- 1Clinical Unit of Infectious Diseases, Microbiology, and Preventive Medicine, University Hospital Virgen del Rocío, CSIC, University of Seville, Seville, Spain
- 2Institute of Biomedicine of Seville, University Hospital Virgen del Rocío, CSIC, University of Seville, Seville, Spain
- 3Department of Medicine, University of Seville, Seville, Spain
Enterobacteriaceae cause different types of community- and hospital-acquired infections. Moreover, the spread of multidrug-resistant Enterobacteriaceae is a public health problem and the World Health Organization pointed them among the pathogens in which the search of new antibiotics is critical. The objective of this study was to analyze the in vitro activity of pentamidine alone and in combination with gentamicin, tobramycin, amikacin, tigecycline, rifampicin, or doripenem against eight clinical strains of carbapenemase-producing and/or colistin-resistant Enterobacteriaceae: five carbapenemase-producing Klebsiella pneumoniae, one carbapenemase-producing Escherichia coli, and two colistin-resistant Enterobacter cloacae. MIC and MBC were determined following standard protocols. MIC results were interpreted for all the antibiotics according to the EUCAST breakpoints but for rifampicin in which the French FSM breakpoint was used. Bactericidal and synergistic activity of pentamidine alone and in combination with antibiotics at concentrations of 1xMIC was measured by time-kill curves. For one selected strain, K. pneumoniae OXA-48/CTX-M-15 time-kill curves were performed also at 1/2xMIC of pentamidine. All studies were performed in triplicate. Pentamidine MIC range was 200–800 μg/mL. The 50, 12.5, 62.5, 87.5, and 62.5% of the strains were susceptible to gentamicin, tobramycin, amikacin, tigecycline, and doripenem, respectively. Only the two E. cloacae strains were susceptible to rifampicin. Pentamidine alone at 1xMIC showed bactericidal activity against all strains, except for the E. cloacae 32 strain. The bactericidal activity of pentamidine alone was also observed in combination. The combinations of pentamidine were synergistic against E. cloacae 32 with amikacin and tobramycin at 24 h and with tigecycline at 8 h. Pentamidine plus rifampicin was the combination that showed synergistic activity against more strains (five out of eight). Pentamidine plus doripenem did not show synergy against any strain. At 1/2xMIC, pentamidine was synergistic with all the studied combinations against the K. pneumoniae OXA-48/CTX-M-15 strain. In summary, pentamidine alone and in combination shows in vitro activity against carbapenemase-producing and/or colistin-resistant Enterobacteriaceae. Pentamidine appears to be a promising option to treat infections caused by these pathogens.
Introduction
Carbapenem-resistance in Enterobacteriaceae is a world health problem that has made the World Health Organization (WHO) to point it as a priority in the list of bacteria for which new antibiotics are urgently needed (World Health Organization, 2017). Moreover, these pathogens have spread globally in the past years, and are associated with carbapenemase production as the most important resistance mechanism (Cantón et al., 2012). Therapeutic options for infections caused by these kinds of pathogens are scarce and colistin is often the only remaining treatment option. However, the appearance of colistin-resistant strains has risen dramatically in the last decade due to antibiotic pressure in both human treatment and its use in agriculture (Kempf et al., 2016; Olaitan et al., 2016). In a recently published study (Hong et al., 2018), the in vitro activity of colistin was analyzed against 356 clinical strains of Enterobacter spp. from eight Korean hospitals, founding that 23.9 and 4.2% of E. cloacae and E. aerogenes strains, respectively, were resistant to colistin.
In this context, clinical experience on the most effective treatment for infections caused by these pathogens is still scarce (Akova et al., 2012). Currently, the majority of clinical studies conclude that the combined treatment with two or more antimicrobials is the better option in terms of increase the survival (Trecarichi and Tumbarello, 2017). Numerous studies, both in vitro and in vivo, have tested the efficacy of antimicrobials combinations against these kinds of pathogens (Pachón-Ibáñez et al., 2018; Wang et al., 2018). Nevertheless, the best combination depends on the susceptibility pattern of the strains and there is no one combination that we could qualify as optimal.
Therefore, the increase in the rates of antimicrobial resistance, the difficulty to find an optimal and effective treatment for infections caused by these pathogens, and the lack of the development of new families of antimicrobials by the pharmaceutical industry (Spellberg and Rex, 2013), make urgent the search for new approaches to combat the problem caused by multi-resistant strains of Gram-negative bacilli (GNB) and, specifically, by carbapenemase-producing and/or colistin-resistant Enterobacteriaceae.
