- 1Graduate School, Heilongjiang University of Chinese Medicine, Harbin, China
- 2The Four Hospital of Heilongjiang University of Chinese Medicine, Heilongjiang University of Chinese Medicine, Harbin, China
- 3Experimental Teaching and Practical Training Center, Heilongjiang University of Chinese Medicine, Harbin, China
- 4First Clinical Medical College, Heilongjiang University of Chinese Medicine, Harbin, China
- 5Research Institute of Chinese Medicine, Heilongjiang University of Chinese Medicine, Harbin, China.
The emergence of the microbiota-gut-brain axis has opened new avenues for improving sleep quality. Recent studies have revealed a close relationship between insomnia and the gut microbiome. Chinese herbal medicines and their active components can alter the relative abundance of sleep-related gut microbiota by reversing dysbiosis in the gut microbiome. Improving sleep quality through the regulation of the gut microbiota using herbal medicine and its active components has become a highly promising therapeutic strategy. This article elucidates how the gut microbiota modulates sleep quality via the intricate communication network of the gut-brain axis. It also reviews the latest research on utilizing herbal medicine and its active components to regulate the gut microbiota for enhancing sleep quality. Additionally, it provides insights into the potential of herbal medicine and its active components in improving sleep quality through the modulation of the gut microbiota.
1 Introduction
Insomnia is a common sleep disorder characterized mainly by poor sleep quality and insufficient sleep duration. It affects approximately 30% of the global population and is correlated with physical and mental consequences such as cognitive decline, anxiety, chronic fatigue, poor concentration, and memory impairment (Rivero-Segura et al., 2025; Zhang et al., 2025). In recent years, an increasing number of studies have shown a close relationship between the gut microbiota and sleep.
As a bridge for communication between the gut microbiota and the brain, the gut-brain axis is a bidirectional regulatory axis involving interactions between the central nervous system (CNS) and the gastrointestinal tract. It also comprises a neuroendocrine network consisting of the CNS, the autonomic nervous system, the hypothalamic-pituitary-adrenal (HPA) axis, and the enteric nervous system (Arzani et al., 2020; Mayer et al., 2015). Gut microbes can participate in regulating the sleep functions of the brain via the gut-brain axis, either directly or indirectly (Wang et al., 2022; Wu J. et al., 2023). Sleep deprivation (SD) has been shown to reduce the Firmicutes-to-Bacteroidetes (F/B) ratio in rats (Yang et al., 2023). Consuming specific probiotics can effectively improve the diversity of the gut microbiota and enhance sleep quality through microbial metabolic products (Lan et al., 2023; Matsuda et al., 2020; Zhu et al., 2023). Therefore, scientifically intervening with the gut microbiota represents a new and effective target for preventing and treating insomnia.
Chinese herbal medicines and their active components serve as unique ‘prebiotics,’ not only providing energy for the activities of the gut microbiota but also improving the gut microenvironment by modulating the intestinal microbiota, thereby affecting various physiological functions of the body (Álvarez et al., 2022; Cheng X. et al., 2024). They not only offer gentle and sustained regulation of the gut microbiota but also exhibit significant drug-like properties, good pharmacokinetics, and low toxicity when it comes to improving sleep quality (Ranteh et al., 2024). Chinese herbal medicines and their active components hold great potential in improving insomnia through the modulation of the gut microbiota.
This paper summarizes the current state of research on the potential of herbal medicines and their active ingredients to improve sleep quality by modulating the gut microbiota. It also provides an overview of recent studies on the mechanisms by which the gut microbiota can be utilized to prevent and treat insomnia.
2 Characteristics of the gut microbiota in patients with insomnia
Insomnia can affect the richness and diversity of the gut microbiota. Compared to the normal group, SD mice exhibit a significant reduction in the abundance of Akkermansia muciniphila, Bacteroides, and Faecalibacterium and a significant increase in the abundance of Aeromonas (Gao et al., 2019; Li N. et al., 2023; Moreno-Indias et al., 2015). Compared to the control group, patients with insomnia show significant differences in the abundance of Collinsella, Adlercreutzia, Erysipelotrichaceae, Clostridiales, Pediococcus, Bacteroides, Staphylococcus, Carnobacterium, Pseudomonas, Odoribacter, Bifidobacterium longum, and Phascolarctobacterium (Barone et al., 2024; Zeng et al., 2024). Similarly, patients with obstructive sleep apnea exhibit significant differences in the abundance of Lactobacillus, Ruminococcaceae, Proteobacteria, Clostridiaceae, Oscillospiraceae, Klebsiella, Desulfovibrionaceae, Bacteroides fragilis, and Faecalibacterium prausnitzii (Ko et al., 2019; Valentini et al., 2020).
Whether it is the alteration of strains associated with inflammation, such as Proteobacteria, Clostridiaceae, Oscillospiraceae, and Klebsiella, or strains related to gut barrier integrity, such as Desulfovibrionaceae, Bacteroides fragilis, and F. prausnitzii, these changes are significantly correlated with parameters related to sleep quality (Valentini et al., 2020). Insomnia not only reduces the gut’s antioxidant capacity, anti-inflammatory cytokine levels, mucin 2 (MUC2), and tight junction protein expression but also increases the levels of pro-inflammatory cytokines, leading to intestinal mucosal damage and increased barrier permeability (Gao et al., 2019; Li L. et al., 2023). When SD animals’ gut microbiota is transferred into normal mice, the donor animals’ gut microbiota is changed, which results in elevated levels of lipopolysaccharides (LPS) and Aeromonas, lowered levels of butyrate and Lachnospiraceae_NK4A136, and increased hippocampal microglia activation and neuronal death (Wang X. et al., 2023). However, pretreatment with Lactiplantibacillus plantarum 124 or A. muciniphila restores the disrupted gut microbiota, reduces oxidative stress, inflammation, and barrier damage in the gut (Li L. et al., 2023), increases acetate and butyrate levels, and prevents synapse loss in microglia-neuron co-cultures stimulated by LPS (Li N. et al., 2023). Thus, disruption of the gut microbiota affects the host’s sleep behavior through mechanisms such as reducing gut barrier integrity and short-chain fatty acid (SCFAs) content and increasing inflammatory mediators that trigger inflammatory responses.
3 Gut microbiota involved in the regulation of insomnia mechanisms
3.1 Hypothalamic-pituitary-adrenal axis
Insomnia activates and leads to dysfunction of the HPA axis (Luo et al., 2024; Wang Z. et al., 2024). Microbes may influence HPA axis activity through inbound neural signaling, SCFAs, epigenetics, gut barrier integrity, or blood-brain barrier (BBB) permeability (Cao C. et al., 2020; Noemi et al., 2024). Therefore, it is suggested that the dysregulation of gut microbiota caused by insomnia may be related to the activation of the HPA axis (Matenchuk et al., 2020).
Disruption of the HPA axis is associated with an increase in pathogenic bacteria (including Enterobacteriaceae, Streptococcaceae, and Veillonellaceae) and a decrease in beneficial bacteria (including Bifidobacterium and Lachnospiraceae) (Jahnke et al., 2021). Certain specific gut microbiota can inhibit HPA axis activation and mediate related brain functions. Escherichia coli and Enterococcus faecalis can lower cortisol levels in the serum of mice, thereby alleviating stress and depressive-anxiety behaviors (Luo et al., 2018; Wu et al., 2021). Analysis indicates that oxymesterone in feces can mediate the negative effect of cortisol on Parabacteroides, while 3-(2,4-cyclopentadien-1-yl)-5α-androstan-17β-ol can mediate the negative effect of cortisol on Aerococcus; mevinolinic acid can also mediate the negative effect of Aerococcus on cortisol (Wang J. et al., 2023). This suggests that signaling between the gut microbiota and the HPA axis is bidirectional (Figure 1).
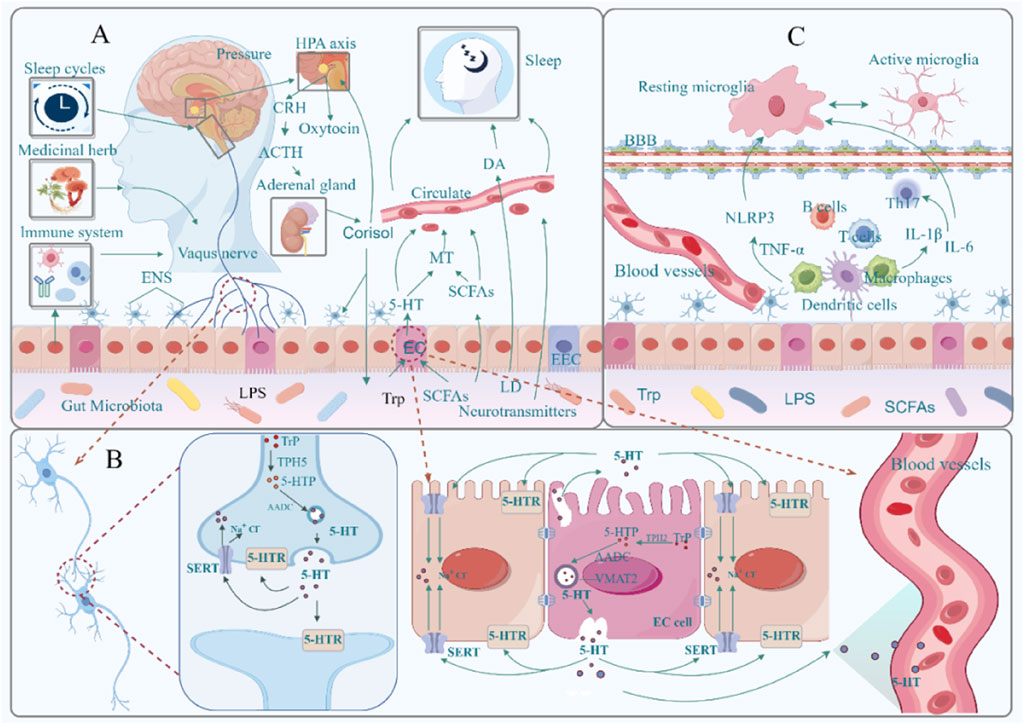
Figure 1. Bidirectional regulatory mechanisms between the gut microbiota and the brain. (A): Microbial endocrine and metabolite pathways, hypothalamic–pituitary–adrenal axis; (B): Microbiota–vagus nerve pathway; (C): Microbiota–immune inflammatory pathway; AANAT, arylalkylamine N-acetyltransferase (a rate-limiting enzyme produced by MT); SCFAs, short-chain fatty acids; 5-HT, 5-hydroxytryptamine; MT, melatonin; DA, dopamine; HPA, hypothalamus-pituitary-adrenal axis; CRH, adrenocorticotropic hormone-releasing hormone; ACTH, adrenocorticotropic hormone; EC, enterochromaffin cells; EEC, intestinal endocrine cell; Trp, tryptophan; 5-HTR, 5-hydroxytryptamine receptor; 5-HTP, 5-hydroxytryptophan; TPH2, tryptophan hydroxylase 2; TPH5, tryptophan hydroxylase 5; SERT, 5-hydroxytryptamine transporter; VMAT2, vesicular monoamine transporter 2; MAO, monoamine oxidase; BBB, blood-brain barrier; LPS, lipopolysaccharide; TNF-α, tumor necrosis factor α; NLRP3, NOD-like receptor thermoprotein domain protein 3; IL-6, interleukin-6; IL-1β, interleukin-1β; Th17, helper T cell 17. Materials provided by Fig Draw (www.fgdraw.com).
3.2 Microbial endocrinological products and metabolites
3.2.1 Melatonin
As a “dark hormone,” melatonin (MT) is crucial for maintaining the body’s normal sleep-wake cycle. Under normal conditions, less than 5% of tryptophan (Trp) in the human body is used for the synthesis of 5-hydroxytryptamine (5-HT) and MT, while the remaining 95% is metabolized through the kynurenine (Kyn) pathway in the liver (Muneer, 2020). Long-term stress can disrupt the balance of Kyn metabolism and endocrine function along the gut-brain axis, leading to dysbiosis in the gut microbiota (Deng et al., 2021). This dysbiosis further stimulates the Kyn pathway, diverting Trp that should be directed towards MT synthesis, thus reducing circulating MT levels (Yan et al., 2023).
In addition to restoring the circadian rhythm equilibrium and regulating the composition of the gut microbiota, exogenous MT supplementation has been shown to raise SCFAs levels and modify communication between the gut microbiota and the brain (Gao et al., 2021; Li et al., 2023a; Li et al., 2023b). Supplementing with MT, for instance, can improve cognitive impairment caused by SD by reducing hippocampal inflammation and neuronal apoptosis, increasing the relative abundance of Lachnospiraceae_NK4A136 and butyrate levels, decreasing the relative abundance of Aeromonas and LPS levels, and regulating the TLR4/NF-κB and MCT1/HDAC3 signaling pathways (Wang X. et al., 2023).
Certain microorganisms in the gut can promote the synthesis of MT. Studies have shown that Lactobacillus reuteri and E. coli can activate the TLR2/4/MyD88/NF-κB signaling pathway, promoting the expression of arylalkylamine N-acetyltransferase (AANAT), which in turn drives the synthesis of MT (Liu et al., 2024). Microbial metabolites such as SCFAs can also mediate the transcription of AANAT by increasing 5-HT levels and promoting the phosphorylation of cAMP response element-binding protein (p-CREB), thereby facilitating MT synthesis (Song et al., 2021) (Figure 1). Notably, these MT molecules originating from the gut may act on MT1 or MT2 receptors in the brain through the circulatory system, thereby regulating sleep behavior in the organism (Li B. et al., 2023).
3.2.2 γ-Aminobutyric acid
γ-Aminobutyric acid (GABA) is a well-known sleep-promoting amino acid. In the intestinal mucosa, there exists a line of entero-neuroendocrine cells: STC-1 and STC-2, which can express mRNA for GABAA receptors and their receptor subtypes (α1, α3, α5, β1, β3, and δ) (Noguchi et al., 2024). Besides the ability of gastrointestinal neuroendocrine cells to synthesize, store, and secrete GABA, certain members of the gut microbiota can also produce GABA.
Lactic acid bacteria and bifidobacteria are key members in the production of GABA. Bifidobacteria colonizing the mucus layer of the gut possess enzymatic mechanisms capable of converting glutamate (Glu), glutamine (Gln), and succinate into GABA. GABA derived from the gut can maintain the intestinal barrier and its function by stimulating Ca2+ signaling, MUC2 release, and proliferation of intestinal stem cells (Engevik et al., 2019; Luck et al., 2021; Zhou et al., 2023). Limosilactobacillus fermentum L18 can secrete high levels of GABA, enhancing the gut barrier by increasing the concentration of connexins and the abundance of beneficial gut bacteria (Kaur et al., 2023). Lactobacillus rhamnosus GG, in addition to protecting the gut barrier, can also enhance brain-derived neurotrophic factor (BDNF) and GABA receptor levels in the hippocampus and amygdala by modulating the gut-brain axis (Zhou et al., 2022). Additionally, studies have shown that the abundance of Bacteroides is positively correlated with the expression of GABA receptor proteins (Bedu-Ferrari et al., 2024; Duan H. et al., 2023).
Relevant research has demonstrated that high doses of GABA produced by Lactobacillus brevis fermentation can raise the relative abundance of good bacteria in the gut and the levels of SCFAs, which in turn can upregulate the GABAergic and 5-HTergic neurotransmitter mRNA and protein expression levels. This will result in a significant increase in theta (θ) and delta (δ) waves and non-rapid eye movement (NREM) sleep (Jeong et al., 2021; Yu et al., 2020). By altering the distribution of gut microbiota and upregulating the production of GABAA receptor proteins in gut tissue, the formula for spleen deficiency and liver qi stagnation enhances the quality of sleep (Duan H. et al., 2023). This has therapeutic benefits for depression and insomnia. It is clear that gut-derived GABA can act as a conduit for information between the brain and the microbiota, helping to regulate sleep behavior via the gut-brain axis and enhancing the organism’s quality of sleep.
