- 1Food Microbiology Division, Food Safety Evaluation Department, National Institute of Food and Drug Safety Evaluation, Cheongju, South Korea
- 2College of Agriculture and Life Sciences, Kangwon National University, Chuncheon, South Korea
- 3School of Food Science, Department of Food Science and Biotechnology, College of Agriculture and Life Sciences, Kyungpook National University, Daegu, South Korea
- 4Department of Bioresource Engineering, McGill University, Montreal, QC, Canada
- 5Department of Food and Nutrition, College of Human Ecology and Kinesiology, Yeungnam University, Gyeongsan, South Korea
Campylobacter spp. are the leading global cause of bacterial colon infections in humans. Enteropathogens are subjected to several stress conditions in the host colon, food complexes, and the environment. Species of the genus Campylobacter, in collective interactions with certain enteropathogens, can manage and survive such stress conditions. The stress-adaptation mechanisms of Campylobacter spp. diverge from other enteropathogenic bacteria, such as Escherichia coli, Salmonella enterica serovar Typhi, S. enterica ser. Paratyphi, S. enterica ser. Typhimurium, and species of the genera Klebsiella and Shigella. This review summarizes the different mechanisms of various stress-adaptive factors on the basis of species diversity in Campylobacter, including their response to various stress conditions that enhance their ability to survive on different types of food and in adverse environmental conditions. Understanding how these stress adaptation mechanisms in Campylobacter, and other enteric bacteria, are used to overcome various challenging environments facilitates the fight against resistance mechanisms in Campylobacter spp., and aids the development of novel therapeutics to control Campylobacter in both veterinary and human populations.
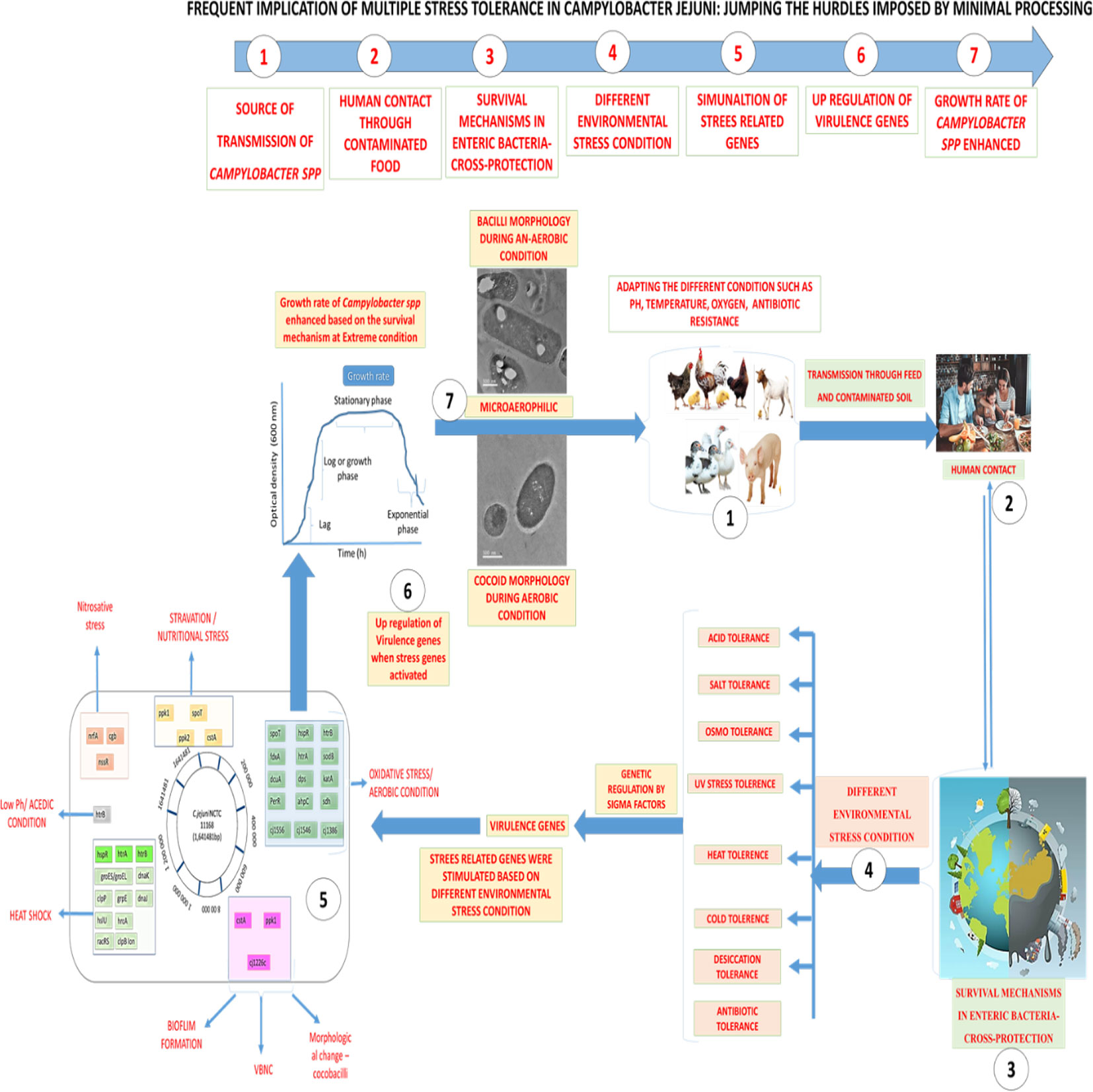
Graphical Abstract Overall flow chart on Stress Tolerance in Campylobacter jejuni at different environment condition 1. Source of transmission of Campylobacter species, 2. Human contact and throughout contaminated food, 3. Survival mechanism in entric-bacterial pathogens, 4. Differental environmental adverse condition, 5. Stimulation of stress related genes involved in sustainability, 6. Up regulation (over expression) of virulence genes, 7. Growth rate and survival of Campylobacter species enhanced.
Introduction
Campylobacter are a Gram-negative, slender, microaerophilic bacteria with a spiral or curved shape (0.2–0.8 mm × 0.5–5 mm). All species of the genus Campylobacter, with the exception of Campylobacter gracilis (nonmotile) and Campylobacter showae (peritrichous flagella), have a single, polar, unsheathed flagellum on one or both sides of the cell. Infection with Campylobacter in humans predominantly occurs through handling and ingestion of Campylobacter-contaminated raw or undercooked meat, raw milk, tap water, chicken salad, and various chicken-containing dishes (Zhao et al., 2003; Jang et al., 2007; Pedersen et al., 2018; Ovesen et al., 2019; The et al., 2019) as illustrated in Figure 1. Most Campylobacter infections involve a mild and self-limiting gastroenteritis, with one to three days of fever and vomiting, followed by abdominal pain with watery or bloody diarrhea for three to seven days (Negretti et al., 2019).
The species Campylobacter jejuni is a zoonotic pathogen that frequently causes acute gastrointestinal infections in humans when undercooked or raw meat or other products are consumed. Fever, vomiting, abdominal pain, and diarrhea are the prevalent symptoms of campylobacteriosis (Altekruse et al., 1999; Gaynor et al., 2005). In some cases, C. jejuni is associated with bacteremia and several post-infectious complications in humans, including immunoreactions and chronic and life-threatening paralysis, such as Guillain–Barré syndrome (GBS) and Miller Fisher syndrome (MFS) (Humphrey et al., 2007; EFSA, 2011).
C. jejuni possesses novel regulatory factors for stress resistance that enable the organism to cause foodborne infections (CDC, 2013). In most pathogens, sigma factor RpoS plays a key role in the stress-resistance mechanisms, but this factor has been reported to be absent in C. jejuni (Allen et al., 2018; Cain et al., 2019). Campylobacter is a foodborne pathogen with high incidence with norovirus, enteropathogenic Escherichia coli, and Salmonella in South Korea (Kim et al., 2017; Wang et al., 2020).
The prevalence of thermophilic Campylobacter for poultry is C. jejuni (6.3%), C. upsaliensis (5.9%), and C. coli (0.7%). Globaly 20.9% C. jejuni are resistant to (fluoro)quinolones. Poultry become colonized shortly after birth; commercial broilers are often particularly colonized with C. jejuni (EFSA, 2010), the major transmission of C. jejuni occurs in small intestinal crypts of poultry within 24 hours (Coward et al., 2008). Campylobacter can reach densities as high as 1 × 108 colony-forming units (CFU/g) in the infected bird’s intestinal mucosa are asymptomatic (Meade et al., 2009). C. jejuni spreads to a small intestine of the gastrointestinal tract, sometimes asymptomatically, after human consumption. The onset of illness is affected by the immune status of the host and the virulence of the Campylobacter strain.
The pathogenesis of C. jejuni foodborne illness involves adhesions, gut-wall invasion, colonization, and ultimately the release of toxins (Bang et al., 2003; Bolton, 2015; Pedersen et al., 2018). Motility of this pathogen is a key factor influencing colonization and survival in the acidic gut environment (Guerry, 2007; Mehat et al., 2018; Negretti et al., 2019). Flagella-oriented genes such as flaA and flaB, and fliF, fliM, and fliY are associated with motility-engaged C. jejuni (Nachamkin et al., 1993; Wassenaar et al., 1993; Carrillo et al., 2004; Sommerlad and Hendrixson, 2007; Lertsethtakarn et al., 2011). Some Gram-negative bacteria secrete a cytolethal distending toxin (CDT) heat-labile exotoxin and able to induce the distension and death of eukaryotic cells, and this has been demonstrated in Campylobacter (Bolton, 2015; Scuron et al., 2016; Pedersen et al., 2018; El-Tawab et al., 2019), which synthesizes this toxin using the genes cdtA, cdtB, and cdtC (Linton et al., 2000; Asakura et al., 2007; Wieczorek et al., 2018). Motility, adherence, invasion, and toxin production are required for cell lysis (Bang et al., 2003). The flagellar guidance of the motility scheme and a chemosensory mechanism that activates flagellar motion result in transmission from the environment to the small bowel (O’Sullivan et al., 2018). Campylobacter has extraordinary motility, particularly in gelatinous or viscous material, as indicated by its single or bipolar flagella and helical filamentous structures. The polar flagellum delivers driving torque and rotating metabolic signals, while corkscrew rotation is possible due to the helical form (Ferrero and Lee, 1988). Mucins and glycoproteins, the predominant components of mucus, are the primary chemical attractants during propagation (Hugdahl et al., 1988; Wadhams and Armitage, 2004; Wuichet et al., 2007; Ellström et al., 2016). Iron acquisition also plays a key role in infection with Campylobacter (Baillon et al., 1999; Bang et al., 2003; Eucker and Konkel, 2012).
The purpose of this review was to examine the mechanisms that enable Campylobacter spp. to survive outside the host environment and remain a threat to public health. A summary of specific survival-based resistance genes is also provided. This information helps identify future pathways to eradicate and control outbreaks of C. jejuni.
General Survival Mechanisms in Enteric Bacteria: Micro-Organism Cross-Protection
An extraordinary characteristic of bacteria is their ability to tolerate extreme environmental conditions or stressors. They not only tolerate ecological stress, but also adapt to different situations such as pressure, temperature, acidity, ultraviolet radiation, dehydration, susceptibility to antibiotics, and salinity. These characteristics raise some questions. Why and how do microbes in these environments survive? What biological mechanisms can we observe from these unique lifestyles? How can we use our understanding or resources to address these conditions, such as pH or temperature, to enhance or slow the growth of microbes?
Micro-organisms commonly face stress or shock during food processing (Ma et al., 2014). Microbes can survive in stressful or adverse environments, and can then tolerate other comparable stressors following the initial stress conditions (Isohanni et al., 2013). Cross-protection capabilities have been identified in Salmonella spp., E. coli, Listeria monocytogenes, and Cronobacter sakazakii (Kim et al., 2012; Spector and Kenyon, 2012; Lapierre et al., 2016; Wieczorek et al., 2018). For C. jejuni, a higher resistance to stress was observed following exposure to previous stressful environments. C. jejuni displayed tolerance or resistance to acid due to acquaintance with acid-aerobic, acidic, and nutrition-deprived stress (Oh et al., 2017), as well as showing oxidative stress cross-protection resulting from acid disturbance (Xu et al., 2019). However, Isohanni and Lyhs (Isohanni et al., 2013) stated that after exposure to heat and cold, C. jejuni did not have any cross-protection capacity, as shown in Figure 2.
Evidence indicates that antimicrobial agents are not used or are used incorrectly for the production of resistance Campylobacter spp. (Pedersen et al., 2018). Patients generally recover from campylobacteriosis without antimicrobial therapy, with treatment based on electrolyte substitution and rehydration. Severe cases can be managed with antibiotics such as tetracycline and macrolides (fluoro) or quinolones, but increases in antibiotic resistance in C. jejuni and C. coli has jeopardized the effectiveness of these therapeutics (Alfredson and Korolik, 2007; Bolinger et al., 2018).
