- 1Department of Microbiology, Immunology, and Infectious Diseases, College of Medicine & Medical Sciences, Arabian Gulf University, Manama, Bahrain
- 2Department of Pathology, Microbiology Section, Salmaniya Medical Complex, Manama, Bahrain
The prevalence of Carbapenem-resistant Klebsiella pneumoniae (CRKP) is currently increasing worldwide, prompting WHO to classify it as an urgent public health threat. CRKP is considered a difficult to treat organism owing to limited therapeutic options. In this study, a total of 24 CRKP clinical isolates were randomly collected from Salmaniya Medical Complex, Bahrain. Bacterial identification and antibiotic susceptibility testing were performed, on MALDI-TOF and VITEK-2 compact, respectively. The isolates were screened for carbapenem resistance markers (blaNDM, blaOXA-23, blaOXA-48 and blaOXA-51) and plasmid-mediated quinolone resistance genes (qnrA, qnrB, and qnrS) by monoplex PCR. On the other hand, only colistin-resistant isolates (n=12) were screened for MCR-1, MCR-2 and MCR-3 genes by monoplex PCR. Moreover, the Genetic environment of blaNDM, integrons analysis, and molecular characterization of plasmids was also performed. Antibiotic susceptibility revealed that all the isolates (100%) were resistant to ceftolozane/tazobactam, piperacillin/tazobactam, 96% resistant to ceftazidime, trimethoprim/sulfamethoxazole, 92% resistant to meropenem, gentamicin and cefepime, 88% resistant to ciprofloxacin, imipenem, and 37% resistant to amikacin. Ceftazidime/avibactam showed the least resistance (12%). 75% (n=12/16) were resistant to colistin and 44% (n=7/16) showed intermediate susceptibility to tigecycline. The detection of resistant determinants showed that the majority (95.8%) of CRKP harbored blaNDM-1, followed by blaOXA-48 (91.6%) blaOXA-51 (45.8%), and blaOXA-23 (41.6%). Sequencing of the blaNDM amplicons revealed the presence of blaNDM-1. Alarmingly, 100% of isolates showed the presence of qnrS. These predominant genes were distributed in various combinations wherein the majority were blaNDM-1 + blaOXA-51+ qnrS + blaOXA-48 (n =10, 41.7%), blaNDM-1 + blaOXA-23+ qnrS + blaOXA-48 (n=8, 33.3%), among others. In conclusion, the resistance rate to most antibiotics is very high in our region, including colistin and tigecycline, and the genetic environment of CRKP is complex with the carriage of multiple resistance markers. Resistance to ceftazidime/avibactam is uncommon and hence can be used as a valuable option for empirical therapy. Molecular data on resistance markers and the genetic environment of CRKP is lacking from this geographical region; this would be the first report addressing the subject matter. Surveillance and strict infection control strategies should be reinforced in clinical settings to curb the emergence and spread of such isolates.
Introduction
The emergence and increase in multidrug-resistant bacteria have become a global health threat (Carlet et al., 2012). The World Health Organization identified antibiotic resistance as one of the top 10 most important global health threats prior to the COVID-19 pandemic. However, there are growing worries that the COVID-19 pandemic would hinder future efforts to combat antibiotic resistance (Choudhury et al., 2022). According to the Centers for Disease Control and Prevention, antibiotic resistance in the US increased by 15% overall between 2019 and the period immediately following the pandemic’s peak in 2020 (Tanne, 2022). Multidrug-resistant Enterobacterales in particular are a leading cause of healthcare-associated infections, which are linked to increased morbidity and death, as well as rising medical expenditures (Friedman et al., 2016). Globally the prevalence of carbapenem-producing Enterobacterales varies significantly (Logan and Weinstein, 2017). Carbapenem-resistant Klebsiella pneumoniae (CRKP), a member of the carbapenem-resistant Enterobacterales (CRE) family, is an emerging cause of healthcare-associated infections worldwide (Band et al., 2018). Worst of all, CRKP can pass on its resistance to other bacteria via horizontal gene transfer, which can include bacterial conjugation, resulting in drug resistance. Two primary mechanisms induce carbapenem resistance. First, CRKP can produce β-lactamases that can hydrolyze cephalosporins, or ESBLs combined with reduced cell wall permeability. Second, the formation of β-lactamases, which can hydrolyze most β-lactams, including carbapenems. Conferring to the Ambler classification, it belongs to class A (KPC, SME, IMI, GES, NMC), class B (NDM, IMP, and VIM family), and class D (OXA-48 like) (Pitout et al., 2015). Because of the capability of hydrolyzing all β-lactam antibiotics except aztreonam, class B enzymes are the most clinically relevant carbapenemases (Elshamy and Aboshanab, 2020).
