- 1The Second Gastroenterology Department, Affiliated Hospital of Shandong University of Traditional Chinese Medicine, Jinan, China
- 2Department of Rehabilitation Medicine, Linyi Maternal and Child Health Center Hospital, Linyi, Shandong, China
- 3Medical Management Department, Affiliated Hospital of Shandong University of Traditional Chinese Medicine, Jinan, China
- 4Department of Traditional Chinese Medicine, Shandong Academy of Occupational Health and Occupational Medicine, Jinan, China
As a highly prevalent gastrointestinal malignant tumor, colorectal cancer poses a serious challenge in terms of increasing morbidity and mortality and late diagnosis due to the invisibility of the disease. Although existing therapies are diverse but limited in efficacy, the mechanism of programmed cell death (PCD) has become a focus of research due to its central role in maintaining body homeostasis and regulating tumor progression. Multimodal cell death pathways, such as apoptosis, autophagy, pyroptosis and ferroptosis, have shown unique advantages in inhibiting the proliferation and migration of colorectal cancer cells and enhancing the sensitivity to chemotherapy by responding to internal and external environmental stimuli. In recent years, natural products have risen to prominence by virtue of their multi-target synergistic effects and chemo-sensitizing properties, and have opened up a new direction for colorectal cancer treatment by precisely regulating the PCD pathway. In this paper, we searched PubMed, Web of Science and CNKI databases for relevant studies in the last 10 years using the keywords (Colorectal cancer) and (programmed cell death) and natural products. This work retrieved 59 studies (55 from the past 5 years and 4 from the past 10 years) to reveal the mechanism of action of natural products targeting PCD, aiming to provide theoretical support and practical guidance for the optimization of clinical therapeutic strategies and the development of innovative drugs.
1 Introduction
Colorectal cancer (CRC) is a malignant tumor originating from the colon and intestinal epithelial cells, the mechanism of which is still unclear and may be related to inflammation, hypoxia, immunity, genetics, and aberrant activation of tumor-associated signaling pathways (Dekker et al., 2019). Clinical symptoms of CRC mainly include abdominal pain, hemorrhage in the stool, intestinal obstruction, abdominal mass etc., and even the appearance of metastatic lesions in other organs such as liver and lung. It has been reported that the incidence and mortality of CRC are increasing worldwide, ranking 3rd in the global cancer incidence rate, accounting for about 10%, and 2nd in the case fatality rate, accounting for about 9.4%, which undoubtedly creates a strong challenge to the global healthcare and economy (Sung et al., 2021).
Currently, surgery, radiotherapy, chemotherapy, immunotherapy, and targeted therapy are the main treatments for CRC, but problems such as the sequelae of surgery, chemotherapy resistance, toxic side effects, high metastasis and recurrence rates seriously affect the patients’ quality of life (Shin et al., 2023). Therefore, it is of great importance to find new therapeutic strategies for the treatment of CRC. Programmed cell death (PCD) is a kind of active and orderly cell death that is regulated by specific intracellular genes in response to a variety of stimuli, and has multiple pathways, including apoptosis, pyroptosis, necroptosis, autophagy, ferroptosis, etc., (Figure 1). The role of PCD is crucial in maintaining homeostasis of normal tissues, and there is a cross-interaction between multiple PCD modes, which together affect cellular outcomes (Yu et al., 2022). A large amount of data suggests that the occurrence and development of CRC are related to the aberrant expression of PCD and affect the regression and prognosis of CRC, which implies that targeting and regulating PCD may be able to bring new hope to CRC patients (Fan et al., 2021).
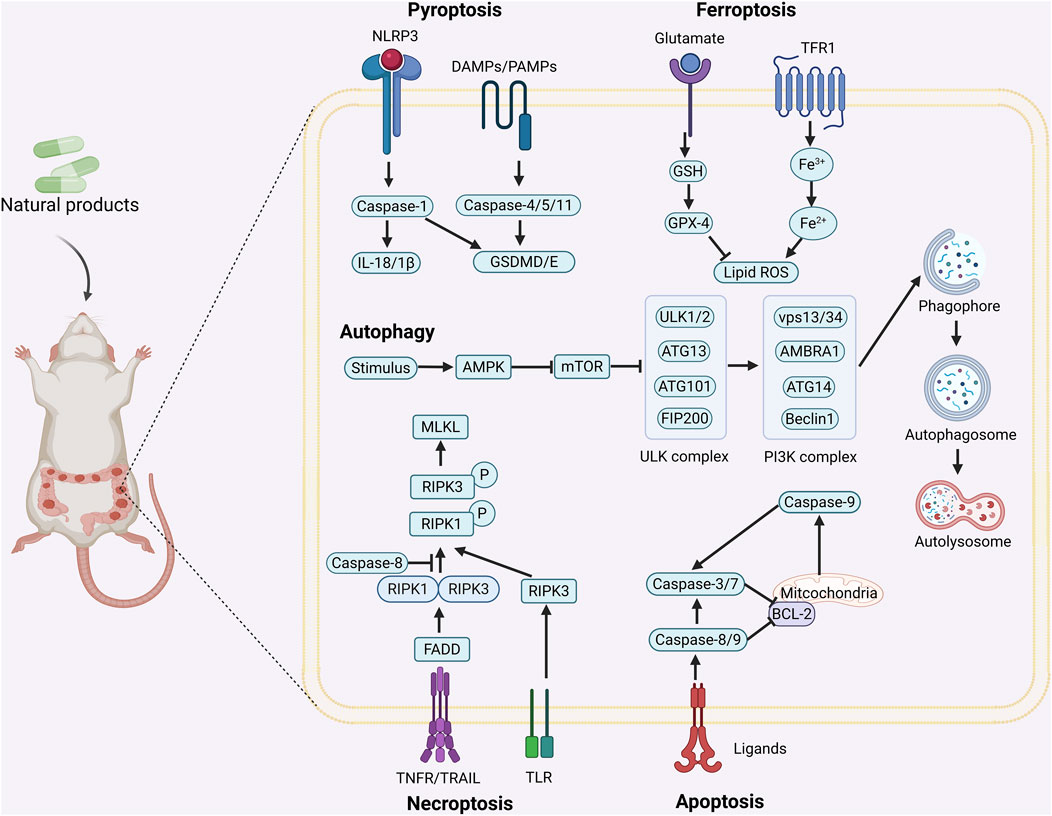
Figure 1. Mechanism of action of natural product-targeted modulation of PCD for the treatment of CRC. Apoptosis is regulated by internal and external pathways, the Bcl-2 family regulates the release of cytochrome c from mitochondria and activates Caspase-9 and Caspase-3/7; the exogenous pathway promotes cell death by activating Caspase-3/7 through Caspase-8. Autophagy is the response of the ULK1 complex (ULK1/Atg13/FIP200) to nutrient deficiency by phosphorylating Beclin1; the latter forms the Vps34-Atg6 complex with Atg14, which drives nucleation of the autophagosome membrane and completes autophagosome formation. Ferroptosis is iron-dependent cell death, where ROS excess triggers lipid peroxidation via Fe2+ catalysis, GSH and GPX-4 antioxidant deficiencies, and Fe2+ to Fe3+ conversion led to cell death. Pyroptosis is triggered by NLRP3 activation of caspase-1/18, which shears GSDMD and forms pores that trigger cell rupture and inflammatory responses. Necroptosis is a receptor-mediated cell death mechanism mediated by RIPK1, RIPK3. RIPK1 binds to FADD to activate caspase-8, which induces apoptosis; under specific conditions, RIPK3 activates MLKL, which leads to rupture of the cell membrane and triggers necroptotic cell death. Created with Biorender.com.
In recent years, natural products have shown great therapeutic potential and have been widely used in the treatment of a variety of oncological diseases, including hepatocellular carcinoma, gastric cancer, and breast cancer (Naeem et al., 2022). These products can exert anti-tumor effects in multiple targets and pathways, while ameliorating the toxic side effects caused by surgical chemotherapy, radiotherapy, targeted therapy and immunotherapy, and prolonging the survival time of patients (Micale et al., 2021). Excitingly, some natural products are candidates for the treatment of CRC, exerting anti-CRC tumor cell viability and proliferation by modulating different forms of PCD to inhibit CRC progression (Islam et al., 2022). Therefore, these natural products may become complementary and alternative therapies for CRC.
2 Natural products targeting programmed death in CRC
2.1 Apoptosis
Apoptosis is an active, orderly process of cell death that occurs under physiological or pathological conditions in order to maintain the homeostasis of its own internal environment through the activation, expression and regulation of a series of genes (Xu et al., 2019). This process involves a variety of morphological and biochemical changes, such as nuclear consolidation, DNA fragmentation, cell membrane remodeling and vesiculation, cellular crumpling, and the formation of apoptotic vesicles. The process can usually take place through three different pathways, namely the exogenous pathway, the endogenous pathway, and the endoplasmic reticulum stress-induced pathway (D'Arcy, 2019).
The exogenous pathway is triggered by the binding of ligands to transmembrane death receptors, and these death ligands mainly include FasL, tumor necrosis factor-α (TNF-α), TRAIL (TNF-related apoptosis-inducing ligand), etc., (Kashyap et al., 2021). In addition, FADD acts as a Fas-associated death domain protein, also known as Mort1. It has the ability to bind specifically to the cytoplasmic region of Fas and plays an interruptive role in apoptotic signaling. When Fas, also known as CD95, binds to the corresponding ligand, FasL, CD95L, the Fas receptor trimerizes and activates, and the activated receptor then binds to FADD, which in turn interacts with caspase-8, activating the latter to form a death-inducing signaling complex. This complex further activates a series of caspase proteins, such as caspase-1/3/7, etc., which promotes the onset of apoptosis in the cells where Fas proteins are located (Ketelut, 2022; Newton et al., 2024).
The endogenous pathway, also known as the mitochondria-mediated apoptosis pathway, is triggered by damage or stress signals within the cell (Kashyap et al., 2021). When a cell is damaged internally, it activates pro-apoptotic proteins in the B-cell lymphoma-2 (Bcl-2) family, such as Bad. These proteins alter the permeability of the outer mitochondrial membrane, resulting in the release of apoptosis-associated proteins, such as cytochrome c, from the mitochondrial membrane gap into the cytoplasm. The released cytochrome C binds to Apaf-1 protein in the cytoplasm to form apoptosome, which in turn activates Caspase-9 and activates downstream effector caspases, such as Caspase-3, to trigger apoptosis.
The endoplasmic reticulum stress pathway refers to the accumulation and aggregation of unfolded proteins in the presence of viral infections and calcium homeostasis disorders, leading to severe endoplasmic reticulum stress. This pathway not only induces the expression of endoplasmic reticulum molecular chaperones such as glucose-regulated proteins GRP78 and GRP94 to produce protective effects, but also independently induces endogenous apoptosis, which ultimately affects the regression of the stressed cells, such as adaptation, injury, or apoptosis (Suraweera et al., 2022; Kaloni et al., 2023).
Numerous data have demonstrated that targeting and regulating apoptosis can effectively inhibit the viability and proliferation of CRC cells, inhibit the development of CRC tumors and enhance the sensitivity of chemotherapy, which is potentially positive for the treatment of CRC (Wang, 2020). It has been reported that Bcl-xL is most frequently amplified in CRC among all cancer types, and the vast majority of CRC exhibits Bcl-xL overexpression and affects CRC survival and progression (Ramesh and Medema, 2020). In addition, the potential prognostic and predictive significance of Bcl2-Associated X (Bax) and Bcl-2 gene expression as well as Bax/Bcl-2 ratio in colorectal cancer has been confirmed (Khodapasand et al., 2015). It has been shown that the nuclear factor kappa-B (NF-κB) signaling pathway plays a key role in apoptosis, tumor promotion, and tumor maintenance, and thus inhibitors of this signaling pathway are useful in CRC treatment (Wang et al., 2009). Notably, p53 shows strong therapeutic potential due to its key function in initiating apoptosis during the cell cycle and in inducing cell arrest and DNA repair in recoverable cells (Yu et al., 2019). In CRC, tumor cell viability, proliferative capacity and angiogenesis can be effectively suppressed by regulating P53 (Malki et al., 2020).
It is not difficult to understand that the pathogenic mechanism of CRC is complex, in addition to the signaling pathways listed above, some other pathogenic mechanisms are also involved, for example, STAT, ROS, β-catenin, AMPK/mTOR, MAPK signaling pathway and so on. The above findings suggest that the occurrence and development of CRC are closely related to apoptosis, and that multiple signaling pathways and targets are involved.
2.1.1 Natural products that regulate apoptosis intervention in CRC
Several experimental data have demonstrated that natural products can inhibit the viability and proliferation of CRC cells by inducing the apoptotic pathway, and reduce the weight and volume of tumors in CRC model mice. This therapeutic pathway is associated with ROS, P53, Bax/Bcl-2, STAT, NF-κB, β-catenin, MAPK, AMPK/mTOR, PI3K/AKT, and JNK. This will provide a reliable theoretical basis for natural products to treat CRC (Figure 2).
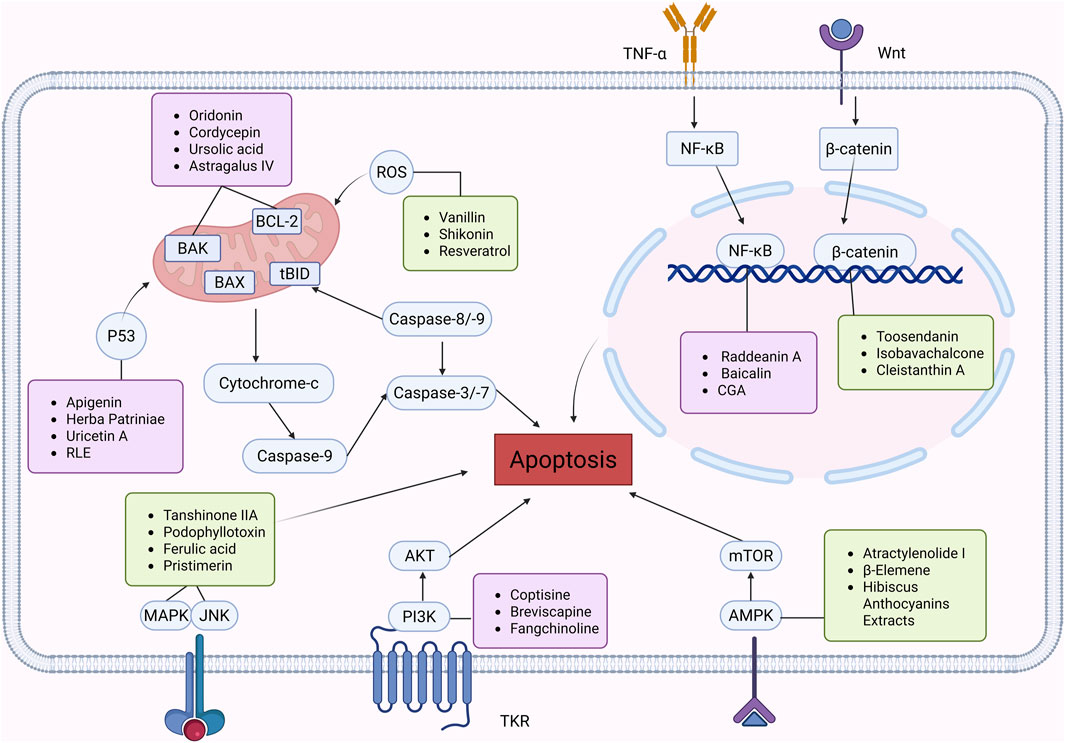
Figure 2. Natural products target ROS, P53, mTOR, NF-κB, β-catenin and other signaling pathways to regulate apoptosis and play a role in intervening CRC. Created with Biorender.com.
2.1.1.1 Targeting the ROS signaling pathway
ROS, as an important signaling molecule in redox state, is crucial for the homeostasis of immune cells and is involved in the pathological process of different diseases, and several data have shown that ROS plays an irreplaceable role in inhibiting the progression of CRC by regulating apoptosis (Gao et al., 2023). In response to the above studies, we identified these products that demonstrate the effectiveness of modulating this pathway.
Vanillin is a natural metabolite extracted from Vanilla planifolia Andrews and is widely used as a food additive. In vitro experiments showed that vanillin significantly inhibited the proliferation of CRC cells and promoted mitochondrial damage and ROS accumulation. In vivo experiments in xenograft mice, vanillin (100 mg/kg) combined with 5-FU (30 mg/kg) significantly inhibited tumor growth, upregulated the expression of ROS and caspase-3/9, induced apoptosis of tumor cells, and improved the sensitivity of 5-FU chemotherapy. This may provide a low-toxicity and highly effective drug candidate for the treatment of CRC (Li et al., 2021). However, unfortunately, long-term toxicity assessment, pharmacokinetic studies, and effects on normal tissues have not been addressed, limiting its clinical translational reference value.
Shikonin (SHI) is derived from the naphthoquinones of the dried roots of Arnebia euchroma (Royle ex Benth.) I.M.Johnst., Lithospermum erythrorhizon Siebold & Zucc. and Arnebia guttata Bunge and was experimentally confirmed to have anti-inflammatory, anti-tumor and promote wound healing properties (Guo et al., 2019). Zhang et al. showed that SHI effectively inhibited the proliferation of HCT116R cells in vitro IC50, produced a synergistic effect (CI = 0.633) when combined with oxaliplatin (32 μM SHI+32 μM OXA), and significantly promoted ROS generation and induced apoptosis through activation of the endoplasmic reticulum stress pathway (up-regulating p-PERK, p-eIF2α, andATF4) and pro-apoptotic mechanisms (elevating Cleaved Caspase-3, Bax, and decreasing Bcl-2), significantly promoted ROS generation and induced apoptosis. In a CRC mouse model, 4 weeks of treatment with SHI (2 mg/kg) in combination with OXA significantly inhibited tumor growth and improved chemosensitivity without observing significant toxicity (Zhang et al., 2023). However, these experiments did not distinguish whether SHI-induced ROS were target-regulated or chemotoxic products, and therefore complementary mitochondrial function tests (e.g., changes in membrane potential) are recommended to validate the specificity of oxidative stress and avoid false-positive results.