Notably, the BBB has very poor permeability to GABA, and whether GABA can cross the BBB to act on receptors derived from the central nervous system remains questionable. However, there are large numbers of GABA receptors present in the enteroendocrine cell lines of the gut (Noguchi et al., 2024). Therefore, it is suggested that the impact of exogenous and gut-derived GABA on brain function may be achieved directly through the enteric nervous system (Tri et al., 2023).
3.2.3 Serotonin
As a key neurotransmitter in the gut-brain axis, 5-hydroxytryptamine (5-HT, serotonin) serves as an important mediator allowing communication between gut microbiota and the brain. Approximately 95% of 5-HT in the body originates from the gastrointestinal (GI) tract (Liu et al., 2021). Within the GI tract, sources of 5-HT include the conversion of Trp to 5-hydroxytryptophan (5-HTP) via tryptophan hydroxylase (TPH) 1 in enterochromaffin (EC) cells and TPH2 in neuronal cells, followed by the transformation into 5-HT through aromatic L-amino acid decarboxylase (AADC) (Layunta et al., 2021); or the induction of 5-HT secretion from EC cells triggered by calcium (Ca2+) influx via noncanonical signaling pathways initiated by interleukin 33 (IL-33) (Chen et al., 2021); and SCFAs promote synthesis by regulating the serotonin transporter (SERT), 5-HT receptors, and inhibiting the conversion of Trp to kynurenine (Buey et al., 2023; Xiao W. et al., 2022).
The variety of the gut microbiota affects 5-HT levels, which in turn impacts the organism’s sleep patterns. Supplementing with Trp and 5-HTP has been shown to improve the diversity of the gut microbiota, increase the amount of SCFAs in the gut, and raise blood levels of 5-HT, all of which improve the quality of sleep for both elderly people and neonates (Chojnacki et al., 2023; Sutanto et al., 2024). After depleting the microbiota with antibiotics, the expression levels of 5-HT decrease, leading to an increase in the onset frequency of REM sleep, frequent transitions between NREM and REM sleep, and ultimately having a negative impact on sleep (Ogawa et al., 2020). For example, Ganoderma lucidum can regulate the gut microbiota and 5-HT-related pathways, increasing serum levels of 5-HT and GABA while decreasing HPA axis hormone levels, effectively alleviating insomnia behavior in anxious mice (J. H. Huang et al., 2022; Yao et al., 2021). Clearly, microbiota can modulate sleep behavior in the brain by influencing the synthesis and release of 5-HT in the gut (Figure 1).
3.2.4 Short-chain fatty acids
SCFAs are the primary metabolites produced by gut bacteria and are believed to be neuromodulatory substances (Gunawan et al., 2024). SCFAs are essential for the gut microbiota’s control of sleep. Through immunological, neurological, and endocrine pathways, the microbiota forms close connections with brain sleep processes; the “gut-brain axis” serves as a link between the two, and SCFAs function as messengers (Figure 1).
Insomnia has been shown to decrease the number of microorganisms that generate SCFAs, such as Faecalibacterium, Roseburia, and Ruminococcaceae, which in turn lowers the amount of SCFAs in the gut (Shimizu et al., 2023; Wu et al., 2022). Elevated gut SCFAs levels have been shown to enhance the quality of sleep (Heath et al., 2020). Relevant studies have demonstrated that elevated SCFAs levels can affect brain development by crossing the blood-brain barrier and regulating the production of 5-HT and DA in the brain (Wang et al., 2020), in addition to helping the brain regulate sleep behavior by increasing 5-HT and MT levels in the gut and inhibiting HPA axis hormone levels (Li B. et al., 2023; Li et al., 2024; Ogawa et al., 2020; Wang J. et al., 2024).
Moreover, SCFAs have the ability to influence ILC3, T cells, and B cells in the gut, which helps to control the intestinal barrier’s immunological balance (Kim, 2021). In addition to counteracting age-related microbiome dysbiosis by attenuating the expression of pro-inflammatory cytokines in microglia, SCFAs also activate colonic NLRP6 inflammasomes, improving damage to the intestinal epithelial barrier and reducing neuroinflammation and neuronal loss in the hippocampus (Vailati-Riboni et al., 2022; Yu et al., 2021). The neural system and certain immunological signaling molecules in the brain can interact to help regulate sleep (Piber et al., 2022).
SCFAs produced by microbiota can affect the activity of the HPA axis. By significantly increasing SCFAs content, it is possible to restore the expression of tight junction genes (OCLN and TJP2) in the hypothalamus and hippocampus and significantly modulate the expression of corticotropin-releasing hormone receptor genes CRF1 and CRF2, thereby alleviating depression and anxiety induced by SD (Chung et al., 2023). Bifidobacterium breve 207-1 can improve sleep quality by significantly increasing SCFAs and GABA levels and overall suppressing HPA axis-related hormones (Li et al., 2024). Acetate, butyrate, and valerate show a negative correlation with HPA-axis-related hormone levels; propionate shows the opposite pattern (Wang J. et al., 2024).
Thus, SCFAs produced by microbiota can profoundly influence the nervous system by improving the gut barrier and the gut immune environment. They achieve the goal of improving insomnia through mechanisms such as increasing the diversity and levels of SCFAs in the gut, stimulating the secretion of sleep-related cytokines, and inhibiting inflammatory responses.
3.2.5 Dopamine
Dopamine (DA), a powerful neurotransmitter that promotes alertness, is essential for controlling sleep-wake cycles (Wu et al., 2024). DA strengthens the gastrointestinal barrier and defense mechanisms in the gastrointestinal tract by stimulating the secretion of distal colon mucus via D5 receptors and improving the secretion of gastrointestinal bicarbonate through D2 receptors and Ca2+-dependent pathways (Feng et al., 2020; Feng et al., 2017; Li et al., 2019).
Additionally, gut microbiota can influence the levels of DA, thereby affecting brain arousal and activity. Relevant studies have indicated that dysbiosis of gut microbiota leads to DA metabolic disorders (characterized by a decrease in homovanillic acid). Supplementation with probiotics has been shown to restore populations of Bacteroides, Blautia, Dialister, F. prausnitzii, and Ruminococcus, which are significantly positively correlated with homovanillic acid levels (Wang et al., 2020). Moreover, E. faecalis and Enterococcus faecium possess tyrosine hydroxylase and dopa decarboxylase activities, enabling them to convert tyrosine into L-DOPA. Further research has demonstrated that transplantation of E. faecalis and E. faecium increases the synthesis of dopa/dopamine within the intestines of PGF mice, facilitating the entry of dopa/dopamine from the gut into the bloodstream, thus elevating dopamine levels in the brain (Wang Y. et al., 2021) (Figure 1). This suggests that modulating the biosynthesis pathway of phenylalanine-tyrosine-dopa-dopamine in gut microbiota could potentially improve brain function. Furthermore, research has demonstrated that the gut microbiota’s SCFAs can directly penetrate the BBB to control the synthesis of 5-HT and DA, which in turn affects brain development (Wang et al., 2020). Thus, gut bacteria can influence the brain’s arousal capacities and functions by modulating the brain’s dopaminergic system through a variety of pathways.
3.3 Microbiota-vagus nerve pathway
Vagal nerve fibers, which receive a variety of signals from the gut and precisely transfer them to the brain, are abundant in the intestinal wall. For instance, the gut microbiota’s metabolism of Trp can trigger enteroendocrine cells’ (EECs’) Trpa1 signaling, which in turn triggers the gut’s vagal nerve system (Ye L. et al., 2021). Through the vagal nerve system, gut bacteria can alter the brain’s GABA receptor expression levels, reducing anxiety and depressive symptoms (Zou et al., 2024). Similar to this, fecal microbiota transplantation can trigger the gut’s vagal nerve system, which results in long-lasting alterations to the brainstem and hippocampus’s 5-HT and DA neurotransmission pathways (Siopi et al., 2023). Through a vagus nerve-dependent mechanism, Lactobacillus reuteri can also alter how oxytocinergic and dopaminergic signals are transmitted in the ventral tegmental area (VTA) (Sgritta et al., 2019). Notably, both dopaminergic circuits in the hippocampus and the VTA play crucial roles in maintaining sleep-wake-related behaviors (Bian W. et al., 2022).
To confirm the involvement of the vagus nerve in information transmission between the gut and the brain, researchers conducted subdiaphragmatic vagotomy experiments. They found that this not only abolishes the therapeutic effects of selective 5-HT reuptake inhibitors but also significantly reduces the activity of nerve fibers showing immunoreactivity to the 5-HT3 receptor in the intestinal mucosa (Glatzle et al., 2002; McVey Neufeld et al., 2019). When 5-HT within the gut binds to densely distributed 5-HT receptors on vagal nerve fibers, the vagus nerve is activated. Neurons then respond to and categorize the 5-HT signals through specific projections before conveying them to the brain (Spencer et al., 2024) (Figure 1).
Research has demonstrated that vagus nerve stimulation can ameliorate depression-like behaviors brought on by SD by lowering the levels of interleukin-1β (IL-1β) and interleukin-6 (IL-6) in peripheral blood and the hippocampus, as well as by preventing astrocyte and microglia activation (Ma et al., 2024). Vagotomy not only alleviates the systemic inflammatory response caused by SD-induced gut microbiota dysbiosis but also weakens the effects of probiotics on neuropsychiatric disorders by reducing the signaling of microbial metabolites along the gut-brain axis (Yun et al., 2020; Zhang et al., 2021). As a result, the vagus nerve allows a variety of gut signals to reach the brain and influence many brain activities.
3.4 Microbe-immune inflammatory pathway
Microbes establish complex interactions with the brain’s sleep regulatory functions through immune-inflammatory pathways (Sgro et al., 2022). SD disrupts the gut barrier and BBB permeability, increases NLRP3 levels, and activates the TLR4/NF-κB signaling pathway in the gut, leading to the transmission of inflammatory signals to the brain, thereby exacerbating neuroinflammation and microglial activation in the brain (J. Sun et al., 2020; Wang Z. et al., 2021; Zhao N. et al., 2023). Restoring disrupted gut microbiota can alleviate gut oxidative stress, inflammatory responses, and barrier damage, thereby improving sleep quality (Lai W. D. et al., 2022; Li L. et al., 2023; Wu Z. et al., 2023). Certain gut microbes can regulate the brain’s sleep functions by mediating the host’s inflammatory response through their metabolites (Chen H. et al., 2023; Yin et al., 2024). For example, trimethylamine N-oxide (TMAO), a metabolite of gut microbiota, can enhance BBB integrity by modulating annexin A1 signaling, protecting the brain from inflammatory damage (Hoyles et al., 2021), and can also affect sleep by promoting NLRP3 activation through the NF-κB signaling pathway (Praveenraj et al., 2022) (Figure 1).
SD triggers gut microbiota dysbiosis, leading to an imbalance in the expression of inflammatory factors such as IL-1β, IL-6, and tumor necrosis factor α (TNF-α) (Zhang M. et al., 2023). Related analyses indicate that IL-1β and TNF-α are positively correlated with Ruminococcus_1 and Ruminococcaceae_UCG-005 in the gut (Yao et al., 2022). NLRP3 is one of the key mediators involved in IL-1β-controlled sleep regulation. Assembly of NLRP3 can activate caspase-1, and activated caspase-1 subsequently cleaves pro-IL-1β into mature IL-1β, which then regulates the CNS control of physiological sleep (Aghelan et al., 2023). TNF-α and IL-1β receptors mediate NF-κB transcription through ligand activation, triggering the transcription of inflammation-related molecules involved in sleep regulation and affecting sleep (Misrani et al., 2024). Although the impact of SD on the BBB is somewhat reversible, restoring normal BBB function remains a lengthy process even after resuming a regular sleep pattern (Axelrod et al., 2023; Puech et al., 2023).
4 Ethnopharmacology
4.1 Overview of traditional therapies
Traditional medicine, particularly traditional Chinese medicine (TCM), has long been used to treat insomnia. According to TCM theory, the onset of insomnia can be attributed to a number of factors, including mental health conditions, dietary choices, excessive work, physical exhaustion, and post-illness debility. These elements can result in a variety of clinical changes, such as liver yang hyperactivity, heart and spleen deficits, and yin deficiency with fire hyperactivity (Qin and Xiao, 2024). Therefore, individualized care and symptom distinction are highly valued in TCM approaches to insomnia treatment. Because treatment strategies are tailored to each patient’s specific etiology and pathophysiology, this ensures individualized therapy.
Chinese herbal medicine formulations are basic therapeutic units within the context of TCM, where various mixtures of medicinal herbs are customized to target particular disease causes. In China, many traditional remedies for insomnia have been preserved and are still in use today. For example, Suanzaoren Decoction is mentioned in the classic book Essential Prescriptions of the Jingui Yaolue (金匮要略), which was put together by the famous doctor Zhang Zhongjing (张仲景) (Rong et al., 2022). Again, one of the oldest pharmacopeias in the world, Taiping Huimin Heji Jufang (太平惠民和剂局方), mentions Guipi Decoction (Zhou, 2016).
Suanzaoren Decoction is composed of five medicinal herbs in the following amounts: 15 g of Ziziphi Spinosae Semen (Suanzaoren, SZR), 9 g of Poria cocos, 9 g of Anemarrhena asphodeloides Bunge, 6 g of Ligusticum chuanxiong, and 3 g of Glycyrrhiza uralensis Fisch. For conditions like restless insomnia, vivid nightmares, palpitations with night sweats, dizziness, and blurred vision caused by hepatic blood insufficiency and rising virtual heat, the major ingredient in this decoction is advised (Kui et al., 2022).
GuiPi Decoction is composed of ten medicinal herbs in the following proportions: 6 g of Panax Ginseng, 3 g of Atractylodes macrocephala, 3 g of Angelica sinensis, 3 g of Poria cocos, 3 g of Astragalus mongholicus Bunge, 3 g of Polygala tenuifolia Willd, 3 g of D. longan Lour (D. longan Lour), 3 g of SZR, 1.5 g of Aucklandia lappa, and 1 g of Processed Glycyrrhiza uralensis Fisch. This decoction is primarily used for its benefits in improving memory, calming palpitations, alleviating irritability and insomnia, and addressing spontaneous sweating and palpitations (Zhou, 2016).
Traditional herbal formulas comprise a diverse array of Chinese medicinal herbs, intended to address the various pathophysiological mechanisms underlying insomnia through comprehensive regulation, thereby achieving synergistic effects across multiple targets. However, the complexity of these formulas, which arises from the inclusion of multiple herbal components, can make it difficult to identify specific active ingredients and their precise mechanisms of action. Additionally, for acute or severe cases of insomnia, Chinese herbal medicine formulas may have a relatively slower onset of action, potentially requiring an extended period before significant therapeutic effects become apparent.
4.2 Trends in modern therapeutic approaches
Promoting TCM internationally is fraught with difficulties as modern medicine develops. The effective components of single herbs have been extensively studied through modern pharmacology, leading to clearer mechanisms of action and easier standardization of production processes, which ensures consistent and stable product quality. Nevertheless, excessive use of some single herbs or their extracts, especially those that include alkaloids or other strong substances, may result in negative side effects or dependence (Rice and Genest, 1965).
Insomnia can be effectively treated using medicinal Chinese herbs that have dual uses as food and medicine. These herbal medicines are appropriate for inclusion in regular diets in addition to their therapeutic benefits (Xin-yuan et al., 2025). They promote long-term conditioning and prevention by efficiently enhancing sleep quality without placing extra strain on the body (Shan et al., 2015). Additionally, the choice of therapeutic foods can be customized to fit specific needs, guaranteeing maximum effectiveness. For instance, in cases where insomnia is attributed to heart-spleen deficiency, herbs such as D. longan Lour. and Ziziphus jujuba Mill are recommended for their blood-nourishing and calming properties (Jing et al., 2023). For individuals experiencing insomnia due to yin deficiency and excessive fire, herbs like Lilium lancifolium Thunb. and lotus (Nelumbo nucifera Gaertn.) seeds are preferred for their ability to nourish yin and reduce heat (Shi et al., 2023).