Acquired Metallo-β-lactamases (MBL) including IMP, VIM, and NDM carbapenemases in Enterobacterales are reported in the literature (Bush and Bradford, 2020). The NDM-1 gene was originally discovered in a Swedish patient (Indian origin) in New Delhi in 2008 (Yong et al., 2009). Since then, NDM-1 producers have been reported in the United Kingdom, Sweden, France, Germany, China, Belgium, Japan, Austria, Australia, Norway, Canada, and the Netherlands (Khan et al., 2017). Since the first blaNDM-1 positive K. pneumoniae isolates were discovered in Nanchang, China in 2013, the disease has spread swiftly throughout the country. In Shanghai, Hunan, Yunnan, and other places, outbreaks of NDM-1 generating K. pneumoniae have been recorded (Zhu et al., 2016). NDM cases are also reported from the Indian sub-continent (Ahmad et al., 2018) and the Middle East (Al-Zahrani and Alsiri, 2018).
Class-D β-lactamase, also known as OXA-type enzymes or oxacillinases, is a group of about 400 genetically distinct enzymes (Khan et al., 2022). Only a limited fraction of the class-D, OXA family functions as a carbapenemase. The common OXA-48, OXA-23, OXA-40, as well as its variations OXA-232, OXA-162, and OXA-181, are among them (Mairi et al., 2018). OXA-48-like enzyme is among the most common carbapenemases in Enterobacterales. Despite the low β-lactamase activity, this enzyme hydrolyzes penicillin and is uninhibited by β-lactamase inhibitors (Pfeifer et al., 2012). Since the discovery of OXA-48 carbapenemase in Turkey in 2001, these strains have been implicated in numerous nosocomial outbreaks around the world (van Duin and Doi, 2017), including Middle East (Shibl et al., 2013), the Mediterranean region (van Duin and Doi, 2017) and European countries (European Centre for Disease Prevention and Control, 2019). OXA-48 K. pneumoniae is endemic to North Africa and the Middle East, while OXA-181 K. pneumoniae is found in India. However, OXA-181 nosocomial epidemics have been reported in Sub-Saharan Africa (Pitout et al., 2019).
Although few researches on CRKP isolates have been undertaken in the Arabian Peninsula, nearly all Gulf Cooperation Council (GCC) countries share similar ESBLs and carbapenemases-producing Enterobacterales, the majority of which were isolated from nosocomial infections (Al-Zahrani and Alsiri, 2018). Furthermore, a review article on gram-negative bacilli producing β-lactamases from GCC states revealed that the most prevalent and widespread β-lactamases genes are NDM-1, and OXA-48 (Zowawi et al., 2013). Owing to the real difficulty of treating CRKP, epidemiological analysis of carbapenemases coding genes in circulating strains is critical for designing strategies to decrease infection outbreaks and creating novel therapeutic techniques (Lombardi et al., 2015). This geographical region lacks exhaustive molecular data on resistance markers and the genetic environment of CRKP. Therefore, this study aimed to analyze the antibiotic resistance profile and genetic environment among clinical CRKP isolates from the Kingdom of Bahrain.
Material and methods
Ethics statement
The protocol of this study was reviewed and approved by the Research Ethics Committee, Arabian Gulf University (AGU) (E012-PI-11/19) and the Ministry of Health (AURS/305/2020).
Bacterial isolates and hospital setting
From December 2020 to June 2021, twenty-four non-duplicate CRKP clinical isolates were included in this study. These were isolated from the blood, urine and endotracheal aspirate, of the patients, admitted to tertiary care hospital (Salmaniya Medical Complex), Kingdom of Bahrain.
Bacterial identification and antimicrobial susceptibility testing
Bacterial species-level identification was confirmed by using a mass spectrometry system (MALDI-TOF Bruker Daltonik GmbH, Bremen, Germany) and antibiotic susceptibility testing of isolates was performed with automated microbiological systems (VITEK-2 compact bioMerieux, Marcy L, Etoile, France). Only the isolates that were identified as K. pneumoniae resistant to carbapenems were included for further molecular analysis.
Amplification of antibiotic-resistant genes by Polymerase chain reaction
For antibiotic-resistant gene detection, whole-cell DNA of strains was prepared from CRKP pure culture. Suspension of each colony was done in 100 µl of nuclease-free sterilized water and incubated at 94°C for 20 min followed by centrifugation at 6,000g at 4°C for 20min. The supernatant was used as a template to perform PCR on a GeneAmp PCR System 9700 (Applied Biosystems, Foster City, CA) using the specific primers as mentioned in Table S1 for the detection of carbapenem-resistance genes (blaKPC, blaNDM, blaOXA-23, blaOXA-48, blaOXA-51), plasmid-mediated quinolone resistance genes (qnrA, qnrB, qnrS) and colistin-resistance plasmid-mediated genes (MCR-1, MCR-2, MCR-3).
