Tracking Fatty Acids From Phytoplankton to Jellyfish Polyps Under Different Stress Regimes: A Three Trophic Levels Experiment
- 1Experimental Ecology (Food webs), GEOMAR Helmholtz Centre for Ocean Research Kiel, Kiel, Germany
- 2Aquatic Ecology, University of Hamburg, Hamburg, Germany
The impacts of biochemicals driving food web processes are under investigation for just the last few decades. In addition, as jellyfish are drawing increasing attentions because of their mass developments and of their potential capacity of driving food web structures and energy flow by “top-down” and “bottom-up” controls. We here show that the provision with the biochemical complex thiamin (vitamin B1) to the common phytoplankton Rhodomonas baltica altered its fatty acid (FA) pattern toward ω3-highly-unsaturated FAs (ω3-HUFA) and that this pattern was further transferred up to the zooplankton consumer, the copepod Acartia tonsa. However, polyps of the Jellyfish Aurelia aurita feeding on A. tonsa only had a low relative ω3-HUFA content, especially due to a reduction in 22:6ω3 (DHA), but elevated levels of 20:4ω6 (ARA). The high proportion of the ω-6 HUFA, ARA in polyps may provide evidence for preferential conversion of ARA in polyps, eventually from DHA in a so far unknown pathway. In contrast to A. tonsa, newly hatched A. salina nauplii used as food for A. aurita polyps were almost devoid of HUFA, but contained high levels of C18 polyunsaturated FAs (C18-PUFA). Consequently, polyps feeding on them contained few HUFA, while high levels of C18-PUFA predominated. This suggests that A. aurita polyps cannot efficiently convert ω3 C18-PUFA to ω3-HUFA. In addition, besides a decrease in saturated FAs, especially an increase in HUFA in A. aurita polyps with decreasing temperature was observed, for which the dietary provision with HUFA seemed to be critical. Altering the FA pattern as a response of temperature reflects an adaptation to seasonal changes and may be related to their life history plasticity.
Introduction
Solar Energy that is fixed by primary producers is transferred to higher trophic levels via predator-prey interactions reallocating biomolecules (such as carbohydrates, lipids, proteins and nucleic acids) through metabolic pathways. The syntheses rates of these essential or semi-essential biomolecules and the transfer efficiencies among food chains will determine the trophic structures of food webs and might regulate “bottom-up” control to higher trophic levels (Hairston and Hairston, 1993). In addition, these bio-compounds are critically important for organisms' fitness by regulating their metabolism, growth, and reproduction, and can serve as indicators of dietary nutritional conditions (Gulati and Demott, 1997; Park et al., 2002, reviewed by Müller-Navarra, 2008). Despite their importance, there are still plenty of open questions regarding the biosynthesis and transfer efficiency of biomolecules in marine food web, including fatty acids (FAs).
FAs in aquatic organisms serve as intermediate energy storage (Lee et al., 2006) but can also be limiting components to support somatic growth (Müller-Navarra et al., 2000) and enhancing immune-competency (Arts and Kohler, 2009). Besides, FAs are structurally and functionally important lipid molecules (Colombo et al., 2016), which, on the one hand, benefit to sustain membrane fluidity and, on the other hand, are also needed as precursors for tissue hormones (Vance and Vance, 2008). Certain highly unsaturated FA (HUFA), such as eicosapentaenoic acid (EPA; 20:5ω3) and docosahexaenoic acid (DHA; 22:6ω3) play critical roles for the fitness of organisms, determining food quality and, thus, carbon energy transfer efficiency to higher trophic levels (Brett and Müller-Navarra, 1997; Müller-Navarra et al., 2004; Persson and Vrede, 2006). For example, it has been shown that fish larvae require a high level of DHA and arachidonic acids (ARA; 20:4ω6) for optimal growth and survival (Izquierdo, 1996; Sargent et al., 1999; Copeman et al., 2002; Yuan et al., 2015). Likewise, another HUFA, the natural dietary content of EPA, was found to be well related to the growth rate and egg production of the freshwater zooplankton Daphnia (Müller-Navarra, 1995; Müller-Navarra et al., 2000).
Despite caveats regarding quantitative assessments (Budge et al., 2006), FAs can be used as trophic markers (FATMs), helping to improve our understanding of so far unknown mass transfer through predator-prey interactions (e.g., Dalsgaard et al., 2003) especially for the nutritionally critical lipids as shown for fish (Kirsch et al., 1998; Budge et al., 2002), and marine mammals (Iverson et al., 1997; Thiemann et al., 2008). FA composition of prey was shown to be involved in the extent of competition in predators and its seasonal succession (Klein Breteler et al., 2005; McMeans et al., 2015). However, although FA tracers were widely used in aquatic food webs from algae via zooplankton to fish, both in freshwater (e.g., Ahlgren et al., 1997; McMeans et al., 2015) and marine systems (Rossi et al., 2006), understanding of FAs transfer in food webs involving jellyfish is still poor.
In recent decades, population increases of jellyfish were reported to cause numerous social-economic and ecological issues (Richardson et al., 2009; Brotz et al., 2012). Although there are indications that they may serve as prey for many top predators such as some fish (Miyajima et al., 2011), penguins (Thiebot et al., 2016), and sea turtles (Carman et al., 2014), on the other hand, they can also heavily prey on fish larvae (Lynam et al., 2005) and mesozooplankton (Behrends and Schneider, 1995), therefore being a competitor for fish. In particular, scyphomedusae exhibit a metagenic life cycle, which involves a sexual pelagic medusae stage and an asexual benthic polyp stage (Arai, 1997). Expansions of polyp populations by asexual reproduction (budding) and success for producing ephyrae larvae (strobilation) determine population recruitment of pelagic medusae. This bentho-pelagic coupling process depends on abiotic factors and also food availability (Lucas et al., 2012). So far, studies have been carried out on energy and nutrient flow in jellyfish by bio-tracers (reviewed by Pitt et al., 2009), but no single study exits which explored FA transfer toward jellyfish (polyps). In order to evaluate whether the FATM concept can be applied as a natural bio-tracer in a food chain involving jellyfish, and, on the other hand, predict fitness of jellyfish, we need to know the extent of the variability in jellyfish FA composition, and the factors driving this variability.
Thus, the object of the present study was to track FAs alteration and transfer in a three-trophic-level food chain, consisting of a primary producer, the phytoplankton Rhodomonas baltica (Karsten, 1898), a primary consumer, the anostracan Artemia salina (Linnaeus, 1758) and copepod Acartia tonsa (Dana, 1849), and polyps of jellyfish Aurelia aurita (Linnaeus, 1758) as predators. There are two primary aims of this study (1) to track FA composition of the predator polyps back to their dietary FA composition, and (2) to assess the effects of environmental temperature and food availability on FA transfer processes.
Materials and Methods
Experiment Set Up
A. aurita polyps were obtained from permanent cultures of the Christian-Albrechts University, Kiel (Germany), originally collected from the North Sea (Helgoland, Germany), and kept at 20°C and salinity of 30 psu. Prior to the experiment, polyps were fed with newly hatched A. salina nauplii and kept in filtered seawater (0.2 μm) exchanged twice a week. To prepare the experiment, polyps were removed carefully from the substrates and were placed individually in 6-well polycarbonate plates. We manipulated three experimental temperatures, 13°C, 20°C, and 27°C, which mimick autumn/spring, average summer, and a heat wave scenario, respectively (Meehl and Tebaldi, 2004; Wiltshire and Manly, 2004; Garrabou et al., 2009). Three food concentrations of high (20 μg C polyp−1 d−1), medium (5 μg C polyp−1 d−1), and low (1.5 μg C polyp−1 d−1) were considered to represent excess, average and starvation food supply (Han, 2009).
