- 1Guangxi Key Laboratory of Forest Ecology and Conservation, College of Forestry, Guangxi University, Nanning, China
- 2School of Biology, St. Andrews University, St. Andrews, United Kingdom
- 3Independent Researcher, Montréal, QC, Canada
The field of predator eavesdropping concentrates on the detection by a predator or parasite of signals that prey direct at conspecifics, and the subsequent evolution by prey to avoid or lessen such detection. Here, we first point out that signaling prey species are often found in mixed-species moving groups or stationary aggregations, and ask the question of how simultaneous signaling, by members of one species or more, might affect predator eavesdropping behavior and the composition of the groups themselves. The detection risk of prey species will be affected by the other species they associate with, and prey should generally avoid joining a group with more detectable species. Yet prey may select to join other species that are preferred by predators, diluting their own risk of attack, as long as that does not lead to substantially greater detection and thereby increased predation. We next review the evidence that prey grouping and collective responses when attacked can confuse predators, leading to lower capture rates. Evidence for this confusion effect mostly involves visually orienting predators. We then ask if a similar phenomenon could occur when animals in a group simultaneously produce acoustic signals and find relevant evidence for predator confusion under such situations in the literature associated with the “cocktail party effect.” As confusion is heightened by similarities among mixed-species group members, this provides a force at ecological or evolutionary timescales to make species that associate in groups, and their signals, more similar to each other. However, heterogeneous mixed-species groups may be favored if species are differentially preferred as prey. We suggest experiments to examine whether the success rates of acoustically orienting predators depend on the group size of their mixed-species prey. More observations on the relative positions of conspecifics and heterospecifics in space, and the temporal association of their signals, will also increase our understanding of the relationship between mixed-species grouping and predator eavesdropping.
Introduction
The field of predator eavesdropping investigates the signaling behavior of prey animals in relation to their predator(s), with an emphasis on the co-evolutionary arms race between these two kinds of actors (Zuk and Kolluru, 1998). Studies to date have typically focused on isolated predator-prey pairs, and more rarely on situations with more than one predator or parasite, such as túngara frogs (Engystomops pustulosus) preyed upon by bats and also attacked by midges (Page et al., 2013). Yet, interactions between predators and prey occur in complex prey communities, in which several prey species are often signaling at the same time and may be grouped together in space. In the túngara frog case, for example, the frogs listen to the calls and silence of other frog species in their vicinity (Phelps et al., 2007). At the same time, other frogs are affected by them—when hourglass frogs (Dendropsophus ebraccatus) call close to túngara frogs, the hourglass frogs attract more midges (Trillo et al., 2016). Prey species are also tied together because eavesdropping predators are often capable of preying on a wide variety of species (Page et al., 2013). This leads to the question of how considering the wider social environment surrounding prey, i.e. the communication network (McGregor and Peake, 2000), might affect our understanding of predator eavesdropping.
Our aim in this article is to explore how the presence of multiple, potentially interacting prey species might affect predator eavesdropping, and conversely how the sensory and cognitive behavior of predators may shape mixed-species groups and aggregations of prey. In the first section, we review common situations in which mixed-species signaling takes place. In the second section, we concentrate on how grouping affects predation. We focus particularly on the “confusion effect,” in which the presence of a large number of prey reduces the ability of the predator to capture a single prey individual (Neill and Cullen, 1974; Krakauer, 1995). The confusion effect has primarily been studied for visually orienting predators (Jeschke and Tollrian, 2007) and not usually in relation to prey signaling. In the third section, therefore, we ask whether the confusion effect might also apply to situations in which multiple species use acoustic signals, a situation referred to as the “cocktail party effect” (Bee and Micheyl, 2008). Overall, our aim is to show how various aspects of grouping can affect and potentially reduce predator eavesdropping, analogous to the way in which prey species develop “private channels” with conspecifics that avoid such detection (Cummings et al., 2003; Nakano et al., 2008). Further, we hope to encourage more studies of predator eavesdropping in mixed-species groups and aggregations.
Signaling in Mixed-Species Groups and Aggregations
Animals often signal when in mixed-species groups of different types and at a variety of scales. Here we will distinguish between moving mixed-species groups, whose existence is entirely due to attraction between species, and stationary mixed-species aggregations, which form in a resource patch or enemy-free space (Powell, 1985; Goodale et al., 2017; Boulay et al., 2019), although interspecific attraction might also play a role (e.g., Ward and Zahavi, 1973; Gu et al., 2017). Aggregations and moving groups also differ in their prevalence across taxa: aggregations, although found in all taxa, are especially common in invertebrates, reptiles, and amphibians, whereas moving groups are predominantly found in fish, mammals, and birds (Goodale et al., 2017; Boulay et al., 2019). Finally, there is a difference in the spacing of individuals between these two kinds of groups. Individuals in moving groups are typically close to each other as they move in the same direction, but aggregations can range greatly in their scale, from groups of carrion insects clustered together on a decaying corpse (Boulay et al., 2019) to groups of seabirds spread over hundreds of square kilometers of ocean (Hunt et al., 1988).
Despite these differences, the benefits of mixed-species groups and aggregations can be quite similar, although the relative proximity of individuals is critical, and benefits will decline as proximity decreases. Benefits of grouping together frequently include increased foraging success and, more rarely, increased resistance to harsh environmental conditions or increased access to conspecific mating partners (Goodale et al., 2017; Boulay et al., 2019). Due to our focus on predator eavesdropping, however, we will primarily focus on benefits related to predation. These include the following: (1) encounter-dilution, a decrease in the probability of being attacked in larger groups; (2) vigilance, the increased detection of predators in larger and/or more diverse groups; (3) confusion, a disruption of the predator's ability to capture prey when many prey flee at the same time (see section Grouping and the Confusion Effect for a detailed discussion of this effect and its interaction with oddity); and (4) defense, the ability for a group of individuals to physically stand up to predators (Beauchamp, 2014; Goodale et al., 2017). These benefits are similar to those that can be found in single-species groups. However, mixed-species groups may be preferred to single-species groups in some situations. For example, competition could be lower, intraspecific social forces (e.g., aggression) could be diminished in mixed-species groups, or a heterospecific could bring a special quality that a conspecific does not have (e.g., it is particularly vigilant or good at finding resources; Sridhar and Guttal, 2018).
