- 1Université de Bordeaux, Bordeaux, France
- 2Centre de Référence Régional des Pathologies Anxieuses et de la Dépression, Pôle de Psychiatrie Générale et Universitaire, Centre Hospitalier Charles Perrens, Bordeaux, France
- 3Bordeaux Population Health, Inserm U1219, Bordeaux, France
- 4NutriNeuro, UMR 1286, INRAE, Bordeaux INP, Bordeaux, France
- 5CHU de Bordeaux, Bordeaux, France
- 6Neurocentre Magendie, Inserm U1215, Bordeaux, France
Background: Pathological anxiety is responsible for major functional impairments and resistance to conventional treatments in anxiety disorders (ADs), posttraumatic stress disorder (PTSD) and major depressive disorder (MDD). Focal neuromodulation therapies such as transcranial magnetic stimulation (TMS), transcranial direct current stimulation (tDCS) and deep brain stimulation (DBS) are being developed to treat those disorders.
Methods: We performed a dimensional systematic review and meta-analysis to assess the evidence of the efficacy of TMS, tDCS and DBS in reducing anxiety symptoms across ADs, PTSD and MDD. Reports were identified through systematic searches in PubMed/Medline, Scopus and Cochrane library (inception to November 2020), followed by review according to the PRISMA guidelines. Controlled clinical trials examining the effectiveness of brain stimulation techniques on generic anxiety symptoms in patients with ADs, PTSD or MDD were selected.
Results: Nineteen studies (RCTs) met inclusion criteria, which included 589 participants. Overall, focal brain activity modulation interventions were associated with greater reduction of anxiety levels than controls [SMD: −0.56 (95% CI, −0.93 to−0.20, I2 = 77%]. Subgroup analyses revealed positive effects for TMS across disorders, and of focal neuromodulation in generalized anxiety disorder and PTSD. Rates of clinical responses and remission were higher in the active conditions. However, the risk of bias was high in most studies.
Conclusions: There is moderate quality evidence for the efficacy of neuromodulation in treating pathological anxiety.
Systematic Review Registration: https://www.crd.york.ac.uk/prospero/display_record.php?RecordID=233084, identifier: PROSPERO CRD42021233084. It was submitted on January 29th, 2021, and registered on March 1st, 2021. No amendment was made to the recorded protocol. A change was applied for the subgroup analyses based on target brain regions, we added the putative nature (excitatory/inhibitory) of brain activity modulation.
Introduction
Pathological anxiety is a hallmark of mental disorders encompassing anxiety disorders, posttraumatic stress disorder and major depressive disorder. Anxiety disorders (ADs), including generalized anxiety disorder (GAD), panic disorder (PD), social anxiety disorder (SAD) and specific phobias (SP), are characterized by a functional impairment due to excessive anxiety. Anxiety is also a core symptom cluster in posttraumatic stress disorder [(PTSD; (1)]. Anxiety is a possible symptom in major depression, and anxious forms of depressive disorders are characterized by higher clinical severity (2–4). Moreover, ADs, PTSD and major depressive disorder (MDD) are highly comorbid internalizing disorders (5). They affect a large population, with lifetime prevalence of 15–20%, 2–7%, and 13–16%, respectively (6, 7), and cause heavy individual and financial burden, summing up to over 150 billion €/year in Europe (8–11). Current therapeutic strategies to manage these conditions rely on pharmacology (antidepressants) and psychotherapy (chiefly cognitive based therapy). However, more than one third of patients with ADs, PTSD or MDD are unresponsive to those conventional treatments (12–15). The intensity of anxiety in MDD is itself predictive of poor therapeutic outcomes (16, 17). As a result, effective treatment of pathological anxiety remains an unmet, pressing need. Key brain regions implicated in ADs, PTSD and MDD are largely overlapping, including the prefrontal cortex and the amygdala (18–21), suggesting that interventions aiming at restoring physiological functioning in this brain network may represent complementary therapeutic options.
In line with this, heavy research efforts are being invested in the development of novel therapeutic tools to control brain activity in ADs, PTSD and MDD, including neuromodulation techniques. Available neuromodulation techniques modify the electrical activity of targeted brain areas with the application of external magnetic (Transcranial Magnetic Stimulation – TMS) or electrical fields (transcranial Direct Current Stimulation- tDCS), or direct intracerebral currents (Deep Brain Stimulation- DBS) (22–24). In TMS, magnetic pulses induce action potentials in local neuronal populations, and their repetition at low or high frequency inhibits or facilitates synaptic transmission and neuronal firing (23). In tDCS, the locally induced electrical field alters membrane polarization and excitability at subthreshold levels, resulting in neuronal firing facilitation dependent on ongoing synaptic activities in areas close to the anode, and inhibition near the cathode (24). The mechanisms of action of DBS remain poorly understood, potentially involving depolarization of axonal fibers, excitation of local neurons (principal neurons and interneurons), or modulation of firing-patterns of neuronal populations (22). In MDD, TMS and tDCS have demonstrated clinical efficacy (25–27) and DBS has shown promising results (28, 29). ADs and PTSD may benefit from TMS and tDCS, as summarized in several recent reviews (30–34) and meta-analyses of the literature (35–37). These neuromodulatory techniques might also provide beneficial effects on MDD-related anxiety. In keeping with their clinical similarities and shared neurobiological substrates, brain stimulation protocols in ADs, PTSD and MDD are similar, with the most frequent procedures consisting in the activation of the left and/or inhibition of the right dorsolateral prefrontal cortex (dlPFC) (26, 27, 34, 35).
Paradoxically, while ADs, PTSD and MDD are neurobiologically related and neurostimulation strategies applied in these disorders are similar, recent quantitative syntheses of the literature have assessed their efficacy on the basis of disorder-specific psychometric measurements (35–37). This categorial approach evaluates symptoms that belong to cognitive, affective, or behavioral domains, with items differing among disorders. Such heterogeneity precludes the global evaluation, and the synthesis of knowledge in neuroscience-based brain stimulation for pathological anxiety. In contrast, a dimensional, transdiagnostic evaluation is critical to evaluate and improve neuroscience-based treatments of pathological anxiety.
To tackle this limitation in the literature, we applied a dimensional approach to evaluate brain stimulation-based treatments for pathological anxiety across related disorders. Systematic review and meta-analysis were performed with the main objective to study the efficacy of spatially targeted brain stimulation techniques on the intensity of generic anxiety symptoms in ADs, PTSD and MDD. The secondary objective was to synthetize available evidence for anxiety reduction with a categorial approach for ADs and PTSD, based on disorder-specific rating scales. This will facilitate comparisons with previous, categorial studies, and will update available evidence.
Methods
Search Strategy
This systematic review was conducted following the PRISMA guidelines (38). Two independent investigators performed the review (FG, TB). A literature search was performed on Pubmed, Scopus, and the Cochrane library, from inception until 31 November 2020. The following terms were used on the three databases: (“brain stimulation” OR “TMS” OR “RTMS” OR “Transcranial Magnetic Stimulation” OR “Theta burst” OR “transcranial direct current stimulation” OR “TDCS” OR “DBS” OR “neuromodulation”) AND (“Anxiety disorders” OR “agoraphobia” OR “social, Anxiety” OR “phobic disorders” OR “Panic disorder” OR “Phobia” OR “stress disorder, post-traumatic” OR “PTSD” OR “anxious symptoms” OR “HAMA” OR “Hamilton anxiety” OR “Anxiety scale” OR “Beck Anxiety Inventory”). Unpublished studies were sought with Google Scholar. After excluding duplicates (both automatically with Excel and manually), titles and abstracts were screened to select potentially eligible articles, which were then studied in their complete forms. Additional articles were identified in previously published reviews and in reference lists of selected studies. Only original articles written in English or French languages were considered eligible for inclusion. Each retrieved record was assessed by two investigators (FG, TB).
Inclusion and Exclusion Criteria
Clinical trials examining the effectiveness of brain stimulation techniques on anxiety symptoms in patients with ADs, PTSD, or MDD were selected in accordance with PICOS (Participants, Intervention, Comparison, Outcomes and Study design) inclusion criteria: (1) clinical trials including adult patients with GAD, PD, PTSD, SP, SAD, or MDD, diagnosed according to DSM-IV, DSM-5 or ICD-10 criteria, without restriction regarding age, concomitant treatment, or date of symptom onset; (2) therapeutic intervention with TMS, tDCS, or DBS, (3) outcome measurement with at least one validated quantitative generic rating scale of anxiety symptoms including the Hamilton Anxiety Rating Scale (HARS), the Beck Anxiety Inventory (BAI), the Hamilton Depression Rating Scale (HAMD-17) anxiety-somatization factor items, or the State-Trait Anxiety Inventory (STAI), (4) controlled designs, both parallel groups or crossover, with or without randomization or blinding.
Studies on patients suffering from bipolar disorders, obsessive-compulsive disorder, schizophrenia, organic psychiatric disorders (DSM-5), or neurological conditions, and pregnant subjects, were not included. Articles corresponding to other stimulation techniques were not included, namely: Alpha-Stim Cranial Electrotherapy Stimulation (CES), Low-Charge Electrotherapy (LCE), rhythmic Low-Field Magnetic Stimulation (LFMS), Magnetic Resonance therapy (MRT), Radio Electric Asymmetric Conveyor brain stimulation (REAC), Transcranial Doppler ultrasound (TCD), Trigeminal Nerve Stimulation (TNS), Transcranial Photobiomodulation (t-PBM), transcranial Random Noise Stimulation (tRNS), and transcutaneous Vagus Nerve Stimulation (tVNS). Articles reporting initial results were not included when data were duplicated in follow-up studies. Excluded articles types were review articles, articles which did not contain original data (protocols, editorials, commentaries, notes), retrospective studies, case reports or series, animal or preclinical studies, and studies on healthy volunteers. Any disagreement in article selection was resolved by discussion between the authors, until a consensus was reached.
Data Extraction
Included articles were reviewed to extract relevant data : article indexing information (authors, year of publication), study design (control, randomization, blinding), sample characteristics (diagnosis, number of patients in each arm of treatment, mean age, gender ratio), brain stimulation protocols (technique, target brain region, target identification method, stimulation and sham systems descriptions, stimulation settings, duration and number of sessions), concomitant therapeutic interventions, symptom measurement tools, dropout rates, and pre- and post-treatment data, including follow-up data (attrition rates, mean score and standard deviation of scale ratings, response and remission definitions and rates, adverse events). Study authors were contacted directly to retrieve data unavailable in original publications (twice in the absence of response to the first request). Data were collected in the form of an electronic spreadsheet (Excel).
Outcomes
The primary outcome was the difference in intensity of anxiety symptoms between the baseline and the end of the treatment period (pre- and post-treatment), measured using validated, generic, quantitative scales of anxiety. Pre-specified secondary outcomes were: (1) the rate of clinically significant response or remission (as defined by study authors), (2) the intensity of anxiety symptoms at the last available follow-up (using the same scales as in the primary outcome analysis), (3) the difference in the severity of stressor-related and anxiety disorders between baseline and the end of the treatment period measured using validated, disorder-specific quantitative scales, (4) the adverse events rates and nature.
Strategy for Data Synthesis (Meta-Analysis)
Analysis was performed using R software (R® 4.1.2). For the treatment and control groups of each study, we extracted (1) baseline/post-treatment mean differences in general anxiety rating scales and/or specific disorder rating scales, and (2) response and remission rates. The change-from-baseline SD was calculated following guidelines of the Cochrane Handbook for Systematic Reviews of Interventions (39).
Where Corr represent the correlation between pre- and post-treatment variance, and was set to 0.5 as suggested by Follman et al. (40).
We calculated between-group standardized mean difference (SMD) and their variance for continuous variables, and Hedges' g to control for small sample bias, as suggested by Harrer et al. (41). Relative Risk and their variance were computed using the “escalc” function of the “epitools” package. The meta-analysis was conducted using a random model with the “rma.mv” function of the “metafor” package. A multi-level model was fitted to account for multiple active or control groups and to control for dependencies between effect sizes. Comparisons were made between active brain stimulation and control conditions. When two validated quantitative scales evaluating the same outcome were available (such as HARS and BAI), results from the scale most frequently found in the other studies were selected for the meta-analysis (see Supplementary Material for details).
Pre-specified subgroup analyses of primary and secondary outcomes were performed for specific disorders (GAD, MDD, PD, PTSD, SAD, SP), stimulation techniques (tDCS, TMS, DBS) and target brain regions. From the pre-registered protocol, we modified the latter subgroup definition by categorizing the putative nature (excitatory/inhibitory) of brain activity modulation. Neuromodulation was considered excitatory for TMS for frequencies ≥ 5 Hz, intermittent theta burst stimulation (iTBS) and for brain regions located below anodes in tDCS. Stimulation was considered inhibitory for low TMS frequencies (mainly 1 Hz) and cathodal tDCS. Multisite neuromodulation protocols were not included in the analysis of discrete target regions. Likewise, when studies were composed of three arms, of which two were active conditions targeting distinct brain regions, individual active arms were included in the corresponding brain target subgroup.
