- 1Department of Pharmacy, The Second Affiliated Hospital, Guangzhou Medical University, Guangzhou, China
- 2Department of Critical Care, The Second Affiliated Hospital, Guangzhou Medical University, Guangzhou, China
- 3Department of Critical Care, Guangzhou Twelfth People’ Hospital, Guangzhou, China
- 4Department of Pharmacy, Guangzhou Institute of Cancer Research, The Affiliated Cancer Hospital, Guangzhou Medical University, Guangzhou, China
Background: The incidence and mortality of severe Gram-positive cocci infections are particularly high in intensive care units (ICUs). Vancomycin remains the treatment of choice for severe infections caused by Gram-positive cocci, particularly methicillin-resistant Staphylococcus aureus (MRSA). Some guidelines recommend therapeutic drug monitoring (TDM) for critically ill patients treated with vancomycin; however, there is currently a lack of evidence to support that TDM improves the mortality rates of these patients. Therefore, we designed this cohort study to compare the impact of monitoring vancomycin blood concentrations on mortality rates in critically ill patients and to provide evidence to support this routine clinical practice.
Methods: Data were extracted from the Medical Information Mart for Intensive Care (MIMIC)-IV database for a retrospective cohort analysis of critically ill patients receiving intravenous vancomycin treatment. The primary outcome was the 28 day mortality rate. The propensity score matching (PSM) method was used to match the baseline characteristics between patients in the TDM group and the non-TDM group. The relationship between 28 day mortality and vancomycin TDM in the critically ill cohort was evaluated using Cox proportional hazards regression analysis and Kaplan-Meier survival curves. Validation of the primary outcomes was conducted by comparing the PSM model and the Cox proportional hazards regression model. The robustness of the conclusion was subsequently verified by subgroup and sensitivity analyses.
Results: Data for 18,056 critically ill patients who met the study criteria were collected from the MIMIC-IV database. Of these, 7,451 patients had at least one record of vancomycin blood concentration monitoring, which we defined as the TDM group. The TDM group exhibited a 28 day mortality rate of 25.7% (1,912/7,451) compared to 16.2% in the non-TDM group (1,723/10,605). After PSM, 4,264 patients were included in each of the TDM and non-TDM groups, with a 28 day mortality rate of 20.0% (1,022/4,264) in the TDM group and 26.4% (1,126/4,264) in the non-TDM group. Multivariate Cox proportional hazards analysis revealed a significantly lower 28 day mortality risk in the TDM group when compared to the non-TDM group (adjusted hazard ratio [HR]: 0.86; 95% confidence interval [CI]: 0.79, 0.93; p < 0.001). Further PSM analyses (adjusted HR: 0.91; 95% CI: 0.84, 0.99; p = 0.033) confirmed the lower risk of mortality in the TDM group. Kaplan-Meier survival analysis revealed a significantly higher survival rate at 28 days for the TDM group (log-rank test, p < 0.001). Subgroup analysis results indicated that patients with sepsis, septic shock, estimated glomerular filtration rate ≤ 60 mL/min/1.73 m2, undergoing renal replacement therapy, using vasoactive drugs, on mechanical ventilation, and those with higher severity scores (Acute Physiology Score III ≥40, Oxford Acute Severity of Illness Score ≥30, Simplified Acute Physiology Score II ≥ 30) significantly benefited from monitoring vancomycin blood concentrations. The results remained unchanged excluding patients staying in ICU for less than 48 h or those infected with MRSA.
Conclusion: This cohort study showed that monitoring vancomycin blood concentrations is associated with a significantly lower 28 day mortality rate in critically ill patients, highlighting the importance of routinely performing vancomycin TDM in these patients.
1 Introduction
Severe infections caused by Gram-positive cocci are prevalent in intensive care units (ICUs), with reported incidence rates ranging from 16.1% to 50% (Vincent et al., 2009; Baykara et al., 2018; Martin et al., 2003; Chen et al., 2023; Tang et al., 2023). In particular, Gram-positive cocci have been isolated from respiratory specimens in more than 50% of cases with ventilator-associated pneumonia (Yoshimura et al., 2022). These infections are often accompanied by high mortality (Hanberger et al., 2011; van Hal et al., 2012). For instance, bacteremia caused by methicillin-resistant Staphylococcus aureus (MRSA) has a 30 day mortality rate of 28%–30.5% (Wang et al., 2010; Yaw et al., 2014). Vancomycin, a glycopeptide antibiotic, has demonstrated efficacy against severe infections caused by Gram-positive cocci, including MRSA (Jeffres, 2017). Furthermore, it has been the treatment of choice for MRSA for decades and remains one of the most frequently used antibiotics in ICUs (Magill et al., 2014). However, due to a narrow therapeutic window, significant inter-individual pharmacokinetic (PK) variability, and dose-dependent nephrotoxicity, some guidelines recommend therapeutic drug monitoring (TDM) for critically ill patients or those with severe MRSA infections treated with vancomycin (Ye et al., 2016; He et al., 2020; Reuter et al., 2022; Abdul-Aziz et al., 2020; Rybak et al., 2020).
In a narrow sense, vancomycin TDM focuses on adjusting dosage based on blood drug concentration measurements, primarily trough levels, to ensure effective treatment while minimizing risk of toxicity. Trough levels, the lowest drug concentration before the next dose, are recommended by the 2009 clinical guideline to be maintained between 10 and 20 mg/L to balance efficacy and toxicity (Rybak et al., 2009). More broadly, vancomycin TDM includes both trough concentration monitoring and the area under the concentration-time curve (AUC), which offers a more comprehensive assessment of drug exposure and correlates better with both efficacy and toxicity. As a result, AUC monitoring has been increasingly incorporated into TDM standards, enabling more precise dose adjustments and individualized treatment strategies, as seen in the 2020 guideline (Rybak et al., 2020). Trough concentration monitoring offers the advantages of simplicity and quick results, as it involves direct measurement. In contrast, traditional AUC monitoring requires multiple blood samples at different time points, followed by nonlinear curve fitting, making it impractical in clinical settings due to the need for repeated sampling (Yamada et al., 2023). Currently, clinical practice primarily uses the peak-trough two-point method and Bayesian software for AUC estimation. The two-point method involves collecting peak and trough concentrations within a dosing interval, followed by calculating AUC using a first-order equation (Meng et al., 2019). The Bayesian method uses a population PK model combined with 1-2 blood concentration measurements to estimate AUC(Rybak et al., 2020; Turner et al., 2018). Thus, regardless of the method, AUC monitoring requires at least 1-2 blood concentration measurements, making blood concentration monitoring essential for accurate AUC estimation. Therefore, as long as blood concentration measurements are available, it is possible to determine if a patient underwent vancomycin TDM, whether through trough level or AUC monitoring.
Emerging evidence supports a specific relationship between nephrotoxicity and both trough concentration (van Hal et al., 2013; Bellos et al., 2020) and the AUC to the minimum inhibitory concentration (AUC/MIC) (Aljefri et al., 2019; Abdelmessih et al., 2022) during the treatment of serious infections caused by Staphylococcus aureus, particularly MRSA. Although vancomycin TDM can effectively reduce the incidence of nephrotoxicity, trough concentrations do not appear to predict clinical efficacy accurately (Lodise et al., 2008; Steinmetz et al., 2015; Prybylski, 2015). A previous systematic review, incorporating data from over 2,000 patients with invasive MRSA infections, found no significant difference in all-cause mortality between patient groups with trough concentrations >10 mg/L compared to those with levels ≥15 mg/L (Steinmetz et al., 2015). Similarly, a previous meta-analysis that included more than 1,600 patients with Staphylococcus aureus bacteremia demonstrated that trough concentrations >15 mg/L did not correlate with reduced rates of treatment failure, duration of bacteremia, or mortality (Prybylski, 2015). It is generally believed that the AUC/MIC ratio is superior to trough concentration in predicting survival outcomes and clinical cure, while this ratio only shows moderate levels of sensitivity and specificity (Lodise et al., 2008; Dalton et al., 2020; Tsutsuura et al., 2021). The multicenter prospective PROVIDE study further elucidated the relationship between initial vancomycin exposure and clinical treatment failure rates in adult patients with MRSA bacteremia, highlighting that higher AUC/MIC ratios do not necessarily translate into a reduced risk of treatment failure but are linked to an increased incidence of acute kidney injury (Lodise et al., 2020). There is still a clear lack of robust data correlating AUC with mortality; furthermore, the available evidence on the impact of vancomycin TDM on mortality rates is highly limited (Flannery et al., 2021; Briassoulis and Briassoulis, 2022). This highlights the necessity for further rigorous studies to clarify these relationships and optimize vancomycin TDM strategies in clinical settings.