5 Herbal medicines and their active components improve sleep quality by modulating gut microbiota
Medicinal foods derived from medicinal Chinese herbs, along with their bioactive compounds, serve as distinctive “prebiotics” that offer several advantages: minimal adverse effects, high safety profiles, enhanced patient compliance, and suitability for long-term consumption (Delzenne and Bindels, 2015). These properties highlight the dual role of these herbs in both nourishment and therapeutic applications, underscoring their unique value. Moreover, they possess the potential to regulate the stability of the gut microbiota, providing a novel perspective on the prevention and treatment of insomnia through modulation of the intestinal microbiome.
5.1 Ziziphi spinosae semen
Ziziphi spinosae semen (Suanzaoren, SZR) refers to the dried mature seeds of Ziziphus jujuba Mill. var. spinosa (Bunge) Hu ex H. F. Chou, a species within the Rhamnaceae family (Commission, 2020). Characterized by its sweet and sour taste with neutral properties, SZR targets the liver, gallbladder, and heart meridians. It is renowned for its functions of “nourishing heart yin, enriching liver blood, calming the mind, and stabilizing the spirit” (Tong et al., 2024), making it particularly effective for treating deficiency-type and chronic insomnia. Historically, SZR has been highly regarded in TCM. Due to its efficacy, both as a single herb and in compound formulations, SZR is widely used in clinical settings for the treatment of insomnia, earning it the accolade of “Eastern Sleep Fruit” (Yong-qing et al., 2024) (Table 1).
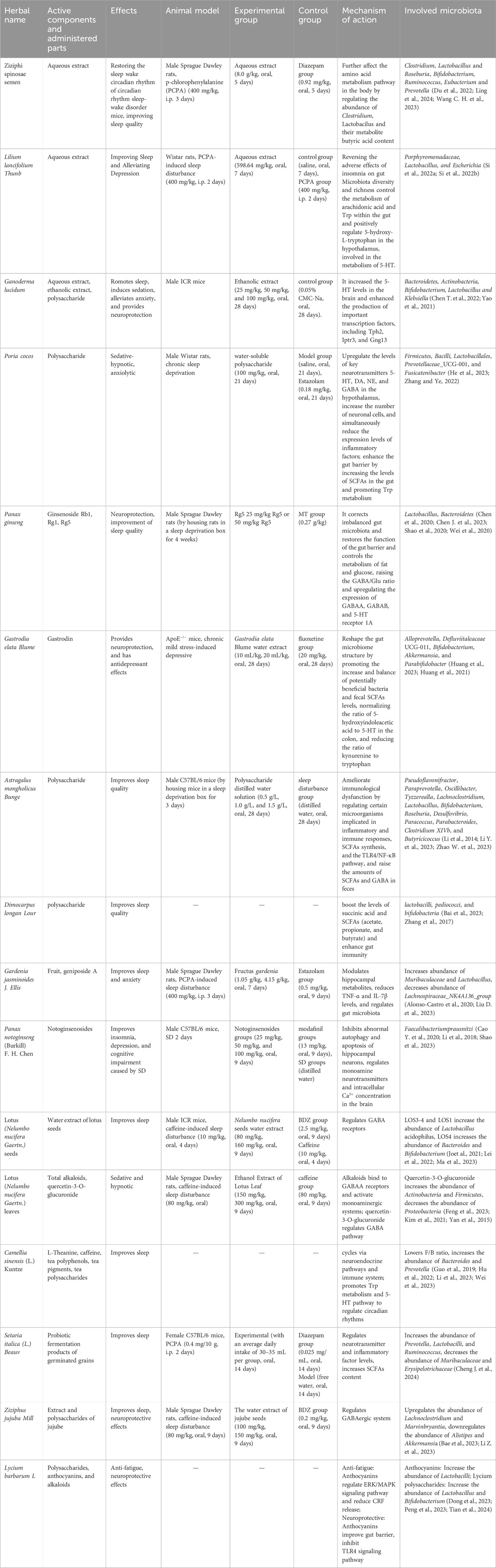
Table 1. By altering the microbiota-gut-brain axis, several Chinese herbal medicines and their active ingredients may enhance the quality of sleep.
Saponins, flavonoids, and alkaloids are the main active components responsible for the sedative and hypnotic effects of SZR (Bian Z. et al., 2022). Previous studies have shown that SZR can regulate physiological processes such as amino acid metabolism, neurotransmitter release, inflammatory factor balance, and HPA axis homeostasis, thereby modulating neural activity and achieving equilibrium in the nervous system to improve sleep (Dong et al., 2021; Liu et al., 2022; Xiao F. et al., 2022).
Recent studies have indicated that SZR can restore the imbalance of gut microbiota caused by insomnia, increasing the relative abundance of beneficial bacteria such as Lactobacillus, Bifidobacterium, Lactococcus, and Eubacterium in rats, while simultaneously reducing the relative abundance of Prevotella (Du et al., 2022; Wang C. H. et al., 2023). The increased abundance of Clostridium and Lactobacillus leads to an increase in the content of their metabolic product, butyrate, which further affects amino acid metabolism pathways in the body, thereby significantly restoring the sleep-wake rhythm in mice with sleep-wake rhythm disorders (Ling et al., 2024). SCFAs can indirectly regulate the levels of 5-HT in both the gut and the brain (Mukherjee et al., 2020; Yu et al., 2020). Moreover, research has shown that the SCFAs increased through microbial metabolism of sour SZR can modulate the expression of representative factors in the TLR4/NF-κB/NLRP3-related signaling pathway in the colon, thereby regulating the microbiota-gut-brain axis and improving insomnia and depressive behaviors in mice (Du et al., 2023; Hua et al., 2021).
It is evident that SZR can regulate the microbiota-gut-brain axis through both the microbiota-neural pathway and the microbiota-immune pathway, thereby achieving control over the organism’s sleep behavior (Figure 2). This may explain how the active components of SZR, which cannot cross the BBB, still manage to improve sleep quality.
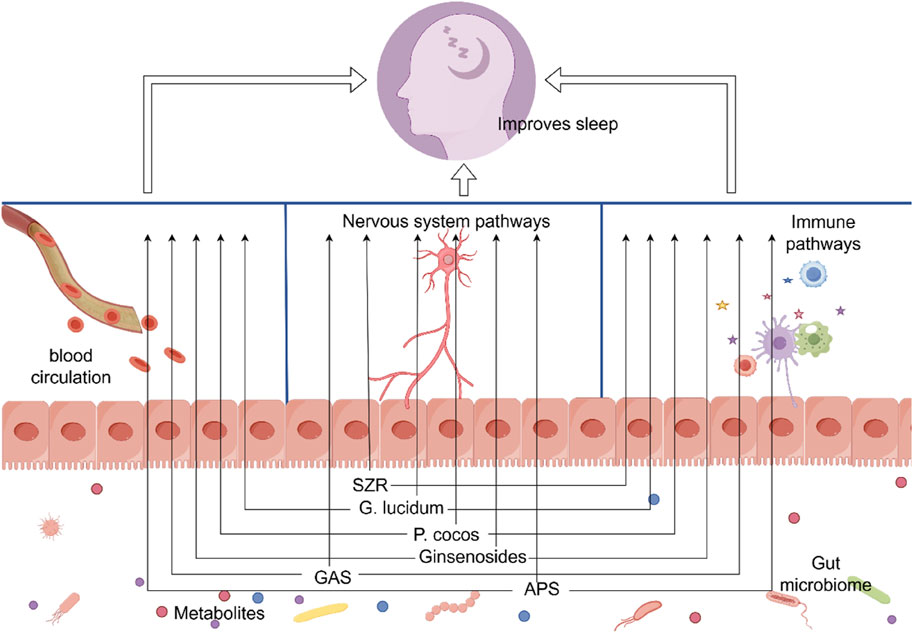
Figure 2. Through altering the gut microbiome, Chinese herbal remedies and their active ingredients can enhance sleep. SZR, ziziphi spinosae semen; G. lucidum, Ganoderma lucidum; P. cocos, Poria cocos; GAS, Gastrodin; APS, Astragalus polysaccharide. Materials provided by FigDraw (www.fgdraw.com).
5.2 Lilium lancifolium Thunb
Lilium lancifolium Thunb (LB) can improve hypothalamic pathology and alleviate insomnia behavior in rats by reducing the levels of HPA axis hormones in the serum, increasing the levels of 5-HT and MT, decreasing the levels of noradrenaline (NE) in the hypothalamus, and upregulating the expression of GABAA receptors and 5-HT1A receptors (Si et al., 2020).
The way that LB regulates the gut flora may have a direct bearing on how well it relieves sleeplessness. In addition to reversing the detrimental effects of insomnia on the diversity, abundance, and fecal metabolic phenotype of the gut microbiota, LB also controls the metabolism of arachidonic acid and Trp, which lowers the expression levels of kynurenine, a chemical linked to mental health issues (Si et al., 2022b). LB significantly modulates the relative abundances of Porphyromonadaceae, Lactobacillus, and Escherichia within the gut and positively regulates 5-hydroxy-L-tryptophan in the hypothalamus, which is involved in the metabolism of 5-HT. With LB intervention, the most important and fundamental pathways are thought to be the serotonergic system and the arachidonic acid metabolic pathway (Si et al., 2022a) (Table 1). Additionally, the decoction of LB and Rehmannia glutinosa (Gaertn.) (LBRD) can lessen neuronal damage linked to depressed behavior by rectifying gut microbiota dysbiosis and avoiding inflammation brought on by compromised gut barriers or BBB leaking. Through the microbiota-gut-brain axis, Lactobacillus, Bifidobacterium, and Lactococcus are shown to be important contributors to the antidepressant effects of LBRD, according to a fecal microbiota transplantation and gut microbiota composition study (Mao et al., 2024).
5.3 Ganoderma lucidum
Research has found that the calming effects of Ganoderma lucidum (G. lucidum) are closely linked to the gut microbiota. When G. lucidum is fermented with Lactobacillus reuteri, it can increase the levels of 5-HT and GABA in the serum and reduce the levels of HPA axis hormones, effectively alleviating insomnia symptoms in anxious mice (Huang et al., 2022). The ethanol extract of G. lucidum also demonstrates a significant impact on the structure of the microbiome. At the phylum level, it increases the abundance of Bacteroidetes and Actinobacteria; at the genus level, it increases the abundance of Bifidobacterium while decreasing the abundance of Lactobacillus and Klebsiella (Chen T. et al., 2022). The hypothalamus’s 5-HT concentrations are positively correlated with Bifidobacterium and Bifidobacterium animalis, which not only raises 5-HT levels there but also increases the production of important transcription factors including Tph2, Iptr3, and Gng13 (Yao et al., 2021). After depleting the gut microbiota with antibiotics, the sleep-promoting effects of the ethanol extract of G. lucidum and the changes in fecal metabolites associated with sleep behavior completely disappear (Yao et al., 2021). This indicates that the gut microbiota plays an indispensable role in the sleep-promoting effects of G. lucidum (Table 1).
Furthermore, polysaccharides derived from G. lucidum have been shown to enhance GABA and 5-HT levels in mice’s brains and enhance the quality of their sleep by lengthening delta waves during NREMS (Ye H. et al., 2021). In addition, polysaccharides from G. lucidum and their hydrolysis products, known as ganoderan peptides, exhibit immunomodulatory activities through various pathways. Specifically, G. lucidum polysaccharides not only reduce the F/B ratio in the gut but also significantly upregulate the expression levels of NF-κBp65, IL-2, and IL-4 in the ileum (Jin et al., 2017); they also significantly enhance the expression of BDNF, inhibit the expression of pro-inflammatory cytokines, and suppress the activation of microglia and astrocytes, thereby exerting an antidepressant effect (Li et al., 2021) (Figure 2). Ganoderan peptides are involved in key pathways such as the folate cycle, biosynthesis and metabolism of fatty acids, and cAMP metabolism (Xie et al., 2023). G. lucidum polysaccharides have significant potential in regulating gut microbiota homeostasis and providing neuroprotection.
5.4 Poria cocos
As one of the preferred core medicines used by ancient people to treat insomnia, Poria cocos (P. cocos) contains polysaccharides and triterpenoids, which are its main active ingredients for calming the mind and inducing tranquility (Lai Y. et al., 2022; Li et al., 2022). Studies have shown that acidic polysaccharides from P. cocos can exert a calming effect by intervening in the gut microbiota and regulating related pathways. These polysaccharides not only increase the abundance of gut microbiota such as Firmicutes, Bacilli, Lactobacillales, Prevotellaceae_UCG-001, and Fusicatenibacter; they also upregulate the levels of key neurotransmitters 5-HT, DA, NE, and GABA in the hypothalamus, increase the number of neuronal cells, and simultaneously reduce the expression levels of inflammatory factors (Zhang and Ye, 2022). Additionally, aqueous extracts and water-soluble polysaccharides from P. cocos can prevent the onset of anxiety effectively by improving gut microbiota imbalances, alleviating metabolic disorders, regulating the levels of gut neuropeptides and neurotransmitters, and inhibiting the TNF-α/NF-κB signaling pathway (Zhang D. et al., 2022; Zhang D. D. et al., 2022) (Figure 2).
Furthermore, polysaccharides from P. cocos can enhance the gut barrier by increasing the levels of SCFAs in the gut and promoting Trp metabolism. They not only promote Trp metabolism by increasing the levels of indole lactate and indole-3-aldehyde (He et al., 2023) (Table 1); they also maintain the integrity and function of the gut barrier by increasing the levels of SCFAs in the gut, regulating the expression of key immune factors such as IL-2, IL-4, IL-6, IL-10, TGF-β, and IFN-γ, and activating the Wnt/β-Catenin signaling pathway (Duan Y. et al., 2023). The levels of Trp and SCFAs, as well as the healthy homeostasis of the gut barrier, are closely linked to the gut microbiota and have significant impacts on the improvement of sleep quality.
5.5 Panax ginseng
Peptides, polysaccharides, and saponins found in Panax ginseng have the ability to cross the blood-brain barrier and regulate the neuroendocrine balance and metabolic environment of the brain (LU et al., 2023). Panax ginseng polysaccharides enhance ginsenoside Rb1 and microbial metabolite exposure by enhancing intestinal absorption and affecting gut microbial metabolism (Shen et al., 2018). Rb1 exerts neuroprotective effects through regulation of Lactobacillus helveticus abundance and GABAA receptor expression (Chen et al., 2020). Ginsenoside Rg1 (Rg1) has potential health-promoting effects on the nervous system. Rg1 can ameliorate morphine-induced gut microbiota dysbiosis (specifically for Bacteroidetes), inhibit gut microbiota-derived tryptophan metabolism, and reduce serotonin, 5-hydroxytryptamine receptor 1B, and 5-hydroxytryptamine receptor 2A levels (Han et al., 2020).
Ginsenoside Rg may affect the gut microbiota and offer neuroprotection through a number of different pathways. Rg1 can keep mice from developing a morphine dependence by regulating the 5-HT neurotransmitter system, preventing the metabolism of Trp produced by the gut microbiota, and improving gut microbiota dysbiosis (particularly with regard to Bacteroidetes) (Chen Z. et al., 2022) (Table 1). Rg5 not only corrects imbalanced gut microbiota and restores the function of the gut barrier, but it also alters the rhythmic characteristics of clock-related proteins and improves the quality of your sleep by controlling the metabolism of fat and glucose, raising the GABA/Glu ratio, and upregulating the expression of GABAA, GABAB, and 5-HT receptor 1A, which in turn affects the GABA and 5-HT neurotransmitter systems (Chen J. B. et al., 2023; Shao et al., 2020; Wei et al., 2020).
