- College of Bioscience and Biotechnology, Hunan Agricultural University, Changsha, China
Osteoporosis is a common chronic metabolic bone disease caused by disturbances in normal bone metabolism and an imbalance between osteoblasts and osteoclasts. Osteoporosis is characterized by a decrease in bone mass and bone density, leading to increased bone fragility. Osteoporosis is usually treated with medications and surgical methods, but these methods often produce certain side effects. Therefore, the use of traditional herbal ingredients for the treatment of osteoporosis has become a focus of attention and a hot topic in recent years. Curcumin, widely distributed among herbs such as turmeric, tulip, and curcuma longa, contains phenolic, terpenoid, and flavonoid components. Modern pharmacological studies have confirmed that curcumin has a variety of functions including antioxidant and anti-inflammatory properties. In addition, curcumin positively regulates the differentiation and promotes the proliferation of osteoblasts, which play a crucial role in bone formation. Multiple studies have shown that curcumin is effective in the treatment of osteoporosis as it interacts with a variety of signaling pathway targets, thereby interfering with the formation of osteoblasts and osteoclasts and regulating the development of osteoporosis. This review summarized the key signaling pathways and their mechanisms of action of curcumin in the prevention and treatment of osteoporosis and analyzed their characteristics and their relationship with osteoporosis and curcumin. This not only proves the medicinal value of curcumin as a traditional herbal ingredient but also further elucidates the molecular mechanism of curcumin’s anti-osteoporosis effect, providing new perspectives for the prevention and treatment of osteoporosis through multiple pathways.
1 Introduction
As the aging population grows, osteoporosis (OP) has emerged as one of the top three major chronic diseases in China (Yu and Xia, 2019). OP is a disease in which bone mass is reduced, microstructure deteriorates and fragility fractures occur. The main reason is the upregulation of osteoclasts, resulting in an imbalance between osteoblasts and osteoclasts, which are the basic cells for bone growth and maintenance because they form bone tissue, and an imbalance between the two can lead to OP (Visconti et al., 2019). Research findings have shown that in the maintenance of the bone metabolic balance, the critical stem cells are BMSC (Wang et al., 2016). Furthermore, the osteogenic conversion of bone marrow mesenchymal stromal cells (BMSCs) is a primary provider of osteoblast precursor cells (Wilkesmann et al., 2020). Osteoblasts are fundamental cells that produce bone, controlling mineralization, and driving bone development (Florencio-Silva et al., 2015). Therefore, a sufficient source of osteoblasts is the key to ensuring bone reconstruction and maintaining bone metabolic balance. OP is often seen to fall in either primary or secondary category and it affects people of all ethnic backgrounds, as well as many older men and women (Romagnoli et al., 2011). Preventing OP calls for reaching a normal peak bone mass, but this requires an individual to nourish their body with a healthy diet comprising calcium, vitamin D in plenty, regular menstrual cycles, and a comprehensive physical workout schedule (Weaver et al., 2016). Menopause triggers significant hormonal fluctuations and localized oxidative inflammation predominantly in women, disrupting the delicate balance maintained by osteoclasts and osteoblasts. This disruption accelerates the process of bone degeneration, ultimately contributing to the development of OP, a condition characterized by weakened bones and increased fracture risk (Baastrup, 2016).
Since ancient times, Chinese herbal medicine has constituted an indispensable and fundamental part of the medical field, carrying with it profound traditional wisdom (Yuan H. et al., 2016). The advancement of modern science and technology has greatly facilitated the in-depth study of Chinese herbal medicine, enabling scientists to accurately analyze its chemical composition and elucidate its complex pharmacological mechanisms (Lihong, 2019). This has resulted in the establishment of a more solid scientific foundation for the application of traditional Chinese herbal medicine. Currently, Chinese herbal medicine is gradually becoming a bridge and intersection point connecting traditional and modern, Eastern and Western medicine (Scheid, 2002). Its wide variety and different efficacy are not only widely used globally for the treatment of all kinds of diseases, but also become a vivid embodiment of the concept of mankind’s pursuit of a harmonious symbiosis with nature. From the long heritage of the ancient classic Shennong’s Classic of Materia Medica to the continuous exploration of modern scientific research, Chinese herbs have consistently played a pivotal role in the history of human medical practice, and continue to contribute to human health and wellbeing in a unique way (Liu et al., 2018). Over time, they have developed a comprehensive theoretical framework and a set of sophisticated practical techniques, which have been passed down from generation to generation through the accumulated wisdom of countless medical practitioners. The essence of Chinese herbal medicine lies in the uniqueness of its “four qi and five flavors” concept, which reveals the subtle regulating effects of the four qualities of cold, heat, warmth, and coolness, as well as the five flavors of acidity, bitterness, sweetness, pungency, and saltiness on the balance of the human body’s internal environment (Zhaoguo et al., 2021). This concept enables the comprehensive goals of disease prevention, treatment, and healthcare to be achieved.
In recent times, turmeric has garnered widespread attention for its potent anti-inflammatory, pain-relieving, and blood circulation-enhancing properties, as documented in reference (Yuandani et al., 2021). Through meticulous clinical research, scientists have successfully identified and isolated key constituents from the turmeric root, including polysaccharides and the bioactive compound curcumin (CUR), as noted in reference (Jiang T. et al., 2021). The discovery that turmeric extracts are capable of inducing both vasoconstriction and vasodilation has significantly expanded our comprehension of turmeric’s multifaceted therapeutic mechanisms. This insight also offers a crucial perspective for the development of medicinal spices rich in curcumin, potentially revolutionizing the field of herbal medicine.
CUR is a bioactive compound extracted from the root of the turmeric plant and has excellent antioxidant and anti-inflammatory properties due to its unique phenolic structure. Several studies have confirmed its effectiveness in combating intracellular reactive oxygen species (ROS) and scavenging free radicals, thereby reducing oxidative stress and enhancing the immune system (Iddir et al., 2020). Therefore, CUR has been widely used in the treatment of cancer, cardiovascular diseases, osteoporosis, etc. The special molecular composition of CUR endows it with unique chemical properties, and the morphological equilibrium of its heptadienone molecules in different pH environments is crucial for its antioxidant and physicochemical properties (Stanić, 2017; Luca et al., 2020; Slika and Patra, 2020).
In addition, CUR has been reported to protect against oxidative damage and promote osteoblast differentiation by attenuating the inhibition of Wnt/βcatenin signaling (Manandhar et al., 2020). CUR can not only reduce the oxidative state of mitochondria but also improve the mitochondrial membrane potential and improve the oxidative stress-induced apoptosis of osteoblasts (Inchingolo et al., 2022). Therefore, CUR promotes the osteogenic differentiation and bone formation of BMSC by interfering with BMSC, osteoblasts, and osteoclasts. In addition, it promotes the growth and specialization of osteoblasts while inhibiting the proliferation and differentiation of osteoclasts, ultimately increasing bone density and improving the microstructure of bone trabeculae. For example, AHMED et al. found that adding CUR to a petri dish helped enhance the osteogenic differentiation of mouse BMSC (Ahmed et al., 2019). Not only that, but CUR can also protect osteoblast function by anti-oxidative stress. According to Li et al., Cur preconditioning reduced the apoptosis of osteoblasts and maintained their differentiation function by eliminating the inhibitory effect of ROS on the GSK3β-Nrf2 signaling pathway (Li et al., 2020a).
Mitogen-activated protein kinase (MAPK) can be switched on by a variety of extracellular stimuli and consists of a conserved tertiary kinase pattern (Bharti et al., 2021). This particular pathway helps regulate various bodily processes, including cell growth, stress response, and inflammation. It is a part of the signal transmission network in cells, regulating gene expression and cytoplasmic activity (Olivares-Bañuelos et al., 2019). In addition, there are specific pathways in the MAPK signaling pathway, such as the ERK1/2 pathway, the c-Jun N-terminal kinase (JNK) pathway, the P38 pathway, and the Extracellular signal-regulated kinase 5 (ERK5) pathway, which have been associated with diseases such as OP and bone formation (Rodríguez-Carballo et al., 2016). Innate immune cells and inflammatory T cells are regulated by nuclear factor-kappa B (NF-κB) through its ability to modulate gene expression. The function of NF-κB is important in the context of different pathogens and infections as it ensures an adequate immune response (Liu et al., 2017). Two Rel family proteins (p50/p65) make up the inactive form which is normally bound to inhibitory proteins (i-κB) in the cytoplasm; the liberation of NF-κB p65/p50 dimerization allows for its transportation into the nucleus. Upon reaching the nucleus, the p65/p50 dimer binds to certain DNA sequences and starts transcription for target genes that are important in different cellular activities such as cell growth (Wang et al., 2015). In a lot of illnesses, NF-κB is highly stimulated in the inflammation zones; this results in the production of pro-inflammatory mediators including cyclooxygenase-2 (COX-2) among others (Al-Harbi et al., 2016). Hence NF-κB has emerged as a target for the progression of anti-inflammatory and anti-cancer drugs. The study of NF-κB and its signaling pathway becomes unavoidable in appreciating its role in OP and devising ways to regulate its functioning efficiently. One of the physiological processes that the pathway for PI3K-AKT is associated with include obesity, diabetes, and cancer. Given its importance and potential for therapeutic intervention, it remains a fascinating and important area of research. Signaling cascades mediated by Phosphoinositide 3-Kinase (PI3K) and Protein kinase B (Akt) regulate key cellular activities and influence tissue-specific functions in adipose tissue, skeletal muscle, liver, brain, and pancreas (Savova et al., 2023). Class I PI3K regulates the production of the vital phosphatidylinositol second messenger that is essential for maintaining a balance in the body, it also contributes to the development of many human illnesses, such as cancer and metabolic ailments (Fruman et al., 2017). This process is initiated by the initiation of tyrosine kinase or G protein-coupled receptors, which leads to the generation of Phosphatidylinositol (Florencio-Silva et al., 2015; Wang et al., 2016; Wilkesmann et al., 2020) trisphosphate (PIP3). This PIP3 further triggers downstream effectors resulting in the amplification of the signal cascade (Oeckinghaus and Ghosh, 2009; Fruman et al., 2017). These signaling pathways have their characteristic targets for OP, and CUR can act on these targets to inhibit or activate the signaling pathway to achieve the purpose of treating OP (Figure 1).
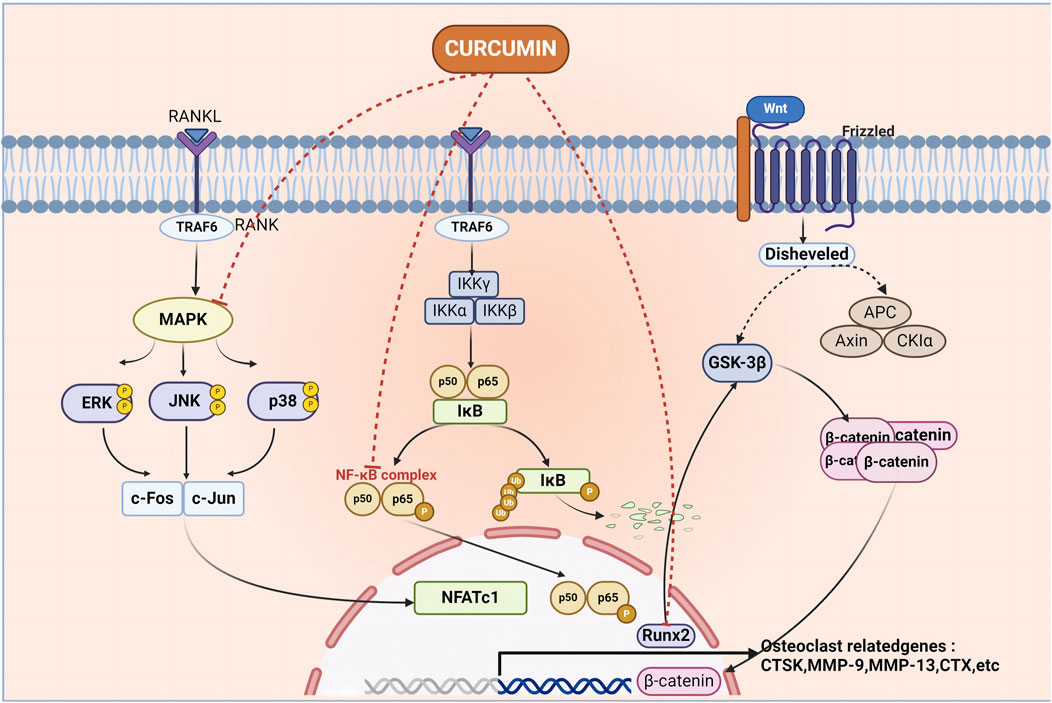
Figure 1. Curcumin acts on multiple pathways to inhibit the formation of osteoclasts, thus achieving the purpose of treating osteoporosis. Curcumin mediates the occurrence of osteoporosis by acting on MAPK, NF-κB, Wnt signaling pathway, MAPK, nuclear factor kappa-B complex, and Runt-related transcription factor 2 molecules.
2 Curcumin
Throughout China’s long history, herbs have consistently held a prominent position in the treatment of human diseases and the maintenance of health. This reflects the profound depth and rich heritage of traditional Chinese medicine (Unschuld, 2018). Chinese herbs, a magnificent cultural legacy, have been used for thousands of years, not only embodying the wisdom and vitality of the ancients but also representing a precious gift from nature. They encompass a vast array of subjects, including plants, animals, and minerals (Hageneder, 2020). Each type of Chinese herbal medicine is a unique chemical treasure trove in nature, containing a variety of pharmacological components such as flavonoids, alkaloids, polysaccharides, volatile oils, etc (Kuzmishyna, 2024). These components exhibit multiple biological activities, including anti-inflammatory, anti-bacterial, anti-viral, enhancement of immunity, and optimization of microcirculation in the body. This highlights the infinite charms of Chinese herbal medicine.
