-S., Baik, T. G., and Jeon, W. K. (2016). Ginkgo biloba L. extract protects against chronic cerebral hypoperfusion by modulating neuroinflammation and the cholinergic system. Phytomedicine 23, 1356–1364. doi:10.1016/j.phymed.2016.07.013
Kim, S.-Y., Kim, Y.-J., Cho, S.-Y., Lee, H.-G., Kwon, S., Park, S.-U., et al. (2023). Efficacy of Artemisia annua Linné in improving cognitive impairment in a chronic cerebral hypoperfusion-induced vascular dementia animal model. Phytomedicine 112, 154683. doi:10.1016/j.phymed.2023.154683
Lee, D., Park, J., Yoon, J., Kim, M.-Y., Choi, H.-Y., and Kim, H. (2012a). Neuroprotective effects of Eleutherococcus senticosus bark on transient global cerebral ischemia in rats. J. Ethnopharmacol. 139, 6–11. doi:10.1016/j.jep.2011.05.024
Lee, K., Jo, I.-Y., Park, S. H., Kim, K. S., Bae, J., Park, J.-W., et al. (2012b). Defatted sesame seed extract reduces brain oedema by regulating aquaporin 4 expression in acute phase of transient focal cerebral ischaemia in rat. Phytother. Res. 26, 1521–1527. doi:10.1002/ptr.4599
Lee, K. M., Bang, J., Kim, B. Y., Lee, I. S., Han, J.-S., Hwang, B. Y., et al. (2015). Fructus mume alleviates chronic cerebral hypoperfusion-induced white matter and hippocampal damage via inhibition of inflammation and downregulation of TLR4 and p38 MAPK signaling. BMC Complement. Altern. Med. 15, 125. doi:10.1186/s12906-015-0652-1
Leung, S. W., Lai, J. H., Wu, J.C.-C., Tsai, Y.-R., Chen, Y.-H., Kang, S.-J., et al. (2020). Neuroprotective effects of emodin against ischemia/reperfusion injury through activating ERK-1/2 signaling pathway. Int. J. Mol. Sci. 21, 2899. doi:10.3390/ijms21082899
Li, D.-J., Li, Y.-H., Yuan, H.-B., Qu, L.-F., and Wang, P. (2017). The novel exercise-induced hormone irisin protects against neuronal injury via activation of the Akt and ERK1/2 signaling pathways and contributes to the neuroprotection of physical exercise in cerebral ischemia. Metabolism 68, 31–42. doi:10.1016/j.metabol.2016.12.003
Li, G., Li, G., Tian, Y., Zhang, Y., Hong, Y., Hao, Y., et al. (2015a). A novel ligustrazine derivative T-VA prevents neurotoxicity in differentiated PC12 cells and protects the brain against ischemia injury in MCAO rats. Int. J. Mol. Sci. 16, 21759–21774. doi:10.3390/ijms160921759
Li, L., Gan, H., Jin, H., Fang, Y., Yang, Y., Zhang, J., et al. (2021b). Astragaloside IV promotes microglia/macrophages M2 polarization and enhances neurogenesis and angiogenesis through PPARγ pathway after cerebral ischemia/reperfusion injury in rats. Int. Immunopharmacol. 92, 107335. doi:10.1016/j.intimp.2020.107335
Li, M., Ma, R. N., Li, L. H., Qu, Y. Z., and Gao, G. D. (2013). Astragaloside IV reduces cerebral edema post-ischemia/reperfusion correlating the suppression of MMP-9 and AQP4. Eur. J. Pharmacol. 715, 189–195. doi:10.1016/j.ejphar.2013.05.022
Li, N., Zhang, X., Zhai, J., Yin, J., Ma, K., Wang, R., et al. (2022). Isoflurane and Netrin-1 combination therapy enhances angiogenesis and neurological recovery by improving the expression of HIF-1α-Netrin-1-UNC5B/VEGF cascade to attenuate cerebral ischemia injury. Exp. Neurol. 352, 114028. doi:10.1016/j.expneurol.2022.114028
Li, R.-P., Wang, Z.-Z., Sun, M.-X., Hou, X.-L., Sun, Y., Deng, Z.-F., et al. (2012b). Polydatin protects learning and memory impairments in a rat model of vascular dementia. Phytomedicine 19, 677–681. doi:10.1016/j.phymed.2012.03.002
Li, S., Dou, B., Shu, S., Wei, L., Zhu, S., Ke, Z., et al. (2021c). Suppressing NK cells by astragaloside IV protects against acute ischemic stroke in mice via inhibiting STAT3. Front. Pharmacol. 12, 802047. doi:10.3389/fphar.2021.802047
Li, S., Wu, C., Zhu, L., Gao, J., Fang, J., Li, D., et al. (2012a). By improving regional cortical blood flow, attenuating mitochondrial dysfunction and sequential apoptosis galangin acts as a potential neuroprotective agent after acute ischemic stroke. Molecules 17, 13403–13423. doi:10.3390/molecules171113403
