- School of Basic Medical Sciences, Chengdu University of Traditional Chinese Medicine, Chengdu, China
Background: Non-alcoholic fatty liver disease (NAFLD) is a common type of chronic liver disease and its incidence is increasing. Its disease progression is closely related to non-alcoholic steatohepatitis and liver fibrosis. Effective treatment is currently lacking. The traditional Chinese medicine ginseng (Panax ginseng) shows unique advantages in NAFLD intervention, but its complex compositional system and molecular mechanism network still need to be systematically analyzed.
Objective: This paper systematically integrates evidence from nearly 20 years of research to elucidate the multi-target pharmacological mechanism of ginseng for the treatment of NAFLD.
Methods: Relevant information was sourced from Pubmed, Web of science, Embase and CNKI databases. Using BioRender and visio to draw biomedical illustrations.
Results: The active ingredients of ginseng contain 2 classes of saponins (tetracyclic triterpene saponins, pentacyclic triterpene saponins and other modified types) and non-saponins. Different cultivation methods, processing techniques and extraction sites have expanded the variety of ginseng constituents and demonstrated different pharmacological activities. Studies have shown that ginseng and its functional components have the ability to regulate lipid metabolism disorders, inflammation, oxidative stress, endoplasmic reticulum stress, insulin resistance, disruption of intestinal flora structure, cell death and senescence. Demonstrates the potential of ginseng for the treatment of NAFLD.
Conclusion: This study reveals for the first time the integrative mechanism of ginseng in the treatment of NAFLD through the tertiary mode of action of “multi-component multi-target multi-pathway”. The multilevel modulatory ability of ginseng provides a new direction for the development of comprehensive therapeutic strategies for NAFLD.
1 Introduction
Non-alcoholic fatty liver disease (NAFLD) is characterized by hepatocellular steatosis and fat accumulation without secondary causes of hepatic fat accumulation, such as heavy alcohol consumption, long-term use of lipotropic drugs, or monogenic genetic disorders (Wei et al., 2024). In recent years, NAFLD has become the most common chronic liver disease worldwide. Epidemiologic data show that the global prevalence of NAFLD is as high as 30%, and the prevalence of NAFLD in overweight and obese groups is even as high as 70% (Lou et al., 2024; Quek et al., 2023). Markov modeling predicts that the disease burden of NAFLD-associated cirrhosis and hepatocellular carcinoma will increase by 115%–130% by 2040, leading to serious challenges for healthcare systems worldwide (Devarbhavi et al., 2023). The pathogenesis of NAFLD begins with nonalcoholic fatty liver, which progresses to non-alcoholic steatohepatitis (NASH) in about 20% of patients, driven by oxidative stress, inflammation, and other pathologic factors. Among patients with NASH, approximately 15%–20% progress to cirrhosis within 30–40 years (Li et al., 2024c; Loomba et al., 2021).
Current clinical management strategies for NAFLD are slightly limited. The common treatment of NAFLD is aimed primarily at alleviating the associated metabolic comorbidities and cardiovascular disease. For example, reducing hyperlipidemia, insulin resistance (IR) and hyperglycemia associated with NAFLD, rather than directly treating the disease itself (Xu et al., 2022). NAFLD patients who do not develop NASH or liver fibrosis have a good prognosis, and their disease can even be reversed (Loomba et al., 2021). First-line therapies are based on lifestyle interventions, but patient compliance is poor, resulting in a high risk of disease progression (Younossi et al., 2023). Pharmacologic therapy is primarily directed at patients who have progressed to NASH and liver fibrosis, but approved drugs (vitamin E and pioglitazone) have limited efficacy. Clinical studies have shown that pioglitazone therapy for NASH has a 47% remission rate, but does not improve fibrosis and may cause weight gain and fluid retention (Paternostro and Trauner, 2022). Although novel agents “glucagon-like peptide-1 receptor agonists and farnesoid X receptor (FXR) agonists” have entered clinical trials, their long-term safety, cost-effectiveness, and efficacy in advanced fibrosis remain controversial (Newsome et al., 2021; Younossi et al., 2022). End-stage patients are dependent on liver transplantation, but donor shortages and postoperative immunosuppressive complications result in 5-year survival rates of only 65% (Terrault et al., 2023). Therefore, the development of novel therapeutic strategies with multi-targeted effects, high safety profile and the ability to block early disease progression is urgent.
Compared with synthetic drugs, traditional Chinese medicine (TCM), as a complementary therapy for liver diseases, has become a new direction for NAFLD drug development due to its wide range of efficacy and multi-component-multi-target properties (Li et al., 2024b; Liu et al., 2022; Zhang and Feng, 2022). Complementary herbal therapies are mostly used clinically for the comprehensive regulation of NAFLD and NASH, among which the hepatoprotective effects of ginseng and its compounds have attracted much attention (Chen et al., 2021). Clinical studies have confirmed that ginseng and its functional components improve metabolic disorders through the following mechanisms: (1) Improve blood glucose and lipid levels by regulating the phosphatidylinositol-3-kinase/protein kinase B (PI3K/AKT) signaling pathway. (2) Inhibiting inflammation-related signaling pathways “nuclear factor kappa-B (NF-κB), mitogen-activated protein kinases, janus kinase 2/signal transducer and activator of transcription (STAT) 5” to reduce inflammation. (3) Scavenges free radicals and reduces cellular damage from oxidative stress (Zhou et al., 2023). Notably, ginseng has shown more comprehensive efficacy than a single synthetic drug in animal models of NAFLD, providing simultaneous improvement in steatosis, IR, and hepatic fibrosis, while demonstrating low toxicity and conferring many benefits (Wang et al., 2021; Yang K. et al., 2023). In this paper, for the first time, we systematically elucidate the multidimensional mechanism of ginseng intervention in NAFLD by integrating nearly 20 years of experimental research evidence, focusing on resolving its key target networks in lipid metabolism regulation, lipotoxic damage repair, cell death and senescence reversal, IR and gut microbiota remodeling (Figure 1). By revealing the component-target-pathway interaction patterns, this study aims to promote the progress of traditional medicine modernization research and provide a theoretical basis for the development of innovative strategies based on the multi-targets of TCM for the treatment of NAFLD.
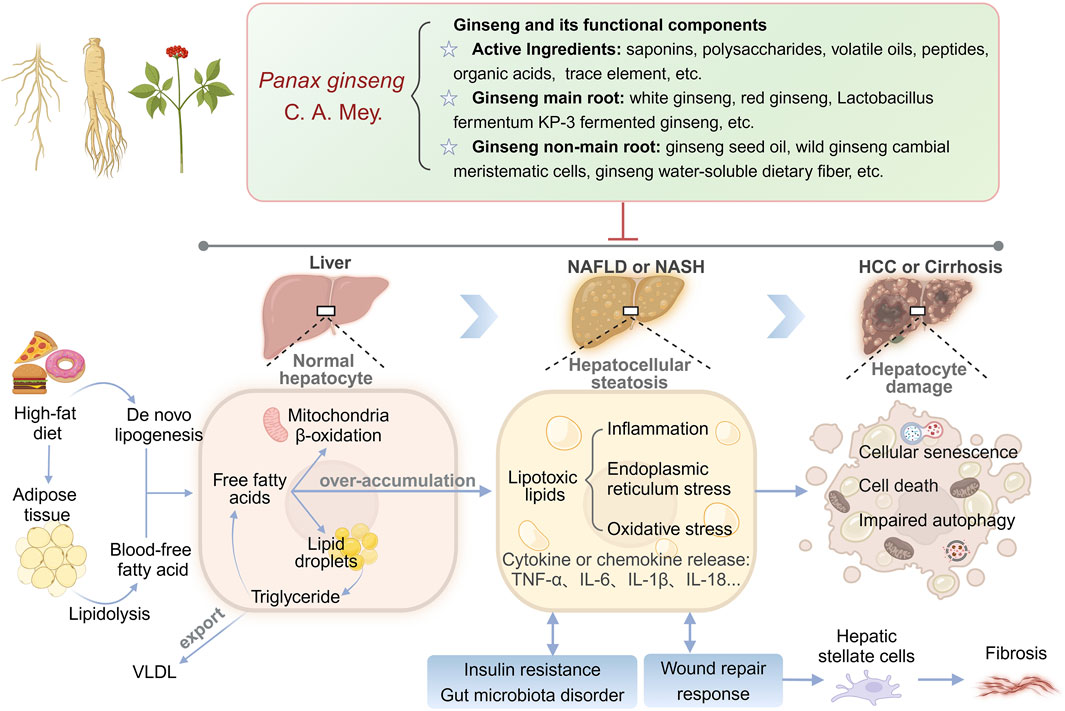
Figure 1. Multi-target mechanisms of ginseng in NAFLD/NASH: from lipid metabolism to hepatocyte damage.
2 Pathogenesis of NAFLD
The pathogenesis of NAFLD is complex. The early “two-hit” hypothesis was considered the central model to explain the progression of NAFLD (Buzzetti et al., 2016). This hypothesis suggests that the first hit is triggered by excessive hepatic lipid deposition, while the second hit is driven by secondary damage, such as inflammation and endoplasmic reticulum stress (ERS), which ultimately leads to NASH and fibrosis. However, with the deepening of the research, the limitations of the “two-hit” model have gradually appeared, and its assumption of linear phasing is difficult to explain the heterogeneity of NAFLD, multi-organ interactions, and the systemic effects of metabolic syndrome. In this context, scholars have proposed the “multiple-hit” hypothesis, which emphasizes that NAFLD is the result of synergistic or superimposed effects of multiple pathological factors in space and time (Buzzetti et al., 2016; Wang et al., 2023). Unlike the “two-hit” model, the “multiple-hit” hypothesis is no longer limited to localized events in the liver, but incorporates extra-hepatic factors (e.g., adipose tissue dysfunction, intestinal flora disruption) into the core mechanistic framework. Excessive lipid accumulation also promotes the production of lipotoxic substances, which in turn activate oxidative stress (OS), inflammation and ERS processes, leading to secondary liver damage and further inducing cell death or senescence (Mahlapuu et al., 2022; Xu et al., 2020). If pathological factors persist, the liver will undergo repeated injury and repair processes and activate hepatic stellate cells, leading to fibroplasia and even cirrhosis (Taru et al., 2024). Inflammatory cytokines, adipokines, dietary, genetic and environmental factors have important effects on IR and gut microbiota homeostasis, and conversely, IR and gut microbiota disorders exacerbate imbalance of lipid metabolism (Chen and Vitetta, 2020; Fujii et al., 2020; Khan et al., 2019; Malesza et al., 2021). Complex pathological mechanisms mutually drive the development of aberrant lipid metabolism in the liver, including excessive nutrient intake, lipotoxicity, activation of the hepatic immune system, OS, ERS, cell death and senescence, IR, disturbances of the gut microbiota, and genetic and epigenetic factors, which are the key risk factors for the development of NAFLD (Buzzetti et al., 2016; Friedman et al., 2018; Huby and Gautier, 2022). Some studies have been reported to demonstrate that if hepatocellular steatosis can be reversed by early intervention through metabolic pathways, the risk causative factors and metabolism-related complications of NAFLD can be mitigated (Loomba et al., 2021). Therefore, we should develop more potential therapeutic approaches to prevent and treat NAFLD.
3 Traditional and modern cognition of ginseng
Ginseng (Latin name: Panax ginseng C.A. Mey.) is a perennial herb in the family araliaceae and is widely used worldwide (Ratan et al., 2021). It is widely known as “the king of herbs, the head of all medicines” in East Asian traditional medicine such as China, Korea and Japan (Potenza et al., 2023). In the famous book Chinese Pharmacopoeia (2020 Edition), there is an exhaustive account of ginseng. It has been used in China for more than 2000 years (Li G. et al., 2024). In modern applications of TCM, ginseng is often used to treat lack of power, shortness of breath, palpitations and insomnia, haemorrhage and life-threatening conditions (Li X. et al., 2022; Ma et al., 2023). It exhibits unique energy-boosting properties that fit the clinical manifestations of fatigue, weakness and abdominal distension and discomfort that are common in NAFLD patients (Lu et al., 2021).
Ginseng has a complex composition including saponins, polysaccharides, volatile oils, peptides, organic acids and trace elements, among others (Li X. et al., 2022; Piao et al., 2020). The pharmacological effects of these components have significant target specificity, and the active ingredients commonly used in current experiments can be broadly categorized into the following 2 groups. (1) Ginsenosides are the core active ingredients of ginseng. According to the structure of the glycosides, they can be categorized into tetracyclic triterpene saponins, pentacyclic triterpene saponins and other modified types. The main saponin currently used in the treatment of NAFLD is a tetracyclic triterpene saponin. It includes protopanaxadiol (PPD), protopanaxatriol (PPT) and PPD/PPT-type saponin derivatives (Table 1). (2) Non-saponin components, such as the polyacetylene alcohol compounds: panaxydol. The above multicomponents target multiple pathological aspects such as lipid metabolism, inflammation, OS and fibrosis through synergistic effects, providing a material basis for the multi-targeting properties of ginseng in the treatment of NAFLD.

Table 1. Classification and representative components of tetracyclic triterpenoid saponins used in NAFLD.
The functional components of ginseng are closely related to the cultivation method, processing technology and extraction site. Those sown in the forest and grown naturally are called forest ginseng, and those harvested after 5-6 years of artificial cultivation are commonly called garden ginseng (Li G. et al., 2024). The long time of ginseng cultivation and the small number of wild plants often lead to expensive prices and limited resources (Li G. et al., 2024). Therefore, researchers have expanded its medicinal value through processing optimization, microbial fermentation technology and multi-site development (Wang et al., 2025). According to the sources and preparation methods, they can be categorized into the following 2 groups. (1) The active ingredient profile is altered by physical or biological transformation using the main root of ginseng as the main body. For example, fresh ginseng washed and dried in the sun is known as sun-dried ginseng, which will retain native saponin components to a greater extent and has the effect of improving lipid metabolism disorders in NAFLD (Li et al., 2022c). Fresh ginseng that has been steamed and dried is called red ginseng (RG), which promotes the conversion of native saponins (Rb1) into rare saponins (Rg3, Rh2 and CK). It has been shown to promote reverse cholesterol transport and anti-inflammatory effects (Li et al., 2022c). Microbial fermentation (Lactobacillus fermentum KP-3 and Monascus ruber) increased the content of secondary ginsenosides, small-molecule peptides, and polysaccharides (Nan et al., 2018; Zhao et al., 2021). Cordyceps militaris fermentation enhances the content of rare ginsenoside (Rg3) (Li et al., 2020). In addition, some studies have used modern techniques to efficiently enrich active ingredients from ginseng roots. For example, targeted enzymatic cleavage of ginsenoside glycosyl groups to generate highly bioavailable glycosides (GBCK25) (Choi N. et al., 2019). The active ingredients generated have the ability to reduce fatty apoptosis and cholesterol synthesis in hepatocytes. (2) Exploiting the pharmacological potential of non-primary root parts of the ginseng plant, including cold-pressed extraction of ginseng seed oil (GSO), in vitro cultivation of wild ginseng meristematic tissue cells, and extraction of water-soluble dietary fibers from ginseng whiskers (Hua et al., 2021; Kim et al., 2018; Lee et al., 2016). These processed ginsengs have a wider range of pharmacological effects, and we summarize the above ginsengs, concoctions and their characteristic extracts into a table (Table 2).
