- 1Evolution, Infection and Genomics, Faculty of Biology, Medicine and Health, Manchester Academic Health Science Centre, The University of Manchester, Manchester, United Kingdom
- 2Faculty of Humanities, Cathie Marsh Institute for Social Research, The University of Manchester, Manchester, United Kingdom
- 3Methods Hub, Department of Sociology, Faculty of Social Sciences, The University of Hong Kong, Hong Kong, Hong Kong SAR, China
Resilience is broadly defined as the ability to maintain or regain functioning in the face of adversity and is influenced by both environmental and genetic factors. The identification of specific genetic factors and their biological pathways underpinning resilient functioning can help in the identification of common key factors, but heterogeneities in the operationalisation of resilience have hampered advances. We conducted a systematic review of genetic variants associated with resilience to enable the identification of general resilience mechanisms. We adopted broad inclusion criteria for the definition of resilience to capture both human and animal model studies, which use a wide range of resilience definitions and measure very different outcomes. Analyzing 158 studies, we found 71 candidate genes associated with resilience. OPRM1 (Opioid receptor mu 1), NPY (neuropeptide Y), CACNA1C (calcium voltage-gated channel subunit alpha1 C), DCC (deleted in colorectal carcinoma), and FKBP5 (FKBP prolyl isomerase 5) had both animal and human variants associated with resilience, supporting the idea of shared biological pathways. Further, for OPRM1, OXTR (oxytocin receptor), CRHR1 (corticotropin-releasing hormone receptor 1), COMT (catechol-O-methyltransferase), BDNF (brain-derived neurotrophic factor), APOE (apolipoprotein E), and SLC6A4 (solute carrier family 6 member 4), the same allele was associated with resilience across divergent resilience definitions, which suggests these genes may therefore provide a starting point for further research examining commonality in resilience pathways.
Introduction
Our understanding of how resilience develops is still not well-established largely due to the heterogeneities in the definition of resilience utilized in the past 50 years. Most studies and concept analyses share some commonality in the definition of resilience as the ability to maintain or regain functioning in the face of adversity [e.g., (1–3)]. This broad definition includes both the ability to recover from a stress-related disorder and the ability to withstand adversity, yet these definitions offer little explanation of the mechanisms by which resilience occurs. While there is currently much ongoing work within resilience research to harmonize the definition and quantification of resilience to allow for significant advances in understanding resilience mechanisms (4–7), there is still extensive previous research that can be utilized for significant advances in the identification of underlying resilience mechanisms.
Traditionally, resilience research has been approached from a psychosocial perspective, often focusing on environmental and social factors that influence resilience (8). This has led to reviews with catalogs of so-called “resilience factors” that may be extrinsic or intrinsic to the individual (e.g., high quality care-giving environment, good communication or social skills, self-efficacy or coping) (9–11), with many current studies focussing on genetic resilience factors including twin studies that suggest genetic heritability of resilience accounts for up to 52% of observed variance (12–14). To date, individual genetic and environmental resilience factors, such as specific candidate genes or demographic predictors, have only been identified as weak predictors of resilient outcomes, explaining only a small proportion of the variance and have proven difficult to replicate (7). Furthermore, many of these factors conceptually overlap and mediate, moderate or correlate with one another (15, 16). For example, an individual's genotype is a factor that may affect resilience by influencing an emotional response to stressors. This emotional response to stressors underpins previously identified resilience factors such as emotional regulation, coping or even problem solving. Thus, mapping an individual's genotype may identify a possible resilience mechanism associated with stress response. Such genotype mapping allows for the identification and understanding of the mediating resilience mechanisms (17).
General Resilience Mechanisms
There are many multifactorial causal pathways that lead to resilient functioning. These pathways involve a complex interplay of biological, social and psychological factors, current experiences and environments, the timing of adversity and experience, and family and community context. However, despite the many factors that may determine if someone is resilient or not, there are potentially fewer distinct biological resilience pathways. Support for this notion comes from psychiatric genetics. For example, a genome-wide association study (GWAS) has indicated that five major psychiatric disorders have a shared risk locus (18), and carrying a “risk” allele of CACNA1C confers risk for bipolar disorder, depression, and schizophrenia (19). Calcium Voltage-Gated Channel Subunit Alpha1 C (CACNA1C) has been functionally implicated in a broad spectrum of neuropsychiatric disorders, and a recent meta-analysis supports a significant association between CACNA1C and major depression disorder (MDD) (20). Similarly, a cross-trait meta-analysis of GWAS identified DCC Netrin 1 Receptor (DCC) as a potential risk gene with a pleiotropic role in attention deficit hyperactivity disorder (ADHD), autism spectrum disorder (ASD), depression, and schizophrenia (21).
There is further support of common risk factors leading to diverse psychiatric endophenotypes from studies showing that phenotypes share common features across diverse forms of psychopathology. However, symptoms belonging to specific disorders in early development may not be predictive of a diagnosis of the same disorder later in development but are a marker of more broad adult mental health problems (22, 23). Indeed, there is a paucity of associations between major psychosocial risk factors and specific disorders (24). This lack of association between risks and diagnostic specificity may be explained by the fact that research hypotheses are based on the assumption that disease processes are disorder specific. Psychological disorders are frequently comorbid, with significant overlaps in symptoms. This suggests that resilience research should engage in a transdiagnostic perspective and identify resilience mechanisms that maintain allo/homeostasis (15), rather than focussing on disorder-specific resilience mechanisms. Allo-/homeostatic systems have evolved to maintain functioning despite adversity, therefore, natural selection is more likely to select for mechanisms ensuring allostasis in the face of all stressors, rather than selecting for disorder-specific stress responses. Thus, biological systems that are protective for specific stressors are predicted to be an extension of a more generalized protection system that contributes to maintaining homeostasis within a broad range of environmental disturbances (25, 26).
This dysfunction-specific rationale (15) combined with evidence for frequent comorbidity of stress-related disorders suggests the existence of general resilience mechanisms and, upon breakdown of these mechanisms, stress negatively affects an array of functional systems concordantly. For example, failure to terminate chronic stress-induced activation of the hypothalamus-pituitary-adrenal (HPA) axis can lead to pathological, long-term dysfunction in multiple brain and body compartments (27, 28). Thus, general resilience mechanisms could be those that support flexible HPA axis deactivation. The existence of a resilience mechanism that helps the individual calibrate stress responses optimally, while remaining flexible enough to deploy resources to alternative coping strategies efficiently, would have important consequences for resilience and stress research.
This dysfunction-specific rationale extends to the idea that protection from multiple diseases may be conferred by a limited number of genetic variants of resilience. This rationale is supported by recent research into genetic variants of longevity in non-agenarians and centenarians, which have shown that long-lived individuals (90+ years) have the same number of genetic variants associated with age-related diseases as the general population, yet have an increased prevalence of a small number of novel protective variants (29–32). These few protective variants, in combination with environmental resilience factors, buffer the effect of disease-associated variants and adverse environmental factors, decreasing morbidity at the end of extreme longevity.
A systematic approach that identifies genetic variants conferring resilience is therefore important for several reasons. First, the ability to map genome and phenome relationships more readily onto individual health trajectories by identifying genetic resilience mechanisms brings a health-related perspective to the current more disease-centric genotyping approaches. Further, identifying genetic variants conferring resilience enables effective pharmacotherapy for novel molecular targets. Underlying genetic variants that are associated with general resilience mechanisms may be targeted and thus used to pharmacologically manipulate dysregulated molecules and pathways associated with developing and sustaining symptom severity of trauma-exposed individuals. For example, targeting general resilience mechanisms involved in brain plasticity and cognition could facilitate a change of perspective of trauma exposure and thus lessen the severity of PTSD symptoms (33). The key challenge lies in identifying the common pathways to resilient functioning representing general resilience mechanisms.
Genetic Variants Conferring Resilience in Humans
Genetic variants, such as allelic variation, can act both as risk and resilience factors. While genetic risk factors increase the likelihood of dysfunction or disease, genetic resilience factors may provide resilience to disease onset or progression (34), or buffer the influence of environmental adversity. However, in the absence of adversity, such genetic resilience factors may appear to have little or no effect. While the role of genetic variants in disease risk has been amply demonstrated (35, 36), much less is known about genetic variants underlying resilience. It is important to note that resilience is not simply the flipside of risk but may co-occur with risk. For example, an individual can experience adversity in a high-risk environment, but also show resilience to a high-risk environment. If exposure and sensitivity to risk factors are high, then the outcome associated with the risk may develop, even in the presence of a resilience factor. Hence the two concepts, risk and resilience, can co-occur. Resilience can also be linked to recovery from stress-related disorders and may not be specific to resisting a specific disease or psychopathology. By focusing on risk factors that predict outcomes requiring diagnostic criteria, studies may not be able to distinguish between individuals who resist developing the disease, and those that recover. Thus, resilience factors may underpin pathways that confer resilience more generally rather than resilience to a particular outcome as defined by current diagnostic criteria.
Most studies on the genetics of resilience in humans have focused thus far on candidate genes with protective variants identified in genes related to serotonergic systems (37), the HPA axis (38), the norepinephrine stress response (39), influencing temporal lobe gray matter volume (40) or amygdala and hippocampal activation to threat (41). While candidate gene approaches have been the mainstay of genomics for over 20 years, increasingly large GWAS have found a lack of robust associations that is seen in candidate gene studies. Among key limitations are lack of statistical power associated with small sample sizes, and potential bias in selecting for candidate genes [e.g., (42–44)]. However, there are some replicable candidate gene x environment (GxE) interactions linked to depression phenotypes within the HPA axis (45) and alcohol use disorder, showing replicability in GWAS with large-effect loci first identified by candidate gene studies (46). Given that resilience is a complex, dynamic process that fundamentally involves both genetic and social/environmental factors, poor replicability across GWAS may be due to the lack of considering both genetic and environmental factors in the study design. While progress on resilience research is advancing, the enormous heterogeneity in the definition of resilience also precludes reproducibility and undermines the robustness of results across studies, ultimately hindering significant advances in the development of effective interventions based on general resilience mechanisms. By focusing on specific genetic variants, we are better able to identify common biological resilience pathways and potential targets for intervention.
Animal Models in the Study of Resilience
The candidate gene approach used in studies on the genetics of human resilience is replicated in genetic animal models of resilience using a targeted approach. Due to ethical reasons, experimental investigations of the genetic effects of resilience, especially regarding the central nervous system, can only be conducted in animals. Many recent animal models have been specifically designed to distinguish between vulnerable and resilient phenotypes, through a variety of approaches.
One approach is to use transgenic models where animal genomes (predominantly rodent) are modified, resulting in knock out (KO) or knock in of specific candidate genes. Several studies using such models have now demonstrated the influence of single genetic variants on stress vulnerability or resilience (47). However, the limitations of using transgenic animal models are important to consider. Transgenic models can provide information about the general function of the specific gene targeted, yet resilience, like many spectrum disorders, is multigenic. Thus, linkage analyses may be a more appropriate approach to detect variants associated with resilience phenotypes [e.g., (48)]. It is also worth noting that genes often play different roles or may potentially even have opposite functions in separate tissues or cell types [e.g., (49)]. Further, the developmental time frame during which gene expression is modulated also needs to be considered, due to the vital influence of developmental timings on the maturation of different parts of the stress system on later stress vulnerability or resilience (50).
Selective breeding of a specific phenotype is another approach used in animal models of resilience. An example of this is the stress reactivity mouse line (51). Starting from a population of outbred mice, two breeding lines were established according to the outcomes of a stress reactivity test. Mice exhibiting hypo- or hyperactivity of the HPA axis, measured via corticosterone levels in blood, were selected for high or low reactivity breeding lines. By the first generation, the breeding line has diverged, allowing for further characterization and investigation of the underlying molecular principles. In this model, the high reactivity breeding line shows cognitive deficits, restlessness, and agitation, which is often seen in melancholic depression (51), and also exhibit analogous neuroendocrine activity and morphometric differences (52). Using such models in genome-wide association studies can lead to the identification of novel genes or networks. Selective breeding also has the potential to identify causal genetic factors using careful research designs, as the phenotypic differences are present before exposure to any stressor.
Similar to the selective breeding approach, the intrinsic heterogeneity of whole populations can be used by grouping animals into subpopulations dependent upon their performance in a behavioral assessment, such as the forced swim test. For example, in a rat model, Wong et al. (53) used chronic restraint stress (CRS) to elicit a depressive-like behavior in the forced swim test, measured by increased floating time/immobility. However, not all animals develop this phenotype, so the cohort is separated into CRS resilient and CRS non-resilient animals, allowing for the identification of genetic differences in these subphenotypes. While this approach has the same advantages as the selective breeding approach, measuring an analogous phenotype with high translatability to human behavior is challenging [e.g., (54)], with some authors arguing that anthropomorphic interpretations of rodents behavior does not accurately model depression or resilience to depression (55).
The main question regarding all animal models of resilience is how they relate to resilience as defined in humans. Since there is no standard definition of resilience in humans, animal models of resilience therefore use different paradigms and outcome variables to measure resilience, analogous to the different definitions and operationalisation of resilience in human studies. Despite the limitations of animal models of resilience, as with the human studies, our broad inclusion criteria enable us to capture the diversity of paradigms used in animal models and aim to identify commonalities in genetic variants underlying resilience.
Differences in Environmental Sensitivity
One of the fundamental concepts of resilience research is that individuals differ in their sensitivity to environmental influences, with increasing evidence that certain genetic variants confer increased environmental sensitivity (56–59). These individual differences in behavioral responses to the environment are seen across species, from zebra finches to mice, non-human primates to humans (60). This concept is traditionally framed within psychology in a diathesis-stress model, where sensitivity is seen as a vulnerability for developing problematic outcomes (diathesis) when faced with adversity (stress) (61). This perspective stipulates that some individuals are disproportionately likely to succumb to the negative effects of contextual stressors. However, this model makes no claims about differences in how resilient or vulnerable individuals develop under favorable conditions. A more recent theoretical framework, the differential susceptibility hypothesis (DSH), suggests individuals differ in environmental sensitivity more generally, not just in the response to negative stressors (62).
In contrast to the diathesis-stress model, which implies a definite and mostly negativity-sensitive group, Belsky et al. describe a group that is sensitive to negative experiences but also positive experiences as “for better AND for worse” (62). The DSH suggests that natural selection drives and maintains these two developmental strategies, which are fundamentally different in susceptibility. The plastic strategy, high susceptibility, is characterized by adaptation to the environment, while the fixed strategy, low susceptibility, reflects little or no reactivity in response to the environment. In resilience research, this theory is important to consider because, on some occasions, those carrying a “protective” allele may function worse than others when not exposed to the risk condition being studied. The DSH also allows for the possibility that some genetic variants may moderate the effects of both positive and negative environments on resilience (63, 64). For example, the same genetic variants that may increase the risk of mental health problems when faced with adversity appear to make individuals more likely to benefit from positive environmental factors. This has important implications for genetic studies of resilience because if individuals are less reactive, i.e., experience little or no psychopathology despite high risk, they could be defined as resilient. Yet a specific reactivity phenotype could be adaptive and confer resilience in some conditions. Very few studies to date capture truly comprehensive measures of the environment, longitudinally and across the complete spectrum from adverse to positive, which may explain why there is such inconsistency in candidate GxE findings (42).
Defining resilience as merely the lack of psychopathology would mean resilience is a passive process, yet most resilience research now points to it being an active, dynamic process (65). Resilience is not only the absence of psychopathology but also positive adaptation (25), although this area is less well-studied. In humans, studies range from measuring self-reported resilience, referring to appraisal, and the concept of functioning resiliently in the face of stress. Despite these being different aspects of resilient phenotypes, the main aim is to find underlying genetic variants in order to identify general resilience mechanisms.
Present Study
To our knowledge, despite the recent advances and reviews on the genetics of resilience (5, 66), no comprehensive, interdisciplinary systematic reviews have been conducted to examine the genetic variants associated with resilience in humans and animals. Due to the complexities of definition, the potential for common resilience mechanisms to share a variety of outcomes, and the importance of animal studies in the search for biological mechanisms of resilience, this review incorporates general inclusion criteria for the definition of resilience in both humans and animal models. It is important to note that we do not seek to develop a novel definition of resilience. Rather, we include all previously published research that have defined resilience in different ways in order to identify general resilience mechanisms and underlying genetic variants.
The main aim of this review is to identify genetic variants associated with resilience. Identifying common genetic variants associated with resilience in humans and animals will provide construct validity to both animal models and human studies of resilience. Identifying genetic variants associated with resilience as a measure of both reactivity and positive adaptation will provide support for the idea of a general resilience mechanism.
Method
This review has been conducted following PRISMA guidelines and guidance by Sagoo et al. (67) on systematic reviews of genetic association studies (67).
A systematic search of EMBASE, Medline, and PubMed was performed in October 2021 with the terms “resilien*” AND “genotype” in search category: title, abstract, and full text. The wildcard operator (*) was used to include all possible suffixes (resilience, resilient, resiliency). In addition, research articles associated with a phenotype of “Resilience, Psychological” in the HuGE (Human Genome Epidemiology) Navigator Phenopedia (68) were included. Published years were not restricted.
To be included in this review, manuscripts had to be written in English, peer reviewed, and report a significant association between a genetic variant and an outcome associated with resilience (either positive adaptation or reactivity). DNA methylation, twin, and heritability studies of resilience were excluded from this review because they do not identify specific genetic variants associated with resilience. Titles and abstracts were initially screened to determine eligibility. If questions remained about the eligibility, the entire manuscript was reviewed to ascertain inclusion. This resulted in 158 studies, including 30 studies identified through references of the records identified through the electronic search. Author 1 conducted the review, with authors 2 and 3 systematically cross-checking the data-extraction process. See Figure 1 for the selection process.
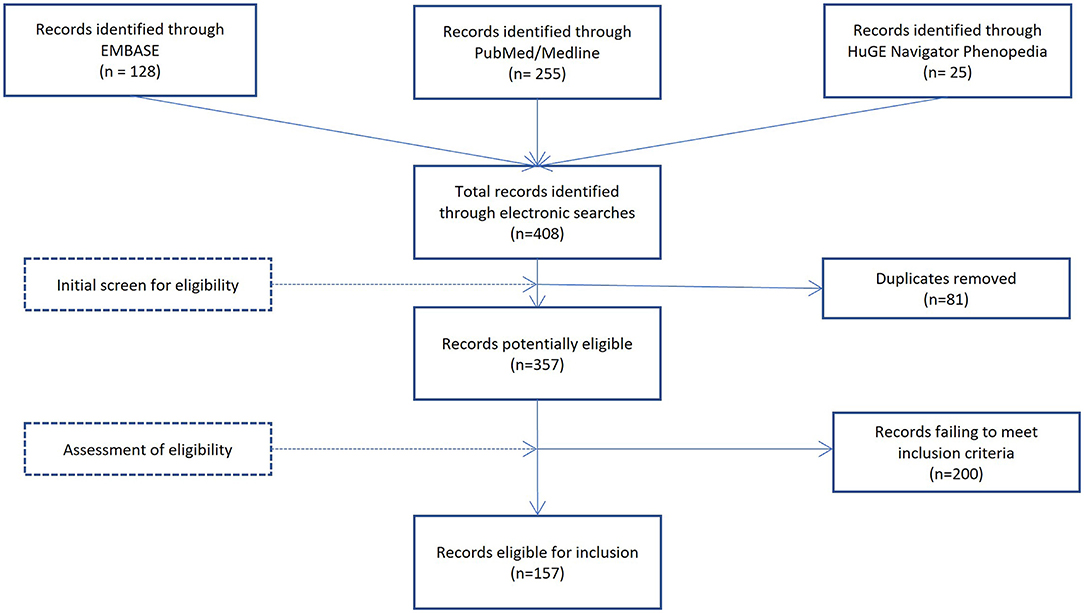
Figure 1. Identification and selection process. The figure shows the sources used for identification and assessment of eligibility of studies for inclusion in this review with number of studies in brackets.
Data Extraction
The following information was extracted:
Human studies: (1) Population characteristics (number of patients vs. controls, age range, sex, adult or child population, important characteristics), (2) resilience measurement, (3) candidate gene, (4) resilient allele (if any), (5) environmental risk factor (if any), (6) key findings.
Animal studies: (1) Species, (2) population characteristics (age, sex, transgenic, KO, subpopulations), (3) candidate gene, (4) environmental stressor (if any), (5) key findings.
All data extraction can be found in Supplementary Table 1.
Results
The systematic searches initially generated 408 titles, that were reduced to 327 after removal of duplicates. An additional 30 studies were identified through reference lists, leaving 357 full-text articles assessed for eligibility. In total, 156 papers were selected for this review. The selected papers were then separated into three areas, (1) animal studies of resilience (n = 28), (2) human studies with resilience measured by positive adaptation (n = 51) and, (3) human studies with resilience measured by level of reactivity (n = 77). A total of 62 candidate genes were associated with resilience. Descriptions of the functional importance for each gene are summarized in Table 1.
