- 1 Bute Medical School, University of St. Andrews, Fife, Scotland, UK
- 2 Autism Research Unit, Department of Psychiatry, University of Cambridge, Cambridge, UK
- 3 Department of Psychiatry, University of Cambridge, Cambridge, UK
Autism spectrum condition (ASC) is recognized as having an inflammatory component. Post-mortem brain samples from patients with ASC display neuroglial activation and inflammatory markers in cerebrospinal fluid, although little is known about the underlying molecular mechanisms. Nuclear factor kappa-light-chain-enhancer of activated B cells (NF-κB) is a protein found in almost all cell types and mediates regulation of immune response by inducing the expression of inflammatory cytokines and chemokines, establishing a feedback mechanism that can produce chronic or excessive inflammation. This article describes immunodetection and immunofluorescence measurements of NF-κB in human post-mortem samples of orbitofrontal cortex tissue donated to two independent centers: London Brain Bank, Kings College London, UK (ASC: n = 3, controls: n = 4) and Autism Tissue Program, Harvard Brain Bank, USA (ASC: n = 6, controls: n = 5). The hypothesis was that concentrations of NF-κB would be elevated, especially in activated microglia in ASC, and pH would be concomitantly reduced (i.e., acidification). Neurons, astrocytes, and microglia all demonstrated increased extranuclear and nuclear translocated NF-κB p65 expression in brain tissue from ASC donors relative to samples from matched controls. These between-groups differences were increased in astrocytes and microglia relative to neurons, but particularly pronounced for highly mature microglia. Measurement of pH in homogenized samples demonstrated a 0.98-unit difference in means and a strong (F = 98.3; p = 0.00018) linear relationship to the expression of nuclear translocated NF-κB in mature microglia. Acridine orange staining localized pH reductions to lysosomal compartments. In summary, NF-κB is aberrantly expressed in orbitofrontal cortex in patients with ASC, as part of a putative molecular cascade leading to inflammation, especially of resident immune cells in brain regions associated with the behavioral and clinical symptoms of ASC.
Introduction
Autism spectrum condition (ASC) is a life-long neurodevelopmental condition characterized by a triad of impairments in social skills, verbal communication, and behavior (Rapin, 1997; Lord et al., 2000). Cognitively, ASC is described as a disorder involving fundamental deficits in central coherence (Frith, 1989), executive function (Ornitz et al., 1993), theory of mind (Baron-Cohen et al., 1985), and empathizing (Baron-Cohen, 2002). Continuing investigations for a neurobiological basis for ASC support the view that genetic, environmental, neurological, and immunological factors contribute to its etiology (Neuhaus et al., 2010). In particular, there is evidence to suggest an association between ASC and neuroinflammation in anterior regions of the neocortex (Pardo et al., 2005; Vargas et al., 2005; Zimmerman et al., 2005), and areas relating to cognitive function appear to be affected by inflammation resulting from activation of microglia and astrocytes (Anderson et al., 2008). In vivo measurements of structural brain changes with magnetic resonance imaging have detected gray matter loss in the orbitofrontal cortex (Hardan et al., 2006; Girgis et al., 2007) and impairment of cognitive functions mediated by the orbitofrontal–amygdala circuit (Loveland et al., 2008) in patients with ASC. Furthermore, markers of oxidative stress are elevated in the orbitofrontal cortex in post-mortem samples of ASC patients (Sajdel-Sulkowska et al., 2011). This region is thus a likely candidate for an underlying cellular mechanism.
Nuclear factor kappa-light-chain-enhancer of activated B cells (NF-κB) is a protein that controls transcription of DNA and is found in almost all cell types (Perkins, 2004). It mediates cellular response to external stressors and is central to the regulation of immune responses by inducing the expression of inflammatory cytokines and chemokines and, in turn, being induced by them (Pahl, 1999; Perkins, 2004). This establishes a positive feedback mechanism (Larsson et al., 2005) which, when NF-κB becomes aberrantly active, has the potential to produce chronic or excessive inflammation associated with several inflammatory diseases (Pahl, 1999). Furthermore, post-mortem studies suggest that NF-κB plays a key role in Alzheimer’s disease (Akiyama et al., 1994) and its possible treatment (Paris et al., 2007), Parkinson’s disease (Block and Hong, 2005) and multiple sclerosis (Glass et al., 2010).
Microglia mediate the immune responses of the central nervous system acting to remove extracellular debris with a similar function to macrophages. Microglial cells have been associated with brain inflammation (Liu and Hong, 2003; Barger, 2005; Kim and Joh, 2006) and pro-inflammatory treatments of microglia acidify cellular lysosomes to a pH ∼5 (Majumdar et al., 2007). Preliminary characterization of this mechanism implicates activation of protein kinase A (PKA) and the activity of chloride channels (Majumdar et al., 2007). The transcriptional activity of NF-κB is stimulated upon phosphorylation of its p65 subunit on serine 276 by PKA (Zhong et al., 1998) and in turn PKA is a downstream target of the transcription factor (Kaltschmidt et al., 2006). With this in mind we postulated that an association may exist between the transcription factor and lysosomal acidity.
This article describes measurement of NF-κB p65 expression levels and pH in post-mortem samples of orbitofrontal cortex from patients with a diagnosis of ASC and control samples from people healthy at the time of death. We hypothesized that concentrations of NF-κB would be elevated in patients and pH would be concomitantly reduced (i.e., acidification), providing evidence for a neuroinflammatory component to ASC.
This hypothesis was initially tested by Western immunodetection of post-mortem brain tissue to measure overall, nuclear and cytosolic NF-κB expression.
Investigations were then focused upon microglial cells due to their role in pro-inflammatory response, as these most strongly mediate aberrant expression of NF-κB. Antigen retrieval and immunofluorescence techniques were used to identify the differential concentrations of intracellular NF-κB in neurons, astrocytes, microglia, and highly activated (i.e., mature or functional) microglia. Immunoreactivity measurements were initially carried out to determine the concentration of NF-κB in the cytoplasm of each cell type as an indication of the availability of inactive NF-κB, and thus its potential for nuclear translocation. The expression of active NF-κB translocated to the cell nuclei was then measured directly, where it binds to DNA and transcribes proteins that result in the production of cytokines as part of an inflammatory response.
Confirmation of the immunodetection and immunofluorescence results in neurons and mature microglia was sought from an independent source of micro-array tissue slides donated from ASC patient and control groups.
Finally, pH was measured in homogenized tissue and compared to the corresponding intracellular NF-κB p65 expression from Western immunodetection. Acridine orange staining allowed measurements of pH localized to lysosomes.
