- Key Laboratory of Animal Parasitology of Ministry of Agriculture, Shanghai Veterinary Research Institute, Chinese Academy of Agricultural Sciences, Shanghai, China
Extracellular vesicles (EVs), are considered as vehicles of cellular communication. Parasites usually release EVs in their excretory-secretory products to modulate host environment. However, little is known about the secretion of EVs by ticks. In this study, we show for the first time that the tick Haemaphysalis longicornis secretes EVs in saliva that resembles exosomes. EVs were purified from pilocarpine induced saliva of partially engorged H. longicornis ticks. Electron microscopy analysis revealed the presence of exosome-like vesicles with a size of 100 nm. Proteomic analysis by LC-MS/MS identified a total of 356 proteins in tick-derived EVs. Proteome data of tick-derived EVs was validated by Western blot analysis. Immunodetection of Hsp70 and GAPDH proteins indicated that the proteomics data of tick-derived EVs were highly reliable. Bioinformatics analysis (Gene Ontology) indicated association of certain biological and molecular functions with proteins which may be helpful during tick development. Likewise, KEGG database revealed involvement of vesicular proteins in proton transport, detoxification, ECM-receptor interaction, ribosome, RNA transport, ABC transporters, and oxidative phosphorylation. The results of this study provide evidence that EVs are being secreted in tick saliva and suggest that tick saliva-derived EVs could play important roles in host-parasite relationships. Moreover, EVs could be a useful tool in development of vaccines or therapeutics against ticks.
Introduction
Haemaphysalis longicornis, also known as bush tick or Asian longhorned tick, belongs to the tick family Ixodidae. Although this tick is native to China, Russia, and Japan, it is now established in Pacific islands including New Zealand, Australia, and the eastern states of the USA (Heath, 2016; Berenbaum, 2018). Haemaphysalis longicornis is an important vector of human disease-causing agents such as thrombocytopenia syndrome virus, Rickettsia japonica, Ehrlichia chaffeensis, Babesia microti, and Anaplasma bovis (Mahara, 1997; Luo et al., 2015; Wu et al., 2017). Likewise, H. longicornis transmits theileriosis to cattle, thereby causing considerable blood loss and death of calves (Heath, 2016). Meanwhile, 25% reduction in dairy products has been observed in Australia and New Zealand. Parthenogenetic reproduction of H. longicornis allows a single female to generate progeny without mating, resulting in massive host infestations (Heath, 2016).
Extracellular vesicles (EVs) are small membrane vesicles derived from the endocytic compartment of cells. EVs have emerged as key players in intercellular communication and can be divided into exosomes, microvesicles, apoptotic bodies, and oncosomes (Devhare and Ray, 2018). Exosomes, formed by the fusion of multivesicular bodies (MVBs) were discovered in 1983 in reticulocytes (Harding et al., 2013). They were first considered as garbage bins being used by cells to discard their waste products (Johnstone, 1992). In some studies, these nano-sized vesicles were also considered as apoptotic blebs, cellular debris, or signs of cell death (Bobrie et al., 2011; Pant et al., 2012). However, the discovery of exosomes as carriers of genetic material (proteins, lipids and miRNAs) and their involvement in cellular communication has opened new horizons. Interaction of exosomes with target cells by binding with the receptors of other cells, fusion with their membranes and releasing their contents into the cytosol of target cells have made them powerful agents of cellular communication (Mathivanan et al., 2010). In addition, exosomes can even be used as vaccine candidates and biomarkers for the diagnosis and treatment of diseases (Zhang et al., 2018).
Parasites use excretory-secretory products to communicate with their host environment. Discovery of EVs within the excretory-secretory products of parasites such as Fasciola hepatica, Echinostoma caproni, Heligmosomoides polygyrus, Dicrocoelium dendriticum, Schistosoma mansoni, Trichuris muris, Echinococcus granulosus, Leishmania amazonensis, Trichomonas vaginalis, Plasmodium vivax, and Trypnosoma cruzi has gained considerable interest over the last few years (Marcilla et al., 2014; Coakley et al., 2015; Barbosa et al., 2018; Eichenberger et al., 2018; Gualdrón-López et al., 2018; Nicolao et al., 2019; RAI and Johnson, 2019). EVs can be purified form secretory products by series of steps by centrifugation, ultracentrifugation, precipitation kits, ExoChip, immunoprecipitation, acoustic nanofilter, size exclusion chromatography (SEC) column purification, and sucrose density gradient techniques (Raposo et al., 1996; Tauro et al., 2012; Kanwar et al., 2014; Lee et al., 2015; Zeringer et al., 2015; Wu et al., 2019). EVs can play a decisive role in parasite-host interactions by transferring their inner contents (virulence factors and effector molecules) from parasites to hosts (Wu et al., 2019). The content of these vesicles consists of a variety of proteins, miRNAs and lipids. Among miRNAs, high abundance of miR-71 and miR-72 has been observed in parasites suggesting their involvement in embryo development, growth, and metabolism of parasite (Chen et al., 2011; Cai et al., 2013). Furthermore, single-stranded DNA, mitochondrial DNA, double-stranded DNA and oncogene amplifications have been identified in microvesicles (Balaj et al., 2011; Thakur et al., 2014). Similarly, proteins like GAPDH, enolase, and Hsp70, usually involved in parasite survival, reproduction, and growth, have been associated within the parasite-derived EVs (Sotillo et al., 2008, 2010). In addition to miRNAs and proteins, lipids are critical components of EVs. Exosomes-like vesicles are highly enriched in an array of lipid species, including sphingomyelin, glycosphingolipids, cholesterol, and ceramide (Skotland et al., 2017; Brzozowski et al., 2018; Chen et al., 2019; Sun et al., 2019). Uptake of lipid contents by the parasites helps them to develop protective mechanisms against host immunity, support parasitic survival, and promote growth (Yesuf and Kenubih, 2019). Therefore, identification of lipid enriched EVs led us to speculate that parasite-derived EVs could provide a mechanism to modulate hosts immune responses.
EVs have been isolated from excretory-secretory products of some parasites but, to the best of our knowledge, ticks have not been studied. Here, we report for the first time that the saliva of the tick H. longicornis secreted exosome-like vesicles. Proteomics analysis of tick-derived EVs revealed the presence of some significant proteins such as GAPDH, heat shock proteins, thioredoxin peroxidase and proteases, which may be used by ticks in modulating host-parasite interactions.
Methods
Ethics Approval and Consent to Participate
All experiments carried out during the study were approved by the Institutional Animal Care and Use Committee of Shanghai Veterinary Research Institute (IACUC No: SHVRI-SOP-1104-003). Rabbits were maintained at the animal house (SHVRI) under normal conditions of regulated temperature (22°C) and light with free access to feed and water. Rabbits were kept in cages in compliance with the guidelines on the Humane Treatment of Laboratory Animals (Ministry of Science and Technology of the People's Republic of China).
Ticks
A colony of H. longicornis ticks (parthenogenetic strain) was collected from Shanghai Wildlife Park, China. The tick colony was established after maintenance of three generations in the Shanghai Veterinary Research Institute, Chinese Academy of Agricultural Sciences, Shanghai, China. After feeding the ticks on New Zealand white rabbits, rearing was done in the laboratory at 25°C (92% humidity) in a dark incubator (Mulenga et al., 1999; Zhou et al., 2006). Finally, the parthenogenetic ticks were used for the collection of saliva.
Collection of Saliva
For infestation, 40 adult ticks were attached per rabbit ear and maintained with the help of ear bags made of cotton cloth. The ear bags were held onto the rabbit ears with the help of surgical stitches and adhesive tape. After attachment, rabbits were placed in cages made of steel. A total of 55 female rabbits (4 months old) were used in this study. After feeding for 4 days, ticks were removed and saliva was collected as previously described (Patton et al., 2012). After washing with sterile distilled water, ticks were attached to glass slides with adhesive tape. Pilocarpine was injected (0.5–1 μl) posterior to fourth coxae in the region of epidermal and anal plates of the tick. Ticks were placed at 37°C in 85% humidity chamber. Saliva was collected with pipette tip after an interval of 20 min. Saliva collected from partially fed ticks was mixed with equal quantity of PBS and stored at −80°C.
Isolation of EVS
For isolation of EVs from saliva of H. longicornis, a protocol described by Abdi et al. (2017) was followed with slight modifications (Supplementary Figure 1). Briefly, saliva was mixed with an equal amount of PBS and centrifuged at 2,600 g for 30 min at 4°C to remove cellular debris. Cell free medium (supernatant) was filtered through 0.22 μm filter (Merck Millipore) to remove contaminating apoptotic bodies and cell debris. Supernatant was centrifuged at 140,000 g for 3 h at 4°C in OptimaTM L-100 XP ultracentrifuge (Beckman Coulter) using an SW 60 (44.5) rotor. Supernatant was removed carefully and pellet was collected. Pellet was washed twice by re-suspending in cold PBS and centrifuging at 150,000 g for 2 h after each wash. A discontinuous gradient was prepared by diluting a stock solution of OptiPrep™ (60% w/v) with 0.25 M sucrose/6 mM EDTA, 60 mM Tris (pH 7.4). The gradient was formed by layering 40, 20, 10, and 5% gradient solutions on top of each other into 4 ml open top thin wall polyallomer (Beckman Coulter). Pellet was loaded on top of gradient and centrifuged at 250,000 g for 18 h. One milliliter fractions were collected from top of the gradient and transferred to 1.5 ml Eppendorf tubes. Weights of the tubes were measured to estimate the density of purified vesicles. A total of 5 fractions were collected, each fraction was diluted in PBS to 4 ml and centrifuged at 150,000 g for 2 h. Resulting pellet was collected and stored at −80°C. Confirmation of EVs within the saliva samples was accessed by electron microscopy.