Resveratrol (RSV) is a non-flavonoid polyphenolic compound isolated and obtained from Reynoutria japonica Houtt., a plant of the Polygonaceae family. It has attracted much attention because of its pharmacological properties such as anti-inflammatory, antioxidant, and anticancer (Ren et al., 2021). Fu et al. used CCK8, apoptosis assay, and Western blot in order to explore the anticancer effects and mechanisms of RSV on HCT116 and SW620 cell lines. The results showed that RSV (0, 6, 12 μg/mL) dose-dependently inhibited CRC cell viability and increased apoptosis and ROS levels, while up-regulating the expression of caspase-3/9 and Bax proteins, down-regulating the expression of Bcl-2. The mechanism of action may be that RSV activates the mitochondrial apoptotic pathway by increasing the release of ROS to exert antitumor activity (Fu et al., 2021). This experiment should further improve the results by supplementing the in vivo experiments, optimizing the concentration gradient, verifying the ROS dependence and analyzing the cellular heterogeneity, so as to provide a more solid theoretical foundation for the clinical application of RSV.
These studies show a potential role for natural products to induce apoptosis to treat CRC and reduce chemotherapeutic drug resistance, but more clinical and high-quality experimental studies are needed to support this idea.
2.1.1.2 Targeting the P53 signaling pathway
p53 is an important tumor suppressor gene in the human body, playing a key role in biological processes such as cell cycle regulation, DNA repair and apoptosis. Studies have shown that restoration of p53 function or activation of the p53 signaling pathway can promote apoptosis of cancer cells and inhibit tumor growth. It has been noted that it has been widely recognized to ameliorate CRC by modulating the p53 pathway to induce apoptosis (Zhao et al., 2023).
Apigenin is a phytoflavonoid compound found in a wide range of fruits and vegetables such as Apium graveolens L which possesses a variety of biological activities, including anti-inflammatory and anticancer properties (Chen et al., 2019). Yang et al. treated HCT116 with apigenin in combination with 5-FU and showed that apigenin significantly increased the Bax/Bcl-2 ratio and upregulated the expression of p53 and ROS in HCT116 and HT29 cells, thereby inhibiting the formation and development of tumor cells. The mechanism may be related to apigenin-mediated activation of the mitochondria-mediated apoptotic pathway by P53, which increases the sensitivity of tumors to 5-FU (Yang et al., 2021). This study was conducted in vitro and lacked in vivo validation to assess the pharmacokinetics, toxicity and antitumor effects of apigenin. In addition, its unique mechanism or clinical translational value in colorectal cancer was not highlighted.
Li confirmed that Herba Patriniae (HP) and its main component Isovitexin can significantly inhibit the progression of CRC. Transcriptome analysis screened nine active components of HP, among which Isovitexin plays a central role by activating the p53 pathway. In in vitro experiments, HP (40–120 μg/mL) and Isovitexin (5–20 μM) dose-dependently induced apoptosis in HCT116 and SW480 cells and blocked the cell cycle into the G0/G1 phase, with concomitant upregulation of p53 and p21, and downregulation of CDK2. In in vivo experiments using an AOM/DSS-induced CRC model, the HP (1.14–4.56 g/kg) dose significantly inhibited tumor formation while upregulating key molecules of the p53/p21 signaling pathway (Li J. et al., 2023). This study presents only positive results and does not mention the ineffectiveness of other active ingredients or pathways, which may lead to selectivity reporting bias.
Uricetin A (UA) is a natural compound derived from the metabolism of ellagitannins by intestinal bacteria and found in Berberis vulgaris L., which has significant anticancer potential in CRC cells (Totiger et al., 2019). In vitro experiments showed that UA inhibited the proliferation of HT29, SW480 and SW620 CRC cell lines in a dose-dependent manner. Treatment with UA (25–100 μM) induced G2/M phase cell cycle arrest, increased pro-apoptotic proteins (p53, p21, cytochrome c, cleaved caspase-3/9), and inhibited anti-apoptotic proteins (Bcl-2, XIAP). In vivo studies have emphasized the safety of UA, with no observed genotoxicity and a protective effect against ulcerative colitis (El-wetidy et al., 2021). The present experimental study found that UA induced ROS generation, but did not verify whether ROS is an upstream signal for apoptosis and cell cycle arrest by antioxidant pretreatment experiments, which may confound correlation and causation.
Zong et al. treated HT29 cells with R. molle leaf methanol extract (RLE) (50, 100, and 200 μg/mL) to clarify the effects of RLE on CRC and the potential molecular mechanisms. In vitro, RLE significantly inhibited HT29 cell viability and migration in a dose- and time-dependent manner at concentrations of 25–200 μg/mL. Bax and p53 were upregulated and Bcl-2 was downregulated. In addition, RLE treatment caused HT29 cell cycle arrest in S phase, which further inhibited cell proliferation. Gas chromatography-mass spectrometry (GC-MS) analysis showed that RLE contained 17 potential anticancer components, which may be the material basis of its anticancer activity (Zong et al., 2021). However, there are some limitations of this study. The study was conducted only in vitro and lacked in vivo experimental validation to assess the efficacy and safety of RLE in vivo. In addition, although gas chromatography-mass spectrometry (GC-MS) analysis identified a variety of potential anticancer components, it did not further identify which components were the main active substances or explore the synergistic effects among them.
In addition, we have identified a number of apoptosis-inducing products (Table 1), which can also play an important role in intervening in the course of CRC. All of these studies suggest that these products are able to induce apoptosis in CRC cells through P53 signaling as an effective pathway.
2.1.1.3 Targeting the STAT signaling pathway
Increasing evidence suggests that STAT-related pathways play an important role in human disease pathology and pharmacological mechanisms. Some studies have found that apoptosis can be induced by regulating the STAT signaling pathway to inhibit tumor angiogenesis, which brings new hope for the treatment of CRC (Ghasemian et al., 2023).
Spica Prunellae is the fruiting spike of the plant drug Prunella vulgaris L. distributed in Northeast Asia, which has significant anticancer activity (Kim et al., 2020). Lin et al. intervened a CRC mouse xenograft model with ethanol extract of Erythrina spicata (EESP) (6 g/kg) for 16 days. In vivo experiments showed that EESP significantly inhibited the growth of transplanted tumors in CRC nude mice. In in vitro experiments, EESP inhibited HT29 cell viability in a dose-dependent manner, with 63% inhibition by 2 mg/mL treatment for 24 h. Mechanistic studies have shown that EESP induces apoptosis and inhibits cell proliferation and angiogenesis through inhibition of STAT3 phosphorylation, upregulation of the pro-apoptotic protein Bax/Bcl-2 ratio, downregulation of the cell cycle proteins Cyclin D1 and CDK4, and reduction of VEGF-A and VEGFR-2 (Lin et al., 2013). The specific composition and content of EESP were not clarified in this experiment, and it should be necessary to analyze the fingerprints by techniques such as LC-MS/MS and verify the synergistic effect of a single component or combination. The current experiment could not confirm the main active substances.
Convallatoxin a natural triterpenoid derived from Adonis amurensis Regel & Radde, has significant anticancer activity (Liu J. et al., 2022). In vitro experiments showed that Convastatin (0–100 nM) significantly downregulated STAT3 phosphorylation levels by inhibiting the interaction of JAK2/STAT3 and mTOR/STAT3 signaling pathways, thereby inhibiting the proliferation, migration and invasion of CRC cells and inducing apoptosis. In in vivo experiments, oral administration of combretastatin at doses of 50 and 150 μg/kg significantly inhibited the growth of HCT116 cell xenograft tumors with low toxicity to normal cells (Zhang et al., 2020). However, the experiment lacked rigor; 30 nM CNT was non-toxic to HUVEC cells in the in vitro assay, but the dose used in the in vivo assay was 150 μg/kg which raises questions about the appropriateness of the concentration used.
In conclusion, these natural products may be a new way to treat CRC by modulating the STAT signaling pathway. However, it is regrettable that there are fewer such products, and in the future, we should make more efforts to explore it.
2.1.1.4 Targeting the Bax/Bcl-2 signaling pathway
Bax and Bcl-2 are the main members of the Bcl-2 family. Bax promotes cell death by permeabilizing the outer mitochondrial membrane in response to different cellular stresses. In contrast, Bcl-2 prevents apoptosis by inhibiting Bax activity. Furthermore, it is exciting to note that modulation of the Bax/Bcl-2 ratio has potentially positive implications for CRC intervention. The results of some studies have shown that this class of natural products can effectively inhibit CRC cell viability and proliferation by down-regulating the anti-apoptotic protein Bcl-2.
Oridonin (Ori) is a diterpenoid isolated from the plant drug Isodon rubescens (Hemsl.) H. Hara. Modern pharmacological studies have shown that Ori has anti-inflammatory and anticancer activities (Liu X. et al., 2020). In vitro experiments showed that Ori inhibited the proliferation of HCT-116, LoVo and other CRC cells and induced apoptosis in a concentration-dependent manner (IC50 values of 10–20 μmol/L). The mechanism of action includes activation of the mitochondrial apoptotic pathway, upregulation of Bim/Bax, downregulation of Bcl-2, and activation of caspase-9/3. In vivo experiments demonstrated that intraperitoneal injection of Ori (10 mg/kg) markedly inhibited the growth of HCT116 and LoVo xenograft tumors and synergistically potentiated the effects of chemotherapeutic agents on drug-resistant CRC cells (Yang et al., 2015). In this study, only two high miR-32 expressing cell lines, HCT-116 and LoVo, were selected as the main subjects, and other low expressing cell lines were not included for comparative validation, which may lead to one-sided conclusions.
Cordycepin is a metabolite extracted from Ophiocordyceps sinensis (Berk.) G.H., which has outstanding effects in anti-inflammatory and anti-tumor effects (Deng et al., 2022). Li et al. treated HCT116 cells with Cordycepin (0, 62.5, 135, 270, and 540 μM) for 24 h. The results showed that cordycepin induced cell death through Bax-dependent mitochondrial apoptotic pathway with a half inhibitory concentration (IC50) of 434 μM. In addition, Cordycepin can induce G1/S phase cell cycle arrest through upregulation of the p53/p21 pathway (Li et al., 2019). However, the study has not yet involved in vivo experimental validation and pharmacokinetic analysis, and its efficacy and safety in animal models need to be further explored.
Ursolic acid (UA) is a natural triterpenoid extracted from the dried root of Chinese kiwifruit Actinidia chinensis Planch, a plant in the kiwifruit family, and has significant anticancer activity (Zhao et al., 2023). Zheng et al. reported that UA inhibited the growth of CRC cells through a multi-target regulatory mechanism, significantly induced apoptosis in RKO cells, blocked the cell cycle in G0/G1 phase, and downregulated the expression of Bcl-2, upregulated the expression of Bax, and activated the caspase-3/8/9 cascade response. In addition, UA can inhibit EMT by up-regulating the epithelial marker E-Cadherin and down-regulating the mesenchymal marker N-Cadherin, thereby inhibiting tumor metastasis. This in vitro study demonstrated that UA has significant anti-tumor effects, which provides an important basis for subsequent in vivo studies. However, this study did not involve in vivo validation and pharmacokinetic analysis, and further studies in animal models are needed to evaluate its efficacy and safety in vivo (Zheng et al., 2021).
Astragalus IV (AS-IV) was extracted from the roots of Astragalus mongholicus Bunge, showing potent biological activities in anti-inflammatory, antioxidant, and anticancer properties (Zhang et al., 2020). Sun et al. treated CRC xenograft mice by AS-IV (20 mg/kg) for 30 days. The results showed that AS-IV dose-dependently inhibited the proliferation of CRC cells and suppressed tumor growth, activated the levels of Caspase-3/7/9, Bax, inhibited the expression of Bcl-2, and promoted the cell cycle arrest at G0 phase (Sun et al., 2019). However, the concentration gradient and positive control group were not set in this experiment, which needs to be further improved in the future.
The above findings suggest that all these products are able to modulate endogenous mitochondrial pathways such as the anti-apoptotic protein Bcl-2 in order to achieve the treatment of CRC, and such drugs may be potential candidates for the treatment of CRC in the future.
2.1.1.5 Targeting the NF-κB signaling pathway
NF-κB is a multifunctional key transcriptional regulator involved in various biological processes, and plays an important role in the process of disease occurrence and development. Therefore, the use of natural products to intervene in NF-κB has become an important measure for the treatment of diseases.
It has been reported that some natural products can mediate NF-κB to regulate apoptosis, which can effectively inhibit CRC progression. For example, Raddeanin A (RA), a triterpenoid isolated from Anemone raddeana Regel, has anti-inflammatory, anticancer, and angiogenesis inhibitory properties (Naz et al., 2020). Wang et al. treated a CRC xenograft mouse model with RA (1.25, 2.5, 5.0 mg/kg) and adriamycin (5 mg/kg) as a positive control for 14 days. The experimental results showed that intraperitoneal injection of RA at doses of 2.5–5.0 mg/kg significantly inhibited the growth of SW480 xenograft tumors. Mechanistic studies showed that RA downregulated the levels of p-LRP6, p-AKT and intranuclear β-catenin and blocked the expression of c-Myc and Cyclin D1. In addition, in vitro experiments showed that RA inhibited the proliferation of SW480, LOVO and other CRC cells in a dose-dependent manner, with IC50 of 1.81–9.65 μM at 24 h (Wang et al., 2018). This provides an important basis for the development of novel CRC therapeutic strategies.
In addition, there are several apoptosis-inducing products (Table 1), which can likewise play an important role in intervening in the course of CRC. The above studies suggest that the NF-κB-related signaling pathway is a meaningful pathway for natural products for CRC therapy.
2.1.1.6 Targeting the β-catenin signaling pathway
β-catenin signaling is the most critical pathway regulating cell invasion, migration, proliferation and differentiation, and its important role in tumor diseases has been well described. Some studies have shown that β-catenin is closely associated with CRC progression. Our discovery of several natural products mediating the β-catenin pathway for the treatment of CRC is of great significance.
Toosendanin (TSN) is a triterpenoid extracted from the dried ripe fruits of the neem plant drug Melia azedarach L. and possesses antioxidant, anti-inflammatory, and anticancer pharmacological activities (Li et al., 2024). Wang et al. demonstrated the efficacy of TSN in vivo in SW480 xenografted nude mice by injecting TSN (0.15, 0.3 mg/kg) for 14 consecutive days in the absence of a positive control group, with daily intraperitoneal injections of 0.15/0.30 mg/kg significantly inhibiting tumor growth (p < 0.01), and reductions in tumor weight and volume correlating with reduced nuclear localization and upregulation of GSK-3β expression in β-catenin. Catenin nuclear localization and upregulation of GSK-3β expression. In vitro studies using SW480 cells demonstrated that TSN (0.125–0.5 μM) inhibited cell proliferation and S-phase cell cycle arrest in a dose- and time-dependent manner (Wang et al., 2015). However, there was no significant difference in tumor volume between the high-dose TSN (0.3 mg/kg) and low-dose (0.15 mg/kg) groups in the in vivo experiments, suggesting that there may be a dose saturation effect or experimental design limitations. Further validation of the dose range or extension of the administration time is needed to confirm the efficacy.
Cleistanthin A (CA) is a natural compound extracted from Phyllanthus taxodiifolius Beille with significant anticancer activity (Pradheepkumar and Shanmugam, 1999). Jearawuttanakul et al. intervened HCT116 and SW480 cells using CA (1 and 10 μM). The experimental results confirmed that CA induced apoptosis in CRC cells, inhibited cell proliferation and viability, and suppressed their invasion and migration ability. Mechanistic studies showed that CA induced apoptosis by inhibiting the Wnt/β-catenin signaling pathway, down-regulating the expression of Survivin and target genes CCND1 and AXIN2, and inhibiting the nuclear translocation and transcriptional activity of β-catenin. In addition, CA significantly inhibited cell migration and invasion within 24 h, and its effects were associated with inhibition of V-type ATPase activity and focal adhesion kinase (FAK) phosphorylation (Jearawuttanakul et al., 2020). The limitations of this study are the lack of in vivo experiments to validate the efficacy and safety of CA, the potential toxicity to normal tissues and the tolerability of long-term treatment have not been clarified and further studies are needed.
In addition, there are several apoptosis-inducing products (Table 1) that can also function by intervening in the course of CRC. The above studies suggest that modulation of the β-catenin signaling pathway may be a new avenue for the treatment of CRC.
2.1.1.7 Targeting the AMPK/mTOR signaling pathway
Atractylenolide I (ALT-1), a natural sesquiterpene lactone from the dried rhizomes of Atractylodes macrocephala Koidz. in the Asteraceae family, is known for its antioxidant, anti-apoptotic, and anti-tumor properties (Liu et al., 2021). Wang et al. intervened CRC xenograft model mice by ALT-1 (25 and 75 mg/kg), administered intraperitoneally for 22 days without a positive control group. ALT-1 significantly upregulated the levels of Bax, Caspase-3 and downregulated the expression of Bcl-2 in a dose-dependent manner, reduced the size and weight of tumors, and inhibited aerobic glycolysis and AKT/mTOR activation, and the effect of 75 mg/kg ALT-1 was more obvious. In addition, in vitro experiments also showed that ALT-1 (0, 80, 150, 200 μM) reduced the viability and proliferation of COLO205 and HCT116 cell lines, and downregulated the phosphorylation of proteins associated with the AKT/mTOR pathway to inhibit the growth of tumor cells (Wang et al., 2020). This study still has some shortcomings, such as the stability and safety of ATL-1 has not been evaluated in this experimental ATL-1. In addition, extensive clinical studies should be conducted to determine its specific efficacy.