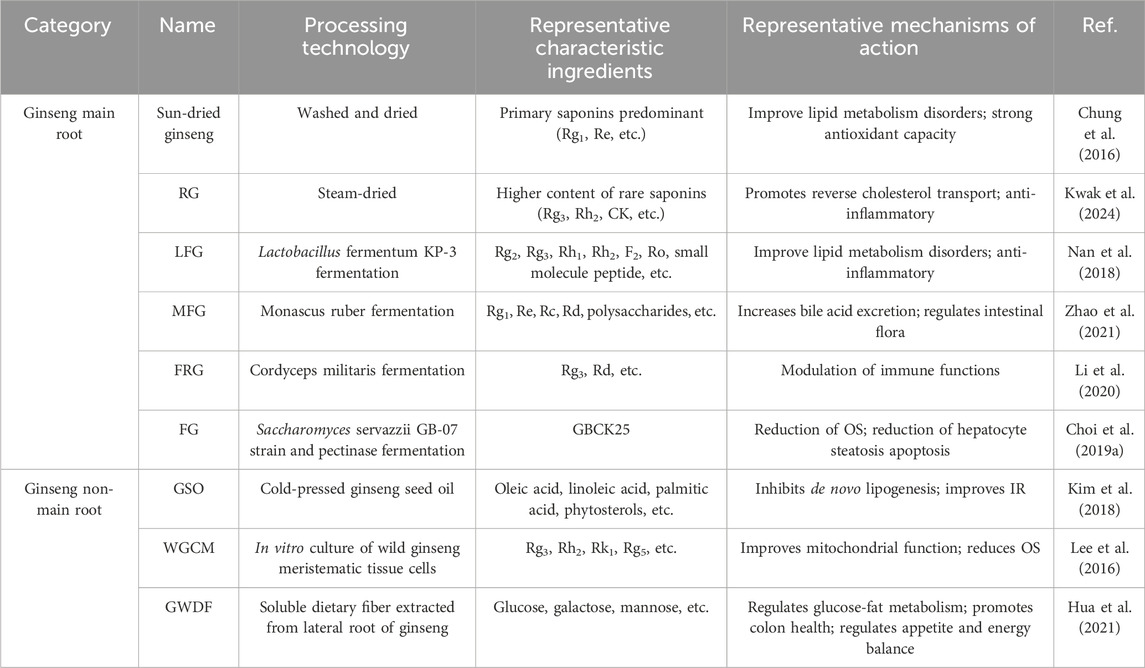
Table 2. Effects of processing parts and processing methods on the composition and mechanism of action of ginseng.
Natural products as supplementation agents are beneficial in promoting glycolipid metabolism and improving hepatocellular steatosis to halt the progression of NAFLD (Cao et al., 2023; Leng et al., 2021). Compared with other traditional medicinal herbs, ginseng exhibits a variety of irreplaceable multidimensional advantages that make it unique in NAFLD intervention. For example, multiple components of ginseng can simultaneously target multiple pathways such as AMP-activated protein kinase (AMPK), peroxisome proliferator-activated receptor (PPAR) and sterol-regulatory element binding protein (SREBP) (Mi et al., 2024). The lipid metabolism pathology network of NAFLD can be improved through the “one drug, multiple effects” model. Mc1 can improve apoptosis and insulin sensitivity, realizing the comprehensive improvement of NAFLD across pathological links, which has more clinical potentials than the herbs with a single mechanism of action (Roh et al., 2020). In addition, ginseng has been used for thousands of years without any serious liver injury, and modern toxicology has confirmed that it has no significant side effects at therapeutic doses (Park et al., 2022). Therefore, ginseng is not only a traditional tonic medicine, but also a multi-target liver metabolism regulator verified by modern science.
4 Pharmacological effects and molecular mechanisms of ginseng against NAFLD
Laboratory studies on ginseng anti-NAFLD have shown that ginseng and its functional components can block multiple underlying pathological mechanisms, including regulating disorders of lipid metabolism in the body, reducing hepatocellular inflammation, ERS, OS, IR, intestinal flora disorders, cellular senescence and death. During the onset of NAFLD, ginseng regulates a variety of hepatic functions such as synthesis, storage, catabolism, transport and secretion, thus exerting a protective effect on the normal structure and function of the liver. A detailed discussion is provided below to facilitate the reader’s access to relevant experimental studies, and we use BioRender and visio to draw biomedical illustrations.
4.1 Lipid metabolism
Excessive accumulation of lipids in hepatocytes is the earliest and most common response to NAFLD (Ebert et al., 2023). After years of research, ginseng and its functional components have been found to directly regulate multiple pathways of lipid metabolism, including the regulation of lipid uptake and transport, increase of lipolysis and decrease of lipid synthesis, etc. The following is a systematic and comprehensive review of the role of ginseng and its functional components in targeting the above lipid metabolism pathways (Table 3).
4.1.1 Regulation of lipid uptake and transportation
As the hub of systemic metabolism, the liver is prone to lipid metabolism disorders in hyperlipidemic and obese states, which directly promote the progression of NAFLD (Mato et al., 2019). Studies have shown that ginseng improves lipid homeostasis through the following mechanisms (Figure 2). Significantly reduces triglyceride (TG), total cholesterol (TC), free fatty acids (FFAs), lipid droplets (LDs), low density lipoprotein (LDL), low density lipoprotein cholesterol (LDL-C), LDL-C to high density lipoprotein cholesterol (HDL-C) ratio (LDL-C/HDL-C) in the liver and serum (Guo et al., 2023; Liang et al., 2021; Meng et al., 2023; Xu et al., 2018). In addition evidence of pathologic improvement showed that model animals raised with ginseng had lighter liver weights and body weights, more aligned hepatocytes, fewer vacuoles, and less steatosis (Meng et al., 2023). Analysis of FFAs using molecular networking showed that RG fed thoroughbred riding horses reduced pro-inflammatory FFAs (C12:0, dodecanoic acid; C14:0, myristric acid; C18:1, oleic acid; C18:2, linoleic acid) (Kwak et al., 2024). The above studies demonstrated some intuitive manifestations of the hypolipidemic properties of ginseng and its functional components.
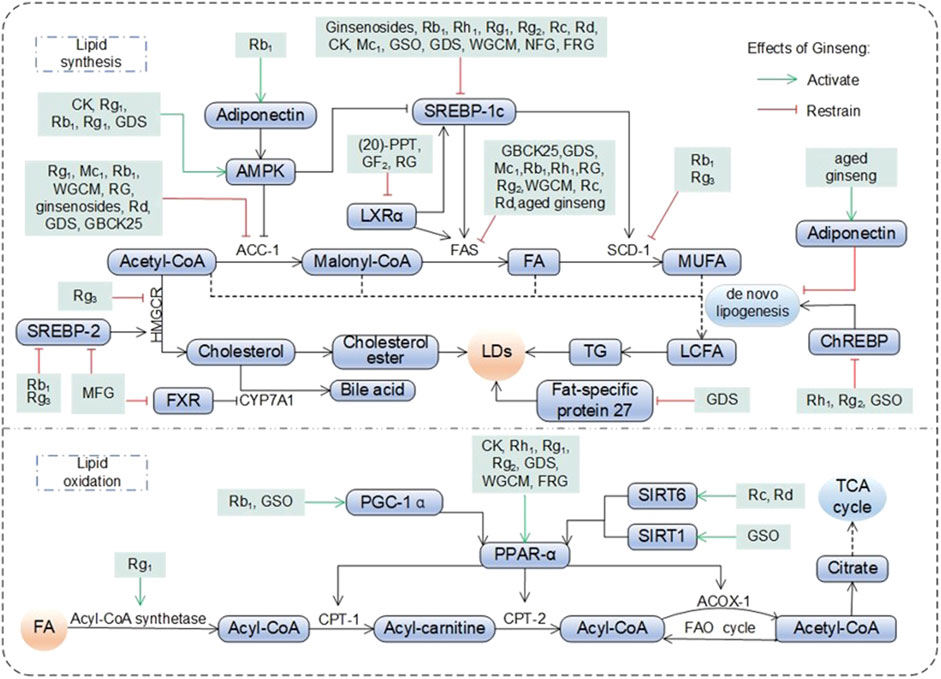
Figure 2. Pharmacological effects and molecular mechanisms of ginseng in regulating lipid metabolism. Fatty acids (FA) entering hepatocytes are broken down into acetyl-CoA by acetyl-CoA synthetase, which enters the mitochondria and participates in the fatty acid oxidation cycle (FAO cycle) or the tricarboxylic acid cycle (TCA cycle) under the promotion of various active substances. Among them, acetyl-CoA also participates in lipid synthesis in the organism, generating long chain fatty acid (LCFA, the process is also known as de novo lipogenesis) under the promotion of AMP-activated protein kinase (AMPK), acetyl-coenzyme A carboxylase 1 (ACC-1), liver X receptor a (LXRa), sterol-regulatory element binding protein (SREBP) 1c, fatty acid synthase (FAS) and stearoyl-coenzyme A desaturase 1 (SCD-1), on the one hand, lipid droplets (LDs) was generated thereafter. On the other hand, cholesterol was generated under the promotion of SREBP-2 and 3-hydroxy-3-methylglutaryl- coenzyme A reductase (HMGCR), which in turn synthesizes LDs, or is broken down by farnesoid X Receptor (FXR) and CYP7A1 into bile acid and excreted. ACOX-1: acyl-coenzyme A oxidase 1; ChREBP: carbohydrate responsive element-binding protein; CK: compound K; CPT: carnitine palmityl transferase; GDS: ginseng diol saponin; GSO: ginseng seed oil; MFG: monascus ruber fermented ginseng: MUFA: monounsaturated fatty acid; NFG: nonfermented ginseng: PGC-1a: peroxisome proliferators-activated receptor y co-activator a; PPAR-a: peroxisome proliferator-activated receptor a; PPT: protopanaxatriol; SIRT: sirtuins; TG: triglyceride; WGCM: wild ginseng cambial meristematic cells.
Aged ginseng reduced appetite and decreased the body’s lipid intake at the source (Chung et al., 2016). Ginseng water-soluble dietary fiber (GWDF) upregulated glucagon-like peptide 1, peptide YY, cholecystokinin and serum gastric hunger hormone levels. It increased satiety, promoted digestion, delayed gastric emptying, decreased food intake and affected glucolipid metabolism in male SD rats (Hua et al., 2021). Aged ginseng and ginsenosides acted as modulators and significantly reversed abnormally high leptin levels. Restores leptin’s appetite-suppressing function by reducing leptin resistance (Chung et al., 2016; Liang et al., 2021).
HDL-C and apolipoprotein A4 (apoA4) have a role in transporting cholesterol from peripheral tissues to the liver for removal. Ginseng and its functional components promotes reverse cholesterol transport into the liver and accelerates cholesterol breakdown and excretion by up-regulating HDL-C and apoA4 (Kim et al., 2018; Park et al., 2018; Xuan et al., 2015). Microsomal triglyceride transfer protein is a lipid transfer protein necessary for the synthesis of very low density lipoprotein in hepatocytes, and its synthesis is upregulated in response to increased TG. Fermented ginseng (FG) and non-fermented ginseng reduces very low density lipoprotein secretion and alleviates hepatic TG accumulation by inhibiting MTP expression (Park et al., 2018). Monascus ruber fermented ginseng (MFG) inhibits FXR receptor, increases cholesterol 7α-hydroxylase (CYP7A1) expression, and promotes bile acid synthesis. Rb1 directly promotes CYP7A1 activity, accelerating the conversion of cholesterol to bile acids and promoting cholesterol excretion (Li et al., 2020; Zhao et al., 2021).
4.1.2 Regulation of lipid synthesis and decomposition
On the one hand, hepatic nascent fatty acid (FA) are esterified to TG and will be stored as LDs in hepatocytes or secreted in other forms into the bloodstream, and on the other hand, it is directly metabolised by the β-oxidation pathway (Badmus et al., 2022). Therefore, an increase in nutrients delivered to the liver and FA synthesis, a decrease in FA oxidation and lipid output will result in an excessive accumulation of fat in the liver (Badmus et al., 2022). Ginseng and its functional components are a potent modulator of lipid metabolism (Figure 2).
Ginseng and its functional components plays a key role in reducing FA synthesis by directly inhibiting the expression of lipid synthesis genes such as SREBP-1 gene (isoforms SREBP-1a and SREBP-1c), fatty acid synthase (FAS) gene, stearoyl-coenzyme A desaturase-1 (SCD-1) gene and acetyl-coenzyme A carboxylase (ACC, ACC-1 and ACC-2 subtypes) 1 (Choi N. et al., 2019; Park et al., 2018; Wang et al., 2021). AMPK is a key sensor of cellular energy status. Rg1 significantly increased AMPK phosphorylation and restored hepatic lipid homeostasis (Xiao et al., 2019). CK and Rg3 inhibited downstream ACC-1 expression and reduced hepatic lipogenesis by activating AMPK phosphorylation (Kim et al., 2013; Lee et al., 2012). In addition, Rb1 and ginseng diol saponin (GDS) significantly inhibited SREBP-1c activity upon activation of AMPK, reducing the activation of downstream targets FAS and SCD-1 (Mi et al., 2024; Shen et al., 2013). GSO, Rh1 and Rg2 block de novo lipogenesis by directly blocking carbohydrate responsive element-binding protein (ChREBP) nuclear translocation and the synthesis of ACC, SCD-1, and FAS. In addition, wild ginseng cambial meristematic cells (WGCM) inhibits DNL by directly reducing the synthesis of the key enzymes ACC, SCD-1, and FAS (Kim et al., 2018; Lee et al., 2016; Wang et al., 2021). Lipocalin is an insulin-sensitive adipocyte-specific cytokine that inhibits de novo lipogenesis. Aged ginseng inhibited de novo lipogenesis and delayed NAFLD progression by upregulating lipocalin expression (Chung et al., 2016).