Early in the food supply chain, C. jejuni is exposed to oxidative and desiccation stresses. Campylobacter are especially susceptible to the former as a processing technique (Humphrey et al., 1995), and in slaughter facilities, survival of Campylobacter in pig, and chicken meat decreases significantly by air-chill-drying the carcass surface (Oosterom et al., 1983). No comparable technique is used during the processing of poultry, and the chilling method initiates the formation of a moist surface that helps bacteria thrive (Butzler and Oosterom, 1991). Due to incomplete oxygen reduction, aerobic respiration generates reactive oxygen species (ROS), including superoxide anions (O2–2) and hydrogen peroxide (H2O2), which can lead to the formation of the extremely poisonous hydroxyl radical (HO). Campylobacter in the chicken or human body can also be subjected to H2O2 or O2 by the immune system to kill the microbes (Melo et al., 2019). The range of enzymes such as catalase, glutathione, cytochrome, peroxidases, peroxiredoxin alkyl hydroperoxide reductase, superoxide dismutase, and other peroxiredoxins are activated in Campylobacter exposed to ROS and these then facilitate long-term aerobic adaptation of the bacteria (Storz and Imlay, 1999) to facilitate long-term aerobic adaptation (Jones et al., 1993; Klancnik et al., 2009). C. jejuni has one catalase, KatA, which supports this process when the cytoplasmic level of H2O2 is high (Bingham-Ramos and Hendrixson, 2008; Melo et al., 2019).
Thermophilic species of Campylobacter, like C. jejuni, multiply at 37 to 42°CC and are unable to grow at temperatures below 30°CC (optimal growth is at 41.5°CC). At different stages of food processing, Campylobacter are exposed to chilled (0–4°CC) and elevated (>37–42°CC) temperatures. Evidence has shown that the response of Campylobacter to colder conditions (Hazeleger et al., 1998; Park, 2002) results in the slowest growth at 30°C. Low temperatures, freezing, and thawing impact different kinds of wastewater (particularly those concerning public health) and their long-term survival of enteric microbes (Zhang et al., 2009; Dasti et al., 2010; Hazeleger et al., 1998). Differences in at least 15 distinct genes were recorded between bacterial-cell and human-body temperatures of 37–42°CC, which is within the range of chicken-body temperatures. Around 48.1% of C. jejuni isolates showed resistance to tetracycline, and subsequent resistance to nalidixic acid (5.5%), ciprofloxacin (5.5%), azithromycin (1.78%), and erythromycin (1.78%) (Narvaez-Bravo et al., 2017). Dasti et al. (2010) reported ciprofloxacin resistance ranging from 4 μg to 32 μg/ml for the minimal inhibitory concentration. Most ciprofloxacin-resistant strains were divided into three major clonal complexes (ST-21, 48, and 353) by multilocus assessment, whereas both antibiotic-resistant strains were uniquely grouped into ST-45.
Other General Survival Mechanisms
The food matrix is one environmental factor that can influence micro-organism survival in the food system (all processes of production, processing, transport, and consumption) (de Oliveira et al., 2019; Farfán et al., 2019). After exposure to stress in the food system, expression of virulence and survival genes increased in Listeria monocytogenes (Olesen et al., 2009; Farfán et al., 2019). Day and Hammack (2019), reported enhanced gene expression under stress tolerance in L. monocytogenes in processed foods like meat and sausage juices compared with a laboratory setting. In contrast, stress-tolerance genes of Lactobacillus sakei were decreased in meat products (Prechtl et al., 2018), chicken meat and juice (Birk et al., 2004). Meat exudate, such as that from poultry meat, contains enzymes, myogens, myoglobin lactic acid, and amino acids (Wang et al., 2013). ‘Chicken juice’ can be used as a food-based model system for investigation of microbial survivability. Birk et al. (2004) recommended using the system to enhance understanding of C. jejuni viability on poultry products. C. jejuni survived longer in chicken juice (due to increased biofilm formation) stored at 5°C and 10°C (Brown et al., 2014). Ligowska et al. (2011) reported that expression of the gene luxS was increased in C. jejuni cultured in chilled poultry-meat juice. This highly conserved gene encodes the enzyme LuxS (S-ribosylhomocysteine lyase), which forms part of a quorum sensing system with autoinducer-2 (AI-2) and regulates gene expression. Differences in the recovery and identification of Campylobacter spp. between meat exudate and carcass rinse sampling methods in poultry have been demonstrated (Simmons et al., 2008; Duffy, 2019), as shown in Figure 3.
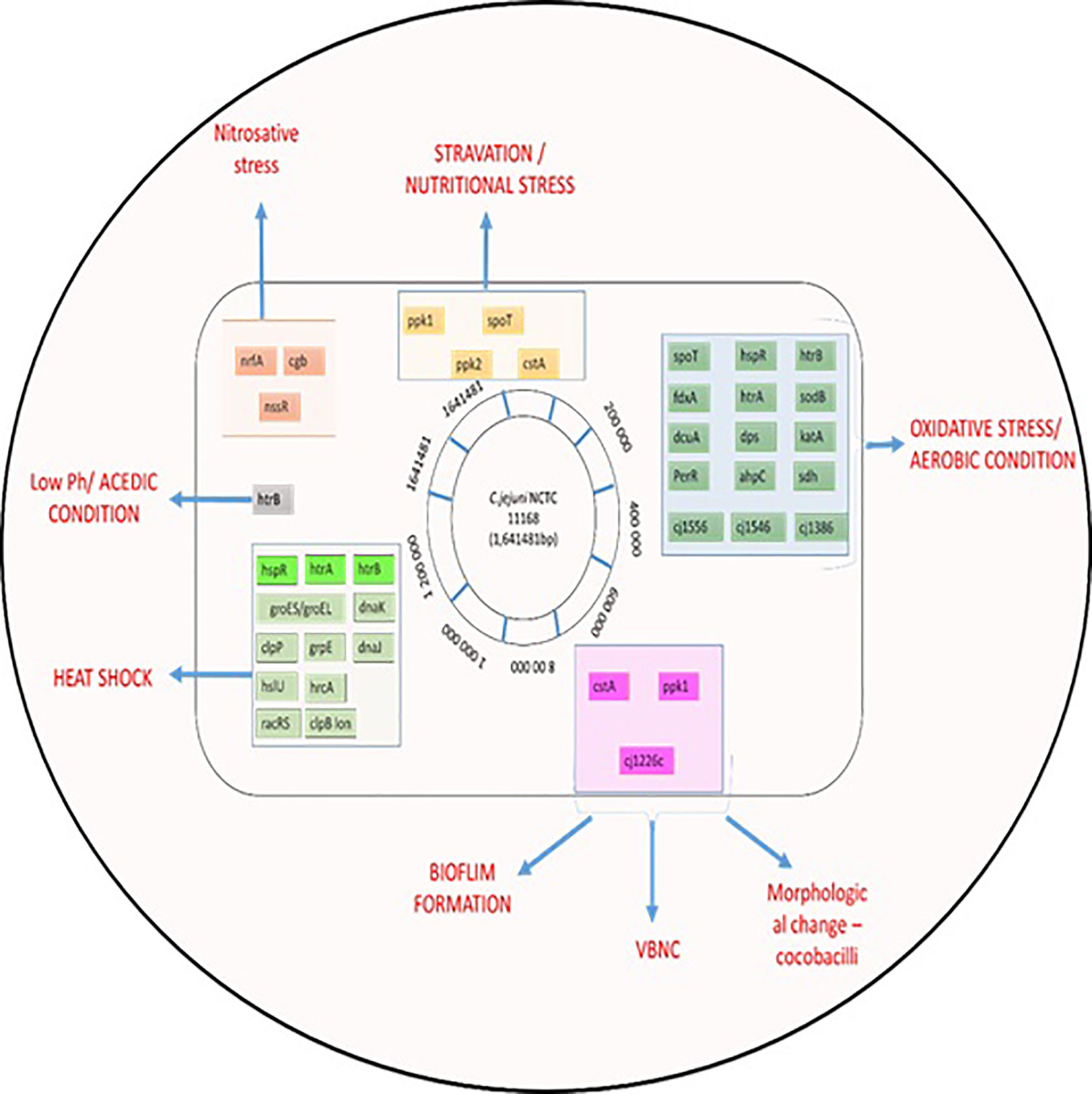
Figure 3 Summary of C. jejuni responses to stresses. The chromosome of C. jejuni NCTC11168 is represented by a black circle on which the location of genes, involved in different stress responses, are shown as colored lines. Genes are colored according to their role; gene names shaded in grey are involved in multiple stress responses.
Previous research has shown that microbes form biofilms during food processing, such as in meat exudate conditions. Species of the genus Salmonella created a biofilm on the surface of stainless steel when cultured in laboratory media or meat exudate (Wang et al., 2013). Differences in the shape and cell density of mature biofilms were observed between food processing and laboratory environments. Longo and Spano (2019) reported the formation of biofilm in L. monocytogenes and species of the genera Pseudomonas and Staphylococcus on meat-treated surfaces, such as polyvinyl chloride, polyurethane, and steel. C. jejuni was more prone to forming biofilms in chicken juice than in a laboratory environment due to high nutrient availability (Brown et al., 2014). Thus, processed foods that contain many macronutrients are easily contaminated by microbes; these foods include the meat juice of chicken and beef, milk protein, and dairy products (Kusumaningrum et al., 2003; Healy et al., 2010).
Viable but Non-Culturable (VBNC) State
Some microbes can endure unfavorable environments, such as nutrient deprivation, desiccation, inadequate pH, and temperature changes (Blanco-Lizarazo et al., 2018; Jin and Riedel–Kruse, 2018). Few microbes are capable of living in these unfavorable environments, but some organisms may enter a VBNC state for subsistence. Microbes in the VBNC state are unable to multiply, and their morphology is transformed into a coccoid shape (Poursina et al., 2018; Jin and Riedel-Kruse, 2018). Bacteria decrease their metabolism in the VBNC state but may retain the virulence capacity to infect a host and cause disease (Oliver, 2010; Fakruddin et al., 2013; Poursina et al., 2018). The VBNC state has been found in several micro-organisms, such as C. jejuni, V. parahaemolyticus, Salmonella ser. Typhi, and Helicobacter pylori (Azevedo et al., 2007; Zeng et al., 2013; Otigbu et al., 2018; Yoon and Lee, 2020). In an unfavorable environment, C jejuni can survive by using the VBNC tactic (Gangaiah et al., 2010; Zeng et al., 2013; Otigbu et al., 2018; Yoon and Lee, 2020). C. jejuni entered the VBNC state when cultured for 18–28 days at 4°C (Jones et al., 1991). Magajna and Schraft (2015) studied the VBNC status of planktonic cells and biofilm cells at 4°C and found that biofilm cells converted to VBNC status quicker than planktonic cells in nutritionally deprived and hostile-temperature environments. The VBNC form of C. jejuni affects CadF expression at 4°C (Otigbu et al., 2018). CadF protein is one of the elements influencing microbial invasion. The VBNC form of Campylobacter has been categorized based on reduced metabolism, augmented production of the degrading enzymes and substrates, and (Chaveerach et al., 2003; Upadhyay et al., 2019). Consequently, microbes can live for longer periods in hostile conditions (Kovacs et al., 2019).
Adaptation to Major Environmental Stresses by Campylobacter spp.
Adaptation by Campylobacter spp. to various stresses such as acidic environment, salt tolerance, thermotolerance (heat and cold), UV stress, osmotolerance, desiccation, biofilm formation, and antibiotic resistance, are explained in detail in Table 1.
Genes Involved in Stress Sensing/Adaptation
Acid-tolerance mechanisms: The adaptive tolerance response (ATR) was identified as the initiator of cross-protection for the survival of microbes under various stressful or unfavorable conditions (Oh et al., 2015), and was also found in foodborne pathogens (Li et al., 2018; Cariri et al., 2019; Mayton et al., 2019). Murphy et al. (2003) discovered an ATR in C. jejuni and a comparable result in the initiation of ATR was observed between stress-exposed and nonexposed organisms when the organism at the mid-exponential stage (8 h) was unable to start an ATR under air- and acidic-stress conditions. Conversely, stationary-phase (48 h) organisms could initiate ATR at pH 4.5 under air and acidic status compared to nonexposed organisms. They displayed acidic cross-protection, which initiated ATR under oxygen or air status. In addition, the ATR initiation of microbes at pH 4.5 varies according to the culture media; this might be due to the different nutrient compositions of the various culture media (Kovacs et al., 2019), -. C. jejuni demonstrated an ATR capacity at pH 4.5 when exposed to aerobic conditions with acidic and nutritional deprivation (Oh et al., 2017). Acidic stress initiated the upregulation of perR genes to counter oxidative disturbance.
Acid shock has a significant biological impact in situations of acidic pH and low (organic) acids. Fatty acids are carboxylic acids generated by fermentation, and include propionate, butyrate, and acetate (Luo et al., 2015; Eguchi and Utsumi, 2016). The fatty acids cause toxicity in their unloaded, protonated form because they may penetrate the plasma membrane, dissociate a proton, and create a lower intracellular pH.
