- 1Hunan Engineering Research Center of Livestock and Poultry Health Care, Colleges of Veterinary Medicine, Hunan Agricultural University, Changsha, China
- 2Changsha Lvye Biotechnology Co., Ltd, Changsha, China
Introduction: Since the comprehensive ban on the addition of antibiotics to livestock and poultry feeds in China, the search for safe and natural antibiotic substitutes has become a hot spot in the animal breeding industry. Areca catechu L (AN), known as the leader among the four southern medicinal herbs, possesses functions such as insecticidal, antibacterial, antiinflammatory, promoting gastrointestinal motility and preventing Alzheimer's disease. Nevertheless, ANE is rarely used as a feed additive in AA broilers, and its specific role remains unclear. This study was conducted to investigate the effects of different levels of areca nut extracts (ANE) on growth performance, slaughter performance and meat quality of AA broiler chickens.
Methods: 128 one-day-old Arbor Acres broilers were randomly divided into eight groups of 16 birds each, housed in three cages with 5-6 birds per cage, with or without ANE supplementation (0, 100, 150, 200, 250, 300, 350, and 400 mg/kg, respectively).
Results: The entire experiment duration was 49 days. Adding 100 and 200 mg/kg ANE to the diet could significantly increase the body weight of broilers at 21 days of age (P ≤ 0.001), and significantly reduce the feed-to-weight ratio from 1 to 21 days of age (P ≤ 0.001). The diet supplemented with 200 mg/kg ANE could significantly increase the average body weight at 49 days of age (P = 0.001). Compared with the control group, the addition of different dosages of ANE in the feed could improve the pH45min, yellowness, and shear force (P ≤ 0.043) of the pectoral and leg muscles of broilers. Additionally, the contents of crude fat and crude protein, which are nutritional components in the pectoral and leg muscles of the ANE-supplemented groups, were to an extent higher than those of the control group (P ≤ 0.036). The addition of different levels of ANE in the diet significantly raised the expression levels of muscle development-related genes, including Myf5, Myf6, MyoD1, IGF-1, and IGF-2 (P ≤ 0.032).
Discussion: In conclusion, An appropriate amount of ANE in the diet has been demonstrated to boost the growth performance and meat quality of broilers, facilitate muscle development, and has no remarkable influence on slaughter performance. the ideal dosage for broilers is 100–200 mg/kg ANE. The findings of this study offer valuable insights into the potential benefits of ANE in poultry production, and provide a basis for further research into the development of ANE as a new feed additive.
1 Introduction
Antibiotics were formerly extensively utilized in livestock production. Nevertheless, due to the chronic addition of antibiotics leading to drug residues, bacterial resistance, and so on (1, 2), many countries have proscribed their employment. This ban represents a major challenge for the entire livestock industry and a significant threat to animal welfare. Therefore, there is an urgent need to discover natural, environmentally friendly and safe feed additives.
Many researchers have come to pay attention to feed additives such as natural plant extracts, intestinal health agents, and digestive enzymes (3, 4). These additives cannot merely improve the immunity and production performance of animals (5), but also alleviate the issue of drug residues in food. Among these, natural plant extracts are one of the prevalent feed additives.
Areca catechu L. is a genus of A. catechu belonging to monocotyledonous palmae, distributed in the tropical and subtropical regions in southern China and other South Asian and Southeast Asian countries (6, 7). A. catechu, the dried seed of Areca palm fruit, is not only one of the commonly used traditional Chinese medicinal materials, but also a traditional leisure food in many regions, and over 600 million people globally frequently chew Areca nut (AN) (6). However, long-term chewing of AN can led to oral submucous fibrosis and, eventually, oral cancer. Hence, the safety issue of AN consumption has aroused widespread concern in society. Whereas, as a traditional medicinal plant (with a medicinal history of more than 2,000 years in China) (8), the physiological activity and medicinal value of AN have been constantly overlooked by the public. Researches have verified that AN possesses a variety of bioactive substances (9), such as alkaloids, phenols, flavonoids, tannins, triterpenes, fatty acids and other functional compounds (10), with multiple physiological activities such as antioxidation, alleviation of fatigue, improvement of gastrointestinal function, treatment of diabetes, restoration of the nervous system, and prevention of cancer. Its main active ingredient, arecoline, has been shown to have antibacterial and anti-inflammatory effects (11), and to stimulate gastrointestinal peristalsis (12). Some studies have found that areca nut extracts (ANE) can be used to eliminate parasites (13), treat gastrointestinal inflammation in dyspepsia (14), and improve the growth performance and intestinal microbiota in Wenchang chickens (15). Nevertheless, ANE is rarely used as a feed additive in AA broilers, and its specific role remains unclear.
Here, this study aims to comprehensively assess the effects of different levels of ANE on growth performance, slaughter performance, meat quality, and muscle nutritional components of AA broilers, with the anticipation of providing referential significance for its utilization as a feed additive.
2 Materials and methods
2.1 Preparation of ANE
The ANE is provided by Changsha Lvye Biotechnology Co., Ltd. (Changsha, China). The preparation process of the extract was as follows: first, mold-contaminated areca nuts and impurities such as dust were removed through manual inspection and sieving. The cleaned nuts were then pulverized and subjected to percolation (with a solvent-to-material ratio of 8:1), followed by concentration and drying to obtain a solid block of ANE. Finally, the solid extract was ground into powder using an extract pulverization unit, then stored at 4°C to maintain its bioactivity until further analysis and incorporation into the experimental diets. The mass of the lyophilized extract was precisely measured to ensure accurate dosage calculations for feed formulation. The active component polyphenols were determined by folin-phenol colorimetry at a content of 10.73%, and arecoline were quantified by high-performance liquid chromatography (HPLC) at a content of 3.07% (16).
2.2 Animals, experimental design, and diets
The experimental procedures complied with the Animal Care and Use Guidelines of China, and were approved by the Animal Care Committee of Hunan Agricultural University (No. 2021090). A total of 128 one-day-old Arbor Acres broilers with an initial body weight of 44.0 ± 1.0 g were randomly divided into 8 groups, with 16 chickens in each group. The chickens were housed in three cages per group, with 5–6 chickens per cage. The cage dimensions for 5 chickens were 0.82 m wide × 1.22 m long × 0.82 m high, and for 6 chickens, the dimensions were 0.82 m wide × 1.46 m long × 0.82 m high. The stocking density was 0.164 m3 per chicken. One group was fed with basic diet, while the remaining testing groups were fed with a basic diet containing 100, 150, 200, 250, 300, 350, and 400 mg/kg ANE. The experimental diets were provided in powdered form and formulated under commission by Changsha Lvye Biotechnology Co., Ltd., China. The feeding experiment was conducted over 49 days, encompassing both the starter stage (1–21 days) and the grower stage (22–49 days). The chicks received a corn-soybean meal diet formulated to meet or exceed the recommended nutrient requirements of broilers. The feed formulation was formulated according to the Poultry Nutrient Requirements (17). Table 1 presents the composition and nutrient levels of the experimental diets.
All the chickens were housed in an environmentally controlled room. During the experimental period, the chickens had free access to food and water. Room temperature was set at 33°C for the first week and reduced by 1°C every 2 days until the birds reached 22°C (18). Light control: 24 h light for 1–3 days, 20~22 h light for 4–7 days and 12 h light on every day thereafter.
2.3 Growth performance
The growth performance of broilers was evaluated by measuring body weight (BW), average daily gain (ADG), average daily feed intake (ADFI), and feed-to-weight ratio (F/G). All birds in each group were individually weighed weekly until slaughter. ADG was calculated for sequential intervals (1–7, 7–14, 14–21, 21–28, 28–35, 35–42, and 42–49 days) and cumulative phases (1–21, 21–49, and 1–49 days). Daily health monitoring was implemented, and deceased birds were promptly removed. Feed intake was monitored daily, with residual feed quantified weekly to determine precise consumption (excluding deceased individuals). Subsequently, ADG and ADFI were adjusted using mortality-adjusted live-day correction factors based on the number of deceased birds and their survival duration (19). The established formulas are as follows (20):
2.4 Serum assay
At the end of the experiment, all chickens were deprived of feed for 12 h, but water was offered ad libitum. Subsequently, blood samples of approximately 8 mL (21) were withdrawn from the wing vein of each chicken and left for 2 h at room temperature, then centrifuged at 3,000 rpm for 10 min and deposited at −20°C until analysis. The serum concentrations of alanine aminotransferase (ALT), aspartate aminotransferase (AST), total protein (TP), albumin (ALB), triglyceride (TG), cholesterol (TC), creatinine (CREA), urea (UREA), uric acid (UA), high-density lipoprotein (HDL-C) and low-density lipoprotein (LDL-C) were measured using a Mindray Animal Automatic Blood Biochemical Analyzer (BS-240VET) (22, 23).