We provided three types of zooplankton prey with different HUFA content, (a) A. salina nauplii (thereafter Art), were hatched from commercial traded eggs (Great Salt Lake, USA), and unfed nauplii were directly used for feeding polyps. (b) copepodites of A. tonsa (thereafter Aca(+)) that were fed with standard R. baltica stock and (c) copepodites of A. tonsa (thereafter as Aca(−)) fed with R. baltica cultures lacking thiamin (vitamin B1). Our standard R. baltica stock (thereafter Rhodo(+)), applied Provasoli's enrichment medium modified by U. Sommer, while in the stock medium of the other phytoplankton treatment (thereafter Rhodo(−)), no thiamin was added. Both R. baltica were cultured at 20°C under 100 μmol photons m−2 s−1 light conditions. To manipulate two different qualities of A. tonsa, newly hatched nauplii were cultured at 20°C, under dark condition, and were fed daily with Rhodo(−) and Rhodo(+), respectively at concentrations adjusted to be above the food limitation level for this copepod species, i.e., >600 μg C L−1 (Kiørboe et al., 1985). Thus, we supplied three food types (i.e., Aca(−), Aca(+), and Art) for feeding polyps, thereafter pol(Aca−), pol(Aca+), and pol(Art), respectively. Overall, we applied a three-factorial design (i.e., temperature, food quality and food quantity) in which each factor had three levels. Each combination of food quality, food quantity, and temperatures at 20°C and 27°C comprised 12 replicates (n = 108, respectively). Given the fact that strobilation might Potentially happen at 13°C and to ensure enough materials for further analysis, each treatment was replicated 18 times for this specific temperature level (n = 162). The experiment lasted for 30 days.
Fatty Acids Analysis
At the end of the experiment, triplicate samples from each treatment of phytoplankton, zooplankton, and polyps were analyzed for FA composition. To prepare FA samples, 10 ml of each phytoplankton treatment was filtered on GF/F filters (Pore size: 0.7 μm). Zooplankton samples were prepared by pooling ca. twenty individuals per each sample. Polyps were put into tin capsules individually. All samples were prepared and stored at −80°C prior to FA analyses.
Samples were analyzed as FA methyl esters (FAMEs) using a gas chromatograph (GC; Thermo Fisher Scientific, Germany). The procedure of preparing FAMEs was modified after Christie (1989) method. In detail, FAs were extracted at −20°C for 12 h with a 1:1:1 (volume) mixture solvent of chloroform/dichloromethane/methanol. 100 μl of FA 19:0 and 21:0 were added to each sample respectively at the beginning of the extraction procedure, and serving as internal standards. In the first cleaning up procedure, we used a 1 mol L−1 potassium chloride solution; the lower liquid phase was collected, and dried sodium sulfate was added to remove possible residual water. Samples were transferred to glass cocoons and 100 μl toluene and 200 μl methanol (supplemented with 1 % concentrated sulphuric acid) were added for esterification, which took place at 50°C for 12 h. Thereafter, FAMEs were cleaned up with a 5 % sodium chloride solution and n-hexane. Upper phases were transferred to new cocoons and evaporated, thereafter the extractive FAMEs were re-dissolved in 100 μl n-hexane for analysis. FAMEs were analyzed using a GC with hydrogen as the carrier gas. Peaks were integrated using the Chromcard software (Thermo Fisher Scientific, Germany), and identified with Supelco 37 FAME mixture (Sigma-Aldrich, USA). In this study, detected FA components including 14:0, 16:0, 18:0, 18:1ω9, 18:2ω6, 18:3ω6, 18:3ω3, 20:0, 20:1ω9, 20:2ω6, 20:4ω6, 20:3ω3, 22:0, 20:5ω3, 22:1ω9, 24:0, 24:1ω9, and 22:6ω3.
Statistics Analysis
FA results were presented as percent of total FAs (% TFA), and single FAs were summed to the respective FA groups, i.e., saturated FA (SFA), monounsaturated FA (MUFA), polyunsaturated FA (PUFA), and HUFA. First, to assess the success of the food quality manipulation of the different zooplankton types, one-way ANOVA was applied to compare FA groups (i.e., SFA, MUFA, PUFA, and HUFA) among phytoplankton or zooplankton treatments. Second, to compare the effect of food types on overall FA composition of A. aurita polyps, permutational multivariate analysis of variance (PERMANOVA, permutation = 999) (Anderson, 2001) on Bray-Curtis similarity matrix was performed. Comparisons of the FA composition of different trophic levels (i.e., phytoplankton, zooplankton and polyps) was restricted to polyps cultured at 20°C and fed high food concentration, because phytoplankton and zooplankton were cultured at 20°C and two types of A. tonsa were fed in excess. All detected FA components and calculated FA groups were included in the PERMANOVA model, respectively. Prior to model fitting, the multivariate homogeneity of FA components and FA groups dispersion from different food quality levels was tested. There was no statistically significantly different dispersion of both FA components data (df = 2, F = 1.03, P = 0.31) and FA groups data (df = 2, F = 1.27, P = 0.27) which met assumption of PERMANOVA tests.
To follow single FAs from phytoplankton via zooplankton to polyps in a controlled setting, we focused on those FAs in organisms of the three experimental trophic levels which contributed most to differences of the polyps' FA composition in each pair-wise comparison, after similarity percentage (SIMPER) analysis, i.e., 16:0, 18:0, 18:1ω9, 18:3ω3 (ALA), 20:4ω6 (ARA), 20:5ω3 (EPA), and 22:6ω3 (DHA). In addition, we also included 18:2ω6 (LIN) in the final analysis which was not included by SIMPER results, but it can be an important intermediate for FA elongation to ARA (Desvilettes and Bec, 2009), similar to ALA as a precursor for EPA and DHA (Vance and Vance, 2008). The selected FA components among different trophic levels and different food quality treatments were tested by ANOVA. At last, a beta regression model extended with precision sub-model (Ferrari and Cribari-Neto, 2004; Cribari-Neto and Zeileis, 2010) was applied to analyze polyps' FA composition under the combination of food quality, food quantity, and temperature. A stepwise backward selection method via an Akaike information criterion (AIC) index was performed to select adequate models.
All statistical test, data explorations, and data visualizations were performed using R software (Version 3.3.3) (R Core Team, 2017). The PERMANOVA tests and beta regressions were performed using R package vegan (Oksanen et al., 2017) and betareg (Cribari-Neto and Zeileis, 2010), respectively. All data visualizations were performed using the R package ggplot2 (Wickham, 2009).
Results
FA Composition of Phytoplankton and Zooplankton Treatments
The supply of thiamin to the growth medium of R. baltica had a positive effect on the relative PUFA, and especially HUFA content (Table 1, Figure 1). Along the experimental food chain, the relative HUFA contents in Aca(−) and Aca(+) differing in the thiamin provision of their food were even more significantly different (Table 1, Figure 1). Compared to the two types of copepods, the share of HUFA in Art (4.2%), was significantly lower than that presented in Aca(+) (48.7%) and even in Aca(−) (32.8%). The differences could be observed in SFA and MUFA, as well (Table 1, Figure 1). In contrast, the relative PUFA content was not that different, PUFA in Art (51.4%) was higher than in Aca(−) (40.1%) but similar to Aca(+) (52.3%).