β-Elemene, a sesquiterpene compound extracted from the dried rhizome of the ginger plant drug Curcuma longa.L., has shown potent antitumor effects (Feng et al., 2024). In vitro experiments, treatment of human CRC cell lines DLD-1 and HT29 with β-Elemene at concentrations ranging from 25 to 600 μmol/L significantly decreased cell viability and induced G2/M phase block. Mechanistic studies showed that β-Elemene activated AMPK phosphorylation by increasing intracellular ROS levels, which in turn inhibited mTOR signaling. In in vivo experiments, intraperitoneal injection of β-Elemene into hormonal nude mice at doses of 25 mg/kg and 50 mg/kg significantly inhibited the growth of HT29 graft tumors (Wang et al., 2022). The present study proposes the ROS/AMPK/mTOR pathway as the central mechanism, but does not exclude the interference of other potential pathways (e.g., PI3K/Akt). For example, whether β-elemene directly regulates AMPK phosphorylation or whether other upstream kinases are involved needs to be further verified by gene silencing or drug intervention experiments.
2.1.1.8 Targeting the MAPK signaling pathway
The MAPK cascade response is a key pathway that regulates a variety of cellular processes such as cell growth, proliferation, differentiation, migration, and apoptosis, and plays a critical role in the outcome and sensitivity of anticancer therapy. Therefore, intervention of MAPK using natural products has emerged as a potential means of treating diseases.
Podophyllotoxin (PT), a lignan compound from the roots and rhizomes of Oncorhynchus mykiss, possesses significant anticancer activity (Motyka et al., 2023). Lee et al. reported that PT (0, 0.1, 0.2, and 0.3 μM) inhibited the proliferation of HCT116 CRC cells in a concentration-dependent manner and activated the p38 MAPK signaling pathway by inducing ROS production, which led to cell cycle arrest in G2/M phase and triggered apoptosis (Lee et al., 2021). However, there are some limitations in this study, such as no in vivo experiments were performed to validate the antitumor effect and safety of PT.
Ferulic acid (FA) is a phenolic compound present in plant medicines such as Angelica sinensis (Oliv.) Diels and Conioselinum anthriscoides (H.Boissieu) Pimenov & Kljuykov, which exhibits antioxidant, anti-oxygen radical, and inhibitory properties against tumor development (Li et al., 2021). Chen et al. intervened CT26 cells with FA (0–400 μM), which inhibited the viability and proliferation ability of CT26 cells, and TUNEL staining showed that FA effectively promoted the overall apoptosis of cancer cells in vivo. In vivo experiments further showed that FA (20, 40, and 80 mg/mL/kg) gavaged BALB/c tumor-bearing mice, and the 80 mg/kg group showed a 41% reduction in tumor volume and a 37% reduction in weight, with no impairment of liver and kidney functions. Mechanistic studies have found that FA downregulates BCL-2 and upregulates BAX through activation of JNK/ERK phosphorylation, while promoting LC3-II expression (Chen et al., 2023). However, this study did not explore the synergistic effect of FA with clinical chemotherapeutic agents. In the future, a large number of clinical trials should be conducted to clarify its true clinical efficacy and synergistic effects.
All of the above studies suggest that such products can inhibit CRC progression through MAPK signaling as an effective pathway.
2.1.1.9 Targeting the JNK signaling pathway
The JNK signaling pathway is a conserved response to multiple internal and external cellular stress signals. In addition, this signaling pathway not only maintains the regulation of apoptosis, growth, proliferation, differentiation, migration, and invasion, but is also a key player in regeneration, tumorigenesis, and other pathologies in a variety of diseases. Therefore, targeting these products to modulate the JNK pathway for the treatment of CRC is of positive significance.
Pristimerin is a natural product of quinone methylated triterpenoids present in Celastrus paniculatus Willd., which has been shown potent anti-cancer effects (Sun et al., 2022). Zhao et al. investigated the mechanism of action of Pristimerin in the treatment of CRC mice induced by injection of HCT116 cells. In vivo experiments showed that Pristimerin (0.125–0.5 mg/kg) significantly inhibited the growth of HCT116 xenograft tumors and the formation of intestinal polyps in APC mice without significant toxicity. The compound induced the expression of the pro-apoptotic protein Noxa through activation of the ROS/ER/JNK pathway. In addition, this experiment was further validated in HCT116 and SW620 cell models, which showed that Pristimerin inhibited cell proliferation and induced apoptosis in a dose-dependent manner (0.4–0.8 μM), thus exerting anti-tumor effects (Zhao et al., 2021). In this study, the in vivo experimental Pristimerin dose was significantly lower than the positive control docetaxel, but the inhibition rate was similar (62.59% vs 60.19%), and there may be an inadequate explanation of the dose-effect relationship.
Garcinone E (GAR E) is a xanthone compound extracted from Garcinia mangostana L., which possesses biological properties such as anti-inflammatory, antioxidant, and anticancer (Mohamed et al., 2022). Li et al. used HT29 and Caco-2 CRC cells as in vitro models. The results showed that GAR E activated the JNK signaling pathway by inducing ROS accumulation, which triggered mitochondrial dysfunction and apoptosis, and blocked the cell cycle into the Sub G1 phase. The specific manifestations of GAR E are elevated Bax/Bcl-2 ratio, activation of PARP and caspase 3/9, and significant increase in JNK1/2 phosphorylation. In vivo studies have further demonstrated that 2.5–5 mg/kg of GAR E significantly inhibited the growth of HT29 xenograft tumors, induced the expression of apoptosis-associated proteins in tumor tissues, and reduced the area of tumor necrosis without causing significant toxicity (Li L. et al., 2023). However, this study still has some limitations, and the in vivo experiments only used a single cell line model, which needs to be extended to more CRC subtypes for validation.
Isolinderalactone (ILL) is a bioactive sesquiterpene lactone derived from Lindera aggregata (Sims) Kosterm. with antitumor properties (Hwang et al., 2019). It was reported that Kwak et al. treated HCT116 and HT29 cell lines with ILL to elucidate the mechanism of action of ILL. The results showed that ILL (3–9 μM) dose-dependently inhibited the proliferation and colony formation of OXA-sensitive and CRC cells through activation of JNK/p38 MAPK signaling, and induced G2/M phase arrest, ROS accumulation, and apoptosis. In vivo validation using a xenograft model further supports the tumor suppressive activity of ILL, although specific dosage details were not explicitly provided (Kwak et al., 2022). Limitations of this study include the lack of in-depth in vivo toxicity analysis and incomplete exploration of chemotherapy combinations.
As described above, TanIIA has potent antitumor activity. Qian et al. experimentally verified the antitumor pharmacological effects of TanIIA (20 mg/kg) by CRC xenograft model mice treated by gavage for 10 days. Tan IIA can mediate the translocation of Bax to the mitochondria, which can lead to mitochondrial damage, activation of Caspse-3/9, ROS levels ultimately leading to mitochondria-dependent apoptosis and inhibiting tumor cell progression. In addition, in SW480 cell assay, CK-8 and colony formation assay showed that cell proliferation and viability were inhibited, flow cytometric analysis showed that Tan IIA induced apoptosis in CRC cells, and western blot analysis showed that the expression of Bax protein was significantly upregulated in Tan IIA group, and the expression of Bcl-2 protein was significantly reduced. Although this experiment verified the inhibitory effect of Tan IIA on CRC cells and xenografted CRC mice (Qian et al., 2023). However, compounds that are structurally similar but do not contain a quinone molecule (e.g., Tanshinone I) were not used as negative controls in this experiment, making it difficult to rule out nonspecific toxicity or PAINS effects. For example, the quinone structure may induce oxidative stress directly rather than through specific signaling pathways.
Chaetocin is a natural metabolite isolated from Trichoderma spp. fungi belonging to the genus Thioketopyrazine, and it possesses a variety of bioactivities and pharmacological functions, such as anticancer, inhibition of cell proliferation or angiogenesis, etc., (Jiang et al., 2021). Wang et al. used Chaetocin (0.5 mg/kg) to treat CRC xenograft model mice for 18 days. The results showed that Chaetocin upregulated the levels of Bax, Caspase-3/9, ROS, JNK and downregulated the expression of Bcl-2 to promote apoptosis to inhibit the tumor volume and weight and to improve the sensitivity of 5-FU drug. Notably, Chaetocin (0–2 μM) was able to inhibit cell growth and cell viability and promote G2/M-phase blockade in in vitro experiments, exerting anticancer effects (Wang et al., 2021). Indicators of drug toxicity (e.g., weight change, liver and kidney function) were not reported in this study to assess the safety of Chaetocin, which is critical for clinical translation.
2.1.1.10 Targeting the PI3K/AKT signaling pathway
The PI3K/AKT pathway is a key signaling cascade involved in the regulation of cell growth, survival, and metabolism, and is also considered one of the most altered molecular pathways in malignant tumors. Therefore, modulation of the PI3K/AKT signaling pathway by these several products for the treatment of CRC might be a new avenue.
Coptisine (COP), an isoquinoline alkaloid in the dried rhizomes of the buttercup plant drug Coptis chinensis Franch., has been shown to possess pharmacological properties such as anti-inflammatory, antioxidant, and anticancer (Lu et al., 2023). Han et al. elucidated the anti-tumor mechanism of action of COP by HCT116 xenografts in CRC model mice without a positive control group. The results showed that COP (50, 100, 150 mg/kg) was able to alleviate tumor growth and tumor weight in CRC model mice. When the COP dose was 150 mg/kg, the effect was more significant, and COP also decreased the levels of Bcl-2, P13K/AKT and upregulated the expression of Caspase-3. The mechanism is related to the inhibition of PI3K/AKT and activation of mitochondria-mediated apoptosis pathway to exert anti-tumor effects. In addition, through experiments, we also found that COP significantly inhibited the viability, proliferation and cell migration of HCT-116 cell line (Han et al., 2018). However, no study related to pharmacological toxicity and clinical trials was conducted in this experiment, and the safety and clinical therapeutic effects are worrisome.
Breviscapine is a flavonoid glycoside extracted from the plant drug Erigeron breviscapus (Vaniot) Hand. -Mazz., and it has various pharmacological applications, such as anti-inflammatory and antitumor activities (Wen et al., 2021). The effects and mechanisms of Breviscapine in CRC were clarified experimentally by Niu et al. Breviscapine (0, 12.5, 25, 50, 100, and 200 μM) was able to effectively inhibit the viability and proliferation of HCT116 and SW480 cell lines in a dose-dependent manner, and limit the migration and invasion of CRC cells. In vivo experiments further showed that Breviscapine (40 mg/kg) was able to inhibit tumor volume and weight, while 5-FU (25 mg/kg) also showed this therapeutic effect and had a synergistic effect with Breviscapine (Niu et al., 2023). However, key factors such as angiogenesis and immune microenvironment were not addressed in this discussion. These factors may interact with the PI3K/AKT pathway, and further studies are needed to fully reveal the antitumor mechanism of Breviscapine.
Fangchinoline (FAN) is an alkaloid extracted from Stephania tetrandra S. Moore, which possesses a variety of biological and pharmacological activities such as anti-inflammatory and anti-tumor (Mérarchi et al., 2018). Jiang et al. experimentally explored the mechanism of action of FAN on CRC. In vivo and ex vivo experiments were used to evaluate its effects. Using MTT, wound healing assay, Transwell and Western blot assays, it was found that FAN (0–9 μM) inhibited cell migration and invasion, suppressed the expression of Caspase-3/9 and Bax, downregulated the level of Bcl-2, and induced apoptosis and G1-phase cell cycle arrest to reverse CRC progression. Moreover, the therapeutic effect of FAN (0.1 and 0.5 mg/mL) was also demonstrated in an in vivo CRC xenograft model mouse, and effectively suppressed the tumor volume and weight, and this experiment elucidated that FAN blocked the PI3K/AKT signaling pathway in order to exert the anti-tumor activity (Jiang et al., 2021). In this study, the FAN dose used for the in vivo experiments was not converted to a human equivalent dose. The difference between this dose and clinically used chemotherapeutic agents (e.g., oxaliplatin) is significant, and the safety window needs to be determined by toxicology studies and pharmacokinetic analysis.
Table 1 lists other natural products that inhibit CRC progression by modulating apoptosis induced by the PI3K/AKT-related signaling pathway. The above studies suggest that modulation of PI3K/AKT signaling by these products is considered an important alternative to inhibit CRC progression and cell cycle arrest.
2.2 Autophagy
Cellular autophagy refers to the transportation of damaged, denatured or senescent proteins and organelles in the cell to the lysosome, which digests and degrades them (Liu et al., 2023). Meanwhile, the energy and small molecules produced by degradation, such as amino acids, are available for reuse by the cell, which is important for the cell to maintain homeostasis of the organism. Cellular autophagy is not only a self-protection mechanism of cells, but also closely related to a variety of physiological and pathological processes. The nature of autophagy can be summarized as intracellular membrane rearrangement, and the process of its occurrence can be divided into four stages: ① the initiation of autophagy: the formation of autophagosome initiation in the cytoplasm, called phagocytosis vesicles; ② the formation of autophagosomes: autophagosomes, through the extension and expansion, ultimately form the double-layer membrane structure wrapped around the cytoplasm and the cell organelles; ③ autophagosomes fused with lysosomes: autophagosomes and lysosomes fused to form autophagolysosomes, which contain a variety of hydrolytic enzymes that can degrade its contents; ④ fusion of autophagosomes: the fusion of autophagosomes with lysosomes is a sign that autophagy is effective, and the degraded products will be released for reuse by the cell (Cao et al., 2021). Several data have shown that cellular autophagy plays an important role in tumor formation and development. Therefore, targeting and regulating cellular autophagy to inhibit tumor formation is considered a promising strategy (Debnath et al., 2023).
The occurrence of cellular autophagy is the joint participation of multiple signaling molecules, which affects the regression and prognosis of the disease. Ma et al. showed that cellular autophagy was regulated by inhibiting the PI3K/AKT/mTOR signaling pathway in order to inhibit cell proliferation, invasion, migration, and epithelial-mesenchymal transition, and to exert an anti-tumor effect (Ma et al. 2020). Bai et al. experimentally confirmed that the effects of larotrectinib (Lar) was able to inhibit the proliferation and migration of CRC cells, and the underlying mechanism was related to the activation of AMPK/mTOR signaling pathway, thus inducing intracellular autophagy (Bo et al., 2024). In addition, Liu et al. showed that atorvastatin calcium (ATO) inhibited the proliferation and migration of HCT116 cells, and the mechanism may be related to the induction of cellular autophagy by regulating the protein expression of LC3 (Liu L. et al., 2024). It has been reported that dexmedetomidine-treated CRC cells have increased LC3 sites and lysosomal structures to induce autophagy and inhibit the proliferation of CRC, which in turn affects the progression of CRC (Hou and Xu, 2024). Therefore, we can find that these target protein molecules are involved in the regulation of autophagy in CRC cells and become promising options for the treatment of CRC.
2.2.1 Regulation of natural products of autophagy intervention in CRC
Autophagy plays a decisive role in all stages of human tumorigenesis, guiding tumorigenesis and promoting tumor progression and metastatic ability. Autophagy regulation is increasingly becoming a new way to treat CRC, and the following products will provide a scientific basis for this (Figure 3).
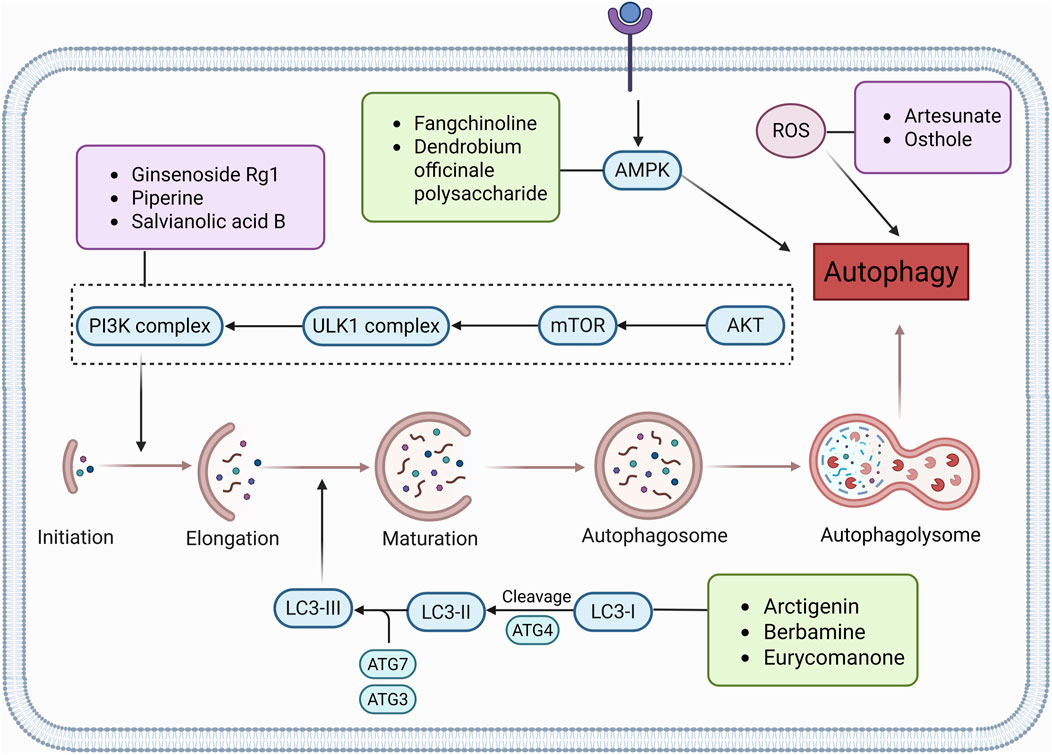
Figure 3. Natural products target ROS, PI3K/AKT, AMPK, and LC3 signaling pathways to regulate autophagy and play a role in intervening in CRC. Created with Biorender.com.
