- 1Institute of Chemical Sciences, Gomal University, Dera Ismail Khan, Pakistan
- 2Natural Products Research Lab (NPRL), Department of Pharmacognosy, Faculty of Pharmacy, Gomal University, Dera Ismail Khan, Pakistan
- 3Department of Pathology, Gomal Medical College, Dera Ismail Khan, Pakistan
- 4Department of Pharmaceutical Chemistry, College of Pharmacy, King Saud University, Riyadh, Saudi Arabia
- 5Faculty of Pharmacy, IBADAT International University, Islamabad, Pakistan
- 6Institute of Pharmaceutical Sciences, University of Veterinary & Animal Sciences (UVAS), Lahore, Pakistan
- 7Faculty of Health and Medical Sciences, University of Azad Jammu and Kashmir Muzaffarabad, Pakistan
- 8Faculty of Pharmacy, Department of Pharmacognosy, The Islamiya University of Bahawalpur, Punjab, Pakistan
- 9 Pakistan Council for Scientific and Industrial Research (PCSIR), Bahawalpur, Peshawar, Pakistan
- 10Centre for Study of Human Health, Emory University, Atlanta, GA, United States
- 11Faculty of Pharmacy and Allied Health Sciences, Iqra University, Islamabad, Pakistan
- 12Department of Life Sciences, Yeungman University, Gyeongsan, South Korea
Introduction: Essential Oils (EOs) are a rich source of secondary metabolites that exhibit various biological activities.
Methods: This study includes GCMS analysis of Pinus roxburghii EO, computational investigation including drug likeness, ADMET properties, molecular docking, and in vitro evaluations for possible antioxidant, antidiabetic, and anti-AGEs actions.
Results: GCMS analysis identified β-pinene (30%) as major component, succeeded by caryophyllene (29.37%), o-xylene (8.98%), aromadendrine (8.29%), and α-himachalene (6.82%). Molecular docking showed significant interaction of transcription regulators 1JIJ with Caryophyllene oxide (ΔG -7.5 (kJ mol-1), 3TOP with α-himachalene (ΔG -6.8 (kJ mol-1) and 4F5S with α-himachalene (ΔG -7.7 (kJ mol-1). The EO exhibited elevated phenolic content (26.3±0.45 mg/G GAE) and considerable antioxidant capacity in DPPH (14.2±0.62), H2O2 (73.3±1.7), and FRAP assays (312±14.6 μg). The antidiabetic assays demonstrated a notable inhibition of α-glucosidase (IC50 0.12 mg/mL) and advanced glycation end products (AGEs) in both non-oxidative (IC50 0.052 mg/mL) and oxidative modes (IC50 1.61 mg/mL). During mechanistic investigations it was observed that EO exerts a protective effect against β-amyloid formation and significantly entraps carbonyl moieties.
Conclusions: It was observed that P. roxburghii EO has notable antidiabetic and anti-AGEs properties and these finding support a good potential for management of diabetes and allied co-morbidities. In future formulation design studies can be helpful for pharmaceutical industry to opt the formulation.
1 Introduction
Essential oils (EOs) are mainly derived from aromatic edible plants and contain a variety of plant secondary metabolites (Maffei et al., 2011). The EOs are stored in secretory ducts, oil cells, or cavities within leaves, stems, flowers, and root parts of aromatic plants (Kiran et al., 2013). The EOs may be found bound to carbohydrates as glycosides, and hydrolysis of the glyosidic bonds is required to liberate them from their major source (Ailli et al., 2023). The EOs comprise terpene hydrocarbons, including mono-, di-, and sesquiterpenes; oxygenated derivatives (aldehydes, ketones, epoxides, alcohols, and esters); and sulfur or nitrogen derivatives (Rehman et al., 2007). These complex mixtures of diverse chemical nature pose several bioactive components that encompass a range of biological activities (Ruxton, 2016). Several investigations have confirmed antibacterial, antioxidant, anti-inflammatory, antibiofilm, antifungal, antidiabetic, and anticancer properties of EOs (Dorman and Deans, 2000; Chaieb et al., 2007; Yap et al., 2013; Saharkhiz et al., 2012; Dagli et al., 2015; You et al., 2022). It has been reported earlier that EOs greatly differ in their compositions, and even the composition of EOs extracted from plants of the same species differs in different geographic locations (Benabdelkader et al., 2011). Due to the small size and high penetration power of secondary metabolites in EOs, researchers are showing keen interest in EOs in the quest for promising drug leads (Herman and Herman, 2015).
Pinus roxburghii Sarg. (Pinus roxburghii; Pinaceae) is a tall tree (up to 30 m) commonly found in Pakistan (Khyber Pakhtunkhwa, Balochistan, and Punjab provinces) that covers a large area of land (Khan et al., 2014; Mehmood et al., 2024). The plant is commonly known as “Chir or Chil Pine” (Amjad et al., 2020). P. roxburghii has long been used on the Asian subcontinent to treat conditions like bronchitis, rheumatic pain (Rodriguez et al., 2021), diaphoresis, giddiness, ulcers, inflammation (Kaushik et al., 2012), snake bites, dermatological and topical illnesses (Labib et al., 2016), and gastrointestinal and liver disorders (Kaushik et al., 2012). Recently, researchers have reported anticancer, antimicrobial, antioxidant, anti-inflammatory, anticonvulsant, analgesic, antiparasitic, and antidiabetic properties of P. roxburghii (Kaushik et al., 2013; Sajid et al., 2018; Zahid et al., 2023). The EO obtained from P. roxburghii has been studied earlier; components including α-pinene, β-phellandrene and β-pinene, δ-3-carene, limonene, and linalool acetate, were identified, and it was proven that this EO can inhibit the activation of the inflammatory transcription factor NF-κB and the expression of the NF-κB-regulated gene (Sajid et al., 2018). Investigators have also reported the antidiabetic potential of P. roxburghii (Kaushik et al., 2014; Kaushik et al., 2015; Saber et al., 2021).
Given the rising global burden of diabetes and oxidative stress-related disorders (WHO. Global burden of disease collaborative network, 2024; Chandimali et al., 2025), there is growing interest in identifying natural therapeutic agents with multi-targeted effects. Although several studies have investigated the pharmacological properties of EOs from other Pinus species, there is a lack of comprehensive research integrating in silico and in vitro approaches to validate the bioactivity of P. roxburghii essential oil (Ayub et al., 2023). This study aims to bridge this gap by systematically evaluating the antioxidant, antidiabetic, and antiglycation potential of P. roxburghii EO. By combining computational docking, molecular dynamics simulations, and in vitro biochemical assays, we provide a holistic assessment of its bioactivity, which could contribute to the development of plant-based therapeutics for metabolic disorders. Given its significant medicinal importance, we investigated P. roxburghii EO for antioxidant, antidiabetic, and anti-AGE (advanced glycation end product) properties using computational and in vitro models to explore its possible applications in diabetes and related co-morbidities. In this study, we provide a holistic assessment of its bioactivity, which could contribute to the development of plant-based therapeutics for metabolic disorders.
2 Materials and methods
2.1 Chemicals and reagents
All chemicals and reagents used were of analytical-grade, including methylglyoxal (MGO), α-glucosidase (Saccharomyces cerevisiae), 2,4,6-Tris (2-pyridyl)-s-triazine (TPTZ), 2,4 dinitrophenyl hydrazine (DNPH), and DPPH (Oxoid, Hampshire, United Kingdom, and Merck, Dorset, United Kingdom). Similarly, sodium azide, D-glucose, and H2O2 were obtained from Daejung (Siheung-si, Korea). The Folin–Ciocalteu’s reagent was purchased from Sigma (Sigma Aldrich, St. Louis, MI, United States).
2.2 Plant collection and authentication and processing
Fresh P. roxburghii leaves were collected from the Takht-e-Suleiman range (31°40′57.66″N 69°56′11.64″E; Balochistan province), and herbarium sheets were prepared. These were further authenticated at the Islamabad herbarium, where the specimen was deposited, and an accession ID was obtained (ISL 36502). The plant material was ground and macerated overnight in water in a flask. Afterward, the EO was extracted through hydrodistillation using a Clevenger apparatus. Sodium sulfide was passed through the collected oil to remove traces of water and stored at 4°C until further use.
