- 1Department of Primatology, Max Planck Institute for Evolutionary Anthropology, Leipzig, Germany
- 2Taï Chimpanzee Project, Centre Suisse de Recherches Scientifiques, Abidjan, Côte d'Ivoire
High dominance status is associated with fitness benefits in many social mammals. Yet, attaining and maintaining a high social status often comes with elevated energetic costs. Dominance rank-related exposure to energetic and psychosocial stressors is predicted to vary depending on the type of breeding system, the means a high rank is acquired and maintained, and the stability of the dominance hierarchy. Using behavioral data and urinary cortisol levels, we investigated whether a high dominance rank is associated with elevated energetic costs in Taï male chimpanzees and whether the relationship between male dominance rank and cortisol levels varies between stable and unstable dominance periods. Additionally, we investigated potential sources of energetic and psychosocial stress linked to competition over dominance status and mating opportunities. We found that higher-ranking males gave more aggressions than lower-ranking males in stable and unstable dominance periods, but that dominance rank and urinary cortisol levels were not associated in either period. Urinary cortisol levels were higher in all males in unstable compared to stable dominance periods, whereas aggression rates showed the reversed pattern, with higher rates in stable periods. Our results indicate that dominance maintenance is not associated with elevated physiological stress for dominant Taï male chimpanzees, and that social instability exposed all males to psychosocial stress, despite lower rates of aggressive interactions. Overall, these findings suggest that male chimpanzees adjust competitive behavior to context dependent conditions, possibly by means of predictability of outcomes of social interactions, and use conflict management strategies, such as avoidance of aggression potentially diminishing the risk of escalation.
Introduction
High dominance status is associated with fitness benefits for males and females in many animal societies (Clutton-Brock, 1988; Lloyd and Rasa, 1989; Cowlishaw and Dunbar, 1991; Pusey, 1997; van Noordwijk and van Schaik, 1999; King and Allainé, 2002; Boesch et al., 2006; Natoli et al., 2007; Rodriguez-Llanes et al., 2009; Majolo et al., 2012). Dominance status has also been associated with health and longevity (Sapolsky, 2005; Blomquist et al., 2011; Archie et al., 2012; Marmot and Sapolsky, 2014; Lewin et al., 2015). For example, dominance status predicted faster wound healing in male baboons (Archie et al., 2012), survival and stronger immune defenses in female spotted hyenas (Watts et al., 2009; Flies et al., 2016), and high socioeconomic status has been related to increased health and longevity in humans (Marmot, 2004). Dominance rank dependent differences in health and survival have to some extent been associated with rank-related differences in exposure to unpredictable and uncontrollable stressors, that when persisting over an extended time might have negative health implications (Sapolsky, 2005; Cohen et al., 2007; Romero et al., 2009). However, the relationship between social status and the extent of stressor exposure varies across social systems, species and even populations (Creel, 2001; Abbott et al., 2003; Creel et al., 2013). Inconsistent findings across different populations of the same species may relate to differences in the stability of the dominance hierarchy, availability of coping or avoidance strategies, as well as differences in analytical methods (Sapolsky, 1983; Creel, 2001; Ostner et al., 2008; Muehlenbein and Watts, 2010; Gesquiere et al., 2011a). Thus, despite various studies examining rank related differences in stressor exposure, more comprehensive studies are needed to disentangle the relationship between social and energetic challenges associated with social status, as well as behavioral causes and consequences of dominance rank in social animals (Creel et al., 2013).
Hypothalamus-Pituitary-Adrenal (HPA) axis activity varies in relation to internal and external challenges that individuals experience on a daily basis (McEwen and Wingfield, 2003). Amongst other means, HPA axis activity can be assessed through the measurement of glucocorticoid hormones (Beehner and Bergman, 2017). To evaluate status dependent differences in social and environmental stressor exposure, many studies have linked dominance status and subordination to variation in glucocorticoid levels (Creel, 2001; Sapolsky, 2005). For example, glucocorticoid levels were negatively correlated with rank in non-human primate species and spotted hyenas in which subordinates frequently receive aggression from higher-ranking individuals (Sapolsky, 1983, 2005; Abbott et al., 2003; Bergman et al., 2005; Setchell et al., 2010). Thus, in accordance with experimental studies (Weiss, 1970; Creel et al., 2013), elevated glucocorticoid levels in subordinates have been related to low predictability and controllability of the social environment (Sapolsky, 2005). However, a negative association between dominance rank and glucocorticoid levels is not a consistent pattern across differing social and mating systems (Creel, 2001; Abbott et al., 2003). For example, dominant individuals have higher glucocorticoid levels than subordinates in several cooperatively breeding species (Creel, 2001). Since dominance is most often associated with predictability and control, higher glucocorticoid levels in dominant individuals have been related to elevated metabolic rates rather than psychosocial factors (Muller and Wrangham, 2004b; Gesquiere et al., 2011a). Accordingly, higher glucocorticoid levels in dominant compared to subordinate individuals are suggested in animal species in which reproductive success is determined by dominance rank, reproductive suppression is uncommon, and where a high rank is gained and maintained through frequent, overt aggression (Creel et al., 2013). Thus, the relationship between dominance and glucocorticoid levels varies depending on the type of breeding system, and by the means a high rank is acquired and maintained (Abbott et al., 2003; Goymann and Wingfield, 2004; Sapolsky, 2005; Creel et al., 2013).
Dominance hierarchies promote social stability (Holekamp and Strauss, 2016), when dyadic dominance relationships are consistent over extended periods (Isbell and Young, 2002), since established formal dominance relationships can reduce the escalation of conflicts of interest (Preuschoft and van Schaik, 2000). However, the formation of dominance relationships and unstable dominance hierarchies induce social instability and thus, reduce the predictability of the social environment (Holekamp and Strauss, 2016). Especially for high-ranking individuals, exposure to energetic and social stressors is elevated during unstable dominance periods due to increased aggression rates and risk of losing benefits related to a high rank position (e.g., reproductive success, priority of access to feeding resources; Sapolsky, 1983; Bergman et al., 2005; Koyama et al., 2017). The suggested increase in social and energetic stressors during such periods is concomitant with elevated glucocorticoid levels in males of several non-human primate species (Sapolsky, 1983; Bergman et al., 2005; Setchell et al., 2010; Gesquiere et al., 2011a; Higham et al., 2013; Mendonça-Furtado et al., 2014). Studies in wild baboons and mandrills found that during stable periods, dominant males had lower glucocorticoid levels than lower-ranking males but found that this relationship was reversed during unstable periods (Sapolsky, 1983; Bergman et al., 2005; Setchell et al., 2010). Conversely, one study in wild male baboons did not find a reversal in the association between dominance status and glucocorticoid levels, but that lower-ranking males had higher glucocorticoid levels than higher-ranking males regardless of hierarchy stability (Gesquiere et al., 2011a). However, males in alpha position showed higher glucocorticoid levels compared to the beta male position in both stable and unstable periods. The latter finding was suggested to be due to higher rates of aggression and mate guarding behavior in alpha males throughout the differing periods of hierarchy stability (Gesquiere et al., 2011a). Similarly, dominant male bearded capuchin monkeys had consistently higher fecal glucocorticoid levels than subordinates in stable and unstable dominance periods (Mendonça-Furtado et al., 2014). However, in seasonally breading rhesus macaques, dominance rank was not associated with glucocorticoid levels during a stable dominance period nor during the mating season, but high-ranking males had higher glucocorticoid levels than lower-ranking males during an unstable dominance period (Higham et al., 2013). Thus, variation in dominance hierarchy stability is another source that affects dominance status related patterns in glucocorticoid levels, potentially leading to a reversal or appearance of the glucocorticoid–dominance status association.
Studies investigating the association between dominance status and glucocorticoid levels in male chimpanzees revealed opposing results. While one study found that dominant males had higher glucocorticoid levels than lower-ranking males (Muller and Wrangham, 2004b), dominance rank was not correlated with fecal glucocorticoid levels in another study in wild male chimpanzees (Muehlenbein and Watts, 2010), nor with urinary glucocorticoid level in young captive chimpanzees during stable dominance periods (Anestis, 2005). Discrepancies between findings in captive and wild chimpanzees might be due to energetic and psychosocial factors that differ tremendously between wild and captive environments (Creel, 2001; Muller and Wrangham, 2005). However, inconsistent findings between the two studies conducted in the wild remain to be determined. Muller and Wrangham (2004b) proposed that elevated aggression rates might be the driving factor influencing elevated urinary glucocorticoid levels in dominant male chimpanzees, since urinary glucocorticoid levels were negatively correlated with food availability and positively with aggression rates (Muller and Wrangham, 2004b). Highest rates of aggression, however, have consistently been shown for dominant male chimpanzees across populations (Muller, 2002; Muehlenbein and Watts, 2010; Sobolewski et al., 2013). Thus, status related differences in aggression rates are unlikely to explain opposing results of the two previous studies. Muller and Wrangham also proposed an alternative hypothesis, which considers that higher glucocorticoid levels in dominant males might be due to constant instability of male dominance hierarchies, due to the fission-fusion nature of chimpanzee social organization (Muller, 2002; Muller and Wrangham, 2004b). Thus, differing results of the previous studies might be due to differences in social dynamics between the respective study groups, for example differences in fission fusion patterns or male group sizes that might affect predictability of social interactions. Furthermore, differences in the relationship between dominance rank and glucocorticoid levels between populations are suggested to vary dependent on dominance rank stability, access to social support, individual personality (Abbott et al., 2003; Anestis, 2005; Sapolsky, 2005; Muehlenbein and Watts, 2010; Wittig et al., 2016), and differences in analytical methods (Gesquiere et al., 2011a; Behringer and Deschner, 2017). Differences in social support systems and individuals' personalities between populations are difficult to assess. However, whether the association between dominance rank and glucocorticoid levels varies between stable and unstable dominance periods is testable when social stability varies within the same study group.
Capitalizing on the occurrence of several rank changes in two adjacent chimpanzee groups, the overall aim of our study was to investigate whether the relationship between male dominance rank and glucocorticoid levels varies between stable and unstable dominance periods. In addition, we used multivariate analysis to advance the understanding of the sources and extent of energetic and psychosocial stressor exposure linked to dominance rank and competition over social status and mating opportunities in wild male chimpanzees. Accordingly, we examined the association between dominance rank, aggression rates and urinary cortisol levels during periods with varying levels of social stability.
We first tested the hypothesis that a high dominance rank is associated with elevated urinary cortisol levels in male chimpanzees due to elevated energy expenditure and/or constant social instability induced by the fission fusion social organization. We predicted a positive association between dominance rank and urinary cortisol levels in stable dominance periods. Experimental and naturalistic studies revealed that both aggressor and victim have elevated cortisol levels after conflicts (Bronson and Eleftheriou, 1964; Wittig et al., 2015). Thus, we predicted that both aggression rates given and received have a positive effect on urinary cortisol levels. However, if higher cortisol levels in dominant individuals are associated with energy expenditure rather than psychosocial factors, we predicted that aggression rates given have a stronger effect on urinary cortisol levels than aggression rates received and dominance rank alone (Georgiev et al., 2014). Alternatively, if both energy expenditure and psychosocial factors contribute to elevated urinary cortisol levels in dominant males, we predicted that aggression rates given and dominance rank affect urinary cortisol levels independently.
Second, we investigated whether unstable dominance periods are associated with elevated urinary cortisol levels and aggression rates in all males. In line with previous research (Sapolsky, 1983; Bergman et al., 2005; Setchell et al., 2010; Gesquiere et al., 2011a; Higham et al., 2013; Mendonça-Furtado et al., 2014), we predicted higher urinary cortisol levels and greater amounts of aggressions given and received for all males during unstable than during stable dominance periods. In addition, based on findings of previous studies (Sapolsky, 1983; Bergman et al., 2005; Setchell et al., 2010; Higham et al., 2013; Mendonça-Furtado et al., 2014), we predicted that urinary cortisol levels and dominance rank are more strongly associated during unstable than during stable dominance periods.
