- 1College of Veterinary Medicine, Jilin University, Changchun, China
- 2Shandong Binzhou Animal Science and Veterinary Medicine Academy, Binzhou, China
Streptococcus suis serotype 2 (SS2) is a zoonotic pathogen that can cause meningitis both in pigs and in human beings. However, the pathogenesis of central nervous system (CNS) infection caused by SS2 have not yet been elucidated. To find the key molecules in cerebrospinal fluid (CSF) needed for the pathogenesis, a SS2 meningoencephalitic pig model and a SS2 non-meningoencephalitic pig model were established in this study. CSF was collected from infected piglets, and protein profiling was performed with label-free proteomics technology. A total of 813 differential proteins, including 52 up-regulated proteins and 761 down-regulated proteins, were found in the CSF of meningoencephalitic pigs compared with both non-meningoencephalitic pigs and healthy pigs. These 813 differential proteins were clustered into three main categories, namely, cellular component, biological process, and molecular function by gene ontology (GO) analysis. The most enriched subclasses of differential proteins in each category were exosome (44.3%), energy pathway (25.0%) and catalytic activity (11.3%), respectively. The most enriched subclasses of upregulated proteins were extracellular (62.1%), protein metabolism (34.5%) and cysteine-type peptidase activity (6.9%), and of downregulated proteins were exosomes (45.0%), energy pathway (24.0%) and catalytic activity (9.4%). Then, the differential proteins were further investigated by using the KEGG database and were found to participate in 16 KEGGs. The most enriched KEGG was citrate cycle (56.6%), and some of these differential proteins are associated with brain diseases such as Huntington's disease (18.6%), Parkinson's disease (23.8%) and Alzheimer's disease (17.6%). Sixteen of the 813 differential proteins, chosen randomly as examples, were further confirmed by enzyme-linked immunosorbent assay (ELISA) to support the proteomic data. To our knowledge, this is the first study to analyze the differential protein profiling of CSF between SS2 meningoencephalitic piglets and non-meningoencephalitic piglets by employing proteomic technology. The discovery and bioinformatics analysis of these differential proteins provides reference data not only for research on pathogenesis of SS2 CNS infection but also for diagnosis and drug therapy research.
Introduction
Streptococcus suis type 2 is a zoonotic pathogen that can cause meningitis, bacteremia, septicemia, endocarditis, and arthritis in pigs and humans. Meningitis is the most serious symptom of S. suis 2 infection and often shows clinical symptoms including vertigo, ataxia, headaches, neck stiffness, fevers, nausea, deafness and so on (Madsen et al., 2002; Mai et al., 2008; van Samkar et al., 2015; Dejace et al., 2017; Sena et al., 2017), and histopathological features including the presence of fibrin, edema and cell infiltration in the meninges and choroid plexus. As a result, this pathogen poses a great threat not only to the swine industry but also to human health.
Obtaining effective and controllable drugs that can pass through the blood-CNS barrier to treat neurological diseases remains a challenge for modern medicine (Coureuil et al., 2017). Understanding the pathogenesis of SS2 meningitis is critical for identifying candidate targets for the diagnosis and therapy of SS2 meningitis. SS2 can cause meningitis due to the interaction between SS2 virulence factors and host molecules. Therefore, not only do the virulence factors from SS2 play an important role in the process of SS2 causing meningitis, but host molecules are also very important for this process.
The blood-CNS barrier, including BCB (blood-CSF barrier) and BBB (blood-brain barrier), is important for maintaining CNS homeostasis. The prerequisite of SS2 causing meningitis is SS2 penetration into the blood-CNS barrier. The blood–CSF barrier is composed of three interfaces: the choroid plexuses (CPs), the pial microvessels and the arachnoid barrier cell layer (Brochner et al., 2015). The CSF is secreted by the CPs, which are villous structures attached to the ventricular ependyma (Strazielle and Ghersi-Egea, 2000). Many studies have reported that some molecules in CSF could be used as potential biomarkers of some brain diseases and bio-targets of therapeutic drugs and pathogenesis research. For example, the porcine cathelicidin PR-39 was found to be significantly increased in CSF of piglets with meningitis and could inhibit neutrophil DNA degradation by bacterial nucleases (de Buhr et al., 2017). Insulin concentrations often found to be decreased in CSF of Alzheimer's disease (AD) patients and could providing insights for AD pathogenesis research (Craft et al., 1998). Comprehensive proteome profiles of different grades of gliomas using CSF could providing insights into disease pathobiology and differentially abundant CSF proteins may serve as potential disease monitoring and prognostic markers for gliomas (Gahoi et al., 2017). The findings of these studies suggested that the discovery of the differential protein in CSF could provide important candidate biological targets for the research of diagnosis, pathogenesis and drug therapy of brain diseases.
Label-free quantification is a method in mass spectrometry (MS). Unlike other methods for protein quantification, label-free quantification does not use a stable isotope to chemically bind to and thus label the protein. This method was widely used to determine the relative amount of proteins in two or more biological samples (Bantscheff et al., 2007; Asara et al., 2008). UniProt is a freely accessible database of protein sequence and functional information, which was built by UniProt consortium that comprises the European Bioinformatics Institute (EBI), the Swiss Institute of Bioinformatics (SIB), and the Protein Information Resource (PIR), many entries being derived from genome sequencing projects. It contains a large amount of information about the biological function of proteins derived from the research literature (O'Donovan et al., 2002; Boeckmann et al., 2003; Wu et al., 2003).
There are no previous reports about the protein profiling of cerebrospinal fluid in meningitic pigs. For this purpose, SS2 meningoencephalitic and non-meningoencephalitic pig models were established in this study. CSF from different pig models was collected and analyzed by protein profiling using label-free proteomics technology. The data of proteomic analyses are useful for research of SS2 CNS infection diagnosis, pathogenesis and therapy.