Electron Microscopy
The pellet was analyzed by electron microscopy at Shanghai Veterinary Research Institute, China. EV sample was fixed 1:1 with 2% glutaraldehyde. A 200 mesh copper grid with carbon-coated formvar film (Agar Scientific, Essex, UK) was incubated onto 5 μL of fixed sample for 30 min. Excess liquid was removed by blotting and grids were allowed to dry at room temperature. Grids were washed with water and stained with phosphotungstic acid for 1 min. After staining, grids were washed with ethanol (70%) followed by four washes with molecular grade water. Finally, the grids were loaded onto a sample holder of a transmission electron microscope (FEI T12 equipped with AMT XR51 CCD camera system) and exposed to 80 kV electron beam for image capture.
SDS-PAGE Analysis and Western Blot
Purified EVs were homogenized with lysis buffer (4% SDS, 1 mM DTT, 150 mM Tris-HCl pH 8.0, protease inhibitor). After 3 min incubation in boiling water, the homogenate was sonicated on ice. The crude extract was then incubated in boiling water again and clarified by centrifugation at 16,000 × g at 25°C for 10 min. The concentration of proteins was determined using a Micro BCA Protein Assay Kit (Thermo Fisher Scientific, Rockford, IL, USA) following the manufacturer's specifications and using BSA (Thermo Fisher Scientific) as a standard. Five micrograms of protein per lane were processed by SDS-PAGE (12% polyacrylamide linear gradient gels; Bio-Rad Laboratories, Hercules, CA, USA) and stained with Coomassie Brilliant blue R-250. Gels were scanned using a Bio-Rad Molecular Imager FX system (Bio-Rad Laboratories).
For western blot analysis, 7 μg of proteins were subjected to 12% SDS-PAGE. It was followed by the transfer of proteins to polyvinylidene fluoride (PVDF) membranes. The membranes were blocked with 5% skim milk diluted in PBS/0.05% Tween (PBST) for 2 h at 37°C. Membranes were washed three times (5 min/washing) with PBST. Blots were incubated overnight at 4°C in rabbit anti-Hsp70 antibody diluted to 1:1,000 in PBST (cat. no. ab79852; Abcam) and rabbit anti-GAPDH antibody diluted to 1:1,000 in PBST (cat. no. ab37168; Abcam). Blotted membranes were washed three times with PBST (10 min/washing) and incubated in the presence of goat anti-rabbit IgG antibody (horseradish peroxidase-conjugated; dilution, 1:2,000; Bethyl Laboratories, Inc., USA) for 1 h at 37°C. Washing with PBST (10 min/washing) was done before visualization of bands. Protein signals were detected with an Enhanced Chemiluminescent Substrate Reagent Kit (NCM Biotech, Sunzhou, China) and were visualized under a Tanon-5200 Chemiluminescent Imaging System (Tanon Science and Technology, Shanghai, China).
Liquid Chromatography Mass Spectrometry (LC-MS/MS)
Purified samples (EVs) dissolved in PBS were diluted in 30 μl SDT buffer (4% SDS, 100 mM DTT, 150 mM Tris-HCl pH 8.0) and boiled for 5 min. The detergents (DTT and other low-molecular-weight components) were removed using 200 μl UA buffer (8 M Urea, 150 mM Tris-HCl pH 8.0) by repeated centrifugation (14,000 × g for 15 min). After centrifugation, the concentrates were mixed with iodoacetamide (IAA, 50 mM IAA in UA) and incubated in darkness for 30 min at room temperature. After 15 min centrifugation, filters were washed three times with 100 μL UA buffer and then 100 μL of dissolution buffer (50 mM triethylammonium bicarbonate, pH 8.5) twice. The tryptic peptides resulting from the digestion were extracted with 0.1% formic acid in 60% acetonitrile. Protein suspension was digested with 2 μg trypsin (Promega, Madison, USA) in 40 μL 25 mM NH4HCO3 overnight at 37°C. The extracts were pooled and completely dried using a vacuum centrifuge.
For protein identification, liquid chromatography-mass spectroscopy assay (LC-MS/MS, Thermo Fisher Scientific) was performed using Q Exactive™ Plus Hybrid Quadrupole-Orbitrap™ Mass Spectrometer coupled on Easy NLC system 1000 (Thermo Fisher Scientific). Five micrograms of proteins were used by LC-MS/MS. Trypsin digested peptides were desalted on Zorbax 300SB-C18 peptide traps (Agilent Technologies, Wilmington, DE, USA) and separated on a C18-reversed phase column (0.15 × 150 mm, Column Technology Inc., Fremont, CA, USA). Mobile phases A (0.1% formic acid in HPLC-grade water) and B (0.1% formic acid in 84% acetonitrile) were delivered using an Easy nLC system (Thermo Fisher Scientific) with a linear gradient of 4–50% B (50 min), 50–100% B (4 min), and 100% B (6 min) at a flow rate of 250 nl/min. A data-dependent method, based on 10 most abundant precursor ions for HCD fragmentation was used to acquire mass spectrometry data. For survey scans (m/z 300–1,800), the target value was determined based on predictive Automatic Gain Control at a resolution of 70,000 at m/z 200 and dynamic exclusion duration of 25 s. The resolution set for the HCD spectra was 17,500 at m/z 200. Normalized collision energy was 27 eV and the under-fill ratio was set as 0.1%.
For in gel protein identification, Ettan™ MDLC controlled by UNICORN™ software (GE Healthcare), was used for desalting and separation of peptides. Peptide mixtures were desalted on RP trap columns and then separated on a C18-reversed phase column. Mobile phase A (0.1% formic acid in HPLC-grade water) and mobile phase B (0.1% formic acid in 84% acetonitrile) were selected. Tryptic peptide mixtures were loaded onto the columns, and separation was done at a flow rate of 2 μL/min by using the linear gradient buffer B described above. LTQ Velos (Thermo Scientific) equipped with a micro-spray interface was connected to the LC setup for eluted peptides detection. Data-dependent MS/MS spectra were obtained simultaneously. Each scan cycle consisted of one full scan mass spectrum (m/z 300–1,800) followed by 20 MS/MS events of the most intense ions with the following dynamic exclusion settings: repeat count 2, repeat duration 30 s, exclusion duration 90 s.
Database Searching and Protein Identification
MaxQuant software (version 1.3.0.5.) suite was used to process Raw MS files. Peak list files were analyzed using Mascot search algorithm (Matrix Science Matrix Science, London, UK; version 2.2) against the UniProtKB Ixodoidea database (downloaded at July 06, 2018, with 190,922 entries) containing both forward and reverse protein sequences. The search parameters were: trypsin enzyme; two missed cleavages; a fragment ion mass tolerance of 0.10 Da; mascot score >20 and peptide tolerance of 20 ppm. Carbamidomethyl of cysteine was specified in Mascot as a fixed modification whereas oxidation of methionine was specified as variable modification. Proteins with at least two peptide (false discovery rate < 0.01) uniquely assigned to the respective sequence were considered.
Gene ontology (GO) is a universally acknowledged functional enrichment database and is generally used to search for enriched GO terms. Differently expressed proteins were classified into GO annotations according to molecular function, biological process, and cellular component. Additionally, KEGG database was mapped to analyse the pathways of the proteins involved.
Statistical Analysis
Right-tailed Fisher's exact test was used to access Gene enrichment of three ontologies (biological processes, cell components, and molecular functions) and KEGG pathway enrichment analysis. GO analyses were performed using DAVID (v6.8; https://david.ncifcrf.gov/) and Cytoscape online software (https://cytoscape.org/) (Shannon et al., 2003; Sherman and Lempicki, 2009; Xing et al., 2016). Unpaired t-tests were used to perform all statistical analyses. P < 0.05 was considered to indicate significant differences. All assays were performed in three replicates.
Results
Haemaphysalis longicornis Saliva Produce Exosome-Like Vesicles
Saliva was collected from partially fed (4 days post-feeding) H. longicornis after injecting with pilocarpine as shown in Figures 1A,B. A total of 2,500 μl of saliva was collected from ~4,000 ticks. EVs, isolated from saliva were observed by electron microscopy. Vesicles appeared within saliva were having a size of 100 nm, which is usually the range of exosomes (Figure 2). Exosomes appeared as typical spherical structures released into the saliva, as previously observed in the case of other parasites (Samoil et al., 2018). To ascertain whether these vesicles were also present in supernatants after ultracentrifugation, we next carried out purification of these structures from supernatants using TEM to visualize them. However, structures resembling EVs were not found in supernatant.
