- Institute of Biological Sciences, Applied Ecology and Phycology, University of Rostock, Rostock, Germany
Mining potash for fertilizer produces vast amounts of highly saline waste that is deposited in potash tailings piles. Rainfall washes the salts out, affecting the surrounding ecosystems. Only salt-tolerant organisms occur close to the piles, since other species cannot survive in these hypersaline conditions. Halophytic plant communities around tailings piles have been well investigated. However, studies exploring the biodiversity of the space behind the vegetation line that separates suitable salinities for plant growth from hostile conditions are rare. On top of the tailings piles, only micro-organisms thrive. This study, for the first time, explored the microalgae and cyanobacteria in biocrusts that inhabit potash tailings pile areas between the vegetation line and the pile body. Two biocrust types were studied: young biocrusts at or close to the tailings piles, and established biocrusts situated in the near surroundings. The estimated abundance of green algae, cyanobacteria and diatoms was studied using a direct microscopy approach, followed by the isolation and establishment of unialgal strains and morphological species determinations. Soil environmental characteristics were analyzed. Soil samples showed ECSat (electrical conductivity) values that drastically exceeded the scales commonly used to describe soil salinity, indicating extremely saline conditions. Indeed, the isolate composition was shaped by soil salinity parameters. Soil ECSat of young biocrusts tended to be higher than in established biocrusts, and filamentous green algae were most abundant. In contrast, established biocrusts tended to have a lower ECSat and were mostly dominated by filamentous cyanobacteria. Algal and cyanobacteria isolate composition differed significantly in young and established biocrusts, although the species number did not. Some of the salt-tolerant algal strains are assumed to be candidates for the formation of artificial biocrusts on the surface of the tailings. Attempts to “green” the piles by the establishment of higher plants to trap rainwater and therefore reduce the salt-output are difficult to apply. Plants require a thick layer of substrate to reduce the salinity, and the substrate easily erodes on the steep slopes. However, microalgae isolated from potash tailings pile areas seem promising, since they can survive on a thinner substrate layer and are already adapted to these hypersaline conditions.
Introduction
Anthropogenic salinization is increasingly affecting ecosystems worldwide. The main drivers are human activities such as extensive agriculture, inadequate water management, mining and the use of de-icing salts. A high ion load, mostly Na+, Ca2+, Mg2+, Cl–, CO32–,HCO3–, and SO42– (Williams, 1987) alters the flora and fauna of wetlands (Herbert et al., 2015) and freshwater systems (Cañedo-Argüelles et al., 2013; Kefford et al., 2016; Kaushal et al., 2018), and further affects the groundwater (Kloppmann et al., 2013) as well as terrestrial habitats such as grasslands (Pan et al., 2015) and farmland (Wang and Li, 2013). In addition to ecological problems, land and water salinization has socio-economic effects (Gorostiza and Saurí, 2019).
One of the main sources of salinization in Europe is potash mining (Cañedo-Argüelles et al., 2013). During the mining process, valuable components (mostly KCl and MgSO4) of the potash ore are separated from unusable salt residues (mostly NaCl), generating liquid and solid salt residues. The brine is piped into streams and the solid overburden is deposited in tailings piles. Further, the pile waste water (up to 330 SA, about 10 times that of marine sea water) leached by rainfall is either collected and discharged into streams or leaches out unhindered. Together, these processes lead to a high Na+ and Cl– output from potash tailings piles into the surrounding environment.
As a consequence, the load of salt ions in water bodies significantly affects the biotic communities, most often described as a decline in aquatic macroinvertebrate and fish diversity (Braukmann and Böhme, 2011; Arle and Wagner, 2013; Ladrera et al., 2017). Terrestrial habitats are also affected by potash tailings piles, and many studies have described the diversity of halophilic fauna and especially flora that replaced native species. Halophytes such as Aster tripolium, Salicornia europaea, and Suaeda maritima (Garve and Garve, 2000; Siefert et al., 2006; Woch and Trzcińska-Tacik, 2015), mainly occur in salt marshes along temperate coastal regions and only rarely inland, are often found next to potash tailings piles, underlining the saline character of these sites. Several habitats that emerged in conjunction with potash mining have even been designated as conservation areas because of the halophilic organisms.
In central Germany, dozens of potash tailings piles, some of them more than 200 m high, form the landscape next to various pile residues from previous smaller-scale mining. These large potash tailings piles are visible from a distance because of their unique appearance and lack of vegetation. Even though halophytes tolerate considerable concentrations of salt, the salinity of bare spoil material is too high to allow halophytes to colonize the piles. Often, a sharp vegetation line can be observed around the piles, following the prevailing salinity gradient. At higher salinities, only micro-organisms are able to thrive, but only a few studies have addressed their occurrence (Eilmus et al., 2007; Korsakova et al., 2013; Olsson et al., 2017). Therefore, the present study provides the first description of terrestrial micro-algal communities on potash tailings pile sites, which have so far been neglected.
Terrestrial algae are ecological key organisms in so-called biological soil crusts (biocrusts). These biocrusts are formed by a consortium of living organisms, which besides algae and cyanobacteria consists of heterotrophic bacteria, fungi, lichens and bryophytes, and their byproducts, creating a topsoil layer of inorganic particles bound together by organic materials. Biocrusts can be characterized as “ecosystem engineers” that form water-stable, aggregated surface layers. These layers have important multi-functional ecological roles in primary production, mineralization, bioweathering, dust trapping, and the stabilization of soils, slopes and entire landscapes, thereby affecting the nutrient and hydrological cycles across several scales (Weber et al., 2016). Consequently, biocrusts have their greatest impact in nutrient- or water-poor habitats that are little influenced by higher plants, often dominating regions with harsh, mostly arid environmental conditions worldwide (Bowker et al., 2010; Weber et al., 2016; Felde et al., 2017).
Biocrusts have been described on mining residues such as abandoned sand-mine areas (Doudle et al., 2011; Williams et al., 2019) and gold-mine tailings (Nyenda et al., 2019), as well as zinc- and lead-mine spoils (Trzcińska and Pawlik-Skowrońska, 2008). Thus, biocrusts can even cope with artificial and toxic conditions. Potash tailings piles, however, have not yet been studied, but various terrestrial algae inhabit other saline ecosystems (Tsujimura et al., 1998; Vinogradova and Darienko, 2008), although comprehensive studies are still rare.
Microalgae and cyanobacteria in biocrusts are well adapted to their often extreme habitats. A key factor for terrestrial phototrophic micro-organisms is water availability, which is strongly influenced by salinity. Although desiccation effects are physiologically closely connected to those of salinity, both stressors reflect two different forms of water deprivation. While under salt stress conditions, algal cells are often still in contact to liquid water of diminished water potential, desiccation leads to strong cellular dehydration. However, both drought and salt stress protective mechanisms work similarly. Desiccation tolerance is given by the formation of water-holding structures such as thick extracellular mucilage layers by the excretion of exopolymeric substances (EPS) and the formation of biofilms, cell colonies and aggregates, Karsten and Holzinger (2012). In turn, EPS have been suggested to have a salt-buffering effect (Chen et al., 2006; Lan et al., 2010; Rossi et al., 2018). Gustavs et al. (2010) showed that some terrestrial algae are able to produce organic osmolytes, which play another important role in desiccation tolerance and in turn is a common mechanism of halotolerant algae to tolerate high salinities (Karsten, 2012). Consequently, terrestrial algae in biocrust communities could have the potential to thrive in the highly saline potash tailings pile sites.
These salt-tolerant microalgae could further be candidates for restoration of the potash tailings piles by artificial biocrust formation. Recent studies reported the successful application of artificial biocrust formation through the inoculation of cyanobacteria in different habitats (e.g., sand dunes) to promote revegetation and restoration of degraded areas (Wang et al., 2009; Doudle et al., 2011; Lan et al., 2014). In the present case, a biocrust cover could potentially trap rainwater and thus reduce the harmful and uncontrolled salt erosion. However, selection of suitable algal isolates for artificial crust formation is crucial for the success of restoration (Román et al., 2018; Giraldo-Silva et al., 2019). Consequently, microalgae and cyanobacteria that are already adapted to the tailings pile conditions might be the most suitable taxa.
Therefore, this study aimed, for the first time, to identify, describe and isolate halotolerant biocrust microalgae and cyanobacteria in the environment nearest to potash tailings piles. Two methodological approaches were used to characterize the algal composition, direct microscopic observation of biocrust material, and a culture-dependent approach including purification and establishment of clonal isolates. The study focused on two different biocrust types that were observed during fieldwork, and that represent the soil salt gradient from the vegetation line to the tailings pile body: first, established biocrusts closer to the vegetation line, and second, young biocrusts found on top or close to the potash tailings. Based on these field observations, we hypothesized that a smaller number of species should occur in young biocrusts, due to the higher salt stress along with a larger number of salt-tolerant taxa compared to established crusts.
Materials and Methods
Study Sites and Terrestrial Algal Communities
The sampling was conducted at five different potash tailings pile sites in Lower Saxony and Saxony-Anhalt, Germany (Table 1 and Figures 1, 2). In both regions the mean annual temperature (1981–2010) is 9.3°C (Dwd, 2018). Saxony-Anhalt is generally drier with a mean annual precipitation of 579 mm (Mule, 2017) than Lower Saxony (787 mm). Three of the selected potash tailings piles derived from potash pits closed in the 1930s. These tailings piles were unprotected for approximately a century, which allowed uncontrolled leaching and a reduction in size (today up to 2 m in height) since their formation.
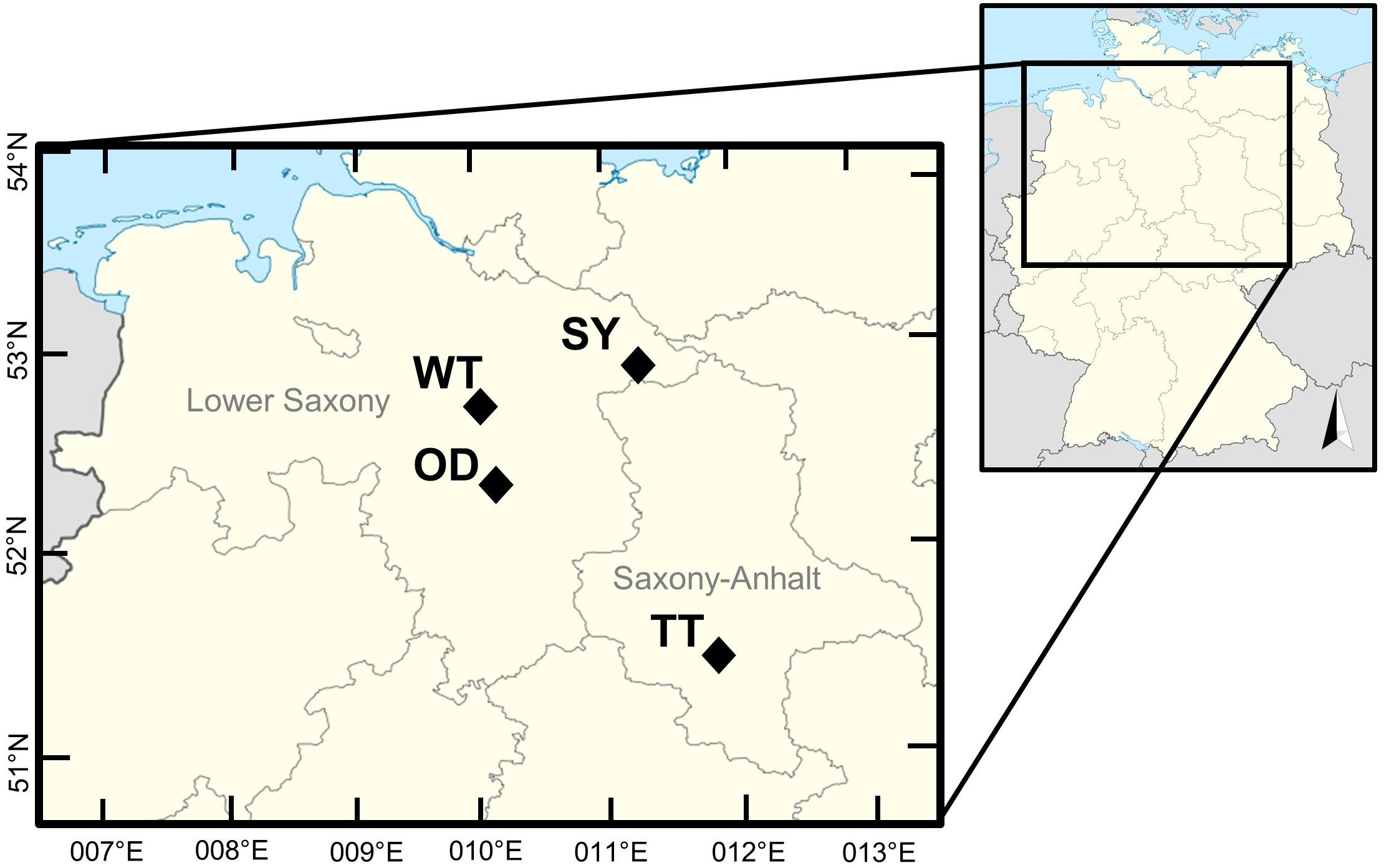
Figure 1. Sampling locations in Lower Saxony and Saxony-Anhalt (OD, Oedesse; SY, Shreyahn; TT, Teutschenthal; WT, Wietze; NN not included).