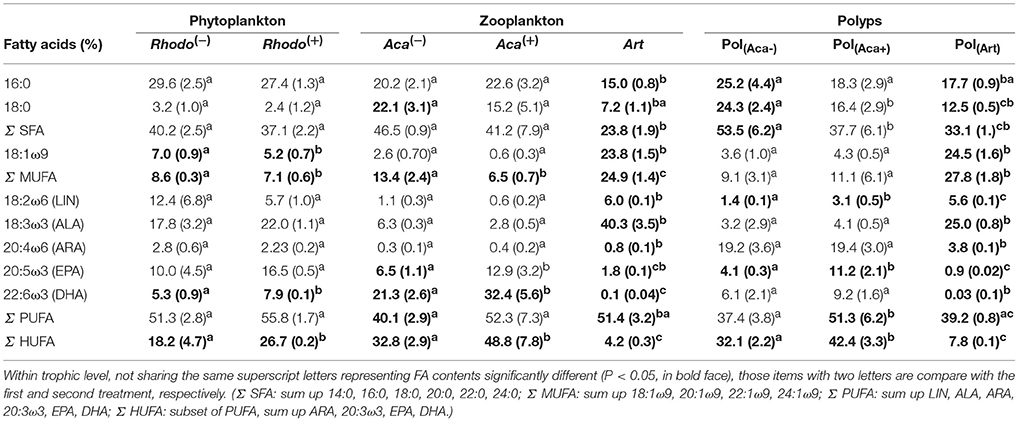
Table 1. FA composition (% total FA, mean ± sd) of organisms from three trophic levels at 20°C treatments.
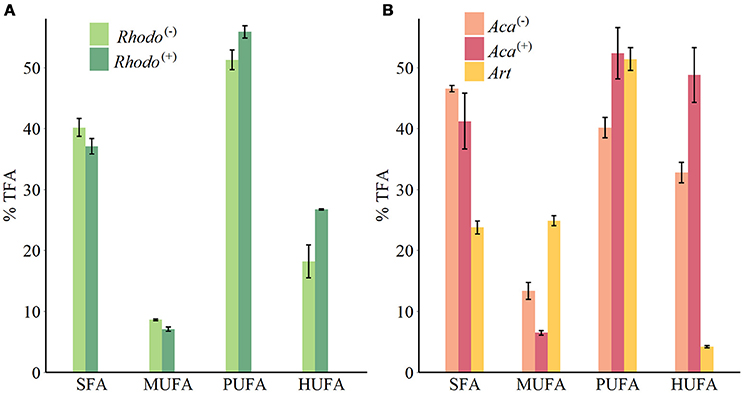
Figure 1. FAs groups composition of phytoplankton (A) and zooplankton (B) (mean ± SE). Rhodo(−): R. baltica cultured in lacking thiamin medium; Rhodo(+): R. baltica cultured in supplied thiamin medium; Aca(−): A. tonsa fed with Rhodo(−); Aca(+): A. tonsa fed with Rhodo(+); Art: newly hatched A. salina nauplii.
Following FA Components Along Different Trophic Levels
The PERMANOVA test (Table 2) showed that FA components and FA groups from polyps were significantly different among the three food quality treatments (Table 1, Figure 2). As showed above, thiamin supplied phytoplankton treatments contributed a higher HUFA content, especially EPA and DHA, which could be tracked at the next trophic level in the experiment. Rhodo(−) contained less DHA compare to Rhodo(+). Alterations in the share of DHA were most pronounced in A. tonsa, following the same pattern as their food. DHA in polyps, however, was not that significantly different (Table 1). On the other hand, the differences in EPA were not significant in two phytoplankton, but significantly different in copepod and polyps (Table 1, Figure 2). Aca(−) contained less EPA than Aca(+), and similarly, pol(Aca−) contained less EPA than pol(Aca+). Moreover, pol(Aca−) contained more 18:0, but less LIN than pol(Aca+). In particular, Figure 2 shows that the relatively high levels of MUFA and PUFA in Art which were also mirrored in pol(Art) were due to the extremely high levels of 18:1ω9 and ALA, respectively.
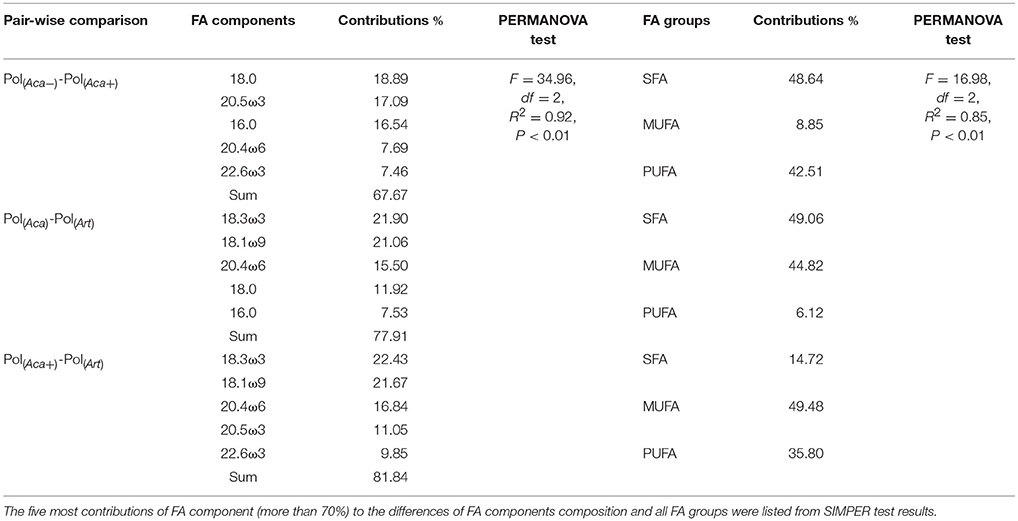
Table 2. Bray-Curtis distances based Non-parametric PERMANOVA and SIMPER test for assemblages of polyps' FA composition.
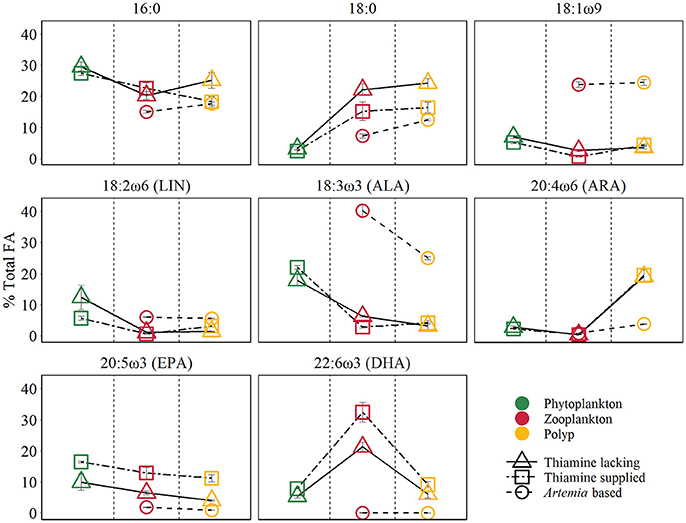
Figure 2. Main FA composition of different trophic levels (mean ±SE). The points colors denote different trophic levels, the points shapes denote different treatments.
Following trophic levels to compare the relative FAs contents among treatments, the differences seemed to be also component-specific (Table 1, Figure 2). For example, Aca(−) and Aca(+) had lower 16:0 but higher 18:0 than their food. These two SFAs contents were not significantly different from zooplankton to polyps, except those increased from Art to pol(Art) (Table 1, Figure 2). In addition, all unsaturated C18-FAs decreased from phytoplankton to zooplankton, either slightly (18:1ω9), moderately (LIN) or sharply (ALA), and their contents were also similar from zooplankton to polyps, with exception of ALA which decreased significantly from Art to pol(Art) (Table 1, Figure 2). Interestingly, ARA content was low in phytoplankton and zooplankton, it was obviously elevated in polyps, even for pol(Art). EPA content decreased smoothly along food chain, while DHA content was significantly high in copepod treatments than their prey and predators. Art and pol(Art) contained extremely low EPA and DHA (Table 1, Figure 2).