Furthermore, the combined use of P. ginseng and SZR can improve the structure of the gut microbiota, promote the proper functioning of the Glu/GABA-Gln metabolic cycle, and increase the synthesis and release of GABA in the hippocampus, thereby significantly improving the sleep state in insomniac rats (Qiao et al., 2022). This suggests that P. ginseng can intervene in the gut microbiota and modulate brain sleep functions through multiple pathways (Figure 2).
5.6 Gastrodia elata Blume
The extraction and isolation of gastrodin (GAS) from Gastrodia elata Blume (G. Blume) have been shown to have a notable impact on illnesses of the central nervous system, including but not limited to sleeplessness, anxiety, depression, cognitive impairment, and ischemic stroke (Zhang et al., 2020). By controlling the expression of inflammatory factors like IL-6 and IL-1β, the activity of B-cell lymphoma-2 (Bcl-2) protein, and the ratio of p-ERK to ERK, GAS not only improves the quality of sleep in mice with insomnia (Long et al., 2021), but it also ameliorates sleep disorders brought on by the deprivation of rapid eye movement sleep (REMS) by modulating the TLR4/NF-κB and Wnt/β-catenin signaling pathways (Liu B. et al., 2023). GAS, therefore, has a lot of potential to enhance the quality of sleep.
Studies already conducted show that GAS can inhibit neuronal apoptosis by regulating the microbiota-gut-brain axis, which improves cognitive impairment and neurodegeneration in AD mice (Zhang Y. et al., 2023). In depressed mice, the aqueous extract of G. elata Blume can reshape the gut microbiome structure by promoting the increase and balance of potentially beneficial bacteria (Alloprevotella, Defluviitaleaceae UCG-011, Bifidobacterium, Akkermansia, and Parabifidobacter) and fecal SCFAs levels, normalizing the ratio of 5-hydroxyindoleacetic acid to 5-HT in the colon, and reducing the ratio of kynurenine to tryptophan (Huang et al., 2023; Huang et al., 2021) (Table 1). The administration of a mixture of antibiotics partially abolishes the neuroprotective effects of GAS in AD mice (Fasina et al., 2022). However, conclusive experimental evidence supporting the notion that GAS can modulate the brain’s sleep function through this microbiota-gut-brain axis remains to be seen.
The effects of G. Blume and its active components on brain function are evident, as they partially target the microbiota-gut-brain axis. Further research is needed to determine the exact processes by which GAS improves sleep quality by altering the gut microbiota in order to enable the more prudent development and usage of G. Blume (Figure 2).
5.7 Astragalus mongholicus Bunge
Rich in flavonoids, polysaccharides, and saponins, Astragalus mongholicus Bunge helps to keep the gut microenvironment stable by controlling the composition, metabolism, and activity of the gut microbiota (Su et al., 2023).
Studies have found that different concentrations of Astragalus Polysaccharides (APS) have a mitigating and protective effect on the spleen and bodily injuries in SD mice (Li et al., 2014). Further research indicates APS can ameliorate immunological dysfunction by regulating certain microorganisms implicated in inflammatory and immune responses, SCFAs synthesis, and the TLR4/NF-κB pathway. For instance, APS can improve the immune function of immunocompromised mice and rats due to decreasing the abundance of Pseudoflavonifractor, Paraprevotella, Oscillibacter, Tyzzerealla, and Lachnoclostridium and increasing the abundance of Lactobacillus, Bifidobacterium, Roseburia, Desulfovibrio, Paracoccus, Parabacteroides, Clostridium XIVb, and Butyricicoccus (Li Y. et al., 2023; Zhao W. et al., 2023). However, in immunocompromised mice with reduced gut microbiota, APS did not improve immunological function (Li Y. et al., 2023) (Table 1, Figure 2).
In order to positively control the gut-brain axis, APS not only increases the relative abundance of Lactobacillus and Bacillus in the gut but also raises the amounts of SCFAs and GABA in feces and improves shrimp immunity (Sun et al., 2023). Additionally, APS can significantly attenuate age-associated disruption of the intestinal barrier, loss of gastrointestinal acid-base balance, reduction in intestinal length, overproliferation of the intestinal stem cells, and sleeping disorders upon aging (Li X. et al., 2023). Therefore, the gut microbiota not only actively aids in the body’s absorption of APS, but it also improves immunological dysfunction by raising gut SCFAs levels and positively regulates the gut-brain axis by encouraging the release of neurotransmitters.
5.8 Dimocarpus longan Lour
Dimocarpus longan Lour. (D. longan Lour.) flesh is one of the herbal remedies commonly used in traditional Chinese medicine to treat insomnia, but there is a dearth of pharmacological activity studies on its potential to improve the quality of sleep. D. longan Lour, which contains GABA, can be used as a natural dietary supplement. Numerous amino acids, such as GABA and Glu, are abundant in longans and are observed to increase in concentration as the fruit ages. Storage at refrigerated temperatures after maturation can increase the GABA content in the fruit (Hu et al., 2021; Zhou et al., 2016). Additionally, by increasing the amount of lactobacilli, pediococci, and bifidobacteria in the stomach, the gut microbiota’s metabolism of longan polysaccharides can boost the levels of succinic acid and SCFAs (acetate, propionate, and butyrate) and enhance gut immunity (Bai et al., 2023; Zhang et al., 2017) (Table 1).
It is evident that, whether as a dietary supplement rich in GABA or by modulating microbial metabolites within the gut, the flesh of D. longan Lour has the potential to regulate the microbiota-gut-brain axis by affecting the synthesis and release of sleep-related neurotransmitters such as GABA, MT, and 5-HT in the gut. This can directly or indirectly modulate the body’s sleep-wake cycle, thereby exerting a potent sleep-promoting effect.
5.9 Other Chinese herbal medicines and their components
Fewer studies have been conducted on herbs like Gardenia jasminoides, Panax notoginseng, and lotus seeds and their effects on gut microbiome interventions to improve sleep quality than on the aforementioned herbs and their active ingredients. In order to determine which herbs and which active ingredients enhance the quality of sleep, this article examines the pertinent literature. It also discusses how these active ingredients affect the gut flora. Through gut microbiome therapies, the ability of these herbs and their active ingredients to enhance sleep quality is assessed. As shown in Table 1.
Studies have shown that Gardenia jasminoides J. Ellis can improve sleep quality and alleviate anxiety symptoms by modulating the gut microbiota and reducing levels of TNF-α and IL-7β (Liu D. et al., 2023). Panax notoginseng saponins, metabolized by gut microbiota, are converted into ginsenoside Rg, which protects hippocampal neurons and regulates neurotransmitter levels in the brain, thereby improving insomnia, depression, and cognitive impairments (Cao Y. et al., 2020; Li et al., 2018; Shao et al., 2023).
Both lotus seeds and lotus leaves can regulate the GABAergic system by modulating the abundance and structure of the gut microbiota, thereby promoting sleep (Jo et al., 2021; Kim et al., 2021; Yan et al., 2015). However, there are significant differences in how they regulate the gut microbiota. Specifically, the oligosaccharide components in lotus seeds can increase the relative abundance of Lactobacillus acidophilus, Bacteroides, and Bifidobacterium (Lei et al., 2022; Ma et al., 2023). Lotus leaves contain flavonoid components that can increase the relative abundance of Firmicutes and Actinobacteria and reduce the abundance of Proteobacteria (Feng et al., 2023). Ziziphus jujuba Mill has the potential to improve sleep quality and provide neuroprotection through modulating the GABAergic system. Specifically, it has been shown to upregulate the abundance of Lachnoclostridium and Marvinbryantia while downregulating the abundance of Alistipes and Akkermansia (Bae et al., 2023; Li Z. et al., 2023). Additionally, Setaria italica (L.) Beauv may increase the abundance of Prevotella, lactic acid bacteria, and Ruminococcus in the gut, thereby increasing the levels of SCFAs and improving sleep quality by regulating neurotransmitter and inflammatory factor levels (Cheng J. et al., 2024). Camellia sinensis (L.) Kuntze may influence neuroendocrine pathways and the immune system by improving the gut microbiota, thereby regulating the sleep-wake cycle (Wei et al., 2023). Lycium barbarum may protect the nervous system by improving the gut barrier and inhibiting TLR4 signaling pathways (Dong et al., 2023; Tian et al., 2024).
The considerable potential of herbs and their active ingredients in modifying the gut microbiota and enhancing sleep quality has already been shown, despite the fact that this field of study is still in its early phases. Not only can more research provide solid support for treating insomnia, but it can also help us develop and use these herbs more sensibly by illuminating the mechanisms by which they interact with the microbiota-gut-brain axis to regulate brain functions related to sleep.
6 Conclusion and perspectives
This article focuses on the research of the microbiota-gut-brain axis, with an emphasis on exploring the pathways and targets for the prevention and treatment of insomnia through the intervention of gut microbiota. It also summarizes recent advances in the study of traditional Chinese herbal medicines and their active components in improving sleep quality by modulating the gut microbiota. Although the specific mechanisms by which gut microbiota participate in sleep regulation have not been fully elucidated, there is compelling evidence that gut microbiota and their metabolites can rapidly transmit relevant information to core brain regions via multiple pathways, including the nervous, endocrine, and immune systems, thereby modulating the brain’s sleep-wake cycle. Traditional Chinese herbal medicines and their active components have demonstrated significant potential in the prevention and treatment of insomnia, with a unique advantage lying in their effective modulation of the gut microbiota. By adjusting the composition of gut microbiota and their metabolites, these herbal medicines and their active ingredients can not only regulate multiple signaling pathways involving neurotransmitters and inflammatory mediators but also optimize physiological functions at several key targets, ultimately achieving improvements in sleep quality.
Despite significant progress in exploring the complex bidirectional interactions between the gut microbiota and insomnia, the intricate interplay between the gut microbiota and the brain, coupled with the multi-component and multi-target nature of Chinese herbal medicines, presents substantial challenges for further research in this field. (1) Model Limitations: Current studies on the relationship between gut microbiota and insomnia predominantly rely on germ-free (GF) or antibiotic-induced microbiota-depleted (AIMD) mouse models. However, these rodent models exhibit significant differences from humans in terms of sleep-wake cycle characteristics, potentially leading to biases when translating findings to human applications. To achieve a more precise and comprehensive understanding of the specific roles and mechanisms of gut microbiota in sleep health and disorders, it is essential to expand research into non-rodent or primate animal models and increase sample sizes to ensure the reliability of conclusions. (2) Complex Mechanisms of Action: The interaction between the gut microbiota and the brain involves multiple pathways, including neural, endocrine, and immune systems. The detailed mechanisms underlying these interactions remain incompletely understood, particularly regarding how Chinese herbal medicine components influence sleep through the “microbiota-gut-brain axis.” To address this, we should strengthen research on specific Chinese herbal medicine components, gut microbiota community structures, and their metabolic products, exploring how these factors jointly regulate brain function and sleep quality. This will deepen our understanding of the mechanisms of the “microbiota-gut-brain axis” and the therapeutic effects of Chinese herbal medicine interventions on this system. (3) Component Diversity and Target Identification: Chinese herbal medicines often contain multiple active components that may influence human health through various pathways. Identifying the key components responsible for improving insomnia and clarifying their exact targets within the microbiota-gut-brain axis is a challenging task. This requires fostering interdisciplinary collaboration among fields such as physiology, pharmacology, molecular biology, microbiology, and neuroscience. Integrating knowledge and technologies from these domains—such as leveraging bioinformatics for big data analysis to reveal complex biological networks—is essential. (4) Personalized Treatment Needs: Given the significant variability in gut microbiota composition among individuals, even the same Chinese herbal medicine herb or its components may exhibit different effects across different people. Therefore, when developing broadly effective treatments, it is crucial to account for this high degree of individual variability. A precision medicine approach—tailoring personalized treatment plans based on an individual’s microbiome profile—is a feasible strategy. However, this necessitates the development of advanced diagnostic tools and technologies to accurately analyze individual microbiota compositions and predict responses to specific herbs or components. (5) Standards and Quality Control: The extraction of active ingredients and formulation processes for Chinese herbal medicines are relatively complex, posing challenges in ensuring consistent product quality and stability. Establishing strict standards for Chinese herbal medicine ingredient extraction, formulation, and quality control systems is critical to ensuring the safety and efficacy of the products. Additionally, enhancing the identification and quantitative analysis of active Chinese herbal medicine components can improve drug consistency and reproducibility. (6) The Importance of Clinical Trials: While preliminary studies suggest that Chinese herbal medicines may improve sleep quality by modulating gut microbiota, these studies are often small-scale and lack rigorous design. The absence of large-scale, multicenter, randomized, double-blind, placebo-controlled clinical trials undermines the persuasiveness and credibility of Chinese herbal medicine efficacy claims. Furthermore, further research is needed on the long-term safety of Chinese herbal medicine use or its combination with other drugs, particularly when it involves modulating brain function via the gut microbiota. Potential adverse effects and side effects require special attention. In the future, rigorously designed large-scale clinical trials are necessary to validate the efficacy of Chinese herbal medicines and their active components in improving sleep through the modulation of the microbiota-gut-brain axis. Long-term follow-up studies should also be considered to assess the sustained effects on chronic insomnia patients and potential issues of dependency or drug resistance.
In the future, by implementing the measures outlined above and conducting multidimensional comparisons between Chinese herbal medicine herbs and existing sleep aids (such as melatonin and benzodiazepines) through high-quality randomized controlled trials, appropriate efficacy indicators, systematic collection and analysis of adverse reactions, exploration of mechanisms of action, and long-term follow-up studies, we can gain a more comprehensive understanding of the advantages and limitations of Chinese herbal medicines compared to conventional sleep aids. This will provide a solid scientific basis for their rational application.
Author contributions
CW: Writing – original draft, Writing – review and editing, Conceptualization, Investigation, Resources. JD: Project administration, Supervision, Writing – review and editing. XS: Data curation, Investigation, Writing – review and editing. FY: Conceptualization, Investigation, Resources, Writing – review and editing. XL: Conceptualization, Investigation, Resources, Writing – review and editing. WS: Conceptualization, Investigation, Resources, Writing – review and editing. XZ: Funding acquisition, Project administration, Supervision, Writing – review and editing.
Funding
The author(s) declare that financial support was received for the research and/or publication of this article. This study was sponsored by the Science and Key Project of “Revealing the List and Taking the Lead” in Heilongjiang Province (2023ZXJ02C02).
Conflict of interest
The authors declare that the research was conducted in the absence of any commercial or financial relationships that could be construed as a potential conflict of interest.
Generative AI statement
The authors declare that no Generative AI was used in the creation of this manuscript.
Publisher’s note
All claims expressed in this article are solely those of the authors and do not necessarily represent those of their affiliated organizations, or those of the publisher, the editors and the reviewers. Any product that may be evaluated in this article, or claim that may be made by its manufacturer, is not guaranteed or endorsed by the publisher.
