- 1Department of Psychiatry, Seoul National University Bundang Hospital, Seongnam, South Korea
- 2Department of Psychiatry, Seoul National University College of Medicine, Seoul, South Korea
- 3Human Rights Center, Hyupsung University, Hwaseong, South Korea
- 4Department of Radiology, Seoul National University Bundang Hospital, Seongnam, South Korea
- 5Department of Radiology, Seoul National University College of Medicine, Seoul, South Korea
- 6Department of Biomedical Informatics, Ajou University School of Medicine, Suwon, South Korea
- 7Office of Biostatistics, Medical Research Collaborating Center, Ajou Research Institute for Innovative Medicine, Ajou University Medical Center, Suwon, South Korea
Objective: This study was performed to investigate altered regional gray matter volume (rGMV) and structural covariance related to somatic symptom disorder (SSD) and longitudinal changes after treatment. Additionally, this study examined the relationships of structural alteration with its phenotypic subtypes.
Methods: Forty-three unmedicated patients with SSD and thirty normal controls completed psychological questionnaires and neurocognitive tests, as well as brain magnetic resonance imaging. Voxel-based morphometry and structural covariances were compared between groups and between subgroups within the SSD group. After 6 months of treatment, SSD patients were followed up for assessments.
Results: Patients with SSD exhibited attenuated structural covariances in the pallidal-cerebellar circuit (FDR < 0.05–0.1), as well as regions in the default mode and sensorimotor network (FDR < 0.2), compared to normal controls. The cerebellar rGMVs were negatively correlated with the severity of somatic symptoms. In subgroup analyses, patients with somatic pain showed denser structural covariances between the bilateral superior temporal pole and left angular gyrus, the left middle temporal pole and left angular gyrus, and the left amygdala and right inferior orbitofrontal gyrus, while patients with headache and dizziness had greater structural covariance between the right inferior temporal gyrus and right cerebellum (FDR < 0.1–0.2). After 6 months of treatment, patients showed improved symptoms, however there was no significant structural alteration.
Conclusion: The findings suggest that attenuated structural covariance may link to dysfunctional brain network and vulnerability to SSD; they also suggested that specific brain regions and networks may contribute to different subtypes of SSD.
Introduction
Somatic symptom disorder (SSD) as defined by the Diagnostic and Statistical Manual of Mental Disorders, Fifth Edition (DSM-5) (1), involves somatic disturbances associated with excessive thoughts and anxiety related to these somatic symptoms that disrupt the activities of daily life. While pain can be the predominant symptom in many patients with SSD, other patients may instead or also experience fatigue, palpitations, headache, nausea, indigestion, breathlessness, and dizziness (2), which have been classified as discrete symptom dimensions. Indeed, SSD shows diagnostic overlap within a broad range of other functional syndromes, including fibromyalgia, irritable bowel syndrome, functional neurological disorder, and somatoform dizziness, with diagnoses primarily based on consensus (3). These diagnoses are presumed to represent a common disease with different subtypes rather than multiple distinct diseases, where clinical phenotypes, diagnostic criteria, etiopathology, and treatment responses are similar among subtypes (3, 4). However, there is little concrete evidence to support this perspective. Moreover, some symptoms as chest pain, breathlessness and dizziness, have been understudied compared to somatic pain (2) and the neural circuits related to specific symptomatic presentations have rarely been investigated. Separate examinations of underlying brain networks for core psychological features of SSD and specific symptoms may produce additional evidence to address perspectives suggesting that SSD represents separate syndromes or subtypes of a single disease.
Previous studies have reported cognitive dysfunctions in psychomotor speed, attention, working memory, and executive functioning in patients with somatic symptoms (5). Other studies also found deficits in cognitive domains including processing speed, attention, memory, and executive functioning in patients with somatic symptom related disorders (6, 7). Recent studies have demonstrated that these cognitive dysfunctions are associated with depression as well as with somatic symptoms (7, 8). Indeed, indicators of emotional dysregulation such as depression, anxiety, anger, and alexithymia also frequently co-occur in patients with somatic symptoms (6, 9, 10), and these affective symptoms are known to affect cognitive functioning (11).
Regarding neurobiological aspects, previous neuroimaging studies on somatization disorder, somatoform disorder, and pain disorder, all of which fall under SSD in the DSM-5, have revealed altered neural circuitries involved in the development of SSD. Patients with SSD have consistently shown changes in the premotor and supplementary motor cortex, middle frontal gyrus (MFG), anterior cingulate cortex (ACC), insula and posterior cingulate cortex (PCC) in both structural and functional neuroimaging studies (12). In structural imaging studies of patients with SSD, morphological alterations and gray matter volume (GMV) changes have been observed in the caudate, ACC, MFG, angular gyrus, pituitary, amygdala, hypothalamus, fusiform gyrus, cuneus, inferior frontal gyrus, PCC and cerebellum (12–14). Previous studies have reported relationships of these brain structures, including the ACC, insula, prefrontal cortex, and amygdala, with psychopathologies that commonly occur in SSD such as alexithymia (15) and somatosensory amplification (2). Functional studies have also revealed disturbance of functional activity in the default mode network (DMN) (16, 17). Moreover, a recent study found aberrantly increased functional connectivity among the DMN, sensorimotor network (SMN), salience network, and dorsal attention network (DAN) in patients with SSD; these findings suggest that deficits in attention and perception are involved in SSD (18). However, few studies have investigated relationships between brain structural alterations, somatic symptoms, and cognitive functioning in patients with SSD. One study reported that a smaller mean GMV in the right MFG was correlated with more severe somatic symptoms and with impaired executive function in patients with somatization disorder (19). Another study reported executive dysfunction and cortical thinning of the prefrontal cortex in patients with chronic pain (20).
The relationships of changes in functional networks including DMN, SMN, salience network, and DAN or patterns caused by regional morphological alterations in patients with SSD remain unknown. Thus, the present study investigated patterns in brain anatomical structures in patients with SSD, by means of structural covariance analysis, which was recently introduced and has not yet been applied in this population (21). Structural covariance analysis can capture co-varied morphological characteristics in GMV or cortical thickness across brain areas, and these covariances partially overlap with functional connectivity because they are related to gray matter development and exhibit functional co-activation with neuroplasticity (21). Brain areas that co-vary in GMVs may be the part of relevant networks that subserve specific behavioral/cognitive symptoms and functions (22). Exploration of such structural covariance in SSD could provide complementary information regarding previous findings of functional magnetic resonance imaging (fMRI) studies, clarifying how inter-regional structures are reorganized with morphological changes and which functional connections and symptoms are interrelated. Symptomatic subtypes of SSD may also be newly differentiated through structural covariance analysis. Additionally, longitudinal investigation of structural brain changes has not been performed previously in patients with SSD.
We hypothesized that (1) unmedicated patients with SSD would show different structural covariance patterns compared to healthy controls, (2) structural covariance patterns would differ according to phenotypic subtypes of SSD, (3) structural alteration would be associated with cognitive functioning and clinical symptoms in patients with SSD, and (4) brain structural alterations would change after treatment. To address these hypotheses, we first compared structural covariances between patients with SSD and healthy controls. In comparing patient groups according to phenotypic differences, we also examined structural covaried regions in association with specific symptoms. Second, we evaluated the associations between individual regional gray matter volume (rGMV) and neurocognitive performance in SSD, which enabled exploration of the involvement of each cognitive function in relation to structural alteration of the brain in patients with SSD. Additionally, we performed follow-up assessments in patients with SSD after 6 months of treatment.
Materials and Methods
Subjects
Forty-three patients with SSD who presented to the Dizziness Center or the Pain Clinic of Seoul National University Bundang Hospital, Republic of Korea, and 30 normal controls were recruited from October 2017 to July 2019. The SSD diagnosis was confirmed by consensus between two clinical psychiatrists following clinical interviews based on the Structured Clinical Interview for DSM-5 Disorders-Clinician Version (23). The normal controls (healthy adults without psychiatric or medical illnesses) were matched for age and sex to the patients with SSD. The inclusion criteria were: (i) adults aged 20–64 years; (ii) confirmed diagnosis of SSD according to the DSM-5 criteria, including two or more somatic symptoms; and (iii) no use of psychotropic medication within the previous 2 months. The exclusion criteria were: (i) presence of new-onset neurological and/or medical disease with physical symptoms within the past 6 months; (ii) presence of any other mental disease, including major depressive disorder, bipolar disorder, a psychotic disorder, an anxiety disorder, a neurocognitive disorder, or a substance use disorder; (iii) history of brain injury and/or brain disease; and (iv) claustrophobia or presence of a non-MRI compatible device. As depressive and anxiety symptoms are common in patients with SSD, patients with such symptoms below the threshold for a clinical diagnosis were not excluded.
