- 1United States Department of Agriculture, Agricultural Research Service, Fort Pierce, FL, United States
- 2Entomology and Nematology Department, Citrus Research and Education Center, University of Florida, Lake Alfred, FL, United States
Citrus volatiles and their breakdown products, such as formic and acetic acids, guide host finding by Asian citrus psyllids (Diaphorina citri). Formic acid elicits psyllid antennal responses and stimulates probing. This study investigated how exposure to vapor phase formic acid induced changes in plant volatile emissions, expression of plant defense genes, and modified psyllid host finding and feeding behaviors on susceptible and resistant citrus cultivars. Chemical analyses of headspace volatiles and behavioral assays were performed before and after exposure of D. citri susceptible and resistant plants to vapor phase formic acid. RT-qPCR elucidated changes in plant defense-associated gene expression following exposure. Electrical Penetration Graph (EPG) measured D. citri feeding behavior on citrus with and without exposure to formic acid. Volatilized formic acid caused a 200–500% increase in release of citrus volatiles 1–3 h after exposure, increased attraction of D. citri to Poncirus trifoliata (resistant) and increased oviposition compared with controls. RT-qPCR showed significant increases in expression of WRKY22, GST1, RAR1, and EDS1 genes following exposure that also reduced phloem, but not xylem, ingestion by adult D. citri. Formic acid exposure caused increased citrus volatile release that modified host finding and feeding behaviors of an important phytopathogen vector.
Introduction
Asian citrus psyllids (Diaphorina citri Kuwayama) vector the bacterial pathogen, “Candidatus Liberibacter asiaticus” (CLas) that causes the devastating citrus greening disease/huanglongbing (HLB). The pathogen is fastidious and phloem-restricted. Disease symptoms include chlorotic leaf mottling, shoot dieback, small misshapen and bitter fruit, and eventual tree decline (Gottwald et al., 2007). Adult D. citri feed on various leafy parts of Rutaceous plants but oviposition and nymphal development require tender young leaves (flush). Psyllids use multiple sensory modalities [vision, olfaction, contact chemoreception, gustation (taste), perception of auditory or vibrational stimuli] to locate host plants and conspecifics. Paris et al. (2017) reported that D. citri responded to a combination of long and short range wavelengths of light. Adult D. citri were strongly attracted to targets consisting of a combination of ultraviolet and yellow or green light. Other studies reported the role of constitutive plant volatiles in host plant selection by members of the Liviidae (Moran and Brown, 1973; Nehlin et al., 1994; Soroker et al., 2004; Cen et al., 2005; Nissinen et al., 2008; Rouseff et al., 2008; Patt and Setamou, 2010; Onagbola et al., 2011; Patt et al., 2011; Poerwanto et al., 2012; Robbins et al., 2012). Volatile stimuli associated with flushing shoots may play an important role in detection, location and evaluation of potential host plants by D. citri (Patt and Setamou, 2010; Sule et al., 2012) while other studies emphasized the limited role of volatile signals in this species (Lapointe et al., 2016). Mann et al. (2012) reported that citrus plants infected with HLB were more attractive to D. citri than healthy plants, possibly due to higher amounts of methyl salicylate released from infected plants.
Plants activate a wide range of defenses in response to wounding, herbivore attack or pathogen infection, resulting in release of volatile organic compounds (VOCs) (Pare and Tumlinson, 1999; Mumm et al., 2008; Erb et al., 2015). Several metabolic pathways may be activated in plants in response to pathogen attack (Liu et al., 2001; Griebel and Zeier, 2010) and wounding. For example, oligosaccharides released from damaged cell walls and oligopeptide systemins play regulatory roles in wound signaling (Bishop et al., 1981; Pearce et al., 1991). Many investigations of plant-pathogen interactions have focused on individual aspects of pathogen-induced plant defense (Dean and Delaney, 2008; Attaran et al., 2009; Griebel and Zeier, 2010) or the responses of insect vectors to infected vs. healthy plants (Mayer et al., 2008a,b; Mauck et al., 2010). Fewer studies have investigated the role of catabolic breakdown products on induced plant volatile release and the subsequent insect interactions. Erb et al. (2015) showed that the release of herbivore-induced indole in maize enhanced induction of defensive volatiles in neighboring maize plants. Rodriguez-Saona et al. (2001) reported that the exogenous application of methyl jasmonate directly and systemically induced emission of volatiles that may serve as odor cues in the searching behavior of natural enemies of herbivorous insects. Salivary sheath secretions by psyllids on citrus leaves were shown to activate plant defenses and increase volatile release by 2 to 10-fold compared with uninfested foliage (Hijaz et al., 2013). Much less has been reported on the relationship between formic acid and plant defense responses. However, the related compound formaldehyde is a known toxicant of plants (Giese et al., 1994), and a detoxification system requiring glutathione-dependent formaldehyde dehydrogenase (FALDH) is necessary (Liu et al., 2001). It has been shown that the level and activity of FALDH were regulated by wounding, salicylic acid (SA) and jasmonate treatment, suggesting an interplay between formaldehyde catabolism and defense-associated pathways (Díaz et al., 2003). Patt et al. (2018) have also reported that the foliar application of methyl jasmonate suppressed the emission of methyl salicylate from CLas-infected trees. However, methyl jasmonate applications enhanced the emission of E-β-ocimene and indole volatiles attractive to many natural enemies of psyllids.
Plant catabolic breakdown products can play a major role in plant-plant signaling or plant-insect interactions. Monoterpenes such as limonene, α-pinene, β-myrcene, linalool, neral, geranial, and β-ocimene are common citrus volatiles found in the atmosphere surrounding citrus orchards (Park et al., 2013). Some monoterpenes such as isomers of β-ocimene are known to be unstable in air (Fahlbusch et al., 2003). Formic acid has long been reported as a naturally occurring carboxylic acid in plants (Tolbert, 1955; Krall and Tolbert, 1957). The enzyme formyltetrahydrofolate synthetase activates formate (the ion of formic acid) formation in the cytoplasm of cells throughout plant tissues (Hiatt, 1965). Formic acid “fed” to plants enters into the pathways of photosynthetic CO2 fixation (Tolbert, 1955). The products of formic acid fixation over short periods of exposure such as those used here include sucrose, phosphate esters of glucose, fructose, amino acids and others (Tolbert, 1955). Plants produce methanol as a byproduct of cell wall metabolism that is emitted at high levels during plant growth (Fall and Benson, 1996; Huve et al., 2007). A series of enzyme-mediated oxidation reactions can occur converting methanol into formaldehyde, formic acid, and ultimately, carbon dioxide (Cossins and Sinha, 1965; Díaz et al., 2003).
Our recent studies reported that the spontaneous oxidative breakdown of citrus volatiles such as ocimene and citral (a mixture of the E-isomer geranial and the Z-isomer neral) produce formic and acetic acids, and these compounds elicit D. citri antennal responses in electroantennographic studies (George et al., 2016). These carboxylic acids are the only elicitors of antennal response we have been able to consistently demonstrate for D. citri and may play a role in host location or selection (George et al., 2016; Lapointe et al., 2016). Our studies identified a phagostimulant blend containing formic acid as the primary component that increases probing activity by citrus psyllids (Lapointe et al., 2016). Psyllid mouthparts including the labial palps and stylets appear to be capable of detecting the presence of formic acid in an inert wax substrate resulting in increased probing frequency and production of longer salivary sheaths. The distal tip of labial palps of D. citri have four basiconic sensilla assumed to be involved in contact chemoreception (Garzo et al., 2012). Adult D. citri produced significantly longer salivary sheaths in probing assays in response to a 3-component phagostimulant blend (formic acid, acetic acid and para-cymene) in wax compared with blank controls (Lapointe et al., 2016). In addition, it was observed that CLas-infected adult D. citri produced more salivary sheaths in response to the formic acid phagostimulant compared to healthy psyllid adults (unpublished). These studies suggest that formic acid may act as an attractant or play a secondary role as an additive or synergistic agent for other odorants and be conditioned by microbe-plant-insect interactions.
In this study, we investigated the effect of formic acid exposure on activation of plant defense genes and the subsequent plant responses. Furthermore, we investigated how exposure to vapor phase formic acid affected volatile release by citrus leaves and modified host selection behavior by D. citri. We also investigated the effect of these plant responses on the insect's host selection, oviposition, nymphal development and adult emergence using preferred and non-preferred host plants. RT-qPCR was used at different time points before and after exposure to formic acid (priming) to assess the expression of defense-related genes in citrus. Electrical penetration graph recordings were performed using adult D. citri to study how the transcriptional reprograming of citrus defense genes correlated with psyllid feeding behavior. Chemical, physiological, molecular and behavioral assays were used to elucidate how formic acid, a citrus volatile degradation byproduct, activates plant defense pathways and causes higher volatile release that modify host finding and feeding behaviors of an important plant disease vector.
Materials and Methods
Asian Citrus Psyllids
Adult D. citri were obtained from a colony established in 2000 at the USDA-ARS U.S. Horticultural Research Laboratory, Fort Pierce, FL. The psyllids were originally collected from citrus trees in the field and subsequently reared in a small air-conditioned insectary (greenhouse) in cages containing orange jasmine, Murraya exotica L. (= M. paniculata auct. non.), until March 2010, when Citrus macrophylla was substituted as the rearing plant. The temperature in the insectary was maintained at ~25°C under a combination of natural sunlight and in some locations supplemental LED lighting. The colony was maintained using procedures similar to those described by Skelley and Hoy (2004), with no infusion of wild types, and the colony was confirmed quarterly to be free of CLas by qPCR (Li et al., 2006). The sex ratio of the colony was ~1:1. All adult psyllids used in the experiments were 8 to 10 days old. Experiments were performed inside a temperature and light controlled environmental walk-in chamber. All experiments were performed at 26°C, 65% relative humidity (RH) under 14:10 light: dark cycle.
