- 1Department of Clinical Korean Medicine, Graduate School, Kyung Hee University, Seoul, Republic of Korea
- 2Department of Korean Internal Medicine, Kyung Hee University College of Korean Medicine, Kyung Hee University Hospital at Gangdong, Seoul, Republic of Korea
- 3Department of Digestive Diseases, College of Korean Medicine, Kyung Hee University, Seoul, Republic of Korea
- 4Division of Digestive Diseases, Department of Korean Internal Medicine, Kyung Hee University Korean Medicine Hospital, Seoul, Republic of Korea
- 5Department of Herbal Pharmacology, College of Korean Medicine, Kyung Hee University, Seoul, Republic of Korea
Background: Bojanggunbi-tang (BGT), a herbal prescription used in traditional Korean medicine, has been used to treat various gastrointestinal (GI) diseases.
Methods: Studies on BGT published until May 2024 were retrieved from the electronic databases of Medline, CENTRAL, Embase, AMED, CNKI, CiNii, Kmbase, KISS, NDSL, and OASIS using GI-related terms. All study types, regardless of the research method or language, were eligible for inclusion. Additional articles on Lonicera japonica, Atractylodes macrocephala, and Alisma canaliculatum, which are key components of BGT, were retrieved from the databases of Medline, CENTRAL, Embase, and Web of Science using GI-specific terms. The basic information, research models, administration methods, evaluation methods, and treatment outcomes of the selected studies were examined subsequently.
Results: Fourteen studies, comprising nine animal studies, one cell-based study, and four human studies, were included in the final analysis. BGT was found to exhibit anti-inflammatory effects, promote restoration of the gastrointestinal mucosa, and regulate GI motility. Analysis of the key herbal components L. japonica, A. macrocephala, and A. canaliculatum revealed that they inhibit inflammatory cytokines and oxidative substances, regulate serotonin and cholinergic pathways, and modulate intestinal microbiota.
Conclusion: This scoping review confirmed the therapeutic potential and mechanisms of action of BGT and its main components, L. japonica, A. macrocephala, and A. canaliculatum, thereby indicating its ability to enhance GI health. Further studies, including randomized clinical trials, must be conducted in the future to confirm these findings.
Scoping review registration: The study was registered in OSF, an international scoping review database: https://doi.org/10.17605/OSF.IO/ATU4S.
1 Introduction
Bojanggunbi-tang (BGT; 補腸健脾湯) is a combination of the herbal prescriptions “Daehwajungeum” and “Sambaek-tang” from ancient Chinese medical literature. It comprises 16 herbs, namely, Lonicera japonica, Atractylodes macrocephala, Paeonia lactiflora, D. lablab, Dioscorea japonica, Crataegus pinnatifida, Poria cocos, Magnolia officinalis, Citrus unshiu, Alisma canaliculatum, Massa medicata, Hordeum vulgare, Zingiber officinale, Aucklandia lappa, Amomum villosum, and Glycyrrhiza uralensis. BGT has been used to treat several diseases, such as acute gastritis, colitis, inflammatory bowel disease (IBD), and gastrointestinal (GI) symptoms (e.g., abdominal pain, indigestion, and diarrhea) in Korea (Joun et al., 1994). Numerous herbs have been used to tonify the spleen and replenish qi (補脾益氣), tonify blood and yin (補血養陰), and induce diuresis to drain dampness (). BGT relieves dampness and improves deficiency syndromes such as spleen deficiency and yin or yang deficiency patterns (Nam et al., 1989). Ryu et al. revealed that BGT was prescribed to over 310,000 patients with IBD or other forms of colitis at a Korean Medical Center between 1994 and 2010 (Ryu et al., 2011). A modified version of BGT (mBGT), comprising the herbs L. japonica, A. macrocephala, and A. canaliculatum, has demonstrated equally favorable effects on colitis (Kim et al., 2021). However, no randomized controlled trial (RCT) or systematic review (SR) has evaluated the efficacy of this formulation, thereby limiting the comprehensive understanding of its effects and mechanisms.
Scoping reviews constitute a flexible and rigorous approach to synthesizing evidence that can address broader research questions (Rodger et al., 2024). Scoping reviews identify different types of evidence available in a particular field, summarize existing evidence, and make recommendations for future research (Tricco et al., 2016). This scoping review summarized the findings of clinical and basic studies on BGT and its main herbal components, L. japonica, A. macrocephala, and A. canaliculatum, which have demonstrated efficacy in previous experimental studies. Furthermore, the present study also analyzed the characteristics and mechanisms of BGT and its constituent herbs as treatments for GI dysfunction to clarify their efficacy and provide insights for future research.
2 Methods
This review adhered to the Preferred Reporting Items for Systematic Reviews and Meta-Analysis (PRISMA) Extension for Scoping Reviews (PRISMA-ScR), a reporting guideline for scoping reviews (Supplementary Table S1). It was also registered in OSF, an international scoping review database (https://doi.org/10.17605/OSF.IO/ATU4S).
Articles related to BGT published up to 2024 were retrieved from the databases of MEDLINE (via PubMed), Cochrane Central Register of Controlled Trials (CENTRAL), EMBASE, Allied and Complementary Medicine Database (AMED), China National Knowledge Infrastructure (CNKI), Citation Information by Nii (CiNii), Korean Medical Database (Kmbase), Korean Studies Information Service System (KISS), National Digital Science Library (NDSL), and Oriental Medicine Advanced Searching Integrated System (OASIS). No restrictions were applied in terms of language or publication dates.
The search terms used to retrieve the articles were as follows: “Bojanggunbi-tang,” “Bojanggunbitang,” “Bojangkunbi-tang,” and “補腸健脾湯.” In addition, the following terms related to GI conditions were also used to retrieve articles: “gastrointestinal,” “gastr*,” “intestin*,” “colon,” “bowel,” “colitis,” and “Crohn” (Supplementary Table S2). All types of studies, including in vitro, in vivo, and clinical studies, that focused on BGT were eligible for inclusion. Fourteen studies, comprising one in vitro study, nine animal studies, and four human studies (Figure 1), were retained after removing duplicates and unrelated studies.
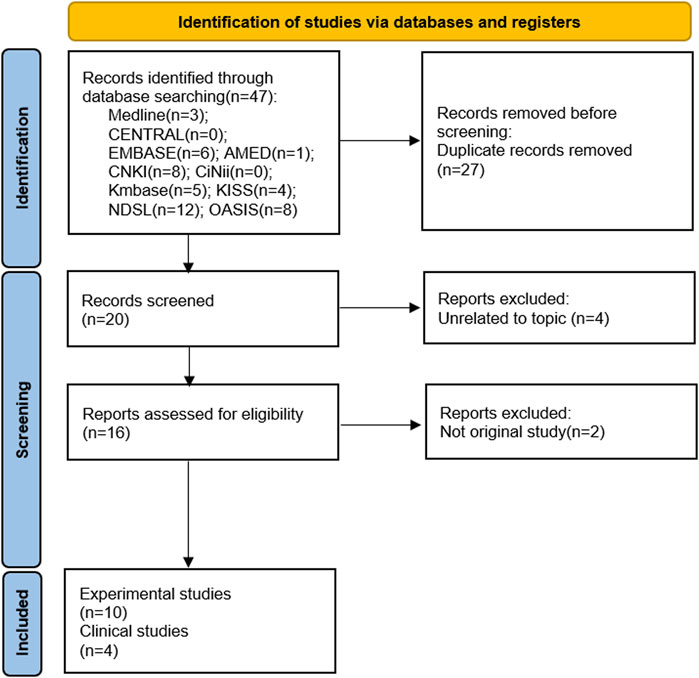
Figure 1. Preferred Reporting Items for Systematic reviews and Meta-Analyses (PRISMA) flow checklist for the study.
Three key herbal components of BGT, namely, L. japonica Thunb. (LJT) and A. macrocephala Koidz. (AMK), and A. canaliculatum A. Braun & C.D.Bouche (AC), were identified through further analysis. An additional search was conducted to retrieve articles related to these herbs from the databases of MEDLINE (via PubMed), CENTRAL, EMBASE, and the Web of Science published until May 2024 to better understand their individual roles. The search terms used to retrieve the articles were as follows: “L. japonica,” “Atractylodes macrocephala,” and “Alisma,” and GI-related terms such as “gastrointestinal,” “gastr*,” “intestin*,” “colon,” “bowel,” “colitis,” and “Crohn” (Supplementary Figure S2). Studies unrelated to GI function or studies evaluating these herbs in combination with other herbs were excluded. This secondary search yielded 11 studies on LJT, 37 studies on AMK, and two studies on AC, which were included in the final analysis (Supplementary Figures S1–S3).
The basic information, research models, administration methods, evaluation methods, and treatment outcomes of each of the selected studies were evaluated. Basic study information included the year of publication and study design. The methods of administering the herbal extracts were analyzed according to the extraction method, concentration, dosage, route of administration, duration, and number of sessions.
3 Results
3.1 Preclinical studies
Several studies have assessed the efficacy of BGT and its modified forms (Table 1). The first study exploring the effects of BGT on the GI tract was conducted by Nam et al. (1989). They measured the levels of gastric juice secretion, acidity, the area of ethanol-induced ulcers, intestinal transportability, and anti-cathartic action to evaluate the effects of BGT. BGT exerted a significant inhibitory effect on gastric juice secretion, reduced acidity, and alleviated ethanol-induced gastric ulcers. Similarly, the study conducted by Joun et al. (1994) revealed that BGT prevented the formation of ulcers induced by pylorus ligation and indomethacin. Furthermore, it exerted inhibitory effects on gastric juice secretion and smooth muscle contractions in the ileum and stomach, reduced free and total acidity, and decreased the transport ability in the large intestine. Ro et al. (2006) demonstrated that BGT inhibited smooth muscle contractions in the ileum, indicating its anti-cathartic effects. They further reported that BGT suppressed transportability in the small and large intestines and prevented diarrhea induced by castor oil, pilocarpine, and barium chloride. Kim et al. (1993) investigated the effects of several traditional herbal medicines used as energy invigorators, including BGT, on serum components and intestinal bacteria. BGT increased the count of lactic acid bacteria in the intestinal flora; furthermore, it regulated the fecal β-glucuronidase activity. Collectively, the findings of these studies indicate that BGT exerts an anti-ulcer effect in the stomach by suppressing the secretion of gastric juice. BGT exhibits anti-diarrheal effects by regulating intestinal transportability and fostering a balance in the intestinal microenvironment. However, studies conducted before 2000 reported conflicting results regarding the transportability and anti-diarrheal effects of BGT, likely due to biases introduced by the experimental methods used. For transportability assessment, the distance traveled by orally administered solutions was calculated through GI tract resection, a method that does not accurately reflect the transportability of each organ. Similarly, the anti-diarrheal effects were subjectively scored based on stool form, leading to a lack of objectivity and reproducibility. In contrast, studies conducted since the 2000s have shown more consistent results by employing more robust and objective measures, such as inflammation markers and histological scores.
Most subsequent studies focused on the efficacy of BGT in the treatment of colitis. Most of these studies employed colitis models created using dextran sulfate sodium (DSS), a substance that is toxic to the gut epithelium. DSS induced acute colitis in mice by compromising the mucosal barrier. Acute colitis in mice exhibits symptoms similar to those of human ulcerative colitis, such as weight loss, colon shortening, diarrhea, bloody stools, and colonic mucosal ulceration (Eggera et al., 2000).
Ryu et al. (2011) examined the effect of BGT on DSS-induced ulcerative colitis and a 2,4,6-trinitrobenzene sulfonic acid (TNBS)-induced CD model. The administration of BGT for 7 days following the induction of colitis revealed the protective effects of BGT against weight loss, colon shortening, and histological changes in the colon, as well as the inhibition of key inflammatory cytokines in the DSS model. BGT prevented colon shortening and improved the survival rate in the TNBS model. Significant improvements in colitis were also observed following the administration of BGT essence and modified BGT (mBGT) in the study by Ko et al., as evidenced by increased colon length, better pathology scores, reduced histological damage, and inhibition of pro-inflammatory cytokines in DSS models (Ko et al., 2017).
