- Department of Chemistry-Biology, University of Sonora, Hermosillo, Mexico
Giardiasis is one of the most common gastrointestinal infections worldwide, mainly in developing countries. The etiological agent is the Giardia lamblia parasite. Giardiasis mainly affects children and immunocompromised people, causing symptoms such as diarrhea, dehydration, abdominal cramps, nausea, and malnutrition. In order to develop an effective vaccine against giardiasis, it is necessary to understand the host-Giardia interactions, the immunological mechanisms involved in protection against infection, and to characterize the parasite antigens that activate the host immune system. In this study, we identify and characterize potential T-cell and B-cell epitopes of Giardia immunogenic proteins by immunoinformatic approaches, and we discuss the potential role of those epitopes to stimulate the host´s immune system. We selected the main immunogenic and protective proteins of Giardia experimentally investigated. We predicted T-cell and B-cell epitopes using immunoinformatic tools (NetMHCII and BCPREDS). Variable surface proteins (VSPs), structural (giardins), metabolic, and cyst wall proteins were identified as the more relevant immunogens of G. lamblia. We described the protein sequences with the highest affinity to bind MHC class II molecules from mouse (I-Ak and I-Ad) and human (DRB1*03:01 and DRB1*13:01) alleles, as well as we selected promiscuous epitopes, which bind to the most common range of MHC class II molecules in human population. In addition, we identified the presence of conserved epitopes within the main protein families (giardins, VSP, CWP) of Giardia. To our knowledge, this is the first in silico study that analyze immunogenic proteins of G. lamblia by combining bioinformatics strategies to identify potential T-cell and B-cell epitopes, which can be potential candidates in the development of peptide-based vaccines. The bioinformatics analysis demonstrated in this study provides a deeper understanding of the Giardia immunogens that bind to critical molecules of the host immune system, such as MHC class II and antibodies, as well as strategies to rational design of peptide-based vaccine against giardiasis.
Introduction
Giardiasis is a highly prevalent foodborne gastrointestinal parasitic infection in developing countries, mainly affecting children and immunocompromised individuals. The clinical manifestations of giardiasis vary from asymptomatic to acute or chronic episodes characterized by severe diarrhea, accompanied with abdominal pain and intestinal lesions that lead to nutrient malabsorption syndrome and weight loss (Eckmann, 2003; Cedillo-Rivera et al., 2009; Ankarklev et al., 2010; Lujan and Svard, 2011; Lopez-Romero et al., 2015). Giardia lamblia is the etiological agent of giardiasis, a binucleated and flagellated protozoan that can infect humans and other mammals. G. lamblia has a simple life cycle, consisting of two different developmental stages defined by specific structural and biochemical features, wherein the cyst is the infective form, whereas the trophozoite is the proliferative form that colonizes the upper tract of small intestine (Lujan, 2006; Cedillo-Rivera et al., 2009; Ankarklev et al., 2010; Lopez-Romero et al., 2015).
The establishment of endoparasitic infections rely on the intricate molecular interaction between each specific stage of the life cycle of parasites and the immune responses of their hosts (Tedla et al., 2019; Smith et al., 2021). Generally, the integration of innate and adaptive immune responses defines the fate of parasitic infections, therefore immunocompetence, immunopolymorphism and immunological memory of the host are important for the resolution of parasitic infections (Lima and Lodoen, 2019; Mukherjee et al., 2019).
Several studies have reported the central role of the immune system in resolution of giardiasis by using different experimental approaches (Li et al., 2004; Ankarklev et al., 2010; Kamda et al., 2012; Dreesen et al., 2014; Grit et al., 2014; Lopez-Romero et al., 2015; Singer, 2016). The mechanism of pathogen clearance mainly depend on the processes mediated by adaptive effector cells, both B and T lymphocytes. Murine models of giardiasis have demonstrated that the establishment of humoral immunity could be implicated in resolution of infection (Singer and Nash, 2000; Eckmann, 2003; Velazquez et al., 2005). In addition, the role of mucosal and circulatory CD4+ T cells has been described as essential to collaborate with the activation of B cells and control murine giardiasis (Singer and Nash, 2000; Lujan, 2011; Singer, 2016). Interestingly, whilst CD4+ T cells are important effectors in giardiasis resolution, CD8+ T lymphocyte responses have been associated to the pathophysiological damage observed during G. lamblia infection, such as enterocyte ultrastructural alterations, representing a paradoxical challenge for immunotherapy against giardiasis (Scott et al., 2004; Lopez-Romero et al., 2015).
The development of effective vaccines against endoparasites is limited, partially due to the complex life-cycle of parasites and the mechanisms that have acquired to successfully overcome some immune responses, such as antigenic variation, and partially to the limitations of classical vaccine design strategies (Skwarczynski and Toth, 2016; Lima and Lodoen, 2019; Moormann et al., 2019; Autheman et al., 2021; Robleda-Castillo et al., 2021). At present, there are no approved vaccines for human use against giardiasis. However, the presence of immunogenic proteins in both, cyst and trophozoite forms of G. lamblia have been described by different approaches. Among the proteins of G. lamblia able to elicit immune responses are the variable surface proteins (VSP), heat shock proteins, lectins, cyst wall proteins (CWP) and cytoskeleton associated proteins, such as giardins and tubulins (Davids et al., 2006; Lopez-Romero et al., 2017; Quintero et al., 2017).
Nowadays, synthetic peptide-based vaccines are designed considering immunodominance, epitope structure, and adjuvants to stimulate and confer protection without the complete protein or pathogen administration (Skwarczynski and Toth, 2016; Malonis et al., 2020). Immunoinformatic analysis have been used to identify immunogenic antigens from medically important protozoa, such as Leishmania, Trypanosoma, and Plasmodium, which have been implemented in multi-peptide vaccines with high efficacy for the control of infection. For the malaria infection, the Mosquirix ™ vaccine is currently in Clinical Trial Phase III (Teh-Poot et al., 2015; Cecilio et al., 2017; Laurens, 2020; Vakili et al., 2020).
Immunoinformatic analysis allows the identification of potential B-cell and T-cell epitopes pursued for the design of new peptide-based vaccine candidates, by combining proteomics and bioinformatics strategies. Potential B-cell epitopes are considered according to their surface accessibility, flexibility and physicochemical characteristics to interact with complementarity-determining regions (CDRs) in the antibody molecule, whereas T-cell lineal peptide epitopes are predicted based on their high-affinity binding to the major histocompatibility complex (MHC) class I and II molecules (Teh-Poot et al., 2015; Goodswen et al., 2017; Robleda-Castillo et al., 2021).
The aim of this study was to identify T-cell and B-cell epitopes within the immunogenic proteins of G. lamblia that induce a potential protective response against giardiasis, using immunoinformatic strategies (Figure 1). In addition, we analyzed and discussed the potential role of those epitopes to stimulate the host’s immune system, providing candidates for the development of peptide-based vaccines.
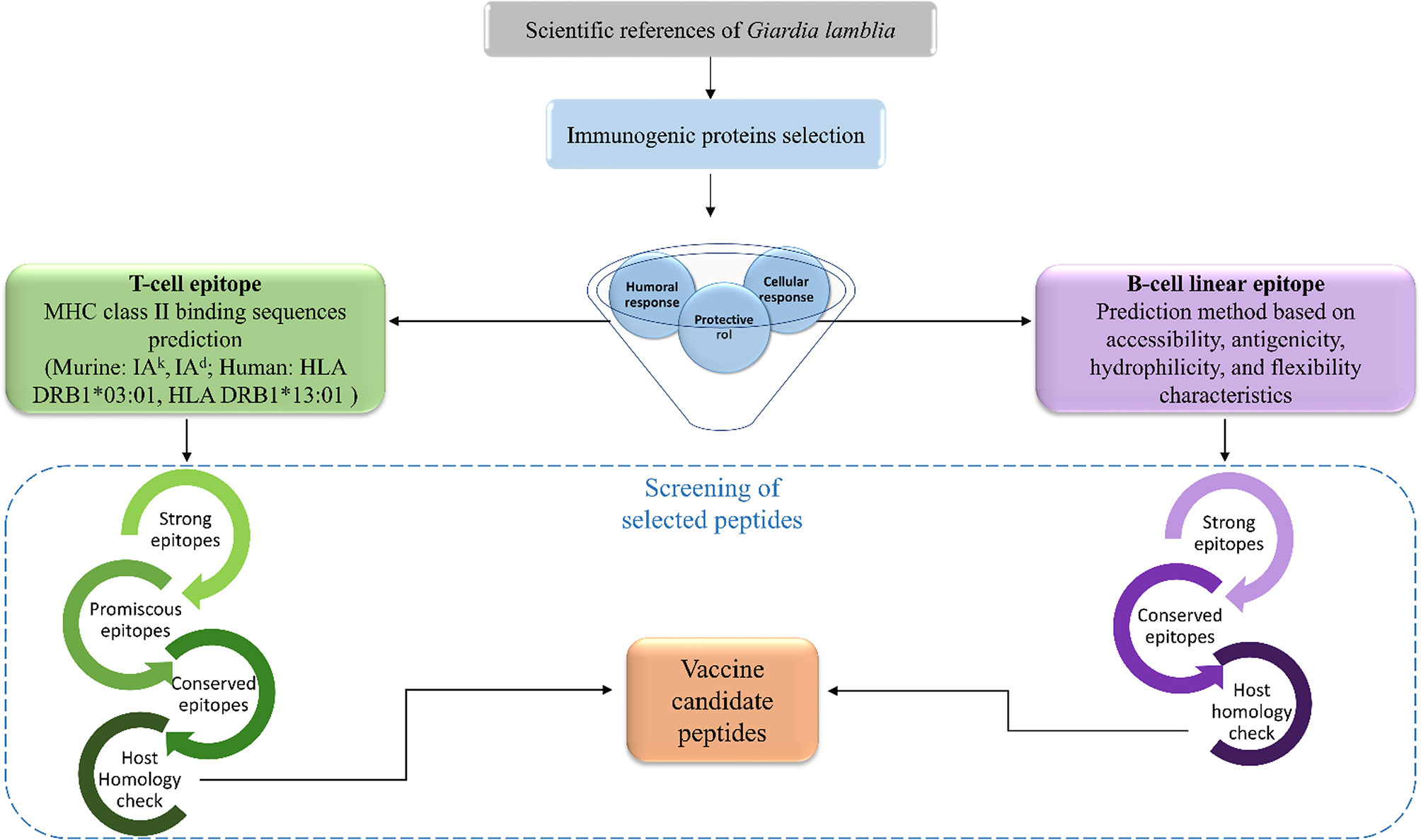
Figure 1 Flowchart of study design. Analysis started from the bibliographic search and selection of G. lamblia proteins reported as immunogenic. Prediction of T- and B-cell epitopes and screening analyses were performed to propose candidate peptides for the vaccine design, such as promiscuous and conservation epitope analysis, and host (human and mouse) homology analysis.