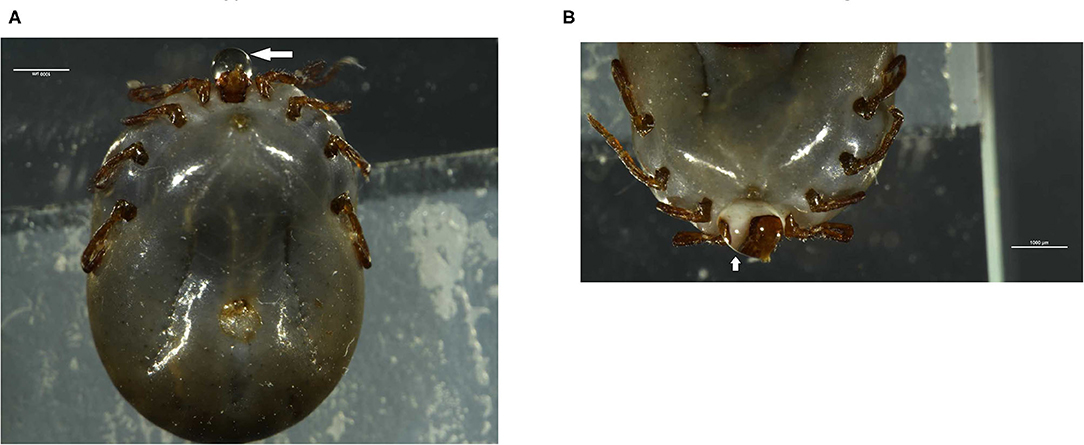
Figure 1. Collection of saliva from partially engorged H. longicornis ticks. (A,B) Ticks were injected with pilocarpine and 0.5–1 μl of saliva was collected. Arrows indicate the saliva secreted by ticks.
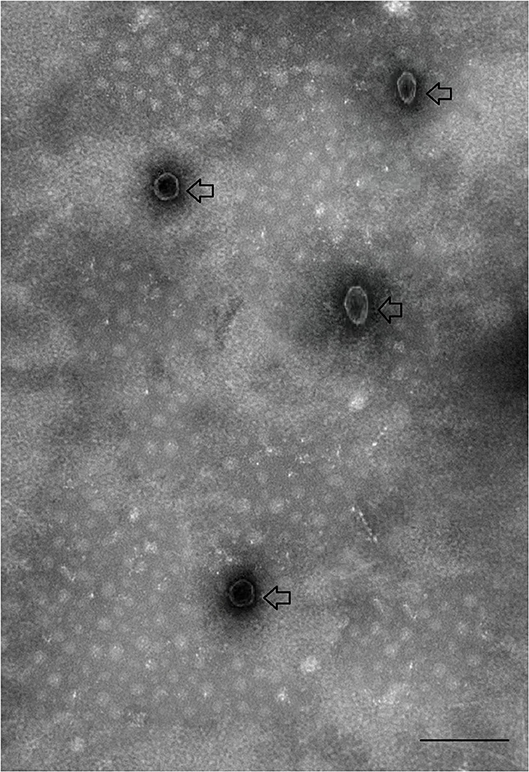
Figure 2. Transmission electron micrograph of exosomes-like vesicles derived from tick saliva. Arrows indicate tick-derived EVs. Scale-bar: 100 nm.
Tick EVs Contain Proteins
Proteomics (LC-MS/MS) was carried out to identify proteins present within purified EVs. SDS-PAGE followed by Coomassie blue staining indicated an enrichment of specific proteins (Figure 3A). Protein identification using MASCOT confirmed the presence of 356 proteins (Supplementary Table 1). Most of the proteins like nuclear proteins (elongation factors and histones), cytoskeletal proteins (actin, tubulin), and stress-related proteins (HSPs) have been identified in previous studies. Proteins have been classified in groups based on function and/or protein families (Table 1). In addition, EVs isolated from tick saliva were found to be enriched with host proteins. MASCOT searches identified the presence of 225 host proteins mainly corresponding to immunoglobulins, histones as well as metabolic proteins (Supplementary Table 2). However, deep analysis revealed slight differences (peptide counts and cover percent) between host proteins and vesicular proteins, e.g., peptide counts for GAPDH from host proteins were 3 while only 2 counts were observed for EVs-derived GAPDH. Similar differences were also observed in other proteins like histones. The presence of host-derived proteins suggests that tick EVs are involved in host-parasite relationships.
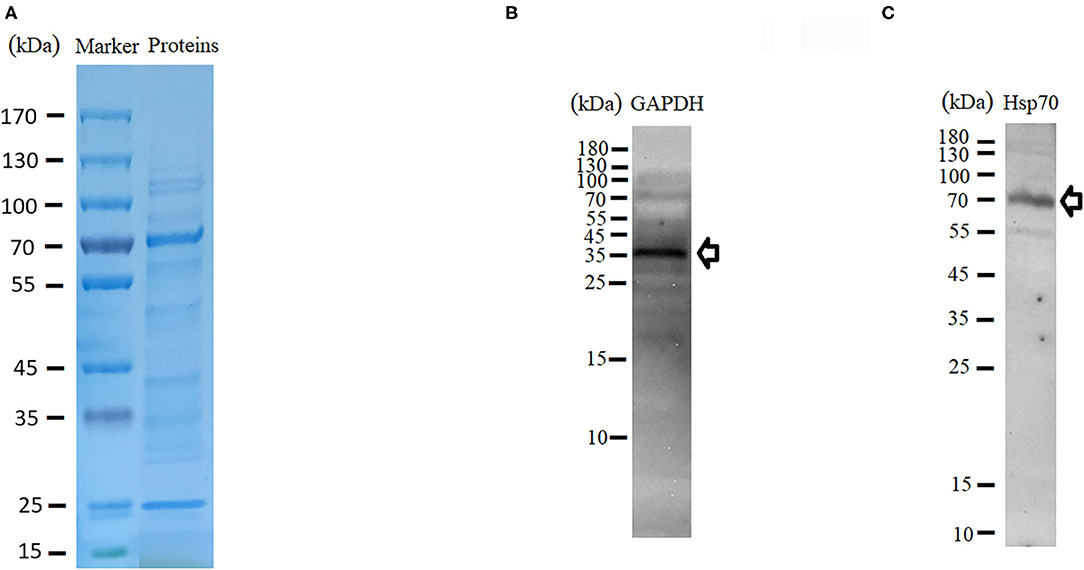
Figure 3. Characterization of protein contents of tick-derived EVs. (A) SDS-PAGE and Coomassie blue stained. (B,C) Validation of GAPDH and Hsp70 through Western blot.
In order to validate proteome data of tick-derived EVs, the supernatant collected during centrifugation was also examined for the presence of proteins within it. LC-MS/MS identified 490 proteins within supernatant, which may be considered as tick saliva proteins (Supplementary Table 3). Presence of these proteins within supernatant suggests that the proteins within pellet are specific to tick EVs. Likewise, western blot analysis was carried out to get further confirmation. For this purpose, HSP70 and GAPDH proteins were selected. Immunodetection of proteins resulted in thick bands of 36 and 70 kDa. The predicted size of GAPDH is 35.6–36 kDa, which matches the observed band (Figure 3B). Similarly, the band observed at 70 kDa matches to Hsp70 (Figure 3C). Validation of the proteins by Western blot indicated that the proteomics data of tick-derived EVs were highly reliable.
Gene ontology (GO) analysis of differentially expressed proteins showed significant enrichment for 142 proteins associated with “Biological process,” 73 associated with “Cellular components,” and 143 associated with “Molecular function” (Figures 4A,B, Supplementary Table 4). In terms of biological process, the P-value (P < 0.05) indicated that ATP hydrolysis coupled proton transport, energy coupled proton transmembrane transport, cellular oxidant detoxification, detoxification, and cellular detoxification were more significant (Figures 5A,B, Supplementary Figure 2A). Analysis of “Cellular components” indicated that most of the proteins were associated with the “cell” category. In addition, further subdivisions revealed that differentially expressed proteins were involved in intracellular parts, cytoplasm, proton-transporting two-sector ATPase complex, and cytoskeleton (Figures 6A,B, Supplementary Figure 2B). Significant “Molecular functions” associated with vesicular proteins were antioxidant activity, peroxidase activity, hydrolase activity, pyrophosphatase activity, and ATPase activity (Figures 7A,B, Supplementary Figure 2C).