Chinese medicine theory profoundly recognizes that the human body is a complex and sophisticated organic system and that the occurrence of diseases is often a reflection of the imbalance of yin and yang in the body and the dysfunction of the internal organs. Therefore, Chinese herbal medicine treatment upholds the principle of “evidence-based treatment”, i.e., based on the patient’s specific condition, physical differences, age and gender characteristics, a careful and detailed consideration, carefully formulated drug combinations, and strive to achieve both symptomatic and curative effects (Hoover, 2021). In addition, the scope of application of Chinese herbs goes far beyond drug treatment, they are also cleverly integrated into food therapy, medicinal meals healthcare products, and other areas of life, becoming an indispensable part of the pursuit of health and enjoyment of life (Hong et al., 2022). In recent years, with the rapid development of science and technology, there has been an explosion of research on the isolation and development of biologically active components from Chinese herbal medicines, which have attracted much attention because of their remarkable pharmacological properties. What is even more gratifying is that the influence of Chinese herbs and their preparations has crossed national borders, and they are not only trusted in China but are also gaining recognition and popularity in Europe and North America as complementary therapeutic means (Rausch et al., 2024). With the help of advanced extraction, separation, and identification technologies, we have been able to explore the pharmacological mechanisms of Chinese herbs and reveal their scientific mysteries, contributing indelibly to the cause of human health and medical progress.
Among them, turmeric is particularly widely used in ancient medicinal formulas, and its medicinal value has been fully reflected in traditional Chinese medicine formulas. For example, turmeric powder paired with other herbs can effectively treat intractable cardiac pain as documented in Sheng Ji Gong Lu (Liu, 2024). This is due to the efficacy of turmeric in activating blood circulation and removing blood stasis, thus relieving heart pain. It is recorded in ancient books that turmeric is used in combination with Citrus aurantium and cinnamon bark to relieve stomach pain due to liver depression and stagnation of qi (McCaskill, 2021). This shows that the combination has unique efficacy in dispersing the liver and regulating qi, moving qi, and relieving pain.
CUR, an extracted component of herbal medicine, is also a highly versatile natural polyphenolic compound that can be isolated from the rhizomes of plants in the Zingaceae and Araceae families, such as Curcuma (C. zedoaria (Berg.) Rosc.), tulip (Curcuma aromatica Salisb.) and others (Jabczyk et al., 2021). In addition, CUR boasts a rich history of widespread consumption as a dietary spice and food coloring, and it is increasingly garnering attention for its diverse pharmacological benefits, primarily its anti-inflammatory and antioxidant properties (Esatbeyoglu et al., 2012a). Its molecular formula is C21H20O6. In varying pH chemical environments, CUR possesses seven carbon atoms on its carbon chain and features two keto groups that can undergo keto-enol tautomerism. Consequently, CUR’s chemical structure is not stable at the physiological pH value (Stanić, 2017). CUR is almost completely insoluble in water, but soluble in organic solvents such as acetone and ethanol, and is fairly stable in the acidic pH of the stomach. From a chemical point of view, the molecule has two similar aromatic ring symmetrys and has conjugated double bonds as effective electron donors to hinder the formation of ROS (Parcheta et al., 2021). Therefore, the main function of CUR is reflected in its functional groups: phenolic groups and diketone structures. These two active functional groups mediate the hydrogen supply reaction of CUR, the Michael addition reaction, and a series of hydrolytic and enzymatic reactions (Figure 2) (Esatbeyoglu et al., 2012b). Despite its therapeutic potential, curcumin’s swift metabolism leads to poor oral bioavailability. Following ingestion, the majority is expelled through bile and feces, with a substantial portion (40%–85%) traversing the gastrointestinal tract unaltered, despite the presence of certain gut microbiota. To enhance its bioavailability, CUR can be co-administered with bromelain (Joshi et al., 2023). Additionally, researchers have proposed an innovative nanotechnology strategy to precisely overcome existing challenges and significantly advance the in-depth study of CUR in both in vitro experiments and in vivo applications (Li and Kataoka, 2020). This strategy exploits the distinctive benefits of nanoparticles, encompassing micelles, liposomes, and nanogels, to markedly enhance the efficacy of CUR through two fundamental mechanisms (Jacob et al., 2024). Primarily, by enhancing solubility, it effectively facilitates the dissolution and distribution of CUR in living organisms. Secondly, by prolonging the blood circulation time and ingeniously blocking unwanted metabolic pathways, it significantly improves the bioavailability of CUR. The second strategy is to significantly enhance the bioavailability of CUR by extending the blood circulation time and subtly blocking unnecessary metabolic pathways. The researchers also found that curcumin’s effectiveness may indeed vary among different populations (Salehi et al., 2019). The study indicates that women may absorb curcumin more effectively than men. Based on area under the plasma concentration-time curve comparisons, the bioavailability of micronized CUR was several times higher in women than in men (the exact value was five times higher in women than in men). This suggests that women may derive higher biological effects from CUR at the same dose. Similarly, for micellar curcumin, bioavailability was significantly higher in females than in males (114 times higher in females than in males).
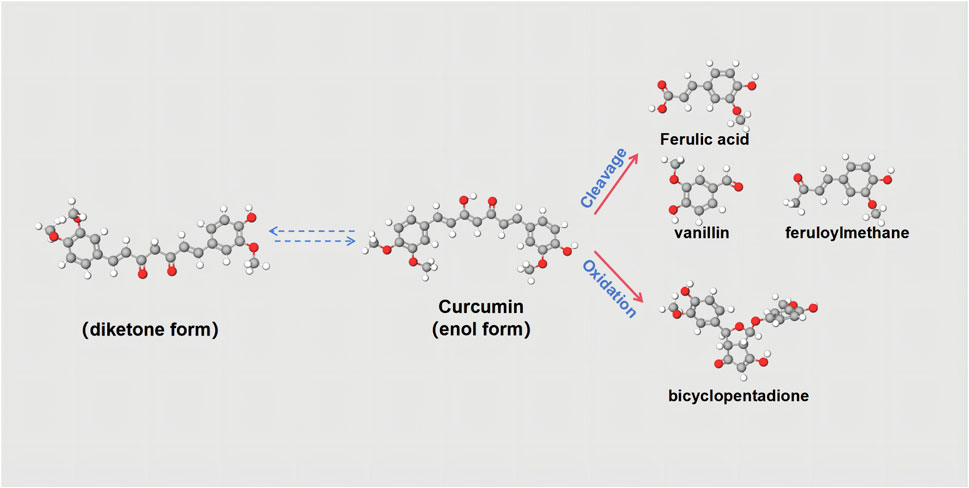
Figure 2. Chemical structures of curcuminoids. Curcumin has keto-enol tautomers, and the two can be converted to each other. Curcumin can be split into Ferulic acid, vanillin, and feruloyl methane. It can also be oxidized to bicyclopentadione.
Derived from natural plants, CUR is a ubiquitous bioactive supplement widely used in the treatment of chronic diseases, including cardiovascular diseases, diabetes, malignancies and osteoarthritis (Table 1). CUR counteracts inflammatory mediators and has the ability to neutralize reactive oxygen species in the body. In addition, it enhances the activity of enzymes such as superoxide dismutase and glutathione peroxidase, thereby strengthening the body’s antioxidant defenses (Sharifi-Rad et al., 2020). CUR is a natural compound that modulates a variety of signaling pathways and possesses both anti-inflammatory and antioxidant properties, and therefore has a positive impact on bone health (Antolin et al., 2024). Recent studies have shown that CUR can influence bone formation by regulating the distinction between osteoblasts (Blair et al., 2017). It promotes the proliferation of osteoblasts and increases the expression of important genes critical for bone formation, including alkaline phosphatase, osteocalcin (OCN), Runt-related transcription factor 2 (Runx2) and various other markers (Yang et al., 2023a). Furthermore, in studies investigating the efficacy of curcumin in the treatment of osteoporosis, researchers have used in vivo experiments to determine the optimal mode of administration and dosage due to the low bioavailability of curcumin. For example, in a related study conducted by Folwarczna et al. in an oestrogen-deficient rat model, it was observed that oral administration of 10 mg/kg/d for 4 weeks did not improve osteoporosis symptoms (Folwarczna, 2013). In contrast, other researchers increased the oral dose of curcumin to 110 mg/kg/d and showed that curcumin significantly enhanced oestrogen-deficiency-induced bone tissue morphology and markedly increased the number of osteoblasts in ovariectomized rats (Jiang Q. et al., 2021). These findings will be summarized in a subsequent review. It can therefore be concluded that the optimal mode of administration of curcumin is daily oral administration via feed and that lower doses may not achieve optimal therapeutic effects. Previous studies have found that the optimal therapeutic dose of curcumin is 110 mg/kg/d. Some findings related to potential side effects or adverse reactions associated with curcumin for the treatment of osteoporosis have emerged from recent studies and reports (Ghahfarrokhi et al., 2023). However, it is important to note that these findings may not be specific to the treatment of osteoporosis, but rather the general application of curcumin as a drug or supplement. For example, curcumin has been shown to potentially cause gastrointestinal disturbances, liver and kidney damage, and an increased risk of bleeding (Liu et al., 2022).
3 The cause and pathogenesis of OP
OP encompasses a range of bone conditions resulting from diverse factors, where the calcification process in bone tissue remains unaffected, calcium salt to the normal ratio of the matrix, and the unit volume of bone tissue reduction is characterized by metabolic bone disease. In most cases of OP, the loss of bone tissue is primarily caused by increased bone resorption. This disparity in the process of bone breakdown and regeneration leads to an increased susceptibility to experiencing fractures, which can significantly impact the quality of life and increase mortality rates in both men and women (Rinonapoli et al., 2021).
The causes of OP can be classified into primary and secondary categories (Figure 3). Based on the established theory, OP is primarily viewed as a bone remodeling disturbance stemming from factors like estrogen insufficiency or the aging process (referred to as primary OP). Conversely, secondary OP arises due to alternative underlying health conditions or medication use that contribute significantly to bone mass depletion (Zhang et al., 2022). Primary OP mainly includes senile and postmenopausal women. Secondary OP includes nutritional, disuse, endocrine, and so on. In women, menopause - the cessation of ovarian function, is one of the main causes of primary OP (Wu D. et al., 2021). The loss of ovarian function is a key factor contributing to bone loss in postmenopausal women, with estrogen believed to play a crucial part in facilitating this procedure (Cheng et al., 2022). Bone remodeling is regulated by estrogen through its control over the synthesis of cytokines and growth factors in bone marrow and bone cells (Crane and Cao, 2014). A number of studies have reported the mechanism of the development of postmenopausal osteoporosis, in which estrogen deficiency in the organism causes inflammatory factors and MicroRNA activation, which leads to disruption of the RANKL-RANK-OPG axis, causing bone loss (Seely et al., 2021). At the same time, estrogen acts as an antioxidant to protect bone against oxidative stress (Shi et al., 2015).
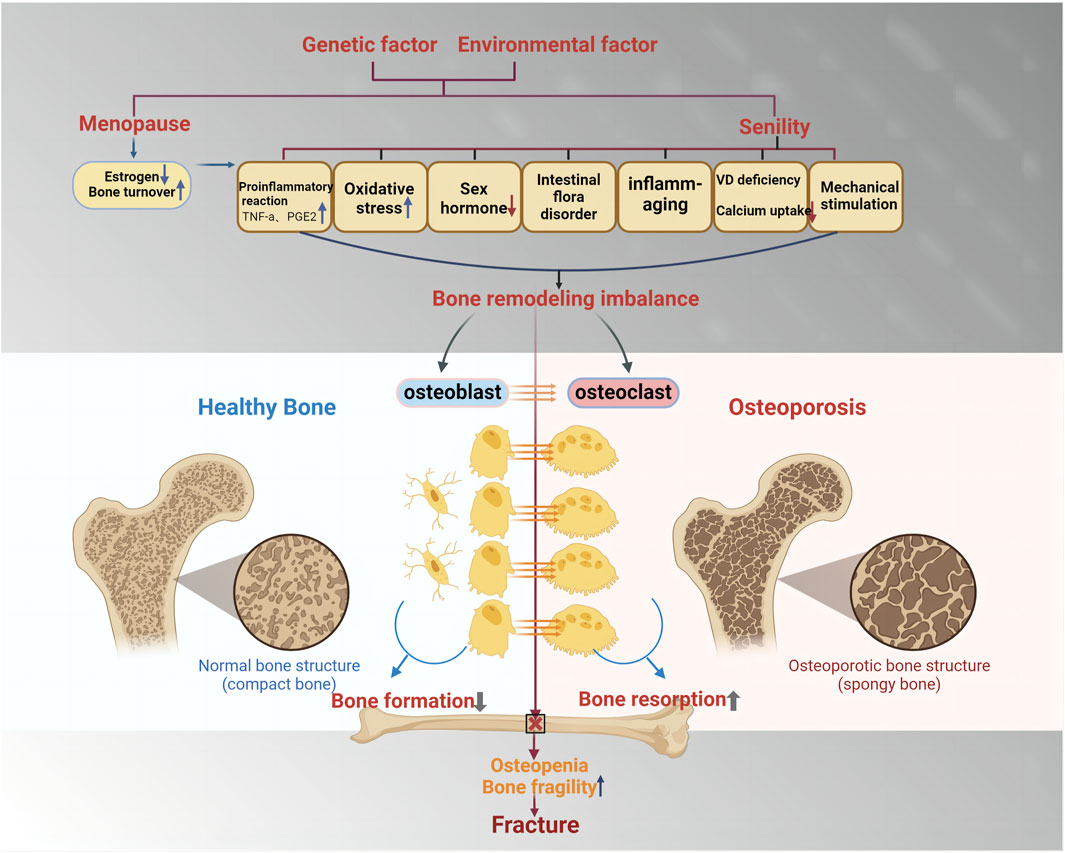
Figure 3. The cause and mechanism of primary osteoporosis. Primary osteoporosis is mainly determined by genetic factors and environmental factors and is divided into two categories: postmenopausal osteoporosis and age-related osteoporosis. Enhanced pro-inflammatory response, oxidative stress, reduced secretion of sex hormones, and intestinal flora disorder will lead to the imbalance of bone reconstruction, the reduction of osteoblast formation, and the increase of osteoclast formation, which will lead to the enhancement of bone absorption and the reduction of bone mass, thus inducing fracture.