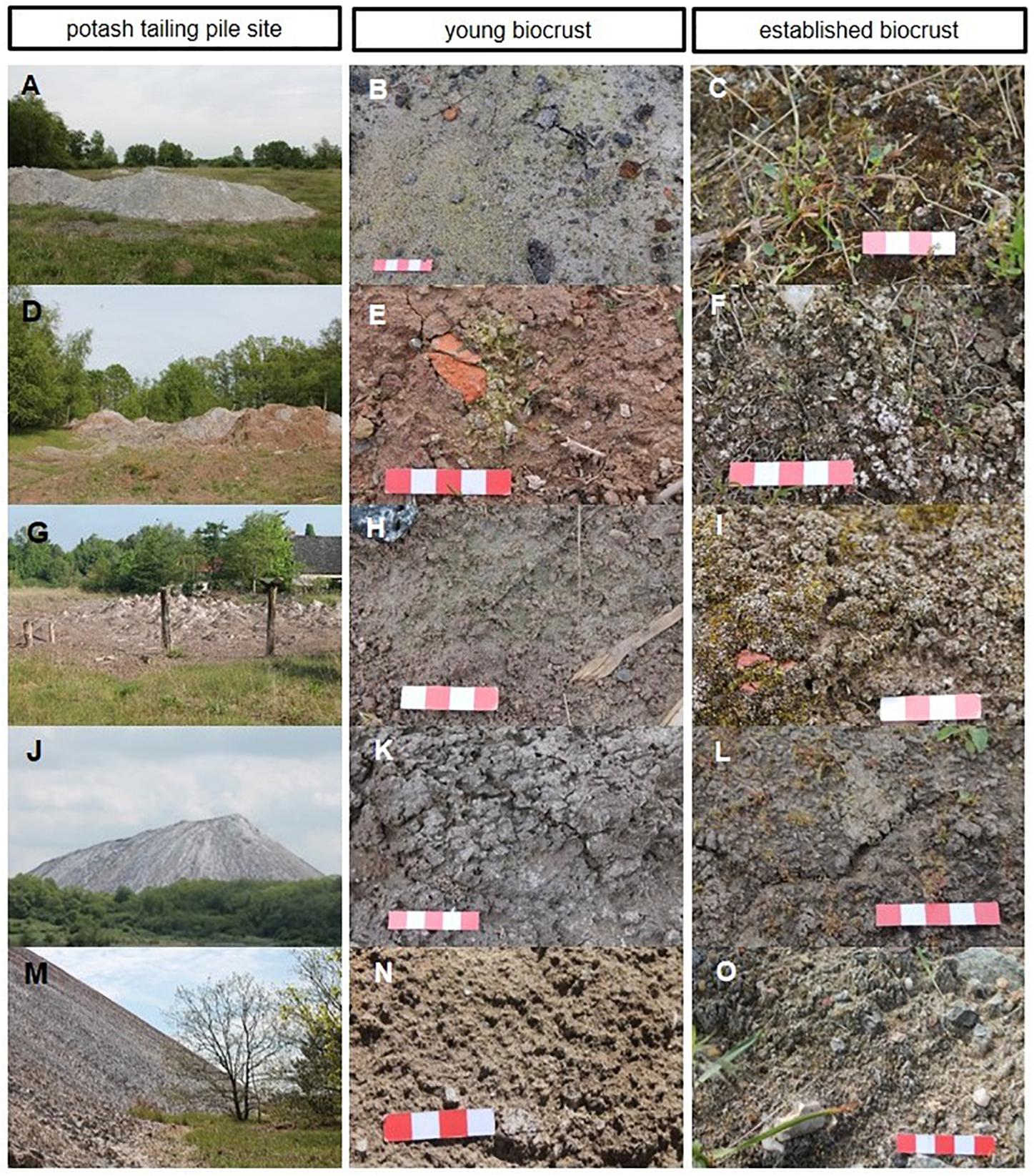
Figure 2. Potash tailings piles sites and the respective young and established terrestrial algal communities. (A–C) Shreyahn; (D–F) Oedesse; (G–I) Wietze; (J–L) Teutschenthal; (M–O) NN; one red or white square of the scale bar indicates 1 cm.
The site in Shreyahn (SY) features three isolated potash tailings pile residues, with a pond in the former pit, and is situated between a small forested area and farmland. It is part of a protected area and the largest “salt lick” of Lower Saxony. Facultative (e.g., Aster tripolium, Glaux maritima, Puccinellia distans, Trifolium fragiferum) and obligate halophytes (Spergularia marina) are present in the surrounding area (Jeckel, 1977). Well-developed biocrust communities including lichens and mosses cover primarily areas closest to of the potash tailings piles and are occasionally interspersed with small halophytes. Young green-algal biocrusts can form smaller patches directly on potash tailings pile material at the pile bottoms.
As in Shreyahn, the sampling site in Klein Oedesse (OD) is a part of a protected area (NSG Schwarzwasserniederung). Located in a woodland tract, the potash tailings pile is adjacent to a shallow pond, with halophytes such as Atriplex longipes, Glaux maritima, Plantago maritima, Sueda maritima, Trifolium fragiferum and others in the surroundings (Garve and Garve, 2000). Sparse, grasses and a juvenile pine tree grow directly on the tailings pile. Well-developed biocrusts could be found around the bottom of the tailings pile. Since the tailings pile is easily accessible, trampling regularly interrupted the biocrust cover. At one spot, a greenish algal cover was observed directly on top of the tailings pile.
In Wietze (WT), an elongated area in the midst of a residential district includes both bare (in the front) and naturally revegetated potash tailings pile residues, indicating a successional gradient. Due to the absence of protected species, this site is not covered by conservation regulations. However, the halophytes Gypsophila scoronerifolia and Hymenolobus procumbens have been reported (Garve and Garve, 2000). Bare spots among the grass-dominated vegetation were occupied by well-developed biocrusts, and a thin and greenish algal crusts was found at the margin of the vegetation line facing the pile body.
In contrast to the sites described above, two of the tailings piles studied are considerably higher, cover larger areas and are generally younger in age (see Table 1). In Teutschenthal (TT) there are two large potash tailings piles, one of them studied here, shaping the district “Teutschenthal-Bahnhof” and creating a large saline area with both facultative (Aster tripolium, Cochlearia danica) and obligate (Salicornia europaea, Suaeda maritima) halophytes (Garve and Garve, 2000). Established biocrusts could be found in front of the vegetation line, some meters distant from the pile body. In addition, a young algal biocrust was situated on flat material at the pile bottom, next to a water catchment basin. At a second large tailings pile site (NN) both young and established biocrusts were found.
Sampling
Biocrust samples were collected with plastic Petri dishes (5.5 cm ø) using a spatula and closed with Parafilm®. In addition, a soil sample of approximately 5 cm× 5 cm× 3 cm from the upper layer of a bare spot close to the respective biocrust was collected and stored in LDPE plastic bags. If no bare spot was available, the biocrust with its adhering soil (3 mm) underneath was removed and the soil below was sampled as described. After sampling, the Petri dishes and plastic bags were opened to air-dry the samples and were then stored closed in a dry, dark place.
Soil Characterization
To determine the electrical conductivity (EC), 10 g air-dried soil (<2 mm) was mixed with 50 ml aqua deion (<5 μS cm–1). After shaking for 1 h followed by 30 min of sedimentation, the EC of the supernatant was measured with an EC meter (Seven MultiTM, Mettler Toledo, Schwarzenbach/Germany, in Lab 731 probe). The measurement values were used to calculate the saturation extract EC, with the following equation:
For pHCaCl2 measurement, 2 g air-dried soil (<2 mm) and 8 ml 0.01 M CaCl2 were mixed, shaken for 5 min, and incubated for 2 h. The pHCaCl2 was measured with a pH meter (Seven Multi, Mettler Toledo, Schwarzenbach/Germany, inLab Expert Pro probe).
To determine tOC, tN and tS, first, inorganic carbon was removed with 10% HCl in the carbonate-containing samples. Then, four replicates of 300 mg of ground soil were each packed tightly into tin boats and analyzed with a Vario El III (Elementar, Langensbold/Germany). The C:N ratio was calculated referring to the molecular mass.
Ion chromatography was conducted to determine cations (Na+, K+, Mg+, Ca2+) and anions (Cl–, SO42–) in aqueous soil extracts. First, 3 g air-dried and ground soil was mixed with 30 ml aqua deion. After 15–18 h of incubation at 70°C in a water bath, samples were filtered through a WhatmanTM GF-6 glass-fiber filter (Whatman/GE Healthcare) and the sample vessel and filtration apparatus were washed with hot aqua deion and added to the sample. After cooling to room temperature, the filtrate was filled with aqua deion to a volume of 50 ml. For anions, samples were analyzed by ion chromatography (Metrohm AG, Switzerland) with chemical suppression, inline dialysis and inline dilution using a Metrohm Metrosep A Supp 5 separation column (eluents: 3.2 mM NaCO3, 1.0 mM NaHCO3; 0.7 ml min–1 flow rate; guard: Metrosep A Supp 4/5). Samples for cation determinations were diluted and acidified with 20 μl 1 M HNO3 before analysis by ion chromatography (Metrohm AG, Switzerland) with inline filtration using a Metrohm Metrospec C 4 – xx/4.0 column (eluents: 1.7 mM HNO3, 0.7 mM C7H5NO4; 0.9 ml min–1 flow rate, guard: Metrosep C4 Guard/4.0). Each test series was calibrated, using internal standards.
Algae Culturing and Identification
Dominant algal groups were identified by means of direct light microscopy. Small pieces collected from the surface of dried biocrust material were mixed with a few drops of tap water, rewetted for 30 min, and observed under an Olympus BX51 light microscope. In order to classify the algal groups, the estimated abundance of diatoms, green algae and cyanobacteria were classified using the following scale: 0 = absent, 1 = observed once, 2 = rare, 3 = occasionally, 4 = frequent, and 5 = abundant (Schulz et al., 2016). For green algae and cyanobacteria, both the filamentous and non-filamentous stages were listed separately as a percentage of identified algae, e.g., 20% unicellular and 80% filamentous green algae. Diatom morphotypes were also identified by direct light microscopy.
For the preparation of enrichment cultures, small pieces of dried biocrusts were placed in a petri dish filled with MBBM + V (Bischoff and Bold, 1963; modified by Starr and Zeiskus, 1993) modified by the addition of 3% NaCl and solidified using 1.5% Difco© agar. After approximately 4 weeks, single colonies were transferred to fresh solid medium. Green algae were placed on solid 3N-BBM + V (Bischoff and Bold, 1963; modified by Starr and Zeiskus, 1993) +3% NaCl, whereas Cyanobacteria were transferred to solid BG-11 (Rippka and Herdmann, 1992) +3% NaCl. This step was repeated until unialgal, clonal isolates were established. Diatoms did not grow in this culture sequence. All cultures were kept at 15–20°C in a 16:8 light:dark cycle and 30 μmol photons m–2 s–1.
For morphological species determination, isolates were observed under an Olympus BX51 light microscope, mainly using the identification key of Ettl and Gärtner (2014). Photomicrographs were taken with the cellSense Entry imaging software (Olympus). The taxon designation was compared with the AlgaeBase database (Guiry and Guiry, 2020) and renamed if necessary, in order to report the latest, taxonomically accepted species and genus names.
A list of taxa was prepared, using the culture-dependent results. In the Supplementary Table S1 it was combined with the culture-independent results but all further and statistical analyses were based on the taxa isolated. The sum of the present taxa of each site reflects the number of taxa per site.
Statistical Analyses
The following calculations were performed with the program R (Version 3.3.2). Analyses of Similarity (ANOSIM) were performed to calculate a correlation between species composition and single categories, i.e., sampling site, biocrust type (young biocrust, established biocrust) and tailings pile age class (recent, older). First, co-correlations between the environmental factors as well as correlations between the main algal groups and environmental factors were calculated by Pearson parametric correlation tests. One-Way Permutational Multivariate Analyses of variance (perMANOVA, Jaccard distance) were performed to test for environmental parameters that significantly affected the composition of isolates. Correlations of single environmental factors with the two biocrust types were analyzed using a Mann-Whitney U test. A non-metric multidimensional scaling of a dissimilarity matrix (Jaccard distance) of the algae presence/absence list was performed using the isoMDS function of the MASS (Venables and Ripley, 2002) package. If not stated otherwise, all calculations were performed using the vegan package (Oksanen et al., 2017) or defaults of R.
Results
Soil Characteristics
In total, ECSat ranged between 49–954 mS cm–1 (Table 2). The median and maximum ECSat of young biocrusts (159 mS cm–1, 954 mS cm–1) were higher than those of established biocrusts (105 mS cm–1, 278 mS cm–1), although this trend was not statistically significant (p = 0.056). The dominant anion was SO42–. Ca2+ was the dominant cation in most samples, although in two soil samples Na+ was present in a higher proportion than Ca2+. The ECSat was positively correlated with the concentrations of Cl–, SO42–, Na+, K+, Mg2+ in the soil. The soil pHCaCl2 ranged from neutral to moderately alkaline (7 and 9). There was no significance difference in soil pHCaCl2 between young and established biocrusts. Further, soil pHCaCl2 was positively correlated with Na+, SO42– and tOC (Pearson correlation, results not shown). Total organic carbon and total nitrogen contents and the ratio between them varied widely among the sites, but no differences between young and established biocrusts could be observed.