As a new treatment strategy, the repurposing of drugs for the treatment of infections caused by these kinds of pathogens seems especially interesting (Younis et al., 2015). This new approach reduces the time, cost, and risk associated with the development of antimicrobial molecules de novo. In addition, its effectiveness has been demonstrated in different medical areas, such as infectious diseases (Debnath et al., 2012). Despite of that several drugs have been recycled for other clinical indications; none has been used for the treatment of bacterial infections.
Pentamidine (in the form of isethionate) is an antiprotozoal agent effective in trypanosomiasis, leishmaniasis, and some fungal infections (Nguewa et al., 2005). To our knowledge, pentamidine has never been used in clinic as antimicrobial agent. Nevertheless, in a recent study Stokes et al. found that pentamidine is able to disturb the outer membrane of GNB, due to the interaction with membrane lipopolysaccharides (Stokes et al., 2017). Moreover, they concluded that pentamidine in combination with antimicrobials typically used for Gram-positive cocci had synergistic activity in vitro against different GNB and in a mice sepsis model by Acinetobacter baumannii.
The aim of this study was to evaluate in vitro the activity of pentamidine alone and in combination with different antimicrobials against clinical strains of carbapenemase-producing and/or colistin-resistant Enterobacteriaceae.
Materials and Methods
Bacterial Strains
Eight clinical strains of carbapenemase-producing and/or colistin-resistant Enterobacteriaceae were studied: (1) five strains of carbapenemase-producing Klebsiella pneumoniae: Kp07, a VIM-1 ST 1603 clone producer from Spain (Miró et al., 2013); Kp21, co-producing VIM-1 and AmpC type beta-lactamase DHA-1 ST 11 clone from Spain (Miró et al., 2013); Kp28, co-producing OXA-48 ST11 clone and the extended spectrum beta-lactamase (ESBL) CTX-M-15 from Spain (Oteo et al., 2015); a Kp29, co-producing KPC-3 ST512 clone with the extended spectrum beta-lactamases TEM-1 and SHV-11 from Spain (López-Cerero et al., 2014); Kp1, a NDM-1 producer from Kenya; (2) Ec271, a Escherichia coli NDM-1 producer from Australia Docobo-Pérez et al., 2012; (3) two strains of Enterobacter spp. from Spain, E. cloacae 32 and E. cloacae 297, both resistant to colistin. Identification of these isolates was confirmed by a Microflex LT-MALDI Biotyper mass spectrometer (Ruiz-Aragón et al., 2018) (Bruker Daltonics GmbH, Bremen, Germany). The presence of carbapenemase genes, and genes coding for other beta-lactamases was confirmed by PCR and sequencing as described previously.
Drugs
All the drugs tested were used as standard laboratory powders (Sigma-Aldrich, Madrid, Spain): pentamidine, aminoglycosides (gentamicin, tobramycin, and amikacin), tigecycline, rifampicin, and doripenem.
Antimicrobial Susceptibility Testing
The minimal inhibitory concentration (MIC) and minimal bactericidal concentration (MBC) values were tested. MICs of antibiotics were determined by broth microdilution as recommended by the (Clinical and Laboratory Standards Institute, 2012), using Mueller Hinton broth II (MHB) (Becton Dickinson & Co., Sparks, MD, United States). MIC results were interpreted according to the European Committee on Antimicrobial Susceptibility Testing (http://www.eucast.org/clinical_breakpoints/) breakpoints for all antibiotics (European Committee on Antimicrobial Susceptibility Testing, 2018), but rifampicin, for which the French Society for Microbiology breakpoint was used (Soussy, 2012). Pentamidine has not susceptibility breakpoints defined.
The MIC value was the lowest concentration of antimicrobials that completely inhibited the bacterial growth. To determine the MBC values, 5-μL aliquots from the wells with no visible growth were spread on agar plates. The MBC value was the lowest concentration at which no colony formation occurred. Heteroresistance in the studied strains was also evaluated by reading the MIC at 24 and 48 h of incubation. Heteroresistance was defined when a fraction of the inoculum was able to grow two dilutions above the MIC value previously determined (Ferreira et al., 2015). All assays were performed in triplicates to ensure reproducibility.
Time-Kill Curves
The concentrations used for pentamidine and the different antimicrobials tested corresponded to the MIC value obtained by microdilution. Moreover, with the Kp28 OXA-48/CTX-M-15 strain the assay was also performed at 1/2xMIC of pentamidine. Experiments were carried out with a starting inoculum of 5 × 105 cfu/mL and the drugs alone or in combination. Tubes were incubated at 37°C, with shaking, and samples were taken at 0, 2, 4, 8, and 24 h, serially diluted and plated (Pournaras et al., 2011; Souli et al., 2011). Bactericidal activities of single drugs or combination were defined as a decrease ≥ 3 log10 cfu/mL from the starting inoculum, bacteriostatic effect was defined as no change respect to the initial bacterial concentration during the 24 h. Synergy was defined as a decrease ≥ 2 log10 cfu/mL for the drugs combination compared with the most active single agent (Pachón-Ibáñez et al., 2018). Experiments were performed three times on separate occasions.