Studies on mBGT (Kim, 2017; Kim et al., 2021) have led to the identification of a more potent and cost-effective combination of BGT. The dried flower buds or flowers of L. japonica Thunb., dried rhizome of A. macrocephala Koidz., and dried rhizome of Alisma orientalis Juz. were prepared as aqueous extracts, and mixed at a ratio of 3:1:1 (the original BGT ratio) and 1:1:1 in a preliminary study conducted by Kim (Kim, 2017) to explore the effects of eight major herbs on BGT. Comparison of these ratios revealed that the 3:1:1 mixture was more effective in preventing colon shortening and improving histological changes. Moreover, the effects of the modified 3:1:1 ratio were superior to those of BGT in terms of reducing colon shortening and improving anatomical and histological scores. Kim et al. (2021) also compared BGT with mBGT at the 3:1:1 ratio and revealed that both formulations improved colon shortening, macroscopic scores, histological damage, clinical scores, and cytokine levels (including the interleukin (IL)-1β, tumor necrosis factor (TNF)-α and IL-17 levels). However, the performance of mBGT was superior to that of BGT, especially in terms of clinical and histological improvements. Thus, mBGT may be a more effective treatment option for patients with colitis.
Two recent studies examined the effects of BGT on the small intestine (Cho et al., 2022; Choi et al., 2023). Cho et al. (2022) used an indomethacin-induced murine colitis model to study the effects of BGT on non-steroidal anti-inflammatory drug (NSAID)-induced small intestinal injury (NSI). The administration of BGT before and after the induction of NSI yielded a reduction in small intestine shortening, ulceration area, and inflammation scores. Choi et al. (2023) explored the regulatory mechanisms of BGT on the interstitial cells of Cajal (ICCs) in the small intestine, the pacemaker cells of the GI tract. The ICC pacemaker potential measured using an electrophysiological method revealed that higher BGT concentrations induced depolarization and decreased the firing frequency in ICCs. Various receptor antagonists used to identify the mechanisms underlying the effects of BGT on ICCs have revealed that BGT acts through the cholinergic receptor muscarinic (CHRM) 3, 5-hydroxytryptamine (5-HT) 3 receptors, and 5-HT4 receptors. Thus, BGT regulates the intracellular Ca2+ levels and activates signaling pathways involving protein kinase C (PKC), mitogen-activated protein kinase (MAPK), the guanylate cycle, and protein kinase G (PKG) through these receptors. CHRM 3 induces smooth muscle contraction and gland secretion (Abrams et al., 2006). Serotonin (5-HT), a monoamine neurotransmitter, regulates intestinal motility. The 5-HT3 and 5-HT4 receptors are key mediators of peristaltic contractions in mammals (Sia et al., 2013). Thus, BGT depolarizes ICCs through the cholinergic and serotonergic pathways, thereby promoting GI movement.
In summary BGT and its modified forms exhibit significant anti-inflammatory and mucosal protective effects throughout the GI tract. Thus, BGT can be used to treat IBD, gastric ulcers, and small intestinal injuries.
3.2 Clinical studies
Four clinical studies, including two case reports, were reviewed in the present study (Table 2). Two retrospective chart reviews analyzed the effectiveness of various herbal medicines, including BGT, in the treatment of irritable bowel syndrome (IBS).
Chang et al. (1985) conducted a retrospective chart review of 116 patients with IBS treated at the Kyung Hee University Korean Medicine Hospital between 1984 and 1985. The primary complaints included lower abdominal discomfort (n = 62), insomnia (n = 55), and abdominal distension (n = 42). Among the eight different prescriptions dispensed, BGT was prescribed in 11.1% of the cases (18 patients). Seventy-six (65.5%) patients reported “improvement,” whereas 25 (21.6%) reported “no change.” Fifteen (12.9%) patients who did not revisit the clinic were labeled as “unknown.” Han et al. (1986) also conducted a chart review of 61 patients with diarrhea-predominant IBS treated at the same hospital between 1985 and 1986. The primary complaints included dyspepsia (n = 32) and abdominal pain (n = 29). Among the three prescriptions dispensed to the patients, BGT was prescribed in 26 cases. Fifteen of these patients “recovered,” whereas eight showed “improvement.”
Two case reports examined the effects of BGT on diarrhea. Yoon (Yoon, 2001) reported the case of a 52-year-old woman with collagenous colitis treated with gamma-BGT. Gami-BGT was administered for a period of 3 months for the management of chronic diarrhea that persisted for 15 years. The frequency of defecation and stool consistency returned to normal after treatment, with the weight of the patient increasing from 55 kg to 57.5 kg. Seo et al. (2004) reported the case of a 16-year-old boy diagnosed with Crohn’s who presented with persistent diarrhea, abdominal pain, rectal bleeding, and anorexia. Although surgery was recommended, the patient refused treatment and opted to continue medication. Gami-BGT was administered three times a day for a period of 2 months. The stool frequency and bloody stools normalized after treatment. Furthermore, the patient reported a decrease in abdominal pain and was able to resume an oral diet.
The findings of these studies indicate the clinical efficacy of BGT and its modified forms in the management of intestinal diseases, particularly diarrhea, including IBS and IBD.
3.3 Herbs
3.3.1 Lonicera japonica Thunb
Ten in vivo studies, three in vitro studies, and one human RCT explored the effects of LJT. Three studies were conducted in both in vivo and in vitro settings (Table 3). Five studies (Lee et al., 2011; Park et al., 2013; Lv et al., 2021; Zhou et al., 2021; Chen et al., 2024) used a DSS-induced colitis model to investigate the effectiveness of LJT. Different concentrations of LJT extract were administered orally for a period of 7–21 d. Notably, symptom relief, alleviation of histological damage, and inhibition of inflammatory cytokines were observed in all five studies. Mechanisms underlying the effect of LJT on colitis were proposed in two of these studies. Park et al. (2013) proposed that LJT extract promotes recovery from colon damage through a cytokine response. LJT extract inhibit interferon (IFN)-γ and IL-17, which are representative T helper cell (Th)1 and Th17 cytokines, respectively. Furthermore, the extract also reduced the levels of Th1/Th17-related cytokines, such as IL-1β, 6, 12, TNF-α, in the colonic mucosa, but not those of IL-10, 23, or transforming growth factor (TGF)-β1. Thus, LJT attenuates colic damage by modulating the Th1/Th17 pathway rather than the regulatory T cell (Treg cell) mechanism. Lv et al. (2021) reported that the LJT extract suppresses the protein expression of cleaved caspase-1, IL-1β, and IL-18 in the colonic macrophages by inhibiting the nucleotide-binding domain-like receptors family pyrin domain containing 3 (NLRP3) inflammasome. This effect was attributed to the enhancer of zeste homolog 2 (EZH2)/autophagy-related protein 5 (ATG5)-mediated autophagy regulation. The findings of these reports demonstrate that the LJT extract exerts anti-inflammatory effects on colitis through mechanisms at the cellular, protein, and gene levels.
The anti-inflammatory effects of LJT in gastritis and peptic ulcer models was demonstrated in the study by Bang et al. (2019). The administration of LJT extract alleviated gastric lesions, decreased the proinflammatory cytokine levels, decreased NF-κB expression, and increased gastric mucus production and antioxidant activity. Sun et al. (2023) reported that the extract exerts antioxidant effects by enhancing the activity of antioxidant enzymes such as superoxide dismutase (SOD), catalase (CAT), and glutathione peroxidase (GSH-Px), and by reducing the malondialdehyde (MDA) levels through the Nrf2 pathway.
The effects of LJT extract on Citrobacter rodentium-induced GI infection were investigated in the study conducted by Minami and Makino (Minami and Makino, 2020). The LJT extract increased the survival rates, enhanced macrophage phagocytic activity, increased the serum inflammatory cytokine levels, and suppressed bacterial colonization in mice. Thus, LJT may be a therapeutic agent for the management of infections of the human bacterial digestive tract.
Zhou et al. (2021) and Chen et al. (2024) evaluated the effect of LJT extract on the intestinal flora in DSS-induced colitis models. The administration of the LJT extract increased the levels of intestinal probiotics, such as Bifidobacterium and Lactobacilli. In contrast, it antagonized pathogenic bacteria, such as Escherichia coli and Enterococcus in a colitis model (Zhou et al., 2021). Similarly, Chen et al. (2024) reported an increase in the levels of Lactobacillus and Romboutsia following the administration of the LJT extract, along with a decrease in the levels of Dubosiella. The administration of the LJT extract also resulted in an increase in the production of total short-chain fatty acids (SCFAs) and gut microbiota-derived metabolites. Furthermore, the LJT extract exhibited significant correlations between specific bacteria and elevated fecal SCFA levels. Notably, fecal microbiota transplantation (FMT) from the LJT-treated group to the colitis group alleviated the symptoms and histological damage. Thus, FMT using LJT-mediated gut microbiota can improve colitis. Sun et al. (2023) also reported the regulatory effect of LJT on the intestinal microbiota in mice subjected to oxidative stress. Notably, LJT improved the microbial diversity, restored the Firmicutes/Bacteroidetes ratio, and increased the relative abundance of beneficial bacteria such as Lactobacillaceae and Bifidobacteriaceae, in addition to reversing the harmful changes induced by D-galactose. In other words, the LJT extract positively modulated the gut microbiota and promoted the production of metabolites, such as SCFAs.
The effect of LJT on GI motility was investigated in two studies (Jung et al., 2014; Nam et al., 2016). Jung et al. (2014) reported that the administration of the LJT extract resulted in a significant increase in the contraction amplitude of the circular muscle in the antrum and the migration percentage of charcoal along the small intestine. Atropine, dopamine, and a selective 5-HT4 receptor antagonist inhibited these effects, indicating that LJT exerts gastroprokinetic effects through cholinergic, dopaminergic, and serotonergic mechanisms. Nam et al. (2016), reported that the LJT extract enhanced the contractile responses of esophageal smooth muscle cells and lower esophageal sphincter (LES) muscle strips and accelerated the GI transit, thereby exerting a prokinetic effect on the GI tract. LJT also enhanced tonic responses in the LES muscle strips induced by carbachol, a cholinergic neurotransmitter that activates acetylcholine receptors. However, LJT-induced esophageal smooth muscle contraction was inhibited by pre-treatment with 5-HT3 and 5-HT4 receptor antagonists. This finding indicates that the cholinergic and serotonergic pathways are involved in LJT-induced contraction.
Only one clinical study was reviewed in the present study. This study by Choi et al. (2020) investigated the efficacy and safety of the administration of the LJT extract to patients with functional dyspepsia (FD). The study participants were divided into two groups: the LJT (46 patients) and placebo (46 patients) groups. A significant improvement in the symptoms and serum antioxidant levels was observed in the LJT group after 8 weeks of treatment. Notably, no treatment-related adverse events were reported.
In conclusion, the LJT extract exerted GI-protective effects in various GI dysfunction models, including IBD, gastritis, gastric ulcers, and GI infections, through its anti-inflammatory, antioxidant, and antibacterial properties. The gastroprokinetic effects exerted through the cholinergic and serotonergic pathways indicate its utility as a treatment for functional GI disorders such as FD.
