- 1College of Pharmacy, Liaoning University of Traditional Chinese Medicine, Dalian, China
- 2Department of Pharmacy, Changzhi Medical College, Changzhi, China
- 3Qimeng Co., LTD, Chifeng, China
Curcumin, a polyphenolic compound derived from the traditional Chinese medicine turmeric, which has a variety of pharmacological effects, including anti-cancer, anti-inflammatory, antioxidant, and antiviral properties. However, its clinical application is hindered by low solubility and bioavailability. To overcome these limitations, researchers have developed various formulations such as nanoformulations, solid dispersions, and microspheres. These advancements have led to improved therapeutic effects and have facilitated the progression of clinical research, primarily focusing on Phase I and Phase II trials for conditions like diabetes, obesity, and metabolic syndrome. In recent years, there has been a noticeable increase in Phase III and IV clinical trials, particularly concerning oral and dental diseases and arthritis. This article reviews recent literature from both domestic and international sources, providing a comprehensive overview of curcumin’s research progress, including its pharmacological mechanisms, formulation developments, and clinical studies.
1 Introduction
Curcumin is a type of polyphenolic compound extracted from the rhizomes of plants in the ginger family. Due to its bright orange-yellow appearance and superior antioxidant capabilities compared to alpha-tocopherol, curcumin is commonly utilized as a dye and culinary spice in many countries, especially in South Asia and India (Hu et al., 2017). Extensive basic research has demonstrated that curcumin possesses various pharmacological effects, including anticancer, anti-inflammatory, and antioxidant properties. However, the low water solubility, poor absorption, and rapid metabolism of curcumin present a challenge to achieving therapeutic blood concentrations after ingestion, thereby limiting its clinical application (Naksuriya et al., 2014). Scholars are currently improving the therapeutic efficacy of curcumin by developing formulations such as nanoformulations, solid dispersions, and liposomes. With the enhancement of its pharmacological effects, there has been an increase in clinical research both domestically and internationally. This article summarizes the pharmacological mechanisms, formulation research progress, and domestic and international clinical research progress of curcumin.
A literature search was conducted on databases including Science Direct, PubMed, Web of Science, Springer, wiley, ACS, RSC, Google Scholar and CNKI, covering publications from the inception of these platforms up to the year 2024. Utilizing “curcumin” as the search term revealed a total of 40,775 articles, including both Chinese and English literature. A total of 25,318 articles were published in English and 15,437 in Chinese. As depicted in Figure 1A, the interest in curcumin research has surged notably in recent years. Research often focuses on pharmacology, metabolism, drug therapy, etc (Figure 1B). The focus of researches are on the antioxidant (13.8%), anticancer (10.4%), and anti-inflammatory (9.3%) attributes of curcumin, alongside the exploration of innovative formulations, including nanoparticles (8%). This review search utilized the following keywords: Curcumin, Pharmacological Effect, Formulation and Clinical research. We have summarized the above content, prioritizing authoritative and valuable literature, and this review compiles a total of 184 relevant documents.
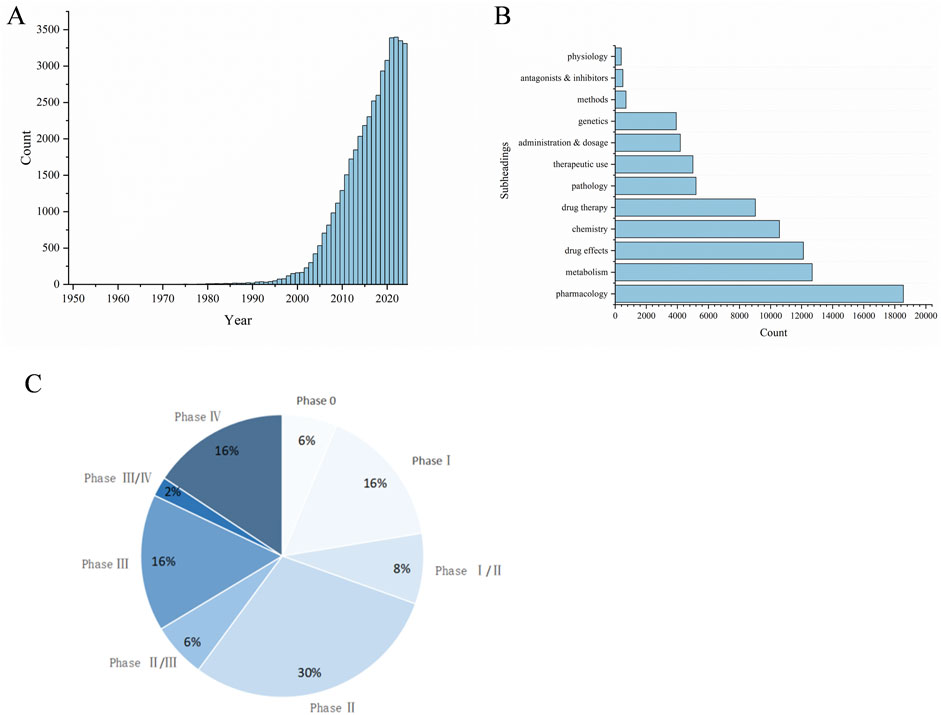
Figure 1. Bibliometric analysis about curcumin. (A) Global trends in publications about Curcumin, (B) Subheadings in publications about Curcumin, (C) Curcumin clinical period distribution.
2 Pharmacological actions and mechanisms of curcumin
2.1 Anti-cancer properties
Curcumin exhibits strong therapeutic potential against various cancers in the blood, oral cavity, skin, lungs, pancreas, and intestines (Adahoun et al., 2017). In normal cells, there is a precise balance between signals that promote growth and those that inhibit growth, leading to orderly proliferation and differentiation only when necessary. However, in cancer cells, this process is completely disrupted, causing uncontrolled cell proliferation. In addition, cancer cells may exhibit metastasis, angiogenesis, and apoptosis inhibition (Hahn and Weinberg, 2002; Sa and Das, 2008). The research indicated that curcumin can effectively inhibit metastasis at various stages of the cascade, including invasion of the primary site, circulation in the blood or lymphatic vessels, extravasation at specific sites, and growth into new lesions (Wu et al., 2021) (Figure 2) (Table 1). Curcumin can target multiple molecular compounds to exert anti-metastatic effects, aciting by inhibiting NF-κB/VEGF signaling pathways to suppress angiogenesis (Huang et al., 2017). Moreover, curcumin regulates growth factors, transcription factors, kinases, enzymes, as well as pro-apoptotic and anti-apoptotic proteins, targeting cancer cell signaling pathways (such as Rb, p23, MAPK, PI3K/Akt, JAK/STAT, Shh and NF-κB), thereby promoting cancer cell apoptosis to achieve anti-tumor effects (Yu et al., 2011; Liang et al., 2014; Liang et al., 2015; Wang et al., 2018; Li et al., 2019; Qiang et al., 2019; Zhang et al., 2020; Shetty et al., 2021; Ham et al., 2022; Jabbari et al., 2022; Mishra et al., 2023). Additionally, curcumin can induce cell cycle arrest by downregulating the mRNA and protein expression of cell cycle protein D1, inhibiting the transition of cells from G (1) to S phase (Cai et al., 2009). This mechanism leads to cell cycle arrest, ultimately suppressing cancer cell growth.
2.2 Anti-inflammatory properties
The anti-inflammatory properties of curcumin are primarily attributable to it capacity to inhibit pro-inflammatory factors. The inflammatory responses are regulated by blocking the expression of pro-inflammatory cytokines such as IL-8, MCP, and MIP through the modulation of signaling pathways, enzymes, and inflammatory mediators (Pontes-Quero et al., 2021) (Figure 3). Wang et al. have demonstrated that curcumin can suppress the expression of inflammatory factors, including NO, IL-6, TNF-a, MDA, IgE, and ICAM-1. Simultaneously, curcumin enhances the levels of IL-10 and SOD, thereby exerting its anti-inflammatory properties and offering a potential therapeutic approach for airway inflammation (Wang et al., 2023). Curcumin has been shown to reduce the production of pro-inflammatory factors, including IL-1, IL-6, IL-8, and TNF-α, by modulating the TLR4/NF-κB/AP-1 signaling pathway. This action contributes to the mitigation of intestinal inflammation in patients suffering from inflammatory bowel disease (Gong et al., 2018). Furthermore, curcumin also inhibits epithelial-mesenchymal transition and inflammatory responses through the suppression of the TLR4/NF-κB and JAK-2/STAT-3 signaling pathways (Wang et al., 2020). Cyclooxygenase-2 (COX-2), lipoxygenase (LOX) and inducible nitric oxide synthase (iNOS) are instrumental in the mediation of the inflammatory process. The inhibitory effects of curcumin on these enzymes play a pivotal role in regulating the inflammatory response (Menon and Sudheer, 2007). Additionally, curcumin has been demonstrated to reduce the number of white blood cells, neutrophils, and eosinophils, exerting a protective effect on the levels of serum inflammatory mediators in various inflammatory diseases, such as phospholipase A2 and total protein (Memarzia et al., 2021).
2.3 Antioxidant properties
Curcumin proficiently eliminates excess reactive oxygen species (ROS) in the body while simultaneously enhancing the activity and expression of antioxidant enzymes, thereby augmenting overall antioxidant capacity (Figure 4). Studies have shown that curcumin can enhance cellular ROS elimination by increasing the activities of catalase, superoxide dismutase, and glutathione peroxidase while decreasing the activities of amylase, α-glucosidase, and β-glucosidase (Balasubramanyam et al., 2003; Lin et al., 2019; Kanwal et al., 2023). It also interferes with protein kinase C activity and inhibits Ca2+ influx. Additionally, curcumin exhibits antioxidant properties by scavenging DPPH and ABTS free radicals and reducing lipid peroxidation through increased catalase activity, thereby alleviating oxidative stress (Chen et al., 2018). The Keap1-Nrf2 pathway is a key antioxidant system, and curcumin is capable of regulating this pathway to maintain the cell’s redox balance and eliminate ROS (Ghareghomi et al., 2021). Furthermore, curcumin may significantly enhance the cell’s antioxidant capacity by suppressing the expression of miR-125b-5p (Lin et al., 2022).
Curcumin enhances the expression of mitochondrial microRNAs, notably let-7d-5p, while concurrently reducing the levels of miR-22-3p. Subsequent investigations indicate that let-7d-5p specifically targets mitochondrial elongation factor 1 (MIEF1), whereas cardiolipin synthase 1 (CRLS1) and malonyl-CoA-acyl carrier protein transacylase (MCAT) are targeted by miR-22-3p. Both let-7d-5p and its target MIEF1, along with miR-22-3p and its targets CRLS1 and MCAT, are instrumental in modulating cellular antioxidant capacity and mitochondrial functionality, with their interactions producing contrasting effects (Li, 2020). Additionally, curcumin may contribute to the maintenance of mitochondrial homeostasis by regulating mitochondrial biogenesis, which supports the stability of the body’s redox system and ultimately mitigates oxidative stress (Chen, 2019).
2.3.1 Gastrointestinal diseases
Oxidative stress in inflammatory bowel disease (IBD) results from an imbalance between oxidants and antioxidants within the affected tissues (Sahoo et al., 2023). Curcumin promotes Parkin-dependent mitophagy by inducing AMPK (AMP-activated protein kinase) and facilitating the nuclear translocation of TFEB (transcription factor EB). This process not only alleviates oxidative stress but also strengthens the integrity of the intestinal barrier and mitochondrial function. Furthermore, curcumin reduces the generation of ROS and mitigates LPS-induced inflammation in vascular smooth muscle cells through the TLR4-MAPK/NF-κB signaling pathway.
2.3.2 Ocular diseases
The accumulation of ROS is commonly linked to a range of retinal diseases, such as cataracts, age-related macular degeneration, uveitis, diabetic retinopathy, retinal tumors, and proliferative vitreoretinopathy (PVR) (Maiuolo et al., 2022). To explore the protective effects of curcumin against oxidative stress-related damage, Raman et al. found that curcumin prevents apoptosis in human retinal epithelial cells. This protection is achieved through several mechanisms, including the inhibition of lipid peroxidation in lens membranes, the induction of antioxidant enzymes, the regulation of calcium homeostasis in the lens, the support of lens chaperones, and the modulation of transcription factor activity within the lens (Raman et al., 2016).
2.3.3 Liver diseases
Curcumin exhibits both preventive and therapeutic effects against oxidative-related liver diseases, such as hepatotoxicity, non-alcoholic fatty liver disease, alcoholic liver disease, liver fibrosis, cirrhosis, and liver injury. These effects are mediated through various cellular signaling pathways. Notably, these include the ERK/p38/MAPK pathway, the Nrf2/ARE/Keap1 signaling cascade in the liver, and the upregulation of detoxification gene expression. Furthermore, Curcumin influences TIMP signaling, the AMPK pathway, lipid metabolism, and the downregulation of Rac1, NOX1, and Rac1-GTP signaling (Farzaei et al., 2018).
2.3.4 Neurodegenerative diseases
The emergence and progression of neurodegenerative diseases (NDD) such as Alzheimer’s disease (AD), Parkinson’s disease (PD), and Huntington’s disease (HD) are intricately associated with oxidative stress. Curcumin plays a significant role in mitigating this stress by inhibiting the oxidation of lipids and proteins, which effectively reduces levels of malondialdehyde, protein carbonyls, thiols, and nitrotyrosine. Furthermore, curcumin enhances the activity of crucial antioxidant enzymes, including superoxide dismutase and glutathione peroxidase, thereby potentially decelerating or ameliorating the trajectory of NDD (Abrahams et al., 2019).
2.3.5 Cardiovascular diseases
Curcumin plays a pivotal role in combating cardiovascular diseases by modulating superoxide dismutase, heme oxygenase-1, and nuclear factor erythroid 2-related factor 2. This modulation improves arterial health, especially by enhancing endothelial function and reducing arterial stiffness, thereby lowering the risk of cardiovascular diseases (CVD) (Fleenor et al., 2019).
2.4 Antiviral properties
Curcumin, the active compound in turmeric, has been demonstrated to possess antiviral properties against both DNA and RNA viruses (Figure 5). Research has indicated that curcumin can inhibit DNA viruses by suppressing the APE1 redox reaction, and it can target RNA viruses by inhibiting viral integrase, proteases, and reducing the activity of Tat and JunD proteins (Mazumder et al., 1995; Si et al., 2007; Zhang H. S. et al., 2011; Ali and Banerjea, 2016). Zhang et al. found that curcumin can disrupt the F-actin in HPIV3, inhibiting the formation of viral inclusion bodies (IB) and suppressing viral replication (Zhang et al., 2021). Additionally, curcumin downregulates PI4KB, preventing its co-localization within the viral IB. Kim et al. demonstrated that curcumin can inhibit the replication of hepatitis C virus by suppressing the PI3K/Akt and SREBP-1 pathways (Kim et al., 2010). Further studies (Thimmulappa et al., 2021) have revealed that curcumin can directly interact with the envelope proteins of enveloped viruses, disrupt the viral envelope, inhibit viral proteases, and modulate host factors such as NF-κB, NRF2 and HMGB1, all of which contribute to its broad-spectrum antiviral activity.