RG reduced the expression of hepatic lipid metabolism-related factors liver X receptors (LXR) α and LXRβ. This process regulates intracellular cholesterol and lipid homeostasis and reduces pro-inflammatory factor production. (Jeong et al., 2018). 20(S)-PPT and GF2 regulated the LXRα by inhibiting the transcription of the adipogenic genes SREBP-1c and FAS to reduce adipogenesis (Kim K. et al., 2024; Oh et al., 2015). Rb1, MFG and Rg3 blocked the SREBP-2/3-hydroxy-3-methylglutaryl-coenzyme A reductase (HMGCR) pathway, while Rg3 directly inhibited the expression of the downstream gene HMGCR (Lee et al., 2012; Li et al., 2020; Zhao et al., 2021). The above processes contribute to the inactivation of key pathways of cholesterol synthesis and reduce cholesterol biosynthesis. GDS targets fat-specific protein, a key protein in lipid droplet synthesis, to reduce its expression and significantly decrease the area of hepatocyte LDs, thereby reducing fat storage (Mi et al., 2024).
During FA oxidation, ginseng and its functional components maintains hepatic lipid homeostasis by stimulating the transcription of PPAR α and PPAR-γ response genes (Liang et al., 2021; Xuan et al., 2015). In addition ginseng and its functional components also synergizes with carnitine palmitoyl transferase (CPT) to accomplish FA oxidation. Examples include the transport and oxidation of acyl-CoA from the cytoplasm to the mitochondrial matrix, and FA oxidation processes involving acyl-CoA synthetase and ester oxygenase (Choi S. Y. et al., 2019; Kim et al., 2013; Mi et al., 2024). One study further found that GSO, Rc and Rd promote PPAR-α-mediated FA oxidation by increasing the expression of sirtuins (SIRT) 1 or SIRT6 proteins (Cui et al., 2023; Kim et al., 2018; Yang Z. et al., 2023). GSO and Rb1 synergistically regulated PPAR-α-mediated FAO by activating peroxisome proliferators-activated receptor γ co-activator α (PGC-1α) (Kim et al., 2018; Shen et al., 2013). STAT5 promotes the binding of PPAR-γ to PPAR response elements and regulates downstream genes related to adipogenesis, lipid metabolism and glucose homeostasis. However, Rg3 blocked STAT5 and PPAR-γ associated target adipogenesis and inhibited the adipogenic process in 3T3-L1 cells (Lee et al., 2017). Fermentation of red ginseng with C. militaris (FRG) reduced adipogenesis and lipid uptake by decreasing the expression of acyl-CoA synthetase long-chain and FA translocase (Choi S. Y. et al., 2019). Bioinformatic experiments and cellular experiments validated that Rf downregulated the methylation level of the DNMT3L gene and reversed the aberrant expression of the adipogenesis-related gene ANXA2 (Chen et al., 2022).
4.2 Lipotoxic injury
The liver converts excess FA to TG for storage in an early adaptive protective response. If FAs are continuously supplied in excess or their processing is impaired, they become substrates for the production of lipotoxic lipids and activate a range of pathological response mechanisms, such as inflammation, ERS and OS, causing hepatocellular damage and accelerating the progression of NAFLD (Geng et al., 2021). Not only do various pathophysiological mechanisms play an important role in the development of NAFLD, but there are complex interactions between the mechanisms themselves. Lipotoxic substances induce hepatocyte stress, injury and even death, leading to chronic inflammatory response and generation of large amounts of reactive oxygen species (ROS) causing OS in hepatocytes (Peiseler et al., 2022). OS can further exacerbate inflammation and induce the endoplasmic reticulum to produce a large number of error proteins, activating the ERS and promoting the progression of NAFLD to cirrhosis and hepatocellular carcinoma (Peiseler et al., 2022). Preventing the production of lipotoxic substances will be the key to treatment. Modern medical research has provided a range of evidence at both the cellular and animal levels to support the idea that inhibition of the process of lipotoxic damage by ginseng and its functional components may be a key target for amelioration of NAFLD, suggesting the potential of ginseng and its functional components as novel therapeutic agents for the prevention and treatment of NAFLD (Table 4; Figure 3).
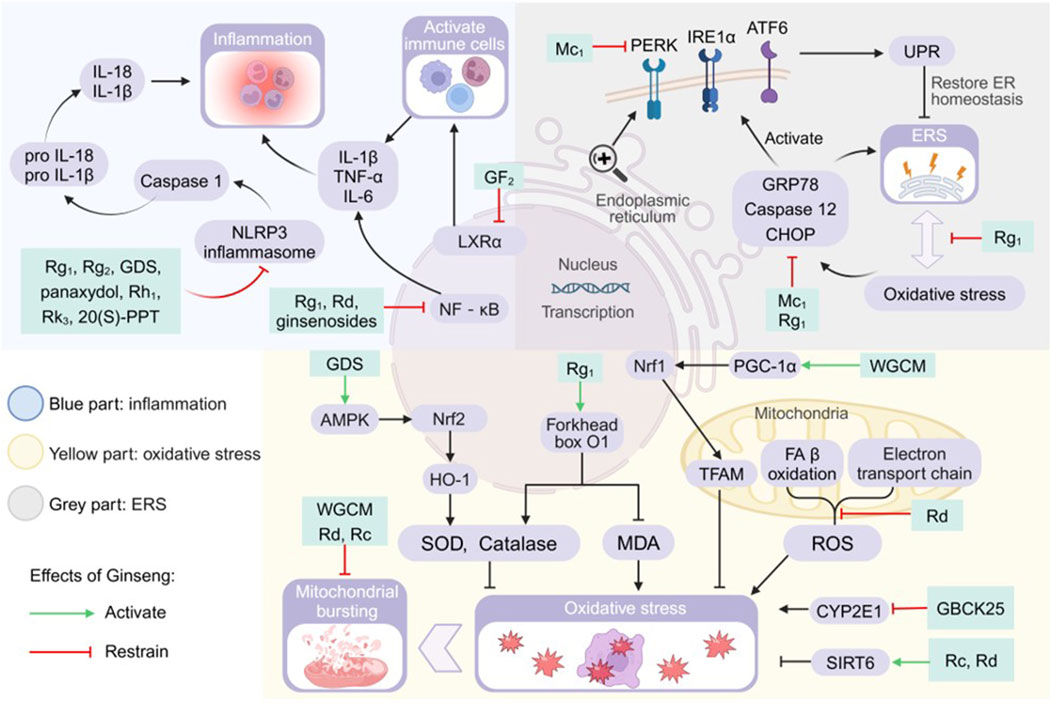
Figure 3. Pharmacological effects and molecular mechanisms of ginseng in ameliorating lipotoxic injury. Blue part: activation of nod-like receptor thermal protein domain associated protein 3 (NLRP3) inflammasome, nuclear factor kappa-B (NF-kB) pathway, and liver X receptor a (LXRa) all promote the release of inflammatory factors or the activation of immune cells, but ginseng and its characteristic extracts have the effect of inhibiting the activation of the above substances or pathways. Gray part: increased glucose-regulated protein78 (GRP78), cysteine-containing aspartate-specific proteases (Caspase) 12 and CCAAT/enhancer binding protein (C/EBP) homologous protein (CHOP) promote endoplasmic reticulum stress (ERS), and they also promote unfolded protein response (UPR) by activating the signaling cascade promoters PRKR-like ER kinase (PERK), inositol-requiring enzyme 1a (IRE1a) and activating transcription factor 6 (ATF6). Ginseng has a mitigating effect on ERS and UPR. Yellow part: the AMP-activated protein kinase/nuclear factor-erythroid 2 related factor 2/heme oxygenase 1 (AMPK/Nrf2/HO-1) pathway, forkhead box 01 genes, mitochondrial transcription factor A (TFAM) and sirtuins (SIRT) 6 promote the production of antioxidants or directly inhibit oxidative stress, and ginseng strengthens their efficacy. Mitochondrial ETC and fatty acid (FA) ẞ oxidation generated reactive oxygen species (ROS) and cytochrome P450 2E1 (CYP2E1) both promoted oxidative stress, and ginseng inhibited their activities. GDS: ginseng diol saponin; IL: interleukin; MDA: malondialdehyde; Nrf1: nuclear respiratory factor 1; PGC-1a: peroxisome proliferators-activated receptor y co-activator a; 20(S)-PPT: 20(S)-protopanaxatriol; SOD: superoxide dismutase; TNF-a: tumor necrosis factor a; WGCM:wild ginseng cambial meristematic cells.
4.2.1 Inflammation
The core pathologic features of NASH and NAFLD encompass lipotoxicity-mediated hepatocellular injury and a chronic inflammatory cascade response. Controlling inflammation is one of the fundamental strategies for treatment (Wiering and Tacke, 2023). In this study, we systematically revealed the molecular mechanisms by which ginseng and its functional components modulate inflammatory signaling networks through multiple targets (Figure 3).
Lipotoxic substances induce hepatocyte damage, leading to abnormal release of alanine aminotransferase (ALT), aspartate aminotransferase (AST), alkaline phosphatase (AKP), and lactate dehydrogenase as a diagnostic indicator of the early stages of the disease. Ginseng and its functional components reduces serum levels of damage markers and this effect is directly related to hepatocyte protection (Kim M. Y. et al., 2024; Xuan et al., 2015). In addition, ginseng and its functional components inhibited the production of various inflammatory factors, such as tumor necrosis factor α (TNF-α), interleukin (IL) 6, IL-1β, IL-18, IL-10, IgA, arginase 1, and C-C motif chemokine ligand (CCL) 2. It also reduced the recruitment and activation of immune cells and improved the inflammatory microenvironment (Kim M. Y. et al., 2024; Wang et al., 2021; Zhao et al., 2021).
Lipid overaccumulation leads to aberrant activation of mammalian target of rapamycin C1, causing macrophage 1 polarisation and elevating levels of CCL2, CCL5, IL-1β, IL-6, inducible nitric oxide sythase and TNF-α. FRG reduced the levels of CCL2, CCL5, IL-1β, IL-6, inducible nitric oxide sythase and TNF-α by inhibiting aberrant activation of mTORC1, which reverses macrophage 1 type to macrophage 2 type polarization. It also increases the levels of the macrophage 2 markers CD163 and IL-10, achieving an anti-inflammatory-promoting repair dynamic balance (Choi S. Y. et al., 2019). RG significantly increased natural killer cells counts in otsuka long-evans tokushima fatty rats, improving their immunity (Hong et al., 2013). In addition, RG reduced the phosphorylation of p38 mitogen-activated protein kinase and decreased the secretion of the inflammatory cytokine IL-1β (Kim et al., 2019). RG attenuates downstream hepatic injury and inflammation by inhibiting the FA binding protein4/c-Jun N-terminal kinase (JNK) pathway and reduces the production of ALT, AST, TNF-α, IL-1β, IL-12a, IL-12b, and IL-17a (Jeong et al., 2018).
In the LXRs regulatory module, GF2 enhances the binding capacity of co-inhibitory factors to LXRα. Reduction of LXRα transcriptional activity improves intracellular lipid homeostasis and correspondingly reduces the number of immune cells and pro-inflammatory factors infiltrating the liver (Kim K. et al., 2024). The body assembles and activates nod-like receptor thermal protein domain associated protein 3 (NLRP3) inflammasome after sensing lipotoxic danger signals, which in turn induces the maturation of cysteine-containing aspartate-specific protease (Caspase) 1 and catalyses the production of pro-inflammatory cytokines IL-18 and IL-1β (Mridha et al., 2017; Yu et al., 2022). Ginseng and its functional components modulated NLRP3 inflammasome-mediated inflammation by blocking the activation of NLRP3 inflammasome and Caspase-1 (Kim M. Y. et al., 2024; Xu et al., 2018). Ginsenosides, Rg1 and Rd reduced the expression of pro-inflammatory factors by directly inhibiting the activation of NF-κB, which is an important nuclear transcriptional regulator of pro-inflammatory genes (Cui et al., 2023; Liang et al., 2021; Xiao et al., 2019; Xu et al., 2018). GDS inhibits nuclear translocation of NF-κB and downregulates transcription of key inflammatory factor genes through activation of AMPK/PPAR-γ (Mi et al., 2024). Another study found that Rc and Rd regulate the production of inflammatory factors TNF-α, IL-6 and IL-1β by increasing SIRT6 protein expression (Cui et al., 2023; Yang Z. et al., 2023). Rg1 specifically upregulates activating transcription factor (ATF) 3/acyl-coenzyme A oxidase (ACOX) 2 to alleviate NAFLD, and how Rg1 regulates NAFLD through these two genes is unknown (Gu et al., 2021). Leukocyte expression of active the MMP9 gene enhanced its ability to infiltrate during the inflammatory process, and Rf regulation of NAFLD inflammation may be related to the MMP9 gene (Chen et al., 2022).
4.2.2 Oxidative stress
The occurrence of OS in the body indicates that the antioxidant system scavenges ROS at a slower rate than the rate of ROS production, which is an important factor in liver injury and NAFLD progression (Kumar et al., 2021). The tricarboxylic acid cycle transfers the generated NADH and FADH2 to oxygen via the electron transport chain, which generates large amounts of ATP and promotes ROS production (Chen et al., 2020). In addition non-electron transport chain sources of ROS, especially the compensatory acceleration of β-oxidation due to hepatic steatosis, appear to generate more ROS and cause OS (Clare et al., 2022). Ginseng improves OS by intervening in the following targets (Figure 3).
