- 1College of Veterinary Medicine, Qingdao Agricultural University, Qingdao, China
- 2Qingdao Center for Animal Disease Control and Prevention, Qingdao, China
Introduction
Senecavirus A (SVA) is an emerging virus, typically inducing a vesicular disease in pigs. Clinical signs are characterized by lethargy and lameness, usually followed by the development of vesicles on the snout, dewclaw or (and) coronary band. The clinical signs are generally indistinguishable from those of other vesicular diseases in pigs (1). SVA-infected cases have been reported in at least 10 countries (2–11), therefore attracting a great deal of attention from the pig industry worldwide.
SVA is the only member of the genus Senecavirus, in the family Picornaviridae (12). The virion is a typical icosahedral particle, encapsulating its own genome that is a positive-sense, single-stranded RNA genome (Figure 1A), approximately 7,300 nt in length, composed of 5' untranslated region (UTR), polyprotein open reading frame (ORF) and 3' UTR. SVA has a length-variable poly(A) tail at the genomic 3' terminus (13). At the 5' terminus, there is no cap structure. Alternatively, a short peptide, VPg, is covalently linked to the 5' terminus, and as the protein primer, plays a crucial role in the synthesis of viral genome.
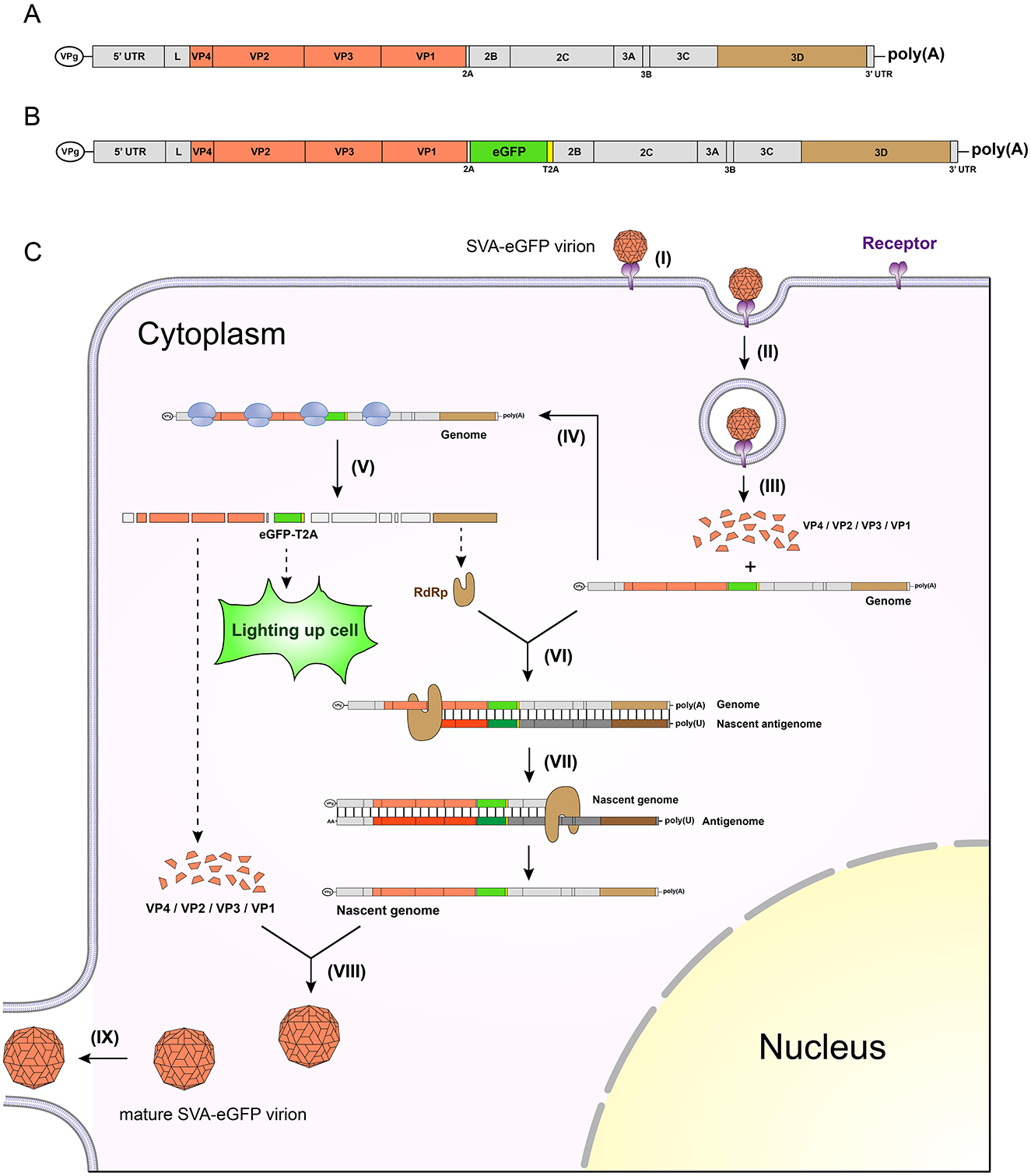
Figure 1. Schematic representations of wild-type genome, chimeric genome, and a single round of replication cycle after SVA-eGFP infection. Wild-type (A) and chimeric (B) SVA genomes. The latter contains an eGFP-T2A fusion fragment, located between the viral 2A and 2B sequences. Genetic elements match their actual lengths in proportion. A single round of viral replication cycle in a cell infected with SVA-eGFP (C). The replication cycle mainly includes nine steps, namely, (I) virus attaching to its receptor, (II) endocytosis-mediated entrance of virion into cell, (III) uncoating of virion, (IV) ribosome-triggered translation of polyprotein, (V) further processing of polyprotein through lysis, (VI) RdRp-initiated replication of nascent antigenome, (VII) replication of nascent genome, (VIII) morphogenesis of virion, and (IX) release of virion.
In an SVA-infected cell, the viral polyprotein precursor is translated and progressively cleaved into 12 proteins: L, VP4, VP2, VP3, VP1, 2A, 2B, 2C, 3A, 3B, 3C and 3D (14). The VP1 to VP4 are structural proteins, which interact with one another, responsible for the viral morphogenesis. The others are non-structural proteins, required for viral replication (15–17). For example, the 2A oligopeptide confers a ribosome “skipping” effect, separating two proteins without needing a proteinase (18).