2.5 Anti-fibrotic properties
The anti-fibrotic effects of curcumin have been demonstrated in a number of organs, including the liver, heart and kidneys. The underlying mechanisms may differ depending on the type of fibrosis. Kong et al. found that curcumin can inhibit hepatic fibrosis by suppressing epithelial-mesenchymal transition (EMT) in hepatocytes and by activating autophagy to inhibit the production of extracellular matrix (Kong et al., 2020). Liu et al. have demonstrated that curcumin can alleviate isoproterenol-induced cardiac hypertrophy and fibrosis by inhibiting autophagy and activating the mTOR pathway (Liu et al., 2018). Wang et al. have also shown that curcumin can attenuate obstructive nephropathy-induced renal interstitial fibrosis by suppressing EMT through the inhibition of the TLR4/NF-κB and PI3K/AKT signaling pathways (Wang et al., 2020).
2.6 Hypoglycemic properties
The primary mechanism by which curcumin exerts its hypoglycemic effects is through the attenuation of insulin resistance. Studies have demonstrated that curcumin can combat insulin resistance by increasing the levels of circulating adiponectin and leptin, activating PPARγ, inhibiting Notch1 signaling, and regulating SREBP target genes (Ding et al., 2016; Balakumar et al., 2023). Furthermore, studies have demonstrated that curcumin can significantly reduce blood glucose and glycated hemoglobin levels, improve glucose homeostasis, and enhance plasma insulin levels (Seo et al., 2008). Research has demonstrated that curcumin reduces α-glucosidase (α-Glu) activity (Liu L. et al., 2024) and inhibits aminopeptidase B activity (Cadel et al., 2019), which is expected to become a potential therapeutic method for the treatment of diabetes. Additionally, experiments have shown that curcumin can ameliorate glucose and lipid metabolism disorders, increase insulin sensitivity, and improve insulin resistance in animal models of type 2 diabetes mellitus (T2DM) (Su et al., 2017).
2.7 Enzyme inhibition activity
Curcumin exhibits significant potential in the treatment of various diseases through the inhibition of multiple enzymes. Research demonstrates that curcumin effectively suppresses steroid sulfatase activity, resulting in decreased levels of active steroid hormones, such as estrogen and androgen, within tissues. This mechanism positions curcumin as a promising therapeutic agent for estrogen-dependent cancers and other related conditions (Balasubramonian and Selcer, 2023). In addition, curcumin inhibits F1-ATPase activity by interfering with the conformational changes of the β subunit’s catalytic site, thereby extending the duration of catalytic activity (Sekiya et al., 2014). It also diminishes the activity of glutathione S-transferase (GST), which may reduce resistance to chemotherapy agents (Hayeshi et al., 2007). Furthermore, curcumin impedes the maturation of proprotein convertase (PC) zymogens, leading to a suppression of PC activity and influencing calcium (Ca2+) uptake into the endoplasmic reticulum (Zhu et al., 2013). Moreover, studies indicate that curcumin lowers the apparent affinity of Na,K-ATPase for potassium (K+) while enhancing its affinity for sodium (Na+) and ATP (Mahmmoud, 2005). Additionally, aminopeptidase B (Ap-B), a Zn2+-aminopeptidase from the M1 family, plays a vital role in the maintenance of glucose homeostasis.
3 Curcumin in silico studies
To facilitate further exploration of the therapeutic potential of curcumin, one can conduct target prediction through the analysis of its physicochemical parameters and structural similarities, thereby evaluating other possible molecular targets. Rahman et al., who conducted docking studies to examine the interactions of curcumin with GSTA1, GSTO1, KEAP1, BACE1, and MAOA (Rahman et al., 2022). Concurrently, Singh et al. investigated curcumin’s interactions with COX-2, 5-LOX, TNF-α, and IL-6 (Singh et al., 2023) to identify potential molecular targets for its antioxidant properties. Computational analyses indicate that curcumin is likely to engage with GSTA1, GSTO1, and KEAP1, thereby enhancing its antioxidant efficacy. In contrast, its binding to BACE1 and MAOA may contribute to the mitigation of oxidative damage (Rahman et al., 2022). Notably, curcumin demonstrates a strong affinity for the enzyme COX-2 (Singh et al., 2023). Throughout the simulation process, curcumin exhibited excellent binding stability and minimal fluctuations when interacting with additional targets, including 5-LOX, TNF-α, and IL-6.
Recent research has docked curcumin with critical compounds of the Wnt/β-catenin signaling pathway, particularly casein kinase-1 (CK1) and glycogen synthase kinase-3 (GSK3B). Findings suggest that curcumin may exert its antimicrobial effects via this pathway (Chopra et al., 2023). Moreover, curcumin has demonstrated effectiveness against Staphylococcus aureus, a common pathogen. Studies indicate that the formation of its biofilm is significantly influenced by the polysaccharide intercellular adhesion (PIA), which is regulated by the icaADBC operon (Khaleghian et al., 2023). This bacterium can express various fibronectin-binding proteins (FnbA) and clumping factor A (ClfA), which facilitate bacterial adhesion to surfaces and contribute to biofilm development. The accessory gene regulator (Agr) system also plays a vital role in regulating numerous pathogenic factors, including biofilm formation. This system, consisting of AgrB, AgrC, AgrA, and AgrD, is crucial for modulating the virulence factors of Staphylococcus aureus. Notably, curcumin exhibits a strong binding affinity for these genes, indicating its potential as a novel therapeutic agent against Staphylococcus aureus.
Furthermore, Patil et al., who investigated the interactions of curcumin with anti-inflammatory targets such as IL-6, TNF-α, NF-κB, and SIRT1. Their findings revealed significant binding interactions with IL-6, TNF-α, and SIRT1, while the interaction with NF-κB was comparatively weaker (Patil et al., 2024). Similarly, Selim et al. performed docking studies with P38α, MAPK, ERK1/2, JNK1, and MK3, which showed favorable binding energies, suggesting these could be potential anti-inflammatory targets for curcumin (Selim et al., 2019). In addition, other researchers have examined curcumin’s interactions with gene targets related to metabolic syndrome, diabetes, and cognitive disorders, reporting promising binding results (Nguyen and Kim, 2022; Vaithiyalingam et al., 2023) (Table 2).
It is essential to recognize that not all docking results are positive. For example, curcumin does not effectively bind to the ATP binding sites of CDK2, CDK4, and CDK6. This suggests that curcumin is unlikely to inhibit the CDK pathway in cancer, indicating that it may influence cancer growth through alternative mechanisms (Ahmed et al., 2017).
4 Research on curcumin formulations
4.1 Nanoparticles
In recent years, there has been a notable increase in the development of biodegradable nanoparticles. These nanoparticles are produced using a variety of materials, including polysaccharides such as chitosan, alginate, and starch (Maghsoudi et al., 2017), as well as proteins such as walnut protein, zein, and sodium caseinate (Li et al., 2022; Liu et al., 2022; Lv et al., 2023), and polymers such as sulfated κ-carrageenan (Nogueira et al., 2022). The curcumin-nanoparticles described above have been demonstrated to improve the solubility, bioavailability, and biological properties of curcumin. It has been demonstrated in studies that polysaccharide-based curcumin nanoparticles, such as those made with chitosan, starch, and alginate, have minimum inhibitory concentrations against Streptococcus mutans that are more than twice as effective as free curcumin (Maghsoudi et al., 2017). Phospholipid and zein-coated curcumin nanoparticles exhibit excellent aqueous dispersibility across a broad pH range (Liu et al., 2022). Additionally, this encapsulation substantially improves the stability of curcumin against heat and enhances its antioxidant properties. In vitro release experiments reveal that these lipid-coated nanoparticles effectively inhibit the leakage of curcumin in the stomach, thereby enhancing its oral delivery efficiency to the small intestine.
In order to further enhance the therapeutic efficacy of curcumin, researchers have explored the possibility of developing targeted nanoparticles. Fan et al. designed a novel PLGA-PEG-B6 peptide-conjugated nanoparticle loaded with curcumin (PLGA-PEG-B6/CUR) and demonstrated its efficacy in enhancing spatial learning and memory in APP/PS1 transgenic mice, as well as reducing the formation and deposition of amyloid-β and tau hyperphosphorylation in the hippocampus (Fan et al., 2018). Hong et al. employed microfluidic-assisted continuous precipitation to prepare enzyme-targeted curcumin-loaded nanoparticles (CUR-P-NPs), which exhibited enhanced cellular uptake, antiproliferative effects on U251 cells, and tumor-targeting abilities compared to curcumin solution and non-targeted curcumin nanoparticles (Hong et al., 2021). However, these nano-targeted materials lack standardized production and quality control measures, which complicates the testing of their efficacy and safety. As a result, these formulations remain in the laboratory stage and face significant hurdles in advancing to clinical trials.
4.2 Nanoemulsions
Nanoemulsions typically consist of an aqueous phase, an oil phase, and surfactants, with the potential inclusion of co-surfactants. Research have demonstrated that nanoemulsification can significantly improve the permeability of curcumin, resulting in a shift from a zero-order release profile to a Higuchi release profile (Rachmawati et al., 2015). Yousef et al. developed a nanoemulsion with biocompatible compounds (surfactant glycerides, lecithin, and co-surfactants ethyl ether and ethanol) to enhance the skin delivery of curcumin (Yousef et al., 2019). This approach offers a novel opportunity for the targeted treatment of psoriasis and skin cancer. It is crucial to evaluate how the parameters of nano-system formulations affect skin permeability. Additionally, it is recommended to address any complications that may arise from non-Newtonian flow behavior. Kumari et al. developed a nanoemulsion that enhanced the dermal penetration and antimicrobial activity of curcumin, thereby suggesting its potential as a topical antimicrobial agent for the treatment of burn wound infections (Kumari and Nanda, 2023). The droplet size of nanoemulsions, which typically ranges from 20 to 500 nm, can influence the bioaccumulation of curcumin. Shen et al. prepared nanoemulsions with varying mean diameters (150 nm and 300 nm), lipid compositions (MCT, corn, and fish oils), and emulsifiers (Tween 80 and whey protein), and observed that the bioaccumulation of curcumin increased with particle size, exhibiting higher levels in corn oil-based nanoemulsions relative to fish oil or MCT-based ones (Shen et al., 2019). Additionally, greater accumulation was noted in whey protein-stabilized nanoemulsions compared to Tween 80-stabilized ones. Several nanoemulsions have already been launched in the field of ophthalmology, showcasing notable benefits in improving drug stability and bioavailability. Consequently, curcumin nanoemulsions show potential for progression into clinical trials as a promising new treatment option for various conditions.
4.3 Nanocrystals
Curcumin nanocrystals are typically composed of the active compound and stabilizers, exhibiting low toxicity and high structural stability. Lizoňová et al. prepared curcumin nanocrystals using a combination of steric and ionic stabilizers (Tween 80, sodium dodecyl sulfate, Poloxamer 188, hydroxypropyl methylcellulose, polyethylene glycol, and phospholipids) via wet milling (Lizoňová et al., 2022). The resulting nanocrystals were found to have colloidal stability in culture media and to be non-toxic to healthy cells. The local application of curcumin nanocrystals is also feasible, as the increased kinetic solubility of nanocrystals can lead to a concentration gradient that promotes passive skin permeation (Pelikh et al., 2021) The dermal penetration and accumulation of curcumin nanocrystals depend on the carrier, permeation enhancers, and particle size. Xiang et al. prepared curcumin nanocrystals with xanthan gum (serving as the carrier) and propylene glycol (acting as the permeation enhancer) (Xiang et al., 2023). The results showed that xanthan gum reduced the follicular penetration and passive skin accumulation of curcumin nanocrystals, whereas propylene glycol enhanced their skin penetration and retention. The results demonstrated that smaller curcumin nanocrystals exhibited higher passive skin permeability compared to larger ones. Furthermore, nanocrystals have the potential to facilitate sustained topical delivery of curcumin by targeting it to hair follicles (Pelikh et al., 2021). Consequently, curcumin nanocrystals exhibit considerable benefits in treating skin disorders, showing potential for progression into clinical trials as an innovative topical therapeutic agent.
4.4 Nano micelles
Nano micelles are thermodynamically stable colloidal aggregates that can passively target drugs to specific sites, enhancing their solubility and bioavailability through improved permeation and retention effects. Desai et al. prepared curcumin cocrystal micelles for nose-to-brain delivery (Desai and Patravale, 2018). The prepared micelles exhibited enhanced antioxidant activity, improved bioavailability, and significant brain distribution, indicating their potential for treatment neurodegenerative diseases. In a study by Tima et al. the thin-film hydration method was employed to prepare curcumin micelles conjugated with an FLT3-specific peptide (FLT3-CUR) (Tima et al., 2019). The FLT3-CUR micelles not only improved solubility but also enhanced anti-leukemic activity. This Curcumin-targeted micelle nanocarrier may be an effective leukemia treatment, especially for FLT3-ITD overexpressing leukemic model. Sun et al. used the thin-film dispersion method to prepare CUR-loaded GAL-PEG-PLA/TPGS micelles, which exhibited sustained-release properties (Sun et al., 2021). The high-GAL-density GPP micelles were advantageous in promoting epithelial cell uptake and improving intestinal permeability. The prepared micelles could improve relative bioavailability while reducing damage to liver and intestinal tissues. However, research on nano-micelles is still in its nascent stages. Their stability necessitates further refinement, and their biocompatibility and safety also require further verification. As a result, they are currently confined to the laboratory phase of investigation.
4.5 Solid dispersions
Solid dispersion is a system where curcumin is dispersed in a carrier in a highly dispersed state, such as molecular, amorphous, or microcrystalline. Research has demonstrated that the use of carriers, such as polyethylene glycol (PEG), polyvinylpyrrolidone (PVP), and Eudragit® EPO (EPO) can increase the solubility, dissolution rate, and bioavailability of curcumin, leading to better therapeutic effects (Huang et al., 2008; Guan, 2009; Gao et al., 2011; Huang et al., 2018; Lu et al., 2018; Zhang et al., 2018; Liang et al., 2019; Lu and Deng, 2019; Shin et al., 2019; Zhang Q. et al., 2019; Yu et al., 2020; Fan et al., 2021; Ren et al., 2022). The extent of improvement varies depending on the carrier used. The combined use of different carriers can also have different effects. For example, the dissolution rate of PVP K30 combined with HPMC is higher than using PVP K30 alone. However, the combination of PVP-based carriers with the surfactant F68, or the combination of polyvinylpyrrolidone and poloxamer, can actually decrease the solubility and bioavailability (Pu et al., 2022; Li et al., 2023). The specific details are summarized in Table 3. In summary, the selection of carrier materials for curcumin solid dispersions is remarkably extensive, and their physicochemical properties and biocompatibility have been rigorously validated. In addition, solid dispersion technology has matured, and many solid dispersion preparations have been marketed. This approach markedly improves the solubility of curcumin while ensuring exceptional stability. As a result, curcumin solid dispersions show great potential for progressing to clinical trials aimed at treating various diseases.