Materials and Methods
Search and Selection of Giardia Immunogenic Proteins
The identification and selection of immunogenic antigens from Giardia was performed on the scientific platform NCBI (PubMed: http://www.ncbi.nlm.nih.gov/pubmed/) by filtering the results to the last 30 years, using several keywords to identify the potential articles, including: Giardia lamblia, immunogenic proteins, protection, immune response, vaccine, variant-surface proteins (VSPs), giardins, and cyst wall proteins (CWPs). Scientific papers were selected based on their evaluations of the humoral and cellular immune response activation by Giardia antigens, as well as in the in vitro and in vivo protection assays. The identified G. lamblia immunogens were categorized according to their functionality and location in the parasite as reported in web site Uniprot (https://www.uniprot.org/) and as reported in publications. The access numbers of the selected immunogens were located in GenBank and GiardiaDB. BLASTp analysis was performed between the assemblages of each protein.
CD4+ T-Cell Epitope Prediction
For MHC-II-binding epitopes, 15-mer long epitopes for each protein were predicted using NetMHCIIpan 3.2 server (http://www.cbs.dtu.dk/services/NetMHCIIpan-3.2/). We selected for T-cell epitopes prediction, the murine MHC class II molecules I-Ak and I-Ad. Those MHC molecules are expressed on the C3H/He and BALB/c mouse models, respectively, which are mouse strains frequently used in giardiasis studies (Belosevic et al., 1984; Venkatesan et al., 1997; Larocque et al., 2003; Lee et al., 2014; Serradell et al., 2019; Garzon et al., 2020). The HLA-DRB1*03:01 and HLA-DRB1*13:01 human MHC class II molecules were selected due to their probable association with susceptibility to infection (AL-Khaliq et al., 2020; El-Beshbishi et al., 2020). The proteins Hen Egg-white Lysozyme (HEL) and ovalbumin (Ova) were used as control antigens for the epitope prediction of MHC class II alleles (I-Ak and I-Ad, respectively). The predicted peptides were classified as strong and weak binders with a threshold percentile rank (% Rank) ≤ 2% and ≤ 10%, respectively. The non-binder peptides (> 10% rank) were not considered in the study. In addition, we performed a host homology analysis. We analyzed the homology of peptides with human proteins sequence (Homo sapiens, taxid:9606) and mouse (Mus musculus, taxid:10090). The immunodominant protein sequences of Giardia were subjected to BLASTp against non-redundant protein sequences (nr) database (Altschul et al., 1990), and complemented with Dynamic Vaxign analysis (Xiang and He, 2009; He et al., 2010). A selection of T-cell and B-cell peptide epitopes were screened in the alignments to identify homologs. A percentage identity > 35% was set as a filter to consider homology in each epitope (Pertsemlidis and Fondon, 2001).
Prediction of Promiscuous Peptides for MHC Class II Alleles
The analyses of epitopes with promiscuous binding to a variety of MHC class II alleles permit a greater chance of the CD4+ T cells stimulation and allow to propose ideal epitopes for a clinically effective vaccine. The identification of T-cell epitopes with promiscuous binding to MHC class II alleles was determined with the TepiTool analysis resource from the IEDB (Paul et al., 2016) (http://tools.iedb.org/tepitool/). The predictions were done by using the consensus method (Wang et al., 2008; Wang et al., 2010) which employs SMM_align, NN_align, Combinatorial library, Sturniolo methods and NetMHCIIpan (Nielsen et al., 2008; Karosiene et al., 2013). A pre-selected reference panel of 26 alleles was employed and only the peptide epitopes binding at least 50% of the alleles were selected as promiscuous (Greenbaum et al., 2011). By default, Tepitool selects the epitopes with a percentile rank ≤ 20 as promiscuous. The input sequences of epitopes were those determined as the strongest binders for murine I-Ak, I-Ad alleles, HLA-DRB1*03:01 and HLA-DRB1*13:01.
B-Cell Epitope Prediction
Linear/continuous B-cell epitopes for secreted or extracellular proteins were identified using BCPred method in BCPREDS server which is based on support vector machine (SVM) that uses string kernels (http://ailab-projects1.ist.psu.edu:8080/bcpred/predict.html) (El-Manzalawy et al., 2008). We used the following parameters for prediction, 80% specificity and a cut-off score > 0.6. Epitopes with a length of 16-mer and 18-mer were selected for the study since most B-cell epitopes are between 15 to 25 long amino acids (Potocnakova et al., 2016), also better accuracy percentages are obtained with peptide windows of 16 amino acids in length (El-Manzalawy et al., 2008).
Epitope Conservation Analysis
To identify the percentage of conservation of the epitopes in the sequences of the proteins classified within the families, giardins, VSPs, and CWPs, the FASTA sequences of proteins were selected for a multi-alignment in T-coffee (https://www.ebi.ac.uk/Tools/msa/tcoffee/) and Boxshade webserver (https://embnet.vital-it.ch/software/BOX_form.html). The conservancies of strong T-cell epitope and B-cell epitopes previously predicted were identify by IEDB epitope conservancy analysis tool (http://tools.iedb.org/conservancy/). The conservancy of epitope sequence was assigned at > 60% for giardins and CWPs, and > 50% for VSPs. Every T-cell and B-cell epitopes that was filtered by the threshold, was subjected to cross-reactivity analysis (mouse and human).
Results
Giardia Immunogenic Proteins Selection
To identify the Giardia immunogens, which have been described in the scientific literature, a screening search (last 30 years) of articles was performed. A total of 29 research articles were selected, wherein 29 proteins with potential high immunogenicity were reported (Table 1). The selected immunogens, mainly belong to WB and GS/M-83 -H7 strains, representative of Giardia A and B assemblages (genetic groups), respectively. The proteins presented a homology (id%) > 78% between assemblages, unlike for VSPs, due the expressed VSPs are different between the trophozoites of assemblages A and B. (Franzén et al., 2009). Proteins were classified based on their location and function (Figure 2). Out of the 29 immunogenic proteins identified, 3 proteins correspond to cyst wall proteins (CWP 1, CWP 2, and CWP 3), 11 proteins are structural proteins located mainly in the ventral disc and cytoskeleton, such as giardins, tubulin, SALP, 21.2 protein, and GHSP-115. In addition, 5 proteins have metabolic functions in Giardia, such as arginine deiminase (ADI), ornithine carbamoyl transferase (OCT), fructose-bisphosphate aldolase (FBA), uridine phosphorylase (UPL), and enolase. Among the intracellular proteins, we also found the Giardia Trophozoite Antigens (GTA-1 and GTA-2) and the binding immunoglobulin protein (BIP). Other immunogens in the study correspond to 7 variants-specific surface proteins (VSPs). Most of the scientific papers (more than 90%) selected during the screening search performed in the present study were focused on evaluating the immunogenicity of Giardia proteins by analyzing the antibody-mediated immune response. Only a few have evaluated its ability to activate cellular immune responses. The immunological assays reported in those papers have been performed using human samples, and animal models susceptible to giardia infection (mice, gerbils, kittens, and puppies). Some of those articles have reported the protective capacity of certain immunogens, such as α-1 giardin, α-11 giardin, 21.2 protein, UPL-1, VSP9B10, VSP1267, VSPH7 and CWP 2 (Larocque et al., 2003; Palm et al., 2003; Serradell et al., 2018; Davids et al., 2019; Serradell et al., 2019).
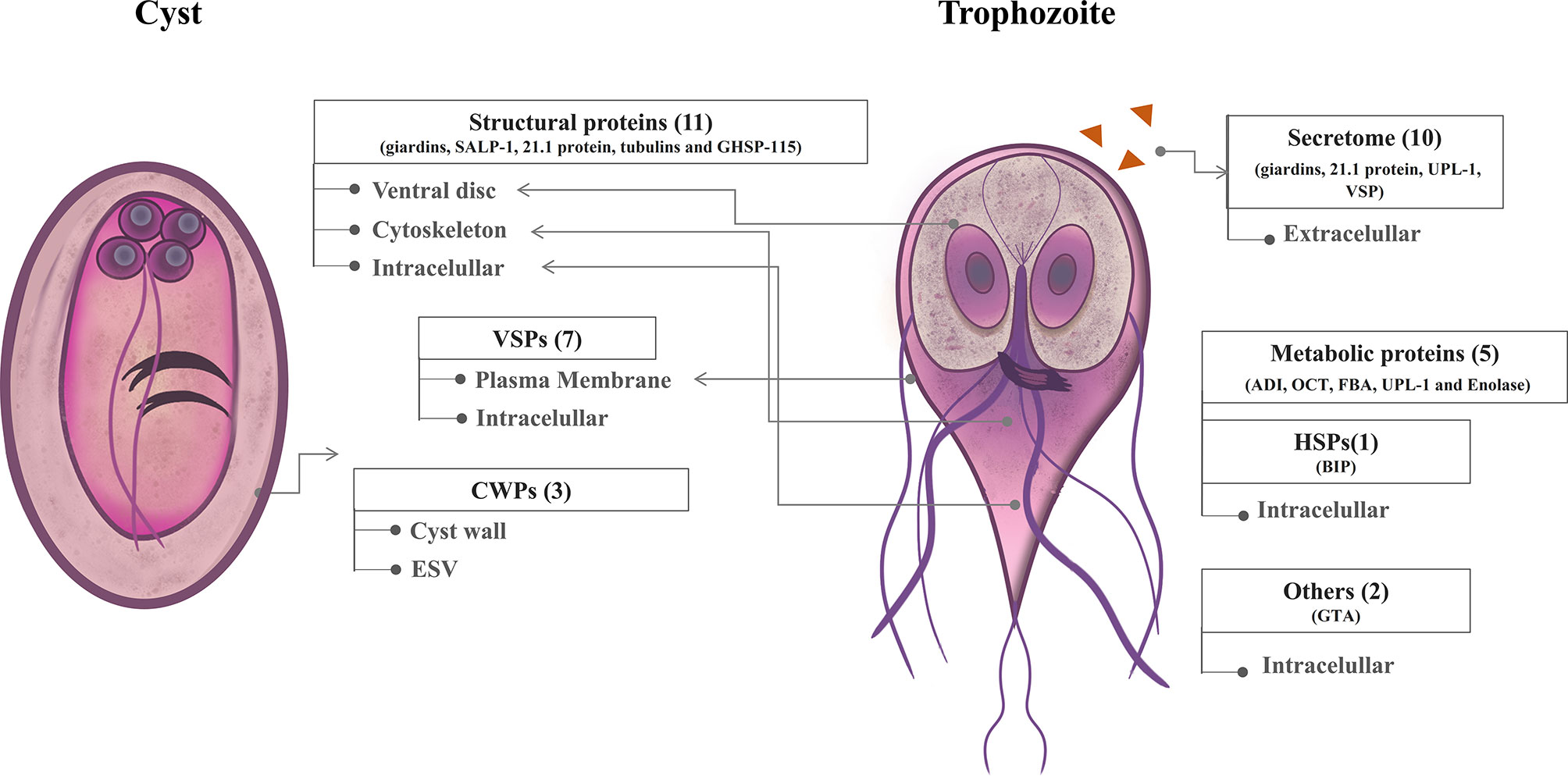
Figure 2 Schematic representation of cellular localization of immunogenic proteins of G. lamblia. A total of 29 proteins have been reported as immunogenic antigens in the cyst and trophozoite of G. lamblia. Immunogenic proteins were classified based on their location and function in structural proteins, metabolic proteins heat shock proteins (HSPs), variable-specific surface proteins (VSPs), and cyst wall proteins (CWPs). Proteins were located in ventral disc, plasma membrane, cytoskeleton, intracellular and secretome/extracellular of the parasite. CWPs can also be found in encystation-specific secretory vesicles (ESV).