Materials and Methods
Ethics Statement
Bama miniature pigs used for infection experiments were purchased from Shandong Binzhou Animal Science Veterinary Medicine Academy. All animal experimental procedures were performed in strict accordance with the Regulations for the Administration of Affairs Concerning Experimental Animals approved by the State Council of the People's Republic of China (1988.11.1).
Bacterial Strains and Culture Conditions
SS2 strains used in this study were isolated from sick pigs. The JZLQ022 strain was isolated from brain tissue of meningitis pig, and the JZLQ001 strain was from lymphonodi mandibulares of arthritis pig. SS2 strains were grown on tryptic soy agar (TSA) plates with 5% newborn bovine serum (CLARK Co. Ltd., Australia) for 10 h at 37°C. Then isolated colonies were transferred into 3 ml of tryptic soy broth (TSB) with 5% newborn bovine serum and incubated for 8 h at 37°C with agitation. The bacterial pellet was obtained by centrifugation and resuspended in phosphate-buffered saline (PBS) with a continuous 10-fold dilution. These dilutions were plated onto TSA plates to accurately determine the CFU per milliliter.
The Establishment of a Porcine Model of SS2 Infection
Eighteen 26-day-old healthy inbred Bama miniature pigs were randomly divided into three groups (n = 6): meningoencephalitis group (200 μL PBS with 5 × 106 CFUs JZLQ022), non-meningoencephalitis group (200 μL PBS with 5 × 106 CFUs JZLQ001) and healthy group (200 μL PBS) by ear intravenous injection. Clinical symptoms including appetite, mental status and body temperature were continuously monitored post infection.
Collection of Samples and Determination of Viable Bacteria in Organs
Heparinized blood was collected 2 h, 4 h, 12 h, 2 d, 3 d, 5 d, and 7 d after infection. The pigs were euthanized with sodium pentobarbital (400 mg/mL at a dose of 500 μL/kg) by ear intravenous injection when pigs were dying. After euthanasia, skin and muscles in the back of neck was cut and foramen magnum was exposed. 10 ml injector was inserted into the foramen magnum keep away from spinal cord to collect the CSF. Brain tissue was collected after opening the cranial cavity. 1 g of brain tissue was made into homogenate with 500 μL PBS and plated onto TSA plates to accurately determine the CFU per gram.
Liquid Chromatography-Mass Spectrometry (LC-MS/MS) Measurements
The CSF samples were added to appropriate doses of lysis buffer (7 M Urea, 2 M Thiourea, 0.1% CHAPS) and vortex blended followed by incubation for 30 min s at 25°C. Then, the sample was centrifuged at 15,000 g for 20 min at 4°C. The supernatant was subpackaged into a 1.5 mL tube. The concentration of total protein in the supernatant was determined by Bradford method (Bradford, 1976). LC-MS/MS was performed at the QLbio Biotechnology Corporation in Beijing using the Thermo Scientific EASY-nLC 1000 System and Thermo Q-Exactive MS system. Raw data were identified by Maxquant software and the Sus scrofa protein database on the Uniprot Website (http://www.uniprot.org/).
Proteomic Data Analysis
Fold changes in proteins were determined according to label-free quantitative (LFQ) intensity between the meningitic group and non-meningitic group or healthy group. Proteins with a difference >1.5-fold or < 0.66-fold, p-values < 0.05 were regarded as differentially abundant proteins. Functional analysis of differential proteins was performed based on the gene ontology (GO) annotations (http://www.geneontology.org/), and the proteins were categorized according to their cellular components, molecular functions and biological processes (http://pantherdb.org/geneListAnalysis.do). The differential proteins were further assigned to the Kyoto Encyclopedia of genes and genomes (KEGG) database (http://kobas.cbi.pku.edu.cn/anno_iden.php).
Validation with Enzyme-Linked Immunosorbent Assay (ELISA) Analyses
Some differential proteins in CSF from differential infected piglets, including 7 up-regulated proteins (CTSL1, CTSZ, SAA, MMP-2, RBP-4, CHGA, and CuZnSOD) and 9 down-regulated proteins (Rock, Clathrin, Vinculin, Arp2/3, RhoA, Bid, CytC, PKC, and ERK), were detected by double-antibody sandwich ELISA kits (Purchased from Shanghai Jin Ma Biological Technology co., LTD).
Results
Establishment of a Pig Model of SS2 Meningoencephalitis
Decreased appetite and depressed emotion were observed both in the two groups of pigs infected with JZLQ022 and JZLQ001, respectively. Hyperpyrexia was monitored, and the body temperature of some pigs in the two groups was up to 40°C at 24 h. Arthritis symptoms, including limp, trouble walking and arthrocele, were observed in some pigs infected with JZLQ001 at 3 d (Supplementary Material Video 1). Trouble walking was also observed in some pigs infected with JZLQ022 at 4 d, and foaming at the mouth and ataxia was observed at 5 d (Supplementary Material Videos 2, 3). By autopsy, conspicuous macroscopic lesions, including lung purulent lesions, spleen swelling and infarction, and kidney nephremia, were observed both in the JZLQ022 and JZLQ001 groups (Figure 1A). In addition, joint effusion was observed in the JZLQ001 group. In accordance with the macroscopic lesions, brain hyperemia and hemorrhage were observed in the JZLQ022 group, but not in the JZLQ001 group (Figure 1A). Collected blood and brain tissues were used for bacterial counting. The JZLQ022 infection group had higher SS2 CFUs than PBS control group and the JZLQ001 group in blood at 2 d, 3 d (Figure 1B) and in brain tissues (Figure 1C). The pig models of meningoencephalitis and non-meningoencephalitis were successfully established.