Heterogeneity between studies was assessed by the Cochran Q test (with p-value < 0.10 considered statistically significant) and quantified by indicator I2 considered low if I2 ≤ 25%; moderate if 25% < I2 < 50%, and high if I2 > 50%.
A meta-regression analysis was conducted to explore potential sources of heterogeneity, using the “rma.mv” package. We assessed the putative moderation effect of the number of sessions of stimulation, the total number of pulses and the motor threshold percentage in TMS studies, the difference in anxiety scores at baseline between active and sham groups (SMD) and the difference between active and sham groups for baseline to post-treatment changes in depression and PTSD scores (SMD). The model was assessed for each factor one by one.
Risk of Bias and Quality Assessment
Publication bias was investigated visually with funnel plots and statistically by applying the Egger's test (with p-value < 0.05 considered statistically significant; R 4.0.4, Metafor package). For each study, risk of bias was assessed using the Risk of Bias tool of the Cochrane Collaboration (39). Risk of bias was assessed by domains for each study and graded as low, uncertain or high. The global risk of bias for each study was estimated as follow: studies assessed with high risk in one domain or intermediate risk in more than three domains were considered as showing a high risk of bias. Studies with a maximum of two intermediate risk items were assigned to low risk of bias. Remaining studies were classified as showing an intermediate risk of bias. Quality of evidence across studies was determined using the GRADE approach (39).
Results
Literature Search Results and Characteristics of Included Studies
A total of 1,586 records were identified through database search (Medline: 588, Scopus: 559, Cochrane: 439). Among those, 234 were duplicate references. Eighty-two articles were retrieved for inclusion assessment. After full-text reading and adding articles found through cross-referencing, 27 studies were included. All were randomized controlled trials (RCTs) (Figure 1). From these, 19 studies fulfilled all eligibility criteria for the primary outcome (42–60) (Tables 1, 2), for a total of 589 participants. The mean age of participants was 40.5 years, and 58.1% were females. Twelve trials assessed TMS interventions (three in GAD, three in PD, one in SP, three in PTSD, two in MDD), five trials tDCS interventions (three in GAD, one in PTSD, one in MDD), and two trials DBS interventions (two in MDD).
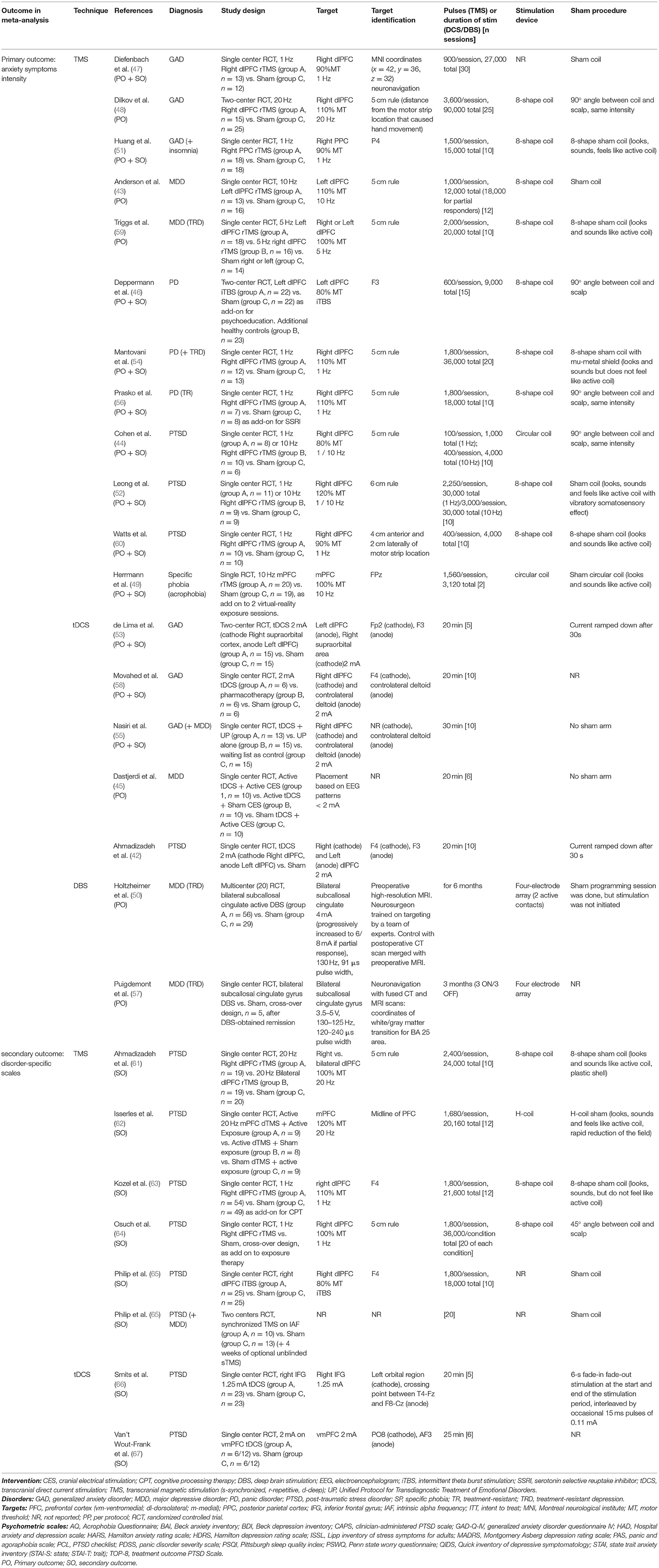
Table 1. Characteristics of included studies (diagnosis, study design, target, stimulation and sham protocols).
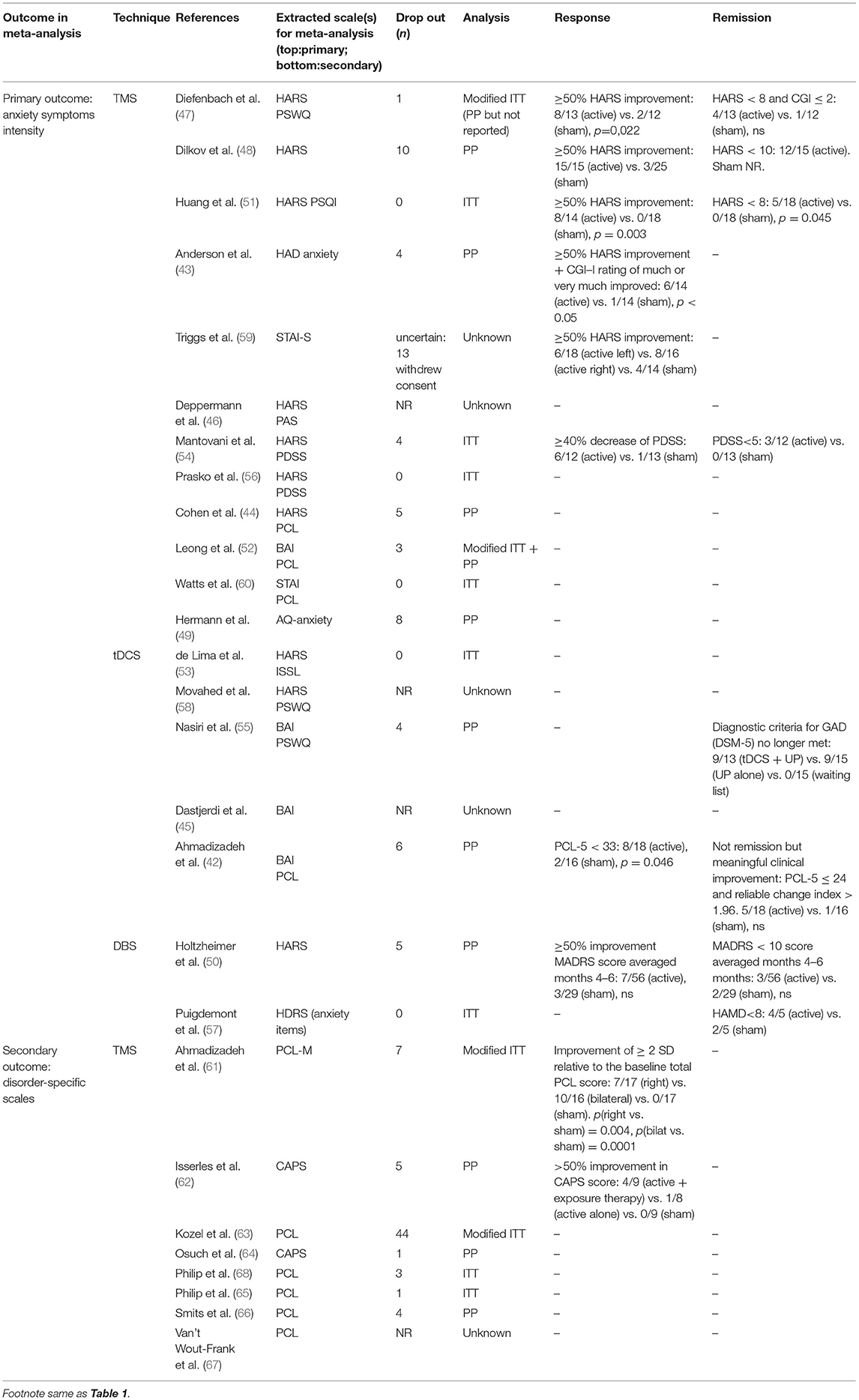
Table 2. Characteristics of included studies (extracted scales, drop out, analysis, response and remission rates).
Twenty-one RCTs were included for the secondary analysis, which was based on disorder-specific scales. Those 21 studies comprised 13 RCTs included in the primary outcome analysis [exclusion of one trial without specific scale measure (48) and five MDD trials (43, 45, 50, 57, 59)], and eight additional RCTs, in which only disorder-specific scores were reported (61–68) (Tables 1, 2). This secondary analysis involved 712 subjects (mean age: 40.3 years; females: 49.9%). Of the 21 studies, 15 trials assessed TMS interventions (two in GAD, three in PD, nine in PTSD, one in SP), and six assessed tDCS interventions (three on GAD symptoms, three on PTSD symptoms). No controlled trial using DBS was found for anxiety or stressor-related disorders. Although not excluded a priori, no article reporting continuous Theta Burst Stimulation (cTBS) protocol was found. Detailed reporting of patients' characteristics, study designs and neurostimulation protocols are provided in the Supplementary Material.
Primary Outcome: Change in Anxiety Symptom Intensity
Main Result
Neurostimulation was associated with a statistically significant reduction in the intensity of anxiety symptoms across ADs, PTSD and MDD [overall SMD, −0.56 (95% CI, −0.93 to −0.20), I2 = 77%], with a medium effect size (SMD > 0.5; Figure 2).