Construction of SVA reverse genetics platform: general methodology
The reverse genetics (RG) technique is a potent tool, whereby a replication-competent RNA virus can be rescued (or recovered) from its genome cDNA clone, facilitating studies on molecular virology, virus-vectored vaccinology, marker-tagged diagnostics and so forth. To data, several groups have independently constructed the RG platform of SVA, using their individual methodologies (19–25), slightly different from one another. We have established an SVA RG platform, demonstrated to be highly efficient in the recovery of viable SVA (26).
The first step, taking our method to develop the SVA RG system as an example, was to construct a cDNA clone of full-length genome, through either chemical synthesis or overlap extension PCR. The cDNA clone was flanked by a T7 promoter and a poly(A) tail at its 5' and 3' ends, respectively. The fusion sequence, T7 promoter-cDNA clone-poly(A) tail, was subcloned into a plasmid for constructing a recombinant one. For virus rescue, BSR-T7/5 cells, which could constitutively express the T7 RNA polymerase, were seeded onto a 6-well plate for incubation overnight at 37°C, followed by transfection with the cDNA clone-containing plasmid. The plasmid-transfected cell monolayer was subsequently cultured at 37°C for 3 d, and then subjected to two freeze-and-thaw cycles to harvest the supernatant for serial blind passages. The passage-5 progeny was analyzed by RT-PCR for detecting whether the virus of interest was successfully rescued.
RG-generated wild-type SVA for omics studies
SVA can be clinically isolated from SVA-infected pigs. An SVA isolate, if not subjected to several rounds of plaque purification, may not be a single SVA clone, even containing other pathogens in the viral stock. If so, an SVA-based omics study would be invaluable. In contrast, RG-generated SVA is a single strain without contamination by other pathogens, required for a comparative study of omics after SVA infection. In our previous study, a wild-type SVA was successfully rescued from its cDNA clone (26). The passage-5 progeny was inoculated into cell monolayers, followed by a series of comparative analyses based on multi-omics, including transcriptomics (27), proteomics (28) and metabolomics (29).
