- 1Department of Biological and Environmental Science, Nanoscience Center, University of Jyväskylä, Jyväskylä, Finland
- 2Department of Biology, University of Turku, Turku, Finland
- 3Division of Clinical Microbiology, Department of Laboratory Medicine, Karolinska Institutet, Stockholm, Sweden
- 4Department of Clinical Microbiology, Karolinska University Hospital, Stockholm, Sweden
Over the past few decades, extensively drug resistant (XDR) resistant Klebsiella pneumoniae has become a notable burden to healthcare all over the world. Especially carbapenemase-producing strains are problematic due to their capability to withstand even last resort antibiotics. Some sequence types (STs) of K. pneumoniae are significantly more prevalent in hospital settings in comparison to other equally resistant strains. This provokes the question whether or not there are phenotypic characteristics that may render certain K. pneumoniae more suitable for epidemic dispersal between patients, hospitals, and different environments. In this study, we selected seven epidemic and non-epidemic carbapenem resistant K. pneumoniae isolates for extensive systematic characterization for phenotypic and genotypic qualities in order to identify potential factors that precede or emerge from epidemic successfulness. Studied characteristics include growth rates and densities in different conditions (media, temperature, pH, resource levels), tolerance to alcohol and drought, inhibition between strains, ability to compensate pH, as well as various genomic features. Overall, there are clear differences between isolates, yet, only drought tolerance was found to notably associate with non-epidemic K. pneumoniae strains. We further report a preliminary study on the potential to control K. pneumoniae ST11 with an antimicrobial component produced by a non-epidemic K. pneumoniae. This component initially restricts bacterial growth, but stable resistance develops rapidly in vitro.
Introduction
Klebsiella pneumoniae is a Gram-negative bacillus causing opportunistic infections outside of the gastrointestinal tract (Podschun and Ullman, 1998). Common conditions include pneumonia, urinary tract infections, wound infections, and less often liver abscess, meningitis, and septicemia. Some of the strains circulating in clinical settings are also showing increasingly virulent phenotypes (Pomakova et al., 2012; Shon et al., 2013). These strains are often characterized by hypermucoviscosity when cultivated on agar plates and they are more resilient against killing by serum or phagocytosis (Catalan-Najera et al., 2017). Moreover, extensively drug-resistant (XDR) among K. pneumoniae is increasing very rapidly compared to many other priority pathogens (World Health Organization, 2014). In particular, infections caused by K. pneumoniae strains which have developed resistance against newer generations of β-lactams, such as carbapenems, can be hazardous and often life-threatening. These carbapenemase genes are found to be abundant in bacteria originating from hospital environments although there are notable regional differences. Yet, sequence typing of the pathogens indicate that resistant K. pneumoniae strains can also disseminate globally between hospitals.
WHO has classified carbapenemase-producing K. pneumoniae as an urgent threat (World Health Organization, 2017). Often, K. pneumoniae isolates are typed by utilizing partial sequences from seven housekeeping genes. These genes are part of the core genome and hence unlikely to be horizontally transferred between different K. pneumoniae strains. As such, sequence typing provides a rudimentary approach to identify genetic similarity among isolates of different sources of origin. It appears that certain sequence types (STs) have been more successful in dispersing between hospitals compared to other equally resistant strains. NDM-1 metallo-β-lactamase producing ST11 and 14 have been noted to be responsible of epidemics in various countries (Yong et al., 2009; Pitout et al., 2015; Samuelsen et al., 2017). Klebsiella pneumoniae carbapenemase (KPC) producing ST258 have even been referred as hyperepidemic clone (Bowers et al., 2015) and its epidemic potential has been further investigated in several meta-analyses (Dautzenberg et al., 2016). ST512, a single-locus variant of ST258, is also highly associated in epidemics globally (Conte et al., 2016). ST147 is also hazardous with numerous virulence and resistance genes (Turton et al., 2018). Despite of the notion that certain STs appear to be more prone for inter-hospital dispersal, it is still unclear what qualities alongside of pathogenicity, if any, may be responsible for this epidemic success. Majority of the surveillance attempts focus on resistance profiles and genotypic features (Giske et al., 2012). Genomic data also accumulates rapidly as whole genome sequences of many strains have become available (Holt et al., 2015). Yet, the phenotypic characteristics of differently successful K. pneumoniae STs are rarely studied in detail, or the phenotypic analysis focus on specific traits such as hypermucoviscousity (Catalan-Najera et al., 2017). Comparison of the phenotypes of epidemic and non-epidemic strains could potentially reveal meaningful interactions between bacteria and their environment that contribute to the epidemic spread of XDR strains.
In this study, we selected 14 carbapenem resistant K. pneumoniae strains isolated from patients hospitalized in USA, Sweden, UK, Greece, or India (Kitchel et al., 2009; Samuelsen et al., 2009; Kitchel et al., 2010; Samuelsen et al., 2011; Vading et al., 2011; Giske et al., 2012; Hasan et al., 2014). Five of these STs are continuously being detected in hospitals in multiple countries and can be considered as epidemiologically successful or epidemic (Table 1). The rest of the STs have made only seldom appearances and have rarely if at all dispersed to other hospitals and may hence be considered as non-epidemic STs. Here, we systematically determined and measured potentially relevant characteristics for these strains in order to reveal differences that may correlate and perhaps partly explain the epidemic success.
Materials and Methods
Strains
All 14 studied Klebsiella pneumoniae strains (see Table 1) were Illumina sequenced at Karolinska Institutet, Sweden. Genome sequences can be found from GenBank under BioProject id PRJNA680903. Sequences of EKP24 and NKP2 were also PacBio sequenced in University of Helsinki, Finland. Sequenced genomes were annotated by Rapid Annotation using Subsystem Technology (RAST, https://rast.nmpdr.org) and both secure and potential protein coding genes were mapped and the distribution of protein families were compared between the strains. Resistance genes were identified with ResFinder (https://cge.cbs.dtu.dk/services/ResFinder/), prophages with Prophage Finder (https://omictools.com/prophage-finder-tool), and CRISPR-regions with CRISPR-finder (https://crispr.i2bc.paris-saclay.fr). An algorithm was written to identify unannotated short open reading frames (ORFs) from the genome files (Supplementary File 1). The algorithm scans the genome for ORFs that have a potential ribosome binding site upstream of the start codon and is not overlapping with annotated genes.