4.6 Liposomes
The formation of liposomes is achieved by the encapsulation of the drug within a lipid bilayer, which is composed of phospholipids and cholesterol. A review of the literature indicated that the formulation of curcumin into liposomes can improve its dissolution rate, bioavailability, and stability (You et al., 2014; Laomeephol et al., 2020; Wang et al., 2021; Xi et al., 2022; Huang et al., 2023). The choice of phospholipids used to prepare the liposomes can affect their storage stability and in vivo bioavailability. For example, curcumin liposomes prepared using milk phospholipids showed better stability under adverse storage conditions (alkaline, oxygen, high temperature, and high humidity) compared to those prepared using krill phospholipids. However, the bioavailability of curcumin-loaded milk phospholipid liposomes was lower than those prepared using krill phospholipids (Wu et al., 2020). Compared to soy phospholipid liposomes, milk fat globule membrane (MFGM) liposomes exhibited higher encapsulation efficiency, smaller particle size, higher absolute zeta potential, and slower in vitro release. MFGM liposomes also showed higher stability under conditions of Fe3+, light, temperature, oxygen, and relative humidity compared to soy phospholipid liposomes (Jin et al., 2016). Although liposomal formulations have demonstrated improvements in various aspects, the results have not fully met expectations. Consequently, researchers have initiated investigations into the potential of coating the liposomes with chitosan, mannitol, or carbopol to further enhance the therapeutic efficacy (Gu and Deng, 2010; Manconi et al., 2017; Li et al., 2018; Li et al., 2020; Xu et al., 2021; Zhou et al., 2021). The details are summarized in Table 4.
Although curcumin liposomes possess the potential to enhance therapeutic efficacy, their development is hindered by several limitations. Firstly, conventional techniques for liposome preparation, such as the thin-film hydration method and ethanol injection, often result in challenges such as low encapsulation efficiency, inconsistent particle size, and the presence of residual organic solvents. Therefore, it is difficult to achieve industrial production. Secondly, the excipients utilized in targeted liposomes, including galactose-modified curcumin liposomes, frequently lack established standards for production and quality control, making it difficult to ensure their safety and efficacy. Lastly, the excipients employed in these liposomes may provoke adverse effects, including immune responses and allergic reactions, as a result of their insolubility and non-biodegradability. Therefore, curcumin liposomes are still only at the laboratory stage at present, and a large amount of basic research is still needed in the future.
4.7 Microspheres and microcapsules
Curcumin-loaded microspheres have been developed for inflammatory treatment. For example, gelatin/silk fibroin (30/70) microspheres encapsulating curcumin have been demonstrated to delay the destruction of joint and synovial tissues, rendering them useful for the local treatment of osteoarthritis (Ratanavaraporn et al., 2017). Curcumin-conjugated chitosan microspheres also exhibit good antimicrobial, antioxidant, and anti-inflammatory activities, which can prevent skin infections (Saranya et al., 2018). Furthermore, curcumin microspheres are often coated with Eudragit® S100 to achieve colon-specific delivery for the treatment of colon cancer. Karade et al. developed Eudragit® S100-coated curcumin microspheres containing ascorbic acid (AA), which improved the stability of curcumin under alkaline conditions and prevented its degradation (Karade and Jadhav, 2018). Zhang et al. prepared Eudragit® S100-coated calcium alginate curcumin microspheres, which showed significantly increased curcumin release in simulated colonic fluid (Zhang L. et al., 2011). The microencapsulation of curcumin can also improve its stability and cell compatibility, thereby enhancing its therapeutic efficacy. For instance, the lotus seed protein-pectin composite microcapsules designed for curcumin significantly enhance its antioxidant properties, photostability, thermal stability, and storage longevity (Su et al., 2022). Research demonstrates that the microencapsulated curcumin provides a sustained, controlled release during the gastric phase, which transitions to a rapid release in the intestinal phase. Following gastrointestinal digestion, the cumulative release rates for curcumin-LSP and curcumin-LSP-pectin particles are found to be 64.3% and 72.4%, respectively. Microspheres and microcapsules can encapsulate drugs within excipients, enabling the modulation of drug release rates for sustained and controlled release. This technology holds significant promise for widespread clinical applications.
4.8 Microemulsions
The typical composition of curcumin microemulsions includes an oil phase (e.g., ethyl oleate), an emulsifier (e.g., polyoxyethylene 40 hydrogenated castor oil), and a co-emulsifier (e.g., polyethylene glycol 400). Zhong et al. developed a liver-targeted curcumin microemulsion by adding N-octadecyllactobionamide as a liver-targeting ligand, achieving a high drug loading of up to 95% (Zhong et al., 2019). Stability experiments have demonstrated that drug-loaded microemulsions exhibit remarkable stability across a range of concentrations, storage times, high-speed centrifugation, and varying Km conditions. This underscores the potential benefits of microemulsions as carriers for controlled-release and targeted drug delivery. In this context, Liu utilized dioctyl sulfosuccinate as an effective charge modifier to formulate a curcumin-targeted self-microemulsion intended for inflammatory conditions (Liu, 2017). By modifying the surface charge of the particles, the negatively charged curcumin self-microemulsion system achieved a high encapsulation efficiency and exhibited a uniform particle size distribution. This design enabled precise targeting of curcumin to the sites of inflammation in colitis, thereby increasing the concentration of the drug on the surface of inflammatory cells in ulcerative colitis. Zhang added a folic acid-polyethylene glycol-cholesterol hemisuccinate ester together with Cremophor EL, which enhanced the absorption of curcumin in the rat colonic mucosa (Zhang, 2012). Furthermore, microemulsions care capable of penetrating the stratum corneum, rendering an optimal choice for topical drug delivery (Luna-Canales et al., 2023). A redox-responsive microemulsion based on the surfactant selenium diol (PSeP) has been demonstrated to significantly improve the solubility, stability, antioxidant capacity, and skin permeability of curcumin in the oil phase, rendering it a promising system for topical applications (Zhang et al., 2023). Therefore, curcumin microemulsion shows considerable advantages in the treatment of skin diseases, promising potential for entry into clinical trials as a novel therapeutic agent for transdermal delivery.
4.9 Microtablets
Curcumin microtablets can be classified into three principal categories: membrane-controlled microtablets, matrix-type microtablets, and matrix-membrane-controlled sustained-release microtablets. Jv et al. prepared membrane-controlled microtablets using chitosan and sodium alginate as the core materials, and then coated them with Eudragit S100 to effectively control the release of curcumin from a self-microemulsion and achieved colon-targeted sustained drug delivery (Jv et al., 2022). Matrix-type microtablets are frequently prepared using microcrystalline cellulose as the matrix material and glyceryl monostearate as the binder. Wang additionally incorporated mucoadhesive materials, such as chitosan and hydroxypropyl methylcellulose K100M (HPMC K100M), into the formulation of gastro-retentive microtablets (Wang, 2019). This prolonged the gastric residence time and increased the contact area between curcumin and the gastric mucosa, thereby enhancing absorption and improving therapeutic efficacy. Wang incorporated HPMC K100M and the effervescent agent sodium bicarbonate to prepare curcumin gastro-floating microtablets (Wang, 2017). These exhibited sustained-release properties and remained buoyant in simulated gastric fluid for more than 8 h. In comparison to traditional curcumin tablets, the gastro-floating microtablets of curcumin demonstrate a remarkable increase in relative bioavailability, achieving a value of 271.23%. Zhao et al. developed matrix-membrane-controlled sustained-release curcumin microtablets using ethylcellulose and microcrystalline cellulose as the matrix materials, carbopol as the retardant, and stearic acid as the binder (Zhao et al., 2015). The microtablets were subsequently coated with a Kollicoat SR30D dispersion containing talc and PEG6000. The sustained-release microtablets demonstrated a drug release of 90.54% within 12 h, in accordance with the Weibull equation. The incorporation of these excipients extends the duration of drug release, improves the medication’s stability and bioavailability, and ultimately facilitates a reduction in both the required dosage and the frequency of administration. Currently, the technology for microtablets is quite mature. Curcumin microtablets can significantly enhance the dissolution rate of curcumin, thereby improving its therapeutic efficacy. Consequently, curcumin microtablets hold promise for entry into clinical trials, poised to become a novel medication for disease treatment.
4.10 Pellets
Curcumin pellets can be classified into immediate-release pellets and sustained-release pellets.Immediate-release pellets are frequently prepared using water-soluble matrices such as polyethylene glycol (PEG). In contrast, sustained-release pellets are typically formulated using lipophilic matrices, including stearic acid and surfactants, such as poloxamer. PEG is a frequently utilised matrix for immediate-release pellets, and the molecular weight of PEG can affect the performance. Wang et al. found that using a lower viscosity PEG4000 resulted in better pellet formation and appearance compared to the higher viscosity PEG6000 (Wang et al., 2013). Hong et al. and Chen et al. used a combination of PEG1000 and PEG6000 (Hong et al., 2013), or PEG4000 and PEG6000 (Chen et al., 2007), respectively, and optimized the formulation by investigating factors, such as drug solution temperature, cooling medium temperature, and matrix ratio to achieve desirable appearance and dissolution characteristics. Fang et al. employed lipophilic matrices, such as glyceryl monostearate and stearic acid, in conjunction with various surfactants, including polyethylene glycol-12-hydroxystearate (Solutol), polyoxyethylene hydrogenated castor oil, and poloxamer, to create sustained-release pellets (Fang et al., 2010). The results indicated that the pellets containing Solutol exhibited significantly better in vitro release performance compared to those with poloxamer or polyoxyethylene hydrogenated castor oil. Research on curcumin pellets has largely stagnated since initial studies conducted over a decade ago, with no recent developments reported in this area. Additionally, the existing pellets have not been subjected to efficacy assessments. As a result, based on available data, progressing curcumin pellets to Phase I clinical trials presents significant challenges.
4.11 Cyclodextrin inclusion complexes
The preparation of curcumin/cyclodextrin polymer (CUR/CDP) inclusion complexes has the potential to improve the solubility and stability of curcumin (Chen et al., 2020). These inclusion complexes have been demonstrated to exhibit enhanced cytotoxicity and apoptotic effects on cancer cells (Roozbehi et al., 2020). It has been demonstrated that CUR/CDP exhibits novel antioxidant activities by scavenging 2,2′-azino-bis(3-ethylbenzothiazoline-6-sulfonic acid) (ABTS) and 1,1-diphenyl-2-picrylhydrazyl (DPPH) free radicals. Furthermore, it has been shown to demonstrates enhanced antiproliferative activity and to induce apoptosis in human malignant melanoma (A375) cells (Chen et al., 2018). Furthermore, CUR/CDP has been demonstrated to enhance the activity of the antioxidant enzyme catalase (CAT), reduce lipid peroxidation, and alleviate oxidative stress, thereby inhibiting cell apoptosis and mitigating liver injury (Chen J. et al., 2022). The incorporation of cyclodextrin inclusion complexes into composite membranes has been demonstrated to enhance the therapeutic efficacy of antimicrobial membranes based on 2,3-dialdehyde cellulose (DAC) or cationic starch and polyvinyl alcohol polymer membranes (MBN). The DAC-composite film exhibits high transparency, yet effectively blocks UV light, which helps delay the degradation of curcumin (Chen et al., 2021). The CUR/CDP incorporated with MBN can specifically bind to target cancer cells, enhancing the cytotoxicity of curcumin against both melanoma and glioblastoma cell lines, and prolonging the cytotoxic effect up to 96 h (Gularte et al., 2020). Cyclodextrin inclusion significantly improves the stability, solubility, and bioavailability of curcumin. Additionally, being a naturally occurring cyclic oligosaccharide, cyclodextrin is notable for its biocompatibility and biodegradability. As a result, complexes formed between curcumin and cyclodextrin demonstrate considerable promise for various clinical applications.
4.12 Gel formulations
Curcumin gel formulations are typically composed of curcumin and an aqueous gel matrix, with the addition of moisturizers, pH adjusters, and permeation enhancers as needed. Liu et al. prepared curcumin gels by incorporating moisturizers and pH adjusters, resulting in good gel formation, spreadability, and thermal and cold resistance (Liu X. Y. et al., 2024). Zhu et al. incorporated gglycyrrhizic acid and polyoxyethylene castor oil (Cremophor EL) into the curcumin gel formulation as permeation enhancers (Zhu et al., 2021). The findings demonstrated that the incorporation of permeation enhancers markedly enhanced the dermal permeation of curcumin, with glycyrrhizic acid exhibiting superior efficacy compared to Cremophor EL at equivalent concentrations. Additionally, gels possess adhesive properties, enabling them to adhere to nasal mucosa, cornea, and conjunctiva, thereby prolonging the drug’s residence time. Chen et al. developed an in-situ nasal gel using poloxamer 407, poloxamer 188 as the gel matrix, and PEG400, 0.02% benzalkonium chloride, and 0.9% sodium chloride (Chen et al., 2013). This gel not only extended the pharmacological effect of curcumin but also significantly enhanced its brain targeting properties. Li et al. prepared a curcumin nano-suspension gel that improved the dissolution of curcumin (Li et al., 2017). In vivo studies demonstrated that this gel formulation could significantly delay the development of lens opacification and improve the therapeutic effect of curcumin on selenium-induced cataracts in rats. Currently, curcumin gel formulations are undergoing phase III clinical trials for the treatment of periodontitis. This suggests that curcumin gel may have the potential to become a new therapeutic option for dental diseases.
4.13 Film and coating formulations
Curcumin film and coating formulations are commonly used for food preservation and wound healing. Han et al. prepared curcumin films using polyvinyl alcohol (PVA 17-88) and deacetylated chitosan as film-forming materials, with glycerol as a plasticizer (Han et al., 2005). The resulting films exhibited favourable film-forming properties and could promote wound healing. Compared to films, coating formulations are more convenient for topical application, as the protective film forms automatically after spraying. The use of permeation enhancers [such as menthol (Chen J. H. et al., 2022) and dimethyl sulfoxide (Chen, 2015)], facilitates the continuous penetration of the active compounds in the film through the skin, thereby ensuring sustained absorption of curcumin. Furthermore, the incorporation of chitosan and its derivatives in the coating formulations can enhance the binding of curcumin to bacteria, thereby improving the antimicrobial activity (Zhang et al., 2024). Cao et al. developed a composite film containing carboxymethyl chitosan, carboxymethyl cellulose, and curcumin, which effectively inhibited the growth of Aspergillus niger and Penicillium, and had a protective effect on food color, making it widely applicable for food preservation (Cao et al., 2022). Thus, curcumin films and coatings demonstrate significant antibacterial properties, offering promising potential for innovative applications in food preservation and wound healing.
