- 1Department of Psychiatry and Psychotherapy, Charité—Universitätsmedizin Berlin, Berlin, Germany
- 2Berlin Institute of Health at Charité—Universitätsmedizin Berlin, BIH Biomedical Innovation Academy, Berlin, Germany
Recent clinical studies have shown that agonists at muscarinic acetylcholine receptors effectively reduce schizophrenia symptoms. It is thus conceivable that, for the first time, a second substance class of procholinergic antipsychotics could become established alongside the usual antidopaminergic antipsychotics. In addition, various basic science studies suggest that there may be a subgroup of schizophrenia in which hypofunction of muscarinic acetylcholine receptors is of etiological importance. This could represent a major opportunity for individualized treatment of schizophrenia if markers can be identified that predict response to procholinergic vs. antidopaminergic interventions. In this perspective, non-response to antidopaminergic antipsychotics, specific symptom patterns like visual hallucinations and strong disorganization, the presence of antimuscarinic antibodies, ERP markers such as mismatch negativity, and radiotracers are presented as possible in vivo markers of muscarinic deficit and thus potentially of response to procholinergic therapeutics. Finally, open questions and further research steps are outlined.
1. Introduction
For many decades, the dopamine theory has been the most influential neurobiological concept in understanding psychosis (1) and it is argued that all currently used antipsychotics work through directly or indirectly changing dopaminergic neurotransmission (2). Although there is converging evidence from different lines of research for the involvement of dopamine in the emergence of psychotic symptoms (1), alterations in other neurotransmitter systems have also been demonstrated, and there is growing agreement that different neurobiological etiologies can underlie psychotic syndromes (3–5).
Firstly, a glutamate theory of schizophrenia has been established mainly based on the observation that antagonists at the glutamatergic NMDA receptor, such as ketamine, phencyclidine, or endogenously formed anti-NMDA antibodies, directly and reliably cause psychotic symptoms and genetic studies showing an increased risk of schizophrenia in individuals with gene variants affecting the glutamate system (6, 7). However, since clinical trials of glutamatergic agents developed based on this theory have yielded only mixed results, no primary glutamatergic drug is to date available for routine clinical use. Currently, studies on glutamatergic drugs focus on compounds targeting specific receptor subtypes as well as on subgroup analyses to identify patient groups with marked glutamatergic alterations and hence potentially increased response to glutamatergic interventions (6, 7).
Secondly, reduced neurotransmission at acetylcholine receptors has been proposed as a non-dopaminergic mechanism underlying schizophrenia symptoms. Acetylcholine receptors are generally divided into nicotinic and muscarinic receptors according to binding ligand, and both have been implicated in the etiology of schizophrenia. Based on the extremely high rate of nicotine use in patients with schizophrenia, which has been interpreted as self-medication of existing deficits, nicotinic acetylcholine receptors, particularly the α7-subtype, have initially become the focus of research interest (8, 9). An important line of research in this context involves sensory gating, i.e., the inhibition of electrophysiological brain responses to (mostly auditory) stimuli played at close temporal intervals after an initial stimulus. Deficits in sensory gating have been frequently reported in schizophrenia patients and linked to attentional deficits (8, 10, 11). Interestingly, agonists at nicotinic receptors (e.g., nicotine) were shown to improve these sensory gating deficits, as well as some of the cognitive deficits, in schizophrenia patients (9, 12, 13). Moreover, post-mortem studies confirmed a reduced density of α7—receptors in different brain regions in deceased schizophrenia patients. Both findings renewed interest in developing nicotinic drugs for schizophrenia, especially for its cognitive deficits, which are insufficiently ameliorated by conventional antipsychotics (8, 9). However, despite promising results in preclinical and phase 1/2 trials, phase 3 trials on nicotinic agonists in schizophrenia have been disappointing due to lack of efficacy and/or tolerability and no primarily nicotinic drug is approved for schizophrenia treatment (12, 13). Current research on nicotinic agents for schizophrenia hence focuses on finding optimal dosage regimes and investigating allosteric modulators (13).
A deficit at muscarinic acetylcholine receptors in at least a proportion of patients with schizophrenia is suggested, for example, by post-mortem studies showing reduced density of muscarinic receptors in patients, by imaging studies with radioligands showing reduced availability of muscarinic receptors, and by the ability of muscarinic antagonists such as scopolamine to elicit schizophrenia-like symptoms in healthy individuals (14, 15). Based on post-mortem studies, it was proposed that approximately one quarter of schizophrenia patients belongs to a subgroup referred to as muscarinic receptor deficit sub-group with schizophrenia (MRDS), which shows a massive and widespread reduction in the density of muscarinic receptors in the CNS (16–18). Based on that, agonists and positive allosteric modulators (PAM) at muscarinic receptors have been investigated as potential schizophrenia treatments [Table 1 for an overview, (22) for further discussion]. Intriguingly, recent successes were reported in phase 2 (20) and phase 3 (21) trials of xanomeline, an agonist at the muscarinic M1 and M4 cholinergic receptors with no direct dopaminergic effect. It should be noted that in these trials, xanomeline was administered combined with trospium, a peripheral muscarinic antagonist, in order to mitigate peripheral cholinergic side effects, but for reasons of simplicity, I will speak of xanomeline as the centrally active compound in the following.
