- Department of Pediatrics, Dalian Municipal Women and Children’s Medical Center (Group), Affiliated to Dalian Medical University, Dalian, Liaoning, China
The role of gut microecology in attention deficit hyperactivity disorder (ADHD) has garnered growing attention. Studies have suggested a potential link between ADHD development and an imbalance in gut microbiota composition. This review aims to analyze the characteristics of the gut microbiota in children with ADHD, explore how changes in the gut microbiota affect ADHD through nervous, neuroendocrine, and immune pathways, and discuss the potential application of microecological agents and fecal microbiota transplantation in the prevention and treatment of ADHD in children. Pubmed, Google Scholar, EBSCO, Scopus and Medline were utilized to conduct searches using the following key terms:Attention Deficit Hyperactivity Disorder OR ADHD AND gut microbiota OR probiotics OR prebiotics OR synbiotics OR fecal microbiota transplantation OR FMT. Studies published in English from all years were included. A thorough review of numerous papers and their references was conducted to identify relevant articles. Sorting and analysis revealed that the gut microbiota of children with ADHD has changed to some extent, and targeting the gut microbiota, using microecological agents or fecal microbiota transplantation, especially in combination with central nervous system stimulants, may provide additional benefits for children with ADHD.
1 Introduction
Attention deficit hyperactivity disorder (ADHD), a prevalent chronic neurodevelopmental disorder in childhood, is primarily characterized by attention deficits and/or hyperactive impulses, disproportionately related to developmental stage (1). Children with ADHD typically exhibit poor academic performance and interpersonal relationships, and the impairment usually persists into adolescence and beyond (2). Specifically, 40-60% of ADHD patients experience partial relief, while 15% still meet diagnostic criteria in adulthood (3). ADHD often co-exists with other neurodevelopmental disorders, such as tic disorders, intellectual disability, autism spectrum disorders (ASD), communication disorders, and specific learning or motor disorders (e.g. developmental coordination disorder, reading ability impairment) (4–6).
In 2012, an epidemiological study found that 6.8% of children and adolescents in Spain had ADHD (7). In 2015, a meta-analysis reported a global ADHD prevalence rate of 7.2% (8). In 2018, the global prevalence of ADHD ranged from 2% to 7%, with an average of about 5% (9). In addition, at least 5% of children have hyperactivity, inattention, and impulsivity that are less severe than the diagnostic criteria for ADHD (9). In the same year, a study by the American National Survey of Children’s Health revealed ADHD diagnoses in 8.4% of children between the ages of 2–17 (10). Another meta-analysis published in 2018 revealed a 5.6% prevalence of ADHD in Chinese children, notably more common in boys (7.7%) compared to girls (3.4%) (11). The latest meta-analysis of global ADHD prevalence in 2023 showed that 7.6% of children between 3 and 12 years and 5.6% of adolescents aged 12 to 18 years were diagnosed with ADHD (12). It can be seen that the prevalence of ADHD in children is high and rising. ADHD not only significantly impacts the quality of life for children and their families, but also imposes a heavy burden on society (13–16).
The underlying cause of ADHD is unknown, but first-degree relatives of ADHD patients have a five to ninefold higher risk of developing ADHD than those without (17). Twin studies show ADHD heritability is about 76% (18). A meta-analysis identified serotonin transporter gene, dopamine transporter gene, and norepinephrine transporter gene as significant positional candidate genes for ADHD status (19, 20). In 2023, a genome-wide association study (GWAS) of ADHD reported 27 significant loci, highlighting 76 potential risk genes that are particularly enriched during early brain development (21). At the same time, a meta-analysis of GWAS showed that approximately 22% of the genetic predisposition was caused by single nucleotide polymorphisms (SNPs) inheritance (22, 23). SNPs are the most frequently occurring genetic variation in the human genome, which are important markers in many studies that link sequence variations to phenotypic changes (24). However, attributing the onset of ADHD entirely to genetic factors cannot explain the high incidence of ADHD in recent years, and attention also needs to be paid to between other factors, such as environmental factors of ADHD.
Environmental factors have a multifaceted impact on ADHD, involving a range of prenatal, perinatal, and postnatal factors. Prenatal factors include maternal stress, substance exposure and environmental toxins. Studies have shown that children whose mothers experienced moderate to severe stress during pregnancy exhibited more severe ADHD symptoms compared to those with less stressful prenatal environments. Mothers who drink or smoke during pregnancy, or who are exposed to lead can interfere with fetal brain development and increase the likelihood of neurobehavioral disorders, and thereby increase the risk of ADHD in their offspring (25, 26). Perinatal factors such as preterm birth, low birth weight and caesarean section are associated with a higher incidence of ADHD in offspring (27). Postnatal factors like breastfeeding, exposure to pollutants and chemicals, allergies and gut microecological disorders can exacerbate ADHD symptoms (28). Shorter breastfeeding periods or lack of exclusive breastfeeding may increase the risk of ADHD symptoms in children (29). Studies have found a high prevalence of allergic comorbidity among children with ADHD (30, 31). Several studies have found that children with allergic diseases have a significantly increased risk of co-occurring ADHD, with risk increasing with the severity and number of allergy symptoms (32, 33). Imbalance of gut microbiota through the gut-brain axis has been implicated in ADHD and is an increasingly studies area (34, 35). In conclusion, environmental factors play a significant role in the development and exacerbation of ADHD symptoms in children.
Regarding ADHD symptom management, behavioral management should be the initial treatment for children <6 with ADHD, medication is recommended as the first-line treatment for ≥6 with ADHD, classified as both stimulants (methylphenidate and amphetamine formulations) and non-stimulants (atomoxetine, viloxazine, clonidine, and guanfacine) (2, 36, 37). Numerous clinical trials have demonstrated the efficacy of stimulants like methylphenidate and amphetamine in reducing ADHD symptoms in short-term treatment (38). A meta-analysis of more than 10,000 children and adolescents found moderate to large effects of methylphenidate and amphetamine based on clinician and teacher assessments of symptom changes (38). However, there are potential side effects of medication, such as insomnia, loss of appetite, nausea, and emotional instability, etc (39). Additionally, 20-35% of children with ADHD poorly respond to treatment (40), and reduced medication adherence can also result from long-term administration tolerance (41). Furthermore, concerns exist about the selection and use of stimulants medications in three areas: the impact of long-term treatment on growth, the likelihood of drug abuse and dependence, and the chance of cardiovascular incidents owing to sympathomimetic properties (2). Thus, the limitations of ADHD medication highlight the importance of continuing to search for new and improved ways to management ADHD.
In recent years, the concept of gut microbiota has taken center stage in scientific research, particularly in understanding its role in the intricate bidirectional communication between the brain and gut. This microscopic ecosystem has been discovered to potentially influence the development of ADHD via multiple distinct pathways (42–44). As understanding of these relationships deepens, the use of microecological agents, which are substances that help to regulate and balance the gut microbiota, has garnered significant attention among researchers and clinicians as a potential therapeutic approach. These agents hold promise as a novel and natural method to regulate gut microbiota imbalances often observed in ADHD patients.
This review examines the gut-brain axis, the characteristics of gut microecology in children with ADHD, the possible mechanisms of gut microecology disorders affecting ADHD and the application of microecological agents in ADHD, with the goal of providing new insights for the prevention and treatment of ADHD.
2 Methods
To investigate the associations between attention deficit hyperactivity disorder, gut microecology, and microecological agents in children, we conducted a comprehensive literature review, which included both clinical and experimental studies. Searchers were carried out utilizing databases like EBSCO, Pubmed, Scopus, Google Scholar, Medline, focusing on the following key terms: gut-brain axis OR (gut microbiota AND central nervous system) OR (gut microbiota AND enteric nervous system AND central nervous system); (attention deficit hyperactivity disorder OR ADHD) AND gut microbiota; (attention deficit hyperactivity disorder OR ADHD) AND (probiotics OR prebiotics OR synbiotics); (attention deficit hyperactivity disorder OR ADHD) AND (fecal microbiota transplantation OR FMT). Research spanning all years were incorporated. The search was limited to published by English.
3 Gut-brain axis
The human gastrointestinal tract harbors a diverse ecosystem, spanning from the oral cavity to the rectum, with a surface area of 150–200 m2 providing ample habitat for microorganisms. The gut content contains a high number of bacteria, ranging from 100,000 to 100 billion per milliliter. Gut microecology has a profound effect on various gastrointestinal and non-gastrointestinal functions. These microorganisms play crucial roles in digestion and absorption of various nutrients, such as proteins, carbohydrates, bile acids, vitamins, and so on (45, 46). Furthermore, gut microbiota has been reported to influence non-gastrointestinal functions, such as brain development, immunity, and maturation of the endocrine system (47–49).
The gut has been found to interact with the brain along what is known as the gut-brain axis (50). This interaction occurs via multiple pathways including neural, immune, endocrine and metabolic. Gut microbiota can produce neurotransmitters directly or indirectly, via the host’s biosynthetic routes, and imbalances in this process can lead to disorders in neurotransmitter and neurodevelopmental function (51, 52). Beneficial bacteria like Bifidobacterium, Lactobacillus, and Bacillus produce various neurotransmitters such as dopamine (DA), norepinephrine (NE), 5-hydroxytryptamine (5-HT), gamma-aminobutyric acid (GABA), acetylcholine (ACh), and histamine, among others (53). The aforementioned neurotransmitters enter the central nervous system (CNS) via the enteric nervous system (ENS) and influence the physiological functions of the brain (54, 55). Research indicates that Bifidobacterium and certain Lactobacillus species like L. plantarum and L. brevis, are known to produce GABA, an inhibitory neurotransmitter crucial for regulating neuronal excitability (56, 57). These bacteria, including Streptococcus, Enterococcus, and Escherichia coli, have been implicated in 5-HT production. 5-HT is primarily synthesized in the gut and plays a significant role in mood regulation (55, 58). Some bacteria, such as Bacillus species, are known to produce DA, which has the ability to reinforce reward-causing behaviors, affect attention, memory, and mood, but Escherichia coli may influence dopamine levels indirectly through their metabolic activities (55, 59). NE is a neurotransmitter involved in attention, arousal, and executive functions. It helps regulate the brain’s ability to focus and maintain attention, which are often impaired in ADHD (60). Several types of gut flora have been identified as capable of producing NE, such as Bacillus mycoides, Bacillus subtilis, Proteus vulgaris, and Escherichia coli (55, 59, 61). In addition, gut microbiota affects the nervous system partially through bacterial metabolites, especially short-chain fatty acids (SCFAs). SCFAs are the main metabolites produced in the colon by bacterial fermentation of dietary fibers and resistant starch (62), which impact neurodevelopment, immune signaling, and the integrity of the blood-brain barrier (BBB) (63). ADHD patients (both children and adults) have significantly lower SCFA levels compared to healthy controls, with medicated individuals showing the most pronounced reductions (64, 65). Lower SCFAs may contribute to pro-inflammatory states, as ADHD patients exhibit elevated immune markers (e.g., sICAM-1, sVCAM-1) alongside SCFA deficits (65). The mechanisms by which short-chain fatty acids affect ADHD include the following three areas: SCFAs influence dopamine, serotonin, and norepinephrine signaling—key pathways disrupted in ADHD (66, 67). SCFAs regulate gut barrier integrity and interact with vagal nerve signaling, indirectly influencing brain function (64, 66). SCFAs like butyrate modulate immune responses and reduce neuroinflammation, which is implicated in ADHD pathophysiology (64, 67).