References
Aghelan, Z., Pashaee, S., Abtahi, S. H., Karima, S., Khazaie, H., Ezati, M., et al. (2023). Natural immunosuppressants as a treatment for chronic insomnia targeting the inflammatory response induced by NLRP3/caspase-1/IL-1β Axis activation: a scooping review. J. Neuroimmune Pharmacol. 18 (3), 294–309. doi:10.1007/s11481-023-10078-7
Alonso-Castro, A. J., Gasca-Martínez, D., Cortez-Mendoza, L. V., Alba-Betancourt, C., Ruiz-Padilla, A. J., and Zapata-Morales, J. R. (2020). Evaluation of the neuropharmacological effects of Gardenin A in mice. Drug Dev. Res. 81 (5), 600–608. doi:10.1002/ddr.21659
Álvarez, S. A., Rocha-Guzmán, N. E., González-Laredo, R. F., Gallegos-Infante, J. A., Moreno-Jiménez, M. R., and Bravo-Muñoz, M. (2022). Ancestral food sources rich in polyphenols, their metabolism, and the potential influence of gut microbiota in the management of depression and anxiety. J. Agric. Food Chem. 70 (4), 944–956. doi:10.1021/acs.jafc.1c06151
Arzani, M., Jahromi, S. R., Ghorbani, Z., Vahabizad, F., Martelletti, P., Ghaemi, A., et al. (2020). Gut-brain Axis and migraine headache: a comprehensive review. J. Headache Pain 21 (1), 15. doi:10.1186/s10194-020-1078-9
Axelrod, S., Li, X., Sun, Y., Lincoln, S., Terceros, A., O'Neil, J., et al. (2023). The Drosophila blood-brain barrier regulates sleep via Moody G protein-coupled receptor signaling. Proc. Natl. Acad. Sci. U. S. A. 120 (42), e2309331120. doi:10.1073/pnas.2309331120
Bae, G. Y., Ahn, Y., Hong, K. B., Jung, E. J., Suh, H. J., and Jo, K. (2023). Sleep-enhancing effect of water extract from jujube (zizyphus jujuba Mill.) seeds fermented by Lactobacillus brevis L32. Foods 12 (15), 2864. doi:10.3390/foods12152864
Bai, Y., Zhou, Y., Li, X., Zhang, R., Huang, F., Fan, B., et al. (2023). Longan pulp polysaccharides regulate gut microbiota and metabolites to protect intestinal epithelial barrier. Food Chem. 422, 136225. doi:10.1016/j.foodchem.2023.136225
Barone, M., Martucci, M., Sciara, G., Conte, M., Medina, L. S. J., Iattoni, L., et al. (2024). Towards a personalized prediction, prevention and therapy of insomnia: gut microbiota profile can discriminate between paradoxical and objective insomnia in post-menopausal women. Epma J. 15 (3), 471–489. doi:10.1007/s13167-024-00369-1
Bedu-Ferrari, C., Biscarrat, P., Pepke, F., Vati, S., Chaudemanche, C., Castelli, F., et al. (2024). In-depth characterization of a selection of gut commensal bacteria reveals their functional capacities to metabolize dietary carbohydrates with prebiotic potential. mSystems 9 (4), e0140123. doi:10.1128/msystems.01401-23
Bian, W. J., Brewer, C. L., Kauer, J. A., and de Lecea, L. (2022). Adolescent sleep shapes social novelty preference in mice. Nat. Neurosci. 25 (7), 912–923. doi:10.1038/s41593-022-01076-8
Bian, Z. H., Zhang, W. M., Tang, J. Y., Fei, Q. Q., Hu, M. M., Chen, X. W., et al. (2022). Effective substance and mechanism of Ziziphi Spinosae Semen extract in treatment of insomnia based on serum metabolomics and network pharmacology. Zhongguo Zhong Yao Za Zhi 47 (1), 188–202. doi:10.19540/j.cnki.cjcmm.20210922.702
Buey, B., Forcén, A., Grasa, L., Layunta, E., Mesonero, J. E., and Latorre, E. (2023). Gut microbiota-derived short-chain fatty acids: novel regulators of intestinal serotonin transporter. Life (Basel) 13 (5), 1085. doi:10.3390/life13051085
Cao, C., Liu, M., Qu, S., Huang, R., Qi, M., Zhu, Z., et al. (2020). Chinese medicine formula Kai-Xin-San ameliorates depression-like behaviours in chronic unpredictable mild stressed mice by regulating gut microbiota-inflammation-stress system. J. Ethnopharmacol. 261, 113055. doi:10.1016/j.jep.2020.113055
Cao, Y., Yang, Y., Wu, H., Lu, Y., Wu, S., Liu, L., et al. (2020). Stem-leaf saponins from Panax notoginseng counteract aberrant autophagy and apoptosis in hippocampal neurons of mice with cognitive impairment induced by sleep deprivation. J. Ginseng Res. 44 (3), 442–452. doi:10.1016/j.jgr.2019.01.009
Chen, H., Shen, J., Li, H., Zheng, X., Kang, D., Xu, Y., et al. (2020). Ginsenoside Rb1 exerts neuroprotective effects through regulation of Lactobacillus helveticus abundance and GABA(A) receptor expression. J. Ginseng Res. 44 (1), 86–95. doi:10.1016/j.jgr.2018.09.002
Chen, H. W., Zhou, R., Cao, B. F., Liu, K., Zhong, Q., Huang, Y. N., et al. (2023). The predictive, preventive, and personalized medicine of insomnia: gut microbiota and inflammation. Epma J. 14 (4), 571–583. doi:10.1007/s13167-023-00345-1
Chen, J. B., Li, M. J., Huo, X. H., Gong, T. J., Han, X., Liu, J. H., et al. (2023). Ginsenoside Rg5 improves sleep by regulating energy metabolism in sleep-deprived rats. Am. J. Chin. Med. 51 (7), 1845–1864. doi:10.1142/s0192415x23500817
Chen, T., Wu, S., Yang, G., Jiang, D., Jiang, Y., and Chen, B. (2022). Effects of Ganoderma resinaceum alcohol extract on sleep and intestinal microbiota in mice. Biotechnol. Bull. 38 (08), 225–232. doi:10.13560/j.cnki.biotech.bull.1985.2021-1470
Chen, Z., Lin, Y., Zhou, Q., Xiao, S., Li, C., Lin, R., et al. (2022). Ginsenoside Rg1 mitigates morphine dependence via regulation of gut microbiota, tryptophan metabolism, and serotonergic system function. Biomed. Pharmacother. 150, 112935. doi:10.1016/j.biopha.2022.112935
Chen, Z., Luo, J., Li, J., Kim, G., Stewart, A., Urban, J. F., et al. (2021). Interleukin-33 promotes serotonin release from enterochromaffin cells for intestinal homeostasis. Immunity 54 (1), 151–163.e6. doi:10.1016/j.immuni.2020.10.014
Cheng, J., Wu, Q., Sun, R., Li, W., Wang, Z., Zhou, M., et al. (2024). Protective effects of a probiotic-fermented germinated grain complex on neurotransmitters and sleep quality in sleep-deprived mice. Front. Microbiol. 15, 1438928. doi:10.3389/fmicb.2024.1438928
Cheng, X., Zhu, Y., Huang, J., Li, Y., Jiang, X., and Yang, Q. (2024). A neutral polysaccharide from Persicaria hydropiper (L.) Spach ameliorates lipopolysaccharide-induced intestinal barrier injury via regulating the gut microbiota and modulating AKT/PI3K/mTOR and MAPK signaling pathways. J. Ethnopharmacol. 320, 117403. doi:10.1016/j.jep.2023.117403
Chojnacki, C., Gąsiorowska, A., Popławski, T., Konrad, P., Chojnacki, M., Fila, M., et al. (2023). Beneficial effect of increased tryptophan intake on its metabolism and mental state of the elderly. Nutrients 15 (4), 847. doi:10.3390/nu15040847
Chung, Y., Wu, J. L., and Huang, W. C. (2023). Effects of prebiotics on intestinal physiology, neuropsychological function, and exercise capacity of mice with sleep deprivation. Food Res. Int. 165, 112568. doi:10.1016/j.foodres.2023.112568
Commission, C. P. (2020). Committee National Pharmacopoeia. Pharmacopoeia of People's Republic of China. Beijing: China Medical Science Press. (in Chinese).
Delzenne, N. M., and Bindels, L. B. (2015). Gut microbiota: Ganoderma lucidum, a new prebiotic agent to treat obesity? Nat. Rev. Gastroenterol. Hepatol. 12 (10), 553–554. doi:10.1038/nrgastro.2015.137
Deng, Y., Zhou, M., Wang, J., Yao, J., Yu, J., Liu, W., et al. (2021). Involvement of the microbiota-gut-brain axis in chronic restraint stress: disturbances of the kynurenine metabolic pathway in both the gut and brain. Gut Microbes 13 (1), 1–16. doi:10.1080/19490976.2020.1869501
Dong, W., Huang, Y., Shu, Y., Fan, X., Tian, X., Yan, Y., et al. (2023). Water extract of goji berries improves neuroinflammation induced by a high-fat and high-fructose diet based on the bile acid-mediated gut-brain axis pathway. Food Funct. 14 (18), 8631–8645. doi:10.1039/d3fo02651e
Dong, Y. J., Jiang, N. H., Zhan, L. H., Teng, X., Fang, X., Lin, M. Q., et al. (2021). Soporific effect of modified Suanzaoren Decoction on mice models of insomnia by regulating Orexin-A and HPA axis homeostasis. Biomed. Pharmacother. 143, 112141. doi:10.1016/j.biopha.2021.112141
Du, H., Liu, J. X., Yan, Y., and Du, C. H. (2022). Mechanism of Suanzaoren Decoction in improving insomnia rats by integrating metabolomics and intestinal flora analysis. Zhongguo Zhong Yao Za Zhi 47 (24), 6741–6752. doi:10.19540/j.cnki.cjcmm.20220902.701
Du, Y., He, B., Wu, B., Yan, T., and Jia, Y. (2023). Suanzaoren decoction improves depressive-like behaviors by regulating the microbiota-gut-brain axis via inhibiting TLR4/NFκB/NLRP3 inflammation signal pathway. J. Chem. Neuroanat. 134, 102349. doi:10.1016/j.jchemneu.2023.102349
Duan H., H., Yu, Q., Ni, Y., Li, J., and Fan, L. (2023). Effect of agaricus bisporus polysaccharides on human gut microbiota during in vitro fermentation: an integrative analysis of microbiome and metabolome. Foods 12 (4), 859. doi:10.3390/foods12040859
Duan Y., Y., Huang, J., Sun, M., Jiang, Y., Wang, S., Wang, L., et al. (2023). Poria cocos polysaccharide improves intestinal barrier function and maintains intestinal homeostasis in mice. Int. J. Biol. Macromol. 249, 125953. doi:10.1016/j.ijbiomac.2023.125953
Engevik, M. A., Luk, B., Chang-Graham, A. L., Hall, A., Herrmann, B., Ruan, W., et al. (2019). Bifidobacterium dentium fortifies the intestinal mucus layer via autophagy and calcium signaling pathways. mBio 10 (3), 010877-e1119. doi:10.1128/mBio.01087-19
Fasina, O. B., Wang, J., Mo, J., Osada, H., Ohno, H., Pan, W., et al. (2022). Gastrodin from Gastrodia elata enhances cognitive function and neuroprotection of AD mice via the regulation of gut microbiota composition and inhibition of neuron inflammation. Front. Pharmacol. 13, 814271. doi:10.3389/fphar.2022.814271
Feng, J., Wang, J., Bu, T., Ge, Z., Yang, K., Sun, P., et al. (2023). Structural, in vitro digestion, and fermentation characteristics of lotus leaf flavonoids. Food Chem. 406, 135007. doi:10.1016/j.foodchem.2022.135007
Feng, X. Y., Yan, J. T., Li, G. W., Liu, J. H., Fan, R. F., Li, S. C., et al. (2020). Source of dopamine in gastric juice and luminal dopamine-induced duodenal bicarbonate secretion via apical dopamine D(2) receptors. Br. J. Pharmacol. 177 (14), 3258–3272. doi:10.1111/bph.15047
Feng, X. Y., Zhang, D. N., Wang, Y. A., Fan, R. F., Hong, F., Zhang, Y., et al. (2017). Dopamine enhances duodenal epithelial permeability via the dopamine D(5) receptor in rodent. Acta Physiol. (Oxf) 220 (1), 113–123. doi:10.1111/apha.12806
Gao, T., Wang, Z., Dong, Y., Cao, J., and Chen, Y. (2021). Melatonin-Mediated colonic microbiota metabolite butyrate prevents acute sleep deprivation-induced colitis in mice. Int. J. Mol. Sci. 22 (21), 11894. doi:10.3390/ijms222111894
Gao, T., Wang, Z., Dong, Y., Cao, J., Lin, R., Wang, X., et al. (2019). Role of melatonin in sleep deprivation-induced intestinal barrier dysfunction in mice. J. Pineal Res. 67 (1), e12574. doi:10.1111/jpi.12574
Glatzle, J., Sternini, C., Robin, C., Zittel, T. T., Wong, H., Reeve, J. R., et al. (2002). Expression of 5-HT3 receptors in the rat gastrointestinal tract. Gastroenterology 123 (1), 217–226. doi:10.1053/gast.2002.34245
Gunawan, S. P., Huang, S. Y., Wang, C. C., Huynh, L. B. P., Nguyen, N. N., Hsu, S. Y., et al. (2024). Sleep deprivation alters pubertal timing in humans and rats: the role of the gut microbiome. Sleep 47 (2), zsad308. doi:10.1093/sleep/zsad308
Guo, T., Song, D., Ho, C. T., Zhang, X., Zhang, C., Cao, J., and Wu, Z. (2019). Omics Analyses of Gut Microbiota in a circadian rhythm disorder mouse model fed with oolong tea polyphenols. J Agric Food Chem. 67 (32), 8847–8854. doi:10.1021/acs.jafc.9b03000
Han, S. K., Joo, M. K., Kim, J. K., Jeung, W., Kang, H., and Kim, D. H. (2020). Bifidobacteria-fermented red ginseng and its constituents ginsenoside rd and protopanaxatriol alleviate anxiety/depression in mice by the amelioration of gut dysbiosis. Nutrients 12 (4), 901. doi:10.3390/nu12040901
He, Y., Gao, W., Wang, X., Lu, W., and Wang, H. (2023). Effects of dietary polysaccharides on tryptophan metabolism in intestinal microbiota based on an in vitro fermentation model. Food Ferment. Industries 49 (15), 8–15. doi:10.13995/j.cnki.11-1802/ts.034677
Heath, A. M., Haszard, J. J., Galland, B. C., Lawley, B., Rehrer, N. J., Drummond, L. N., et al. (2020). Association between the faecal short-chain fatty acid propionate and infant sleep. Eur. J. Clin. Nutr. 74 (9), 1362–1365. doi:10.1038/s41430-019-0556-0