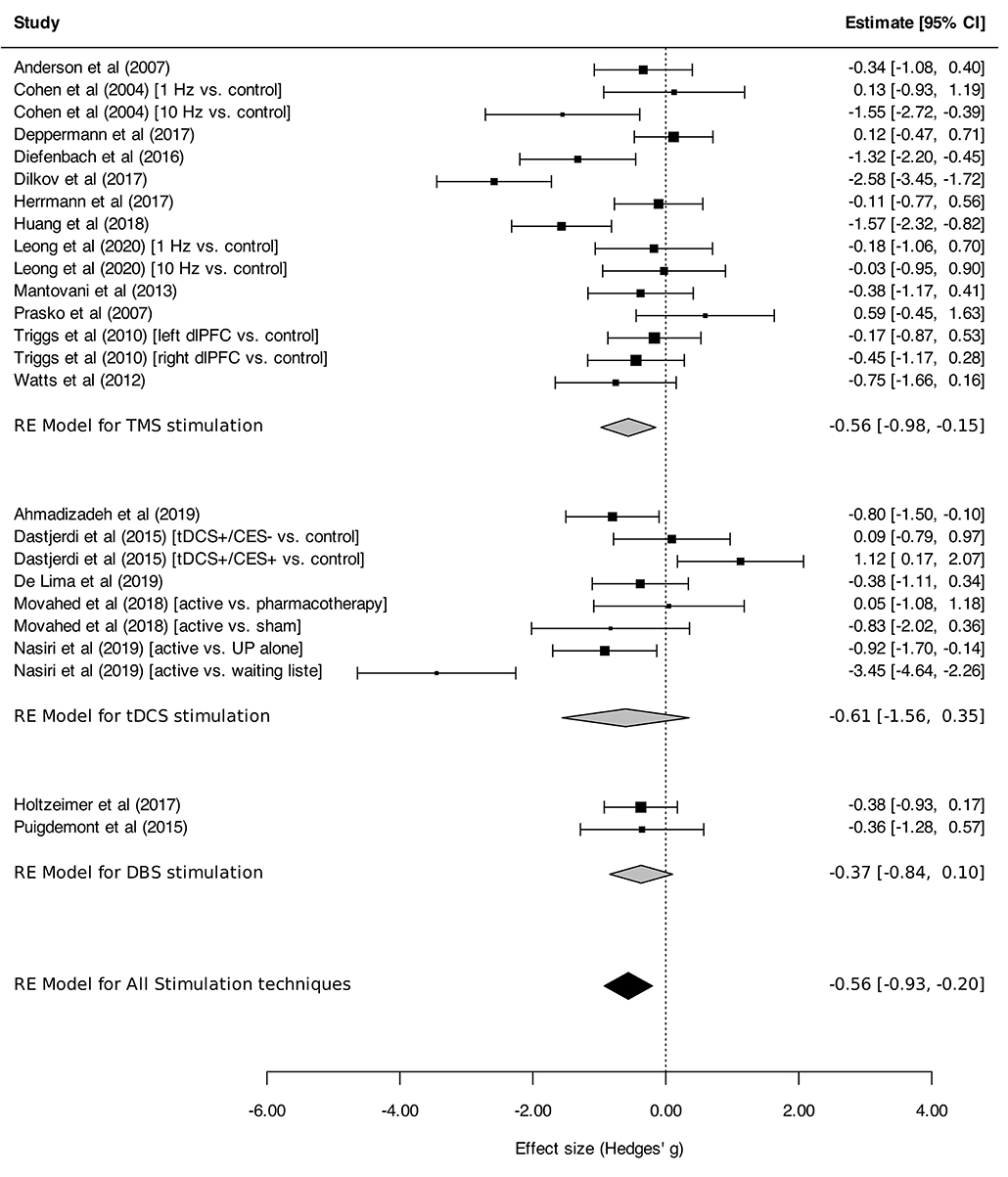
Figure 2. Change in the anxiety symptoms intensity in anxiety, stressor-related disorders and MDD, subgroups by stimulation technique. Forest plot displaying effect estimates and confidence intervals for both individual studies and overall meta-analyses. Each study is represented by a block at the point estimate of the intervention SMD, with a horizontal line extending on each side representing the confidence interval. The area of the block is proportional to the weight assigned to that study in the meta-analysis. When comparisons were made between three arms, the corresponding conditions, active or control (preceded with “vs”), are indicated between brackets. Statistics: TMS: heterogeneity Sigma12 = 0.00, Sigma22 = 0.48; Q(df = 14) = 50.8 (p < 0.0001); I2 = 74%; test for overall effect: Z = −2.68 (p = 0.007); tDCS: heterogeneity Sigma12 = 0.53, Sigma22 = 0.79; Q(df = 7) = 39.6 (p < 0.0001); I2 = 86%; test for overall effect: Z = −1.24 (p = 0.21); DBS: heterogeneity Sigma12 = 0.00, Sigma22 = 0.00; Q(df = 1) = 0.001 (p < 0.97); I2 = 0%; test for overall effect: Z = −1.54 (p = 0.12); Total: heterogeneity Sigma12 = 0.20, Sigma22 = 0.37; Q(df = 24) = 90.8 (p < 0.0001); I2 = 77%; test for overall effect: Z = −3.0599 (p = 0.002); test for subgroup differences: F(df1 = 2, df2 = 22) = 0.05, p = 0.95.
Subgroup Analyses
Regarding stimulation techniques, results in the TMS subgroup showed moderate reduction in anxiety symptom severity [SMDTMS: −0.56 (95% CI, −0.98 to −0.15), I2 = 74%]. Similar results were obtained in tDCS and DBS subgroups, with a tendency toward decline of anxiety symptom intensity, although without reaching statistical significance [SMDtDCS : −0.61 (95% CI, −1.56 to 0.35), I2 = 86%; SMDDBS: −0.37 (95% CI, −0.84 to 0.10), I2 = 0%; Figure 2]. Subgroup analyses performed by disorder showed effects on anxiety in favor of neurostimulation in GAD and PTSD [SMDGAD: −1.36 (95% CI, −2.12 to −0.60), I2 = 82%; SMDPTSD: −0.52 (95% CI, −0.94 to −0.10), I2 = 20%; Figure 3]. In contrast, no beneficial effect of neurostimulation was found in PD [SMDPD: 0.05 (95% CI, −0.38 to 0.48), I2 = 0%), in SP (SMDSP: −0.11 (95% CI, −0.77 to 0.56), or in MDD (SMDMDD: −0.16 (95% CI, −0.52 to 0.21), I2 = 30%]. As for target brain region subgroups, the vast majority of protocols targeted the dlPFC (left dlPFC excitation: four RCTs, right dlPFC excitation: four RCTs, right dlPFC inhibition: eight RCTs). Right dlPFC inhibition was significantly associated with a reduction of anxiety levels across disorders [SMD: −0.66 (95% CI, −1.32 to −0.00), I2 = 78%], as was right dlPFC excitation but without strictly reaching significance in this case [SMD: −1.14 (95% CI, −2.29 to 0.01), I2 = 85%]. No evidence for therapeutic benefit was found for left dlPFC excitation [SMD: −0.16 (95% CI, −0.50 to 0.18), I2 = 0%]. A single study targeted the right posterior parietal cortex (PPC), with positive results [SMD: −1.57 [95% CI, −2.31 to −0.81; (51)] (Figure 4). Other trials applied bilateral dlPFC (42), ventromedial PFC (49) or bilateral cingulate cortex (50, 57). The ventromedial PFC study was not included in this analysis because the side of the stimulation was not specified (49). Stimulation protocols and results are reported in Tables 3, 4.
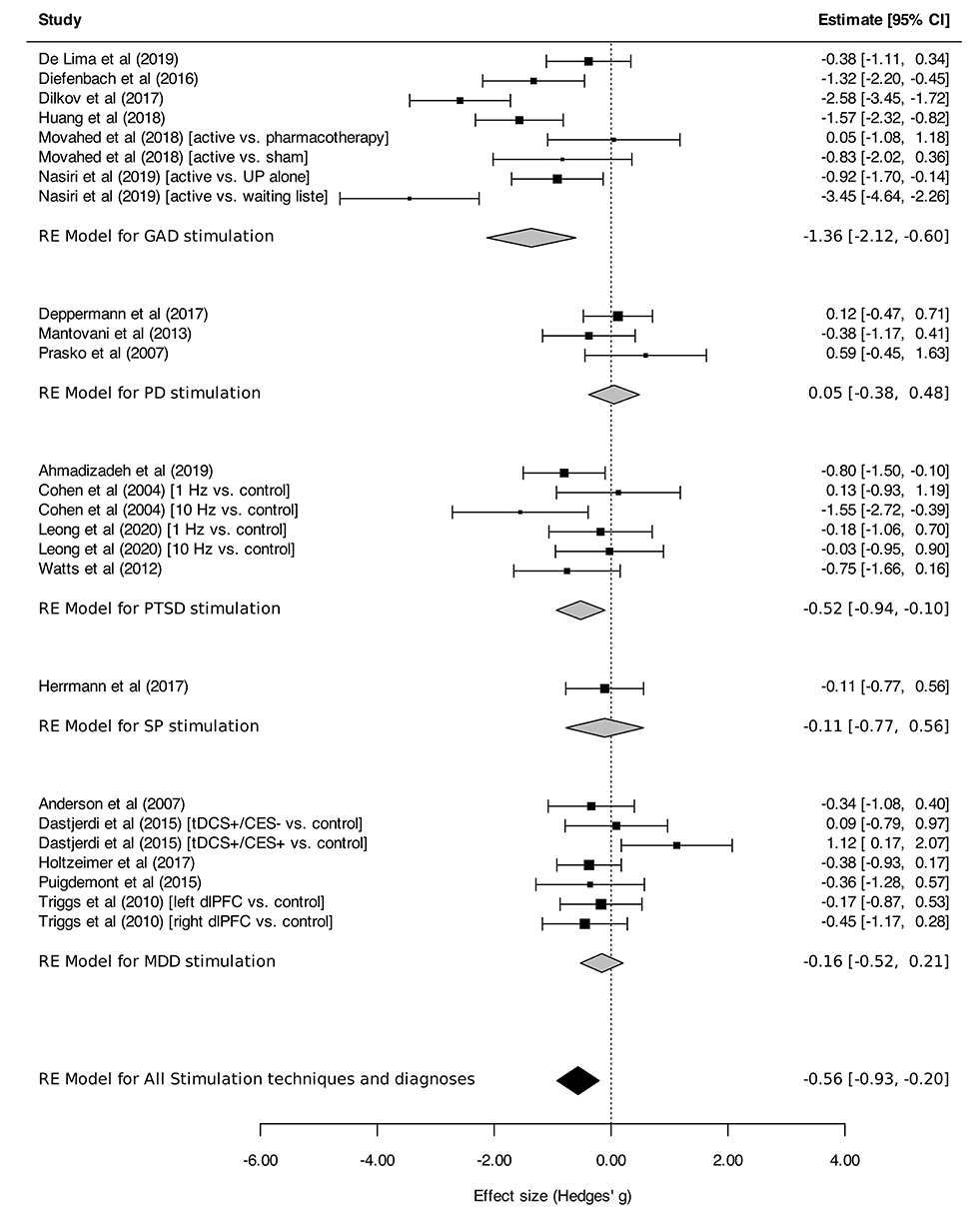
Figure 3. Change in the anxiety symptoms intensity in anxiety, stressor-related disorders and MDD, subgroups by disorder. Statistics: GAD: heterogeneity Sigma12 = 0.00, Sigma22 = 0.97; Q(df = 7) = 34.64, p < 0.0001); I2 = 82%; test for overall effect: Z = −3.52 (p = 0.0004); PD: heterogeneity Sigma12 = 0.00, Sigma22 = 0.00; Q(df = 2) = 2.23, p = 0.33); I2 = 00%; test for overall effect: Z = 0.24 (p = 0.81); PTSD: heterogeneity Sigma12 = 0.00, Sigma22 = 0.05; Q(df = 5) = 7.01, p = 0.22); I2 = 20%; test for overall effect: Z = −2.44 (p = 0.22); SP: heterogeneity : not applicable; test for overall effect: Z = −0.32 (p = 0.75); MDD: heterogeneity Sigma12 = 0.00, Sigma22 = 0.05; Q(df = 5) = 7.01, p = 0.22); I2 = 20%; test for overall effect: Z = −2.44 (p = 0.22); Total: heterogeneity Sigma12 = 0.06, Sigma22 = 0.00; Q(df = 6) = 8.94, p-val = 0.18); I2 = 30%; test for overall effect: Z = −3.0599 (p = 0.002); test for subgroup differences: F(df1 = 4, df2 = 20) = 3.69, p = 0.02.
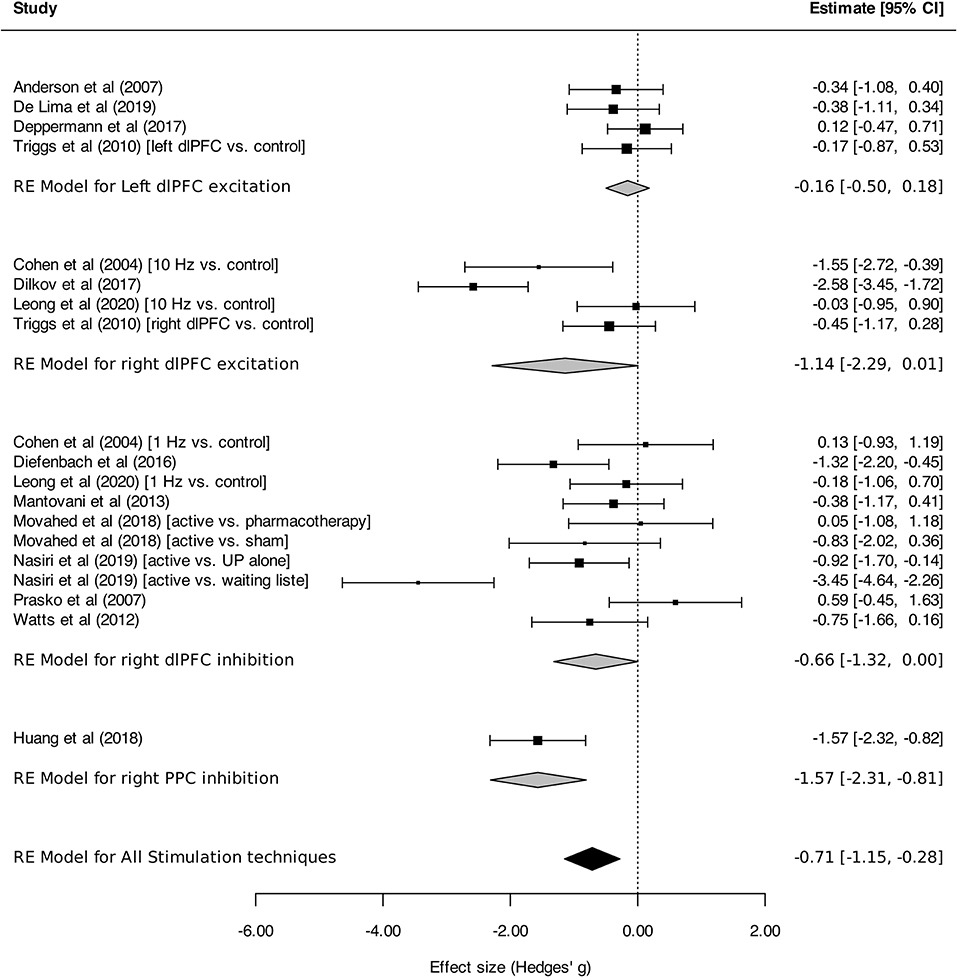
Figure 4. Change in the anxiety symptoms intensity in anxiety, stressor-related disorders and MDD, subgroups by target brain region. Statistics: left dlPFC excitation: heterogeneity Sigma12 = 0.00, Sigma22 = 0.00; Q(df = 3) = 1.44, p = 0.70; I2 = 00%; test for overall effect: Z = −0.91 (p = 0.36); right dlPFC excitation: heterogeneity Sigma12 = 0.57, Sigma22 = 0.57; Q(df = 3) = 20.21, p = 0.0002; test for overall effect: Z = −1.95 (p = 0.0002; I2 = 85%); right dlPFC inhibition: heterogeneity Sigma12 = 0.15, Sigma22 = 0.68; Q(df = 9) = 34.66, p < 0.0001; I2 = 78%; test for overall effect: Z = −1.95 (p = 0.05); right PPC (posterior parietal cortex) inhibition: heterogeneity : not applicable; test for overall effect: Z = −4.09 (p < 0.0001).
