- 1Psychology Department, Psychophysiology Lab, University of Arizona, Tucson, AZ, United States
- 2Openwater, San Francisco, CA, United States
Introduction: Up to 50% of individuals fail to respond to current depression treatments. Repetitive negative thought and default mode network hyperconnectivity are central in depression and can potentially be targeted using novel neuromodulation techniques. This community-based study assessed whether a treatment using non-invasive transcranial focused ultrasound targeting the default mode network can decrease depression symptoms and repetitive negative thought, and improve quality of life.
Methods: Study recruitment began in August 2023 and ended in February 2024. Twenty individuals aged 18 – 50 were enrolled from among 247 screened. Exclusion criteria included history of psychosis/mania, acute suicidality, MRI contraindications, pregnancy, and medical and neurological factors that may complicate diagnosis or brain function. Participants completed up to three weeks of transcranial ultrasound (11 sessions) targeting the anterior medial prefrontal cortex; ten minutes per session. Depression severity (Beck Depression Inventory – II and the Hamilton Depression Rating Scale), repetitive negative thought (Perseverative Thinking Questionnaire), and quality of life (World Health Organization Quality of Life Scale) were outcomes.
Results: This sample was young (mean 30.4 years ± 10.0), predominantly female (75%), with moderate to severe depression and high comorbidity. Fifty percent of participants endorsed current psychiatric medication use. Ten percent of subjects dropped out of the study due to time constraints. Significant decreases in depression were observed over the course of treatment on self-report, 10.9 (p < 0.001, CI = -13.55, -7.92) and interview depression ratings, 4.2 (p < 0.001, CI = -5.85, -2.62), as well as significant decreases in repetitive negative thought, 8.4 (p <0.001, CI = -10.55, -6.03). Improvements in physical and psychological well-being were also observed over the course of treatment, 7.2 (p < 0.001, CI = 3.64, 10.63) and 11.2 (p < 0.001, CI = 7.79, 14.49), respectively, as well as improvements in environment satisfaction, 5.0 (p =0.001, CI = 2.24, 7.56).
Discussion: Non-invasive transcranial focused ultrasound holds promise as a treatment for depression holds promise as a treatment for depression, however, future work including control arms is required to ascertain its causal role in depression.
Clinical trial registration: https://clinicaltrials.gov/study/NCT06320028intr=Ultrasound&cond=depression&locStr=Arizona&country=United%20States&state=Arizona&rank=1, identifier NCT06320028.
Introduction
Depression is a leading cause of disability (1), affecting 21 million adults and significantly diminishing quality of life (2). Major Depressive Disorder (MDD) is typically recurrent (3–5), and impairment is compounded with subsequent episodes (6). Critically, current interventions are not effective for certain profiles of depression (7, 8).
In conjunction with depressed mood and related symptoms, Repetitive Negative Thought (RNT) has been identified as a maintaining factor in depression (9), as well as a predictor of depression improvements (8). The brain’s Default Mode Network (DMN), which has greater connectivity during self-referential processing [e.g., mind-wandering (10, 11)] and, in particular, negative self-referential processing [e.g., RNT (12)], is also shown to play an important role in depression. Studies have identified that greater DMN connectivity (e.g., hyperconnectivity) has been associated with greater depression severity and RNT (13, 14). Together, these findings highlight the mechanistic roles that RNT and DMN hyperconnectivity play in the development and maintenance of depression.
Because roughly 50% of depressed individuals are treatment-resistant to traditional treatments (7, 15), more effective interventions are needed, ideally those deriving from a better mechanistic understanding of depression. DMN connectivity has been altered (e.g., using transcranial magnetic stimulation (TMS), psychedelics, meditation) in various clinical populations (16, 17), with the goal of improving treatment approaches. A novel neuromodulation technique, non-invasive Transcranial Focused Ultrasound Stimulation (tFUS), holds promise in the treatment of depression (18, 19).
Unlike other noninvasive methods (TMS and transcranial electrical stimulation (TES) using direct (tDCS) or alternating (tACS) current), tFUS uses low-intensity ultrasound involving a focused nonthermal ultrasound beam, which safely passes through the skull (20) to exert electro-mechanical effects on target neurons, including the ability to induce excitatory and inhibitory effects depending on the ultrasound parameters used (21, 22). tFUS also presents advantages beyond other non-invasive neuromodulation techniques (e.g., TMS) due to its ability to target deeper brain regions with greater precision (22), without side effects (e.g., skin irritation, local pain) that can accompany techniques like TMS (23).
Limited research supports tFUS as a treatment for depression. Resnik and colleagues examined tFUS targeting the right inferior frontal gyrus, a component of the executive control network, on symptoms of depression; those engaging in a five-day treatment regime experienced a decrease in worry (18) compared to those receiving sham. Additionally, Sanguinetti and colleagues also found that tFUS decreased negatively-valanced emotions and altered DMN connectivity (19). These findings provide the foundation for further exploring the use of tFUS as a treatment for depression.
The present study aimed to assess whether treatment using tFUS delivered to the anterior medial prefrontal cortex (amPFC), a hub of the DMN (11), can decrease depression symptoms and RNT, improve quality of life, and whether changes in depression severity are mirrored by changes in RNT.
Methods
The Institutional Review Board of the University of Arizona approved the experimental protocol (IRB approval number: STUDY00002019). All participants signed an informed consent document before participation. Participants were recruited from August 2023 to February 2024.
Clinical Trial Registration number: 019782-00001, https://clinicaltrials.gov/study/NCT06320028intr=Ultrasound&cond=depression&locStr=Arizona&country=United%20States&state=Arizona&rank=1 identifier, NCT06320028.
Participants
Individuals with a current major depressive episode, assessed using the Structured Clinical Interview for the DSM-5 (SCID-5) (24) were enrolled. They also experienced clinically significant RNT, characterized by a total score on the Perseverative Thinking Questionnaire (PTQ) (25) above the 75% percentile (≥37).
The SCID-5 is a gold-standard, semi-structured clinical interview tool used to assess psychiatric disorders recognized by the Diagnostic and Statistical Manual of Mental Disorders, 5th edition (DSM-5) (26), including modules assessing current episode and history of depression, mania and psychosis, substance-use, anxiety-related disorders, and posttraumatic stress (24). The interrater reliability of the SCID-5 has been extensively validated, with published kappa coefficients ranging from 0.66 to 0.83 across various diagnostic modules (24), indicating good agreement on categorical judgements between raters. The PTQ is a self-report measure consisting of 15-items measuring the degree of negative thinking patterns (e.g., The same thought keeps going through my mind, Thoughts intrude into my mind) using a Likert scale of 0 (never) to 4 (almost always) for each question (25). Validation studies indicate that PTQ is a highly reliable measure of RNT (α =0.95) (25).
Participants were ages 18 – 50, right-handed, English-speaking, and without any neurological symptoms or symptoms of mania/psychosis. Additional exclusion criteria included: history of head injury with loss of consciousness; uncorrected vision and/or hearing impairment that would interfere with study participation; current or history of brain or mental illness judged likely to interfere with testing, including drug and/or alcohol dependence; a diagnosed sleep disorder (e.g., Insomnia); current drug, alcohol or prescription drug intoxication; history of epilepsy; history of diagnosed migraines; metal implants in head or face, including permanent dental retainers; history of cardiac problems that could impact brain function (e.g., atrial fibrillation); and current active suicidal ideation necessitating immediate treatment. During the consent process, participants were instructed to maintain their current medication and psychotherapy regimens and not make any changes for the duration of their study participation.
Overview of ultrasound treatment protocol
Eligible participants completed up to three weeks of ultrasound treatment. Before treatment, they completed an MRI session, a clinical interview, and self-report surveys. The first week of ultrasound involved five sessions within a seven-day period. Participants completed the same baseline assessments after completing week 1, and if they did not meet early remission criteria (defined below), they continued tFUS treatment for for two more weeks, three sessions per week, each within a seven-day period. Participants completed the same series of assessments after week 3. Participants completed a subset of the symptom outcome measures after completing week 1 and week 3 (weekly), and some after each tFUS session (daily).