4.14 Vesicles
Curcumin vesicles are typically composed of surfactants and cholesterol, with common non-ionic surfactants being Span and Tween. Wang prepared curcumin vesicles by dissolving Span 80, cholesterol, and curcumin in ethanol, evaporating the solvent, and then hydrating the mixture with pH 6.5 PBS (Wang, 2017). The results indicated that the bioavailability of curcumin was significantly improved compared to a curcumin suspension. Lv formulated curcumin vesicles by dissolving Span 60, cholesterol, and curcumin in a chloroform: ethanol (4:1) solution, evaporating the solvent, and then hydrating with ultrapure water (Lv, 2022). After transferrin modification, these vesicles could effectively target and treat glioblastoma, with good safety profiles in vivo. Hao et al. prepared curcumin vesicles using Tween 60 and Tween 80, and found that the Tween-60 vesicles were more effective in improving the UV absorption, fluorescence emission intensity, binding constant, and free radical scavenging ability of curcumin compared to the Tween-80 vesicles (Hao et al., 2021). While curcumin vesicles have been shown to improve the effectiveness of curcumin, the existing research on their distribution, metabolism, and clearance within the body is still limited. Additionally, the long-term safety of these vesicles has yet to be established, which is why they are not yet suitable for advancement to clinical trial phases.
4.15 Tablet formulations
Tablets represent a traditional and common dosage form, with relatively low production costs and simple manufacturing processes. Zheng et al. prepared curcumin sustained-release tablets using a direct compression method with a single-punch tablet press (Zheng et al., 2015). They used sodium dodecyl sulfate as a solubilizer, anhydrous lactose as a pore-forming agent, talc and magnesium stearate as lubricants, and corn starch as a filler. Carbopol and HPMC K4M were used in combination as the sustained-release materials, resulting in an 80% drug release in 8 h. Zhao et al. combined phospholipid complexation and PVP K30 solid dispersion techniques to prepare a compound curcumin solid dispersion tablet (Zhao et al., 2014). This tablet formulation could promote drug disintegration, increase gastrointestinal absorption, slow down the elimination rate, and inhibit the distribution, thereby improving the bioavailability of curcumin. Furthermore, tablets can be combined with various innovative technologies, including solid dispersion and cyclodextrin inclusion. This synergy not only improves the dissolution and bioavailability of curcumin but also enhances portability and ease of use. As such, these formulations are poised to become the favored choice in clinical trials.
5 Clinical research on curcumin
As the solubility and bioavailability of curcumin have improved, clinical research on curcumin has begun to gain momentum. The majority of existing clinical studies are primarily focused on Phase I and Phase II (Figure 1C). Phase I clinical trials are primarily concerned with the assessment of the drug’s safety and tolerability. Dose escalation studies have demonstrated that oral doses of curcumin as high as 12 g/d do not result in significant toxicity in the short term (Liu et al., 2013). Furthermore, numerous trials have demonstrated the safety and efficacy of curcumin in the treatment of diabetes, osteoarthritis, cancer, and inflammatory diseases. A survey conducted up to 2023 revealed that the majority of curcumin clinical trials, 22%, are related to metabolic abnormalities associated with obesity and insulin resistance. This is followed by musculoskeletal diseases (MSK, 17%), neurological diseases (NEURO, 11%), gastrointestinal diseases (GI, excluding non-alcoholic fatty liver, 10%), and cancer (CA, 9%) (Panknin et al., 2023). For further details, please refer to Table 5. In a clinical trial for T2DM, Adibian M et al. administered 1,500 mg of curcumin daily for 10 consecutive weeks (Adibian et al., 2019). The results demonstrated that curcumin reduced total cholesterol levels and inflammatory markers; In a study conducted by Hodaei et al., a dosage of the same medication was found to result in a reduction in fasting blood sugar and body weight in patients (Hodaei et al., 2019). The addition of a small amount of piperine (PIP) to curcumin has been demonstrated to lower the atherogenic lipid index in the serum, as well as to improve blood sugar and liver parameters in patients with T2DM (Panahi et al., 2017; Panahi et al., 2018). A daily supplementation of 70 mg of curcumin for 8 weeks has been demonstrated to improve liver fat content and body mass index in patients with non-alcoholic fatty liver disease (Rahmani et al., 2016). Several clinical studies have demonstrated that curcumin exhibits significant therapeutic efficacy against major metabolic complications associated with the development and progression of metabolic disorders, including obesity, T2DM, and non-alcoholic fatty liver disease. These findings highlight the potent anti-inflammatory and antioxidant properties of curcumin.
Osteoarthritis is the most prevalent musculoskeletal disease treated with curcumin, with Atabaki M et al. found that curcumin can reduce Visual Analog Scale (VAS) scores, C-reactive protein (CRP), CD4 and CD8 T cells, Th17 cells, and B cell frequencies to alleviate osteoarthritis (Atabaki et al., 2020). However, the therapeutic effects of curcumin on rheumatoid arthritis, which is also classified as a musculoskeletal disorder, have not demonstrated significant efficacy (Javadi et al., 2019). The addition of boswellic acid can reduce pain-related symptoms in patients (Haroyan et al., 2018). Furthermore, curcumin has demonstrated efficacy in the treatment of various neurological disorders, including migraines and severe depression. A daily intake of 80 mg of curcumin resulted in a significant reduction in the gene expression and plasma levels of IL-17 in migraine patients after 2 months, leading to an improvement in migraines (Djalali et al., 2020); while a daily intake of 160 mg of curcumin was found to result in a discernible improvement in mood after 8 weeks (Lopresti et al., 2014). Clinical trials indicate that curcumin, due to its exceptional antioxidant and anti-inflammatory properties, can ameliorate the symptoms of gastrointestinal barrier damage during Helicobacter pylori gastric infection and exertional heat stress (Judaki et al., 2017; Szymanski et al., 2018). Furthermore, clinical trials have indicated that curcumin, when used in combination with other drugs or treatments, can enhance treatment efficacy and reduce the side effects of other drugs. The combination of curcumin, high taurine (100 μM) and vitamin D3 (50 nM) is employed in the treatment of diabetic retinopathy (Filippelli et al., 2020). Studies have demonstrated that while each of the agents in question has a relatively weak anti-inflammatory effect when used in isolation, their combined use can result in a significant alteration of the average levels of soluble mediators of inflammation and retinal damage. In a series of clinical trials, Howells LM and colleagues demonstrated that curcumin is a safe and tolerable adjunctive medication for FOLFOX chemotherapy in patients with metastatic colorectal cancer (Howells et al., 2019).
In recent years, there has been a notable increase in the number of clinical registrations for curcumin at Phase III and Phase IV. India has emerged as the primary country responsible for these registrations. These registrations are mainly focused on oral and dental diseases, arthritis, and diabetes (Table 6). The primary research subjects within the field of oral and dental diseases are oral mucositis and periodontitis. India has consecutively registered clinical trials related to oral mucositis in 2017 (CTRI/2017/01/007732, CTRI/2017/08/009461, CTRI/2017/09/009674), 2020 (CTRI/2020/10/028666), 2021 (NCT04896164, CTRI/2021/01/030470), 2022 (CTRI/2022/08/044967), and 2023 (CTRI/2023/07/055584). Additionally, nearly half of these trials originate from the Maulana Azad Institute of Dental Sciences, which indirectly indicates the potential therapeutic effects of curcumin in clinical treatment of this disease. However, further extensive clinical verification and refinement are still required to establish a solid data foundation for clinical application. In 2017, India registered a clinical trial for the use of daily curcumin tablets at 500 mg for a continuous 6-week period (CTRI/2017/11/010494), followed by the registration of a clinical trial in 2019 for the application of curcumin gel for a continuous 1-year period (CTRI/2019/04/018697). Furthermore, in 2022, a clinical trial for the use of curcumin-containing toothpaste for a continuous 7-month period (CTRI/2022/07/043703) to treat periodontitis was also registered. In July 2019, the University of Ains Sham registered a clinical trial for the adjunctive treatment of curcumin paste (NCT04032132), followed by the registration in August of the same year for a clinical trial using curcumin-simvastatin-EDTA (NCT04044417) to treat periodontitis. The employment of diverse dosage forms and administration methods in Phase IV clinical trials will permit a multidimensional exploration of the actual effects of curcumin on periodontitis in clinical practice. This may facilitate the earlier application of the findings in clinical settings. In summary, curcumin represents a promising novel therapeutic agent for the treatment of oral and dental diseases. Since 2018, there has been a notable increase in the number of clinical trials for musculoskeletal diseases such as arthritis and rheumatoid arthritis that have reached Phase III and Phase IV. India registered the use of curcumin-pip tablets for the treatment of rheumatoid arthritis and early knee osteoarthritis in 2018 (CTRI/2018/04/013279) and 2022 (CTRI/2022/07/043621) respectively. Following a 4-year interval, the same medication was registered for the treatment of different types of arthriti. This led us to speculate that curcumin-pip tablets may have certain clinical efficacy for rheumatoid arthritis. Furthermore, experts have successively conducted clinical trials on diabetes and its complications in multiple countries including Thailand (NCT01052025), Egypt (NCT04528212), Mexico (NCT03262363, NCT03917784), and Iran (NCT02529969) to explore the therapeutic effects of curcumin on diabetes. Over the past 5 years, there have been only two Phase III and Phase IV clinical trials related to cancer treatment (CTRI/2023/02/050028, CTRI/2019/03/018265). A review of clinical trials conducted between 2005 and 2015 revealed a number of studies related to colorectal cancer and pancreatic cancer in Israel (NCT00295035, NCT00486460), prostate cancer in the United States (NCT02064673), and In India, clinical trials related to squamous cell head and neck cancer and advanced cervical cancer (CTRI/2008/091/000195, CTRI/2008/091/000131) have yet to yield clear clinical outcomes. Moreover, no favourable clinical results have been reported. This leads us to hypothesize that the role of curcumin in clinical cancer treatment is minimal or non-existent.
6 Conclusion
This article provides a comprehensive review of the research advancements related to curcumin, focusing on three key areas: pharmacological effects, formulation studies, and clinical applications. Previous reviews of curcumin have predominantly focused on specific diseases or formulations, with few examining its overall pharmacological effects, formulation research, and clinical applications comprehensively, such as Hao et al. (2023). Firstly, this review presented a comprehensive overview of the pharmacological mechanisms of curcumin. However, they fell short in establishing a link between these mechanisms and clinical outcomes. Secondly, this review highlighted poor bioavailability as a fundamental obstacle to clinical application and suggested enhancements through methodologies such as nanotechnology and solid dispersions. Nevertheless, this review did not provide more details on the challenges of implementing these formulations in clinical settings, providing only a singular example. Compared to the review by Hao et al., this article not only encapsulates the primary pharmacological effects of curcumin but also connects curcumin’s effects to clinical outcomes. This would provide more context for the potential therapeutic benefits. Furthermore, this article summarizesthe results of molecular docking of curcumin, potentially aiding further exploration of the compound’s therapeutic properties. Additionally, it underscores the progress in research on various curcumin formulations, evaluating their feasibility/infeasibility within clinical settings.
Curcumin exhibits a multitude of biological activities, including anticancer, anti-inflammatory, antioxidant, and antibacterial effects. Extensive research has been conducted to explore its mechanisms of action, establishing a theoretical foundation for future clinical trials. In addition, some researchers have utilized network pharmacology to identify curcumin’s targets across various diseases. By selecting core targets and conducting molecular docking studies, they have found that a binding energy of less than −5.0 kcal/mol indicates a strong interaction between curcumin and these targets, suggesting their potential as therapeutic candidates. Computational simulations can also be employed to predict curcumin’s therapeutic potential for different diseases, which may help mitigate the costs associated with traditional trial-and-error approaches. However, it is important to note that the accuracy of these simulations can vary due to potential biases in the underlying databases. Therefore, further animal studies and clinical observations are essential for validation.
A comprehensive review of curcumin’s pharmacological effects reveals that it possesses a diverse range of beneficial properties. When taken at a daily dosage of 12 g, curcumin shows good tolerability and does not appear to cause significant toxicity in the short term. However, its clinical application is limited by challenges such as low solubility, poor bioavailability, and a short half-life. To address these challenges, researchers both domestically and internationally have explored various formulation technologies to create innovative dosage forms, including nano-targeted formulations (such as liposomes, nanoparticles, and micelles), nanoemulsions, and nanocrystals.
Despite these advancements, current clinical trials predominantly focus on tablets or capsules. This phenomenon is worthy of our thought. Here are our views on this phenomenon. Firstly, most existing nano-targeted formulations rely on the Enhanced Permeability and Retention (EPR) effect for cancer treatment, which has demonstrated significant efficacy in animal models. However, EPR effect was not found in human (Sharifi et al., 2022). Thus, it is difficult to apply nano-targeted formulations in clinical practice. Secondly, the lack of standardized production and quality control protocols for these nano-targeted materials complicates the effective assessment of their safety and efficacy. Thirdly, excipients such as mesoporous silica, due to their insolubility, non-biodegradability, and inadequate biocompatibility, may accumulate in tissues and organs, thereby impeding excretion and potentially leading to long-term toxicity. Fourthly, the field of nano-toxicology has not kept pace with the rapid advancements in nano-formulations. In-depth studies on the toxicity mechanism of nano-materials are needed to guide the design and modification of nano-materials to reduce their toxicity and improve their biocompatibility and safety. Fifthly, although contemporary nano-targeting agents exhibit favorable physicochemical properties and pharmacological effects, the incorporation of nano-targeted materials can lead to a range of adverse effects following oral administration. For example, alterations in the composition of the human gut microbiota may contribute to obesity, metabolic syndrome, and an increased incidence of other chronic inflammatory diseases. Additionally, the stability of nano-formulations is poor, rendering them prone to aggregation, sedimentation, leakage, and structural degradation during storage and transportation, which adversely impacts their physicochemical properties and therapeutic efficacy. For example, nano-emulsions may have problems such as particle size growth, gelation, and drug precipitation during storage. In summary, nano-targeted formulations prepared under the existing conditions are difficult to meet the standards of national pharmacopoeia of various countries. Additionally, the intricate preparation processes and advanced technologies required result in significantly higher manufacturing costs compared to conventional dosage forms such as tablets and capsules. Thus, these formulations are limited to the laboratory stage. Consequently, the clinical application of nano-formulations remains challenging.
Furthermore, there are still some deficiencies in curcumin research that need to be strengthened in subsequent studies. Firstly, the drug loading capacity of the existing preparations is generally not high. The low drug loading capacity leads to insufficient drug concentration, making it difficult to achieve the expected therapeutic effect. However, to achieve the expected therapeutic effect, patients may need to increase the frequency or dosage of medication, which raise the risk of side effects. Secondly, Phase III trials are pivotal in assessing the approval of curcumin for market release, while Phase IV trials play a vital role in ongoing surveillance after approval. Together, these stages safeguard the safety, efficacy, and judicious clinical application of medications. Phase IV clinical trials not only confirm the findings of pre-market studies of curcumin but also address any biases from earlier trials, fill in gaps of missing information, and provide a solid foundation for clinical application. However, the present clinical studies in Phase III and Phase IV are limited, and the treatment of diseases is mainly focused on dental diseases, arthritis, and diabetes. A large number of cell tests and animal experiments indicate that curcumin has other therapeutic effects. However, there is a lack of substantial Phase III and Phase IV clinical evidence to demonstrate its therapeutic effects in the human body. Furthermore, the existing clinical studies mostly involve oral administration, with very few involving topical application.
