- School of Dentistry, Faculty of Medicine, Pontificia Universidad Catolica de Chile, Santiago, Chile
Orofacial pain, encompassing sensory and emotional discomfort in the facial and oral regions, is a multifaceted condition that significantly impacts patients’ quality of life. This review focuses on the role of Transient Receptor Potential Vanilloid 1 (TRPV1) channels in modulating orofacial pain and new ligands targeting this receptor. TRPV1 channels act as key mediators of nociception, responding to stimuli such as temperature, pH changes, and capsaicin molecules. Recent advancements in TRPV1-targeted therapeutics, including natural, synthetic, and protein-based molecules, offer promising strategies for pain management. This review analyzed studies related to TRPV1-mediated pain inhibition, including seven clinical trials and preclinical investigations. The compounds studied in these works demonstrated pain relief, although adverse effects were reported. TRPV1-targeted molecules represent a novel avenue for developing innovative pharmacological interventions, addressing the limitations of current therapies, and improving patient outcomes in managing orofacial pain.
1 Introduction
Orofacial pain corresponds to unpleasant sensory and emotional experiences associated with the soft tissue of the head, face, and oral cavity (Labanca et al., 2023a). The term includes different local conditions, from dental to temporomandibular, neuropathic, burning mouth, chronic idiopathic facial pain, and headaches (da Silva Araújo Júnior et al., 2021). The assessment, diagnosis, and management of orofacial pain may be associated with high healthcare costs, difficult access, and poor quality of life (Shueb et al., 2015). Even though oral diseases display different symptoms, many patients seek dental advice due to mouth and facial pain (Badel et al., 2019) (Rotpenpian and Yakkaphan, 2021a).
The thermal and mechanical sensitivity of the oral mucosa differs from elsewhere in the body. The oral mucous membrane has Aδ-fibers and unmyelinated C-fibers innervations that are polymodal sensory afferents encoding nociception (Setty and David, 2014; Hossain et al., 2019). However, the trigeminal pain system has singularities (Setty and David, 2014). After a tissue injury, inflammatory mediators’ expression and proinflammatory cytokines are increased, activating the transient receptor potential (TRP) ion channels to provide the excitatory trigger in neurons (Bíró and Szallasi, 2012; Chung et al., 2011). Thermo-TRPs are temperature-dependent ion channels first described in the late ‘90s (Cesare and McNaughton, 1996; Caterina et al., 1997).
Several TRP channels are thermal sensors corresponding to a subgroup of ten channels that are expressed in sensory nerve endings and skin cells (Caterina, 2007). Recently, it was discovered that TRPC5 is essential for inflammatory tooth pain and, together with TRPA1, can explain cold tooth pain (Bernal et al., 2021). This emphasizes the crucial role of the TRP family in sensing orofacial pain (Luo et al., 2021). TRPV1 is the most popular member of the TRP family, and its ability to respond to capsaicin underscores the receptor as a pharmacological target (Rosenbaum and Simon, 2007). Understanding the capsaicin binding site and mechanism of action contributes to both the pharmacological and genetic basis of pain sensation (Julius, 2013). The bindings of agonist compounds are sufficient to produce conformational modifications in the channel, transforming the non-conducting “close state” to a conducting “open state” (Henderson, 2013). Experimental data suggest that most drugs, both agonists and antagonists, act on TRPV1 near the binding site of capsaicin (Yang and Zheng, 2017). TRPV1’s sensory activation and modulation by agonists and antagonists will improve our comprehension of how different molecular detectors -within a single ion channel protein-interact with the channel’s gate and propose new drug formulations (Carnevale and Rohacs, 2016). This review summarizes the principal advances in discovering new molecules targeting TRPV1 and their complex roles in signaling orofacial pain diseases.
2 TRPV1 involvement in inflammatory dental pain
Dental caries is an ecological and non-communicable disease caused by acidic by-products from the bacterial fermentation of free sugars (Giacaman et al., 2022). It is the most common cause of orofacial pain caused by inflammatory reactions (Peres et al., 2019). If caries progresses and destroys hard tissue, it spreads to the pulp, causing inflammation, infection, and posterior tooth loss (Labanca et al., 2023b). Inflammation of the dental pulp or pulpitis is associated with thermal and mechanical hypersensitivity, for example, with the application of heat and cold stimuli (Gibbs et al., 2011a). Applying heat and cold stimuli evokes dental pain by activating the dental nociceptors in the dental pulp, which oversees the family of TRP channels (Gibbs et al., 2011b). The dental primary afferent neurons transmit the stimuli from the inferior alveolar nerve, a branch of the trigeminal nerve, specifically the mandibular branch (Rotpenpian and Yakkaphan, 2021a; Huff et al., 2023). After receiving excessive, uncontrollable inflammation due to pulpitis or a thermal stimulus, first-order neurons in the nerve will receive increased pain signals that arrive at the trigeminal ganglia and then are sent to the second-order neurons in the trigeminal nucleus caudalis. After that, the third-order neurons in the thalamus will receive the signals, and pain modulation occurs (Figure 1) (Rotpenpian and Yakkaphan, 2021a).
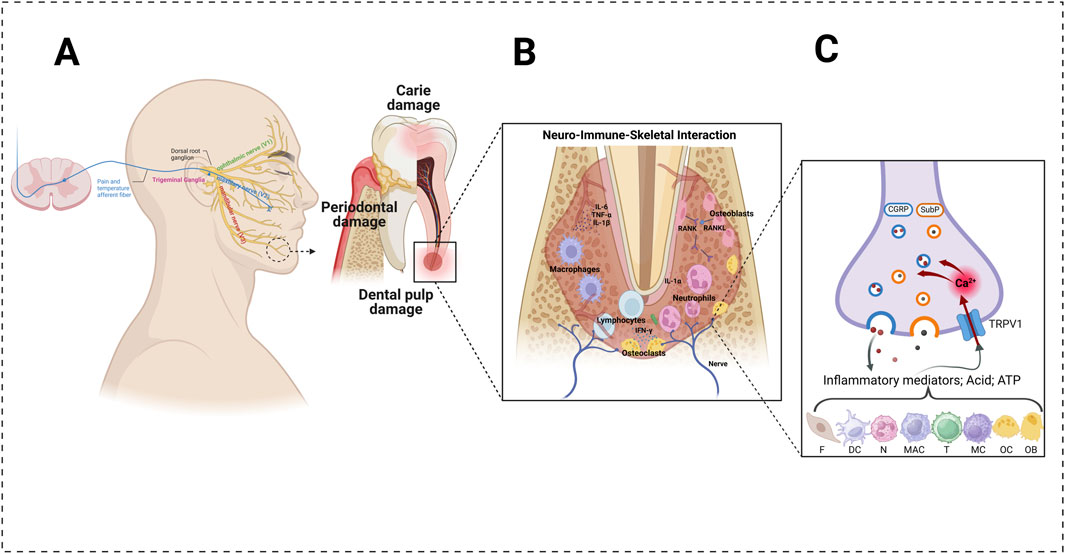
Figure 1. Involvement of the TRPV1 channel in the transduction of dental pain under inflammatory conditions. (A) Anatomy of the trigeminal afferents (left) and mandibular molar under the action of odontopathogenic factors (right). The alveolar bone undergoes resorption under infection. (B) In dental infection-inflammatory conditions, the various structures of the dentine–pulp–skeletal complex (e.g., odontoblasts, fibroblasts, dendritic cells, resident mast cells, neutrophils, monocytes, and T-lymphocytes) detect invading pathogens, leading to the initiation of an immune response. (C) Pro-inflammatory cytokines (e.g., interleukin-1β, tumor necrosis factor), inflammatory mediators (e.g., prostaglandin E2, bradykinin), and reactive oxygen metabolites (e.g., hydrogen peroxide, 8-hydroxy-2′-deoxyguanosine) can activate and sensitize the transient receptor potential vanilloid subtype 1 (TRPV1). Its activation induces calcium influx into nerve terminals and triggers the exocytosis of vesicles containing calcitonin gene-related peptide (CGRP) and substance P (SubP). The concerted activation of TRPV1 can generate an action potential, which is transmitted to the brain and leads to nociception. (F) fibroblast; DC: dendritic cell; MC: mast cell; MAC: macrophage; T: T-lymphocyte; N: neutrophil; OC: osteoclastic cell; OB: osteoblastic cell. Created with BioRender.com.
Recently, some investigators have discovered that TRP channels have been implicated in the transduction of external stimuli into pain signals, and these receptors have been detected in odontoblast and dental primary afferent neurons (Chung et al., 2011; Nilius and Szallasi, 2014). Gibbs et al. evaluated the percentage of rat trigeminal neurons that expressed TRPV1 and TRPV2 receptors; they observed that TRPV1 is expressed in 25% of the neuronal cell bodies in the trigeminal ganglia (TG) and TRPV2 in 15%. In the dental pulp, it is different; TRPV1 is expressed in 17% of the neurons and TRPV2 in about 50%, indicating that TRPV2 dominates the innervation compared with TRPV1 (Gibbs et al., 2011a). Another study suggests that TRPV1 is expressed in pulpal afferents in rat models (Chung et al., 2011). Furthermore, the expression of TRPV1 was observed in pulp inflammation evocated by pulp exposure; in the investigation of Cha et al., they observed that TRPV1 expression was significantly higher than the controls, which indicates that increased nociception signals in TG (Cha et al., 2020). Similar results have been observed by Chung et al., which established that applying lipopolysaccharide, a product of gram-negative bacteria, in the dentinal surface upregulates the expression of TRPV1 in mouse TG (Chung et al., 2011). Hossain et al. investigated the mechanism of the pain’s transduction. They observed that TRP channels act as polymodal receptors involved in the transduction of various external stimuli, and its mechanism consists of an external stimulus that activates and opens the TRP channels expressed in odontoblasts which produce the increase of intracellular Ca2+ concentration, so it transmits the nociceptor stimuli. Moreover, these odontoblasts can release ATP and glutamate, which act as agonists on the TRPV1 receptors of the adjacent nerve fibers of dental pulp afferents neurons and activate the sensory nerves of the dental pulp (Hossain et al., 2019). Purinergic receptors, primarily activated by ATP, and glutamatergic receptors, stimulated by glutamate, play a pivotal role in modulating TRPV1 activity. This influence can occur both directly, through dynamic interactions between receptors, and indirectly, by engaging complex intracellular signaling pathways (Jin et al., 2004).
