- 1School of Pharmacy, Chengdu University of Traditional Chinese Medicine, Chengdu, China
- 2College of Pharmacy and Biological Engineering, Chengdu University, Chengdu, China
- 3International Association of Quality Research in Chinese Medicine, Macau, China
- 4State Key Laboratory of Quality Research in Chinese Medicine, Institute of Chinese Medical Sciences, University of Macau, Macau, China
- 5Sichuan Key Laboratory of Medical American Cockroach, Chengdu, China
Periplaneta americana, a magic medicinal insect being present for over 300 million years, exhibits desirable therapeutic outcome for gastrointestinal ulcer treatment. Nowadays, P. americana ethanol extract (PAE) has been shown to ameliorate ulcerative colitis (UC) by either single-use or in combination with other therapeutic agents in clinics. However, its underlying mechanisms are still seldom known. Herein, we investigated the anti-UC activity of PAE by alleviating intestinal inflammation and regulating the disturbed gut microbiota structure in dextran sulfate sodium (DSS)-induced UC rats. Based on multiple constitute analyses by HPLC for quality control, PAE was administrated to DSS-induced UC rats by oral gavage for 2 weeks. The anti-UC effect of PAE was evaluated by inflammatory cytokine production, immunohistochemical staining, and gut microbiota analysis via 16S rRNA sequencing. As a result, PAE remarkably attenuated DSS-induced UC in rats. The colonic inflammatory responses manifested as decreased colonic atrophy, intestinal histopathology scores and inflammatory cytokines. In addition, PAE improved the intestinal barrier function via activating Keap1/Nrf-2 pathway and promoting the expressions of tight junction proteins. It was observed that the UC rats showed symptoms of gut microbial disturbance, i.e., the increased Firmicutes/Bacteroidetes ratio and the significantly decreased probiotics such as Lactobacillus, Roseburia, and Pectobacterium, which were negatively correlated with these detected pro-inflammatory cytokines (secreted by immune CD4+ T cells, and including IFN-γ, TNF-α, IL-6, IL-8, IL-17, IL-1β). Besides, PAE administration regulated the abnormal intestinal microbial composition and made it similar to that in normal rats. Therefore, PAE could attenuate the DSS-induced UC in rats, by means of ameliorating intestinal inflammation, improving intestinal barrier function, and regulating the disturbed gut microbiota, especially improving beneficial intestinal flora growth, modulating the flora structure, and restoring the intestinal-immune system.
Introduction
Ulcerative colitis (UC) is a representative chronic inflammatory bowel disease (IBD) with high morbidity worldwide, characterized by recurrent remission and relapse (Danese and Fiocchi, 2011). It is reported that the incidence of UC ranges from 35 to 100 per 100,000 people. Moreover, a global epidemiology study has reported that compared to United States and United Kingdom, Southern Europe and Asia have a more rapid growth rate of UC incidence (Hanauer, 2006; Molodecky et al., 2012). Typical symptoms include frequent bowel movement, bloody diarrhea, abdominal pain, urgency, tenesmus, and some common complications such as proctitis, rectal abscess, enterobrosis, and colon cancer (Feuerstein and Cheifetz, 2014). However, despite the increased global attention, the etiology and pathogenesis of UC remain unclear, making it one of the most challenging gastrointestinal diseases. Until now, 5-aminosalicylic acids and corticosteroids, which could regulate the imbalance between pro-inflammatory cytokines and anti-inflammatory cytokines, have been known as the important agents for UC treatment (Huang et al., 2017b). However, some potential side-effects of these drugs, such as anti-antibody reaction, allergy, infection and mutagenesis, could be brought by long-term use and therefore compromise their clinical applications (Renna et al., 2014; Gu et al., 2017). Thus, to investigate the effective anti-UC drugs with higher drug safety is of great significance and urgency.
Complementary and alternative medicines show the potentials of being effective, low-cost treatment approaches with high safety. Some plant-derived compounds such as artemisinin, taxol, camptothecin are the representative examples of natural’s gifts to medicine at present. Epidemiological surveys suggest that, the majority of patients with IBD receive traditional medicines (Kong et al., 2005; Guo et al., 2017). Some natural products such as berberine (Habtemariam, 2016), sophocarpidine (Wang et al., 2012), indigo naturalis (Wang Y. et al., 2017), andrographolide (Liu et al., 2014), and curcumin (Sreedhar et al., 2016) also exhibit efficacy in treating UC. In addition, the medicinal insects, whose species are more than twice as many as medical plants, have also received extensive research interests. Periplaneta americana, more widely known as American cockroach, was first recorded in an ancient Chinese pharmacopeia “Shen Nong Ben Cao Jing.” As one of the largest and oldest insect groups being present for over 300 million years, this miraculous insect has also been employed as a traditional Chinese medicine for over 2,000 years, for its functions of activating blood circulation, dissipating blood stasis, promoting digestion, and inducing diuresis. Modern studies have revealed its various bioactivities (Srivastava et al., 2011; Zhang et al., 2013; Huang et al., 2017a; Shen et al., 2017; Zhao et al., 2017) such as gastric protection, wound healing, antitumor activity, immune enhancement, liver protection, and antiviral effect. P. americana has been developed into clinical drugs under the approval of China Food and Drug Administration (CFDA), such as “Kangfuxin oral liquid (Z51021834),” “Xinmainong injection (Z20060443),” and “Ganlong capsule (Z20050113).” Particularly, Kangfuxin oral liquid, which is prepared by the ethanol extract of P. americana, has been widely demonstrated for its wound healing effect in peptic ulcer treatment (Chen et al., 2016; Shen et al., 2017). In view of its significant role in gastric protection, Li et al. (2016) has been demonstrated for the therapeutic effects of P. americana in UC rats induced by dinitrochlorobenzene and acetic acid, which are attributed to the anti-inflammation function and fibroblasts viability. Previous studies (He et al., 2012; Zhang H. C. et al., 2017) have also indicated that, the anti-inflammation activity in UC model are related to the regulation of inflammation cytokines including iNOS, MPO, IL-4, IL-10, EGF, MUC2, and IL-6. Moreover, the mucositis amelioration of Kangfuxin liquid was also observed in Randomized Phase III Clinical Study (Luo et al., 2016). However, to date, the chemical constitutes and the underlying anti-UC mechanisms of P. americana have not been well described.
Several signal transduction cascades and transcription factors are involved in the pathological inflammatory process, such as NF-κB, MAPK, JAK-STAT, and Keap1/Nrf2/ARE (Ahmed et al., 2017) signaling pathways. At present, an increasing number of researches (Yang et al., 2016; Ahmed et al., 2017) have begun to focus on the role of Nrf2/Keap1 pathway in the inflammation-associated pathogenesis, as well as the suppression of pro-inflammatory signaling pathways. Besides, previous studies have demonstrated the decreased expression of Nrf2 in dextran sulfate sodium (DSS)-induced UC model and the important role of Nrf2 signaling pathway in UC treatment by some chemo-preventive agents (Dae Park et al., 2017; Seo et al., 2017; Liu et al., 2018; Tan and Zheng, 2018).
Up to now, although the development of IBD has been confirmed to be related to various factors including immune dysregulation, genetic disorder and barrier dysfunction, the pathogenesis of IBD still remains unclear. Gut microflora has been recorded as the highest cell density in any ecosystem, with a profound and crucial influence on human health. And the close relationship between gut microflora dysfunction and IBD has been widely acknowledged (Ohkusa and Koido, 2015). According to relevant reports, fecal bacteria from healthy donors are expected to have therapeutic effects on patients with IBD (Anderson et al., 2012). In particular, gut microflora is crucial for the protection of intestinal mucosa, and microbiota dysbiosis could lead to mucosal injury. Studies (Tao et al., 2017; Wang K. et al., 2017; Wang Y. et al., 2017) have indicated that the coexistence between the host and gut microbiota is beneficial for modulating the intestinal mucosal immune system. Gut microbiota is considered to be relevant to intestinal local inflammation and mucosal immune system. It is well known that antimicrobial drugs could generally improve IBD by modulating the host microbiota (McIlroy et al., 2017). The anti-inflammation activity of P. americana on gastric protection has been reported in previous studies. However, whether the anti-UC effect of P. americana is involved in its gut microbiota regulation activity still remains unclear. In this study, we evaluated the anti-UC activity of ethanol extracts of P. americana (PAE), which were provided by GoodDoctor Pharmaceutical Co., Ltd., and widely used as the preparation materials for Kangfuxin liquid, in a dextran sodium sulfate-induced UC rat model that mimicked many histopathological and immune characteristics of human intestinal inflammation. Meanwhile, the PAEs were characterized by means of high performance liquid chromatography (HPLC) quantification for quality control. Eventually, the anti-UC effects and mechanisms of the PAEs related to anti-inflammation, intestinal barrier improvement, and gut microbiota regulation were evaluated.
Materials and Methods
Chemicals and Materials
P. americana ethanol extracts (PAE) were extracted from P. americana provided by Good Agricultural Practice (GAP) breeding base of GoodDoctor Pharmaceutical Co., Ltd. (Sichuan, China), while P. americana water extracts were obtained by water extraction following the steps below: after being degreased with petroleum ether, P. americana crude powders (100 g), obtained from the Good Agricultural Practice (GAP) breeding base of GoodDoctor Pharmaceutical Co., Ltd. (Sichuan, China), was soaked for 2 h, extracted with water for 10 times, boiled for 30 min, and finally collected by freeze-drying after filtration. Both ethanol and water extracts of P. americana were dissolved in DMSO for cell experiments and then suspended in 0.5% CMC-Na solution for animal test, respectively. HPLC-grade acetonitrile and water used in this study were purchased from Fisher Scientific UK.
HPLC Analysis
Chromatographic analysis was performed on an Agilent 1260 series HPLC system equipped with a diode array detector (DAD), using a Zorbax XDB C18 (4.6 mm × 250 mm, 5 μm) at a column temperature of 25°C. The flow rate and injection volume were 1 ml/min and 10 μl, respectively. The methanol–water (2.5:97.5) system was employed as the mobile phase for quantitative determination of multiple standard compounds, i.e., guanosine, uridine, inoside, cytidine, hypoxanthine, thymine, adenine, cytosine, and uracil. All these standard compounds were dissolved together by 3% methanol to form a mixed standard solution. The optimized detection wavelength was 254 nm.
Anti-inflammatory Effect of PAE in vitro
RAW 264.7 macrophage cell line, obtained from American Type Culture Collection (ATCC; Manassas, VA, United States), was cultured in RPMI-1640 medium supplemented with 10% FBS, 100 U/ml penicillin and 100 μg/ml streptomycin. All cells were incubated in a humidified 5% CO2 incubator at 37°C, and plated in 96-well plates at densities of 1 × 104 cells/well. The concentrations of non-toxic PAE in RAW 264.7 were evaluated by MTT assay described in supplementary materials in the first place, and the effects of PAE on the pro-inflammatory cytokine production in RAW 264.7 cells were then explored. After 24 h adherence, the cells were pre-incubated in a FBS-free medium containing a series of amounts of PAE for 2 h, and then treated with 1 μg/ml of LPS stimulation for 12 h. After then, cell culture supernatants were collected and assayed for NO production by reacting with Griess reagent (Hu et al., 2017) and the amounts of inflammatory cytokines, including TNF-α, IL-1β, PGE2, were calculated by an enzyme-linked immunosorbent assay (ELISA) kit for rats (Ge et al., 2017) (R&D Systems, Inc., Minneapolis, MN, United States). Meanwhile, the cell viability was assessed by incubating with MTT regent for 4 h, and the absorbance of DMSO solution containing formazan crystal was measured at 570 nm (Zhang J. et al., 2017).
Anti-UC Effects in DSS-Induced UC Rats
In view of the high reproducibility in experimental implementation and the high similarity with the features of clinical UC, the DSS-induced UC model is widely recognized and frequently used to evaluate the anti-UC effects involved in the anti-inflammatory approaches, in which cases, repeated administration of DSS could result in the disruption of the colonic mucosal architecture and long-term chronic inflammation. Herein, the UC rat model was established by oral administration of 5% DSS (w/v) dissolved in drinking water for seven consecutive days. The UC rats were randomly divided into four groups (n = 8) with various treatments for 14 consecutive days, i.e., the model group, the high-dose PAE group, the low-dose PAE group, and the P. americana water extract group (W-E). More specifically, rats in model group were given only saline by gavage administration once per day. UC rats in high- and low-dose PAE groups were administrated with 200 mg/kg and 100 mg/kg of PAE, respectively. Since water decoction is a traditional preparation approach of Chinese medicine, W-Es (200 mg/kg) of P. americana herein were obtained and evaluated to determine whether they could alleviate UC-model rats and serve as the counterpart of PAE. During the administration of various agents, 3% DSS (w/v) was given to rats to avoid self-cure. Apart from these UC rats, rats without DSS treatment were only given saline in the control group.
