- 1School of Clinical Medicine, Chengdu University of Traditional Chinese Medicine, Chengdu, Sichuan, China
- 2Department of Infectious Diseases, Hospital of Chengdu University of Traditional Chinese Medicine, Chengdu, Sichuan, China
- 3Department of Integrated Traditional Chinese and Western Medicine Oncology, The Fourth Hospital of Hebei Medical University, Shijiazhuang, Hebei, China
- 4Clinical Research Center, Shijiazhuang Fifth Hospital, Shijiazhuang, Hebei, China
Background: The global prevalence of non-alcoholic fatty liver disease (NAFLD) has surged, largely driven by modern lifestyle changes and dietary shifts. As these factors profoundly impact human health, exploring effective therapeutic strategies for NAFLD has become a pressing medical concern. Previous studies have suggested that ginsenosides may offer a potential treatment approach for NAFLD by reducing oxidative stress and controlling inflammation. However, its efficacy and safety remain unclear. Therefore, the aim of this systematic review and meta-analysis is to evaluate the role of ginsenosides in the treatment of NAFLD.
Methods: We searched for relevant studies published through September 2024, including databases such as PubMed, Embase, Web of Science, China National Knowledge Infrastructure (CNKI), and Wanfang Data. The SYstematic Review Center for Laboratory animal Experimentation (SYRCLE) Animal Experiment Bias Risk Assessment Tool was used to evaluate the quality of the literature. Subsequently, Review Manager (RevMan, version 5.3) and STATA 15 software was utilized for data analysis.
Results: Finally, 30 studies involving a total of 604 animals were included in the analysis. The results showed that, compared with the model group, ginsenosides significantly reduces total cholesterol (TC), triglycerides (TG), alanine aminotransferase (ALT), aspartate aminotransferase (AST), low-density lipoprotein cholesterol (LDL), body weight, liver weight, liver index, serum insulin, tumor necrosis factor-α (TNF-α), interleukin-1 (IL-1), interleukin-6 (IL-6) and NAFLD Activity Score (NAS). Due to the high heterogeneity, we conducted subgroup analyses of the main results ALT, AST, TC and TG by animal strains, modeling methods, administration methods, ginsenoside dosages and types of ginsenosides. The results showed that the heterogeneity of TC may be derived from differences in modeling methods. The results showed that the heterogeneity of TC may be derived from differences in modeling methods.
Conclusion: In this study, we summarized the molecular mechanism of ginsenosides in regulating NAFLD, mainly focusing on inhibiting inflammation and oxidative stress, improving insulin sensitivity, and regulating intestinal flora. Preclinical evidence indicates that ginsenosides represent a novel therapeutic avenue for NAFLD. The mechanism of ginsenosides in treating NAFLD may involve anti-inflammation, antioxidation, improving insulin resistance, and regulating intestinal flora. However, the inclusion of studies with low methodological quality and the existence of publication bias may undermine the validity of the results. To fully elucidate the mechanisms underlying the therapeutic effects of ginsenosides, future research should employ more rigorous experimental designs and conduct comprehensive investigations.
Systematic Review Registration: https://www.crd.york.ac.uk/PROSPERO/.
Introduction
Non-alcoholic fatty liver disease (NAFLD) has emerged as a rapidly spreading global health issue, afflicting approximately one-fourth of the adult population worldwide (Guo et al., 2022). As the hepatic manifestation of metabolic syndrome, NAFLD encompasses a continuum of liver disorders, ranging from simple steatosis to more severe conditions such as non-alcoholic steatohepatitis (NASH), fibrosis, cirrhosis, and even hepatocellular carcinoma (HCC) (Huby and Gautier, 2022). Currently, there are no approved drugs for the treatment of NAFLD and NASH, and there are other drugs clinically available for the treatment of NAFLD and NASH-related problems, which increases the need to understand the pathogenesis and progression of NAFLD and NASH to develop new treatment strategies. Recently, research has increasingly focused on identifying novel therapies for NAFLD/NASH.
Ginseng is a rhizomatous plant and has long been used as a traditional herbal medicine for treating various physiological and pathological conditions (Lü et al., 2009). Ginseng contains different types of bioactive components such as ginsenosides, polysaccharides, etc. (Attele et al., 1999). Among these, ginsenosides (i.e., steroidal saponins) have been identified as the principal bioactive compounds responsible for modulating numerous biological and physiological processes in the human body. Accumulating evidence indicates that ginsenosides exert potent pharmacological effects in various diseases by suppressing oxidative stress, inflammation, and fibrosis (Zhao et al., 2023; Yi, 2021). An increasing number of studies have shown that ginseng and ginsenosides play pharmacological roles in the pathogenesis and progression of NAFLD and NASH. Ginsenoside Rg3 has been reported to reduce liver inflammation and fibrosis by regulating the NF-κB signaling pathway, thereby preventing the progression of NAFLD to NASH (Lee et al., 2020). Ginsenoside Rg3 reduces insulin resistance and lipotoxicity associated with obesity through the STAT5-PPARγ pathway (Lee et al., 2017). In addition, ginsenoside Rg1 protects the liver from age-related fatty liver disease by maintaining hepatic orkhead box protein O1 (FOXO1) activity nd reducing inflammation (Qi et al., 2020). Given the promising efficacy of ginsenosides, researchers have increasingly focused on exploring their applications in NAFLD treatment. Although there is sufficient evidence detailing the effects of ginsenosides on many signaling pathways and cellular functions in NAFLD, an overall view of their mechanism of action remains elusive. Therefore, this systematic review aims to synthesize existing research reports, summarizing the key findings on the therapeutic effects of ginsenosides in NAFLD, as well as their pharmacological actions and potential underlying mechanisms.
Materials and methods
Literature search and review strategy
This report is registered in PROSPERO (NO. CRD42024611305). Five databases, PubMed, Web of Science, Embase, China National Knowledge Infrastructure (CNKI) and Wanfang Data were searched from the establishment of the database to September 2024. Searches were conducted using MeSH terms and free-text words appropriately adapted for each database. The medical subject terms (MeSH) and free terms used for database searches are “Non-alcoholic Fatty Liver Disease” or “Non-alcoholic Fatty Liver Disease” or “Fatty Liver, Nonalcoholic” or “Fatty Livers, Nonalcoholic” or “Liver, Nonalcoholic Fatty” or “Livers, Nonalcoholic Fatty” or “Nonalcoholic Fatty Liver” or “Nonalcoholic Fatty Livers” or “NAFLD” or “Nonalcoholic Fatty Liver Disease” or “Nonalcoholic Steatohepatitis” or “Nonalcoholic Steatohepatitides” or “Steatohepatitides, Nonalcoholic” or “Steatohepatitis, Nonalcoholic” and “Ginsenosides” or “Ginsenoside” or “Panaxosides” or “Sanchinosides”.
Inclusion and exclusion criteria
The following inclusion criteria should be met: 1) the experiment was based on the NAFLD/NASH model only; 2) only the ginsenoside group was included or it received ginsenoside; 3) the included studies consist of a model group and ginsenoside group; 4) the primary endpoints were as follows: total cholesterol (TC), triglycerides (TG), alanine aminotransferase (ALT), aspartate aminotransferase (AST), high-density lipoprotein cholesterol (HDL), low-density lipoprotein cholesterol (LDL), NAFLD Activity Score (NAS), and the secondary endpoints were as follows: animal body weight, liver weight, liver index, serum insulin, tumor necrosis factor-α (TNF-α), interleukin-1 (IL-1), interleukin-6 (IL-6).
The exclusion criteria of this study were as follows: 1) Animal experiments are not included; 2) Review and/or meta-analysis; 3) Randomized controlled trials or clinical studies; 4) Ginseng extracts or mixtures, experimental group without ginsenosides and NAFLD/NASH or other ginsenosides and NAFLD/NASH measures; 5) Insufficient primary and secondary outcome data; 6) comment, conference, editorial, letter, reply; 7) Irrelevant or duplicate publication.