2.5 Slaughter performance
After blood sampling, the chickens (all chickens from each replicate) were killed by cervical dislocation. The slaughter weight, half-empty weight, full-empty weight, pectoral muscle weight, leg muscle weight, abdominal fat weight, and skin and subcutaneous fat weight were determined in accordance with the Poultry Production Performance Terminology and Measurement Calculation Method (NY/T 823-2020). The slaughter rate, half-empty weight rate, full-empty weight rate, pectoral muscle rate, leg muscle rate, abdominal fat rate and skin and subcutaneous fat rate of each chicken were calculated based on the weight (24).
2.6 Meat color and PH measurement
Meat color was ascertained using a CR-400 colorimeter. Twenty grams of each chicken was employed to establish the Opto value 45 min after slaughter. Each meat sample was measured five times, and the mean value was obtained. The pH of intrathoracic muscles was gauged 45 min after slaughter (pH45min) using a carcass meat quality pH-Star meter (Matthaus, Germany). Each meat sample was measured five times, and the average was taken. The samples were then placed in sealed bags, labeled, and stored in a 4°C refrigerator for 24 h. The pH was measured again after 24 h (pH24h) (25, 26).
2.7 Muscle cooking loss measurement
To measure cooking loss, muscle samples were dried with paper and weighed 45 min after slaughter (W1). The samples were placed in a plastic bag and cooked in a hot water bath until the temperature reached 70°C by using a thermometer inserted in the central part of the muscle. The cooked samples were then placed under running water to cool them to room temperature. They were blotted dry and weighed once again (W2). Water loss was calculated as the percentage of the initial weight (27).
2.8 Dripping loss measurement
By adopting the natural evaporation method, the muscle was initially weighed (W1). Then it was enclosed in a self-sealed bag to make sure that the bag did not adhere to the meat. After 24 h in the refrigerator at 4°C, the samples were removed and reweighed (W2) (27, 28). The dripping loss was calculated as follows:
2.9 Shearing force measurement
Fresh chicken breasts were collected, and external fat and connective tissue were removed. The samples were then cut into 5 × 2 × 2 cm cubes, and the muscle fibers were gauged using a digital-explicit muscle tenderness meter (C-LM3B, Tenovo International Co., Ltd., Beijing, China). Each sample was measured five times, and the average was acquired (29, 30).
2.10 Relative weight of organs
After dissection, the liver, spleen, heart, kidneys and bursa of Fabricius were removed and weighed, and the relative weight was expressed as a percentage to the individual BW (31).
2.11 Determination of crude protein content
One gram of muscle was taken, weighted (m), and, placed in a digestion tube. Copper and potassium sulfate were added as catalysts, and sulfuric acid was also added. The muscle was digested using the graphite digestion apparatus (SH220F, Shandong Haineng Technology). After the digested muscle was cooled to room temperature, a K9840 automatic nitrogen determination analyzer (Shandong Haineng Technology) was employed for detection. The blank sample receiving solution was titrated with 0.1 mol/L hydrochloric acid standard titration solution (c) until the color changed, and the volume of hydrochloric acid used for titration (V1) and the titrated volume of the blank sample after detection (V2) was recorded. The crude protein content of the muscle was calculated (32, 33). The calculation formula is as follows:
2.12 Determination of crude fat content
This Soxhlet extraction protocol followed the method of Hopkins et al. (34). Six gram of muscle was taken and weighed (m), which was disposed of in a freeze dryer (SCIENTZ-10 N/A, Ningbo Xin zhi Biology). The initial weight of the Soxhlet extractor cup (m1) was recorded. It was then wrapped in filter paper and, placed in the extraction cup for repeated extraction and rinsed with petroleum ether (30–60°C). After cooling to room temperature, the extraction cup was dried at 105°C and the final weight (m2) was recorded. Then the intramuscular fat content was calculated (35). The calculation formula was as follows:
2.13 Quantitative real-time polymerase chain reaction (RT-PCR)
Total RNA was extracted from the muscles by means of TRIzol reagent, in accordance with the manufacturer's protocol. The concentrations of extracted RNA were measured by employing a Nanodrop 2,000 spectrophotometer (Thermo Fisher Scientific Inc., Ottawa, ON, Canada). Complementary DNA (cDNA) was generated from RNA with the assistance of an iScriptTM cDNA synthesis kit (Hunan Aikerui Biological Engineering Co., Ltd, Changsha, China). Quantitative real-time polymerase chain reaction (RT-PCR) of genes was carried out using the NCBI primer tool (https://www.ncbi.nlm.nih.gov/). The genes included IGF1, IGF2, Myod1, Myf5, Myf6, MyoG, and β-actin. Table 2 shows the primers used in this study, which were developed by Shengong Biological Engineering Co., Ltd (Shanghai, China). The cycling conditions were 95°C for 30 s, 40 cycles at 95°C for 5 s, and 60°C for 30 s. Relative gene expression was calculated by means of the 2−ΔΔCT method (36).
2.14 Statistical analysis
SPSS version 26.0 (IBM SPSS, Chicago, IL, USA) and GraphPad Prism version 9.0 (GraphPad Software, San Diego, USA) were used for statistical analysis. Homogeneity of variance test and one-way ANOVA were carried out for data conforming to normal distribution; the LSD method was utilized for the post-test of neat variance, and the Tamheni T2 was adopted for irregularity. The test level where P < 0.05 was considered to be significant, and P < 0.01 was referred to highly significant. All values were presented as mean ± SE.
3 Results
3.1 Effect of ANE on growth performance in broilers
As outlined in Table 3, Broilers fed diets supplemented with 100, 200, and 400 mg/kg ANE exhibited significant increases in body weight of 15.7%, 15.9%, and 15.0%, respectively, compared to the control group at 21 days of age (P ≤ 0.002). In comparison to the control group, all treatment groups, with the exception of the 150 mg/kg ANE group, enhanced the ADG during 1–21 days, especially 100 and 200 mg/kg (P < 0.001). All ANE treatment groups lowered the F/G ratio of broilers within 1–21 days (P ≤ 0.02). No notable disparities were discernible among all the groups concerning feed intake, average daily weight, and F/G ratio within 22–49 days (P > 0.05). However, Dietary supplementation with 200 mg/kg ANE significantly improved both final body weight (9.2% increase) at day 49 and ADG (9.5% increase) throughout the 1–49 day period compared to the control group (P ≤ 0.45). These findings demonstrate that dietary ANE supplementation significantly enhanced broiler growth performance, with optimal effects achieved at 100 and 200 mg/kg doses.
3.2 Effect of ANE on serum biochemical indices in broilers
As illustrated in Table 4, there were no significant disparities among all ANE- supplemented groups in the levels of ALT, AST, TP, ALB, TG, TC, CREA, HDL-C, LDL-C when compared to the control group (P > 0.05). In contrast to the control group, UREA contents were lower in the 200, 300, and 350 mg/kg ANE addition groups (P ≤ 0.035). UA activity was increased in the 400 mg/kg ANE addition group as opposed to the control group (P = 0.008).
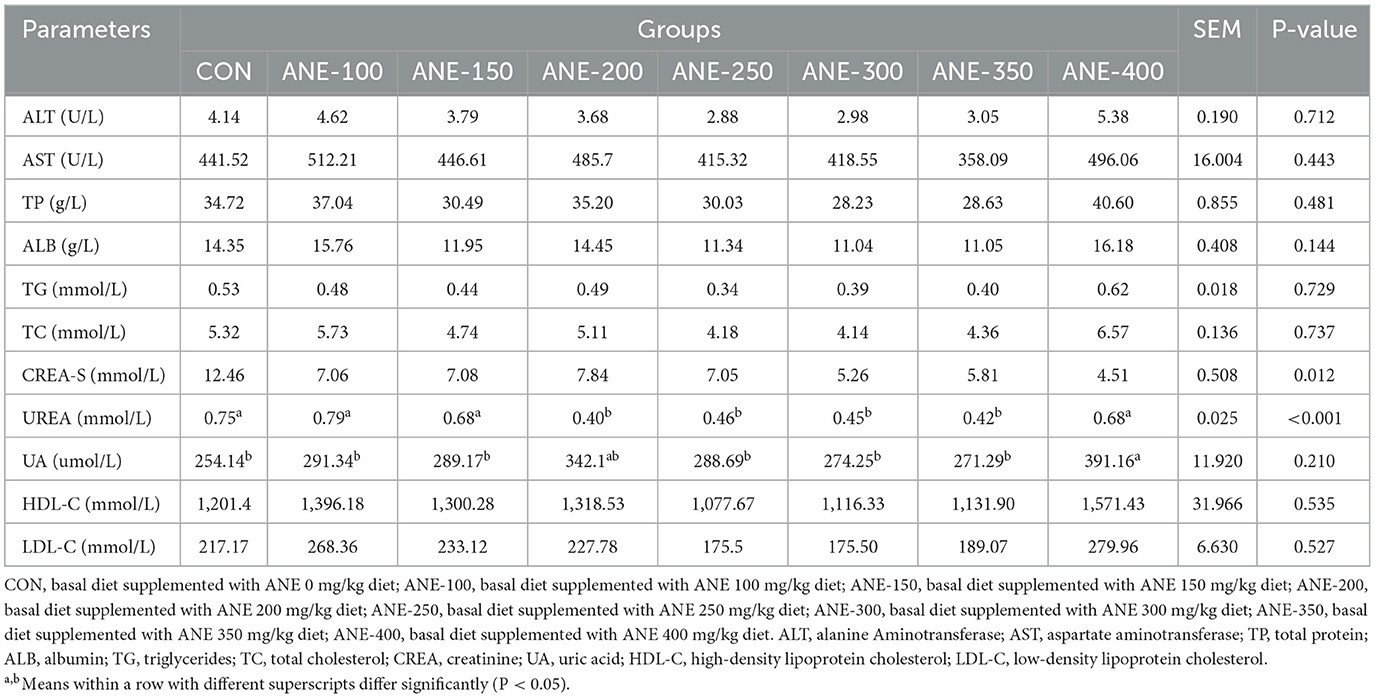
Table 4. Effects of different levels of ANE on serum biochemical indicators in Arbor Acres broilers.