T- Cell and B-Cell Epitopes From Giardia Immunogenic Proteins
The cellular and humoral immune responses have an important role in the clearance of giardiasis. CD4+ helper T lymphocytes are involved in the activation of the effector mechanisms against Giardia. CD4+ cells are activated by dendritic cells, as well as by B lymphocytes through the MHC II-peptide presentation, for this reason, we initially identified T-cell epitopes from Giardia immunogenic proteins. T- cell epitopes that had an affinity to the murine MHC class II I-Ak and I-Ad molecules, as well as to the human MHC class II HLA-DRB1*03: 01 and HLA-DRB1*13: 01 were identified. We used the NetMHCIIpan server for T-cell epitope prediction. Out of the 29 proteins that were subjected to prediction, a total of 354 strong binder peptides and 1,298 weak binder peptides were predicted (Figure 3). The subsequent analyzes were focused on strong peptides. We recorded the first 5 epitopes of each protein with the highest affinity to the MHC class II molecules I-Ak, I-Ad, HLA-DRB1 * 03: 01 and HLA-DRB1 * 13: 01 (Tables S1, S2). Then, we selected the 20 peptide epitopes with the strongest binding affinity to each MHC class II molecule analyzed (Tables 2, 3). The strong binders showed a similar percentile rank to the main immunodominant epitope (48-63) of the hen egg-white lysozyme (HEL) (Nelson et al., 1992; Velazquez et al., 2002) and to the peptide (323-339) of ovalbumin (OVA) (McFarland et al., 1999) (Table S3). Both peptide sequences have a high affinity binding to I-Ak and I-Ad alleles, respectively. Due to the high affinity with MHC class II molecules and the capacity to activate the cellular immune response, the binding registers of HEL and OVA peptides have been highly characterized and used as study models (McFarland et al., 1999; Bevaart et al., 2004; Dissanayake et al., 2005; Lovitch and Unanue, 2005; Landais et al., 2009; Strong and Unanue, 2011). Several sequences of giardins, UPL-1, ADI, GTA-1 and enolase showed high binding affinity for murine and human MHC II alleles (Tables 2, 3). Additionally, a criterion for selection of T-cell epitopes was that they should be promiscuous. Since MHC class II alleles have different binding specificities, selection of peptides that bind to several MHC variants can allow the designing of vaccines to achieve a broad allelic coverage and protect against infection. We used the cut-off values of Tepitool to be binding to ≥ 50% of the MHC class II alleles more frequently in the world population, we found that 26 peptides sequences were highly promiscuous epitopes (Table 4). These data were used as screening for subsequent analyzes.
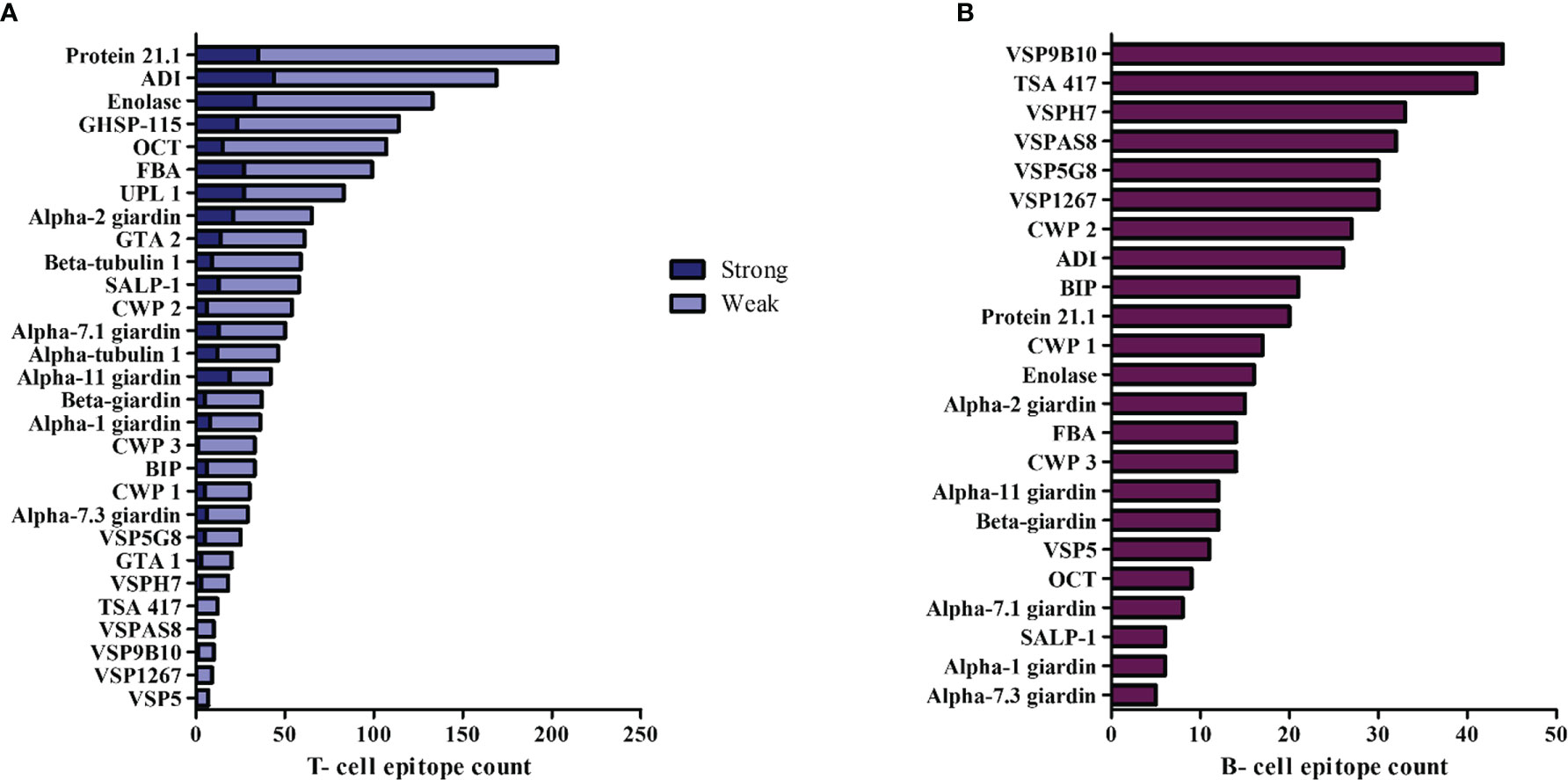
Figure 3 Distribution of T-cell and B- cell epitopes from G. lamblia immunogens. (A) T-cell epitope count for each immunogenic protein. The total count of the T- cell epitopes (strong and weak binders) was performed by the prediction in NetMHCII of MHC class II (I-Ak, I-Ad, DRB1*03:01, DRB1*13:01). (B) B-cell epitope count for each immunogenic protein. Epitope prediction of length 16 and 18 amino acids was conducted in BCPRED.
B-cell linear epitopes of Giardia immunogens were identified by using BCPRED tool. A total of 535 B-cell epitopes were identified (Figure 3). The analysis showed that the VSP membrane proteins presented a higher number of B-cell epitopes than other intracellular or cytoskeletal proteins such as giardins. The B-cell epitopes with the highest score were selected, a total of 32 epitopes were identified with a score > 0.970 (Table 5). Some B-cell epitopes of proteins, such as β-giardin, tubulins and VSPs obtained the maximum score issued by the BCPRED algorithm. Only in certain sequences a high homology with the proteins of human and mouse were observed, as was the case with BIP. Some predicted epitope sequences present dipeptides of proline/glycine (PG) and glutamine/proline (QP or PQ) which have been identified frequently in B-cell epitopes that induce an IgA antibody response, as well as dipeptide of alanine/serine (AG), glycine/proline (GP) and tryptophan/lysine (WK) in epitopes that activate an IgG antibody response (Gupta et al., 2013).
Giardins, VSPs, and CWPs Have Conserved T-Cell and B-Cell Epitopes
Among the immunogenic proteins identified on Giardia, there are three families highly characterized in the parasite, giardins, VSPs, and CWPs. It was of our interest to know whether those Giardia protein families conserved the predicted T-cells and B-cells epitopes. A multiple alignment of those three protein families was carried out and the epitopes that had > 60% conservation for the giardins and CWPs, and > 50% conservation for the VSPs were located. Giardins present 11 T-cell and 10 B-cell conserved epitopes (Table 6). The T-cell epitopes 3, 7, and 9 have amino acid residues shared with the B-cell epitopes 2, 6, and 4, respectively (Figure 4A). Regarding the VSPs, we identified 5 T-cell and 6 B-cell conserved epitopes (Table 7), which are found at the C-terminal amino acid residues. The number 1 T-cell epitope was conserved in seven proteins. In addition, the T-cell epitope 3 was the only one that overlaps with the numbers 2 and 3 of B-cell epitopes (Figure 4B). In the CWP family, we identified 8 and 7 T-cell and B- cell conserved epitopes, respectively (Table 8). Several T-cell and B-cell epitopes overlap in CWPs as T-cell epitope 1 (159-173 aa) with B-cell epitope 4 (164-181 aa) (Figure 4C).