DNA sequencing
At Genoscreen Lab, PCR-generated fragments were sequenced (Campus Institut Pasteur de, France). Using the Clustal omega tool (https://www.ebi.ac.uk/Tools/msa/clustalo/), the derived protein sequence was aligned with blaNDM variants to verify the amino acid substitution in the query sequence for known variants. Additionally, online BLAST software (http://www.ncbi.nlm.nih.gov/BLAST/) was used to analyze the similarities between the amplified nucleotide sequence and the deduced protein sequences and was confirmed as blaNDM-1. Under the following accession numbers, these sequences have been added to the GenBank nucleotide database: ON506904, ON506905, ON506906, ON506907, ON506908, ON506909, ON506910, ON506911, ON506912, ON506913, ON506914, ON506915, ON506916, ON506917, ON506918, ON493161, ON493162, ON493163, ON755345 and ON755346 accessible at the National Center of Biotechnology Information website (http://www.ncbi.nlm.nih.gov).
Isolation and separation of plasmid DNA
Plasmid DNA was isolated from CRKP clinical isolates using the Qiagen Plasmid Mini Kit following the manufacturer’s instructions, which included steps 1 and 2 of clearing a bacterial lysate, adsorbing DNA onto the QIAprep membrane, and steps 3 washing and elution of plasmid. The isolated Plasmid DNA samples were then electrophoresed in 0.9% agarose gel stained with ethidium bromide (0.5 μg/ml) and visualized under an ultraviolet Gel documentation azure Biosystem C-200.
Genetic environment analysis
The genes present upstream and downstream of blaNDM, was identified by the Genetic Environment analysis as described previously (Poirel et al., 2011b).
Integron analysis