Third, we investigated whether urinary cortisol levels and aggression rates are elevated when sexually receptive females are present. Competition over mating opportunities is considered to be high in male chimpanzees (Goodall, 1986; Georgiev et al., 2014). We therefore predicted higher urinary cortisol levels and numbers of aggressions given and received, when sexually receptive females are present compared to when absent (Muller and Wrangham, 2004b). Furthermore, reproductive skew has been associated with higher glucocorticoid levels in dominants in non-human primates, due to elevated mating effort by dominant individuals (Fichtel et al., 2007; Setchell et al., 2010). Reproductive success is also highest for dominant male chimpanzees across populations (Surbeck et al., 2017). Thus, we predicted a stronger association between urinary cortisol levels and dominance rank, as well as numbers of aggressions given and dominance rank when sexually receptive females are present compared to when absent.
Materials and Methods
Data Collection and Subjects
We collected data from males from two habituated chimpanzee groups at the Taï National Park, Côte D'Ivoire (5°45′N, 7°07′W) (Wittig, 2018), between October 2013 and May 2014 and September 2014 and May 2015. We conducted all-day focal animal sampling (Altmann, 1974) on all males >10 years (South: N = 5, age = 11–20 years; East: N = 5, age = 11 to circa 37 years) for a total of 1,785 observation hours in East group and 1,654 in South group. During focal follows, we (AP & LS) collected urine samples (see below) and recorded all social interactions and changes in activity continuously, using CyberTracker software (v3.389; http://www.cybertracker.org/). Social interactions were any affiliative or aggressive interaction between two or more individuals, including copulations and vocalizations. Aggressive interactions included directed contact aggressions (attacks; e.g., bites, hits), non-contact aggressions (e.g., chases, charges and displays), threats (e.g., arm waves), and non-directed displays (Nishida et al., 1999; Wittig and Boesch, 2003b). Chases and attacks are considered as high intensity aggressions in chimpanzees (Muller and Wrangham, 2004a), while threats, charges and displays can be classified as low intensity aggressions, however, threats have a lower intensity than charges and displays (Wittig and Boesch, 2003b). Since chimpanzees live in a fission–fusion social system, with fluid changes in subgroup (party) size, composition, and duration (Nishida, 1968), we continuously updated the party composition and size, defined as all adult and sub adult individuals above the age of nine within visual range of the focal individual. All occurrences of hunts, border patrols, and intergroup encounters were recorded ad libitum.
Dominance Ranks and Dominance Rank Changes
We determined dominance ranks for the males of both groups separately, using uni-directional submissive pant grunt vocalizations (Wittig and Boesch, 2003a), by applying a likelihood-based adaptation of the Elo rating approach (Neumann et al., 2011; Foerster et al., 2016), described in detail in (Mielke et al., 2017) (Supplementary Material). We increased the density of our pant grunt data by pooling the pant grunt dataset collected by the Taï Chimpanzee Project's researchers and field assistants. Inter-observer reliability between researchers were >90% and data from field assistants were only included once they had more than 80% overlap with a trained reference observer when collecting data simultaneously (Mielke et al., 2017, 2018). Ordinal ranks were standardized daily between 0 and 1, with 1 being the highest rank.
During the study period, several rank changes occurred in both groups, which at times affected all the males of the study groups. We detected rank changes either through direct observation of escalated aggression between males, resulting in reversals in the pant grunt vocalizations or through the observation of a reversal in the submissive vocalizations, which are cases when the preceding aggression was most likely not observed. Two of the rank changes concerned the alpha male position of the South group, and one of these alpha male changes resulted in an extended period of dominance instability that resulted in rank changes among all males in the group. Altogether, we observed seven rank changes between males in the South group and five between the East group males. The maximum number of rank changes among East group males within a short time period were three rank changes that happened to occur all within a week. However, the alpha male position remained stable in the East group throughout the study period. For each day that a dominance rank change was observed and confirmed post-hoc, we assigned the preceding and the following week as a 2 weeks period of rank instability (rank instability). For the second alpha male change observed in the South group which resulted in an extended period of dominance rank uncertainty among three of the five males, we assigned the period of rank instability starting 1 week before the observed escalated aggression (16th December 2014) until 1 week after all dominance ranks were settled (5th May 2015). Thus, the longest period of rank instability assigned for South group males lasted almost 5 months (Figure S1), while the longest period for East group males lasted only 3 weeks (Figure S2). Overall, we identified three rank instability periods for South group and four for East group. We categorized each period according to the number of rank changes that occurred among the males, creating periods of instability with varying levels of expected intensities. We considered periods with no dominance rank changes as a period of “none” rank instability, a rank change between two males as a “low” rank instability and rank changes between at least three males, or an alpha male change as a period of “high” rank instability. This resulted in three “low” and one “high” rank instability periods for East group males and one “low” and two “high” for South group.
Overall, 1,717 pant grunts were observed between males during the data collection period. During stable dominance periods (“none” and “low” rank instability, an average of 1 pant grunt (median with 0 as first and 2 as third quartile) were observed per focal day between the East group males (N = 473) and an average of 1 pant grunt (median with 0 as first and 3 as third quartile) between the South group males (N = 358). During the extended period of dominance instability in South group the daily pant grunt rate between males dropped to an average of 0 pant grunts (with 0 and 1 as first and third quartiles; see Supplementary Material for further information). In comparison, the average daily pant grunt rate between males was 1 pant grunt (0.5 as first and 3 as third quartile) during the unstable dominance period (“high” rank instability) in the East group.
Female Parity and Sexual Swellings
An additional source of social instability is the presence of sexually receptive females with a high fertilization potential, which in chimpanzees are parous females with fully tumescent sexual swellings (Muller and Wrangham, 2004a; Muller et al., 2006; Emery Thompson and Wrangham, 2008; Sobolewski et al., 2013; Georgiev et al., 2014). Previous studies have shown that the presence of fully tumescent parous females induces male-male competition, but not the presence of fully tumescent nulliparous females (Muller and Wrangham, 2004b; Sobolewski et al., 2013). Nulliparous females are not particularly attractive to males when they are fully tumescent (Muller et al., 2006), due to their low fertilization potential (Emery Thompson and Wrangham, 2008), and males prefer mating with parous and older females than with nulliparous ones (Muller et al., 2006). Thus, to relate changes in urinary cortisol levels and numbers of aggressions given and received to competition over sexually receptive females, we only considered fully tumescent parous but not nulliparous females. In addition, parous Taï females resume cycling after average lactational amenorrhea periods of 21.6–28.8 months (Deschner and Boesch, 2007), which is also the time that has been estimated for the start of the weaning process (Fahy et al., 2014), and the shortest observed interbirth interval in Taï is 3.3 years (Lemoine et al., unpublished data). Thus, we assumed that sexual swellings of females with infants younger than 24 months are most likely not yet part of a regular menstrual cycle and hence, that the presence of such females would not induce male-male competition. We therefore only considered fully tumescent parous females with an infant older than 24 months. Using detailed information on the occurrence of ovulation during female swelling cycles would have been a better approach; however, this data was not available.
To account for the presence of fully tumescent parous females, we created three variables that differ in their expected competition intensities. First, we created a variable that accounted for the presence or absence of fully tumescent parous females on each focal day. This allowed us to account for male-male competition induced by the presence of fully tumescent parous females in general, and made our data comparable to previous chimpanzee studies. Second, the intensity of male-male competition is expected to vary dependent on the overlap of periods with potentially receptive females present. Since monopolization potential is influenced by the number of male competitors and the number of fully tumescent parous females (Boesch et al., 2006; Ostner et al., 2008), the intensity of male-male competition is predicted to be lower when two or more fully tumescent parous females are present (Mitani et al., 1996), and also when they are absent. Thus, we created a second variable that accounted for the number of fully tumescent parous females present on each focal day, to investigate whether competition intensities vary with the number of fully tumescent parous females present. Third, parous female chimpanzees experience a variable number of swelling cycles (average 11.7–19.4 cycles in Taï females) before conception (Deschner and Boesch, 2007). However, male mating effort and aggression rates are highest when fully tumescent parous females are close to conception, which indicates that males have to some extend the ability to assess the profitability of mating attempts (Emery Thompson and Wrangham, 2008). Thus, we created a third variable that only accounted for the presence or absence of fully tumescent parous females close to conception on each focal day. We classified fully tumescent parous females as “potentially fertile females” when their sexual swelling occurred within 6 months prior to conception. Since swelling phases during which an ovulation occurs do not always lead to conception (Deschner et al., 2003), this measure includes the presence of potentially fertile females that conceived (N = 4) and those that did not conceive (N = 3) during the data collection period, and can include the same female over more than one swelling cycle. Out of the three variables accounting for the presence of sexually receptive females, we expected that this third variable would reflect the periods with the highest male-male competition intensities. A female's conceptive cycle was estimated by subtracting an average gestation length of 228 days from the birth date of the respective infant (Nissen and Yerkes, 1943).
For all three variables, we did not consider that a male was in the presence of a sexually receptive female when the mother of a focal individual was the only female that had a sexual swelling at a given focal day (Surbeck et al., 2012). There were only two cases in the dataset were the mother of the focal individual was the only parous female with a sexual swelling. For these 2 days, we modified the presence of fully tumescent parous females to ‘no’ and changed the number of fully tumescent females present to zero.
Fruit Availability
We calculated a fruit availability index on a monthly basis separately for East and South group following a previously established index for Taï chimpanzees (Anderson et al., 2002, 2005). In brief, we calculated the index using data on the phenology of fruiting trees, tree density and tree basal area of the 74 most frequently eaten species for the chimpanzees. Monthly phenology data was obtained from the TCP long-term database. The fruit availability index FAm, for month m is defined as follows:
Dk = the density of species k across the study area
Bk = the mean basal area (measured by trunk diameter at breast height) of species k across the study area
Pkm = the percentage of trees of species k with mature fruits across the study area in month m.
Urine Sample Collection and Urinary Cortisol Analysis
We systematically recorded and when possible collected every urination of the focal subjects during each focal follow (mean ± SD = 1.1 ± 0.7 per observation hour). Sampling was carried out with disposable plastic pipettes from leaf litter into 2 ml cryo vials. The samples were stored in a thermos can with frozen cool packs during the day and transferred into liquid nitrogen within 12 h of collection upon arrival at camp. We shipped the samples to the Laboratory of Endocrinology at the Max Plank Institute for Evolutionary Anthropology (MPI-EVA) in Leipzig, Germany, on dry ice and stored them at −80°C until extraction.
We extracted 1,581 urine samples and measured urinary cortisol with liquid chromatography–tandem mass spectrometry (LC-MS/MS) and MassLynx (version 4.1; QuanLynx-Software), using a method adapted from Hauser et al. (2008) (see Supplementary Material). To control for variation in urine concentration, we measured creatinine levels in all urine samples and expressed urinary cortisol values as ng/mg creatinine (Bahr et al., 2000).
Statistical Analysis
Urinary Cortisol Model (Model 1)
We fitted a Linear Mixed Model (LMM) to investigate whether a focal individual's dominance rank, aggression rates given and received, rank instability (i.e., none, low, high) and the presence of fully tumescent parous females had an effect on urinary cortisol levels. The LMM was fitted with Gaussian error structure (Baayen, 2008), using the functions lmer of the R package lme4 (Bates et al., 2015). Log-transformed urinary cortisol levels (cortisol ng/mg creatinine) of each urine sample collected during a focal follow day was the response of the model. One of the key terms in the model was the interaction between rank instability and the focal individual's dominance rank, to investigate whether the association between dominance rank and urinary cortisol levels differed between stable and unstable dominance periods. Further key terms were aggression rates given and received, and the interaction between dominance rank and the presence of fully tumescent parous females. This latter interaction is likely due to highest mating effort and energy expenditure predicted for dominant males when fully tumescent parous females are present. Aggression rates given and received were an accumulative measure per urine sample, while all other fixed effects had one value per day. We calculated accumulative aggression rates per urine sample corrected for the observation time between the start of a focal follow day and the time of the urination. We log-transformed aggression rates since both aggression rates given and received had a strong positive skew.