RG-mediated point mutation for unveiling SVA characteristics
It has been widely reported that one single point mutation can even extensively change the picornaviral characteristics (30–33). Site-directed mutagenesis (SDM) can be used for modifying a wild-type SVA cDNA clone to reconstruct a mutant. If able to be rescued from the mutant, the SDM-modified SVA can be compared with its wild-type counterpart concerning some viral features. For example, two SVA variants were constructed via SDM to introduce different mutated sites in their RdRps (S460L alone and I212V-S460L in combination), consequently suggesting both variants that had lower recombination capacity than the wild-type strain (34). More recently, Zhao et al. (13) used the RG tool with method of structural biology to optimize novel oncolytic SVA mutants: viral receptor-associated SVA-S177A, and viral antigenic peptide-related SVA-S177A/P60S, both of which showed not only higher infectivity but also lower immunogenicity than the wild-type strain did (35).
Fluorescent marker-tagged SVA facilitating viral studies
Picornavirus is capable to accommodate a foreign sequence in its genome. The shorter the foreign sequence is, the stronger the accommodation capability is. Fluorescent protein and luciferase sequences are widely used as tracking markers inserted into wild-type SVA cDNA clones to rescue marker-tagged viruses for antiviral screening, virus neutralization test, oncolytic analysis, etc (19, 25, 36, 37). These fluorescent markers include enhanced green fluorescent protein (eGFP), red fluorescent protein, NanoLuc® luciferase (NLuc), Gaussia luciferase, and so on.
eGFP-tagged SVA
The eGFP is most broadly used for constructing fluorescent marker-tagged picornaviruses. An eGFP-tagged SVA (SVA-eGFP) has a chimeric genome that generally contains an eGFP ORF fused with a Thosea asigna virus 2A (T2A) sequence (Figure 1B). The SVA-eGFP virion can enter a susceptible cell via an endocytic pathway, followed by a series of processes to encode the eGFP and finally to generate mature virions in the cell, as schematically shown in Figure 1C. Notably, the eGFP is expressed as the eGFP-T2A fusion pattern, which can “light up” the cell through observation using a fluorescence microscope, indicating that the cell has been infected with SVA-eGFP.
Viral titration based on TCID50 assay is involved in observing cytopathic effect (CPE) for calculating a titer value. However, a few reports have shown that some SVA strains hardly induce the typical CPE on a cell monolayer (7, 38, 39), therefore inconducive to the viral titration. One of the advantages in use of SVA-eGFP is facilitating the viral titration, because fluorescence-emitted wells in the TCID50 assay indicate that their corresponding cell monolayers have been infected with SVA-eGFP. The accuracy of titer measurement through identifying fluorescent wells is much higher than that through observing the CPE formation.
The conventional virus neutralization test (VNT) is generally considered as a gold standard for recognizing virus-specific antibodies, but unfortunately is a labor- and time-consuming method. If a wild-type SVA is used for VNT, the prerequisite is that the wild-type SVA is able to induce obvious CPE on a 96-well plate. In contrast, if SVA-eGFP is used, CPE would be unnecessary for VNT readings, because the SVA-eGFP can function as a virus-infected indicator to imply which wells are infected or not. Fluorescence-free wells suggest that neutralizing antibodies have completely neutralized SVA-eGFP at a certain dilution of viral suspension. More importantly, 2 days are enough for the incubation of 96-well plate to recognize a fluorescent phenotype after SVA infection, whereas the wild-type SVA-based VNT need at least 4 days. Therefore, use of SVA-eGFP contributes to VNT for clinical sera (19, 25).
Another SVA-eGFP-related advantage is favorable to determine whether a replication-competent virus is successfully rescued from its cDNA clone. If there is no green fluorescence with serial blind passages, commonly three passages after plasmid transfection, it can be concluded that the viable SVA-eGFP fails to be rescued. This is very important especially for a large number of genetically modified SVA-eGFP cDNA clones that need to be analyzed for uncovering their own recovery abilities. For example, Meng et al. (40) constructed a series of 36 different cDNA clones, independently transfected into cell monolayers in an attempt to rescue genetically modified SVA-eGFPs. The green fluorescence was demonstrated to facilitate the fast screening for some SVA-eGFP cDNA clones without ability of virus recovery (40).