In vitro Selection of Resistant Mutants
Time–kill curves were used. Strains elected strains were incubated with drugs at concentrations 1 × MIC, and Kp28 OXA-48/CTX-M-15 also at pentamidine concentration of 1/2 × MIC. Furthermore, the possible combinations of pentamidine plus the different studied antimicrobials were tested. Tubes with 20 mL of MHB with an inoculum of 5 × 105 cfu/mL of each one of the strains were used. Tubes with the bacterial inoculum and without drugs were used as growth controls. The bacterial growth was counted at 0 and 24 h after incubation at 37°C. Ten-fold dilutions were made and 100 μL was plated on sheep blood agar and incubated for 24 h at 37°C. For the detection of resistant mutants, the MIC of each one of the studied drugs was carried out in triplicate for a maximum of five colonies at each time-point.
Results
MIC/MBC and Heteroresistance
Individual MIC/MBC of each drug tested for the different clinical strains are shown in Table 1. All the strains were multidrug-resistant (MDR) (Magiorakos et al., 2012) except Kp28 OXA-48/CTX-M-15 which was resistant to rifampicin and fosfomycin. Heteroresistance was observed with tigecycline for the Kp1 NDM-1 (1 mg/L) and Kp21 VIM-1/DHA-1 strains (1 mg/L) and with doripenem for E. cloacae 32 (1 mg/L). The antibiotic susceptibility profiles are included in the Supplementary material.
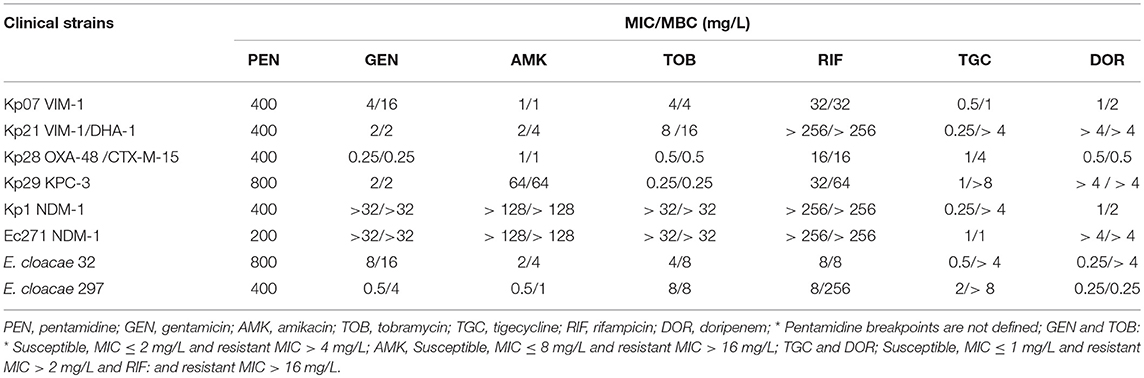
Table 1. MIC/MBC of the different drugs for the eight carbapenemase-producing and/or colistin-resistant Enterobacteriaceae clinical strains.
Time-Kill Curves
The bactericidal activity of the drugs alone is shown in Table 2. Pentamidine alone at 1xMIC was bactericidal from 2 to 24 h against six of the strains and from 4 to 24 h against Kp21 VIM-1/DHA-1; however, pentamidine alone was no bactericidal against E. cloacae 32. Doripenem showed bactericidal activity against three strains, and gentamycin, tobramycin, and amikacin were bactericidal against two strains each. Tigecycline alone was the only antimicrobial that showed not bactericidal effect against any strain.