As a control predictor, we added the time of the day a urine sample was collected, to account for diurnal variation in cortisol secretion (Muller and Lipson, 2003). We furthermore controlled for group identity, average male party size (Sobolewski, 2012), log-transformed age (Sapolsky and Altmann, 1991; Goncharova and Lapin, 2002; Gesquiere et al., 2011b) and monthly fruit availability (Muller and Wrangham, 2004b). To control for seasonal variation in cortisol levels we converted Julian dates into a circular variable and included its sine and cosine into the model (Stolwijk et al., 1999; Wessling et al., 2018). As random effects, we included the identity of the focal individual, and date nested in group. To avoid confounding effects of group-level cooperative behaviors on urinary cortisol levels (Samuni et al., 2019), we excluded all urine samples from a focal follow that were collected <2 h before, and all samples after, hunts, patrols or intergroup encounters (N = 469). We also excluded all urine samples from focal follows that were <2 h in duration (N = 33) and samples that were not collected during focal follows (N = 96). The remaining sample size for the model was 983 urine samples, collected from 10 focal individuals on 189 unique group days. Of the 983 urine samples included in this analysis, 692 were collected during “none,” 64 during “low” and 227 during “high” rank instability periods; 457 samples were collected when fully tumescent parous females were present, 526 when they were absent. East group contributed 456 urine samples with an average of 91 ± 30 (mean ± SD) samples per focal and South group 527 urine samples with an average of 105 ± 17 (mean ± SD) samples per individual.
Aggressive interactions occurred more often in the morning than in the afternoon in our data set, showing a weak negative correlation between the number of aggressions observed and time of the day (r = −0.2). If aggressions rates are confounded with the time of the day, the known biological diurnal decline in cortisol levels may lead to an elevated type I error rate (false positive) for the effects of aggressions. Thus, we used data simulations to investigate whether the effect of aggression rates on urinary cortisol levels can be determined independent from the expected diurnal decline in cortisol (Supplementary Material). The results of the simulations indicate that the effects of aggression rates and day time on urinary cortisol levels can be determined independently, even in the presence of higher numbers of aggressions in the morning (Supplementary Material).
Aggression Models (Models 2.1 and 2.2)
To investigate whether dominance rank, unstable dominance periods and the presence of fully tumescent parous females influenced a focal individual's number of aggressions given (actor) or received (receiver), we fitted two Generalized Linear Mixed Models (GLMM) with Poisson error structure and log link function (McCullagh and Nelder, 1989). The response was the number of aggressions given (model 2.1) or received (model 2.2) per focal day. The key terms for each of the models were the interaction between rank instability and a focal individual's dominance rank, as well as the interaction between the presence of fully tumescent parous females and dominance rank during individual focal follow days.
Since aggression rates are influenced by male party size (Muller, 2002) and food availability (Muller and Wrangham, 2004b), we controlled for the average male party size and fruit availability in each GLMM. Additionally, we controlled for the occurrence of group hunts, patrols and intergroup encounters (group-level cooperation, yes/no) on a focal follow day, since the occurrence of group-level cooperative behavior might influence daily aggression rates. We furthermore controlled for observation effort by including the log-transformed observation hours per focal follow day as an offset term (McCullagh and Nelder, 1989) into the models. Random effects were the identity of the focal individual, and date nested in group. We excluded all data for which focal follows lasted <2 h (N = 37). The remaining sample size for the model was 460 focal follow days, collected from 10 focal individuals on 410 unique group days.
Accounting for the Presence of Sexually Receptive Females (Model 1, 2a, 2b)
The three variables accounting for the presence of sexually receptive females (presence and number of fully tumescent parous and potentially fertile females) were highly correlated. We therefore investigated each of them in a separate model and additionally fitted the cortisol and aggression models (1, 2a,b) with the respective variables by exchanging them one at a time (described above for fully tumescent parous females). In the result section we present the overall model results only for the model including the fully tumescent parous female variable with the strongest effect on urinary cortisol levels or aggressions given and received. We furthermore report the estimates and statistics for models with the other two variables in the respective result sections, however, the overall model results of the other two models are presented in the Supplementary Material. We predicted that the effect of the number of fully tumescent parous females present, and its interaction with dominance rank on urinary cortisol levels and aggressions given is stronger when only one fully tumescent parous female is present, when the competition of males over the one female is expected to be highest. Thus, we included the number of fully tumescent parous females linear and squared and their interactions with dominance rank into the cortisol and aggression models. The squared term accounts for the possibility that urinary cortisol levels and aggression rates are highest in the presence of only one fully tumescent female, as opposed to the absence or presence of multiple fully tumescent parous females, for which we predicted no or a weaker effect, respectively.
Model Fitting and Inference
We z-transformed all covariates to a mean of zero and a standard deviation (SD) of one (Schielzeth, 2010). In all models we included random slopes when a fixed effect had at least two unique cases with at least two observations per level of the random effect, in order to keep type I error rate at the nominal 5% (Schielzeth and Forstmeier, 2009; Barr et al., 2013).
The fit of each full model was compared with that of a respective null model which did not include the key terms but which was otherwise identical to the respective full model (Forstmeier and Schielzeth, 2011). The significance of the full-null model comparison was derived using a likelihood ratio test (R function anova with argument test set to “Chisq”; Dobson and Barnett, 2008). P-values for individual fixed effects were derived with likelihood ratio tests comparing the full with a respective reduced model (R function drop1; Barr et al., 2013). Post-hoc pairwise comparisons of urinary cortisol levels or number of aggressions given and received among factors with more than two levels that had a significant effect in the respective model were calculated using the function glht of the R package multcomp (Bretz et al., 2016), without an adjustment of P-values. All analysis were conducted in R version 3.4.2 (R Core Team, 2016). In accordance with our predictions, we removed interactions and squared terms from the model when interactions or squared terms that concerned test predictors had no significant effect on urinary cortisol levels or aggressions given and received, to evaluate the effect of the respective test main effect independent from an interaction or a squared term. Effect sizes for the models were derived for the entirety of fixed effects (R2m) and for the entirety of fixed and random effects (R2c; Nakagawa and Schielzeth, 2013). See Supplementary Material for model assumption, stability and collinearity checks, and model formulas (Tables S1.1, S1.2).
Descriptive Information on Aggression Data
Average accumulative aggression rates given per urine sample were lower in East group males (0.71) than in South group males (0.93), while accumulative aggression rates received per urine sample were comparable between the two groups (East: 0.19, South: 0.17; see Table 1 for aggression rates calculated per observation hours per focal individual).
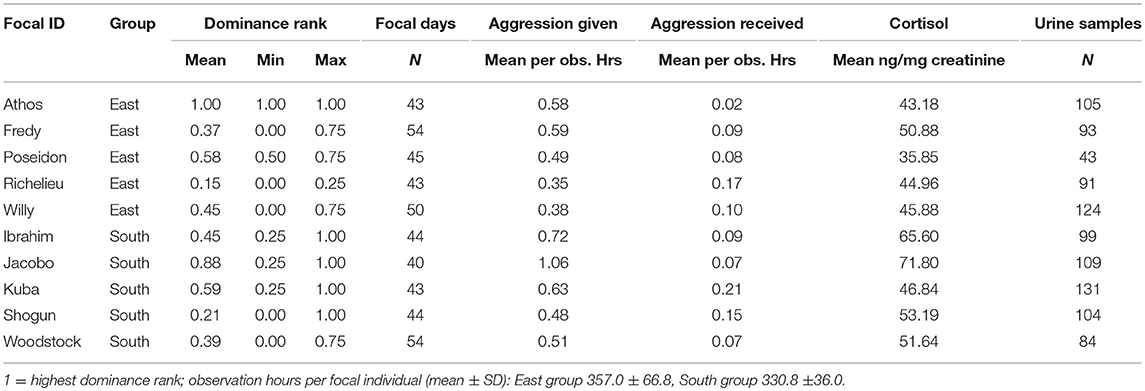
Table 1. Dominance rank, number of focal days and urine samples, aggression rates given and received, and average male chimpanzee urinary cortisol levels per focal individual.
We observed 2,307 aggressive interactions during the data collection period. Displays were the most common form of aggression, while attack and chases were rare events, each representing only 6% of all the aggressions (Table 2). We observed 1,957 aggressions given by the focal individuals (aggressor). The majority of these aggressions were non-directed displays (N = 727), and aggressions directed at adult females (N = 416). Aggressions directed to adult males (N = 263) and simultaneously directed at adult males and females (N = 92) were relatively rare, while aggressions directed at non-adults were more common (N = 354). For a few cases the identity or sex of the recipient of the aggression could not be determined (N = 102). Males had a mean rate of aggressions given per day of 0.35 (with 0.12 and 0.67 as first and third quartile) in the East group and 0.46 (with 0.19 and 0.91 as first and third quartile) in the South group. We recorded 350 aggressions that a focal individual received (victim) from males (N = 318), from females (N = 26), from coalitions between males and females (N = 5), and one was from unknown aggressor. Males had a mean rate of aggressions received per day of 0 (with 0 and 0.12 as first and third quartile) in the East group and 0 (with 0 and 0.14 as first and third quartile) in the South group. Aggression intensities did not differ much between unstable dominance periods and when potentially fertile or fully tumescent parous females were present (Table 2), however the proportion of aggressions categorized as attacks was lower during “high” (3%) compared to “low” (7%) and no (“none,” 6%) rank instability periods.
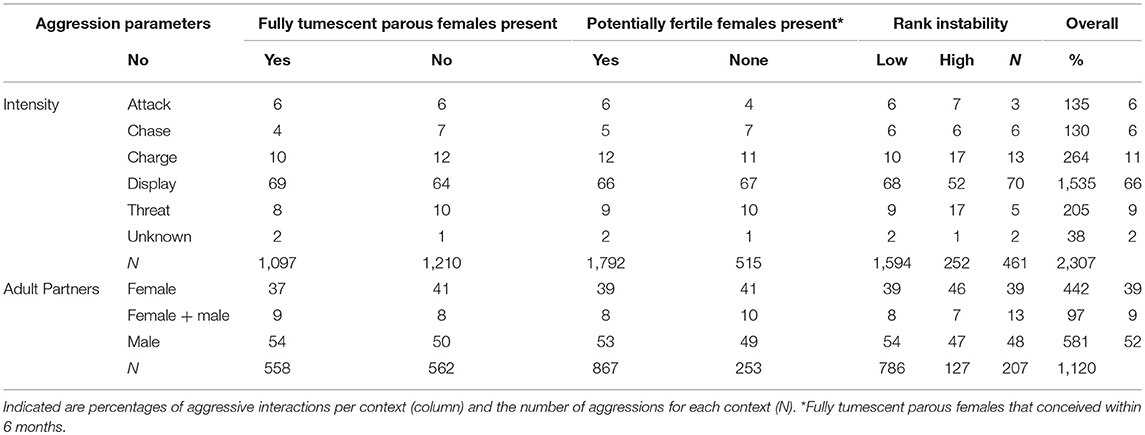
Table 2. Intensities of all aggressions observed for focal males during the data collection period (N = 2307), and sex of opponents for a subset of aggressions between adults (N = 1120), presented in relation to the presence and absence of fully tumescent parous and potentially fertile females, and rank instability.
Results
Cortisol Models
Effects of Dominance Rank, Aggression Rates, Rank Instability, and Presence of Fully Tumescent Parous Females on Male Urinary Cortisol Levels (Model 1)
The full-null model comparisons of the models including the presence (χ2 = 15.83, df = 9, P = 0.071; Table S2) or number of fully tumescent parous females were not significant (χ2 = 16.81, df = 11, P = 0.113; Table S3). However, the combination of key terms of the model including the presence of potentially fertile females explained more of the variation in urinary cortisol levels than the model lacking these terms (full-null model comparison: χ2 = 17.88, df = 9, P = 0.037). The interaction between rank instability and dominance rank (χ2 = 1.46, df = 2, P = 0.483; Figure S3), and the interaction between dominance rank and presence of potentially fertile females (χ2 = 1.22, df = 1, P = 0.289) did not have a significant effect on urinary cortisol levels. A reduced model fitted without the interactions revealed that rank instability had a significant effect on urinary cortisol levels (Table 3). Urinary cortisol levels were significantly higher during periods of “high” rank instability compared to no (“none”) rank instability (Estimate = 0.39, SE = 0.11, z-value = 3.74, P < 0.001), and also compared to the ‘low’ rank instability periods (Estimate = 0.33, SE = 0.15, z-value = 2.62, P = 0.024; Figure 1). Urinary cortisol levels did not differ between periods of “low” and no (“none”) rank instability (Estimate = 0.07, SE = 0.12, z-value = 0.57, P = 0.569). A focal male's dominance rank was not significantly associated with urinary cortisol levels, nor aggression rates given or received. The presence of potentially fertile females tended to have a positive effect on urinary cortisol levels. The strongest predictors of urinary cortisol levels were the control terms daytime and seasonality. Overall, the fixed effects explained 47% (R2m = 0.47) of the variation in urinary cortisol levels, while fixed and random effects together explained 79% R2c = 0.79).