Growth Experiments
Growth densities to each strain were measured in temperature of +37°C and room temperature with 230 rpm shaking or without shaking. Cells were grown overnight in 5 ml of LB media (+37°C, 230 rpm) and then transferred into 5 ml of fresh LB media in 1:5,000 ratio. Fresh cultures were grown in experimental settings of +37°C and 230 rpm or RT and 0 rpm for 20 h and growth densities were calculated as colony forming units (cfu)/ml. As a standard initial liquid culture for the growth curve experiments all the strains were cultured in 5 ml of 100% LB, +37°C, and 230 rpm overnight and then transferred into experimental settings. In order to test the effect of shaking, the initiating cultures were prepared then transferred into 5 ml of LB in 1:100 ratio and grown in experimental settings of +37°C and 230 rpm and +37°C and 0 rpm. In growth curve experiments the effect of different media and varying concentrations and compositions of nutrients on growth was determined for each strain. One hundred percent LB was used throughout the experiments unless mentioned otherwise. Growth curves were measured in 10 and 1% LB, 100% BHI, and 100% of pure DMEM by diluting the initial culture in 1:100. Growth curves were measured at +37°C, 595 nm wavelength with Multiscan FC (Thermo Scientific) for 20 h in 5 min intervals and maximum growth and average growth rate were calculated.
Survival in Acidic pH and Compensation Capacity
Bacterial cells’ ability to tolerate acidic surrounding pH and capability to compensate it by metabolism was measured for each strain in pH 3–7. Initial cultures were grown in 5 ml LB pH 7 at +37°C and 210 rpm overnight. Each strain was then transferred into 5 ml LB of either pH 3, pH 4, pH 5, pH 6, or pH 7 in 1:100 ratio and cultured in +37°C and 210 rpm. In pH 5–7 cultures were grown for 90 h and growth densities were calculated by plating in 16, 24, and 90 h. Then 1.5 ml of culture was filtered through 0.2 μm and supernatant pH was measured with Basic pH Meter (Denver Instruments) in 24 and 90 h. Cultures in pH 3–4 were shortened into 24 h experiment and growth densities were calculated in 16 and 24 h and supernatant pH was measured in 24 h. Growth curves were measured by diluting the initial pH 7 cultures in 1:100 ratio into LB pH of 3–7 and growth curves were measured at +37°C, 595 nm wavelength for 20 h in 5 min intervals.
Cross-Strain Interactions
In aim to study the dynamics all the strains were cultured separately, and their metabolic products secreted into surroundings were tested against other strains in cross-strain inhibition experiments. Cross-strain interactions were tested by collecting the media after overnight culturing at +37°C and 210 rpm. Overnight cultures were centrifuged first with 7,000 × g for 4 min, and the supernatant was centrifuged again with 10,000 × g for 1 min. Each strain was cross-plated with all the supernatants (overnight, +37°C), and the inhibition of the growth of each strain was observed.
Supernatant Inhibition and Prophages
A 4-week evolutionary experiment was designed to study appearance, persistence, and reversibility of putative colicin E3 resistance in sensitive EKP24 strain. For the first 2 weeks EKP24 was cultured with (n = 5) and without (n = 5) colicin E3 in 10% LB media supplemented with either NKP2 (containing colicin E3) or EKP24 (not containing colicin E3) supernatant filtrate (0.2 μm) in 1:4 ratio. Ten percent LB media was supplemented with 25 μg/ml of kanamycin and 150 μg/ml of ampicillin. After 2 weeks EKP24 cultured with the presence of colicin E3 were divided into two sets of samples (both n = 5). Other set was continued with colicin E3 exposure as described earlier. In the other set of samples, colicin E3 containing supernatant was replaced with EKP24 supernatant. Cultures were refreshed in 1:100 ratio three times and samples stored once a week with glycerol at −80°C. Development and persistence of colicin E3 resistance was determined by plating. Samples were taken at the beginning of the experiment, before division of colicin E3 exposed EKP24, and at the end of the experiment and were used for DNA extraction. DNA was isolated with DNeasy Blood & Tissue Kit (Qiagen) and sequenced with Illumina HiSeq. The observed reads were mapped to original PacBio-sequenced genome of NKP2 (described above) in order to detect the genetic variants. The variants developed under the exposure of colicin E3 were identified by filtering out those variants that were already present in the beginning of the experiment. The genetic analysis was performed with CLC Genomics Workbench v11 (Qiagen).
Interactions between the putative colicin E3 producing NKP2 and susceptible EKP24 bacterial cells were also observed with confocal microscopy. Then 200 μl of 1% LB-agar was placed into a chamber of eight-chambered ibidi® ibiTreat μ-Slide (Ibidi GmbH) covered with CID lid for µ-dishes (Ibidi GmbH), and 3 μl of NKP2 and EKP24 were injected under the agar onto opposite sides. Encounter of the two strains was visualized with Nikon AR1 laser scanning confocal microscope with 60× water immersion objective and using Galvano scanner.
Production of putative colicin E3 was further studied by growing NKP2 strain in different medias. NKP2 was grown in LB concentrations of 100, 10, and 1%, LB without tryptone, 100% Shieh (Song et al., 1988) and in 100% DMEM in +37°C and 200–230 rpm overnight. NKP2 cultures were filtered through 0.2 μm filter and colicin E3 presence was determined by plating the supernatant with susceptible EKP24 strain.
Alcohol Exposure
All studied strains were exposed to multiple concentrations of ethanol (20, 50, 75, and 90%) and their ability to survive the exposure were measured with spectroscopy. In 30 s exposure experiment all the strains were first grown in 5 ml of LB (+37°C, 230 rpm, overnight) and then transferred in 1:10 ratio into fresh LB and left to grow overnight at +37°C on 96-well plate 100 μl per well to form biofilm. On the following day media was gently removed and replaced with 200 μl of ethanol in concentrations of 20, 50, 75, and 90%. After 30 s incubation in RT ethanol was replaced with 100 μl of LB and growth at +37°C was measured in 595 nm wavelength for 20 h in 5 min intervals. In ethanol evaporation experiment, cultures for 96-well plate were prepared as described earlier but volume was lowered into 35 μl per well. Ninety-six-plate cultures were incubated overnight at +37°C without the lid to let the excess media evaporate. Dried biofilms were exposed to 50 μl of ethanol (either 20, 50, 75, or 90%) and left to fully evaporate before addition of 200 μl of LB per well. Growth curve measurements was performed as earlier described.
Drought Tolerance
The capability to survive over long-lasting drought in a room air humidity was tested by culturing the strains on 96-well plate by transferring overnight grown culture (5 ml LB, +37°C, 210 rpm) in 1:10 ratio to LB. One hundred microliters per well was used with four replicates of each strain. Plates were incubated at +37°C for 3 days in order to grow biofilm. After 3 days, plates were relocated to RT and lids were removed to ensure total evaporation of media. After 12 days, 2 months and 6 months in drought, 200 μl of fresh LB was added into each well and the growth curves were measured at +37°C, 595 nm wavelength, 20 h in 5 min intervals.