3.3.2 Atractylodes macrocephala Koidz
Twenty-four in vivo and 19 in vitro studies investigated the effects of AMK. Notably, six studies conducted both in vivo and in vitro experiments (Table 4). Eight in vivo studies (Feng et al., 2020; Li et al., 2021; Ren et al., 2021a; Kai et al., 2022; Yang et al., 2022; Cheng et al., 2023; Hu et al., 2023; Li et al., 2023) and two in vitro studies (Zong et al., 2021; Li et al., 2023) evaluated the effects of AMK on colitis. AMK inhibited weight loss, colon shortening, and overexpression of pro-inflammatory cytokines in all in vivo studies in addition to promoting the recovery of intestinal tissue damage, gut microbiota, and its metabolites. Yang et al. (2022) demonstrated the anti-inflammatory effects of AMK through the regulation of the Th17/Treg cell balance in colitis, which may be mediated by inhibiting the IL-6/STAT3 signaling pathway, as indicated by the expression levels of inflammatory cytokines. Cheng et al. (2023) identified the mechanisms related to gut microbiota metabolism and discovered 56 AMK-related metabolites involved in maintaining intestinal homeostasis. Ascorbate and aldarate, arginine and proline, tryptophan, galactose, and amino sugar and nucleotide sugar metabolisms were the five most affected metabolic pathways. Hu et al. (2023) examined the effects of AMK on tryptophan metabolism and revealed that AMK treatment increased the levels of tryptophan metabolites, particularly those of aryl hydrocarbon receptor (AhR) ligands, in the feces and plasma. This finidng indicates that the beneficial effects of AMK on colitis are associated with the modulation of tryptophan metabolism. AMK polysaccharides decreased the apoptosis rate and increased the viability of intestinal epithelial cells in the in vitro study by Zong et al. (2021). This finding indicates that AMK enhanced the proliferation and survival of intestinal epithelial cells. AMK also upregulates the expression of tight junction proteins, thereby contributing to barrier formation. Microarray data indicates that the ITSN1-OT1 signaling pathway regulates the ability of AMK to restore intestinal barrier function by blocking the nuclear import of phosphorylated STAT2. Li et al. (2021) also reported that AMK enhanced the expression of tight junction proteins, such as ZO-1 and Occludin, indicating strengthened intestinal barrier integrity. These findings are comparable with those of the standard ulcerative colitis (UC) treatment with 5-Aminosalicylic acid. AMK alleviates colitis by strengthening barrier integrity through the enhanced expression of tight junction proteins. AMK and its polysaccharides demonstrated similar effects in TNBS-induced colitis, LPS-induced enteritis, and DSS-induced colitis models. Ren et al. (2021a) evaluated the therapeutic effects of Atractylenolide (ATL)-3, an AMK polysaccharide, on TNBS-induced colitis in mice. ATL-3 significantly alleviated colitis symptoms by reducing inflammation, lowering oxidative stress, and improving intestinal microbiota balance. Li et al. (2021) investigated the effects of AMK on LPS-induced enteritis and reported that AMK reduces intestinal inflammation by lowering the levels of inflammatory markers. Furthermore, it maintains the balance of the intestinal microbiota and improves the structural stability of the intestinal mucosa. The findings of these studies indicate that AMK exerts significant anti-inflammatory and protective effects against enteritis induced by various methods. Thus, AMK is a promising therapeutic agent for the treatment of IBD that supports overall gut health and stability.
Twenty studies examined the effect of AMK in digestive tract disease models, including diarrhea, constipation, IBS, gastritis, gastric ulcer, and GI cancer. The effects of AMK extract on diarrhea were evaluated in three studies (Kim et al., 2005; Wang et al., 2022; Chenxing et al., 2024). AMK extract alleviated symptoms and reduced the levels of inflammatory cytokines in all three studies. Wang et al. (2022) demonstrated the effects of AMK polysaccharides on improving watery stools and the restoration of the intestinal flora in a senna-induced diarrhea model. These findings suggest that AMK polysaccharides has a positive effect on gut microbiota balance, which is essential for GI health. Chenxing et al. (2024) reported that the administration of AMK extract significantly improved mucosal integrity, increased GI hormone levels, and enhanced the intestinal propulsion rate. Thus, the AMK extract may strengthen the structural and functional health of the gastrointestinal system, thereby contributing to diarrhea relief. Furthermore, AMK extract stimulated the expression of aquaporins, epithelial tight junction proteins, and mRNA, which play crucial roles in water reabsorption from the colon and reinforcement of intestinal epithelial integrity. These effects underscore the role of AMK in promoting water balance and supporting the epithelial barrier in the intestine, contributing to its anti-diarrheal properties.
Three studies (Jia et al., 2022; Yang et al., 2023; Qin et al., 2024) investigated the effects of AMK extract in a constipation model, with a focus on symptom relief and the underlying biochemical pathways. AMK extract alleviated constipation by increasing fecal water content and enhancing the GI transit rate in all three studies. Analysis of the effect of AMK on tryptophan metabolism revealed its role in regulating GI hormone and gut neurotransmitter levels, including 5-HT. Yang et al. (2023) reported that AMK-treated mice exhibited an increase in the count of beneficial gut flora and SCFAs. Furthermore, they reported significant changes in 30 metabolites and 15 metabolic pathways, including tryptophan metabolism, unsaturated fatty acid biosynthesis, and primary bile acid biosynthesis. These metabolites produced by the intestinal microorganisms stimulate tryptophan metabolism, leading to the synthesis of 5-HT, which is associated with improved gut motility. Qin et al. (2024) identified 14 altered metabolites and four metabolic pathways (i.e., tryptophan metabolism, taurine and hypotaurine metabolism, tyrosine metabolism, and primary bile acid biosynthesis) regulated by AMK treatment that were correlated with constipation indicators. This study also highlighted the ability of AMK to modulate key enzymes in the tryptophan metabolic pathway, such as tryptophan hydroxylase, monoamine oxidase, and indoleamine-2,3-dioxygenase. These findings indicate that tryptophan metabolism plays a crucial role in relieving constipation through AMK. Collectively, the findings of these studies provide evidence that AMK extract may alleviate constipation by modulating the gut microbiota, metabolic pathways, and neurotransmitter levels, especially through the regulation of the metabolism of tryptophan, thereby contributing to increased 5-HT synthesis and enhanced GI function.
Choi et al. (2024a) used a mouse model of zymosan-induced IBS to explore the effects of AMK on IBS and revealed that AMK effectively reduced colonic inflammation, normalized colon length, and improved stool consistency. Thus, AMK yielded results comparable with those of established IBS treatments such as amitriptyline and sulfasalazine. The findings of this study suggest the potential of AMK as a therapeutic agent for IBS, primarily through the modulation of ion channels, particularly TRPV1, which is involved in reducing the visceral hypersensitivity associated with IBS.
Amin et al. (2022) and Yang et al. (2020) investigated the effects of AMK in a gastritis model, with a focus on its anti-inflammatory and protective mechanisms. Yang et al. (2020) examined ATL, a polysaccharide component of AMK, and reported its ability to reduce the expression of pro-inflammatory cytokines IL-6 and IL-1β, suggesting a targeted anti-inflammatory action. Amin et al. (2022) conducted in vivo and in vitro studies. The in vivo study revealed that the administration of the AMK extract decreased gastric tissue damage and immune cell infiltration, highlighting its protective role against gastric injury. The in vitro study revealed that the AMK extract inhibited the upregulation of inflammatory mediators, including nitric oxide (NO) and prostaglandin E2 (PGE2), and downregulated the expression of related inflammatory genes and proteins. Furthermore, AMK extract also suppressed nuclear translocation of the NF-κB subunit p50 and increased the phosphorylation of IκBα and Akt. These findings indicate that its anti-inflammatory and antioxidant effects are mediated through the modulation of the Akt/IκBα/NF-κB signaling pathway.
Two studies (Zeng et al., 2023; Zhai et al., 2023) investigated the effects of AMK in an ethanol-induced gastric ulcer model and demonstrated the ability of AMK to alleviate gastric ulcers, reduce inflammatory markers, and improve the balance of intestinal flora. Zeng et al. (2023) measured the levels of oxidative stress markers and reported that AMK reduced the MDA levels, a marker of oxidative stress, and increased the levels of antioxidant enzymes such as SOD and GSH-Px. Furthermore, AMK normalized the expression of matrix metalloproteinase-9 (MMP-9), tissue inhibitor of metalloproteinase-1 (TIMP-1), and NF-κB proteins. These findings indicate that AMK alleviates ulcers by restoring balance in the NF-κB-MMP-9/TIMP-1 pathway. Zhai et al. (2023) explored the mechanism of the anti-ulcer effects of AMK and revealed that it inhibited the activation of the NLRP3 inflammasome, a key trigger of NF-κB activation that leads to inflammatory damage. The inhibition of the NF-κB/NLRP3 axis was central to the protective effects of AMK against ethanol-induced gastric ulcers. Pepsin activity, H+-K+-ATPase enzymatic activity (an indicator of gastric acid secretion), and the levels of malondialdehyde (MDA) and the mucosal protective factors PGE2 and MUC5AC were also quantified. AMK protected the gastric mucosa by reducing inflammation and oxidative stress, inhibiting H+-K+-ATPase and pepsin activities, and enhancing the levels of PGE2 and mucin. Collectively, these findings suggest that the AMK extract can potentially treat gastritis and gastric ulcers by modulating oxidative stress, inflammatory pathways, and gastric mucosa protective mechanisms, particularly through the NF-κB-related pathways.
The anti-cancer effects of AMK were examined by nine studies (Ma et al., 2014; Shim et al., 2015; Tian and Yu, 2017; Feng et al., 2019; Chan et al., 2020; Sun et al., 2022; Zhang et al., 2022; Chen et al., 2023; Choi et al., 2024b) using colon, colorectal, and gastric cancer models. Chen et al. (2023) explored the cytotoxic and apoptotic effects of AMK polysaccharides on colon cancer cells. Shim et al. (2015) explored the anti-cancer properties of Atractylochromene, a compound present in AMK, with a specific target on colon cancer through the inhibition of the Wnt/β-catenin signaling pathway. Atractylochromene suppressed the nuclear translocation of β-catenin and galectin-3 in SW-480 colon cancer cells, thereby reducing the expression of cyclin D1, a gene involved in cancer cell proliferation, ultimately resulting in decreased growth of the cancer cells. Chan et al. (2020) investigated the anti-tumor potential of ATL-1 in the human colon adenocarcinoma cell line HT-29 and revealed that ATL-1 induced apoptosis through a mitochondria-dependent pathway by modulating pro-apoptotic (Bax/Bak) and anti-apoptotic (Bcl-2) proteins. This resulted in the activation of a caspase cascade essential for apoptosis without inducing necrosis. The findings of these studies underscore the potential of AMK in colon cancer treatment through the inhibition of cancer cell proliferation and the promotion of mitochondria-mediated apoptosis. Feng et al. (2019) investigated the role of AMK polysaccharides in colorectal cancer and reported that AMK enhances the phagocytosis of cancer cells by promoting the migration of bone marrow-derived macrophages (BMDMs) and increased mRNA expression of inflammatory cytokines and NO. Furthermore, AMK specifically activates toll-like receptor 4 (TLR4), which plays a pivotal role in the activation of macrophages. Notably, TLR4 and MyD88 inhibitors suppressed the anti-tumor effect of AMK, indicating that AMK promotes anti-cancer immunity via the TLR4/MyD88 pathway. This finding is further supported by the in vivo results in TLR4-deficient mice. Sun et al. (2022) reported that the application of ATL-1 to HCT-116 colorectal cancer cells reduced cell viability and motility, induced apoptosis, and enhanced oxaliplatin chemotherapy efficacy through the downregulation of the PDK1/FoxO1 pathway. This led to increased apoptosis and decreased tumor-promoting behaviors. In vitro and in vivo models were used by Zhang et al. (2022) to evaluate the anticancer effects of ATL-3 on colorectal cancer. ATL-3 significantly inhibited tumor growth and upregulated the expression of apoptotic markers, such as p53, in the in vivo model. ATL-3 induced apoptosis in cancer cells through the Bax/Bcl-2 signaling pathway and by activating caspases in the in vitro model. Thus, AMK and its components, ATL-1 and ATL-3, exhibit promising anti-tumor effects in colorectal cancer by leveraging immune activation and apoptotic modulation, thereby potentially enhancing chemotherapy outcomes. Choi et al. (2024b) observed that the AMK extract induced apoptosis by reducing cell growth and increasing ROS generation, revealing mitochondrial pathway involvement through altered membrane potential, caspase activity, and B-cell lymphoma protein levels. Ma et al. (2014) investigated the effect of ATL-1 on gastric cancer cells, with a particular focus on cancer stem cell characteristics. ATL-1 inhibited gastric cancer cell proliferation and induced apoptosis by targeting the Notch signaling pathway, a critical regulator of cancer progression. Notably, ATL-1 decreased the expression of Notch1 and associated proteins such as Jagged1, Hes1, and Hey1, thereby reducing cancer cell growth. ATL-1 also specifically targeted gastric cancer stem-like cells (GCSLCs) by impairing their colony formation and self-renewal capabilities, suggesting its potential to serve as a therapeutic agent by targeting pathways essential for tumor growth and maintenance. Tian and Yu (2017) explored the effects of ATL-2 on the gastric carcinoma cell lines HGC-27 and AGS and demonstrated that ATL-2 inhibits cell proliferation and migration by inducing apoptosis through the mitochondrial pathway, specifically by increasing the Bax/Bcl-2 ratio and suppressing the Akt/ERK signaling pathways. Collectively, these studies underscore the potential of AMK extract and its components to serve as therapeutic agents for GI cancers. The cytotoxic and apoptotic effects of AMK in various cancer models were achieved through immune modulation, apoptosis induction, and pathway-specific inhibition. These findings suggest its potential as a therapeutic option for GI cancer treatment.
