- Department of Pharmacology, Qingdao University School of Pharmacy, Qingdao, China
Background: Alcohol use disorder (AUD) and post-traumatic stress disorder (PTSD) exhibit a significant degree of comorbidity. Nevertheless, the specific effects and underlying mechanisms by which alcohol, as a risk factor, contributes to the development of PTSD-ike phenotypes remain poorly understood. Both chronic alcohol consumption and exposure to traumatic stress can lead to synaptic damage in the hippocampus, potentially serving as a neurobiological basis for the exacerbation of PTSD induced by alcohol.
Methods: In this study, an animal model was established by allowing mice to voluntarily consume alcohol for 2 weeks, followed by exposure to a single prolonged stress combined with foot shock (SPS&FS). Subsequently, the mice received an intraperitoneal injection of rolipram (1 mg/kg), and behavioral, biochemical, and morphological analyses were performed.
Results: The findings revealed that individuals with early alcohol exposure exhibited more pronounced deficits in fear extinction during the fear extinction task (FET) and displayed higher levels of anxiety-like behavior in both the open field test (OFT) and the elevated plus maze test (EPM). Activation of cAMP-PKA signaling enhanced the downregulation of brain-derived neurotrophic factor (BDNF) and tyrosine kinase receptor B (TrkB), upregulated the expression of PSD95, synaptophysin, AMPA, and NMDA receptor subtypes, and reversed the impairment of CA1 synaptic function and dendritic structure in the hippocampus.
Conclusion: Activation of the cAMP-PKA pathway facilitated fear extinction in PTSD mice with early alcohol exposure, alleviated anxiety-like behavior, attenuated symptoms of AUD following ethanol relapses. These findings suggest that modulating hippocampal synaptic plasticity by activating the cAMP-PKA pathway may represent a promising therapeutic approach for attenuating alcohol-exacerbated PTSD-like behaviors.
1 Introduction
Post-traumatic stress disorder (PTSD) is a complex psychiatric condition triggered by traumatic events, characterized primarily by persistent intrusive fear memories that are difficult to extinguish (Maercker et al., 2022; Bryant et al., 2023; Yanpallewar et al., 2022; Messent, 2013). This leads to the long-term maintenance of anxiety-related symptoms in PTSD patients (Wen et al., 2022). Currently, enhancing fear extinction is a pivotal focus in PTSD treatment research; however, the precise neurobiological mechanisms underlying this process have yet to be fully elucidated. Long-term alcohol exposure has been identified as a significant risk factor for the development of PTSD (Logrip et al., 2012). Clinical studies conducted on military personnel revealed that the prevalence of PTSD was 9.8%, whereas the prevalence of PTSD among individuals with a history of alcohol consumption was significantly higher, reaching 27.5% (Stein et al., 2017). Clinical evidence demonstrates that alcohol exposure impairs the extinction of fear memories, thereby exacerbating negative emotional states such as anxiety-like behaviors and promoting the development of PTSD (McDevitt-Murphy et al., 2017; Wen et al., 2022). This impairment in emotional regulation further reinforces an individual’s psychological dependence on alcohol, forming a “negative reinforcement” reward mechanism that ultimately leads to excessive drinking behavior and contributes to the development of AUD (Weiss et al., 2021). This creates a vicious cycle between PTSD and alcohol use disorder (AUD), severely compromising patients’ mental health and social functioning (Leeies et al., 2010).
Prolonged exposure (PE) therapy has been developed to mitigate trauma-related fear and anxiety in patients with PTSD through the process of fear extinction (Back et al., 2019; Hunt et al., 2023). The amygdala, hippocampus, and prefrontal cortex are all involved in the regulation of fear memories (Izquierdo et al., 2016). Among these regions, the hippocampus is considered particularly critical for fear memory extinction (Lacagnina et al., 2019). Neuroimaging studies consistently demonstrate significantly reduced hippocampal activation patterns in individuals with severe AUD and those diagnosed with PTSD (Wang Z. et al., 2023). Additionally, a notable reduction in hippocampal volume has been observed, which may be associated with impaired synaptic plasticity in this region (Harnett et al., 2020; Garcia, 2002). Consequently, enhancing synaptic function in the hippocampus may improve the efficacy of extinction learning, which is crucial for alleviating the symptoms of PTSD.
Brain-derived neurotrophic factor (BDNF) is a crucial regulator of synaptic plasticity in the brain (Peters et al., 2010). Activation of BDNF and its receptor tyrosine kinase receptor B (TrkB) can mitigate stress-induced impairments in fear memory extinction, thereby ameliorating anxiety-like behaviors associated with PTSD (Shimoda et al., 2024; Sur and Lee, 2022). The BDNF-mediated effect is closely linked to glutamatergic neurotransmission, particularly through the modulation of AMPA and NMDA receptors, which play essential roles in enhancing synaptic plasticity and regulating learning and memory processes, including hippocampal-dependent fear memory extinction (Park et al., 2021; Radulovic et al., 2019). Furthermore, the levels of PSD95 are strongly correlated with long-term potentiation (LTP). Its upregulation may facilitate synaptic remodeling, thereby potentially promoting fear memory extinction (Suzuki et al., 2018; Li et al., 2018; Ziółkowska et al., 2023). Meanwhile, synaptophysin, a presynaptic vesicular membrane protein, exhibits dynamic expression patterns that are likely to reflect alterations in synaptic transmission efficacy. These changes may contribute to either the suppression or extinguishing of fear memories (Cousin, 2021; Popova et al., 2014).
Cyclic adenosine monophosphate (cAMP), a pivotal second messenger, facilitates LTP by activating protein kinase A (PKA) and is essential for synaptic transmission, neuronal excitability, neuroplasticity, and neuroprotection (Shahoha et al., 2022; Heckman et al., 2018). These findings suggest that pharmacological modulation of hippocampal synaptic function via PKA signaling pathway activation may represent a promising therapeutic strategy for alcohol-induced PTSD-like behaviors.
The present study aims to investigate the neural mechanisms by which alcohol exposure exacerbates PTSD-like behaviors and to further validate whether activating the cAMP-PKA signaling pathway can restore synaptic plasticity impairment, thereby ameliorating PTSD and AUD-associated behaviors. By elucidating this mechanism, this study will provide a theoretical foundation for developing novel intervention strategies for comorbid PTSD and AUD.
2 Materials and methods
2.1 Animals
The experimental protocol employed adult male C57BL/6J mice (purchased from Beijing Weitong Lihua Animal Laboratory Co., Ltd.) aged between 6 and 8 weeks, with body weights ranging from 20 to 25 g. Environmental parameters in the animal facility were strictly controlled, with ambient temperature maintained at 24°C ± 2°C and relative humidity 40%–50%. A standardized 12 h light/dark cycle was implemented. Individual housing was provided for each mouse, ensuring ad libitum access to food and water supply. Animal procedures were approved by the Committee of Animal Experimental Ethics of Qingdao University, China (approval number: QDU-AEC-2025099).
2.2 SPS&FS procedure
The experimental protocol for inducing PTSD-like behavior in murine models was conducted using a standardized combination of prolonged stress and plantar electrical stimulation, as previously described (Xi et al., 2023; Stein et al., 2017; Xi et al., 2021; 2023; Lou et al., 2022; Wang et al., 2022). The procedure was executed in the following sequence: Initially, mice were subjected to confinement in a restraint apparatus for 2 h, after which they were immediately transferred to an acrylic chamber (50 cm × 30 cm) containing water maintained at a temperature of 22°C–24°C for a 20 min forced swim session. Subsequently, the mice were exposed to ether vapor until the onset of unconsciousness (duration <1 min), and this procedure was repeated twice consecutively. After an additional 15 min rest interval, the subjects were administered a single electric shock (2 mA, 2 s) within a designated apparatus and returned to their respective home cages.
2.3 Two-bottle choice test (2-BC)
A continuous two-bottle choice paradigm was employed to evaluate murine ethanol consumption patterns over 24 h (Wei et al., 2024). The mice were individually housed in cages equipped with two plastic bottles, offering a choice between an ethanol solution and water. The rodents were granted unrestricted access to both beverages. The alcohol concentration systematically increased from 10% to 15% and subsequently stabilized at 20%. To mitigate potential location-based preferences, the water bottle positions were alternated every 24 h. Both ethanol and water were replenished daily at fixed times to ensure consistency in fluid availability and concentration.
2.4 Conditioned place preference (CPP)
The ethanol-induced conditioned place preference (CPP) experiment was conducted using a three-compartment environmental conditioning apparatus, which primarily comprised two distinct chambers (Lei et al., 2024; Lu et al., 2017; Pati et al., 2019; Sharifi et al., 2022; Potula et al., 2023). One of these is black with a relatively smooth floor and is designated as the unconditioned side. The opposing chamber featured a black-and-white striped pattern with a circular texture on the floor and was designated as the conditioned side. These two compartments were delineated using a smaller intermediary box. Throughout the training phase, an isolation door was inserted into the middle compartment to partition the two chambers, thereby creating an entirely separate environment. The experimental protocol for the CPP was segmented into three stages: baseline preference assessment (day 1), conditioning phase (days 2–9), and post-conditioning test (day 10).
During the pre-conditioning phase (day 1), the divider was removed to permit unrestricted access to both chambers, allowing mice to freely explore the apparatus for 20 min. Behavioral tracking was performed using a camera recording system to quantify the time spent in each chamber, enabling the calculation of baseline preference scores for both conditioned and unconditioned sides. Animals exhibiting a preference of >800 s for either compartment were excluded from subsequent statistical analysis, to ensure balanced baseline preferences (Wang J. et al., 2023).
The conditioning phase (days 2–9) consisted of eight consecutive days of training. On alternate days (days 2, 4, 6, and 8), the mice received intraperitoneal injections of saline and were confined to the unconditioned chamber for 30 min. Conversely, on the intervening days (days 3, 5, 7, and 9), the mice were administered ethanol (2 g/kg, i.p.) and restricted to the conditioned chamber for an equivalent duration. This alternating schedule resulted in four pairings for each treatment condition. The control animals received saline injections during all the conditioning sessions.
On the post-conditioning test day (day 10), the isolation doors on both sides were removed, and the mice were allowed unrestricted access to both chambers for 20 min. The time spent in each chamber was recorded to assess the place’s preference.