Solid Phase Micro Extraction (SPME) Sampling of Citrus Volatiles Following the Formic Acid Exposure
The effect of formic acid in headspace over citrus plants at low (~1 ppm) concentrations, achieved by allowing 50 μL of pure formic acid to volatilize in a 49 L glass bell jar container, was investigated with Solid Phase Micro Extraction (SPME). Although both formic and acetic acids are more prevalent in the canopies of citrus trees than their pre-cursor products (Park et al., 2013), little information exists about their ambient concentrations in nature. Fifty Microliters of formic acid was used to expose plants because this dose optimally increased volatile release without burning/wilting damage to the plants in preliminary experiments that tested doses ranging from 10 to 1000 μL. At a 1,000 μL exposure dose, plants wilted or showed phytotoxic symptoms. The exposure was limited to 1 h to prevent damage to plants. C. macrophylla plants grown in 3.79 L pots were watered 24 h prior to the start of each experiment. The exposed soil surface of pots was covered with stretched parafilm to minimize contamination by volatiles from the potting mix. Potted plants were introduced individually into a 49 L glass jar (Analytical Research Systems, Gainesville, FL, USA) and sealed. Initial SPME sampling was conducted for 1 h in the empty jar by exposing a 50 mm Carboxen/PDMS-/DVB fiber to the headspace for 1 h through overhead sampling ports. Plants were then introduced into jars for 1 h and the unexposed plants were subsequently sampled by SPME. Thereafter, jars were briefly opened to pipette 50 μL of formic acid (>95%, CAS: 64-18-6, Sigma-Aldrich, USA) into 10 × 100 mm glass Petri dishes (KIMAX, USA) at the bottom of the treatment glass jars and re-sealed. Headspace sampling using SPME was performed on blank jars equilibrated for 1 h, unexposed plants maintained in jars for 1 h, and exposed plants 1 and 19 h following introduction of formic acid. Once the sampling was completed, the fiber was retracted into the sampling device, and promptly desorbed with Gas Chromatography-Mass Spectrometry (GC-MS) for analysis.
Another set of experiments was performed to investigate the breakdown of formic acid after exposure to air. Formic acid and water were placed in jars as described above, but without plants. SPME sampling was performed at 0, 1, 2, and 19 h after exposure to measure breakdown of formic acid in empty glass jars. All the experiments were performed in a temperature and humidity controlled walk-in chamber under controlled conditions of 26°C, 60% RH and continuous fluorescent light (F40T12/C50 natural light, Philips, USA).
GC-MS Analysis
Each sample was analyzed by GC-MS using the Thermo DSQII equipped with a 30 m × 0.25 mm x 0.25 μ film DB5-MS column and helium carrier gas flowing at a constant rate of 1.0 mL/min. The PTV injector was operated in the CT-splitless mode at 250°C. After 2 min, the split and purge vents were opened with the split vent flow at 20 mL/min and the purge vent flow at 3.5 mL/min. The column oven program was started at 10°C with a 4-min hold, then temperature was increased at 4°C /min to 280°C with a 10-min hold. The mass spectrometer was scanned for the first 5.5 min from m/z 10 to 200, then for 5.5 min to 40 min, from m/z 40 to 400, and 40 min from m/z 50 to 550. Throughout the analysis, the MS source was maintained at 220°C and the GC transfer line at 300°C.
Behavioral Choice Assays Using Psyllid Resistant or Susceptible Citrus Following Formic Acid Exposure
Behavioral choice assays were used to investigate the preference of D. citri adults, and subsequent development of nymphs on psyllid susceptible and resistant host plants following exposure to vapor phase formic acid. Host selection, oviposition, nymphal development, and adult emergence were studied on moderately resistant P. trifoliata accession “Towne-G” (CRC 3207) and highly susceptible C. macrophylla (Hall et al., 2015) following formic acid exposure. Potted plants (with 1–2 flush shoots, 30–40 cm tall) in plastic cone-shaped containers were exposed to 50 μL of formic acid headspace volatiles for an hour inside a 49 L sealed glass jar. Unexposed (control) plants were kept in the sealed glass jar for an hour with 50 μL of water.
After 1 h exposure to formic acid (exposed) or water control (unexposed), plants were arranged in pairs and placed without contacting each other in screened cages (60 × 60 × 60 cm, BugDorm 2, BioQuip Products Inc., Rancho Dominguez, CA, USA). These cages were placed under grow lights in an environmental chamber (26°C, 65% relative humidity, 14:10 L:D).
Three different choice experiments were performed to study the effect of formic acid exposure on Asian citrus psyllid host choice and adult development: (1) C. macrophylla vs. exposed P. trifoliata; (2) C. macrophylla vs. exposed C. macrophylla; and (3) P. trifoliata vs. exposed P. trifoliata. Fifty D. citri adults (~1:1 male:female ratio) were introduced into each cage containing two plants (one exposed, one unexposed) and allowed to feed and oviposit for 72 h. Experiments were replicated four times. The number of psyllids on each plant was recorded at 3, 21, and 72 h after introduction of the psyllids. The number of nymphs and their nymphal instars were recorded 12 d after introduction of adults. Cages were checked for adult emergence from day 15 onwards; newly emerged adults were removed daily using an aspirator. Adult host choice was subjected to analysis of variance (ANOVA) followed by Tukey's HSD test (α = 0.05). Nymphal development and cumulative adult emergence data were log transformed and analyzed by ANOVA followed by Tukey's HSD test (α = 0.05).
Electrical Penetration Graph (EPG) Recordings on Formic Acid Exposed C. macrophylla Leaves
Plants
EPG studies were performed on young, fully expanded leaves of C. macrophylla seedlings. Selected citrus plants with flush shoots were washed and watered 24 h prior to the EPG experiment. Plants were exposed to 50 μL of formic acid or water in glass jars for an hour as described above. Psyllids were tethered and constrained to the adaxial (upper) leaf surface. The remaining leaf area was covered with blue tape (Fisherbrand, USA). The length of the gold wire tether was adjusted to constrain psyllid movement and feeding to the 1.5 cm2 area surrounding the midribs of leaves (George et al., 2017).
Electrical Penetration Graph Recordings
EPG recordings were obtained using a DC-monitor, GIGA-8 model (EPG-Systems, Wageningen, Netherlands) (Tjallingii and Esch, 1993), adjusted to 50x gain. The analog signal was digitized through a DI-710 board, and displayed using Windaq Lite ver. 2.40 software (Dataq Instruments Inc. Akron, OH, USA) on a Dell desktop computer. The EPG monitoring system was housed in a grounded Faraday cage in an environmentally controlled room under continuous lighting conditions. Temperature was set to 26°C with 60–65% RH. Adult D. citri were collected 4 h prior to the start of the experiment each day and were starved inside collection vials. Psyllids were placed into a freezer (−4°C) to immobilize them for 1 min and then held by a plastic pipette tip connected to a gentle vacuum supply under a dissecting microscope. Psyllids were attached to a 1.5 cm long 25 μm-diam. gold wire (Sigmund Cohn Corp., Mt. Vernon, NY) by a droplet of silver conducting paint (Ladd Research Industries, Burlington, VT) applied to the pronotum. The other end of gold wire lead was attached to a copper electrode (3 cm × 1 mm diameter) connected to the EPG probe. The tethered insect was placed on the adaxial surface of formic acid exposed or unexposed C. macrophylla leaf. To complete the electrical circuit, a reference copper electrode (10 cm × 2 mm) was inserted into the soil medium at the base of the citrus plant. Subsequent insect behavior was not noticeably affected by the tethering procedure (George et al., 2017; George and Lapointe, 2019). The feeding behaviors of ten individual D. citri adults each on formic acid exposed and unexposed C. macrophylla plants were monitored for 21 h.
Characterization of EPG waveforms was accomplished by visually identifying and annotating waveform patterns based on comparison to prior histological studies (Bonani et al., 2010). A waveform browser (Dataq Instruments Inc., Akron, OH) was used to annotate waveforms. The number and duration of waveform bouts were tabulated in an electronic spreadsheet. The waveforms were visually inspected for frequency patterns and annotated as non-probing (Np), intercellular probing (C), phloem penetration (D), phloem salivation (E1), phloem ingestion (E2) or xylem ingestion (G) (Bonani et al., 2010; George et al., 2017; George and Lapointe, 2019). Earlier studies reported no significant differences in probing and feeding behavior of male and female D. citri with respect to leaf maturity or leaf surface (Luo et al., 2015; George et al., 2017). The number of waveform bouts (count), mean duration and total duration (count x mean duration) for individual waveforms were analyzed by Kruskal-Wallis test (α = 0.05) using JMP without differentiating insect sex (v. 10, SAS Inc, Cary, NC, USA).
The Effect of Formic Acid on the Expression of Citrus Defense-Associated Genes
To investigate the effect of formic acid exposure on citrus plant defenses and signaling pathways, we performed gene expression analysis following formic acid exposure. The genes investigated were selected based on previous investigations of citrus defenses against a canker pathogen (Shi et al., 2015), representing key genes in signaling and pathways that may share similarity to defense against herbivory insects (Taylor et al., 2004; Maffei et al., 2007). These genes included marker genes for pathogen-associated molecular pattern (PAMP)-triggered immunity (PTI) (WRKY22 and SGT1) (Asai et al., 2002), PTI and effector-triggered immunity (ETI) perception and signaling (RAR1 and EDS1) (Torp and Jorgensen, 1986; Parker et al., 1996), salicylic acid (SA) receptors (NPR2 and NPR3) (Zhang et al., 2006; Fu et al., 2012), SA metabolism (PAL1 and ICS1) (MauchMani and Slusarenko, 1996; Wildermuth et al., 2001), and jasmonic acid (JA) signaling (COI1 and JAR1) (Staswick and Tiryaki, 2004; Yan et al., 2009). The effect of formic acid on the expression of citrus defense-associated genes was measured during a time course from 1 to 21 h after exposure.