Diabetes is the most common secondary cause of surgery. Several researchers have found an association between the management of blood glucose levels and the likelihood of fractures, as well as increased bone fragility in diabetic patients (Napoli et al., 2017). Studies have shown that people with diabetes have a significantly higher risk of fracture than the general population (Starup-Linde et al., 2017). In a comprehensive Nurses’ Health Study, the incidence of hip fracture in patients diagnosed with T1DM was found to be six times higher than the overall incidence of hip fracture observed in the general population in this particular study, which had an average age of 65 years (Vilaca et al., 2020). Persistent high blood sugar, a hallmark of diabetes, triggers the generation of advanced glycation end-products (AGEs) (Khalid et al., 2022). These molecules can cause significant alterations in the structure of type I collagen and other vital biological components that are central to preserving the structural integrity of bone, consequently impeding the osteogenic process. Research has demonstrated that the receptor for AGEs (RAGE) suppresses osteoblast proliferation by disrupting multiple signaling pathways, such as the PI3 kinase, extracellular signal-regulated kinase (ERK), and Wnt pathways (Zhou et al., 2023). Furthermore, RAGE plays a role in enhancing the development of osteoclasts, which can result in disordered bone formation. The aggregation of AGEs upon their binding to RAGE forms cross-links with bone matrix proteins, which could impact the rigidity and breaking point of the bone (Burr and Allen, 2019). It has been observed that individuals with diabetes often exhibit considerably lower levels of alkaline phosphatase, a critical enzyme for bone mineralization. A reduction in the activity of alkaline phosphatase is typically detected following the diagnosis of diabetes. In consequence, diabetes adversely influences bone metabolism, leading to the weakening of cellular functions and the deterioration of the extracellular matrix. This can manifest in the form of bone loss, an altered bone microarchitecture, a reduction in bone turnover, and an increased susceptibility to fractures even under low-impact conditions (Kemmler and von Stengel, 2019). Additionally, the development of mineral and vitamin D deficiencies can be attributed to gastrointestinal dysfunction, malnutrition, and malabsorption, ultimately leading to deterioration of bone health (Minisola et al., 2021). Notably, patients with chronic liver disease are at a higher risk of fracture due to hepatic osteodystrophy. Clinical conditions associated with wasting osteoporosis include spinal cord injuries as well as other neurological and neuromuscular disorders, post-fracture immobilization, and prolonged bed rest (either actual or simulated) (Ponzano, 2022). This results in immediate bone loss in the trabecular and cortical regions of the bone, a relative increase in bone resorption, and a decrease in bone formation. Thus, disuse is considered to be one of the main factors contributing to rapid bone loss and OP. In addition, alterations in osteoblastic pathways are thought to play a crucial role in the bone loss associated with wasted bone.
Diabetes is the most common secondary cause of OP. Some researchers have found that there exists a relationship between the management of blood sugar levels and the likelihood of experiencing fractures and that people with diabetes have increased bone fragility (Napoli et al., 2017). Studies have shown that people with diabetes have a significantly higher risk of fracture compared to the general population (Wallander et al., 2017). The occurrence of hip fractures among individuals diagnosed “with T1DM in a comprehensive Nurses” Health study was found to be sixfold greater compared to the overall prevalence of hip fractures observed within the general population sample of this particular investigation, who had an average age of 65 years (Ballane et al., 2014). Therefore, bone metabolism is negatively affected by diabetes, leading to impaired cell function and degradation of the extracellular matrix, which leads to bone loss, changes in bone microstructure, reduced bone turnover, and susceptibility to low-trauma fractures (Asadipooya and Uy, 2019). Furthermore, the occurrence of mineral and vitamin D deficiencies can be attributed to gastrointestinal disorders, malnutrition, and malabsorption, ultimately resulting in the deterioration of bone health. It is worth noting that individuals with chronic liver disease face an elevated risk of fractures due to hepatic bone dystrophy (Sobh et al., 2022). Clinical conditions linked to disuse OP encompass spinal cord injury, along with other neurological and neuromuscular disorders, immobilization following fractures, and prolonged periods of bed rest (whether actual or simulated) (Brent et al., 2021). This results in immediate loss of bone in trabeculae and cortical compartments, along with a relative increase in bone resorption and a decrease in bone formation (Seeman, 2003). Hence, disuse is considered one of the primary factors leading to rapid bone loss and OP. Additionally, alterations in osteocyte pathways are believed to play a crucial role in bone loss associated with bone disuse (Rolvien and Amling, 2022).
Initial investigations have indicated that bone loss begins shortly after injury in patients with spinal cord injury and nerve/neuromuscular disorders. During the first year, subregional bone sites may experience bone loss rates of up to 2%–4% per month (Poole et al., 2015). Nevertheless, disuse OP encompasses not only overall systemic bone loss but also localized bone loss in various conditions. For example, in a group of post-stroke patients, the lower limbs impacted by the stroke showed reduced mineral and geometric properties in comparison to the unaffected upper limbs (Kazakia et al., 2014).
From a mechanism point of view, an important factor that causes OP is oxidative stress, which usually refers to an excess of free radicals, an imbalance between free radical oxidants and antioxidants, resulting in cell damage that affects its contents (Manolagas, 2010). In addition, signaling pathways such as NF-κB are activated to produce downstream cytokines. As the oxidizer continues or increases, the entire physiological system is affected. Changes in cells and their organelles alter cell function by inhibiting or activating various cellular pathways (Liu and Tang, 2020).
The bone mineral density seems to be influenced by the consumption of phenols, as they function as scavengers of free radicals and safeguard against oxidative damage to bone cells (Chisari et al., 2019). Polyphenols target a range of molecular mechanisms and signaling pathways, including mTOR, NF-κB, and Wnt/β-catenin. Furthermore, they interact involving intracellular signaling cascades, including PI3K, PKB/Akt, tyrosine kinases, protein kinase C (PKC), and MAPKs (Maleki Dana et al., 2021). To date, a multitude of investigations have confirmed that curcumin plays a pivotal role in modulating the signaling pathways implicated in the pathogenesis of osteoporosis.
4 Mechanism of action of CUR against OP: Based on multiple pathways
The Wnt signaling pathway is highly conserved in biological evolution and is essential for core processes such as organism construction, cell proliferation, differentiation, and polarity determination (Habib and Acebrón, 2022). There are 19 Wnt proteins in mammals that differ in sequence but share lipid modifications, secreted glycoproteins, and conserved cysteine residues, features that enable enhanced function via Frizzled receptors and the LGR5/6 complex (Martin-Orozco et al., 2019). Wnt signaling is classified into classical (β-catenin-dependent) and non-classical categories. Aberrant expression of the core transcription factor β-catenin is closely associated with a variety of diseases, especially tumor formation (Wu Z-L. et al., 2021; Zhu et al., 2022). In the Wnt/β-catenin pathway, β-catenin accumulates and enters the nucleus to regulate gene expression. In addition, several components of the Wnt pathway are strongly associated with the development of cancer and degenerative diseases (SMA, 2020). In addition, hereditary bone mass abnormalities are associated with specific mutations in LRP5, Wnt5a, ROR2, and DVL1, and these abnormalities not only affect the skeletal system but also spread to other parts of the body (Table 2). These findings not only reveal the strong connection between the Wnt signaling pathway and human health but also deepen our knowledge of the signaling mechanisms.
Furthermore, the Wnt signaling pathway is critical for OP, as it regulates the growth, differentiation, and apoptosis of mesenchymal stem cells (MSCs), driving the differentiation of bone marrow progenitor cells to osteoblasts and influencing bone formation and growth (Amjadi-Moheb and Akhavan-Niaki, 2019). This process is achieved by inhibiting adipocyte differentiation-associated transcription factors and enhancing osteoblast differentiation-associated transcription factor expression, balancing adipogenesis and osteogenesis. In the absence of β-catenin, cells may develop into chondrocytes rather than osteoblasts (Yuan X. et al., 2016). Wnt signaling also promotes osteoblast formation and inhibits anisocytosis, in contrast to PPARγ. In some cases, increased Wnt signaling reduces osteoclast genesis and bone resorption by elevating osteoblast protein expression in osteoblasts and inhibiting the binding of RANKL to osteoclast precursors using bone-protecting aggrecan, which inhibits osteoclast differentiation and activity and reduces bone resorption (Srisubin, 2021).
NF-κB is a key factor in the regulation of immune cells, affecting survival, activation, and differentiation, especially innate immune and inflammatory T cells. It is bound by p50/p65 to IκB in an inactive complex (Baldwin, 1996). signals such as TNF activate IKK, which degrades IκB and releases NF-κB into nuclear regulatory genes (Hayden and Ghosh, 2014). Continued activation may lead to aberrant cell proliferation. NF-κB is important in inflammation, promoting pro-inflammatory factors and COX-2 expression, with potential as an anti-inflammatory and anti-cancer drug. In osteoporosis, NF-κB regulates osteogenic and osteoclast function (Deng et al., 2024). Upon activation, p50/p65 enters the nucleus to promote transcription, especially in the RANKL-RANK environment to induce osteoclast formation, and with c-Fos to form AP-1, which is critical for osteoclast development (Qu et al., 2022). It was also found that inhibition of the NF-κB signaling pathway suppressed RANKL-induced osteoclast differentiation. In addition, the cytokine TNF-α promotes RANK-RANKL binding through activation of the NF-κB pathway, which in turn promotes osteoclast genesis (Amin et al., 2020). The pathogenesis of OP is closely related to inflammation and oxidative stress, in which NF-κB plays a central role, responding to oxidative stress by decreasing SOD expression and increasing MDA synthesis (Neganova et al., 2021). ROS accumulation also enhances NF-κB through phosphorylation activation, leading to the upregulation of inflammatory cytokines and NLRP3 inflammasome synthesis (Sho and Xu, 2019). AP-1, a downstream factor of NF-κB, is responsible for regulating oxidative stress-induced pro-inflammatory cytokine production (Lee et al., 2017). Notably, the NF-κB signaling pathway is regulated by estrogen and affects ERα and β activity (Chen et al., 2018). In menopausal women, estrogen deficiency leads to increased secretion of pro-inflammatory cytokines such as TNF-α and IL-6, which in turn may induce OP (Salamanna et al., 2018).
After a long period of extensive research, the PI3K-AKT pathway is also a compelling area of study due to its multiple functions. This signaling pathway involves key proteins such as PI3K and Akt, which play critical roles in mediating growth factor signaling, organismal growth, and regulation of fundamental cellular processes (He et al., 2021). In addition, the PI3K-AKT pathway affects cell differentiation, metabolic activity, cytoskeletal organization, and key processes such as apoptosis and cancer cell survival (He et al., 2021). Thus, this pathway is intricately linked to a range of health problems such as osteoporosis. Osteoblast growth and differentiation are regulated by PI3K/AKT and osteoblast signaling pathways involved in the OP process (Ponzetti and Rucci, 2021). It was found that reducing p-PI3K and p-AKT expression inhibited PI3K/AKT pathway activation in osteoblasts. In OP model rats, although the mRNA levels of PI3K, PDK1, and Akt were stable, the expression of phosphorylated proteins was significantly decreased (Jantan et al., 2021). LY294002, a PI3K inhibitor, blocked PI3K/Akt signaling in osteoblasts and inhibited cell proliferation, ALP activity, calcium accumulation, and expression of osteogenic markers, indicating that the PI3K/Akt pathway is essential for osteoblast function and mineralized bone formation is critical (Zhou et al., 2019). Also, LY294002 promoted the mRNA expression of Caspase-3 and Caspase-9. Dexamethasone, as a synthetic glucocorticoid, downregulated p-PI3K and p-AKT, upregulated GSK-3β expression, inhibited osteoblast proliferation and induced apoptosis, which was useful for the treatment of glucocorticoid-induced OP (Deng et al., 2019). In contrast, IL-37 activated the PI3K/AKT pathway, promoting bone formation-related gene expression, mineral deposition, and ALP function in MSCs. PI3K/AKT inhibitors partially reversed the enhancing effect of IL-37 on osteogenic differentiation of MSCs (Ye et al., 2019).
Signaling between the cell surface and the nucleus is handled by a group of protein kinases, MAPK, which can be activated by external factors such as hormones, stress, and adhesion, and are key to cellular communication (Jarouliya and Keservani, 2019). MAPK gets its name from its activation by mitogens such as growth factors (Yue and López, 2020). Across the evolutionary spectrum, from yeast to Homo sapiens, the MAPK cascade maintains a consistent three-tiered kinase configuration. This includes the presence of upstream kinases like MAP4K and downstream effectors such as MAPKAPK (Rahman, 2018). The pathway is activated by a three-order enzymatic cascade from MAP3K, MAPKK to MAPK, which regulates cell growth, differentiation, stress adaptation, and inflammation. The MAPK signaling pathway includes ERK1/2, JNK, P38, and ERK5, which respond to different stimuli and are involved in a variety of cellular processes (Guo et al., 2020). Factors related to OP such as RANKL, OPG, PTHBMP, TGF-B, IL-1, IL6, TNF-a, and estrogen are associated with the MAPK signaling pathway. RANKL promotes osteoclast differentiation through the activation of RANK, which then activates signaling pathways such as NF-KBNFATc1, AP-1, MAPK, and so on. Estrogen, on the other hand, promotes osteoblast proliferation and inhibits osteoclast apoptosis through activation of the MAPK pathway, such as ERK1/2, P38, and JNK, and plays an anti-OP role (Su et al., 2024).
The therapeutic potential of curcumin for osteoporosis is well-documented and is supported by a substantial body of evidence derived from a variety of biological mechanisms and a considerable number of long-term studies. All of these studies have yielded positive results, and some have progressed to the clinical trial stage. However, there is still a paucity of relevant clinical research data. Consequently, there has been a notable surge in scientific interest in curcumin, largely due to its remarkable capacity to safeguard bone health and its vast range of therapeutic applications. Findings from preclinical and a limited number of clinical studies indicate that curcumin exerts a profound influence on the activities of osteoblasts and osteoclasts. By fostering bone formation while impeding the development of osteoclasts, curcumin plays a pivotal role in promoting bone health (Yang et al., 2023b).