2.2.1.1 Targeting the ROS signaling pathway
As mentioned above, natural products targeting ROS can regulate apoptosis to inhibit CRC tumor progression. Excitingly, targeting ROS to induce cellular autophagy also exerts such an inhibitory effect.
Artesunate, a derivative extracted from the above-ground part of Artemisia annua L., family Asteraceae, has been reported to possess antioxidant and antitumor pharmacological properties (Kong et al., 2019). Huang et al. experimentally confirmed that Artesunate inhibited CRC cells by regulating the ROS-related signaling pathway and inducing cellular autophagy. In SW480 and HCT116 cells, Artesunate (1, 2, 4, and 8 μM) treatment led to mitochondrial dysfunction, greatly promoted mitochondrial ROS production, upregulated the expression of p-IRE1α, LC3B, Beclin1, and Atg3, and led to the cell cycle arrest in G0/G1 phase to inhibit cell proliferation. In in vivo experiments, the results similarly demonstrated the ability of Artesunate (30 and 60 mg/kg) to significantly inhibit tumor size and cell viability and proliferation in CT26-derived tumor model mice in vivo (Huang et al., 2022). In this experiment, changes in body weight and organ indices in mice indicated only low toxicity, but no long-term toxicity data were provided, limiting the drug safety assessment.
Osthole is derived from the umbelliferae plant drug serpentine Cnidium monnieri (L.) Cuss. was shown to have anti-tumor activity. (Sun et al., 2021). Song et al. found in Osthole intervention in CRC model mice that Osthole (50 mg/kg) induced autophagic flux damage leading to CRC cell death, reduced the number and size of tumor cells and enhanced chemotherapeutic 5-FU sensitivity. In addition, Osthole (0, 5, 10, 20, and 50 μM) significantly upregulated the levels of ROS and Beclin1, downregulated the ratio of LC3B-I/LC3B-II, disrupted mitochondrial homeostasis, decreased ATP production, and increased the level of calcium ions in HCT116 and HT29 cells, effectively inhibiting CRC cell viability and proliferation (Song et al., 2024). This study did not investigate the synergistic mechanism of Osthole with existing drugs (e.g., 5-FU) and only reported no significant synergistic effect.
Table 2 lists other natural products that inhibit CRC progression by modulating ROS-related signaling pathway-induced cellular autophagy. These results suggest that the modulation of autophagy-related targets can effectively inhibit the viability of CRC cells and tumor growth, and may become a new avenue for CRC treatment in the future.
2.2.1.2 Targeting the mTOR/PI3K/AKT signaling
mTOR/PI3K/AKT is a major regulator of cellular metabolism and plays an important role in treating the course of CRC through multiple signaling pathways in order to regulate cell growth and proliferation. The following natural products can better respond to it.
Ginsenoside Rg1 is a triterpenoid saponin compound from the dried roots and rhizomes of the Panax ginseng C.A. Mey. from the family Wujiaceae, with excellent pharmacological properties such as anti-inflammatory, immunomodulatory, and so on (Cheng et al., 2022). Liu et al. used Ginsenoside Rg1 (100 mg/kg) to intervene in CRC xenograft model mice for 14 days. 5-FU (20 mg/kg) was used as a positive control group. Western blot results showed that Ginsenoside Rg1 upregulated the levels of autophagy marker proteins LC3 and Beclin-1, and downregulated the p-Akt, p-mTOR and p-p70S6k protein expression. Meanwhile, Ginsenoside Rg1 inhibited tumor growth and proliferation in CRC model mice in vivo. In addition, this experiment also revealed that Ginsenoside Rg1 (80, 160, and 320 μmol/L) was able to inhibit the viability of CT260 cells through Ginsenoside Rg1-induced CT26 cell line, and further indicated that Ginsenoside Rg1 exerted its antitumor effects through the Akt/mTOR/p70S6K signaling pathway to induce cell autophagy (Liu R. et al., 2024). This study was based on the mouse CT26 cell line and a nude mouse model and did not include human colorectal cancer cells or clinical samples for validation. In addition, the pharmacokinetics (e.g., bioavailability, metabolites) of Rg1 were not addressed, which may affect the assessment of its feasibility as a drug candidate.
Piperine, an alkaloid molecule extracted from Piper nigrum L. and Piper longum L., has been shown to have pharmacological activities such as anti-inflammatory and anticancer (Haq et al., 2021). Xia et al. intervened in CRC xenografts by Piperine (20 mg/kg) in mice for 27 days without a positive control group. The results of the study showed that Piperine was able to inhibit the volume and weight of tumors in vivo in CRC model mice, increased the levels of LC3, LC3-II, ROS, and Beclin1, and decreased the levels of p-AKT and mTOR. It was further observed by HCT116 and SW480 in vitro experiments that Piperine (0, 25, 50, 100, and 150 μM) inhibited CRC cell viability and proliferative capacity in a dose-dependent manner (Xia et al., 2024). The low water solubility and in vivo pharmacokinetic properties of piperine were not systematically studied in this experiment, which may limit its practical application. In addition, no coadministration experiments with existing chemotherapeutic agents (e.g., 5-FU) have been conducted to assess the potential for synergistic effects.
Salvianolic acid B (Sal B) is an active compound in the dried roots and rhizomes of Salvia miltiorrhiza Bge. Modern pharmacological studies have shown that Sal B has anti-inflammatory and anti-tumor properties (Fu et al., 2024). In vitro experiments with HCT116 and HT29 cells showed that Sal B was dose-dependent cytotoxic at a concentration of 200 μM. Mechanistically, Sal B inhibited the AKT/mTOR signaling axis and decreased the phosphorylation of AKT, mTOR and p70S6K, while activating the phosphorylation of ULK1. Intraperitoneal injection of 80 mg/kg significantly reduced tumor growth without systemic toxicity. Co-administration with the autophagy inhibitor 3-MA (20 mg/kg) attenuated anti-tumor activity, confirming the pro-death effect of autophagy (Jing et al., 2016). Despite these findings, this study did not explore the effects of Sal B in a metastatic CRC model, nor did it assess its synergistic effects with conventional chemotherapy such as 5-FU or OXA.
Polyphyllin II (PPII), a natural steroidal saponin present in Paris yunnanensis Franch., has been shown to have potent anticancer activity against a wide range of cancers (Jiao et al., 2022). Li et al. showed that PPII (1, 2, 3, and 4 μM) inhibited the proliferation of colorectal cancer cells, induced G2/M-phase cycle blockade, and activated autophagy process in a dose-dependent manner. The mechanism includes downregulation of PAK1, blocking of AKT/mTOR signaling pathway, resulting in increased levels of LC3B-II and decreased phosphorylation of PI3K/AKT/mTOR pathway. Experiments in xenografted CRC mice showed that PPII (50 mg/kg/day) significantly inhibited the growth of transplanted tumors without significant toxicity (Li et al., 2022). Although the current study reveals the mechanism by which PPII exerts antitumor effects through autophagy regulation, further optimization of in vivo delivery strategies and exploration of its combination therapy with targeted drugs are needed.
Scoparone (Scop) is a natural bioactive substance extracted from the plant drug Artemisia capillaris Thunb, which has been proved to have anti-inflammatory and anticancer properties in modern pharmacology (Liu Y. et al., 2020). Huang et al. explored the mechanism of action of Scop on CRC cells by HT29 and HCT116 cell lines. The results showed that Scop (0, 100, 200, and 300 μM) inhibited the activity and proliferation of CRC cells in a dose-dependent manner, and western blot showed that Scop upregulated the expression of LC3-II, ATG5, and Beclin1, and lowered the levels of P62, PAK1, AKT, and mTOR, which was related to the inhibition of AKT/mTOR signaling pathway to promote the cell proliferation. mTOR signaling pathway to promote cellular autophagy. In addition, Scop (50 mg/kg) was able to significantly inhibit the volume and weight of tumors in xenograft CRC model mice in vivo (Huang et al., 2023). However, autophagosome formation was not directly observed by electron microscopy in this study, which may affect the complete assessment of autophagic flow. In addition, the experiment of cell viability recovery after inhibition of autophagy did not exclude the interference of other cell death pathways (e.g., apoptosis), which needs to be further verified.
Table 2 lists other natural products that inhibit CRC progression by modulating cellular autophagy induced by the mTOR/PI3K/AKT-related signaling pathway. Thus, mTOR/PI3K/AKT becomes a potential target for natural products that inhibit CRC tumor growth.
2.2.1.3 Targeting the AMPK signaling pathway
AMPK is a key regulator of energy metabolism and plays a critical role in the control of cell growth and regulation of cellular autophagy. The results of several studies have demonstrated the ability of natural products to modulate AMPK signaling, thereby affecting the prevention and management of CRC.
Dendrobium officinale polysaccharide (DOP) is an active compound extracted from orchid medicines, and modern pharmacological studies have demonstrated the antitumor effects of DOP. Modern pharmacological studies have demonstrated that DOP has significant anti-tumor effects (Wang et al., 2022). Zhang et al. intervened CT26 cells using DOP (200, 400, and 800 μg/mL) to elucidate the mechanism of action. The results showed that DOP inhibited the proliferation of CT26 and HCT116 cells in a dose-dependent manner in the concentration range of 0–800 μg/mL, induced ROS generation, disrupted MMP, and inhibited ATP production, which in turn activated the AMPK/mTOR pathway. DOP-induced cytotoxicity, excessive autophagy and mitochondrial dysfunction were reversed after ROS removal using NAC in the experiments, validating the critical role of ROS in this process (Zhang et al., 2021). No in vivo experiments were performed in this study, so the efficacy and safety of DOP in vivo could not be fully assessed.
2.2.1.4 Targeting the LC3-related protein signaling pathway
LC3-associated protein is a marker of autophagosome formation, and its dysregulation is inextricably linked to the progression of a variety of diseases, while it has also become the centerpiece of mammalian autophagy monitoring. Some studies have found that regulation of autophagy-related protein LC3 can inhibit CRC progression. Fortunately, we found them in natural products.
Berbamine (BE), a quinoline alkaloid extracted from Berberis vulgaris L., possesses a variety of pharmacological activities, including anti-inflammatory and anticancer (Farooqi et al., 2022). Mou et al. treated the HT29 cell line with different doses of BE (0, 7, 14, and 28 μM) to pass CCK8, Western Blot, and wound healing assays to analyze the action mechanism of BE on CRC. The experimental results showed that BE significantly inhibited the migration of HT29 cells at a concentration of just 14 μM, and Western blot detected the upregulation of Bax and Caspase-3/9 expression, the downregulation of Bcl-2 expression, and a significant increase in the expression of LC3B-II, ATG5, ATG12, and Beclin-1 (Mou et al., 2019) This study provides a theoretical basis for BE as a potential therapeutic agent for CRC, but its limitations are the lack of in vivo animal experimental validation and the unexplored differences in the effects of this drug in other colon cancer cell lines.
Eurycomanone (EN) is a diterpenoid extracted from Eurycoma longifolia Jack, a natural medicine widely distributed in Southeast Asia, and modern pharmacological research studies have shown that EN has anti-inflammatory and anti-tumor properties (Xu et al., 2024). Ye et al. used EN (0, 8, 16, 24, and 32 μM) to induce HCT116, SW620 cell lines. The results showed that the IC50 of EN on HCT116, SW620 and other CRC cell lines was 20.9–35.8 μM, which significantly reduced cell viability, clone formation ability and migration ability, and decreased the phosphorylation level of vascular endothelial growth factor receptor 2 (VEGFR2). In vivo experiments showed that 10 mg/kg of EN inhibited autophagy through activation of the mTOR signaling pathway, reduced tumor volume by 58.8%, downregulated the expression of the proliferation marker Ki-67, upregulated the level of the autophagy substrate p62, and inhibited vascular endothelial growth factor (VEGF)-induced angiogenesis, reducing lumen formation and endothelial cell migration (Ye et al., 2022). However, this study still has some shortcomings, such as the lack of validation of the efficacy of EN in other solid tumors.
Table 2 lists other natural products. Thus, modulating autophagy by targeting LC3-related signaling pathways offers a promising strategy for CRC treatment.
2.3 Ferroptosis
Ferroptosis is a type of cell death induced by lipid peroxidation of highly expressed unsaturated fatty acids in the cell membrane catalyzed by divalent iron or ester oxygenase (Zeng et al., 2023). The main features can be characterized as follows: ① Iron-dependent: the occurrence of ferroptosis is dependent on the accumulation of intracellular iron, which leads to a decrease in glutathione peroxidase activity and an increase in lipid peroxidation through the Fenton reaction; ②Lipid peroxidation: lipid peroxidation of unsaturated fatty acids occurs in response to divalent iron or esteroxygenase; ③Impairment of the antioxidant system: Glutathione peroxidase 4 (GPX4), as the core enzyme of the antioxidant system, its activity is reduced in ferroptosis, leading to a decrease in cellular antioxidant capacity; ④ cell death: cell death occurs as a result of lipid peroxidation and impaired antioxidant system (Mou et al., 2019). Several data support that the occurrence of Ferroptosis is closely related with the expression of GPX4, NFE2-related factor 2 (Nrf2) and other key signaling molecules (Liu X. et al., 2022). GPX4, a monomeric glutathione peroxidase with a catalytic role in the reduction of lipid peroxides, plays an important role in the process of ferroptosis and protects cells and tissues from free radicals damage by inhibiting lipid peroxidation. In contrast, deletion or dysfunction of GPX4 can lead to the accumulation of intracellular peroxides, which induces the onset of cellular ferroptosis and causes cell death (Liang et al., 2023). Nrf2 is considered to be a major regulator of antioxidant responses, and many pathological conditions are associated with an imbalance in redox homeostasis. In addition, Nrf2 levels are directly correlated with ferroptosis sensitivity, and upregulation of Nrf2 expression inhibits ferroptosis, whereas decreasing Nrf2 levels enhances the sensitivity of cancer cells to pro-ferroptosis agents (Dodson et al., 2019).
Notably, a large number of studies have validated the critical role of ferroptosis in inhibiting CRC progression, including the inhibition of tumor proliferation and metastasis (Wang et al., 2022). Therefore, ferroptosis is considered to be an important tumor suppressor mechanism and a potential target for CRC therapy. Sui et al. demonstrated through cell experiment that ferroptosis inducers upregulated the expression of ROS in HCT116, LoVo, and HT29 cells, and at the same time, reduced the level of GPX4 in order to inhibit the activity and proliferation of CRC cells, and exerted anti-tumor effect (Sui et al., 2018). In an experiment with CRC xenograft model mice, cetuximab enhanced RSL3-induced ferroptosis by inhibiting the Nrf2/HO-1 axis to suppress the weight and volume of CRC tumor cells, which has potential positive significance for the treatment of CRC (Yang et al., 2021). The above studies suggest that ferroptosis has great potential in the treatment of CRC, thus providing a new avenue for the treatment of CRC.
2.3.1 Natural products regulating ferroptosis intervention in CRC
A large amount of scientific evidence has proved that Ferroptosis plays an important role in the occurrence and development of CRC, and it may be an effective way to treat CRC. The following products have demonstrated the therapeutic effects of CRC by targeting and regulating important signaling pathways related to Ferroptosis (Figure 4).
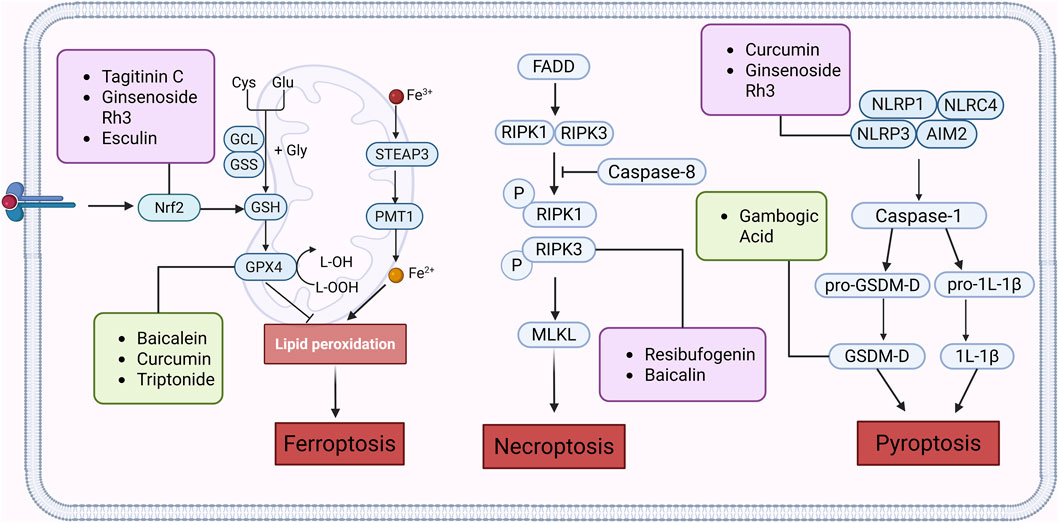
Figure 4. Natural products play a role in intervening in CRC by targeting signalling pathways such as Nrf2, GPX4, NLRP3, RIPK1, and RIPK3 and regulating different forms of cell death mechanisms such as Ferroptosis, Pyroptosis and Necroptosis. Created with Biorender.com.
2.3.1.1 Targeting the Nrf2 signaling pathway
Nrf2 is considered to be a major cellular defense mechanism and an important regulator of cell survival, protecting cells and tissues from various toxicants and carcinogens by increasing the expression of many cytoprotective genes. Some studies have shown that targeting and regulating the Nrf2 signaling pathway by natural products has positive implications for the treatment of CRC.