The weight loss, stool consistency and occult/gross bleeding of all rats were recorded every 3 days throughout the experiment. Disease activity index (DAI) scores were calculated based on the previously described evaluation standard (Cho et al., 2011), to assess the extent of UC. After 14 days of administration, all rats were sacrificed by isoflurane inhalation, and their colon samples were collected and exampled. Part of the colon segments were fixed by 4% paraformaldehyde, embedded in paraffin and then cut into 4 μm thick sections. These sections were stained with hematoxylin and eosin (HE) and alcian blue, respectively, in accordance with the standard procedures for histopathological analysis. Additionally, the remaining colon segments were weighted and homogenized with 0.1 M phosphate buffer (pH 7.4). The homogenate suspension was collected by centrifugation at 5,000 rpm for 15 min. The amounts of inflammation-associated cytokines including IFN-γ, IL-17, IL-8, IL-6, IL-1β, and TNF-α were determined using ELISA kits according to the manufacturers’ instructions.
Intestinal Barrier Function Activities
The intestinal barrier functions were evaluated via Keap1 and Nrf-2 mRNA analysis by quantitative reverse transcription PCR (qRT-PCR) and the expressions of tight junction proteins (ZO-1, occludin, and claudin-1) were via Western blot analysis. Total RNA of colon tissue was extracted by TRIzol reagents according to the manufacturer’s instructions (Invitrogen, United States). The PCR reaction mixture contained 2 × qPCR mix, 7.5 μM PCR primer, 2.5 μl reverse transcription product and 8.0 μl ddH2O. The RT quantitative reaction was performed as follows: precycling at 95°C for 10 min, then 40 cycles of denaturation at 95°C for 15 s and annealing at 60°C for 60 s. Primers for Keap1, Nrf2, and GAPDH were listed in Table 1. Fluorescence was measured at the end of each annealing step, and the melting curves were monitored to identify the specificity of the PCR products. The 2−ΔΔCt method was used to determine the mRNA expression levels of Keap1 and Nrf-2 relative to the control gene GAPDH.
The colon segments were homogenized with RIPA buffer containing protease inhibitor (1:100). After protein concentration was determined by BCR protein assay kit, Western blot assay was performed as described (Kang et al., 2017). The primary antibodies against ZO-1, occludin and claudin-1 were then incubated overnight at 4°C. GAPDH antibody was used as an internal control to ascertain the equal loading of proteins. The obtained chemiluminescence bands were analyzed with ImageJ software.
Furthermore, intestinal epithelial permeability in vivo was determined via FITC probe based on the previously described method (Moussaoui et al., 2014). Briefly, UC model rats were employed and administrated with saline, high-dose PAE, low-dose PAE, and W-E for 14 consecutive days. After then, these UC rats and normal rats were fasted overnight and given fluorescein isothiocyanate (FITC)-dextran solution (4 kDa, 600 mg/kg) by gavage. Their blood samples were collected from retinal veins 4 h after administration and then centrifuged at 3,000 × g and 4°C for 10 min. Serum levels of FITC were measured at 480 and 520 nm using a microplate reader.
Effects on Gut Microbiota
In light of the anti-UC effects of high-dose PAE, whether PAE could regulate gut microbiota was investigated. Primarily, total genomic DNAs from the feces of rats in various groups were extracted using DNeasy PowerSoil Kit (QIAGEN) according to the descriptions. DNA concentration and quality were checked using a NanoDrop Spectrophotometer. Bacterial 16S rRNA gene sequences (V4 region) were amplified by PCR instrument (Applied Biosystems® Gene Amp® PCR System 9700) using primer 5′→3′ (Caporaso et al., 2011): 515F (5′-GTGCCAGCMGCCGCGGTAA-3′) and 806R (5′-GGACTACHVGGGTWTCTAAT-3′). The amplification procedures were conducted at 94°C for 1 min in the first place, and then followed by 30 cycles (denaturation at 94°C for 20 s, annealing at 54°C for 30 s, and elongation at 72°C for 30 s) and a final extension at 72°C for 5 min. PCR reactions were performed in triplicate: 25 μl mixture containing 1x PCR buffer, 1.5 mM MgCl2, each deoxynucleoside triphosphate at 0.4 μM, each primer at 1.0 μM, 0.5 U of KOD-Plus-Neo (TOYOBO) and 10 ng template DNA. Amplicons were extracted from 2% agarose gels, purified with the OMEGA Gel Extraction Kit (Omega Bio-Tek, United States) and then quantified with Qubit @ 2.0 Fluorometer (Thermo Fisher Scientific, United States).
MiSeq Illumina sequencing was further processed on the sequencing reaction (Illumina Inc., San Diego, CA, United States) for paired-end reads to establish a DNA library using the TruSeq DNA PCR-Free Sample Prep Kit (FC-121-3001/3003) as per the standard protocols. The paired-end reads were merged by FLASH and then assigned to each sample according to the unique barcodes, so as to get rid of the low-quality tags (length < 200 bp, more than two ambiguous base ‘N,’ or the average base quality score < 30). High-quality tags were clustered into operational taxonomic units (OTUs) using UPARSE (Edgar, 2013) algorithm in QIIME software based on a 97% sequence similarity, and these OTUs were further subjected to analysis using database of Greengenes by PyNAST algorithm. Alpha and Beta diversities and principal coordinate analysis (PCoA) were analyzed by QIIME, Mothur, and R software. LEfSe analysis were done using Python LEfSe package (Segata et al., 2011).
Statistical Analysis
The experimental data were analyzed by GraphPad Prism 6.0 software and presented as mean ± SD. The values of various groups were evaluated by one-way ANOVA and difference test. P < 0.05, P < 0.01, and P < 0.001, calculated using SPSS software version 17.0., were considered statistically significant.
Results
HPLC Quantitative Analysis
Given the complex composition of herbal extracts, the quantitative analysis was of great importance for the quality control of PAE herein. The amounts of nine physiological small molecules, i.e., guanosine, uridine, inosine, cytidine, hypoxanthine, thymine, adenine, cytosine, and uracil, in PAE were determined by HPLC. These nucleotides, nucleosides and nucleobases were deemed to be the potential active components in some natural products such as Cordyceps sinensis (Yuan et al., 2008; Yang et al., 2010) and Sipunculus nudus (Ge et al., 2016). As shown in Figure 1A, these nine compounds in mixed standard substances were analyzed, with a satisfied degree of separation and methodological investigation being obtained. The standard curves and linear ranges of these compounds were as follows: cytimidine: y = 132.64x-3.1286 (r2 = 0.9999, 0.311∼6.22 mg⋅ml−1), uracil: y = 216.56x+ 0.3047 (r2 = 1, 0.432∼8.64 mg⋅ml−1), cytidine: y = 79.1x-0.1193 (r2 = 1, 0.354∼7.08 mg⋅ml−1), hypoxanthine: y = 153.25x-18.512 (r2 = 0.9996, 0.392∼7.84 mg⋅ml−1), uridine: y = 22.569x-1.056 (r2 = 1, 0.389∼7.78 mg⋅ml−1), thymine: y = 28.056x+ 2.0681 (r2 = 0.9990, 0.272∼5.44 mg⋅ml−1), adenine: y = 4.1931x+ 3.7809 (r2 = 0.9997, 0.363∼7.26 mg⋅ml−1), inosine: y = 100.13x+ 40.147 (r2 = 0.9993, 0.302∼6.04 mg⋅ml−1), guanosine: y = 15.581x + 12.32 (r2 = 0.9992, 0.304∼6.08 mg⋅ml−1). Figure 1B provides clear evidence that the concentrations of these nine compounds in PAE sample could be determined. Accordingly, based on the external standard method, the amounts of these nine compounds, i.e., cytimidine, uracil, cytidine, hypoxanthine, uridine, thymine, adenine, inosine, and guanosine, in PAE were calculated and the results were 6.165, 0.724, 1.375, 2.466, 1.422, 0.451, 2.885, 2.373, and 1.737 mg/g, respectively. This result indicated that the multicomponent quantification method could provide a definite quality control approach for PAE.
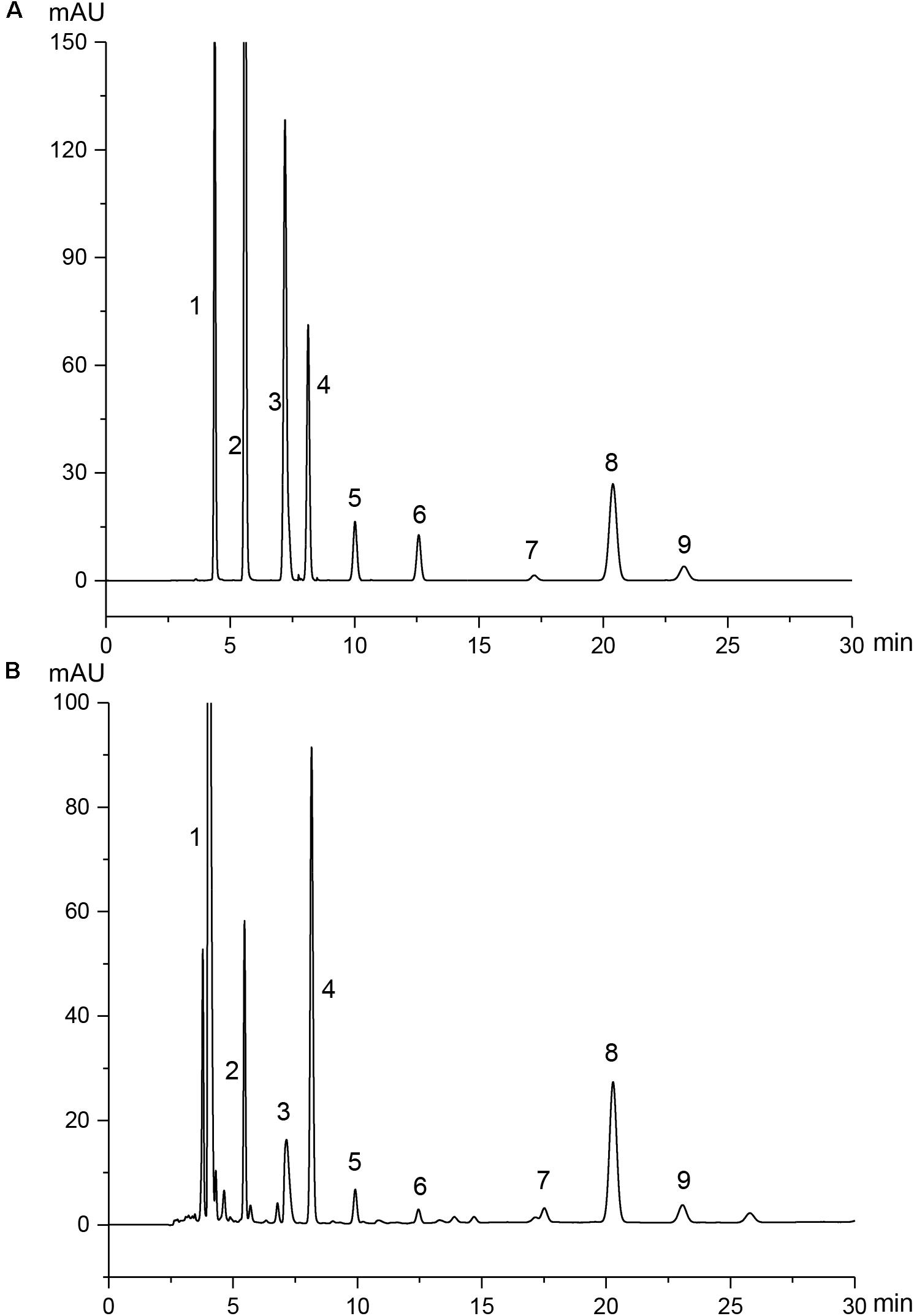
FIGURE 1. HPLC chromatograms of mixed standard substances (A) and PAE samples (B). Peaks 1∼9 are derived from cytimidine, uracil, cytidine, hypoxanthine, uridine, thymine, adenine, inosine, and guanosine, respectively.