Materials and Methods
Tissue Samples
The UK cohort consisted of seven samples of fixed orbitofrontal cortex sections from four control and three ASC donors obtained from the Medical Research Council’s London Brain Bank (Institute of Psychiatry, King’s College London). Samples were age, sex, and post-mortem interval (PMI) matched (Table 1). Protein extraction from formalin fixed tissue was performed according to Shi et al. (2006), where tissue sections were placed in 50 μl of 20 mM Tris–HCl buffer pH 8 with 2% SDS plus pepstatin 10 μg/ml and heated to remove formalin cross-links.
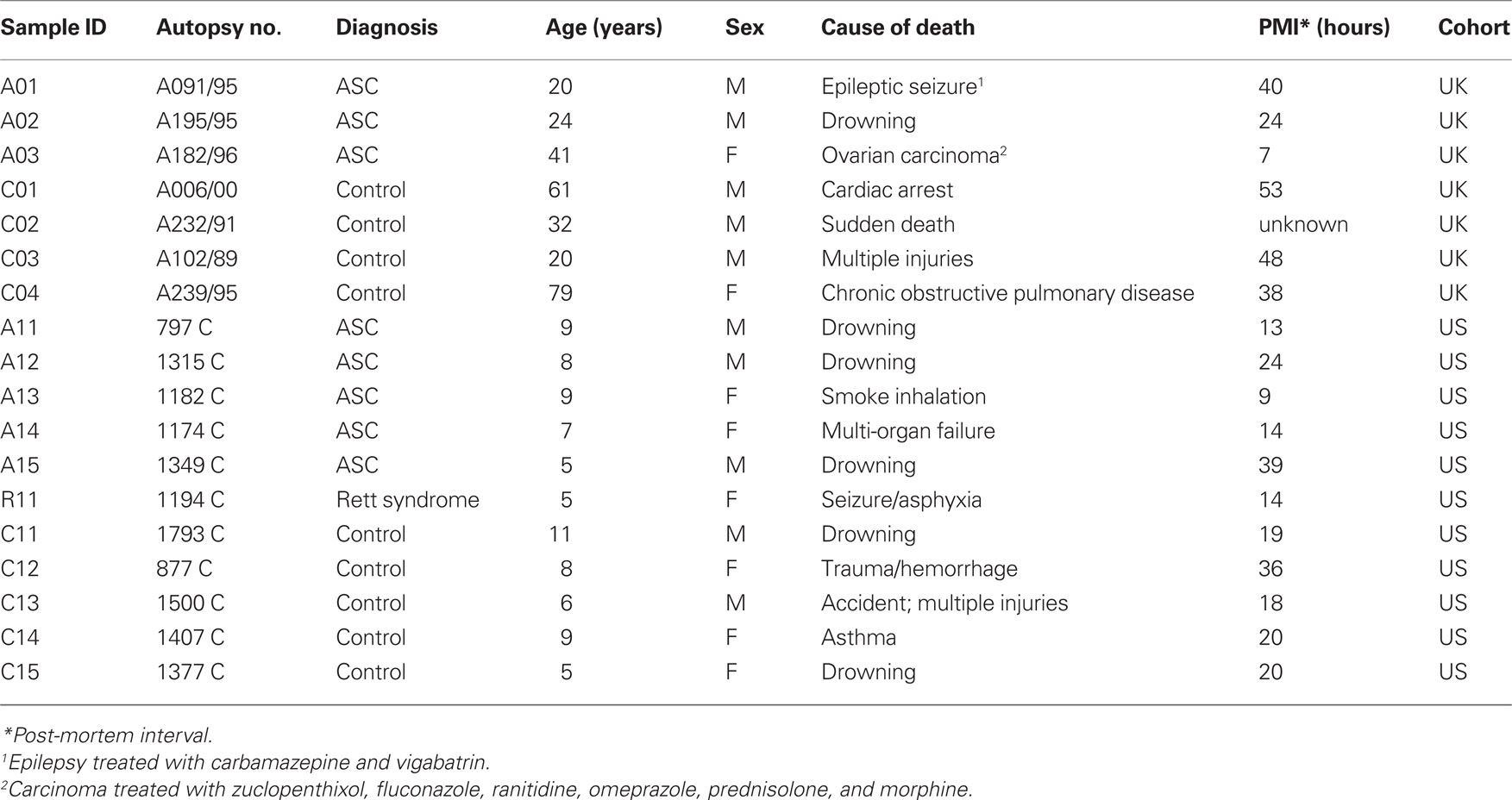
Table 1. Demographic information on tissue donors for the UK (London Brain Bank) and US (Autism Tissue Program/Harvard Brain Tissue Resource Center) cohorts.
Samples of the US cohort were sections from six ASC patients and five age, sex, and PMI matched control donors obtained as tissue micro-arrays (Eberhart et al., 2006) from the Autism Tissue Program (Harvard Brain Tissue Resource Center, Boston). One patient had a diagnosis of Rett syndrome, a neurodevelopmental disorder classified as an ASC. The age-at-death of donors was considerably younger (5–11 years) than the UK cohort (20 –79 years; Table 1).
Tissue Separation
Tissue samples from the UK cohort were processed for nuclear and cytosolic extraction using two separation buffers. The tissue was homogenized in 10 volumes of buffer 1 (Tris 10 mM, NaH2PO4 20 mM, EDTA 1 mM, pH 7.8 PMSF 0.1 mM, pepstatin 10 μg/ml, and leupeptin 10 μg/ml). Homogenate was incubated for 20 min and osmolarity restored by adding 1/20 volume of KCl 2.4 M, 1/40 volume of NaCl 1.2 M, 1/5 volume sucrose 1.25 M. Samples were spun for 5 min at 3,500 rpm, supernatant removed and pellet resuspended on 0.6 M sucrose and spun for a further 10 min at 10,000 rpm.
Subsequently, the supernatant was diluted in buffer 2 (imidazole 30 mM, KCl 120 mM, NaCl 30 mM, NaH2PO4, sucrose 250 mM pH 6.8, protease inhibitors pepstatin 10 μg/ml and leupeptin 10 μg/ml) and spun again at 3,500 rpm for 15 min. The resultant pellets contained the remaining nuclear proteins, and the supernatants the cytosolic proteins.
Western Immunodetection
Protein samples run on SDS-polyacrylamide gels were electroblotted to nitrocellulose membranes (Schleicher & Schuell Bioscience GmbH, Germany), blocked with 5% non-fat dry milk in phosphate buffered saline with 0.1% Tween-20 (PBST), and probed with a 1:1,000 dilution of anti-p65 antibody (Santa Cruz Biotechnology). PBST washed membranes were then incubated with HRP-conjugated goat anti-rabbit antisera (Sigma Ltd, UK), and developed with enhanced chemiluminescence reagents (Pierce Ltd, UK). Signal was detected using a LAS 3000 image analyzer (Fujifilm, Japan) and bands quantified using ImageJ software.