Ginsenoside Rh3 (GRh3) is an active compound extracted from the dried roots and rhizomes of the plant drug P. ginseng C.A. Mey. Which has been shown to possess a wide range of benefits including anti-inflammatory, antioxidant and anticarcinogenic properties (Yang et al., 2022). Wu et al. found that GRh3 had a dose-dependent inhibitory effect on the proliferation of HT29, HCT116 and other CRC cells in the concentration range of 10–160 μM. In in vivo experiments, GRh3 (20 mg/kg/d) was administered by gavage, and the tumor volume of the administered mice was reduced by more than 40% compared with that of the control group after 21 days, and there was no obvious liver or kidney toxicity. Mechanistic studies confirmed that GRh3 induces thermal apoptosis and ferroptosis through the Stat3/p53/Nrf2 signaling axis (Wu et al., 2023). This trial did not explore the potential effects of long-term dosing on normal tissues and lacked studies on the combined effects with clinically used chemotherapeutic agents.
Esculin is the main active ingredient in the stem bark of Fraxinus chinensis subsp. rhynchophylla (Hance) A.E.Murray., which has a variety of pharmacological effects, including anti-inflammatory, antioxidant, and antitumor (Cai and Cai, 2023). Ji et al. used Esculin (20, 40, 80 μM) to treat HCT116 cell line. CCK-8 assay and Annexin V/PI staining showed that Esculin inhibited cell viability and proliferation in a dose-dependent manner, upregulated the protein levels of Malondialdehyde (MDA), ROS, PKR, PERK, p-eIF2α, COX2 and ACSL4, and downregulated the expression of GSH, SOD and FTH1. In addition, an AOM/DSS-induced in vivo CRC mouse model was used to validate the potential of Esculin to inhibit tumorigenesis and to elucidate its underlying mechanisms. The experimental results further suggested that Esculin (20 and 40 mg/kg) may inhibit CRC and progression by inducing apoptosis and ferroptosis in vivo (Ji et al., 2024). Survival analysis was not performed in this study, and efficacy was assessed only by tumor number and colon length, lacking direct evidence of survival benefit.
Table 3 lists other natural products that inhibit CRC progression by modulating ferroptosis induced by Nrf2-related signaling pathways. The above studies suggest that modulation of Nrf2 signaling by these products is considered an effective strategy to inhibit CRC progression.
2.3.1.2 Targeting the GPX4 signaling pathway
The GPX4 signaling pathway is an antioxidant defense system against ferroptosis in normal cells, which maintains cellular redox homeostasis and specifically converts lipid peroxides to nontoxic lipocalciferol in a GSH-dependent manner to abrogate the oxidative activity of lipid peroxides, thereby preventing cellular ferroptosis. Mediating the GPX4-related pathway has been reported to effectively intervene in CRC progression. Notably, the ability of the following natural products to inhibit CRC cell progression was achieved by modulating GPX4 targets.
As described above, Baicalein was able to mediate the NF-κB signaling pathway to induce apoptosis to inhibit the viability and proliferation of CRC cells. Excitingly, Baicalein was also able to regulate ferroptosis to inhibit the progression of CRC tumor cells. Lai et al. showed that Baicalein (7.5–30 μM) dose-dependently decreased the expression of GPX4, GSH, and upregulated the levels of ROS, MDA, leading to a decrease MMP in HCT116 and DLD1 cell lines, which ultimately led to the phenomenon of ferroptosis. Furthermore, in CRC model mice, Baicalein (10 and 20 mg/kg) inhibited tumor cell growth in a dose-dependent manner. The mechanism is related to the fact that Baicalein induces ferroptosis through inducing the JAK2/STAT3/GPX4 axis to exert anti-CRC, providing a theoretical basis for its use as a therapeutic candidate for colorectal cancer (Lai et al., 2024). However, in vivo experiments have only used a subcutaneous graft tumor model, which does not mimic common clinical metastases. Furthermore, the lack of combination studies with other iron-death inducers limits the assessment of their synergistic potential.
Curcumin (CUR), an active compound in the dried rhizomes of the plant drug Curcuma longa.L. of the ginger family, is of interest because of its anti-inflammatory, antioxidant, and immunomodulatory, antitumor properties (Kotha and Luthria, 2019). As reported by Ming, both CUR (50, 100, and 200 mg/kg) and positive control cisplatin were able to inhibit the growth of tumors in CRC model mice in vivo, and reduce the activity and proliferation capacity of tumor cells. In addition, PCR, Western Blot and immunohistochemical analyses showed that CUR was able to enhance the levels of ROS, MDA, Fe2+, and decreased the expression of GSH and GPX4 epitopes, which was the same as the results of the in vitro experiments of SW620 cell line induced by CUR (10, 20, 40, and 80 μM). The therapeutic mechanism may be that CUR regulates the GSH/GPX4 axis to induce ferroptosis, thus exerting an inhibitory effect on CRC (Ming et al., 2024). However, the optimal concentration experiment and the specific concentration of cisplatin were not performed.
Triptonide (TN), a diterpenoid epoxide present in the botanical drug Rehmannia glutinosa (Gaertn.) Libosch. ex-DC., has been shown to have a wide range of biological properties including anti-inflammatory, antioxidant, and anticancer properties (Song et al., 2023). Wang et al. showed that the inhibitory effect of TN in HCT116 and LoVo cell lines to reveal its mechanism of action. The experimental results showed that TN (25, 50, and 100 μM) inhibited the proliferation of CRC cells and acted through ferroptosis pathway. Western blot assay showed that TN dose-dependently upregulated the levels of ROS, MDA, and Fe2+, and decreased the epitope protein expression of GSH and GPX4. Meanwhile, in vivo experiments in CRC model mice revealed that TN (5 mg/kg) inhibited tumor volume and weight in heterozygous tumor-bearing mice. In addition, this effect was associated with inhibition of the SLC7A11/GPX4 axis (Wang et al., 2024). However, this experiment was only studied at the animal and cellular level, and in the future, it needs to be evaluated in clinical trials for its safety and action effects.
The above findings suggest that these results confirm the potential role of natural product-induced ferroptosis in the treatment of CRC and that more studies are needed to support this idea.
2.4 Pyroptosis
Pyroptosis, which occurs predominantly in phagocytes, is a programmed cellular necrosis mediated by gasdermin proteins, dependent on inflammatory caspases and GSDMs, that forms pores in the cell membrane and releases intracellular contents to cause an intense inflammatory response (Loveless et al., 2021). Cellular pyroptosis mechanisms mainly include classical and non-classical pathways. In the classical cellular pyroptosis pathway, NLR pyrin domain-containing 3 (NLRP3), NLRC4, AIM2, Pyrin and other inflammatory vesicles are activated and cleave Pro-Caspase-1 to form active Caspase-1, which can cleave GSDMD protein to form active N-terminal and C-terminal, and the N-terminal end contributes to the perforation of cell membrane and cell death. At the same time, Caspase-1 can also process pro-IL-1β to form active IL-1β, which is released extracellularly to amplify inflammatory responses.
In addition, the Caspase-4/5/11-dependent mode of cell death is referred to as nonclassical pathway cellular pyroptosis (Vasudevan et al., 2023). In the nonclassical pathway of cellular pyroptosis, LPS can directly bind to Caspase-4/5/11, and activated Caspase-4/5/11 can cleave GSDMD proteins, and the N-terminal end of GSDMD proteins can both mediate cellular pyroptosis and activate NLRP3 inflammatory vesicles to activate Caspase-1, which ultimately produces Interleukin-1β (IL-1β) and release it to the outside of the membrane, causing an inflammatory response (Li R. et al., 2023).
In tumor immunotherapy, it has been reported that inducing tumor cell pyroptosis can activate the immune system and enhance the anti-tumor effect (Rao et al., 2022). Some studies have shown that targeting and regulating cellular pyroptosis is a promising approach for the treatment of CRC (Zhou et al., 2023). The experimental results of Guan et al. showed that targeting HDAC2-mediated cellular pyroptosis could enhance the sensitivity of CRC antitumor drug therapy, reduce drug resistance, and exert antitumor effects (Guan et al., 2024). In addition, Xie et al. demonstrated in vivo and ex vivo that simvastatin could induce pyroptosis through the ROS/caspase-1/GSDMD pathway, inhibit the proliferation of CRC cells, and suppress the growth and weight of tumors, inhibiting the progression of CRC disease (Xie et al., 2023). Therefore, targeting and regulating pyroptosis-related signaling pathways to treat CRC may be an effective strategy.
2.4.1 Natural products that regulate pyroptosis intervention in CRC
NLRP3 and GSDMD have received much attention for being key regulatory proteins during the pyroptosis response, and thus regulation of this target is of profound significance in the treatment of CRC. Excitingly, we found several substances in some natural products that induce GSDMD/NLRP3 to inhibit the inflammatory infiltration and progression of CRC cells (Figure 4).
Gambogic Acid (GA), an active compound secreted in the form of dried resin by the plant Garcinia gummi-gutta (L.) N. Robson, was demonstrated to have a significant anticancer effect (Liu B. et al., 2020). Xu et al. used GA (0–4 μmol/L) to treat HCT116 and CT26 cell lines to elucidate the potential anticancer effects. It was found that GA reduced CRC cell viability and proliferative capacity in a dose- and time-dependent manner, and the formation of pyroptotic vesicles was observed by transmission electron microscopy. In in vivo experiments, GA (2.8 mg/kg) was able to suppress the volume and weight of solid tumors in CRC model mice, and Western blot assay revealed that GA upregulated the expression of GSDME-N, suggesting that GA-mediated GSDME-dependent pyroptosis is involved in GA-triggered cell death in vivo (Xu et al., 2022). In this experiment, the difference in efficacy between the high-dose group and the low-dose group was significant, but the basis for dose selection was not stated. The IC50 of CT26 cells was 1 μmol/L in vitro, while the high dose was 8 mg/kg in vivo, so there may be a problem of the reasonableness of the dose conversion.
Strikingly, CUR not only mediates GPX4-regulated ferroptosis to inhibit CRC cell progression, but also regulates cellular pyroptosis to exert anti-cancer effects against CRC tumors. Dal et al. used CUR to evaluate the anti-cancer mechanism on four different CRC cell lines (HCT116, LoVo, HT29, and SW480). The in vitro results showed that CUR (1, 5, 25, 50, and 100 μM) was able to reduce cell viability and proliferation, decrease the levels of IL-1β, IL-18, as well as increase the SubG1 phase to promote apoptosis and inhibit the development of CRC cells in all tested cell lines. In addition, in terms of cellular pyroptosis, it was found that the NLRP3 inflammatory vesicle component was elevated in SW480, HCT116 cell lines, albeit to a lesser extent in the latter, and no NLRP3 inflammatory vesicle activation was observed in LoVo and HT29 cells. It is possible that this result is due to the ineffectiveness of CUR on the NLRP3 inflammatory vesicle complex in this cell line, and in the future, we should need to further investigate other inflammatory vesicle complexes to confirm the different effects of CUR on CRC (Dal and Aru, 2023).
As described above, Ginsenoside Rh3 (GRh3) has shown great therapeutic potential in anti-inflammatory, anti-tumor, etc. Wu et al. treated (HT29, HCT116, SW620, DLD1, and RKO) cell lines with GRh3 (0, 10, 20, 40, 80, and 160 μM). The experimental results showed that GRh3 was able to inhibit the viability and proliferation of all five cell types in a dose- and time-dependent manner. In addition, the experiment to further determine whether the drug could inhibit the growth of tumor cells in vivo, a CRC xenograft mouse model was suggested and treated with GRh3 (20 mg/kg) intervention for 21 days without a positive control group. The results showed that GRh3 could significantly inhibit the size and weight of tumors in vivo, and western blot assay revealed that GRh3 could inhibit the reduction of the levels of IL-1β, IL-18, NLRP3, and reduce the inflammatory infiltration, and exert anti-tumor effects. Meanwhile HE staining showed that GRh3 could significantly inhibit CRC in vivo and in vitro without damaging the organism (Wu et al., 2023). The findings would have been more adequate if the experiment could have established a positive control group and a concentration gradient.
2.5 Necroptosis
Necroptosis, also known as programmed necrosis, is a form of inflammatory cell death mediated and regulated by RIPKI and RIPK3 kinases (Yan et al., 2021). Under normal conditions, signaling by TNF-α, FASL, and TRAIL recruits RIPK1 kinase to a complex of inhibitors of apoptosis (cIAPs) at the plasma membrane, leading to a cascade reaction that activates NF-κB and promotes cell survival. When this process is blocked, RIPK1 forms a secondary complex with factors such as activated caspase- 8, which induces apoptosis (Tong et al., 2022). Under conditions such as cellular stress, caspase- 8 activity is inhibited, leading to the formation of necrotic vesicles, and the regulatory function of RIPK1 is switched from apoptosis to necrotic apoptosis, while RIPK3 is recruited to necrotic vesicles and subsequently phosphorylated by RIPK1. Activated RIPK3 then phosphorylates MLKL and translocates to the plasma membrane to form a pore complex. This complex disrupts membrane integrity, enhances membrane permeability, releases inflammatory mediators, and ultimately leads to cell death (Chen et al., 2022).
There is more evidence that necrotic apoptosis plays a key role in the regulation of cancer biology, including cancer development, metastasis, and immunity (Khoury et al., 2020). Moreover, necroptosis has a bidirectional regulatory effect on cancer: on the one hand, it promotes cancer metastasis and cancer progression; on the other hand, it also prevents tumor development when apoptosis is impaired (Ye et al., 2023).
The view that receptor-interacting protein kinase 3 (RIPK3) expression is reported to be crucial in CRC, as its expression may affect the response to chemotherapy and, consequently, colorectal tumorigenesis and progression (Huang et al., 2023). Bozec et al. found that CRC model mice in the presence of RIPK3 deletion were more likely to exhibit higher levels of heterotopic hyperplasia and progress to highly differentiated intramucosal adenocarcinomas, promoting tumor formation (Bozec et al., 2016). Experimental data from Chen et al. demonstrated that 5-FU mediated the PUMA/RIPK3 signaling pathway to regulate necrotic apoptosis in order to enhance tumor cell killing and anti-tumorimmune responses, thus improving CRC treatment, thereby improving the treatment of CRC (Chen et al., 2024). The above studies suggest that necroptosis regulated by RIPK3-related signaling pathway can inhibit the progression of CRC and bring new hope for the treatment of CRC.
2.5.1 Natural products that regulate necro-apoptotic in CRC
Interestingly, some products have been shown to regulate RIPK1/RIPK3/MLKL-related signaling target protein molecules to inhibit necroptosis and thus inhibit the progression and metastasis of CRC cells (Figure 4).
Resibufogenin (RBG) is an active compound extracted from the venom of Bufo toad, and has been shown to have significant anti-inflammatory and anti-tumor activity in modern pharmacology (Zhang and Jian, 2024). Han et al. treated SW480 and HCT-116 cell lines with RBG (0.1, 1, 2.5, 5, and 10 μM) and found that RBG reduced the activity and proliferation of CRC cells and inhibited the migration of the cells. In vivo experiments further confirmed that RBG (5 and 10 mg/kg) dose-dependently inhibited the weight and volume of tumors in CRC model mice, and western blot results showed that RBG was able to upregulate the levels of PYGL, GLUD1, GLUL, RIPK3, and MLKL. In addition, this experiment also confirmed the ability of RBG to inhibit the phenomenon of liver metastasis by immunohistochemistry and fluorescence imaging (Han et al., 2018). In the present study, although a role for necrotic apoptosis was demonstrated by RIPK3 knockdown experiments, the potential contribution of other cell death pathways (e.g., ferroptosis or pyroptosis) was not completely excluded.
Yang et al. used Baicalin (100 μmol/L) to intervene in CT26.WT cells for 72 h. The experimental results showed that after Baicalin treatment, the survival rate of CT26. WT cells decreased significantly with the prolongation of the action time, and DAPI staining showed that the nuclei of the cells were densely stained and fragmented; necrotic apoptosis was observed by transmission electron microscopy, with cell swelling, mitochondrial swelling, and exudation of the contents, and necrotic apoptosis was observed. Meanwhile, baicalin significantly upregulated the expression levels of RIPK3 mRNA and protein (P < 0.01), while z-VAD-fmk attenuated this effect. Mechanistic studies revealed that RIPK3 is a key marker protein for necrotic apoptosis and plays an important role in baicalein-induced cell death (Yang et al.). However, there are still some shortcomings in this experiment, such as the experiment was only conducted in vitro and lacked in vivo experimental validation to comprehensively assess the antitumor effect and safety of baicalin in the whole animal model.
3 Conclusion
CRC is a common malignant tumor characterized by uncontrolled division and survival of abnormal cells in the colon or rectum. With the improvement of living standards, the incidence of CRC tends to be younger, and the morbidity and mortality rates are high. At this stage, clinical treatments for CRC include preoperative radiotherapy and systemic therapy for downstaging, endoscopic and surgical local resection, local ablative therapy for metastasis, extensive surgery for localized and metastatic disease, palliative chemotherapy, immunotherapy, and targeted therapies, etc. The above treatments can lead to an improvement in the survival of CRC patients. However, the survival of CRC patients is still threatened, and the need to further explore new therapeutic means is an urgent issue at this stage.
In recent years, natural products have received widespread attention due to their natural origin, multi-target effects, and low toxicity. Natural products from plants, marine organisms, microorganisms and other sources have demonstrated significant anti-tumor activity in a variety of cancers. A large number of studies have shown that natural products can exert anti-CRC effects by targeting the programmed death pathway to inhibit tumor cell proliferation, induce apoptosis, inhibit angiogenesis, and modulate the immune microenvironment, among other pathways. Therefore, this paper summarizes the therapeutic potential exhibited by different forms of PCD in the treatment of CRC. In addition, we found that there are fewer natural products targeting ferroptosis, pyroptosis, and necroptosis pathways, which should be further explored in the future to expand the options of natural products for the treatment of CRC.