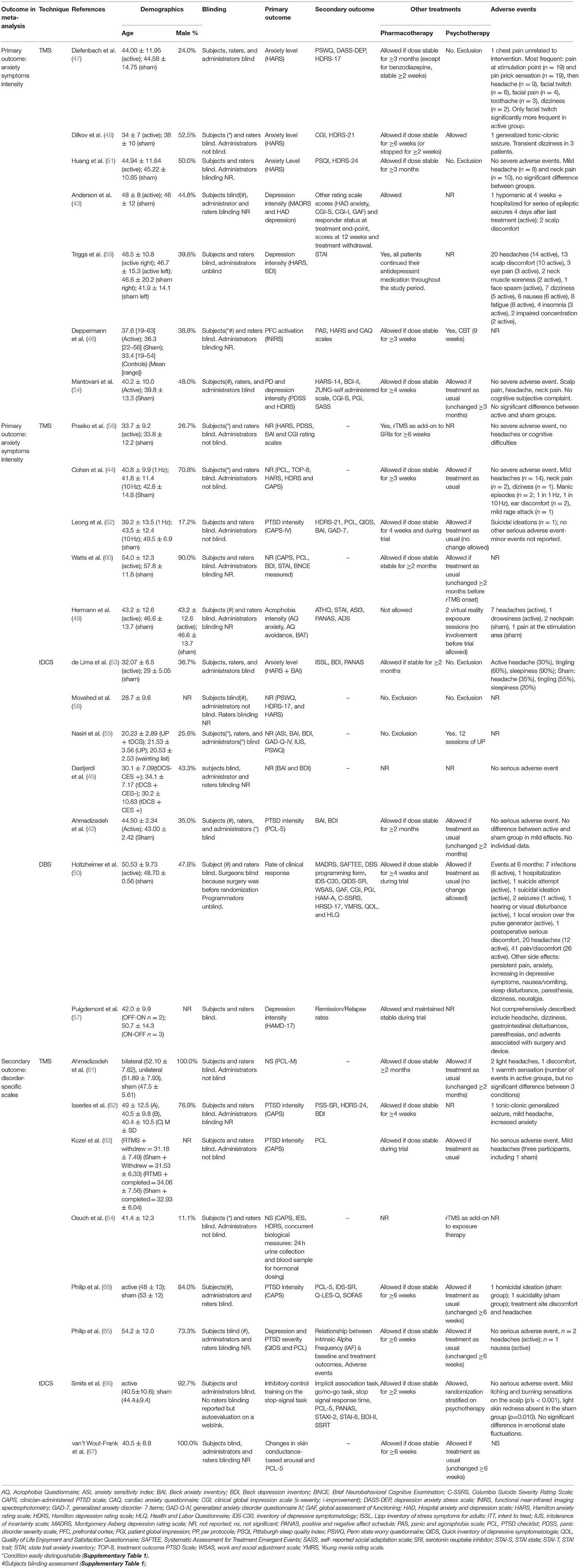
Table 3. Characteristics of included studies (socio-demographic data, blinding protocols, primary and secondary outcomes, other treatments, adverse events).
Secondary Outcomes
Rates of Clinically Significant Response and Remission
Meta-analysis was performed on response and remission rates, as defined by the authors. Criteria were alternatively defined on the basis of generic or disorder-specific scales (see Table 2). Overall, neurostimulation was associated with higher response rates than control interventions [RR: 3.91 (95% CI, 2.15–7.13), I2 = 31%]. This result was found in TMS studies [RR: 4.86 (95% CI, 2.39–9.89), I2 = 39%] but was not statistically significant for tDCS [RR: 3.56 (95% CI, 0.88–14.35)] or DBS [RR: 1.21 (95% CI, 0.34–4.33); Figure 5]. Anxiety remission rates were higher after neurostimulation overall [RR: 2.11 (95%CI, 1.02–4.36), I2 = 33%], and also after TMS [RR: 5.85 (95% CI, 1.39–24.56), I2 = 0%], but not after tDCS [RR: 3.63 (95% CI, 0.22–59.97), I2 = 76%], and DBS [RR: 1.49 (95% CI, 0.57–3.91), I2 = 0%; Figure 6].
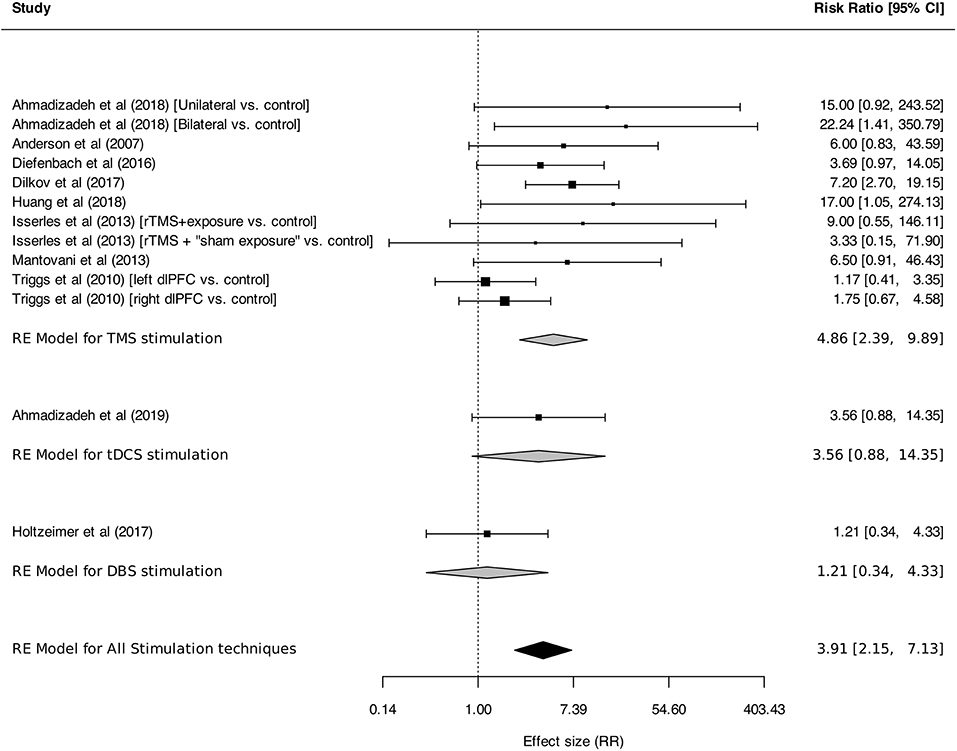
Figure 5. Response rates for anxiety, stressor-related disorders and MDD, subgroups by stimulation technique. Statistics: TMS: heterogeneity Sigma12 = 0.42, Sigma22 = 0.00, Q(df = 10) = 13.36, p = 0.20; I2 = 39%; test for overall effect: Z = 4.35 (p < 0.0001); tDCS: heterogeneity: not applicable. DBS: heterogeneity: not applicable. Total: heterogeneity Sigma12 = 0.36, Sigma22 = 0.00, Q(df = 12) = 15.84, p = 0.20; I2 = 37%; test for overall effect: Z = 4.45 (p < 0.0001); F(df1 = 2, df2 = 22) = 0.05, p = 0.95.
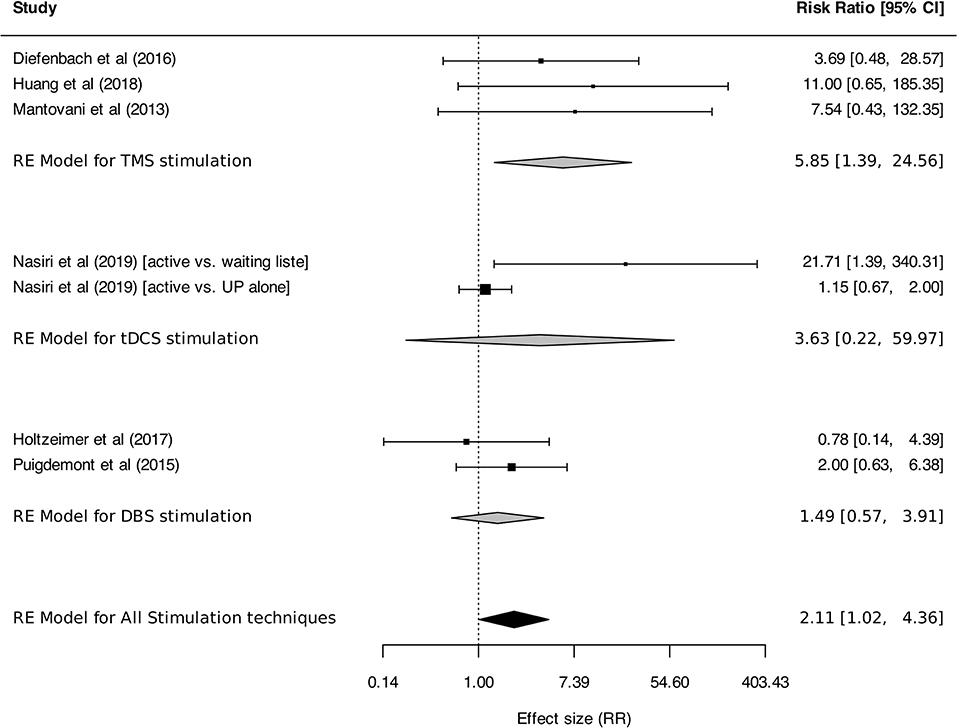
Figure 6. Remission rates for anxiety, stressor-related disorders and MDD, subgroups by stimulation technique. Statistics: TMS: heterogeneity Sigma12 = 0.00, Sigma22 = 0.00, Q(df = 2) = 0.42, p = 0.81; I2 = 0%; test for overall effect: Z = 2.41 (p = 0.02); tDCS: heterogeneity Sigma12 = 0.00, Sigma22 = 3.28, Q(df = 1) = 4.20, p-val = 0.04; I2 = 76%; test for overall effect: Z = 2.41 (p = 0.02); DBS: heterogeneity Chi2 = 0.79, df = 1 (p = 0.37); I2 = 0%; test for overall effect: Z = 0.90 (p = 0.37); Total: heterogeneity Sigma12 = 0.00, Sigma22 = 0.29, Q(df = 6) = 9.15, p = 0.17; I2 = 33%; test for overall effect: Z = 2.01 (p = 0.04); test for subgroup differences: F(df1 = 2, df2 = 4) = 0.97, p = 0.45.
Follow-Up Results
Data of follow-up visits were not submitted to meta-analysis because of a high heterogeneity in the length of follow-up periods, data reporting and attrition rates (see Table 4 for detailed reporting).
For TMS, three studies stopped follow-up visits after 2–4 weeks (44, 51, 56), 7 after 1–3 months (43, 47–49, 52, 59, 60), and one at 6 months (54). Four studies showed persistent differences between active and sham groups at the end of follow-up in favor of neurostimulation (47, 48, 51, 54) and two reported data suggesting the persistence of differences between groups, although statistics were not presented (52, 59). There was no statistically significant difference between groups in two trials (43, 49). Finally, one article reported incomplete data (60).
In tDCS studies, follow-up visits were also conducted at different time points: 1 week (53), 4 weeks (42), 2 months (58), and 3 months (55). Three studies described a persistently significant difference between active and sham groups (42, 55, 58). One trial presented within-group comparisons, but no statistics were provided for between-group comparisons at the end of follow-up (53).
In DBS studies, all subjects underwent an unblinded treatment phase following the endpoint evaluation. Thereafter, they received an active stimulation for six consecutive months (50), or until loss to follow-up (57). Values of HARS were similar between treatment groups at 6 months follow-up in both studies.