Hoyles, L., Pontifex, M. G., Rodriguez-Ramiro, I., Anis-Alavi, M. A., Jelane, K. S., Snelling, T., et al. (2021). Regulation of blood-brain barrier integrity by microbiome-associated methylamines and cognition by trimethylamine N-oxide. Microbiome 9 (1), 235. doi:10.1186/s40168-021-01181-z
Hu, S., Luo, L., Bian, X., Liu, R. H., Zhao, S., Chen, Y., Sun, K., Jiang, J., Liu, Z., Zeng, L., et al. (2022). Pu-erh tea restored circadian rhythm disruption by regulating tryptophan metabolism. J Agric Food Chem. 70 (18), 5610–5623. doi:10.1021/acs.jafc.2c01883
Hu, W., Wang, B., Ali, M. M., Chen, X., Zhang, J., Zheng, S., et al. (2021). Free amino acids profile and expression analysis of core genes involved in branched-chain amino acids metabolism during fruit development of longan (Dimocarpus longan Lour.) cultivars with different aroma types. Biol. (Basel) 10 (8), 807. doi:10.3390/biology10080807
Hua, Y., Guo, S., Xie, H., Zhu, Y., Yan, H., Tao, W. W., et al. (2021). Ziziphus jujuba Mill. Var. spinosa (Bunge) Hu ex H. F. Chou seed ameliorates insomnia in rats by regulating metabolomics and intestinal flora composition. Front. Pharmacol. 12, 653767. doi:10.3389/fphar.2021.653767
Huang, H. S., Lin, Y. E., Panyod, S., Chen, R. A., Lin, Y. C., Chai, L. M. X., et al. (2023). Anti-depressive-like and cognitive impairment alleviation effects of Gastrodia elata Blume water extract is related to gut microbiome remodeling in ApoE(-/-) mice exposed to unpredictable chronic mild stress. J. Ethnopharmacol. 302 (Pt B), 115872. doi:10.1016/j.jep.2022.115872
Huang, J. H., Li, Y., Zhang, S., Zou, Y., Zheng, Q. W., Lin, J. F., et al. (2022). Amelioration effect of water extract from Ganoderma resinaceum FQ23 solid-state fermentation fungal substance with high-yield ergothioneine on anxiety-like insomnia mice. Food Funct. 13 (24), 12925–12937. doi:10.1039/d2fo01847k
Huang, Y. J., Choong, L. C., Panyod, S., Lin, Y. E., Huang, H. S., Lu, K. H., et al. (2021). Gastrodia elata Blume water extract modulates neurotransmitters and alters the gut microbiota in a mild social defeat stress-induced depression mouse model. Phytother. Res. 35 (9), 5133–5142. doi:10.1002/ptr.7091
Jahnke, J. R., Roach, J., Azcarate-Peril, M. A., and Thompson, A. L. (2021). Maternal precarity and HPA axis functioning shape infant gut microbiota and HPA axis development in humans. PLoS One 16 (5), e0251782. doi:10.1371/journal.pone.0251782
Jeong, A. H., Hwang, J., Jo, K., Kim, S., Ahn, Y., Suh, H. J., et al. (2021). Fermented gamma aminobutyric acid improves sleep behaviors in fruit flies and rodent models. Int. J. Mol. Sci. 22 (7), 3537. doi:10.3390/ijms22073537
Jin, M., Zhu, Y., Shao, D., Zhao, K., Xu, C., Li, Q., et al. (2017). Effects of polysaccharide from mycelia of Ganoderma lucidum on intestinal barrier functions of rats. Int. J. Biol. Macromol. 94 (Pt A), 1–9. doi:10.1016/j.ijbiomac.2016.09.099
Jing, Y., Meng-yun, H., Wen-bin, D., and Hou-ping, X. (2023). Study on medication law of TCM in the treatment of insomnia due to deficiency of both heart and spleen based on data mining. Inn. Mong. J. Traditional Chin. Med. 42 (11), 115–118. doi:10.16040/j.cnki.cn15-1101.2023.11.054
Jo, K., Kim, S., Hong, K. B., and Suh, H. J. (2021). Nelumbo nucifera promotes non-rapid eye movement sleep by regulating GABAergic receptors in rat model. J. Ethnopharmacol. 267, 113511. doi:10.1016/j.jep.2020.113511
Kaur, S., Sharma, P., Mayer, M. J., Neuert, S., Narbad, A., and Kaur, S. (2023). Beneficial effects of GABA-producing potential probiotic Limosilactobacillus fermentum L18 of human origin on intestinal permeability and human gut microbiota. Microb. Cell Fact. 22 (1), 256. doi:10.1186/s12934-023-02264-2
Kim, C. H. (2021). Control of lymphocyte functions by gut microbiota-derived short-chain fatty acids. Cell Mol. Immunol. 18 (5), 1161–1171. doi:10.1038/s41423-020-00625-0
Kim, S., Hong, K. B., Jo, K., and Suh, H. J. (2021). Quercetin-3-O-glucuronide in the ethanol extract of Lotus leaf (Nelumbo nucifera) enhances sleep quantity and quality in a rodent model via a GABAergic mechanism. Molecules 26 (10), 3023. doi:10.3390/molecules26103023
Ko, C. Y., Liu, Q. Q., Su, H. Z., Zhang, H. P., Fan, J. M., Yang, J. H., et al. (2019). Gut microbiota in obstructive sleep apnea-hypopnea syndrome: disease-related dysbiosis and metabolic comorbidities. Clin. Sci. (Lond) 133 (7), 905–917. doi:10.1042/cs20180891
Kui, Z., Zhe, X., Xueping, X., Xin, S., Chenxu, P., and Yuhong, B. (2022). Modern research evolvements of Suanzaoren Decoction in treating insomnia by regulating circadian rhythm. Tianjin J. Traditional Chin. Med. 39 (11), 1483–1489. doi:10.11656/j.issn.1672-1519.2022.11.21
Lai, W. D., Tung, T. H., Teng, C. Y., Chang, C. H., Chen, Y. C., Huang, H. Y., et al. (2022). Fish oil ameliorates neuropsychiatric behaviors and gut dysbiosis by elevating selected microbiota-derived metabolites and tissue tight junctions in rats under chronic sleep deprivation. Food Funct. 13 (5), 2662–2680. doi:10.1039/d2fo00181k
Lai, Y., Yu, H., Deng, H., Fang, Q., Lei, H., Liu, L., et al. (2022). Three main metabolites from Wolfiporia cocos (F. A. Wolf) Ryvarden and Gilb regulate the gut microbiota in mice: a comparative study using microbiome-metabolomics. Front. Pharmacol. 13, 911140. doi:10.3389/fphar.2022.911140
Lan, Y., Lu, J., Qiao, G., Mao, X., Zhao, J., Wang, G., et al. (2023). Bifidobacterium breve CCFM1025 improves sleep quality via regulating the activity of the HPA Axis: a randomized clinical trial. Nutrients 15 (21), 4700. doi:10.3390/nu15214700
Layunta, E., Buey, B., Mesonero, J. E., and Latorre, E. (2021). Crosstalk between intestinal serotonergic system and pattern recognition receptors on the microbiota-gut-brain Axis. Front. Endocrinol. (Lausanne) 12, 748254. doi:10.3389/fendo.2021.748254
Lei, Y., Zhang, Y., Wang, Q., Zheng, B., Miao, S., and Lu, X. (2022). Structural characterization and in vitro analysis of the prebiotic activity of oligosaccharides from lotus (Nelumbo nucifera Gaertn.) seeds. Food Chem. 388, 133045. doi:10.1016/j.foodchem.2022.133045
Li, A., Kou, R., Lui, H., Chen, M., Wang, J., Liu, Q., et al. (2023). Multi-omics analyses reveal relationships among polyphenol-rich oolong tea consumption, gut microbiota, and metabolic profile: A pilot study. Food Chem. 426, 136653. doi:10.1016/j.foodchem.2023.136653
Li, B., Hsieh, Y. R., Lai, W. D., Tung, T. H., Chen, Y. X., Yang, C. H., et al. (2023). Melatonin ameliorates neuropsychiatric behaviors, gut microbiome, and microbiota-derived metabolites in rats with chronic sleep deprivation. Int. J. Mol. Sci. 24 (23), 16820. doi:10.3390/ijms242316820
Li, H., Xiao, J., Li, X., Chen, H., Kang, D., Shao, Y., et al. (2018). Low cerebral exposure cannot hinder the neuroprotective effects of Panax notoginsenosides. Drug Metab. Dispos. 46 (1), 53–65. doi:10.1124/dmd.117.078436
Li, H., Xiao, Y., Han, L., Jia, Y., Luo, S., Zhang, D., et al. (2021). Ganoderma lucidum polysaccharides ameliorated depression-like behaviors in the chronic social defeat stress depression model via modulation of Dectin-1 and the innate immune system. Brain Res. Bull. 171, 16–24. doi:10.1016/j.brainresbull.2021.03.002
Li, J., Li, Y., Zhao, J., Li, L., Wang, Y., Chen, F., et al. (2024). Effects of Bifidobacterium breve 207-1 on regulating lifestyle behaviors and mental wellness in healthy adults based on the microbiome-gut-brain axis: a randomized, double-blind, placebo-controlled trial. Eur. J. Nutr. 63, 2567–2585. doi:10.1007/s00394-024-03447-2
Li, L., Wu, L., Jiang, T., Liang, T., Yang, L., Li, Y., et al. (2023). Lactiplantibacillus plantarum 124 modulates sleep deprivation-associated markers of intestinal barrier dysfunction in mice in conjunction with the regulation of gut microbiota. Nutrients 15 (18), 4002. doi:10.3390/nu15184002
Li, L., Zuo, Z. T., and Wang, Y. Z. (2022). The traditional usages, chemical components and pharmacological activities of wolfiporia cocos: a review. Am. J. Chin. Med. 50 (2), 389–440. doi:10.1142/s0192415x22500161
Li, N., Tan, S., Wang, Y., Deng, J., Wang, N., Zhu, S., et al. (2023). Akkermansia muciniphila supplementation prevents cognitive impairment in sleep-deprived mice by modulating microglial engulfment of synapses. Gut Microbes 15 (2), 2252764. doi:10.1080/19490976.2023.2252764
Li, S., Qiao, E., Zhang, C., Jin, G., and Gu, Y. (2014). The effect of Astragalus polysaccharides on the spleen tissue structure of mice subjected to continuous sleep deprivation. J. Hyg. Res. 43 (05), 838–841. doi:10.19813/j.cnki.weishengyanjiu.2014.05.027
Li, W., Wang, Z., Cao, J., Dong, Y., and Chen, Y. (2023a). Melatonin improves skin barrier damage caused by sleep restriction through gut microbiota. J. Pineal Res. 75 (1), e12874. doi:10.1111/jpi.12874
Li, W., Wang, Z., Cao, J., Dong, Y., and Chen, Y. (2023b). Melatonin improves the homeostasis of mice gut microbiota rhythm caused by sleep restriction. Microbes Infect. 25 (6), 105121. doi:10.1016/j.micinf.2023.105121
Li, X., Yang, S., Wang, S., Shi, Y., Dai, Y., Zhang, X., et al. (2023). Regulation and mechanism of Astragalus polysaccharide on ameliorating aging in Drosophila melanogaster. Int. J. Biol. Macromol. 234, 123632. doi:10.1016/j.ijbiomac.2023.123632
Li, Y., Zhang, Y., Zhang, X. L., Feng, X. Y., Liu, C. Z., Zhang, X. N., et al. (2019). Dopamine promotes colonic mucus secretion through dopamine D(5) receptor in rats. Am. J. Physiol. Cell Physiol. 316 (3), C393-C403–c403. doi:10.1152/ajpcell.00261.2017
Li, Y., Zheng, J., Wang, Y., Yang, H., Cao, L., Gan, S., et al. (2023). Immuno-stimulatory activity of Astragalus polysaccharides in cyclophosphamide-induced immunosuppressed mice by regulating gut microbiota. Int. J. Biol. Macromol. 242 (Pt 2), 124789. doi:10.1016/j.ijbiomac.2023.124789
Li, Z., Wu, M., Wei, W., An, Y., Li, Y., Wen, Q., et al. (2023). Fingerprinting evaluation and gut microbiota regulation of polysaccharides from jujube (Ziziphus jujuba Mill.) fruit. Int. J. Mol. Sci. 24 (8), 7239. doi:10.3390/ijms24087239
Ling, H., Shengmei, Z., Yu, T., Xiangping, P., Yan, Y., and Chenhui, D. (2024). Clostridium, Lactobacillus and Roseburia rhythm disturbance in mice model based on metabolomics and gut microbiota. Chin. Traditional Herb. Drugs 55 (18), 6208–6225. doi:10.7501/j.issn.0253-2670.2024.18.011
Liu, B., Fan, L., Wang, Y., Wang, H., Yan, Y., Chen, S., et al. (2024). Gut microbiota regulates host melatonin production through epithelial cell MyD88. Gut Microbes 16 (1), 2313769. doi:10.1080/19490976.2024.2313769
Liu, B., Li, F., Xu, Y., Wu, Q., and Shi, J. (2023). Gastrodin improves cognitive dysfunction in REM sleep-deprived rats by regulating TLR4/NF-κB and wnt/β-catenin signaling pathways. Brain Sci. 13 (2), 179. doi:10.3390/brainsci13020179
Liu, D., Wang, Q., Li, Y., Yuan, Z., Liu, Z., Guo, J., et al. (2023). Fructus gardeniae ameliorates anxiety-like behaviors induced by sleep deprivation via regulating hippocampal metabolomics and gut microbiota. Front. Cell Infect. Microbiol. 13, 1167312. doi:10.3389/fcimb.2023.1167312
Liu, J. X., Li, J. H., Du, C. H., and Yan, Y. (2022). Metabonomic study of biochemical changes in serum of PCPA-induced insomnia rats after treatment with Suanzaoren Decoction. Zhongguo Zhong Yao Za Zhi 47 (6), 1632–1641. doi:10.19540/j.cnki.cjcmm.20211204.701
Liu, N., Sun, S., Wang, P., Sun, Y., Hu, Q., and Wang, X. (2021). The mechanism of secretion and metabolism of gut-derived 5-hydroxytryptamine. Int. J. Mol. Sci. 22 (15), 7931. doi:10.3390/ijms22157931
Long, P., Hu, X., Hu, Q., Tao, L., Zhou, X., Shen, X., et al. (2021). Sedative and hypnotic effects of gastrodin on PCPA-induced insomnia mice. Pharmacol. Clin. Chin. Materia Medica 37 (05), 33–38. doi:10.13412/j.cnki.zyyl.2021.05.007
Luck, B., Horvath, T. D., Engevik, K. A., Ruan, W., Haidacher, S. J., Hoch, K. M., et al. (2021). Neurotransmitter profiles are altered in the gut and brain of mice mono-associated with Bifidobacterium dentium. Biomolecules 11 (8), 1091. doi:10.3390/biom11081091