Morphological Characterization
Morphological characteristics of the colonies were analyzed. In order to get single colonies, all strains were cultured in 5 ml LB in +37°C, 210 rpm overnight. Cultures were diluted into 10−6 in water and plated onto LB-agar plates. Plates were incubated at +37°C for overnight and the colonies were photographed. Strains EKP5, EKP3 and EKP22 were found to produce translucent colonies along with the traditional colonies. These translucent colonies were further cultivated by transferring one colony to a fresh LB-agar plate and incubated at +37°C overnight or transferred into liquid culture of 5 ml LB and cultured at +37°C and 210 rpm overnight before plating in 10−6 dilution onto the new LB-plates. Growth densities were calculated from these plates. From the same liquid culture used for the growth density definition, 1:100 dilutions were made into LB and growth curves were measured in +37°C, 595 nm wavelength, 20 h in 5 min intervals.
Statistical Analyses
To explore if epidemic or non-epidemic strains can be characterized by their capabilities, we performed discriminant analysis using MASS package (Venables & Ripley, 2002) in R (version. 3.3.2). Effects of individual variables on discriminant function were tested by regressing predicted values against original variables. Overall performance of discriminant function was addressed by Bayesian logistic regression of epidemic status against predicted values of discriminant function using Stan with R (McElreath, 2016).
Results
Selection of Strains and Genomic Analysis
We selected 14 K. pneumoniae strains for detailed phenotypic and genomic analysis in an attempt to identify characteristics that may potentially associate with epidemic STs. The strains were abbreviated either as EKP or NKP for Epidemic and Non-epidemic K. pneumoniae, respectively, and the strain number was derived from an internal naming system. The strains and their key genomic traits are listed in Table 1. Note that two epidemic STs are represented twice (ST14 and ST11), but their genetic features differ from one another and were thus selected for phenotypic studies in order to evaluate whether the phenotypes of different strains of a single ST are similar. Epidemic and non-epidemic STs are not grouping together when the genomic regions used for sequence typing are used to infer genetic relationship (Figure 1). As such, the epidemic strains do not appear to share a common ancestor that diverged from non-successful strains. Therefore, epidemic success is not likely to be linked to a single vertically inherited (genetic) trait, which evolved once. This however does not exclude the possibility that traits preceding epidemic spread are transferred horizontally between strains of different STs. Intriguingly, strains EKP3 and EKP22 (both ST14) and EKP8 and EKP24 (both ST11) are phenotypically different regardless of the same ST (Supplementary Figure 1), sometimes being even the opposite phenotypic extremes out of all strains. Colony morphologies of all strains are highly similar (Supplementary Figure 2).
The genomes of all the strains were annotated and their overall gene contents compared. Based on the annotation, epidemic and non-epidemic strains appear to be generally uniform metabolically and functionally, hence providing no apparent genome-level design differences to explain epidemic qualities (Table 2). Neither the presence or absence of CRISPR system or the number of CRISPR loci appear to be linked with epidemic successfulness. Also, the number of mobile elements such as prophages or plasmids do not associate specifically with either group. The most obvious potentially explanatory features, i.e. virulence genes and antibiotic resistance genes, are similar between epidemic and non-epidemic K. pneumoniae despite of differences among individual strains (Tables 1 and 2). We further speculated that some generally overlooked features such as short open reading frames (ORFs) of length 30 to 150 nucleotides could possibly be linked with epidemic qualities. These genes are rarely identified as coding regions with automated annotation algorithms despite of the fact that they are sometimes transcribed and translated and may reflect recent adaptations to new life strategies or specific conditions (that may be related to epidemic spread). We prepared an algorithm to extract all short ORFs which are preceded by a (near-)perfect ribosome biding site and which do not overlap with existing annotated ORFs (Python code is available in Supplementary File 1). On average, approximately 200 unannotated ORFs were extracted from the sequences. Yet, while putative short genes are common, their count is similar in epidemic and non-epidemic strains (data not shown).
Phenotypic Qualities of the Strains
It is possible that epidemic spread selects for or is preceded by specific phenotypic traits. These traits may not necessarily be linked with any particular genetic feature as there may be several mutational pathways to acquire the quality and hence, they may be difficult to identify with genetic or genomic comparisons. As such, we listed a number of measurable phenotypes that may be linked with epidemic success. The traits, their speculated association with epidemic dispersal, and the variables used in this study are listed in Table 3. The original data from measurements is available in Supplementary File 2. Supplementary Figure 1 summarizes the results for epidemic and non-epidemic strains.
We carried out a discriminant analysis for the measured phenotypic data. The analysis associated several phenotypic measurements with discriminant function. The discriminant function is a combination of linear effects of variables that give best separation of the data to distinct classes, in this study epidemic and non-epidemic strains. This approach revealed highly significant (posterior values did not overlap with zero) likelihood for a given trait to belong to either epidemic or non-epidemic group (resolved with Bayesian logistic regression between predicted values of discriminant function and epidemic status, Bayesian R2 = 45%, Figure 2). Regressing predicted values of discriminant function against original variables indicated especially strong role of various measurements of drought tolerance in discriminant function (Figure 3) and non-epidemic strains with high discriminant function score were clearly more drought tolerant.