Li, S., Zhang, Y., and Yang, L. (2007). Improving effect of Ginkgolide B on mitochondrial respiration of ischemic neuron after cerebral thrombosis in tree shrews. Chin. Med. J. (Engl.) 120, 1529–1533. doi:10.1097/00029330-200709010-00012
Li, W.-X., Deng, Y.-Y., Li, F., Liu, B., Liu, H.-Y., Shi, J.-S., et al. (2015b). Icariin, a major constituent of flavonoids from Epimedium brevicornum, protects against cognitive deficits induced by chronic brain hypoperfusion via its anti-amyloidogenic effect in rats. Pharmacol. Biochem. Behav. 138, 40–48. doi:10.1016/j.pbb.2015.09.001
Li, Y., Xiang, L., Wang, C., Song, Y., Miao, J., and Miao, M. (2021a). Protection against acute cerebral ischemia/reperfusion injury by Leonuri Herba Total Alkali via modulation of BDNF-TrKB-PI3K/Akt signaling pathway in rats. Biomed. Pharmacother. 133, 111021. doi:10.1016/j.biopha.2020.111021
Li, Y., Yang, Q., Shi, Z.-H., Zhou, M., Yan, L., Li, H., et al. (2019). The anti-inflammatory effect of feiyangchangweiyan capsule and its main components on pelvic inflammatory disease in rats via the regulation of the NF-κB and BAX/BCL-2 pathway. Evid. Based Complement. Altern. Med. 2019, 9585727. doi:10.1155/2019/9585727
Li, Z., Zhang, M., Yang, L., Fan, D., Zhang, P., Zhang, L., et al. (2024). Sophoricoside ameliorates cerebral ischemia-reperfusion injury dependent on activating AMPK. Eur. J. Pharmacol. 971, 176439. doi:10.1016/j.ejphar.2024.176439
Liang, K., Ye, Y., Wang, Y., Zhang, J., and Li, C. (2014). Formononetin mediates neuroprotection against cerebral ischemia/reperfusion in rats via downregulation of the Bax/Bcl-2 ratio and upregulation PI3K/Akt signaling pathway. J. Neurol. Sci. 344, 100–104. doi:10.1016/j.jns.2014.06.033
Lin, M., Sun, W., Gong, W., Zhou, Z., Ding, Y., and Hou, Q. (2015). Methylophiopogonanone A protects against cerebral ischemia/reperfusion injury and attenuates blood-brain barrier disruption in vitro. PLoS One 10, e0124558. doi:10.1371/journal.pone.0124558
Lin, Y., Zhang, J.-C., Fu, J., Chen, F., Wang, J., Wu, Z.-L., et al. (2013). Hyperforin attenuates brain damage induced by transient middle cerebral artery occlusion (MCAO) in rats via inhibition of TRPC6 channels degradation. J. Cereb. Blood Flow. Metab. 33, 253–262. doi:10.1038/jcbfm.2012.164
Ling, Y., Jin, L., Ma, Q., Huang, Y., Yang, Q., Chen, M., et al. (2021). Salvianolic acid A alleviated inflammatory response mediated by microglia through inhibiting the activation of TLR2/4 in acute cerebral ischemia-reperfusion. Phytomedicine 87, 153569. doi:10.1016/j.phymed.2021.153569
Liu, B., Li, Y., Han, Y., Wang, S., Yang, H., Zhao, Y., et al. (2021a). Notoginsenoside R1 intervenes degradation and redistribution of tight junctions to ameliorate blood-brain barrier permeability by Caveolin-1/MMP2/9 pathway after acute ischemic stroke. Phytomedicine 90, 153660. doi:10.1016/j.phymed.2021.153660
Liu, B., Zhao, T., Li, Y., Han, Y., Xu, Y., Yang, H., et al. (2022). Notoginsenoside R1 ameliorates mitochondrial dysfunction to circumvent neuronal energy failure in acute phase of focal cerebral ischemia. Phytother. Res. 36, 2223–2235. doi:10.1002/ptr.7450
Liu, C., Chen, Y., Lu, C., Chen, H., Deng, J., Yan, Y., et al. (2019c). Betulinic acid suppresses Th17 response and ameliorates psoriasis-like murine skin inflammation. Int. Immunopharmacol. 73, 343–352. doi:10.1016/j.intimp.2019.05.030
Liu, C., Liu, N.-N., Zhang, S., Ma, G.-D., Yang, H.-G., Kong, L.-L., et al. (2021b). Salvianolic acid A prevented cerebrovascular endothelial injury caused by acute ischemic stroke through inhibiting the Src signaling pathway. Acta Pharmacol. Sin. 42, 370–381. doi:10.1038/s41401-020-00568-2
Liu, D.-D., Yuan, X., Chu, S.-F., Chen, C., Ren, Q., Luo, P., et al. (2019b). CZ-7, a new derivative of Claulansine F, ameliorates 2VO-induced vascular dementia in rats through a Nrf2-mediated antioxidant responses. Acta Pharmacol. Sin. 40, 425–440. doi:10.1038/s41401-018-0078-7
Liu, J., Jiang, X., Zhang, Q., Lin, S., Zhu, J., Zhang, Y., et al. (2017). Neuroprotective effects of Kukoamine A against cerebral ischemia via antioxidant and inactivation of apoptosis pathway. Neurochem. Int. 107, 191–197. doi:10.1016/j.neuint.2016.12.024