These developments make it seem possible that in the near future, a second substance class of muscarinic antipsychotics may be established alongside current antidopaminergic antipsychotics. This would lend new urgent importance to the question of whether there is an “antimuscarinic subtype” in schizophrenia and, particularly, how to identify it in clinical settings since this subtype might show non-response to antidopaminergic medication and response to procholinergic treatment. This perspective is intended to present several potential in vivo markers of muscarinic deficit in schizophrenia that ideally in the future could be used for personalized therapy planning and help in deciding whether to initiate treatment primarily with a dopamine antagonist or a muscarinic acetylcholine agonist.
2. Possible biomarkers for muscarinic dysfunction in schizophrenia
The following sections describe markers that could indicate a muscarinic deficit in patients with schizophrenia. A summary is provided in Table 2.
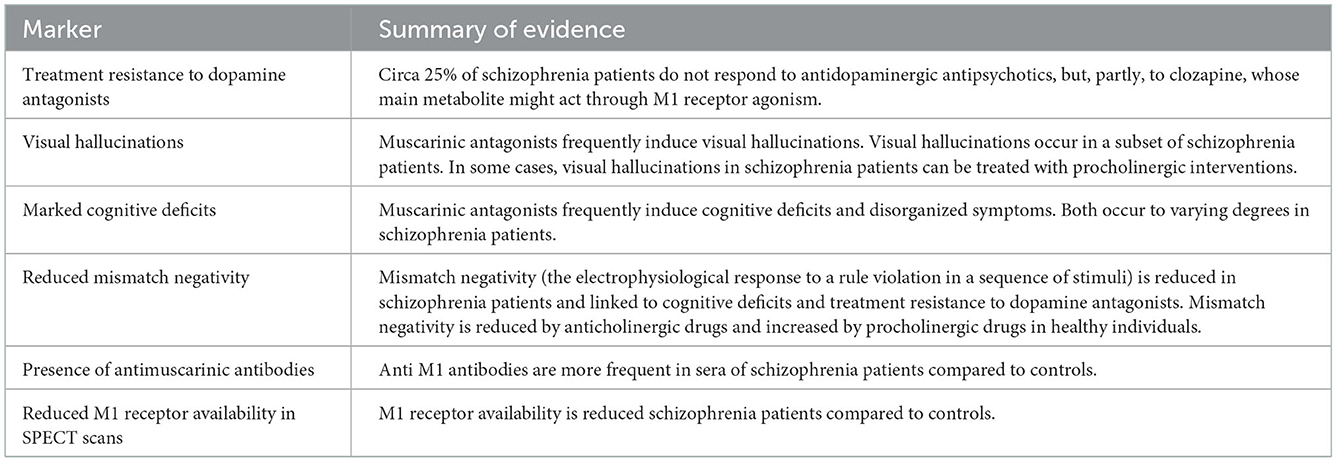
Table 2. Possible markers of muscarinic deficits in schizophrenia and brief summary of the evidence.
2.1. Non-response to dopaminergic antipsychotics
About 20% of schizophrenia patients show no improvement at all when treated treated with common antipsychotics (23). Currently, clozapine is the most effective antipsychotic for patients with non-response to antidopaminergic agents (24). Its superiority over all other antipsychotics, as well as its particular efficacy (25) in non-responders to antidopaminergic antipsychotics hints to a unique non-dopaminergic mechanism of action of clozapine. In this context, it is crucial to note that N-desmethylclozapine, the major metabolite of clozapine, exhibits unique agonism at the M1 receptor (26, 27), potentially making it the first representative of the “procholinergic” antipsychotics (28). Its specific efficacy in non-responders to antidopaminergic antipsychotics together with the procholinergic property of clozapine's active metabolite make it conceivable that a proportion of patients unresponsive to antidopaminergic antipsychotics in fact have a muscarinic deficit underlying their symptoms, which is partially revised by N-desmethylclozapine. If this were true, the relative efficacy of other procholinergic antipsychotics such as xanomeline could be particularly high in patients with treatment resistance to antidopaminergic antipsychotics.
2.2. Specific pattern of symptoms
A hypothetical subtype of schizophrenia with a predominant underlying muscarinic deficit might show specific symptom patterns compared with other subtypes. Information about these clinical correlates of a muscarinic deficit may be provided by the symptoms elicited by anticholinergic agents. First and foremost here are cognitive impairments, which are caused by anticholinergics (29) and are also present in a large proportion of patients with schizophrenia (30). Moreover, intoxications with muscarine receptor antagonists induce psychotic states with specific characteristics which are only present in a minority of schizophrenia patients, like visual hallucinations and strong disorganization (31). Interestingly, case reports suggest that visual hallucinations in some schizophrenia patients can successfully be treated with acetylcholine esterase inhibitors, suggesting an underlying cholinergic deficit (32). From this, it can be hypothesized that patients with marked cognitive deficits and disorganization and with visual hallucinations might specifically benefit from procholinergic treatment.