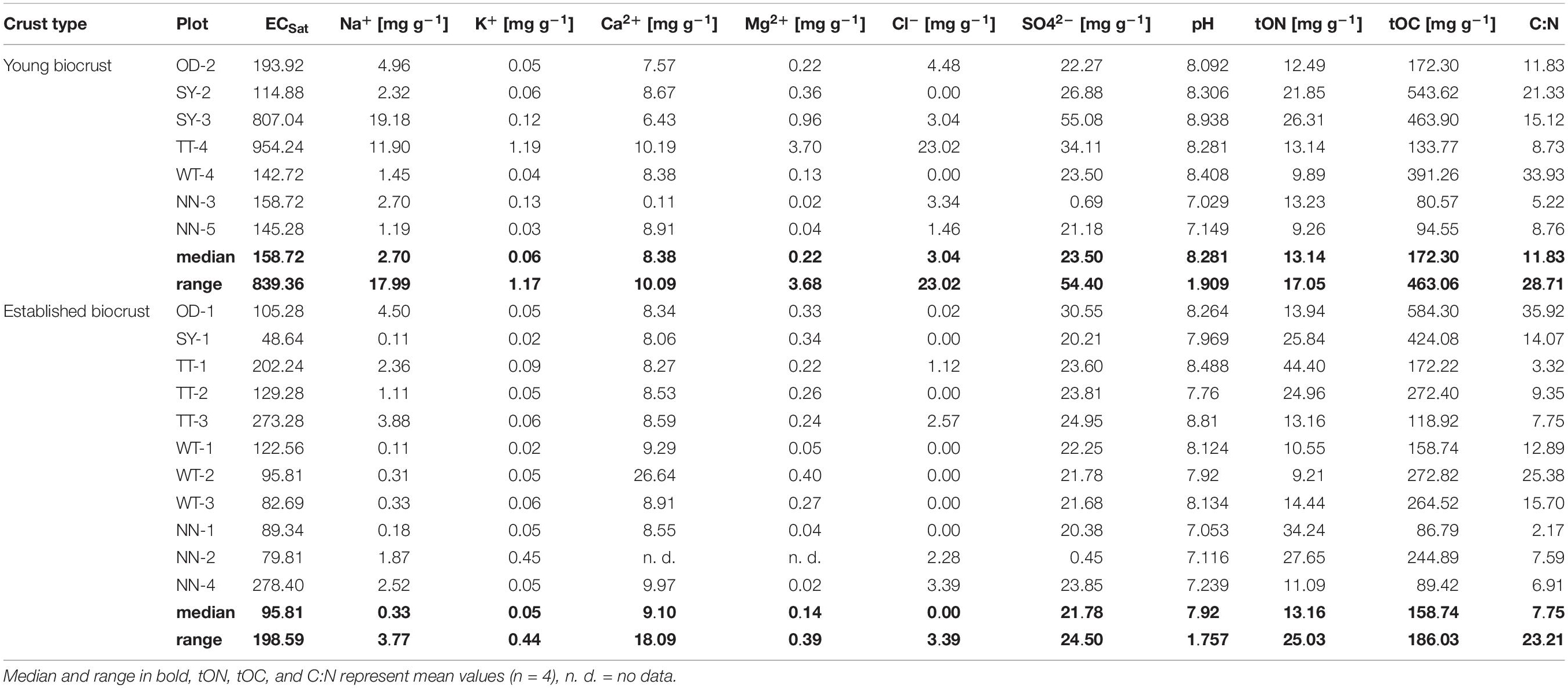
Table 2. Characterization of soil samples from young and established biocrusts in potash tailings pile areas (OD: Oedesse; SY: Shreyahn; TT: Teutschenthal; WT: Wietze; NN: not further described).
ECSat tended to differ, and the concentrations of Na+ differed significantly between the soil of young and established biocrusts (Table 3).
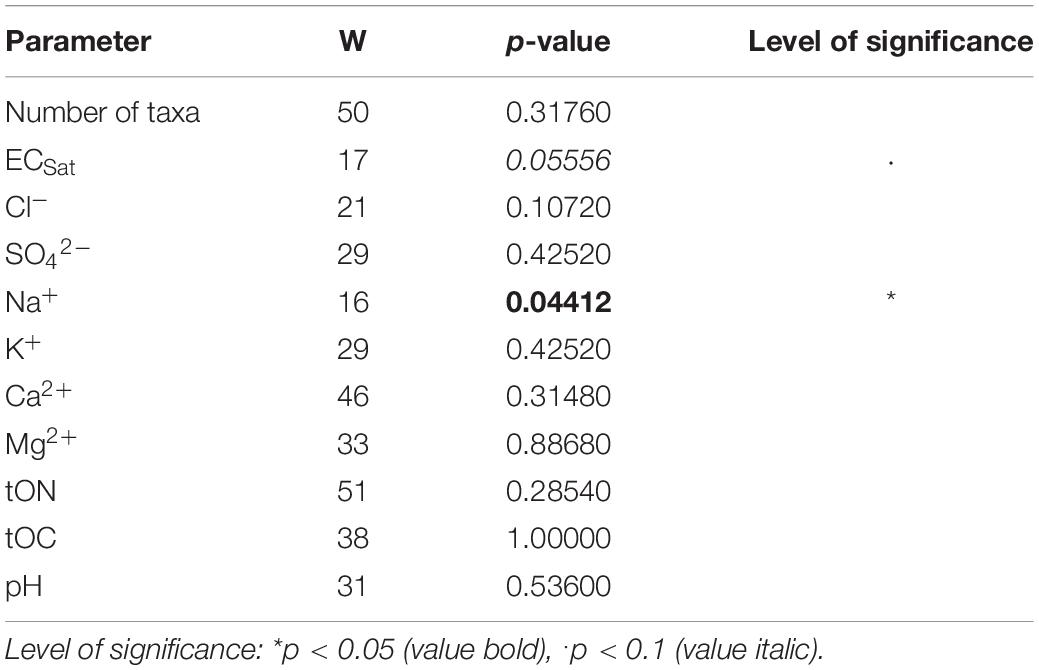
Table 3. Results of screening for significant differences between young and established biocrusts regarding number of algae taxa (culture-dependent approach) and environmental parameters, by Man-Whitney U Test.
Estimation of Major Algal Group Composition and Abundance
The composition and abundance of major algal groups in young and established biocrusts was estimated by direct microscopy (Figure 3, details in Figures 4, 5). Diatoms (Figure 4) were generally rare to absent. Most of the established biocrust samples were dominated by cyanobacteria; green algae were more abundant than cyanobacteria in only four samples, and one sample showed an equal distribution of all three algal groups. Regarding the organization level, mostly unicellular green algae were observed, whereas filamentous green algae were present in five of 11 samples. Most cyanobacteria in established biocrusts were filamentous, and unicellular cyanobacteria were observed in only three samples. In contrast, all samples from young biocrusts were dominated by green algae, and two of these samples contained a larger share of filamentous organisms than of non-filamentous. The maximum cyanobacteria frequency was “occasional,” two samples lacked cyanobacteria. In four samples of young biocrusts, diatoms occurred; in one sample they were rare, and in the other samples, diatoms were observed only once.
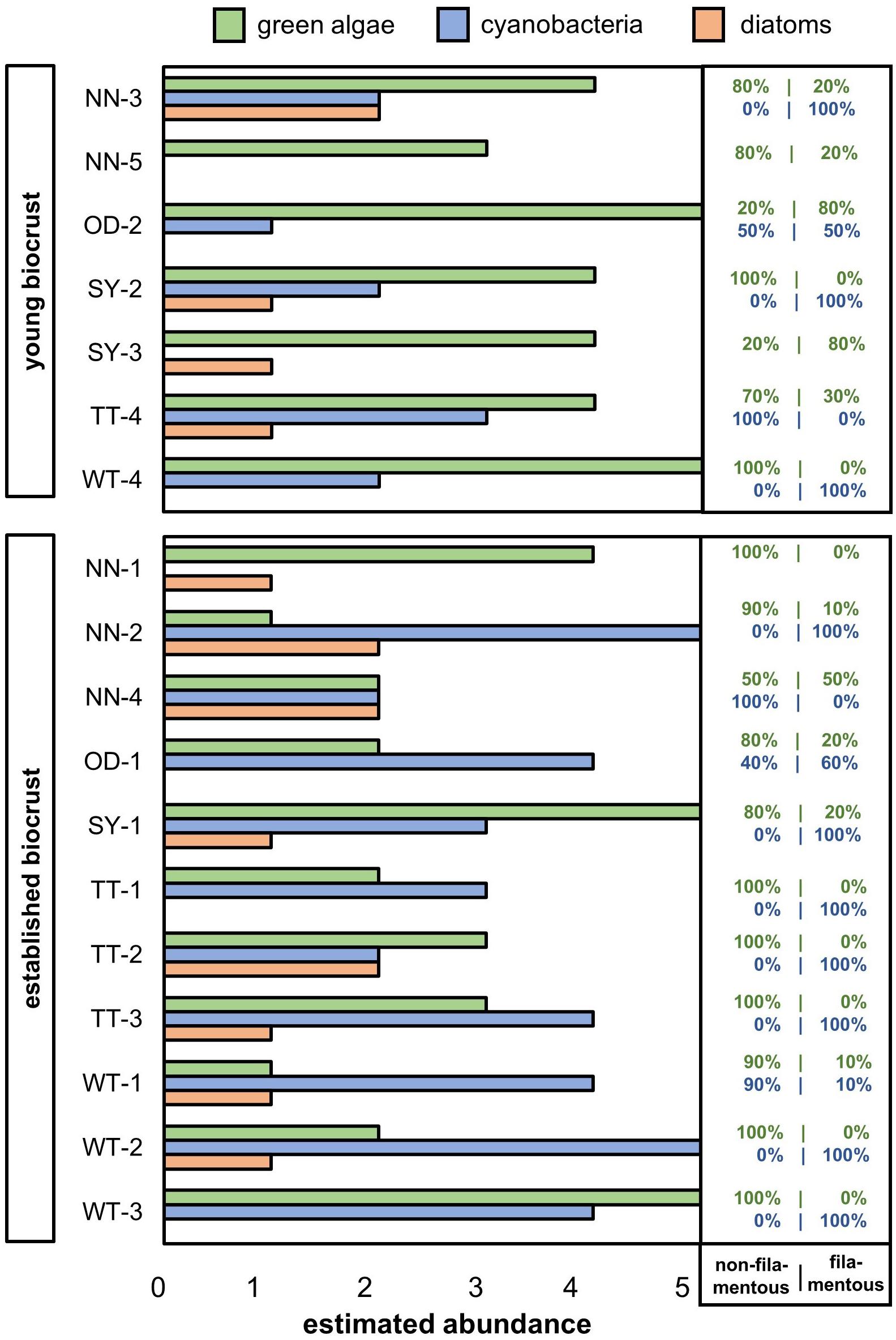
Figure 3. Estimated abundance of major algal groups in young and established biocrusts from potash tailings pile sites (OD, Oedesse; SY, Shreyahn; TT, Teutschenthal; WT, Wietze; NN, not further described) determined by direct microscopy of biocrust material. The estimated percentage of filamentous and non-filamentous green algae (green) and cyanobacteria (blue) are noted on the right; all observed diatoms were non-filamentous.
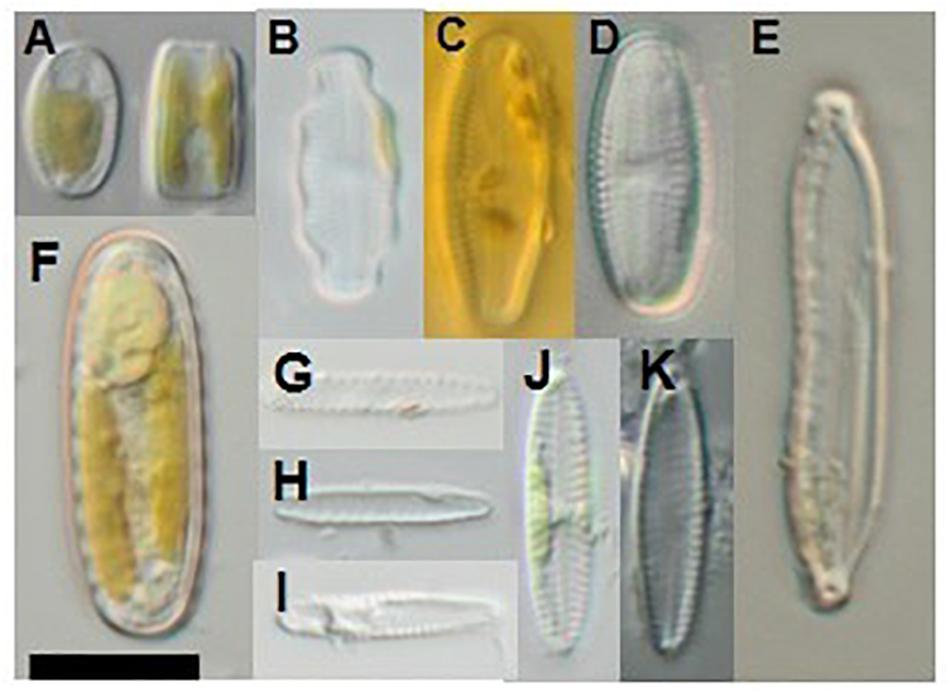
Figure 4. Diatom morphotypes observed directly in rewetted biocrust material. (A) Cymbellaceae; (B) Luticola cf. nivalis; (C,D) Luticola sp.; (E) Hantzschia sp.; (F) Surirella sp.; (G–K) Navicula sp.; scale bar indicates 10 μm.