Several studies have investigated the effects of AMK in various GI dysfunction models. For instance, Song et al. (2014), Song et al. (2015), Song et al. (2017), and Ren et al. (2021b) examined the effects of AMK extracts and their compounds on the migration and mucosal repair of intestinal epithelial cells, emphasizing their potential for the treatment of GI injuries. AMK extract accelerates the migration of intestinal epithelial cell during early mucosal repair by modulating the membrane potential and cytosolic Ca2+ levels in a polyamine-dependent manner, particularly by upregulating Kv1.1 channel mRNA and protein expression. The follow-up study conducted by Song et al. (2015) further revealed that the administration of AMK extract increased the expression of Rho mRNA and non-muscle myosin 2 protein and promoted stress fiber formation. These processes are all mediated by polyamine signaling, underscoring the role of polyamines in facilitating cell migration into the wound area. Song et al. (2017) also investigated the effect of ATL-1 on rat-derived IEC-6 cells and reported that ATL-1 enhanced epithelial cell migration and proliferation by increasing the polyamine and intracellular calcium levels, which are critical for mucosal healing, thereby supporting the use of ATL-1 as a potential agent for GI repair. Ren et al. (2021b) compared four active ingredients of AMK and identified ATL-3 as the most effective compound for promoting intestinal epithelial repair. ATL-3 exerts significant effects on cell migration, proliferation, and anti-inflammatory responses by increasing the intracellular calcium levels and upregulating the key proteins and cytokines associated with cellular repair and inflammation reduction. Thus, AMK and its components are promising candidates for therapeutic applications in gastrointestinal mucosal injuries, as they can potentially enhance epithelial healing by increasing polyamine and intracellular calcium levels.
Xu et al. (2023) examined the cellular mechanisms of ATL-1, a major bioactive component of AMK, in a model replicating the oxidative stress-induced colonic mucosal epithelial cell dysfunction observed in IBS. ATL-1 effectively alleviated oxidative stress-induced dysfunction by improving the cell viability and reducing apoptosis. Treatment with ATL-1 suppresses the expression of miR-34a-5p induced by oxidative stress, which directly targets lactate dehydrogenase A (LDHA), a key enzyme in glucose metabolism. ATL-1 restored glucose metabolism through the modulation of the miR-34a-5p pathway, thereby reducing colonic epithelial cell injury and maintaining metabolic homeostasis.
Xie et al. (2013) examined the effects of AMK on enteric mucosal immunity in a mice model injected with a foot-and-mouth disease vaccine. AMK polysaccharides enhanced the serum-specific IgG response and improved gut mucosal immunity as evidenced by the increase in the total SIgA concentration, elevated mRNA expression of TGF-β, IL-6, and TNF-α, increase in the IgA+ cell area, and a higher number of intraepithelial lymphocytes in the duodenum. Thus, AMK enhances the local mucosal immune response.
In summary, AMK and its active compounds demonstrate therapeutic potential in various GI disorders, including colitis (IBD), diarrhea, constipation, IBS, gastritis, gastric ulcers, and GI cancers. AMK restores intestinal function through its anti-inflammatory and antioxidant effects in patients with inflammatory and functional GI disorders. Furthermore, it promotes mucosal healing, and exerts a positive influence on the gut microbiota, particularly through the modulation of tryptophan metabolism, a pathway implicated in IBD and IBS. AMK exerts protective effects through the NF-κB-related pathways in gastric disorders such as gastritis and gastric ulcers, thereby reducing inflammation and oxidative stress while enhancing gastric mucosal defenses. Extensive studies using GI cancer models have revealed that AMK compounds, especially ATL-1 and ATL-3, inhibit tumor growth through the TLR4/MyD88 pathways and mitochondrial signaling. In addition, they enhance immune responses and synergize with chemotherapy by targeting cancer cell apoptosis and proliferation. In vitro studies underscored the role of AMK in epithelial repair and barrier function enhancement, suggesting its efficacy in restoring GI mucosal integrity. Thus, AMK holds promise as a multifaceted therapeutic agent that supports GI health, modulates inflammation, enhances epithelial repair, and provides anticancer benefits. It is particularly valuable for the treatment of GI cancers and broader gastrointestinal diseases.
3.3.3 Alisma canaliculatum A.Braun & C.D.Bouche
The two studies on ‘A. canaliculatum A. Braun & C.D.Bouche (AC) included in this review comprised one in vivo study and one in vitro study (Table 5). Kim et al. (2018) reported the anti-inflammatory effects of the AC extract in gastritis and colitis models in in vitro models. The anti-inflammatory mechanism was mediated through the suppression of NF-κB/AP-1 signaling pathways. The AC extract inhibited the activation of NF-κB and AP-1, which play crucial roles in inflammatory responses, at the molecular level. The extract also inhibited the upstream signaling enzymes associated with NF-κB (Src and Syk) and AP-1 (TAK1 and MMK4/7). These findings indicate that the suppression of these enzymes mediates the anti-inflammatory potential of AC extract by targeting the NF-κB/AP-1 pathways.
Kwon et al. (2021) reported the anti-cancer effects of the AC extract on AGS gastric cancer cells. The extract suppressed the proliferation of AGS cell and induced apoptosis. Furthermore, it activated caspase-3 and caspase-9 and increased the phosphorylation of p38, indicating that AC extract-induced apoptosis involves the mitochondrial pathways and MAPK cascades.
Thus, AC exhibited anti-inflammatory and anti-cancer effects in the GI disease model, indicating its potential as a therapeutic agent.
4 Discussion
Nine in vivo, one in vitro, and four human studies were analyzed in the present study to understand the research trends in BGT. The findings of the present study confirm that BGT and its modified forms exhibit anti-inflammatory effects, promote recovery of the GI mucosa, and regulate GI motility. BGT has been widely used for the treatment of diarrhea in Korea. Nevertheless, studies on the effects of BGT remain limited despite its effectiveness, making it difficult to understand the effects and mechanisms of action despite its efficacy. Therefore, the present study summarized all available studies on BGT and analyzed the effects of its key components to clarify its therapeutic potential and mechanisms.
4.1 Review of the main results
Studies on the efficacy of BGT have primarily focused on IBD, including UC and Crohn’s disease (CD), which are characterized by chronic relapsing intestinal inflammation (Zhang and Li, 2014). The pathogenesis of IBD involves the activation of T cells, monocytes, and macrophages (Fujino et al., 2003). TNF-α, IL-1β, IL-6, IFN-γ, IL-10, IL-12, and IL-17 are pro-inflammatory cytokines that exacerbate mucosal inflammation in IBD. Recent studies on the treatment strategies for IBD have focused on reducing inflammation through the regulation of pro-inflammatory cytokines (Abraham et al., 2017). The present study revealed that BGT exerts an anti-inflammatory effect by reducing the levels of cytokines, especially those of TNF-α, IL-1β, and IL-17, which is consistent with these findings.
TNF-α plays a key role in the development of several chronic inflammatory disorders, including IBD. Notably, elevated TNF-α levels have been detected in the serum of patients with UC and CD. Biologic therapies targeting TNF-α administered intravenously or subcutaneously have transformed the treatment of IBD and emerged as the most effective agents for achieving and maintaining disease remission (Souza et al., 2023). Infliximab, an anti-TNF-α medication, has achieved and maintained remission in patients with moderate-to-severe IBD (Hanauer et al., 2002).
IL-1β, an agonistic ligand of the IL-1 family, is involved in the innate immune response. Furthermore, it serves as a key mediator of inflammation in various human disorders, including IBD (Garlanda et al., 2013). It recruits and activates immune cells within the gut mucosa, thereby promoting pro-inflammatory responses (Aggeletopoulou et al., 2024). Elevated IL-1β expression has also been observed in patients with IBD (McAlindon et al., 1998), and its levels have been positively correlated with the severity of mucosal inflammation (Al-Sadi et al., 2008). IL-1β also influences downstream T-cell responses, such as the activation of Th17 cells, which produce IL-17 (Aggeletopoulou et al., 2024).
IL-17 induces various inflammatory mediators, particularly those involved in the proliferation, maturation, and recruitment of neutrophils (Witowski et al., 2004). Notably, IL-17 also stimulates the production of IL-1β and TNF-α in macrophages, which amplify the inflammatory response. Elevated IL-17 levels have also been detected in the colorectal tissue and serum of patients with IBD (Fujino et al., 2003).
BGT has demonstrated anti-carcinogenic effects in various diarrheal models (Joun et al., 1994; Ro et al., 2006). Diarrhea, defined as the excretion of liquid-like stool owing to abnormally accelerated peristalsis in the small and large intestines, is attributed to impaired digestion. Thus, accelerated intestinal motility is a key factor involved in the development of diarrhea (Yamamoto et al., 1993). BGT suppresses transport in the small and large intestines. Furthermore, it inhibits the contraction of ileal smooth muscle to exert an anti-cathartic effect. However, the results of the studies vary. Four clinical studies indicated that BGT alleviates the symptoms of diarrhea in patients with IBS and IBD. Notably, BGT depolarized and reduced the pacemaker potential frequency in ICCs to generate “slow waves,” i.e., regular contractions of the GI smooth muscles, in an in vitro study (Choi et al., 2023). GI tract motor dysfunction is a leading cause of GERD and FD in Korea (Noh et al., 2010; Sinn et al., 2010). These findings indicate the utility of BGT in regulating GI motility. While the studies suggest that BGT has the potential to alleviate symptoms of GI motility disorders, such as IBS and FD, the limitations of these studies highlight the need for further research. The clinical research was constrained by small sample sizes, a lack of objective assessment tools, and the absence of RCTs. Therefore, well-designed clinical studies are essential to confirm these promising findings.
The effect of BGT on the intestinal microflora was evaluated in the study conducted by Kim et al. (1993). The administration of BGT increased the count of lactic acid bacteria in the gut and modulated β-glucuronidase activation in the antibiotic-pre-treated group. The levels of β-glucuronidase, a lysosomal enzyme involved in the breakdown of β-D-glucuronides, are elevated in necrotic areas and body fluids of patients with cancer (Awolade et al., 2020). These findings indicate that BGT may act as a prebiotic and aid in maintaining intestinal homeostasis.
Some studies have examined the anti-ulcer effects of BGT using peptic ulcer models induced by ethanol, indomethacin, or pylorus ligation (Nam et al., 1989; Joun et al., 1994). Notably, BGT inhibited ulcer formation and reduced gastric acid secretion and acidity in these studies. These anti-ulcer effects may be attributed to the suppression of gastric juice secretion or the reduction of gastric acidity.
Despite the emergence of these promising findings, the limited number of studies has led to the requirement to conduct further studies exploring individual herbs present in BGT to explore their effects and mechanisms. mBGT, a new, simple, and effective formulation that includes only L. japonica, A. macrocephala, and A. orientalis, the most potent herbs from BGT, was developed in recent years (Kim, 2017; Kim et al., 2021). The key herbs present in mBGT were analyzed in the present study.