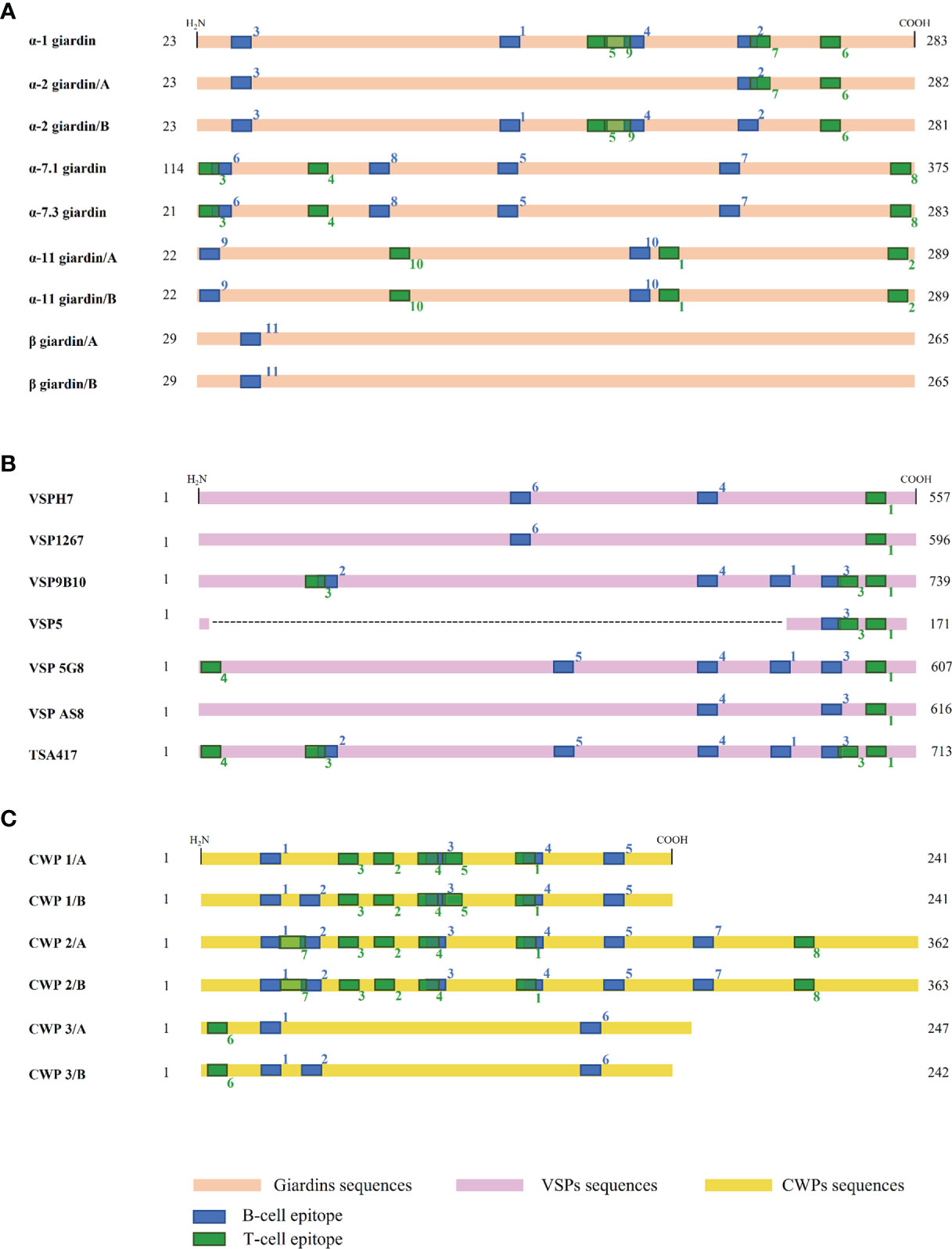
Figure 4 Giardins, VSPs, and CWPs conserved T-cell and B-cell epitopes. Epitope conservation analysis with a cutoff > 60% conservancy for giardins (A) and VSPs (B), a cutoff > 50% conservancy for CWPs (C). The regions shown in green, and blue are T-cell and B-cell epitopes, respectively. Conserved epitopes shown in this figure are found in Tables 6–8.
Discussion
Vaccine development has evolved over the years since Edward Jenner introduced the smallpox vaccine in 1796. Nowadays, in order to generate specific and safe vaccines with fewer side effects, extensive research is needed to design vaccines. In the initial phases, it is necessary to understand pathophysiology of infection, the pathogen-host relationship, as well as also to identify and characterize the immunodominant antigens that can generate immunity. Each research focused on those aspects supports the design of effective and safe vaccines for the population. At present, there is no vaccine for human giardiasis. Therefore, in this study, several T-cell and B-cell epitopes of G. lamblia immunogens were identified, which presented different immunogenic characteristics, some T-cell epitopes were promiscuous with strong binding affinity to MHC class II molecules, epitopes without homology to the hosts and conserved among protein families.
The G. lamblia antigens shown in Table 1 are molecules that have been experimentally characterized as immunogens. In addition to the physicochemical properties, other characteristics contribute to the immunogenicity of a molecule. i) Foreignness of the immunogen: there must be a degree of phylogenetic difference between the candidate molecule and the host to avoid self-reactivity (Crumpton, 1974). In the present study, several amino acid regions of G. lamblia immunogens (tubulin, enolase, BIP, CWP and VSP) showed some degree of homology with human and mouse molecules, ubiquitous proteins in eukaryotic cells. Although, those peptides could potentially cause an autoimmune or allergenic reaction, suggesting the necessity to do additional studies to evaluate the safety of those predicted peptides. ii) Exposure to the immune system: Giardia is a non-invasive parasite of the intestinal mucosa, therefore extracellular proteins play an important role in stimulating the immune system (Troeger et al., 2007; Cotton et al., 2011). The antigen location is crucial for easy recognition by the immune response, which is why surface proteins have been targets for vaccine development (Serradell et al., 2016; Abdi et al., 2019; Uwase et al., 2020). Giardia proteins that are located in the cytoskeleton, ventral disc, membrane, and proteins of secretome (proteins with an asterisk in Table 1) can have greater accessibility for the immune system, activating an efficient antibody-mediated response, as well as antigen uptake and presentation by antigen-presenting cells (Kaufmann and Hess, 1999; Foged et al., 2005; Mora and Telford, 2010). iii) Chemical stability and conservation of proteins: Giardins are a large group of structural proteins that are divided into alpha, beta, and gamma, there are 21 genes for alpha-giardins that are conserved in assemblages A and B (Feliziani et al., 2011). CWP 1, CWP 2, and CWP 3 have around 60% identity in a sequence of 26 kDa, as well as conserved regions rich in leucine and cysteine (Lujan et al., 1995; Sun et al., 2003; Abdul-Wahid and Faubert, 2004). VSPs also have multiple cysteine domains, which also make them resistant to reduction by proteases (Nash et al., 1991; Papanastasiou et al., 1997). Besides, the three families of proteins mentioned above have been found expressed in the early stages of the encystation/excystation of G. lamblia (McCaffery et al., 1994; Hehl et al., 2000; Carranza et al., 2002; Weiland et al., 2003).
The characterization of immune responses induced by Giardia immunogens has mainly focused on IgA and IgG humoral response, perhaps due to the accessibility and feasibility of in vitro immunological assays, together with the evaluation in experimental animals. Infected mice with G. lamblia have demonstrated the establishment of humoral immunity around the third to fifth week post-infection, which could be implicated in the resolution of infection (Singer and Nash, 2000; Eckmann, 2003; Velazquez et al., 2005). We identified 24 immunodominant B-cell epitopes from immunogenic proteins of Giardia by bioinformatic analysis. Several studies have demonstrated the high immunogenicity of excretory/secretory proteins of Giardia (Palm et al., 2003; Hanevik et al., 2011; Jiménez et al., 2014). The metabolic proteins ADI, OCT, and enolase were recognized by serum from patients with acute giardiasis (Palm et al., 2003). In other microorganisms, the immunological role of those proteins has been evaluated. Enolase has a protective role in candidiasis (Montagnoli et al., 2004). OCT activates an antibody response in Streptococcus suis infection, and it is involved in reducing pathogenicity factors (Wang et al., 2020). VSPs are highly expressed on the membrane of Giardia trophozoite and are involved in the antigenic variation of the parasite. Although the mechanisms that induce antigenic switching are unknown, it is hypothesized that anti-VSP antibodies could stimulate the VSP switching. Several studies indicate the high effectiveness of VSPs to activate an antibody-mediated response in infected humans and animals (Stäger et al., 1998; Hjøllo et al., 2018; Serradell et al., 2018), as well as the effector mechanisms of anti-VSP antibodies against trophozoites, such as cytotoxicity, opsonization, and neutralization (Nash and Aggarwal, 1986; Stäger et al., 1997; Rivero et al., 2010).
The clearance of Giardia infection requires humoral and cellular immune mechanisms. In Giardia, there is little research focused on characterizing the cellular response, however, it is known that CD4+ T lymphocytes play an important role in infection. CD4-deficient mice treated with an anti-CD4 antibody could not clear the infection, as well as CD4+ T cells deficiency is related to chronic giardiasis (Heyworth et al., 1987; Singer and Nash, 2000). In this study, 26 epitopes are proposed to activate CD4+ cells due to their high affinity to several MHC class II molecules. First, four MHC class II alleles were chosen, the MHC class II I-Ak and I-Ad that are expressed in mice widely used as model for giardiasis, as well as the HLA-DRB1 * 03: 01 and HLA-DRB1 * 13: 01 alleles that are related to an increased risk of G. lamblia infection (AL-Khaliq et al., 2020; El-Beshbishi et al., 2020). MHC class II molecules are expressed in dendritic cells and B lymphocytes, which are chemoattracted by trophozoite-stimulated epithelial cells (Roxström-Lindquist et al., 2005). Dendritic cells pre-stimulated with Giardia antigens can confer IL-6-dependent protection, which has been related to B-lymphocyte growth and T-cell differentiation (Weaver et al., 2006; Kamda et al., 2012). Proinflammatory chemokines, including TNF-α, and B lymphocyte activating interleukins, such as IL-4 and IL-5 belong to the chemokine profile described in Giardia infection (Cotton et al., 2015; Serradell et al., 2018). Additionally, an increase in IL-17 producing CD4+ cells from infected patients with Giardia (Saghaug et al., 2015). Interleukin IL-17 has been associated with IgA production and infection control (Dann et al., 2015). Although we focused the analysis on the strong epitopes classified by the NetMHCII algorithm, we did not disregard sequences with low affinity to MHC class II for future tests, due peptides with a low binding affinity can activate effective T-cell response, as the HEL 20-35 peptide (Nelson et al., 1992; Velazquez et al., 2002).
In this study, we identified conserved epitopes among giardins, VSPs, and CWPs. T-cell and B-cell epitopes overlap in some amino acid residues. Responses between B and T cells are closely linked for the development of an effective immune response. B cells as an antigen presenting cell can recognize antigens through the BCR, as well as present T-dependent antigens through the binding of peptides to MHC class II molecules. T-helper cells recognize peptide-MHC class II complex and send activation signals to the B cell (Shimoda and Koni, 2007; Akkaya et al., 2019). Those pathways promote processes such as the isotype switch, affinity maturation and immunological memory, necessary in the development of protective immune responses. Does it remain to be evaluated whether these epitopes can be generated naturally? Are they resistant to antigen processing? if the antibodies generated are limited to the course of the infection or will it be a long-lived response?. In the analysis of multiple alignments between the proteins showed several semi-conserved epitopes in the three protein families (giardins, CWPs and VSPs), although we understand that changes in amino acids can reduce the binding affinity with MHC class II and immunoglobulins, these regions can be targets for a vaccine that protects by dampening the variations that occur in the parasite throughout its life cycle.
The proteins described in this study have been proven to be immunogenic, however, only few of them have been evaluated in protection assays. Prior to consider an immunogen as a vaccine candidate, it is crucial to demonstrate its protective capacity by using experimental models. The proteins α-1 giardin, α-11 giardin, 21.2 protein, UPL-1, VSP9B10, VSP1267, VSPH7 and CWP 2 have shown to induce a protective immune response against infection by G. lamblia (Table 1) when administered orally or intraperitoneally. Mice and Mongolian gerbils were commonly used as animal models in protection assays, although other Giardia-susceptible animals, such as cats and dogs have also been used (Serradell et al., 2016). Currently, there is no human or dog effective vaccine against G. lamblia. In 1999, Fort Dodge Animal Health developed a vaccine based on killed disrupted trophozoites (GiardiaVax), which attenuated giardiasis symptoms, produced antibodies, and reduced the shedding cysts to 30% and 5% in vaccinated kitten and puppies respectively (Olson et al., 2000). GiardiaVax was also tested on Meriones unguiculatus, showing protection in 33% of the mice at the third day post-infection, the rest of the vaccinated group cleared the infection at seventh day (Jiménez-Cardoso et al., 2002). However, other studies differ in the vaccine efficacy. It was reported that the Giardia parasite persisted by week 28 in vaccinated cats with three doses of Giardiavax (Stein et al., 2003), as well as in dogs, no differences were found in the elimination of cysts between the control and vaccinated group (Anderson et al., 2004). First-generation vaccines, such as the whole pathogen vaccine are characterized by generating no or low cell-mediated response and can also generate adverse effects such as hypersensitivity (Jiskoot et al., 2019). In recent years, protection strategies for clinically relevant pathogens have been focused on the peptide- and epitope-based vaccines (Table S4). Initially, in silico analysis facilitate the identification of T- and B-cell epitopes, and which can significantly reduce time and cost of research. Peptide-based vaccines can generate an effective and targeted immune response if the proper adjuvants and delivery system are considered. In gastrointestinal infections such as giardiasis several mucosal adjuvants can be used, such as choleric toxin, which increase the permeability of the intestinal epithelium promoting the antigen-uptake by immune cells (Rhee et al., 2012).