Data extraction
All the searched articles were imported into EndNote X9, and duplicate articles were removed. Two researchers independently conducted literature collection according to the inclusion and exclusion criteria. Initially, titles and abstracts were selected to exclude irrelevant articles. After the preliminary screening, potentially eligible articles underwent full-text screening for final determination.
Two reviewers extracted the following information from the included studies: 1) Basic data: last name, first name of the initial author, and publication year; 2) Characteristics of experimental animals, including animal type, gender, sample size, age, and weight; 3) Modeling method and criteria for successful modeling; 4) Treatment information: administration method, source, duration, and dose of the intervention drug; 5) Outcome measures: analysis of indicators such as TC, TG, ALT, AST, HDL, LDL, animal body weight, liver weight, liver index, serum insulin, TNF-α, IL-1, IL-6 and NAFLD Activity Score (NAS). All outcome measures are continuous data; therefore, the mean and standard deviation are plotted for each intervention group. If the results are presented only in chart form, we try to contact the authors for more details. In the same experiment, if there were multiple doses in treatment group, the highest dose group was chosen. If the results were acquired at multiple time points, only the data of the last time point is collected for analysis. If there is no response, the GetData Graph Digitizer software is used for graphic quantification.
Quality evaluation
The SYstematic Review Center for Laboratory animal Experimentation (SYRCLE) risk of bias assessment tool was used to assess methodological quality. The assessment includes ten items (Guo et al., 2022): sequence generation (Huby and Gautier, 2022). baseline characteristics (Lü et al., 2009). allocation concealment (Attele et al., 1999). random housing (Zhao et al., 2023). blinding of experimenters (Yi, 2021). random outcome assessment (Lee et al., 2020). blinding of outcome assessors (Lee et al., 2017). incomplete outcome data (Qi et al., 2020). selective outcome reporting (Cui et al., 2023). other sources of bias. In addition, the assessment score for each part is yes (low risk of bias), no (high risk of bias), and unclear (the risk of bias is insufficient to be evaluated from the reported details). In case of disagreement, it is resolved through consultation with the corresponding author.
Statistical analysis
Data analysis was performed using Review Manager (RevMan, version 5.3) and STATA 15 software. Since the variable type data in this report is continuous, standardized mean differences (SMD) and 95% confidence intervals (CI) are used to represent the effect size. For certain outcomes that are fairly standard (AST, ALT, TC, TG, HDL and LDL), using weighted mean differences (MD) could make the results more clinically interpretable. Heterogeneity between studies was evaluated using I-squared (I2). A fixed-effects model is used to combine effect sizes for I2 ≤ 50%. I2 > 50% is considered to represent great heterogeneity, and a random effects model is used to combine effect sizes. Due to the high heterogeneity, we conducted subgroup analyses of the main results ALT, AST, TC and TG by ginsenoside dosages (≤40 mg/kg and >40 mg/kg, according to the median dose used in the included studies), modeling methods (HFD, HSFD and HSFD and CCl4), types of ginsenosides (protopanaxatriol (PPT), protopanaxadiol (PPD) and other), animal strains (mice and rats), administration methods (intraperitoneal injection and gavage). Sensitivity analysis is performed when there is a significant deviation in individual results. If there are at least 10 studies for each outcome, funnel plots and Egger’s test are used to assess potential publication bias. The Trim-and-fill method is used when there is publication bias. This study did not conduct a sensitivity analysis based on the quality of the studies, mainly because the included studies had excessively high heterogeneity in terms of design, samples, and measurement methods, making it difficult to adopt a unified standard for quality evaluation.
Results
Study inclusion
According to our search strategy, a total of 402 relevant studies were identified. Among them, there were 61 in PubMed, 120 in Embase, 65 in Web of Science, 84 from CNKI and 72 from Wanfang. After removing duplicate documents, 164 documents were retained. By reading the titles, abstracts, and full texts, finally, according to our exclusion criteria, 30 studies were included for further analysis (Qi et al., 2020; Cui et al., 2023; Gu et al., 2021; Guo et al., 2023; Hou et al., 2020; Huang et al., 2017; Li and Fan, 2024; Li et al., 2024; Liang et al., 2021; Shi et al., 2024; Song et al., 2020; Xu YS. et al., 2018; Yang SM. et al., 2023; Yao, 2020; Zhang et al., 2024; Zhang et al., 2022; Cui, 2024; Gao et al., 2023; Han et al., 2019; He et al., 2021; Hong et al., 2018; Jiang, 2021; Liang et al., 2023; Liao and Xu, 2016; Peng et al., 2015; Ruan et al., 2020; Wang et al., 2021; Xiao Y. et al., 2019; Xie et al., 2023; Xu, 2018). The flow chart is shown in Figure 1.
Study characteristics
A total of 30 studies were included in this research, which suggests that the protective effect of ginsenosides on NAFLD has attracted extensive attention in recent years. A total of 604 animals were included in these studies, with 304 in the model group and 300 in the ginsenoside group. The animal species utilized were rats and mice. Among them, 10 studies used rats (Gu et al., 2021; Hou et al., 2020; Gao et al., 2023; Jiang, 2021; Liang et al., 2023; Liao and Xu, 2016; Peng et al., 2015; Ruan et al., 2020; Xiao Y. et al., 2019; Xie et al., 2023), while 20 studies used all mice. In all studies, the weight of rats was 115–220g, and the weight of mice was 14–25 g. However, 10 studies did not report the animal weight or merely indicated the animals’ age in weeks. In terms of gender, only two studies (Xu YS. et al., 2018; Xu, 2018) utilized female mice, while the remaining 28 studies opted for male mice. The experimental group was given ginsenosides, and the model group was given normal saline or others. In terms of the modeling methods, three study (Guo et al., 2023; Li et al., 2024; Yang SM. et al., 2023) used HSFD combined with carbon tetrachloride (CCl4) for modeling, 16 studies used high-fat diet (HFD) for modeling, and the remaining 11 studies used high-sucrose and high-fat diet (HSFD) for modeling. The ginsenoside dosages in these studies varied across multiple levels, ranging from 1 to 200 mg/kg/day. The maximum dose was 200 mg/kg and lasted for a total of 12 weeks. The detailed information of the included studies is listed in Supplementary Table 1 and Figure 2.
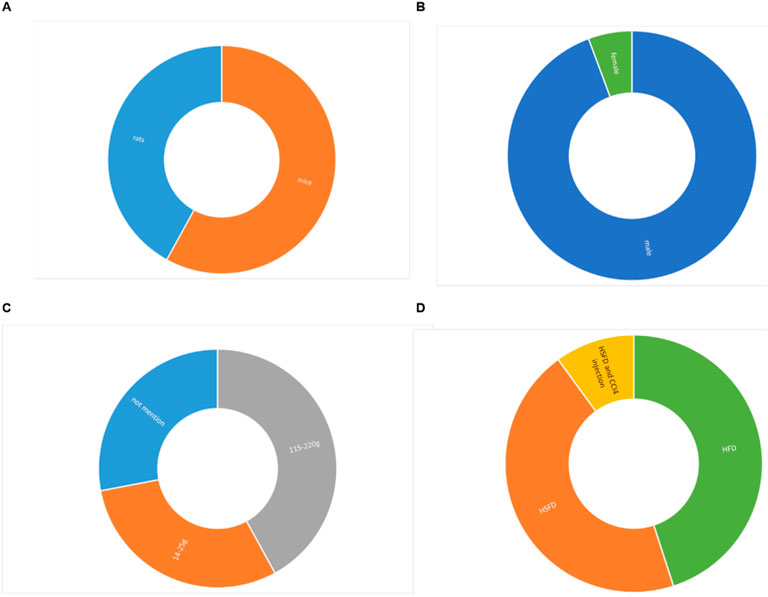
Figure 2. Study characteristics of studies. (A). Animal strains, (B). Animal sex, (C). Weight, (D). Modeling method.