Symptom outcome measures and adverse event tracking
Before any ultrasound intervention sessions, participants completed baseline surveys: Beck Depression Inventory-II (BDI-II) (27), PTQ (25), Hamilton Depression Rating Scale (HDRS) (28), the World Health Organization Quality of Life Scale (WHOQOL-BREF) (29), and the Columbia Suicide Severity Rating Scale (CSSRS) (30).
The BDI-II is a self-report measure consisting of 21 items measuring current, key symptoms of depression (e.g., sadness, loss of interest, suicidality) using a Likert response scale from 0 to 4 (e.g., 0 – I do not feel sad; 4 – I am so unhappy I cannot stand it) (27). The Hamilton Depression Rating Scale (HDRS) is a 17-item interview administered by a clinician to assess current key depression symptoms (e.g., depressed mood, pathological guilt, Suicide) on a Likert scale of 0 to 4 (e.g., 0 – absent; 4 – severe: Patient reports virtually only these feeling states in verbal and non-verbal communication, or depressed almost every day and missed three or more days of work or reports suicidal ideation for three or more days) (28). Both the BDI-II and HDRS have excellent published reliability [BDI-II α = 0.93 (27) and HDRS interrater reliability = 0.90 (28)]. As previously mentioned, the PTQ is a highly reliable measure of RNT (α =0.95) (25).
The WHOQOL-BREF is a 26-item self-report measure assessing four aspects of quality of life (QOL): physical well-being, psychological well-being, social satisfaction, and environment satisfaction (29). This measure uses a Likert scale of 1 to 5 for each question (e.g., How would you rate your quality of life? 1 – very poor; 5 – very good). The WHOQOL-BREF is a reliable measure of QOL with published alpha coefficients ranging from 0.66 – 0.8 across the four domains of QOL (29).
The CSSRS is an assessment tool for evaluating the severity of suicidal ideation and behaviors, measuring key aspects such as the intensity and frequency of suicidal thoughts, associated intent, and types of behaviors (e.g., actual, aborted, or interrupted attempts) (30). It includes both “yes or no” questions (e.g., “Have you wished you were dead or wished you could go to sleep and not wake up?”) and scaled questions (e.g., "When you have the thoughts how long do they last?": 1 - easily able to control thoughts; 5 - more than 8 hours/persistent or continuous). In prior work, the CSSRS demonstrates 100% sensitivity and specificity for identifying actual and interrupted attempts, and 99.4% specificity and 100% sensitivity for identifying aborted attempts, demonstrating high accuracy in identification while minimizing false positives (30). This measure was used in the present study to track changes in suicidal ideation throughout treatment.
These measures were re-administered following the conclusion of treatment after 1 week and 3 weeks (if applicable) of ultrasound sessions to assess weekly changes in symptoms. In addition to being administered before and after treatment, the BDI-II and PTQ were administered after each ultrasound session to assess daily symptom progression.
Before each ultrasound session, subjects were asked whether they experienced adverse events that may be due to the ultrasound. For reported events, the onset and duration of the event were noted, the severity was rated, and the relationship to study procedure was assessed. After each ultrasound session, participants completed a sensation questionnaire to assess sensations subjects may have experienced from the ultrasound, including: itching, heat/burning, tingling, vibrating/pulsing, sound, tension, and pain. Before beginning each subsequent ultrasound session (e.g., at the beginning of the next session) and acutely after completion of the sensation questionnaire, subjects were asked whether they experienced any sensations or other issues during the ultrasound session. For reported events, further probing would determine whether an adverse event related to the study occurred. If related to the study, the onset and duration of the event were noted, the severity rated, and the relationship to study procedure assessed. Additionally, SWI MRI images were collected at baseline and after treatment conclusion to provide an objective index of whether ultrasound may have created any damage to neurons or vasculature (see MRI scans section for more detail).
Early remission, remission, and response criteria
To meet early remission criteria following week 1, participants must have a BDI-II score of < 13 and a HDRS score of < 8, and a PTQ score of < 18. If any of these criteria were not met, the participant continued treatment for two additional weeks.
After completion of the treatment protocol (i.e., after week 1 or week 3), remission (defined above) and response were assessed, with a decrease of scores below 50% of baseline considered a response as commonly used in previous treatment literature (15, 31).
MRI scans
Scanning sessions included a T1 weighted structural scan, PETRA short TE scan (skull density), twelve-minute BOLD functional resting-state scan, and Susceptibility Weighted Image (SWI) before beginning ultrasound treatment, after one week of treatment, and after three weeks of treatment (if applicable). The PETRA scans were used for localization and targeting and the SWI images were assessed by board-certified neurologists to assess micro-hemorrhaging. Other MRI acquisitions are not analyzed here and will be reported in a separate paper.
Ultrasound session procedures, device specifications, and targeting precision
After localization and placement of the ultrasound device, each ultrasound session took ten minutes to complete. Participants were instructed to sit quietly, keeping their eyes open. After the ultrasound treatment was complete, the participant sat quietly for another 20 minutes, with eyes open or closed and letting their thoughts come and go.
tFUS was delivered using a custom Neuromodulation device (32) consisting of 128 element ultrasound array (Openwater) with the steerable ultrasound beam having the following parameters: acoustic frequency = 400 kHz, pulse duration = 5 ms, pulse repetition rate (PRR) = 10 Hz, a maximal spatial peak/temporal average acoustic intensity = 670 mW/cm2, peak negative pressure 820 kPa. The ultrasound probe was secured by a custom-designed headset created by Openwater. Localite Neuronavigation Software (TMS Navigator 3.3 adapted for ultrasound device) and hardware registered the position of the probe with respect to the patient’s structural MRI, providing information to develop a novel electronically-steered, stereotactic tFUS treatment plan to the personalized target for each participant’s left anterior-medial prefrontal cortex [amPFC; MNI Coordinates -5, 45, -3 (10, 33, 34)]. This target was selected because this region was defined by resting-state connectivity, showing high between-node centrality as a DMN hub and showing a large main effect of self-relevancy in task-related paradigms (10).
The ultrasound array in the custom headset was affixed at the general location of the amPFC target (MNI coordinates: -5, 45, -3) with precise targeting achieved by electronic steering within limits that meet safety parameters for ultrasound exposure (32) (Figure 1). A multi-foci, radial pattern approach was used that distributed the delivered energy in five sub-foci within 5mm from each other (which is the width of the focus in the nominal place, as defined by the -6dB pressure region). The K-Wave modeled peak energy delivery relative to the target location was highly accurate, with the -3dB centroid location of the focus falling within 1.0 +/- 1.1mm of the data measured with a hydrophone in a water tank (.02 +/-.276 mm in the lateral-axial plane, and.87 +/- 1.2mm in the axial direction). The actual pressure values estimated in K-wave and measured in the water tank agreed within 3.6 +- 1.2% within the -6dB contours. For a detailed description of translating MNI coordinates of our target into participant native space, as well as more information about the modeling approach, please see Bawiec et al. (2024) (32).
