- 1Department of Pharmacy, The Affiliated Hospital of Guangdong Medical University, Zhanjiang, China
- 2School of Ocean and Tropical Medicine, Guangdong Medical University, Zhanjiang, Guangdong, China
- 3School of Chemistry and Environmental Science, Guangdong Ocean University, Zhanjiang, China
- 4Guangdong Jianliyuan Medical Technology Co., Ltd., Zhanjiang, China
Objective: Preventing exposure to hazardous drugs is crucial for healthcare workers to avoid health risks. To mitigate healthcare workers’ risks when handling hazardous drugs, we developed a novel closed-system drug-transfer device (CSTD) called CSTD(JLY). This CSTD has an automatic pressure-relief structure. Hence, it can significantly decrease the resistance in the push/pull of a piston rod if an operator transfers drugs, thereby reducing the burden on the hands of the operator during drug transfer.
Methods: We investigated the closed performance of the novel CSTD (JLY) by comparing it with the performance of a syringe. We selected a simulation drug (fluorescein sodium), commonly used drugs (lansoprazole, nimodipine, and tropisetron), and a commonly used anti-cancer agent (cyclophosphamide) to conduct exposure evaluation.
Results: Compared with a syringe, CSTD(JLY) could reduce drug leakage significantly. Our novel CSTD with an automatic pressure-relief structure had superior closed performance. CSTD(JLY) could solve the problem of liquid-drug leakage during drug transfer.
Conclusion: This feature could reduce the exposure risk healthcare workers and patients.
1 Introduction
Concentrated drug transfer at pharmacy intravenous admixture services (PIVAS) is an important measure for hospitals to improve the safety, efficiency, and normalization of drug treatment. However, the safety at PIVAS faces multiple challenges, especially for the transfer of anti-cancer drugs (ACDs).
Most ACDs are cytotoxic preparations with teratogenic, carcinogenic, and mutagenic effects. They cause damage to normal human tissues and organs, especially to bone marrow, the digestive tract and reproductive system, while killing or inhibiting tumor cells (Moretti et al., 2011; Vainio, 1982). Moreover, nurses or pharmacists participating in the compounding, storage, or transport of ACDs are exposed to the leakage of toxic drugs (Brechtelsbauer, 2023; Zhu et al., 2023). Numerous studies have demonstrated that long-term and frequent exposure to ACDs may have far-reaching effects owing to accumulation within the body. Such accumulation can result in occupational hazards to healthcare workers: bone-marrow suppression, leukopenia, hair loss, gastrointestinal symptoms, skin allergies, corneal damage, damage to chromosomes and DNA, and menstrual abnormalities (Sorsa et al., 1985; Sorsa and Anderson, 1996).
The operation used most frequently at a PIVAS is drug transfer by a syringe (Sessink et al., 2011), which has the advantage of low cost, but the operator must exert significant force to transfer the liquid drug from a vial. Long-term operation can cause tenosynovitis in the hand (Nimunkar et al., 2017). More importantly, syringe use has a poor closed performance. In particular, a liquid drug can overflow during drug transfer, so drug leakage is possible (Fox et al., 2023; Yang et al., 2023; Hao et al., 2022). Studies have demonstrated a prevalence of contamination of 0.42% in the suction of liquid drugs through regular operation by a syringe (Miyake et al., 2013). A PIVAS can achieve the transfer of toxic drugs for chemotherapy in a zonal, concentrated manner in a negative-pressure environment using a “biological safety cabinet”. This strategy greatly alleviates the risk of healthcare workers being exposed to ACDs. However, drug transfer using a syringe at a PIVAS increases the probability of ACD exposure (Alavattam et al., 2020; Marler-Hausen et al., 2020; Amichay et al., 2021).
In 2004, the National Institute for Occupational Safety and Health (NIOSH) detailed a drug-transfer device that mechanically prohibits the transfer of environmental contaminants into the system and the escape of hazardous drug or vapor concentrations outside a closed-system drug-transfer device (CSTD) (Bartel et al., 2018). Compared with use of a syringe in conventional transfer of a drug, a CSTD can prohibit external environmental agents from contaminating drugs or drugs leaking into the environment (Harrison et al., 2006). This device makes the entire process of drug transfer complete in a relatively closed condition. This device reduces direct exposure of healthcare workers to chemotherapy drugs, as well as improving the safety and efficiency of drug transfer. With a CSTD, drug transfer can be undertaken without a biological safety cabinet, so the convenience of drug transfer is improved. Studies (Harrison et al., 2006) have shown that, in an appropriate environment, a CSTD can stop drugs being contaminated within 168 h.
Multiple institutions, including the NIOSH, International Society of Oncology Pharmacy Practitioners, American Society of Health-System Pharmacists, United States Pharmacopoeia, and Oncology Nursing Society, have recommended use of a CSTD in the management of hazardous drugs. Some countries and regions have mandated the use of a CSTD by medical institutions through legislation (Ezquer-Garin et al., 2019; Chan and Lim, 2016).
Dr. Sessink developed a CSTD in 1999 called PhaSeal®, which was trialed for 1 year in Ängelholm Hospital in Sweden. PhaSeal prevented environmental contamination during drug transfer (Sessink et al., 1999). PhaSeal was popularized in 30 hospitals in the USA from 2004 to 2010, and comparative analysis was conducted for surface contamination of the ACD cyclophosphamide. Compared with the standard method of drug transfer, the method using the CSTD PhaSeal caused the surface contamination of cyclophosphamide to decrease from 95% to 13% (Sessink et al., 2013). Favier et al. (2012) compared the environmental contamination before and after the introduction of PhaSeal into the pharmacies of two hospitals. When PhaSeal was used for drug transfer, contamination of the work environment decreased by 93%. Clark and Sessink (2013) conducted sampling by zone at 12 locations, and studied the contamination by cyclophosphamide and 5-fluorouracil in a hospital after use of the CSTD EquaShield®. Contamination by cyclophosphamide and 5-fluorouracil was not found in any of the pharmacies, infusion rooms, or cancer center office. Bartel et al. (2018) reported on the effectiveness of the CSTD Halo® used in ACD transfer and administration processes on the reduction in surface contamination by sampling at 13 locations in oncology hospitals in the USA. At least one marked ACD was detected in 66.7% of samples (104 out of 156 samples) before use of the CSTD. The percentage of samples contaminated decreased to 5.8% after use of the CSTD (9 out of 156 samples). In the administrative zones, the percent contamination was 78% (61 out of 78 samples) before use of the CSTD, and only 2.6% after use of the CSTD. In addition, 26 participants provided scores for the ease of use of the CSTD: all of them expressed that they were “quite satisfied” or “very satisfied” with the CSTD. Yu et al. (Gerding et al., 2022) used PhaSeal in China to evaluate the effect of a CSTD used at a PIVAS on the cyclophosphamide contaminant residue in a work environment. They took samples from 19 positions at worktops, transfer trolleys, and platforms for leaving cabins in three biological safety cabinets. When the conventional method of drug transfer was used, the median value of all monitoring points was 1.30 ng/cm2 before cleaning and decontamination, and 0.22 ng/cm2 after cleaning and decontamination. After the CSTD had been used for drug transfer, the median value of all monitoring points was 0.06 ng/cm2. Therefore, use of a CSTD can reduce the leakage of hazardous drugs during compounding and transfer.
The global manufacturers producing CSTDs are mainly BD Medical, Equashield, and ICU Medical. These three manufacturers collectively hold >80% of the market share. North America accounts for ∼75% of the market share, followed by Europe and Japan (∼20%) (Alavattam et al., 2020; Tang et al., 2022; Hilliquin et al., 2019).
CSTDs have not been popularized widely in the Chinese market, but some Chinese enterprises have seen business opportunities and developed them. From the perspective of product design, if a CSTD for the transfer of ACDs is developed and launched, it will have a broad market space (Hao et al., 2022; Piccardo et al., 2021; Wilkinson et al., 2024). However, owing to high cost of the CSTD (such as BD Medical, Equashield, and ICU Medical, etc.), it cannot be fully popularized in most regions in the world.