To prevent interference from olfactory cues, the apparatus was thoroughly cleaned with a 75% ethanol solution between trials, a blower device was used to accelerate the dissipation of alcohol odor. Behavioral testing began after 30 min to ensure alcohol odor dissipation and prevent experimental interference.
2.5 Experimental procedures and drug administration
Following the procurement of experimental animals, all mice underwent a 7-day acclimatization period in the housing facility under standard conditions. The mice were equally divided into five groups (n = 9 per group): Control, Control + EtOH, SPS & FS, SPS & FS + EtOH, and SPS & FS + EtOH + ROL. Control, SPS & FS, SPS & FS + EtOH, and SPS & FS + EtOH + ROL for the assessment of behavioral, Western blot, and Golgi staining; Specifically, six mice were randomly selected from each group for Western blot analysis. The remaining three mice in each group were preserved with intact whole brains for Golgi staining. To ensure sufficient sample size and reliable Golgi staining results, an additional two mice per group were included under identical experimental conditions specifically dedicated to Golgi staining analysis (n = 5 per group). Control, Control + EtOH, SPS & FS + EtOH, SPS & FS + EtOH + ROL for the detection of 2-BC and CPP.
For pharmacological intervention, mice in the treatment group received daily intraperitoneal injections of rolipram (1 mg/kg; Cat: HY-16900; MCE), whereas control animals were administered an equivalent volume of vehicle solution following the same injection protocol.
2.6 Behavioral tests
In our animal behavioral experiments, we strictly adhered to a double-blind protocol to minimize potential experimenter bias and ensure objective data collection.
2.6.1 Open field test (OFT)
The OFT was conducted following established protocols (Zhao et al., 2024). The open field test was conducted using a white cube-shaped apparatus (50 cm × 50 cm × 50 cm). Each mouse was placed in a corner at the start of the test and recorded for 10 min using a camera located above the box. To eliminate potential olfactory cues between trials, the apparatus was thoroughly cleaned with a 75% ethanol solution. Behavioral data were analyzed using the VisuTrack automated tracking system (Xin Ruan Information Technology Co., Ltd., Shanghai, China).
2.6.2 Elevated plus maze test (EPM)
The EPM test was performed as previously described. The EPM consisted of a cross-shaped platform with two open arms and two closed arms (25 cm × 8 cm × 12 cm), elevated 50 cm above the ground. At the beginning of the experiment, the mice were placed in the central area facing the closed arm and allowed to explore freely for 5 min, and the activity was recorded using a camera located above the box. The time and number of entries in the open and closed arms were recorded.
2.6.3 Fear extinction task (FET)
The FET test was performed as previously described (Gao et al., 2023). The fear extinction experiment was conducted using a combination of a neutral tone (CS) and a plantar shock (US), wherein the CS condition (1 kHz, 90 dB) remained constant, whereas the US condition (1 mA, 2 s) was administered via a metal mesh at the base of the fear chamber. The experiment comprised four stages. The initial stage involved an adaptation period on day one, during which the mice were placed in the apparatus for 5 min without any stimulation to eliminate the influence of unfamiliar environments. On day two, the mice were again placed in the fear chamber for 5 min with simultaneous application of both CS and US. US was administered three times, with intervals between each repetition lasting approximately 73–74 s. On day three, mice freely explored the fear chamber for 15 min under only CS conditions, and their freezing time was recorded at 3-min intervals. Finally, on day four, the freezing time of the mice in the fear chamber under CS conditions was recorded for 5 min to evaluate the regression of their fear memory.
2.7 Golgi staining and sholl analysis
Golgi staining was conducted utilizing the Golgi staining kit (Catalog No. G1152, Servicebio Technology, Wuhan, China). The mice were placed in a gas anesthesia chamber containing isoflurane and anesthetized until the absence of pain reflexes, followed by decapitation. The excised brain was rinsed with double-distilled water, subsequently immersed in a pre-prepared staining solution (comprising equal proportions of liquid A and liquid B), stored in the dark at room temperature for 14 days. Thereafter, it was transferred to solution C for 7 days before being sectioned into 100-micron coronal slices using an oscillating microslicer. The sections were mounted on gelatin slides and incubated in a mixture of D and E for 10 min. Following cleansing with double-distilled water, dehydration was performed using a series of ethanol concentrations, followed by three xylene washes prior to cover glass application. Subsequently, the Fiji software was used to trace and analyze the dendrites of pyramidal neurons in the CA1 region of the hippocampus. Sholl analysis in Fiji involved plotting concentric circles at equidistant intervals around the neuronal soma as a reference point to quantify the number of dendrites intersecting each circle. Add total dendrite length and branch count were also recorded.
2.8 Western blot
In the experimental protocols, after the mice were anesthetized, the hippocampal tissue was dissected under ice bath conditions, and then the tissue was typically subjected to lysis in RIPA buffer supplemented with protease and phosphatase inhibitors (manufactured by Shenzhen Solaibao Technology Co., Ltd.), and the protein concentration was determined using the BCA protein content determination kit (product number: ZJ102, produced by Nanjing Epizan Biotechnology Co., Ltd.). Subsequently, the proteins were separated via SDS-PAGE and transferred to a PVDF membrane. The membrane was then incubated in a 5% non-denatured milk powder solution at room temperature, followed by incubation with the primary antibody at 4°C overnight. The membrane was then washed four times with TBST for 5 min each. Thereafter, the membrane was incubated with the secondary antibody (Goat Anti-Rabbit/Mouse IgG, product number: ZB-2305, dilution ratio: 1:5,000, manufactured by Zhongshan Jinqiao Biotechnology Co., LTD.) at room temperature for 2 h and subjected to further washing with TBST buffer. Finally, protein visualization was conducted using the AI-800 imaging system (General Electric Company, United States), and the optical density values of each band were quantitatively analyzed using ImageJ software.
Primary antibody with the following proteins: anti-p-PKA (Ser133, 5661, 1:1,000, Cell Signaling Technology); anti-PKA (ab32514, 1:1,000, Abcam); anti-p-CREB (Ser133, ab32096, 1:1,000, Abcam); manti-CREB (ab32515, 1:1,000, Abcam); anti-BDNF (ab108319, 1:1,000, Abcam); anti-p-TrkB (ab229908, 1:1,000, Abcam); anti-TrkB (ab187041, 1:2,000, Abcam); anti-PSD95 (20665-1-AP, 1:1,000, Proteintech); anti-Synaptophysin (36406S, 1:1,000, Cell Signaling Technology); anti-NR2A (ab124913, 1:1,000, Abcam); anti-NR2B (ab65783, 1; 1,000, Abcam); anti-GluA1 (13185S, 1:1,000, Cell Signaling Technology); anti-GluA2 (13607S, 1:1,000, Cell Signaling Technology); anti-β-tubulin (AC021, 1:5,000, ABclonal); anti-GAPDH (60004-1-Ig, 1:5,000, Proteintech).
2.9 Statistical analysis
The experimental data were analyzed using GraphPad Prism 9.0 software, and statistical plots were expressed as mean ± SEM. For normally distributed data, independent sample t-test were used for two groups, and one-way or two-way ANOVA for four groups. Only significant comparisons are labeled in the figures. Statistical significance was set at P < 0.05.
3 Results
3.1 Activation of the cAMP-PKA ameliorated the deficits in fear extinction and anxiety-like behaviors in PTSD mice with alcohol exposure
To investigate the impact of early alcohol consumption on PTSD-like behavior and the effects of cAMP-PKA activation on fear extinction, we employed rolipram, a selective PDE4 inhibitor that upregulates cAMP-PKA pathway activity, developed the following experimental protocol (Figure 1A). The results showed that SPS&FS mice with prior alcohol exposure exhibited significantly impaired fear extinction, characterized by prolonged freezing time and reduced extinction coefficient [Figure 1B: F (3, 160) = 18.32, P < 0.0001; Figure 1C: F (3, 32) = 12.29, P < 0.0001; Figure 1D: F (3, 32) = 11.39, P < 0.0001]. Rolipram administration effectively enhanced fear memory extinction by reducing freezing time and increasing the extinction coefficient (Figures 1B–D). SPS&FS mice with prior alcohol exposure also exhibited more severe anxiety-like behavior, as evidenced by a significant decrease in entries [Figure 1F: F (3, 32) = 8.919, P = 0.0002; Figure 1G: F (3, 32) = 13.54, P < 0.0001] and time spent in the central area during the OFT and in the open arms during the EPM test [Figure 1I: F (3, 32) = 11.40, P < 0.0001; Figure 1J: F (3, 32) = 8.555, P = 0.0003]. Rolipram effectively alleviated anxiety-related phenotypes (Figures 1E–J). These findings highlight that alcohol exposure exacerbates fear extinction deficits and anxiety-like behavior in PTSD mice, with the significant ameliorating effects of rolipram on these behaviors.