Luciferase-tagged SVA
Luciferases are proteins with enzymatic activity, which can catalyze the oxidation of substrate (commonly luciferin), causing the emission of photons. Luciferase is another fluorescent marker, widely used for the construction of recombinant viruses using RG technique. Different from fluorescent proteins, on the one hand, luciferases do not need the excitation light for lighting up cells but require appropriate luciferins; on the other hand, luciferase-induced fluorescence can be quantified using a microplate reader, whereas fluorescent protein-induced fluorescence cannot be done.
There are various types of luciferases with their own characteristics. NLuc is a novel luciferase, shorter, smaller and “brighter” than the other two common ones, firefly and Renilla reniformis luciferases. NLuc is a promising fluorescent marker to construct chimeric viruses for imaging assay in vitro or in vivo (41–44). The NLuc-tagged SVA (SVA-NLuc) is able of efficiently expressing NLuc, which as a bright indicator has been demonstrated to facilitate greatly the SVA-mediated oncolytic analysis in vitro (37), whereas there has unfortunately been no further study on an in vivo test.
The luminescence intensity is roughly proportional to the titer of luciferase-tagged SVA in cells. Such a quantifiable feature contributes to the high-throughput screening of anti-SVA drugs (36, 45, 46). For example, Wang et al. (23) used the SVA-NLuc to analyze a drug library composed of 136 natural products, and rapidly screened out five efficient anti-SVA products, namely, monensin sodium salt, progesterone, hypophyllanthin, 4-hydroxyderricin, and 2-methoxyestrone (45). In addition, its quantitative trait significantly shortens the period of conventional method for determining an SVA growth curve (37).
Drawback of marker-tagged SVAs: genetic instability with passaging
As mentioned above, marker-tagged SVAs greatly facilitate studies of SVA, whereas unfortunately, foreign sequences have been demonstrated to be genetically unstable in recombinant SVAs. We found that SVA-eGFP-induced fluorescence was gradually weakening in intensity with viral passaging, and RT-PCR demonstrated that the eGFP sequence was consecutively deleted from the chimeric SVA genome (19). The eGFP ORF is 720 bp in length, longer than the NLuc ORF (516 bp). In theory, the longer a marker is, the more unstable its sequence is. Indeed, we found that NLuc was genetically more stable than eGFP with passaging (37), whereas, as a foreign fragment, the NLuc sequence was still deleted gradually from the SVA-NLuc genome with passaging. Such an unstable feature limits the application of marker-tagged SVAs, resulting in only low-passage progenies that can be used.
Development of novel SVA vaccine using RG technique
Because a foreign sequence is unstable in a chimeric SVA genome, SVA has no potential in developing a virus-vectored vaccine. In addition, different SVA strains are prone to genetic recombination with each other (47). Therefore, live vaccines cannot be used in preventing SVA infection. Conventional inactivated vaccines have been widely reported to be safe and efficient against SVA infection (39, 48–52). Interestingly, Fan et al. (53) used the RG tool to construct an engineering His-tagged SVA, further processed into a novel inactivated vaccine. In this study, one 6 × His tag was fused to the C-terminal of VP1, and could be displayed on the surface of SVA virion. The 6 × His tag was so short that it was relatively stable in the chimeric SVA with passaging, and more importantly, allowed one-step purification of SVA antigens through Ni2+ affinity columns (53). Such an engineering strain provided a novel method of concentrating SVA antigens for the production of inactivated vaccines.
Future challenges for developing RG system of SVA
Numerous groups have established their own RG systems for SVA recovery (19–25), opening up new avenues for in-depth dissection of SVA molecular mechanisms, whereas some technique challenges still remain to be addressed. The first one is how a full-length cDNA clone is rapidly, efficiently constructed. We found that many genetically unstable fragments were possibly prone to being deleted from a longer sequence via the conventional method, restriction enzyme-based cloning, for constructing an SVA cDNA clone. Chemical synthesis, albeit costly, is more efficient than the conventional method in constructing a full-length cDNA clone. The second challenge is rescue of a strain that can induce obvious CPEs on a cell monolayer. We previously rescued different SVA strains from their own cDNA clones. Unfortunately, SVA-induced CPE was almost invisible during initial passaging in vitro (26), therefore limiting the application of SVA as a model virus. The third challenge is how a risk-free SVA strain is rescued as a model virus for use in vivo. In other words, we should use the RG technique to facilitate our studies on SVA, rather than to create some putative risks in bio-safety.