We wished to reduce potential occupational hazards to healthcare workers in the transfer of ACDs. Our research team developed a novel, dual-barreled CSTD called “CSTD(JLY)” in 2015. We obtained Chinese invention patent authorizations for relevant technologies in 2020 (Xie et al., 2021; Chen et al., 2020; Chen et al., 2023a; Chen et al., 2023b) and applied for European invention patents (Chen, 2024).
To investigate the performance of this novel CSTD (JLY), we chose several commonly used drugs to conduct exposure evaluation of CSTD(JLY). Our study could solve the problem of leakage upon the transfer of liquid drugs, thereby reducing the exposure risk for healthcare workers and patients.
2 Experimental
2.1 Materials
Fluorescein sodium, lansoprazole, nimodipine, tropisetron, cyclophosphamide, and physiologic (0.9%) saline, which were all analytical reagents, were purchased from Shanghai Aladdin Biochemical Technology (Shanghai, China).
The CSTD used in experiments, CSTD(JLY), was provided by Guangdong Jianliyuan Medical Technology (Guangdong, China), and its main structure is shown in Figure 1. Figure 1A shows CSTD(JLY) with a needle in a sheath. Figure 1B shows CSTD(JLY) with the needle sheath rotated by 90°. The components of CSTD(JLY) are shown in Figure 1.
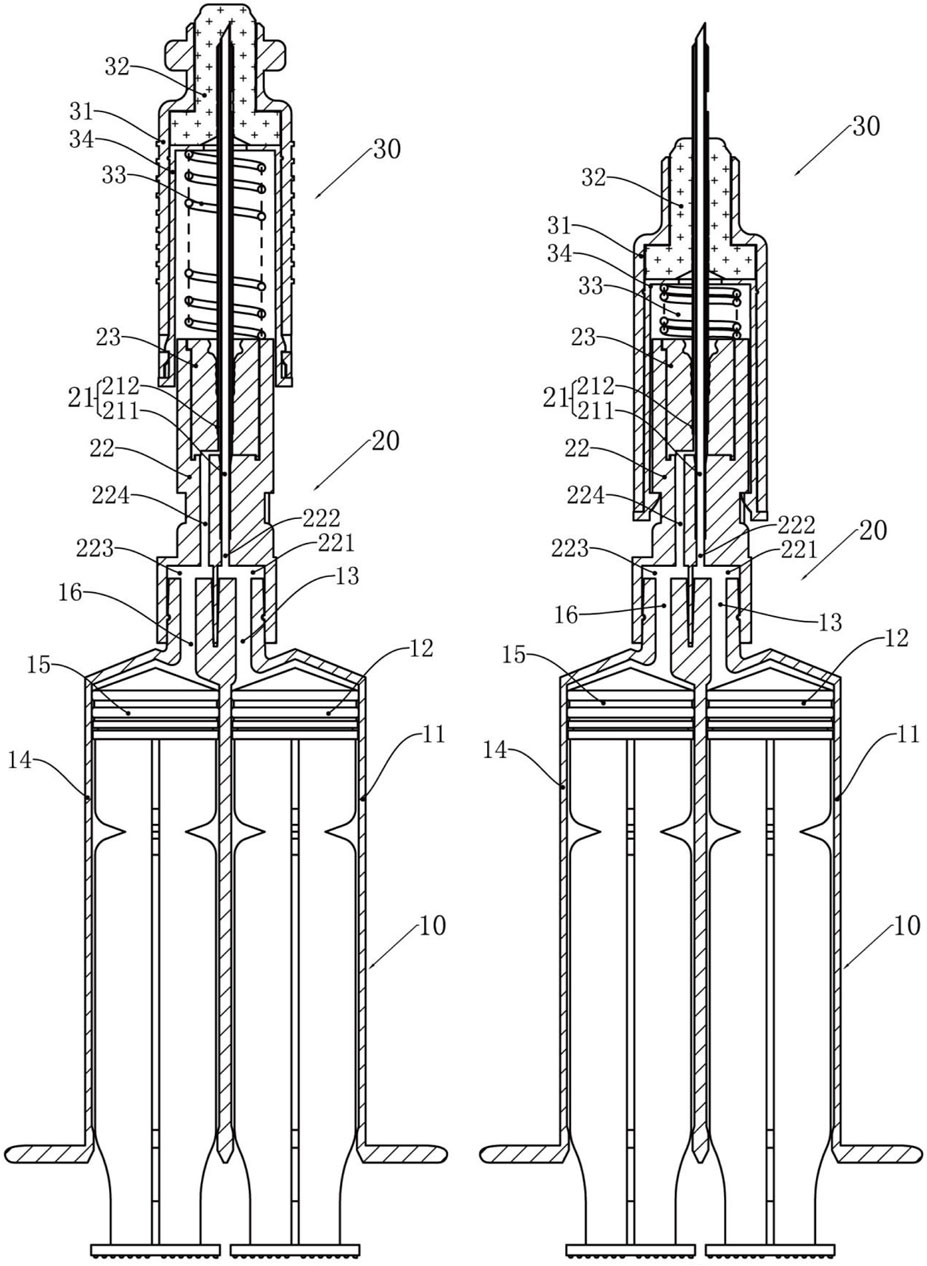
Figure 1. A dual-barrel CSTD called “CSTD(JLY)” (schematic). 10 = double-chamber syringe. 11 = first syringe barrel. 12 = first piston rod. 13 = first joint. 14 = second syringe barrel. 15 = second piston rod. 16 = second joint. 20 = transfer needle assembly. 21 = transfer needles. 211 = inner columnar channel. 212 = side annular channel. 22 = rear end of needle seat. 221 = first connection hole. 222 = first channel. 223 = second connection hole. 224 = second channel. 23 = needle penetrating. 30 = syringe needle sealing assembly. 31 = sheath. 32 = closing member. 33 = elastic member. 34 = inner sleeve.
2.2 Methods
All experiments were conducted at 25°C and 1 atmosphere of pressure. Weigh 1.00 g of the drug and put it into the vial, inject 10 mL of normal saline into the vial and dissolve the drug evenly, then seal it with a sealing cap. The concentration of all drugs was 0.100 g/mL and the volume was 10 mL.
In Experiment I, drug transfer was simulated in a laboratory. The gliding performance of the piston rod in the drug-transfer device during push/pull for drug transfer was determined with a tensile testing machine (CH-983C; Shengwu New Materials (Shenzhen), Shenzhen, China). A syringe and CSTD(JLY) were compared for differences. All data were presented in this study as the mean ± standard deviation (SD) of three independent experiments.
In Experiment II, drug transfer was simulated in a laboratory. A syringe and CSTD(JLY) were compared for differences in percent drug leakage during the transfer of fluorescein sodium (FS). The drugs in the infusion bottle, drug-transfer device, puncture outfit, and vial were collected separately. Collect the liquid directly from the infusion bottle, and then add 10 mL of solvent to flush the infusion bottle after collection, and finally collect it into the liquid medicine. This part serves as the final drug utilization part. Rinse the dispenser and the cillin bottle with 10 mL of solvent, respectively, and collect the residue as the dispenser and the residue of the Cillin bottle.FS content (mg/mL) in all of the parts exposed to the drug were determined with a high-performance liquid chromatography (HPLC) instrument (LC-40; Shimadzu, Beijing, China) to indirectly investigate FS leakage. The quality of the drug was calculated. Finally, mass loss is used to represent. In addition, FS leakage was directly shown by photography under irradiation by an ultraviolet lamp.
In Experiment III, the transfer of three drugs (lansoprazole, nimodipine, tropisetron) was simulated in a laboratory. A syringe and CSTD(JLY) were compared for differences in drug leakage during drug transfer. The drugs in the infusion bottle, drug-transfer device, puncture outfit, and vial were collected separately. Collect the liquid directly from the infusion bottle, and then add 10 mL of solvent to flush the infusion bottle after collection, and finally collect it into the liquid medicine. This part serves as the final drug utilization part. Rinse the dispenser and the cillin bottle with 10 mL of solvent, respectively, and collect the residue as the dispenser and the residue of the Cillin bottle. The drug content in all of the parts exposed to the drug were determined with a HPLC instrument (LC-40) to investigate drug leakage. The quality of the drug was calculated. Finally, mass loss is used to represent.