Liu, L., Vollmer, M. K., Ahmad, A. S., Fernandez, V. M., Kim, H., and Doré, S. (2019a). Pretreatment with Korean red ginseng or dimethyl fumarate attenuates reactive gliosis and confers sustained neuroprotection against cerebral hypoxic-ischemic damage by an Nrf2-dependent mechanism. Free Radic. Biol. Med. 131, 98–114. doi:10.1016/j.freeradbiomed.2018.11.017
Liu, L., Zhang, X., Wang, L., Yang, R., Cui, L., Li, M., et al. (2010). The neuroprotective effects of Tanshinone IIA are associated with induced nuclear translocation of TORC1 and upregulated expression of TORC1, pCREB and BDNF in the acute stage of ischemic stroke. Brain Res. Bull. 82, 228–233. doi:10.1016/j.brainresbull.2010.04.005
Liu, X., Fan, L., Li, J., Bai, Z., Wang, Y., Liu, Y., et al. (2023). Mailuoning oral liquid attenuates convalescent cerebral ischemia by inhibiting AMPK/mTOR-associated apoptosis and promoting CREB/BDNF-mediated neuroprotection. J. Ethnopharmacol. 317, 116731. doi:10.1016/j.jep.2023.116731
Luan, P., Xu, J., Ding, X., Cui, Q., Jiang, L., Xu, Y., et al. (2020). Neuroprotective effect of salvianolate on cerebral ischaemia-reperfusion injury in rats by inhibiting the Caspase-3 signal pathway. Eur. J. Pharmacol. 872, 172944. doi:10.1016/j.ejphar.2020.172944
Luo, T., Li, N., He, Y., Weng, S., Wang, T., Zou, Q., et al. (2015). Emodin inhibits human sperm functions by reducing sperm [Ca(2+)]i and tyrosine phosphorylation. Reprod. Toxicol. 51, 14–21. doi:10.1016/j.reprotox.2014.11.007
Ma, R., Lu, D., Xie, Q., Yuan, J., Ren, M., Li, Y., et al. (2023). l-Borneol and d-Borneol promote transdifferentiation of astrocytes into neurons in rats by regulating Wnt/Notch pathway to exert neuroprotective effect during recovery from cerebral ischaemia. Phytomedicine 109, 154583. doi:10.1016/j.phymed.2022.154583
Ma, R., Xie, Q., Li, H., Guo, X., Wang, J., Li, Y., et al. (2021). l-Borneol exerted the neuroprotective effect by promoting angiogenesis coupled with neurogenesis via ang1-VEGF-BDNF pathway. Front. Pharmacol. 12, 641894. doi:10.3389/fphar.2021.641894
Mahmood, Q., Wang, G.-F., Wu, G., Wang, H., Zhou, C.-X., Yang, H.-Y., et al. (2017). Salvianolic acid A inhibits calpain activation and eNOS uncoupling during focal cerebral ischemia in mice. Phytomedicine 25, 8–14. doi:10.1016/j.phymed.2016.12.004
Mancuso, C., and Santangelo, R. (2017). Panax ginseng and Panax quinquefolius: from pharmacology to toxicology. Food Chem. Toxicol. 107, 362–372. doi:10.1016/j.fct.2017.07.019
Mao, X.-N., Zhou, H.-J., Yang, X.-J., Zhao, L.-X., Kuang, X., Chen, C., et al. (2017). Neuroprotective effect of a novel gastrodin derivative against ischemic brain injury: involvement of peroxiredoxin and TLR4 signaling inhibition. Oncotarget 8, 90979–90995. doi:10.18632/oncotarget.18773
Mei, N., Guo, X., Ren, Z., Kobayashi, D., Wada, K., and Guo, L. (2017a). Review of Ginkgo biloba-induced toxicity, from experimental studies to human case reports. J. Environ. Sci. Health C Environ. Carcinog. Ecotoxicol. Rev. 35, 1–28. doi:10.1080/10590501.2016.1278298
Mei, N., Guo, X., Ren, Z., Kobayashi, D., Wada, K., and Guo, L. (2017b). Review of Ginkgo biloba-induced toxicity, from experimental studies to human case reports. J. Environ. Sci. Health C Environ. Carcinog. Ecotoxicol. Rev. 35, 1–28. doi:10.1080/10590501.2016.1278298
Nazari, S., Rameshrad, M., and Hosseinzadeh, H. (2017). Toxicological effects of Glycyrrhiza glabra (licorice): a review. Phytother. Res. 31, 1635–1650. doi:10.1002/ptr.5893
Niu, H.-M., Ma, D.-L., Wang, M.-Y., Chen, X.-P., Zhang, Li, Li, Y.-L., et al. (2020). Epimedium flavonoids protect neurons and synapses in the brain via activating NRG1/ErbB4 and BDNF/Fyn signaling pathways in a chronic cerebral hypoperfusion rat model. Brain Res. Bull. 162, 132–140. doi:10.1016/j.brainresbull.2020.06.012