The gut-brain axis signal transduction mechanism involves two primary barriers: the intestinal barrier and BBB. The intestinal barrier serves as the first line of defense between the human body and the intestinal lumen, and its function impacts the transport of substances between the body and the intestinal lumen, as well as the invasion of pathogens (68). Disturbance of gut microbiota increases intestinal permeability by disrupting mucosal surface biofilms, leading to lipopolysaccharide translocation and hyperactivation of the immune system. This results in elevated levels of pro-inflammatory cytokines (69). Excessive release of pro-inflammatory cytokines is detrimental to the CNS (70). SCFAs, including propionic, butyric, and acetic acids, are end products of bacterial fermentation and function as signaling molecules with anti-inflammatory properties. They also protect colonic epithelial cells (71). Numerous studies indicate that SCFAs maintain barrier integrity, protect the BBB from oxidative stress, and promote tight junction protein expression (72, 73).
Gut microbiota also modulates the permeability and integrity of the BBB. The BBB plays an important role in regulating the exchange between the cerebrospinal fluid and the circulatory system, helping to maintain the stability of the internal environment of the CNS (74). The gut microbiota influences the permeability of BBB by regulating the expression of tight junction proteins (75). Research on mice indicates a heightened permeability of the BBB in germ-free (GF) compared to normal mice (76, 77). Subsequent studies have demonstrated that treatment with sodium butyrate can effectively restore tight junction proteins and reduce BBB permeability in GF mice (78).
An increasing number of studies suggest a close relationship between alterations in gut microbiota diversity and composition and various central nervous system diseases, including ADHD, ASD, obsessive-compulsive disorder, Alzheimer’s disease, Parkinson’s disease, and multiple sclerosis (79, 80). Numerous studies have demonstrated that the composition of the gut microbiome in individuals with various psychiatric disorders differs from that of healthy individuals (81, 82). By meticulously regulating disordered gut microbiota, it is possible to witness a notable improvement in neurological functioning to a certain extent (83). In summary, gut microbiota is crucial in maintaining the balance between health and disease, particularly in relation to brain function and behavior.
4 Gut microbiota characteristics of children with ADHD
Recent studies have explored the relationship between childhood ADHD and the gut microbiome. Among these, two studies have linked the composition of the gut microbiome in infancy to the development of ADHD in children. In 2015, Pärtty et al. showed that babies with higher levels of Bifidobacterium in their gut microbiota had a lower risk of developing ADHD in the future (84). In 2023, Cassidy-Bushrow et al. published a birth cohort study showing that early life gut microbiome characteristics were linked to ADHD development by age 10. The gut microbiota of children with ADHD at 6 months of age was different, characterized by a lack of lactic acid bacteria like Lactobacillales and Enterococcaceae (85). These two prospective studies indicate that focusing on the microecological composition of the gut early in life is crucial for the development of ADHD. Concurrently, an increasing number of scientists are conducting cross-sectional studies to examine the changes in gut microbiota of children with ADHD.
In 2017, Aarts et al. analyzed the gut microbiota of ADHD patients and controls using 16S rRNA. They found a decrease in the abundance of Firmicutes and an increase of Actinobacteria and Bifidobacterium in ADHD patients (86). In 2018, Prehn-Kristensen et al, discovered a notable decrease in gut microbiota diversity in boys with ADHD, and the composition varied greatly between patients and controls (87). ADHD children exhibited elevated levels of Bacteroidaceae, Neisseriaceae and Neisseria spec have been identified as potential indicators of ADHD in adolescents. However, the study’s limitation lies in its inability to exclude the drug’s effects on the gut microbiota (87). Jiang et al. demonstrated a significant reduction in Faecalibacterium in the gut microbiota of children with ADHD, which was negatively associated with symptoms reported by parents (88). In 2019, Stevens et al. found a strong association between the relative ADHD symptom scores and abundance of Bifidobacterium (89). In 2020, Wang et al. reported elevated gut microbiota diversity in children with ADHD, along with decreased relative abundance of Bacteroides coprocola and increased relative abundance of Bacteroides ovatus, Sutterella stercoricanis, and Bacteroides uniformis (79). In 2020, Szopinska-Tokov et al. continued to expand the sample size based on the study by Aarts et al. and seven genera of gut microbiota were found to be correlated with ADHD symptom scores in patients with ADHD (86, 90).
In 2022, Wang et al. revealed that children with ADHD had higher levels of Agathobacter, Anaistipes and Lachnospiraceae UCG-010, lower levels of plasma TNF-α levels than healthy children, and a negative correlation between TNF-α levels and ADHD symptom scores and gut microbiota diversity (91). In the same year, Lee et al. discovered that Agathobacter in the gut microbiota was linked to withdrawal and depressive symptoms in ADHD children, while Ruminococcus gnavus was associated with rule-breaking behavior (92). In 2023, Bundgaard-Nielsen et al. found that the relative abundance of Eggerthella was higher in children with ADHD, in agreement with the results of Aarts (86). The relative abundance of Colobacterium spp、Cholera spp and Hodgkinella spp was low, which is consistent with the findings of Prehn-Kristensen (86, 87, 93).
All these studies noted differences in the gut microbiota composition in children with ADHD based on 16S rRNA, but their results may be limited by differences in population and dietary patterns. Metagenomic technology has advanced, enabling an increasing number of ADHD studies to utilize it, thereby improving the accuracy of results.
In 2020, Wan et al. found no significant differences in alpha diversity in gut microbiota of children with ADHD and healthy children, but significant differences in the composition of the microbiota. Compared to healthy children, ADHD had substantially reduced abundances of Faecalibacterium and Veillonellaceae, and markedly increased abundances of Odoribacter and Enterococcus. At species level, the abundance of Ruminocococockus gnavus, Faecalibacterium prausnitzii and Lachnospiraceae bacterium in children with ADHD decreased significantly, while the abundance of Paraprevotella xylaniphila, Odoribacter spanchnicus, Veillonella parvula, and Bacteroides caccae increased significantly (94). The reduced abundance of Faecalibacterium observed in this study is consistent with the findings of Jiang et al. (88).
In 2022, Li et al. through metagenomic technology from 207 fecal samples that the children’s gut microbiome with ADHD was more abundant in Prevotella (amnii, buccae and copri) and Bifidobacterium (breve and bifidum) (95). This result is consistent with the findings of Aarts (66). In addition, the presence of distinguishable gut microbiota patterns in ADHD subgroups predicts that more accurate gut microbiota information can be obtained through subgroup analysis. It was further shown that Bacteroides ovatus supplementation improved spatial working memory deficit and reversed EEG rhythm in ADHD rats (95). In 2023, Stiernborg et al. investigated the impact of neuroleptics on the ADHD gut microbiome and found that children with drug-treated ADHD had significantly different β-diversity, lower functional gut microbiota, and lower abundance of the strain Bacteroides stercoris CL09T03C01 and bacterial genes encoding an enzyme in vitamin B12 synthesis, as compared to the control group (96). In 2023, Wang et al. discovered variations in β-diversity between children with ADHD and healthy controls by high-throughput next-generation sequencing, with significantly increased abundance of Ascomycetes and Candida in children with ADHD (97). In 2024, Wang et al. found that the gut microbiota of children with ADHD was characterized by enrichment of Anaerostipes_hadrus, Lachnospira, and Phascolarcto - bacterum_ faecium, which might help identify potential biomarkers of ADHD (98). Changes in the gut microbiome of children with ADHD are presented in Supplementary Table 1.
Although the above studies of the gut microbiome in children with ADHD vary due to differences in populations inclusion, sample sizes, and testing methods, leading to diverse findings and even contradictory conclusions, upon more detailed analysis, there has been some evidence of altered gut microbiota in children with ADHD using different testing methods (Figure 1). Therefore, it is important to explore the possible mechanisms by which gut microecology affects ADHD.
5 Possible mechanism of gut microbiota imbalance affecting ADHD
The gut microbiota comprises trillions of microorganisms residing in the human intestine, including bacteria, archaea, fungi, viruses, protozoa, and other organisms, that exist in the human intestinal environment (67, 99). The gut microecology provides the host with various functions, such as the production of vitamins, absorption of calcium ions, resistance against pathogens, and enhancement of immune function (100, 101). Growing evidence suggests that gut microecology affects brain development and function (102–104). Gut microecological disorders may directly or indirectly affect neural function through neural, metabolic, immune pathways and vice versa (105).
5.1 Nerve pathway
Control of the intestine is by the autonomic nervous system (ANS) and ENS. The vagus nerve, the 10th cranial nerve, is a major component of the ANS and plays a critical role in emotion regulation (106), and stress regulation by affecting the hypothalamic-pituitary-adrenal (HPA) axis (49). Mouse models had demonstrated that activation of gastrointestinal vagal afferents influenced rewarding behavior (67), while vagotomy attenuated aggressive behavior in ADHD rats (107). Gut microbiota can modulate host emotional and behavioral responses via vagal afferents. Pathogenic infections such as Campylobacter jejuni and Citrobacter aminopropionate induced anxiety-like behaviors in animal models (108). Long-term intake of Lactobacillus rhamnosus GG (LGG) can regulate the mRNA level of GABA receptors via the vagus nerve, thereby alleviating anxiety symptoms in mice (109).
Recent studies have also reported potential mechanisms for the interaction between the gut microbiota and ENS. ENS is a complex network of neurons and glial cells that communicate with CNS through afferent neurons that carry sensory information along the spinal cord and vagus nerve pathways. The ENS also controls secretion and motility in the gastrointestinal tract, which contributes to the maintenance of the intestinal barrier integrity (110). ENS dysfunction is associated not only with a variety of gastrointestinal problems, but also with an increasing number of CNS illnesses, such as ASD (111). It has been shown that ENS changes occur in the early stages of some neurological diseases, even prior to overt CNS involvement (112). ENS maturation and function are influenced by gut microecology, which may involve activation of pattern recognition receptors, including Toll-like receptors and 5-HT expression (113, 114). Antibiotic-mediated gut microbiota disturbance leads to reduced intestinal glial cell number, ENS structural and functional changes in mice (115). A different study likewise found that GF mice’s myenteric plexus’s AH neurons were less excitable, leading to decreased gastrointestinal motility, indicating gut microbiota is crucial for ENS development (116). Animal experiments further showed that restoring gut microbiota could effectively improve the intestinal function of antibiotic-induced mice and increase the number of intestinal glial cells and neurons, while supplementation of SCFAs could restore neuronal loss caused by antibiotics (117). The irregular excitability of the ENS caused by gut microbiota imbalance was also significantly reduced after supplementation with Bifidobacterium longum (118). The above studies indicate that gut microecology may further alter host mood and behavior patterns by affecting the ANS and/or ENS, which is of great significance for understanding gut-brain axis interaction, especially ADHD pathogenesis.
5.2 Neuroendocrine pathway
ADHD-related brain networks are innervated by neurotransmitters such as DA, NA, GABA and 5-HT et al. Impaired reward sensitivity, issues with attention and working memory, and poor behavioral regulation (hyperactivity and impulsivity) can all result from disorders involving these neurotransmitters (60, 119–122). The synthesis and control of multiple neurotransmitters are concurrently linked to the gut flora. The gut microbiota communicates with CNS by producing neurotransmitter precursors/neurotransmitters or metabolites. Among them, DA, 5-HT, GABA and SCFAs are crucial in the development of disorders affecting the neurological system (16, 95). DA receptors, particularly D1 and D2, are involved in signaling reward circuits, learning, and memory. A higher density of the DA transporter in ADHD patients can lead to altered dopamine levels in the synaptic cleft, affecting cognitive processes (123). 5-HT influences mood and impulse control. Increasing serotonin levels can help manage ADHD symptoms related to impulsivity and hyperactivity (124, 125). GABA is the primary inhibitory neurotransmitter, helping to reduce neuronal excitability. Lower GABA levels have been linked to increased impulsivity in ADHD (126). SCFAs, produced by gut microbiota, play a role in the gut-brain axis. They influence glucose metabolism and immune regulation, potentially impacting brain function (64, 127).