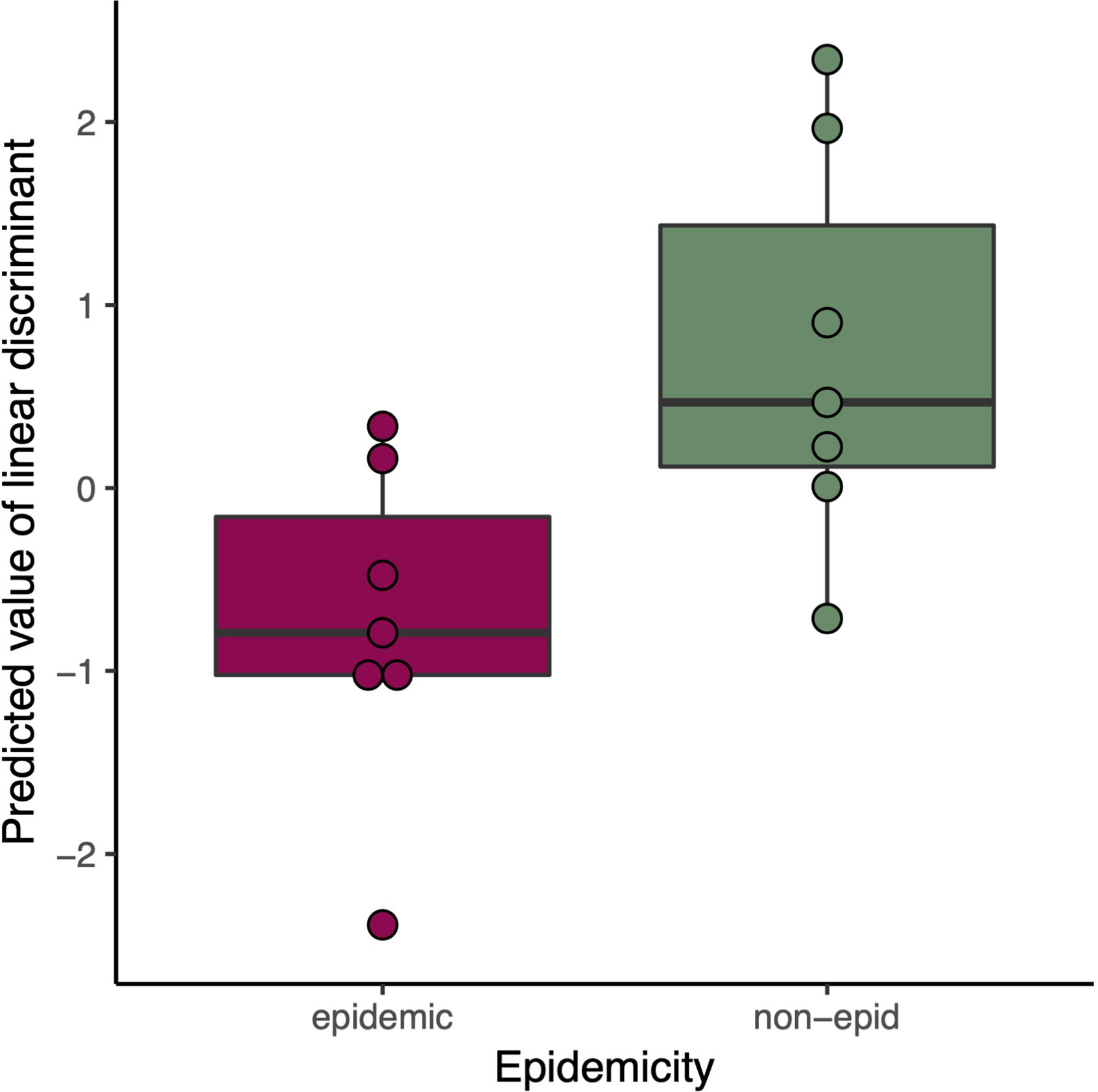
Figure 2 Predicted Linear discriminant values in epidemic (red) and non-epidemic (green) K. pneumoniae strains.
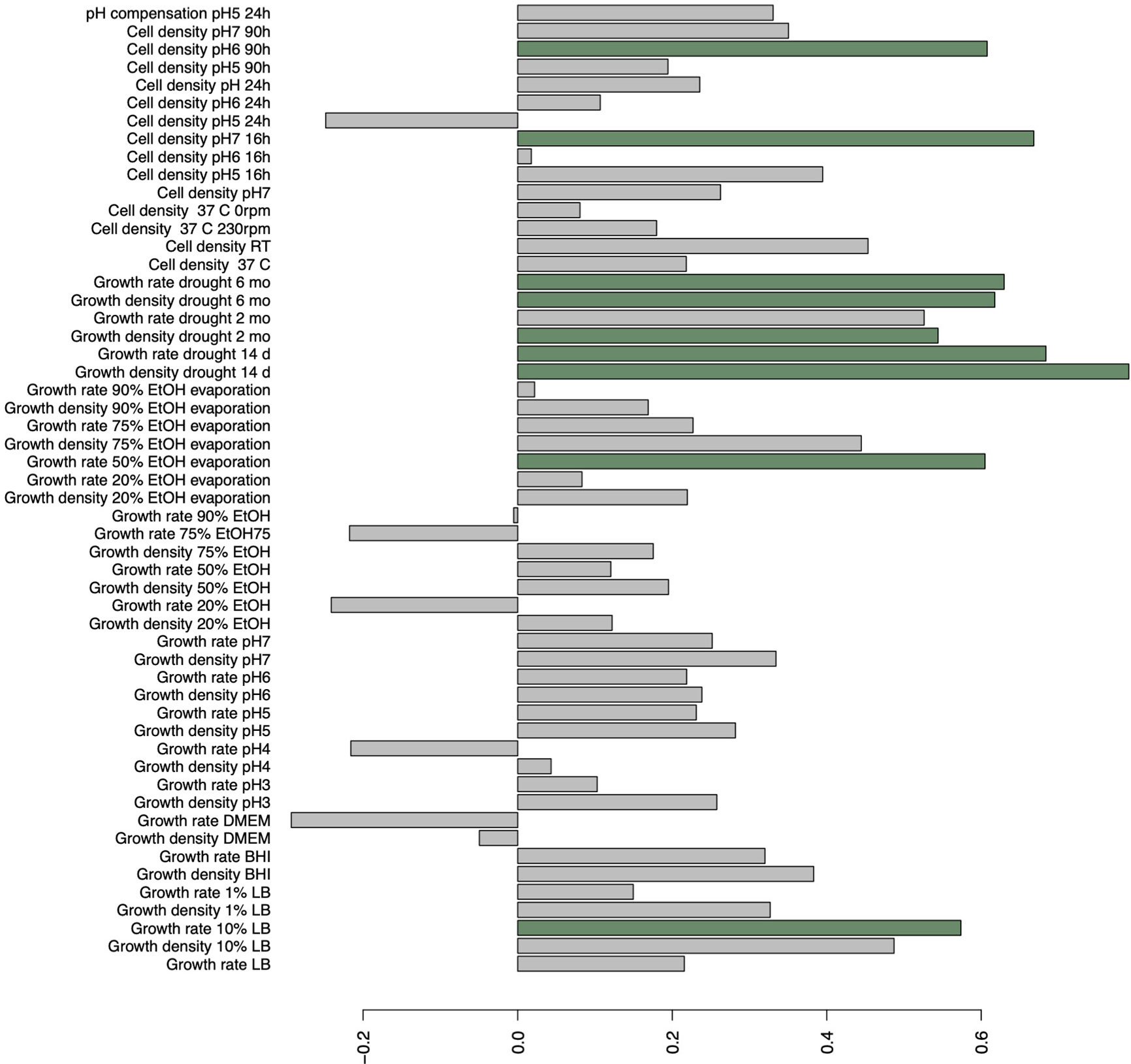
Figure 3 Correlations of original variables on discriminant function explaining the best linear combinations of variables in explaining epidemic status of strains. Bars highlighted with green indicate significant (p < 0.05) correlations.