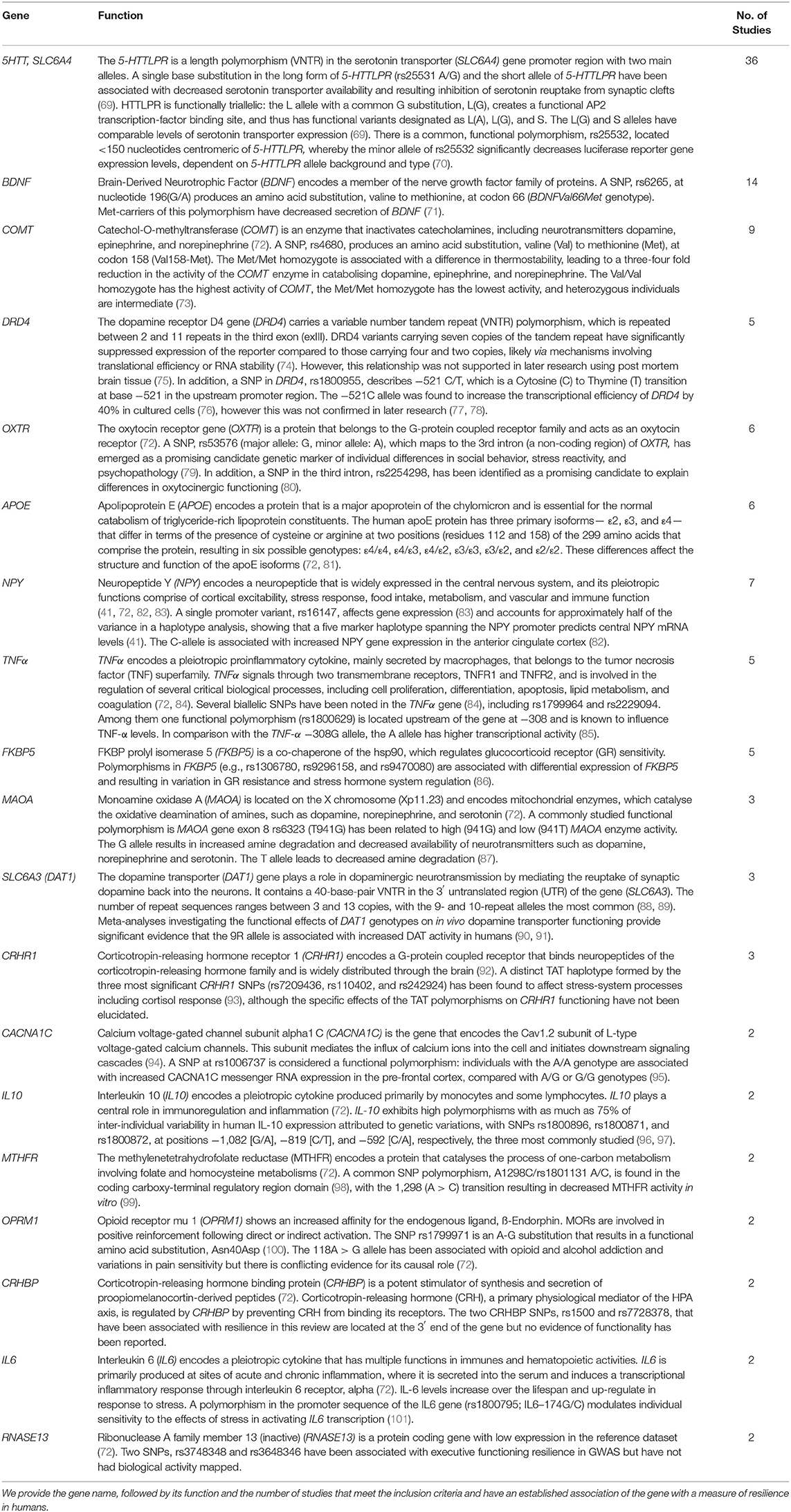
Table 1. Gene functions for each gene identified in the systematic review with two or more studies reporting association with resilience.
Alleles Associated With Resilience as Measured by Positive Adaptation (N = 51)
Resilience is measured using a number of different instruments and measurement tools as highlighted in Table 2.
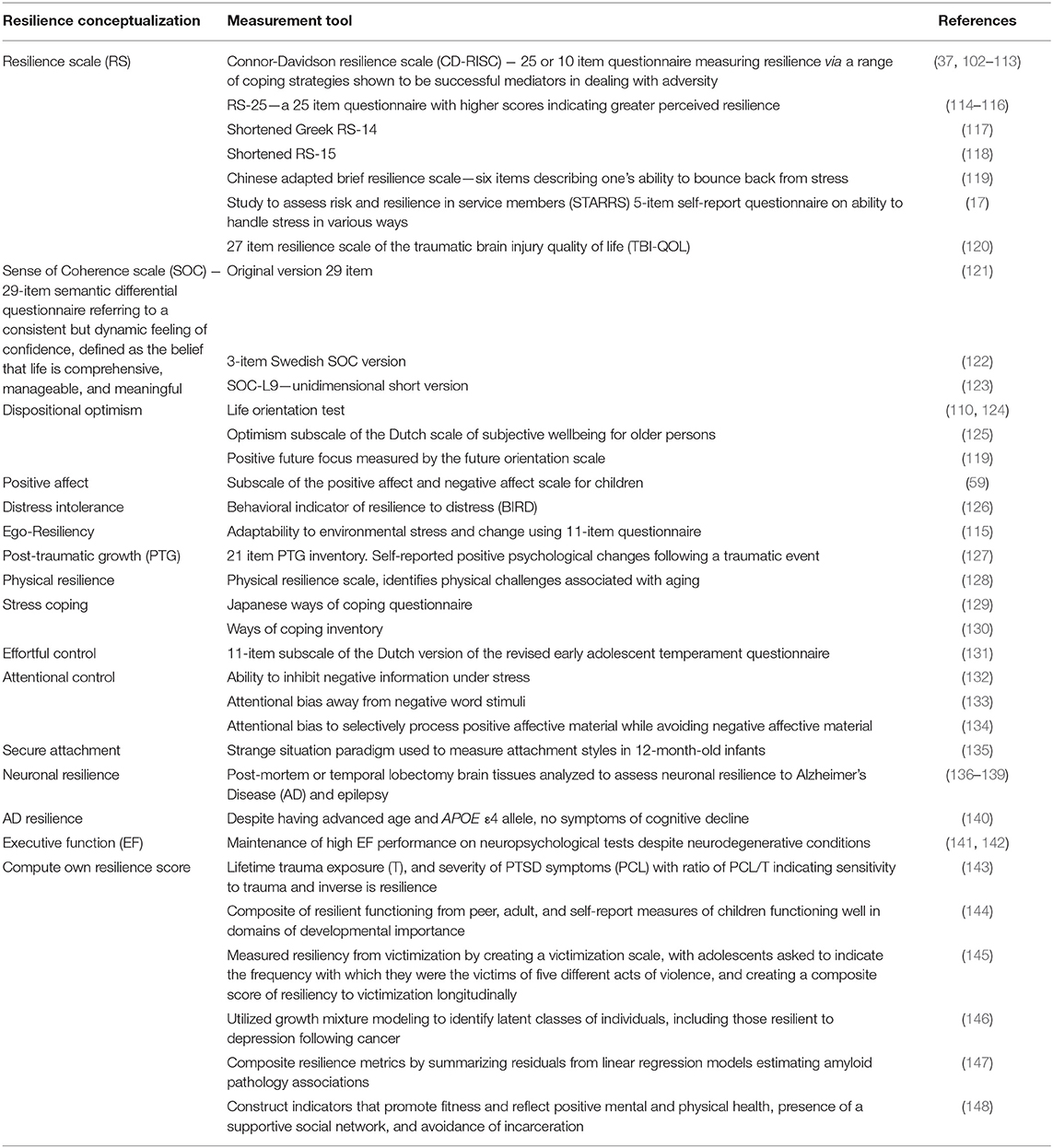
Table 2. Operationalisation of resilience as a form of positive adaptation and measurement tools utilized.
Below we discuss, in order of highest frequency, the genes and their function that were identified in two or more studies as being associated with resilience to assess whether consensus is reached on which alleles are associated with resilience.
Serotonin Transporter (5-HTT, SLC6A4)
The most frequently investigated polymorphism (n = 18) was the 5-HTTLPR (serotonin transporter linked polymorphic region) which is located in the promoter of the human serotonin transporter gene (SLC6A4). Out of these studies, 12 found the L′/L′ allele to be associated with resilience (37, 59, 102, 114, 117, 126, 130–134, 144) and four studies found the S or S′ allele to be associated with resilience (103, 104, 123, 145), and one study found that only haplotypes of the serotonin transporter were associated with resilience, the single genetic variants were not (115). There are a several factors that might contribute to these inconsistent findings.
First, the studies used different allelic variation classification. The A 44 bp deletion/insertion results in a short (S) or a long (L) allele, creating a biallelic classification of S/L alleles (149). The rs25531 is a SNP in the L allele, which leads to an A-G polymorphism. This provides a functional triallelic polymorphism as S, LG, and LA. The LG allele is functionally equivalent to the S allele, having the same expression levels, both of which are lower than LA (69). Consequently, the L′/L′ can be reclassified as equal to LA/LA; L′/S′ = LA/S and LA/LG; and S′/S′ = S/S & LG/S and LG/LG. This is the triallelic classification system. 12 of the studies excluded the SNP rs25531 from their analyses (37, 102, 103, 114, 115, 117, 126, 130, 132, 134, 144, 145), and five studies genotyped for rs25531 and recoded accordingly (59, 104, 123, 131, 133). One study found a significant association with the biallelic classification system, but not with the triallelic (37), and another study excluded the results of the triallelic system due to a low number of participants carrying the LG allele (102).
A second factor contributing to inconsistencies in findings between studies are differences in the developmental stage at which effects were studied. Eight of the studies investigated children or adolescents, while ten investigated adults. Within the studies that did use the triallelic classification system, those that found that the L/L or L′/L′ genotype of 5-HTTLPR contributed to resilience, investigated children/adolescents (59, 131, 133), while those that found the S/S or S′/S′ genotype investigated adults (104, 123). This could be explained by the evolutionary genetic theory of antagonistic pleiotropy (150), which was first proposed as an explanation for multiple effects of a gene with opposing effects on fitness depending on the developmental stage. Notably, genes enhancing reproductive success in early life are predicted to become deleterious in later life. This opposite effect in young vs. old participants should be interpreted with caution because, although there is some evidence emerging of the age-genotype interaction [e.g., (151, 152)], the evidence is limited.
A third factor that may explain discrepancies in consensus are sex differences in the 5-HTTLPR polymorphism. Previous research from both animals and humans suggests that males and females carrying the short 5-HTTLPR allele respond to environmental stress factors in opposite directions (153, 154). A recent review of sex differences in affective disorders found the S allele to be differentially associated with an increased risk of depression, anxiety, and internalizing behavior in women, and increased risk of aggressiveness, conduct disorder, and externalizing behavior in men (155). The two studies that investigated female-only populations (114, 132) found the L allele to be associated with resilience, while one study investigating males only found the S/S homozygous genotype to be associated with resilience.
Brain-Derived Neurotrophic Factor
The second most frequently investigated gene was Brain-Derived Neurotrophic Factor (BDNF) (n = 5). All five of the studies reviewed investigated SNP rs6265 in exon 11 of the BDNF gene (105, 116, 122, 129, 131). Of the four studies, three found Val/Val homozygous genotype to be associated with resilience, while two studies found the Met allele to be associated with resilience. This inconsistency in results could, again, be due to a number of confounds. Allele frequencies of the BDNF Val66Met polymorphism are dependent on ethnic background: frequency of the Met allele among Caucasians is 25–32% but is more common in Asians at 40–50% (156). Of the five studies, Aizawa et al. (129) and Kang et al. (105) both studied Asian populations, Nederhof et al. (131) and Surtees et al. (122) both studied European Caucasians, and Peters et al. (116) studied Brazilian nationals. Sex differences in the populations studied may also result in inconsistent findings. A recent review found some action mechanisms or functions of BDNF vary according to sex and encourage considering sex influences when drawing conclusions from studies (157). One study reviewed only observed a significant association between resilience and the Val allele in males (105).
Two of the studies assessed polygenic resilience with a gene-gene interaction effect. One study suggests a sex-dependent result, where the BDNF Val66Met polymorphism modulates the effects of the Val158Met polymorphism of COMT on resilience, but only in males (105). The other study investigated the gene-gene interaction between BDNF Val66Met polymorphism and 5-HTTLPR, specifically investigating resilience depending on the genotype of the other gene variant (131).
Oxytocin Receptor Gene
Three studies investigated the rs53576 polymorphism in the oxytocin receptor gene (OXTR). Cicchetti and Rogosch (144) found the impact of child maltreatment on resilient functioning was decreased in children with the GG genotype when compared to GA or AA genotypes, assuming the dominant effect of the A allele. Saphire-Bernstein et al. (124) reported a link between the GG genotype and higher levels of optimism, mastery, and self-esteem, suggesting that the influence of this OXTR polymorphism on psychological resources may primarily mediate the effects of OXTR on depressive symptoms.
In contrast, Bradley et al. (158) reported that, regardless of family environment, adults with the AA genotype had higher levels of positive affect and resilient coping scores. The authors reported that GG and AG genotypes were more influenced positively and negatively by the family environment, in keeping with the differential susceptibility hypothesis.
One study investigated the OXTR variant rs2254298 on psychological resilience as measured by CD-RISC (106). The authors found a dose-dependent effect of the A allele in ethnically Korean adolescents and young adults, with highest resilience in carriers of the GG genotype, decreasing by 3.84 on the CD-RISC scale with a one-copy increase in the A allele. An OXTR haplotype containing the rs2254298 A allele was also associated with lower CD-RISC scores.
Apolipoprotein E
Four studies investigated Apolipoprotein E (APOE). The presence of the ε4 allele is a strong genetic risk factor for Alzheimer's disease (AD), whereas the ε2 allele is protective against AD. Two of the studies found both the ε3 and ε2 allele to be associated with resilience (136, 143), and the other study found ε3 allele to be associated with resilience (137). However, this study did not genotype for the APOE ε2 allele, so there is consensus amongst the studies reviewed about which allele is associated with resilience despite the studies using different instruments to measure resilience and associating the allele with different outcomes: AD, PTSD, and epilepsy. A fourth study found US veterans with mild traumatic brain injuries (TBIs) had significantly better scores on the resilience scale of the TBI- Quality of Life measure (120), but again, the study did not genotype for ε2 or ε3 isoforms of APOE so it cannot be included in the consensus of the APOE ε2 allele conferring resilience.
Catechol-O-Methyltransferase
Three studies investigated catechol-O-methyltransferase (COMT). All studies investigated a functional polymorphism on rs3680, Val158Met (105, 107, 126), yet again the results were inconsistent. Of the two studies which used the same instrument to measure resilience, the CD-RISC, van Rooij et al. (107) found childhood trauma load was associated with increased inhibition-related hippocampal activation in Val/Val carriers, with the relationship between childhood trauma and resilience mediated by hippocampal activation. This implies that Val/Val carriers may develop a mechanism to cope with early adversity by relying more on contextual information processed in the hippocampal region in order to regulate behavior. However, Kang et al. (105) found a conflicting, polygenic, and sex-dependent result. Males with homozygous Val allele of BDNF and COMT Met-present genotype were found to have higher resilience than BDNF Met carriers. Amstadter et al. (126) also found associated resilience with the Met allele. This inconsistency could again be attributed to sex differences: van Rooij et al. (107) only studied women, while Kang et al. (105) only found a significant association with resilience in males. COMT enzyme activity and the neurochemistry and behavior of COMT knock out mice are both markedly sexually dimorphic (159). In addition, the Val158Met polymorphism shows sexual dimorphism in predisposition to psychiatric disorders (159).
Results could also be conflicting due to the different ethnic groups analyzed by the researchers. COMT Val/Met allele frequencies differ across ethnic groups, with higher heterozygosity in European populations than other geographical regions (160).
Further the assumed mode of inheritance in each study may contribute to inconsistencies in results. Both Kang et al. (105) and van Rooij et al. (107) assumed dominance of the Met allele and grouped the sample into subjects with the Val/Val genotype vs. Val/Met and Met/Met. By contrast, Amstadter et al. (126) assumed dominance of the Val allele (Met/Met vs. Val/Met and Val/Val). Due to this difference in modes of inheritance, it is unclear whether heterozygotes of rs4680 in COMT (Val/Met carriers) would be associated with increased or decreased resilience.
Dopamine Receptor D4
The human dopamine receptor D4 gene (DRD4) was investigated by three studies (108, 135, 144). Das et al. (108) found an interaction effect of childhood adversity, the DRD4 7-repeat allele, and resilience. A decline in resilience was associated with increased childhood adversity in individuals who lacked the 7-repeat allele, therefore suggesting that the 7-repeat allele is protective. Conflicting results come from Gervai's et al. (135) study, which analyzed a haplotype of the 7-repeat allele and the −521C/T promoter polymorphism of DRD4 which found an absence of the T.7 haplotype acts as a resilience factor. Cicchetti and Rogosch (144) studied the effects of the −521C/T promoter polymorphism of DRD4 (rs1800955) and revealed another interaction effect where maltreated children with CC or CT genotypes were protected from the significantly detrimental effect of extensive maltreatment, assuming the dominant effect of the C allele (CC and CT vs. TT). This is in some way consistent with Gervai et al. (135).
Tumor Necrosis Factor α
Two studies investigated tumor necrosis factor α (TNFα) (113, 146). Bruenig et al. (113) examined a SNP (rs1800629) in the promoter region of TNFα, which has been identified with potential functional consequences for gene transcription. While no significant effect was observed between CD-RISC and genotype, there was a significant correlation between resilience and decreased TNFα serum levels. Dunn et al. (146) found two SNPs to be significantly associated with a resilient latent class: rs2229094 and rs1800629. Individuals who possess the A rare allele for rs1800629 had higher odds of belonging to the resilient class, while individuals who possess the T allele in rs2229094 had higher odds of belonging to the resilient class.
Interleukin-10
Interleukin-10 (IL10), was associated with resilience in two studies (110, 146). Rana et al. (110) found the SNP rs1800896 in IL10 to be associated with optimism in an exploratory multi-locus polygenic analysis with two other SNPs: rs6323 of MAOA and rs1800792 of Fibrinogen Gamma Chain (FGG). Dunn et al. (146) found participants who possessed a G allele of SNP rs1518111 of IL10 to have higher odds of belonging to the resilient latent class. Both studies used a strategy of selecting high-probability candidate genes to analyse. While scientifically justifiable, selecting genes from a list of “usual suspects” introduces bias, and many genetic variants identified by GWAS have included genes previously not thought to be involved in the target disease etiology (161).
Ribonuclease a Family Member 13
Two studies, although of the same population, found two significant genome-wide associations between SNPs (rs3748348 and rsrs3648346) of Ribonuclease A Family Member 13 (RNASE13), and executive functioning resilience (141, 142), suggesting a cognitive basis for resilience. Pathway-based analysis by gene set enrichment analysis implicated EF resilience with genetic pathways involving dendritic/neuron spine, presynaptic membrane, and post-synaptic density (142). RNASE13 has been linked to susceptibility for aortic aneurysm in Japanese individuals (162).
Monoamine Oxidase A
Two studies investigated monoamine oxidase A (MAOA) genetic variants (110, 163). In addition, Azadmarzabadi et al. (111) examined mRNA levels of MAOA and found that MAOA expression is downregulated in individuals with low stress resilience, as measured by the CD-RISC. Rana et al. (110) found SNP rs6323 in MAOA to be associated with both resilience and optimism in an exploratory multi-locus polygenic analysis. Tikkanen et al. (163) found alcoholic violent offenders carrying the low MAOA activity genotype (3-repeat allele) had no increase in risk of reoffending when exposed to the independent predictors of recidivism, heavy drinking and childhood physical abuse. Significant associations have previously been reported between MAOA and anger-related traits (164).
Alleles Associated With Resilience as Operationalised by Levels of Reactivity (N = 77)
The 77 studies that found alleles associated with resilience via an array of measures that associated with some level of reactivity to the environment are shown in Table 3. Despite these wide ranges of operationalising resilience, they will be examined together with the goal of finding shared biological pathways.