Sociodemographic data, psychological variables, neurocognitive functioning, and brain MRI data were collected from all participants in an initial assessment. For patients with SSD, treatment including pharmacological and non-pharmacological therapy was provided after the initial assessment. After 6 months, follow-up assessments of the patient group were performed to evaluate symptom and brain structural changes. Participants in the normal control group did not receive any specific intervention or undergo a follow-up assessment. The flow chart of study protocol is presented in Figure 1.
Clinical and Neurocognitive Measures
After providing an informed consent, participants were asked to complete the following questionnaires: the Korean version of the Somatization Subscale in Symptom Checklist-90R (SCL-90-R-SOM) (24), Patient Health Questionnaire-15 (PHQ-15) (25), Beck Depression Inventory-II (BDI-II) (26), Beck Anxiety Inventory (BAI) (27), State–Trait Anger Expression Inventory (STAXI) (28), and Toronto Alexithymia Scale-20 (TAS-20) (29). For subgroup comparisons, we recruited patients in two symptom subgroups according to their main symptoms. Organ subtype classifications, previously suggested for inclusion as functional somatic syndromes and somatoform disorders were referenced (3). According to that classification, patients were sorted into two subtypes: subtype 1 (general symptoms; n = 22), and subtype 2 (musculoskeletal symptoms; n = 21). Additionally, neurocognitive functions were assessed using the Korean version of the Computerized Neurocognitive Function Test (CNT4.0, Maxmedica Inc., Seoul, Korea) (30) as follows: Auditory Verbal Learning Test (AVLT), Trail-Making Test (TMT), Wisconsin Card Sorting Test (WCST), Digit Span Test, Continuous Performance Test (CPT), and Verbal Fluency Test (VFT). We analyzed these measures as well as initial demographics (i.e., age and sex) using independent two sample t-tests (for continuous variables) and chi-square tests (for categorical variables). In the SSD group, clinical, neurocognitive, and brain structural variables were compared between pre- and post- treatment using the paired sample t-tests. Additionally, Pearson and Spearman correlation analyses were used to investigate the relationships between changes in the rGMV (delta; Δ = Post – Pre), neurocognitive functioning (Δ) and the clinical index (Δ). Statistical analyses were performed using the MATLAB software (MathWorks, Sherborn, MA, United States).
Voxel-Based Morphometry Analysis
All MRI brain scans were obtained using a 3T Philips Achieva (Philips Healthcare, Inc., Best, Netherlands) with an 8-channel SENSE head coil at SNUBH. T1-weighted images were obtained using a fast field-echo three-dimensional imaging sequence with the following parameters: repetition time (TR), 8.1 ms; echo time (TE), 4.6 ms; flip angle (FA), 8°; field-of-view, 240 mm × 240 mm; acquisition matrix, 240 × 240; slice thickness, 1 mm; number of excitations, 1; scan time, 4 m 7 s.
To extract regional volumes from the entire brain, a Voxel-Based Morphometry (VBM) analysis was performed using the VBM-DARTEL procedure (SPM12; Wellcome Trust Centre for Neuroimaging, London, United Kingdom) (31), which provides clearer segmentation of tissues and better registration compared to a previously optimized VBM method (31).
No motion artifacts or other abnormalities were found on the T1-weighted images, which were inspected by a well-trained physician. The procedure for preprocessing T1-weighted images involved the following steps: (i) manual reorientation to the anterior commissure, (ii) gray matter segmentation based on a standard tissue probability map provided in SPM12, (iii) creation of a study-specific template, spatial normalization of individual images to the DARTEL template, and adjustment for volume signal changes during spatial normalization, and (iv) spatial smoothing of gray matter partitions using an 8 mm full-width at half-maximum Gaussian kernel. After preprocessing, rGMV was obtained by averaging the values in each area from 116 regions, which were defined using the Automated Anatomical Labeling Atlas (32).
Structural Covariance Analysis
In each group, we conducted partial correlation analyses between the rGMVs of two regions based on cross-sectional VBM data [covariates of age, sex, and total intra-cranial volume (TIV)] as a measure of structural covaried patterns (Figure 2A). Then, group-level inferences were applied using Fisher’s r-to-z transformation. For this, each correlation value was transformed to a normally distributed value, ZNC or ZSSD = 0.5 × [log(1 + R) – log(1-R)], and compared with Z = (ZNC - ZSSD)/√[1/(NNC-3-M) + 1/(NSSD-3-M)], where NNC, NSSD, and M represent sample sizes for each group and the number of covariates used in the partial correlation analysis. Structural covariances were compared between the SSD group and normal controls (Figure 2B). The SSD group were further grouped as subtype 1 and subtype 2, and structural covariances and regional volume differences were compared between the two subtypes (Figure 2B). The rGMV data were correlated with the questionnaire data using partial correlation analysis, including the BDI-II and BAI scores as covariates, as well as age, sex, and TIV (Figure 2C).
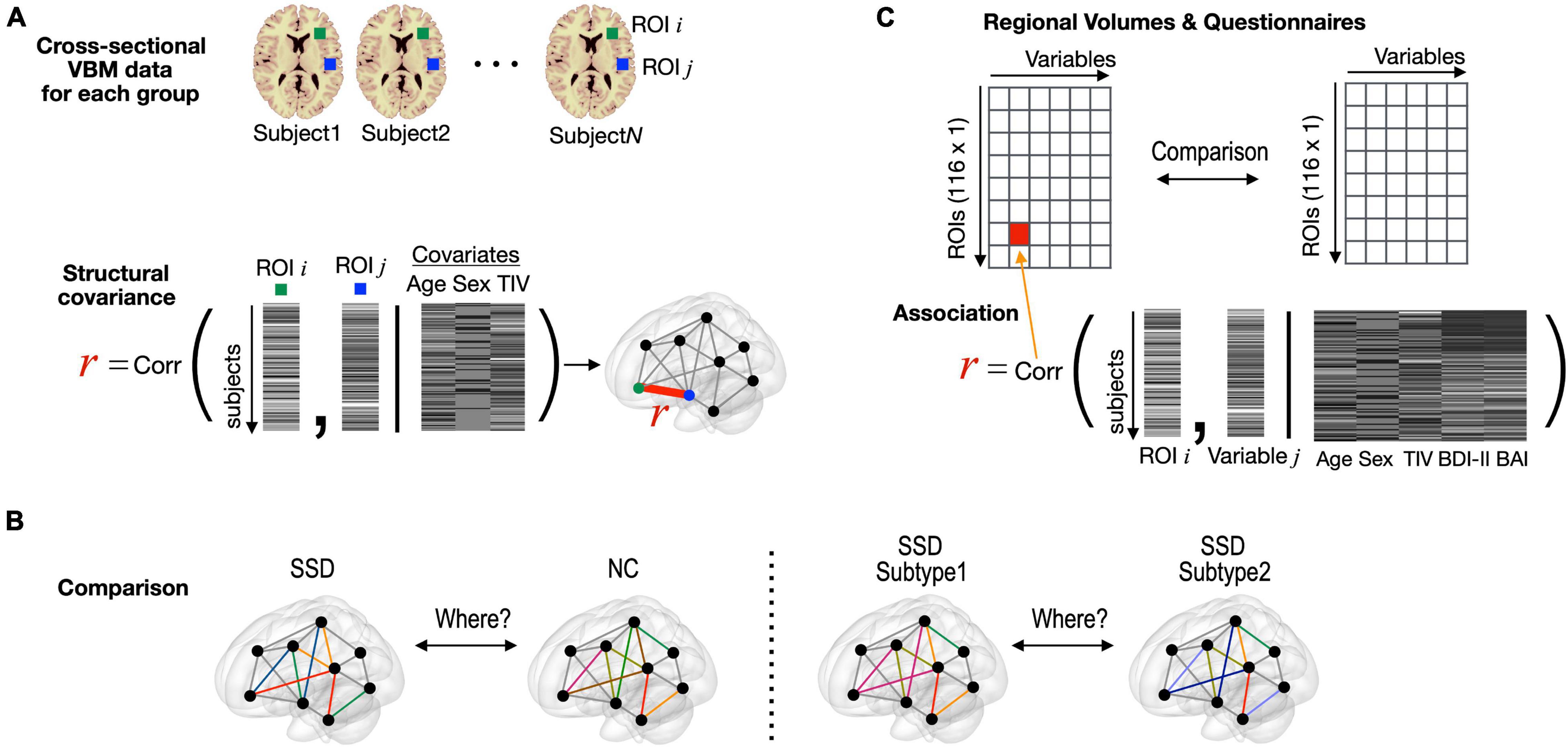
Figure 2. Statistical procedures used in this study. (A) Structural covariance between two regions was defined as partial correlation between two regional volumes (covariates: age, sex, and TIV), which were obtained from cross-sectional VBM data for each group. (B) Structural covariance was compared edge-by-edge between the entire SSD and normal control groups and between the subtype 1 and subtype 2 groups within SSD. (C) Partial correlation analysis with rGMV data and clinical variable. TIV, total intra-cranial volume; VBM, voxel-based morphometry; SSD, somatic symptom disorder; NC, normal control; ROI, region of interest; BDI-II, Beck Depression Inventory-II; BAI, Beck Anxiety Inventory.