Detection and characterization of class 1, 2 and 3 integrons in CRKP isolates were investigated by amplification of integrase genes including intI1, intI2, and intI3 by using specific primers mentioned in Table S1.
Results
Distribution and antibiotic resistance pattern of the isolates
Blood provided the majority of the CRKP clinical isolates (n=17, 70.8%), followed by urine (n=5, 20.8%) and endotracheal aspirate (n=2, 8.3%). Antibiotic susceptibility revealed that all the isolates (n=24, 100%) were resistant to ceftolozane/tazobactam and piperacillin/tazobactam, 96% (n=23) resistant to ceftazidime and trimethoprim/sulfamethoxazole, 92% (n=22) showed resistance to meropenem, gentamicin and cefepime, 88% (n=21) resistant to ciprofloxacin, imipenem, and 37% (n=9) resistant to amikacin. Ceftazidime/avibactam showed the least resistance (n=3, 12%). Among the 16 isolates tested for colistin and tigecycline resistance, 75% (n=12) were resistant to colistin and 44% (n=7) showed intermediate susceptibility to tigecycline (Figure 1).
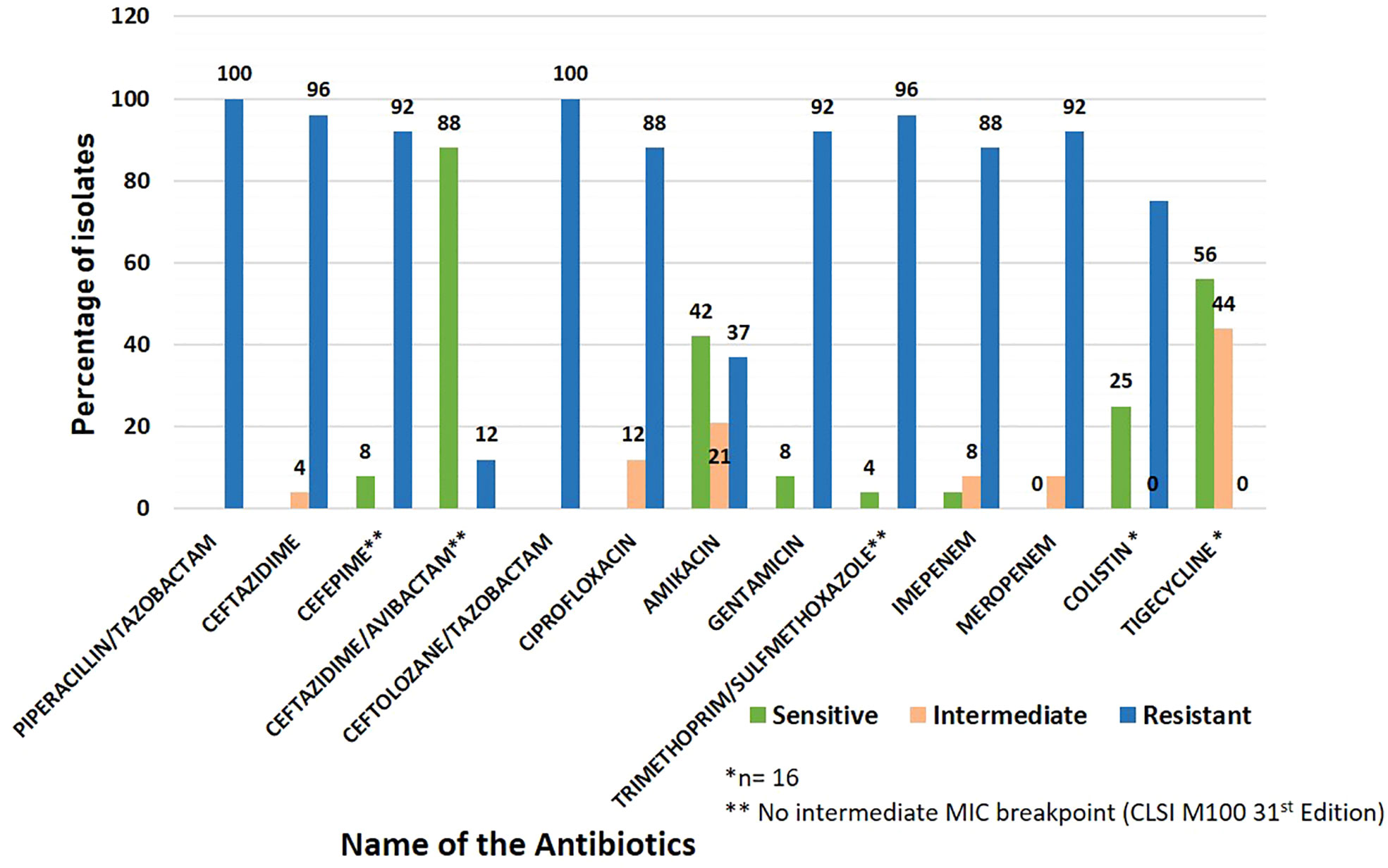
Figure 1 Antibiotic susceptibility pattern in carbapenem-resistant Klebsiella pneumoniae (CRKP) clinical isolates included in the study.
Minimum inhibitory concentrations
The CRKP clinical isolates showed high Minimum inhibitory concentrations (MICs) against frequently used antibiotics in clinical settings vis-à-vis Amikacin, Cefepime, Ceftazidime, Ceftolozane/tazobactam, Ciprofloxacin, Gentamicin, Meropenem, Piperacillin/tazobactam, and Trimethoprim/sulfamethoxazole as shown in Table 1.
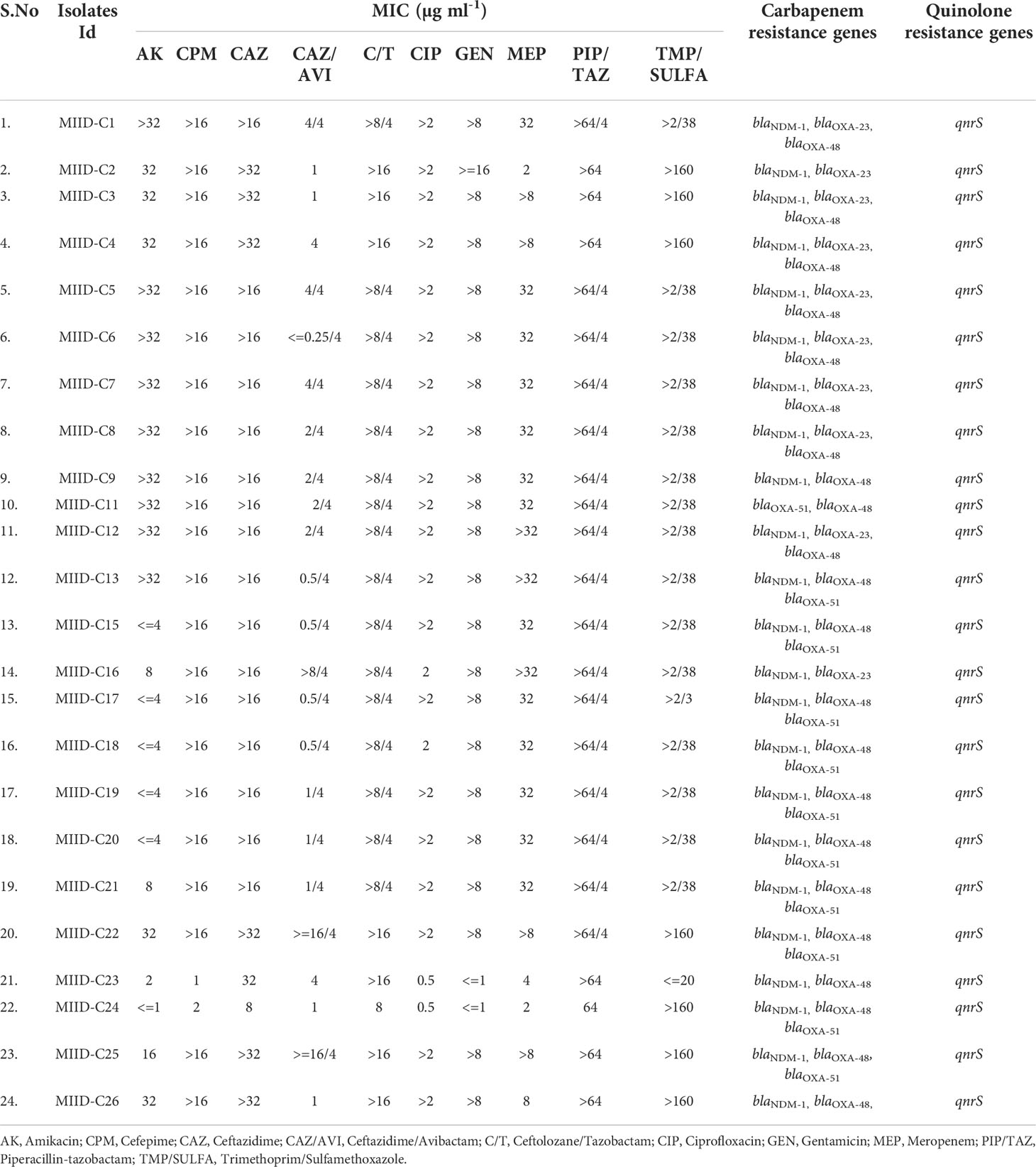
Table 1 Minimum Inhibitory Concentrations (MICs) distribution of respective antibiotics in carbapenem-resistant Klebsiella pneumoniae (CRKP) clinical isolates tested in the study.