Park, S. J., Nam, K. W., Lee, H. J., Cho, E. Y., Koo, U., and Mar, W. (2009). Neuroprotective effects of an alkaloid-free ethyl acetate extract from the root of Sophora flavescens Ait. against focal cerebral ischemia in rats. Phytomedicine 16, 1042–1051. doi:10.1016/j.phymed.2009.03.017
Peng, D., Wang, Y.-X., Huang, T.-H., Luo, D., Qiao, L.-J., Wang, Q., et al. (2022a). Ligustilide improves cognitive impairment via regulating the SIRT1/ire1α/XBP1s/CHOP pathway in vascular dementia rats. Oxid. Med. Cell. Longev. 2022, 6664990. doi:10.1155/2022/6664990
Peng, T., Li, S., Liu, L., Yang, C., Farhan, M., Chen, L., et al. (2022b). Artemisinin attenuated ischemic stroke induced cell apoptosis through activation of ERK1/2/CREB/BCL-2 signaling pathway in vitro and in vivo. Int. J. Biol. Sci. 18, 4578–4594. doi:10.7150/ijbs.69892
Pengyue, Z., Tao, G., Hongyun, H., Liqiang, Y., and Yihao, D. (2017). Breviscapine confers a neuroprotective efficacy against transient focal cerebral ischemia by attenuating neuronal and astrocytic autophagy in the penumbra. Biomed. Pharmacother. 90, 69–76. doi:10.1016/j.biopha.2017.03.039
Powers, W. J. (2020). Acute ischemic stroke. N. Engl. J. Med. 383, 252–260. doi:10.1056/NEJMcp1917030
Qi, Z., Yan, F., Shi, W., Zhang, C., Dong, W., Zhao, Y., et al. (2014). AKT-related autophagy contributes to the neuroprotective efficacy of hydroxysafflor yellow A against ischemic stroke in rats. Transl. Stroke Res. 5, 501–509. doi:10.1007/s12975-014-0346-x
Qin, C., Yang, S., Chu, Y.-H., Zhang, H., Pang, X.-W., Chen, L., et al. (2022). Signaling pathways involved in ischemic stroke: molecular mechanisms and therapeutic interventions. Signal Transduct. Target Ther. 7, 215. doi:10.1038/s41392-022-01064-1
Qin, X., Li, L., Lv, Q., Yu, B., Yang, S., He, T., et al. (2012). Underlying mechanism of protection from hypoxic injury seen with n-butanol extract of Potentilla anserine L. in hippocampal neurons. Neural Regen. Res. 7, 2576–2582. doi:10.3969/j.issn.1673-5374.2012.33.002
Rao, V. L., Bowen, K. K., and Dempsey, R. J. (2001). Transient focal cerebral ischemia down-regulates glutamate transporters GLT-1 and EAAC1 expression in rat brain. Neurochem. Res. 26, 497–502. doi:10.1023/a:1010956711295
Reilly, M., and Fitzgerald, G. A. (1993). Cellular activation by thromboxane A2 and other eicosanoids. Eur. Heart J. 14 (Suppl. K), 88–93.
Rodrigues, F. T. S., de Sousa, C. N. S., Ximenes, N. C., Almeida, A. B., Cabral, L. M., Patrocínio, C. F. V., et al. (2017). Effects of standard ethanolic extract from Erythrina velutina in acute cerebral ischemia in mice. Biomed. Pharmacother. 96, 1230–1239. doi:10.1016/j.biopha.2017.11.093
Saleem, S., Zhuang, H., Biswal, S., Christen, Y., and Doré, S. (2008). Ginkgo biloba extract neuroprotective action is dependent on heme oxygenase 1 in ischemic reperfusion brain injury. Stroke 39, 3389–3396. doi:10.1161/STROKEAHA.108.523480
Seo, H.-W., Ha, T.-Y., Ko, G., Jang, A., Choi, J.-W., Lee, D.-H., et al. (2023). Scutellaria baicalensis attenuated neurological impairment by regulating programmed cell death pathway in ischemic stroke mice. Cells 12, 2133. doi:10.3390/cells12172133
Shi, R., Zheng, C.-B., Wang, H., Rao, Q., Du, T., Bai, C., et al. (2020). Gastrodin alleviates vascular dementia in a 2-VO-vascular dementia rat model by altering amyloid and tau levels. Pharmacology 105, 386–396. doi:10.1159/000504056
Shi, Y.-H., Zhang, X.-L., Ying, P.-J., Wu, Z.-Q., Lin, L.-L., Chen, W., et al. (2021). Neuroprotective effect of astragaloside IV on cerebral ischemia/reperfusion injury rats through sirt1/mapt pathway. Front. Pharmacol. 12, 639898. doi:10.3389/fphar.2021.639898
Song, J., Zhang, W., Wang, J., Yang, H., Zhou, Q., Wang, H., et al. (2019). Inhibition of FOXO3a/BIM signaling pathway contributes to the protective effect of salvianolic acid A against cerebral ischemia/reperfusion injury. Acta Pharm. Sin. B 9, 505–515. doi:10.1016/j.apsb.2019.01.010
Sun, T., Liu, B., and Li, P. (2015). Nerve protective effect of asiaticoside against ischemia-hypoxia in cultured rat cortex neurons. Med. Sci. Monit. 21, 3036–3041. doi:10.12659/MSM.894024