Additionally, spontaneous pain in symptomatic pulpitis is due to the sensitization of dental pulp afferent neurons that produce hyperexcitability of the nerves (Hossain et al., 2019). This hyperexcitability is caused by the upregulation of the TRPs in these zones, which is seen in carious human teeth (Chung et al., 2011). This can also be explained because, under this inflammatory state, the threshold for the activation of TRP channels may decrease and cause hypersensitivity of the tooth to external stimuli (Hossain et al., 2019).
Some clinical studies have observed that TRPV1 acts as a mechanoreceptor in tooth pain, but it is controversial (De Petrocellis and Moriello, 2013). The influx of calcium through TRPV1 can significantly influence its activity, potentially modulating its sensitivity and interactions with other ion channels (Darré and Domene, 2015). Additionally, TRPV1 activation by low pH or TRPA1 activation can inhibit Piezo channels (Borbiro et al., 2015). This suggests that calcium influx through various pathways may modulate Piezo channel activity, highlighting the intricate crosstalk between these ion channels in sensory signaling. This mechanism explains that during inflammation, the extravasation of fluid from blood vessels can elevate the pulp pressure, which can excite the mechanoreceptors on the nerve fibers of the pulp and lead to spontaneous pain (Hossain et al., 2019).
3 Non-odontogenic orofacial pain
Orofacial pain (OP) is often associated with dental pathologies; nonetheless, the origin can be related to non-odontogenic disorders such as migraine, cancer, trigeminal neuralgias, and temporomandibular joint (TMJ) (Rotpenpian and Yakkaphan, 2021b). An estimated 25% of the population has experienced some form of OP, with the age range of 18–25 being the most common for this type of pain (Bender, 2018). The temporomandibular disorder includes the TMJ, masticatory muscles, and associated structures (Leite-Panissi et al., 2023a). Pain in the joint can be produced by disc disorders, diseases such as degenerative joint disease, systemic arthritis, osteonecrosis, neoplasm, and osteochondritis dissecans; fractures and congenital disorders can also produce it (Ahmad and Schiffman, 2016). Inflammation in the joint can lead to increased TRPV1, activated by heat, low pH, and capsaicin (Leite-Panissi et al., 2023a). It has been demonstrated that many TMJ TRPV-1 immunoreactive nerves are marked with a neuropeptide related to the calcitonin gene, which participates in nociception mechanisms and inflammation of this region (Leite-Panissi et al., 2023b) The activation of the TRPV1 channel can cause somatic inflammation both via peripheral sensitization and central sensitization (Park, 2015). There has been a great pharmaceutical effort to develop small-molecule inhibitors of TRP channels; however, there has been limited success (Park, 2015).
Another disorder that causes orofacial pain is trigeminal neuralgia (TN), which is described as a chronic neuropathic pain disorder characterized by recurrent, paroxysmal episodes of short-lasting severe intensity electric shock-like pain along the sensory distribution of the trigeminal nerve (Khawaja and Scrivani, 2023a). This type of pain causes a poor quality of life, and in some extreme cases, suicide has been attributed to the disorder (Cruccu et al., 2020). TN is a rare condition, with a prevalence of 0.03%–0.3%, and has an annual incidence of 4.3–8 per 100,000 with an average age of 53–57 years, with women the most affected by the disorder (Khawaja and Scrivani, 2023a). On the other hand, trigeminal neuropathic pain is associated with trigeminal nerve injury, which leads to chronic pain such as aching and burning (Urano et al., 2012a). It has been shown that TRPV1 afferents are involved in mechanical hyperalgesia in mice following a chronic constriction injury of the infraorbital nerve (Wang et al., 2020a). Inhibition of TRPV1 by injecting an antagonist of the channel attenuated mechanical hyperalgesia and ongoing pain (Wang et al., 2020a). Trigeminal neuropathic pain has also been associated with heat hyperalgesia, and as a form of treatment, capsazepine has been investigated, showing partial inhibition of heat hyperalgesia in a dose-dependent manner (Urano et al., 2012b).
As we mentioned before, OP can be caused by various non-odontogenic pathologies. One of them is cancer. Cancer is a disease that arises not from foreign substances invading our bodies but from the human cells, which transform into pathological organisms (Hausman, 2019). OP can be caused by regional or distant cancer (Romero-Reyes and Salvemini, 2016). Cancer in the orofacial region produces not only spontaneous pain but also affects the function of the region, having a negative impact on the quality of life of the patient (Romero-Reyes and Salvemini, 2016). The prevalence of pain in all cancer types is around 40%; nonetheless, pain in head and neck cancer has a prevalence of 70%, which increases to 90% in advanced cases (Khawaja and Scrivani, 2023b). TRPV1 channel has been linked to inflammation and calcium signaling (Bujak et al., 2019). It has been studied that chronic inflammation is associated with tumorigenesis, and aberrant calcium signaling promotes metastasis, proliferation, and cancer cell survival (Bujak et al., 2019). TRPV1 either promotes or inhibits cell death, depending on the type of cancer cell (Li et al., 2021). TRPV1 agonist/antagonist has been demonstrated to affect cell proliferation, cell death, and metastasis by activating TRPV1 channels and increasing intracellular calcium levels (Li et al., 2021). Migraine is another disease that causes OP, affecting more than 10% of the general population (Iannone et al., 2022a). TRPV1 contributes to this pain because it is expressed on dural nerve fibers of trigeminal origin in humans, which releases calcitonin gene-related peptide (CGRP) (Iannone et al., 2022b). CGRP is a member of the calcitonin family, in which levels in the cranial circulation are elevated during migraine attacks, and an intravenous infusion of CGRP causes migraine-like headaches. It has been demonstrated that capsaicin, a selective agonist of TRPV1, can be applied topically in the areas innervated by the trigeminal nerve, helping to improve the effects of cluster headaches and migraine (Dussor et al., 2014). This will occur due to capsaicin desensitization and, therefore, the reduced ability to release CGRP (De Logu et al., 2019a). TRPA1 is one of the primary transducers linked to the CGRP release, causing local inflammation, neuronal sensitization, and, therefore, pain (De Logu et al., 2019b).
Many patients who come in for a consult to treat pain in the orofacial region are wrongly diagnosed with toothache when they present a chronic OP condition (Renton, 2020). This can cause unnecessary surgery or giving wrong advice to the patient (Renton, 2020). To avoid inconsistent diagnosis, the clinician should first rule out odontogenic causes that could be causing the pain (Ando et al., 2022). Once this has been ruled out, the clinician should focus on non-odontogenic causes of OP, such as musculoskeletal, neurovascular, and neuropathic types of pain (Ando et al., 2022). It is essential that dentists maintain the possibilities open, expanding into examination techniques and consulting with another specialist if needed (De Laat, 2020). OP is an issue that is gaining more and more importance because it represents both a medical and social problem (Dubner et al., 2016). Inflammation causes the activation of many receptors on the terminals of afferent TG neurons, one of the main ones being TRPV1, which is expressed in orofacial tissues (Dubner, 2016). As mentioned before, TRPV1 is linked to many non-odontogenic disorders; it's because of this that it is important to keep all the possibilities open, knowing about all the possible causes of OP (De Laat, 2020).
4 Peripheral pathology
The international classification of orofacial pain mentions lesions or disorders affecting teeth and supporting areas as the main determinants of pain (Labanca et al., 2023c). Some viral infections of the gingival tissues can be ulcerated and painful to touch; this is increased by eating or drinking acidic, hot, or cold food or drinks, which also causes pain (Dubner, 2016). The oral mucosa also involves orofacial pain and implicated lesions such as ulcers, erosions, and vesicles (Rotpenpian and Yakkaphan, 2021b). One infection agent is herpes simplex virus (HSV). HSV is a neurotropic virus that becomes latent after infection, and it's located in the sensory ganglia (Kitagawa et al., 2013; Takasaki et al., 2000).
Kitagawa et al. (2013) demonstrated that herpes simplex virus (HSV) infection induces allodynia and hyperalgesia in a mouse model. To better understand the role of TRPV1 in arthritic and postherpetic pain, they examined the effects of JST-653, a selective TRPV1 antagonist. Their findings suggest that TRPV1 plays a significant role in the development of pain associated with these conditions, highlighting its potential as a therapeutic target for managing virus-induced and chronic inflammatory pain. Notably, the administration of JST-653 effectively attenuated mechanical hyperalgesia in the HSV-inoculated mice, providing strong evidence for TRPV1’s involvement in mediating pain hypersensitivity.
Orofacial pain can also be attributed to a lesion or disease of the cranial nerves (Rotpenpian and Yakkaphan, 2021b). The classification includes trigeminal neuropathic pain attributed to the varicella-zoster virus; it consists of a unilateral facial pain of less than 3 months duration that is distributed in one or more branches of the trigeminal nerve and is associated with clinical signs as acute herpes zoster (Classification of Chronic Pain, 2011). The reactivation of the varicella-zoster virus causes herpes zoster, and it causes acute herpetic pain (Takasaki et al., 2000). Sang et al. observed the expression of TRPV1 in skin herpes zoster lesions with immunofluorescent staining, which shows that it was increased and mainly present on epidermal keratinocytes compared with control skin, so they conclude that this receptor contributes to acute zoster pain and that there is a combination between nociceptive and neuropathic pain due to peripheral nerve sensitization (Han et al., 2016). Guedon & cols. inoculated rats with varicella-zoster virus, and through qPCR analysis of host gene expression, they observed a greater expression of TRPV1 in rats infected with the virus (Guedon et al., 2015). This is an important public health problem because only 30%-50% of patients can relieve their symptoms (Guedon et al., 2015).