Immunofluorescence
Tissue samples were fixed in formalin and embedded in paraffin blocks. Slides were deparaffinized in xylene three times, each for 5 min, then hydrated gradually through graded alcohols: washed in 100% ethanol twice for 10 min each, then 95% ethanol twice for 10 min each and finally washed in deionized water for 1 min with stirring. Sections were cut 5 μm thick and mounted onto these pre-treated slides.
Antigen retrieval was carried out in a pressure microwave where slides were covered in 10 mM sodium citrate buffer pH 6.0. After cooling for 20 min, sections were blocked in 10% normal goat block for 15 min and placed in anti-p65 NF-κB antibody 1:200 (Santa Cruz Biotechnology Inc., Santa Cruz, CA, USA) overnight at 4°C. Sections were washed continuously for 5 min and placed in HRP-conjugated goat anti-rabbit antisera 1:200 (Molecular Probes Inc., Eugene, OR, USA) for 30 min at room temperature.
Sections were then stained with either: anti-Beta III Tubulin (1:10,000), a microtubule element antibody targeted exclusively in neurons; anti-GFAP (1:250), an intermediate filament (IF) protein antibody specific for astrocytes; anti-CD11b (1:250), a complement component 3-receptor 3-subunit antibody sensitive to microglia (Ford et al., 1995; Becher and Antel, 1996); or anti-CD11c (1:250), a type I transmembrane protein antibody found on highly activated microglia (Hughes et al., 2003). This was followed by a final 5 min wash and slides were mounted with 4′,6-diamidino-2-phenylindole (DAPI) with Vectashield (Vector Labs Ltd, UK) to identify cell nuclei.
One hundred cells of each type (neurons, astrocytes, and microglia) from each sample were selected at random, blinded from group (i.e., ASC or control). Cells were graded for immunoreactivity according to the intensity of antibody signal within the cell on an integer scale of 0–3 (Schmidt and Bankole, 1965). For each cell type, the percentage of cells with weak (scale 1) and strong (scale 3) intensity was calculated.
Antigen positive cells were also counted in each sample to quantify the intensity of anti-p65 signal in the nucleus, providing a measurement of nuclear translocation of NF-κB p65 and thus the active state of the molecule within each cell type.
Measurement of pH
Equal volumes of neural tissue were homogenized using a mortar and pestle in 10 volumes of deionized water at 4°C. The pH of the homogenate was measured using a MeterLab PHM201 pH meter (Radiometer Analytical, Villeurbanne Cedex, France) calibrated with two standards for pH 4 and 7. Measurements were made eight times over 4 days and averaged to yield a final value.
Using the protocol described by Morgan and Galione (2007) and Lee et al. (1983), the pH of lysosomes was measured. Tissue sections were simultaneously loaded with 10 μM acridine orange and 1 μM Lysotracker Red DND-99 for 15–20 min at room temperature, at which time the fluorescence had reached equilibrium; that is, the dyes were present throughout the rest of the experiment. Acridine orange responds rapidly and profoundly to changes in pH, whereas Lysotracker Red responds only relatively slowly and remains essentially fixed throughout the experiment. Results are expressed as the ratios of the acridine orange/Lysotracker Red signals such that an increase in the ratio reflects an increase in pH.
Statistical Analysis
Small sample sizes precluded valid formal between-group (i.e., ASC vs. control) statistical tests. Nevertheless, in many cases there was no overlap in the values obtained for each group. Unless indicated, all data are expressed as mean ± standard error across the samples. Statistical testing of within-group correlation was undertaken using SPSS (v17, SPSS Inc.), with the level for significance set at p < 0.05.
Results
Expression of NF-κB P65 in Neural Tissue: UK Cohort
Western immunodetection of neural tissue samples analyzed for overall NF-κB p65 expression is shown in Figure 1A. Densitometry demonstrated a 2.9-fold increase of NF-κB p65 expression in ASC samples; Figure 1B.
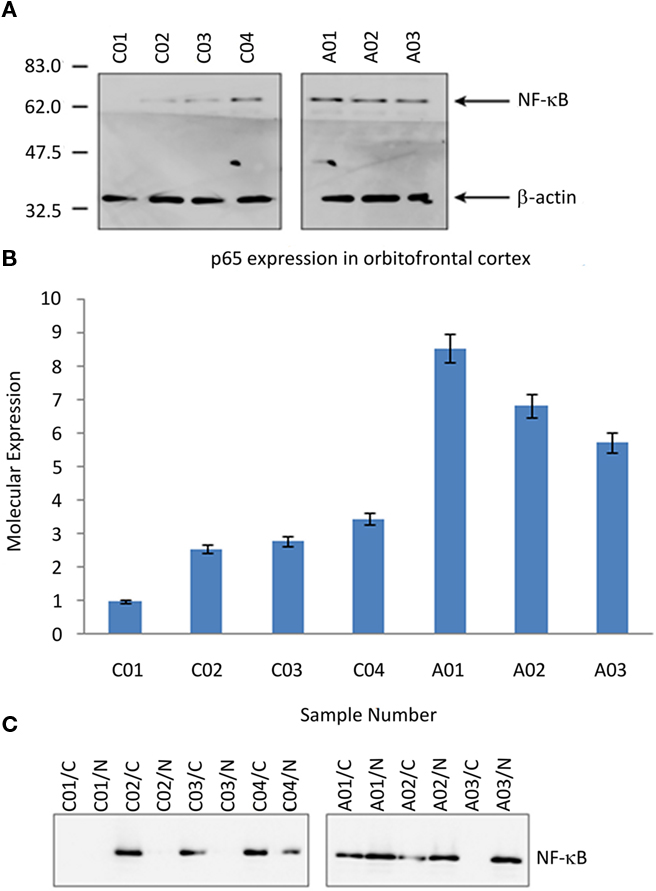
Figure 1. NF-κB in post-mortem tissue from the orbitofrontal cortex from ASC and control donors. (A) Image of Western blot probed with the anti-NF-κB p65 and anti-β-actin antibodies (loading control). Molecular mass markers are shown in kDa. (B) Relative expression of NF-κB p65 subunit, normalized to the lowest value recorded (Study ID C01). (C) Image of fractioned samples probed with anti-NF-κB p65. Sample IDs suffixed “/N” are the nuclear component, and suffixed “/C” the cytosolic component. Sample IDs prefixed “A” are from ASC donors, prefixed “C” from control donors.
Nuclear translocation of NF-κB p65 is predominately associated with the activation of the transcription factor. Separated tissue lysates were used to determine the subcellular location of p65 expression. In control tissue NF-κB p65 was mainly located within the cellular cytoplasm, whereas in tissue samples from ASC patients the expression was predominately within the nucleus. Western immunodetection of neural tissues analyzed for overall NF-κB p65 expression for the UK cohort is shown in Figure 1C.