All statistical analyses were performed using the MATLAB-based custom software (MathWorks, Sherborn, MA, United States). For inferences regarding structural covariance, several thresholds of the false discovery rate (FDR) = 0.05, 0.1, 0.15, and 0.2 were considered significant for addressing multiple-comparison issues (FDR < 0.05 indicated significance) (33). FDR thresholding controls the expected proportion of false positives only among brain areas exhibiting significant values (33). FDR control levels in the 0.1–0.2 range are considered practically acceptable, and several neuroimaging studies have applied them (34, 35).
Results
Demographic and Clinical Characteristics of the Participants
The demographic and clinical data are presented in Table 1. No significant differences in demographic characteristics were observed between the groups. Patients with SSD had significantly higher scores on questionnaires and exhibited poorer performance than the normal controls on the AVLT, CPT, and VFT (Table 1). Finally, all 22 patients in the subtype 1 group had dizziness with additional symptom as headache or concentration difficulties. All of 21 patients in the subtype 2 group had musculoskeletal pain including limb pain or backache or moving pain with additional symptom as pain on another place or tingling sensation. Three patients in the subtype 2 group had previously been diagnosed with fibromyalgia.
Comparison of Structural Covariance Between Somatic Symptom Disorder and Normal Control Group
Although the SSD group did not exhibit any significant changes in regional volume, it exhibited disrupted structures in multiple brain regions (i.e., changed structural covariances), compared with the normal control group. In the current study, structural covariances, which were centered at the bilateral pallidum and related to the cerebellum, were significantly decreased in the SSD group, compared with the normal control group (Figure 3 and Supplementary Table 1; FDR < 0.05–0.2). In detail, the regional volume of the bilateral pallidum showed altered connections with the cerebellar lobes including the bilateral crus 2, 7b, and 8 lobes, as well as the vermis 8 and 9 regions (Figures 3B,C and Supplementary Table 1). Reduced covariance was also found between the left Heschl’s gyrus and left superior temporal gyrus (STG), the right superior temporal pole (TPsup) and left dorsolateral superior frontal gyrus (SFGdor), and the right precuneus and right middle occipital gyrus (MOG) in patients with SSD.
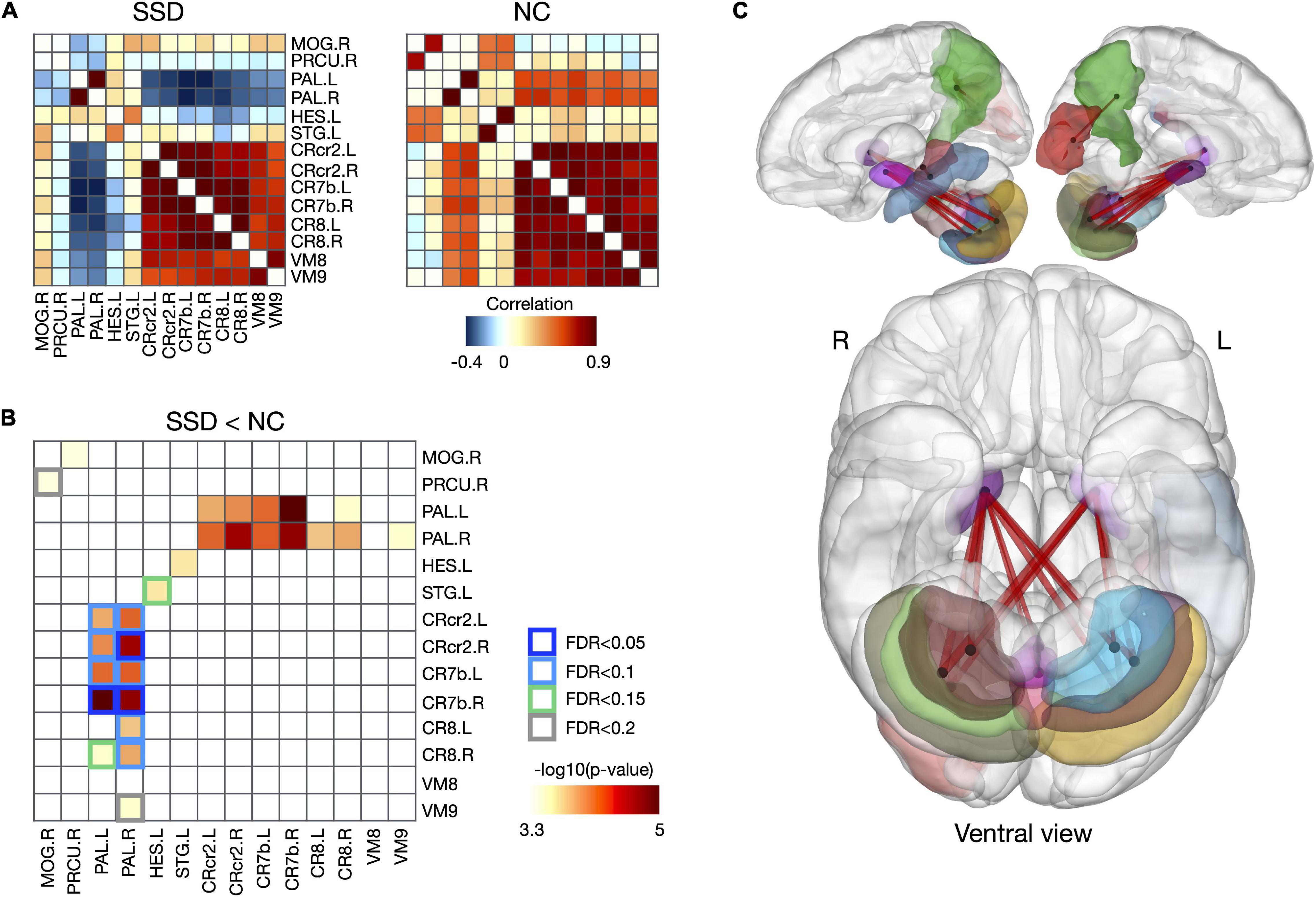
Figure 3. Disrupted structural covariance connectivity in patients with SSD. (A) Group-averaging structural covariance connectivity for SSD and NC group. (B) Reduced structural covariances and relating regions in patients with SSD, and (C) their 3D rendering. SSD, somatic symptom disorder; NC, normal control; MOG, middle occipital gyrus; PRCU, precuneus; PAL, pallidum; HES, Heschl’s gyrus; STG, superior temporal gyrus; CRcr2, cerebellar crus 2; CR7b, cerebellar lobe 7b; CR8, cerebellar lobe 8; VM8, vermis 8; VM 9, vermis 9; L, left; R, right.
Correlations Between Regional Gray Matter Volumes and Clinical Variables
Figure 4 shows the associations of psychological/neurocognitive variables with the rGMVs of multiple brain regions after controlling for age, sex, TIV, and BDI-II and BAI scores (P < 0.01, uncorrected) for each group; 43 significant differences were found between the SSD and normal control groups, and an association study was conducted for regions showing significance. As shown in Figure 4B, the SCL-90-R-SOM scores were negatively correlated with the rGMVs of right cerebellar lobe 3 and bilateral cerebellar lobes 4–5 in patients with SSD. Regarding the cognitive tests, TMT-A response time was negatively correlated with the rGMV of the right pallidum, while the CPT overt response score was negatively correlated with the rGMV of the left medial orbitofrontal gyrus (OFGmed), and the CPT response time was negatively correlated with the rGMV of the bilateral supramarginal gyrus (SMG) in patients with SSD.
