Decreased Frequencies of Gamma/Delta T Cells Expressing Th1/Th17 Cytokine, Cytotoxic, and Immune Markers in Latent Tuberculosis-Diabetes/Pre-Diabetes Comorbidity
- 1National Institutes of Health-NIRT-International Center for Excellence in Research, Chennai, India
- 2Indian Council of Medical Research-National Institute for Research in Tuberculosis (ICMR-NIRT), Chennai, India
- 3Laboratory of Parasitic Diseases, National Institute of Allergy and Infectious Diseases, National Institutes of Health, Bethesda, MD, United States
Antigen-specific gamma-delta (γδ) T cells are important in exhibiting anti-mycobacterial immunity, but their role in latent tuberculosis (LTB) with diabetes mellitus (DM) or pre-DM (PDM) and non-DM comorbidities have not been studied. Thus, we have studied the baseline, mycobacterial (PPD, WCL), and positive control antigen-stimulated γδ T cells expressing Th1 (IFNγ, TNFα, IL-2) and Th17 (IL-17A, IL-17F, IL-22) cytokine as well as cytotoxic (perforin [PFN], granzyme [GZE B], granulysin [GNLSN]) and immune (GMCSF, PD-1, CD69) markers in LTB (DM, PDM, NDM) comorbidities by flow cytometry. In the unstimulated (UNS) condition, we did not observe any significant difference in the frequencies of γδ T cells expressing Th1 and Th17 cytokine, cytotoxic, and immune markers. In contrast, upon PPD antigen stimulation, the frequencies of γδ T cells expressing Th1 (IFNγ, TNFα) and Th17 (IL-17F, IL-22) cytokine, cytotoxic (PFN, GZE B, GNLSN), and immune (CD69) markers were significantly diminished in LTB DM and/or PDM individuals compared to LTB NDM individuals. Similarly, upon WCL antigen stimulation, the frequencies of γδ T cells expressing Th1 (TNFα) and Th17 (IL-17A, IL-22) cytokine, cytotoxic (PFN), and immune (PD-1, CD69) markers were significantly diminished in LTB DM and/or PDM individuals compared to LTB NDM individuals. Finally, upon P/I stimulation we did not observe any significant difference in the γδ T cell frequencies expressing cytokine, cytotoxic, and immune markers between the study populations. The culture supernatant levels of IFNγ, TNFα, and IL-17A cytokines were significantly increased in LTB DM and PDM after stimulation with Mtb antigens compared to LTB NDM individuals. Therefore, diminished γδ T cells expressing cytokine, cytotoxic, and other immune markers and elevated levels of cytokines in the supernatants is a characteristic feature of LTB PDM/DM co-morbidities.
Introduction
Tuberculosis, caused by Mycobacterium tuberculosis (Mtb), affects around 10 million people and causes 1.4 million deaths worldwide in the year 2019 (World Health Organization, 2020). Latent tuberculosis (LTB) is defined as an asymptomatic clinical state without any infection or not transmissible, whereas active TB is defined by the presence of clinical symptoms and affects different parts of the body (Barry et al., 2009). Globally, one-quarter of the world’s population is infected with LTB (Dheda et al., 2016). In addition to this, type 2 diabetes mellitus (DM) comorbidity enhances the risk of TB development by three times (Restrepo, 2016). Hence, a higher risk of active TB and reactivation of LTB infection has long been well-known to be linked with the risk of DM (Siddiqui et al., 2016). In India, DM prevalence is calculated to reach 123.5 million by 2040 (International Diabetes Federation, 2015). Similarly, 5%–10% of the pre-diabetes mellitus (PDM) or intermediate hyperglycemic individuals become diabetic every year depending on the inhabitants and geographical location (Forouhi et al., 2007; Abdul-Ghani and DeFronzo, 2009). PDM prevalence is shown to be as high as 25% of individuals among active TB (Viswanathan et al., 2012).
Type 1 (IFNγ and TNFα), type 17 (IL-17), IL-1, and IL-12 family cytokines are thought to be crucial for host protection against pulmonary tuberculosis (PTB) (O’Garra et al., 2013; Mayer-Barber and Sher, 2015). TB DM comorbidity is associated with enhanced plasma and antigen-specific levels of pro-inflammatory cytokines (Restrepo et al., 2008; Kumar et al., 2013). LTB DM is associated with decreased systemic and Mtb antigen-specific levels of type 1 and type 17 cytokines (Kumar et al., 2014). The previous study has also shown that LTB PDM was characterized by decreased antigen-specific frequencies of T helper (Th)1/T cytotoxic (Tc)1 and Th17/Tc17 cells (Kumar et al., 2017). Likewise, non-conventional T cells play a crucial function in protecting against TB disease, especially in early infection stages (De Libero and Mori, 2014; Huang, 2016; Meermeier et al., 2018). Among them, γδ T cells are innate-like T lymphocytes that comprise a small segment (1%–5%) of the circulating T lymphocytes and play a vital role in triggering host cell-mediated immune responses (Hiromatsu et al., 1992; Ribeiro et al., 2015). Antigen-specific γδ T cells constitute a primary innate immune defense that could play an important function in anti-mycobacteria-mediated immunity. They are also able to recognize Mtb antigens by responding to Bacille Calmette–Guerin (BCG) vaccination, inhibiting mycobacterial growth, and providing protective immunity upon adoptive transfer (Balbi et al., 1993; Zhang et al., 2006; Brandes et al., 2009; Price and Hope, 2009). Recently, upon moderate-dose TB challenge, the expansion of Vγ2Vδ2 T cells was observed and correlated with decreased TB infection and disease pathology in non-human primates (NHPs) vaccinated with attenuated HMBPP-producing Listeria monocytogenes (Shen et al., 2019).
Recent data suggest that reduced frequencies in γδ T cells are associated with reduced survival rate and increased lung injury upon H5N1 respiratory infection (Dong et al., 2018). It was also previously described that influenza-affected individuals require γδ T cells for effective immunity and maintenance of lung homeostasis (Xi-zhi et al., 2018; Sabbaghi et al., 2020). γδ T cell exhaustion results in impaired host defense to lung infections by different pathogens like Klebsiella pneumonia, Streptococcus pneumoniae, and Staphylococcus aureus (Moore et al., 2000; Kirby et al., 2007; Cho et al., 2010). Although γδ T cells are considered to be the primary orchestrator of immune responses in TB disease and DM known to increase the clinical disease severity and affect treatment response, the mechanism of DM or PDM comorbidities in altering the disease severity and γδ T cell-induced correlates of protective immunological response in LTB disease is not known. Especially, the roles of γδ T cell immune responses in LTB DM, pre-DM (PDM), and non-DM (NDM) comorbidity are not characterized.
Hence, we measured the frequencies of γδ T cells expressing Th1 (IFNγ, TNFα, IL-2) and Th17 (IL-17A, IL-17F, IL-22) cytokines, cytotoxic markers [perforin (PFN), granulysin (GNLSN), granzyme (GZE) B], and other immune markers (GMCSF, PD-1, CD69) using immune phenotyping by flow cytometry. In addition, we measured the Mtb antigen-stimulated supernatants of IFNγ, TNFα, and IL-17A cytokines between the LTB comorbidities. We reveal that γδ T cells expressing Th1, Th17, cytotoxic, and immune markers were significantly diminished in LTB DM and/or PDM compared to LTB NDM-coinfected individuals. The antigen-stimulated supernatant levels of IFNγ, TNFα, and IL-17A cytokines were significantly increased between the study individuals. Overall, our data suggest that diminished frequencies of γδ T cells expressing cytokines and cytotoxic markers and elevated cytokines in the supernatants are an important characteristic feature in LTB DM and PDM comorbidities.
Materials and Methods
Study Group
The study was sanctioned by the National Institute of Research in Tuberculosis (NIRT) Internal Ethics Committee (NIRTIEC2011013), and informed written consent was obtained from all study individuals. A group of 60 patients were recruited with LTB disease and separated into three different arms (20 individuals in each arm) based on their diabetes status as follows: diabetes mellitus (DM), pre-DM (PDM), and non-DM (NDM) (Table 1). LTB positivity was defined by both tuberculin skin test (TST) and QuantiFERON-TB GOLD in tube ELISA. These individuals did not have any active tuberculosis pulmonary symptoms and possessed normal chest radiographs. The TST positive was expressed as an induration at the site of tuberculin inoculation of at least 12 mm in diameter to minimize false positivity occurrence owing to contact with environmental mycobacteria. Type 2 DM (HbA1c levels > 6.5%, random blood glucose > 200 mg/dl), PDM (HbA1c levels >5.7% to <6.4%, random blood glucose 140–199 mg/dl), and NDM (HbA1c levels <5.7% random blood glucose <140 mg/dl) were detected on the basis of glycated hemoglobin (HbA1c) levels and random blood glucose according to the criteria given by the American Diabetes Association. The study individuals were BCG vaccinated, HIV negative, and not under the usage of any steroids and did not have any signs or symptoms of related lung or systemic disease.