In the future, polymers combination and drugs combination can be considered to enhance the efficacy of the drug and improve patient adherence through. It is also essential to develop excipients that demonstrate good biocompatibility and pose no biological toxicity, thereby minimizing side effects. Given that turmeric is a medicinal botanical drug with dietary uses, curcumin can be made into medicinal cuisine or food products to improve adherence, particularly among pediatric patients. In the future, the preparation process of curcumin should be improved, including the preparation of organic agent-free preparations and the use of biodegradable materials (polysaccharides, proteins, etc.). Furthermore, specific excipients and dosage forms can be tailored and developed based on the various types of diseases, for enhancing the rational application of formulations. For example, in treating gastric-related conditions, gastro-retentive formulations can prolong the drug’s retention in the stomach, thereby enhancing therapeutic outcomes. In conclusion, it is essential to enhance the quality control and safety assessment of pharmaceutical excipients, while conducting in-depth research on the interaction mechanisms between excipients and drugs. Furthermore, continuous innovation and development of novel pharmaceutical excipients will provide robust support for the advancement and innovation of curcumin formulations. Concurrently, the therapeutic potential of curcumin can be increased by exploring innovative targeted drug delivery strategies, improved formulation systems, and effective combination therapies. These modalities can promote its timely application in the treatment of various diseases and provide new avenues for therapeutic approaches.
Author contributions
MH: Conceptualization, Visualization, Writing–original draft. CZ: Funding acquisition, Supervision, Writing–review and editing. TW: Investigation, Writing–original draft. HH: Investigation, Writing–original draft.
Funding
The author(s) declare that financial support was received for the research and/or publication of this article. The National Natural Science Foundation of China (No. 81503257); Inner Mongolia Major science and technology project (No. 2021ZD0017); Liaoning Provincial Science and Technology Programme Joint Programme (Applied Basic Research Project) (2023JH2/101700206); Liaoning University of Traditional Chinese Medicine-Natural Science University Key Project (2021LZY047); Liaoning Provincial Department of Education university basic scientific research project reserve project (LJ212410162055); National Key Research and Development Program (No. 2018YFC1706903).
Conflict of interest
Author CZ was employed by Qimeng Co., LTD.
The remaining authors declare that the research was conducted in the absence of any commercial or financial relationships that could be construed as a potential conflict of interest.
Generative AI statement
The author(s) declare that no Generative AI was used in the creation of this manuscript.
Publisher’s note
All claims expressed in this article are solely those of the authors and do not necessarily represent those of their affiliated organizations, or those of the publisher, the editors and the reviewers. Any product that may be evaluated in this article, or claim that may be made by its manufacturer, is not guaranteed or endorsed by the publisher.
References
Abrahams, S., Haylett, W. L., Johnson, G., Carr, J. A., and Bardien, S. (2019). Antioxidant effects of curcumin in models of neurodegeneration, aging, oxidative and nitrosative stress: a review. Neuroscience 406, 1–21. doi:10.1016/j.neuroscience.2019.02.020
Adahoun, M. A., Al-Akhras, M. H., Jaafar, M. S., and Bououdina, M. (2017). Enhanced anti-cancer and antimicrobial activities of curcumin nanoparticles. Artif. Cells Nanomed Biotechnol. 45 (1), 98–107. doi:10.3109/21691401.2015.1129628
Adibian, M., Hodaei, H., Nikpayam, O., Sohrab, G., Hekmatdoost, A., and Hedayati, M. (2019). The effects of curcumin supplementation on high-sensitivity C-reactive protein, serum adiponectin, and lipid profile in patients with type 2 diabetes: a randomized, double-blind, placebo-controlled trial. Phytother. Res. 33 (5), 1374–1383. doi:10.1002/ptr.6328
Ahmed, M., Abdul Qadir, M., Imtiaz Shafiq, M., Muddassar, M., Hameed, A., Nadeem Arshad, M., et al. (2017). Curcumin: synthesis optimization and in silico interaction with cyclin dependent kinase. Acta Pharm. 67 (3), 385–395. doi:10.1515/acph-2017-0023
Ali, A., and Banerjea, A. C. (2016). Curcumin inhibits HIV-1 by promoting Tat protein degradation. Sci. Rep. 6, 27539. doi:10.1038/srep27539
Atabaki, M., Shariati-Sarabi, Z., Tavakkol-Afshari, J., and Mohammadi, M. (2020). Significant immunomodulatory properties of curcumin in patients with osteoarthritis; a successful clinical trial in Iran. Int. Immunopharmacol. 85, 106607. doi:10.1016/j.intimp.2020.106607
Balakumar, P., Venkatesan, K., Abdulla Khan, N., Raghavendra, N. M., Venugopal, V., Bharathi, D. R., et al. (2023). Mechanistic insights into the beneficial effects of curcumin on insulin resistance: opportunities and challenges. Drug Discov. Today 28 (7), 103627. doi:10.1016/j.drudis.2023.103627
Balasubramanyam, M., Koteswari, A. A., Kumar, R. S., Monickaraj, S. F., Maheswari, J. U., and Mohan, V. (2003). Curcumin-induced inhibition of cellular reactive oxygen species generation: novel therapeutic implications. J. Biosci. 28 (6), 715–721. doi:10.1007/bf02708432
Balasubramonian, B., and Selcer, K. W. (2023). The phytochemical curcumin inhibits steroid sulfatase activity in rat liver tissue and NIH-3T3 mouse fibroblast cells. Steroids 191, 109163. doi:10.1016/j.steroids.2022.109163
Cadel, S., Darmon, C., Désert, A., Mahbouli, M., Piesse, C., Ghélis, T., et al. (2019). The effects of curcumin, mangiferin, resveratrol and other natural plant products on aminopeptidase B activity. Biochem. Biophys. Res. Commun. 512 (4), 832–837. doi:10.1016/j.bbrc.2019.02.143
Cai, X. Z., Wang, J., Li, X. D., Wang, G. L., Liu, F. N., Cheng, M. S., et al. (2009). Curcumin suppresses proliferation and invasion in human gastric cancer cells by downregulation of PAK1 activity and cyclin D1 expression. Cancer Biol. Ther. 8 (14), 1360–1368. doi:10.4161/cbt.8.14.8720
Cao, L. Y., Chen, C., Zhou, Z., Dou, S. Y., Sun, Y., Zhu, B. W., et al. (2022). Fabrication of a carboxymethyl chitosan/oxidized carboxymethylcellulose/curcumin composite film and its application in the film-coating preservation of strawberry. Mod. Food Sci. Technol. 38 (12), 247–254. doi:10.13982/j.mfst.1673-9078.2022.12.0101
Chen, J., Cao, X., Qin, X., Liu, H., Chen, S., Zhong, S., et al. (2020). Proteomic analysis of the molecular mechanism of curcumin/β-cyclodextrin polymer inclusion complex inhibiting HepG2 cells growth. J. Food Biochem. 44 (2), e13119. doi:10.1111/jfbc.13119
Chen, J., Chen, W. D., and Cui, Y. J. (2007). Study on the preparation process of curcumin drops. China Pharm. Clin. (02), 135–137.
Chen, J., Li, J., Fan, T., Zhong, S., Qin, X., Li, R., et al. (2022a). Protective effects of curcumin/cyclodextrin polymer inclusion complex against hydrogen peroxide-induced LO2 cells damage. Food Sci. Nutr. 10 (5), 1649–1656. doi:10.1002/fsn3.2787
Chen, J., Qin, X., Zhong, S., Chen, S., Su, W., and Liu, Y. (2018). Characterization of curcumin/cyclodextrin polymer inclusion complex and investigation on its antioxidant and antiproliferative activities. Molecules 23 (5), 1179. doi:10.3390/molecules23051179
Chen, J. H. (2015). Preparation of curcumin film and observation of its effect on promoting wound healing in animals. Master’s thesis. Nanjing: Nanjing Agricultural University.
Chen, J. H., Dai, W., Cai, Y., Liu, Y. P., Qi, Y. C., Fang, G. Y., et al. (2022b). Study on the pharmacokinetics of curcumin in the curcumin film in rats. Animal Husb. & Veterinary Med. 54 (11), 51–56.
Chen, L. (2019). Establishment of cell and animal detection models for curcumin antioxidativity. Master’s thesis. Xiamen: Jimei University.
Chen, L., Dong, Q., Shi, Q., Du, Y., Zeng, Q., Zhao, Y., et al. (2021). Novel 2,3-dialdehyde cellulose-based films with photodynamic inactivation potency by incorporating the β-cyclodextrin/curcumin inclusion complex. Biomacromolecules 22 (7), 2790–2801. doi:10.1021/acs.biomac.1c00165
Chen, X., Yang, H. B., Hu, Y. Q., Yang, Y. L., Zhi, F., Tang, W. G., et al. (2013). Enhanced brain targeting of curcumin by intranasal administration of a thermosensitive poloxamer hydrogel. Pharm. Clin. Res. 21 (01), 9–12. doi:10.13664/j.cnki.pcr.2013.01.001
Chopra, H., Bibi, S., Mohanta, Y. K., Kumar Mohanta, T., Kumar, S., Singh, I., et al. (2023). In vitro and in silico characterization of curcumin-loaded chitosan-PVA hydrogels: antimicrobial and potential wound healing activity. Gels 9 (5), 394. doi:10.3390/gels9050394
Chuah, A. M., Jacob, B., Jie, Z., Ramesh, S., Mandal, S., Puthan, J. K., et al. (2014). Enhanced bioavailability and bioefficacy of an amorphous solid dispersion of curcumin. Food Chem. 156, 227–233. doi:10.1016/j.foodchem.2014.01.108
Cruz-Correa, M., Shoskes, D. A., Sanchez, P., Zhao, R., Hylind, L. M., Wexner, S. D., et al. (2006). Combination treatment with curcumin and quercetin of adenomas in familial adenomatous polyposis. Clin. Gastroenterol. Hepatol. 4 (8), 1035–1038. doi:10.1016/j.cgh.2006.03.020
Desai, P. P., and Patravale, V. B. (2018). Curcumin cocrystal micelles-multifunctional nanocomposites for management of neurodegenerative ailments. J. Pharm. Sci. 107 (4), 1143–1156. doi:10.1016/j.xphs.2017.11.014
Ding, L., Li, J., Song, B., Xiao, X., Zhang, B., Qi, M., et al. (2016). Curcumin rescues high fat diet-induced obesity and insulin sensitivity in mice through regulating SREBP pathway. Toxicol. Appl. Pharmacol. 304, 99–109. doi:10.1016/j.taap.2016.05.011
Djalali, M., Abdolahi, M., Hosseini, R., Miraghajani, M., Mohammadi, H., and Djalali, M. (2020). The effects of nano-curcumin supplementation on Th1/Th17 balance in migraine patients: a randomized controlled clinical trial. Complement. Ther. Clin. Pract. 41, 101256. doi:10.1016/j.ctcp.2020.101256
Fan, S., Zheng, Y., Liu, X., Fang, W., Chen, X., Liao, W., et al. (2018). Curcumin-loaded PLGA-PEG nanoparticles conjugated with B6 peptide for potential use in Alzheimer's disease. Drug Deliv. 25 (1), 1091–1102. doi:10.1080/10717544.2018.1461955
Fan, W., Zhang, X., Zhu, W., and Di, L. (2020). The preparation of curcumin sustained-release solid dispersion by hot-melt Extrusion-Ⅱ. Optimization of preparation process and evaluation in vitro and in vivo. J. Pharm. Sci. 109 (3), 1253–1260. doi:10.1016/j.xphs.2019.11.020
Fan, W., Zhang, X., Zhu, W., Zhang, X., and Di, L. (2021). Preparation of curcumin-eudragit(®) E PO solid dispersions with gradient temperature through hot-melt extrusion. Molecules 26 (16), 4964. doi:10.3390/molecules26164964
Fang, Y., Xiang, B., Pan, Z. H., and Cao, D. Y. (2010). Studies on preparation and dissolution test in vitro of sustained-release dropping pills of curcumin. J. Chin. Med. Mater 33 (01), 111–114. doi:10.13863/j.issn1001-4454.2010.01.041
Farzaei, M. H., Zobeiri, M., Parvizi, F., El-Senduny, F. F., Marmouzi, I., Coy-Barrera, E., et al. (2018). Curcumin in liver diseases: a systematic review of the cellular mechanisms of oxidative stress and clinical perspective. Nutrients 10 (7), 855. doi:10.3390/nu10070855
Filippelli, M., Campagna, G., Vito, P., Zotti, T., Ventre, L., Rinaldi, M., et al. (2020). Anti-inflammatory effect of curcumin, homotaurine, and vitamin D3 on human vitreous in patients with diabetic retinopathy. Front. Neurol. 11, 592274. doi:10.3389/fneur.2020.592274
Fleenor, B. S., Carlini, N. A., and Campbell, M. S. (2019). Curcumin and arterial function in health and disease: impact on oxidative stress and inflammation. Curr. Opin. Clin. Nutr. Metab. Care 22 (6), 459–464. doi:10.1097/mco.0000000000000598
Funamoto, M., Sunagawa, Y., Katanasaka, Y., Miyazaki, Y., Imaizumi, A., Kakeya, H., et al. (2016). Highly absorptive curcumin reduces serum atherosclerotic low-density lipoprotein levels in patients with mild COPD. Int. J. Chron. Obstruct Pulmon Dis. 11, 2029–2034. doi:10.2147/copd.S104490
Gao, K., Zhang, B., and Zhao, F. (2023). Antibiofilm effect of curcumin against Staphylococcus aureus surface wound biofilm-associated infection: in vitro and in silico. Appl. Biochem. Biotechnol. 195 (9), 5329–5337. doi:10.1007/s12010-022-03844-5
Gao, Z. K., Zhang, X. L., and Wang, J. D. (2011). Preparation of curcumin solid dispersion and inclusion complex. J. Chin. Med. Mater 34 (11), 1784–1788. doi:10.13863/j.issn1001-4454.2011.11.050
Ghareghomi, S., Rahban, M., Moosavi-Movahedi, Z., Habibi-Rezaei, M., Saso, L., and Moosavi-Movahedi, A. A. (2021). The potential role of curcumin in modulating the master antioxidant pathway in diabetic hypoxia-induced complications. Molecules 26 (24), 7658. doi:10.3390/molecules26247658
Gong, Z., Zhao, S., Zhou, J., Yan, J., Wang, L., Du, X., et al. (2018). Curcumin alleviates DSS-induced colitis via inhibiting NLRP3 inflammsome activation and IL-1β production. Mol. Immunol. 104, 11–19. doi:10.1016/j.molimm.2018.09.004
Gu, J. J., and Deng, Y. J. (2010). Preparation of curcumin liposomes and its oral pharmacokinetics in rats. J. Chengdu Med. Coll. 5 (02), 97–100.