How do mixed-species moving groups and stationary aggregations relate to predator eavesdropping? Most studies on this topic focus on sexual signals, which are fundamental to animal fitness, and thus are strongly selected to persist even in the face of predation. Sexual advertisement is not usually a key feature of moving mixed-species groups, perhaps because of the risk of hybridization (e.g., Herzing and Elliser, 2013). However, moving groups tend to be constantly noisy, and hence conspicuous to predators, because of the need for group members to keep in contact as they move (Goodale and Beauchamp, 2010; Pagani-Núñez et al., 2018). Group members are also constantly exchanging information, including about predation via alarm calls (Goodale et al., 2010; Magrath et al., 2015). For this reason, we will return to discussing moving groups in sections Grouping and the Confusion Effect and The Cocktail Party Effect: Is There an Auditory Analog of the Confusion Effect?, where we investigate whether predators can be confused when facing large groups and, possibly, by multiple individuals simultaneously signaling within them.
In mixed-species aggregations, in contrast, sexual advertisement is common. In a few cases, mixed-species leks have been described (e.g., Gibson et al., 2002, in birds; and Srygley and Penz, 1999, in butterflies), whose main function appears to be sexual attraction. More frequently, different species at resource-based aggregations sexually advertise at the same time, forming what is known as a “mixed-species chorus” (Phelps et al., 2007; Nityananda and Bee, 2011). Indeed, the term “mixed-species chorus” can be used to describe simultaneous signaling of many animals in any habitat patch, such as crickets in a grassland (Schmidt et al., 2013) or birds in a forest singing at dawn (Luther, 2009). Because the audience for a sexual signal includes only conspecifics, heterospecific signals are simply considered noise and interference in such contexts. Hence, studies on these phenomena often focus on how species avoid overlapping (and hence competition) in time or acoustic characteristics (Cody and Brown, 1969; Hödl, 1977; Chek et al., 2003; Schmidt et al., 2013), although some recent work has shown that the expected partitioning may not always occur (Tobias et al., 2014).
Yet it is important to remember that despite some interference costs, animals may also gain from grouping with heterospecifics if they are sufficiently close to each other to accrue dilution, vigilance, confusion, or defensive benefits. For example, Rana frog species can aggregate together (at a scale of usually more than 1 but < 10 meters apart; Given, 1990). Although this is not a tight group, individuals might benefit from dilution if predators search over wide areas (tens of meters or greater). For example, frogs could monitor each other's vocalizations to assess predation risk (Phelps et al., 2007) and perhaps benefit from some acoustic confusion of the predator (see section The Cocktail Party Effect: Is There an Auditory Analog of the Confusion Effect?). It is indeed possible that some aggregating animals may prefer to be closest to heterospecifics. Conspecifics may tend to be widely dispersed due to the requirements of territoriality, whereas heterospecifics could be closer without creating mating competition. However, a potential cost of having heterospecifics as the closest neighbors could be a risk of hybridization, at least in cases where the neighbor is from a closely related species with incomplete reproductive barriers. For these reasons, future work should give more attention to the relative distances between signaling and non-signaling animals of the same and different species (Table 1, point 1).
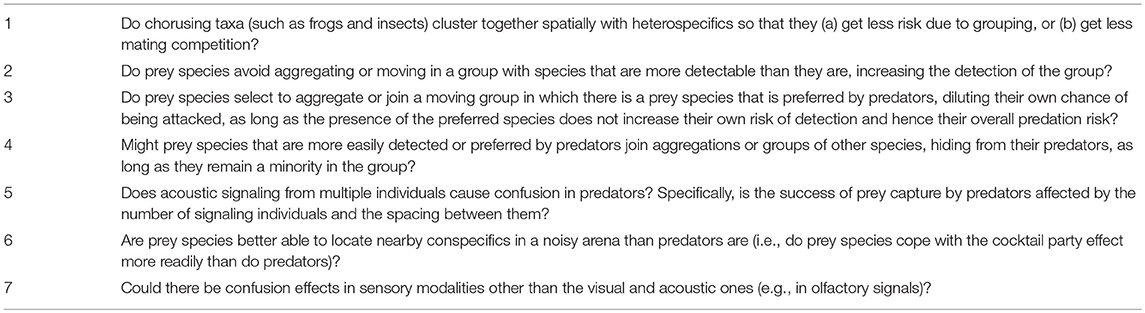
Table 1. Fruitful directions for future research on how the social environment of prey species influences predator eavesdropping.
The benefits and costs of grouping need not be symmetric between two or more interacting species. As mentioned above, Trillo et al. (2016) recently studied the hourglass frog, which calls in mixed-species choruses with túngara frogs. They found that hourglass frogs closely associated with calling túngara frogs were approached by more parasitic midges. In contrast, the association affected neither the detection of frogs by predatory bats, nor the approach by midges to túngara frogs. One possible explanation for this result is that midges are especially good at detecting túngara frog calls (Bernal et al., 2006). The presence of a highly detectable species could thus increase detection risk for the whole aggregation, and future work should test for such “collateral damage” (sensu Trillo et al., 2016) in other systems. A related hypothesis, which also invites future investigations, is that less detectable species should avoid grouping with more easily detected ones (Table 1, point 2).
Another kind of asymmetry between prey species occurs if one species is preferred by predators because it is easier to attack and consume or because it provides more nutritional reward. Other prey species might prefer to associate with it, because once a predator finds the group, it will attack its preferred prey first, thus lowering the risk for other members (although prey species should not join such a group if the lowered risk of attack is outweighed by a greater risk of detection). Indeed, some species in mixed-species moving groups have been shown to prefer to associate with vulnerable prey species (in ungulates: Fitzgibbon, 1990; in fish: Mathis and Chivers, 2003). Again, evidence for such a “shadow of safety” (sensu Trillo et al., 2016) effect should be looked for in different systems and in both mixed-species moving groups and aggregations (Table 1, point 3). Paula A. Trillo and colleagues are currently developing a mathematical model describing how predators attack mixed-species groups, which incorporates this kind of asymmetry between prey species (i.e in predators' preferences), as well as asymmetries in detectability, discussed above.
A final (admittedly hypothetical) scenario is also possible: a prey species that is more easily detected or preferred by predators might try to hide in a group of less preferred prey (Table 1, point 4). Here, the prey might lower its own risk of predation by masking its detection or diluting its risk of attack, as long as it remains sufficiently rare in the group to avoid attracting additional predators. This behavior would work best if the prey species looked similar to the other group members. Otherwise, it could not hide well and might be preferentially targeted by the predator due to an “oddity effect” (see section Grouping and the Confusion Effect).