In Experiment IV, the ACD cyclophosphamide was transferred in a biological safety cabinet at the PIVAS of the Affiliated Hospital of Guangdong Medical University (Guangdong, China). A syringe and CSTD(JLY) were compared for differences in drug leakage during drug transfer. The drugs in the infusion bottle, drug-transfer device, puncture outfit, and vial were collected separately. Collect the liquid directly from the infusion bottle, and then add 10 mL of solvent to flush the infusion bottle after collection, and finally collect it into the liquid medicine. This part serves as the final drug utilization part. Rinse the dispenser and the cillin bottle with 10 mL of solvent, respectively, and collect the residue as the dispenser and the residue of the Cillin bottle. The drug content in all of parts exposed to the drug were determined with a HPLC instrument (LC-40) to investigate drug leakage. The quality of the drug was calculated. Finally, mass loss is used to represent.
In Experiments II–IV, all data were presented in this study as the mean ± standard deviation (SD) of six independent experiments, percent leakage was calculated using the following formula:
Leakage = 100% − Yield in infusion bottle − Residual rate in drug-transfer device and puncture outfit − Residual rate in vial.
Which m0 is the original quality of the drug before dispensing, m1 is the mass of the drug in the infusion bottle, m2 is the mass of the drug in drug-transfer device and puncture outfit, m3 is the mass of the drug in vial.
2.3 Statistical analysis
All data were presented in this study as the mean ± standard deviation (SD) of three to six independent experiments. Analysis of variance (ANOVA) was performed, and Tukey’s test was used to determine the significance of the differences between data with p < 0.05 as statistically significant.
3 Results and discussion
3.1 Structural characteristics of CSTD(JLY)
Figure 2 shows the various parts used in drug transfer by CSTD(JLY), including the dual-barrel drug-transfer device, air filter, Luer/Spike connector, and vial connector. They were designed for ACDs to ensure no leakage during drug transfer (Chen et al., 2020; Chen et al., 2023a; Chen et al., 2023b; Chen, 2024). A detailed description based on drug transfer by CSTD(JLY) is shown below.
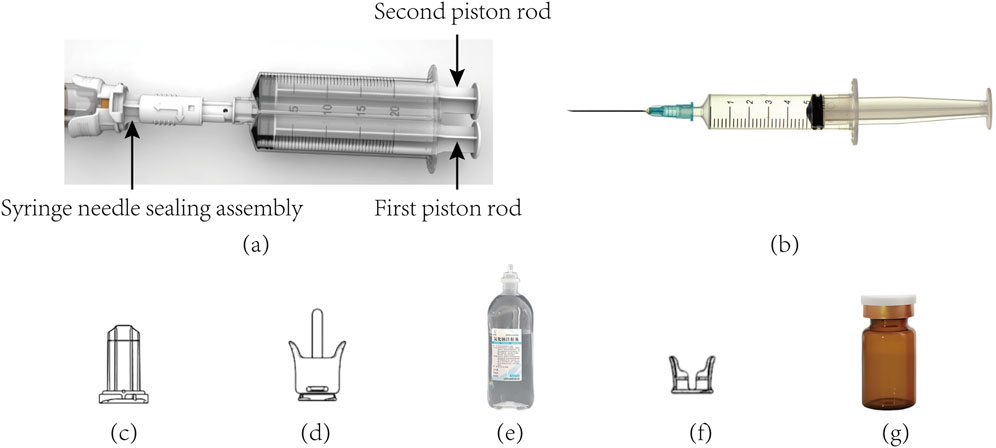
Figure 2. Photographs of the various parts involved in drug transfer by CSTD(JLY). (a) CSTD(JLY) (b) PS (c) air filter (d) luer/spike connector (e) infusion bottle (f) vial connector (g) vial.
3.1.1 System preparation and air storage
First, all parts of CSTD(JLY) were assembled correctly and in good operating condition (Figures 1, 2). The air filter was connected with the syringe needle sealing assembly (SNSA). The first piston rod was pulled outwards to store air in the first syringe barrel, making preparations for subsequent air replenishment and pressure relief. After air storage had been completed, CSTD(JLY) was disconnected from the air filter.
3.1.2 Suction of solvent
First, The Luer/Spike connector was inserted into the infusion bottle. The other end was connected firmly with the SNSA.
Second, the needle sheath was rotated by 90° (Figure 1). This action caused the needle to pierce the sealing assembly and stretch into the infusion bottle. At this moment, the needle sealing assembly came into close contact with the infusion bottle, maintaining a closed state during liquid suction.
Third, the second piston rod was pulled to suck one part of the solvent (e.g., 0.9% saline) into the second syringe barrel from the infusion bottle through a closed channel. At this moment, negative pressure was generated in the infusion bottle, which can result in leakage. Thus, the air stored in the first syringe barrel was replenished automatically to the infusion bottle until a balance in air pressure was reached, ensuring that the liquid was strictly closed within this system during liquid suction.
3.1.3 Dissolution of drug powder and suction of a liquid drug
First, after solvent suction had been completed, the SNSA was rotated by 90° so that the needle retracted into the SNSA (Figure 1). Then, CSTD(JLY) was disconnected with the Luer/Spike connector. At this moment, the infusion bottle maintained a closed connection with the Luer/Spike connector.
Second, one end of the vial connector was connected with the vial and the other end was connected with the SNSA. At this moment, the front-end face (with sealing rubber) of the SNSA was closely connected with the rubber opening of the vial, so that the whole system was closed. CSTD(JLY) was rotated by 90°, causing the needle to pierce the sealing assembly and stretch into the vial. Then, the second piston rod was pushed to inject the solvent into the vial, which was used to dissolve the drug powder.
Third, after the drug powder in the vial had dissolved fully, the liquid drug was sucked into the second syringe barrel. At this moment, negative pressure was generated in the vial, which can result in leakage. Thus, the air stored in the first syringe barrel was replenished automatically to the vial, ensuring that the liquid was strictly closed within this system in the entire process.
3.1.4 Transfer of liquid drug
First, after suction of the liquid drug had been completed, the SNSA was rotated by 90° so that the needle retracted into the SNSA. Then, the drug-transfer device was rotated reversely so that CSTD(JLY) was disconnected with the vial connector.
Second, the SNSA was connected with the Luer/Spike connector on the infusion bottle. At this moment, the front-end face (with sealing rubber) of the SNSA was closely connected to the rubber opening of the Luer/Spike connector. The dual-barrel drug-transfer device was rotated, causing the needle to pierce the sealing assembly and stretch into the infusion bottle. Then, the second piston rod was pushed to transfer the liquid drug to the infusion bottle. At this moment, positive pressure was generated in the infusion bottle, which can result in leakage. However, air pressure can cause automatic discharge of air into the first syringe barrel, ensuring that the liquid was strictly closed within this system in the whole process.
3.1.5 Drug transfer by a syringe
At present, drug transfer by syringe is used mainly clinically. A syringe is inserted into the infusion bottle and sucks the solvent, and then the solvent is injected into the vial. After the drug powder is dissolved, the liquid drug in the vial is sucked and injected into the infusion bottle. There is no strict sealing or timely positive/negative pressure relief in each operation, so leakage of a liquid drug is serious.
Compared with a syringe, if CSTD(JLY) is used for drug transfer, the transfer needle is reset automatically and resealed after each operation in the entire process. The connection in each step of drug transfer reaches a strict closed state, and positive/negative pressure in the vial and infusion bottle is relieved in time. This process ensures that exposure to and contamination of a liquid drug does not occur. Hence, the safety of ACD transfer is greatly increased, which alleviates the risk to healthcare workers.
3.2 Gliding performance of CSTD(JLY) and a syringe
The gliding performance of a drug-transfer device directly affects the safety and effectiveness of drug transfer. A drug-transfer device with good performance should ensure neither injection difficulty owing to too great a resistance nor incorrect delivery of a drug owing to too low a resistance during push/pull. Good gliding performance can ensure smoothness during use, thus reducing the leakage caused during drug transfer and enhancing user comfort.
In this experiment, two sizes (10 mL, 30 mL) of CSTD(JLY) were investigated for gliding performances during pull and push, with comparison with a syringe being conducted (Figure 3; Table 1).