Isolation of Peripheral Blood Mononuclear Cell Isolation and Thawing
Peripheral blood mononuclear cells (PBMCs) were isolated from the collected peripheral blood samples using Ficoll-Paque (GE Life Sciences) density gradient centrifugation for 30 min at 400 × g at 4°C, and the cells were stored at -80°C using fetal bovine serum (FBS) and dimethyl sulfoxide (DMSO). The PBMC cells were thawed in 37°C in a water bath using a thawing [Roswell Park Memorial Institute 1640 (nine parts), RPMI-FBS (one part)] medium.
Mycobacterial Antigens and Stimulation
The antigens used are purified protein derivative (PPD; Statens Serum Institut, 10 μg/ml), whole-cell lysate (WCL 1 μg/ml), and phorbol 12-myristate 13-acetate (P)/ionomycin (P/I; Calbiochem, 12.5 and 125 ng/ml). The thawed PBMC cells were either unstimulated or stimulated with mycobacterial (Mtb) antigens and incubated for 18 h at 37°C in 5% CO2. FastImmune™ brefeldin A (10 μg/ml) was added after 2 h of post-incubation. Once incubation was completed, the cells were transferred into sterile falcon tubes, washed with PBS, and dissolved using a permeabilization buffer, and cell staining was performed. The cultured PBMC supernatants were collected and used for ELISA.
PBMC Staining and Flow Cytometry
The thawed cells were washed with PBS first and PBS/1% BSA later, then stained with surface [anti-CD3-AmCyan clone-SK7 (also known as Leu-4) (ASR), catalog no.: 339197, BD Biosciences], anti-CD69 (PB, anti-human, clone-FN50, catalog no: 310920, BioLegend), and anti-CD279 (PD-1) (PB, anti-human, clone-EH12.2H7, catalog no: 329916, BioLegend)] antibodies for 30–60 min at 4°C in the dark. Next, the cells were washed and permeabilized with 2 ml of 1× BD Perm/Wash buffer (BD Biosciences) and stained with intracellular cytokines for an additional 2 h at 4°C before washing (1× permeabilization buffer) and acquisition. The intracellular antibodies used in the study are as follows: γδ TCR (PE-Cyanine7, anti-human, clone-B1, BioLegend, catalog no: 331222), αβ TCR (PerCp/Cyanine5.5, anti-human, clone-IP26, BioLegend, catalog no: 306724), TNFα (FITC, mouse anti-human, clone-6401.1111 [RUO-(GMP)] catalog no: 340511, BD Pharmingen), IFNγ (PE, mouse anti-human, clone-B27 catalog no: 562016, BD Pharmingen), IL-2 (APC, human monoclonal antibody, clone-MQ1-17H12, catalog no: 17-7029-41, eBioscience™), IL-17A (FITC, anti-human, clone-CZ8-23G1, catalog no: 130-120-550, Miltenyi Biotec), IL-22 [PE, monoclonal antibody, clone-22URPI, catalog no: 12-7229-42, Invitrogen (eBioscience)], IL-17F (Alexa Fluor 647, mouse anti-human, clone-O33-782, catalog no: 561333, BD Biosciences), perforin (FITC, anti-human, clone-delta G9, catalog no: 130-096-668, Miltenyi Biotec), granzyme B [Alexa Fluor 647, mouse anti-human, clone-GB11, catalog no: BDB560212, Invitrogen (eBioscience)], granulysin [PE, anti-human, clone-DH2, catalog no: 12-8828-42, Invitrogen (eBioscience)], and granulocyte macrophage-colony-stimulating factor [GM-CSF] [pacific blue (PB), anti-human, clone-BVD2-21C11, catalog no: 502314, BioLegend]. For multicolor flow cytometry analysis, the stained PBMCs (CD3+γδ+ T cells) were acquired using an eight-color FACSCanto II flow cytometer with FACSDiva software v.6 (Becton Dickinson and Company, Cockeysville, MD). The lymphocyte gating was set using forward vs. side scatter, and 50,000 gated lymphocyte events were acquired. The gating strategy for γδ T cells expressing Th1 and Th17 cytokines and cytotoxic and immune markers was determined by FMO and exemplified as frequencies (unstimulated) and net frequencies (antigen stimulation).
ELISA
We measured the unstimulated, Mtb antigen (PPD, WCL), and P/I-stimulated levels of IFNγ, TNFα, and IL-17A cytokines using ELISA (DuoSet Kits R&D Systems). The detection limit of IFNγ is 9.375–600 pg/ml, that of TNFα is 15.625–1,000 pg/ml, and that of IL-17A is 15.625–1,000 pg/ml.
Data Analysis
The cytokine and cytotoxic marker frequencies of γδ T cell subsets were analyzed using FlowJo 3 (version 10, [Treestar, Ashland, OR]) software. The statistical analysis was carried out using GraphPad Prism (version 9) (GraphPad Software, Inc., San Diego, CA). Geometric means (GM) were used to measure the central tendency, and intergroup comparisons were analyzed by the non-parametric Kruskal–Wallis test. The Mtb antigen and P/I stimulation cytokine levels were represented as net cytokine values. For heatmap analysis, the frequencies of cytokines and cytotoxic and immune markers were normalized and the data are shown using GraphPad Prism with p < 0.05 (ANOVA) considered as statistically significant. The smallest value in each data set is defined as 0%, and the highest value in the dataset is defined as 100%.
Results
LTB DM/PDM Comorbidities Are Associated With Reduced γδ T Cells Expressing Th1 Cytokines
The gating strategy and representative plots of γδ T cells expressing Th1 (IFNγ, IL-2, TNFα) and Th17 (IL-17A, IL-17F, IL-22) cytokines as well as cytotoxic [perforin (PFN), granzyme (GZE) B, granulysin (GNLSN)] and immune (GMCSF, PD-1, CD69) markers are shown (Supplementary Figure 1 and Supplementary Figures 2A–C). We determined the baseline (unstimulated, UNS) and Mtb antigen (PPD, WCL) and positive (P/I) control-stimulated frequencies of γδ T cells expressing Th1 cytokines in LTB (NDM, PDM, and DM) comorbid individuals using multicolor flow cytometry (Figure 1). In UNS stimulation, the frequencies of γδ T expressing Th1 cytokines were not significantly different in LTB coinfected individuals with NDM, PDM, and DM comorbidities. In contrast, the frequencies of γδ T cells expressing Th1 cytokines were significantly reduced in PPD [IFNγ (geometric mean (GM) of DM is 0.568 versus (vs.) GM of PDM is 1.590 vs. GM of NDM is 1.510), TNFα (GM of DM is 0.402 vs. GM of PDM is 0.337 vs. GM of NDM is 0.906)] and WCL [TNFα (GM of DM is 0.439 vs. GM of PDM is 0.449 vs. GM of NDM is 0.913)] antigen stimulation in LTB DM and PDM individuals compared to LTB NDM individuals. Finally, upon P/I stimulation, the frequencies of γδ T cells expressing Th1 cytokines were not significantly different among LTB DM, PDM, and NDM individuals (Figure 1). Therefore, LTB PDM and DM comorbid individuals are associated with reduced Th1 expressing cytokine frequencies.