FA Composition of Polyps Under Different Temperature and Food Conditions
The FA composition of polyps was significantly affected by temperature, prey FA composition and food availability (Table 3, Figure 3). Increasing temperature led to high level of SFA which consequently caused relatively lower levels of unsaturated FAs (UFA, i.e., MUFA, PUFA, HUFA) (Table 3, Figure 3). However, this effect was not very strong for those polyps which had a low FA content anyway, e.g., for MUFA in pol(Aca−) and pol(Aca+), and HUFA in pol(Art). Food type also had effects on FA composition of polyps. The FA composition of pol(Art) were significantly different from that of pol(Aca−) and pol(Aca+), while the latter two treatments had a similar FA groups composition (Table 3, Figure 3). Interestingly, when offered prey at higher abundance, polyps had a lower SFA content, but higher UFA content (Table 3, Figure 3).
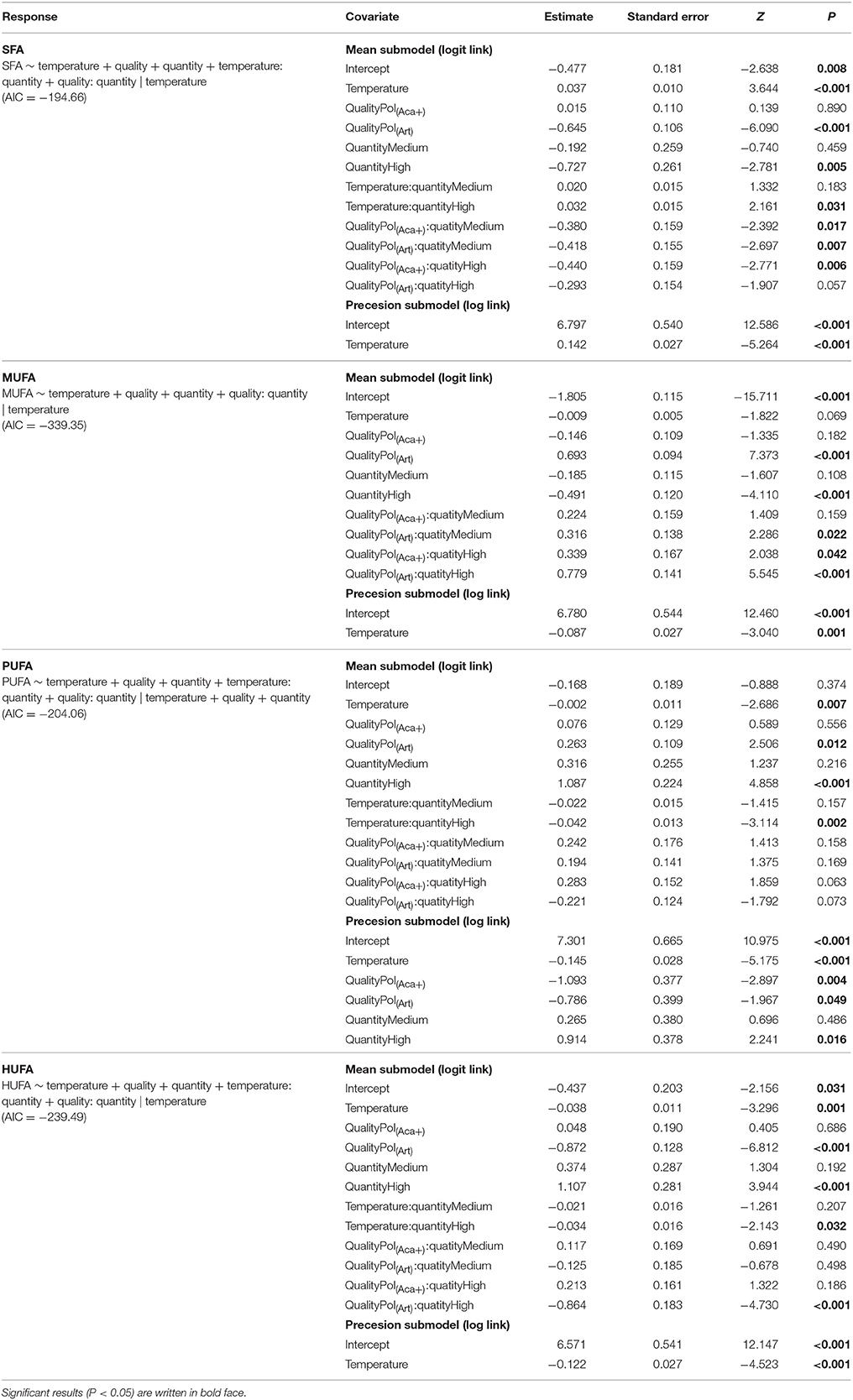
Table 3. Beta regression coefficients of FA groups composition of polyps under temperature, food quality and food quantity effects.
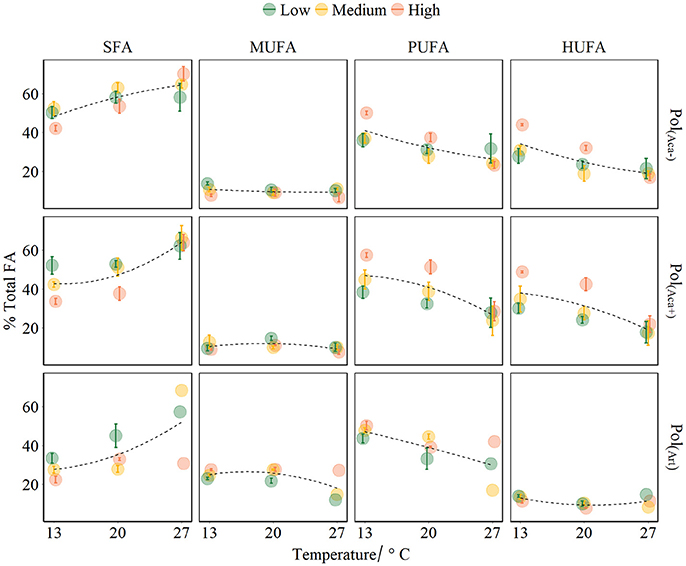
Figure 3. FA groups of polyps affected by temperature, food quality and food quantity treatments (mean ± SE). The points colors denote food different concentrations. Fitted curves using method “loess” (span = 2) from R package ggplot2. Pol(Aca−): polyps fed with Aca(−); Pol(Aca+): polyps fed with Aca(+); Pol(Art): polyps fed with Art.
Interaction effects of these three factors dependent on the FA groups. For example, the SFA content of pol(Art) were significant lower than pol(Aca−) [ANOVA, F(1, 4) = 60.6, P < 0.001] and pol(Aca+) [ANOVA, F(1, 4) = 20, P < 0.001] at low temperature, but similar at the highest temperature [ANOVA, F (1, 4) = 2.5, P = 0.2 and F (1, 4) = 2.1, P = 0.2]. Overall, the relative HUFA content in polyps was affected by the interaction of temperature-quantity and quality-quantity. No effect of temperature-prey FA composition and their three-way interactions could be detected (Table 3, Figure 3). In particular, the HUFA content of polyps cultured at 20°C, seems to be similarly affected by either medium concentration of food rich in HUFA (Aca(+)) or by high food concentration of moderate HUFA content food [Aca(−)] [ANOVA, F(1, 4) = 1.45, P = 0.3]. Likewise, low concentrations of HUFA-rich food (Aca(+)) contributed more than high concentrations of HUFA-poor food (Art) [ANOVA, F(1, 4) = 100.6, P < 0.001].
