- 1School of Clinical Medicine, Shandong Second Medical University, Weifang, Shandong, China
- 2Department of General Medicine, Affiliated Hospital of Shandong Second Medical University, Weifang, Shandong, China
- 3Department of Radiotherapy, Affiliated Hospital of Shandong Second Medical University, Weifang, Shandong, China
Cisplatin is a highly effective broad-spectrum anticancer drug, but its severe ototoxicity limits its clinical application. Cisplatin ototoxicity is mainly manifested as irreversible hearing loss, and its mechanism involves various pathways such as DNA damage, oxidative stress, inflammatory response, mitochondrial dysfunction, and ferroptosis. In recent years, natural polyphenols have shown great potential in combating cisplatin ototoxicity due to their powerful antioxidant, anti-inflammatory and anti-apoptotic properties.A variety of polyphenolic compounds, such as resveratrol, curcumin, quercetin, etc., can effectively attenuate the damage of cisplatin on Corti organs, spiral ganglion neurons and vascular striatum by scavenging free radicals, inhibiting the release of inflammatory factors, and regulating the expression of apoptosis-related proteins. In addition, some polyphenols can enhance the anti-tumour effect while antagonizing ototoxicity.Although polyphenols show good application prospects in the prevention and treatment of cisplatin ototoxicity, there are still some problems that need to be solved, such as the low bioavailability of polyphenols, the mechanism of action has not yet been fully elucidated, the optimal dosing regimen has not yet been determined, whether there is any superimposed effect of combining the various types of polyphenols, and whether the oral polyphenols can exert an otoprotective effect through the regulation of the intestinal flora through the intestinal-auricular axis.This study provides new insights into polyphenols as potential drug candidates for CIO by summarising the cytotoxic mechanisms of cisplatin and the mechanism of action of polyphenols targeting these mechanisms in order to retard the progression of CIO. It provides new ideas and approaches for the next step focusing on the development of highly effective and low-toxic polyphenols for clinical control of cisplatin ototoxicity.
1 Introduction
Cisplatin is a chemotherapeutic agent that is widely used as an antitumor agent for the treatment of various types of cancers, such as ovarian, prostate, testicular, lung, nasopharyngeal, esophageal, lymphoma, squamous cell carcinoma of the head and neck, and osteogenic sarcoma (Tang et al., 2024; Wang L. et al., 2023). However, its prevalent effects of renal damage, neurotoxicity and hearing impairment have severely limited the clinical use of cisplatin (Fetoni et al., 2022; Crona et al., 2017). Cisplatin-induced ototoxicity (CIO) is characterised by hair cell death through the formation of DNA adducts, mitochondrial dysfunction, oxidative stress and inflammation, ultimately leading to apoptosis, necrotic apoptosis, pyroptosis, or ferroptosis (Tan and Vlajkovic, 2023). The ototoxicity of cisplatin is permanent sensorineural hearing loss due to the major damage to the spiral apparatus (Corti organ), spiral ganglion neurons (SGN) and vascular striae (Rybak et al., 2019; Anfuso et al., 2022) and the lack of regenerative capacity of mammalian hair cells and SGN.
Given the key role of oxidative stress in CIO, antioxidants have been used as otoprotective agents in a large number of studies targeting the prevention of cisplatin-induced hearing loss, such as N-acetylcysteine, sodium thiosulfate, amphotericin, vitamins, statins, dexamethasone, D-methionine, and ginkgo biloba extract (Rose et al., 2024). However, most of the experimental tests have been conducted in animal models and in vitro experiments, and only a few clinical trials have been conducted. In these studies, polyphenolic compounds demonstrated potent antioxidant and anti-inflammatory properties.
Polyphenols are natural bioactive compounds, mostly from herbs, fruits, vegetables and medicinal plants. Recent studies strongly support the role of polyphenols in the prevention of diseases, especially aging, cancer, cardiovascular and neurodegenerative diseases (Xiang et al., 2023; Dalgaard et al., 2019; Grabska-Kobyłecka et al., 2023; Zhou et al., 2016). Polyphenols can prevent ototoxicity of cisplatin by improving cellular antioxidant homeostasis, modulating signaling pathways, mitigating inflammatory responses, and ameliorating the disruption of cisplatin’s intra-auricular environment in a dose- and time-dependent manner (Maiuolo et al., 2022).Moreover, after studies of natural compounds with anti-tumor properties, polyphenols have emerged as strong chemotherapeutic sensitization candidates (Jakobušić Brala et al., 2023; Abotaleb et al., 2020; Bouyahya et al., 2022; Ko et al., 2017), inhibiting the oncogenic transformation of normal cells through the regulation of relevant genes, tumor growth and development, angiogenesis and metastasis, downregulation of various oncogenic-related molecules and upregulation of tumor suppressor proteins, modulation of reactive oxygen species levels in cells to regulate cell proliferation, survival and apoptosis, and also transformation of the gut microbiota to acquire bioactivity-promoting properties to inhibit cancer development and progression (Anantharaju et al., 2016).Polyphenol combination therapy significantly improves anticancer efficacy and reduces drug resistance and chemotherapeutic toxicity through multi-target synergy. The application of nanocarriers can significantly improve the water solubility and stability of polyphenols, thus enhancing their selective killing ability on tumor cells (Vladu et al., 2022; Li et al., 2016).Therefore, the chemotherapy regimen of cisplatin combined with polyphenols has the potential to become a new option for cancer treatment in the future.
Current research in the field of ototoxicity protection is still dominated by experimental animal models, and in this paper, “polyphenols,” “flavonoids,” “anthocyanin,” “flavonols,” “flavones” “flavanones,” “flavan-3-ols,” “isoflavones,” “stilbenes,” “phenolic acids,” “lignans,” “cisplatin-induced ototoxicity” as search terms in PubMed, a total of 79 papers were retrieved and combined with de-duplication to screen 55 preclinical studies in which polyphenols were used as the primary intervention. We analyzed the protective mechanism of polyphenols against cisplatin ototoxicity in these studies, and before that, we comprehensively summarized and reviewed the cytotoxic mechanism of cisplatin. In this way, we hope to provide new insights into polyphenols as potential drug candidates for CIO.
2 Mechanism of cisplatin ototoxicity
Cisplatin enters the cochlea, mainly through the blood-strial barrier and the blood-perilymph barrier (Sung et al., 2024). After entering the cochlea, cisplatin is taken up into cells by diffusive and passive transport, copper transporter protein 1 (Ctr1) and organic cation transporter protein 2 (OCT2), endocytosis, and toxicity through DNA adduct formation, oxidative stress, mitochondrial dysfunction, inflammatory response, and ferroptosis (Elmorsy et al., 2024).
2.1 Cisplatin-induced DNA damage and apoptosis
Once cisplatin enters the cell it can be hydrolyzed to form toxic aqua-cisplatin complexes and form intra-and interstrand crosslinks with DNA (Johnstone et al., 2016), the formation of such DNA adducts induces structural distortions of the DNA helix, which in turn leads to DNA damage, which activates multiple signaling pathways, one of which is the activation of the ATM-Chk2-p53 pathway (Benkafadar et al., 2017). Ataxia Telangiectasia Mutated (ATM) is a protein kinase that is activated in response to DNA double-strand breaks (DSBs). ATM can activate p53 either through activation of ATM checkpoint kinase 2 (Chk2) or directly. p53 activation activates the downstream Bcl family of proteins and the transcriptional activator of transcription 1 (STAT1). P53 activates the downstream Bcl family of pro-apoptotic proteins (Bax, Bak, Bim, etc.) and reduces the number of anti-apoptotic proteins (Bcl-xl, Bcl-2, etc.), which triggers the release of Cyt-c from the mitochondria, and ultimately activates the downstream cytosolic asparagine caspases that cause apoptosis (Cederroth et al., 2024). Activated STAT1 promotes the transcription of inflammatory genes such as COX-2, iNOS, and TNF-α, leading to an inflammatory response (Mukherjea et al., 2011). (See Figure 1)
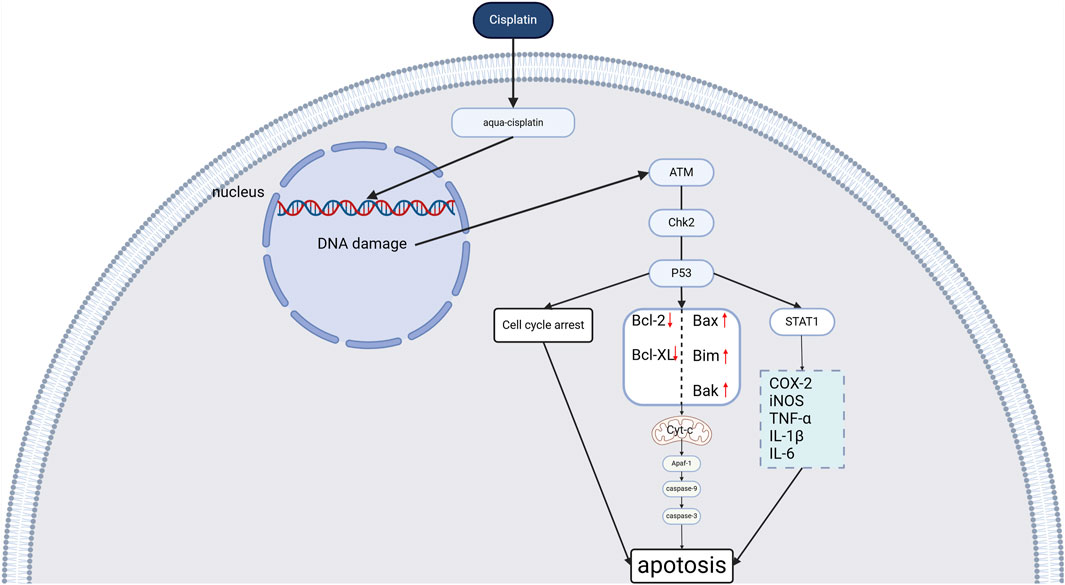
Figure 1. Cisplatin-induced cochlear DNA damage (Created in https://BioRender.com).
2.2 Cisplatin induces oxidative stress
The cochlea’s high metabolic rate results in its production of abundant reactive oxygen species (ROS), so it requires an active antioxidant defense system [glutathione (GSH), oxidized glutathione (GSSG), and antioxidant enzymes (superoxide dismutase (SOD), catalase (CAT), glutathione peroxidase (GPx), and glutathione reductase (GR)] to achieve a dynamic oxidant-antioxidant balance to maintain healthy hearing. However, when cisplatin acts, it can induce oxidative stress by generating reactive oxygen species (ROS) and depleting cellular antioxidants.
Cisplatin is able to bind directly to the sulfhydryl groups of antioxidant enzymes in the cochlea, leading to the inactivation of the enzymes, and it also decreases the levels of glutathione and NADPH (Nicotinamide Adenine Dinucleotide Phosphate), both of which are essential for the activity of the enzyme glutathione peroxidase (Gündoğdu et al., 2019). Together, these changes lead to a decrease in antioxidant enzyme activity in the cochlea and an increase in the amount of ROS in the cells. Cisplatin activates NOX (nicotinamide adenine dinucleotide phosphate oxidase) (especially the NOX3 isoform) (Ramkumar et al., 2021), xanthine oxidase (XO) (Tan and Vlajkovic, 2023), and increases the production of superoxide radicals. In addition, mitochondrial dysfunction is another important mechanism leading to ROS production.