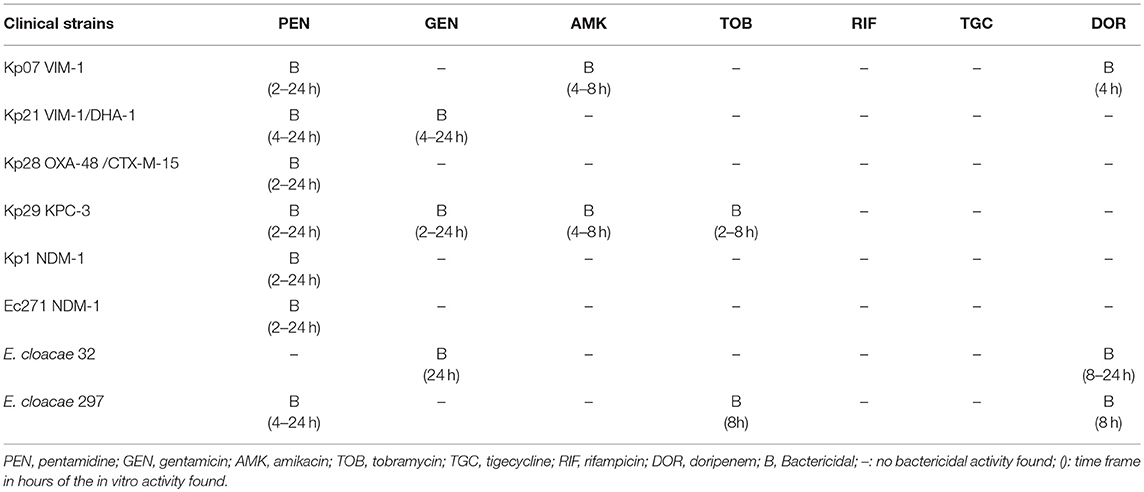
Table 2. Bactericidal activity of drugs alone against eight of carbapenemase-producing and/or colistin-resistant Enterobacteriaceae clinical strains.
The in vitro activity of pentamidine in combination with antimicrobials is shown in Table 3. The bactericidal activity of pentamidine alone was also observed in combination. The combinations of pentamidine were synergistic against E. cloacae 32 with amikacin, tobramycin and rifampicin at 24 h. Pentamidine plus rifampicin was the combination that showed synergistic activity against more strains: Kp21 VIM-1/DHA-1, Kp29 KPC-3 and E. cloacae 297 at 2 h and E. cloacae 32 at 24 h. Pentamidine plus doripenem did not show synergy against any strain.
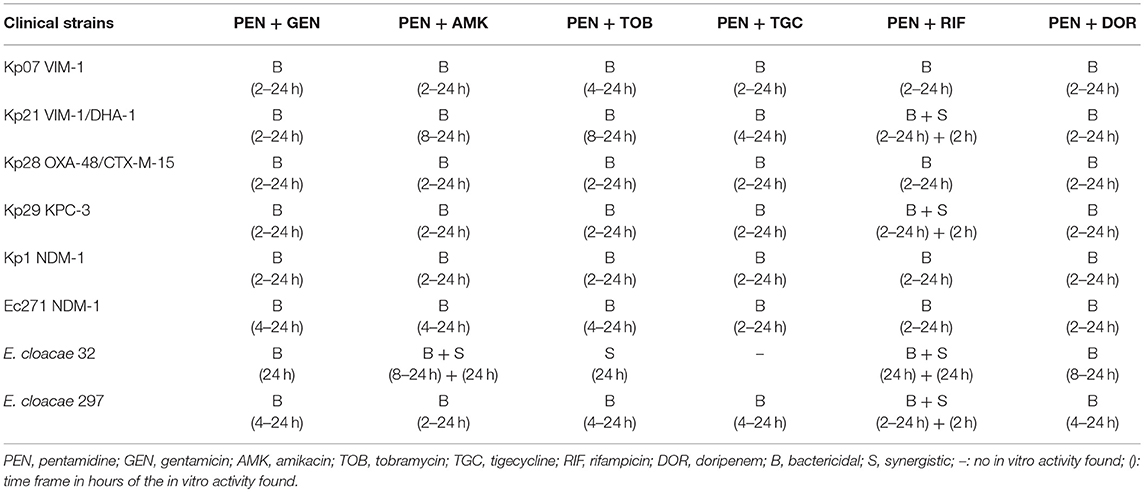
Table 3. In vitro activity of pentamidine in combination with antimicrobials against eight of carbapenemase-producing and/or colistin-resistant Enterobacteriaceae clinical strains.
The activity of pentamidine at 1/2xMIC in combination with antimicrobials against Kp28 OXA-48/CTX-M-15 is showed in Figure 1. Pentamidine 1/2xMIC plus antimicrobials showed synergism at 24 h, but the combination with tobramycin due to the high bactericidal activity of tobramycin at 24 h.