Sampling of leaf tissues was performed at different time intervals following exposure to formic acid. One leaf per plant (n = 3 plants) was collected at 1, 2, 3, 6, and 21 h after formic acid exposure or control treatments. Leaves collected before formic acid exposure were considered as 0 h samples. Samples were put in liquid nitrogen immediately after collection and stored at −80°C for RNA isolation, cDNA synthesis and gene expression analysis. Total RNA was extracted using TriZol reagent (Invitrogen, Carlsbad, CA, USA) according to manufacturer's instructions, followed by DNase treatment and clean up with RNeasy Plant Mini Kit (Qiagen, Gaithersburg, MD, USA). cDNA synthesis was performed using QuantiTect Reverse Transcription kit (Qiagen) from 1 μg of RNA. SYBR green reagent (Thermo Fisher Scientific, Waltham, MA) was used for RT-qPCR following manufacture protocols with primers published previously (Shi et al., 2015) amplifying from 10 ng of cDNA. Comparative Ct (ΔΔCt) method was applied to generate relative quantification (RQ) values from three biological replicates from each treatment at each time point. Amplification of citrus glyceraldehyde-3-phosphate dehydrogenase C2 (GAPC2) was used for normalization (Mafra et al., 2012). Statistical significance was calculated (paired samples, Kruskal-Wallis in R, α = 0.05) (The R Foundation for Statistical Computing, Vienna, Austria).
Results
Citrus Volatile Profiles Following the Formic Acid Exposure
Formic acid exposure caused a 100–200% increase in the peak areas of limonene, methyl anthranilate, E-β-ocimene, citronellal, and β-myrcene plant volatiles within an hour after exposure (Figure 1A). Citrus volatiles such as neral and geranial showed a 500–700% increase in peak area within an hour following the 1-h exposure to formic acid. A significant increase in the amount of Υ-terpinene was also observed after the 1-h formic acid exposure (Figure 1B). Formic acid was not detected in the headspace samples of plant volatiles from the control jar. However, formic acid was easily detected in the SPME sampling 1-h after exposing the blank jar with 50 μL of the acid. The concentration of formic acid vapor appeared as a pulse, peaking at 1 h after introduction and then quickly disappearing over the subsequent 2 h leaving negligible amounts in the jars by 19 h (Figure 1A, dotted line).
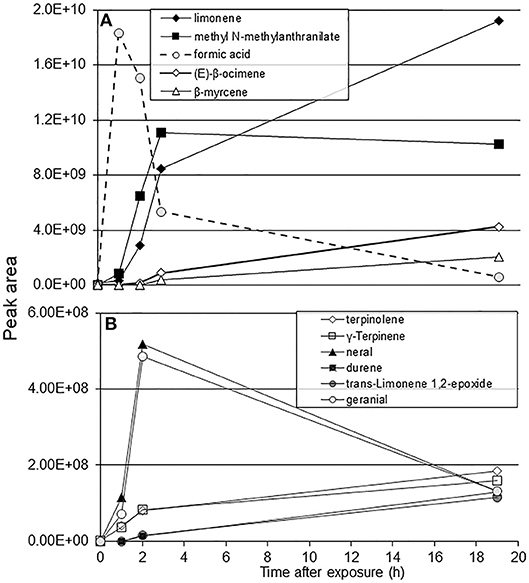
Figure 1. SPME-GC/MS analysis of C. macrophylla volatile peaks following exposure to 50 μL of formic acid for 1 h. (A) peak area of major components; (B) peak area of additional citrus volatiles.
Psyllid Behavioral Response to Resistant or Susceptible Citrus Following Formic Acid Exposure
Formic acid exposure of susceptible C. macrophylla caused significant increase in volatile release and in turn attracted significantly more psyllids (P = 0.02) to exposed (25 ± 5) than unexposed plants (12 ± 3) after 3 h. The arrestment effect of the volatiles was not statistically significant at 21 and 72 h (Figure 2A). Significantly more psyllids selected formic acid exposed P. trifoliata than control P. trifoliata plants at 3 h (P = 0.006) and 21 h after exposure (P = 0.03) (Figure 2B). No significant difference was observed in psyllid choice at 72 h after formic acid exposure. In the cage containing P. trifoliata plants exposed to formic acid vapor vs. unexposed C. macrophylla, no differences were observed in the number of psyllids that settled on the P. trifoliata plants (19 ± 10) vs. C. macrophylla plants (14 ± 6) after 3 h (P = 0.4) (Figure 2C). As the volatile release began to diminish, the arrestment effect of formic acid faded away and psyllids began moving from trifoliate plants to C. macrophylla plants. By 72 h, significantly more psyllids were found on C. macrophylla plants than exposed trifoliate plants [F(1,7) = 3.18; P = 0.03, n = 4] (Figure 2C).
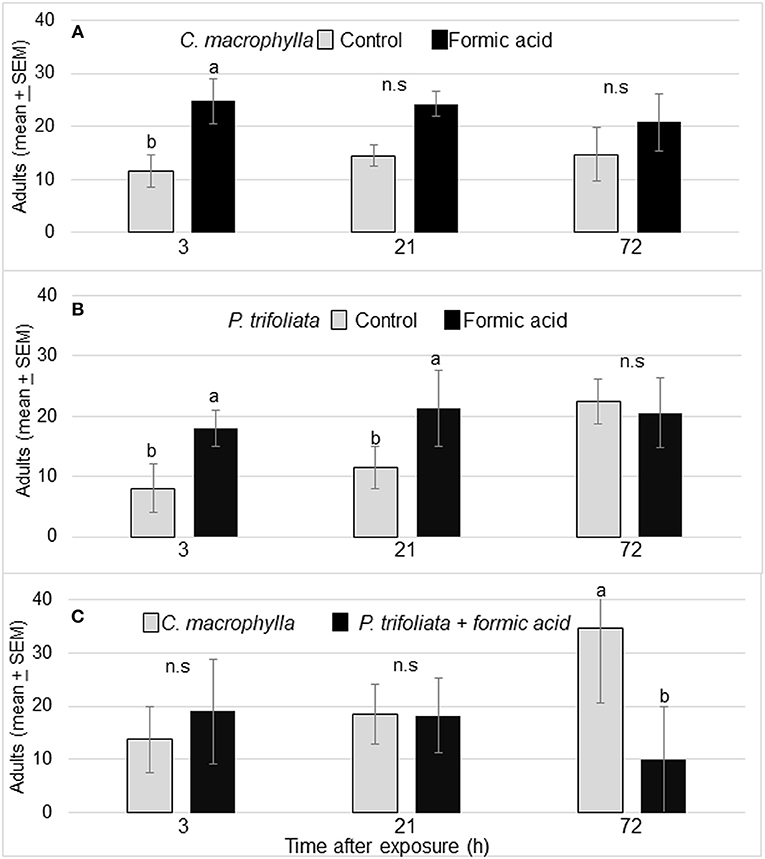
Figure 2. (A) Mean (± SEM) no. of D. citri on control and formic acid-exposed C. macrophylla at 3, 21, and 72 h after exposure. (B) Mean (± SEM) number of psyllids on exposed P. trifoliata (Towne-G) vs. unexposed P. trifoliata plants. (C) Mean number (± SEM) of D. citri adults on C. macrophylla vs. P. trifoliata (Towne-G) plants exposed to formic acid. Means followed by the same letters do not differ by Tukey's HSD following a significant ANOVA (α = 0.05, n = 4).
The higher volatile release associated with formic acid exposure attracted more psyllids to the formic acid exposed plants than unexposed plants (Figure 2). This attraction toward formic acid exposed plants was also reflected in the oviposition behavior of psyllids on these plants. Significantly more nymphs were observed on exposed than unexposed trifoliate plants [F(1,7) = 11.9; P = 0.01, n = 4] after 12 d (Figure 3A). In cages containing C. macrophylla and exposed P. trifoliata, significantly more nymphs were observed on C. macrophylla than P. trifoliata [F(1,7) = 6.9; P = 0.03] (Figure 3A). Even though more nymphs were observed on exposed compared to unexposed P. trifoliata plants, the antibiosis and antixenosis associated with P. trifoliata resulted in delayed nymphal development and reduced adult emergence. Only 23 out of 114 total nymphs (20%) emerged as adults after 23 days on P. trifoliata (Figure 3B). Psyllid nymphs developed more successfully on C. macrophylla plants, and 661 out of 781 (85%) total nymphs emerged as adults. No adults emerged from the exposed trifoliate plants that were assayed as a choice with C. macrophylla (Figure 3B).
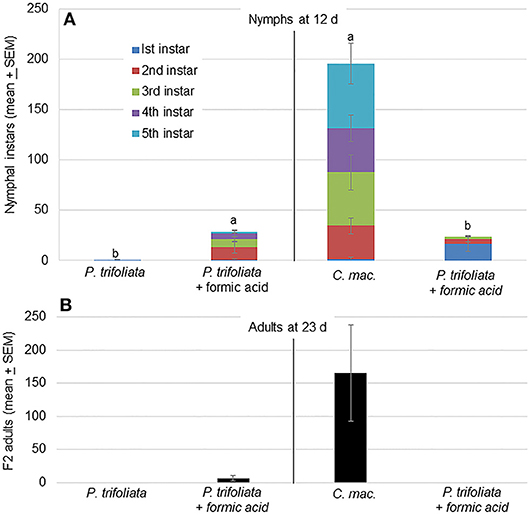
Figure 3. (A) Mean (± SEM, n = 4) number of D. citri nymphal instars on individual plants in two treatment cages 12 d after introduction of psyllids. Significantly more nymphs were located on P. trifoliata exposed to vapor phase formic acid compared with the number on untreated (control) plants [F(1,5) = 11.9; P = 0.01]. In a separate test, more nymphs were observed on untreated C. macrophylla plants compared with the number on P. trifoliata plants exposed to formic acid [F(1,5) = 10.8; P = 0.03]. Treatments followed by the same letters are not significantly different by Tukey's HSD (α = 0.05) following a significant ANOVA. (B) Number of F2 adults present on plants 23 d after start of the experiment.
Feeding Behavior of Psyllids on Formic Acid Exposed C. macrophylla Leaves
Waveforms produced by D. citri adults during the 21 h recording periods were typical for psyllids and readily classified according to the work of Bonani et al. (2010), for the following feeding behaviors: non-probing (Np), intercellular probing (C), phloem penetration (D), phloem salivation (E1), phloem ingestion (E2), or xylem ingestion (G). The EPG recordings showed that phloem penetration (waveform D) and phloem ingestion (waveform E2) were significantly reduced on formic acid exposed leaves compared to those on unexposed leaves (Table 1). The number of bouts (P = 0.04), mean duration (P = 0.03), and total duration (P = 0.03) of phloem ingestion were significantly lower on exposed plants than control plants. But, the total duration of non-probing activities (Np) were significantly increased on exposed plants as compared to controls (P = 0.03), which corresponded to significantly lower feeding activities (Figure 4, Table 1). Psyllids spent more than half of their time on leaves in non-probing activities (56%) on exposed plants compared to control plants (34%) (Figure 4). Less than 1% of the time was spend in phloem feeding activities (waveform E1+E2) on exposed plants compared to 17% on control plants. No difference was observed in the overall time spent in xylem feeding (waveform G) on exposed (16%) vs. control plants (17%) (Table 1, Figure 4).