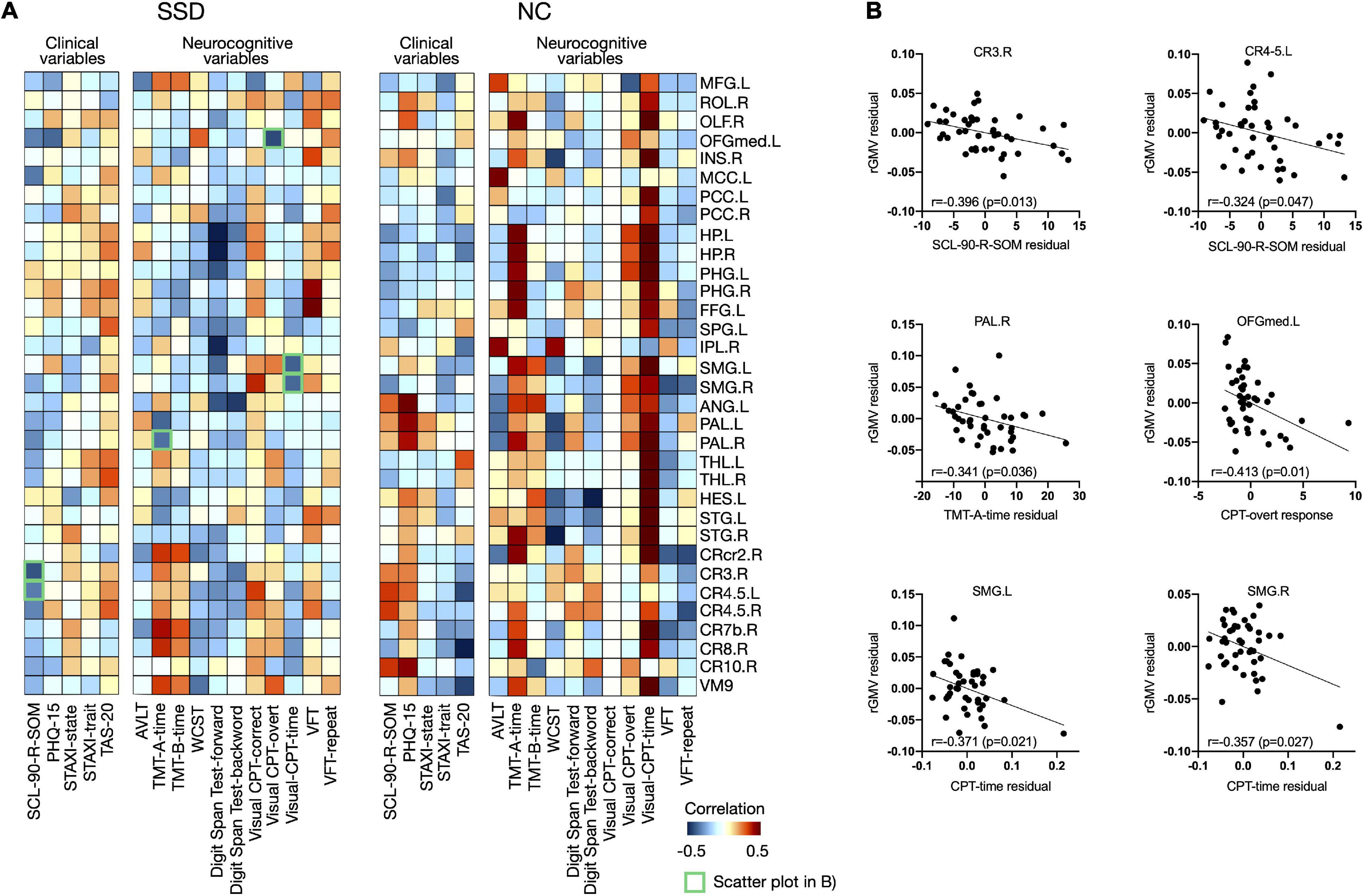
Figure 4. (A) Associations between clinical/neurocognitive variables and rGMVs in the whole brain for each group and (B) remarkable negative correlations among them, presented as scatter plots, are marked as green boxes in panel (A). rGMV, regional gray matter volume; SSD, somatic symptom disorder; NC, normal control; MFG, middle frontal gyrus; ROL, Rolandic gyrus; OLF, olfactory cortex; OFGmed, medial orbitofrontal gyrus; INS, insula; MCC, middle cingulate cortex; PCC, posterior cingulate cortex; HP, hippocampus; PHG, parahippocampal gyrus; FFG, fusiform gyrus; SPG, superior parietal gyrus; IPL, inferior parietal lobule; SMG, supramarginal gyrus; ANG, angular gyrus; PAL, pallidum; THL, thalamus; HES, Heschl’s gyrus; STG, superior temporal gyrus; CRcr2, cerebellar crus 2; CR3, cerebellar lobe 3; CR4.5, cerebellar lobes 4–5; CR7b, cerebellar lobe 7b; CR8, cerebellar lobe 8; CR10, cerebellar lobe 10; VM9, vermis 9; L, left; R, right; SCL-90-R-SOM, Somatization subscale from Symptom Checklist-90-Revised; PHQ-15, Patient Health Questionnaire-15; STAXI, State-Trait Anger Expression Inventory; TAS-20, Toronto Alexithymia Scale-20; AVLT, Auditory Verbal Learning Test; TMT, Trail-Making Test; WCST, Wisconsin Card Sorting Test; CPT, Continuous Performance Test; VFT, Verbal Fluency Test.
Comparison of Structural Covariance Between Somatic Symptom Disorder Subgroups
Patients with SSD subtype 2 showed decreased rGMV at the left MFG, left inferior orbitofrontal gyrus (OFGinf), bilateral Rolandic gyri, right Heschl’s gyrus, right middle temporal gyrus (MTG), bilateral cerebellar crus 1, 2, 7b, 8, and 9, and left cerebellar region 10, compared to patients with SSD subtype 1 (FDR < 0.2; Figure 5A).
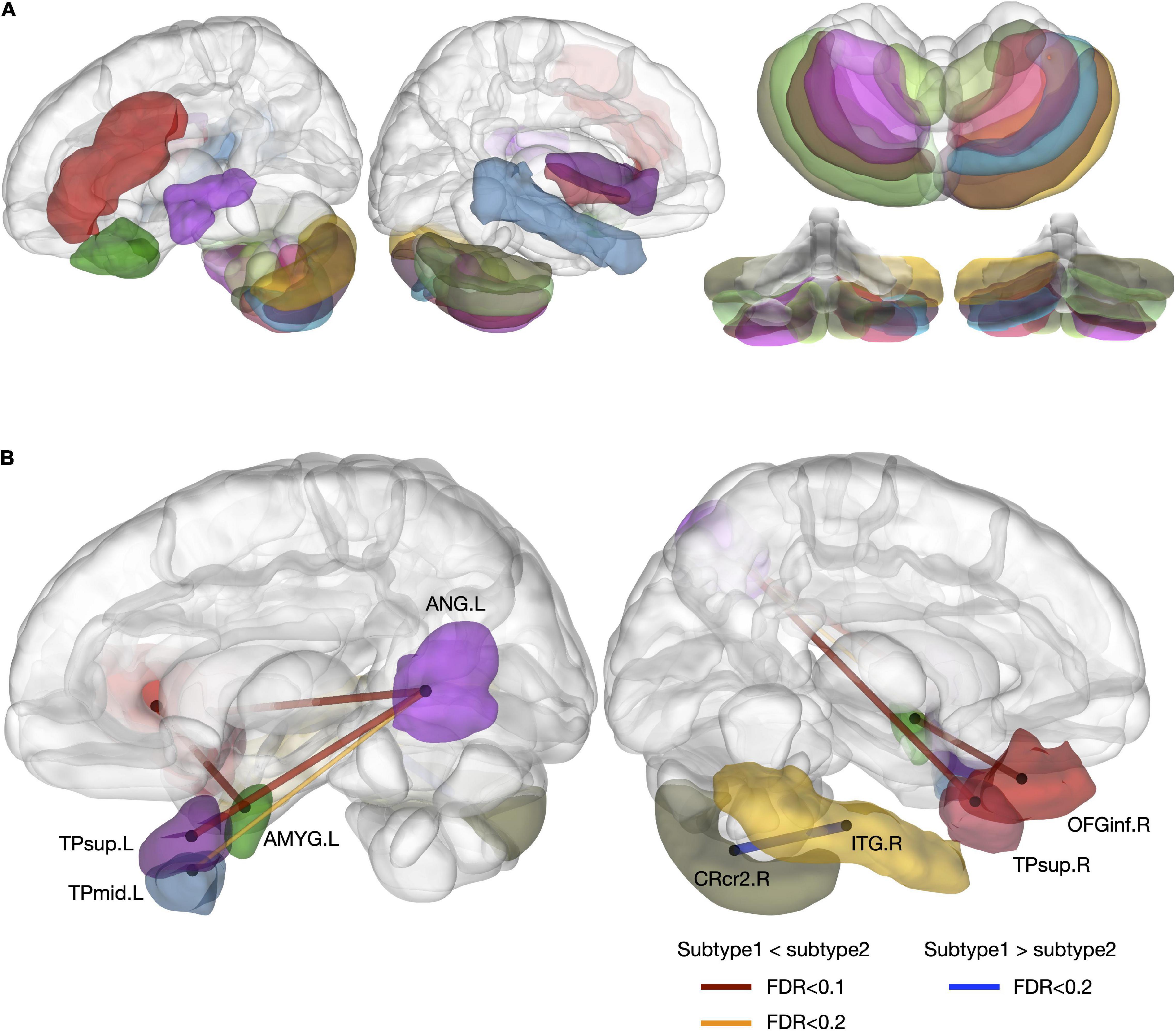
Figure 5. Differences in regional volumes and structural covariance networks between SSD subtypes. (A) Regional volume difference was reduced in subtype 2, compared to subtype 1 (FDR < 0.2). (B) Different structural covariance patterns between the two subtypes (FDR < 0.2). TPsup, superior temporal pole; AMYG, amygdala; ANG, angular gyrus; CRcr2, cerebellar crus 2; ITG, inferior temporal gyrus; OFGinf, inferior orbitofrontal gyrus; L, left; R, right.