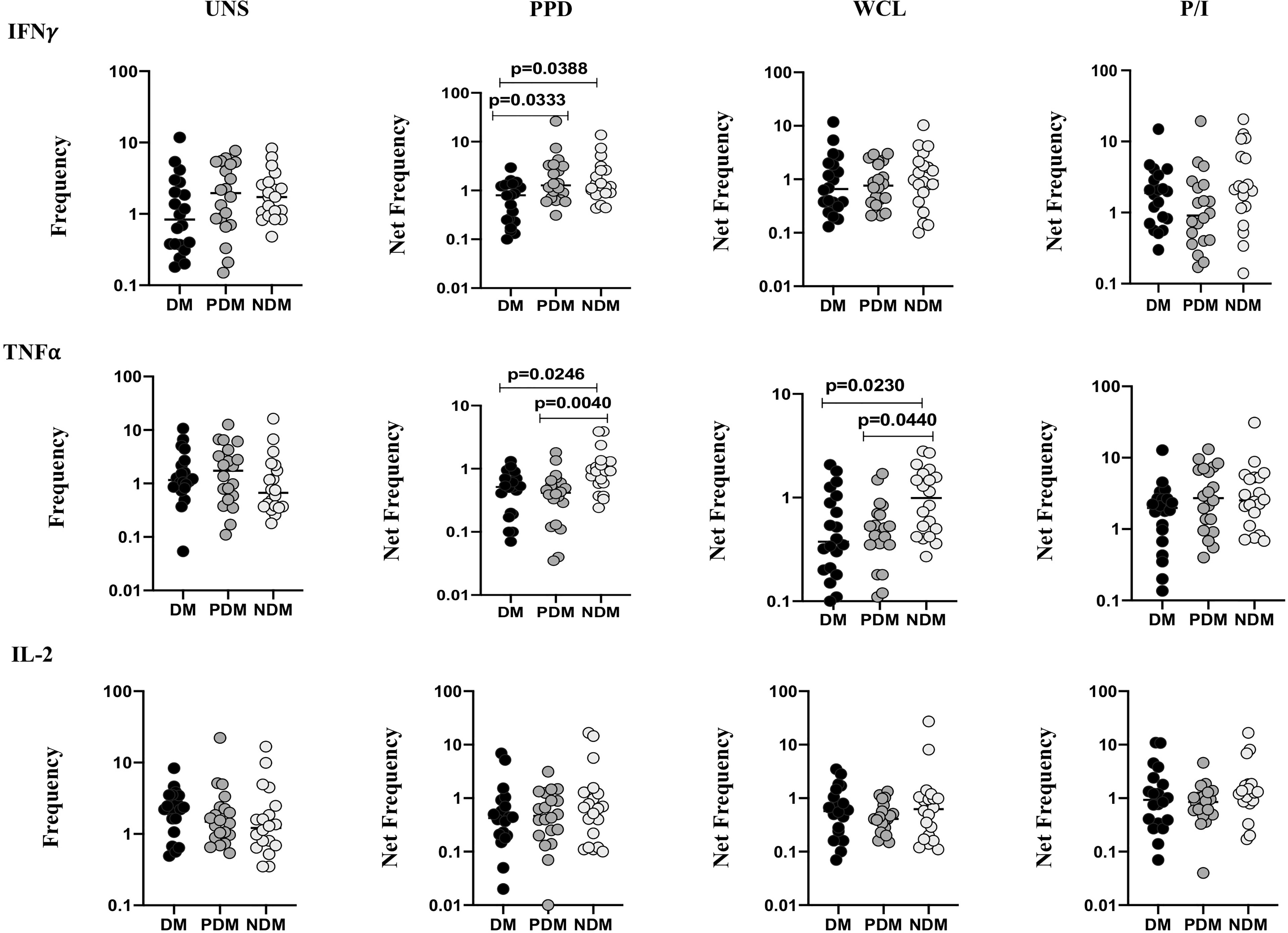
Figure 1 Decreased frequencies of γδ T cells expressing Th1 cytokines in LTB comorbidities. Peripheral blood mononuclear cells (PBMCs) were either untreated or treated with Mtb or positive control antigens for 18 h. The absolute (unstimulated, UNS) and antigen-stimulated (PPD, WCL, P/I) net frequencies of Th1 (IFNγ, IL-2, TNFα) cytokines were shown in LTB DM (n = 20), LTB PDM (n = 20), and LTB NDM (n = 20) groups. Geometric mean values were represented using bars, and every circle denotes a single individual. Kruskal–Wallis test with multiple Dunn’s comparison was used to determine the p values.
LTB DM/PDM Comorbidities Are Associated With Reduced γδ T Cells Expressing Th17 Cytokines
We determined the baseline (UNS) and mycobacterial antigen (PPD, WCL) and positive (P/I) control antigen-stimulated frequencies of γδ T cells expressing Th17 (IL-17A, IL-17F, IL-22) cytokines in LTB (NDM, PDM, and DM) comorbid individuals using multicolor flow cytometry (Figure 2). In UNS stimulation, the frequencies of γδ T cells expressing Th17 cytokines did not significantly differ between LTB individuals with NDM, PDM, and DM comorbidities. In contrast, the frequencies of γδ T cells expressing Th17 cytokines were significantly reduced in PPD [IL-17F (GM of DM is 0.449 vs. GM of PDM is 0.410 vs. GM of NDM is 1.022), IL-22 (GM of DM is 0.342 vs. GM of PDM is 0.162 vs. GM of NDM is 0.848)] and WCL [IL-17A (GM of DM is 0.356 vs. GM of PDM is 0.402 vs. GM of NDM is 0.807), IL-22 (GM of DM is 0.486 vs. GM of PDM is 0.302 vs. GM of NDM is 1.329)] antigen stimulation in LTB PDM and DM individuals compared to LTB NDM individuals. Finally, upon P/I stimulation, the frequencies of γδ T cells expressing Th17 cytokines did not exhibit a significant difference between the study population (Figure 2). Thus, γδ T cells expressing Th17 expressing cytokine frequencies were significantly diminished in LTB PDM and DM comorbid individuals.
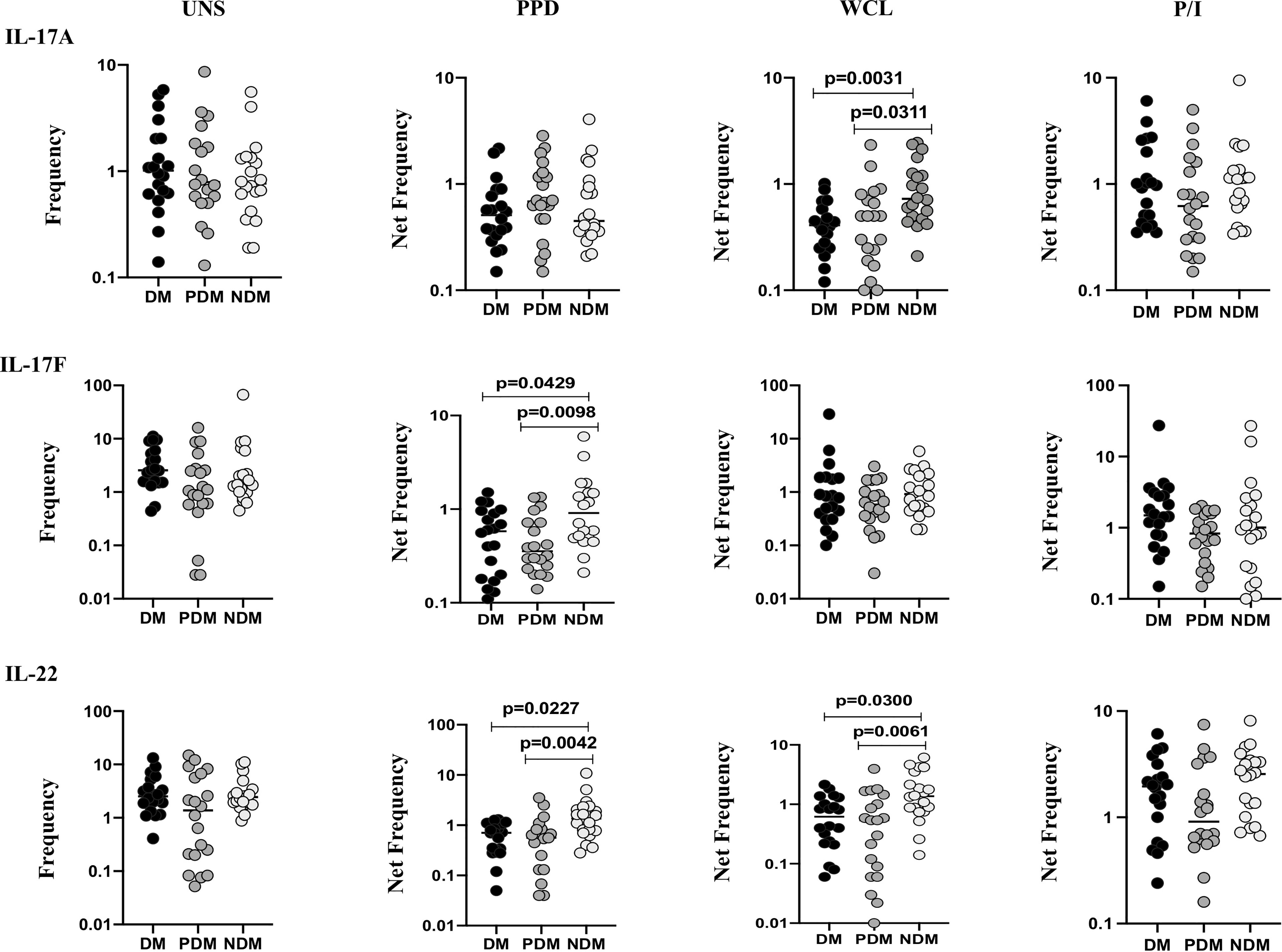
Figure 2 Decreased frequencies of γδ T cells expressing Th17 cytokines in LTB comorbidities. PBMCs were either untreated or treated with Mtb or positive control antigens for 18 h. The absolute (unstimulated, UNS) and antigen-stimulated (PPD, WCL, P/I) net frequencies of Th17 (IL-17A, IL-17F, IL-22) cytokines were shown in LTB DM (n = 20), LTB PDM (n = 20), and LTB NDM (n = 20) groups. Geometric mean values were represented using bars, and every circle denotes a single individual. Kruskal–Wallis test with multiple Dunn’s comparison was used to determine the p values.