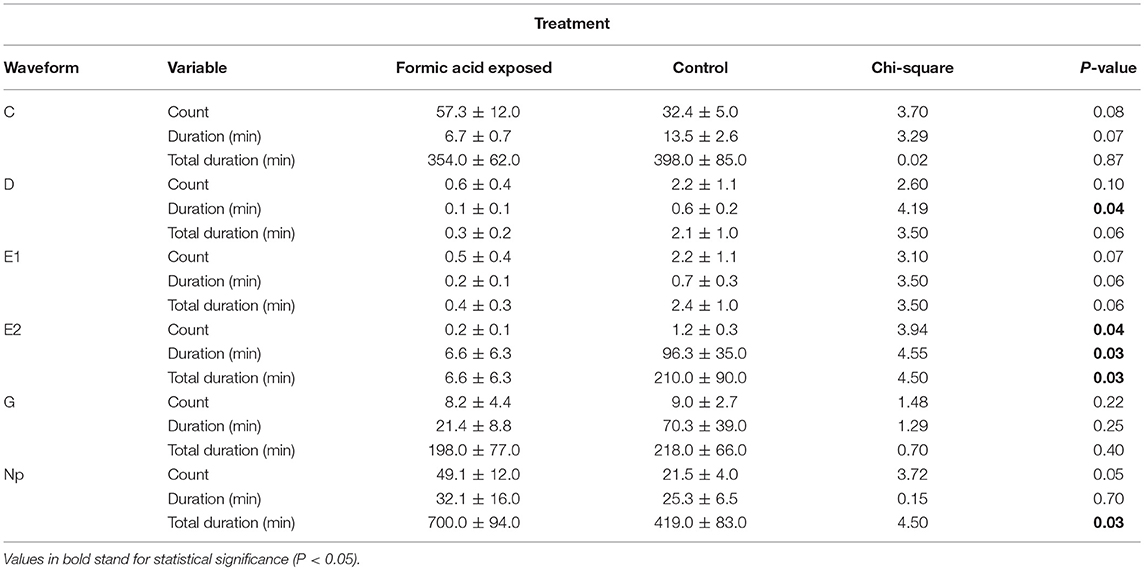
Table 1. Frequency, mean duration and total duration of xylem and phloem feeding activities of Diaphorina citri adults on young C. macrophylla leaves, following formic acid exposure.
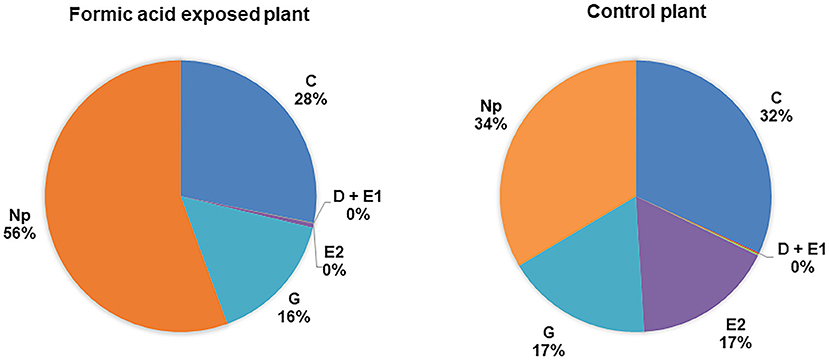
Figure 4. Percentage of total duration for each feeding activity (waveform) by D. citri adults on C. macrophylla plants exposed to formic acid vapor. Phloem ingestion (E2) was rarely observed on exposed plants compared with control plants. Psyllids spent more time on non-probing activities on exposed than control plants (P = 0.04, n = 10). No difference was observed in the duration of xylem feeding (G) performed by psyllids on exposed and control plants (P = 0.24, n = 10).
Expression of Citrus Defense-Associated Genes Following Formic Acid Exposure
Chemical analysis of headspace volatiles following the formic acid exposure showed a higher rate of citrus volatile release, which influenced host selection behaviors of psyllids. Formic acid exposure also caused significant reduction in phloem feeding behaviors of psyllids and caused more non-probing activities. We hypothesized that formic acid exposure could activate various general plant defense genes associated with wounding or plant signaling pathways, which could be associated with increased volatile emissions.
Formic acid treatment significantly increased expression of: WRKY22 and GST1 at 1h after the treatment (Figures 5A,B), RAR1 from 1 to 2 h, and EDS1 from 2 to 3 h after treatment (Figures 5C,D); NPR2 from 1 to 2 h, and NPR3 at 1 h after the treatment (Figures 5E,F). Formic acid exposure also upregulated the expression of PAL1 at 6 h, but downregulated the expression of ICS1 at 21 h after treatment (Figures 5G,H). For the JA pathway genes, formic acid decreased expression of COI1 and JAR1 at 21 h after treatment (Figures 5I,J).
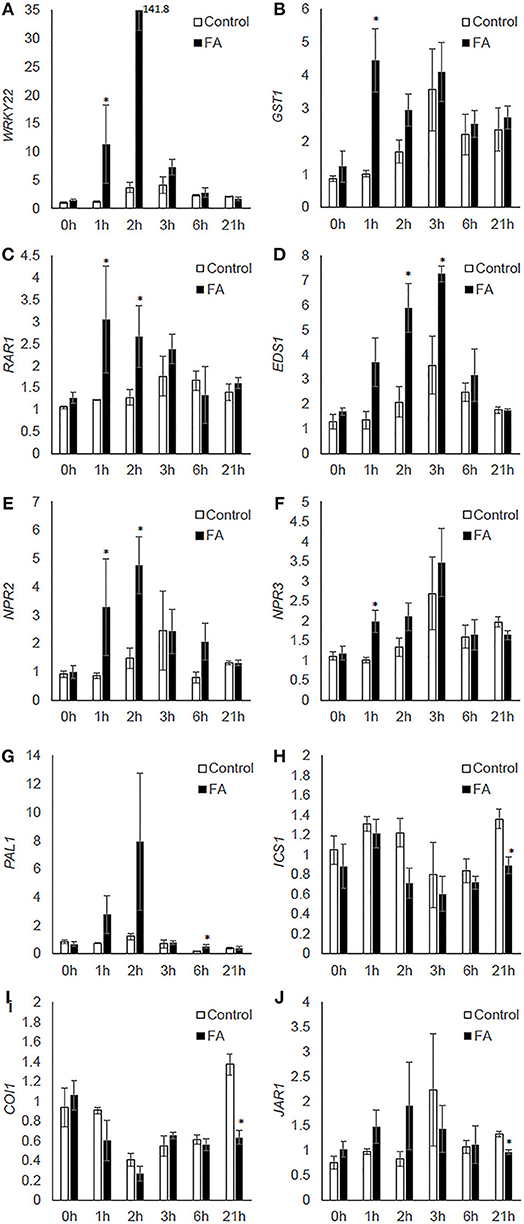
Figure 5. The effect of formic acid on the transcriptional levels of citrus defense-associated genes (A–J) at 1, 2, 3, 6, and 21 h after treatment. Leaf samples were collected at each time point from three formic acid-exposed C. macrophylla plants (FA) and three untreated controls (control). Leaves collected prior to treatments were used as 0 h reference. The relative quantification of each gene was calculated by normalization with citrus endogenous gene GAPDH and referenced to one randomly selected 0 h sample. An asterisk indicates significant difference between treatment and control at each time point (Kruskal-Wallis, α = 0.05). Bars are means ± SEM (n = 3).
Discussion
Volatile organic compounds (VOCs) and their oxidative breakdown products act as important semiochemicals in plant-insect interactions. Herbivorous insects use these chemical cues to differentiate between host and non-host plants (Li et al., 2016), and to locate feeding and oviposition sites. Previously, we reported that citrus volatiles such as β-ocimene and citral undergo oxidative breakdown in air and produce a number of smaller molecules including formic and acetic acids (George et al., 2016). Electroantennographic studies showed that formic and acetic acids elicit psyllid antennal responses (George et al., 2016), and act as phagostimulants that increase psyllid probing/feeding behavior (Lapointe et al., 2016). Other studies reported that photochemical oxidation of VOCs in general can lead to formation of a wide range of gaseous reaction or aerosol products (Bergström et al., 2014; Jud et al., 2016). These oxidative products may have ecological consequences for plant-insect interactions, when plant surfaces are exposed by gaseous contact or direct deposition. Earlier studies have shown that exposure of plants to methyl vinyl ketone and macroclein up-regulated plant defense genes (Alméras et al., 2003; Karl et al., 2010). Very few studies have investigated potential effects of oxidation products on plant-insect interactions. Li et al. (2016) reported disruption of larval host orientation by diamondback moths (Plutella xylostella) to cabbage (Brassica oleraceae) resulting from ozone-mediated degradation of cabbage volatiles.
Plants can respond to volatiles with modified emission of VOCs quantitatively and/or qualitatively affecting the orientation of insect herbivores to those modified VOC profiles or to degradation products of the induced emissions. Under the conditions of our study, brief (1 h) exposure to vapor phase formic acid increased release of citrus VOCs such as limonene, β-ocimene and myrcene. George et al. (2016) reported that formic and acetic acids are produced following oxidative breakdown of major citrus volatiles such as β-ocimene and citral. Oxidation of several molecules including acetaldehyde and formaldehyde produces acetic and formic acids (Oikawa and Lerdau, 2013; Park et al., 2013). Studies have measured acetic and formic acid present in the air above citrus orchards (Park et al., 2013). Degradation of VOCs is a common phenomenon in the air above citrus groves, and these breakdown products could play a role in perpetuating volatile production from citrus.