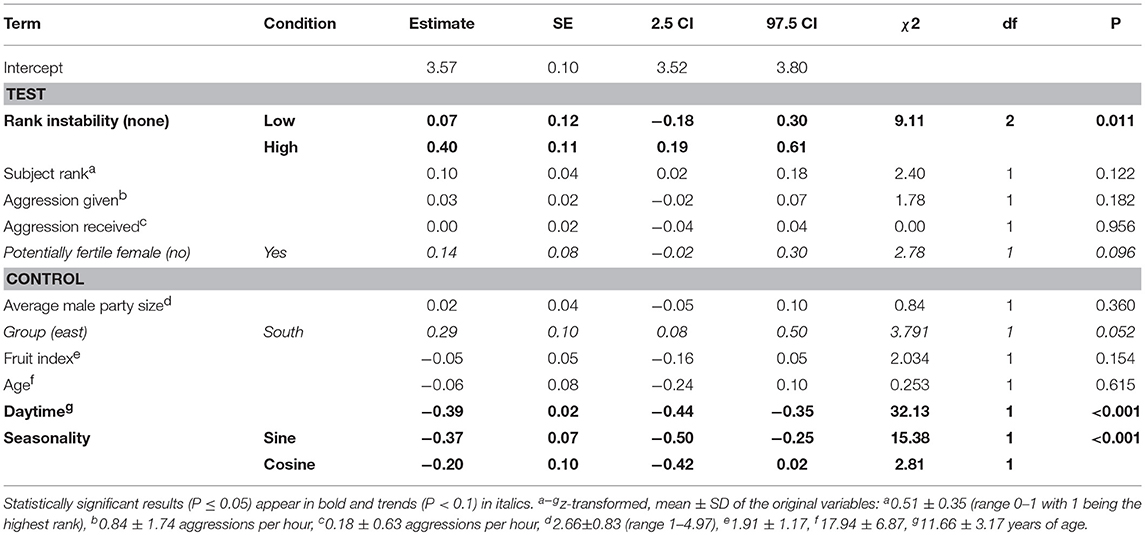
Table 3. Effect of dominance rank, aggression rates, rank instability and presence of potentially fertile females on male chimpanzees' urinary cortisol levels (model 1).
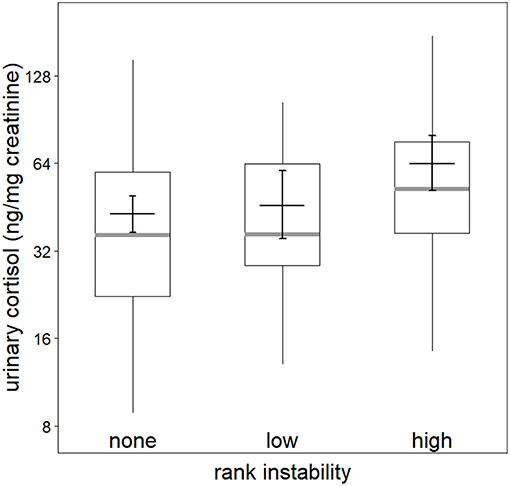
Figure 1. Effect of rank instability on urinary cortisol levels (logarithmic scale). Indicated are the median (gray bar), the fitted model and its 95% confidence intervals (black bar and error bars; based on presence of potentially fertile females and group identity manually dummy coded and centered). Boxes indicate quartiles (25 and 75%) and vertical lines represent quantiles (2.5 and 97.5%).
Aggression Models
Effects of Dominance Rank, Rank Instability, and Presence of Fully Tumescent Parous Females on the Number of Aggressions Given by Focal Males (Model 2.1)
The combination of the key terms of the model including the number (full-null model comparison: χ2 = 23.91, df = 9, P = 0.004) or presence of fully tumescent parous females (full-null model comparison: χ2 = 19.45, df = 7, P = 0.007; Table S4) explained more of the variation in numbers of aggressions given than the models lacking these terms. The full-null model comparison of the model accounting for the presence of potentially fertile females was not significant (χ2 = 12.08, df = 7, P = 0.098; Table S5).
The interaction between rank instability and dominance rank (χ2 = 0.49, df = 2, P = 0.783), as well as the interaction between dominance rank and number (squared term: χ2 = 0.23, df = 1, P = 0.629, linear term: χ2 = 0.34, df = 1, P = 0.557) or presence of fully tumescent parous females (χ2 = 0.87, df = 1, P = 0.351; Table S4) were not significant. A reduced model not including the interactions revealed a non-significant effect of the number of fully tumescent parous females as squared term (χ2 = 0.23, df = 1, P = 0.629). Thus, we additionally removed the squared term from the model and fitted a second reduced model to investigate the effect of number of fully tumescent parous females as linear term. The latter model revealed that rank instability had a significant effect on aggression rates given (Table 4, Figure 2A). Compared to no (“none”) rank instability, the number of aggressions given was significantly lower during “high” rank instability periods (Estimate = −0.34, SE = 0.12, z-value = −2.84, P = 0.005). The amount of aggressions given did not differ between “low” and “high” (Estimate = 0.28, SE = 0.18, z-value = 1.53, P = 0.126), nor between “low” and no (“none”) rank instability periods (Estimate = −0.06, SE = 0.16, z-value = −0.37, P = 0.710). High-ranking males gave significantly more aggressions than lower-ranking males (Figure 2B) and the number of aggressions given significantly increased with an increasing number of fully tumescent parous females (Table 4, Figure 2C), as well as in the presence of fully tumescent parous females (Estimate = 0.32, SE = 0.10, χ2 = 7.96, df = 1, P = 0.005; Table S4). South group males gave higher numbers of aggressions than East group males and both fruit availability and average male party size had a strong, positive effect on the number of aggressions given. The fixed effects explained 16% (R2m = 0.16) of the variation in the number of aggressions given, while fixed and random effect together explained 85% (R2c = 0.85).
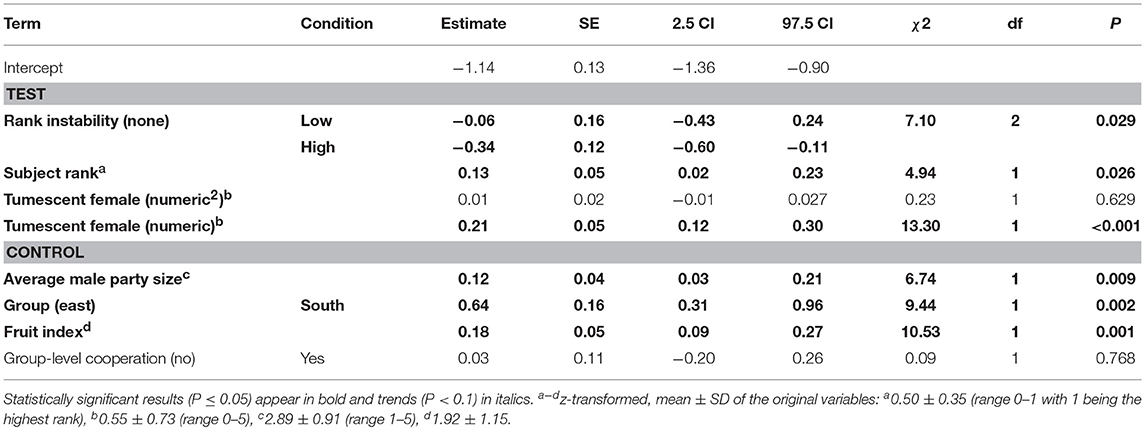
Table 4. Effect of rank instability, subject rank and number of fully tumescent parous females present (tumescent female) on the number of aggressions given by male chimpanzees (model 2.1).
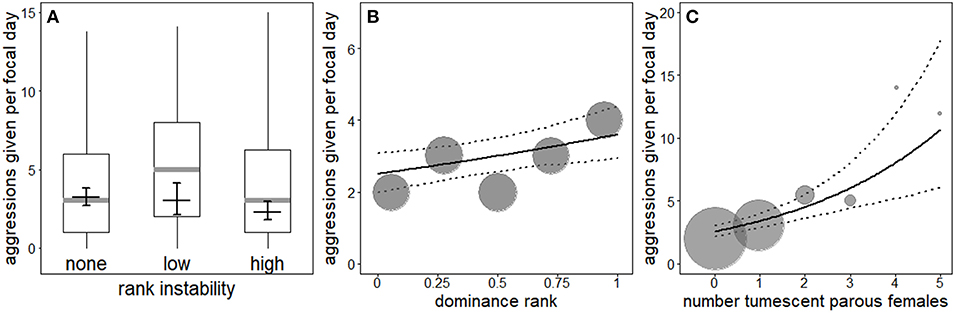
Figure 2. Effect of rank instability on the number of aggressions given by males (A). Indicated are the median (gray horizontal line), the fitted model and its 95% confidence intervals (black line with error bars; based on group identity and group-level cooperation manually dummy coded and centered). Boxes indicate quartiles (25 and 75%), and vertical lines represent quantiles (2.5 and 97.5%). Effect of dominance rank (B) and number of fully tumescent parous females (C) on the number of aggressions given. The solid and dotted lines indicate the fitted model and its 95% confidence intervals, respectively (based on rank instability, group identity and group-level cooperation manually dummy coded and then centered). The area of the symbols is proportionate to the number of observations for the respective value of the predictor.
Effects of Dominance Rank, Rank Instability, and Presence of Fully Tumescent Parous Females on the Number of Aggressions Males Received (Model 2.2)
The key terms in the models including the number (full-null model comparison: χ2 = 22.29, df = 9, P = 0.008) or presence of fully tumescent parous females (full-null model comparison: χ2 = 18.73, df = 7, P = 0.009; Table S6), or the presence of potentially fertile females (full-null model comparison: χ2 = 15.92, df = 7, P = 0.026; Table S7) explained more of the variation in the numbers of aggression received than the models lacking these key terms. The interaction between dominance rank and number (squared term: χ2 = 0.21, df = 1, P = 0.651, linear term: χ2 = 0.57, df = 1, P = 0.450) or presence (χ2 = 0.77, df = 1, P = 0.381) of fully tumescent parous females, or presence of potentially fertile females (χ2 = 0.63, df = 1, P = 0.428) was not significant.
A reduced model, fitted without the interaction between dominance rank and number of fully tumescent parous females, revealed that the number of fully tumescent females as squared term had no significant effect on aggressions received (χ2 = 0.85, df = 1, P = 0.356). Thus, we removed the squared term and fitted another reduced model without the squared term. The latter model revealed that low-ranking, but not high-ranking individuals received significantly less aggressions during “high” rank instability, compared to “low” and no (“none”) rank instability periods (Table 5, Figure 3A). The number of fully tumescent parous females significantly increased the number of aggressions a focal individual received (Table 5, Figure 3B), and the presence of fully tumescent parous females tended to have a positive effect on the number of aggressions received (Estimate = 0.26, SE = 0.15, χ2 = 2.94, df = 1, P = 0.086, Table S6). The presence of potentially fertile females had no effect on the number of aggressions received (Estimate = −0.08, SE = 0.20, χ2 = 0.17, df = 1, P = 0.683, Table S7). In addition, consistent with the results for aggressions given, South group males received more aggressions than East group males and both average male party size and fruit availability had a positive effect on the number of aggressions received. The fixed effects explained 16% (R2m = 0.16) of the variation of aggressions received, while fixed and random effect together explained 42% (R2c = 0.42).