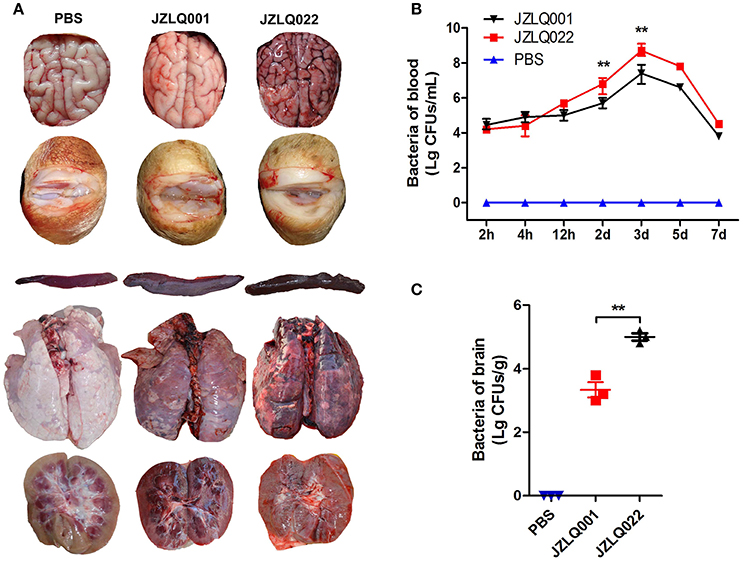
Figure 1. Establishment of SS2 meningoencephalitis and non-meningoencephalitis pig models. (A) Autopsy results of brain and arthrosis; (Control: pig infected with PBS representing the healthy group; JZLQ001: pig infected with the JZLQ001 strain representing the non-meningoencephalitic group; JZLQ022: pig infected with the JZLQ022 strain representing the meningoencephalitic group). (B) Bacteria counts in blood collected at different time points after infection with SS2 (n = 6; **p < 0.01). (C) Bacteria counts in brain tissue collected at different time points after infection with SS2 (n = 3; **p < 0.01).
Comprehensive Analysis of Proteomic Data
The CSF of pigs from the three treatment groups was collected and analyzed by label-free proteomics technology at the QL-bio Biotechnology Limited Company (Beijing, China). Each sample was analyzed in triplicate. A total of 3456 proteins were authenticated with LC-MS/MS technology and calculated by iBAQ and LFQ algorithms with Maxquant software. The notes of these proteins can be found in Uniprot database. The analysis results show good reproducibility based on the heat-map (Figure 2A) and similarity (the more approximate the value is to 1, the higher the similarity between the two sets of data will be) (Figure 2B). Most molecules in CSF from the meningitic group present lower detection concentrations (green) compared with the other two groups, and a small number of molecules present high detection concentrations (red) (Figure 2A).
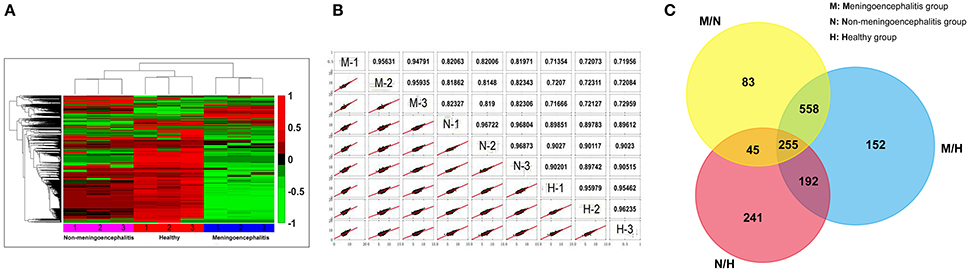
Figure 2. Comprehensive analytic results of proteomic data. (A) The heat-map of proteomics results. (Red, 1: up-regulated proteins; Green, −1: down-regulated proteins; Black, 0: Unchanged proteins). (B) Repetitive analysis of proteomic data. (M, meningoencephalitic group; N, non-meningoencephalitic group; H, Healthy group). (C) Venn diagram of the number of differential proteins among the three treatment groups. M/N, Differential proteins between the meningoencephalitic group and non-meningoencephalitic group; M/H, Differential proteins between the meningoencephalitic group and healthy group; N/H, Differential proteins between the non-meningoencephalitic group and healthy group.
Comparison of the changes in proteins between pairs of groups resulted in some similarities and considerable differences. The Venn diagram shows the distribution of the proteins between each pair of groups. Differentially expressed proteins were detected between each pair of groups. Among these proteins, 255 proteins were differentially distributed for all three comparisons (meningoencephalitis vs. non-meningoencephalitis, M/N; meningoencephalitis vs. healthy, M/H; non-meningoencephalitis vs. healthy, N/H). A total of 447 (255 plus 192) co-differential proteins were found when analyzing M/H against N/H. These 447 proteins may provide hints for SS2 infection related research. A total of 813 (255 plus 558) co-differential proteins were found when analyzing M/N against M/H (Figure 2C). These 813 proteins may provide hints for SS2 meningitis related research and are called 813CDPs in the following.
GO Analysis and Classification of the 813CDPs
The 813CDPs were clustered into three main categories, namely, biological process, cellular component, and molecular function, by gene ontology (GO) analysis. The top 10 most enriched GO terms were selected in each category. The 10 GO terms in cellular component contained exosomes (44.3%), lysosome (38.8%), mitochondrion (30.8%), cytoplasm (65.5%), centrosome (17.5%), cytosol (23.4%), cytoskeleton (8.9%), nucleolus (15.8%), mitochondrial matrix (3.2%), and microtubule (3.9%) (Figure 3A). The 10 GO terms in cellular biological process contained energy pathway (25%), metabolism (25%), protein metabolism (12.5%), transport (10.7%), cell growth and/or maintenance (9.4%), protein folding (0.6%), hormone metabolism (0.3%), lipid metabolism (0.8%), regulation of exocytosis (0.3%) and electron transport (0.3%) (Figure 3B). The 10 GO terms in molecular function contained catalytic activity (11.2%), GTPase activity (4.8), chaperone activity (3.2%), ATPase activity (2.7%), structural constitute of cytoskeleton (2.9%), transporter activity (6.8%), ligase activity (2%), calcium ion binding (2.6%), and hydrolase activity (2.6%) (Figure 3C). The 813CDPs contain 52 up-regulated proteins (Table 1, Supplementary Material Data Sheet 1) and 761 down-regulated proteins (Supplementary Material Data Sheet 2). The most enriched subclasses of upregulated proteins were extracellular (62.1%), protein metabolism (34.5%) and cysteine-type peptidase activity (6.9%), and of downregulated proteins were exosomes (45.0%), energy pathway (24.0%), and catalytic activity (9.4%) (Supplementary Material Data Sheet 1). The 52 up-regulated proteins contain 27 characterized proteins and 25 uncharacterized proteins (Table 1).