Poncirus trifoliata cultivars and their hybrids are primarily used as citrus rootstocks, but also show promise in scion breeding as sources for resistance to D. citri and other important citrus pests/diseases (Lapointe et al., 1999). Behavioral studies using P. trifoliata genotypes have reported varying levels of resistance to colonization by D. citri (Richardson and Hall, 2013). Hall et al. (2015) reported reductions in oviposition by D. citri and subsequent nymphal infestation on P. trifoliata accessions such as Towne-G, compared with that on Citrus sinensis. George et al. (2018) showed that host plant resistance in P. trifoliata is mediated by antibiosis and antixenosis that reduced oviposition and delayed nymphal development. The results from the current behavioral assays are congruent with earlier findings indicating that oviposition by and nymphal development of D. citri are reduced on P. trifoliata compared with those on C. macrophylla (George and Lapointe, 2019). Our results indicate increased attraction of D. citri adults to C. macrophylla plants that were exposed to formic acid. This effect also occurred with normally D. citri-resistant P. trifoliata (Figures 2A,B). Formic acid-exposed P. trifoliata attracted more D. citri compared with unexposed P. trifoliata. In fact, formic acid-exposed P. trifoliata were as attractive to D. citri as untreated C. macrophylla, a preferred host (Figure 2C). These results suggest that formic acid induces release of citrus volatiles that influence D. citri host selection behavior. By exposing P. trifoliata to vapor phase formic acid, a plant genotype normally resistant to D. citri colonization was rendered more susceptible. The effect was ephemeral and reversible, persisting over a period of hours during which the emitted volatile profile was modified. These findings may have practical implications such as the development of trap cropping strategies and attract-and-kill devices for psyllid control.
Earlier studies have shown that increased release of (S)-linalool by transgenic tobacco resulted in decreased oviposition by Helicoverpa armigera, even though it did not affect larval development (McCallum et al., 2011). In contrast, we observed increased attraction and oviposition by psyllids to plants emitting higher amount of volatiles. More D. citri nymphs survived to the adult stage on P. trifoliata exposed to formic acid than on unexposed P. trifoliata counterparts (Figures 3A,B). Resistance factors associated with P. trifoliata plants affected nymphal development and resulted in reduced adult emergence compared with C. macrophylla. In cages containing untreated C. macrophylla and formic acid-exposed trifoliate plants, we observed that more psyllids settled on P. trifoliata plants than the preferred host during the initial 3 h, but the effect was transient and adult D. citri returned to the preferred C. macrophylla plant by 72 h after exposure (Figure 2C). This resulted in greater nymph and adult development on C. macrophylla compared with that on P. trifoliata (Figures 3A,B). Therefore, the direct plant defense that renders P. trifoliata resistant to D. citri was not neutralized by exposure to formic acid. These results indicate a significant but transient increase in VOCs, and the up-regulation of certain genes typically associated with indirect defense observed within the first 6 h following exposure of P. trifoliata to formic acid. However, this plant response cannot be considered as inducible defense with respect to the D. citri herbivore, which counter-intuitively appeared to exploit the quantitative increase in overall volatile production as evidenced by increased attraction to exposed plants, not unlike what occurs among certain hemipteran insect phytopathogen vectors in response to pathogen-infected host plants (Mauck et al., 2010).
Formic acid exposure of citrus plants may have also caused chemical wounding of plant tissues activating plant responses, displayed as upregulation of general defense genes observed in our RT-qPCR analysis. Following herbivore attack, injury or wounding, plants have the capacity to activate those cells competent for activation of defense mechanisms, which largely depends on transcriptional activation of specific genes (Howe and Jander, 2008). Following wounding, plants produce reactive oxygen species (ROS) including superoxide anion (Doke et al., 1991) and hydrogen peroxide (Orozco-Cardenas and Ryan, 1999) in the damaged tissue. These injury or wound-activated responses that occur in a time window ranging from minutes to several hours function to heal damaged tissues and prevent further damage (Leon et al., 2001). Also, wounding can cause activation of ion channels at the plasma membrane and reversible protein phosphorylation within minutes. These wound-induced responses are rapid and could lead to an oxidative burst and consequent expression of defense-related genes (Savatin et al., 2014). Following these primary signals, secondary wound-related signals activate different defense functions in systemic tissues, which may include oxylipins and jasmonic acid (JA) (Leon et al., 2001; Schaller, 2001). Further studies have reported that jasmonic acid regulates the production of, or acts as, a mobile wound signal (Schilmiller and Howe, 2005). These primary and secondary signaling activities could have contributed to physiological and metabolic changes in our citrus plants, which resulted in increased volatile release in the first 1–3 h following the formic acid exposure. As shown in the gene expression studies, the effects lasted only 1–3 h after exposure after which plants began to re-assumed pre-exposure volatile release profiles. It is possible that the formic acid exposure caused increased VOCs as a response to wounding damage, which affected host selection and oviposition of psyllids. Given that formic acid in vapor phase disappeared quickly in our exposure chambers, it is also possible that it settled onto citrus leaf surfaces, affecting exposed plants both in vapor phase and during contact after settling. Such contact effects may have occurred in the absence of visible wounding to the plants.
In addition to acting as chemical cues for host finding by herbivores, plant volatiles also recruit natural enemies (Pare and Tumlinson, 1999). Induced plant defenses also include innate immunity triggered by the perception of insect elicitors (Pare et al., 2005), formation of physical barriers such as cell wall lignification (Hanley et al., 2007), production of defensive proteins and secondary metabolites, and phytohormone-mediated defense such as by salicylic and jasmonic acids. Using RT-qPCR, we assessed the expression of citrus defense-associated genes to investigate the effect of formic acid volatiles on citrus defense. Significant up-regulations of WRKY22, GST1, RAR1, EDS1, NPR2, NPR3, and PAL1 were observed within the first 6 h of treatment (Figure 5). This time course was consistent with the rapid increase of volatile release (Figures 1A,B), suggesting a possible causal relationship between these enhanced gene expressions and chemical changes induced by formic acid. Among these genes, WRKY22 and GST1 were established as defense marker genes inducible by plant pathogens or derived elicitors (Asai et al., 2002). Involvement of RAR1 was initially identified in ETI-mediated resistance to powdery mildew in barley (Torp and Jorgensen, 1986) and then found to have roles in development, basal and systemic acquired resistance (SAR) (Fu et al., 2009). Similar to RAR1, EDS1 is involved in various types of plant resistance responses (Parker et al., 1996; Yun et al., 2003; Navarro et al., 2004). NPR2 and NPR3, homologs of Arabidopsis NPR3 and NPR4, respectively, are receptors of salicylic acid, which is essential for plant activation of SAR and ETI (Fu et al., 2012). PAL1 encodes an enzyme for the production of trans-cinnamic acid, a key precursor for biosynthesis of salicylic acid, lignin (cell wall-based defense), and antimicrobial metabolites (Bate et al., 1994; MauchMani and Slusarenko, 1996; Mishina and Zeier, 2007). In the current investigation, increased expression of these genes suggests exposure of citrus to formic acid caused a rapid initiation of defenses similar to those induced by phytopathogens and influenced the outcome of citrus-psyllid interactions (Figure 4 and Table 1). Several hours after formic acid exposure, downregulation of ICS1, COI1, and JAR1 (21 h after the treatment) was observed (Figure 5). ICS1 is another enzyme catalyzing a different pathway of salicylic acid synthesis (Strawn et al., 2007). COI1 and JAR1 are jasmonic acid defense pathway genes involved in the signal perception (receptor) (Yan et al., 2009) and activation (Staswick and Tiryaki, 2004), respectively. The downregulation of these genes may be a later effect of formic acid exposure in citrus where pathway modifications contributed to the influence on psyllid feeding behavior.
The feeding behavior of adult D. citri measured by electrical penetration graph recordings on plants previously exposed to vapor phase formic acid differed from that of D. citri on unexposed plants. Significant reductions of the number of phloem ingestion bouts, mean duration of individual bouts and total duration of phloem ingestion were observed on formic acid-exposed leaves compared to control leaves (Figure 4 and Table 1). We observed an association between upregulation of citrus defense-associated genes and reduced phloem ingestion, suggesting that defenses activated in citrus by formic acid may have diminished psyllid feeding. The citrus genes selected for analysis were mostly related to defense signaling, and hence may not encode protein products that specifically differentiate between types of psyllid-feeding activities. It is likely that certain secondary metabolic pathways or downstream protein products regulated by these defense-signaling events were activated upon exposure to formic acid and contributed to reduced feeding. Phloem tissue consists of living conductive cells equipped to activate defense mechanisms such as induction of defensive proteins and secondary metabolites that may mediate psyllid feeding. It is also possible that formic acid induced a systemic wound signaling effect in citrus plants that elevated metabolic activity associated with plant defenses mediating phloem feeding by psyllids as these metabolites and signaling molecules are transported through the phloem (Ruiz-Medrano et al., 2001; Zhao et al., 2005). It is interesting to speculate how plant cells perceive formic acid molecules and activate self-protection against insects: is there a receptor protein specifically binding formic acid that turns on signal transduction events? Or does formic acid diffuse into plant cells and serve as a substrate for defense pathways? These hypotheses are worthy of further investigation. Moreover, in a related investigation, we have observed that treatment of citrus leaves with chitin (a plant defense elicitor derived from the insect cuticle) induced the expression of citrus defense-associated genes in a highly similar manner to formic acid exposure, and resulted in greatly reduced psyllid phloem and xylem feeding durations (unpublished data). These results further support the hypothesis that host defense affected the observed changes in psyllid feeding behavior. If so, the citrus-related defense genes investigated here could serve as transcriptional markers for induction of psyllid resistance in citrus.