An adaptive tolerance response to aerobic + acid conditions in C. jejuni (Oh et al., 2019) was shown to induce a global stress response mechanism (S.H Kim, unpublished data). An adaptive tolerance response (ATR) produced as a result of sub-chronic stress adaptive response and offers protection against subsequent lethal stress exposure (Noreen, 2019). We have defined an ATR in C. jejuni previously. The mediation of acid and oxygen concentration, makes them to adopt improved survival mechanism against lethal pH (Taylor et al., 2017). De novo protein synthesis was necessary for the initiation of ATR in C.jejuni, which implies enhanced protein synthesis occurred during the induction phase. During the induction of an ATR to acid stress, analysis of protein expression profiles demonstrated a global cellular response (S.H Kim, unpublished data). Based on MALDI-TOF mass spectrometry different Protein expressed during induction of the ATR in C.jejuni, which revealed that the majority of proteins were involved in modification, repair and biosynthesis.
The ATR in C. jejuni has been shown to incorporate up-regulation of generic stress proteins involved in protein defense or breakdown, such as the heat-shock response based on universal chaperones DnaK and GroEL, which are among the most highly conserved protein-coding genes known to be involved (Tang et al., 2017). Chaperone proteins may be involved in aerobic + acid denaturation or damage repair of proteins. Chaperone based GroEL and DnaK heat shock protein (HSPs) have been described as caused under acid conditions in Salmonella typhimurium (Ghazaei, 2017), which plays a major role after mild stress, either in the prevention of subsequent DNA damage or in the repair of already damaged DNA. The reported protein response were found to be closely associated with following pathogens such as S. typhimurium (Ghazaei, 2017), Escherichia coli (Burt et al., 2007) and Acinetobacter baumannii (Cardoso et al., 2010). This global reaction, in C. jejuni, which induced various mechanisms of survival and offers an initial insight into mechanisms that contribute to resistance of aerobic + acid susceptibility.
ATR-related RpoS: Transcription controller σs, encoded by the rpoS gene (RNA polymerase sigma factor), is a replacement sigma factor, the amount of which increases dramatically during any permanent stage of the microbes. The increase in σs concentration and gene expression is known to influence acid-shock proteins, such as high osmolality, low pH, hydration, and oxidation in cell survival (Ferreira et al., 2001). Sudden increases in cell acidification also cause strong increases in rpoS levels. Mutants that are defective in rpoS or that generate low concentrations of rpoS are highly susceptible to acidic conditions.
Salt-Tolerance Mechanisms
Sodium chloride (NaCl) is one of the most used preservatives in the food industry. C. jejuni is highly responsive to high osmolarity compared to most other enteric microbes (Feng et al., 2018; Kovacs et al., 2019). C. jejuni is unable to multiply with ≥2% NaCl at 42°C, but can multiply in the presence of 0.5% to 1.5% NaCl at 42°C (Gomes et al., 2018). Lake et al. (2019) reported that C. jejuni could tolerate 7.5% sodium chloride (NaCl) in media at 4°C better than at 22–30°C as measured using bioluminescence. In microarray analysis, Zhao et al. (2019) found that C. jejuni had augmented expression of oxidative-stress genes and heat-shock genes after exposure to hyperosmotic conditions.
Genetic Regulation by Sigma Factors
C. jejuni has a genome size of 1.4 Kbp, coding for approximately 1731 genes. In contrast to other environmental and food pathogens that have several gene-regulation processes occurring via sigma factors, C. jejuni has only three sigma factors (Wösten et al., 1998; Parkhill et al., 2000; Carrillo et al., 2004), and no recorded extracytoplasmic-function (ECF) sigma factors. The three sigma variables account for most operations related to gene regulation. Sigma 70 or RpoD is the housekeeping sigma factor that regulates most C. jejuni promoters. The other two sigma factors, sigma 28 (FilA, Filament A) and sigma 54 (RpoN), regulate 44 different genes that are mostly related to flagellar synthesis and protein secretion (Studholme and Dixon, 2003; Porcelli et al., 2013). The regulatory mechanisms and nucleic-base composition of the sigma-factor promoters were detailed by Petersen et al. (2003). Major promoters recognized by C. jejuni sigma subunits have the –10 element, whereas there is no consensus for the –35 element. The regulatory roles of RpoN in C. jejuni under various stress conditions were shown were shown using RpoN mutation and complementation in a study by Hwang et al. (2011). FilA is thought to regulate motility as well as the virulence of C. jejuni (Carrillo et al., 2004). Thorough genomic research into these mutant strains is required to elucidate the intricacies of gene regulation among the three sigma variables in this uncommon pathogen. Furthermore, how the lack of conservation of the –35 element contributes to optimal transcription in vivo remains to be determined. Morphological differences may exist, such as the conversion of a spiral bacterium to a coccus-/rod-shaped bacterium under osmotic and cold stress (Carrillo et al., 2004; Hwang et al., 2011). Even if C. jejuni is regarded as a pathogen transmitted via meat and poultry, it is not very tolerant to several nonoptimal conditions, particularly desiccation and osmotic stress.
Role of Osmolytes in Cryotolerance
Compared with Salmonella spp. and E. coli-like enteric bacteria, little is known about the mechanisms that enable survival of Campylobacter spp. under various environmental and stress conditions. A previous study found that C. jejuni’s ability to influence gene expression after exposure to environmental stress was a barrier to comparison with other bacteria (Park, 2002). Rapid temperature decreases cause bacteria to express a distinct set of proteins, and this response is known as cold shock. These proteins are predominantly nucleases, helicases, and ribosome-related elements that communicate with and bind to RNA and DNA. Cold-shock proteins induce a membrane adaptation, cold signal sensing, and translation-device alteration (Ultee et al., 2019). Ultee et al. (2019) reported motility for oxygen consumption, protein synthesis, and C. jejuni survival capacity at 4°C. Lu et al. (2011) revealed that C. jejuni survive at in low-temperature. This indicates that C. jejuni may produce a cold-shock effect that influences low-temperature gene expression to 4°C. CspA is the main cold-shock protein in C. jejuni, and functions as an RNA chaperone to enhance more effective cold-shock protein translation (Parkhill et al., 2000; Giuliodori et al., 2010). It is not yet clear how C. jejuni respond to or regulate the expression of genes during cold shocks. Based on documented studies, the cold-shock reaction is presented as a complex system of genes that are regulated by the same stimulus, where post-transcriptional conditions are essential. C. jejuni poses problems to food security and public health in the food-processing industry, since it survives for several months at 4°C. C. jejuni declined by about 1 log cfu/ml when stored at 4°C for seven days (Guévremont et al., 2015; Lake et al., 2019). Oxidative stress can upregulate cold-shock protein expression, which can extend the life span of C. jejuni in hypothermal conditions (Karki et al., 2019).
Survival During Ultraviolet (UV) Stress
VBNC refers to a state in which conventional culture on enhanced agar media does not detect microbial cells, although it remains feasible to resuscitate the microbes under preferential circumstances. This unique survival strategy has been shown to exist in nature (Salma et al., 2013). More than 60 different bacterial species have been found to be VBNC, including both Gram-negative (e.g., E. coli, S. enterica, C. jejuni, H. pylori, Pseudomonas aeruginosa, and species of the genera Legionella and Vibrio) and Gram-positive (e.g., species of the genus Enterococcus, Micrococcus luteus, and L. monocytogenes) species (Salma et al., 2013). Following a severe dose of UV (0.192 J/cm2), no viable Campylobacter cells were identified from the original level of 7 log cfu/ml in the liquid media (skimmed milk exposed to UV and diluted 1:4 with extreme rehabilitation diluents) (Xiong, 2009). Substantial variability of up to 4 log cfu/ml was observed in the susceptibility of Campylobacter isolates following UV treatment. In UV-treated (0.192 J/cm) fresh chicken fillet, C. jejuni, was decreased by 0.76 cfu/g, whereas, a reduction in C. jejuni of up to 3.97 log cfu/cm was attained with UV treatment of packaging and surface materials. These data indicated that Campylobacter is UV-prone, but concerning differentials occurred among the studied isolates. Overall, UV application could help improve the microbiological quality of raw chicken and remove contamination of related surfaces and packaging (Haughton et al., 2011).
Investigations were conducted concerning organism survival in rivers, coastal waters, and sewage to investigate the natural and artificial habitats of C. jejuni with UV-B light (280–315 nm) (Hénault-Ethier et al., 2016; García-Peña et al., 2017; Otigbu et al., 2018). Another research project in conjunction with these revealed that C. jejuni was susceptible to UV-C light (254 nm). UV sensitivity was greater than that of other microbes (Butler et al., 1987). The application of UV-C radiation to decrease C. jejuni in chicken breast also attracted interest (Rodrigues et al., 2019), as well as in broiler meat (Zhuang et al., 2019) and ready-to-eat ham (Yang et al., 2017). UV-light techniques have been extensively explored for reducing micro-organisms, including Campylobacter, in foodstuffs (Rodrigues et al., 2019; Zhuang et al., 2019).
UV irradiation achieved a maximal reduction of C. jejuni on broiler meat and broiler skin of 0.7 and 0.8 log, respectively. The maximal decrease by UV irradiation on broiler carcasses (254 nm, 32.9 m W/s per square inch) was 0.4 log, and the combination of UV and activated oxygen also achieved a 0.4 log reduction in C. jejuni. The primary sanitation method for C. jejuni in broiler carcasses cannot rely on UV irradiation alone or in conjunction with activated oxygen. However, application of these methods in conjunction with other sanitization techniques, as well as the adequate processing and sanitation of processing plants, may be more efficient than the use of these processes to reduce C. jejuni on broiler carcass surfaces (Isohanni and Lyhs, 2009). UV irradiation was less efficient at removing C. jejuni on broiler meat and skin than on agar plates. It reduces C. jejuni on grilled skin a little more effectively than on meat. Dry meat undergo ultraviolet radiation has low invasive capacity, and the cutting edges of food perhaps produced shade that interfered with UV irradiation (Rodrigues et al., 2019). The fibers could be isolated by swabbing the surfaces and allowing the swabs to absorb humidity from below the meat layer. After flaming, the skin did not appear to have changed much, and bacteria could not have crossed the threshold skin into the meat. Wong et al. (1998) also indicated that gram positive bacteria were more efficiently reduced by UV irradiation. However, the effects of UV irradiation can differ considerably in C. jejuni isolates from different origins and at different growth stages (Yaun et al., 2003).
Oxidative Stress and Aerotolerance
Campylobacter does not usually grow in environments of atmospheric oxygen (air) due to it being microaerophilic and requiring 5–10% carbon dioxide (CO2) (Frirdich et al., 2019). Campylobacter can tolerate oxidative stress even after exposure towards aerobic conditions (Kim et al., 2015). Microaerophilic environment generates favorable growth conditions for C. jejuni (Geng et al., 2019). Karki et al. (2019) found that subcultures of C. jejuni could develop colonies on blood agar at 4, 37, and 42°C in air conditions. This exposure to aerobic conditions leads to the transformation of both the cell morphology and the pattern of the external membrane proteins. Their results indicated that the bacterial cells had high survivability in aerobic conditions compared to microaerobic conditions. Geng et al. (2019) reported that subcultures of C. jejuni from both sterile chicken mince and stream water developed colonies at 5, 25, and 37°C on blood agar, and that cells were more likely to survive when cultured in a microaerophilic than an aerobic environment.
In comparison with microaerobic conditions owing to oxidative pressure, C. jejuni showed external structural changes in the form of coccoid morphology (Oh et al., 2015), and the inner ATP synthesis of C. jejuni decreased with oxidative stress (Cain et al., 2019). Under microaerophilic environments, C. jejuni may develop better than under oxygenic conditions at a cell concentration of <105 cfu/ml (Kaakoush et al., 2007).
C. jejuni Heat-Shock Response
Heating is one of the sanitizing techniques used for food preservation in the food sector. Heat treatment readily reduces the survival of C. jejuni relative to other enteric micro-organisms. For C. coli, decimal reduction times (D-values) were 381, 89, 21.9, and 5.7 s at 49.9, 55.4, 60.0, and 62.5°C, respectively, in phosphate buffer saline (PBS) (Habib et al., 2010; Upadhyay et al., 2019). Treatment of C. jejuni at 55°C for 3 min, decreased the density by 2–3 log cfu/ml (Kovacs et al., 2019). Heat treatment caused C. jejuni to lose its invasion capacity, and upregulate transcriptional factor HrcA for acid shock (Xu et al., 2019).
Desiccation Tolerance
Tolerance to desiccation in Campylobacter spp. was first reported by Fernandez et al. (1985) in several biotypes of C. coli and C. jejuni subjected to 2–8 hours of exposure. The RpoN sigma factor does not significantly contribute to the tolerance to osmotic shock or desiccation, whereas tolerance of cold or refrigeration temperatures can be directly correlated with bacterial survival capacity in cold environments (Burgess et al., 2016). The extreme sensitivity to desiccation and poor tolerance to heat and drying established that blowing hot air was an efficient method to prevent carrying dormant C. jejuni from poultry to human hosts in commercial settings (Berrang et al., 2011). Such methods could be applied to farms to prevent pathogenic carriers through poultry.