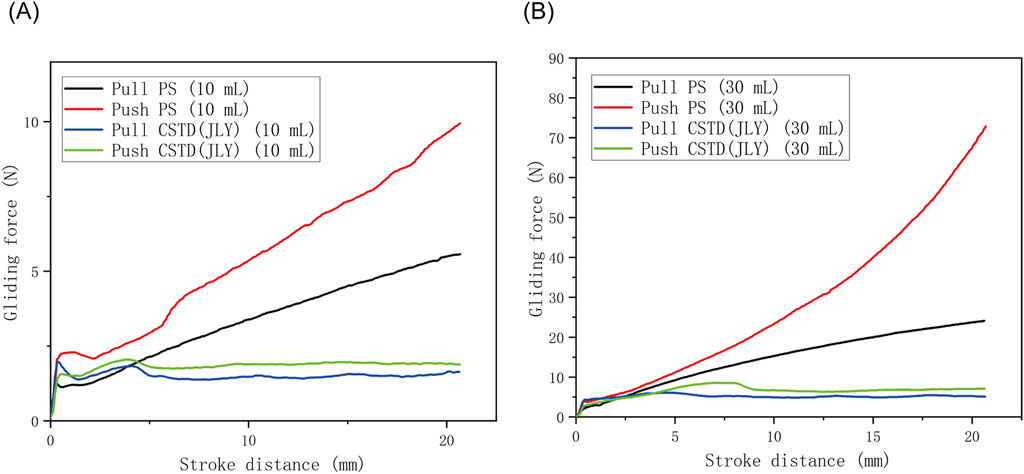
Figure 3. Comparison of push/pull gliding performance between a syringe (PS) and CSTD(JLY) of sizes (A) 10 mL and (B) 30 mL.
According to Figure 3, in a push/pull stroke of 0–21 mm, the maximum gliding force in a push/pull process of a 10-mL drug-transfer device was smaller than that in the push/pull process of a 30-mL drug-transfer device. Additionally, for both devices, the push gliding force tended to be larger than the pull gliding force. The push/pull gliding force of CSTD(JLY) tended to be stable after 5 mm of gliding.
The maximum gliding force in the push/pull of a syringe was far greater than that in the push/pull of CSTD(JLY) (Table 1). With an increase in the push/pull stroke, the gliding force exhibited a linear ascending trend, reaching 5.5–76.35 N at 21 mm, which was about 3–9-times the gliding force (1.7–8.6 N) of CSTD(JLY). The latter had a relatively even gliding force, maintained at ∼2 N (10 mL) and ∼6 N (30 mL). The air pressure generated in the push/pull of piston rod in one of the syringe barrels is transferred to the other syringe barrel (this is why air storage is required; see Section 3.1.1). Hence, the air pressure is balanced and the force used by the operator is small and even during the entire push/pull process.
These results suggested that CSTD(JLY) could decrease the resistance in the push/pull of the piston rod by the operator during drug transfer. This phenomenon greatly reduced the flowing pressure of the liquid drug, thus preventing the risk of liquid-drug leakage. If a syringe is used, the operator must use a large force repeatedly in the entire operation to complete drug transfer. This action causes muscle fatigue and joint pain in the hands, which can result in tenosynovitis.
3.3 Closed performance of CSTD(JLY)
We chose several commonly used clinical drugs to conduct exposure evaluation for CSTD(JLY) and a syringe.
3.3.1 Closed performance of CSTD(JLY) for FS transfer
The average yield of drug in an infusion bottle was 96.17% upon drug transfer by CSTD(JLY) and 92.64% in the drug-transfer process by a syringe (Figure 4A). Compared to the syringe, drug utilization upon drug transfer by CSTD(JLY) was significantly higher than that upon drug transfer by a syringe. During drug transfer by CSTD(JLY), the drug transfer and puncture outfit were flushed separately repeatedly to collect the drugs from them. The collected drugs were added together to obtain a residual sample for CSTD(JLY). Figure 4B reveals that the average percent residual was 0.73% in upon drug transfer by CSTD(JLY) and 0.33% upon drug transfer by a syringe, and the difference was not significant. Hence, the average percent residual was equivalent between these two drug-transfer devices. Figure 4C reveals that the percent residual in a vial was 2.44% upon drug transfer by CSTD(JLY) and 2.96% upon drug transfer by a syringe, and the difference was not significant. Hence, the average residual in a vial was equivalent between these two drug-transfer methods. As shown in Figure 4D, the average percent leakage of a sample upon drug transfer by CSTD(JLY) (0.67%) was significantly lower than that upon drug transfer by a syringe (4.06%).
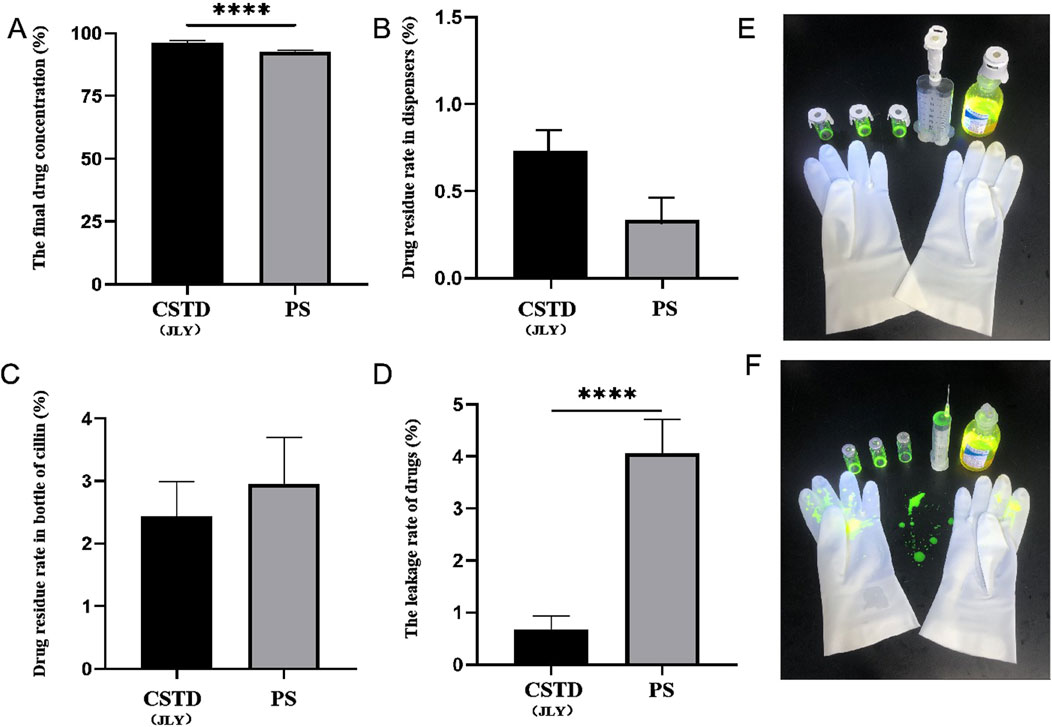
Figure 4. Closed performance of CSTD(JLY) and a syringe (PS) for the transfer of fluorescein sodium (FS): (A) Final concentration of FS in an infusion bottle; (B) Percent residual of FS in the drug-transfer device; (C) Percent residual of FS in a vial; (D) Percent leakage of FS; (E) FS leakage after transfer by CSTD(JLY); (F) FS leakage after transfer by a PS; Statistically significant differences from the syringe group were indicated as ****p < 0.0001.
Figure 4E shows photographs under irradiation by a ultraviolet lamp when the analyst wore gloves to transfer FS using CSTD(JLY). The upper part shows three vials, one CSTD(JLY), and one infusion bottle, and the lower part shows one pair of gloves. FS is yellowish-green under irradiation. Figure 4E reveals no FS leakage after drug transfer by CSTD(JLY).
Figure 4F shows photographs under irradiation by a ultraviolet lamp when the analyst wore gloves to transfer FS using by a syringe. The upper part shows three vials, one syringe, and one infusion bottle, and the lower part shows one pair of gloves. Figure 4F reveals serious leakage FS on the gloves and experimental-table surface after drug transfer by a syringe.
