- 1School of Biology and Biological Engineering, South China University of Technology, Guangzhou, China
- 2BGI-Shenzhen, Shenzhen, China
- 3BGI Education Center, University of Chinese Academy of Sciences, Shenzhen, China
- 4School of Future Technology, University of Chinese Academy of Sciences, Beijing, China
- 5Lanzhou University, Lanzhou, China
Migraine is a very common, multifactorial, and recurrent central nervous system disorder that causes throbbing headache, photophobia, phonophobia, nausea, and disability. Migraine occurs more often in females, and its complex physiopathology is not yet fully understood. An increasing number of gastrointestinal disorders have been linked to the occurrence of migraine suggesting that gut microbiota might play a pivotal role in migraine through the gut–brain axis. In the present work, we performed a metagenome-wide association study (MWAS) to determine the relationship between gut microbiota and migraine by analyzing 108 shotgun-sequenced fecal samples obtained from elderly women who suffer from migraine and matched healthy controls. Notably, the alpha diversity was significantly decreased in the migraine group at species, genus, and Kyoto Encyclopedia of Genes and Genomes (KEGG) orthologous levels. Firmicutes, especially the “unfriendly” Clostridium spp., were significantly enriched in the migraine group. Conversely, the healthy controls held more beneficial microorganisms, such as Faecalibacterium prausnitzii, Bifidobacterium adolescentis, and Methanobrevibacter smithii. For functional modules, the migraine group was enriched in gut–brain modules (GBMs) including kynurenine degradation and γ-aminobutyric acid (GABA) synthesis. However, the healthy controls held higher gut metabolic modules (GMMs) including glycolysis, homoacetogenesis, and GBMs including quinolinic acid degradation and S-adenosyl methionine (SAM) synthesis. The differences in gut microbiota composition and function between the migraine and healthy groups provided new information as well as novel therapeutic targets and strategies for migraine treatment, which could help to improve the early diagnosis of the disease, as well as the long-term prognosis and the life quality of patients suffering from migraine.
Introduction
Migraine can cause a severe unilateral throbbing headache or a pulsing sensation, which are typically accompanied by nausea, vomiting, and extreme sensitivity to light and sound, thereby adversely affecting daily activities (MacGregor, 2017). Constipation and mood changes also frequently occur together with migraine (Vacca, 2019). Migraines usually begin in adolescence or early adulthood, it is three times more common in women (17%) than in men (5–8%), and it accounts for over 90% of patients with recurrent headache (MacGregor, 2017). Multiple factors, including inheritance (Ulrich et al., 1999), hormones (Sacco et al., 2012), dietary habits (Nazari and Eghbali, 2012), mental stress (Lipton et al., 2014), and gastrointestinal disorders (Camara-Lemarroy et al., 2016), have been reported as triggers of migraine. For the prognosis, some patients remit, some experience recurrent episodes, and in others the condition evolves into a chronic and more refractory state.
Human gut microbiome, which is considered as the second genome and brain of human body (Li et al., 2014), is thought to be closely related to migraine (Gonzalez et al., 2016). The recent gut–brain axis theory (Carabotti et al., 2015) has proposed a bidirectional communication between the central and enteric nervous systems, linking the emotional and cognitive centers of the brain with the peripheral intestinal functions. Studies have found that irritable bowel syndrome (IBS) occurs in over half of migraine patients (Lau et al., 2014), and inflammatory bowel disease (IBD) patients are 2.7 times more likely to have migraines (Dimitrova et al., 2013). The fact that both IBD and IBS are severe gut disorders associated with gut permeability and inflammation caused by gut microbes through the gut–brain axis (Aggarwal et al., 2012) suggests an important role of gut bacteria in migraine. In an uncontrolled observational study on 1,020 patients, researchers found that multispecies probiotic formulations can reduce the intensity and the frequency of migraine attacks (Straube et al., 2018). However, another randomized placebo-controlled study conducted on 63 patients showed that the use of multispecies probiotics, compared with placebo, does not significantly affect intestinal permeability or inflammation (de Roos et al., 2017). These paradoxical conclusions need further confirmation.
The diagnosis of a migraine is based on signs and symptoms (Bartleson and Cutrer, 2010), such as with or without aura, duration, unilateral, pulsating headache, inability to work, nausea and vomiting, photophobia, and phonophobia. Diagnostic uncertainty has been associated with diagnostic variation (physicians giving different diagnoses to the same patient), over-testing, suboptimal management, more hospitalizations and referrals, and increased health care expenditure. In contrast to traditional pharmacotherapy, which is marked by unavoidable and often dangerous side effects (e.g., analgesics might cause nervous disorders; Do et al., 2019), dietary intervention is thought to be a promising safe therapeutic strategy to prevent migraine through the regulation of gut microbes (Camara-Lemarroy et al., 2016). Both the ketogenic diet (Gross et al., 2019) and the traditional Chinese medicinal plant Gastrodia elata, which is also used as food in China (Hua et al., 2019), have been reported to regulate gut microbiota and to promote remission from migraine. The few publications available, which are based on probiotic treatment, however, are with different conclusions about the effects of probiotics on migraine in humans (de Roos et al., 2017; Straube et al., 2018).
In this work, we performed a metagenome-wide association study (MWAS) based on shotgun-sequenced fecal samples obtained from 108 elderly women consisting of 54 migraineurs and 54 healthy subjects in order to shed some light over the connections between gut microbiota composition and function and migraine. The study aimed at discovering differences in gut microbiota that could help to design strategies based on the modulation of the gut microecology that could improve the long-term prognosis of migraine and provide a guidance for early diagnosis and management of this recurrent disease.
Materials and Methods
Statement of Human Rights
The data used in this study were obtained from a previous study (Xie et al., 2016) whose ethics statement was approved by the local ethics committee, and an informed consent was collected from each subject. All the procedures followed were in accordance with the ethical standards of the responsible committee on human experimentation (BGI-Shenzhen, China, Ethics Approval No. BGI-IRB 14074).
Materials
The 108 stool samples, from 54 migraineurs and 54 matched 201 healthy controls, were shotgun sequenced during a former study (Table S1) (Xie et al., 2016). All the samples and clinical indexes were collected by Prof. Spector's group at King's College London. Subjects were excluded if they had a history of chronic serious infection, any current infection, and any type of malignant cancer; individuals who had received antibiotic treatment within 1 month before participating in this study were also excluded.
Quality Control and Host Genome Filtering
The raw reads that had 50% low-quality bases (quality ≤ 20) or more than five ambiguous bases were excluded. The remaining reads were mapped to the human genome (hg19) by SOAP v2.22 (-m 100 -x 600 -v 7 -p 6 -l 30 -r 1 -M 4 -c 0.95), and the matching reads were removed (Fang et al., 2018). The high-quality non-human reads were defined as clean reads.