Discussion
Jellyfish are drawing increasingly attentions because of a preceived incidence of mass developments and of their potential capacity of driving food webs structure (West et al., 2009) and their importance in global biogeochemical cycles (Vargas et al., 2002; Lebrato et al., 2013). Yet little empirical studies can be found in the literature on the dynamic of essential or semi-essential biomolecules (e.g., FAs) in gelatinous zooplankton and their transfer along food chains dominated by jellyfish. Our study provided the first insights into the dynamic of critical biochemical substances such as vitamin B1 and ω3-HUFA in a system encompassing a common phytoplankton, zooplankton, and jellyfish.
FA Composition of Phytoplankton and Zooplankton
Our results could clearly show that the provision of phytoplankton R. baltica with thiamin (vitamin B1) had a significant effect on its FA composition, and especially HUFA content. Differences between both thiamin treatments, suggest that the conversion from ALA to DHA is dependent on thiamin provision in this VB1 auxotrophic phytoplankton. Thiamin deficiency resulted in high MUFA and low HUFA relative content in R. baltica. It is known that thiamin plays a critical role in energy metabolism (Lonsdale, 2006). Thiamin diphosphate (TPP) which serves as a cofactor of enzymatic reactions to form acetyl CoA in glucose catabolism is the link between thiamin metabolism and FA synthesis (Lonsdale, 2006). Acetyl CoA is a main source to biosynthesize FAs (Wakil, 1960). Thus, an adequate supply of thiamin can improve an organisms' capacity of synthesizing FAs (Boxer and Stetten, 1944). For example, in a medical trial, FA synthesis of thiamin-deficient treatments was only 13% of trials receiving thiamin (Volpe and Marasa, 1978). However, in aquatic sciences, the association between thiamin supply and the level of FA synthesis has not been well studied yet. Keeping in mind that ca. 22% of eukaryotic algae are thiamin auxotroph and that HUFA may limit secondary production, our finding may have important implications in critical roles of regulating both food web structure and dynamics due to the role of phytoplankton as primary producer, and zooplankton as link to higher trophic levels (Arrigo, 2005; Finkel et al., 2009; Steinberg and Landry, 2017). The level of nutrient supply (i.e., N, P, thiamin etc.) and synthesized biochemical molecules (i.e., fatty acids, amino acids, etc.) determines phytoplankton abundance and prey quality for zooplankton. Effects of zooplankton are potentially transmitted up to higher trophic levels and control their performances indirectly (i.e., growth rate, reproduction, etc.). Therefore, there are abundant potentials for further progress in determining the importance of vitamins in general, and thiamin, in particular, to shape marine food web under future ocean scenarios.
Moreover, when feeding on Rhodo(−), Aca(−) also had strongly reduced DHA and EPA contents. Thus, the relative HUFA content could be followed up to the next trophic level, i.e., the common herbivorous zooplankton A. tonsa, was highly diet dependent. However, also contrasting patterns were found for other FA components. For example, Aca(−) and Aca(+) had lower 16:0 but higher 18:0 than their food, indicating that they had likely elongated 16:0 to 18:0. Furthermore, in both treatments, relative DHA contents in A. tonsa increased relative to levels in their food while ALA levels decreased, indicating some conversion from ALA via EPA to DHA as shown by Ridgway and Mcleod (2015). From the differences between both thiamin treatments, we suggest that the conversion from ALA to DHA seems to be dependent on thiamin provision in the B1 auxotrophic phytoplankton. However, for a more mechanistic explanation on how certain FA components are regulated by thiamin supply, further studies are needed which specifically should explore the association of thiamin with HUFA. This is not only critical for understanding FA pathways but also their transfer in food webs with practical implications for aquaculture (Woodbury, 1943). We also emphasize the great difference in the DHA content between A. tonsa and A. salina. The latter is an often-used food source for young fish in aquaculture settings. Our finding suggests that natural food, even when co-limited by vitamin B1, is of higher food quality than commercially used A. salina food supply.
FA Composition of Polyps Affected by Dietary FA
FA composition of polyps mostly reflected that of their diet, except for a much lower DHA and a higher relative ARA content in polyps than in A. tonsa. DHA could have been converted to ARA, but this would imply a biochemical pathway not related to the ALA-EPA pathway (Bell and Tocher, 2009), but transforming an ω3-HUFA into an ω6-HUFA. In contrast, it seems that ω3-HUFA (EPA and DHA) in polyps were retrieved from the diet, which is most probably true for the other specific FAs, such as 16:0, 18:0, 18:1ω9, and LIN. A lower relative ALA content of pol(Art) compared to their diet may indicate that some of the ALA provided by Art were converted by the polyp, but not to ω3-HUFA as those levels were stable and below the levels when feeding on copepod. However, polyps feeding on ALA-rich Art had still a manifold higher ALA content themselves compared to polyps feeding on both copepod diet, indicating low conversion in jellyfish polyps.
In our study, the ω6-HUFA ARA was found to be a major HUFA component of polyps, which may have implications for the jellyfish life history and food web processes. Unlike the ω3-HUFA EPA and DHA, for which beneficial effects to resist environmental stress in fish (Sargent et al., 1999), enhance growth and reproduction in zooplankton (Sundbom and Vrede, 1997; Abrusán et al., 2007; Müller-Navarra, 2008), the functional importance of ARA in aquatic food webs is less documented with exceptions of few studies from fishery (Yuan et al., 2015). ARA concentration in fish tissue seems to be diet dependent, and functionally regulating fish larval growth, survival, immune function and resistance to stress (reviewed by Bell and Sargent, 2003). For example, recent studies showed that an adequate ARA supply (independently or combined with DHA) were positively associated with survival and growth of fish larvae (Yuan et al., 2015; Rombenso et al., 2016). Therefore, dietary ARA-deficiency might impact the nutritional state and performances of fish larvae in the field. If ARA is similarly important for the performance of polyps (i.e., survival, growth, and asexual reproduction) this may determine pelagic dynamics of medusae retrieved by strobilation (Lucas et al., 2012). The emerging question is whether this ability of biosynthesizing ω-6 in polyps will be beneficial in the competition with other benthic organisms, and for the medusae stage competing with fish larvae for common prey, making them resistant to nutritional imbalance and cope with insufficient food conditions. Above that, ARA or the ARA/DHA ratio may serve as a trophic marker for cnidarians when studying trophic interactions between polyps and their predators, for example, in nudibranchs.
FA Composition of Polyps Affected by Temperature and Food Conditions
In line with the membrane fluidity concept (Singer and Nicolson, 1972; Pruitt, 1990), polyps had a high level of PUFA at the lower experimental temperatures, representing autumn/spring conditions, while containing more SFA at the higher temperature, reflecting a heat wave scenario. Thus, low-temperature-dependent retention of PUFA might be an adaptation of polyps to maintain their physiological activities while overwintering. In contrast, heat waves lead to enhanced SFA in cellular lipids. Higher melting points of SFA (Knothe and Dunn, 2009) may help organisms to resist cellular heat injury. Thus, cnidarians have the ability to control their cellular lipids' saturation degree, which is beneficial for the acclimatization to temperature shifts, and may ensure the plasticity of jellyfish polyps' life history traits.