3.3 Effect of ANE on the relative organ weights of broilers
Table 5 presents the relative organ weights of broilers following dietary supplementation with ANE. The relative liver weights of all ANE-added groups were lower than those of the control group, among which the 150, 200, and 400 mg/kg ANE added-groups decreased significantly (P ≤ 0.046). Additionally, in comparison with the control group, the relative weight of the bursa of Fabricius in the 350 mg/kg ANE addition group decreased markedly (P = 0.04). However, no significant difference were observed among all groups in the relative weights of the heart, spleen or kidneys (P > 0.05).
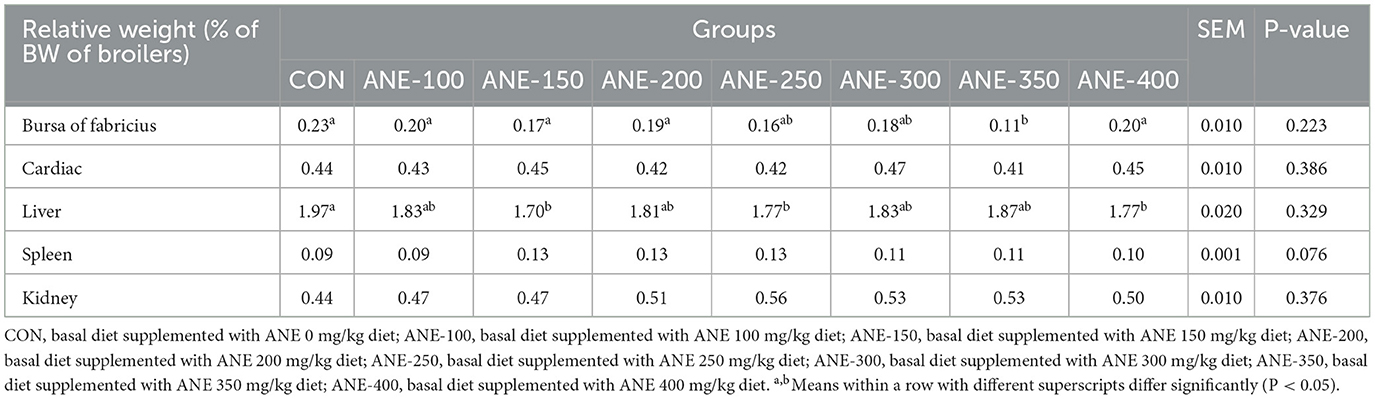
Table 5. Effects of different levels of ANE on the relative weight of organs in Arbor Acres broilers.
3.4 Effect of ANE on slaughtering performance of broilers
As depicted in Table 6, there were no significant differences among all groups in slaughter rate, half-eviscerated rate, full-eviscerated rate, pectoral muscle rate, leg muscle rate, abdominal fat rate, skin and subcutaneous fat rate (P > 0.05), indicating that the addition of ANE in the diet had no significant impact on the slaughter performance of broilers.
3.5 Effect of ANE on meat quality of broilers
In Table 7, in contrast to the control group, the pectoral muscle pH45min was found to be distinctly elevated in each ANE dosage group, reaching their highest at a dietary concentration of 200 mg/kg ANE (P ≤ 0.023), while the pH24h values in all groups were observed to decrease, yet these differences were not found to be significant (P > 0.05). The drip loss rate and cooking loss rate of the pectoral muscle presented no significant discrepancy compared to the control group (P > 0.05). In comparison with the control group, as the ANE dosage increased, the meat color L* gradually rose, and the meat color a* progressively decreased. Additionally, the b* values of the 250, 300, and 400 mg/kg ANE addition groups were significantly lower than those of the control group (P ≤ 0.034). Compared to the control group, the pectoral muscle shear force in the 100, 150, 300, and 350 mg/kg ANE addition groups significantly enhanced (P ≤ 0.043).
As shown in Table 8, the pH45min and pH24h values of the leg muscle in the 200 and 400 mg/kg ANE supplementation groups were remarkably higher than those of the control group (P ≤ 0.01). The drip loss and cooking loss exhibited no remarkable differences between the ANE addition groups and the control group (P > 0.05). In contrast to the control group, the meat color L* in the 200, 250, and 300 mg/kg ANE addition groups were higher, and the meat color b* in the 400 mg/kg ANE addition group was considerably lower (P ≤ 0.05). There was no notable difference in the a* values between the ANE treatment groups and the control group (P > 0.05). Additionally, the shear force of leg muscles showed no significant discrepancy in the ANE addition groups in comparison to the control group (P > 0.05), except that the 150 mg/kg group decreased conspicuously. These findings suggested that dietary ANE supplementation significantly improved pH and shear force values, ultimately enhancing meat quality parameters.
3.6 Effect of ANE on nutrient composition in muscle of broilers
Table 9 shows the effect of ANE on nutrient composition in muscle of broilers, when compared with the control group, the crude fat content in the pectoral muscle of broilers markedly rose after the addition of ANE (P ≤ 0.001), among which the 100 mg/kg group had the largest increase extent, approaching approximately 2.8 times that of the control group, while the crude fat content in the leg muscle exhibited a significant increase in the 150 and 350 mg/kg ANE addition groups.
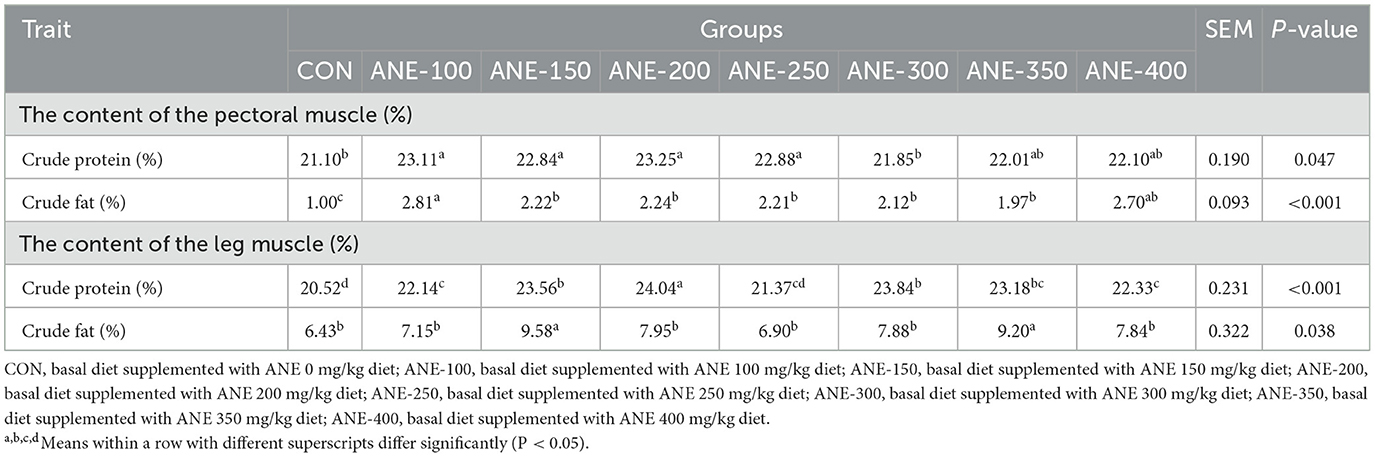
Table 9. Effects of different levels of ANE on the nutrient composition in the muscle of Arbor Acres broilers.