Biofilm Formation and Stress Adaptation
Extracellular polysaccharide (EPS) accumulation leads to biofilm formation by microbes, biofilm formation could allow additional species to accumulate on surfaces (Simoes and Simões, 2013; Maes et al., 2019). EPSs compressed of nucleic acids, polysaccharides, proteins, phospholipids, and teichoic acids to form biofilms (Miao et al., 2019). Many factors stimulate biofilm formation, including temperature, NaCl, pH, compounds of food, and type of surface (Arnold and Silvers, 2000; Nguyen et al., 2006; Speranza et al., 2011; Vázquez-Sánchez et al., 2013; Mavri et al., 2016; Whitehouse et al., 2018; Longo and Spano, 2019; Xu et al., 2019). Biofilms can form on dairy-product-handling machinery and nutrition-handling surfaces (Miao et al., 2019), and can therefore contribute to the occurrence of foodborne diseases and create a public health issue (Maes et al., 2019; Miao et al., 2019). There are numerous reports on foodborne diseases in relation to biofilm development (Metselaar et al., 2015; Mavri et al., 2016; Whitehouse et al., 2018; Ma et al., 2019). Microbes in biofilms are more resistant to antibiotics than plankton cells are (Stewart and Costerton, 2001; Olsen, 2015). C. jejuni preconditions define their environment for growth, and Surface attachment and biofilm generation are vital tools for environmental stability (Dykes et al., 2003), as shown in Figure 4.
C. jejuni can generate biofilms in liquid media as a monospecies (Sałamaszyńska-Guz et al., 2018) in aerobic conditions (Ovesen et al., 2019) C. jejuni can form biofilms both as a monospecies and as a combination of microbes (The et al., 2019) and nutritional components (Bronnec et al., 2016). Sałamaszyńska-Guz et al. (2018) showed that the aggregating and pellicle form of C. jejuni that forms at 30–37°C in a microaerobic environment allows the bacteria to survive under aerobic conditions. Ovesen et al. (2019) demonstrated that C. jejuni easily creates biofilms, and that flagellar motility aggravated biofilm production. It currently reads as though it is the report of Ovesen et al., 2019 stated that C. jejuni could acclimate to develop a biofilm linked to CsrA under aerobic conditions (Askoura et al., 2016; Ye et al., 2019). Therefore, CsrA mutation leads to inhibition of biofilm formation (Fields and Thompson, 2008). C. jejuni can also contribute to biofilm formation in combination with other microbes under a microaerobic environment, but the combination is specific to the microbes and the environment (The et al., 2019), for example the poultry environment is an example of this specific environment/microbe combination. The biofilm formation capacity of C. jejuni depends on culture media, oxidative stress, temperature, and interspecies composition (Bronnec et al., 2016). Protein generation, quorum sensing, and flagellar sensing also influence the capacity of C. jejuni to generate biofilms, as shown in Table 1.
Antibiotic Susceptibility of C. jejuni
Antibiotics are typically used to fight against bacterial infections (Pedersen et al., 2018), and possess different mechanisms to kill or inhibit bacteria. For example, quinolones, such as nalidixic acid, dysregulate DNA synthesis in microbial cells (Jacoby, 2005), whereas macrolides, including erythromycin, bind to ribosomes in the microbes, blocking elongation of the peptide loop (Arsic et al., 2018). Severe cases of campylobacteriosis require adequate treatment with antibiotics (Wieczorek and Osek, 2013), usually a fluoroquinolone and macrolide combination (Devi et al., 2019). Improper and frequent antibiotic use has led to increased antibiotic resistance in Campylobacter, which is a public health issue. Consequently, the fluoroquinolone and macrolide efficacy can fail to overcome the antibiotic resistance of Campylobacter (Pedersen et al., 2018; Bolinger et al., 2018; Silvan et al., 2018; Devi et al., 2019). The continuous usage of antibiotics such as tetracycline, ciprofloxacin, and erythromycin leads to the development of resistance in enteropathogens; specific resistance genes to these antibiotics were identified in C. jejuni isolates (Wirz et al., 2010), and comparable trends in C. coli were reported in Canada (Devi et al., 2019). Zwe et al. (2018) found that C. jejuni isolated from ducks in Singapore was resistant to ciprofloxacin (86.7%), nalidixic acid (84.4%), and erythromycin (11.1%) (Devi et al., 2019). The development of antibiotic resistance in Campylobacter means the treatment regime of campylobacteriosis will involve other antibiotics, like gentamycin (Aarestrup and Engberg, 2001; Pedersen et al., 2018).
Conclusion
Campylobacter use a range of approaches for environmental and genomic survival, and molecular studies have facilitated a better understanding of these processes. Genetic modifications within the species C. jejuni have been significantly targeted, and genome sequencing for this species has been completed. Epidemiological studies and phenotypical analyses found variations in the incidence of strains of C. jejuni, or environmental circumstances between strains of C. jejuni. It has been easier to understand mechanisms that affect C. jejuni persistence by examining the transformation of this important pathogen in natural settings, such as soil and water, and combining connections with environmental changes. However, the reported differences in various strains of C. jejuni highlight the constraints of drawing generalized conclusions from individual strain research.
The multiple stress responses of Campylobacter spp. may facilitate survival in extreme environmental conditions, in addition to increasing resistance to subsequent traumatic conditions, which might enhance acquisition of virulence genes. Our review demonstrates the contribution of stress-tolerance responses to the resistance and pathogenicity of C. jejuni. Minor factors involved in stress management based on stress-responsible protein production are also involved in the activation and up- or down-regulation of virulence genes, and may contribute to the pathogenesis of C. jejuni. This finding is based on reported studies validated in different isolates of C. jejuni in response to stress adaptation, therefore caution should be taken in segregating and characterizing strains of C. jejuni. Gram-negative microaerophilic bacteria like H. pylori and C. jejuni are extremely common, and are human gastrointestinal pathogens. Only by combining these separate strands can the role of environmental survival in transmitting these important pathogens be fully understood.
Required Future Research to Fill Current Knowledge Gaps
Major gaps in current research on stress responses on C. jejuni, so far, researchers have predominantly focused on antibiotic resistance and oxidative stress in C. jejuni. However, various other stress conditions and specific survival-mechanism-based evolutionary adaptation methods exist to overcome modern preservative conditions, such as acidity, alkalinity, osmotic imbalance, freezing, high temperatures, UV light, and dryness (reduced water content). Future research should concentrate on understanding the genetic make-up of C. jejuni that helps this organism survive various environmental conditions. Identification of these evolutionary adaptive mechanisms and specific signaling pathways will assist future researchers in developing effective methods to overcome the adaptive mechanism(s) of C. jejuni. Furthermore, understanding C. jejuni stress-oriented genes and their specific expression mechanisms based on environmental stressors have implications in biofilm interactions and their signaling mechanism(s), and in practical terms this could help with current technological hurdles in the food system.
Author Contributions
The manuscript was written in detail and sectioned for specialized discussion with the respective authors in the field of research. Designing the outline of the review manuscript (Multiple stress tolerance in Campylobacter jejuni), visualization, and conceptualization—S-HK, RC, D-HO. Cross-protection and other general survival mechanisms towards environmental stress—SR. Genes involved in stress sensing/adaptation, acid tolerance mechanisms, protective mechanisms, systems for resistance to acid (AR1) or repressed by oxidants or glucose—AP. System of acid resistance 2 (AR2)/dependent on glutamate, system of acid resistance 3 (AR3)/arginine, ATR-reliant RpoS—EP, HJ, S-BH. Salt tolerance mechanisms—AP, RC. Genetic regulation by sigma factors—RC, S-HK, EB-M. Role of osmolytes in cryotolerance—RC, EB-M, FE, KB. C. jejuni heat-shock response—RC, S-HK. Desiccation tolerance—W-SB, AP. Biofilm formation and stress adaptation—RC, SR. Antibiotic susceptibility of C. jejuni—S-HK. All authors contributed to the article and approved the submitted version.
Funding
Article processing charges have been covered by the Korea Research Fellowship Program through the National Research Foundation of Korea (NRF) funded by the Ministry of Science, in Young Researchers Program [2018007551].
Conflict of Interest
The authors declare that the research was conducted in the absence of any commercial or financial relationships that could be construed as a potential conflict of interest.
References
Aarestrup F. M., Engberg J. (2001). Antimicrobial resistance of thermophilic Campylobacter. Vet. Res. 32, 311–321. doi: 10.1051/vetres:2001127
Alfredson D. A., Korolik V. (2007). Antibiotic resistance and resistance mechanisms in Campylobacter jejuni and Campylobacter coli. FEMS Microbiol. Lett. 277, 123–132. doi: 10.1111/j.1574-6968.2007.00935.x
Allen R. C., Angst D. C., Hall A. R. (2018). Resistance gene carriage predicts growth in the absence of antibiotics for natural and clinical Escherichia coli. Appl. Environ. Microbiol. 85, 02111–02118. doi: 10.1128/AEM.02111-18
Altekruse S. F., Stern N. J., Fields P. II, Swerdlow D. L. (1999). Campylobacter jejuni—an emerging foodborne pathogen. Emerg. Infect. Dis. 5, 28–35. doi: 10.3201/eid0501.990104
Arnold J. W., Silvers S. (2000). Comparison of poultry processing equipment surfaces for susceptibility to bacterial attachment and biofilm formation. Poult. Sci. 79, 1215–1221. doi: 10.1093/ps/79.8.1215
Arsic B., Barber J., Čikoš A., Mladenovic M., Stankovic N., Novak P. (2018). 16-membered macrolide antibiotics: a review. Int. J. Antimicrob. Agents 51, 283–298. doi: 10.1016/j.ijantimicag.2017.05.020