Catecholamines, including DA and NA, are key neurotransmitters that influence mood, behavior, and a host of other processes in the central and peripheral nervous systems (128–130). The gut microbiome has the capacity to create DA, NA, and their precursors (131). Asano et al. found that the intestinal lumen of GF mice had lower levels of free DA and NA than those of specific pathogen-free (SPF) animals. The levels of DA and NA in the intestinal lumen significantly increased in GF mice after receiving fecal transplantation from SPF mice, suggesting that gut microbiota is involved in the generation of intestinal catecholamines (132). In addition, Prevotella and Bacteroides in the intestine can indirectly affect DA bioavailability by directly affecting DA transporters or changing the level of intestinal peptide hormones (133). Oral administration of Lactobacillus plantarum PS128 can improve DA-related neurological diseases (134). Aarts et al. discovered a negative correlation between cyclohexadienyl dehydratase and reward anticipatory responses, as well as a significant increase in the relative abundance of the Bifidobacterium genus in fecal samples from ADHD patients. This genus is involved in the synthesis of the dopaminergic precursor, phenylalanine (86). An increasing amount of evidence indicates that the gut bacteria may be involved in the production and breakdown of NA. It has shown that E. coli K-12, Bacillus spp and Saccharomyces spp can produce NA (55). In the brain, NA inhibits the transcription of inflammatory genes and increases the level of brain-derived neurotrophic factor produced by microglia and astrocytes, thus further promoting neuronal survival and neuroprotection (135).
GABA is critical to the pathophysiology of ADHD. Children with ADHD have been shown to have lower GABA levels, and GABA imbalances are associated with reduced ability to focus on high-difficulty tasks (136, 137). Bifidobacterium, Lactobacillus and Escherichia coli have been documented to generate GABA (138, 139). By raising GABA levels, early Lactobacillus intervention can lower the incidence of ADHD in later life (84, 140).
The neurotransmitter 5-HT is crucial for neuronal transmission and is implicated in the etiology of ADHD. Children with ADHD exhibit lower 5-HT levels compared to healthy individuals, and the primary symptoms of ADHD, such as impulsivity, hyperactivity, and inattention, are linked to these low levels of 5-HT (141). Although there is no evidence that 5-HT levels in the gut are directly related to ADHD brain function, approximately 90% of 5-HT in the body is produced by Enterochromaffin cells, and the gut microbiota can alter the utilization of the availability of tryptophan, a 5-HT precursor (142, 143). Tryptophan can cross the BBB and affect 5-HT synthesis in the central nervous system (144). Clinical studies have demonstrated that ADHD patients have lower 5-HT concentrations, consistent with observations in GF mice, and tryptophan supplementation can alleviate ADHD-related symptoms (145).
SCFAs are gut microbiota products that degrade cellulose and dietary fibers (146). High-dose propionic acid administration during pregnancy alters social behavior in rat pups during the neonatal period and adolescence (147). Studies have found lower plasma and fecal SCFA levels in ADHD patients compared to healthy controls. Propionic acid levels have been negatively correlated with ADHD symptom severity, suggesting its potential as a biomarker (64, 127). Butyrate possesses anti-inflammatory properties, inhibiting pro-inflammatory cytokines synthesis such as IL-6 and IL-12, and inducing anti-inflammatory cytokine IL-10 release (148). Another study revealed SCFAs improved reduced microglia activity in GF mice (149). Psychostimulant medications, commonly used to treat ADHD, may alter gut microbiota composition and SCFA levels, potentially affecting the gut-brain axis and treatment outcomes (64).
The HPA axis is the key to neuroendocrine transmission and stress response systems, and one study found abnormal HPA axis function in severely hyperactive ADHD children (150). Another study finds delayed HPA axis response to stress linked to ADHD impulsive behavior (131). The HPA axis’s growth and regulation can be influenced by gut bacteria (49). The HPA axis of GF mice is over-responsive to restraint stress, and early administration of Bifidobacterium is effective in reversing the excessive HPA stress response in GF mice (151).
The above studies suggest that gut microbiota impacts the nervous system by influencing neurotransmitters or SCFAs, and that this pathway may also be an important way in which disordered gut microbiota impacts ADHD.
5.3 Immune pathway
The gut microbiota plays a critical role in regulating the host immune system and mitigating inflammation and immune-mediated illnesses (152–154). Dysbiosis, or alterations in the gut microbiota, can disrupt tight junction protein expression and trigger neuroinflammatory responses (78, 155), impairing both intestinal barrier and blood-brain barrier (BBB) function (156).
Disruption of the BBB is a proposed mechanism for neurological disorders including ADHD (157, 158). Microglia are critical for regulating BBB integrity and function. The brain’s immune system is primarily composed of microglia. Microglia participate in neuroinflammatory processes and brain homeostasis regulation. Their development and maturation depend highly on gut microbiota (42, 159). It has been shown that reduced gut microbiota diversity can cause microglia dysfunction linked to neurodegenerative diseases or behavioral disorders, via neuroinflammation (42).
There is evidence that people with ADHD have a significantly increased risk of atopic dermatitis, allergic rhinitis, eczema, and autoimmune diseases (32). Individuals with atopic disorders have a significantly increased risk of developing ADHD in the future, ranging from 30-50%, compared to non-atopic children (32). A meta-analysis finds mild inflammation in peripheral cytokine levels in patients with ADHD (160). ADHD children had higher levels of circulating pro-inflammatory cytokines, including TNF-α, IL-16, and IL-13 (161, 162). Another study found that behavior in ADHD patients was associated with inflammatory markers, with IL-13 levels linked to inattention and IL-16 levels linked to hyperactivity (161). The possible mechanisms by which gut microbiota affects ADHD through various pathways are shown in Figure 2.
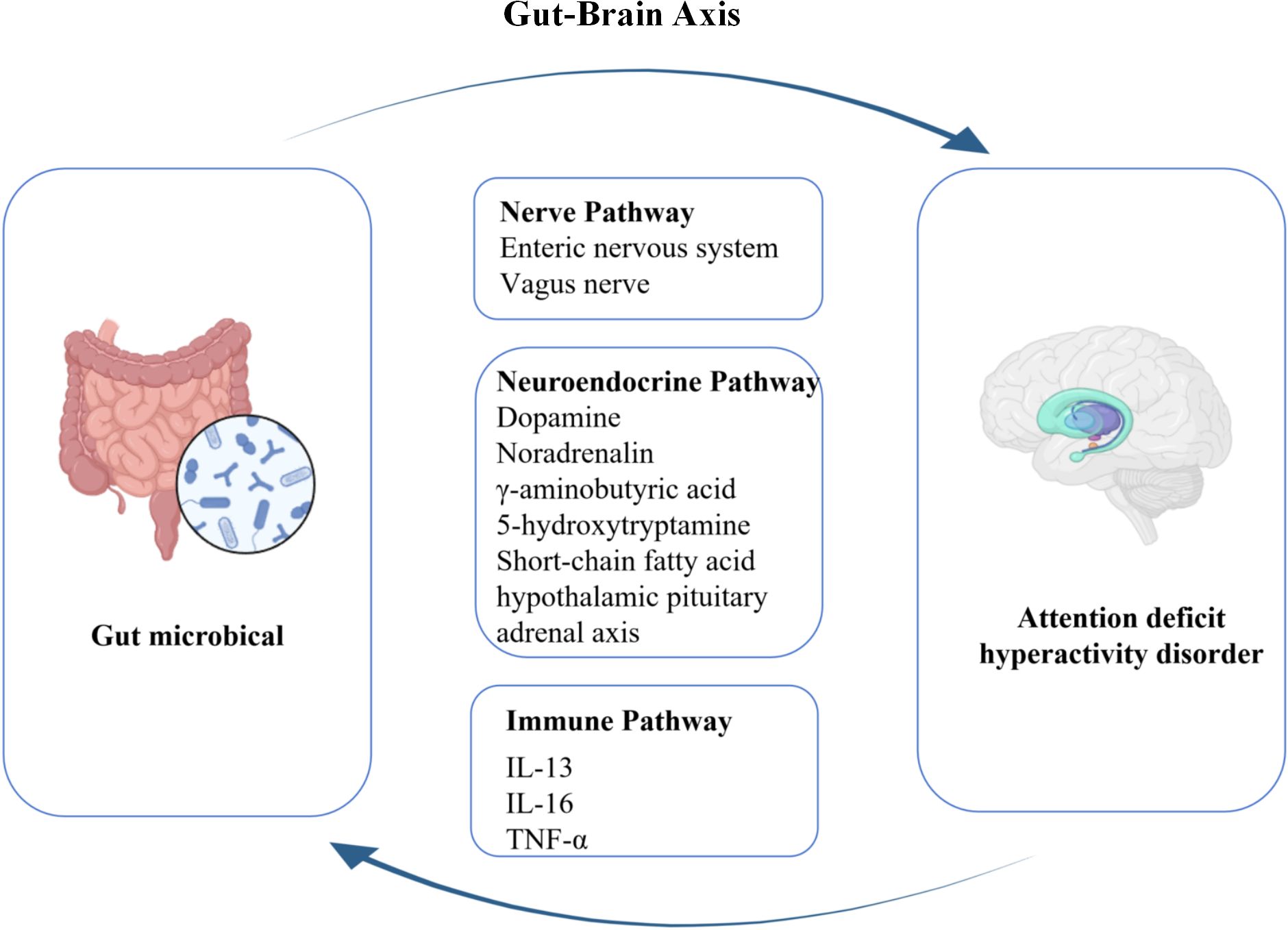
Figure 2. The possible mechanisms of gut microbiota in influencing ADHD via the gut-brain axis. IL, Interleukin; TNF-α, Tumor Necrosis Factor-α.
6 Microecological agent in ADHD
According to the disruption of the gut microbiota in ADHD children, the gut microbiota affects neurodevelopmental disorders, especially ADHD, through the gut-brain axis. Thus, targeting the gut microbiota has become a cutting-edge treatment strategy for ADHD. Currently, the common types of microecological agents are probiotics, prebiotics and synbiotics.
6.1 Probiotics
Probiotics are microorganisms that benefit the host and improve or restore gut microbiota. Currently, the clinical applications of probiotics mainly include inflammatory bowel disease, lactose intolerance, allergies and neurological diseases (163). Research indicates probiotics may positively impact Alzheimer’s disease and Parkinson’s disease by modulating the gut-brain axis, neurotransmitter production, and reducing neuroinflammation, as well as alleviating anxiety, depression and cognitive disorders. They may also have clinical benefits for children with ADHD (164).
In 2015, Pärtty et al. published the first study on probiotics for preventing ADHD, which showed that early LGG treatment decreased the incidence of ASD and ADHD by the age of 13 (84). In 2017, Bazinet et al. conducted a double-blind, randomized, placebo-controlled research to investigate the impact of probiotic supplementation on children’s anxiety, ADHD, and memory (165). Probiotics contained Bifidobacterium longum R0175 and Lactobacillus helveticus R0052, the treatment course was 13 weeks. A total of 48 children with ADHD (6–15 years old) participated in the study, which showed that probiotic supplementation did not significantly improve symptoms in children with ADHD as a whole. However, after excluding children treated with stimulants, those who were not treated with medication showed a reduction in hyperactivity and inattentiveness, and an improvement in memory functioning (165). This suggests that the effects of probiotics on ADHD may be influenced by other medications.
In 2020, Kumperščak et al. published a randomized, double-blind, placebo-controlled trial enrolling 32 children and adolescents with ADHD (aged 4–17 years), examining changes in ADHD symptoms, the Child Self-Report of the Pediatric Quality of Life Inventory™, and serum cytokine levels in the two groups before LGG treatment and after 3 months of treatment (166). The study showed a significant improvement in The Quality of Life scores after 3 months of LGG supplementation in children with ADHD, whereas there was no substantial change in the group receiving a placebo. Nonetheless, there were no variations between the two groups’ hardcore symptoms, mood, or behavior. TNF-α and IL-12 p70, two pro-inflammatory serum cytokines, were only lower in the probiotic group (166). The findings imply that LGG supplementation might be advantageous, but more thorough investigation with a longer observation period and more participants is necessary due to the variations in inflammatory cytokine levels.