2.3. Mismatch negativity
Mismatch negativity (MMN) refers to the electrophysiological response to a rule violation (e.g., an irregular pitch) in a sequence of (in most studies auditory) stimuli. A reduction of MMN in patients with schizophrenia compared to controls has now been shown in many studies and is considered one of the most promising biomarkers for predicting the risk of conversion to clinical psychosis in people at high risk and the response to specific therapies (33–35). In addition to the established deficits in higher cognitive processes, schizophrenia patients also show disturbances in basal sensory processes, such as pitch recognition (36). These sensory deficits are associated with difficulties in detecting linguistic subtleties such as irony or emotional coloring and consequently linked to the psychosocial functional impairments that are often prognostic in schizophrenia (36, 37). Measures of MMN are correlated to the magnitude of these sensory processing deficits (38). Consistent with that, recent studies revealed that reduced MMN is particularly present in a subgroup of patients with marked cognitive deficits and poor social functioning (34, 39, 40) and predicts poor response to therapy with common antipsychotics (41, 42). Studies on the neurophysiological substrate of MMN reductions have demonstrated associations to hypofunction of the glutamatergic NMDA receptor (43). However, a reduction in MMN through an antimuscarinic drug (scopolamine as compared to glycopyrrolate, a peripheral anticholinergic without effects in the CNS) has also been demonstrated in healthy individuals (44). Importantly, it was shown in a recent preregistered trial that only biperiden, a muscarinic acetylcholine receptor antagonist, but not amisulpride, a dopamine D2/D3 receptor antagonist, galantamine, an acetylcholinesterase inhibitor increasing acetylcholine levels, nor levodopa, a dopamine precursor increasing dopamine levels, changed mismatch negativity in healthy individuals (45, 46). Taken together, these findings suggest that muscarinic deficits entail reduced mismatch negativity, possibly through dysfunctional modulation of NMDA-dependent neurotransmission (4, 47). This would be consistent with the finding that response to antidopaminergic drugs is weaker in patients with strong MMN reductions. If reduced MMN indeed indicated a cholinergic deficit, it could be hypothesized that it predicts good response to procholinergic treatment.
2.4. Antimuscarinic antibodies
The exact pathomechanism underlying a potential cholinergic deficit in schizophrenia is unclear, with post-mortem studies suggesting reduced receptor density (15). Interestingly, antibodies targeting M1 receptors can also be found significantly more often in the sera of schizophrenia patients compared to controls, although this comparison ]based on few rather small studies (48). Within schizophrenia patients, one study reported significant correlations between M1 antibodies and specific negative symptoms (poverty of speech) (49), which are in turn predictive of poor outcomes under usual treatments (50). The exact effect of these antibodies on muscarinic receptors has yet to be elucidated and agonistic or antagonistic impacts are possible as well as an antibody-mediated destruction of receptors or receptor-expressing cells (48). Although data are still sparse, it seems possible that antimuscarinic antibodies play an etiologic role in a subset of patients. Their presence could accordingly be a predictive marker for a good response to promuscarinic treatment.
2.5. Neuroimaging
The muscarinic M1 receptor availability can be determined in vivo using radioactive tracers, such as 123I-IQNB for SPECT (51). In schizophrenia, a reduced M1 receptor availability compared to healthy controls was reported (52). With respect to associations between M1 receptor availability and specific schizophrenia symptoms, results have been mixed, with reports that lower receptor availability is related to increased positive symptoms (52) and, conversely, to increased cognitive deficits and negative symptoms but not positive symptoms (53). The sensitivity of in vivo assessments of M1 receptor status might be further increased with newly developed PET tracers for the M1 receptor such as 11C-LSN3172176 (54). Neuroimaging with radioligands thus has the potential to determine the extent of muscarinic deficit in schizophrenia and potentially to predict the benefit of procholinergic treatment. Along similar lines, the striatal dopamine synthesis capacity assessed via F-DOPA-PET has been used to objectify a hyperdopaminergic state in schizophrenia patients (55). Recent research indeed showed that absence of hyperdopaminergia according to F-DOPA-PET predicted subsequent non-response to antipsychotics (56). Neuroimaging markers could thus potentially inform therapy assignment by providing primary procholinergic medication to patients who lack an increase in striatal dopamine synthesis but have a reduction in muscarinic receptor availability.
3. Discussion
The current success of agonists at muscarinic acetylcholine receptors in the treatment of schizophrenia makes it seem possible that in the future a second substance class of procholinergic antipsychotics will be established alongside the primarily antidopaminergic antipsychotics. In this case, it would be important for individualized treatment to find specific predictors of response to procholinergic (compared with antidopaminergic) antipsychotics. Possible candidates for such predictors include treatment resistance to antidopaminergic treatment, the presence of severe cognitive impairment and/or visual hallucinations, reduced mismatch negativity, the presence of antimuscarinic antibodies, and reduced availability of muscarinic receptors on radionucleotide imaging.