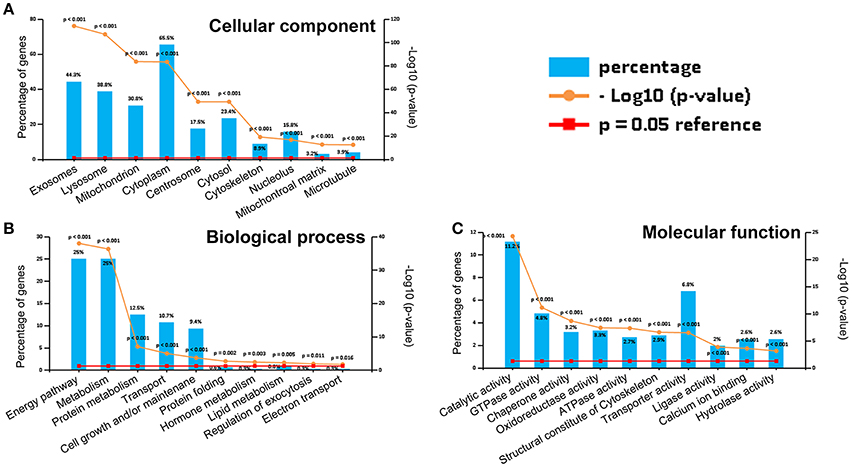
Figure 3. Gene ontology (GO) analysis of 813CDPs. (A) Percentage and p-value of proteins in cellular component. (B) Percentage and p-value of proteins in biological process. (C) Percentage and p-value of proteins in molecular function.
Analysis of Highly Enriched Kyoto Encyclopedia of Genes and Genomes (KEGG)
The 813CDPs were further investigated by using the KEGG database and were found to participate in 16 KEGGs (Figure 4, Supplementary Material Data Sheet 3). The most enriched KEGG was citrate cycle (56.6%). Interestingly, 49 of 813CDPs are associated with neurological diseases such as Huntington's disease (18.6%), Parkinson's disease (23.8%) and Alzheimer's disease (17.6%). And 23 of 49 differential proteins (genes name: ATP5A1, ATP5B, ATP5F1, COX5B, COX6B, CYCS, LOC100524613, LOC100525869, LOC733678, NDUFA10, NDUFA12, NDUFA8, NDUFA9, NDUFB10, NDUFB4, NDUFC2, NDUFS1, NDUFS2, NDUFS3, NDUFS7, NDUFV1, SDHA, SDHB) are co-exsit in KEGGs of the three neurological diseases (Figure 5). The other enriched KEGGs were Oxidative phosphorylation (25.8%), Collecting duct acid secretion (30.7%), Synaptic vesicle cycle (44.05%), Phagosome (14.8%), Central carbon metabolism in cancer (21%), Pyruvate metabolism (27%), Glycosis/Gluconeogenesis (29.2%), Gap junction (17.8%), Long-term depression (20.3%), Aminoacyl-tRNA biosynthesis (19.1%), Valine, leucine and isoleucine degradation (22.4%) and Endocrine and other-factor regulated calcium reabsorption (33.3%) (Figure 4).
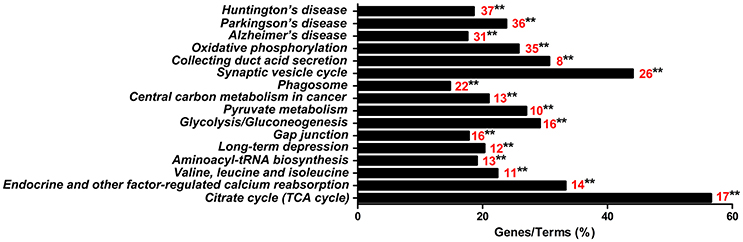
Figure 4. KEGG analysis of the 813 differential proteins. Red number indicates the number of proteins associated with each pathway (**p < 0.01).
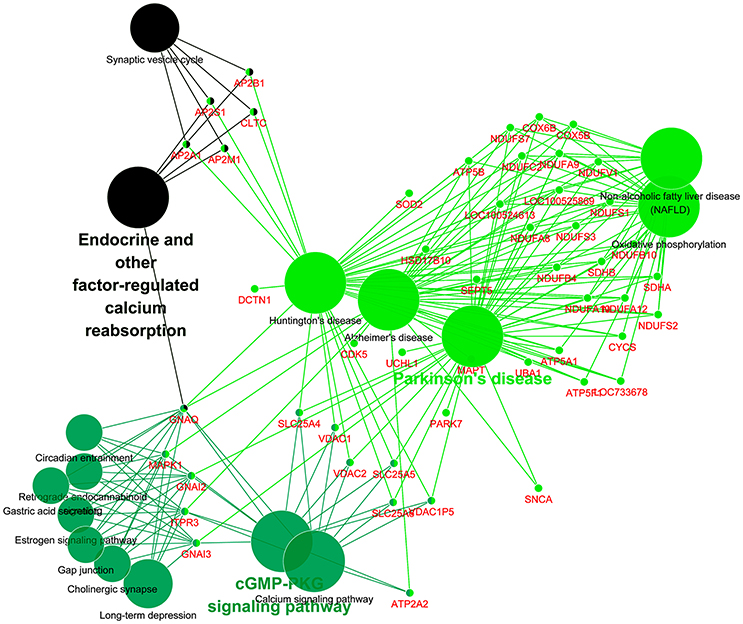
Figure 5. KEGG analysis of differential proteins involved in neurological diseases. Each small dot represents a gene, and each large dot represents a pathway.