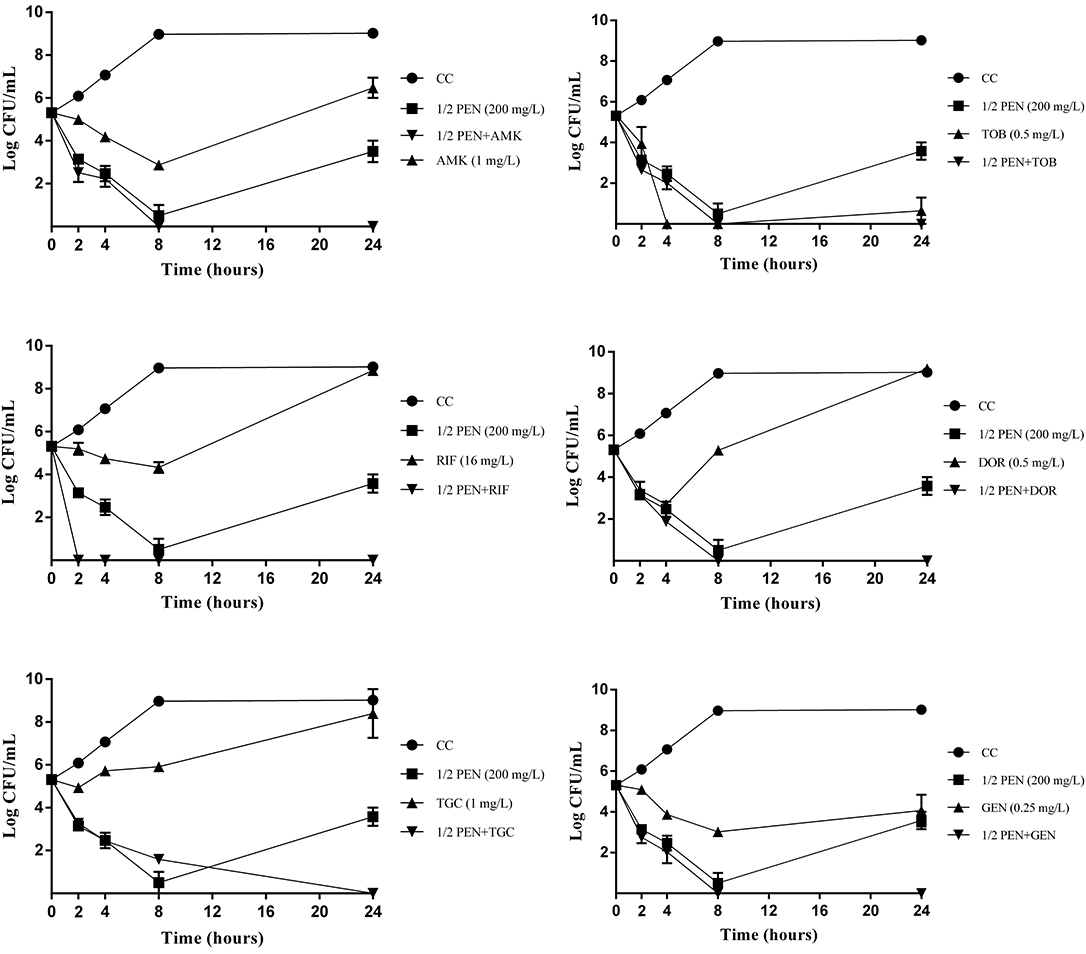
Figure 1. Time-kill curves for pentamidine (PEN) at 1/2xMIC in combination with antimicrobials against the clinical strain Kp28 OXA-48/CTX-M-15. CC: growth control, filled circles; PEN (1/2xMIC), filled squares; antimicrobials (1xMIC), filled triangles, combination of PEN + antimicrobial, filled inverted triangles.
In vitro Selection of Resistant Mutants
The high bactericidal activity of pentamidine in combination with antimicrobials, achieving bacterial concentrations close to 0 log cfu/mL did not allow to analyze the selection of resistant mutants after 24 h incubation.
Discussion
This study evaluates the use of pentamidine as antimicrobial agent against clinical strains of carbapenemase-producing and/or colistin-resistant Enterobacteriaceae, finding a strong in vitro activity both with pentamidine alone and combined with other antimicrobials, as aminoglycosides, tigecycline, doripenem, and rifampicin. Additionally, pentamidine was synergistic against selected strains in combination with some of these antimicrobials, especially when was studied combined with rifampicin.
Pentamidine showed a MIC rage against eight clinical strains of carbapenemase-producing and/or colistin-resistant Enterobacteriaceae from 200 to 800 mg/L. Due to its use as an antiprotozoal agent (Nguewa et al., 2005), no susceptibility breakpoints for pentamidine are defined. However, the MIC values obtained are in accordance to those reported analyzing the in vitro activity of pentamidine and five pentamidine analogs against a E. coli strain, with MIC values ranging from 100 to > 200 mg/L (Stokes et al., 2017). Moreover, we found that pentamidine at MIC concentration is bactericidal against seven of the eight tested strains; furthermore, at 1/2xMIC was bactericidal against the Kp28 OXA-48/CTX-M-15 producer strain.