Greater structural covariance was detected between the bilateral TPsup and left angular gyrus, the left middle temporal pole (TPmid) and left angular gyrus, and the left amygdala and right OFGinf in patients with SSD subtype 2. In contrast, structural connectivity was greater between the right inferior temporal gyrus (ITG) and right cerebellar crus 2 in patients with SSD subtype 1 (FDR < 0.1–0.2; Figure 5B).
Longitudinal Follow-Up
After 6 months of treatment, 23 patients (53.5%) underwent follow-up assessments; 3 received only non-pharmacological treatment and 20 received both pharmacological and non-pharmacological treatments (Supplementary Table 2). Table 2 shows the initial and follow-up clinical variables and cognitive functioning. The mean SCL-90-R-SOM (p = 0.001), PHQ-15 (p < 0.001), and BAI (p = 0.001) scores had significantly decreased at the 6-month follow-up assessment. There were significant changes in performance on the AVLT (p = 0.003) and VFT (p = 0.014). Spearman correlation analyses showed a significant association between Δ PHQ-15 and Δ VLT (r = −0.465, p = 0.025).
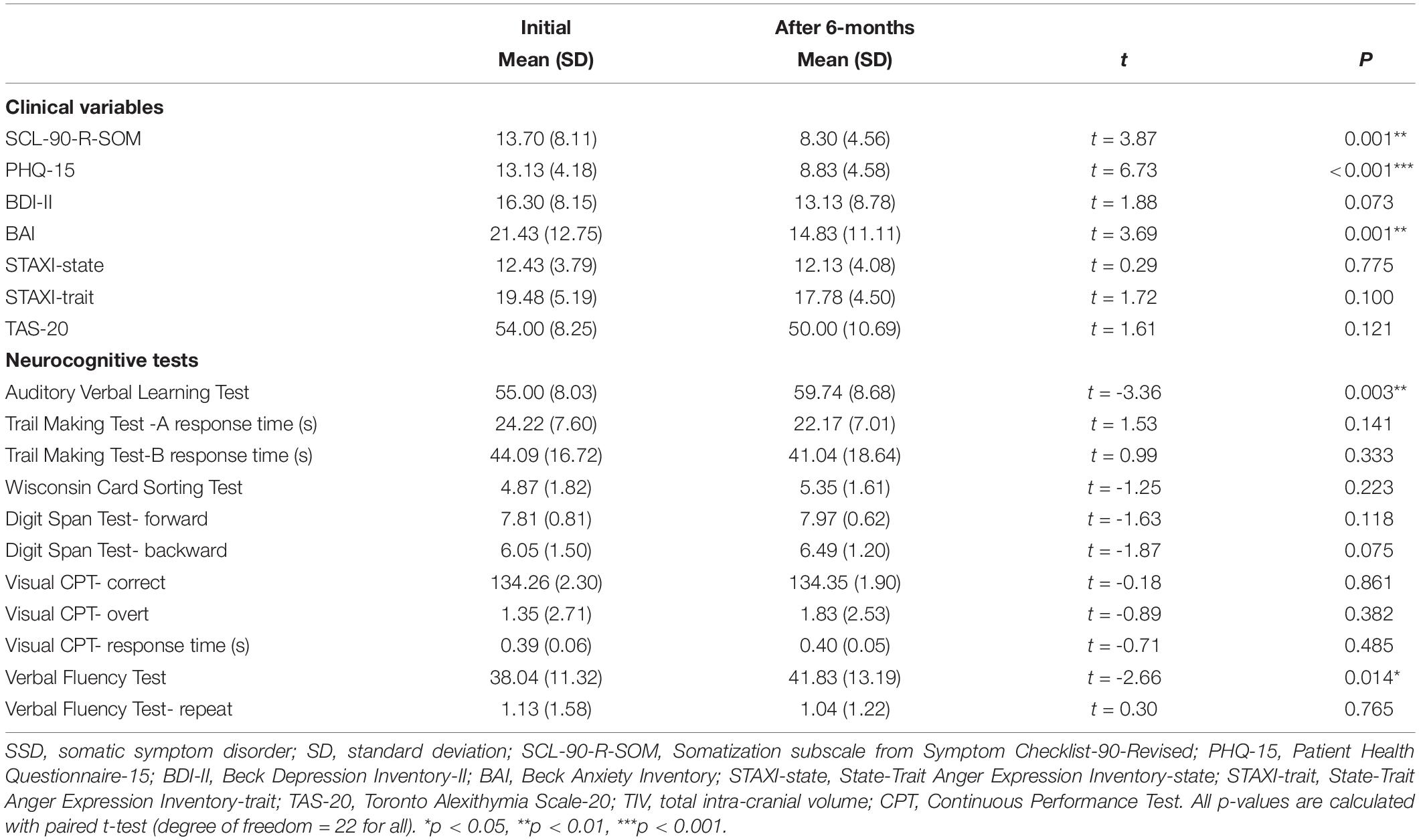
Table 2. The change of psychological and neurocognitive variables after 6 months in patients with SSD (N = 23).
However, regarding the rGMV and structural covariance, there was no significant changes in pre- and post-treatment comparisons. Also, correlations analyses revealed no significant association between Δ clinical variable and Δ rGMV.
Discussion
Reduced Structural Covariances in Patients With Somatic Symptom Disorder
In this study, we found altered structural covariances between cortical and subcortical regions in patients with SSD. Notably, the cerebellar-pallidal circuit showed the largest reduction in structural covariance in patients with SSD. Although the cerebellum has not been considered a central region in SSD, some studies have suggested cerebellar involvement in pain and somatization disorders (16, 19, 36). Other fMRI studies reported that activity in the cerebellar lobules was related to pain processing (37, 38), suggesting that the cerebellar network may be involved in the processing of chronic affective pain. According to a literature review, the pallidum was a key node in subcortical networks underlying somatosensory amplification, which plays a role in somatic symptoms (2). Guo et al. (39) were the first to report decreased functional connectivity of the pallidum in patients with somatization disorder. In line with previous findings, our study suggested that the set of reduced structural links underlying morphological alteration occurred solely at the cerebellar-pallidal circuit which has been associated with clinically relevant dysfunctions in SSD.
Functional imaging studies of SSD have also revealed involvement of various damaged networks, such as the DMN, SMN, salience network, and DAN (18). Consistent with these findings, regions showing reduced structural covariance were part of the DMN (TPsup and SFGdor) and SMN (Heschl’s gyrus and STG) in our study. Previous studies have suggested a role for the DMN in the loss of top-down regulation in neural mechanisms of SSD (17, 18). And interactions among the SMN, DMN and salience network appear to be associated with sensory processing, adjusted by attentional and emotional control in SSD (18, 40). Previous research reported abnormal functional connectivity in the bilateral MOG of patients with SSD and suggested that activity in this region may be associated with aversive experiences and somatic symptoms (36) and the dorsolateral prefrontal cortex (DLPFC) including SFGdor is thought to subserve top-down attentional control (41) and the inhibitory control of pain processing (42). Although the present results could not explain how these reduced covariances between regions are related to the mechanisms of SSD, results suggest the existence of covarying structural links that were previously demonstrated as core functional networks in SSD.