Rg1, WGCM, Rh1 and Rg2 reduced abnormally elevated levels of ROS and malondialdehyde (MDA) and increased the vigour of antioxidants such as superoxide dismutase (SOD), catalase, glutamate dehydrogenase and glutathione (Hou et al., 2022; Lee et al., 2016; Wang et al., 2021). Further studies revealed that GDS supplementation activated AMPK and further activated the nuclear factor-erythroid 2 related factor 2/heme oxygenase 1 signalling pathway, elevating the level of SOD and enhancing the antioxidant capacity of hepatocytes (Mi et al., 2024). Rg1 elevated nuclear FOXO1 protein levels and subsequently targeted to increase SOD and CAT expression and decrease MDA levels (Qi et al., 2020).
Rd repairs abnormal mitochondrial morphology, ameliorates REDOX disorder, and reduces ROS generation due to electron leakage from ETC (Cui et al., 2023). WGCM elevated the levels of mitochondrial biogenesis related factors, such as PGC-1α, nuclear respiratory factor 1 and mitochondrial transcription factor A, which inhibited mitochondrial OS and promoted mitochondrial biogenesis (Lee et al., 2016). SIRT6 protein attenuated the mitochondrial stress and ameliorated redox deficits in the organism, and ginseng Rc and Rd improved OS status in normal mice better than in SIRT6-deficient mice (Cui et al., 2023; Yang Z. et al., 2023). Rg1 attenuated OS injury-induced cellular senescence and mitigated hepatic OS by improving biological processes such as cellular matrix composition, membrane receptors, and cellular responses to the outside (Hou et al., 2022). GBCK25 inhibited cytochrome P450 2E1 expression and blocked the lipid peroxidation chain reaction, while down-regulating JNK phosphorylation and attenuating OS-associated cell injury (Choi N. et al., 2019).
4.2.3 Endoplasmic reticulum stress
The accumulation of large amounts of FFAs in fatty liver promotes the production of lipotoxic substances causing ER structural disruption and decrease in number, and ERS occurs in hepatocytes in order to restore homeostasis of the internal environment (Lebeaupin et al., 2018; Lei et al., 2023). During this process, the ER will trigger the unfolded protein response (UPR) to restore the protein homeostasis of the ER, and if the ERS remains at a high level for a long period of time, the terminal UPR programme will trigger cell death (Senft and Ronai, 2015; Zhang et al., 2022). Lipotoxicity induced ERS-OS interaction network constitutes the core pathology axis. We outline the evidence that ginseng intervention in NAFLD is associated with ERS and discuss possible points of intervention (Figure 3).
ERS and OS are both adaptive responses to NAFLD in the early stages of the body, and become damaging factors when they exceed a certain limit, and they form a two-way positive feedback between them. Rg1 was found to achieve synergistic hepatoprotection through dual regulation of ERS-OS cross-talk (Xu et al., 2018). Furthermore, Rg1 significantly reduced OS-induced ERS marker levels, suah as CCAAT/enhancer binding protein (C/EBP) homologous protein (CHOP), glucose-regulated protein78 (GRP78) and Caspase-12. It also attenuated NLRP3 inflammasome-mediated associated inflammation. Binding of the ER chaperone protein GRP78 to unfolded or misfolded proteins will activate ERS sensors, including inositol-requiring enzyme 1α, PRKR-like ER kinase, and ATF6, which are the initiators of the major signalling cascade of the UPR and the survival mechanism of the ERS (Xu et al., 2018). UPR promotes restoration of ER homeostasis, but failure of restoration induces increased synthesis of pro-apoptotic proteins and triggers cell death. Mc1 reduced the expression levels of GRP78 and CHOP proteins, which inhibited ERS and UPR activation, and Mc1 has not been found to ameliorate ERS through activation of the AMPK signalling pathway (Roh et al., 2020).
4.3 Other ways
Recent reports have suggested that the pathogenesis of NAFLD is ‘multiple-hit’ and that the causative factors are unlikely to be the same in all patients, so that co-morbidities of multiple factors are necessary for the development of NAFLD (Wang et al., 2023). Many molecular pathways that promote the development of NAFLD, such as early IR, gut microbiota and metabolites, are involved in hepatocyte lipotoxic stress and injury, and further contribute to hepatocyte senescence and death, stellate cell activation and fibrosis (Wang et al., 2023). These pathways and mechanisms ultimately lead to the transition of NAFLD to NASH and possibly to end-stage liver disease (Loomba et al., 2021). The mechanisms by which ginseng affects these deficiencies are unclear and are corresponding summarised in this paper (Table 5).
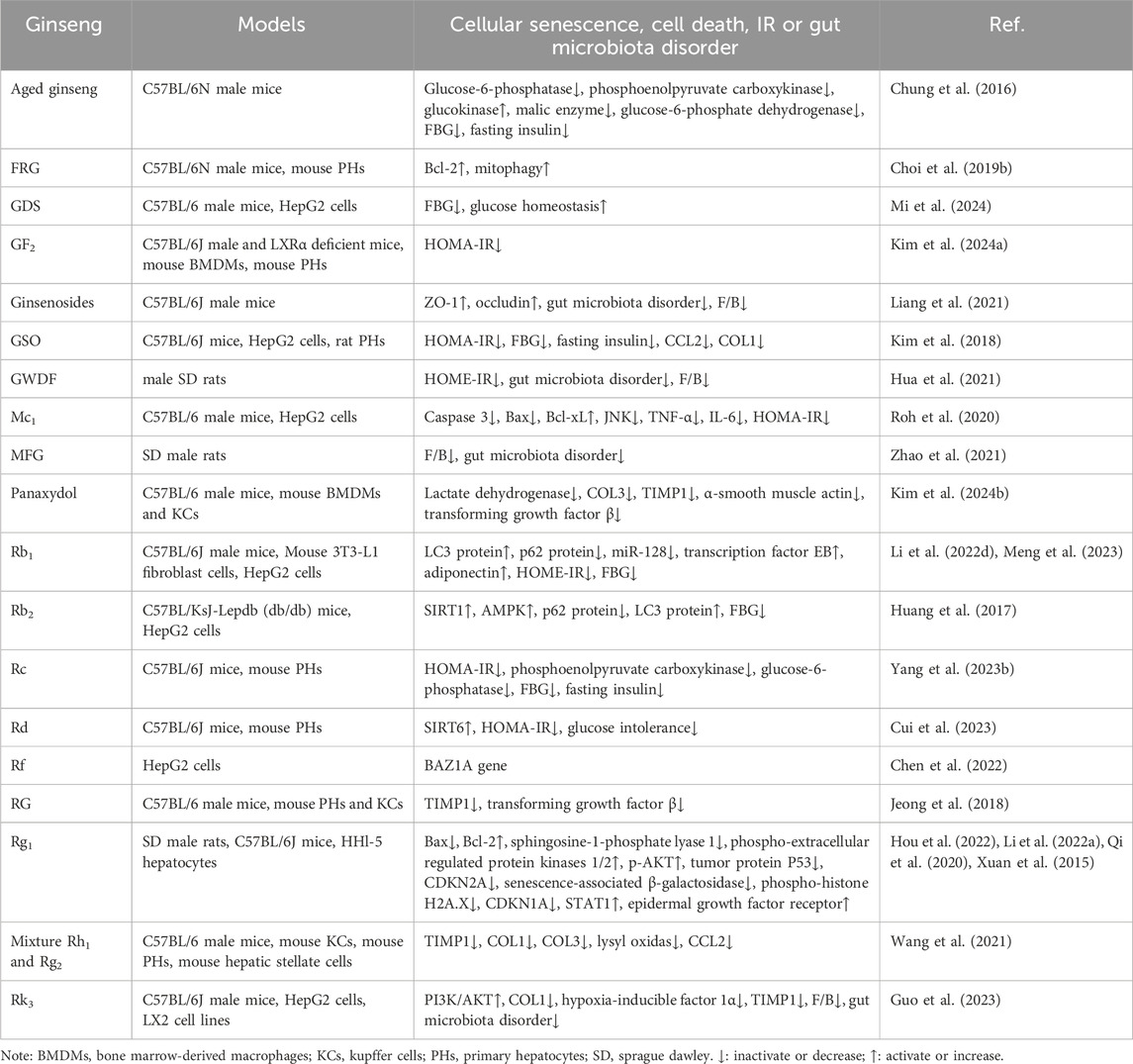
Table 5. Summary of cellular senescence, cell death, IR and gut microbiota disorder by which ginseng ameliorates NAFLD.
4.3.1 Cellular senescence and death
Hepatocyte death (apoptosis, pyroptosis, impaired autophagy) and fibrosis are key components of NAFLD progression to NASH (Shojaie et al., 2020). Hepatic injury factors such as fat deposition, inflammation, OS and ERS are involved in the pathological changes and accelerate the process of hepatocyte death and fibrosis. Ginseng significantly ameliorates a variety of cell death and aging-related pathological processes through the following multi-targeted mechanisms (Figure 4).
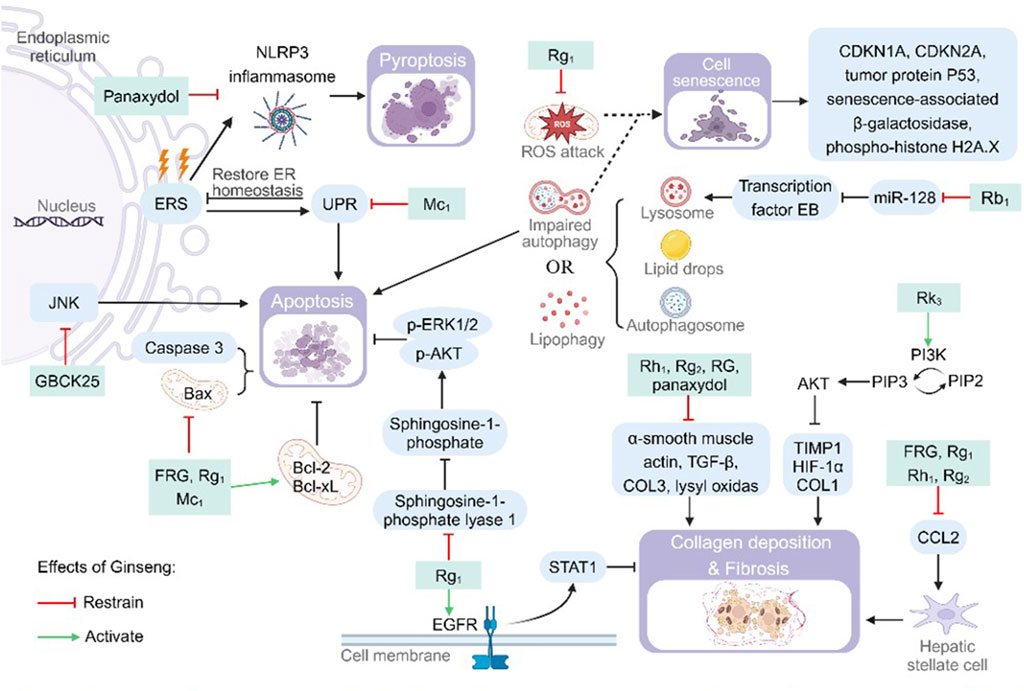
Figure 4. Pharmacological effects and molecular mechanisms of ginseng in reducing cellular senescence and death. Pathways or substances such as c-Jun N-terminal kinase (JNK), Bcl2-associated X (Bax), cysteine-containing aspartate-specific proteases (Caspase) 3 and sphingosine-1-phosphate lyase 1 have apoptosis-promoting effects, but ginseng and its characteristic metabolites inhibit the above processes. Endoplasmic reticulum stress (ERS) promotes cellular pyroptosis by upregulating nod-like receptor thermal protein domain associated protein 3 (NLRP3) inflammasome synthesis or apoptosis by promoting the unfolded protein response (UPR), but ginseng inhibits this process. Lysosomes, autophagosomes, and lipid droplets interact to cause lipophagy, and impairment of this process promotes cellular senescence. Ginseng attenuates cellular senescence by inhibiting miR-128 to increase the number of lysosomes or by inhibiting mitochondrial damage. Ginseng inhibits the expression of substances associated with collagen (COL) deposition and fibrosis, such as a-smooth muscle actin, transforming growth factor B (TGF-B), COL3 and lysyl oxidas, or inhibits liver fibrosis by activating the epidermal growth factor receptor (EGFR) and protein kinase B (PI3K/AKT) pathways. Bcl-2: B-cell lymphoma-2; Bcl-XL: B-cell lymphoma-extra large; CDKN: cyclin-dependent kinase inhibitor; ER: endoplasmic reticulum; HIF-1a: hypoxia-inducible factor 1a; p-AKT: phospho-protein kinase B; p-ERK: phospho-extracellular regulated protein kinases; PI3K: phosphatidylinositol-3-kinase; PIP2: phosphatidylinositol bisphosphate; PIP3: phosphatidylinositol trisphosphate; ROS: reactive oxygen species; STAT: signal transducer and activator of transcription; TIMP1: tissue inhibitor of metalloproteinase 1.
Upon stimulation of the organism from internal or external factors, pro-Caspase-3, the common downstream effector part of multiple apoptotic pathways, will be cleaved to the activator Caspase-3 and activate the cascade reaction of apoptosis (McComb et al., 2019). FRG, Rg1 and Mc1 significantly downregulated the expression levels of pro-apoptotic proteins Bcl-2-associated X (Bax) and Caspase-3, while up-regulating the expression levels of anti-apoptotic proteins B-cell lymphoma-2 (Bcl-2) and B-cell lymphoma-extra large (Bcl-xL) to reduce hepatocyte apoptosis (Choi S. Y. et al., 2019; Li G. et al., 2022; Roh et al., 2020). Supplementation of Mc1 lowered reduced CHOP and Bax activity. This process avoids prolonged high levels of ERS, which activates apoptosis induced by the terminal UPR program (Roh et al., 2020). Rg1 reduces sphingosine-1-phosphate cleavage by inhibiting sphingosine-1-phosphate lyase 1. Enhanced sphingosine-1-phosphate/p-AKT survival signaling in the liver and promoted hepatocyte survival (Li G. et al., 2022). GBCK25 reduced FFAs-induced steatotic apoptosis in hepatocytes by inhibiting the activation of the JNK pathway (Choi N. et al., 2019). Activation of the NLRP3 inflammasome promotes pro-Caspase-1 cleavage and activation. Activated Caspase-1 promotes both the maturation and release of IL-1β and IL-18, as well as being involved in the process of cellular pyroptosis. Panaxydol inhibited NLRP3 inflammatory vesicle activation (ASC oligomerization) and synthesis of lactate dehydrogenase (LDH), a marker of cellular cell death. The reduction of Caspase-1 activity and LDH content reversed the Caspase-1-dependent pathway of cellular cell death (Kim M. Y. et al., 2024). Lipophagy is the interaction of LC3 protein on the autophagosome membrane with envelope proteins on LDs to encapsulate LDs in autophagosomes for degradation. Abnormal lipophagy or degradation of autophagosomes (generation of p62 protein) will cause cell death. Rb1 and Rb2 increased autophagic vesicle survival by increasing LC3 protein and decreasing p62 protein content. Rb2 accelerated LD degradation by activating AMPK/SIRT1, upregulating LC3 protein and decreasing p62 protein (Huang et al., 2017; Meng et al., 2023). Rb1 enhances the transcription of transcription factor EB nuclear translocation and its downstream lysosome-associated genes by inhibiting miR-128. This resulted in increased lysosomal degradation of autophagic lipids and alleviated palmitic acid-induced autophagic flux block (Meng et al., 2023).