Reactive oxygen species (ROS) and their derived superoxide radicals can trigger cytotoxic effects through multiple mechanisms: first, excessive generation of ROS induces lipid peroxidation and leads to depletion of intracellular antioxidant enzyme levels, exacerbating the disruption of redox homeostasis; second, ROS induces the release of Cyt-c from the mitochondria, which in turn activates the caspase-9 and caspase-3 cascade reaction inducing apoptosis (Rybak et al., 2019); furthermore, ROS interacting with nitric oxide can form peroxynitrite (ONOO-), leading to protein inactivation; meanwhile, ROS generate reactive aldehydes such as 4-hydroxynonenal (4-HNE) through the formation of hydroxyl radicals and a series of reactions, which in turn trigger calcium ion endocytosis (Callejo et al., 2015).
However, signaling pathways that antagonize oxidative stress also exist in cells. Nrf2 is a major regulator of the cellular oxidative stress response and maintenance of redox homeostasis (Ma, 2013), and is regulated by signaling pathways such as NF-κB, Notch, PI3K-AKT, and Brca-1 (Li et al., 2023a), with the PI3K/AKT signaling pathway being a sensory hair cell important regulator for survival against oxidative stress (Kucharava et al., 2019). Activated Nrf2 promotes the gene expression of several antioxidant enzymes and proteins, such as superoxide dismutase (SOD), glutathione S-transferase (GST), glutathione peroxidase (GPx), heme oxygenase-1 (HO-1), and NAD(P)H as a means of protecting the cells from oxidative stress and maintaining the dynamic balance between oxidation and antioxidant protection. Activation of NF-κB also activates the downstream JNK pathway, leading to the production of ROS, which may cause further damage to the OHC (Wang X. et al., 2023).
2.3 Cisplatin induces mitochondrial dysfunction
When cisplatin enters the mitochondria, it acts directly on the inner mitochondrial membrane, leading to the impairment of the function of the electron transport chain, the reduction of ATP production and the induction of ROS generation (Elmorsy et al., 2024), and the inhibition of the efficient antioxidant defence system composed of glutathione (GSH) and enzymes, such as superoxide dismutase (SOD) and glutathione peroxidase (GPx), in the interstitial space of the mitochondrial membrane. Meanwhile, large amounts of ROS trigger mtDNA mutations when acting on mitochondrial DNA, protein oxidation when acting on respiratory chain proteins, and lipid peroxidation when acting on mitochondrial membranes (Tan and Song, 2023),these damages further lead to mitochondrial dysfunction, which in turn prompts more ROS production, forming a positive feedback loop (Tan and Vlajkovic, 2023).
Dysfunctional mitochondria release into the cytoplasm, it binds to with Apaf-1, triggering caspase-9, caspase-3 related cascade reactions that lead to apoptosis (Santucci et al., 2019; Lu et al., 2022).Anti-apoptotic proteins such as Bcl-2 in the BCL-2 family and Bax and other pro-apoptotic members of the BCL-2 family balance and maintain normal mitochondrial function (Li et al., 2023b), and pro-apoptotic Bcl-2 family members induce the release of Cyt-c, which is inhibited by anti-apoptotic members. In addition, when mitochondria are damaged, apoptosis-inducing factor (AIF) is translocated from the mitochondria to the nucleus, where it may mediate chromatin condensation and large-scale DNA fragmentation by binding to DNA (Hong et al., 2004), leading to cell death (See Figure 2).
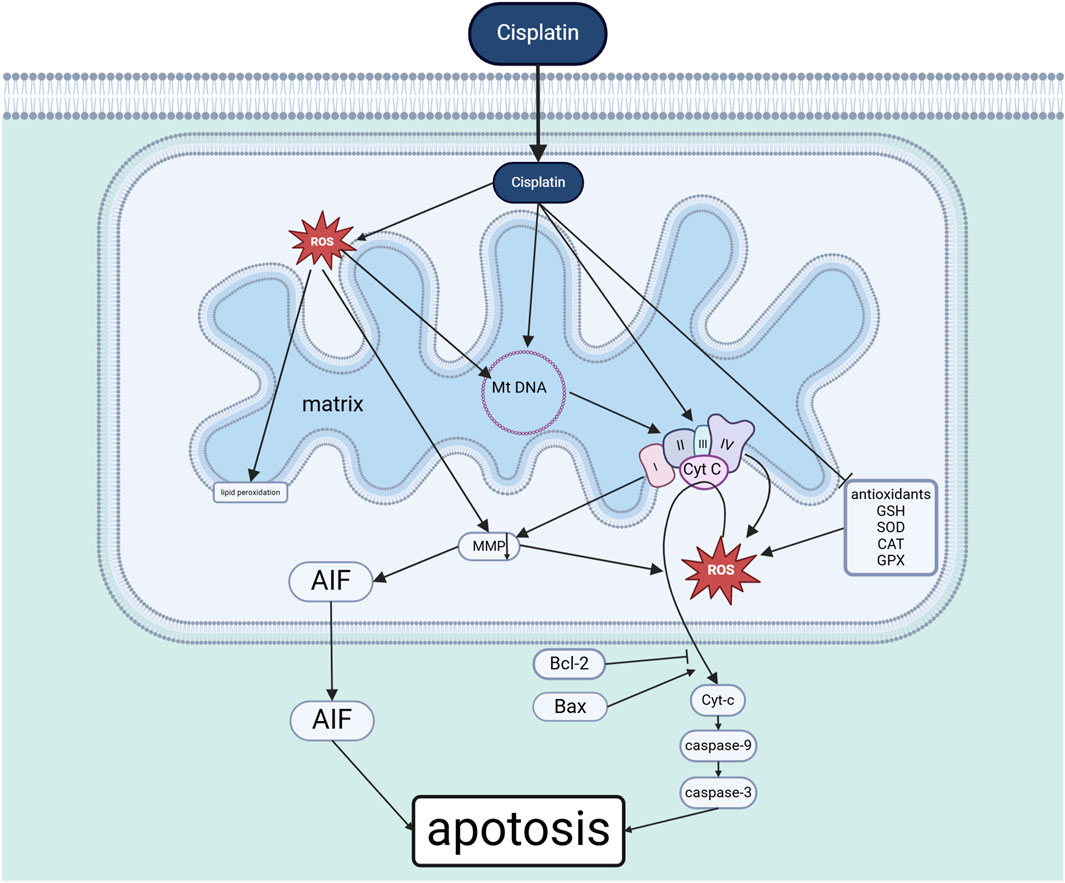
Figure 2. Cisplatin-induced cochlear mitochondrial dysfunction (Created in https://BioRender.com).
2.4 Cisplatin induces inflammation
The mechanism of cellular damage caused by cisplatin is inextricably linked to inflammation. The entry of cisplatin into the inner ear leads to the formation of inner ear cellular damage products (DAMPs), which, together with cisplatin, bind to toll-like receptors (TLRs) (Babolmorad et al., 2021) and activate downstream signaling, producing transcription factors (e.g., nuclear factor kappa B (NF-κB), activator protein-1 (AP-1), and interferon regulatory factor 3 (IRF3)), which in turn regulate the expression and secretion of proinflammatory signaling molecules. NF-κB induces cytokines such as tumor necrosis factor α (TNF-α), interleukin-1β (IL-1β), and interleukin-6 (IL-6) to bind to their respective receptors, which initiates and prolongs the progression of inflammation and contributes to the generation of ROS (Gong et al., 2020). Among them, TNF-α and IL-1β can activate NF-κB and thus promote the secretion of excess pro-inflammatory cytokines, forming a positive feedback and amplifying the inflammatory response cascade, which ultimately leads to more OHC damage (Wang L. et al., 2023).
Cisplatin also acts on the STAT family, inducing the protein expression of transcriptional activator of transcription-1 (STAT1) and down-regulating the expression of transcriptional activator of transcription-3 (STAT3) in the cochlea (Bhatta et al., 2019). STAT1 leads to the expression of various pro-inflammatory mediators, such as cyclooxygenase 2 (COX-2), iNOS, TNF-α, IL-1β, and IL-6, which further exacerbate the inflammation in the cochlea (Tan and Vlajkovic, 2023). However, STAT3 inhibits the cellular inflammatory response and promotes cell survival (Ramkumar et al., 2021). Increased ROS also activate STAT1 triggering the inflammatory process in the cochlea (Kaur et al., 2011), and the binding of inflammatory cytokines to their receptors further leads to an increase in ROS production in OHC cells.ROS and inflammatory responses reinforce each other, forming a positive feedback loop that further exacerbates OHC damage (See Figure 3).
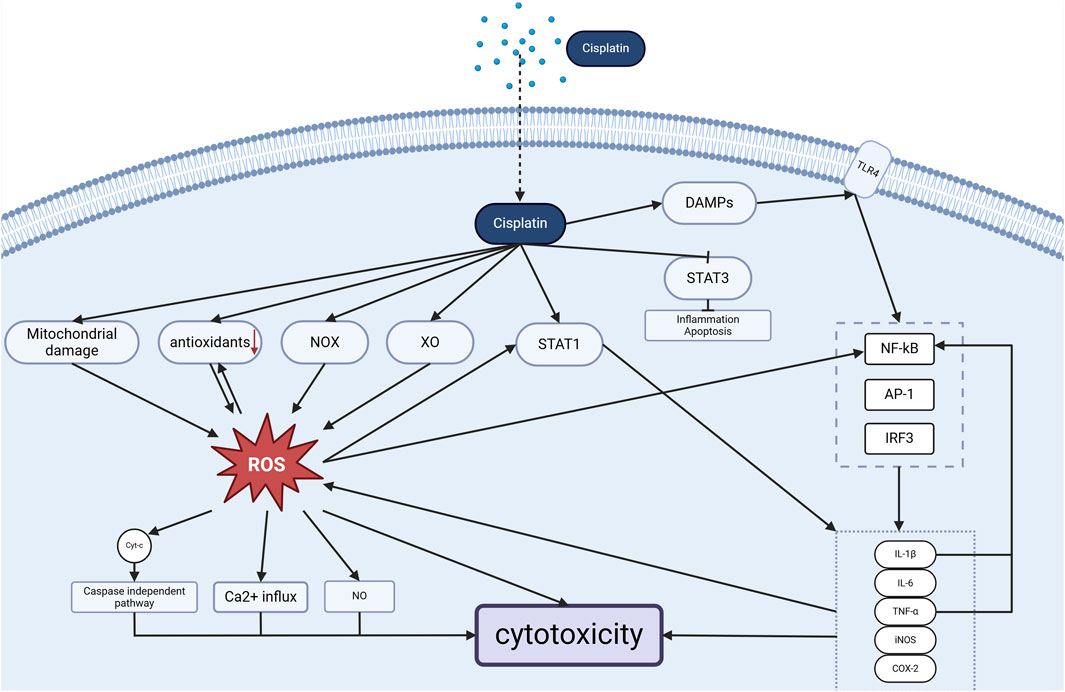
Figure 3. Cisplatin-induced cochlear Oxidative stress and inflammation (Created in https://BioRender.com).