Study quality
As shown in Table 1 and Supplementary Figure 1, we conducted a strict assessment of the quality of the included studies. The quality scores of all the studies fell within the range of four–five points. Among the 30 included studies, 28 studies reported the use of random grouping methods. The basic characteristics of animals between study groups were balanced in all studies. However, with respect to allocation concealment, seven studies failed to report any details regarding this aspect, and 21 studies were unclear. Concerning the blinding of experimenters, it remained ambiguous in 17 studies as to whether the experimenters were blinded during the conduct of the experiments. 27 studies did not report random for outcome assessment, and 22 studies did not report blinding of outcome assessors. In addition, no incomplete experimental data or selective reporting was found. These studies had no biases from other sources.
Effect of ginsenoside treatment on NAFLD
Primary outcome measure
Analysis of lipid content
A total of 25 studies reported the effect of ginsenosides on TC levels. The pooled results showed that compared with the model group, ginsenoside group significantly decreased TC levels [n = 510, MD = -0.93 [-1.11, −0.76], P < 0.05; heterogeneity: I2 = 96%, P < 0.00001] (Figure 3).
A total of 28 studies reported the effect of ginsenosides on TG levels. The pooled results showed that compared with the model group, ginsenoside group significantly decreased TG levels [n = 562, MD = −0.44 [-0.56, −0.33], P < 0.05; heterogeneity: I2 = 99%, P < 0.00001] (Figure 4).
A total of 17 studies reported the effect of ginsenosides on HDL levels. The pooled results showed that there was no significant difference in HDL levels between the two groups [n = 352, MD = 0.11 [-0.00, 0.23], P = 0.05; heterogeneity: I2 = 95%, P < 0.00001] (Figure 5).
A total of 18 studies reported the effect of ginsenosides on LDL levels. The pooled results showed that two studies (Yao, 2020; Xu et al., 2018a) showed no significant difference in LDL levels between the two groups. The remaining studies indicated that compared with the model group, ginsenoside group significantly decreased LDL levels [n = 360, MD = −0.25 [-0.32, −0.19], P < 0.05; heterogeneity: I2 = 97%, P < 0.00001] (Figure 6).
Histological changes
A total of five studies reported the effect of ginsenosides on NAS score. The pooled results showed that compared with the model group, ginsenoside group significantly reduced NAS score [n = 90, SMD = −6.78 [-8.95, −4.61], P < 0.05; heterogeneity: I2 = 69%, P < 0.00001] (Figure 7). Due to the limited research data, more studies are needed on the results related to histology or inflammatory markers to interpret and evaluate these results more accurately.
Analysis of liver function
A total of 27 studies reported the effect of ginsenosides on ALT. The pooled results showed that compared with the model group, ginsenoside group significantly reduced ALT levels [n = 550, MD = −30.01 [-34.94, −25.08], P < 0.05; heterogeneity: I2 = 98%, P < 0.00001] (Figure 8).
A total of 27 studies reported the effect of ginsenosides on AST. The pooled results showed that compared with the model group, ginsenoside group significantly reduced AST levels [n = 550, MD = −47.44 [-58.63, −36.26], P < 0.05; heterogeneity: I2 = 99%, P < 0.00001] (Figure 9).
A total of 23 studies reported the effect of ginsenosides on body weight. The pooled results showed that compared with the model group, ginsenoside group significantly reduced body weight [n = 409, SMD = −3.00 [-3.76, −2.23], P < 0.05; heterogeneity: I2 = 84%, P < 0.00001] (Figure 10).
A total of 13 studies reported the effect of ginsenosides on liver weight. The pooled results showed that compared with the model group, ginsenoside group significantly reduced liver weight [n = 232, SMD = −2.51 [-3.43, −1.59], P < 0.05; heterogeneity: I2 = 84%, P < 0.00001] (Figure 11).
A total of 11 studies reported the effect of ginsenosides on liver index. The pooled results showed that compared with the model group, ginsenoside group significantly reduced liver index [n = 211, SMD = −1.78 [-2.56, −1.00], P < 0.05; heterogeneity: I2 = 80%, P < 0.00001] (Figure 12).
A total of seven studies reported the effect of ginsenosides on serum insulin. The pooled results showed that compared with the model group, ginsenoside group significantly reduced serum insulin levels [n = 124, SMD = −3.79 [-5.38, −2.19], P < 0.05; heterogeneity: I2 = 85%, P < 0.00001] (Figure 13). Except for HDL, other indicators have been reduced by ginsenosides.
Inflammation-related indicators
A total of seven studies reported the effect of ginsenosides on IL-1. The pooled results showed that compared with the model group, ginsenoside group significantly reduced the expression level of IL-1 [n = 163, SMD = −3.65 [-5.23, −2.07], P < 0.05; heterogeneity: I2 = 89%, P < 0.00001] (Figure 14).
A total of eight studies reported the effect of ginsenosides on IL-6. The pooled results showed that compared with the model group, ginsenoside group significantly reduced the expression level of IL-6 [n = 204, SMD = −3.72 [-5.02, −2.43], P < 0.05; heterogeneity: I2 = 87%, P < 0.00001] (Figure 15).
A total of nine studies reported the effect of ginsenosides on TNF-α. The pooled results showed that compared with the model group, ginsenoside group significantly reduced the expression level of TNF-α (n = 186, SMD = −4.22 [-5.51, −2.93], P < 0.05; heterogeneity: I2 = 81%, P < 0.00001) (Figure 16).
Publication bias
To further explore publication bias, for each outcome with a minimum of 10 studies, funnel plots and Egger’s test were employed to evaluate any possible systematic biases in the publication of research findings. The results indicated the presence of publication bias for the relevant indicators, as shown in Figures 17, 18 and Supplementary Figures 2, 3. Subsequently, the trim-and-fill method was utilized to assess the influence of this publication bias on the overall results. Based on the random effects model, the results of the trim-and-fill method show that these missing study data do not affect the stability of the results (Figures 19, 20).
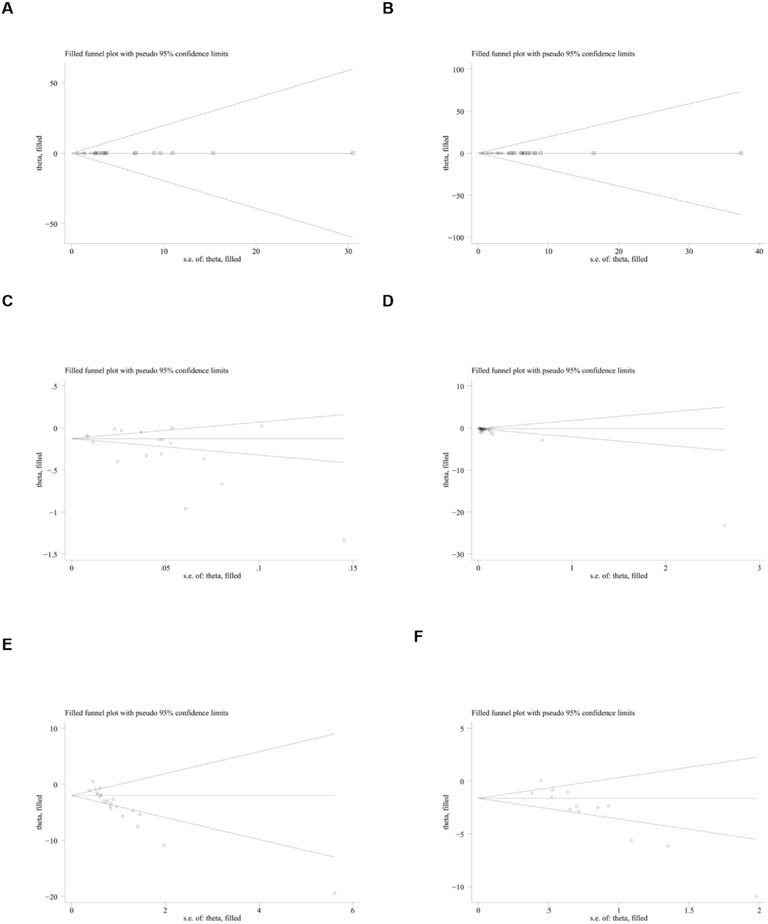
Figure 19. Results of trim and fll method. (A) ALT, (B) AST, (C) LDL, (D) TG, (E) body weight, (F) liver weight.