In ICUs, vancomycin is predominantly administered empirically by clinicians based on the severity of infection, the prevalence of local pathogens, and patterns of antibiotic resistance without results generated by pathogen culture (Jones et al., 2020; Cowley et al., 2019). This practice has generated clinical debate about whether routine TDM of vancomycin is necessary for all critically ill patients (Darko et al., 2003). In particular, we need to know whether routine TDM can reduce the mortality rate of these patients. Although some guidelines advocate the use of TDM during vancomycin therapy in critical settings, these recommendations are based on limited clinical and safety data and do not consider the costs associated with vancomycin TDM. Currently, there is insufficient evidence to evaluate the impact of this practice on mortality and cost-effectiveness (Ye et al., 2016; He et al., 2020; Reuter et al., 2022; Abdul-Aziz et al., 2020; Rybak et al., 2009). In the updated 2020 vancomycin TDM guideline, the Therapeutic Drug Monitoring Committee of the Chinese Pharmacological Society reported that despite recommendations for TDM in critically ill patients, these were classified as low-quality evidence due to the lack of supportive data (He et al., 2020). Some previous studies reported that routine vancomycin TDM may consume significant time and healthcare resources, and may potentially lack cost-effectiveness (Jeffres, 2017; Darko et al., 2003). Consequently, in the present study, we utilized a large dataset from the MIMIC-IV database to investigate the impact of monitoring vancomycin blood concentrations on mortality rates in critically ill patients. Our hypothesis was that monitoring vancomycin blood concentrations would reduce mortality. If our hypothesis is proven, our findings would provide the most direct evidence to support the routine implementation of vancomycin TDM in critically ill patients.
2 Materials and methods
2.1 Data source
The Medical Information Mart for Intensive Care IV (MIMIC-IV) database, hosted at PhysioNet, is a publicly accessible, single-center repository that includes data relating to 730,141 ICU admissions at the Beth Israel Deaconess Medical Center in the United States, spanning from 2008 to 2019 (Johnson et al., 2023). Researcher Huaidong Peng (certification number: 59679596) and colleagues utilized the database to extract a wide range of clinical data, including patient demographics, vital signs, laboratory tests, comorbidities, severity of illness scores, therapeutic interventions, and specifics regarding vancomycin administration and TDM information. The use of the MIMIC-IV database was authorized by the Institutional Review Boards of the Massachusetts Institute of Technology and Beth Israel Deaconess Medical Center. Our study was conducted in accordance with the Declaration of Helsinki, and due to the anonymization of participant data and its standardized format, additional approval from the ethics committee was deemed unnecessary.
2.2 Study population
All patients recorded in the MIMIC-IV database were considered eligible for inclusion in this study. For those with multiple ICU admissions, only the data from their first ICU stay were analyzed. We included patients who began intravenous vancomycin treatment either before or after ICU admission. Vancomycin TDM was defined as having at least one blood vancomycin concentration measurement during the ICU stay, irrespective of whether it was a trough, peak, or random concentration. Patients receiving vancomycin TDM during their ICU hospitalization were designated as the experimental group (the TDM group), while those administered vancomycin without subsequent TDM formed the control group (the non-TDM group). Additionally, the analysis was limited to adult patients aged 18 years and older. The patient enrollment process for this study is depicted in Figure 1.
2.3 Covariates and outcome
We used Structured Query Language to systematically extract patient data from the database. The extracted data included a range of variables: basic demographic details for hospital admission registration such as gender, age, and race; vital signs, including heart rate, mean arterial pressure, respiratory rate, temperature, and SpO2; laboratory tests, including white blood cell count (WBC), hemoglobin, hematocrit, platelets, creatinine, blood urea nitrogen (BUN), finger glucose, potassium, and bicarbonate; comorbidities, including hypertension, congestive heart failure, chronic obstructive pulmonary disease (COPD), liver disease, diabetes, renal disease, and malignant cancer; severity of illness scores, including the Acute Physiology Score (APS) III, Simplified Acute Physiology Score (SAPS) II, Sequential Organ Failure Assessment (SOFA) score, Charlson Comorbidity Index, and Oxford Acute Severity of Illness Score (OASIS); therapeutic interventions such as renal replacement therapy (RRT), vasoactive drugs, and mechanical ventilation; and vancomycin administration and TDM information. The estimated glomerular filtration rate (eGFR) was calculated using the Chronic Kidney Disease Epidemiology Collaboration (CKD-EPI) formula, which adjusts for serum creatinine while accounting for the patient’s gender, age, and creatinine levels (Inker et al., 2021).
The primary outcome of this study was 28 day mortality. Secondary outcomes included ICU mortality, hospital mortality, 60 day mortality, and 90 day mortality.
2.4 Statistical analysis
We addressed missing data using K-Nearest Neighbors imputation (Faisal and Tutz, 2022), detailed information relating to missing data is provided in Supplementary Table 1. Continuous variables are reported as either mean ± standard deviation or median with interquartile range (IQR), while categorical variables are reported as frequency (percentage). The conformity of continuous variables to the normal distribution was tested using the Shapiro-Wilk test or Kolmogorov-Smirnov test. For continuous variables that conformed to a normal distribution, we used the Student’s t-test to compare means. For non-normally distributed continuous variables, the Wilcoxon’s rank-sum test was applied. For categorical variables, we used Pearson’s chi-squared test or Fisher’s exact test, as appropriate.
Cox proportional hazards regression analyses were used to investigate the independent association between vancomycin TDM and 28 day mortality, yielding hazard ratios (HRs) and 95% confidence intervals (CIs). Survival distributions were estimated by Kaplan-Meier analysis, and differences were assessed by the log-rank test. To balance baseline characteristics between the TDM and non-TDM groups, we implemented propensity score matching (PSM) using a 1:1 nearest neighbor matching algorithm with a caliper width of 0.1. The variables listed in Table 1 were used to generate the propensity score. The primary outcome was further validated using the PSM model, which applied the estimated propensity scores as weights. The results were then compared to those derived from the Cox proportional hazards regression model.
All statistical analyses were conducted using R statistical software, version 3.3.2 (http://www.R-project.org, The R Foundation) and Free Statistics software, version 1.9 (https://www.clinicalscientists.cn/freestatistics/). A two-tailed test approach was adopted, and p < 0.05 was considered statistically significant.
2.5 Subgroup analysis and sensitivity analysis
To evaluate the robustness of our findings, we conducted subgroup analyses stratified by gender, age, race, sepsis, septic shock, eGFR, RRT, the use of vasoactive drugs, mechanical ventilation, APS III, OASIS, and SAPS II. Furthermore, to delineate independent associations, we performed distinct sensitivity analyses. Sensitivity analysis was performed after excluding patients with ICU hospitalization time less than 48 h or diagnosed with MRSA infection. These analyses aimed to ensure the reliability and applicability of our results across diverse patient groups and clinical scenarios.