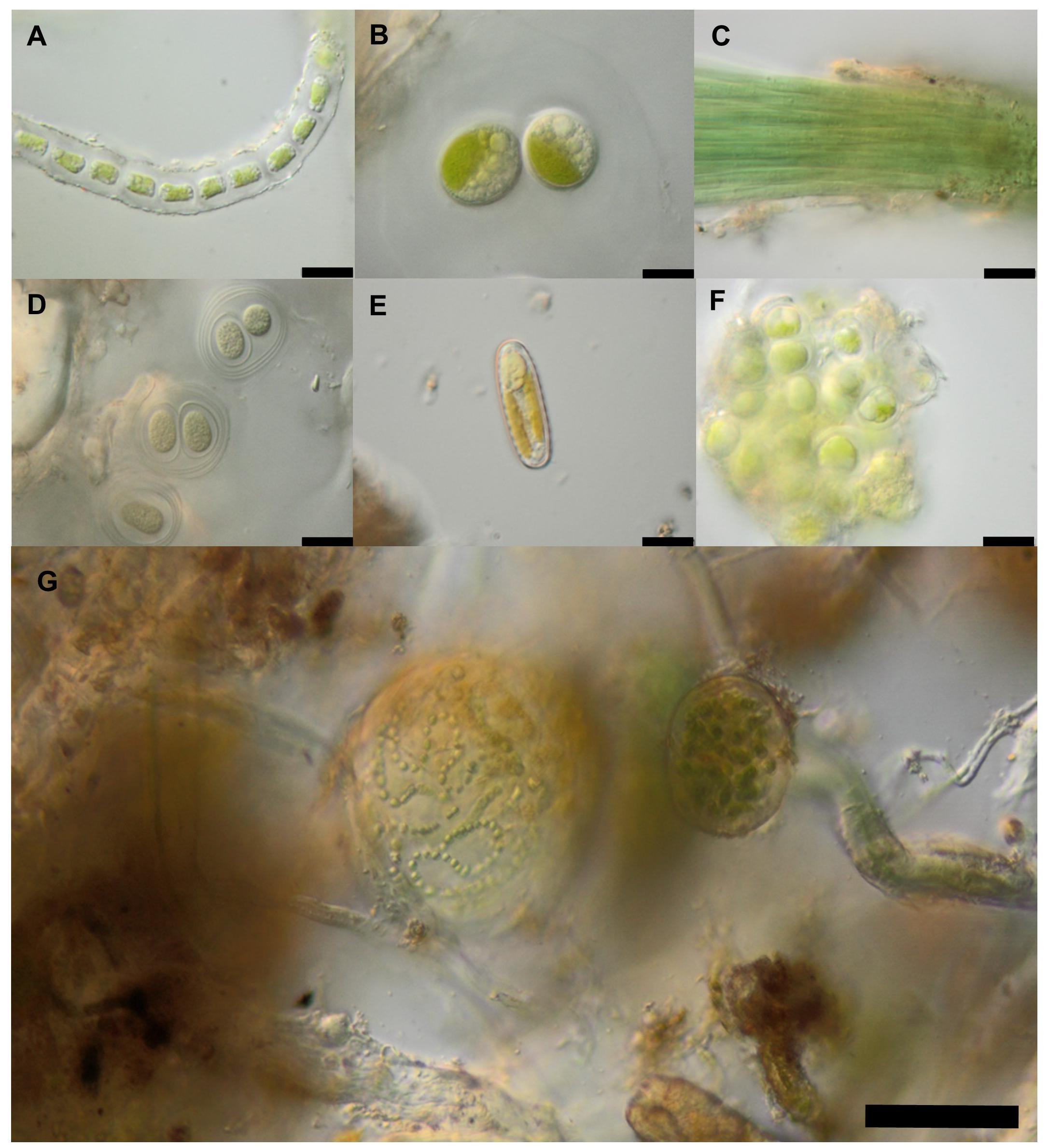
Figure 5. Microphotographs of direct microscopy observations of biocrust material. (A) filament of Ulothrix-like algae; (B) Mesotaenium cf. chlamydospora; (C) Microcoleus vaginatus; (D) Gloeocapsa sp.; (E) Surirella sp.; (F) Apatococcus lobatus; (G) Nostoc sp. colonies and Micocoleus vaginatus filaments between soil particles (brownish). Scale bars indicates 10 μm (A–F) and 50 μm (G).
Identification of Diatoms
Direct observations of dry biocrust material revealed in total six different morphotypes of diatoms: Navicula sp., Hantzschia sp., Surirella sp., Luticola sp. and Luticola cf. nivalis (Figure 4). Only Surirella sp. occurred in the cultures.
Identification of Green Algae and Cyanobacteria
Number of Taxa
In total, 73 algal and cyanobacterial taxa were identified by enrichment culturing and subsequent morphological identification. Up to 17 taxa were identified per site (Figure 6). Each established biocrusts sample contained on average 11 taxa and each young biocrust sample 10. There was no significant difference in species number between both biocrust types. In total, the two biocrust types had 25 taxa in common, whereas 32 taxa were only found in established biocrusts and 16 in young biocrusts, respectively.
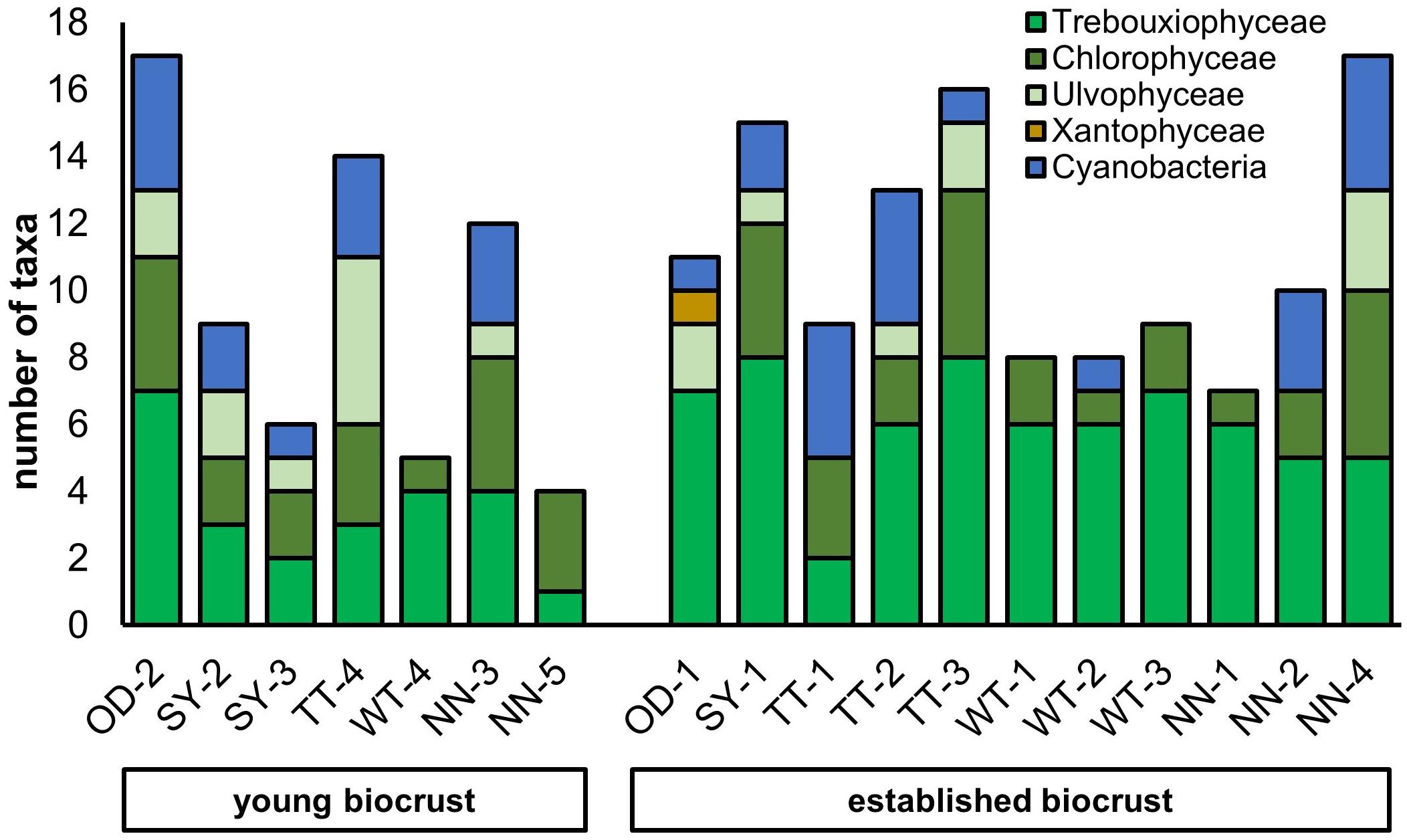
Figure 6. Number of taxa isolated from biocrust samples (OD, Oedesse; SY, Shreyahn; TT, Teutschenthal; WT, Wietze; NN, not further described) grouped by biocrust type divided in algae classes and cyanobacteria.
Overall, the majority of isolated species were green algae whereas cyanobacteria were clearly less diverse. In five samples, no cyanobacteria were isolated. In regard to green algae, most algae belonged to the class Trebouxiophyceae, followed by Chlorophyceae. Interestingly, Ulvophyceae were most frequent in young biocrusts and were rarely found in established biocrusts. For Xanthophyceae, one taxon was recorded.
Algal and Cyanobacterial Isolate Composition
The isolate composition of green algae and cyanobacteria was determined by the culture-dependent approach (Figure 7). The most frequent green alga taxa during culturing were Stichococcus bacillaris, Nannochloris sp., Bracteacoccus minor, and Diplosphaera chodatii. Besides S. bacillaris, other species of this genus, S. allas and S. exiguus, were each found in several samples. Other frequent taxa were Planophila terrestris, Spongiochloris excentrica, Myrmecia bisecta and Tetracystis sp. Two filamentous green algae genera were observed, Ulothrix and Pseudoclonium, both of members of Ulvophyceae. Leptolyngbya sp., and Nostoc sp. were frequent filamentous cyanobacteria isolates.
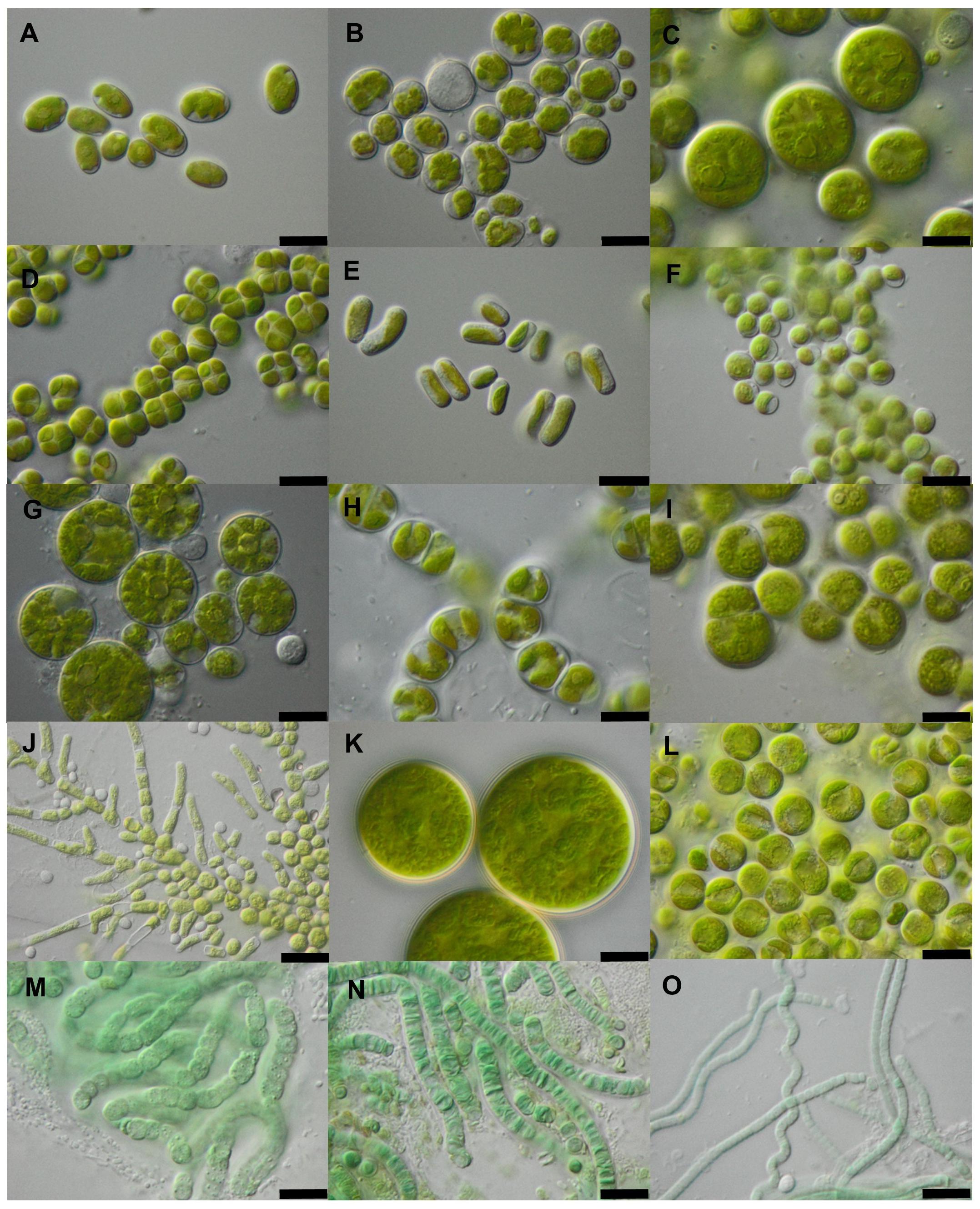
Figure 7. Green algae and cyanobacteria isolated from biocrusts in potash tailings pile areas. (A) Chloroidium ellipsoideum (WT-1); (B) Parietochloris cohaerens (NN-4); (C) Spongiochloris excentrica (TT-5); (D) Diplosphaera chodatii (NN-5); (E) Stichococcus allas (NN-4); (F) Chlorella sp. 1 (SY-1); (G) Borodinellopsis texensis; (H) Ulothrix aequalis (TT-3); (I) Planophila laetevirens (OD-2); (J) Pseudendoclonium sp. (TT-5); (K) Bracteacoccus minor (OD-3); (L) Myrmecia bisecta (TT-3); (M) Nostoc sp. (SY-1); (N) Pseudophormidium edaphicum (NN-8); (O) Leptolyngbya sp. (NN-5). Scale bars indicate 10 μm (A–N), and 20 μm (O).