4.1 Mechanism of curcumin regulating Wnt pathway in osteoporosis
Turmeric root contains a naturally occurring compound called CUR, whose pharmacological effects have been extensively studied, and curcumin has antioxidant and anti-inflammatory properties (Ahmad et al., 2020). CUR, a bioactive compound, engages in direct interactions with a range of molecular entities such as COX-2, DNA polymerase, lipoxygenase (LOX), glycogen synthase kinase-3β (GSK-3β), and cytokines including tumor necrosis factor-alpha (TNF-α) (Grover et al., 2021). Furthermore, it modulates the activity of various transcription factors in an indirect manner, encompassing NF-κB, activator protein 1 (AP-1), β-cyclin, signal transducers and activators of transcription (STAT), and peroxisome proliferator-activated receptor gamma (PPARγ). The multifaceted regulatory influence of curcumin on these molecular targets underscores its robust anti-inflammatory properties, suggesting its potential as a therapeutic agent in the treatment of inflammation-associated disorders (Chainoglou and Hadjipavlou-Litina, 2019). For instance, many studies have shown that the typical WNT/β-catenin pathway stimulates inflammation and that ROS activates the typical WNT/β-catenin pathway through oxidation and inactivation of nuclear oxyreducing proteins (a redox-sensitive regulator) under NOX stimulation, thereby stimulating the oncogenic process (Vallée et al., 2019a). CUR exerts its influence on the Wnt signaling pathway by reducing the activity of β-catenin, thereby downregulating the expression of genes targeted by β-catenin (Li et al., 2021). Computational studies suggest that curcumin may impede the recruitment of axin to the cell membrane, which is crucial for preserving the integrity of β-catenin destruction complexes in the normal cellular context. This action prevents the accumulation of β-cyclin in the nucleus, thus hindering its interaction with lymphocyte enhancer factor/T-cell-specific transcription factor (Lef/Tcf) complexes and dampening the transcriptional activation of target genes (Hosseinzadeh et al., 2018). Consequently, this leads to the suppression of hepatocellular carcinoma cell proliferation and the induction of programmed cell death, or apoptosis. This shows that curcumin has a significant role in the treatment of inflammation and cancer by regulating the Wnt signaling pathway.
CUR has the potential to affect the Wnt signaling pathway, which is important in the treatment of OP. CUR appears to inhibit this pathway, resulting in an anti-osteoporotic effect on the organism (Yang et al., 2023b). Some researchers found that curcumin gavage inhibited ovxinduced EZH2 mRNA levels in mandible and femur, and ovx-induced upregulation of the number of EZH2-positive cells was reduced (Toyokawa et al., 2019). In contrast, CUR gavage restored ovx-mediated downregulation of β-Catenin and Runx2 mRNA levels (Faienza et al., 2024). The protective influence of curcumin against OP is likely attributed to its capacity to suppress the transcriptional activity of EZH2, which in turn leads to a diminished activation of the Wnt/β-Catenin signaling pathway (Brockmueller et al., 2023). In addition, CUR alters downstream effectors of the Wnt signaling pathway, including c-Myc and cell cycle protein D1 (Vallée et al., 2019b). Deletion of the mouse β-catenin gene was found to promote the differentiation of MSCs to adipocytes, while hindering the differentiation of osteoblasts. This implies that the regulation of bone formation is largely influenced by the Wnt/β-catenin pathway (Song et al., 2012). Chen et al. showed that CUR has the potential to enhance nuclear translocation of β-catenin (Chen et al., 2016b). This was primarily achieved by increasing the enzymatic activity of GSK3β phosphorylated glycogen synthase, resulting in beneficial effects on bone health. Furthermore, it has been observed that the intake of curcumin, at a rate of 100 mg/kg/d, substantially alleviated the decrease in bone mineral density and the loss of bone mineral in rats subjected to conditions that mimic glucocorticoid-induced osteoporosis (Chen et al., 2016b). This result was attributed to the modulation of the Wnt/β-catenin pathway and the alleviation of impaired osteoblast differentiation in the in vitro setting. In addition, it has been found that glucocorticoid (GC)-induced Wnt/β-catenin mRNA expression levels were significantly downregulated in OP model rats, whereas Cur intervention increased serum OCN levels and decreased C-terminal peptide of type I collagen (Yang et al., 2023a). The expression of genes pivotal to osteoblast differentiation and function, such as ALP, Runx2, and osterix (Osx), was found to be elevated. ALP and OCN serve as indicators of the bone formation process, in contrast to C-telopeptide of type I collagen, which is a biomarker for bone resorption (Tang et al., 2020). It is evident that CUR can promote bone formation and inhibit bone resorption through the regulation of the Wnt/βcatenin signaling pathway (Chen et al., 2016c).
4.2 Regulation of NF-κB signaling pathway by curcumin in osteoporosis
In conclusion, curcumin shows promise in the therapeutic management of osteoporosis (OP) by targeting the regulation of the NF-κB/IL-6 signalling pathway, a mechanism that significantly inhibits inflammatory processes (Yang et al., 2023a). This highlights the critical role of curcumin in alleviating bone health challenges. Additionally, curcumin has been shown to be beneficial in addressing diabetes-induced osteoporosis by positively affecting key signaling pathways such as NF-κB and transforming growth factor β1 (TGF-β1) (Zamanian et al., 2024), which are essential for protecting bone health. Osteoporosis is a common complication in diabetic patients, with a higher prevalence in the diabetic stage. In particular, CUR successfully reversed the overexpression of inflammatory cytokines such as TNF-α, IL-1β, IL-6 and chemokines such as MCP-1 in diabetic samples, which strongly demonstrated its anti-inflammatory efficacy against hyperglycemias-induced lesions (Kong et al., 2021). In a hyperglycemic environment, CUR pretreatment not only promotes differentiation induction of BMSCs, but also significantly enhances angiogenesis (Wang et al., 2012; Zhang et al., 2023), providing a new perspective for the treatment of diabetes-induced osteoporosis. Notably, CUR inhibits the NF-κB pathway, thereby preventing the decline in bone mineral density (BMD) common in diabetic patients (JiaQiang et al., 2021). This discovery has the potential to revolutionize the treatment of osteoporosis. In vivo experiments further confirmed that daily CUR treatment at 100 mg/kg effectively prevented bone loss and promoted angiogenesis in diabetes-induced osteoporosis (Yang et al., 2023a). Given the strong link between osteoporosis and oxidative stress, it was found that CUR not only has antioxidant and anti-inflammatory effects but also attenuates oxidative stress and promotes bone formation by modulating the MDA/GSH ratio, as demonstrated by the mouse OVX model (JiaQiang et al., 2021). Low concentration of CUR-protected osteoblasts under oxidative stress conditions, reduced the levels of inflammatory factors such as RANKL and IL-6 and reduced the secretion of inflammatory cytokines such as IL-6 and RANKL by inhibiting the phosphorylation of P65 in the NF-κB signaling pathway, which in turn promoted bone formation (Li et al., 2020b).
In addition, CUR reduced the intranuclear expression of NF-κB p65 by inhibiting the phosphorylation of IκBα and its degradation, thereby inhibiting NF-κB activation, which plays a key role in the inhibition of osteoclast genesis in rheumatoid arthritis patients. A large number of studies have consistently shown that CUR reverses biological abnormalities in inflammatory and oxidative processes by decreasing the activity of NF-κB transcription factors, as well as reducing the expression of p-p65 and the transcription of its phosphorylated factors (Zhong et al., 2016).
In summary, the activity of the NF-κB signaling pathway, a key pathway regulating the expression of IL-6 and RANKL, is tightly regulated by CUR, which in turn affects the osteogenesis process in preosteoblasts. CUR, by virtue of its dual antioxidant and anti-inflammatory effects, promotes bone formation by inhibiting the phosphorylation of P65, and exhibits inhibitory effects on osteoclasts in in vivo experiments, reducing bone resorption, and may have directly promoted osteoblast activity and bone formation. This series of findings highlights the centrality of the NF-κB signaling pathway in the mechanism of action of CUR.
4.3 Curcumin interferes with osteoporosis and PI3K-AKT signaling pathway
The PI3K/AKT signaling pathway, as a well-recognized anti-apoptotic and pro-survival signal transduction pathway, is of great importance. Numerous studies have shown that CUR exhibits significant therapeutic potential in the areas of inflammation alleviation, neurological disorders and anti-cancer by upregulating the expression of PI3K and AKT proteins (Hamzehzadeh et al., 2018). Especially, in the study of osteoporosis model rats, although the mRNA expression levels of PI3K, PDK1 and Akt remained stable in bone tissues, the protein expression of their phosphorylated forms was significantly decreased, a finding that reveals key signaling changes during osteoporosis pathology (Wang et al., 2023). Further exploration revealed that when PI3K activation was blocked, the mRNA expression of cell proliferation ability, ALP activity, calcium accumulation, and osteogenesis-related genes such as OCN, Osterix, and Runx2 were suppressed, whereas the mRNA expression of apoptosis-related genes, Caspase-3 and Caspase-9, increased accordingly, highlighting the central role of the PI3K/AKT pathway in the central role of the PI3K/AKT pathway in maintaining bone health (Aimaiti et al., 2020). It is particularly exciting that curcumin was shown in another study to be able to elevate the protein and mRNA levels of ALP, COL1 and RUNX2, and activate the PI3K/AKT/Nrf2 signaling pathway, which effectively promotes the osteogenic differentiation of human periodontal stem cells, providing a new strategy for bone regeneration therapy (Xiong et al., 2020). In addition, CUR has demonstrated its unique efficacy in the treatment of osteoarthritis by precisely intervening in the PI3K-AKT signaling pathway, which protects joint health by inhibiting the PI3K/AKT/mTOR signaling cascade, promoting autophagic response, reducing joint inflammation and restoring joint homeostasis (Song et al., 2022). Although activation of the PI3K/AKT/mTOR pathway has a positive effect on chondrocyte proliferation and differentiation and reduces apoptosis, there is a dearth of research on the specific mechanisms and effects of curcumin in treating OP through this pathway. However, there have been preliminary studies such as the work of Riva et al. By administering high-dose curcumin (1000 mg/day) orally to 57 healthy subjects with low BMD for a period of 24 weeks, not only did the subjects confirm good tolerance of CUR, but also positive changes in BMD were observed (Riva et al., 2017). In response to the current status of OP as a common complication of spinal cord injury and the lack of treatment options, the Hatefi team’s study was equally encouraging. They found that 6 months of CUR supplementation (at a dose of 110 mg/kg) significantly elevated BMD parameters and effectively reduced biomarker levels of bone loss in spinal cord injury patients, providing strong evidence for slowing the process of OP (Kheiridoost et al., 2022). In summary, CUR, as an active ingredient in natural herbs, has shown the first signs of its ability to treat OP by modulating signaling pathways. Although the specific mechanism and data on curcumin’s treatment of osteoporosis through the PI3K pathway are yet to be enriched, the potential it exhibits undoubtedly opens up a broad prospect for future in-depth research and clinical application.
4.4 Curcumin interferes with osteoporosis and MAPK signaling pathway
To date, various experiments on the effects of curcumin on p38 MAPKs and related diseases have been successively conducted (Peng et al., 2021b). Some researchers found that curcumin induced p38 MAPK phosphorylation, which increased apoptosis and facilitated tumour suppression in retinoblastoma cells (Shehzad and Lee, 2013). Curcumin positively affects glucose uptake in L6 myotubular cells through activation of p38 MAPKs (Ferraro et al., 2014). Curcumin inhibits COX-2 expression by suppressing p38 MAPK activation in human keratinocytes. In ovarian cancer, curcumin upregulated the phosphorylation of p38 MAPK, thereby promoting apoptosis (Wu et al., 2022). The role of curcumin in reducing inflammation by inhibiting the MAPK pathway has been validated in a variety of models and experiments. In a study of middle cerebral artery occlusion in rats, we found that curcumin reduces inflammation by inhibiting the TLR4/p38/MAPK pathway (Huang et al., 2018). In a separate investigation, mice were subjected to colitis through the administration of dinitrobenzene sulfonic acid. Within this context, curcumin demonstrated its efficacy by suppressing the activation of the p38 MAPK and NF-κB signaling cascades, which resulted in a noticeable decrease in the levels of the pro-inflammatory cytokine interleukin-1 beta (IL-1β). Furthermore, curcumin’s anti-inflammatory properties were also evident in its capacity to mitigate hepatic inflammation and cell death (Ma et al., 2023). This was achieved through the modulation of inflammatory signaling pathways, notably the p38 MAPK pathway, consequently diminishing the expression of cytokines implicated in inflammation, such as IL-1β (Ashrafizadeh et al., 2020). In an experimental asthmatic condition replicated in mice, curcumin exerts regulatory effects on secretory phospholipase A2 function, consequently leading to a diminution in the concentrations of the pro-inflammatory mediators Cox-2 and Prostaglandin D2 (Memarzia et al., 2022). CUR and its nano formulation have demonstrated the ability to mitigate and potentially avert the process of programmed cell death by impeding the signaling pathways of Toll-like receptor 4 (TLR4) and NF-κB, as well as MAPK cascade. In a rodent model of periodontal disease, the topical application of curcumin in nanoparticle form has been observed to curtail inflammatory responses by inhibiting the initiation of NF-κB and p38 MAPK pathways (Zambrano et al., 2018). In human intestinal microvascular endothelial cells exposed to vascular endothelial growth factor, the presence of curcumin has been shown to curtail the process of angiogenesis. This effect is achieved through the suppression of COX-2 expression at both the transcriptional and translational levels, thereby hindering the synthesis of prostaglandin E2. Additionally, curcumin exerts its anti-angiogenic influence by impeding the activation of the JNK and p38 MAPK signaling pathways (Saberi-Karimian et al., 2019). In the spectrum of inflammatory responses observed in inflammatory bowel disease, the engagement of endothelial cell adhesion molecules stands out as a crucial component. Studies have confirmed that curcumin inhibits vascular cell adhesion molecule expression by affecting Akt, NF-κB, and p38 MAPK, and reduces TNF-α, IL-1β, and lipopolysaccharides, ultimately leading to a reduction in inflammation (Patel et al., 2020).