Additionally it has been proposed that although ginseng lowered Bax levels, it also elevated Bcl-2 during modeling of the disease. Because the Bax/Bcl-2 changes during treatment were slight, it is possible that the improvement in the course of Rg1 treatment for NAFLD was not cell death (Qi et al., 2020). In addition, impaired autophagy and free radical attack on mitochondria both contribute to cellular senescence. Rg1-treated mitochondria showed reduced swelling and vacuolization, more intact cytoplasmic matrix, and restoration of cellular autophagy. The expression of the key signals tumor protein P53, senescence-associated β-galactosidase, the nuclear damage marker phospho-histone H2A.X, and cyclin-dependent kinase inhibitor (CDKN) 1A/CDKN2A was decreased in the early stage of senescence (Hou et al., 2022; Qi et al., 2020). Validated by bioinformatic experiments and cellular experiments, Rf was found to inhibit aging-related phenotypes by affecting the BAZ1A gene (Chen et al., 2022). Hepatocyte injury or necrosis activates the body to repair damaged cells, and it causes hepatic fibroproliferation when repair persists. Panaxydol, Rg1, Rh1 and Rg2 was found to be effective in reducing the size of fibrotic areas, the number of inflammatory foci in the periportal and pericentric areas. Decreased the levels of liver fibrosis markers collagen (COL) 1, COL3, lysyl oxidas, tissue inhibitor of metalloproteinase 1 (TIMP1), α-smooth muscle actin, transforming growth factor β and hypoxia-inducible factor 1α (Kim M. Y. et al., 2024; Wang et al., 2021; Xu et al., 2018). In addition, Rk3 reduced the expression of hypoxia-inducible factor 1α, COL1 and hepatic TIMP1 through activation of the PI3K/AKT signaling pathway, and attenuated COL deposition and fibrosis in hepatocytes (Guo et al., 2023). FRG, Rg1, Rh1 and Rg2 inhibit high CCL2 expression. Reduced hepatic fibrosis and even cirrhosis formation by blocking CCL2 hepatic stellate cell activation (Choi S. Y. et al., 2019; Qi et al., 2020; Wang et al., 2021). Rg1 promotes the expression of downstream negative regulators of hepatic fibrosis and increases extracellular matrix degradation by regulating the epidermal growth factor receptor/STAT1 axis (Hou et al., 2022).
4.3.2 Insulin resistance
As a hub of systemic metabolic regulation, hepatic lipotoxicity injury (inflammation/OS/ERS) triggers systemic IR through multiple cascade reactions, which is manifested by (1) decreased glucose disposal in non-hepatic tissues (including adipose tissues and muscles). (2) dysregulation of lipolysis leading to aberrant lipid release. (3) impaired hepatic glycogen storage, which causes metabolic abnormalities and exacerbates systemic IR (Sakurai et al., 2021). In this study, we systematically elucidated the action network of ginseng in ameliorating disorders of glucolipid metabolism through multi-target synergism (Figure 5).
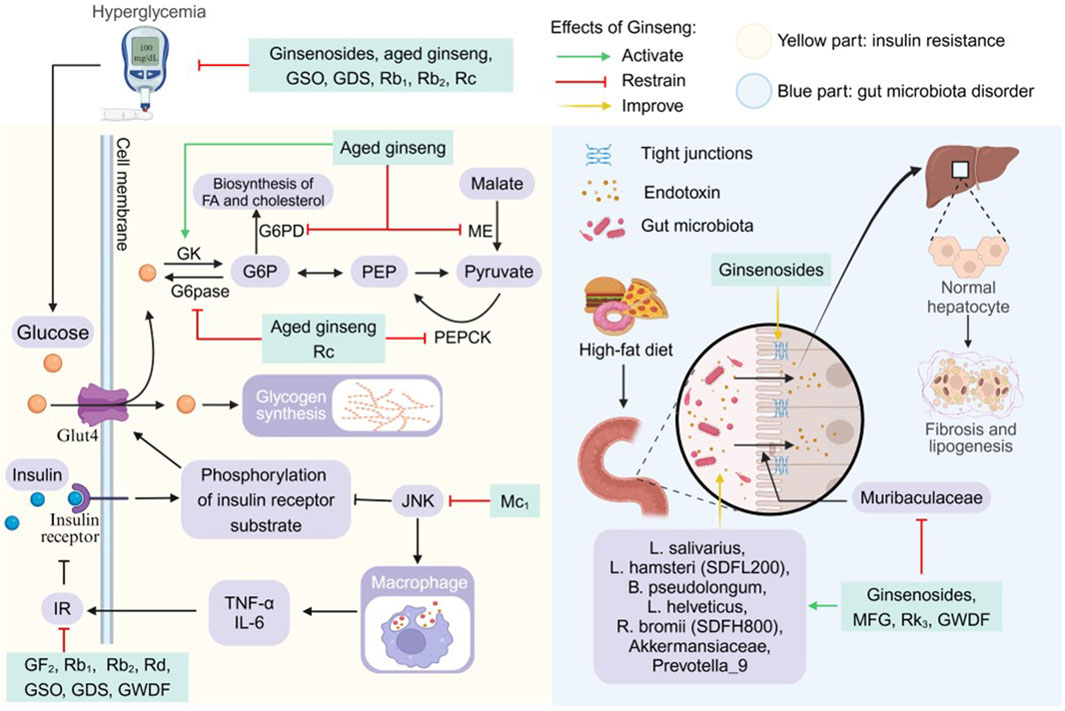
Figure 5. Pharmacological effects and molecular mechanisms of ginseng in ameliorating insulin resistance and gut microbiota disorders. Blue part: ginseng improved gut microbiota disorders and impaired intestinal vascular barrier induced by high-fat diet, and attenuated hepatic lipid deposition and hepatic fibrosis. Gray part: JNK reduces insulin sensitivity by inhibiting phosphorylation of insulin receptor substrateand promoting the production of inflammatory cytokines, but ginseng inhibits c-Jun N-terminal kinase (JNK) activation and insulin resistance (IR). Ginseng promotes the expression of glucokinase (GK), which activates glucose uptake and utilization, and inhibits glucose and lipid synthesis by inhibiting the activity of glucose-6- phosphatase (G6pase), phosphoenolpyruvate carboxykinase (PEPCK), malic enzyme (ME), and glucose-6-phosphate dehydrogenase (G6PD). FA: fatty acid; G6P: glucose-6-phosphate; GDS: ginseng diol saponin; Glu4: glucose transporter type 4; GSO: ginseng seed oil; GWDF: ginseng water-soluble dietary fiber; IL-6; interleukin 6; MFG: monascus ruber fermented ginseng; PEP: phosphoenolpyruvate; TNF-a: tumor necrosis factor a.
Ginseng and its functional components reduced fasting blood glucose (FBG) levels, fasting insulin and homeostatic model assessment for insulin resistance index (HOMA-IR) in animals of NAFLD disease model and improved glucose homeostasis, glucose tolerance and insulin tolerance in the organism (Cui et al., 2023; Kim K. et al., 2024; Li et al., 2022d). JNK elevates levels of TNF-α and IL-6 during obesity-driven macrophage activation, subsequently triggering IR. Mc1 inhibited the above process. In addition Mc1 restored insulin receptor substrate 1 tyrosine phosphorylation levels and increased insulin sensitivity in the process of driving down JNK phosphorylation levels (Roh et al., 2020). In regulating glucose metabolic activities, aged ginseng activates glucose uptake and utilization by decreasing insulin, FBG levels and increasing glucokinase expression. It also inhibits the synthesis of pyruvate, FAs and cholesterol by inhibiting malic enzyme and glucose-6-phosphate dehydrogenase enzyme activities (Chung et al., 2016). In addition both Rc and aged ginseng reduce the expression of glucose-6-phosphatase and phosphoenolpyruvate carboxykinase. This process reduces gluconeogenesis, decreases the body’s blood glucose level and improves glucose intolerance (Chung et al., 2016; Yang Z. et al., 2023). Rb1 improvement of IR and glucose tolerance is partially dependent on lipocalin. Specifically, it improves systemic glucose metabolism, insulin homeostasis, FA oxidation, and hepatic insulin sensitivity after activation of AMPK using lipocalin (Li et al., 2022d).
4.3.3 Gut microbiota disorder
The microbial community in the human gastrointestinal tract has been shown to be involved in a variety of physiopathological processes in the gut, and a unique gut microbiome signature exists for NAFLD (Aron-Wisnewsky et al., 2020; Vallianou et al., 2021). The intestinal microecological disorder of NAFLD is characterized by the imbalance of “flora-intestinal-hepatic axis”, and the present study systematically reveals that ginseng can improve the metabolic abnormalities by regulating the homeostasis of intestinal flora in a multi-dimensional way (Figure 5) (Hsu and Schnabl, 2023).
High-fat diets increases intestinal permeability and exposes the liver to endotoxins, and a disturbed gut microbiota also disrupts the intestinal vascular barrier, enhancing intestinal permeability and bacterial lipopolysaccharide leakage into the circulation (Kobayashi et al., 2022; Mouries et al., 2019). Ginsenosides treatment increased the tight junction proteins ZO-1 and occludin levels in a dose-dependent manner, attenuating microecological dysregulation-mediated intestinal leakage and metabolic endotoxaemia (Liang et al., 2021). Qualitative and quantitative abnormalities of the gut microbiota are involved in the pathogenesis of NAFLD and NASH and promote hepatic lipogenesis and fibrosis (Hu et al., 2020; Kolodziejczyk et al., 2019). The study show that GWDF significantly increased the abundances of biomarkers such as L. salivarius, L. hamsteri (SDFL200), B. pseudolongum, L. helveticus, and R. bromii (SDFH800), and show a significant prebiotic effect. MFG and Rk3 selectively promoted colonisation by the probiotic bacteria Akkermansiaceae and Prevotella_9 and reduce the relative abundance of Muribaculaceae (Guo et al., 2023; Hua et al., 2021; Zhao et al., 2021). GWDF, ginsenosides, MFG and Rk3 had the effect of decreasing the Firmicutes and Bacteroidetes ratio, increasing the concentration of fecal short-chain FA, the abundance and diversity of intestinal flora species, and ameliorating the imbalance of gut microbiota induced by high-fat diets (Guo et al., 2023; Hua et al., 2021; Liang et al., 2021; Zhao et al., 2021). The above results demonstrated that ginseng has a three-in-one action model of “flora reconstruction, barrier repair and metabolic regulation”.
5 Conclusion and outlook
The burden caused by NAFLD is rising and treating NAFLD and its comorbidities is an important clinical challenge. Targeting lipid metabolism is gaining attention as a potential therapeutic target, and herbal medicine has been widely used as a complementary therapy for a long time. Ginseng and its functional components have hepatoprotective effects on patients and experimental animals, but ginseng itself has numerous components and complex targets and pathways, so finding safe and effective components and sorting out the mechanism of action deserve in-depth exploration. In this paper, we present a systematic review of the main features of ginseng in improving lipid metabolism in NAFLD through multiple components, pathways and targets.
In summary, ginseng and its functional components was found to help slow the progression of non-alcoholic fatty liver to NASH. Increased lipid uptake and synthesis, decreased catabolism and excretion, inflammation, ERS, OS, IR, cellular senescence, cell death and gut microbiota disorder are common pathogenic pathways. Modern studies have shown that ginseng and its functional components works by targeting AMPK, PPAR/PGC-1α, adipocytokine, FXR, LXR, SIRT, PI3K/AKT, JNK/insulin receptor substrate, sphingosine-1-phosphate/AKT/extracellular regulated protein kinases, nuclear factor-erythroid 2 related factor 2/heme oxygenase 1, PGC-1α/nuclear respiratory factor 1/mitochondrial transcription factor A, epidermal growth factor receptor, NLRP3 and NF-κB mechanisms to exert lipid-lowering and alleviate hepatic lipotoxicity. In addition, it can also affect the dynamics of lipid synthesis, oxidation and excretion by biometabolic pathways such as lipid de novo lipogenesis, β-oxidation, tricarboxylic acid cycle, gluconeogenesis, UPR, and mitochondrial biogenesis. This “network targeting-pharmacological” action characterizes the nature of NAFLD, which is characterized by multiple organ interactions and multiple pathologies.
At the level of mechanism research, we note that there is a cognitive bias in the current academic community that emphasizes on component mechanisms but not on systematic evaluation. Although ginseng has been shown to reduce hepatic cholesterol accumulation by activating FXR, it is puzzling that ginseng has been shown to promote cholesterol synthesis by up-regulating HMGCR expression (Li et al., 2020). It is worth noting that, compared with the compensatory metabolic escape phenomenon that often occurs with single-target chemical drugs. Ginsenosides may be more conducive to maintaining the long-term stability of glucose-lipid metabolism homeostasis by improving IR through the bi-directional regulation of insulin receptor substrate PI3K/AKT and JNK signaling (Roh et al., 2020). This paradoxical phenomenon suggests that there may be antagonistic effects among the complex components of Chinese medicines, and that relying solely on single component studies may result in misjudgment of the overall efficacy. Therefore, we strongly suggest that future studies should establish a three-dimensional evaluation system of “chemical component-biological effect-clinical phenotype,” and pay special attention to the key regulatory effects of non-saponin components such as ginseng polysaccharides and volatile oils on the intestinal flora-liver axis.