Muscarinic acetylcholine receptors can be divided into 5 subtypes (M1–M5), with mainly agonists at M1 and M4 subtypes being considered candidates in schizophrenia treatment, whereas stimulation of M2 and M3 subtypes is related to gastrointestinal and other peripheral side effects. Animal research furthermore suggests that enforcing stimulation at M4 receptors through positive allosteric modulators (PAM) particularly improves proxy markers of positive schizophrenia symptoms such as hyperlocomotion or impaired prepulse inhibition induced by dopamine agonists or NMDA antagonists (28, 57). In contrast, PAM at M1 receptors have mainly been investigated for cognition-enhancing potentials across different neuropsychiatric disorders including schizophrenia. For instance, it could be shown that PAM at M1 receptors improve performance in tasks designed to assess cognitive ability (e.g. novel object recognition task) in schizophrenia mouse models (28, 57). While xanomeline is an M1 and M4 preferring but not fully specific muscarinic agonist, these results highlight that possibly in the future specific schizophrenia symptom clusters could be addressed with selective modulators. In this case, treatment could be guided by both predominant symptoms (positive vs. cognitive symptoms) and possibly by investigations with subtype-specific PET tracers (58).
A crucial question in attempts to find biomarkers for response to muscarinic agonists concerns the precise cause-effect relationship between muscarinic deficit and psychotic symptoms, specifically the question, if muscarinic deficiency causes psychosis through dopaminergic or dopamine-independent pathways. Obviously, the hope to identify distinct predictors for the response to antidopaminergic vs. procholinergic treatment rests on the assumption that there are dissociable etiologies behind psychotic syndromes (schematically, a hyperdopaminergic and a hypomuscarinergic etiology, respecetively). A variety of complex interactions between muscarinergic and dopaminergic neurotransmission have been established (59), leading to the hypothesis that muscarinic agonists ultimately work through modifying dopamine release (14). This hypothesis is supported for example by the finding that psychotic symptoms in M1 receptor knockout mice are related to increased dopamine levels in the nucleus accumbens (60). Conversely, substantial parts of the evidence summarized above like the existence of a distinguishable subgroup with marked deficiency in muscarinic receptors, the non-response to antidopaminergic drugs in a proportion of patients, and the discovery that non-responding patients show no alterations in the dopamine system according to PET studies speak for the existence of patient subgroup where psychotic symptoms are mainly caused by dopamine-independent mechanisms (3). Accordingly, it has been hypothesized that different subtypes of schizophrenia pathophysiologically involve a disturbance in the dopaminergic vs. cholinergic modulation of glutamate receptors, which ultimately causes the development of psychotic symptoms (4). This would indeed imply a primarily dopaminergic vs. a primarily cholinergic etiology, and thus the hope that (a proportion of) patients with inadequate response to dopaminergic interventions could benefit from muscarinergic agents. Fittingly, a potential synergism of antidopaminergic and promuscarinic interventions is suggested by animal studies showing enhanced efficacy of classical antipsychotics by additional administration of M1 receptor PAM (61). Results from the ongoing ARISE trial, which is evaluating the efficacy of xanomeline-trospium as add-on therapy in 400 patients with inadequate response to antidopaminergic antipsychotics such as risperone, paliperone, and aripiprazole and is scheduled to be completed in March 2024 (62), may soon provide new insights into this debate: Adding a second antipsychotic to a first antipsychotic has generally not been shown to be effective in randomized trials (63) and is not a recommended strategy in the case of non-response (64), which may be because all current antipsychotics ultimately act through dopaminergic mechanisms and therefore no synergistic effect occurs when combining multiple agents. Hence, xanomeline proving effective as an add-on treatment would be (tentative) evidence that it indeed possesses a distinct non-dopaminergic mechanism of action.
The most rigorous way of testing the capacity of the suggested biomarkers to identify those schizophrenia patients who will likely benefit more from a procholinergic as compared to an antidopaminergic treatment would be to test their specific ability to predict individual outcome of a clinical trial of, for example xanomeline-trospium compared with amisulpride treatment. A specific predictor, as compared to a general prognostic factor, is a pretreatment variable (e.g., a quantification of the markers listed above), whose influence on the treatment outcome (e.g., on the reduction in a schizophrenia severity score such as the positive and negative syndrome scale) differs between treatments (65). For instance, it could be hypothesized that presence of antimuscarinic antibodies predicts good treatment outcome in the xanomeline arm, but bad outcome in the amisulpride arm of a trial. Statistically, this would be captured in a significant interaction effect between presence of antimuscarinic antibodies and treatment in a predictive model (65). A study testing such treatment-predictor interactions would be quite effortful, as all potential markers would need to be collected at baseline and the detection of treatment-by-variable interactions requires relatively large sample sizes (66). However, if successful, i.e., if one or more markers prove to be specific predictors of the outcome of procholinergic or antidopaminergic treatment, it would represent a real milestone in the individualized treatment of schizophrenia.