3.3.2 Closed performance of CSTD(JLY) for the transfer of lansoprazole
Lansoprazole was transferred by CSTD(JLY) and a syringe separately (Figure 5). The average yield of a sample in an infusion bottle upon drug transfer by CSTD(JLY) was 95.69%, significantly higher than that of a sample in an infusion bottle during drug transfer by a syringe (90.61%) (Figure 5A). In drug transfer by CSTD(JLY), the drug-transfer device and puncture outfit were flushed separately repeatedly to collect drugs from them. The collected drugs were added together to obtain a residual sample in the drug-transfer device of CSTD(JLY). Figure 5B reveals that the average percent residual in drug transfer by CSTD(JLY) was 0.72%, significantly lower than that in drug transfer by a syringe (1.96%). Figure 5C shows that the average percent residual of a sample in the vial upon drug transfer by CSTD(JLY) (1.96%) did not exhibit a significant difference from that of a sample in the vial upon drug transfer by a syringe. Hence, the average percent residual of a sample in a vial was equivalent between these two methods of drug transfer. Figure 5D demonstrates that the average percent leakage of a sample upon drug transfer by CSTD(JLY) (1.63%) was significantly lower than that of a sample upon drug transfer by a syringe (4.86%).
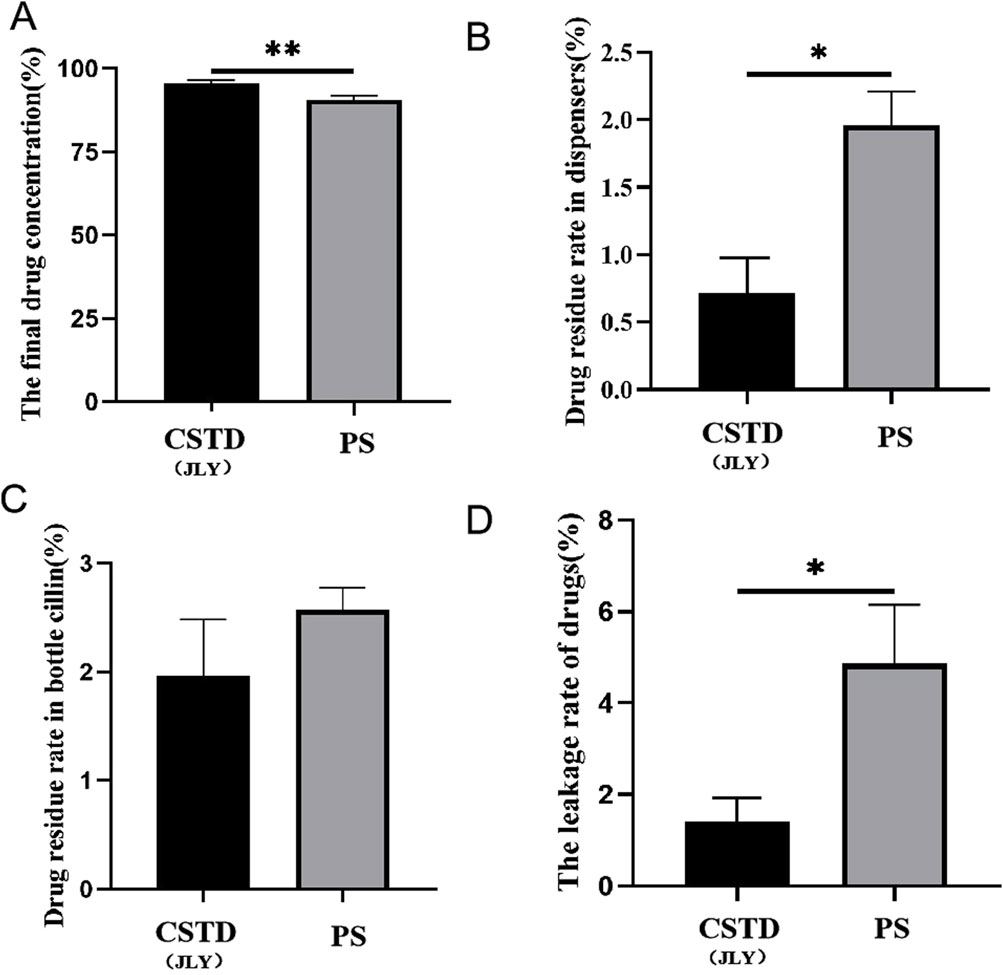
Figure 5. Closed performance of CSTD(JLY) and a syringe (PS) for the transfer of lansoprazole: (A) Final concentration of lansoprazole in an infusion bottle; (B) Percent residual of lansoprazole in the drug-transfer device; (C) Percent residual of lansoprazole in a vial; (D) Percent leakage of lansoprazole; Statistically significant differences from the syringe group were indicated as *p < 0.05 and **p < 0.01.
3.3.3 Closed performance of CSTD(JLY) for the transfer of nimodipine
Nimodipine was transferred by CSTD(JLY) and a syringe separately (Figure 6). The average yield of a sample in an infusion bottle upon drug transfer by CSTD(JLY) was 95.180%, significantly higher than that of a sample in an infusion bottle upon drug transfer by a syringe (87.185%) (Figure 6A). The average percent residual in the drug-transfer device upon drug transfer by CSTD(JLY) was 0.290%, which was not significantly different from that in the drug-transfer device upon drug transfer by a syringe (0.430%) (Figure 6B). The percent residual of a sample in a vial upon drug transfer by CSTD(JLY) (3.805%) exhibited no significant difference from that of a sample in a vial upon drug transfer by a syringe (4.175%). Hence, the percent residual of a sample in a vial was equivalent between these two methods of drug transfer (Figure 6C). Figure 6D reveals that the average percent leakage of a sample upon drug transfer by CSTD(JLY) was 0.725%, significantly lower than that of a sample upon drug transfer by a syringe (6.87%), which indicated that use of CSTD(JLY) could reduce drug leakage significantly.
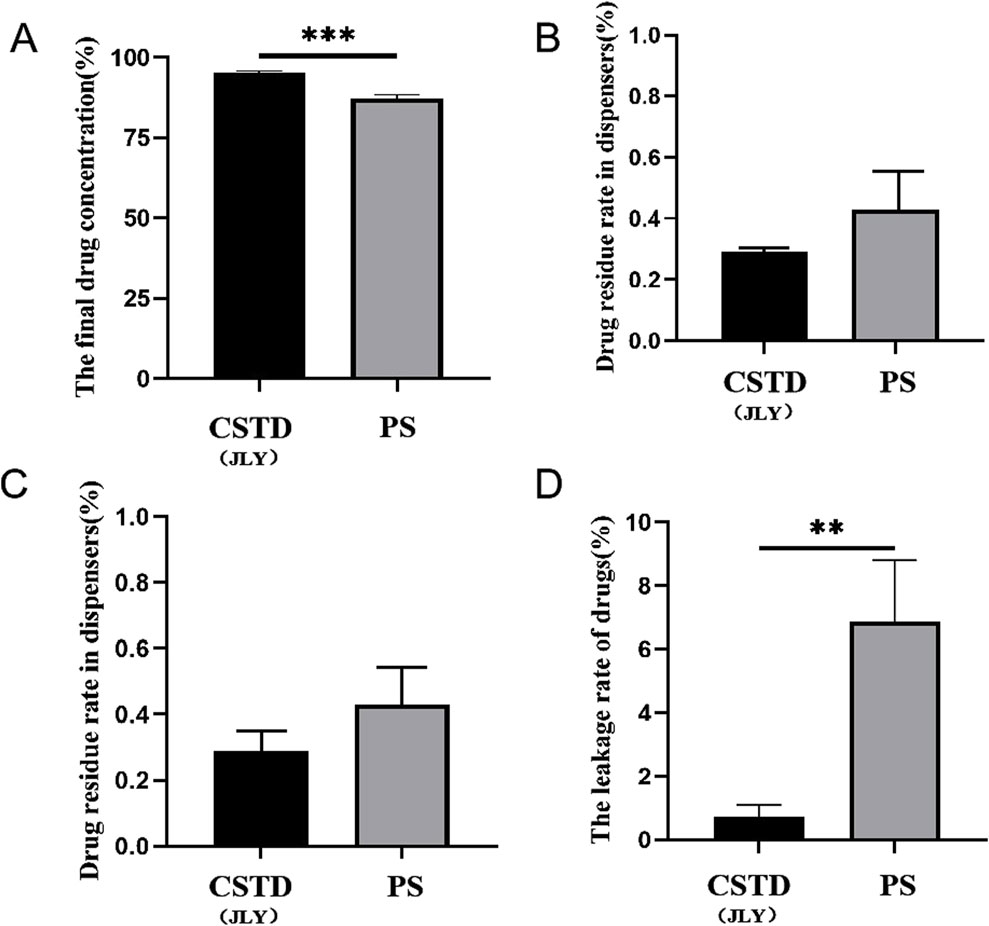
Figure 6. Closed performance of CSTD(JLY) and a syringe (PS) for the transfer of nimodipine: (A) Final concentration of nimodipine in an infusion bottle; (B) Percent residual of nimodipine in the drug-transfer device; (C) Percent residual rate of nimodipine in a vial; (D) Percent leakage of nimodipine; Statistically significant differences from the syringe group were indicated as **p < 0.01 and ***p < 0.001.