Some traumas can cause chronic pain, and when they occur in the trigeminal nerve, they generate unilateral or bilateral facial persisting for more than 3 months (International Classification of Orofacial Pain, 2020). The origin of the trauma can be accidents, orthognathic surgery, or dental procedures (Kämmerer et al., 2024). It has been observed that TRPV1 plays an essential role in the development and maintaining of hyperalgesia induced by trigeminal neuropathic pain (J, 2005).
5 New molecules and possibilities
In clinical practice, various medications are used to treat pain, including opioids, nonsteroidal anti-inflammatory drugs (NSAIDs), antidepressants, antiepileptics, local anesthetics, muscle relaxants, and calcium channel blockers (Leeuw and Klasser, 2018; Khan et al., 2019a). However, these medications often provide unsatisfactory pain relief, may cause severe side effects, and can even lead to addiction (Furlan et al., 2006; Cavalli et al., 2019; Payne, 2000; Labianca et al., 2012). A logical approach to minimizing these side effects is to focus on peripheral pain receptors, where the pain originates. Among these receptors, TRPV1 stands out, as its activation can transmit and amplify pain through mechanisms that include increased neuronal excitability, the release of inflammatory mediators, and changes in neural plasticity (Gunthorpe and Szallasi, 2008). Therefore, TRPV1 has become an attractive target for developing new molecules to modulate this receptor (Jara-Oseguera et al., 2008).
Current studies are focused on molecules that act as agonists or antagonists of TRPV1 (LS and P, 2008; Premkumar and Sikand, 2008; Wong and Gavva, 2009). An agonist molecule initially binds to the receptor, activating it. This activation generates action potential, often perceived as itching, stinging, or burning sensations. However, when applied repeatedly or at high concentrations, it induces a long-lasting effect known as ‘desensitization’ of the TRPV1+ fibers. One example of a molecule used in this context is capsaicin (Arora et al., 2021). This compound has been utilized in clinical pain management, such as in cases of postherpetic neuralgia, HIV-related neuropathy, painful diabetic neuropathy, and chemotherapy-induced peripheral neuropathy (Derry et al., 2017).
A systematic review by Darry et al. (2017) evaluated the use of topical capsaicin for chronic neuropathic pain in clinical trials on postherpetic neuralgia, painful HIV-related neuropathy, and painful diabetic neuropathy. In cases of postherpetic neuralgia, patients reported feeling ‘much’ or ‘very much’ improved with high-concentration capsaicin (8%) compared to an ‘active’ placebo (0.04% topical capsaicin) after use for 8–12 weeks. In the case of painful HIV-related neuropathy, the studies included in the systematic review showed average reductions in pain intensity with the use of an 8% topical capsaicin patch for 2–12 weeks compared to the control. For painful diabetic peripheral neuropathy, the sole study reported 30% and 50% reductions in pain intensity over 2 to 8 and 2–12 weeks, respectively, when using 8% topical capsaicin compared to baseline (Derry et al., 2017).
Several studies have explored the use of capsaicin in orofacial pain management, mainly targeting conditions such as burning mouth syndrome (BMS) (Militão et al., 2021), oral neuropathic pain and trigeminal neuralgia (Epstein et al., 1994), oral mucositis (Berger et al., 1995a), and temporomandibular joint disorder (TMD) (Campbell et al., 2017). Ricken et al. (2021) demonstrated that a 180-day treatment with 0.025% capsaicin gel in BMS patients led to symptom reduction or complete remission and improved quality of life. In the study by Epstein et al. (1994), 31.6% of patients achieved complete remission of neuropathic pain, and an additional 31.6% reported partial remission. In contrast, topical treatments for trigeminal neuralgia showed no significant improvement. For oral mucositis, Berger et al. (1995b) (administered capsaicin in a candy (taffy) form, resulting in substantial pain relief for 11 patients undergoing cancer therapy. Regarding TMD, treatment with a high-dose (8%) capsaicin patch yielded significantly lower pain levels in TMD patients during the week following application compared to controls. Additionally, patients experienced a decreased thermal pain threshold 2 hours post-application, though it returned to baseline after 1 week.
On the other hand, TRPV1 antagonists block the receptor to relieve pain and itch sensations by inhibiting receptor activation and preventing nociceptive signals from traveling from peripheral sites to the central nervous system. Several novel small-molecule antagonists have been developed to prevent the activation of both native and heterologous TRPV1 channels (Table 1). These antagonists include BCTC, AMG9810, and SB-705498 (Gavva et al., 2005; Pomonis et al., 2003; Gibson et al., 2014). In orofacial pain studies, we identified two clinical trials that evaluated the antinociceptive activity of the molecules ABT-102 (Rowbotham et al., 2011a) and AZD1386 (Quiding et al., 2013a).
ABT-102 (Rowbotham et al., 2011a) was administered in doses of 4 mg, 2 mg, or 1 mg, given orally twice daily to male and female participants with no history of orofacial pain, oral trauma, or recent medication use that could interfere with the outcomes. Evaluations focused on the effects of ABT-102 on thermal sensation and induced pain. Assessments included quantitative thermosensory testing (QTT) performed on the abdominal skin and tongue, an oral liquid test (OLT) using heated water, prolonged thermal stimulation (LTS), and a hot water bath test (WBT). Additionally, adverse events such as core body temperature, vital signs, and laboratory tests were monitored. The main results from the Phase 1 clinical study indicated dose-dependent modulation of thermosensation, particularly in parameters such as the heat pain threshold (HPT) and OLT. ABT-102 significantly increased heat pain thresholds in both cutaneous and oral regions at all tested doses (1 mg, 2 mg, and 4 mg, twice daily), with the most pronounced effects observed 5 h after administration.
Regarding safety, adverse events were reported by 70.8% of participants receiving ABT-102, compared to 58.3% in the placebo group. The most common adverse events included increased body temperature, headache, nausea, feeling hot, and abdominal discomfort, all classified as mild or moderate. No serious adverse events, deaths, or clinically significant laboratory, ECG, or vital sign parameter changes were observed (Table 1) (Rowbotham et al., 2011b).
AZD1386 (Quiding et al., 2013b) was evaluated for its antinociceptive activity in patients undergoing surgical removal of at least one impacted mandibular third molar and, when indicated, the ipsilateral upper third molar. After surgery, patients who requested pain relief within 6 h were randomized to receive one of three treatments: 95 mg AZD1386 (solution), placebo (solution/capsule), or 500 mg naproxen (capsule). AZD1386 demonstrated rapid and significant pain relief compared to placebo, particularly in the first few hours after administration. Pain relief onset was faster with AZD1386 (median of 0.3 h for first perceptible relief) compared to placebo (1.3 h) and naproxen (0.8 h). Although AZD1386 showed efficacy in early pain relief measures (pain intensity difference PID% within the first hour), the primary outcome (weighted pain intensity difference over 8 h SPID%) was not significantly different from placebo. Approximately 85% of patients treated with AZD1386 reported perceptible pain relief, but about 80% still required rescue analgesics, comparable to the placebo group. Naproxen demonstrated superior efficacy to AZD1386, with fewer patients needing rescue analgesics (48%).
Regarding safety, there were no early discontinuations or serious adverse events. A slight increase in body temperature was observed with AZD1386 use (maximum of 38.1°C in two patients), but it had no significant clinical impact. Additionally, laboratory and ECG parameters showed no clinically relevant changes (Table 1) (Quiding et al., 2013b).
Civamide (cis-8-methyl-N-vanillyl-6-nonenamide), a TRPV1 agonist and neuronal calcium channel blocker, has been studied for orofacial pain management due to its ability to inhibit the neuronal release of excitatory neurotransmitters acting as a calcium channel blocker. A clinical trial (ClinicalTrials.gov ID: NCT01886313) (Study Details | Civamide Nasal) registered in March 2014 evaluated Civamide Nasal Spray 0.01% [20 µg/dose (10 µL per nostril, twice daily)] for 6 weeks in patients diagnosed with postherpetic neuralgia of the trigeminal nerve. The trial included 11 participants: 6 in the Civamide group and 5 in the placebo group. At baseline, mean pain scores were 6.4 (±0.84) for the Civamide group and 5.2 (±1.02) for the placebo group; by week 6, scores instantly decreased to 5.3 (±1.24) and 3.1 (±2.13), respectively. Thus, the small sample size limited the study’s statistical power to detect significant differences between groups. The more frequently reported adverse effects were site burning, irritation, sneezing, and pruritus after Civamide use.
The study (ClinicalTrials.gov ID: NCT00281684) used a third molar extraction model to test the efficacy of the TRPV1 antagonist SB705498 in controlling pain after surgery. A total of 145 participants were divided into four groups: placebo (n = 37), SB705498 at 400 mg (n = 36) and 1,000 mg (n = 34), and co-codamol (n = 38). Pain intensity was assessed using the visual analog scale (VAS) and verbal rating Scale (VRS) over 10 h post-treatment. The data from VAS demonstrated that co-codamol and SB705498 400 mg outperformed better than placebo and SB705498 1,000 mg. VRS scores supported these findings, with Co-Codamol showing the most remarkable efficacy (0.5 ± 1.18), followed by SB705498 1,000 mg (0.6 ± 0.53), SB705498 400 mg (0.8 ± 1.04), and placebo (1.1 ± 0.83). SB705498 was well-tolerated for both doses, with the highest frequency of side effects in the 1,000 mg group. Common adverse effects included headache, fever, warmth sensation, and mild gastrointestinal symptoms. The authors reported no serious treatment-related adverse effects.
Thus, preclinical studies investigating new molecules targeting TRPV1 to treat orofacial pain are presented here (Table 2) (Arora et al., 2021; Bai et al., 2018; Santos et al., 2022; Park et al., 2009; Melo et al., 2017; de Oliveira et al., 2022a; Guo et al., 2019; Gualdani et al., 2015; Hitomi et al., 2016; Kun et al., 2012; de la Rosa-Lugo et al., 2017; Damasceno et al., 2016; Wang et al., 2020b; Biggs et al., 2008a; Ando et al., 2020; Yeon et al., 2010; Wu et al., 2010; Anderson et al., 2014; Soares et al., 2019; Wanasuntronwong et al., 2022; Neubert et al., 2008; Lee et al., 2018; Bai et al., 2021; Rocha et al., 2022a). It identified 24 studies that used different animal models, with rats (n = 14) and mice (n = 9) being the most frequently employed species. The Zebrafish model for nociception evaluation was tested in a few (n = 3) studies, but it is considered a recent model but may answer a variety of tools to study pain and associated behavior (Leite-Panissi et al., 2023b; de Oliveira et al., 2022b; Rocha et al., 2022b). One (n = 1) study evaluated the efficacy of SB-750364 TRPV1 antagonist on injury-induced discharge in the lingual nerve in a ferret model. The data revealed a spontaneous activity reduction in fine filaments from terminal nerves in 61% (Biggs et al., 2008b).