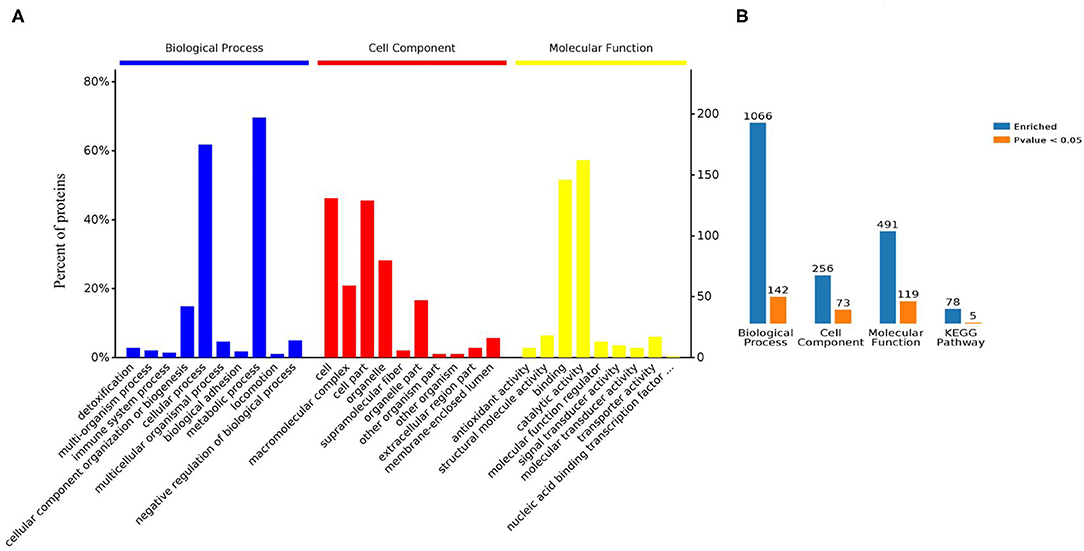
Figure 4. Bioinformatic analyses of the proteins derived from tick EVs. (A) Enrichment analysis by biological process, cell component, and molecular function. (B) Enriched GO terms and KEGG pathways analysis of differently expressed proteins: blue: enriched; orange: significantly enriched.
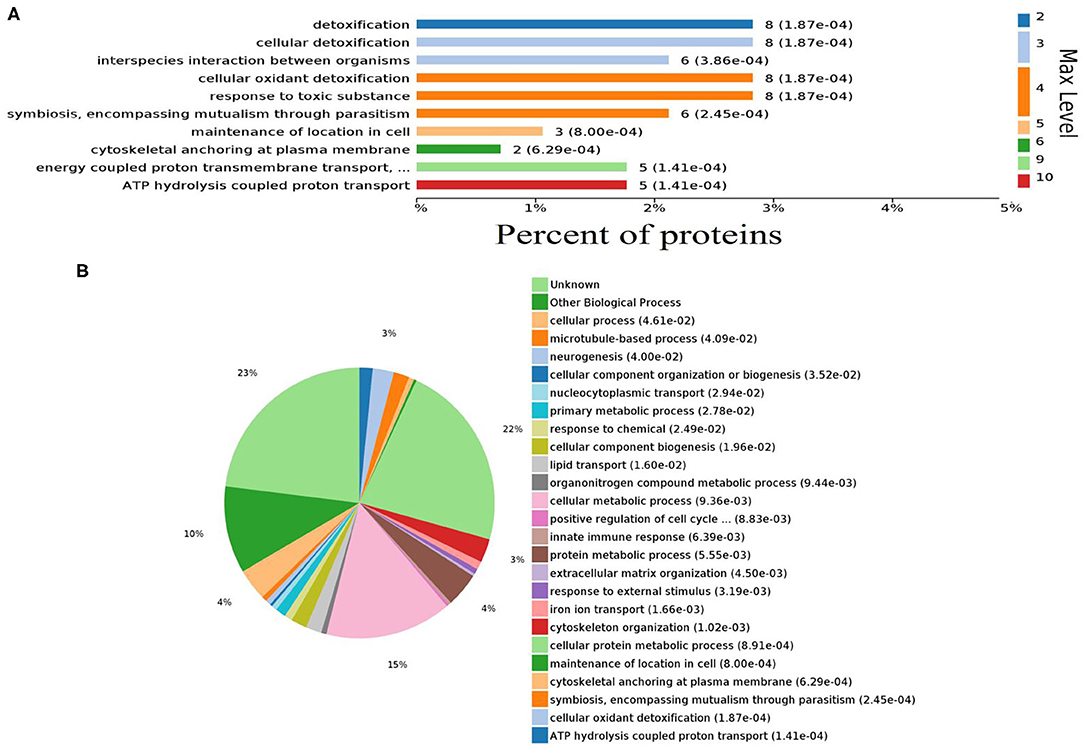
Figure 5. Abundantly represented gene ontology biological process terms. (A) Bar chart showing the percentage of proteins involved in biological process. (B) Pie chart distribution of enriched biological processes.
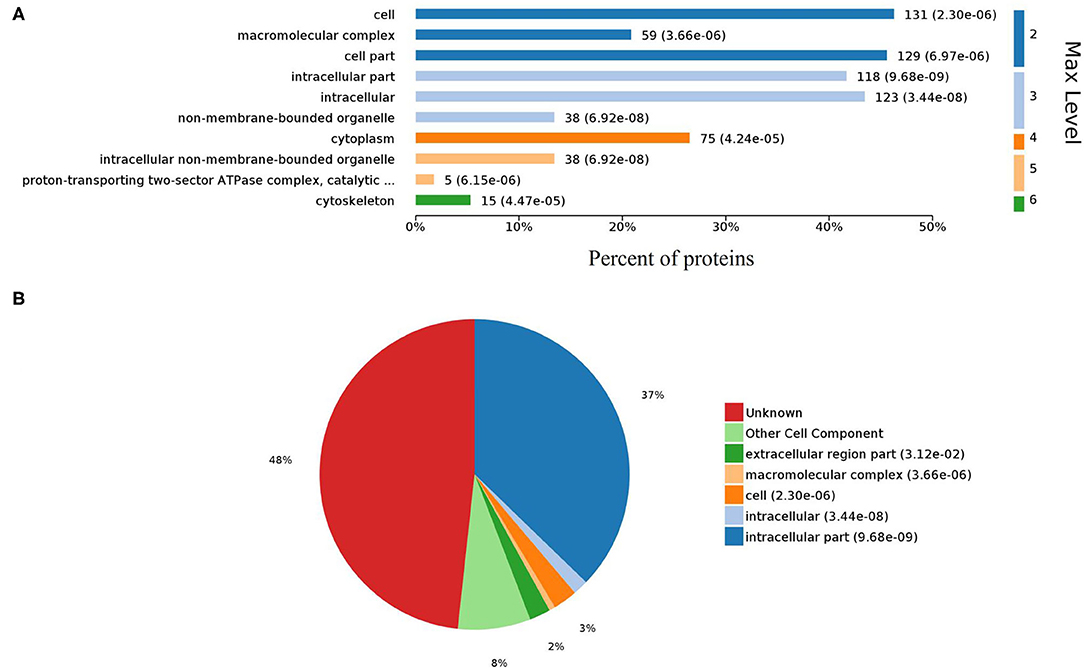
Figure 6. Gene Ontology analysis of cellular components. (A) Bar chart representing the levels of cell components. (B) Pie chart showing the classification of cell components.
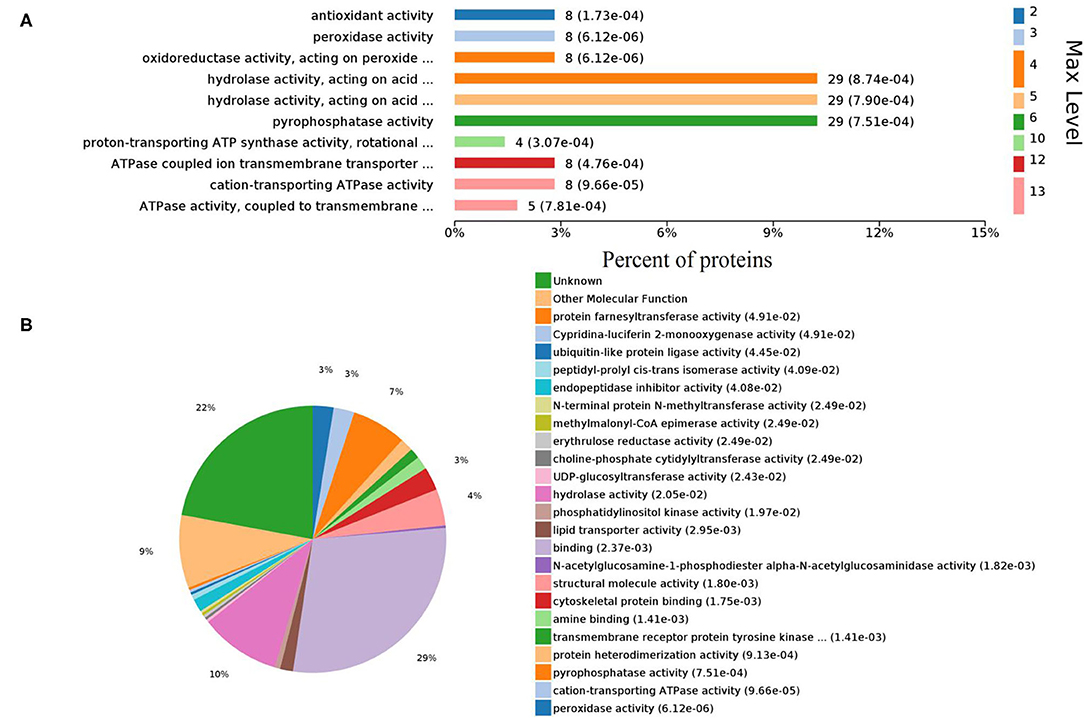
Figure 7. Enriched GO terms of molecular functions. (A) Different levels of molecular functions. (B) Pie chart denoting the distribution summary of molecular functions.