3 Results
3.1 Patient characteristics
A total of 18,056 patients who received intravenous vancomycin were enrolled in our cohort from the MIMIC-IV database. The mean age of the patients was 66.0 ± 16.0 years, and 58.7% were male (n = 10,599). The overall 28 day mortality rate was 20.1% (3,635/18,056). Of these, 7,451 patients (41.3%) underwent at least one round of vancomycin blood concentration monitoring, while 10,605 (58.7%) patients did not (Table 1). Table 1 presents the clinical information of both the non-TDM and TDM groups before and after PSM. When considering all enrolled patients, those in the TDM group were older, had higher WBC counts, lower eGFR levels, higher BUN levels, and a higher proportion of comorbidities such as heart failure, COPD, liver disease, and kidney disease when compared to the non-TDM group (all p < 0.001). The incidence of sepsis and septic shock was higher in the TDM group, and all five severity scores were higher than those in the non-TDM group (all p < 0.001). Additionally, there was a higher proportion of patients with definitive MRSA infections, as well as more patients requiring RRT, mechanical ventilation, and vasoactive drug treatment (all p < 0.001). These data suggest that patients in the TDM group were in more severe condition than those in the non-TDM group.
After performing PSM, the characteristics of 4,264 patients were successfully matched between the two groups. The standardized mean differences (SMD) of all variables after PSM were less than 10%, indicating good quality of the matched samples (Table 1). After minimizing the interference of confounders through PSM, monitoring vancomycin blood concentrations was identified as the main factor.
3.2 Primary outcome
Table 2 presents the mortality data arising from our analysis. According to the MIMIC-IV database, the 28 day mortality rate for critically ill patients treated with vancomycin was 20.1% (3,635/18,056). Notably, the 28 day mortality rate in the TDM group was 25.7% (1,912/7,451), which was significantly higher than 16.2% (1,723/10,605) in the non-TDM group (p < 0.001). After PSM, the overall 28 day mortality rate was 25.2% (2,148/8,528). However, within the matched cohort, the 28 day mortality rate of the TDM group was significantly lower than that of the non-TDM group (20.0% vs 26.4%, p = 0.009).
3.3 Secondary outcomes
ICU mortality, hospital mortality, 60 day mortality, and 90 day mortality, all showed patterns that were similar to the 28 day mortality results (Table 2). After PSM, all of these mortality metrics shifted from being higher in the TDM group than the non-TDM group before PSM to being significantly lower in the TDM group after PSM, with all differences were significant (p < 0.05).
3.4 Association between vancomycin TDM and 28 day mortality
In extended multivariable Cox regression models, we made adjustments using various covariates, and the changes in the HRs are detailed in Table 3. After incorporating all covariates, multivariate Cox proportional hazards regression analysis revealed an adjusted HR of 0.86 (95% CI: 0.79, 0.93; p < 0.001), indicating a significant reduction in hazard. This finding was consistent with the post-matched cohort results, where PSM (adjusted HR: 0.91; 95% CI: 0.84, 0.99; p = 0.033) showed that monitoring vancomycin blood concentrations was significantly associated with reduced 28 day mortality among critically ill patients (Table 4). This robust statistical evidence highlights the efficacy of vancomycin TDM in improving survival outcomes in this vulnerable patient population.
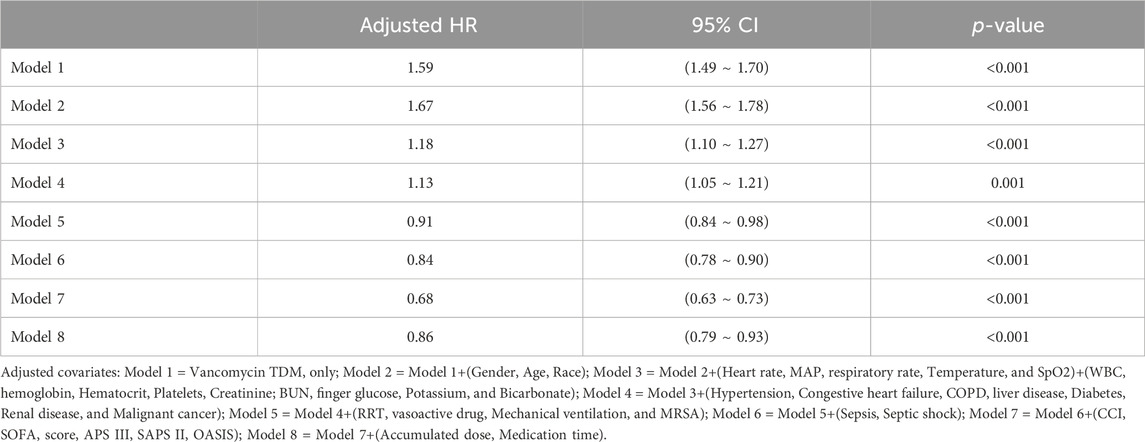
Table 3. Association between TDM and 28 day mortality using Cox proportional hazards regression analysis.
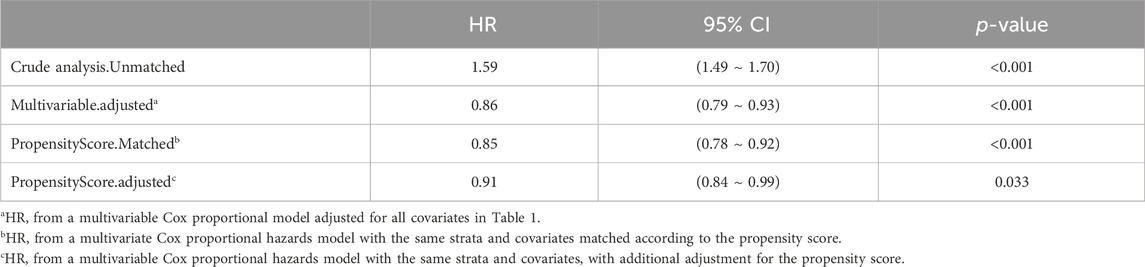
Table 4. The association between vancomycin TDM and 28 day mortality, as determined by analyses incorporating multiple models.
A Kaplan-Meier survival curve also demonstrated that the TDM group had a lower 28 day mortality rate (log-rank test: p < 0.001; Figure 2). Results from the 60 day and 90 day survival curves were consistent with those from the 28 day results (detailed results are available in Supplementary Images 1, 2).
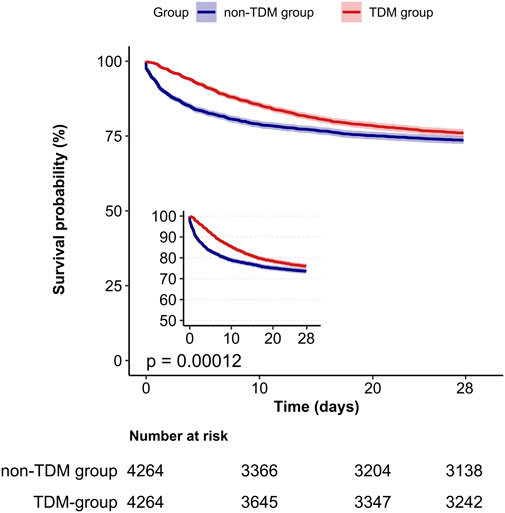
Figure 2. Kaplan-Meier survival curves for 28-day mortality in critically ill patients from the TDM group and the non-TDM group.
3.5 Subgroup analysis
Next, we stratified our cohort into various demographic and clinical subgroups based on gender, age, race, sepsis, septic shock, eGFR, RRT, the use of vasoactive drugs, mechanical ventilation, APS III, OASIS, and SAPS II. The impact of monitoring vancomycin blood concentrations on 28 day mortality was investigated and the results were visualized as a forest plot (Figure 3). Subgroup analyses indicated a general negative correlation between monitoring vancomycin blood concentrations and 28 day mortality among critically ill patients. The results showed lower 28 day mortality in several subgroups, including males (HR: 0.76; 95% CI: 0.67, 0.85), females (HR: 0.85; 95% CI: 0.75, 0.97), patients younger than 65 years (HR: 0.63; 95% CI: 0.54, 0.74), those aged 65 years or older (HR: 0.90; 95% CI: 0.81, 1.00), White individuals (HR: 0.81; 95% CI: 0.72, 0.90), non-White individuals (HR: 0.79; 95% CI: 0.69, 0.91), patients with sepsis (HR: 0.79; 95% CI: 0.73, 0.87), those with septic shock (HR: 0.73; 95% CI: 0.66, 0.82), patients with eGFR <15 mL/min/1.73 m2 (HR: 0.51; 95% CI: 0.41, 0.65), those with eGFR between 15 and 60 mL/min/1.73 m2 (HR: 0.76; 95% CI: 0.68, 0.86), patients undergoing RRT (HR: 0.46; 95% CI: 0.32, 0.65), those receiving vasoactive drugs (HR: 0.73; 95% CI: 0.65, 0.81), those on mechanical ventilation (HR: 0.79; 95% CI: 0.71, 0.88), and patients with APS III scores ≥40 (HR: 0.77; 95% CI: 0.70, 0.84), OASIS scores ≥30 (HR: 0.77; 95% CI: 0.70, 0.84), or SAPS II scores ≥30 (HR: 0.78; 95% CI: 0.71, 0.85). However, interactions were identified between age, septic shock, RRT, vasoactive drugs, vasoactive drugs, APS III, OASIS, and SAPS II (p for interaction <0.05) (Figure 3).