3.3.4 Closed performance of CSTD(JLY) for the transfer of tropisetron
Tropisetron was transferred by CSTD(JLY) and a syringe separately (Figure 7). The average yield of a sample in an infusion bottle upon drug transfer by CSTD(JLY) was 97.52%, significantly higher than that of a sample in an infusion bottle upon drug transfer by a syringe (87.93%) (Figure 7A). The average percent residual in the drug-transfer device upon drug transfer by CSTD(JLY) was 0.39%, which was not significantly different from that in the drug-transfer device upon drug transfer by a syringe (0.91%) (Figure 7B). The percent residual of a sample in a vial upon drug transfer by CSTD(JLY) (1.903%) exhibited no significant difference from that of a sample in a vial upon drug transfer by a syringe (3.813%). Hence, the percent residual of a sample in a vial was equivalent between these two methods of drug transfer (Figure 7C). Figure 7D reveals that the average percent leakage of a sample upon drug transfer by CSTD(JLY) was 0.187%, significantly lower than that of a sample upon drug transfer by a syringe (7.347%), which indicated that drug transfer by CSTD(JLY) could reduce drug leakage significantly.
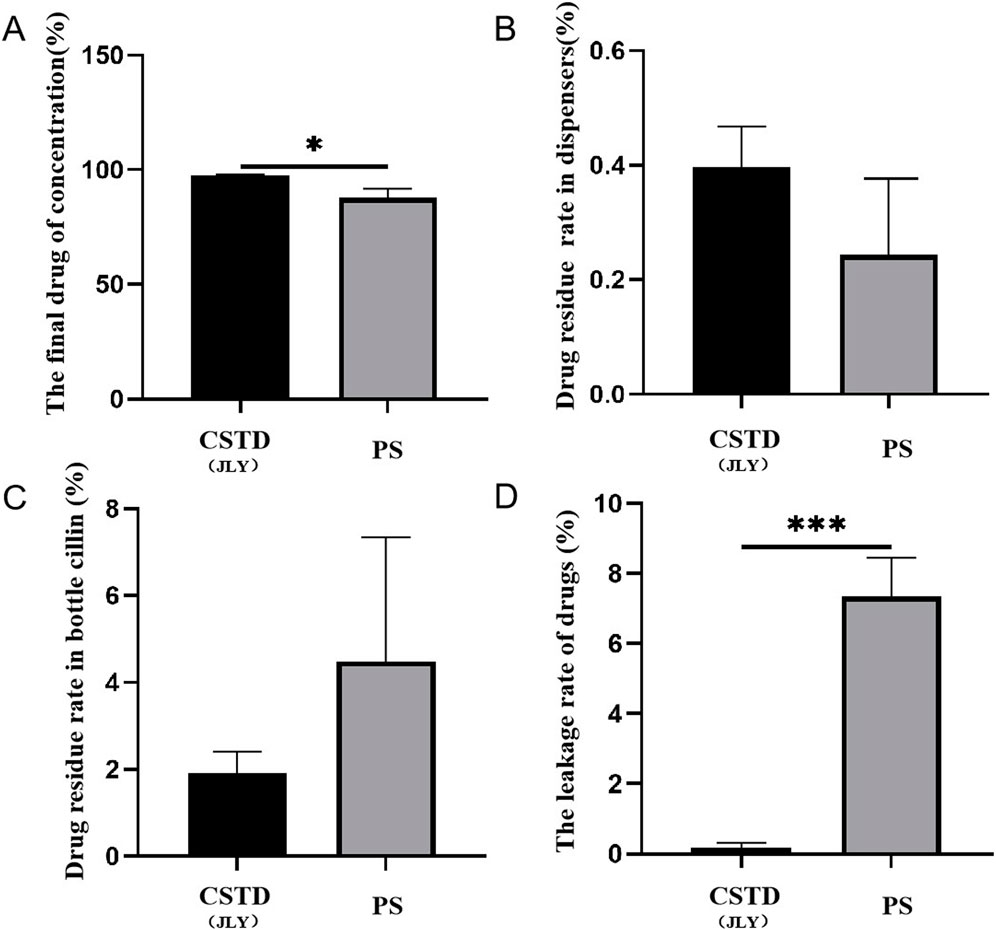
Figure 7. Closed performance of CSTD(JLY) and a syringe (PS) for the transfer of tropisetron: (A) Final concentration of tropisetron in an infusion bottle; (B) Percent residual of tropisetron in the drug- transfer device; (C) Percent residual of tropisetron in a vial; (D) Percent leakage of tropisetron; Statistically significant differences from the syringe group were indicated as *p < 0.05, **p < 0.01 and ***p < 0.001.
3.3.5 Closed performance of CSTD(JLY) for the transfer of cyclophosphamide
Cyclophosphamide is a commonly used ACD. If healthcare workers are exposed to this hazardous agent, they may suffer asthma, birth defects, miscarriage, or cancer. The transfer of cyclophosphamide by CSTD(JLY) and a syringe were simulated in a biological safety cabinet in a PIVAS of the Affiliated Hospital of Guangdong Medical University. The residue of cyclophosphamide was analyzed by HPLC, and percent leakage of cyclophosphamide was calculated.
Figure 8A reveals that when CSTD(JLY) was used to transfer cyclophosphamide to an infusion bottle, the yield of cyclophosphamide within the infusion bottle was 96.81%, significantly higher than that of cyclophosphamide upon drug transfer by a syringe (87.73%). The average percent residual in the drug-transfer device upon drug transfer by CSTD(JLY) was 0.27%, significantly lower than that in the drug transfer-device upon drug transfer by a syringe (0.56%) (Figure 8B). The percent residual in a vial upon drug transfer by CSTD(JLY) was 1.49%, significantly lower than that in a vial upon drug transfer by a syringe (2.44%) (Figure 8C). Figure 8D demonstrates that the average percent leakage of a sample upon drug transfer by CSTD(JLY) was 1.45%, significantly lower than that of a sample upon drug transfer by a syringe (9.27%), which indicates that drug transfer by CSTD(JLY) could reduce drug leakage significantly. It is consistent with the literature report that using the CSTD PhaSeal can significantly reduce the leakage of cyclophosphamide, compared with the standard method of drug transfer (Sessink et al., 2013; Favier et al., 2012; Clark and Sessink, 2013).