Nostoc sp. was only present in established biocrusts and absent in young biocrusts. Likewise, Parietochloris cohaerens and Diplosphaera chodatii were found in all established biocrust sample sites whereas the latter only occurred once in young biocrusts. Chloroidium ellipsoideum and Stichococcus sp. 1 were also characteristic of established biocrusts. Chlorella vulgaris was more often found in young than in established biocrust samples.
Some of the morphologically identified taxa could only be observed by direct microscopy (Supplementary Table S1). This includes members of the genera Mesotaenium and Gloeocapsa. In addition, Microcoleus vaginatus was often observed by direct microscopy (Figure 5) but was mainly absent in the subsequent enrichment culture; in some samples this was the case for Nostoc sp. as well.
Dissimilarities in the presence of algal isolates between samples are shown in the nMDS plot (Figure 8). First, no clustering of samples from similar sites could be observed, indicating no site-specific isolate compositions; accordingly, this fact was proved to be non-significant (Table 4). Grouping the sites by tailings pile age (recent, older) also indicated no significant differences. However, grouping the samples by biocrust type revealed a distinct division, in the lower left of the graph for young and the upper right for established biocrusts. Analysis of similarity confirmed a significant difference in the isolate composition between the young and established biocrusts (Table 4).
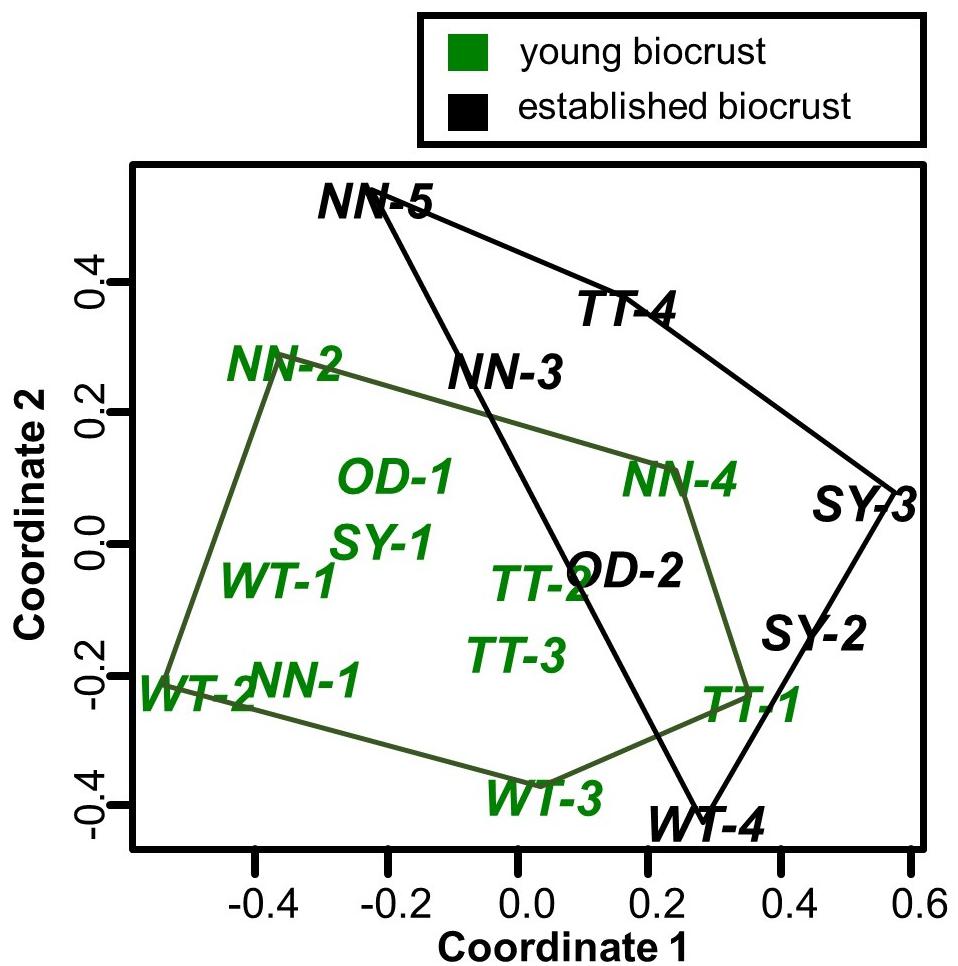
Figure 8. nMDS plot showing the present morphotaxa composition of green algae and cyanobacteria isolated from young and established biocrusts sampled in potash tailings pile environments (OD, Oedesse; SY, Shreyahn; TT, Teutschenthal; WT, Wietze; NN, not further described) polygons show the biocrust types; stress = 0.179 and Nostoc sp. were prominent cyanobacteria taxa.
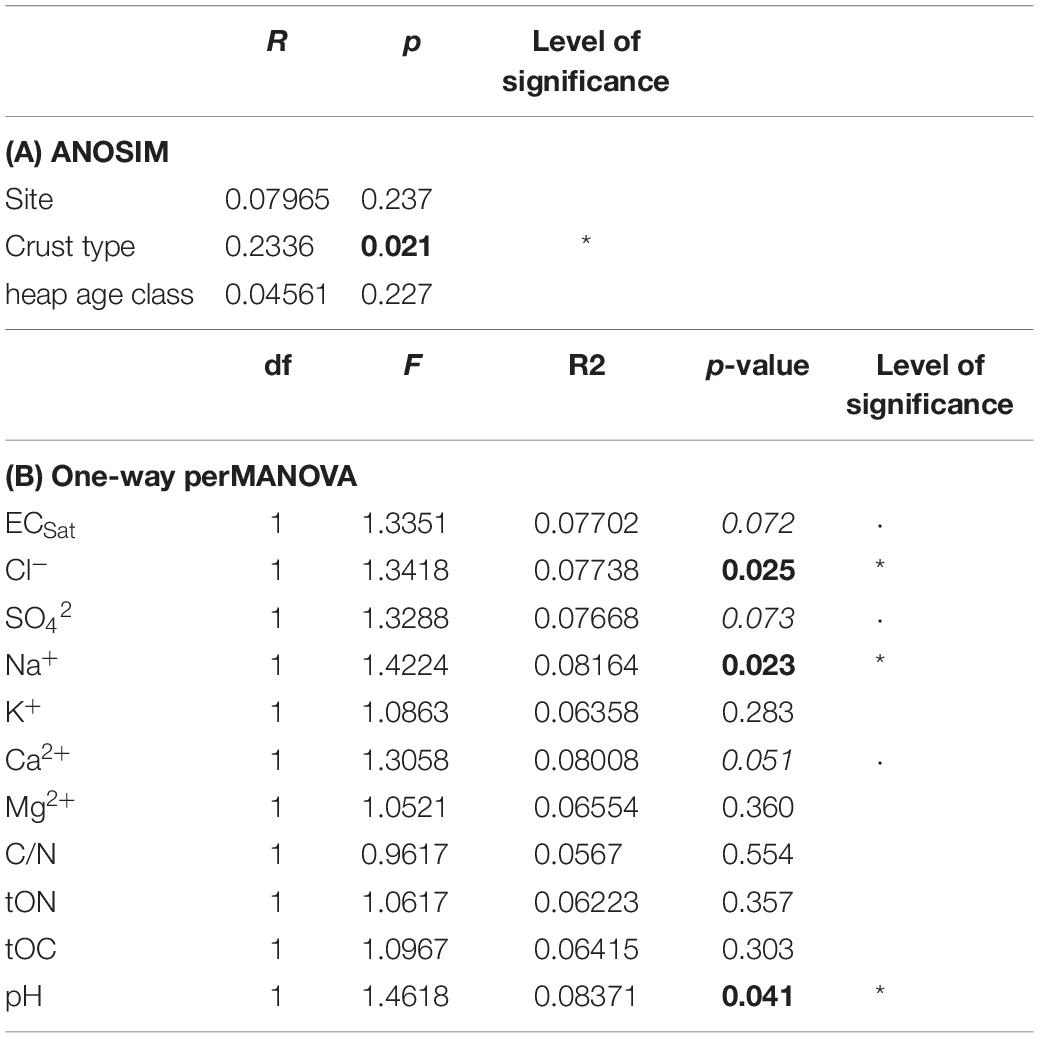
Table 4. (A) Analyses of similarity to reveal significant differences of the present algal isolate composition between the sites, biocrust type (young, established) and potash tailings pile age classes. (B) Influence of abiotic conditions on algal isolate composition tested by PerManova; given are: F-value, R2-value (which corresponds to the variation in the isolate composition explained by the respective factor) and level of significance: *p < 0.05 (values in bold), ⋅p < 0.1 (values italic); df: degrees of freedom.
Algal and Cyanobacterial Composition and Soil Characteristics
The major algal groups, cyanobacteria, green algae and diatoms that were studied with direct microscopy, showed no correlation between their estimated abundance and the ECSat of the soil. However, significantly more filamentous green algae were found with increasing ECSat of the soil. In contrast, filamentous cyanobacteria showed a negative trend. Further, ECSat and SO42– concentrations of the soil tended to influence the total composition of algal isolates, whereas Na+ and Cl– concentrations and pHCaCl2 of the soil were found to significantly affect the isolate composition (Table 4). All other tested concentrations of ions, tOC and tON in the soil did not correlate with the present algal and cyanobacteria isolate composition.
Discussion
Soil Characteristics Reflect Extremely Saline Environments
Soils with an ECSat of >16 mS cm–1 are defined as very highly saline soils (Whitney, 1988). According to this definition, the soils in the present study can be regarded as “extremely saline” soils (Table 2). Until now, only one study has recorded terrestrial algae in soils with an ECSat up to 260 mS cm–1 (Tsujimura et al., 1998). Other studies on biocrusts on saline soils reported only sites with ECSat values <30 mS cm–1 (e.g., Fox et al., 2009; Kidron, 2016; Sandoval Pérez et al., 2016; Schulz et al., 2016; Zhou et al., 2016; Ghiloufi et al., 2017; Kakeh et al., 2018). In the present study, however, much higher ranges of ECSat (up to 950 mS cm–1) were documented, emphasizing the extreme soil salinities of the sampling sites.
Na+ and Cl– were not the prominent ions measured in the aqueous soil extracts of the present study. The main component of fresh potash mining residues, however, is NaCl. This distinct difference is explained by the lower sorption of these ions and consequently by their rapid leaching. The most dominant anion in the soil solution was SO42–. Likewise, SO42– was the main ion in a nickel-mining seepage with very high EC (Van Dam et al., 2014). A high sulfate content in soils typically indicates gypsum or anhydrite. Besides addressing the absolute amounts of ions, which also mirrors the EC, the ion ratios are crucial for physiological processes in cells. According to Albrecht (1975) the optimal cation balance for plant growth in agricultural soils is: Ca 60–75%, Mg 10–20%, K 2–5%, Na 0.5%, H 10% and other cations 5%. Thus, there was a strong deficiency of Mg and K in the tested soil samples, whereas Na had a considerably higher share. Taken together, these findings indicate the extreme, chemically unbalanced and rather artificial soil conditions due to potash mining, which thus comprise unique habitats for organisms.
Bare potash tailings pile material is considered to be very low in organic matter. Since this material is a residue from a mined mineral, it is not comparable to soils on the earth’s surface that were formed by complex biological processes, leading to enrichment of organic matter over long periods. The soil samples of young biocrusts collected at or very close to the pile body did not differ from established biocrusts in tOC. This might be caused by the suppression of fungal growth in alkaline soils, which can cause a significant decrease of the soil microbial biomass (Weyman-Kaczmarkowa and Pędziwilk, 2000). However, in a broad study that analyzed biocrust samples of several countries in Europe, tOC was also found to vary strongly (Williams et al., 2018), which led the authors to the conclusion that tOC, among other soil parameters, is not suitable to describe crust developmental stages.