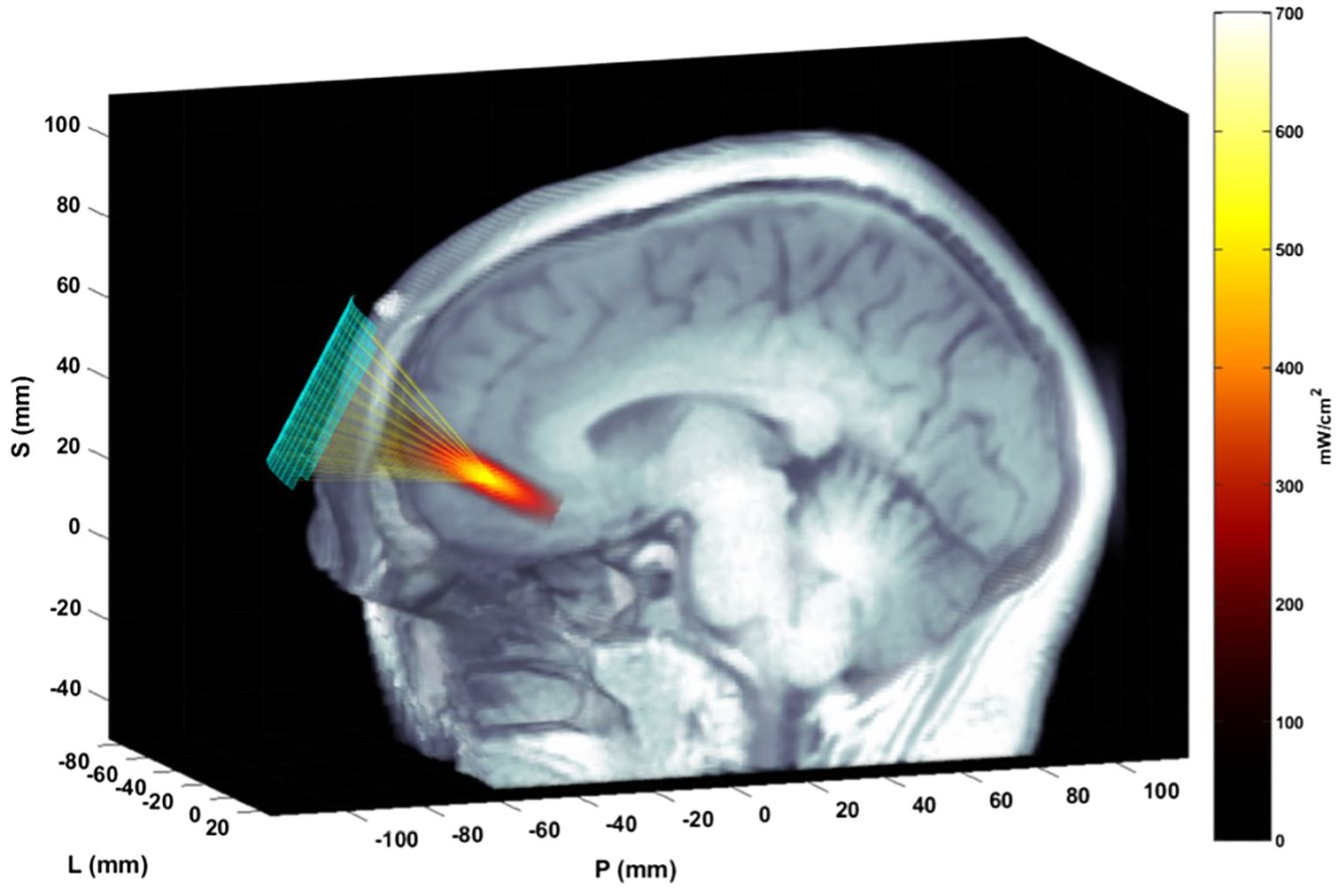
Figure 1. Ultrasound focusing to the amPFC. The matrix array transducer is positioned on the forehead and focuses sound through the skull and to the target. The transducer position is measured with the Localite TMSNavigator Neuronavigation system (Localite GmbH, Bonn, Germany). A focal spot, modeled based on the computed time delays using the ultrasound simulation package K-Wave, is overlain on the MRI image, representing the pulse-averaged spatial distribution of applied acoustic intensity.
Statistical analysis
For all statistical analyses, an alpha of 0.05 was employed and significance tests were two-tailed. Analyses were conducted in R studio (version: 2023.09.1 + 494) (35).
Seven Multi-level Models (MLM), which can account for missing data and within-subject variability, were used to assess change in the main outcomes of interest: depression symptoms (BDI-II and HDRS), RNT (PTQ), and four subscales reflecting QOL (WHOQOL-BREF physical well-being, psychological well-being, social satisfaction, and environment satisfaction subscales).
In each model, “time” was specified as the independent variable, modeling the average change in symptoms across timepoints. A random intercept was specified to account for within-subject variation in baseline symptoms.
Full information maximum likelihood estimation was applied to each model to handle missing data from three subjects who did not complete post 3 assessments. A Satterthwaite degrees of freedom adjustment was applied to each model to account for the small sample size.
Given that “time” was already scaled from 0 to 2 (baseline = 0; week 1 = 1; week 3 = 2) centering was not required. This scaling represents the progression of assessment timepoints. Bootstrap confidence intervals (CIs), a non-parametric approach that resamples the data to estimate the distribution of the model parameters, were used to assess the robustness of the results. Bootstrapping is ideal for small sample sizes and data with considerable variability.
Two linear regression models assessed the relationship between change in depression symptoms and change in RNT. Model one assessed the relationship between change in self-report depression symptoms (BDI-II) and change in RNT (PTQ) and model two assessed the relationship between change in clinical interview depression ratings (HDRS) and change in RNT (PTQ). Change scores for the BDI-II, HDRS, and PTQ were calculated as baseline minus post, with greater change values indicating a greater decrease in depression symptoms and RNT.
Results
Sample characteristics
From among 386 individuals initially contacted, 247 completed the initial pre-screen web-based survey. Eighty-six potential participants completed a phone screen to confirm responses on the pre-screen survey related to eligibility, and 35 completed the SCID for DSM-5 to confirm a diagnosis of current depression and an absence of mania/psychosis. Twenty participants were enrolled in the study (CONSORT diagram in Figure 2). Participant demographics are presented in Table 1. This relatively young (mean 30.4 years ± 10.0) and predominantly female (75%) sample had moderate to severe depression (BDI-II = 38.9 ± 9.3, HDRS = 19.9 ± 6.3, PTQ = 144.4 ± 6.2). The sample was also highly comorbid, and more than half had early onset depression (before the age of 13). Fifty percent of participants were currently taking medication related to their anxiety and/or depression during the intervention.
Thirty percent of participants did not provide race and ethnicity information. For the 70% of participants that did complete demographic information, 45% of participants identified as White, 10% Black, 5% Chinese, 5% Middle Eastern, and 5% Indian. Seventy percent of participants identified as non-Hispanic. Additionally, 45% of participants were employed part-time and 15% employed part-time, 15% were students, and 25% of participants were unemployed at the time of study enrollment.
Adverse events
Dropout rate, as one index of the acceptability of tFUS treatment, was low: 10% (2/10) did not complete treatment, discontinuing after week 1 of treatment due to lack of symptom improvement. Dropout was not due to adverse events.
No serious adverse events were reported. Reported sensations (itching, heat/burning, tingling, vibrating/pulsing, sound, tension, and pain) are presented in Table 2; for aversive sensations, the modal and median endorsement was 0 (no sensation). All means were below 2.2 on the 10-point scale. For pain and tension specifically, individual reports attributed the pain and tension to the tightness of the headset, not the ultrasound itself. Additionally, none of the participants endorsed suicidal ideation posing imminent risk to self. One subject reported a transient increase, compared to baseline, in suicidal ideation during the post 3 assessment due to a “relationship breakup” unrelated to study procedures.
SWI images acquired at baseline before tFUS sessions and again after week 1 and week 3 were read by two board-certified neuroradiologists. SWI images are sensitive to vascular micro-hemorrhages. All 20 scans per timepoint were determined to be normal with no findings on SWI, indicating that there were no microhemorrhages resulting from tFUS delivery. Three participants’ baseline SWI readings revealed nonspecific white matter hyperintensities which may be seen with chronic microangiopathic ischemic changes and decreased susceptibility which may be related to microhemorrhages. With no change in the pre and post treatment MRI scans of these presumed microhemorrhages, they were deemed chronic.
Depression symptoms and RNT
For the BDI-II and HDRS, respectively, 60% and 45% of all 20 participants met response criteria. Thirty-five percent (7/20) met remission criteria for both the BDI-II and HDRS. Significant decreases in depression severity and RNT were observed (Figure 3). Depression symptoms, characterized by the BDI-II and HDRS total scores, significantly decreased by 10.9 (p < 0.001, CI = -13.55, -7.92) and 4.2 (p < 0.001, CI = -5.85, -2.62), respectively, across time. RNT, characterized by PTQ total scores, also significantly decreased by 8.4 (p <0.001, CI = -10.55, -6.03), across time.