Author contributions
LZ: Writing – original draft, Conceptualization. ZL: Writing – original draft, Conceptualization. YL: Software, Writing – review & editing. FL: Funding acquisition, Writing – review & editing. SY: Investigation, Writing – review & editing.
Funding
The author(s) declare that financial support was received for the research and/or publication of this article. This work was supported by the National Program of Undergraduate Innovation and Entrepreneurship (Grant No. 202310435039), and the enterprise grant from Qingdao Haijia Agricultural Development Co., Ltd (Heng 20240060).
Acknowledgments
We gratefully thank other members in our group for their discussion in this paper.
Conflict of interest
The authors declare that the research was conducted in the absence of any commercial or financial relationships that could be construed as a potential conflict of interest.
The authors declare that this study received funding from the Qingdao Haijia Agricultural Development Co., Ltd. The funder had the following involvement in the study: data collection.
Generative AI statement
The author(s) declare that no Gen AI was used in the creation of this manuscript.
Publisher's note
All claims expressed in this article are solely those of the authors and do not necessarily represent those of their affiliated organizations, or those of the publisher, the editors and the reviewers. Any product that may be evaluated in this article, or claim that may be made by its manufacturer, is not guaranteed or endorsed by the publisher.
References
1. Leme RA, Alfieri AF, Alfieri AA. Update on senecavirus infection in pigs. Viruses. (2017) 9:170. doi: 10.3390/v9070170
2. Pasma T, Davidson S, Shaw SL. Idiopathic vesicular disease in swine in Manitoba. Can Vet J. (2008) 49:84–5.
3. Singh K, Corner S, Clark S, Scherba G, Fredrickson R. Seneca valley virus and vesicular lesions in a pig with idiopathic vesicular disease. J Vet Sci Technol. (2012) 3:1–3. doi: 10.4172/2157-7579.1000123
4. Leme RA, Zotti E, Alcantara BK, Oliveira MV, Freitas LA, Alfieri AF, et al. Senecavirus A: an emerging vesicular infection in brazilian pig herds. Transbound Emerg Dis. (2015) 62:603–11. doi: 10.1111/tbed.12430
5. Sun D, Vannucci F, Knutson TP, Corzo C, Marthaler DG. Emergence and whole-genome sequence of senecavirus A in Colombia. Transbound Emerg Dis. (2017) 64:1346–9. doi: 10.1111/tbed.12669
6. Navarro-López R, Perez-de la Rosa J, Rocha-Martínez M, Hernández G, Hernández M, Solís-Hernández M, et al. First detection and genetic characterization of senecavirus A in pigs from Mexico. J Swine Health Prod. (2023) 31:289–94. doi: 10.54846/jshap/1358
7. Bennett B, Urzúa-Encina C, Pardo-Roa C, Ariyama N, Lecocq C, Rivera C, et al. First report and genetic characterization of seneca valley virus (SVV) in Chile. Transbound Emerg Dis. (2022) 69:e3462–e8. doi: 10.1111/tbed.14747
8. Wu Q, Zhao X, Bai Y, Sun B, Xie Q, Ma J. The first identification and complete genome of senecavirus A affecting pig with idiopathic vesicular disease in China. Transbound Emerg Dis. (2017) 64:1633–40. doi: 10.1111/tbed.12557
9. Saeng-Chuto K, Rodtian P, Temeeyasen G, Wegner M, Nilubol D. The first detection of senecavirus A in pigs in Thailand, 2016. Transbound Emerg Dis. (2018) 65:285–8. doi: 10.1111/tbed.12654
10. Arzt J, Bertram MR, Vu LT, Pauszek SJ, Hartwig EJ, Smoliga GR, et al. First detection and genome sequence of senecavirus A in Vietnam. Microbiol Resour Announc. (2019) 8:e01247–18. doi: 10.1128/MRA.01247-18