2.3 GC-MS analysis
EO component analysis was performed by using GC-MS (Shimadzu GC 2010; Japan) with a pre-installed autosampler (AOC-20i) and a capillary column (dimensions, 30 m × 0.25 mm id, 0.25-μm film thickness, a DB-5 MS column). The DB-5 MS capillary column was chosen for its high thermal stability, non-polar nature, and ability to efficiently separate volatile and semi-volatile compounds, particularly terpenoids and oxygenated components in P. roxburghii essential oil. The temperature of the system oven was initially adjusted to range from 45°C to 90°C, increasing at a rate of 2°C (per minute) and then increasing at a rate of 3°C (per minute) from 91°C to 240°C. After reaching 240°C, temperature was maintained for 5 min. The temperatures of the injector (240°C) and detector (280°C) were maintained at predetermined levels. The temperature program was optimized with a gradual increase (45–240°C) to allow efficient separation of highly volatile monoterpenes at lower temperatures, mid-range oxygenated terpenes at moderate temperatures, and heavier semi-volatiles at the final hold (240°C for 5 min). This process was followed to ensure the accurate identification and resolution of bioactive compounds. An aliquot of EO (0.5 μL) was injected, and helium (1 mL/min) was utilized as a carrier gas to load the sample. The GCMS-QP 2010 Plus (Shimadzu, Japan) instrument was used to analyze and identify GC-MS components. The device operated in electron ionization (EI) mode at 70 eV. Mass units ranging from 35 to 500 AMU were observed. Compounds such as limonene, carvacrol, thymol, α-pinene, β-pinene, β-myrcene, and sabinene were used as a compound mix for identification along with the NIST mass spectrum library (Aziz et al., 2024).
2.4 Molecular docking
Potential interactions of ligands with the amino acid residues in the target protein’s active pocket were observed through molecular docking investigations. CAStp (version 3.0) tool was used for the determination of active sites. The 3D structure of compounds was retrieved from the PubChem database, while the target structures such as the receptor for advanced glycation end products (RAGE) (Devi et al., 2016), 3TOP (the C-terminal subunit of human maltase-glucoamylase (MGAM) in complex with the inhibitor acarbose) (Rafey et al., 2022), and 4F5S (the crystal structure of bovine serum albumin) (Soliman et al., 2022) were downloaded from the Protein Data Bank (PDB). Stepwise procedures were used to prepare the target proteins for docking studies, including the addition of charges, extraction of co-crystallized ligands, and the addition of water. Molecular docking was performed using the Lamarckian approach with AutoDock Vina (version 1.2.x (2021–present) (Trott and Olson, 2010). Discovery Studio (version 2019), LigPlot +(version 2.2), and PyMOL (version 2.3.4) were then used to further analyze each pose for interaction analysis. All generated poses were evaluated based on free energy and RMSD values using the following formula:
where N is the number of atoms in the ligand; xi, yi, and zi are the coordinates of atom i in the docked pose; and xi,ref, yi,ref, and zi,ref are the coordinates of atom i in the reference pose (e.g., the lowest-energy conformation or crystallographic pose).
The RMSD values less than ≤ 2.0 Å indicated a stable binding pose (Fusani et al., 2020).
2.5 Total phenolic contents
The Folin–Ciocalteu method was used to determine the total phenolic contents (TPCs) (Chlopicka et al., 2012). Precisely, 0.3 mL of the sample (varying concentrations) was placed in an aliquot, allowed to react with 2.25 mL of Folin’s reagent, and then left undisturbed for 15 min. Subsequently, 2.25 mL of Na2CO3 (6%) was added and incubated for a further half-hour. Using a UV spectrophotometer, absorbance was determined at 725 nm. The results were expressed as gallic acid equivalent mg (GAE/g) of extract using a gallic acid standard curve with a range of 0–200 μg/mL for standardization.
2.6 Antioxidant activities
2.6.1 DPPH assay
Standard procedure was employed to determine the antioxidant activity of the samples (Chlopicka et al., 2012). In brief, 0.5 mL of newly prepared DPPH solution in methanol (0.1 mM) was mixed with the test sample (0.5 mL, different concentrations) and incubated for 30 min (in a dark area to prevent the influence of the influence). Subsequently, absorbance of the reaction mixture was recorded at 517 nm using a UV spectrophotometer (Shimadzu UV-1700; Japan). The control group contained DPPH solution in ethanol with same concentrations without the test sample. Ascorbic acid was used as the standard, and the results were presented as follows:
The outcomes were expressed as
where Aa is the absorbance of the sample, Ab is the absorbance of the control, and ascorbic acid was used as the standard drug.
2.6.2 Hydrogen peroxide assay
The measurement of hydrogen peroxide (H2O2)-based antioxidant activity was carried out using a modified method (Hussen and Endalew, 2023). In brief, 400 µL of the test sample and 600 µL of the H2O2 stock solution (2 mM) were combined, and after 10 min, the reaction solution was vortexed, followed by the measurement of absorbance at 230 nm using a UV spectrophotometer (Shimadzu UV-1700; Japan). The control group contained H2O2 solution in ethanol with the same concentrations without the test sample. The results were expressed using the following equation, where ascorbic acid is considered the reference standard.
where Aa is the absorbance of the sample, Ab is the absorbance of the control, and ascorbic acid was used as the standard drug.
2.6.3 FRAP assay
The ferrous ion reduction power was determined using a modified approach (Song et al., 2023). In brief, fresh FRAP reagent was prepared by combining ferric chloride (20 mM) and TPTZ (10 mM) in a defined ratio (10:1:1) with acetate buffer (300 mM). After reacting with 3 mL of the FRAP reagent, the tested sample (100 µL) was left in a dark area for 30 min. The UV–Vis spectrophotometer (Shimadzu UV-1700; Japan) was then used to record absorbance at 593 nm. The µg equivalents were used to express the results of the FeSO4 standard curve, which was further used to determine the ferrous reduction capacity, serving as the control.
2.7 Antidiabetic assays
2.7.1 α-glucosidase inhibition assay
A modified α-glucosidase inhibition assay was used to record the antidiabetic potential of the test samples (Fatmawati et al., 2023). In brief, the test sample (4 mg–0.039 mg/mL; 50 µL) was mixed with α-glucosidase (Saccharomyces cerevisiae) (0.2 U/mL in 0.1 M phosphate buffer at pH 6.8) and incubated for 10 min at 37°C. Subsequently, 100 µL of p-nitrophenyl-α-D-glucopyranoside (0.29 mM) was added to the reaction mixture that had already been incubated, and it was kept at 37°C for 30 min. After adding Na2CO3 (200 mM; 50 µL) to terminate the reaction, the optical density (at 400 nm) was measured. The reaction mixture without the test sample, operating at similar conditions, served as the control. Acarbose served as the reference standard, and % inhibition was determined as follows:
where Aa is the absorbance of the sample, Ab is the absorbance of the control, and acarbose was used as the standard drug. For α-glucosidase, the inhibition criteria were defined as follows: strong inhibition, IC50 ≤ 0.02 mg/mL; moderate inhibition, IC50 0.02–0.5 mg/mL; and weak inhibition, IC50 > 0.5 mg/mL (criteria based on comparison with standard drug acarbose).
2.8 Advance glycation end product assay
2.8.1 BSA-glucose assay
A modified protocol was used to perform AGE assay (non-oxidative mode) (Anwar et al., 2023). In brief, 50 mM of sodium azide (0.02%) was already present in the phosphate buffer (pH 7.4); then, 135 µL of D-glucose (500 mM), 135 µL of BSA (10 mg/mL) and 30 µL of test samples (varying concentrations) were added to the mixture. The mixture was then incubated for a week at 37°C. Subsequently, unreactive contaminants were eliminated, and the additional reaction was terminated by adding trichloroacetic acid (TCA) to the reaction mixture. The spectrofluorometer (FLUOstar Omega®, BMG Lab Tech, Aylesbury, United Kingdom) was used to measure the fluorescence intensity (Emission: 370:440 nm and 335:385 nm, respectively). The reaction mixture without the test sample, operating at similar conditions, served as the control. Aminoguanidine was used as the standard in the study, and the following formula was used to calculate the percentage of inhibition:
where Fa is the florescence of the test sample, Fb is the fluorescence of the control, and IC50 values were calculated using a linear plot. Rutin was used as the standard drug. For AGE experiments, the inhibition criteria were defined as follows: significant inhibition, IC50 ≤ standard inhibitor; moderate inhibition, IC50 between 1 and 2 times the IC50 value of the standard; and weak inhibition, IC50 > 2 times the IC50 of rutin.
2.8.2 BSA-MGO (methylglyoxal) assay
A modified protocol was used to perform AGE assay (oxidative mode) (Anwar et al., 2023). In brief, methylglyoxal (135 μL, 5.75 mM) in the phosphate buffer solution (pH 7.4, 50 mM) with sodium azide (0.02%) was mixed with BSA (135 μL, 10 mg/mL). This solution was combined with 30 μL of test extracts, which were then kept at 37°C for a week. A spectrofluorometer (FLUOstar Omega®, BMG Lab Tech, Aylesbury, United Kingdom) was used to measure the fluorescence intensity (excitation: emission at 370:440 nm and 335:385 nm). The reaction mixture without the test sample, operating at similar conditions, served as the control. Aminoguanidine was used as the standard, and the percentage of inhibition was calculated as follows:
where Fa is the florescence of the test sample, Fb is the fluorescence of the control, and IC50 values were calculated using a linear plot. Rutin was used as the standard drug.