To date, ginseng is considered an edible and medicinal plant with a long history of medicinal use and a wide range of pharmacological effects. Ginseng and its functional components blocks the above pathogenesis of NAFLD through multi-components, multi-pathways, multi-targets and multi-levels, and has hepatoprotective effects on experimental animals. To summarise the relevant studies, the most relevant components of ginseng are ginsenosides, whose pharmacological mechanisms focus on the regulation of lipid metabolism and the subsequent reduction of lipotoxicity injury, and therefore we consider them to be the typical mechanisms of ginseng in the treatment of NAFLD. A study using network-based approaches to investigate the therapeutic effects and key mechanisms of ginseng revealed similar results (Kim Y. W. et al., 2024). Although the methods of these two studies are different, we adopt the attitude of seeking common ground while reserving differences, and believe that the results of the two studies can confirm and complement each other, verifying the rationality of ginseng in treating liver diseases. However, there are some slight differences between the two studies, such as network pharmacology found that regulation of protein function may be a key core target of ginseng in the treatment of liver-related diseases, but the experimental data on this aspect are slightly insufficient. From the perspective of clinical practice, we believe that ginseng has important application value because of its “both symptoms and root causes.” It can rapidly reduce hepatic TG deposition through acute activation of AMPK, and can also regulate the secretion of adipokines to realize the long-term benefit of metabolic memory. This dual-phase regulation advantage is extremely rare among existing chemical drugs, and we look forward to exploring it in more relevant studies in the future.
Taken together, these findings demonstrate the effectiveness of ginseng and its functional components in terms of key mechanisms against NAFLD. However, there are still some unknowns to be confirmed in this study. Firstly there are a few studies that showed discrepancies in the results, for example, ginseng reduced cholesterol transport by decreasing the activity of LDL receptor and increased the expression of HMGCR thereby increasing cholesterol synthesis (Li et al., 2020). We need more high-quality experiments to strengthen the level of evidence from controversial experiments. Secondly, in view of the shortcomings of the current quality control system, the current evaluation standard of saponin content as a single quality control index has seriously lagged behind the progress of basic research. We believe that a multidimensional quality control model should be constructed based on the correlation feature of “component-pathway-function,” which includes the assessment of bioavailability of metabolome and the detection of metabolic transformation ability of intestinal flora. For example, emphasizing ginseng’s absorption, disposition, metabolism, excretion and biosynthesis will remedy the shortcomings of the current quality control methods in order to better achieve the effect of comprehensive evaluation of the quality of TCM (Li X. et al., 2022). This innovative quality control concept may lead to a paradigm shift in TCM modernization research. Finally, there are still many important unexplored signalling molecules or pathways to be added in modern research. At the translational medicine level, for example, breakthroughs in the cutting-edge area of macrophage polarization regulation may lead to clinical benefits (Loomba et al., 2021). In genetic medicine, individualized intervention studies of ginseng for people with mutations in patatin-like phospholipase domain containing protein 3 may provide new ideas to address the therapeutic dilemma of genetically susceptible NAFLD (Park et al., 2023). It is also of interest that the regulation of the mitochondrial quality control network by the SIRT family has not yet been fully elucidated in ginseng studies, which may be an important bridge connecting lipotoxic injury to cellular aging mechanisms (Xu et al., 2024). However, the role of ginseng in this regard has been slightly underreported and more work needs to be done in these area.
Overall, the practice of ginseng functional components in the treatment of NAFLD is derived from the TCM theory, and its efficacy has been further verified by modern studies. We summarise the relevant studies and confirm that ginseng and functional components achieve therapeutic effects on NAFLD through multi-components, multi-targets, and multi-pathways. We believe that ginseng and its functional components play an ameliorative role in NAFLD, which has a complex pathogenesis, through multi-targets, and have significant pharmacological effects on NAFLD, reducing hepatic lipid accumulation by regulating lipid uptake and transport, increasing lipid catabolism, and decreasing lipid synthesis and other related mechanisms. In addition, ginseng ameliorates hepatic steatosis and hepatocyte injury by inhibiting lipotoxicity-related factors and pathways to attenuate hepatocyte inflammation, ERS, OS, cell death, cellular senescence and gut microbiota disorders. All these can promote the understanding and application of ginseng to compensate for the lack of NAFLD drugs and the development of green and natural medicines, so that more NAFLD patients can be helped.
Author contributions
PX: Investigation, Visualization, Writing – original draft, Writing – review and editing. ZY: Formal Analysis, Investigation, Writing – review and editing. XL: Formal Analysis, Investigation, Writing – review and editing. QF: Supervision, Writing – review and editing. YS: Conceptualization, Funding acquisition, Writing – review and editing.
Funding
The author(s) declare that financial support was received for the research and/or publication of this article. The study was supported by the National Natural Science Foundation of China (Grant no. 82204956), Natural Science Foundation of Sichuan (Grant no. 2024NSFSC1856), Science and Technology Project of Sichuan Province (Grant no. 2022YFS0409), and the Chengdu University of Traditional Chinese Medicine 2024 Joint University-Institute Innovation Fund (LHJJ202402016).
Acknowledgments
The authors would like to thank all the participants in this study. All figures are created with BioRender and Visio.
Conflict of interest
The authors declare that the research was conducted in the absence of any commercial or financial relationships that could be construed as a potential conflict of interest.
Generative AI statement
The authors declare that no Generative AI was used in the creation of this manuscript.
Publisher’s note
All claims expressed in this article are solely those of the authors and do not necessarily represent those of their affiliated organizations, or those of the publisher, the editors and the reviewers. Any product that may be evaluated in this article, or claim that may be made by its manufacturer, is not guaranteed or endorsed by the publisher.
References
Aron-Wisnewsky, J., Vigliotti, C., Witjes, J., Le, P., Holleboom, A. G., Verheij, J., et al. (2020). Gut microbiota and human NAFLD: disentangling microbial signatures from metabolic disorders. Nat. Rev. Gastroenterol. Hepatol. 17 (5), 279–297. doi:10.1038/s41575-020-0269-9
Badmus, O. O., Hillhouse, S. A., Anderson, C. D., Hinds, T. D., and Stec, D. E. (2022). Molecular mechanisms of metabolic associated fatty liver disease (MAFLD): functional analysis of lipid metabolism pathways. Clin. Sci. (Lond) 136 (18), 1347–1366. doi:10.1042/cs20220572
Buzzetti, E., Pinzani, M., and Tsochatzis, E. A. (2016). The multiple-hit pathogenesis of non-alcoholic fatty liver disease (NAFLD). Metabolism 65 (8), 1038–1048. doi:10.1016/j.metabol.2015.12.012
Cao, Y., Fang, X., Sun, M., Zhang, Y., Shan, M., Lan, X., et al. (2023). Preventive and therapeutic effects of natural products and herbal extracts on nonalcoholic fatty liver disease/nonalcoholic steatohepatitis. Phytother. Res. 37 (9), 3867–3897. doi:10.1002/ptr.7932
Chen, J., and Vitetta, L. (2020). Gut microbiota metabolites in NAFLD pathogenesis and therapeutic implications. Int. J. Mol. Sci. 21 (15), 5214. doi:10.3390/ijms21155214
Chen, L., Wang, L., Ao, W., Chen, Y., Li, S., Huang, Z., et al. (2022). Bioinformatics study of the potential therapeutic effects of ginsenoside Rf in reversing nonalcoholic fatty liver disease. Biomed. Pharmacother. 149, 112879. doi:10.1016/j.biopha.2022.112879
Chen, M., Xie, Y., Gong, S., Wang, Y., Yu, H., Zhou, T., et al. (2021). Traditional Chinese medicine in the treatment of nonalcoholic steatohepatitis. Pharmacol. Res. 172, 105849. doi:10.1016/j.phrs.2021.105849
Chen, Z., Tian, R., She, Z., Cai, J., and Li, H. (2020). Role of oxidative stress in the pathogenesis of nonalcoholic fatty liver disease. Free Radic. Biol. Med. 152, 116–141. doi:10.1016/j.freeradbiomed.2020.02.025
Choi, N., Kim, J. W., Jeong, H., Shin, D. G., Seo, J. H., Kim, J. H., et al. (2019a). Fermented ginseng, GBCK25, ameliorates steatosis and inflammation in nonalcoholic steatohepatitis model. J. Ginseng Res. 43 (2), 196–208. doi:10.1016/j.jgr.2017.10.002
Choi, S. Y., Park, J. S., Shon, C. H., Lee, C. Y., Ryu, J. M., Son, D. J., et al. (2019b). Fermented Korean red ginseng extract enriched in Rd and Rg3 protects against non-alcoholic fatty liver disease through regulation of mTORC1. Nutrients 11 (12), 2963. doi:10.3390/nu11122963
Chung, S. I., Nam, S. J., Xu, M., Kang, M. Y., and Lee, S. C. (2016). Aged ginseng (Panax ginseng Meyer) reduces blood glucose levels and improves lipid metabolism in high fat diet-fed mice. Food Sci. Biotechnol. 25 (1), 267–273. doi:10.1007/s10068-016-0039-1
Clare, K., Dillon, J. F., and Brennan, P. N. (2022). Reactive oxygen species and oxidative stress in the pathogenesis of MAFLD. J. Clin. Transl. Hepatol. 10 (5), 939–946. doi:10.14218/jcth.2022.00067
Cui, T., Xiao, X., Pan, Z., Tang, K., Zhong, Y., Chen, Y., et al. (2023). Harnessing the therapeutic potential of ginsenoside Rd for activating SIRT6 in treating a mouse model of nonalcoholic fatty liver disease. ACS Omega 8 (32), 29735–29745. doi:10.1021/acsomega.3c04122
Devarbhavi, H., Asrani, S. K., Arab, J. P., Nartey, Y. A., Pose, E., and Kamath, P. S. (2023). Global burden of liver disease: 2023 update. J. Hepatol. 79 (2), 516–537. doi:10.1016/j.jhep.2023.03.017
Ebert, T., Widman, L., Stenvinkel, P., and Hagström, H. (2023). Increased risk for microvascular outcomes in NAFLD-A nationwide, population-based cohort study. J. Intern Med. 294 (2), 216–227. doi:10.1111/joim.13673
Friedman, S. L., Neuschwander-Tetri, B. A., Rinella, M., and Sanyal, A. J. (2018). Mechanisms of NAFLD development and therapeutic strategies. Nat. Med. 24 (7), 908–922. doi:10.1038/s41591-018-0104-9
Fujii, H., Kawada, N., and Japan Study Group Of Nafld, J.-N. (2020). The role of insulin resistance and diabetes in nonalcoholic fatty liver disease. Int. J. Mol. Sci. 21 (11), 3863. doi:10.3390/ijms21113863
Geng, Y., Faber, K. N., de Meijer, V. E., Blokzijl, H., and Moshage, H. (2021). How does hepatic lipid accumulation lead to lipotoxicity in non-alcoholic fatty liver disease? Hepatol. Int. 15 (1), 21–35. doi:10.1007/s12072-020-10121-2
Gu, D., Yi, H., Jiang, K., Fakhar, S. H., Shi, J., He, Y., et al. (2021). Transcriptome analysis reveals the efficacy of ginsenoside-Rg1 in the treatment of nonalcoholic fatty liver disease. Life Sci. 267, 118986. doi:10.1016/j.lfs.2020.118986
Guo, M., Zhu, C., Fu, R., Ma, X., Duan, Z., and Fan, D. (2023). Ginsenoside Rk3 regulates nonalcoholic steatohepatitis by modulation of intestinal flora and the PI3K/AKT signaling pathway in C57bl/6 mice. J. Agric. Food Chem. 71 (24), 9370–9380. doi:10.1021/acs.jafc.3c00789
Hong, S. H., Suk, K. T., Choi, S. H., Lee, J. W., Sung, H. T., Kim, C. H., et al. (2013). Anti-oxidant and natural killer cell activity of Korean red ginseng (Panax ginseng) and urushiol (Rhus vernicifera Stokes) on non-alcoholic fatty liver disease of rat. Food Chem. Toxicol. 55, 586–591. doi:10.1016/j.fct.2013.01.022