Efficacy by Disorder as Measured With Disorder-Specific Scales
Figure 7 presents the efficacy of neurostimulation on reducing the severity of anxiety and stressor-related disorders, assessed by disorder-specific scales. Significant reduction of symptom severity was found for neurostimulation in the whole sample of trials [SMD, −0.89 (95%CI, −1.29 to −0.50), I2 = 83%]. However, in the subgroup analysis, a significant reduction of symptom intensity in favor of neurostimulation was only found for GAD [SMD, −0.98 (95% CI, −1.93 to −0.03), I2 = 86%] and PTSD [SMD, −0.98 (95% CI, −1.52 to −0.44), I2 = 83%].
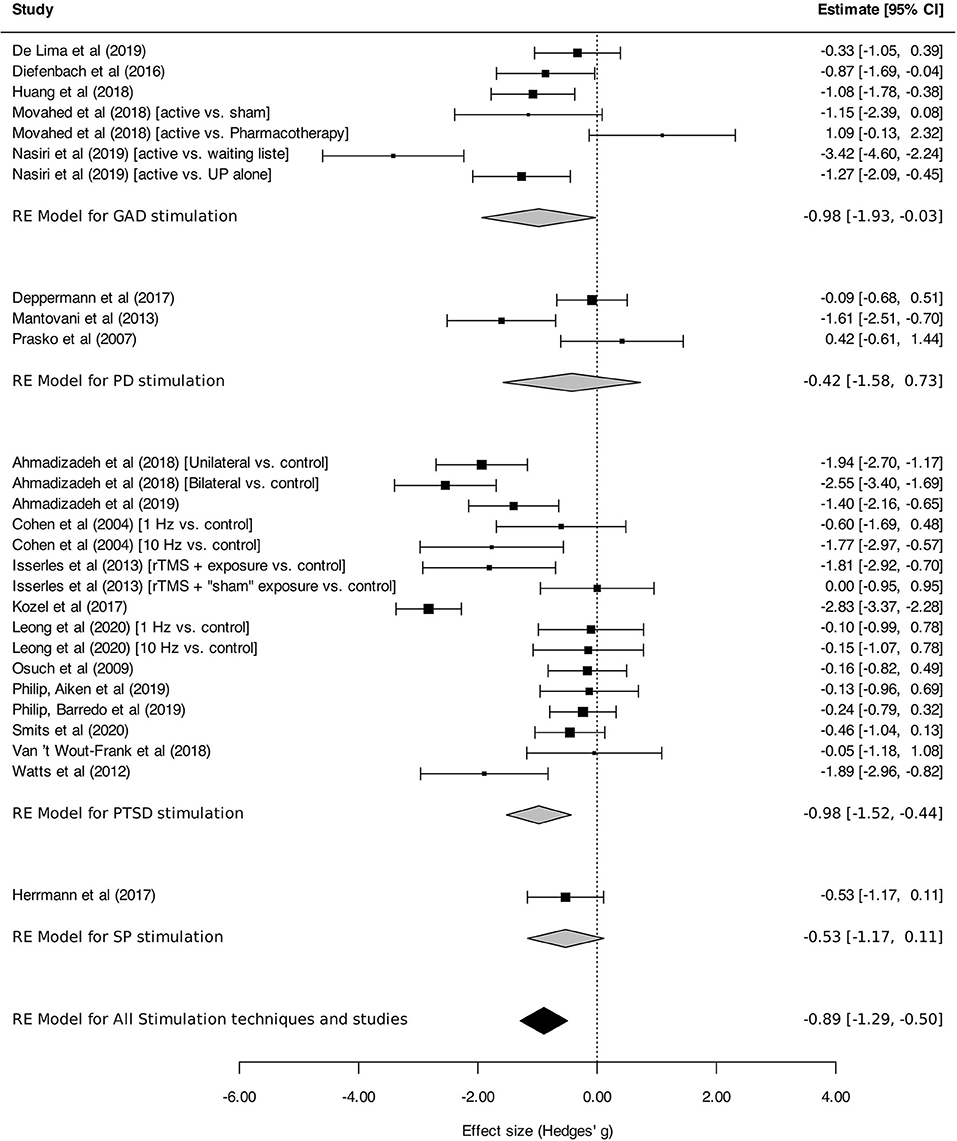
Figure 7. Change in the severity of anxiety, stressor-related disorders, assessed by disorder-specific scale. Statistics: GAD: heterogeneity Sigma12 = 0.19, Sigma22 = 1.13; Q(df = 6) = 31.04, p < 0.0001; I2 = 86%; test for overall effect: Z = −2.02 (p = 0.04); PD: heterogeneity Sigma12 = 0.42; Sigma22 = 0.42; Q(df = 2) = 10.22, p = 0.006; I2 = 82%; test for overall effect: Z = −0.72 (p = 0.47); PTSD: heterogeneity Sigma12 = 0.51; Sigma22 = 0.32; Q(df = 15) = 103.78, p < 0.0001; I2 = 83%; test for overall effect: Z = −3.52 (p = 0.0004); SP: heterogeneity : not applicable; test for overall effect: Z = −1.63 (p = 0.10); Total: heterogeneity Sigma12 = 0.25, Sigma22 = 0.57; Q(df = 26) = 153.87, p < 0.0001; I2 = 83%; test for overall effect: Z = –4.40 (p < 0.0001); test for subgroup differences: F(df1 = 3, df2 = 23) = 0.29, p = 0.83.
Adverse Events
Data regarding adverse events were mostly reported irrespective of intervention groups (active or control). They are therefore reported in a descriptive manner (see Supplementary Material for a more detailed reporting). For TMS studies, serious adverse events comprised three cases of generalized tonic-clonic seizures in active groups (43, 48, 62), two suicidal ideation episodes in the active group (52), one homicidal ideation emergence, one hospitalization for suicidality during follow-up [both receiving sham stimulation; (68)], two manic and one hypomanic episodes [all receiving active stimulation; (43, 44)], and one chest pain occurrence in the active group, which was considered to be unrelated to the intervention (47). No serious adverse event was reported for tDCS. Other adverse events were mild, including: (i) TMS: headaches, pain at the stimulation point, neck, face (when specified, there was no significant difference between active and sham conditions for these side effects), dizziness/nausea, ipsilateral facial twitch, and ear discomfort; (ii) tDCS: headache, tingling, sleepiness, mild itching or burning sensations on the scalp and light skin redness. Only sleepiness, sensations on the scalp and skin redness were more frequently reported in active groups.
Side effects were reported in only one of the two DBS studies (50). Serious adverse events at 6 months were infections (six patients in the active stimulation group, one sham) related to the study device or surgery, hospitalization (one active) for unspecified reason, suicide attempt (one active), suicidal ideation (one active), seizures (one active, one sham), hearing or visual disturbances (one active), local erosion over the pulse generator (one active), serious postoperative discomfort (8). The most frequent adverse events were mild, including headache, acute pain/discomfort, persistent pain, anxiety, increase in depressive symptom intensity, nausea/vomiting, sleep disturbances, paresthesia, and dizziness (50).
Meta-Regression Analysis
In the meta-regression analysis, the difference in pre/post-treatment changes of depression scores between active and sham arms (Coefficient = 0.86, p = 0.0003) significantly accounted for the heterogeneity [heterogeneity test QE(df = 19) = 19.6, p = 0.42]. No statistically significant link was found for other covariates, namely: the baseline anxiety scores, the changes in PTSD scores, the gender ratio and the mean age of participants, the total number of sessions during the protocol and, in the TMS studies, the total number of pulses and the percentage of motor threshold did not explain results heterogeneity. Statistical characteristics for each factor are reported in the Table 5.
Risk of Bias and Quality Assessment
Publication Bias
Visual inspection of the funnel plot of studies included for primary outcome analysis revealed an asymmetric distribution of studies, indicating that publication bias may have occurred (Figure 8A). However, Egger's test was not indicative of a statistically significant bias (p = 0.46). There was no evidence of publication bias for studies included for the analysis of disorders-specific scales (Figure 8B; p = 0.59).
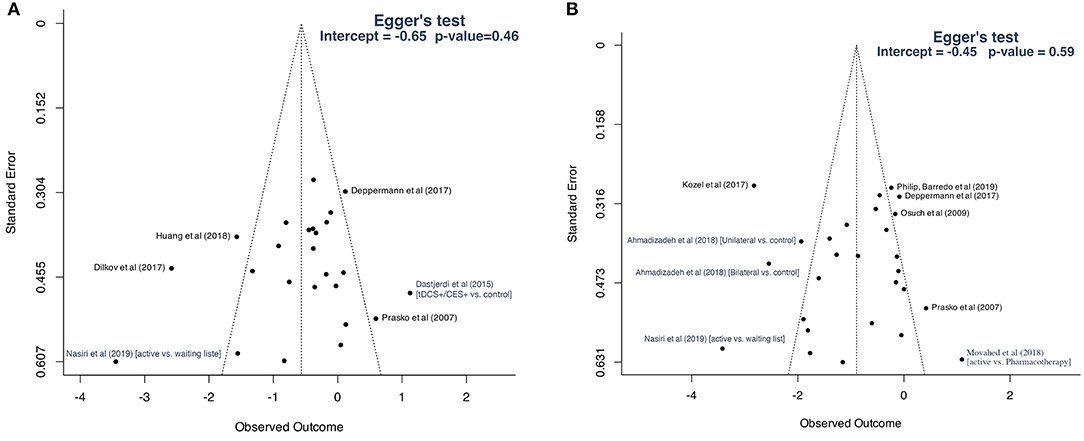
Figure 8. Funnel plots: (A) studies included for primary outcome; (B) studies included for secondary analysis based on disorder-specific scales.
Risk of Bias in Methodology
Detailed evaluation of the risk of bias is reported in Supplementary Table 1. The majority of studies were considered at high risk of bias, both for the studies included for primary outcome analysis (14/19; 73.7%) and for studies included for disorder-specific scales (16/21; 76.2%). The remaining studies were at low (3/19, 15.8%; 3/21, 14.3%, respectively) or intermediate risk of bias (2/19, 10.5%; 2/21, 9.5%, respectively). Contrary to what was initially planned in the registered protocol, no sensitivity analysis was conducted in the meta-analysis, because of the insufficient number of studies demonstrating a low risk of bias.
Limitations in blinding, especially neurostimulation blinding (10/19 and 11/21, respectively), and attrition bias (9/19 and 11/21, respectively), accounted for almost the totality of the high risk of bias. Conversely, outcome blinding or selective reporting of results were generally considered at low risk of bias. In most cases, methods of random sequence generation and randomization concealment were not reported. Only six of the 27 studies applied and reported appropriate randomization (48, 50, 52, 57, 63, 66). Overall, according to the GRADE guidelines (39), evidence from this study was considered of moderate quality.
Discussion
The treatment of pathological anxiety in ADs, PTSD and MDD is faced with high levels of resistance. Focal brain stimulation is a promising therapeutic avenue. However, there is currently a lack of synthesis of results from neuromodulatory interventions for anxiety across those disorders. This review and meta-analysis summarized available evidence regarding the efficacy of focal brain stimulation on pathological anxiety, with a dimensional perspective. Results are globally in favor of a positive effect of neurostimulation at the end of interventions across ADs, PTSD and MDD, with a medium effect size, and a moderate quality of evidence. Active neuromodulation protocols also resulted in greater response and/or remission rates than control conditions. These results suggest that focal brain stimulation may be an interesting option for the treatment of pathological anxiety. However, long-term efficacy data were insufficient to conduct a meta-analytic study, being scarce and heterogeneous. Nevertheless, follow-up data were qualitatively in favor of a prolonged favorable effect of neurostimulation.
The meta-analysis purposely gathered results from heterogeneous conditions and protocols, which were investigated in subgroup analyses. Regarding stimulation techniques, both TMS and tDCS showed a tendency toward an improvement of anxiety symptom intensity and resulted in greater response and/or remission rates than control conditions immediately after treatment. Mild adverse events typical of the stimulation techniques were reported. DBS showed a tendency toward an improvement of anxiety symptom intensity immediately after treatment. However, the meta-analysis was limited to two studies on MDD, which included patients with moderate baseline anxiety levels. This analysis is not sufficient to determine the therapeutic potential of DBS. As a result, at present, only TMS and tDCS appear as potential neuromodulatory options for the treatment of pathological anxiety.
As for categorically defined disorders, positive effects were found in PTSD and GAD subgroups, on the basis of psychometric scores measuring either generic anxiety or disorder-specific scores. Positive effects were also found for MDD-related anxiety. In contrast, there was no evidence for positive impact of brain stimulation in PD, and the only trial on patients with SP showed no positive effects. No study was found for SAD. Overall, our results indicate that in categorial terms, PTSD and GAD may benefit from non-invasive brain stimulation.