Validation strategies for the effectiveness of a peptide-based vaccine can be completed with additional in silico and experimental assays. In several viral pathogens, IFN-γ response activation is evaluated, due to the importance of this cytokine in effector mechanisms. Additionally, 3-D modeling and molecular docking are performed for the multi-epitopes vaccine constructs. All subunit- and epitope-based vaccines shown in Table S4 have high protection efficacy in their respective diseases, as well as induced specific humoral and cellular responses. The advances in vaccines design of parasites show methodological strategies for the antigens characterization that can be implemented in Giardia studies. Likewise, Giardia shares some characteristics with other protozoa. Giardia presents antigenic variation, characteristic of the differential expression of variable surface proteins (VSP). Plasmodium and Trypanosoma are other parasites that express variable surface antigens (Borst and Ulbert, 2001; Kyes et al., 2007). Heat shock proteins are highly conserved molecules, in Leishmania which have been described as immunomodulatory proteins as well as have been used as components of vaccines (Lopez-Romero et al., 2017). Although there is little research on the immunological characterization of Giardia HSPs, studies have described the BIP protein as an immunogenic protein (Lee et al., 2014). We believe that more studies are needed to analyze the similarities among immunogenic antigens of Giardia and other pathogens, as well as the immune responses that may activate.
Our study is restricted by limiting immunoinformatic analyses to G. lamblia immunogenic proteins. At present, proteins are the molecules most characterized at the immunological level, however, different types of antigens may contribute to elicit immune responses during G. lamblia infection. Trophozoites of G. lamblia are able to activate innate immune responses, such as the complement system through the lectin pathway, after the recognition of surface N-acetylglucosamine (GlcNAc) by mannose-binding lectin (MBL). Interestingly, specific surface glycoconjugate antigens, glycosylphosphatidylinositol (GPI) and lipophosphoglycan (LPG) have been described as important inducers of immune responses during parasitic infection with Trypanosoma spp, Leishmania spp, Plasmodium falciparum, Cryptosporidium y Entamoeba histolytica (Ropert and Gazzinelli, 2000; Priest et al., 2003; Wong-Baeza et al., 2010). Based on this information, it is necessary to address future analyses at the molecular basis under the immune responses elicited by GlcNAc and other glycoconjugate antigens present in trophozoites and cysts of G. lamblia, in addition, the immunogenic role of post-translational modifications, such as glycosylation in VSPs, should be fully analyzed.
The present study describes a global approach to the identification of immunodominant and protective antigens of Giardia, being the first study to determine potential T-cell and B-cell immunogenic epitopes predicted by immunoinformatic tools as candidates for a vaccine against Giardia infection. For effective vaccine development against Giardia, it is necessary to consider several factors: i) the inclusion of conserved and variable protein sequences from the most common Giardia assemblages in humans (A and B); ii) the activation of the immune mechanisms of innate and adaptive response, considering the relationship between the parasite, gut mucosal immune system, microbiota, and the tolerogenic environment.: iii) the use of proper adjuvants; iv) administration routes to guarantee an immune response in mucosa. For future studies, in vitro and in vivo assays are required to verify the effectiveness and protective role of T-cell and B-cell epitopes in giardiasis. These results obtained in the present study suggest that experimental administration of a multi-epitope vaccine constructed on basis of immunoinformatic approach could provide an effective prophylactic strategy against Giardia.
Data Availability Statement
The original contributions presented in the study are included in the article/Supplementary Material. Further inquiries can be directed to the corresponding author.
Author Contributions
TG performed and is involved in immunoinformatic analyses, wrote the manuscript, and prepared all figures. GL-R and DO-T performed in silico assays and analyzed the data. EA contributed to the writing and editing of the manuscript. AG-E contributed to the writing and editing of the manuscript. RR-Z contributed to the library searches and assembling relevant literature. CV designed and supervised the project, revised the manuscript, and was responsible for the funding. All authors contributed to the article and approved the submitted version.
Funding
National Council for Science and Technology of Mexico (CONACyT, CB2017-2018 A1-S-21831).
Conflict of Interest
The authors declare that the research was conducted in the absence of any commercial or financial relationships that could be construed as a potential conflict of interest.
Publisher’s Note
All claims expressed in this article are solely those of the authors and do not necessarily represent those of their affiliated organizations, or those of the publisher, the editors and the reviewers. Any product that may be evaluated in this article, or claim that may be made by its manufacturer, is not guaranteed or endorsed by the publisher.
Acknowledgments
The authors TG and DO-T would like to express their gratitude to CONACyT for the scholarship granted, also EA express his gratitude for posdoctoral fellowship, Estancias Posdoctorales por México, 2020, CONACyT. In addition, we appreciate the support by Lucila Rascon, laboratory technical assistant.
Supplementary Material
The Supplementary Material for this article can be found online at: https://www.frontiersin.org/articles/10.3389/fcimb.2021.769446/full#supplementary-material
References
Abdi, R. D., Dunlap, J. R., Gillespie, B. E., Ensermu, D. B., Almeida, R. A., Dego, O. K. (2019). Comparison of Staphylococcus Aureus Surface Protein Extraction Methods and Immunogenicity. Heliyon 5 (10), e02528. doi: 10.1016/j.heliyon.2019.e02528
Abdul-Wahid, A., Faubert, G. M. (2004). Similarity in Cyst Wall Protein (CWP) Trafficking Between Encysting Giardia Duodenalis Trophozoites and CWP-Expressing Human Embryonic Kidney-293 Cells. Biochem. Biophys. Res. Commun. 324 (3), 1069–1080. doi: 10.1016/j.bbrc.2004.09.167
Abdul-Wahid, ,.A., Faubert, G. (2008). Characterization of the Local Immune Response to Cyst Antigens During the Acute and Elimination Phases of Primary Murine Giardiasis. Int. J. Parasitol. 38 (6), 691–703. doi: 10.1016/j.ijpara.2007.10.004
Akkaya, M., Kwak, K., Pierce, S. K. (2019). B Cell Memory: Building Two Walls of Protection Against Pathogens. Nat. Rev. Immunol. 20, 4, 229–238. doi: 10.1038/s41577-019-0244-2
AL-Khaliq, I. M. A., Ghadban, M. M., Karimau, K. A. A. L. H. (2020). Influence of Human Leukocyte Antigen Hla-Drb1 on Susceptibility To Giardia Lamblia Infection of Iraqi Patients. Biochem. Cell. Arch. 20 (2), 5513–5516.
Altschul, S. F., Gish, W., Miller, W., Myers, E. W., Lipman, D. J. (1990). Basic Local Alignment Search Tool. J. Mol. Biol. 215 (3), 403–410. doi: 10.1016/S0022-2836(05)80360-2
Anderson, K. A., Brooks, A. S., Morrison, A. L., Reid-Smith, R. J., Martin, S.W., Benn, D. M., et al. (2004). Impact of Giardia Vaccination on Asymptomatic Giardia Infections in Dogs at a Research Facility. Can. Vet. J. 45 (11), 924–930.
Ankarklev, J., Jerlström-Hultqvist, J., Ringqvist, E., Troell, K., Svärd, S. G. (2010). Behind the Smile: Cell Biology and Disease Mechanisms of Giardia Species. Nat. Rev. Microbiol. 8 (6), 413–422. doi: 10.1038/nrmicro2317
Autheman, D., Crosnier, C., Clare, S., Goulding, D. A., Brandt, C., Harcourt, K., et al. (2021). An Invariant Trypanosoma Vivax Vaccine Antigen Induces Protective Immunity. Nature 595, 96–100. doi: 10.1038/s41586-021-03597-x
Bae, S. S., Kim, J., Kim, T. S., Yong, T. S., Park, S. J. (2009). Giardia Lamblia: Immunogenicity and Intracellular Distribution of GHSP-115, a Member of the Giardia Head-Stalk Family of Proteins. Exp. Parasitol. 122 (1), 11–16. doi: 10.1016/j.exppara.2009.01.005
Belosevic, M., Faubert, G. M., Skamene, E., MacLean, J. D. (1984). Susceptibility and Resistance of Inbred Mice to Giardia Muris. Infect. Immun. 44 (2), 282–286. doi: 10.1128/iai.44.2.282-286.1984
Bevaart, L., Van Ojik, H. H., Sun, A. W., Sulahian, T. H., Leusen, J. H.W., Weiner, G. J., et al. (2004). CpG Oligodeoxynucleotides Enhance Fcγri-Mediated Cross Presentation by Dendritic Cells. Int. Immunol. 16 (8), 1091–1098. doi: 10.1093/intimm/dxh110
Bienz, M., Dai, W. J., Welle, M., Gottstein, B., Müller, N. (2003). Interleukin-6-Deficient Mice Are Highly Susceptible to Giardia Lamblia Infection But Exhibit Normal Intestinal Immunoglobulin A Responses Against the Parasite. Infect. Immun. 71 (3), 1569–1573. doi: 10.1128/IAI.71.3.1569-1573.2003
Bienz, M., Wittwer, P., Zimmermann, V., Müller, N. (2001). Molecular Characterisation of a Predominant Antigenic Region of Giardia Lamblia Variant Surface Protein H7. Int. J. Parasitol. 31 (8), 827–832. doi: 10.1016/S0020-7519(01)00182-5
Borst, P., Ulbert, S. (2001). Control of VSG Gene Expression Sites. Mol. Biochem. Parasitol. 114, 17–27. doi: 10.1016/S0166-6851(01)00243-2
Cabrera-Licona, A., Solano-González, E., Fonseca-Liñán, R., Bazán-Tejeda, M. L., Argüello-García, R., Bermúdez-Cruz, R. M., et al. (2017). Expression and Secretion of the Giardia Duodenalis Variant Surface Protein 9B10A by Transfected Trophozoites Causes Damage to Epithelial Cell Monolayers Mediated by Protease Activity. Exp. Parasitol. 179, 49–64. doi: 10.1016/j.exppara.2017.06.006
Carranza, P. G., Feltes, G., Ropolo, A., Quintana, S. M.C., Touz, M. C., Luján, H. D. (2002). Simultaneous Expression of Different Variant-Specific Surface Proteins in Single Giardia Lamblia Trophozoites During Encystation. Infect. Immun. 70 (9), 5265–5268. doi: 10.1128/IAI.70.9.5265-5268.2002
Cecilio, P., Pérez-Cabezas, B., Fernández, L., Moreno, J., Carrillo, E., Requena, J. M., et al. (2017). Pre-Clinical Antigenicity Studies of an Innovative Multivalent Vaccine for Human Visceral Leishmaniasis. PloS Negl. Trop. Dis. 11 (11), e0005951. doi: 10.1371/journal.pntd.0005951
Cedillo-Rivera, R., Leal, Y. A., Yépez-Mulia, L., Gómez-Delgado, A., Ortega-Pierres, G., Tapia-Conyer, R., et al. (2009). Seroepidemiology of Giardiasis in Mexico. Am. J. Trop. Med. Hyg. 80 (1), 6–10. doi: 10.4269/ajtmh.2009.80.6
Cotton, J., Amat, C., Buret, A. (2015). Disruptions of Host Immunity and Inflammation by Giardia Duodenalis: Potential Consequences for Co-Infections in the Gastro-Intestinal Tract. Pathogens 4 (4), 764–792. doi: 10.3390/pathogens4040764
Cotton, J. A., Beatty, J. K., Buret, A. G. (2011). Host Parasite Interactions and Pathophysiology in Giardia Infections. Int. J. Parasitol. 41 (9), 925–933. doi: 10.1016/j.ijpara.2011.05.002
Crumpton, M. J. (1974). “CHAPTER 1 - Protein Antigens: The Molecular Bases of Antigenicity and Immunogenicity,”. The antigens. Academic Press, p. 1–78. doi: 10.1016/B978-0-12-635502-4.50008-4.