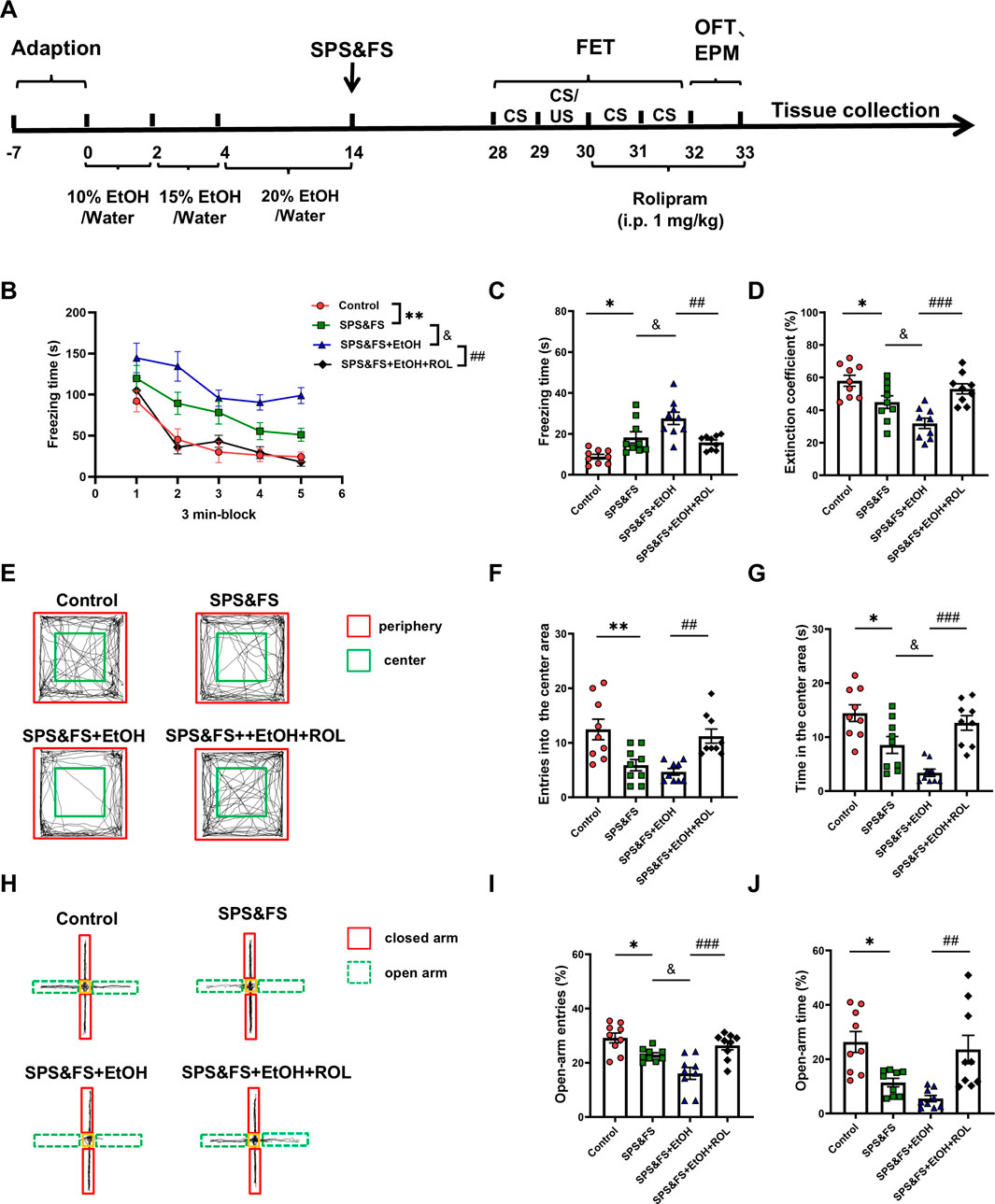
Figure 1. Activation of the cAMP-PKA ameliorated the deficits in fear extinction and anxiety-like behaviors in PTSD mice with alcohol exposure. (A) Flow chart of the experiment. (B) Freezing time during the re-exposure phase of FET. (C) Freezing time in the 24-h post-fear test. (D) The extinction coefficient measured in the FET test. (E) Representative tracking plot from the OFT. (F, G) Number of entries and time spent in the center area. (H) Representative track plot of the EPM test. (I, J) Number of entries and time spent in the open arms during the EPM test (n = 9 mice per group). All data are presented as mean ± SEM. Data were analyzed using contrast analyses following one-way or two-way ANOVA. *p < 0.05, **p < 0.01 vs. control; &p < 0.05 vs. SPS&FS; ##p < 0.01, ###p < 0.001 vs. SPS&FS + EtOH.
3.2 Activation of cAMP-PKA ameliorates the impairment of hippocampal BDNF-TrkB signaling in PTSD mice with alcohol exposure
In the central nervous system, PKA activation promotes CREB phosphorylation, influencing the transcription of target genes, including BDNF. This process contributes to the extinction of fear memory. To determine the role of the PKA signaling pathway in alcohol-exacerbated PTSD-like behavior, we detected changes in PKA, CREB, BDNF, and TrkB protein levels using Western blotting. The results showed that SPS&FS combined with alcohol consumption decreased the phosphorylation levels of PKA and CREB. Notably, rolipram treatment significantly increased the phosphorylation levels of PKA and CREB [Figure 2A: p-PKA, F (3, 20) = 18.34, P < 0.0001; PKA, F (3, 20) = 0.4219, P = 0.7393; Figure 2B: p-CREB, F (3, 20) = 16.05, P < 0.0001; CREB, F (3, 20) = 2.593, P = 0.0811]. Additionally, alcohol exposure in SPS&FS mice led to a significant decrease in BDNF and TrkB phosphorylation levels. Administration of rolipram effectively restored these molecular deficits [Figure 2C: BDNF, F (3, 20) = 14.45, P < 0.0001; Figure 2D: p-TrkB, F (3, 20) = 19.57, P < 0.0001; TrkB, F (3, 20) = 0.2584, P = 0.8545]. These findings suggest that rolipram administration facilitates fear extinction and attenuates anxiety-like behavior by potentiating the PKA/CREB/BDNF/TrkB signaling pathway.
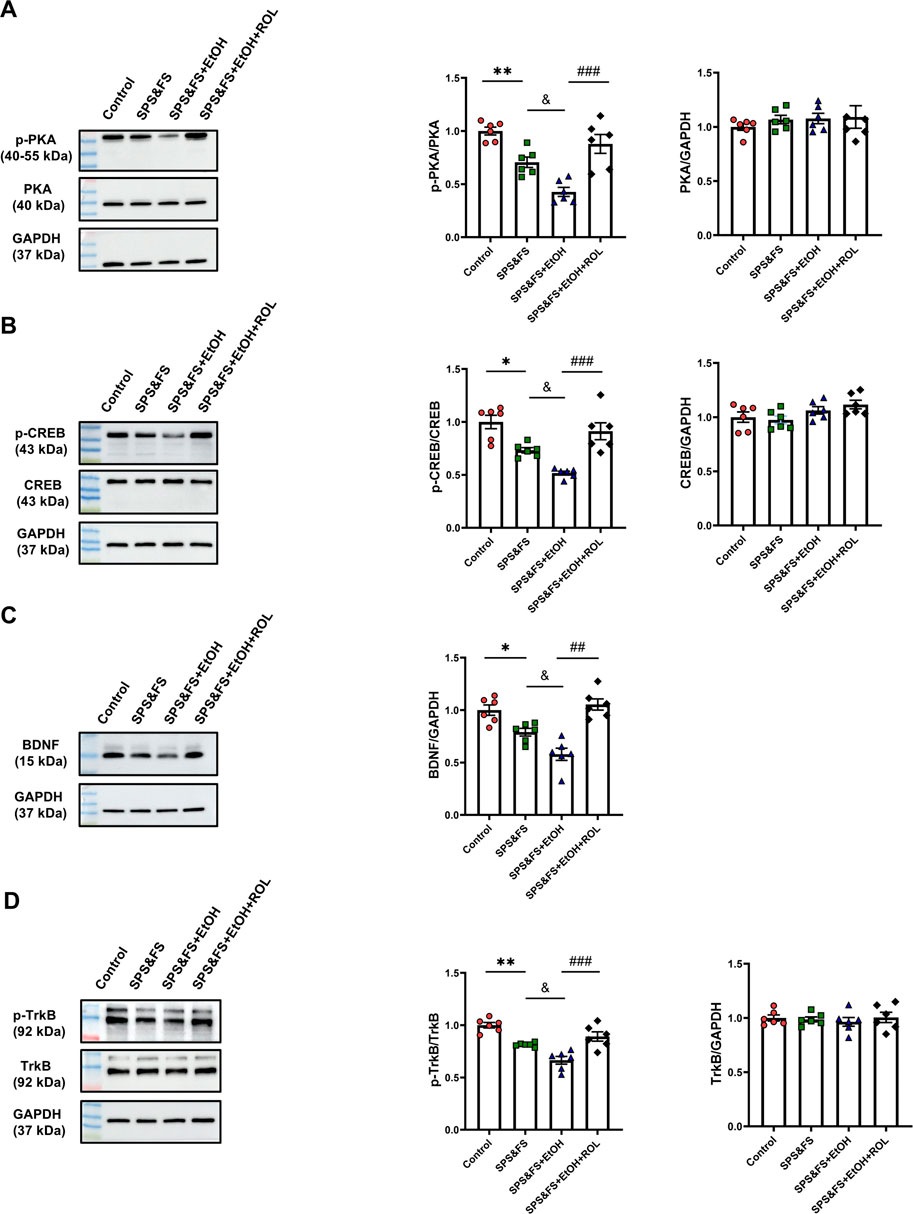
Figure 2. Activation of cAMP-PKA ameliorates the impairment of hippocampal BDNF-TrkB signaling in PTSD mice with alcohol exposure. (A) Protein expression of p-PKA, and PKA were detected by Western blot analysis. (B) Protein expression of p-CREB, and CREB was detected by Western blot analysis. (C) Protein expression of BDNF was detected by Western blot analysis. (D) Protein expression of p-TrkB, and PKA were detected by Western blot analysis (n = 6 mice per group). All data are presented as mean ± SEM. Data were analyzed using contrast analyses following one way ANOVA. *p < 0.05, **p < 0.01 vs. control; &p < 0.05 vs. SPS&FS; ##p < 0.01, ###p < 0.001 vs. SPS&FS + EtOH.
3.3 Activation of the cAMP-PKA mitigated the reduction in synaptic plasticity proteins in the hippocampus of PTSD mice with alcohol exposure
Considering the key regulatory role of BDNF in synaptic plasticity, we further investigated the expression of other key synaptic structure-related proteins to provide a more comprehensive elucidation of the underlying molecular mechanisms involved. The results showed that the expression of PSD95 and synaptophysin was significantly decreased in SPS&FS mice after exposure to alcohol. Rolipram treatment significantly attenuated these impairments [Figure 3A: PSD95, F (3, 20) = 17.19, P < 0.0001; synaptophysin, F (3, 20) = 11.49, P = 0.0001]. In addition, we studied the expression of AMPA and NMDA receptor subunits. The data showed that NR2A expression was downregulated, whereas NR2B expression was upregulated in SPS&FS mice with early alcohol consumption. These phenotypic changes were reversed by the administration of rolipram [Figure 3B: NR2A, F (3, 20) = 17.50, P < 0.0001; NR2B, F (3, 20) = 9.738, P = 0.0004]. GluA1 and GluA2 are the two main subunits of the AMPA receptor. In alcohol-exposed SPS&FS mice, the protein levels of GluA1 were significantly reduced, whereas those of GluA2 remained unchanged. Rolipram treatment effectively restored GluA1 expression to normal levels [Figure 3C: GluA1, F (3, 20) = 15.28, P < 0.0001; GluA2, F (3, 20) = 0.7103, P = 0.5572]. These findings provide further evidence that modulating synaptic plasticity via PKA contributes to the improvement of PTSD-like behaviors.