Also, the relationship between psyllids and formic acid may have additional ecological significance. It is interesting to speculate with regard to the role of formic acid in the context of possible mutualism between formicine ants and phloem-feeding hemipterans, such as D. citri. Several studies have reported mutualistic behavior between formicines and hemipterans, through which ants benefit by feeding on high quality honeydew while the hemipterans receive protection from parasitoids and predators (Tena et al., 2013). The metapleural (unique to ants) and other glands of many ant species are highly acidic due in large part to formic and other carboxylic acids (Hermann and Blum, 1981; Yek and Mueller, 2011). Ant gland secretions range from weakly bacteriostatic to strongly bactericidal and have been implicated in defense and, in the case of Attines (fungus farming ants), maintenance of the fungal garden (Bot et al., 2002). Formicinae produce formic acid in their venom glands (Dufour glands) deployed as a spray from their acidopores when threatened. This defense serves to ward off predators and parasitoids from ants or their mutualistic partners such as aphids, scale insects and psyllids. The spray or dry deposition of formic acid on leaf surfaces may help to keep predators away and function to alert psyllids to the presence of ants. This could possibly explain why psyllid antennae detect formic acid as a host cue or one related to protection from predators and parasitoids. Deposition of formic acid by ants could conceivably serve to orient psyllids to hosts or portions thereof occupied by ants. Given the ability of formic acid to modify both the behavior of D. citri and the profile of volatile organic compounds emitted by citrus leaves, it is logical to investigate the role of formic acid in the tritrophic interaction between the plant, insect parasite and ants as mutualists, a topic we hope to pursue in future studies.
We used behavioral, chemo-ecological, physiological and molecular techniques to investigate the role of oxidative breakdown products of host volatiles on host-vector interactions involving a major plant disease vector. We have shown how exposure of citrus plants to formic acid can activate citrus metabolic pathways resulting in a modified volatile profile emitted by the plant. This induced volatile release in turn modified the host-finding behavior and patterns of vascular content ingestion by the Asian citrus psyllid, vector of the single most devastating citrus disease worldwide. Formic acid exposure rendered the resistant P. trifoliata acceptable for feeding and oviposition. Our RT-qPCR studies described transcriptional changes in genes associated with plant defense pathways although many other concomitant metabolic pathways may have also been affected. This induction of susceptibility and release of volatiles supports a hypothesis previously presented by our group that the absence of volatile cues may confer resistance to colonization of P. trifoliata by D. citri compared with susceptible citrus genotypes (Robbins et al., 2012; George and Lapointe, 2019). Formic acid is a highly reactive and ubiquitous component of biological systems. Our work is a start toward understanding how this small molecule and citrus volatiles in general are capable of modulating the relationship between a plant and an important phytopathogen vector.
Data Availability
All datasets generated for this study are included in the manuscript and the supplementary files.
Author Contributions
JG, QS, SL, and LS conceived and designed the experiments. JG and QS performed the experiments. JG, QS, and SL analyzed the data. JG, QS, SL, LS, and ES wrote the paper. All authors commented on and approved the final version of the manuscript.
Conflict of Interest Statement
The authors declare that the research was conducted in the absence of any commercial or financial relationships that could be construed as a potential conflict of interest.
The reviewer, JP, declared a shared affiliation, with no collaboration, with several of the authors, JG, QS, ES, and SL, to the handling editor at the time of review.
Acknowledgments
We acknowledge Dr. Rocco Alessandro for performing the SPME and GC-MS analysis of headspace volatiles. In addition, we acknowledge Larry Markle, Itze Cabral, James Fralix, Anna Sara Hill (USDA-ARS, Fort Pierce) for their contributions and technical support to this research project. This article reports the results of research only. Mention of a trademark or proprietary product is solely for the purpose of providing specific information and does not constitute a guarantee or warranty of the product by the U.S. Department of Agriculture and does not imply its approval to the exclusion of other products that may also be suitable. USDA is an equal opportunity provider and employer. The research was partially funded by a grant (#853) from the Citrus Research & Development Foundation.
References
Alméras, E., Stolz, S., Vollenweider, S., Reymond, P., Mene-Saffrane, L., and Farmer, E. E. (2003). Reactive electrophile species activate defense gene expression in Arabidopsis. Plant J. 34, 205–216. doi: 10.1046/j.1365-313X.2003.01718.x
Asai, T., Tena, G., Plotnikova, J., Willmann, M. R., Chiu, W. L., Gomez-Gomez, L., et al. (2002). MAP kinase signalling cascade in Arabidopsis innate immunity. Nature 415, 977–983. doi: 10.1038/415977a
Attaran, E., Zeier, T. E., Griebel, T., and Zeier, J. (2009). Methyl salicylate production and jasmonate signaling are not essential for systemic acquired resistance in Arabidopsis. Plant Cell 21, 954–971. doi: 10.1105/tpc.108.063164
Bate, N. J., Orr, J., Ni, W., Meromi, A., Nadler-Hassar, T., Doerner, P. W., et al. (1994). Quantitative relationship between phenylalanine ammonia-lyase levels and phenylpropanoid accumulation in transgenic tobacco identifies a rate-determining step in natural product synthesis. Proc. Natl Acad. Sci. U.S.A. 91, 7608–7612. doi: 10.1073/pnas.91.16.7608
Bergström, R., Hallquist, M., Simpson, D., Wildt, J., and Mentel, T. F. (2014). Biotic stress: A significant contributor to organic aerosol in Europe? Atmos. Chem. Phys. 14, 603–647. doi: 10.5194/acp-14-13643-2014
Bishop, P. D., Makus, D. J., Pearce, G., and Ryan, C. A. (1981). Proteinase inhibitor-inducing factor activity in tomato leaves resides in oligosaccharides enzymatically released from cell walls. Proc. Natl Acad. Sci. U.S.A. 78, 3536–3540. doi: 10.1073/pnas.78.6.3536
Bonani, J. P., Fereres, A., Garzo, E., Miranda, M. P., Appezzato-Da-Gloria, B., and Lopes, J. R. S. (2010). Characterization of electrical penetration graphs of the Asian citrus psyllid, Diaphorina citri, in sweet orange seedlings. Entomol. Exp. Appl. 134, 35–49. doi: 10.1111/j.1570-7458.2009.00937.x
Bot, A. N. M., Ortius-Lechner, D., Finster, K., Maile, R., and Boomsma, J. J. (2002). Variable sensitivity of fungi and bacteria to compounds produced by the metapleural glands of leaf-cutting ants. Insectes Sociaux. 49, 363–370. doi: 10.1007/PL00012660
Cen, Y. J., Ye, J. M., Xu, C. B., and Feng, A. W. (2005). The taxis of Diaphorina citri to the volatile oils extracted from non-host plants. J. S. China Agric. Univ. 26, 41–44. Available online at: http://europepmc.org/abstract/CBA/612109
Cossins, E. A., and Sinha, S. K. (1965). The utilization of carbon-1 compounds by plants. II. the formation and metabolism of formate by higher plant tissues. Can. J. Biochem. 43, 685–698. doi: 10.1139/o65-079
Dean, J. V., and Delaney, S. P. (2008). Metabolism of salicylic acid in wild type, ugt74f1 and ugt74f2 glucosyltransferase mutants of Arabidopsis thaliana. Physiol. Plant. 132, 417–425. doi: 10.1111/j.1399-3054.2007.01041.x
Díaz, M., Achkor, H., Titarenko, E., and Martínez, M. C. (2003). The gene encoding glutathione-dependent formaldehyde dehydrogenase/GSNO reductase is responsive to wounding, jasmonic acid and salicylic acid. FEBS Lett. 543, 136–139. doi: 10.1016/S0014-5793(03)00426-5
Doke, N., Miura, Y., Chai, H. B., and Kawakita, K. (1991). “Involvement of active oxygen in induction of plant defense response against infection and injury,” in Current topics in plant physiology, Vol. 6, eds E. Pell and K. Stephen (Rockville, MD: American Society of Plant Physiologists), 84–96.
Erb, M., Veyrat, N., Robert, A. M. C., Xu, H., Frey, M., Ton, J., et al. (2015). Indole is an essential herbivore-induced volatile priming signal in maize. Nat. Commun. 6:6273. doi: 10.1038/ncomms7273
Fahlbusch, K. G., Hammerschmidt, F. J., Panten, J., PickenHagen, W., Schatkowski, D., Bauer, K., et al. (2003). Flavors and Fragrances. Ullmann's Encyclopedia of Industrial Chemistry. Weinheim: John Wiley and Sons.
Fall, R., and Benson, A. A. (1996). Leaf methanol–the simplest natural product from plants. Trends Plant Sci. 9, 296–301. doi: 10.1016/S1360-1385(96)88175-0
Fu, D. Q., Ghabrial, S., and Kachroo, A. (2009). GmRAR1 and GmSGT1 are required for Basal, R gene-mediated and systemic acquired resistance in soybean. Mol. Plant-Microbe Interact. 22, 86–95. doi: 10.1094/MPMI-22-1-0086
Fu, Z. Q., Yan, S. P., Saleh, A., Wang, W., Ruble, J., Oka, N., et al. (2012). NPR3 and NPR4 are receptors for the immune signal salicylic acid in plants. Nature 486, 228–232. doi: 10.1038/nature11162
Garzo, E., Bonani, J. P., Lopes, J. S., and Fereres, A. (2012). Morphological description of the mouthparts of the Asian citrus psyllid, Diaphorina citri Kuwayama (Hemiptera: Psyllidae). Arthropod. Struct. Dev. 41, 79–86. doi: 10.1016/j.asd.2011.07.005
George, J., Ammar, E., Hall, D. G., Shatters, R. G., and Lapointe, S. L. (2018). Prolonged phloem ingestion by Diaphorina citri nymphs compared to adults is correlated with increased acquisition of citrus greening pathogen. Sci. Rep. 8:10352. doi: 10.1038/s41598-018-28442-6
George, J., Ammar, E.-D., Hall, D. G., and Lapointe, S. L. (2017). Sclerenchymatous ring as a barrier to phloem feeding by Asian citrus psyllid: Evidence from electrical penetration graph and visualization of stylet pathways. PLoS ONE 12:e0173520. doi: 10.1371/journal.pone.0173520
George, J., and Lapointe, S. L. (2019). Host plant resistance associated with Poncirus trifoliata influences the oviposition, larval development and adult emergence of Asian citrus psyllids, Diaphorina citri. Pest Manag. Sci. 75, 279–285. doi: 10.1002/ps.5113
George, J., Robbins, P. S., Alessandro, R. T., Stelinski, L. L., and Lapointe, S. L. (2016). Formic and acetic acids in degradation products of plant volatiles elicit olfactory and behavioral responses from an insect vector. Chem. Senses 41, 325–338. doi: 10.1093/chemse/bjw005
Giese, M., Bauer-Doranth, U., Langebartels, C., and Sandermann, H. Jr. (1994). Detoxification of formaldehyde by the spider plant (Chlorophytum comosum L.) and by soybean (Glycine max L.) cell-suspension cultures. Plant Physiol. 104, 1301–1309. doi: 10.1104/pp.104.4.1301
Gottwald, T. R., Graca, J. V., and Bassanezi, R. B. (2007). Citrus huanglongbing: the pathogen, its epidemiology and impact. Plant Health Prog. doi: 10.1094/PHP-2007-0906-01-RV
Griebel, T., and Zeier, J. (2010). A role for beta-sitosterol to stigmasterol conversion in plant-pathogen interactions. Plant J. 63, 254–268. doi: 10.1111/j.1365-313X.2010.04235.x
Hall, D. G., George, J., and Lapointe, S. L. (2015). Further investigations on colonization of Poncirus trifoliata by the Asian citrus psyllid. Crop Prot. 72, 112–118. doi: 10.1016/j.cropro.2015.03.010
Hanley, M. E., Lamont, B. B., Fairbanks, M. M., and Rafferty, C. M. (2007). Plant structural traits and their role in anti-herbivore defence. Perspect. Plant Ecol. 8, 157–178. doi: 10.1016/j.ppees.2007.01.001
Hermann, H. R., and Blum, M. S. (1981). Defensive Mechanisms in the Social Hymenoptera, Social Insects, Vol 2, New York, NY: Academic Press, 77–197.