Subgroup and sensitivity analysis
Due to the high heterogeneity, we conducted subgroup analyses of the main results ALT, AST, TC and TG by animal strains, modeling methods, administration methods, ginsenoside dosages and types of ginsenosides. The results showed that the heterogeneity of TC may be derived from differences in modeling methods (Supplementary Table 2). For ALT, AST, HDL, LDL, TC, TG, body weight, IL-1, IL-6, liver index, liver weight, serum insulin, TNF-α and NAS score sensitivity analysis was performed by removing one study at each stage. The results show that removing one study has no significant impact on the size of the overall effect. The results are shown in Figures 21–23.
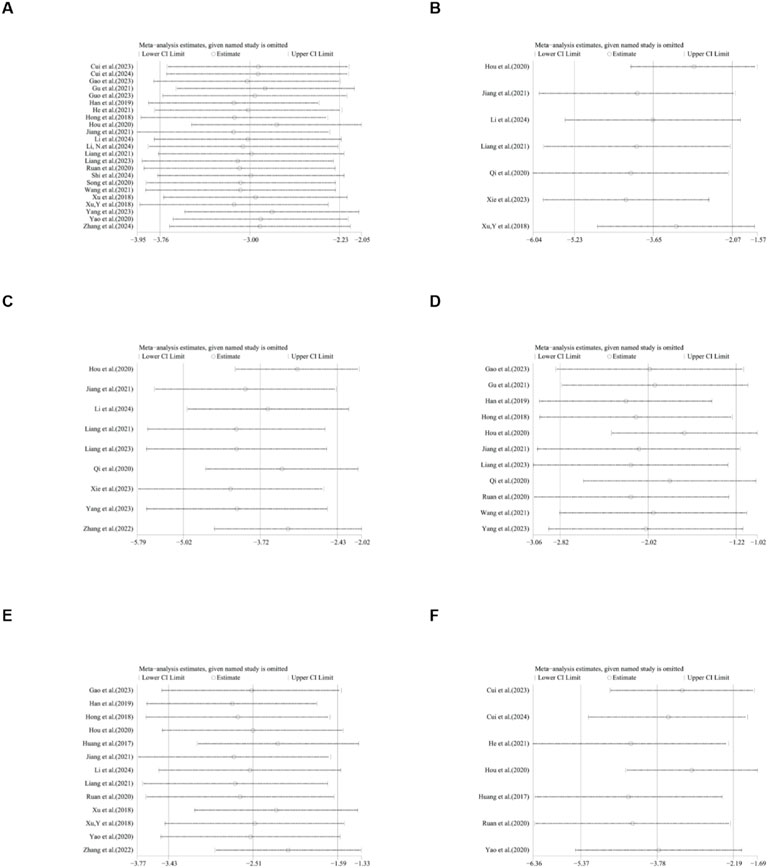
Figure 22. Results of Sensitivity analysis. (A) body weight, (B) IL-1, (C) IL-6, (D) liver index, (E) liver weight, (F) serum insulin.
Discussion
Our research conducted a quantitative assessment of the pharmacological effects of ginsenosides in preclinical animal models of NAFLD. A total of 30 papers were included in this research, involving a total of 604 animals. In this study, we found that ginsenosides can reduce lipid-related indicators including TC, TG, and LDL, while having no significant effect on HDL. Moreover, ginsenosides play a protective role in liver function. They decrease the levels of ALT and AST. Additionally, ginsenosides have an inhibitory effect on inflammatory factors. The levels of IL-1, IL-6 and TNF-α were found to be reduced after ginsenoside treatment. They led to a decrease in body weight, liver weight, liver index and serum insulin. Due to the high heterogeneity, we conducted subgroup analyses of the main results ALT, AST, TC and TG by animal strains, modeling methods, administration methods, ginsenoside dosages and types of ginsenosides. The results showed that the heterogeneity of TC may be derived from differences in modeling methods. Sensitivity analysis was performed by removing one study at each stage. The results show that removing one study has no significant impact on the size of the overall effect.
In this review, we conducted a meta-analysis of preclinical trials of ginsenosides in the treatment of NAFLD. The purpose is to evaluate the lipid-lowering effect and antioxidant and anti-inflammatory properties of ginsenosides in the treatment of NAFLD. Current evidence shows that ginsenosides can effectively control the progression of NAFLD through antioxidant and anti-inflammatory mechanisms. This meta-analysis is based on animal experiments, so it cannot represent the results of clinical trials, but it still has certain reference value and guiding significance for future experiments.
The clinical transformation strategies of ginsenosides: Although this study confirmed the significant efficacy of ginsenosides on NAFLD in animal models, translating them into clinical applications still faces key challenges in bioavailability. Bioavailability is an obstacle to the conversion of ginsenosides for human use, which is quite appropriate, as many ginsenosides have low oral bioavailability or require intestinal microbiota conversion (such as in the case of compound K). Interestingly, the new drug delivery system may offer better solubility, oral absorption rate and bioavailability. A series of nano-delivery systems have been developed to enhance efficiency and reduce related adverse reactions (Kim et al., 2018). It is worth noting that the ginsenoside compound K prepared with polymer micelles has good biodegradability and biocompatibility, and its anti-tumor effect is stronger than that of free compound K (Yang et al., 2017). In order to enhance the bioavailability level, Rb1 has been encapsulated into nano-RB1 using polymer nano-capsules, thereby significantly inhibiting the activities of NF-κB and NLRP3 inflammasomes (Liu et al., 2020). However, as far as we know, although progress has been made in the development of traditional Chinese medicine, there is still no compound K preparation available for patients. Currently, compound K capsules are undergoing clinical trials for the treatment of rheumatoid arthritis (Chen L. et al., 2017).
Comparison of other intervention measures: In this study, ginsenoside intervention demonstrated good therapeutic potential. Compared with other intervention methods, the effect of ginsenosides is unique. Dietary management is a very promising strategy for intervening in NAFLD. panaxadiol saponin component (PDS-C) can significantly alleviate the liver function, liver steatosis and blood lipid levels of mice with NAFLD, and reduce oxidative stress and inflammation. Supplementation of PDS-C reduces insulin resistance and glucose homeostasis in mice with NAFLD, although its therapeutic effect is not as obvious as that of metformin (Mi et al., 2024). Compound K and metformin reduces glucose intolerance and hepatic steatosis in rats fed with HFD through AMPK activation (Hwang et al., 2018).