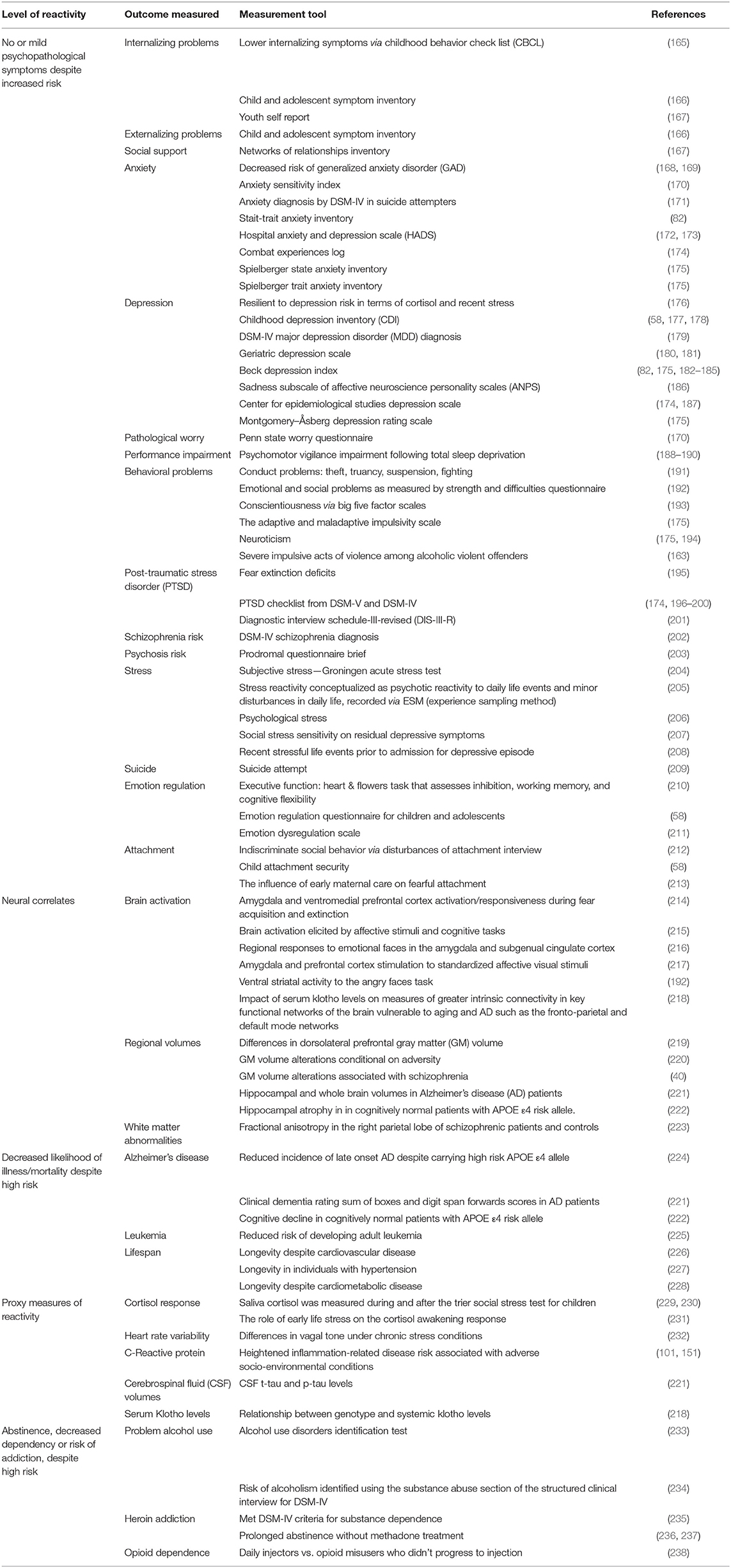
Table 3. Operationalisation of resilience as measure of reactivity, the outcome measured, and measurement tool utilized.
Serotonin Transporter (5-HTT, SLC6A4)
The most frequently investigated polymorphism associated with the operationalisation of resilience as levels of reactivity, was again the 5-HTTLPR (n = 18). The environmental measures included stressful life events, stroke, war zone combat, victimization, racial discrimination, child abandonment, interferon-alpha treatment, and neuroticism. Of these 16 studies, 13 found the L allele to be associated with resilience (132, 148, 165, 166, 174, 180, 182, 191, 194, 196, 212, 214, 216), whereas three studies found the S allele to be associated with resilience (177, 197, 203), and two studies found the SL heterozygous allele to be associated with resilience (176, 220).
Apolipoprotein E
Two studies investigated APOE, both concerning the severity of PTSD symptoms among veterans exposed to combat trauma (198, 201). Both studies found that absence of the ε4 allele was associated with significantly less severity of PTSD symptoms than carriers of the ε4 allele.
Brain-Derived Neurotrophic Factor
Eight studies investigated the BDNF Val66Met polymorphism (172, 173, 178, 179, 200, 203, 212, 219) in environmental measures of childhood adversity, childhood abandonment, traumatic life events, newly diagnosed diabetes, and physical exercise. Of the eight studies, 4 associated the Met allele with resilience (178, 179, 203, 212), and the other three found Val/Val or Val/Met genotype to be associated with resilience (172, 173, 200, 219). Interestingly, the four studies that found the Met allele to be associated with resilience all studied children or young adults. Min et al. (172) found a gender-specific effect with the Val/Met genotype in males appearing to be resilient against the higher levels of anxiety associated with exposure to childhood maltreatment. Mata et al. (178), also found gender-specific effects where being physically active was protective for girls with a BDNF met allele against the development of depressive symptoms, but not for girls with a Val/Val genotype—examining how physical activity contributes to resilience to psychopathology.
Catechol-O-Methyltransferase
Five studies investigated COMT polymorphisms, and all studies reported the Val allele as associated with resilience, reaching consensus (186, 204, 215, 217, 229). Of the five studies, two studies were fMRI studies assessing the effects of COMT Val158Met genotype on emotional stimuli processing. They found the presence of a Val158 allele results in an increase in emotional resilience against negative mood states via decreased neuronal activation to unpleasant stimuli (215, 217). Two studies used psychological measures; subjective stress experience (204), and sadness score (186), and one study assessed resilience via cortisol response levels (229).
Corticotropin-Releasing Hormone Receptor 1
Five studies investigated the interaction between child abuse/maltreatment and polymorphisms in the corticotropin-releasing hormone receptor 1 gene (CHRH1). CRHR1 is a major regulator of neuroendocrine, autonomic, and behavioral responses to stress, and its ligand, CRH, has been associated with mood and anxiety disorders (239). The studies found significant GxE interactions with multiple individual SNPs, as well as with a common three allele haplotype, the TAT haplotype, formed by SNPs rs7209436, rs110402, and rs242924. Bradley et al. (240) found that carriers of the TAT haplotype were found to be protected from the adverse impact of severe childhood abuse, as measured by the Childhood Trauma Questionnaire (CTQ). In an extended sample of the Bradley et al. study, Heim et al. (241) confirmed the protective effect of the rs110402 A-allele against the negative emotional consequences of childhood abuse in the male subsample only. They hypothesize that this effect may not be due to sex differences but due to the differences in the abuse experienced, with physical abuse most commonly experienced by men, and sexual abuse most commonly experienced by women. Although, Polanczyk et al. (242) only replicated the protective effect of the TAT haplotype in a sample of women. The second sample in Polanczyk's et al. study consisted of men and women, and no significant result was found. The authors suggest that this difference is attributable to the different types of measurement of maltreatment between the samples, with maltreatment reported via the CTQ more likely to elicit emotional memories, and the hypothesized link between CRHR1's protective effect is related to its function in the consolidation of memories of emotionally arousing experiences.
Grabe et al. (183) identified a previously unreported SNP, rs17689882, in which the minor allele (A) had a protective effect against depressive symptoms among individuals who had experienced moderate to severe neglect. Laucht et al. (184) replicated this result, finding the minor A allele as protective.
Dopamine Transporter 1
Two studies investigated GxE interactions associated with the dopamine transporter 1 gene (DAT1, SLC6A3). Felten et al. (186) found carriers of the homozygous 9-repeat allele showed dramatically reduced sadness scores in a sample of healthy Caucasians. In contrast, Satterfield et al. (188) found that subjects homozygous for the 10-repeat allele were resilient to the build-up of cognitive performance impairment due to total sleep deprivation.
FKBP Prolyl Isomerase 5
Three studies investigated GxE interactions associated with the glucocorticoid receptor co-chaperone, FKBP5, which modulates glucocorticoid receptor sensitivity. Two of the three studies examined the moderating effects of FKBP5 rs3800373 on suicide risk (209) and attachment security and depressive symptoms (58). Roy et al. (209) found that individuals that lacked either major homozygote were resilient to the effects of childhood trauma (suicide attempt prevalence 0.24). Similarly, Borelli et al. (58) found that minor C allele homozygotes had the most optimal psychological profiles in positive caregiving contexts, but the most maladaptive outcomes in adverse caregiving contexts. This is supportive of the DSH. Buchmann et al. (230) investigated the interaction between the FKBP5 rs1360780 genotype, childhood adversity, and cortisol response. The authors found a blunted cortisol response to environmental challenges, as observed in homozygous carriers of the 9-repeat allele, indicative of an enhanced biological resilience to healthy individuals with a history of childhood adversity.
Interleukin-6
Two studies investigated how the IL6 rs1800795 polymorphism interacts with adverse environmental factors to promote chronic inflammation. The first study found that older adults with a C allele of the polymorphism are protected from the increased risk of inflammation-related disease and mortality shown in individuals with the G allele of the polymorphism and high socioeconomic adversity exposure (101). The second study identified a protective effect of the G allele in adolescents, which desensitizes individual physiology to the pro-inflammatory effects of adverse socioenvironmental conditions (151). This age-dependent antagonistic pleiotropy suggests that genetic resilience is dependent on the developmental environment that interacts with social conditions.
Neuropeptide Y
Six studies investigated GxE interactions associated with the polymorphism rs16147 of Neuropeptide Y. Five of the six studies associated individuals homozygous for the T allele with resilience (82, 119, 168, 232). Sommer et al. (82) found that childhood adversity was significantly associated with increased depression and anxiety for homozygote carriers of the C allele, but homozygote carriers of the T allele were protected. Amstadter et al. (168) found the TT genotype interacts with impact level of hurricane exposure to be protective against anxiety. Chang et al. (232) reported an interaction between chronic high stress, the TT genotype, and high cardiac vagal tone, a physiological measure that is gaining support as a marker of resilience by capturing the integration of the varying regulatory mechanisms of the autonomic nervous system (243). Gan et al. (119) reported two separate studies within the same paper. Study 1 found that T allele carrier earthquake survivors reported consistent levels of resilience in low, moderate and high trauma exposure. Study 2 found T allele carriers who reported a high number of early stressful life events were associated with a greater positive future focus.
Donner et al. (169), in contrast to the other studies, found no GxE interaction effect with rs16147, childhood adversity, and anxiety susceptibility, but instead found risk haplotypes significantly associated with anxiety that carry the T-allele of the SNP.
Resnick et al. (118) found an association between NPY and psychological resilience following hip fracture but they did not report allelic variation.
Opioid Receptor μ 1
Two studies investigated the A118G (SNP rs1799971) polymorphism of the μ-opioid receptor gene (OPRM1). Levran et al. (236) assessed whether opioid-related genetic variants contribute to reduced vulnerability to relapse to heroin in persons without agonist treatment. The authors found that carrying at least one copy of the G allele is significantly higher in subjects with long term abstinence from heroin, suggesting this genotype may blunt the endogenous stress response and contribute to resilience against relapse.
Troisi et al. (213) found that G allele carriers had similarly high scores on fearful attachment, regardless of the quality of maternal care. By comparison, AA genotype carriers were more affected by early experience with those recalling high levels of maternal care exhibiting less fearful attachment behavior, and those recalling low levels of maternal care exhibiting high levels of fearful attachment. This is again in line with the DSH and suggests G allele carriers have a decreased sensitivity to adverse rearing environments.
Oxytocin Receptor
Three studies investigated the interactions between polymorphisms in OXTR and environments on a range of outcomes. Two of the studies investigated the rs53576 polymorphism in OXTR, and both studies associated A allele carriers with resilience. Bradley's et al. (211) findings suggest that A allele carriers are more resilient to the adverse effects of severe childhood adversity by being protected against emotional dysregulation and disorganized attachment. Hostinar et al. (167) replicated this finding, showing maltreated adolescent A-carriers exhibit the same level of psychological symptoms as non-maltreated adolescents, supporting resilience research that the experience of maltreatment does not deterministically lead to psychopathology.
Loth et al. (192) investigated the rs237915 SNP of OXTR and found that CC homozygotes were more resilient against the effect of stressful life experiences than other genotypes, partly mediated by genotype-dependent sensitivity to the reinforcement values of negative social cues.
Tumor Necrosis Factor α
Two studies investigated the TNFα G308A polymorphism on performance impairment during total sleep deprivation (TSD) (189, 190). Skeiky et al. corroborated Satterfield and colleagues' earlier findings that indicated that the A allele at the TNFα 308 locus (SNP rs1800629) is associated with resilience to psychomotor vigilance performance impairment during TSD, in comparison with the more common G allele.
Animal Models of Resilience (N = 28)
28 Studies of Animal Models of Resilience met the Inclusion Criteria (Supplementary File 1). Of the 28 Studies, 20 Studies Used Transgenic Mouse Populations, five Studies Used Subpopulations, and three Used Selective Breeding. The Findings of Animal Genes With Relevant Human Homologs Are Discussed Below.
Opioid Receptor, Mu 1
Briand et al. (244) used a transgenic mouse model, with C57BL/6 mice possessing the functionally equivalent SNP in the mouse Oprm1 gene (A112G) as the human OPRM1 A118G SNP. To determine the role of this polymorphism in behavioral interactions and to investigate the functional mechanisms underlying this behavior, the authors used a social defeat paradigm. The authors found that G-allele carriers were resilient to the deleterious consequences of stress, as measured by positive social interactions and reduced anhedonia. This is consistent with the human studies of OPRM1 also investigated in this review, which found G-allele carriers are resilient against relapse to heroin use (236) and show decreased levels of fearful attachment in low maternal care environments (213). An increase in brain activation in stress and reward circuitry is also evident in resilient Oprm1 G/G mice in Briand's et al. (244) study, thus suggesting that modeling this SNP in mice provides construct validity for this model of resilience These models are in direct contrast with results in a recent meta-analysis which found no moderating effect of OPRM1 A118G, on response to naltrexone treatment in individuals with alcohol use disorder (245).
Neuropeptide Y
Cohen et al. examined the Npy-ergic system and its association with behavioral responses to stress in a rat model of PTSD (246). The authors classified subpopulations of Sprague-Dawley rats based on their performance in elevated plus maze and acoustic startle response tests using a pre-set cut-off criterion after exposure to predator-scent stress. The study found protective qualities of Npy. Notably, an extreme reduction in Npy expression in selected brain regions of the animals severely affected by the stressor. This behavioral disruption was reversed by administering exogenous Npy directly into the hippocampus of the stressed animals 1 h post-exposure. While the study did not examine genetic variation associated with NPY polymorphisms, the results are still worth noting due to homologous findings in humans.
Calcium Channel, Voltage-Dependent, L Type, Alpha 1C Subunit
CACNA1C has been identified as one of the strongest genetic risk factors for the development of affective disorders in both GWAS and candidate gene studies, [e.g., (18, 19, 247)]. Three of the 18 animal models examined this gene in mouse models using different approaches.
Terrillion et al. (248) used a subpopulation approach, classifying male wild-type or conditional Cacna1c knockout mice on a C57BL/6 background into resilient or susceptible based on their performance in a chronic social defeat test. They found expression of Cacna1c in the nucleus accumbens (NAc) is unchanged in resilient mice compared with control mice not exposed to social stress. Susceptible mice show a decrease in Cacna1c expression in the NAc after the social defeat paradigm, suggesting normal Cacna1c function in the NAc is crucial for resiliency to social stressors.
Dedic et al. (249) used a transgenic mouse model to assess the impact of Cacna1c deletion at different stages of development (See Supplementary Table 1 for genetic background and breeding timelines). If embryonic Cacna1c deletion in forebrain glutamatergic neurons occurs, then endophenotypes associated with psychiatric disorders manifest themselves. If the deletion of Cacna1c from forebrain glutamatergic neurons occurs in adulthood, the opposite effect occurs, inducing improved cognitive flexibility, strengthened synaptic plasticity, and increased stress resilience.
Michels et al. (250) examined a gene-stress interaction using immortalized mouse hippocampal cells, a well-established model system that reflects a common cellular response to environmental stress. In contrast with Terrillion's et al. (248) work, the authors show that reduced Cacna1c expression mediates neuroprotective effects against oxidative stress, predominantly at the level of mitochondria. This implies that reduced CACNA1C expression converges to control mitochondrial function, resulting in cellular resilience against oxidative stress. There is a growing body of evidence that major psychiatric illnesses are associated with impaired cellular resilience and synaptic dysfunction at various levels (251). These results suggest that alterations in mitochondrial function could affect critical cellular processes, dampening synaptic plasticity, and contributing to the pathophysiology of psychiatric disorders.
Decreased or increased CACNA1C expression levels have been associated with the SNP rs1006737, the same SNP investigated in studies included in this review. This, and Dedic's work, suggests that alterations in CACNA1C expression may be developmental-stage, brain-region stage, cell-type or sex-specific.
Deleted in Colorectal Carcinoma
Manitt et al. used a transgenic mouse model to examine the netrin-1 guidance cue receptor gene, deleted in colorectal cancer (dcc), and resilience (252). The dcc haploinsufficient male mice were maintained on a BL/6 background and bred with wild-type BL/6 female mice. Using dcc loss-of-function transgenic mice, the study found reduced dcc expression confers resilience against developing neuroanatomical, neurochemical, and behavioral traits associated with mental disorders involving medial prefrontal cortex (mPFC) dysfunction. Significantly, these protective phenotypes are only present in adult but not pre-pubertal mice and most likely result from selective alterations in the reorganization of mPFC dopamine circuitry. One human study found in this review examined how DCC contributes to the risk of schizophrenia (202). The authors found that individuals heterozygous at one SNP, rs2270954, had decreased risk of schizophrenia. Further studies have demonstrated that microRNA regulation of DCC by miR-218, a posttranscriptional repressor of DCC, maybe a switch of susceptibility vs. resilience to stress-related disorders (253). Together, these studies suggest that DCC could be a promising candidate gene that contributes to the genetic basis of resilience.
FK506-Binding Protein
Three studies investigated transgenic mouse models of Fkbp5, a co-chaperone protein of the Hsp90 complex that regulates the glucocorticoid receptor. FKBP5 SNPs have been associated with psychiatric disorders including depression, PTSD, bipolar disorder, and anxiety (254). FKBP51, encoded by the FKBP5 gene, and FKBP52, encoded by the FKBP4 gene, are components of the chaperone-receptor heterocomplex and differentially regulate the glucocorticoid receptors or mineralocorticoid receptors. Hartmann et al. (255) characterized heterozygous Fkbp52 knockout mice kept on a mixed 129SvJ × C57BL/6 background, under basal and chronic social defeat stress conditions (CSDS). Fkbp52 heterozygous knockout mice demonstrated stress resilience, such as reduced basal corticosterone levels and more active stress-coping behavior in the forced swim test (FST) following CSDS. This mimics the phenotype of Fkbp51 deficient mice in the FST, as found by O'Leary et al. (254). O'Leary et al. tested how Fkbp5 deletion in aged mice affects behavior, given that FKBP51 levels increase with age. They found that aged Fkbp51 deficient mice displayed enhanced active stress-coping behavior following the acute severe stress exposure of the FST, similar to levels of control mice treated with antidepressants. This antidepressant behavior in the Fkbp5−/− did not affect cognition or other basic motor functions, and reduced corticosterone levels following stress were also observed.
Kwon et al. (256) exposed wild-type and Fkbp5 knock-out mice to chronic restraint stress. They found Fkbp5-deficient mice were resilient to the depressive-like behavior exhibited by wild-type mice following chronic restraint stress. Further RNA sequencing analyses revealed a module of co-expressed genes, M55, that was downregulated in the WT group and restored in the KO group. Gene ontology enrichment analysis of M55 revealed biological functions involved with inflammatory response, gland morphogenesis and nervous system development.
Together, these findings suggest that absence of FKBP5 and regulation of the immune response results in a decrease of corticosterone levels and HPA-axis activity, which increases resilience to depressive phenotypes.
Leucine-Rich Repeat Kinase 2
Depression has been reported to be more common in patients with Parkinson's disease (PD) than in the general population, often appearing earlier than the onset of motor symptoms (257). The G2019S mutation in LRRK2 causes late-onset PD, but it is unclear how this mutation impacts depression-related behaviors (258). Matikainen-Ankney et al. (258) addressed this by subjecting Lrrk2-G2019S knock-in and wild-type mice to CSDS. Mice used in the study were congenic on C57BL/6NTac background. The study showed that young adult G2019S knock-in mice were highly resilient to CSDS, failing to exhibit social avoidance compared to wild-type mice. There were no behavioral differences between the genotypes found in the absence of CSDS.
Apolipoprotein E
Given the well-documented research on the APOE ε4 genotype association with genetic risk for Alzheimer's Disease (AD), and human imaging studies suggesting APOE ε4 is associated with brain structures linked to cognitive decline, Kulkarni et al. (259) assessed brain structure and function in APOE ε4 knock-in rats. Male and female WT and human APOE ε4 knock in Sprague Dawley rats were studied for changes in brain structure and behavioral tests. There was evidence of sex differences in brain structure and function in the APOE ε4 knock in rats with the data suggesting female carriers are more resilient to cognitive/emotional problems possibly due to altered brain volumes and enhanced connectivity.
Discussion
Our review of 157 articles revealed 62 genes that were empirically associated with resilience in humans and animal models (Supplementary Table 1). Despite the complexities of defining the concept of resilience, enormous heterogeneity in the way resilience is defined, operationalised and measured, and the way empirical resilience research is designed and conducted, we found that several genetic variants are consistently associated with resilience (Table 4).