Talman, A. M., Clain, J., Duval, R., Ménard, R., and Ariey, F. (2019). Artemisinin bioactivity and resistance in malaria parasites. Trends Parasitol. 35, 953–963. doi:10.1016/j.pt.2019.09.005
Tan, Z., Yang, G., Qiu, J., Yan, W., Liu, Y., Ma, Z., et al. (2022). Quercetin alleviates demyelination through regulating microglial phenotype transformation to mitigate neuropsychiatric symptoms in mice with vascular dementia. Mol. Neurobiol. 59, 3140–3158. doi:10.1007/s12035-021-02712-3
Teixeira, L. L., Alencar, H. M. N. da S., Ferreira, L. O., Rodrigues, J. C. M., de Souza, R. D., Celestino Pinto, L., et al. (2023). Oral treatment with the extract of euterpe oleracea mart. Improves motor dysfunction and reduces brain injury in rats subjected to ischemic stroke. Nutrients 15, 1207. doi:10.3390/nu15051207
Tiang, N., Ahad, M. A., Murugaiyah, V., and Hassan, Z. (2020). Xanthone-enriched fraction of Garcinia mangostana and α-mangostin improve the spatial learning and memory of chronic cerebral hypoperfusion rats. J. Pharm. Pharmacol. 72, 1629–1644. doi:10.1111/jphp.13345
Tu, L., Wang, Y., Chen, D., Xiang, P., Shen, J., Li, Y., et al. (2018). Protective effects of notoginsenoside R1 via regulation of the PI3K-Akt-mTOR/JNK pathway in neonatal cerebral hypoxic-ischemic brain injury. Neurochem. Res. 43, 1210–1226. doi:10.1007/s11064-018-2538-3
Wan, Q., Ma, X., Zhang, Z.-J., Sun, T., Xia, F., Zhao, G., et al. (2017). Ginsenoside reduces cognitive impairment during chronic cerebral hypoperfusion through brain-derived neurotrophic factor regulated by epigenetic modulation. Mol. Neurobiol. 54, 2889–2900. doi:10.1007/s12035-016-9868-4
Wan, Y.-S., You, Y., Ding, Q.-Y., Xu, Y.-X., Chen, H., Wang, R.-R., et al. (2022). Triptolide protects against white matter injury induced by chronic cerebral hypoperfusion in mice. Acta Pharmacol. Sin. 43, 15–25. doi:10.1038/s41401-021-00637-0
Wang, C., Chen, H., Jiang, H.-H., Mao, B.-B., and Yu, H. (2021). Total flavonoids of chuju decrease oxidative stress and cell apoptosis in ischemic stroke rats: network and experimental analyses. Front. Neurosci. 15, 772401. doi:10.3389/fnins.2021.772401
Wang, C., Liu, Y., Liu, X., Zhang, Y., Yan, X., Deng, X., et al. (2023a). Scutellarin alleviates ischemic brain injury in the acute phase by affecting the activity of neurotransmitters in neurons. Molecules 28, 3181. doi:10.3390/molecules28073181
Wang, C., Zhang, D., Ma, H., and Liu, J. (2007). Neuroprotective effects of emodin-8-O-beta-D-glucoside in vivo and in vitro. Eur. J. Pharmacol. 577, 58–63. doi:10.1016/j.ejphar.2007.08.033
Wang, J., Zhang, Y., Zhang, M., Sun, S., Zhong, Y., Han, L., et al. (2022). Feasibility of catalpol intranasal administration and its protective effect on acute cerebral ischemia in rats via anti-oxidative and anti-apoptotic mechanisms. Drug Des. devel. Ther. 16, 279–296. doi:10.2147/DDDT.S343928
Wang, L., Liu, C., Wang, L., and Tang, B. (2023b). Astragaloside IV mitigates cerebral ischaemia-reperfusion injury via inhibition of P62/Keap1/Nrf2 pathway-mediated ferroptosis. Eur. J. Pharmacol. 944, 175516. doi:10.1016/j.ejphar.2023.175516
Wang, S., Li, M., Guo, Y., Li, C., Wu, L., Zhou, X.-F., et al. (2017a). Effects of Panax notoginseng ginsenoside Rb1 on abnormal hippocampal microenvironment in rats. J. Ethnopharmacol. 202, 138–146. doi:10.1016/j.jep.2017.01.005
Wang, T., Wang, C., Wu, Q., Zheng, K., Chen, J., Lan, Y., et al. (2017b). Evaluation of tanshinone IIA developmental toxicity in zebrafish embryos. Molecules 22, 660. doi:10.3390/molecules22040660
Wang, X., Zhu, W., Xing, M., Zhu, H., Chen, E., and Zhou, J. (2023c). Matrine disrupts Nrf2/GPX4 antioxidant system and promotes hepatocyte ferroptosis. Chem. Biol. Interact. 384, 110713. doi:10.1016/j.cbi.2023.110713
Wang, Y., Shen, Y., Liu, Z., Gu, J., Xu, C., Qian, S., et al. (2019). Dl-NBP (Dl-3-N-butylphthalide) treatment promotes neurological functional recovery accompanied by the upregulation of white matter integrity and HIF-1α/VEGF/Notch/Dll4 expression. Front. Pharmacol. 10, 1595. doi:10.3389/fphar.2019.01595