LTB DM/PDM Comorbidities Are Associated With Reduced γδ T Cell Expressing Cytotoxic Markers
To determine the γδ T cells expressing cytotoxic markers in LTB individuals with NDM, PDM, and DM comorbidities, we used multicolor flow cytometry to delineate the baseline (UNS) and Mtb antigen-specific (PPD, WCL) stimulated frequencies expressing cytotoxic (perforin (PFN), granzyme B (GZE B), and granulysin (GNLSN) markers) (Figure 3). In UNS condition, the frequencies of γδ T cells expressing cytotoxic markers were not significantly altered in LTB PDM and LTB DM individuals compared to LTB NDM individuals. In contrast, net frequencies of γδ T cells expressing cytotoxic markers were significantly reduced upon PPD [PFN (GM of DM is 0.574 vs. GM of PDM is 0.295 vs. GM of NDM is 0.739), GZE B (GM of DM is 0.533 vs. GM of PDM is 0.403 vs. GM of NDM is 1.313], GNLSN [GM of DM is 0.316 vs. GM of PDM is 0.358 vs. GM of NDM is 0.793)] and WCL [PFN (GM of DM is 0.507 vs. GM of PDM is 0.265 vs. GM of NDM is 1.377)] antigen stimulation in LTB DM and LTB PDM individuals compared to LTB NDM individuals. Finally, upon P/I stimulation, frequencies of γδ T cells expressing cytotoxic markers were not altered in LTB DM and LTB PDM when compared to LTB NDM individuals (Figure 3). Therefore, γδ T cells expressing cytotoxic marker frequencies were significantly diminished in LTB DM and PDM individuals.
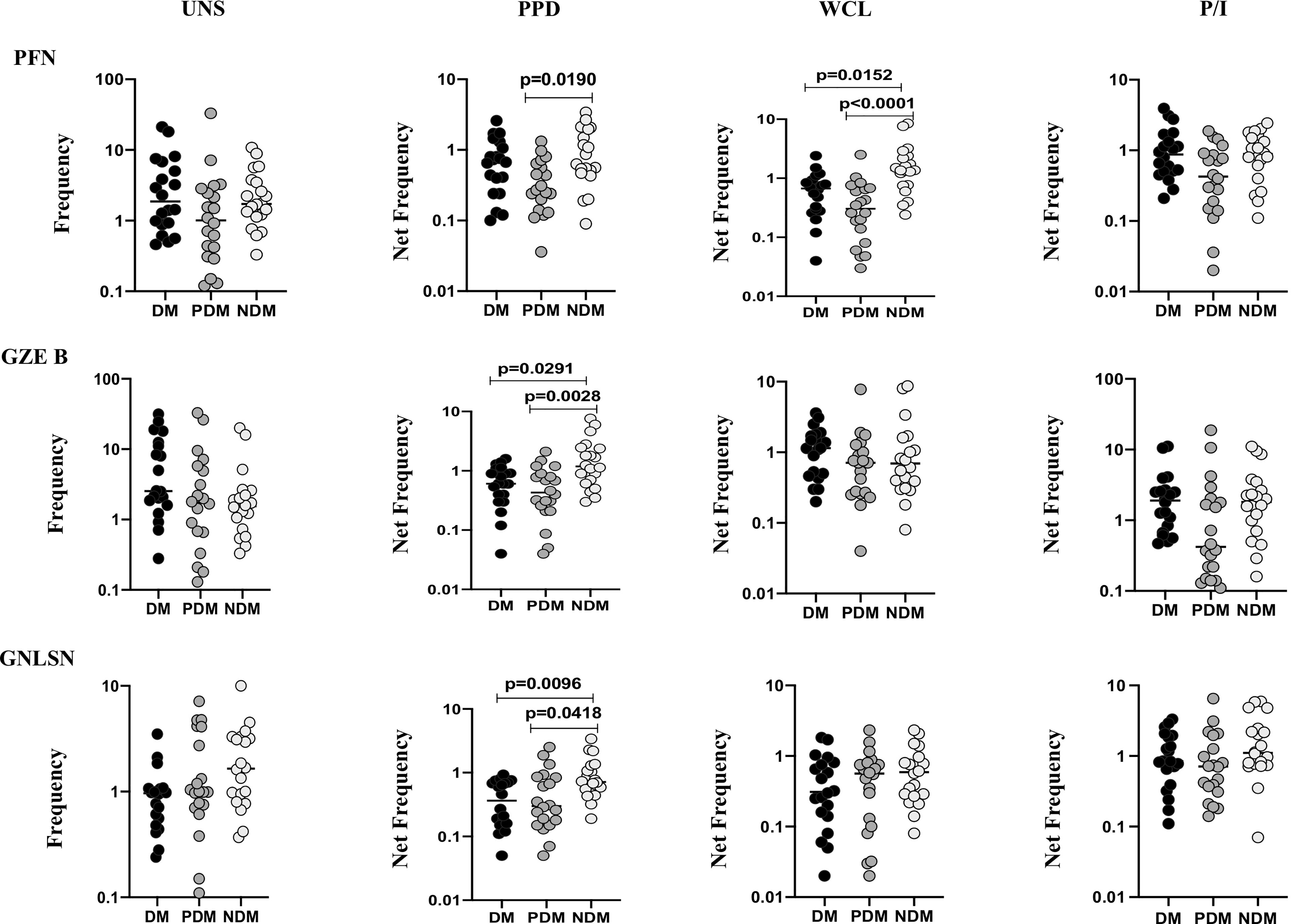
Figure 3 Decreased frequencies of γδ T cells expressing cytotoxic markers in LTB comorbidities. PBMCs were either untreated or treated with Mtb or positive control antigens for 18 h. The absolute (unstimulated, UNS) and antigen-stimulated (PPD, WCL, P/I) net frequencies of cytotoxic (PFN, GZEB, GNLYSN) markers were shown in LTB DM (n = 20), LTB PDM (n = 20), and LTB NDM (n = 20) groups. Geometric mean values were represented using bars, and every circle denotes a single individual. Kruskal–Wallis test with multiple Dunn’s comparison were used to determine the p values.
LTB DM/PDM Comorbidities Are Associated With Reduced γδ T Cell Expressing Immune Markers
To determine the γδ T cells expressing immune markers in LTB individuals with NDM, PDM, and DM comorbidities, we used multicolor flow cytometry to delineate the UNS and Mtb antigen-specific (PPD, WCL) stimulated frequencies expressing immune (GMCSF, PD-1, CD69) markers (Figure 4). In UNS conditions, the frequencies of γδ T cells expressing immune markers were not significantly altered [except GMCSF (GM of DM is 1.317 vs. GM of NDM is 1.437), DM vs. NDM] in LTB PDM and LTB DM individuals compared to LTB NDM individuals. In contrast, net frequencies of γδ T cells expressing immune markers were significantly reduced upon PPD [CD69 (GM of DM is 0.468 vs. GM of PDM is 0.374 vs. GM of NDM is 1.253)] and WCL [PD-1 (GM of DM is 0.469 vs. GM of PDM is 0.329 vs. GM of NDM is 0.855), CD69 (GM of DM is 0.775 vs. GM of PDM is 0.464 vs. GM of NDM is 1.393)] antigen stimulation in LTB DM and LTB PDM individuals compared to LTB NDM individuals. Finally, upon P/I stimulation, frequencies of γδ T cells expressing immune markers were not altered in LTB DM and LTB PDM when compared to LTB NDM individuals (Figure 4). Therefore, γδ T cells expressing immune marker frequencies were significantly diminished in LTB DM and PDM individuals.