The flowers of LJT, commonly known as Jin Yin Hua (金銀花), Ren Dong (忍冬), or Japanese honeysuckle, are widely used in traditional Chinese medicine to treat exopathogenic wind-heat, epidemic febrile diseases, sores, carbuncles, and infectious diseases (Shang et al., 2011). Numerous studies have reported that these therapeutic effects of LJT are similar to those of BGT. Studies on colitis have demonstrated the anti-inflammatory effects of LJT and its underlying mechanisms of action. Analysis of the cytokine levels and T cell populations has provided insights into the modulation of the Th1/Th17 pathway by LJT through the inhibition of the NLRP3 inflammasome. The NLRP3 inflammasome, which is present in the mucosal macrophages and dendritic cells, plays a crucial role in inflammation regulation by modulating the activation of pro-inflammatory cytokines such as IL-1β and IL-18. These cytokines drive gut inflammation and tissue damage. Furthermore, they promote the differentiation of naïve T cells into Th17 cells, which contribute to the development and persistence of inflammation (Coccia et al., 2012; Prossomariti et al., 2018). LJT increases the secretion of mucosal protective factors; reduces oxidative stress; and downregulates NF-κB expression, a key factor in mucosal inflammation, thereby protecting the gastric mucosa (Bang et al., 2019). LJT extract enhances the abundance of probiotics, reduces pathogenic bacteria, and promotes the production of gut microbiota-derived metabolites such as SCFAs. FMT further validated these effects. Previous studies have explored the effects of LJT on GI motility. LJT improves gastric emptying and GI transit by enhancing the contraction of circular muscles in the antrum, esophageal smooth muscle cells, and lower esophageal sphincter muscle. Thus, the gastroprokinetic effects of LJT are mediated through the cholinergic and serotonergic pathways, as supported by neurotransmitter analysis and electric stimulation. LJT extract alleviated symptoms of FD and reduced the levels of 8-OhdG, a biomarker of oxidative DNA damage, in a clinical study. These findings suggest that the gastroprokinetic effects of LJT, consequently those of BGT, could aid in the treatment of GI motility disorders, including FD.
Atractylodes macrocephala Koidz. (AMK), also known as “Baizhu (白朮)” in traditional Chinese medicine, has been used to treat various conditions, including spleen hypofunction, loss of appetite, abdominal distension, diarrhea, dizziness, and heart palpitation, for thousands of years (Shu et al., 2017). AMK rhizome exhibits various biological activities, such as enhanced GI function and anti-tumor, anti-inflammatory, anti-aging, anti-oxidant, anti-osteoporotic, anti-bacterial, and neuroprotective effects (Zhu et al., 2018). The studies included in this review provide evidence for these therapeutic properties. The significant anti-inflammatory effects exerted by AMK in patients with various GI disorders such as colitis, gastritis, and gastric ulcer can be largely attributed to its ability to regulate key cytokine pathways. AMK inhibits pro-inflammatory cytokines such as TNF-α, IL-6, and IL-1β. In addition, it reduces immune cell infiltration in the inflamed tissues, thereby decreasing local inflammation. NF-κB and IL-6/STAT3, which play pivotal roles in the chronic inflammation of the GI tract, suppress key pathways to mediate these anti-inflammatory actions. AMK components such as ATL-1 and ATL-3 modulate oxidative stress and cytokine production by downregulating these pathways, thereby preventing further tissue damage, and promoting the recovery of the inflamed GI tissues. AMK also has a positive effect on the composition of the gut microbiota, which plays a vital role in host physiology. This effect is mediated by metabolites produced by microbes or derived from the transformation of host or environmental molecules (Devlin et al., 2016). AMK treatment increased the abundance of beneficial gut microbiota and the production of SCFAs in models of colitis and other GI disorders. Specific studies have highlighted the effect of AMK on the metabolism of tryptophan, which produces metabolites linked to anti-inflammatory responses and mucosal healing. Alterations in the metabolism of tryptophan play an active role in the pathogenesis of IBD. Dysfunction in the production of serotonin has been linked to the development of IBS (Agus et al., 2018). Cheng et al. (2023) proposed that AMK may act as a prebiotic for UC by modulating the gut microbiota metabolism, particularly the metabolism of tryptophan. Hu et al. (2023), reported that AMK significantly increased the levels of AhR ligands in a DSS-induced colitis model, indicating that AMK ameliorates IBD by regulating the metabolism of tryptophan. Similarly, AMK normalized the levels of tryptophan metabolites (such as 5-HT, indole, and kynurenic acid) and the expression of related enzymes (such as tryptophan hydroxylase, monoamine oxidase, and IDO) in constipation models (Yang et al., 2023; Qin et al., 2024). AMK modulates microbial metabolites and pathways, such as tryptophan and bile acid metabolism, to support a balanced microbiome, which is essential for managing conditions such as colitis and constipation. This finding further underscores its therapeutic potential. The ability of AMK to enhance the integrity of the intestinal barrier is a key mechanism that supports GI health. AMK polysaccharides increase the expression of tight junction proteins, such as ZO-1, Occludin, and Claudin-1, to strengthen the intestinal epithelial barrier in patients with conditions such as colitis and diarrhea. Barrier reinforcement reduces gut permeability and protects the gut from harmful pathogens and toxins. AMK also increases the production of mucins, such as MUC2, which coat the gut lining, thereby exerting protective effects and supporting epithelial repair. The ability of AMK to maintain the intestinal barrier aids in symptomatic relief. Moreover, it supports long-term GI health by mitigating gut dysfunction and inflammation. AMK and its active compounds, ATL-1 and ATL-3, have demonstrated promising anti-cancer effects in GI cancer models by inhibiting tumor growth and proliferation through the induction of apoptosis and modulation of the immune system. AMK activates TLR4, leading to enhanced immune cell activity against tumors through the MyD88 pathway. Moreover, the effect of AMK on signaling pathways such as Wnt/β-catenin, TLR4/MyD88, and mitochondrial pathways suppress cancer cell proliferation, increase cancer cell apoptosis, and improve responses to chemotherapy agents. Thus, AMK exhibits significant potential as a complementary therapeutic agent for GI cancers owing to its ability to target cancer cell growth and enhance immune responses. In summary, the therapeutic effects of AMK in GI disorders stem from multifaceted mechanisms, including anti-inflammatory, microbiota-modulatory, barrier-enhancing, and anti-tumor activities. These properties suggest that AMK is a promising agent for the management of functional and inflammatory GI disorders, as well as GI cancers, thereby providing comprehensive support for GI health and stability.
Rhizoma Alismatis (澤瀉), the dried rhizome of the plant A. canaliculatum A. Braun & C.D.Bouche (AC) from the Alismataceae family, is commonly used in traditional Chinese medicine to treat conditions such as dysuria, edema, nephropathy, hyperlipidemia, diabetes, and inflammation (Tian et al., 2014). Studies on AC remain limited; however, it exhibits anti-inflammatory effects in gastritis and colitis models through NF-κB-related mechanisms and anti-tumor effects in gastric cancer models through mitochondrial pathways. These therapeutic effects were similar to those observed for AMK, contributing to our understanding of the potential benefits of BGT.
Quality assessment of the data was conducted in accordance with the Animal Research: Reporting of In Vivo Experiments (ARRIVE) 2.0 guidelines (Percie du Sert et al., 2020) using a 21-item checklist. A total of 43 articles that met the eligibility criteria were included in the study. The ARRIVE scores ranged from 0 to 100, based on whether the specific items were reported. The bar graph displays all the scores assigned to each of the 43 studies, from the lowest (33%) to the highest (86%) (Supplementary Figure S4). The mean checklist score was 69%, which falls into the medium adherence category (50%–79%). The items that received the highest scores included the number of animals used, the size of control and experimental groups, and the definition of experimental outcomes. Conversely, the least frequently reported items were related to housing and husbandry, animal care and monitoring, protocol registration, and generalizability (Supplementary Figure S5). Our study found that the quality of reporting for key items that influence the interpretation of preclinical studies was generally poor. Notably, details crucial for ensuring the reproducibility of preclinical studies, such as animal housing, husbandry, and anesthetics, were often underreported, potentially impacting study results. This highlights a limitation in the overall quality of research adhering to the ARRIVE guidelines and suggests a broader shortfall in ethical considerations within current animal research. Therefore, it is essential for future in vivo studies to not only comprehensively implement the ARRIVE guidelines but also to address these ethical gaps. This will facilitate the effective translation of preclinical research findings into clinical therapies while ensuring adherence to ethical principles and promoting the conduct of high-quality animal research.
4.2 Strengths and limitations of the study
This study had certain limitations that must be addressed. First, studies on BGT are limited. The lack of high-quality evidence, such as those obtained through RCTs or SRs, and the limited number of experimental studies made gaining a precise understanding of the efficacy and mechanisms of BGT challenging. Additionally, a significant limitation arises from the relying on preclinical study outcomes as the sole predictors of clinical efficacy. While preclinical models provide valuable insights into initial safety and mechanisms, they often fail to replicate the complex interactions present in human physiology. This discrepancy underscores the need for caution when extrapolating these findings to clinical settings. Therefore, it is crucial to advance toward meticulously designed clinical trials that build upon preclinical research. Such trials should incorporate robust methodologies, diverse patient populations, and comprehensive endpoints to adequately evaluate the therapeutic potential and safety profile of interventions like BGT. Only through this rigorous translational approach can the true clinical benefits be ascertained, thereby enhancing the reliability and applicability of the research outcomes. Second, confirming the interactions among the three primary herbal components analyzed in this review was difficult. Herbal medicines are characterized by the synergistic effects arising from a combination of multiple herbs. The effects of the individual herbal components were evaluated in the present review; however, whether the same mechanisms would be retained when the three herbs or additional components are combined remains uncertain. Further studies, including experimental studies and large-scale clinical studies involving diverse populations, must be conducted in this regard to elucidate the mechanisms of action of BGT. In conclusion, addressing the limitations identified in this study is crucial to substantiate the clinical efficacy of BGT and its role within the broader therapeutic spectrum. Achieving this will require well-structured preclinical and clinical studies designed to elucidate the complex interactions and mechanisms of BGT. Such research should include diverse populations to comprehensively evaluate the therapeutic potential of BGT.
Despite these limitations, the present study also has several strengths. Our primary objective was to provide a comprehensive synthesis of current knowledge regarding BGT and its effects on the GI tract. By summarizing existing studies, we aimed to identify gaps in the literature and suggest areas for future research, thereby offering value to both practitioners and researchers. To the best of our knowledge, this is the first scoping review to comprehensively synthesize the effects and mechanisms of BGT on GI function. The findings of this scoping review extend the therapeutic potential of BGT, which has primarily been studied in the context of colitis, to a broader range of GI symptoms. Additionally, by analyzing the effects and mechanisms of the main herbal components of BGT, we could infer the mechanisms and efficacy of BGT, which is widely used in clinical settings. The study attempted to assess the quality of in vivo studies using the ARRIVE Tool. This review uniquely integrates traditional and modern pharmacological insights, bridging the historical uses of BGT with contemporary scientific understanding. By focusing on the key components of BGT, our study highlights the synergistic effects of its herbal constituents, offering novel perspectives that contribute to the existing body of knowledge and underscore the therapeutic potential of this traditional formulation. Furthermore, this review visualized and presented the diverse mechanisms identified in the studies using diagrams, thereby enhancing the clarity and understanding of the findings.
5 Conclusion
BGT and its key herbal components, L. japonica Thunb., A. macrocephala Koidz., and A. canaliculatum A. Braun & C.D.Bouche, are multifaceted therapeutic agents for the management of GI disorders. The anti-inflammatory, microbiota-modulatory, intestinal barrier-enhancing, and anti-tumor effects of BGT exhibit promise for the management of functional and inflammatory GI conditions, including diarrhea, IBD, and GI cancers. BGT and its active components can address multiple facets of GI health and disease by modulating key inflammatory cytokines, supporting microbiome balance, reinforcing the intestinal barrier, and targeting cancer-related pathways (Figure 2). These findings underscore the therapeutic versatility of BGT, indicating its potential for broader clinical applications as an effective natural intervention for GI health and stability. Nevertheless, further studies, particularly clinical studies, must be conducted in the future to deepen our understanding of the underlying mechanisms and optimize the clinical use of BGT.