In terms of natural products against CRC, we clearly recognize that: (1) natural products usually have complex chemical structures and are able to intervene in cancer cell proliferation, apoptosis, invasion, and metastasis through multi-targeting actions. For example, CUR can target the AMPK/mTOR pathway to induce apoptosis in order to develop the migration and proliferation ability of CRC cells. (2) The multi-target mechanism reduces the possibility of cancer cells becoming resistant to a single drug target. (3) Natural products are generally derived from plants, microorganisms or marine organisms, which are highly biocompatible and have lower toxic side effects than chemotherapeutic drugs such as 5-FU or oxaliplatin. (4) Many natural products have strong antioxidant and anti-inflammatory properties that can improve the pro-inflammatory microenvironment of CRC. Natural products can enhance the sensitivity of chemotherapeutic drugs or reverse tumor resistance. (5) Certain natural products have the potential to prevent CRC and can be used as a means of primary prevention of cancer.
Although the above illustrates the outstanding contribution of natural products in the treatment of CRC, there are still some shortcomings, such as: (1) natural products tend to have lower absorption, bioavailability and half-life. (2) Compared with conventional chemotherapeutic agents, natural products usually have weaker anticancer potency, so single use may not be sufficient for complete tumor suppression. (3) The specific mechanisms are often complex and not fully defined, which may limit their widespread clinical use. (4) Natural products may interact with other anticancer drugs or conventional medications (e.g., anticoagulants, anti-inflammatory drugs, etc.), affecting therapeutic efficacy or increasing the risk of adverse reactions. (5) Despite showing significant effects in in vitro and animal studies, there are a limited number of high-quality clinical trials for natural products and the evidence base is weak.
Of concern, their clinical application also faces many challenges, especially low bioavailability and poor solubility. The complex structure of natural products and the fact that most of them are fat-soluble and difficult to be effectively absorbed limit their clinical efficacy. Therefore, improving water solubility and bioavailability has become an important task in modern drug development. Currently, scientists are trying to improve the drug delivery system of natural products through nanotechnology, liposome encapsulation and molecular modification. For example, nanoparticle carriers can improve the solubility and stability of drugs, and chemical modifications can help to increase the solubility of drugs in the bloodstream, thereby improving bioavailability and therapeutic efficacy. These approaches open up new possibilities for the clinical application of natural products. The successful modification of camptothecin is a classic example of natural product drug development. Originally derived from the Mappia nimmoniana (J.Graham) Byng & Stull, camptothecin has strong antitumor activity, but its low water solubility and instability have limited its clinical application. By optimizing the molecular structure, scientists developed Irinotecan, a modified drug with significant improvements in solubility, stability and bioavailability, which is widely used in the treatment of colorectal cancer (Mollica et al., 2012; Huang et al., 2022). The transformation of camptothecin to irinotecan demonstrates the great potential of natural products in drug development, proving that structural modifications and delivery system innovations can break through the biological limitations of natural products and advance their clinical applications. This case provides valuable experience for the research and application of other natural products and opens up new directions in cancer therapy.
Future research should focus on the following areas: (1) Further explore the mechanism of action of natural compounds through multi-omics techniques (e.g., genomics, metabolomics). (2) Conduct high-quality clinical trials to verify the safety and efficacy of natural compounds. (3) Explore the use of natural compounds in combination with chemotherapeutic drugs or immunotherapy to enhance the effect of comprehensive treatment. (4) Optimize the structure of natural compounds to improve bioavailability and stability. In conclusion, natural compounds are expected to provide safe, effective and versatile therapeutic options for CRC treatment, but their development and application strategies need to be continuously optimized.
Author contributions
YZ: Conceptualization, Investigation, Software, Writing – original draft, Writing – review and editing. NF: Conceptualization, Data curation, Formal Analysis, Project administration, Validation, Writing – review and editing. CL: Conceptualization, Data curation, Investigation, Software, Writing – review and editing. ZL: Conceptualization, Data curation, Formal Analysis, Investigation, Methodology, Project administration, Supervision, Validation, Writing – review and editing.
Funding
The author(s) declare that no financial support was received for the research and/or publication of this article.
Conflict of interest
The authors declare that the research was conducted in the absence of any commercial or financial relationships that could be construed as a potential conflict of interest.
Generative AI statement
The authors declare that no Generative AI was used in the creation of this manuscript.
Publisher’s note
All claims expressed in this article are solely those of the authors and do not necessarily represent those of their affiliated organizations, or those of the publisher, the editors and the reviewers. Any product that may be evaluated in this article, or claim that may be made by its manufacturer, is not guaranteed or endorsed by the publisher.
References
Bhardwaj, M., Cho, H. J., Paul, S., Jakhar, R., Khan, I., Lee, S. J., et al. (2017). Vitexin induces apoptosis by suppressing autophagy in multi-drug-resistant colorectal cancer cells. Oncotarget 9 (3), 3278–3291. doi:10.18632/oncotarget.22890
Bozec, D., Iuga, A. C., Roda, G., Dahan, S., and Yeretssian, G. (2016). Critical function of the necroptosis adaptor RIPK3 in protecting from intestinal tumorigenesis. Oncotarget 7 (29), 46384–46400. doi:10.18632/oncotarget.10135
Cai, T., and Cai, B. (2023). Pharmacological activities of esculin and esculetin: a review. Med. Baltim. 102 (40), e35306. doi:10.1097/MD.0000000000035306
Cao, W., Li, J., Yang, K., and Cao, D. (2021). An overview of autophagy: mechanism, regulation and research progress. Bull. Cancer 108 (3), 304–322. doi:10.1016/j.bulcan.2020.11.004
Chen, D., Ermine, K., Wang, Y. J., Chen, X., Lu, X., Wang, P., et al. (2024). PUMA/RIP3 mediates chemotherapy response via necroptosis and local immune activation in colorectal cancer. Mol. Cancer Ther. 23 (3), 354–367. doi:10.1158/1535-7163.MCT-23-0162
Chen, S., Zhao, D., Luan, C., Zheng, J., Liu, W., Feng, Z., et al. (2023). Ferulic acid induces autophagy and apoptosis in colon cancer CT26 cells via the MAPK pathway. Molecules 28 (16), 6014. doi:10.3390/molecules28166014
Chen, X., Xu, H., Yu, X., Wang, X., Zhu, X., and Xu, X. (2019). Apigenin inhibits in vitro and in vivo tumorigenesis in cisplatin-resistant colon cancer cells by inducing autophagy, programmed cell death and targeting m-TOR/PI3K/Akt signalling pathway. J. BUON 24 (2), 488–493.
Chen, Y., Ren, W., Wang, Q., He, Y., Ma, D., and Cai, Z. (2022). The regulation of necroptosis by ubiquitylation. Apoptosis 27 (9-10), 668–684. doi:10.1007/s10495-022-01755-8
Cheng, H., Liu, J., Zhang, D., Wang, J., Tan, Y., Feng, W., et al. (2022). Ginsenoside Rg1 alleviates Acute ulcerative colitis by modulating Gut Microbiota and Microbial Tryptophan metabolism. Front. Immunol. 13, 817600. doi:10.3389/fimmu.2022.817600
Dai, W., Dai, Y. G., Ren, D. F., and Zhu, D. W. (2023). Dieckol, a natural polyphenolic drug, inhibits the proliferation and migration of colon cancer cells by inhibiting PI3K, AKT, and mTOR phosphorylation. J. Biochem. Mol. Toxicol. 37 (5), e23313. doi:10.1002/jbt.23313
Dal, Z., and Aru, B. (2023). The role of curcumin on apoptosis and NLRP3 inflammasome-dependent pyroptosis on colorectal cancer in vitro. Turk J. Med. Sci. 53 (4), 883–893. doi:10.55730/1300-0144.5652
D'Arcy, M. S. (2019). Cell death: a review of the major forms of apoptosis, necrosis and autophagy. Cell Biol. Int. 43 (6), 582–592. doi:10.1002/cbin.11137
Debnath, J., Gammoh, N., and Ryan, K. M. (2023). Autophagy and autophagy-related pathways in cancer. Nat. Rev. Mol. Cell Biol. 24 (8), 560–575. doi:10.1038/s41580-023-00585-z
Dekker, E., Tanis, P. J., Vleugels, J. L. A., Kasi, P. M., and Wallace, M. B. (2019). Colorectal cancer. Lancet 394, 1467–1480. doi:10.1016/S0140-6736(19)32319-0
Deng, Q., Li, X., Fang, C., Li, X., Zhang, J., Xi, Q., et al. (2022). Cordycepin enhances anti-tumor immunity in colon cancer by inhibiting phagocytosis immune checkpoint CD47 expression. Int. Immunopharmacol. 107, 108695. doi:10.1016/j.intimp.2022.108695
Dodson, M., Castro-Portuguez, R., and Zhang, D. D. (2019). Nrf2 plays a critical role in mitigating lipid peroxidation and ferroptosis. Redox Biol. 23, 101107. doi:10.1016/j.redox.2019.101107
El-Wetidy, M. S., Ahmad, R., Rady, I., Helal, H., Rady, M. I., Vaali-Mohammed, M. A., et al. (2021). Urolithin A induces cell cycle arrest and apoptosis by inhibiting Bcl-2, increasing p53-p21 proteins and reactive oxygen species production in colorectal cancer cells. Cell Stress Chaperones 26 (3), 473–493. doi:10.1007/s12192-020-01189-8
Fan, A., Wang, B., Wang, X., Nie, Y., Fan, D., Zhao, X., et al. (2021). Immunotherapy in colorectal cancer: current achievements and future perspective. Int. J. Biol. Sci. 17 (14), 3837–3849. doi:10.7150/ijbs.64077
Farooqi, A. A., Wen, R., Attar, R., Taverna, S., Butt, G., and Xu, B. (2022). Regulation of cell-signaling pathways by Berbamine in different cancers. Int. J. Mol. Sci. 23 (5), 2758. doi:10.3390/ijms23052758
Feng, Y., A, Q., Zhao, Z., Wu, M., Yang, C., Liang, W., et al. (2024). Beta-elemene: a phytochemical with promise as a drug candidate for tumor therapy and adjuvant tumor therapy. Biomed. Pharmacother. 172, 116266. doi:10.1016/j.biopha.2024.116266
Fu, Y., Ye, Y., Zhu, G., Xu, Y., Sun, J., Wu, H., et al. (2021). Resveratrol induces human colorectal cancer cell apoptosis by activating the mitochondrial pathway via increasing reactive oxygen species. Mol. Med. Rep. 23 (3), 170. doi:10.3892/mmr.2020.11809
Fu, Y., Zhou, X., Wang, L., Fan, W., Gao, S., Zhang, D., et al. (2024). Salvianolic acid B attenuates liver fibrosis by targeting Ecm1 and inhibiting hepatocyte ferroptosis. Redox Biol. 69, 103029. doi:10.1016/j.redox.2024.103029
Gao, Y., Wang, Y., Wang, X., Ma, J., Wei, M., Li, N., et al. (2023). FABP4 regulates cell proliferation, stemness, apoptosis, and glycolysis in colorectal cancer via modulating ROS/ERK/mTOR pathway. Discov. Med. 35 (176), 361–371. doi:10.24976/Discov.Med.202335176.37
Gao, Z., Jiang, J., Hou, L., and Ji, F. (2022). Lysionotin induces ferroptosis to suppress development of colorectal cancer via promoting Nrf2 degradation. Oxid. Med. Cell Longev. 2022, 1366957. doi:10.1155/2022/1366957
Geng, Y. D., Zhang, L., Wang, G. Y., Feng, X. J., Chen, Z. L., Jiang, L., et al. (2020). Xanthatin mediates G2/M cell cycle arrest, autophagy and apoptosis via ROS/XIAP signaling in human colon cancer cells. Nat. Prod. Res. 34 (18), 2616–2620. doi:10.1080/14786419.2018.1544976
Ghasemian, A., Omear, H. A., Mansoori, Y., Mansouri, P., Deng, X., Darbeheshti, F., et al. (2023). Long non-coding RNAs and JAK/STAT signaling pathway regulation in colorectal cancer development. Front. Genet. 14, 1297093. doi:10.3389/fgene.2023.1297093
Guan, X., Liu, R., Wang, B., Xiong, R., Cui, L., Liao, Y., et al. (2024). Inhibition of HDAC2 sensitises antitumour therapy by promoting NLRP3/GSDMD-mediated pyroptosis in colorectal cancer. Clin. Transl. Med. 14 (6), e1692. doi:10.1002/ctm2.1692
Guo, C., He, J., Song, X., Tan, L., Wang, M., Jiang, P., et al. (2019). Pharmacological properties and derivatives of shikonin-A review in recent years. Pharmacol. Res. 149, 104463. doi:10.1016/j.phrs.2019.104463
Han, B., Jiang, P., Li, Z., Yu, Y., Huang, T., Ye, X., et al. (2018). Coptisine-induced apoptosis in human colon cancer cells (HCT-116) is mediated by PI3K/Akt and mitochondrial-associated apoptotic pathway. Phytomedicine 48, 152–160. doi:10.1016/j.phymed.2017.12.027
Han, Q., Ma, Y., Wang, H., Dai, Y., Chen, C., Liu, Y., et al. (2018). Resibufogenin suppresses colorectal cancer growth and metastasis through RIP3-mediated necroptosis. J. Transl. Med. 16 (1), 201. doi:10.1186/s12967-018-1580-x
Haq, I. U., Imran, M., Nadeem, M., Tufail, T., Gondal, T. A., and Mubarak, M. S. (2021). Piperine: a review of its biological effects. Phytother. Res. 35 (2), 680–700. doi:10.1002/ptr.6855
Hou, X., and Xu, G. (2024). Effects of dexmedetomidine on proliferation and autophagy of human colon cancer cells. J. Clin. Anesthesiol. 40 (07), 735–740.