It is difficult to determine whether the biocrust emerged due to a previous input of organic matter from litter, or whether the organic carbon was accumulated predominantly from the photosynthetic activity of algae and cyanobacteria in the biocrust itself. Most likely a combination of both processes occurs, because biocrusts are known to strongly contribute to the C pools of soils (Sancho et al., 2017). Organic matter covering the tailings piles lowers the salinity of the surface and acts as a kind of buffer layer, which could be crucial for the establishment of a biocrust in such an extreme saline habitat.
Rich Algal and Cyanobacteria Isolate Composition
Methodological Considerations
Morphological identification based on the culture-dependent approach was especially challenging in this study, because some of the isolates may have an irregular morphology due to the saline culture medium, and most descriptions from the literature are based on isolates cultured in non-saline media. For this reason, several morphotypes could not be identified at species level. Due to the salt stressor, cells of one species could be shaped differently and be assigned to a different morphotype, thus leading to overestimation of the true biodiversity. In contrast, cells could lose special characteristics such as pyrenoid morphology due to the stressor, which might cause underestimation of the true biodiversity. This effect, termed phenotypic plasticity, has been described for both green algae and cyanobacteria subject to several stressors, such as temperature (Neustupa et al., 2008; Soares et al., 2013) as well as salinity (Darienko et al., 2015).
Organisms observed with direct microscopy could not be completely rewetted, which impeded species determination. Some taxa may have been present only as resting stages, which are often impossible to identify. For secure species identification, it is proposed to use polyphasic approaches including morphological, genetic and ecophysiological observations (Darienko et al., 2010), preferably in combination with methods that are not culture-dependent. Identification of diatoms was also challenging. In view of these difficulties, morphotypes were defined only to the highest taxon level possible using the methods in this study. For more precise species determination, diatoms must be observed on permanent slides or further details evaluated with scanning electron microscopy. However, diatoms were not a focus of this study, as they were comparatively rare at the study sites and are generally not regarded as crust-initiating organisms.
The morphological species determination provides a first insight of algae and cyanobacteria communities of biocrusts in potash tailings pile areas. For a more precise description of the taxon composition, a polyphasic approach is recommended.
Number of Morphologically Identified Taxa
In total, 73 microalgal and cyanobacterial taxa were observed with the culture-dependent approach, and six diatom morphotypes were identified by direct microscopy. This number is similar to the results of another study of microalgae and cyanobacteria in salt-affected soils that reported 74 species (Vinogradova and Darienko, 2008). In temperate regions, a lower taxa richness was observed for biocrusts in forests (Glaser et al., 2018) and sand dunes (Schulz et al., 2016). On the one hand, it was rather surprising to find more species in this extreme habitat than in forests or dunes, where the edaphic parameters are less challenging for algae. On the other hand, in the potash-tailings pile areas biocrusts face lower competitive pressure by higher plants, which could explain the relatively high number of taxa. Concerning biocrust types, there was no difference in species number between young and established biocrusts, which agrees with a study along a transect of a glacier forefield that also reported no differences between young and established biocrusts (Borchhardt et al., 2019). In contrast, a study in a non-saline region showed an increase in species number concerning green algae and cyanobacteria in biocrusts during their succession (Zhang et al., 2018). In summary, our hypothesis that the species number in young biocrusts is lower than in established biocrusts was not confirmed. This result underlines that even biocrusts of early successional stages can represent diverse algal communities, which is especially remarkable for the extreme environment of potash tailings piles.
Major Algal Group Estimated Composition Differed Between Biocrust Types
Filamentous microalgae can be regarded as crust-initiating, as they interweave soil particles and thus stabilize the surface. This form of organization seemed to be important for biocrusts on these tailings piles, as we found filamentous taxa in all samples. In young biocrusts this function was mainly accomplished by green algae, whereas in established biocrusts cyanobacteria were the most abundant filamentous organisms. In arid regions, cyanobacteria are generally regarded as the first organisms that occur during biocrust succession, followed by green algae (Belnap, 2006). This succession pattern seems to be more variable in temperate regions, since both green algae and cyanobacteria dominated biocrusts appear in habitats strongly disturbed by humans (Szyja et al., 2018). In our study, cyanobacteria were notably less abundant in young biocrusts compared to filamentous green algae, which might be explained by the specific environmental conditions. Interestingly, another study in temperate regions on mine dumps, from lignite mining, also found a low abundance of cyanobacteria in initial biocrusts, in this case attributed to very low pH values (Lukešová, 2001). Cyanobacteria are normally missing in habitats with an acid pH range (Brock, 1973). However, all soil samples of the present study were within the pHCaCl2 range preferred by cyanobacteria. There was no difference between pHCaCl2 and biocrust types, whereas the estimated abundance of cyanobacteria was much higher in established biocrusts. Thus, the pHCaCl2 cannot explain the lack of cyanobacteria in young biocrusts. The estimated abundance of filamentous green algae showed a positive correlation with ECSat. In contrast, the estimated abundance of filamentous cyanobacteria identified by direct microscopy tended to indicate a negative correlation with ECSat. Tsujimura et al. (1998) found a similar trend for cyanobacteria but only for Nostocales, whereas Oscillatoriales showed a negative trend. However, this subdivision was not evaluated with respect to estimated abundance in the present study.
Algal Isolate Composition Is Shaped by Soil Salinity and pH
The most crucial environmental factor in potash tailings pile areas is salinity. The concentrations of Na+ and Cl– in the soil solution had a significant effect on algal isolate composition, which can be explained by the pronounced toxicity of both ions to all living cells (Kirst, 1990 and literature cited therein). In contrast, Ca2+ is not toxic and is important as a messenger in salt-tolerance processes (Kirst, 1990 and literature cited therein), and therefore this cation could be important for the composition of green algal and cyanobacterial isolates. K+ is compatible with the cell metabolism and did not affect the composition of green-algal and cyanobacterial isolates. However, the ratio between the toxic Na+ to the nutrient K+ is important, since K+ is likely exchanged with the toxic Na+ in cells, as reported for cyanobacterial cells by Hagemann (2011). Even though SO42– was the prominent ion, there was no significant effect on the composition of algal and cyanobacterial isolates, since it was not the growth-limiting nutrient. In a study on Chlorella sp. increased SO42– concentrations showed lower toxicity than Cl– (Van Dam et al., 2014). This further explains why Cl– is more important for the composition of algal and cyanobacterial isolates. Generally, the lack of a significant effect of ECSat on algal isolate composition underlines the importance of individual ions. Salinity could also explain the differences in the composition of algal isolates between young and established biocrusts, since the concentration of Na+ in the soil solution was significantly different and ECSat also tended to differ between biocrust types. In conclusion, species found in young biocrusts may tolerate higher soil salinities. Still, there might be differently halotolerant species among the microalgae. The three dimensions of a biocrust offer numerous microhabitats with distinct environmental conditions. Less salt-tolerant organisms could be situated in the middle layer, sheltered by salt-tolerant and polysaccharide excreting algae. It would be very interesting to evaluate the salt tolerance range of the taxa isolated here in future and to compare the response patterns of isolates from both young and established biocrusts.
Another factor that significantly affected the composition of algal isolates was soil pHCaCl2. Different results regarding pHCaCl2 and algal community composition have been reported. Some studies showed a significant effect of pH (Castillo-Monroy et al., 2016) and others found no correlation (Schulz et al., 2016; Glaser et al., 2018). Soil pH is crucial for the availability of plant nutrients. Potassium and sulfur are easily bioavailable in slightly acidic to strongly alkaline soils, whereas other nutrients such as nitrogen, phosphorus and some trace elements show a lower bioavailability in alkaline soils. Especially phosphorus has decreasing bioavailability with increasing alkalinity of soils. Several soil samples in this study were alkaline, which leads to decreased bioavailability of several other nutritional elements (e.g., Ca2+, Mg2+, K+, Fe2+). This may explain why the taxa diversity depends on pH.
Present Taxa Mirror Extreme Soil Salinity
Most diatom morphotypes identified by direct microscopy are known terrestrial taxa. Luticola nivalis was previously found in biocrust samples (Kidron et al., 2012; Borchhardt et al., 2017), and several species of Hantzschia sp., Luticola sp. and Navicula sp. were members of biocrusts in sand dunes (Schulz et al., 2016).
Some taxa of green algae identified in the present study have been reported from salt-affected soils. This includes taxa such as the filamentous Dilabifilum/Pseudendoclonium-like algae (Vinogradova and Darienko, 2008), Tetracystis (Kirkwood and Henley, 2006; Vinogradova and Darienko, 2008), Chlorella (Shubert and Starks, 1980; Vinogradova and Darienko, 2008) and Borodin ellipsis as well as Diplosphaera chodatii, Chloroidium ellipsoideum and Stichococcus bacillaris (Vinogradova and Darienko, 2008). Some of these algae are known to produce osmotically active substances such as sucrose (Chlorella vulgaris, Chloroidium ellipsoideum), ribitol (Chloroidium ellipsoideum) and sorbitol (Stichococcus sp.), which indicates their adaption to osmotic stress (Darienko et al., 2010). In conclusion, we found several species that are typical of salt-affected environments, which supports our hypothesis that microalgae in biocrusts of potash tailings piles tolerate high salinities.
The genus Klebsormidium was present in none of the studied samples. In general, Klebsormidium is a common member of biocrusts worldwide (Glaser et al., 2018; Samolov et al., 2019). Klebsormidium species tolerate desiccation (Holzinger et al., 2011; Karsten et al., 2014a, b) but only moderate osmotic stress (Karsten and Rindi, 2010; Kaplan et al., 2012). Klebsormidium sp. cultured in 30 SA showed a drastically reduced growth rate than in lower salinities (Karsten and Rindi, 2010). Therefore, members of this genus might not tolerate hypersaline conditions. Further, Klebsormidium often is observed under low pH soil conditions (Lukešová, 2001; Škaloud et al., 2014), and the neutral or slightly alkaline soil pHCaCl2 of the potash tailings pile sites did not provide favorable conditions. Taken together, the high soil salinity together with the measured soil pHCaCl2 could explain the absence of the otherwise cosmopolitan Klebsormidium.
In extremely saline ecosystems such as salt lakes and salt flats, Dunaliella is the only chlorophyte genus found that can tolerate salinities up to 170 SA (DasSarma and Arora, 2001). This taxon was also described in soil samples (Arif, 1992; Kirkwood and Henley, 2006; Buchheim et al., 2010). In this study, Dunaliella was observed in brine drains at one potash tailings pile site (unpublished data). However, this genus was absent in both biocrust types, which may indicate that it is unlikely to live in the biocrust community.
Several algae occurred mainly in one crust type, in either the young or established biocrusts. For example, the filamentous cyanobacterium Nostoc sp. occurred in most of the samples from established biocrusts and was absent in young biocrusts. Generally, the presence of Nostoc sp. in the highly saline potash tailings pile areas is not surprising since it has been recorded from several salt affected habitats (Polderman, 1974; Marshall, 1982; Arif, 1992; Tsujimura et al., 1998). Tsujimura et al. (1998) reported that the abundance of Nostocales taxa was not negatively affected by soil EC, even in higher ranges. Therefore, the higher median ECSat of young biocrust soil samples cannot explain the absence of Nostoc sp. In contrast, filamentous Ulothrix or Ulothrix-like algae were clearly more abundant in young biocrusts, as detected by direct microscopy. Previous studies have also reported Ulothrix in initial biocrust stages (Zhao et al., 2008; Gypser et al., 2016). Many species of this genus are freshwater algae, whereas Ulothrix tenerrima was recorded in saline lakes in China (Wen et al., 2005) and U. subflaccida and U. pseudoflaccida were found in salt marshes (Polderman, 1974). The soil-stabilizing effect of the filamentous green alga Ulothrix in young biocrusts could be functionally replaced by filamentous cyanobacteria such as Nostoc sp. in established biocrusts. One may speculate that the carbon input is higher in mature biocrusts than in younger biocrusts. Therefore, in order to maintain the C:N homeostasis, N could become the limiting nutrient for organism growth. This in turn would strengthen the niche for N-fixing organisms such as Nostoc.
Conclusion and Outlook
Biocrusts occured under the extremely saline soil conditions of potash tailings pile areas and contained a diverse microalgal community including green algae, cyanobacteria and diatoms.
We identified several taxa that have been described previously in saline environments. Thus, our hypothesis that biocrusts from potash tailings piles would contain typical species of salt-affected habitats was confirmed. Further, the isolate composition (culture-dependent approach) differed between young and established biocrusts. The former showed a higher estimated abundance (culture-independent approach) of filamentous green algae such as Ulothrix whereas the latter exhibited a higher estimated abundance of filamentous cyanobacteria. We conclude that the occurrence of filamentous organisms is crucial for the establishment of a biocrust, but the taxa that provide this trait vary depending on the biocrust type. Our hypothesis that the number of microalgal and cyanobacterial species would be lower in young biocrusts was not confirmed, underlining the existence of a diverse algal community, even in early successional stages. pH and soil salinity, in particular Na+ and Cl–, affected algal isolate composition. Especially the latter effect is not surprising since potash tailings pile areas are shaped by the salt output of the salty dump material, which also results in communities of halophilic vascular plants.
Morphological species identification provided a first idea of the algal isolate composition in these extremely saline environments, but the results should be better supported by using a polyphasic approach in the future. Nevertheless, with this approach we were able to establish several clonal cultures, which could be used for greening the potash tailings piles.