Among the molecules investigated, capsaicin and resiniferatoxin (RTX) were extensively evaluated, appearing in multiple studies (2 articles each). Most of the substances tested were of natural origin, such as eucalyptol, oleanolic acid, curcumin, menthol, capsaicin, eugenol, and cannabidiol, reflecting the interest in bioactive plant compounds and their antinociceptive properties. In addition, synthetic compounds such as ADM_12 and SB-750364 and emerging therapies, including stem cells derived from dental pulp (SHED) and Lentivirus containing a TRPV1 shRNA sequence, were also addressed, exploring new intervention possibilities for orofacial pain. Pain induction models varied from chemical stimuli, such as capsaicin, formalin, and glutamate, to neuropathic models involving nerve injuries or transections, such as chronic infraorbital nerve constriction. Most studies reported promising results, demonstrating that the evaluated molecules significantly reduced nociceptive or hyperalgesia behaviors, modulating the TRPV1 receptor. These data highlight the potential of new therapeutic approaches for orofacial pain conditions mediated by this receptor. Figure 2 shows all molecules and proteins studied in the articles included in this review.
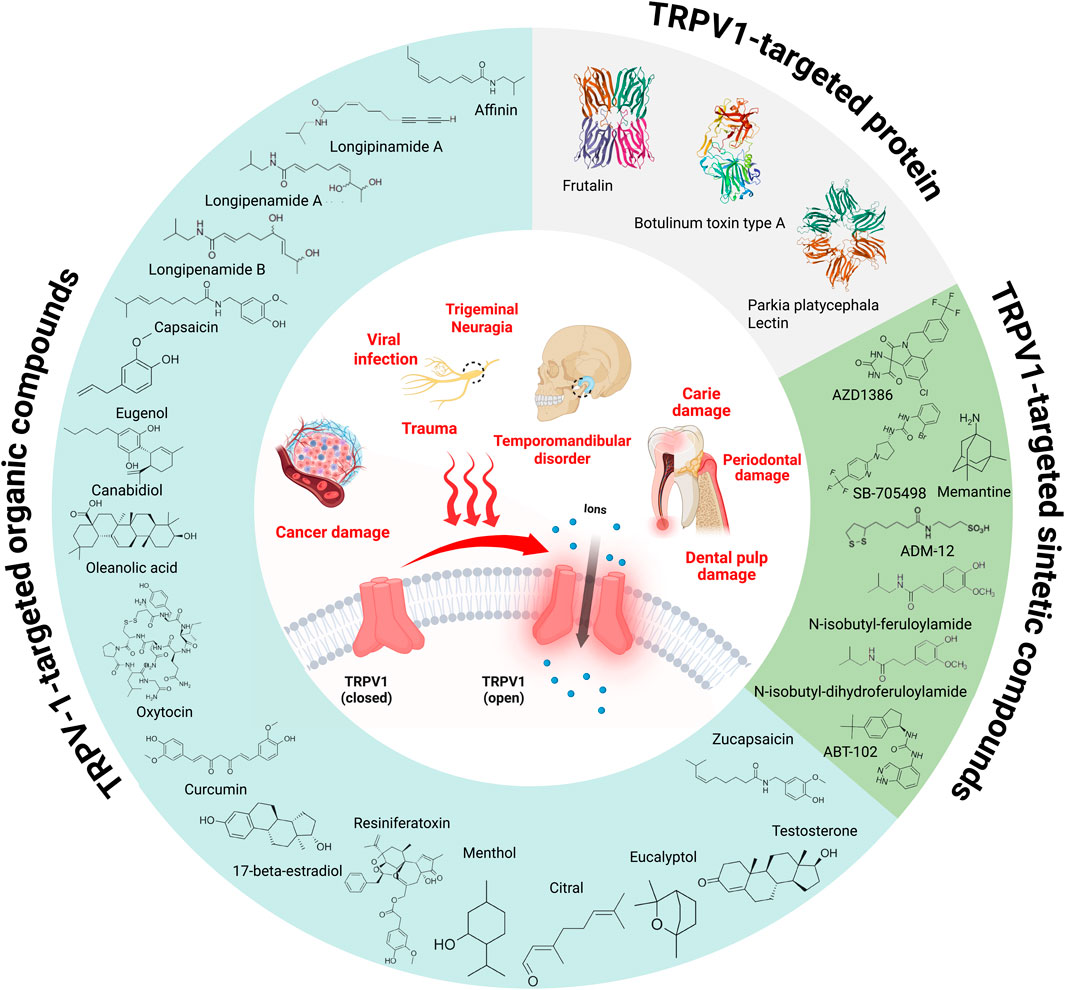
Figure 2. Schematic representation of orofacial factors contributing to TRPV1 activation (inner circle) alongside chemical and protein molecules studied in the articles included in this review. Chemical structures were drawn using Marvin - Chemical Drawing Software, while protein structures were retrieved from the RCSB Protein Data Bank (RCSB PDB). The figure was created with BioRender.com.
6 Review highlights and discussion
Patients undergoing orofacial pain often face significant expenses, averaging £333 per 6-month period, including treatment fees, medication, and travel costs. Furthermore, the indirect costs to employers are even more pronounced, with absenteeism contributing an average of £1,242 per person over the same period (Breckons et al., 2018). Recent findings also emphasize the variability of financial impacts based on the severity of persistent orofacial pain. Patients classified with high-graded chronic pain scale (GCPS) status experience a £366 increase in healthcare costs within 6 months compared to those with lower GCPS levels (Durham et al., 2016). These findings underscore the multifaceted impact of orofacial pain, which extends beyond physical suffering to include significant social consequences.
Diagnosing and managing chronic orofacial pain remains a significant clinical challenge due to its multifaceted nature and the often-ambiguous overlap of symptoms with other conditions (Classification of Chronic Pain, 2011; Diatchenko et al., 2005). Chronic orofacial pain is notably complex because of its multifactorial etiology (Classification of Chronic Pain, 2011). As previously discussed, this type of pain can stem from various underlying issues, including inflammatory dental pain, non-odontogenic orofacial pain, and peripheral pathologies (Giacaman et al., 2022; Khan et al., 2019b).
Currently, one of the most pressing challenges in orofacial pain management lies in the use of medications (Abd-Elsayed et al., 2024). Despite advancements, the limitations in pain control are evident, with a notable incidence of adverse reactions that pose substantial risks to patients (Häggman-Henrikson et al., 2017). The use of analgesics, for example, is fraught with concerns regarding addiction, tolerance, and hypersensitivity reactions (Furlan et al., 2006; Cavalli et al., 2019; Payne, 2000; Labianca et al., 2012). Furthermore, these medications often result in additional side effects, such as gastrointestinal bleeding and an increased risk of cardiovascular events (Cavalli et al., 2019; Porreca and Ossipov, 2009). Anti-inflammatory therapies, while commonly employed, frequently offer only limited relief, necessitating supplementary treatments to achieve satisfactory pain management (Hersh et al., 2020). Given these challenges, there is a growing interest in targeted therapeutic approaches aimed at addressing the root cause of pain. Emerging research focuses on identifying molecules that can directly modulate nociceptive pathways, particularly those involving terminal regions where pain originates (Gunthorpe and Szallasi, 2008). A promising study area involves targeting receptors such as TRPV1, which are implicated in the transmission and modulation of pain signals (Wang et al., 2020b; Zhang et al., 2021; Luo et al., 2018). These novel strategies hold the potential to overcome the limitations of traditional therapies, offering a more precise and practical approach to managing chronic orofacial pain (Rotpenpian and Yakkaphan, 2021b; Dubner, 2016).
According to the articles in this review, the various molecules studied with TRPV1 activity demonstrated antinociceptive activity in preclinical and clinical trials (Jara-Oseguera et al., 2008). However, during these tests, adverse effects on body temperature (Tb), primarily hyperthermia, were repeatedly observed (Rowbotham et al., 2011b; Quiding et al., 2013c). Due to these side effects, clinical trials and the subsequent advancement toward the commercialization of these drugs have been delayed or halted (De Petrocellis and Moriello, 2013). One potential solution is to develop molecules that act on TRPV1 with sufficient analgesic efficacy but without altering Tb, such as compounds that do not block the activation of hTRPV1 by protons and temperature but remain potent blockers of capsaicin (CAP) or those with low blocking potency for protons and heat while maintaining high CAP-blocking potency (Garami et al., 2020). Another alternative is the combination of TRPV1 antagonists with antipyretic drugs, though this approach requires further research, as the temperature alteration linked to TRPV1 is not mediated by cyclooxygenase (Engström et al., 2013). A promising alternative might involve blocking sympathetic activation, as Garami et al. (2020) suggested.
Another point worth discussing is the predominant use of male animals in research studies (Santos et al., 2022; Park et al., 2009; Guo et al., 2019; Hitomi et al., 2016; Kun et al., 2012; Ando et al., 2020; Yeon et al., 2010; Anderson et al., 2014; Wanasuntronwong et al., 2022; Lee et al., 2018; Bai et al., 2021; Hwang et al., 2020). This approach raises controversy, given that women report orofacial pain more frequently than men (Häggman-Henrikson et al., 2020). Orofacial pain is approximately twice as prevalent in adult women (20–70 years) compared to men in the same age range, with an odds ratio (OR) of 2.58 (95% CI: 2.48–2.68). Furthermore, women exhibit a significantly higher risk of developing orofacial pain (OR 2.37) and persistent pain (OR 2.56) (Häggman-Henrikson et al., 2020). Temporomandibular disorders (TMD) are also more common in women, who are 2.2 times more likely to experience them (OR = 2.24).