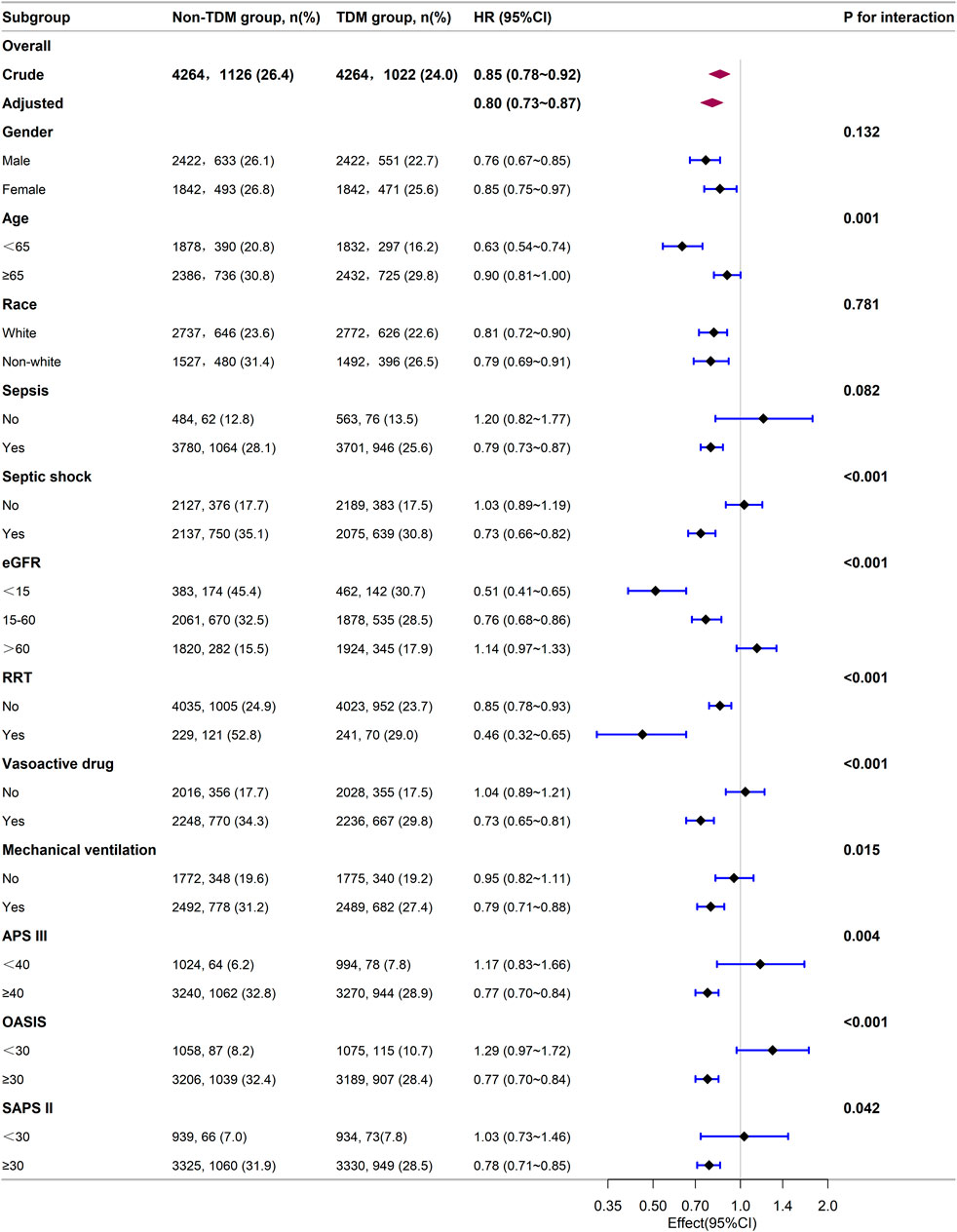
Figure 3. Subgroup analysis of the relationship between vancomycin TDM and 28-day mortality, as visualized by a forest plot.
3.6 Sensitivity analysis
There were 18,056 patients in the entire cohort. After excluding 6,058 patients whose ICU stay was less than 48 h, 11,998 patients remained for final analysis. Our findings indicated that vancomycin TDM was associated with a lower risk of 28 day mortality (multivariable Cox proportional model, adjusted HR: 0.87; 95% CI: 0.79, 0.95; p = 0.002). Furthermore, after excluding 1,307 patients with positive microbiological cultures for MRSA infection, the association between vancomycin TDM and 28 day mortality remained significant (multivariable Cox proportional model, adjusted HR: 0.84; 95% CI: 0.78, 0.92; p < 0.001) (Table 5).
4 Discussion
In this large retrospective cohort study, we found that patients in the TDM group exhibited more severe conditions and a higher 28 day mortality rate compared to those in the non-TDM group. After balancing the baseline characteristics of patients between the two groups using PSM, the mortality rate in the TDM group was lower than that in the non-TDM group. After including all covariates, the multivariate Cox model suggested that vancomycin TDM is a protective factor that can reduce the 28 day mortality rate among critically ill patients. Both the Cox proportional hazards regression analysis model and the PSM model corroborated this finding. Kaplan-Meier survival curves identified a lower 28 day mortality rate in the TDM group. Collectively, these results demonstrated that monitoring vancomycin blood concentrations was associated with a lower 28 day mortality rate in critically ill patients.
Typically, the severity of illness in critically ill patients correlates positively with both the duration of ICU admission and mortality rate (Knaus et al., 1991). In patients treated with vancomycin, increased disease severity not only prolongs hospitalization but also increases the need for TDM (Abdul-Aziz et al., 2020; Wong et al., 2014). Consequently, critically ill patients undergoing vancomycin TDM may exhibit higher mortality rates due to their more severe condition, while those not undergoing TDM, reflecting milder conditions, may present lower mortality rates. Our findings corroborate this hypothesis. If vancomycin TDM indeed mitigates the risk of mortality in critically ill patients, the severity of the underlying conditions might obscure these beneficial effects. Our analysis using multivariate Cox proportional hazards regression and PSM model robustly demonstrated that, after adjusting for covariates reflecting disease severity, monitoring vancomycin blood concentrations was associated with a significant reduction in the 28 day mortality rate in critically ill patients. Although monitoring drug concentrations alone does not constitute a complete TDM process, it is a fundamental component of TDM. It provides the basis for individualized vancomycin dosing, allowing clinicians to optimize therapy based on patient-specific PK and PD characteristics. Drug concentration monitoring enables timely dose adjustments, achieving therapeutic levels, reducing toxicity, and improving patient outcomes. Therefore, drug concentration monitoring represents an essential part of TDM and significantly reflects the application of TDM in clinical practice.