Acquisition of Gene Abundance and Taxonomic Profiles From Metagenomic Samples
The clean reads were aligned against the latest 11.4 M human gut microbial gene catalog (Xie et al., 2016) through SOAP v2.22 (-m 100 -x 600 -v 7 -p 6 -l 30 -r 1 -M 4 -c 0.9) to generate the gene abundance profile. To obtain the taxonomic profiles, metaphlan2 (Truong et al., 2015) (–input_type fastq –ignore_viruses –nproc 6) was used to generate phyla, genera, and species profiles from the clean reads.
Calculation of Gut Microbiome Functional Profiles
Putative amino acid sequences were translated from the gene catalog (Xie et al., 2016) and aligned against the proteins or domains in the Kyoto Encyclopedia of Genes and Genomes (KEGG) databases (release 79.0, with animal and plant genes removed) using BLASTP (v2.26, default parameter, except -m 8 -e 1e-5 -F -a 6 -b 50). Each protein was assigned to a KEGG orthologous (KO) group on the basis of the highest scoring annotated hit(s) containing at least one segment pair scoring over 60 bits. The relative abundance profile of KOs was determined by summing the relative abundance of genes from each KO using the mapped reads per sample (Xie et al., 2016). The abundance of each gut metabolic module (GMM) (-a 2 -d GMM.v1.07.txt -s average) and gut neuroactive module (GBM) (default parameter) were calculated as shown in the former article (Vieira-Silva et al., 2016; Valles-Colomer et al., 2019).
Permutational Multivariate Analysis of Variance of the Effect of Related Factors on Gut Microbiome
To evaluate the effects of the clinical and lifestyle factors on the microbiome, we performed the permutational multivariate analysis of variance (PERMANOVA) of the gene abundance of the samples. The Bray–Curtis distance and 9,999 permutations in R (3.2.5, vegan package) were used.
Richness and Diversity Analysis
Alpha diversity (within samples) at species, genus, and KO levels of the two groups was quantified by the Shannon index on the basis of the relative gene abundance profile.
Differential Analysis of the Gut Microbiome Between the Two Groups
MWAS was used to investigate the differences in taxon composition between fecal microbiomes of healthy controls and migraineurs. To investigate the specific differences in the gut microbiome composition and function between the migraine group and the healthy controls, first, the top 15 species, the top 10 genera, and the top 5 phyla of each group were selected according to their average relative abundances, and each of the taxon was compared by Wilcoxon rank-sum test to compare their differences between two groups (p < 0.05). Second, the significantly different species, genera, phyla (Table S4), GMMs (Table S5), and GBMs (Table S6) between two groups were tested by Wilcoxon rank-sum test (p < 0.05). Third, the significantly changed species analyzed above were further analyzed by Spearman's rank correlation (p < 0.05) according to their relative abundances in all samples. Then the software Cytoscape 3.4.0 was used to visualize the co-occurrence network of these species.
Results
Characterization of the Gut Microbiomes
To investigate the characteristics of gut microbiome in migraine patients, a metagenomic shotgun-sequencing study was performed on a total of 108 fecal samples from 54 individuals with migraine and 54 healthy controls with matched age and body mass index (BMI) (Figure S1). After removal of low-quality and human DNA reads, an average of 7.27 gigabase pairs per sample were aligned to a gut microbiome gene catalog comprising 11.4 million genes (Xie et al., 2016), achieving an average of 77.6 ± 1.6% matched reads per sample (Table S2).
PERMANOVA revealed significant differences in the gut microbiome of the two groups (p = 0.0066, R2 = 0.014, Table S1b). The alpha diversity was evidently decreased in the migraine group at both genus (p = 0.036, Wilcoxon rank-sum test, Figure 1A) and species (p = 0.048, Wilcoxon rank-sum test, Figure 1B) levels, whereas the species richness was not significantly different in the two groups at either level (genus, p = 0.64; species, p = 1.0; Wilcoxon rank-sum test, Figures S2A,B). Similarly, the KO analysis showed an evident decrease of the alpha diversity in the migraine group (p = 0.045, Wilcoxon rank-sum test, Figure S2C), whereas the difference in species richness between the two groups was not significant (p = 0.085, Wilcoxon rank-sum test, Figure S2D). The data used in Figure 1 and Figure S1 are included in Table S3.
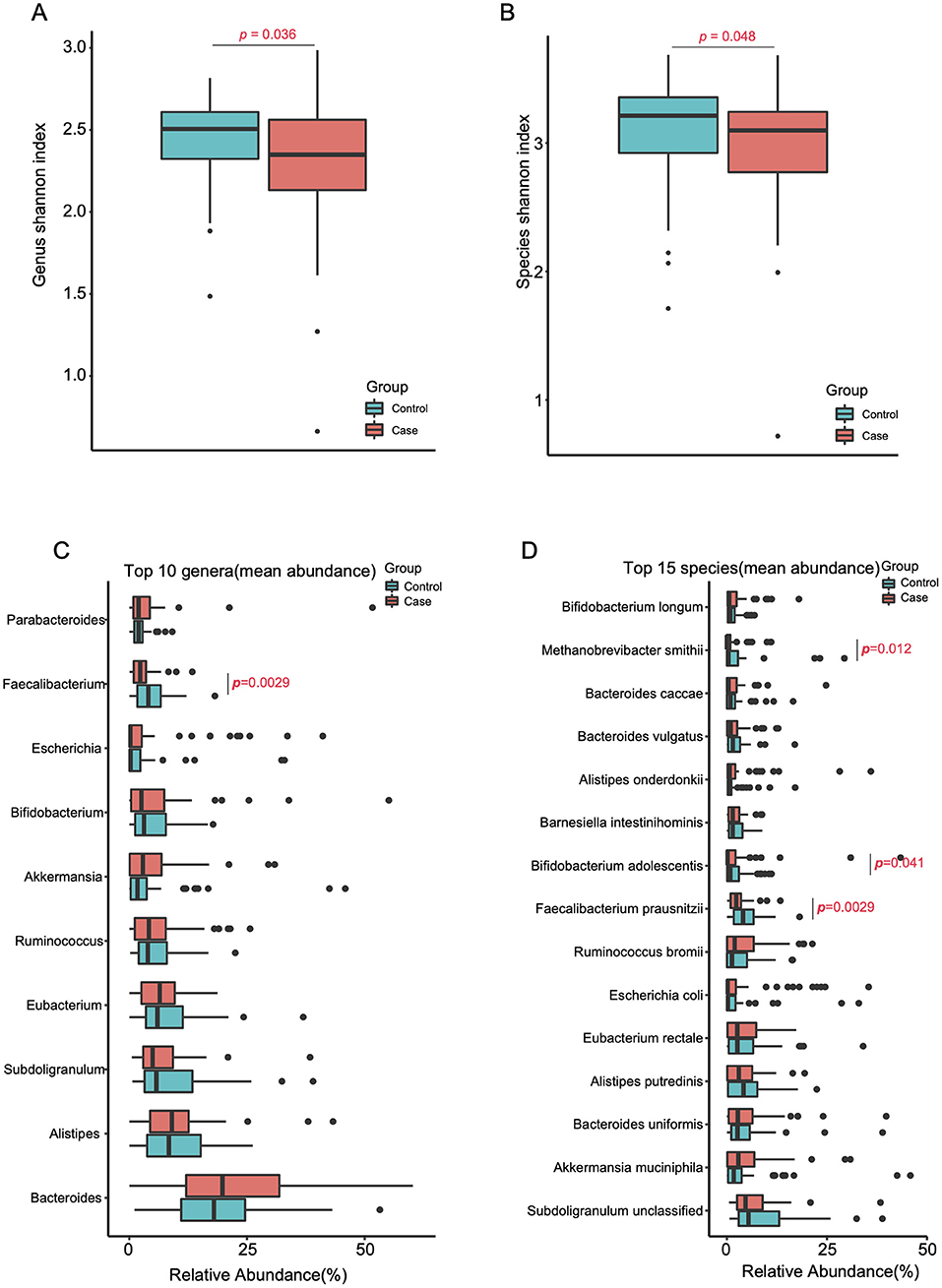
Figure 1. Reduced gut microbial diversity in migraineurs. Alpha-diversity (Shannon index) at the genus (A) and species (B) levels of the two cohorts (Tested by two-tailed Wilcoxon-rank sum test). The top 10 genera (C) and top 15 species (D) (mean relative abundance higher than 3.09 and 1.83%, respectively) in the migraine patients and the control individuals (two-tailed Wilcoxon-rank sum test, Tables S4B,C).