Guan, H. (2009). Study on the content of curcumin solution-filled hard capsules and curcumin solid dispersion. Master’s thesis. Shenyang: Shenyang Pharmaceutical University.
Gularte, M. S., Quadrado, R. F. N., Pedra, N. S., Soares, M. S. P., Bona, N. P., Spanevello, R. M., et al. (2020). Preparation, characterization and antitumor activity of a cationic starch-derivative membrane embedded with a β-cyclodextrin/curcumin inclusion complex. Int. J. Biol. Macromol. 148, 140–152. doi:10.1016/j.ijbiomac.2020.01.104
Gupte, P. A., Giramkar, S. A., Harke, S. M., Kulkarni, S. K., Deshmukh, A. P., Hingorani, L. L., et al. (2019). Evaluation of the efficacy and safety of Capsule Longvida(®) Optimized Curcumin (solid lipid curcumin particles) in knee osteoarthritis: a pilot clinical study. J. Inflamm. Res. 12, 145–152. doi:10.2147/jir.S205390
Hahn, W. C., and Weinberg, R. A. (2002). Rules for making human tumor cells. N. Engl. J. Med. 347 (20), 1593–1603. doi:10.1056/NEJMra021902
Ham, I. H., Wang, L., Lee, D., Woo, J., Kim, T. H., Jeong, H. Y., et al. (2022). Curcumin inhibits the cancer-associated fibroblast-derived chemoresistance of gastric cancer through the suppression of the JAK/STAT3 signaling pathway. Int. J. Oncol. 61 (1), 85. doi:10.3892/ijo.2022.5375
Han, G., Liu, Y. M., Xia, Z. L., Li, W. N., and Liu, X. P. (2005). Study on the preparation of wound healing membrane with curcumin and chitosan. J. Chin. Med. Mater (12), 1108–1109. doi:10.13863/j.issn1001-4454.2005.12.027
Hao, M., Chu, Y., Lei, J., Yao, Z., Wang, P., Chen, Z., et al. (2023). Pharmacological mechanisms and clinical applications of curcumin: update. Aging Dis. 14 (3), 716–749. doi:10.14336/ad.2022.1101
Hao, X. K., Zhen, Y. Q., Wang, Q., Zhang, Y. K., Xu, H. X., Xia, X. Y., et al. (2021). Entrapment effect of tween surfactant niosomes on curcumin. Chemistry 84 (11), 1243–1247. doi:10.14159/j.cnki.0441-3776.2021.11.017
Haroyan, A., Mukuchyan, V., Mkrtchyan, N., Minasyan, N., Gasparyan, S., Sargsyan, A., et al. (2018). Efficacy and safety of curcumin and its combination with boswellic acid in osteoarthritis: a comparative, randomized, double-blind, placebo-controlled study. BMC Complement. Altern. Med. 18 (1), 7. doi:10.1186/s12906-017-2062-z
Hayeshi, R., Mutingwende, I., Mavengere, W., Masiyanise, V., and Mukanganyama, S. (2007). The inhibition of human glutathione S-transferases activity by plant polyphenolic compounds ellagic acid and curcumin. Food Chem. Toxicol. 45 (2), 286–295. doi:10.1016/j.fct.2006.07.027
Heshmati, J., Moini, A., Sepidarkish, M., Morvaridzadeh, M., Salehi, M., Palmowski, A., et al. (2021). Effects of curcumin supplementation on blood glucose, insulin resistance and androgens in patients with polycystic ovary syndrome: a randomized double-blind placebo-controlled clinical trial. Phytomedicine 80, 153395. doi:10.1016/j.phymed.2020.153395
Hodaei, H., Adibian, M., Nikpayam, O., Hedayati, M., and Sohrab, G. (2019). The effect of curcumin supplementation on anthropometric indices, insulin resistance and oxidative stress in patients with type 2 diabetes: a randomized, double-blind clinical trial. Diabetol. Metab. Syndr. 11, 41. doi:10.1186/s13098-019-0437-7
Hong, W., Gao, Y., Lou, B., Ying, S., Wu, W., Ji, X., et al. (2021). Curcumin-loaded hybrid nanoparticles: microchannel-based preparation and antitumor activity in a mouse model. Int. J. Nanomedicine 16, 4147–4159. doi:10.2147/ijn.S303829
Hong, Y., He, W., Cao, Y., and Fu, J. Y. (2013). Preparation of curcumin guttate pills. Chin. J. Clin. Rational Drug Use 6(02), 43–44. doi:10.15887/j.cnki.13-1389/r.2013.02.001
Howells, L. M., Iwuji, C. O. O., Irving, G. R. B., Barber, S., Walter, H., Sidat, Z., et al. (2019). Curcumin combined with FOLFOX chemotherapy is safe and tolerable in patients with metastatic colorectal cancer in a randomized phase IIa trial. J. Nutr. 149 (7), 1133–1139. doi:10.1093/jn/nxz029
Hu, R. W., Carey, E. J., Lindor, K. D., and Tabibian, J. H. (2017). Curcumin in hepatobiliary disease: pharmacotherapeutic properties and emerging potential clinical applications. Ann. Hepatol. 16 (6), 835–841. doi:10.5604/01.3001.0010.5273
Huang, F., Yao, Y., Wu, J., Liu, Q., Zhang, J., Pu, X., et al. (2017). Curcumin inhibits gastric cancer-derived mesenchymal stem cells mediated angiogenesis by regulating NF-κB/VEGF signaling. Am. J. Transl. Res. 9 (12), 5538–5547.
Huang, R., Lu, X. Y., Han, J. W., Li, J. S., and Di, L. Q. (2018). Preparation and bioavailability of curcumin/piperine solid dispersions. Chin. Tradit. Herb. Drugs 49 (19), 4528–4534.
Huang, S., Xu, D., Zhang, L., Hao, L., Jia, Y., Zhang, X., et al. (2023). Therapeutic effects of curcumin liposomes and nanocrystals on inflammatory osteolysis: in vitro and in vivo comparative study. Pharmacol. Res. 192, 106778. doi:10.1016/j.phrs.2023.106778
Huang, X. W., Xu, J. H., Wu, G. H., and Wen, C. X. (2008). Pharmacokinetics of curcumin solid dispersion in mice. Chin. Pharmacol. Bull. (11), 1525–1527.
Jabbari, N., Ghoran, S. H., Semsari, H., Hussen, B. M., and Babaei, E. (2022). Gemini curcumin suppresses gastric cancer AGS cell proliferation through modulation of lncRNA CCAT2 and c-myc genes. Turk J. Pharm. Sci. 19 (3), 239–245. doi:10.4274/tjps.galenos.2021.03502
Javadi, M., Khadem Haghighian, H., Goodarzy, S., Abbasi, M., and Nassiri-Asl, M. (2019). Effect of curcumin nanomicelle on the clinical symptoms of patients with rheumatoid arthritis: a randomized, double-blind, controlled trial. Int. J. Rheum. Dis. 22 (10), 1857–1862. doi:10.1111/1756-185x.13688
Jin, H. H., Lu, Q., and Jiang, J. G. (2016). Curcumin liposomes prepared with milk fat globule membrane phospholipids and soybean lecithin. J. Dairy Sci. 99 (3), 1780–1790. doi:10.3168/jds.2015-10391
Judaki, A., Rahmani, A., Feizi, J., Asadollahi, K., and Hafezi Ahmadi, M. R. (2017). Curcumin in combination with triple therapy regimes ameliorates oxidative stress and histopathologic changes in chronic gastritis-associated helicobacter pylori infection. Arq. Gastroenterol. 54 (3), 177–182. doi:10.1590/s0004-2803.201700000-18
Jv, J. R., Dai, J. D., Zhu, X. Y., Guo, P. C., and Wu, S. Y. (2022). Study on the prescription of curcumin self-microemulsion colon inflammation-targeted pellets. J. Chang. Univ. Chin. 38 (09), 977–982. doi:10.13463/j.cnki.cczyy.2022.09.009
Kanwal, Q., Ahmed, M., Hamza, M., Ahmad, M., Atiq Ur, R., Yousaf, N., et al. (2023). Curcumin nanoparticles: physicochemical fabrication, characterization, antioxidant, enzyme inhibition, molecular docking and simulation studies. RSC Adv. 13 (32), 22268–22280. doi:10.1039/d3ra01432k
Karade, P. G., and Jadhav, N. R. (2018). Colon targeted curcumin microspheres laden with ascorbic acid for bioavailability enhancement. J. Microencapsul. 35 (4), 372–380. doi:10.1080/02652048.2018.1501111
Khaleghian, M., Sahrayi, H., Hafezi, Y., Mirshafeeyan, M., Moghaddam, Z. S., Farasati Far, B., et al. (2023). In silico design and mechanistic study of niosome-encapsulated curcumin against multidrug-resistant Staphylococcus aureus biofilms. Front. Microbiol. 14, 1277533. doi:10.3389/fmicb.2023.1277533
Kim, K., Kim, K. H., Kim, H. Y., Cho, H. K., Sakamoto, N., and Cheong, J. (2010). Curcumin inhibits hepatitis C virus replication via suppressing the Akt-SREBP-1 pathway. FEBS Lett. 584 (4), 707–712. doi:10.1016/j.febslet.2009.12.019
Kong, D., Zhang, Z., Chen, L., Huang, W., Zhang, F., Wang, L., et al. (2020). Curcumin blunts epithelial-mesenchymal transition of hepatocytes to alleviate hepatic fibrosis through regulating oxidative stress and autophagy. Redox Biol. 36, 101600. doi:10.1016/j.redox.2020.101600
Kumari, M., and Nanda, D. K. (2023). Potential of Curcumin nanoemulsion as antimicrobial and wound healing agent in burn wound infection. Burns 49 (5), 1003–1016. doi:10.1016/j.burns.2022.10.008
Laomeephol, C., Ferreira, H., Kanokpanont, S., Neves, N. M., Kobayashi, H., and Damrongsakkul, S. (2020). Dual-functional liposomes for curcumin delivery and accelerating silk fibroin hydrogel formation. Int. J. Pharm. 589, 119844. doi:10.1016/j.ijpharm.2020.119844
Li, K., Zhang, Y., Hao, X., Xie, D., Wang, C., Zhang, H., et al. (2022). Improved stability and in vitro anti-arthritis bioactivity of curcumin-casein nanoparticles by ultrasound-driven encapsulation. Nutrients 14 (23), 5192. doi:10.3390/nu14235192
Li, K. L., Li, Y., Zhao, J., Chen, R., and Zhang, J. Q. (2020). Release characteristics and pharmacokinetic properties of chitosan coated curcumin ethosomes. Chin. Pharm. J. 55 (11), 919–924.
Li, W. Z., Zhang, Y. J., Zhong, W., Wang, J., Sun, M. Y., and Wang, Z. Y. (2023). Preparation and evaluation of curcumin binary carrier solid dispersion. Chin. J. Hosp. Pharm. 43 (16), 1821–1827. doi:10.13286/j.1001-5213.2023.16.09
Li, X., He, S., Tian, Y., Weiss, R. M., and Martin, D. T. (2019). Synergistic inhibition of GP130 and ERK signaling blocks chemoresistant bladder cancer cell growth. J. Cell. Signal. 63, 109381. doi:10.1016/j.cellsig.2019.109381
Li, X., Liu, Y. L., Zhang, P., Ji, M. M., Zhang, J., Luo, Y., et al. (2017). Therapeutical effect of nanosuspension-based gel of curcumin on selenium-induced cataract in rats. Chin. J. Exp. Tradit. Med. Formulae 23 (22), 9–13. doi:10.13422/j.cnki.syfjx.2017220009
Li, Y., Zhao, J., Yu, Z. S., Zeng, M., and Zhang, J. Q. (2018). Release characteristics in vitro and pharmacokinetics of chitosan coated curcumin liposomes in rats. Chin. Pharmacol. Bull. 34 (06), 810–814.
Li, Y. Y. (2020). MicroRNA and its target genes Mediate the antioxidant Activity of curcumin. Master’s thesis. Xiamen: Jimei University.
Liang, Q., Wang, Y. R., and Deng, Y. Y. (2019). Effect of HPMCAS/curcumin amorphous solid dispersion in enhancing dissolution and chemical stability of curcumin. Zhongguo Zhong Yao Za Zhi 44 (15), 3305–3311. doi:10.19540/j.cnki.cjcmm.20190516.301
Liang, T., Zhang, X., Xue, W., Zhao, S., Zhang, X., and Pei, J. (2014). Curcumin induced human gastric cancer BGC-823 cells apoptosis by ROS-mediated ASK1-MKK4-JNK stress signaling pathway. Int. J. Mol. Sci. 15 (9), 15754–15765. doi:10.3390/ijms150915754
Liang, Z., Xie, W., Wu, R., Geng, H., Zhao, L., Xie, C., et al. (2015). Inhibition of tobacco smoke-induced bladder MAPK activation and epithelial-mesenchymal transition in mice by curcumin. Int. J. Clin. Exp. Pathol. 8 (5), 4503–4513.
Lin, L., Chen, X., Sun, X., Xiao, B., Li, J., Liu, J., et al. (2022). MiR-125b-5p is targeted by curcumin to regulate the cellular antioxidant capacity. Free Radic. Res. 56 (9-10), 640–650. doi:10.1080/10715762.2022.2162393
Lin, X., Bai, D., Wei, Z., Zhang, Y., Huang, Y., Deng, H., et al. (2019). Curcumin attenuates oxidative stress in RAW264.7 cells by increasing the activity of antioxidant enzymes and activating the Nrf2-Keap1 pathway. PLoS One 14 (5), e0216711. doi:10.1371/journal.pone.0216711
Liu, J., Chen, S., Lv, L., Song, L., Guo, S., and Huang, S. (2013). Recent progress in studying curcumin and its nano-preparations for cancer therapy. Curr. Pharm. Des. 19 (11), 1974–1993. doi:10.2174/1381612811319110003
Liu, L., Wang, Z., Yap, P. L., Zhang, Q., Ni, Y., and Losic, D. (2024a). Inhibition of α-glucosidase activity by curcumin loaded on ZnO@rGO nanocarrier for potential treatment of diabetes mellitus. Luminescence 39 (1), e4668. doi:10.1002/bio.4668
Liu, L. H. (2017). Preparation and evaluation of curcumin anti-inflammatory targeted self-microemulsion. Master’s thesis. Beijing: Beijing University of Chinese Medicine.