Clinical Symptoms and Neurocognitive Functioning in Somatic Symptom Disorder
In the present study, regional volumes of several regions were related to clinical and cognitive dysfunctions in patients with SSD. SCL-90-R-SOM scores were negatively correlated with the rGMVs of the right cerebellar lobe 3 and left cerebellar lobes 4–5; thus, a smaller GMV of the regional cerebellum was associated with more severe somatic symptoms. This result of the involvement of the cerebellum and its association with somatic symptoms in SSD as shown in fibromyalgia (43) support the presence of common neural involvement as well as similar clinical symptoms in these diagnoses. On cognitive tests, patients with SSD showed significantly poorer performance than did the normal controls on the tests of verbal functioning and sustained attention. Positive associations of the left OFGmed and bilateral SMG GMV with sustained attentional capacity, as measured by the CPT, were observed in this study. The OFGmed and SMG were shown to be involved in selective attention (44), and previous studies demonstrated impaired attention and attentional bias in SSD patients, who tend to be hypervigilant to somatic sensations, rendering them less responsive to external stimuli (45). Impairment in these regions could reduce the capacity for sustained attention, as observed in patients with SSD. Correlation analyses indicated that patients with a smaller rGMV of the right pallidum performed worse on the TMT-A, which measures attention and psychomotor speed. Performance on the TMT-A mostly reflects motor skills, which involve basal ganglia–thalamocortical circuits (46); this may explain the link between TMT-A performance and pallidum volume. In summary, these results suggest that the right pallidum, left OFGmed and SMG may be relevant structural regions related to neurocognitive functioning in patients with SSD.
Specific Structural Regions in Somatic Symptom Disorder Subtypes
In our study, patients with pain exhibited lower GMV in the left MFG, left OFGinf, bilateral Rolandic gyrus, right Heschl’s gyrus, right MTG, and cerebellum, compared to patients with dizziness. Moreover, significant structural connectivity was observed between the bilateral TPsup and left angular gyrus, left TPmid and left angular gyrus, and left amygdala and right OFGinf in patients with pain. These regions are mainly involved in the DMN (i.e., the MFG, OFG, MTG, and angular gyrus), salience network (i.e., the amygdala), and SMN (i.e., the Heschl’s gyrus, Rolandic gyrus, and cerebellum), which are key damaged functional networks consistently observed in SSD and pain disorders (16, 18). These regions are known to be involved in memory and emotional tagging of sensory perception, suggesting that emotional responses to pain inputs may alter the intensity and experience of pain symptoms (47). Meanwhile, patients with dizziness exhibited greater structural connectivity between the right ITG and right cerebellum in the dominant hemisphere (with respect to vestibular functioning) in right-handed individuals. The ITG is involved in visual perception, language, and memory previous studies reported the relationship of the ITG to alexithymia and somatization disorder (39, 48). Increased structural connectivity between the ITG and cerebellum would enhance the perception and memory of dizziness, which might underlie the maladaptive cortical plasticity and memory consolidation seen in patients. We included patients with dizziness in this study, which is a relatively understudied symptom compared to pain in SSD. Furthermore, dizziness has been placed at the interface of functional neurological disorder and SSD; these diagnoses are defined using similar characteristics, and share some pathophysiology (49). Our findings suggest that specific structural alterations are involved in specific symptom presentations in SSD that differ from the neural circuits common to core psychological symptoms of SSD. The present results elaborate further studies related to subtyping of SSD to understand diagnostic clarification and interventions more fully.
Longitudinal Changes After Treatment
After 6 months of treatment, patients with SSD showed a significantly improved performance on verbal memorization and verbal fluency as well as reduced somatic symptoms compared with initial assessment. The changes of cognitive functioning after treatment may reflect improved clinical status. Specifically, the score on VFT of the patients was significantly lower at initial assessment compared with the normal controls but recovered after treatment. The correlation between the changes of PHQ-15 score and VFT score further support that specific cognitive functioning is associated with the disease state. Although the data on neurocognitive dysfunction in SSD are inconsistent, the neurocognitive functions of attention and verbal memory have repeatedly been suggested to be possible neurobiological markers that can be impaired in patients with SSD (7, 8). In a previous work, we also introduced that neurocognitive dysfunctions are subtle, but certain cognitive functions may be related to the somatic symptoms and clinical improvements of patients with SSD (50).
In this study, there was no significant differences in the GMVs between patients with SSD and normal controls, though the GMVs of several regions were associated with clinical symptom severity. Our results may suggest candidate regions relevant to somatic symptom and cognitive functioning rather than specific structural alterations involved in the development of SSD. Results of the previous structural studies in SSD were inconsistent, which have reported morphological alterations in the caudate, ACC, MFG, angular gyrus, pituitary, amygdala, hypothalamus, fusiform gyrus, cuneus, inferior frontal gyrus, PCC and cerebellum (12–14). However, a recent review pointed that previous studies of SSD were rarely account for the influence of psychiatric comorbidities, thus observed structural changes may be related to comorbid psychiatric disorders rather than to SSD itself (51). Indeed, a previous study found no volumetric differences between patients with SSD and normal controls when controlling for depression and anxiety (52, 53). These discrepancies may also be present due to heterogenous population with various symptoms, differences in disease duration and the impact of inconsistent treatments. Additionally, we found no significant changes of the GMV and structural covariance in patients with SSD after treatment regardless of clinical improvement. These findings may support that SSD is related to altered brain networks rather than altered regional structures. Previous studies have suggested that pain causes network dysfunctions rather than structural abnormalities (54, 55). A recent study revealed altered structural covariance network but no differences in structural volumes in patients with headache compared with normal controls (56). Another longitudinal study reported that there was no longitudinal GMV alterations in patients with migraine, which suggests that migraine is not a structural disease and the use of network analyses on functional imaging may show rather robust findings in migraine (57). Therefore, further studies using combined neuroimaging modalities may clarify how structural and functional networks are related and engaged in SSD.
Taken together, we can assume that SSD is a disease with dysfunctions of complex brain networks rather than defects of regional structures, and the structural covariance rather than regional volume may capture these alterations and altered structural covariance may interact with a risk or vulnerability trait rather than a disease state. Longer period of observational study with a larger sample size is needed to examine relationships among these factors.
This study has several limitations. First, we could not completely rule out effects of depression and anxiety on clinical symptoms or cognitive functioning, although the BDI-II and BAI scores were used as covariates in the analyses. Also, we did not include factors related to social functioning, which are associated with clinical symptoms. Second, due to the modest sample size we could not perform correlation analyses between clinical variables and imaging data within subgroups. In our study, correlations between structural covariances and clinical variables were not significant after FDR correction, and we presented them with uncorrected p-values. This may also be due to the restricted sample size and we recommend future studies with larger sample sizes. Third, all images were preprocessed and analyzed by independent researchers who were blinded to the patients’ clinical data. However, investigators were not blinded to group allocation for performing neurocognitive tests and statistical comparisons. These may have led to assessor bias. Lastly, though this longitudinal study was preliminary a drop-out rate was relatively high, and the follow-up assessments were performed on only SSD patients; thus, it was not possible to examine a group × time interaction.
Conclusion
To the best of our knowledge, this study is the first to explore structural alterations and their relationships with organ symptoms and neurocognitive functioning of SSD using structural covariance analysis. Also, this is the first longitudinal structural MRI study of SSD. Ethno-genetic studies have suggested that Asians more frequently exhibit somatic symptoms compared to members of Western cultures, and patients in Asian countries more often complain of breathlessness and dizziness (58). The present investigation of brain structural patterns in SSD in this population may also provide additional understanding of the cross-cultural specificity of this disorder. This study demonstrated structural alterations in the pallidal-cerebellar circuit, as well as in the DMN and SMN, in patients with SSD. Correlations were observed between rGMVs and clinical/neurocognitive variables in patients with SSD, which suggests the involvement of structural alterations in SSD. Meanwhile, structural alterations in GMV were subtle in patients with SSD despite their experiencing definite discomfort from somatic symptoms. This might suggest the presence of neural alterations at the level of “functional” rather than “structural” disruptions in SSD that are relevant to “dysfunctional” rather than “impaired” states. Our results regarding alterations in structural covariance other than in rGMVs may support this and suggest the utility of structural covariance analysis combined with functional imaging to explore attenuated structural alterations in this population. Notably, our longitudinal observation of structural covariance in clinically improved patients suggest that attenuated structural covariance may reflect the risk or vulnerability to SSD rather than clinical courses. Additionally, subtype analyses suggested that specific neural networks and brain regions may contribute to the presentation of organ phenotypic symptoms among various somatic symptoms in SSD but this hypothesis should be tested with detailed experiments in further studies. Also, further studies including other SSD subtypes, such as patients with gastrointestinal and cardiopulmonary symptoms, may facilitate the elucidation of brain networks underpinning the subtypes.