Data availability statement
The original contributions presented in the study are included in the article/supplementary material, further inquiries can be directed to the corresponding author.
Author contributions
The author confirms being the sole contributor of this work and has approved it for publication.
Funding
HS was participant in the BIH Charité Digital Clinician Scientist Program funded by the Charité—Universitätsmedizin Berlin and the Berlin Institute of Health at Charité (BIH).
Conflict of interest
The author declares that the research was conducted in the absence of any commercial or financial relationships that could be construed as a potential conflict of interest.
Publisher's note
All claims expressed in this article are solely those of the authors and do not necessarily represent those of their affiliated organizations, or those of the publisher, the editors and the reviewers. Any product that may be evaluated in this article, or claim that may be made by its manufacturer, is not guaranteed or endorsed by the publisher.
References
1. Howes OD, Kapur S. The dopamine hypothesis of schizophrenia: version III—the final common pathway. Schizophr Bull. (2009) 35:549–62. doi: 10.1093/schbul/sbp006
2. Seeman P. Targeting the Dopamine D2 receptor in schizophrenia. Expert Opin Ther Targets. (2006) 10:515–31. doi: 10.1517/14728222.10.4.515
3. Howes OD, Kapur S, A. Neurobiological hypothesis for the classification of schizophrenia: type A (Hyperdopaminergic) and type B (normodopaminergic). Br J Psychiatry. (2014) 205:1–3. doi: 10.1192/bjp.bp.113.138578
4. Stephan KE, Friston KJ, Frith CD. Dysconnection in schizophrenia: from abnormal synaptic plasticity to failures of self-monitoring. Schizophr Bull. (2009) 35:509–27. doi: 10.1093/schbul/sbn176
5. Keshavan MS, Nasrallah HA, Tandon R. Schizophrenia, “Just the Facts” 6. Moving ahead with the schizophrenia concept: from the elephant to the mouse. Schizophr Res. (2011) 127:3–13. doi: 10.1016/j.schres.2011.01.011
6. Uno Y, Coyle JT. Glutamate hypothesis in schizophrenia. Psychiatr Clin Neurosci. (2019) 73:204–15. doi: 10.1111/pcn.12823
7. Moghaddam B, Javitt D. From revolution to evolution: the glutamate hypothesis of schizophrenia and its implication for treatment. Neuropsychopharmacology. (2012) 37:4–15. doi: 10.1038/npp.2011.181
8. Adler LE, Olincy A, Waldo M, Harris JG, Griffith J, Stevens K, et al. Schizophrenia, sensory gating, and nicotinic receptors. Schizophr Bull. (1998) 24:189–202. doi: 10.1093/oxfordjournals.schbul.a033320
9. Martin LF, Freedman R. Schizophrenia and the alpha7 nicotinic acetylcholine receptor. Int Rev Neurobiol. (2007) 78:225–46. doi: 10.1016/S0074-7742(06)78008-4
10. Potter D, Summerfelt A, Gold J, Buchanan RW. Review of clinical correlates of P50 sensory gating abnormalities in patients with schizophrenia. Schizophr Bull. (2006) 32:692–700. doi: 10.1093/schbul/sbj050
11. Patterson JV, Hetrick WP, Boutros NN, Jin Y, Sandman C, Stern H, et al. P50 Sensory gating ratios in schizophrenics and controls: a review and data analysis. Psychiatr Res. (2008) 158:226–47. doi: 10.1016/j.psychres.2007.02.009
12. Terry AV Jr. Callahan PM. Alpha7 nicotinic acetylcholine receptors as therapeutic targets in schizophrenia: update on animal and clinical studies and strategies for the future. Neuropharmacology. (2020) 170:108053. doi: 10.1016/j.neuropharm.2020.108053
13. Tregellas JR, Wylie KP. Alpha7 nicotinic receptors as therapeutic targets in schizophrenia. Nicotine Tob Res. (2019) 21:349–56. doi: 10.1093/ntr/nty034
14. Raedler TJ, Bymaster FP, Tandon R, Copolov D, Dean B. Towards a muscarinic hypothesis of schizophrenia. Mol Psychiatry. (2007) 12:232–46. doi: 10.1038/sj.mp.4001924
15. Scarr E, Dean B. Muscarinic receptors: do they have a role in the pathology and treatment of schizophrenia? J Neurochem. (2008) 107:1188–95. doi: 10.1111/j.1471-4159.2008.05711.x
16. Scarr E, Cowie TF, Kanellakis S, Sundram S, Pantelis C, Dean B. Decreased cortical muscarinic receptors define a subgroup of subjects with schizophrenia. Mol Psychiatry. (2009) 14:1017–23. doi: 10.1038/mp.2008.28
17. Scarr E, Craig JM, Cairns MJ, Seo MS, Galati JC, Beveridge NJ, et al. Decreased cortical muscarinic M1 receptors in schizophrenia are associated with changes in gene promoter methylation, MRNA and gene targeting microrna. Transl Psychiatry. (2013) 3:e230. doi: 10.1038/tp.2013.3
18. Gibbons AS, Scarr E, Boer S, Money T, Jeon WJ, Felder C, et al. Widespread decreases in cortical muscarinic receptors in a subset of people with schizophrenia. Int J Neuropsychopharmacol. (2013) 16:37–46. doi: 10.1017/S1461145712000028
19. Cerevel Therapeutics. Topline results for Cvl-231 in phase 1B clinical trial in patients with schizophrenia (2021). Available online at: https://investors.cerevel.com/news-releases/news-release-details/cerevel-therapeutics-announces-positive-topline-results-cvl-231/ (accessed December 7, 2022).