2.5 Cisplatin induced iron death
ferroptosis is a ROS-dependent form of cell death with two major biochemical features: iron accumulation and lipid peroxidation (Tang et al., 2021). Two major signaling pathways, nuclear factor erythroid 2-related factor 2 (NRF2) and glutathione peroxidase 4 (GPX4), play important roles in ferroptosis. Normally, the transcription factor Nrf2 and its inhibitory protein KEAP1 are tightly bound to inhibit activity, but in the presence of ROS, a conformational change in KEAP1 results in the release of NRF2, which binds to the antioxidant response element (ARE) and activates the downstream genes heme oxygenase-1 (HO-1), glutathione peroxidase 4 (GPX4), quinone oxidoreductase1(NQO1), thioredoxin reductase 1 (SRXN1), and transcription of solute carrier family 7 member 11 (SLC7A11), which enhance cellular antioxidant defenses and inhibit ferroptosis (Wang et al., 2022; Honkura et al., 2016). Cisplatin exacerbates hair cell damage through a dual mechanism: on the one hand, it activates nuclear receptor coactivator 4 (NCOA4)-mediated ferritinophagy, which prompts ferritin to release free Fe2+, generating a large amount of reactive oxygen species (ROS) via the Fenton reaction and inducing a lipid peroxidation chain reaction (Dai et al., 2024); on the other hand, inhibition of the antioxidant defense pathway of the transcription factor Nrf2 leads to reduced glutathione (GSH) synthesis and decreased activity of antioxidant enzymes (e.g., GPX4), further weakening cellular scavenging of free radicals (Lv et al., 2025). GPX4 was the first central inhibitor of ferroptosis to be discovered, and GPX4 uses glutathione to protect cells from ferroptosis through elimination of phospholipid peroxides to protect cells from ferroptosis (Stockwell et al., 2020) (Figure 4).
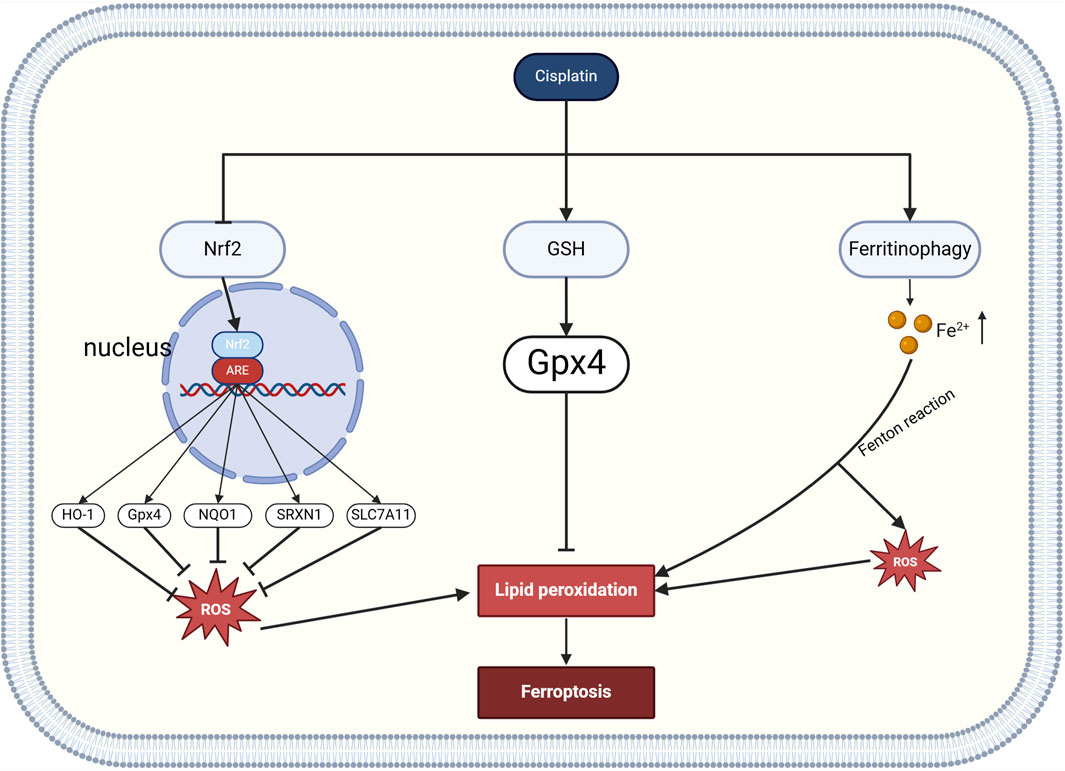
Figure 4. cisplatin-induced cochlear ferroptosis (Created in https://BioRender.com).
3 Polyphenols antagonise the mechanism of cisplatin ototoxicity
Polyphenols are a large group of natural products, the most common natural antioxidants, characterised by the presence of multiple phenolic units in their structure. There are approximately 8,000 known polyphenol compounds, of which more than 4,000 belong to flavonoids (Cheynier, 2005). In this context, the present study systematically combed natural polyphenols (both flavonoids and non-flavonoids) with ototoxic antagonistic effects in preclinical studies and reviewed the possible mechanisms of prevention of ototoxicity by these natural compounds (Figure 5 and Table 1).
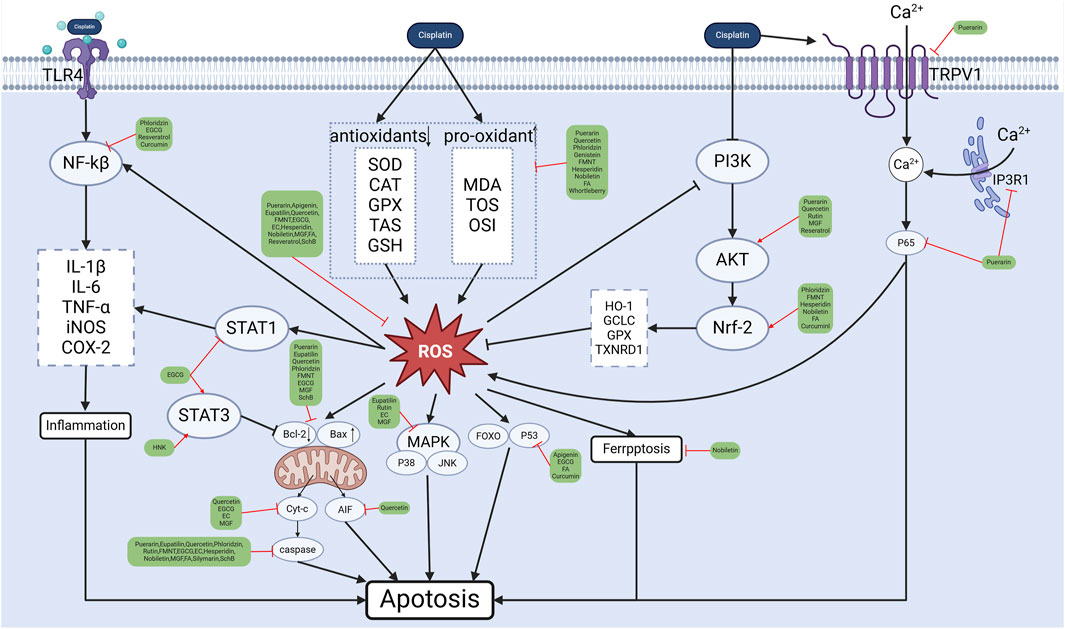
Figure 5. Polyphenols’ protective mechanism against ototoxicity. (Created in https://BioRender.com).
Clinical evidence for polyphenolic natural products in the field of hearing protection is still limited, and Ginkgo biloba Linn (Ginkgoaceae) leaves extract (GBE) is one of the more well-studied polyphenolic agents, with active ingredients including Ginkgo flavonoids, Quercetin, Ginkgolides, Organic acids, and other components. In a randomized, double-blind, prospective clinical trial of 15 cancer patients, the GBE intervention significantly protected the mean distortion product otoacoustic emissions (DPOAEs) of the patients. DPOAEs) mean amplitude and signal-to-noise ratio (Dias et al., 2015). Notably, two systematic reviews and Meta-analyses further validated the clinical value of GBE-assisted treatment for sudden sensorineural hearing loss (SSNHL), showing that the combined intervention significantly increased the clinical recovery rate and improved the pure tone average (Si et al., 2022; Yuan et al., 2023). Doluperine capsules, a novel combination agent, demonstrated excellent hearing protection in patients with type 2 diabetes mellitus combined with SSNHL by targeting inflammatory and oxidative stress pathways, and its core ingredient curcumin, the most bioavailable polyphenol in the turmeric plant, may provide therapeutic benefits through a multi-targeted mechanism of action (Tajdini et al., 2023).
3.1 Flavonoids
Flavonoids have a general structural backbone of C6-C3-C6 in which two C6 units (ring A and ring B) are phenolic in nature. Due to variations in hydroxylation pattern and chromophore ring (ring C), they can be further classified into different subgroups such as flavonoids, flavanones, isoflavonoids, flavanols, flavan-3-ols and anthocyanins. Flavonoids ameliorate ototoxic drug-induced hearing impairment by acting as ROS scavengers or inhibitors of JNK, MAPK, and other apoptotic signaling pathways, and by inhibiting aldose reductase activity, xanthine oxidase (Liao et al., 2024), common natural flavonoid mechanisms of action are described below.
3.1.1 Puerarin
Puerarin, one of the major components of Pueraria lobata, has been reported to have a wide range of pharmacological activities, including anti-inflammatory, antioxidant and anti-apoptotic effects. Puerarin reduces oxidative stress, lowers MDA levels, inhibits lipid peroxidation, protects cell membrane integrity, and inhibits cisplatin-induced damage to HEI-OC1 auditory cells by neutralizing superoxide anion, hydroxyl radical, hydrogen peroxide, and DPPH radicals (Yu et al., 2010). Puerarin can also inhibit cisplatin-induced hair cell injury by modulating apoptosis-related proteins (e.g., Bax and cleaved caspase-3) to attenuate the mitochondrial apoptotic pathway, reduce ROS accumulation and activate the Akt signaling pathway (Xu et al., 2022). Another study showed that Puerarin could block cisplatin-induced activation of TRPV1 and IP3R1, prevent intracellular calcium overload, and inhibit p65 to reduce excessive ROS production, thereby ameliorating cisplatin-induced ototoxicity and blocking apoptosis (Lin et al., 2024).
3.1.2 Apigenin
Apigenin is a natural flavonoid found in a variety of foods and beverages with antioxidant, anti-inflammatory and anti-tumour properties. Apigenin inhibits cisplatin-induced mitochondrial ROS accumulation and protects HC from cisplatin-induced damage by inhibiting apoptosis-related signalling pathways such as p53 and FoxO (Kong et al., 2024).
3.1.3 Eupatilin
Eupatilin is a pharmacologically active flavonoid found mainly in the genus Artemisia.Eupatilin reduces intracellular and mitochondrial ROS levels, inhibits the p38/JNK pathway, and reduces cisplatin-induced apoptosis of HEI-OC1 cells; it reduces cisplatin-induced hair cell loss, and protects auditory hair cells (Lu et al., 2022).
3.1.4 Quercetin (QU)
QU is a widely used natural flavonoid compound that can be an effective antioxidant against cisplatin ototoxicity.QU scavenges a variety of free radicals such as peroxides and superoxides, as well as inhibits xanthine oxidase, blocks lipid peroxidation, chelates transition metals, and reduces cytosolic calcium uptake (Gündoğdu et al., 2019). It has been demonstrated that QU increases the number of hair cells, protects mitochondria, reduces apoptosis and maintains hair cell ultrastructure (Lee S. H. et al., 2015). Moreover, QU can activate the PI3K/AKT signalling pathway, inhibit cisplatin-induced oxidative stress, protect mitochondrial function, reduce apoptosis of the mitochondrial pathway in pericytes, maintain the integrity of the endothelial barrier, and attenuate hearing loss (Huang et al., 2024).