The KEGG database is a collection of various pathways, representing the molecular interactions and reaction networks. To identify the pathways involved, we mapped the KEGG database and found that identified proteins were enriched in 78 pathways (Figure 8A, Supplementary Table 5). Further analysis of the P-values (P < 0.05: Figures 8B,C) revealed that differentially expressed proteins were mainly involved in ECM-receptor interaction, ribosome, RNA transport, ABC transporters, and oxidative phosphorylation.
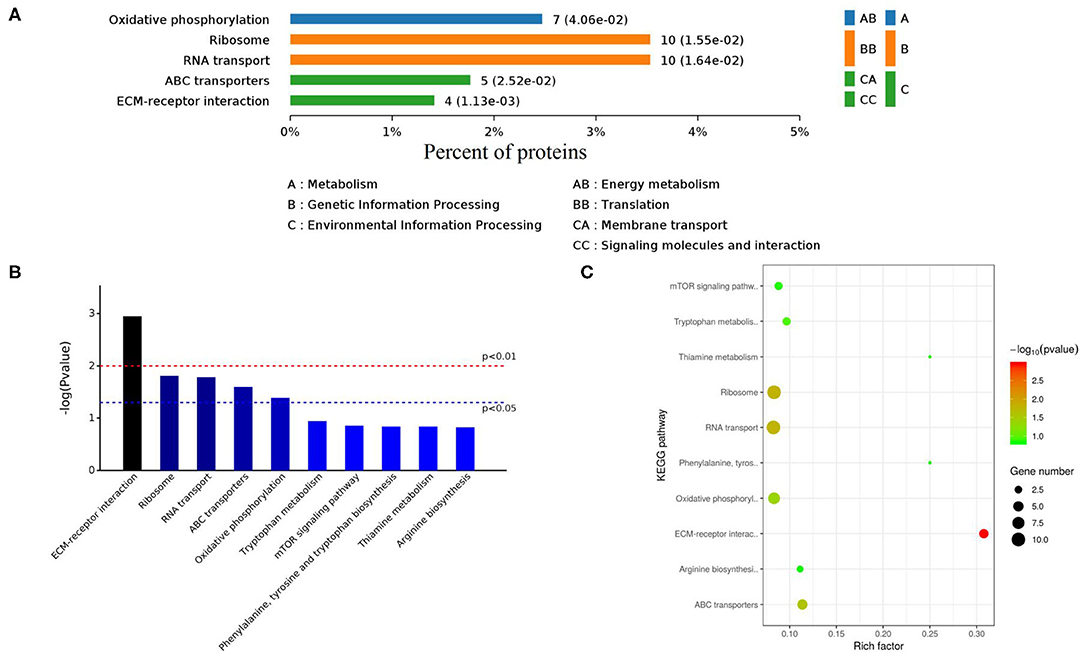
Figure 8. KEGG enrichment pathways of differently expressed proteins. (A) Classes of enriched KEGG pathways. (B) Distribution of KEGG pathways (Top 10). (C) Scattered plot of differently expressed proteins. X-axis indicates the rich factor; Y-axis indicates the name of KEGG pathway. The dot size means the protein number and dot color indicates the q-value.
Discussion
EVs are known to transfer intracellular information from one cell or tissue to other (Nawaz et al., 2019; Wu et al., 2019). The information (proteins, miRNAs) transferred by EVs are thought to mediate cellular activity and pathways in recipient cells (Zhu et al., 2016). Recent studies revealed that parasites such as Schistosoma japonicum, Leshmania infantum, Toxoplasma gondii, and Fasciola hepatica release EVs in their excretory-secretory products (Cwiklinski et al., 2015; Zhu et al., 2016; Li et al., 2018; Marshall et al., 2018). In the context of ticks, Zhou et al. (2018) demonstrated that tick embryonic cell line Ixodes scapularis ISE6 is also capable of secreting extracellular vesicles including exosomes. However, it remained unknown whether ticks such as H. longicornis, a major agent causing severe pathology of theileriosis, could secrete vesicles in saliva as well. Here, we isolated vesicles from saliva of H. longicornis. Transmission electron microscopy was carried out to examine EVs. Consistent with previous reports, we demonstrated that H. longicornis-derived EVs are similar in size (100 nm) and shape to other parasite-derived exosomes.
LC-MS/MS showed a wide variety of proteins within tick-derived EVs. The major protein groups included structural proteins, metabolic proteins, nuclear proteins, transporters, enzymes, and some proteins for which no homologs (Table 1). Structural/cytoskeletal proteins such as myosin, tropomyosin, actin, and tubulin alpha chain found in our study have been recorded with high scores previously in parasite-derived EVs such as B. malayi, S. japonicum and L. infantum (Atayde et al., 2015; Zamanian et al., 2015; Zhu et al., 2016). Actin, representing 1.1% of proteins identified, has been known to play immunogenic role in Echinostoma caproni (Sotillo et al., 2010). Likewise, myosin proteins (1.7%) localized beneath the plasma membrane exhibit biophysical properties required to generate fast movements in parasites such as T. gondii and other apicomplexans (Sibley et al., 1998; Herm-Götz et al., 2002). The presence of structural proteins suggests that they may be associated with the production of vesicles (Wubbolts et al., 2003).
The vitellogenin group of proteins, representing 4.5% of proteins identified in our study, have not been previously identified in EVs of other parasites. Vitellogenin (Vg), also considered as female-specific protein, is synthesized as a high molecular-mass precursor in ovaries, gut, and fat body of ixodid ticks (Rosell and Coons, 1992; Thompson et al., 2007; Boldbaatar et al., 2010). After its release into the haemolymph, Vg is taken up by oocytes through receptor mediated endocytosis, and is then accumulated in yolk granules.Vg is considered as a source for embryo development and egg formation during tick reproduction (Xavier et al., 2018). Antioxidant property of Vg, diminishing heme-induced lipid peroxidation has been reported. Importantly, silencing of Vg in ticks feeding on sheep resulted in reduced engorgement and oviposition rates (Esteves et al., 2017). Likewise, the protein ferritin identified within the tick EVs, has been known to play crucial role in blood-feeding and reproduction in H. longicornis and Ixodes ricinus (Hajdusek et al., 2009; Galay et al., 2013). Moreover, insect ferritin was also implicated in iron transport, immune response and oxidative stress (Orino et al., 2001; Ong et al., 2006). As these proteins have been identified in saliva of ticks, therefore, it has been believed that tick saliva is beneficial for reproduction and blood-feeding of ticks. However, the association of these particular proteins with tick EVs still requires further confirmation.
Some other proteins like heat-shock proteins (HSPs), thioredoxin peroxidase, metalloprotease, glyceraldehyde-3-phosphate dehydrogenase, and glutathione S-transferase have also been identified in the context of parasite-derived EVs. HSPs play key roles in differentiation, adaptation, and protection of parasites from killing mechanisms of hosts such as low pH and reactive oxygen metabolites (Johnson and Brown, 2009). Moreover, heat-shock proteins (HSP70) are thought to induce transformation of promastigote stage of Leishmania donovani to its amastigote stage (Wiesgigl and Clos, 2001). Thioredoxin peroxidase, an antioxidant enzyme, is also identified in parasite-derived exosomes (Tzelos et al., 2016). It has been proposed that these vesicle-derived enzymes direct the immune system of host toward Th2 immune response, which is thought to be favorable for parasite development within the host (Robinson et al., 2010; Dalton et al., 2013). In addition to the proteins described above, MS spectra of tick-derived EVs were also analyzed for the presence of host proteins within these EVs. These host proteins have been known to be present in saliva of tick species and parasite-derived EVs (Buck et al., 2014; Kim et al., 2016; Samoil et al., 2018). In recent studies, it has been proposed that the host proteins like fibrinogen, serum albumin, and serotransferrin are likely associated with the events toward tick feeding (Kim et al., 2016). Therefore, presence of such proteins within tick-derived EVs clearly implicates these structures in host-parasite communication processes. However, their role in tick EVs requires further investigation.
Moreover, the proteome of H. longicornis tick saliva-derived EVs showed similarity with proteins identified from tick saliva (Supplementary Table 6). Proteins such as enolase, histones, heat shock proteins, lipocalin, thioredoxin, and vitellogenin have been identified within saliva of Ornithodoros moubata, Ixodes scapularis, Rhipicephalus sanguineus, Amblyomma americanum, Dermacentor andersoni, and Haemaphysalis longicornis. (Díaz-MartÍN et al., 2013; Oliveira et al., 2013; Mudenda et al., 2014; Tirloni et al., 2015; Ren et al., 2019). Due to the similarty in proteomes, it has been proposed that ticks could use these nano-sized vesicles to produce saliva (Díaz-MartÍN et al., 2013). Therefore, the findings of current study gave further confirmaton to assumptions derived in previous studies.
Gene Ontology (GO) database determines the functional annotation of gene products with a vocabulary of ontological terms describing their biological processes, molecular functions, and cellular components of the cell (Ashburner et al., 2000; Consortium, 2004; Thomas, 2017). GO data revealed that the possible outcomes of vesicular proteins were hydrolysis coupled proton transport, energy coupled proton transmembrane transport, and detoxification. “Biological process” indicated role of proteins in proton transport as well as in removal of harmful toxins accumulated within the body. In “cell component” category, highly enriched category was found to be “cell.” This analysis revealed that major biological as well as metabolic functions occur within cell. “Molecular function” analysis indicated that proteins were mainly involved in binding and catalytic activity. However, other significant functions associated with proteins were the transfer of ions across the membranes as well as inhibition of oxidation. KEGG pathways analysis showed that proteins regulated several pathways associated with ribosome biosynthesis (ribosome), transport of RNA species (RNA transport pathway), and regulation of oxidative pathway as well as generation of ATPs (oxidative phosphorylation). Bioinformatics analysis of vesicular proteins revealed that proteins are associated with certain biological as well as molecular functions which may be beneficial for reproduction and survival of ticks.