20. Brannan SK, Sawchak S, Miller AC, Lieberman JA, Paul SM, Breier A. Muscarinic cholinergic receptor agonist and peripheral antagonist for schizophrenia. N Engl J Med. (2021) 384:717–26. doi: 10.1056/NEJMoa2017015
21. Karuna Therapeutics. Topline results: phase 3 emergent-2 trial of karxt in schizophrenia (2022). Available online at: https://investors.karunatx.com/static-files/be83d1a8-35a7-43b6-b842-b7140ba9a51d (accessed December 7, 2022).
22. Yohn SE, Weiden PJ, Felder CC, Stahl SM. Muscarinic acetylcholine receptors for psychotic disorders: bench-side to clinic. Trends Pharmacol Sci. (2022) 43:1098–112. doi: 10.1016/j.tips.2022.09.006
23. Samara MT, Nikolakopoulou A, Salanti G, Leucht S. How Many Patients with Schizophrenia Do Not Respond to Antipsychotic Drugs in the Short Term? An Analysis Based on Individual Patient Data from Randomized Controlled Trials. Schizophr Bull. (2019) 45:639–46. doi: 10.1093/schbul/sby095
24. Siskind D, McCartney L, Goldschlager R, Kisely S, Clozapine V. First- and second-generation antipsychotics in treatment-refractory schizophrenia: systematic review and meta-analysis Br J Psychiatr. (2016) 209:385–92. doi: 10.1192/bjp.bp.115.177261
25. Agid O, Arenovich T, Sajeev G, Zipursky RB, Kapur S, Foussias G, et al. An algorithm-based approach to first-episode schizophrenia: response rates over 3 prospective antipsychotic trials with a retrospective data analysis. J Clin Psychiatry. (2011) 72:1439–44. doi: 10.4088/JCP.09m05785yel
26. Sur C, Mallorga PJ, Wittmann M, Jacobson MA, Pascarella D, Williams JB, et al. N-desmethylclozapine, an allosteric agonist at muscarinic 1 receptor, potentiates n-methyl-d-aspartate receptor activity. Proc Natl Acad Sci U S A. (2003) 100:13674–9. doi: 10.1073/pnas.1835612100
27. Weiner DM, Meltzer HY, Veinbergs I, Donohue EM, Spalding TA, Smith TT, et al. The role of M1 muscarinic receptor agonism of n-desmethylclozapine in the unique clinical effects of clozapine. Psychopharmacology (Berl). (2004) 177:207–16. doi: 10.1007/s00213-004-1940-5
28. Foster DJ, Bryant ZK, Conn PJ. Targeting muscarinic receptors to treat schizophrenia. Behav Brain Res. (2021) 405:113201. doi: 10.1016/j.bbr.2021.113201
29. Campbell N, Boustani M, Limbil T, Ott C, Fox C, Maidment I, et al. The cognitive impact of anticholinergics: a clinical review. Clin Interv Aging. (2009) 4:225–33. doi: 10.2147/cia.s5358
30. Fioravanti M, Carlone O, Vitale B, Cinti ME, Clare L, A. Meta-analysis of cognitive deficits in adults with a diagnosis of schizophrenia. Neuropsychol Rev. (2005) 15:73–95. doi: 10.1007/s11065-005-6254-9
31. Lakstygal AM, Kolesnikova TO, Khatsko SL, Zabegalov KN, Volgin AD, Demin KA, et al. Dark classics in chemical neuroscience: atropine, scopolamine, and other anticholinergic deliriant hallucinogens. ACS Chem Neurosci. (2019) 10:2144–59. doi: 10.1021/acschemneuro.8b00615
32. Patel SS, Attard A, Jacobsen P, Shergill S. Acetylcholinesterase inhibitors (Achei's) for the treatment of visual hallucinations in schizophrenia: a case report. BMC Psychiatry. (2010) 10:68. doi: 10.1186/1471-244X-10-68
33. Luck SJ, Mathalon DH, O'Donnell BF, Hamalainen MS, Spencer KM, Javitt DC, et al. A roadmap for the development and validation of event-related potential biomarkers in schizophrenia research. Biol Psychiatry. (2011) 70:28–34. doi: 10.1016/j.biopsych.2010.09.021
34. Light GA, Swerdlow NR. Future clinical uses of neurophysiological biomarkers to predict and monitor treatment response for schizophrenia. Ann N Y Acad Sci. (2015) 1344:105–19. doi: 10.1111/nyas.12730
35. Erickson MA, Ruffle A, Gold JM, A. Meta-analysis of mismatch negativity in schizophrenia: from clinical risk to disease specificity and progression. Biol Psychiatry. (2016) 79:980–7. doi: 10.1016/j.biopsych.2015.08.025
36. Javitt DC, Sweet RA. Auditory dysfunction in schizophrenia: integrating clinical and basic features. Nat Rev Neurosci. (2015) 16:535–50. doi: 10.1038/nrn4002