In 2021, Ahmadvand et al. included 34 children with ADHD (8–12 years) in a randomized, double-blind, placebo-controlled trial (167). All children were randomly assigned to receive either probiotic supplement (n=17) or placebo (n=17) for 8 weeks on top of Ritalin, a stimulant for the treatment of ADHD. The probiotics included Bifidobacterium bifidum, Lactobacillus fermentum, Lactobacillus acidophilus, and Lactobacillusreuteri. At the start of the trial and after 8 weeks of treatment, clinical symptoms were documented using the Hamilton Anxiety Rating Scale (HAM-A), Children’s Depression Inventory (CDI), and rating scale of ADHD (ADHD-RS). In addition, blood samples were taken at the start and eight weeks to assess metabolic information. The results showed that the addition of probiotics positively affected the levels of ADHD-RS, HAM-A, C-reactive protein in serum and total antioxidant capacity, while there was no effect on CDI and other metabolic profiles (167). This study was the first clinical study to explore the effect of probiotic supplementation on children with ADHD who were undergoing Ritalin treatment. Unfortunately, the research was not able to evaluate changes in gut microbiota and its gene expression related to inflammation and oxidative stress to clarify the pathogenesis.
In 2022, Wang et al. used Bifidobacterium bifidum (Bf-688) for the first time as ADHD supplementation (168). 30 ADHD children, ages 4 to 16, completed this single-arm, open-label trial, and all subjects received Bf-688 daily for 8 weeks. Fecal samples were collected for gut microbiota testing at baseline, the 4th and 8th weeks. At the 4th and 8th weeks of Bf-688 supplementation, the children with ADHD showed improvements in their symptoms of inattention and hyperactivity/impulsivity, as well as a rise in their weight and BMI. Gut microbiota testing revealed a significant decrease in the ratio of Firmicutes to Bacteroidetes (F/B) from baseline to week 8. Throughout the course of the 8-week treatment, Firmicutes significantly declined while Proteobacteria greatly rose. Bacteroidota considerably declined and Shigella greatly rose after Bf-688 was stopped for four weeks. The authors concluded that Bf-688 significantly improved clinical symptoms in children with ADHD by affecting the gut microbiota’s makeup. However, the research was a single-arm trial, ADHD symptom scoring was relatively subjective, and the sample size was insufficient for performing a subgroup analysis, so the results need further verification.
In 2022, Ghanaatgar et al. used compound probiotics as a supplement to Ritalin for the treatment of ADHD (169). 38 children between the ages of 6 and 12 finished the double-blind, randomized, placebo-controlled study. The probiotic capsules used in the study contained 14 strains and were administered for 8 weeks. The Conners Parent Rating Scale–short version (CPRS–RS) and the Clinical Global Impression–Severity (CGI–S) scale were collected from all subjects before the intervention, at the conclusion of four weeks and eight weeks. When compared to the placebo group, the compound probiotic group’s CPRS-RS and CGI-S outcomes were noticeably better. This study clearly showed that compound probiotics, as a supplement to Ritalin, could provide clinical benefits to children with ADHD. However, the study lacked gut microbiota testing results and was not able to follow up long-term after treatment. As a result, care should be taken when extrapolating the findings to the whole ADHD population. Detailed clinical studies on probiotics for the treatment of ADHD are shown in Table 1.
In 2024, Yin et al. found in an animal research that Bifidobacterium animalis subsp. lactis A6 (BAA6) could ameliorate hyperactivity symptoms and spatial memory deficits in ADHD rats, increase the number of neurons in the hippocampus, elevate neurotransmitters levels such as DA, NA and ACh in brain tissue, improve brain-derived neurotrophic factor and reduce glutamate (170). Lactobacillus, Romboutsia, Blautia, and Turicibacter were significantly enriched in the intestinal tract of ADHD rats after BAA6 intervention (170). This study shows that BAA6 has great potential as a dietary supplement that may improve memory dysfunction associated with ADHD through the gut-brain axis.
6.2 Prebiotics/synbiotics
Non-digestible components of food called prebiotics specifically increase the action of probiotics that are already in the intestines. When combined, probiotics and prebiotics form synbiotics. While there is a lack of clinical or basic research on prebiotics as a treatment for ADHD. In 2020, Skott et al. published a randomized, double-blind, controlled trial to evaluate the impact of Synbiotic 2000 on symptoms of autism, ADHD, and functional impairment (171). For 9 weeks, 182 children and adults received either Synbiotic 2000 or a placebo daily. The outcomes showed that both Synbiotic 2000 and the placebo reduced ADHD symptoms. There were no significant differences in ADHD functioning or autistic symptoms between the two groups. Although no clear specific effects of Synbiotic 2000 were observed, an examination of patients with elevated levels of soluble vascular cell adhesion molecule-1, suggested that Synbiotic 2000 might improve autism symptoms in children and regulate mood in adults with ADHD.
7 Fecal microbiota transplantation
FMT is the process of transferring healthy donor feces into a patient (receiver) in order to balance the gut microbiota, mend the intestinal mucosal barrier, manage inflammation, and adjust the immune system. Studies have demonstrated that transplantation of fecal microbiota from patients with different mental illnesses into GF mice results in abnormal behavior (172–174). Another study found that transplanting the fecal microbiota of ADHD patients into mice not only altered the composition of gut microbiota, but also their brain structure, functions and behavioral (175).
There are currently no studies investigating FMT for children with ADHD. The only case report on FMT for adult ADHD was published by Hooi et al. in 2022 (176). A 22-year-old woman who experienced multiple episodes of severe diarrhea due to a recurrent Clostridioides difficile infection. Following TMT treatment, comorbid ADHD and anxiety symptoms were alleviated, with the CGI–S dropping from 5 points before treatment to 2 points. Fecal samples were subjected to metagenomic sequencing both before and after FMT, and the results revealed that a total of 106 bacteria were elevated or colonized and 59 bacteria were reduced or eradicated after treatment. Three prominent engrafted species that have been negatively associated with ADHD in the past include Faecalibacterium prausnitzii, Lactobacillus ruminis, and Lactobacillus rogosae. Important species linked to the pathophysiology of ADHD, including Bacteroides ovatus, Bacteroides uniformis, Enterococcus avium, Enterococcus gallinarum, Fusobacterium ulcerans, Bifidobacterium adolescentis, Bifidobacterium animalis, Bifidobacterium breve and Bifidobacterium longum, were all found to be diminished after FMT. Although the patient in this report was a 22-year-old adult female, this case provides hope for the future of FMT treatment for children with ADHD.
The studies mentioned above show that microecological agents have demonstrated positive therapeutic effects in the prevention and treatment of ADHD: supplementing with LGG in early life can significantly reduce the risk of ADHD、compound probiotics as a Ritalin supplement can dramatically improve the symptoms in children with ADHD、the advantages of FMT for adult ADHD may open new directions for the treatment of children with ADHD in the future.
8 Conclusion
In summary, there is an alteration in the gut microbiome of children with ADHD, and the gut microbiota can affect ADHD through neural, endocrine, and immune pathways. Therefore, targeting the gut microbiota, with microecological agents or FMT, especially in combination with central nervous system stimulants, may provide additional benefits for children with ADHD. Future, large-scale, randomized, controlled clinical trials of microecological agent or FMT are needed to explore their effectiveness and safety for children with ADHD.
Author contributions
YL: Writing – original draft, Writing – review & editing, Supervision, Validation. PZ: Writing – original draft, Writing – review & editing, Conceptualization, Formal Analysis, Software. HS: Formal Analysis, Funding acquisition, Supervision, Validation, Writing – review & editing.
Funding
The author(s) declare that financial support was received for the research and/or publication of this article. This work was supported by the Liaoning Provincial Doctoral Research Start-up Fund of China (2023010655-JH3/101).
Conflict of interest
The authors declare that the research was conducted in the absence of any commercial or financial relationships that could be construed as a potential conflict of interest.
Generative AI statement
The author(s) declare that no Generative AI was used in the creation of this manuscript.
Publisher’s note
All claims expressed in this article are solely those of the authors and do not necessarily represent those of their affiliated organizations, or those of the publisher, the editors and the reviewers. Any product that may be evaluated in this article, or claim that may be made by its manufacturer, is not guaranteed or endorsed by the publisher.
Supplementary material
The Supplementary Material for this article can be found online at: https://www.frontiersin.org/articles/10.3389/fpsyt.2025.1588135/full#supplementary-material
Abbreviations
ADHD, Attention deficit hyperactivity disorder; ASD, Autism spectrum disorders; GWAS, Genome-wide association study; DA, Dopamine; NE, Norepinephrine; 5-HT, 5-hydroxytryptamine; GABA, Gamma-aminobutyric acid; Ach, Acetylcholine; CNS, Central nervous system; ENS, Enteric nervous system; SCFAs, Short-chain fatty acids; BBB, blood-brain barrier; GF, Germ-free; ANS, Autonomic nervous system; HPA, Hypothalamic-pituitary-adrenal; LGG, Lactobacillus rhamnosus GG; SPF, Specific pathogen-free; IL, Interleukin; TNF-α, Tumor necrosis factor-α; HAM-A, Hamilton anxiety rating scale; CDI, Children’s depression inventory; ADHD-RS, Rating scale of ADHD; Bf-688, Bifidobacterium bifidum; F/B, Firmicutes to Bacteroidetes; CPRS–RS, Conners parent rating scale–short version; CGI–S, Clinical global impression–severity; Hs-CRP, High-sensitivity C-reactive protein; TAC, Total antioxidant capacity; BAA6, Bifidobacterium animalis subsp. lactis A6; FMT, Fecal microbiota transplantation.
References
1. Diagnostic and statistical manual of mental disorders: DSM-5™. 5th ed. Arlington, VA, US: American Psychiatric Publishing, Inc. (2013).