To the best of our knowledge, we report for the first time that the tick H. longicornis is also capable of secreting exosome-like vesicles. However, some limitations have been associated with the present study. This study deals with ticks, and saliva is the main excretory source of ticks, however, it is quite difficult to collect enough amount of saliva to be used separately for all the standard techniques. In addition, EVs isolated from saliva were just enough to be used for SDS-PAGE, electron microscopy, BCA, Western blot, and LC-MS/MS. Therefore, characterization of exosomes by nano-particle tracking analysis is not provided. At this stage, we would like to present the interesting results at an early stage to other researchers. Further categorization of tick-derived EVs is required to improve knowledge about the proteins within EVs and their associated functions.
Conclusions
In summary, the present study constitutes the first analysis of secretion of EVs from ticks. The present study indicates that EVs may be a useful pathway for ticks for the transfer of genetic materials to host cells, thereby helping ticks in modulating host immune responses. However, better understanding of how EVs increase tick attachment to host skin as well as modulation of host immune responses will be helpful in pathogenesis and development of therapeutics and vaccine against ticks.
Data Availability Statement
The datasets generated for this study can be found in the ProteomeXchange (accession number PXD020300).
Ethics Statement
The animal study was reviewed and approved by Institutional Animal Care and Use Committee of Shanghai Veterinary Research Institute.
Author Contributions
JZ conceived and designed the experiments. MN performed the experiments. HZ, JC, and YZ completed the data analysis. MM, IH, MH, and ZH contributed reagents, materials, analysis, and tools. JZ and MN wrote the paper. All authors read and approved the final manuscript.
Funding
This work was supported by a grant (2018YFC0840403) from The National Key Research and Development Programme of China. The funders had no role in study design, data collection and analysis, decision to publish, or preparation of the manuscript.
Conflict of Interest
The authors declare that the research was conducted in the absence of any commercial or financial relationships that could be construed as a potential conflict of interest.
Acknowledgments
We are thankful to Huang Jingwei, Wang Yanan, and Xu Zhengmao for their valuable support during the experimental work. We also thank Shanghai Hoogen Biotechnology Co. Ltd. for providing technical assistance.
Supplementary Material
The Supplementary Material for this article can be found online at: https://www.frontiersin.org/articles/10.3389/fcimb.2020.542319/full#supplementary-material
Supplementary Figure 1. Schematic representation of steps followed to purify tick EVs.
Supplementary Figure 2. Histograms representing significantly enriched (P ≤ 0.05) biological processes (A), cellular components (B) and molecular functions (C).
Supplementary Table 1. Protein content of tick-derived EVs identified using MASCOT search engine.
Supplementary Table 2. Host proteins identified in purified EVs.
Supplementary Table 3. Proteins present in supernatant of tick-derived EVs.
Supplementary Table 4. Details of Biological processes, cellular components and molecular functions.
Supplementary Table 5. KEGG Pathway analysis of proteins identified from tick-saliva derived EVs.
Supplementary Table 6. Common proteins between tick saliva and tick-derived EVs.
References
Abdi, A., Yu, L., Goulding, D., Rono, M. K., Bejon, P., Choudhary, J., et al. (2017). Proteomic analysis of extracellular vesicles from a Plasmodium falciparum Kenyan clinical isolate defines a core parasite secretome. Wellcome Open Res. 2, 50. doi: 10.12688/wellcomeopenres.11910.1
Ashburner, M., Ball, C. A., Blake, J. A., Botstein, D., Butler, H., Cherry, J. M., et al. (2000). Gene ontology: tool for the unification of biology. Nat. Genet. 25, 25–29. doi: 10.1038/75556
Atayde, V. D., Aslan, H., Townsend, S., Hassani, K., Kamhawi, S., and Olivier, M. (2015). Exosome secretion by the parasitic protozoan Leishmania within the sand fly midgut. Cell Rep. 13, 957–967. doi: 10.1016/j.celrep.2015.09.058
Balaj, L., Lessard, R., Dai, L., Cho, Y.-J., Pomeroy, S. L., Breakefield, X. O., et al. (2011). Tumour microvesicles contain retrotransposon elements and amplified oncogene sequences. Nat. Commun. 2, 1–9. doi: 10.1038/ncomms1180
Barbosa, F. M. C., Dupin, T. V., Toledo, M. D. S., Reis, N. F. D. C., Ribeiro, K., Cronemberger-Andrade, A., et al. (2018). Extracellular vesicles released by Leishmania (Leishmania) amazonensis promote disease progression and induce the production of different cytokines in macrophages and B-1 Cells. Front. Microbiol. 9:3056. doi: 10.3389/fmicb.2018.03056
Bobrie, A., Colombo, M., Raposo, G., and Théry, C. (2011). Exosome secretion: molecular mechanisms and roles in immune responses. Traffic 12, 1659–1668. doi: 10.1111/j.1600-0854.2011.01225.x
Boldbaatar, D., Umemiya-Shirafuji, R., Liao, M., Tanaka, T., Xuan, X., and Fujisaki, K. (2010). Multiple vitellogenins from the Haemaphysalis longicornis tick are crucial for ovarian development. J. Insect. Physiol. 56, 1587–1598. doi: 10.1016/j.jinsphys.2010.05.019
Brzozowski, J. S., Jankowski, H., Bond, D. R., Mccague, S. B., Munro, B. R., Predebon, M. J., et al. (2018). Lipidomic profiling of extracellular vesicles derived from prostate and prostate cancer cell lines. Lipids Health Dis. 17:211. doi: 10.1186/s12944-018-0854-x
Buck, A., Coakley, G., Simbari, F., Mcsorley, H., Quintana, J. F., Le Bihan, T., et al. (2014). Exosomes secreted by nematode parasites transfer small RNAs to mammalian cells and modulate innate immunity. Nat. Commun. 5:5488. doi: 10.1038/ncomms6488
Cai, P., Piao, X., Hao, L., Liu, S., Hou, N., Wang, H., et al. (2013). A deep analysis of the small non-coding RNA population in Schistosoma japonicum eggs. PLoS ONE 8:e64003. doi: 10.1371/journal.pone.0064003
Chen, M., Ai, L., Xu, M., Chen, S., Zhang, Y., Guo, J., et al. (2011). Identification and characterization of microRNAs in Trichinella spiralis by comparison with Brugia malayi and Caenorhabditis elegans. Parasitol. Res. 109, 553–558. doi: 10.1007/s00436-011-2283-x
Chen, S., Datta-Chaudhuri, A., Deme, P., Dickens, A., Dastgheyb, R., Bhargava, P., et al. (2019). Lipidomic characterization of extracellular vesicles in human serum. J. Circulat. Biomarkers 8:1849454419879848. doi: 10.1177/1849454419879848
Coakley, G., Maizels, R. M., and Buck, A. H. (2015). Exosomes and other extracellular vesicles: the new communicators in parasite infections. Trends Parasitol. 31, 477–489. doi: 10.1016/j.pt.2015.06.009
Consortium, G. O. (2004). The Gene Ontology (GO) database and informatics resource. Nucleic Acids Res. 32, D258–D261. doi: 10.1093/nar/gkh036
Cwiklinski, K., de la torre-escudero, E., Trelis, M., Bernal, D., Dufresne, P. J., Brennan, G. P., et al. (2015). The extracellular vesicles of the helminth pathogen, Fasciola hepatica: biogenesis pathways and cargo molecules involved in parasite pathogenesis. Mol. Cell. Proteomics 14, 3258–3273. doi: 10.1074/mcp.M115.053934
Dalton, J. P., Robinson, M. W., Mulcahy, G., O'neill, S. M., and Donnelly, S. (2013). Immunomodulatory molecules of Fasciola hepatica: candidates for both vaccine and immunotherapeutic development. Vet. Parasitol. 195, 272–285. doi: 10.1016/j.vetpar.2013.04.008
Devhare, P. B. and Ray, R. B. (2018). Extracellular vesicles: novel mediator for cell to cell communications in liver pathogenesis. Mol. Aspects Med. 60, 115–122. doi: 10.1016/j.mam.2017.11.001