While inflammation and oxidative stress are the keys to osteoporosis, based on the above mechanism of action of curcumin mediating the MAPK pathway to reduce inflammation and anti-oxidative stress to treat various diseases, it can be surmised that curcumin based on the MAPK pathway to treat OP also has similar targets and mechanisms. It turns out that this is indeed the case. A comprehensive study showed that taking CUR significantly increased BMD and alleviated bone loss in postmenopausal women with OP. In a 12-month clinical trial that was randomized and double-blinded, involving 60 postmenopausal women, the concurrent administration of curcumin at a dosage of 110 mg daily and alendronate at 5 mg daily was observed to notably enhance BMD and more effectively diminish the levels of bone resorption markers compared to the use of either drug alone (Khanizadeh et al., 2018). The potential synergistic effect of CUR and alendronate indicates a promising approach for both the amelioration and prevention of OP in postmenopausal women. Furthermore, a study by Khanizadeh and colleagues, involving a 6-month randomized, triple-blind trial with 120 participants, demonstrated that the concurrent application of nano cellular curcumin integrated with black seed oil notably decreased serum levels of bone turnover biomarkers. These markers include ALP, osteocalcin, and bridging proteins, thereby potentially mitigating the risk of osteoporosis among this demographic (Kim et al., 2011). Several studies have shown that CUR positively affects the P38 MAPK signaling pathway, resulting in anti-inflammatory, neuroprotective and apoptotic effects (Shamsnia et al., 2023). Curcumin has demonstrated the ability to decrease the phosphorylation of p38 MAPK, a mechanism that positions it as a promising therapeutic agent for osteoporosis management, and a study conducted by Chen and colleagues demonstrated that CUR has a protective effect on osteoblasts, preventing DeX-induced apoptosis. This was achieved by inhibiting the expression of pro-apoptotic proteins and enhancing the ERK pathway (Figure 4) (Chen et al., 2016d). These findings suggest that CUR is an effective drug for the treatment of glucocorticoid-induced OP. Heo et al. also found that CUR was able to impede the differentiation of BMMs to osteoblasts by inhibiting RANKL-induced expression of the osteoclast transcription factors c-Fos and NFAT1 (Heo et al., 2014). In addition, it activated the downstream MAPK pathway (Yang et al., 2023a). The findings indicated that the in vitro application of curcumin in a mouse model led to a significant suppression of osteoclast genesis and the maturation of osteoclasts. Consequently, curcumin has the potential to be utilized in the therapeutic management of osteoporosis by modulating the MAPK signaling pathway.
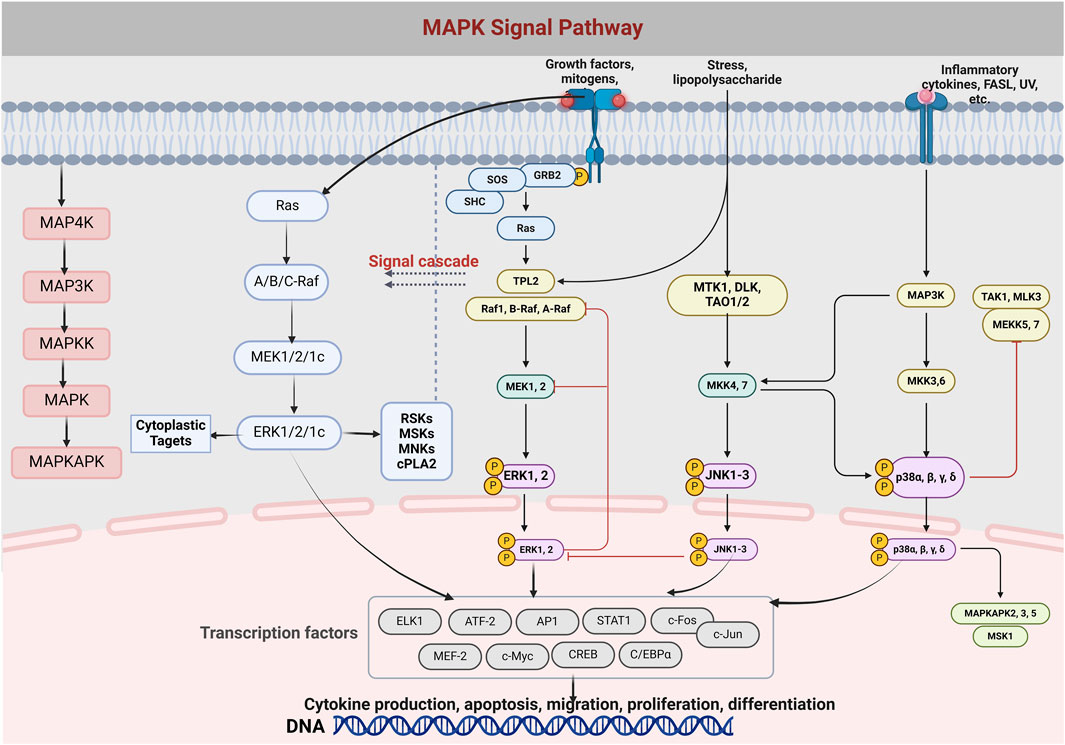
Figure 4. The diagram depicts the formation and differentiation of the MAPK/Erk signaling pathway and the cascade of MAPK. MAPK, mitogen-activated protein excitation, MAPK exists in the cytoplasm and can be translocated to the nucleus to catalyze the phosphorylation of dozens of cytoplasmic proteins and many nuclear transcription factors.
5 Conclusion and perspectives
In recent years, a comprehensive review of various signaling pathways implicated in OP, when integrated with the therapeutic mechanisms of curcumin, has revealed its multifaceted role in bone health. CUR demonstrates the capacity to mitigate inflammation within bone tissues, curb the differentiation and proliferation of osteoclasts, foster the growth of osteoblasts, and reduce oxidative stress. This is achieved through a coordinated regulation of multiple signaling pathways, including but not limited to NF-κB, Wnt/β-catenin, PI3K/Akt, and MAPK. Our study and summary provide evidence and reference for the treatment of OP through multiple signaling pathways mediated by CUR, demonstrating a mechanism characterized by multi-target, multi-pathway and multi-level interactions. Although relevant studies have confirmed that CUR, as a component of Chinese herbal medicine, can treat OP by regulating related proteins and has received much attention and made some progress in recent years, there are still many challenges and problems that need to be solved. One of the main challenges is the low bioavailability of curcumin, which leads to a decrease in the therapeutic effect of the compound. Therefore, researchers are trying to improve the clinical application of curcumin through nanotechnology. In addition, although some controlled in vitro experiments have demonstrated the potential of curcumin in the treatment of osteoporosis, these experiments have not clearly demonstrated that the effects of the compound on bone-forming and bone-resorbing cells can be manifested in actual bone tissue. Moreover, research on the mechanism of action of curcumin in the treatment of osteoporosis is still predominantly confined to preclinical animal and cellular experiments, which lack sufficient clinical data support. Consequently, it is imperative to conduct more high-quality clinical studies in the future to provide further evidence that curcumin can effectively treat osteoporosis through multiple signaling pathways. Finally, curcumin has therapeutic effects on osteoporosis due to its anti-inflammatory effects, but its specific anti-inflammatory mechanisms and targets of action have not been fully clarified as well as studies on related signaling pathways are limited. Therefore, further studies are needed to investigate the common mechanism of action and targets of CUR and other polyphenols based on osteoporosis-related signaling pathways. This will better utilize the advantages of multi-targets, multi-levels and multi-pathways of natural products and provide assistance for more in-depth related studies.
Author contributions
KW: Conceptualization, Data curation, Formal Analysis, Funding acquisition, Investigation, Methodology, Project administration, Resources, Software, Supervision, Validation, Visualization, Writing–original draft, Writing–review and editing.
Funding
The author(s) declare that financial support was received for the research, authorship, and/or publication of this article. This research was supported by Hunan Provincial Science and Technology Department (2019TP2004).
Acknowledgments
The figures were draw in Biorender website (https://www.biorender.com/) and MolView website (https://molview.org/).
Conflict of interest
The author declares that the research was conducted in the absence of any commercial or financial relationships that could be construed as a potential conflict of interest.
Publisher’s note
All claims expressed in this article are solely those of the authors and do not necessarily represent those of their affiliated organizations, or those of the publisher, the editors and the reviewers. Any product that may be evaluated in this article, or claim that may be made by its manufacturer, is not guaranteed or endorsed by the publisher.
References
Ahmad, R. S., Hussain, M. B., Sultan, M. T., Arshad, M. S., Waheed, M., Shariati, M. A., et al. (2020). Biochemistry, safety, pharmacological activities, and clinical applications of turmeric: a mechanistic review. Evid. Based Complement. Altern. Med. 2020, 7656919. Epub 2020/05/27. doi:10.1155/2020/7656919
Ahmed, M. F., El-Sayed, A. K., Chen, H., Zhao, R., Yusuf, M. S., Zuo, Q., et al. (2019). Comparison between curcumin and all-trans retinoic acid in the osteogenic differentiation of mouse bone marrow mesenchymal stem cells. Exp. Ther. Med. 17 (5), 4154–4166. Epub 2019/04/17. doi:10.3892/etm.2019.7414
Aimaiti, A., Wahafu, T., Keremu, A., Yicheng, L., and Li, C. (2020). Strontium ameliorates glucocorticoid inhibition of osteogenesis via the Erk signaling pathway. Biol. trace Elem. Res. 197 (2), 591–598. doi:10.1007/s12011-019-02009-6
Al-Harbi, N. O., Imam, F., Al-Harbi, M. M., Ansari, M. A., Zoheir, K. M., Korashy, H. M., et al. (2016). Dexamethasone attenuates Lps-induced acute lung injury through inhibition of Nf-Κb, Cox-2, and pro-inflammatory mediators. Immunol. Investig. 45 (4), 349–369. doi:10.3109/08820139.2016.1157814
Amin, N., Boccardi, V., Taghizadeh, M., and Jafarnejad, S. (2020). Probiotics and bone disorders: the role of Rankl/Rank/Opg pathway. Aging Clin. Exp. Res. 32, 363–371. doi:10.1007/s40520-019-01223-5
Amjadi-Moheb, F., and Akhavan-Niaki, H. (2019). Wnt signaling pathway in osteoporosis: epigenetic regulation, interaction with other signaling pathways, and therapeutic promises. J. Cell Physiol. 234 (9), 14641–14650. Epub 2019/01/30. doi:10.1002/jcp.28207
Antolin, C. N. C., Ferreyra, MdC. C., Moustafa, W. H. H., Peyruchaud, O., and Aguirre, P. C. (2024). Curcumin and Amaranth as potential anti-inflammatory and protective agents in bone and joint diseases. Funct. Foods Health Dis. 14 (7), 487–502. doi:10.31989/ffhd.v14i7.1386
Asadipooya, K., and Uy, E. M. (2019). Advanced glycation end products (ages), receptor for ages, diabetes, and bone: review of the literature. J. Endocr. Soc. 3 (10), 1799–1818. Epub 2019/09/19. doi:10.1210/js.2019-00160
Ashrafizadeh, M., Ahmadi, Z., Mohammadinejad, R., Farkhondeh, T., and Samarghandian, S. (2020). Curcumin activates the Nrf2 pathway and induces cellular protection against oxidative injury. Curr. Mol. Med. 20 (2), 116–133. doi:10.2174/1566524019666191016150757
Baastrup, C. I. (2016). The acute bone atrophy and its roentgen picture. Acta Radiol. 57 (10), e152–e209. doi:10.1177/0284185116661444
Baldwin, A. S. (1996). The Nf-kappa B and I kappa B proteins: new discoveries and insights. Annu. Rev. Immunol. 14, 649–683. doi:10.1146/annurev.immunol.14.1.649
Ballane, G., Cauley, J. A., Luckey, M. M., and Fuleihan, G. E. H. (2014). Secular trends in hip fractures worldwide: opposing trends east versus west. J. bone mineral Res. 29 (8), 1745–1755. doi:10.1002/jbmr.2218
Bharti, J., Sahil, S., Mehta, S., Ahmad, S., Singh, B., Padhy, A. K., et al. (2021). Mitogen-activated protein kinase, plants, and heat stress. Harsh Environ. plant Resil. Mol. Funct. aspects, 323–354. doi:10.1007/978-3-030-65912-7_13
Blair, H. C., Larrouture, Q. C., Li, Y., Lin, H., Beer-Stoltz, D., Liu, L., et al. (2017). Osteoblast differentiation and bone matrix formation in vivo and in vitro. Tissue Eng. Part B Rev. 23 (3), 268–280. doi:10.1089/ten.TEB.2016.0454
Brent, M. B., Brüel, A., and Thomsen, J. S. (2021). A systematic review of animal models of disuse-induced bone loss. Calcif. Tissue Int. 108 (5), 561–575. doi:10.1007/s00223-020-00799-9
Brockmueller, A., Samuel, S. M., Mazurakova, A., Büsselberg, D., Kubatka, P., and Shakibaei, M. (2023). Curcumin, Calebin a and Chemosensitization: How are they linked to Colorectal cancer? Life Sci. 318, 121504. doi:10.1016/j.lfs.2023.121504
Chainoglou, E., and Hadjipavlou-Litina, D. (2019). Curcumin analogues and derivatives with anti-proliferative and anti-inflammatory activity: structural characteristics and molecular targets. Expert Opin. drug Discov. 14 (8), 821–842. doi:10.1080/17460441.2019.1614560
Chen, H.-H., Chen, S.-P., Zheng, Q.-L., Nie, S.-P., Li, W.-J., Hu, X.-J., et al. (2018). Genistein promotes proliferation of human cervical cancer cells through estrogen receptor-mediated Pi3k/Akt-Nf-Κb pathway. J. Cancer 9 (2), 288–295. doi:10.7150/jca.20499
Chen, Z., Xue, J., Shen, T., Ba, G., Yu, D., and Fu, Q. (2016a). Curcumin alleviates glucocorticoid-induced osteoporosis by protecting osteoblasts from apoptosis in vivo and in vitro. Clin. Exp. Pharmacol. Physiol. 43 (2), 268–276. Epub 2015/10/31. doi:10.1111/1440-1681.12513
Chen, Z., Xue, J., Shen, T., Ba, G., Yu, D., and Fu, Q. (2016d). Curcumin alleviates glucocorticoid-induced osteoporosis by protecting osteoblasts from apoptosis in vivo and in vitro. Clin. Exp. Pharmacol. Physiology 43 (2), 268–276. doi:10.1111/1440-1681.12513
Chen, Z., Xue, J., Shen, T., Mu, S., and Fu, Q. (2016b). Curcumin alleviates glucocorticoid-induced osteoporosis through the regulation of the wnt signaling pathway. Int. J. Mol. Med. 37 (2), 329–338. doi:10.3892/ijmm.2015.2432
Chen, Z., Xue, J., Shen, T., Mu, S., and Fu, Q. (2016c). Curcumin alleviates glucocorticoid-induced osteoporosis through the regulation of the wnt signaling pathway. Int. J. Mol. Med. 37 (2), 329–338. Epub 2015/12/18. doi:10.3892/ijmm.2015.2432
Cheng, C.-H., Chen, L.-R., and Chen, K.-H. (2022). Osteoporosis due to hormone imbalance: an overview of the effects of estrogen deficiency and glucocorticoid overuse on bone turnover. Int. J. Mol. Sci. 23 (3), 1376. doi:10.3390/ijms23031376