Our results support the general concept that organisms can adapt to temperature shifts by alterating the UFA: SFA ratio. For example, phytoplankton were found to increase the level of lipid unsaturation significantly when temperature decreased (Thompson, 1996; Jiang and Gao, 2004). This pattern was confirmed as well as for zooplankton (Werbrouck et al., 2016) and fish (Laurel et al., 2012). Above that, our study addresses that the UFA: SFA regulation in polyps is connected with the FAs obtained from the food; either of high nutritional value at low concentration or at high rations to compensate for a nutritional inferior quality. Comparing HUFA in polyps feeding on A. tonsa with those feeding on A. salina may reveal A. salina to be inferior to A. tonsa, especially at low temperatures because of the lower HUFA content. In addition, A. tonsa is dominating zooplankton in most coastal waters and it fulfills the polyps essential FAs requirements. The capacities of the polyp to biochemically regulate the degree of unsaturation, enable seasonal adaption and show the plasticity of a potentially important life history trait for jellyfish polyps. As a next step, it would be essential to know whether HUFA are transferred to jellyfish's medusae stage as an important link to the pelagic food web.
Conclusions
In this study, we followed the FA composition in a three-level food chain encompassing the common marine plankton R. baltica (as primary producer) and A. tonsa (as a primary consumer) and the polyps of jellyfish A. aurita. We found vitamin B1 induced alterations of the FA, especially HUFA composition at the primary producer level being transferred to the primary consumer level. Further up the food chain, HUFA of jellyfish polyps were influenced by the diet with similar relative levels for EPA, but strongly reduced DHA levels when feeding on A. tonsa. In contrast, ARA levels increased in the expense of DHA, making a conversion likely. However, this would biochemically imply a conversion of a ω3-HUFA to a ω6-HUFA in jellyfish polyps. Whether ARA can even be used as a potentially FATM for jellyfish needs to be clarified in the future. Comparing FA composition of A. aurita polyps feeding on A. tonsa with those feeding on A. salina, revealed that A. salina was inferior because of a much lower HUFA content than in A. tonsa. As the high relative content of C18-PUFA in A. salina was mirrored in the polyps feeding on them, it suggests that conversion along the ω3 route is only minor in jellyfish polyps. In addition, our results on jellyfish polyps toward temperature changes are consistent with other studies which found low temperature induced high content of UFA, while high temperature is connected with an elevation of SFA. We could show that the degree of HUFA increase at decreasing temperatures is connected to the dietary provision with HUFA, Therefore, more efforts are needed to investigate the functions of FAs and other biomolecules related to jellyfish life history traits, and including the pelagic medusae stage.
Data Availability
The data that support the findings of this study are publicly available online in PANGAEA repository (www.pangaea.de).
Author Contributions
XC and JJ designed the experiment and XC implement the experiment and analyzed fatty acids samples. XC, JJ, US, and DM-N analyzed data, discussed the results, and wrote the paper.
Conflict of Interest Statement
The authors declare that the research was conducted in the absence of any commercial or financial relationships that could be construed as a potential conflict of interest.
Acknowledgments
We would like to thank Sarah Kaehlert for her kind helps during the time when we carried out this experiment, and Thomas Hansen for his support of fatty acids samples analysis. We also thank Achim Zeileis and Kriste Makareviciute for their suggestions and discussions of statistics. We also thank two reviewers for their constructive comments and suggestions on this paper. We gratefully acknowledge the funding supports from China Scholarship Council.
References
Abrusán, G., Fink, P., and Lampert, W. (2007). Biochemical limitation of resting egg production in Daphnia. Limnol. Oceanogr. 52, 1724–1728. doi: 10.4319/lo.2007.52.4.1724
Ahlgren, G., Goedkoop, W., Markensten, H., and Boberg, M. (1997). Seasonal variations in food quality for pelagic and benthic invertebrates in Lake Erken–the role of fatty acids. Freshwat. Biol. 38, 555–570. doi: 10.1046/j.1365-2427.1997.00219.x
Anderson, M. J. (2001). A new method for non-parametric multivariate analysis of variance. Austral Ecol. 26, 32–46. doi: 10.1111/j.1442-9993.2001.01070.pp.x
Arrigo, K. R. (2005). Marine microorganisms and global nutrient cycles. Nature 437, 349–355. doi: 10.1038/nature04159
Arts, M. T., and Kohler, C. C. (2009). “Health and condition in fish: the influence of lipids on membrane competency and immune response,” in Lipids in Aquatic Ecosystems, eds M. T. Arts, M. T. Brett, and M. J. Kainz (New York, NY: Springer), 237–256.
Behrends, G., and Schneider, G. (1995). Impact of Aurelia aurita medusae (Cnidaria, Scyphozoa) on the standing stock and community composition of mesozooplankton in the Kiel Bight (western Baltic Sea). Mar. Ecol. Prog. Ser. 127, 39–45. doi: 10.3354/meps127039
Bell, J. G., and Sargent, J. R. (2003). Arachidonic acid in aquaculture feeds: current status and future opportunities. Aquaculture 218, 491–499. doi: 10.1016/S0044-8486(02)00370-8
Bell, M. V., and Tocher, D. R. (2009). “Biosynthesis of polyunsaturated fatty acids in aquatic ecosystems: general pathways and new directions,” in Lipids in Aquatic Ecosystems, eds M. T. Arts, M. T. Brett, and M. J. Kainz (New York, NY: Springer), 211–236.
Boxer, G. E., and Stetten, D. Jr. (1944). The role of thiamine in the synthesis of fatty acids from carbohydrate precursors. J. Biol. Chem. 153, 607–616.
Brett, M. T., and Müller-Navarra, D. C. (1997). The role of highly unsaturated fatty acids in aquatic foodweb processes. Freshwat. Biol. 38, 483–499. doi: 10.1046/j.1365-2427.1997.00220.x
Brotz, L., Cheung, W. W., Kleisner, K., Pakhomov, E., and Pauly, D. (2012). Increasing jellyfish populations: trends in large marine ecosystems. Hydrobiologia 690, 3–20. doi: 10.1007/s10750-012-1039-7
Budge, S. M., Iverson, S. J., Bowen, W. D., and Ackman, R. G. (2002). Among-and within-species variability in fatty acid signatures of marine fish and invertebrates on the Scotian Shelf, Georges Bank, and southern Gulf of St. Lawrence. Can. J. Fish. Aquat. Sci. 59, 886–898. doi: 10.1139/f02-062
Budge, S. M., Iverson, S. J., and Koopman, H. N. (2006). Studying trophic ecology in marine ecosystems using fatty acids: a primer on analysis and interpretation. Mar. Mamm. Sci. 22, 759–801. doi: 10.1111/j.1748-7692.2006.00079.x
Carman, V. G., Botto, F., Gaitán, E., Albareda, D., Campagna, C., and Mianzan, H. (2014). A jellyfish diet for the herbivorous green turtle Chelonia mydas in the temperate SW Atlantic. Mar. Biol. 161, 339–349. doi: 10.1007/s00227-013-2339-9
Colombo, S., Parrish, C., and Whiticar, M. (2016). Fatty acid stable isotope signatures of molluscs exposed to finfish farming outputs. Aquaculture Environ. Interact. 8, 611–617. doi: 10.3354/aei00202
Copeman, L., Parrish, C., Brown, J., and Harel, M. (2002). Effects of docosahexaenoic, eicosapentaenoic, and arachidonic acids on the early growth, survival, lipid composition and pigmentation of yellowtail flounder (Limanda ferruginea): a live food enrichment experiment. Aquaculture 210, 285–304. doi: 10.1016/S0044-8486(01)00849-3
Cribari-Neto, F., and Zeileis, A. (2010). Beta regression in R. J. Stat. Softw. 34, 1–24. doi: 10.18637/jss.v034.i02
Dalsgaard, J., St John, M. S., Kattner, G., Müller-Navarra, D., and Hagen, W. (2003). Fatty acid trophic markers in the pelagic marine environment. Adv. Mar. Biol. 46, 225–340. doi: 10.1016/S0065-2881(03)46005-7
Desvilettes, C., and Bec, A. (2009). “Formation and transfer of fatty acids in aquatic microbial food webs: role of heterotrophic protists,” in Lipids in Aquatic Ecosystems, eds M. T. Arts, M. T. Brett, and M. J. Kainz (New York, NY: Springer), 25–42.