2.8.3 β-amyloid protection
For the determination of β-amyloid protection, a Congo red solution (0.139 mg/2 mL) was made for this experiment, and 25 µL of it was mixed with 25 µL of the glycated sample (BSA + Glucose). The mixture was then incubated at 30°C for 20 min. Following incubation, 50 µL of distilled water was added to the mixture, and absorbance at the 530 nm wavelength was measured using a spectrophotometer. By comparing the test findings with the control, the tested samples’ protective role was ultimately determined. For interpretation, if the absorbance of the glycated sample is less than that of the control (BSA + glucose), it is reported as protective (Miroliaei et al., 2017).
2.8.4 Free carbonyl group estimation
The presence of a carbonyl group in glycated samples was detected using modified methods, as described earlier (Anwar et al., 2023). A solution of DNPH (2,4 dinitrophenyl hydrazine) with a concentration of 10 mM (mM) was produced using 2.5 M (M) hydrochloric acid (HCl). The glycated sample (500 µL) was subjected to incubation with a solution of DNPH (500 µL) for a duration of 1 h at room temperature. This was then followed by precipitation using 1.0 mL of the TCA solution with a concentration of 20%. The solid was rinsed with a mixture of ethanol and ethyl acetate in equal volumes (1:1 v/v, 1 mL), and the resulting solid was dissolved in urea (1 mL, 6 M). The absorbance of the solution was measured at a wavelength of 365 nm. The concentration of protein carbonyl groups was determined using the molar extinction coefficient (ɛ at 365 nm = 21 mM-1cm−1) and reported as nM/mg of protein.
2.9 Statistical analysis
The biological activity studies were conducted in three independent trials, and the result was expressed as ±SD. A one-way analysis of variance (ANOVA) was conducted, followed by a post hoc Tukey test, with a significance level (p value) of less than 0.05.
3 Results
3.1 GC-MS analysis
The GC-MS spectra of P. roxburghii were quite complex, and a total of 24 compounds were identified (Table 2). Results revealed the presence of a significant amount of β-pinene (30.08%), followed by caryophyllene oxide (29.37%), O-xylene (8.98%), and aromadendrene (8.29%) (Figure 1). Other compounds such as methylbenzene, ethylbenzene, α-thujone, camphene, (+)-sabinene, amyl vinyl carbinol, α-phellandrene, o-cymene, limonene, L-pinocarveol, p-cymen-8-ol, α-terpineol, citronellyl formate, (+)-carvone, α-himachalene, and β-farnesene (Tables 1, 2; Figure 2).
3.2 Drug-likeness ADMET analysis
The GC-MS spectra of P. roxburghii EO showed a large concentration of β-pinene, caryophyllene oxide, aromadendrene, o-xylene, α-phellandrene, and α-himachalene. We, therefore, decided to analyze these compounds for in silico characterization. The drug-likeness score of all components showed deviation from the set value (−1 to +1); however, values were only a slight different from the range in the case of α-phellandrene (−1.23) and α-himachalene (−1.24). Lipinski’s rule was followed by all compounds except aromadendrene, which showed a violation in the case of Log p (5.22) (Lipinski et al., 1997); however, still, it was only a minor deviation (Tables 1, 2; Figure 3, 4). The ADMET analysis of P. roxburghii indicated that all tested compounds showed low absorption from the oral route except caryophyllene oxide (high) and that all tested compounds were able to cross blood–brain barrier (BBB) except α-himachalene (Table 4). None of the molecules affected P-gp substrate and were mostly metabolized in the liver. Detailed ADMET results are mentioned in Table 4.
3.3 Molecular docking
For molecular docking, target proteins 3CJJ, 3TOP, and 3F5S were selected for ligand docking. Based on the relative abundance of components in P. roxburghii EO, β-pinene, caryophyllene oxide, and α-himachalene were selected for molecular docking investigations. Based on the findings, it was evident that the docking complex in 3CJJ and caryophyllene oxide was stabilized by both H-bonding and hydrophobic interactions, whereas in all other tested molecules, hydrophobic forces were mainly observed (Table 5; Figure 5). During docking with 3TOP and 4F5S receptors, hydrophobic forces played a dominant role in protein folding (Table 5; Figures 6, 7).
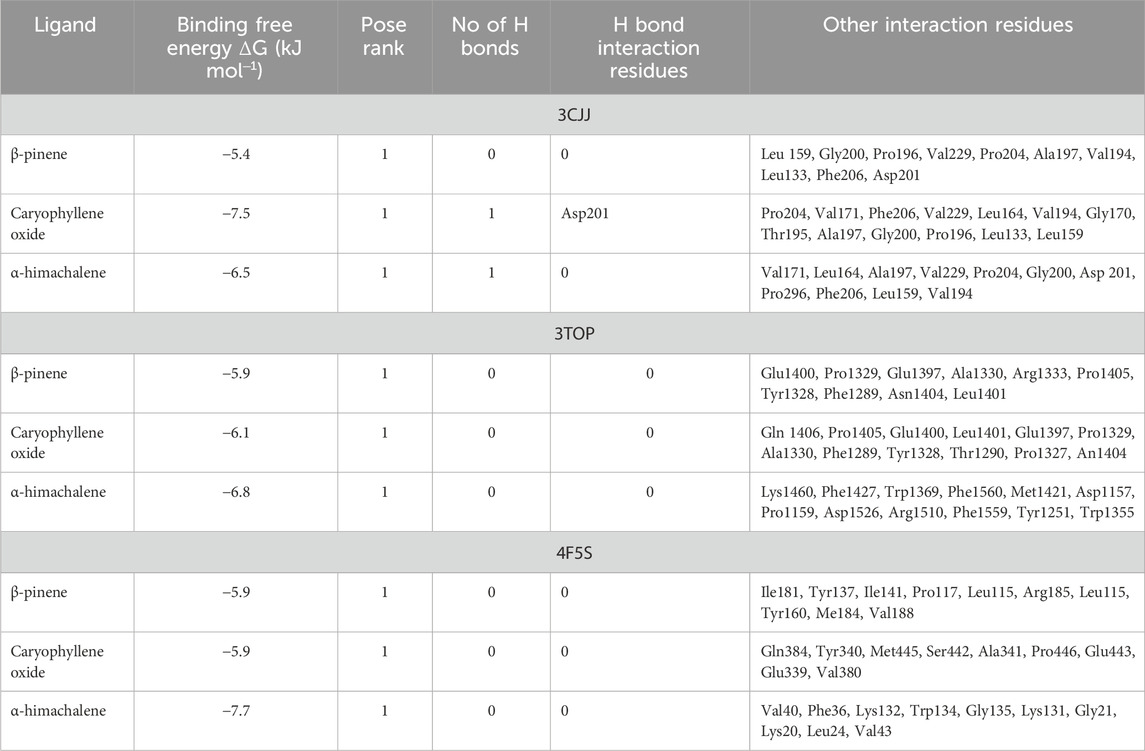
Table 5. Docking score and interaction analysis of major components in the P. roxburghii essential oil.