Liu, R., Zhang, H. B., Yang, J., Wang, J. R., Liu, J. X., and Li, C. L. (2018). Curcumin alleviates isoproterenol-induced cardiac hypertrophy and fibrosis through inhibition of autophagy and activation of mTOR. Eur. Rev. Med. Pharmacol. Sci. 22 (21), 7500–7508. doi:10.26355/eurrev_201811_16291
Liu, X. Y., Chen, X. P., Jiao, L. R., Chen, L., Feng, F., Chen, L., et al. (2022). Fabrication and characterization of lipid coated curcumin/zein nanoparticles. Food Ferment Ind. 48 (05), 130–135. doi:10.13995/j.cnki.11-1802/ts.027492
Liu, X. Y., Wang, Y. T., Tai, G. X., Pan, H. Y., and Zhang, J. (2024b). Optimization of curcumin gel formulation by response surface methodology and estimation of its stability. J. Changshu Inst. Technol. 38 (02), 51–56. doi:10.16101/j.cnki.cn32-1749/z.2024.02.009
Lizoňová, D., Hládek, F., Chvíla, S., Baláž, A., Staňková, Š., and Štěpánek, F. (2022). Surface stabilization determines macrophage uptake, cytotoxicity, and bioactivity of curcumin nanocrystals. Int. J. Pharm. 626, 122133. doi:10.1016/j.ijpharm.2022.122133
Lopresti, A. L., Maes, M., Maker, G. L., Hood, S. D., and Drummond, P. D. (2014). Curcumin for the treatment of major depression: a randomised, double-blind, placebo controlled study. J. Affect Disord. 167, 368–375. doi:10.1016/j.jad.2014.06.001
Lu, J. L., and Deng, L. N. (2019). Preparation of Co-amorphous curcumin-tryptophan and its pharmacokinetic study in rats. China Pharm. 30 (17), 2348–2354.
Lu, X. Y., Han, J. W., Li, W., and Li, J. S. (2018). Preparation and in vitro evaluation of curcumin/piperine amorphous solid dispersion. Res. Pract. Chin. Med. 32 (03), 49–53. doi:10.13728/j.1673-6427.2018.03.013
Luna-Canales, I. C., Delgado-Buenrostro, N. L., Chirino, Y. I., Nava-Arzaluz, G., Piñón-Segundo, E., Martínez-Cruz, G., et al. (2023). Curcumin-loaded microemulsion: formulation, characterization, and in vitro skin penetration. Drug Dev. Ind. Pharm. 49 (1), 42–51. doi:10.1080/03639045.2023.2182121
Lv, J., Zhou, X., Wang, W., Cheng, Y., and Wang, F. (2023). Solubilization mechanism of self-assembled walnut protein nanoparticles and curcumin encapsulation. J. Sci. Food Agric. 103 (10), 4908–4918. doi:10.1002/jsfa.12559
Lv, J. Q. (2022). Preparation, evaluation and glioma-targeted therapy of curcumin nanoliposomes. Master’s thesis. Tianjin: Tianjin University of Science and Technology.
Maghsoudi, A., Yazdian, F., Shahmoradi, S., Ghaderi, L., Hemati, M., and Amoabediny, G. (2017). Curcumin-loaded polysaccharide nanoparticles: optimization and anticariogenic activity against Streptococcus mutans. Mater Sci. Eng. C Mater Biol. Appl. 75, 1259–1267. doi:10.1016/j.msec.2017.03.032
Mahmmoud, Y. A. (2005). Curcumin modulation of Na,K-ATPase: phosphoenzyme accumulation, decreased K+ occlusion, and inhibition of hydrolytic activity. Br. J. Pharmacol. 145 (2), 236–245. doi:10.1038/sj.bjp.0706185
Maiuolo, J., Bulotta, R. M., Oppedisano, F., Bosco, F., Scarano, F., Nucera, S., et al. (2022). Potential properties of natural nutraceuticals and antioxidants in age-related eye disorders. Life (Basel) 13 (1), 77. doi:10.3390/life13010077
Manconi, M., Manca, M. L., Valenti, D., Escribano, E., Hillaireau, H., Fadda, A. M., et al. (2017). Chitosan and hyaluronan coated liposomes for pulmonary administration of curcumin. Int. J. Pharm. 525 (1), 203–210. doi:10.1016/j.ijpharm.2017.04.044
Mazumder, A., Raghavan, K., Weinstein, J., Kohn, K. W., and Pommier, Y. (1995). Inhibition of human immunodeficiency virus type-1 integrase by curcumin. Biochem. Pharmacol. 49 (8), 1165–1170. doi:10.1016/0006-2952(95)98514-a
Memarzia, A., Khazdair, M. R., Behrouz, S., Gholamnezhad, Z., Jafarnezhad, M., Saadat, S., et al. (2021). Experimental and clinical reports on anti-inflammatory, antioxidant, and immunomodulatory effects of Curcuma longa and curcumin, an updated and comprehensive review. Biofactors 47 (3), 311–350. doi:10.1002/biof.1716
Menon, V. P., and Sudheer, A. R. (2007). Antioxidant and anti-inflammatory properties of curcumin. Adv. Exp. Med. Biol. 595, 105–125. doi:10.1007/978-0-387-46401-5_3
Mishra, A. P., Singh, P., Yadav, S., Nigam, M., Seidel, V., and Rodrigues, C. F. (2023). Role of the dietary phytochemical curcumin in targeting cancer cell signalling pathways. Plants (Basel) 12 (9), 1782. doi:10.3390/plants12091782
Naksuriya, O., Okonogi, S., Schiffelers, R. M., and Hennink, W. E. (2014). Curcumin nanoformulations: a review of pharmaceutical properties and preclinical studies and clinical data related to cancer treatment. Biomaterials 35 (10), 3365–3383. doi:10.1016/j.biomaterials.2013.12.090
Nguyen, H. D., Jo, W. H., Hoang, N. H. M., and Kim, M. S. (2022). Curcumin-attenuated TREM-1/DAP12/NLRP3/caspase-1/IL1B, TLR4/NF-κB pathways, and tau hyperphosphorylation induced by 1,2-diacetyl benzene: an in vitro and in silico study. Neurotox. Res. 40 (5), 1272–1291. doi:10.1007/s12640-022-00535-1
Nguyen, H. D., and Kim, M. S. (2022). The protective effects of curcumin on metabolic syndrome and its components: in-silico analysis for genes, transcription factors, and microRNAs involved. Arch. Biochem. Biophys. 727, 109326. doi:10.1016/j.abb.2022.109326
Nogueira, L. F. B., Cruz, M. A. E., Tovani, C. B., Lopes, H. B., Beloti, M. M., Ciancaglini, P., et al. (2022). Curcumin-loaded carrageenan nanoparticles: fabrication, characterization, and assessment of the effects on osteoblasts mineralization. Colloids Surf. B Biointerfaces 217, 112622. doi:10.1016/j.colsurfb.2022.112622
Panahi, Y., Khalili, N., Sahebi, E., Namazi, S., Reiner, Ž., Majeed, M., et al. (2017). Curcuminoids modify lipid profile in type 2 diabetes mellitus: a randomized controlled trial. Complement. Ther. Med. 33, 1–5. doi:10.1016/j.ctim.2017.05.006
Panahi, Y., Khalili, N., Sahebi, E., Namazi, S., Simental-Mendía, L. E., Majeed, M., et al. (2018). Effects of curcuminoids plus piperine on glycemic, hepatic and inflammatory biomarkers in patients with type 2 diabetes mellitus: a randomized double-blind placebo-controlled trial. Drug Res. (Stuttg) 68 (7), 403–409. doi:10.1055/s-0044-101752
Panknin, T. M., Howe, C. L., Hauer, M., Bucchireddigari, B., Rossi, A. M., and Funk, J. L. (2023). Curcumin supplementation and human disease: a scoping review of clinical trials. Int. J. Mol. Sci. 24 (5), 4476. doi:10.3390/ijms24054476
Patil, R., Telang, G., Aswar, U., and Vyas, N. (2024). Comparative analyses of anti-inflammatory effects of Resveratrol, Pterostilbene and Curcumin: in-silico and in-vitro evidences. Silico Pharmacol. 12 (1), 38. doi:10.1007/s40203-024-00211-6
Pelikh, O., Eckert, R. W., Pinnapireddy, S. R., and Keck, C. M. (2021). Hair follicle targeting with curcumin nanocrystals: influence of the formulation properties on the penetration efficacy. J. Control Release 329, 598–613. doi:10.1016/j.jconrel.2020.09.053
Pontes-Quero, G. M., Benito-Garzón, L., Pérez Cano, J., Aguilar, M. R., and Vázquez-Lasa, B. (2021). Amphiphilic polymeric nanoparticles encapsulating curcumin: antioxidant, anti-inflammatory and biocompatibility studies. Mater Sci. Eng. C Mater Biol. Appl. 121, 111793. doi:10.1016/j.msec.2020.111793
Pu, L. L., Gao, J., and Lai, X. R. (2022). Preparation and in vitro evaluation of solid dispersion of Curcumae Longae Rhizoma extract. Chin. Tradit. Herb. Drugs 53 (01), 99–106.
Qiang, Z., Meng, L., Yi, C., Yu, L., Chen, W., and Sha, W. (2019). Curcumin regulates the miR-21/PTEN/Akt pathway and acts in synergy with PD98059 to induce apoptosis of human gastric cancer MGC-803 cells. J. Int. Med. Res. 47 (3), 1288–1297. doi:10.1177/0300060518822213
Rachmawati, H., Budiputra, D. K., and Mauludin, R. (2015). Curcumin nanoemulsion for transdermal application: formulation and evaluation. Drug Dev. Ind. Pharm. 41 (4), 560–566. doi:10.3109/03639045.2014.884127
Rahman, M. A., Shuvo, A. A., Bepari, A. K., Hasan Apu, M., Shill, M. C., Hossain, M., et al. (2022). Curcumin improves D-galactose and normal-aging associated memory impairment in mice: in vivo and in silico-based studies. PLoS One 17 (6), e0270123. doi:10.1371/journal.pone.0270123
Rahmani, S., Asgary, S., Askari, G., Keshvari, M., Hatamipour, M., Feizi, A., et al. (2016). Treatment of non-alcoholic fatty liver disease with curcumin: a randomized placebo-controlled trial. Phytother. Res. 30 (9), 1540–1548. doi:10.1002/ptr.5659
Raman, T., Ramar, M., Arumugam, M., Nabavi, S. M., and Varsha, M. K. (2016). Cytoprotective mechanism of action of curcumin against cataract. Pharmacol. Rep. 68 (3), 561–569. doi:10.1016/j.pharep.2015.12.012
Ratanavaraporn, J., Soontornvipart, K., Shuangshoti, S., Shuangshoti, S., and Damrongsakkul, S. (2017). Localized delivery of curcumin from injectable gelatin/Thai silk fibroin microspheres for anti-inflammatory treatment of osteoarthritis in a rat model. Inflammopharmacology 25 (2), 211–221. doi:10.1007/s10787-017-0318-3
Ren, L. L., Chen, J., Li, X. J., Qu, L. S., and Cheng, K. M. (2022). Optimization of efficient preparation technology of curcumin solid dispersion. Food Drug 24 (04), 300–303.