Huang, Q., Liu, X., Wang, H., Liu, X., Zhang, Q., Li, K., et al. (2022). A nanotherapeutic strategy to overcome chemoresistance to irinotecan/7-ethyl-10-hydroxy-camptothecin in colorectal cancer. Acta Biomater. 137, 262–275. doi:10.1016/j.actbio.2021.10.034
Huang, S., Lin, L., Ma, Y., Zhu, Q., and Weng, N. (2023). Scoparone induces autophagic cell death via the PAK1/AKT axis in colorectal cancer. Eur. J. Pharmacol. 959, 176091. doi:10.1016/j.ejphar.2023.176091
Huang, Y., Yang, W., Yang, L., Wang, T., Li, C., Yu, J., et al. (2023). Nrf2 inhibition increases sensitivity to chemotherapy of colorectal cancer by promoting ferroptosis and pyroptosis. Sci. Rep. 13 (1), 14359. doi:10.1038/s41598-023-41490-x
Huang, Z., Gan, S., Zhuang, X., Chen, Y., Lu, L., Wang, Y., et al. (2022). Artesunate inhibits the cell growth in colorectal cancer by promoting ROS-dependent cell senescence and autophagy. Cells 11 (16), 2472. doi:10.3390/cells11162472
Hwang, J. Y., Park, J. H., Kim, M. J., Kim, W. J., Ha, K. T., Choi, B. T., et al. (2019). Isolinderalactone regulates the BCL-2/caspase-3/PARP pathway and suppresses tumor growth in a human glioblastoma multiforme xenograft mouse model. Cancer Lett. 443, 25–33. doi:10.1016/j.canlet.2018.11.027
Islam, M. R., Akash, S., Rahman, M. M., Nowrin, F. T., Akter, T., Shohag, S., et al. (2022). Colon cancer and colorectal cancer: prevention and treatment by potential natural products. Chem. Biol. Interact. 368, 110170. doi:10.1016/j.cbi.2022.110170
Jearawuttanakul, K., Khumkhrong, P., Suksen, K., Reabroi, S., Munyoo, B., Tuchinda, P., et al. (2020). Cleistanthin A induces apoptosis and suppresses motility of colorectal cancer cells. Eur. J. Pharmacol. 889, 173604. doi:10.1016/j.ejphar.2020.173604
Ji, X., Chen, Z., Lin, W., Wu, Q., Wu, Y., Hong, Y., et al. (2024). Esculin induces endoplasmic reticulum stress and drives apoptosis and ferroptosis in colorectal cancer via PERK regulating eIF2α/CHOP and Nrf2/HO-1 cascades. J. Ethnopharmacol. 328, 118139. doi:10.1016/j.jep.2024.118139
Jiang, F., Ren, S., Chen, Y., Zhang, A., Zhu, Y., Zhang, Z., et al. (2021). Fangchinoline exerts antitumour activity by suppressing the EGFR-PI3K/AKT signalling pathway in colon adenocarcinoma. Oncol. Rep. 45 (1), 139–150. doi:10.3892/or.2020.7857
Jiang, H., Li, Y., Xiang, X., Tang, Z., Liu, K., Su, Q., et al. (2021). Chaetocin: a review of its anticancer potentials and mechanisms. Eur. J. Pharmacol. 910, 174459. doi:10.1016/j.ejphar.2021.174459
Jiao, Y., Xin, M., Xu, J., Xiang, X., Li, X., Jiang, J., et al. (2022). Polyphyllin II induced apoptosis of NSCLC cells by inhibiting autophagy through the mTOR pathway. Pharm. Biol. 60 (1), 1781–1789. doi:10.1080/13880209.2022.2120021
Jing, Z., Fei, W., Zhou, J., Zhang, L., Chen, L., Zhang, X., et al. (2016). Salvianolic acid B, a novel autophagy inducer, exerts antitumor activity as a single agent in colorectal cancer cells. Oncotarget 7 (38), 61509–61519. doi:10.18632/oncotarget.11385
Kaloni, D., Diepstraten, S. T., Strasser, A., and Kelly, G. L. (2023). BCL-2 protein family: attractive targets for cancer therapy. Apoptosis 28 (1-2), 20–38. doi:10.1007/s10495-022-01780-7
Kashyap, D., Garg, V. K., and Goel, N. (2021). Intrinsic and extrinsic pathways of apoptosis: role in cancer development and prognosis. Adv. Protein Chem. Struct. Biol. 125, 73–120. doi:10.1016/bs.apcsb.2021.01.003
Ketelut-Carneiro, N., and Fitzgerald, K. A. (2022). Apoptosis, pyroptosis, and necroptosis-Oh My! The many ways a cell can Die. J. Mol. Biol. 434 (4), 167378. doi:10.1016/j.jmb.2021.167378
Khodapasand, E., Jafarzadeh, N., Farrokhi, F., Kamalidehghan, B., and Houshmand, M. (2015). Is Bax/Bcl-2 ratio considered as a prognostic marker with age and tumor location in colorectal cancer? Iran. Biomed. J. 19 (2), 69–75. doi:10.6091/ibj.1366.2015
Khoury, M. K., Gupta, K., Franco, S. R., and Liu, B. (2020). Necroptosis in the Pathophysiology of disease. Am. J. Pathol. 190 (2), 272–285. doi:10.1016/j.ajpath.2019.10.012
Kim, A., Lee, S. Y., Seo, C. S., and Chung, S. K. (2020). Prunellae Spica extract suppresses Teratoma formation of Pluripotent stem cells through p53-mediated apoptosis. Nutrients 12 (3), 721. doi:10.3390/nu12030721
Kong, Z., Liu, R., and Cheng, Y. (2019). Artesunate alleviates liver fibrosis by regulating ferroptosis signaling pathway. Biomed. Pharmacother. 109, 2043–2053. doi:10.1016/j.biopha.2018.11.030
Kotha, R. R., and Luthria, D. L. (2019). Curcumin: biological, Pharmaceutical, Nutraceutical, and Analytical Aspects. Molecules 24 (16), 2930. doi:10.3390/molecules24162930
Kwak, A. W., Park, J. W., Lee, S. O., Lee, J. Y., Seo, J. H., Yoon, G., et al. (2022). Isolinderalactone sensitizes oxaliplatin-resistance colorectal cancer cells through JNK/p38 MAPK signaling pathways. Phytomedicine 105, 154383. doi:10.1016/j.phymed.2022.154383
Lai, J. Q., Zhao, L. L., Hong, C., Zou, Q. M., Su, J. X., Li, S. J., et al. (2024). Baicalein triggers ferroptosis in colorectal cancer cells via blocking the JAK2/STAT3/GPX4 axis. Acta Pharmacol. Sin. 45 (8), 1715–1726. doi:10.1038/s41401-024-01258-z
Lee, S. O., Joo, S. H., Kwak, A. W., Lee, M. H., Seo, J. H., Cho, S. S., et al. (2021). Podophyllotoxin Induces ROS-Mediated Apoptosis and Cell Cycle Arrest in Human Colorectal Cancer Cells via p38 MAPK Signaling. Biomol Ther (Seoul) 29 (6): 658–666. doi:10.4062/biomolther.2021.143
Li, D., Li, Y., Jiang, X., Liu, W., and Zhao, Q. (2022). Evodiamine: a Privileged structure with Broad-ranging biological activities. Mini Rev. Med. Chem. 22 (21), 2680–2701. doi:10.2174/1389557522666220404090835
Li, S. Z., Ren, J. W., Fei, J., Zhang, X. D., Du, R. L., et al. (2019). Cordycepin induces Bax-dependent apoptosis in colorectal cancer cells. Mol. Med. Rep. 19 (2): 901–908. doi:10.3892/mmr.2018.9717
Li, D., Rui, Y. X., Guo, S. D., Luan, F., Liu, R., and Zeng, N. (2021). Ferulic acid: a review of its pharmacology, pharmacokinetics and derivatives. Life Sci. 284, 119921. doi:10.1016/j.lfs.2021.119921
Li, G., Kong, B., Tong, Q., Li, Y., Chen, L., Zeng, J., et al. (2021). Vanillin downregulates NNMT and attenuates NNMT-related resistance to 5-fluorouracil via ROS-induced cell apoptosis in colorectal cancer cells. Oncol. Rep. 45 (6), 110. doi:10.3892/or.2021.8061
Li, J., Shang, L., Zhou, F., Wang, S., Liu, N., Zhou, M., et al. (2023). Herba Patriniae and its component Isovitexin show anti-colorectal cancer effects by inducing apoptosis and cell-cycle arrest via p53 activation. Biomed. Pharmacother. 168, 115690. doi:10.1016/j.biopha.2023.115690
Li, J. K., Sun, H. T., Jiang, X. L., Chen, Y. F., Zhang, Z., Wang, Y., et al. (2022). Polyphyllin II induces protective autophagy and apoptosis via inhibiting PI3K/AKT/mTOR and STAT3 signaling in colorectal cancer cells. Int. J. Mol. Sci. 23 (19), 11890. doi:10.3390/ijms231911890
Li, L., Dickinson, M. S., Coers, J., and Miao, E. A. (2023). Pyroptosis in defense against intracellular bacteria. Semin. Immunol. 69, 101805. doi:10.1016/j.smim.2023.101805
Li, R., Zheng, C., Shiu, P. H., Rangsinth, P., Wang, W., Kwan, Y. W., et al. (2023). Garcinone E triggers apoptosis and cell cycle arrest in human colorectal cancer cells by mediating a reactive oxygen species-dependent JNK signaling pathway. Biomed. Pharmacother. 162, 114617. doi:10.1016/j.biopha.2023.114617
Li, S., Xiong, Q., Shen, Y., Lin, J., Zhang, L., Wu, Y., et al. (2024). Toosendanin: upgrade of an old agent in cancer treatment. Chin. J. Nat. Med. 22 (10), 887–899. doi:10.1016/S1875-5364(24)60693-X
Liang, D., Feng, Y., Zandkarimi, F., Wang, H., Zhang, Z., Kim, J., et al. (2023). Ferroptosis surveillance independent of GPX4 and differentially regulated by sex hormones. Cell 186 (13), 2748–2764.e22. doi:10.1016/j.cell.2023.05.003
Lin, W., Zheng, L., Zhuang, Q., Zhao, J., Cao, Z., Zeng, J., et al. (2013). Spica prunellae promotes cancer cell apoptosis, inhibits cell proliferation and tumor angiogenesis in a mouse model of colorectal cancer via suppression of stat3 pathway. BMC Complement. Altern. Med. 13, 144. doi:10.1186/1472-6882-13-144
Liu, B., Deng, X., Jiang, Q., Li, G., Zhang, J., Zhang, N., et al. (2020). Scoparone improves hepatic inflammation and autophagy in mice with nonalcoholic steatohepatitis by regulating the ROS/P38/Nrf2 axis and PI3K/AKT/mTOR pathway in macrophages. Biomed. Pharmacother. 125, 109895. doi:10.1016/j.biopha.2020.109895
Liu, J., Kang, R., and Tang, D. (2022). Signaling pathways and defense mechanisms of ferroptosis. FEBS J. 289 (22), 7038–7050. doi:10.1111/febs.16059
Liu, L., Liu, Y., Chen, Y., Wang, Li, Xie, R., Zhongcheng, M. O., et al. (2024). Downregulation of MTMR3 by atorvastatin calcium suppresses autophagy and inhibits proliferation and migration of colorectal cancer HCT116 cells. Chin. J. Surg. Integr. 30 (06), 910–918.
Liu, P., Zhao, G., Zhang, L., Gong, Y., and Gu, Y. (2021). Atractylenolide I inhibits antibiotic-induced dysbiosis of the intestinal microbiome. Ann. Transl. Med. 9 (20), 1539. doi:10.21037/atm-21-4656
Liu R., R., Zhang, B., Zou, S., Cui, L., Lin, L., and Li, L. (2024). Ginsenoside Rg1 induces autophagy in colorectal cancer through inhibition of the Akt/mTOR/p70S6K pathway. J. Microbiol. Biotechnol. 34 (4), 774–782. doi:10.4014/jmb.2310.10043
Liu, S., Yao, S., Yang, H., Liu, S., and Wang, Y. (2023). Autophagy: regulator of cell death. Cell Death Dis. 14 (10), 648. doi:10.1038/s41419-023-06154-8
Liu, X., Geng, Z., Ding, X., Lou, Y., and Zhang, X. (2022). Convallatoxin suppresses osteosarcoma cell proliferation, migration, invasion, and enhances osteogenic differentiation by downregulating parathyroid hormone receptor 1 (PTHR1) expression and inactivating Wnt/β-catenin pathway. Bioengineered 13 (5), 13280–13292. doi:10.1080/21655979.2022.2080363
Liu X., X., Xu, J., Zhou, J., and Shen, Q. (2020). Oridonin and its derivatives for cancer treatment and overcoming therapeutic resistance. Genes Dis. 8 (4), 448–462. doi:10.1016/j.gendis.2020.06.010
Liu, Y., Chen, Y., Lin, L., and Li, H. (2020). Gambogic acid as a candidate for cancer therapy: a review. Int. J. Nanomedicine 15, 10385–10399. doi:10.2147/IJN.S277645
Loveless, R., Bloomquist, R., and Teng, Y. (2021). Pyroptosis at the forefront of anticancer immunity. J. Exp. Clin. Cancer Res. 40 (1), 264. doi:10.1186/s13046-021-02065-8
Lu, Q., Tang, Y., Luo, S., Gong, Q., and Li, C. (2023). Coptisine, the Characteristic Constituent from Coptis chinensis, exhibits significant therapeutic potential in treating cancers, metabolic and inflammatory diseases. Am. J. Chin. Med. 51 (8), 2121–2156. doi:10.1142/S0192415X2350091X
Luo, Q., Jia, L., Huang, C., Qi, Q., Jahangir, A., Xia, Y., et al. (2022). Polyphyllin, I promotes autophagic cell death and apoptosis of colon cancer cells via the ROS-inhibited AKT/mTOR pathway. Int. J. Mol. Sci. 23 (16), 9368. doi:10.3390/ijms23169368
Ma, Q., Liu, L., Zhao, S., Huang, Z., Li, C., Jiang, S., et al. (2022). Biosynthesis of vanillin by different microorganisms: a review. World J. Microbiol. Biotechnol. 38 (3), 40. doi:10.1007/s11274-022-03228-1
Ma, Z., Lou, S., and Jiang, Z. (2020). PHLDA2 regulates EMT and autophagy in colorectal cancer via the PI3K/AKT signaling pathway. Aging (Albany NY) 12 (9), 7985–8000. doi:10.18632/aging.103117
Malki, A., ElRuz, R. A., Gupta, I., Allouch, A., Vranic, S., and Al Moustafa, A. E. (2020). Molecular mechanisms of colon cancer progression and metastasis: recent Insights and Advancements. Int. J. Mol. Sci. 22 (1), 130. doi:10.3390/ijms22010130
Mérarchi, M., Sethi, G., Fan, L., Mishra, S., Arfuso, F., and Ahn, K. S. (2018). Molecular targets modulated by Fangchinoline in tumor cells and Preclinical models. Molecules 23 (10), 2538. doi:10.3390/molecules23102538
Micale, N., Molonia, M. S., Citarella, A., Cimino, F., Saija, A., Cristani, M., et al. (2021). Natural product-based Hybrids as potential candidates for the treatment of cancer: focus on curcumin and Resveratrol. Molecules 26 (15), 4665. doi:10.3390/molecules26154665
Ming, T., Lei, J., Peng, Y., Wang, M., Liang, Y., Tang, S., et al. (2024). Curcumin suppresses colorectal cancer by induction of ferroptosis via regulation of p53 and solute carrier family 7 member 11/glutathione/glutathione peroxidase 4 signaling axis. Phytother. Res. 38 (8), 3954–3972. doi:10.1002/ptr.8258
Mohamed, G. A., Ibrahim, S. R. M., Hareeri, R. H., Binmahfouz, L. S., Bagher, A. M., Abdallah, H. M., et al. (2022). Garcinone E Mitigates oxidative inflammatory response and protects against experimental Autoimmune hepatitis via modulation of Nrf2/HO-1, NF-κB and TNF-α/JNK Axis. Nutrients 15 (1), 16. doi:10.3390/nu15010016
Mollica, A., Stefanucci, A., Feliciani, F., Cacciatore, I., Cornacchia, C., and Pinnen, F. (2012). Delivery methods of camptothecin and its hydrosoluble analogue irinotecan for treatment of colorectal cancer. Curr. Drug Deliv. 9 (2), 122–131. doi:10.2174/156720112800234558
Motyka, S., Jafernik, K., Ekiert, H., Sharifi-Rad, J., Calina, D., Al-Omari, B., et al. (2023). Podophyllotoxin and its derivatives: potential anticancer agents of natural origin in cancer chemotherapy. Biomed. Pharmacother. 158, 114145. doi:10.1016/j.biopha.2022.114145
Mou, L., Liang, B., Liu, G., Jiang, J., Liu, J., Zhou, B., et al. (2019). Berbamine exerts anticancer effects on human colon cancer cells via induction of autophagy and apoptosis, inhibition of cell migration and MEK/ERK signalling pathway. J. BUON 24 (5), 1870–1875.
Mou, Y., Wang, J., Wu, J., He, D., Zhang, C., Duan, C., et al. (2019). Ferroptosis, a new form of cell death: opportunities and challenges in cancer. J. Hematol. Oncol. 12 (1), 34. doi:10.1186/s13045-019-0720-y
Naeem, A., Hu, P., Yang, M., Zhang, J., Liu, Y., Zhu, W., et al. (2022). Natural products as anticancer agents: current Status and future perspectives. Molecules 27 (23), 8367. doi:10.3390/molecules27238367
Naz, I., Ramchandani, S., Khan, M. R., Yang, M. H., and Ahn, K. S. (2020). Anticancer potential of Raddeanin A, a natural triterpenoid isolated from Anemone raddeana Regel. Molecules 25 (5), 1035. doi:10.3390/molecules25051035
Newton, K., Strasser, A., Kayagaki, N., and Dixit, V. M. (2024). Cell death. Cell 187 (2), 235–256. doi:10.1016/j.cell.2023.11.044
Niu, P., Liu, F., Lei, F., Peng, J., Wang, Y., Zhao, J., et al. (2023). Breviscapine regulates the proliferation, migration, invasion, and apoptosis of colorectal cancer cells via the PI3K/AKT pathway. Sci. Rep. 13 (1), 9674. doi:10.1038/s41598-023-33792-x
Pradheepkumar, C. P., and Shanmugam, G. (1999). Anticancer potential of cleistanthin A isolated from the tropical plant Cleistanthus collinus. Oncol. Res. 11 (5), 225–232.