Differences of the Gut Microbiome Between Two Groups
To investigate the differences in highly abundant bacteria in the gut of the two groups, we chose the top 15 species, top 10 genera, and top 5 phyla. Interestingly, the migraine group showed significantly higher levels of the phylum Firmicutes (p = 0.0023, Figure S3) and a reduction in the level of the beneficial genus Faecalibacterium (p = 0.0029, Figure 1C) relative to the control group. At the species level (Figure 1D), Faecalibacterium prausnitzii (p=0.0029), Bifidobacterium adolescentis (p=0.041), and Methanobrevibacter smithii (p = 0.012) were significantly enriched in the healthy controls.
To further illustrate the differences between the two cohorts, 21 and 22 significantly enriched species (Figure 2, p < 0.05, false discovery rate [FDR] = 0.14, Table S4) were identified for the migraine group and the healthy controls, respectively. The species enriched in the migraine group were Blautia hydrogenotrophica, Clostridium asparagiforme, Clostridium clostridioforme, Clostridium bolteae, Clostridium citroniae, Clostridium hathewayi, Clostridium ramosum, Clostridium spiroforme, Clostridium symbiosum, Eggerthella lenta, Flavonifractor plautii, Lachnospiraceae bacterium, and Ruminococcus gnavus. B. hydrogenotrophica metabolizes H2/CO2 to acetate and contributes to the breakdown of plant polysaccharides and proteins in the host (Bernalier et al., 1996). Except for C. bolteae, an autism-associated bacterium (Pequegnat et al., 2013), all the other Clostridium spp. enriched in the migraine group have been reported to be correlated with solid tissue inflammation (Elsayed and Zhang, 2004b), infection (Finegold et al., 2005), and bacteremia (Elsayed and Zhang, 2004a). E. lenta and F. plautii can cause bacteremia (Wong et al., 2014) and bloodstream infection (Berger et al., 2018), respectively. R. gnavus, which degrades mucin (Crost et al., 2016), is enriched in IBD patients (Hall et al., 2017) and can cause bacteremia (Hansen et al., 2013).
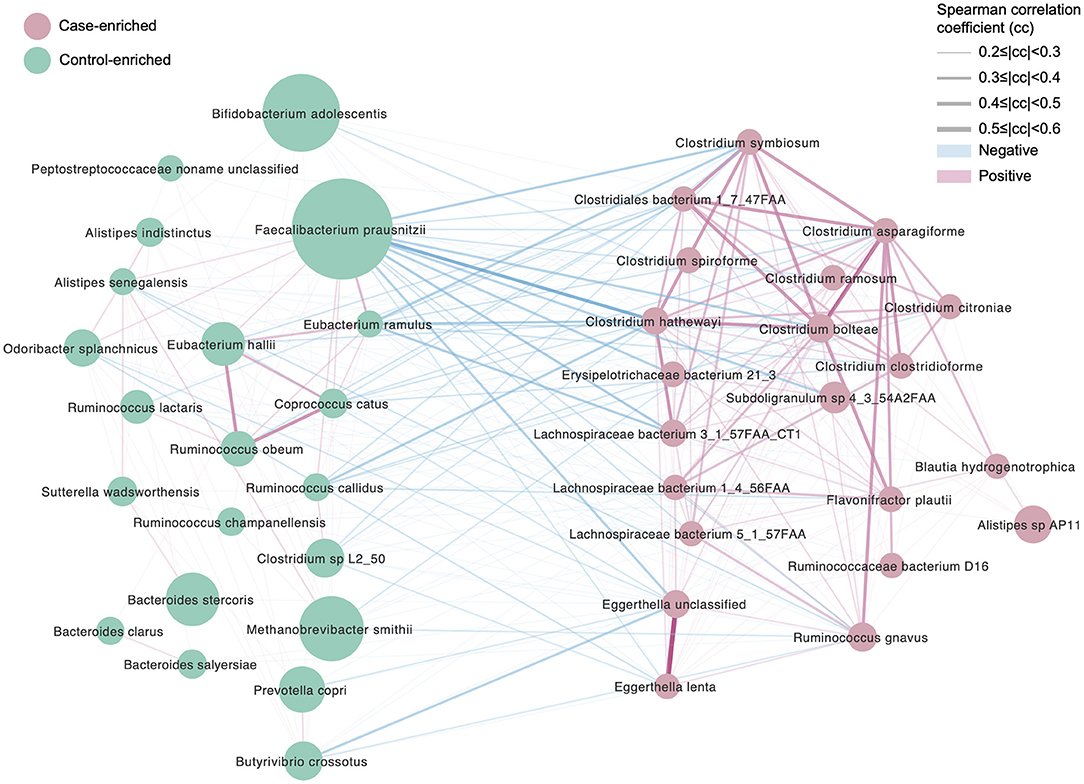
Figure 2. Differentially enriched species, control vs. migraine. The orientation of enrichment was determined by two-tailed Wilcoxon-rank sum test (U statistic of two-tail Wilcoxon rank-sum test, p < 0.05, FDR< 0.29, Table S4C). The size of the nodes is consistent with the relative abundance of the species. Species were colored according to the extent of enrichment. The edges between the nodes indicate positive (red) and negative (blue) Spearman's correlation, respectively, the line width represents the correlation coefficient calculated for the samples under comparison.