We also want to emphasize that in all the various scenarios discussed above, the question of whether the group persists (i.e., whether the other species move away) may be influenced by traits of the species other than their detectability or attraction to predators. For example, a species' mobility will determine if it can move away from other species without being followed, and its relative dominance (often correlated with size) will determine whether it can put an end to groups that are not beneficial to it, or stay in groups that are not beneficial to other group members. The idea of escaping from a group is similar to the phenomenon in which some host fish can “punish” cheating cleaning fish by simply ending their association with them (Johnstone and Bshary, 2002). But escape may not always be possible for slow or subordinate species.
We conclude this section by emphasizing that conspicuous mixed-species moving groups are easy targets for predators that eavesdrop on the behavior of their prey. Also, sexual advertisements will often occur in aggregations of individuals of both the same and different species. Therefore, the evolutionary processes that reduce detection by predators will also be influenced by other species signaling in the same environment. To understand the relationships between prey species, we need to know whether they share predators and which species predators prefer in addition to their relative mobility and dominance. Further, it would seem important in the future to gather more data on not only the synchrony of species' signaling in mixed-species choruses (e.g., Tobias et al., 2014) but also on the spatial positioning of individuals of the same and different species. We hypothesize that (a) prey should avoid grouping with more detectable species; (b) they should prefer grouping with species that are more preferred by predators, as long as the presence of such species does not increase their own detection; and (c) the more detectable or preferred species might try to hide in a group of less vulnerable species.
Grouping and the Confusion Effect
Having discussed the different situations in which heterospecifics may be signaling together, we now turn to how grouping itself can affect predator eavesdropping. Specifically, in this section we will look at the so-called “confusion effect” experienced by a predator that needs to select one individual to attack from a large group of prey, a process we will refer to as “prey targeting.” In many ways, predator eavesdropping and prey targeting are quite distinct processes. The simplest kind of predator eavesdropping involves an isolated predator-prey dyad, with the predator, far away from the prey, using the prey's signaling to detect and then approach it (Figure 1A). In contrast, we will define prey targeting as occurring after the predator has detected a group of prey and is now close to it; here the escaping prey are usually not signaling (Figure 1B).
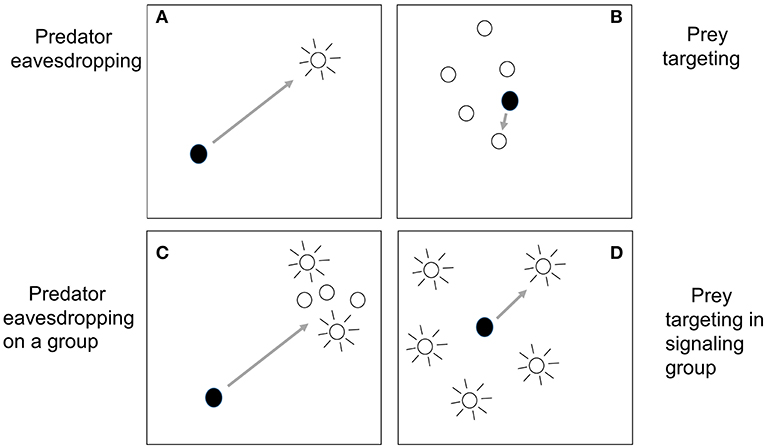
Figure 1. How the simplest cases of predator eavesdropping (A) differ from prey targeting (B) is clear. In predator eavesdropping, a distant predator detects a signaling prey, and subsequently approaches, whereas in prey targeting, the predator has already detected a tight group of prey, is close to it, and now selects one individual to attack. However, other situations may be more of a blend of both processes. In (C), predator eavesdropping on a group, a distant predator approaches a group as a whole after detecting the signaling of some of its members. Hence, the signaling of some group members influences the predation risk of others; see section Signaling in Mixed-Species Groups and Aggregations for a discussion of this situation. In (D), prey targeting in a signaling group, the predator is close to or inside a group in which multiple individuals are signaling. In the widely dispersed group shown in this panel, the predator must travel a long distance to attack one prey, close to the distances traveled in predator eavesdropping. In section The Cocktail Party Effect: Is There an Auditory Analog of the Confusion Effect?, we ask if a situation such as (D) might result in a confusion effect, as occurs in the simpler case of prey targeting.
Yet despite the apparently strong distinction between predator eavesdropping and prey targeting, in some situations they can blend together. First, as argued in the last section, the prey may not be isolated and the eavesdropping predator may detect from afar some or all of the group members and subsequently approach (Figure 1C). In this situation, all group members may be signaling, or only some, and the signaling of one group member may lead the predator to discover other group members that it would have otherwise been less able to detect, as studied by Trillo et al. (2016) and discussed in the last section. We could call this situation “predator eavesdropping on a group.” Second, there may be situations in which the predator has approached close to or within a group in which multiple individuals are signaling (Figure 1D). While the predator's proximity to the group as a whole might be reminiscent of prey targeting, the distances between group members may be large, so that the predator is still far from any one individual, as in predator eavesdropping. This might occur, for example, when a parasitic fly is inside a field with many calling crickets (Zuk et al., 2006), or when a hawk that has approached a bird group hears many alarm calls from different individuals around it (Perrins, 1968). We refer to this situation as “prey targeting within a signaling group,” and there is the potential for the predator's attack behavior to be influenced by the signals. We will return to this idea in section The Cocktail Party Effect: Is There an Auditory Analog of the Confusion Effect?, where we ask whether the predator could be confused by multiple signals after first discussing the confusion effect in more simple situations.
The idea that predators might be confused by the rapid motion of their prey was discussed long ago (Grinnell, 1903; Miller, 1922), and a small literature has developed to address the issue experimentally (Milinski, 1977; Jeschke and Tollrian, 2007). Jeschke and Tollrian (2007) summarized 25 studies, with 16 of these positively demonstrating a confusion effect. All but three of these studies focused on visually orienting predators, with the few exceptions involving tactile orientation. These studies demonstrate that the magnitude of the confusion effect can be large. In a laboratory study on fish, for example, Landeau and Terborgh (1986) found that predators caught 100% of prey when the prey were solitary, but only 13% when the prey were in groups of 5. Other laboratory studies on fish have shown that this effect is a result of the predator being less able to target an individual prey item (Ioannou et al., 2008). Another set of studies have used neural network modeling and an experimental setup in which humans try to capture moving objects on a computer screen. Again, capture rate decreases as the number of potential targets increases. This effect can occur when prey move independently of each other (Ruxton et al., 2007), but may be even more prominent when prey coordinate their movement following an attack (Ioannou et al., 2012). Finally, recent work has focused on how the confusion effect can be enhanced by striping patterns on the prey (e.g., Hogan et al., 2016).