Notably, ADs, PTSD and MDD were pooled in the main analysis, as a consequence of the dimensional approach of the study. This strategy is in line with the National Institute of Mental Health research domain criteria initiative, which organizes mental disorders in constructs, e.g., the negative valence system, and allows a multi-level integration of neuroscientific findings to identify biological targets for treatment (69). The choice of the disorders included was guided by a line of arguments pointing toward similarities in neurobiological bases. From a phenomenological perspective, they are internalizing disorders that share the highest levels of comorbidities and a great number of reciprocal risk interactions (5). In agreement with this, their susceptibility profiles and potential genetic risk factors also overlap (5). Moreover, brain activation patterns identified in ADs, MDD and PTSD are similar, implicating a fronto-limbic network (18, 21). On the contrary, obsessive-compulsive disorder was not included, as it is known to follow distinct, cortico–striato–thalamo–cortical pathogenic mechanisms (70). Likewise, somatic and dissociative disorders (1) were considered too different in their expression and pathophysiology, and were not included. Interestingly, the finding of an association between anxious et depressive symptoms reduction in the meta-regression analysis may further suggest the existence of common biological underpinnings, or, at least, of close interactions between the two phenomena.
Regarding the relative efficacy of brain stimulation depending on target brain regions, both right dlPFC inhibition and excitation tended to alleviate anxiety symptoms, with a larger effect size for right dlPFC activation. Left dlPFC activation had no global effect. These findings are overall consistent with the negative correlation identified between pathological anxiety levels and the resting state connectivity strength between right dlPFC and amygdala (71). However, other authors reported similar correlations, but with left dlPFC (72, 73). It should be emphasized that the classification of the net effect (excitatory vs. inhibitory) of brain stimulation is derived from simple observations of motor cortex responses, that may not directly translate to PFC. Moreover, the net effect of brain stimulation is dependent on a number of factors, including stimulation parameters (duration, intensity etc.), variability in subjects' intrinsic cortical excitability, and ongoing pharmacological treatments (74). Thus, the “excitatory” or “inhibitory” classification should be interpreted with caution. Future preclinical studies should specifically investigate this issue in the PFC. Moreover, it should be reminded that our analysis pooled various stimulation techniques and protocols. Therapeutic effects of focal brain stimulation also depend on the accurate placement of stimulation devices above targeted brain regions. In most studies included in the meta-analysis, target area was determined by scalp distances anterior to regions where motor responses were obtained. This placement method likely resulted in imprecise, heterogenous targeting. Indeed, it has been demonstrated that results obtained with such positioning methods differ from those obtained with gold standard, brain imaging-based stimulation device positioning (75, 76). Future clinical trials should aim to optimize stimulation parameters, and may primarily investigate the right dlPFC as a target, as our results would suggest.
Results of the present study are generally in agreement with, and extend results from previous reviews of the literature, including narrative reviews (31, 32, 34) and meta-analyses (35–37, 77, 78). Among narrative reviews, the most closely related to our work systematically reviewed the effectiveness of non-invasive brain stimulation for the treatment of anxiety disorders across RCTs and open-label studies (34). Interestingly, no clear evidence was reported in this work for a positive effect of brain stimulation in SP, on a larger number of trials than reported in our meta-analysis [including open-label studies; (34)]. This suggests that current neuromodulation strategies have no efficacy in SP. Contrary to our results, Vicario et al. (34) suggested that neuromodulation may improve PD, and that left dlPFC stimulation is more efficacious. However, the majority of studies included in the review were not sham-controlled, possibly accounting for those differences with our results.
Three meta-analyses were recently conducted for non-invasive brain stimulation in PTSD (36), rTMS in GAD (37), and rTMS in ADs (35), reaching similar conclusions to the present review. Evaluating the efficacy of non-invasive brain stimulation in PTSD across RCTs revealed a strong positive therapeutic effect of TMS (36). Cui et al. reported a positive effect for rTMS on GAD, based on a much larger study sample size than reported here (n = 21 vs. 3). This discrepancy is due to the inclusion of 19/21 studies published in Chinese language by Cui et al. (37). Most consisted in inhibitory stimulation of the right dlPFC. Cirillo et al. reported a reduction in clinical severity after TMS, both in GAD and in PTSD, using disorder-specific scales, and suggested that right dlPFC excitatory stimulation may be superior to targeting left dlPFC (35). Despite reaching conclusions consistent with those obtained in our meta-analysis, these studies were restricted to single diagnostic categories, and/or stimulation techniques, and/or disorder-specific instruments.
Prior to our work, another transdiagnosis meta-analysis had been published. Trevizol and colleagues conducted a meta-analysis investigating the effects of TMS on generic anxiety scores across ADs, PTSD and OCD, and found no evidence for a positive effect, including PD, PTSD and OCD subgroups (77). Most RCTs (8/14) reported interventions on OCD, possibly accounting for differences between conclusions from this and our work. Four of those negative OCD studies targeted the dlPFC, further supporting the distinct pathophysiology of OCD, and its exclusion from neurobiologically-based dimensional studies of anxiety. Differences for the PTSD subgroup may be partly accounted for by the publication after 2016 of studies included in our review. During the conduct of our study -i.e., after the protocol was pre-registered- a closely related work has been released by Vergallito et al. (78). Using both disorder-specific and generic anxiety scores, they found that rTMS and tDCS reduced anxiety levels across ADs. This main finding is in agreement with our primary and secondary analyses. Depression scores were also lower in intervention vs. control groups (78). In addition to our analysis, one study was included for which we could not obtain original results despite contacting the authors (79). In contrast with Vergallito et al., we ranked risk of bias as high in a majority of studies, whenever, the sham stimulation induced sounds and/or bodily sensations that might have been readily identified by intervention administrators or subjects. Our risk of bias classification is conservative, attributing high risk of bias if intervention administrators were not blinded, or if participants could guess the intervention group. For instance, we conservatively ranked 90° TMS coil placement as a high risk of performance bias. This classification may be mitigated by a meta-analysis reporting that blinding assessment was satisfactory in MDD participants receiving sham TMS with either sham coils or angled coil placement (80). However, in one study included here, while angled TMS placement was used and blinding quality was measured, patients guessed better than chance their allocation group (46). Notably, Vergallito et al. restricted the analysis to ADs as defined in DSM-5. As demonstrated by changes between the 4th and 5th versions of the manual, diagnostic categories are a moving target, and an obstacle for neurobiologically-informed research. Instead, similarities in the anxiety phenomenon across ADs, PTSD and MDD call for a dimensional evaluation, which constitutes the originality and relevance of the present study.
Limitations
First despite performing an extensive search of literature databases and applying PRISMA recommendations, a limited number of studies were included, with relatively small sample sizes [except for two studies measuring disorder-specific symptoms; (63, 66)]. Second, the primary outcome of the meta-analysis (generic anxiety scales results) was the explicit primary outcome in only 5/19 of the studies included. Third, therapeutic efficacy was evaluated at the end of brain modulation trials, while long-lasting reduction of symptoms intensity is warranted. Fourth, as pharmacological treatments were often administered concomitantly with brain stimulation, the relative contribution of the two strategies and their interactions remain to be clarified. Fifth, although only RCTs were analyzed, the risk of bias was high in a majority of studies, including the three studies showing the strongest positive effects (42, 48, 55). This was mostly due to randomization methods (insufficiently described), attrition, and insufficient blinding. Moreover, we could not entirely rule out that publication bias had occurred.
Future Perspectives
Larger-sized, multicentered studies with pre-specified primary outcomes are warranted to fully assess the potential efficacy of TMS, tDCS and DBS in pathological anxiety. Trials designed to demonstrate long-lasting therapeutic responses and remissions will be critical in order to support the application of brain stimulation strategies in daily practice. In this respect, the adoption of standard follow-up intervals will tremendously help reproducibility and generalizability of future findings. The relative contributions and interactions between medication, psychotherapy and neuromodulation will need to be clarified. In a dimensional view, generic anxiety scales should be used to assess outcomes, and inclusion criteria might even be defined in a dimensional manner. Care should also be taken to apply and report adequate randomization and blinding procedures, including measurements of blinding assessment. Moreover, sham conditions will need to be hardly distinguishable from active brain stimulation (81, 82).
Alternative brain targets should be investigated. It is likely that targeting dlPFC to treat pathological anxiety has been empirically carried forward -at least in part- from usual clinical practice in MDD [as indicated by (47)], and not necessarily informed by neuroscientific findings. Indeed, there is some, but limited evidence for the implication of dlPFC in pathological anxiety (71–73) and in the preclinical model of fear conditioning (83). In contrast, the anxiety circuit comprises key brain regions such as the medial PFC, the amygdala, and the insula (10, 84) as demonstrated by a recent meta-analysis of fMRI data (18). Their neuromodulation might provide greater therapeutic outcomes than targeting dlPFC. However, those key nodes are located deeply within the brain. Their modulation will require the application of invasive or novel non-invasive DBS techniques [e.g., H-coil TMS: e.g., (62, 85); interference-based deep tDCS: (86); transcranial ultrasounds: (87)].
Finally, several research lines aim to develop brain stimulation procedures in a precision medicine framework. In the spatial domain, neuromodulated regions could be targeted selectively in different patient “clusters” distinguished by symptoms and neurobiological (e.g., imaging) dimensions [e.g., (88)]. In the temporal domain, instead of delivering continuous patterns of regular pulses, stimulation paradigms could adapt to ongoing, pathological brain activities to restore physiological functioning [(i.e., “closed-loop” stimulation; e.g., (89)]. Overall, it is likely that empirical brain modulation will move toward precise, neurobiological data-based techniques.
Conclusion
Current evidence suggests that focal brain stimulation may be beneficial to alleviate pathological anxiety across ADs, PTSD and MDD. Additional, large-scale, RCTs measuring long-term effects of neuromodulation on anxiety are warranted. Future research will expand and refine our knowledge in the perspective of offering precision medicine neuromodulation tools to manage pathological anxiety in the context of high treatment resistance levels.
Data Availability Statement
The original contributions presented in the study are included in the article/Supplementary Material. Further inquiries can be directed to the corresponding author/s.
Author Contributions
FG, FS, BA, and TB: study design. FG and TB: data collection and manuscript drafting. FG, FS, AS, and TB: data analysis. FG, FS, AS, BA, and TB: revisions and approval. All authors contributed to the article and approved the submitted version.
Funding
This work was funded by a CCA Inserm Bettencourt grant to Thomas Bienvenu (R20005GS).
Conflict of Interest
Unrelated to brain stimulation techniques, BA received speaker's honoraria and/or a travel allowance from Lundbeck, Sanofi, Janssen-Cilag, and Eli Lilly. He has served on the advisory board of Janssen-Cilag.
The remaining authors declare that the research was conducted in the absence of any commercial or financial relationships that could be construed as a potential conflict of interest.
Publisher's Note
All claims expressed in this article are solely those of the authors and do not necessarily represent those of their affiliated organizations, or those of the publisher, the editors and the reviewers. Any product that may be evaluated in this article, or claim that may be made by its manufacturer, is not guaranteed or endorsed by the publisher.
Acknowledgments
Professor Marie Tournier is acknowledged for her inputs at the early stage of this study. Scientists who provided data from their studies are deeply thanked for their contributions, in particular Professor Maria J. Portella for re-analyzing data from Puigdemont et al. (57). The authors thank Ekaterina Escoffier for her efforts in retrieving missing full text articles. TB is the recipient of a CCA-Inserm Bettencourt clinician-scientist fellowship from Fondation Bettencourt-Schueller.
Supplementary Material
The Supplementary Material for this article can be found online at: https://www.frontiersin.org/articles/10.3389/fpsyt.2022.910897/full#supplementary-material
References
1. American Psychiatric Association. DSM-5: Diagnostic and Statistical Manual of Mental Disorders. 5th ed. Arlington, VA: American Psychiatric Association (2013).