Chisari, E., Shivappa, N., and Vyas, S. (2019). Polyphenol-rich foods and osteoporosis. Curr. Pharm. Des. 25 (22), 2459–2466. Epub 2019/07/25. doi:10.2174/1381612825666190722093959
Crane, J. L., and Cao, X. (2014). Bone marrow mesenchymal stem cells and TGF-β signaling in bone remodeling. J. Clin. investigation 124 (2), 466–472. doi:10.1172/JCI70050
Deng, S., Dai, G., Chen, S., Nie, Z., Zhou, J., Fang, H., et al. (2019). Dexamethasone induces osteoblast apoptosis through ROS-PI3K/AKT/GSK3β signaling pathway. Biomed. & Pharmacother. 110, 602–608. doi:10.1016/j.biopha.2018.11.103
Deng, T.-T., Ding, W.-Y., Lu, X.-X., Zhang, Q.-H., Du, J.-X., Wang, L.-J., et al. (2024). Pharmacological and mechanistic aspects of quercetin in osteoporosis. Front. Pharmacol. 15, 1338951. doi:10.3389/fphar.2024.1338951
de Sousa Guardiano Reis, P. C., Alves, A. G. P., Guillo, L. A., de Sousa, M. A. N., Trindade, N. R., and Silva, M. S. (2022). Curcumin supplementation reduces blood glucose and serum lipids of Brazilian women with high waist circumference: a randomized clinical trial. Arch. Endocrinol. Metab. 66 (6), 800–807. Epub 2022/09/27. doi:10.20945/2359-3997000000513
Esatbeyoglu, T., Huebbe, P., Ernst, I. M., Chin, D., Wagner, A. E., and Rimbach, G. (2012a). Curcumin--from molecule to biological function. Angew. Chem. Int. Ed. Engl. 51 (22), 5308–5332. Epub 2012/05/09. doi:10.1002/anie.201107724
Esatbeyoglu, T., Huebbe, P., Ernst, I. M. A., Chin, D., Wagner, A. E., and Rimbach, G. (2012b). Curcumin—from molecule to biological function. Angew. Chem. Int. Ed. 51 (22), 5308–5332. doi:10.1002/anie.201107724
Faienza, M. F., Giardinelli, S., Annicchiarico, A., Chiarito, M., Barile, B., Corbo, F., et al. (2024). Nutraceuticals and functional foods: a comprehensive review of their role in bone health. Int. J. Mol. Sci. 25 (11), 5873. doi:10.3390/ijms25115873
Fan, D., Lu, J., Yu, N., Xie, Y., and Zhen, L. (2022). Curcumin prevents diabetic osteoporosis through promoting osteogenesis and angiogenesis coupling via Nf-Κb signaling. Evid. Based Complement. Altern. Med. 2022, 4974343. Epub 2022/11/18. doi:10.1155/2022/4974343
Ferraro, E., Giammarioli, A. M., Chiandotto, S., Spoletini, I., and Rosano, G. (2014). Exercise-induced skeletal muscle remodeling and metabolic adaptation: redox signaling and role of autophagy. Antioxidants & redox Signal. 21 (1), 154–176. doi:10.1089/ars.2013.5773
Florencio-Silva, R., Sasso, G. RdS., Sasso-Cerri, E., Simões, M. J., and Cerri, P. S. (2015). Biology of bone tissue: structure, function, and factors that influence bone cells. BioMed Res. Int. 2015, 421746. doi:10.1155/2015/421746
Folwarczna, J. (2013). Curcumin and its potential effects on the development of postmenopausal osteoporosis. Nutr. diet menopause, 165–180. doi:10.1007/978-1-62703-373-2_13
Fruman, D. A., Chiu, H., Hopkins, B. D., Bagrodia, S., Cantley, L. C., and Abraham, R. T. (2017). The Pi3k pathway in human disease. Cell 170 (4), 605–635. Epub 2017/08/13. doi:10.1016/j.cell.2017.07.029
Ghahfarrokhi, S. H., Heidari-Soureshjani, S., Talebi-Boroujeni, P., and Sherwin, C. M. (2023). Effect and mechanism of curcumin on bone loss and osteoporosis: a systematic review. Curr. Tradit. Med. 9 (6), 145–154. doi:10.2174/2215083809666221024090809
Giordano, A., and Tommonaro, G. (2019). Curcumin and cancer. Nutrients 11 (10), 2376. Epub 2019/10/09. doi:10.3390/nu11102376
Gong, Y., Slee, R. B., Fukai, N., Rawadi, G., Roman-Roman, S., Reginato, A. M., et al. (2001). Ldl receptor-related protein 5 (Lrp5) affects bone accrual and eye development. Cell 107 (4), 513–523. doi:10.1016/s0092-8674(01)00571-2
Grover, M., Behl, T., Sachdeva, M., Bungao, S., Aleya, L., and Setia, D. (2021). Focus on multi-targeted role of curcumin: a boon in therapeutic paradigm. Environ. Sci. Pollut. Res. 28, 18893–18907. doi:10.1007/s11356-021-12809-w
Gunes, H., Gulen, D., Mutlu, R., Gumus, A., Tas, T., and Topkaya, A. E. (2016). Antibacterial effects of curcumin: an in vitro minimum inhibitory concentration study. Toxicol. industrial health 32 (2), 246–250. doi:10.1177/0748233713498458
Guo, Y. J., Pan, W. W., Liu, S. B., Shen, Z. F., Xu, Y., and Hu, L. L. (2020). Erk/mapk signalling pathway and tumorigenesis. Exp. Ther. Med. 19 (3), 1997–2007. doi:10.3892/etm.2020.8454
Habib, S. J., and Acebrón, S. P. (2022). Wnt signalling in cell division: from mechanisms to tissue engineering. Trends Cell Biol. 32 (12), 1035–1048. doi:10.1016/j.tcb.2022.05.006
Hageneder, F. (2020). The living Wisdom of trees: a guide to the natural hHistory, symbolism and healing power of trees: watkins mMedia lLimited.
Hamzehzadeh, L., Atkin, S. L., Majeed, M., Butler, A. E., and Sahebkar, A. (2018). The versatile role of curcumin in cancer prevention and treatment: a focus on Pi3k/Akt pathway. J. Cell. physiology 233 (10), 6530–6537. doi:10.1002/jcp.26620
Hayden, M. S., and Ghosh, S. (2014). Regulation of Nf-Κb by Tnf family cytokines. Seminars in immunology (Elsevier).
He, Y., Sun, M. M., Zhang, G. G., Yang, J., Chen, K. S., Xu, W. W., et al. (2021). Targeting Pi3k/Akt signal transduction for cancer therapy. Signal Transduct. Target. Ther. 6 (1), 425. doi:10.1038/s41392-021-00828-5
Heo, D. N., Ko, W.-K., Moon, H.-J., Kim, H.-J., Lee, S. J., Lee, J. B., et al. (2014). Inhibition of osteoclast differentiation by gold nanoparticles functionalized with cyclodextrin curcumin complexes. ACS nano 8 (12), 12049–12062. doi:10.1021/nn504329u
Hong, H., Wee, K., and Soh, S. B. (2022). Chinese medicine for health: holistic healing, inner harmony and herbal recipes. World Sci., 288. doi:10.1142/9789811263200_0007
Hoover, K. E. (2021). The ambivalent promise of integrative medicine: reforming integration discourse and practices. Pennsylvania, United States: University of Pittsburgh.
Hosseinzadeh, A., Javad-Moosavi, S. A., Reiter, R. J., Hemati, K., Ghaznavi, H., and Mehrzadi, S. (2018). Idiopathic pulmonary fibrosis (Ipf) signaling pathways and protective roles of melatonin. Life Sci. 201, 17–29. doi:10.1016/j.lfs.2018.03.032
Huang, L., Chen, C., Zhang, X., Li, X., Chen, Z., Yang, C., et al. (2018). Neuroprotective effect of curcumin against cerebral ischemia-reperfusion via mediating autophagy and inflammation. J. Mol. Neurosci. 64, 129–139. doi:10.1007/s12031-017-1006-x
Iddir, M., Brito, A., Dingeo, G., Fernandez Del Campo, S. S., Samouda, H., La Frano, M. R., et al. (2020). Strengthening the immune system and reducing inflammation and oxidative stress through diet and nutrition: considerations during the Covid-19 crisis. Nutrients 12 (6), 1562. doi:10.3390/nu12061562
Inchingolo, A. D., Inchingolo, A. M., Malcangi, G., Avantario, P., Azzollini, D., Buongiorno, S., et al. (2022). Effects of resveratrol, curcumin and Quercetin supplementation on bone metabolism-A systematic review. Nutrients 14 (17), 3519. doi:10.3390/nu14173519
Jabczyk, M., Nowak, J., Hudzik, B., and Zubelewicz-Szkodzińska, B. (2021). Curcumin and its potential impact on microbiota. Nutrients 13 (6), 2004. Epub 2021/07/03. doi:10.3390/nu13062004
Jacob, S., Kather, F. S., Morsy, M. A., Boddu, S. H., Attimarad, M., Shah, J., et al. (2024). Advances in nanocarrier systems for overcoming formulation challenges of curcumin: current insights. Nanomaterials 14 (8), 672. doi:10.3390/nano14080672
Jantan, I., Haque, M. A., Arshad, L., Harikrishnan, H., Septama, A. W., and Mohamed-Hussein, Z.-A. (2021). Dietary polyphenols suppress chronic inflammation by modulation of multiple inflammation-associated cell signaling pathways. J. Nutr. Biochem. 93, 108634. doi:10.1016/j.jnutbio.2021.108634
Jarouliya, U., and Keservani, R. K. (2019) “Protein function as cell surface and nuclear receptor in human diseases,” in Medicinal chemistry with pharmaceutical product development. Apple Academic Press, 1–32.
Jenkins, Z. A., Kogelenberg, Mv, Morgan, T., Jeffs, A. R., Fukuzawa, R., Pearl, E. J., et al. (2009). Germline mutations in Wtx cause a Sclerosing skeletal dysplasia but do not predispose to tumorigenesis. Nat. Genet. 41, 95–100. doi:10.1038/ng.270
Jiang, Q., Lei, Y. H., Krishnadath, D. C., Zhu, B. Y., and Zhou, X. W. (2021b). Curcumin regulates EZH2/Wnt/β-Catenin pathway in the mandible and femur of ovariectomized osteoporosis rats. Kaohsiung J. Med. Sci. 37 (6), 513–519. doi:10.1002/kjm2.12346
Jiang, T., Ghosh, R., and Charcosset, C. (2021a). Extraction, purification and applications of curcumin from plant materials-a comprehensive review. Trends food Sci. & Technol. 112, 419–430. doi:10.1016/j.tifs.2021.04.015
JiaQiang, X., Gao, R., Liang, W., Wu, C., Li, F., Huang, S., et al. (2021). Oxidative stress reducing osteogenesis reversed by curcumin via Nf-Κb signaling and had a role in anti-osteoporosis.
Joshi, P., Bisht, A., Paliwal, A., Dwivedi, J., and Sharma, S. (2023). Recent updates on clinical developments of curcumin and its derivatives. Phytotherapy Res. 37 (11), 5109–5158. doi:10.1002/ptr.7974
Kazakia, G. J., Tjong, W., Nirody, J. A., Burghardt, A. J., Carballido-Gamio, J., Patsch, J. M., et al. (2014). The influence of disuse on bone microstructure and mechanics assessed by Hr-Pqct. Bone 63, 132–140. Epub 2014/03/08. doi:10.1016/j.bone.2014.02.014
Kemmler, W., and von Stengel, S. (2019) “The role of exercise on fracture reduction and bone strengthening,” in Muscle and exercise physiology. Elsevier, 433–455.
Khalid, M., Petroianu, G., and Adem, A. (2022). Advanced glycation end products and diabetes mellitus: mechanisms and perspectives. Biomolecules 12 (4), 542. doi:10.3390/biom12040542
Khanizadeh, F., Rahmani, A., Asadollahi, K., and Ahmadi, M. R. H. (2018). Combination therapy of curcumin and alendronate modulates bone turnover markers and enhances bone mineral density in postmenopausal women with osteoporosis. Arch. Endocrinol. Metab. 62 (4), 438–445. Epub 2018/10/12. doi:10.20945/2359-3997000000060
Kheiridoost, H., Shakouri, S. K., Shojaei-Zarghani, S., Dolatkhah, N., and Farshbaf-Khalili, A. (2022). Efficacy of nanomicelle curcumin, nigella sativa oil, and their combination on bone turnover markers and their safety in postmenopausal women with primary osteoporosis and Osteopenia: a triple-blind randomized controlled trial. Food Sci. & Nutr. 10 (2), 515–524. doi:10.1002/fsn3.2674
Kim, W. K., Ke, K., Sul, O. J., Kim, H. J., Kim, S. H., Lee, M. H., et al. (2011). Curcumin protects against Ovariectomy-induced bone loss and decreases Osteoclastogenesis. J. Cell Biochem. 112 (11), 3159–3166. Epub 2011/07/07. doi:10.1002/jcb.23242
Kong, M., Xie, K., Lv, M., Li, J., Yao, J., Yan, K., et al. (2021). Anti-inflammatory Phytochemicals for the treatment of diabetes and its complications: Lessons learned and future promise. Biomed. & Pharmacother. 133, 110975. doi:10.1016/j.biopha.2020.110975
Lammi, L., Arte, S., Somer, M., Jarvinen, H., Lahermo, P., Thesleff, I., et al. (2004). Mutations in Axin2 cause familial tooth agenesis and predispose to colorectal cancer. Am. J. Hum. Genet. 74 (5), 1043–1050. Epub 2004/03/26. doi:10.1086/386293
Lee, S.-B., Lee, W. S., Shin, J.-S., Jang, D. S., and Lee, K. T. (2017). Xanthotoxin suppresses LPS-induced expression of iNOS, COX-2, TNF-α, and IL-6 via AP-1, NF-κB, and JAK-STAT inactivation in RAW 264.7 macrophages. Int. Immunopharmacol. 49, 21–29. doi:10.1016/j.intimp.2017.05.021
Li, J., and Kataoka, K. (2020). Chemo-physical strategies to advance the in vivo functionality of targeted nanomedicine: the next generation. J. Am. Chem. Soc. 143 (2), 538–559. doi:10.1021/jacs.0c09029
Li, S., Zhou, G., Liu, W., Ye, J., Yuan, F., and Zhang, Z. (2021). Curcumol inhibits lung adenocarcinoma growth and metastasis via inactivation of Pi3k/Akt and wnt/Β-catenin pathway. Oncol. Res. 28 (7-8), 685–700. doi:10.3727/096504020X15917007265498
Li, W., Suwanwela, N. C., and Patumraj, S. (2016). Curcumin by down-regulating Nf-Kb and elevating Nrf2, reduces brain Edema and neurological dysfunction after cerebral I/R. Microvasc. Res. 106, 117–127. doi:10.1016/j.mvr.2015.12.008
Li, X., Chen, Y., Mao, Y., Dai, P., Sun, X., Zhang, X., et al. (2020a). Curcumin protects osteoblasts from oxidative stress-induced dysfunction via GSK3β-Nrf2 signaling pathway. Front. Bioeng. Biotechnol. 8, 625. Epub 2020/07/03. doi:10.3389/fbioe.2020.00625
Li, X., Chen, Y., Mao, Y., Dai, P., Sun, X., Zhang, X., et al. (2020b). Curcumin protects osteoblasts from oxidative stress-induced dysfunction via GSK3β-Nrf2 signaling pathway. Front. Bioeng. Biotechnol. 8, 625. doi:10.3389/fbioe.2020.00625
Lihong, L. (2019). Classical Chinese medicine. Hong Kong, China: The Chinese University of Hong Kong Press.