Wang, Y.-H., Chern, C.-M., Liou, K.-T., Kuo, Y.-H., and Shen, Y.-C. (2019). Ergostatrien-7,9(11),22-trien-3β-ol from Antrodia camphorata ameliorates ischemic stroke brain injury via downregulation of p65NF-κ-B and caspase 3, and activation of Akt/GSK3/catenin-associated neurogenesis. Food Funct. 10, 4725–4738. doi:10.1039/c9fo00908f
Wang, Z., Liu, T., Gan, L., Wang, T., Yuan, X., Zhang, B., et al. (2010). Shikonin protects mouse brain against cerebral ischemia/reperfusion injury through its antioxidant activity. Eur. J. Pharmacol. 643, 211–217. doi:10.1016/j.ejphar.2010.06.027
Wu, R., Liang, Y., Xu, M., Fu, K., Zhang, Y., Wu, L., et al. (2021). Advances in chemical constituents, clinical applications, pharmacology, pharmacokinetics and toxicology of Erigeron breviscapus. Front. Pharmacol. 12, 656335. doi:10.3389/fphar.2021.656335
Wu, S., Huang, R., Zhang, R., Xiao, C., Wang, L., Luo, M., et al. (2023). Gastrodin and gastrodigenin improve energy metabolism disorders and mitochondrial dysfunction to antagonize vascular dementia. Molecules 28, 2598. doi:10.3390/molecules28062598
Xie, Q., Lu, D., Yuan, J., Ren, M., Li, Y., Wang, J., et al. (2023). l-borneol promotes neurovascular unit protection in the subacute phase of transient middle cerebral artery occlusion rats: p38-MAPK pathway activation, anti-inflammatory, and anti-apoptotic effect. Phytother. Res. 37, 4166–4184. doi:10.1002/ptr.7878
Xiong, D., Deng, Y., Huang, B., Yin, C., Liu, B., Shi, J., et al. (2016). Icariin attenuates cerebral ischemia-reperfusion injury through inhibition of inflammatory response mediated by NF-κB, PPARα and PPARγ in rats. Int. Immunopharmacol. 30, 157–162. doi:10.1016/j.intimp.2015.11.035
Xu, D., Kong, T., Shao, Z., Liu, M., Zhang, R., Zhang, S., et al. (2021). Orexin-A alleviates astrocytic apoptosis and inflammation via inhibiting OX1R-mediated NF-κB and MAPK signaling pathways in cerebral ischemia/reperfusion injury. Biochim. Biophys. Acta Mol. Basis Dis. 1867, 166230. doi:10.1016/j.bbadis.2021.166230
Xuying, W., Jiangbo, Z., Yuping, Z., Xili, M., Yiwen, Z., Tianbao, Z., et al. (2010). Effect of astragaloside IV on the general and peripartum reproductive toxicity in Sprague-Dawley rats. Int. J. Toxicol. 29, 505–516. doi:10.1177/1091581810376840
Yang, B., Sun, Y., Lv, C., Zhang, W., and Chen, Y. (2020). Procyanidins exhibits neuroprotective activities against cerebral ischemia reperfusion injury by inhibiting TLR4-NLRP3 inflammasome signal pathway. Psychopharmacol. (Berl.) 237, 3283–3293. doi:10.1007/s00213-020-05610-z
Yang, L.-X., Zhang, X., and Zhao, G. (2016). Ginsenoside Rd attenuates DNA damage by increasing expression of DNA glycosylase endonuclease VIII-like proteins after focal cerebral ischemia. Chin. Med. J. (Engl.) 129, 1955–1962. doi:10.4103/0366-6999.187851
Yang, M.-Y., Song, Z.-Y., Gan, H.-L., Zheng, M.-H., Liu, Q., Meng, X.-T., et al. (2022b). Non-clinical safety evaluation of salvianolic acid A: acute, 4-week intravenous toxicities and genotoxicity evaluations. BMC Pharmacol. Toxicol. 23, 83. doi:10.1186/s40360-022-00622-1
Yang, X., Zheng, T., Hong, H., Cai, N., Zhou, X., Sun, C., et al. (2018). Neuroprotective effects of Ginkgo biloba extract and Ginkgolide B against oxygen-glucose deprivation/reoxygenation and glucose injury in a new in vitro multicellular network model. Front. Med. 12, 307–318. doi:10.1007/s11684-017-0547-2
Yang, Y., Song, J., Liu, N., Wei, G., Liu, S., Zhang, S., et al. (2022a). Salvianolic acid A relieves cognitive disorder after chronic cerebral ischemia: involvement of Drd2/Cryab/NF-κB pathway. Pharmacol. Res. 175, 105989. doi:10.1016/j.phrs.2021.105989
Yao, Z.-H., Wang, J., Yuan, J.-P., Xiao, K., Zhang, S.-F., Xie, Y.-C., et al. (2021). EGB761 ameliorates chronic cerebral hypoperfusion-induced cognitive dysfunction and synaptic plasticity impairment. Aging (Albany NY) 13, 9522–9541. doi:10.18632/aging.202555