Verification of a Subset of Differential Proteins by ELISA
According to the previous reports, some differential proteins were selected to be identified by ELISA (n = 6). The detection results for 7 up-regulated proteins (CTSL1, CTSZ, SAA, MMP-2, RBP-4, CHGA, CuZnSOD) and 9 down-regulated proteins (Rock, Clathrin, Vinculin, Arp2/3, RhoA, Bid, CytC, PKC, ERK) are in accord with the results of proteomic analysis (Figure 6).
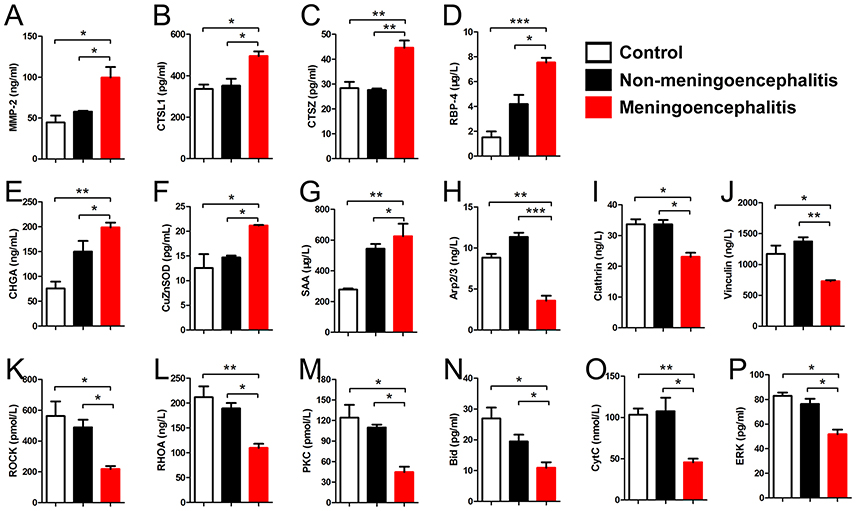
Figure 6. Detection results of differential proteins in the meningitis group by ELISA. (A–G) Special up-regulated proteins in meningoencephalitis group. (H–P) Special down-regulated proteins in meningoencephalitis group. *p < 0.05; **p < 0.01; ***p < 0.001.
Discussion
The SS2 meningoencephalitic pig model was successfully established in this study, and differential protein profiling of CSF in meningoencephalitic, non-meningoencephalitic and healthy pigs was analyzed by label-free proteomic analysis technology. A total of 813 co-differential proteins were found when analyzing the meningoencephalitic group with the non-meningitic group against the meningoencephalitic group with the healthy group. Seven up-regulated proteins and 9 down-regulated proteins of these 813 co-differential proteins were further confirmed by ELISA to support the proteomic data. The data provided important hints for research associated with SS2 meningoencephalitis.
The JZLQ022 strain was isolated from brain tissue of meningoencephalitis pig, and the JZLQ001 strain was from lymphonodi mandibulares of arthritis pig. In our pre-experiments, we found that JZLQ022 mainly cause meningitis and JZLQ001 mainly causes arthritis in pigs. Therefore, two SS2 strains, JZLQ022 and JZLQ001, were used to build the meningoencephalitic pig model and non-meningoencephalitic pig model respectively. The model used in this study was a hemorrhagic meningoencephalitis based on results of autopsy (Figure 1A), JZLQ022 strain not only induced meningitis, but also induced severe meningoencephalitis and hemorrhagic encephalitis by crossing BBB and/or BCB. So the differences between the two strains should be analyzed in the future which would provide important implications on mechanism of SS2 across BBB and/or BCB.
Seven up-regulated proteins (CTSL1, CTSZ, SAA, MMP-2, RBP-4, CHGA, and CuZnSOD) in the CSF of meningoencephalitic piglets compared with both non-meningoencephalitic and healthy piglets were found and confirmed by ELISA. One of these proteins, matrix metalloproteinase-2 (MMP-2), is also called 72 kDa type IV collagenase or gelatinase A (Devarajan et al., 1992). There are no previous reports about the relationship between levels of CSF MMP-2 and bacterial meningitis. However, previous reports show that increased levels of serum MMP-2 may reflect the degree of damage to the BCB in bacterial meningitis (Kanoh et al., 2008). Analysis of MMP levels in the CSF indicates early tumor recurrence and can be used to diagnose CNS tumors, such as malignant astrocytomas, brain metastases, and carcinomatous meningitis (Friedberg et al., 1998). Cathepsin Z (CTSZ) is a member of the cysteine cathepsin protease family (Turk et al., 2012) and cathepsin L1 (CTSL1) is a lysosomal cysteine protease that plays a major role in intracellular protein catabolism. There are no reports on the role of CTSZ and CTSL1 in SS meningitis. However, cathepsin B in CSF from patients has been reported to be associated with neurologic diseases (Nagai et al., 2000). Superoxide dismutase [Cu-Zn], also known as superoxide dismutase 1 or SOD1, is one of three superoxide dismutases in humans. It is implicated in apoptosis and amyotrophic lateral sclerosis (Rosen, 1993). Superoxide dismutase [Cu-Zn] levels were markedly elevated in various neurological diseases, such as bacterial meningitis and encephalitis, and their varied magnitudes may be associated with the underlying diseases (Yoshida et al., 1994). Retinol binding protein 4 (RBP4) is a protein that is encoded in humans by the RBP4 gene (Rocchi et al., 1989). Susceptibility to diet-induced obesity and glucose intolerance in the APP (SWE)/PSEN1 (A246E) mouse model of Alzheimer's disease is associated with increased RBP4 (Mody et al., 2011). Inflammation-dependent cerebral deposition of serum amyloid, a protein, was found in a mouse model of amyloidosis. Inflammation plays an important role in the process of amyloid deposition, and inhibition of inflammatory cascades may attenuate amyloidogenic processes, such as Alzheimer's disease (Guo et al., 2002). Chromogranin A, also called parathyroid secretory protein 1, is a member of the granin family of neuroendocrine secretory proteins, which is encoded by the CHGA gene (Helman et al., 1988). Elevated levels of Chromogranin A in CSF from patients were associated with Astrocytes of Multiple Sclerosis White Matter Lesions (van Luijn et al., 2016).