37. Revheim N, Corcoran CM, Dias E, Hellmann E, Martinez A, Butler PD, et al. Reading deficits in schizophrenia and individuals at high clinical risk: relationship to sensory function, course of illness, and psychosocial outcome. Am J Psychiatry. (2014) 171:949–59. doi: 10.1176/appi.ajp.2014.13091196
38. Naatanen R, Kahkonen S. Central auditory dysfunction in schizophrenia as revealed by the mismatch negativity (MMN) and its magnetic equivalent MMNM: a review. Int J Neuropsychopharmacol. (2009) 12:125–35. doi: 10.1017/S1461145708009322
39. Naatanen R, Kujala T, Kreegipuu K, Carlson S, Escera C, Baldeweg T, et al. The mismatch negativity: an index of cognitive decline in neuropsychiatric and neurological diseases and in ageing. Brain. (2011) 134(Pt 12):3435-53. doi: 10.1093/brain/awr064
40. Wu G, Tang X, Gan R, Zeng J, Hu Y, Xu L, et al. Automatic auditory processing features in distinct subtypes of patients at clinical high risk for psychosis: forecasting remission with mismatch negativity. Hum Brain Mapp. (2022). doi: 10.1002/hbm.26021
41. Kim M, Kim T, Hwang WJ, Lho SK, Moon S, Lee TY, et al. Forecasting prognostic trajectories with mismatch negativity in early psychosis. Psychol Med (2021) 2:1–11. doi: 10.1017/S0033291721003068
42. Nakajima S, Higuchi Y, Tateno T, Sasabayashi D, Mizukami Y, Nishiyama S, et al. Duration mismatch negativity predicts remission in first-episode schizophrenia patients. Front Psychiatry. (2021) 12:777378. doi: 10.3389/fpsyt.2021.777378
43. Harms L, Parras GG, Michie PT, Malmierca MS. The role of glutamate neurotransmission in mismatch negativity (mmn), a measure of auditory synaptic plasticity and change-detection. Neuroscience. (2021) 456:106–13. doi: 10.1016/j.neuroscience.2020.01.046
44. Pekkonen E, Hirvonen J, Jaaskelainen IP, Kaakkola S, Huttunen J. Auditory sensory memory and the cholinergic system: implications for alzheimer's disease. Neuroimage. (2001) 14:376–82. doi: 10.1006/nimg.2001.0805
45. Schobi D, Homberg F, Frassle S, Endepols H, Moran RJ, Friston KJ, et al. Model-based prediction of muscarinic receptor function from auditory mismatch negativity responses. Neuroimage. (2021) 237:118096. doi: 10.1016/j.neuroimage.2021.118096
46. Weber LA, Tomiello S, Schobi D, Wellstein KV, Mueller D, Iglesias S, et al. Auditory mismatch responses are differentially sensitive to changes in muscarinic acetylcholine vs. dopamine receptor function. Elife. (2022) 11. doi: 10.7554/eLife.74835
47. Gu Q. Neuromodulatory transmitter systems in the cortex and their role in cortical plasticity. Neuroscience. (2002) 111:815–35. doi: 10.1016/s0306-4522(02)00026-x
48. Ryan AE, Mowry BJ, Kesby JP, Scott JG, Greer JM. Is there a role for antibodies targeting muscarinic acetylcholine receptors in the pathogenesis of schizophrenia? Aust N Z J Psychiatry. (2019) 53:1059–69. doi: 10.1177/0004867419864438
49. Jones AL, Mowry BJ, McLean DE, Mantzioris BX, Pender MP, Greer JM. Elevated levels of autoantibodies targeting the m1 muscarinic acetylcholine receptor and neurofilament medium in sera from subgroups of patients with schizophrenia. J Neuroimmunol. (2014) 269:68–75. doi: 10.1016/j.jneuroim.2014.02.008
50. Wilcox J, Winokur G, Tsuang M. Predictive value of thought disorder in new-onset psychosis. Compr Psychiatry. (2012) 53:674–8. doi: 10.1016/j.comppsych.2011.12.002
51. Eckelman WC. Imaging of muscarinic receptors in the central nervous system. Curr Pharm Des. (2006) 12:3901–13. doi: 10.2174/138161206778559678
52. Raedler TJ, Knable MB, Jones DW, Urbina RA, Gorey JG, Lee KS, et al. In vivo determination of muscarinic acetylcholine receptor availability in schizophrenia. Am J Psychiatry. (2003) 160:118–27. doi: 10.1176/appi.ajp.160.1.118
53. Bakker G, Vingerhoets C, Boucherie D, Caan M, Bloemen O, Eersels J, et al. Relationship between muscarinic M1 receptor binding and cognition in medication-free subjects with psychosis. Neuroimage Clin. (2018) 18:713–9. doi: 10.1016/j.nicl.2018.02.030