The Expressions of Pro-inflammatory Factors Were Down-Regulated in LPS-Stimulated RAW 264.7 Cells
Macrophages are the major source of pro-inflammatory cytokines. In order to investigate the inhibition effects of PAE on inflammatory factor expression, the amounts of NO, TNF-α, IL-Iβ, and PGE2 in LPS-stimulated RAW 264.7 cells were determined by Griess reaction and ELISA assay. The potential cytotoxicity of PAE on RAW 264.7 cells was first evaluated by MTT assay, indicating that PAE (0.25, 0.5, and 1 μg/ml) was not significantly cytotoxic after 24 h incubation (Supplementary Figure S1). As is well known, the NO amount in RAW 284.7 cell culture medium could be dramatically increased by the simulation of LPS. Whether PAE could attenuate LPS-induced NO production in RAW 264.7 macrophages was then examined. The significant reduction in NO production induced by the LPS-simulated macrophages was due to the addition of PAE in a concentration-dependent manner (Figure 2A). Subsequently, the changes of inflammatory factor production were evaluated based on its non-toxicity against macrophage cells. As shown in Figures 2B–D, compared with the blank control or PAE group, LPS induced a more significant increase in the production of pro-inflammatory cytokines (by 2.34-fold for TNF-α, 4.37-fold for IL-Iβ, and 2.44-fold for PGE2). However, the pretreatment of PAE with various concentrations could significantly inhibit the LPS-induced production. These results indicated that PAE could remarkably alleviate the inflammatory reaction by inhibiting the production of nitric oxide and pro-inflammatory cytokines.
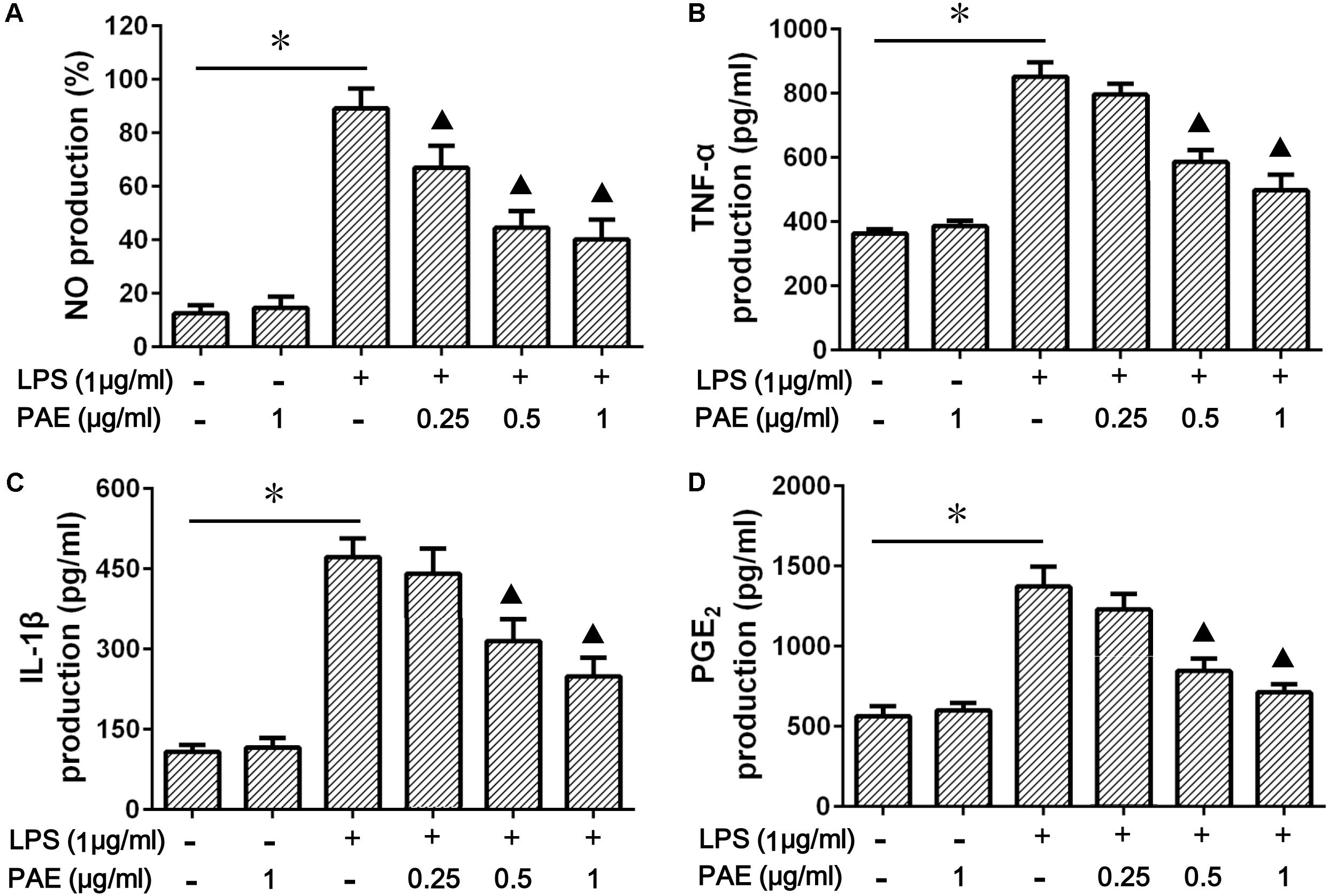
FIGURE 2. Effects of PAE on the production of NO (A), TNF-α (B), IL-Iβ (C), and PGE2 (D) in LPS-stimulated RAW 264.7 macrophages. RAW 264.7 cells were pre-incubated in FBS-free medium containing a series of amounts of PAE for 2 h, and then co-treated with 1 μg/ml of LPS stimulation for 12 h. ∗P < 0.05 blank control group or PAE vs. LPS-stimulated group, P < 0.05 LPS + PAE groups vs. LPS-stimulated group (n = 6 per group).
PAE Attenuated DSS-Induced UC in Rats
Preliminary experiments have shown that continuous administration of 5% DSS in drinking water for more than five consecutive days could induce various symptoms, such as diarrhea and even hematochezia, which exhibit similarities with human UC. However, if the 5% DSS is replaced with fresh water, these symptoms in rats would gradually disappear after 7 days. Therefore, to avoid the self-cure of UC rats after the termination of 5% DSS administration, 3% DSS was used to keep the pathological condition during the administration of PAE agents herein (Figure 3A). The body weight changes of the rats throughout the experiment clearly reflected their physiological status. Figure 3B shows a significant body weight loss of DSS-treated UC rats at day 21, in comparison with the control group. However, rats in PAE-H group exhibited a less distinct weight loss compared with the model group. In view of these representative clinical features, the DAI was used to evaluate the therapeutic activity of PAE. As shown in Figure 3C, DAI scores of rats treated with DSS were significantly increased in comparison with those of the control group. However, DAI scores of PAE-H group remarkably dropped, indicating the improvement effect of high-dose PAE on UC-related pathological states. In addition to the severe synechia, hyperemia, edema, the colon length of rats treated with DSS was apparently shortened. Figures 3D,E show the colon shortening of rats treated with DSS. Given all that, high-dose PAE could significantly ameliorate the colon shortening.
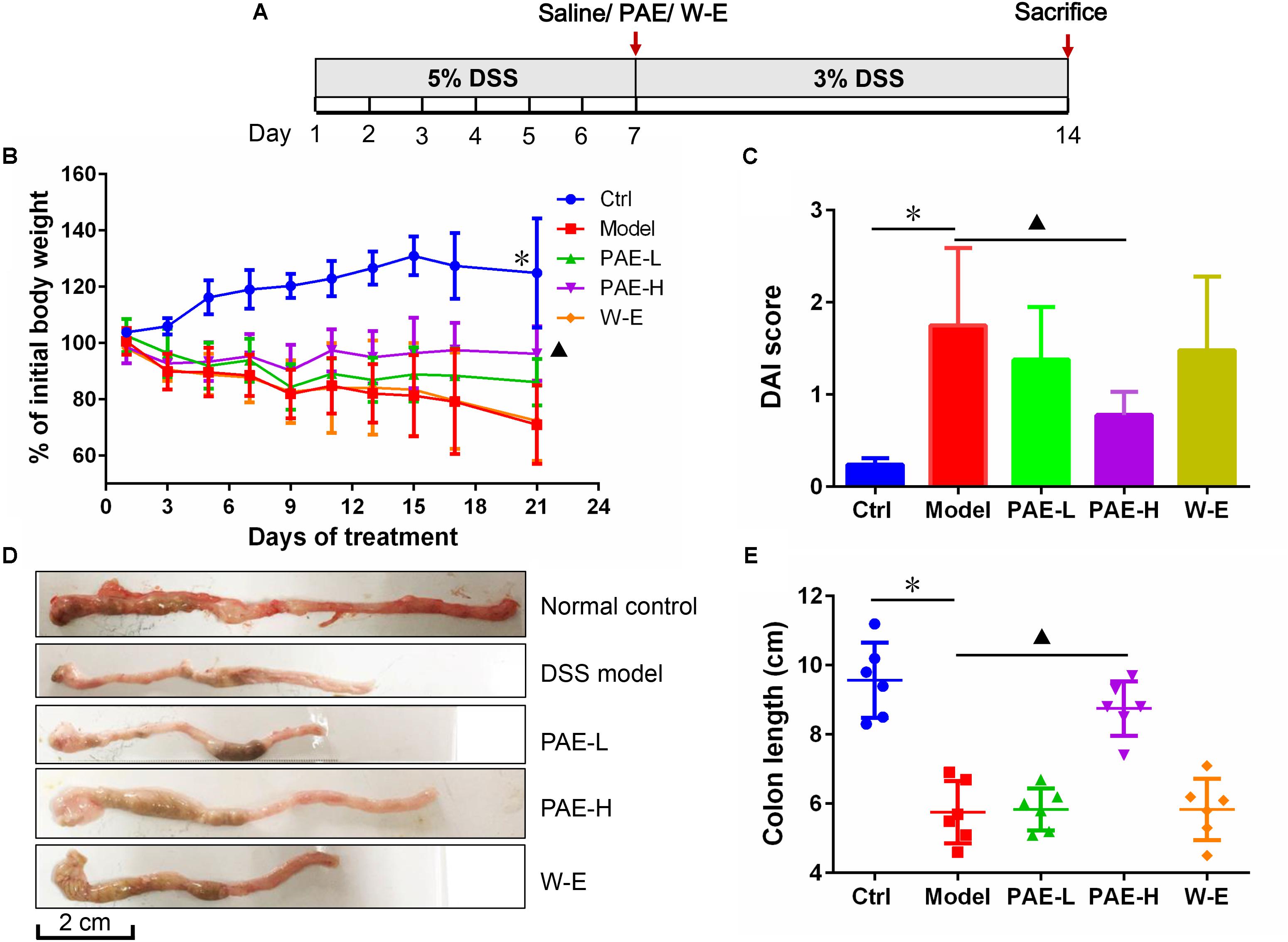
FIGURE 3. Effects of PAE treatment on the of DSS-induced UC rats after 14 days of continuous gavage. (A) The medication regimen. (B) Rats’ body weight changes from day 1 to day 21 throughout the experiment. (C) DAI scores of rats in various groups. (D) Representative photographs of rats’ colons in various groups. (E) Colon length of rats in various groups. ∗P < 0.05 untreated control group vs. DSS-induced UC model group, P < 0.05 model group vs. PAE-H group (n = 6 per group).
PAE Ameliorated the Histopathological Changes in Colon Tissues
Based on the above-mentioned findings, DSS could induce severe inflammation of colon tissues. The histopathological screening of rats’ colon sections by HE-staining revealed no necrosis or inflammation in the control group (Figures 4A,B). Rats in the DSS model group showed colon tissue injuries, including crypt distortion, goblet cell loss, severe epithelia damage, and mucosal inflammatory cell infiltration (Chen et al., 2015). However, the administration of high-dose PAE could significantly protect colon crypt structure and reduce histologic inflammation. Moreover, it was hypothesized that PAE could protect the intestinal mucosa from damages by reinforcing its self-repair. To further demonstrate this hypothesis, alcian blue staining was conducted to investigate the intestinal mucosa changes after PAE administration during the DSS treatment. Figures 4C,D show that DSS could remarkably decrease the expressions of mucins, which were involved in the repair of colon mucosa, compared with the control group. Nevertheless, both high- and low-dose PAEs could increase the mucin expressions, showing much more positive blue cells in both PAE-H and PAE-L groups. These results suggested that PAE could inhibit colonic inflammation and promote the restoration of intestinal mucosa.