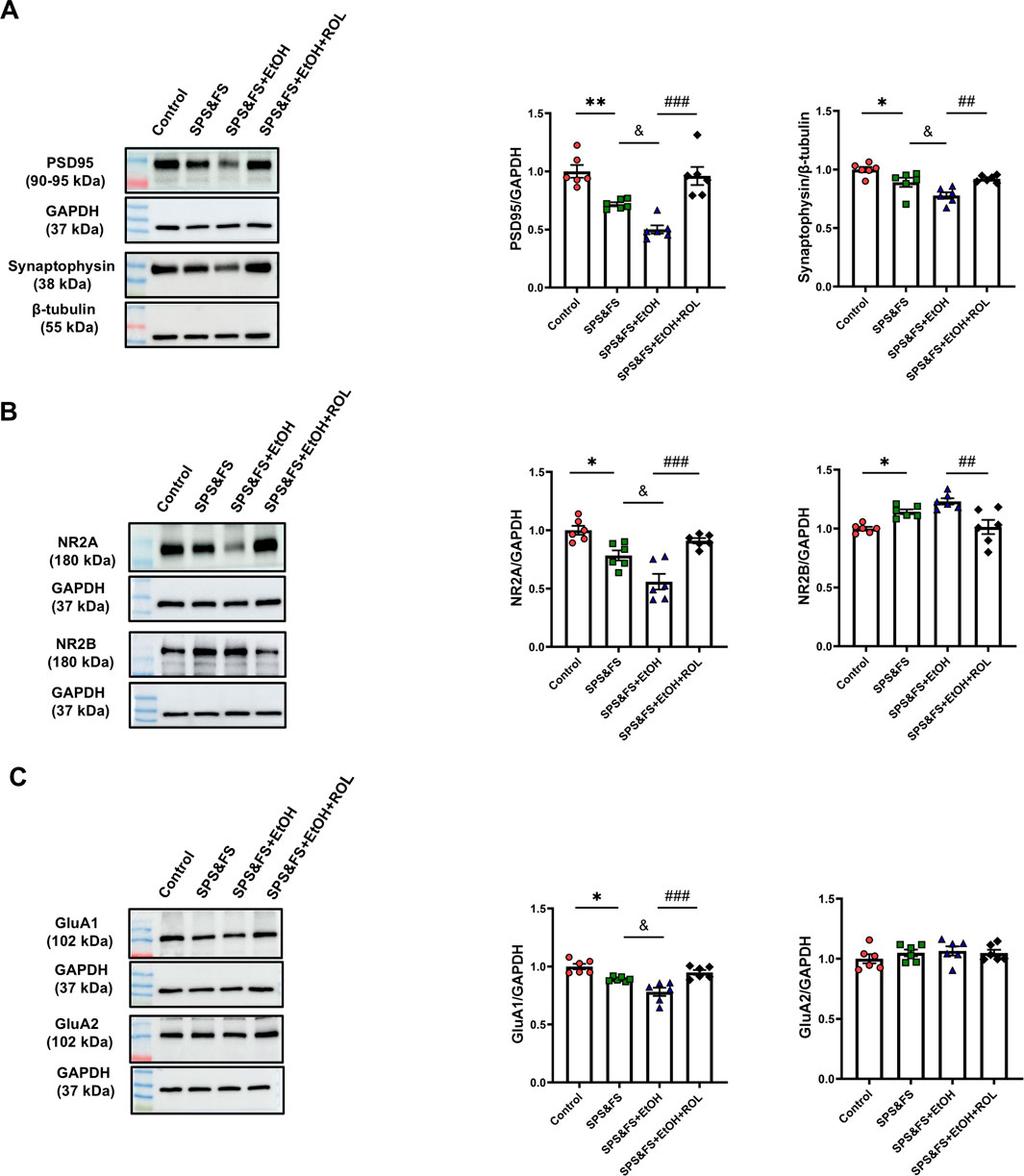
Figure 3. Activation of the cAMP-PKA mitigated the reduction in synaptic plasticity proteins in the hippocampus of PTSD mice with alcohol exposure. (A) Protein expression of PSD95, and Synaptophysin was detected by Western blot analysis. (B) Protein expression of NR2A, and NR2B were detected by Western blot analysis. (C) Protein expression of GluA1, and GluA2 was detected by Western blot analysis (n = 6 mice per group). All data are presented as mean ± SEM. Data were analyzed using contrast analyses following one-way or two-way ANOVA. *p < 0.05, **p < 0.01 vs. control; &p < 0.05 vs. SPS&FS; ##p < 0.01, ###p < 0.001 vs. SPS&FS + EtOH.
3.4 Activation of the cAMP-PKA ameliorated the impairment of dendritic structure in hippocampal CA1 pyramidal cells in PTSD mice with alcohol exposure
We detected changes in synaptic proteins at the molecular level and subsequently examined the morphological structure of the synapse. The CA1 subregion of the hippocampus, which is critical for long-term potentiation and structural plasticity, was stained using the Golgi technique to visualize the complexity of dendrites. Representative images and reconstructed dendritic structures are shown in Figures 4A, B. Concentric circles spaced at 20 μm intervals were drawn, and the number of intersections between the concentric circles and dendrites was calculated as a parameter to evaluate the complexity of the dendritic tree. Sholl analysis revealed that the number of intersections between the dendrites and concentric circles varied at different radial distances from the cell body [Figure 4C: F (3, 684) = 16.35, P < 0.0001]. Notably, the number of dendritic intersections was significantly reduced in alcohol-exposed SPS&FS mice. This reduction was markedly attenuated by rolipram treatment, leading to the restoration of levels comparable to those in the control group. Rolipram also effectively prevented the alcohol-induced reduction in total dendrite length and overall dendritic numbers [Figure 4D; total dendrite length, F (3, 76) = 9.149, P < 0.0001; dendritic numbers, F (3, 76) = 12.81, P < 0.0001]. These results suggest that rolipram treatment ameliorated the impairment of dendritic structures in the hippocampal CA1 region of PTSD mice with alcohol exposure.
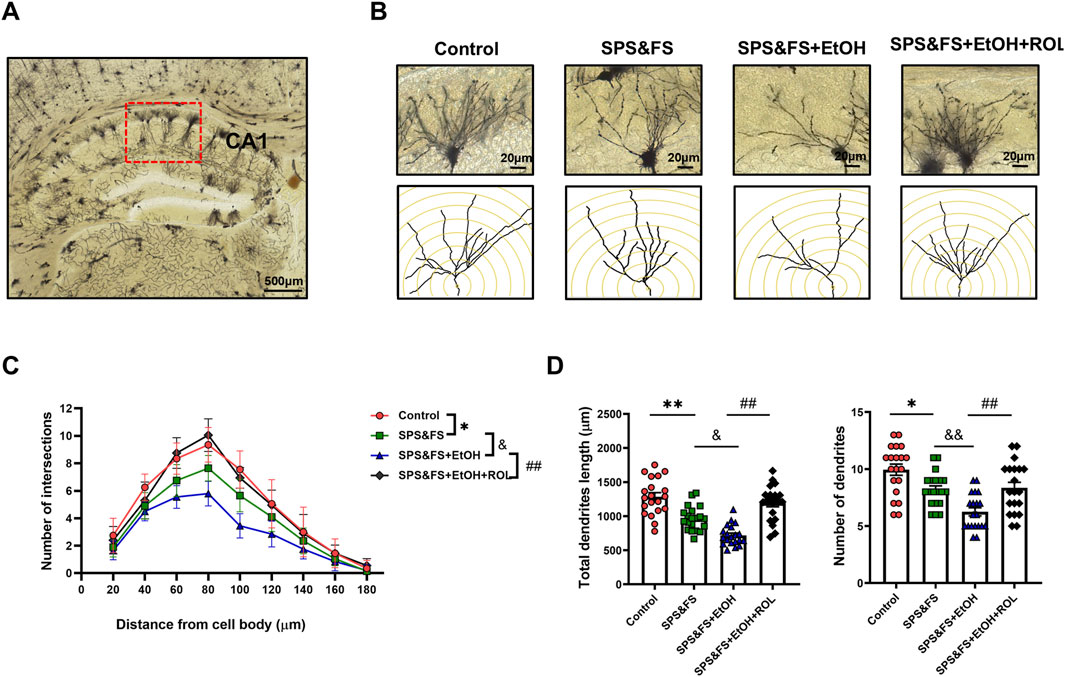
Figure 4. Activation of the cAMP-PKA ameliorated the impairment of dendritic structure in hippocampal CA1 pyramidal cells in PTSD mice with alcohol re-exposure. (A) Representative images of the hippocampal CA1 region labeled using Golgi staining. Scale bar = 500 μm. (B) Representative images of neuronal dendrites. Scale bar = 20 μm. (C) Sholl analysis of the hippocampal CA1 neurons revealed the alterations of basal dendritic intersections at distinct distances from soma (n = 20 cells/five mice per group). (D) Total dendritic length and dendritic numbers of neurons (n = 9 mice per group). All data are presented as mean ± SEM. Data were analyzed using contrast analyses following one-way ANOVA. *p < 0.05, **p < 0.01 vs. control; &p < 0.05, &&p < 0.01 vs. SPS&FS; ##p < 0.01, vs. SPS&FS + EtOH.