Hiatt, A. J. (1965). Formic acid activation in plants. I. Purification, properties and distribution of formyltetrahydrofolate synthetase. Plant Physiol. 40, 184–188. doi: 10.1104/pp.40.1.184
Hijaz, F., El-Shesheny, I., and Killiny, N. (2013). Herbivory by the insect Diaphorina citri induces greater change in citrus plant volatile profile than does infection by the bacterium, Candidatus Liberibacter asiaticus. Plant Signal. Behav. 8:e25677. doi: 10.4161/psb.25677
Howe, G. A., and Jander, G. (2008). Plant immunity to insect herbivores. Ann. Rev. Plant Biol. 59:41–66. doi: 10.1146/annurev.arplant.59.032607.092825
Huve, K., Christ, M. M., Kleist, E., Uerlings, R., Niinemets, U., Walter, A., et al. (2007). Simultaneous growth and emission measurements demonstrate an interactive control of methanol release by leaf expansion and stomata. J. Exp. Bot. 58, 1783–1793. doi: 10.1093/jxb/erm038
Jud, W., Vanzo, E., Li, Z., Ghirardo, A., Zimmer, I., Sharkey, T. D., et al. (2016). Effects of heat and drought stress on post-illumination bursts of volatile organic compounds in isoprene-emitting and non-emitting poplar. Plant. Cell. Environ. 39, 1204–1215. doi: 10.1111/pce.12643
Karl, T., Harley, P., Emmons, L., Thornton, B., Guenther, A., Basu, C., et al. (2010). Efficient atmospheric cleansing of oxidized organic trace gases by vegetation. Science 330, 816–819. doi: 10.1126/science.1192534
Krall, A. R., and Tolbert, N. E. (1957). A comparison of the light dependent metabolism of carbon monoxide by barley leaves with that of formaldehyde, formate and carbon dioxide. Plant Physiol. 32, 321–326. doi: 10.1104/pp.32.4.321
Lapointe, S. L., George, J., and Hall, D. G. (2016). A phagostimulant blend for the Asian citrus psyllid. J. Chem. Ecol. 42, 941–951. doi: 10.1007/s10886-016-0745-4
Lapointe, S. L., Shapiro, J. P., and Bowman, K. D. (1999). Identification of sources of plant resistance to Diaprepes abbreviatus (Coleoptera: Curculionidae) by three bioassays. J. Econ. Entomol. 92, 999–1004. doi: 10.1093/jee/92.4.999
Leon, J., Rojo, E., and Sanchez-Serrano, J. J. (2001). Wound signaling in plants. J. Exp. Bot. 52, 1–9. doi: 10.1093/jexbot/52.354.1
Li, T., Blande, J. D., and Holopainen, J. K. (2016). Atmospheric transformation of plant volatiles disrupts host plant finding. Sci. Rep. 6:33851. doi: 10.1038/srep33851
Li, W. B., Hartung, J. S., and Levy, L. (2006). Quantitative real-time PCR for detection and identification of Candidatus Liberibacter species associated with citrus huanglongbing. J. Microbiol. Methods 66, 104–115. doi: 10.1016/j.mimet.2005.10.018
Liu, L., Hausladen, A., Zeng, M., Que, L., Heitman, J., and Stamler, J. S. (2001). A metabolic enzyme for S-nitrosothiol conserved from bacteria to humans. Nature 410, 490–494. doi: 10.1038/35068596
Luo, X., Yen, A. L., Powell, K. S., Wu, F., Wang, Y., and Zeng, L. (2015). Feeding behavior of Diaphorina citri (Hemiptera:Liviidae) and its acquisition of Candidatus Liberibacter asiaticus, on huanglongbing-infected Citrus reticulata leaves of several maturity stages. Fla. Entomol. 98, 186–192. doi: 10.1653/024.098.0132
Maffei, M. E., Mithöfer, A., and Boland, W. (2007). Before gene expression: early events in plant-insect interaction. Trends Plant Sci. 12, 310–316. doi: 10.1016/j.tplants.2007.06.001
Mafra, V., Kubo, K. S., Alves-Ferreira, M., Ribeiro-Alves, M., Stuart, R. M., Boava, L. P., et al. (2012). Reference genes for accurate transcript normalization in citrus genotypes under different experimental conditions. PLoS ONE 7:e31263. doi: 10.1371/journal.pone.0031263
Mann, R. S., Ali, J. G., Hermann, S. L., Tiwari, S., Pelz-Stelinski, K. S., Alborn, H. T., et al. (2012). Induced release of a plant-defense volatile 'deceptively' attracts insect vectors to plants infected with a bacterial pathogen. PLoS Pathog. 8:e1002610. doi: 10.1371/journal.ppat.1002610
MauchMani, B., and Slusarenko, A. J. (1996). Production of salicylic acid precursors is a major function of phenylalanine ammonia-lyase in the resistance of arabidopsis to Peronospora parasitica. Plant Cell 8, 203–212. doi: 10.1105/tpc.8.2.203
Mauck, K., De Moraes, C., and Mescher, M. (2010). Deceptive chemical signals induced by a plant virus attracts insect vectors to inferior hosts. Proc. Natl. Acad. Sci. U.S.A. 107, 3600–3605. doi: 10.1073/pnas.0907191107
Mayer, C. J., Vilcinskas, A., and Gross, J. (2008a). Phytopathogen lures its insect vector by altering host plant odor. J. Chem. Ecol. 34, 1045–1049. doi: 10.1007/s10886-008-9516-1
Mayer, C. J., Vilcinskas, A., and Gross, J. (2008b). Pathogen-induced release of plant allomone manipulates vector insect behavior. J. Chem. Ecol. 34, 1518–1522. doi: 10.1007/s10886-008-9564-6
McCallum, E., Cunningham, J. G., Lucker, J., Zalucki, M. P., De Voss, J. J., and Botella, J. R. (2011). Increased plant volatile production affects oviposition, but not larval development, in the moth Helicoverpa Armigera. J. Exp. Biol. 214, 3672–3677. doi: 10.1242/jeb.059923
Mishina, T. E., and Zeier, J. (2007). Pathogen-associated molecular pattern recognition rather than development of tissue necrosis contributes to bacterial induction of systemic acquired resistance in Arabidopsis. Plant J. 50, 500–513. doi: 10.1111/j.1365-313X.2007.03067.x
Moran, V. C., and Brown, R. P. (1973). The antennae, host plant chemoreception and probing activity of the citrus psylla, Trioza erytreae (Del Guerico) (Homoptera: Psyllidae). J. Entomol. Soc. S. Africa 36, 191–202.