Furthermore, the crude protein contents in the pectoral muscles of broilers were enhanced in the 100, 150, 200, and 250 mg/kg ANE supplementation groups as compared to the control group (P ≤ 0.018). Except for the 250 mg/kg ANE addition group, the crude protein contents in the leg muscles were elevated in all other testing groups in contrast to the control, especially 200 mg/kg (P ≤ 0.036). The results demonstrate that ANE supplementation significantly increased intramuscular nutrient content, thereby improving meat quality parameters.
3.7 Effect of ANE on growth and development-related genes in broilers
As the expression results of genes related to muscle development (Table 10) demonstrated, in the pectoral muscle, the level of IGF-1 gene was higher in the 100 and 300 mg/kg ANE addition groups in comparison with the control group (P ≤ 0.032), and the expressions of gene IGF-2 in the group with the addition of 200 and 300 mg/kg ANE and the Myod1 in the 100 mg/kg ANE addition group were markedly enhanced compared with the control group (P ≤ 0.002). Nevertheless, the level of the Myf5 gene was considerably elevated in all the ANE supplementation groups as against the control group (P ≤ 0.001), and the expression of the Myf6 gene was prominently declined in the 200 and 300 mg/kg ANE addition groups (P ≤ 0.012); while there was no significant discrepancy in the levels of MYOG gene in the ANE addition groups in comparison to the control group (P > 0.05). Furthermore, in the leg muscles of broilers, there were no notable differences in the levels of IGF-1, Myod1, and Myf5 between the ANE treatment groups and the control group (P > 0.05). However, the expression of the IGF-2 gene in the 100 and 300 mg/kg ANE supplementation group was significantly increased as opposed to the control group (P ≤ 0.032); the levels of the Myf6 gene in the 100, 200, and 300 mg/kg ANE addition group and MYOG in the 400 mg/kg ANE supplementation group were markedly enhanced compared to the control group (P ≤ 0.017). Those findings indicated that ANE could promote the muscle growth and development of AA broilers. Notably, lower ANE doses demonstrated superior efficacy in the measured parameters.
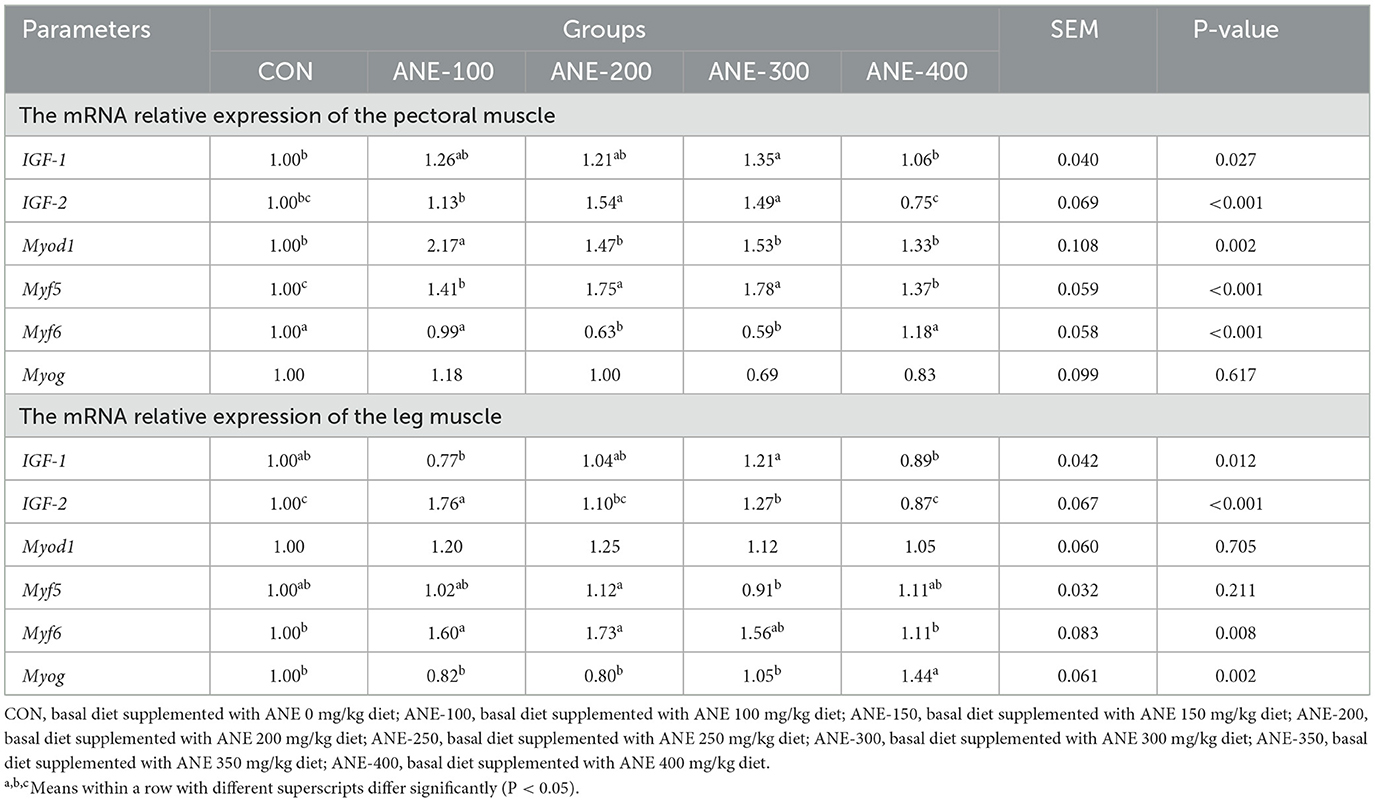
Table 10. Effects of different levels of ANE on the mRNA relative expression of growth and development related genes in the muscles.
4 Discussion
Since the ban on antibiotics, poultry diseases have gradually occurred, severely affecting animal welfare. As the consumption of processed products has increased dramatically, consumers are more attentive to quality of meat (37). It is noteworthy that the decline in animal welfare can have a negative impact on poultry growth performance and meat quality. AN, a natural plant with a 2,000-year history of edible and medicinal use, contains valuable bioactive constituents such as alkaloids, polyphenols, polysaccharides, and fatty acids. It has been conclusively demonstrated to possess a repertoire of bioactive functions, including anti-inflammatory, antioxidant, and anti-parasitic activities, etc. (38), thereby emerging as a potential feed additive in poultry nutrition. To determine the optimal dosage of ANE that could simultaneously enhance animal growth and improve meat quality, a series of experimental groups were established. Each group consisted of 16 chickens housed and fed in 3 cages. Such an experimental design not only meets statistical significance requirements, but also complies with the requirements and regulations of the Animal Welfare Act. The augmented body weight of broilers supplemented with ANE in the present study accords with the findings of Wang (15), who reported that ANE treatment significantly enhanced the growth and decreased the FCR in Wenchang chickens. Additionally, we noted that in the early growth stage of broilers, in comparison to the control group, the ADG rose by 16.8% and 18%, respectively, when 100 and 200 mg/kg ANE were added to the diet. The 18.2% ADG improvement in the 200 mg/kg ANE group exceeds reported effects of oregano essential oil (12–15% ADG gain) (39) and aligns with bacitracin methylene disalicylate (20–22% gain) (40). However, based on the outcome yielded in this investigation, there was no significant impact on the ADFI, which is consistent with Lee's findings (41). Previous studies have demonstrated that arecoline can stimulate the sympathetic nervous system, boost salivary secretion and gastrointestinal peristalsis, and assist in improving digestive function (12). Our study revealed that higher weight of AA broilers at the age of 1–21 days was achieved with ANE supplementation, resulting in a remarkedly lower F/G ratio. The reason for this alteration might be that the ANE possesses the function of promoting digestion (7), indicating that ANE has potential as a feed additive. Poultry meat production performance is mainly evaluated by its slaughter rate and eviscerating rate (42). The superior meat quality is mainly manifested in that the slaughter rate exceeds 80%, and the eviscerating rate surpasses 60%. The results of our experiment demonstrated that the supplementation of ANE resulted in a slaughter rate of over 90% and an eviscerating rate exceeding 70% in broilers. These findings suggest that the appropriate supplementation of ANE can effectively improve the meat quality of broilers.
The excessive fat deposition in broilers, particularly the increase of abdominal fat, may be a contributing factor to the declined feed conversion ratio and meat quality (43, 44). The current study indicated that dietary ANE could reduce the abdominal fat rate of broilers to a certain degree, which may be closely related to arecoline, the main component of ANE. This finding is in line with that of Xu et al. (45), who observed the promotion of lipid metabolism in mice. It is manifested by the increased serum TC, TG, and liver TC levels and the reduction of abdominal fat accumulation.