Regarding specific diagnoses, women are 2.08 times more likely to develop muscular disorders, while the prevalence of disc displacement is 1.6 times higher in women. Additionally, conditions such as arthralgia, arthritis, or arthrosis are 2.09 times more likely to occur in women than in men (Bueno et al., 2018). These findings underscore the importance of considering gender differences in future studies, as data obtained exclusively from men cannot be validly generalized to women. Although several molecules with antinociceptive activity targeting TRPV1 have been proposed to manage orofacial pain, some limitations still prevent these molecules from being effectively made available to the population. Therefore, further studies are needed to understand their mechanisms of action better, minimize adverse effects such as alterations in body temperature, and ensure their long-term safety and efficacy.
7 Conclusion
In summary, we have reviewed a large body of evidence on TRPV1 in orofacial pain, mainly focused on the advances in discovering new molecules targeting TRPV1 and their contributions to pain behaviors. Orofacial pain is one of the most debilitating human diseases and represents a social and economic burden for our society. Managing orofacial pain is still a significant challenge for healthcare professionals, and the potential for work-related disability further contributes to a considerable economic strain on the healthcare system.
Author contributions
AC: Supervision, Writing – original draft, Writing – review and editing. NC: Conceptualization, Writing – original draft, Writing – review and editing. FE: Conceptualization, Writing – original draft, Writing – review and editing. BB: Conceptualization, Funding acquisition, Supervision, Writing – original draft, Writing – review and editing.
Funding
The author(s) declare that financial support was received for the research and/or publication of this article. The research leading to these results received funding from ANID (Agencia Nacional de Investigación y Desarrollo) under grant agreement FONDECYT de Iniciación #11230490 to BB and a postdoctoral fellowship from VRI-UC to ACMA.
Conflict of interest
The authors declare that the research was conducted in the absence of any commercial or financial relationships that could be construed as a potential conflict of interest.
Generative AI statement
The author(s) declare that no Generative AI was used in the creation of this manuscript.
Publisher’s note
All claims expressed in this article are solely those of the authors and do not necessarily represent those of their affiliated organizations, or those of the publisher, the editors and the reviewers. Any product that may be evaluated in this article, or claim that may be made by its manufacturer, is not guaranteed or endorsed by the publisher.
References
Abd-Elsayed, A., Stark, C. W., Topoluk, N., Isaamullah, M., Uzodinma, P., Viswanath, O., et al. (2024). A brief review of complex regional pain syndrome and current management. Ann. Med. 56, 2334398. doi:10.1080/07853890.2024.2334398
Ahmad, M., and Schiffman, E. L. (2016). Temporomandibular joint disorders and orofacial pain. Dent. Clin. North Am. 60, 105–124. doi:10.1016/j.cden.2015.08.004
Anderson, E. M., Jenkins, A. C., Caudle, R. M., and Neubert, J. K. (2014). The effects of a co-application of menthol and capsaicin on nociceptive behaviors of the rat on the operant orofacial pain assessment device. PLoS One 9, e89137. doi:10.1371/journal.pone.0089137
Ando, A., Han, P. P., and Patel, S. (2022). Thinking outside the tooth: diagnosis and management of patients with neuropathic orofacial pain. Dent. Clin. North Am. 66, 229–244. doi:10.1016/j.cden.2022.01.003
Ando, M., Hayashi, Y., Hitomi, S., Shibuta, I., Furukawa, A., Oto, T., et al. (2020). Oxytocin-dependent regulation of trps expression in trigeminal ganglion neurons attenuates orofacial neuropathic pain following infraorbital nerve injury in rats. Int. J. Mol. Sci. 21, 9173–9190. doi:10.3390/ijms21239173
Arora, V., Campbell, J. N., and Chung, M. K. (2021). Fight fire with fire: neurobiology of capsaicin-induced analgesia for chronic pain. Pharmacol. Ther. 220, 107743. doi:10.1016/j.pharmthera.2020.107743
Badel, T., Zadravec, D., Kes, V. B., Smoljan, M., Lovko, S. K., Zavoreo, I., et al. (2019). Orofacial pain – diagnostic and therapeutic challenges. Acta Clin. Croat. 58, 82–89. doi:10.20471/acc.2019.58.s1.12
Bai, X., Zhang, X., Wang, C., Liu, Y., Liu, X., Fan, Y., et al. (2021). Stem cells from human exfoliated deciduous teeth attenuate trigeminal neuralgia in rats. Stem Cells Int. 2021, 8819884. doi:10.1155/2021/8819884
Bai, X., Zhang, X., and Zhou, Q. (2018). Effect of testosterone on TRPV1 expression in a model of orofacial myositis pain in the rat. J. Mol. Neurosci. 64, 93–101. doi:10.1007/s12031-017-1009-7
Bender, S. D. (2018). Orofacial pain: where we are and where we are going. Dent. Clin. North Am. 62, ix–x. doi:10.1016/j.cden.2018.08.001
Berger, A., Henderson, M., Nadoohnan, W., Duff, V., Cooper, D., Saberski, L., et al. (1995a). Oral capsaicin provides temporary relief for oral mucositis pain secondary to chemotherapy/radiation therapy, J. Pain Symptom Manage., vol. 10. 243, 248. doi:10.1016/0885-3924(94)00130-D
Berger, A., Henderson, M., Nadoolman, W., Duffy, V., Cooper, D., Saberski, L., et al. (1995b). Oral capsaicin provides temporary relief for oral mucositis pain secondary to chemotherapy/radiation therapy. J. Pain Symptom Manage 10, 243–248. doi:10.1016/0885-3924(94)00130-D
Bernal, L., Sotelo-Hitschfeld, P., König, C., Sinica, V., Wyatt, A., Winter, Z., et al. (2021). Odontoblast TRPC5 channels signal cold pain in teeth. Sci. Adv. 7, eabf5567. doi:10.1126/sciadv.abf5567
Biggs, J. E., Yates, J. M., Loescher, A. R., Clayton, N. M., Robinson, P. P., and Boissonade, F. M. (2008a). Effect of SB-750364, a specific TRPV1 receptor antagonist, on injury-induced ectopic discharge in the lingual nerve. Neurosci. Lett. 443, 41–45. doi:10.1016/j.neulet.2008.06.088
Biggs, J. E., Yates, J. M., Loescher, A. R., Clayton, N. M., Robinson, P. P., and Boissonade, F. M. (2008b). Effect of SB-750364, a specific TRPV1 receptor antagonist, on injury-induced ectopic discharge in the lingual nerve. Neurosci. Lett. 443, 41–45. doi:10.1016/J.NEULET.2008.06.088
Borbiro, I., Badheka, D., and Rohacs, T. (2015). Activation of TRPV1 channels inhibits mechanosensitive Piezo channel activity by depleting membrane phosphoinositides. Sci. Signal 8, ra15. doi:10.1126/SCISIGNAL.2005667
Breckons, M., Shen, J., Bunga, J., Vale, L., and Durham, J. (2018). DEEP study: indirect and out-of-pocket costs of persistent orofacial pain. J. Dent. Res. 97, 1200–1206. doi:10.1177/0022034518773310
Bueno, C. H., Pereira, D. D., Pattussi, M. P., Grossi, P. K., and Grossi, M. L. (2018). Gender differences in temporomandibular disorders in adult populational studies: a systematic review and meta-analysis. J. Oral Rehabil. 45, 720–729. doi:10.1111/joor.12661
Bujak, J. K., Kosmala, D., Szopa, I. M., Majchrzak, K., and Bednarczyk, P. (2019). Inflammation, cancer and immunity—implication of TRPV1 channel. Front. Oncol. 9, 1087. doi:10.3389/fonc.2019.01087
Campbell, B. K., Fillingim, R. B., Lee, S., Brao, R., Price, D. D., and Neubert, J. K. (2017). Effects of high-dose capsaicin on TMD subjects: a randomized clinical study. JDR Clin. Trans. Res. 2, 58–65. doi:10.1177/2380084416675837
Carnevale, V., and Rohacs, T. (2016). TRPV1: a target for rational drug design. Pharm. (Basel) 9, 52. doi:10.3390/ph9030052
Caterina, M. J. (2007). Transient receptor potential ion channels as participants in thermosensation and thermoregulation. Am. J. Physiology-Regulatory, Integr. Comp. Physiology 292, R64–R76. doi:10.1152/ajpregu.00446.2006
Caterina, M. J., Schumacher, M. A., Tominaga, M., Rosen, T. A., Levine, J. D., and Julius, D. (1997). The capsaicin receptor: a heat-activated ion channel in the pain pathway. Nature 389, 816–824. doi:10.1038/39807
Cavalli, E., Mammana, S., Nicoletti, F., Bramanti, P., and Mazzon, E. (2019). The neuropathic pain: an overview of the current treatment and future therapeutic approaches. Int. J. Immunopathol. Pharmacol. 33, 2058738419838383. doi:10.1177/2058738419838383
Cesare, P., and McNaughton, P. (1996). A novel heat-activated current in nociceptive neurons and its sensitization by bradykinin. Proc. Natl. Acad. Sci. 93, 15435–15439. doi:10.1073/pnas.93.26.15435
Cha, M., Sallem, I., Jang, H. W., and Jung, I. Y. (2020). Role of transient receptor potential vanilloid type 1 in the trigeminal ganglion and brain stem following dental pulp inflammation. Int. Endod. J. 53, 62–71. doi:10.1111/iej.13204
Chung, M. K., Lee, J., Duraes, G., and Ro, J. Y. (2011). Lipopolysaccharide-induced pulpitis up-regulates TRPV1 in trigeminal ganglia. J. Dent. Res. 90, 1103–1107. doi:10.1177/0022034511413284