Besides the robust bactericidal effect found with pentamidine alone, more important is that its combinations with the different antimicrobials tested potentiates the effect of these antimicrobials alone against the clinical strains using the time-kill assay. The in vitro activity observed with pentamidine in combination with antibiotics suggests there is strong possibility to repurpose it for antibacterial use against these difficult to treat MDR GNB (Pachón-Ibáñez et al., 2018; Rodríguez-Baño et al., 2018). These results are in accordance to the ones reported by Stokes et al. in which they found that the combination of pentamidine with rifampicin, novobiocin, erythromycin, or vancomycin potentiated the antimicrobials alone against a wild-type E. coli strain using chequerboard broth microdilution assays (Stokes et al., 2017). It is noteworthy that synergistic activity was observed when pentamidine was combined with amikacin, tobramycin, tigecycline, and/or rifampicin against the colistin-resistant E. cloacae 32, strain against which pentamidine alone did not show bactericidal activity. We would also like to mention, that no more synergistic effect with pentamidine in combination was observed due to the excellent bactericidal activity found with pentamidine alone at MIC concentration.
Pentamidine plus rifampicin was the combination that showed synergism against more of the tested strains (five out of eight). This excellent activity was also pointed out in the Stokes et al. study, in which pentamidine synergized with rifampicin against a wide phylogenetic distribution of antibiotic-resistant strains, including naturally polymyxin-resistant Serratia species (Stokes et al., 2017). The combination of rifampicin with other antimicrobials has been proved to be useful, both in vitro and in vivo, against other MDR GNB as Acinetobacter baumannii (Pachón-Ibáñez et al., 2010), as other example of repurposing a drug, such as rifampicin, previously used in staphylococcal and mycobacterial infections.
In summary, these results suggest that pentamidine, alone or in combination, may be a new alternative for the treatment of infections caused by carbapenemase-producing and/or colistin-resistant Enterobacteriaceae. To investigate further the possible usefulness of pentamidine new data from pharmacokinetics and pharmacodynamics and in vivo efficacy in experimental models of infection, including the dosage and safety, are required.
Author Contributions
MP-I has planned and coordinated the experiments, analyzed the results, and written the manuscript. GL-H and TC-C had performed the in vitro experiments. YS had reviewed the manuscript. RÁ-M, EC-M, and JP had reviewed the manuscript and the experiments.
Funding
This work was supported by Plan Nacional de I+D+i 2013-2016 and Instituto de Salud Carlos III, Subdirección General de Redes y Centros de Investigación Cooperativa, Ministerio de Economía, Industria y Competitividad, Spanish Network for Research in Infectious Diseases (REIPI RD16/0016/0009)—co-financed by European Development Regional Fund A way to achieve Europe, Operative program Intelligent Growth 2014-2020.
Conflict of Interest Statement
The authors declare that the research was conducted in the absence of any commercial or financial relationships that could be construed as a potential conflict of interest.
Supplementary Material
The Supplementary Material for this article can be found online at: https://www.frontiersin.org/articles/10.3389/fcimb.2018.00363/full#supplementary-material
References
Akova, M., Daikos, G. L., Tzouvelekis, L., and Carmeli, Y. (2012). Interventional strategies and current clinical experience with carbapenemase-producing Gram-negative bacteria. Clin. Microbiol. Infect. 18, 439–448. doi: 10.1111/j.1469-0691.2012.03823.x
Cantón, R., Akóva, M., Carmeli, Y., Giske, C. G., Glupczynski, Y., Gniadkowski, M., et al. (2012). Rapid evolution and spread of carbapenemases among Enterobacteriaceae in Europe. Clin. Microbiol. Infect. 18, 413–431. doi: 10.1111/j.1469-0691.2012.03821.x
Clinical and Laboratory Standards Institute (2012). Methods for Dilution Antimicrobial Susceptibility Tests for Bacteria That Grow Aerobically; Approved Standard—Ninth Edition. CLSI document M07-A9. Wayne, PA: Clinical and Laboratory Standards Institute.
Debnath, A., Parsonage, D., Andrade, R. M., He, C., Cobo, E. R., Hirata, K., et al. (2012). A high-throughput drug screen for Entamoeba histolytica identifies a new lead and target. Nat. Med. 18, 956–960. doi: 10.1038/nm.2758
Docobo-Pérez, F., Nordmann, P., Dominguez-Herrera, J., Lopez-Rojas, R., Smani, Y., Poirel, L., et al. (2012). Efficacies of colistin and tigecycline in mice with experimental pneumonia due to NDM-1-producing strains of Klebsiella pneumoniae and Escherichia coli. Int. J. Antimicrob. Agents 39, 251–254. doi: 10.1016/j.ijantimicag.2011.10.012
European Committee on Antimicrobial Susceptibility Testing (2018). European Society of Clinical Microbiology and Infectious Diseases. Clinical Breakpoints. EUCAST.