Cross-Inhibition and Antibacterial Potential of a Putative Colicin
We further studied the cross-strain inhibition given that the epidemic successfulness could emerge from the ability of epidemic strains to suppress non-epidemic strains during spread between hospitals or hosts (Figure 4). The inhibition, when detected, was determined with a dilution series to be either due to molecular activity or prophage induction (diluted phages produce distinct plaques unlike inhibiting molecules). Pairwise inhibition was infrequent and no general pattern between epidemic and non-epidemic strains was identifiable.
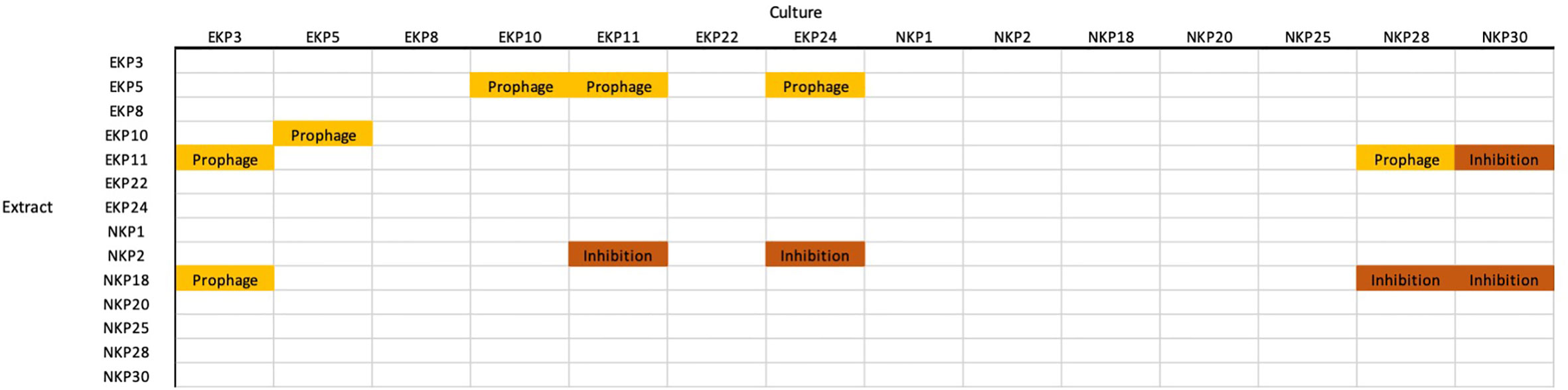
Figure 4 Cross-strain inhibition. “Prophage” indicates that the strain used for generating the extract produces a phage into the medium. “Inhibition” is a non-viral extract that inhibited the growth of the culture strain.
As a curiosity, we selected one cross-inhibiting strain pair for more detailed analysis. NKP2 produces a component into its medium that inhibits EKP24 (ST11). Given the wide dispersal of ST11 K. pneumoniae and its association with NDM-1 encoding plasmids and hypervirulence (Gu et al., 2017), the inhibiting factor could provide a possible way to control these strains. However, the strain used in this study is not hypervirulent and therefore assessing direct applicability against hypervirulent strains was not conducted. Genomic comparison of NKP2 and EKP24 revealed the presence of genes for Colicin E3 in NKP2 that were absent from EKP24. Hence, Colicin E3 was hypothesized to be the inhibiting molecule. Co-culturing of these strains in the same medium demonstrates that EKP24 is unable to multiply. We further studied the adaptation of EKP24 to the continuous presence of the hypothesized Colicin E3 by serially culturing EKP24 in the presence of NKP2 medium extract for 4 weeks (n = 5). These cultures were refreshed three times a week. After 2 weeks, we removed the selection from five additional replicates. Resistance to NKP2 medium (with hypothesized Colicin E3) emerged already during the first culture transfer, and it remained stable even after the removal of selection. Re-sequencing of three putative Colicin E3 resistant samples revealed a prevalent mutation in Aerobactin siderophore receptor IutA. This mutation was absent in a culture that was not exposed to NKP2 extract. IutA has been shown to serve as a receptor for cloacin DF13 (Van Tiel-Menkveld et al., 1982), which is homologous to Colicin E6 and E3 (Akutsu et al., 1989). As such, we hypothesize that NKP2 extract rapidly selected for IutA mutants. Also, it is worth noting that NKP2 medium was not observed to inhibit the growth of the other ST11 strain EKP8, hence showing narrow activity. Altogether, the hypothesized Colicin E3 does not appear to provide efficient antimicrobial activity against drug-resistant K. pneumoniae strains even when the targeted strain is initially sensitive to the colicin.
Discussion
K. pneumoniae has become one of the priority drug-resistant pathogens in hospital settings worldwide. Some K. pneumoniae STs are more prevalent compared to others, which provokes the question whether there are qualities in these genetically related groups that have made them more potent for dispersal. Here, we studied multiple phenotypic and genotypic characteristics of seven epidemic and non-epidemic carbapenem resistant K. pneumoniae isolates that emerged from different parts of the world. None of the specific genetic qualities associated uniformly with epidemic or non-epidemic strains. Overall, this again suggests that sequence typing is not an optimal approach for inferring qualities of individual pathogens. In other words, there are K. pneumoniae strains that are relatively different from one another for their specific characteristics while still grouping together in ST-analyses. Phenotypic characterization revealed indication that, while genetic differences were minute based on ST-analyses, phenotypic differences that separate epidemic and non-epidemic K. pneumoniae strains do exist. Such apparently controversial result could emerge if phenotypes are strongly dictated by genetic differences other than indicated by core genome-based ST-analyses or even by epigenetic modifications (Casadesus and Low, 2006).