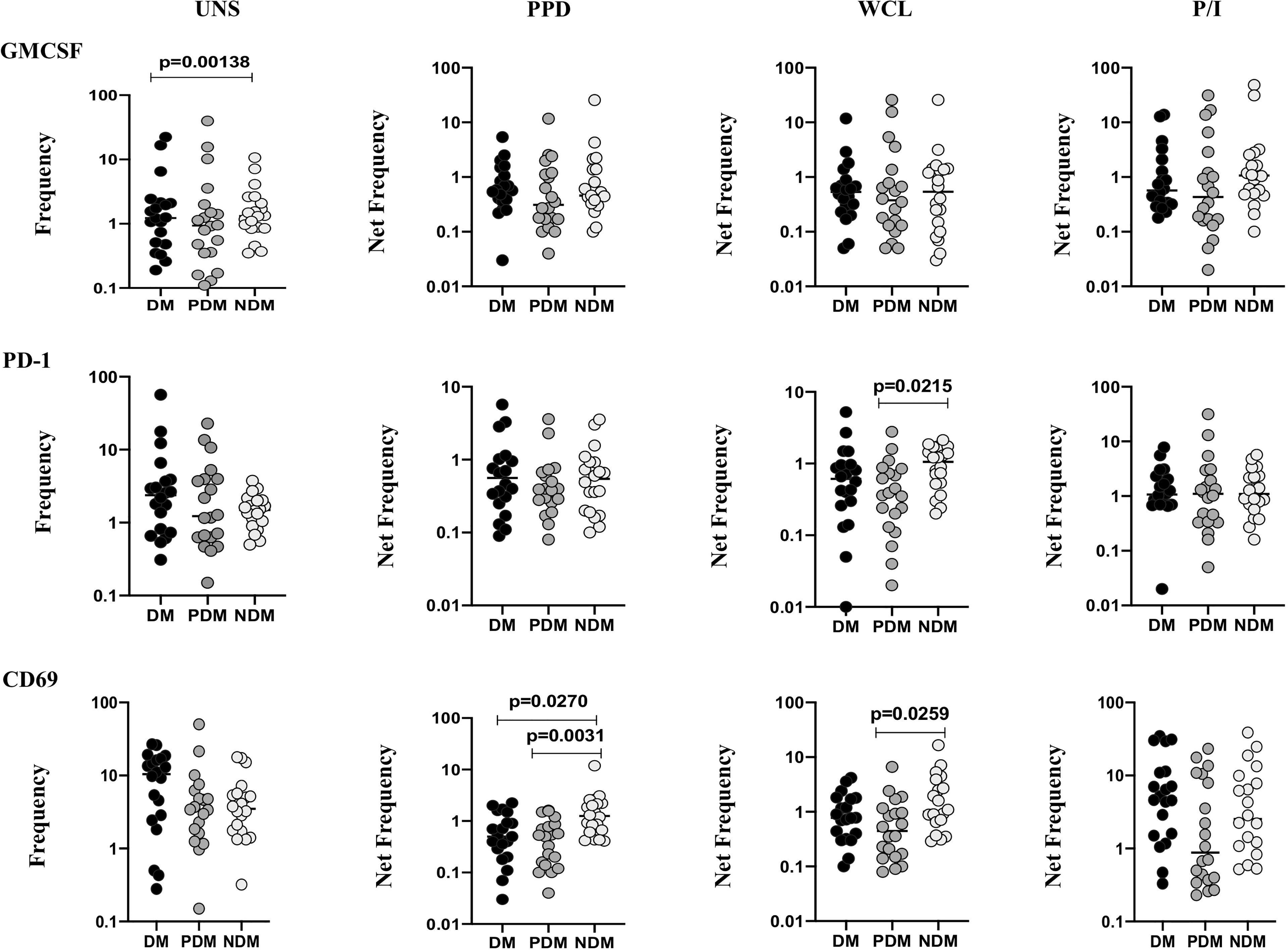
Figure 4 Decreased frequencies of γδ T cells expressing immune markers in LTB comorbidities. PBMCs were either untreated or treated with Mtb or positive control antigens for 18 h. The absolute (unstimulated, UNS) and antigen-stimulated (PPD, WCL, P/I) net frequencies of immune (GMCSF, PD-1, CD69) markers were shown in LTB DM (n = 20), LTB PDM (n = 20), and LTB NDM (n = 20) groups. Geometric mean values were represented using bars, and every circle denotes a single individual. Kruskal–Wallis test with multiple Dunn’s comparison was used to determine the p values.
Elevated PBMC Culture Supernatant Levels of Cytokines in LTB Comorbidities
The UNS, Mtb (PPD, WCL) antigen, and P/I-stimulated supernatant levels of IFNγ, TNFα, and IL-17A cytokines in LTB (NDM, PDM, DM) comorbidities are shown (Figure 5). As shown in Figures 5A–C, the UNS levels of IFNγ, TNFα, and IL-17A cytokines did not significantly differ between the LTB coinfected (NDM, PDM, DM) individuals. However, after stimulation with Mtb antigens, IFNγ [GM of NDM is 6.723 vs. GM of PDM is 6.011 vs. GM of DM is 95.29 pg/ml in PPD and GM of NDM is 5.243 vs. GM of PDM is 5.293 vs. GM of DM is 48.99 pg/ml in WCL] supernatant levels were significantly increased in LTB DM and LTB PDM compared to LTB NDM individuals (Figure 5A). Similarly, upon stimulation with Mtb antigens, TNFα [GM of NDM is 2.304 vs. GM of DM is 7.403 pg/ml in PPD] supernatant levels were significantly increased in LTB DM compared to LTB NDM individuals (Figure 5B). Finally, IL-17A [GM of NDM is 0.52 vs. GM of PDM is 1.521 vs. GM of DM is 1.274 pg/ml in PPD and GM of NDM is 0.475 vs. GM of PDM is 1.863 vs. GM of DM is 1.195 pg/ml in WCL] supernatant levels were significantly increased in LTB PDM and LTB DM compared to LTB NDM individuals (Figure 5C). We did not observe any significant difference upon P/I stimulation between the study individuals (Figures 5A–C). Hence, LTB-coinfected individuals were associated with increased supernatant levels of IFNγ, TNFα, and IL-17A cytokines.
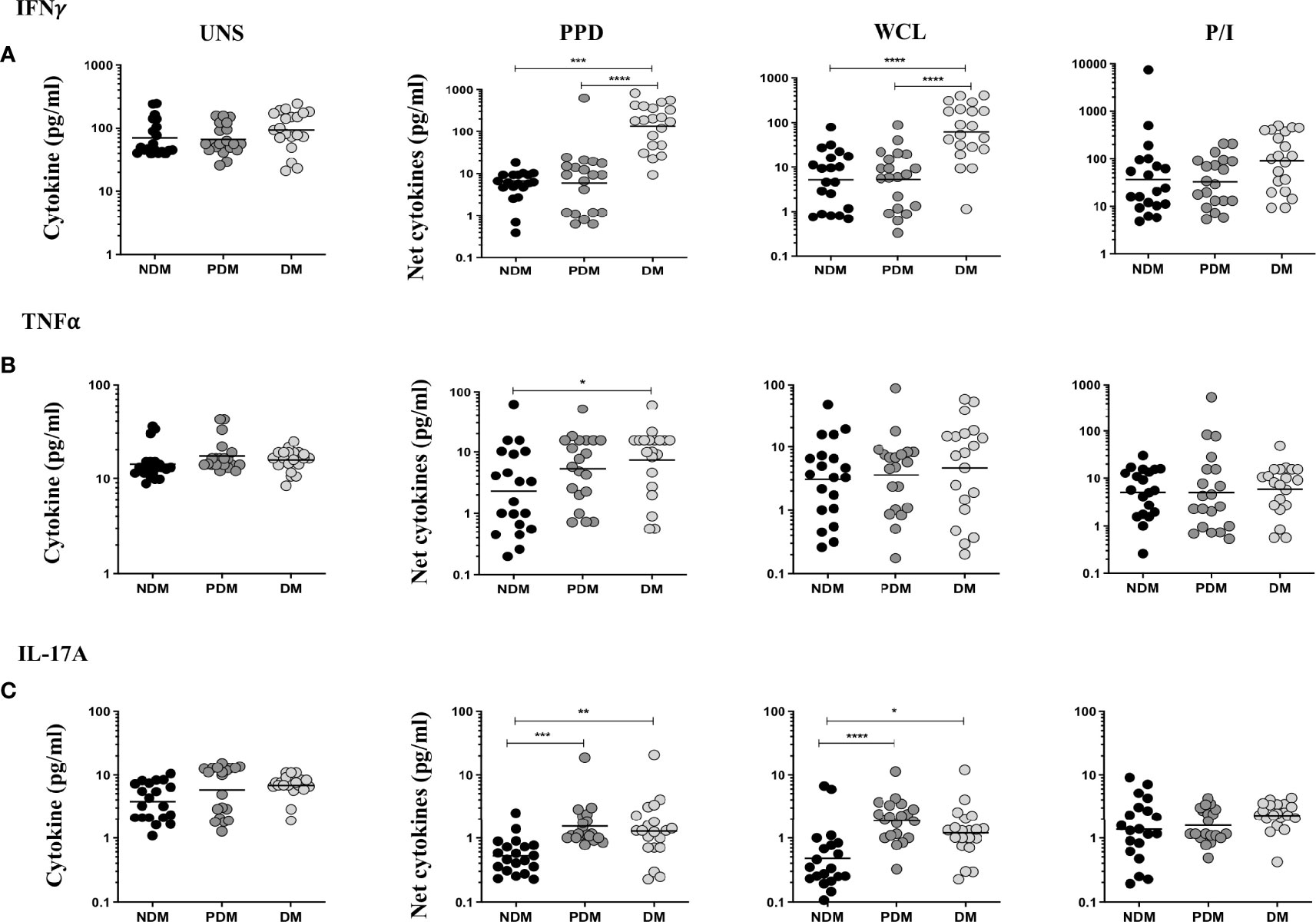
Figure 5 LTB (DM and PDM) coinfection is associated with increased antigen-specific supernatant levels of (A) IFNγ, (B) TNFα, and (C) IL-17A cytokines. PBMCs were cultured with no antigen (UNS), stimulated with Mtb (PPD, WCL), and positive (P/I) control antigens for 18 h in LTB NDM (n = 20), LTB PDM (n = 20), and LTB DM (n = 20) individuals. We measured the net cytokine levels by subtracting the antigen-induced cytokine or cytotoxic and immune markers from their baseline levels for each individual. Geometric mean values were represented using bars, and every circle denotes a single individual. p values were analyzed by Kruskal–Wallis test.