11. Sushila M, Kanisht B, Deepika C, Monika P, Vijay K, Vinay GJ, et al. Detection and genomic characterization of senecavirus from Indian Pigs. Indian J Anim Res. (2023) 57:1344–50. doi: 10.18805/IJAR.B-4722
12. Oliveira TES, Leme RA, Agnol AMD, Gerez JR, Pelaquim IF, Miyabe FM, et al. Seneca valley virus induces immunodepressionin suckling piglets by selective apoptosis of B lymphocytes. Microb Pathog. (2021) 158:105022. doi: 10.1016/j.micpath.2021.105022
13. Zhao D, Li Y, Li Z, Zhu L, Sang Y, Zhang H, et al. Only fourteen 3′-end poly(A)s sufficient for rescuing senecavirus A from its cDNA clone, but inadequate to meet requirement of viral replication. Virus Res. (2023) 328:199076. doi: 10.1016/j.virusres.2023.199076
14. Liu F, Wang Q, Huang Y, Wang N, Shan H. A 5-year review of senecavirus A in China since its emergence in 2015. Front Vet Sci. (2020) 7:567792. doi: 10.3389/fvets.2020.567792
15. Choudhury SM, Ma X, Zeng Z, Luo Z, Li Y, Nian X, et al. Senecavirus a 3D interacts with NLRP3 to induce IL-1β production by activating NF-κB and ion channel signals. Microbiol Spectr. (2022) 10:e0209721. doi: 10.1128/spectrum.02097-21
16. Liu H, Li K, Chen W, Yang F, Cao W, Zhang K, et al. Senecavirus A 2B protein suppresses type I interferon production by inducing the degradation of MAVS. Mol Immunol. (2022) 142:11–21. doi: 10.1016/j.molimm.2021.12.015
17. Zhao K, Guo XR, Liu SF, Liu XN, Han Y, Wang LL, et al. 2B and 3C proteins of senecavirus A antagonize the antiviral activity of DDX21 via the caspase-dependent degradation of DDX21. Front Immunol. (2022) 13:951984. doi: 10.3389/fimmu.2022.951984
18. de Lima JGS, Lanza DCF. 2A and 2A-like sequences: distribution in different virus species and applications in biotechnology. Viruses. (2021) 13:2160. doi: 10.3390/v13112160
19. Liu F, Huang Y, Wang Q, Shan H. Construction of eGFP-tagged senecavirus A for facilitating virus neutralization test and antiviral assay. Viruses. (2020) 12:E283. doi: 10.3390/v12030283
20. Zhang X, Lu J, Deng T, Zhao P, Peng Z, Chen L, et al. Development of an improved dual-promoter-based reverse genetics system for emerging Senecavirus A. J Virol Methods. (2020) 286:113973. doi: 10.1016/j.jviromet.2020.113973
21. Fernandes MHV, de Lima M, Joshi LR, Diel DG. A virulent and pathogenic infectious clone of Senecavirus A. J Gen Virol. (2021) 102:001643. doi: 10.1099/jgv.0.001643
22. Chen Z, Yuan F, Li Y, Shang P, Schroeder R, Lechtenberg K, et al. Construction and characterization of a full-length cDNA infectious clone of emerging porcine Senecavirus A. Virology. (2016) 497:111–24. doi: 10.1016/j.virol.2016.07.003
23. Wang H, Mo Y, Liu W, Niu C, He Q, Ren T, et al. Construction and characterization of a full-length infectious clone of an emerging senecavirus A strain. Arch Virol. (2024) 169:25. doi: 10.1007/s00705-023-05951-y
24. Wang M, Mou C, Chen M, Chen Z. Infectious recombinant senecavirus A expressing novel reporter proteins. Appl Microbiol Biotechnol. (2021) 105:2385–97. doi: 10.1007/s00253-021-11181-6
25. Huang W, Chen Y, Xu T, Xiong T, Lv Y, Liu D, et al. Development and characterization of a recombinant Senecavirus A expressing enhanced green fluorescent protein. Front Microbiol. (2024) 15:1443696. doi: 10.3389/fmicb.2024.1443696
26. Liu F, Huang Y, Wang Q, Li J, Shan H. Rescue of senecavirus A to uncover mutation profiles of its progenies during 80 serial passages in vitro. Vet Microbiol. (2021) 253:108969. doi: 10.1016/j.vetmic.2020.108969
27. Li Y, Chu H, Jiang Y, Li Z, Wang J, Liu F. Comparative transcriptomics analysis on senecavirus A-infected and non-infected cells. Front Vet Sci. (2024) 11:1431879. doi: 10.3389/fvets.2024.1431879