Data Availability Statement
The raw data supporting the conclusions of this article will be made available by the authors, without undue reservation.
Ethics Statement
The studies involving human participants were reviewed and approved by the Institutional Review Board of Seoul National University Bundang Hospital (B1710426302). The patients/participants provided their written informed consent to participate in this study.
Author Contributions
HP: funding acquisition, conceptualization, data curation, and writing – preparation of the original draft. YJ: data curation and analysis of data. LS: data curation and analysis of imaging data. I-YY: conceptualization and methodology. BP: methodology, statistical analysis, and figures. All authors contributed to the article and approved the submitted version.
Funding
This study was supported by a National Research Foundation of Korea (NRF) grant funded by the Korea government (NRF-2017R1C1B2012733 and NRF-2020R1A2C1102105).
Conflict of Interest
The authors declare that the research was conducted in the absence of any commercial or financial relationships that could be construed as a potential conflict of interest.
Publisher’s Note
All claims expressed in this article are solely those of the authors and do not necessarily represent those of their affiliated organizations, or those of the publisher, the editors and the reviewers. Any product that may be evaluated in this article, or claim that may be made by its manufacturer, is not guaranteed or endorsed by the publisher.
Supplementary Material
The Supplementary Material for this article can be found online at: https://www.frontiersin.org/articles/10.3389/fpsyt.2022.817527/full#supplementary-material
References
1. American Psychiatric Association. Diagnostic and Statistical Manual of Mental Disorders (DSM-5§). Washington, DC: American Psychiatric Publishing (2013).
2. Perez DL, Barsky AJ, Vago DR, Baslet G, Silbersweig DA. A neural circuit framework for somatosensory amplification in somatoform disorders. J Neuropsychiatry Clin Neurosci. (2015) 27:e40–50. doi: 10.1176/appi.neuropsych.13070170
3. Fink P, Schröder A. One single diagnosis, bodily distress syndrome, succeeded to capture 10 diagnostic categories of functional somatic syndromes and somatoform disorders. J Psychosom Res. (2010) 68:415–26. doi: 10.1016/j.jpsychores.2010.02.004
4. Rief W, Broadbent E. Explaining medically unexplained symptoms-models and mechanisms. Clin Psychol Rev. (2007) 27:821–41. doi: 10.1016/j.cpr.2007.07.005
5. Hall NM, Kuzminskyte R, Pedersen AD, Ørnbøl E, Fink P. The relationship between cognitive functions, somatization and behavioural coping in patients with multiple functional somatic symptoms. Nordic J Psychiatry. (2011) 65:216–24. doi: 10.3109/08039488.2010.528024
6. de Vroege L, Koppenol I, Kop WJ, Riem MM, van der Feltz-Cornelis CM. Neurocognitive functioning in patients with conversion disorder/functional neurological disorder. J Neuropsychol. (2021) 15:69–87. doi: 10.1111/jnp.12206
7. de Vroege L, Timmermans A, Kop WJ, van der Feltz-Cornelis CM. Neurocognitive dysfunctioning and the impact of comorbid depression and anxiety in patients with somatic symptom and related disorders: a cross-sectional clinical study. Psychol Med. (2018) 48:1803–13. doi: 10.1017/s0033291717003300
8. Inamura K, Shinagawa S, Nagata T, Tagai K, Nukariya K, Nakayama K. Cognitive dysfunction in patients with late-life somatic symptom disorder: a comparison according to disease severity. Psychosomatics. (2015) 56:486–94. doi: 10.1016/j.psym.2014.10.004
9. Liu L, Cohen S, Schulz MS, Waldinger RJ. Sources of somatization: exploring the roles of insecurity in relationships and styles of anger experience and expression. Soc Sci Med. (2011) 73:1436–43. doi: 10.1016/j.socscimed.2011.07.034
10. Koh KB, Kim DK, Kim SY, Park JK, Han M. The relation between anger management style, mood and somatic symptoms in anxiety disorders and somatoform disorders. Psychiatry Res. (2008) 160:372–9. doi: 10.3349/ymj.2008.49.1.46
11. Hill NL, Mogle J, Wion R, Munoz E, DePasquale N, Yevchak AM, et al. Subjective cognitive impairment and affective symptoms: a systematic review. Gerontologist. (2016) 56:e109–27. doi: 10.1093/geront/gnw091
12. Boeckle M, Schrimpf M, Liegl G, Pieh C. Neural correlates of somatoform disorders from a meta-analytic perspective on neuroimaging studies. Neuroimage. (2016) 11:606–13. doi: 10.1016/j.nicl.2016.04.001
13. Yildirim H, Atmaca M, Sirlier B, Kayali A. Pituitary volumes are reduced in patients with somatization disorder. Psychiatry Invest. (2012) 9:278. doi: 10.4306/pi.2012.9.3.278
14. Delvecchio G, Rossetti MG, Caletti E, Arighi A, Galimberti D, Basilico P, et al. The neuroanatomy of somatoform disorders: a magnetic resonance imaging study. Psychosomatics. (2019) 60:278–88. doi: 10.1016/j.psym.2018.07.005
15. Goerlich KS. The multifaceted nature of alexithymia–a neuroscientific perspective. Front Psychol. (2018) 9:1614. doi: 10.3389/fpsyg.2018.01614
16. Wang H, Guo W, Liu F, Chen J, Wu R, Zhang Z, et al. Clinical significance of increased cerebellar default-mode network connectivity in resting-state patients with drug-naive somatization disorder. Medicine. (2016) 95:e4043. doi: 10.1097/md.0000000000004043
17. Su Q, Yao D, Jiang M, Liu F, Jiang J, Xu C, et al. Dissociation of regional activity in default mode network in medication-naive, first-episode somatization disorder. PLoS One. (2014) 9:e99273. doi: 10.1371/journal.pone.0099273
18. Kim SM, Hong JS, Min KJ, Han DH. Brain functional connectivity in patients with somatic symptom disorder. Psychosom Med. (2019) 81:313–8. doi: 10.1097/psy.0000000000000681
19. Li R, Liu F, Su Q, Zhang Z, Zhao J, Wang Y, et al. Bidirectional causal connectivity in the cortico-limbic-cerebellar circuit related to structural alterations in first-episode, drug-naive somatization disorder. Front Psychiatry. (2018) 9:162. doi: 10.3389/fpsyt.2018.00162
20. Lee D-H, Lee KJ, Cho KIK, Noh EC, Jang JH, Kim YC, et al. Brain alterations and neurocognitive dysfunction in patients with complex regional pain syndrome. J Pain. (2015) 16:580–6. doi: 10.1016/j.jpain.2015.03.006
21. Alexander-Bloch A, Giedd JN, Bullmore E. Imaging structural co-variance between human brain regions. Nat Rev Neurosci. (2013) 14:322–36. doi: 10.1038/nrn3465
22. Zielinski BA, Gennatas ED, Zhou J, Seeley WW. Network-level structural covariance in the developing brain. Proc Natl Acad Sci USA. (2010) 107:18191–6. doi: 10.1073/pnas.1003109107
23. First MB, Williams JB, Karg RS, Spitzer RL. Structured Clinical Interview for DSM-5 Disorders: SCID-5-CV Clinician Version. Washington, DC: American Psychiatric Association Publishing (2016).
24. Kim K, Kim J, Won H. Korean Manual of Symptom Checklist-90-Revision. Seoul: Jung Ang Juk Sung Publisher (1984). p. 8–10.
25. Han C, Pae CU, Patkar AA, Masand PS, Kim KW, Joe SH, et al. Psychometric properties of the patient health questionnaire–15 (PHQ–15) for measuring the somatic symptoms of psychiatric outpatients. Psychosomatics. (2009) 50:580–5. doi: 10.1016/s0033-3182(09)70859-x
26. Lim SY, Lee EJ, Jeong SW, Kim HC, Jeong CH, Jeon TY, et al. The validation study of beck depression scale 2 in Korean version. Anxiety Mood. (2011) 7:48–53. doi: 10.1037/t05261-000
28. Chon KK, Hahn DW, Lee CH, Spielberger C. Korean adaptation of the state-trait anger expression inventory: anger and blood pressure. Korean J Health Psychol. (1997) 2:60–78.