Notably, OPRM1, NPY, CACNA1C, DCC, APOE, and FKBP5 had both animal and human variants linked to measures of resilience. This supports the idea of shared biological pathways of resilience. The A118G polymorphism in OPRM1 stands out because in all studies carriers of the G-allele were classified as resilient despite completely different environmental measures and outcomes. This suggests that the A118G polymorphism in OPRM1 could be a novel therapeutic target for further investigation. A recent meta-analysis and systematic review indicates that G allele carriers of OPRM1 A118G required more opioid analgesia in pain management (260), and COMT haplotypes are also associated with pain sensitivity and opioid efficacy (261). Pain perception and opioid response are complex traits and might involve interactions between these or multiple genes (262), important for future gene network research. Evidence suggests that variation in OPRM1, as measured by the A118G polymorphism, is associated with individual differences in rejection sensitivity (263), suggesting an overlap between physical and social pain. A systematic review found pain perception to be a resilience factor directly salient to physical illness (16).
DCC also stands out as a promising candidate gene that contributes to the genetic basis of resilience. In addition to the results of the candidate gene studies included in this review, in both human and animal studies that identified associations between variation in DCC and resilience, DCC also shows well-established associations with schizophrenia (264), MDD (265) and cross-disorder risk (21), intelligence (266), cognitive ability and educational attainment (267), noting that the latter two measures have wide support as individual resilience factors. These genome-wide significant findings set DCC apart from those that are mainly supported by small candidate gene studies (regarded as problematic in the field). Thus, the association between DCC and resilience warrants further investigation.
The rs16147 SNP in human NPY reported in this review influences hypothalamic–pituitary–adrenal (HPA)-axis responsiveness to acute psychosocial stress (268). Further studies in humans have demonstrated that genetic variations in the NPY locus, especially in the promoter region, substantially mediate NPY release, with low NPY expression predicting lower resilience (41, 269). This suggests that NPY is a particularly plausible candidate for modulating effects of environmental stress exposure on resilience as supported by the rat model in this review and other animal models (270, 271). Higher NPY plasma levels have also been found in veterans resilient to PTSD (272), potentially representing a biological correlate of resilience to, or recovery from, the adverse effects of stress. Recent systematic reviews and meta-analyses have found NPY variation to interact with childhood trauma to influence anxiety sensitivity (273) and the risk of obesity (274). Resilience, specifically emotional and family resilience, are protective factors against obesity in children (275, 276), suggesting interaction effects between NPY, resilience and obesity.
What inferences can we make about resilience by these genetic association results? Although not all studies reach consensus on alleles associated with resilience, sex differences are an important consideration for several reasons. First, COMT enzyme activity and the neurochemistry and behavior of Comt knock out mice are both markedly sexually dimorphic (159). Sex effects were also found in BDNF and mouse models of APOE. Clinical evidence supported by rodent and human studies suggests that females are more vulnerable to drug addiction than males (277). It is further important to consider sex effects within environmental exposure measures. For example, males will be more likely to be exposed to physical abuse, and females will be more likely to be exposed to sexual abuse. Some of the studies investigated in this systematic review had only male participants or female participants [e.g., (107, 132)]. For the results of these studies to be generalized, they need to include both sexes, or be investigated separately.
Another important consideration in these candidate gene studies is sample size. The sample size of the human studies ranged from n = 12 to n = 7,335 so the vast majority were severely underpowered (278, 279) suggesting high false discovery rates for the positive associations reported here. Despite this, the consensus of the genetic variants repeatedly associated with resilience, in its many forms, warrants further investigation. We would particularly recommend whole genome data analyses of resilience, where resilience is quantified using a residuals approach, as recommended by emerging frameworks [e.g., (6, 280)].
Genetic Associations of Resiliency Phenotypes
Due to the vast array of resiliency phenotypes, as shown in Tables 2, 3, we analyzed the current literature to assess if the resiliency phenotypes in our systematic review were found to be associated with overlapping candidate genes in recent meta-analyses, GWAS or systematic reviews. We used the resiliency phenotypes where resilience was operationalised as positive adaptation.
Longevity
Longevity was used as a resilience phenotype in three studies (226–228) and has also been previously associated with individuals with protective variants, buffering the effect of disease-associated variants and adverse environmental factors, decreasing morbidity at the end of extreme longevity (29–32). A recent review and meta-analysis confirmed the association of five polymorphisms that were significantly associated with exceptional longevity: ACE rs4340, APOE ε2/3/4, FOXO3A rs2802292, the KLOTHO KL-VS variant and IL6 rs1800795 (281). Of these five polymorphisms, three had genetic associations with resilience within our review, with resilience conceptualized as decreased sensitivity to trauma (143), neuronal resilience to AD (137), epilepsy (136), elevated serum klotho levels that promote a resilient brain (218), and desensitized physiology to pro-inflammatory effects (101, 151).
Sense of Coherence
Sense of coherence, referring to a consistent but dynamic feeling of confidence, defined as the belief that life is comprehensive, manageable and meaningful, was operationalised as a resilience phenotype in three studies (121–123). The first GWA analysis recently conducted for SOC yielded a significant association, rs74920024 on chromosome 2 (282). The association locus is close to Glycerol-3-Phosphate Dehydrogenase 2 (GPD2), a gene encoding a protein that localizes to the inner mitochondrial membrane and is responsible for the conversion of glycerol-3-phosphate to dihydroxyacetone phosphate. While there is no overlap of this gene with candidate genes found in this review, GPD2 has been previously associated with large GWAS's on cognitive ability (283) and educational attainment from the Social Science Genetic Consortium 2018 GWAS (267), and cognitive aspects of educational attainment from a large GWAS meta-analysis combining a cognitive consortium and human intelligence GWAS (284). While these traits have not been used as a proxy for resilience within this review, they have wide support as individual level resilience factors [e.g., (285–287)], with high cognitive skills in childhood predicting resilience to adversity in adolescence (288).
Coping
Two studies within this review operationalised resilience as stress coping (129, 130). Çarkaxhiu Bulut et al. (130), evaluated the SLC64A 5-HTTLPR polymorphism and resilience components on the development of psychopathology in adolescent sexual abuse cases, while Aizawa et al. (129) examined genetic association of BDNF and variation in stress-coping style. A recent systematic review of genetic influences on coping (289) found multiple associations between SLC64A and Adrenoceptor Beta 2 (ADRB2) and coping phenotypes. One important finding of their review that echoes the sentiments within our own review, is the lack of consensus regarding instruments for coping phenotypes. Again, despite the lack of consensus of instruments within the reviews, there is overlap on the candidate genes associated with coping and resiliency phenotypes. A study included in this review found a novel association of a SNP within the promoter region of the ADRB2 gene that was associated with relative resilience to childhood adversity (199). Previous research has revealed links between ADRB2 and resting blood pressure (BP) and regulation of BP in stressful situations (290, 291). This genetic association of ADRB2 found in both coping and resiliency phenotypes is interesting given the known links between stress and risk factors for cardiovascular disease.
Attachment
Gervai et al. (135) examined the link between DRD4 gene polymorphism and infant attachment and found that not carrying the T.7 haplotype of the DRD4 acts as a resilience factor in the optimal development of early attachment. Within a recent systematic review of gene-environment interactions on infant and child attachment (292), DRD4 was associated with the interaction between maternal sensitivity and parenting style in five studies, with contradictory results. While there was also a lack of consensus of which DRD4 allele was associated with resilience in the studies meeting our selection criteria in this review, later research found the DRD4 7-repeat allele to be protective against the risk of a dysfunction in emotional regulation associated with birthweight (293). This is consistent with Das's et al. (108) finding but contradictory to Gervai's et al. (135) and Cicchetti and Rogosch's (144). While these associations are suggestive of reoccurring patterns of associations, ultimately, there is insufficient evidence to meaningfully comment on the direct influence of DRD4 on attachment or resilience, a position reiterated in a later systematic review of the genetics of adult attachment (294).
Post-traumatic Growth
While the conceptualization of resilience in the literature is highly variable, the relationship between PTG and resilience is not clear. Some researchers equate resilience with PTG (295), while others assume it is a form of resilience (296). Only one study included in this review met the inclusion criteria (127) and examined the association between PTG, PTSD and genetic variants. The study found a GxE association between PTG and Regulator of G Protein Signaling 2 (RGS2) (rs4606), driven by disaster exposure. However, a recent systematic review found no gene expression changes associated with PTG (297), again highlighting a focus on pathogenic research, which fails to adequately measure or acknowledge positive post-trauma outcomes, whether they be conceptualized at resilience or PTG.
Assessing the Validity of Animal Models of Resilience
Future work focusing on shared biological pathways between the candidate genes highlighted in this review may reveal common biological mechanisms of resilience. Revealing these mechanisms would bring us closer to understanding the process of adapting to aversive stimuli and could reveal new therapeutic targets for symptom reduction. Animal models of disease therefore provide an ethically and economically viable experimental platform to examine these common biological mechanisms of resilience and identify biomarkers that may facilitate the development of resilience to adversity, or reduce the symptoms of post-adversity outcomes. It is therefore crucial that future animal models of resilience are judged by specific validity criteria. While currently no validity criteria for animal models of resilience exist, animal models of psychiatric disorders use validity criteria following Willner (298). He defined three specific multidimensional criteria that must be met for an animal model of a psychiatric disorder to be considered relevant for human pathology: face validity, predictive validity and construct validity (298). The first criterion, face validity, is considered as the degree of phenomenological similarity between the model and the phenotype being modeled. This phenomenological identity encompasses behavioral and cognitive aspects only, not just their physiological or neural bases. For resilience, face validity would be whether the animal can avoid some of the deleterious behavioral effects of chronic stress and is thus less prone to exhibit maladaptive behavioral traits. This would mimic diagnostic criteria such as those in DSM-5, where criteria are generally behavioral or cognitive, and a threshold for diagnosis is when such symptoms cause significant impairment in the individual's ability to function.
The second criterion, predictive validity, corresponds to human-animal correlations of therapeutic outcomes. While the criterion of predictive validity initially relied on a pharmacological correlation (i.e., the animal model shows the same response to a specific treatment as humans), a more recent paper by the same author (299), extended the criterion to include the response to all available treatments, not just pharmacological treatments. For example, within the depression model, this means not just pharmacological antidepressants, but also electroconvulsive therapy. Extending to models of resilience, there are behavioral treatment options for enhancing resilience in humans and animals using non-pharmacological treatments, such as stress inoculation (300), exposure therapy/fear extinction (301) and cognitive behavioral therapy (302). There is limited research on predictive validity of pharmacological correlations of animal models of resilience. However, using the chronic social defeat stress paradigm, Wilkinson et al. (303) found a significant overlap in genes that are regulated in resilience phenotypes and those that are regulated by chronic antidepressant treatment of susceptible individuals, with Holanda et al. (304) repeating this finding with acute and prolonged swimming stress. This suggests that resilient individuals may be less vulnerable to the adverse effects of chronic stress by exhibiting the same changes in specific expression profiles in response to stress as those induced by antidepressants. Experimentally, evaluating which genetic variants determine an animal's capacity for resilience could allow for predictive validity of animal models via new treatment options for stress-related disorders.
Construct validity, the third criterion, refers to the assumption that the behavior of the animal model is homologous to the behavior seen in humans. Ideally, construct validity in animal models of resilience would be achieved by replicating in an animal the etiologic processes that create a resilient phenotype in humans. One of the biggest challenges for establishing construct validity within animal models is the absence of reliable genetic markers of resilience (305). Since OPRM1, NPY, CACNA1C, DCC, and FKBP5 had both animal and human variants linked to measures of resilience this provides some construct validity for these animal models of resilience. The OPRM1 mouse model of resilience is the strongest candidate to provide construct validity of resilience because in all studies, carriers of the G-allele were considered resilient. By incorporating animal models in the study of resilience in this review, we maximized construct validity of animal models of resilience by focusing on homologous resilience alleles that underlie general resilience mechanisms in both humans and animals.
Genetic Background in Model Systems
Similarly to how consideration of ethnicity is important in human studies (156, 160), the genetic background (e.g., mouse or rat strain) of animal models of resilience is critical in determining the response to stressors. Increasing evidence suggests that genotype-phenotype relationships cannot be reliably inferred by studying a singular genetic background, with genetic background dramatically altering experimental conclusions (306, 307). An example of this is the Cacna1c+/− phenotype. Our review found three animal studies reporting a positive association with Cacna1c and an outcome associated with resilience (248–250). Yet research on F1 crosses between male C57BL/6J mice heterozygous for null alleles of Cacna1c and wild-type females from 30 inbred laboratory strains, found the majority of null phenotypes were not generalisable (307). Phenotypic responses strongly interacted with the genetic background of the mouse strain, with some responses drawing diametrically opposed conclusions on the same allele. The choice of genetic background in animal models of resilience is critically important because genetic mutations in some strains can lead to the disruption of genes crucial in certain biological processes (308). In our review, Briand et al. (244) found G-allele carriers of C57BL/6 mice possessing the functionally equivalent SNP in the mouse Oprm1 gene (A112G) as the human OPRM1 A118G SNP, resilient to the deleterious consequences of stress. Future animal studies modeling this SNP in this mouse model should carefully select the background: DBA/2J possess coding and non-coding variants in Oprm1 and therefore exhibit weaker morphine preference and response compared with C57BL/6J (309, 310). Known mutations in genes that are relevant to the phenotype-of-interest, in this case human G-allele carriers of OPRM1 resilient to heroin relapse (237), must be avoided in order to reduce unwanted bias. These results do not negate the invaluable contributions of mouse genetics to biomedical science, but they highlight that in order to reveal the functional biological networks underpinning resilience, we must broaden our focus beyond single genotype models and instead leverage the opportunities available using advanced genetic models such as recombinant inbred strains (311, 312).
Developmental and Epigenetic Aspects
The developmental time frame during which gene expression is modulated needs to be considered due to the vital influence of developmental timings on maturation of different parts of the stress system on later stress vulnerability or resilience (50). As seen in Table 4, both SLC6A4 and BDNF have alleles associated with resilience when the populations are either adults or children. This can be explained by age-dependent antagonistic pleiotropy, suggesting that genetic resilience is dependent on the interaction between developmental stage and social conditions. This is again supported by an animal model in this review showing the opposite impact of Cacna1c deletion on psychopathology and cognitive flexibility at different stages of development (249), with a recent review also showing gene expression in tissues is time dependent (313).
The consideration of developmental timeframe effects is of paramount importance in the DSH framework. One view of the DSH could be that genetic variants are not predefined as resilience or even risk factors, but often they determine both risk and resilience dependent on the current context of gene-environment interaction. This conceptualization could also offer significant insight into the many discrepancies in this review regarding which allele is associated with resilience. According to the DSH, specific polymorphisms would be characterized by differential environmental sensitivity, enhancing positive outcomes in positive environments, and elevating vulnerability in adverse environments. Indeed, five studies investigated within this review report findings consistent with the DSH (58, 144, 168, 212, 213).
When investigating underlying biological mechanisms of resilience, epigenetic mechanisms such as DNA and histone modifications, and non-coding RNAs are critical in regulating cellular function and systemic physiology through their impact on gene expression (312). Such mechanisms are emerging as key mediators of environmental factors (313–315), providing a potential pathway through which individuals modify their individual responsiveness to a stressor, which may in turn contribute to individual resilience (316). Many epigenomic modifications are dynamic and reversible and may also provide targets for intervention strategies. For example, a recent systematic review of DNA methylation studies in PTSD, PTG and resilience found that high resilience was associated with a blunted immune response (297). While our aim for this review was to identify genetic variants associated with resilience, the incorporation of epigenetic variants and markers will be an important consideration for future research into resilience factors.
Conclusion
Despite limitations linked to the complexities of defining resilience, the vast array of measurement instruments, outcomes, and populations investigated, 62 candidate genes that met the inclusion criteria have been identified, and 22 studies reach consensus on which allele confers resilience. Based on these findings, future work should focus on shared biological pathways between these candidate genes, which may reveal common biological mechanisms of resilience. This would support the omnigenic model (317): genetic variants underlie biological processes, not diagnoses, and the same processes play a role in different manifestations of resilience. We recommend longitudinal studies of genetic variants of OPRM1, OXTR, CRHR1, COMT, BDNF, APOE, and SLC6A4 in human and animal models with consideration of developmental timing, type and severity of stressor, sex and ethnicity.
Data Availability Statement
The original contributions presented in the study are included in the article/Supplementary Material, further inquiries can be directed to the corresponding author.
Author Contributions
SC defined the research question, performed the systematic review and analyzed the articles. TC and RH systematically crosschecked the review. SC wrote the manuscript. Substantive edits and revisions were conducted by RH and SC. All authors read and approved the final manuscript.
Funding
SC was supported by ESRC grant ES/P000347/1 Soc-B (Social-Biological) Center for Doctoral Training: UCL-Manchester-Essex Consortium.
Conflict of Interest
The authors declare that the research was conducted in the absence of any commercial or financial relationships that could be construed as a potential conflict of interest.
Publisher's Note
All claims expressed in this article are solely those of the authors and do not necessarily represent those of their affiliated organizations, or those of the publisher, the editors and the reviewers. Any product that may be evaluated in this article, or claim that may be made by its manufacturer, is not guaranteed or endorsed by the publisher.
Supplementary Material
The Supplementary Material for this article can be found online at: https://www.frontiersin.org/articles/10.3389/fpsyt.2022.840120/full#supplementary-material
References
1. Fletcher D, Sarkar M. Psychological resilience: a review and critique of definitions, concepts, and theory. Euro Psychol. (2013) 18:12. doi: 10.1027/1016-9040/a000124
2. Garcia-Dia MJ, DiNapoli JM, Garcia-Ona L, Jakubowski R, O'Flaherty D. Concept analysis: resilience. Arch Psychiatr Nurs. (2013) 27:264–270. doi: 10.1016/j.apnu.2013.07.003
3. Windle G. What is resilience? A review and concept analysis. Rev Clin Gerontol. (2011) 21:152–69. doi: 10.1017/S0959259810000420
4. Bonanno GA, Diminich ED. Annual research review: positive adjustment to adversity–trajectories of minimal-impact resilience and emergent resilience. J Child Psychol Psychiatry. (2013) 54:378–401. doi: 10.1111/jcpp.12021
5. Choi KW, Stein MB, Dunn EC, Koenen KC, Smoller JW. Genomics and psychological resilience: a research agenda. Mol Psychiatry. (2019) 24:1770–8. doi: 10.1038/s41380-019-0457-6
6. Ioannidis K, Askelund AD, Kievit RA, Van Harmelen AL. The complex neurobiology of resilient functioning after childhood maltreatment. BMC Medicine. (2020) 18:42. doi: 10.1186/s12916-020-1490-7
7. Kalisch R, Baker DG, Basten U, Boks MP, Bonanno GA, Brummelman E, et al. The resilience framework as a strategy to combat stress-related disorders. Nat Hum Behav. (2017) 1:784–90. doi: 10.1038/s41562-017-0200-8
8. Cicchetti D, Rogosch FA. Adaptive coping under conditions of extreme stress: Multilevel influences on the determinants of resilience in maltreated children. New Dir Child Adolesc Dev. (2009) 2009:47–59. doi: 10.1002/cd.242
9. Johnson JL, Wiechelt SA. Introduction to the special issue on resilience. Subst Use Misuse. (2004) 39:657–70. doi: 10.1081/JA-120034010
10. Khambati N, Mahedy L, Heron J, Emond A. Educational and emotional health outcomes in adolescence following maltreatment in early childhood: a population-based study of protective factors. Child Abuse Neglect. (2018) 81:343–53. doi: 10.1016/j.chiabu.2018.05.008
11. Werner EE, Smith RS. Journeys From Childhood to Midlife: Risk, Resilience, and Recovery. New York, NY: Cornell University Press (2001).