Asakura M., Samosornsuk W., Taguchi M., Kobayashi K., Misawa N., Kusumoto M., et al. (2007). Comparative analysis of cytolethal distending toxin (cdt) genes among Campylobacter jejuni, C. coli and C. fetus strains. Microb. Pathog. 42, 174–183. doi: 10.1016/j.micpath.2007.01.005
Askoura M., Sarvan S., Couture J.-F., Stintzi A. (2016). The Campylobacter jejuni ferric uptake regulator promotes acid survival and cross-protection against oxidative stress. Infect. Immun. 84, 1287–1300. doi: 10.1128/IAI.01377-15
Auesukaree C., Damnernsawad A., Kruatrachue M., Pokethitiyook P., Boonchird C., Kaneko Y., et al. (2009). Genome-wide identification of genes involved in tolerance to various environmental stresses in Saccharomyces cerevisiae. J. Appl. Genet. 50, 301–310. doi: 10.1007/BF03195688
Avila-Ramirez C., Tinajero-Trejo M., Davidge K. S., Monk C. E., Kelly D. J., Poole R. K. (2013). Do globins in microaerophilic Campylobacter jejuni confer nitrosative stress tolerance under oxygen limitation? Antioxid. Redox Signal. 18, 424–431. doi: 10.1089/ars.2012.4750
Azevedo N. F., Almeida C., Cerqueira L., Dias S., Keevil C. W., Vieira M. J. (2007). Coccoid form of Helicobacter pylori as a morphological manifestation of cell adaptation to the environment. Appl. Environ. Microbiol. 73, 3423–3427. doi: 10.1128/AEM.00047-07
Baillon M. L. A., van Vliet A. H., Ketley J. M., Constantinidou C., Penn C. W. (1999). An iron-regulated alkyl hydroperoxide reductase (AhpC) confers aerotolerance and oxidative stress resistance to the microaerophilic pathogen Campylobacter jejuni. J. Bacteriol. 181, 4798–4804. doi: 10.1128/JB.181.16.4798-4804.1999
Bang D. D., Nielsen E. M., Scheutz F., Pedersen K., Handberg K., Madsen M. (2003). PCR detection of seven virulence and toxin genes of Campylobacter jejuni and Campylobacter coli isolates from Danish pigs and cattle and cytolethal distending toxin production of the isolates. J. Appl. Microbiol. 94, 1003–1014. doi: 10.1046/j.1365-2672.2003.01926.x
Berrang M. E., Hofacre C. L., Meinersmann R. J. (2011). Forced hot air to dry feces and kill bacteria on transport cage flooring1. J. Appl. Poult. Res. 20, 567–572. doi: 10.3382/japr.2011-00391
Bingham-Ramos L. K., Hendrixson D. R. (2008). Characterization of two putative cytochrome c peroxidases of Campylobacter jejuni involved in promoting commensal colonization of poultry. Infect. Immun. 76, 1105–1114. doi: 10.1128/IAI.01430-07
Birk T., Ingmer H., Andersen M. T., Jørgensen K., Brøndsted L. (2004). Chicken juice, a food based model system suitable to study survival of Campylobacter jejuni. Lett. Appl. Microbiol. 38, 66–71. doi: 10.1046/j.1472-765x.2003.01446.x
Blanco-Lizarazo C. M., Sotelo-Díaz I., Arjona-Roman J. L., Llorente-Bousquets A., Miranda-Ruvalcaba R. (2018). Effect of starter culture and low concentrations of sodium nitrite on fatty acids, color, and Escherichia coli behavior during salami processing. Int. J. Food Sci. 2018, 5934305. doi: 10.1155/2018/5934305
Bolinger H. K., Zhang Q., Miller W. G., Kathariou S. (2018). Lack of evidence for erm (B) infiltration into erythromycin-resistant Campylobacter coli and Campylobacter jejuni from commercial turkey production in Eastern North Carolina: a major turkey-growing region in the United States. Foodborne Pathog. Dis. 15, 698–700. doi: 10.1089/fpd.2018.2477
Bolton D. J. (2015). Campylobacter virulence and survival factors. Food Microbiol. 48, 99–108. doi: 10.1016/j.fm.2014.11.017
Bronnec V., Turoňová H., Bouju A., Cruveiller S., Rodrigues R., Demnerova K., et al. (2016). Adhesion, biofilm formation, and genomic features of Campylobacter jejuni Bf, an atypical strain able to grow under aerobic conditions. Front. Microbiol. 7, 1002. doi: 10.3389/fmicb.2016.01002
Brown H. L., Reuter M., Salt L. J., Cross K. L., Betts R. P. (2014). A.H.M. van Vliet, Chicken juice enhances surface attachment and biofilm formation of Campylobacter jejuni. Appl. Environ. Microbiol. 80, 7053–7060. doi: 10.1128/AEM.02614-14
Burgess C. M., Gianotti A., Gruzdev N., Holah J., Knøchel S., Lehner A., et al. (2016). The response of foodborne pathogens to osmotic and desiccation stresses in the food chain. Int. J. Food Microbiol. 221, 37–53. doi: 10.1016/j.ijfoodmicro.2015.12.014
Burt S. A., van der Zee R., Koets A. P., de Graaff A. M., van Knapen F., Gaastra W., et al. (2007). Carvacrol induces heat shock protein 60 and inhibits synthesis of flagellin in Escherichia coli O157: H7. Appl. Environ. Microbiol. 73 (14), 4484–4490. doi: 10.1128/AEM.00340-07
Butler R. C., Lund V., Carlson D. A. (1987). Susceptibility of Campylobacter jejuni and Yersinia enterocolitica to UV radiation. Appl. Environ. Microbiol. 53, 375–378. doi: 10.1128/AEM.53.2.375-378.1987
Butzler J. P., Oosterom J. (1991). Campylobacter: pathogenicity and significance in foods. Int. J. Food Microbiol. 12, 1–8. doi: 10.1016/0168-1605(91)90043-O
Cain J. A., Dale A. L., Niewold P., Klare W. P., Man L., White M. Y., et al. (2019). Proteomics reveals multiple phenotypes associated with N-linked glycosylation in Campylobacter jejuni. Mol. Cell. Proteom. 18, 715–734. doi: 10.1074/mcp.RA118.001199
Cardoso K., Gandra R. F., Wisniewski E. S., Osaku C. A., Kadowaki M. K., Felipach-Neto V., et al. (2010). DnaK and GroEL are induced in response to antibiotic and heat shock in Acinetobacter baumannii. J. Med. Microbiol. 59 (9), 1061–1068. doi: 10.1099/jmm.0.020339-0
Cariri M. L., de Melo A. N. F., Mizzi L., Ritter A. C., Tondo E., de Souza E. L., et al. (2019). Quantitative assessment of tolerance response to stress after exposure to oregano and rosemary essential oils, carvacrol and 1, 8-cineole in Salmonella Enteritidis 86 and its isogenic deletion mutants Δdps, ΔrpoS and ΔompR. Food Res. Int. 122, 679–687. doi: 10.1016/j.foodres.2019.01.046
Carrillo C. D., Taboada E., Nash J. H., Lanthier P., Kelly J., Lau P. C., et al. (2004). Genome-wide expression analyses of Campylobacter jejuni NCTC11168 reveals coordinate regulation of motility and virulence by flhA. J. Biol. Chem. 279, 20327–20338. doi: 10.1074/jbc.M401134200
Centers for Disease Control and Prevention(CDC) (2013). Multistate outbreak of Campylobacter jejuni infections associated with undercooked chicken livers—northeastern United State. MMWR Morb. Mortal. Wkly. Rep. 62, 874–876.
Chaveerach P., ter Huurne A. A. H. M., Lipman L. J. A., van Knapen F. (2003). Survival and resuscitation of ten strains of Campylobacter jejuni and Campylobacter coli under acid conditions. Appl. Environ. Microbiol. 69, 711–714. doi: 10.1128/AEM.69.1.711-714.2003
Chen A. P., Wang G. L., Qu Z. L., Lu C. X., Liu N., Wang F., et al. (2007). Ectopic expression of ThCYP1, a stress-responsive cyclophilin gene from Thellungiella halophila, confers salt tolerance in fission yeast and tobacco cells. Plant Cell Rep. 26, 237–245. doi: 10.1007/s00299-006-0238-y
Chon M. T., Ingmer H., Mulholland F., Jørgensen K., Wells J. M., Brøndsted L. (2007). Contribution of conserved ATP-dependent proteases of Campylobacter jejuni to stress tolerance and virulence. Appl. Environ. Microbiol. 73, 7803–7813. doi: 10.1128/AEM.00698-07
Coward C., van Diemen P. M., Conlan A. J., Gog J. R., Stevens M. P., Jones M. A., et al. (2008). Competing isogenic Campylobacter strains exhibit variable population structures in vivo. Appl. Environ. Microbiol. 74, 3857–3867. doi: 10.1128/AEM.02835-07
Dasti J. II, Tareen A. M., Lugert R., Zautner A. E., Groß U. (2010). Campylobacter jejuni: A brief overview on pathogenicity-associated factors and disease-mediating mechanisms. Int. J. Med. Microbiol. 300, 205–211. doi: 10.1016/j.ijmm.2009.07.002
Day J. B., Hammack T. S. (2019). Bio-Plex suspension array immuno-detection of Listeria monocytogenes from cantaloupe and packaged salad using virulence protein inducing activated charcoal enrichment media. Food Microbiol. 84, 103225. doi: 10.1016/j.fm.2019.05.009
de Oliveira M. G., Rizzi C., Galli V., Lopes G. V., Haubert L., Dellagostin O. A., et al. (2019). Presence of genes associated with adhesion, invasion, and toxin production in Campylobacter jejuni isolates and effect of temperature on their expression. Can. J. Microbiol. 65, 253–260. doi: 10.1139/cjm-2018-0539
Devi A., Mahony T. J., Wilkinson J. M., Vanniasinkam T. (2019). Antimicrobial susceptibility of clinical isolates of Campylobacter jejuni from New South Wales, Australia. J. Glob. Antimicrob. Resist. 16, 76–80. doi: 10.1016/j.jgar.2018.09.011
Duffy L. (2019). Campylobacter through poultry processing: selection and survival. The University of Queensland, PhD Thesis, pp. 1–331. doi: /10.14264/uql.2019.26
Dykes G. A., Sampathkumar B., Korber D. R. (2003). Planktonic or biofilm growth affects survival, hydrophobicity and protein expression patterns of a pathogenic Campylobacter jejuni strain. Int. J. Food Microbiol. 89, 1–10. doi: 10.1016/S0168-1605(03)00123-5
EFSA (2010). Scientific opinion on quantification of the risk posed by broiler meat to human campylobacteriosis in the EU. EFSA J. 8, 200. doi: 10.3389/fmicb.2011.00200
EFSA (2011). Scientific opinion on Campylobacter in broiler meat production: control options and performance objectives and/or targets at different stages of the food chain. EFSA J. 9, 2105–2246. doi: 10.2903/j.efsa.2011.2105
Eguchi Y., Utsumi R. (2016). “Two component Systems in Sensing and Adapting to Acid Stress in Escherichia Coli,” in Stress and environmental regulation of gene expression and adaptation in bacteria. Ed. de Brujin F. J. (Hoboken, NJ: Wiley), 927–934.
Einsle O. (2011). Structure and function of formate-dependent cytochrome c nitrite reductase, NrfA. Methods Enzymol. 496, 399–422. doi: 10.1016/B978-0-12-386489-5.00016-6
Ellström P., Hansson I., Nilsson A., Rautelin H., Olsson Engvall E. (2016). Lipooligosaccharide locus classes and putative virulence genes among chicken and human Campylobacter jejuni isolates. BMC Microbiol. 16, 116. doi: 10.1186/s12866-016-0740-5
Elvers K. T., Wu G., Gilberthorpe N. J., Poole R. K., Park S. F. (2004). Role of an inducible single-domain hemoglobin in mediating resistance to nitric oxide and nitrosative stress in Campylobacter jejuni and Campylobacter coli. J. Bacteriol. 186, 5332–5341. doi: 10.1128/JB.186.16.5332-5341.2004
El-Tawab A., Awad A., Elhofy F., Hotzel H., Sobhy M. (2019). Phenotypic and genotypic chactracterization of Campylobacter isolated from poultry. Benha V. M. J. 37 (2), 33–36.