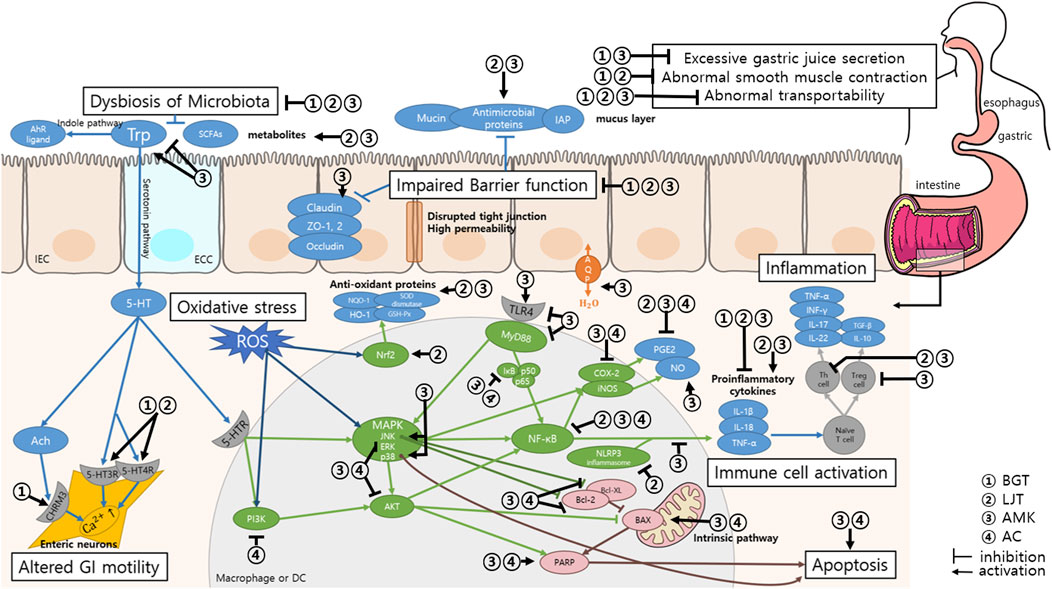
Figure 2. Summary of the mechanisms of action of Bojanggunbi-tang and its composing herbs on GI function. Changes in gut microbiota lead to reduced production of SCFAs and impaired Trp metabolism, contributing to disruptions in the serotonin pathway and intestinal barrier function. BGT and its components regulate this imbalance by promoting the growth of beneficial microbiota and modulating Trp metabolism. BGT and its components can regulate GI motility: LJT through the serotonergic pathways and BGT through the cholinergic and serotonergic pathways. Dysbiosis and oxidative stress impair intestinal barrier function, leading to decreased levels of tight junction proteins and increased gut permeability, which exacerbates inflammation. BGT and its components strengthen the intestinal barrier by promoting mucosal recovery, increasing the number of goblet cells, and regulating tight junction proteins. BGT reduces inflammation by modulating pro-inflammatory cytokines (TNF-α, IL-1β, and IL-17) and inhibiting key inflammatory pathways, such as NF-κB, TLR4, and the NLRP3 inflammasome. LJT and AMK regulate immune responses by controlling the Th1/Th17 pathways to achieve a balance between pro-inflammatory and anti-inflammatory cytokines. BGT components also exhibit antioxidant properties that enhance the activity of proteins such as Nrf2 and inhibit oxidative stress pathways (MAPK and NF-κB), thereby contributing to reduced ROS and apoptosis. Intrinsic apoptosis pathway, triggered by inflammation and oxidative stress, leads to cellular damage in the GI mucosa. BGT and its components, particularly AMK, inhibit mitochondrial pathways (BAX/Bcl-2), preventing excessive apoptosis and promoting tissue recovery. Abbreviations: BGT, Bojanggunbi-tang; LJT, Lonicera japonica Thunb.; AMK, Atractylodes macrocephala Koidz.; AC, Alisma canaliculatum A.Braun & C.D.Bouche; AhR, Aryl hydrocarbon receptor; Trp, Tryptophan; SCFAs, Short-chain fatty acids; IAP:, IEC, Intestinal epithelial cell; ECC, Enterochromaffin cell; ZO, Zonula occludens; AQP, Aquaporin; 5-HT, 5-hydroxytryptamine; Ach, Acetylcholine; 5-HTR, 5-HT receptor; CHRM2, Cholinergic receptor muscarinic 2; ROS, Reactive oxygen species; NQO-1, NAD(P)H: Quinone oxidoreductase-1; SOD, Superoxide dismutase; HO-1, Heme oxygenase-1; GSH-Px, Glutathione peroxidase; Nrf2, Nuclear factor erythroid-2-related factor 2; MAPK, Mitogen-activated protein kinase; JNK, c-Jun N-terminal kinase; ERK, extracellular signal-regulated kinase; p38, p38 mitogen-activated protein kinases; AKT, Serine/Threonine protein kinase; TLR4, Toll-like receptor 4; MyD88, Myeloid differentiation primary response gene 88; IκB, Inhibitor of NF-κB; NF-κB, Nuclear transcription factor-κB; COX-2, Cyclolxygenase-2; iNOS, inducible NO synthase; PGE2, Prostaglandin E2; NO, Nitric oxide; NLRP3, nucleotide-binding domain-like receptors family pyrin domain containing 3; IL, Interleukin; INF, Interferon; TGF, Transforming growth factor; Th cell, helper T cell; Treg cell, regulatory T cell; Bcl-2, B-cell lymphoma-2; Bcl-xL, B-cell lymphoma-extra-large; BAX, Bcl-2-associated X protein; PARP, Poly ADP ribose polymerase.
Data availability statement
The original contributions presented in the study are included in the article/Supplementary Material, further inquiries can be directed to the corresponding author.
Author contributions
CP: Writing–original draft. MK: Data curation, Formal Analysis, Writing–review and editing. J-WP: Conceptualization, Investigation, Methodology, Resources, Writing–review and editing. JK: Formal Analysis, Investigation, Methodology, Writing–review and editing. YB: Conceptualization, Writing–review and editing. S-JK: Data curation, Investigation, Methodology, Resources, Writing–review and editing.
Funding
The author(s) declare that financial support was received for the research, authorship, and/or publication of this article. This research was supported by the National Research Foundation of Korea (NRF) grant funded by the Korean Government (MSIT) (No. 2022R1C1C1004937).
Conflict of interest
The authors declare that the research was conducted in the absence of any commercial or financial relationships that could be construed as a potential conflict of interest.
Generative AI statement
The author(s) declare that no Generative AI was used in the creation of this manuscript.
Publisher’s note
All claims expressed in this article are solely those of the authors and do not necessarily represent those of their affiliated organizations, or those of the publisher, the editors and the reviewers. Any product that may be evaluated in this article, or claim that may be made by its manufacturer, is not guaranteed or endorsed by the publisher.
Supplementary material
The Supplementary Material for this article can be found online at: https://www.frontiersin.org/articles/10.3389/fphar.2025.1543194/full#supplementary-material
References
Abraham, C., Dulai, P. S., Vermeire, S., and Sandborn, W. J. (2017). Lessons learned from trials targeting cytokine pathways in patients with inflammatory bowel diseases. Gastroenterology 152 (2), 374–388. doi:10.1053/j.gastro.2016.10.018
Abrams, P., Andersson, K. E., Buccafusco, J. J., Chapple, C., de Groat, W. C., Fryer, A. D., et al. (2006). Muscarinic receptors: their distribution and function in body systems, and the implications for treating overactive bladder. Br. J. Pharmacol. 148 (5), 565–578. doi:10.1038/sj.bjp.0706780
Aggeletopoulou, I., Kalafateli, M., Tsounis, E. P., and Triantos, C. (2024). Exploring the role of IL-1β in inflammatory bowel disease pathogenesis. Front. Med. (Lausanne) 11, 1307394. doi:10.3389/fmed.2024.1307394
Agus, A., Planchais, J., and Sokol, H. (2018). Gut microbiota regulation of tryptophan metabolism in health and disease. Cell Host & Microbe 23 (6), 716–724. doi:10.1016/j.chom.2018.05.003
Al-Sadi, R., Ye, D., Dokladny, K., and Ma, T. Y. (2008). Mechanism of IL-1beta-induced increase in intestinal epithelial tight junction permeability. J. Immunol. 180 (8), 5653–5661. doi:10.4049/jimmunol.180.8.5653
Amin, A., Hossen, M. J., Fu, X.-Q., Chou, J.-Y., Wu, J.-Y., Wang, X.-Q., et al. (2022). Inhibition of the Akt/NF-κB pathway is involved in the anti-gastritis effects of an ethanolic extract of the rhizome of Atractylodes macrocephala. J. Ethnopharmacol. 293, 115251. doi:10.1016/j.jep.2022.115251
Awolade, P., Cele, N., Kerru, N., Gummidi, L., Oluwakemi, E., and Singh, P. (2020). Therapeutic significance of β-glucuronidase activity and its inhibitors: a review. Eur. J. Med. Chem. 187, 111921. doi:10.1016/j.ejmech.2019.111921
Bang, B. W., Park, D., Kwon, K. S., Lee, D. H., Jang, M. J., Park, S. K., et al. (2019). BST-104, a water extract of Lonicera japonica, has a gastroprotective effect via antioxidant and anti-inflammatory activities. J. Med. Food 22 (2), 140–151. doi:10.1089/jmf.2018.4231
Chan, K. W. K., Chung, H. Y., and Ho, W. S. (2020). Anti-tumor activity of Atractylenolide I in human colon adenocarcinoma in vitro. Molecules 25 (1), 212. doi:10.3390/molecules25010212
Chang, I. G., Ryu, K. W., Park, D. W., and Ryu, B. H. (1985). Clinical review of irritable bowel syndrome. J. Korean Med. 6 (2), 30–36.