Lima, C. F., Pereira-Wilson, C., and Rattan, S. I. (2011). Curcumin induces Heme Oxygenase-1 in normal human skin fibroblasts through redox signaling: relevance for anti-aging intervention. Mol. Nutr. & food Res. 55 (3), 430–442. doi:10.1002/mnfr.201000221
Liu, H. (2024). Traditional Chinese medicine for visceral pain. Visceral pain: from bench to bedside. Springer, 119–166.
Liu, N., and Tang, M. (2020). Toxic effects and involved molecular pathways of nanoparticles on cells and subcellular organelles. J. Appl. Toxicol. 40 (1), 16–36. doi:10.1002/jat.3817
Liu, S., Liu, J., He, L., Liu, L., Cheng, B., Zhou, F., et al. (2022). A comprehensive review on the benefits and problems of curcumin with respect to human health. Molecules 27 (14), 4400. doi:10.3390/molecules27144400
Liu, T., Zhang, L., Joo, D., and Sun, S.-C. (2017). Nf-κb signaling in inflammation. Signal Transduct. Target. Ther. 2 (1), 17023. doi:10.1038/sigtrans.2017.23
Liu, W., Lu, L., Ma, C., Yan, C., Zhao, Z., Mohammadtursun, N., et al. (2018). The evolution of traditional Chinese medicine as a disciplinary concept and its essence throughout history. Traditional Med. Mod. Med. 1 (03), 171–180. doi:10.1142/s257590001810002x
Luca, S. V., Macovei, I., Bujor, A., Miron, A., Skalicka-Woźniak, K., Aprotosoaie, A. C., et al. (2020). Bioactivity of dietary polyphenols: the role of metabolites. Crit. Rev. Food Sci. Nutr. 60 (4), 626–659. doi:10.1080/10408398.2018.1546669
Ma, Y., Fei, Y., Ding, S., Jiang, H., Fang, J., and Liu, G. (2023). Trace metal elements: a bridge between host and intestinal microorganisms. Sci. China Life Sci. 66 (9), 1976–1993. doi:10.1007/s11427-022-2359-4
Maleki Dana, P., Sadoughi, F., Mansournia, M. A., Mirzaei, H., Asemi, Z., and Yousefi, B. (2021). Targeting wnt signaling pathway by polyphenols: implication for aging and age-related diseases. Biogerontology 22 (5), 479–494. Epub 2021/09/05. doi:10.1007/s10522-021-09934-x
Manandhar, S., Kabekkodu, S. P., and Pai, K. S. R. (2020). Aberrant canonical wnt signaling: phytochemical based modulation. Phytomedicine 76, 153243. doi:10.1016/j.phymed.2020.153243
Manolagas, S. C. (2010). From estrogen-centric to aging and oxidative stress: a revised perspective of the pathogenesis of osteoporosis. Endocr. Rev. 31 (3), 266–300. doi:10.1210/er.2009-0024
Martin-Orozco, E., Sanchez-Fernandez, A., Ortiz-Parra, I., and Ayala-San Nicolas, M. (2019). Wnt signaling in tumors: the way to evade drugs and immunity. Front. Immunol. 10, 2854. Epub 2020/01/11. doi:10.3389/fimmu.2019.02854
McCaskill, L. D. (2021). The use of essential oils in traditional Chinese veterinary medicine: small animal practice. Am. J. Traditional Chin. Veterinary Med. 67, 67–78. doi:10.59565/vgcp9123
Memarzia, A., Saadat, S., Behrouz, S., and Boskabady, M. H. (2022). Curcuma longa and curcumin affect respiratory and allergic disorders, experimental and clinical evidence: a comprehensive and updated review. Biofactors 48 (3), 521–551. doi:10.1002/biof.1818
Minisola, S., Colangelo, L., Pepe, J., Diacinti, D., Cipriani, C., and Rao, S. D. (2021). Osteomalacia and vitamin D status: a clinical update 2020. J. Bone Mineral Res. Plus 5 (1), e10447. doi:10.1002/jbm4.10447
Napoli, N., Chandran, M., Pierroz, D. D., Abrahamsen, B., Schwartz, A. V., Ferrari, S. L., et al. (2017). Mechanisms of diabetes mellitus-induced bone fragility. Nat. Rev. Endocrinol. 13 (4), 208–219. doi:10.1038/nrendo.2016.153
Neganova, M., Liu, J., Aleksandrova, Y., Klochkov, S., and Fan, R. (2021). Therapeutic influence on important targets associated with chronic inflammation and oxidative stress in cancer treatment. Cancers 13 (23), 6062. doi:10.3390/cancers13236062
Oeckinghaus, A., and Ghosh, S. (2009). The Nf-Kappab family of transcription factors and its regulation. Cold Spring Harb. Perspect. Biol. 1 (4), a000034. Epub 2010/01/13. doi:10.1101/cshperspect.a000034
Olivares-Bañuelos, T. N., Chí-Castañeda, D., and Ortega, A. (2019). Glutamate transporters: gene expression regulation and signaling properties. Neuropharmacology 161, 107550. doi:10.1016/j.neuropharm.2019.02.032
Pan, R., Qiu, S., Lu, D.-x, and Dong, J. (2008). Curcumin improves learning and memory ability and its neuroprotective mechanism in mice. Chin. Med. J. 121 (09), 832–839. doi:10.1097/00029330-200805010-00015
Parcheta, M., Świsłocka, R., Orzechowska, S., Akimowicz, M., Choińska, R., and Lewandowski, W. (2021). Recent developments in effective antioxidants: the structure and antioxidant properties. Materials 14 (8), 1984. doi:10.3390/ma14081984
Patel, S. S., Acharya, A., Ray, R., Agrawal, R., Raghuwanshi, R., and Jain, P. (2020). Cellular and molecular mechanisms of curcumin in prevention and treatment of disease. Crit. Rev. food Sci. Nutr. 60 (6), 887–939. doi:10.1080/10408398.2018.1552244
Peng, Y., Ao, M., Dong, B., Jiang, Y., Yu, L., Chen, Z., et al. (2021a). Anti-inflammatory effects of curcumin in the inflammatory diseases: status, limitations and countermeasures. Drug Des. Devel Ther. 15, 4503–4525. Epub 2021/11/11. doi:10.2147/dddt.S327378
Peng, Y., Ao, M., Dong, B., Jiang, Y., Yu, L., Chen, Z., et al. (2021b). Anti-inflammatory effects of curcumin in the inflammatory diseases: status, limitations and countermeasures. Drug Des. Dev. Ther. 15, 4503–4525. doi:10.2147/DDDT.S327378
Ponzano, M. (2022). Risk assessment and interventions for individuals at risk of osteoporotic fractures.
Ponzetti, M., and Rucci, N. (2021). Osteoblast differentiation and signaling: established concepts and emerging topics. Int. J. Mol. Sci. 22 (13), 6651. doi:10.3390/ijms22136651
Poole, K. E., Treece, G. M., Gee, A. H., Brown, J. P., McClung, M. R., Wang, A., et al. (2015). Denosumab rapidly increases cortical bone in key locations of the femur: a 3d bone mapping study in women with osteoporosis. J. Bone Mineral Res. 30 (1), 46–54. doi:10.1002/jbmr.2325
Qu, Z., Zhang, B., Kong, L., Gong, Y., Feng, M., Gao, X., et al. (2022). Receptor activator of nuclear factor-Κb Ligand-mediated osteoclastogenesis signaling pathway and related therapeutic natural compounds. Front. Pharmacol. 13, 1043975. doi:10.3389/fphar.2022.1043975
Rahman, A. (2018). Molecular mechanism of apoptosis signal-regulating kinase I oligomerization and auto-activation. Cookeville, TN, United States: Tennessee Technological University.
Rausch, H., Schröder, S., Friedemann, T., Cameron, S., Kuchta, K., and Konrad, M. (2024). History and present of European traditional herbal medicine (Phytotherapy). History, present and prospect of world traditional medicine. World Sci., 131–234. doi:10.1142/9789811282171_0003
Rinonapoli, G., Ruggiero, C., Meccariello, L., Bisaccia, M., Ceccarini, P., and Caraffa, A. (2021). Osteoporosis in men: a review of an underestimated bone condition. Int. J. Mol. Sci. 22 (4), 2105. doi:10.3390/ijms22042105
Riva, A., Togni, S., Giacomelli, L., Franceschi, F., Eggenhoffner, R., Feragalli, B., et al. (2017). Effects of a curcumin-based supplementation in asymptomatic subjects with low bone density: a preliminary 24-Week supplement study. Eur. Rev. Med. & Pharmacol. Sci. 21 (7), 1684–1689.
Rodríguez-Carballo, E., Gámez, B., and Ventura, F. (2016). P38 Mapk signaling in osteoblast differentiation. Front. Cell Dev. Biol. 4, 40. Epub 2016/05/21. doi:10.3389/fcell.2016.00040
Rolvien, T., and Amling, M. (2022). Disuse osteoporosis: clinical and mechanistic insights. Calcif. Tissue Int. 110 (5), 592–604. Epub 2021/03/20. doi:10.1007/s00223-021-00836-1
Romagnoli, E., Fiacco, R. D., Russo, S., Piemonte, S., Fidanza, F., Colapietro, F., et al. (2011). Secondary osteoporosis in men and women: clinical challenge of an Unresolved issue. J. Rheumatology 38 (8), 1671–1679. doi:10.3899/jrheum.110030
Saberi-Karimian, M., Katsiki, N., Caraglia, M., Boccellino, M., Majeed, M., and Sahebkar, A. (2019). Vascular endothelial growth factor: an important molecular target of curcumin. Crit. Rev. food Sci. Nutr. 59 (2), 299–312. doi:10.1080/10408398.2017.1366892
Salamanna, F., Borsari, V., Contartese, D., Aldini, N. N., and Fini, M. (2018). Link between estrogen deficiency osteoporosis and susceptibility to bone metastases: a way towards precision medicine in cancer patients. Breast 41, 42–50. doi:10.1016/j.breast.2018.06.013
Salehi, B., Stojanović-Radić, Z., Matejić, J., Sharifi-Rad, M., Kumar, N. V. A., Martins, N., et al. (2019). The therapeutic potential of curcumin: a review of clinical trials. Eur. J. Med. Chem. 163, 527–545. doi:10.1016/j.ejmech.2018.12.016
Savova, M. S., Mihaylova, L. V., Tews, D., Wabitsch, M., and Georgiev, M. I. (2023). Targeting Pi3k/Akt signaling pathway in obesity. Biomed. & Pharmacother. 159, 114244. doi:10.1016/j.biopha.2023.114244
Scheid, V. (2002). Chinese medicine in contemporary China: plurality and synthesis. Durham, NC, United States: Duke University Press.
Seely, K. D., Kotelko, C. A., Douglas, H., Bealer, B., and Brooks, A. E. (2021). The human gut microbiota: a key mediator of osteoporosis and osteogenesis. Int. J. Mol. Sci. 22 (17), 9452. doi:10.3390/ijms22179452
Seeman, E. (2003). Reduced bone formation and increased bone resorption: rational targets for the treatment of osteoporosis. Osteoporos. Int. 14 (3), 2–8. doi:10.1007/s00198-002-1340-9
Shamsnia, H. S., Roustaei, M., Ahmadvand, D., Butler, A. E., Amirlou, D., Soltani, S., et al. (2023). Impact of curcumin on P38 mapk: therapeutic implications. Inflammopharmacology 31 (5), 2201–2212. Epub 2023/07/27. doi:10.1007/s10787-023-01265-2
Sharifi-Rad, J., Rayess, Y. E., Rizk, A. A., Sadaka, C., Zgheib, R., Zam, W., et al. (2020). Turmeric and its major compound curcumin on health: bioactive effects and safety Profiles for food, Pharmaceutical, biotechnological and medicinal applications. Front. Pharmacol. 11, 01021. doi:10.3389/fphar.2020.01021
Shehzad, A., and Lee, Y. S. (2013). Molecular mechanisms of curcumin action: signal transduction. Biofactors 39 (1), 27–36. doi:10.1002/biof.1065
Shi, C., Wu, J., Yan, Q., Wang, R., and Miao, D. (2015). Bone marrow ablation demonstrates that estrogen plays an important role in osteogenesis and bone turnover via an antioxidative mechanism. Bone 79, 94–104. doi:10.1016/j.bone.2015.05.034
Sho, T., and Xu, J. (2019). Role and mechanism of Ros scavengers in alleviating Nlrp3-mediated inflammation. Biotechnol. Appl. Biochem. 66 (1), 4–13. doi:10.1002/bab.1700
Si, X., Wang, Y., Wong, J., Zhang, J., McManus, B. M., and Luo, H. (2007). Dysregulation of the Ubiquitin-Proteasome system by curcumin suppresses Coxsackievirus B3 replication. J. virology 81 (7), 3142–3150. doi:10.1128/JVI.02028-06
Slika, L., and Patra, D. (2020). A short review on chemical properties, stability and nano-technological advances for curcumin delivery. Expert Opin. drug Deliv. 17 (1), 61–75. doi:10.1080/17425247.2020.1702644
Sma, A. N. (2020). The canonical wnt signaling (Wnt/β-Catenin pathway): a potential target for cancer prevention and therapy. Iran. Biomed. J. 24 (5), 269–280. Epub 2020/05/21. doi:10.29252/ibj.24.5.264
Sobh, M. M., Abdalbary, M., Elnagar, S., Nagy, E., Elshabrawy, N., Abdelsalam, M., et al. (2022). Secondary osteoporosis and metabolic bone diseases. J. Clin. Med. 11 (9), 2382. Epub 2022/05/15. doi:10.3390/jcm11092382
Song, L., Liu, M., Ono, N., Bringhurst, F. R., Kronenberg, H. M., and Guo, J. (2012). Loss of wnt/β-catenin signaling causes cell fate shift of preosteoblasts from osteoblasts to adipocytes. J. Bone Mineral Res. 27 (11), 2344–2358. doi:10.1002/jbmr.1694
Song, Y., Meng, Z., Zhang, S., Li, N., Hu, W., and Li, H. (2022). Mir-4739/Itga10/Pi3k signaling regulates differentiation and apoptosis of osteoblast. Regen. Ther. 21, 342–350. Epub 2022/09/27. doi:10.1016/j.reth.2022.08.002
Srisubin, T. (2021). Laponite hydrogel scaffolds containing graphene and phosphonate moieties for bone tissue engineering. United Kingdom: The University of Manchester.