2. Fava M, Alpert JE, Carmin CN, Wisniewski SR, Trivedi MH, Biggs MM, et al. Clinical correlates and symptom patterns of anxious depression among patients with major depressive disorder in STAR*D. Psychol Med. (2004) 34:1299–308. doi: 10.1017/S0033291704002612
3. Hasin DS, Sarvet AL, Meyers JL, Saha TD, Ruan WJ, Stohl M, et al. Epidemiology of adult DSM-5 major depressive disorder and its specifiers in the United States. JAMA Psychiatry. (2018) 75:336. doi: 10.1001/jamapsychiatry.2017.4602
4. Fava M, Graves LM, Benazzi F, Scalia MJ, Ionescu IA, Alpert JE, et al. A cross-sectional study of the prevalence of cognitive and physical symptoms during long-term antidepressant treatment. J Clin Psychiatry. (2006) 67:1754–9. doi: 10.4088/JCP.v67n1113
5. Kalin NH. The critical relationship between anxiety and depression. AJP. (2020) 177:365–7. doi: 10.1176/appi.ajp.2020.20030305
6. Alonso J, Angermeyer MC, Bernert S, Bruffaerts R, Brugha TS, Bryson H, et al. Prevalence of mental disorders in Europe: results from the European Study of the Epidemiology of Mental Disorders (ESEMeD) project. Acta Psychiatr Scand Suppl. (2004) 109:21–7. doi: 10.1111/j.1600-0047.2004.00325.x
7. Kessler RC, Berglund P, Demler O, Jin R, Merikangas KR, Walters EE. Lifetime prevalence and age-of-onset distributions of DSM-IV disorders in the National Comorbidity Survey Replication. Arch Gen Psychiatry. (2005) 62:593. doi: 10.1001/archpsyc.62.6.593
8. Olesen J, Gustavsson A, Svensson M, Wittchen H-U, Jönsson B, on on behalf of the CDBE2010 study group and the European Brai Council. The economic cost of brain disorders in Europe: Economic cost of brain disorders in Europe. Eur J Neurol. (2012) 19:155–62. doi: 10.1111/j.1468-1331.2011.03590.x
9. Gustavsson A, Svensson M, Jacobi F, Allgulander C, Alonso J, Beghi E, et al. Cost of disorders of the brain in Europe 2010. Eur Neuropsychopharmacol. (2011) 21:718–79. doi: 10.1016/j.euroneuro.2011.08.008
10. Penninx BW, Pine DS, Holmes EA, Reif A. Anxiety disorders. Lancet. (2021) 397:914–27. doi: 10.1016/S0140-6736(21)00359-7
11. Wittchen HU, Jacobi F, Rehm J, Gustavsson A, Svensson M, Jönsson B, et al. The size and burden of mental disorders and other disorders of the brain in Europe 2010. Eur Neuropsychopharmacol. (2011) 21:655–79. doi: 10.1016/j.euroneuro.2011.07.018
12. Bisson JI, Roberts NP, Andrew M, Cooper R, Lewis C. Psychological therapies for chronic post-traumatic stress disorder (PTSD) in adults. Cochr Database Syst Rev. (2013) 2013:CD003388. doi: 10.1002/14651858.CD003388.pub4
13. Bystritsky A. Treatment-resistant anxiety disorders. Mol Psychiatry. (2006) 11:805–14. doi: 10.1038/sj.mp.4001852
14. Rush AJ, Trivedi MH, Wisniewski SR, Nierenberg AA, Stewart JW, Warden D, et al. Acute and longer-term outcomes in depressed outpatients requiring one or several treatment steps: a STAR*D Report. Am J Psychiatry. (2006) 13:1905–17. doi: 10.1176/ajp.2006.163.11.1905
15. Stein DJ, Ipser JC, Seedat S, Sager C, Amos T. Pharmacotherapy for post traumatic stress disorder (PTSD). Cochr Database Syst Rev. (2006) 2006:CD002795. doi: 10.1002/14651858.CD002795.pub2
16. Papakostas GI, Perlis RH, Scalia MJ, Petersen TJ, Fava M. A Meta-analysis of early sustained response rates between antidepressants and placebo for the treatment of major depressive disorder. J Clin Psychopharmacol. (2006) 26:56–60. doi: 10.1097/01.jcp.0000195042.62724.76
17. Smits JAJ, Minhajuddin A, Thase ME, Jarrett RB. Outcomes of acute phase cognitive therapy in outpatients with anxious versus nonanxious depression. Psychother Psychosom. (2012) 81:153–60. doi: 10.1159/000334909
18. Chavanne AV, Robinson OJ. The overlapping neurobiology of induced and pathological anxiety: a meta-analysis of functional neural activation. AJP. (2021) 178:156–64. doi: 10.1176/appi.ajp.2020.19111153
19. Etkin A, Schatzberg AF. Common abnormalities and disorder-specific compensation during implicit regulation of emotional processing in generalized anxiety and major depressive disorders. AJP. (2011) 168:968–78. doi: 10.1176/appi.ajp.2011.10091290
20. Gray JP, Müller VI, Eickhoff SB, Fox PT. Multimodal abnormalities of brain structure and function in major depressive disorder: a meta-analysis of neuroimaging studies. AJP. (2020) 177:422–34. doi: 10.1176/appi.ajp.2019.19050560
21. Janiri D, Moser DA, Doucet GE, Luber MJ, Rasgon A, Lee WH, et al. Shared neural phenotypes for mood and anxiety disorders a meta-analysis of 226 task-related functional imaging studies. FOC. (2021) 19:256–63. doi: 10.1176/appi.focus.19206
22. Ashkan K, Rogers P, Bergman H, Ughratdar I. Insights into the mechanisms of deep brain stimulation. Nat Rev Neurol. (2017) 13:548–54. doi: 10.1038/nrneurol.2017.105
23. Hallett M. Transcranial magnetic stimulation: a primer. Neuron. (2007) 55:187–99. doi: 10.1016/j.neuron.2007.06.026
24. Stagg CJ, Antal A, Nitsche MA. Physiology of transcranial direct current stimulation. J ECT. (2018) 34:144–52. doi: 10.1097/YCT.0000000000000510
25. Brunoni AR, Chaimani A, Moffa AH, Razza LB, Gattaz WF, Daskalakis ZJ, et al. Repetitive transcranial magnetic stimulation for the acute treatment of major depressive episodes: a systematic review with network meta-analysis. JAMA Psychiatry. (2017) 74:143. doi: 10.1001/jamapsychiatry.2016.3644
26. Mutz J, Edgcumbe DR, Brunoni AR, Fu CHY. Efficacy and acceptability of non-invasive brain stimulation for the treatment of adult unipolar and bipolar depression: a systematic review and meta-analysis of randomised sham-controlled trials. Neurosci Biobehav Rev. (2018) 92:291–303. doi: 10.1016/j.neubiorev.2018.05.015
27. Razza LB, Palumbo P, Moffa AH, Carvalho AF, Solmi M, Loo CK, et al. A systematic review and meta-analysis on the effects of transcranial direct current stimulation in depressive episodes. Depress Anxiety. (2020) 37:594–608. doi: 10.1002/da.23004
28. Kisely S, Li A, Warren N, Siskind D. A systematic review and meta-analysis of deep brain stimulation for depression. Depress Anxiety. (2018) 35:468–80. doi: 10.1002/da.22746
29. Wu Y, Mo J, Sui L, Zhang J, Hu W, Zhang C, et al. Deep brain stimulation in treatment-resistant depression: a systematic review and meta-analysis on efficacy and safety. Front Neurosci. (2021) 15:655412. doi: 10.3389/fnins.2021.655412
30. Iannone A, Cruz APDM, Brasil-Neto JP, Boechat-Barros R. Transcranial magnetic stimulation and transcranial direct current stimulation appear to be safe neuromodulatory techniques useful in the treatment of anxiety disorders and other neuropsychiatric disorders. Arq Neuropsiquiatr. (2016) 74:829–35. doi: 10.1590/0004-282X20160115
31. Rodrigues PA, Zaninotto AL, Neville IS, Hayashi CY, Brunoni AR, Teixeira MJ, et al. Transcranial magnetic stimulation for the treatment of anxiety disorder. Neuropsychiatr Dis Treat. (2019) 15:2743. doi: 10.2147/NDT.S201407
32. Sagliano L, Atripaldi D, De Vita D, D'Olimpio F, Trojano L. Non-invasive brain stimulation in generalized anxiety disorder: a systematic review. Progr Neuropsychopharmacol Biol Psychiatry. (2019) 93:31–8. doi: 10.1016/j.pnpbp.2019.03.002
33. Stein DJ, Fernandes Medeiros L, Caumo W, Torres IL. Transcranial direct current stimulation in patients with anxiety: current perspectives. NDT Volume. (2020) 16:161–9. doi: 10.2147/NDT.S195840
34. Vicario CM, Salehinejad MA, Felmingham K, Martino G, Nitsche MA. A systematic review on the therapeutic effectiveness of non-invasive brain stimulation for the treatment of anxiety disorders. Neurosci Biobehav Rev. (2019) 96:219–31. doi: 10.1016/j.neubiorev.2018.12.012
35. Cirillo P, Gold AK, Nardi AE, Ornelas AC, Nierenberg AA, Camprodon J, et al. Transcranial magnetic stimulation in anxiety and trauma-related disorders: a systematic review and meta-analysis. Brain Behav. (2019) 9:e01284. doi: 10.1002/brb3.1284
36. Kan RLD, Zhang BBB, Zhang JJQ, Kranz GS. Non-invasive brain stimulation for posttraumatic stress disorder: a systematic review and meta-analysis. Transl Psychiatry. (2020) 10:168. doi: 10.1038/s41398-020-0851-5
37. Cui H, Jiang L, Wei Y, Li W, Li H, Zhu J, et al. Efficacy and safety of repetitive transcranial magnetic stimulation for generalised anxiety disorder: A meta-analysis. Gen Psych. (2019) 32:e100051. doi: 10.1136/gpsych-2019-100051
38. Page MJ, McKenzie JE, Bossuyt PM, Boutron I, Hoffmann TC, Mulrow CD, et al. The PRISMA 2020 statement: an updated guideline for reporting systematic reviews. Int J Surg. (2021) 88:105906. doi: 10.1016/j.ijsu.2021.105906
39. Higgins JPT, Thomas J, Chandler J, Cumpston M, Li T, Page MJ, et al. Cochrane Handbook for Systematic Reviews of Interventions. Wiley (2019). p. 694. doi: 10.1002/9781119536604
40. Follmann D, Elliott P, Suh IL, Cutler J. Variance imputation for overviews of clinical trials with continuous response. J Clin Epidemiol. (1992) 45:769–73. doi: 10.1016/0895-4356(92)90054-Q
41. Harrer M, Cuijpers P, Furukawa TA, Ebert DD. Doing Meta-Analysis With R: A Hands-on Guide. New York, NY: Chapman and Hall/CRC (2021).
42. Ahmadizadeh MJ, Rezaei M, Fitzgerald PB. Transcranial direct current stimulation (tDCS) for post-traumatic stress disorder (PTSD): a randomized, double-blinded, controlled trial. Brain Res Bull. (2019) 153:273–8. doi: 10.1016/j.brainresbull.2019.09.011
43. Anderson IM, Delvai NA, Ashim B, Ashim S, Lewin C, Singh V, et al. Adjunctive fast repetitive transcranial magnetic stimulation in depression. Br J Psychiatry. (2007) 190:533–4. doi: 10.1192/bjp.bp.106.028019
44. Cohen H, Kaplan Z, Kotler M, Kouperman I, Moisa R, Grisaru N. Repetitive transcranial magnetic stimulation of the right dorsolateral prefrontal cortex in posttraumatic stress disorder: a double-blind, placebo-controlled study. AJP. (2004) 161:515–24. doi: 10.1176/appi.ajp.161.3.515
45. Dastjerdi G, Mirhoseini H, Mohammadi E. Investigating the synergistic effects of transcranial direct current stimulation and cranial electrical stimulation in treatment of major depression in a double blinded controlled trial. Biomed Pharmacol J. (2015) 8:1267–74. doi: 10.13005/bpj/885
46. Deppermann S, Vennewald N, Diemer J, Sickinger S, Haeussinger FB, Dresler T, et al. Neurobiological and clinical effects of fNIRS-controlled rTMS in patients with panic disorder/agoraphobia during cognitive-behavioural therapy. NeuroImage Clin. (2017) 16:668–77. doi: 10.1016/j.nicl.2017.09.013
47. Diefenbach GJ, Bragdon LB, Zertuche L, Hyatt CJ, Hallion LS, Tolin DF, et al. Repetitive transcranial magnetic stimulation for generalised anxiety disorder: a pilot randomised, double-blind, sham-controlled trial. Br J Psychiatry. (2016) 209:222–8. doi: 10.1192/bjp.bp.115.168203
48. Dilkov D, Hawken ER, Kaludiev E, Milev R. Repetitive transcranial magnetic stimulation of the right dorsal lateral prefrontal cortex in the treatment of generalized anxiety disorder: a randomized, double-blind sham controlled clinical trial. Progr Neuropsychopharmacol Biol Psychiatry. (2017) 78:61–5. doi: 10.1016/j.pnpbp.2017.05.018
49. Herrmann MJ, Katzorke A, Busch Y, Gromer D, Polak T, Pauli P, et al. Medial prefrontal cortex stimulation accelerates therapy response of exposure therapy in acrophobia. Brain Stimul. (2017) 10:291–7. doi: 10.1016/j.brs.2016.11.007
50. Holtzheimer PE, Husain MM, Lisanby SH, Taylor SF, Whitworth LA, McClintock S, et al. Subcallosal cingulate deep brain stimulation for treatment-resistant depression: a multisite, randomised, sham-controlled trial. Lancet Psychiatry. (2017) 4:839–49. doi: 10.1016/S2215-0366(17)30371-1
51. Huang Z, Li Y, Bianchi MT, Zhan S, Jiang F, Li N, et al. Repetitive transcranial magnetic stimulation of the right parietal cortex for comorbid generalized anxiety disorder and insomnia: a randomized, double-blind, sham-controlled pilot study. Brain Stimul. (2018) 11:1103–9. doi: 10.1016/j.brs.2018.05.016
52. Leong K, Chan P, Ong L, Zwicker A, Willan S, Lam RW, et al. A Randomized sham-controlled trial of 1-Hz and 10-Hz Repetitive Transcranial Magnetic Stimulation (rTMS) of the Right Dorsolateral Prefrontal Cortex in Civilian Post-traumatic Stress Disorder: Un essai randomisé contrôlé simulé de stimulation magnétique transcrânienne repetitive (SMTr) de 1 Hz et 10 Hz du cortex préfrontal dorsolatéral droit dans le trouble de stress post-traumatique chez des civils. Can J Psychiatry. (2020) 65:770–8. doi: 10.1177/0706743720923064
53. de Lima AL, Braga FMA, da Costa RMM, Gomes EP, Brunoni AR, Pegado R. Transcranial direct current stimulation for the treatment of generalized anxiety disorder: a randomized clinical trial. J Affect Disord. (2019) 259:31–7. doi: 10.1016/j.jad.2019.08.020
54. Mantovani A, Aly M, Dagan Y, Allart A, Lisanby SH. Randomized sham controlled trial of repetitive transcranial magnetic stimulation to the dorsolateral prefrontal cortex for the treatment of panic disorder with comorbid major depression. J Affect Disord. (2013) 144:153–9. doi: 10.1016/j.jad.2012.05.038
55. Nasiri F, Mashhadi A, Bigdeli I, Chamanabad AG, Ellard KK. Augmenting the unified protocol for transdiagnostic treatment of emotional disorders with transcranial direct current stimulation in individuals with generalized anxiety disorder and comorbid depression: a randomized controlled trial. J Affect Disord. (2020) 262:405–13. doi: 10.1016/j.jad.2019.11.064
56. Praško J, Záleský R, Bareš M, Horáček J, Kopeček M, Novák T, et al. The effect of repetitive transcranial magnetic stimulation (rTMS) add on serotonin reuptake inhibitors in patients with panic disorder: a randomized, double blind sham controlled study. Neuro Endocrinol Lett. (2007) 28:33–8.