Díaz-MartÍN, V., Manzano-Román, R., Valero, L., Oleaga, A., Encinas-Grandes, A., and Pérez.-Sánchez, R. (2013). An insight into the proteome of the saliva of the argasid tick Ornithodoros moubata reveals important differences in saliva protein composition between the sexes. J. Proteomics 80, 216–235. doi: 10.1016/j.jprot.2013.01.015
Eichenberger, R. M., Talukder, M. H., Field, M. A., Wangchuk, P., Giacomin, P., Loukas, A., et al. (2018). Characterization of Trichuris muris secreted proteins and extracellular vesicles provides new insights into host–parasite communication. J. Extracell. Vesicles 7:1428004. doi: 10.1080/20013078.2018.1428004
Esteves, E., Maruyama, S. R., Kawahara, R., Fujita, A., Martins, L. A., Righi, A. A., et al. (2017). Analysis of the salivary gland transcriptome of unfed and partially fed Amblyomma sculptum ticks and descriptive proteome of the saliva. Front. Cell. Infect. Microbiol. 7:476. doi: 10.3389/fcimb.2017.00476
Galay, R. L., Aung, K. M., Umemiya-Shirafuji, R., Maeda, H., Matsuo, T., Kawaguchi, H., et al. (2013). Multiple ferritins are vital to successful blood feeding and reproduction of the hard tick Haemaphysalis longicornis. J. Exp. Biol. 216, 1905–1915. doi: 10.1242/jeb.081240
Gualdrón-López, M., Flannery, E. L., Kangwanrangsan, N., Chuenchob, V., Fernandez-Orth, D., Segui-Barber, J., et al. (2018). Characterization of Plasmodium vivax proteins in plasma-derived exosomes from malaria-infected liver-chimeric humanized mice. Front. Microbiol. 9:1271. doi: 10.3389/fmicb.2018.01271
Hajdusek, O., Sojka, D., Kopacek, P., Buresova, V., Franta, Z., Sauman, I., et al. (2009). Knockdown of proteins involved in iron metabolism limits tick reproduction and development. Proc. Natl. Acad. Sci. U.S.A. 106, 1033–1038. doi: 10.1073/pnas.0807961106
Harding, C. V., Heuser, J. E., and Stahl, P. D. (2013). Exosomes: looking back three decades and into the future. J. Cell Biol. 200, 367–371. doi: 10.1083/jcb.201212113
Heath, A. (2016). Biology, ecology and distribution of the tick, Haemaphysalis longicornis Neumann (Acari: Ixodidae) in New Zealand. N. Z. Vet. J. 64, 10–20. doi: 10.1080/00480169.2015.1035769
Herm-Götz, A., Weiss, S., Stratmann, R., Fujita-Becker, S., Ruff, C., Meyhöfer, E., et al. (2002). Toxoplasma gondii myosin A and its light chain: a fast, single-headed, plus-end-directed motor. EMBO J. 21, 2149–2158. doi: 10.1093/emboj/21.9.2149
Johnson, J. L., and Brown, C. (2009). Plasticity of the Hsp90 chaperone machine in divergent eukaryotic organisms. Cell Stress Chaperones 14, 83–94. doi: 10.1007/s12192-008-0058-9
Johnstone, R. (1992). Maturation of reticulocytes: formation of exosomes as a mechanism for shedding membrane proteins. Biochem. Cell Biol. 70, 179–190. doi: 10.1139/o92-028
Kanwar, S. S., Dunlay, C. J., Simeone, D. M., and Nagrath, S. (2014). Microfluidic device (ExoChip) for on-chip isolation, quantification and characterization of circulating exosomes. Lab. Chip 14, 1891–1900. doi: 10.1039/C4LC00136B
Kim, T. K., Tirloni, L., Pinto, A. F., Moresco, J., Yates III, J. R., da Silva Vaz, I., Jr et al. (2016). Ixodes scapularis tick saliva proteins sequentially secreted every 24 h during blood feeding. PLoS Negl. Trop. Dis. 10:e0004323. doi: 10.1371/journal.pntd.0004323
Lee, K., Shao, H., Weissleder, R., and Lee, H. (2015). Acoustic purification of extracellular microvesicles. ACS Nano 9, 2321–2327. doi: 10.1021/nn506538f
Li, Y., Liu, Y., Xiu, F., Wang, J., Cong, H., He, S., et al. (2018). Characterization of exosomes derived from Toxoplasma gondii and their functions in modulating immune responses. Int. J. Nanomed. 13:467. doi: 10.2147/IJN.S151110
Luo, L.-M., Zhao, L., Wen, H.-L., Zhang, Z.-T., Liu, J.-W., Fang, L.-Z., et al. (2015). Haemaphysalis longicornis ticks as reservoir and vector of severe fever with thrombocytopenia syndrome virus in China. Emerging Infect. Dis. 21, 1770–1776. doi: 10.3201/eid2110.150126
Mahara, F. (1997). Japanese spotted fever: report of 31 cases and review of the literature. Emerging Infect. Dis. 3:105. doi: 10.3201/eid0302.970203
Marcilla, A., Martin-Jaular, L., Trelis, M., de Menezes-Neto, A., Osuna, A., Bernal, D., et al. (2014). Extracellular vesicles in parasitic diseases. J. Extracell. Vesicles 3:25040. doi: 10.3402/jev.v3.25040
Marshall, S., Kelly, P. H., Singh, B. K., Pope, R. M., Kim, P., Zhanbolat, B., et al. (2018). Extracellular release of virulence factor major surface protease via exosomes in Leishmania infantum promastigotes. Parasit. Vectors 11:355. doi: 10.1186/s13071-018-2937-y
Mathivanan, S., Ji, H., and Simpson, R. J. (2010). Exosomes: extracellular organelles important in intercellular communication. J. Proteomics 73, 1907–1920. doi: 10.1016/j.jprot.2010.06.006
Mudenda, L., Pierlé, S. A., Turse, J. E., Scoles, G. A., Purvine, S. O., Nicora, C. D., et al. (2014). Proteomics informed by transcriptomics identifies novel secreted proteins in Dermacentor andersoni saliva. Int. J. Parasitol. 44, 1029–1037. doi: 10.1016/j.ijpara.2014.07.003
Mulenga, A., Sugimoto, C., Sako, Y., Ohashi, K., Musoke, A., Shubash, M., et al. (1999). Molecular characterization of a Haemaphysalis longicornis tick salivary gland-associated 29-kilodalton protein and its effect as a vaccine against tick infestation in rabbits. Infect. Immun. 67, 1652–1658. doi: 10.1128/IAI.67.4.1652-1658.1999
Nawaz, M., Malik, M. I., Hameed, M., and Zhou, J. (2019). Research progress on the composition and function of parasite-derived exosomes. Acta Trop. 196, 1–192. doi: 10.1016/j.actatropica.2019.05.004
Nicolao, M. C., Rodrigues, C. R., and Cumino, A. C. (2019). Extracellular vesicles from Echinococcus granulosus larval stage: Isolation, characterization and uptake by dendritic cells. PLoS Negl. Trop. Dis. 13:e0007032. doi: 10.1371/journal.pntd.0007032
Oliveira, C., Anatriello, E., de Miranda-Santos, I., Francischetti, I., Sá-Nunes, A., Ferreira, B., et al. (2013). Proteome of Rhipicephalus sanguineus tick saliva induced by the secretagogues pilocarpine and dopamine. Ticks Tick Borne Dis. 4, 469–477. doi: 10.1016/j.ttbdis.2013.05.001
Ong, S. T., Ho, J. Z. S., Ho, B., and Ding, J. L. (2006). Iron-withholding strategy in innate immunity. Immunobiology 211, 295–314. doi: 10.1016/j.imbio.2006.02.004
Orino, K., Lehman, L., Tsuji, Y., Ayaki, H., Torti, S. V., and Torti, F. M. (2001). Ferritin and the response to oxidative stress. Biochem. J. 357, 241–247. doi: 10.1042/bj3570241
Pant, S., Hilton, H., and Burczynski, M. E. (2012). The multifaceted exosome: biogenesis, role in normal and aberrant cellular function, and frontiers for pharmacological and biomarker opportunities. Biochem. Pharmacol. 83, 1484–1494. doi: 10.1016/j.bcp.2011.12.037
Patton, T. G., Dietrich, G., Brandt, K., Dolan, M. C., Piesman, J., and Gilmore, R. D. Jr. (2012). Saliva, salivary gland, and hemolymph collection from Ixodes scapularis ticks. J. Vis. Exp. 60, e3894. doi: 10.3791/3894
RAI, A. K., and Johnson, P. J. (2019). Trichomonas vaginalis extracellular vesicles are internalized by host cells using proteoglycans and caveolin-dependent endocytosis. Proc.Natl. Acad. Sci. U.S.A. 116, 21354–21360. doi: 10.1073/pnas.1912356116
Raposo, G., Nijman, H. W., Stoorvogel, W., Liejendekker, R., Harding, C. V., Melief, C., et al. (1996). B lymphocytes secrete antigen-presenting vesicles. J. Exp. Med. 183, 1161–1172. doi: 10.1084/jem.183.3.1161
Ren, S., Zhang, B., Xue, X., Wang, X., Zhao, H., Zhang, X., et al. (2019). Salivary gland proteome analysis of developing adult female Haemaphysalis longicornis ticks: molecular motor and TCA cycle-related proteins play an important role throughout development. Parasit. Vectors 12:613. doi: 10.1186/s13071-019-3864-2
Robinson, M., Hutchinson, A., Dalton, J., and Donnelly, S. (2010). Peroxiredoxin: a central player in immune modulation. Parasite Immunol. 32, 305–313. doi: 10.1111/j.1365-3024.2010.01201.x