Expression of NF-κB P65 in Neurons, Astrocytes, and Microglia: UK Cohort
The immunoreactivity intensity from neurons stained by anti-Beta III Tubulin in tissue from ASC patients predominately scored in the range 2–3 (78%), whilst neurons in tissue from the control group had the majority of fluorescence scores in the range 0–1 (69%; Table 2). There was an increase in nuclear translocation of NF-κB p65 in neurons, within tissue from ASC patients (21.67 ± 1.50%) compared to controls (17.00 ± 1.41%; Figure 2A). Examples of CD11c, p65, and DAPI staining are shown Figure 3D.
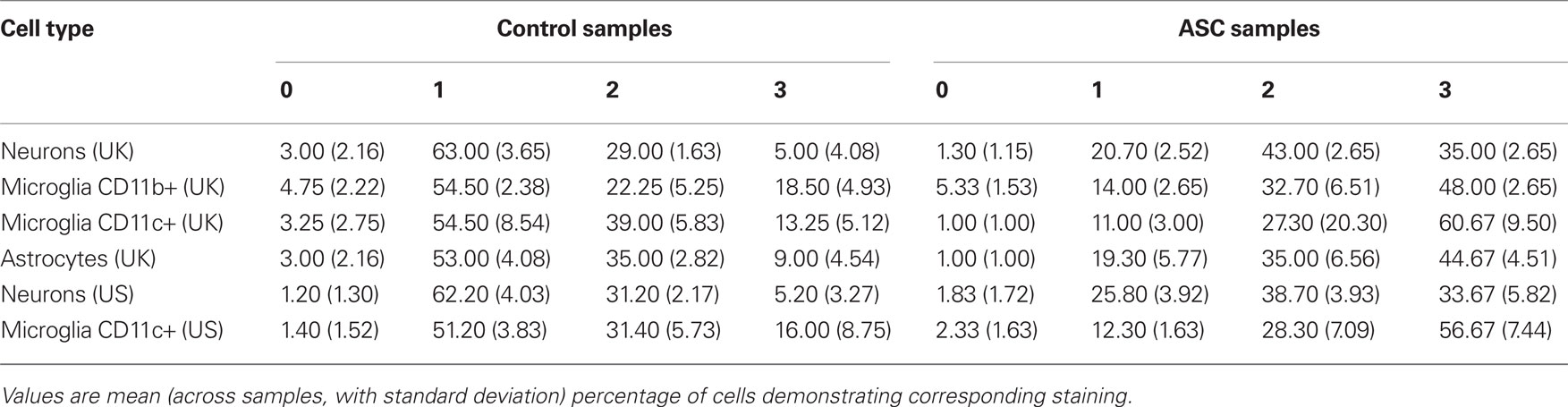
Table 2. Immunoreactivities of each cell type on an integer scale of 0 (weakest) to 3 (strongest) intensities.
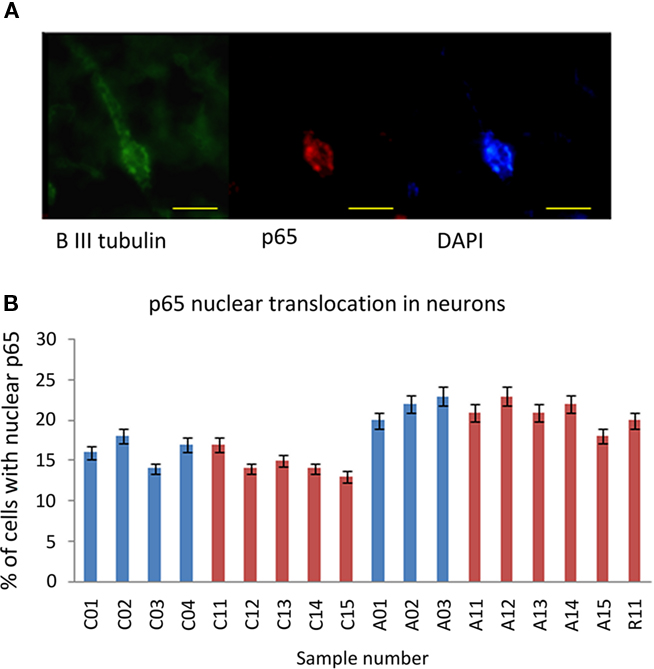
Figure 2. NF-κB p65 expression in neurons. (A) A representative image of a neuron stained with anti-β-III Tubulin (green), anti-p65 (red), and DAPI (blue). Scale bar = 10 μm. (B) Percentage of neurons with anti-p65 nuclear staining for each sample. Sample IDs prefixed “A” are from ASC donors, prefixed “C” from control donors.
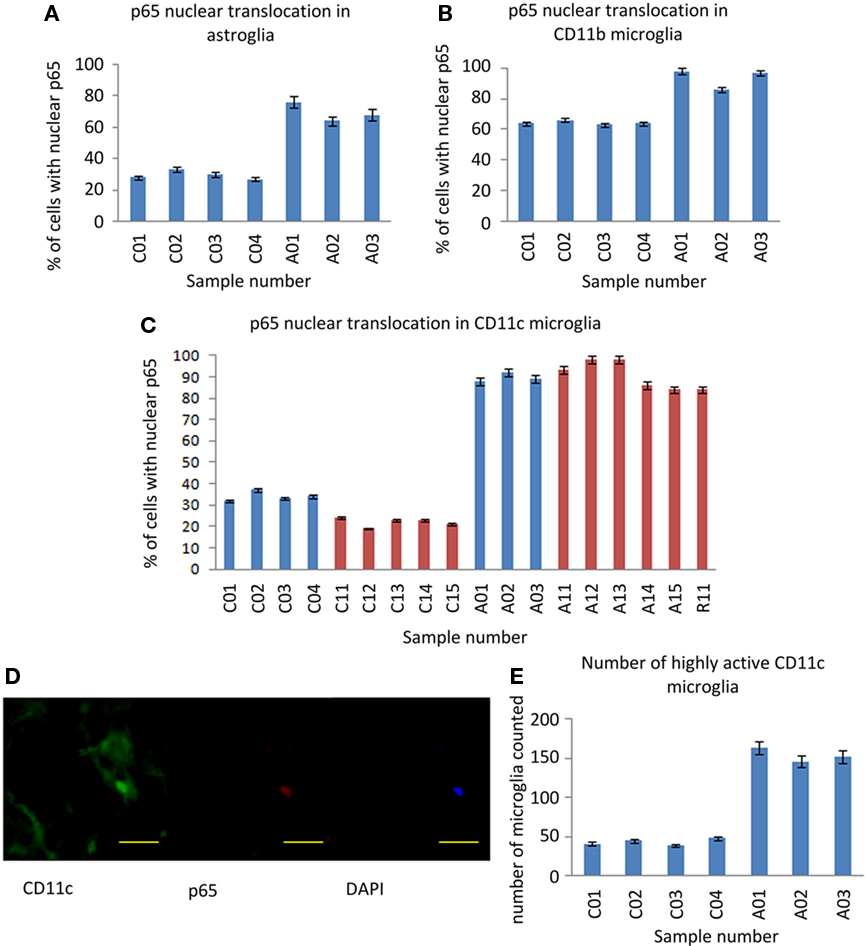
Figure 3. NF-κB p65 expression in astrocytes and microglia. (A) Percentage of GFAP stained astrocytes with anti-p65 nuclear staining. (B) Percentage of CD11b stained microglia with anti-p65 nuclear staining. (C) Percentage of CD11c stained (highly activated) microglia with anti-p65 nuclear staining. (D) A representative microglial cell with nuclear NF-κB p65 staining with anti-CD11c (green), anti-p65 (red), and DAPI (blue). Scale bar = 20 μm. (E) The number of CD11c positive microglia found in 20 fields of random sampling. Blue bars represent samples from the UK cohort, red bars samples from the US cohort. Sample IDs prefixed “A” are from ASC donors, prefixed “C” from control donors.