While most of the studied factors could not explicitly help explain epidemic qualities, the strong association of drought tolerance with epidemically non-successful strains could give some insights on the dissemination. All the isolates in this study were able to withstand 6 months of dryness. Interestingly, non-epidemic strains generally recovered faster and into higher density after the drought, which presents the possibility that non-epidemic strains may have qualities that provide them opportunities to cause infections in specific cases, for example, after long-term residence on surfaces (Kramer et al., 2006). Bacteria have multiple ways to protect itself during the drought. For example, effective biofilm formation is a major protection mechanism in bacterial species incapable of endosporulation (Sunde et al., 2009; Reza et al., 2019) or cyst formation (Malinich and Bauer, 2018). Nosocomial carbapenem resistant K. pneumoniae infections are mainly acquired by two routes, either in person-to-person contact (Casewell and Phillips, 1977) or via contaminated surfaces and instrumentation (Jarvis et al., 1985; Revdiwala et al., 2012). Also, a gut carriage of carbapenem resistant Klebsiella have been associated with an elevated infection risk (Tischendorf et al., 2016; Gorrie et al., 2017). It is possible that drought tolerant strains are more likely to remain viable on inanimate surfaces, from which they are only rarely transferred to the patient. On the other hand, the epidemically successful strains could be more adapted to human host and tolerated as the colonizing pathogens. These might be two different strategies for the same species to survive in the shared environment and results of evolutionary trade-offs between inside host and outside host conditions (e.g. Mikonranta et al., 2015; Ashrafi et al., 2018). However, the reasons why certain STs seem to develop higher tolerance towards dryness remains unclear.
K. pneumoniae has also noted to be able to grow in acidic pH and even capable to compensate acidic environment of phagosomes (Cano et al., 2015). All the strains in this study tolerated decreasing pH up to 4. In pH 3 no viable dividing cells were detected and for that reason no pH compensation was observed either. In the viable cultures with initial pH of 4, cells were able to increase the pH in the surrounding media by 1.04–1.62 in 24 h. Strain EKP10 was an exception as it was barely able to grow in pH 4 and could not compensate the low pH. Altogether, long-term exposure to environments that have pH of 3 or below serves as a potential barrier for K. pneumoniae viability. Increasing tolerance towards the alcohol-based hand rubs and sanitizers have been observed in multiple species of XDR bacteria. It has been suggested as one possible explanation to the increasing emergence of these pathogens in hospital environments (Pidot et al., 2018). The K. pneumoniae isolates utilized in this study, however, were not able to withstand ethanol at concentrations 50% (v/v) or above.
Overall, different K. pneumoniae strains vary in their phenotypic characteristics and hence may cause infections via differing routes. This highlights the importance of exerting precautionary steps in healthcare. Namely, these include early recognition of both carriers and infected patients (Borer et al., 2012; Gorrie et al., 2017; Liu et al., 2019), and controlling the spread of pathogens with apparently still effective hand hygiene and disinfection of inanimate surfaces (Kramer et al., 2006; Borer et al., 2012; Gorrie et al., 2017). Multiple studies also focus on novel approaches to prevent infections by coating endotracheal tubes and catheters with new antibacterial agents (Caratto et al., 2017; Bjorling et al., 2018; Seitz et al., 2019). Such multifrontal measures to control K. pneumoniae is likely to be necessary for tempering both epidemic and non-epidemic strains.
In conclusion, we were able to detect one specific quality that associated with epidemic status of K. pneumoniae isolates. Drought tolerance and recovery from dryness of up to 6 months significantly associated with the non-epidemic strains. Thus, we need to consider the possibility that non-epidemic strains may have unique advantages in specific conditions that let them occasionally show up as local epidemics in surveillance studies. Additionally, some non-epidemic isolates inhibited the growth of epidemic strains. This may allow these strains to cause infections instead of their epidemic contemporaries. However, it is possible although unlikely that pure coincidence may be behind the epidemic success of certain K. pneumoniae STs. The initial establishment, instead of any specific characteristic, may have provided certain strains chances to acquire new virulence factors and resistance elements, hence furthering their spread.
Data Availability Statement
All data is available either as Supplementary Material or in GenBank.
Author Contributions
KK and MJ conceived and planned the experiments. KK, RP, and MJ carried out the experiments. KK assembled and curated the results. MJ prepared the script for finding ORFs. TK conducted statistical analyses. A-MÖ-G and CG collected the bacterial strains. KK, MJ, RP, and A-MÖ-G conducted genetic analyses. All authors contributed to the interpretation of the results. First draft of the manuscript was written by KK and MJ and other authors provided critical feedback and helped shape the research, analysis, and the final manuscript. All authors contributed to the article and approved the submitted version.
Funding
Authors wish to acknowledge funding from the Academy of Finland (grants no.252411, no.297049 and no. 336518 to MJ and no. 322204 to RP), Emil Aaltonen Foundation and Jane and Aatos Erkko Foundation.
Conflict of Interest
The authors declare that the research was conducted in the absence of any commercial or financial relationships that could be construed as a potential conflict of interest.
Supplementary Material
The Supplementary Material for this article can be found online at: https://www.frontiersin.org/articles/10.3389/fcimb.2021.599924/full#supplementary-material
Supplementary Figure 1 | Summary of all measurements.
Supplementary File 1 | Phyton script for short ORFs.
Supplementary File 2 | Original measurement data.