12. Amstadter AB, Myers JM, Kendler KS. Psychiatric resilience: longitudinal twin study. Br J Psychiatry. (2014) 205:275–80. doi: 10.1192/bjp.bp.113.130906
13. Boardman JD, Blalock CL, Button TM. Sex differences in the heritability of resilience. Twin Res Hum Genet. (2008) 11:12–27. doi: 10.1375/twin.11.1.12
14. Waaktaar T, Torgersen S. Genetic and environmental causes of variation in trait resilience in young people. Behav Genet. (2012) 42:366–77. doi: 10.1007/s10519-011-9519-5
15. Kalisch R, Muller MB, Tuscher O. A conceptual framework for the neurobiological study of resilience. Behav Brain Sci. (2015) 38:e92. doi: 10.1017/S0140525X1400082X
16. Stewart DE, Yuen T. A systematic review of resilience in the physically ill. Psychosomatics. (2011) 52:199–209. doi: 10.1016/j.psym.2011.01.036
17. Stein MB, Choi KW, Jain S, Campbell-Sills L, Chen CY, Gelernter J, et al. Genome-wide analyses of psychological resilience in U. S. army soldiers. Am J Med Genet Part B Neuropsychiatr Genet. (2019) 180:310–9. doi: 10.1002/ajmg.b.32730
18. Cross-Disorder Group of the Psychiatric Genomics Consortium. Identification of risk loci with shared effects on five major psychiatric disorders: a genome-wide analysis. Lancet. (2013) 381:1371–9. doi: 10.1016/S0140-6736(12)62129-1
19. Green EK, Grozeva D, Jones I, Jones L, Kirov G, Caesar S, et al. The bipolar disorder risk allele at cacna1c also confers risk of recurrent major depression and of schizophrenia. Mol Psychiatry. (2010) 15:1016–22. doi: 10.1038/mp.2009.49
20. Rao S, Yao Y, Zheng C, Ryan J, Mao C, Zhang F, et al. Common variants in cacna1c and mdd susceptibility: a comprehensive meta-analysis. Am J Med Genet Part B Neuropsychiatr Genet. (2016) 171:896–903. doi: 10.1002/ajmg.b.32466
21. Wu Y, Cao H, Baranova A, Huang H, Li S, Cai L, et al. Multi-trait analysis for genome-wide association study of five psychiatric disorders. Transl Psychiatry. (2020) 10:209. doi: 10.1038/s41398-020-00924-0
22. Fisher HL, Caspi A, Poulton R, Meier MH, Houts R, Harrington H, et al. Specificity of childhood psychotic symptoms for predicting schizophrenia by 38 years of age: a birth cohort study. Psychol Med. (2013) 43:2077–86. doi: 10.1017/S0033291712003091
23. Lahey BB, Applegate B, Hakes JK, Zald DH, Hariri AR, Rathouz PJ. Is there a general factor of prevalent psychopathology during adulthood? J Abnorm Psychol. (2012) 121:971. doi: 10.1037/a0028355
24. McMahon SD, Grant KE, Compas BE, Thurm AE, Ey S. Stress and psychopathology in children and adolescents: is there evidence of specificity? J Child Psychol Psychiatry. (2003) 44:107–33. doi: 10.1111/1469-7610.00105
25. Davydov DM, Stewart R, Ritchie K, Chaudieu I. Resilience and mental health. Clin Psychol Rev. (2010) 30:479–95. doi: 10.1016/j.cpr.2010.03.003
26. Russo SJ, Murrough JW, Han MH, Charney DS, Nestler EJ. Neurobiology of resilience. Nat Neurosci. (2012) 15:1475. doi: 10.1038/nn.3234
27. De Kloet E. About stress hormones and resilience to psychopathology. J Neuroendocrinol. (2008) 20:885–92. doi: 10.1111/j.1365-2826.2008.01707.x
28. McEwen BS, Stellar E. Stress and the individual: mechanisms leading to disease. Arch Intern Med. (1993) 153:2093–101. doi: 10.1001/archinte.153.18.2093
29. Sebastiani P, Bae H, Sun FX, Andersen SL, Daw EW, Malovini A, et al. Meta-analysis of genetic variants associated with human exceptional longevity. Aging. (2013) 5:653. doi: 10.18632/aging.100594
30. Sebastiani P, Gurinovich A, Bae H, Andersen S, Malovini A, Atzmon G, et al. Four genome-wide association studies identify new extreme longevity variants. J Gerontol Ser A Biomed Sci Med Sci. (2017) 72:1453–64. doi: 10.1093/gerona/glx027
31. Sebastiani P, Sun F, Andersen SL, Lee J, Wojczynski MK, Sanders JL, et al. Families enriched for exceptional longevity also have increased health-span: findings from the long life family study. Front Public Health. (2013) 1:38. doi: 10.3389/fpubh.2013.00038
32. Stevenson M, Bae H, Schupf N, Andersen S, Zhang Q, Perls T, et al. Burden of disease variants in participants of the long life family study. Aging. (2015) 7:123–32. doi: 10.18632/aging.100724
33. Horn SR, Feder A. Understanding resilience and preventing and treating ptsd. Harv Rev Psychiatry. (2018) 26:158–74. doi: 10.1097/HRP.0000000000000194
34. Rutter M. Implications of resilience concepts for scientific understanding. Ann N Y Acad Sci. (2006) 1094:1–12. doi: 10.1196/annals.1376.002
35. Adeyemo A, Rotimi C. Genetic variants associated with complex human diseases show wide variation across multiple populations. Public Health Genomics. (2010) 13:72–9. doi: 10.1159/000218711
36. Visscher PM, Wray NR, Zhang Q, Sklar P, McCarthy MI, Brown MA, et al. 10 years of gwas discovery: biology, function, and translation. Am J Hum Genet. (2017) 101:5–22. doi: 10.1016/j.ajhg.2017.06.005
37. Stein MB, Campbell-Sills L, Gelernter J. Genetic variation in 5httlpr is associated with emotional resilience. Am J Med Genet Part B Neuropsychiatr Genet. (2009) 150:900–6. doi: 10.1002/ajmg.b.30916
38. Binder EB, Bradley RG, Liu W, Epstein MP, Deveau TC, Mercer KB, et al. Association of fkbp5 polymorphisms and childhood abuse with risk of posttraumatic stress disorder symptoms in adults. JAMA. (2008) 299:1291–305. doi: 10.1001/jama.299.11.1291
39. Neumeister A, Charney DS, Belfer I, Geraci M, Holmes C, Sharabi Y, et al. Sympathoneural adrenomedullary functional effects of α2c- adrenoreceptor gene polymorphism in healthy humans. Pharmacogen Genomics. (2005) 15:143–9. doi: 10.1097/01213011-200503000-00002
40. Benedetti F, Poletti S, Radaelli D, Bernasconi A, Cavallaro R, Falini A, et al. Temporal lobe grey matter volume in schizophrenia is associated with a genetic polymorphism influencing glycogen synthase kinase 3-β activity. Genes Brain Behav. (2010) 9:365–71. doi: 10.1111/j.1601-183X.2010.00566.x
41. Zhou Z, Zhu G, Hariri AR, Enoch MA, Scott D, Sinha R, et al. Genetic variation in human npy expression affects stress response and emotion. Nature. (2008) 452:997–1001. doi: 10.1038/nature06858
42. Border R, Johnson EC, Evans LM, Smolen A, Berley N, Sullivan PF, et al. No support for historical candidate gene or candidate gene-by-interaction hypotheses for major depression across multiple large samples. Am J Psychiatry. (2019) 176:376–87. doi: 10.1176/appi.ajp.2018.18070881
43. Duncan LE, Keller MC. A critical review of the first 10 years of candidate gene-by-environment interaction research in psychiatry. Am J Psychiatry. (2011) 168:1041–9. doi: 10.1176/appi.ajp.2011.11020191
44. Johnson EC, Border R, Melroy-Greif WE, de Leeuw CA, Ehringer MA, Keller MC. No evidence that schizophrenia candidate genes are more associated with schizophrenia than noncandidate genes. Biol Psychiatry. (2017) 82:702–8. doi: 10.1016/j.biopsych.2017.06.033
45. Gonda X, Petschner P, Eszlari N, Baksa D, Edes A, Antal P, et al. Genetic variants in major depressive disorder: from pathophysiology to therapy. Pharmacol Ther. (2019) 194:22–43. doi: 10.1016/j.pharmthera.2018.09.002
46. Walters RK, Polimanti R, Johnson EC, McClintick JN, Adams MJ, Adkins AE, et al. Transancestral gwas of alcohol dependence reveals common genetic underpinnings with psychiatric disorders. Nat Neurosci. (2018) 21:1656–69. doi: 10.1038/s41593-018-0275-1
47. Scharf SH, Schmidt MV. Animal models of stress vulnerability and resilience in translational research. Curr Psychiatry Rep. (2012) 14:159–65. doi: 10.1007/s11920-012-0256-0
48. Ashbrook DG, Cahill S, Hager R. A cross-species systems genetics analysis links apbb1ip as a candidate for schizophrenia and prepulse inhibition. Front Behav Neurosci. (2019) 13:266. doi: 10.3389/fnbeh.2019.00266
49. Refojo D, Schweizer M, Kuehne C, Ehrenberg S, Thoeringer C, Vogl AM, et al. Glutamatergic and dopaminergic neurons mediate anxiogenic and anxiolytic effects of crhr1. Science. (2011) 333:1903–7. doi: 10.1126/science.1202107
50. Lupien SJ, McEwen BS, Gunnar MR, Heim C. Effects of stress throughout the lifespan on the brain, behaviour and cognition. Nat Rev Neurosci. (2009) 10:434. doi: 10.1038/nrn2639
51. Touma C, Bunck M, Glasl L, Nussbaumer M, Palme R, Stein H, et al. Mice selected for high versus low stress reactivity: a new animal model for affective disorders. Psychoneuroendocrinology. (2008) 33:839–62. doi: 10.1016/j.psyneuen.2008.03.013
52. Heinzmann J-M, Kloiber S, Ebling-Mattos G, Bielohuby M, Schmidt MV, et al. Mice selected for extremes in stress reactivity reveal key endophenotypes of major depression: a translational approach. Psychoneuroendocrinology. (2014) 49:229–43. doi: 10.1016/j.psyneuen.2014.07.008
53. Wong ML, Arcos-Burgos M, Liu S, Velez JI, Yu C, Baune BT, et al. The phf21b gene is associated with major depression and modulates the stress response. Mol Psychiatry. (2017) 22:1015–25. doi: 10.1038/mp.2016.174
54. Murray KN, Edye ME, Manca M, Vernon AC, Oladipo JM, Fasolino V, et al. Evolution of a maternal immune activation (mia) model in rats: early developmental effects. Brain Behav Immunity. (2019) 75:48–59. doi: 10.1016/j.bbi.2018.09.005
55. Molendijk ML, de Kloet ER. Coping with the forced swim stressor: current state-of-the-art. Behav Brain Res. (2019) 364:1–10. doi: 10.1016/j.bbr.2019.02.005
56. Belsky J. The differential susceptibility hypothesis: sensitivity to the environment for better and for worse. JAMA Pediatr. (2016) 170:321–2. doi: 10.1001/jamapediatrics.2015.4263
57. Belsky J, Hartman S. Gene-environment interaction in evolutionary perspective: differential susceptibility to environmental influences. World Psychiatry. (2014) 13:87. doi: 10.1002/wps.20092
58. Borelli JL, Smiley PA, Rasmussen HF, Gomez A, Seaman LC, Nurmi EL. Interactive effects of attachment and fkbp5 genotype on school-aged children's emotion regulation and depressive symptoms. Behav Brain Res. (2017) 325:278–89. doi: 10.1016/j.bbr.2016.07.035
59. Hankin BL, Nederhof E, Oppenheimer CW, Jenness J, Young JF, Abela JRZ, et al. Differential susceptibility in youth: evidence that 5-httlpr x positive parenting is associated with positive affect ‘for better and worse'. Transl Psychiatry. (2011) 1:e44. doi: 10.1038/tp.2011.44
60. Wolf M, Van Doorn GS, Weissing FJ. Evolutionary emergence of responsive and unresponsive personalities. Proc Natl Acad Sci USA. (2008) 105:15825–30. doi: 10.1073/pnas.0805473105
61. Zuckerman M. Vulnerability to Psychopathology: A Biosocial Model. Washington, DC: American Psychological Association (1999). doi: 10.1037/10316-000
62. Belsky J, Bakermans-Kranenburg MJ, Van IJzendoorn MH. For better and for worse: differential susceptibility to environmental influences. Curr Dir Psychol Sci. (2007) 16:300–4. doi: 10.1111/j.1467-8721.2007.00525.x
63. Keers R, Coleman JR, Lester KJ, Roberts S, Breen G, Thastum M, et al. A genome-wide test of the differential susceptibility hypothesis reveals a genetic predictor of differential response to psychological treatments for child anxiety disorders. Psychother Psychosom. (2016) 85:146–58. doi: 10.1159/000444023
64. Pluess M. Individual differences in environmental sensitivity. Child Dev Perspect. (2015) 9:138–43. doi: 10.1111/cdep.12120
65. Stainton A, Chisholm K, Kaiser N, Rosen M, Upthegrove R, Ruhrmann S, et al. Resilience as a multimodal dynamic process. Early Interv Psychiatry. (2019) 13:725–32. doi: 10.1111/eip.12726
66. Maul S, Giegling I, Fabbri C, Corponi F, Serretti A, Rujescu D. Genetics of resilience: Implications from genome-wide association studies and candidate genes of the stress response system in posttraumatic stress disorder and depression. Am J Med Gen Part B Neuropsychiatr Genet. (2020) 183:77–94. doi: 10.1002/ajmg.b.32763
67. Sagoo GS, Little J, Higgins JP. Systematic reviews of genetic association studies. PLoS Med. (2009) 6:e1000028. doi: 10.1371/journal.pmed.1000028
68. Yu W, Clyne M, Khoury MJ, Gwinn M. Phenopedia and genopedia: disease-centered and gene-centered views of the evolving knowledge of human genetic associations. Bioinformatics. (2009) 26:145–6. doi: 10.1093/bioinformatics/btp618
69. Hu XZ, Lipsky RH, Zhu G, Akhtar LA, Taubman J, Greenberg BD, et al. Serotonin transporter promoter gain-of-function genotypes are linked to obsessive-compulsive disorder. Am J Hum Genet. (2006) 78:815–26. doi: 10.1086/503850
70. Wendland JR, Moya PR, Kruse MR, Ren-Patterson RF, Jensen CL, Timpano KR, et al. A novel, putative gain-of-function haplotype at slc6a4 associates with obsessive-compulsive disorder. Hum Mol Genet. (2008) 17:717–23. doi: 10.1093/hmg/ddm343
71. Egan MF, Kojima M, Callicott JH, Goldberg TE, Kolachana BS, Bertolino A, et al. The bdnf val66met polymorphism affects activity-dependent secretion of bdnf and human memory and hippocampal function. Cell. (2003) 112:257–69. doi: 10.1016/S0092-8674(03)00035-7
72. NCBI Resource Coordinators. Database resources of the national center for biotechnology information. Nucleic Acids Res. (2016) 44:D7–19. doi: 10.1093/nar/gkv1290
73. Lachman HM, Papolos DF, Saito T, Yu YM, Szumlanski CL, Weinshilboum RM. Human catechol-o-methyltransferase pharmacogenetics: description of a functional polymorphism and its potential application to neuropsychiatric disorders. Pharmacogenet Genom. (1996) 6:243–250. doi: 10.1097/00008571-199606000-00007
74. Schoots O, Van Tol HHM. The human dopamine d4 receptor repeat sequences modulate expression. Pharmacogenom J. (2003) 3:343–8. doi: 10.1038/sj.tpj.6500208
75. Simpson J, Vetuz G, Wilson M, Brookes KJ, Kent L. The drd4 receptor exon 3 vntr and 5' snp variants and mrna expression in human post-mortem brain tissue. Am J Med Genet B Neuropsychiatr Genet. (2010) 153b:1228–33. doi: 10.1002/ajmg.b.31084
76. Okuyama Y, Ishiguro H, Nankai M, Shibuya H, Watanabe A, Arinami T. Identification of a polymorphism in the promoter region of drd4associated with the human novelty seeking personality trait. Mol Psychiatry. (2000) 5:64. doi: 10.1038/sj.mp.4000563
77. Kereszturi E, Kiraly O, Barta C, Molnar N, Sasvari-Szekely M, Csapo Z. No direct effect of the−521 c/t polymorphism in the human dopamine d4 receptor gene promoter on transcriptional activity. BMC Mol Biol. (2006) 7:18. doi: 10.1186/1471-2199-7-18
78. Tei S, Mitsuhashi H, Ishiura S. Data describing the effect of drd4 promoter polymorphisms on promoter activity. Data Brief. (2016) 7:1112–7. doi: 10.1016/j.dib.2016.03.084
79. Sippel LM, Han S, Watkins LE, Harpaz-Rotem I, Southwick SM, Krystal JH, et al. Oxytocin receptor gene polymorphisms, attachment, and ptsd: results from the national health and resilience in veterans study. J Psychiatr Res. (2017) 94:139–47. doi: 10.1016/j.jpsychires.2017.07.008
80. Meyer-Lindenberg A, Domes G, Kirsch P, Heinrichs M. Oxytocin and vasopressin in the human brain: social neuropeptides for translational medicine. Nat Rev Neurosci. (2011) 12:524–38. doi: 10.1038/nrn3044
81. Weisgraber KH, Rall S, Mahley R. Human e apoprotein heterogeneity. Cysteine-arginine interchanges in the amino acid sequence of the apo-e isoforms. J Biol Chem. (1981) 256:9077–83. doi: 10.1016/S0021-9258(19)52510-8
82. Sommer WH, Lidström J, Sun H, Passer D, Eskay R, Parker SC, et al. Human npy promoter variation rs16147: T> c as a moderator of prefrontal npy gene expression and negative affect. Hum Mutat. (2010) 31:E1594–608. doi: 10.1002/humu.21299
83. Buckland PR, Hoogendoorn B, Coleman SL, Guy CA, Smith SK, O'Donovan MC. Strong bias in the location of functional promoter polymorphisms. Hum Mutat. (2005) 26:214–23. doi: 10.1002/humu.20207
84. Richardson A, Sisay-Joof F, Ackerman H, Usen S, Katundu P, Taylor T, et al. Nucleotide diversity of the tnf gene region in an african village. Genes Immunity. (2001) 2:343–8. doi: 10.1038/sj.gene.6363789
85. Wilson AG, Symons JA, McDowell TL, McDevitt HO, Duff GW. Effects of a polymorphism in the human tumor necrosis factor α promoter on transcriptional activation. Proc Natl Acad Sci USA. (1997) 94:3195–9. doi: 10.1073/pnas.94.7.3195
86. Binder EB. The role of fkbp5, a co-chaperone of the glucocorticoid receptor in the pathogenesis and therapy of affective and anxiety disorders. Psychoneuroendocrinology. (2009) 34:S186–95. doi: 10.1016/j.psyneuen.2009.05.021
87. Hotamisligil G, Breakefield X. Human monoamine oxidase a gene determines levels of enzyme activity. Am J Hum Genet. (1991) 49:383.