Heatmap Analysis of γδ T Cells Expressing Cytokines and Cytotoxic and Immune Markers in LTB Comorbidities
We performed the heatmap analysis of γδ T cells expressing Th1 (IFNγ, IL-2, TNFα) and Th17 (IL-17A, IL-17F, IL-22) cytokines as well as cytotoxic (PFN, GZE B, GNLSN) and immune (GMCSF, PD-1, CD69) markers in LTB DM, LTB PDM, and LTB NDM comorbidities (Figures 6A–C). Our data show that the expression of cytokines and cytotoxic and immune markers were significantly different between the LTB (DM, PDM, NDM) comorbidities.
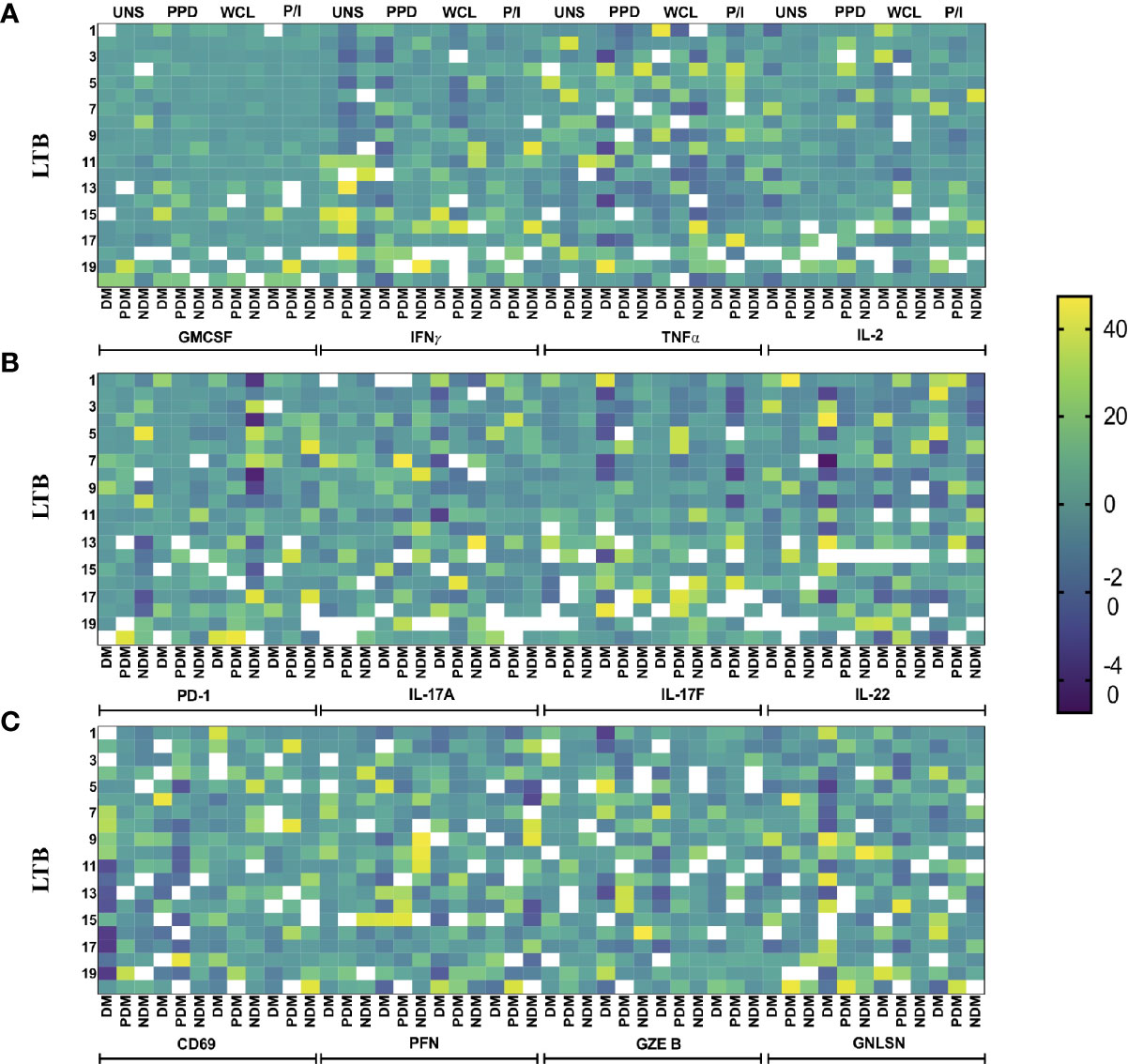
Figure 6 Heatmap analysis of γδ T cells. The cytokine and cytotoxic and immune marker frequencies were normalized across all LTB groups, and the expression data are shown. Each column indicates a single person. We show the heatmap expression of (A) GMCSF and Th1 cytokines, (B) PD-1 and Th17 cytokines, and (C) PD-1 and cytotoxic markers in LTB patients coinfected with DM (n = 20), PDM (n = 20), and NDM (n = 20) individuals upon UNS, Mtb (PPD, WCL), and positive antigen (P/I) control stimulation with continuous color shading.
Discussion
γδ T cells mainly contribute to anti-mycobacterial response built by other immune cells (Li and Wu, 2008; Brandes et al., 2009; Price and Hope, 2009). The activity of γδ T cells against Mtb occurred mostly via secreted cytokines, effector cytotoxic molecules, and chemotaxis. They were able to generate various cytokines that function against TB disease especially Th1, Th2, Th17 and regulatory cytokines (García et al., 1997; Tsukaguchi et al., 1999; Wesch et al., 2001). The role of γδ T cells expressing cytokine, cytotoxic, and other immune markers in LTB (NDM, PDM, and DM) comorbidities has not been studied and therefore was examined in the present study. Our data illustrate that γδ T cells expressing Th1 and Th17 cytokines as well as cytotoxic and immune markers were significantly decreased in LTB DM and/or LTB PDM compared to LTB NDM individuals.
γδ T cells can produce Th1 (TNFα, IFNγ) cytokines which are crucial for immune protection against viruses and intracellular microbes (O’Brien et al., 2009; Bonneville et al., 2010). Our previous studies have shown that antigen-specific Th1 responses in CD4+ T cells were reduced in LTB DM and LTB PDM comorbidities (Kumar et al., 2017). Our findings suggest that the frequencies of γδ T cells expressing Th1 cytokines (mainly IFNγ, TNFα) are also decreased in the LTB DM and LTB PDM groups compared to the LTB NDM group. The decreased frequencies might contribute to compromised immune protection in LTB individuals. Upon Mtb infection, γδ T cells activate Th17 cytokines and could proficiently control the effector role of other immune cells (Song et al., 2016). Specifically, IL-17-expressing γδ T cells could be involved in Mtb-mediated immune response associated with disease pathology in PTB disease (Umemura et al., 2007; van de Veerdonk et al., 2010). Similarly, IL-22 is produced by a wide range of cells, which includes Th1, Th17, γδ T cells, and innate immune cells like innate lymphoid cells (ILCs) (Toussirot et al., 2018; Cella et al., 2019). Our earlier study has shown that antigen-specific Th17 responses in CD4+ T cells were reduced in LTB DM and LTB PDM comorbidities (Kumar et al., 2017). Our current study demonstrates diminished frequencies of γδ T cells expressing IL-17 family cytokines in LTB DM or LTB PDM comorbidity. Thus, diminished protective cytokine production by γδ T cells could also contribute to diminished immune protection in these comorbidities.
γδ T cells confer to the primary immunity against Mtb disease by the production of cytokines and chemokines as well as stimulating cytotoxic molecules (Dieli et al., 2003; Qin et al., 2009). The important feature of cytotoxic markers (perforin, granzyme B, granulysin) is to lyse the Mtb-affected cells directly (Stenger, 2001). Our results exhibit that γδ T cell frequencies expressing perforin, granzyme B, granulysin, and perforin were significantly reduced upon PPD and WCL antigen stimulation in LTB (DM and PDM) coinfected individuals compared to LTB NDM individuals. This is in line with our previous study showing that CD8+ T cells expressing cytotoxic (perforin, granzyme B, CD107a) marker frequencies were diminished significantly in the LTB DM group when compared to the LTB NDM group (Kumar et al., 2015). In addition to the above cytokines and cytotoxic markers, we also studied immune (GMCSF, PD-1, CD69) markers and both PD-1 and CD69 were associated with significantly reduced frequencies in LTB DM and/or PDM compared to LTB NDM individuals. Thus, activation and cytotoxic marker expression are diminished in LTB DM and LTB PDM comorbidity.
We also show that the PBMC-stimulated supernatants of IFNγ, TNFα, and IL-17A cytokines were significantly increased in the DM and/or PDM comorbidities, indicating that the comorbidities might influence the disease pathogenesis in LTB individuals. Our study has certain limitations by showing only the baseline frequencies of LTB-coinfected individuals and not any follow-up frequencies of cytokines and cytotoxic markers upon treatment for DM. The other limitation is the sample size used in the study is small. Also, we did not examine the frequencies of γδ T cells expressing Th2 cytokines in LTB DM comorbidity in this study. Overall, our data reveal that DM and PDM comorbidities were characterized by decreased Th1, Th17, cytotoxic, and other immune markers and possibly associated with reduced immune protection in LTB infection.