3.1.5 Phloridzin and rhizodendron
Phloridzin and rhizoposide are the main flavonoid structural compounds of apple with antioxidant and anticancer effects (Un et al., 2021). Phloridzin inhibits mitochondrial dysfunction and caspase activation through activation of HO-1 expression induced by the Nrf2 and JNK pathway nuclei; and inhibits cisplatin-induced apoptosis in HEI-OC1 cells (Choi et al., 2011).
3.1.6 Rutin
Rutin belongs to the vitamin P family and is a natural flavonol glycoside. Rutin protects hair cells and spiral ganglion neurons from cisplatin-induced ototoxicity by reducing ROS production after cisplatin exposure, activating PI3K/AKT signalling and inhibiting the JNK/p38/MAPK signalling pathway (Zheng et al., 2022).
3.1.7 Genistein (genistein/strongylisoflavone, GST)
GST is a phytoestrogen found in large quantities in soybeans, GST increases the levels of antioxidant enzymes and decreases the levels of oxidant parameters, preventing cisplatin ototoxicity (Tan et al., 2022).
3.1.8 Formononetin (FMNT)
FMNT is a natural flavonoid found in large quantities in herbs such as Angelica sinensis and Astragalus membranaceus.FMNT reduces ROS overload by activating the PI3K/AKT-Nrf2 signalling pathway and its downstream antioxidant genes, decreases the c-caspase-3/caspase-3 ratio (Li et al., 2023a) and inhibits apoptosis ameliorating cisplatin-induced hair cell death.
3.1.9 Epigallocatechin-3-gallate (EGCG) and epicatechin
EGCG, a polyphenol abundant in green tea extract, is a STAT1 inhibitor.EGCG prevents cisplatin cytotoxicity through anti-apoptotic and antioxidant effects (Cho et al., 2014a); counteracts NO-induced ototoxicity by effectively inhibiting the activation of caspase-3, NF-κB, and preventing the destruction of hair cells in the organ of Corti, both in vitro and ex vivo (Kim et al., 2012); and protects auditory neurons from oxidative damage induced by H2O2 attack (Xie et al., 2004).EGCG acts through phosphatidylinositol 3-kinase (PI3K)/Akt signalling in cochlear NSCs to promote cell growth and neuronal differentiation, which can be used for the treatment of hearing loss (Zhang et al., 2016), and has an important protective mechanism against cochlear oxidative damage. Failing to provide additional protection against cisplatin toxicity after knockdown of the STAT1 gene in mice (Schmitt et al., 2009); EGCG reduced cisplatin-induced ROS generation and ERK1/2 and STAT1 activity, but retained STAT3 and Bcl-xL activity, allowing cisplatin to continue its anti-tumour effects (Borse et al., 2017). These studies suggest that EGCG is an ideal otoprotective agent for the treatment of cisplatin-induced hearing loss and may not compromise its antitumour efficacy.
Epicatechin (EC) is a minor component of green tea. It prevents cisplatin-induced ototoxicity by blocking ROS production and inhibiting changes in mitochondrial membrane potential (MMP) (Kim et al., 2008). EC inhibits cisplatin activation of JNK, ERK, Cyt-c and caspase-3 (Lee et al., 2010).
3.1.10 Hesperidin and nobiletin
Hesperidin and hesperetin are natural flavonoid compounds found mainly in the peel of citrus fruits. Hesperidin is a potent natural antioxidant that increases antioxidant enzymes and reduces oxidants to prevent ototoxicity (Kara et al., 2016; Başoğlu et al., 2012). In addition, Hesperidin activates the Nrf2/NQO1 pathway, decreases ROS levels, and enhances the antioxidant capacity of hair cells and HEI-OC1 cells, thereby attenuating cisplatin-induced oxidative damage (Lou et al., 2024). Nobiletin is a polymethoxyflavonoid with antioxidant and anti-apoptotic properties. The protective function of nobiletin against cisplatin-induced ototoxicity has been attributed to the activation of autophagy and the activation of NRF2/GPX4, which in turn inhibits the onset of ferroptosis (Song et al., 2024).
3.1.11 Mangiferin (MGF) and pycnogenol
Extracted from the genus Mangiferin, MGF protects against cisplatin-associated ototoxicity by down-regulating ROS accumulation, restoring mitochondrial function, and inhibiting apoptosis in in vitro (HEI-OC1 cells and cochlear hair cells) and in vivo (zebrafish larvae and C57BL/6 J mice) models and, the molecular mechanism of which may be attributed to its inhibition of the ROS-MAPK-caspase-3 signalling pathway (Lu et al., 2024). Pycnogenol prevents cisplatin-induced cochlear apoptosis and is protective against cisplatin ototoxicity (Eryilmaz et al., 2016).
3.1.12 Silymarin
Silymarin, a lipophilic extract from the seeds of Silybum marianum, is protective against ototoxicity by inhibiting the expression of caspase-3 and PARP in cisplatin-induced cleavage through mechanisms such as scavenging of free radicals, reduction of ROS formation, and inhibition of fatty acid peroxidation (Cho et al., 2014b).
3.2 Non-flavonoids
3.2.1 Ferulic acid (FA)
FA belongs to the phenolic acid family and is abundant in fruits and vegetables.FA acts as a potent antioxidant by up-regulating the cytoprotective system for scavenging free radicals and enhancing cellular stress response (Mancuso and Santangelo, 2014).FA inhibits cisplatin-induced cytotoxicity by blocking ROS formation and inducing endogenous antioxidant production, which in turn inhibits cisplatin-induced cytotoxicity (Jo et al., 2019). It exhibits antioxidant and otoprotective activity in the cochlea by up-regulating the Nrf-2/HO-1 pathway, while down-regulating p53 phosphorylation.Notably, this action of FA exhibits a biphasic response: it is pro-oxidant at lower concentrations and antioxidant at higher concentrations (Paciello et al., 2020). This unique concentration-dependent effect provides a richer direction for thinking about the application and research of ferulic acid in related fields.
3.2.2 Resveratrol
Resveratrol belongs to the group of stilbenes and is widely found in a variety of plants such as berries, grapes and nuts. Animal experiments have confirmed the protective effect of resveratrol against cisplatin-induced ototoxicity (Yumusakhuylu et al., 2012; Simsek et al., 2019). With progressive research, it has been found that resveratrol significantly reduces cisplatin-induced increase in ROS (Lee S. K. et al., 2015), thus inhibiting cisplatin-induced cytotoxicity. The auriculoprotective effect of low-dose RV may be mediated by the anti-inflammatory effects of NFκB, IL6, and IL1β, as well as the antioxidant effects of CYP1A1 and the intracytoplasmic receptor for advanced glycation endproducts (RAGE).However, high doses of RV were not otoprotective in rats with cisplatin-induced hearing loss (Lee et al., 2020). In experiments targeting a mouse model of hearing loss and HEI-OC1 cells, resveratrol was found to upregulate miR-455-5p, which in turn downregulated phosphatase and tensin homolog (PTEN) and activated the phosphatidylinositol 3-kinase-protein kinase B (PI3K-Akt) signaling pathway in order to counteract cisplatin ototoxicity (Liu et al., 2021). Further studies demonstrated that growth arrest-specific transcript 5 (GAS5) could act as a molecular sponge for miR-455-5p and regulate miR-455-5p/PTEN expression to counteract cisplatin-induced apoptosis and ROS production (Wu et al., 2024).
3.2.3 Schisandrin B (SchB)
SchB is one of the most prevalent and potent naturally occurring lignan monomers in Schisandrin B. It has a variety of pharmacological effects, including antioxidant, anti-inflammatory, and anti-apoptotic effects. In cisplatin-induced mouse experiments, treatment with SchB was able to enhance the survival of cochlear hair cells and effectively prevent apoptosis, as well as reduce cisplatin-induced ROS production (Li et al., 2024).
3.2.4 Polyphenol
Curcumin is a phytochemical isolated from turmeric that has the ability to enhance the antitumour activity of cisplatin and reduce its side effects (Abadi et al., 2022), can directly scavenge free radicals and also enhance endogenous antioxidant enzyme activity by activating the Nrf2 pathway and inducing HO-1 expression to achieve antioxidant effects, which in turn reduce hearing damage (Fetoni et al., 2014).When curcumin is administered together with cisplatin, it exhibits different mechanisms of action depending on the cellular environment and dose, exerting dual antioxidant and pro-oxidant effects (Arwanda et al., 2023). Curcumin may enhance the anti-tumour effect of cisplatin by promoting a significant increase in the pro-apoptotic expression of Bax, inhibiting STAT3 phosphorylation, nuclear translocation of Nrf-2, and expression of NF-κB; and activating the Nrf-2/HO-1 signalling pathway, inhibiting p53 phosphorylation, expression of NF-κB, and enhancing endogenous antioxidant defence mechanisms to counteract cisplatin-induced ototoxicity (Paciello et al., 2020; Fetoni et al., 2015).The above studies have confirmed that curcumin has a significant dose-effect relationship, and there is an optimal concentration window--it can synergistically enhance the anticancer efficacy of cisplatin and minimize its toxic side effects.
Honokiol (HNK) is a multifunctional polyphenol derived from an Asian herb (Magnolia bark), HNK prevents cisplatin ototoxicity in vitro and in vivo and does not interfere with cisplatin treatment in homozygous mice (Tan et al., 2020). This is attributed to the direct activation of the protein sirtuin 3 (SIRT3) by HNK, which promotes ROS reduction and detoxification.
Ecklonia Cava Polyphenol (ECP) extract is a polyphenolic compound taken from brown algae living in the Far Eastern seas. It has a protective effect against cisplatin-induced cell death by reducing particularly reactive oxygen radicals and increasing the levels of enzymes such as catalase and glutathione peroxidase (Düzenli et al., 2016).
Caffeic acid, the active component of honey bee propolis extract, reduced intracellular ROS production through free radical scavenging activity; and decreased the expression of caspase-3, inhibited apoptosis, and ultimately attenuated cisplatin-induced hair cell loss in the HEI-OC1 cell line (Choi et al., 2014).
Pomegranate extract (PE) is rich in polyphenols and exhibits strong antioxidant activity (Sahin et al., 2025). Oral administration of PE has been shown to have a protective effect on the cochlea against cisplatin toxicity in rats (Yazici et al., 2012).
Anthocyanins is an extract of Vaccinium myrtillus, which presents antioxidant effects at high doses, thus reducing oxidative stress indices and preventing cell degeneration and protecting hearing from cisplatin-induced ototoxicity (Özdemir et al., 2019).