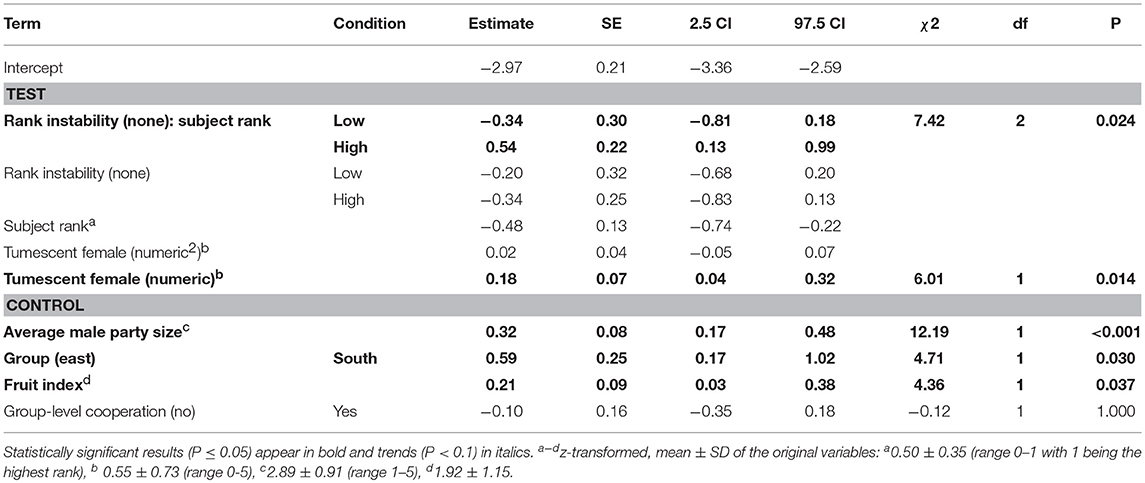
Table 5. Effect of rank instability, subject rank and number of fully tumescent parous females (tumescent female) present on the number of aggressions males received (model 2.2).
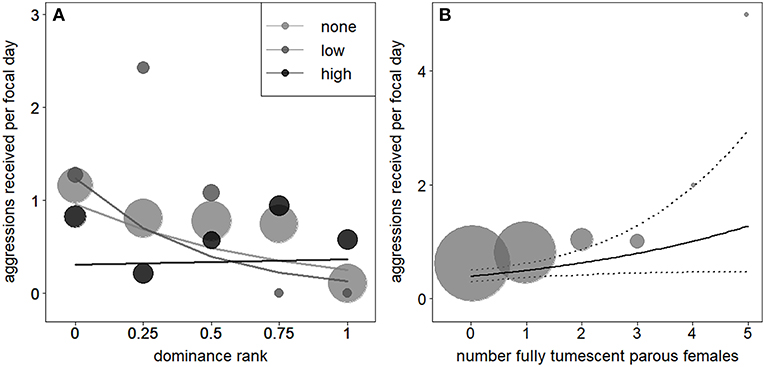
Figure 3. Effect of rank instability and subject rank (1 highest rank) on number of aggressions males received (A). The lines show the fitted model (based on group identity and group-level cooperation manually dummy coded and then centered). Effect of the number of fully tumescent parous females (B) on the number of aggressions given. The solid line indicates the fitted model (based on rank instability, group identity, and group-level cooperation manually dummy coded and then centered). The dotted lines represent the model's 95% confidence limits. In (A,B), larger point areas depict a larger number of observations.
Discussion
Our study provides new insights into the association between dominance status and exposure to energetic and psychosocial stressors in Taï male chimpanzees during socially stable and unstable periods. As predicted, we found that higher-ranking males gave significantly more aggressions than lower-ranking males in stable and unstable dominance periods. However, in line with one of the previous studies in male chimpanzees (Muehlenbein and Watts, 2010), we did not find an association between dominance rank and urinary cortisol levels in stable nor in unstable dominance periods. Additionally, in contrast to our predictions, individual variation in aggression rates given and received were not significantly associated with urinary cortisol levels. Thus, a clear difference in aggression rates given and received across dominance rank positions did not result in similar dominance rank related glucocorticoid profiles. Accordingly, while dominance has been predicted to be associated with elevated physiological stress in male chimpanzees (Muller and Wrangham, 2004a), our results suggest that a high dominance status is not associated with elevated exposure to psychosocial and/or energetic stressors in our study groups.
In accordance with our predictions and previous studies on non-human primates, we found that urinary cortisol levels were higher in all males during unstable compared to stable dominance periods, and that urinary cortisol levels tended to be higher in the presence of potentially fertile females compared to when absent. Unexpectedly, however, aggression rates given and received were lower during periods of rank instability. Thus, higher urinary cortisol levels of males during social instability might be due to the exposure to psychosocial rather than energetic stressors. These results are in line with findings of a recent study conducted in the same chimpanzee groups and study period (Samuni et al., 2019), as well as with another study in male chimpanzees (Wittig et al., 2015), that both found support for an association between urinary glucocorticoid levels and exposure to psychosocial more than energetic stressors. Overall, our results indicate that all males, independent of dominance status, were affected by social instability and therefore support previous empirical work (Sapolsky, 1992, 2005), by showing that social uncertainty is a potent psychosocial stressor.
Dominance Status, Aggression Rates, and Urinary Cortisol Levels During Stable Dominance Periods
Dominant individuals are expected to have higher glucocorticoid levels than subordinates in social animal species in which dominance status is maintained through repeated and frequent physical reassertion, in which reproductive success is strongly related to dominance status and subordinates have the possibility to avoid or cope with intimidation from dominants (Sapolsky, 2005; Creel et al., 2013). Several studies, especially in cooperatively breeding species (Creel et al., 2013), but also in non-human primates (Barrett et al., 2002; Muller and Wrangham, 2004b; Gesquiere et al., 2011a), support this prediction. Most of these predictions are suitable as well for male chimpanzees, though, dominant individuals do not monopolize reproduction exclusively (Boesch et al., 2006; Surbeck et al., 2017). However, in our study, urinary cortisol levels were not correlated with dominance rank. Despite frequent aggressive displays and overall higher aggression rates given, dominant individual's cortisol levels were not significantly higher than in subordinates. In line with findings of studies in African wild dogs and gray wolves (Creel, 2005), male sifakas (Fichtel et al., 2007), and bonobos (Surbeck et al., 2012) differences in aggression rates alone did not explain status specific glucocorticoid profiles or the absence of such in our study groups.
A lack of a dominance rank-glucocorticoid level association during periods of rank stability was also found in wild male tufted capuchin monkeys, redfronted lemurs, and long-tailed and rhesus macaques (Lynch et al., 2002; Ostner et al., 2008; Girard-Buttoz et al., 2009; Higham et al., 2013). Some of these studies also found a dissociation between aggression rates and glucocorticoid levels, indicating that aggression rates and glucocorticoid levels are linked under some but not all circumstances. For example, when aggressions are generally of low intensity, a correlation between aggression rates and glucocorticoid levels is unlikely. Accordingly, it is possible that aggressions were generally of low intensity in our study groups, and that therefore variation in aggression rates did not predict cortisol levels. Indeed, low-intensity aggressions such as directed and non-directed displays and charges were the most common form of aggression, while high-intensity aggressions, namely chases and attacks, were relatively rare. However, displays and charges were also the most common form of aggressions in other chimpanzee populations and attack rates were higher in our study (26 observation hours per attack) than reported for males in other chimpanzee groups (Kanyawara: 32 observation hours per attack, Gombe: 33–68 observation hours per attack; summarized in Muller, 2002). Thus, differences in aggression intensities are unlikely to explain why aggression rates were not associated with urinary cortisol levels in our study. However, it is possible that attacks in our study groups are not comparable to attacks in other populations, since injury rates are generally low. During the data collection period (16 months per group), seven injuries were recorded for South group males, the most severe injury being the loss of an ear. Five injuries were recorded for East group males, all of these injuries were cuts. Previous studies indicate that the intensity of intergroup aggression is much lower in western compared to eastern chimpanzees (Boesch et al., 2008), and that rates of both between and within community killings in Taï are among the lowest recorded across 18 habituated communities (Wilson et al., 2014). Thus, within-group aggression intensities might also be lower for Taï than for other chimpanzee populations, potentially explaining why aggressions given and received are not associated with psychosocial stress for males in our study groups. However, since comparative data on injury rates are currently lacking, we cannot assess this possibility and suggest further investigation. Furthermore, while urinary glucocorticoid levels increased after single aggressive interactions in Budongo chimpanzees (Wittig et al., 2015), whether aggressive interactions are generally stressful for chimpanzees and whether aggression rates are associated with glucocorticoid levels in other chimpanzee populations needs yet to be investigated.
Dominance Status and Urinary Cortisol Levels During Stable and Unstable Dominance Periods
Differences in social stability have been proposed to potentially underlie inconsistent findings of the dominance rank-glucocorticoid level relationship in chimpanzees (Muehlenbein and Watts, 2010) and other non-human primate species (Sapolsky, 2005). However, our results do not support this suggestion, since dominance rank did not predict urinary cortisol levels differently in unstable dominance periods. Thus, inconsistent findings between chimpanzee populations might primarily depend on group specific social dynamics, the level of risk associated with aggression and/or differences in male group sizes rather than being dependent on social instability. For example, male group size (N = 5) was relatively small in our two study groups, compared to 11 males in the study of Muller and Wrangham (2004b). Predictability of male social behavior might be higher for dominant males in groups with a small number of males, suggesting that social unpredictability might not be a relevant threat during stable periods for dominant males in our study groups. However, small male group sizes might not per se induce low male status competition, considering the short tenure length of the second alpha male in the South group during our data collection period. Additionally, rank-dependent differences in cortisol levels might be less pronounced in groups with shallow male hierarchies, when males have similar resource holding potential (Kaburu and Newton-Fisher, 2015). Further studies across chimpanzee populations with differing male group sizes and steepness of the hierarchies (Kaburu and Newton-Fisher, 2015) are therefore needed before general conclusions can be drawn.
It is also possible that we did not find a significant effect of dominance rank on urinary cortisol levels, due to between individual differences in cortisol levels that might be independent of dominance rank. A recent study in male chimpanzees found that adult males are relatively consistent in their cortisol secretion patterns and that individuals differ consistently from each other in average cortisol levels and in the shape of their circadian urinary cortisol rhythm (Sonnweber et al., 2018). Consistent differences between males were independent of dominance rank (Sonnweber et al., 2018), which suggests that early life experiences (e.g., Pryce et al., 2011), genetic determinants (e.g., Zera et al., 2007), and/or differences in personalities (e.g., Anestis, 2005) might underlie this variation. In addition, while our finding that during “high” rank instability periods cortisol levels increased in all males, indicates that all males were affected by social instability, it is likely that not all males were exposed to the same risk levels. Hence, it might be that high-ranking males that experienced rank challenges had stronger HPA axis responses than males that were not directly challenged (Sapolsky, 1992; Higham et al., 2013), such as the alpha male in the East group. While our data did not allow investigating the latter possibility, to examine changes in cortisol levels of male chimpanzees in relation to expected differences in risk levels during unstable dominance periods entails a promising topic for future studies.
Aggression Rates and Urinary Cortisol Levels During Unstable Dominance Periods
In line with previous studies in non-human primates (Sapolsky, 1992; Bergman et al., 2005; Setchell et al., 2010; Higham et al., 2013), we found that urinary cortisol levels were higher in all males during unstable compared to stable dominance periods. However, in contrast to our predictions, the number of aggressions given and received was lower during unstable than during stable periods. On the one hand, this result supports our finding that aggression rates are not associated with urinary cortisol levels in males of our study groups and suggests that periods of elevated male-male competition are potent psychosocial stressors, even in the absence of elevated frequencies of aggressive interactions. On the other hand, this result raises the question why, in contrast to previous studies in chimpanzees (Goodall, 1986; Kaburu et al., 2013; Kalan and Boesch, 2018), aggression rates were lower during periods of unstable dominance hierarchy. One possibility is that overall rates of aggression might be reduced, while intensity of aggressive interactions increased. However, our observations do not support this suggestion since the proportion of aggressions that were attacks was two times lower during periods of “high” when compared to periods of no (“none”) rank instability, and the proportion of chases was unchanged. Both attacks and chases are considered as high intensity aggressions in chimpanzees (Muller and Wrangham, 2004a). Furthermore, the proportion of aggressions between males was lower during periods of “high” than during periods of no (“none”) rank instability. Moreover, during periods of “high” rank instability, all males independent of dominance rank gave fewer aggressions than during stable periods (“none”), and lower-ranking but not higher-ranking males received less aggression. This shows that aggression rates given and received were reduced during periods of “high” rank instability. However, the number of aggression received did not change for high-ranking males, which indicates that low- and high-ranking males received similar rates of aggression during unstable periods. Nevertheless, besides overall reduced aggression rates, the proportion of aggressions directed up the hierarchy was higher during unstable (South: 35%; East: 18%) than during stable periods (South: 13%; East: 14%).