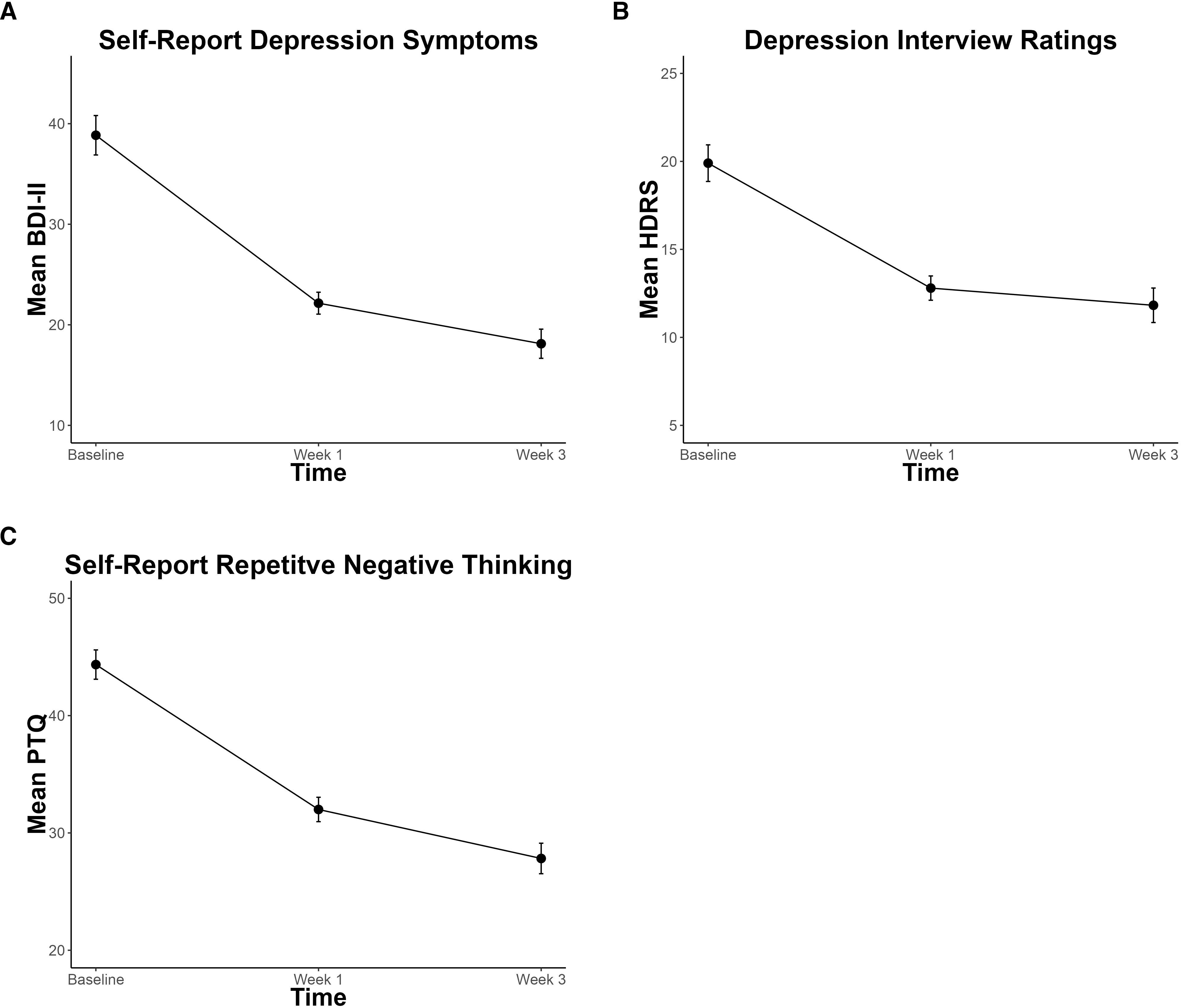
Figure 3. Significant decreases in depression symptoms and repetitive negative thought over the course of non-invasive Transcranial Focused Ultrasound Treatment, assessed by (A) Beck-Depression Inventory – II (BDI-II), (B) Hamilton Depression Rating Scale (HDRS), and (C) Perseverative Thinking Questionnaire (PTQ). Error bars represent within-participant standard error.
There was a significant positive relationship between change in depression and change in RNT (Figure 4), for both the BDI-II self-report (R2 = 0.67, F = 36.84 (1, 18), p < 0.001, CI = 0.76, 1.57) and HDRS interview ratings (R2 = 0.37, F =10.59 (1, 18), p = 0.004, CI = 0.17, 0.79).
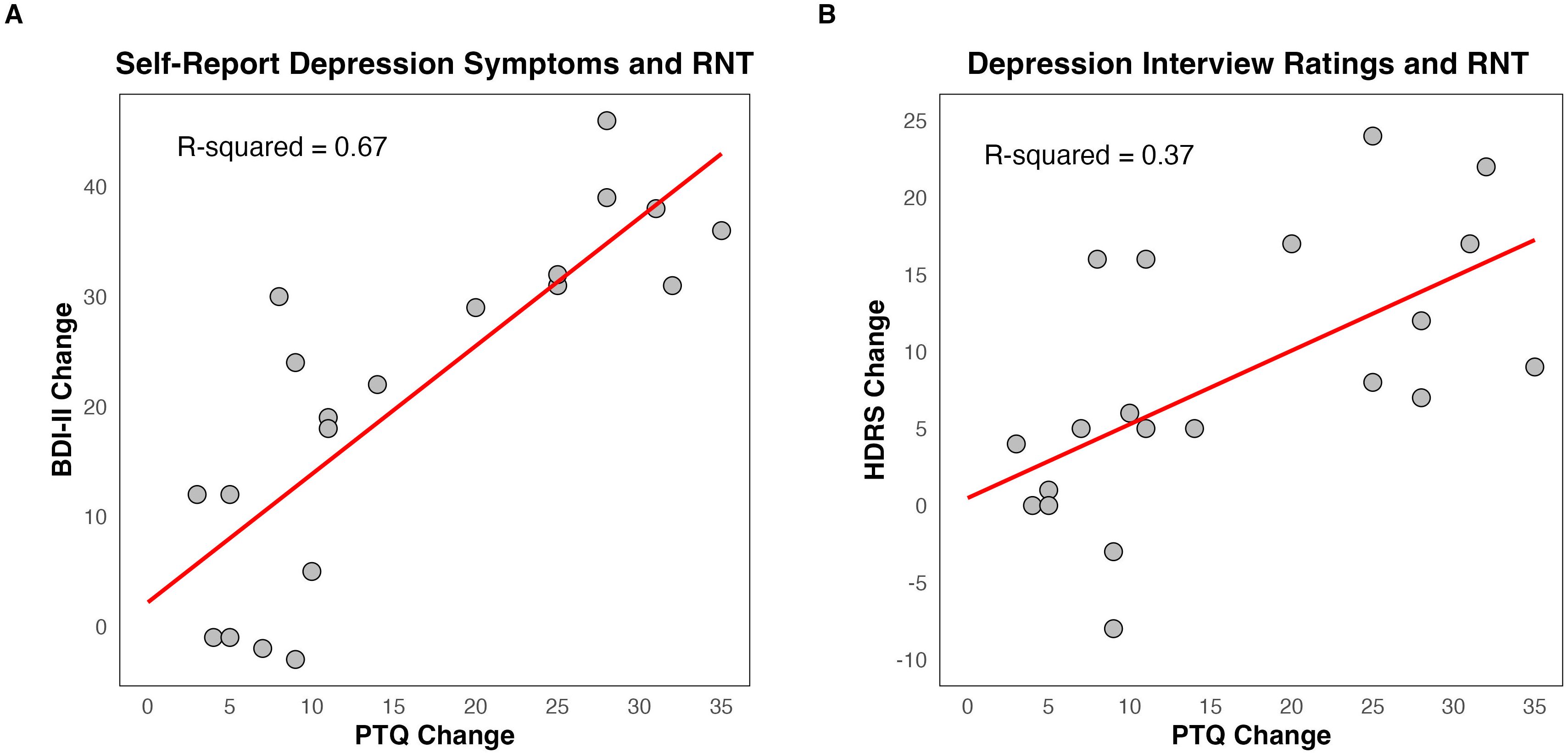
Figure 4. Relationship between change in depression symptoms and change in repetitive negative thought (RNT). (A) Change in Beck Depression Inventory – II (BDI-II) and change in Perseverative Thinking Questionnaire (PTQ). (B) Change in Hamilton Depression Rating Scale (HDRS) and change in PTQ. The scatter plot represents a linear regression containing the R-squared value to assess the strength of the relationship and the red line to visualize the linear fit. Change scores for BDI-II, HDRS, and PTQ were computed as baseline minus post scores, meaning greater positive numbers reflect a greater decrease in depression symptoms and RNT.