4 Discussion
The protective mechanism of polyphenols against cisplatin ototoxicity is mainly to build two major protective barriers in normal tissues. The first is the antioxidant barrier: polyphenols establish antioxidant defense by activating the Nrf2 signaling pathway, and Nrf2 upregulates the expression of HO-1, SOD-1, NQO-1 and other oxidative genes, so as to increase the number of antioxidant enzymes (SOD/CAT/GPX/GSH), and to neutralize cisplatin-induced reactive oxygen species (ROS) and reactive nitrogen species (RNS). Reactive ROS and RNS to reduce ROS accumulation, mitochondrial damage, inflammatory response and ferroptosis, and polyphenols also regulate the Nrf2 by activating the PI3K/AKT signaling pathway. The second is the inflammation-apoptosis dual inhibition barrier: blocking the NF-κB/p38-MAPK/COX-2/iNOS inflammatory cascade, attenuating the inflammatory response through the dual regulation of STAT3/STAT1 and inhibiting the JNK/p53-mediated pro-apoptotic signaling and regulating the balance of Bcl-2/Bax/caspase-3, which is the most effective way to reduce the inflammatory response in mitochondria and ferroptosis. (Zhang et al., 2025; Maiuolo et al., 2022; Li et al., 2023b; Stankovic et al., 2020; Gill et al., 2024).
Polyphenols face significant bioavailability bottlenecks when administered orally, and their pharmacokinetic properties make it difficult to reach therapeutic window concentrations in inner ear hair cells and auditory nerves (Osakabe et al., 2023), a limitation that significantly restricts their potential application in the prevention and treatment of CIO.However, reactive oxygen species present a dual role in the pathological process of CIO: as a key pathogenic factor mediating cisplatin ototoxicity and as a pharmacological basis for its tumor cell-killing effects, and this paradoxical property makes the construction of an inner-ear-targeted delivery system a central challenge for synergistic chemotherapy with polyphenolic compounds.
Recent breakthroughs in nano-delivery systems have provided innovative strategies to address this challenge (Thakur et al., 2024). Nano-embedded curcumin not only significantly improves cell survival and attenuates the ototoxicity of cisplatin through enhanced bioavailability and targeted delivery (Salehi et al., 2014), but also synergistically enhances the anticancer efficacy of cisplatin in combination with cisplatin while improving curcumin bioavailability (Sandhiutami et al., 2021). In addition resveratrol has low bioavailability due to its poor water solubility, low stability and rapid metabolism. Some studies have demonstrated that different types of resveratrol nanoparticles can significantly improve its therapeutic potential in a variety of diseases such as neurodegenerative diseases, cancer, diabetes, etc. (Chung et al., 2020).EGCG is a promising natural compound that enhances the efficacy of chemotherapy and reduces side effects through multiple pathways, and combining it with nanotechnology can break through the limitations of poor bioavailability and targeting to significantly enhance the therapeutic effect (Wang X. et al., 2023). Tetrahedral DNA nanostructures (TDNs) as carriers loaded with antioxidant EGCG can efficiently penetrate the biological barrier, sustained release of drugs and antioxidant effects, and significantly improve the noise-induced hearing loss, which provides a novel delivery system for the treatment of inner ear diseases (Chen et al., 2022).
Different types of polyphenols may have different mechanisms in preventing cisplatin ototoxicity, and may act synergistically in enhancing its anticancer activity to reduce side effects.EGCG has antioxidant, anti-inflammatory, and inhibitory effects on Caspase-1 and NF-κB, and curcumin regulates the Bcl-2/BAX ratio, inhibits the mitochondrial apoptotic pathway, and reduces Caspase-3 activation. The superimposition of these two effects enhances the anti-apoptotic effect and reduces the dosage requirement of a single component, providing a potential solution for adjuvant therapy to cisplatin chemotherapy, reducing side effects and improving the quality of life of patients (Primadewi et al., 2023). Potential interactions between polyphenols and common drugs used in cisplatin combination chemotherapy also need to be noted. One study demonstrated that curcumin combined with paclitaxel treatment was superior to paclitaxel-placebo combination therapy in terms of ORR and physical performance after 12 weeks of treatment, and there were also no major safety concerns or reduction in quality of life (Saghatelyan et al., 2020).EGCG synergistically enhances the efficacy of drugs such as 5 - Fluorouracil and cisplatin, and reduces cardiac, renal, and other organ EGCG may synergistically enhance the efficacy of drugs such as 5-fluorouracil and cisplatin and reduce the toxicity in the heart and kidney, but some drugs (e.g., bortezomib, sunitinib) may antagonize EGCG, but clinical evidence is limited, and further validation of their safety and efficacy is needed (Lecumberri et al., 2013).
Despite their low bioavailability, oral polyphenols can modulate the intestinal flora through their antimicrobial properties, increasing the proportion of beneficial bacteria in the gut and reducing pathogenic bacteria (Scazzocchio et al., 2020). Improvement in the balance of intestinal flora helps to maintain the integrity of the intestinal barrier, reduces the influx of harmful substances into the body, and inhibits inflammatory responses (Liu et al., 2024). It has been reported that polyphenols ameliorate certain diseases by modulating the intestinal flora (Da C Pinaffi-Langley et al., 2024; Sarubbo et al., 2023). At the same time, we have come to recognize the association between the gut-inner ear axis and hearing loss (Guo et al., 2024; Yin et al., 2023). Based on this, the topic of whether orally administered polyphenols can ameliorate hearing loss by modulating the intestinal flora is of significant research value.
5 Conclusion
Cisplatin is a highly effective and broad-spectrum anticancer drug, but its severe ototoxicity limits its clinical application, and its mechanism involves various pathways such as DNA damage, oxidative stress, inflammatory response, mitochondrial dysfunction, and ferroptosis. Polyphenols show promise in mitigating cisplatin-induced damage to Corti organs, spiral ganglion neurons, and vascular striatum by scavenging free radicals, inhibiting inflammatory factors, and regulating apoptosis-related proteins. However, several challenges remain, including low bioavailability, an incomplete understanding of their mechanisms, uncertainties about the optimal dosing regimen, and the potential interactions among different polyphenols. Further studies should explore the optimal strategy for the combined application of polyphenols and cisplatin to provide new ideas and methods for clinical prevention and treatment of cisplatin ototoxicity.
5.1 Future perspectives
Cisplatin, as a commonly used chemotherapeutic agent, is highly effective, but the problem of CIO seriously affects patients’ quality of life. Polyphenols have shown impressive dual potentials in preventing CIO and enhancing the efficacy of chemotherapy, and are expected to be a key factor in improving the status of cisplatin chemotherapy. However, polyphenols still face many obstacles in moving from basic research to clinical application.
Currently, most of the relevant studies remain in the preclinical stage, and the lack of key pharmacokinetic data for clinical application, such as effective dose, bioavailability and other information, restricts the development of rational drug regimens. In terms of drug delivery strategies, the emergence of nano drug delivery systems holds promise for improving the oral utilization of polyphenols. However, this system still has large optimization aspects in terms of shape, size, and targeted delivery efficiency, which is still far from the ideal state of precision and efficiency. In addition, many important issues need to be further explored, such as the mechanism of the superimposed effect and its safety when polyphenols are applied in combination, the possible interactions with other chemotherapeutic agents such as 5 - fluorouracil, paclitaxel analogs, gemcitabine, pemetrexed, etc., as well as whether oral polyphenols have an impact on ototoxicity by regulating the intestinal flora.
Addressing the above issues has immeasurable clinical value in improving the comprehensive efficacy of cisplatin chemotherapy and effectively reducing the risk of ototoxicity. It will not only bring better treatment experience for patients, but also promote new breakthroughs in the field of anti-cancer therapy.
Author contributions
SH: Software, Writing – original draft. JS: Methodology, Writing – original draft. WL: Methodology, Validation, Writing – original draft. JL: Conceptualization, Writing – review and editing. HY: Investigation, Software, Writing – review and editing. SW: Project administration, Supervision, Writing – review and editing. YC: Writing – review and editing.
Funding
The author(s) declare that no financial support was received for the research and/or publication of this article.
Conflict of interest
The authors declare that the research was conducted in the absence of any commercial or financial relationships that could be construed as a potential conflict of interest.
Generative AI statement
The author(s) declare that no Generative AI was used in the creation of this manuscript.
Publisher’s note
All claims expressed in this article are solely those of the authors and do not necessarily represent those of their affiliated organizations, or those of the publisher, the editors and the reviewers. Any product that may be evaluated in this article, or claim that may be made by its manufacturer, is not guaranteed or endorsed by the publisher.