Dann, S. M., Manthey, C. F., Le, C., Miyamoto, Y., Gima, L., Abrahim, A., et al. (2015). IL-17A Promotes Protective IgA Responses and Expression of Other Potential Effectors Against the Lumen-Dwelling Enteric Parasite Giardia. Exp. Parasitol. 156, 68–78. doi: 10.1016/j.exppara.2015.06.003
Davids, B. J., Liu, C. M., Hanson, E. M., Le, C. H.Y., Ang, J., Hanevik, K., et al. (2019). Identification of Conserved Candidate Vaccine Antigens in the Surface Proteome of Giardia Lamblia. Infect. Immun. 87 (6), 1–14. doi: 10.1128/IAI.00219-19
Davids, B. J., Palm, J.E.D., Housley, M. P., Smith, J. R., Andersen, Y. S., Martin, M. G., et al. (2006). Polymeric Immunoglobulin Receptor in Intestinal Immune Defense Against the Lumen-Dwelling Protozoan Parasite Giardia. J. Immunol. 177 (9), 6281–6290. doi: 10.4049/jimmunol.177.9.6281
Dissanayake, S. K., Tuera, N., Ostrand-Rosenberg, S. (2005). Presentation of Endogenously Synthesized MHC Class II-Restricted Epitopes by MHC Class II Cancer Vaccines Is Independent of Transporter Associated With Ag Processing and the Proteasome. J. Immunol. 174 (4), 1811–1819. doi: 10.4049/jimmunol.174.4.1811
Dreesen, L., De Bosscher, K., Grit, G., Staels, B., Lubberts, E., Bauge, E., et al. (2014). Giardia Muris Infection in Mice Is Associated With a Protective Interleukin 17a Response and Induction of Peroxisome Proliferator-Activated Receptor Alpha. Infect. Immun. 82 (8), 3333–3340. doi: 10.1128/IAI.01536-14
Eckmann, L. (2003). Mucosal Defences Against Giardia. Parasite Immunol 25, 259–270. doi: 10.1046/j.1365-3024.2003.00634.x
El-Beshbishi, S., Elblihy, A., Atia, R., Megahed, A., Auf, F. (2020). Human Leukocyte Antigen Class-II DRB1 Alleles and Giardia Lamblia Infection in Children: A Case-Control Study. Asian Pac. J. Trop. Med. 13 (2), 56–61. doi: 10.4103/1995-7645.275413
El-Manzalawy, Y., Dobbs, D., Honavar, V. (2008). Predicting Linear B-Cell Epitopes Using String Kernels. J. Mol. Recognit. 21 (4), 243–255. doi: 10.1002/jmr.893
Feliziani, C., Merino, M. C., Rivero, M. R., Hellman, U., Pistoresi-Palencia, M. C., Rápolo, A. S. (2011). Immunodominant Proteins α-1 Giardin and β-Giardin Are Expressed in Both Assemblages A and B of Giardia Lamblia. BMC Microbiol. 11 (1), 233. doi: 10.1186/1471-2180-11-233
Feng, X. M., Zheng, W. Y., Zhang, H. M., Shi, W. Y., Li, Y., Cui, B. J., et al. (2016). Vaccination With Bivalent DNA Vaccine of A1-Giardin and Cwp2 Delivered by Attenuated Salmonella Typhimurium Reduces Trophozoites and Cysts in the Feces of Mice Infected With Giardia Lamblia. PloS One 11 (6), 1–16. doi: 10.1371/journal.pone.0157872
Foged, C., Brodin, B., Frokjaer, S., Sundblad, A. (2005). Particle Size and Surface Charge Affect Particle Uptake by Human Dendritic Cells in an In vitro Model. Int. J. Pharm. 298 (2), 315–322. doi: 10.1016/j.ijpharm.2005.03.035
Franzén, O., Jerlström-Hultqvist, J., Castro, E., Sherwood, E., Ankarklev, J., Reiner, D. S., et al. (2009). Draft Genome Sequencing of Giardia Intestinalis Assemblage B Isolate GS: Is Human Giardiasis Caused by Two Different Species? PloS Pathog. 5 (8), e1000560. doi: 10.1371/journal.ppat.1000560.
Garzon, T., Valencia, L., Dominguez, V., Rascon, L., Quintero, J., Garibay-Escobar, A., et al. (2020). Differential Antibody Responses to Giardia Lamblia Strain Variants Expressing Dissimilar Levels of an Immunogenic Protein. Parasite Immunol. 42 (10), 1–11. doi: 10.1111/pim.12767
Goodswen, S. J., Kennedy, P. J., Ellis, J. T. (2017). On the Application of Reverse Vaccinology to Parasitic Diseases: A Perspective on Feature Selection and Ranking of Vaccine Candidates. Int. J. Parasitol. 47 (12), 779–790. doi: 10.1016/j.ijpara.2017.08.004
Greenbaum, J., Sidney, J., Chung, J., Brander, C., Peters, B., Sette, A. (2011). Functional Classification of Class II Human Leukocyte Antigen (HLA) Molecules Reveals Seven Different Supertypes and a Surprising Degree of Repertoire Sharing Across Supertypes. Immunogenetics 63 (6), 325–335. doi: 10.1007/s00251-011-0513-0
Grit, G. H., Van Coppernolle, S., Devriendt, B., Geurden, T., Dreesen, L., Hope, J., et al. (2014). Evaluation of Cellular and Humoral Systemic Immune Response Against Giardia Duodenalis Infection in Cattle. Vet. Parasitol. 202 (3–4), 145–155. doi: 10.1016/j.vetpar.2014.03.012
Gupta, S., Ansari, H. R., Gautam, A., Raghava, G. P.S. (2013). Identification of B-Cell Epitopes in an Antigen for Inducing Specific Class of Antibodies. Biol. Direct 8 (1), 1–15. doi: 10.1186/1745-6150-8-27
Hanevik, K., Kristoffersen, E., Svard, S., Bruserud, O., Ringqvist, E., Sørnes, S., et al. (2011). Human Cellular Immune Response Against Giardia Lamblia 5 Years After Acute Giardiasis. J. Infect. Dis. 204 (11), 1779–1786. doi: 10.1093/infdis/jir639
Hehl, A. B., Marti, M., Köhler, P. (2000). Stage-Specific Expression and Targeting of Cyst Wall Protein-Green Fluorescent Protein Chimeras in Giardia. Mol. Biol. Cell 11 (5), 1789–1800. doi: 10.1091/mbc.11.5.1789
He, Y., Xiang, Z., Mobley, H. L.T. (2010). Vaxign: The First Web-Based Vaccine Design Program for Reverse Vaccinology and Applications for Vaccine Development. J. Biomed. Biotechnol. 2010, 15. doi: 10.1155/2010/297505
Heyworth, M. F., Carlson, J. R., Ermak, T. H. (1987). Clearance of Giardia Muris Infection Requires Helper/Inducer T Lymphocytes. J. Exp. Med. 165 (6), 1743–1748. doi: 10.1084/jem.165.6.1743
Hjøllo, T., Bratland, E., Steinsland, H., Radunovic, M., Langeland, N., Hanevik, K. (2018). Longitudinal Cohort Study of Serum Antibody Responses Towards Giardia Lamblia Variant-Specific Surface Proteins in a Non-Endemic Area. Exp. Parasitol. 191 (May), 66–72. doi: 10.1016/j.exppara.2018.06.005
Jenikova, G., Hruz, P., Andersson, M. K., Tejman-Yarden, N., Ferreira, P. C.D., Andersen, Y. S., et al. (2011). A1-Giardin Based Live Heterologous Vaccine Protects Against Giardia Lamblia Infection in a Murine Model. Vaccine 29 (51), 9529–9537. doi: 10.1016/j.vaccine.2011.09.126
Jiménez-Cardoso, E., Eligio-García, L., Cortés-Campos, A. (2002). Evaluación De La Capacidad Inmunogénica De La Vacuna Giardia-Vax, Utilizando Un Modelo Experimental De Giardiasis En Gerbos (Meriones Unguiculatus). Vet. Mex. 33 (1), 49–54. doi: 10.21753/vmoa.33.001.60
Jiménez, J. C., Fontaine, J., Creusy, C., Fleurisse, L., Grzych, J. M., Capron, M., et al. (2014). Antibody and Cytokine Responses to Giardia Excretory/Secretory Proteins in Giardia Intestinalis-Infected BALB/c Mice. Parasitol. Res. 113 (7), 2709–2718. doi: 10.1007/s00436-014-3927-4
Jiskoot, W., Kersten, G. F.A., Mastrobattista, E., Slütter, B. (2019). “Chapter 22-Vaccines,” in Pharmaceutical Biotechnology: Fundamentals and Applications. Eds. Crommelin, D. J. A., Sindelar, R. D., Meibohm, B., Springer. 281–304.