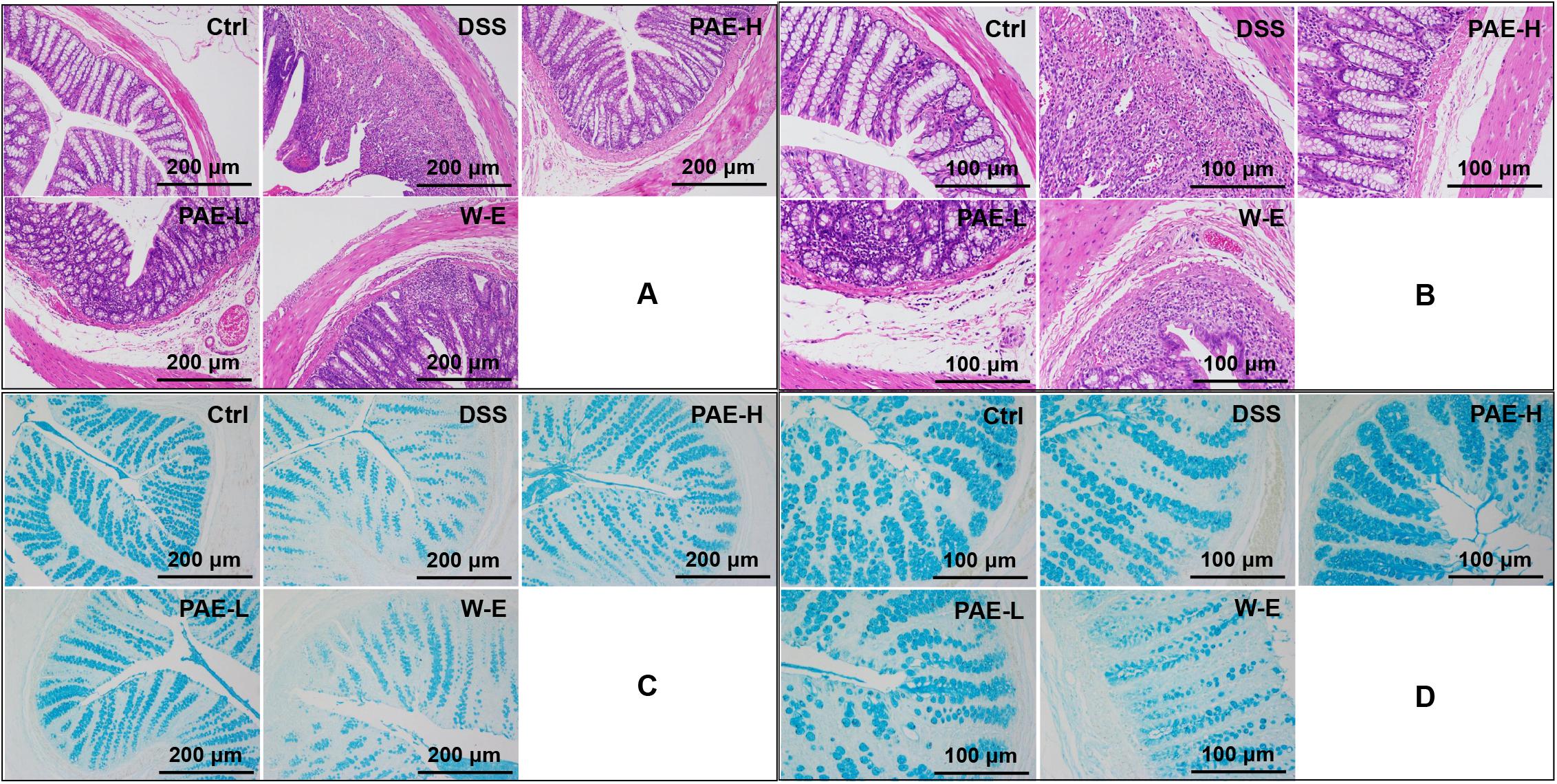
FIGURE 4. Effects of PAE on DSS-induced UC rats by HE staining and alcian blue staining of colorectum sections (A and C with magnification ×100, B and D with magnification ×200).
PAE Reduced the Expressions of Inflammatory Cytokines in the Colorectum Tissues of UC Rats
In the intestinal mucosal immune system, neutrophils and macrophages are responsible for inflammatory cytokine secretion, which could disrupt epithelial integrity and cause colon injury (Grisham and Yamada, 1992). The production of pro-inflammatory cytokines was significantly increased in DSS-induced model group compared with the control group. However, both PAE and water extract reduced the abnormally increased amounts of pro-inflammatory cytokines (Figure 5). It has been widely demonstrated that the increased amounts of TNF-α, IL-6, and IL-1β were related with the inflamed mucosa and superficial ulcers in UC rats (Pavlick et al., 2002). Additionally, IFN-γ, IL-8, and IL-17 also served as the pro-inflammatory cytokines to evaluate the UC status (Qu et al., 2017). As a result, high-dose PAE significantly diminished these raised pro-inflammatory cytokines, with the most potent efficacy. These results suggested that PAE could regulate the expressions of pro-inflammatory cytokines, providing an evidence for the remission of epithelial cell necrosis, edema, and neutrophil infiltration.
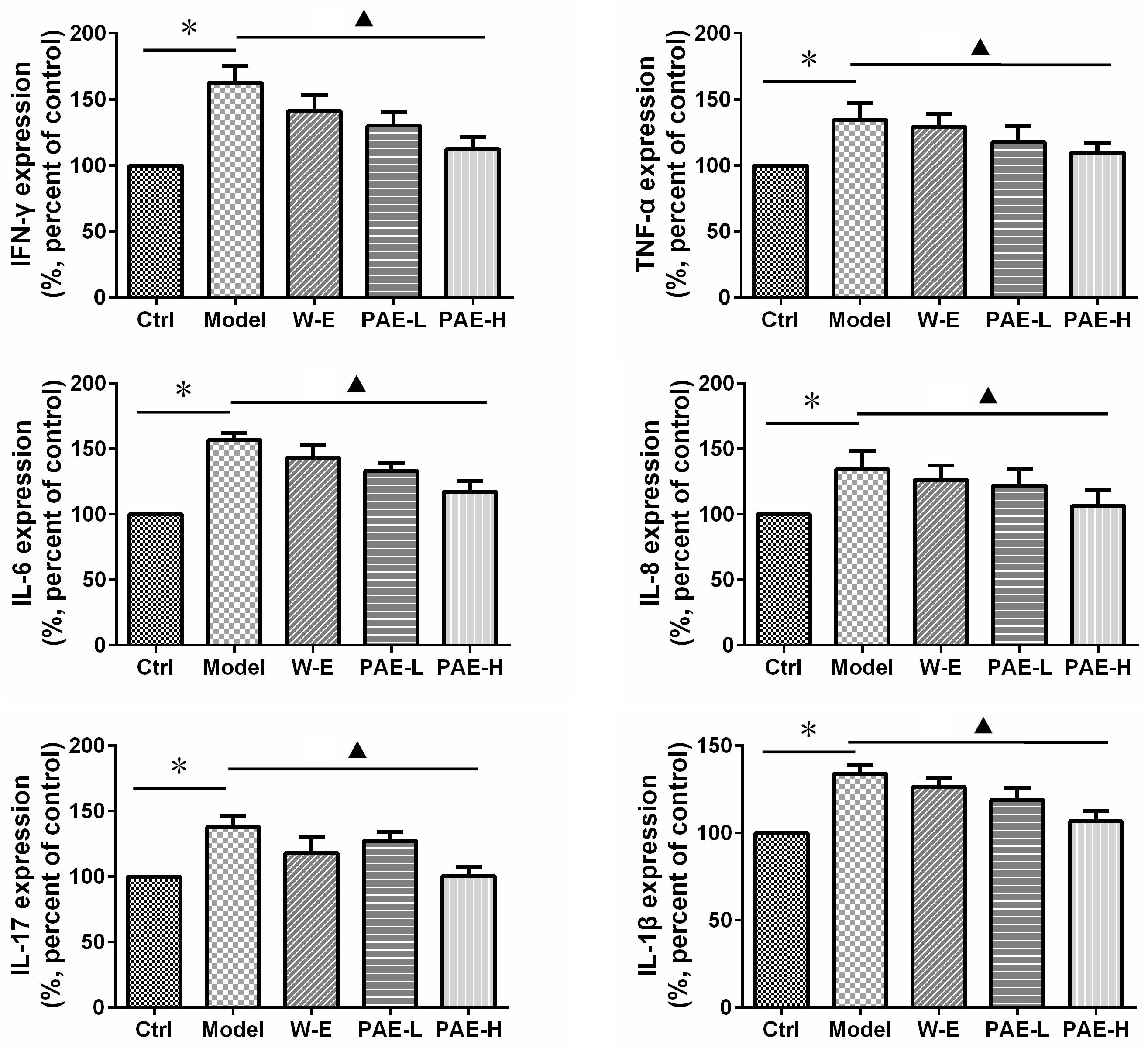
FIGURE 5. Effects of PAE on the amounts of inflammatory cytokines, including IFN-γ, TNF-α, IL-6, IL-8, IL-17, IL-1β, in the colorectum tissues of DSS-induced UC rats by ELISA measurement. ∗P < 0.05 untreated control group vs. DSS-induced UC model group, P < 0.05 model group vs. PAE-H group (n = 6 per group).
PAE Improved the Intestinal Mucosal Barrier Function by Keap1/Nrf-2 mRNA and the Expressions of Tight Junction Proteins
Substantial evidence indicates that UC is associated with oxidative stress, which may play a significant role in its etiologies (Patlevic et al., 2016). It has been substantially demonstrated that nuclear factor (erythroid derived 2)-like 2 (Nrf2), a redox-sensitive transcription factor, as well as its tight interact with Kelch-like ECH-associated protein 1 (Keap1) could promote the antioxidant activity and provide therapeutic benefits in inflammation and associated disorders. Keap1/Nrf-2 signaling pathway plays a key role in the protection of intestinal cells against oxidative stress (Yang N. et al., 2017). Therefore, the mRNA expressions of Keap1 (Figure 6A) and Nrf-2 (Figure 6B) were measured by real-time quantitative PCR. As shown in the previous figures, the Keap1 and Nrf-2 mRNA expressions of rats in the UC model group were down-regulated, compared with normal rats. However, the administration of high-dose PAE significantly increased the mRNA expressions of both Keap1 and Nrf-2, indicating that PAE could activate Keap1/Nrf-2 signaling pathway to resist the intestinal mucosal injury.
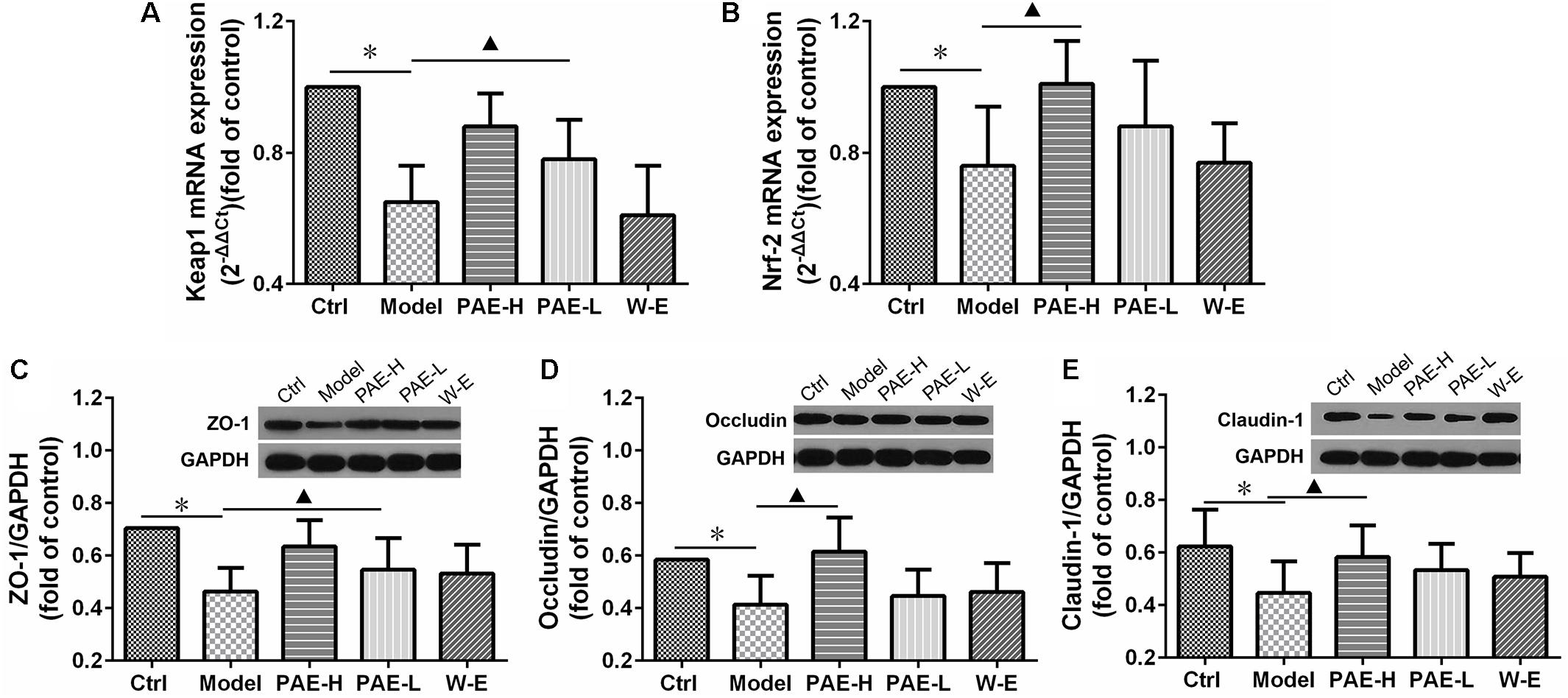
FIGURE 6. Effects of PAE on the intestinal mucosal barrier function. mRNA expressions of Keap1 (A) and Nrf-2 (B) determined by real-time quantitative PCR analysis. Expressions of ZO-1 (C), occludin (D), and claudin-1 (E) over GAPDH determined by Western blot analysis. ∗P < 0.05 untreated control group vs. DSS-induced UC model group, P < 0.05 model group vs. PAE-treated group (n = 6 per group).