Hou, J., Ma, R., Zhu, S., and Wang, Y. (2022). Revealing the therapeutic targets and mechanism of ginsenoside Rg1 for liver damage related to anti-oxidative stress using proteomic analysis. Int. J. Mol. Sci. 23 (17), 10045. doi:10.3390/ijms231710045
Hsu, C. L., and Schnabl, B. (2023). The gut-liver axis and gut microbiota in health and liver disease. Nat. Rev. Microbiol. 21 (11), 719–733. doi:10.1038/s41579-023-00904-3
Hu, H., Lin, A., Kong, M., Yao, X., Yin, M., Xia, H., et al. (2020). Intestinal microbiome and NAFLD: molecular insights and therapeutic perspectives. J. Gastroenterol. 55 (2), 142–158. doi:10.1007/s00535-019-01649-8
Hua, M., Fan, M. L., Li, Z. M., Sha, J. Y., Li, S. S., and Sun, Y. S. (2021). Ginseng soluble dietary fiber can regulate the intestinal flora structure, promote colon health, affect appetite and glucolipid metabolism in rats. J. Funct. Foods 83, 104534. doi:10.1016/j.jff.2021.104534
Huang, Q., Wang, T., Yang, L., and Wang, H. Y. (2017). Ginsenoside Rb2 alleviates hepatic lipid accumulation by restoring autophagy via induction of Sirt1 and activation of AMPK. Int. J. Mol. Sci. 18 (5), 1063. doi:10.3390/ijms18051063
Huby, T., and Gautier, E. L. (2022). Immune cell-mediated features of non-alcoholic steatohepatitis. Nat. Rev. Immunol. 22 (7), 429–443. doi:10.1038/s41577-021-00639-3
Jeong, H., Kim, J. W., Yang, M. S., Park, C., Kim, J. H., Lim, C. W., et al. (2018). Beneficial effects of Korean red ginseng in the progression of non-alcoholic steatohepatitis via FABP4 modulation. Am. J. Chin. Med. 46, 1581–1607. doi:10.1142/s0192415x18500817
Khan, R. S., Bril, F., Cusi, K., and Newsome, P. N. (2019). Modulation of insulin resistance in nonalcoholic fatty liver disease. Hepatology 70 (2), 711–724. doi:10.1002/hep.30429
Kim, G. W., Jo, H. K., and Chung, S. H. (2018). Ginseng seed oil ameliorates hepatic lipid accumulation in vitro and in vivo. J. Ginseng Res. 42 (4), 419–428. doi:10.1016/j.jgr.2017.04.010
Kim, J. C., Jeon, J. Y., Yang, W. S., Kim, C. H., and Eom, D. W. (2019). Combined amelioration of ginsenoside (Rg1, Rb1, and rg3)-enriched Korean red ginseng and probiotic lactobacillus on non-alcoholic fatty liver disease. Curr. Pharm. Biotechnol. 20 (3), 222–231. doi:10.2174/1389201020666190311143554
Kim, K., Kim, M. H., Kang, J. I., Baek, J. I., Jeon, B. M., Kim, H. M., et al. (2024a). Ginsenoside F2 restrains hepatic steatosis and inflammation by altering the binding affinity of liver X receptor coregulators. J. Ginseng Res. 48 (1), 89–97. doi:10.1016/j.jgr.2023.10.001
Kim, M. S., Lee, K. T., Iseli, T. J., Hoy, A. J., George, J., Grewal, T., et al. (2013). Compound K modulates fatty acid-induced lipid droplet formation and expression of proteins involved in lipid metabolism in hepatocytes. Liver Int. 33 (10), 1583–1593. doi:10.1111/liv.12287
Kim, M. Y., Jeong, B., Lee, G. S., Jeon, H., Yang, Y. M., Yang, H., et al. (2024b). Panaxydol extracted from Panax ginseng inhibits NLRP3 inflammasome activation to ameliorate NASH-induced liver injury. Int. Immunopharmacol. 128, 111565. doi:10.1016/j.intimp.2024.111565
Kim, Y. W., Bak, S. B., Song, Y. R., Kim, C. E., and Lee, W. Y. (2024c). Systematic exploration of therapeutic effects and key mechanisms of Panax ginseng using network-based approaches. J. Ginseng Res. 48 (4), 373–383. doi:10.1016/j.jgr.2024.01.005
Kobayashi, T., Iwaki, M., Nakajima, A., Nogami, A., and Yoneda, M. (2022). Current research on the pathogenesis of NAFLD/NASH and the gut-liver Axis: gut microbiota, dysbiosis, and leaky-gut syndrome. Int. J. Mol. Sci. 23 (19), 11689. doi:10.3390/ijms231911689
Kolodziejczyk, A. A., Zheng, D., Shibolet, O., and Elinav, E. (2019). The role of the microbiome in NAFLD and NASH. EMBO Mol. Med. 11 (2), e9302. doi:10.15252/emmm.201809302
Kumar, S., Duan, Q., Wu, R., Harris, E. N., and Su, Q. (2021). Pathophysiological communication between hepatocytes and non-parenchymal cells in liver injury from NAFLD to liver fibrosis. Adv. Drug Deliv. Rev. 176, 113869. doi:10.1016/j.addr.2021.113869
Kwak, Y. B., Yoo, H. H., and Yoon, J. (2024). The impact of the administration of red ginseng (Panax ginseng) on lipid metabolism and free fatty acid profiles in healthy horses using a molecular networking approach. Front. Vet. Sci. 11, 1285000. doi:10.3389/fvets.2024.1285000
Lebeaupin, C., Vallée, D., Hazari, Y., Hetz, C., Chevet, E., and Bailly-Maitre, B. (2018). Endoplasmic reticulum stress signalling and the pathogenesis of non-alcoholic fatty liver disease. J. Hepatol. 69 (4), 927–947. doi:10.1016/j.jhep.2018.06.008
Lee, J. B., Yoon, S. J., Lee, S. H., Lee, M. S., Jung, H., Kim, T. D., et al. (2017). Ginsenoside Rg3 ameliorated HFD-induced hepatic steatosis through downregulation of STAT5-PPARγ. J. Endocrinol. 235 (3), 223–235. doi:10.1530/joe-17-0233
Lee, S., Lee, M. S., Kim, C. T., Kim, I. H., and Kim, Y. (2012). Ginsenoside Rg3 reduces lipid accumulation with AMP-Activated Protein Kinase (AMPK) activation in HepG2 cells. Int. J. Mol. Sci. 13 (5), 5729–5739. doi:10.3390/ijms13055729
Lee, S. B., Cho, H. I., Jin, Y. W., Lee, E. K., Ahn, J. Y., and Lee, S. M. (2016). Wild ginseng cambial meristematic cells ameliorate hepatic steatosis and mitochondrial dysfunction in high-fat diet-fed mice. J. Pharm. Pharmacol. 68 (1), 119–127. doi:10.1111/jphp.12487
Lei, N., Song, H., Zeng, L., Ji, S., Meng, X., Zhu, X., et al. (2023). Persistent lipid accumulation leads to persistent exacerbation of endoplasmic reticulum stress and inflammation in progressive NASH via the ire1α/TRAF2 complex. Molecules 28 (7), 3185. doi:10.3390/molecules28073185
Leng, Y. R., Zhang, M. H., Luo, J. G., and Zhang, H. (2021). Pathogenesis of NASH and promising natural products. Chin. J. Nat. Med. 19 (1), 12–27. doi:10.1016/s1875-5364(21)60002-x
Li, F., Wu, Z., and Sui, X. (2020). Biotransformation of ginsenoside Rb1 with wild Cordyceps sinensis and Ascomycota sp. and its antihyperlipidemic effects on the diet-induced cholesterol of zebrafish. J. Food Biochem. 44 (6), e13192. doi:10.1111/jfbc.13192
Li, G., Wang, H., Dong, Z. Q., Wang, Y. P., Wang, T., Yao, X., et al. (2024a). Development situation and trends of Ginseng Radix et Rhizoma industry in China. Zhongguo Zhong Yao Za Zhi 49 (17), 4818–4828. doi:10.19540/j.cnki.cjcmm.20240615.102
Li, G., Xie, H., Cao, X., Ma, C., Li, Y., and Chen, L. (2022a). Ginsenoside Rg1 exerts anti-apoptotic effects on non-alcoholic fatty liver cells by downregulating the expression of SGPL1. Mol. Med. Rep. 25 (5), 178. doi:10.3892/mmr.2022.12694
Li, X., Liu, J., Zuo, T. T., Hu, Y., Li, Z., Wang, H. D., et al. (2022b). Advances and challenges in ginseng research from 2011 to 2020: the phytochemistry, quality control, metabolism, and biosynthesis. Nat. Prod. Rep. 39 (4), 875–909. doi:10.1039/d1np00071c
Li, X., Xu, X., Tao, S., Su, Y., Wen, L., Wang, D., et al. (2024b). Gut microbes combined with metabolomics reveal the protective effects of Qijia Rougan decoction against CCl(4)-induced hepatic fibrosis. Front. Pharmacol. 15, 1347120. doi:10.3389/fphar.2024.1347120
Li, X., Zhou, L., Xu, X., Liu, X., Wu, W., Feng, Q., et al. (2024c). Metabolic reprogramming in hepatocellular carcinoma: a bibliometric and visualized study from 2011 to 2023. Front. Pharmacol. 15, 1392241. doi:10.3389/fphar.2024.1392241
Li, Y., Yang, B., Guo, W., Zhang, P., Zhang, J., Zhao, J., et al. (2022c). Classification of three types of ginseng samples based on ginsenoside profiles: appropriate data normalization improves the efficiency of multivariate analysis. Heliyon 8 (12), e12044. doi:10.1016/j.heliyon.2022.e12044
Li, Y., Zhang, S., Zhu, Z., Zhou, R., Xu, P., Zhou, L., et al. (2022d). Upregulation of adiponectin by Ginsenoside Rb1 contributes to amelioration of hepatic steatosis induced by high fat diet. J. Ginseng Res. 46 (4), 561–571. doi:10.1016/j.jgr.2021.10.005
Liang, W., Zhou, K., Jian, P., Chang, Z., Zhang, Q., Liu, Y., et al. (2021). Ginsenosides improve nonalcoholic fatty liver disease via integrated regulation of gut microbiota, inflammation and energy homeostasis. Front. Pharmacol. 12, 622841. doi:10.3389/fphar.2021.622841
Liu, C., Huang, R., Yu, H., Gong, Y., Wu, P., Feng, Q., et al. (2022). Fuzheng Xiaozheng prescription exerts anti-hepatocellular carcinoma effects by improving lipid and glucose metabolisms via regulating circRNA-miRNA-mRNA networks. Phytomedicine 103, 154226. doi:10.1016/j.phymed.2022.154226
Loomba, R., Friedman, S. L., and Shulman, G. I. (2021). Mechanisms and disease consequences of nonalcoholic fatty liver disease. Cell 184 (10), 2537–2564. doi:10.1016/j.cell.2021.04.015
Lou, T. W., Yang, R. X., and Fan, J. G. (2024). The global burden of fatty liver disease: the major impact of China. Hepatobiliary Surg. Nutr. 13 (1), 119–123. doi:10.21037/hbsn-23-556
Lu, B., Wang, D., Xie, D., Wu, C., and Sun, M. (2023). 20(S)-Protopanaxatriol ameliorates MAFLD by inhibiting NLRP3 inflammasome. Eur. J. Pharmacol. 940, 175468. doi:10.1016/j.ejphar.2022.175468
Lu, G., Liu, Z., Wang, X., and Wang, C. (2021). Recent advances in Panax ginseng C.A. Meyer as a herb for anti-fatigue: an effects and mechanisms review. Foods 10 (5), 1030. doi:10.3390/foods10051030
Ma, R., Yang, P., Jing, C., Fu, B., Teng, X., Zhao, D., et al. (2023). Comparison of the metabolomic and proteomic profiles associated with triterpene and phytosterol accumulation between wild and cultivated ginseng. Plant Physiol. Biochem. 195, 288–299. doi:10.1016/j.plaphy.2023.01.020
Mahlapuu, M., Caputo, M., Xia, Y., and Cansby, E. (2022). GCKIII kinases in lipotoxicity: roles in NAFLD and beyond. Hepatol. Commun. 6 (10), 2613–2622. doi:10.1002/hep4.2013
Malesza, I. J., Malesza, M., Walkowiak, J., Mussin, N., Walkowiak, D., Aringazina, R., et al. (2021). High-fat, western-style diet, systemic inflammation, and gut microbiota: a narrative review. Cells 10 (11), 3164. doi:10.3390/cells10113164
Mato, J. M., Alonso, C., Noureddin, M., and Lu, S. C. (2019). Biomarkers and subtypes of deranged lipid metabolism in non-alcoholic fatty liver disease. World J. Gastroenterol. 25 (24), 3009–3020. doi:10.3748/wjg.v25.i24.3009
McComb, S., Chan, P. K., Guinot, A., Hartmannsdottir, H., Jenni, S., Dobay, M. P., et al. (2019). Efficient apoptosis requires feedback amplification of upstream apoptotic signals by effector caspase-3 or -7. Sci. Adv. 5 (7), eaau9433. doi:10.1126/sciadv.aau9433
Meng, Z., Lu, J., Ge, G., Wang, G., Zhang, R., Li, Y., et al. (2023). Ginsenoside Rb1 induces autophagic lipid degradation via miR-128 targeting TFEB. Food Funct. 14 (1), 240–249. doi:10.1039/d2fo02719d
Mi, A., Hu, Q., Liu, Y., Zhao, Y., Shen, F., Lan, J., et al. (2024). Hepatoprotective efficacy and interventional mechanism of the panaxadiol saponin component in high-fat diet-induced NAFLD mice. Food Funct. 15 (2), 794–808. doi:10.1039/d3fo03572g
Mouries, J., Brescia, P., Silvestri, A., Spadoni, I., Sorribas, M., Wiest, R., et al. (2019). Microbiota-driven gut vascular barrier disruption is a prerequisite for non-alcoholic steatohepatitis development. J. Hepatol. 71 (6), 1216–1228. doi:10.1016/j.jhep.2019.08.005
Mridha, A. R., Wree, A., Robertson, A. A. B., Yeh, M. M., Johnson, C. D., Van Rooyen, D. M., et al. (2017). NLRP3 inflammasome blockade reduces liver inflammation and fibrosis in experimental NASH in mice. J. Hepatol. 66 (5), 1037–1046. doi:10.1016/j.jhep.2017.01.022
Nan, B., Liu, Y. L., You, Y., Li, W. C., Fan, J. J., Wang, Y. S., et al. (2018). Protective effects of enhanced minor ginsenosides in Lactobacillus fermentum KP-3-fermented ginseng in mice fed a high fat diet. Food Funct. 9 (11), 6020–6028. doi:10.1039/c8fo01056k
Newsome, P. N., Buchholtz, K., Cusi, K., Linder, M., Okanoue, T., Ratziu, V., et al. (2021). A placebo-controlled trial of subcutaneous semaglutide in nonalcoholic steatohepatitis. N. Engl. J. Med. 384 (12), 1113–1124. doi:10.1056/NEJMoa2028395
Oh, G. S., Yoon, J., Lee, G. G., Oh, W. K., and Kim, S. W. (2015). 20(S)-protopanaxatriol inhibits liver X receptor α-mediated expression of lipogenic genes in hepatocytes. J. Pharmacol. Sci. 128 (2), 71–77. doi:10.1016/j.jphs.2015.05.007
Park, C. H., Kim, M., Woo, M., Lee, K. H., An, B. K., Yokozawa, T., et al. (2018). Comparison of the effects of nonfermented and fermented Panax ginseng root against hypertriglycemia in high-fat diet-fed mice. J. Med. Food 21 (4), 317–321. doi:10.1089/jmf.2017.4035