Comparison with relevant literature: A meta-analysis by Yang et al. (2023a) examined the efficacy of ginseng in animal studies of NAFLD (including 41 studies). Although this study focused on the overall formulation of ginseng (rather than just isolated ginsenosides), it reported similar significant reductions in lipid parameters and transaminases after treatment with ginseng. And HDL has also significantly increased (Yang K. et al., 2023). This study quantitatively evaluated the efficacy of ginsenosides in NAFLD, complementing the meta-analysis of 41 studies included by Yang et al. (2023b). This study indicates that the whole ginseng preparation can significantly increase HDL levels, while no similar effect was observed in this study. This difference may stem from the complexity of the components, while purified ginsenosides lack such synergistic effects. In addition, Yang et al.‘s research covered different ginseng varieties and administration routes, while this study focused on a single purified component, more clearly revealing the direct mechanism of action of ginsenosides. This suggests that future studies can explore the strategy of “combined administration of ginsenosides and non-ginsenosides components” to integrate the advantages of both and provide a more comprehensive treatment plan for NAFLD.
However, this study has some inevitable limitations. First, some studies did not provide detailed baseline characteristics. At the same time, given the insufficient methodological quality of some studies, the results of this study should be interpreted with caution, and more qualitative studies are needed in the future. The absence of such baseline data makes it more difficult to confirm whether each group is comparable before treatment, although these studies usually use the same species of animals. Second, there is publication bias in this study, which may be related to the quality of the included literature. Animal experiments that produce negative results contrary to its hypothesis are less likely to be reported. More data (and perhaps more consistent experimental designs among studies) are needed to accurately identify the sources of heterogeneity. Due to the high heterogeneity, we conducted subgroup analyses of the main results ALT, AST, TC and TG by animal strains, modeling methods, administration methods, ginsenoside dosages and types of ginsenosides. Only the heterogeneity results of TC suggest that it may stem from the differences in modeling methods. Moreover, the data in some studies are not provided directly as raw data but are obtained indirectly through data extraction tools, which may lead to data measurement bias. In addition, many of the included studies conducted hematoxylin-eosin (H&E) staining tests on liver histology, but only a few of the five studies reported quantitative histological results that could be used for combined analysis. Therefore, this meta-analysis mainly focused on biochemical results. While the amelioration of hepatic enzyme levels and normalization of lipid profiles suggest clinical benefits in NAFLD management, conclusive quantitative validation of steatosis regression or fibrosis attenuation remains insufficiently characterized through current diagnostic modalities. Furthermore, due to the relatively small sample size of the research and the certain complexity of the research data. Multiple comparisons significantly increase the probability of Type I error (false positive error). Moreover, excessive comparisons reduce the statistical test power, increasing the risk of Type II error (false negative error) and potentially overlooking real differences. The complexity of multiple comparison results also complicates data analysis and conclusion drawing, interfering with the accurate determination of the true relationships among various factors. In this study, sensitivity analysis based on study quality was not conducted due to several constraints. First, the included studies exhibited high data heterogeneity, with significant variations in study subject characteristics, interventions, and measurement methods. Second, data missing was prevalent, rendering a reliable quality assessment system unfeasible. Third, the absence of a universally accepted quality evaluation standard in the field, along with varying assessment tools, precluded consistent quality grading. Finally, the diversity in study designs, such as grouping, sample size, and observation period, reduced comparability among studies, making it difficult to isolate the impact of quality on results. Collectively, these factors rendered sensitivity analysis based on study quality infeasible in this research. Moreover, the lack of key information in some studies led to significant uncertainties in the quality assessment. There is minimal evidence of the efficacy of ginsenosides in the treatment of NAFLD in vitro and in vivo experiments. Therefore, clinical examinations are needed. However, due to concerns about bioavailability, the transition from preclinical to clinical research has been delayed.
The protective effect of ginsenosides in NAFLD
Based on the findings of this review, ginsenosides have demonstrated efficacy across a diverse range of NAFLD and NASH models. Through a comprehensive meta-analysis of the included studies, we have constructed a mechanistic diagram (Figure 24).
Anti-inflammation and antioxidation
In the absence of other chronic liver diseases and alcoholism, NAFLD is characterized by a fat accumulation in the liver that exceeds 5%. NAFLD ranges from simple steatosis to NASH, which is characterized by liver lesions, inflammation, and fibrosis (Cobbina and Akhlaghi, 2017). Elevated reactive oxidative stress, lipid peroxidation, inflammation, and fibrosis lead to tissue damage and play an important role in the development and progression of NAFLD (Pereira et al., 2022). Nuclear factor-κB (NF-κB) is the main regulator of the inflammatory response and shows an antagonistic relationship in controlling inflammation (de Gregorio et al., 2020). Ginsenoside Rg1 has been shown to suppress the activity of NF-κB signaling pathway, thereby preventing the production of pro-inflammatory cytokines. The activation of NOD-like receptor protein 3 (NLRP3) inflammasome occurs in NAFLD. NLRP3 inflammasome blockade can alleviate liver inflammation and fibrosis in experimental NASH in mice (Mridha et al., 2017). Specifically, Rg1 has been shown to inhibit the activation of the NLRP3 inflammasome, leading to a significant reduction in the production of pro-inflammatory cytokines, such as IL-1β and IL-18 (Xu YS. et al., 2018). Moreover, Rg1 maintains the activity of forkhead box O1 (FOXO1) in the liver, which not only enhances its antioxidant capacity but also suppresses inflammation and promotes metabolic homeostasis. Rg1 attenuates NAFLD progression in D-galactose-induced murine models through targeted hepatoprotection, effectively mitigating steatotic accumulation, inflammatory cascades, and hepatocellular damage (Qi et al., 2020). Rg1 reduces ALT/AST levels and pro-inflammatory cytokines, and effectively reduces hepatic steatosis and inflammation, which may be related to the AMP-activated protein kinase (AMPK)/NF-κB pathway (Xiao Q. et al., 2019). Mitochondria are the primary source of reactive oxygen species (ROS) in cells. The intimate connection between mitochondria and the endoplasmic reticulum (ER) via the mitochondria-associated membrane means that ROS generated by mitochondria can trigger ER stress (Burgos-Morón et al., 2019). Studies have shown that ginsenoside Rg1 alleviates NAFLD by inhibiting lipid peroxidation and endoplasmic reticulum stress in HFD-induced NAFLD mice. In db/db mice with NAFLD, ginsenoside Re exerts its protective effects possibly through the inhibition of oxidative stress and the upregulation of peroxisome proliferator - activated receptor γ (PPARγ) expression (Jiang et al., 2021).
Regulating intestinal flora
Intestinal flora imbalance plays a key role in the pathogenesis of NAFLD through its metabolites. Restoration of the intestinal microbiota and supplementation of symbiotic bacterial metabolites offer potential therapeutic strategies for this condition (Chen and Vitetta, 2020). Ginsenosides can regulate the composition and diversity of the intestinal flora and promote the metabolic homeostasis of the microbial community (Zheng et al., 2021). Metabolites of the intestinal flora, including short-chain fatty acids, tryptophan and its derivatives, play a crucial role in regulating intestinal and systemic immune homeostasis (Li et al., 2023). Ginsenoside extract (GE) exerts multiple beneficial effects, such as regulating the intestinal microbiota, enhancing the intestinal barrier function, restoring energy balance, and alleviating metabolic inflammation. In addition, GE may become a potential drug for preventing NAFLD by integrating prebiotic, anti-inflammatory and energy-regulating effects (Liang et al., 2021). Ginsenoside Rk3 treatment significantly changed the abundance of short-chain fatty acids. These changes are related to beneficial changes in the species and composition of the intestinal microbiota. Ginsenoside Rk3 helps to clarify the interaction between the host and microorganisms, positioning it as a promising candidate for the treatment of NASH (Guo et al., 2023). After Rh4 treatment, the degree of hepatic steatosis, the level of lobular inflammation and bile acids in liver tissue are reduced. At the same time, Rh4 treatment significantly increases the levels of intestinal short-chain fatty acid and bile acids, and accompanied by complementary diversity and composition of the intestinal microbiota (Yang et al., 2023a). The analysis performed by 16 S rRNA sequencing revealed that Rg5 intervention induced changes in the composition of the intestinal microbiota, promoted an increase in beneficial bacteria such as Bacteroides and Akkermansia, and at the same time reduced the relative abundance of harmful bacteria, taking Olsenella as an example (Shi et al., 2024).