Qian, J., Cao, Y., Zhang, J., Li, L., Wu, J., Yu, J., et al. (2023). Tanshinone IIA alleviates the biological Characteristics of colorectal cancer via activating the ROS/JNK signaling pathway. Anticancer Agents Med. Chem. 23 (2), 227–236. doi:10.2174/1871520622666220421093430
Ramesh, P., and Medema, J. P. (2020). BCL-2 family deregulation in colorectal cancer: potential for BH3 mimetics in therapy. Apoptosis 25 (5-6), 305–320. doi:10.1007/s10495-020-01601-9
Ranjbary, A. G., Bagherzadeh, A., Sabbaghi, S. S., Faghihi, A., Karimi, D. N., Naji, S., et al. (2023). Chlorogenic acid induces apoptosis and cell-cycle arrest in colorectal cancer cells. Mol. Biol. Rep. 50 (12), 9845–9857. doi:10.1007/s11033-023-08854-y
Rao, Z., Zhu, Y., Yang, P., Chen, Z., Xia, Y., Qiao, C., et al. (2022). Pyroptosis in inflammatory diseases and cancer. Theranostics 12 (9), 4310–4329. doi:10.7150/thno.71086
Ren, B., Kwah, M. X., Liu, C., Ma, Z., Shanmugam, M. K., Ding, L., et al. (2021). Resveratrol for cancer therapy: challenges and future perspectives. Cancer Lett. 515, 63–72. doi:10.1016/j.canlet.2021.05.001
Saiprasad, G., Chitra, P., Manikandan, R., and Sudhandiran, G. (2014). Hesperidin induces apoptosis and triggers autophagic markers through inhibition of Aurora-A mediated phosphoinositide-3-kinase/Akt/mammalian target of rapamycin and glycogen synthase kinase-3 beta signalling cascades in experimental colon carcinogenesis. Eur. J. Cancer 50 (14), 2489–2507. doi:10.1016/j.ejca.2014.06.013
Shin, A. E., Giancotti, F. G., and Rustgi, A. K. (2023). Metastatic colorectal cancer: mechanisms and emerging therapeutics. Trends Pharmacol. Sci. 44 (4), 222–236. doi:10.1016/j.tips.2023.01.003
Song, J., Ham, J., Park, W., Song, G., and Lim, W. (2024). Osthole impairs mitochondrial metabolism and the autophagic flux in colorectal cancer. Phytomedicine 125, 155383. doi:10.1016/j.phymed.2024.155383
Song, J., He, G. N., and Dai, L. (2023). A comprehensive review on celastrol, triptolide and triptonide: Insights on their pharmacological activity, toxicity, combination therapy, new dosage form and novel drug delivery routes. Biomed. Pharmacother. 162, 114705. doi:10.1016/j.biopha.2023.114705
Sui, X., Zhang, R., Liu, S., Duan, T., Zhai, L., Zhang, M., et al. (2018). RSL3 drives ferroptosis through GPX4 Inactivation and ROS production in colorectal cancer. Front. Pharmacol. 9, 1371. doi:10.3389/fphar.2018.01371
Sun, M., Sun, M., and Zhang, J. (2021). Osthole: an overview of its sources, biological activities, and modification development. Med. Chem. Res. 30 (10), 1767–1794. doi:10.1007/s00044-021-02775-w
Sun, P., Liu, Y., Wang, Q., and Zhang, B. (2019). Astragaloside IV inhibits human colorectal cancer cell growth. Front. Biosci. Landmark Ed. 24 (3), 597–606. doi:10.2741/4738
Sun, Y. S., Wang, Z. W., Gao, Z., Zhao, W., Thakur, K., Zhong, Q., et al. (2022). Proanthocyanidin oligomers extract from hawthorn mediates cell cycle arrest, apoptosis, and lysosome vacuolation on HCT116 cells. Curr. Res. Food Sci. 5, 904–917. doi:10.1016/j.crfs.2022.05.009
Sung, H., Ferlay, J., Siegel, R. L., Laversanne, M., Soerjomataram, I., Jemal, A., et al. (2021). Global cancer Statistics 2020: GLOBOCAN Estimates of incidence and mortality worldwide for 36 cancers in 185 Countries. CA Cancer J. Clin. 71 (3), 209–249. doi:10.3322/caac.21660
Suraweera, C. D., Banjara, S., Hinds, M. G., and Kvansakul, M. (2022). Metazoans and intrinsic apoptosis: an Evolutionary analysis of the Bcl-2 family. Int. J. Mol. Sci. 23 (7), 3691. doi:10.3390/ijms23073691
Tong, X., Tang, R., Xiao, M., Xu, J., Wang, W., Zhang, B., et al. (2022). Targeting cell death pathways for cancer therapy: recent developments in necroptosis, pyroptosis, ferroptosis, and cuproptosis research. J. Hematol. Oncol. 15 (1), 174. doi:10.1186/s13045-022-01392-3
Totiger, T. M., Srinivasan, S., Jala, V. R., Lamichhane, P., Dosch, A. R., Gaidarski, A. A., et al. (2019). Urolithin A, a novel natural compound to target PI3K/AKT/mTOR pathway in Pancreatic cancer. Mol. Cancer Ther. 18 (2), 301–311. doi:10.1158/1535-7163.MCT-18-0464
Vasudevan, S. O., Behl, B., and Rathinam, V. A. (2023). Pyroptosis-induced inflammation and tissue damage. Semin. Immunol. 69, 101781. doi:10.1016/j.smim.2023.101781
Wang, G., Feng, C. C., Chu, S. J., Zhang, R., Lu, Y. M., Zhu, J. S., et al. (2015). Toosendanin inhibits growth and induces apoptosis in colorectal cancer cells through suppression of AKT/GSK-3β/β-catenin pathway. Int. J. Oncol. 47 (5), 1767–1774. doi:10.3892/ijo.2015.3157
Wang, G. Y., Zhang, L., Geng, Y. D., Wang, B., Feng, X. J., Chen, Z. L., et al. (2022). β-Elemene induces apoptosis and autophagy in colorectal cancer cells through regulating the ROS/AMPK/mTOR pathway. Chin. J. Nat. Med. 20 (1), 9–21. doi:10.1016/S1875-5364(21)60118-8
Wang, H. (2020). MicroRNAs and apoptosis in colorectal cancer. Int. J. Mol. Sci. 21 (15), 5353. doi:10.3390/ijms21155353
Wang, H., Wen, C., Chen, S., Li, W., Qin, Q., He, L., et al. (2021). ROS/JNK/C-Jun pathway is involved in Chaetocin induced colorectal cancer cells apoptosis and Macrophage phagocytosis Enhancement. Front. Pharmacol. 12, 729367. doi:10.3389/fphar.2021.729367
Wang, H. Y., Ge, J. C., Zhang, F. Y., Zha, X. Q., Liu, J., Li, Q. M., et al. (2022). Dendrobium officinale polysaccharide promotes M1 polarization of TAMs to inhibit tumor growth by targeting TLR2. Carbohydr. Polym. 292, 119683. doi:10.1016/j.carbpol.2022.119683
Wang, K., Huang, W., Sang, X., Wu, X., Shan, Q., Tang, D., et al. (2020). Atractylenolide I inhibits colorectal cancer cell proliferation by affecting metabolism and stemness via AKT/mTOR signaling. Phytomedicine 68, 153191. doi:10.1016/j.phymed.2020.153191
Wang, S., Liu, Z., Wang, L., and Zhang, X. (2009). NF-kappaB signaling pathway, inflammation and colorectal cancer. Cell Mol. Immunol. 6 (5), 327–334. doi:10.1038/cmi.2009.43
Wang, W., Zhao, X., Zhou, J., and Li, H. (2024). A novel antitumor mechanism of triptonide in colorectal cancer: inducing ferroptosis via the SLC7A11/GPX4 axis. Funct. Integr. Genomics 24 (4), 126. doi:10.1007/s10142-024-01402-2
Wang, Y., Bao, X., Zhao, A., Zhang, J., Zhang, M., Zhang, Q., et al. (2018). Raddeanin A inhibits growth and induces apoptosis in human colorectal cancer through downregulating the Wnt/β-catenin and NF-κB signaling pathway. Life Sci. 207, 532–549. doi:10.1016/j.lfs.2018.06.035
Wang, Y., Zhang, Z., Sun, W., Zhang, J., Xu, Q., Zhou, X., et al. (2022). Ferroptosis in colorectal cancer: potential mechanisms and effective therapeutic targets. Biomed. Pharmacother. 153, 113524. doi:10.1016/j.biopha.2022.113524
Wen, L., He, T., Yu, A., Sun, S., Li, X., Wei, J., et al. (2021). Breviscapine: a review on its Phytochemistry, pharmacokinetics and therapeutic effects. Am. J. Chin. Med. 49 (6), 1369–1397. doi:10.1142/S0192415X21500646
Wu, R., Zhao, J., Wei, P., Tang, M., Ma, Z., Zhao, Y., et al. (2023). Piper nigrum extract inhibits the growth of human colorectal cancer HT29 cells by inducing p53-mediated apoptosis. Pharm. (Basel) 16 (9), 1325. doi:10.3390/ph16091325
Wu, Y., Pi, D., Zhou, S., Yi, Z., Dong, Y., Wang, W., et al. (2023). Ginsenoside Rh3 induces pyroptosis and ferroptosis through the Stat3/p53/NRF2 axis in colorectal cancer cells. Acta Biochim. Biophys. Sin. (Shanghai) 55 (4), 587–600. doi:10.3724/abbs.2023068
Xiang, X., Tian, Y., Hu, J., Xiong, R., Bautista, M., Deng, L., et al. (2021). Fangchinoline exerts anticancer effects on colorectal cancer by inducing autophagy via regulation AMPK/mTOR/ULK1 pathway. Biochem. Pharmacol. 186:114475. doi:10.1016/j.bcp.2021.114475
Xia, J., Guo, P., Yang, J., Zhang, T., Pan, K., and Wei, H. (2024). Piperine induces autophagy of colon cancer cells: Dual modulation of AKT/mTOR signaling pathway and ROS production. Biochem. Biophys. Res. Commun. 728, 150340. doi:10.1016/j.bbrc.2024.150340
Xie, W., Peng, M., Liu, Y., Zhang, B., Yi, L., and Long, Y. (2023). Simvastatin induces pyroptosis via ROS/caspase-1/GSDMD pathway in colon cancer. Cell Commun. Signal 21 (1), 329. doi:10.1186/s12964-023-01359-y
Xu, H., Zhang, D., Wei, R., Zhou, Y., Dai, G., Li, J., et al. (2022). Gambogic acid induces pyroptosis of colorectal cancer cells through the GSDME-dependent pathway and Elicits an antitumor immune response. Cancers (Basel) 14 (22), 5505. doi:10.3390/cancers14225505
Xu, W., Wang, Z., Liu, T., Ma, X., Jiao, M., Zhao, W., et al. (2024). Eurycomanone inhibits osteosarcoma growth and metastasis by suppressing GRP78 expression. J. Ethnopharmacol. 335, 118709. doi:10.1016/j.jep.2024.118709
Xu, X., Lai, Y., and Hua, Z. C. (2019). Apoptosis and apoptotic body: disease message and therapeutic target potentials. Biosci. Rep. 39 (1), BSR20180992. doi:10.1042/BSR20180992
Yan, J., Wan, P., Choksi, S., and Liu, Z. G. (2021). Necroptosis and tumor progression. Trends Cancer 8 (1), 21–27. doi:10.1016/j.trecan.2021.09.003
Yang, A., Wu, B., He, W., Bicheng, H. U., Huang, H., Lei, X. U., et al. (2019). Effect of baicalin on the necroptosis of mouse colon cancer in vitro. Her. Med. 38 (02), 167–172.
Yang, C., Song, J., Hwang, S., Choi, J., Song, G., and Lim, W. (2021). Apigenin enhances apoptosis induction by 5-fluorouracil through regulation of thymidylate synthase in colorectal cancer cells. Redox Biol. 47, 102144. doi:10.1016/j.redox.2021.102144
Yang, J., Jiang, H., Wang, C., Yang, B., Zhao, L., Hu, D., et al. (2015). Oridonin triggers apoptosis in colorectal carcinoma cells and suppression of microRNA-32 expression augments oridonin-mediated apoptotic effects. Biomed. Pharmacother. 72, 125–134. doi:10.1016/j.biopha.2015.04.016
Yang, J., Mo, J., Dai, J., Ye, C., Cen, W., Zheng, X., et al. (2021). Cetuximab promotes RSL3-induced ferroptosis by suppressing the Nrf2/HO-1 signalling pathway in KRAS mutant colorectal cancer. Cell Death Dis. 12 (11), 1079. doi:10.1038/s41419-021-04367-3
Yang, T., Xu, W., Wei, X., Zhang, Z., Sun, Y., Liu, H., et al. (2022). Determination of ginsenoside Rh3 in rat plasma by LC-MS/MS and its application to a pharmacokinetic study. Biomed. Chromatogr. 36 (2), e5268. doi:10.1002/bmc.5268
Ye, G., Xu, M., Shu, Y., Sun, X., Mai, Y., Hong, Y., et al. (2022). A Quassinoid diterpenoid Eurycomanone from Eurycoma longifolia Jack exerts anti-cancer effect through autophagy inhibition. Molecules 27 (14), 4398. doi:10.3390/molecules27144398
Ye, K., Chen, Z., and Xu, Y. (2023). The double-edged functions of necroptosis. Cell Death Dis. 14 (2), 163. doi:10.1038/s41419-023-05691-6
Yu, G., Luo, H., Zhang, N., Wang, Y., Li, Y., Huang, H., et al. (2019). Loss of p53 sensitizes cells to Palmitic acid-induced apoptosis by reactive oxygen species accumulation. Int. J. Mol. Sci. 20 (24), 6268. doi:10.3390/ijms20246268
Yu, Y. Q., Gamez-Belmonte, R., Patankar, J. V., Liebing, E., and Becker, C. (2022). The role of programmed necrosis in colorectal cancer. Cancers (Basel) 14 (17), 4295. doi:10.3390/cancers14174295
Zeng, F., Nijiati, S., Tang, L., Ye, J., Zhou, Z., and Chen, X. (2023). Ferroptosis detection: from approaches to applications. Angew. Chem. Int. Ed. Engl. 62 (35), e202300379. doi:10.1002/anie.202300379
Zhang, H., and Jian, B. (2024). Resibufogenin: an emerging therapeutic compound with Multifaceted pharmacological effects - a comprehensive review. Med. Sci. Monit. 30, e942783. doi:10.12659/MSM.942783
Zhang, J., Wu, C., Gao, L., Du, G., and Qin, X. (2020). Astragaloside IV derived from Astragalus membranaceus: a research review on the pharmacological effects. Adv. Pharmacol. 87, 89–112. doi:10.1016/bs.apha.2019.08.002
Zhang, K., Zhou, X., Wang, J., Zhou, Y., Qi, W., Chen, H., et al. (2021). Dendrobium officinale polysaccharide triggers mitochondrial disorder to induce colon cancer cell death via ROS-AMPK-autophagy pathway. Carbohydr. Polym. 264, 118018. doi:10.1016/j.carbpol.2021.118018
Zhang, R., Yu, Q., Lu, W., Shen, J., Zhou, D., Wang, Y., et al. (2019). Grape seed procyanidin B2 promotes the autophagy and apoptosis in colorectal cancer cells via regulating PI3K/Akt signaling pathway. Onco Targets Ther. 12, 4109–4118. doi:10.2147/OTT.S195615
Zhang, Z., Shen, C., Zhou, F., and Zhang, Y. (2023). Shikonin potentiates therapeutic efficacy of oxaliplatin through reactive oxygen species-mediated intrinsic apoptosis and endoplasmic reticulum stress in oxaliplatin-resistant colorectal cancer cells. Drug Dev. Res. 84 (3), 542–555. doi:10.1002/ddr.22044
Zhang, Z. H., Li, M. Y., Wang, Z., Zuo, H. X., Wang, J. Y., Xing, Y., et al. (2020). Convallatoxin promotes apoptosis and inhibits proliferation and angiogenesis through crosstalk between JAK2/STAT3 (T705) and mTOR/STAT3 (S727) signaling pathways in colorectal cancer. Phytomedicine 68, 153172. doi:10.1016/j.phymed.2020.153172
Zhao, H., Tang, S., Tao, Q., Ming, T., Lei, J., Liang, Y., et al. (2023). Ursolic acid suppresses colorectal cancer by down-regulation of Wnt/β-catenin signaling pathway activity. J. Agric. Food Chem. 71 (9), 3981–3993. doi:10.1021/acs.jafc.2c06775
Zhao, Q., Bi, Y., Guo, J., Liu, Y., Zhong, J., Liu, Y., et al. (2021). Effect of pristimerin on apoptosis through activation of ROS/endoplasmic reticulum (ER) stress-mediated noxa in colorectal cancer. Phytomedicine 80, 153399. doi:10.1016/j.phymed.2020.153399
Zhao, Y., Ma, R., Wang, C., Hu, R., Wu, W., Sun, X., et al. (2023). CAPG interference induces apoptosis and ferroptosis in colorectal cancer cells through the P53 pathway. Mol. Cell Probes 71, 101919. doi:10.1016/j.mcp.2023.101919
Zheng, J. L., Wang, S. S., Shen, K. P., Chen, L., Peng, X., Chen, J. F., et al. (2021). Ursolic acid induces apoptosis and anoikis in colorectal carcinoma RKO cells. BMC Complement. Med. Ther. 21 (1), 52. doi:10.1186/s12906-021-03232-2
Zhou, P., Zhang, S., Wang, M., and Zhou, J. (2023). The induction mechanism of ferroptosis, necroptosis, and pyroptosis in inflammatory Bowel disease, colorectal cancer, and intestinal injury. Biomolecules 13 (5), 820. doi:10.3390/biom13050820
Zhu, M. L., Zhang, P. M., Jiang, M., Yu, S. W., and Wang, L. (2020). Myricetin induces apoptosis and autophagy by inhibiting PI3K/Akt/mTOR signalling in human colon cancer cells. BMC Complement. Med. Ther. 20 (1), 209. doi:10.1186/s12906-020-02965-w
Zong, L., Yang, Y., Zhang, J., Dai, L., Luo, Y., Yang, J., et al. (2021). Rhododendron molle G. Don extract induces apoptosis and inhibits migration in human colorectal cancer cells and potential anticancer components analysis. Molecules 26 (10), 2990. doi:10.3390/molecules26102990
Glossary
ALT-1 Atractylenolide I
ATG Arctigenin
AS-IV Astragaloside IV
ATO atorvastatin caleium
Bax Bcl2-Associated X
Bcl-2 B-cell lymphoma-2
BE Berbamine
CA Cleistanthin A
CGA Chlorogenic acid
COP Coptisine
CRC Colorectal cancer
CUR Curcumin
DOP Dendrobium officinale polysaccharide
EESP ethanol extract of Spica Prunellae
EN Eurycomanone
EVO Evodiamine
FA Ferulic acid
Fan Fangchinoline
GA Gambogic Acid
GAR E Garcinone E
GPX4 Glutathione peroxidase 4
GRh3 Ginsenoside Rh3
GSH Glutathione
Has Hibiscus Anthocyanins Extracts
HP Herba patriniae
IBC Isobavachalcone
IL-1β Interleukin-1β
LC3 light chain 3
MDA Malondialdehyde
MLKL Mixed lineage kinase domain-like protein
mTOR mammalian target of rapamycin
NF-κB Nuclear factor kappa-B
NLRP3 NLR pyrin domain-containing 3
Nrf2 NFE2-related factor 2
Ori Oridonin
PCD programmed cell death
PI3K Phosphatidylinositol 3-kinase
PPII Polyphyllin II
PT Podophyllotoxin
RA Raddeanin A
RBG Resibufogenin
RIPK receptor-interacting protein kinase
RSV Resveratrol
SHI Shikonin
SOD Superoxidedismutase
TanIIA Tanshinone IIA
TC Tagitinin C
TN triptonide)
TNF-α tumor necrosis factor-α
TRAIL TNF-related apoptosis-inducing ligand
TSN Toosendanin
UA Urolithin A
Keywords: CRC, PCD, natural product, mechanism, Chinese medicine, drug resistance
Citation: Zheng Y, Feng N, Li C and Li Z (2025) Natural products target programmed cell death signaling mechanisms to treat colorectal cancer. Front. Pharmacol. 16:1565332. doi: 10.3389/fphar.2025.1565332
Received: 23 January 2025; Accepted: 08 April 2025;
Published: 24 April 2025.
Edited by:
Michael Heinrich, University College London, United KingdomReviewed by:
Suwisit Manmuan, Burapha University, ThailandHector Esquer, University of Colorado, United States
Copyright © 2025 Zheng, Feng, Li and Li. This is an open-access article distributed under the terms of the Creative Commons Attribution License (CC BY). The use, distribution or reproduction in other forums is permitted, provided the original author(s) and the copyright owner(s) are credited and that the original publication in this journal is cited, in accordance with accepted academic practice. No use, distribution or reproduction is permitted which does not comply with these terms.
*Correspondence: Zuoqiang Li, YWxyYW1pQDE2My5jb20=