88. Mitchell RJ, Howlett S, Earl L, White NG, McComb J, Schanfield MS, et al. Distribution of the 3' vntr polymorphism in the human dopamine transporter gene in world populations. Hum Biol. (2000) 72:295–304. Available online at: https://pubmed.ncbi.nlm.nih.gov/10803661/
89. VanNess SH, Owens MJ, Kilts CD. The variable number of tandem repeats element in dat1 regulates in vitro dopamine transporter density. BMC Genet. (2005) 6:55. doi: 10.1186/1471-2156-6-55
90. Costa A, Riedel M, Müller U, Möller HJ, Ettinger U. Relationship between slc6a3 genotype and striatal dopamine transporter availability: a meta-analysis of human single photon emission computed tomography studies. Synapse. (2011) 65:998–1005. doi: 10.1002/syn.20927
91. Faraone SV, Spencer TJ, Madras BK, Zhang-James Y, Biederman J. Functional effects of dopamine transporter gene genotypes on in vivo dopamine transporter functioning: a meta-analysis. Mol Psychiatry. (2014) 19:880–9. doi: 10.1038/mp.2013.126
92. Steckler T, Holsboer F. Corticotropin-releasing hormone receptor subtypes and emotion. Biol Psychiatry. (1999) 46:1480–508. doi: 10.1016/S0006-3223(99)00170-5
93. Sumner JA, McLaughlin KA, Walsh K, Sheridan MA, Koenen KC. Crhr1 genotype and history of maltreatment predict cortisol reactivity to stress in adolescents. Psychoneuroendocrinology. (2014) 43:71–80. doi: 10.1016/j.psyneuen.2014.02.002
94. Davis SE, Bauer EP. L-type voltage-gated calcium channels in the basolateral amygdala are necessary for fear extinction. J Neurosci. (2012) 32:13582–6. doi: 10.1523/JNEUROSCI.0809-12.2012
95. Bigos KL, Mattay VS, Callicott JH, Straub RE, Vakkalanka R, Kolachana B, et al. Genetic variation in cacna1c affects brain circuitries related to mental illness. Arch Gen Psychiatry. (2010) 67:939–45. doi: 10.1001/archgenpsychiatry.2010.96
96. Ouma C, Davenport GC, Were T, Otieno MF, Hittner JB, Vulule JM, et al. Haplotypes of il-10 promoter variants are associated with susceptibility to severe malarial anemia and functional changes in il-10 production. Hum Genet. (2008) 124:515–24. doi: 10.1007/s00439-008-0578-5
97. Westendorp RG, Langermans JA, Huizinga TW, Elouali AH, Verweij CL, Boomsma DI, et al. Genetic influence on cytokine production and fatal meningococcal disease. Lancet. (1997) 349:170–3. doi: 10.1016/S0140-6736(96)06413-6
98. Homberger A, Linnebank M, Winter C, Willenbring H, Marquardt T, Harms E, et al. Genomic structure and transcript variants of the human methylenetetrahydrofolate reductase gene. Euro J Hum Genet. (2000) 8:725–9. doi: 10.1038/sj.ejhg.5200522
99. van der Put NM, Gabreëls F, Stevens EM, Smeitink JA, Trijbels FJ, Eskes TK, et al. A second common mutation in the methylenetetrahydrofolate reductase gene: an additional risk factor for neural-tube defects? Am J Hum Genet. (1998) 62:1044–51. doi: 10.1086/301825
100. Bond C, LaForge KS, Tian M, Melia D, Zhang S, Borg L, et al. Single-nucleotide polymorphism in the human mu opioid receptor gene alters β-endorphin binding and activity: possible implications for opiate addiction. Proc Nat Acad Sci USA. (1998) 95:9608–13. doi: 10.1073/pnas.95.16.9608
101. Cole SW, Arevalo JM, Takahashi R, Sloan EK, Lutgendorf SK, Sood AK, et al. Computational identification of gene–social environment interaction at the human il6 locus. Proc Natl Acad Sci USA. (2010) 107:5681–6. doi: 10.1073/pnas.0911515107
102. O'Hara R, Marcus P, Thompson WK, Flournoy J, Vahia I, Lin X, et al. 5-httlpr short allele, resilience, and successful aging in older adults. Am J Geriatr Psychiatry. (2012) 20:452–6. doi: 10.1097/JGP.0b013e31823e2d03
103. Carli V, Mandelli L, Zaninotto L, Roy A, Recchia L, Stoppia L, et al. A protective genetic variant for adverse environments? The role of childhood traumas and serotonin transporter gene on resilience and depressive severity in a high-risk population. Euro Psychiatry. (2011) 26:471–8. doi: 10.1016/j.eurpsy.2011.04.008
104. Graham DP, Helmer DA, Harding MJ, Kosten TR, Petersen NJ, Nielsen DA. Serotonin transporter genotype and mild traumatic brain injury independently influence resilience and perception of limitations in veterans. J Psychiatr Res. (2013) 47:835–42. doi: 10.1016/j.jpsychires.2013.02.006
105. Kang JI, Kim SJ, Song YY, Namkoong K, An SK. Genetic influence of comt and bdnf gene polymorphisms on resilience in healthy college students. Neuropsychobiology. (2013) 68:174–80. doi: 10.1159/000353257
106. Kim HW, Kang JI, An SK, Kim SJ. Oxytocin receptor gene variants are associated with emotion recognition and resilience, but not with false-belief reasoning performance in healthy young korean volunteers. CNS Neurosci Ther. (2019) 25:519–26. doi: 10.1111/cns.13075
107. van Rooij SJH, Stevens JS, Ely TD, Fani N, Smith AK, Kerley KA, et al. Childhood trauma and comt genotype interact to increase hippocampal activation in resilient individuals. Front Psychiatry. (2016) 7:156. doi: 10.3389/fpsyt.2016.00156
108. Das D, Cherbuin N, Tan X, Anstey KJ, Easteal S. Drd4-exoniii-vntr moderates the effect of childhood adversities on emotional resilience in young-adults. PLoS ONE. (2011) 6:e20177. doi: 10.1371/journal.pone.0020177
109. Bruenig D, Morris CP, Mehta D, Harvey W, Lawford B, Young RM, et al. Nitric oxide pathway genes (nos1ap and nos1) are involved in ptsd severity, depression, anxiety, stress and resilience. Gene. (2017) 625:42–8. doi: 10.1016/j.gene.2017.04.048
110. Rana BK, Darst BF, Bloss C, Shih PA, Depp C, Nievergelt CM, et al. Candidate snp associations of optimism and resilience in older adults: exploratory study of 935 community-dwelling adults. Am J Geriatr Psychiatry. (2014) 22:997–1006.5. doi: 10.1016/j.jagp.2014.03.009
111. Azadmarzabadi E, Haghighatfard A, Mohammadi A. Low resilience to stress is associated with candidate gene expression alterations in the dopaminergic signalling pathway. Psychogeriatrics. (2018) 18:190–201. doi: 10.1111/psyg.12312
112. Terock J, Van der Auwera S, Hannemann A, Janowitz D, Homuth G, Teumer A, et al. Interaction of childhood trauma with rs1360780 of the fkbp5 gene on trait resilience in a general population sample. J Psychiatr Res. (2019) 116:104–11. doi: 10.1016/j.jpsychires.2019.06.008
113. Bruenig D, Mehta D, Morris CP, Harvey W, Lawford B, Young RM, et al. Genetic and serum biomarker evidence for a relationship between tnfalpha and ptsd in vietnam war combat veterans. Compr Psychiatry. (2017) 74:125–33. doi: 10.1016/j.comppsych.2017.01.015
114. Defrancesco M, Liebaert J, Kemmler G, Aigner F, Niederstatter H, Parson W, et al. Psychosocial state after bariatric surgery is associated with the serotonin-transporter promoter polymorphism. Eat Weight Disord. (2013) 18:311–6. doi: 10.1007/s40519-013-0045-8
115. Taylor ZE, Sulik MJ, Eisenberg N, Spinrad TL, Silva KM, Lemery-Chalfant K, et al. Development of ego-resiliency: relations to observed parenting and polymorphisms in the serotonin transporter gene during early childhood. Soc Dev. (2014) 23:433–50. doi: 10.1111/sode.12041
116. Peters RB, Xavier J, Mondin TC, Cardoso TA, Ferreira FB, Teixeira L, et al. Bdnf val66met polymorphism and resilience in major depressive disorder: the impact of cognitive psychotherapy. Braz J Psychiatry. (2020) 43:22–8. doi: 10.1590/1516-4446-2019-0726
117. Delis F, Carvalho AF, Poulia N, Bozidis P, Paika V, Ntountoulaki E, et al. Resilience mediates the influence of a polymorphism in the serotonin transporter gene on the relationship between the burden of chronic illness and depression. Psychother Psychosom. (2017) 86:305–7. doi: 10.1159/000478020
118. Resnick B, Klinedinst NJ, Yerges-Armstrong L, Magaziner J, Orwig D, Hochberg MC, et al. Genotype, resilience and function and physical activity post hip fracture. Int J Orthopaed Trauma Nurs. (2019) 34:36–42. doi: 10.1016/j.ijotn.2019.03.005
119. Gan Y, Chen Y, Han X, Yu NX, Wang L. Neuropeptide y gene × environment interaction predicts resilience and positive future focus. Appl Psychol Health Wellbeing. (2019) 11:438–58. doi: 10.1111/aphw.12162
120. Lange RT, Merritt VC, Brickell TA, Dalgard CL, Soltis AR, Hershaw J, et al. Apolipoprotein e e4 is associated with worse self-reported neurobehavioral symptoms following uncomplicated mild traumatic brain injury in U.S. military service members. Behav Brain Res. (2021) 415:113491. doi: 10.1016/j.bbr.2021.113491
121. Strohmaier J, Amelang M, Hothorn LA, Witt SH, Nieratschker V, Gerhard D, et al. The psychiatric vulnerability gene cacna1c and its sex-specific relationship with personality traits, resilience factors and depressive symptoms in the general population. Mol Psychiatry. (2013) 18:607–13. doi: 10.1038/mp.2012.53
122. Surtees PG, Wainwright N, Willis-Owen S, Sandhu MS, Luben R, Day NE, et al. The brain-derived neurotrophic factor val66met polymorphism is associated with sense of coherence in a non-clinical community sample of 7335 adults. J Psychiatr Res. (2007) 41:707–10. doi: 10.1016/j.jpsychires.2006.05.015
123. Reinelt E, Barnow S, Stopsack M, Aldinger M, Schmidt CO, John U, et al. Social support and the serotonin transporter genotype (5-httlpr) moderate levels of resilience, sense of coherence, and depression. Am J Med Genet Part B Neuropsychiatr Genet. (2015) 168:383–91. doi: 10.1002/ajmg.b.32322
124. Saphire-Bernstein S, Way BM, Kim HS, Sherman DK, Taylor SE. Oxytocin receptor gene (oxtr) is related to psychological resources. Proc Natl Acad Sci USA. (2011) 108:15118–22. doi: 10.1073/pnas.1113137108
125. Klok MD, Giltay EJ, Van der Does AJ, Geleijnse JM, Antypa N, Penninx BW, et al. A common and functional mineralocorticoid receptor haplotype enhances optimism and protects against depression in females. Transl Psychiatry. (2011) 1:e62. doi: 10.1038/tp.2011.59
126. Amstadter AB, Daughters SB, MacPherson L, Reynolds EK, Danielson CK, Wang F, et al. Genetic associations with performance on a behavioral measure of distress intolerance. J Psychiatr Res. (2012) 46:87–94. doi: 10.1016/j.jpsychires.2011.09.017
127. Dunn EC, Solovieff N, Lowe SR, Gallagher PJ, Chaponis J, Rosand J, et al. Interaction between genetic variants and exposure to hurricane katrina on post-traumatic stress and post-traumatic growth: a prospective analysis of low income adults. J Affect Disord. (2014) 152–4:243–9. doi: 10.1016/j.jad.2013.09.018
128. Resnick B, Klinedinst NJ, Yerges-Armstrong L, Choi EY, Dorsey SG. The impact of genetics on physical resilience and successful aging. J Aging Health. (2015) 27:1084–104. doi: 10.1177/0898264315577586
129. Aizawa S, Ishitobi Y, Masuda K, Inoue A, Oshita H, Hirakawa H, et al. Genetic association of the transcription of neuroplasticity-related genes and variation in stress-coping style. Brain Behav. (2015) 5:e00360. doi: 10.1002/brb3.360
130. Çarkaxhiu Bulut G, Rodopman Arman A, Güney I, Gültepe P. Evaluation of 5-httlpr gene polymorphism and resilience components on the development of psychopathology in adolescent sexual abuse cases. Noro Psikiyatri Arsivi. (2017) 54:234–8. doi: 10.5152/npa.2016.12528
131. Nederhof E, Bouma E, Riese H, Laceulle O, Ormel J, Oldehinkel A. Evidence for plasticity genotypes in a gene–gene–environment interaction: the trails study. Genes Brain Behav. (2010) 9:968–73. doi: 10.1111/j.1601-183X.2010.00637.x
132. Markus CR, De Raedt R. Differential effects of 5-httlpr genotypes on inhibition of negative emotional information following acute stress exposure and tryptophan challenge. Neuropsychopharmacology. (2011) 36:819–26. doi: 10.1038/npp.2010.221
133. Kwang T, Wells TT, McGeary JE, Swann WB Jr, Beevers CG. Association of the serotonin transporter promoter region polymorphism with biased attention for negative word stimuli. Depres Anxiety. (2010) 27:746–51. doi: 10.1002/da.20708
134. Fox E, Ridgewell A, Ashwin C. Looking on the bright side: biased attention and the human serotonin transporter gene. Proc R Soc Biol Sci. (2009) 276:1747–51. doi: 10.1098/rspb.2008.1788
135. Gervai J, Nemoda Z, Lakatos K, Ronai Z, Toth I, Ney K, et al. Transmission disequilibrium tests confirm the link between drd4 gene polymorphism and infant attachment. Am J Med Genet Part B Neuropsychiatr Genet. (2005) 132B:126–30. doi: 10.1002/ajmg.b.30102
136. Aboud O, Mrak RE, Boop FA, Griffin WS. Epilepsy: neuroinflammation, neurodegeneration, apoe genotype. Acta Neuropathol Commun. (2013) 1:41. doi: 10.1186/2051-5960-1-41
137. Aboud O, Mrak RE, Boop F, Griffin ST. Apolipoprotein epsilon 3 alleles are associated with indicators of neuronal resilience. BMC Med. (2012) 10:35. doi: 10.1186/1741-7015-10-35
138. Felsky D, Xu J, Chibnik LB, Schneider JA, Knight J, Kennedy JL, et al. Genetic epistasis regulates amyloid deposition in resilient aging. Alzheimers Dement. (2017) 13:1107–16. doi: 10.1016/j.jalz.2017.01.027
139. Li Z, Farias FHG, Dube U, Del-Aguila JL, Mihindukulasuriya KA, Fernandez MV, et al. The tmem106b ftld-protective variant, rs1990621, is also associated with increased neuronal proportion. Acta Neuropathol. (2020) 139:45–61. doi: 10.1007/s00401-019-02066-0
140. Ridge PG, Karch CM, Hsu S, Arano I, Teerlink CC, Ebbert MTW, et al. Linkage, whole genome sequence, and biological data implicate variants in rab10 in alzheimer's disease resilience. Genome Med. (2017) 9:100. doi: 10.1186/s13073-017-0486-1
141. Mukherjee S, Kim S, Gibbons LE, Nho K, Risacher SL, Glymour MM, et al. Genetic architecture of resilience of executive functioning. Brain Imaging Behav. (2012) 6:621–33. doi: 10.1007/s11682-012-9184-1
142. Mukherjee S, Kim S, Ramanan VK, Gibbons LE, Nho K, Glymour MM, et al. Gene-based gwas and biological pathway analysis of the resilience of executive functioning. Brain Imaging Behav. (2014) 8:110–8. doi: 10.1007/s11682-013-9259-7
143. James LM, Engdahl BE, Georgopoulos AP. Apolipoprotein e: the resilience gene. Exp Brain Res. (2017) 235:1853–9. doi: 10.1007/s00221-017-4941-4
144. Cicchetti D, Rogosch FA. Gene x environment interaction and resilience: effects of child maltreatment and serotonin, corticotropin releasing hormone, dopamine, oxytocin genes. Dev Psychopathol. (2012) 24:411–27. doi: 10.1017/S0954579412000077
145. Beaver KM, Mancini C, DeLisi M, Vaughn MG. Resiliency to victimization: the role of genetic factors. J Interpers Viol. (2011) 26:874–98. doi: 10.1177/0886260510365860
146. Dunn LB, Aouizerat BE, Langford DJ, Cooper BA, Dhruva A, Cataldo JK, et al. Cytokine gene variation is associated with depressive symptom trajectories in oncology patients and family caregivers. Euro J Oncol Nurs. (2013) 17:346–53. doi: 10.1016/j.ejon.2012.10.004
147. Dumitrescu L, Mahoney ER, Mukherjee S, Lee ML, Bush WS, Engelman CD, et al. Genetic variants and functional pathways associated with resilience to alzheimer's disease. Brain. (2020) 143:2561–75. doi: 10.1093/brain/awaa209
148. Gibbons FX, Roberts ME, Gerrard M, Li Z, Beach SR, Simons RL, et al. The impact of stress on the life history strategies of african american adolescents: cognitions, genetic moderation, and the role of discrimination. Dev Psychol. (2012) 48:722. doi: 10.1037/a0026599
149. Heils A, Teufel A, Petri S, Stöber G, Riederer P, Bengel D, et al. Allelic variation of human serotonin transporter gene expression. J Neurochem. (1996) 66:2621–4. doi: 10.1046/j.1471-4159.1996.66062621.x
150. Williams GC. Pleiotropy, natural selection, and the evolution of senescence. Evolution. (1957) 11:398–411. doi: 10.1111/j.1558-5646.1957.tb02911.x
151. Cole SW, Arevalo JM, Manu K, Telzer EH, Kiang L, Bower JE, et al. Antagonistic pleiotropy at the human il6 promoter confers genetic resilience to the pro-inflammatory effects of adverse social conditions in adolescence. Dev Psychol. (2011) 47:1173–80. doi: 10.1037/a0023871
152. Ungewitter E, Scrable H. Antagonistic pleiotropy and p53. Mech Ageing Dev. (2009) 130:10–7. doi: 10.1016/j.mad.2008.06.002
153. Barr CS, Newman TK, Schwandt M, Shannon C, Dvoskin RL, Lindell SG, et al. Sexual dichotomy of an interaction between early adversity and the serotonin transporter gene promoter variant in rhesus macaques. Proc Natl Acad Sci USA. (2004) 101:12358. doi: 10.1073/pnas.0403763101
154. Sjöberg RL, Leppert J, Öhrvik J, Nilsson KW, Lindström L, Oreland L, et al. Development of depression: sex and the interaction between environment and a promoter polymorphism of the serotonin transporter gene. Int J Neuropsychopharmacol. (2006) 9:443–9. doi: 10.1017/S1461145705005936
155. Gressier F, Calati R, Serretti A. 5-httlpr and gender differences in affective disorders: a systematic review. J Affect Disord. (2016) 190:193–207. doi: 10.1016/j.jad.2015.09.027
156. Verhagen M, Van Der Meij A, Van Deurzen P, Janzing J, Arias-Vasquez A, Buitelaar J, et al. Meta-analysis of the bdnf val66met polymorphism in major depressive disorder: effects of gender and ethnicity. Mol Psychiatry. (2010) 15:260. doi: 10.1038/mp.2008.109
157. Chan CB, Ye K. Sex differences in brain-derived neurotrophic factor signaling and functions. J Neurosci Res. (2017) 95:328–35. doi: 10.1002/jnr.23863
158. Bradley B, Davis TA, Wingo AP, Mercer KB, Ressler KJ. Family environment and adult resilience: contributions of positive parenting and the oxytocin receptor gene. Euro J Psychotraumatol. (2013) 18:4. doi: 10.3402/ejpt.v4i0.21659
159. Harrison PJ, Tunbridge EM. Catechol-o-methyltransferase (comt): a gene contributing to sex differences in brain function, and to sexual dimorphism in the predisposition to psychiatric disorders. Neuropsychopharmacology. (2007) 33:3037. doi: 10.1038/sj.npp.1301543
160. Palmatier MA, Kang AM, Kidd KK. Global variation in the frequencies of functionally different catechol-o-methyltransferase alleles. Biol Psychiatry. (1999) 46:557–67. doi: 10.1016/S0006-3223(99)00098-0
161. Dick DM, Agrawal A, Keller MC, Adkins A, Aliev F, Monroe S, et al. Candidate gene–environment interaction research: reflections and recommendations. Perspect Psychol Sci. (2015) 10:37–59. doi: 10.1177/1745691614556682
162. Yamada Y, Sakuma J, Takeuchi I, Yasukochi Y, Kato K, Oguri M, et al. Identification of egflam, spatc1l and rnase13 as novel susceptibility loci for aortic aneurysm in japanese individuals by exome-wide association studies. Int J Mol Med. (2017) 39:1091–100. doi: 10.3892/ijmm.2017.2927
163. Tikkanen R, Ducci F, Goldman D, Holi M, Lindberg N, Tiihonen J, et al. Maoa alters the effects of heavy drinking and childhood physical abuse on risk for severe impulsive acts of violence among alcoholic violent offenders. Alcohol Clin Exp Res. (2010) 34:853–60. doi: 10.1111/j.1530-0277.2010.01157.x
164. Antypa N, Giegling I, Calati R, Schneider B, Hartmann AM, Friedl M, et al. Maoa and maob polymorphisms and anger-related traits in suicidal participants and controls. Eur Arch Psychiatry Clin Neurosci. (2013) 263:393–403. doi: 10.1007/s00406-012-0378-8
165. Agnafors S, Svedin CG, Oreland L, Bladh M, Comasco E, Sydsjo G. A biopsychosocial approach to risk and resilience on behavior in children followed from birth to age 12. Child Psychiatry Hum Dev. (2017) 48:584–96. doi: 10.1007/s10578-016-0684-x
166. Cline JI, Belsky J, Li Z, Melhuish E, Lysenko L, McFarquhar T, et al. Take your mind off it: coping style, serotonin transporter linked polymorphic region genotype (5-httlpr), and children's internalizing and externalizing problems. Dev Psychopathol. (2015) 27 (4 Pt. 1):1129–43. doi: 10.1017/S0954579415000723