Improving insulin resistance
NAFLD is intricately linked to hepatic insulin resistance. The accumulation of hepatic diglycerides activates protein kinase C - ε (PKC - ε), which in turn impairs insulin receptor activation and insulin-stimulated glycogen synthesis (Yang SM. et al., 2023). In rats fed a HFD, significant elevations are observed in liver function markers, blood lipid levels, glucose intolerance, and insulin resistance (Chen XJ. et al., 2017). Ginsenoside Mc1 exerts a protective effect on ER stress-induced apoptotic damage, insulin resistance and lipogenesis in palmitate-treated hepatocytes and livers of diet-induced obesity (DIO) mice. Mc1 may be a potential therapeutic strategy for preventing NAFLD in obese and insulin-resistant patients (Roh et al., 2020). Ginsenoside Rg3 reduces obesity-induced insulin resistance and lipotoxicity through signal transducer and activator of transcription 5 (STAT5)-PPAR-γ (Lee et al., 2017). Ginsenoside Rg1 prevents liver insulin resistance by maintaining insulin signal sensitivity and is a promising alternative drug (Mo et al., 2019). HCV core protein binding protein 6 (HCBP6) plays a role in regulating lipolysis and fatty acid oxidation. In addition, ginsenoside Rh2 upregulates the expression of HCBP6. As a result, administration of ginsenoside Rh2 reduces HFD-induced fatty liver and glucose tolerance (Lu et al., 2020).
Conclusion
This systematic preclinical assessment demonstrates that ginsenosides effectively reduce body weight, liver weight, and other pathological manifestations associated with NAFLD. Mechanistically, ginsenosides significantly decrease lipid accumulation, alleviate insulin resistance, and inhibit inflammatory responses and oxidative stress. These findings underscore the unique therapeutic potential of ginsenosides in the treatment of NAFLD and NASH. However, future research involving more rigorously designed experimental models is essential for a more comprehensive and cautious interpretation of these conclusions.
Data availability statement
The original contributions presented in the study are included in the article/Supplementary Material, further inquiries can be directed to the corresponding authors.
Author contributions
LH: Conceptualization, Data curation, Formal Analysis, Writing – original draft. QP: Conceptualization, Writing – original draft. SL: Conceptualization, Writing – original draft. XH: Funding acquisition, Writing – original draft. HY: Formal Analysis, Writing – original draft.
Funding
The author(s) declare that financial support was received for the research and/or publication of this article. The present study was financially supported by Science and Technology Program of Hebei (223777156D) and Clinical Medical School Graduate Research Innovation Practice Project (2023KCY06); National Natural Science Foundation of China (No. 81973840 and No. 81273748).
Acknowledgments
The authors acknowledge using Biorender (https://app.biorender.com/) to create the schemata (Figure 24).
Conflict of interest
The authors declare that the research was conducted in the absence of any commercial or financial relationships that could be construed as a potential conflict of interest.
Generative AI statement
The author(s) declare that no Generative AI was used in the creation of this manuscript.
Publisher’s note
All claims expressed in this article are solely those of the authors and do not necessarily represent those of their affiliated organizations, or those of the publisher, the editors and the reviewers. Any product that may be evaluated in this article, or claim that may be made by its manufacturer, is not guaranteed or endorsed by the publisher.
Supplementary material
The Supplementary Material for this article can be found online at: https://www.frontiersin.org/articles/10.3389/fphar.2025.1564852/full#supplementary-material
References
Attele, A. S., Wu, J. A., and Yuan, C. S. (1999). Ginseng pharmacology: multiple constituents and multiple actions. Biochem. Pharmacol. 58 (11), 1685–1693. doi:10.1016/s0006-2952(99)00212-9
Burgos-Morón, E., Abad-Jiménez, Z., Marañón, A. M., Iannantuoni, F., Escribano-López, I., López-Domènech, S., et al. (2019). Relationship between oxidative stress, Er stress, and inflammation in type 2 diabetes: the battle continues. J. Clin. Med. 8 (9), 2019. doi:10.3390/jcm8091385
Chen, J., and Vitetta, L. (2020). Gut microbiota metabolites in nafld pathogenesis and therapeutic implications. Int. J. Mol. Sci. 21 (15), 5214–5307/29. doi:10.3390/ijms21155214
Chen, L., Zhou, L., Wang, Y., Yang, G., Huang, J., Tan, Z., et al. (2017a). Food and sex-related impacts on the pharmacokinetics of a single-dose of ginsenoside compound K in healthy subjects. Front. Pharmacol. 8, 636. doi:10.3389/fphar.2017.00636
Chen, X. J., Liu, W. J., Wen, M. L., Liang, H., Wu, S. M., Zhu, Y. Z., et al. (2017b). Ameliorative effects of compound K and ginsenoside Rh1 on non-alcoholic fatty liver disease in rats. Sci. Rep. 7, 41144. doi:10.1038/srep41144
Cobbina, E., and Akhlaghi, F. (2017). Non-alcoholic fatty liver disease (nafld) - pathogenesis, classification, and effect on drug metabolizing enzymes and transporters. Drug metab. Rev. 49 (2), 197–211. doi:10.1080/03602532.2017.1293683
Cui, T. (2024). Research on the activation of sirt6 by ginsenoside rd to improve non-alcoholic fatty liver disease induced by high-fat diet. Guangzhou University of Chinese Medicine. (In Chinese).
Cui, T. Q., Xiao, X. X., Pan, Z. S., Tang, K. J., Zhong, Y. D., Chen, Y. J., et al. (2023). Harnessing the therapeutic potential of ginsenoside rd for activating Sirt6 in treating a mouse model of nonalcoholic fatty liver disease. Acs Omega 8 (32), 29735–29745. doi:10.1021/acsomega.3c04122
de Gregorio, E., Colell, A., Morales, A., and Marí, M. (2020). Relevance of sirt1-nf-κb Axis as therapeutic target to ameliorate inflammation in liver disease. Int. J. Mol. Sci. 21 (11), 3858. doi:10.3390/ijms21113858
Gao, X., Zhang, L., Yang, Y., Luo, J., Jiang, K., Li, S., et al. (2023). Effect of ginsenoside Rg1 on non-alcoholic fatty liver disease and intestinal microbiota in rats. J. Chongqing Med. Univ. 48 (04), 381–389. (In Chinese). doi:10.13406/j.cnki.cyxb.003212
Gu, D. S., Yi, H. A., Jiang, K. R., Fakhar, S. H., Shi, J., He, Y. S., et al. (2021). Transcriptome analysis reveals the efficacy of ginsenoside-rg1 in the treatment of nonalcoholic fatty liver disease. Life Sci. 267, 118986. doi:10.1016/j.lfs.2020.118986
Guo, M. D., Zhu, C. H., Fu, R. Z., Ma, X. X., Duan, Z. G., and Fan, D. D. (2023). Ginsenoside Rk3 regulates nonalcoholic steatohepatitis by modulation of intestinal flora and the pi3k/akt signaling pathway in C57bl/6 mice. J. Agric. Food Chem. 71 (24), 9370–9380. doi:10.1021/acs.jafc.3c00789
Guo, X., Yin, X., Liu, Z., and Wang, J. (2022). Non-alcoholic fatty liver disease (nafld) pathogenesis and natural products for prevention and treatment. Int. J. Mol. Sci. 23 (24), 15489–15524. doi:10.3390/ijms232415489
Han, X., Zhang, F., Liu, C., Zhang, H., Gu, L., Liu, Y., et al. (2019). Therapeutical effect of ginsenoside Rb1 on non-alcoholic fatty liver disease of mice. Shandong Med. J. 59 (08), 37–40. (In Chinese).