Chen, J., Xu, W., Liu, Y., Liang, X., Chen, Y., Liang, J., et al. (2024). Lonicera japonica Thunb. and its characteristic component chlorogenic acid alleviated experimental colitis by promoting Lactobacillus and fecal short-chain fatty acids production. Food Front. 5 (4), 1583–1602. doi:10.1002/fft2.412
Chen, T., Zheng, X., Ouyang, L., Han, Z., Yang, Y., Gu, L., et al. (2023). Six polyacetylenes from Atractylodes macrocephala Koidz and their anti-colon cancer activity. Fitoterapia 167, 105490. doi:10.1016/j.fitote.2023.105490
Cheng, H., Zhang, D., Wu, J., Liu, J., Tan, Y., Feng, W., et al. (2023). Atractylodes macrocephala Koidz. volatile oil relieves acute ulcerative colitis via regulating gut microbiota and gut microbiota metabolism. Front. Immunol. 14, 1127785. doi:10.3389/fimmu.2023.1127785
Chenxing, W., Jie, S., Yajuan, T., Ting, L., Yuying, Z., Suhong, C., et al. (2024). The rhizomes of Atractylodes macrocephala Koidz improve gastrointestinal health and pregnancy outcomes in pregnant mice via modulating intestinal barrier and water-fluid metabolism. J. Ethnopharmacol. 326, 117971. doi:10.1016/j.jep.2024.117971
Cho, M. J., Bae, J. H., Park, J. H., Bu, Y. M., Lee, B. J., Park, J. W., et al. (2022). Protective effect of the traditional Korean herbal prescription, Bojangunbi-tang, on non-steroidal anti-inflammatory drug-induced small bowel injury. Pharmacogn. Mag. 18 (77), 183–187. doi:10.4103/pm.pm_333_21
Choi, N. R., Choi, W. G., Lee, J. H., Park, J., Kim, Y. T., Das, R., et al. (2024a). Atractylodes macrocephala Koidz alleviates symptoms in zymosan-induced irritable bowel syndrome mouse model through TRPV1, NaV1.5, and NaV1.7 channel modulation. Nutrients 16 (11), 1683. doi:10.3390/nu16111683
Choi, N. R., Choi, W. G., Zhu, A., Park, J., Kim, Y. T., Hong, J., et al. (2024b). Exploring the therapeutic effects of Atractylodes macrocephala Koidz against human gastric cancer. Nutrients 16 (7), 965. doi:10.3390/nu16070965
Choi, N. R., Jeong, H. j., Choi, W. G., Park, J. W., Ko, S. J., and Kim, B. J. (2023). A study on the effects of muscarinic and serotonergic regulation by bojanggunbi-tang on the pacemaker potential of the interstitial cells of cajal in the murine small intestine. Int. J. Med. Sci. 20 (8), 1000–1008. doi:10.7150/ijms.83986
Choi, Y., Kim, N., Noh, G. T., Lee, J. Y., and Lee, D. H. (2020). The efficacy and safety of GCWB104 (flos Lonicera extract) in functional dyspepsia: a single-center, randomized, double-blind, placebo-controlled study. Gut Liver 14 (1), 67–78. doi:10.5009/gnl19283
Coccia, M., Harrison, O. J., Schiering, C., Asquith, M. J., Becher, B., Powrie, F., et al. (2012). IL-1β mediates chronic intestinal inflammation by promoting the accumulation of IL-17A secreting innate lymphoid cells and CD4(+) Th17 cells. J. Exp. Med. 209 (9), 1595–1609. doi:10.1084/jem.20111453
Devlin, A. S., Marcobal, A., Dodd, D., Nayfach, S., Plummer, N., Meyer, T., et al. (2016). Modulation of a circulating uremic solute via rational genetic manipulation of the gut microbiota. Cell Host & Microbe 20 (6), 709–715. doi:10.1016/j.chom.2016.10.021
Eggera, B., Bajaj-Elliottb, M., MacDonaldb, T. T., Inglina, R., Eysseleinc, V. E., and Büchlera, M. W. (2000). Characterisation of acute murine dextran sodium sulphate colitis: cytokine profile and dose dependency. Digestion 62 (4), 240–248. doi:10.1159/000007822
Feng, W., Liu, J., Tan, Y., Ao, H., Wang, J., and Peng, C. (2020). Polysaccharides from Atractylodes macrocephala Koidz. Ameliorate ulcerative colitis via extensive modification of gut microbiota and host metabolism. Food Res. Int. 138, 109777. doi:10.1016/j.foodres.2020.109777
Feng, Z., Yang, R., Wu, L., Tang, S., Wei, B., Guo, L., et al. (2019). Atractylodes macrocephala polysaccharides regulate the innate immunity of colorectal cancer cells by modulating the TLR4 signaling pathway. OncoTargets Ther. 12, 7111–7121. doi:10.2147/ott.S219623
Fujino, S., Andoh, A., Bamba, S., Ogawa, A., Hata, K., Araki, Y., et al. (2003). Increased expression of interleukin 17 in inflammatory bowel disease. Gut 52 (1), 65–70. doi:10.1136/gut.52.1.65
Garlanda, C., Dinarello, C. A., and Mantovani, A. (2013). The interleukin-1 family: back to the future. Immunity 39 (6), 1003–1018. doi:10.1016/j.immuni.2013.11.010
Han, G. E., Ryu, B. H., Park, D. W., and Chang, I. G. (1986). Clinical study of diarrhea in irritable colon syndorme. J. Korean Med. 7 (2), 201–206.
Hanauer, S. B., Feagan, B. G., Lichtenstein, G. R., Mayer, L. F., Schreiber, S., Colombel, J. F., et al. (2002). Maintenance infliximab for Crohn's disease: the ACCENT I randomised trial. Lancet 359 (9317), 1541–1549. doi:10.1016/s0140-6736(02)08512-4
Hu, P., Yan, X., Zeng, Y., Jiang, Z., Liu, J., and Feng, W. W. (2023). An UPLC-MS/MS method for targeted analysis of microbial and host tryptophan metabolism after administration of polysaccharides from Atractylodes macrocephala Koidz. in ulcerative colitis mice. J. Pharm. Biomed. Analysis 235, 115585. doi:10.1016/j.jpba.2023.115585
Jia, M. X., Yu, M., Qin, L. L., Jia, H. M., and Zou, Z. M. (2022). Ameliorative effect of polysaccharides from raw Atractylodes macrocephala on constipation induced by loperamide in rats. Chin. Traditional Herb. Drugs 53 (24), 7808–7815. doi:10.7501/j.issn.0253-2670.2022.24.018
Joun, J. H., Ryu, B. H., Park, D. W., and Ryu, K. W. (1994). Experimental studies on the effects of Bojanggunbitang. J. Korean Med. 15 (1), 83–99.
Jung, D. H., Choi, E. J., Jeon, H. H., Lee, Y. H., and Hyojin, P. (2014). Effects of GC7101, a novel prokinetic agent on gastric motor function: ex vivo study. J. Neurogastroenterol. Motil. 20 (4), 469–474. doi:10.5056/jnm14010
Kai, L., Zong, X., Jiang, Q., Lu, Z., Wang, F., Wang, Y., et al. (2022). Protective effects of polysaccharides from Atractylodes macrocephalae Koidz. against dextran sulfate sodium induced intestinal mucosal injury on mice. Int. J. Biol. Macromol. 195, 142–151. doi:10.1016/j.ijbiomac.2021.12.042
Kim, H. G. (2017). Study on a newly develped herbal prescription derived from Bojanggunbi-tang for inflammatory bowel disease. (dissertation). Kyung Hee University, Seoul, Republic of Korea.
Kim, H. G., Kim, M.-Y., and Cho, J. Y. (2018). Alisma canaliculatum ethanol extract suppresses inflammatory responses in LPS-stimulated macrophages, HCl/EtOH-induced gastritis, and DSS-triggered colitis by targeting Src/Syk and TAK1 activities. J. Ethnopharmacol. 219, 202–212. doi:10.1016/j.jep.2018.03.022
Kim, H. G., Ko, S. J., Hong, B. g., Kim, J. s., Lee, B. j., Joo, H. j., et al. (2021). Anti-colitic effects of the modified Bojanggunbi-tang on dextran sulfate sodium-induced mouse colitis model. Pak. J. Pharm. Sci. 34 (6), 2159–2165. doi:10.36721/PJPS.2021.34.6.REG.2159-2165.1
Kim, N. J., Kim, D. H., Park, J. B., and Hong, N. D. (1993). Effect of combined preparations of crude drugs used as energy invigorator on serum components and intestinal bacteria in mice. Korean J. Pharmacogn. 24 (3), 235–243.
Kim, S. H., Jung, H. N., Lee, K. Y., Kim, J., Lee, J. C., and Jang, Y. S. (2005). Suppression of TH2-type immune response-mediated allergic diarrhea following oral administration of traditional Korean medicine: Atractylodes macrocephala Koidz. Immunopharmacol. Immunotoxicol. 27 (2), 331–343. doi:10.1081/IPH-200067950
Ko, S. J., Kim, H. G., Kuo, B., Bu, Y. M., Kim, J. S., and Park, J. W. (2017). The protective effect of bojanggunbi-tang essence on murine colitis. Gastroenterology 152 (5S1), 735–736. doi:10.1016/s0016-5085(17)32556-8
Kwon, M. J., Kim, J. N., Park, J., Kim, Y. T., Lee, M. J., and Kim, B. J. (2021). Alisma canaliculatum extract affects AGS gastric cancer cells by inducing apoptosis. Int. J. Med. Sci. 18 (10), 2155–2161. doi:10.7150/ijms.55212
Lee, K. H., Whang, K.-S., and Rhee, K.-H. (2011). Effects of Lonicera japonica Thunb. on dextran sulfate sodium-induced experimental colitis in mice. J. Med. Plants Res. 5 (22), 5437–5443. doi:10.5897/JMPR.9000529
Lemons, J. M. S., Narrowe, A. B., Liu, L., Firrman, J., Mahalak, K. K., and Van den Abbeele, P. (2023). Impact of Baizhu, Daqingye, and Hehuanhua extracts on the human gut microbiome. Front. Cell. Infect. Microbiol. 13, 1298392. doi:10.3389/fcimb.2023.1298392
Li, L., He, Y., Wang, N., Li, Y., Du, Y., He, N., et al. (2023). Atractylone in the Atractylodes macrocephala rhizoma essential oil and its anti-inflammatory activity. Mol. Basel, Switz. 28 (21), 7340. doi:10.3390/molecules28217340
Li, W., Xiang, X., Li, B., Wang, Y., Qian, L., Tian, Y., et al. (2021). PAMK relieves LPS-induced enteritis and improves intestinal flora disorder in Goslings. Evidence-based Complementary Altern. Med. 2021, 9721353. doi:10.1155/2021/9721353
Lv, Q., Xing, Y., Liu, J., Dong, D., Liu, Y., Qiao, H., et al. (2021). Lonicerin targets EZH2 to alleviate ulcerative colitis by autophagy-mediated NLRP3 inflammasome inactivation. Acta Pharm. Sin. B 11 (9), 2880–2899. doi:10.1016/j.apsb.2021.03.011
Ma, L., Mao, R. R., Shen, K., Zheng, Y. H., Li, Y. Q., Liu, J. W., et al. (2014). Atractylenolide I-mediated Notch pathway inhibition attenuates gastric cancer stem cell traits. Biochem. Biophysical Res. Commun. 450 (1), 353–359. doi:10.1016/j.bbrc.2014.05.110
McAlindon, M. E., Hawkey, C. J., and Mahida, Y. R. (1998). Expression of interleukin 1 beta and interleukin 1 beta converting enzyme by intestinal macrophages in health and inflammatory bowel disease. Gut 42 (2), 214–219. doi:10.1136/gut.42.2.214
Minami, M., and Makino, T. (2020). Effects of Lonicera japonica flower bud extract on Citrobacter rodentium-induced digestive tract infection. Med. (Basel) 7 (9), 52. doi:10.3390/medicines7090052
Nam, S. M., Choi, D. Y., Ahn, C. B., and Yim, J. H. (1989). Effects of bojangkunbitang mixed with gaja on function of gastrointestinal tract to rats and mice. J. Korean Med. 10 (2), 140–152.
Nam, Y., Lee, J. M., Wang, Y., Ha, H. S., and Sohn, U. D. (2016). The effect of Flos Lonicerae Japonicae extract on gastro-intestinal motility function. J. Ethnopharmacol. 179, 280–290. doi:10.1016/j.jep.2015.12.056
Noh, Y. W., Jung, H. K., Kim, S. E., and Jung, S. A. (2010). Overlap of erosive and non-erosive reflux diseases with functional gastrointestinal disorders according to Rome III criteria. J. Neurogastroenterol. Motil. 16 (2), 148–156. doi:10.5056/jnm.2010.16.2.148
Park, J. W., Bae, H., Lee, G., Hong, B. G., Yoo, H. H., Lim, S. J., et al. (2013). Prophylactic effects of Lonicera japonica extract on dextran sulphate sodium-induced colitis in a mouse model by the inhibition of the Th1/Th17 response. Br. J. Nutr. 109 (2), 283–292. doi:10.1017/s0007114512001122
Percie du Sert, N., Ahluwalia, A., Alam, S., Avey, M. T., Baker, M., Browne, W. J., et al. (2020). Reporting animal research: explanation and elaboration for the ARRIVE guidelines 2.0. PLOS Biol. 18 (7), e3000411. doi:10.1371/journal.pbio.3000411
Prossomariti, A., Sokol, H., and Ricciardiello, L. (2018). Nucleotide-binding domain leucine-rich repeat containing proteins and intestinal microbiota: pivotal players in colitis and colitis-associated cancer development. Front. Immunol. 9, 1039. doi:10.3389/fimmu.2018.01039
Qin, L.-L., Yu, M., Yang, P., and Zou, Z.-M. (2024). The rhizomes of Atractylodes macrocephala relieve loperamide-induced constipation in rats by regulation of tryptophan metabolism. J. Ethnopharmacol. 322, 117637. doi:10.1016/j.jep.2023.117637
Ren, Y., Jiang, W., Luo, C., Zhang, X., and Huang, M. (2021a). Atractylenolide III ameliorates TNBS-induced intestinal inflammation in mice by reducing oxidative stress and regulating intestinal flora. Chem. Biodivers. 18 (8), e2001001. doi:10.1002/cbdv.202001001
Ren, Y., Jiang, W., Luo, C., Zhang, X., and Huang, M. (2021b). The promotive effect of the active ingredients of Atractylodes macrocephala on intestinal epithelial repair through activating Ca2+ pathway. Nat. Product. Commun. 16 (11), 1934578X2110403. doi:10.1177/1934578X211040357
Ro, W. S., Kim, J. S., Yoon, S. H., and Ryu, B. H. (2006). Experimental studies on the anti-cathartic effects of dolichoris semen, terminaliae fructus and bojanggunbi-tang. J. Korean Med. 27 (2), 356–370.