References
Abadi, A. J., Mirzaei, S., Mahabady, M. K., Hashemi, F., Zabolian, A., Hashemi, F., et al. (2022). Curcumin and its derivatives in cancer therapy: potentiating antitumor activity of cisplatin and reducing side effects. Phytotherapy Res. PTR 36 (1), 189–213. doi:10.1002/ptr.7305
Abotaleb, M., Liskova, A., Kubatka, P., and Büsselberg, D. (2020). Therapeutic potential of plant phenolic acids in the treatment of cancer. Biomolecules 10 (2), 221. doi:10.3390/biom10020221
Anantharaju, P. G., Gowda, P. C., Vimalambike, M. G., and Madhunapantula, S. V. (2016). An overview on the role of dietary phenolics for the treatment of cancers. Nutr. J. 15 (1), 99. doi:10.1186/s12937-016-0217-2
Anfuso, C. D., Cosentino, A., Agafonova, A., Zappalà, A., Giurdanella, G., Trovato Salinaro, A., et al. (2022). Pericytes of stria Vascularis are targets of cisplatin-induced ototoxicity: new insights into the molecular mechanisms involved in blood-labyrinth barrier breakdown. Int. J. Mol. Sci. 23 (24), 15790. doi:10.3390/ijms232415790
Arwanda, M. D., Haryuna, T. S., Adriztina, I., and Khalid, K. (2023). Curcumin prevents ototoxicity induced by cisplatin as evaluated with OAE. Iran. J. otorhinolaryngology 35 (129), 189–197. doi:10.22038/IJORL.2023.71786.3452
Babolmorad, G., Latif, A., Domingo, I. K., Pollock, N. M., Delyea, C., Rieger, A. M., et al. (2021). Toll-like receptor 4 is activated by platinum and contributes to cisplatin-induced ototoxicity. EMBO Rep. 22 (5), e51280. doi:10.15252/embr.202051-280
Başoğlu, M. S., Eren, E., Aslan, H., Bingölballı, A. G., Oztürkcan, S., and Katılmış, H. (2012). Prevention of cisplatin ototoxicity: efficacy of micronized flavonoid fraction in a Guinea pig model. Int. J. Pediatr. otorhinolaryngology 76 (9), 1343–1346. doi:10.1016/j.ijporl.2012.06.003
Benkafadar, N., Menardo, J., Bourien, J., Nouvian, R., François, F., Decaudin, D., et al. (2017). Reversible p53 inhibition prevents cisplatin ototoxicity without blocking chemotherapeutic efficacy. EMBO Mol. Med. 9 (1), 7–26. doi:10.15252/emmm.201606230
Bhatta, P., Dhukhwa, A., Sheehan, K., Al Aameri, R. F. H., Borse, V., Ghosh, S., et al. (2019). Capsaicin protects against cisplatin ototoxicity by changing the STAT3/STAT1 ratio and activating cannabinoid (CB2) receptors in the cochlea. Sci. Rep. 9 (1), 4131. doi:10.1038/s41598-019-40425-9
Borse, V., Al Aameri, R. F. H., Sheehan, K., Sheth, S., Kaur, T., Mukherjea, D., et al. (2017). Epigallocatechin-3-gallate, a prototypic chemopreventative agent for protection against cisplatin-based ototoxicity. Cell death and Dis. 8 (7), e2921. doi:10.1038/cddis.2017.314
Bouyahya, A., Omari, N. E., Bakrim, S., Hachlafi, N. E., Balahbib, A., Wilairatana, P., et al. (2022). Advances in dietary phenolic compounds to improve chemosensitivity of anticancer drugs. Cancers 14 (19), 4573. doi:10.3390/cancers14194573
Callejo, A., Sedó-Cabezón, L., Juan, I. D., and Llorens, J. (2015). Cisplatin-induced ototoxicity: effects, mechanisms and protection strategies. Toxics 3 (3), 268–293. doi:10.3390/toxics3030268
Cederroth, C. R., Dyhrfjeld-Johnsen, J., and Canlon, B. (2024). Pharmacological approaches to hearing loss. Pharmacol. Rev. 76 (6), 1063–1088. doi:10.1124/pharmrev.124.001195
Chen, Y., Gu, J., Liu, Y., Xu, K., Song, J., Wang, X., et al. (2022). Epigallocatechin gallate-loaded tetrahedral DNA nanostructures as a novel inner ear drug delivery system. Nanoscale 14 (22), 8000–8011. doi:10.1039/d1nr07921b
Cheynier, V. (2005). Polyphenols in foods are more complex than often thought. Am. J. Clin. Nutr. 81 (1 Suppl. l), 223S–229S. doi:10.1093/ajcn/81.1.223S
Cho, S. I., Lee, J. E., and Do, N. Y. (2014a). Protective effect of silymarin against cisplatin-induced ototoxicity. Int. J. Pediatr. otorhinolaryngology 78 (3), 474–478. doi:10.1016/j.ijporl.2013.12.024
Cho, S. I., Lee, J. H., Park, J. H., and Do, N. Y. (2014b). Protective effect of (-)-epigallocatechin-3-gallate against cisplatin-induced ototoxicity. J. laryngology otology 128 (4), 350–355. doi:10.1017/S0022215114000553
Choi, B. M., Chen, X. Y., Gao, S. S., Zhu, R., and Kim, B. R. (2011). Anti-apoptotic effect of phloretin on cisplatin-induced apoptosis in HEI-OC1 auditory cells. Pharmacol. Rep. 63, 708–716. doi:10.1016/s1734-1140(11)70582-5
Choi, J., Kim, S. H., Rah, Y. C., Chae, S. W., Lee, J. D., Lee, B. D., et al. (2014). Effects of caffeic acid on cisplatin-induced hair cell damage in HEI-OC1 auditory cells. Int. J. Pediatr. otorhinolaryngology 78 (12), 2198–2204. doi:10.1016/j.ijporl.2014.10.013
Chung, I.-M., Subramanian, U., Thirupathi, P., Venkidasamy, B., Samynathan, R., Gangadhar, B. H., et al. (2020). Resveratrol nanoparticles: a promising therapeutic advancement over native resveratrol. Processes 8 (4), 458. doi:10.3390/pr8040458
Crona, D. J., Faso, A., Nishijima, T. F., McGraw, K. A., Galsky, M. D., and Milowsky, M. I. (2017). A systematic review of strategies to prevent cisplatin-induced nephrotoxicity. Oncol. 22 (5), 609–619. doi:10.1634/theoncologist.2016-0319
Da C Pinaffi-Langley, A. C., Tarantini, S., Hord, N. G., and Yabluchanskiy, A. (2024). Polyphenol-derived microbiota metabolites and cardiovascular health: a concise review of human studies. Antioxidants Basel, Switz. 13 (12), 1552. doi:10.3390/antiox13121552
Dai, D., Chen, C., Lu, C., Guo, Y., Li, Q., and Sun, C. (2024). Apoptosis, autophagy, ferroptosis, and pyroptosis in cisplatin-induced ototoxicity and protective agents. Front. Pharmacol. 15, 1430469. doi:10.3389/fphar.2024.1430469
Dalgaard, F., Bondonno, N. P., Murray, K., Bondonno, C. P., Lewis, J. R., Croft, K. D., et al. (2019). Associations between habitual flavonoid intake and hospital admissions for atherosclerotic cardiovascular disease: a prospective cohort study. Lancet. Planet. health 3 (11), e450–e459. doi:10.1016/S2542-5196(19)30212-8
Dias, M. A., Sampaio, A. L., Venosa, A. R., Meneses, E. deA., and Oliveira, C. A. (2015). The chemopreventive effect of Ginkgo biloba extract 761 against cisplatin ototoxicity: a pilot study. Int. tinnitus J. 19 (2), 12–19. doi:10.5935/0946-5448.20150003
Düzenli, U., Olgun, Y., Aktaş, S., Pamukoğlu, A., and Altun, Z. (2016). Effect of ecklonia Cava polyphenol extract in house ear institute-organ of Corti 1 cells against cisplatin ototoxicity: a preliminary study. Turkish archives otorhinolaryngology 54 (4), 141–145. doi:10.5152/tao.2016.1974
Elmorsy, E. A., Saber, S., Hamad, R. S., Abdel-Reheim, M. A., El-Kott, A. F., AlShehri, M. A., et al. (2024). Advances in understanding cisplatin-induced toxicity: molecular mechanisms and protective strategies. Eur. Fed. Pharm. Sci. 203, 106939. doi:10.1016/j.ejps.2024.106939
Eryilmaz, A., Eliyatkin, N., Demirci, B., Basal, Y., Kurt Omurlu, I., Gunel, C., et al. (2016). Protective effect of Pycnogenol on cisplatin-induced ototoxicity in rats. Pharm. Biol. 54 (11), 2777–2781. doi:10.1080/13880209.2016.1177093
Fetoni, A. R., Eramo, S. L., Paciello, F., Rolesi, R., Podda, M. V., Troiani, D., et al. (2014). Curcuma longa (curcumin) decreases in vivo cisplatin-induced ototoxicity through heme oxygenase-1 induction. Otology and Neurotol. 35 (5), e169–e177. doi:10.1097/MAO.0000000000000302
Fetoni, A. R., Paciello, F., Mezzogori, D., Rolesi, R., Eramo, S. L., Paludetti, G., et al. (2015). Molecular targets for anticancer redox chemotherapy and cisplatin-induced ototoxicity: the role of curcumin on pSTAT3 and Nrf-2 signalling. Br. J. cancer 113 (10), 1434–1444. doi:10.1038/bjc.2015.359
Fetoni, A. R., Paciello, F., and Troiani, D. (2022). Cisplatin chemotherapy and cochlear damage: otoprotective and chemosensitization properties of polyphenols. Antioxidants and redox Signal. 36 (16-18), 1229–1245. doi:10.1089/ars.2021.0183
Gill, N. B., Dowker-Key, P. D., Hedrick, M., and Bettaieb, A. (2024). Unveiling the role of oxidative stress in cochlear hair cell death: prospective phytochemical therapeutics against sensorineural hearing loss. Int. J. Mol. Sci. 25 (8), 4272. doi:10.3390/ijms25084272
Gong, T., Liu, L., Jiang, W., and Zhou, R. (2020). DAMP-sensing receptors in sterile inflammation and inflammatory diseases. Nat. Rev. Immunol. 20 (2), 95–112. doi:10.1038/s41577-019-0215-7
Grabska-Kobyłecka, I., Szpakowski, P., Król, A., Książek-Winiarek, D., Kobyłecki, A., Głąbiński, A., et al. (2023). Polyphenols and their impact on the prevention of neurodegenerative diseases and development. Nutrients 15 (15), 3454. doi:10.3390/nu15153454
Gündoğdu, R., Erkan, M., Aydın, M., Sönmez, M. F., Vural, A., Kökoğlu, K., et al. (2019). Assessment of the effectiveness of quercetin on cisplatin-induced ototoxicity in rats. J. Int. Adv. otology 15 (2), 229–236. doi:10.5152/iao.2019.5902
Guo, Z., Wu, Y., Chen, B., Kong, M., Xie, P., Li, Y., et al. (2024). Superparamagnetic iron oxide nanoparticle regulates microbiota-gut-inner ear axis for hearing protection. Natl. Sci. Rev. 11 (6), nwae100. doi:10.1093/nsr/nwae100
Hong, S. J., Dawson, T. M., and Dawson, V. L. (2004). Nuclear and mitochondrial conversations in cell death: PARP-1 and AIF signaling. Trends Pharmacol. Sci. 25 (5), 259–264. doi:10.1016/j.tips.2004.03.005
Honkura, Y., Matsuo, H., Murakami, S., Sakiyama, M., Mizutari, K., Shiotani, A., et al. (2016). NRF2 is a key target for prevention of noise-induced hearing loss by reducing oxidative damage of cochlea. Sci. Rep. 6, 19329. doi:10.1038/srep19329
Huang, T. L., Jiang, W. J., Zhou, Z., Shi, T. F., Yu, M., Yu, M., et al. (2024). Quercetin attenuates cisplatin-induced mitochondrial apoptosis via PI3K/Akt mediated inhibition of oxidative stress in pericytes and improves the blood labyrinth barrier permeability. Chemico-biological Interact. 393, 110939. doi:10.1016/j.cbi.2024.110939
Jakobušić Brala, C., Karković Marković, A., Kugić, A., Torić, J., and Barbarić, M. (2023). Combination chemotherapy with selected polyphenols in preclinical and clinical studies-an update overview. Mol. Basel, Switz. 28 (9), 3746. doi:10.3390/molecules28093746