The quality of meat is mainly appraised by gauging its pH value, meat color, drip loss, cooking loss, and shearing force (46). The pH of muscles generally ranges between 7 and 7.5, which is determined by the amount of lactic acid present. Excessive accumulation of lactic acid in muscles leads to a decrease in pH, lowering its quality. Generally, the greater the pH value in the meat, the better the tenderness. A rapid drop in pH will result in paler and less fresh meat (47). Studies have shown that muscle glycogen, pH, and related meat quality traits are both regulated by genetics and nutrition (48). The chicken with thinner pectoral muscles is characterized by a higher pH in the breast (49). At 45 min after slaughter, our study revealed that ANE increased the pH of muscles. Particularly, the pH of pectoral muscles significantly increased in the presence of ANE compared to the control group. At 24 h post-slaughter, the pH of leg muscles remained higher than that of the control group, while there was no alteration in the pectoral muscles. It indicated that the appropriate dose of ANE supplementation guarantees the freshness of broiler meat. Meat color is the most visual indicator of physiological and biochemical changes in muscle. The meat color index is comprised of three values: L*, a*, and b*. These values are influenced by myoglobin and hemoglobin, especially the redox degree of myoglobin (50). The L* value reflects brightness, while the b* value represents the oxidation level of meat fat and the extent of bacterial growth (51). The present findings illustrate that supplementation with ANE results in a notable increase in the L* value of pectoral and leg muscles, thereby enhancing the quality of the meat. This may be related to the flavonoids present in ANE (52). Furthermore, the current study showed that the decreased redness a* and yellowness b* values in comparison with the control group signified that the oxidation of muscle fat was inhibited upon supplementation with ANE, thereby improving meat quality. These results might be associated with the polyphenols present in ANE (53). Additionally, the ethanol extract of ANE has been demonstrated in numerous studies to possess potent antioxidant properties, and the polyphenols epicatechin and syringic acid in ANE play an important role (54). A smaller shearing force indicates greater muscle tenderness, while drip loss and cooking loss reflect the ability of the muscle to retain water in different environments (55, 56). In comparison to the control group, the ANE supplementation group reduced the drip loss of broilers, though no significant distinction was observed. And the addition of ANE resulted in an increase in shearing force observed in the pectoral muscle, while a decrease was moted in the leg muscle. The elevated shearing force values of beef, pork, and chicken are attributed to the presence of fat cells surrounding the muscle fibers (56). However, the shearing force of cooked meat is reduced by the accumulation of fat cells around the muscle (57), and more in-depth study is required to clarify the mechanism.
The relative weights of internal organs, in particular the liver and spleen, are commonly utilized indicators for evaluating immune status and physiological health in poultry (58). In the present study, the relative liver weights were significantly lower in broilers supplemented with ANE compared to the control group. This finding may be related to the antioxidant function of ANE (7). However, ANE supplementation didn't alter the relative weights of other examined organs, including the kidneys and spleen, consistent with the findings by Li et al. (59). This suggests that the supplementation of ANE did not adversely affect the overall normal health and wellbeing of the broilers during the course of the research. In addition, our findings align with Sari et al. (60), who reported that a dose of 1,000 mg/kg oral ANE was the most efficacious in the cellular immune model, and there were no adverse effects on the liver and kidney functions. Nevertheless, further research is needed to ascertain the safety limit of ANE for hepatic and renal functions.
Serum biochemical indicators are crucial indicators for reflecting the material metabolism and functional alterations of certain tissues and organs of animals (61). The high content of TP enhances the metabolism of the body, leading to the growth and development of animals (60). Our current research discovered a higher serum TP in the 100 mg/kg ANE addition group compared to the control group, indicating that protein synthesis can be promoted through ANE supplementation, resulting in a promoting effect on the growth and development of the body. Similar studies showed that the levels of TP and ALB in the blood of broilers were increased by triterpenoids contained in rosemary extract (62). Typically, the lipid metabolism in the body can be reflected by the content of TC in serum. The greater the content of TC in serum, the higher the occurrence of cardiovascular, cerebrovascular and kidney diseases (63). The present study discovered that ANE supplementation in broilers could reduce serum TC, which aligns with Hu et al. (64), who reported that the level of TC can be declined by the plant extract berberine. UA is the end product of purine metabolism, and also a biomarker in kidney disease. Low UA facilitates protein digestion and absorption, stabilizing the body's physiological function (65). Rosebrough et al. (66) found that the plasma UA content of broilers can be augmented by the reduced dietary energy ratio. Our study demonstrated that the level of UA in serum increased significantly with the addition of 400 mg/kg ANE. It signified that catabolism of proteins might be altered, meaning there was a burden on the kidneys of broilers with this dose. Nevertheless, the current study indicated that ANE supplementation induced no significant impact on the serum levels of ALT, AST, CREA and UREA in broilers, indicating the absence of toxic or adverse effects at the administered dose. This finding is consistent with the hepatoprotective properties of ANE on hepatic biomarkers reported by Pithayanukul et al. (67).
As we all know, protein and fat levels are two of the most crucial factors influencing meat quality. Furthermore, they serve as indicative markers of the nutritional profile of meat (68). Moderate levels of fat cannot only enhance the tenderness of meat, but also be related to the juiciness and flavor of meat. Generally, the lower the crude fat (CF) in muscle, the poorer the fat deposition capacity and meat flavor will be (69). Keeping the intramuscular fat content within 2% to 3% can promise the meat flavor to be the best (70). The findings of the present study showed that the intramuscular fat content could be significantly increased by the addition of ANE, especially with the 100 mg/kg ANE supplementation. The intramuscular fat content of the pectoral muscle was observed to reach 2.81%, which was 1.8 times higher than that of the control group. Additionally, the intramuscular fat content of the leg muscle was observed to reach 9.58% with 150 mg/kg ANE supplementation, which was 48.9% higher compared to the control group. The observed increase in intramuscular fat content suggested that the meat flavor of broilers could be improved with ANE supplementation. A previous study (68) has reported that the higher the crude protein in muscle, the greater the content, indicating high nutritional value. The current study revealed that the crude protein content in the pectoral muscle and the leg muscle was significantly increased, which indicated that the muscle quality and nutritional value could be significantly improved with ANE supplementation.
Muscle growth is a dynamic process involving anabolism and catabolism. Insulin-like growth factors (IGFs) are a group of polypeptides that contribute to muscle growth. Most of them are synthesized in the liver and released into the bloodstream to act on target cells, thereby promoting growth and regulating metabolism (71). IFG-1 is a peptide hormone mainly produced by the liver and stimulated by growth hormone (GH). IFG-1 increases the total number of cells in muscle with adequate protein, resulting in facilitating the growth of new muscle cells (52). Additionally, IFG-1 is associated with connective tissue generation and also plays an important role in glucose metabolism along with insulin (72). IIFG-2 is an embryonic growth factor and a major growth-promoting hormone during pregnancy, which regulates cell proliferation, growth, migration, differentiation, and survival (73). In our study, the mRNA expressions of IFG-1 and IFG-2 were conspicuously higher in the pectoral and leg muscles with ANE supplementation, except for the 400 mg/kg ANE addition group, compared to the control group. It indicated that Igfs did not play a crucial role with 400 mg/kg ANE supplementation. Davis (74) discovered that myogenic regulatory factors are important regulatory elements in the process of myogenesis. They play a vital role in the development and occurrence of muscles, as well as in guiding the differentiation of muscle satellite cells and skeletal muscle regeneration. Skeletal myogenesis is regulated by four myogenic regulatory factors (Mrfs), including Myf5, Myod, Myog, and Mrf4 (75). Mrfs coordinate multiple periods of the muscle lineage, such as various stages of skeletal muscle development, from myoblast proliferation and cell cycle exit to myoblast fusion and myotube maturation (76). Among them, Myod1, Myf5, Myog, and Myf6 exert a crucial role in regulating muscle growth and development. Our study demonstrated that the mRNA expression of Myod1 and Myf5 in pectoral and leg muscles were significantly augmented, suggesting that myogenic cell typing and proliferation were enhanced. Moreover, early skeletal muscle development was facilitated by ANE supplementation. Myod primarily assumes an important part in the process of muscle differentiation and is expressed at the end of differentiation. As a transcription activator, it promotes the transcription of muscle-specific target genes. It is also significant for muscle differentiation, cell cycle exit and muscle atrophy (77). Myf6 transcribes and regulates a broad spectrum of muscle factors and muscle-secreted proteins in skeletal muscle fibers, resulting in the establishment of ligand-receptor interactions between muscle stem cells and their related muscle fibers (78). Nevertheless, the mRNA expression of Myf6 in pectoral muscles was markedly decreased with 200 and 300 mg/kg ANE supplementation. This indicated that the process of muscle differentiation and maturation was faster at the end of the period. Previous research revealed that two polymorphic loci in the 5′ regulatory region of the Myog gene have notable impacts on the muscle fiber density, muscle weight, leg muscle weight and leg muscle development rate of chickens (79). This might be the reason why the Myog mRNA expression in the pectoral muscle is contrary to that in the leg muscle in our study. The early transcription factors of Myod1 and Myf5 mRNA expression are not significantly higher than that of Myf6 in leg muscles. This indicated that the early development of leg muscles was more rapid and the mature stage of muscle fibers was more evident. However, it was still shown that ANE could boost the expression of genes related to muscle growth and development, thereby exerting a promoting role in muscle growth and development. It can, therefore, be concluded that ANE exerts a beneficial effect on broilers. The present study demonstrated that ANE promoted growth performance, prevented pH from dropping in muscles, and raised the flesh color value of pectoral muscle, as well as the crude fat and crude protein content in the muscle of broilers.