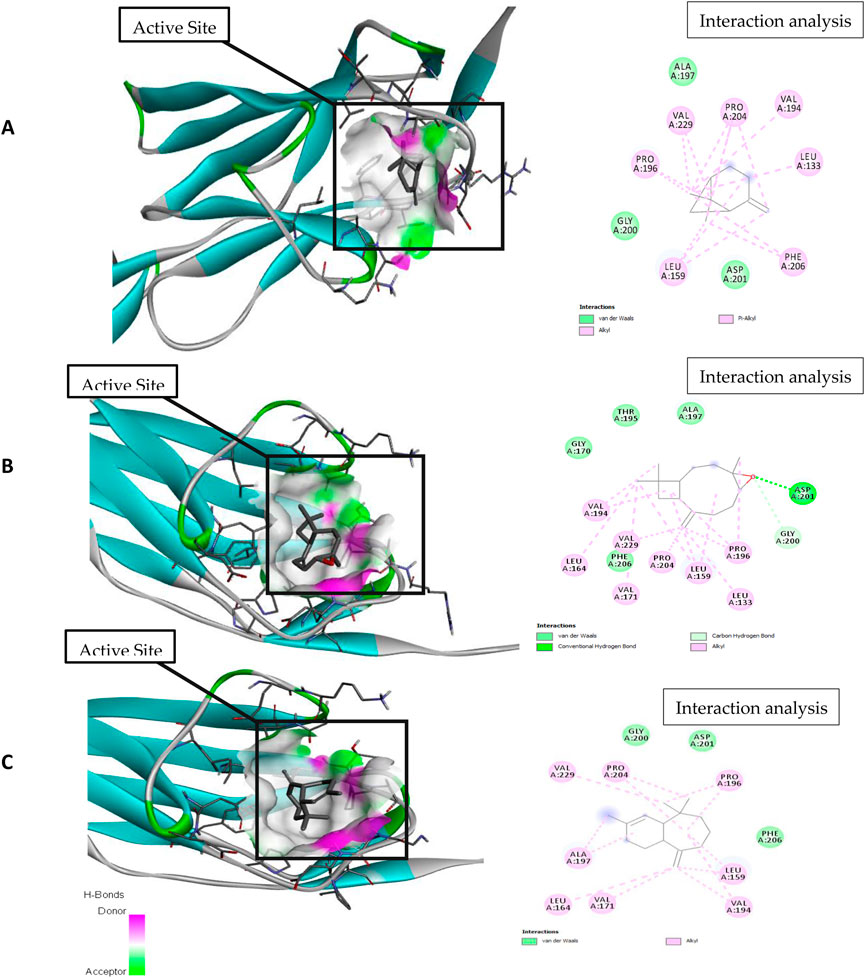
Figure 5. Molecular docking and interaction analysis of β-pinene (A), caryophyllene oxide (B), and α-himachalene (C) with 3CJJ from the P. roxburghii essential oil.
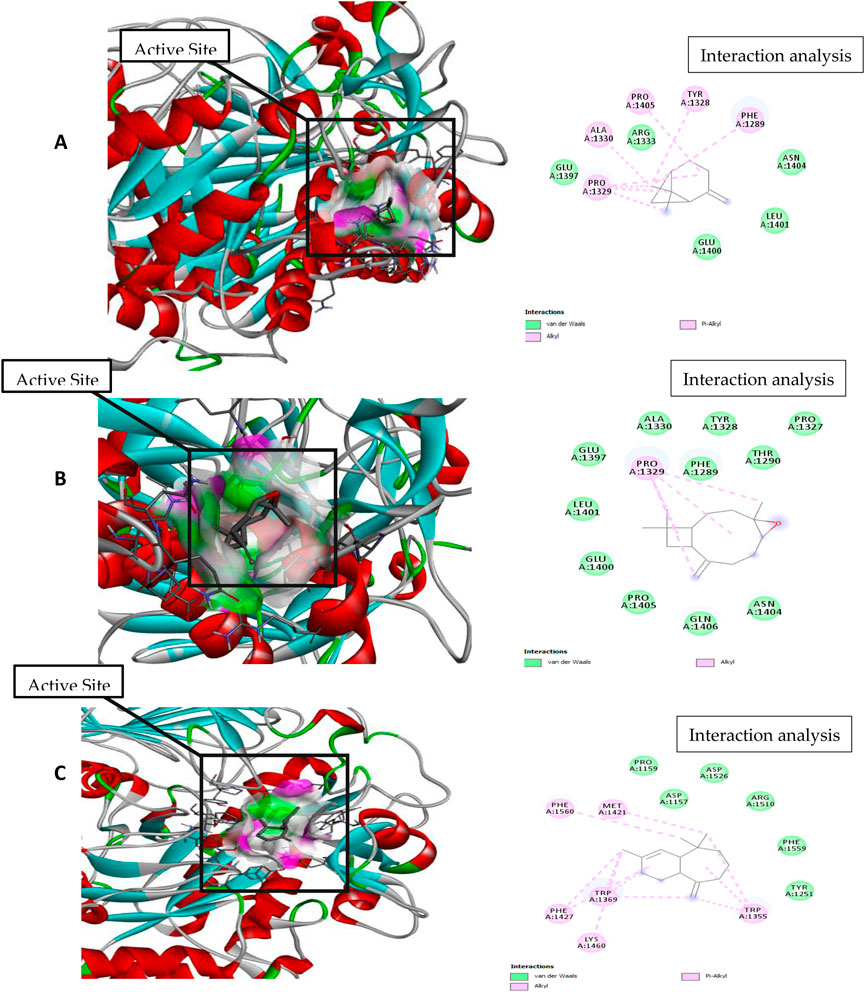
Figure 6. Molecular docking and interaction analysis of β-pinene (A), caryophyllene oxide (B), and α-himachalene (C) with 3TOP from the P. roxburghii essential oil.
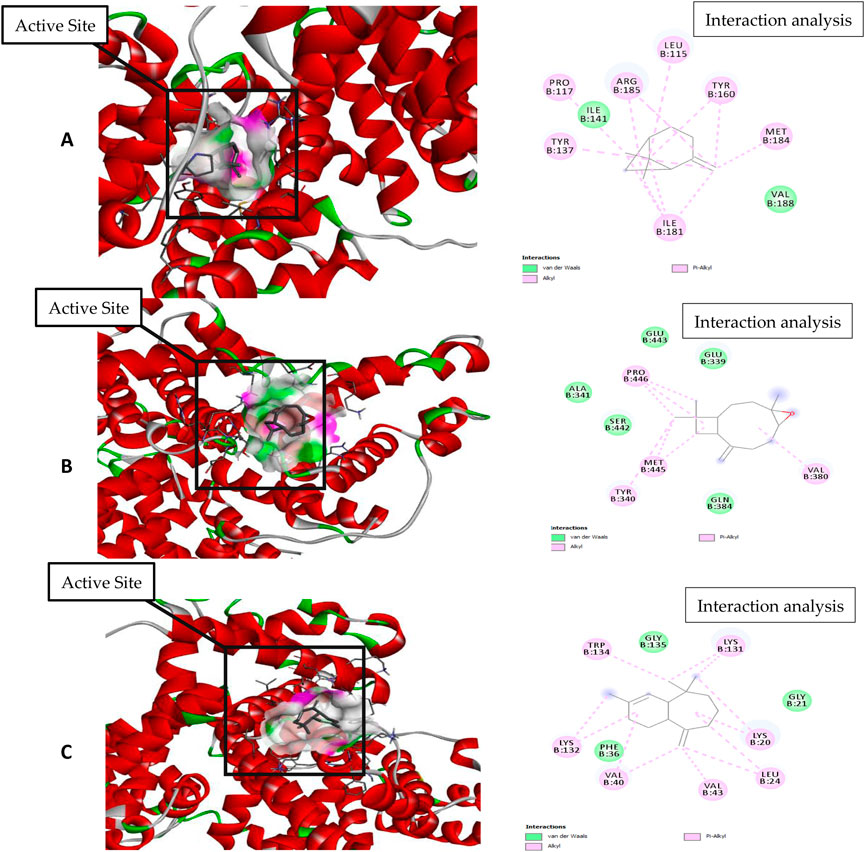
Figure 7. Molecular docking and interaction analysis of β-pinene (A), caryophyllene oxide (B), and α-himachalene (C) with 4F5S from the P. roxburghii essential oil.
3.4 Biological evaluation
The P. roxburghii EO was assayed for the presence of phenolic compounds using the Folin–Ciocalteu reagent. The gallic acid equivalents (mg/g GAE) were calculated using the gallic acid calibration curve. It was noticed that P. roxburghii (0.263 ± 0.45 mg/g GAE) possessed slight phenolic contents (Table 6). Further significantly high antioxidant potential in all assays, including FRAP (312 ± 14.6 µg), DPPH (IC50 14.2 ± 0.62 μg/mL), and H2O2 (73.3 ± 1.7 inhibition) assays, was recorded (Table 6). The P. roxburghii EO inhibition of α-glucosidase (IC50 0.12 mg/mL) was considered moderate after comparison with the standard drug acarbose (IC50 0.0014 mg/mL), which is eight times less potent (Table 7). In AGE assays, P. roxburghii EO showed inhibition in both non-oxidative (BSA-glucose; IC50 0.052 mg/mL) and oxidative modes (BSA-MGO; IC50 1.61 mg/mL). These findings were considered significant (BSA Glucose) and moderate (BSA-MGO) based on the criteria set compared with the standard used (rutin) (Table 7). Furthermore, during mechanistic studies, the EOs were analyzed for their potential role in the protection of β-amyloid sheet formation and fructosamine entrapment. The P. roxburghii EOs were able to possess a moderate protective effect against β-amyloid formation (Table 8). The DTNB molar extinction coefficient value (ɛ at 365 nm = 21 mM−1 cm−1) was used for free carbonyl entrapment (µM), and it was observed that the P. roxburghii EO S. presented moderate entrapment of carbonyls (Figure 8).