Reduced aggression rates and lower aggression intensity during unstable dominance periods might be part of a conflict management strategy that aims to avoid escalation (Wittig and Boesch, 2003b). Social animals are able to monitor costs and benefits associated with contest competition and adjust their fighting behavior accordingly (Parker, 1974; Hsu et al., 2006; Arnott and Elwood, 2008). For example, when aggressive interactions are more likely to escalate and the risk of injury is overtly increased, the costs of physical aggression might be higher than the potential benefits of the contested resource (Hsu et al., 2006; Arnott and Elwood, 2008). In such situations, it might be adaptive to avoid or retreat from aggressive interactions overall (Parker, 1974; Clutton-Brock et al., 1979; Hsu et al., 2006, 2008). Furthermore, when competitors have equal fighting abilities, low intensity aggressions, such as displays, might allow contest competition without disproportionally elevated costs, since contests are longer and often escalate between opponents with similar fighting abilities (Enquist and Leimar, 1983; Jonart et al., 2007). Reduced aggression rates during “high” rank instability periods and higher percentages of low intensity aggressions such as displays and charges therefore suggest that males avoided escalation. However, a previous study in the South group found higher aggression rates during a period of unstable dominance hierarchy compared to before and after (Kalan and Boesch, 2018), thus, aggression rates are not always reduced during periods of unstable dominance hierarchy in Taï. This indicates that males might adjust competitive behavior to social circumstances and previous experience (Hsu et al., 2006).
Dominance Status, Aggression Rates, and Urinary Cortisol Levels in Relation to Mate Competition
Across chimpanzee populations reproductive success is highest for dominant males. However, reproductive skew is only moderate indicating that alternative mating strategies exist for lower ranking males (Boesch et al., 2006; Inoue et al., 2008; Newton-Fisher et al., 2009; Wroblewski et al., 2009). Since sexually-receptive females are rare, due to the prolonged interbirth intervals, and unpredictably distributed in time, male-male competition over mating opportunities is considered to be high in chimpanzees (Goodall, 1986; Georgiev et al., 2014). Furthermore, mating effort and competition are expected to be higher for dominant males, especially when mating can be monopolized (Mitani et al., 1996; Georgiev et al., 2014). However, in contrast to our predictions, dominance rank was not associated with urinary cortisol levels nor with aggressions given or received when fully tumescent parous or potentially fertile females were present nor when only one fully tumescent parous female was present. This indicates that mating effort and associated energetic costs might not be higher for dominant males in our study groups, which is in line with a previous study in male chimpanzees that also did not find an association between dominance rank and costs of mating effort (Georgiev et al., 2014).
Male chimpanzees prefer mating with parous and older females than with nulliparous females (Muller et al., 2006). Thus, males compete more over the access to fully tumescent parous than over nulliparous females. Accordingly, aggression rates, as well as testosterone and glucocorticoid levels increase in males when fully tumescent parous females are present (Muller and Wrangham, 2004a,b; Sobolewski et al., 2013; Georgiev et al., 2014). In support of results of previous studies, we found higher numbers of aggressions given and a corresponding trend for aggressions received when fully tumescent parous females were present compared to their absence. The presence of multiple fully tumescent parous females significantly increased aggressions given and received, while aggressions given or received did not change in the presence of potentially fertile females. Furthermore, the number and presence of fully tumescent parous females had no effect on urinary cortisol levels, yet, urinary cortisol levels tended to be higher when potentially fertile females were present. The latter results are consistent with our findings that variation in aggression rates given and received is not associated with urinary cortisol levels and furthermore indicate that competition induced by the presence of fully tumescent parous females is not associated with energetic or psychosocial stressor exposure for males in our study groups.
Competition between males is most intense when fully tumescent parous females are close to conception (Deschner et al., 2004; Emery Thompson and Wrangham, 2008). However, we found that the number of aggressions given and received did not differ in relation to the presence or absence of potentially fertile females, but that cortisol levels tended to be higher when potentially fertile females were present. These results further suggest that Taï male chimpanzees do not increase aggressive behavior in periods with elevated male-male competition potential, when the risk of escalation of aggressive conflicts is at its highest. The trend for higher urinary cortisol levels in the presence of potentially fertile females might be associated with elevated social tension between males. This might be further driven by reduced feeding time and increased energy expenditure (Georgiev et al., 2014), since males often allocate all their time into mating effort, and constantly monitor and follow the sexually receptive female, even in the absence of a chance to copulate (Georgiev et al., 2014; Preis pers. obs.).
Conclusions
Altogether, our results indicate that dominance maintenance is not associated with elevated physiological stress for dominant males in our study groups, or alternatively that dominant males are able to counterbalance energetically demanding behavior with energy intake or other coping mechanisms. Thus, the benefits of a high dominance status come at a low cost for Taï male chimpanzees, when considering challenges associated with the direct social and natural environment. However, it might also be that individual HPA regulation determines whether a male is able to acquire and maintain dominance (Beehner and Bergman, 2017), for example only males with a low HPA axis responsiveness. Furthermore, our study revealed a consistent pattern, that periods with potentially high male-male competition over status and mating opportunities were associated with higher urinary cortisol levels in all males irrespective of male rank, while periods with potentially low male-male competition were associated with higher aggression rates. These results indicate that aggression rates are not always a good indicator of competition intensities and that avoidance of aggression and other non-aggressive forms of competitive behavior (Surbeck et al., 2012) need to be considered. This variation in the frequency and intensity of aggressive behaviors during periods of social instability indicates that male chimpanzees adjust competitive behaviors to context dependent conditions, and possibly depending on the predictability of outcomes of social interactions. When the risk of escalation is high, avoidance of aggressive behaviors likely is an adaptive conflict management strategy used by Taï male chimpanzees, potentially diminishing the risk of injury.
Data Availability
The datasets generated for this study are available on request to the corresponding author.
Ethics Statement
Permissions to conduct the research was granted by the Ministries of Research and Environment of Ivory Coast and Office Ivorien des Parcs et Reserves. Methods were approved by the Ethikrat der Max-Planck-Gesellschaft.
Author Contributions
AP carried out the field data collection, endocrine laboratory work, design of the study, data analysis, and drafted the manuscript. LS carried out the field data collection. TD, CC, and RMW participated in the design of the study and revising the manuscript.
Funding
The Max Planck Society, the Leakey Foundation (AP), the Minerva Foundation (LS), and the European Research Council (ERC) under the European Union's Horizon 2020 research and innovation program (Grant Agreement No. 679787) awarded to CC funded this research.
Conflict of Interest Statement
The authors declare that the research was conducted in the absence of any commercial or financial relationships that could be construed as a potential conflict of interest.
Acknowledgments
We thank the Ivorian Ministry of Environment and Forests and Ministry of Higher Education and Scientific Research, the Office Ivoirien des Parcs et Reserves of Côte d'Ivoire, and the Centre Suisse de Recherches Scientifiques. We thank Anne-Sophie Crunchant for fieldwork support, Sylvain Lemoine and all staff members of the Taï Chimpanzee Project for assistance in the field, Alexander Mielke for helpful comments and providing the R code to calculate ELO ranks, Christophe Boesch for his continued support and advice and Roger Mundry for statistical counsel and helpful comments. We thank Emma I. K. Vitikainen and Ben T. Hirsch for their helpful comments.
Supplementary Material
The Supplementary Material for this article can be found online at: https://www.frontiersin.org/articles/10.3389/fevo.2019.00107/full#supplementary-material
References
Abbott, D. H., Keverne, E. B., Bercovitch, F. B., Shively, C. A., Mendoza, S. P., Saltzman, W., et al. (2003). Are subordinates always stressed? A comparative analysis of rank differences in cortisol levels among primates. Hormones Behav. 43, 67–82. doi: 10.1016/S0018-506X(02)00037-5
Altmann, J. (1974). Observational study of behavior: Sampling methods. Behaviour, 49 (3–4), 227–266. doi: 10.1163/156853974X00534
Anderson, D. P., Nordheim, E. V., Boesch, C., and Moermond, T. C. (2002). “Factors influencing fission-fusion grouping in chimpanzees in the Taï National Park, Côte d'Ivoire,” in Behavioural Diversity in Chimpanzees and Bonobos, eds C. Boesch, G. Hohmann, and L. Marchant (Cambridge: Cambridge University Press), 90–101.
Anderson, D. P., Nordheim, E. V., Moermond, T. C., Gone Bi, Z. B., and Boesch, C. (2005). Factors influencing tree phenology in Tai National Park, Cote d'Ivoire1. Biotropica 37, 631–640. doi: 10.1111/j.1744-7429.2005.00080.x
Anestis, S. F. (2005). Behavioral style, dominance rank, and urinary cortisol in young chimpanzees (Pan troglodytes). Behaviour 142, 1245–1268. doi: 10.1163/156853905774539418
Archie, E. A., Altmann, J., and Alberts, S. C. (2012). Social status predicts wound healing in wild baboons. Proc. Natl. Acad. Sci. U.S.A. 109, 9017–9022. doi: 10.1073/pnas.1206391109
Arnott, G., and Elwood, R. W. (2008). Information gathering and decision making about resource value in animal contests. Anim. Behav. 76, 529–542. doi: 10.1016/j.anbehav.2008.04.019
Baayen, R. H. (2008). Analyzing Linguistic Data: A Practical Introduction to Statistics Using R. Cambridge: Cambridge University Press.
Bahr, N. I., Palme, R., Möhle, U., Hodges, J. K., and Heistermann, M. (2000). Comparative aspects of the metabolism and excretion of cortisol in three individual nonhuman primates. Gen. Comp. Endocrinol. 117, 427–438. doi: 10.1006/gcen.1999.7431
Barr, D. J., Levy, R., Scheepers, C., and Tily, H. J. (2013). Random effects structure for confirmatory hypothesis testing: keep it maximal. J. Mem. Lang. 68, 255–278. doi: 10.1016/j.jml.2012.11.001
Barrett, G. M., Shimizu, K., Bardi, M., Asaba, S., and Mori, A. (2002). Endocrine correlates of rank, reproduction, and female-directed aggression in male japanese macaques (Macaca fuscata). Horm. Behav. 42, 85–96. doi: 10.1006/hbeh.2002.1804
Bates, D., Mächler, M., Bolker, B., and Walker, S. (2015). Fitting linear mixed-effects models using lme4. J. Stat. Softw. 67:i01. doi: 10.18637/jss.v067.i01
Beehner, J. C., and Bergman, T. J. (2017). The next step for stress research in primates: to identify relationships between glucocorticoid secretion and fitness. Horm. Behav. 91, 68–83. doi: 10.1016/j.yhbeh.2017.03.003
Behringer, V., and Deschner, T. (2017). Non-invasive monitoring of physiological markers in primates. Horm. Behav. 91, 3–18. doi: 10.1016/j.yhbeh.2017.02.001
Bergman, T. J., Beehner, J. C., Cheney, D. L., Seyfarth, R. M., and Whitten, P. L. (2005). Correlates of stress in free-ranging male chacma baboons, Papio hamadryas ursinus. Anim. Behav. 70, 703–713. doi: 10.1016/j.anbehav.2004.12.017
Blomquist, G. E., Sade, D. S., and Berard, J. D. (2011). Rank-related fitness differences and their demographic pathways in semi-free-ranging rhesus macaques (Macaca mulatta). Int. J. Primatol. 32, 193–208. doi: 10.1007/s10764-010-9461-z
Boesch, C., Crockford, C., Herbinger, I., Wittig, R., Moebius, Y., and Normand, E. (2008). Intergroup conflicts among chimpanzees in Taï National Park: lethal violence and the female perspective. Am. J. Primatol. 70, 519–532. doi: 10.1002/ajp.20524
Boesch, C., Kohou, G., Néné, H., and Vigilant, L. (2006). Male competition and paternity in wild chimpanzees of the Taï forest. Am. J. Phys. Anthropol. 130, 103–115. doi: 10.1002/ajpa.20341
Bretz, F., Hothorn, T., and Westfall, P. (2016). Multiple Comparisons Using R. Boca Raton, FL: CRC Press.