Between-group differences in the immunoreactivity of astrocytes stained by anti-GFAP were observed with 80% of cells from ASC samples scoring 2–3 compared to 56% of cells from control samples scoring 0–1; Table 2. Differences in nuclear NF-κB p65 expression between ASC and control tissue samples were observed. On average 88.00 ± 4.00% of astrocytes in tissue from ASC patients demonstrated nuclear localization of the transcription factor compared to 33.00 ± 3.16% in control samples; Figure 3A.
Differences in immunoreactivity for CD11b positive microglia were also observed, with 81% of cells from ASC samples scoring 2–3 compared to 59% of cells from control samples scoring 0–1; Table 2. The between-group difference in nuclear translocation in these cells was also more pronounced than in neurons. In CD11b positive microglia, 93.67 ± 3.00% of cells from samples from ASC patients expressed nuclear NF-κB p65 compared to 64.25 ± 1.26% from controls; Figure 3B.
Similarly, CD11c positive (highly active, mature) microglia in samples from ASC donors had raised levels of immunoreactivity with 88% of cells from ASC samples scoring in the range 2–3 compared to 58% of cells from control samples scoring in the range 0–1; Table 2. Nuclear NF-κB p65 expression in CD11c positive microglia from ASC samples was 89.67 ± 2.08% of cells and 34.00 ± 2.16% from controls; Figure 3C. Furthermore, using 20 visual fields randomly selected blind to group, the number of active CD11c positive cells present was 3.75 times greater in tissue from ASC donors; Figure 3E.
In summary, all cell types demonstrated increased extranuclear and nuclear translocated NF-κB p65 expression in samples of brain tissue from ASC donors relative to samples from matched controls. These between-groups differences were increased in astrocytes and microglia relative to neurons, but particularly pronounced for highly active microglia identified by anti-CD11c staining.
Expression of NF-κB P65 in Neurons and Microglia: US Cohort
Immunoreactivity measurements of samples from the US cohort stained by anti-Beta III Tubulin for neuronal identification revealed that 69% of cells from ASC samples scored 2–3, whilst 63% of cells from control samples scored 0–1; Table 2. Nuclear translocation of NF-κB p65 occurred in 20.83 ± 1.72% of cells in ASC samples compared to 14.60 ± 1.52% controls; Figure 2B.
Immunoreactivity analysis of CD11c positive microglia resulted in 85% of cells from ASC samples scoring 2–3, whilst 53% of cells from control samples scored 0–1; Table 2. Nuclear translocation occurred in 90.50 ± 6.66% of cells in ASC samples compared to 22.00 ± 2.00% from controls; Figure 3C.
Due to limited sample volumes, processing and analysis for astrocytes and CD11b positive microglia was not undertaken.
These data confirmed results derived from the UK cohort, with differences in nuclear translocation of NF-κB p65 in neurons 6.23% points higher for ASC samples from the UK cohort and 4.67% points higher from the US cohort. In CD11c positive, highly activated microglia, between-group differences in nuclear translocation of NF-κB p65 were similarly elevated in the US cohort (68.50% points higher in ASC samples) and the UK cohort (55.67% points higher).
Differences in pH and Relationship to NF-κB P65 Expression: UK Cohort
Measurement of homogenized tissue yielded a 0.92-unit pH between-group difference (Figure 4A), decreased in ASC samples relative to control samples from the UK cohort. The relationship between pH and NF-κB p65 expression was explored by linear regression and a highly significant effect observed [F(1,5) = 98.3; p = 0.00018; Figure 4B].
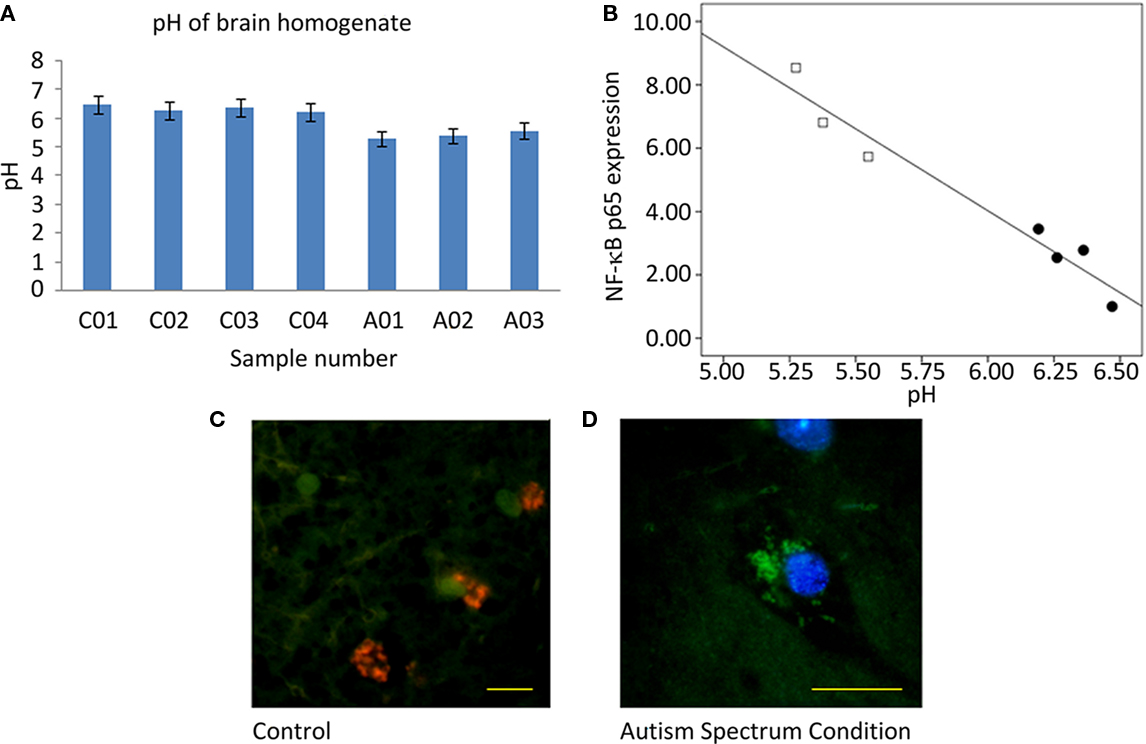
Figure 4. Measurement of pH. (A) Graph of pH of homogenized tissue samples. (B) Plot of normalized overall expression of NF-κB p65 from quantification of Western blot vs. pH of homogenized samples with a superimposed line of linear regression. Filled circles are samples from ASC donors, open circles samples from control donors. (C) Immunofluorescent image from a tissue sample from control donor (×63 objective, scale bar = 20 μm) and (D) from a sample from ASC donor (×100 objective, scale bar = 20 μm). Images are pseudocolored and show the ratio of acridine orange/Lysotracker Red fluorescence. Green hues represent low lysomic pH, and red hues represent high lysomic pH.