Park, J., Zhao, Y., Zhang, F., Zhang, S., Kwong, A. C., Zhang, Y., et al. (2023). IL-6/STAT3 axis dictates the PNPLA3-mediated susceptibility to non-alcoholic fatty liver disease. J. Hepatol. 78 (1), 45–56. doi:10.1016/j.jhep.2022.08.022
Park, S. K., Kim, S. W., Seo, H. W., Hyun, S. H., Kyung, J. S., Youn, S. H., et al. (2022). Long-term evaluation of safety and biological effects of Korean Red Ginseng (Panax Ginseng): a long-term in vivo study. BMC Complement. Med. Ther. 22 (1), 284. doi:10.1186/s12906-022-03736-5
Paternostro, R., and Trauner, M. (2022). Current treatment of non-alcoholic fatty liver disease. J. Intern Med. 292 (2), 190–204. doi:10.1111/joim.13531
Peiseler, M., Schwabe, R., Hampe, J., Kubes, P., Heikenwälder, M., and Tacke, F. (2022). Immune mechanisms linking metabolic injury to inflammation and fibrosis in fatty liver disease - novel insights into cellular communication circuits. J. Hepatol. 77 (4), 1136–1160. doi:10.1016/j.jhep.2022.06.012
Piao, X., Zhang, H., Kang, J. P., Yang, D. U., Li, Y., Pang, S., et al. (2020). Advances in saponin diversity of Panax ginseng. Molecules 25 (15), 3452. doi:10.3390/molecules25153452
Potenza, M. A., Montagnani, M., Santacroce, L., Charitos, I. A., and Bottalico, L. (2023). Ancient herbal therapy: a brief history of Panax ginseng. J. Ginseng Res. 47 (3), 359–365. doi:10.1016/j.jgr.2022.03.004
Qi, R., Jiang, R., Xiao, H., Wang, Z., He, S., Wang, L., et al. (2020). Ginsenoside Rg1 protects against d-galactose induced fatty liver disease in a mouse model via FOXO1 transcriptional factor. Life Sci. 254, 117776. doi:10.1016/j.lfs.2020.117776
Quek, J., Chan, K. E., Wong, Z. Y., Tan, C., Tan, B., Lim, W. H., et al. (2023). Global prevalence of non-alcoholic fatty liver disease and non-alcoholic steatohepatitis in the overweight and obese population: a systematic review and meta-analysis. Lancet Gastroenterol. Hepatol. 8 (1), 20–30. doi:10.1016/s2468-1253(22)00317-x
Ratan, Z. A., Haidere, M. F., Hong, Y. H., Park, S. H., Lee, J. O., Lee, J., et al. (2021). Pharmacological potential of ginseng and its major component ginsenosides. J. Ginseng Res. 45 (2), 199–210. doi:10.1016/j.jgr.2020.02.004
Roh, E., Hwang, H. J., Kim, J. W., Hong, S. H., Kim, J. A., Lee, Y. B., et al. (2020). Ginsenoside Mc1 improves liver steatosis and insulin resistance by attenuating ER stress. J. Ethnopharmacol. 259, 112927. doi:10.1016/j.jep.2020.112927
Sakurai, Y., Kubota, N., Yamauchi, T., and Kadowaki, T. (2021). Role of insulin resistance in MAFLD. Int. J. Mol. Sci. 22 (8), 4156. doi:10.3390/ijms22084156
Senft, D., and Ronai, Z. A. (2015). UPR, autophagy, and mitochondria crosstalk underlies the ER stress response. Trends Biochem. Sci. 40 (3), 141–148. doi:10.1016/j.tibs.2015.01.002
Shen, L., Xiong, Y., Wang, D. Q., Howles, P., Basford, J. E., Wang, J., et al. (2013). Ginsenoside Rb1 reduces fatty liver by activating AMP-activated protein kinase in obese rats. J. Lipid Res. 54 (5), 1430–1438. doi:10.1194/jlr.M035907
Shojaie, L., Iorga, A., and Dara, L. (2020). Cell death in liver diseases: a review. Int. J. Mol. Sci. 21 (24), 9682. doi:10.3390/ijms21249682
Taru, V., Szabo, G., Mehal, W., and Reiberger, T. (2024). Inflammasomes in chronic liver disease: hepatic injury, fibrosis progression and systemic inflammation. J. Hepatol. 81 (5), 895–910. doi:10.1016/j.jhep.2024.06.016
Terrault, N. A., Francoz, C., Berenguer, M., Charlton, M., and Heimbach, J. (2023). Liver transplantation 2023: status report, current and future challenges. Clin. Gastroenterol. Hepatol. 21 (8), 2150–2166. doi:10.1016/j.cgh.2023.04.005
Vallianou, N., Christodoulatos, G. S., Karampela, I., Tsilingiris, D., Magkos, F., Stratigou, T., et al. (2021). Understanding the role of the gut microbiome and microbial metabolites in non-alcoholic fatty liver disease: current evidence and perspectives. Biomolecules 12 (1), 56. doi:10.3390/biom12010056
Wang, C., Tang, X., Yan, F., Wang, Y., Wang, X., Zhao, D., et al. (2025). Different processing methods affect the chemical composition and in vitro anti-tumor activity of ginseng. J. Food Sci. 90 (1), e17639. doi:10.1111/1750-3841.17639
Wang, F., Park, J. S., Ma, Y., Ma, H., Lee, Y. J., Lee, G. R., et al. (2021). Ginseng saponin enriched in Rh1 and Rg2 ameliorates nonalcoholic fatty liver disease by inhibiting inflammasome activation. Nutrients 13 (3), 856. doi:10.3390/nu13030856
Wang, Y. D., Wu, L. L., Qi, X. Y., Wang, Y. Y., Liao, Z. Z., Liu, J. H., et al. (2023). New insight of obesity-associated NAFLD: dysregulated “crosstalk” between multi-organ and the liver? Genes Dis. 10 (3), 799–812. doi:10.1016/j.gendis.2021.12.013
Wei, S., Wang, L., Evans, P. C., and Xu, S. (2024). NAFLD and NASH: etiology, targets and emerging therapies. Drug Discov. Today 29 (3), 103910. doi:10.1016/j.drudis.2024.103910
Wiering, L., and Tacke, F. (2023). Treating inflammation to combat non-alcoholic fatty liver disease. J. Endocrinol. 256 (1), e220194. doi:10.1530/joe-22-0194
Xiao, Q., Zhang, S., Yang, C., Du, R., Zhao, J., Li, J., et al. (2019). Ginsenoside Rg1 ameliorates palmitic acid-induced hepatic steatosis and inflammation in HepG2 cells via the AMPK/NF-κB pathway. Int. J. Endocrinol. 2019, 7514802. doi:10.1155/2019/7514802
Xu, G., Quan, S., Schell, J., Gao, Y., Varmazyad, M., Sreenivas, P., et al. (2024). Mitochondrial ACSS1-K635 acetylation knock-in mice exhibit altered metabolism, cell senescence, and nonalcoholic fatty liver disease. Sci. Adv. 10 (20), eadj5942. doi:10.1126/sciadv.adj5942
Xu, H., Zhao, Q., Song, N., Yan, Z., Lin, R., Wu, S., et al. (2020). AdipoR1/AdipoR2 dual agonist recovers nonalcoholic steatohepatitis and related fibrosis via endoplasmic reticulum-mitochondria axis. Nat. Commun. 11 (1), 5807. doi:10.1038/s41467-020-19668-y
Xu, X., Poulsen, K. L., Wu, L., Liu, S., Miyata, T., Song, Q., et al. (2022). Targeted therapeutics and novel signaling pathways in non-alcohol-associated fatty liver/steatohepatitis (NAFL/NASH). Signal Transduct. Target Ther. 7 (1), 287. doi:10.1038/s41392-022-01119-3
Xu, Y., Yang, C., Zhang, S., Li, J., Xiao, Q., and Huang, W. (2018). Ginsenoside Rg1 protects against non-alcoholic fatty liver disease by ameliorating lipid peroxidation, endoplasmic reticulum stress, and inflammasome activation. Biol. Pharm. Bull. 41 (11), 1638–1644. doi:10.1248/bpb.b18-00132
Xuan, P., De-Bin, H., Mi-Ya, Y., and Shi-Jun, P. (2015). Ginsenoside Rg1 improves liver function by regulating fat metabolism in rats with non-alcoholic fatty liver disease. Chin. J. Pathophysiol. 31 (5), 864–870. doi:10.3892/mmr.2022.12694
Yang, K., Kim, H. H., Shim, Y. R., and Song, M. J. (2023a). The efficacy of Panax ginseng for the treatment of nonalcoholic fatty liver disease: a systematic review and meta-analysis of preclinical studies. Nutrients 15 (3), 721. doi:10.3390/nu15030721
Yang, Z., Yu, Y., Sun, N., Zhou, L., Zhang, D., Chen, H., et al. (2023b). Ginsenosides Rc, as a novel SIRT6 activator, protects mice against high fat diet induced NAFLD. J. Ginseng Res. 47 (3), 376–384. doi:10.1016/j.jgr.2020.07.005
Younossi, Z. M., Stepanova, M., Nader, F., Loomba, R., Anstee, Q. M., Ratziu, V., et al. (2022). Obeticholic acid impact on quality of life in patients with nonalcoholic steatohepatitis: REGENERATE 18-month interim analysis. Clin. Gastroenterol. Hepatol. 20 (9), 2050–2058.e12. doi:10.1016/j.cgh.2021.07.020
Younossi, Z. M., Zelber-Sagi, S., Henry, L., and Gerber, L. H. (2023). Lifestyle interventions in nonalcoholic fatty liver disease. Nat. Rev. Gastroenterol. Hepatol. 20 (11), 708–722. doi:10.1038/s41575-023-00800-4
Yu, L., Hong, W., Lu, S., Li, Y., Guan, Y., Weng, X., et al. (2022). The NLRP3 inflammasome in non-alcoholic fatty liver disease and steatohepatitis: therapeutic targets and treatment. Front. Pharmacol. 13, 780496. doi:10.3389/fphar.2022.780496
Zhang, J., and Feng, Q. (2022). Pharmacological effects and molecular protective mechanisms of Astragalus polysaccharides on nonalcoholic fatty liver disease. Front. Pharmacol. 13, 854674. doi:10.3389/fphar.2022.854674
Zhang, J., Guo, J., Yang, N., Huang, Y., Hu, T., and Rao, C. (2022). Endoplasmic reticulum stress-mediated cell death in liver injury. Cell Death Dis. 13 (12), 1051. doi:10.1038/s41419-022-05444-x
Zhao, C., Qu, Q., Yang, F., Li, Z., Yang, P., Han, L., et al. (2021). Monascus ruber fermented Panax ginseng ameliorates lipid metabolism disorders and modulate gut microbiota in rats fed a high-fat diet. J. Ethnopharmacol. 278, 114300. doi:10.1016/j.jep.2021.114300
Zhou, G., Wang, C. Z., Mohammadi, S., Sawadogo, W. R., Ma, Q., and Yuan, C. S. (2023). Pharmacological effects of ginseng: multiple constituents and multiple actions on humans. Am. J. Chin. Med. 51 (5), 1085–1104. doi:10.1142/s0192415x23500507
Glossary
ACC acetyl-coenzyme A carboxylase
ACOX acyl-coenzyme A oxidase
AKP alkaline phosphatase
AKT protein kinase B
ALT alanine aminotransferase
AMPK AMP-activated protein kinase
AST aspartate aminotransferase
ATF activating transcription factor
Bax Bcl-2-associated X
Bcl-2 B-cell lymphoma-2
Bcl-XL B-cell lymphoma-extra large
Caspase cysteine-containing aspartate-specific protease
CCL C-C motif chemokine ligand
CDKN cyclin-dependent kinase inhibitor
CHOP CCAAT/enhancer binding protein (C/EBP) homologous protein
ChREBP carbohydrate responsive element-binding protein
COL collagen
CPT carnitine palmitoyl transferase
CYP7A1 cholesterol 7α-hydroxylase
ERS endoplasmic reticulum stress
FA fatty acid
FAS fatty acid synthase
FBG fasting blood-glucose
FFAs free fatty acids
FG fermented ginseng
FRG fermentation of red ginseng with C. militaris
FXR farnesoid X receptor
GDS ginseng diol saponin
GRP78 glucose-regulated protein78
GSO ginseng seed oil
GWDF ginseng water-soluble dietary fiber
HDL-C high density lipoprotein cholesterol
HMGCR 3-hydroxy-3-methylglutaryl-coenzyme A reductase
HOMA-IR insulin resistance index
IL interleukin
IR insulin resistance
JNK c-Jun N-terminal kinase
LDL low density lipid protein
LDL-C low density lipoprotein cholesterol
LDs lipid droplets
LFG lactobacillus fermentum KP-3 fermented ginseng
LXR liver X receptor
MDA malondialdehyde
MFG monascus ruber fermented ginseng
NAFLD non-alcoholic fatty liver disease
NASH non-alcoholic steatohepatitis
NF-κB nuclear factor kappa-B
NLRP3 nod-like receptor thermal protein domain associated protein 3
Nrf2 nuclear factor-erythroid 2 related factor 2
PGC-1α peroxisome proliferators-activated receptor γ co-activator α
PI3K phosphatidylinositol-3-kinase
PPAR peroxisome proliferator-activated receptor
PPD protopanaxadiol
PPT protopanaxatriol
RG red ginseng
ROS reactive oxygen species
SCD-1 stearoyl-coenzyme A desaturase-1
SIRT sirtuins
SOD superoxide dismutase
SREBP sterol-regulatory element binding protein
STAT signal transducer and activator of transcription
TC total cholesterol
TCM traditional Chinese medicine
TG triglyceride
TIMP tissue inhibitor of metalloproteinase 1
TNF-α tumor necrosis factor α
UPR unfolded protein response
WGCM wild ginseng cambial meristematic cells
Keywords: ginseng (Panax ginseng), non-alcoholic fatty liver disease (NAFLD), lipid metabolism, lipotoxic injury, multi-target pharmacological mechanism
Citation: Xiao P, Ye Z, Li X, Feng Q and Su Y (2025) Ginseng and its functional components in non-alcoholic fatty liver disease: therapeutic effects and multi-target pharmacological mechanisms. Front. Pharmacol. 16:1540255. doi: 10.3389/fphar.2025.1540255
Received: 05 December 2024; Accepted: 28 March 2025;
Published: 09 April 2025.
Edited by:
Yu-Jie Liu, Shanxi University of Chinese Medicine, ChinaReviewed by:
Qingsong Qu, Beijing University of Chinese Medicine, ChinaFangtong Li, Changchun University of Chinese Medicine, China
Copyright © 2025 Xiao, Ye, Li, Feng and Su. This is an open-access article distributed under the terms of the Creative Commons Attribution License (CC BY). The use, distribution or reproduction in other forums is permitted, provided the original author(s) and the copyright owner(s) are credited and that the original publication in this journal is cited, in accordance with accepted academic practice. No use, distribution or reproduction is permitted which does not comply with these terms.
*Correspondence: Quansheng Feng, ZmVuZ3FzMTE4QDE2My5jb20=; Yue Su, c3V5dWVAY2R1dGNtLmVkdS5jbg==