Nine down-regulated proteins (Rock, Clathrin, Vinculin, Arp2/3, RhoA, Bid, CytC, PKC, ERK) in the CSF of meningitic piglets compared with both non-meningitic and healthy piglets were found and further confirmed by ELISA. Rho/Rock and PKC signaling pathway have been reported to mediated pathogen breaking through the BBB. For example, globotriaosylceramide (Gb3), which interacts with SS2 Fhb to mediate SS2 penetration into the BBB, needs the activation of the Rho/ROCK signaling pathway (Kong et al., 2017). PKC mediates the penetration of Escherichia coli K1 and some fungi species in bacterial meningitis (Sukumaran and Prasadarao, 2002; Kim et al., 2012; Salmeri et al., 2012). Vinculin is associated with focal adhesion and adherens junctions (Xu et al., 1998) which are important for maintenance of BBB function. The loss of vinculin impacts a variety of cell functions; it can disrupt the formation of the complex, and prevents cell adhesion and spreading. The absence of vinculin demonstrates a decrease in cell spreading (Goldmann and Ingber, 2002). However, there are no reports about the relationship between clathrin/vinculin and meningitis. Cytoskeleton rearrangement is important for the maintenance of BCB and BBB function. The Arp2/3 complex is a seven-subunit protein complex that plays a major role in the regulation of the actin cytoskeleton and is found in most actin cytoskeleton-containing eukaryotic cells (Veltman and Insall, 2010). Arp2/3 complex-regulated actin rearrangement mediates methamphetamine-induced occludin endocytosis (Park et al., 2013). Previous reports indicate that apoptosis is involved in bacterial meningitis (Xu et al., 2017a,b). In this study, Bid and CytC were down-regulated in the CSF of meningitic piglets compared with both non-meningitic and healthy piglets. Bid, the BH3 interacting-domain death agonist, is a pro-apoptotic member of the Bcl-2 protein family (Wang et al., 1996). Cytochrome c (cytC) is an electron-transfer protein that possesses a wide range of properties and functions in a large number of different redox processes (Moore et al., 1986). Bid and CytC are important regulators of apoptosis (Chipuk et al., 2012). This suggests that apoptosis may play a role in the process of SS2 breakthrough BCB or BBB.
Furthermore, 23 differential proteins (genes name: ATP5A1, ATP5B, ATP5F1, COX5B, COX6B, CYCS, LOC100524613, LOC100525869, LOC733678, NDUFA10, NDUFA12, NDUFA8, NDUFA9, NDUFB10, NDUFB4, NDUFC2, NDUFS1, NDUFS2, NDUFS3, NDUFS7, NDUFV1, SDHA, SDHB) which are involved in brain diseases such as Huntington's disease, Parkinson's disease, and Alzheimer's disease (Figure 5) were selected. This suggests that there may be a common mechanism between these brain diseases and meningitis. But the role of these proteins in process of SS2 meningitis need to be further investigated.
The present work is the first study, to our knowledge, to analyze the differential protein profiling of CSF in SS2 meningoencephalitic pigs compared with both non-meningoencephalitic pigs and healthy pigs. The data provide a theoretical basis for diagnosis, pathogenesis and drug therapy research of SS2 CNS infection. Further studies need to clarify the role of these differential proteins in the process of SS2 meningoencephalitis, though some of these proteins have been reported to be associated with neurological diseases.
Author Contributions
Designed the experiments: LL. Performed the experiments: HL, LJ, YS, RZ, WG, SL, and GQ. Conducted the analysis: HL, LJ, HJ, and JW. Provided the analysis tools and technical support: JG, CS, XF, and WH. Wrote and revised the manuscript: HL and LJ. All authors read and approved the final manuscript.
Funding
This study was supported by the National Key R&D Program of China (2017YFD0500204).
Conflict of Interest Statement
The authors declare that the research was conducted in the absence of any commercial or financial relationships that could be construed as a potential conflict of interest.
Supplementary Material
The Supplementary Material for this article can be found online at: https://www.frontiersin.org/articles/10.3389/fcimb.2018.00035/full#supplementary-material
References
Asara, J. M., Christofk, H. R., Freimark, L. M., and Cantley, L. C. (2008). A label-free quantification method by MS/MS TIC compared to SILAC and spectral counting in a proteomics screen. Proteomics 8, 994–999. doi: 10.1002/pmic.200700426
Bantscheff, M., Schirle, M., Sweetman, G., Rick, J., and Kuster, B. (2007). Quantitative mass spectrometry in proteomics: a critical review. Anal. Bioanal. Chem. 389, 1017–1031. doi: 10.1007/s00216-007-1486-6
Boeckmann, B., Bairoch, A., Apweiler, R., Blatter, M. C., Estreicher, A., Gasteiger, E., et al. (2003). The SWISS-PROT protein knowledgebase and its supplement TrEMBL in 2003. Nucleic Acids Res. 31, 365–370. doi: 10.1093/nar/gkg095
Bradford, M. M. (1976). A rapid and sensitive method for the quantitation of microgram quantities of protein utilizing the principle of protein-dye binding. Anal. Biochem. 72, 248–254. doi: 10.1016/0003-2697(76)90527-3
Brochner, C. B., Holst, C. B., and Mollgard, K. (2015). Outer brain barriers in rat and human development. Front. Neurosci. 9:75. doi: 10.3389/fnins.2015.00075
Chipuk, J. E., McStay, G. P., Bharti, A., Kuwana, T., Clarke, C. J., Siskind, L. J., et al. (2012). Sphingolipid metabolism cooperates with BAK and BAX to promote the mitochondrial pathway of apoptosis. Cell 148, 988–1000. doi: 10.1016/j.cell.2012.01.038
Coureuil, M., Lecuyer, H., Bourdoulous, S., and Nassif, X. (2017). A journey into the brain: insight into how bacterial pathogens cross blood-brain barriers. Nat. Rev. Microbiol. 15, 149–159. doi: 10.1038/nrmicro.2016.178
Craft, S., Peskind, E., Schwartz, M. W., Schellenberg, G. D., Raskind, M., and Porte, D. J. (1998). Cerebrospinal fluid and plasma insulin levels in Alzheimer's disease: relationship to severity of dementia and apolipoprotein E genotype. Neurology 50, 164–168. doi: 10.1212/WNL.50.1.164
de Buhr, N., Reuner, F., Neumann, A., Stump-Guthier, C., Tenenbaum, T., Schroten, H., et al. (2017). Neutrophil extracellular trap formation in the Streptococcus suis-infected cerebrospinal fluid compartment. Cell. Microbiol. 19:e12649. doi: 10.1111/cmi.12649
Dejace, J., Bagley, P., and Wood, E. (2017). Streptococcus suis meningitis can require a prolonged treatment course. Int. J. Infect. Dis. 65, 34–36. doi: 10.1016/j.ijid.2017.09.011
Devarajan, P., Johnston, J. J., Ginsberg, S. S., Van Wart, H. E., and Berliner, N. (1992). Structure and expression of neutrophil gelatinase cDNA. Identity with type IV collagenase from HT1080 cells. J. Biol. Chem. 267, 25228–25232.