Luo, Y., Yu, L., Zhang, P., Lin, W., Xu, H., Dou, Z., et al. (2024). Larger hypothalamic subfield volumes in patients with chronic insomnia disorder and relationships to levels of corticotropin-releasing hormone. J. Affect Disord. 351, 870–877. doi:10.1016/j.jad.2024.02.023
Luo, Y., Zeng, B., Zeng, L., Du, X., Li, B., Huo, R., et al. (2018). Gut microbiota regulates mouse behaviors through glucocorticoid receptor pathway genes in the hippocampus. Transl. Psychiatry 8 (1), 187. doi:10.1038/s41398-018-0240-5
Lu, Z., Zheng, Q., Cheung, S., Han, Q., and Liang, Q. (2023). Research progress on Ginseng Radix et Rhizoma in requlation of neurotransmitter metabolism. Chin. Traditional Herb. Drugs 54 (21), 7260–7272. doi:10.7501/j.issn.0253-2670.2023.21.034
Ma, S. N., Liu, X. H., and Cai, W. S. (2024). Preventive noninvasive vagal nerve stimulation reduces insufficient sleep-induced depression by improving the autonomic nervous system. Biomed. Pharmacother. 173, 116344. doi:10.1016/j.biopha.2024.116344
Ma, X., Zheng, Z., Wang, Q., Zuo, J., Ju, J., Zheng, B., et al. (2023). The modulation effect of lotus (Nelumbo nucifera Gaertn.) seeds oligosaccharides with different structures on intestinal flora and action mode of growth effects on Bifidobacterium in vivo and in vitro. Food Chem. 419, 136057. doi:10.1016/j.foodchem.2023.136057
Mao, Q., Zhang, H., Zhang, Z., Lu, Y., Pan, J., Guo, D., et al. (2024). Co-decoction of Lilii bulbus and Radix Rehmannia Recens and its key bioactive ingredient verbascoside inhibit neuroinflammation and intestinal permeability associated with chronic stress-induced depression via the gut microbiota-brain axis. Phytomedicine 129, 155510. doi:10.1016/j.phymed.2024.155510
Matenchuk, B. A., Mandhane, P. J., and Kozyrskyj, A. L. (2020). Sleep, circadian rhythm, and gut microbiota. Sleep. Med. Rev. 53, 101340. doi:10.1016/j.smrv.2020.101340
Matsuda, Y., Ozawa, N., Shinozaki, T., Wakabayashi, K. I., Suzuki, K., Kawano, Y., et al. (2020). Ergothioneine, a metabolite of the gut bacterium Lactobacillus reuteri, protects against stress-induced sleep disturbances. Transl. Psychiatry 10 (1), 170. doi:10.1038/s41398-020-0855-1
Mayer, E. A., Tillisch, K., and Gupta, A. (2015). Gut/brain axis and the microbiota. J. Clin. Invest 125 (3), 926–938. doi:10.1172/jci76304
McVey Neufeld, K. A., Bienenstock, J., Bharwani, A., Champagne-Jorgensen, K., Mao, Y., West, C., et al. (2019). Oral selective serotonin reuptake inhibitors activate vagus nerve dependent gut-brain signalling. Sci. Rep. 9 (1), 14290. doi:10.1038/s41598-019-50807-8
Misrani, A., Tabassum, S., Zhang, Z. Y., Tan, S. H., and Long, C. (2024). Urolithin A prevents sleep-deprivation-induced neuroinflammation and mitochondrial dysfunction in young and aged mice. Mol. Neurobiol. 61 (3), 1448–1466. doi:10.1007/s12035-023-03651-x
Moreno-Indias, I., Torres, M., Montserrat, J. M., Sanchez-Alcoholado, L., Cardona, F., Tinahones, F. J., et al. (2015). Intermittent hypoxia alters gut microbiota diversity in a mouse model of sleep apnoea. Eur. Respir. J. 45 (4), 1055–1065. doi:10.1183/09031936.00184314
Muneer, A. (2020). Kynurenine pathway of tryptophan metabolism in neuropsychiatric disorders: pathophysiologic and therapeutic considerations. Clin. Psychopharmacol. Neurosci. 18 (4), 507–526. doi:10.9758/cpn.2020.18.4.507
Mukherjee, A., Lordan, C., Ross, R. P., and Cotter, P. D. (2020). Gut microbes from the phylogenetically diverse genus Eubacterium and their various contributions to gut health. Gut Microbes 12 (1), 1802866. doi:10.1080/19490976.2020.1802866
Noemi, C. N., Bob, P., and Bókkon, I. (2024). Long-term implicit epigenetic stress information in the enteric nervous system and its contribution to developing and perpetuating IBS. Curr. Neuropharmacol. 22 (13), 2100–2112. doi:10.2174/1570159x22666240507095700
Noguchi, H., Ikenaga, T., Ueno, S., Kohashi, C., Matsumura, Y., Kakumoto, Y., et al. (2024). Effect of single oral coingestion of GABA and malic acid on postprandial GLP-1, glucose, and insulin responses in healthy volunteers: a randomized, double-blind, placebo-controlled, crossover study. Mol. Nutr. Food Res. 68 (7), e2300610. doi:10.1002/mnfr.202300610
Ogawa, Y., Miyoshi, C., Obana, N., Yajima, K., Hotta-Hirashima, N., Ikkyu, A., et al. (2020). Gut microbiota depletion by chronic antibiotic treatment alters the sleep/wake architecture and sleep EEG power spectra in mice. Sci. Rep. 10 (1), 19554. doi:10.1038/s41598-020-76562-9
Piber, D., Cho, J. H., Lee, O., Lamkin, D. M., Olmstead, R., and Irwin, M. R. (2022). Sleep disturbance and activation of cellular and transcriptional mechanisms of inflammation in older adults. Brain Behav. Immun. 106, 67–75. doi:10.1016/j.bbi.2022.08.004
Praveenraj, S. S., Sonali, S., Anand, N., Tousif, H. A., Vichitra, C., Kalyan, M., et al. (2022). The role of a gut microbial-derived metabolite, trimethylamine N-oxide (TMAO), in neurological disorders. Mol. Neurobiol. 59 (11), 6684–6700. doi:10.1007/s12035-022-02990-5
Puech, C., Badran, M., Runion, A. R., Barrow, M. B., Cataldo, K., and Gozal, D. (2023). Cognitive impairments, neuroinflammation and blood-brain barrier permeability in mice exposed to chronic sleep fragmentation during the daylight period. Int. J. Mol. Sci. 24 (12), 9880. doi:10.3390/ijms24129880
Qiao, T., Wang, Y., Liang, K., Zheng, B., Ma, J., Li, F., et al. (2022). Effects of the Radix Ginseng and Semen Ziziphi Spinosae drug pair on the GLU/GABA-GLN metabolic cycle and the intestinal microflora of insomniac rats based on the brain-gut axis. Front. Pharmacol. 13, 1094507. doi:10.3389/fphar.2022.1094507
Qin, Y., and Xiao, J. (2024). Research progress in traditional Chinese medicine diagnosis and treatment of insomnia. Hebei J. Traditional Chin. Med. 46 (09), 1580–1584. doi:10.3969/j.issn.1002-2619.2024.09.037
Ranteh, O., Tedasen, A., Rahman, M. A., Ibrahim, M. A., and Sama-Ae, I. (2024). Bioactive compounds from Ocimum tenuiflorum and Poria cocos: a novel natural Compound for insomnia treatment based on A computational approach. Comput. Biol. Med. 175, 108491. doi:10.1016/j.compbiomed.2024.108491
Rice, W. B., and Genest, K. (1965). Acute toxicity of extracts of morning-glory seed in mice. Nature 207 (994), 302–303. doi:10.1038/207302b0
Rivero-Segura, N. A., Cuartas, J. D. R., Garcia-delaTorre, P., Sanchez-Garcia, S., Ramirez-Aldana, R., and Gomez-Verjan, J. C. (2025). Insomnia accelerates the epigenetic clocks in older adults. Geroscience doi:10.1007/s11357-025-01608-7
Rong, Z., Zheng, C., and Luping, L. (2022). Treating 69 cases of sub-health insomnia by suanzaoren tang and Guipi pills from synopsis of golden chamber. West. J. Traditional Chin. Med. 35 (10), 90–93.
Sgritta, M., Dooling, S. W., Buffington, S. A., Momin, E. N., Francis, M. B., Britton, R. A., et al. (2019). Mechanisms underlying microbial-mediated changes in social behavior in mouse models of autism spectrum disorder. Neuron 101 (2), 246–259. doi:10.1016/j.neuron.2018.11.018
Sgro, M., Kodila, Z. N., Brady, R. D., Reichelt, A. C., Mychaisuk, R., and Yamakawa, G. R. (2022). Synchronizing our clocks as we age: the influence of the brain-gut-immune axis on the sleep-wake cycle across the lifespan. Sleep 45 (3), zsab268. doi:10.1093/sleep/zsab268
Shan, F., Huang, L., Guo, J., and Chen, M. (2015). History and development of “one root of medicine and food”. Chin. Bull. Life Sci. 27 (8), 97–105. doi:10.13376/j.cbls/2015146
Shao, J., Zheng, X., Qu, L., Zhang, H., Yuan, H., Hui, J., et al. (2020). Ginsenoside Rg5/Rk1 ameliorated sleep via regulating the GABAergic/serotoninergic signaling pathway in a rodent model. Food Funct. 11 (2), 1245–1257. doi:10.1039/c9fo02248a
Shao, L., Wang, L., Shi, Y. Y., Zhang, W., Tan, L. W., Wan, J. B., et al. (2023). Biotransformation of the saponins in Panax notoginseng leaves mediated by gut microbiota from insomniac patients. J. Sep. Sci. 46 (6), e2200803. doi:10.1002/jssc.202200803
Shen, H., Gao, X. J., Li, T., Jing, W. H., Han, B. L., Jia, Y. M., et al. (2018). Ginseng polysaccharides enhanced ginsenoside Rb1 and microbial metabolites exposure through enhancing intestinal absorption and affecting gut microbial metabolism. J. Ethnopharmacol. 216, 47–56. doi:10.1016/j.jep.2018.01.021
Shi, X., Chen, L., Li, W., and Wang, R. (2023). Research progress in the prevention and treatment of insomnia with classical prescriptions. Zhong Nan Da Xue Xue Bao Yi Xue Ban. 48 (10), 1494–1505. doi:10.11817/j.issn.1672-7347.2023.230200
Shimizu, Y., Yamamura, R., Yokoi, Y., Ayabe, T., Ukawa, S., Nakamura, K., et al. (2023). Shorter sleep time relates to lower human defensin 5 secretion and compositional disturbance of the intestinal microbiota accompanied by decreased short-chain fatty acid production. Gut Microbes 15 (1), 2190306. doi:10.1080/19490976.2023.2190306
Si, Y., Chen, X., Guo, T., Wei, W., Wang, L., Zhang, F., et al. (2022a). Comprehensive 16S rDNA sequencing and LC-MS/MS-Based metabolomics to investigate intestinal flora and metabolic profiles of the serum, hypothalamus and Hippocampus in p-chlorophenylalanine-induced insomnia rats treated with Lilium brownie. Neurochem. Res. 47 (3), 574–589. doi:10.1007/s11064-021-03466-z
Si, Y., Wang, L., Lan, J., Li, H., Guo, T., Chen, X., et al. (2020). Lilium davidii extract alleviates p-chlorophenylalanine-induced insomnia in rats through modification of the hypothalamic-related neurotransmitters, melatonin and homeostasis of the hypothalamic-pituitary-adrenal axis. Pharm. Biol. 58 (1), 915–924. doi:10.1080/13880209.2020.1812674
Si, Y., Wei, W., Chen, X., Xie, X., Guo, T., Sasaki, Y., et al. (2022b). A comprehensive study on the relieving effect of Lilium brownii on the intestinal flora and metabolic disorder in p-chlorphenylalanine induced insomnia rats. Pharm. Biol. 60 (1), 131–143. doi:10.1080/13880209.2021.2019283
Siopi, E., Galerne, M., Rivagorda, M., Saha, S., Moigneu, C., Moriceau, S., et al. (2023). Gut microbiota changes require vagus nerve integrity to promote depressive-like behaviors in mice. Mol. Psychiatry 28 (7), 3002–3012. doi:10.1038/s41380-023-02071-6
Song, L., He, M., Sun, Q., Wang, Y., Zhang, J., Fang, Y., et al. (2021). Roseburia hominis increases intestinal melatonin level by activating p-CREB-AANAT pathway. Nutrients 14 (1), 117. doi:10.3390/nu14010117
Spencer, N. J., Kyloh, M. A., Travis, L., and Hibberd, T. J. (2024). Identification of vagal afferent nerve endings in the mouse colon and their spatial relationship with enterochromaffin cells. Cell Tissue Res. 396 (3), 313–327. doi:10.1007/s00441-024-03879-6
Su, M., Tang, T., Tang, W., Long, Y., Wang, L., and Liu, M. (2023). Astragalus improves intestinal barrier function and immunity by acting on intestinal microbiota to treat T2DM: a research review. Front. Immunol. 14, 1243834. doi:10.3389/fimmu.2023.1243834
Sun, J., Wu, J., Hua, F., Chen, Y., Zhan, F., and Xu, G. (2020). Sleep deprivation induces cognitive impairment by increasing blood-brain barrier permeability via CD44. Front. Neurol. 11, 563916. doi:10.3389/fneur.2020.563916
Sun, S., Bian, C., Zhou, N., Shen, Z., and Yu, M. (2023). Dietary Astragalus polysaccharides improve the growth and innate immune response of giant freshwater prawn Macrobrachium rosenbergii: insights from the brain-gut axis. Int. J. Biol. Macromol. 243, 125158. doi:10.1016/j.ijbiomac.2023.125158
Sutanto, C. N., Xia, X., Heng, C. W., Tan, Y. S., Lee, D. P. S., Fam, J., et al. (2024). The impact of 5-hydroxytryptophan supplementation on sleep quality and gut microbiota composition in older adults: a randomized controlled trial. Clin. Nutr. 43 (3), 593–602. doi:10.1016/j.clnu.2024.01.010
Tian, X., Dong, W., Zhou, W., Yan, Y., Lu, L., Mi, J., et al. (2024). The polysaccharides from the fruits of Lycium barbarum ameliorate high-fat and high-fructose diet-induced cognitive impairment via regulating blood glucose and mediating gut microbiota. Int. J. Biol. Macromol. 258 (Pt 2), 129036. doi:10.1016/j.ijbiomac.2023.129036
Tong, Q., Feifei, G., Ning, L., Wenjing, L., Hui, R., Xiaomin, c., et al. (2024). Research progress on the chemical composition, pharmacological action, and clinical application of Ziziphi spinosae semen. China Pharm. 28 (09), 98–108.