167. Hostinar CE, Cicchetti D, Rogosch FA. Oxytocin receptor gene polymorphism, perceived social support, and psychological symptoms in maltreated adolescents. Dev Psychopathol. (2014) 26:465–77. doi: 10.1017/S0954579414000066
168. Amstadter AB, Koenen KC, Ruggiero KJ, Acierno R, Galea S, Kilpatrick DG, et al. Npy moderates the relation between hurricane exposure and generalized anxiety disorder in an epidemiologic sample of hurricane-exposed adults. Depress Anxiety. (2010) 27:270–5. doi: 10.1002/da.20648
169. Donner J, Sipila T, Ripatti S, Kananen L, Chen X, Kendler KS, et al. Support for involvement of glutamate decarboxylase 1 and neuropeptide y in anxiety susceptibility. Am J Med Genet Part B Neuropsychiatr Genet. (2012) 159:316–27. doi: 10.1002/ajmg.b.32029
170. Berking A-C, Thiel C, Schiele MA, Baumann C, Kalisch R, et al. An investigation of genetic variability of DNA methyltransferases dnmt3a and 3b does not provide evidence for a major role in the pathogenesis of panic disorder and dimensional anxiety phenotypes. J Neural Transm. (2020) 127:1527–37. doi: 10.1007/s00702-020-02206-x
171. Janelidze S, Suchankova P, Ekman A, Erhardt S, Sellgren C, Samuelsson M, et al. Low il-8 is associated with anxiety in suicidal patients: genetic variation and decreased protein levels. Acta Psychiatr Scand. (2015) 131:269–78. doi: 10.1111/acps.12339
172. Min JA, Lee HJ, Lee SH, Park YM, Kang SG, Chae JH. Gender-specific effects of brain-derived neurotrophic factor val66met polymorphism and childhood maltreatment on anxiety. Neuropsychobiology. (2013) 67:6–13. doi: 10.1159/000342384
173. Ryu JS, Lee YM, Kim YS, Kang S, Park JS, Ahn CW, et al. Association between bdnf polymorphism and depressive symptoms in patients newly diagnosed with type 2 diabetes mellitus. Yonsei Med J. (2021) 62:359–65. doi: 10.3349/ymj.2021.62.4.359
174. Telch MJ, Beevers CG, Rosenfield D, Lee HJ, Reijntjes A, Ferrell RE, et al. 5-httlpr genotype potentiates the effects of war zone stressors on the emergence of ptsd, depressive and anxiety symptoms in soldiers deployed to iraq. World Psychiatry. (2015) 14:198–206. doi: 10.1002/wps.20215
175. Vaht M, Kiive E, Veidebaum T, Harro J. A functional vesicular monoamine transporter 1 (vmat1) gene variant is associated with affect and the prevalence of anxiety, affective, and alcohol use disorders in a longitudinal population-representative birth cohort study. Int J Neuropsychopharmacol. (2016) 19:pyw013. doi: 10.1093/ijnp/pyw013
176. Ancelin ML, Scali J, Norton J, Ritchie K, Dupuy AM, Chaudieu I, et al. Heterogeneity in hpa axis dysregulation and serotonergic vulnerability to depression. Psychoneuroendocrinology. (2017) 77:90–4. doi: 10.1016/j.psyneuen.2016.11.016
177. Banny AM, Cicchetti D, Rogosch FA, Oshri A, Crick NR. Vulnerability to depression: a moderated mediation model of the roles of child maltreatment, peer victimization, and serotonin transporter linked polymorphic region genetic variation among children from low socioeconomic status backgrounds. Dev Psychopathol. (2013) 25:599–614. doi: 10.1017/S0954579413000047
178. Mata J, Thompson RJ, Gotlib IH. Bdnf genotype moderates the relation between physical activity and depressive symptoms. Health Psychol. (2010) 29:130–3. doi: 10.1037/a0017261
179. Cruz-Fuentes CS, Benjet C, Martinez-Levy GA, Perez-Molina A, Briones-Velasco M, Suarez-Gonzalez J. Bdnf met66 modulates the cumulative effect of psychosocial childhood adversities on major depression in adolescents. Brain Behav. (2014) 4:290–7. doi: 10.1002/brb3.220
180. Kohen R, Cain KC, Mitchell PH, Becker K, Buzaitis A, Millard SP, et al. Association of serotonin transporter gene polymorphisms with poststroke depression. Arch Gen Psychiatry. (2008) 65:1296–302. doi: 10.1001/archpsyc.65.11.1296
181. Lenze EJ, Shardell M, Ferrell RE, Orwig D, Yu-Yahiro J, Hawkes W, et al. Association of serotonin-1a and 2a receptor promoter polymorphisms with depressive symptoms and functional recovery in elderly persons after hip fracture. J Affect Disord. (2008) 111:61–6. doi: 10.1016/j.jad.2008.02.005
182. Lotrich FE, Ferrell RE, Rabinovitz M, Pollock BG. Risk for depression during interferon-alpha treatment is affected by the serotonin transporter polymorphism. Biol Psychiatry. (2009) 65:344–8. doi: 10.1016/j.biopsych.2008.08.009
183. Grabe HJ, Schwahn C, Appel K, Mahler J, Schulz A, Spitzer C, et al. Childhood maltreatment, the corticotropin-releasing hormone receptor gene and adult depression in the general population. Am J Med Genet Part B Neuropsychiatr Gen. (2010) 153B:1483–93. doi: 10.1002/ajmg.b.31131
184. Laucht M, Treutlein J, Blomeyer D, Buchmann AF, Schmidt MH, Esser G, et al. Interactive effects of corticotropin-releasing hormone receptor 1 gene and childhood adversity on depressive symptoms in young adults: findings from a longitudinal study. Euro Neuropsychopharmacol. (2013) 23:358–67. doi: 10.1016/j.euroneuro.2012.06.002
185. Vinkers CH, Joels M, Milaneschi Y, Gerritsen L, Kahn RS, Penninx BW, et al. Mineralocorticoid receptor haplotypes sex-dependently moderate depression susceptibility following childhood maltreatment. Psychoneuroendocrinology. (2015) 54:90–102. doi: 10.1016/j.psyneuen.2015.01.018
186. Felten A, Montag C, Markett S, Walter NT, Reuter M. Genetically determined dopamine availability predicts disposition for depression. Brain Behav. (2011) 1:109–18. doi: 10.1002/brb3.20
187. Saad S, Dunn LB, Koetters T, Dhruva A, Langford DJ, Merriman JD, et al. Cytokine gene variations associated with subsyndromal depressive symptoms in patients with breast cancer. Eur J Oncol Nurs. (2014) 18:397–404. doi: 10.1016/j.ejon.2014.03.009
188. Satterfield BC, Wisor JP, Schmidt MA, Van Dongen HPA. Time-on-task effect during sleep deprivation in healthy young adults is modulated by dopamine transporter genotype. Sleep. (2017) 40:zsx167. doi: 10.1093/sleep/zsx167
189. Satterfield BC, Wisor JP, Field SA, Schmidt MA, Van Dongen HPA. Tnfalpha g308a polymorphism is associated with resilience to sleep deprivation-induced psychomotor vigilance performance impairment in healthy young adults. Brain Behavior Immunity. (2015) 47:66–74. doi: 10.1016/j.bbi.2014.12.009
190. Skeiky L, Brager AJ, Satterfield BC, Petrovick M, Balkin TJ, Capaldi VF, et al. Tnfα g308a genotype, resilience to sleep deprivation, and the effect of caffeine on psychomotor vigilance performance in a randomized, double-blind, placebo-controlled, crossover study. Chronobiol Int. (2020) 37:1461–4. doi: 10.1080/07420528.2020.1821044
191. Brody GH, Beach SR, Chen YF, Obasi E, Philibert RA, Kogan SM, et al. Perceived discrimination, serotonin transporter linked polymorphic region status, and the development of conduct problems. Dev Psychopathol. (2011) 23:617–27. doi: 10.1017/S0954579411000046
192. Loth E, Poline JB, Thyreau B, Jia T, Tao C, Lourdusamy A, et al. Oxytocin receptor genotype modulates ventral striatal activity to social cues and response to stressful life events. Biol Psychiatry. (2014) 76:367–76. doi: 10.1016/j.biopsych.2013.07.043
193. Martel MM, Nikolas M, Jernigan K, Friderici K, Nigg JT. Diversity in pathways to common childhood disruptive behavior disorders. J Abnorm Child Psychol. (2012) 40:1223–36. doi: 10.1007/s10802-012-9646-3
194. Verschoor E, Markus CR. Effects of acute psychosocial stress exposure on endocrine and affective reactivity in college students differing in the 5-httlpr genotype and trait neuroticism. Stress. (2011) 14:407–19. doi: 10.3109/10253890.2010.548886
195. Deslauriers J, Acheson DT, Maihofer AX, Nievergelt CM, Baker DG, Geyer MA, et al. Comt val158met polymorphism links to altered fear conditioning and extinction are modulated by ptsd and childhood trauma. Depress Anxiety. (2018) 35:32–42. doi: 10.1002/da.22678
196. Kolassa IT, Ertl V, Eckart C, Glockner F, Kolassa S, Papassotiropoulos A, et al. Association study of trauma load and slc6a4 promoter polymorphism in posttraumatic stress disorder: evidence from survivors of the rwandan genocide. J Clin Psychiatry. (2010) 71:543–7. doi: 10.4088/JCP.08m04787blu
197. Hemmings SM, Martin LI, Klopper M, van der Merwe L, Aitken L, de Wit E, et al. Bdnf val66met and drd2 taq1a polymorphisms interact to influence ptsd symptom severity: a preliminary investigation in a south african population. Prog Neuro Psychopharmacol Biol Psychiatry. (2013) 40:273–80. doi: 10.1016/j.pnpbp.2012.10.011
198. Mota NP, Han S, Harpaz-Rotem I, Maruff P, Krystal JH, Southwick SM, et al. Apolipoprotein e gene polymorphism, trauma burden, and posttraumatic stress symptoms in U.S. military veterans: results from the national health and resilience in veterans study. Depres Anxiety. (2018) 35:168–77. doi: 10.1002/da.22698
199. Liberzon I, King AP, Ressler KJ, Almli LM, Zhang P, Ma ST, et al. Interaction of the adrb2 gene polymorphism with childhood trauma in predicting adult symptoms of posttraumatic stress disorderadrb2 gene polymorphism, childhood trauma, and ptsdadrb2 gene polymorphism, childhood trauma, and ptsd. JAMA Psychiatry. (2014) 71:1174–82. doi: 10.1001/jamapsychiatry.2014.999
200. Pitts BL, Whealin JM, Harpaz-Rotem I, Duman RS, Krystal JH, Southwick SM, et al. Bdnf val66met polymorphism and posttraumatic stress symptoms in U.S. Military veterans: protective effect of physical exercise. Psychoneuroendocrinology. (2019) 100:198–202. doi: 10.1016/j.psyneuen.2018.10.011
201. Lyons MJ, Genderson M, Grant MD, Logue M, Zink T, McKenzie R, et al. Gene-environment interaction of apoe genotype and combat exposure on ptsd. Am J Med Gen Part B Neuropsychiatr Genet. (2013) 162:762–9. doi: 10.1002/ajmg.b.32154
202. Grant A, Fathalli F, Rouleau G, Joober R, Flores C. Association between schizophrenia and genetic variation in dcc: a case-control study. Schizophr Res. (2012) 137:26–31. doi: 10.1016/j.schres.2012.02.023
203. Nestor PG, Choate Hasler V, O'Donovan K, Lapp HE, Boodai SB, Hunter R. Psychiatric risk and resilience: plasticity genes and positive mental health. Brain Behav. (2021) 11:e02137. doi: 10.1002/brb3.2137
204. Jabbi M, Kema IP, van der Pompe G, te Meerman GJ, Ormel J, den Boer JA. Catechol-o-methyltransferase polymorphism and susceptibility to major depressive disorder modulates psychological stress response. Psychiatr Genet. (2007) 17:183–93. doi: 10.1097/YPG.0b013e32808374df
205. Peerbooms O, Rutten BP, Collip D, Lardinois M, Lataster T, Thewissen V, et al. Evidence that interactive effects of comt and mthfr moderate psychotic response to environmental stress. Acta Psychiatr Scand. (2012) 125:247–56. doi: 10.1111/j.1600-0447.2011.01806.x
206. Lin X, Liu J, Fu P, Zeng X, Qin J, Tang Z, et al. Associations between gene polymorphisms and psychological stress in the guangxi minority region of china. Med Sci Monit. (2018) 24:6680–7. doi: 10.12659/MSM.910432
207. van Winkel M, Peeters F, van Winkel R, Kenis G, Collip D, Geschwind N, et al. Impact of variation in the bdnf gene on social stress sensitivity and the buffering impact of positive emotions: replication and extension of a gene–environment interaction. Euro Neuropsychopharmacol. (2014) 24:930–8. doi: 10.1016/j.euroneuro.2014.02.005
208. Benedetti F, Radaelli D, Poletti S, Locatelli C, Dallaspezia S, Lorenzi C, et al. Association of the c (– 1019) g 5-ht1a promoter polymorphism with exposure to stressors preceding hospitalization for bipolar depression. J Affect Disord. (2011) 132:297–300. doi: 10.1016/j.jad.2011.02.024
209. Roy A, Hodgkinson CA, Deluca V, Goldman D, Enoch MA. Two hpa axis genes, crhbp and fkbp5, interact with childhood trauma to increase the risk for suicidal behavior. J Psychiatr Res. (2012) 46:72–9. doi: 10.1016/j.jpsychires.2011.09.009
210. Weikum WM, Brain U, Chau CMY, Grunau RE, Boyce WT, Diamond A, et al. Prenatal serotonin reuptake inhibitor (sri) antidepressant exposure and serotonin transporter promoter genotype (slc6a4) influence executive functions at 6 years of age. Front Cell Neurosci. (2013) 7:180. doi: 10.3389/fncel.2013.00180
211. Bradley B, Westen D, Mercer KB, Binder EB, Jovanovic T, Crain D, et al. Association between childhood maltreatment and adult emotional dysregulation in a low-income, urban, african american sample: moderation by oxytocin receptor gene. Dev Psychopathol. (2011) 23:439–52. doi: 10.1017/S0954579411000162
212. Drury SS, Gleason MM, Theall KP, Smyke AT, Nelson CA, Fox NA, et al. Genetic sensitivity to the caregiving context: the influence of 5httlpr and bdnf val66met on indiscriminate social behavior. Physiol Behav. (2012) 106:728–35. doi: 10.1016/j.physbeh.2011.11.014
213. Troisi A, Frazzetto G, Carola V, Di Lorenzo G, Coviello M, Siracusano A, et al. Variation in the mu-opioid receptor gene (oprm1) moderates the influence of early maternal care on fearful attachment. Soc Cogn Affect Neurosci. (2012) 7:542–7. doi: 10.1093/scan/nsr037
214. Hermann A, Kupper Y, Schmitz A, Walter B, Vaitl D, Hennig J, et al. Functional gene polymorphisms in the serotonin system and traumatic life events modulate the neural basis of fear acquisition and extinction. PLoS ONE. (2012) 7:e44352. doi: 10.1371/journal.pone.0044352
215. Heinz A, Smolka MN. The effects of catechol o-methyltransferase genotype on brain activation elicited by affective stimuli and cognitive tasks. Rev Neurosci. (2006) 17:359–67. doi: 10.1515/REVNEURO.2006.17.3.359
216. O'Nions EJP, Dolan RJ, Roiser JP. Serotonin transporter genotype modulates subgenual response to fearful faces using an incidental task. J Cogn Neurosci. (2011) 23:3681–93. doi: 10.1162/jocn_a_00055
217. Smolka MN, Schumann G, Wrase J, Grusser SM, Flor H, Mann K, et al. Catechol-o-methyltransferase val158met genotype affects processing of emotional stimuli in the amygdala and prefrontal cortex. J Neurosci. (2005) 25:836–42. doi: 10.1523/JNEUROSCI.1792-04.2005
218. Yokoyama JS, Marx G, Brown JA, Bonham LW, Wang D, Coppola G, et al. Systemic klotho is associated with klotho variation and predicts intrinsic cortical connectivity in healthy human aging. Brain Imaging Behav. (2017) 11:391–400. doi: 10.1007/s11682-016-9598-2
219. Kim SN, Kang DH, Yun JY, Lee TY, Jung WH, Jang JH, et al. Impact of the bdnf val66met polymorphism on regional brain gray matter volumes: relevance to the stress response. Psychiatry Invest. (2013) 10:173–9. doi: 10.4306/pi.2013.10.2.173
220. Ancelin ML, Carrière I, Artero S, Maller JJ, Meslin C, Dupuy AM, et al. Structural brain alterations in older adults exposed to early-life adversity. Psychoneuroendocrinology. (2021) 129:105272. doi: 10.1016/j.psyneuen.2021.105272
221. Pillai JA, Bebek G, Khrestian M, Bena J, Bergmann CC, Bush WS, et al. Tnfrsf1b gene variants and related soluble tnfr2 levels impact resilience in alzheimer's disease. Front Aging Neurosci. (2021) 13:638922. doi: 10.3389/fnagi.2021.638922
222. Porter T, Burnham SC, Dore V, Savage G, Bourgeat P, Begemann K, et al. Kibra is associated with accelerated cognitive decline and hippocampal atrophy in apoe epsilon4-positive cognitively normal adults with high abeta-amyloid burden. Sci Rep. (2018) 8:2034. doi: 10.1038/s41598-018-20513-y
223. Fullerton JM, Klauser P, Lenroot RK, Shaw AD, Overs B, Heath A, et al. Differential effect of disease-associated st8sia2 haplotype on cerebral white matter diffusion properties in schizophrenia and healthy controls. Transl Psychiatry. (2018) 8:21. doi: 10.1038/s41398-017-0052-z
224. Ayers KL, Mirshahi UL, Wardeh AH, Murray MF, Hao K, Glicksberg BS, et al. A loss of function variant in casp7 protects against alzheimer's disease in homozygous apoe epsilon4 allele carriers. BMC Genom. (2016) 17 (Suppl. 2):445. doi: 10.1186/s12864-016-2725-z
225. Liu P, Zhang M, Xie X, Jin J, Holman CD. Polymorphisms of 5,10-methylenetetrahydrofolate reductase and thymidylate synthase, dietary folate intake, and the risk of leukemia in adults. Tumour Biol. (2016) 37:3265–75. doi: 10.1007/s13277-015-4168-6
226. Donlon TA, Chen R, Masaki KH, Willcox BJ, Morris BJ. Association with longevity of phosphatidylinositol 3-kinase regulatory subunit 1 gene variants stems from protection against mortality risk in men with cardiovascular disease. Gerontology. (2021) 68:162–70. doi: 10.1159/000515390
227. Donlon TA, Chen R, Masaki KH, Willcox DC, Allsopp RC, Willcox BJ, et al. Association of growth hormone receptor gene variant with longevity in men is due to amelioration of increased mortality risk from hypertension. Aging. (2021) 13:14745–67. doi: 10.18632/aging.203133
228. Morris BJ, Chen R, Donlon TA, Masaki KH, Willcox DC, Allsopp RC, et al. Lifespan extension conferred by mitogen-activated protein kinase kinase kinase 5 (map3k5) longevity-associated gene variation is confined to at-risk men with a cardiometabolic disease. Aging. (2021) 13:7953–74. doi: 10.18632/aging.202844
229. Armbruster D, Mueller A, Strobel A, Lesch KP, Brocke B, Kirschbaum C. Children under stress - comt genotype and stressful life events predict cortisol increase in an acute social stress paradigm. Int J Neuropsychopharmacol. (2012) 15:1229–39. doi: 10.1017/S1461145711001763
230. Buchmann AF, Holz N, Boecker R, Blomeyer D, Rietschel M, Witt SH, et al. Moderating role of fkbp5 genotype in the impact of childhood adversity on cortisol stress response during adulthood. Euro Neuropsychopharmacol. (2014) 24:837–45. doi: 10.1016/j.euroneuro.2013.12.001
231. Klaus K, Butler K, Gutierrez H, Durrant SJ, Pennington K. Interactive effects of early life stress and cacna1c genotype on cortisol awakening response. Biol Psychol. (2018) 136:22–8. doi: 10.1016/j.biopsycho.2018.05.002
232. Chang HA, Fang WH, Chang TC, Huang SY, Chang CC. Association of neuropeptide y promoter polymorphism (rs16147) with perceived stress and cardiac vagal outflow in humans. Sci Rep. (2016) 6:31683. doi: 10.1038/srep31683
233. Brown-Rice KA, Scholl JL, Fercho KA, Pearson K, Kallsen NA, Davies GE, et al. Neural and psychological characteristics of college students with alcoholic parents differ depending on current alcohol use. Prog Neuropsychopharmacol Biol Psychiatry. (2018) 81:284–96. doi: 10.1016/j.pnpbp.2017.09.010
234. Enoch MA, Hodgkinson CA, Shen PH, Gorodetsky E, Marietta CA, Roy A, et al. Gabbr1 and slc6a1, two genes involved in modulation of gaba synaptic transmission, influence risk for alcoholism: results from three ethnically diverse populations. Alcohol Clin Exp Res. (2016) 40:93–101. doi: 10.1111/acer.12929
235. Enoch MA, Hodgkinson CA, Yuan Q, Shen PH, Goldman D, Roy A. The influence of gabra2, childhood trauma, and their interaction on alcohol, heroin, cocaine dependence. Biol Psychiatry. (2010) 67:20–7. doi: 10.1016/j.biopsych.2009.08.019
236. Levran O, Peles E, Randesi M, da Rosa JC, Adelson M, Kreek MJ. The mu-opioid receptor nonsynonymous variant 118a>g is associated with prolonged abstinence from heroin without agonist treatment. Pharmacogenomics. (2017) 18:1387–91. doi: 10.2217/pgs-2017-0092
237. Levran O, Peles E, Randesi M, Correa da Rosa J, Shen PH, Rotrosen J, et al. Genetic variations in genes of the stress response pathway are associated with prolonged abstinence from heroin. Pharmacogenomics. (2018) 19:333–41. doi: 10.2217/pgs-2017-0179
238. Nelson EC, Agrawal A, Heath AC, Bogdan R, Sherva R, Zhang B, et al. Evidence of cnih3 involvement in opioid dependence. Mol Psychiatry. (2016) 21:608–14. doi: 10.1038/mp.2015.102
239. Reul JM, Holsboer F. Corticotropin-releasing factor receptors 1 and 2 in anxiety and depression. Curr Opin Pharmacol. (2002) 2:23–33. doi: 10.1016/S1471-4892(01)00117-5