He, W., Yang, R., and Luo, T. (2021). Effect of ginsenoside Rg1 on liver fibrosis in mice with non-alcoholic fatty liver disease. J. Chin. Med. Mater. 44 (05), 1208–1212. (In Chinese). doi:10.13863/j.issn1001-4454.2021.05.032
Hong, Y., Gu, X., Xu, J., Lin, Y., and Si, Q. (2018). The effect and mechanism of ginsenoside rb2 on lipid metabolism in the liver of mice with hyperlipidemic fatty liver. J. Wenzhou Med. Univ. 48 (05), 338–341+49. (In Chinese).
Hou, Y. H., Gu, D. S., Peng, J. Z., Jiang, K. R., Li, Z. G., Shi, J., et al. (2020). Ginsenoside Rg1 regulates liver lipid factor metabolism in nafld model rats. Acs Omega 5 (19), 10878–10890. doi:10.1021/acsomega.0c00529
Huang, Q., Wang, T., Yang, L., and Wang, H. Y. (2017). Ginsenoside Rb2 alleviates hepatic lipid accumulation by restoring autophagy via induction of Sirt1 and activation of ampk. Int. J. Mol. Sci. 18 (5), 1063. doi:10.3390/ijms18051063
Huby, T., and Gautier, E. L. (2022). Immune cell-mediated features of non-alcoholic steatohepatitis. Nat. Rev. Immunol. 22 (7), 429–443. doi:10.1038/s41577-021-00639-3
Hwang, Y. C., Oh, D. H., Choi, M. C., Lee, S. Y., Ahn, K. J., Chung, H. Y., et al. (2018). Compound K attenuates glucose intolerance and hepatic steatosis through ampk-dependent pathways in type 2 diabetic oletf rats. Korean J. Intern. Med. 33 (2), 347–355. doi:10.3904/kjim.2015.208
Jiang, K. (2021). Study on the molecular mechanism of ginsenoside rg1 regulating early liver fibrosis in non-alcoholic fatty liver disease. Kunming Medical University. (In Chinese).
Jiang, Y., Sui, D., Li, M., Xu, H., Yu, X., Liu, J., et al. (2021). Ginsenoside re improves inflammation and fibrosis in hepatic tissue by upregulating pparγ expression and inhibiting oxidative stress in Db/Db mice. Evidence-based complementary Altern. Med. 2021, 9003603. doi:10.1155/2021/9003603
Kim, H., Lee, J. H., Kim, J. E., Kim, Y. S., Ryu, C. H., Lee, H. J., et al. (2018). Micro-/Nano-Sized delivery systems of ginsenosides for improved systemic bioavailability. J. Ginseng Res. 42 (3), 361–369. doi:10.1016/j.jgr.2017.12.003
Lee, J. B., Yoon, S. J., Lee, S. H., Lee, M. S., Jung, H., Kim, T. D., et al. (2017). Ginsenoside Rg3 ameliorated hfd-induced hepatic steatosis through downregulation of stat5-pparγ. J. Endocrinol. 235 (3), 223–235. doi:10.1530/joe-17-0233
Lee, J. H., Oh, J. Y., Kim, S. H., Oh, I. J., Lee, Y. H., Lee, K. W., et al. (2020). Pharmaceutical efficacy of gypenoside lxxv on non-alcoholic steatohepatitis (nash). Biomolecules 10 (10), 1426. doi:10.3390/biom10101426
Li, C. Y., and Fan, D. J. (2024). Ginsenoside R3 alleviates non-alcoholic fatty liver disease by regulating the PPARγ/miR-103-3p pathway. Trop. J. Pharm. Res. 23 (8), 1239–1247. doi:10.4314/tjpr.v23i8.2
Li, F., Wang, S., Chao, X., and Wang, S. (2023). Editorial: recent advances in novel therapeutic molecules and targets for inflammatory diseases. Front. Pharmacol. 13, 1121821. doi:10.3389/fphar.2022.1121821
Li, N., Zhu, C. H., Fu, R. Z., Ma, X. X., Duan, Z. G., and Fan, D. D. (2024). Ginsenoside Rg5 inhibits lipid accumulation and hepatocyte apoptosis via the Notch1 signaling pathway in nash mice. Phytomedicine 124, 155287. doi:10.1016/j.phymed.2023.155287
Liang, W., Zhou, K., Jian, P., Chang, Z., Zhang, Q., Liu, Y., et al. (2021). Ginsenosides improve nonalcoholic fatty liver disease via integrated regulation of gut microbiota, inflammation and energy homeostasis. Front. Pharmacol. 12, 622841. doi:10.3389/fphar.2021.622841
Liang, Y., Fu, J., Shi, Y., Gao, X., Lu, F., and Liu, S. (2023). Integrating network pharmacology and experimental verification to explore mechanism of ginsenoside Rb in treating metabolic associated fatty liver disease. Drug Eval. Res. 46 (12), 2580–2591. (In Chinese).
Liao, W., and Xu, D. (2016). Study on the improvement effect of ginsenoside rg1 on fatty acid β -oxidation in rats with non-alcoholic fatty liver disease. Chongqing Med. J. 45 (09), 1179–1182. (In Chinese). doi:10.3969/j.issn.1671-8348.2016.09.009
Liu, Y., Zhu, H., Zhou, W., and Ye, Q. (2020). Anti-inflammatory and anti-gouty-arthritic effect of free ginsenoside Rb1 and nano ginsenoside Rb1 against msu induced gouty arthritis in experimental animals. Chemico-biological Interact. 332, 109285. doi:10.1016/j.cbi.2020.109285
Lu, H., Yuan, X., Zhang, Y., Han, M., Liu, S., Han, K., et al. (2020). Hcbp6 deficiency exacerbates glucose and lipid metabolism disorders in non-alcoholic fatty liver mice. Biomed. and Pharmacother. 129, 110347. doi:10.1016/j.biopha.2020.110347
Lü, J. M., Yao, Q., and Chen, C. (2009). Ginseng compounds: an update on their molecular mechanisms and medical applications. Curr. Vasc. Pharmacol. 7 (3), 293–302. doi:10.2174/157016109788340767
Mi, A., Hu, Q., Liu, Y., Zhao, Y., Shen, F., Lan, J., et al. (2024). Hepatoprotective efficacy and interventional mechanism of the panaxadiol saponin component in high-fat diet-induced nafld mice. Food Funct. 15 (2), 794–808. doi:10.1039/d3fo03572g
Mo, J., Zhou, Y., Yang, R., Zhang, P., He, B., Yang, J., et al. (2019). Ginsenoside Rg1 ameliorates palmitic acid-induced insulin resistance in Hepg2 cells in association with modulating akt and jnk activity. Pharmacol. Rep. P. R. 71 (6), 1160–1167. doi:10.1016/j.pharep.2019.07.004
Mridha, A. R., Wree, A., Robertson, A. A. B., Yeh, M. M., Johnson, C. D., Van Rooyen, D. M., et al. (2017). Nlrp3 inflammasome Blockade Reduces liver inflammation and fibrosis in experimental nash in mice. J. Hepatol. 66 (5), 1037–1046. doi:10.1016/j.jhep.2017.01.022
Peng, X., Huang, D., Yan, M., and Peng, S. (2015). Ginsenoside Rg1 improves liver function by regulating fat metabolism in rats with non-alcoholic fatty liver disease. Chin. J. Pathophysiol. 31 (05), 864–870. (In Chinese).