It is pertinent to note that only a limited number of studies have investigated the clinical outcomes of vancomycin dose adjustments under TDM guidance compared to those without such interventions (Ye et al., 2013; Fernández de Gatta et al., 1996; Iwamoto et al., 2003; Liu et al., 2024; Welty and Copa, 1994; Marella et al., 2020; Cardile et al., 2015). Predominantly, these investigations were derived from small-scale, single-disease studies, and non-randomized controlled trials. For instance, Machado et al. (2017) investigated the effect of antimicrobial TDM, including agents such as imipenem, meropenem, piperacillin, and vancomycin, on the prognosis of burns patients but found no evidence of prognostic improvement. A systematic review and meta-analysis investigated the advantages of vancomycin TDM and demonstrated a significant improvement in clinical efficacy (HR: 2.62; 95% CI: 1.34, 5.11; p = 0.005) and a marked reduction in nephrotoxicity (HR: 0.25; 95% CI: 0.13, 0.48; p < 0.0001), albeit without mortality data (Ye et al., 2013). In another study, Fernández de Gatta et al. (1996) found that vancomycin TDM reduced nephrotoxicity in patients with hematological malignancies; however, the sample sizes analyzed in this study were small. Another retrospective analysis of 184 MRSA infections, classified into TDM and non-TDM groups, did not report mortality outcomes (Iwamoto et al., 2003). A previous study by Huanhuan et al. compared patients with postoperative intracerebral hemorrhage who received TDM to those who did not; the analysis found no significant differences between the two groups in terms of 14 day mortality rate and the length of hospital stay (Liu et al., 2024). Welty et al. further reported that vancomycin TDM was associated with a lower incidence of nephrotoxicity, a shorter treatment duration, a reduced total dose, and a shorter hospital stay, but not mortality rates (Welty and Copa, 1994). In another study, Marella et al. compared the efficacy of vancomycin TDM in critically ill adult patients undergoing extracorporeal membrane oxygenation and found no significant difference in mortality rates between the TDM group (62.3%) and the non-TDM group (68.4%) (Marella et al., 2020). Cardile et al. found that TDM successfully achieved the initial target trough concentration of vancomycin for Gram-positive cocci infections more rapidly, particularly for MRSA infections; however, there was no significant difference between the groups in terms of treatment failure and in-hospital mortality rates (Cardile et al., 2015). To our knowledge, our present study is the first to use large-scale data to confirm that monitoring vancomycin blood concentrations can significantly reduce mortality rates in critically ill patients when compared to the administration of vancomycin without monitoring. The strength of our study lies in the provision of detailed mortality data, a large sample size, and consistent results.
Vancomycin TDM provides substantial benefits for critically ill patients in several key aspects. First, attaining therapeutic levels of vancomycin in ICUs remains a significant challenge. Previous evidence indicated that 40%–55.8% of ICU patients do not attain adequate initial trough concentrations post-administration, potentially leading to therapeutic failure (Mahmoodian et al., 2016; Obara et al., 2016; Alshehri et al., 2020; Bakke et al., 2017). The implementation of TDM for vancomycin enables clinicians to tailor pharmacotherapy more precisely, ensuring the timely attainment of therapeutic targets (Bakke et al., 2017; Truong et al., 2018; Flannery et al., 2020) and enhancing the likelihood of treatment success (Ye et al., 2013; Shahrami et al., 2016). Many studies have investigated the relationship between mortality rates and trough concentrations or AUC/MIC within a specific range (Alshehri et al., 2020; Li et al., 2022; Chen et al., 2024). If the trough concentrations or AUC/MIC ratios fall outside of these therapeutic ranges, dose adjustments can be effectively employed to align with established targets, thereby mitigating the risk of mortality (Steinmetz et al., 2015; Casapao et al., 2015; Lodise et al., 2014). Furthermore, both trough concentrations and AUC can predict potential nephrotoxicity. When high trough concentrations or AUC are detected, adjustments in dosage and administration can prevent drug-related nephrotoxicity (Hall et al., 2024; Ishigo et al., 2024), thereby reducing patient mortality (Aljefri et al., 2019; Abdelmessih et al., 2022; Li et al., 2022; Chen et al., 2024). Although TDM increases medical costs, dosage adjustment remains cost-effective for some critically ill patients (Ye et al., 2016; Fernández de Gatta et al., 1996). These considerations clearly demonstrate the necessity of implementing vancomycin TDM in critical care settings.
Our subgroup analysis showed that patients diagnosed with sepsis or septic shock had better survival outcomes from monitoring vancomycin blood concentrations compared to those without such diagnoses. Patients receiving RRT, vasopressor drugs, or mechanical ventilation showed significantly greater survival benefits from monitoring vancomycin blood concentrations compared to those who did not. Compared to patients with eGFR ≥60 mL/min/1.73 m2, those with eGFR <60 mL/min/1.73 m2 gained survival benefits from monitoring vancomycin blood concentrations, especially those with eGFR <15 mL/min/1.73 m2, while patients with eGFR ≥60 mL/min/1.73 m2 did not show survival benefits. Additionally, patients with higher severity scores (APS III ≥40, OASIS ≥30, SAPS II ≥ 30) benefited from vancomycin TDM, while those with lower severity scores did not. These findings suggest that only critically ill patients with severe conditions benefit from monitoring vancomycin blood concentrations. This indicates that monitoring vancomycin blood concentrations is especially important for critically ill patients, probably because precise dosing is necessary to balance efficacy and toxicity in severe cases. These findings are crucial for guiding clinical decisions regarding the implementation of TDM in critically ill patients receiving vancomycin.
Due to time constraints, a short ICU stay might preclude the possibility of conducting vancomycin TDM, even if it is needed. When excluding patients with an ICU stay of less than 48 h, our findings mirrored those of the entire cohort, further confirming the survival benefits of monitoring vancomycin blood concentrations for critically ill patients. Previously, most research on precise clinical guidance relating to vancomycin TDM focused on infections caused by MRSA (Steinmetz et al., 2015; Lodise et al., 2020; Bakke et al., 2017; Casapao et al., 2015; Lodise et al., 2014), whereas vancomycin was often used empirically in ICU settings (Cowley et al., 2019; Chow et al., 2020; Bostwick et al., 2019). To investigate the impact of monitoring vancomycin blood concentrations on mortality during empirical use, we excluded those with confirmed MRSA infections. Our results demonstrated clear survival benefits for severely ill patients without confirmed MRSA infection.
This study has several limitations that need to be considered. First, as a retrospective study, the non-randomized grouping of patients introduced inherent baseline disparities. To address potential selection and ascertainment biases, we used PSM to mitigate these disparities. However, residual confounding factors might have influenced prognostic outcomes for critically ill patients. Second, although our findings indicated that monitoring vancomycin blood concentrations reduced mortality in critically ill patients, our analysis did not consider cost-effectiveness. This leaves the economic feasibility of routine implementation for all critically ill patients uncertain. Third, monitoring vancomycin blood concentrations is not equivalent to completing the full TDM process; it merely represents a foundational step. Furthermore, due to the limitations of our data sources, we were unable to determine which patients underwent AUC monitoring and which only had trough levels measured. Moreover, it is unclear who led the TDM implementation, who interpreted the results, and how the treatment regimen was adjusted based on the monitoring results. Additionally, blood concentration monitoring may serve as a surrogate marker for overall higher quality of care, and the influence of other unknown variables on patient outcomes cannot be excluded. Fourth, due to the complex clinical scenarios of critically ill patients and the prevalence of infections, a definitive causal relationship between monitoring vancomycin blood concentrations and patient outcomes in critical care settings has yet to be established. Therefore, our findings should be interpreted with caution.
5 Conclusion
This cohort study showed that monitoring vancomycin blood concentrations is associated with a significantly lower 28 day mortality rate in critically ill patients, with greater survival benefits observed in those with more severe conditions, underscoring the importance of routine vancomycin TDM in these patients.
Data availability statement
The original contributions presented in the study are included in the article/Supplementary Material, further inquiries can be directed to the corresponding authors.
Ethics statement
The studies involving human subjects were approved, and the requirement for additional ethical approval was waived by the Clinical Research Ethics Committee of the Second Affiliated Hospital of Guangzhou Medical University. This waiver was granted because the MIMIC-IV database had already received ethical approval from the institutional review boards (IRBs) at Beth Israel Deaconess Medical Center and the Massachusetts Institute of Technology. The studies were conducted in accordance with local legislation and institutional requirements. Additionally, the ethics committee/institutional review board waived the requirement for written informed consent from participants or their legal guardians/next of kin, as the studies adhered to local legal and institutional guidelines and the database does not contain protected health information.