Classification of Chronic Pain (2011). Revis. - Int. Assoc. Study Pain (IASP). doi:10.1097/j.pain.0000000000001390
Cruccu, G., Di Stefano, G., and Truini, A. (2020). Trigeminal neuralgia. N. Engl. J. Med. 383, 754–762. doi:10.1056/NEJMra1914484
Damasceno, MBMV, De Melo Júnior, JDMA, Santos, SAAR, Melo, L. T. M., Leite, L. H. I., Vieira-Neto, A. E., et al. (2016). Frutalin reduces acute and neuropathic nociceptive behaviours in rodent models of orofacial pain. Chem. Biol. Interact. 256, 9–15. doi:10.1016/j.cbi.2016.06.016
Darré, L., and Domene, C. (2015). Binding of capsaicin to the TRPV1 ion channel. Mol. Pharm. 12, 4454–4465. doi:10.1021/acs.molpharmaceut.5b00641
da Silva Araújo Júnior, C. A., Rebelo Vieira, J. M., Rebelo, M. A. B., Herkrath, F. J., Herkrath, A. P. C. de Q., de Queiroz, A. C., et al. (2021). The influence of change on sense of coherence on dental services use among adolescents: a two-year prospective follow-up study. BMC Oral Health 21, 1–10. doi:10.1186/S12903-021-02026-9/TABLES/4
De Laat, A. (2020). Differential diagnosis of toothache to prevent erroneous and unnecessary dental treatment. J. Oral Rehabil. 47, 775–781. doi:10.1111/joor.12946
de la Rosa-Lugo, V., Acevedo-Quiroz, M., Déciga-Campos, M., and Rios, M. Y. (2017). Antinociceptive effect of natural and synthetic alkamides involves TRPV1 receptors. J. Pharm. Pharmacol. 69, 884–895. doi:10.1111/jphp.12721
De Logu, F., Landini, L., Janal, M. N., Li Puma, S., De Cesaris, F., Geppetti, P., et al. (2019a). Migraine-provoking substances evoke periorbital allodynia in mice. J. Headache Pain 20, 18. doi:10.1186/S10194-019-0968-1
De Logu, F., Nassini, R., Landini, L., and Geppetti, P. (2019b). Pathways of CGRP release from primary sensory neurons. Handb. Exp. Pharmacol., vol. 255, Springer New York LLC; 65–84. doi:10.1007/164_2018_145
de Oliveira, L. G., Santos, SAAR, dos Santos Silva, R. R., Teixeira, C. S., and Campos, A. R. (2022a). Parkia platycephala lectin (PPL) inhibits orofacial nociception responses via TRPV1 modulation. Molecules 27, 7506. doi:10.3390/molecules27217506
de Oliveira, L. G., Santos, SAAR, dos Santos Silva, R. R., Teixeira, C. S., and Campos, A. R. (2022b). Parkia platycephala lectin (PPL) inhibits orofacial nociception responses via TRPV1 modulation. Molecules 27, 7506. doi:10.3390/MOLECULES27217506
De Petrocellis, L., and Moriello, A. S. (2013). Modulation of the TRPV1 channel: current clinical trials and recent patents with focus on neurological conditions. Recent Pat. CNS Drug Discov. 8, 180–204. doi:10.2174/1574889808666131209124012
Derry, S., Rice, A. S. C., Cole, P., Tan, T., and Moore, R. A. (2017). Topical capsaicin (high concentration) for chronic neuropathic pain in adults. Cochrane Database Syst. Rev. 2017, CD007393. doi:10.1002/14651858.CD007393.pub4
Diatchenko, L., Slade, G. D., Nackley, A. G., Bhalang, K., Sigurdsson, A., Belfer, I., et al. (2005). Genetic basis for individual variations in pain perception and the development of a chronic pain condition. Hum. Mol. Genet. 14, 135–143. doi:10.1093/hmg/ddi013
Dubner, R. (2016). Emerging research on orofacial pain. J. Dent. Res. 95, 1081–1083. doi:10.1177/0022034516661704
Dubner, R., Hargreaves, K. M., and Ruparel, S. (2016). Role of oxidized lipids and TRP channels in orofacial pain and inflammation. J. Dent. Res. 95, 1117–1123. doi:10.1177/0022034516653751
Durham, J., Shen, J., Breckons, M., Steele, J. G., Araujo-Soares, V., Exley, C., et al. (2016). Healthcare cost and impact of persistent orofacial pain: the DEEP study cohort. J. Dent. Res. 95, 1147–1154. doi:10.1177/0022034516648088
Dussor, G., Yan, J., Xie, J. Y., Ossipov, M. H., Dodick, D. W., and Porreca, F. (2014). Targeting TRP channels for novel migraine therapeutics. ACS Chem. Neurosci. 5, 1085–1096. doi:10.1021/cn500083e
Engström, R. L., Wilhelms, D. B., Eskilsson, A., Vasilache, A. M., Elander, L., Engblom, D., et al. (2013). Acetaminophen reduces lipopolysaccharide-induced fever by inhibiting cyclooxygenase-2. Neuropharmacology 71, 124–129. doi:10.1016/j.neuropharm.2013.03.012
Epstein, J. B., Marcoe, J. H., and Columbia, B. (1994). Topical application of capsaicin for treatment of oral neuropathic pain and trigeminal neuralgia. Oral Surg. Oral Med. Oral Pathol. 77, 135–140. doi:10.1016/0030-4220(94)90275-5
Furlan, A. D., Sandoval, J. A., Mailis-Gagnon, A., and Tunks, E. (2006). Opioids for chronic noncancer pain: a meta-analysis of effectiveness and side effects. CMAJ Can. Med. Assoc. J. 174, 1589–1594. doi:10.1503/cmaj.051528
Garami, A., Shimansky, Y. P., Rumbus, Z., Vizin, R. C. L., Farkas, N., Hegyi, J., et al. (2020). Hyperthermia induced by transient receptor potential vanilloid-1 (TRPV1) antagonists in human clinical trials: insights from mathematical modeling and meta-analysis. Pharmacol. Ther. 208, 107474. doi:10.1016/j.pharmthera.2020.107474
Gavva, N. R., Tamir, R., Qu, Y., Klionsky, L., Zhang, T. J., Immke, D., et al. (2005). AMG 9810 [(E)-3-(4-t-butylphenyl)-N-(2,3-dihydrobenzo[b] [1,4] dioxin-6-yl)acrylamide], a novel vanilloid receptor 1 (TRPV1) antagonist with antihyperalgesic properties. J. Pharmacol. Exp. Ther. 313, 474–484. doi:10.1124/jpet.104.079855
Giacaman, R. A., Fernandez, C. E., Munoz-Manriquez, N., Echeverria, C., Valdes, S., Castro, R. J., et al. (2022). Understanding dental caries as a non-communicable and behavioral disease: management implications. Front. Oral Health 3, 764479. doi:10.3389/froh.2022.764479
Gibbs, J. L., Melnyk, J. L., and Basbaum, A. I. (2011a). Differential TRPV1 and TRPV2 channel expression in dental pulp. J. Dent. Res. 90, 765–770. doi:10.1177/0022034511402206
Gibbs, J. L., Melnyk, J. L., and Basbaum, A. I. (2011b). Differential TRPV1 and TRPV2 channel expression in dental pulp. J. Dent. Res. 90, 765–770. doi:10.1177/0022034511402206
Gibson, R. A., Robertson, J., Mistry, H., McCallum, S., Fernando, D., Wyres, M., et al. (2014). A randomised trial evaluating the effects of the TRPV1 antagonist SB705498 on pruritus induced by histamine, and cowhage challenge in healthy volunteers. PLoS One 9, e100610. doi:10.1371/journal.pone.0100610
Gualdani, R., Ceruti, S., Magni, G., Merli, D., Di Cesare Mannelli, L., Francesconi, O., et al. (2015). Lipoic-based TRPA1/TRPV1 antagonist to treat orofacial pain. ACS Chem. Neurosci. 6, 380–385. doi:10.1021/cn500248u
Guedon, J. M. G., Yee, M. B., Zhang, M., Harvey, S. A. K., Goins, W. F., and Kinchington, P. R. (2015). Neuronal changes induced by varicella zoster virus in a rat model of postherpetic neuralgia. Virology 482, 167–180. doi:10.1016/J.VIROL.2015.03.046
Gunthorpe, M. J., and Szallasi, A. (2008). Peripheral TRPV1 receptors as targets for drug development: new molecules and mechanisms, Curr. Pharm. Des., vol. 14. 32, 41. doi:10.2174/138161208783330754
Guo, R., Zhou, Y., Long, H., Shan, D., Wen, J., Hu, H., et al. (2019). Transient receptor potential Vanilloid 1-based gene therapy alleviates orthodontic pain in rats. Int. J. Oral Sci. 11, 11. doi:10.1038/s41368-019-0044-3
Häggman-Henrikson, B., Alstergren, P., Davidson, T., Högestätt, E. D., Östlund, P., Tranaeus, S., et al. (2017). Pharmacological treatment of oro-facial pain - health technology assessment including a systematic review with network meta-analysis. J. Oral Rehabil. 44, 800–826. doi:10.1111/joor.12539
Häggman-Henrikson, B., Liv, P., Ilgunas, A., Visscher, C. M., Lobbezoo, F., Durham, J., et al. (2020). Increasing gender differences in the prevalence and chronification of orofacial pain in the population. Pain 161, 1768–1775. doi:10.1097/j.pain.0000000000001872
Han, S. B., Kim, H., Hyun, C. S., Lee, J. D., Chung, J. H., and Kim, H. S. (2016). Transient receptor potential vanilloid-1 in epidermal keratinocytes may contribute to acute pain in herpes zoster. Acta Derm. Venereol. 96, 319–322. doi:10.2340/00015555-2247
Henderson, R. (2013). Structural biology: ion channel seen by electron microscopy. Nature 504, 93–94. doi:10.1038/504093a
Hersh, E. V., Moore, P. A., Grosser, T., Polomano, R. C., Farrar, J. T., Saraghi, M., et al. (2020). Nonsteroidal anti-inflammatory drugs and opioids in postsurgical dental pain. J. Dent. Res. 99, 777–786. doi:10.1177/0022034520914254
Hitomi, S., Ono, K., Yamaguchi, K., Terawaki, K., Imai, R., Kubota, K., et al. (2016). The traditional Japanese medicine hangeshashinto alleviates oral ulcer-induced pain in a rat model. Arch. Oral Biol. 66, 30–37. doi:10.1016/j.archoralbio.2016.02.002
Hossain, M. Z., Bakri, M. M., Yahya, F., Ando, H., Unno, S., and Kitagawa, J. (2019). The role of transient receptor potential (TRP) channels in the transduction of dental pain. Int. J. Mol. Sci. 20, 526–531. doi:10.3390/ijms20030526
Huff, T., Weisbrod, L. J., and Daly, D. T. (2023). “Neuroanatomy,” in Cranial nerve, 5. Trigeminal).