29. Lee Y, Rim H, Lee J. Development and validation of a Korean version of the 20-item Toronto alexithymia scale (TAS-20K). J Korean Neuropsychiatr Assoc. (1996) 35:888–99.
30. Kwon JS, Lyoo IK, Hong KS, Yeon BK, Ha KS. Development and standardization of the computerized memory assessment for Korean adults. J Korean Neuropsychiatr Assoc. (2002) 41:347–62.
31. Ashburner J. A fast diffeomorphic image registration algorithm. J Neuroimage. (2007) 38:95–113. doi: 10.1016/j.neuroimage.2007.07.007
32. Tzourio-Mazoyer N, Landeau B, Papathanassiou D, Crivello F, Etard O, Delcroix N, et al. Automated anatomical labeling of activations in SPM using a macroscopic anatomical parcellation of the MNI MRI single-subject brain. Neuroimage. (2002) 15:273–89. doi: 10.1006/nimg.2001.0978
33. Genovese CR, Lazar NA, Nichols T. Thresholding of statistical maps in functional neuroimaging using the false discovery rate. Neuroimage. (2002) 15:870–8. doi: 10.1006/nimg.2001.1037
34. Gordon BA, McCullough A, Mishra S, Blazey TM, Su Y, Christensen J, et al. Cross-sectional and longitudinal atrophy is preferentially associated with tau rather than amyloid β positron emission tomography pathology. Alzheimers Dement. (2018) 10:245–52. doi: 10.1016/j.dadm.2018.02.003
35. Jung K-I, Park MH, Park B, Kim SY, Kim YO, Kim B-N, et al. Cerebellar gray matter volume, executive function, and insomnia: gender differences in adolescents. J Sci Rep. (2019) 9:1–9. doi: 10.1038/s41598-018-37154-w
36. Li Q, Xiao Y, Li Y, Li L, Lu N, Xu Z, et al. Altered regional brain function in the treatment-naive patients with somatic symptom disorder: a resting-state fMRI study. Brain Behav. (2016) 6:e00521. doi: 10.1002/brb3.521
37. Helmchen C, Mohr C, Erdmann C, Petersen D, Nitschke MF. Differential cerebellar activation related to perceived pain intensity during noxious thermal stimulation in humans: a functional magnetic resonance imaging study. Neurosci Lett. (2003) 335:202–6. doi: 10.1016/s0304-3940(02)01164-3
38. Moulton EA, Schmahmann JD, Becerra L, Borsook D. The cerebellum and pain: passive integrator or active participator? Brain Res Rev. (2010) 65:14–27. doi: 10.1016/j.brainresrev.2010.05.005
39. Guo W, Liu F, Chen J, Wu R, Li L, Zhang Z, et al. Anatomical distance affects cortical-subcortical connectivity in first-episode, drug-naive somatization disorder. J Affect Disord. (2017) 217:153–8. doi: 10.1016/j.jad.2017.04.008
40. Zhao Z, Huang T, Tang C, Ni K, Pan X, Yan C, et al. Altered resting-state intra-and inter-network functional connectivity in patients with persistent somatoform pain disorder. PLoS One. (2017) 12:e0176494. doi: 10.1371/journal.pone.0176494
41. Wiech K, Ploner M, Tracey I. Neurocognitive aspects of pain perception. Trends Cogn Sci. (2008) 12:306–13. doi: 10.1016/j.tics.2008.05.005
42. Seminowicz DA, Moayedi M. The dorsolateral prefrontal cortex in acute and chronic pain. J Pain. (2017) 18:1027–35. doi: 10.1016/j.jpain.2017.03.008
43. Kim H, Kim J, Loggia ML, Cahalan C, Garcia RG, Vangel MG, et al. Fibromyalgia is characterized by altered frontal and cerebellar structural covariance brain networks. Neuroimage. (2015) 7:667–77. doi: 10.1016/j.nicl.2015.02.022
44. Yoncheva YN, Zevin JD, Maurer U, McCandliss BD. Auditory selective attention to speech modulates activity in the visual word form area. Cereb Cortex. (2010) 20:622–32. doi: 10.1093/cercor/bhp129
45. Rief W, Isaac M. The future of somatoform disorders: somatic symptom disorder, bodily distress disorder or functional syndromes? Curr Opin Psychiatry. (2014) 27:315–9. doi: 10.1097/YCO.0000000000000089
46. DeLong M, Wichmann T. Changing views of basal ganglia circuits and circuit disorders. Clin EEG Neurosci. (2010) 41:61–7. doi: 10.1177/155005941004100204
47. Blaizot X, Mansilla F, Insausti A, Constans J, Salinas-Alaman A, Pro-Sistiaga P, et al. The human parahippocampal region: I. Temporal pole cytoarchitectonic and MRI correlation. Cerebral Cortex. (2010) 20:2198–212. doi: 10.1093/cercor/bhp289
48. Su Q, Yao D, Jiang M, Liu F, Jiang J, Xu C, et al. Increased functional connectivity strength of right inferior temporal gyrus in first-episode, drug-naive somatization disorder. Aust N Z J Psychiatry. (2015) 49:74–81. doi: 10.1177/0004867414553949
49. Bègue I, Adams C, Stone J, Perez DL. Structural alterations in functional neurological disorder and related conditions: a software and hardware problem? Neuroimage. (2019) 22:101798.
50. Jang YE, Jang Y, Choi HY, Park HY. The relationships between cognitive control and psychological symptoms in patients with somatic symptom disorder: a pilot longitudinal study. Cogn Neuropsychiatry. (2021) 26:242–56. doi: 10.1080/13546805.2021.1923470
51. Rossetti MG, Delvecchio G, Calati R, Perlini C, Bellani M, Brambilla P. Structural neuroimaging of somatoform disorders: a systematic review. Neurosci Biobehav Rev. (2020) 122:66–78. doi: 10.1016/j.neubiorev.2020.12.017
52. Hsu MC, Harris RE, Sundgren PC, Welsh RC, Fernandes CR, Clauw DJ, et al. No consistent difference in gray matter volume between individuals with fibromyalgia and age-matched healthy subjects when controlling for affective disorder. PAIN. (2009) 143:262–7. doi: 10.1016/j.pain.2009.03.017
53. Perez DL, Williams B, Matin N, LaFrance WC, Costumero-Ramos V, Fricchione GL, et al. Corticolimbic structural alterations linked to health status and trait anxiety in functional neurological disorder. J Neurol Neurosurg Psychiatry. (2017) 88:1052–9. doi: 10.1176/appi.neuropsych.13070170
54. Yu CX, Ji TT, Song H, Li B, Han Q, Li L, et al. Abnormality of spontaneous brain activities in patients with chronic neck and shoulder pain: a resting-state fMRI study. J Int Med Res. (2017) 45:182–92. doi: 10.1177/0300060516679345
55. Naegel S, Holle D, Desmarattes N, Theysohn N, Diener H-C, Katsarava Z, et al. Cortical plasticity in episodic and chronic cluster headache. Neuroimage. (2014) 6:415–23. doi: 10.1016/j.nicl.2014.10.003
56. Lee DA, Lee H-J, Kim HC, Park KM. Alterations of the structural covariance network in the hypothalamus of patients with cluster headache. J Neurol. (2021) 269:316–22. doi: 10.1007/s00415-021-10629-z
57. Mehnert J, Schulte L, May A. No grey matter alterations in longitudinal data of migraine patients. Brain. (2020) 143:e93. doi: 10.1093/brain/awaa300
Keywords: gray matter volume (GMV), magnetic resonance imaging, medically unexplained symptoms (MUS), structural covariance (SC), subtype
Citation: Park HY, Jang YE, Sunwoo L, Yoon I-Y and Park B (2022) A Longitudinal Study on Attenuated Structural Covariance in Patients With Somatic Symptom Disorder. Front. Psychiatry 13:817527. doi: 10.3389/fpsyt.2022.817527
Received: 23 November 2021; Accepted: 04 April 2022;
Published: 17 May 2022.
Edited by:
Wenbin Guo, Central South University, ChinaReviewed by:
Howard Schneider, Sheppard Clinic North, CanadaKonstantinos Kalafatakis, Queen Mary University of London, United Kingdom
Copyright © 2022 Park, Jang, Sunwoo, Yoon and Park. This is an open-access article distributed under the terms of the Creative Commons Attribution License (CC BY). The use, distribution or reproduction in other forums is permitted, provided the original author(s) and the copyright owner(s) are credited and that the original publication in this journal is cited, in accordance with accepted academic practice. No use, distribution or reproduction is permitted which does not comply with these terms.
*Correspondence: Bumhee Park, YmhwYXJrQGFqb3UuYWMua3I=