Kamda, J. D., Nash, T. E., Singer, S. M. (2012). Giardia Duodenalis: Dendritic Cell Defects in IL-6 Deficient Mice Contribute to Susceptibility to Intestinal Infection. Exp. Parasitol. 130 (3), 288–291. doi: 10.1016/j.exppara.2012.01.003
Karosiene, E., Rasmussen, M., Blicher, T., Lund, O., Buus, S., Nielsen, M. (2013). NetMHCIIpan-3.0, a Common Pan-Specific MHC Class II Prediction Method Including All Three Human MHC Class II Isotypes, HLA-DR, HLA-DP and HLA-DQ. Immunogenetics 65 (10), 711–724. doi: 10.1007/s00251-013-0720-y
Kaufmann, S. H.E., Hess, J. (1999). Impact of Intracellular Location of and Antigen Display by Intracellular Bacteria: Implications for Vaccine Development. Immunol. Lett. 65 (1–2), 81–84. doi: 10.1016/S0165-2478(98)00128-X
Kyes, S. A., Kraemer, S. M., Smith, J. D. (2007). Antigenic Variation in Plasmodium Falciparum: Gene Organization and Regulation of the Var Multigene Family. Eukaryot. Cell 6, 1511–1520. doi: 10.1128/EC.00173-07
Landais, E., Romagnoli, P. A., Corper, A. L., Shires, J., Altman, J. D., Wilson, I. A., et al. (2009). New Design of MHC Class II Tetramers to Accommodate Fundamental Principles of Antigen Presentation. J. Immunol. 183 (12), 7949–7957. doi: 10.4049/jimmunol.0902493
Larocque, R., Nakagaki, K., Lee, P., Abdul-Wahid, A., Faubert, G. M. (2003). Oral Immunization of BALB/c Mice With Giardia Duodenalis Recombinant Cyst Wall Protein Inhibits Shedding of Cysts. Infect. Immun. 71 (10), 5662–5669. doi: 10.1128/IAI.71.10.5662-5669.2003
Laurens, M. B. (2020). RTS,S/AS01 Vaccine (MosquirixTM): An Overview. Hum. Vaccin. Immunother. 16 (3), 480–489. doi: 10.1080/21645515.2019.1669415
Lee, P., Abdul-Wahid, A., Faubert, G. M. (2009). Comparison of the Local Immune Response Against Giardia Lamblia Cyst Wall Protein 2 Induced by Recombinant Lactococcus Lactis and Streptococcus Gordonii. Microbes Infect. 11 (1), 20–28. doi: 10.1016/j.micinf.2008.10.002
Lee, H. Y., Kim, J., Noh, H. J., Kim, H. P., Park, S. J. (2014). Giardia Lamblia Binding Immunoglobulin Protein Triggers Maturation of Dendritic Cells via Activation of TLR4-MyD88-P38 and ERK1/2 MAPKs. Parasite Immunol. 36 (12), 627–646. doi: 10.1111/pim.12119
Lima, T. S., Lodoen, M. B. (2019). Mechanisms of Human Innate Immune Evasion by Toxoplasma Gondii. Front. Cell. Infect. Microbiol. 9 (MAR), 1–8. doi: 10.3389/fcimb.2019.00103
Li, E., Zhou, P., Petrin, Z., Singer, S. M. (2004). Mast Cell-Dependent Control of Giardia Lamblia Infections in Mice. Infect. Immun. 72 (11), 6642–6649. doi: 10.1128/IAI.72.11.6642-6649.2004
Lopez-Romero, G., Garzon, T., Rascon, R., Valdez, A., Quintero, J., Arvizu-Flores, A. A., et al. (2017). Characterization of BIP Protein of G. Lamblia as a Potential Immunogen in a Mouse Infection Model. Immunobiology 222 (8–9), 884–891. doi: 10.1016/j.imbio.2017.05.008
Lopez-Romero, G., Quintero, J., Astiazarán-García, H., Velazquez, C. (2015). Host Defences Against Giardia Lamblia. Parasite Immunol. 37 (8), 394–406. doi: 10.1111/pim.12210
Lovitch, S. B., Unanue, E. R. (2005). Conformational Isomers of a Peptide-Class II Major Histocompatibility Complex. Immunol. Rev. 207, 293–313. doi: 10.1111/j.0105-2896.2005.00298.x
Lujan, H. D. (2011). Mechanisms of Adaptation in the Intestinal Parasite Giardia Lamblia. Essays Biochem. 51 (1), 177–191. doi: 10.1042/bse0510177
Lujan, H. D., Mowatt, M. R., Conrad, J. T., Bowers, B., Nash, T. E. (1995). Identification of a Novel Giardia Lamblia Cyst Wall Protein With Leucine- Rich Repeats: Implications for Secretory Granule Formation and Protein Assembly Into the Cyst Wall. J. Biol. Chem. 270 (49), 29307–29313. doi: 10.1074/jbc.270.49.29307
Ma’ayeh, S. Y., Liu, J., Peirasmaki, D., Hörnaeus, K., Lind, S. B., Grabherr, M., et al. (2017). Characterization of the Giardia Intestinalis Secretome During Interaction With Human Intestinal Epithelial Cells: The Impact on Host Cells. PLoS Negl. Trop. Dis. 11 (12), e0006120. doi: 10.1371/journal.pntd.0006120
Malonis, R. J., Lai, J. R., Vergnolle, O. (2020). Peptide-Based Vaccines: Current Progress and Future Challenges. Chem. Rev. 120 (6), 3210–3229. doi: 10.1021/acs.chemrev.9b00472
McCaffery, J.M., Faubert, G. M., Gillin, F. D. (1994). Giardia Lamblia: Traffic of a Trophozoite Variant Surface Protein and a Major Cyst Wall Epitope During Growth, Encystation, and Antigenic Switching. Exp. Parasitol. 79 (3), 236–249. doi: 10.1006/expr.1994.1087
McFarland, B. J., Sant, A. J., Lybrand, T. P., Beeson, C. (1999). Ovalbumin(323-339) Peptide Binds to the Major Histocompatibility Complex Class II I-A(d) Protein Using Two Functionally Distinct Registers. Biochemistry 38 (50), 16663–16670. doi: 10.1021/bi991393l
Montagnoli, C., Sandini, S., Bacci, A., Romani, L., La Valle, R. (2004). Immunogenicity and Protective Effect of Recombinant Enolase of Candida Albicans in a Murine Model of Systemic Candidiasis. Med. Mycol. 42 (4), 319–324. doi: 10.1080/13693780310001644653
Moormann, A. M., Nixon, C. E., Forconi, C. S. (2019). Immune Effector Mechanisms in Malaria: An Update Focusing on Human Immunity. Parasite Immunol. 41 (8), 1–14. doi: 10.1111/pim.12628
Mora, M., Telford, J. L. (2010). Genome-Based Approaches to Vaccine Development. J. Mol. Med. 88 (2), 143–147. doi: 10.1007/s00109-009-0574-9
Mukherjee, S., Huda, S., Sinha Babu, S. P. (2019). Toll-Like Receptor Polymorphism in Host Immune Response to Infectious Diseases: A Review. Scand. J. Immunol. 90 (1), 1–18. doi: 10.1111/sji.12771
Nash, T. E., Aggarwal, A. (1986). Cytotoxicity of Monoclonal Antibodies to a Subset of Giardia Isolates. J. Immunol. 136 (7), 2628 LP–2632.
Nash, T. E., Merritt, J. W., Conrad, J. T. (1991). Isolate and Epitope Variability in Susceptibility of Giardia Lamblia to Intestinal Proteases. Infect. Immun. 59 (4), 1334–1340. doi: 10.1128/iai.59.4.1334-1340.1991
Nelson, C. A., Roof, R. W., McCourt, D. W., Unanue, E. R. (1992). Identification of the Naturally Processed Form of Hen Egg White Lysozyme Bound to the Murine Major Histocompatibility Complex Class II Molecule I-Ak. Proc. Natl. Acad. Sci. USA 89 (16), 7380–7383. doi: 10.1073/pnas.89.16.7380
Nielsen, M., Lundegaard, C., Blicher, T., Peters, B., Sette, A., Justesen, S., et al. (2008). Quantitative Predictions of Peptide Binding to Any HLA-DR Molecule of Known Sequence: NetMHCIIpan. PloS Comput. Biol. 4 (7), 1–10. doi: 10.1371/journal.pcbi.1000107
Olson, M. E., Ceri, H., Morck, D. W. (2000). Giardia Vaccination. Parasitol. Today 16 (5), 213–217. doi: 10.1016/S0169-4758(99)01623-3
Palm, J.E.D., Weiland, M. E.-L., Griffiths, W. J., Ljungström, I., Svärd, S. G. (2003). Identification of Immunoreactive Proteins During Acute Human Giardiasis. J. Infect. Dis. 187 (12), 1849–1859. doi: 10.1086/375356
Papanastasiou, P., Bruderer, T., Li, Y., Bommeli, C., Köhler, P. (1997). Primary Structure and Biochemical Properties of a Variant-Specific Surface Protein of Giardia1Note: The Nucleotide Sequence Information Reported in This Paper Has Been Submitted to the EMBL Data Library With the Accession No. Z83743.1. Mol. Biochem. Parasitol. 86 (1), 13–27. doi: 10.1016/S0166-6851(97)02836-3
Paul, S., Sidney, J., Sette, A., Peters, B. (2016). TepiTool: A Pipeline for Computational Prediction of T Cell Epitope Candidates. Curr. Protoc. Immunol. 114, 18.19.1–18.19.24. doi: 10.1002/cpim.12
Pertsemlidis, A., Fondon, J. W. (2001). Having a BLAST With Bioinformatics (and Avoiding BLASTphemy). Genome Biol. 2 (10), reviews2002.1. doi: 10.1186/gb-2001-2-10-reviews2002
Potocnakova, L., Bhide, M., Borszekova Pulzova, L. (2016). An Introduction to B-Cell Epitope Mapping and In Silico Epitope Prediction. J. Immunol. Res. 2016, 11. doi: 10.1155/2016/6760830
Priest, J. W., Mehlert, A., Arrowood, M. J., Riggs, M. W., Ferguson, M. A. J. (2003). Characterization of a Low Molecular Weight Glycolipid Antigen From Cryptosporidium Parvum. J. Biol. Chem. 278 (52), 52212–52222. doi: 10.1074/jbc.M306835200
Quintero, J., Valdez, A., Samaniego, B., Lopez-Romero, G., Astiazaran-Garcia, H., Rascon, L., et al. (2017). Isolation and Partial Characterization of an Immunogenic Antigen of Giardia Lamblia. Parasitol. Int. 66 (3), 324–330. doi: 10.1016/j.parint.2017.01.007
Radunovic, M., Klotz, C., Saghaug, C. S., Brattbakk, H. R., Aebischer, T., Langeland, N., et al. (2017). Genetic Variation in Potential Giardia Vaccine Candidates Cyst Wall Protein 2 and A1-Giardin. Parasitol. Res. 116 (8), 2151–2158. doi: 10.1007/s00436-017-5516-9
Reiner, D. S., Gillin, F. D. (1991). Human Secretory and Serum Antibodies Recognize Environmentally Induced Antigens of Giardia Lamblia. Infect. Immun. 60 (2), 637–643. doi: 10.1128/iai.60.2.637-643.1992
Rhee, J. H., Lee, S. E., Kim, S. Y. (2012). Mucosal Vaccine Adjuvants Update. Clin. Exp. Vaccine Res. 1 (1), 50–63. doi: 10.7774/cevr.2012.1.1.50
Rivero, F. D., Saura, A., Prucca, C. G., Carranza, P. G., Torri, A., Lujan, H. D. (2010). Disruption of Antigenic Variation Is Crucial for Effective Parasite Vaccine. Nat. Med. 16 (5), 551–557. doi: 10.1038/nm.2141