Data Availability Statement
The original contributions presented in the study are included in the article/Supplementary Material. Further inquiries can be directed to the corresponding author.
Ethics Statement
The studies involving human participants were reviewed and approved by the National Institute of Research in Tuberculosis (NIRT) Internal Ethics Committee. The patients/participants provided their written informed consent to participate in this study.
Author Contributions
Conceived and designed the experiments: GK and SB. Performed the experiments: GK, NP, and KM. Analyzed the data: GK. Contributed materials/reagents/analysis tools: PM and SB. Wrote the paper: GK and SB. All authors contributed to the article and approved the submitted version.
Funding
The author (GK) thank the financial support from the DBT-RA Program in Biotechnology and Life Sciences. The funding was supported by the Division of Intramural Research, National Institute of Allergy and Infectious Diseases (NIAID), NIH. The funders have no role in the study design, data collection or analysis, data interpretation, or manuscript writing.
Conflict of Interest
The authors declare that the research was conducted in the absence of any commercial or financial relationships that could be construed as a potential conflict of interest.
Publisher’s Note
All claims expressed in this article are solely those of the authors and do not necessarily represent those of their affiliated organizations, or those of the publisher, the editors and the reviewers. Any product that may be evaluated in this article, or claim that may be made by its manufacturer, is not guaranteed or endorsed by the publisher.
Acknowledgments
The authors thank NIH-NIRT-ICER and the staff members of the Department of Clinical Research, NIRT, Government Stanley Hospital, Government General Hospital, and Government Kilpauk Medical Hospital, Chennai, Tamil Nadu, for providing the assistance in patient recruitment for this study.
Supplementary Material
The Supplementary Material for this article can be found online at: https://www.frontiersin.org/articles/10.3389/fcimb.2021.756854/full#supplementary-material
Supplementary Figure 1 | Gating strategy of γδ T cells expressing Th1, Th17 cytokines, cytotoxic and immune markers. Single cells were gated from lymphocytes and further gated on CD3+ T cell population and γδ T cells were gated based on positive γδ TCR expression and the frequencies of different cytokines, cytotoxic and immune markers were shown.
Supplementary Figure 2 | Representative plots for γδ T cells expressing (A) Th1 cytokines and GMCSF, (B) Th17 cytokines and PD-1, (C) cytotoxic markers and CD69 upon UNS, Mtb (PPD, WCL) antigen stimulation and positive antigen (P/I) control stimulation.
References
Abdul-Ghani, M. A., DeFronzo, R. A. (2009). Pathophysiology of Prediabetes. Curr. Diab Rep. 9 (3), 193–199. doi: 10.1007/s11892-009-0032-7
Balbi, B., Valle, M. T., Oddera, S., Giunti, D., Manca, F., Rossi, G. A., et al. (1993). T-Lymphocytes With γδ+Vδ2+ Antigen Receptors are Present in Increased Proportions in a Fraction of Patients With Tuberculosis or With Sarcoidosis. Am. Rev. Respir. Dis. 148 (6), 1685–1690. doi: 10.1164/ajrccm/148.6_Pt_1.1685
Barry, C. E., 3rd, Boshoff, H. I., Dartois, V., Dick, T., Ehrt, S., Flynn, J., et al. (2009). The Spectrum of Latent Tuberculosis: Rethinking the Biology and Intervention Strategies. Nat. Rev. Microbiol. 7 (12), 845–855. doi: 10.1038/nrmicro2236
Bonneville, M., O’Brien, R. L., Born, W. K. (2010). Gammadelta T Cell Effector Functions: A Blend of Innate Programming and Acquired Plasticity. Nat. Rev. Immunol. 10 (7), 467–478. doi: 10.1038/nri2781
Brandes, M., Willimann, K., Bioley, G., Levy, N., Eberl, M., Luo, M., et al. (2009). Cross-Presenting Human Gammadelta T Cells Induce Robust CD8+ Alpha Beta T Cell Responses. Proc. Natl. Acad. Sci. U.S.A. 106 (7), 2307–2312. doi: 10.1073/pnas.0810059106
Cella, M., Gamini, R., Sécca, C., Collins, P. L., Zhao, S., Peng, V., et al. (2019). Subsets of ILC3–ILC1-Like Cells Generate a Diversity Spectrum of Innate Lymphoid Cells in Human Mucosal Tissues. Nat. Immunol. 20 (8), 980–991. doi: 10.1038/s41590-019-0425-y
Cho, J. S., Pietras, E. M., Garcia, N. C., Ramos, R. I., Farzam, D. M., Monroe, H. R., et al. (2010). IL-17 is Essential for Host Defense Against Cutaneous Staphylococcus Aureus Infection in Mice. J. Clin. Invest 120, 1762–1773. doi: 10.1172/JCI40891
De Libero, G., Mori, L. (2014). The T-Cell Response to Lipid Antigens of Mycobacterium Tuberculosis. Front. Immunol. 5, 219. doi: 10.3389/fimmu.2014.00219
Dheda, K., Barry, C. E., 3rd, Maartens, G. (2016). Tuberculosis. Lancet 387 (10024), 1211–1226. doi: 10.1016/S0140-6736(15)00151-8
Dieli, F., Ivanyi, J., Marsh, P., Williams, A., Naylor, I., Sireci, G., et al. (2003). Characterization of Lung γδ T Cells Following Intranasal Infection With Mycobacterium Bovis Bacillus Calmette-Guérin. J. Immunol. 170 (1), 463–469. doi: 10.4049/jimmunol.170.1.463
Dong, P., Ju, X., Yan, Y., Zhang, S., Cai, M., Wang, H., et al. (2018). γδ T Cells Provide Protective Function in Highly Pathogenic Avian H5N1 Influenza a Virus Infection. Front. Immunol. 9, 2812. doi: 10.3389/fimmu.2018.02812
Forouhi, N. G., Luan, J., Hennings, S., Wareham, N. J. (2007). Incidence of Type 2 Diabetes in England and its Association With Baseline Impaired Fasting Glucose: The Ely Study 1990–2000. Diabet Med. 24 (2), 200–207. doi: 10.1111/j.1464-5491.2007.02068.x
García, V. E., Sieling, P. A., Gong, J., Barnes, P. F., Uyemura, K., Tanaka, Y., et al. (1997). Single-Cell Cytokine Analysis of γδ T Cell Responses to Nonpeptide Mycobacterial Antigens. J. Immunol. 159 (3), 1328–1335.
Hiromatsu, K., Yoshikai, Y., Matsuzaki, G., Ohga, S., Muramori, K., Matsumoto, K., et al. (1992). A Protective Role of γδ T Cells in Primary Infection With Listeria Monocytogenes in Mice. J. Exp. Med. 175 (1), 49–56. doi: 10.1084/jem.175.1.49
Huang, S. (2016). Targeting Innate-Like T Cells in Tuberculosis. Front. Immunol. 7, 594. doi: 10.3389/fimmu.2016.00594
International Diabetes Federation (2015). IDF Diabetes Atlas, International Diabetes Federation. 6th edition. (Brussels, Belgium: Brussels International Diabetes Federation 2015).