Friedberg, M. H., Glantz, M. J., Klempner, M. S., Cole, B. F., and Perides, G. (1998). Specific matrix metalloproteinase profiles in the cerebrospinal fluid correlated with the presence of malignant astrocytomas, brain metastases, and carcinomatous meningitis. Cancer-Am. Cancer Soc. 82, 923–930. doi: 10.1002/(SICI)1097-0142(19980301)82:5<923::AID-CNCR18>3.0.CO;2-2
Gahoi, N., Malhotra, D., Moiyadi, A., Varma, S. G., Gandhi, M. N., and Srivastava, S. (2017). Multi-pronged proteomic analysis to study the glioma pathobiology using cerebrospinal fluid samples. Proteomics Clin. Appl. 1700056. doi: 10.1002/prca.201700056
Goldmann, W. H., and Ingber, D. E. (2002). Intact vinculin protein is required for control of cell shape, cell mechanics, and rac-dependent lamellipodia formation. Biochem. Biophys. Res. Commun. 290, 749–755. doi: 10.1006/bbrc.2001.6243
Guo, J. T., Yu, J., Grass, D., de Beer, F. C., and Kindy, M. S. (2002). Inflammation-dependent cerebral deposition of serum amyloid a protein in a mouse model of amyloidosis. J. Neurosci. 22, 5900–5909.
Helman, L. J., Ahn, T. G., Levine, M. A., Allison, A., Cohen, P. S., Cooper, M. J., et al. (1988). Molecular cloning and primary structure of human chromogranin A (secretory protein I) cDNA. J. Biol. Chem. 263, 11559–11563.
Kanoh, Y., Ohara, T., Kanoh, M., and Akahoshi, T. (2008). Serum matrix metalloproteinase-2 levels indicate blood-CSF barrier damage in patients with infectious meningitis. Inflammation 31, 99–104. doi: 10.1007/s10753-007-9054-y
Kim, J. C., Crary, B., Chang, Y. C., Kwon-Chung, K. J., and Kim, K. J. (2012). Cryptococcus neoformans activates RhoGTPase proteins followed by protein kinase C, focal adhesion kinase, and ezrin to promote traversal across the blood-brain barrier. J. Biol. Chem. 287, 36147–36157. doi: 10.1074/jbc.M112.389676
Kong, D., Chen, Z., Wang, J., Lv, Q., Jiang, H., Zheng, Y., et al. (2017). Interaction of factor H-binding protein of Streptococcus suis with globotriaosylceramide promotes the development of meningitis. Virulence, 8, 1290–1302. doi: 10.1080/21505594.2017.1317426
Madsen, L. W., Svensmark, B., Elvestad, K., Aalbaek, B., and Jensen, H. E. (2002). Streptococcus suis serotype 2 infection in pigs: new diagnostic and pathogenetic aspects. J. Comp. Pathol. 126, 57–65. doi: 10.1053/jcpa.2001.0522
Mai, N. T., Hoa, N. T., Nga, T. V., Linh, L. D., Chau, T. T., Sinh, D. X., et al. (2008). Streptococcus suis meningitis in adults in Vietnam. Clin. Infect. Dis. 46, 659–667. doi: 10.1086/527385
Mody, N., Agouni, A., McIlroy, G. D., Platt, B., and Delibegovic, M. (2011). Susceptibility to diet-induced obesity and glucose intolerance in the APP (SWE)/PSEN1 (A246E) mouse model of Alzheimer's disease is associated with increased brain levels of protein tyrosine phosphatase 1B (PTP1B) and retinol-binding protein 4 (RBP4), and basal phosphorylation of S6 ribosomal protein. Diabetologia 54, 2143–2151. doi: 10.1007/s00125-011-2160-2
Moore, G. R., Pettigrew, G. W., and Rogers, N. K. (1986). Factors influencing redox potentials of electron transfer proteins. Proc. Natl. Acad. Sci. U.S.A. 83, 4998–4999. doi: 10.1073/pnas.83.14.4998
Nagai, A., Murakawa, Y., Terashima, M., Shimode, K., Umegae, N., Takeuchi, H., et al. (2000). Cystatin, C., and cathepsin B in CSF from patients with inflammatory neurologic diseases. Neurology 55, 1828–1832. doi: 10.1212/WNL.55.12.1828
O'Donovan, C., Martin, M. J., Gattiker, A., Gasteiger, E., Bairoch, A., and Apweiler, R. (2002). High-quality protein knowledge resource: SWISS-PROT and TrEMBL. Brief. Bioinformatics 3, 275–284. doi: 10.1093/bib/3.3.275
Park, M., Kim, H. J., Lim, B., Wylegala, A., and Toborek, M. (2013). Methamphetamine-induced occludin endocytosis is mediated by the Arp2/3 complex-regulated actin rearrangement. J. Biol. Chem. 288, 33324–33334. doi: 10.1074/jbc.M113.483487
Rocchi, M., Covone, A., Romeo, G., Faraonio, R., and Colantuoni, V. (1989). Regional mapping of RBP4 to 10q23—q24 and RBP1 to 3q21—q22 in man. Somat. Cell Mol. Genet. 15, 185–190. doi: 10.1007/BF01535081
Rosen, D. R. (1993). Mutations in Cu/Zn superoxide dismutase gene are associated with familial amyotrophic lateral sclerosis. Nature 362, 59–62.