The species significantly enriched in the controls were Bacteroides clarus, Bacteroides intestinalis, Bacteroides salyersiae, Bacteroides stercoris, Butyrivibrio crossotus, Clostridium sp. L2_50, Coprococcus catus, Eubacterium hallii, Eubacterium ramulus, Odoribacter splanchnicus, Peptostreptococcaceae noname unclassified, Prevotella copri, Ruminococcus callidus, Ruminococcus champanellensis, Ruminococcus obeum, and Sutterella wadsworthensis. In this list, at least one bacterium regarded as “unfriendly” can be found: O. splanchnicus, which has been isolated from the crevicular spaces of dogs with periodontitis (Hardham et al., 2008). However, many more species regarded as beneficial were found in the healthy subjects compared with the migraineurs. B. clarus is significantly decreased in colorectal cancer (Watanabe et al., 2010; Liang et al., 2017), and its enrichment in controls may be beneficial. S. wadsworthensis protects against IBD (Wexler et al., 1996); C. catus ferments fructose, lactate, and pyruvate to short-chain fatty acids (SCFAs) (Holdeman and Moore, 1974); E. hallii contributes to the intestinal propionate formation (Engels et al., 2016) and improves insulin sensitivity (Udayappan et al., 2016); E. ramulus produces propionic acid and dihydroxyphenylacetic acid (Schneider and Blaut, 2000; Braune et al., 2001); R. obeum plays an important role in the recovery process from Vibrio cholerae infection (Lawson and Finegold, 2015). In addition, species involved in polysaccharide degradation were also enriched in the healthy controls. These were B. intestinalis, which degrades arabinoxylan for energy acquisition (Wang et al., 2016); B. crossotus, which metabolizes polysaccharides into simpler sugars (Kelly et al., 2010); and R. callidus and R. champanellensis, which degrade various plant hemicelluloses and cellulose (Chassard et al., 2012).
Functional Alterations in the Gut Microbiome of Migraineurs
Significantly different GMMs and GBMs between two groups were analyzed (p < 0.05, Wilcoxon rank-sum test). Seven significantly changed GMMs between two groups were observed (Figure 3A), and glutamate degradation II is the only GMM that is significantly higher in the migraine group. Serine degradation, homoacetogenesis, glycerol degradation, mannose degradation, glycolysis (preparatory phase), and pyruvate:ferredoxin oxidoreductase were the six GMMs found to be enriched in the healthy controls. Serine is a major energy and SCFAs contributor in the human body and can be degraded to pyruvate by Escherichia coli (Su et al., 1989). Homoacetogenesis produces acetate by consuming hydrogen (Ni et al., 2011). Higher glycerol degradation can reduce the triglycerides synthesis and phospholipids synthesis. Mannose, which has been reported to impair tumor growth and to enhance chemotherapy, can be degraded into fructose, which is then catabolized to lactate, a precursor of SCFAs (Gonzalez et al., 2018). Glycolysis (preparatory phase) consumes energy to convert glucose into two 3-carbon molecules (Kathagen et al., 2013). Pyruvate:ferredoxin oxidoreductase is a key enzyme in metabolism that catalyzes pyruvate to acetyl-CoA and CO2 (Furdui and Ragsdale, 2000). Taken together, these results indicated that the gut microbiota of the healthy controls were more active in energy metabolism and SCFA synthesis, which might be beneficial in maintaining their health.
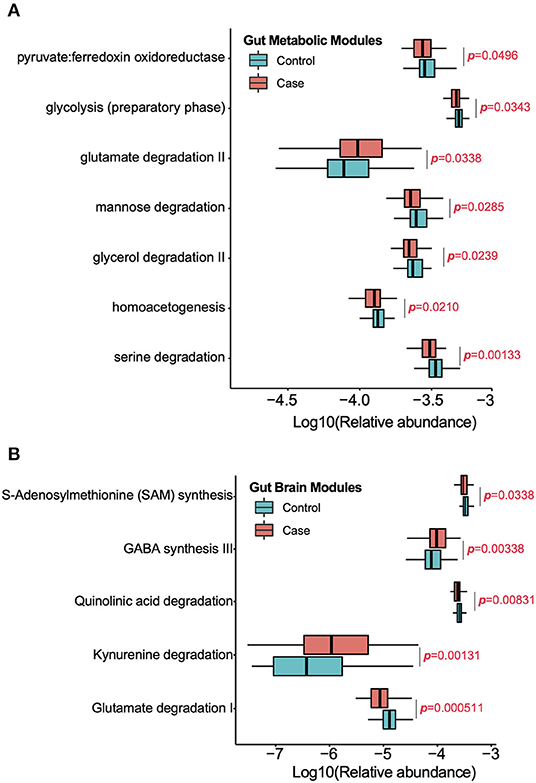
Figure 3. Significantly enriched GMMs (A) and GBMs (B) in each group were analyzed by Wilcoxon rank sum test (p < 0.05). The Y-axis is the significantly enriched GMMs and GBMs in two groups and X-axis is the logarithm of relative abundance for each GMM and GBM. P-value was labeled.
Five significantly changed GBMs between two groups were observed (Figure 3B). Glutamate degradation I, quinolinic acid degradation, and S-adenosyl methionine (SAM) synthesis were predominant in the control group, which meant that there was a decrease in the levels of glutamate and quinolinic acid while an increase in those of the SAM in the healthy controls. Glutamate is a neurotransmitter in the healthy brain. Quinolinic acid is an endogenous N-methyl-D-aspartate (NMDA) receptor agonist and possesses neuroactive activity (Heyes et al., 1992). SAM is a major methyl donor in the brain. Conversely, kynurenine degradation and γ-aminobutyric acid (GABA) synthesis III were enriched in the migraine group. Kynurenine is a metabolite of tryptophan and can be degraded to quinolinic acid and kynurenic acid, an NMDA antagonist, and is thought to be involved in the pathophysiology and pathogenesis of schizophrenia. GABA is the chief inhibitory neurotransmitter in the developmentally mature mammalian central nervous system.