240. Bradley RG, Binder EB, Epstein MP, Tang Y, Nair HP, Liu W, et al. Influence of child abuse on adult depression: moderation by the corticotropin-releasing hormone receptor gene. Arch Gen Psychiatry. (2008) 65:190–200. doi: 10.1001/archgenpsychiatry.2007.26
241. Heim C, Bradley B, Mletzko T, Deveau TC, Musselmann DL, Nemeroff CB, et al. Effect of childhood trauma on adult depression and neuroendocrine function: sex-specific moderation by crh receptor 1 gene. Front Behav Neurosci. (2009) 3:41. doi: 10.3389/neuro.08.041.2009
242. Polanczyk G, Caspi A, Williams B, Price TS, Danese A, Sugden K, et al. Protective effect of crhr1 gene variants on the development of adult depression following childhood maltreatment: replication and extensionmaltreatment/crhr1 variant effects on depression. Arch Gen Psychiatry. (2009) 66:978–85. doi: 10.1001/archgenpsychiatry.2009.114
243. Damanti S, Rossi PD, Cesari M. Heart rate variability: a possible marker of resilience. Euro Geriatr Med. (2019) 10:529–30. doi: 10.1007/s41999-019-00192-2
244. Briand LA, Hilario M, Dow HC, Brodkin ES, Blendy JA, Berton O. Mouse model of oprm1 (a118g) polymorphism increases sociability and dominance and confers resilience to social defeat. J Neurosci. (2015) 35:3582–90. doi: 10.1523/JNEUROSCI.4685-14.2015
245. Hartwell EE, Feinn R, Morris PE, Gelernter J, Krystal J, Arias AJ, et al. Systematic review and meta-analysis of the moderating effect of rs1799971 in oprm1, the mu-opioid receptor gene, on response to naltrexone treatment of alcohol use disorder. Addiction. (2020) 115:1426–37. doi: 10.1111/add.14975
246. Cohen H, Liu T, Kozlovsky N, Kaplan Z, Zohar J, Mathé AA. The neuropeptide y (npy)-ergic system is associated with behavioral resilience to stress exposure in an animal model of post-traumatic stress disorder. Neuropsychopharmacology. (2011) 37:350. doi: 10.1038/npp.2011.230
247. Lotan A, Fenckova M, Bralten J, Alttoa A, Dixson L, Williams RW, et al. Neuroinformatic analyses of common and distinct genetic components associated with major neuropsychiatric disorders. Front Neurosci. (2014) 8:331. doi: 10.3389/fnins.2014.00331
248. Terrillion CE, Francis TC, Puche AC, Lobo MK, Gould TD. Decreased nucleus accumbens expression of psychiatric disorder risk gene cacna1c promotes susceptibility to social stress. Int J Neuropsychopharmacol. (2017) 20:428–33. doi: 10.1093/ijnp/pyw112
249. Dedic N, Pohlmann ML, Richter JS, Mehta D, Czamara D, Metzger MW, et al. Cross-disorder risk gene cacna1c differentially modulates susceptibility to psychiatric disorders during development and adulthood. Mol Psychiatry. (2018) 23:533–43. doi: 10.1038/mp.2017.133
250. Michels S, Ganjam GK, Martins H, Schratt GM, Wöhr M, Schwarting RKW, et al. Downregulation of the psychiatric susceptibility gene cacna1c promotes mitochondrial resilience to oxidative stress in neuronal cells. Cell Death Discov. (2018) 4:54. doi: 10.1038/s41420-018-0061-6
251. Manji H, Kato T, Di Prospero NA, Ness S, Beal MF, Krams M, et al. Impaired mitochondrial function in psychiatric disorders. Nat Rev Neurosci. (2012) 13:293–307. doi: 10.1038/nrn3229
252. Manitt C, Eng C, Pokinko M, Ryan RT, Torres-Berrio A, Lopez JP, et al. Dcc orchestrates the development of the prefrontal cortex during adolescence and is altered in psychiatric patients. Transl Psychiatry. (2013) 3:e105. doi: 10.1038/tp.2013.105
253. Torres-Berrio A, Lopez JP, Bagot RC, Nouel D, Dal Bo G, Cuesta S, et al. Dcc confers susceptibility to depression-like behaviors in humans and mice and is regulated by mir-218. Biol Psychiatry. (2017) 81:306–15. doi: 10.1016/j.biopsych.2016.08.017
254. O'Leary JC III, Dharia S, Blair LJ, Brady S, Johnson AG, et al. A new anti-depressive strategy for the elderly: ablation of fkbp5/fkbp51. PLoS ONE. (2011) 6:e24840. doi: 10.1371/journal.pone.0024840
255. Hartmann J, Wagner KV, Dedic N, Marinescu D, Scharf SH, Wang XD, et al. Fkbp52 heterozygosity alters behavioral, endocrine and neurogenetic parameters under basal and chronic stress conditions in mice. Psychoneuroendocrinology. (2012) 37:2009–21. doi: 10.1016/j.psyneuen.2012.04.017
256. Kwon J, Kim YJ, Choi K, Seol S, Kang HJ. Identification of stress resilience module by weighted gene co-expression network analysis in fkbp5-deficient mice. Mol Brain. (2019) 12:99. doi: 10.1186/s13041-019-0521-9
257. Ishihara L, Brayne C. A systematic review of depression and mental illness preceding parkinson's disease. Acta Neurol Scand. (2006) 113:211–20. doi: 10.1111/j.1600-0404.2006.00579.x
258. Matikainen-Ankney BA, Kezunovic N, Menard C, Flanigan ME, Zhong Y, Russo SJ, et al. Parkinson's disease-linked lrrk2-g2019s mutation alters synaptic plasticity and promotes resilience to chronic social stress in young adulthood. J Neurosci. (2018) 38:9700–11. doi: 10.1523/JNEUROSCI.1457-18.2018
259. Kulkarni P, Grant S, Morrison TR, Cai X, Iriah S, Kristal BS, et al. Characterizing the human apoe epsilon 4 knock-in transgene in female and male rats with multimodal magnetic resonance imaging. Brain Res. (2020) 1747:147030. doi: 10.1016/j.brainres.2020.147030
260. Yu Z, Wen L, Shen X, Zhang H. Effects of the oprm1 a118g polymorphism (rs1799971) on opioid analgesia in cancer pain: a systematic review and meta-analysis. Clin J Pain. (2019) 35:77–86. doi: 10.1097/AJP.0000000000000636
261. Diatchenko L, Slade GD, Nackley AG, Bhalang K, Sigurdsson A, Belfer I, et al. Genetic basis for individual variations in pain perception and the development of a chronic pain condition. Hum Mol Genet. (2005) 14:135–43. doi: 10.1093/hmg/ddi013
262. Landau R, Liu SK, Blouin JL, Carvalho B. The effect of oprm1 and comt genotypes on the analgesic response to intravenous fentanyl labor analgesia. Anesth Analg. (2013) 116:386–91. doi: 10.1213/ANE.0b013e318273f2c7
263. Way BM, Taylor SE, Eisenberger NI. Variation in the mu-opioid receptor gene (oprm1) is associated with dispositional and neural sensitivity to social rejection. Proc Natl Acad Sci USA. (2009) 106:15079–84. doi: 10.1073/pnas.0812612106
264. Trubetskoy V, Pardiñas AF, Qi T, Panagiotaropoulou G, Awasthi S, Bigdeli TB, et al. Mapping genomic loci implicates genes and synaptic biology in schizophrenia. Nature. (2022) 604:502–8. doi: 10.1038/s41586-022-04434-5
265. Wray NR, Ripke S, Mattheisen M, Trzaskowski M, Byrne EM, Abdellaoui A, et al. Genome-wide association analyses identify 44 risk variants and refine the genetic architecture of major depression. Nat Genet. (2018) 50:668–81. doi: 10.1038/s41588-018-0090-3
266. Savage JE, Jansen PR, Stringer S, Watanabe K, Bryois J, De Leeuw CA, et al. Genome-wide association meta-analysis in 269,867 individuals identifies new genetic and functional links to intelligence. Nat Genet. (2018) 50:912–9. doi: 10.1038/s41588-018-0152-6
267. Lee JJ, Wedow R, Okbay A, Kong E, Maghzian O, Zacher M, et al. Gene discovery and polygenic prediction from a genome-wide association study of educational attainment in 1.1 million individuals. Nat Genet. (2018) 50:1112–21. doi: 10.1038/s41588-018-0147-3
268. Witt SH, Buchmann AF, Blomeyer D, Nieratschker V, Treutlein J, Esser G, et al. An interaction between a neuropeptide y gene polymorphism and early adversity modulates endocrine stress responses. Psychoneuroendocrinology. (2011) 36:1010–20. doi: 10.1016/j.psyneuen.2010.12.015
269. Zhang K, Rao F, Pablo Miramontes-Gonzalez J, Hightower CM, Vaught B, Chen Y, et al. Neuropeptide y (npy): genetic variation in the human promoter alters glucocorticoid signaling, yielding increased npy secretion and stress responses. J Am Coll Cardiol. (2012) 60:1678–89. doi: 10.1016/j.jacc.2012.06.042
270. Lindell SG, Schwandt ML, Sun H, Sparenborg JD, Bjork K, Kasckow JW, et al. Functional npy variation as a factor in stress resilience and alcohol consumption in rhesus macaques. Arch Gen Psychiatry. (2010) 67:423–31. doi: 10.1001/archgenpsychiatry.2010.23
271. Thorsell A, Michalkiewicz M, Dumont Y, Quirion R, Caberlotto L, Rimondini R, et al. Behavioral insensitivity to restraint stress, absent fear suppression of behavior and impaired spatial learning in transgenic rats with hippocampal neuropeptide y overexpression. Proc Natl Acad Sci USA. (2000) 97:12852. doi: 10.1073/pnas.220232997
272. Yehuda R, Brand S, Yang RK. Plasma neuropeptide y concentrations in combat exposed veterans: relationship to trauma exposure, recovery from ptsd, and coping. Biol Psychiatry. (2006) 59:660–3. doi: 10.1016/j.biopsych.2005.08.027
273. Womersley JS, Martin L, van der Merwe L, Seedat S, Hemmings SMJ. Genetic variation in neuropeptide y interacts with childhood trauma to influence anxiety sensitivity. Anxiety Stress Cop. (2021) 34:450–64. doi: 10.1080/10615806.2021.1876225
274. Zain SM, Mohamed Z, Jalaludin MY, Fauzi F, Hamidi A, Zaharan NL. Comprehensive evaluation of the neuropeptide-y gene variants in the risk of obesity: a case-control study and meta-analysis. Pharmacogenet Genom. (2015) 25:501–10. doi: 10.1097/FPC.0000000000000164
275. Foster BA, Weinstein K. Moderating effects of components of resilience on obesity across income strata in the national survey of children's health. Acad Pediatr. (2019) 19:58–66. doi: 10.1016/j.acap.2018.08.012
276. Heerman WJ, Samuels LR, González Peña T, van Wyk C, Mayberry LS, Lounds Taylor J, et al. Family resilience and childhood obesity among children exposed to adverse childhood experiences in a national survey. Obesity Sci Pract. (2022) 8:3–11. doi: 10.1002/osp4.497
277. Zlebnik NE. Females pay a higher price for addiction. Neuropsychopharmacology. (2019) 44:1179–81. doi: 10.1038/s41386-019-0373-0
278. Burton PR, Hansell AL, Fortier I, Manolio TA, Khoury MJ, Little J, et al. Size matters: Just how big is big? Quantifying realistic sample size requirements for human genome epidemiology. Int J Epidemiol. (2009) 38:263–73. doi: 10.1093/ije/dyn147
279. Munaf ò MR, Gage SH. Improving the reliability and reporting of genetic association studies. Drug Alcohol Depend. (2013) 132:411–3. doi: 10.1016/j.drugalcdep.2013.03.023
280. Kalisch R, Köber G, Binder H, Ahrens KF, Basten U, Chmitorz A, et al. A generic solution for the operationalization and measurement of resilience and resilience processes in longitudinal observations: rationale and basic design of the marp and lora studies. PsyArXiv Preprints. (2020). doi: 10.31234/osf.io/jg238
281. Revelas M, Thalamuthu A, Oldmeadow C, Evans TJ, Armstrong NJ, Kwok JB, et al. Review and meta-analysis of genetic polymorphisms associated with exceptional human longevity. Mech Ageing Dev. (2018) 175:24–34. doi: 10.1016/j.mad.2018.06.002
282. Silventoinen K, Vuoksimaa E, Volanen S-M, Palviainen T, Rose RJ, et al. The genetic background of the associations between sense of coherence and mental health, self-esteem and personality. Soc Psychiatry Psychiatr Epidemiol. (2022) 57:423–33. doi: 10.1007/s00127-021-02098-6
283. Lam M, Trampush JW, Yu J, Knowles E, Davies G, Liewald DC, et al. Large-scale cognitive gwas meta-analysis reveals tissue-specific neural expression and potential nootropic drug targets. Cell Rep. (2017) 21:2597–613. doi: 10.1016/j.celrep.2017.11.028
284. Demange PA, Malanchini M, Mallard TT, Biroli P, Cox SR, Grotzinger AD, et al. Investigating the genetic architecture of noncognitive skills using gwas-by-subtraction. Nat Genet. (2021) 53:35–44. doi: 10.1038/s41588-020-00754-2
285. Bennett S, Farrington DP, Huesmann LR. Explaining gender differences in crime and violence: the importance of social cognitive skills. Aggress Viol Behav. (2005) 10:263–88. doi: 10.1016/j.avb.2004.07.001
286. Harpur LJ, Polek E, van Harmelen AL. The role of timing of maltreatment and child intelligence in pathways to low symptoms of depression and anxiety in adolescence. Child Abuse Neglect. (2015) 47:24–37. doi: 10.1016/j.chiabu.2015.05.019
287. Pargas RCM, Brennan PA, Hammen C, Le Brocque R. Resilience to maternal depression in young adulthood. Dev Psychol. (2010) 46:805–14. doi: 10.1037/a0019817
288. Cahill S, Hager R, Chandola T. The validity of the residuals approach to measuring resilience to adverse childhood experiences. Child Adolesc Psychiatry Ment Health. (2022) 16:18. doi: 10.1186/s13034-022-00449-y
289. Dunn SH, Conley YP. A systematic review of genetic influences on coping. Biol Res Nurs. (2015) 17:87–93. doi: 10.1177/1099800414527340
290. Busjahn A, Freier K, Faulhaber HD, Li GH, Rosenthal M, Jordan J, et al. Beta-2 adrenergic receptor gene variations and coping styles in twins. Biol Psychol. (2002) 61:97–109. doi: 10.1016/S0301-0511(02)00054-6
291. Li GH, Faulhaber HD, Rosenthal M, Schuster H, Jordan J, Timmermann B, et al. B-2 adrenergic receptor gene variations and blood pressure under stress in normal twins. Psychophysiology. (2001) 38:485–9. doi: 10.1111/1469-8986.3830485
292. Golds L, de Kruiff K, MacBeth A. Disentangling genes, attachment, and environment: a systematic review of the developmental psychopathology literature on gene–environment interactions and attachment. Dev Psychopathol. (2020) 32:357–81. doi: 10.1017/S0954579419000142
293. Wazana A, Moss E, Jolicoeur-Martineau A, Graffi J, Tsabari G, Lecompte V, et al. The interplay of birth weight, dopamine receptor d4 gene (drd4), and early maternal care in the prediction of disorganized attachment at 36 months of age. Dev Psychopathol. (2015) 27 (4 Pt. 1):1145–61. doi: 10.1017/S0954579415000735
294. Erkoreka L, Zumarraga M, Arrue A, Zamalloa MI, Arnaiz A, Olivas O, et al. Genetics of adult attachment: an updated review of the literature. World J Psychiatry. (2021) 11:530–42. doi: 10.5498/wjp.v11.i9.530
295. Westphal M, Bonanno GA. Posttraumatic growth and resilience to trauma: different sides of the same coin or different coins? Appl Psychol. (2007) 56:417–27. doi: 10.1111/j.1464-0597.2007.00298.x
296. Johnson RJ, Hobfoll SE, Hall BJ, Canetti-Nisim D, Galea S, Palmieri PA. Posttraumatic growth: action and reaction. Appl Psychol. (2007) 56:428–36. doi: 10.1111/j.1464-0597.2007.00296.x
297. Mehta D, Miller O, Bruenig D, David G, Shakespeare-Finch J. A systematic review of DNA methylation and gene expression studies in posttraumatic stress disorder, posttraumatic growth, and resilience. J Traum Stress. (2020) 33:171–80. doi: 10.1002/jts.22472
298. Willner P. The validity of animal models of depression. Psychopharmacology. (1984) 83:1–16. doi: 10.1007/BF00427414
299. Willner P, Mitchell PJ. Animal models of depression: a diathesis/stress approach. Biol Psychiatry. (2002) 30:701–26. doi: 10.1002/0470854871.chxviii1
300. Lyons DM, Parker KJ, Schatzberg AF. Animal models of early life stress: implications for understanding resilience. Dev Psychobiol. (2010) 52:402–10. doi: 10.1002/dev.20429
301. Paredes D, Morilak DA. A rodent model of exposure therapy: the use of fear extinction as a therapeutic intervention for ptsd. Front Behav Neurosci. (2019) 13:46. doi: 10.3389/fnbeh.2019.00046
302. Joyce S, Shand F, Tighe J, Laurent SJ, Bryant RA, Harvey SB. Road to resilience: a systematic review and meta-analysis of resilience training programmes and interventions. BMJ Open. (2018) 8:e017858. doi: 10.1136/bmjopen-2017-017858
303. Wilkinson MB, Xiao G, Kumar A, LaPlant Q, Renthal W, Sikder D, et al. Imipramine treatment and resiliency exhibit similar chromatin regulation in the mouse nucleus accumbens in depression models. J Neurosci. (2009) 29:7820–32. doi: 10.1523/JNEUROSCI.0932-09.2009
304. Holanda VAD, Oliveira MC, Da Silva Junior ED, Calo G, Ruzza C, Gavioli EC. Blockade of nociceptin/orphanin fq signaling facilitates an active copying strategy due to acute and repeated stressful stimuli in mice. Neurobiol Stress. (2020) 13:100255. doi: 10.1016/j.ynstr.2020.100255
305. Nestler EJ, Hyman SE. Animal models of neuropsychiatric disorders. Nat Neurosci. (2010) 13:1161–9. doi: 10.1038/nn.2647
306. Neuner SM, Heuer SE, Huentelman MJ, O'Connell KM, Kaczorowski CC. Harnessing genetic complexity to enhance translatability of alzheimer's disease mouse models: a path toward precision medicine. Neuron. (2019) 101:399–411.e5. doi: 10.1016/j.neuron.2018.11.040
307. Sittig LJ, Carbonetto P, Engel KA, Krauss KS, Barrios-Camacho CM, Palmer AA. Genetic background limits generalizability of genotype-phenotype relationships. Neuron. (2016) 91:1253–9. doi: 10.1016/j.neuron.2016.08.013
308. Li H, Auwerx J. Mouse systems genetics as a prelude to precision medicine. Trends Genet. (2020) 36:259–72. doi: 10.1016/j.tig.2020.01.004
309. Berrettini WH, Ferraro TN, Alexander RC, Buchberg AM, Vogel WH. Quantitative trait loci mapping of three loci controlling morphine preference using inbred mouse strains. Nat Genet. (1994) 7:54–8. doi: 10.1038/ng0594-54
310. Li H, Wang X, Rukina D, Huang Q, Lin T, Sorrentino V, et al. An integrated systems genetics and omics toolkit to probe gene function. Cell Systems. (2018) 6:90–102.4. doi: 10.1016/j.cels.2017.10.016
311. Ashbrook DG, Arends D, Prins P, Mulligan MK, Roy S, Williams EG, et al. A platform for experimental precision medicine: the extended bxd mouse family. Cell Syst. (2021) 12:235–47.e9. doi: 10.1016/j.cels.2020.12.002
312. Dai Z, Ramesh V, Locasale JW. The evolving metabolic landscape of chromatin biology and epigenetics. Nat Rev Genet. (2020) 21:737–53. doi: 10.1038/s41576-020-0270-8
313. Woods RM, Lorusso JM, Potter HG, Neill JC, Glazier JD, Hager R. Maternal immune activation in rodent models: a systematic review of neurodevelopmental changes in gene expression and epigenetic modulation in the offspring brain. Neurosci Biobehav Rev. (2021) 129:389–421. doi: 10.1016/j.neubiorev.2021.07.015
314. Kofink D, Boks MP, Timmers HM, Kas MJ. Epigenetic dynamics in psychiatric disorders: environmental programming of neurodevelopmental processes. Neurosci Biobehav Rev. (2013) 37:831–45. doi: 10.1016/j.neubiorev.2013.03.020
315. Lewis CR, Olive MF. Early life stress interactions with the epigenome: potential mechanisms driving vulnerability towards psychiatric illness. Behav Pharmacol. (2014) 25:341. doi: 10.1097/FBP.0000000000000057
316. Feder A, Nestler EJ, Charney DS. Psychobiology and molecular genetics of resilience. Nat Rev Neurosci. (2009) 10:446–57. doi: 10.1038/nrn2649
Keywords: resilience, genotype, systematic review, positive adaptation, adversity, gene-environment, animal models
Citation: Cahill S, Chandola T and Hager R (2022) Genetic Variants Associated With Resilience in Human and Animal Studies. Front. Psychiatry 13:840120. doi: 10.3389/fpsyt.2022.840120
Received: 20 December 2021; Accepted: 19 April 2022;
Published: 20 May 2022.
Edited by:
Francis J. McMahon, National Institutes of Health (NIH), United StatesReviewed by:
Zsofia Nemoda, Semmelweis University, HungaryDagmar Bruenig, Queensland University of Technology, Australia
Copyright © 2022 Cahill, Chandola and Hager. This is an open-access article distributed under the terms of the Creative Commons Attribution License (CC BY). The use, distribution or reproduction in other forums is permitted, provided the original author(s) and the copyright owner(s) are credited and that the original publication in this journal is cited, in accordance with accepted academic practice. No use, distribution or reproduction is permitted which does not comply with these terms.
*Correspondence: Stephanie Cahill, c3RlcGhhbmllLmNhaGlsbEBtYW5jaGVzdGVyLmFjLnVr