Author contributions
HP: Data curation, Formal Analysis, Investigation, Validation, Writing–original draft, Methodology, Writing–review and editing, Project administration, Visualization. YO: Data curation, Formal Analysis, Investigation, Methodology, Validation, Writing–original draft, Project administration, Software. RZ: Project administration, Validation, Visualization, Data curation, Writing–original draft, Formal Analysis, Investigation, Methodology. RW: Supervision, Writing–original draft, Methodology. DW: Supervision, Writing–review and editing, Methodology. QY: Supervision, Writing–review and editing, Data curation, Methodology, Project administration, Software, Validation. XL: Supervision, Writing–review and editing, Data curation, Methodology, Project administration, Resources, Validation, Funding acquisition.
Funding
The author(s) declare that financial support was received for the research, authorship, and/or publication of this article. This work was supported by the Medical Scientific Research Foundation of Guangdong Province (No. B2024150), Guangzhou Health Science and Technology Project (No. 20251A011077) and the Hospital Pharmacy Research Foundation of Guangdong Province (No. 2023KJ16).
Acknowledgments
The authors express their deep gratitude to all the researchers who built and maintained the MIMIC IV database.
Conflict of interest
The authors declare that the research was conducted in the absence of any commercial or financial relationships that could be construed as a potential conflict of interest.
Publisher’s note
All claims expressed in this article are solely those of the authors and do not necessarily represent those of their affiliated organizations, or those of the publisher, the editors and the reviewers. Any product that may be evaluated in this article, or claim that may be made by its manufacturer, is not guaranteed or endorsed by the publisher.
Supplementary material
The Supplementary Material for this article can be found online at: https://www.frontiersin.org/articles/10.3389/fphar.2024.1458600/full#supplementary-material
References
Abdelmessih, E., Patel, N., Vekaria, J., Crovetto, B., SanFilippo, S., Adams, C., et al. (2022). Vancomycin area under the curve versus trough only guided dosing and the risk of acute kidney injury: systematic review and meta-analysis. Pharmacotherapy 42 (9), 741–753. doi:10.1002/phar.2722
Abdul-Aziz, M. H., Alffenaar, J. C., Bassetti, M., Bracht, H., Dimopoulos, G., Marriott, D., et al. (2020). Antimicrobial therapeutic drug monitoring in critically ill adult patients: a Position Paper<sub/>. Intensive Care Med. 46 (6), 1127–1153. doi:10.1007/s00134-020-06050-1
Aljefri, D. M., Avedissian, S. N., Rhodes, N. J., Postelnick, M. J., Nguyen, K., and Scheetz, M. H. (2019). Vancomycin area under the curve and acute kidney injury: a meta-analysis. Clin. Infect. Dis. 69 (11), 1881–1887. doi:10.1093/cid/ciz051
Alshehri, N., Ahmed, A. E., Yenugadhati, N., Javad, S., Al Sulaiman, K., H, M. A.-D., et al. (2020). Vancomycin in ICU patients with gram-positive infections: initial Trough Levels and mortality. Ther. Clin. Risk Manag. 16, 979–987. doi:10.2147/tcrm.S266295
Bakke, V., Sporsem, H., Von der Lippe, E., Nordøy, I., Lao, Y., Nyrerød, H. C., et al. (2017). Vancomycin levels are frequently subtherapeutic in critically ill patients: a prospective observational study. Acta Anaesthesiol. Scand. 61 (6), 627–635. doi:10.1111/aas.12897
Baykara, N., Akalın, H., Arslantaş, M. K., Hancı, V., Çağlayan, Ç., Kahveci, F., et al. (2018). Epidemiology of sepsis in intensive care units in Turkey: a multicenter, point-prevalence study. Crit. Care 22 (1), 93. doi:10.1186/s13054-018-2013-1
Bellos, I., Daskalakis, G., and Pergialiotis, V. (2020). Relationship of vancomycin trough levels with acute kidney injury risk: an exposure-toxicity meta-analysis. J. Antimicrob. Chemother. 75 (10), 2725–2734. doi:10.1093/jac/dkaa184
Bostwick, A. D., Jones, B. E., Paine, R., Goetz, M. B., Samore, M., and Jones, M. (2019). Potential impact of hospital-acquired pneumonia guidelines on empiric antibiotics. An evaluation of 113 veterans affairs medical centers. Ann. Am. Thorac. Soc. 16 (11), 1392–1398. doi:10.1513/AnnalsATS.201902-162OC
Briassoulis, P., and Briassoulis, G. (2022). Bayesian models for therapeutic monitoring of vancomycin in the critically ill: what should they predict? Crit. Care Med. 50 (1), e100–e101. doi:10.1097/ccm.0000000000005228
Cardile, A. P., Tan, C., Lustik, M. B., Stratton, A. N., Madar, C. S., Elegino, J., et al. (2015). Optimization of time to initial vancomycin target trough improves clinical outcomes. Springerplus 4, 364. doi:10.1186/s40064-015-1146-9
Casapao, A. M., Lodise, T. P., Davis, S. L., Claeys, K. C., Kullar, R., Levine, D. P., et al. (2015). Association between vancomycin day 1 exposure profile and outcomes among patients with methicillin-resistant Staphylococcus aureus infective endocarditis. Antimicrob. Agents Chemother. 59 (6), 2978–2985. doi:10.1128/aac.03970-14
Chen, J., Lin, J., Weng, J., Ju, Y., and Li, Y. (2024). Association between trough serum vancomycin concentration and vancomycin-associated acute kidney injury and 30-day mortality in critically ill elderly adults. BMC Infect. Dis. 24 (1), 330. doi:10.1186/s12879-024-09227-x
Chen, X., Zhou, Y., Luo, L., Peng, X., and Xiang, T. (2023). A predictive model for the identification of the risk of sepsis in patients with Gram-positive bacteria in the intensive care unit. J. Thorac. Dis. 15 (9), 4896–4913. doi:10.21037/jtd-23-1133
Chow, T. C. H., Li, J. Y. S., Wong, J. C. L., Poon, F. M. H., Lam, H. S., Lam, T. T., et al. (2020). Vancomycin prescribing practices and therapeutic drug monitoring for critically ill neonatal and pediatric patients: a survey of physicians and pharmacists in Hong Kong. Front. Pediatr. 8, 538298. doi:10.3389/fped.2020.538298
Cowley, M. C., Ritchie, D. J., Hampton, N., Kollef, M. H., and Micek, S. T. (2019). Outcomes associated with de-escalating therapy for methicillin-resistant Staphylococcus aureus in culture-negative nosocomial pneumonia. Chest 155 (1), 53–59. doi:10.1016/j.chest.2018.10.014
Dalton, B. R., Rajakumar, I., Langevin, A., Ondro, C., Sabuda, D., Griener, T. P., et al. (2020). Vancomycin area under the curve to minimum inhibitory concentration ratio predicting clinical outcome: a systematic review and meta-analysis with pooled sensitivity and specificity. Clin. Microbiol. Infect. 26 (4), 436–446. doi:10.1016/j.cmi.2019.10.029
Darko, W., Medicis, J. J., Smith, A., Guharoy, R., and Lehmann, D. E. (2003). Mississippi mud no more: cost-effectiveness of pharmacokinetic dosage adjustment of vancomycin to prevent nephrotoxicity. Pharmacotherapy 23 (5), 643–650. doi:10.1592/phco.23.5.643.32199
Faisal, S., and Tutz, G. (2022). Nearest neighbor imputation for categorical data by weighting of attributes. Inf. Sci. 592, 306–319. doi:10.1016/j.ins.2022.01.056