Discussion
Migraine susceptibility is multifactorial with genetic, hormonal, and environmental factors. The physiopathology of migraine is complex and still not fully understood. Recent reports demonstrate an increased frequency of gastrointestinal disorders, such as Helicobacter pylori infection, IBS, gastroparesis, hepatobiliary disorders, celiac disease, and alterations in the microbiota have been linked to the occurrence of migraine (Camara-Lemarroy et al., 2016). However, several case–control studies based on probiotic treatment have different conclusions (de Roos et al., 2017; Straube et al., 2018). Up to now, the precise characteristics and changes of gut microbiome in migraine were not fully elucidated. In this study, we presented the first set of evidence obtained from human cohorts for a significant gut microbiome dysbiosis from both compositional and functional aspects in migraine.
Significant differences in the gut microbiota composition were observed in our study. First, the migraine group showed a significantly lower alpha diversity at species, genus, and KO levels than did the healthy controls, which might be caused by a significant depletion in some highly abundant bacteria in migraine, such as Faecalibacterium, F. prausnitzii, B. adolescentis, and M. smithii. F. prausnitzii is a major producer of butyrate (Machiels et al., 2014), B. adolescentis exhibits strain-specific effects in the alleviation of constipation (Wang et al., 2017), and M. smithii, a predominant archaeon in the human gut, can affect the specificity and efficiency of dietary polysaccharides to influence host calorie harvest and adiposity (Samuel et al., 2007). Notably, Firmicutes, the main bacterial phylum in the gut, was significantly enriched in migraine. Second, some species thought to be detrimental to human health, especially Clostridium spp., were significantly enriched in migraineurs. Third, the controls held more beneficial microorganisms, such as B. adolescentis, F. prausnitzii, and Bacteroides intestinalis, suggesting that the control subjects held a healthier gut microenvironment than did migraineurs. Notably, some “unfriendly” species, such as Odoribacter splanchnicus and Prevotella copri, were also elevated in controls, suggesting the likelihood of susceptibility to intestinal inflammation (Hardham et al., 2008) and arthritis (Scher et al., 2013) in the seemingly healthy control subjects.
From a functional point of view, significant changes in GMMs and GBMs between two groups were observed. Interestingly, the migraineurs had less GMMs and GBMs enriched than did the controls. The healthy controls held more modules related to substrates metabolism, glycolysis (preparatory phase), and SCFA production, whereas glutamate degradation II is the only significantly changed GMM enriched in migraine. These results suggested that migraineurs might suffer from metabolic dysfunctions and insufficient SCFA synthesis.
For GBMs, kynurenine degradation and GABA synthesis III were significantly higher in the migraine group. The higher kynurenine degradation module observed in the migraine group suggested the potential presence of elevated concentration of its catabolites, that is, the neuroexcitatory quinolinic acid and neuroinhibitory kynurenic acid, which can cause diseases in the nervous and immune systems (Heyes et al., 1992). The higher GABA synthesis observed in the migraine group indicated the potential presence of higher levels of GABA in the brain, which might be beneficial for health. Oral GABA administration was reported to relieve anxiety (Abdou et al., 2006), improve mood (Sakashita et al., 2019), and reduce symptoms of premenstrual syndrome (Rapkin and Akopians, 2012). In addition, GABA also supports the physiologic adjustment of pituitary gland function and controls growth hormone secretion from the pituitary gland (Acs et al., 1987), promotes muscle protein synthesis (Olarescu et al., 2000), stabilizes blood pressure (Ma et al., 2015), and relieves pain (Jasmin et al., 2004). On the contrary, the higher capability of glutamate degradation I was observed in the healthy group. Glutamate is the principal excitatory neurotransmitter in the healthy brain and provides energy for normal brain function, whose depletion is a common feature of many neuropsychiatric conditions such as schizophrenia (Zhou and Danbolt, 2014). In addition, higher quinolinic acid degradation was observed in the control group. Quinolinic acid is an NMDA antagonist, has a potent neurotoxic effect, and may be involved in many psychiatric disorders and neurodegenerative processes in the brain. These GBM changes reminded us that the healthy volunteers might also have a risk in getting mental disorders, although they have no significant signs. Higher synthesis of SAM in controls indicated higher levels of SAM, an antidepressant with few side effects (Young and Shalchi, 2005). The functional redundancy, which was reported by Kang et al. (2015), was also found in both migraine group and healthy controls in our study, which meant that the gut microbiome can self-regulate to relieve symptoms when their hosts suffered from migraine. In addition, changes in some unhealthy gut microbiome composition and function in the healthy group might also indicate that migraine may still happen in seemingly healthy people in the future.
In summary, our results revealed a significant decrease in species diversity and metabolic functions in the gut microbiota of migraine sufferers, which highlighted the importance of maintaining species diversity to improve the gut microecosystem stability. Additionally, the monitoring of harmful bacteria such as Clostridium spp. could be a new strategy for early diagnosis and timely prevention of migraine. In addition, proper probiotics could be supplemented to migraineurs to treat their intestinal dysbiosis or prevent them from gut disorders, which may reduce the occurrence of migraine attacks. Our findings revealed that gut microbiota can be a potential target for migraine management and offered not only novel promising treatment strategies but also an important functional basis for future research on this disease.
Data Availability Statement
The datasets analyzed in this study can be found in the European Bioinformatics Institute (EBI) with the accession ID ERP010708 or in the China National Genebank (CNGB) with a program ID CNPhis0000107.
Ethics Statement
The studies involving human participants were reviewed and approved by Research Health Authority NRES Committee London - Westminster. The patients/participants provided their written informed consent to participate in this study.
Author Contributions
QW and JC conceived and designed the study. QW and AW were in charge of data analysis and graphics presentation. JC wrote the paper. QW and ZL reviewed and revised the manuscript. All authors read and approved the manuscript.
Funding
The TwinsUK resource was funded by the Wellcome Trust 105022/Z/14/Z and by the National Institute for Health Research (NIHR) BioResource Clinical Research Facility and Biomedical Research Center based at Guy's and St. Thomas' NHS Foundation Trust and King's College London. Prof. Spector was a NIHR Senior Investigator.
Conflict of Interest
JC and QW are/were employed by BGI-Shenzhen.
The remaining authors declare that the research was conducted in the absence of any commercial or financial relationships that could be construed as a potential conflict of interest.
Acknowledgments
We are grateful to Prof. Spector at King's College London for sample and clinical index collection.