Quality of life
Physical and psychological well-being significantly improved by 7.2 (p < 0.001, CI = 3.64, 10.63) and 11.2 (p < 0.001, CI = 7.79, 14.49) and environment satisfaction improved by 5.0 (p < 0.001, CI = 2.24, 7.56), across time (Figure 5). No significant improvements in social satisfaction were observed (p = 0.15, CI = -0.87, 6.61).
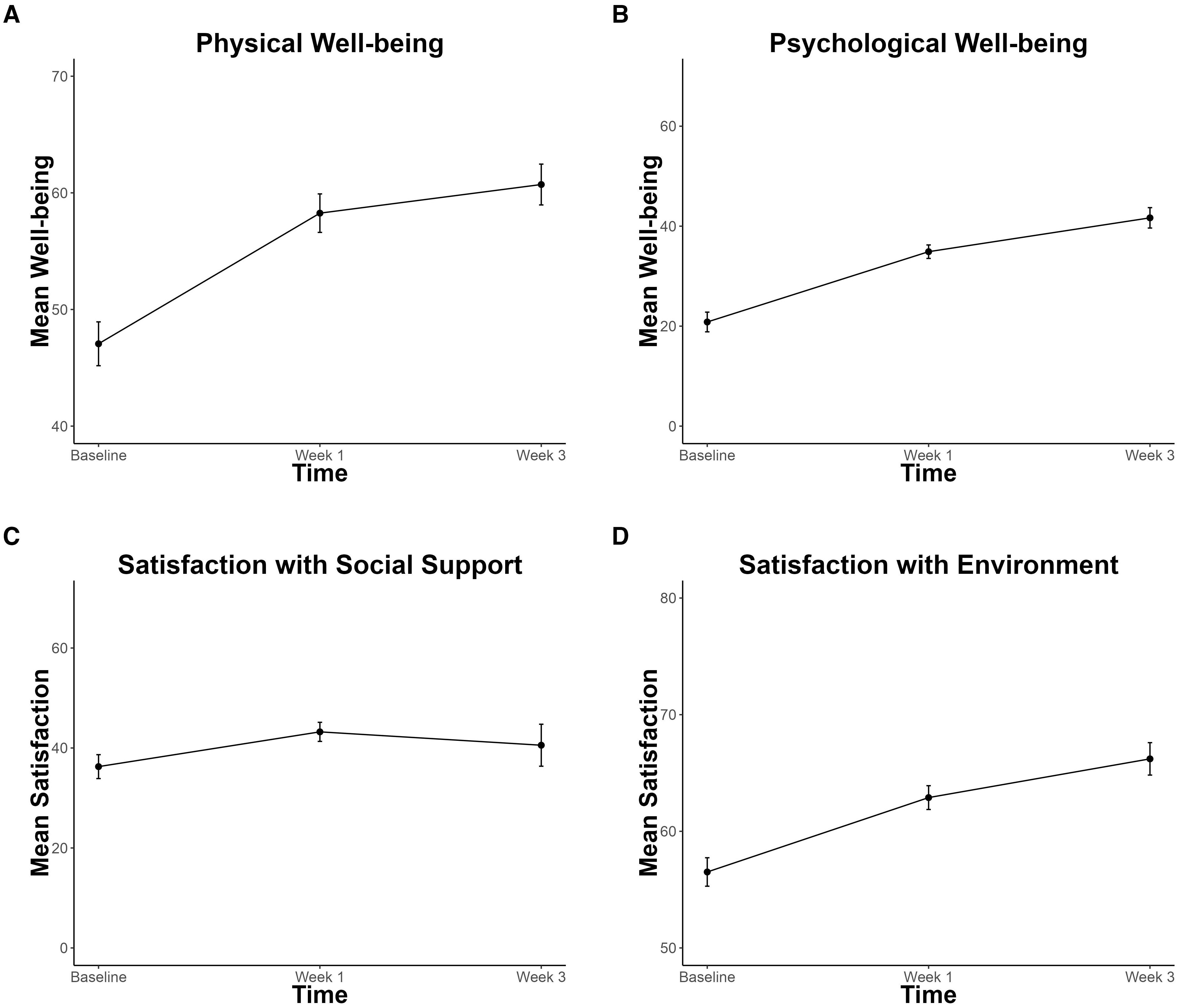
Figure 5. Improvements in Quality of Life. (A) Physical Well-being (B) Psychological Well-being (C) Social Satisfaction (D) Environment Satisfaction subscales of the World Health Organization Quality of Life Scale (WHOQOL-BREF). Significant improvement is found for panels (A, B, D). Error bars represent within-participant standard error.
Discussion
Adverse events
Transcranial focused ultrasound treatment for depression using a novel, electronically-steered, stereotactic approach was successfully delivered without serious adverse events. Participants only reported transient, mild to moderate discomfort (e.g., tension and pain) which is similar to sensations experienced in many neuromodulation treatments for depression, such as rTMS (36). Unlike TMS or tDCS, where the source of the pain and discomfort is largely due to the delivery of the magnetic stimulation itself (e.g., skin irritation, local pain) (23), several participants identified the source of the pain and tension to be from the headset. Unlike other neuromodulation techniques, such as TMS, where up to 22.6% of participants experienced headaches from the active treatment (37), there were no reports of headaches related to tFUS delivery.
On average, previous neuromodulation techniques experience a 4.5% dropout rate due to stimulation-related adverse events (38, 39). In the present study, zero percent of participants dropped out due to tFUS-related adverse events and only 10% of participants dropped out due to lack of positive effects of the treatment, which is also significantly better than dropout rates in traditional clinical depression trials, such as individual psychotherapy and pharmaceuticals with up to one-third drop out prior to treatment completion (40–42). Overall, these findings support the notion that not only is tFUS comparably safe to novel interventions such as TMS and tDCS, it may also have fewer side effects and lower dropout compared to other neuromodulation techniques.
Decreases in depression symptoms and RNT
There was a significant, observed decrease in depressed mood and RNT in individuals with current major depression over the course of treatment in just three weeks. For the BDI-II and HDRS, respectively, 60% and 45% of participants met response criteria. These percentages are comparable to traditional treatments for depression, such as antidepressants and psychotherapy (45 – 55%) in samples without substantial comorbidity; (43). The rates in the current study were achieved despite substantial comorbidity, a known poor prognostic sign (44).
A potential advantage of tFUS compared to traditional interventions is the rapidity of response: the response rate of 45-60% and remission rate of 35% occurred after just three weeks of treatment, which exceeds what has been found in rTMS interventions for depression with remission rates of as little as 18.6% and up to 30% after up to six weeks of treatment involving more sessions (36, 45). The response from tFUS also occurred with fewer sessions (11) than traditional cognitive behavioral therapy (46) [~12 – 20 sessions, once or twice per week (47)]. Given the open-label design without a control group, it is not possible to infer that tFUS is the causal reason participants experienced decreases in depression symptoms and RNT at this time. This study, however, shows initial promise for the application of tFUS for treating MDD with the potential to offer a more rapid response than traditional treatments.