While the current findings are promising, further investigations are still needed to elucidate the metabolic mechanisms underlying ANE's effects in poultry and identify the primary active constituents responsible for its biological activity. Moreover, it is imperative to expand sample sizes through on-farm trials in commercial poultry faccilities to validate efficacy under real-world production conditions, while concurrently, conducting longitudinal safety assessments to establish a robust foundation for regulatory approval of ANE as a novel feed additive.
5 Conclusion
This study discovered that ANE exerted positive effects on AA broilers. An appropriate amount of ANE in the diet has been demonstrated to boost the growth performance and meat quality of broilers, facilitate muscle development, and has no remarkable influence on slaughter performance. These results can provide further evidence using ANE as a feed additive in broiler production. Considering both the experimental results and economic feasibility, it is recommended that the optimal dosage for broilers is 100–200 mg/kg ANE.
Data availability statement
The datasets presented in this study can be found in online repositories. The names of the repository/repositories and accession number(s) can be found in the article/supplementary material.
Ethics statement
The animal study was approved by the Animal Care and Use Guidelines of China and Animal Care Committee of Hunan Agricultural University (No. 2021090). The study was conducted in accordance with the local legislation and institutional requirements.
Author contributions
JX: Writing – original draft, Data curation, Formal analysis, Methodology, Visualization. ML: Data curation, Investigation, Visualization, Writing – original draft. RongL: Investigation, Writing – review & editing. ZZ: Investigation, Writing – review & editing. YM: Investigation, Writing – review & editing. RT: Conceptualization, Formal analysis, Validation, Writing – review & editing. LZ: Conceptualization, Formal analysis, Validation, Writing – review & editing. JW: Supervision, Validation, Writing – review & editing. LW: Supervision, Validation, Writing – review & editing. RongfL: Conceptualization, Methodology, Software, Supervision, Writing – review & editing.
Funding
The author(s) declare that financial support was received for the research and/or publication of this article.
Acknowledgments
We would appreciate Changsha Lvye Biotechnology Co., Ltd for providing areca nut extracts samples. And we would like to thank the support of the Key Research and Development Plan of Hunan Province (No. 2020NK2032).
Conflict of interest
ML, LW, and RongfL were employed by Changsha Lvye Biotechnology Co., Ltd.
The remaining authors declare that the research was conducted in the absence of any commercial or financial relationships that could be construed as a potential conflict of interest.
Generative AI statement
The author(s) declare that no Gen AI was used in the creation of this manuscript.
Publisher's note
All claims expressed in this article are solely those of the authors and do not necessarily represent those of their affiliated organizations, or those of the publisher, the editors and the reviewers. Any product that may be evaluated in this article, or claim that may be made by its manufacturer, is not guaranteed or endorsed by the publisher.
References
1. Geerts N, De Vooght L, Passaris I, Delputte P, Van den Bergh B, Cos P. Antibiotic tolerance indicative of persistence is pervasive among clinical streptococcus pneumoniae isolates and shows strong condition dependence. Microbiol Spectr. (2022) 10:e0270122. doi: 10.1128/spectrum.02701-22
2. Khataei MM, Epi SBH, Lood R, Spégel P, Yamini Y, Turner C. A review of green solvent extraction techniques and their use in antibiotic residue analysis. J Pharm Biomed Anal. (2022) 209:114487. doi: 10.1016/j.jpba.2021.114487
3. Hasted TL, Sharif S, Boerlin P, Diarra MS. Immunostimulatory potential of fruits and their extracts in poultry. Front Immunol. (2021) 12:641696. doi: 10.3389/fimmu.2021.641696
4. Witte W. Selective pressure by antibiotic use in livestock. Int J Antimicrob Agents. (2000) 16:S19–24. doi: 10.1016/S0924-8579(00)00301-0
5. Movahhedkhah S, Rasouli B, Seidavi A, Mazzei D, Laudadio V, Tufarelli V. Summer savory (Satureja hortensis L.) extract as natural feed additive in broilers: effects on growth, plasma constituents, immune response, and ileal microflora. Animals. (2019) 9:87. doi: 10.3390/ani9030087
6. Oliveira NG, Ramos DL, Dinis-Oliveira RJ. Genetic toxicology and toxicokinetics of arecoline and related areca nut compounds: an updated review. Arch Toxicol. (2021) 95:375–93. doi: 10.1007/s00204-020-02926-9
7. Peng W, Liu YJ, Wu N, Sun T, He XY, Gao YX, et al. Areca catechu L. (Arecaceae): a review of its traditional uses, botany, phytochemistry, pharmacology and toxicology. J Ethnopharmacol. (2015) 164:340–56. doi: 10.1016/j.jep.2015.02.010
8. Liu PF, Chang YF. The controversial roles of areca nut: medicine or toxin? Int J Mol Sci. (2023) 24:8996. doi: 10.3390/ijms24108996
9. Agostini-Costa TDS. Bioactive compounds and health benefits of some palm species traditionally used in Africa and the Americas - a review. J Ethnopharmacol. (2018) 224:202–29. doi: 10.1016/j.jep.2018.05.035
10. Chen X, He Y, Deng Y. Chemical composition, pharmacological, and toxicological effects of betel nut. Evid Based Complement Alternat Med. (2021) 2021:1808081. doi: 10.1155/2021/1808081
11. Bekhbat M, Treadway MT, Goldsmith DR, Woolwine BJ, Haroon E, Miller AH, et al. Gene signatures in peripheral blood immune cells related to insulin resistance and low tyrosine metabolism define a sub-type of depression with high CRP and anhedonia. Brain Behav Immun. (2020) 88:161–5. doi: 10.1016/j.bbi.2020.03.015
12. Kaushal M, Mishra AK, Raju BS, Ihsan R, Chakraborty A, Sharma J, et al. Betel quid chewing as an environmental risk factor for breast cancer. Mutat Res. (2010) 703:143–8. doi: 10.1016/j.mrgentox.2010.08.011
13. Wang D, Zhou L, Li W, Zhou H, Hou G. Anticoccidial effects of areca nut (Areca catechu L.) extract on broiler chicks experimentally infected with Eimeria tenella. Exp Parasitol. (2018) 184:16–21. doi: 10.1016/j.exppara.2017.11.002
14. Salehi B, Konovalov DA, Fru P, Kapewangolo P, Peron G, Ksenija MS, et al. Areca catechu-from farm to food and biomedical applications. Phytother Res. (2020) 34:2140–58. doi: 10.1002/ptr.6665
15. Wang S, Wang H, Jiang Q, Dai J, Dai W, Kang X, et al. Supplementation of dietary areca nut extract modulates the growth performance, cecal microbiota composition, and immune function in Wenchang chickens. Front Vet Sci. (2023) 10:1278312. doi: 10.3389/fvets.2023.1278312
16. Cox S, Piatkov I, Vickers ER, Ma G. High-performance liquid chromatographic determination of arecoline in human saliva. J Chromatogr A. (2004) 1032:93–5. doi: 10.1016/j.chroma.2003.11.076
17. China Feed Database. Tables of feed composition and nutritive values in China (2020) (in Chinese). Available online at: https://www.chinafeeddata.org.cn/admin/Login/slcfb (accessed March 21, 2022).