57. Puigdemont D, Portella M, Pérez-Egea R, Molet J, Gironell A, Diego-Adeliño J, et al. A randomized double-blind crossover trial of deep brain stimulation of the subcallosal cingulate gyrus in patients with treatment-resistant depression: a pilot study of relapse prevention. J Psychiatry Neurosci. (2015) 40:224–31. doi: 10.1503/jpn.130295
58. Movahed FS, Goradel JA, Pouresmali A, Mowlaie M. Effectiveness of transcranial direct current stimulation on worry, anxiety, and depression in generalized anxiety disorder: a randomized, single-blind pharmacotherapy and sham-controlled clinical trial. Iran J Psychiatry Behav Sci. (2018) 12:e11071. doi: 10.5812/ijpbs.11071
59. Triggs WJ, Ricciuti N, Ward HE, Cheng J, Bowers D, Goodman WK, et al. Right and left dorsolateral pre-frontal rTMS treatment of refractory depression: a randomized, sham-controlled trial. Psychiatry Res. (2010) 178:467–74. doi: 10.1016/j.psychres.2010.05.009
60. Watts BV, Landon B, Groft A, Young-Xu Y. A sham controlled study of repetitive transcranial magnetic stimulation for posttraumatic stress disorder. Brain Stimul. (2012) 5:38–43. doi: 10.1016/j.brs.2011.02.002
61. Ahmadizadeh M-J, Rezaei M. Unilateral right and bilateral dorsolateral prefrontal cortex transcranial magnetic stimulation in treatment post-traumatic stress disorder: a randomized controlled study. Brain Res Bull. (2018) 140:334–40. doi: 10.1016/j.brainresbull.2018.06.001
62. Isserles M, Shalev AY, Roth Y, Peri T, Kutz I, Zlotnick E, et al. Effectiveness of deep transcranial magnetic stimulation combined with a brief exposure procedure in post-traumatic stress disorder – a pilot study. Brain Stimul. (2013) 6:377–83. doi: 10.1016/j.brs.2012.07.008
63. Kozel FA, Motes MA, Didehbani N, DeLaRosa B, Bass C, Schraufnagel CD, et al. Repetitive TMS to augment cognitive processing therapy in combat veterans of recent conflicts with PTSD: a randomized clinical trial. J Affect Disord. (2018) 229:506–14. doi: 10.1016/j.jad.2017.12.046
64. Osuch EA, Benson BE, Luckenbaugh DA, Geraci M, Post RM, McCann U. Repetitive TMS combined with exposure therapy for PTSD: a preliminary study. J Anxiety Disord. (2009) 23:54–9. doi: 10.1016/j.janxdis.2008.03.015
65. Philip NS, Aiken EE, Kelley ME, Burch W, Waterman L, Holtzheimer PE. Synchronized transcranial magnetic stimulation for posttraumatic stress disorder and comorbid major depression. Brain Stimul. (2019) 12:1335–7. doi: 10.1016/j.brs.2019.06.010
66. Smits FM, Geuze E, Schutter DJLG, van Honk J, Gladwin TE. Effects of tDCS during inhibitory control training on performance and PTSD, aggression and anxiety symptoms: a randomized-controlled trial in a military sample. Psychol Med. (2021) 24:1–11. doi: 10.1017/S0033291721000817
67. van 't Wout-Frank M, Shea MT, Larson VC, Greenberg BD, Philip NS. Combined transcranial direct current stimulation with virtual reality exposure for posttraumatic stress disorder: feasibility and pilot results. Brain Stimul. (2019) 12:41–3. doi: 10.1016/j.brs.2018.09.011
68. Philip NS, Barredo J, Aiken E, Larson V, Jones RN, Shea MT, et al. Theta-burst transcranial magnetic stimulation for posttraumatic stress disorder. AJP. (2019) 176:939–48. doi: 10.1176/appi.ajp.2019.18101160
69. Insel T, Cuthbert B, Garvey M, Heinssen R, Pine DS, Quinn K, et al. Research domain criteria (RDoC): toward a new classification framework for research on mental disorders. AJP. (2010) 167:748–51. doi: 10.1176/appi.ajp.2010.09091379
70. Stein DJ, Costa DL, Lochner C, Miguel EC, Reddy YC, Shavitt RG, et al. Obsessive–compulsive disorder. Nat Rev Dis Prim. (2019) 5:1–21. doi: 10.1038/s41572-019-0102-3
71. Etkin A, Prater KE, Schatzberg AF, Menon V, Greicius MD. Disrupted amygdalar subregion functional connectivity and evidence of a compensatory network in generalized anxiety disorder. Arch Gen Psychiatry. (2009) 66:1361–72. doi: 10.1001/archgenpsychiatry.2009.104
72. Ironside M, Browning M, Ansari TL, Harvey CJ, Sekyi-Djan MN, Bishop SJ, et al. Effect of prefrontal cortex stimulation on regulation of amygdala response to threat in individuals with trait anxiety: a randomized clinical trial. JAMA Psychiatry. (2019) 76:71–8. doi: 10.1001/jamapsychiatry.2018.2172
73. Bishop S, Duncan J, Brett M, Lawrence AD. Prefrontal cortical function and anxiety: controlling attention to threat-related stimuli. Nat Neurosci. (2004) 7:184–8. doi: 10.1038/nn1173
74. Lefaucheur JP, Aleman A, Baeken C, Benninger DH, Brunelin J, Di Lazzaro V, et al. Evidence-based guidelines on the therapeutic use of repetitive transcranial magnetic stimulation (rTMS): an update (2014–2018). Clin Neurophysiol. (2020) 131:474–528. doi: 10.1016/j.clinph.2019.11.002
75. Cash RFH, Weigand A, Zalesky A, Siddiqi SH, Downar J, Fitzgerald PB, et al. Using brain imaging to improve spatial targeting of transcranial magnetic stimulation for depression. Biol Psychiatry. (2021) 90:689–700. doi: 10.1016/j.biopsych.2020.05.033
76. Schönfeldt-Lecuona C, Lefaucheur J-P, Cardenas-Morales L, Wolf RC, Kammer T, Herwig U. The value of neuronavigated rTMS for the treatment of depression. Neurophysiol Clin. (2010) 40:37–43. doi: 10.1016/j.neucli.2009.06.004
77. Trevizol AP, Shiozawa P, Sato IA, Sachdev P, Sarkhel S, Cook I, et al. Transcranial magnetic stimulation for anxiety symptoms: an updated systematic review and meta-analysis. Abnorm Behav Psychol. (2016) 2:108. doi: 10.4172/2472-0496.1000108
78. Vergallito A, Gallucci A, Pisoni A, Punzi M, Caselli G, Ruggiero GM, et al. Effectiveness of noninvasive brain stimulation in the treatment of anxiety disorders: a meta-analysis of sham or behaviour-controlled studies. J Psychiatry Neurosci. (2021) 46:E592–614. doi: 10.1503/jpn.210050
79. Notzon S, Deppermann S, Fallgatter A, Diemer J, Kroczek A, Domschke K, et al. Psychophysiological effects of an iTBS modulated virtual reality challenge including participants with spider phobia. Biol Psychol. (2015) 112:66–76. doi: 10.1016/j.biopsycho.2015.10.003
80. Berlim MT, Broadbent HJ, Van den Eynde F. Blinding integrity in randomized sham-controlled trials of repetitive transcranial magnetic stimulation for major depression: a systematic review and meta-analysis. Int J Neuropsychopharmacol. (2013) 16:1173–81. doi: 10.1017/S1461145712001691
81. Duecker F, Sack AT. Rethinking the role of sham TMS. Front. Psychol. (2015) 6:210. doi: 10.3389/fpsyg.2015.00210
82. Fonteneau C, Mondino M, Arns M, Baeken C, Bikson M, Brunoni AR, et al. Sham tDCS: a hidden source of variability? Reflections for further blinded, controlled trials. Brain Stimul. (2019) 12:668–73. doi: 10.1016/j.brs.2018.12.977
83. Marković V, Vicario CM, Yavari F, Salehinejad MA, Nitsche MA. A systematic review on the effect of transcranial direct current and magnetic stimulation on fear memory and extinction. Front. Hum. Neurosci. (2021) 15:655947. doi: 10.3389/fnhum.2021.655947
84. Dejean C, Courtin J, Rozeske RR, Bonnet MC, Dousset V, Michelet T, et al. Neuronal circuits for fear expression and recovery: recent advances and potential therapeutic strategies. Biol Psychiatry. (2015) 78:298–306. doi: 10.1016/j.biopsych.2015.03.017
85. Legrand M, Troubat R, Brizard B, Le Guisquet A-M, Belzung C, El-Hage W. Prefrontal cortex rTMS reverses behavioral impairments and differentially activates c-Fos in a mouse model of post-traumatic stress disorder. Brain Sti1mul. (2019) 12:87–95. doi: 10.1016/j.brs.2018.09.003
86. Grossman N, Bono D, Dedic N, Kodandaramaiah SB, Rudenko A, Suk H-J, et al. Noninvasive deep brain stimulation via temporally interfering electric fields. Cell. (2017) 169:1029–41.e16. doi: 10.1016/j.cell.2017.05.024
87. Wang P, Zhang J, Yu J, Smith C, Feng W. Brain modulatory effects by low-intensity transcranial ultrasound stimulation (TUS): a systematic review on both animal and human studies. Front Neurosci. (2019) 13:696. doi: 10.3389/fnins.2019.00696
88. Drysdale AT, Grosenick L, Downar J, Dunlop K, Mansouri F, Meng Y, et al. Resting-state connectivity biomarkers define neurophysiological subtypes of depression. Nat Med. (2017) 23:28–38. doi: 10.1038/nm.4246
Keywords: DBS (deep brain stimulation), tDCS – transcranial direct current stimulation, rTMS (repetitive transcranial magnetic stimulation), anxiety, meta-analysis
Citation: Gay F, Singier A, Aouizerate B, Salvo F and Bienvenu TCM (2022) Neuromodulation Treatments of Pathological Anxiety in Anxiety Disorders, Stressor-Related Disorders, and Major Depressive Disorder: A Dimensional Systematic Review and Meta-Analysis. Front. Psychiatry 13:910897. doi: 10.3389/fpsyt.2022.910897
Received: 01 April 2022; Accepted: 27 May 2022;
Published: 01 July 2022.
Edited by:
Martin J. Herrmann, Julius Maximilian University of Würzburg, GermanyReviewed by:
Carmelo Mario Vicario, University of Messina, ItalyMoussa Antoine Chalah, GHU Psychiatrie et Neurosciences, France
Alessandra Vergallito, University of Milano-Bicocca, Italy
Copyright © 2022 Gay, Singier, Aouizerate, Salvo and Bienvenu. This is an open-access article distributed under the terms of the Creative Commons Attribution License (CC BY). The use, distribution or reproduction in other forums is permitted, provided the original author(s) and the copyright owner(s) are credited and that the original publication in this journal is cited, in accordance with accepted academic practice. No use, distribution or reproduction is permitted which does not comply with these terms.
*Correspondence: Thomas C. M. Bienvenu, dGhvbWFzLmJpZW52ZW51QHUtYm9yZGVhdXguZnI=; Florian Gay, Zmxvcmlhbi5nYXlAdS1ib3JkZWF1eC5mcg==
†ORCID: Florian Gay orcid.org/0000-0002-5157-5408
Francesco Salvo orcid.org/0000-0003-3210-7171
Allison Singier orcid.org/0000-0003-3151-6299
Thomas C. M. Bienvenu orcid.org/0000-0002-7416-0504