Eucker T. P., Konkel M. E. (2012). The cooperative action of bacterial fibronectin-binding proteins and secreted proteins promote maximal Campylobacter jejuni invasion of host cells by stimulating membrane ruffling. Cell. Microbiol. 14, 226–238. doi: 10.1111/j.1462-5822.2011.01714.x
Fakruddin M., Mannan K. S. B., Andrews S. (2013). Viable but nonculturable bacteria: food safety and public health perspective. ISRN Microbiol. 2013, 703813. doi: 10.1155/2013/703813
Farfán M., Lártiga N., Benavides M. B., Alegria-Morán R., Sáenz L., Salcedo C., et al. (2019). Capacity to adhere to and invade human epithelial cells, as related to the presence of virulence genes in, motility of, and biofilm formation of Campylobacter jejuni strains isolated from chicken and cattle. Can. J. Microbiol. 65, 126–134. doi: 10.1139/cjm-2018-0503
Feng J., Ma L., Nie J., Konkel M. E., Lu X. (2018). Environmental stress-induced bacterial lysis and extracellular DNA release contribute to Campylobacter jejuni biofilm formation. Appl. Environ. Microbiol. 84, e02068–e02017. doi: 10.1128/AEM.02068-17
Fernández H., Vergara M., Tapia F. (1985). Dessication resistance in thermotolerant Campylobacter species. Infection 13, 197. doi: 10.1007/BF01642813
Ferreira A., O’Byrne C. P., Boor K. J. (2001). Role of ςB in heat, ethanol, acid, and oxidative stress resistance and during carbon starvation in Listeria monocytogenes. Appl. Environ. Microbiol. 67, 4454–4457. doi: 10.1128/AEM.67.10.4454-4457.2001
Ferrero R. L., Lee A. (1988). Motility of Campylobacter jejuni in a viscous environment: comparison with conventional rod-shaped bacteria. J. Gen. Microbiol. 134, 53–59. doi: 10.1099/00221287-134-1-53
Fields J. A., Thompson S. A. (2008). Campylobacter jejuni CsrA mediates oxidative stress responses, biofilm formation, and host cell invasion. J. Bacteriol. 190, 3411–3416. doi: 10.1128/JB.01928-07
Frirdich E., Biboy J., Pryjma M., Lee J., Huynh S., Parker C. T., et al. (2019). The Campylobacter jejuni helical to coccoid transition involves changes to peptidoglycan and the ability to elicit an immune response. Mol. Microbiol. 112, 280–301. doi: 10.1111/mmi.14269
Gangaiah D., Liu Z., Arcos J., Kassem I. I., Sanad Y., Torrelles J. B., et al. (2010). Polyphosphate kinase 2: a novel determinant of stress responses and pathogenesis in Campylobacter jejuni. PloS One 5, e12142. doi: 10.1371/journal.pone.0012142
García-Peña F. J., Llorente M. T., Serrano T., Ruano M. J., Belliure J., Benzal J., et al. (2017). Isolation of Campylobacter spp. from three species of antarctic penguins in different geographic locations. EcoHealth 14, 78–87. doi: 10.1007/s10393-016-1203-z
Gaynor E. C., Wells D. H., MacKichan J. K., Falkow S. (2005). The Campylobacter jejuni stringent response controls specific stress survival and virulence-associated phenotypes. Mol. Microbiol. 56, 8–27. doi: 10.1111/j.1365-2958.2005.04525.x
Geng Y., Liu G., Liu L., Deng Q., Zhao L., Sun X. X., et al. (2019). Real-time recombinase polymerase amplification assay for the rapid and sensitive detection of Campylobacter jejuni in food samples. J. Microbiol. Methods 157, 31–36. doi: 10.1016/j.mimet.2018.12.017
Gerth U., Krüger E., Derré I., Msadek T., Hecker M. (1998). Stress induction of the Bacillus subilis clpP gene encoding a homologue of the proteolytic component of the Clp protease and the involvement of ClpP and ClpX in stress tolerance. Mol. Microbiol. 28, 787–802. doi: 10.1046/j.1365-2958.1998.00840.x
Ghazaei C. (2017). Role and mechanism of the Hsp70 molecular chaperone machines in bacterial pathogens. J. Med. Microbiol. 66 (3), 259–265. doi: 10.1099/jmm.0.000429
Giuliodori A. M., di Pietro F., Marzi S., Masquida B., Wagner R., Romby P., et al. (2010). The cspA mRNA is a thermosensor that modulates translation of the cold-shock protein CspA. Mol. Cell 37, 21–33. doi: 10.1016/j.molcel.2009.11.033
Gomes C. N., Passaglia J., Vilela F. P., da Silva F. M. P., Duque S. S., Falcao J. P. (2018). High survival rates of Campylobacter coli under different stress conditions suggest that more rigorous food control measures might be needed in Brazil. Food Microbiol. 73, 327–333. doi: 10.1016/j.fm.2018.02.014
Graillot V., Dormoy I., Dupuy J., Shay J. W., Huc L., Mirey G., et al. (2016). Genotoxicity of cytolethal distending toxin (CDT) on isogenic human colorectal cell lines: potential promoting effects for colorectal carcinogenesis. Front. Cell. Infect. Microbiol. 6, 34. doi: 10.3389/fcimb.2016.00034
Guerry P. (2007). Campylobacter flagella: not just for motility. Trends Microbiol. 15, 456–461. doi: 10.1016/j.tim.2007.09.0
Guévremont E., Lamoureux L., Ward P., Villeneuve S. (2015). Survival of Campylobacter jejuni on fresh spinach stored at 4 °C or 12 °C. Food Control 50, 736–739. doi: 10.1016/j.foodcont.2014.10.023
Habib I., Uyttendaele M., de Zutter L. (2010). Survival of poultry-derived Campylobacter jejuni of multilocus sequence type clonal complexes 21 and 45 under freeze, chill, oxidative, acid and heat stresses. Food Microbiol. 27, 829–834. doi: 10.1016/j.fm.2010.04.009
Haughton P. N., Lyng J. G., Morgan D. J., Cronin D. A., Fanning S., Whyte P. (2011). Efficacy of high-intensity pulsed light for the microbiological decontamination of chicken, associated packaging, and contact surfaces. Foodborne Pathog. Dis. 8, 109–117. doi: 10.1089/fpd.2010.0640
Hazeleger W. C., Wouters J. A., Rombouts F. M., Abee T. (1998). Physiological activity of Campylobacter jejuni far below the minimal growth temperature. Appl. Environ. Microbiol. 64, 3917–3922. doi: 10.1128/AEM.64.10.3917-3922.1998
Healy B., Cooney S., O’Brien S., Iversen C., Whyte P., Nally J., et al. (2010). Cronobacter (Enterobacter sakazakii): an opportunistic foodborne pathogen. Foodborne Pathog. Dis. 7, 339–350. doi: 10.1089/fpd.2009.0379
Hénault-Ethier L., Martin V. J., Gélinas Y. (2016). Persistence of Escherichia coli in batch and continuous vermicomposting systems. Waste Manage. 56, 88–99. doi: 10.1016/j.wasman.2016.07.033
Hugdahl M. B., Beery J. T., Doyle M. P. (1988). Chemotactic behavior of Campylobacter jejuni. Infect. Immun. 56, 1560–1566. doi: 10.1128/IAI.56.6.1560-1566.1988
Humphrey T., Mason M., Martin K. (1995). The isolation of Campylobacter jejuni from contaminated surfaces and its survival in diluents. Int. J. Food Microbiol. 26, 295–303. doi: 10.1016/0168-1605(94)00135-s
Humphrey T., O’Brien S., Madsen M. (2007). Campylobacters as zoonotic pathogens: a food production perspective. Int. J. Food Microbiol. 117, 237–257. doi: 10.1016/j.ijfoodmicro.2007.01.006
Hwang S., Jeon B., Yun J., Ryu S. (2011). Roles of RpoN in the resistance of Campylobacter jejuni under various stress conditions. BMC Microbiol. 11, 207. doi: 10.1186/1471-2180-11-207
Isohanni P. M., Lyhs U. (2009). Use of ultraviolet irradiation to reduce Campylobacter jejuni on broiler meat. Poult. Sci. 88, 661–668. doi: 10.3382/ps.2008-00259
Isohanni P., Huehn S., Aho T., Alter T., Lyhs U. (2013). Heat stress adaptation induces cross-protection against lethal acid stress conditions in Arcobacter butzleri but not in Campylobacter jejuni. Food Microbiol. 34, 431–435. doi: 10.1016/j.fm.2013.02.001
Jacoby G. A. (2005). Mechanisms of resistance to quinolones. Clin. Infect. Dis. 41, S120–S126. doi: 10.1086/428052
Jang K. I., Kim M. G., Ha S. D., Kim K. S., Lee K. H., Chung D. H., et al. (2007). Morphology and adhesion of Campylobacter jejuni to chicken skin under varying conditions. J. Microbiol. Biotechnol. 17, 202–206.
Jin X., Riedel-Kruse I. H. (2018). Biofilm Lithography enables high-resolution cell patterning via optogenetic adhesin expression. Proc. Natl. Acad. Sci. U. S. A. 115, 3698–3703. doi: 10.1073/pnas.1720676115
Jones D. M., Sutcliffe E. M., Curry A. (1991). Recovery of viable but non-culturable Campylobacter jejuni. J. Gen. Microbiol. 137, 2477–2482. doi: 10.1099/00221287-137-10-2477
Jones D. M., Sutcliffe E. M., Rios R., Fox A. J., Curry A. (1993). Campylobacter jejuni adapts to aerobic metabolism in the environment. J. Med. Microbiol. 38, 145–150. doi: 10.1099/00222615-38-2-145
Kaakoush N. O., Miller W. G., de Reuse H., Mendz G. L. (2007). Oxygen requirement and tolerance of Campylobacter jejuni. Res. Microbiol. 158, 644–650. doi: 10.1016/j.resmic.2007.07.009
Kang G. Z., Li G. Z., Liu G. Q., Xu W., Peng X. Q., Wang C. Y., et al. (2013). Exogenous salicylic acid enhances wheat drought tolerance by influence on the expression of genes related to ascorbate-glutathione cycle. Biol. Plantarum 57 (4), 718–724. doi: 10.1007/s10535-013-0335-z
Karki A. B., Wells H., Fakhr M. K. (2019). Retail liver juices enhance the survivability of Campylobacter jejuni and Campylobacter coli at low temperatures. Sci. Rep. 9, 2733. doi: 10.1038/s41598-018-35820-7
Kim J. S., Artymovich K. A., Hall D. F., Smith E. J., Fulton R., Bell J., et al. (2012). Passage of Campylobacter jejuni through the chicken reservoir or mice promotes phase variation in contingency genes Cj0045 and Cj0170 that strongly associates with colonization and disease in a mouse model. Microbiology 158, 1304–1316. doi: 10.1099/mic.0.057158-0
Kim J. C., Oh E., Kim J., Jeon B. (2015). Regulation of oxidative stress resistance in Campylobacter jejuni, a microaerophilic foodborne pathogen. Front. Microbiol. 6, 751. doi: 10.3389/fmicb.2015.00751
Kim S. H., Park C., Lee E. J., Bang W. S., Kim Y. J., Kim J. S. (2017). Biofilm formation of Campylobacter strains isolated from raw chickens and its reduction with DNase I treatment. Food Control. 71, 94–100. doi: 10.1016/j.foodcont.2016.06.038
Klancnik A., Guzej B., Jamnik P., Vuckovic D., Abram M., Mozina S. S. (2009). Stress response and pathogenic potential of Campylobacter jejuni cells exposed to starvation. Res. Microbiol. 160, 345–352. doi: 10.1016/j.resmic.2009.05.002
Kovacs J. K., Felso P., Horvath G., Schmidt J., Dorn A., Abraham H., et al. (2019). Stress response and virulence potential modulating effect of peppermint essential oil in Campylobacter jejuni. BioMed. Res. Int. 2019, 2971741. doi: 10.1155/2019/2971741
Kusumaningrum H. D., Riboldi G., Hazeleger W. C., Beumer R. R. (2003). Survival of foodborne pathogens on stainless steel surfaces and cross-contamination to foods. Int. J. Food Microbiol. 85, 227–236. doi: 10.1016/S0168-1605(02)00540-8
Lake I. R., Colón-González F. J., Takkinen J., Rossi M., Sudre B., Dias J. G., et al. (2019). Exploring Campylobacter seasonality across Europe using The European Surveillance System (TESSy), 2008 to 2016. Euro Surveill. 24, 1800028. doi: 10.2807/1560-7917.ES.2019.24.13.180028
Lapierre L., Gatica M. A., Riquelme V., Vergara C., Yanez J. M., San Martin B., et al. (2016). Characterization of antimicrobial susceptibility and its association with virulence genes related to adherence, invasion, and cytotoxicity in Campylobacter jejuni and Campylobacter coli isolates from animals, meat, and humans. Microb. Drug Resist. 22, 432–444. doi: 10.1089/mdr.2015.0055
Laranjo M., Oliveira S. (2011). Tolerance of Mesorhizobium type strains to different environmental stresses. Antonie Van Leeuwenhoek. 99, 651–662. doi: 10.1007/s10482-010-9539-9
Lertsethtakarn P., Ottemann K. M., Hendrixson D. R. (2011). Motility and chemotaxis in Campylobacter and Helicobacter. Annu. Rev. Microbiol. 65, 389–410. doi: 10.1146/annurev-micro-090110-102908
Li X., Kim M.-J., Yuk H.-G. (2018). Influence of 405 nm light-emitting diode illumination on the inactivation of Listeria monocytogenes and Salmonella spp. on ready-to-eat fresh salmon surface at chilling storage for 8 h and their susceptibility to simulated gastric fluid. Food Control 88, 61–68. doi: 10.1016/j.foodcont.2018.01.002
Ligowska M., Cohn M. T., Stabler R. A., Wren B. W., Brondsted L. (2011). Effect of chicken meat environment on gene expression of Campylobacter jejuni and its relevance to survival in food. Int. J. Food Microbiol. 145, S111–S115. doi: 10.1016/j.ijfoodmicro.2010.08.027
Lin A. E., Krastel K., Hobb R. II, Thompson S. A., Cvitkovitch D. G., Gaynor E. C. (2009a). Atypical roles for Campylobacter jejuni amino acid ATP binding cassette transporter components PaqP and PaqQ in bacterial stress tolerance and pathogen-host cell dynamics. Infect. Immun. 77 (11), 4912–4924. doi: 10.1128/IAI.00571-08
Lin A. E., Krastel K., Hobb R. II, Thompson S. A., Cvitkovitch D. G., Gaynor E. C. (2009b). Atypical roles for Campylobacter jejuni AA-ABC transporter components PaqP and PaqQ in bacterial stress tolerance and pathogen-host cell dynamics. Infect. Immun. 7 (11). doi: 10.1128/IAI.00571-08
Linton D., Gilbert M., Hitchen P. G., Dell A., Morris H. R., Wakarchuk W. W., et al. (2000). Phase variation of a beta-1,3 galactosyltransferase involved in generation of the ganglioside GM1-like lipo-oligosaccharide of Campylobacter jejuni. Mol. Microbiol. 37, 501–514. doi: 10.1046/j.1365-2958.2000.02020.x
Longo A., Spano G. (2019). “Stress responses of LAB,” in Food molecular microbiology. Eds. Paramithiotis S., Patra J. K. (UK: CRC Press), 164–182.
Lu X., Liu Q., Wu D., Al-Qadiri H. M., Al-Alami N. I., Kang D. H., et al. (2011). Using of infrared spectroscopy to study the survival and injury of Escherichia coli O157:H7, Campylobacter jejuni and Pseudomonas aeruginosa under cold stress in low nutrient media. Food Microbiol. 28, 537–546. doi: 10.1016/j.fm.2010.11.002
Luo C., Lü F., Shao L., He P. (2015). Application of eco-compatible biochar in anaerobic digestion to relieve acid stress and promote the selective colonization of functional microbes. Water Res. 68, 710–718. doi: 10.1016/j.watres.2014.10.052
Ma L., Wang Y., Shen J., Zhang Q., Wu C. (2014). Tracking Campylobacter contamination along a broiler chicken production chain from the farm level to retail in China. Int. J. Food Microbiol. 181, 77–84. doi: 10.1016/j.ijfoodmicro.2014.04.023
Ma Z., Bumunang E. W., Stanford K., Bie X., Niu Y. D., McAllister T. A. (2019). Biofilm formation by shiga toxin-producing Escherichia coli on stainless steel coupons as affected by temperature and incubation time. Microorganisms 7:95. doi: 10.3390/microorganisms7040095
Maes S., Heyndrickx M., Vackier T., Steenackers H., Verplaetse A., Reu K. (2019). Identification and spoilage potential of the remaining dominant microbiota on food contact surfaces after cleaning and disinfection in different food industries. J. Food Prot. 82, 262–275. doi: 10.4315/0362-028X.JFP-18-226
Magajna B. A., Schraft H. (2015). Campylobacter jejuni biofilm cells become viable but non-culturable (VBNC) in low nutrient conditions at 4 °C more quickly than their planktonic counterparts. Food Control. 50, 45–50. doi: 10.1016/j.foodcont.2014.08.022
Master S. S., Springer B., Sander P., Boettger E. C., Deretic V., Timmins G. S. (2002). Oxidative stress response genes in Mycobacterium tuberculosis: role of ahpC in resistance to peroxynitrite and stage-specific survival in macrophages. Microbiology 148, 3139–3144. doi: 10.1099/00221287-148-10-3139
Mavri A., Ribič U., Možina S. S. (2016). “The biocide and antibiotic resistance in Campylobacter jejuni and Campylobacter coli,” in Emerging and traditional technologies for safe, healthy and quality food. Eds. Nedovič V., Raspor P., Levič J., Šaponjac V. T., Barbosa-Cánovas G. V.(Springer, Cham: International Publishing Switzerland), 269–283.