An important pattern found repeatedly in empirical and theoretical evaluations of the confusion effect is that it is lessened by differences among the group members. In other words, there is an “oddity effect” in which individuals that look different from the majority of the group are captured at a higher rate. This result has been shown particularly in fish. In the experiment of Landeau and Terborgh (1986), an odd fish (one individual dyed blue out of eight normally silvered fish in total) was more often captured than when prey types were evenly distributed (four blue fish, four natural fish). Theodorakis (1989) showed similar results for body size; fish of a size class that constitutes a minority of the group were targeted more often. This phenomenon probably explains why fish with odd phenotypes leave schools when predation pressure increases (Wolf, 1985) and why rare fish appear to be captured more often in coral reefs (Almany et al., 2007). The same effect has been shown in experiments with humans analyzed through neural networks: the confusion effect is less pronounced in heterogeneous groups (Tosh et al., 2006; Ruxton et al., 2007).
The oddity effect may explain why different species in mixed-species groups often have similar phenotypes. Moynihan (1968) was among the first to notice similarities among the participants in avian mixed-species groups and argued that species might become morphologically and behaviorally similar to facilitate intra-group communication. Barnard (1979) viewed this idea as a group-selectionist argument and suggested that similarities were caused instead by the oddity effect. Lost in the ensuing discussion was the question of whether there really are similarities within mixed-species groups beyond what we would expect from shared ancestry or shared habitat. Beauchamp and Goodale (2011) reviewed cases of putative plumage mimicry in avian groups and found support for the idea: in 14/22 cases, two species in mixed-species groups were ranked by human observers as more alike than other species that are either closely related or live in similar habitats. Meta-analyses of mixed-species avian groups have also found that birds of similar sizes tend to associate together (Sridhar et al., 2012; Mammides et al., 2018), and similar patterns have been shown in the fish literature: fish groups are often strongly size-assorted (Krause et al., 1996), and schooling among fish with similar appearances has also been described (Pereira et al., 2011). In many cases such resemblances may be examples of ecological assortment, although some of the most striking examples suggest mimicry (Beauchamp and Goodale, 2011).
The oddity effect may be complicated by interactions with conspicuousness and size. Tosh et al. (2007) found through neural network analyses that cryptic prey were actually safer when rare in groups mostly composed of conspicuous prey. Rodgers et al. (2013) supported this result in lab studies with Daphnia. Later, Rodgers et al. (2015) found that larger Daphnia were always preferentially attacked, even when constituting the majority of a group, perhaps because they were more obvious and profitable. Here again asymmetries between species come into play. If one species is less preferred than another it may be selected to remain distinguishable from those more preferred.
To summarize this section, one benefit of grouping is that a large number of fleeing individuals can distract the predator from targeting a single prey. The confusion effect is lessened in groups that are more heterogeneous. Species that look alike may be selected to associate together, and there may be a force over evolutionary time toward phenotypic convergence. However, some heterogeneity in mixed-species groups can occur when a species joins others more preferred by shared predators.
The Cocktail Party Effect: Is There an Auditory Analog of the Confusion Effect?
In drawing a comparison between predator eavesdropping and prey targeting, as we did in section Grouping and the Confusion Effect on the confusion effect, one large difference still remains: predator eavesdropping implies that prey signaling increases predator detection, but prey targeting need not involve prey signaling at all. One can then ask whether there is any evidence that synchronous signaling by members of a group or aggregation (diagrammatized in Figure 1D) produces confusion (Table 1, point 5). This question leads us from the visual to the auditory modality, where signaling can more easily be turned on and off. More specifically, it leads to the phenomenon known as the cocktail party effect: how can people concentrate on one person's speech in a crowded room when everyone is talking at the same time (Bronkhorst, 2000)?
As the above definition of the cocktail party effect implies, much of the work in this field has concentrated on humans, and some reviews emphasize the uniqueness of speech and speech recognition (Bronkhorst, 2000). However, the universal qualities of the problem have been emphasized by Bee and Micheyl (2008) in their article “‘The Cocktail party problem’: What is it? How can it be solved? And why should animal behaviorists study it.” They convincingly argued that many animals produce their signals under noisy conditions, and especially in environments with many other conspecific and/or heterospecific signalers. Bee and Micheyl (2008) and Hulse (2002) fit the cocktail party effect in the wider field of auditory scene analysis (Bregman, 1990), wherein animals take in sounds from a complex auditory environment and assign them to distinct sources.
The key to coping with the cocktail party effect, or performing auditory scene analysis, is finding similarities between sounds. Commonalties between sounds lead to their “integration” and assignment to one sound source, whereas differences among sounds lead to “segregation” (Bee and Micheyl, 2008). There are two components to auditory scene analysis: one is the parsing of sounds in a temporal sequence (sequential segregation), and the other is the parsing of sounds made at one time, but at different frequencies (i.e., perceived to be at different pitch, known as simultaneous segregation). Sequential segregation, of greater interest for this article, can be performed by looking at differences in frequency, in repetitive characteristics of the sound over time, in common modulation patterns over time, and in differences of spatial position (Bee and Micheyl, 2008; Farris and Ryan, 2011). A famous experimental paradigm known as “two-frequency spatial segregation tests” (see Figure 1 in Itatani and Klump, 2017) has been used to demonstrate sequential segregation by humans. If the frequency between two sounds presented from the same location is not very different, the human listener perceives one sound source, but if the frequency difference is greater, the listener perceives two sound sources. Ingenious experiments with animals have translated this paradigm to other species. For example, taking advantage of frog's phonotaxis to a speaker that broadcasts calls at a species-specific rate, or in a species-specific order, scientists can demonstrate that the frogs are able to segregate certain calls separated to a certain degree by frequency or spatial position, but not others separated by less than this threshold (Farris and Ryan, 2011; Nityananda and Bee, 2011).