Yu, H., Li, W., Cao, X., Wang, X., Zhao, Y., Song, L., et al. (2019). Echinocystic acid, a natural plant extract, alleviates cerebral ischemia/reperfusion injury via inhibiting the JNK signaling pathway. Eur. J. Pharmacol. 861, 172610. doi:10.1016/j.ejphar.2019.172610
Yu, H., Liu, P., Tang, H., Jing, J., Lv, X., Chen, L., et al. (2016). Oleuropein, a natural extract from plants, offers neuroprotection in focal cerebral ischemia/reperfusion injury in mice. Eur. J. Pharmacol. 775, 113–119. doi:10.1016/j.ejphar.2016.02.027
Yu, L., Zhang, Y., Chen, Q., He, Y., Zhou, H., Wan, H., et al. (2022). Formononetin protects against inflammation associated with cerebral ischemia-reperfusion injury in rats by targeting the JAK2/STAT3 signaling pathway. Biomed. Pharmacother. 149, 112836. doi:10.1016/j.biopha.2022.112836
Yuan, L., Sun, S., Pan, X., Zheng, L., Li, Y., Yang, J., et al. (2020). Pseudoginsenoside-F11 improves long-term neurological function and promotes neurogenesis after transient cerebral ischemia in mice. Neurochem. Int. 133, 104586. doi:10.1016/j.neuint.2019.104586
Zhang, C., Liu, X., Xu, H., Hu, G., Zhang, X., Xie, Z., et al. (2020a). Protopanaxadiol ginsenoside Rd protects against NMDA receptor-mediated excitotoxicity by attenuating calcineurin-regulated DAPK1 activity. Sci. Rep. 10, 8078. doi:10.1038/s41598-020-64738-2
Zhang, J., Liu, M., Huang, M., Chen, M., Zhang, D., Luo, L., et al. (2019). Ginsenoside F1 promotes angiogenesis by activating the IGF-1/IGF1R pathway. Pharmacol. Res. 144, 292–305. doi:10.1016/j.phrs.2019.04.021
Zhang, J., Wu, C., Gao, L., Du, G., and Qin, X. (2020b). Astragaloside IV derived from Astragalus membranaceus: a research review on the pharmacological effects. Adv. Pharmacol. 87, 89–112. doi:10.1016/bs.apha.2019.08.002
Zhang, P., Xu, J., Cui, Q., Lin, G., Wang, F., Ding, X., et al. (2023b). Multi-pathway neuroprotective effects of a novel salidroside derivative SHPL-49 against acute cerebral ischemic injury. Eur. J. Pharmacol. 949, 175716. doi:10.1016/j.ejphar.2023.175716
Zhang, Q.-Y., Wang, Z.-J., Sun, D.-M., Wang, Y., Xu, P., Wu, W.-J., et al. (2017a). Novel therapeutic effects of leonurine on ischemic stroke: new mechanisms of BBB integrity. Oxid. Med. Cell. Longev. 2017, 7150376. doi:10.1155/2017/7150376
Zhang, S., Song, Z., Shi, L., Zhou, L., Zhang, J., Cui, J., et al. (2021). A dandelion polysaccharide and its selenium nanoparticles: structure features and evaluation of anti-tumor activity in zebrafish models. Carbohydr. Polym. 270, 118365. doi:10.1016/j.carbpol.2021.118365
Zhang, S., Yu, Y., Sheng, M., Chen, X., Wu, Q., Kou, J., et al. (2023c). Ruscogenin timing administration mitigates cerebral ischemia-reperfusion injury through regulating circadian genes and activating Nrf2 pathway. Phytomedicine 120, 155028. doi:10.1016/j.phymed.2023.155028
Zhang, W., Song, J.-K., Yan, R., Li, L., Xiao, Z.-Y., Zhou, W.-X., et al. (2018). Diterpene ginkgolides protect against cerebral ischemia/reperfusion damage in rats by activating Nrf2 and CREB through PI3K/Akt signaling. Acta Pharmacol. Sin. 39, 1259–1272. doi:10.1038/aps.2017.149
Zhang, W., Zhang, P., Xu, L.-H., Gao, K., Zhang, J.-L., Yao, M.-N., et al. (2024). Ethanol extract of Verbena officinalis alleviates MCAO-induced ischaemic stroke by inhibiting IL17A pathway-regulated neuroinflammation. Phytomedicine 123, 155237. doi:10.1016/j.phymed.2023.155237
Zhang, W., Zhang, Q., Deng, W., Li, Y., Xing, G., Shi, X., et al. (2014). Neuroprotective effect of pretreatment with ganoderma lucidum in cerebral ischemia/reperfusion injury in rat hippocampus. Neural Regen. Res. 9, 1446–1452. doi:10.4103/1673-5374.139461
Zhang, Y., Zhang, X., Cui, L., Chen, R., Zhang, C., Li, Y., et al. (2017b). Salvianolic Acids for Injection (SAFI) promotes functional recovery and neurogenesis via sonic hedgehog pathway after stroke in mice. Neurochem. Int. 110, 38–48. doi:10.1016/j.neuint.2017.09.001
Zhang, Y.-C., Gan, F.-F., Shelar, S. B., Ng, K.-Y., and Chew, E.-H. (2013). Antioxidant and Nrf2 inducing activities of luteolin, a flavonoid constituent in Ixeris sonchifolia Hance, provide neuroprotective effects against ischemia-induced cellular injury. Food Chem. Toxicol. 59, 272–280. doi:10.1016/j.fct.2013.05.058