Bronson, F. H., and Eleftheriou, B. E. (1964). Chronic physiological effects of fighting in mice. Gen. Comp. Endocrinol. 4, 9–14. doi: 10.1016/0016-6480(64)90033-4
Clutton-Brock, T. H. (1988). Reproductive Success: Studies of Individual Variation in Contrasting Breeding Systems. University of Chicago, IL: Chicago Press.
Clutton-Brock, T. H., Albon, S. D., Gibson, R. M., and Guinness, F. E. (1979). The logical stag: adaptive aspects of fighting in red deer (Cervus elaphus L.). Anim. Behav. 27, 211–225. doi: 10.1016/0003-3472(79)90141-6
Cohen, S., Janicki-Deverts, D., and Miller, G. E. (2007). Psychological stress and disease. JAMA 298:1685. doi: 10.1001/jama.298.14.1685
Cowlishaw, G., and Dunbar, R. I. (1991). Dominance rank and mating success in male primates. Anim. Behav. 41, 1045–1056.
Creel, S. (2001). Social dominance and stress hormones. Trends Ecol. Evol. 16, 491–497. doi: 10.1016/S0169-5347(01)02227-3
Creel, S. (2005). Dominance, aggression, and glucocorticoid levels in social carnivores. J. Mammal. 86, 255–264. doi: 10.1644/BHE-002.1
Creel, S., Dantzer, B., Goymann, W., and Rubenstein, D. R. (2013). The ecology of stress: effects of the social environment. Funct. Ecol. 27, 66–80. doi: 10.1111/j.1365-2435.2012.02029.x
Deschner, T., and Boesch, C. (2007). Can the patterns of sexual swelling cycles in female taï chimpanzees be explained by the cost-of-sexual-attraction hypothesis? Int. J. Primatol. 28, 389–406. doi: 10.1007/s10764-007-9120-1
Deschner, T., Heistermann, M., Hodges, K., and Boesch, C. (2003). Timing and probability of ovulation in relation to sex skin swelling in wild West African chimpanzees, Pan troglodytes verus. Anim. Behav. 66, 551–560. doi: 10.1006/anbe.2003.2210
Deschner, T., Heistermann, M., Hodges, K., and Boesch, C. (2004). Female sexual swelling size, timing of ovulation, and male behavior in wild West African chimpanzees. Horm. Behav. 46, 204–215. doi: 10.1016/j.yhbeh.2004.03.013
Dobson, A. J., and Barnett, A. (2008). An Introduction to Generalized Linear Models. New York, NY: CRC Press.
Emery Thompson, M., and Wrangham, R. W. (2008). Male mating interest varies with female fecundity in Pan troglodytes schweinfurthii of Kanyawara, Kibale National Park. Int. J. Primatol. 29, 885–905. doi: 10.1007/s10764-008-9286-1
Enquist, M., and Leimar, O. (1983). Evolution of fighting behaviour: decision rules and assessment of relative strength. J. Theor. Biol. 102, 387–410. doi: 10.1016/0022-5193(83)90376-4
Fahy, G. E., Richards, M. P., Fuller, B. T., Deschner, T., Hublin, J.-J., and Boesch, C. (2014). Stable nitrogen isotope analysis of dentine serial sections elucidate sex differences in weaning patterns of wild chimpanzees (Pan troglodytes): weaning in Chimpanzees. Am. J. Phys. Anthropol. 153, 635–642. doi: 10.1002/ajpa.22464
Fichtel, C., Kraus, C., Ganswindt, A., and Heistermann, M. (2007). Influence of reproductive season and rank on fecal glucocorticoid levels in free-ranging male Verreaux's sifakas (Propithecus verreauxi). Horm. Behav. 51, 640–648. doi: 10.1016/j.yhbeh.2007.03.005
Flies, A. S., Mansfield, L. S., Flies, E. J., Grant, C. K., and Holekamp, K. E. (2016). Socioecological predictors of immune defences in wild spotted hyenas. Funct. Ecol. 30, 1549–1557. doi: 10.1111/1365-2435.12638
Foerster, S., Franz, M., Murray, C. M., Gilby, I. C., Feldblum, J. T., Walker, K. K., et al. (2016). Chimpanzee females queue but males compete for social status. Sci. Rep. 6, 1–11. doi: 10.1038/srep35404
Forstmeier, W., and Schielzeth, H. (2011). Cryptic multiple hypotheses testing in linear models: overestimated effect sizes and the winner's curse. Behav. Ecol. Sociobiol. 65, 47–55. doi: 10.1007/s00265-010-1038-5
Georgiev, A. V., Russell, A. F., Emery Thompson, M., Otali, E., Muller, M. N., and Wrangham, R. W. (2014). The foraging costs of mating effort in male chimpanzees (Pan troglodytes schweinfurthii). Int. J. Primatol. 35, 725–745. doi: 10.1007/s10764-014-9788-y
Gesquiere, L. R., Learn, N. H., Simao, M. C. M., Onyango, P. O., Alberts, S. C., and Altmann, J. (2011a). Life at the top: rank and stress in wild male baboons. Science 333, 357–360. doi: 10.1126/science.1207120
Gesquiere, L. R., Onyango, P. O., Alberts, S. C., and Altmann, J. (2011b). Endocrinology of year-round reproduction in a highly seasonal habitat: environmental variability in testosterone and glucocorticoids in baboon males. Am. J. Phys. Anthropol. 144, 169–176. doi: 10.1002/ajpa.21374
Girard-Buttoz, C., Heistermann, M., Krummel, S., and Engelhardt, A. (2009). Seasonal and social influences on fecal androgen and glucocorticoid excretion in wild male long-tailed macaques (Macaca fascicularis). Physiol. Behav. 98, 168–175. doi: 10.1016/j.physbeh.2009.05.005
Goncharova, N. D., and Lapin, B. A. (2002). Effects of aging on hypothalamic–pituitary–adrenal system function in non-human primates. Mech. Ageing Dev. 123, 1191–1201. doi: 10.1016/S0047-6374(02)00012-X
Goymann, W., and Wingfield, J. C. (2004). Allostatic load, social status and stress hormones: the costs of social status matter. Anim. Behav. 67, 591–602. doi: 10.1016/j.anbehav.2003.08.007
Hauser, B., Deschner, T., and Boesch, C. (2008). Development of a liquid chromatography-tandem mass spectrometry method for the determination of 23 endogenous steroids in small quantities of primate urine. J. Chromatogr. B 862, 100–112. doi: 10.1016/j.jchromb.2007.11.009
Higham, J. P., Heistermann, M., and Maestripieri, D. (2013). The endocrinology of male rhesus macaque social and reproductive status: a test of the challenge and social stress hypotheses. Behav. Ecol. Sociobiol. 67, 19–30. doi: 10.1007/s00265-012-1420-6
Holekamp, K. E., and Strauss, E. D. (2016). Aggression and dominance: an interdisciplinary overview. Curr. Opin. Behav. Sci. 12, 44–51. doi: 10.1016/j.cobeha.2016.08.005
Hsu, Y., Earley, R. L., and Wolf, L. L. (2006). Modulation of aggressive behaviour by fighting experience: mechanisms and contest outcomes. Biol. Rev. 81, 33–74. doi: 10.1017/S146479310500686X
Hsu, Y., Lee, S.-P., Chen, M.-H., Yang, S.-Y., and Cheng, K.-C. (2008). Switching assessment strategy during a contest: fighting in killifish Kryptolebias marmoratus. Anim. Behav. 75, 1641–1649. doi: 10.1016/j.anbehav.2007.10.017
Inoue, E., Inoue-Murayama, M., Vigilant, L., Takenaka, O., and Nishida, T. (2008). Relatedness in wild chimpanzees: influence of paternity, male philopatry, and demographic factors. Am. J. Phys. Anthropol. 137, 256–262. doi: 10.1002/ajpa.20865
Isbell, L. A., and Young, T. P. (2002). Ecological models of female social relationships in primates: similarities, disparities, and some directions for future clarity. Behaviour 139, 177–202. doi: 10.1163/156853902760102645
Jonart, L. M., Hill, G. E., and Badyaev, A. V. (2007). Fighting ability and motivation: determinants of dominance and contest strategies in females of a passerine bird. Anim. Behav. 74, 1675–1681. doi: 10.1016/j.anbehav.2007.03.012
Kaburu, S. S. K., Inoue, S., and Newton-Fisher, N. E. (2013). Death of the alpha: within-community lethal violence among chimpanzees of the mahale mountains National Park: coalitional lethal violence in chimpanzees. Am. J. Primatol. 75, 789–797. doi: 10.1002/ajp.22135
Kaburu, S. S. K., and Newton-Fisher, N. E. (2015). Egalitarian despots: hierarchy steepness, reciprocity and the grooming-trade model in wild chimpanzees, Pan troglodytes. Anim. Behav. 99, 61–71. doi: 10.1016/j.anbehav.2014.10.018
Kalan, A. K., and Boesch, C. (2018). Re-emergence of the leaf clip gesture during an alpha takeover affects variation in male chimpanzee loud calls. PeerJ 6:e5079. doi: 10.7717/peerj.5079
King, W. J., and Allainé, D. (2002). Social, maternal, and environmental influences on reproductive success in female Alpine marmots (Marmota marmota). Can. J. Zool. 80, 2137–2143. doi: 10.1139/z02-205
Koyama, N. F., Ronkainen, K., and Aureli, F. (2017). Durability and flexibility of chimpanzee grooming patterns during a period of dominance instability. Am. J. Primatol. 79:e22713. doi: 10.1002/ajp.22713
Lewin, N., Treidel, L. A., Holekamp, K. E., Place, N. J., and Haussmann, M. F. (2015). Socioecological variables predict telomere length in wild spotted hyenas. Biol. Lett. 11:20140991. doi: 10.1098/rsbl.2014.0991
Lloyd, P. H., and Rasa, O. A. E. (1989). Status, reproductive success and fitness in Cape mountain zebra (Equus zebra zebra). Behav. Ecol. Sociobiol. 25, 411–420.
Lynch, J. W., Ziegler, T. E., and Strier, K. B. (2002). Individual and seasonal variation in fecal testosterone and cortisol levels of wild male tufted capuchin Monkeys, Cebus apella nigritus. Horm. Behav. 41, 275–287. doi: 10.1006/hbeh.2002.1772
Majolo, B., Lehmann, J., de Bortoli Vizioli, A., and Schino, G. (2012). Fitness-related benefits of dominance in primates. Am. J. Phys. Anthropol. 147, 652–660. doi: 10.1002/ajpa.22031
Marmot, M. G. (2004). The Status Syndrome: How Social Standing Affects our Health and Longevity. London: Bloomsbury.
Marmot, M. G., and Sapolsky, R. (2014). “Of baboons and men: social circumstances, biology, and the social gradient in health,” in Sociality, Hierarchy, Health: Comparative Biodemography: Papers from a Workshop (Washington, DC).
McEwen, B. S., and Wingfield, J. C. (2003). The concept of allostasis in biology and biomedicine. Horm. Behav. 43, 2–15. doi: 10.1016/S0018-506X(02)00024-7
Mendonça-Furtado, O., Edaes, M., Palme, R., Rodrigues, A., Siqueira, J., and Izar, P. (2014). Does hierarchy stability influence testosterone and cortisol levels of bearded capuchin monkeys (Sapajus libidinosus) adult males? A comparison between two wild groups. Behav. Proc. 109, 79–88. doi: 10.1016/j.beproc.2014.09.010
Mielke, A., Preis, A., Samuni, L., Gogarten, J. F., Wittig, R. M., and Crockford, C. (2018). Flexible decision-making in grooming partner choice in sooty mangabeys and chimpanzees. R. Soc. Open Sci. 5:172143. doi: 10.1098/rsos.172143
Mielke, A., Samuni, L., Preis, A., Gogarten, J. F., Crockford, C., and Wittig, R. M. (2017). Bystanders intervene to impede grooming in Western chimpanzees and sooty mangabeys. R. Soc. Open Sci. 4:171296. doi: 10.1098/rsos.171296
Mitani, J. C., Gros-Louis, J., and Richards, A. F. (1996). Sexual dimorphism, the operational sex ratio, and the intensity of male competition in polygynous primates. Am. Nat. 147, 966–980.