28. Liu F, Ni B, Wei R. Comparative proteomic profiling: cellular metabolisms are mainly affected in senecavirus A-inoculated cells at an early stage of infection. Viruses. (2021) 13:1036. doi: 10.3390/v13061036
29. Liu F, Ni B, Wei R. Senecavirus A- and non-infected cells at early stage of infection: comparative metabolomic profiles. Front Cell Infect Microbiol. (2022) 11:736506. doi: 10.3389/fcimb.2021.736506
30. Vignuzzi M, Stone JK, Arnold JJ, Cameron CE, Andino R. Quasispecies diversity determines pathogenesis through cooperative interactions in a viral population. Nature. (2006) 439:344–8. doi: 10.1038/nature04388
31. Pfeiffer JK, Kirkegaard K. A single mutation in poliovirus RNA-dependent RNA polymerase confers resistance to mutagenic nucleotide analogs via increased fidelity. Proc Natl Acad Sci USA. (2003) 100:7289–94. doi: 10.1073/pnas.1232294100
32. Zeng J, Wang H, Xie X, Yang D, Zhou G, Yu L. An increased replication fidelity mutant of foot-and-mouth disease virus retains fitness in vitro and virulence in vivo. Antiviral Res. (2013) 100:1–7. doi: 10.1016/j.antiviral.2013.07.008
33. Abdelnabi R, Morais ATS, Leyssen P, Imbert I, Beaucourt S, Blanc H, et al. Understanding the mechanism of the broad-spectrum antiviral activity of favipiravir (T-705): key role of the F1 motif of the viral polymerase. J Virol. (2017) 91:e00487–17. doi: 10.1128/JVI.00487-17
34. Li C, Wang H, Shi J, Yang D, Zhou G, Chang J, et al. Senecavirus-specific recombination assays reveal the intimate link between polymerase fidelity and RNA recombination. J Virol. (2019) 93:e00576–19. doi: 10.1128/JVI.00576-19
35. Zhao Z, Cao L, Sun Z, Liu W, Li X, Fang K, et al. A structure-guided genetic modification strategy: developing seneca valley virus therapy against nonsensitive nonsmall cell lung carcinoma. J Virol. (2023) 97:e0045923. doi: 10.1128/jvi.00459-23
36. Wang H, Mo Y, Liu W, He Q, Ren T, Ouyang K, et al. Construction and characterization of recombinant senecavirus A expressing secreted luciferase for antiviral screening. J Virol Methods. (2024) 327:114932. doi: 10.1016/j.jviromet.2024.114932
37. Liu F, Wang Q, Huang Y, Wang N, Shan H. Rescue of NanoLuc luciferase-expressing senecavirus A with oncolytic activity. Virus Res. (2021) 292:198232. doi: 10.1016/j.virusres.2020.198232
38. Joshi LR, Mohr KA, Clement T, Hain KS, Myers B, Yaros J, et al. Detection of the emerging picornavirus senecavirus A in pigs, mice, and houseflies. J Clin Microbiol. (2016) 54:1536–45. doi: 10.1128/JCM.03390-15
39. Wang B, Gao F, Hu R, Huyan H, Wang G, Cao Z, et al. Development and evaluation of inactivated vaccines incorporating a novel Senecavirus A strain-based Immunogen and various adjuvants in mice. Front Vet Sci. (2024) 11:1376678. doi: 10.3389/fvets.2024.1376678
40. Meng H, Wang Q, Liu M, Li Z, Hao X, Zhao D, et al. The 5′-end motif of senecavirus A cDNA clone is genetically modified in 36 different ways for uncovering profiles of virus recovery. Front Microbiol. (2022) 13:957849. doi: 10.3389/fmicb.2022.957849
41. Baker C, Xie X, Zou J, Muruato A, Fink K, Shi PY. Using recombination-dependent lethal mutations to stabilize reporter flaviviruses for rapid serodiagnosis and drug discovery. EBioMedicine. (2020) 57:102838. doi: 10.1016/j.ebiom.2020.102838
42. Kanai Y, Kawagishi T, Matsuura Y, Kobayashi T. In vivo live imaging of oncolytic mammalian orthoreovirus expressing nanoluc luciferase in tumor xenograft mice. J Virol. (2019) 93:e00401–19. doi: 10.1128/JVI.00401-19