Hwang, S. M., Lee, K., Im, S. T., Go, E. J., Kim, Y. H., and Park, C. K. (2020). Co-application of eugenol and QX-314 elicits the prolonged blockade of voltage-gated sodium channels in nociceptive trigeminal ganglion neurons. Biomolecules 10, 1513–1514. doi:10.3390/biom10111513
Iannone, L. F., De Logu, F., Geppetti, P., and De Cesaris, F. (2022a). The role of TRP ion channels in migraine and headache. Neurosci. Lett. 768, 136380. doi:10.1016/j.neulet.2021.136380
Iannone, L. F., De Logu, F., Geppetti, P., and De Cesaris, F. (2022b). The role of TRP ion channels in migraine and headache. Neurosci. Lett. 768, 136380. doi:10.1016/J.NEULET.2021.136380
International Classification of Orofacial Pain (2020). International classification of orofacial pain, 1st edition (ICOP). Cephalalgia 40, 129–221. doi:10.1177/0333102419893823
Jara-Oseguera, A., Simon, S. A., and Rosenbaum, T. (2008). TRPV1: on the road to pain relief. Curr. Mol. Pharmacol. 1, 255–269. doi:10.2174/1874467210801030255
Jin, Y. H., Bailey, T. W., Li, B. Y., Schild, J. H., and Andresen, M. C. (2004). Purinergic and vanilloid receptor activation releases glutamate from separate cranial afferent terminals in nucleus tractus solitarius. J. Neurosci. 24, 4709–4717. doi:10.1523/JNEUROSCI.0753-04.2004
J, S. (2005). Topical analgesics in neuropathic pain. Curr. Pharm. Des. 11, 2995–3004. doi:10.2174/1381612054865019
Julius, D. (2013). TRP channels and pain. Annu. Rev. Cell Dev. Biol. 29, 355–384. doi:10.1146/annurev-cellbio-101011-155833
Kämmerer, P. W., Heimes, D., Hartmann, A., Kesting, M., Khoury, F., Schiegnitz, E., et al. (2024). Clinical insights into traumatic injury of the inferior alveolar and lingual nerves: a comprehensive approach from diagnosis to therapeutic interventions. Clin. Oral Investig. 28, 216. doi:10.1007/S00784-024-05615-4
Khan, J., Zusman, T., Wang, Q., and Eliav, E. (2019a). Acute and chronic pain in orofacial trauma patients. Dent. Traumatol. 35, 348–357. doi:10.1111/edt.12493
Khan, J., Zusman, T., Wang, Q., and Eliav, E. (2019b). Acute and chronic pain in orofacial trauma patients. Dent. Traumatol. 35, 348–357. doi:10.1111/edt.12493
Khawaja, S. N., and Scrivani, S. J. (2023a). Trigeminal neuralgia. Dent. Clin. North Am. 67, 99–115. doi:10.1016/j.cden.2022.07.008
Khawaja, S. N., and Scrivani, S. J. (2023b). Head and neck cancer-related pain. Dent. Clin. North Am. 67, 129–140. doi:10.1016/j.cden.2022.07.010
Kitagawa, Y., Tamai, I., Hamada, Y., Usui, K., Wada, M., Sakata, M., et al. (2013). Orally administered selective TRPV1 antagonist, JTS-653, attenuates chronic pain refractory to non-steroidal anti-inflammatory drugs in rats and mice including post-herpetic pain. J. Pharmacol. Sci. 122, 128–137. doi:10.1254/JPHS.12276FP
Kun, J., Helyes, Z., Perkecz, A., Ban, A., Polgar, B., Szolcsanyi, J., et al. (2012). Effect of surgical and chemical sensory denervation on non-neural expression of the transient receptor potential vanilloid 1 (TRPV1) receptors in the rat. J. Mol. Neurosci. 48, 795–803. doi:10.1007/s12031-012-9766-9
Labanca, M., Gianò, M., Franco, C., and Rezzani, R. (2023a). Orofacial pain and dentistry management: guidelines for a more comprehensive evidence-based approach. Diagnostics 13, 1–17. doi:10.3390/diagnostics13172854
Labanca, M., Gianò, M., Franco, C., and Rezzani, R. (2023b). Orofacial pain and dentistry management: guidelines for a more comprehensive evidence-based approach. Diagnostics 13, 2854. doi:10.3390/diagnostics13172854
Labanca, M., Gianò, M., Franco, C., and Rezzani, R. (2023c). Orofacial pain and dentistry management: guidelines for a more comprehensive evidence-based approach. Diagnostics 13, 2854. doi:10.3390/DIAGNOSTICS13172854
Labianca, R., Sarzi-Puttini, P., Zuccaro, S. M., Cherubino, P., Vellucci, R., and Fornasari, D. (2012). Adverse effects associated with non-opioid and opioid treatment in patients with chronic pain. Clin. Drug Investig. 32, 53–63. doi:10.2165/11630080-000000000-00000
Lee, Y. H., Huang, Y. F., Chou, H. H., Lin, W. T., Yang, H. W., and Lin-Shiau, S. Y. (2018). Studies on a novel regimen for management of orofacial pain and morphine tolerance. J. Dent. Sci. 13, 131–137. doi:10.1016/j.jds.2017.08.006
Leeuw, R., and Klasser, G. D. (2018). Orofacial pain: guidelines for assessment, diagnosis, and management. vol. os17. 6th. doi:10.1308/135576110791013929
Leite-Panissi, C. R. A., De Paula, B. B., Neubert, J. K., and Caudle, R. M. (2023a). Influence of TRPV1 on thermal nociception in rats with temporomandibular joint persistent inflammation evaluated by the operant orofacial pain assessment device (OPAD). J. Pain Res. 16, 2047–2062. doi:10.2147/JPR.S405258
Leite-Panissi, C. R. A., De Paula, B. B., Neubert, J. K., and Caudle, R. M. (2023b). Influence of TRPV1 on thermal nociception in rats with temporomandibular joint persistent inflammation evaluated by the operant orofacial pain assessment device (OPAD). J. Pain Res. 16, 2047–2062. doi:10.2147/JPR.S405258
Li, L., Chen, C., Chiang, C., Xiao, T., Chen, Y., Zhao, Y., et al. (2021). The impact of trpv1 on cancer pathogenesis and therapy: a systematic review. Int. J. Biol. Sci. 17, 2034–2049. doi:10.7150/ijbs.59918
Ls, P., and P, S. (2008). TRPV1: a target for next generation analgesics. Curr. Neuropharmacol. 6, 151–163. doi:10.2174/157015908784533888
Luo, J., Bavencoffe, A., Yang, P., Feng, J., Yin, S., Qian, A., et al. (2018). Zinc inhibits TRPV1 to alleviate chemotherapy-induced neuropathic pain. J. Neurosci. 38, 474–483. doi:10.1523/JNEUROSCI.1816-17.2017
Luo, Y., Suttle, A., Zhang, Q., Wang, P., and Chen, Y. (2021). Transient receptor potential (TRP) ion channels in orofacial pain. Mol. Neurobiol. 58, 2836–2850. doi:10.1007/s12035-021-02284-2
Melo, J. J. de M. de A. de, Damasceno, M. de B. M. V., Santos, SAAR, Barbosa, T. M., Araújo, J. R. C., Vieira-Neto, A. E., et al. (2017). Acute and neuropathic orofacial antinociceptive effect of eucalyptol. Inflammopharmacology 25, 247–254. doi:10.1007/s10787-017-0324-5
Militão, R. C., Natalia Souza de Péder, S., Suemi Kamikawa, D., Pieralisi, N., Chicarelli, M., and de Souza Tolentino, E. (2021). Evaluation of a Protocol For Topical Application of Capsaicine Gel 0.025 % in the Management of Burning Mouth Syndrome Correlating its Impact on Quality of Life Evaluación de un Protocolo de Aplicación Tópica de Capsaicina Gel 0,025 % en el Tratamiento del Síndrome de Boca Ardiente Correlacionando su Impacto con la Calidad de Vida, 15.