2. Posner J, Polanczyk GV, and Sonuga-Barke E. Attention-deficit hyperactivity disorder. Lancet (London England). (2020) 395:450–62. doi: 10.1016/S0140-6736(19)33004-1
3. Faraone SV, Biederman J, and Mick E. The age-dependent decline of attention deficit hyperactivity disorder: a meta-analysis of follow-up studies. psychol Med. (2006) 36:159–65. doi: 10.1017/S003329170500471X
4. Lichtenstein P, Carlström E, Råstam M, Gillberg C, and Anckarsäter H. The genetics of autism spectrum disorders and related neuropsychiatric disorders in childhood. Am J Psychiatry. (2010) 167:1357–63. doi: 10.1176/appi.ajp.2010.10020223
5. Jensen CM and Steinhausen HC. Comorbid mental disorders in children and adolescents with attention-deficit/hyperactivity disorder in a large nationwide study. Attention deficit hyperactivity Disord. (2015) 7:27–38. doi: 10.1007/s12402-014-0142-1
6. Ahuja A, Martin J, Langley K, and Thapar A. Intellectual disability in children with attention deficit hyperactivity disorder. J Pediatr. (2013) 163:890–5.e1. doi: 10.1016/j.jpeds.2013.02.043
7. Catalá-López F, Peiró S, Ridao M, Sanfélix-Gimeno G, Gènova-Maleras R, Catalá MA, et al. Prevalence of attention deficit hyperactivity disorder among children and adolescents in Spain: a systematic review and meta-analysis of epidemiological studies. BMC Psychiatry. (2012) 12:168. doi: 10.1186/1471-244X-12-168
8. Thomas R, Sanders S, Doust J, Beller E, and Glasziou P. Prevalence of attention-deficit/hyperactivity disorder: a systematic review and meta-analysis. Pediatrics. (2015) 135:e994–1001. doi: 10.1542/peds.2014-3482
9. Sayal K, Prasad V, Daley D, Ford T, and Coghill D. ADHD in children and young people: prevalence, care pathways, and service provision. Lancet Psychiatry. (2018) 5:175–86. doi: 10.1016/S2215-0366(17)30167-0
10. Danielson ML, Bitsko RH, Ghandour RM, Holbrook JR, Kogan MD, Blumberg SJ, et al. Prevalence of parent-reported ADHD diagnosis and associated treatment among U.S. Children and adolescents, 2016. J Clin Child Adolesc Psychol. (2018) 47:199–212. doi: 10.1080/15374416.2017.1417860
11. Li SM, Feng W, Fang F, Dong XH, Zhang ZJ, Yang QQ, et al. Prevalence of attention deficit and hyperactivity disorder in children in China: a systematic review and Meta-analysis. Chin J Epidemiol. (2018) 39:993–8. doi: 10.3760/cma.j.issn.0254-6450.2018.07.024
12. Salari N, Ghasemi H, Abdoli N, Rahmani A, Shiri MH, Hashemian AH, et al. The global prevalence of ADHD in children and adolescents: a systematic review and meta-analysis. Ital J Pediatr. (2023) 49:48. doi: 10.1186/s13052-023-01456-1
13. Spencer TJ, Biederman J, and Mick E. Attention-deficit/hyperactivity disorder: diagnosis, lifespan, comorbidities, and neurobiology. J Pediatr Psychol. (2007) 32:631–42. doi: 10.1093/jpepsy/jsm005
14. Wehmeier PM, Schacht A, and Barkley RA. Social and emotional impairment in children and adolescents with ADHD and the impact on quality of life. J Adolesc Health. (2010) 46:209–17. doi: 10.1016/j.jadohealth.2009.09.009
15. Pelham WE, Foster EM, and Robb JA. The economic impact of attention-deficit/hyperactivity disorder in children and adolescents. J Pediatr Psychol. (2007) 32:711–27. doi: 10.1093/jpepsy/jsm022
16. Sonuga-Barke EJ, Koerting J, Smith E, McCann DC, and Thompson M. Early detection and intervention for attention-deficit/hyperactivity disorder. Expert Rev Neurother. (2011) 11:557–63. doi: 10.1586/ern.11.39
17. Faraone SV, Biederman J, and Monuteaux MC. Toward guidelines for pedigree selection in genetic studies of attention deficit hyperactivity disorder. Genet Epidemiol. (2000) 18:1–16. doi: 10.1002/(SICI)1098-2272(200001)18:1<1::AID-GEPI1>3.0.CO;2-X
18. Thapar A, Cooper M, Eyre O, and Langley K. What have we learnt about the causes of ADHD? J Child Psychol psychiatry Allied disciplines. (2013) 54:3–16. doi: 10.1111/j.1469-7610.2012.02611.x
19. Gizer IR, Ficks C, and Waldman ID. Candidate gene studies of ADHD: a meta-analytic review. Hum Genet. (2009) 126:51–90. doi: 10.1007/s00439-009-0694-x
20. Faraone SV, Perlis RH, Doyle AE, Smoller JW, Goralnick JJ, Holmgren MA, et al. Molecular genetics of attention-deficit/hyperactivity disorder. Biol Psychiatry. (2005) 57:1313–23. doi: 10.1016/j.biopsych.2004.11.024
21. Demontis D, Walters GB, Athanasiadis G, Walters R, Therrien K, Nielsen TT, et al. Genome-wide analyses of ADHD identify 27 risk loci, refine the genetic architecture and implicate several cognitive domains. Nat Genet. (2023) 55:198–208. doi: 10.1038/s41588-022-01285-8
22. Demontis D, Walters RK, Martin J, Mattheisen M, Als TD, Agerbo E, et al. Discovery of the first genome-wide significant risk loci for attention deficit/hyperactivity disorder. Nat Genet. (2019) 51:63–75. doi: 10.1038/s41588-018-0269-7
23. Brikell I, Burton C, Mota NR, and Martin J. Insights into attention-deficit/hyperactivity disorder from recent genetic studies. psychol Med. (2021) 51:2274–86. doi: 10.1017/S0033291721000982
24. Kim S and Misra A. SNP genotyping: technologies and biomedical applications. Annu Rev Biomed Eng. (2007) 9:289–320. doi: 10.1146/annurev.bioeng.9.060906.152037
25. Froehlich TE, Anixt JS, Loe IM, Chirdkiatgumchai V, Kuan L, Gilman RC, et al. Update on environmental risk factors for attention-deficit/hyperactivity disorder. Curr Psychiatry Rep. (2011) 13:333–44. doi: 10.1007/s11920-011-0221-3
26. Yusuf Ali A, Inyang B, Koshy FS, George K, Poudel P, Chalasani R, et al. Elements that influence the development of attention deficit hyperactivity disorder (ADHD) in children. Cureus. (2022) 14:e27835. doi: 10.7759/cureus.27835
27. Amiri S, Malek A, Sadegfard M, and Abdi S. Pregnancy-related maternal risk factors of attention-deficit hyperactivity disorder: a case-control study. ISRN Pediatr. (2012) 2012:458064. doi: 10.5402/2012/458064
28. Lebeña A, Faresjö Å, Jones MP, Bengtsson F, Faresjö T, Ludvigsson J, et al. Early environmental predictors for attention-deficit hyperactivity disorder (ADHD), autism spectrum disorder (ASD) and their co-occurrence: The prospective ABIS-Study. Sci Rep. (2024) 14:14759. doi: 10.1038/s41598-024-65067-4
29. Soled D, Keim SA, Rapoport E, Rosen L, and Adesman A. Breastfeeding is associated with a reduced risk of attention-deficit/hyperactivity disorder among preschool children. J Dev Behav pediatrics: JDBP. (2021) 42:9–15. doi: 10.1097/DBP.0000000000000854
30. Chen MH, Su TP, Chen YS, Hsu JW, Huang KL, Chang WH, et al. Comorbidity of allergic and autoimmune diseases among patients with ADHD. J attention Disord. (2017) 21:219–27. doi: 10.1177/1087054712474686
31. Miyazaki C, Koyama M, Ota E, Swa T, Mlunde LB, Amiya RM, et al. Allergic diseases in children with attention deficit hyperactivity disorder: a systematic review and meta-analysis. BMC Psychiatry. (2017) 17:120. doi: 10.1186/s12888-017-1281-7
32. Schans JV, Çiçek R, De Vries TW, Hak E, and Hoekstra PJ. Association of atopic diseases and attention-deficit/hyperactivity disorder: A systematic review and meta-analyses. Neurosci Biobehav Rev. (2017) 74:139–48. doi: 10.1016/j.neubiorev.2017.01.011
33. Lin YT, Chen YC, Gau SS, Yeh TH, Fan HY, Hwang YY, et al. Associations between allergic diseases and attention deficit hyperactivity/oppositional defiant disorders in children. Pediatr Res. (2016) 80:480–5. doi: 10.1038/pr.2016.111
34. Dam SA, Mostert JC, Szopinska-Tokov JW, Bloemendaal M, Amato M, Arias-Vasquez A, et al. The role of the gut-brain axis in attention-deficit/hyperactivity disorder. Gastroenterol Clinics North America. (2019) 48:407–31. doi: 10.1016/j.gtc.2019.05.001
35. Mitrea L, Nemeş SA, Szabo K, Teleky BE, and Vodnar DC. Guts imbalance imbalances the brain: A review of gut microbiota association with neurological and psychiatric disorders. Front Med. (2022) 9. doi: 10.3389/fmed.2022.813204
36. Wolraich ML, Hagan JF Jr., Allan C, Chan E, Davison D, Earls M, et al. Clinical practice guideline for the diagnosis, evaluation, and treatment of attention-deficit/hyperactivity disorder in children and adolescents. Pediatrics. (2019) 144:e20192528. doi: 10.1542/peds.2019-2528
37. Faraone SV, Bellgrove MA, Brikell I, Cortese S, Hartman CA, Hollis C, et al. Attention-deficit/hyperactivity disorder. Nat Rev Dis Primers. (2024) 10:11. doi: 10.1038/s41572-024-00495-0
38. Cortese S, Adamo N, Del Giovane C, Mohr-Jensen C, Hayes AJ, Carucci S, et al. Comparative efficacy and tolerability of medications for attention-deficit hyperactivity disorder in children, adolescents, and adults: a systematic review and network meta-analysis. Lancet Psychiatry. (2018) 5:727–38. doi: 10.1016/S2215-0366(18)30269-4
39. Chang Z, Ghirardi L, Quinn PD, Asherson P, D'Onofrio BM, Larsson H, et al. Risks and benefits of attention-deficit/hyperactivity disorder medication on behavioral and neuropsychiatric outcomes: A qualitative review of pharmacoepidemiology studies using linked prescription databases. Biol Psychiatry. (2019) 86:335–43. doi: 10.1016/j.biopsych.2019.04.009
40. Childress AC and Sallee FR. Attention-deficit/hyperactivity disorder with inadequate response to stimulants: approaches to management. CNS Drugs. (2014) 28:121–9. doi: 10.1007/s40263-013-0130-6
41. Charach A and Fernandez R. Enhancing ADHD medication adherence: challenges and opportunities. Curr Psychiatry Rep. (2013) 15:371. doi: 10.1007/s11920-013-0371-6
42. Morais LH, Schreiber HLT, and Mazmanian SK. The gut microbiota-brain axis in behaviour and brain disorders. Nat Rev Microbiol. (2021) 19:241–55. doi: 10.1038/s41579-020-00460-0
43. Wang Y and Kasper LH. The role of microbiome in central nervous system disorders. Brain behavior Immun. (2014) 38:1–12. doi: 10.1016/j.bbi.2013.12.015
44. Cryan JF and Dinan TG. Mind-altering microorganisms: the impact of the gut microbiota on brain and behaviour. Nat Rev Neurosci. (2012) 13:701–12. doi: 10.1038/nrn3346
45. Rajilić-Stojanović M. Function of the microbiota. Best Pract Res Clin Gastroenterol. (2013) 27:5–16. doi: 10.1016/j.bpg.2013.03.006
46. Ullah H, Arbab S, Chang C, Bibi S, Muhammad N, Rehman SU, et al. Gut microbiota therapy in gastrointestinal diseases. Front Cell Dev Biol. (2025) 13. doi: 10.3389/fcell.2025.1514636