Supplementary Material
The Supplementary Material for this article can be found online at: https://www.frontiersin.org/articles/10.3389/fcimb.2019.00470/full#supplementary-material
References
Abdou, A. M., Higashiguchi, S., Horie, K., Kim, M., Hatta, H., and Yokogoshi, H. (2006). Relaxation and immunity enhancement effects of gamma-aminobutyric acid (GABA) administration in humans. Biofactors 26, 201–208. doi: 10.1002/biof.5520260305
Acs, Z., Szabo, B., Kapocs, G., and Makara, G. B. (1987). gamma-Aminobutyric acid stimulates pituitary growth hormone secretion in the neonatal rat. A superfusion study. Endocrinology 120, 1790–1798. doi: 10.1210/endo-120-5-1790
Aggarwal, M., Puri, V., and Puri, S. (2012). Serotonin and CGRP in migraine. Ann. Neurosci. 19, 88–94. doi: 10.5214/ans.0972.7531.12190210
Bartleson, J. D., and Cutrer, F. M. (2010). Migraine update. Diagnosis and treatment. Minn. Med. 93, 36–41.
Berger, F. K., Schwab, N., Glanemann, M., Bohle, R. M., Gartner, B., and Groesdonk, H. V. (2018). Flavonifractor (Eubacterium) plautii bloodstream infection following acute cholecystitis. IDCases 14:e00461. doi: 10.1016/j.idcr.2018.e00461
Bernalier, A., Willems, A., Leclerc, M., Rochet, V., and Collins, M. D. (1996). Ruminococcus hydrogenotrophicus sp. nov., a new H2/CO2-utilizing acetogenic bacterium isolated from human feces. Arch. Microbiol. 166, 176–183. doi: 10.1007/s002030050373
Braune, A., Gutschow, M., Engst, W., and Blaut, M. (2001). Degradation of quercetin and luteolin by Eubacterium ramulus. Appl. Environ. Microbiol. 67, 5558–5567. doi: 10.1128/AEM.67.12.5558-5567.2001
Camara-Lemarroy, C. R., Rodriguez-Gutierrez, R., Monreal-Robles, R., and Marfil-Rivera, A. (2016). Gastrointestinal disorders associated with migraine: a comprehensive review. World J. Gastroenterol. 22, 8149–8160. doi: 10.3748/wjg.v22.i36.8149
Carabotti, M., Scirocco, A., Maselli, M. A., and Severi, C. (2015). The gut-brain axis: interactions between enteric microbiota, central and enteric nervous systems. Ann. Gastroenterol. 28, 203–209.
Chassard, C., Delmas, E., Robert, C., Lawson, P. A., and Bernalier-Donadille, A. (2012). Ruminococcus champanellensis sp. nov., a cellulose-degrading bacterium from human gut microbiota. Int. J. Syst. Evol. Microbiol. 62, 138–143. doi: 10.1099/ijs.0.027375-0
Crost, E. H., Tailford, L. E., Monestier, M., Swarbreck, D., Henrissat, B., Crossman, L. C., et al. (2016). The mucin-degradation strategy of Ruminococcus gnavus: the importance of intramolecular trans-sialidases. Gut Microbes 7, 302–312. doi: 10.1080/19490976.2016.1186334
de Roos, N. M., van Hemert, S., Rovers, J. M. P., Smits, M. G., and Witteman, B. J. M. (2017). The effects of a multispecies probiotic on migraine and markers of intestinal permeability-results of a randomized placebo-controlled study. Eur. J. Clin. Nutr. 71, 1455–1462. doi: 10.1038/ejcn.2017.57
Dimitrova, A. K., Ungaro, R. C., Lebwohl, B., Lewis, S. K., Tennyson, C. A., Green, M. W., et al. (2013). Prevalence of migraine in patients with celiac disease and inflammatory bowel disease. Headache 53, 344–355. doi: 10.1111/j.1526-4610.2012.02260.x
Do, T. P., Guo, S., and Ashina, M. (2019). Therapeutic novelties in migraine: new drugs, new hope? J. Headache Pain 20:37. doi: 10.1186/s10194-019-0974-3
Elsayed, S., and Zhang, K. (2004a). Bacteremia caused by Clostridium symbiosum. J. Clin. Microbiol. 42, 4390–4392. doi: 10.1128/JCM.42.9.4390-4392.2004
Elsayed, S., and Zhang, K. (2004b). Human infection caused by Clostridium hathewayi. Emerg Infect. Dis. 10, 1950–1952. doi: 10.3201/eid1011.040006
Engels, C., Ruscheweyh, H. J., Beerenwinkel, N., Lacroix, C., and Schwab, C. (2016). The common gut microbe Eubacterium hallii also contributes to intestinal propionate formation. Front. Microbiol. 7:713. doi: 10.3389/fmicb.2016.00713
Fang, C., Zhong, H., Lin, Y., Chen, B., Han, M., Ren, H., et al. (2018). Assessment of the cPAS-based BGISEQ-500 platform for metagenomic sequencing. Gigascience 7, 1–8. doi: 10.1093/gigascience/gix133
Finegold, S. M., Song, Y., Liu, C., Hecht, D. W., Summanen, P., Kononen, E., et al. (2005). Clostridium clostridioforme: a mixture of three clinically important species. Eur. J. Clin. Microbiol. Infect. Dis. 24, 319–324. doi: 10.1007/s10096-005-1334-6
Furdui, C., and Ragsdale, S. W. (2000). The role of pyruvate ferredoxin oxidoreductase in pyruvate synthesis during autotrophic growth by the Wood-Ljungdahl pathway. J. Biol. Chem. 275, 28494–28499. doi: 10.1074/jbc.M003291200
Gonzalez, A., Hyde, E., Sangwan, N., Gilbert, J. A., Viirre, E., and Knight, R. (2016). Migraines are correlated with higher levels of nitrate-, nitrite-, and nitric oxide-reducing oral microbes in the American Gut Project Cohort. mSystems 1:e00105–00116. doi: 10.1128/mSystems.00105-16
Gonzalez, P. S., O'Prey, J., Cardaci, S., Barthet, V. J. A., Sakamaki, J. I., Beaumatin, F., et al. (2018). Mannose impairs tumour growth and enhances chemotherapy. Nature 563, 719–723. doi: 10.1038/s41586-018-0729-3