Stanić, Z. (2017). Curcumin, a compound from natural sources, a true scientific challenge–a review. Plant Foods Hum. Nutr. 72, 1–12. doi:10.1007/s11130-016-0590-1
Starup-Linde, J., Frost, M., Vestergaard, P., and Abrahamsen, B. (2017). Epidemiology of fractures in diabetes. Calcif. tissue Int. 100, 109–121. doi:10.1007/s00223-016-0175-x
Su, Z., Yao, B., Liu, G., and Fang, J. (2024). Polyphenols as potential preventers of osteoporosis: a comprehensive review on antioxidant and anti-inflammatory effects, molecular mechanisms, and signal pathways in bone metabolism. J. Nutr. Biochem. 123, 109488. doi:10.1016/j.jnutbio.2023.109488
Tang, J., Xie, J., Chen, W., Tang, C., Wu, J., Wang, Y., et al. (2020). Runt-related transcription factor 1 is required for murine osteoblast differentiation and bone formation. J. Biol. Chem. 295 (33), 11669–11681. Epub 2020/06/24. doi:10.1074/jbc.RA119.007896
Toyokawa, G., Takada, K., Tagawa, T., Hamamoto, R., Yamada, Y., Shimokawa, M., et al. (2019). A positive correlation between the Ezh2 and Pd-L1 expression in resected lung adenocarcinomas. Ann. Thorac. Surg. 107 (2), 393–400. doi:10.1016/j.athoracsur.2018.08.056
Unschuld, P. U. (2018). Traditional Chinese medicine: heritage and adaptation. Columbia University Press.
Vallée, A., Lecarpentier, Y., and Vallée, J.-N. (2019a). Targeting the canonical WNT/β-Catenin pathway in cancer treatment using non-steroidal anti-inflammatory drugs. Cells 8 (7), 726. doi:10.3390/cells8070726
Vallée, A., Lecarpentier, Y., and Vallée, J.-N. (2019b). Curcumin: a therapeutic strategy in cancers by inhibiting the canonical WNT/β-catenin pathway. J. Exp. & Clin. Cancer Res. 38 (1), 323. doi:10.1186/s13046-019-1320-y
van Amerongen, R., Bowman, A. N., and Nusse, R. (2012). Developmental stage and time dictate the fate of Wnt/β-catenin-responsive stem cells in the mammary gland. Cell Stem Cell 11 (3), 387–400. Epub 2012/08/07. doi:10.1016/j.stem.2012.05.023
Vilaca, T., Schini, M., Harnan, S., Sutton, A., Poku, E., Allen, I. E., et al. (2020). The risk of hip and non-vertebral fractures in type 1 and type 2 diabetes: a systematic review and meta-analysis update. Bone 137, 115457. doi:10.1016/j.bone.2020.115457
Visconti, R., Iversen, T., and Cottrell, J. (2019). A review of dysregulated osteoblast and osteoclast coupling in bone disease and failure. Bone Res. 7, 200. doi:10.35248/2572-4916.19.7.201
Wallander, M., Axelsson, K. F., Nilsson, A. G., Lundh, D., and Lorentzon, M. (2017). Type 2 diabetes and risk of hip fractures and non-skeletal fall injuries in the elderly: a study from the fractures and fall injuries in the elderly cohort (frailco). J. bone mineral Res. 32 (3), 449–460. doi:10.1002/jbmr.3002
Wang, C., Meng, H., Wang, X., Zhao, C., Peng, J., and Wang, Y. (2016). Differentiation of bone marrow mesenchymal stem cells in osteoblasts and adipocytes and its role in treatment of osteoporosis. Med. Sci. Monit. 22, 226–233. Epub 2016/01/23. doi:10.12659/msm.897044
Wang, J., Li, G., Wang, Z., Zhang, X., Yao, L., Wang, F., et al. (2012). High glucose-induced expression of inflammatory cytokines and reactive oxygen species in cultured astrocytes. Neuroscience 202, 58–68. doi:10.1016/j.neuroscience.2011.11.062
Wang, W., Nag, S. A., and Zhang, R. (2015). Targeting the Nfκb signaling pathways for breast cancer prevention and therapy. Curr. Med. Chem. 22 (2), 264–289. doi:10.2174/0929867321666141106124315
Wang, Z., Zhang, H., Li, Q., Zhang, L., Chen, L., Wang, H., et al. (2023). Long non-coding Rna Kcnq1ot1 alleviates postmenopausal osteoporosis by modulating Mir-421-3p/Mtor Axis. Sci. Rep. 13 (1), 2333. doi:10.1038/s41598-023-29546-4
Weaver, C. M., Gordon, C. M., Janz, K. F., Kalkwarf, H. J., Lappe, J. M., Lewis, R., et al. (2016). The national osteoporosis foundation's position statement on peak bone mass development and lifestyle factors: a systematic review and implementation recommendations. Osteoporos. Int. 27 (4), 1281–1386. Epub 2016/02/10. doi:10.1007/s00198-015-3440-3
White, J. J., Mazzeu, J. F., Hoischen, A., Jhangiani, S., Gambin, T., Alcino, M. C., et al. (2015). Dvl1 frameshift mutations clustering in the penultimate exon cause autosomal-dominant robinow syndrome. Am. J. Hum. Genet. 96 (4), 612–622. doi:10.1016/j.ajhg.2015.02.015
Wilkesmann, S., Fellenberg, J., Nawaz, Q., Reible, B., Moghaddam, A., Boccaccini, A. R., et al. (2020). Primary osteoblasts, osteoblast precursor cells or osteoblast-like cell lines: which human cell types are (most) suitable for characterizing 45s5-bioactive glass? J. Biomed. Mater. Res. Part A 108 (3), 663–674. doi:10.1002/jbm.a.36846
Wu, D., Cline-Smith, A., Shashkova, E., Perla, A., Katyal, A., and Aurora, R. (2021a). T-cell mediated inflammation in postmenopausal osteoporosis. Front. Immunol. 12, 687551. Epub 2021/07/20. doi:10.3389/fimmu.2021.687551
Wu, M.-F., Huang, Y.-H., Chiu, L.-Y., Cherng, S.-H., Sheu, G.-T., and Yang, T.-Y. (2022). Curcumin induces apoptosis of chemoresistant lung cancer cells via Ros-regulated P38 Mapk phosphorylation. Int. J. Mol. Sci. 23 (15), 8248. doi:10.3390/ijms23158248
Wu, Z.-L., Xie, Q.-Q., Liu, T.-C., Yang, X., Zhang, G.-Z., and Zhang, H.-H. (2021b). Role of the wnt pathway in the formation, development, and degeneration of intervertebral discs. Pathology - Res. Pract. 220, 153366. doi:10.1016/j.prp.2021.153366
Xiong, Y., Zhao, B., Zhang, W., Jia, L., Zhang, Y., and Xu, X. (2020). Curcumin promotes osteogenic differentiation of periodontal ligament stem cells through the Pi3k/Akt/Nrf2 signaling pathway. Iran. J. basic Med. Sci. 23 (7), 954–960. doi:10.22038/IJBMS.2020.44070.10351
Yang, S., Sun, Y., Kapilevich, L., Zhang, X., and Huang, Y. (2023a). Protective effects of curcumin against osteoporosis and its molecular mechanisms: a recent review in preclinical trials. Front. Pharmacol. 14, 1249418. Epub 2023/10/04. doi:10.3389/fphar.2023.1249418
Yang, S., Sun, Y., Kapilevich, L., Zhang, Xa, and Huang, Y. (2023b). Protective effects of curcumin against osteoporosis and its molecular mechanisms: a recent review in preclinical trials. Front. Pharmacol. 14, 1249418. doi:10.3389/fphar.2023.1249418
Ye, C., Zhang, W., Hang, K., Chen, M., Hou, W., Chen, J., et al. (2019). Extracellular Il-37 promotes osteogenic differentiation of human bone marrow mesenchymal stem cells via activation of the Pi3k/Akt signaling pathway. Cell death & Dis. 10 (10), 753. doi:10.1038/s41419-019-1904-7
Yu, F., and Xia, W. (2019). The epidemiology of osteoporosis, associated fragility fractures, and management gap in China. Archives Osteoporos. 14 (1), 32. doi:10.1007/s11657-018-0549-y
Yuan, H., Ma, Q., Ye, L., and Piao, G. (2016a). The traditional medicine and modern medicine from natural products. Molecules 21 (5), 559. doi:10.3390/molecules21050559
Yuan, X., Liu, H., Huang, H., Liu, H., Li, L., Yang, J., et al. (2016b). The key role of canonical wnt/β-catenin signaling in cartilage chondrocytes. Curr. drug targets 17 (4), 475–484. doi:10.2174/1389450116666150825112623
Yuandani, J. I., Rohani, A. S., and Sumantri, I. B. (2021). Immunomodulatory effects and mechanisms of curcuma species and their bioactive compounds: a review. Front. Pharmacol. 12, 643119. doi:10.3389/fphar.2021.643119
Yue, J., and López, J. M. (2020). Understanding Mapk signaling pathways in apoptosis. Int. J. Mol. Sci. 21 (7), 2346. doi:10.3390/ijms21072346
Zamanian, M. Y., Alsaab, H. O., Golmohammadi, M., Yumashev, A., Jabba, A. M., Abid, M. K., et al. (2024). Nf-κb pathway as a molecular target for curcumin in diabetes Mellitus treatment: focusing on oxidative stress and inflammation. Cell Biochem. Funct. 42 (4), e4030. doi:10.1002/cbf.4030
Zambrano, L. M., Brandao, D. A., Rocha, F. R., Marsiglio, R. P., Longo, I. B., Primo, F. L., et al. (2018). Local administration of curcumin-loaded nanoparticles effectively inhibits inflammation and bone resorption associated with experimental periodontal disease. Sci. Rep. 8 (1), 6652. doi:10.1038/s41598-018-24866-2
Zhang, C., Li, H., Li, J., Hu, J., Yang, K., and Tao, L. (2023). Oxidative stress: a common pathological state in a high-risk population for osteoporosis. Biomed. & Pharmacother. 163, 114834. doi:10.1016/j.biopha.2023.114834
Zhang, W., Gao, R., Rong, X., Zhu, S., Cui, Y., Liu, H., et al. (2022). Immunoporosis: role of immune system in the pathophysiology of different types of osteoporosis. Front. Endocrinol. (Lausanne) 13, 965258. Epub 2022/09/24. doi:10.3389/fendo.2022.965258
Zhaoguo, L., Qing, W., Yurui, X., Zhaoguo, L., Qing, W., and Yurui, X. (2021). Key concepts in traditional Chinese medicine Ii. Springer.
Zhong, W., Qian, K., Xiong, J., Ma, K., Wang, A., and Zou, Y. (2016). Curcumin alleviates lipopolysaccharide induced sepsis and liver failure by suppression of oxidative stress-related inflammation via Pi3k/Akt and Nf-Κb related signaling. Biomed. & Pharmacother. 83, 302–313. doi:10.1016/j.biopha.2016.06.036
Zhou, H., Jiao, G., Dong, M., Chi, H., Wang, H., Wu, W., et al. (2019). Orthosilicic acid accelerates bone formation in human osteoblast-like cells through the Pi3k–Akt–Mtor pathway. Biol. trace Elem. Res. 190, 327–335. doi:10.1007/s12011-018-1574-9
Zhou, J., Liu, S., Bi, S., Kong, W., Qian, R., Xie, X., et al. (2023). The rage signaling in osteoporosis. Biomed. & Pharmacother. 165, 115044. doi:10.1016/j.biopha.2023.115044
Keywords: curcumin, osteoporosis, herbal medicine, mechanism, pharmacological effect, pathway
Citation: Wang K (2024) The potential therapeutic role of curcumin in osteoporosis treatment: based on multiple signaling pathways. Front. Pharmacol. 15:1446536. doi: 10.3389/fphar.2024.1446536
Received: 10 June 2024; Accepted: 29 July 2024;
Published: 08 August 2024.
Edited by:
Hongbo Li, Shaanxi University of Science and Technology, ChinaReviewed by:
Qingshan Shen, Nanyang Institute of Technology, ChinaXiao Han, Howard Hughes Medical Institute (HHMI), United States
Zhanyi Yang, Northeast Agricultural University, China
Copyright © 2024 Wang. This is an open-access article distributed under the terms of the Creative Commons Attribution License (CC BY). The use, distribution or reproduction in other forums is permitted, provided the original author(s) and the copyright owner(s) are credited and that the original publication in this journal is cited, in accordance with accepted academic practice. No use, distribution or reproduction is permitted which does not comply with these terms.
*Correspondence: Keyu Wang, d2FuZ2tleXUwMzI0QDE2My5jb20=