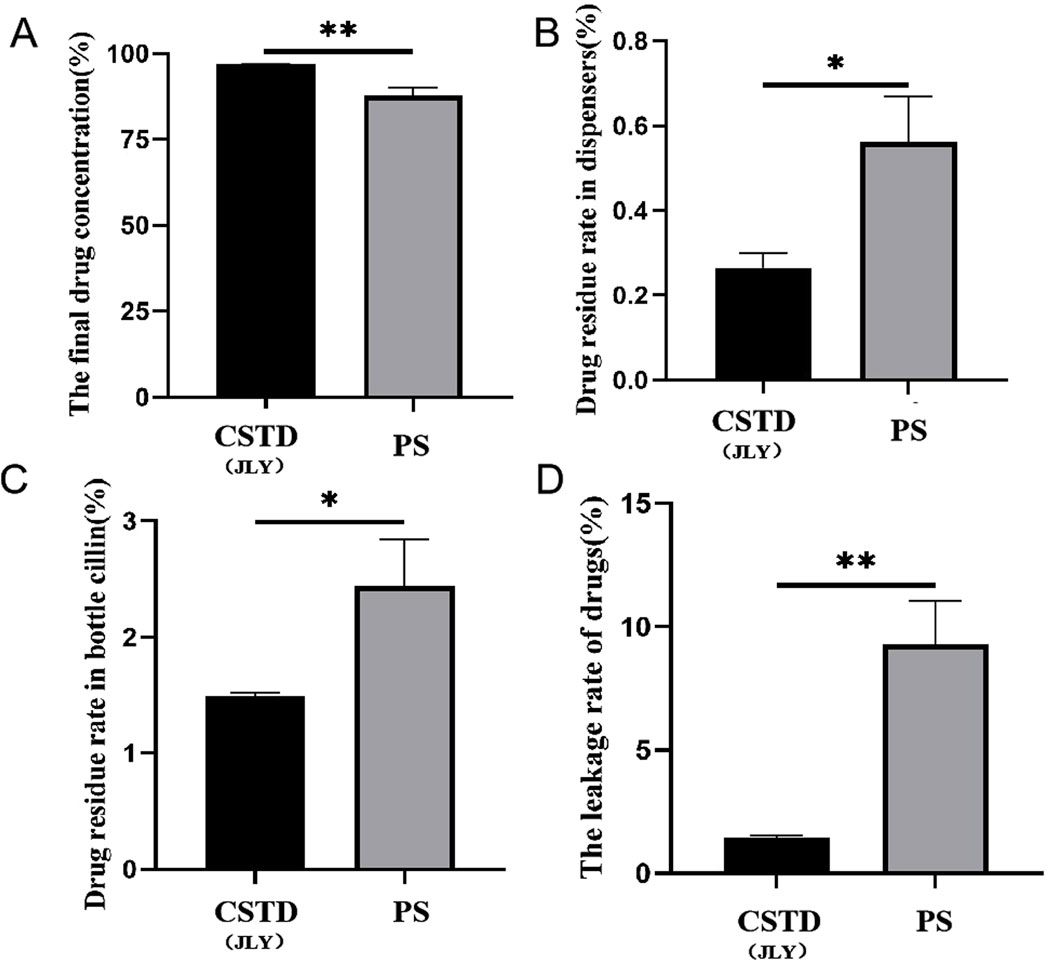
Figure 8. Closed performance of CSTD(JLY) and a syringe (PS) for the transfer of cyclophosphamide: (A) Final concentration of cyclophosphamide in an infusion bottle; (B) Percent residual of cyclophosphamide in the drug-transfer device; (C) Percent residual of cyclophosphamide in a vial; (D) Percent leakage of cyclophosphamide; Statistically significant differences from the syringe group were indicated as *p < 0.05, **p < 0.01 and ***p < 0.001.
4 Conclusion
Compared with drug transfer by a syringe, drug transfer by CSTD(JLY) could increase the yield of drug significantly and reduce drug leakage greatly. The main reason is that CSTD(JLY) has a special structure that enables automatic pressure relief. This feature provides the most crucial function of achieving superior closed performance and relieving pressure during drug transfer.
CSTD(JLY) can decrease the resistance in the push/pull of the piston rod significantly when an operator transfers drugs, thereby reducing the burden on the hands of the operator during drug transfer. There are many interfaces in the CSTD(JLY) system to ensure that the system is highly sealed during the drug transfer process. CSTD(JLY) could prohibit the leakage of FS, common clinical drugs, and common ACDs. CSTD(JLY) could solve the problem of drug leakage during drug transfer, thereby reducing the exposure risk for healthcare workers and patients.
Data availability statement
The original contributions presented in the study are included in the article/supplementary material, further inquiries can be directed to the corresponding authors.
Author contributions
LX: Investigation, Project administration, Writing – original draft. GC: Investigation, Project administration, Writing – original draft. QO: Data curation, Writing – original draft. WQ: Methodology, Writing – review and editing. XX: Validation, Writing – original draft. XC: Validation, Writing – original draft. LL: Data curation, Writing – original draft. SL: Methodology, Writing – review and editing. RC: Conceptualization, Supervision, Writing – review and editing. RL: Methodology, Writing – review and editing. ZQ: Conceptualization, Supervision, Writing – review and editing.
Funding
The author(s) declare that financial support was received for the research and/or publication of this article. This study was supported by Project of Traditional Chinese Medical of Guangdong Province (B2024217).
Conflict of interest
Authors RC and RL were employed by Guangdong Jianliyuan Medical Technology Co., Ltd.
The remaining authors declare that the research was conducted in the absence of any commercial or financial relationships that could be construed as a potential conflict of interest.
Generative AI statement
The author(s) declare that no Generative AI was used in the creation of this manuscript.
Publisher’s note
All claims expressed in this article are solely those of the authors and do not necessarily represent those of their affiliated organizations, or those of the publisher, the editors and the reviewers. Any product that may be evaluated in this article, or claim that may be made by its manufacturer, is not guaranteed or endorsed by the publisher.
References
Alavattam, S., Zamiri, C., Goswami, S., Weiser, S., Cram, M., Christian, T., et al. (2020). Challenges of using closed system transfer devices with biological drug products: an industry perspective. J. Pharm. Sci. 109, 22–29. doi:10.1016/j.xphs.2019.10.042
Amichay, M., Shimon, O., and Raveh, E. (2021). Prevention of coronavirus contamination from the environment using an air-cleaning closed system drug-transfer device. Pharm. Pract. (Granada) 4, 2576. doi:10.18549/PharmPract.2021.4.2576
Bartel, S. B., Tyler, T. G., and Power, L. A. (2018). Multicenter evaluation of a New closed system drug-transfer device in reducing surface contamination by antineoplastic hazardous drugs. Am. J. Health-Syst. Pharm. 75 (4), 199–211. doi:10.2146/ajhp160948
Brechtelsbauer, E. (2023). Identification and reduction of hazardous drug surface contamination through the use of a novel closed-system transfer device coupled with a point-of-care hazardous drug detection system. Am. J. Health. Syst. Pharm. 80, 435–444. doi:10.1093/ajhp/zxac336
Chan, H. K., and Lim, Y. M. (2016). Cost analysis of using a closed-system transfer device (CSTD) for antineoplastic drug preparation in a Malaysian government-funded hospital. Asian pac. J. Cancer Prev. 17 (11), 4951–4957. doi:10.22034/APJCP.2016.17.11.4951
Chen, R. Z. (2024). Inventor; Guangdong Jianliyuan Medical Technology Co., Ltd., assignee. Closed type medicine liquid transfer device and system with double-chamber internal circulation. European Patent Application, Publication No., EP4464300A1.
Chen, R. Z., Luo, R. Q., Xie, S. G., Chen, W. Q., Tang, B. H., Zhen, L. Y., et al. (2023b). Inventor; Guangdong Jianliyuan Medical Technology Co., Ltd., assignee. A disposable dual-chamber closed system drug transfer device. Chin. Pat. Pat. No. CN307928845S.
Chen, R. Z., Xie, S. G., Chen, W. Q., and Luo, R. Q. (2020). Inventor; Guangdong Jianliyuan Medical Technology Co., Ltd., assignee. A closed drug transfer and transfer needle and its application in drug compounding and transfer. Chin. Pat. Pat. No. CN111346008A.
Chen, R. Z., Xie, S. G., Chen, W. Q., and Luo, R. Q. (2023a). Inventor; Guangdong jianliyuan medical Technology Co., ltd., assignee. A closed needle structure. Chinese Patent. Patent No.: CN112999465B.