Rosell, R., and Coons, L. B. (1992). The role of the fat body, midgut and ovary in vitellogenin production and vitellogenesis in the female tick, Dermacentor variabilis. Int. J. Parasitol. 22, 341–349. doi: 10.1016/S0020-7519(05)80012-8
Samoil, V., Dagenais, M., Ganapathy, V., Aldridge, J., Glebov, A., Jardim, A., et al. (2018). Vesicle-based secretion in schistosomes: analysis of protein and microRNA (miRNA) content of exosome-like vesicles derived from Schistosoma mansoni. Sci. Rep. 8, 1–16. doi: 10.1038/s41598-018-21587-4
Shannon, P., Markiel, A., Ozier, O., Baliga, N. S., Wang, J. T., Ramage, D., et al. (2003). Cytoscape: a software environment for integrated models of biomolecular interaction networks. Genome Res. 13, 2498–2504. doi: 10.1101/gr.1239303
Sherman, B. T., and Lempicki, R. A. (2009). Systematic and integrative analysis of large gene lists using DAVID bioinformatics resources. Nat. Protoc. 4:44. doi: 10.1038/nprot.2008.211
Sibley, L. D., Håkansson, S., and Carruthers, V. B. (1998). Gliding motility: an efficient mechanism for cell penetration. Curr. Biol. 8, R12–R14. doi: 10.1016/S0960-9822(98)70008-9
Skotland, T., Sandvig, K., and Llorente, A. (2017). Lipids in exosomes: current knowledge and the way forward. Prog. Lipid Res. 66, 30–41. doi: 10.1016/j.plipres.2017.03.001
Sotillo, J., Valero, L., Sanchez del Pino, M., Fried, B., Esteban, J., Marcilla, A., et al. (2008). Identification of antigenic proteins from Echinostoma caproni (Trematoda) recognized by mouse immunoglobulins M, A and G using an immunoproteomic approach. Parasite Immunol. 30, 271–279. doi: 10.1111/j.1365-3024.2007.01019.x
Sotillo, J., Valero, M. L., Del Pino, M. M. S., Fried, B., Esteban, J. G., Marcilla, A., et al. (2010). Excretory/secretory proteome of the adult stage of Echinostoma caproni. Parasitol. Res. 107, 691–697. doi: 10.1007/s00436-010-1923-x
Sun, Y., Saito, K., and Saito, Y. (2019). Lipid profile characterization and lipoprotein comparison of extracellular vesicles from human plasma and serum. Metabolites 9:259. doi: 10.3390/metabo9110259
Tauro, B. J., Greening, D. W., Mathias, R. A., Ji, H., Mathivanan, S., Scott, A. M., et al. (2012). Comparison of ultracentrifugation, density gradient separation, and immunoaffinity capture methods for isolating human colon cancer cell line LIM1863-derived exosomes. Methods 56, 293–304. doi: 10.1016/j.ymeth.2012.01.002
Thakur, B. K., Zhang, H., Becker, A., Matei, I., Huang, Y., Costa-Silva, B., et al. (2014). Double-stranded DNA in exosomes: a novel biomarker in cancer detection. Cell Res. 24, 766–769. doi: 10.1038/cr.2014.44
Thomas, P. D. (2017). “The gene ontology and the meaning of biological function,” in The Gene Ontology Handbook, eds C. Dessimoz, and N. kunca (New York, NY: Humana Press), 15–24. doi: 10.1007/978-1-4939-3743-1_2
Thompson, D. M., Khalil, S. M., Jeffers, L. A., Sonenshine, D. E., Mitchell, R. D., Osgood, C. J., et al. (2007). Sequence and the developmental and tissue-specific regulation of the first complete vitellogenin messenger RNA from ticks responsible for heme sequestration. Insect. Biochem. Mol. Biol. 37, 363–374. doi: 10.1016/j.ibmb.2007.01.004
Tirloni, L., Islam, M. S., kim, T. K., Diedrich, J. K., Yates, J. R., Pinto, A. F., et al. (2015). Saliva from nymph and adult females of Haemaphysalis longicornis: a proteomic study. Parasit. Vectors 8:338. doi: 10.1186/s13071-015-0918-y
Tzelos, T., Matthews, J. B., Buck, A. H., Simbari, F., Frew, D., Inglis, N. F., et al. (2016). A preliminary proteomic characterisation of extracellular vesicles released by the ovine parasitic nematode, Teladorsagia circumcincta. Vet. Parasitol. 221, 84–92. doi: 10.1016/j.vetpar.2016.03.008
Wiesgigl, M., and Clos, J. (2001). Heat shock protein 90 homeostasis controls stage differentiation in Leishmania donovani. Mol. Biol. Cell. 12, 3307–3316. doi: 10.1091/mbc.12.11.3307
Wu, J., Cao, J., Zhou, Y., Zhang, H., Gong, H., and Zhou, J. (2017). Evaluation on infectivity of Babesia microti to domestic animals and ticks outside the Ixodes genus. Front. Microbiol. 8:1915. doi: 10.3389/fmicb.2017.01915
Wu, Z., Wang, L., Li, J., Wang, L., Wu, Z., and Sun, X. (2019). Extracellular vesicle-mediated communication within host-parasite interactions. Front. Immunol. 9:3066. doi: 10.3389/fimmu.2018.03066
Wubbolts, R., Leckie, R. S., Veenhuizen, P. T., Schwarzmann, G., Möbius, W., Hoernschemeyer, J., et al. (2003). Proteomic and biochemical analyses of human B cell-derived exosomes Potential implications for their function and multivesicular body formation. J. Biol. Chem. 278, 10963–10972. doi: 10.1074/jbc.M207550200
Xavier, M. A., Tirloni, L., Pinto, A. F., Diedrich, J. K., Yates, J. R., Mulenga, A., et al. (2018). A proteomic insight into vitellogenesis during tick ovary maturation. Sci. Rep, 8, 1–14. doi: 10.1038/s41598-018-23090-2
Xing, Z., Chu, C., Chen, L., and Kong, X. (2016). The use of gene ontology terms and KEGG pathways for analysis and prediction of oncogenes. Biochim. et Biophys. Acta 1860, 2725–2734. doi: 10.1016/j.bbagen.2016.01.012
Yesuf, M., and Kenubih, A. (2019). A review on the role of lipid in selected apicomplexan, anaerobic, kinetoplastid and intestinal parasitic infections. World 9, 129–134. doi: 10.36380/scil.2019.wvj17
Zamanian, M., Fraser, L. M., Agbedanu, P. N., Harischandra, H., Moorhead, A. R., Day, T. A., et al. (2015). Release of small RNA-containing exosome-like vesicles from the human filarial parasite Brugia malayi. PLoS Neglect. Trop. Dis. 9:e0004069. doi: 10.1371/journal.pntd.0004069
Zeringer, E., Barta, T., Li, M., and Vlassov, A. V. (2015). Strategies for isolation of exosomes. Cold Spring Harb. Protoc. (2015) 2015, 319–23. doi: 10.1101/pdb.top074476
Zhang, W., Jiang, X., Bao, J., Wang, Y., Liu, H., and Tang, L. (2018). Exosomes in pathogen infections: a bridge to deliver molecules and link functions. Front. Immunol. 9:90. doi: 10.3389/fimmu.2018.00090
Zhou, J., Gong, H., Zhou, Y., Xuan, X., and Fujisaki, K. (2006). Identification of a glycine-rich protein from the tick Rhipicephalus haemaphysaloides and evaluation of its vaccine potential against tick feeding. Parasitol. Res. 100, 77–84. doi: 10.1007/s00436-006-0243-7
Zhou, W., Woodson, M., Neupane, B., Bai, F., Sherman, M. B., Choi, K. H., et al. (2018). Exosomes serve as novel modes of tick-borne flavivirus transmission from arthropod to human cells and facilitates dissemination of viral RNA and proteins to the vertebrate neuronal cells. PLoS Pathog 14:e1006764. doi: 10.1371/journal.ppat.1006764
Keywords: exosomes, extracellular vesicles, saliva, proteomics, Haemaphysalis longicornis
Citation: Nawaz M, Malik MI, Zhang H, Hassan IA, Cao J, Zhou Y, Hameed M, Hussain Kuthu Z and Zhou J (2020) Proteomic Analysis of Exosome-Like Vesicles Isolated From Saliva of the Tick Haemaphysalis longicornis. Front. Cell. Infect. Microbiol. 10:542319. doi: 10.3389/fcimb.2020.542319
Received: 12 March 2020; Accepted: 11 September 2020;
Published: 22 October 2020.
Edited by:
Ana Claudia Torrecilhas, Federal University of São Paulo, BrazilReviewed by:
Elisa Azuara-Liceaga, Universidad Autónoma de la Ciudad de México, MexicoPatricia Xander, Federal University of São Paulo, Brazil
Copyright © 2020 Nawaz, Malik, Zhang, Hassan, Cao, Zhou, Hameed, Hussain Kuthu and Zhou. This is an open-access article distributed under the terms of the Creative Commons Attribution License (CC BY). The use, distribution or reproduction in other forums is permitted, provided the original author(s) and the copyright owner(s) are credited and that the original publication in this journal is cited, in accordance with accepted academic practice. No use, distribution or reproduction is permitted which does not comply with these terms.
*Correspondence: Jinlin Zhou, amlubGluemhvdUBzaHZyaS5hYy5jbg==