54. Naganawa M, Nabulsi N, Henry S, Matuskey D, Lin SF, Slieker L, et al. First-in-human assessment of (11)C-Lsn3172176, an M1 muscarinic acetylcholine receptor pet radiotracer. J Nucl Med. (2021) 62:553–60. doi: 10.2967/jnumed.120.246967
55. McCutcheon R, Beck K, Jauhar S, Howes OD. Defining the locus of dopaminergic dysfunction in schizophrenia: a meta-analysis and test of the mesolimbic hypothesis. Schizophr Bull. (2018) 44:1301–11. doi: 10.1093/schbul/sbx180
56. Veronese M, Santangelo B, Jauhar S, D'Ambrosio E, Demjaha A, Salimbeni H, et al. A Potential biomarker for treatment stratification in psychosis: evaluation of an [(18)F] fdopa pet imaging approach. Neuropsychopharmacology. (2021) 46:1122–32. doi: 10.1038/s41386-020-00866-7
57. Moran SP, Maksymetz J, Conn PJ. Targeting muscarinic acetylcholine receptors for the treatment of psychiatric and neurological disorders. Trends Pharmacol Sci. (2019) 40:1006–20. doi: 10.1016/j.tips.2019.10.007
58. Ozenil M, Aronow J, Millard M, Langer T, Wadsak W, Hacker M, et al. Update on pet tracer development for muscarinic acetylcholine receptors. pharmaceuticals (basel) (2021) 14:6. doi: 10.3390/ph14060530
59. Lester DB, Rogers TD, Blaha CD. Acetylcholine-dopamine interactions in the pathophysiology and treatment of CNS disorders. CNS Neurosci Ther. (2010) 16:137–62. doi: 10.1111/j.1755-5949.2010.00142.x
60. Gerber DJ, Sotnikova TD, Gainetdinov RR, Huang SY, Caron MG, Tonegawa S. Hyperactivity, elevated dopaminergic transmission, and response to amphetamine in M1 muscarinic acetylcholine receptor-deficient mice. Proc Natl Acad Sci U S A. (2001) 98:15312–7. doi: 10.1073/pnas.261583798
61. Choy KH, Shackleford DM, Malone DT, Mistry SN, Patil RT, Scammells PJ, et al. Positive allosteric modulation of the muscarinic M1 receptor improves efficacy of antipsychotics in mouse glutamatergic deficit models of behavior. J Pharmacol Exp Ther. (2016) 359:354–65. doi: 10.1124/jpet.116.235788
62. Clinicaltrials.gov. A study to assess efficacy and safety of adjunctive karxt in subjects with inadequately controlled symptoms of schizophrenia (Arise) (2021). Available online at: https://clinicaltrials.gov/ct2/show/NCT05145413 (accessed December 7, 2022).
63. Galling B, Roldan A, Hagi K, Rietschel L, Walyzada F, Zheng W, et al. Antipsychotic augmentation vs. monotherapy in schizophrenia: systematic review, meta-analysis and meta-regression analysis. World Psychiatry. (2017) 16:77–89. doi: 10.1002/wps.20387
64. Shimomura Y, Kikuchi Y, Suzuki T, Uchida H, Mimura M, Takeuchi H. Antipsychotic treatment strategies for acute phase and treatment resistance in schizophrenia: a systematic review of the guidelines and algorithms. Schizophr Res. (2021) 236:142–55. doi: 10.1016/j.schres.2021.07.040
65. Lipkovich I, Dmitrienko A. B. R D' Agostino S tutorial in biostatistics: data-driven subgroup identification and analysis in clinical trials. Stat Med. (2017) 36:136–96. doi: 10.1002/sim.7064
Keywords: schizophrenia, psychosis, muscarinic receptor, personalized medicine, acetylcholine, antipsychotics, biomarker
Citation: Stuke H (2023) Markers of muscarinic deficit for individualized treatment in schizophrenia. Front. Psychiatry 13:1100030. doi: 10.3389/fpsyt.2022.1100030
Received: 16 November 2022; Accepted: 20 December 2022;
Published: 09 January 2023.
Edited by:
Elisabetta C. Del Re, Harvard Medical School, United StatesReviewed by:
Verner Knott, University of Ottawa, CanadaJoshua Kantrowitz, Columbia University, United States
Copyright © 2023 Stuke. This is an open-access article distributed under the terms of the Creative Commons Attribution License (CC BY). The use, distribution or reproduction in other forums is permitted, provided the original author(s) and the copyright owner(s) are credited and that the original publication in this journal is cited, in accordance with accepted academic practice. No use, distribution or reproduction is permitted which does not comply with these terms.
*Correspondence: Heiner Stuke, aGVpbmVyLnN0dWtlQGNoYXJpdGUuZGU=