18. Zhu La AT, Wen Q, Xiao Y, Hu D, Liu D, Guo Y, et al. A new bacillus velezensis strain CML532 improves chicken growth performance and reduces intestinal clostridium perfringens colonization. Microorganisms. (2024) 12:771. doi: 10.3390/microorganisms12040771
19. Kim JH, Jung H, Pitargue FM, Han GP, Choi HS, Kil DY. Effect of dietary calcium concentrations in low non-phytate phosphorus diets containing phytase on growth performance, bone mineralization, litter quality, and footpad dermatitis incidence in growing broiler chickens. Asian-Australas J Anim Sci. (2017) 30:979–83. doi: 10.5713/ajas.17.0112
20. Alkhalf A, Alhaj M, Al-Homidan I. Influence of probiotic supplementation on blood parameters and growth performance in broiler chickens. Saudi J Biol Sci. (2010) 17:219–25. doi: 10.1016/j.sjbs.2010.04.005
21. Kelly LM, Alworth LC. Techniques for collecting blood from the domestic chicken. Lab Anim. (2013) 42:359–61. doi: 10.1038/laban.394
22. Wang J, Qu J, Liu S, Xu Q, Li X, Zhu Y, et al. Tannic acid ameliorates systemic glucose and lipid metabolic impairment induced by low-dose T-2 toxin exposure. J Agric Food Chem. (2023) 71:12574–86. doi: 10.1021/acs.jafc.3c02934
23. Zhang L, Li X, Liu X, Wu X, Xu Q, Qu J, et al. High-carbohydrate diet consumption poses a more severe liver cholesterol deposition than a high-fat and high-calorie diet in mice. Int J Mol Sci. (2023) 24:14700. doi: 10.3390/ijms241914700
24. Ye M, Chao X, Ye C, Guo L, Fan Z, Ma X, et al. EGR1 mRNA expression levels and polymorphisms are associated with slaughter performance in chickens. Poult Sci. (2025) 104:104533. doi: 10.1016/j.psj.2024.104533
25. Allen CD, Russell SM, Fletcher DL. The relationship of broiler breast meat color and pH to shelf-life and odor development. Poult Sci. (1997) 76:1042–6. doi: 10.1093/ps/76.7.1042
26. Huanhong K, Lumsangkul C, Arjin C, Sirilun S, Tangpao T, Wang YL, et al. Dietary supplementation of coffee pulp extract enhances growth performance and intestinal morphology in broiler chicken. Poult Sci. (2025) 104:104873. doi: 10.1016/j.psj.2025.104873
27. Chaiwang N, Marupanthorn K, Krutthai N, Wattanakul W, Jaturasitha S, Arjin C, et al. Assessment of nucleic acid content, amino acid profile, carcass, and meat quality of Thai native chicken. Poult Sci. (2023) 102:103067. doi: 10.1016/j.psj.2023.103067
28. Li W, Kai L, Wei W, Fan Y, Wang Y, Lu Z. Dietary metabolizable energy and crude protein levels affect Taihe silky fowl growth performance, meat quality, and cecal microbiota during fattening. Poult Sci. (2024) 103:104363. doi: 10.1016/j.psj.2024.104363
29. Chaiwang N, Bunmee T, Arjin C, Wattanakul W, Krutthai N, Mekchay S, et al. Effect of deep bedding floor and fermented feed supplement on productive performance, carcase, meat quality and fatty acid profile of crossbred pigs. Ital J Anim Sci. (2021) 20:479–88. doi: 10.1080/1828051X.2021.1893133
30. Norkeaw R, Arjin C, Sartsook A, Hnokaew P, Chaiwang N, Mekchay S, et al. Supplementation of thymol powder or green tea extract along with linseed in pig diet increases oxidative stability in blood and pork. Ital J Anim Sci. (2023) 22:309–20. doi: 10.1080/1828051X.2023.2188885
31. Cai G, Mao N, Gu P, Zhu T, He J, Peng S, et al. Effects of alhagi honey polysaccharides as feed supplement on intestine function and microbiome, immune function, and growth performance in chicken. Int J Mol Sci. (2022) 23:14332. doi: 10.3390/ijms232214332
32. Benedict RC. Determination of nitrogen and protein content of meat and meat products. J Assoc Off Anal Chem. (1987) 70:69–74. doi: 10.1093/jaoac/70.1.69a
33. Vinklárková B, Chromý V, Šprongl L, Bittová M, Rikanová M, Ohnútková I, et al. The Kjeldahl method as a primary reference procedure for total protein in certified reference materials used in clinical chemistry. II selection of direct kjeldahl analysis and its preliminary performance parameters. Crit Rev Anal Chem. (2015) 45:112–8. doi: 10.1080/10408347.2014.892821
34. Hopkins DL, Clayton EH, Lamb TA, van de Ven RJ, Refshauge G, Kerr MJ, et al. The impact of supplementing lambs with algae on growth, meat traits and oxidative status. Meat Sci. (2014) 98:135–41. doi: 10.1016/j.meatsci.2014.05.016
35. Thiex NJ, Anderson S, Gildemeister B. Crude fat, hexanes extraction, in feed, cereal grain, and forage (Randall/Soxtec/submersion method): collaborative study. J AOAC Int. (2003) 86:899–908. doi: 10.1093/jaoac/86.5.899
36. Zhang L, Li X, Liu X, Wang X, Li X, Cheng X, et al. Purified diet versus whole food diet and the inconsistent results in studies using animal models. Food Funct. (2022) 13:4286–301. doi: 10.1039/D1FO04311K
37. Li X, Zhang D, Ren C, Bai Y, Ijaz M, Hou C, et al. Effects of protein posttranslational modifications on meat quality: a review. Compr Rev Food Sci Food Saf. (2021) 20:289–331. doi: 10.1111/1541-4337.12668
38. Sun H, Yu W, Li H, Hu X, Wang X. Bioactive components of areca nut: an overview of their positive impacts targeting different organs. Nutrients. (2024) 16:695. doi: 10.3390/nu16050695
39. Zhang LY, Peng QY, Liu YR, Ma QG, Zhang JY, Guo YP, et al. Effects of oregano essential oil as an antibiotic growth promoter alternative on growth performance, antioxidant status, and intestinal health of broilers. Poult Sci. (2021) 100:101163. doi: 10.1016/j.psj.2021.101163
40. Li J, Xiao Y, Fan Q, Yang H, Yang C, Zhang G, et al. Dietary bacitracin methylene disalicylate improves growth performance by mediating the gut microbiota in broilers. Antibiotics. (2022) 11:818. doi: 10.3390/antibiotics11060818
41. Lee J, Gunawardhana ND, Jang S, Choi YH, Illeperuma RP, Kim A, et al. Effect of areca nut on helicobacter pylori-induced gastric diseases in mice. J Microbiol Biotechnol. (2016) 26:1817–23. doi: 10.4014/jmb.1606.06043
42. Dong J, Qiu H, Gao S, Hou L, Liu H, Zhu L, et al. A combination of selenium and Bacillus subtilis improves the quality and flavor of meat and slaughter performance of broilers. Front Vet Sci. (2023) 10:1259760. doi: 10.3389/fvets.2023.1259760
43. Luo N, Shu J, Yuan X, Jin Y, Cui H, Zhao G, et al. Differential regulation of intramuscular fat and abdominal fat deposition in chickens. BMC Genomics. (2022) 23:308. doi: 10.1186/s12864-022-08538-0
44. Ye M, Fan Z, Xu Y, Luan K, Guo L, Zhang S, et al. Exploring the association between fat-related traits in chickens and the RGS16 gene: insights from polymorphism and functional validation analysis. Front Vet Sci. (2023) 10:1180797. doi: 10.3389/fvets.2023.1180797
45. Xu M, Su S, Jiang S, Li W, Zhang Z, Zhang J, et al. Short-term arecoline exposure affected the systemic health state of mice, in which gut microbes played an important role. Ecotoxicol Environ Saf. (2023) 259:115055. doi: 10.1016/j.ecoenv.2023.115055
46. Deng S, Liu R, Li C, Xu X, Zhou G. Meat quality and flavor compounds of soft-boiled chickens: effect of Chinese yellow-feathered chicken breed and slaughter age. Poult Sci. (2022) 101:102168. doi: 10.1016/j.psj.2022.102168
47. Le Bihan-Duval E, Debut M, Berri CM, Sellier N, Santé-Lhoutellier V, Jégo Y, et al. Chicken meat quality: genetic variability and relationship with growth and muscle characteristics. BMC Genet. (2008) 9:53. doi: 10.1186/1471-2156-9-53
48. Yalçin S, Özkul H, Özkan S, Gous R, Yaşa I, Babacanoglu E. Effect of dietary protein regime on meat quality traits and carcase nutrient content of broilers from two commercial genotypes. Br Poult Sci. (2010) 51:621–8. doi: 10.1080/00071668.2010.520302
49. Sibut V, Le Bihan-Duval E, Tesseraud S, Godet E, Bordeau T, Cailleau-Audouin E, et al. Adenosine monophosphate-activated protein kinase involved in variations of muscle glycogen and breast meat quality between lean and fat chickens. J Anim Sci. (2008) 86:2888–96. doi: 10.2527/jas.2008-1062
50. Mir NA, Rafiq A, Kumar F, Singh V, Shukla V. Determinants of broiler chicken meat quality and factors affecting them: a review. J Food Sci Technol. (2017) 54:2997–3009. doi: 10.1007/s13197-017-2789-z