Gross, E. C., Klement, R. J., Schoenen, J., D'Agostino, D. P., and Fischer, D. (2019). Potential protective mechanisms of ketone bodies in migraine prevention. Nutrients 11:E811. doi: 10.3390/nu1104081
Hall, A. B., Yassour, M., Sauk, J., Garner, A., Jiang, X., Arthur, T., et al. (2017). A novel Ruminococcus gnavus clade enriched in inflammatory bowel disease patients. Genome Med. 9:103. doi: 10.1186/s13073-017-0490-5
Hansen, S. G., Skov, M. N., and Justesen, U. S. (2013). Two cases of Ruminococcus gnavus bacteremia associated with diverticulitis. J. Clin. Microbiol. 51, 1334–1336. doi: 10.1128/JCM.03382-12
Hardham, J. M., King, K. W., Dreier, K., Wong, J., Strietzel, C., Eversole, R. R., et al. (2008). Transfer of Bacteroides splanchnicus to Odoribacter gen. nov. as Odoribacter splanchnicus comb. nov., and description of Odoribacter denticanis sp. nov., isolated from the crevicular spaces of canine periodontitis patients. Int. J. Syst. Evol. Microbiol. 58, 103–109. doi: 10.1099/ijs.0.63458-0
Heyes, M. P., Saito, K., Crowley, J. S., Davis, L. E., Demitrack, M. A., Der, M., et al. (1992). Quinolinic acid and kynurenine pathway metabolism in inflammatory and non-inflammatory neurological disease. Brain 115, 1249–1273. doi: 10.1093/brain/115.5.1249
Holdeman, L. V., and Moore, W. E. C. (1974). New genus, coprococcus, twelve new species, and emended descriptions of four previously described species of bacteria from human feces. Int. J. Syst. Evol. Microbiol. 24, 260–277. doi: 10.1099/00207713-24-2-260
Hua, Z. Y., Li, H. M., Sun, J. H., Huo, H. R., Li, X. Q., Huang, L. Q., et al. (2019). [Effect of fresh Gastrodia elata on gut microbiota in mice]. Zhongguo Zhong Yao Za Zhi 44, 1004–1009. doi: 10.19540/j.cnki.cjcmm.2019.0018
Jasmin, L., Wu, M. V., and Ohara, P. T. (2004). GABA puts a stop to pain. Curr. Drug Targets CNS Neurol. Disord. 3, 487–505. doi: 10.2174/1568007043336716
Kang, S., Ma, W., Li, F. Y., Zhang, Q., Niu, J., Ding, Y., et al. (2015). Functional redundancy instead of species redundancy determines community stability in a typical steppe of inner mongolia. PLoS ONE 10:e0145605. doi: 10.1371/journal.pone.0145605
Kathagen, A., Schulte, A., Balcke, G., Phillips, H. S., Martens, T., Matschke, J., et al. (2013). Hypoxia and oxygenation induce a metabolic switch between pentose phosphate pathway and glycolysis in glioma stem-like cells. Acta Neuropathol. 126, 763–780. doi: 10.1007/s00401-013-1173-y
Kelly, W. J., Leahy, S. C., Altermann, E., Yeoman, C. J., Dunne, J. C., Kong, Z., et al. (2010). The glycobiome of the rumen bacterium Butyrivibrio proteoclasticus B316(T) highlights adaptation to a polysaccharide-rich environment. PLoS ONE 5:e11942. doi: 10.1371/journal.pone.0011942
Lau, C. I., Lin, C. C., Chen, W. H., Wang, H. C., and Kao, C. H. (2014). Association between migraine and irritable bowel syndrome: a population-based retrospective cohort study. Eur. J. Neurol. 21, 1198–1204. doi: 10.1111/ene.12468
Lawson, P. A., and Finegold, S. M. (2015). Reclassification of Ruminococcus obeum as Blautia obeum comb. nov. Int. J. Syst. Evol. Microbiol. 65, 789–793. doi: 10.1099/ijs.0.000015
Li, J., Jia, H., Cai, X., Zhong, H., Feng, Q., Sunagawa, S., et al. (2014). An integrated catalog of reference genes in the human gut microbiome. Nat. Biotechnol. 32, 834–841. doi: 10.1038/nbt.2942
Liang, Q., Chiu, J., Chen, Y., Huang, Y., Higashimori, A., Fang, J., et al. (2017). Fecal bacteria act as novel biomarkers for noninvasive diagnosis of colorectal cancer. Clin. Cancer Res. 23, 2061–2070. doi: 10.1158/1078-0432.CCR-16-1599
Lipton, R. B., Buse, D. C., Hall, C. B., Tennen, H., Defreitas, T. A., Borkowski, T. M., et al. (2014). Reduction in perceived stress as a migraine trigger: testing the “let-down headache” hypothesis. Neurology 82, 1395–1401. doi: 10.1212/WNL.0000000000000332
Ma, P., Li, T., Ji, F., Wang, H., and Pang, J. (2015). Effect of GABA on blood pressure and blood dynamics of anesthetic rats. Int. J. Clin. Exp. Med. 8, 14296–14302.
Machiels, K., Joossens, M., Sabino, J., De Preter, V., Arijs, I., Eeckhaut, V., et al. (2014). A decrease of the butyrate-producing species Roseburia hominis and Faecalibacterium prausnitzii defines dysbiosis in patients with ulcerative colitis. Gut 63, 1275–1283. doi: 10.1136/gutjnl-2013-304833
Nazari, F., and Eghbali, M. (2012). Migraine and its relationship with dietary habits in women. Iran J. Nurs. Midwifery Res. 17(2 Suppl 1), S65–S71.
Ni, B. J., Liu, H., Nie, Y. Q., Zeng, R. J., Du, G. C., Chen, J., et al. (2011). Coupling glucose fermentation and homoacetogenesis for elevated acetate production: experimental and mathematical approaches. Biotechnol. Bioeng. 108, 345–353. doi: 10.1002/bit.22908
Olarescu, N. C., Gunawardane, K., Hansen, T. K., Moller, N., and Jorgensen, J. O. L. (2000). “Normal physiology of growth hormone in adults,” in Endotext, eds K. R. Feingold, B. Anawalt, A. Boyce, G. Chrousos, K. Dungan, A. Grossman, J. M. Hershman, G. Kaltsas, C. Koch, P. Kopp, M. Korbonits, R. McLachlan, J. E. Morley, M. New, L. Perreault, J. Purnell, R. Rebar, F. Singer, D.L. Trence, A. Vinik, and D. P. Wilson (South Dartmouth, MA).