Kirby, A. C., Newton, D. J., Carding, S. R., Kaye, P. M. (2007). Evidence for the Involvement of Lung-Specific Gammadelta T Cell Subsets in Local Responses to Streptococcus Pneumoniae Infection. Eur. J. Immunol. 37, 3404–3413. doi: 10.1002/eji.200737216
Kumar, N. P., George, P. J., Kumaran, P., Dolla, C. K., Nutman, T. B., Babu, S. (2014). Diminished Systemic and Antigen-Specific Type 1, Type 17, and Other Proinflammatory Cytokines in Diabetic and Prediabetic Individuals With Latent Mycobacterium Tuberculosis Infection. J. Infect. Dis. 210 (10), 1670–1678. doi: 10.1093/infdis/jiu329
Kumar, N. P., Moideen, K., Dolla, C. K., Nutman, T. B., Babu, S. (2017). Prediabetes Is Associated With the Modulation of Antigen-Specific Th1/Tc1 and Th17/Tc17 Responses in Latent Mycobacterium tuberculosis infection. PloS One 12 (5), e0178000. doi: 10.1371/journal.pone.0178000
Kumar, N. P., Sridhar, R., Banurekha, V. V., Jawahar, M. S., Fay, M. P., Nutman, T. B. (2013). Type 2 Diabetes Mellitus Coincident With Pulmonary Tuberculosis Is Associated With Heightened Systemic Type 1, Type 17, and Other Proinflammatory Cytokines. Ann. Am. Thorac. Soc. 10 (5), 441–449. doi: 10.1513/AnnalsATS.201305-112OC
Kumar, N. P., Sridhar, R., Nair, D., Banurekha, V. V., Nutman, T. B., Babu, S. (2015). Type 2 Diabetes Mellitus is Associated With Altered CD8(+) T and Natural Killer Cell Function in Pulmonary Tuberculosis. Immunol 144 (4), 677–686. doi: 10.1111/imm.12421
Li, L., Wu, C. Y. (2008). CD4+CD25+Treg Cells Inhibit Human Memory γδ T Cells to Produce IFN-γ in Response to M. Tuberculosis Antigen ESAT-6. Blood 111 (12), 5629–5636. doi: 10.1182/blood-2008-02-139899
Mayer-Barber, K. D., Sher, A. (2015). Cytokine and Lipid Mediator Networks in Tuberculosis. Immunol. Rev. 264 (1), 264–275. doi: 10.1111/imr.12249
Meermeier, E. W., Harriff, M. J., Karamooz, E., Lewinsohn, D. M. (2018). MAIT Cells and Microbial Immunity. Immunol. Cell. Biol. 96 (6), 607–617. doi: 10.1111/imcb.12022
Moore, T. A., Moore, B. B., Newstead, M. W., Standiford, T. J. (2000). Gamma Delta-T Cells are Critical for Survival and Early Proinflammatory Cytokine Gene Expression During Murine Klebsiella Pneumonia. J. Immunol. 165, 2643–2650. doi: 10.4049/jimmunol.165.5.2643
O’Brien, R. L., Roark, C. L., Born, W. K. (2009). IL-17-Producing Gammadelta T Cells. Eur. J. Immunol. 39 (3), 662–666. doi: 10.1002/eji.200839120
O’Garra, A., Redford, P. S., McNab, F. W., Bloom, C. I., Wilkinson, R. J., Berry, M. P. (2013). The immune response in tuberculosis. Annu. Rev. Immunol. 31, 475–527. doi: 10.1146/annurev-immunol-032712-095939
Price, S. J., Hope, J. (2009). Enhanced Secretion of Interferon-γ by Bovine γδ T Cells Induced by Coculture With Mycobacterium Bovis-Infected Dendritic Cells: Evidence for Reciprocal Activating Signals. Immunol 126 (2), 201–208. doi: 10.1111/j.1365-2567.2008.02889.x
Qin, G., Herold, M. J., Kimmel, B., Muller, I., Rincon-Orozco, B., Kunzmann, V., et al. (2009). Phosphoantigen-Expanded Human Gammadelta T Cells Display Potent Cytotoxicity Against Monocyte-Derived Macrophages Infected With Human and Avian Influenza Viruses. J. Infect. Dis. 200 (6), 858–865. doi: 10.1086/605413
Restrepo, B. I. (2016). Diabetes and Tuberculosis. Microbiol. Spectr 4 (6). doi: 10.1128/microbiolspec.TNMI7-0023-2016
Restrepo, B. I., Fisher-Hoch, S. P., Pino, P. A., Salinas, A., Rahbar, M. H., Mora, F., et al. (2008). Tuberculosis in Poorly Controlled Type 2 Diabetes: Altered Cytokine Expression in Peripheral White Blood Cells. Clin. Infect. Dis. 47 (5), 634–641. doi: 10.1086/590565
Ribeiro, S. T., Ribot, J. C., Silva-Santos, B. (2015). Five Layers of Receptor Signaling in Gamma Delta T-Cell Differentiation and Activation. Front. Immunol. 6, 15. doi: 10.3389/fimmu.2015.00015
Sabbaghi, A., Miri, S.M., Keshavarz, M., Mahooti, M., Zebardast, A., Ghaemi, A. (2020). Role of γδ T Cells in Controlling Viral Infections With a Focus on Influenza Virus: Implications for Designing Novel Therapeutic Approaches. Virol. J. 17, 174. doi: 10.1186/s12985-020-01449-0
Shen, L., Frencher, J., Huang, D., Wang, W., Yang, E., Chen, C. Y., et al. (2019). Immunization of Vγ2vδ2 T Cells Programs Sustained Effector Memory Responses That Control Tuberculosis in Nonhuman Primates. Proc. Natl. Acad. Sci. U.S.A. 116 (13), 6371–6378. doi: 10.1073/pnas.1811380116
Siddiqui, A. N., Khayyam, K. U., Sharma, M. (2016). Effect of Diabetes Mellitus on Tuberculosis Treatment Outcome and Adverse Reactions in Patients Receiving Directly Observed Treatment Strategy in India: A Prospective Study. Biomed. Res. Int. 2016, 7273935. doi: 10.1155/2016/7273935
Song, X., He, X., Li, X., Qian, Y. (2016). The Roles and Functional Mechanisms of Interleukin-17 Family Cytokines in Mucosal Immunity. Cell. Mol. Immunol. 13 (4), 418–431. doi: 10.1038/cmi.2015.105
Stenger, S. (2001). Cytolytic T Cells in the Immune Response to Mycobacterium Tuberculosis. Scand. J. Infect. Dis. 33 (7), 483–487. doi: 10.1080/00365540110026584
Toussirot, É, Laheurte, C., Gaugler, B., Gabriel, D., Saas, P. (2018). Increased IL-22- and IL- 17A-Producing Mucosal-Associated Invariant T Cells in the Peripheral Blood of Patients With Ankylosing Spondylitis. Front. Immunol. 9, 1610. doi: 10.3389/fimmu.2018.01610
Tsukaguchi, K., De Lange, B., Boom, W. H. (1999). Differential Regulation of IFN-γ, TNF-α, and IL-10 Production by CD4+αβTCR+ T Cells and Vδ2 γδ T Cells in Response to Monocytes Infected With Mycobacterium Tuberculosis-H37Ra. Cell. Immunol. 194 (1), 12–20. doi: 10.1006/cimm.1999.1497
Umemura, M., Yahagi, A., Hamada, S., Begum, M. D., Watanabe, H., Kawakami, K., et al. (2007). IL-17-Mediated Regulation of Innate and Acquired Immune Response Against Pulmonary Mycobacterium Bovis Bacille Calmette-Guerin Infection. J. Immunol. 178 (6), 3786–3796. doi: 10.4049/jimmunol.178.6.3786
van de Veerdonk, F. L., Teirlinck, A. C., Kleinnijenhuis, J., Kullberg, B. J., van Crevel, R., van der Meer, J. W., et al. (2010). Mycobacterium Tuberculosis Induces IL-17A Responses Through TLR4 and Dectin-1 and is Critically Dependent on Endogenous IL-1. J. Leukoc Biol. 88 (2), 227–232. doi: 10.1189/jlb.0809550
Viswanathan, V., Kumpatla, S., Aravindalochanan, V., Rajan, R., Chinnasamy, C., Srinivasan, R., et al. (2012). Prevalence of Diabetes and Pre-Diabetes and Associated Risk Factors Among Tuberculosis Patients in India. PloS One 7 (7), e41367. doi: 10.1371/journal.pone.0041367
Wesch, D., Glatzel, A., Kabelitz, D. (2001). Differentiation of Resting Human Peripheral Blood γδ T Cells Toward Th1- or Th2-Phenotype. Cell. Immunol. 212 (2), 110–117. doi: 10.1006/cimm.2001.1850
World health organization (2020) Global Tuberculosis Report (WHO). Available at: https://apps.who.int/iris/bitstream/handle/10665/336069/9789240013131-eng.pdf.
Xi-zhi, J. G., Dash, P., Crawford, J. C., Allen, E. K., Zamora, A. E., Boyd, D. F., et al. (2018). Lung γδ T Cells Mediate Protective Responses During Neonatal Influenza Infection That are Associated With Type 2 Immunity. Immun 49 (3), 531–544. doi: 10.1016/j.immuni.2018.07.011
Keywords: γδ T cells, Th1/Th17 cytokines, cytotoxic and immune markers, latent tuberculosis, diabetes mellitus, pre-diabetes
Citation: Kathamuthu GR, Kumar NP, Moideen K, Menon PA and Babu S (2021) Decreased Frequencies of Gamma/Delta T Cells Expressing Th1/Th17 Cytokine, Cytotoxic, and Immune Markers in Latent Tuberculosis-Diabetes/Pre-Diabetes Comorbidity. Front. Cell. Infect. Microbiol. 11:756854. doi: 10.3389/fcimb.2021.756854
Received: 11 August 2021; Accepted: 01 October 2021;
Published: 26 October 2021.
Edited by:
Ran Wang, University of Queensland, AustraliaReviewed by:
Surya Prakash Pandey, University of Pittsburgh, United StatesPaulo J. G. Bettencourt, Catholic University of Portugal, Portugal
Copyright © 2021 Kathamuthu, Kumar, Moideen, Menon and Babu. This is an open-access article distributed under the terms of the Creative Commons Attribution License (CC BY). The use, distribution or reproduction in other forums is permitted, provided the original author(s) and the copyright owner(s) are credited and that the original publication in this journal is cited, in accordance with accepted academic practice. No use, distribution or reproduction is permitted which does not comply with these terms.
*Correspondence: Gokul Raj Kathamuthu, gokul.r@nirt.res.in