Fernández de Gatta, M. D., Calvo, M. V., Hernández, J. M., Caballero, D., San Miguel, J. F., and Domínguez-Gil, A. (1996). Cost-effectiveness analysis of serum vancomycin concentration monitoring in patients with hematologic malignancies. Clin. Pharmacol. Ther. 60 (3), 332–340. doi:10.1016/s0009-9236(96)90060-0
Flannery, A. H., Delozier, N. L., Effoe, S. A., Wallace, K. L., Cook, A. M., and Burgess, D. S. (2020). First-dose vancomycin pharmacokinetics versus empiric dosing on area-under-the-curve target attainment in critically ill patients. Pharmacotherapy 40 (12), 1210–1218. doi:10.1002/phar.2486
Flannery, A. H., Landmesser, K. B., and Mynatt, R. P. (2021). New ways to skin a cat or still a cat chasing its tail? Bayesian vancomycin monitoring in the ICU. Crit. Care Med. 49 (10), 1844–1847. doi:10.1097/ccm.0000000000005121
Hall, N. M., Brown, M. L., Edwards, W. S., Oster, R. A., Cordell, W., and Stripling, J. (2024). Model-informed precision dosing improves outcomes in patients receiving vancomycin for gram-positive infections. Open Forum Infect. Dis. 11 (1), ofae002. doi:10.1093/ofid/ofae002
Hanberger, H., Walther, S., Leone, M., Barie, P. S., Rello, J., Lipman, J., et al. (2011). Increased mortality associated with methicillin-resistant Staphylococcus aureus (MRSA) infection in the intensive care unit: results from the EPIC II study. Int. J. Antimicrob. Agents 38 (4), 331–335. doi:10.1016/j.ijantimicag.2011.05.013
He, N., Su, S., Ye, Z., Du, G., He, B., Li, D., et al. (2020). Evidence-based guideline for therapeutic drug monitoring of vancomycin: 2020 update by the division of therapeutic drug monitoring, Chinese pharmacological society. Clin. Infect. Dis. 71 (Suppl. 4), S363–s371. doi:10.1093/cid/ciaa1536
Inker, L. A., Eneanya, N. D., Coresh, J., Tighiouart, H., Wang, D., Sang, Y., et al. (2021). New creatinine- and cystatin C-based equations to estimate GFR without race. N. Engl. J. Med. 385 (19), 1737–1749. doi:10.1056/NEJMoa2102953
Ishigo, T., Matsumoto, K., Yoshida, H., Tanaka, H., Ibe, Y., Fujii, S., et al. (2024). Relationship between nephrotoxicity and area under the concentration-time curve of vancomycin in critically ill patients: a multicenter retrospective study. Microbiol. Spectr. 12, e0373923. doi:10.1128/spectrum.03739-23
Iwamoto, T., Kagawa, Y., and Kojima, M. (2003). Clinical efficacy of therapeutic drug monitoring in patients receiving vancomycin. Biol. Pharm. Bull. 26 (6), 876–879. doi:10.1248/bpb.26.876
Jeffres, M. N. (2017). The whole price of vancomycin: toxicities, troughs, and time. Drugs 77 (11), 1143–1154. doi:10.1007/s40265-017-0764-7
Johnson, A. E. W., Bulgarelli, L., Shen, L., Gayles, A., Shammout, A., Horng, S., et al. (2023). MIMIC-IV, a freely accessible electronic health record dataset. Sci. Data 10 (1), 1. doi:10.1038/s41597-022-01899-x
Jones, B. E., Ying, J., Stevens, V., Haroldsen, C., He, T., Nevers, M., et al. (2020). Empirical anti-MRSA vs standard antibiotic therapy and risk of 30-day mortality in patients hospitalized for pneumonia. JAMA Intern Med. 180 (4), 552–560. doi:10.1001/jamainternmed.2019.7495
Knaus, W. A., Wagner, D. P., Draper, E. A., Zimmerman, J. E., Bergner, M., Bastos, P. G., et al. (1991). The Apache III prognostic system. Risk prediction of hospital mortality for critically ill hospitalized adults. Chest 100 (6), 1619–1636. doi:10.1378/chest.100.6.1619
Li, L., Zhang, L., Li, S., Xu, F., Li, L., Li, S., et al. (2022). Effect of first trough vancomycin concentration on the occurrence of aki in critically ill patients: a retrospective study of the MIMIC-IV database. Front. Med. (Lausanne) 9, 879861. doi:10.3389/fmed.2022.879861
Liu, H., Yang, H., Guo, X., Bai, Y., and SiRi, G. (2024). Clinical benefits of therapeutic drug monitoring of vancomycin therapy in patients with postoperative intracerebral hemorrhage: a retrospective cohort study. Eur. J. Hosp. Pharm. 31 (3), 240–246. doi:10.1136/ejhpharm-2022-003455
Lodise, T. P., Drusano, G. L., Zasowski, E., Dihmess, A., Lazariu, V., Cosler, L., et al. (2014). Vancomycin exposure in patients with methicillin-resistant Staphylococcus aureus bloodstream infections: how much is enough? Clin. Infect. Dis. 59 (5), 666–675. doi:10.1093/cid/ciu398
Lodise, T. P., Graves, J., Evans, A., Graffunder, E., Helmecke, M., Lomaestro, B. M., et al. (2008). Relationship between vancomycin MIC and failure among patients with methicillin-resistant Staphylococcus aureus bacteremia treated with vancomycin. Antimicrob. Agents Chemother. 52 (9), 3315–3320. doi:10.1128/aac.00113-08
Lodise, T. P., Rosenkranz, S. L., Finnemeyer, M., Evans, S., Sims, M., Zervos, M. J., et al. (2020). The emperor's new clothes: PRospective observational evaluation of the association between initial VancomycIn exposure and failure rates among ADult HospitalizEd patients with methicillin-resistant Staphylococcus aureus bloodstream infections (PROVIDE). Clin. Infect. Dis. 70 (8), 1536–1545. doi:10.1093/cid/ciz460
Machado, A. S., Oliveira, M. S., Sanches, C., Silva Junior, C. V. D., Gomez, D. S., Gemperli, R., et al. (2017). Clinical outcome and antimicrobial therapeutic drug monitoring for the treatment of infections in acute burn patients. Clin. Ther. 39 (8), 1649–1657. doi:10.1016/j.clinthera.2017.06.008
Magill, S. S., Edwards, J. R., Beldavs, Z. G., Dumyati, G., Janelle, S. J., Kainer, M. A., et al. (2014). Prevalence of antimicrobial use in US acute care hospitals, May-September 2011. Jama 312 (14), 1438–1446. doi:10.1001/jama.2014.12923
Mahmoodian, A., Abbasi, S., and Farsaei, S. (2016). A new approach to Vancomycin utilization evaluation: a cross-sectional study in intensive care unit. J. Res. Pharm. Pract. 5 (4), 279–284. doi:10.4103/2279-042x.192453
Marella, P., Roberts, J., Hay, K., and Shekar, K. (2020). Effectiveness of vancomycin dosing guided by therapeutic drug monitoring in adult patients receiving extracorporeal membrane oxygenation. Antimicrob. Agents Chemother. 64 (9), e01179. doi:10.1128/aac.01179-20
Martin, G. S., Mannino, D. M., Eaton, S., and Moss, M. (2003). The epidemiology of sepsis in the United States from 1979 through 2000. N. Engl. J. Med. 348 (16), 1546–1554. doi:10.1056/NEJMoa022139
Meng, L., Wong, T., Huang, S., Mui, E., Nguyen, V., Espinosa, G., et al. (2019). Conversion from vancomycin trough concentration-guided dosing to area under the curve-guided dosing using two sample measurements in adults: implementation at an academic medical center. Pharmacotherapy 39 (4), 433–442. doi:10.1002/phar.2234
Obara, V. Y., Zacas, C. P., Carrilho, C. M., and Delfino, V. D. (2016). Currently used dosage regimens of vancomycin fail to achieve therapeutic levels in approximately 40% of intensive care unit patients. Rev. Bras. Ter. Intensiva 28 (4), 380–386. doi:10.5935/0103-507x.20160071
Prybylski, J. P. (2015). Vancomycin Trough concentration as a predictor of clinical outcomes in patients with Staphylococcus aureus bacteremia: a meta-analysis of observational studies. Pharmacotherapy 35 (10), 889–898. doi:10.1002/phar.1638