Jo, E. R., Youn, C. K., Jun, Y., and Cho, S. I. (2019). The protective role of ferulic acid against cisplatin-induced ototoxicity. Int. J. Pediatr. otorhinolaryngology 120, 30–35. doi:10.1016/j.ijporl.2019.02.001
Johnstone, T. C., Suntharalingam, K., and Lippard, S. J. (2016). The next generation of platinum drugs: targeted Pt(II) agents, nanoparticle delivery, and Pt(IV) prodrugs. Chem. Rev. 116 (5), 3436–3486. doi:10.1021/acs.chemrev.5b00597
Kara, M., Türkön, H., Karaca, T., Güçlü, O., Uysal, S., Türkyılmaz, M., et al. (2016). Evaluation of the protective effects of hesperetin against cisplatin-induced ototoxicity in a rat animal model. Int. J. Pediatr. otorhinolaryngology 85, 12–18. doi:10.1016/j.ijporl.2016.03.019
Kaur, T., Mukherjea, D., Sheehan, K., Jajoo, S., Rybak, L. P., and Ramkumar, V. (2011). Short interfering RNA against STAT1 attenuates cisplatin-induced ototoxicity in the rat by suppressing inflammation. Cell death and Dis. 2 (7), e180. doi:10.1038/cddis.2011.63
Kim, C. H., Kang, S. U., Pyun, J., Lee, M. H., Hwang, H. S., and Lee, H. (2008). Epicatechin protects auditory cells against cisplatin-induced death. Apoptosis Int. J. Program. cell death 13 (9), 1184–1194. doi:10.1007/s10495-008-0242-5
Kim, S. J., Lee, J. H., Kim, B. S., So, H. S., Park, R., Myung, N. Y., et al. (2012). (-)-Epigallocatechin-3-gallate protects against NO-induced ototoxicity through the regulation of caspase- 1, caspase-3, and NF-κB activation. PloS one 7 (9), e43967. doi:10.1371/journal.pone.0043967
Ko, J. H., Sethi, G., Um, J. Y., Shanmugam, M. K., Arfuso, F., Kumar, A. P., et al. (2017). The role of resveratrol in cancer therapy. Int. J. Mol. Sci. 18 (12), 2589. doi:10.3390/ijms18122589
Kong, S., Xiao, Y., Chen, L., Jin, Y., Qiao, R., Xu, K., et al. (2024). Apigenin attenuates cisplatin-induced hair cell damage in the zebrafish lateral line. Food Chem. Toxicol. 194, 115099. doi:10.1016/j.fct.2024.115099
Kucharava, K., Sekulic-Jablanovic, M., Horvath, L., Bodmer, D., and Petkovic, V. (2019). Pasireotide protects mammalian cochlear hair cells from gentamicin ototoxicity by activating the PI3K-Akt pathway. Cell death and Dis. 10 (2), 110. doi:10.1038/s41419-019-1386-7
Lecumberri, E., Dupertuis, Y. M., Miralbell, R., and Pichard, C. (2013). Green tea polyphenol epigallocatechin-3-gallate (EGCG) as adjuvant in cancer therapy. Clin. Nutr. Edinb. Scotl. 32 (6), 894–903. doi:10.1016/j.clnu.2013.03.008
Lee, C. H., Kim, K. W., Lee, S. M., and Kim, S. Y. (2020). Dose-dependent effects of resveratrol on cisplatin-induced hearing loss. Int. J. Mol. Sci. 22 (1), 113. doi:10.3390/ijms22010113
Lee, J. S., Kang, S. U., Hwang, H. S., Pyun, J. H., Choung, Y. H., and Kim, C. H. (2010). Epicatechin protects the auditory organ by attenuating cisplatin-induced ototoxicity through inhibition of ERK. Toxicol. Lett. 199 (3), 308–316. doi:10.1016/j.toxlet.2010.09.013
Lee, S. H., Kim, H. S., An, Y. S., Chang, J., Choi, J., and Im, G. J. (2015a). Protective effect of resveratrol against cisplatin-induced ototoxicity in HEI-OC1 auditory cells. Int. J. Pediatr. otorhinolaryngology 79 (1), 58–62. doi:10.1016/j.ijporl.2014.11.008
Lee, S. K., Oh, K. H., Chung, A. Y., Park, H. C., Lee, S. H., Kwon, S. Y., et al. (2015b). Protective role of quercetin against cisplatin-induced hair cell damage in zebrafish embryos. Hum. and Exp. Toxicol. 34 (11), 1043–1052. doi:10.1177/0960327114567766
Li, W., Guo, Y., Zhang, C., Wu, R., Yang, A. Y., Gaspar, J., et al. (2016). Dietary phytochemicals and cancer chemoprevention: a perspective on oxidative stress, inflammation, and epigenetics. Chem. Res. Toxicol. 29 (12), 2071–2095. doi:10.1021/acs.chemrestox.6b00413
Li, Y., Liu, Z., Chen, J., Wang, R., An, X., Tian, C., et al. (2024). Schisandrin B protect inner hair cells from cisplatin by inhibiting celluar oxidative stress and apoptosis. Toxicol. vitro Int. J. Publ. Assoc. BIBRA 99, 105852. doi:10.1016/j.tiv.2024.105852
Li, Y., Wu, J., Yu, H., Lu, X., and Ni, Y. (2023b). Formononetin ameliorates cisplatin-induced hair cell death via activation of the PI3K/AKT-Nrf2 signaling pathway. Heliyon 10 (1), e23750. doi:10.1016/j.heliyon.2023.e23750
Li, Y., Zhang, T., Song, Q., Gao, D., Li, Y., Jie, H., et al. (2023a). Cisplatin ototoxicity mechanism and antagonistic intervention strategy: a scope review. Front. Cell. Neurosci. 17, 1197051. doi:10.3389/fncel.2023.1197051
Liao, Y., Mao, H., Gao, X., Lin, H., Li, W., Chen, Y., et al. (2024). Drug screening identifies aldose reductase as a novel target for treating cisplatin-induced hearing loss. Free Radic. Biol. and Med. 210, 430–447. doi:10.1016/j.freeradbiomed.2023.11.025
Lin, Y., Liang, R., Xie, K., Ma, T., Zhang, J., Xu, T., et al. (2024). Puerarin inhibits cisplatin-induced ototoxicity in mice through regulation of TRPV1-dependent calcium overload. Biochem. Pharmacol. 220, 115962. doi:10.1016/j.bcp.2023.115962
Liu, Y., Fang, M., Tu, X., Mo, X., Zhang, L., Yang, B., et al. (2024). Dietary polyphenols as anti-aging agents: targeting the hallmarks of aging. Nutrients 16 (19), 3305. doi:10.3390/nu16193305
Liu, Y., Wu, H., Zhang, F., Yang, J., and He, J. (2021). Resveratrol upregulates miR-455-5p to antagonize cisplatin ototoxicity via modulating the PTEN-PI3K-AKT axis. Biochem. cell Biol. = Biochimie Biol. Cell. 99 (3), 385–395. doi:10.1139/bcb-2020-0459
Lou, J., Wu, F., He, W., Hu, R., Cai, Z., Chen, G., et al. (2024). Hesperidin activates Nrf2 to protect cochlear hair cells from cisplatin-induced damage. Redox Rep. Commun. free Radic. Res. 29 (1), 2341470. doi:10.1080/13510002.2024.2341470
Lu, X., Deng, T., Dong, H., Han, J., Yu, Y., Xiang, D., et al. (2022). Novel application of eupatilin for effectively attenuating cisplatin-induced auditory hair cell death via mitochondrial apoptosis pathway. Oxidative Med. Cell. Longev. 2022, 1090034. doi:10.1155/2022/1090034
Lu, X., Yin, N., Chen, C., Zhou, Y., Ji, L., Zhang, B., et al. (2024). Mangiferin alleviates cisplatin-induced ototoxicity in sensorineural hearing loss. Biomed. and Pharmacother. = Biomedecine and Pharmacother. 178, 117174. doi:10.1016/j.biopha.2024.117174
Lv, X., Yang, C., Li, X., Liu, Y., Yang, Y., Jin, T., et al. (2025). Ferroptosis and hearing loss: from molecular mechanisms to therapeutic interventions. J. enzyme inhibition Med. Chem. 40 (1), 2468853. doi:10.1080/14756366.2025.2468853
Ma, Q. (2013). Role of nrf2 in oxidative stress and toxicity. Annu. Rev. Pharmacol. Toxicol. 53, 401–426. doi:10.1146/annurev-pharmtox-011112-140320
Maiuolo, J., Musolino, V., Gliozzi, M., Carresi, C., Oppedisano, F., Nucera, S., et al. (2022). The employment of genera Vaccinium, citrus, olea, and CynaraPolyphenols for the reduction of selected anti-cancer drug side effects. Nutrients 14 (8), 1574. doi:10.3390/nu14081574
Mancuso, C., and Santangelo, R. (2014). Ferulic acid: pharmacological and toxicological aspects. Food Chem. Toxicol. 65, 185–195. doi:10.1016/j.fct.2013.12.024
Mukherjea, D., Jajoo, S., Sheehan, K., Kaur, T., Sheth, S., Bunch, J., et al. (2011). NOX3 NADPH oxidase couples transient receptor potential vanilloid 1 to signal transducer and activator of transcription 1-mediated inflammation and hearing loss. Antioxidants and redox Signal. 14 (6), 999–1010. doi:10.1089/ars.2010.3497
Osakabe, N., Modafferi, S., Ontario, M. L., Rampulla, F., Zimbone, V., Migliore, M. R., et al. (2023). Polyphenols in inner ear neurobiology, health and disease: from bench to clinics. Med. Kaunas. Lith. 59 (11), 2045. doi:10.3390/medicina59112045
Özdemir, D., Özgür, A., Kalkan, Y., Terzi, S., Tümkaya, L., Yılmaz, A., et al. (2019). The protective effects of whortleberry extract against cisplatin-induced ototoxicity in rats. Braz. J. otorhinolaryngology 85 (1), 55–62. doi:10.1016/j.bjorl.2017.10.009
Paciello, F., Fetoni, A. R., Mezzogori, D., Rolesi, R., Di Pino, A., Paludetti, G., et al. (2020). The dual role of curcumin and ferulic acid in counteracting chemoresistance and cisplatin-induced ototoxicity. Sci. Rep. 10 (1), 1063. doi:10.1038/s41598-020-57965-0
Primadewi, N., Kariosentono, H., Probandari, A., and Wiboworini, B. (2023). The effect of combination between green tea extract and curcumin extract from Mt. Lawu on BAX, bcl-2 and caspase-3 in cisplatin-induced rat models. Pharmacogn. J. 15 (2), 370–374. doi:10.5530/pj.2023.15.57
Ramkumar, V., Mukherjea, D., Dhukhwa, A., and Rybak, L. P. (2021). Oxidative stress and inflammation caused by cisplatin ototoxicity. Antioxidants Basel, Switz. 10 (12), 1919. doi:10.3390/antiox10121919
Rose, O., Croonenberg, T., Clemens, S., Hinteregger, T., Eppacher, S., Huber-Cantonati, P., et al. (2024). Cisplatin-induced hearing loss, oxidative stress, and antioxidants as a therapeutic strategy-A state-of-the-art review. Antioxidants Basel, Switz. 13 (12), 1578. doi:10.3390/antiox13121578
Rybak, L. P., Mukherjea, D., and Ramkumar, V. (2019). Mechanisms of cisplatin-induced ototoxicity and prevention. Seminars Hear. 40 (2), 197–204. doi:10.1055/s-0039-1684048
Saghatelyan, T., Tananyan, A., Janoyan, N., Tadevosyan, A., Petrosyan, H., Hovhannisyan, A., et al. (2020). Efficacy and safety of curcumin in combination with paclitaxel in patients with advanced, metastatic breast cancer: a comparative, randomized, double-blind, placebo-controlled clinical trial. Phytomedicine Int. J. phytotherapy Phytopharm. 70, 153218. doi:10.1016/j.phymed.2020.153218
Sahin, K., Sahin Aktura, S., Bahceci, I., Mercantepe, T., Tumkaya, L., Topcu, A., et al. (2025). Is Punica granatum efficient against sepsis? A comparative study of amifostine versus pomegranate. Life Basel, Switz. 15 (1), 78. doi:10.3390/life15010078
Salehi, P., Akinpelu, O. V., Waissbluth, S., Peleva, E., Meehan, B., Rak, J., et al. (2014). Attenuation of cisplatin ototoxicity by otoprotective effects of nanoencapsulated curcumin and dexamethasone in a Guinea pig model. Otological Soc. Am. Neurotol. 35 (7), 1131–1139. doi:10.1097/MAO.0000000000000403