Salmeri, M., Motta, C., Mastrojeni, S., Amodeo, A., Anfuso, C. D., Giurdanella, G., et al. (2012). Involvement of PKCalpha-MAPK/ERK-phospholipase A(2) pathway in the Escherichia coli invasion of brain microvascular endothelial cells. Neurosci. Lett. 511, 33–37. doi: 10.1016/j.neulet.2012.01.031
Sena, E. S., Carvalho, D. A. J., Abrunhosa, J., Almeida, E. S. C., and Arshad, Q. (2017). Pig's ear: streptococcus suis Meningitis and its associated inner ear implications. IDCases 10, 55–57. doi: 10.1016/j.idcr.2017.08.015
Strazielle, N., and Ghersi-Egea, J. F. (2000). Choroid plexus in the central nervous system: biology and physiopathology. J. Neuropathol. Exp. Neurol. 59, 561–574. doi: 10.1093/jnen/59.7.561
Sukumaran, S. K., and Prasadarao, N. V. (2002). Regulation of protein kinase C in Escherichia coli K1 invasion of human brain microvascular endothelial cells. J. Biol. Chem. 277, 12253–12262. doi: 10.1074/jbc.M110740200
Turk, V., Stoka, V., Vasiljeva, O., Renko, M., Sun, T., Turk, B., et al. (2012). Cysteine cathepsins: from structure, function and regulation to new frontiers. Biochim. Biophys. Acta 1824, 68–88. doi: 10.1016/j.bbapap.2011.10.002
van Luijn, M. M., van Meurs, M., Stoop, M. P., Verbraak, E., Wierenga-Wolf, A. F., Melief, M. J., et al. (2016). Elevated expression of the cerebrospinal fluid disease markers chromogranin, a., and clusterin in astrocytes of multiple sclerosis white matter lesions. J. Neuropathol. Exp. Neurol. 75, 86–98. doi: 10.1093/jnen/nlv004
van Samkar, A., Brouwer, M. C., Schultsz, C., van der Ende, A., and van de Beek, D. (2015). Streptococcus suis meningitis: a systematic review and meta-analysis. PLoS Negl. Trop. Dis. 9:e0004191. doi: 10.1371/journal.pntd.0004191
Veltman, D. M., and Insall, R. H. (2010). WASP family proteins: their evolution and its physiological implications. Mol. Biol. Cell 21, 2880–2893. doi: 10.1091/mbc.E10-04-0372
Wang, K., Yin, X. M., Chao, D. T., Milliman, C. L., and Korsmeyer, S. J. (1996). BID: a novel BH3 domain-only death agonist. Genes Dev. 10, 2859–2869. doi: 10.1101/gad.10.22.2859
Wu, C. H., Yeh, L. S., Huang, H., Arminski, L., Castro-Alvear, J., Chen, Y., et al. (2003). The protein information resource. Nucleic Acids Res. 31, 345–347. doi: 10.1093/nar/gkg040
Xu, D., Lian, D., Wu, J., Liu, Y., Zhu, M., Sun, J., et al. (2017a). Brain-derived neurotrophic factor reduces inflammation and hippocampal apoptosis in experimental Streptococcus pneumoniae meningitis. J. Neuroinflammation 14:156. doi: 10.1186/s12974-017-0930-6
Xu, D., Lian, D., Zhang, Z., Liu, Y., Sun, J., and Li, L. (2017b). Brain-derived neurotrophic factor is regulated via MyD88/NF-kappaB signaling in experimental Streptococcus pneumoniae meningitis. Sci. Rep. 7:3545. doi: 10.1038/s41598-017-03861-z
Xu, W., Baribault, H., and Adamson, E. D. (1998). Vinculin knockout results in heart and brain defects during embryonic development. Development 125, 327–337.
Keywords: Streptococcus suis, meningoencephalitis, blood-CSF barrier, cerebrospinal fluid, proteomics
Citation: Liu H, Jia L, Guo W, Sun Y, Zhu R, Li S, Qu G, Jiang H, Wang J, Gu J, Sun C, Feng X, Han W and Lei L (2018) Differential Protein Profiling of Cerebrospinal Fluid in Piglets with Severe Meningoencephalitis Caused by Streptococcus suis Type 2 Compared to Controls. Front. Cell. Infect. Microbiol. 8:35. doi: 10.3389/fcimb.2018.00035
Received: 26 September 2017; Accepted: 25 January 2018;
Published: 09 February 2018.
Edited by:
Margaret E. Bauer, School of Medicine, Indiana University Bloomington, United StatesCopyright © 2018 Liu, Jia, Guo, Sun, Zhu, Li, Qu, Jiang, Wang, Gu, Sun, Feng, Han and Lei. This is an open-access article distributed under the terms of the Creative Commons Attribution License (CC BY). The use, distribution or reproduction in other forums is permitted, provided the original author(s) and the copyright owner are credited and that the original publication in this journal is cited, in accordance with accepted academic practice. No use, distribution or reproduction is permitted which does not comply with these terms.
*Correspondence: Liancheng Lei, bGVpbGNAamx1LmVkdS5jbg==; bGVpbGlhbmNoZW5nQDE2My5jb20=
†Co-first author.