Aberrant pH was localized to subcellular compartments by immunofluorescence. Low pH observed in homogenized tissue from ASC samples appears to be a result of a reduced pH in the lysosomal compartments of cells. Tissue from ASC patients had lysosomes that fluoresced green whereas that from controls fluoresced orange; Figures 4C,D.
Discussion
An emerging focus of research into ASC has suggested neuroinflammation as an underlying biological model, with evidence from irregular cytokine profiles in the cerebrospinal fluid of children with ASC (Zimmerman et al., 2005), together with activated astrocyte and microglia in post-mortem brain tissue (Pardo et al., 2005). Nevertheless, the underlying molecular events remain unclear.
In this article, for the first time to our knowledge, we report the aberrant expression of a pro-inflammatory transcription factor, NF-κB, in samples donated to the London Brain Bank (UK cohort) and Harvard Brain Tissue Resource Center (US cohort). This discovery could play a major role in refining diagnostic tests and therapeutic interventions for ASC. Excess NF-κB p65 expression was observed in cytosolic, but predominantly nuclear compartments in ASC samples (Figure 1). These relative increases were subsequently localized to neurons, astrocytes, and microglia, but were particularly pronounced in highly activated (CD11c positive) microglia. Furthermore, nuclear translocation of NF-κB suggests activation of the molecule.
NF-κB induces the expression of inflammatory cytokines and chemokines and, in turn, is induced by them (Barnes and Karin, 1997; Pahl, 1999). This establishes a positive feedback mechanism (Perkins, 2004), which has the potential, when NF-κB becomes aberrantly active, to produce the chronic or excessive inflammation associated with several inflammatory diseases (Barnes and Karin, 1997; Mattson et al., 2000; Mattson and Meffert, 2006; Memet, 2006).
Primarily in neurons, NF-κB is activated in order to provide a protective function. A small, 6% point difference between ASC and control groups suggests the presence of extensive stress on neurons in ASC is unlikely. For confirmation, the cell morphology of neurons was screened (Mpoke and Wolfe, 2003) for signs of apoptosis or necrosis to assess the relative rates of cell death. There were minimal, if any, differences between-groups, an observation that concurs with work by Hausmann et al. (2004) who reported that apoptosis was not detected in non-traumatically injured brain tissue when the PMI was less than 72 h. The samples reported in the study fall into this category (Table 1). Although needing to be confirmed at the molecular level, this may well be a key finding as it demonstrates the potential reversibility of the condition, something not commonly observed in many neurological disorders where there is high irreversible cell death.
The elevated nuclear translocation in ASC samples (Figure 3) supports previous work on astrocyte and microglia activation in the condition (Pardo et al., 2005; Vargas et al., 2005; Zimmerman et al., 2005; Anderson et al., 2008). The activation of microglia induces an array of cellular events which accumulate to reduce neural function. This is potentially of interest more widely as previous studies have identified a potential link between low pH of homogenized tissue and learning disabilities (Rae et al., 2003) as well as Alzheimer’s disease (Majumdar et al., 2007).
Confirmation of the immunofluorescence results was obtained from an independent set of samples from the Autism Tissue Program at the Harvard Brain Tissue Resource Center (US cohort). Close correspondence in magnitude and direction of between-group differences with the UK cohort was observed. However, it is worthy of note that samples from the US cohort were donated by people very much younger than the UK cohort. Thus, as well as validating the results from the UK cohort, the observation of aberrant expression of NF-κB can be extended to cover an age range from 5 to 40 years.
While the origin of inflammatory signaling in ASC remains undetermined, genetic or epigenetic factors are mechanisms which can subsequently up-regulate the NF-κB signaling cascade. Animals subject to prenatal immunological challenges during early gestation subsequently displayed marked learning deficits (Meyer et al., 2006) and morphological brain changes post-natally (Li et al., 2009). Extracellular detection of pathogens by toll-like receptors leads to signaling pathways resulting in over-expression of NF-κB. Theoretically, this would allow for the range of environmental stimuli which are associated with the condition to act on a central node of the inflammatory component of the condition. Supported by the increase in NF-κB expression at the protein level, an inherited component is most likely why the chronic inflammatory state maintains throughout adulthood.
The ∼1 unit pH difference observed in homogenate brain tissue from controls and ASC patients (Figure 4A) appears to be a result of increased lysosomal activity (Figures 4C,D). Coupled to the highly significant linear relationship between pH and NF-κB (Figure 4B), the inference is that acidification does not influence cognitive function directly, but is a consequence of neuroinflammation.
Consistent observations of pH reduction in brain tissue from patients with schizophrenia have unclear origins, with medication and cause of death effects suggested in addition to it reflecting features of the disorder (Lipska et al., 2006; Halim et al., 2008). Thus, there remains the possibility that reductions in pH represent agonal artifact, and indeed ante mortem hypoxia and long terminal phases as well as gender, are known to lead to pH reductions in post-mortem brain tissue (Monoranu et al., 2009), although there is no correlation with PMI and age at death. In this study donors were matched on all these quantities (Table 1). The linear modeling between NF-κB concentrations and pH is highly significant and furthermore is located in the lysosomes (Figure 4) Nevertheless, a post-mortem change in pH from chemical cascades involving NF-κB cannot be excluded. Should further experimentation confirm the relationship between these cellular markers of inflammation and pH, then this may be a potential biomarker for diagnosis and response to therapeutic interventions. Measurements of in vivo intracellular pH can be achieved non-invasively with phosphorous-31 magnetic resonance spectroscopy (Pettegrew et al., 1988) or magnetization transfer techniques (Sun et al., 2007).