43. Chiem K, Rangel-Moreno J, Nogales A, Martinez-Sobrido L. A luciferase-fluorescent reporter influenza virus for live imaging and quantification of viral infection. J Vis Exp. (2019) 150:e59890. doi: 10.3791/59890-v
44. Anindita PD, Sasaki M, Nobori H, Sato A, Carr M, Ito N, et al. Generation of recombinant rabies viruses encoding NanoLuc luciferase for antiviral activity assays. Virus Res. (2016) 215:121–8. doi: 10.1016/j.virusres.2016.02.002
45. Wang X, Sha Z, Chi T, Cui J, Zheng H, Chen F, et al. Screening and action pathway of compounds antagonistic senecavirus a in vitro from natural product library (in Chinese). China Anim Husb Vet Med. (2024) 51:3155–65. doi: 10.16431/j.cnki.1671-7236.2024.07.039
46. Guo X, Zhao K, Liu X, Lei B, Zhang W, Li X, et al. Construction and generation of a recombinant senecavirus a stably expressing the nanoluc luciferase for quantitative antiviral assay. Front Microbiol. (2021) 12:745502. doi: 10.3389/fmicb.2021.745502
47. Li Y, Liu T, Zhang Y, Duan X, Liu F. RNA recombination: non-negligible factor for preventing emergence or reemergence of Senecavirus A. Front Vet Sci. (2024) 11:1357179. doi: 10.3389/fvets.2024.1357179
48. Barbosa AO, Gava D, Tochetto C, Ribeiro LC, Bastos APA, Morés MAZ, et al. Immunogenicity of an inactivated senecavirus A vaccine with a contemporary Brazilian strain in mice. Vaccines. (2024) 12:845. doi: 10.3390/vaccines12080845
49. Zhang J, Wang P, Li Z, Xie Y, Jin N, Zhang H, et al. Adjuvant screening of the senecavirus A inactivated vaccine in mice and evaluation of its immunogenicity in pigs. BMC Vet Res. (2024) 20:82. doi: 10.1186/s12917-024-03949-5
50. Buckley A, Lager K. Efficacy of an inactivated Senecavirus A vaccine in weaned pigs and mature sows. Vaccine. (2022) 40:1747–54. doi: 10.1016/j.vaccine.2022.02.018
51. Li N, Qiao QL, Guo HF, Wang BY, Huang Q, Wang Z, et al. Evaluation of immunogenicity and protective efficacy of a novel senecavirus A strain-based inactivated vaccine in mice. Res Vet Sci. (2021) 142:133–40. doi: 10.1016/j.rvsc.2021.12.010
52. Yang F, Zhu Z, Liu H, Cao W, Zhang W, Wei T, et al. Evaluation of antibody response in sows after vaccination with senecavirus A vaccine and the effect of maternal antibody transfer on antibody dynamics in offspring. Vaccines. (2021) 9:1066. doi: 10.3390/vaccines9101066
Keywords: Senecavirus A, reverse genetics, cDNA clone, rescue, fluorescent marker
Citation: Zhu L, Li Z, Li Y, Liu F and Yang S (2025) Using reverse genetics tool for study of Senecavirus A: pros and cons. Front. Vet. Sci. 12:1546709. doi: 10.3389/fvets.2025.1546709
Received: 17 December 2024; Accepted: 14 March 2025;
Published: 02 April 2025.
Edited by:
Simon Paul Graham, The Pirbright Institute, United KingdomReviewed by:
Jiangwei Song, Beijing Academy of Agricultural and Forestry Sciences, ChinaLikangli Li, Chinese Academy of Agricultural Sciences, China
Copyright © 2025 Zhu, Li, Li, Liu and Yang. This is an open-access article distributed under the terms of the Creative Commons Attribution License (CC BY). The use, distribution or reproduction in other forums is permitted, provided the original author(s) and the copyright owner(s) are credited and that the original publication in this journal is cited, in accordance with accepted academic practice. No use, distribution or reproduction is permitted which does not comply with these terms.
*Correspondence: Fuxiao Liu, bGF1ZGF3bkAxMjYuY29t; Shengnan Yang, MjYwMDU0NTI1QHFxLmNvbQ==
†These authors have contributed equally to this work