These studies have shown that animals are able to cope with the cocktail party effect so that they can recognize and localize conspecific calls with high accuracy and little error. For example, some chorusing insects such as crickets have highly tuned frequency selectivity and “neuronal gain control” that provoke strong responses to conspecific calls (Schmidt and Römer, 2011). Tree frogs, a model taxon for the cocktail party effect, rely not only on such “matched frequency filtering” (Bee, 2015) but show many abilities to sequentially segregate sound based on spatial positioning (Bee and Riemersma, 2008) and amplitude modulation patterns (Lee et al., 2017). If prey species can deal with the cocktail party effect more effectively and quickly than predators, they could reach their mating targets while predators remain confused. Given that selection for the localization of a mate is very strong, it is likely the solution of a prey species might be better than a predator's, which usually needs to localize many different prey species. However, experiments are needed to further test this idea (Table 1, point 6).
The fact that similarities between signals impede the segregation of different sound sources makes it seem plausible that predators can be confused when multiple prey individuals are signaling simultaneously in a group. However, is there any evidence for this hypothesis? Echo-locating bats, which must hear sounds reflected off objects, struggle in cluttered arenas, and change their echolocation patterns in response, demonstrating that many sounds from different sources can be distracting (Moss and Surlykke, 2010). Similarly, a bat that hunts using auditory cues was shown to switch to echolocation in a noisy habitat, again indicating that multiple sources of noise can degrade the sensory pathway for prey detection (Gomes et al., 2016). Concurrent sources of noise can also interfere with the neuronal mapping of sound in the acoustically orienting barn owl, Tyto alba (Keller and Takahashi, 2005). However, more concrete evidence for a confusion effect due to group signaling is as yet missing. To test this hypothesis most directly, one should look at the effectiveness of predator attacks when prey groups contain different numbers of calling individuals. A confusion effect would be demonstrated if increased group sizes led to more targeting and localization errors.
Using the analogy to the confusion effect in the visual modality, the cocktail party effect might provide a selective force making the signals of heterospecifics that call together more similar. For example, researchers reported long ago similarities between the alarm calls of different bird species in mixed-species flocks (Marler, 1955; Jurisevic and Sanderson, 1994). This observation has been attributed to the different prey species all making signals that predatory hawks find hard to detect (Klump et al., 1986; Jones and Hill, 2001) and to similarities between species in their auditory physiology (Henry et al., 2016). However, it is also possible that alarm calls have been selected to be similar because similar sounds coming simultaneously from different places disrupt a predator's localization abilities (Grinnell, 1903; Perrins, 1968). Further, calls might sound similar at long distances from which they are heard by predators but still have subtle differences detectable at close range by other prey (Ruxton, 2009). Note that convergence seems particularly likely for alarm and contact calls in mixed-species moving groups since these types of signals should be under weaker divergent selection than sexual advertisement calls. Also, differences among prey in the degree to which they are preferred by predators may have similar effects as those discussed in section Grouping and the Confusion Effect. That is, in mixed-species aggregations or moving groups, if a predator prefers a certain prey species, other species might be selected to differentiate themselves, even if the overall attack rate on the group thereby increases due to a weakening of the confusion effect by an oddity effect.
In summary for this section, investigations of the cocktail party effect and ways of coping with it imply that predators can be confused by similar sounds coming from multiple locations. Hence, the cocktail party effect may cause an auditory confusion effect. If this is true, then prey may not need to make their calls less conspicuous. Generally, one way for prey to avoid predator eavesdropping is to group together and have a more effective solution to the cocktail party effect than the predator. However, direct tests of this hypothesis require data on capture rates in groups of different sizes for acoustically orienting predators. We note in closing that confusion effects may not be confined to visual or auditory domains but could also be found in other modalities (e.g., olfactory confusion?), although the lack of a large body of literature on how humans and non-human animals parse different sources of signals in such modalities makes the ideas more speculative (Table 1, point 7).
Conclusions
Ultimately, the field of predator eavesdropping aims to understand how prey can maximize communication with one another while minimizing detection by predators. In this contribution, we point out that prey are often associated with other prey species, and thus that their detection risk may be affected by how well the predator detects the other species. We expect prey to avoid grouping with more detectable species. Other expectations include that prey should select groupings with species that are more preferred by predators as long as this does not lead to them being more easily detected, and for more detectable or preferred prey to attempt to hide in groups that include larger numbers of other prey. We also discuss how prey detection can be disrupted when predators get confused, just as when they do not sense prey, and how this confusion effect is affected by group size and composition. Because similarity increases confusion, frequent participants in mixed-species groups might be selected to be more similar in their phenotypic attributes and signaling. At the same time, heterogeneity may still be favored in groups if predators prefer one prey species in the mix, making it beneficial for the other species to appear different. Other situations favoring heterogeneous mixed-species groups could arise if species are vigilant for predators that are dangerous for companion species although not for themselves (Rasa, 1983; Ridley et al., 2007), or if one species provides protection for the other (Quinn and Ueta, 2008).
Given the degree to which animal species participate in different kinds of groups or aggregations, we hope that the field of predator eavesdropping will continue to expand toward fully understanding the social environment in which signaling takes place. In section The Cocktail Party effect: Is There an Auditory Analog of the Confusion Effect?, we argue that it would be worthwhile to manipulate group size in chorusing animals and determine the success rate of acoustically orienting predators. But observational data can also be useful to determine inter-individual distances between conspecifics, at what scale conspecifics are grouped together, where heterospecifics are positioned relative to them, how often different species signal at the same time, the degree to which they share predators, and their relative dominance and mobility. We believe exploring this rich set of variables will uncover further complexity in how predators influence prey communication systems.
Author Contributions
EG, GR, and GB conceived the article collectively. EG wrote the first draft, and then EG, GR, and GB worked together on subsequent revisions.
Funding
EG is grateful for the support of the Special Talents Recruitment Program of Guangxi University, and National Science Foundation of China grants 31560119 and 31770424. The funding organizations played no role in the development of the ideas in this article, or in the preparation, review, or approval of the manuscript.
Conflict of Interest Statement
The authors declare that the research was conducted in the absence of any commercial or financial relationships that could be construed as a potential conflict of interest.
Acknowledgments
EG thanks the Animal Behavior Society for help attending the How Enemies Shape Communication Systems symposium. We also thank Ximena Bernal and Rachel Page for their invitation to the symposium, they and the other participants for their discussion and encouragement, and Dr. Bernal, Michael Kopp and Jeffrey Lucas for their constructive comments that helped improve the manuscript.