Conclusion
To summarize: NF-κB is aberrantly expressed in the orbitofrontal cortex as indicated by measurements on post-mortem tissue from ASC patients, and particularly in highly activated microglia. This region is a locus of abnormal function in ASC that underlies the abnormal development of social and cognitive skills (Sabbagh, 2004). This is the first discovery of its kind that identifies a potential mechanism for neuroinflammation in ASC through increased expression of this pro-inflammatory molecule and the significant involvement of resident immune cells. The connection of this result to changes in intracellular acidity indicates an investigation of pH across the entire brain parenchyma in living patients.
Whilst evidence of causal link remains to be established, the idea that the induction of inflammation via the NF-κB signaling cascade is observed in regions of the neocortex associated with behavioral and clinical symptoms of ASC gives credence and impetus to interventions focusing on this potential therapeutic target.
Conflict of Interest Statement
The authors declare that the research was conducted in the absence of any commercial or financial relationships that could be construed as a potential conflict of interest.
Acknowledgments
This work was supported by grants to Adam Young from Archimedes Pharmaceuticals, the University of St Andrews, and the Pathological Society of Great Britain and Ireland. We are grateful to J. Pickett and C. Eberhart at the Autism Tissue Program and the Harvard University, and C. Troakes at King’s College London Brain Bank, Institute of Psychiatry for providing samples. We thank Dr G. Cramb for his helpful comments and assistance. Finally, we extend our gratitude to the families of the donors for supporting the tissue collection programs.
References
Akiyama, H., Nishimura, T., Kondo, H., Ikeda, K., Hayashi, Y., and Mcgeer, P. L. (1994). Expression of the receptor for macrophage colony stimulating factor by brain microglia and its upregulation in brains of patients with Alzheimer’s disease and amyotrophic lateral sclerosis. Brain Res. 639, 171–174.
Anderson, M. P., Hooker, B. S., and Herbert, M. R. (2008). Bridging from cells to cognition in autism pathophysiology: biological pathways to defective brain function and plasticity. Am. J. Biochem. Biotechnol. 4, 167–176.
Barger, S. W. (2005). Vascular consequences of passive Abeta immunization for Alzheimer’s disease. Is avoidance of “malactivation” of microglia enough? J. Neuroinflammation 2, 2.
Barnes, P. J., and Karin, M. (1997). Nuclear factor-kappaB: a pivotal transcription factor in chronic inflammatory diseases. N. Engl. J. Med. 336, 1066–1071.
Baron-Cohen, S., Leslie, A. M., and Frith, U. (1985). Does the autistic child have a “theory of mind”? Cognition 21, 37–46.
Becher, B., and Antel, J. P. (1996). Comparison of phenotypic and functional properties of immediately ex vivo and cultured human adult microglia. Glia 18, 1–10.
Block, M. L., and Hong, J. S. (2005). Microglia and inflammation-mediated neurodegeneration: multiple triggers with a common mechanism. Prog. Neurobiol. 76, 77–98.
Eberhart, C. G., Copeland, J., and Abel, T. W. (2006). Brief report: S6 ribosomal protein phosphorylation in autistic frontal cortex and cerebellum: a tissue array analysis. J. Autism Dev. Disord. 36, 1131–1135.
Ford, A. L., Goodsall, A. L., Hickey, W. F., and Sedgwick, J. D. (1995). Normal adult ramified microglia separated from other central nervous system macrophages by flow cytometric sorting. Phenotypic differences defined and direct ex vivo antigen presentation to myelin basic protein-reactive CD4+ T cells compared. J. Immunol. 154, 4309–4321.
Frith, U. (1989). A new look at language and communication in autism. Br. J. Disord. Commun. 24, 123–150.
Girgis, R. R., Minshew, N. J., Melhem, N. M., Nutche, J. J., Keshavan, M. S., and Hardan, A. Y. (2007). Volumetric alterations of the orbitofrontal cortex in autism. Prog. Neuropsychopharmacol. Biol. Psychiatry 31, 41–45.
Glass, C. K., Saijo, K., Winner, B., Marchetto, M. C., and Gage, F. H. (2010). Mechanisms underlying inflammation in neurodegeneration. Cell 140, 918–934.
Halim, N. D., Lipska, B. K., Hyde, T. M., Deep-Soboslay, A., Saylor, E. M., Herman, M., Thakar, M. J., Verma, A., and Kleinman, J. E. (2008). Increased lactate levels and reduced pH in postmortem brains of schizophrenics: medication confounds. J. Neurosci. Methods 169, 208–213.
Hardan, A. Y., Girgis, R. R., Lacerda, A. L., Yorbik, O., Kilpatrick, M., Keshavan, M. S., and Minshew, N. J. (2006). Magnetic resonance imaging study of the orbitofrontal cortex in autism. J. Child Neurol. 21, 866–871.
Hausmann, R., Biermann, T., Wiest, I., Tubel, J., and Betz, P. (2004). Neuronal apoptosis following human brain injury. Int. J. Legal Med. 118, 32–36.
Hughes, E. H., Schlichtenbrede, F. C., Murphy, C. C., Sarra, G. M., Luthert, P. J., Ali, R. R., and Dick, A. D. (2003). Generation of activated sialoadhesin-positive microglia during retinal degeneration. Invest. Ophthalmol. Vis. Sci. 44, 2229–2234.
Kaltschmidt, B., Ndiaye, D., Korte, M., Pothion, S., Arbibe, L., Prullage, M., Pfeiffer, J., Lindecke, A., Staiger, V., Israel, A., Kaltschmidt, C., and Memet, S. (2006). NF-kappaB regulates spatial memory formation and synaptic plasticity through protein kinase A/CREB signaling. Mol. Cell. Biol. 26, 2936–2946.
Kim, Y. S., and Joh, T. H. (2006). Microglia, major player in the brain inflammation: their roles in the pathogenesis of Parkinson’s disease. Exp. Mol. Med. 38, 333–347.
Larsson, H. J., Eaton, W. W., Madsen, K. M., Vestergaard, M., Olesen, A. V., Agerbo, E., Schendel, D., Thorsen, P., and Mortensen, P. B. (2005). Risk factors for autism: perinatal factors, parental psychiatric history, and socioeconomic status. Am. J. Epidemiol. 161, 916–925; discussion 926–928.
Lee, H. C., Johnson, C., and Epel, D. (1983). Changes in internal pH associated with initiation of motility and acrosome reaction of sea urchin sperm. Dev. Biol. 95, 31–45.
Li, Q., Cheung, C., Wei, R., Hui, E. S., Feldon, J., Meyer, U., Chung, S., Chua, S. E., Sham, P. C., Wu, E. X., and McAlonan, G. M. (2009). Prenatal immune challenge is an environmental risk factor for brain and behavior change relevant to schizophrenia: evidence from MRI in a mouse model. PLoS ONE 4, e6354. doi: 10.1371/journal.pone.0006354
Lipska, B. K., Deep-Soboslay, A., Weickert, C. S., Hyde, T. M., Martin, C. E., Herman, M. M., and Kleinman, J. E. (2006). Critical factors in gene expression in postmortem human brain: focus on studies in schizophrenia. Biol. Psychiatry 60, 650–658.