Tri, B. D., Shashni, B., Matsui, H., and Nagasaki, Y. (2023). Designing poly(gamma-aminobutyric acid)-based nanoparticles for the treatment of major depressive disorders. J. Control Release 360, 110–121. doi:10.1016/j.jconrel.2023.06.021
Vailati-Riboni, M., Rund, L., Caetano-Silva, M. E., Hutchinson, N. T., Wang, S. S., Soto-Díaz, K., et al. (2022). Dietary fiber as a counterbalance to age-related microglial cell dysfunction. Front. Nutr. 9, 835824. doi:10.3389/fnut.2022.835824
Valentini, F., Evangelisti, M., Arpinelli, M., Di Nardo, G., Borro, M., Simmaco, M., et al. (2020). Gut microbiota composition in children with obstructive sleep apnoea syndrome: a pilot study. Sleep. Med. 76, 140–147. doi:10.1016/j.sleep.2020.10.017
Wang, C. H., Han, R., Li, Z., Heng, Y.-R., Chen, Y.-N., Pei, X.-P., et al. (2023). The mechanism of ziziphi spinosae semen and fried ziziphi spinosae semen to improve insomnia based on integrating metabolomics and intestinal flora. Acta Pharm. Sin. 58 (07), 1940–1951. doi:10.16438/j.0513-4870.2022-1327
Wang, J., Liu, S., Xie, Y., and Xu, C. (2023). Association analysis of gut microbiota-metabolites-neuroendocrine changes in male rats acute exposure to simulated altitude of 5500 m. Sci. Rep. 13 (1), 9225. doi:10.1038/s41598-023-35573-y
Wang, J., Xin, J., Xu, X., Chen, W., Lv, Y., Wei, Y., et al. (2024). Bacopaside I alleviates depressive-like behaviors by modulating the gut microbiome and host metabolism in CUMS-induced mice. Biomed. Pharmacother. 170, 115679. doi:10.1016/j.biopha.2023.115679
Wang, X., Wang, Z., Cao, J., Dong, Y., and Chen, Y. (2023). Gut microbiota-derived metabolites mediate the neuroprotective effect of melatonin in cognitive impairment induced by sleep deprivation. Microbiome 11 (1), 17. doi:10.1186/s40168-022-01452-3
Wang, Y., Li, N., Yang, J. J., Zhao, D. M., Chen, B., Zhang, G. Q., et al. (2020). Probiotics and fructo-oligosaccharide intervention modulate the microbiota-gut brain axis to improve autism spectrum reducing also the hyper-serotonergic state and the dopamine metabolism disorder. Pharmacol. Res. 157, 104784. doi:10.1016/j.phrs.2020.104784
Wang, Z., Wang, Z., Lu, T., Chen, W., Yan, W., Yuan, K., et al. (2022). The microbiota-gut-brain axis in sleep disorders. Sleep. Med. Rev. 65, 101691. doi:10.1016/j.smrv.2022.101691
Wang Y., Y., Tong, Q., Ma, S. R., Zhao, Z. X., Pan, L. B., Cong, L., et al. (2021). Oral berberine improves brain dopa/dopamine levels to ameliorate Parkinson's disease by regulating gut microbiota. Signal Transduct. Target Ther. 6 (1), 77. doi:10.1038/s41392-020-00456-5
Wang Z., Z., Chen, W. H., Li, S. X., He, Z. M., Zhu, W. L., Ji, Y. B., et al. (2021). Gut microbiota modulates the inflammatory response and cognitive impairment induced by sleep deprivation. Mol. Psychiatry 26 (11), 6277–6292. doi:10.1038/s41380-021-01113-1
Wang Z., Z., Li, D., Chen, M., Yu, X., Chen, C., Chen, Y., et al. (2024). A comprehensive study on the regulation of Compound Zaoren Granules on cAMP/CREB signaling pathway and metabolic disorder in CUMS-PCPA induced insomnia rats. J. Ethnopharmacol. 332, 118401. doi:10.1016/j.jep.2024.118401
Wei, Y., Xu, J., Miao, S., Wei, K., Peng, L., Wang, Y., et al. (2023). Recent advances in the utilization of tea active ingredients to regulate sleep through neuroendocrine pathway, immune system and intestinal microbiota. Crit. Rev. Food Sci. Nutr. 63 (25), 7598–7626. doi:10.1080/10408398.2022.2048291
Wei, Y., Yang, H., Zhu, C., Deng, J., and Fan, D. (2020). Hypoglycemic effect of ginsenoside Rg5 mediated partly by modulating gut microbiota dysbiosis in diabetic db/db mice. J. Agric. Food Chem. 68 (18), 5107–5117. doi:10.1021/acs.jafc.0c00605
Wu, J., Lu, Y., Cai, X., Chen, Y., Shen, Z., and Lyv, Q. (2022). Gut microbiota dysbiosis in 4- to 6-year-old children with obstructive sleep apnea-hypopnea syndrome. Pediatr. Pulmonol. 57 (9), 2012–2022. doi:10.1002/ppul.25967
Wu, J., Zhang, B., Zhou, S., Huang, Z., Xu, Y., Lu, X., et al. (2023). Associations between gut microbiota and sleep: a two-sample, bidirectional Mendelian randomization study. Front. Microbiol. 14, 1236847. doi:10.3389/fmicb.2023.1236847
Wu, M., Zhang, X., Feng, S., Freda, S. N., Kumari, P., Dumrongprechachan, V., et al. (2024). Dopamine pathways mediating affective state transitions after sleep loss. Neuron 112 (1), 141–154.e8. doi:10.1016/j.neuron.2023.10.002
Wu, W. L., Adame, M. D., Liou, C. W., Barlow, J. T., Lai, T. T., Sharon, G., et al. (2021). Microbiota regulate social behaviour via stress response neurons in the brain. Nature 595 (7867), 409–414. doi:10.1038/s41586-021-03669-y
Wu, Z., Liu, L., Li, L., Cao, X., Jia, W., Liao, X., et al. (2023). Oral nano-antioxidants improve sleep by restoring intestinal barrier integrity and preventing systemic inflammation. Natl. Sci. Rev. 10 (12), nwad309. doi:10.1093/nsr/nwad309
Xiao, F., Shao, S., Zhang, H., Li, G., Piao, S., Zhao, D., et al. (2022). Neuroprotective effect of Ziziphi Spinosae Semen on rats with p-chlorophenylalanine-induced insomnia via activation of GABA(A) receptor. Front. Pharmacol. 13, 965308. doi:10.3389/fphar.2022.965308
Xiao, W., Li, J., Gao, X., Yang, H., Su, J., Weng, R., et al. (2022). Involvement of the gut-brain axis in vascular depression via tryptophan metabolism: a benefit of short chain fatty acids. Exp. Neurol. 358, 114225. doi:10.1016/j.expneurol.2022.114225
Xie, J., Lin, D., Li, J., Zhou, T., Lin, S., and Lin, Z. (2023). Effects of Ganoderma lucidum polysaccharide peptide ameliorating cyclophosphamide-induced immune dysfunctions based on metabolomics analysis. Front. Nutr. 10, 1179749. doi:10.3389/fnut.2023.1179749
Xin-yuan, S., Ya-ping, Z., Kang-meng, S., Chun-nian, H., and Pei-gen, X. (2025). Review, revision and prospect of the list of food and medicinal substances in China. China J. Chin. Materia Medica, 1–12. doi:10.19540/j.cnki.cjcmm.20241015.601
Yan, M. Z., Chang, Q., Zhong, Y., Xiao, B. X., Feng, L., Cao, F. R., et al. (2015). Lotus leaf alkaloid extract displays sedative-hypnotic and anxiolytic effects through GABAA receptor. J. Agric. Food Chem. 63 (42), 9277–9285. doi:10.1021/acs.jafc.5b04141
Yan, T., Shi, L., Liu, T., Zhang, X., Yang, M., Peng, W., et al. (2023). Diet-rich in wheat bran modulates tryptophan metabolism and AhR/IL-22 signalling mediated metabolic health and gut dysbacteriosis: a novel prebiotic-like activity of wheat bran. Food Res. Int. 163, 112179. doi:10.1016/j.foodres.2022.112179
Yang, D. F., Huang, W. C., Wu, C. W., Huang, C. Y., Yang, Y. S. H., and Tung, Y. T. (2023). Acute sleep deprivation exacerbates systemic inflammation and psychiatry disorders through gut microbiota dysbiosis and disruption of circadian rhythms. Microbiol. Res. 268, 127292. doi:10.1016/j.micres.2022.127292
Yao, C., Wang, Z., Jiang, H., Yan, R., Huang, Q., Wang, Y., et al. (2021). Ganoderma lucidum promotes sleep through a gut microbiota-dependent and serotonin-involved pathway in mice. Sci. Rep. 11 (1), 13660. doi:10.1038/s41598-021-92913-6
Yao, Z. Y., Li, X. H., Zuo, L., Xiong, Q., He, W. T., Li, D. X., et al. (2022). Maternal sleep deprivation induces gut microbial dysbiosis and neuroinflammation in offspring rats. Zool. Res. 43 (3), 380–390. doi:10.24272/j.issn.2095-8137.2022.023
Ye, H., Li, Q., Zhang, Y., Yang, S., Liu, J., and Luo, J. (2021). Compared with the effect of sleep improvement by different extractsfrom Ganoderma lucidum fruit bodies in mice. Sci. Technol. Food Industry 42 (17), 350–356. doi:10.13386/j.issn1002-0306.2020090275
Ye, L., Bae, M., Cassilly, C. D., Jabba, S. V., Thorpe, D. W., Martin, A. M., et al. (2021). Enteroendocrine cells sense bacterial tryptophan catabolites to activate enteric and vagal neuronal pathways. Cell Host Microbe 29 (2), 179–196.e9. doi:10.1016/j.chom.2020.11.011
Yin, C., Zhang, M., Jin, S., Zhou, Y., Ding, L., Lv, Q., et al. (2024). Mechanism of Salvia miltiorrhiza Bunge extract to alleviate Chronic Sleep Deprivation-Induced cognitive dysfunction in rats. Phytomedicine 130, 155725. doi:10.1016/j.phymed.2024.155725
Yong-qing, M., Chang, X., Qiao-feng, L., zheng, X., Jin-sheng, Z., and cheng-yu, w. (2024). Evidence mapping of clinical studies on 23 commonly used Chinese patent medicines for treating insomnia. China J. Chin. Materia Medica 49 (14), 3952–3962. doi:10.19540/j.cnki.cjcmm.20240410.501
Yu, L., Han, X., Cen, S., Duan, H., Feng, S., Xue, Y., et al. (2020). Beneficial effect of GABA-rich fermented milk on insomnia involving regulation of gut microbiota. Microbiol. Res. 233, 126409. doi:10.1016/j.micres.2020.126409
Yu, R., Wen, S., Wang, Q., Wang, C., Zhang, L., Wu, X., et al. (2021). Mulberroside A repairs high fructose diet-induced damage of intestinal epithelial and blood-brain barriers in mice: a potential for preventing hippocampal neuroinflammatory injury. J. Neurochem. 157 (6), 1979–1991. doi:10.1111/jnc.15242
Yun, S. W., Kim, J. K., Lee, K. E., Oh, Y. J., Choi, H. J., Han, M. J., et al. (2020). A probiotic Lactobacillus gasseri alleviates Escherichia coli-induced cognitive impairment and depression in mice by regulating IL-1β expression and gut microbiota. Nutrients 12 (11), 3441. doi:10.3390/nu12113441
Zeng, H., Xu, J., Zheng, L., Zhan, Z., Fang, Z., Li, Y., et al. (2024). Traditional Chinese herbal formulas modulate gut microbiome and improve insomnia in patients with distinct syndrome types: insights from an interventional clinical study. Front. Cell Infect. Microbiol. 14, 1395267. doi:10.3389/fcimb.2024.1395267
Zhang, D., Li, H., Luo, X., Liu, D., Wei, Q., and Ye, X. (2022). Integrated 16S rDNA, metabolomics, and TNF-α/NF-κB signaling pathway analyses to explain the modulatory effect of Poria cocos aqueous extract on anxiety-like behavior. Phytomedicine 104, 154300. doi:10.1016/j.phymed.2022.154300
Zhang, D., and Ye, X. (2022). Mechanism of Poria cocos acidic polysaccharide in quietin g the spirit based on intestinal flora and metabonomics. China J. Traditional Chin. Med. Pharm. 37 (05), 2575–2583.
Zhang, J., Yang, G., Wen, Y., Liu, S., Li, C., Yang, R., et al. (2017). Intestinal microbiota are involved in the immunomodulatory activities of longan polysaccharide. Mol. Nutr. Food Res. 61 (11). doi:10.1002/mnfr.201700466
Zhang, M., Du, Y., Chen, L., Tang, Q., Liu, C., Li, N., et al. (2025). Genome-wide, integrative analysis implicates exosome-derived MicroRNA dysregulation in chronic insomnia. Sleep, zsaf051. doi:10.1093/sleep/zsaf051
Zhang, M., Zhang, M., Kou, G., and Li, Y. (2023). The relationship between gut microbiota and inflammatory response, learning and memory in mice by sleep deprivation. Front. Cell Infect. Microbiol. 13, 1159771. doi:10.3389/fcimb.2023.1159771
Zhang, Y., Xie, B., Chen, X., Zhang, J., and Yuan, S. (2021). A key role of gut microbiota-vagus nerve/spleen axis in sleep deprivation-mediated aggravation of systemic inflammation after LPS administration. Life Sci. 265, 118736. doi:10.1016/j.lfs.2020.118736
Zhang, Z. L., Gao, Y. G., Zang, P., Gu, P. P., Zhao, Y., He, Z. M., et al. (2020). Research progress on mechanism of gastrodin and p-hydroxybenzyl alcohol on central nervous system. Zhongguo Zhong Yao Za Zhi 45 (2), 312–320. doi:10.19540/j.cnki.cjcmm.20190730.401
Zhang D. D., D. D., Li, H. J., Zhang, H. R., and Ye, X. C. (2022). Poria cocos water-soluble polysaccharide modulates anxiety-like behavior induced by sleep deprivation by regulating the gut dysbiosis, metabolic disorders and TNF-α/NF-κB signaling pathway. Food Funct. 13 (12), 6648–6664. doi:10.1039/d2fo00811d
Zhang Y., Y., Chen, Y., Yuan, S., Yu, Q., Fu, J., Chen, L., et al. (2023). Effect of gastrodin against cognitive impairment and neurodegeneration in APP/PS1 mice via regulating gut microbiota-gut-brain axis. Exp. Brain Res. 241 (6), 1661–1673. doi:10.1007/s00221-023-06632-7
Zhao, N., Chen, Q. G., Chen, X., Liu, X. T., Geng, F., Zhu, M. M., et al. (2023). Intestinal dysbiosis mediates cognitive impairment via the intestine and brain NLRP3 inflammasome activation in chronic sleep deprivation. Brain Behav. Immun. 108, 98–117. doi:10.1016/j.bbi.2022.11.013
Zhao, W., Duan, C., Liu, Y., Lu, G., Lyu, Q., Liu, X., et al. (2023). Modulating effects of Astragalus polysaccharide on immune disorders via gut microbiota and the TLR4/NF-κB pathway in rats with syndrome of dampness stagnancy due to spleen deficiency. J. Zhejiang Univ. Sci. B 24 (7), 650–662. doi:10.1631/jzus.B2200491
Zhou, B., Jin, G., Pang, X., Mo, Q., Bao, J., Liu, T., et al. (2022). Lactobacillus rhamnosus GG colonization in early life regulates gut-brain axis and relieves anxiety-like behavior in adulthood. Pharmacol. Res. 177, 106090. doi:10.1016/j.phrs.2022.106090
Zhou, J., He, L., Liu, M., Guo, X., Du, G., Yan, L., et al. (2023). Sleep loss impairs intestinal stem cell function and gut homeostasis through the modulation of the GABA signalling pathway in Drosophila. Cell Prolif. 56 (9), e13437. doi:10.1111/cpr.13437
Zhou, M., Ndeurumio, K. H., Zhao, L., and Hu, Z. (2016). Impact of precooling and controlled-atmosphere storage on γ-aminobutyric acid (GABA) accumulation in longan (Dimocarpus longan Lour.) fruit. J. Agric. Food Chem. 64 (33), 6443–6450. doi:10.1021/acs.jafc.6b01738
Zhou, S. (2016). Handbook of prescriptions commonly used by general practitioners. Changsha: Hunan Science and Technology Press.
Zhu, R., Fang, Y., Li, H., Liu, Y., Wei, J., Zhang, S., et al. (2023). Psychobiotic Lactobacillus plantarum JYLP-326 relieves anxiety, depression, and insomnia symptoms in test anxious college via modulating the gut microbiota and its metabolism. Front. Immunol. 14, 1158137. doi:10.3389/fimmu.2023.1158137
Zou, Q., Han, S., Liang, J., Yan, G., Wang, Q., Wang, Y., et al. (2024). Alleviating effect of vagus nerve cutting in Salmonella-induced gut infections and anxiety-like behavior via enhancing microbiota-derived GABA. Brain Behav. Immun. 119, 607–620. doi:10.1016/j.bbi.2024.04.034
Glossary
CNS Central nervous system
HPA Hypothalamic-pituitary-adrenal
SD Sleep deprivation
F/B Firmicutes-to-Bacteroidetes
MUC2 Mucin 2
LPS Lipopolysaccharides
SCFAs Short-chain fatty acids
BBB Blood-brain barrier
CORT Corticosterone
CRF Corticotropin-releasing factor
GABA γ-Aminobutyric acid
MT Melatonin
Trp Tryptophan
5-HT 5-Hydroxytryptamine
Kyn Kynurenine
AANAT N-acetyltransferase
Glu Glutamate
Gln Glutamine
NREM Non-rapid eye movement
REMS Rapid eye movement sleep
GI Gastrointestinal
5-HTTP 5-hydroxytryptophan
TPH Tryptophan hydroxylase
TCM Traditional Chinese medicine
EC Enterochromaffin
EEC Enteroendocrine
AADC Aromatic L-amino acid decarboxylase
IL-33 Interleukin 33
SERT Serotonin transporter
DA Dopamine
VTA Ventral tegmental area
TMAO Trimethylamine N-oxide
TNF-α Tumor necrosis factor α
NLRP3 NOD-like receptor thermoprotein domain protein 3
LB Lilium lancifolium Thunb
LBRD Lilium lancifolium Thunb and Rehmannia glutinosa (Gaertn.) Libosch.
GAS Gastrodin
Keywords: Chinese herbal medicines and their active components, microbiota-gutbrain axis, insomnia, improving sleep quality, Gut Microbiota
Citation: Wu C, Dou J, Song X, Yang F, Liu X, Song W and Zhang X (2025) Gut microbiota: a new target for the prevention and treatment of insomnia using Chinese herbal medicines and their active components. Front. Pharmacol. 16:1572007. doi: 10.3389/fphar.2025.1572007
Received: 06 February 2025; Accepted: 18 April 2025;
Published: 06 May 2025.
Edited by:
Dae-Wui Yoon, Jungwon University, Republic of KoreaReviewed by:
Yingying Yue, Hubei University of Chinese Medicine, ChinaDa-Been Lee, Korea University, Republic of Korea
Copyright © 2025 Wu, Dou, Song, Yang, Liu, Song and Zhang. This is an open-access article distributed under the terms of the Creative Commons Attribution License (CC BY). The use, distribution or reproduction in other forums is permitted, provided the original author(s) and the copyright owner(s) are credited and that the original publication in this journal is cited, in accordance with accepted academic practice. No use, distribution or reproduction is permitted which does not comply with these terms.
*Correspondence: Xiwu Zhang, MTQ5NzcyMTA1QHFxLmNvbQ==
†These authors have contributed equally to this work and share first authorship