Antimicrobial resistance genes detection
All the isolates subjected to PCR assays to detect resistant determinants to β-lactam antibiotics (carbapenems and cephalosporins) showed that the majority of CRKP harbored blaNDM-1 (95.8%; 23/24), followed by blaOXA-48 (91.6%; 22/24) blaOXA-51 (45.8%; 11/24), and blaOXA-23 (41.6%; 10/24). None of our isolates showed the presence of blaKPC gene. Sequencing of the blaNDM amplicons revealed the confirmation of blaNDM-1. Alarmingly, 100% of isolates showed the presence of qnrS. These predominant genes were distributed in various combinations: blaNDM-1 + blaOXA-51+qnrS + blaOXA-48 (n =10, 41.7%), blaNDM-1 + blaOXA-23+ qnrS + blaOXA-48 (n=8, 33.3%), blaNDM-1 + qnrS+ blaOXA-48 (n=3, 12.5%), blaNDM-1+ blaOXA-23 + qnrS (n=2, 4.2%), blaOXA-51 + qnrS+ blaOXA-48 (n=1, 4.2%). None of the colistin-resistant isolates showed the presence of plasmid-mediated MCR genes that we screened. Here probably other resistance mechanisms are likely to have a role in colistin resistance. No correlation was found between various gene combinations and MIC values of carbapenem.
Plasmid profiling
Plasmid profiling was carried out on each of the CRKP isolates to gather an insight on similarity (or dissimilarity) in types/number of plasmids carried by these isolates. The number of plasmids carried in these isolates were as follows: Eight isolates (MIID-C9, MIID-C15, MIID-C17, MIID-C18, MIID-C19, MIID-C20, MIID-C21 and MIID-C22) carried seven plasmids; two isolates (MIID-5 and MIID-8) carried six plasmids; eight isolates (MIID-C1, MIID-C3, MIID-C4, MIID-C6, MIID-C7, MIID-C11, MIID-C12 and MIID-C13) carried five plasmids; two isolates (MIID-24 and MIID-25) carried four plasmids; three isolates (MIID-2, MIID-23 and MIID-26) carried two plasmids; and one isolate (MIID-16) carried single plasmid, respectively. The plasmid sizes ranged approximately from 1.0 to 18.1 kb, with the most prevalent plasmid being approximately 1.5 kb in size and found in all of the CRKP clinical isolates (Table 2; Figure 2).