Improvements in quality of life
Physical and psychological functioning, as well as satisfaction with one’s environment, significantly increased over the course of treatment. This extends previous clinical intervention work where quality of life is not commonly considered a main outcome in treatments for depression (48, 49). Additionally, certain treatments (e.g., antidepressants) fail to lead to greater improvements in quality of life compared to controls (50), which prompts an important re-evaluation of what “improvement” means when developing and validating treatment protocols. It will be critical in future work to assess sustained changes in quality of life resulting from tFUS for depression, as well as treatments for depression generally.
The lack of improvement in social satisfaction after tFUS suggests the potential for future tFUS studies to augment tFUS with interventions that are known to improve social relationships and support, such as interpersonal psychotherapy and cognitive behavioral therapy (51), as a multimodal package that addresses the full dimensionality of improving QOL. Despite the promise of tFUS on quality of life in depressed individuals based on these findings, future work with control arms is needed to ascertain the causal role of tFUS in depression.
Impact of tFUS on the DMN
tFUS is a novel neuromodulation technique that holds promise as a tool that can directly modulate brain function with precision (22). Although the direct immediate impact of tFUS on functional connectivity was not assessed in the present study, it is hypothesized that the tFUS parameters used in this open-label case series (pulse repetition rate = 10Hz, acoustic frequency = 400kHz) promoted an inhibitory effect on brain connectivity. Low pulse repetition frequency of tFUS coupled with lower acoustic frequency have been shown to have an inhibitory effect on brain activity by weakening neural firing patterns (52–55). Lord and colleagues demonstrated that targeting the other major hub of the DMN, the posterior cingulate cortex (PCC), using similar inhibitory tFUS parameters (pulse repetition frequency = 10.526Hz, acoustic frequency = 500kHz) in a healthy sample had an inhibitory effect on DMN connectivity, where there was observed decrease in connectivity between the amPFC and PCC (56). The precise mechanism of how the delivery of ultrasound energy translates to changes in neural activity, however, remains a matter of some debate (21), and more research is needed to confirm its inhibitory effects on neural function.
Role of RNT and the DMN in depression
There was a significant, positive relationship between the change in depression symptoms and change in RNT, wherein those with greater decreases in RNT experienced greater decreases in depression symptoms. These findings support previous literature identifying the relationship between RNT and depression (8, 9), however, future work requiring larger sample sizes and a control group should aim to apply more sophisticated models coupled with longitudinal datasets to assess a predictive relationship between RNT and depression.
Our results also provide preliminary support regarding the DMN’s role in depression and RNT, as we were successfully able to decrease symptoms while directly targeting a major hub of the DMN. Although the casual relationship between DMN connectivity, depression symptoms, and RNT was not assessed in the present study, it is hypothesized that through directly inhibiting DMN function, resulting in a decrease in functional connectivity within the DMN, participants are experiencing decreases in RNT and depression symptoms. It is critical that future research, namely randomized clinical trials, aim to assess the causal relationship between changes in DMN connectivity, RNT, and depression symptoms, as well as the temporal relationship between change in RNT and change in DMN connectivity throughout the course of tFUS treatment. Further evidence will include resting-state functional connectivity MRI analysis to assess whether changes in DMN connectivity track changes in depression symptoms and RNT.
Limitations and future directions
The present study provides important, preliminary evidence for the potential use of tFUS as a novel, targeted intervention for depression. A critical limitation is that this study was an open-label unblinded trial with a relatively small sample size and, as such, the present study was not able to assess the causal role of tFUS targeting the amPFC in depression treatment. To assess whether there is a causal relationship between tFUS delivery and a decrease in depression symptoms and RNT, a randomized controlled trial with active and sham ultrasound is needed to control for nonspecific factors and minimize the impact of a placebo effect.
Limitations related to the delivery of tFUS include choosing a target (amPFC) that requires traversing a region with thicker skull density compared to other potential DMN targets (e.g., PCC) and, as a result, delivering less energy to the target due to dispersion of the tFUS signal. However, we are confident that some energy was delivered, and although we cannot infer causality without a control group, we also observed decreases in depression symptoms in the present study. It is, therefore, unclear whether targeting the amPFC is the most potent approach for modulating DMN connectivity in relation to decreasing depression symptoms, and future work incorporating control arms is needed to dissect the differential impact of targeting different hubs of the DMN (56). An empirical question that also still remains is whether engaging in tasks or activities acutely after ultrasound delivery amplify or attenuate effects (57). Future work should aim to understand the optimal protocol for neuromodulation delivery (TMS, TDCS, tFUS). Finally, future work should employ cognitive measures that may relate to symptom improvement and DMN targeting (58–60). Despite these limitations, the present findings provide a strong foundation for the implementation of tFUS as a treatment for depression with pronounced and rapid observed anti-depressant effects over the course of treatment, suggesting the promise of a randomized clinical trial.
Data availability statement
The raw data supporting the conclusions of this article will be made available by the authors, without undue reservation.
Ethics statement
The studies involving humans were approved by The Human Subjects Protection Program (HSPP). The studies were conducted in accordance with the local legislation and institutional requirements. The participants provided their written informed consent to participate in this study.
Author contributions
JS: Conceptualization, Data curation, Formal analysis, Investigation, Methodology, Project administration, Software, Supervision, Visualization, Writing – original draft, Writing – review & editing. JD-F: Data curation, Methodology, Supervision, Writing – review & editing. KA: Project administration, Writing – review & editing. CB: Conceptualization, Investigation, Methodology, Resources, Software, Supervision, Writing – review & editing. PH: Investigation, Resources, Software, Visualization, Writing – original draft, Writing – review & editing. SO: Investigation, Resources, Software, Writing – review & editing. SK: Resources, Writing – review & editing. AA: Conceptualization, Resources, Supervision, Writing – review & editing. JA: Conceptualization, Funding acquisition, Methodology, Resources, Supervision, Validation, Writing – original draft, Writing – review & editing, Investigation, Software, Visualization.
Funding
The author(s) declare that financial support was received for the research and/or publication of this article. John Allen received an investigator-initiated grant from Openwater.
Acknowledgments
The authors are indebted to Diheng Zhang, Kelly Chen, Logan Blair, and Sarah Lass for their assistance with treatment sessions and study logistics. The authors wish to thank Jessica Andrews-Hanna for her role in target selection. Jessica Schachtner and John Allen had full access to all study data and take responsibility for the integrity of the data and the accuracy of the data analysis. Jessica Schachtner, affiliated with the University of Arizona, is responsible for the data analysis conducted in this manuscript.
Conflict of interest
JA received an investigator-initiated grant from Openwater. SK and PH are full-time employees of Openwater. AA, CB, & SO were full-time employees of Openwater during parts of the study. The Openwater team supported the success of the study by ensuring the ultrasound equipment was functioning properly. The Openwater team was involved in the review of the manuscript, providing their expertise in describing the ultrasound technology and providing (Figure 1). The remaining authors affiliated with the University of Arizona, JS, JF, KA, and JA declare that the research was conducted in the absence of any commercial or financial relationships that could be construed as a potential conflict of interest.
Publisher’s note
All claims expressed in this article are solely those of the authors and do not necessarily represent those of their affiliated organizations, or those of the publisher, the editors and the reviewers. Any product that may be evaluated in this article, or claim that may be made by its manufacturer, is not guaranteed or endorsed by the publisher.
References
1. World Health Organization. Depression (2024). Available online at: https://www.who.int/health-topics/depression (Accessed April 17, 2024).
2. Depression - National Institute of Mental Health (NIMH) (2024). Available online at: https://www.nimh.nih.gov/health/topics/depression (Accessed April 17, 2024).