Since potash tailings piles pose an ongoing environmental problem that alters the adjacent ecosystems, a vegetation cover of the pile body would be appropriate to trap rainwater, thus preventing uncontrolled leakage of the highly saline brine. However, vascular plants as a natural cover are difficult to install, as they often have deep roots and thus need a thick layer of substrate, which easily erodes on the steep flanks of the tailings pile. Biocrusts, in contrast, would only establish in the upper millimeters, which indicates the potential of biocrusts to have a positive effect on rain-water trapping. Therefore, microalgal isolates derived from biocrusts of potash tailings pile areas could offer an alternative solution for the envisaged vegetation cover. Ulothrix is of particular interest in this regard, since this genus was growing in young biocrusts at or very close to the potash tailings piles. The high diversity of algae in biocrusts existing under extreme saline conditions is a promising finding for the idea of greening potash tailings piles. Further studies should evaluate the potential for establishing artificial biocrusts on this extremely saline mining-waste material.
Data Availability Statement
All datasets generated for this study are included in the article/Supplementary Material.
Author Contributions
UK and KG developed the idea. VS collected the data that was further analyzed by KG and VS. VS wrote the first draft of manuscript which was edited by UK and KG.
Funding
This project was funded by the Deutsche Bundesstiftung Umwelt (DBU) and the Gesellschaft für Chemische Technik und Biotechnologie e.V. (DECHEMA), Max-Buchner-Forschungsstipendium (MBFSt 3583).
Conflict of Interest
The authors declare that the research was conducted in the absence of any commercial or financial relationships that could be construed as a potential conflict of interest.
The reviewer, LB-W, declared a past co-authorship with one of the authors, UK, to the handling Editor.
Acknowledgments
Many thanks to the nature conservation authority of the Peine and Lüchow-Dannenberg districts, as well as the Wietze community, the GESA (Gesellschaft zur Entwicklung und Sanierung von Altstandorten MBH) and PD Dr. Albrecht Palm for granting permission and/or access to the sampling sites. We would like to thank, in particular, Dr. Ramona Kern as well as Dr. Matthias Kockx, Dr. Alexandra Wölk, and Christopher Lubs for their contribution to the sampling. Further, we thank Dr. Tatiana Mikhailyuk for her introduction to morphological identification of microalgae. We also thank Evelin Bolzman in the group of Prof. Bernd Lennartz for performing the ion chromatography analyses and also Dr. Christel Baum for advice on interpreting soil data. Determination of diatom taxa was strongly supported by Anh Tu Van and Kana Kuriyama, who, we thank most cordially.
Supplementary Material
The Supplementary Material for this article can be found online at: https://www.frontiersin.org/articles/10.3389/fevo.2020.00046/full#supplementary-material
References
Arif, I. A. (1992). Algae from the saline soils of Al-Shiggah in Al-Qaseem, Saudi Arabia. J. Arid Environ. 22, 333–338. doi: 10.1016/s0140-1963(18)30575-5
Arle, J., and Wagner, F. (2013). Effects of anthropogenic salinisation on the ecological status of macroinvertebrate assemblages in the Werra River (Thuringia, Germany). Hydrobiologia 701, 129–148. doi: 10.1007/s10750-012-1265-z
Belnap, J. (2006). The potential roles of biological soil crusts in dryland hydrologic cycles. Hydrol. Process. 20, 3159–3178. doi: 10.1002/hyp.6325
Bischoff, H. W., and Bold, H. C. (1963). Some Soil Algae from Enchanted Rock and Related Algal Species. Austin, TX: University of Texas Publication, 1–95.
Borchhardt, N., Baum, C., Thiem, D., Köpcke, T., Karsten, U., Leinweber, P., et al. (2019). Soil microbial phosphorus turnover and identity of algae and fungi in biological soil crusts along a transect in a glacier foreland. Eur. J. Soil Biol. 91, 9–17. doi: 10.1016/j.ejsobi.2018.12.006
Borchhardt, N., Schiefelbein, U., Abarca, N., Boy, J., Mikhailyuk, T., Sipman, H. J. M., et al. (2017). Diversity of algae and lichens in biological soil crusts of Ardley and King George islands, Antarctica Antarctic Sci. 29, 229–237. doi: 10.1017/s0954102016000638
Bowker, M. A., Soliveres, S., and Maestre, F. T. (2010). Competition increases with abiotic stress and regulates the diversity of biological soil crusts. J. Ecol. 98, 551–560. doi: 10.1111/j.1365-2745.2010.01647.x
Braukmann, U., and Böhme, D. (2011). Salt pollution of the middle and lower sections of the river Werra (Germany) and its impact on benthic macroinvertebrates. Limnologica 41, 113–124. doi: 10.1016/j.limno.2010.09.003
Brock, T. D. (1973). Lower pH Limit for the existence of blue-green algae: evolutionary and ecological implications. Science 179, 480–483. doi: 10.1126/science.179.4072.480
Buchheim, M. A., Kirkwood, A. E., Buchheim, J. A., Verghese, B., and Henley, W. J. (2010). Hypersaline soil supports a diverse community of Dunaliella (Chlorophyceae). J. Phycol. 46, 1038–1047. doi: 10.1111/j.1529-8817.2010.00886.x
Cañedo-Argüelles, M., Kefford, B. J., Piscart, C., Prat, N., Schäfer, R. B., and Schulz, C. J. (2013). Salinisation of rivers: an urgent ecological issue. Environ. Pollut. 173, 157–167. doi: 10.1016/j.envpol.2012.10.011
Castillo-Monroy, A. P., Benítez, Á., Reyes-Bueno, F., Donoso, D. A., and Cueva, A. (2016). Biocrust structure responds to soil variables along a tropical scrubland elevation gradient. J. Arid Environ. 124, 31–38. doi: 10.1016/j.jaridenv.2015.06.015
Chen, L., Li, D., Song, L., Hu, C., Wang, G., and Liu, Y. (2006). Effects of salt stress on carbohydrate metabolism in desert soil alga. J. Integr. Plant Biol. 48, 914–919. doi: 10.1111/j.1744-7909.2006.00291.x
Darienko, T., Gustavs, L., Eggert, A., Wolf, W., and Pröschold, T. (2015). Evaluating the species boundaries of green microalgae (Coccomyxa, Trebouxiophyceae, Chlorophyta) using integrative taxonomy and DNA barcoding with further implications for the species identification in environmental samples. PLoS One 10:e0127838. doi: 10.1371/journal.pone.0127838
Darienko, T., Gustavs, L., Mudimu, O., Menendez, C. R., Schumann, R., Karsten, U., et al. (2010). Chloroidium, a common terrestrial coccoid green alga previously assigned to Chlorella (Trebouxiophyceae, Chlorophyta). Eur. J. Phycol. 45, 79–95. doi: 10.1080/09670260903362820
DasSarma, S., and Arora, P. (2001). Halophiles. Encyclopedia of Life Sciences. London: Nature Publishishing Group, 1–9.
Doudle, S., Williams, W., and Galea, V. (2011). “Improving rehabilitation outcomes using biocrusts,” in Eigth International Heavy Minerals Conference (Carlton, VIC: Australasian Institute for Mining and Metallurgy), 85–97.
Eilmus, S., Rösch, C., and Bothe, H. (2007). Prokaryotic life in a potash-polluted marsh with emphasis on N-metabolizing microorganisms. Environ. Pollut. 146, 478–491. doi: 10.1016/j.envpol.2006.07.008
Ettl, H., and Gärtner, G. (2014). Syllabus der Boden-, Luft-und Flechtenalgen. Berlin: Springer-Verlag.
Felde, V. J. M. N. L., Chamizo, S., Felix-Henningsen, P., and Drahorad, S. L. (2017). What stabilizes biological soil crusts in the negev desert? Plant Soil 429, 9–18. doi: 10.1007/s11104-017-3459-7
Fox, S. J. C., Mills, A. J., and Poch, R. M. (2009). Micromorphology of surface crusts in the Knersvlakte, South Africa. J. Mount. Sci. 6, 189–196. doi: 10.1007/s11629-009-1036-0
Garve, E., and Garve, V. (2000). Halophyten an kalihalden in deutschland und frankreich (Elsass). Tuexenia 20, 375–417.
Ghiloufi, W., Büdel, B., and Chaieb, M. (2017). Effects of biological soil crusts on a Mediterranean perennial grass (Stipa tenacissima L.). Plant Biosys. 151, 158–167.
Giraldo-Silva, A., Nelson, C., Bargter, N. N., and Garcia-Pichel, F. (2019). Nursing biocrusts: isolation, cultivation, and fitness test of indigenous cyanobacteria. Restor. Ecol. 27, 793–803. doi: 10.1111/rec.12920
Glaser, K., Baumann, K., Leinweber, P., Mikhailyuk, T., and Karsten, U. (2018). Algal richness in BSCs in forests under different management intensity with some implications for P cycling. Biogeosciences 15, 4181–4192. doi: 10.5194/bg-15-4181-2018
Gorostiza, S., and Saurí, D. (2019). Naturalizing pollution: a critical social science view on the link between potash mining and salinization in the Llobregat river basin, northeast Spain. Philos. Trans. R. Soc. B 374:20180006. doi: 10.1098/rstb.2018.0006
Guiry, M. D., and Guiry, G. M. (2020). AlgaeBase. Galway: World-wide electronic publication, National University of Ireland.
Gustavs, L., Eggert, A., Michalik, D., and Karsten, U. (2010). Physiological and biochemical responses of green microalgae from different habitats to osmotic and matric stress. Protoplasma 243, 3–14.
Gypser, S., Herppich, W. B., Fischer, T., Lange, P., and Veste, M. (2016). Photosynthetic characteristics and their spatial variance on biological soil crusts covering initial soils of post-mining sites in Lower Lusatia, NE Germany. Flora – Morphol. Distribut. Funct. Ecol. Plants 220, 103–116. doi: 10.1016/j.flora.2016.02.012
Hagemann, M. (2011). Molecular biology of cyanobacterial salt acclimation. FEMS Microbiol. Rev. 35, 87–123. doi: 10.1111/j.1574-6976.2010.00234.x
Herbert, E. R., Boon, P., Burgin, A. J., Neubauer, S. C., Franklin, R. B., Ardon, M., et al. (2015). A global perspective on wetland salinization: ecological consequences of a growing threat to freshwater wetlands. Ecosphere 6, 1–43.
Holzinger, A., Lütz, C., and Karsten, U. (2011). Desiccation stress causes structural and ultrastructural alterations in the aeroterrestrial green alga Klebsormidium crenulatum (Klebsormidiophyceae, Streptophyta) isolated from an alpine soil crust. J. Phycol. 47, 591–602. doi: 10.1111/j.1529-8817.2011.00980.x
Jeckel, G. (1977). Flora und Vegetation des NSG “Salzfloragebiet bei Schreyahn” in NE-Niedersachsen. Mitteilungen Floristisch-soziologischen Arbeitsgemeinschaft 19, 241–251.
Kakeh, J., Gorji, M., Sohrabi, M., Tavili, A., and Pourbabaee, A. A. (2018). Effects of biological soil crusts on some physicochemical characteristics of rangeland soils of Alagol, Turkmen Sahra, NE Iran. Soil Tillage Res. 181, 152–159. doi: 10.1016/j.still.2018.04.007
Kaplan, F., Lewis, L. A., Wastian, J., and Holzinger, A. (2012). Plasmolysis effects and osmotic potential of two phylogenetically distinct alpine strains of Klebsormidium (Streptophyta). Protoplasma 249, 789–804. doi: 10.1007/s00709-011-0324-z
Karsten, U. (2012). “Seaweed acclimation to salinity and desiccation stress,” in Seaweed Biology: Novel Insights into Ecophysiology, Ecology and Utilization. Ecological Studies 219, eds C. Wiencke and K. Bischof (Berlin: Springer-Verlag), 87–107. doi: 10.1007/978-3-642-28451-9_5
Karsten, U., Herburger, K., and Holzinger, A. (2014a). Dehydration, temperature, and light tolerance in members of the aeroterrestrial green algal genus Interfilum (Streptophyta) from biogeographically different temperate soils. J. Phycol. 50, 804–816. doi: 10.1111/jpy.12210
Karsten, U., and Holzinger, A. (2012). Light, temperature, and desiccation effects on photosynthetic activity, and drought-induced ultrastructural changes in the green alga Klebsormidium dissectum (Streptophyta) from a high alpine soil crust. Microb. Ecol. 63, 51–63. doi: 10.1007/s00248-011-9924-6
Karsten, U., Holzinger, A., Karsten, U., and Holzinger, A. (2014b). Green algae in alpine biological soil crust communities: acclimation strategies against ultraviolet radiation and dehydration. Biodivers. Conserv. 23, 1845–1858. doi: 10.1007/s10531-014-0653-2
Karsten, U., and Rindi, F. (2010). Ecophysiological performance of an urban strain of the aeroterrestrial green alga Klebsormidium sp. (Klebsormidiales, Klebsormidiophyceae). Eur. J. Phycol. 45, 426–435. doi: 10.1080/09670262.2010.498587
Kaushal, S. S., Likens, G. E., Pace, M. L., Utz, R. M., Haq, S., Gorman, J., et al. (2018). Freshwater salinization syndrome on a continental scale. Proc. Natl. Acad. Sci. U.S.A. 115, E574–E583.