Robleda-Castillo, R., Ros-Lucas, A., Martinez-Peinado, N., Alonso-Padilla, J. (2021). An Overview of Current Uses and Future Opportunities for Computer-Assisted Design of Vaccines for Neglected Tropical Diseases. Adv. Appl. Bioinform. Chem. 14, 25–47. doi: 10.2147/AABC.S258759
Ropert, C., Gazzinelli, R. T. (2000). Signaling of Immune System Cells by Glycosylphosphatidylinositol (GPI) Anchor and Related Structures Derived From Parasitic Protozoa. Curr. Opin. Microbiol. 3 (4), 395–403. doi: 10.1016/S1369-5274(00)00111-9
Roxström-Lindquist, K., Ringqvist, E., Palm, D., Svärd, S. (2005). Giardia Lamblia-Induced Changes in Gene Expression in Differentiated Caco-2 Human Intestinal Epithelial Cells. Infect. Immun. 73 (12), 8204–8208. doi: 10.1128/IAI.73.12.8204-8208.2005
Saghaug, C. S., Sørnes, S., Peirasmaki, D., Svärd, S., Langeland, N., Hanevik, K. (2015). Human Memory CD4+ T Cell Immune Responses Against Giardia Lamblia. Clin. Vaccine Immunol. 23 (1), 11–18. doi: 10.1128/CVI.00419-15
Scott, K. G.E., Yu, L. C.H., Buret, A. G. (2004). Role of CD8+ and CD4+ T Lymphocytes in Jejunal Mucosal Injury During Murine Giardiasis. Infect. Immun. 72 (6), 3536–3542. doi: 10.1128/IAI.72.6.3536-3542.2004
Serradell, M. C., Gargantini, P. R., Saura, A., Oms, S. R., Rupil, L. L., Berod, L., et al. (2018). Cytokines, Antibodies, and Histopathological Profiles During Giardia Infection and Variant-Specific Surface Protein-Based Vaccination. Infect. Immun. 86 (6), e00773–e00717. doi: 10.1128/IAI.00773-17
Serradell, M. C., Rupil, L. L., Martino, R. A., Prucca, C. G., Carranza, P. G., Saura, A., et al. (2019). Efficient Oral Vaccination by Bioengineering Virus-Like Particles With Protozoan Surface Proteins. Nat. Commun. 10 (1), 361. doi: 10.1038/s41467-018-08265-9
Serradell, M. C., Saura, A., Rupil, L. L., Gargantini, P. R., Faya, M. I., Furlan, P. J., et al. (2016). Vaccination of Domestic Animals With a Novel Oral Vaccine Prevents Giardia Infections, Alleviates Signs of Giardiasis and Reduces Transmission to Humans. NPJ Vaccines 1, 16018. doi: 10.1038/npjvaccines.2016.18
Shimoda, M., Koni, P. A. (2007). MHC-Restricted B-Cell Antigen Presentation in Memory B-Cell Maintenance and Differentiation. Crit. Rev. Immunol. 27 (1), 47–60. doi: 10.1615/CritRevImmunol.v27.i1.40
Singer, S. M. (2016). Control of Giardiasis by Interleukin-17 in Humans and Mice - Are the Questions All Answered? Clin. Vaccine Immunol. 23 (1), 2–5. doi: 10.1128/CVI.00648-15
Singer, S. M., Nash, T. E. (2000). T-Cell-Dependent Control of Acute Giardia Lamblia Infections in Mice. Infect. Immun. 68 (1), 170–175. doi: 10.1128/IAI.68.1.170-175.2000
Skwarczynski, M., Toth, I. (2016). Peptide-Based Synthetic Vaccines. Chem. Sci. 7 (2), 842–854. doi: 10.1039/C5SC03892H
Smith, N. C., Sinden, R. E., Ramakrishnan, C. (2021). Editorial: Get Over the Gut: Apicomplexan Parasite Interaction, Survival and Stage Progression in Vertebrate and Invertebrate Digestive Tracts 11, 680555. doi: 10.3389/fcimb.2021.680555
Stäger, S., Felleisen, R., Gottstein, B., Müller, N. (1997). Giardia Lamblia Variant Surface Protein H7 Stimulates a Heterogeneous Repertoire of Antibodies Displaying Differential Cytological Effects on the Parasite. Mol. Biochem. Parasitol. 85 (1), 113–124. doi: 10.1016/S0166-6851(96)02818-6
Stäger, S., Gottstein, B., Sager, H., Jungi, T. W., Müller, N. (1998). Influence of Antibodies in Mother’s Milk on Antigenic Variation of Giardia Lamblia in the Murine Mother-Offspring Model of Infection. Infect. Immun. 66 (4), 1287–1292. doi: 10.1128/IAI.66.4.1287-1292.1998
Stein, J. E., Radecki, S. V., Lappin, M. R. (2003). Efficacy of Giardia Vaccination in the Treatment of Giardiasis in Cats. J. Am. Vet. Med. Assoc. 222 (11), 1548–1551. doi: 10.2460/javma.2003.222.1548
Strong, B. S.I., Unanue, E. R. (2011). Presentation of Type B Peptide-MHC Complexes From Hen Egg White Lysozyme by TLR Ligands and Type I IFNs Independent of H2-DM Regulation. J. Immunol. (Baltimore Md.: 1950) 187 (5), 2193–2201. doi: 10.4049/jimmunol.1100152
Sun, C. H. J., McCaffery, M., Reiner, D. S., Gillin, F. D. (2003). Mining the Giardia Lamblia Genome for New Cyst Wall Proteins. J. Biol. Chem. 278 (24), 21701–21708. doi: 10.1074/jbc.M302023200
Tedla, M. G., Every, A. L., Scheerlinck, J. P. Y. (2019). Investigating Immune Responses to Parasites Using Transgenesis. Parasit. Vectors 12 (1), 1–14. doi: 10.1186/s13071-019-3550-4
Teh-Poot, C., Tzec-Arjona, E., Martínez-Vega, P., Jesus Ramirez-Sierra, M., Rosado-Vallado, M., Dumonteil, E. (2015). From Genome Screening to Creation of Vaccine Against Trypanosoma Cruzi by Use of Immunoinformatics. J. Infect. Dis. 211 (2), 258–266. doi: 10.1093/infdis/jiu418
Téllez, A., Palm, D., Weiland, M., Alemán, J., Winiecka-Krusnell, J., Linder, E., et al. (2005). Secretory Antibodies Against Giardia Intestinalis in Lactating Nicaraguan Women. Parasite Immunol. 27 (5), 163–169. doi: 10.1111/j.1365-3024.2005.00758.x
Troeger, H., Joerg Epple, H., Schneider, T., Wahnschaffe, U., Ullrich, R., Burchard, G. D., et al. (2007). Effect of Chronic Giardia Lamblia Infection on Epithelial Transport and Barrier Function in Human Duodenum. Gut 56 (3), 328–335. doi: 10.1136/gut.2006.100198
Uwase, J., Chu, R., Kassegne, K., Lei, Y., Shen, F., Fu, H., et al. (2020). Immunogenicity Analysis of Conserved Fragments in Plasmodium Ovale Species Merozoite Surface Protein 4. Malaria J. 19 (1), 1–11. doi: 10.1186/s12936-020-03207-7
Vakili, B., Nezafat, N., Zare, B., Erfani, N., Akbari, M., Ghasemi, Y., et al. (2020). A New Multi-Epitope Peptide Vaccine Induces Immune Responses and Protection Against Leishmania Infantum in BALB/c Mice. Med. Microbiol. Immunol. 209 (1), 69–79. doi: 10.1007/s00430-019-00640-7
Velazquez, C., Beltran, M., Ontiveros, N., Rascon, L., Figueroa, D. C., Granados, A. J., et al. (2005). Giardia Lamblia Infection Induces Different Secretory and Systemic Antibody Responses in Mice. Parasite Immunol. 27 (9), 351–356. doi: 10.1111/j.1365-3024.2005.00793.x
Velazquez, C., Vidavsky, I., Der Van Drift, K., Gross, M. L., Unanue, E. R. (2002). Chemical Identification of a Low Abundance Lysozyme Peptide Family Bound to I-A K Histocompatibility Molecules. J. Biol. Chem. 277 (45), 42514–42522. doi: 10.1074/jbc.M202316200
Venkatesan, P., Finch, R. G., Wakelin, D. (1997). A Comparison of Mucosal Inflammatory Responses to Giardia Muris in Resistant B10 and Susceptible BALB/c Mice. Parasite Immunol. 19 (3), 137–143. doi: 10.1046/j.1365-3024.1997.d01-189.x
Wang, P., Sidney, J., Dow, C., Mothé, B., Sette, A., Peters, B. (2008). A Systematic Assessment of MHC Class II Peptide Binding Predictions and Evaluation of a Consensus Approach. PloS Comput. Biol. 4 (4), e1000048. doi: 10.1371/journal.pcbi.1000048
Wang, P., Sidney, J., Kim, Y., Sette, A., Lund, O., Nielsen, M., et al. (2010). Peptide Binding Predictions for HLA DR, DP and DQ Molecules. BMC Bioinformatics 11 (1), 568. doi: 10.1186/1471-2105-11-568
Wang, Y., Yi, L., Sun, L. Y., Liu, Y. C., Wen, W. Y., Li, X. K., et al. (2020). Identification and Characterization of a Streptococcus Suis Immunogenic Ornithine Carbamoytransferase Involved in Bacterial Adherence. J. Microbiol. Immunol. Infect. 53 (2), 234–239. doi: 10.1016/j.jmii.2018.05.004
Weaver, C. T., Harrington, L. E., Mangan, P. R., Gavrieli, M., Murphy, K. M. (2006). Th17: An Effector CD4 T Cell Lineage With Regulatory T Cell Ties. Immunity 24 (6), 677–688. doi: 10.1016/j.immuni.2006.06.002
Weiland, M. E.L., Palm, J.E.D., Griffiths, W. J., McCaffery, J.M., Svärd, S. G. (2003). Characterisation of Alpha-1 Giardin: An Immunodominant Giardia Lamblia Annexin With Glycosaminoglycan-Binding Activity. Int. J. Parasitol. 33 (12), 1341–1351. doi: 10.1016/S0020-7519(03)00201-7
Wong-Baeza, I., Alcántara-Hernández, M., Mancilla-Herrera, I., Ramírez-Saldívar, I., Arriaga-Pizano, L., Ferat-Osorio, E., et al. (2010). The Role of Lipopeptidophosphoglycan in the Immune Response to Entamoeba Histolytica. J. Biomed. Biotechnol. 2010, 254521. doi: 10.1155/2010/254521
Keywords: immunogenic, epitope, protection, vaccine, immunoinformatic
Citation: Garzon T, Ortega-Tirado D, Lopez-Romero G, Alday E, Robles-Zepeda RE, Garibay-Escobar A and Velazquez C (2021) “Immunoinformatic Identification of T-Cell and B-Cell Epitopes From Giardia lamblia Immunogenic Proteins as Candidates to Develop Peptide-Based Vaccines Against Giardiasis”. Front. Cell. Infect. Microbiol. 11:769446. doi: 10.3389/fcimb.2021.769446
Received: 02 September 2021; Accepted: 08 October 2021;
Published: 27 October 2021.
Edited by:
Maria E. Francia, Institut Pasteur de Montevideo, UruguayCopyright © 2021 Garzon, Ortega-Tirado, Lopez-Romero, Alday, Robles-Zepeda, Garibay-Escobar and Velazquez. This is an open-access article distributed under the terms of the Creative Commons Attribution License (CC BY). The use, distribution or reproduction in other forums is permitted, provided the original author(s) and the copyright owner(s) are credited and that the original publication in this journal is cited, in accordance with accepted academic practice. No use, distribution or reproduction is permitted which does not comply with these terms.
*Correspondence: Carlos Velazquez, dmVsYXoyQHVuaXNvbi5teA==; orcid.org/0000-0002-3728-7848