3.5 Activation of the cAMP-PKA attenuated alcohol consumption and preference in PTSD mice with alcohol exposure
To evaluate the effects of SPS&FS traumatic stress on AUD-related behaviors and the regulatory role of the PKA signaling pathway. We conducted 2-BC and CPP experiments at later stages (Figure 5A). Prior to the application of SPS&FS stimulation, all mice exhibited comparable levels of alcohol consumption and preference, with no significant distinctions observed between the experimental groups [Supplementary Figure S1A: alcohol intake, F (2, 120) = 1.086, P = 0.3409; alcohol preference, F (2, 120) = 2.321, P = 0.1025; total liquid, F (2, 12) = 0.9157, P = 0.4265]. The comparison between SPS&FS mice and non-stressed mice showed that the comorbidities exacerbated excessive drinking behavior, characterized by increased alcohol intake and preference, while total fluid consumption remained unaffected [Figure 5B: F (2, 12) = 2.874, P = 0.0955]. However, treatment with rolipram significantly attenuated this stress-induced escalation in alcohol consumption [Figure 5B: alcohol intake, F (2, 130) = 22.41, P < 0.0001; alcohol preference, F (2, 120) = 23.10, P < 0.0001]. To facilitate a more precise observation of alcohol consumption, we used a paired t-test to compare alcohol intake and preference in the same group of mice before and after exposure to SPS&FS stress, as well as before and after rolipram administration. The analysis revealed a substantial and statistically significant increase in alcohol consumption in mice following exposure to SPS&FS stress [Figure 5C: Alcohol intake, t (8) = 2.851, P = 0.0214; Alcohol preference, t (8) = 3.794, P = 0.0053]. Notably, the administration of rolipram effectively prevented any further escalation in alcohol intake or preference in stress-exposed mice [Figure 5D: alcohol intake, t (8) = 0.8249, P = 0.4333; alcohol preference, t (8) = 0.2298, P = 0.8240].
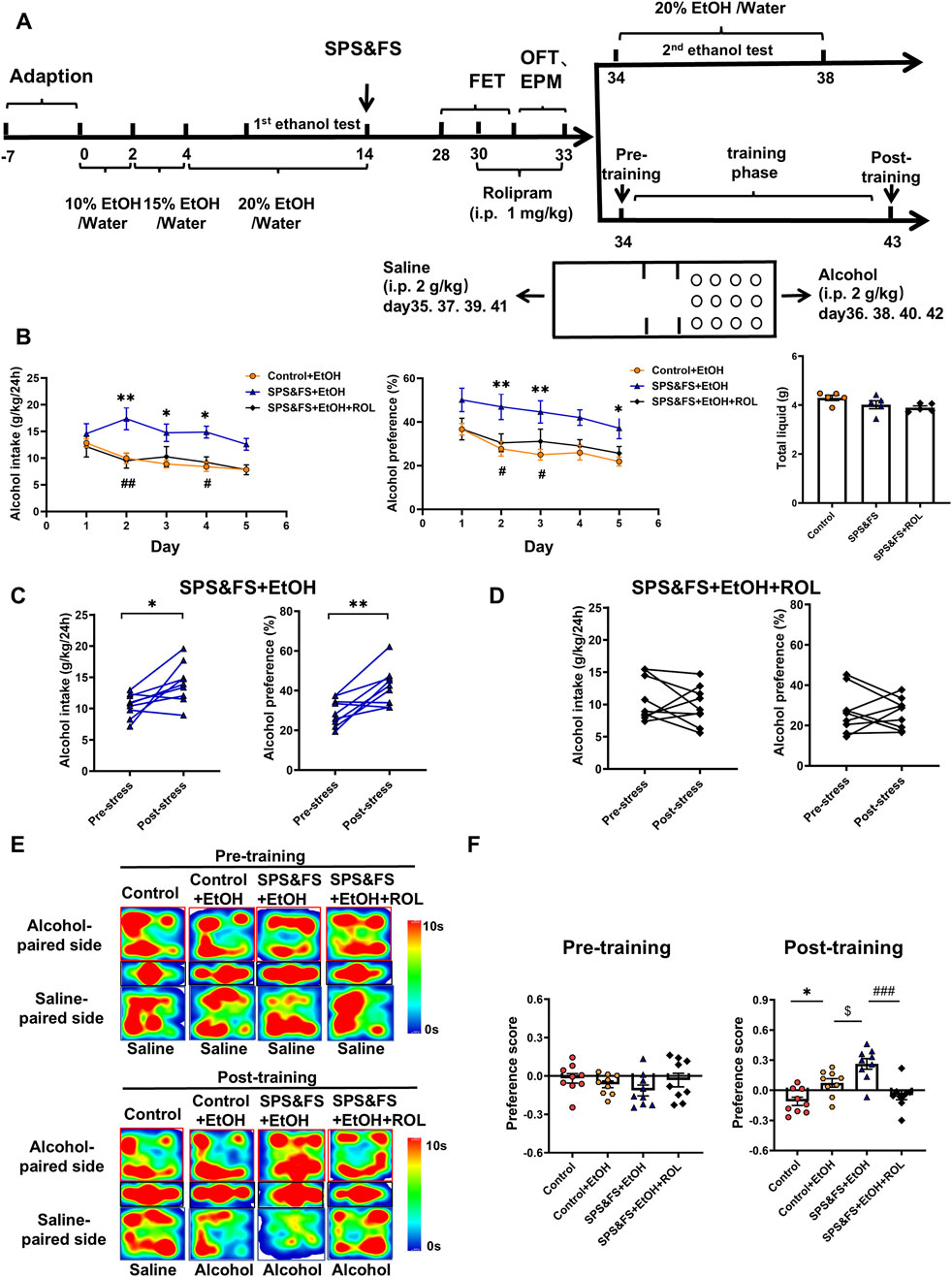
Figure 5. Activation of the cAMP-PKA attenuated alcohol consumption and preference in PTSD mice with alcohol exposure. (A) Flow chart of the experiment. (B) Alcohol intake, alcohol preference and total liquid were measured during rolipram treatment. (C) Paired t-tests were used to detect the changes in alcohol intake and preference in the same mice before and after exposure to stress. (D) Paired t-tests were conducted to compare alcohol intake and preference in the same mice before and after exposure to stress, following rolipram treatment. (E) Heatmap representation of the mouse movement trajectory during the CPP test. (F) Preference scores in CPP test were detected. All data are presented as mean ± SEM. Data were analyzed using contrast analyses following one-way or two-way ANOVA. *p < 0.05, **p < 0.01 vs. control; $p < 0.05 vs. control + EtOH; #p < 0.05, ##p < 0.01, ###p < 0.001 vs. SPS&FS + EtOH.
The alcohol-induced CPP assay was used to assess alcohol preference in SPS&FS mice both before and after rolipram administration. After training, environmental cues elicited alcohol craving and seeking. During the pre-training process, the mice in each group did not show a clear preference for either side of the box. After training, stressed mice showed a marked preference for the alcohol-paired side, spending significantly more time there and achieving higher preference scores. After PKA activation by rolipram, the stressed mice showed a significant reduction in preference and time spent on the alcohol-paired side, indicating a decrease in alcohol preference after PTSD stress [Figures 5E, F: Pre-training, F (3, 32) = 1.065, P = 0.3778; Post-training, F (3, 32) = 13.14, P < 0.0001].
4 Discussion
The interaction between alcohol and stress is complex, with stress serving as the primary driver of relapse following abstinence (Breese et al., 2011; Fein, 2013). Alcohol significantly affects various learning and memory processes, including fear conditioning (Alijan-Pour et al., 2012). Furthermore, chronic substance use exacerbates arousal levels, anxiety, and sensitivity of neurobiological stress systems, thereby elevating the risk of PTSD development (Sinha, 2024). Consequently, alcohol use may substantially increase individual susceptibility to PTSD. In this study, we found that individuals with early alcohol exposure showed more pronounced fear extinction deficits and higher anxiety levels, and that the administration of rolipram to activate the cAMP-PKA signaling pathway effectively ameliorated these impairments. Our study confirmed that activating the PKA pathway to regulate synaptic plasticity can alleviate fear extinction deficits and reduce alcohol preference following traumatic stress. Rolipram treatment reversed the downregulation of BDNF and TrkB, increased the expression of PSD95 and synaptophysin, improved the abnormal expression of AMPA and NMDA receptor subtypes, and rescued damage to synaptic structures (Figure 6). This study provides crucial insights into the modulation of synaptic plasticity in the hippocampus through cAMP-PKA pathway activation, providing potential therapeutic strategies for PTSD/AUD treatment.
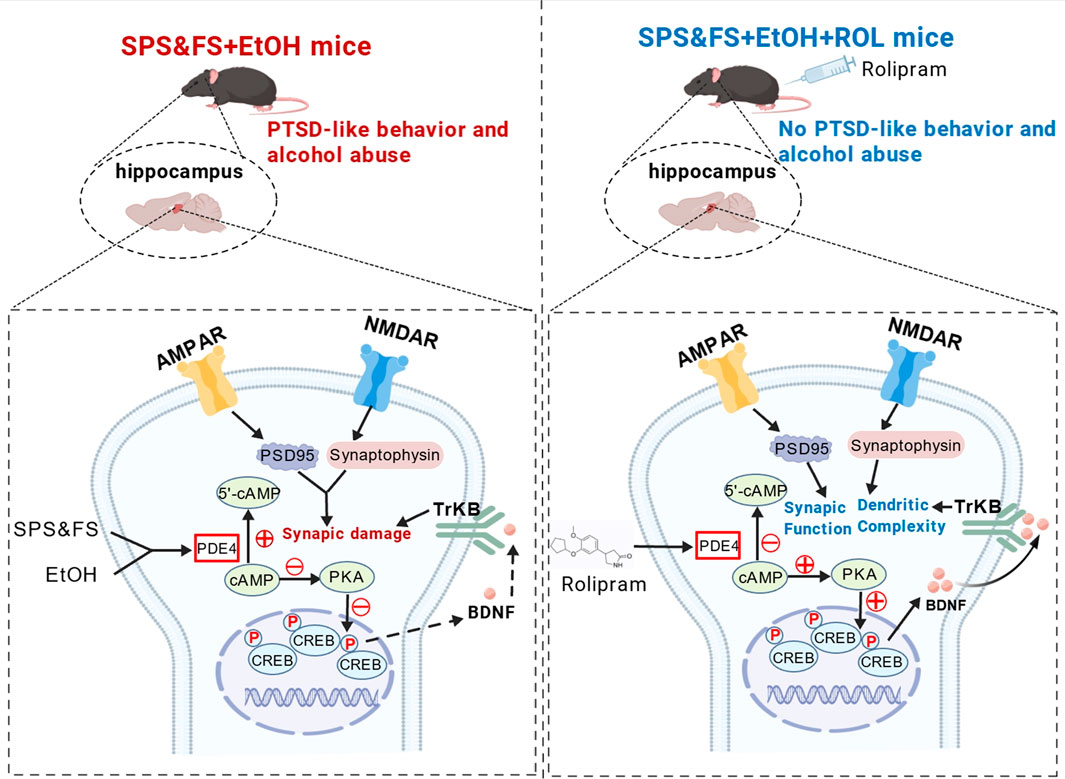
Figure 6. Mechanistic illustration of rolipram-induced cAMP-PKA activation in the therapeutic of PTSD-like behaviors exacerbated by alcohol exposure. Alcohol exposure together with later SPS&FS trauma stimulation promoted cAMP hydrolysis, downregulated the activity of PKA-CREB-BDNF-TrkB signaling, resulting in aberrant expression of the key synaptic protein AMPA with NMDA receptor subunits, PSD95 and Synaptophysin, and this synaptic impairment exacerbated fear and anxiety-like behavior in PTSD mice to induce AUD-related behaviors. Following Rolipram administration, the PKA-CREB-BDNF-TrkB signaling pathway was activated, leading to the upregulation and downregulation of synaptic-associated proteins. This activation enhanced synaptic structure and function, as well as dendritic spine complexity, thereby ameliorating fear extinction deficits and anxiety-like behaviors in PTSD mice with alcohol exposure. Additionally, it reduced ethanol intake and alcohol preference in mice during subsequent alcohol re-.exposure.