Clark, B. A., and Sessink, P. J. (2013). Use of a closed system drug-transfer device eliminates surface contamination with antineoplastic agents. J. Oncol. Pharm. Pract. 19 (2), 99–104. doi:10.1177/1078155212468367
Ezquer-Garin, C., Ferriols-Lisart, R., and Alós-Almiñana, M. (2019). Stability of mycophenolate mofetil in polypropylene 5% dextrose infusion bags and chemical compatibility associated with the use of the Equashield® closed-system transfer device. Biomed. Chromatogr. 33, e4529. doi:10.1002/bmc.4529
Favier, B., Labrosse, H., Gilles-Afchain, L., Cropet, C., Perol, D., Chaumard, N., et al. (2012). The PhaSeal® system: impact of its use on workplace contamination and duration of chemotherapy preparation. J. Oncol. Pharm. Pract. 18 (1), 37–45. doi:10.1177/1078155211401454
Fox, J., Rumsey, A., Rojek, R., Rensing, K., Gasama, H., Grimes-Jenkins, L., et al. (2023). The impact on central line-associated bloodstream infection rates following the introduction of a closed system transfer device in oncology wards. Infect. Prev. Pract. 5, 100268. doi:10.1016/j.infpip.2023.100268
Gerding, J., Anhäuser, L., Eickmann, U., and Nienhaus, A. (2022). A simple approach to assess the cancer risk of occupational exposure to genotoxic drugs in healthcare settings. J. Occup. Med. Toxicol. 17 (1), 8. doi:10.1186/s12995-022-00349-z
Hao, M. L., Wang, T., Zhu, J. Q., Song, Y. J., Gong, T. J., Zou, L. K., et al. (2022). Evaluation of external contamination on the vial surfaces of some hazardous drugs that commonly used in Chinese hospitals and comparison between environmental contamination generated during robotic compounding by IV dispensing robot vs. Manual compounding in biological safety cabinet. J. Oncol. Pharm. Pract. 28 (7), 1487–1498. doi:10.1177/10781552211023571
Harrison, B. R., Peters, B. G., and Bing, M. R. (2006). Comparison of surface contamination with cyclophosphamide and fluorouracil using a closed-system drug transfer device versus standard preparation techniques. Am. J. Health Syst. Pharm. 63 (18), 1736–1744. doi:10.2146/ajhp050258
Hilliquin, D., Tanguay, C., Gagné, S., Caron, N. J., and Bussières, J. F. (2019). Cross-sectional evaluation of surface contamination with antineoplastic drugs in Canadian health care centres. Can. J. Hosp. Pharm. 72 (5), 377–384. doi:10.4212/cjhp.v72i5.2931
Marler-Hausen, T., Holt, C., Headley, C., and Sessink, P. (2020). Use of a closed-system drug transfer device reduces contamination with doxorubicin during bolus injection. Br. J. Nurs. 29 (10), S15–S21. doi:10.12968/bjon.2020.29.10.S15
Miyake, T., Iwamoto, T., Tanimura, M., and Okuda, M. (2013). Impact of closed-system drug transfer device on exposure of environment and healthcare provider to cyclophosphamide in Japanese hospital. SpringerPlus 2, 273. doi:10.1186/2193-1801-2-273
Moretti, M., Bonfiglioli, R., Feretti, D., Pavanello, S., Mussi, F., Grollino, M. G., et al. (2011). A study protocol for the evaluation of occupational mutagenic/carcinogenic risks in subjects exposed to antineoplastic drugs: a multicentric project. BMC Public Health 11, 195. doi:10.1186/1471-2458-11-195
Nimunkar, A. J., Chun, K. S., Phung, N., Wreksoatmodjo, K., Yen, T. Y., and Radwin, R. G. (2017). Reducing thumb extensor risk in laboratory rat gavage. Appl. Ergon. 58, 151–155. doi:10.1016/j.apergo.2016.06.008
Piccardo, M. T., Forlani, A., and Izzotti, A. (2021). Effectiveness of closed system drug transfer devices in reducing leakage during antineoplastic drugs compounding. Int. J. Environ. Res. Public Health 18 (15), 7957. doi:10.3390/ijerph18157957
Sessink, P. J., Trahan, J., and Coyne, J. W. (2013). Reduction in surface contamination with cyclophosphamide in 30 US hospital pharmacies following implementation of a closed-system drug transfer device. Hosp. Pharm. 48 (3), 204–212. doi:10.1310/hpj4803-204
Sessink, P. J. M., Connor, T. H., Jorgenson, J. A., and Tyler, T. G. (2011). Reduction in surface contamination with antineoplastic drugs in 22 hospital pharmacies in the US following implementation of a closed-system drug transfer device. J. Oncol. Pharm. Pract. 17 (1), 39–48. doi:10.1177/1078155210361431
Sessink, P. J. M., Rolf, M.-A. E., and Rydén, N. S. (1999). Evaluation of the PhaSeal hazardous drug containment system. Hos. Pharm. 34 (11), 1311–1317. doi:10.1177/001857879903401110
Sorsa, M., and Anderson, D. (1996). Monitoring of occupational exposure to cytostatic anticancer agents. Mutat. Res. 355 (1-2), 253–261. doi:10.1016/0027-5107(96)00031-0
Sorsa, M., Hemminki, K., and Vainio, H. (1985). Occupational exposure to anticancer drug—potential and real hazards. Mutat. Res. 154 (2), 135–149. doi:10.1016/0165-1110(85)90024-7
Tang, Y. W., Che, X. T., Wang, Y. L., Ye, X., Cao, W. L., and Wang, Y. (2022). Evaluation of closed system transfer devices in preventing chemotherapy agents contamination during compounding process—a single and comparative study in China. Front. Public Health 10, 827835. doi:10.3389/fpubh.2022.827835
Vainio, H. (1982). Inhalation anesthetics, anticancer drugs and sterilants as chemical hazards in hospitals. Scand. J. Work Environ. Health 8 (2), 94–107. doi:10.5271/sjweh.2483
Wilkinson, A. S., Walker, K. E., Ozolina, L., Machníková, R., Johnson, A. J., Bhogal, N., et al. (2024). Integrity performance assessment of a closed system transfer device syringe adaptor lock as a terminal closure for Luer-Lock syringes. Eur. J. Hosp. Pharm. 31, 50–56. doi:10.1136/ejhpharm-2021-003148
Xie, S. G., Chen, R. Z., Chen, W. Q., and Lin, P. Z. (2021). Inventor; Guangdong Jianliyuan Medical Technology Co., Ltd., assignee. A closed system drug transfer device and its application in drug compounding and transfer. Chin. Pat. Pat. No.:CN111346009B.
Yang, C., Ni, X., Zhang, L., and Peng, L. (2023). Intravenous compounding robots in pharmacy intravenous admixture services: a systematic review. Med. Baltim. 102 (19), e33476. doi:10.1097/MD.0000000000033476
Keywords: closed performance, automatic pressure-relief, hazardous drugs, closed-system drugtransfer device, occupational protection, CSTD
Citation: Xie L, Chen G, Ouyang Q, Quan W, Xie X, Chen X, Li L, Li S, Chen R, Luo R and Qiu Z (2025) A high-efficiency automatic pressure-relief drug transfer device for anticancer medications with superior closed performance. Front. Pharmacol. 16:1579771. doi: 10.3389/fphar.2025.1579771
Received: 19 February 2025; Accepted: 02 April 2025;
Published: 25 April 2025.
Edited by:
Talha Bin Emran, Brown University, United StatesReviewed by:
Mohammad Nurul Amin, Atish Dipankar University of Science and Technology, BangladeshMohammad Rashedul Islam, University of Chittagong, Bangladesh
Rashu Barua, New York University, United States
Preethi Jayakumar, Northwell Health, United States
Md Hasif Sinha, University of Louisville, United States
Md Saqline Mostaq, University of Louisiana at Monroe, United States
Copyright © 2025 Xie, Chen, Ouyang, Quan, Xie, Chen, Li, Li, Chen, Luo and Qiu. This is an open-access article distributed under the terms of the Creative Commons Attribution License (CC BY). The use, distribution or reproduction in other forums is permitted, provided the original author(s) and the copyright owner(s) are credited and that the original publication in this journal is cited, in accordance with accepted academic practice. No use, distribution or reproduction is permitted which does not comply with these terms.
*Correspondence: Qianqian Ouyang, b3V5YW5ncWlhbnFpYW5AZ2RtdS5lZHU=; Zhiqing Qiu, MTM3MDI4NzE0NzJAMTYzLmNvbQ==