Furthermore, the protein expressions of ZO-1, occludin and claudin-1 were measured by Western blot analysis to evaluate the tight junction integrity of the colon (Zhang L. C. et al., 2017). As shown in Figures 6C,D,E, the amounts of all these proteins were decreased in UC rats compared with the normal rats in the control group, indicating that the tight junction integrity was compromised. However, PAE promoted the expressions of these proteins remarkably, indicating its improvement on the tight junction structure and promotion on the intestinal mucosal barrier function. Comparatively speaking, the water extract could not up-regulate the mRNA expressions of Keap1/Nrf-2 and tight junction-related proteins, which was in accord with its negative anti-UC activity as mentioned above.
PAE Modulated Gut Microbiota in Ulcerative Colitis Rats
The expression of 16S rRNA gene sequence was used to evaluate the amelioration of high-dose PAE on DSS-induced UC. Totally, the mean values of 10141 effective sequences in each sample were collected to generate 1,825 OTUs, based on a similarity of at least 97%. The rarefaction curve plateau (Figure 7A) with the current sequencing indicates that most of the diversities have already been captured in all samples. It appeared that UC model rats possessed the least number of species, compared with the normal rats and the UC rats administrated with high-dose PAE. As shown in Figure 7B, Shannon index was used to indicate the alpha diversity of microbial communities, showing a significant decrease of the alpha diversity due to DSS treatment and a remarkable increase of microbial diversity due to the administration of PAE-H. As shown in the Venn diagram (Figure 7C), 746 OTUs (96.84%) overlapped among these three groups, 34 OTUs (0.54%) between the control and model groups, 73 OTUs (0.22%) between the DSS and PAE-H groups, and 302 OTUs (1.56%) between the control and PAE-H groups. PCA (Figure 7D) and PCoA (Figure 7E) analyses based on the weighted UniFrac distance matrices suggested that the gut microbiota in three groups were significantly diversified. Although the administration of high-dose PAE could not completely reverse the gut microbiota to those of the control group, PAE still presented the function of regulating abnormal gut microbiota in DSS-induced UC rats. The system clustering tree in Figure 7F provides evidences of a significant difference existing among the three groups, with a higher similarity shown in the microbiota communities between the PAE-H group and the control group.
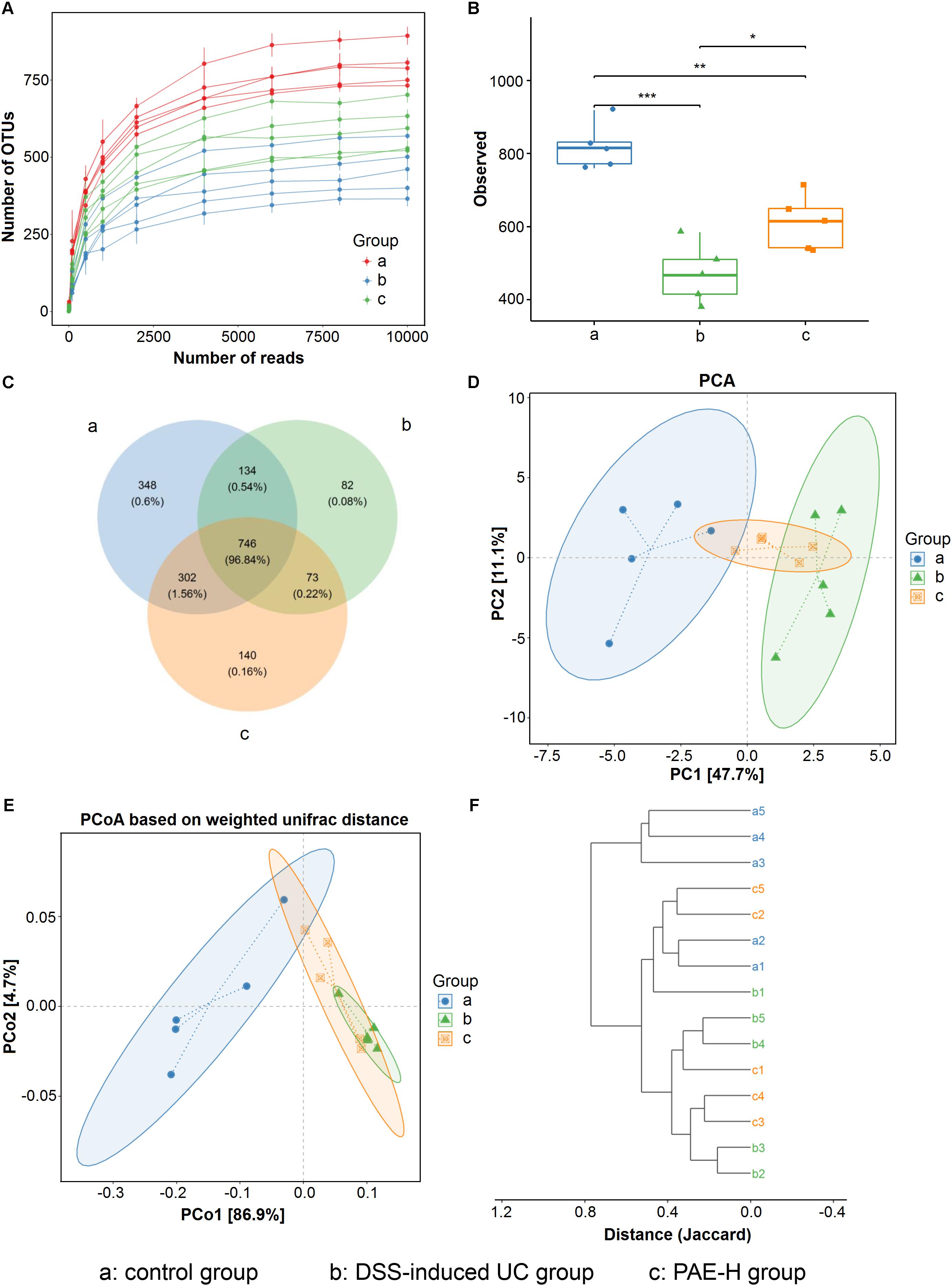
FIGURE 7. PAE regulation on the disturbed gut microbiota in DSS-induced UC rats. (A) Rarefaction curves determined at the 97% similarity level. (B) Alpha diversity analyzed by Shannon diversity index. (C) Venn diagram of OTUs in the three groups. (D) PCA analysis of variation. (E) PCoA analysis of variation based on the weighted UniFrac distance. (F) Cluster dendrogram of the three groups based on Jaccard distance. Significant difference among two group was set as ∗P < 0.05, ∗∗P < 0.01, ∗∗∗P < 0.001 (n = 6 per group).
Histograms were used to reflect the differences among various groups on species and relative abundance of intestinal microbiota. At the phyla level (Figure 8A), Firmicutes, Bacteroidetes, Proteobacteria, and Actinobacteria were the predominant and most numerous species in all groups. The significantly increased Bacteroidetes and decreased Firmicutes acted as the representative characters of the DSS-induced UC rat model. The result was accordant with the rising Firmicutes/Bacteroidetes ratio of UC rats in a previous report (Tao et al., 2017; Yang Y. et al., 2017). However, after the administration of PAE-H, the abnormal microbiota community structure became similar to that of the untreated control group. The detailed information about the regulation effect of PAE on gut microbiota of UC rats was provided in bar plots at genus level (Figure 8B). As shown in the figure, 12 genera were identified in all samples. The most remarkable change on microbiota abundance in UC rats was Lactobacillus, in comparison with normal rats. Lactobacillus, a well-known probiotic (Curro et al., 2017; Amer et al., 2018), exhibited beneficial effects on inflammatory bowel disorders by stimulating immune cells, depressing pro-inflammatory cytokine secretion, and inducting anti-inflammatory cytokines. Specifically, the increase in the abundance of Lactobacillus was observed in PAE-H group, indicating that PAE could help to protect intestinal tract and alleviate intestinal inflammation by increasing the probiotics.
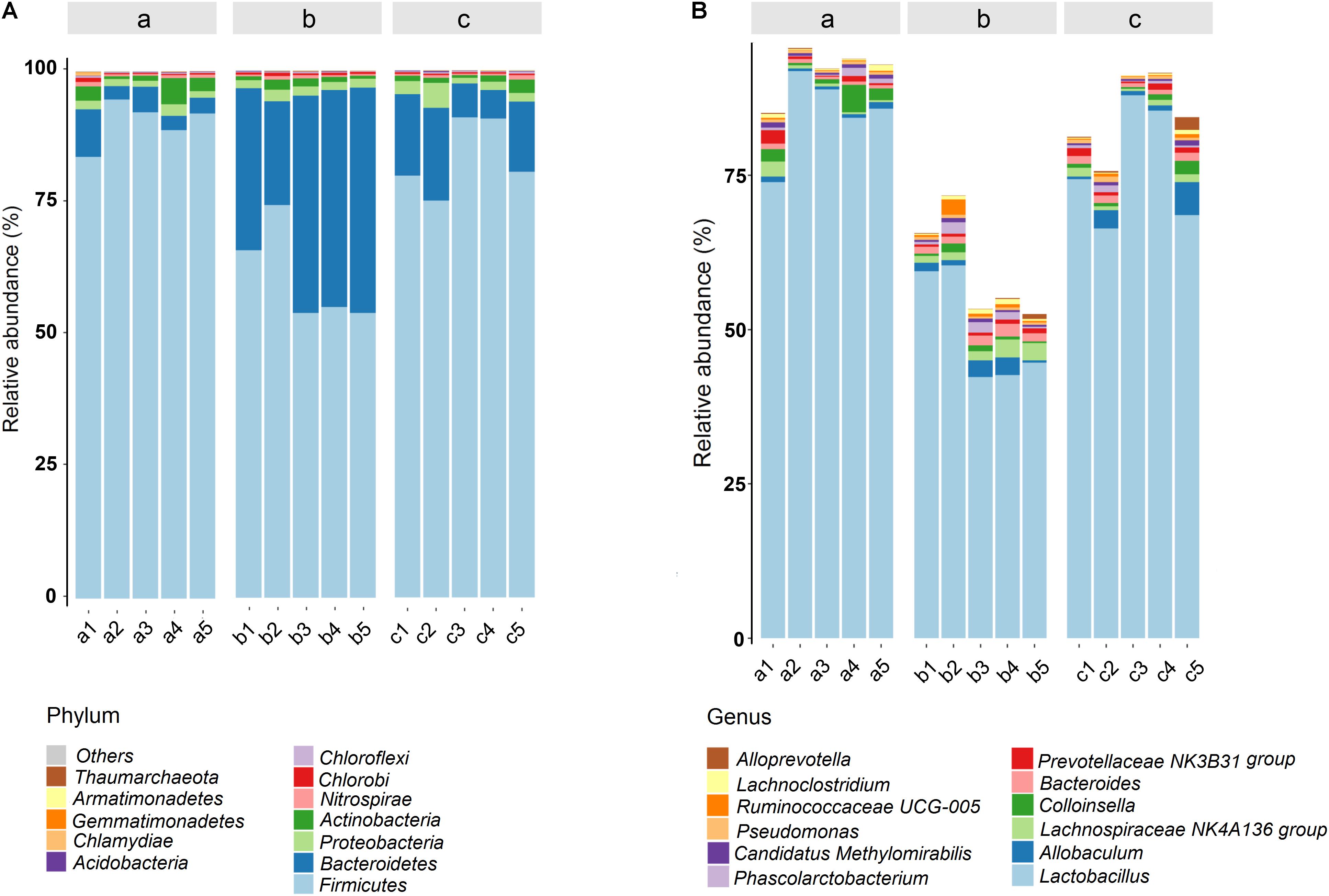
FIGURE 8. Gut microbial community structures of rats from various groups. Microbial community bar plot by phylum (A) and genus (B). a: normal rats serve as the control group; b: UC rats induced by DSS; c: UC rats treated with high-dose PAE (n = 6 per group).