Mumm, R., Posthumus, M. A., and Dicke, M. (2008). Significance of terpenoids in induced indirect plant defence against herbivorous arthropods. Plant Cell. Environ. 31, 575–585. doi: 10.1111/j.1365-3040.2008.01783.x
Navarro, L., Zipfel, C., Rowland, O., Keller, I., Robatzek, S., Boller, T., et al. (2004). The transcriptional innate immune response to flg22 interplay and overlap with Avr gene-dependent defense responses and bacterial pathogenesis. Plant Physiol. 135, 1113–1128. doi: 10.1104/pp.103.036749
Nehlin, G., Valterova, I., and Borg-Karlson, A. K. (1994). Use of conifer volatiles to reduce injury caused by carrot psyllid, Trioza apicalis, Förster (Homoptera: Psylloidea). J. Chem. Ecol. 20, 771–783. doi: 10.1007/BF02059612
Nissinen, A., Kristoffersen, L., and Anderbrant, O. (2008). Physiological state of female and light intensity affect the host-plant selection of carrrot psyllid, Trioza apicalis (Hemiptera: Triozidae). Eur. J. Entomol. 105, 227–232. doi: 10.14411/eje.2008.032
Oikawa, P. Y., and Lerdau, M. T. (2013). Catabolism of volatile organic compounds influences plant survival. Trends Plant Sci. 18, 695–703. doi: 10.1016/j.tplants.2013.08.011
Onagbola, E. O., Rouseff, R. L., Smoot, J. M., and Stelinski, L. L. (2011). Guava leaf volatiles and dimethyl disulphide inhibit response of Diaphorina citri Kuwayama to host plant volatiles. J. Appl. Entomol. 135, 404–414. doi: 10.1111/j.1439-0418.2010.01565.x
Orozco-Cardenas, M., and Ryan, C. A. (1999). Hydrogen peroxideis generated sytemically in plant leaves by wounding and systemin via the octaddecanoid pathway. Proc. Natl Acad. Sci. U.S.A. 96, 6553–6557. doi: 10.1073/pnas.96.11.6553
Pare, P. W., Farag, M. A., Krishnamachari, V., Zhang, H. M., Ryu, C. M., and Kloepper, J. W. (2005). Elicitors and priming agents initiate plant defense responses. Photosynth. Res. 85, 149–159. doi: 10.1007/s11120-005-1001-x
Pare, P. W., and Tumlinson, J. H. (1999). Plant volatiles as a defense against insect herbivores. Plant Physiol. 121, 325–332. doi: 10.1104/pp.121.2.325
Paris, T. M., Allan, S. A., Udell, B. J., and Stansly, P. A. (2017). Evidence of behavior-based utilization by the Asian citrus psyllid of a combination of UV and green or yellow wavelengths. PLoS ONE 12:e0189228. doi: 10.1371/journal
Park, J. H., Goldstein, A. H., Timkovsky, J., Fares, S., Weber, R., Karlik, J., et al. (2013). Active atmosphere-ecosystem exchange of the vast majority of detected volatile organic compounds. Science 341, 643–647. doi: 10.1126/science.1235053
Parker, J. E., Holub, E. B., Frost, L. N., Falk, A., Gunn, N. D., and Daniels, M. J. (1996). Characterization of eds1, a mutation in Arabidopsis suppressing resistance to Peronospora parasitica specified by several different RPP genes. Plant Cell 8, 2033–2046. doi: 10.1105/tpc.8.11.2033
Patt, J. M., Meikle, W. M., Mafra-neto, A., Setamou, M., Mangan, R., Yang, C., et al. (2011). Multimodal cues drive host-plant assessment in Asian citrus psyllid (Diaphorina citri). Environ. Entomol. 40, 1494–1502. doi: 10.1603/EN11149
Patt, J. M., Robbins, P. S., Niedz, R., McCollum, G., and Alessandro, R. (2018). Exogenous application of the plant signalers methyl jasmonate and salicylic acid induces changes in volatile emissions from citrus foliage and influences the aggregation behavior of Asian citrus psyllid (Diaphorina citri), vector of Huanglongbing. PLoS ONE 13:e0193724. doi: 10.1371/journal.pone.0193724
Patt, J. M., and Setamou, M. (2010). Responses of the Asian citrus psyllid to volatiles emitted by the flushing shoots of its rutaceous host plants. Environ. Entomol. 39, 618–624. doi: 10.1603/EN09216
Pearce, G., Strydom, D., Johnson, S., and Ryan, C. A. (1991). A polypeptide from tomato leaves induces wound-inducible proteinase inhibitor proteins. Science 253, 895–898. doi: 10.1126/science.253.5022.895
Poerwanto, M. E., Trisyono, Y. A., Beattie, G. A. C., Subandiyah, S., Martono, E., and Holford, P. (2012). Olfactory responses of the Asiatic citrus psyllid (Diaphorina citri) to mineral oil-treated mandarin leaves. Am. J. Agri. Biol. Sci. 7, 50–55. doi: 10.3844/ajabssp.2012.50.55
Richardson, M. L., and Hall, D. G. (2013). Resistance of Poncirus and Citrus x Poncirus germplasm to the Asian citrus psyllid. Crop Sci. 53, 183–188. doi: 10.2135/cropsci2012.02.0091
Robbins, P. S., Alessandro, R. T., Stelinski, L. L., and Lapointe, S. L. (2012). Volatile profiles of young leaves of Rutaceae spp. varying in susceptibility to the Asian citrus psyllid (Hemiptera: Psyllidae). Fla. Entomol. 95, 774–776. doi: 10.1653/024.095.0331
Rodriguez-Saona, C., Crafts-Brandner, S. J., Par,é, P. W., and Henneberry, T. J. (2001). Exogenous methyl jasmonate induces volatile emissions in cotton plants. J. Chem. Ecol. 27, 679–695. doi: 10.1023/A:1010393700918
Rouseff, R. L., Onagbola, E. O., Smoot, J. M., and Stelinski, L. L. (2008). Sulfur volatiles in guava (Psidium guajava L.) leaves: possible defense mechanism. J. Agri. Food Chem. 56, 8905–8910. doi: 10.1021/jf801735v
Ruiz-Medrano, R., Xoconostle-Cazares, B., and Lucas, W. J. (2001). The phloem as a conduit for inter-organ communication. Curr. Opin. Plant Biol. 4, 202–209. doi: 10.1016/S1369-5266(00)00162-X
Savatin, D. V., Gramegna, G., Modesti, V., and Cervone, F. (2014). Wounding in the plant tissue: the defense of a dangerous passage. Front. Plant Sci. 5:e470. doi: 10.3389/fpls.2014.00470
Schaller, F. (2001). Enzymes of the biosynthesis of octadecanoid-derived signalling molecules. J. Exp. Bot. 52, 11–23.
Schilmiller, L. A., and Howe, G. A. (2005). Systemic signaling in the wound response. Curr. Opin. Plant Biol. 8, 369–377. doi: 10.1016/j.pbi.2005.05.008
Shi, Q., Febres, V. J., Jones, J. B., and Moore, G. A. (2015). Responsiveness of different citrus genotypes to the Xanthomonas citri ssp citri-derived pathogen-associated molecular pattern (PAMP) flg22 correlates with resistance to citrus canker. Mol. Plant Pathol. 16, 507–520. doi: 10.1111/mpp.12206
Skelley, L. H., and Hoy, M. A. (2004). A synchronous rearing method for the Asian citrus psyllid and its parasitoids in quarantine. Biol. Control 29, 14–23. doi: 10.1016/S1049-9644(03)00129-4
Soroker, V., Talebaev, S., Hararai, A. R., and Wesley, S. D. (2004). The role of chemical cues in host and mate location in the pear psylla Cacopsylla bidens (Homoptera: Psyllidae). J. Insect Behav. 17, 613–626. doi: 10.1023/B:JOIR.0000042544.35561.1c
Staswick, P. E., and Tiryaki, I. (2004). The oxylipin signal jasmonic acid is activated by an enzyme that conjugates it to isoleucine in Arabidopsis. Plant Cell 16, 2117–2127. doi: 10.1105/tpc.104.023549
Strawn, M. A., Marr, S. K., Inoue, K., Inada, N., Zubieta, C., and Wildermuth, M. C. (2007). Arabidopsis isochorismate synthase functional in pathogen-induced salicylate biosynthesis exhibits properties consistent with a role in diverse stress responses. J. Biol. Chem. 282, 5919–5933. doi: 10.1074/jbc.M605193200
Sule, H., Rita, M., Omar, D., and Kah-Wei, A. H. (2012). Response of Diaphorina citri Kuwayama (Hemiptera: Psyllidae) to volatiles emitted from leaves of two rutaceous plants. J. Agri. Sci. 4, 152–159. doi: 10.5539/jas.v4n6p152
Taylor, J. E., Hatcher, P. E., and Paul, N. D. (2004). Crosstalk between plant responses to pathogens and herbivores: a view from the outside in. J. Exp. Bot. 55, 159–168. doi: 10.1093/jxb/erh053
Tena, A., Hoddle, C. D., and Hoddle, M. S. (2013). Competition between honeydew producers in an ant–hemipteran interaction may enhance biological control of an invasive pest. Bull. Entomol. Res. 103, 714–723. doi: 10.1017/S000748531300045X
Tjallingii, W., and Esch, T. H. (1993). Fine structure of aphid stylet routes in plant tissues in correlation with EPG signals. Physiol. Entomol. 18, 317–328. doi: 10.1111/j.1365-3032.1993.tb00604.x
Torp, J., and Jorgensen, J. H. (1986). Modification of barley powdery mildew resistance gene Ml-A12 by induced mutation. Can. J. Gene. Cytol. 28, 725–731. doi: 10.1139/g86-102
Wildermuth, M. C., Dewdney, J., Wu, G., and Ausubel, F. M. (2001). Isochorismate synthase is required to synthesize salicylic acid for plant defense. Nature 414, 562–565. doi: 10.1038/35107108
Yan, J. B., Zhang, C., Gu, M., Bai, Z. Y., Zhang, W. G., Qi, T. C., et al. (2009). The Arabidopsis Coronatine Insensitive1 protein is a jasmonate receptor. Plant Cell 21, 2220–2236. doi: 10.1105/tpc.109.065730
Yek, S. H., and Mueller, U. G. (2011). The metapleural gland of ants. Biol. Rev. 86, 774–791. doi: 10.1111/j.1469-185X.2010.00170.x
Yun, B. W., Atkinson, H. A., Gaborit, C., Greenland, A., Read, N. D., Pallas, J. A., et al. (2003). Loss of actin cytoskeletal function and EDS1 activity, in combination, severely compromises non-host resistance in Arabidopsis against wheat powdery mildew. Plant J. 34, 768–777. doi: 10.1046/j.1365-313X.2003.01773.x
Zhang, Y. L., Cheng, Y. T., Qu, N., Zhao, Q. G., Bi, D. L., and Li, X. (2006). Negative regulation of defense responses in Arabidopsis by two NPR1 paralogs. Plant J. 48, 647–656. doi: 10.1111/j.1365-313X.2006.02903.x
Keywords: citrus, Diaphorina citri, electrical penetration graph, formic acid, induced volatiles, Poncirus trifoliata, host plant resistance, Asian citrus psyllid
Citation: George J, Shi Q, Stelinski LL, Stover E and Lapointe SL (2019) Host Selection, Oviposition and Feeding by a Phytopathogen Vector, Diaphorina citri (Hemiptera: Liviidae), Modulated by Plant Exposure to Formic Acid. Front. Ecol. Evol. 7:78. doi: 10.3389/fevo.2019.00078
Received: 19 November 2018; Accepted: 04 March 2019;
Published: 29 March 2019.
Edited by:
Claude Becker, Gregor Mendel Institute of Molecular Plant Biology (GMI), AustriaReviewed by:
Xinnian Zeng, South China Agricultural University, ChinaJoseph Patt, United States Department of Agriculture, United States
Copyright © 2019 George, Shi, Stelinski, Stover and Lapointe. This is an open-access article distributed under the terms of the Creative Commons Attribution License (CC BY). The use, distribution or reproduction in other forums is permitted, provided the original author(s) and the copyright owner(s) are credited and that the original publication in this journal is cited, in accordance with accepted academic practice. No use, distribution or reproduction is permitted which does not comply with these terms.
*Correspondence: Justin George, Z2VvcmdlanVzdGluZUBnbWFpbC5jb20=