Sandhiutami, N. M. D., Arozal, W., Louisa, M., Rahmat, D., and Wuyung, P. E. (2021). Curcumin nanoparticle enhances the anticancer effect of cisplatin by inhibiting PI3K/AKT and JAK/STAT3 pathway in rat ovarian carcinoma induced by DMBA. Front. Pharmacol. 11, 603235. doi:10.3389/fphar.2020.603235
Santucci, R., Sinibaldi, F., Cozza, P., Polticelli, F., and Fiorucci, L. (2019). Cytochrome c: an extreme multifunctional protein with a key role in cell fate. Int. J. Biol. Macromol. 136, 1237–1246. doi:10.1016/j.ijbiomac.2019.06.180
Sarubbo, F., Moranta, D., Tejada, S., Jiménez, M., and Esteban, S. (2023). Impact of gut microbiota in brain ageing: polyphenols as beneficial modulators. Antioxidants Basel, Switz. 12 (4), 812. doi:10.3390/antiox12040812
Scazzocchio, B., Minghetti, L., and D'Archivio, M. (2020). Interaction between gut microbiota and curcumin: a new key of understanding for the health effects of curcumin. Nutrients 12 (9), 2499. doi:10.3390/nu12092499
Schmitt, N. C., Rubel, E. W., and Nathanson, N. M. (2009). Cisplatin-induced hair cell death requires STAT1 and is attenuated by epigallocatechin gallate. J. Neurosci. official J. Soc. Neurosci. 29 (12), 3843–3851. doi:10.1523/JNEUROSCI.5842-08.2009
Si, X., Yu, Z., Ren, X., Huang, L., and Feng, Y. (2022). Efficacy and safety of standardized Ginkgo biloba L. leaves extract as an adjuvant therapy for sudden sensorineural hearing loss: a systematic review and meta-analysis. J. Ethnopharmacol. 282, 114587. doi:10.1016/j.jep.2021.114587
Simsek, G., Taş, B. M., Muluk, N. B., Azman, M., and Kılıç, R. (2019). Comparison of the protective efficacy between intratympanic dexamethasone and resveratrol treatments against cisplatin-induced ototoxicity: an experimental study. Eur. Fed. Oto-Rhino-Laryngological 276 (12), 3287–3293. doi:10.1007/s00405-019-05635-x
Song, W., Zhang, L., Cui, X., Wang, R., Ma, J., Xu, Y., et al. (2024). Nobiletin alleviates cisplatin-induced ototoxicity via activating autophagy and inhibiting NRF2/GPX4-mediated ferroptosis. Sci. Rep. 14 (1), 7889. doi:10.1038/s41598-024-55614-4
Stankovic, J. S. K., Selakovic, D., Mihailovic, V., and Rosic, G. (2020). Antioxidant supplementation in the treatment of neurotoxicity induced by platinum-based chemotherapeutics—a review. Int. J. Mol. Sci. 21 (20), 7753. doi:10.3390/ijms21207753
Stockwell, B. R., Jiang, X., and Gu, W. (2020). Emerging mechanisms and disease relevance of ferroptosis. Trends cell Biol. 30 (6), 478–490. doi:10.1016/j.tcb.2020.02.009
Sung, C. Y. W., Hayase, N., Yuen, P. S. T., Lee, J., Fernandez, K., Hu, X., et al. (2024). Macrophage depletion protects against cisplatin-induced ototoxicity and nephrotoxicity. Sci. Adv. 10 (30), eadk9878. doi:10.1126/sciadv.adk9878
Tajdini, A., Karimi Yazdi, A., Ravand, H., and Sahebi, L. (2023). The use of herbal medicine in sudden sensorineural hearing loss in diabetic patients. Iran. J. otorhinolaryngology 35 (129), 207–215. doi:10.22038/IJORL.2023.69813.3368
Tan, M., Toplu, Y., Varan, E., Sapmaz, E., Özhan, O., Parlakpınar, H., et al. (2022). The effect of genistein on cisplatin induced ototoxicity and oxidative stress. Braz. J. otorhinolaryngology 88 (1), 105–111. doi:10.1016/j.bjorl.2021.07.001
Tan, W. J. T., and Song, L. (2023). Role of mitochondrial dysfunction and oxidative stress in sensorineural hearing loss. Hear. Res. 434, 108783. doi:10.1016/j.heares.2023.108783
Tan, W. J. T., and Vlajkovic, S. M. (2023). Molecular characteristics of cisplatin-induced ototoxicity and therapeutic interventions. Int. J. Mol. Sci. 24 (22), 16545. doi:10.3390/ijms242216545
Tan, X., Zhou, Y., Agarwal, A., Lim, M., Xu, Y., Zhu, Y., et al. (2020). Systemic application of honokiol prevents cisplatin ototoxicity without compromising its antitumor effect. Am. J. cancer Res. 10 (12), 4416–4434.
Tang, D., Chen, X., Kang, R., and Kroemer, G. (2021). Ferroptosis: molecular mechanisms and health implications. Cell Res. 31 (2), 107–125. doi:10.1038/s41422-020-00441-1
Tang, D., Wang, X., Wu, J., Li, Y., Li, C., Qiao, X., et al. (2024). Cinchonine and cinchonidine alleviate cisplatin-induced ototoxicity by regulating PI3K-AKT signaling. CNS Neurosci. and Ther. 30 (2), e14403. doi:10.1111/cns.14403
Thakur, N. S., Rus, I., Herbert, A., Zallocchi, M., Chakrabarty, B., Joshi, A. D., et al. (2024). Crosslinked-hybrid nanoparticle embedded in thermogel for sustained co-delivery to inner ear. J. nanobiotechnology 22 (1), 482. doi:10.1186/s12951-024-02686-z
Un, H., Ugan, R. A., Gurbuz, M. A., Bayir, Y., Kahramanlar, A., Kaya, G., et al. (2021). Phloretin and phloridzin guard against cisplatin-induced nephrotoxicity in mice through inhibiting oxidative stress and inflammation. Life Sci. 266, 118869. doi:10.1016/j.lfs.2020.118869
Vladu, A. F., Ficai, D., Ene, A. G., and Ficai, A. (2022). Combination therapy using polyphenols: an efficient way to improve antitumoral activity and reduce resistance. Int. J. Mol. Sci. 23 (18), 10244. doi:10.3390/ijms231810244
Wang, L., Li, P., and Feng, K. (2023a). EGCG adjuvant chemotherapy: current status and future perspectives. Eur. J. Med. Chem. 250, 115197. doi:10.1016/j.ejmech.2023.115197
Wang, W., Ma, P., Gao, W., Lu, P., Ding, X., Chen, J., et al. (2022). Nrf2 knockout affected the ferroptosis signaling pathway against cisplatin-induced hair cell-like HEI-OC1 cell death. Oxidative Med. Cell. Longev. 2022, 2210733. doi:10.1155/2022/2210733
Wang, X., Zhou, Y., Wang, D., Wang, Y., Zhou, Z., Ma, X., et al. (2023b). Cisplatin-induced ototoxicity: from signaling network to therapeutic targets. Biomed. and Pharmacother. = Biomedecine and Pharmacother. 157, 114045. doi:10.1016/j.biopha.2022.114045
Wu, W., Li, Y., He, J., Yang, J., and Liu, Y. (2024). Resveratrol shields against cisplatin-induced ototoxicity through epigenetic lncRNA GAS5 modulation of miR-455-5p/PTEN pathway. Int. Immunopharmacol. 138, 112464. doi:10.1016/j.intimp.2024.112464
Xiang, Y., Xu, H., Chen, H., Tang, D., Huang, Z., Zhang, Y., et al. (2023). Tea consumption and attenuation of biological aging: a longitudinal analysis from two cohort studies. Lancet regional health. West. Pac. 42, 100955. doi:10.1016/j.lanwpc.2023.100955
Xie, D., Liu, G., Zhu, G., Wu, W., and Ge, S. (2004). (-)-Epigallocatechin-3-gallate protects cultured spiral ganglion cells from H2O2-induced oxidizing damage. Acta oto-laryngologica 124 (4), 464–470. doi:10.1080/00016480410018278
Xu, B., Li, J., Chen, X., and Kou, M. (2022). Puerarin attenuates cisplatin-induced apoptosis of hair cells through the mitochondrial apoptotic pathway. Biochimica biophysica acta. Mol. cell Res. 1869 (4), 119208. doi:10.1016/j.bbamcr.2021.119208
Yazici, Z. M., Meric, A., Midi, A., Arınc, Y. V., Kahya, V., and Hafız, G. (2012). Reduction of cisplatin ototoxicity in rats by oral administration of pomegranate extract. Eur. Fed. Oto-Rhino-Laryngological 269 (1), 45–52. doi:10.1007/s00405-011-1582-2
Yin, Q., Shi, G., and Zhu, L. (2023). Association between gut microbiota and sensorineural hearing loss: a Mendelian randomization study. Front. Microbiol. 14, 1230125. doi:10.3389/fmicb.2023.1230125
Yu, H. H., Jung, S. Y., Shin, M. K., Park, R., So, H. S., and You, Y. O. (2010). Pueraria thunbergiana inhibits cisplatin-induced damage of HEI-OC1 auditory cells through scavenging free radicals. Phytotherapy Res. PTR 24 (6), 834–839. doi:10.1002/ptr.3027
Yuan, C., Zhang, H., Sun, C., and Zhang, K. (2023). Efficacy and safety of Ginkgo biloba extract as an adjuvant in the treatment of Chinese patients with sudden hearing loss: a meta-analysis. Pharm. Biol. 61 (1), 610–620. doi:10.1080/13880209.2023.2190782
Yumusakhuylu, A. C., Yazici, M., Sari, M., Binnetoglu, A., Kosemihal, E., Akdas, F., et al. (2012). Protective role of resveratrol against cisplatin induced ototoxicity in Guinea pigs. Int. J. Pediatr. otorhinolaryngology 76 (3), 404–408. doi:10.1016/j.ijporl.2011.12.021
Zhang, X., Qian, J., Wei, B., and Zhang, B. (2025). Flavonoids as modulators of Nrf2 signaling pathway in alleviating cisplatin-induced organ toxicity. Yangtze Med. 9, 52–77. doi:10.4236/ym.2025.91006
Zhang, Y., He, Q., Dong, J., Jia, Z., Hao, F., and Shan, C. (2016). Effects of epigallocatechin-3-gallate on proliferation and differentiation of mouse cochlear neural stem cells: involvement of PI3K/Akt signaling pathway. Eur. J. Pharm. Sci. 88, 267–273. doi:10.1016/j.ejps.2016.03.017
Zheng, S., Liu, C., Tang, D., Zheng, Z., Yan, R., Wu, C., et al. (2022). The protective effect of rutin against the cisplatin-induced cochlear damage in vitro. Neurotoxicology 90, 102–111. doi:10.1016/j.neuro.2022.03.005
Keywords: antagonistic effect, polyphenols, cisplatin, signalling pathway, apoptosis
Citation: Han S, Sun J, Li W, Li J, Yu H, Wang S and Chi Y (2025) Studies on the mechanism of ototoxic action of cisplatin and the antagonistic effect of polyphenolic compounds. Front. Pharmacol. 16:1586243. doi: 10.3389/fphar.2025.1586243
Received: 02 March 2025; Accepted: 09 April 2025;
Published: 25 April 2025.
Edited by:
Johnson O. Oladele, Royal Scientific Research Institute, NigeriaReviewed by:
Temidayo Adigun, Chrisland University, NigeriaOluwaseyi Okoro, Ministry of Health and Wellness, Jamaica
Copyright © 2025 Han, Sun, Li, Li, Yu, Wang and Chi. This is an open-access article distributed under the terms of the Creative Commons Attribution License (CC BY). The use, distribution or reproduction in other forums is permitted, provided the original author(s) and the copyright owner(s) are credited and that the original publication in this journal is cited, in accordance with accepted academic practice. No use, distribution or reproduction is permitted which does not comply with these terms.
*Correspondence: Yuhua Chi, Y2hpeXVodWFkaW5nQDE2My5jb20=