Table 7. Advance glycation end products and α-glucosidase inhibitory potential of the P. roxburghii essential oil.

Table 8. Detection of the protective role of the P. roxburghii essential oil against β-amyloid formation.
4 Discussions
The GC-MS analysis of P. roxburghii EO confirmed the presence of cyclic monoterpenes, including β-pinene (abundant terpenoids). Earlier investigations have suggested a bit of diversity in components and their concentrations (Satyal et al., 2013; Labib et al., 2017; Ayub et al., 2025). The essential oil composition varies in general due to certain environmental factors (such as climate, soil, and altitude), plant development stages, and extraction tissue (Eaissi et al., 2011; Benomari et al., 2023). Furthermore, harvesting time and post-harvest processing can also alter the concentration of volatile components through oxidative or enzymatic activities (Sun et al., 2022).
The most abundant component identified from GC-MS analysis was further used in computational assays including drug-likeness, ADMET, and docking analysis. The drug-likeness software generally uses algorithms that specifically determine whether the chemical entity under question poses physical and chemical properties to be considered a drug molecule (Bickerton et al., 2012) Based on Lipinki’s rule and drug-likeness scores (molinspiration tool), it was evident that aromadendrin showed a slight deviation (+1 to −1), whereas higher drug-likeness scores were recorded with other tested compounds. The ADMET analysis of P. roxburghii EO showed interesting results, and all tested compounds showed lower absorbance rates from the oral route except for caryophyllene oxide (high). Likewise, all compounds were able to cross the BBB except α-himachalene (Figure 4). Compounds with BBB permeability can potentially influence central nervous system (CNS) functions, highlighting the importance of evaluating their neurological safety profiles (Velásquez-Jiménez et al., 2021; Isabel et al., 2024). Based on the existing literature, these compounds have not shown significant adverse CNS effects in previous experimental studies; however, further in vivo assays are recommended for comprehensive safety evaluation. Similarly, none of the tested molecules were P-gp substrate (responsible for exclusion or transport of drugs), which means that none of these molecules can be actively removed from cells by P-glycoprotein. Metabolism in the liver is mainly regulated by CYP450 enzymes, and drug molecules that serve as substrate for these enzymes will be metabolized in the liver and vice versa (Han et al., 2019). It is obvious from our in silico analysis that few of the compounds acted as substrates, and others were inhibitors of these enzymes, which shows that these molecules may not be metabolized in the liver. Log kp is an indicator of skin permeation, and the more negative the log kp (with Kp in cm/s), the lower the skin permeation of the tested molecule (Daina et al., 2017). Based on the findings of our in silico analysis, it was evident that all tested molecules had low skin permeation.
In docking studies for 3CJJ, caryophyllene oxide showed the best complex formation with the target protein, exhibiting a high free energy [−7.5 ΔG (kJ mol‒1)]. This interaction was stabilized by H-bond formation through Asp201. The protein folding was protected by hydrophobic interactions that occurred through Van der Waal’s π-alkyl and alkyl interactions through Leu 159, Gly200, Pro196, Val229, Pro204, Ala197, Val194, Leu133, Phe206, and Asp201 amino acid residues. α-himachalene was also able to form a strong interaction with 3CJJ with high free energy [−6.5 ΔG (kJ mol‒1)]. The complex formation was mainly supported through hydrophobic interactions (Van der Waal’s and alkyl interactions) involving Val171, Leu164, Ala197, Val229, Pro204, Gly200, Asp 201, Pro296, Phe206, Leu159, and Val194 amino acid residues (Table 1; Figure 1). Finally, β-pinene docking with 3CJJ showed a stable complex formation with significantly high free energy [−5.4 ΔG (kJ mol‒1)]. In this study, no H-bond formation occurred between the ligand and receptor proteins, and mainly hydrophobic interactions were observed with Leu 159, Gly200, Pro196, Val229, Pro204, Ala197, Val194, Leu133, Phe206, and Asp201.
In docking studies for 3TOP, α-himachalene showed the best docking with the target protein, exhibiting a high free energy [−6.8 ΔG (kJ mol‒1)]. In this study, protein folding was protected by hydrophobic interactions that occurred through Van der Waal’s π-alkyl and alkyl interactions, including 12 amino acids, namely, Lys1460, Phe1427, Trp1369, Phe1560, Met1421, Asp1157, Pro1159, Asp1526, Arg1510, Phe1559, Tyr1251, and Trp1355 amino acids. Caryophyllene oxide was also able to show a strong interaction with receptors with high free energy [−6.1 ΔG (kJ mol‒1)]. The complex formation was mainly supported through hydrophobic interactions (Van der Waal’s and alkyl interactions) involving 12 amino acids, namely, Gln 1406, Pro 1405, Glu 1400, Leu 1401, Glu 1397, Pro 1329, Ala 1330, Phe 1289, Tyr 1328, Thr 1290, Pro 1327, and An 1404, amino acid residues. Similarly, β-pinene docking with 3TOP showed a stable complex formation with significantly high free energy [−5.9 ΔG (kJ mol‒1)]. In this study, no H-bond formation occurred between the ligand and receptor proteins, and mainly hydrophobic attachments were recorded with Glu1400, Pro1329, Glu1397, Ala1330, Arg1333, Pro1405, Tyr1328, Phe1289, Asn1404, and Leu1401. The interaction of the bovine serum albumin receptor 5NN8 with the major components of P. roxburghii EO was studied. β-pinene showed the best docking with the target protein with a high free energy [−5.5 ΔG (kJ mol‒1)]. Protein folding was protected by hydrophobic interactions that were formed through Van der Waal’s π-alkyl and alkyl interactions, including five amino acids, namely, Glu430, Leu431, Arg385, Met427, and Val388. Similarly, α-himachalene interacted with receptors with a high free energy [−5.4 ΔG (kJ mol‒1)]. In this case, neighboring amino acids included Arg168, Leu167, Thr151, Pro186, Ala150, Thr149, and Asn140 (Table 5; Figure 7). β-pinene docking with 5NN8 also showed a stable complex formation with a significantly high free energy [−4.7 ΔG (kJ mol‒1)]. The complex is mainly supported by hydrophobic interactions that involve Asp163, Ile164, Arg190, Lys162, Asp243, Asp185, and Asn188 (Table 5; Figure 7).
The P. roxburghii EOs were observed as rich in phenolic contents, and this was due to the presence of diverse monoterpenes as we noticed and reported earlier (Labaib et al., 2017; Ayub et al., 2023). High phenolic contents are mainly associated with strong antioxidant potential since these compounds have a strong affinity for free radicals and deactivate them, thereby reducing oxidative stress (Aryal et al., 2019). The EOs were, therefore, assessed for antioxidant potential through different analytical mechanisms, including FRAP, H2O2, and DPPH assays. It was observed that P. roxburghii EO exhibited oxidative stress-lowering capacity. Similar findings were reported in earlier investigations on essential oils in different geographical locations (Djerrad et al., 2015; Mehmood et al., 2024; Ancuceanu et al., 2024). Various pathological conditions trigger excessive production of ROS, which are the main reason for the increase in oxidative stress (Phaniendra et al., 2015). Reports have suggested that elevated oxidative burden unfavorably affects various cellular functions, thereby leading to diseased conditions (García-Sánchez et al., 2020). Similar to the case of diabetes mellitus, high oxidative stress results in impaired β-cell function, leading to disordered glucose regulation in the body (Caturano et al., 2023). Thus, the antioxidative potential of P. roxburghii EO can be useful for the management of diabetes due to significant antioxidant effects.
Among various mechanisms of diabetes mellitus, the consideration of the α-glucosidase enzyme in the intestinal lumen is very important. We investigated four EOs for potential α-glucosidase inhibition, and moderate α-glucosidase inhibition was recorded in the case of P. roxburghii EO. Earlier investigations have reported a significant α-glucosidase inhibition but slightly lower than that of our investigation, which is mainly attributed to geographical diversities (Maurya et al., 2022) and differences in terpenoid composition. The terpenoid contents mainly contribute toward diverse biological activities, including α-glucosidase (Masyita et al., 2022). Among these, mono-terpenoids are very important since they have very low affinity for water (hydrophobic) and thereby deactivate enzymes, especially α-glucosidase, thus playing a key role in the absorption of carbohydrates from gut mucosa (Kozioł et al., 2014).