47. Kelsey CM, Prescott S, Mcculloch JA, Trinchieri G, Valladares TL, Dreisbach C, et al. Gut microbiota composition is associated with newborn functional brain connectivity and behavioral temperament. Brain behavior Immun. (2021) 91:472–86. doi: 10.1016/j.bbi.2020.11.003
48. Gensollen T, Iyer SS, Kasper DL, and Blumberg RS. How colonization by microbiota in early life shapes the immune system. Sci (New York NY). (2016) 352:539–44. doi: 10.1126/science.aad9378
49. Sudo N. Microbiome, HPA axis and production of endocrine hormones in the gut. Adv Exp Med Biol. (2014) 817:177–94. doi: 10.1007/978-1-4939-0897-4_8
50. Gershon MD and Margolis KG. The gut, its microbiome, and the brain: connections and communications. J Clin Invest. (2021) 131:e143768. doi: 10.1172/JCI143768
51. Ullah H, Arbab S, Tian Y, Liu CQ, Chen Y, Qijie L, et al. The gut microbiota-brain axis in neurological disorder. Front Neurosci. (2023) 17. doi: 10.3389/fnins.2023.1225875
52. Sittipo P, Choi J, Lee S, and Lee YK. The function of gut microbiota in immune-related neurological disorders: a review. J Neuroinflamm. (2022) 19:154. doi: 10.1186/s12974-022-02510-1
53. Yang J, Fu X, Liao X, and Li Y. Effects of gut microbial-based treatments on gut microbiota, behavioral symptoms, and gastrointestinal symptoms in children with autism spectrum disorder: A systematic review. Psychiatry Res. (2020) 293:113471. doi: 10.1016/j.psychres.2020.113471
54. Dicks LMT. Gut bacteria and neurotransmitters. Microorganisms. (2022) 10:1838. doi: 10.3390/microorganisms10091838
55. Miri S, Yeo J, Abubaker S, and Hammami R. Neuromicrobiology, an emerging neurometabolic facet of the gut microbiome? Front Microbiol. (2023) 14. doi: 10.3389/fmicb.2023.1098412
56. Braga JD, Thongngam M, and Kumrungsee T. Gamma-aminobutyric acid as a potential postbiotic mediator in the gut-brain axis. NPJ Sci Food. (2024) 8:16. doi: 10.1038/s41538-024-00253-2
57. Chen Y, Xu J, and Chen Y. Regulation of neurotransmitters by the gut microbiota and effects on cognition in neurological disorders. Nutrients. (2021) 13:2099. doi: 10.3390/nu13062099
58. Barandouzi ZA, Lee J, Del Carmen Rosas M, Chen J, Henderson WA, Starkweather AR, et al. Associations of neurotransmitters and the gut microbiome with emotional distress in mixed type of irritable bowel syndrome. Sci Rep. (2022) 12:1648. doi: 10.1038/s41598-022-05756-0
59. Strandwitz P. Neurotransmitter modulation by the gut microbiota. Brain Res. (2018) 1693:128–33. doi: 10.1016/j.brainres.2018.03.015
60. Del Campo N, Chamberlain SR, Sahakian BJ, and Robbins TW. The roles of dopamine and noradrenaline in the pathophysiology and treatment of attention-deficit/hyperactivity disorder. Biol Psychiatry. (2011) 69:e145–57. doi: 10.1016/j.biopsych.2011.02.036
61. Kasarello K, Cudnoch-Jedrzejewska A, and Czarzasta K. Communication of gut microbiota and brain via immune and neuroendocrine signaling. Front Microbiol. (2023) 14. doi: 10.3389/fmicb.2023.1118529
62. Silva YP, Bernardi A, and Frozza RL. The role of short-chain fatty acids from gut microbiota in gut-brain communication. Front Endocrinol. (2020) 11. doi: 10.3389/fendo.2020.00025
63. Ahmed H, Leyrolle Q, Koistinen V, Kärkkäinen O, Layé S, Delzenne N, et al. Microbiota-derived metabolites as drivers of gut-brain communication. Gut Microbes. (2022) 14:2102878. doi: 10.1080/19490976.2022.2102878
64. Boonchooduang N, Louthrenoo O, Likhitweerawong N, Kunasol C, Thonusin C, Sriwichaiin S, et al. Impact of psychostimulants on microbiota and short-chain fatty acids alterations in children with attention-deficit/hyperactivity disorder. Sci Rep. (2025) 15:3034. doi: 10.1038/s41598-025-87546-y
65. Yang LL, Stiernborg M, Skott E, Xu J, Wu Y, Landberg R, et al. Effects of a synbiotic on plasma immune activity markers and short-chain fatty acids in children and adults with ADHD-A randomized controlled trial. Nutrients. (2023) 15:1293. doi: 10.3390/nu15051293
66. Shirvani-Rad S, Ejtahed HS, Ettehad Marvasti F, Taghavi M, Sharifi F, Arzaghi SM, et al. The role of gut microbiota-brain axis in pathophysiology of ADHD: A systematic review. J attention Disord. (2022) 26:1698–710. doi: 10.1177/10870547211073474
67. Checa-Ros A, Jeréz-Calero A, Molina-Carballo A, Campoy C, and Muñoz-Hoyos A. Current evidence on the role of the gut microbiome in ADHD pathophysiology and therapeutic implications. Nutrients. (2021) 13:249. doi: 10.3390/nu13010249
68. Takiishi T, Fenero CIM, and Câmara NOS. Intestinal barrier and gut microbiota: Shaping our immune responses throughout life. Tissue barriers. (2017) 5:e1373208. doi: 10.1080/21688370.2017.1373208
69. An L, Wirth U, Koch D, Schirren M, Drefs M, Koliogiannis D, et al. The role of gut-derived lipopolysaccharides and the intestinal barrier in fatty liver diseases. J gastrointestinal Surg. (2022) 26:671–83. doi: 10.1007/s11605-021-05188-7
70. Góralczyk-Bińkowska A, Szmajda-Krygier D, and Kozłowska E. The microbiota-gut-brain axis in psychiatric disorders. Int J Mol Sci. (2022) 23:11245. doi: 10.3390/ijms231911245
71. Martin-Gallausiaux C, Marinelli L, Blottière HM, Larraufie P, and Lapaque N. SCFA: mechanisms and functional importance in the gut. Proc Nutr Soc. (2021) 80:37–49. doi: 10.1017/S0029665120006916
72. Xu Q, Zhang R, Mu Y, Song Y, Hao N, Wei Y, et al. Propionate ameliorates alcohol-induced liver injury in mice via the gut-liver axis: focus on the improvement of intestinal permeability. J Agric Food Chem. (2022) 70:6084–96. doi: 10.1021/acs.jafc.2c00633
73. Hoyles L, Snelling T, Umlai UK, Nicholson JK, Carding SR, Glen RC, et al. Microbiome-host systems interactions: protective effects of propionate upon the blood-brain barrier. Microbiome. (2018) 6:55. doi: 10.1186/s40168-018-0439-y
74. Kadry H, Noorani B, and Cucullo L. A blood-brain barrier overview on structure, function, impairment, and biomarkers of integrity. Fluids barriers CNS. (2020) 17:69. doi: 10.1186/s12987-020-00230-3
75. Alaish SM, Smith AD, Timmons J, Greenspon J, Eyvazzadeh D, Murphy E, et al. Gut microbiota, tight junction protein expression, intestinal resistance, bacterial translocation and mortality following cholestasis depend on the genetic background of the host. Gut Microbes. (2013) 4:292–305. doi: 10.4161/gmic.24706
76. Parker A, Fonseca S, and Carding SR. Gut microbes and metabolites as modulators of blood-brain barrier integrity and brain health. Gut Microbes. (2020) 11:135–57. doi: 10.1080/19490976.2019.1638722
77. Mou Y, Du Y, Zhou L, Yue J, Hu X, Liu Y, et al. Gut microbiota interact with the brain through systemic chronic inflammation: implications on neuroinflammation, neurodegeneration, and aging. Front Immunol. (2022) 13. doi: 10.3389/fimmu.2022.796288
78. Braniste V, Al-Asmakh M, Kowal C, Anuar F, Abbaspour A, Tóth M, et al. The gut microbiota influences blood-brain barrier permeability in mice. Sci Trans Med. (2014) 6:263ra158. doi: 10.1126/scitranslmed.3009759
79. Wang LJ, Yang CY, Chou WJ, Lee MJ, Chou MC, Kuo HC, et al. Gut microbiota and dietary patterns in children with attention-deficit/hyperactivity disorder. Eur Child Adolesc Psychiatry. (2020) 29:287–97. doi: 10.1007/s00787-019-01352-2
80. Doroszkiewicz J, Groblewska M, and Mroczko B. The role of gut microbiota and gut-brain interplay in selected diseases of the central nervous system. Int J Mol Sci. (2021) 22:10028. doi: 10.3390/ijms221810028
81. Nikolova VL, Smith MRB, Hall LJ, Cleare AJ, Stone JM, Young AH, et al. Perturbations in gut microbiota composition in psychiatric disorders: A review and meta-analysis. JAMA Psychiatry. (2021) 78:1343–54. doi: 10.1001/jamapsychiatry.2021.2573
82. Mcguinness AJ, Davis JA, Dawson SL, Loughman A, Collier F, O'Hely M, et al. A systematic review of gut microbiota composition in observational studies of major depressive disorder, bipolar disorder and schizophrenia. Mol Psychiatry. (2022) 27:1920–35. doi: 10.1038/s41380-022-01456-3
83. Loh JS, Mak WQ, Tan LKS, Ng CX, Chan HH, Yeow SH, et al. Microbiota–gut–brain axis and its therapeutic applications in neurodegenerative diseases. Signal Transduction Targeted Ther. (2024) 9:37. doi: 10.1038/s41392-024-01743-1
84. Pärtty A, Kalliomäki M, Wacklin P, Salminen S, and Isolauri E. A possible link between early probiotic intervention and the risk of neuropsychiatric disorders later in childhood: a randomized trial. Pediatr Res. (2015) 77:823–8. doi: 10.1038/pr.2015.51
85. Cassidy-Bushrow AE, Sitarik AR, Johnson CC, Johnson-Hooper TM, Kassem Z, Levin AM, et al. Early-life gut microbiota and attention deficit hyperactivity disorder in preadolescents. Pediatr Res. (2023) 93:2051–60. doi: 10.1038/s41390-022-02051-6
86. Aarts E, Ederveen THA, Naaijen J, Zwiers MP, Boekhorst J, Timmerman HM, et al. Gut microbiome in ADHD and its relation to neural reward anticipation. PloS One. (2017) 12:e0183509. doi: 10.1371/journal.pone.0183509
87. Prehn-Kristensen A, Zimmermann A, Tittmann L, Lieb W, Schreiber S, Baving L, et al. Reduced microbiome alpha diversity in young patients with ADHD. PloS One. (2018) 13:e0200728. doi: 10.1371/journal.pone.0200728
88. Jiang HY, Zhou YY, Zhou GL, Li YC, Yuan J, Li XH, et al. Gut microbiota profiles in treatment-naïve children with attention deficit hyperactivity disorder. Behav Brain Res. (2018) 347:408–13. doi: 10.1016/j.bbr.2018.03.036
89. Stevens AJ, Purcell RV, Darling KA, Eggleston MJF, Kennedy MA, Rucklidge JJ, et al. Human gut microbiome changes during a 10 week Randomised Control Trial for micronutrient supplementation in children with attention deficit hyperactivity disorder. Sci Rep. (2019) 9:10128. doi: 10.1038/s41598-019-46146-3
90. Szopinska-Tokov J, Dam S, Naaijen J, Konstanti P, Rommelse N, Belzer C, et al. Correction: Szopinska-Tokov et al. Investigating the Gut Microbiota Composition of Individuals with Attention-Deficit/Hyperactivity Disorder and Association with Symptoms. Microorganisms. (2020) 8:406. doi: 10.3390/microorganisms8030406
91. Wang LJ, Li SC, Li SW, Kuo HC, Lee SY, Huang LH, et al. Gut microbiota and plasma cytokine levels in patients with attention-deficit/hyperactivity disorder. Trans Psychiatry. (2022) 12:76. doi: 10.1038/s41398-022-01844-x