3. Burcusa SL, Iacono WG. Risk for recurrence in depression. Clin Psychol Rev. (2007) 27:959–85. doi: 10.1016/j.cpr.2007.02.005
4. Judd LL, Paulus MJ, Schettler PJ, Akiskal HS, Endicott J, Leon AC, et al. Does incomplete recovery from first lifetime major depressive episode herald a chronic course of illness? Am J Psychiatry. (2000) 157(9):1501–4. doi: 10.1176/appi.ajp.157.9.1501
5. Solomon DA, Keller MB, Leon AC, Mueller TI, Lavori PW, Shea MT, et al. Multiple recurrences of major depressive disorder. Am J Psychiatry. (2000) 157:229–33. doi: 10.1176/appi.ajp.157.2.229
6. Kessler RC, Avenevoli S, Ries Merikangas K. Mood disorders in children and adolescents: an epidemiologic perspective. Biol Psychiatry. (2001) 49:1002–14. doi: 10.1016/S0006-3223(01)01129-5
7. Gaynes BN, Lux L, Gartlehner G, Asher G, Forman-Hoffman V, Green J, et al. Defining treatment-resistant depression. Depress Anxiety. (2020) 37:134–45. doi: 10.1002/da.22968
8. Kertz SJ, Koran J, Stevens KT, Björgvinsson T. Repetitive negative thinking predicts depression and anxiety symptom improvement during brief cognitive behavioral therapy. Behav Res Ther. (2015) 68:54–63. doi: 10.1016/j.brat.2015.03.006
9. Taylor MM, Snyder HR. Repetitive negative thinking shared across rumination and worry predicts symptoms of depression and anxiety. J Psychopathol Behav Assess. (2021) 43:904–15. doi: 10.1007/s10862-021-09898-9
10. Andrews-Hanna JR, Reidler JS, Sepulcre J, Poulin R, Buckner RL. Functional-anatomic fractionation of the brain’s default network. Neuron. (2010) 65:550–62. doi: 10.1016/j.neuron.2010.02.005
11. Buckner RL, Andrews-Hanna JR, Schacter DL. The brain’s default network. Ann N Y Acad Sci. (2008) 1124:1–38. doi: 10.1196/nyas.2008.1124.issue-1
12. Nejad AB, Fossati P, Lemogne C. Self-referential processing, rumination, and cortical midline structures in major depression. Front Hum Neurosci. (2013) 7:666. doi: 10.3389/fnhum.2013.00666
13. Sheline YI, Price JL, Yan Z, Mintun MA. Resting-state functional MRI in depression unmasks increased connectivity between networks via the dorsal nexus. Proc Natl Acad Sci U S A. (2010) 107:11020–5. doi: 10.1073/pnas.1000446107
14. Shi H, Wang X, Yi J, Zhu X, Zhang X, Yang J, et al. Default mode network alterations during implicit emotional faces processing in first-episode, treatment-naive major depression patients. Front Psychol. (2015) 6:1198. doi: 10.3389/fpsyg.2015.01198
15. Rush AJ, Trivedi MH, Wisniewski SR, Nierenberg AA, Stewart JW, Warden D, et al. Acute and longer-term outcomes in depressed outpatients requiring one or several treatment steps: a STAR*D report. Am J Psychiatry. (2006) 163:1905–17. doi: 10.1176/ajp.2006.163.11.1905
16. Gattuso JJ, Perkins D, Ruffell S, Lawrence AJ, Hoyer D, Jacobson LH, et al. Default mode network modulation by psychedelics: A systematic review. Int J Neuropsychopharmacol. (2023) 26:155–88. doi: 10.1093/ijnp/pyac074
17. Marchetti I, Koster EHW, Sonuga-Barke EJ, De Raedt R. The default mode network and recurrent depression: A neurobiological model of cognitive risk factors. Neuropsychol Rev. (2012) 22:229–51. doi: 10.1007/s11065-012-9199-9
18. Reznik SJ, Sanguinetti JL, Tyler WJ, Daft C, Allen JJB. A double-blind pilot study of transcranial ultrasound (TUS) as a five-day intervention: TUS mitigates worry among depressed participants. Neurol Psychiatry Brain Res. (2020) :37:60–6. doi: 10.1016/j.npbr.2020.06.004
19. Sanguinetti JL, Hameroff S, Smith EE, Sato T, Daft CMW, Tyler WJ, et al. Transcranial focused ultrasound to the right prefrontal cortex improves mood and alters functional connectivity in humans. Front Hum Neurosci. (2020) 14:52. doi: 10.3389/fnhum.2020.00052
20. Kubanek J. Neuromodulation with transcranial focused ultrasound. Neurosurg Focus. (2018) 44:E14. doi: 10.3171/2017.11.FOCUS17621
21. Dell’Italia J, Sanguinetti JL, Monti MM, Bystritsky A, Reggente N. Current state of potential mechanisms supporting low intensity focused ultrasound for neuromodulation. Front Hum Neurosci. (2022) 16:872639. doi: 10.3389/fnhum.2022.872639
22. Fini M, Tyler WJ. Transcranial focused ultrasound: a new tool for non-invasive neuromodulation. Int Rev Psychiatry Abingdon Engl. (2017) 29:168–77. doi: 10.1080/09540261.2017.1302924
23. Taylor R, Galvez V, Loo C. Transcranial magnetic stimulation (TMS) safety: a practical guide for psychiatrists. Australas Psychiatry. (2018) 26:189–92. doi: 10.1177/1039856217748249
24. First MB, Williams JBW, Karg RS, Spitzer RL. Structured clinical Interview for DSM-5 Disorders-Clinician Version SCID-5-CV. Arlington, VA: American Psychiatric Association (2016). Available at: https://www.appi.org/Products/Interviewing/Structured-Clinical-Interview-for-DSM-5-Disorders (Accessed February 18, 2024).
25. Ehring T, Zetsche U, Weidacker K, Wahl K, Schönfeld S, Ehlers A. The Perseverative Thinking Questionnaire (PTQ): Validation of a content-independent measure of repetitive negative thinking. J Behav Ther Exp Psychiatry. (2011) 42:225–32. doi: 10.1016/j.jbtep.2010.12.003
26. American Psychiatric Association. Diagnostic and statistical manual of mental disorders, 5th ed. (2022) American Psychiatric Association. Maine. doi: 10.1176/appi.books.9780890425787
27. Dozois DJA, Dobson KS, Ahnberg JL. A psychometric evaluation of the Beck Depression Inventory–II. Psychol Assess. (1998) 10:83–9. doi: 10.1037/1040-3590.10.2.83
28. Hamilton M. A rating scale for depression. J Neurol Neurosurg Psychiatry. (1960) 23:56–62. doi: 10.1136/jnnp.23.1.56
29. The World Health Organization quality of life assessment (WHOQOL). World Health Organization Group. Soc Sci Med. (1995) 41:1403–9. doi: 10.1016/0277-9536(95)00112-K
30. Posner K, Brown GK, Stanley B, Brent DA, Yershova KV, Oquendo MA, et al. The columbia–suicide severity rating scale: initial validity and internal consistency findings from three multisite studies with adolescents and adults. Am J Psychiatry. (2011) 168:1266–77. doi: 10.1176/appi.ajp.2011.10111704
31. Rush AJ, Kraemer HC, Sackeim HA, Fava M, Trivedi MH, Frank E, et al. Report by the ACNP task force on response and remission in major depressive disorder. Neuropsychopharmacology. (2006) 31:1841–53. doi: 10.1038/sj.npp.1301131
32. Bawiec CR, Hollender PJ, Ornellas SB, Schachtner JN, Dahill-Fuchel JF, Konecky SD, et al. A wearable, steerable, transcranial low-intensity focused ultrasound system. J Ultrasound Med. (2024) 44(2):239–61. doi: 10.1002/jum.16600
33. Fox MD, Snyder AZ, Vincent JL, Corbetta M, Van Essen DC, Raichle ME. The human brain is intrinsically organized into dynamic, anticorrelated functional networks. Proc Natl Acad Sci. (2005) 102:9673–8. doi: 10.1073/pnas.0504136102
34. Kaiser RH, Andrews-Hanna JR, Wager TD, Pizzagalli DA. Large-scale network dysfunction in major depressive disorder: A meta-analysis of resting-state functional connectivity. JAMA Psychiatry. (2015) 72:603–11. doi: 10.1001/jamapsychiatry.2015.0071
35. RStudio Team. RStudio: Integrated Development for R. Boston, MA: RStudio (2020). Available at: http://www.rstudio.com/ (Accessed February 18, 2024).