Ferrari, S., and Cribari-Neto, F. (2004). Beta regression for modelling rates and proportions. J. Appl. Stat. 31, 799–815. doi: 10.1080/0266476042000214501
Finkel, Z. V., Beardall, J., Flynn, K. J., Quigg, A., Rees, T. A. V., and Raven, J. A. (2009). Phytoplankton in a changing world: cell size and elemental stoichiometry. J. Plankton Res. 32, 119–137. doi: 10.1093/plankt/fbp098
Garrabou, J., Coma, R., Bensoussan, N., Bally, M., Chevaldonné, P., Cigliano, M., et al. (2009). Mass mortality in Northwestern Mediterranean rocky benthic communities: effects of the 2003 heat wave. Global Change Biol. 15, 1090–1103. doi: 10.1111/j.1365-2486.2008.01823.x
Gulati, R., and Demott, W. (1997). The role of food quality for zooplankton: remarks on the state-of-the-art, perspectives and priorities. Freshwat. Biol. 38, 753–768. doi: 10.1046/j.1365-2427.1997.00275.x
Hairston, N. G. Jr., and Hairston, N. G. Sr. (1993). Cause-effect relationships in energy flow, trophic structure, and interspecific interactions. Am. Nat. 142, 379–411. doi: 10.1086/285546
Han, C. H. (2009). Feeding Ecology of the Common Jellyfish Aurelia aurita s.l. in different life stages. PhD Thesis, Hiroshima University, Hiroshima, Japan.
Iverson, S. J., Frost, K. J., and Lowry, L. F. (1997). Fatty acid signatures reveal fine scale structure of foraging distribution of harbor seals and their prey in Prince William Sound, Alaska. Mar. Ecol. Prog. Ser. 51, 255–271. doi: 10.3354/meps151255
Izquierdo, M. (1996). Essential fatty acid requirements of cultured marine fish larvae. Aquacult. Nutr. 2, 183–191. doi: 10.1111/j.1365-2095.1996.tb00058.x
Jiang, H., and Gao, K. (2004). Effects of lowering temperature during culture on the production of polyunsaturated fatty acids in the marine diatom Phaeodactylum tricornutum (Bacillariophyceae). J. Phycol. 40, 651–654. doi: 10.1111/j.1529-8817.2004.03112.x
Kiørboe, T., Møhlenberg, F., and Hamburger, K. (1985). Bioenergetics of the planktonic copepod Acartia tonsa: relation between feeding, egg production and respiration, and composition of specific dynamic action. Mar. Ecol. Prog. Ser. 26, 85–97. doi: 10.3354/meps026085
Kirsch, P. E., Iverson, S. J., Bowen, W. D., Kerr, S. R., and Ackman, R. G. (1998). Dietary effects on the fatty acid signature of whole Atlantic cod (Gadus morhua). Can. J. Fish. Aquat. Sci. 55, 1378–1386. doi: 10.1139/f98-019
Klein Breteler, W. C. M., Schogt, N., and Rampen, S. (2005). Effect of diatom nutrient limitation on copepod development: role of essential lipids. Mar. Ecol. Prog. Ser. 291, 125–133. doi: 10.3354/meps291125
Knothe, G., and Dunn, R. O. (2009). A comprehensive evaluation of the melting points of fatty acids and esters determined by differential scanning calorimetry. J. Am. Oil Chem. Soc. 86, 843–856. doi: 10.1007/s11746-009-1423-2
Laurel, B. J., Copeman, L. A., and Parrish, C. C. (2012). Role of temperature on lipid/fatty acid composition in Pacific cod (Gadus macrocephalus) eggs and unfed larvae. Mar. Biol. 159, 2025–2034. doi: 10.1007/s00227-012-1989-3
Lebrato, M., Mendes, P. D. J., Steinberg, D. K., Cartes, J. E., Jones, B. M., Birsa, L. M., et al. (2013). Jelly biomass sinking speed reveals a fast carbon export mechanism. Limnol. Oceanogr. 58, 1113–1122. doi: 10.4319/lo.2013.58.3.1113
Lee, R., Hagen, W., and Kattner, G. (2006). Lipid storage in marine zooplankton. Mar. Ecol. Progr. Ser. 307, 273–306. doi: 10.3354/meps307273
Lonsdale, D. (2006). A review of the biochemistry, metabolism and clinical benefits of thiamin (e) and its derivatives. Evid. Based Complement. Alternat. Med. 3, 49–59. doi: 10.1093/ecam/nek009
Lucas, C. H., Graham, W. M., and Widmer, C. (2012). Jellyfish life histories: role of polyps in forming and maintaining scyphomedusa populations. Adv. Mar. Biol. 63:133. doi: 10.1016/B978-0-12-394282-1.00003-X
Lynam, C. P., Heath, M. R., Hay, S. J., and Brierley, A. S. (2005). Evidence for impacts by jellyfish on North Sea herring recruitment. Mar. Ecol. Prog. Ser. 298, 157–167. doi: 10.3354/meps298157
McMeans, B. C., Koussoroplis, A. M., and Kainz, M. J. (2015). Effects of seasonal seston and temperature changes on lake zooplankton fatty acids. Limnol. Oceanogr. 60, 573–583. doi: 10.1002/lno.10041
Meehl, G. A., and Tebaldi, C. (2004). More intense, more frequent, and longer lasting heat waves in the 21st century. Science 305, 994–997. doi: 10.1126/science.1098704
Miyajima, Y., Masuda, R., Kurihara, A., Kamata, R., Yamashita, Y., and Takeuchi, T. (2011). Juveniles of threadsail filefish, Stephanolepis cirrhifer, can survive and grow by feeding on moon jellyfish Aurelia aurita. Fish. Sci. 77, 41–48. doi: 10.1007/s12562-010-0305-8
Müller-Navarra, D. C. (1995). Biochemical versus mineral limitation in Daphnia. Limnol. Oceanogr. 40, 1209–1214. doi: 10.4319/lo.1995.40.7.1209
Müller-Navarra, D. C. (2008). Food web paradigms: the biochemical view on trophic interactions. Int. Rev. Hydrobiol. 93, 489–505. doi: 10.1002/iroh.200711046
Müller-Navarra, D. C., Brett, M. T., Liston, A. M., and Goldman, C. R. (2000). A highly unsaturated fatty acid predicts carbon transfer between primary producers and consumers. Nature 403, 74–77. doi: 10.1038/47469
Müller-Navarra, D. C., Brett, M. T., Park, S., Chandra, S., Ballantyne, A. P., Zorita, E., et al. (2004). Unsaturated fatty acid content in seston and tropho-dynamic coupling in lakes. Nature 427, 69–72. doi: 10.1038/nature02210
Oksanen, J., Blanchet, F. G., Friendly, M., Kindt, R., Legendre, P., McGlinn, D., et al. (2017). Vegan: Community Ecology Package. R package version 2.4-5. Available online at: https://CRAN.R-project.org/package=vegan.