Ferreira, G. F., Santos, J. R., Costa, M. C., Holanda, R. A., Denadai, A. M., Freitas, G. J., et al. (2015). Heteroresistance to itraconazole alters the morphology and increases the virulence of Cryptococcus gattii. Antimicrob. Agents Chemother. 59, 4600–4609. doi: 10.1128/AAC.00466-15
Hong, Y. K., Lee, J. Y., and Ko, K. S. (2018). Colistin resistance in Enterobacter spp. isolates in Korea. J. Microbiol. 56, 435–440. doi: 10.1007/s12275-018-7449-0
Kempf, I., Jouy, E., and Chauvin, C. (2016). Colistin use and colistin resistance in bacteria from animals. Int. J. Antimicrob. Agents 48, 598–606. doi: 10.1016/j.ijantimicag.2016.09.016
López-Cerero, L., Egea, P., Gracia-Ahufinger, I., Gonzalez-Padilla, M., Rodriguez-Lopez, F., Rodriguez-Bano, J., et al. (2014). Characterisation of the first ongoing outbreak due to KPC-3-producing Klebsiella pneumoniae (ST512) in Spain. Int. J. Antimicrob. Agents 44, 538–540. doi: 10.1016/j.ijantimicag.2014.08.006
Magiorakos, A. P., Srinivasan, A., Carey, R. B., Carmeli, Y., Falagas, M. E., Giske, C. G., et al. (2012). Multidrug-resistant, extensively drug-resistant and pandrug-resistant bacteria: an international expert proposal for interim standard definitions for acquired resistance. Clin Microbiol Infect. 18, 268–281. doi: 10.1111/j.1469-0691.2011.03570.x
Miró, E., Aguero, J., Larrosa, M. N., Fernandez, A., Conejo, M. C., Bou, G., et al. (2013). Prevalence and molecular epidemiology of acquired AmpC beta-lactamases and carbapenemases in Enterobacteriaceae isolates from 35 hospitals in Spain. Eur. J. Clin. Microbiol. Infect. Dis. 32, 253–259. doi: 10.1007/s10096-012-1737-0
Nguewa, P. A., Fuertes, M. A., Cepeda, V., Iborra, S., Carrion, J., Valladares, B., et al. (2005). Pentamidine is an antiparasitic and apoptotic drug that selectively modifies ubiquitin. Chem. Biodivers. 2, 1387–1400. doi: 10.1002/cbdv.200590111
Olaitan, A. O., Morand, S., and Rolain, J. M. (2016). Emergence of colistin-resistant bacteria in humans without colistin usage: a new worry and cause for vigilance. Int. J. Antimicrob. Agents 47, 1–3. doi: 10.1016/j.ijantimicag.2015.11.009
Oteo, J., Ortega, A., Bartolome, R., Bou, G., Conejo, C., Fernandez-Martinez, M., et al. (2015). Prospective multicenter study of carbapenemase-producing Enterobacteriaceae from 83 hospitals in Spain reveals high in vitro susceptibility to colistin and meropenem. Antimicrob. Agents Chemother. 59, 3406–3412. doi: 10.1128/AAC.00086-15
Pachón-Ibáñez, M. E., Docobo-Perez, F., Lopez-Rojas, R., Domínguez-Herrera, J., Jimenez-Mejias, M. E., García-Curiel, A., et al. (2010). Efficacy of rifampin and its combinations with imipenem, sulbactam, and colistin in experimental models of infection caused by imipenem-resistant Acinetobacter baumannii. Antimicrob Agents Chemother. 54, 1165–1172. doi: 10.1128/AAC.00367-09
Pachón-Ibáñez, M. E., Labrador-Herrera, G., Cebrero-Cangueiro, T., Diaz, C., Smani, Y., Del Palacio, J. P., et al. (2018). Efficacy of colistin and its combination with rifampin in vitro and in experimental models of infection caused by carbapenemase-producing clinical isolates of Klebsiella pneumoniae. Front. Microbiol. 9:912. doi: 10.3389/fmicb.2018.00912
Pournaras, S., Vrioni, G., Neou, E., Dendrinos, J., Dimitroulia, E., Poulou, A., et al. (2011). Activity of tigecycline alone and in combination with colistin and meropenem against Klebsiella pneumoniae carbapenemase (KPC)-producing Enterobacteriaceae strains by time-kill assay. Int. J. Antimicrob. Agents 37, 244–247. doi: 10.1016/j.ijantimicag.2010.10.031