Pequegnat, B., Sagermann, M., Valliani, M., Toh, M., Chow, H., Allen-Vercoe, E., et al. (2013). A vaccine and diagnostic target for Clostridium bolteae, an autism-associated bacterium. Vaccine 31, 2787–2790. doi: 10.1016/j.vaccine.2013.04.018
Rapkin, A. J., and Akopians, A. L. (2012). Pathophysiology of premenstrual syndrome and premenstrual dysphoric disorder. Menopause Int. 18, 52–59. doi: 10.1258/mi.2012.012014
Sacco, S., Ricci, S., Degan, D., and Carolei, A. (2012). Migraine in women: the role of hormones and their impact on vascular diseases. J Headache Pain 13, 177–189. doi: 10.1007/s10194-012-0424-y
Sakashita, M., Nakamura, U., Horie, N., Yokoyama, Y., Kim, M., and Fujita, S. (2019). Oral supplementation using gamma-aminobutyric acid and whey protein improves whole body fat-free mass in men after resistance training. J. Clin. Med. Res. 11, 428–434. doi: 10.14740/jocmr3817
Samuel, B. S., Hansen, E. E., Manchester, J. K., Coutinho, P. M., Henrissat, B., Fulton, R., et al. (2007). Genomic and metabolic adaptations of Methanobrevibacter smithii to the human gut. Proc. Natl. Acad. Sci. U.S.A. 104, 10643–10648. doi: 10.1073/pnas.0704189104
Scher, J. U., Sczesnak, A., Longman, R. S., Segata, N., Ubeda, C., Bielski, C., et al. (2013). Expansion of intestinal Prevotella copri correlates with enhanced susceptibility to arthritis. Elife 2:e01202. doi: 10.7554/eLife.01202.028
Schneider, H., and Blaut, M. (2000). Anaerobic degradation of flavonoids by Eubacterium ramulus. Arch. Microbiol. 173, 71–75. doi: 10.1007/s002030050010
Straube, A., Muller, H., Stiegelbauer, V., and Frauwallner, A. (2018). [Migraine prophylaxis with a probiotic. Results of an uncontrolled observational study with 1,020 patients]. MMW Fortschr Med. 160(Suppl. 5), 16–21. doi: 10.1007/s15006-018-1052-5
Su, H. S., Lang, B. F., and Newman, E. B. (1989). L-serine degradation in Escherichia coli K-12: cloning and sequencing of the sdaA gene. J. Bacteriol. 171, 5095–5102. doi: 10.1128/JB.171.9.5095-5102.1989
Truong, D. T., Franzosa, E. A., Tickle, T. L., Scholz, M., Weingart, G., Pasolli, E., et al. (2015). MetaPhlAn2 for enhanced metagenomic taxonomic profiling. Nat. Methods 12, 902–903. doi: 10.1038/nmeth.3589
Udayappan, S., Manneras-Holm, L., Chaplin-Scott, A., Belzer, C., Herrema, H., Dallinga-Thie, G. M., et al. (2016). Oral treatment with Eubacterium hallii improves insulin sensitivity in db/db mice. NPJ Biofilms Microbiomes 2:16009. doi: 10.1038/npjbiofilms.2016.9
Ulrich, V., Gervil, M., Kyvik, K. O., Olesen, J., and Russell, M. B. (1999). The inheritance of migraine with aura estimated by means of structural equation modelling. J. Med. Genet. 36, 225–227.
Vacca, V. M. Jr. (2019). Migraine in adults: a head start. Nurs. Manage. 49, 22–29. doi: 10.1097/01.NURSE.0000554607.72406.6e
Valles-Colomer, M., Falony, G., Darzi, Y., Tigchelaar, E. F., Wang, J., Tito, R. Y., et al. (2019). The neuroactive potential of the human gut microbiota in quality of life and depression. Nat. Microbiol. 4, 623–632. doi: 10.1038/s41564-018-0337-x
Vieira-Silva, S., Falony, G., Darzi, Y., Lima-Mendez, G., Garcia Yunta, R., Okuda, S., et al. (2016). Species-function relationships shape ecological properties of the human gut microbiome. Nat. Microbiol. 1:16088. doi: 10.1038/nmicrobiol.2016.88
Wang, K., Pereira, G. V., Cavalcante, J. J., Zhang, M., Mackie, R., and Cann, I. (2016). Bacteroides intestinalis DSM 17393, a member of the human colonic microbiome, upregulates multiple endoxylanases during growth on xylan. Sci. Rep. 6:34360. doi: 10.1038/srep34360
Wang, L., Hu, L., Xu, Q., Yin, B., Fang, D., Wang, G., et al. (2017). Bifidobacterium adolescentis exerts strain-specific effects on constipation induced by loperamide in BALB/c mice. Int. J. Mol. Sci. 18:318. doi: 10.3390/ijms18020318
Watanabe, Y., Nagai, F., Morotomi, M., Sakon, H., and Tanaka, R. (2010). Bacteroides clarus sp. nov., Bacteroides fluxus sp. nov. and Bacteroides oleiciplenus sp. nov., isolated from human faeces. Int. J. Syst. Evol. Microbiol. 60, 1864–1869. doi: 10.1099/ijs.0.015107-0
Wexler, H. M., Reeves, D., Summanen, P. H., Molitoris, E., McTeague, M., Duncan, J., et al. (1996). Sutterella wadsworthensis gen. nov., sp. nov., bile-resistant microaerophilic Campylobacter gracilis-like clinical isolates. Int. J. Syst. Bacteriol. 46, 252–258. doi: 10.1099/00207713-46-1-252
Wong, D., Aoki, F., and Rubinstein, E. (2014). Bacteremia caused by Eggerthella lenta in an elderly man with a gastrointestinal malignancy: a case report. Can. J. Infect. Dis. Med. Microbiol. 25, e85–e86. doi: 10.1155/2014/802481
Xie, H., Guo, R., Zhong, H., Feng, Q., Lan, Z., Qin, B., et al. (2016). Shotgun metagenomics of 250 adult twins reveals genetic and environmental impacts on the gut microbiome. Cell Syst. 3, 572–584 e573. doi: 10.1016/j.cels.2016.10.004
Young, S. N., and Shalchi, M. (2005). The effect of methionine and S-adenosylmethionine on S-adenosylmethionine levels in the rat brain. J. Psychiatry Neurosci. 30, 44–48.
Keywords: migraine, gut microbiota, elderly women, metagenome-wide association study, structural characterization, functional modules
Citation: Chen J, Wang Q, Wang A and Lin Z (2020) Structural and Functional Characterization of the Gut Microbiota in Elderly Women With Migraine. Front. Cell. Infect. Microbiol. 9:470. doi: 10.3389/fcimb.2019.00470
Received: 09 June 2019; Accepted: 20 December 2019;
Published: 29 January 2020.
Edited by:
Elisa L. Hill-Yardin, RMIT University, AustraliaReviewed by:
Jennifer Louise Wood, Swinburne University of Technology, AustraliaSteve Petrovski, La Trobe University, Australia
Copyright © 2020 Chen, Wang, Wang and Lin. This is an open-access article distributed under the terms of the Creative Commons Attribution License (CC BY). The use, distribution or reproduction in other forums is permitted, provided the original author(s) and the copyright owner(s) are credited and that the original publication in this journal is cited, in accordance with accepted academic practice. No use, distribution or reproduction is permitted which does not comply with these terms.
*Correspondence: Qi Wang, d2FuZ3FpMUBnZW5vbWljcy5jbg==; Zhanglin Lin, emhhbmdsaW5saW5Ac2N1dC5lZHUuY24=
†These authors have contributed equally to this work