Extensive clinical research has consistently identified alcohol consumption as a significant barrier to recovery in patients with various psychiatric disorders. Early exposure to alcohol not only increases susceptibility to PTSD, but also exacerbates its severity (Possemato et al., 2022; McFarlane et al., 2009). While existing studies have predominantly focused on the influence of stress on subsequent alcohol consumption patterns, emerging evidence suggests that early alcohol exposure exacerbates the traumatic phenotype in mouse models of PTSD (Anderson et al., 2016; Gonzalez et al., 2021; Barkell et al., 2022). However, the precise neural mechanisms underlying this phenomenon remain poorly understood. To address this critical gap, we established a novel model by sequentially exposing mice to ethanol followed by traumatic stimulation. Comprehensive behavioral analyses revealed that PTSD mice with prior alcohol exposure exhibited significant deficits in fear memory extinction and pronounced anxiety-like behaviors, thereby validating the detrimental effects of ethanol exposure on stress response.
Traumatic memory represents a central component in the pathophysiology of PTSD, with extinction serving as a fundamental mechanism for attenuating trauma-associated memories (Kida, 2019). Emerging evidence suggests that enhancing the fear extinction process through pharmacological assistance can significantly improve the effect of PE therapy and effectively shorten the duration of treatment (Maren and Holmes, 2016). This underscores the need for further investigation into the regulatory mechanisms underlying fear resolution and the identification of more potent therapeutic targets. Recent experimental studies emphasize the importance of enhanced hippocampal neurogenesis in facilitating the extinction of fear memories. Building on this finding, we investigated the potential implications of hippocampal synaptic plasticity in the context of alcohol exposure, which exacerbates PTSD-like behaviors.
The cAMP-PKA pathway plays a crucial role in fear extinction, mainly by modulating hippocampal synaptic plasticity via BDNF/TrkB signaling (Gao et al., 2023; Ji et al., 2023; Chen et al., 2024). TrkB, the specific receptor for BDNF, is widely distributed in the hippocampal dendritic spines and pyramidal neurons. The binding of BDNF to TrkB initiates persistent signaling in dendrites and axons, thereby regulating dendritic development (Qiu et al., 2020). Disruption of the BDNF/TrkB signaling pathway leads to a substantial decrease in both early and late LTP at CA1 hippocampal synapses, ultimately impairing the memory function associated with synaptic activity (Moya-Alvarado et al., 2023). In our study, we observed a significant downregulation of key proteins in the PKA/BDNF/TrkB pathway. Golgi staining revealed reduced dendritic complexity and length in hippocampal CA1, underscoring the critical role of this pathway in the pathophysiology of alcohol exposure-induced exacerbation of PTSD-like behaviors, as well as its contribution to fear extinction deficits and anxiety-like behaviors.
PSD95, a critical scaffold protein essential for the organization and function of the postsynaptic density complex, plays a pivotal role in synaptic plasticity (Zeng et al., 2016). Synaptophysin, a synaptic vesicle membrane protein, is another key regulator of synaptic activity (Marco-Manclus et al., 2022). Together with key glutamate-gated ion channels, such as NMDAR and AMPAR, they regulate synaptic plasticity and mediate neurodegenerative and neuroprotective processes in the brain. NR2A, a predominant subunit of NMDARs, is predominantly localized at synaptic sites, where it facilitates CREB activation and subsequent expression of plasticity-related genes (Scherzer et al., 1998; Law et al., 2003; Ji et al., 2017). In contrast, NR2B subunits are primarily expressed in extra synaptic regions, where their activation leads to sustained CREB dephosphorylation and transcriptional inactivation (Gong et al., 2020). Our analysis revealed significant downregulation of PSD95, synaptophysin, and NR2A and upregulation of NR2B expression in SPS&FS mice exposed to alcohol. Rolipram treatment effectively attenuated these reductions and restored the protein levels. Regarding AMPA receptor subunits, we focused on GluA1 and GluA2, which are the two principal components of this receptor complex (Stockwell et al., 2024). Alcohol-exposed SPS&FS mice exhibited significant reductions in GluA1 protein levels, whereas GluA2 expression remained unchanged. Rolipram treatment effectively restored GluA1 expression to normal levels.
Stress-induced ethanol consumption in mice is mediated by PTSD-related anxiety and alcohol exposure (Pucci et al., 2019; Khantzian, 1997). Our study revealed that mice with PTSD and early alcohol consumption displayed elevated anxiety-like behavior. Sequential alcohol exposure, trauma induction, and subsequent alcohol reintroduction significantly increased ethanol consumption and dependence, as evidenced by the CPP test. Rolipram-induced activation of the PKA pathway effectively mitigated these behavioral and physiological effects, highlighting the role of alcohol in stress-related consumption.
In conclusion, this study demonstrates that activation of the PKA signaling pathway regulates the expression of AMPA and NMDA receptor subunits, enhances synaptic structure and function in the hippocampus, and improves the fear response and drinking behavior in exacerbated PTSD-like behaviors due to alcohol exposure. These findings suggest that synaptic plasticity may be the underlying neural mechanism for the comorbidity of alcohol exposure and exacerbated PTSD-like behaviors. This could explain the intensification of PTSD-like behavior after early alcohol consumption. Therefore, targeting synaptic plasticity via the PKA pathway may represent a promising therapeutic strategy for treating comorbid PTSD and AUD.
5 Conclusion
Prior alcohol exposure exacerbates fear extinction deficits and intensifies anxiety-like behaviors in murine models of PTSD disorder. These detrimental effects can be effectively mitigated through the activation of the PKA signaling pathway, which enhances hippocampal synaptic function and reduces alcohol consumption and preference following a traumatic stress. Consequently, therapeutic modulation of synaptic plasticity through the PKA pathway may represent a promising novel intervention strategy for treating comorbid PTSD and AUD in the future.
6 Limitations
The relationship between early alcohol exposure and PTSD in female mice was not explored. 2) Our study focused only on the hippocampus and did not consider the role played by other brain regions. In addition to the hippocampus, the prefrontal cortex is also involved in the control of fear memory, and the cerebral cortex and hippocampus may cooperate to control fear. 3) Rolipram’s modulation of synaptic plasticity may involve multiple pathways, future studies could benefit from targeted genetic or optogenetic manipulations to confirm cAMP-PAK signaling pathway specificity.
Data availability statement
The raw data supporting the conclusion of this article will be made available by the authors, without undue reservation.
Ethics statement
The animal study was approved by Committee of Animal Experimental Ethics of Qingdao University. The study was conducted in accordance with the local legislation and institutional requirements.
Author contributions
SZ: Data curation, Investigation, Methodology, Resources, Supervision, Validation, Visualization, Writing – original draft, Writing – review and editing. WZ: Data curation, Investigation, Resources, Software, Supervision, Writing – original draft. ZW: Investigation, Supervision, Visualization, Writing – original draft. XC: Formal Analysis, Methodology, Software, Writing – original draft. FZ: Investigation, Supervision, Visualization, Writing – original draft, Writing – review and editing. HZ: Conceptualization, Funding acquisition, Investigation, Project administration, Supervision, Writing – original draft, Writing – review and editing.
Funding
The author(s) declare that financial support was received for the research and/or publication of this article. This work was supported by research grants from Taishan Scholar Young Talent Program (Grant No. tsqn202312172), the Key Project by Qingdao Bureau of Sciences and Technology (Grant No. 22-3-3-hygg-25-hy), the National Natural Science Foundation of China (Grant No. 82301679), the China Postdoctoral Science Foundation (Grant No. 2024T170449), the Natural Science Foundation of Shandong Province (Grant No. ZR2023QH444), the Key Program of Brain Science and Brain-Like Intelligence Technology of the China Ministry of Science and Technology (Grant No. STI2030-Major Projects 2021ZD0202900), and the Qingdao Postdoctoral Applied Research Project (Grant No. QDBSH20230102104).
Conflict of interest
The authors declare that the research was conducted in the absence of any commercial or financial relationships that could be construed as a potential conflict of interest.
Generative AI statement
The author(s) declare that no Generative AI was used in the creation of this manuscript.
Publisher’s note
All claims expressed in this article are solely those of the authors and do not necessarily represent those of their affiliated organizations, or those of the publisher, the editors and the reviewers. Any product that may be evaluated in this article, or claim that may be made by its manufacturer, is not guaranteed or endorsed by the publisher.
Supplementary material
The Supplementary Material for this article can be found online at: https://www.frontiersin.org/articles/10.3389/fphar.2025.1592187/full#supplementary-material
References
Alijan-Pour, J., Abrari, K., Lashkar Bluki, T., Ghorbanian, M. T., Goudarzi, I., and Elahdadi Salmani, M. (2012). Ethanol disrupts reactivated contextual conditioned fear memory: behavioral and histological perspectives. Cell J. 13, 265–274.