Zhang, Z., Shu, X., Cao, Q., Xu, L., Wang, Z., Li, C., et al. (2023a). Compound from magnolia officinalis ameliorates white matter injury by promoting oligodendrocyte maturation in chronic cerebral ischemia models. Neurosci. Bull. 39, 1497–1511. doi:10.1007/s12264-023-01068-z
Zhao, G.-C., Yuan, Y.-L., Chai, F.-R., and Ji, F.-J. (2017). Effect of Melilotus officinalis extract on the apoptosis of brain tissues by altering cerebral thrombosis and inflammatory mediators in acute cerebral ischemia. Biomed. Pharmacother. 89, 1346–1352. doi:10.1016/j.biopha.2017.02.109
Zhao, P., Zhou, R., Zhu, X.-Y., Hao, Y.-J., Li, N., Wang, J., et al. (2015). Matrine attenuates focal cerebral ischemic injury by improving antioxidant activity and inhibiting apoptosis in mice. Int. J. Mol. Med. 36, 633–644. doi:10.3892/ijmm.2015.2260
Zhao, Y., Shi, X., Wang, J., Mang, J., and Xu, Z. (2021). Betulinic acid ameliorates cerebral injury in middle cerebral artery occlusion rats through regulating autophagy. ACS Chem. Neurosci. 12, 2829–2837. doi:10.1021/acschemneuro.1c00198
Zheng, M., Zhou, M., Chen, M., Lu, Y., Shi, D., Wang, J., et al. (2021). Neuroprotective effect of daidzein extracted from pueraria lobate radix in a stroke model via the akt/mTOR/BDNF channel. Front. Pharmacol. 12, 772485. doi:10.3389/fphar.2021.772485
Zhou, D., Cen, K., Liu, W., Liu, F., Liu, R., Sun, Y., et al. (2021b). Xuesaitong exerts long-term neuroprotection for stroke recovery by inhibiting the ROCKII pathway, in vitro and in vivo. J. Ethnopharmacol. 272, 113943. doi:10.1016/j.jep.2021.113943
Zhou, M., Li, D., Li, L., Zhao, P., Yue, S., Li, X., et al. (2021a). Post-stroke treatment of storax improves long-term outcomes of stroke in rats. J. Ethnopharmacol. 280, 114467. doi:10.1016/j.jep.2021.114467
Zhou, Y., Li, H., Lu, L., Fu, D., Liu, A., Li, J., et al. (2014). Ginsenoside Rg1 provides neuroprotection against blood brain barrier disruption and neurological injury in a rat model of cerebral ischemia/reperfusion through downregulation of aquaporin 4 expression. Phytomedicine 21, 998–1003. doi:10.1016/j.phymed.2013.12.005
Zhu, J.-D., Wang, J.-J., Zhang, X.-H., Yu, Y., and Kang, Z.-S. (2018). Panax ginseng extract attenuates neuronal injury and cognitive deficits in rats with vascular dementia induced by chronic cerebral hypoperfusion. Neural Regen. Res. 13, 664–672. doi:10.4103/1673-5374.230292
Zhu, Y. Z., Wu, W., Zhu, Q., and Liu, X. (2018). Discovery of Leonuri and therapeutical applications: from bench to bedside. Pharmacol. Ther. 188, 26–35. doi:10.1016/j.pharmthera.2018.01.006
Zong, W., Zeng, X., Chen, S., Chen, L., Zhou, L., Wang, X., et al. (2019). Ginsenoside compound K attenuates cognitive deficits in vascular dementia rats by reducing the Aβ deposition. J. Pharmacol. Sci. 139, 223–230. doi:10.1016/j.jphs.2019.01.013
Glossary
Keywords: natural herbal extract, herbs, cerebral ischemia, blood-brain barrier, flavonoids
Citation: Yang J, Yu B and Zheng J (2024) Natural herbal extract roles and mechanisms in treating cerebral ischemia: A systematic review. Front. Pharmacol. 15:1424146. doi: 10.3389/fphar.2024.1424146
Received: 27 April 2024; Accepted: 03 July 2024;
Published: 02 August 2024.
Edited by:
Nouria Lakhdar-Ghazal, Mohammed V University, MoroccoReviewed by:
Ahmed Elsayed Noreldin, Damanhour University, EgyptYuxiang Fei, China Pharmaceutical University, China
Copyright © 2024 Yang, Yu and Zheng. This is an open-access article distributed under the terms of the Creative Commons Attribution License (CC BY). The use, distribution or reproduction in other forums is permitted, provided the original author(s) and the copyright owner(s) are credited and that the original publication in this journal is cited, in accordance with accepted academic practice. No use, distribution or reproduction is permitted which does not comply with these terms.
*Correspondence: Jian Zheng, emhlbmdqaWFuQGNtdS5lZHUuY24=