Roozbehi, S., Dadashzadeh, S., Mirshahi, M., Sadeghizadeh, M., and Sajedi, R. H. (2020). Targeted anticancer prodrug therapy using dextran mediated enzyme-antibody conjugate and β-cyclodextrin-curcumin inclusion complex. Int. J. Biol. Macromol. 160, 1029–1041. doi:10.1016/j.ijbiomac.2020.05.225
Sa, G., and Das, T. (2008). Anti cancer effects of curcumin: cycle of life and death. Cell Div. 3, 14. doi:10.1186/1747-1028-3-14
Sahoo, D. K., Heilmann, R. M., Paital, B., Patel, A., Yadav, V. K., Wong, D., et al. (2023). Oxidative stress, hormones, and effects of natural antioxidants on intestinal inflammation in inflammatory bowel disease. Front. Endocrinol. (Lausanne) 14, 1217165. doi:10.3389/fendo.2023.1217165
Sangouni, A. A., Taghdir, M., Mirahmadi, J., Sepandi, M., and Parastouei, K. (2022). Effects of curcumin and/or coenzyme Q10 supplementation on metabolic control in subjects with metabolic syndrome: a randomized clinical trial. Nutr. J. 21 (1), 62. doi:10.1186/s12937-022-00816-7
Saraf-Bank, S., Ahmadi, A., Paknahad, Z., Maracy, M., and Nourian, M. (2019). Effects of curcumin on cardiovascular risk factors in obese and overweight adolescent girls: a randomized clinical trial. Sao Paulo Med. J. 137 (5), 414–422. doi:10.1590/1516-3180.2018.0454120419
Saranya, T. S., Rajan, V. K., Biswas, R., Jayakumar, R., and Sathianarayanan, S. (2018). Synthesis, characterisation and biomedical applications of curcumin conjugated chitosan microspheres. Int. J. Biol. Macromol. 110, 227–233. doi:10.1016/j.ijbiomac.2017.12.044
Sekiya, M., Hisasaka, R., Iwamoto-Kihara, A., Futai, M., and Nakanishi-Matsui, M. (2014). A unique mechanism of curcumin inhibition on F1 ATPase. Biochem. Biophys. Res. Commun. 452 (4), 940–944. doi:10.1016/j.bbrc.2014.09.027
Selim, N. M., Elgazar, A. A., Abdel-Hamid, N. M., El-Magd, M. R. A., Yasri, A., Hefnawy, H. M. E., et al. (2019). Chrysophanol, physcion, hesperidin and curcumin modulate the gene expression of pro-inflammatory mediators induced by LPS in HepG2: in silico and molecular studies. Antioxidants (Basel) 8 (9), 371. doi:10.3390/antiox8090371
Seo, K. I., Choi, M. S., Jung, U. J., Kim, H. J., Yeo, J., Jeon, S. M., et al. (2008). Effect of curcumin supplementation on blood glucose, plasma insulin, and glucose homeostasis related enzyme activities in diabetic db/db mice. Mol. Nutr. Food Res. 52 (9), 995–1004. doi:10.1002/mnfr.200700184
Sharifi, M., Cho, W. C., Ansariesfahani, A., Tarharoudi, R., Malekisarvar, H., Sari, S., et al. (2022). An updated review on EPR-based solid tumor targeting nanocarriers for cancer treatment. Cancers (Basel) 14 (12), 2868. doi:10.3390/cancers14122868
Shen, P., Zhang, R., McClements, D. J., and Park, Y. (2019). Nanoemulsion-based delivery systems for testing nutraceutical efficacy using Caenorhabditis elegans: demonstration of curcumin bioaccumulation and body-fat reduction. Food Res. Int. 120, 157–166. doi:10.1016/j.foodres.2019.02.036
Shetty, N. P., Prabhakaran, M., and Srivastava, A. K. (2021). Pleiotropic nature of curcumin in targeting multiple apoptotic-mediated factors and related strategies to treat gastric cancer: a review. Phytother. Res. 35 (10), 5397–5416. doi:10.1002/ptr.7158
Shin, M. S., Yu, J. S., Lee, J., Ji, Y. S., Joung, H. J., Han, Y. M., et al. (2019). A hydroxypropyl methylcellulose-based solid dispersion of curcumin with enhanced bioavailability and its hepatoprotective activity. Biomolecules 9 (7), 281. doi:10.3390/biom9070281
Si, X., Wang, Y., Wong, J., Zhang, J., McManus, B. M., and Luo, H. (2007). Dysregulation of the ubiquitin-proteasome system by curcumin suppresses coxsackievirus B3 replication. J. Virol. 81 (7), 3142–3150. doi:10.1128/jvi.02028-06
Singh, A., Soni, U., Varadwaj, P. K., Misra, K., and Rizvi, S. I. (2023). Anti-inflammatory effect of curcumin in an accelerated senescence model of Wistar rat: an in vivo and in-silico study. J. Biomol. Struct. Dyn. 43, 1459–1470. doi:10.1080/07391102.2023.2291832
Song, I. S., Cha, J. S., and Choi, M. K. (2016). Characterization, in vivo and in vitro evaluation of solid dispersion of curcumin containing d-α-Tocopheryl polyethylene glycol 1000 succinate and mannitol. Molecules 21 (10), 1386. doi:10.3390/molecules21101386
Su, L. Q., Wang, Y. D., and Chi, H. Y. (2017). Effect of curcumin on glucose and lipid metabolism, FFAs and TNF-α in serum of type 2 diabetes mellitus rat models. Saudi J. Biol. Sci. 24 (8), 1776–1780. doi:10.1016/j.sjbs.2017.11.011
Su, Y., Chen, Y., Zhang, L., Adhikari, B., Xu, B., Li, J., et al. (2022). Synthesis and characterization of lotus seed protein-based curcumin microcapsules with enhanced solubility, stability, and sustained release. J. Sci. Food Agric. 102 (6), 2220–2231. doi:10.1002/jsfa.11560
Sun, S., Du, X., Fu, M., Khan, A. R., Ji, J., Liu, W., et al. (2021). Galactosamine-modified PEG-PLA/TPGS micelles for the oral delivery of curcumin. Int. J. Pharm. 595, 120227. doi:10.1016/j.ijpharm.2021.120227
Szymanski, M. C., Gillum, T. L., Gould, L. M., Morin, D. S., and Kuennen, M. R. (2018). Short-term dietary curcumin supplementation reduces gastrointestinal barrier damage and physiological strain responses during exertional heat stress. J. Appl. Physiol. 124 (2), 330–340. doi:10.1152/japplphysiol.00515.2017
Tao, L., Zhang, X., Xue, W., Zhao, S., Xiang, Z., and Pei, J. (2014). Curcumin induced human gastric cancer BGC-823 cells apoptosis by ROS-mediated ASK1-MKK4-JNK stress signaling pathway. Int. J. Mol. Sci. 15 (9), 15754–15765. doi:10.3390/ijms150915754
Thambamroong, T., Seetalarom, K., Saichaemchan, S., Pumsutas, Y., and Prasongsook, N. (2022). Efficacy of curcumin on treating cancer anorexia-cachexia syndrome in locally or advanced head and neck cancer: a double-blind, placebo-controlled randomised phase IIa trial (CurChexia). J. Nutr. Metab. 2022, 5425619. doi:10.1155/2022/5425619
Thimmulappa, R. K., Mudnakudu-Nagaraju, K. K., Shivamallu, C., Subramaniam, K. J. T., Radhakrishnan, A., Bhojraj, S., et al. (2021). Antiviral and immunomodulatory activity of curcumin: a case for prophylactic therapy for COVID-19. Heliyon 7 (2), e06350. doi:10.1016/j.heliyon.2021.e06350
Tima, S., Okonogi, S., Ampasavate, C., Berkland, C., and Anuchapreeda, S. (2019). FLT3-specific curcumin micelles enhance activity of curcumin on FLT3-ITD overexpressing MV4-11 leukemic cells. Drug Dev. Ind. Pharm. 45 (3), 498–505. doi:10.1080/03639045.2018.1562462
Vaithiyalingam, M., Sumathi, D. L., and Sabarathinam, S. (2023). Isolation and in silico study of curcumin from curcuma longa and its anti-diabetic activity. Appl. Biochem. Biotechnol. 195 (2), 947–957. doi:10.1007/s12010-022-04173-3
Wang, J. Y., Wang, X., Wang, X. J., Zheng, B. Z., Wang, Y., Wang, X., et al. (2018). Curcumin inhibits the growth via Wnt/β-catenin pathway in non-small-cell lung cancer cells. Eur. Rev. Med. Pharmacol. Sci. 22 (21), 7492–7499. doi:10.26355/eurrev_201811_16290
Wang, M. F. (2019). Preparation and evaluation of a gastro-adhesive drug delivery system of curcumin. Master’s thesis. Qingdao: Qingdao University of Science and Technology.
Wang, X., Liao, Z., Zhao, G., Dong, W., Huang, X., Zhou, X., et al. (2023). Curcumin nanocrystals self-stabilized Pickering emulsion freeze-dried powder: development, characterization, and suppression of airway inflammation. Int. J. Biol. Macromol. 245, 125493. doi:10.1016/j.ijbiomac.2023.125493
Wang, X. X. (2017). Preparation and bioavailability study of curcumin microcapsules and vesicles. Master’s thesis. Qingdao: Qingdao University of Science and Technology.
Wang, Y., Ding, R., Zhang, Z., Zhong, C., Wang, J., and Wang, M. (2021). Curcumin-loaded liposomes with the hepatic and lysosomal dual-targeted effects for therapy of hepatocellular carcinoma. Int. J. Pharm. 602, 120628. doi:10.1016/j.ijpharm.2021.120628
Wang, Y. H., Fan, X. Q., and Hou, L. X. (2013). Forming process and quality evaluation of curcumin dripping pills. China Pharm. 24 (31), 2929–2931.
Wang, Z., Chen, Z., Li, B., Zhang, B., Du, Y., Liu, Y., et al. (2020). Curcumin attenuates renal interstitial fibrosis of obstructive nephropathy by suppressing epithelial-mesenchymal transition through inhibition of the TLR4/NF-кB and PI3K/AKT signalling pathways. Pharm. Biol. 58 (1), 828–837. doi:10.1080/13880209.2020.1809462
Wu, Q., Ou, H., Shang, Y., Zhang, X., Wu, J., and Fan, F. (2021). Nanoscale formulations: incorporating curcumin into combination strategies for the treatment of lung cancer. Drug Des. Devel Ther. 15, 2695–2709. doi:10.2147/dddt.S311107
Wu, Y., Mou, B., Song, S., Tan, C. P., Lai, O. M., Shen, C., et al. (2020). Curcumin-loaded liposomes prepared from bovine milk and krill phospholipids: effects of chemical composition on storage stability, in-vitro digestibility and anti-hyperglycemic properties. Food Res. Int. 136, 109301. doi:10.1016/j.foodres.2020.109301
Xi, N., Wang, Y. H., Wang, L. S., and Jia, H. P. (2022). Preparation of mitochondrial targeting curcumin TPP-PEG-PLGA iposomes and study on promoting hepatoma cell apoptosis. Chin. Pharm. J. 57 (17), 1438–1446.
Xiang, H., Xu, S., Zhang, W., Li, Y., Zhou, Y., and Miao, X. (2023). Skin permeation of curcumin nanocrystals: effect of particle size, delivery vehicles, and permeation enhancer. Colloids Surf. B Biointerfaces 224, 113203. doi:10.1016/j.colsurfb.2023.113203
Xu, J., Jiang, Y., Dong, Y. Y., Zhao, L. Y., Zhang, W. M., and Zhang, D. S. (2023). Preparation and in vivo pharmacokinetics of curcumin self-micellizing solid dispersions. Chin. Tradit. Pat. Med. 45 (05), 1397–1403.
Xu, W. J., Li, Z. Y., and Chen, X. T. (2021). Pharmacokinetics and bioavailability of curcumin-proliposomes in rats. Tradit. Chin. Med. Rehabil. 12 (09), 63–66. doi:10.19787/j.issn.1008-1879.2021.09.021
You, J., Dai, D. P., He, W. J., Li, G., Song, S. C., Wei, Y. H., et al. (2014). Preparation of curcumin-loaded long-circulating liposomes and its pharmacokinetics in rats. China J. Chin. Mater. Med. 39 (07), 1238–1242.
Yousef, S. A., Mohammed, Y. H., Namjoshi, S., Grice, J. E., Benson, H. A. E., Sakran, W., et al. (2019). Mechanistic evaluation of enhanced curcumin delivery through human skin in vitro from optimised nanoemulsion formulations fabricated with different penetration enhancers. Pharmaceutics 11 (12), 639. doi:10.3390/pharmaceutics11120639
Yu, J. Y., Kim, J. A., Joung, H. J., Ko, J. A., and Park, H. J. (2020). Preparation and characterization of curcumin solid dispersion using HPMC. J. Food Sci. 85 (11), 3866–3873. doi:10.1111/1750-3841.15489
Yu, L. L., Wu, J. G., Dai, N., Yu, H. G., and Si, J. M. (2011). Curcumin reverses chemoresistance of human gastric cancer cells by downregulating the NF-κB transcription factor. Oncol. Rep. 26 (5), 1197–1203. doi:10.3892/or.2011.1410
Yu, L.-L., Wu, J.-G., Dai, N., Yu, H. G., and Si, J. M. (2011). Curcumin reverses chemoresistance of human gastric cancer cells by downregulating the NF-κB transcription factor. Oncol. Rep. 26, 1197–1203. doi:10.3892/or.2011.1410
Zhang, C., Zhang, K., Zang, G., Chen, T., Lu, N., Wang, S., et al. (2021). Curcumin inhibits replication of human parainfluenza virus type 3 by affecting viral inclusion body formation. Biomed. Res. Int. 2021, 1807293. doi:10.1155/2021/1807293
Zhang, H. S., Ruan, Z., and Sang, W. W. (2011a). HDAC1/NFκB pathway is involved in curcumin inhibiting of Tat-mediated long terminal repeat transactivation. J. Cell Physiol. 226 (12), 3385–3391. doi:10.1002/jcp.22691
Zhang, L. (2012). Study on folic acid-modified curcumin colon-targeted self-microemulsion drug delivery system. Master’s thesis. Shandong: Shandong University.
Zhang, L., Cao, F., Ding, B., Li, Q., Xi, Y., and Zhai, G. (2011b). Eudragit® S100 coated calcium pectinate microspheres of curcumin for colon targeting. J. Microencapsul. 28 (7), 659–667. doi:10.3109/02652048.2011.604436
Zhang, P. M., Wang, W. X., Sun, J. F., Chen, Z. Z., Ma, Q. Y., and Wang, J. (2024). Antibacterial effect and mechanism of chitosan/curcumin composite photodynamic coating on Staphylococcus aureus. Food Sci. 45 (06), 233–243.
Zhang, Q., Polyakov, N. E., Chistyachenko, Y. S., Khvostov, M. V., Frolova, T. S., Tolstikova, T. G., et al. (2018). Preparation of curcumin self-micelle solid dispersion with enhanced bioavailability and cytotoxic activity by mechanochemistry. Drug Deliv. 25 (1), 198–209. doi:10.1080/10717544.2017.1422298
Zhang, Q., Suntsova, L., Chistyachenko, Y. S., Evseenko, V., Khvostov, M. V., Polyakov, N. E., et al. (2019a). Preparation, physicochemical and pharmacological study of curcumin solid dispersion with an arabinogalactan complexation agent. Int. J. Biol. Macromol. 128, 158–166. doi:10.1016/j.ijbiomac.2019.01.079
Zhang, X., Zhang, C., Ren, Z., Zhang, F., Xu, J., Zhang, X., et al. (2020). Curcumin affects gastric cancer cell migration, invasion and cytoskeletal remodeling through gli1-β-catenin. Cancer Manag. Res. 12, 3795–3806. doi:10.2147/cmar.S244384
Zhang, X. Y., Xu, Y., Di, L. Q., Fan, W. L., and Li, L. (2019b). Preparation and in vitro evaluation of curcumin solid dispersions. J. Nanjing Univ. Nat. Sci. 55 (03), 511–517. doi:10.13232/j.cnki.jnju.2019.03.019
Zhang, Y., Mu, M., Zhou, Y., Xie, H., and Zhao, S. (2023). Redox-responsive microemulsion: fabrication and application to curcumin encapsulation. J. Colloid Interface Sci. 647, 384–394. doi:10.1016/j.jcis.2023.05.129
Zhao, W., Liu, R., and Zhang, Y. J. (2015). Preparation and formulation optimization of curcumin sustained-release mini-pill. Lishizhen Med. Mater Med. Res. 26 (09), 2140–2143.
Zhao, Z. M., Gao, H. H., Zhao, J. Y., Huang, X. J., Yao, N., Zeng, X. H., et al. (2014). Pharmacokinetics of compound curcumin phytosome-pvp dispersible tablets in rabbits. J. Jiangxi Univ. Chin. Med. 26 (06), 62–64.
Zheng, S. H., Li, X., Zhou, L., Yang, F. L., Chen, Q. H., Zhang, J. Y., et al. (2015). Preparation and release study of curcumin sustained-release tablets. Northwest Pharm. J. 30 (01), 65–67.
Zhong, X. Q., Deng, L. N., Guo, H. Y., Bai, X. N., Han, X. Y., and Zhang, Y. (2019). Preparation and property of novel liver targeting microemulsion. Cent. South Pharm. 17 (04), 507–512.
Zhou, W., Cheng, C., Ma, L., Zou, L., Liu, W., Li, R., et al. (2021). The Formation of chitosan-coated rhamnolipid liposomes containing curcumin: stability and in vitro digestion. Molecules 26 (3), 560. doi:10.3390/molecules26030560
Zhu, J., Bultynck, G., Luyten, T., Parys, J. B., Creemers, J. W., Van de Ven, W. J., et al. (2013). Curcumin affects proprotein convertase activity: elucidation of the molecular and subcellular mechanism. Biochim. Biophys. Acta 1833 (8), 1924–1935. doi:10.1016/j.bbamcr.2013.04.002
Keywords: curcumin, formulation, pharmacological effect, clinical research, review
Citation: Hao M, Zhang C, Wang T and Hu H (2025) Pharmacological effects, formulations, and clinical research progress of curcumin. Front. Pharmacol. 16:1509045. doi: 10.3389/fphar.2025.1509045
Received: 10 October 2024; Accepted: 03 March 2025;
Published: 17 March 2025.
Edited by:
Rajeev K. Singla, Sichuan University, ChinaReviewed by:
Hatice Kızıltaş, Yüzüncü Yıl University, TürkiyeAo Lu, Chongqing Medical University, China
Copyright © 2025 Hao, Zhang, Wang and Hu. This is an open-access article distributed under the terms of the Creative Commons Attribution License (CC BY). The use, distribution or reproduction in other forums is permitted, provided the original author(s) and the copyright owner(s) are credited and that the original publication in this journal is cited, in accordance with accepted academic practice. No use, distribution or reproduction is permitted which does not comply with these terms.
*Correspondence: Chungang Zhang, emhhbmdjaHVuZ2FuZ3BoYXJtQG91dGxvb2suY29t
†These authors have contributed equally to this work and share first authorship