Muehlenbein, M. P., and Watts, D. P. (2010). The costs of dominance: testosterone, cortisol and intestinal parasites in wild male chimpanzees. Biopsychosoc. Med. 4:21. doi: 10.1186/1751-0759-4-21
Muller, M. N. (2002). “Agonistic relations among Kanyawara chimpanzees,” in Behavioural Diversity in Chimpanzees and Bonobos, eds C. Boesch, G. Hohmann, and L. Marchant (Cambridge: Cambridge University Press), 112–124. doi: 10.1017/CBO9780511606397.012
Muller, M. N., and Lipson, S. F. (2003). Diurnal patterns of urinary steroid excretion in wild chimpanzees. Am. J. Primatol. 60, 161–166. doi: 10.1002/ajp.10103
Muller, M. N., Thompson, M. E., and Wrangham, R. W. (2006). Male chimpanzees prefer mating with old females. Curr. Biol. 16, 2234–2238. doi: 10.1016/j.cub.2006.09.042
Muller, M. N., and Wrangham, R. W. (2004a). Dominance, aggression and testosterone in wild chimpanzees: a test of the “challenge hypothesis.” Anim. Behav. 67, 113–123. doi: 10.1016/j.anbehav.2003.03.013
Muller, M. N., and Wrangham, R. W. (2004b). Dominance, cortisol and stress in wild chimpanzees (Pan troglodytes schweinfurthii). Behav. Ecol. Sociobiol. 55, 332–340. doi: 10.1007/s00265-003-0713-1
Muller, M. N., and Wrangham, R. W. (2005). Testosterone and energetics in wild chimpanzees (Pan troglodytes schweinfurthii). Am. J. Primatol. 66, 119–130. doi: 10.1002/ajp.20132
Nakagawa, S., and Schielzeth, H. (2013). A general and simple method for obtaining R 2 from generalized linear mixed-effects models. Methods Ecol. Evol. 4, 133–142. doi: 10.1111/j.2041-210x.2012.00261.x
Natoli, E., Schmid, M., Say, L., and Pontier, D. (2007). Male reproductive success in a social group of urban feral cats (Felis catus L.). Ethology 113, 283–289. doi: 10.1111/j.1439-0310.2006.01320.x
Neumann, C., Duboscq, J., Dubuc, C., Ginting, A., Irwan, A. M., Agil, M., et al. (2011). Assessing dominance hierarchies: validation and advantages of progressive evaluation with Elo-rating. Anim. Behav. 82, 911–921. doi: 10.1016/j.anbehav.2011.07.016
Newton-Fisher, N. E., Thompson, M. E., Reynolds, V., Boesch, C., and Vigilant, L. (2009). Paternity and social rank in wild chimpanzees (Pan troglodytes) from the Budongo Forest, Uganda. Am. J. Phys. Anthropol. 142, 417–428. doi: 10.1002/ajpa.21241
Nishida, T. (1968). The social group of wild chimpanzees in the Mahali Mountains. Primates 9, 167–224.
Nishida, T., Kano, T., Goodall, J., McGrew, W. C., and Nakamura, M. (1999). Ethogram and ethnography of Mahale chimpanzees. Anthropol. Sci. 107, 141–188.
Nissen, H. W., and Yerkes, R. M. (1943). Reproduction in the chimpanzee: report on forty-nine births. Anat. Rec. 86, 567–578.
Ostner, J., Kappeler, P., and Heistermann, M. (2008). Androgen and glucocorticoid levels reflect seasonally occurring social challenges in male redfronted lemurs (Eulemur fulvus rufus). Behav. Ecol. Sociobiol. 62, 627–638. doi: 10.1007/s00265-007-0487-y
Parker, G. A. (1974). Assessment strategy and the evolution of fighting behaviour. J. Theor. Biol. 47, 223–243. doi: 10.1016/0022-5193(74)90111-8
Preuschoft, S., and van Schaik, C. P. (2000). “Dominance and communcation: conflict management in various social setting,” in Natural Conflict Resolution (Berkeley, CA: University of California Press), 77–105.
Pryce, C. R., Aubert, Y., Maier, C., Pearce, P. C., and Fuchs, E. (2011). The developmental impact of prenatal stress, prenatal dexamethasone and postnatal social stress on physiology, behaviour and neuroanatomy of primate offspring: studies in rhesus macaque and common marmoset. Psychopharmacology 214, 33–53. doi: 10.1007/s00213-010-1989-2
Pusey, A. (1997). The influence of dominance rank on the reproductive success of female chimpanzees. Science 277, 828–831. doi: 10.1126/science,.277.5327.828
R Core Team (2016). R: A Language and Environment for Statistical Computing. Vienna: R Foundation for Statistical Computing.
Rodriguez-Llanes, J. M., Verbeke, G., and Finlayson, C. (2009). Reproductive benefits of high social status in male macaques (Macaca). Anim. Behav. 78, 643–649. doi: 10.1016/j.anbehav.2009.06.012
Romero, L. M., Dickens, M. J., and Cyr, N. E. (2009). The reactive scope model — A new model integrating homeostasis, allostasis, and stress. Horm. Behav. 55, 375–389. doi: 10.1016/j.yhbeh.2008.12.009
Samuni, L., Preis, A., Deschner, T., Wittig, R. M., and Crockford, C. (2019). Cortisol and oxytocin show independent activity during chimpanzee intergroup conflict. Psychoneuroendocrinology 104, 165–173. doi: 10.1016/j.psyneuen.2019.02.007
Sapolsky, R. M. (1983). Endocrine aspects of social instability in the olive baboon (Papio anubis). Am. J. Primatol. 5, 365–379. doi: 10.1002/ajp.1350050406
Sapolsky, R. M. (1992). Cortisol concentrations and the social significance of rank instability among wild baboons. Psychoneuroendocrinology 17, 701–709. doi: 10.1016/0306-4530(92)90029-7
Sapolsky, R. M. (2005). The influence of social hierarchy on primate health. Science 308, 648–652. doi: 10.1126/science.1106477
Sapolsky, R. M., and Altmann, J. (1991). Incidence of hypercortisolism and dexamethasone resistance increases with age among wild baboons. Biol. Psychiatry 30, 1008–1016.
Schielzeth, H. (2010). Simple means to improve the interpretability of regression coefficients: interpretation of regression coefficients. Methods Ecol. Evol. 1, 103–113. doi: 10.1111/j.2041-210X.2010.00012.x
Schielzeth, H., and Forstmeier, W. (2009). Conclusions beyond support: overconfident estimates in mixed models. Behav. Ecol. 20, 416–420. doi: 10.1093/beheco/arn145
Setchell, J. M., Smith, T., Wickings, E. J., and Knapp, L. A. (2010). Stress, social behaviour, and secondary sexual traits in a male primate. Horm. Behav. 58, 720–728. doi: 10.1016/j.yhbeh.2010.07.004
Sobolewski, M. E. (2012). The Hormonal Correlates of Male Chimpanzee Social Behavior. Ph.D. Thesis, University of Michigan (Ann Arbor, MI).
Sobolewski, M. E., Brown, J. L., and Mitani, J. C. (2013). Female parity, male aggression, and the challenge hypothesis in wild chimpanzees. Primates 54, 81–88. doi: 10.1007/s10329-012-0332-4
Sonnweber, R., Araya-Ajoy, Y. G., Behringer, V., Deschner, T., Tkaczynski, P., Fedurek, P., et al. (2018). Circadian rhythms of urinary cortisol levels vary between individuals in wild male chimpanzees: a reaction norm approach. Front. Ecol. Evol. 6:85. doi: 10.3389/fevo.2018.00085
Stolwijk, A. M., Straatman, H., and Zielhuis, G. A. (1999). Studying seasonality by using sine and cosine functions in regression analysis. J. Epidemiol. Commun. Health 53, 235–238. doi: 10.1136/jech.53.4.235
Surbeck, M., Deschner, T., Weltring, A., and Hohmann, G. (2012). Social correlates of variation in urinary cortisol in wild male bonobos (Pan paniscus). Horm. Behav. 62, 27–35. doi: 10.1016/j.yhbeh.2012.04.013
Surbeck, M., Langergraber, K. E., Fruth, B., Vigilant, L., and Hohmann, G. (2017). Male reproductive skew is higher in bonobos than chimpanzees. Curr. Biol. 27, R640–R641. doi: 10.1016/j.cub.2017.05.039
van Noordwijk, M. A., and van Schaik, C. P. (1999). The effects of dominance rank and group size on female lifetime reproductive success in wild long-tailed macaques, Macaca fascicularis. Primates 40, 105–130.
Watts, H. E., Tanner, J. B., Lundrigan, B. L., and Holekamp, K. E. (2009). Post-weaning maternal effects and the evolution of female dominance in the spotted hyena. Proc. R. Soc. B Biol. Sci. 276, 2291–2298. doi: 10.1098/rspb.2009.0268
Weiss, J. M. (1970). Somatic effects of predictable and unpredictable shock. Psychosom. Med. 32, 397–408.
Wessling, E. G., Deschner, T., Mundry, R., Pruetz, J. D., Wittig, R. M., and Kühl, H. S. (2018). Seasonal variation in physiology challenges the notion of chimpanzees (Pan troglodytes verus) as a forest-adapted species. Front. Ecol. Evol. 6:60. doi: 10.3389/fevo.2018.00060
Wilson, M. L., Boesch, C., Fruth, B., Furuichi, T., Gilby, I. C., Hashimoto, C., et al. (2014). Lethal aggression in Pan is better explained by adaptive strategies than human impacts. Nature 513:414. doi: 10.1038/nature13727
Wittig, R. M. (2018). “Taï chimpanzees,” in Encyclopedia of Animal Cognition and Behavior, eds J. Vonk and T. Shackelford (Cham: Springer International Publishing), 1–7.
Wittig, R. M., and Boesch, C. (2003a). Food competition and linear dominance hierarchy among female chimpanzees of the Taï National Park. Int. J. Primatol. 24, 847–867. doi: 10.1023/A:1024632923180
Wittig, R. M., and Boesch, C. (2003b). The choice of post-conflict interactions in wild chimpanzees (Pan troglodytes). Behaviour 140, 1527–1559. doi: 10.1163/156853903771980701
Wittig, R. M., Crockford, C., Weltring, A., Deschner, T., and Zuberbühler, K. (2015). Single aggressive interactions increase urinary glucocorticoid levels in wild male chimpanzees. PLoS ONE 10:e0118695. doi: 10.1371/journal.pone.0118695
Wittig, R. M., Crockford, C., Weltring, A., Langergraber, K. E., Deschner, T., and Zuberbühler, K. (2016). Social support reduces stress hormone levels in wild chimpanzees across stressful events and everyday affiliations. Nat. Commun. 7:13361. doi: 10.1038/ncomms13361
Wroblewski, E. E., Murray, C. M., Keele, B. F., Schumacher-Stankey, J. C., Hahn, B. H., and Pusey, A. E. (2009). Male dominance rank and reproductive success in chimpanzees, Pan troglodytes schweinfurthii. Anim. Behav. 77, 873–885. doi: 10.1016/j.anbehav.2008.12.014
Keywords: glucocorticoids, rank maintenance, rank instability, mating effort, conflict management
Citation: Preis A, Samuni L, Deschner T, Crockford C and Wittig RM (2019) Urinary Cortisol, Aggression, Dominance and Competition in Wild, West African Male Chimpanzees. Front. Ecol. Evol. 7:107. doi: 10.3389/fevo.2019.00107
Received: 08 January 2019; Accepted: 18 March 2019;
Published: 05 April 2019.
Edited by:
Marian Yi-ling Wong, University of Wollongong, AustraliaReviewed by:
Emma I. K. Vitikainen, University of Helsinki, FinlandBen T. Hirsch, James Cook University, Australia
Copyright © 2019 Preis, Samuni, Deschner, Crockford and Wittig. This is an open-access article distributed under the terms of the Creative Commons Attribution License (CC BY). The use, distribution or reproduction in other forums is permitted, provided the original author(s) and the copyright owner(s) are credited and that the original publication in this journal is cited, in accordance with accepted academic practice. No use, distribution or reproduction is permitted which does not comply with these terms.
*Correspondence: Anna Preis, YW5uYV9wcmVpc0BldmEubXBnLmRl
Roman M. Wittig, d2l0dGlnQGV2YS5tcGcuZGU=
†These authors have contributed equally to this work