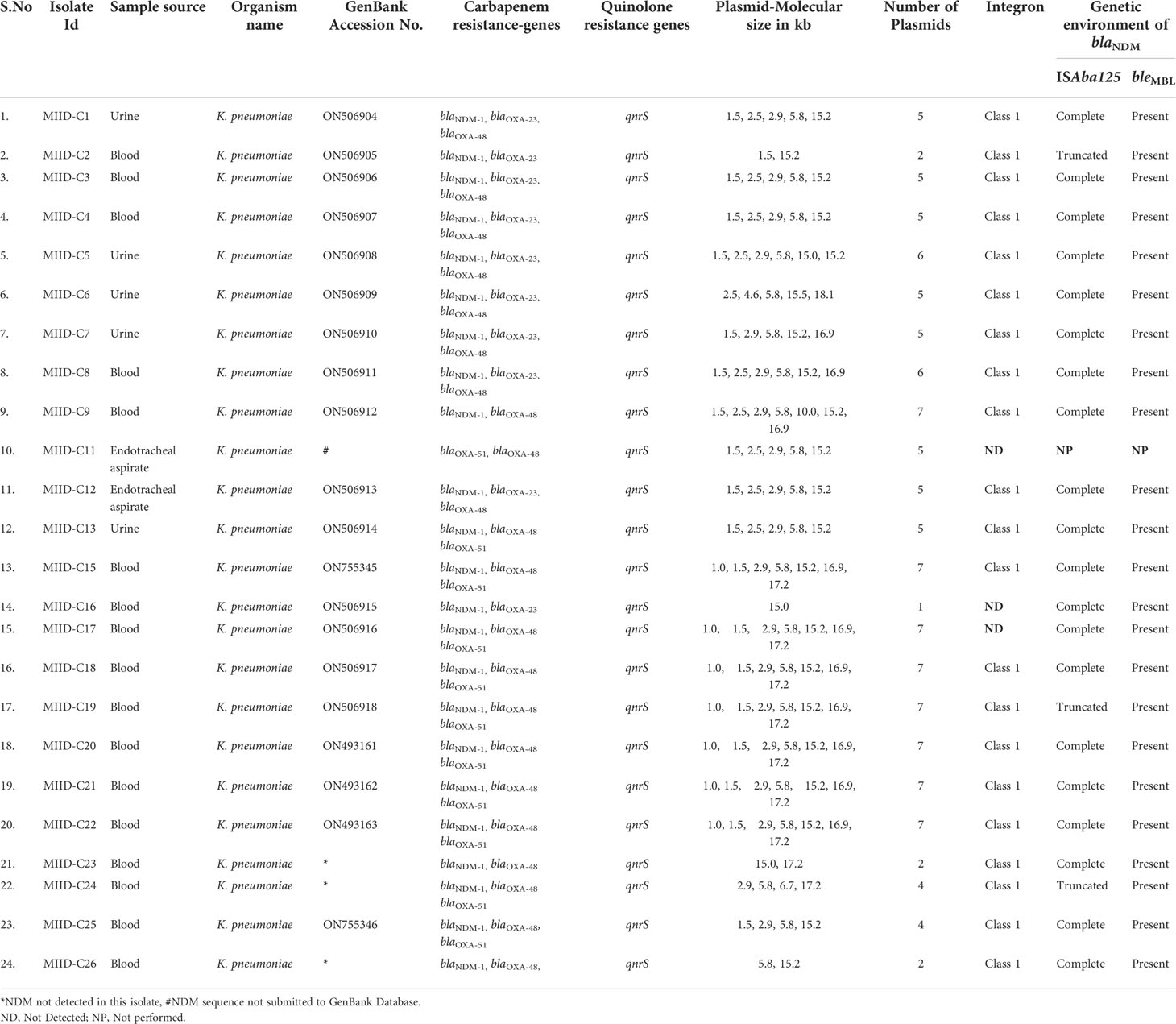
Table 2 Genetic characterization of carbapenem-resistant Klebsiella pneumoniae (CRKP) clinical isolates.
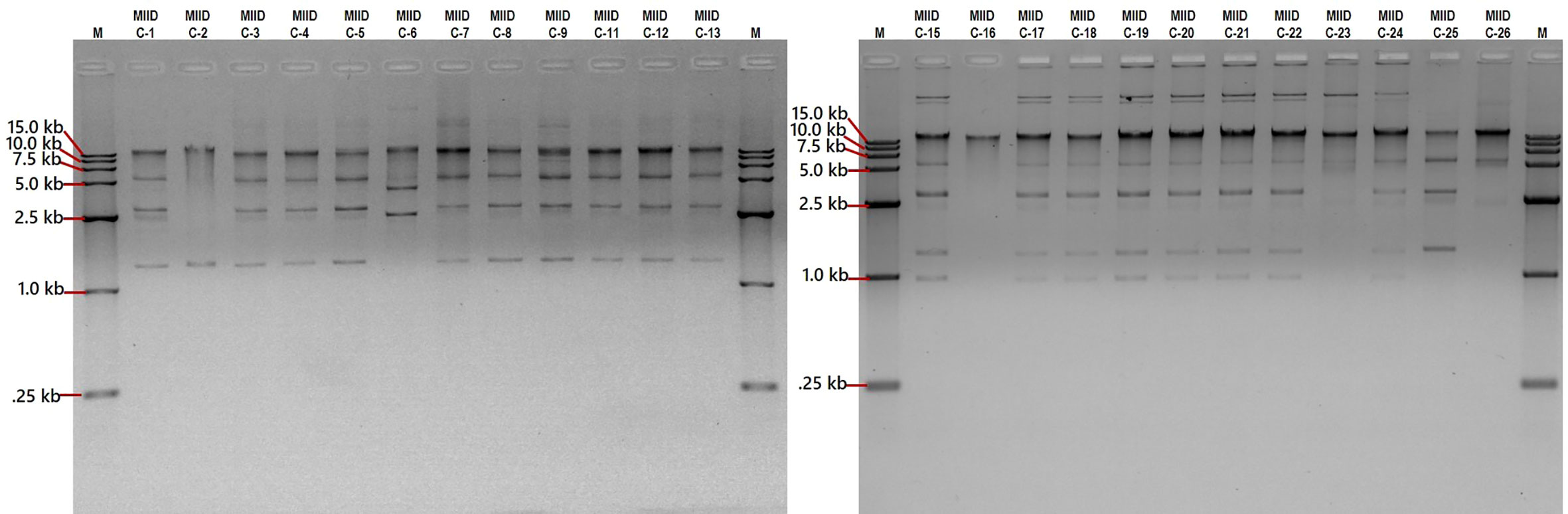
Figure 2 Separation of plasmid DNA molecular weight on 0.9% agarose gel stained with ethidium bromide. Lanes M, DNA marker 15.0 kb (cat. no. 239135), lanes MIID-C1 to MIID-26 CRKP clinical isolates carrying different sizes of plasmids.