Persistent hyperglycemia generally leads to the development of several micro and macrovascular complications, primarily due to the production of advanced glycation end products (AGEs) through non-enzymatic pathways (Lee et al., 2022). Thus, an ideal drug lead should not only reduce the development of hyperglycemia but also slow down AGE formation. We thus investigated EO for its anti-AGE activity and inhibition mechanisms. A significant moderate inhibition of AGEs in both oxidative and non-oxidative modes was observed, and to the best of our knowledge, no previous data are available on the same essential oil. Despite this, several investigations have confirmed AGE inhibitory potential of essential oils rich in monoterpenoids including α-pinene, β-pinene, and caryophyllene oxide (Thomas and Essien, 2020; Yadav et al., 2023; El et al., 2024), and this was mainly correlated with its high terpenoid contents and strong antioxidant potential in scavenging free radicals (Khan et al., 2020; Rhizlan et al., 2024).
The AGE formation may also result in protein dysfunction due to interference with β-sheets of the protein (Lannuzzi et al., 2014). The β-amyloid assay was performed, as described earlier, and the test sample was considered as having a “protective effect” if its absorbance was less than that of the control, i.e., BSA + Glucose. Protective means very good antiglycation properties, and moderate means moderate protection against glycation. As obvious, the EO exhibited lower absorbance compared to the control. Likewise, it showed significant carbonyl entrapment. Thus, a drug candidate with a protective effect against β-amyloid formation and carbonyl entrapment is desirable for maintaining protein function. During persistent hyperglycemia, free carbonyls and their intermediates are generated, which are the main promoters of AGE formation (Schalkwijk et al., 1999).
With these findings, the P. roxburghii EO can be further investigated for in vivo validation. However, the volatile nature of essential oils and geographical variations can limit its effectiveness. We, therefore, propose formulation design and synergy studies with other compounds, which can be very helpful for the pharmaceutical industry.
5 Conclusion
The major compounds of P. roxburghii EO were docked against selected transcriptional regulators 3CJJ, 3 TOP, and 4F5S, revealing significant interactions within the active pockets. The findings of this investigation confirmed the significant antioxidant potential of P. roxburghii EO, which is rich in terpenoid moieties. The EO significantly contributed to the inhibition of α-glucosidase and AGEs through β-amyloid protective effects and free carbonyl entrapment. It was, therefore, concluded that P. roxburghii EO can be effectively employed for the management of diabetes and its associated complications. These findings can be helpful in future formulation design and will provide a potential integrated approach for diabetes and its comorbidities.
Data availability statement
The original contributions presented in the study are included in the article/supplementary material, further inquiries can be directed to the corresponding author.
Author contributions
RN: investigation, methodology, software, and writing – original draft. SI: data curation, investigation, project administration, supervision, and writing – original draft. RB: methodology, software, and writing – original draft. HA: formal analysis, funding acquisition, investigation, methodology, supervision, and writing – original draft. AA: investigation, methodology, software, and writing – original draft. SC: data curation, investigation, methodology, software, and writing – original draft. QA: investigation, methodology, validation, and writing – original draft. MW: Data curation, Investigation, Software, Writing – original draft MA: data curation, formal analysis, methodology, software, and writing – original draft. SI: investigation, methodology, software, and writing – original draft. MR: investigation, methodology, software, and writing – original draft. AA: conceptualization, funding acquisition, investigation, project administration, software, supervision, writing – original draft, and writing – review and editing.
Funding
The author(s) declare that financial support was received for the research and/or publication of this article. The authors extend their appreciation to the Researchers Supporting Project, King Saud University, Riyadh, Saudi Arabia, for funding this work through (grant number RSPD2025R566).
Conflict of interest
The authors declare that the research was conducted in the absence of any commercial or financial relationships that could be construed as a potential conflict of interest.
Generative AI statement
The author(s) declare that no Generative AI was used in the creation of this manuscript.
Publisher’s note
All claims expressed in this article are solely those of the authors and do not necessarily represent those of their affiliated organizations, or those of the publisher, the editors and the reviewers. Any product that may be evaluated in this article, or claim that may be made by its manufacturer, is not guaranteed or endorsed by the publisher.
References
Ailli, A., Handaq, N., Touijer, H., Gourich, A. A., Drioiche, A., Zibouh, K., et al. (2023). Phytochemistry and biological activities of essential oils from six aromatic medicinal plants with cosmetic properties. Antibiotics 12 (4), 721. doi:10.3390/antibiotics12040721
Amjad, M. S., Zahoor, U., Bussmann, R. W., Altaf, M., Gardazi, S. M. H., and Abbasi, A. M. (2020). Ethnobotanical survey of the medicinal flora of harighal, azad jammu and kashmir, Pakistan. J. Ethnobiol. Ethnomed. 16 (1), 65. doi:10.1186/s13002-020-00417-w
Ancuceanu, R., Anghel, A. I., Hovaneț, M. V., Ciobanu, A. M., Lascu, B. E., and Dinu, M. (2024). Antioxidant activity of essential oils from Pinaceae species. Antioxidants 13 (3), 286. doi:10.3390/antiox13030286
Anwar, L., Ali, S. A., Khan, S., Uzairullah, M. M., Mustafa, N., Ali, U. A., et al. (2023). Fenugreek seed ethanolic extract inhibited formation of advanced glycation end products via scavenging reactive carbonyl intermediates. Heliyon 9 (6), e16866. doi:10.1016/j.heliyon.2023.e16866
Aryal, S., Baniya, M. K., Danekhu, K., Kunwar, P., Gurung, R., and Koirala, N. (2019). Total phenolic content, flavonoid content and antioxidant potential of wild vegetables from western Nepal. Plants 8 (4), 96. doi:10.3390/plants8040096
Ayub, M. A., Amin, H. I. M., Waseem, R., Amin, K. Y. M., Hanif, M. A., Hussain, A., et al. (2025). Short path molecular distillation of the essential oil from Pinus roxburghii oleoresin affords volatile fractions with powerful antioxidant and antimicrobial activities comparable with common synthetic agents and antimicrobials. Heliyon 11 (3), e42282. doi:10.1016/j.heliyon.2025.e42282
Ayub, S., Malak, N., Cossío-Bayúgar, R., Nasreen, N., Khan, A., Niaz, S., et al. (2023). In vitro and in silico protocols for the assessment of anti-tick compounds from Pinus roxburghii against Rhipicephalus (Boophilus) microplus ticks. Anim. (Basel) 13 (8), 1388. doi:10.3390/ani13081388
Aziz, M. I., Hasan, M. M., Ullah, R., Bari, A., Khan, M. A., Hasnain, S. Z. U., et al. (2024). Potential role of Citrus bergamia flower essential oils against oral pathogens. BMC Complement. Med. Ther. 24 (1), 157. doi:10.1186/s12906-024-04457-7
Benabdelkader, T., Zitouni, A., Guitton, Y., Jullien, F., Maitre, D., Casabianca, H., et al. (2011). Essential oils from wild populations of Algerian Lavandula stoechas L.: composition, chemical variability, and in vitro biological properties. Chem. Biodivers. 8, 937–953. doi:10.1002/cbdv.201000301
Benomari, F. Z., Sarazin, M., Chaib, D., Pichette, A., Boumghar, H., Boumghar, Y., et al. (2023). Chemical variability and chemotype concept of essential oils from Algerian wild plants. Molecules 28 (11), 4439. doi:10.3390/molecules28114439
Bickerton, G. R., Paolini, G. V., Besnard, J., Muresan, S., and Hopkins, A. L. (2012). Quantifying the chemical beauty of drugs. Nat. Chem. 4 (2), 90–98. doi:10.1038/nchem.1243
Caturano, A., D'Angelo, M., Mormone, A., Russo, V., Mollica, M. P., Salvatore, T., et al. (2023). Oxidative stress in type 2 diabetes: impacts from pathogenesis to lifestyle modifications. Curr. Issues Mol. Biol. 45 (8), 6651–6666. doi:10.3390/cimb45080420
Chaieb, K., Zmantar, T., Ksouri, R., Hajlaoui, H., Mahdouani, K., Abdelly, C., et al. (2007). Antioxidant properties of the essential oils of Eugenia caryophyllata and its antifungal activity against a large number of clinical Candida species. Mycoses 50, 403–406. doi:10.1111/j.1439-0507.2007.01391.x
Chandimali, N., Bak, S. G., Park, E. H., Lim, H. J., Won, Y. S., Kim, E. K., et al. (2025). Free radicals and their impact on health and antioxidant defenses: a review. Cell Death Discov. 11, 19. doi:10.1038/s41420-024-02278-8
Chlopicka, J., Pasko, P., Gorinstein, S., Jedryas, A., and Zagrodzki, P. (2012). Total phenolic and total flavonoid content, antioxidant activity and sensory evaluation of pseudocereal breads. LWT Food Sci. Technol. 46 (2), 548–555. doi:10.1016/j.lwt.2011.11.009
Dagli, N., Dagli, R., Mahmoud, R. S., and Baroudi, K. (2015). Essential oils, their therapeutic properties, and implication in dentistry: a review. J. Int. Soc. Prev. Community Dent. 5 (5), 335–340. doi:10.4103/2231-0762.165933