92. Lee MJ, Lai HC, Kuo YL, and Chen VC. Association between gut microbiota and emotional-behavioral symptoms in children with attention-deficit/hyperactivity disorder. J personalized Med. (2022) 12:1634. doi: 10.3390/jpm12101634
93. Bundgaard-Nielsen C, Lauritsen MB, Knudsen JK, Rold LS, Larsen MH, Hindersson P, et al. Children and adolescents with attention deficit hyperactivity disorder and autism spectrum disorder share distinct microbiota compositions. Gut Microbes. (2023) 15:2211923. doi: 10.1080/19490976.2023.2211923
94. Wan L, Ge WR, Zhang S, Sun YL, Wang B, Yang G, et al. Case-control study of the effects of gut microbiota composition on neurotransmitter metabolic pathways in children with attention deficit hyperactivity disorder. Front Neurosci. (2020) 14. doi: 10.3389/fnins.2020.00127
95. Li Y, Sun H, Huang Y, Yin A, Zhang L, Han J, et al. Gut metagenomic characteristics of ADHD reveal low Bacteroides ovatus-associated host cognitive impairment. Gut Microbes. (2022) 14:2125747. doi: 10.1080/19490976.2022.2125747
96. Stiernborg M, Debelius JW, Yang LL, Skott E, Millischer V, Giacobini M, et al. Bacterial gut microbiome differences in adults with ADHD and in children with ADHD on psychostimulant medication. Brain behavior Immun. (2023) 110:310–21. doi: 10.1016/j.bbi.2023.03.012
97. Wang LJ, Li SC, Yeh YM, Lee SY, Kuo HC, Yang CY, et al. Gut mycobiome dysbiosis and its impact on intestinal permeability in attention-deficit/hyperactivity disorder. J Child Psychol psychiatry Allied disciplines. (2023) 64:1280–91. doi: 10.1111/jcpp.13779
98. Wang N, Wang H, Bai Y, Zhao Y, Zheng X, Gao X, et al. Metagenomic analysis reveals difference of gut microbiota in ADHD. J attention Disord. (2024) 28:872–9. doi: 10.1177/10870547231225491
99. De Vos WM, Tilg H, Van Hul M, and Cani PD. Gut microbiome and health: mechanistic insights. Gut. (2022) 71:1020–32. doi: 10.1136/gutjnl-2021-326789
100. Rieder R, Wisniewski PJ, Alderman BL, and Campbell SC. Microbes and mental health: A review. Brain behavior Immun. (2017) 66:9–17. doi: 10.1016/j.bbi.2017.01.016
101. Thursby E and Juge N. Introduction to the human gut microbiota. Biochem J. (2017) 474:1823–36. doi: 10.1042/BCJ20160510
102. Tillisch K. The effects of gut microbiota on CNS function in humans. Gut Microbes. (2014) 5:404–10. doi: 10.4161/gmic.29232
103. Douglas-Escobar M, Elliott E, and Neu J. Effect of intestinal microbial ecology on the developing brain. JAMA Pediatr. (2013) 167:374–9. doi: 10.1001/jamapediatrics.2013.497
104. Al-Asmakh M, Anuar F, Zadjali F, Rafter J, and Pettersson S. Gut microbial communities modulating brain development and function. Gut Microbes. (2012) 3:366–73. doi: 10.4161/gmic.21287
105. Belzer C and De Vos WM. Microbes inside–from diversity to function: the case of Akkermansia. ISME J. (2012) 6:1449–58. doi: 10.1038/ismej.2012.6
106. Breit S, Kupferberg A, Rogler G, and Hasler G. Vagus nerve as modulator of the brain-gut axis in psychiatric and inflammatory disorders. Front Psychiatry. (2018) 9. doi: 10.3389/fpsyt.2018.00044
107. Hida H. The importance of vagus nerve afferent in the formation of emotions in attention-deficit hyperactivity disorder model rat. Brain Nerve = Shinkei kenkyu no shinpo. (2016) 68:633–9. doi: 10.11477/mf.1416200454
108. Lyte M, Li W, Opitz N, Gaykema RP, and Goehler LE. Induction of anxiety-like behavior in mice during the initial stages of infection with the agent of murine colonic hyperplasia Citrobacter rodentium. Physiol Behav. (2006) 89:350–7. doi: 10.1016/j.physbeh.2006.06.019
109. Bravo JA, Forsythe P, Chew MV, Escaravage E, Savignac HM, Dinan TG, et al. Ingestion of Lactobacillus strain regulates emotional behavior and central GABA receptor expression in a mouse via the vagus nerve. Proc Natl Acad Sci U.S.A. (2011) 108:16050–5. doi: 10.1073/pnas.1102999108
110. Furness JB. The enteric nervous system and neurogastroenterology. Nat Rev Gastroenterol Hepatol. (2012) 9:286–94. doi: 10.1038/nrgastro.2012.32
111. Wang X, Tang R, Wei Z, Zhan Y, Lu J, Li Z, et al. The enteric nervous system deficits in autism spectrum disorder. Front Neurosci. (2023) 17. doi: 10.3389/fnins.2023.1101071
112. Montanari M, Imbriani P, Bonsi P, Martella G, and Peppe A. Beyond the microbiota: understanding the role of the enteric nervous system in Parkinson’s disease from mice to human. Biomedicines. (2023) 11:1560. doi: 10.3390/biomedicines11061560
113. Heiss CN and Olofsson LE. The role of the gut microbiota in development, function and disorders of the central nervous system and the enteric nervous system. J Neuroendocrinol. (2019) 31:e12684. doi: 10.1111/jne.2019.31.issue-5
114. Hyland NP and Cryan JF. Microbe-host interactions: Influence of the gut microbiota on the enteric nervous system. Dev Biol. (2016) 417:182–7. doi: 10.1016/j.ydbio.2016.06.027
115. Caputi V, Marsilio I, Filpa V, Cerantola S, Orso G, and Bistoletti M. Antibiotic-induced dysbiosis of the microbiota impairs gut neuromuscular function in juvenile mice. Br J Pharmacol. (2017) 174:3623–39. doi: 10.1111/bph.v174.20
116. Mcvey Neufeld KA, Mao YK, Bienenstock J, Foster JA, and Kunze WA. The microbiome is essential for normal gut intrinsic primary afferent neuron excitability in the mouse. Neurogastroenterol Motil. (2013) 25:183–e88. doi: 10.1111/nmo.12049
117. Vicentini FA, Keenan CM, Wallace LE, Woods C, Cavin JB, Flockton AR, et al. Intestinal microbiota shapes gut physiology and regulates enteric neurons and glia. Microbiome. (2021) 9:210. doi: 10.1186/s40168-021-01165-z
118. Bercik P, Park AJ, Sinclair D, Khoshdel A, Lu J, Huang X, et al. The anxiolytic effect of Bifidobacterium longum NCC3001 involves vagal pathways for gut-brain communication. Neurogastroenterol Motil. (2011) 23:1132–9. doi: 10.1111/j.1365-2982.2011.01796.x
119. Hayes DJ, Jupp B, Sawiak SJ, Merlo E, Caprioli D, Dalley JW, et al. Brain γ-aminobutyric acid: a neglected role in impulsivity. Eur J Neurosci. (2014) 39:1921–32. doi: 10.1111/ejn.2014.39.issue-11
120. Banerjee E and Nandagopal K. Does serotonin deficit mediate susceptibility to ADHD? Neurochemistry Int. (2015) 82:52–68. doi: 10.1016/j.neuint.2015.02.001
121. Plichta MM and Scheres A. Ventral-striatal responsiveness during reward anticipation in ADHD and its relation to trait impulsivity in the healthy population: a meta-analytic review of the fMRI literature. Neurosci Biobehav Rev. (2014) 38:125–34. doi: 10.1016/j.neubiorev.2013.07.012
122. Makris N, Biederman J, Monuteaux MC, and Seidman LJ. Towards conceptualizing a neural systems-based anatomy of attention-deficit/hyperactivity disorder. Dev Neurosci. (2009) 31:36–49. doi: 10.1159/000207492
123. Da Silva BS, Grevet EH, Silva LCF, Ramos JKN, Rovaris DL, Bau CHD, et al. An overview on neurobiology and therapeutics of attention-deficit/hyperactivity disorder. Discover Ment Health. (2023) 3:2. doi: 10.1007/s44192-022-00030-1
124. Oades RD, Lasky-Su J, Christiansen H, Faraone SV, Sonuga-Barke EJ, Banaschewski T, et al. The influence of serotonin- and other genes on impulsive behavioral aggression and cognitive impulsivity in children with attention-deficit/hyperactivity disorder (ADHD): Findings from a family-based association test (FBAT) analysis. Behav Brain functions: BBF. (2008) 4:48. doi: 10.1186/1744-9081-4-48
125. Worbe Y, Savulich G, Voon V, Fernandez-Egea E, and Robbins TW. Serotonin depletion induces ‘waiting impulsivity’ on the human four-choice serial reaction time task: cross-species translational significance. Neuropsychopharmacology. (2014) 39:1519–26. doi: 10.1038/npp.2013.351
126. Edden RA, Crocetti D, Zhu H, Gilbert DL, and Mostofsky SH. Reduced GABA concentration in attention-deficit/hyperactivity disorder. Arch Gen Psychiatry. (2012) 69:750–3. doi: 10.1001/archgenpsychiatry.2011.2280
127. Yang LL, Stiernborg M, Skott E, Gillberg T, Landberg R, Giacobini M, et al. Lower plasma concentrations of short-chain fatty acids (SCFAs) in patients with ADHD. J Psychiatr Res. (2022) 156:36–43. doi: 10.1016/j.jpsychires.2022.09.042
128. Cortese S. The neurobiology and genetics of Attention-Deficit/Hyperactivity Disorder (ADHD): what every clinician should know. Eur J paediatric neurology: EJPN. (2012) 16:422–33. doi: 10.1016/j.ejpn.2012.01.009
129. Blum K, Chen AL, Braverman ER, Comings DE, Chen TJ, Arcuri V, et al. Attention-deficit-hyperactivity disorder and reward deficiency syndrome. Neuropsychiatr Dis Treat. (2008) 4:893–918. doi: 10.2147/ndt.s2627
130. Kalenik A, Kardaś K, Rahnama A, Sirojć K, and Wolańczyk T. Gut microbiota and probiotic therapy in ADHD: A review of current knowledge. Prog Neuropsychopharmacol Biol Psychiatry. (2021) 110:110277. doi: 10.1016/j.pnpbp.2021.110277
131. Hong HJ, Shin DW, Lee EH, Oh YH, and Noh KS. Hypothalamic-pituitary-adrenal reactivity in boys with attention deficit hyperactivity disorder. Yonsei Med J. (2003) 44:608–14. doi: 10.3349/ymj.2003.44.4.608
132. Asano Y, Hiramoto T, Nishino R, Aiba Y, Kimura T, Yoshihara K, et al. Critical role of gut microbiota in the production of biologically active, free catecholamines in the gut lumen of mice. Am J Physiol Gastrointestinal liver Physiol. (2012) 303:G1288–95. doi: 10.1152/ajpgi.00341.2012
133. Hamamah S, Aghazarian A, Nazaryan A, Hajnal A, and Covasa M. Role of microbiota-gut-brain axis in regulating dopaminergic signaling. Biomedicines. (2022) 10:436. doi: 10.3390/biomedicines10020436
134. Liao JF, Cheng YF, You ST, Kuo WC, Huang CW, Chiou JJ, et al. Lactobacillus plantarum PS128 alleviates neurodegenerative progression in 1-methyl-4-phenyl-1,2,3,6-tetrahydropyridine-induced mouse models of Parkinson’s disease. Brain behavior Immun. (2020) 90:26–46. doi: 10.1016/j.bbi.2020.07.036
135. O’Donnell J, Zeppenfeld D, Mcconnell E, Pena S, and Nedergaard M. Norepinephrine: a neuromodulator that boosts the function of multiple cell types to optimize CNS performance. Neurochemical Res. (2012) 37:2496–512. doi: 10.1007/s11064-012-0818-x
136. Hu Y, Chen X, Gu H, and Yang Y. Resting-state glutamate and GABA concentrations predict task-induced deactivation in the default mode network. J neuroscience: Off J Soc Neurosci. (2013) 33:18566–73. doi: 10.1523/JNEUROSCI.1973-13.2013