References
Almany, G. R., Peacock, L. F., Syms, C., McCormick, M. I., and Jones, G. P. (2007). Predators target rare prey in coral reef fish assemblages. Oecologia 152, 751–761. doi: 10.1007/s00442-007-0693-3
Barnard, C. J. (1979). Predation and the evolution of social mimicry in birds. Am. Nat. 113, 613–618. doi: 10.1086/283419
Beauchamp, G. (2014). Social Predation: How Group Living Benefits Predators and Prey. London: Academic Press.
Beauchamp, G., and Goodale, E. (2011). Plumage mimicry in avian mixed-species flocks: more or less than meet the eye? Auk 128, 487–496. doi: 10.1525/auk.2011.11016
Bee, M. A. (2015). Treefrogs as animal models for research on auditory scene analysis and the cocktail party problem. Int. J. Psychophysiol. 95, 216–237. doi: 10.1016/j.ijpsycho.2014.01.004
Bee, M. A., and Micheyl, C. (2008). The “Cocktail Party Problem”: what is it? How can it be solved? And why should animal behaviorists study it? J. Comp. Psychol. 122, 235–251. doi: 10.1037/0735-7036.122.3.235
Bee, M. A., and Riemersma, K. K. (2008). Does common spatial origin promote the auditory grouping of temporally separated signal elements in grey treefrogs? Anim. Behav. 76, 831–843. doi: 10.1016/j.anbehav.2008.01.026
Bernal, X. E., Rand, A. S., and Ryan, M. J. (2006). Acoustic preferences and localization performance of blood-sucking flies (Corethrella coquillett) to túngara frog calls. Behav. Ecol. 17, 709–715. doi: 10.1093/beheco/arl003
Boulay, J., Aubernon, C., Ruxton, G. D., Hédouin, V., Deneubourg, J.-L., and Charabidzé, D. (2019). Mixed-species aggregations in arthropods. Insect Sci. 26, 2–19. doi: 10.1111/1744-7917.12502
Bregman, A. S. (1990). Auditory Scene Analysis: The Perceptual Organization of Sound. Cambridge, MA: MIT Press. doi: 10.7551/mitpress/1486.001.0001
Bronkhorst, A. W. (2000). The cocktail party phenomenon: a review of speech intelligibility in multiple-talker conditions. Acustica/Acta Acust. 86, 117–128.
Chek, A. A., Bogart, J. P., and Lougheed, S. C. (2003). Mating signal partitioning in multi-species assemblages: a null model test using frogs. Ecol. Lett. 6, 235–247. doi: 10.1046/j.1461-0248.2003.00420.x
Cody, M. L., and Brown, J. H. (1969). Song asynchrony in neighboring bird species. Nature 222, 778–780. doi: 10.1038/222778b0
Cummings, M. E., Rosenthal, G. G., and Ryan, M. J. (2003). A private ultraviolet channel in visual communication. Proc. R. Soc. B. 270, 897–904. doi: 10.1098/rspb.2003.2334
Farris, H. E., and Ryan, M. J. (2011). Relative comparisons of call parameters enable auditory grouping in frogs. Nat. Commun. 2:410. doi: 10.1038/ncomms1417
Fitzgibbon, C. D. (1990). Mixed-species grouping in Thomson's and Grant's gazelles: the antipredator benefits. Anim. Behav. 39, 1116–1126. doi: 10.1016/S0003-3472(05)80784-5
Gibson, R. M., Aspbury, A. S., and McDaniel, L. L. (2002). Active formation of mixed–species grouse leks: a role for predation in lek evolution? Proc. R. Soc. B. 269, 2503–2507. doi: 10.1098/rspb.2002.2187
Given, M. F. (1990). Spatial distribution and vocal interaction in Rana clamitans and R. virgatipes. J. Herpetol. 24, 377–382. doi: 10.2307/1565053
Gomes, D. G. E., Page, R. A., Geipel, I., Taylor, R. C., Ryan, M. J., and Halfwerk, W. (2016). Bats perceptually weight prey cues across sensory systems when hunting in noise. Science 353, 1277–1280. doi: 10.1126/science.aaf7934
Goodale, E., and Beauchamp, G. (2010). The relationship between leadership and gregariousness in mixed-species bird flocks. J. Avian Biol. 41, 99–103. doi: 10.1111/j.1600-048X.2009.04828.x
Goodale, E., Beauchamp, G., Magrath, R., Nieh, J. C., and Ruxton, G. D. (2010). Interspecific information transfer influences animal community structure. Trends Ecol. Evol. 25, 354–361. doi: 10.1016/j.tree.2010.01.002
Goodale, E., Beauchamp, G., and Ruxton, G. D. (2017). Mixed-species Animal Groups: Behavior, Community Structure and Conservation. London: Academic Press.