Daina, A., Michielin, O., and Zoete, V. (2017). SwissADME: a free web tool to evaluate pharmacokinetics, drug-likeness and medicinal chemistry friendliness of small molecules. Sci. Rep. 7, 42717. doi:10.1038/srep42717
Devi, A. M., Gopinath, G., Bandaru, S., Sankeshi, V., Mangalarapu, M., Sudha, N. S., et al. (2016). Virtual screening of RAGE inhibitors using molecular docking. Bioinformation 12 (3), 124–130. doi:10.6026/97320630012124
Djerrad, Z., Kadik, L., and Djouahri, A. (2015). Chemical variability and antioxidant activities among Pinus halepensis Mill. essential oils provenances, depending on geographic variation and environmental conditions. Ind. Crops Prod. 74, 440–449. doi:10.1016/j.indcrop.2015.05.049
Dorman, H. J., and Deans, S. G. (2000). Antimicrobial agents from plants: antibacterial activity of plant volatile oils. J. Appl. Microbiol. 88, 308–316. doi:10.1046/j.1365-2672.2000.00969.x
Eaissi, A., Medini, H., Simmonds, M., Lynen, F., Farhat, F., Chemli, R., et al. (2011). Variation in volatile leaf oils of seven Eucalyptus species harvested from Zerniza Arboreta (Tunisia). Chem. Biodivers. 8, 362–372. doi:10.1002/cbdv.201000103
El, H. N., Kandsi, F., Elbouzidi, A., Lafdil, F. Z., Nouioura, G., Abdallah, E. M., et al. (2024). Extraction of bioactive compound-rich essential oil from Cistus ladanifer L. by microwave-assisted hydrodistillation: GC-MS characterization, in vitro pharmacological activities, and molecular docking. Separations 11 (7), 199. doi:10.3390/separations11070199
Fatmawati, S., Auwaliyah, F., Yuliana, , Hasanah, N., Putri, D. A., Kainama, H., et al. (2023). Antioxidant and α-glucosidase inhibitory activities of compound isolated from Stachytarpheta jamaicensis (L.) Vahl. leaves. Sci. Rep. 13, 18597. doi:10.1038/s41598-023-45357-z
Fusani, L., Palmer, D. S., Somers, D. O., and Wall, I. D. (2020). Exploring ligand stability in protein crystal structures using binding pose metadynamics. J. Chem. Inf. Model. 60 (3), 1528–1539. doi:10.1021/acs.jcim.9b00843
García-Sánchez, A., Miranda-Díaz, A. G., and Cardona-Muñoz, E. G. (2020). The role of oxidative stress in physiopathology and pharmacological treatment with pro- and antioxidant properties in chronic diseases. Oxid. Med. Cell. Longev. 2020, 2082145. doi:10.1155/2020/2082145
Han, Y., Zhang, J., Hu, C. Q., Zhang, X., Ma, B., and Zhang, P. (2019). In silico ADME and toxicity prediction of ceftazidime and its impurities. Front. Pharmacol. 10, 434. doi:10.3389/fphar.2019.00434
Herman, A., and Herman, A. P. (2015). Essential oils and their constituents as skin penetration enhancers for transdermal drug delivery: a review. J. Pharm. Pharmacol. 67 (4), 473–604. doi:10.1111/jphp.12334
Hussen, E. M., and Endalew, S. A. (2023). In vitro antioxidant and free-radical scavenging activities of polar leaf extracts of Vernonia amygdalina. BMC Complement. Med. Ther. 23 (1), 146. doi:10.1186/s12906-023-03923-y
Isabel, U. V., Belén, A. D. L. R. M., and Elena, G. B. (2024). A new frontier in neuropharmacology: recent progress in natural products research for blood–brain barrier crossing. Curr. Res. Biotechnol. 8, 100235. doi:10.1016/j.crbiot.2024.100235
Kaushik, D., Kumar, A., Kaushik, P., and Rana, A. C. (2012). Analgesic and anti-inflammatory activity of Pinus roxburghii Sarg. Adv. Pharmacol. Sci. 2012, 245431. doi:10.1155/2012/245431
Kaushik, P., Kaushik, D., and Khokra, S. L. (2013). Ethnobotany and phytopharmacology of Pinus roxburghii Sargent: a plant review. J. Integr. Med. 11 (6), 371–376. doi:10.3736/jintegrmed2013053
Kaushik, P., Khokra, S., and Kaushik, D. (2015). Evaluation of antidiabetic potential of Pinus roxburghii bark extract in alloxan-induced diabetic rats. J. Pharmacogn. Nat. Prod. 1 (1), 2–5. doi:10.4172/2472-0992.1000105
Kaushik, P., Lal, K. S., Rana, A. C., and Kaushik, D. (2014). Pharmacophore modeling and molecular docking studies on Pinus roxburghii as a target for diabetes mellitus. Adv. Bioinforma. 2014, 903246. doi:10.1155/2014/903246
Khan, M., Liu, H., Wang, J., and Sun, B. (2020). Inhibitory effect of phenolic compounds and plant extracts on the formation of advance glycation end products: a comprehensive review. Food Res. Int. 130, 108933. doi:10.1016/j.foodres.2019.108933
Khan, N., Ali, K., and Shaukat, S. (2014). Phytosociology, structure and dynamics of Pinus roxburghii associations from Northern Pakistan. J. For. Res. 25 (3), 511–521. doi:10.1007/s11676-014-0490-x
Kiran, C. R., Chakka, A. K., Amma, K. P., Menon, A. N., Kumar, M. M., and Venugopalan, V. V. (2013). Influence of cultivar and maturity at harvest on the essential oil composition, oleoresin and [6]-gingerol contents in fresh ginger from northeast India. J. Agric. Food Chem. 61, 4145–4154. doi:10.1021/jf400095y
Kozioł, A., Stryjewska, A., Librowski, T., Sałat, K., Gaweł, M., Moniczewski, A., et al. (2014). An overview of the pharmacological properties and potential applications of natural monoterpenes. Mini Rev. Med. Chem. 14 (14), 1156–1168. doi:10.2174/1389557514666141127145820
Labib, R. M., Radhakrishnan, S. V. S., and Ross, S. A. (2016). Phytochemical and biological investigation of Pinus roxburghii. Planta Med. 82, PC74. doi:10.1055/s-0036-1578776
Labib, R. M., Youssef, F. S., Ashour, M. L., Abdel-Daim, M. M., and Ross, S. A. (2017). Chemical composition of Pinus roxburghii bark volatile oil and validation of its anti-inflammatory activity using molecular modeling and bleomycin-induced inflammation in albino mice. Molecules 22 (9), 1384. doi:10.3390/molecules22091384
Lannuzzi, C., Irace, G., and Sirangelo, L. (2014). Differential effects of glycation on protein aggregation and amyloid formation. Front. Mol. Biosci. 1, 9. doi:10.3389/fmolb.2014.00009
Lee, J., Yun, J. S., and Ko, S. H. (2022). Advanced glycation end products and their effect on vascular complications in type 2 diabetes mellitus. Nutrients 14 (15), 3086. doi:10.3390/nu14153086
Lipinski, C. A., Lombardo, F., Dominy, B. W., and Feeney, P. J. (1997). Experimental and computational approaches to estimate solubility and permeability in drug discovery and development settings. Adv. Drug Deliv. Rev. 23 (1–3), 3–25. doi:10.1016/s0169-409x(96)00423-1
Maffei, M. E., Gertsch, J., and Appendino, G. (2011). Plant volatiles: production, function and pharmacology. Nat. Prod. Rep. 28, 1359–1380. doi:10.1039/c1np00021g
Masyita, A., Mustika, S. R., Dwi Astuti, A., Yasir, B., Rahma, R. N., Emran, T. B., et al. (2022). Terpenes and terpenoids as main bioactive compounds of essential oils, their roles in human health and potential application as natural food preservatives. Food Chem. 13, 100217. doi:10.1016/j.fochx.2022.100217
Maurya, A. K., Vashisath, S., Aggarwal, G., Yadav, V., and Agnihotri, V. K. (2022). Chemical diversity and α-glucosidase inhibitory activity in needles essential oils of four Pinus species from Northwestern Himalaya, India. Chem. Biodivers. 19 (12), e202200428. doi:10.1002/cbdv.202200428
Mehmood, S., Abbasi, A. M., Hussain, H., Khan, S. A., Almutairi, H. H., Ismail, A. M., et al. (2024). Phytochemical profile, antioxidant and antibacterial activities analysis of crude extract and essential oil of Pinus roxburghii and Pinus wallichiana: in vitro and in silico analyses. Cogent Food Agric. 10 (1), 2403648. doi:10.1080/23311932.2024.2403648
Miroliaei, M., Aminjafari, A., Ślusarczyk, S., Nawrot-Hadzik, I., Rahimmalek, M., and Matkowski, A. (2017). Inhibition of glycation-induced cytotoxicity, protein glycation, and activity of proteolytic enzymes by extract from Perovskia atriplicifolia roots. Pharmacogn. Mag. 13 (Suppl. 3), S676–S683. doi:10.4103/pm.pm_559_16
Phaniendra, A., Jestadi, D. B., and Periyasamy, L. (2015). Free radicals: properties, sources, targets, and their implication in various diseases. Indian J. Clin. biochem. 30 (1), 11–26. doi:10.1007/s12291-014-0446-0
Rafey, A., Amin, A., Kamran, M., Aziz, M. I., Athar, V., Niaz, S. I., et al. (2022). Evaluation of major constituents of medicinally important plants for anti-inflammatory, antidiabetic and AGEs inhibiting properties: in vitro and simulatory evidence. Molecules 27 (19), 6715. doi:10.3390/molecules27196715
Rehman, S. U., Ahmad, M. M., Kazmi, Z. H., and Raza, M. S. (2007). Physico-chemical variations in essential oils of Citrus reticulata. J. Food Sci. Technol. 44, 353–356. doi:10.1021/acsomega.1c00895
Rhizlan, A., Amine, E., Ouassou, H., Elrherabi, A., Berraaouan, A., Legssyer, A., et al. (2024). In vitro study on antioxidant and antiglycation activities, and molecular docking of Moroccan medicinal plants for diabetes. Curr. Tradit. Med. 10 (7), 201–214. doi:10.2174/2215083810666230831154738
Rodriguez, S., Skeet, K., Mehmetoglu-Gurbuz, T., Goldfarb, M., Karri, S., Rocha, J., et al. (2021). Phytochemicals as an alternative or integrative option, in conjunction with conventional treatments for hepatocellular carcinoma. Cancers 13 (22), 5753. doi:10.3390/cancers13225753
Ruxton, C. (2016). Tea: hydration and other health benefits. Prim. Health Care 26, 34–42. doi:10.7748/phc.2016.e1162
Satyal, P., Paudel, P., Raut, J., Deo, A., Dosoky, N.S., and Setzer, W.N. (2013). Volatile constituents of Pinus roxburghii from Nepal. Pharma. Res. 5, (1), 43.