Park, S., Brett, M. T., Müller-Navarra, D. C., and Goldman, C. R. (2002). Essential fatty acid content and the phosphorus to carbon ratio in cultured algae as indicators of food quality for Daphnia. Freshwat. Biol. 47, 1377–1390. doi: 10.1046/j.1365-2427.2002.00870.x
Persson, J., and Vrede, T. (2006). Polyunsaturated fatty acids in zooplankton: variation due to taxonomy and trophic position. Freshwat. Biol. 51, 887–900. doi: 10.1111/j.1365-2427.2006.01540.x
Pitt, K., Connolly, R., and Meziane, T. (2009). Stable isotope and fatty acid tracers in energy and nutrient studies of jellyfish: a review. Hydrobiologia 616, 119–132. doi: 10.1007/s10750-008-9581-z
Pruitt, N. L. (1990). Adaptations to temperature in the cellular membranes of crustacea: membrane structure and metabolism. J. Therm. Biol. 15, 1–8. doi: 10.1016/0306-4565(90)90040-O
R Core Team (2017). R: A Language and Environment for Statistical, Computing. Available online at: https://www.R-project.org/
Richardson, A. J., Bakun, A., Hays, G. C., and Gibbons, M. J. (2009). The jellyfish joyride: causes, consequences and management responses to a more gelatinous future. Trends Ecol. Evol. 24, 312–322. doi: 10.1016/j.tree.2009.01.010
Ridgway, N., and Mcleod, R. (2015). Biochemistry of Lipids, Lipoproteins and Membranes. Boston, MA: Elsevier.
Rombenso, A. N., Trushenski, J. T., Jirsa, D., and Drawbridge, M. (2016). Docosahexaenoic acid (DHA) and arachidonic acid (ARA) are essential to meet LC-PUFA requirements of juvenile California Yellowtail (Seriola dorsalis). Aquaculture 463, 123–134. doi: 10.1016/j.aquaculture.2016.05.004
Rossi, S., Sabates, A., Latasa, M., and Reyes, E. (2006). Lipid biomarkers and trophic linkages between phytoplankton, zooplankton and anchovy (Engraulis encrasicolus) larvae in the NW Mediterranean. J. Plankt. Res. 28, 551–562. doi: 10.1093/plankt/fbi140
Sargent, J., Mcevoy, L., Estevez, A., Bell, G., Bell, M., Henderson, J., et al. (1999). Lipid nutrition of marine fish during early development: current status and future directions. Aquaculture 179, 217–229. doi: 10.1016/S0044-8486(99)00191-X
Singer, S. J., and Nicolson, G. L. (1972). The fluid mosaic model of the structure of cell membranes. Science 175, 720–731. doi: 10.1126/science.175.4023.720
Steinberg, D. K., and Landry, M. R. (2017). Zooplankton and the ocean carbon cycle. Ann. Rev. Mar. Sci. 9, 413–444. doi: 10.1146/annurev-marine-010814-015924
Sundbom, M., and Vrede, T. (1997). Effects of fatty acid and phosphorus content of food on the growth, survival and reproduction of Daphnia. Freshwat. Biol. 38, 665–674. doi: 10.1046/j.1365-2427.1997.00235.x
Thiebot, J.-B., Ito, K., Raclot, T., Poupart, T., Kato, A., Ropert-Coudert, Y., et al. (2016). On the significance of Antarctic jellyfish as food for Adélie penguins, as revealed by video loggers. Mar. Biol. 163:108. doi: 10.1007/s00227-016-2890-2
Thiemann, G. W., Iverson, S. J., and Stirling, I. (2008). Polar bear diets and arctic marine food webs: insights from fatty acid analysis. Ecol. Monogr. 78, 591–613. doi: 10.1890/07-1050.1
Thompson, G. A. (1996). Lipids and membrane function in green algae. Biochim. Biophys. Acta Lipids Lipid Metab. 1302, 17–45. doi: 10.1016/0005-2760(96)00045-8
Vance, D. D., and Vance, J. E. (2008). Biochemistry of Lipids, Lipoproteins and Membranes. Amsterdam: Elsevier.
Vargas, C. A., Tönnesson, K., Sell, A., Maar, M., Møller, E. F., Zervoudaki, T., et al. (2002). Importance of copepods versus appendicularians in vertical carbon fluxes in a Swedish fjord. Mar. Ecol. Prog. Ser. 241, 125–138. doi: 10.3354/meps241125
Volpe, J. J., and Marasa, J. C. (1978). A role for thiamine in the regulation of fatty acid and cholesterol biosynthesis in cultured cells of neural origin. J. Neurochem. 30, 975–981. doi: 10.1111/j.1471-4159.1978.tb12389.x
Wakil, S. J. (1960). Mechanism of fatty acid synthesis. J. Lipid Res. 2, 1–24. doi: 10.1093/ajcn/8.5.630
Werbrouck, E., Tiselius, P., Van Gansbeke, D., Cervin, G., Vanreusel, A., and De Troch, M. (2016). Temperature impact on the trophic transfer of fatty acids in the congeneric copepods Acartia tonsa and Acartia clausi. J. Sea Res. 112, 41–48. doi: 10.1016/j.seares.2016.03.001
West, E. J., Pitt, K. A., Welsh, D. T., Koop, K., and Rissik, D. (2009). Top-down and bottom-up influences of jellyfish on primary productivity and planktonic assemblages. Limnol. Oceanogr. 54, 2058–2071. doi: 10.4319/lo.2009.54.6.2058
Wiltshire, K. H., and Manly, B. F. (2004). The warming trend at Helgoland Roads, North Sea: phytoplankton response. Helgol. Mar. Res. 58, 269–273. doi: 10.1007/s10152-004-0196-0
Woodbury, L. A. (1943). Vitamin B1 deficiency in hatchery reared rainbow trout. Transact. Am. Fish. Soc. 72, 30–34.
Yuan, Y., Li, S., Mai, K., Xu, W., Zhang, Y., and Ai, Q. (2015). The effect of dietary arachidonic acid (ARA) on growth performance, fatty acid composition and expression of ARA metabolism-related genes in larval half-smooth tongue sole (Cynoglossus semilaevis). Br. J. Nutr. 113, 1518–1530. doi: 10.1017/S0007114515000781
Keywords: biomolecules, thiamin, fatty acids, Aurelia aurita, polyp, food chain, food quality
Citation: Chi X, Javidpour J, Sommer U and Mueller-Navarra D (2018) Tracking Fatty Acids From Phytoplankton to Jellyfish Polyps Under Different Stress Regimes: A Three Trophic Levels Experiment. Front. Ecol. Evol. 6:118. doi: 10.3389/fevo.2018.00118
Received: 21 March 2018; Accepted: 23 July 2018;
Published: 17 August 2018.
Edited by:
Martin Kainz, Danube University Krems, AustriaReviewed by:
Davi Castro Tavares, Leibniz Centre for Tropical Marine Research (LG), GermanyMariano Ordano, CONICET Unidad Ejecutora Lillo (UEL), Argentina
Copyright © 2018 Chi, Javidpour, Sommer and Mueller-Navarra. This is an open-access article distributed under the terms of the Creative Commons Attribution License (CC BY). The use, distribution or reproduction in other forums is permitted, provided the original author(s) and the copyright owner(s) are credited and that the original publication in this journal is cited, in accordance with accepted academic practice. No use, distribution or reproduction is permitted which does not comply with these terms.
*Correspondence: Xupeng Chi, xchi@geomar.de
Jamileh Javidpour, jjavid@geomar.de