Neubert, J. K., King, C., Malphurs, W., Wong, F., Weaver, J. P., Jenkins, A. C., et al. (2008). Characterization of mouse orofacial pain and the effects of lesioning TRPV1-expressing neurons on operant behavior. Mol. Pain 4, 43. doi:10.1186/1744-8069-4-43
Nilius, B., and Szallasi, A. (2014). Transient receptor potential channels as drug targets: from the science of basic research to the art of medicine. Pharmacol. Rev. 66, 676–814. doi:10.1124/pr.113.008268
Park, C. K. (2015). Maresin 1 inhibits TRPV1 in temporomandibular joint-related trigeminal nociceptive neurons and TMJ inflammation-induced synaptic plasticity in the trigeminal nucleus. Mediat. Inflamm. 2015, 275126. doi:10.1155/2015/275126
Park, C. K., Kim, K., Jung, S. J., Kim, M. J., Ahn, D. K., Hong, S. D., et al. (2009). Molecular mechanism for local anesthetic action of eugenol in the rat trigeminal system. Pain 144, 84–94. doi:10.1016/j.pain.2009.03.016
Payne, R. (2000). Limitations of NSAIDs for pain management: toxicity or lack of efficacy? J. Pain, 1 (3), Churchill Livingstone Inc., 14–18. doi:10.1054/jpai.2000.16611
Peres, M. A., D Macpherson, L. M., Weyant, R. J., Daly, B., Venturelli, R., Mathur, M. R., et al. (2019). Oral diseases: a global public health challenge. Oral Dis. a Glob. public health Chall. 394, 249–260. doi:10.1016/S0140-6736(19)31146-8
Pomonis, J. D., Harrison, J. E., Mark, L., Bristol, D. R., Valenzano, K. J., and Walker, K.N-(4-Tertiarybutylphenyl)-4-(3-cholorphyridin-2-yl)tetrahydropyrazine-1 (2H)-carbox-amide (BCTC) (2003). N-(4-Tertiarybutylphenyl)-4-(3-cholorphyridin-2-yl)tetrahydropyrazine -1(2H)-carbox-amide (BCTC), a novel, orally effective vanilloid receptor 1 antagonist with analgesic properties: II. in vivo characterization in rat models of inflammatory and neuropathic pain. J. Pharmacol. Exp. Ther. 306, 387–393. doi:10.1124/jpet.102.046268
Porreca, F., and Ossipov, M. H. (2009). Nausea and vomiting side effects with opioid analgesics during treatment of chronic pain: mechanisms, implications, and management options. Pain Med. 10, 654–662. doi:10.1111/j.1526-4637.2009.00583.x
Premkumar, L. S., and Sikand, P. (2008). TRPV1: a target for next generation analgesics. Curr. Neuropharmacol. 6, 151–163. doi:10.2174/157015908784533888
Quiding, H., Jonzon, B., Svensson, O., Webster, L., Reimfelt, A., Karin, A., et al. (2013a). TRPV1 antagonistic analgesic effect: a randomized study of AZD1386 in pain after third molar extraction. Pain 154, 808–812. doi:10.1016/J.PAIN.2013.02.004
Quiding, H., Jonzon, B., Svensson, O., Webster, L., Reimfelt, A., Karin, A., et al. (2013b). TRPV1 antagonistic analgesic effect: a randomized study of AZD1386 in pain after third molar extraction. Pain 154, 808–812. doi:10.1016/J.PAIN.2013.02.004
Quiding, H., Jonzon, B., Svensson, O., Webster, L., Reimfelt, A., Karin, A., et al. (2013c). TRPV1 antagonistic analgesic effect: a randomized study of AZD1386 in pain after third molar extraction. Pain 154, 808–812. doi:10.1016/j.pain.2013.02.004
Rocha, B. R., Lima Veras, P. J., de Oliveira, L. G., Vieira-Neto, A. E., Sessle, B. J., Villaça Zogheib, L., et al. (2022a). Botulinum toxin promotes orofacial antinociception by modulating TRPV1 and NMDA receptors in adult zebrafish. Toxicon 210, 158–166. doi:10.1016/j.toxicon.2022.02.005
Rocha, B. R., Lima Veras, P. J., de Oliveira, L. G., Vieira-Neto, A. E., Sessle, B. J., Villaça Zogheib, L., et al. (2022b). Botulinum toxin promotes orofacial antinociception by modulating TRPV1 and NMDA receptors in adult zebrafish. Toxicon 210, 158–166. doi:10.1016/J.TOXICON.2022.02.005
Romero-Reyes, M., and Salvemini, D. (2016). Cancer and orofacial pain. Med. Oral Patol. Oral Cir. Bucal 21, e665–e671. doi:10.4317/medoral.21515
Rotpenpian, N., and Yakkaphan, P. (2021a). Review of literatures: physiology of orofacial pain in dentistry. ENeuro 8, 565–578. doi:10.1523/ENEURO.0535-20.2021
Rotpenpian, N., and Yakkaphan, P. (2021b). Review of literatures: physiology of orofacial pain in dentistry. ENeuro 8, ENEURO.0535–20.2021. doi:10.1523/ENEURO.0535-20.2021
Rowbotham, M. C., Nothaft, W., Duan, W. R., Wang, Y., Faltynek, C., McGaraughty, S., et al. (2011a). Oral and cutaneous thermosensory profile of selective TRPV1 inhibition by ABT-102 in a randomized healthy volunteer trial. Pain 152, 1192–1200. doi:10.1016/J.PAIN.2011.01.051
Rowbotham, M. C., Nothaft, W., Duan, W. R., Wang, Y., Faltynek, C., McGaraughty, S., et al. (2011b). Oral and cutaneous thermosensory profile of selective TRPV1 inhibition by ABT-102 in a randomized healthy volunteer trial. Pain 152, 1192–1200. doi:10.1016/j.pain.2011.01.051
Santos, SAAR, Vidal Damasceno, M. de B. M., Magalhães, F. E. A., Sessle, B. J., de Oliveira, B. A., Batista, F. L. A., et al. (2022). Transient receptor potential channel involvement in antinociceptive effect of citral in orofacial acute and chronic pain models. EXCLI J. 21, 869–887. doi:10.17179/excli2022-5042
Setty, S., and David, J. (2014). Classification and epidemiology of orofacial pain, 15, 24. doi:10.1007/978-3-319-01875-1_3
Shueb, S. S., Nixdorf, D. R., John, M. T., Alonso, B. F., and Durham, J. (2015). What is the impact of acute and chronic orofacial pain on quality of life? J. Dent. 43, 1203–1210. doi:10.1016/j.jdent.2015.06.001
Soares, I. C. R., Santos, SAAR, Coelho, R. F., Alves, Y. A., Vieira-Neto, A. E., Tavares, K. C. S., et al. (2019). Oleanolic acid promotes orofacial antinociception in adult zebrafish (Danio rerio) through TRPV1 receptors. Chem. Biol. Interact. 299, 37–43. doi:10.1016/j.cbi.2018.11.018
Civamide Nasal (2000). Study Details Civamide nasal solution for postherpetic neuralgia of the trigeminal nerve ClinicalTrials.gov n.d. Available online at: https://clinicaltrials.gov/study/NCT01886313 (Accessed January 27, 2025).
Takasaki, I., Andoh, T., Shiraki, K., and Kuraishi, Y. (2000). Allodynia and hyperalgesia induced by herpes simplex virus type-1 infection in mice. Pain 86, 95–101. doi:10.1016/S0304-3959(00)00240-2
Urano, H., Ara, T., Fujinami, Y., and Yukihiro Hiraoka, B. (2012a). Aberrant TRPV1 expression in heat hyperalgesia associated with trigeminal neuropathic pain. Int. J. Med. Sci. 9, 690–697. doi:10.7150/IJMS.4706
Urano, H., Ara, T., Fujinami, Y., and Yukihiro Hiraoka, B. (2012b). Aberrant TRPV1 expression in heat hyperalgesia associated with trigeminal neuropathic pain. Int. J. Med. Sci. 9, 690–697. doi:10.7150/ijms.4706
Wanasuntronwong, A., Kaewsrisung, S., Rotpenpian, N., Arayapisit, T., Pavasant, P., and Supronsinchai, W. (2022). Efficacy and mechanism of the antinociceptive effects of cannabidiol on acute orofacial nociception induced by Complete Freund’s Adjuvant in male Mus musculus mice. Arch. Oral Biol. 144, 105570. doi:10.1016/j.archoralbio.2022.105570
Wang, S., Bian, C., Yang, J., Arora, V., Gao, Y., Wei, F., et al. (2020a). Ablation of TRPV1+ afferent terminals by capsaicin mediates long-lasting analgesia for trigeminal neuropathic pain. ENeuro 7, ENEURO.0118–20.2020. doi:10.1523/ENEURO.0118-20.2020
Wang, S., Bian, C., Yang, J., Arora, V., Gao, Y., Wei, F., et al. (2020b). Ablation of TRPV1+ afferent terminals by capsaicin mediates long-lasting analgesia for trigeminal neuropathic pain. ENeuro 7, ENEURO.0118–20.2020. doi:10.1523/ENEURO.0118-20.2020
Wong, G. Y., and Gavva, N. R. (2009). Therapeutic potential of vanilloid receptor TRPV1 agonists and antagonists as analgesics: recent advances and setbacks. Brain Res. Rev. 60, 267–277. doi:10.1016/J.BRAINRESREV.2008.12.006
Wu, Y. W., Bi, Y. P., Kou, X. X., Xu, W., Ma, L. Q., Wang, K. W., et al. (2010). 17-β-Estradiol enhanced allodynia of inflammatory temporomandibular joint through upregulation of hippocampal TRPV1 in ovariectomized rats. J. Neurosci. 30, 8710–8719. doi:10.1523/JNEUROSCI.6323-09.2010
Yang, F., and Zheng, J. (2017). Understand spiciness: mechanism of TRPV1 channel activation by capsaicin. Protein Cell 8, 169–177. doi:10.1007/s13238-016-0353-7
Yeon, K. Y., Kim, S. A., Kim, Y. H., Lee, M. K., Ahn, D. K., Kim, H. J., et al. (2010). Curcumin produces an antihyperalgesic effect via antagonism of TRPV1. J. Dent. Res. 89, 170–174. doi:10.1177/0022034509356169
Keywords: pain, orofacial pain, toothache, facial neuralgia, TRPV cation Channels, vanilloid Receptor
Citation: Andrade ACM, Molina Esquivel N, Goldschmied Rossel F and Benso B (2025) TRPV1-target drugs for the treatment of orofacial pain. Front. Pharmacol. 16:1568109. doi: 10.3389/fphar.2025.1568109
Received: 28 January 2025; Accepted: 01 April 2025;
Published: 24 April 2025.
Edited by:
Ramzi Mothana, King Saud University, Saudi ArabiaReviewed by:
Enoch Luis, National Council of Science and Technology (CONACYT), MexicoCristian Acosta, CONICET Mendoza, Argentina
Copyright © 2025 Andrade, Molina Esquivel, Goldschmied Rossel and Benso. This is an open-access article distributed under the terms of the Creative Commons Attribution License (CC BY). The use, distribution or reproduction in other forums is permitted, provided the original author(s) and the copyright owner(s) are credited and that the original publication in this journal is cited, in accordance with accepted academic practice. No use, distribution or reproduction is permitted which does not comply with these terms.
*Correspondence: Bruna Benso, YnJ1bmEuYmVuc29AdWMuY2w=