Saber, F. R., Mohsen, E., El-Hawary, S., Eltanany, B. M., Elimam, H., Sobeh, M., et al. (2021). Chemometric-enhanced metabolic profiling of five Pinus species using HPLC-MS/MS spectrometry: correlation to in vitro anti-aging, anti-Alzheimer, and antidiabetic activities. J. Chromatogr. B 1177, 122759. doi:10.1016/j.jchromb.2021.122759
Saharkhiz, M. J., Motamedi, M., Zomorodian, K., Pakshir, K., Miri, R., and Hemyari, K. (2012). Chemical composition, antifungal and antibiofilm activities of the essential oils of Mentha piperita L. ISRN Pharm. 2012, 718645. doi:10.5402/2012/718645
Sajid, A., Manzoor, Q., Iqbal, M., Tyagi, A. K., Sarfraz, R. A., and Sajid, A. (2018). Pinus Roxburghii essential oil anticancer activity and chemical composition evaluation. EXCLI J. 17, 233–245. doi:10.17179/excli2016-670
Schalkwijk, C. G., Posthuma, N., ten Brink, H. J., ter Wee, P. M., and Teerlink, T. (1999). Induction of 1,2-dicarbonyl compounds, intermediates in the formation of advanced glycation end-products, during heat-sterilization of glucose-based peritoneal dialysis fluids. Perit. Dial. Int. 19 (4), 325–333. doi:10.1177/089686089901900408
Soliman, E., Ibrahim, M. M., El-Khouly, M. E., El-Mehasseb, I., Ramadan, A. E. M., Mahfouz, M. E., et al. (2022). BSA interaction, molecular docking, and antibacterial activity of zinc(II) complexes containing the sterically demanding biomimetic N3S2 ligand: the effect of structure flexibility. Molecules 27 (11), 3543. doi:10.3390/molecules27113543
Song, W., Xu, Z., Gao, P., and Liu, X. (2023). Chemical composition and in vitro antioxidant activity and anti-acetylcholinesterase activity of essential oils from Tadehagi triquetrum (L.) Ohashi. Molecules 28 (6), 2734. doi:10.3390/molecules28062734
Sun, J., Sun, P., Kang, C., Zhang, L., Guo, L., and Kou, Y. (2022). Chemical composition and biological activities of essential oils from six Lamiaceae folk medicinal plants. Front. Plant Sci. 13, 919294. doi:10.3389/fpls.2022.919294
Thomas, P. S., and Essien, E. E. (2020). Antiglycation, antioxidant, and cytotoxic activities of Uvaria chamae root and essential oil composition. Nat. Prod. Res. 34 (6), 880–883. doi:10.1080/14786419.2018.1504048
Trott, O., and Olson, A. J. (2010). AutoDock Vina: improving the speed and accuracy of docking with a new scoring function, efficient optimization, and multithreading. J. Comput. Chem. 31 (2), 455–461. doi:10.1002/jcc.21334
Velásquez-Jiménez, D., Corella-Salazar, D. A., Zuñiga-Martínez, B. S., Domínguez-Avila, J. A., Montiel-Herrera, M., Salazar-López, N. J., et al. (2021). Phenolic compounds that cross the blood–brain barrier exert positive health effects as central nervous system antioxidants. Food Funct. 12 (21), 10356–10369. doi:10.1039/d1fo02017j
WHO. Global burden of disease collaborative network (2024). Global burden of disease study 2021 results. Institute for Health Metrics and Evaluation. Available online at: https://vizhub.healthdata.org/gbd-results/.
Yadav, N., Palkhede, J. D., and Kim, S. Y. (2023). Anti-glucotoxicity effect of phytoconstituents via inhibiting MGO-AGEs formation and breaking MGO-AGEs. Int. J. Mol. Sci. 24 (8), 7672. doi:10.3390/ijms24087672
Yap, P. S., Lim, S. H., Hu, C. P., and Yiap, B. C. (2013). Combination of essential oils and antibiotics reduce antibiotic resistance in plasmid-conferred multidrug resistant bacteria. Phytomedicine 20, 710–713. doi:10.1016/j.phymed.2013.02.013
You, Z., Li, Y., Zhang, K., Zheng, X., Wong, V. K. W., and Liu, W. (2022). Inhibitory effect of plant essential oils on α-glucosidase. Food Sci. Biotechnol. 31 (12), 1593–1602. doi:10.1007/s10068-022-01145-5
Zahid, N., Khan, S. A., Al-Hamaid, F. M., Shah, M. M., Farraj, D. A. A., Elshikh, M. S., et al. (2023). In vitro and in silico validation of antibacterial potential of Pinus roxburghii and Cedrus deodara leaves’ extract against human pathogenic bacteria. J. King Saud. Univ. Sci. 35 (2), 102518. doi:10.1016/j.jksus.2022.102518
Keywords: oxidative stress, monoterpenes, α-glucosidase, AGEs, β-amyloid
Citation: Naz Qaisrani R, Iram Niaz S, Bano R, Abuelizz HA, Alvi AM, Chaman S, Aziz Q, Wazir MA, Akram M, Ishtiaq S, Ramzan M and Amin A (2025) Integrative in silico and in vitro analysis of Pinus roxburghii essential oil: unveiling its antioxidant, antidiabetic, and antiglycation potential. Front. Pharmacol. 16:1554562. doi: 10.3389/fphar.2025.1554562
Received: 02 January 2025; Accepted: 20 March 2025;
Published: 29 April 2025.
Edited by:
Wenlong Sun, Shandong University of Technology, ChinaReviewed by:
Mariia I. Shanaida, Ternopil State Medical University, UkraineMohamed Addi, Mohamed Premier University, Morocco
Copyright © 2025 Naz Qaisrani, Iram Niaz, Bano, Abuelizz, Alvi, Chaman, Aziz, Wazir, Akram, Ishtiaq, Ramzan and Amin. This is an open-access article distributed under the terms of the Creative Commons Attribution License (CC BY). The use, distribution or reproduction in other forums is permitted, provided the original author(s) and the copyright owner(s) are credited and that the original publication in this journal is cited, in accordance with accepted academic practice. No use, distribution or reproduction is permitted which does not comply with these terms.
*Correspondence: Adnan Amin, YWRuYW4uYW1pbnBoYXJtYWN5QGdtYWlsLmNvbQ==