Reuter, S. E., Stocker, S. L., Alffenaar, J. C., Baldelli, S., Cattaneo, D., Jones, G., et al. (2022). Optimal practice for vancomycin therapeutic drug monitoring: position statement from the anti-infectives committee of the international association of therapeutic drug monitoring and clinical toxicology. Ther. Drug Monit. 44 (1), 121–132. doi:10.1097/ftd.0000000000000944
Rybak, M. J., Le, J., Lodise, T. P., Levine, D. P., Bradley, J. S., Liu, C., et al. (2020). Therapeutic monitoring of vancomycin for serious methicillin-resistant Staphylococcus aureus infections: a revised consensus guideline and review by the American society of health-system pharmacists, the infectious diseases society of America, the pediatric infectious diseases society, and the society of infectious diseases pharmacists. Am. J. Health Syst. Pharm. 77 (11), 835–864. doi:10.1093/ajhp/zxaa036
Rybak, M. J., Lomaestro, B. M., Rotschafer, J. C., Moellering, R. C., Craig, W. A., Billeter, M., et al. (2009). Vancomycin therapeutic guidelines: a summary of consensus recommendations from the infectious diseases society of America, the American society of health-system pharmacists, and the society of infectious diseases pharmacists. Clin. Infect. Dis. 49 (3), 325–327. doi:10.1086/600877
Shahrami, B., Najmeddin, F., Mousavi, S., Ahmadi, A., Rouini, M. R., Sadeghi, K., et al. (2016). Achievement of vancomycin therapeutic goals in critically ill patients: early individualization may Be beneficial. Crit. Care Res. Pract. 2016, 1245815. doi:10.1155/2016/1245815
Steinmetz, T., Eliakim-Raz, N., Goldberg, E., Leibovici, L., and Yahav, D. (2015). Association of vancomycin serum concentrations with efficacy in patients with MRSA infections: a systematic review and meta-analysis. Clin. Microbiol. Infect. 21 (7), 665–673. doi:10.1016/j.cmi.2015.04.003
Tang, A., Shi, Y., Dong, Q., Wang, S., Ge, Y., Wang, C., et al. (2023). Prognostic differences in sepsis caused by gram-negative bacteria and gram-positive bacteria: a systematic review and meta-analysis. Crit. Care 27 (1), 467. doi:10.1186/s13054-023-04750-w
Truong, J., Smith, S. R., Veillette, J. J., and Forland, S. C. (2018). Individualized pharmacokinetic dosing of vancomycin reduces time to therapeutic trough concentrations in critically ill patients. J. Clin. Pharmacol. 58 (9), 1123–1130. doi:10.1002/jcph.1273
Tsutsuura, M., Moriyama, H., Kojima, N., Mizukami, Y., Tashiro, S., Osa, S., et al. (2021). The monitoring of vancomycin: a systematic review and meta-analyses of area under the concentration-time curve-guided dosing and trough-guided dosing. BMC Infect. Dis. 21 (1), 153. doi:10.1186/s12879-021-05858-6
Turner, R. B., Kojiro, K., Shephard, E. A., Won, R., Chang, E., Chan, D., et al. (2018). Review and validation of bayesian dose-optimizing software and equations for calculation of the vancomycin area under the curve in critically ill patients. Pharmacotherapy 38 (12), 1174–1183. doi:10.1002/phar.2191
van Hal, S. J., Jensen, S. O., Vaska, V. L., Espedido, B. A., Paterson, D. L., and Gosbell, I. B. (2012). Predictors of mortality in Staphylococcus aureus bacteremia. Clin. Microbiol. Rev. 25 (2), 362–386. doi:10.1128/cmr.05022-11
van Hal, S. J., Paterson, D. L., and Lodise, T. P. (2013). Systematic review and meta-analysis of vancomycin-induced nephrotoxicity associated with dosing schedules that maintain troughs between 15 and 20 milligrams per liter. Antimicrob. Agents Chemother. 57 (2), 734–744. doi:10.1128/aac.01568-12
Vincent, J. L., Rello, J., Marshall, J., Silva, E., Anzueto, A., Martin, C. D., et al. (2009). International study of the prevalence and outcomes of infection in intensive care units. Jama 302 (21), 2323–2329. doi:10.1001/jama.2009.1754
Wang, J. T., Wang, J. L., Fang, C. T., Chie, W. C., Lai, M. S., Lauderdale, T. L., et al. (2010). Risk factors for mortality of nosocomial methicillin-resistant Staphylococcus aureus (MRSA) bloodstream infection: with investigation of the potential role of community-associated MRSA strains. J. Infect. 61 (6), 449–457. doi:10.1016/j.jinf.2010.09.029
Welty, T. E., and Copa, A. K. (1994). Impact of vancomycin therapeutic drug monitoring on patient care. Ann. Pharmacother. 28 (12), 1335–1339. doi:10.1177/106002809402801201
Wong, G., Sime, F. B., Lipman, J., and Roberts, J. A. (2014). How do we use therapeutic drug monitoring to improve outcomes from severe infections in critically ill patients? BMC Infect. Dis. 14, 288. doi:10.1186/1471-2334-14-288
Yamada, Y., Niwa, T., Ono, Y., Yamada, S., Niwa, K., Yasue, M., et al. (2023). Comparison of the incidence of vancomycin-associated nephrotoxicity following the change from trough-guided dosing to AUC-guided doing using trough-only data. J. Antimicrob. Chemother. 78 (12), 2933–2937. doi:10.1093/jac/dkad333
Yaw, L. K., Robinson, J. O., and Ho, K. M. (2014). A comparison of long-term outcomes after meticillin-resistant and meticillin-sensitive Staphylococcus aureus bacteraemia: an observational cohort study. Lancet Infect. Dis. 14 (10), 967–975. doi:10.1016/s1473-3099(14)70876-x
Ye, Z. K., Chen, Y. L., Chen, K., Zhang, X. L., Du, G. H., He, B., et al. (2016). Therapeutic drug monitoring of vancomycin: a guideline of the division of therapeutic drug monitoring, Chinese pharmacological society. J. Antimicrob. Chemother. 71 (11), 3020–3025. doi:10.1093/jac/dkw254
Ye, Z. K., Tang, H. L., and Zhai, S. D. (2013). Benefits of therapeutic drug monitoring of vancomycin: a systematic review and meta-analysis. PLoS One 8 (10), e77169. doi:10.1371/journal.pone.0077169
Yoshimura, J., Yamakawa, K., Ohta, Y., Nakamura, K., Hashimoto, H., Kawada, M., et al. (2022). Effect of gram stain-guided initial antibiotic therapy on clinical response in patients with ventilator-associated pneumonia: the GRACE-VAP randomized clinical trial. JAMA Netw. Open 5 (4), e226136. doi:10.1001/jamanetworkopen.2022.6136
Keywords: vancomycin, therapeutic drug monitoring, critically ill patients, mortality, mimic iv
Citation: Peng H, Ou Y, Zhang R, Wang R, Wen D, Yang Q and Liu X (2024) Monitoring vancomycin blood concentrations reduces mortality risk in critically ill patients: a retrospective cohort study using the MIMIC-IV database. Front. Pharmacol. 15:1458600. doi: 10.3389/fphar.2024.1458600
Received: 02 July 2024; Accepted: 31 October 2024;
Published: 14 November 2024.
Edited by:
Gregory Peterson, University of Tasmania, AustraliaReviewed by:
Jan Hartinger, Charles University, CzechiaJuan He, Shanghai Jiao Tong University, China
Copyright © 2024 Peng, Ou, Zhang, Wang, Wen, Yang and Liu. This is an open-access article distributed under the terms of the Creative Commons Attribution License (CC BY). The use, distribution or reproduction in other forums is permitted, provided the original author(s) and the copyright owner(s) are credited and that the original publication in this journal is cited, in accordance with accepted academic practice. No use, distribution or reproduction is permitted which does not comply with these terms.
*Correspondence: Qilin Yang, eWFuZ3FpbGluQGd6aG11LmVkdS5jbg==; Xiaorui Liu, bGl1eGlhb3J1aUBnemhtdS5lZHUuY24=
†These authors share first authorship