References
Akutsu A., Masaki H., Ohta T. (1989). Molecular structure and immunity specificity of colicin E6, an evolutionary intermediate between E-group colicins and cloacin DF13. J. Bacteriol. 171, 6430–6436. doi: 10.1128/JB.171.12.6430-6436.1989
Ashrafi M., Novak-Frazer L., Bates M., Baguneid M., Alonso-Rasgado T., Xia G., et al. (2018). Validation of biofilm formation on human skin wound models and demonstration of clinically translatable bacteria-specific volatile signatures. Sci. Rep. 8, 9431. doi: 10.1038/s41598-018-27504-z
Bjorling G., Johansson D., Bergstrom L., Strekalovsky A., Sanchez J., Frostell C., et al. (2018). Evaluation of central venous catheters coated with a noble metal alloy-A randomized clinical pilot study of coating durability, performance and tolerability. J. Biomed. Mater. Res. B Appl. Biomater. 106, 2337–2344. doi: 10.1002/jbm.b.34041
Borer A., Saidel-Odes L., Eskira S., Nativ R., Riesenberg K., Livshiz-Riven I., et al. (2012). Risk factors for developing clinical infection with carbapenem-resistant Klebsiella pneumoniae in hospital patients initially only colonized with carbapenem-resistant K pneumoniae. Am. J. Infect. Control 40, 421–425. doi: 10.1016/j.ajic.2011.05.022
Bowers J. R., Kitchel B., Driebe E. M., MacCannell D. R., Roe C., Lemmer D., et al. (2015). Genomic Analysis of the Emergence and Rapid Global Dissemination of the Clonal Group 258 Klebsiella pneumoniae Pandemic. PloS One 10, e0133727. doi: 10.1371/journal.pone.0133727
Cano V., March C., Insua J. L., Aguilo N., Llobet E., Moranta D., et al. (2015). Klebsiella pneumoniae survives within macrophages by avoiding delivery to lysosomes. Cell. Microbiol. 17, 1537–1560. doi: 10.1111/cmi.12466
Caratto V., Ball L., Sanguineti E., Insorsi A., Firpo I., Alberti S., et al. (2017). Antibacterial activity of standard and N-doped titanium dioxide-coated endotracheal tubes: an in vitro study. Rev. Bras. Ter. Intensiva. 29, 55–62. doi: 10.5935/0103-507X.20170009
Casadesus J., Low D. (2006). Epigenetic gene regulation in the bacterial world. Microbiol. Mol. Biol. Rev. 70, 830–856. doi: 10.1128/MMBR.00016-06
Casewell M., Phillips I. (1977). Hands as route of transmission for Klebsiella species. Br. Med. J. 2, 1315–1317. doi: 10.1136/bmj.2.6098.1315
Catalan-Najera J. C., Garza-Ramos U., Barrios-Camacho H. (2017). Hypervirulence and hypermucoviscosity: two different but complementary Klebsiella spp. phenotypes? Virulence 8, 1–13. doi: 10.1080/21505594.2017.1317412
Conte V., Monaco M., Giani T., D’Ancona F., Moro M. L., Arena F., et al. (2016). Molecular epidemiology of KPC-producing Klebsiella pneumoniae from invasive infections in Italy: increasing diversity with predominance of the ST512 clade II sublineage. J. Antimicrob. Chemother. 71, 3386–3391. doi: 10.1093/jac/dkw337
Dautzenberg M. J., Haverkate M. R., Bonten M. J., Bootsma M. C. (2016). Epidemic potential of Escherichia coli ST131 and Klebsiella pneumoniae ST258: a systematic review and meta-analysis. BMJ Open 6, e009971–2015-009971. doi: 10.1136/bmjopen-2015-009971
Giske C. G., Froding I., Hasan C. M., Turlej-Rogacka A., Toleman M., Livermore D., et al. (2012). Diverse sequence types of Klebsiella pneumoniae contribute to the dissemination of blaNDM-1 in India, Sweden, and the United Kingdom. Antimicrob. Agents Chemother. 56, 2735–2738. doi: 10.1128/AAC.06142-11
Gorrie C. L., Mirceta M., Wick R. R., Edwards D. J., Thomson N. R., Strugnell R. A., et al. (2017). Gastrointestinal Carriage Is a Major Reservoir of Klebsiella pneumoniae Infection in Intensive Care Patients. Clin. Infect. Dis. 65, 208–215. doi: 10.1093/cid/cix270
Gu D., Dong N., Zheng Z., Lin D., Huang M., Wang L., et al. (2017). A fatal outbreak of ST11 carbapenem-resistant hypervirulent Klebsiella pneumoniae in a Chinese hospital: a molecular epidemiological study. Lancet Infect. Dis. 18, 37–46. doi: 10.1016/S1473-3099(17)30489-9
Hasan C. M., Turlej-Rogacka A., Vatopoulos A. C., Giakkoupi P., Maatallah M., Giske C. G. (2014). Dissemination of blaVIM in Greece at the peak of the epidemic of 2005-2006: clonal expansion of Klebsiella pneumoniae clonal complex 147. Clin. Microbiol. Infect. 20, 34–37. doi: 10.1111/1469-0691.12187
Holt K. E., Wertheim H., Zadoks R. N., Baker S., Whitehouse C. A., Dance D., et al. (2015). Klebsiella pneumoniae, an urgent threat. Proc. Natl. Acad. Sci. 112, E3574–E3581. doi: 10.1073/pnas.1501049112
Jarvis W. R., Munn V. P., Highsmith A. K., Culver D. H., Hughes J. M. (1985). The epidemiology of nosocomial infections caused by Klebsiella pneumoniae. Infect. Control. 6, 68–74. doi: 10.1017/S0195941700062639
Kitchel B., Rasheed J. K., Patel J. B., Srinivasan A., Navon-Venezia S., Carmeli Y., et al. (2009). Molecular epidemiology of KPC-producing Klebsiella pneumoniae isolates in the United States: clonal expansion of multilocus sequence type 258. Antimicrob. Agents Chemother. 53, 3365–3370. doi: 10.1128/AAC.00126-09
Kitchel B., Rasheed J. K., Endimiani A., Hujer A. M., Anderson K. F., Bonomo R. A., et al. (2010). Genetic factors associated with elevated carbapenem resistance in KPC-producing Klebsiella pneumoniae. Antimicrob. Agents Chemother. 54, 4201–4207. doi: 10.1128/AAC.00008-10
Kramer A., Schwebke I., Kampf G. (2006). How long do nosocomial pathogens persist on inanimate surfaces? A systematic review. BMC Infect. Dis. 6, 130–2334-6-130. doi: 10.1186/1471-2334-6-130
Liu Q., Liu L., Li Y., Chen X., Yan Q., Liu W. E. (2019). Fecal Carriage and Epidemiology of Carbapenem-Resistant Enterobacteriaceae Among Hospitalized Patients in a University Hospital. Infect. Drug Resist. 12, 3935–3942. doi: 10.2147/IDR.S233795
Malinich E. A., Bauer C. E. (2018). Transcriptome analysis of Azospirillum brasilense vegetative and cyst states reveals large-scale alterations in metabolic and replicative gene expression. Microb. Genom. 4, e000200. doi: 10.1099/mgen.0.000200
McElreath R. (2016). Statistical Rethinking. A Bayesian Course with Examples in R and Stan (Boca Raton, Florida, USA: CRC Press).