To figure out whether the changes in gut microbial of UC rats were correlated to the intestinal inflammatory symptoms, the relationship between the 40 microflora genera with high abundance in all samples and those above-mentioned six pro-inflammatory cytokines, were analyzed using Spearman’s correlation coefficient, based on the hierarchical clustering and Heatmap. As shown in Figure 9, apart from IL-17, all the other pro-inflammatory cytokines exhibited significant correlations with these predominant microflora genera. Particularly, Lactobacillus exhibited a significantly negative correlation with inflammatory factors IL-6, TNF-α, and IFN-γ, indicating that an increase in the abundance of Lactobacillus would help to suppress the production of these inflammatory factors. On the contrary, the pathogenic bacteria Bacteroides exhibited a significantly positive correlation with IL-6, TNF-α, and IFN-γ. The results manifested that the consecutive administration of PAE could significantly alleviate the intestinal inflammation (shown in Figure 5), which was greatly associated with the increase of Lactobacillus and the down-regulation of Bacteroides in UC rats.
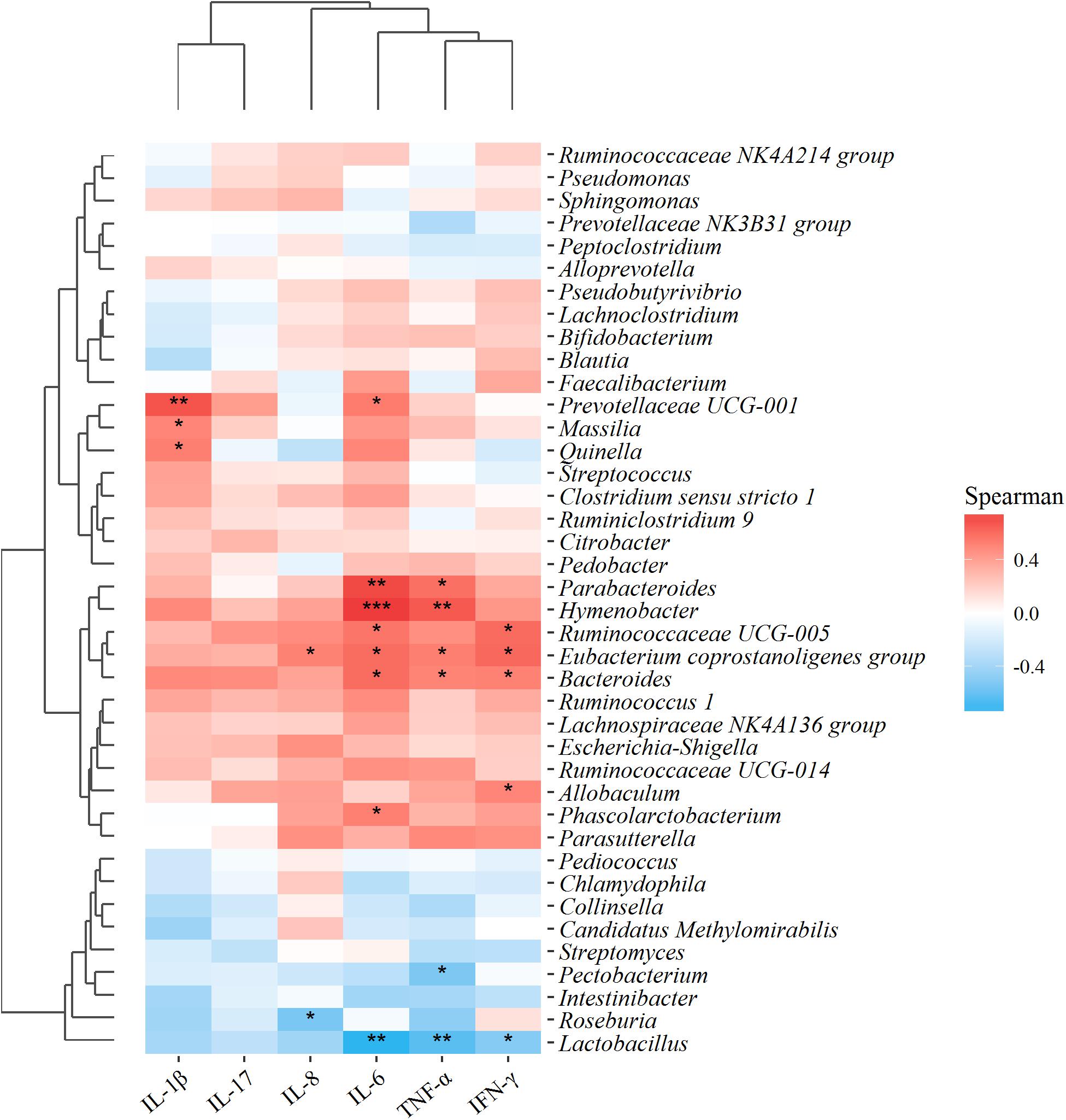
FIGURE 9. Correlation analysis between 40 microflora genera with high abundance in all samples and environmental variables, i.e., the above-mentioned six pro-inflammatory cytokines. Red and blue blocks represent the positive and negative correlations, respectively. Gradation of color indicates the correlation degree. ∗∗∗P < 0.001; ∗∗P < 0.01; ∗P < 0.05 (n = 6 per group).
Discussion
It is known that the pathogenic manifestations of UC include defects in epithelial barrier, immune response, leukocyte recruitment and colonic microflora, but the underlying specific etiologic causes and mechanisms still remain unknown (Lynch and Hsu, 2017). In particular, UC frequently leads to intestinal epithelium injury, resulting in the generation of endogenous and exogenous antigens and the bacteria translocation via the portal venous system. Intestinal barrier defects are common in IBD patients and believed to increase the uptake of luminal antigens across the intestinal epithelium, which in turn would trigger the immune system and the development of mucosal inflammation (Vancamelbeke et al., 2017). Given the limited therapeutic effect of the current approaches focusing on immune response, improvement of intestinal barrier function in intestinal epithelial cells may provide new avenues for UC treatment characterized by changes in barrier function. Tight junctions and adherence junctions are primarily responsible for the restriction and modulation of intestinal permeability. Samak et al. (2015) found that DSS treatment could increase tyrosine phosphorylation of occludin and ZO-1 in Caco-2 cells, indicating the disruption of tight junctions. A previous study (Lahey et al., 2017) has demonstrated the promotion of tight junction, composed of various transmembrane proteins such as occluding, claudin family proteins and intracellular zona occludens (ZO) family proteins, could help to alleviate UC symptoms. In present study, representative proteins of tight junctions, i.e., ZO-1, occludin and claudin-1, were evaluated, showing that DSS treatment could down-regulate the tight junction proteins in colons of UC rats (Figure 5). Additionally, the intestinal epithelial tight junction serves a key role in protecting against inflammation, and the disrupted tight junction is a main cause of intestinal barrier dysfunction and inflammation. Therefore, whether PAE could regulate intestinal permeability in vivo was further evaluated using FITC-dextran. As shown in Supplementary Figure S2, DSS-induced UC model could significantly exacerbate intestinal permeability and induce a higher amount of FITC-dextran in serum compared with the control rats. However, the raised serum level of FITC in UC rats was remarkably reduced by the administration of high-dose PAE. The results were in line with the fact that PAE could regulate the expressions of tight junction-associated proteins. Besides, oral administration of PAE could restore the disturbed intestinal barrier function, by partly improving the tight junctions and up-regulating their associated proteins in the inflamed colon tissues.
Furthermore, based on the recognized relationship between IBD and intestinal immunity, more researches focusing on the regulation of intestinal microbiome associated with UC have been carried out, with the intestinal microflora being recorded as the highest intestinal immune system. Particularly, the intestinal microbiota has been demonstrated as one of the critical factors to influence nutrient metabolism and immune response, and keep the host healthy in various intestinal diseases (Zhang M. et al., 2017). The microbial diversity has been found in IBD patients and healthy individuals, with the diversity, stability and clusters in IBD patients being notably reduced. According to a previous report (Rajilic-Stojanovic et al., 2007), the gut microbiota mainly consists of Firmicutes, Bacteroidetes, Actinobacteria, Proteobacteria, and Fusobacteria, among which, Bacteroidetes and Firmicutes predominate and account for ∼90% of the total gut microbiota. However, based on direct gene sequencing technology by real time PCR, the proportions of these commensal bacteria could vary greatly in IBD patients. Microbial dysbiosis is generally found in UC patients, which is characterized by a reduction of bacterial diversity and an increase in the ratio of Bacteroidetes/Firmicutes. The intestinal bacteria, such as Bacteroides, Eubacterium, and Lactobacillus, could be reduced in IBD (Ott et al., 2004). The decreased ratio of Firmicutes/Bacteroidetes was found in TNBS-induced colitis rats (Tao et al., 2017). In our present study, based on the 16S rRNA gene sequence analysis of samples from the normal group, the DSS-induced UC group and sequential PAE treatment groups, the predominant intestinal bacteria profiles were greatly diversified. Gut microbiota community in various group at class, order and family level, respectively, were exhibited in Supplementary Figure S3. Figures 7, 8 show that the DSS-induced UC is often accompanied by shifts in gut microbiota structure, with a significant decrease of intestinal bacterial diversity, a reduction of Firmicutes and an increase of Bacteroidetes amounts, which have also been found in a previous study (Yeom et al., 2016). However, when compared with the UC rats treated with PAE, a much higher abundance of order Lactobacillales showed up, with the well-known probiotic properties. It has been reported that the strains of Lactobacillus subspecies could reduce mucosal permeability, prevent colitis onset, and alleviate inflammatory reaction (Madsen et al., 1999). The activity of PAE to increase Lactobacillales in UC rats would further verify its intestinal barrier improvement and anti-inflammation effects.
To evaluate the intestinal immunity regulation effect, multivariate direct gradients were used to analyze the relationship between inflammatory factors/cytokines in colonic epithelial tissue samples and intestinal microflora (Figure 9). As expected, protective bacteria such as Pectobacterium, Roseburia, and Lactobacillus were significantly negatively correlated with inflammatory cytokines such as IL-8, IL-6, TNF-α, and IFN-γ, while aggressive bacteria such as Prevotellaceae UCG-001, Massilia, Bacteroides, Parabacteroides, Hymenobacter, and Ruminococcaceae UCG-005 were positively correlated with pro-inflammatory cytokines such as IL-1β, IL-8, IL-6, TNF-α and IFN-γ. More importantly, these evaluated inflammatory cytokines were secreted by helper T cells, also called CD4+ T cells, which served an important part in the immune system (Gaboriau-Routhiau et al., 2009). CD4+ T cells were also confirmed as an important pathogenesis of IBD, meaning that the imbalance of maintenance-regulated factor/cytokine expressions in CD4+ T cells might be one of the main mechanisms of disease. To be more specific, IFN-γ, IL-1β, and TNF-α were secreted by Th1, IL-6 by Th2, and IL-17 by Th17. In UC rats, both Th1/Th2 and Th17/Treg were up-regulated due to the remarkably increased effects on pro-inflammatory factors (Figure 5). Nevertheless, the unbalanced Th1/Th2 and Th17/Treg ratios could be reduced by PAE treatment, indicating the anti-inflammation and intestinal immunity regulation effects of PAE.