Mayton H. M., Marcus I. M., Walker S. L. (2019). Escherichia coli O157:H7 and Salmonella Typhimurium adhesion to spinach leaf surfaces: Sensitivity to water chemistry and nutrient availability. Food Microbiol. 78, 134–142. doi: 10.1016/j.fm.2018.10.002
Meade K. G., Narciandi F., Cahalane S., Reiman C., Allan B., O’Farrelly C. (2009). Comparative in vivo infection models yield insights on early host immune response to Campylobacter in chickens. Immunogenetics 61, 101–110. doi: 10.1007/s00251-008-0346-7
Mehat J. W., Park S. F., van Vliet A. H. M., La Ragione R. M. (2018). CapC, a novel autotransporter and virulence factor of Campylobacter jejuni. Appl. Environ. Microbiol. 84, e01032–e01018. doi: 10.1128/AEM.01032-18
Melo R. T., Grazziotin A. L., Valadares Júnior E. C., Prado R. R., Mendonça E. P., Monteiro G. P., et al. (2019). Evolution of Campylobacter jejuni of poultry origin in Brazil. Food Microbiol. 82, 489–496. doi: 10.1016/j.fm.2019.03.009
Metselaar K. I., Ibusquiza P. S., Camargo A. R. O., Krieg M., Zwietering M. H., den Besten H. M. W., et al. (2015). Performance of stress resistant variants of Listeria monocytogenes in mixed species biofilms with Lactobacillus plantarum. Int. J. Food Microbiol. 213, 24–30. doi: 10.1016/j.ijfoodmicro.2015.04.021
Miao J., Lin S., Soteyome T., Peters B. M., Li Y., Chen H., et al. (2019). Biofilm formation of Staphylococcus aureus under food heat processing conditions: first report on CML production within biofilm. Sci. Rep. 9, 1312. doi: 10.1038/s41598-018-35558-2
Monk C. E., Pearson B. M., Mulholland F., Smith H. K., Poole R. K. (2008). Oxygen- and NssR-dependent globin expression and enhanced iron acquisition in the response of Campylobacter to nitrosative stress. J. Biol. Chem. 283, 28413–28425. doi: 10.1074/jbc.M801016200
Mühlig A., Behr J., Scherer S., Müller-Herbst S. (2014). Stress response of Salmonella enterica serovar typhimurium to acidified nitrite. Appl. Environ. Microbiol. 80, 6373–6382. doi: 10.1128/AEM.01696-14
Murphy C., Carroll C., Jordan K. N. (2003). Induction of an adaptive tolerance response in the foodborne pathogen, Campylobacter jejuni. FEMS Microbiol. Lett. 223, 89–93. doi: 10.1016/S0378-1097(03)00348-3
Nachamkin I., Yang X. H., Stern N. J. (1993). Role of Campylobacter jejuni flagella as colonization factors for three-day-old chicks: analysis with flagellar mutants. Appl. Environ. Microbiol. 59, 1269–1273. doi: 10.1128/AEM.59.5.1269-1273.1993
Narvaez-Bravo C., Taboada E. N., Mutschall S. K., Aslam M. (2017). Epidemiology of antimicrobial resistant Campylobacter spp. isolated from retail meats in Canada. Int. J. Food Microbiol. 253, 43–47.
Negretti N. M., Clair G., Talukdar P. K., Gourley C. R., Huynh S., Adkins J. N., et al. (2019). Campylobacter jejuni demonstrates conserved proteomic and transcriptomic responses when co-cultured with human INT 407 and Caco-2 epithelial cells. Front. Microbiol. 10, 755. doi: 10.3389/fmicb.2019.00755
Nguyen H. T. T., Corry J. E. L., Miles C. A. (2006). Heat resistance and mechanism of heat inactivation in thermophilic campylobacters. Appl. Environ. Microbiol. 72, 908–913. doi: 10.1128/AEM.72.1.908-913.2006
Noreen Z. (2019). Role of Type VI Secretion System in Stress Adaptations and Developing Control Strategies for Campylobacter jejuni (Doctoral dissertation (Islamabad: COMSATS University).
Oh E., McMullen L., Jeon B. (2015). Impact of oxidative stress defense on bacterial survival and morphological change in Campylobacter jejuni under aerobic conditions. Front. Microbiol. 6, 295. doi: 10.3389/fmicb.2015.00295
Oh J. Y., Kwon Y. K., Wei B., Jang H. K., Lim S. K., Kim C. H., et al. (2017). Epidemiological relationships of Campylobacter jejuni strains isolated from humans and chickens in South Korea. J. Microbiol. 55, 13–20. doi: 10.1007/s12275-017-6308-8
Oh E., Andrews K. J., McMullen L. M., Jeon B. (2019). Tolerance to stress conditions associated with food safety in Campylobacter jejuni strains isolated from retail raw chicken. Sci. Rep. 9 (1), 1–9. doi: 10.1038/s41598-019-48373-0
Olesen I., Vogensen F. K., Jespersen L. (2009). Gene transcription and virulence potential of Listeria monocytogenes strains after exposure to acidic and NaCl stress. Foodborne Pathog. Dis. 6, 669–680. doi: 10.1089/fpd.2008.0243
Oliver J. D. (2010). Recent findings on the viable but nonculturable state in pathogenic bacteria. FEMS Microbiol. Rev. 34, 415–425. doi: 10.1111/j.1574-6976.2009.00200.x
Olsen I. (2015). Biofilm-specific antibiotic tolerance and resistance. Eur. J. Clin. Microbiol. Infect. Dis. 34, 877–886. doi: 10.1007/s10096-015-2323-z
Oosterom J., de Wilde G. J. A., de Boer E., de Blaauw L. H., Karman H. (1983). Survival of Campylobacter jejuni during poultry processing and pig slaughtering. J. Food Prot. 46, 702–706. doi: 10.4315/0362-028X-46.8.702
O’Sullivan L., Lucid A., Neve H., Franz C. M., Bolton D., McAuliffe O., et al. (2018). Comparative genomics of Cp8viruses with special reference to Campylobacter phage vB_CjeM_los1, isolated from a slaughterhouse in Ireland. Arch. Virol. 163 (8), 2139–2154.
Otigbu A. C., Clarke A. M., Fri J., Akanbi E. O., Njom H. A. (2018). Antibiotic sensitivity profiling and virulence potential of Campylobacter jejuni isolates from estuarine water in the Eastern Cape Province, South Africa. Int. J. Environ. Res. Public Health 15, 925. doi: 10.3390/ijerph15050925
Ovesen S., Durack J., Kirk K. F., Nielsen H. L., Nielsen H., Lynch S. V. (2019). Motility and biofilm formation of the emerging gastrointestinal pathogen Campylobacter concisus differs under microaerophilic and anaerobic environments. Gut Microbes. 10, 34–44. doi: 10.1080/19490976.2018.1472201
Park S. F. (2002). The physiology of Campylobacter species and its relevance to their role as foodborne pathogens. Int. J. Food Microbiol. 74, 177–188. doi: 10.1016/s0168-1605(01)00678-x
Parkhill J., Wren B. W., Mungall K., Ketley J. M., Churcher C., Basham D., et al. (2000). The genome sequence of the food-borne pathogen Campylobacter jejuni reveals hypervariable sequences. Nature 403, 665–668. doi: 10.1038/35001088
Parsell D. A., Lindquist S. (1993). The function of heat-shock proteins stress tolerance: degradation and reactivation of damaged proteins. Annu. Rev. Genet. 27, 437–496. doi: 10.1146/annurev.ge.27.120193.002253
Pedersen S. K., Wagenaar J. A., Vigre H., Roer L., Mikoleit M., Aidara-Kane A., et al. (2018). Proficiency of WHO global foodborne infections network external quality assurance system participants in identification and susceptibility testing of thermotolerant Campylobacter spp. from 2003 to 2012. J. Clin. Microbiol. 56, e01066–e01018. doi: 10.1128/JCM.01066-18
Petersen L., Larsen T. S., Ussery D. W., On S. L., Krogh A. (2003). RpoD promoters in Campylobacter jejuni exhibit a strong periodic signal instead of a -35 box. J. Mol. Biol. 326, 1361–1372. doi: 10.1016/S0022-2836(03)00034-2
Pittman M. S., Elvers K. T., Lee L., Jones M. A., Poole R. K., Park S. F., et al. (2007). Growth of Campylobacter jejuni on nitrate and nitrite: electron transport to NapA and NrfA via NrfH and distinct roles for NrfA and the globin Cgb in protection against nitrosative stress. Mol. Microbiol. 63, 575–590. doi: 10.1111/j.1365-2958.2006.05532.x
Poli V. F., Thorsen L., Olesen I., Wik M. T., Jespersen L. (2012). Differentiation of the virulence potential of Campylobacter jejuni strains by use of gene transcription analysis and a Caco-2 assay. Int. J. Food Microbiol. 155, 60–68. doi: 10.1016/j.ijfoodmicro.2012.01.019
Porcelli I., Reuter M., Pearson B. M., Wilhelm T., van Vliet A. H. M. (2013). Parallel evolution of genome structure and transcriptional landscape in the Epsilonproteobacteria. BMC Genomics 14, 616. doi: 10.1186/1471-2164-14-616
Poursina F., Fagri J., Mirzaei N., Safaei H. G. (2018). Overexpression of spoT gene in coccoid forms of clinical Helicobacter pylori isolates. Folia Microbiol. 63, 459–465. doi: 10.1007/s12223-017-0557-0
Prechtl R. M., Janßen D., Behr J., Ludwig C., Küster B., Vogel R. F., et al. (2018). Sucrose-induced proteomic response and carbohydrate utilization of Lactobacillus sakei TMW 1.411 during dextran formation. Front. Microbiol. 9, 2796. doi: 10.3389/fmicb.2018.02796
Rodrigues B., Alvares T., Costa M., Sampaio G., de L. Torre C. A. L., Panzenhagen P., et al (2019). Combined effect of modified atmosphere package and short-wave ultraviolet does not affect Proteus mirabilis growth on Rainbow Trout Fillets (Oncorhynchus mykiss). J. Food Nutr. Res. 7, 342–346. doi: 10.12691/jfnr-7-5-2
Sałamaszyńska-Guz A., Rose S., Lykkebo C. A., Taciak B., Bącal P., Uśpieński T., et al. (2018). Biofilm formation and motility are promoted by Cj0588-directed methylation of rRNA in Campylobacter jejuni. Front. Cell. Infect. Microbiol. 7, 533. doi: 10.3389/fcimb.2017.00533
Salma M., Rousseaux S., Sequeira-Le Grand A., Divol B., Alexandre H. (2013). Characterization of the viable but nonculturable (VBNC) state in Saccharomyces cerevisiae. PloS One 8, e77600. doi: 10.1371/journal.pone.0077600
Sarabhai S., Harjai K., Sharma P., Capalash N. (2015). Ellagic acid derivatives from Terminalia chebula Retz. increase the susceptibility of Pseudomonas aeruginosa to stress by inhibiting polyphosphate kinase. J. Appl. Microbiol. 118, 817–825. doi: 10.1111/jam.12733
Scuron M. D., Boesze-Battaglia K., Dlakic M., Shenker B. J. (2016). The cytolethal distending toxin contributes to microbial virulence and disease pathogenesis by acting as a tri-perditious toxin. Front. Cell. Infect. Microbiol. 6, 168. doi: 10.3389/fcimb.2016.00168
Silvan J. M., Zorraquin-Pena I., de Llano D. G., Moreno-Arribas M. V., Martinez-Rodriguez A. J. (2018). Antibacterial activity of glutathione-stabilized silver nanoparticles against Campylobacter multidrug-resistant strains. Front. Microbiol. 9, 458. doi: 10.3389/fmicb.2018.00458
Simmons M., Hiett K. L., Stern N. J., Frank J. F. (2008). Comparison of poultry exudate and carcass rinse sampling methods for the recovery of Campylobacter spp. subtypes demonstrates unique subtypes recovered from exudate. J. Microbiol. Methods 74, 89–93. doi: 10.1016/j.mimet.2008.03.007
Simões L. C., Simões M. (2013). Biofilms in drinking water: problems and solutions. RSC Adv. 3, 2520–2533. doi: 10.1039/c2ra22243d
Sommerlad S. M., Hendrixson D. R. (2007). Analysis of the roles of FlgP and FlgQ in flagellar motility of Campylobacter jejuni. J. Bacteriol. 189, 179–186. doi: 10.1128/JB.01199-06
Spector M. P., Kenyon W. J. (2012). Resistance and survival strategies of Salmonella enterica to environmental stresses. Food Res. Int. 45, 455–481. doi: 10.1016/j.foodres.2011.06.056
Speranza B., Corbo M. R., Sinigaglia M. (2011). Effects of nutritional and environmental conditions on Salmonella sp. biofilm formation. J. Food Sci. 76, M12–M16. doi: 10.1111/j.1750-3841.2010.01936.x
Stewart P. S., Costerton J. W. (2001). Antibiotic resistance of bacteria in biofilms. Lancet 358, 135–138. doi: 10.1016/S0140-6736(01)05321-1
Storz G., Imlay J. A. (1999). Oxidative stress. Curr. Opin. Microbiol. 2, 188–194. doi: 10.1016/s1369-5274(99)80033-2
Studholme D. J., Dixon R. (2003). Domain architectures of σ54-dependent transcriptional activators. J. Bacteriol. 185, 1757–1767. doi: 10.1128/JB.185.6.1757-1767.2003
Svensson S. L., Frirdich E., Gaynor E. C. (2008). “Chapter 32: Survival strategies of Campylobacter jejuni: stress responses, the viable but nonculturable state, and biofilms,” in Campylobacter, 3rd ed. Eds. Nachamkin I., Szymanski C. M., Blaser M. J. (Washington, DC: ASM Press), 571–590.