Mikonranta L., Mappes J., Laakso J., Ketola T. (2015). Within-host evolution decreases virulence in an opportunistic bacterial pathogen. BMC Evol. Biol. 15, 165. doi: 10.1186/s12862-015-0447-5
Pidot S. J., Gao W., Buultjens A. H., Monk I. R., Guerillot R., Carter G. P., et al. (2018). Increasing tolerance of hospital Enterococcus faecium to handwash alcohols. Sci. Transl. Med. 10, eaar6115. doi: 10.1126/scitranslmed.aar6115
Pitout J. D., Nordmann P., Poirel L. (2015). Carbapenemase-Producing Klebsiella pneumoniae, a Key Pathogen Set for Global Nosocomial Dominance. Antimicrob. Agents Chemother. 59, 5873–5884. doi: 10.1128/AAC.01019-15
Podschun R., Ullmann U. (1998). Klebsiella spp. as nosocomial pathogens: epidemiology, taxonomy, typing methods, and pathogenicity factors. Clin. Microbiol. Rev. 11 (4), 589–603. doi: 10.1128/CMR.11.4.589
Pomakova D. K., Hsiao C. B., Beanan J. M., Olson R., MacDonald U., Keynan Y., et al. (2012). Clinical and phenotypic differences between classic and hypervirulent Klebsiella pneumonia: an emerging and under-recognized pathogenic variant. Eur. J. Clin. Microbiol. Infect. Dis. 31, 981–989. doi: 10.1007/s10096-011-1396-6
Revdiwala S., Rajdev B. M., Mulla S. (2012). Characterization of bacterial etiologic agents of biofilm formation in medical devices in critical care setup. Crit. Care Res. Pract. 2012, 945805. doi: 10.1155/2012/945805
Reza A., Sutton J. M., Rahman K. M. (2019). Effectiveness of Efflux Pump Inhibitors as Biofilm Disruptors and Resistance Breakers in Gram-Negative (ESKAPEE) Bacteria. Antibiotics (Basel) 8, 10.3390/antibiotics8040229. doi: 10.3390/antibiotics8040229
Samuelsen O., Naseer U., Tofteland S., Skutlaberg D. H., Onken A., Hjetland R., et al. (2009). Emergence of clonally related Klebsiella pneumoniae isolates of sequence type 258 producing plasmid-mediated KPC carbapenemase in Norway and Sweden. J. Antimicrob. Chemother. 63, 654–658. doi: 10.1093/jac/dkp018
Samuelsen O., Toleman M. A., Hasseltvedt V., Fuursted K., Leegaard T. M., Walsh T. R., et al. (2011). Molecular characterization of VIM-producing Klebsiella pneumoniae from Scandinavia reveals genetic relatedness with international clonal complexes encoding transferable multidrug resistance. Clin. Microbiol. Infect. 17, 1811–1816. doi: 10.1111/j.1469-0691.2011.03532.x
Samuelsen O., Overballe-Petersen S., Bjornholt J. V., Brisse S., Doumith M., Woodford N., et al. (2017). Molecular and epidemiological characterization of carbapenemase-producing Enterobacteriaceae in Norwato 2014. PloS One 12, e0187832. doi: 10.1371/journal.pone.0187832
Seitz A. P., Schumacher F., Baker J., Soddemann M., Wilker B., Caldwell C. C., et al. (2019). Sphingosine-coating of plastic surfaces prevents ventilator-associated pneumonia. J. Mol. Med. (Berl.) 97, 1195–1211. doi: 10.1007/s00109-019-01800-1
Shon A. S., Bajwa R. P., Russo T. A. (2013). Hypervirulent (hypermucoviscous) Klebsiella pneumoniae: a new and dangerous breed. Virulence 4, 107–118. doi: 10.4161/viru.22718
Song Y. L., Fryer J. L., Rohovec J. S. (1988). Comparison of six media for the cultivation of Flexibacter columnaris. Fish Pathol 23, 91–94.
Sunde E. P., Setlow P., Hederstedt L., Halle B. (2009). The physical state of water in bacterial spores. Proc. Natl. Acad. Sci. U.S.A. 106, 19334–19339. doi: 10.1073/pnas.0908712106
Tischendorf J., de Avila R. A., Safdar N. (2016). Risk of infection following colonization with carbapenem-resistant Enterobactericeae: A systematic review. Am. J. Infect. Control. 44, 539–543. doi: 10.1016/j.ajic.2015.12.005
Turton J. F., Payne Z., Coward A., Hopkins K. L., Turton J. A., Doumith M., et al. (2018). Virulence genes in isolates of Klebsiella pneumoniae from the UK during 2016, including among carbapenemase gene-positive hypervirulent K1-ST23 and ‘non-hypervirulent’ types ST147, ST15 and ST383. J. Med. Microbiol. 67, 118–128. doi: 10.1099/jmm.0.000653
Vading M., Samuelsen O., Haldorsen B., Sundsfjord A. S., Giske C. G. (2011). Comparison of disk diffusion, Etest and VITEK2 for detection of carbapenemase-producing Klebsiella pneumoniae with the EUCAST and CLSI breakpoint systems. Clin. Microbiol. Infect. 17, 668–674. doi: 10.1111/j.1469-0691.2010.03299.x
Van Tiel-Menkveld C. J., Mentjox-Vervuust J. K., Oudega B., Degraaf F. K. (1982). Siderophore production by Enterobacter cloacae and a common receptor protein for the uptake of aerobactin and cloacin DFl3. J. Bacteriol. 150, 490–497. doi: 10.1128/JB.150.2.490-497.1982
Venables W. N., Ripley B. D. (2002). Modern Applied Statistics with S. 4th ed. (New York: Springer).
World Health Organisation [WHO] (2014). Antimicrobial Resistance: Global Report on Surveillance (Geneva: WHO).
World Health Organization [WHO] (2017). Media Centre. News Release. WHO publishes list of bacteria for which new antibiotics are urgently needed. (Geneva, Switzerland: WHO).
Yong D., Toleman M. A., Giske C. G., Cho H. S., Sundman K., Lee K., et al. (2009). Characterization of a new metallo-beta-lactamase gene, bla(NDM-1), and a novel erythromycin esterase gene carried on a unique genetic structure in Klebsiella pneumoniae sequence type 14 from India. Antimicrob. Agents Chemother. 53, 5046–5054. doi: 10.1128/AAC.00774-09
Keywords: XDR Klebsiella pneumoniae, extended-spectrum beta-lactamase, epidemic, antibiotic resistance, virulence
Citation: Koskinen K, Penttinen R, Örmälä-Odegrip A-M, Giske CG, Ketola T and Jalasvuori M (2021) Systematic Comparison of Epidemic and Non-Epidemic Carbapenem Resistant Klebsiella pneumoniae Strains. Front. Cell. Infect. Microbiol. 11:599924. doi: 10.3389/fcimb.2021.599924
Received: 28 August 2020; Accepted: 08 January 2021;
Published: 23 February 2021.
Edited by:
Rodnei Dennis Rossoni, Sao Paulo State University, BrazilReviewed by:
Tatiana Amabile De Campos, University of Brasilia, BrazilJonatas Rafael De Oliveira, Anhembi Morumbi University, Brazil
Copyright © 2021 Koskinen, Penttinen, Örmälä-Odegrip, Giske, Ketola and Jalasvuori. This is an open-access article distributed under the terms of the Creative Commons Attribution License (CC BY). The use, distribution or reproduction in other forums is permitted, provided the original author(s) and the copyright owner(s) are credited and that the original publication in this journal is cited, in accordance with accepted academic practice. No use, distribution or reproduction is permitted which does not comply with these terms.
*Correspondence: Matti Jalasvuori, bWF0dGkuamFsYXN2dW9yaUBqeXUuZmk=