Gu, H., Chen, J., Ewing, H., Liu, X., Zhao, J., and Goodale, E. (2017). Heterospecific attraction to the vocalizations of birds in mass-fruiting trees. Behav. Ecol. Sociobiol. 71:82. doi: 10.1007/s00265-017-2312-6
Henry, K. S., Gall, M. D., Vélez, A., and Lucas, J. R. (2016). “Avian auditory processing at four different scales: variation among species, seasons, sexes, and individuals,” in Psychological Mechanisms in Animal Communication, eds M. A. Bee and C. T. Miller (New York, NY: Spring International Publishing), 17–55. doi: 10.1007/978-3-319-48690-1_2
Herzing, D. L., and Elliser, C. R. (2013). Directionality of sexual activities during mixed-species encounters between Atlantic spotted dolphin (Stenella frontalis) and Bottlenose dolphins (Tursops truncatus). Int. J. Comp. Psyc. 26, 124–134. Available online at: https://escholarship.org/uc/item/3np7z795
Hödl, W. (1977). Call differences and calling site segregation in anuran species from Central Amazonian floating meadows. Oecologia 28, 351–363. doi: 10.1007/BF00345990
Hogan, B. G., Cuthill, I. C., and Scott-Samuel, N. E. (2016). Dazzle camouflage, target tracking, and the confusion effect. Behav. Ecol. 27, 1547–1551. doi: 10.1093/beheco/arw081
Hulse, S. H. (2002). Auditory scene analysis in animal communication. Adv. Stud. Behav. 31, 163–200. doi: 10.1016/S0065-3454(02)80008-0
Hunt, G. L. Jr., Harrison, N. M., Hamner, W. M., and Obst, B. S. (1988). Observations of a mixed-species flock of birds foraging on euphausiids near St. Matthew Island, Bering Sea. Auk 105, 345–349. doi: 10.2307/4087500
Ioannou, C. C., Guttal, V., and Couzin, I. D. (2012). Predatory fish select for coordinated collective motion in virtual prey. Science 337, 1212–1215. doi: 10.1126/science.1218919
Ioannou, C. C., Tosh, C. R., Neville, L., and Krause, J. (2008). The confusion effect - from neural networks to reduced predation risk. Behav. Ecol. 19, 126–130. doi: 10.1093/beheco/arm109
Itatani, N., and Klump, G. M. (2017). Animal models for auditory streaming. Philos. T. R. Soc. B 372:20160112. doi: 10.1098/rstb.2016.0112
Jeschke, J. M., and Tollrian, R. (2007). Predator swarming: which predators become confused and why? Anim. Behav. 74, 387–393. doi: 10.1016/j.anbehav.2006.08.020
Johnstone, R. A., and Bshary, R. (2002). From parasitism to mutualism: partner control in asymmetric interactions. Ecol. Lett. 5, 634–639. doi: 10.1046/j.1461-0248.2002.00358.x
Jones, K. J., and Hill, W. L. (2001). Auditory perception of hawks and owls for passerine alarm calls. Ethology 107, 717–726. doi: 10.1046/j.1439-0310.2001.00698.x
Jurisevic, M. A., and Sanderson, K. J. (1994). Alarm vocalizations in Australian birds–convergent characteristics and phylogenetic differences. Emu 94, 67–77. doi: 10.1071/MU9940067
Keller, C. H., and Takahashi, T. T. (2005). Localization and identification of concurrent sounds in the owl's auditory space map. J. Neurosci. 25, 10446–10461. doi: 10.1523/JNEUROSCI.2093-05.2005
Klump, G. M., Kretzschmar, E., and Curio, E. (1986). The hearing of an avian predator and its avian prey. Behav. Ecol. Sociobiol. 18, 317–323. doi: 10.1007/BF00299662
Krakauer, D. C. (1995). Groups confuse predators by exploiting perceptual bottlenecks: a connectionist model of the confusion effect. Behav. Ecol. Sociobiol. 36, 421–429. doi: 10.1007/BF00177338
Krause, J., Godin, J.-G. J., and Brown, D. (1996). Size-assortiveness in multi-species fish shoals. J. Fish Biol. 49, 221–225. doi: 10.1111/j.1095-8649.1996.tb00018.x
Landeau, L., and Terborgh, J. (1986). Oddity and the confusion effect in predation. Anim. Behav. 34, 1372–1380. doi: 10.1016/S0003-3472(86)80208-1
Lee, N., Ward, J. L., Velez, A., Micheyl, C., and Bee, M. A. (2017). Frogs exploit statistical regularities in noisy acoustic scenes to solve cocktail-party-like problems. Curr. Biol. 27, 743–750. doi: 10.1016/j.cub.2017.01.031
Luther, D. (2009). The influence of the acoustic community on songs of birds in a neotropical rain forest. Behav. Ecol. 20, 864–871. doi: 10.1093/beheco/arp074
Magrath, R. D., Haff, T. M., Fallow, P. M., and Radford, A. N. (2015). Eavesdropping on heterospecific alarm calls: from mechanisms to consequences. Biol. Rev. 90, 560–586. doi: 10.1111/brv.12122
Mammides, C., Chen, J., Goodale, U. M., Kotagama, S. W., and Goodale, E. (2018). Measurement of species associations in mixed-species bird flocks across environmental and human disturbance gradients. Ecosphere 9:e02324. doi: 10.1002/ecs2.2324
Mathis, A., and Chivers, D. P. (2003). Overriding the oddity effect in mixed-species aggregations: group choice by armored and nonarmored prey. Behav. Ecol. 14, 334–339. doi: 10.1093/beheco/14.3.334
McGregor, P. K., and Peake, T. M. (2000). Communication networks: social environments for receiving and signalling behaviour. Acta Ethol. 2, 71–81. doi: 10.1007/s102110000015
Milinski, M. (1977). Do all members of a swarm suffer the same predation? Z. Tierpsychol. 45, 373–388. doi: 10.1111/j.1439-0310.1977.tb02027.x
Miller, R. C. (1922). The significance of the gregarious habit. Ecology 3, 122–126. doi: 10.2307/1929145
Moss, C. F., and Surlykke, A. (2010). Probing the natural scene by echolocation in bats. Front. Behav. Neurosci. 4:33. doi: 10.3389/fnbeh.2010.00033
Moynihan, M. (1968). Social mimicry: character convergence versus character displacement. Evolution 22, 315–331. doi: 10.1111/j.1558-5646.1968.tb05900.x
Nakano, R., Skals, N., Takanashi, T., Surlykke, A., Koike, T., and Yoshida, K., et al. (2008). Moths produce extremely quiet ultrasonic courtship songs by rubbing specialized scales. Proc. Natl. Acad. Sci. U.S.A. 105, 11812–11817. doi: 10.1073/pnas.0804056105
Neill, S. R. S. J., and Cullen, J. M. (1974). Experiments on whether schooling by their prey affects the hunting behavior of cephalopods and fish predators. J. Zool. 172, 549–569. doi: 10.1111/j.1469-7998.1974.tb04385.x
Nityananda, V., and Bee, M. A. (2011). Finding your mate at a cocktail party: frequency separation promotes auditory stream segregation of concurrent voices in multi-species frog choruses. PLoS ONE 6:e21191. doi: 10.1371/journal.pone.0021191
Pagani-Núñez, E., Xia, X., Beauchamp, G., He, R., Husson, J. H. D., and Liang, D., et al. (2018). Are vocal characteristics related to leadership patterns in mixed-species bird flocks? J. Avian Biol. 2018:e01674. doi: 10.1111/jav.01674
Page, R. A., Ryan, M. J., and Bernal, X. E. (2013). “Be loved, be prey, be eaten,” in Animal Behavior Case Studies: Integration and Application of Animal Behavior, ed. K. Yasakawa (Santa Barbara, CA: ABC-CLIO Editorial), 123–154.