Conclusion
In view of the recognized efficacy of Kangfuxin liquid, a famous commercial product made from Periplaneta americana extract, in the clinical treatment of gastrointestinal ulcers, the suppression effect of P. americana on experimental UC and the related mechanisms were investigated in the present study. Nine physiological small molecules in PAE, including cytimidine, uracil, cytidine, hypoxanthine, uridine, thymine, adenine, inosine, and guanosine, were simultaneously determined by HPLC as the quality control approach. According to the DSS-induced UC model, the administration of PAE could prominently ameliorate the intestinal damage and the associated DAI. The anti-UC mechanism of PAE could assist to inhibit inflammatory cytokines production in colon, resist oxidative stress, preserve intestinal barrier integrity and regulate the disturbed gut microbiota structure. Specifically, PAE could decrease a series of inflammatory factors such as TNF-α and IL-1β in RAW 264.7 macrophage and in colons of UC rats. The activation of Keap1/Nrf-2 pathway and the protection effect of tight junction integrity of PAE were observed. Interestingly, DSS treatment could result in significant dysbacteriosis in UC rats in comparison with normal rats, with the significantly increased Bacteroidetes and decreased Firmicutes being the representative characteristics. Nevertheless, the Firmicutes/Bacteroidetes ratio could be down-regulated by the administration of PAE. It could also increase the amounts of probiotics like Lactobacillus, which could help to modulate inflammatory bowel disorder, depress pro-inflammatory cytokine secretion, and improve intestinal barrier function. These results suggested that PAE, a complementary and alternative medicine, could be a potential pharmaceutical candidate to ameliorate UC.
Ethics Statement
Adult male Wistar rats (180–220 g) were purchased from the Laboratory Animal Services Center, Chengdu, China. Animals in our experiments were conducted in accordance with internationally accepted guide lines for the use and care of laboratory animals, as well as those for animal experimentation issued and approved by the administrative committee of laboratory animals in Chengdu University of Traditional Chinese Medicine. Animals were housed under a standard environment condition with a temperature of 20–25°C and a 12 h dark/light cycle, and allowed free access to sterilized water and standard food.
Author Contributions
XM and XL contributed to the animal experiments. YH and XZ contributed to the quality control by HPLC. JZ and YW contributed to data analysis and manuscript writing. CF and FG contributed to the conception and experiment design.
Funding
This work was supported by grants from Key Program of Science and Technology Innovation Project of Sichuan Province (Grant No. 2017RZ0042), Postdoctoral Science Foundation of Sichuan Province, and National Project of Traditional Chinese Medicine Standardization (Grant No. ZYBZH-C-SC-53).
Conflict of Interest Statement
The authors declare that the research was conducted in the absence of any commercial or financial relationships that could be construed as a potential conflict of interest.
Acknowledgments
The authors acknowledge with thanks the financial support and P. americana extract samples from GoodDoctor Pharmaceutical Co., Ltd. and the great help from Dr. Jingjing Li and Dr. Fei Gao from Li Ka Shing, Faculty of Medicine, Hong Kong University.
Supplementary Material
The Supplementary Material for this article can be found online at: https://www.frontiersin.org/articles/10.3389/fphar.2018.00944/full#supplementary-material
References
Ahmed, S. M., Luo, L., Namani, A., Wang, X. J., and Tang, X. (2017). Nrf2 signaling pathway: pivotal roles in inflammation. Biochim. Biophys. Acta 1863, 585–597. doi: 10.1016/j.bbadis.2016.11.005
Amer, M., Nadeem, M., Nazir, S. U. R., Fakhar, M., Abid, F., Ain, Q. U., et al. (2018). Probiotics and their use in inflammatory bowel disease. Altern. Ther. Health Med. 24, 16–23.
Anderson, J. L., Edney, R. J., and Whelan, K. (2012). Systematic review: faecal microbiota transplantation in the management of inflammatory bowel disease. Aliment. Pharmacol. Ther. 36, 503–516. doi: 10.1111/j.1365-2036.2012.05220.x
Caporaso, J. G., Lauber, C. L., Walters, W. A., Berg-Lyons, D., Lozupone, C. A., Turnbaugh, P. J., et al. (2011). Global patterns of 16S rRNA diversity at a depth of millions of sequences per sample. Proc. Natl. Acad. Sci. U.S.A. 108(Suppl. 1), 4516–4522. doi: 10.1073/pnas.1000080107
Chen, G., Yang, Y., Liu, M., Teng, Z., Ye, J., Xu, Y., et al. (2015). Banxia xiexin decoction protects against dextran sulfate sodium-induced chronic ulcerative colitis in mice. J. Ethnopharmacol. 166, 149–156. doi: 10.1016/j.je.2015.03.027
Chen, P., Shen, Y., Shi, H., Ma, X., Lin, B., Xiao, T., et al. (2016). Gastroprotective effects of Kangfuxin-against ethanol-induced gastric ulcer via attenuating oxidative stress and ER stress in mice. Chem. Biol. Interact. 260, 75–83. doi: 10.1016/j.cbi.2016.10.021
Cho, E. J., Shin, J. S., Noh, Y. S., Cho, Y. W., Hong, S. J., Park, J. H., et al. (2011). Anti-inflammatory effects of methanol extract of Patrinia scabiosifolia in mice with ulcerative colitis. J. Ethnopharmacol. 136, 428–435. doi: 10.1016/j.jep.2010.04.047
Curro, D., Ianiro, G., Pecere, S., Bibbo, S., and Cammarota, G. (2017). Probiotics, fibre and herbal medicinal products for functional and inflammatory bowel disorders. Br. J. Pharmacol. 174, 1426–1449. doi: 10.1111/bph.13632
Dae, Park D, Yum, H. W., Zhong, X., Kim, S. H., Kim, S. H., Kim, D. H., et al. (2017). Perilla frutescens extracts protects against dextran sulfate sodium-induced murine colitis: NF-kappaB, STAT3, and Nrf2 as putative targets. Front. Pharmacol. 8:482. doi: 10.3389/fphar.2017.00482
Danese, S., and Fiocchi, C. (2011). Ulcerative colitis. N. Engl. J. Med. 365, 1713–1725. doi: 10.1056/NEJMra1102942
Edgar, R. C. (2013). UPARSE: highly accurate OTU sequences from microbial amplicon reads. Nat. Methods 10, 996–998. doi: 10.1038/nmeth.2604
Feuerstein, J. D., and Cheifetz, A. S. (2014). Ulcerative colitis: epidemiology, diagnosis, and management. Mayo Clin. Proc. 89, 1553–1563. doi: 10.1016/j.mayocp.2014.07.002
Gaboriau-Routhiau, V., Rakotobe, S., Lécuyer, E., Mulder, I., Lan, A., Bridonneau, C., et al. (2009). The key role of segmented filamentous bacteria in the coordinated maturation of gut helper T cell responses. Immunity 31, 677–689. doi: 10.1016/j.immuni.2009.08.020
Ge, H., Tang, H., Liang, Y., Wu, J., Yang, Q., Zeng, L., et al. (2017). Rhein attenuates inflammation through inhibition of NF-kappaB and NALP3 inflammasome in vivo and in vitro. Drug Des. Devel. Ther. 11, 1663–1671. doi: 10.2147/dddt.s133069
Ge, Y., Tang, Y., Guo, S., Liu, X., Zhu, Z., Zhang, L., et al. (2016). Simultaneous quantitation of free amino acids, nucleosides and nucleobases in Sipunculus nudus by ultra-high performance liquid chromatography with triple quadrupole mass spectrometry. Molecules 21:408. doi: 10.3390/molecules21040408
Grisham, M. B., and Yamada, T. (1992). Neutrophils, nitrogen oxides, and inflammatory bowel disease. Ann. N. Y. Acad. Sci. 664, 103–115. doi: 10.1111/j.1749-6632.1992.tb39753.x
Gu, P., Zhu, L., Liu, Y., Zhang, L., Liu, J., and Shen, H. (2017). Protective effects of paeoniflorin on TNBS-induced ulcerative colitis through inhibiting NF-kappaB pathway and apoptosis in mice. Int. Immunopharmacol. 50, 152–160. doi: 10.1016/j.intimp.2017.06.022
Guo, B. J., Bian, Z. X., Qiu, H. C., Wang, Y. T., and Wang, Y. (2017). Biological and clinical implications of herbal medicine and natural products for the treatment of inflammatory bowel disease. Ann. N. Y. Acad. Sci. 1401, 37–48. doi: 10.1111/nyas.13414
Habtemariam, S. (2016). Berberine and inflammatory bowel disease: a concise review. Pharmacol. Res. 113, 592–599. doi: 10.1016/j.phrs.2016.09.041
Hanauer, S. B. (2006). Inflammatory bowel disease: epidemiology, pathogenesis, and therapeutic opportunities. Inflamm. Bowel Dis. 12(Suppl. 1), S3–S9. doi: 10.1097/01.MIB.0000195385.19268.68
He, X. J., Xi, Z. X., Yuan, H., Lu, Y., and Bai, X. H. (2012). Effects of American cockroach extract on the expression of MUC2 and IL-6 in the colonic mucosa of rats with ulcerative colitis. J. Weifang Med. Coll. 34, 59–62.
Hu, Y., Zhang, J., Zou, L., Fu, C., Li, P., and Zhao, G. (2017). Chemical characterization, antioxidant, immune-regulating and anticancer activities of a novel bioactive polysaccharide from Chenopodium quinoa seeds. Int. J. Biol. Macromol. 99, 622–629. doi: 10.1016/j.ijbiomac.2017.03.019
Huang, Y. F., Li, L. J., Gao, S. Q., Chu, Y., Niu, J., Geng, F. N., et al. (2017a). Evidence based anti-osteoporosis effects of Periplaneta americana L on osteoblasts, osteoclasts, vascular endothelial cells and bone marrow derived mesenchymal stem cells. BMC Complement Altern. Med. 17:413. doi: 10.1186/s12906-017-1917-7
Huang, Y. F., Zhou, J. T., Qu, C., Dou, Y. X., Huang, Q. H., Lin, Z. X., et al. (2017b). Anti-inflammatory effects of Brucea javanica oil emulsion by suppressing NF-kappaB activation on dextran sulfate sodium-induced ulcerative colitis in mice. J. Ethnopharmacol. 198, 389–398. doi: 10.1016/j.jep.2017.01.042
Kang, S., Kim, W., Jeong, S., Lee, Y., Nam, J., Lee, S., et al. (2017). Oxidized 5-aminosalicylic acid activates Nrf2-HO-1 pathway by covalently binding to Keap1: implication in anti-inflammatory actions of 5-aminosalicylic acid. Free Radic. Biol. Med. 108, 715–724. doi: 10.1016/j.freeradbiomed.2017.04.366
Kong, S. C., Hurlstone, D. P., Pocock, C. Y., Walkington, L. A., Farquharson, N. R., Bramble, M. G., et al. (2005). The Incidence of self-prescribed oral complementary and alternative medicine use by patients with gastrointestinal diseases. J. Clin. Gastroenterol. 39, 138–141. doi: 10.1097/01.mcg.0000150177.73706.8c
Lahey, K. A., Ronaghan, N. J., Shang, J., Dion, S. P., Désilets, A., Leduc, R., et al. (2017). Signaling pathways induced by serine proteases to increase intestinal epithelial barrier function. PLoS One 12:e0180259. doi: 10.1371/journal.pone.0180259
Li, N., Lu, R., Yu, Y., Lu, Y., Huang, L., Jin, J., et al. (2016). Protective effect of Periplaneta americana extract in ulcerative colitis rats induced by dinitrochlorobenzene and acetic acid. Pharm. Biol. 54, 2560–2567. doi: 10.3109/13880209.2016.1170862
Liu, D., Huo, X., Gao, L., Zhang, J., Ni, H., and Cao, L. (2018). NF-kappaB and Nrf2 pathways contribute to the protective effect of Licochalcone A on dextran sulphate sodium-induced ulcerative colitis in mice. Biomed. Pharmacother. 102, 922–929. doi: 10.1016/j.biopha.2018.03.130
Liu, W., Guo, W., Guo, L., Gu, Y., Cai, P., Xie, N., et al. (2014). Andrographolide sulfonate ameliorates experimental colitis in mice by inhibiting Th1/Th17 response. Int. Immunopharmacol. 20, 337–345. doi: 10.1016/j.intimp.2014.03.015
Luo, Y., Feng, M., Fan, Z., Zhu, X., Jin, F., Li, R., et al. (2016). Effect of kangfuxin solution on chemo/radiotherapy-induced mucositis in nasopharyngeal carcinoma patients: a multicenter, prospective randomized phase III clinical study. Evid. Based Complement Alternat. Med. 2016:8692343. doi: 10.1155/2016/8692343
Lynch, W. D., and Hsu, R. (2017). Colitis, Ulcerative in StatPearls Treasure Island (FL). London: StatPearls Publishing LLC.