Pereira, E., Araujo, B. P., Rodrigues, K. L., Silvares, R. R., Martins, C. S. M., Flores, E. E. I., et al. (2022). Simvastatin improves microcirculatory function in nonalcoholic fatty liver disease and downregulates oxidative and ale-rage stress. Nutrients 14 (3), 716–803/13. doi:10.3390/nu14030716
Qi, R., Jiang, R., Xiao, H., Wang, Z., He, S., Wang, L., et al. (2020). Ginsenoside Rg1 protects against D-galactose induced fatty liver disease in a mouse model via Foxo1 transcriptional factor. Life Sci. 254:117776. doi:10.1016/j.lfs.2020.117776
Roh, E., Hwang, H. J., Kim, J. W., Hong, S. H., Kim, J. A., Lee, Y. B., et al. (2020). Ginsenoside Mc1 improves liver steatosis and insulin resistance by attenuating Er stress. J. Ethnopharmacol. 259, 112927. doi:10.1016/j.jep.2020.112927
Ruan, J., Xiao, T., Chen, J., Meng, X., Song, H., and Wang, B. (2020). Research of mechanisms of ginsenosides adjusting glycolipid metabolism in nonalcoholic fatty liver disease rats. Chin. Archives Traditional Chin. Med. 38 (08), 101–106+269. (In Chinese). doi:10.13193/j.issn.1673-7717.2020.08.025
Shi, Y., Chen, J., Qu, D., Sun, Q., Yu, Y., Zhang, H., et al. (2024). Ginsenoside rg(5) activates the lkb1/ampk/mtor signaling pathway and modifies the gut microbiota to alleviate nonalcoholic fatty liver disease induced by a high-fat diet. Nutrients 16 (6), 842–903/28. doi:10.3390/nu16060842
Song, B., Sun, Y., Chu, Y. F., Wang, J., Zheng, H. W., Liu, L. L., et al. (2020). Ginsenoside Rb1 alleviated high-fat-diet-induced hepatocytic apoptosis via peroxisome proliferator-activated receptor Γ. Biomed Res. Int. 2020, 2315230. doi:10.1155/2020/2315230
Wang, K., Hu, H., Xu, Y., Ding, R., Wang, Y., and Duan, Z. (2021). Effect of ginsenoside Rb2 on nonalcoholic fatty liver disease in mice and its mechanism. J. Trop. Med. 21 (02), 125–129+43+0. (In Chinese). doi:10.3969/j.issn.1672-3619.2021.02.001
Xiao, Q., Zhang, S., Yang, C., Du, R., Zhao, J., Li, J., et al. (2019b). Ginsenoside Rg1 ameliorates palmitic acid-induced hepatic steatosis and inflammation in Hepg2 cells via the ampk/nf-κb pathway. Int. J. Endocrinol. 2019, 7514802. doi:10.1155/2019/7514802
Xiao, Y., Hou, Y., Yin, X., Kang, F., Li, S., Yang, S., et al. (2019a). Ginsenoside rg1 intervenes in the apoptosis of hepatocytes in rat models of non-alcoholic fatty liver disease. Chin. J. Tissue Eng. Res. 23 (03), 384–390. (In Chinese). doi:10.3969/j.issn.2095-4344.0597
Xie, J., Li, D., Liu, C., and Xiao, X. (2023). The intervention effect of ginsenoside Rg1 on rats with non-alcoholic fatty liver disease. Chin. Tradit. Pat. Med. 45 (11), 3799–3802. (In Chinese).
Xu, Y. (2018). Study on the improvement effect and mechanism of ginsenoside Rg1 on non-alcoholic fatty liver disease in mice. Chongqing Medical University. (In Chinese).
Xu, Y., Yang, C., Zhang, S., Li, J., Xiao, Q., and Huang, W. (2018a). Ginsenoside Rg1 protects against non-alcoholic fatty liver disease by ameliorating lipid peroxidation, endoplasmic reticulum stress, and inflammasome activation. Biol. and Pharm. Bull. 41 (11), 1638–1644. doi:10.1248/bpb.b18-00132
Yang, K., Kim, H. H., Shim, Y. R., and Song, M. J. (2023b). The efficacy of panax ginseng for the treatment of nonalcoholic fatty liver disease: a systematic review and meta-analysis of preclinical studies. Nutrients 15 (3), 721–802/12. doi:10.3390/nu15030721
Yang, L., Zhang, Z., Hou, J., Jin, X., Ke, Z., Liu, D., et al. (2017). Targeted delivery of ginsenoside compound K using tpgs/peg-pcl mixed micelles for effective treatment of lung cancer. Int. J. nanomedicine 12, 7653–7667. doi:10.2147/ijn.S144305
Yang, S. M., Duan, Z. G., Zhang, S., Fan, C. Y., Zhu, C. H., Fu, R. Z., et al. (2023a). Ginsenoside Rh4 improves hepatic lipid metabolism and inflammation in a model of nafld by targeting the gut liver Axis and modulating the fxr signaling pathway. Foods 12 (13), 2492. doi:10.3390/foods12132492
Yao, Y. (2020). Ginsenosides reduce body weight and ameliorate hepatic steatosis in high fat diet-induced obese mice via endoplasmic reticulum stress and P-Stat3/Stat3 signaling. Mol. Med. Rep. 21 (3), 1059–1070. doi:10.3892/mmr.2020.10935
Yi, Y. S. (2021). New mechanisms of ginseng saponin-mediated anti-inflammatory action via targeting canonical inflammasome signaling pathways. J. Ethnopharmacol. 278, 114292. doi:10.1016/j.jep.2021.114292
Zhang, J., Duan, M., Wu, S., Jiang, S., Hu, S., Chen, W., et al. (2024). Comprehensive pharmacological and experimental study of ginsenoside re as a potential therapeutic agent for non-alcoholic fatty liver disease. Biomed. Pharmacother. 177, 116955. doi:10.1016/j.biopha.2024.116955
Zhang, J. J., Ma, X. X., and Fan, D. D. (2022). Ginsenoside ck ameliorates hepatic lipid accumulation via activating the lkb1/ampk pathway in vitro and in vivo. Food and Funct. 13 (3), 1153–1167. doi:10.1039/d1fo03026d
Zhao, F., Lu, M., and Wang, H. (2023). Ginsenoside Rg1 ameliorates chronic intermittent hypoxia-induced vascular endothelial dysfunction by suppressing the formation of mitochondrial reactive oxygen species through the calpain-1 pathway. J. ginseng Res. 47 (1), 144–154. doi:10.1016/j.jgr.2022.07.006
Keywords: ginsenoside, animal model, NAFLD, preclinical studies, meta-analysis
Citation: Hao L, Peng Q, Li S, Hu X and Yan H (2025) Novel insights from meta-analysis: the efficacy of ginsenosides in non-alcoholic fatty liver disease. Front. Pharmacol. 16:1564852. doi: 10.3389/fphar.2025.1564852
Received: 22 January 2025; Accepted: 12 May 2025;
Published: 27 May 2025.
Edited by:
Wei Guo, University of North Carolina at Greensboro, United StatesReviewed by:
Hong Zou, Chinese Academy of Sciences (CAS), ChinaMohamed Hany, Alexandria University, Egypt
Copyright © 2025 Hao, Peng, Li, Hu and Yan. This is an open-access article distributed under the terms of the Creative Commons Attribution License (CC BY). The use, distribution or reproduction in other forums is permitted, provided the original author(s) and the copyright owner(s) are credited and that the original publication in this journal is cited, in accordance with accepted academic practice. No use, distribution or reproduction is permitted which does not comply with these terms.
*Correspondence: Xiaoyu Hu, eGlhb3l1aHUyMDIyMDZAMTYzLmNvbQ==; Huimin Yan, eWFuaG0yNTM4QDE2My5jb20=
†These authors have contributed equally to this work