Liu, B., and Hong, J. S. (2003). Role of microglia in inflammation-mediated neurodegenerative diseases: mechanisms and strategies for therapeutic intervention. J. Pharmacol. Exp. Ther. 304, 1–7.
Lord, C., Cook, E. H., Leventhal, B. L., and Amaral, D. G. (2000). Autism spectrum disorders. Neuron 28, 355–363.
Loveland, K. A., Bachevalier, J., Pearson, D. A., and Lane, D. M. (2008). Fronto-limbic functioning in children and adolescents with and without autism. Neuropsychologia 46, 49–62.
Majumdar, A., Cruz, D., Asamoah, N., Buxbaum, A., Sohar, I., Lobel, P., and Maxfield, F. R. (2007). Activation of microglia acidifies lysosomes and leads to degradation of Alzheimer amyloid fibrils. Mol. Biol. Cell 18, 1490–1496.
Mattson, M. P., Culmsee, C., Yu, Z., and Camandola, S. (2000). Roles of nuclear factor kappaB in neuronal survival and plasticity. J. Neurochem. 74, 443–456.
Mattson, M. P., and Meffert, M. K. (2006). Roles for NF-kappaB in nerve cell survival, plasticity, and disease. Cell Death Differ. 13, 852–860.
Memet, S. (2006). NF-kappaB functions in the nervous system: from development to disease. Biochem. Pharmacol. 72, 1180–1195.
Meyer, U., Feldon, J., Schedlowski, M., and Yee, B. K. (2006). Immunological stress at the maternal-foetal interface: a link between neurodevelopment and adult psychopathology. Brain Behav. Immun. 20, 378–388.
Monoranu, C. M., Apfelbacher, M., Grunblatt, E., Puppe, B., Alafuzoff, I., Ferrer, I., Al-Saraj, S., Keyvani, K., Schmitt, A., Falkai, P., Schittenhelm, J., Halliday, G., Kril, J., Harper, C., McLean, C., Riederer, P., and Roggendorf, W. (2009). pH measurement as quality control on human post mortem brain tissue: a study of the BrainNet Europe consortium. Neuropathol. Appl. Neurobiol. 35, 329–337.
Morgan, A. J., and Galione, A. (2007). NAADP induces pH changes in the lumen of acidic Ca2+ stores. Biochem. J. 402, 301–310.
Mpoke, S., and Wolfe, J. (2003). Differential staining of apoptotic nuclei in living cells: application to macronuclear elimination in tetrahymena. J. Histochem. 45, 675–683.
Neuhaus, E., Beauchaine, T. P., and Bernier, R. (2010). Neurobiological correlates of social functioning in autism. Clin. Psychol. Rev.
Ornitz, E. M., Lane, S. J., Sugiyama, T., and de Traversay, J. (1993). Startle modulation studies in autism. J. Autism Dev. Disord. 23, 619–637.
Pahl, H. L. (1999). Activators and target genes of Rel/NF-kappaB transcription factors. Oncogene 18, 6853–6866.
Pardo, C. A., Vargas, D. L., and Zimmerman, A. W. (2005). Immunity, neuroglia and neuroinflammation in autism. Int. Rev. Psychiatry 17, 485–495.
Paris, D., Patel, N., Quadros, A., Linan, M., Bakshi, P., Ait-Ghezala, G., and Mullan, M. (2007). Inhibition of Abeta production by NF-kappaB inhibitors. Neurosci. Lett. 415, 11–16.
Pettegrew, J. W., Withers, G., Panchalingam, K., and Post, J. F. M. (1988). Considerations for brain pH assessment by 31P NMR. Magn. Reson. Imaging 6, 135–142.
Rae, C., Scott, R. B., Lee, M., Simpson, J. M., Hines, N., Paul, C., Anderson, M., Karmiloff-Smith, A., Styles, P., and Radda, G. K. (2003). Brain bioenergetics and cognitive ability. Dev. Neurosci. 25, 324–331.
Sabbagh, M. A. (2004). Understanding orbitofrontal contributions to theory-of-mind reasoning: implications for autism. Brain Cogn. 55, 209–219.
Sajdel-Sulkowska, E. M., Xu, M., McGinnis, W., and Koibuchi, N. (2011). Brain region-specific changes in oxidative stress and neurotrophin levels in autism spectrum disorders (ASD). Cerebellum 10, 43–48.
Schmidt, E. L., and Bankole, R. O. (1965). Specificity of immunofluorescent staining for study of Aspergillus flavus in soil. Appl. Microbiol. 13, 673–679.
Shi, S. R., Liu, C., Balgley, B. M., Lee, C., and Taylor, C. R. (2006). Protein extraction from formalin-fixed, paraffin-embedded tissue sections: quality evaluation by mass spectrometry. J. Histochem. Cytochem. 54, 739–743.
Sun, P. Z., Zhou, J. Y., Sun, W. Y., Huang, J., and van Zijl, P. C. M. (2007). Detection of the ischemic penumbra using pH-weighted MRI. J. Cereb. Blood Flow Metab. 27, 1129–1136.
Vargas, D. L., Nascimbene, C., Krishnan, C., Zimmerman, A. W., and Pardo, C. A. (2005). Neuroglial activation and neuroinflammation in the brain of patients with autism. Ann. Neurol. 57, 67–81.
Zhong, H., Voll, R. E., and Ghosh, S. (1998). Phosphorylation of NF-kappa B p65 by PKA stimulates transcriptional activity by promoting a novel bivalent interaction with the coactivator CBP/p300. Mol. Cell 1, 661–671.
Keywords: NF-κB, autism spectrum condition, brain, inflammation, orbitofrontal cortex, pH
Citation: Young AMH, Campbell E, Lynch S, Suckling J and Powis SJ (2011) Aberrant NF-kappaB expression in autism spectrum condition: a mechanism for neuroinflammation. Front. Psychiatry 2:27. doi: 10.3389/fpsyt.2011.00027
Received: 10 December 2010;
Accepted: 03 May 2011;
Published online: 13 May 2011.
Edited by:
Lin He, Shanghai Jiao Tong University, ChinaReviewed by:
Gráinne Mcalonan, University of Hong Kong, Hong KongElizabeth Maria Sajdel-Sulkowska, Harvard Medical School, Brigham and Women’s Hospital, USA
Copyright: © 2011 Young, Campbell, Lynch, Suckling and Powis. This is an open-access article subject to a non-exclusive license between the authors and Frontiers Media SA, which permits use, distribution and reproduction in other forums, provided the original authors and source are credited and other Frontiers conditions are complied with.
*Correspondence: John Suckling, Department of Psychiatry, University of Cambridge, Herchel Smith Building, Robinson Way, Cambridge CB2 0SZ, UK. e-mail:anMzNjlAY2FtLmFjLnVr