Anderson, R. I., Lopez, M. F., and Becker, H. C. (2016). Forced swim stress increases ethanol consumption in C57BL/6J mice with a history of chronic intermittent ethanol exposure. Psychopharmacol. Berl. 233, 2035–2043. doi:10.1007/s00213-016-4257-2
Back, S. E., Killeen, T., Badour, C. L., Flanagan, J. C., Allan, N. P., Ana, E. S., et al. (2019). Concurrent treatment of substance use disorders and PTSD using prolonged exposure: a randomized clinical trial in military veterans. Addict. Behav. 90, 369–377. doi:10.1016/j.addbeh.2018.11.032
Barkell, G. A., Parekh, S. V., Paniccia, J. E., Martin, A. J., Reissner, K. J., Knapp, D. J., et al. (2022). Chronic ethanol consumption exacerbates future stress-enhanced fear learning, an effect mediated by dorsal hippocampal astrocytes. Alcohol Clin. Exp. Res. 46, 2177–2190. doi:10.1111/acer.14963
Breese, G. R., Sinha, R., and Heilig, M. (2011). Chronic alcohol neuroadaptation and stress contribute to susceptibility for alcohol craving and relapse. Pharmacol. Ther. 129, 149–171. doi:10.1016/j.pharmthera.2010.09.007
Bryant, R. A., Nickerson, A., Morina, N., and Liddell, B. (2023). Posttraumatic stress disorder in refugees. Annu. Rev. Clin. Psychol. 19, 413–436. doi:10.1146/annurev-clinpsy-080921-080359
Chen, L., Zhang, Y., Wang, Z., Zhang, Z., Wang, J., Zhu, G., et al. (2024). Activation of GPER1 by G1 prevents PTSD-like behaviors in mice: illustrating the mechanisms from BDNF/TrkB to mitochondria and synaptic connection. CNS Neurosci. Ther. 30, e14855. doi:10.1111/cns.14855
Cousin, M. A. (2021). Synaptophysin-dependent synaptobrevin-2 trafficking at the presynapse-Mechanism and function. J. Neurochem. 159, 78–89. doi:10.1111/jnc.15499
Fein, G. (2013). Lifetime and current mood and anxiety disorders in short-term and long-term abstinent alcoholics. Alcohol Clin. Exp. Res. 37, 1930–1938. doi:10.1111/acer.12170
Gao, F., Wang, J., Yang, S., Ji, M., and Zhu, G. (2023). Fear extinction induced by activation of PKA ameliorates anxiety-like behavior in PTSD mice. Neuropharmacology 222, 109306. doi:10.1016/j.neuropharm.2022.109306
Garcia, R. (2002). Stress, synaptic plasticity, and psychopathology. Rev. Neurosci. 13, 195–208. doi:10.1515/revneuro.2002.13.3.195
Gong, X., Xu, L., Fang, X., Zhao, X., Du, Y., Wu, H., et al. (2020). Protective effects of grape seed procyanidin on isoflurane-induced cognitive impairment in mice. Pharm. Biol. 58, 200–207. doi:10.1080/13880209.2020.1730913
Gonzalez, S. T., Marty, V., Spigelman, I., Reise, S. P., and Fanselow, M. S. (2021). Impact of stress resilience and susceptibility on fear learning, anxiety, and alcohol intake. Neurobiol. Stress 15, 100335. doi:10.1016/j.ynstr.2021.100335
Harnett, N. G., Goodman, A. M., and Knight, D. C. (2020). PTSD-related neuroimaging abnormalities in brain function, structure, and biochemistry. Exp. Neurol. 330, 113331. doi:10.1016/j.expneurol.2020.113331
Heckman, P. R. A., Blokland, A., Bollen, E. P. P., and Prickaerts, J. (2018). Phosphodiesterase inhibition and modulation of corticostriatal and hippocampal circuits: clinical overview and translational considerations. Neurosci. Biobehav Rev. 87, 233–254. doi:10.1016/j.neubiorev.2018.02.007
Hunt, C., Park, J., Bomyea, J., and Colvonen, P. J. (2023). Sleep efficiency predicts improvements in fear extinction and PTSD symptoms during prolonged exposure for veterans with comorbid insomnia. Psychiatry Res. 324, 115216. doi:10.1016/j.psychres.2023.115216
Izquierdo, I., Furini, C. R. G., and Myskiw, J. C. (2016). Fear memory. Physiol. Rev. 96, 695–750. doi:10.1152/physrev.00018.2015
Ji, L.-L., Peng, J.-B., Fu, C.-H., Tong, L., and Wang, Z.-Y. (2017). Sigma-1 receptor activation ameliorates anxiety-like behavior through NR2A-CREB-BDNF signaling pathway in a rat model submitted to single-prolonged stress. Mol. Med. Rep. 16, 4987–4993. doi:10.3892/mmr.2017.7185
Ji, M., Zhang, Z., Gao, F., Yang, S., Wang, J., Wang, X., et al. (2023). Curculigoside rescues hippocampal synaptic deficits elicited by PTSD through activating cAMP-PKA signaling. Phytother. Res. 37, 759–773. doi:10.1002/ptr.7658
Khantzian, E. J. (1997). The self-medication hypothesis of substance use disorders: a reconsideration and recent applications. Harv Rev. Psychiatry 4, 231–244. doi:10.3109/10673229709030550
Kida, S. (2019). Reconsolidation/destabilization, extinction and forgetting of fear memory as therapeutic targets for PTSD. Psychopharmacology 236, 49–57. doi:10.1007/s00213-018-5086-2
Lacagnina, A. F., Brockway, E. T., Crovetti, C. R., Shue, F., McCarty, M. J., Sattler, K. P., et al. (2019). Distinct hippocampal engrams control extinction and relapse of fear memory. Nat. Neurosci. 22, 753–761. doi:10.1038/s41593-019-0361-z
Law, A. J., Weickert, C. S., Webster, M. J., Herman, M. M., Kleinman, J. E., and Harrison, P. J. (2003). Expression of NMDA receptor NR1, NR2A and NR2B subunit mRNAs during development of the human hippocampal formation. Eur. J. Neurosci. 18, 1197–1205. doi:10.1046/j.1460-9568.2003.02850.x
Leeies, M., Pagura, J., Sareen, J., and Bolton, J. M. (2010). The use of alcohol and drugs to self-medicate symptoms of posttraumatic stress disorder. Depress Anxiety 27, 731–736. doi:10.1002/da.20677
Lei, J., Zhang, P., Li, T., Cui, C., Li, M., Yang, X., et al. (2024). Alternating bilateral sensory stimulation alleviates alcohol-induced conditioned place preference via a superior colliculus-VTA circuit. Cell Rep. 43, 114383. doi:10.1016/j.celrep.2024.114383
Li, L.-P., Dustrude, E. T., Haulcomb, M. M., Abreu, A. R., Fitz, S. D., Johnson, P. L., et al. (2018). PSD95 and nNOS interaction as a novel molecular target to modulate conditioned fear: relevance to PTSD. Transl. Psychiatry 8, 155. doi:10.1038/s41398-018-0208-5
Logrip, M. L., Zorrilla, E. P., and Koob, G. F. (2012). Stress modulation of drug self-administration: implications for addiction comorbidity with post-traumatic stress disorder. Neuropharmacology 62, 552–564. doi:10.1016/j.neuropharm.2011.07.007
Lou, C., Fang, M., Ye, S., Fang, Z., Amin, N., and Chen, Y. (2022). Fluoxetine protects against inflammation and promotes autophagy in mice model of post-traumatic stress disorder. Behav. Brain Res. 433, 114004. doi:10.1016/j.bbr.2022.114004
Lu, G.-L., Yau, H.-J., and Chiou, L.-C. (2017). Conditioned place preference training prevents hippocampal depotentiation in an orexin-dependent manner. J. Biomed. Sci. 24, 69. doi:10.1186/s12929-017-0378-0
Maercker, A., Cloitre, M., Bachem, R., Schlumpf, Y. R., Khoury, B., Hitchcock, C., et al. (2022). Complex post-traumatic stress disorder. Lancet 400, 60–72. doi:10.1016/S0140-6736(22)00821-2
Marco-Manclus, P., Ávila-González, D., Paredes, R., and Portillo, W. (2022). Sexual experience in female mice involves synaptophysin-related plasticity in the accessory olfactory bulb. Physiology and Behav. 244, 113649. doi:10.1016/j.physbeh.2021.113649
Maren, S., and Holmes, A. (2016). Stress and fear extinction. Neuropsychopharmacology 41, 58–79. doi:10.1038/npp.2015.180
McDevitt-Murphy, M. E., Luciano, M. T., Tripp, J. C., and Eddinger, J. E. (2017). Drinking motives and PTSD-related alcohol expectancies among combat veterans. Addict. Behav. 64, 217–222. doi:10.1016/j.addbeh.2016.08.029
McFarlane, A. C., Browne, D., Bryant, R. A., O’Donnell, M., Silove, D., Creamer, M., et al. (2009). A longitudinal analysis of alcohol consumption and the risk of posttraumatic symptoms. J. Affect Disord. 118, 166–172. doi:10.1016/j.jad.2009.01.017
Messent, P. (2013). DSM-5. Clin. Child. Psychol. Psychiatry 18, 479–482. doi:10.1177/1359104513502138
Moya-Alvarado, G., Tiburcio-Felix, R., Ibáñez, M. R., Aguirre-Soto, A. A., Guerra, M. V., Wu, C., et al. (2023). BDNF/TrkB signaling endosomes in axons coordinate CREB/mTOR activation and protein synthesis in the cell body to induce dendritic growth in cortical neurons. Elife 12, e77455. doi:10.7554/eLife.77455
Park, P., Georgiou, J., Sanderson, T. M., Ko, K.-H., Kang, H., Kim, J.-I., et al. (2021). PKA drives an increase in AMPA receptor unitary conductance during LTP in the hippocampus. Nat. Commun. 12, 413. doi:10.1038/s41467-020-20523-3
Pati, D., Pina, M. M., and Kash, T. L. (2019). Ethanol-induced conditioned place preference and aversion differentially alter plasticity in the bed nucleus of stria terminalis. Neuropsychopharmacology 44, 1843–1854. doi:10.1038/s41386-019-0349-0