Genetic environment of the blaNDM
In all 23 NDM-1 expressing CRKP isolates, the bleomycin resistance gene (bleMBL) was identified downstream of the blaNDM-1 gene by PCR-based genetic environment study of the blaNDM gene. The complete ISAba125 sequence was found upstream of blaNDM-1 in twenty isolates (MIID-C1, MIID-C3, MIID-C4, MIID-C5, MIID-C6, MIID-C7, MIID-C8, MIID-C9, MIID-C12, MIID-C13, MIID-C15, MIID-C16, MIID-C17, MIID-C18, MIID-C20, MIID-C21, MIID-C22, MIID-C23, MIID-C25 and MIID-C26). Furthermore, three blaNDM-1 carrying Klebsiella pneumoniae isolates (MIID-C2, MIID-C19 and MIID-C24) had truncated ISAba125, upstream of blaNDM-1 as shown in Table 2 and Figure 3.
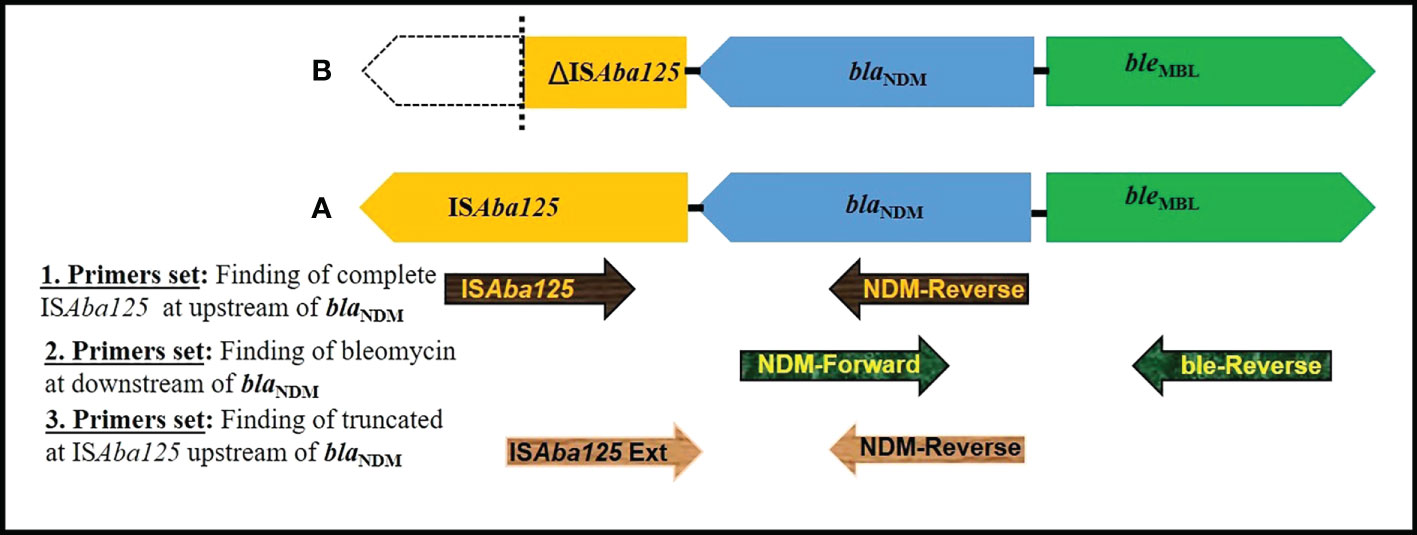
Figure 3 Schematic representation of genetic elements surrounding blaNDM. (A) In MIID-C1, MIID-C3, MIID-C4, MIID-C5, MIID-C6, MIID-C7, MIID-C8, MIID-C9, MIID-C12, MIID-C13, MIID-C15, MIID-C16, MIID-C17, MIID-C18, MIID-C20, MIID-C21, MIID-C22, MIID-C23, MIID-C25 and MIID-C26 complete element of ISAba125 at upstream and bleomycin gene at downstream to blaNDM was found. (B) (MIID-C2, MIID-C19 and MIID-C24, truncated ISAba125 at upstream and bleomycin gene downstream to blaNDM was found. Arrow indicates the position of primer (used of primers as mentioned in Supplementary Table S1).