36. Miron JP, Jodoin VD, Lespérance P, Blumberger DM. Repetitive transcranial magnetic stimulation for major depressive disorder: basic principles and future directions. Ther Adv Psychopharmacol. (2021) 11:20451253211042696. doi: 10.1177/20451253211042696
37. Wang WL, Wang SY, Hung HY, Chen MH, Juan CH, Li CT. Safety of transcranial magnetic stimulation in unipolar depression: A systematic review and meta-analysis of randomized-controlled trials. J Affect Disord. (2022) 301:400–25. doi: 10.1016/j.jad.2022.01.047
38. Moffa AH, Brunoni AR, Fregni F, Palm U, Padberg F, Blumberger DM, et al. Safety and acceptability of transcranial direct current stimulation for the acute treatment of major depressive episodes: Analysis of individual patient data. J Affect Disord. (2017) 221:1–5. doi: 10.1016/j.jad.2017.06.021
39. O’Reardon JP, Solvason HB, Janicak PG, Sampson S, Isenberg KE, Nahas Z, et al. Efficacy and safety of transcranial magnetic stimulation in the acute treatment of major depression: A multisite randomized controlled trial. Biol Psychiatry. (2007) 62:1208–16. doi: 10.1016/j.biopsych.2007.01.018
40. Cooper AA, Conklin LR. Dropout from individual psychotherapy for major depression: A meta-analysis of randomized clinical trials. Clin Psychol Rev. (2015) 40:57–65. doi: 10.1016/j.cpr.2015.05.001
41. Elkin I, Shea MT, Watkins JT, Imber SD, Sotsky SM, Collins JF, et al. National institute of mental health treatment of depression collaborative research program: general effectiveness of treatments. Arch Gen Psychiatry. (1989) 46:971–82. doi: 10.1001/archpsyc.1989.01810110013002
42. MaChado M, Iskedjian M, Ruiz I, Einarson TR. Remission, dropouts, and adverse drug reaction rates in major depressive disorder: a meta-analysis of head-to-head trials. Curr Med Res Opin. (2006) 22:1825–37. doi: 10.1185/030079906X132415
43. Khan A, Faucett J, Lichtenberg P, Kirsch I, Brown WA. A systematic review of comparative efficacy of treatments and controls for depression. PloS One. (2012) 7:e41778. doi: 10.1371/journal.pone.0041778
44. Kraus C, Kadriu B, Lanzenberger R, Zarate CA Jr., Kasper S. Prognosis and improved outcomes in major depression: a review. Transl Psychiatry. (2019) 9:1–17. doi: 10.1038/s41398-019-0460-3
45. Berlim MT, van den Eynde F, Tovar-Perdomo S, Daskalakis ZJ. Response, remission and drop-out rates following high-frequency repetitive transcranial magnetic stimulation (rTMS) for treating major depression: a systematic review and meta-analysis of randomized, double-blind and sham-controlled trials. Psychol Med. (2014) 44:225–39. doi: 10.1017/S0033291713000512
46. Bruijniks SJE, Lemmens LHJM, Hollon SD, Peeters FPML, Cuijpers P, Arntz A, et al. The effects of once- versus twice-weekly sessions on psychotherapy outcomes in depressed patients. Br J Psychiatry. (2020) 216:222–30. doi: 10.1192/bjp.2019.265
48. Hofmann SG, Curtiss J, Carpenter JK, Kind S. Effect of treatments for depression on quality of life: a meta-analysis*. Cognit Behav Ther. (2017) 46:265–86. doi: 10.1080/16506073.2017.1304445
49. Kolovos S, Kleiboer A, Cuijpers P. Effect of psychotherapy for depression on quality of life: meta-analysis. Br J Psychiatry. (2016) 209:460–8. doi: 10.1192/bjp.bp.115.175059
50. Almohammed OA, Alsalem AA, Almangour AA, Alotaibi LH, Yami MSA, Lai L. Antidepressants and health-related quality of life (HRQoL) for patients with depression: Analysis of the medical expenditure panel survey from the United States. PloS One. (2022) 17:e0265928. doi: 10.1371/journal.pone.0265928
51. Gaines AN, Constantino MJ, Coyne AE, Atkinson LR, Bagby RM, Ravitz P, et al. Change in satisfaction with social support as a common outcome in interpersonal psychotherapy and cognitive behavioral therapy for depression. J Psychother Integr. (2023) 33:457–64. doi: 10.1037/int0000303
52. Fomenko A, Neudorfer C, Dallapiazza RF, Kalia SK, Lozano AM. Low-intensity ultrasound neuromodulation: An overview of mechanisms and emerging human applications. Brain Stimulat. (2018) 11:1209–17. doi: 10.1016/j.brs.2018.08.013
53. Dalecki D. Mechanical bioeffects of ultrasound. Annu Rev BioMed Eng. (2004) 6:229–48. doi: 10.1146/annurev.bioeng.6.040803.140126
54. Blackmore J, Shrivastava S, Sallet J, Butler CR, Cleveland RO. Ultrasound neuromodulation: A review of results, mechanisms and safety. Ultrasound Med Biol. (2019) 45:1509–36. doi: 10.1016/j.ultrasmedbio.2018.12.015
55. Kuhn T, Spivak NM, Dang BH, Becerra S, Halavi SE, Rotstein N, et al. Transcranial focused ultrasound selectively increases perfusion and modulates functional connectivity of deep brain regions in humans. Front Neural Circuits. (2023) 17:1120410. doi: 10.3389/fncir.2023.1120410
56. Lord B, Sanguinetti JL, Ruiz L, Miskovic V, Segre J, Young S, et al. Transcranial focused ultrasound to the posterior cingulate cortex modulates default mode network and subjective experience: an fMRI pilot study. Front Hum Neurosci. (2024) 18:1392199. doi: 10.3389/fnhum.2024.1392199
57. Ziebell P, Rodrigues J, Forster A, Sanguinetti JL, Allen JJ, Hewig J. Inhibition of midfrontal theta with transcranial ultrasound explains greater approach versus withdrawal behavior in humans. Brain Stimul Basic Transl Clin Res Neuromodulation. (2023) 16:1278–88. doi: 10.1016/j.brs.2023.08.011
58. Vatansever D, Menon DK, Manktelow AE, Sahakian BJ, Stamatakis EA. Default mode network connectivity during task execution. NeuroImage. (2015) 122:96–104. doi: 10.1016/j.neuroimage.2015.07.053
59. Sambataro F, Visintin E, Doerig N, Brakowski J, Holtforth MG, Seifritz E, et al. Altered dynamics of brain connectivity in major depressive disorder at-rest and during task performance. Psychiatry Res Neuroimaging. (2017) 259:1–9. doi: 10.1016/j.pscychresns.2016.11.001
Keywords: mood disorder, transcranial ultrasonic neuromodulation, repetitive negative thinking (RNT), depression, default mode network
Citation: Schachtner JN, Dahill-Fuchel JF, Allen KE, Bawiec CR, Hollender PJ, Ornellas SB, Konecky SD, Achrol AS and Allen JJB (2025) Transcranial focused ultrasound targeting the default mode network for the treatment of depression. Front. Psychiatry 16:1451828. doi: 10.3389/fpsyt.2025.1451828
Received: 19 June 2024; Accepted: 26 February 2025;
Published: 04 April 2025.
Edited by:
Andrea Fiorillo, University of Campania Luigi Vanvitelli, ItalyReviewed by:
Gregory Fonzo, The University of Texas at Austin, United StatesGiulio Longo, Marche Polytechnic University, Italy
Copyright © 2025 Schachtner, Dahill-Fuchel, Allen, Bawiec, Hollender, Ornellas, Konecky, Achrol and Allen. This is an open-access article distributed under the terms of the Creative Commons Attribution License (CC BY). The use, distribution or reproduction in other forums is permitted, provided the original author(s) and the copyright owner(s) are credited and that the original publication in this journal is cited, in accordance with accepted academic practice. No use, distribution or reproduction is permitted which does not comply with these terms.
*Correspondence: Jessica N. Schachtner, am5zY2hhY2h0bmVyQGFyaXpvbmEuZWR1; am5zY2hhY2h0bmVyQGRvbnMudXNmY2EuZWR1