Pereira, P. H. C., Feitosa, J. L. L., and Ferreira, B. P. (2011). Mixed-species schooling behavior and protective mimicry involving coral reef fish from the genus Haemulon (Haemulidae). Neotrop. Ichthyol. 9, 741–746. doi: 10.1590/S1679-62252011005000037
Perrins, C. (1968). The purpose of the high-intensity alarm calls in small passerines. Ibis 110, 200–201. doi: 10.1111/j.1474-919X.1968.tb00441.x
Phelps, S. M., Rand, A. S., and Ryan, M. J. (2007). The mixed-species chorus as public information: túngara frogs eavesdrop on a heterospecific. Behav. Ecol. 18, 108–114. doi: 10.1093/beheco/arl063
Powell, G. V. N. (1985). Sociobiology and adaptive significance of interspecific foraging flocks in the Neotropics. Ornithol. Monogr. 36, 713–732. doi: 10.2307/40168313
Quinn, J. L., and Ueta, M. (2008). Protective nesting associations in birds. Ibis 150, 146–167. doi: 10.1111/j.1474-919X.2008.00823.x
Rasa, O. A. E. (1983). Dwarf mongoose and hornbill mutualism in the Taru Desert, Kenya. Behav. Ecol. Sociobiol. 12, 181–190. doi: 10.1007/BF00290770
Ridley, A. R., Child, M. F., and Bell, M. B. V. (2007). Interspecific audience effects on the alarm-calling behaviour of a kleptoparasitic bird. Biol. Lett. 3, 589–591. doi: 10.1098/rsbl.2007.0325
Rodgers, G. M., Downing, B., and Morrell, L. J. (2015). Prey body size mediates the predation risk associated with being “odd”. Behav. Ecol. 26, 242–246. doi: 10.1093/beheco/aru185
Rodgers, G. M., Kimbell, H., and Morrell, L. J. (2013). Mixed-phenotype grouping: the interaction between oddity and crypsis. Oecologia 172, 59–68. doi: 10.1007/s00442-012-2473-y
Ruxton, G. D. (2009). Non-visual crypsis: a review of the empirical evidence for camouflage to senses other than vision. Philos. Trans. R. Soc. B 364, 549–557. doi: 10.1098/rstb.2008.0228
Ruxton, G. D., Jackson, A. L., and Tosh, C. R. (2007). Confusion of predators does not rely on specialist coordinated behavior. Behav. Ecol. 18, 590–596. doi: 10.1093/beheco/arm009
Schmidt, A. K. D., Roemer, H., and Riede, K. (2013). Spectral niche segregation and community organization in a tropical cricket assemblage. Behav. Ecol. 24, 470–480. doi: 10.1093/beheco/ars187
Schmidt, A. K. D., and Römer, H. (2011). Solutions to the cocktail party problem in insects: selective filters, spatial release from masking and gain control in tropical crickets. PLoS ONE 6:e28593. doi: 10.1371/journal.pone.0028593
Sridhar, H., and Guttal, V. (2018). Friendship across species borders: factors that facilitate and constrain heterospecific sociality. Philos. Trans. R. Soc. B 373:20170014. doi: 10.1098/rstb.2017.0014
Sridhar, H., Srinivasan, U., Askins, R. A., Canales-Delgadillo, J. C., Chen, C.-C., and Ewert, D. N., et al. (2012). Positive relationships between association strength and phenotypic similarity characterize the assembly of mixed-species bird flocks worldwide. Am. Nat. 180, 777–790. doi: 10.1086/668012
Srygley, R. B., and Penz, C. M. (1999). Lekking in neotropical owl butterflies, Caligo illioneus and C. oileus (Lepidoptera: Brassolinae). J. Insect Behav. 12, 81–103. doi: 10.1023/A:1020981215501
Theodorakis, C. W. (1989). Size segregation and the effects of oddity on predation risk in minnow schools. Anim. Behav. 38, 496–502. doi: 10.1016/S0003-3472(89)80042-9
Tobias, J. A., Planque, R., Cram, D. L., and Seddon, N. (2014). Species interactions and the structure of complex communication networks. Proc. Natl. Acad. Sci. U.S.A. 111,1020–1025. doi: 10.1073/pnas.1314337111
Tosh, C. R., Jackson, A. L., and Ruxton, G. D. (2006). The confusion effect in predatory neural networks. Am. Nat. 167, E52–E65. doi: 10.1086/499413
Tosh, C. R., Jackson, A. L., and Ruxton, G. D. (2007). Individuals from different-looking animal species may group together to confuse shared predators: simulations with artificial neural networks. Proc. R. Soc. B. 274, 827–832. doi: 10.1098/rspb.2006.3760
Trillo, P. A., Bernal, X. E., Caldwell, M. S., Halfwerk, W. H., Wessel, M. O., and Page, R. A. (2016). Collateral damage or a shadow of safety? The effects of signaling heterospecific neighbours on the risks of parasitism and predation. Proc. R. Soc. B. 283:20160343. doi: 10.1098/rspb.2016.0343
Ward, P., and Zahavi, A. (1973). The importance of certain assemblages of birds as “information-centres” for food-finding. Ibis 115, 517–534. doi: 10.1111/j.1474-919X.1973.tb01990.x
Wolf, N. G. (1985). Odd fish abandon mixed-species groups when threatened. Behav. Ecol. Sociobiol. 17, 47–52. doi: 10.1007/BF00299428
Zuk, M., and Kolluru, G. R. (1998). Exploitation of sexual signals by predators and parasitoids. Q. Rev. Biol. 73, 415–438. doi: 10.1086/420412
Keywords: animal sociality, cocktail party problem, communication networks, confusion effect, group living, mixed-species animal groups, mixed-species choruses, predator eavesdropping
Citation: Goodale E, Ruxton GD and Beauchamp G (2019) Predator Eavesdropping in a Mixed-Species Environment: How Prey Species May Use Grouping, Confusion, and the Cocktail Party Effect to Reduce Predator Detection. Front. Ecol. Evol. 7:141. doi: 10.3389/fevo.2019.00141
Received: 19 October 2018; Accepted: 09 April 2019;
Published: 08 May 2019.
Edited by:
Ximena E. Bernal, Purdue University, United StatesReviewed by:
Jeffrey Lucas, Purdue University, United StatesMichael Kopp, Aix-Marseille Université, France
Copyright © 2019 Goodale, Ruxton and Beauchamp. This is an open-access article distributed under the terms of the Creative Commons Attribution License (CC BY). The use, distribution or reproduction in other forums is permitted, provided the original author(s) and the copyright owner(s) are credited and that the original publication in this journal is cited, in accordance with accepted academic practice. No use, distribution or reproduction is permitted which does not comply with these terms.
*Correspondence: Eben Goodale, ZWJlbmdvb2RhbGVAZ3h1LmVkdS5jbg==; ZWJlbi5nb29kYWxlQG91dGxvb2suY29t