Svensson S. L., Davis L. M., Mackichan J. K., Allan B. J., Pajaniappan M., Thompson S. A., et al. (2009). The CprS sensor kinase of the zoonotic pathogen Campylobacter jejuni influences biofilm formation and is required for optimal chick colonization. Mol. Microbiol. 71, 253–272. doi: 10.1111/j.1365-2958.2008.06534.x
Tang Y., Cawthraw S., Bagnall M. C., Gielbert A. J., Woodward M. J., Petrovska L. (2017). Identification of temperature regulated factors of Campylobacter jejuni and their potential roles in virulence. AIMS Microbiol. 3 (4), 885. doi: 10.3934/microbiol.2017.4.885
Taylor A. J., Zakai S. A., Kelly D. J. (2017). The periplasmic chaperone network of Campylobacter jejuni: evidence that SalC (Cj1289) and PpiD (Cj0694) are involved in maintaining outer membrane integrity. Front. Microbiol. 8, 531. doi: 10.3389/fmicb.2017.00531
The A. H. T., Lee S. M., Dykes G. A. (2019). Association of some Campylobacter jejuni with Pseudomonas aeruginosa biofilms increases attachment under conditions mimicking those in the environment. PloS One 14, e0215275. doi: 10.1371/journal.pone.0215275
Ultee E., Ramijan K., Dame R. T., Briegel A., Claessen D. (2019). Stress-induced adaptive morphogenesis in bacteria. Adv. Microb. Physiol. 74, 97–141. doi: 10.1016/bs.ampbs.2019.02.001
Upadhyay A., Arsi K., Upadhyaya I., Donoghue A. M., Donoghue D. J. (2019). “Natural and environmentally friendly strategies for controlling Campylobacter jejuni colonization in poultry, survival in poultry products and infection in humans,” in Food safety in poultry meat production. Eds. Venkitanarayanan K., Thakur S., Ricke S. C. (CT, USA: Springer Nature Switzerland), 67–93.
Vázquez-Sánchez D., Habimana O., Holck A. (2013). Impact of food-related environmental factors on the adherence and biofilm formation of natural Staphylococcus aureus isolates. Curr. Microbiol. 66, 110–121. doi: 10.1007/s00284-012-0247-8
Völker U., Mach H., Schmid R., Hecker M. (1992). Stress proteins and cross-protection by heat shock and salt stress in Bacillus subtilis. J. Gen. Microbiol. 138, 2125–2135. doi: 10.1099/00221287-138-10-2125
Wadhams G. H., Armitage J. P. (2004). Making sense of it all: bacterial chemotaxis. Nat. Rev. Mol. Cell Biol. 5, 1024–1037. doi: 10.1038/nrm1524
Wang G., Clark C. G., Taylor T. M., Pucknell C., Barton C., Price L., et al. (2002). Colony multiplex PCR assay for identification and differentiation of Campylobacter jejuni, C. coli, C. lari, C. upsaliensis, and C. fetus subsp. fetus. J. Clin. Microbiol. 40, 4744–4747. doi: 10.1128/JCM.40.12.4744-4747.2002
Wang H., Ding S., Wang G., Xu X., Zhou G. (2013). In situ characterization and analysis of Salmonella biofilm formation under meat processing environments using a combined microscopic and spectroscopic approach. Int. J. Food Microbiol. 167, 293–302. doi: 10.1016/j.ijfoodmicro.2013.10.005
Wang C., Zhou H., Guo F., Yang B., Su X., Lin J., et al. (2020). Oral immunization of chickens with Lactococcus lactis expressing cjaA temporarily reduces Campylobacter jejuni colonization. Foodborne Pathog. Dis. 17 (6), 366–372.
Wassenaar T. M., van der Zeijst B. A., Ayling R., Newell D. G. (1993). Colonization of chicks by motility mutants of Campylobacter jejuni demonstrates the importance of flagellin A expression. J. Gen. Microbiol. 139, 1171–1175. doi: 10.1099/00221287-139-6-1171
Whitehouse C. A., Zhao S., Tate H. (2018). Antimicrobial resistance in Campylobacter species: mechanisms and genomic epidemiology. Adv. Appl. Microbiol. 103, 1–47. doi: 10.1016/bs.aambs.2018.01.001
Wieczorek K., Osek J. (2013). Antimicrobial resistance mechanisms among Campylobacter. BioMed. Res. Int. 2013, 340605. doi: 10.1155/2013/340605
Wieczorek K., Wolkowicz T., Osek J. (2018). Antimicrobial resistance and virulence-associated traits of Campylobacter jejuni isolated from poultry food chain and humans with diarrhea. Front. Microbiol. 9, 1508. doi: 10.3389/fmicb.2018.01508
Wirz S. E., Overesch G., Kuhnert P., Korczak B. M. (2010). Genotype and antibiotic resistance analyses of Campylobacter isolates from ceca and carcasses of slaughtered broiler flocks. Appl. Environ. Microbiol. 76 (19), 6377–6386. doi: 10.1128/AEM.00813-10
Wong E., Linton R. H., Gerrard D. E. (1998). Reduction of Escherichia coli and Salmonella senftenbergon pork skin and pork muscle using ultraviolet light. Food Microbiol. 15, 415–423. doi: 10.1006/fmic.1998.0185
Wösten M. M. S. M., Boeve M., Koot M. G. A., van Nuenen A. C., van der Zeijst B. A. M. (1998). Identification of Campylobacter jejuni promoter sequences. J. Bacteriol. 180, 594–599. doi: 10.1128/JB.180.3.594-599.1998
Wuichet K., Alexander R. P., Zhulin I. B. (2007). Comparative genomic and protein sequence analyses of a complex system controlling bacterial chemotaxis. Methods Enzymol. 422, 1–31. doi: 10.1016/S0076-6879(06)22001-9
Xiong J. (2009). Survival of Animal-derived Campylobacter Strains in Raw and Pasteurized Milk, and the Roles of Capsule in Campylobacter Survival in vitro, and in Chick Colonization. MS Thesis, 2009-09-10, University of North Carolina. Available at: http://www.lib.ncsu.edu/resolver/1840.16/1045
Xu G., Li C., Yao Y. (2009). Proteomics analysis of drought stress-responsive proteins in Hippophae rhamnoides L. Plant Mol. Biol. Rep. 27 (2), 153–161. doi: 10.1007/s11105-008-0067-y
Xu T., Yu M., Liu J., Lin H., Liang J., Zhang X. H. (2019). Role of RpoN from Labrenzia aggregata LZB033 (Rhodobacteraceae) in formation of flagella and biofilms, motility, and environmental adaptation. Appl. Environ. Microbiol. 85, e02844–e02818. doi: 10.1128/AEM.02844-18
Yang S., Sadekuzzaman M., Ha S.-D. (2017). Reduction of Listeria monocytogenes on chicken breasts by combined treatment with UV-C light and bacteriophage ListShield. LWT 86, 193–200. doi: 10.1016/j.lwt.2017.07.060
Yaun B. R., Sumner S. S., Eifert J. D., Marcy J. E. (2003). Response of Salmonella and Escherichia coli O157:H7 to UV energy. J. Food Prot. 66, 1071–1073. doi: 10.4315/0362-028x-66.6.1071
Ye B., He S., Zhou X., Cui Y., Zhou M., Shi X. (2019). Response to acid adaptation in Salmonella enterica serovar enteritidis. J. Food Sci. 84, 599–605. doi: 10.1111/1750-3841.14465
Yoon J. H., Lee S. Y. (2020). Characteristics of viable-but-nonculturable Vibrio parahaemolyticus induced by nutrient-deficiency at cold temperature. Crit. Rev. Food Sci. Nutr. 60, 1302–1320. doi: 10.1080/10408398.2019.1570076
Zeng X., Xu F., Lin J. (2013). Specific TonB-ExbB-ExbD energy transduction systems required for ferric enterobactin acquisition in Campylobacter. FEMS Microbiol. Lett. 347, 83–91. doi: 10.1111/1574-6968.12221
Zhang L., Man S. M., Day A. S., Leach S. T., Lemberg D. A., Dutt S., et al. (2009). Detection and isolation of Campylobacter species other than C. jejuni from children with Crohn’s disease. J. Clin. Microbiol. 47, 453–455. doi: 10.1128/JCM.01949-08
Zhao T., Ezeike G. O., Doyle M. P., Hung Y. C., Howell R. S. (2003). Reduction of Campylobacter jejuni on poultry by low-temperature treatment. J. Food Prot. 66, 652–655. doi: 10.4315/0362-028x-66.4.652
Zhao Z., Peng T., Oh J. I., Glaeser J., Weber L., Li Q., et al. (2019). A response regulator of the OmpR family is part of the regulatory network controlling the oxidative stress response of Rhodobacter sphaeroides. Environ. Microbiol. Rep. 11, 118–128. doi: 10.1111/1758-2229.12718
Zhichang Z., Wanrong Z., Jinping Y., Jianjun Z., Xufeng L. Z. L., Yang Y. (2010). Over-expression of Arabidopsis DnaJ (Hsp40) contributes to NaCl-stress tolerance. Afr. J. Biotechnol. 9, 972–978. doi: 10.5897/AJB09.1450
Zhuang H., Rothrock M. J. Jr., Hiett K. L., Lawrence K. C., Gamble G. R., Bowker B. C., et al. (2019). In-package air cold plasma treatment of chicken breast meat: treatment time effect. J. Food Qual. 2019, 1837351. doi: 10.1155/2019/1837351
Keywords: Campylobacter, stress, resistance mechanisms, stress adaptation, enteric bacteria
Citation: Kim S-H, Chelliah R, Ramakrishnan SR, Perumal AS, Bang W-S, Rubab M, Daliri EB-M, Barathikannan K, Elahi F, Park E, Jo HY, Hwang S-B and Oh DH (2021) Review on Stress Tolerance in Campylobacter jejuni. Front. Cell. Infect. Microbiol. 10:596570. doi: 10.3389/fcimb.2020.596570
Received: 19 August 2020; Accepted: 03 December 2020;
Published: 04 February 2021.
Edited by:
Alessandra Piccirillo, University of Padua, ItalyJon Audia, University of South Alabama, United States
Copyright © 2021 Kim, Chelliah, Ramakrishnan, Perumal, Bang, Rubab, Daliri, Barathikannan, Elahi, Park, Jo, Hwang and Oh. This is an open-access article distributed under the terms of the Creative Commons Attribution License (CC BY). The use, distribution or reproduction in other forums is permitted, provided the original author(s) and the copyright owner(s) are credited and that the original publication in this journal is cited, in accordance with accepted academic practice. No use, distribution or reproduction is permitted which does not comply with these terms.
*Correspondence: Se-Hun Kim, aG9vbnllNzdAbmF2ZXIuY29t; Ramachandran Chelliah, cmFtYWNoYW5kcmFuODY1QGdtYWlsLmNvbQ==; Deog Hwan Oh, ZGVvZ2h3YUBrYW5nd29uLmFjLmty
†These authors have contributed equally to this work and share first authorship