137. Naaijen J, Bralten J, Poelmans G, IMAGE consortium, Glennon JC, Franke B, et al. Glutamatergic and GABAergic gene sets in attention-deficit/hyperactivity disorder: association to overlapping traits in ADHD and autism. Trans Psychiatry. (2017) 7:e999. doi: 10.1038/tp.2016.273
138. Barrett E, Ross RP, O’Toole PW, Fitzgerald GF, and Stanton C. γ-Aminobutyric acid production by culturable bacteria from the human intestine. J Appl Microbiol. (2012) 113:411–7. doi: 10.1111/j.1365-2672.2012.05344.x
139. Feehily C and Karatzas KA. Role of glutamate metabolism in bacterial responses towards acid and other stresses. J Appl Microbiol. (2013) 114:11–24. doi: 10.1111/j.1365-2672.2012.05434.x
140. Janik R, Thomason LAM, Stanisz AM, Forsythe P, Bienenstock J, Stanisz GJ, et al. Magnetic resonance spectroscopy reveals oral Lactobacillus promotion of increases in brain GABA, N-acetyl aspartate and glutamate. NeuroImage. (2016) 125:988–95. doi: 10.1016/j.neuroimage.2015.11.018
141. Oades RD. Role of the serotonin system in ADHD: treatment implications. Expert Rev Neurother. (2007) 7:1357–74. doi: 10.1586/14737175.7.10.1357
142. Yano JM, Yu K, Donaldson GP, Shastri GG, Ann P, Ma L, et al. Indigenous bacteria from the gut microbiota regulate host serotonin biosynthesis. Cell. (2015) 161:264–76. doi: 10.1016/j.cell.2015.02.047
143. Kennedy PJ, Cryan JF, Dinan TG, and Clarke G. Kynurenine pathway metabolism and the microbiota-gut-brain axis. Neuropharmacology. (2017) 112:399–412. doi: 10.1016/j.neuropharm.2016.07.002
144. Clarke G, Grenham S, Scully P, Fitzgerald P, Moloney RD, Shanahan F, et al. The microbiome-gut-brain axis during early life regulates the hippocampal serotonergic system in a sex-dependent manner. Mol Psychiatry. (2013) 18:666–73. doi: 10.1038/mp.2012.77
145. Gao J, Xu K, Liu H, Liu G, Bai M, Peng C, et al. Impact of the gut microbiota on intestinal immunity mediated by tryptophan metabolism. Front Cell infection Microbiol. (2018) 8. doi: 10.3389/fcimb.2018.00013
146. Hu J, Lin S, Zheng B, and Cheung PCK. Short-chain fatty acids in control of energy metabolism. Crit Rev Food Sci Nutr. (2018) 58:1243–9. doi: 10.1080/10408398.2016.1245650
147. Foley KA, Macfabe DF, Vaz A, Ossenkopp KP, and Kavaliers M. Sexually dimorphic effects of prenatal exposure to propionic acid and lipopolysaccharide on social behavior in neonatal, adolescent, and adult rats: implications for autism spectrum disorders Int J Dev Neurosci. (2014) 39:68–78. doi: 10.1016/j.ijdevneu.2014.04.001
148. Elce A, Amato F, Zarrilli F, Calignano A, Troncone R, Castaldo G, et al. Butyrate modulating effects on pro-inflammatory pathways in human intestinal epithelial cells. Beneficial Microbes. (2017) 8:841–7. doi: 10.3920/BM2016.0197
149. Erny D, Hrabě De Angelis AL, Jaitin D, Wieghofer P, Staszewski O, David E, et al. Host microbiota constantly control maturation and function of microglia in the CNS. Nat Neurosci. (2015) 18:965–77. doi: 10.1038/nn.4030
150. Kaneko M, Hoshino Y, Hashimoto S, Okano T, and Kumashiro H. Hypothalamic-pituitary-adrenal axis function in children with attention-deficit hyperactivity disorder. J Autism Dev Disord. (1993) 23:59–65. doi: 10.1007/BF01066418
151. Sudo N, Chida Y, Aiba Y, Sonoda J, Oyama N, Yu XN, et al. Postnatal microbial colonization programs the hypothalamic-pituitary-adrenal system for stress response in mice. J Physiol. (2004) 558:263–75. doi: 10.1113/jphysiol.2004.063388
152. Sherman MP, Zaghouani H, and Niklas V. Gut microbiota, the immune system, and diet influence the neonatal gut-brain axis. Pediatr Res. (2015) 77:127–35. doi: 10.1038/pr.2014.161
153. Maynard CL, Elson CO, Hatton RD, and Weaver CT. Reciprocal interactions of the intestinal microbiota and immune system. Nature. (2012) 489:231–41. doi: 10.1038/nature11551
154. Ullah H, Arbab S, Tian Y, Chen Y, Liu CQ, Li Q, et al. Crosstalk between gut microbiota and host immune system and its response to traumatic injury. Front Immunol. (2024) 15. doi: 10.3389/fimmu.2024.1413485
155. Kelly JR, Kennedy PJ, Cryan JF, Dinan TG, Clarke G, Hyland NP, et al. Breaking down the barriers: the gut microbiome, intestinal permeability and stress-related psychiatric disorders. Front Cell Neurosci. (2015) 9. doi: 10.3389/fncel.2015.00392
156. Felger JC and Treadway MT. Inflammation effects on motivation and motor activity: role of dopamine. Neuropsychopharmacology. (2017) 42:216–41. doi: 10.1038/npp.2016.143
157. Leffa DT, Torres ILS, and Rohde LA. A review on the role of inflammation in attention-deficit/hyperactivity disorder. Neuroimmunomodulation. (2018) 25:328–33. doi: 10.1159/000489635
158. Pollak TA, Drndarski S, Stone JM, David AS, McGuire P, Abbott NJ, et al. The blood-brain barrier in psychosis. Lancet Psychiatry. (2018) 5:79–92. doi: 10.1016/S2215-0366(17)30293-6
159. Cryan JF, O’riordan KJ, Cowan CSM, Sandhu KV, Bastiaanssen TFS, Boehme M, et al. The microbiota-gut-brain axis. Physiol Rev. (2019) 99:1877–2013. doi: 10.1152/physrev.00018.2018
160. Anand D, Colpo GD, Zeni G, Zeni CP, and Teixeira AL. Attention-deficit/hyperactivity disorder and inflammation: what does current knowledge tell us? A systematic review. Front Psychiatry. (2017) 8. doi: 10.3389/fpsyt.2017.00228
161. Oades RD, Myint AM, Dauvermann MR, Schimmelmann BG, and Schwarz MJ. Attention-deficit hyperactivity disorder (ADHD) and glial integrity: an exploration of associations of cytokines and kynurenine metabolites with symptoms and attention. Behav Brain functions: BBF. (2010) 6:32. doi: 10.1186/1744-9081-6-32
162. Verlaet AA, Noriega DB, Hermans N, and Savelkoul HF. Nutrition, immunological mechanisms and dietary immunomodulation in ADHD. Eur Child Adolesc Psychiatry. (2014) 23:519–29. doi: 10.1007/s00787-014-0522-2
163. Limketkai BN, Akobeng AK, Gordon M, and Adepoju AA. Probiotics for induction of remission in Crohn’s disease. Cochrane Database systematic Rev. (2020) 7:Cd006634.
164. Lee SHF, Ahmad SR, Lim YC, and Zulkipli IN. The use of probiotic therapy in metabolic and neurological diseases. Front Nutr. (2022) 9. doi: 10.3389/fnut.2022.887019
165. Bazinet PA. Effects of probiotics on memory, ADHD, and anxiety in children. Acadia University (2017).
166. Kumperscak HG, Gricar A, Ülen I, and Micetic-Turk D. A pilot randomized control trial with the probiotic strain lactobacillus rhamnosus GG (LGG) in ADHD: children and adolescents report better health-related quality of life. Front Psychiatry. (2020) 11. doi: 10.3389/fpsyt.2020.00181
167. Ahmadvand A, Sepehrmanesh Z, Shahzeidi A, Ali Mansournia M, and Ghaderi A. Clinical and metabolic reaction to probiotic supplement in children suffering attention-deficit hyperactivity disorder: A randomized, double-blind, placebo-controlled experiment. Int Arch Health Sci. (2021) 8:90–6. doi: 10.4103/iahs.iahs_112_20
168. Wang LJ, Yang CY, Kuo HC, Chou WJ, Tsai CS, Lee SY, et al. Effect of bifidobacterium bifidum on clinical characteristics and gut microbiota in attention-deficit/hyperactivity disorder. J personalized Med. (2022) 12:227. doi: 10.3390/jpm12020227
169. Ghanaatgar M, Taherzadeh S, Ariyanfar S, Razeghi Jahromi S, Martami F, Mahmoudi Gharaei J, et al. Probiotic supplement as an adjunctive therapy with Ritalin for treatment of attention-deficit hyperactivity disorder symptoms in children: a double-blind placebo-controlled randomized clinical trial. Nutr Food Sci. (2022) 53:19–34. doi: 10.1108/NFS-12-2021-0388
170. Yin X, Liu W, Feng H, Huang J, Wang Q, Zhang Q, et al. Bifidobacterium animalis subsp. lactis A6 attenuates hippocampal damage and memory impairments in an ADHD rat model. Food Funct. (2024) 15:2668–78. doi: 10.1039/D3FO04665F
171. Skott E, Yang LL, Stiernborg M, Söderström Å, Rȕegg J, Schalling M, et al. Effects of a synbiotic on symptoms, and daily functioning in attention deficit hyperactivity disorder - A double-blind randomized controlled trial. Brain behavior Immun. (2020) 89:9–19. doi: 10.1016/j.bbi.2020.05.056
172. Kelly JR, Borre Y, O' Brien C, Patterson E, El Aidy S, Deane J, et al. Transferring the blues: Depression-associated gut microbiota induces neurobehavioural changes in the rat. J Psychiatr Res. (2016) 82:109–18. doi: 10.1016/j.jpsychires.2016.07.019
173. Sharon G, Cruz NJ, Kang DW, Gandal MJ, Wang B, Kim YM, et al. Human gut microbiota from autism spectrum disorder promote behavioral symptoms in mice. Cell. (2019) 177:1600–18.e17. doi: 10.1016/j.cell.2019.05.004
174. Zhu F, Guo R, Wang W, Ju Y, Wang Q, Ma Q, et al. Transplantation of microbiota from drug-free patients with schizophrenia causes schizophrenia-like abnormal behaviors and dysregulated kynurenine metabolism in mice. Mol Psychiatry. (2020) 25:2905–18. doi: 10.1038/s41380-019-0475-4
175. Tengeler AC, Dam SA, Wiesmann M, Naaijen J, van Bodegom M, Belzer C, et al. Gut microbiota from persons with attention-deficit/hyperactivity disorder affects the brain in mice. Microbiome. (2020) 8:44. doi: 10.1186/s40168-020-00816-x
Keywords: ADHD, gut microbiota, microecological agents, children, gut-brain axes
Citation: Liu Y, Zhang P and Sun H (2025) A narrative review of research advances in gut microbiota and microecological agents in children with attention deficit hyperactivity disorder (ADHD). Front. Psychiatry 16:1588135. doi: 10.3389/fpsyt.2025.1588135
Received: 05 March 2025; Accepted: 28 April 2025;
Published: 23 May 2025.
Edited by:
Diogo Alpuim Costa, CUF Oncologia, PortugalReviewed by:
Laura Mitrea, University of Agricultural Sciences and Veterinary Medicine of Cluj-Napoca, RomaniaHanif Ullah, Sichuan University, China
Copyright © 2025 Liu, Zhang and Sun. This is an open-access article distributed under the terms of the Creative Commons Attribution License (CC BY). The use, distribution or reproduction in other forums is permitted, provided the original author(s) and the copyright owner(s) are credited and that the original publication in this journal is cited, in accordance with accepted academic practice. No use, distribution or reproduction is permitted which does not comply with these terms.
*Correspondence: Yang Liu, bGl1eWRsZmV6eEAxNjMuY29t
†These authors have contributed equally to this work