Kefford, B. J., Buchwalter, D., Cañedo-Argüelles, M., Davis, J., Duncan, R. P., Hoffmann, A., et al. (2016). Salinized rivers: degraded systems or new habitats for salt-tolerant faunas? Biol. Lett. 12, 20151072. doi: 10.1098/rsbl.2015.1072
Kidron, G. J. (2016). Linking surface and subsurface properties of biocrusted and non-biocrusted habitats of fine-grained fluvial sediments (playas) from the Negev Desert. J. Hydrol. Hydromech. 64, 141–149. doi: 10.1515/johh-2016-0006
Kidron, G. J., Barinova, S., and Vonshak, A. (2012). The effects of heavy winter rains and rare summer rains on biological soil crusts in the Negev Desert. Catena 95, 6–11. doi: 10.1016/j.catena.2012.02.021
Kirkwood, A. E., and Henley, W. J. (2006). Algal community dynamics and halotolerance in a Terrestrial, hypersaline environment. J. Phycol. 42, 537–547. doi: 10.1111/j.1529-8817.2006.00227.x
Kirst, G. O. (1990). Salinity tolerance of eukaryotic marine algae. Ann. Rev. Plant Biol. 41, 21–53. doi: 10.1146/annurev.pp.41.060190.000321
Kloppmann, W., Bourhane, A., and Schomburgk, S. (2013). Groundwater salinization in France. Procedia Earth Planet. Sci. 7, 440–443. doi: 10.1016/j.proeps.2013.03.225
Korsakova, E. S., Anan’ina, L. N., Nazarov, A. V., Bachurin, B. A., and Plotnikova, E. G. (2013). Diversity of bacteria of the family Halomonadaceae at the mining area of the Verkhnekamsk salt deposit. Microbiology 82, 249–252. doi: 10.1134/s0026261713020070
Ladrera, R., Cañedo-Argüelles, M., and Prat, N. (2017). Impact of potash mining in streams: the Llobregat basin (northeast Spain) as a case study. J. Limnol. 76, 343–354.
Lan, S., Wu, L., Zhang, D., Hu, C., and Liu, Y. (2010). Effects of drought and salt stresses on man-made cyanobacterial crusts. Eur. J. Soil Biol. 46, 381–386. doi: 10.1016/j.ejsobi.2010.08.002
Lan, S., Zhang, Q., Wu, L., Liu, Y., Zhang, D., and Hu, C. (2014). Artificially accelerating the reversal of desertification: cyanobacterial inoculation facilitates the succession of vegetation communities. Environ. Sci. Technol. 48, 307–315. doi: 10.1021/es403785j
Lukešová, A. (2001). Soil algae in brown coal and lignite post-mining areas in central Europe (Czech Republic and Germany). Restor. Ecol. 9, 341–350. doi: 10.1046/j.1526-100x.2001.94002.x
Marshall, H. G. (1982). Phytoplankton composition from two saline lakes in San Salvador, Bahamas. Bull. Mar. Sci. 32, 351–353.
Neustupa, J., Št’astný, J., and Hodač, L. (2008). Temperature-related phenotypic plasticity in the green microalga Micrasterias rotata. Aquat. Microb. Ecol. 51, 77–86. doi: 10.3354/ame01184
Nyenda, T., Jacobs, S. M., Gwenzi, W., and Muvengwi, J. (2019). Biological crusts enhance fertility and texture of gold mine tailings. Ecol. Eng. 135, 54–60. doi: 10.1016/j.ecoleng.2019.03.007
Oksanen, J., Kindt, F. G. B., Michael Friendly, R., Legendre, P., McGlinn, D., Minchin, P. R., et al. (2017). vegan: Community Ecology Package. R Packag. Version 2.4-2. Available online at: https://cran.r-project.org
Olsson, B. E., Korsakova, E. S., Anan’ina, L. N., Pyankova, A. A., Mavrodi, O. V., Plotnikova, E. G., et al. (2017). Draft genome sequences of strains Salinicola socius SMB35T, Salinicola sp. MH3R3-1 and Chromohalobacter sp. SMB17 from the Verkhnekamsk potash mining region of Russia. Stand. Genomic Sci. 12:39.
Pan, C., Zhao, H., Feng, Q., Liu, J., Liu, L., Cai, Y., et al. (2015). Temporal variations of ground-dwelling arthropods in relation to grassland salinization. Eur. J. Soil Biol. 68, 25–32. doi: 10.1016/j.ejsobi.2015.03.003
Polderman, P. J. G. (1974). The algae of saline areas near Vlissingen (The Netherlands). Acta Bot. Neerlandica 23, 65–79. doi: 10.1111/j.1438-8677.1974.tb00921.x
Rippka, R., and Herdmann, H. (1992). Pasteur collection of cyanobacterial strains in axenic culture. institut pasteur, paris, France. Catal. Strains 1, 1–103.
Román, J. R., Roncero-Ramos, B., Chamizo, S., Rodríguez-Caballero, E., and Cantón, Y. (2018). Restoring soil functions by means of cyanobacteria inoculation: importance of soil conditions and species selection. Land Degrad. Dev. 29, 3184–3193. doi: 10.1002/ldr.3064
Rossi, F., Mugnai, G., and De Philippis, R. (2018). Complex role of the polymeric matrix in biological soil crusts. Plant Soil 429, 19–34. doi: 10.1007/s11104-017-3441-4
Rowell, D. L. (1997). Bodenkunde Untersuchungsmethoden und ihre Anwendungen. Berlin Heidelberg. New York, NY: Springer.
Samolov, E., Mikhailyuk, T., Lukešová, A., Glaser, K., Büdel, B., and Karsten, U. (2019). Usual alga from unusual habitats: biodiversity of Klebsormidium (Klebsormidiophyceae, Streptophyta) from the phylogenetic superclade G isolated from biological soil crusts. Mol. Phylogenet. Evol. 133, 236–255. doi: 10.1016/j.ympev.2018.12.018
Sancho, L. G., Belnap, J., Colesie, C., Raggio, J., and Weber, B. (2017). “Carbon budgets of biological soil crusts at micro-, meso-, and global scales,” in Biological SoilCrusts: An Organizing Principle in Drylands, eds B. Weber, B. Büdel, and J. Belnap (Cham: Springer), 287–304. doi: 10.1007/978-3-319-30214-0_15
Sandoval Pérez, A. L., Camargo-Ricalde, S. L., Montaño, N. M., García-Oliva, F., Alarcón, A., Montaño-Arias, S. A., et al. (2016). Biocrusts, inside and outside resource islands of Mimosa luisana (Leguminosae), improve soil carbon and nitrogen dynamics in a tropical semiarid ecosystem. Eur. J. Soil Biol. 74, 93–103. doi: 10.1016/j.ejsobi.2016.03.006
Schulz, K., Mikhailyuk, T., Dreßler, M., Leinweber, P., and Karsten, U. (2016). Biological soil crusts from coastal dunes at the baltic sea: cyanobacterial and algal biodiversity and related soil properties. Microb. Ecol. 71, 178–193. doi: 10.1007/s00248-015-0691-7
Shubert, L. E., and Starks, T. L. (1980). Soil-algal relationships from surface mined soils. Br. Phycol. J. 15, 417–428. doi: 10.1080/00071618000650421
Siefert, B., Büchel, G., and Lebküchner-Neugebauer, J. (2006). Potash mining waste pile Sollstedt (Thuringia): investigations of the spreading of waste solutes in the Roethian Karst. Grundwasser 11, 99–110.
Škaloud, P., Lukešová, A., Malavasi, V., Ryšánek, D., Hrčková, K., and Rindi, F. (2014). Molecular evidence for the polyphyletic origin of low pH adaptation in the genus Klebsormidium (Klebsormidiophyceae, Streptophyta). Plant Ecol. Evol. 147, 333–345. doi: 10.5091/plecevo.2014.989
Soares, M. C. S., Lürling, M., and Huszar, V. L. M. (2013). Growth and temperature-related phenotypic plasticity in the cyanobacterium Cylindrospermopsis raciborskii. Phycol. Res. 61, 61–67. doi: 10.1111/pre.12001
Starr, R. C., and Zeiskus, J. A. (1993). UTEX – The culture collection of algae at the University of Texas at Austin. J. Phycol. 29, 1–106. doi: 10.1111/j.0022-3646.1993.00001.x
Szyja, M., Büdel, B., and Colesie, C. (2018). Ecophysiological characterization of early successional biological soil crusts in heavily human-impacted areas. Biogeosciences 15, 1919–1931. doi: 10.5194/bg-15-1919-2018
Trzcińska, M., and Pawlik-Skowrońska, B. (2008). Soil algal communities inhabiting zinc and lead mine spoils. J. Appl. Phycol. 20, 341–348. doi: 10.1007/s10811-007-9259-3
Tsujimura, S., Tsujimura, S., Nakahara, H., Kosaki, T., Ishida, N., Iskakov, A. R., et al. (1998). Distribution of soil algae in salinized irrigation land in the arid region of Central Asia: II. a case study of 25-year-old bakbakty farm in the flood plain of the river Ili, Kazakstan. Soil Sci. Plant Nutr. 44, 67–76. doi: 10.1080/00380768.1998.10414427
Van Dam, R. A., Harford, A. J., Lunn, S. A., and Gagnon, M. M. (2014). Identifying the Cause of toxicity of a saline mine water. PLoS One 9:e106857. doi: 10.1371/journal.pone.0106857
Venables, W. N., and Ripley, B. D. (2002). Modern Applied Statistics with S. Statistics and Computing, fourth ed. Berlin: Springer.
Vinogradova, O. N., and Darienko, T. M. (2008). Algae of azovo-syvashsky national nature park (Ukraine). Int. J. Algae 10, 1–15.
Wang, W., Liu, Y., Li, D., Hu, C., and Rao, B. (2009). Feasibility of cyanobacterial inoculation for biological soil crusts formation in desert area. Soil Biol. Biochem. 41, 926–929. doi: 10.1016/j.soilbio.2008.07.001
Wang, Y., and Li, Y. (2013). Land exploitation resulting in soil salinization in a desert-oasis ecotone. Catena 100, 50–56. doi: 10.1016/j.catena.2012.08.005
Weber, B., Büdel, B., and Belnap, J. (2016). Biological Soil Crusts: An Organizing Principle in Drylands. Cham: Springer.
Wen, Z., Mian-Ping, Z., Xian-Zhong, X., Xi-Fang, L., Gan-Lin, G., and Zhi-Hui, H. (2005). Biological and ecological features of saline lakes in northern Tibet, China. Hydrobiologia 541, 189–203. doi: 10.1007/s10750-004-5707-0
Weyman-Kaczmarkowa, W., and Pędziwilk, Z. (2000). The development of fungi as affected by pH and type of soil, in relation to the occurrence of bacteria and soil fungistatic activity. Microbiol. Res. 155, 107–112. doi: 10.1016/S0944-5013(00)80045-2
Whitney, D. A. (1988). “Soil salinity,” in Recommended Chemical Soil Test Procedures for the North Central Region, Vol. 221, (West Lafayette, IN: North Central Regional Research Publication), 59–60.
Williams, L., Jung, P., Zheng, K., Maier, S., Peer, T., Grube, M., et al. (2018). Assessing recovery of biological soil crusts across a latitudinal gradient in Western Europe. Restor. Ecol. 26, 543–554. doi: 10.1111/rec.12579
Williams, W., Schneemilch, M., Chilton, A., Williams, S., and Neilan, B. (2019). Microbial biobanking cyanobacteria-rich topsoil facilitates mine rehabilitation. Biogeosciences 16, 2189–2204. doi: 10.5194/bg-16-2189-2019
Williams, W. D. (1987). Salinization of Rivers and streams: an important environ- mental hazard. Ambio 16, 180–185.
Woch, M. W., and Trzcińska-Tacik, H. (2015). High occurrence of rare inland halophytes on post-mining sites in western Ukraine. Nordic J. Bot. 33, 101–108. doi: 10.1111/njb.00607
Zhang, Y., Duan, P., Zhang, P., and Li, M. (2018). Variations in cyanobacterial and algal communities and soil characteristics under biocrust development under similar environmental conditions. Plant Soil 429, 241–251. doi: 10.1007/s11104-017-3443-2
Zhao, J., Zhang, B., and Zhang, Y. (2008). Chlorophytes of biological soil crusts in gurbantunggut desert, Xinjiang Autonomous Region, China. Front. Biol. China 3, 40–44. doi: 10.1007/s11515-008-0007-0
Keywords: biocrusts, secondary salinization, salt tolerance, green algae, cyanobacteria, diatoms
Citation: Sommer V, Karsten U and Glaser K (2020) Halophilic Algal Communities in Biological Soil Crusts Isolated From Potash Tailings Pile Areas. Front. Ecol. Evol. 8:46. doi: 10.3389/fevo.2020.00046
Received: 30 July 2019; Accepted: 13 February 2020;
Published: 06 March 2020.
Edited by:
Maik Veste, Brandenburg University of Technology Cottbus-Senftenberg, GermanyReviewed by:
RongLiang Jia, Chinese Academy of Sciences, ChinaLaura Briegel-Williams, University of Kaiserslautern, Germany
Copyright © 2020 Sommer, Karsten and Glaser. This is an open-access article distributed under the terms of the Creative Commons Attribution License (CC BY). The use, distribution or reproduction in other forums is permitted, provided the original author(s) and the copyright owner(s) are credited and that the original publication in this journal is cited, in accordance with accepted academic practice. No use, distribution or reproduction is permitted which does not comply with these terms.
*Correspondence: Karin Glaser, a2FyaW4uZ2xhc2VyQHVuaS1yb3N0b2NrLmRl