Madsen, K. L., Doyle, J. S., Jewell, L. D., Tavernini, M. M., and Fedorak, R. N. (1999). Lactobacillus species prevents colitis in interleukin 10 gene-deficient mice. Gastroenterology 116, 1107–1114. doi: 10.1016/S0016-5085(99)70013-2
McIlroy, J., Ianiro, G., Mukhopadhya, I., Hansen, R., and Hold, G. L. (2017). Review article: the gut microbiome in inflammatory bowel disease-avenues for microbial management. Aliment. Pharmacol. Ther. 47, 26–42. doi: 10.1111/apt.14384
Molodecky, N. A., Soon, I. S., Rabi, D. M., Ghali, W. A., Ferris, M., Chernoff, G., et al. (2012). Increasing incidence and prevalence of the inflammatory bowel diseases with time, based on systematic review. Gastroenterology 142, 46.e42–54.e42; quiz e30. doi: 10.1053/j.gastro.2011.10.001
Moussaoui, N., Braniste, V., Ait-Belgnaoui, A., Gabanou, M., Sekkal, S., Olier, M., et al. (2014). Changes in intestinal glucocorticoid sensitivity in early life shape the risk of epithelial barrier defect in maternal-deprived rats. PLoS One 9:e88382. doi: 10.1371/journal.pone.0088382
Ohkusa, T., and Koido, S. (2015). Intestinal microbiota and ulcerative colitis. J. Infect. Chemother. 21, 761–768. doi: 10.1016/j.jiac.2015.07.010
Ott, S. J., Musfeldt, M., Wenderoth, D. F., Hampe, J., Brant, O., Fölsch, U. R., et al. (2004). Reduction in diversity of the colonic mucosa associated bacterial microflora in patients with active inflammatory bowel disease. Gut 53, 685–693. doi: 10.1136/gut.2003.025403
Patlevic, P., Vaskova, J., Svorc, P. Jr., Vasko, L., and Svorc, P. (2016). Reactive oxygen species and antioxidant defense in human gastrointestinal diseases. Integr. Med. Res. 5, 250–258. doi: 10.1016/j.imr.2016.07.004
Pavlick, K. P., Laroux, F. S., Fuseler, J., Wolf, R. E., Gray, L., Hoffman, J., et al. (2002). Role of reactive metabolites of oxygen and nitrogen in inflammatory bowel disease. Free Radic. Biol. Med. 33, 311–322. doi: 10.1016/S0891-5849(02)00853-5
Qu, C., Yuan, Z. W., Yu, X. T., Huang, Y. F., Yang, G. H., Chen, J. N., et al. (2017). Patchouli alcohol ameliorates dextran sodium sulfate-induced experimental colitis and suppresses tryptophan catabolism. Pharmacol. Res. 121, 70–82. doi: 10.1016/j.phrs.2017.04.017
Rajilic-Stojanovic, M., Smidt, H., and De Vos, W. M. (2007). Diversity of the human gastrointestinal tract microbiota revisited. Environ. Microbiol. 9, 2125–2136. doi: 10.1111/j.1462-2920.2007.01369.x
Renna, S., Cottone, M., and Orlando, A. (2014). Optimization of the treatment with immunosuppressants and biologics in inflammatory bowel disease. World J. Gastroenterol. 20, 9675–9690. doi: 10.3748/wjg.v20.i29.9675
Samak, G., Chaudhry, K. K., Gangwar, R., Narayanan, D., Jaggar, J. H., and Rao, R. (2015). Calcium/Ask1/MKK7/JNK2/c-Src signalling cascade mediates disruption of intestinal epithelial tight junctions by dextran sulfate sodium. Biochem. J. 465, 503–515. doi: 10.1042/bj20140450
Segata, N., Izard, J., Waldron, L., Gevers, D., Miropolsky, L., Garrett, W. S., et al. (2011). Metagenomic biomarker discovery and explanation. Genome Biol. 12:R60. doi: 10.1186/gb-2011-12-6-r60
Seo, H., Oh, J., Hahn, D., Kwon, C. S., Lee, J. S., and Kim, J. S. (2017). Protective effect of glyceollins in a mouse model of dextran sulfate sodium-induced colitis. J. Med. Food 20, 1055–1062. doi: 10.1089/jmf.2017.3960
Shen, Y., Sun, J., Niu, C., Yu, D., Chen, Z., Cong, W., et al. (2017). Mechanistic evaluation of gastroprotective effects of Kangfuxin on ethanol-induced gastric ulcer in mice. Chem. Biol. Interact. 273, 115–124. doi: 10.1016/j.cbi.2017.06.007
Sreedhar, R., Arumugam, S., Thandavarayan, R. A., Karuppagounder, V., and Watanabe, K. (2016). Curcumin as a therapeutic agent in the chemoprevention of inflammatory bowel disease. Drug Discov. Today 21, 843–849. doi: 10.1016/j.drudis.2016.03.007
Srivastava, D., Gaur, S. N., Arora, N., and Singh, B. P. (2011). Clinico-immunological changes post-immunotherapy with Periplaneta americana. Eur. J. Clin. Invest. 41, 879–888. doi: 10.1111/j.1365-2362.2011.02480.x
Tan, Y., and Zheng, C. (2018). Effects of alpinetin on intestinal barrier function, inflammation and oxidative stress in dextran sulfate sodium-induced ulcerative colitis mice. Am. J. Med. Sci. 355, 377–386. doi: 10.1016/j.amjms.2018.01.002
Tao, J. H., Duan, J. A., Jiang, S., Feng, N. N., Qiu, W. Q., and Ling, Y. (2017). Polysaccharides from Chrysanthemum morifolium ramat ameliorate colitis rats by modulating the intestinal microbiota community. Oncotarget 8, 80790–80803. doi: 10.18632/oncotarget.20477
Vancamelbeke, M., Vanuytsel, T., Farré, R., Verstockt, S., Ferrante, M., Van, Assche G, et al. (2017). Genetic and transcriptomic bases of intestinal epithelial barrier dysfunction in inflammatory bowel disease. Inflamm. Bowel Dis. 23, 1718–1729. doi: 10.1097/mib.0000000000001246
Wang, K., Jin, X., You, M., Tian, W., Le, Leu RK, Topping, D. L., et al. (2017). Dietary propolis ameliorates dextran sulfate sodium-induced colitis and modulates the gut microbiota in rats fed a western diet. Nutrients 9:E875. doi: 10.3390/nu9080875
Wang, X. J., Deng, H. Z., Jiang, B., and Yao, H. (2012). The natural plant product sophocarpine ameliorates dextran sodium sulfate-induced colitis in mice by regulating cytokine balance. Int. J. Colorectal. Dis. 27, 575–581. doi: 10.1007/s00384-011-1352-z
Wang, Y., Liu, L., Guo, Y., Mao, T., Shi, R., and Li, J. (2017). Effects of indigo naturalis on colonic mucosal injuries and inflammation in rats with dextran sodium sulphate-induced ulcerative colitis. Exp. Ther. Med. 14, 1327–1336. doi: 10.3892/etm.2017.4701
Yang, F. Q., Li, D. Q., Feng, K., Hu, D. J., and Li, S. P. (2010). Determination of nucleotides, nucleosides and their transformation products in Cordyceps by ion-pairing reversed-phase liquid chromatography-mass spectrometry. J. Chromatogr. A 1217, 5501–5510. doi: 10.1016/j.chroma.2010.06.062
Yang, N., Xia, Z., Shao, N., Li, B., Xue, L., Peng, Y., et al. (2017). Carnosic acid prevents dextran sulfate sodium-induced acute colitis associated with the regulation of the Keap1/Nrf2 pathway. Sci. Rep. 7:11036. doi: 10.1038/s41598-017-11408-5
Yang Q., Chen G., Yang Y., Cai X., Pang Z., Hu C., et al. (2016). Formononetin ameliorates DSS-induced ulcerative colitis in mice through induction of Nrf2 in colons. J. Chin. Pharm. Sci. 25, 178–188. doi: 10.5246/jcps.2016.03.021
Yang, Y., Chen, G., Yang, Q., Ye, J., Cai, X., Tsering, P., et al. (2017). Gut microbiota drives the attenuation of dextran sulphate sodium-induced colitis by Huangqin decoction. Oncotarget 8, 48863–48874. doi: 10.18632/oncotarget.16458
Zhang, J., Zheng, Y., Xie, X., Wang, L., Su, Z., Wang, Y., et al. (2017). Cleavable multifunctional targeting mixed micelles with sequential ph-triggered tat peptide activation for improved antihepatocellular carcinoma efficacy. Mol. Pharm. 14, 3644–3659. doi: 10.1021/acs.molpharmaceut.7b00404
Zhang, L. C., Wang, Y., Tong, L. C., Sun, S., Liu, W. Y., Zhang, S., et al. (2017). Berberine alleviates dextran sodium sulfate-induced colitis by improving intestinal barrier function and reducing inflammation and oxidative stress. Exp. Ther. Med. 13, 3374–3382. doi: 10.3892/etm.2017.4402
Zhang, M., Sun, K., Wu, Y., Yang, Y., Tso, P., and Wu, Z. (2017). Interactions between intestinal microbiota and host immune response in inflammatory bowel disease. Front. Immunol. 8:942. doi: 10.3389/fimmu.2017.00942
Yeom, Y., Kim, B. S., Kim, S. J., and Kim, Y. (2016). Sasa quelpaertensis leaf extract regulates microbial dysbiosis by modulating the composition and diversity of the microbiota in dextran sulfate sodium-induced colitis mice. BMC Complement Altern. Med. 16:481. doi: 10.1186/s12906-016-1456-7
Yuan, J. P., Zhao, S. Y., Wang, J. H., Kuang, H. C., and Liu, X. (2008). Distribution of nucleosides and nucleobases in edible fungi. J. Agric. Food Chem. 56, 809–815. doi: 10.1021/jf0719205
Zhang, H., Wei, L., Zhang, Z., Liu, S., Zhao, G., Zhang, J., et al. (2013). Protective effect of Periplaneta americana extract on intestinal mucosal barrier function in patients with sepsis. J. Tradit. Chin. Med. 33, 70–73. doi: 10.1016/S0254-6272(13)60103-X
Zhang, H. C., Zhang, C. G., Geng, F. N., Shen, Y. M., Liu, B., et al. (2017). Study on the therapeutic effect of Periplaneta americana extract ento-a on oxazolone induced ulcerative colitis in rats. J. Chin. Med. Mater. 40, 1420–1424.
Keywords: ulcerative colitis, Periplaneta americana, 16S rRNA, gut microbiota, intestinal immunity
Citation: Ma X, Hu Y, Li X, Zheng X, Wang Y, Zhang J, Fu C and Geng F (2018) Periplaneta americana Ameliorates Dextran Sulfate Sodium-Induced Ulcerative Colitis in Rats by Keap1/Nrf-2 Activation, Intestinal Barrier Function, and Gut Microbiota Regulation. Front. Pharmacol. 9:944. doi: 10.3389/fphar.2018.00944
Received: 10 January 2018; Accepted: 02 August 2018;
Published: 22 August 2018.
Edited by:
Lyndy Joy McGaw, University of Pretoria, South AfricaReviewed by:
William Chi-Shing Tai, Hong Kong Polytechnic University, Hong KongJian Li, Beijing University of Chinese Medicine, China
Emmanuel Mfotie Njoya, Chengdu Institute of Biology (CAS), China
Copyright © 2018 Ma, Hu, Li, Zheng, Wang, Zhang, Fu and Geng. This is an open-access article distributed under the terms of the Creative Commons Attribution License (CC BY). The use, distribution or reproduction in other forums is permitted, provided the original author(s) and the copyright owner(s) are credited and that the original publication in this journal is cited, in accordance with accepted academic practice. No use, distribution or reproduction is permitted which does not comply with these terms.
*Correspondence: Jinming Zhang, emhhbmdqaW5taW5nMTk4N0AxMjYuY29t Chaomei Fu, Y2hhb21laWZ1QDEyNi5jb20= Funeng Geng, aGFveWlzaGVuZ2dmbkAxMjYuY29t
†These authors have contributed equally to this work