51. Cao R, Yan L, Xiao S, Hou B, Zhou X, Wang W, et al. Effects of different low-temperature storage methods on the quality and processing characteristics of fresh beef. Foods. (2023) 12. doi: 10.3390/foods12040782
52. Wang Z, Zhao F, Wei P, Chai X, Hou G, Meng Q. Phytochemistry, health benefits, and food applications of sea buckthorn (Hippophae rhamnoides L): a comprehensive review. Front Nutr. (2022) 9:1036295. doi: 10.3389/fnut.2022.1036295
53. Ma J, DU X, Zhao A, Wang Z, Guo Q, Qin N, et al. Anti-hypoxic pharmacological effects of betelnut polyphenols. Zhong Nan Da Xue Xue Bao Yi Xue Ban. (2022) 47:512–20. doi: 10.11817/j.issn.1672-7347.2022.210582
54. Ji X, Guo J, Pan F, Kuang F, Chen H, Guo X, et al. Structural elucidation and antioxidant activities of a neutral polysaccharide from arecanut (Areca catechu L.). Front Nutr. (2022) 9:853115. doi: 10.3389/fnut.2022.853115
55. Piórkowska K, Żukowski K, Nowak J, Połtowicz K, Ropka-Molik K, Gurgul A. Genome-wide RNA-Seq analysis of breast muscles of two broiler chicken groups differing in shear force. Anim Genet. (2016) 47:68–80. doi: 10.1111/age.12388
56. Roy BC, Bruce HL. Contribution of intramuscular connective tissue and its structural components on meat tenderness-revisited: a review. Crit Rev Food Sci Nutr. (2024) 64:9280–310. doi: 10.1080/10408398.2023.2211671
57. Devatkal SK, Vishnuraj MR, Kulkarni VV, Kotaiah T. Carcass and meat quality characterization of indigenous and improved variety of chicken genotypes. Poult Sci. (2018) 97:2947–56. doi: 10.3382/ps/pey108
58. Heckert RA, Estevez I, Russek-Cohen E, Pettit-Riley R. Effects of density and perch availability on the immune status of broilers. Poult Sci. (2002) 81:451–7. doi: 10.1093/ps/81.4.451
59. Li K, Wang C, Zhao Z, Wu Z, Wu Z, Tian X, et al. A comparison for the effects of raw, smoked, and smoked and brined areca nut extracts on the immune and inflammatory responses in the Kunming mice. J Food Biochem. (2020) 44:e13319. doi: 10.1111/jfbc.13319
60. Sari LM, Hakim RF, Mubarak Z, Andriyanto A. Analysis of phenolic compounds and immunomodulatory activity of areca nut extract from aceh, Indonesia, against Staphylococcus aureus infection in sprague-dawley rats. Vet World. (2020) 13:134–40. doi: 10.14202/vetworld.2020.134-140
61. Chen X, Horn N, Cotter PF, Applegate TJ. Growth, serum biochemistry, complement activity, and liver gene expression responses of pekin ducklings to graded levels of cultured aflatoxin B1. Poult Sci. (2014) 93:2028–36. doi: 10.3382/ps.2014-03904
62. Tang S, Yin B, Xu J, Bao E. Rosemary reduces heat stress by inducing CRYAB and HSP70 expression in broiler chickens. Oxid Med Cell Longev. (2018) 2018:7014126. doi: 10.1155/2018/7014126
63. Wu X, Chen H, Gao X, Gao H, He Q, Li G, et al. Natural herbal remedy wumei decoction ameliorates intestinal mucosal inflammation by inhibiting Th1/Th17 cell differentiation and maintaining microbial homeostasis. Inflamm Bowel Dis. (2022) 28:1061–71. doi: 10.1093/ibd/izab348
64. Hu S, Wang J, Liu E, Zhang X, Xiang J, Li W, et al. Protective effect of berberine in diabetic nephropathy: a systematic review and meta-analysis revealing the mechanism of action. Pharmacol Res. (2022) 185:106481. doi: 10.1016/j.phrs.2022.106481
65. Fathallah-Shaykh SA, Cramer MT. Uric acid and the kidney. Pediatr Nephrol. (2014) 29:999–1008. doi: 10.1007/s00467-013-2549-x
66. Rosebrough RW, McMurtry JP, Vasilatos-Younken R. In vitro lipid metabolism, growth and metabolic hormone concentrations in hyperthyroid chickens. Br J Nutr. (1992) 68:667–76. doi: 10.1079/BJN19920124
67. Pithayanukul P, Nithitanakool S, Bavovada R. Hepatoprotective potential of extracts from seeds of Areca catechu and nutgalls of Quercus infectoria. Molecules. (2009) 14:4987–5000. doi: 10.3390/molecules14124987
68. Cosgrove M, Flynn A, Kiely M. Consumption of red meat, white meat and processed meat in Irish adults in relation to dietary quality. Br J Nutr. (2005) 93:933–42. doi: 10.1079/BJN20051427
69. Ribeiro T, Lordelo MM, Costa P, Alves SP, Benevides WS, Bessa RJ, et al. Effect of reduced dietary protein and supplementation with a docosahexaenoic acid product on broiler performance and meat quality. Br Poult Sci. (2014) 55:752–65. doi: 10.1080/00071668.2014.971222
70. Fernandez X, Monin G, Talmant A, Mourot J, Lebret B. Influence of intramuscular fat content on the quality of pig meat - 1. Composition of the lipid fraction and sensory characteristics of m longissimus lumborum. Meat Sci. (1999) 53:59–65. doi: 10.1016/S0309-1740(99)00037-6
71. Nipkow M, Wirthgen E, Luft P, Rebl A, Hoeflich A, Goldammer T. Characterization of igf1 and igf2 genes during maraena whitefish (Coregonus maraena) ontogeny and the effect of temperature on embryogenesis and igf expression. Growth Horm IGF Res. (2018) 40:32–43. doi: 10.1016/j.ghir.2018.04.003
72. Santilli F, Simeone PG, Guagnano MT, Leo M, Maccarone MT, Di Castelnuovo A, et al. Effects of liraglutide on weight loss, fat distribution, and beta-cell function in obese subjects with prediabetes or early type 2 diabetes. Diabetes Care. (2017) 40:1556–64. doi: 10.2337/dc17-0589
73. Buchanan CM, Phillips AR, Cooper GJ. Preptin derived from proinsulin-like growth factor II (proIGF-II) is secreted from pancreatic islet beta-cells and enhances insulin secretion. Biochem J. (2001) 360:431–9. doi: 10.1042/bj3600431
74. Davis RL, Weintraub H, Lassar AB. Expression of a single transfected cDNA converts fibroblasts to myoblasts. Cell. (1987) 51:987–1000. doi: 10.1016/0092-8674(87)90585-X
75. Nederveen JP, Fortino SA, Baker JM, Snijders T, Joanisse S, McGlory C, et al. Consistent expression pattern of myogenic regulatory factors in whole muscle and isolated human muscle satellite cells after eccentric contractions in humans. J Appl Physiol. (1985) 127:1419–26. doi: 10.1152/japplphysiol.01123.2018
76. Codato R, Perichon M, Divol A, Fung E, Sotiropoulos A, Bigot A, et al. The SMYD3 methyltransferase promotes myogenesis by activating the myogenin regulatory network. Sci Rep. (2019) 9:17298. doi: 10.1038/s41598-019-53577-5
77. Shen X, Cui C, Tang S, Han S, Zhang Y, Xia L, et al. MyoG-enhanced circGPD2 regulates chicken skeletal muscle development by targeting miR-203a. Int J Biol Macromol. (2022) 222:2212–24. doi: 10.1016/j.ijbiomac.2022.10.013
78. Lazure F, Blackburn DM, Corchado AH, Sahinyan K, Karam N, Sharanek A, et al. Myf6/MRF4 is a myogenic niche regulator required for the maintenance of the muscle stem cell pool. EMBO Rep. (2020) 21:e49499. doi: 10.15252/embr.201949499
Keywords: areca nut extract, broiler, growth performance, slaughtering performance, meat quality
Citation: Xu J, Li M, Li R, Zhang Z, Ma Y, Tao R, Zou L, Wang J, Wen L and Li R (2025) Effect of areca nut extracts on growth performance, slaughtering performance, and meat quality of broiler chickens. Front. Vet. Sci. 12:1579415. doi: 10.3389/fvets.2025.1579415
Received: 19 February 2025; Accepted: 08 April 2025;
Published: 28 April 2025.
Edited by:
Adrian Macri, University of Agricultural Sciences and Veterinary Medicine of Cluj-Napoca, RomaniaReviewed by:
Wojciech Wójcik, Warsaw University of Life Sciences, PolandKulisara Marupanthorn, Chiang Mai Rajabhat University, Thailand
Goran Kiš, University of Zagreb, Croatia
Copyright © 2025 Xu, Li, Li, Zhang, Ma, Tao, Zou, Wang, Wen and Li. This is an open-access article distributed under the terms of the Creative Commons Attribution License (CC BY). The use, distribution or reproduction in other forums is permitted, provided the original author(s) and the copyright owner(s) are credited and that the original publication in this journal is cited, in accordance with accepted academic practice. No use, distribution or reproduction is permitted which does not comply with these terms.
*Correspondence: Rongfang Li, bHJmMDQwOEAxMjYuY29t; Lixin Wen, c2Z3bHg4MDE1QHNpbmEuY29t
†These authors have contributed equally to this work