Peters, J., Dieppa-Perea, L. M., Melendez, L. M., and Quirk, G. J. (2010). Induction of fear extinction with hippocampal-infralimbic BDNF. Science 328, 1288–1290. doi:10.1126/science.1186909
Popova, D., Ágústsdóttir, A., Lindholm, J., Mazulis, U., Akamine, Y., Castrén, E., et al. (2014). Combination of fluoxetine and extinction treatments forms a unique synaptic protein profile that correlates with long-term fear reduction in adult mice. Eur. Neuropsychopharmacol. 24, 1162–1174. doi:10.1016/j.euroneuro.2014.04.002
Possemato, K., Silander, N., Bellete, N., Emery, J. B., De Stefano, L., and Pigeon, W. (2022). Characteristics of posttraumatic nightmares and their relationship to PTSD severity among combat veterans with PTSD and hazardous alcohol use. J. Nerv. Ment. Dis. 210, 223–226. doi:10.1097/NMD.0000000000001422
Potula, R., Gentile, T. A., Meissler, J. J., Shekarabi, A., Wiah, S., Farkas, D. J., et al. (2023). Purinergic P2X7 receptor antagonist inhibits methamphetamine-induced reward, hyperlocomotion, and cortical IL-7A levels in mice: a role for P2X7/IL-17A crosstalk in methamphetamine behaviors? Brain Behav. Immun. 107, 47–52. doi:10.1016/j.bbi.2022.09.012
Pucci, M., Micioni Di Bonaventura, M. V., Wille-Bille, A., Fernández, M. S., Maccarrone, M., Pautassi, R. M., et al. (2019). Environmental stressors and alcoholism development: focus on molecular targets and their epigenetic regulation. Neurosci. Biobehav Rev. 106, 165–181. doi:10.1016/j.neubiorev.2018.07.004
Qiu, L.-L., Pan, W., Luo, D., Zhang, G.-F., Zhou, Z.-Q., Sun, X.-Y., et al. (2020). Dysregulation of BDNF/TrkB signaling mediated by NMDAR/Ca2+/calpain might contribute to postoperative cognitive dysfunction in aging mice. J. Neuroinflammation 17, 23. doi:10.1186/s12974-019-1695-x
Radulovic, J., Ren, L. Y., and Gao, C. (2019). N-Methyl D-aspartate receptor subunit signaling in fear extinction. Psychopharmacol. Berl. 236, 239–250. doi:10.1007/s00213-018-5022-5
Scherzer, C. R., Landwehrmeyer, G. B., Kerner, J. A., Counihan, T. J., Kosinski, C. M., Standaert, D. G., et al. (1998). Expression of N-methyl-D-aspartate receptor subunit mRNAs in the human brain: hippocampus and cortex. J. Comp. Neurol. 390, 75–90. doi:10.1002/(sici)1096-9861(19980105)390:1<75::aid-cne7>3.0.co;2-n
Shahoha, M., Cohen, R., Ben-Simon, Y., and Ashery, U. (2022). cAMP-dependent synaptic plasticity at the hippocampal mossy fiber terminal. Front. Synaptic Neurosci. 14, 861215. doi:10.3389/fnsyn.2022.861215
Sharifi, A., Karimi-Haghighi, S., Shabani, R., Asgari, H. R., Ahadi, R., and Haghparast, A. (2022). Cannabidiol impairs the rewarding effects of methamphetamine: involvement of dopaminergic receptors in the nucleus accumbens. Prog. Neuro-Psychopharmacology Biol. Psychiatry 113, 110458. doi:10.1016/j.pnpbp.2021.110458
Shimoda, R., Amaya, Y., Okamoto, M., Soya, S., Soya, M., Koizumi, H., et al. (2024). Accelerated fear extinction by regular light-intensity exercise: a possible role of hippocampal BDNF-TrkB signaling. Med. Sci. Sports Exerc 56, 221–229. doi:10.1249/MSS.0000000000003312
Sinha, R. (2024). Stress and substance use disorders: risk, relapse, and treatment outcomes. J. Clin. Invest 134, e172883. doi:10.1172/JCI172883
Stein, M. B., Campbell-Sills, L., Gelernter, J., He, F., Heeringa, S. G., Nock, M. K., et al. (2017). Alcohol misuse and Co-occurring mental disorders among new soldiers in the U.S. Army. Alcohol Clin. Exp. Res. 41, 139–148. doi:10.1111/acer.13269
Stockwell, I., Watson, J. F., and Greger, I. H. (2024). Tuning synaptic strength by regulation of AMPA glutamate receptor localization. Bioessays 46, e2400006. doi:10.1002/bies.202400006
Sur, B., and Lee, B. (2022). Ginsenoside Rg3 modulates spatial memory and fear memory extinction by the HPA axis and BDNF-TrkB pathway in a rat post-traumatic stress disorder. J. Nat. Med. 76, 821–831. doi:10.1007/s11418-022-01636-z
Suzuki, T., Kametani, K., Guo, W., and Li, W. (2018). Protein components of post-synaptic density lattice, a backbone structure for type I excitatory synapses. J. Neurochem. 144, 390–407. doi:10.1111/jnc.14254
Wang, J., Gao, F., Cui, S., Yang, S., Gao, F., Wang, X., et al. (2022). Utility of 7,8-dihydroxyflavone in preventing astrocytic and synaptic deficits in the hippocampus elicited by PTSD. Pharmacol. Res. 176, 106079. doi:10.1016/j.phrs.2022.106079
Wang J., J., Liang, M., Shang, Q., Qian, H., An, R., Liu, H., et al. (2023). Psilocin suppresses methamphetamine-induced hyperlocomotion and acquisition of conditioned place preference via D2R-mediated ERK signaling. CNS Neurosci. Ther. 29, 831–841. doi:10.1111/cns.14054
Wang Z., Z., Luo, C., Zhou, E. W., Sandhu, A. F., Yuan, X., Williams, G. E., et al. (2023). Molecular toxicology and pathophysiology of comorbid alcohol use disorder and post-traumatic stress disorder associated with traumatic brain injury. Int. J. Mol. Sci. 24, 8805. doi:10.3390/ijms24108805
Wei, R., Zong, F., Dong, J., Zhao, W., Zhang, F., Wang, W., et al. (2024). Identification of phosphodiesterase-7A (PDE7A) as a novel target for reducing ethanol consumption in mice. Int. J. Neuropsychopharmacol. 27, pyae032. doi:10.1093/ijnp/pyae032
Weiss, N. H., Goncharenko, S., Raudales, A. M., Schick, M. R., and Contractor, A. A. (2021). Alcohol to down-regulate negative and positive emotions: extending our understanding of the functional role of alcohol in relation to posttraumatic stress disorder. Addict. Behav. 115, 106777. doi:10.1016/j.addbeh.2020.106777
Wen, Z., Seo, J., Pace-Schott, E. F., and Milad, M. R. (2022). Abnormal dynamic functional connectivity during fear extinction learning in PTSD and anxiety disorders. Mol. Psychiatry 27, 2216–2224. doi:10.1038/s41380-022-01462-5
Xi, K., Huang, X., Liu, T., Liu, Y., Mao, H., Wang, M., et al. (2021). Translational relevance of behavioral, neural, and electroencephalographic profiles in a mouse model of post-traumatic stress disorder. Neurobiol. Stress 15, 100391. doi:10.1016/j.ynstr.2021.100391
Xi, K., Xiao, H., Huang, X., Yuan, Z., Liu, M., Mao, H., et al. (2023). Reversal of hyperactive higher-order thalamus attenuates defensiveness in a mouse model of PTSD. Sci. Adv. 9, eade5987. doi:10.1126/sciadv.ade5987
Yanpallewar, S., Tomassoni-Ardori, F., Palko, M. E., Hong, Z., Kiris, E., Becker, J., et al. (2022). TrkA-cholinergic signaling modulates fear encoding and extinction learning in PTSD-like behavior. Transl. Psychiatry 12, 111. doi:10.1038/s41398-022-01869-2
Zeng, M., Shang, Y., Araki, Y., Guo, T., Huganir, R. L., and Zhang, M. (2016). Phase transition in postsynaptic densities underlies formation of syaptic complexes and synaptic plasticity. Cell 166, 1163–1175.e12. doi:10.1016/j.cell.2016.07.008
Zhao, W., Zhao, S., Wei, R., Wang, Z., Zhang, F., Zong, F., et al. (2024). cGAS/STING signaling pathway-mediated microglial activation in the PFC underlies chronic ethanol exposure-induced anxiety-like behaviors in mice. Int. Immunopharmacol. 134, 112185. doi:10.1016/j.intimp.2024.112185
Keywords: cAMP-PKA signaling, alcohol exposure, PTSD, fear memory extinction, synaptic plasticity
Citation: Zhao S, Zhao W, Wang Z, Chen X, Zong F and Zhang H (2025) The regulation and mechanism of the cAMP-PKA pathway on PTSD-like behaviors exacerbated by alcohol exposure. Front. Pharmacol. 16:1592187. doi: 10.3389/fphar.2025.1592187
Received: 12 March 2025; Accepted: 08 May 2025;
Published: 16 May 2025.
Edited by:
Marcelo F. Lopez, Medical University of South Carolina, United StatesReviewed by:
Carla Cannizzaro, University of Palermo, ItalyPaola Palombo, Federal University of São Paulo, Brazil
Copyright © 2025 Zhao, Zhao, Wang, Chen, Zong and Zhang. This is an open-access article distributed under the terms of the Creative Commons Attribution License (CC BY). The use, distribution or reproduction in other forums is permitted, provided the original author(s) and the copyright owner(s) are credited and that the original publication in this journal is cited, in accordance with accepted academic practice. No use, distribution or reproduction is permitted which does not comply with these terms.
*Correspondence: Fangjiao Zong, em9uZ2ZqQHFkdS5lZHUuY24=; Hanting Zhang, aHR6aGFuZ0BxZHUuZWR1LmNu
†These authors have contributed equally to this work