Rodríguez-Baño, J., Gutiérrez-Gutiérrez, B., Machuca, I., and Pascual, A. (2018). Treatment of infections caused by extended-spectrum-beta-lactamase-, ampc-, and carbapenemase-producing enterobacteriaceae. Clin. Microbiol. Rev. 31, e00079–e00017. doi: 10.1128/CMR.00079-17
Ruiz-Aragón, J., Ballestero-Téllez, M., Gutiérrez-Gutiérrez, B., de Cueto, M., Rodríguez-Baño, J., and Pascual, Á. (2018). Direct bacterial identification from positive blood cultures using matrix-assisted laser desorption/ionization time-of-flight (MALDI-TOF) mass spectrometry: A systematic review and meta-analysis. Enferm. Infecc. Microbiol. Clin. 36, 484–492. doi: 10.1016/j.eimc.2017.08.012
Souli, M., Galani, I., Boukovalas, S., Gourgoulis, M. G., Chryssouli, Z., Kanellakopoulou, K., et al. (2011). In vitro interactions of antimicrobial combinations with fosfomycin against KPC-2-producing Klebsiella pneumoniae and protection of resistance development. Antimicrob. Agents Chemother. 55, 2395–2397. doi: 10.1128/AAC.01086-10
Soussy, C. J. (2012). Antibiogram Committee of the French Society for Microbiology, Recommendations. Available online at: http://www.sfm-microbiologie.org/UserFiles/files/casfm/CASFM_2012.pdf
Spellberg, B., and Rex, J. H. (2013). The value of single-pathogen antibacterial agents. Nat. Rev. Drug Discov. 12:963. doi: 10.1038/nrd3957-c1
Stokes, J. M., MacNair, C. R., Ilyas, B., French, S., Cote, J. P., Bouwman, C., et al. (2017). Pentamidine sensitizes Gram-negative pathogens to antibiotics and overcomes acquired colistin resistance. Nat. Microbiol. 2:17028. doi: 10.1038/nmicrobiol.2017.28
Trecarichi, E. M., and Tumbarello, M. (2017). Therapeutic options for carbapenem-resistant Enterobacteriaceae infections. Virulence 8, 470–484. doi: 10.1080/21505594.2017.1292196
Wang, J., He, J. T., Bai, Y., Wang, R., and Cai, Y. (2018). Synergistic activity of colistin/fosfomycin combination against carbapenemase-Producing Klebsiella pneumoniae in an in vitro pharmacokinetic/pharmacodynamic model. Biomed Res. Int. 2018:5720417. doi: 10.1155/2018/5720417
World Health Organization (2017). Global Priority List of Antibiotic-resistant Bacteria to Guide Research, Discovery, and Development of New Antibiotics. Available online at: http://www.who.int/medicines/publications/global-priority-list-antibiotic-resistant-bacteria/en/
Keywords: Enterobacteriaceae, colistin-resistant, carbapenemase producers, pentamidine, in vitro activity
Citation: Cebrero-Cangueiro T, Álvarez-Marín R, Labrador-Herrera G, Smani Y, Cordero-Matía E, Pachón J and Pachón-Ibáñez ME (2018) In vitro Activity of Pentamidine Alone and in Combination With Aminoglycosides, Tigecycline, Rifampicin, and Doripenem Against Clinical Strains of Carbapenemase-Producing and/or Colistin-Resistant Enterobacteriaceae. Front. Cell. Infect. Microbiol. 8:363. doi: 10.3389/fcimb.2018.00363
Received: 13 August 2018; Accepted: 01 October 2018;
Published: 18 October 2018.
Edited by:
Maria Tomas, Complexo Hospitalario Universitario A Coruña, SpainReviewed by:
Robson Souza Leão, Rio de Janeiro State University, BrazilNaybi Muñoz, Centro de Investigación Científica de Yucatán, Mexico
Copyright © 2018 Cebrero-Cangueiro, Álvarez-Marín, Labrador-Herrera, Smani, Cordero-Matía, Pachón and Pachón-Ibáñez. This is an open-access article distributed under the terms of the Creative Commons Attribution License (CC BY). The use, distribution or reproduction in other forums is permitted, provided the original author(s) and the copyright owner(s) are credited and that the original publication in this journal is cited, in accordance with accepted academic practice. No use, distribution or reproduction is permitted which does not comply with these terms.
*Correspondence: Jerónimo Pachón, pachon@us.es