Rodger, D., Admani, A., and Thomas, M. (2024). What is a scoping review? Evid. Based Nurs. 27 (3), 84–85. doi:10.1136/ebnurs-2024-103969
Ryu, B. H., Ro, W. S., Park, J. W., Bu, Y. M., Lee, B. J., Lim, S. J., et al. (2011). Bojanggunbi-tang, a traditional Korean herbal prescription, ameliorates colonic inflammation induced by dextran sulfate sodium and 2,4,6-trinitrobenzene sulfonic acid in mice. J. Ethnopharmacol. 135 (2), 582–585. doi:10.1016/j.jep.2011.03.004
Seo, S. H., Jeong, J. O., Lee, E. H., Choi, I. S., Park, S. E., Kim, W. I., et al. (2004). A case study of suspected crohn's disease treated with bojanggunbi-tang. J. Korean Med. 25 (3), 648.
Shang, X., Pan, H., Li, M., Miao, X., and Ding, H. (2011). Lonicera japonica Thunb.: ethnopharmacology, phytochemistry and pharmacology of an important traditional Chinese medicine. J. Ethnopharmacol. 138 (1), 1–21. doi:10.1016/j.jep.2011.08.016
Shim, A. R., Dong, G. Z., Lee, H. J., and Ryu, J. H. (2015). Atractylochromene is a repressor of Wnt/β-catenin signaling in colon cancer cells. Biomol. Ther. 23 (1), 26–30. doi:10.4062/BIOMOLTHER.2014.095
Shu, Y.-T., Kao, K.-T., and Weng, C.-S. (2017). In vitro antibacterial and cytotoxic activities of plasma-modified polyethylene terephthalate nonwoven dressing with aqueous extract of Rhizome Atractylodes macrocephala. Mater. Sci. Eng. C 77, 606–612. doi:10.1016/j.msec.2017.03.291
Sia, T. C., Whiting, M., Kyloh, M., Nicholas, S. J., Oliver, J., Brookes, S. J., et al. (2013). 5-HT3 and 5-HT4 antagonists inhibit peristaltic contractions in Guinea-pig distal colon by mechanisms independent of endogenous 5-HT. Front. Neurosci. 7, 136. doi:10.3389/fnins.2013.00136
Sinn, D. H., Lim, S. W., Kim, K. M., Son, H. J., Jae, J. K., Rhee, J. C., et al. (2010). The speed of eating and functional dyspepsia in young women. Gut Liver 4 (2), 173–178. doi:10.5009/gnl.2010.4.2.173
Song, H. P., Hou, X. Q., Li, R. Y., Yu, R., Li, X., Zhou, S. N., et al. (2017). Atractylenolide I stimulates intestinal epithelial repair through polyamine-mediated Ca2+ signaling pathway. Phytomedicine 28, 27–35. doi:10.1016/j.phymed.2017.03.001
Song, H.-P., Li, R.-L., Chen, X., Wang, Y.-Y., Cai, J.-Z., Liu, J., et al. (2014). Atractylodes macrocephala Koidz promotes intestinal epithelial restitution via the polyamine—voltage-gated K+ channel pathway. J. Ethnopharmacol. 152 (1), 163–172. doi:10.1016/j.jep.2013.12.049
Song, H.-P., Li, R.-L., Zhou, C., Cai, X., and Huang, H.-Y. (2015). Atractylodes macrocephala Koidz stimulates intestinal epithelial cell migration through a polyamine dependent mechanism. J. Ethnopharmacol. 159, 23–35. doi:10.1016/j.jep.2014.10.059
Souza, R. F., Caetano, M. A. F., Magalhães, H. I. R., and Castelucci, P. (2023). Study of tumor necrosis factor receptor in the inflammatory bowel disease. World J. Gastroenterology 29 (18), 2733–2746. doi:10.3748/wjg.v29.i18.2733
Sun, W., Zhu, J., Qin, G., Huang, Y., Cheng, S., Chen, Z., et al. (2023). Lonicera japonica polysaccharides alleviate D-galactose-induced oxidative stress and restore gut microbiota in ICR mice. Int. J. Biol. Macromol. 245, 125517. doi:10.1016/j.ijbiomac.2023.125517
Sun, Y., Liu, Y., Cai, Y., Han, P. P., Hu, S., and Cao, L. J. (2022). Atractylenolide I inhibited the development of malignant colorectal cancer cells and enhanced oxaliplatin sensitivity through the PDK1-FoxO1 axis. J. Gastrointest. Oncol. 13 (5), 2382–2392. doi:10.21037/jgo-22-910
Tian, S., and Yu, H. (2017). Atractylenolide II inhibits proliferation, motility and induces apoptosis in human gastric carcinoma cell lines HGC-27 and AGS. Molecules 22 (11), 1886. doi:10.3390/molecules22111886
Tian, T., Chen, H., and Zhao, Y.-Y. (2014). Traditional uses, phytochemistry, pharmacology, toxicology and quality control of Alisma orientale (Sam.) Juzep: a review. J. Ethnopharmacol. 158, 373–387. doi:10.1016/j.jep.2014.10.061
Tricco, A. C., Lillie, E., Zarin, W., O’Brien, K., Colquhoun, H., Kastner, M., et al. (2016). A scoping review on the conduct and reporting of scoping reviews. BMC Med. Res. Methodol. 16 (1), 15. doi:10.1186/s12874-016-0116-4
Wang, R., Zhou, G., Wang, M., Peng, Y., and Li, X. (2014). The metabolism of polysaccharide from Atractylodes macrocephala Koidz and its effect on intestinal microflora. Complement. Altern. Med. 2014, 926381. doi:10.1155/2014/926381
Wang, Z. Q., Wu, Q. Y., Ye, R. L., Nabi, F., Shang, Y. F., Ali, S., et al. (2022). Protective effects of Atractylodes macrocephala polysaccharide on iIELs and the IL-6, TNF-α mRNA level in E. coli induced diarrheal mice. Pak. J. Zoology 54 (5), 2193–2201. doi:10.17582/journal.pjz/20200316150354
Witowski, J., Książek, K., and Jörres, A. (2004). Interleukin-17: a mediator of inflammatory responses. Cell. Mol. Life Sci. 61 (5), 567–579. doi:10.1007/s00018-003-3228-z
Xie, F., Sakwiwatkul, K., Zhang, C., Wang, Y., Zhai, L., and Hu, S. (2013). Atractylodis macrocephalae Koidz. polysaccharides enhance both serum IgG response and gut mucosal immunity. Carbohydr. Polym. 91 (1), 68–73. doi:10.1016/j.carbpol.2012.07.083
Xu, R., Liu, X., Tian, M., and Chen, D. (2023). Atractylodes-I overcomes the oxidative stress-induced colonic mucosal epithelial cells dysfunction to prevent irritable bowel syndrome via modulating the miR-34a-5p-LDHA signaling pathway. Curr. Mol. Med. 23 (8), 825–833. doi:10.2174/1566524022666220811161111
Yamamoto, K., Takase, H., Abe, K., Saito, Y., and Suzuki, A. (1993). Pharmacological studies on antidiarrheal effects of a preparation containing berberine and geranii herba. Nihon Yakurigaku Zasshi 101 (3), 169–175. doi:10.1254/fpj.101.3_169
Yang, H., Wu, C., Chen, L., Chang, X., Luo, G., Wu, K., et al. (2023). A. macrocephala polysaccharide induces alterations to gut microbiome and serum metabolome in constipated mice. Microb. Pathog. 178, 106084. doi:10.1016/j.micpath.2023.106084
Yang, M., Zhang, Q., Taha, R., Abdelmotalab, M. I., Wen, Q., Yuan, Y., et al. (2022). Polysaccharide from Atractylodes macrocephala Koidz. ameliorates DSS-induced colitis in mice by regulating the Th17/Treg cell balance. Front. Immunol. 13, 1021695. doi:10.3389/fimmu.2022.1021695
Yang, S., Zhang, J., Yan, Y., Yang, M., Li, C., Li, J., et al. (2020). Network pharmacology-based strategy to investigate the pharmacologic mechanisms of Atractylodes macrocephala Koidz. For the treatment of chronic gastritis. Front. Pharmacol. 10, 1629. doi:10.3389/fphar.2019.01629
Yoon, S. H. (2001). A case of 15 Years-diarrhea reccedded with bojanggyunbitang in collagenous colitis. J. Orient. chronic Dis. 7 (1), 78–81.
Zeng, L., Shen, L., Fan, Y., Luo, Q., Hong, R., Sun, X., et al. (2023). Atractylodes processing products protect against gastric ulcers in rats by influencing the NF-κB-MMP-9/TIMP-1 regulatory mechanism and intestinal flora. Chem. Biodivers. 20 (6), e202300068. doi:10.1002/cbdv.202300068
Zhai, C., Lu, F., Du, X., Zhang, M., Zhang, Y., Ma, Y., et al. (2023). Green carbon dots derived from Atractylodes macrocephala: a potential nanodrug for treating alcoholic gastric ulcer. Colloids Surfaces B Biointerfaces 230, 113492. doi:10.1016/j.colsurfb.2023.113492
Zhang, D., Li, X., Song, D., Chen, S., Zhang, Z., Cao, S., et al. (2022). Atractylenolide III induces apoptosis by regulating the Bax/Bcl-2 signaling pathway in human colorectal cancer HCT-116 Cells in vitro and in vivo. Anti-Cancer Drugs 33 (1), 30–47. doi:10.1097/CAD.0000000000001136
Zhang, Y. Z., and Li, Y. Y. (2014). Inflammatory bowel disease: pathogenesis. World J. Gastroenterology 20 (1), 91–99. doi:10.3748/wjg.v20.i1.91
Zhou, X., Lu, Q., Kang, X., Tian, G., Ming, D., and Yang, J. (2021). Protective role of a new polysaccharide extracted from Lonicera japonica Thunb in mice with ulcerative colitis induced by dextran sulphate sodium. BioMed Res. Int. 2021, 8878633. doi:10.1155/2021/8878633
Zhu, B., Zhang, Q.-l., Hua, J.-w., Cheng, W.-l., and Qin, L.-p. (2018). The traditional uses, phytochemistry, and pharmacology of Atractylodes macrocephala Koidz.: a review. J. Ethnopharmacol. 226, 143–167. doi:10.1016/j.jep.2018.08.023
Keywords: Bojanggunbi-tang, herbal medicine, gastrointestinal, Lonicera japonica, Atractylodes macrocephala, Alisma canaliculatum
Citation: Park C, Kim M, Park J-W, Kim J, Bu Y and Ko S-J (2025) Effect of Bojanggunbi-tang and its primary constituent herbs on the gastrointestinal tract: a scoping review. Front. Pharmacol. 16:1543194. doi: 10.3389/fphar.2025.1543194
Received: 11 December 2024; Accepted: 18 February 2025;
Published: 12 March 2025.
Edited by:
Haiyang Wu, First Affiliated Hospital of Zhengzhou University, ChinaReviewed by:
Lei Gao, Houston Methodist Research Institute, United StatesWenzhi Wang, Baylor College of Medicine, United States
Copyright © 2025 Park, Kim, Park, Kim, Bu and Ko. This is an open-access article distributed under the terms of the Creative Commons Attribution License (CC BY). The use, distribution or reproduction in other forums is permitted, provided the original author(s) and the copyright owner(s) are credited and that the original publication in this journal is cited, in accordance with accepted academic practice. No use, distribution or reproduction is permitted which does not comply with these terms.
*Correspondence: Seok-Jae Ko, a29rb2tva28xMTlAZGF1bS5uZXQ=