Integron analysis
All CRKP clinical isolates carry class 1 integron except three isolates (MIID-C11, MIID-C16 and MIID-C17). The class 2 and class 3 integrons were not found in any CRKP isolates as shown in Table 2.
Discussion
The multidrug-resistant strain of K. pneumoniae, a common nosocomial pathogen that regularly causes difficult-to-treat infections globally, is a serious public health concern (Cao et al., 2014). Hospital patients experience higher rates of morbidity and mortality because of rapid CRKP spread, which is the primary cause of treatment failure (Al-Zahrani and Alsiri, 2018). CRKP has also been reported in most of the Gulf Cooperation Council countries (Zowawi et al., 2013). The results of the current CRKP isolates are worrying. All the isolates were multidrug resistant and molecular analysis revealed double or triple carbapenemase gene combinations (NDM-1, OXA-48, OXA-51, OXA-23) with co-existence of qnrS gene. All these isolates carried one to seven number of plasmids varying in size from 1.0 to 18.1 kb. The genetic environment of the NDM-1 carrying isolates revealed majority with downstream of bleMBL while three isolates had truncated ISAba125, upstream of blaNDM-1.
These isolates were highly resistant to most of the commonly used antibiotics including ceftolozane/tazobactam but least resistance to ceftazidime/avibactam and tigecycline. In the United Arab Emirates, ceftolozane-tazobactam and ceftazidime-avibactam were reported as effective alternatives for treating bacteria that produce ESBL and carbapenemase enzymes (Alatoom et al., 2017). Despite being effective against many gram-negative infections, ceftolozane/tazobactam does not show clinically significant potency against Enterobacterales that produce carbapenemase or are carbapenem-resistant (Sutherland and Nicolau, 2015). Accordingly, all the isolates in our study were resistant to ceftolozane/tazobactam. This was in agreement with Alatoom et al. from UAE where their isolates positive for both NDM-1 and OXA-48 showed 100% resistance to ceftolozane/tazobactam (Alatoom et al., 2017). Similarly, Sader et al. also found that all CRKPs were ceftolozane/tazobactam resistant (Sader et al., 2021). Another study from China also reported high resistance of CRKP to ceftolozane/tazobactam (98.1%) (Yin et al., 2019). Contrary to the current study, a study from the United States (Sutherland and Nicolau, 2017) reported 94% ceftolozane/tazobactam susceptibility which was comparable to the 93% previously reported by Farrell and colleagues (Farrell et al., 2014).
Avibactam is a broad-spectrum non-β-lactam β-lactamase inhibitor that inhibits a variety of serine β-lactamases (Bush, 2015). The addition of avibactam to ceftazidime improves ceftazidime effectiveness against common gram-negative bacteria, including the majority of those that produce β-lactamase enzymes and are resistant to carbapenem drugs. In the present study ceftazidime-avibactam had better activity against all the CRKP isolates whereas a study from UAE reported 45% susceptibility to ceftazidime-avibactam (Alatoom et al., 2017). Higher susceptibility rates were also reported by Flamm et al. (Flamm et al., 2014) and Lagace-Wiens et al. (Lagacé-Wiens et al., 2014). In a different trial, Mutter et al. observed good activity of ceftazidime/avibactam against carbapenem-resistant Enterobacterales without carbapenemase synthesis. Ceftazidime-avibactam is also reported to exhibit strong in vitro action against Enterobacterales that produce KPC enzymes and may be potent additions to the arsenal of antimicrobial drugs already in use (Kazmierczak et al., 2016). Hence this drug combination can be used as a valuable option for empirical therapy. This also further underscores the requirement for regional studies to assess the impact of novel medications on regional MDR isolates because the prevalence and types of carbapenemase vary among geographical locations (Alatoom et al., 2017).
Since tigecycline and colistin are the drugs of last resort used to treat CRKP infections, the emergence of CRKP strains that also exhibit resistance to these drugs has become a significant clinical challenge (Zhang et al., 2018). Globally, incidences of colistin-resistant CRKP have been recorded as colistin use has increased. A therapeutic issue brought on by the advent of colistin-resistant in CRKP poses a risk of sending patients and physicians back to the “pre-antibiotic period” (Rojas et al., 2017). The isolates tested for colistin and tigecycline resistance in this study demonstrated that 75% were resistant to colistin and 44% showed intermediate susceptibility to tigecycline. While Saeed et al. from Bahrain found that 0.06% of CRE isolates had combination resistance to both colistin and tigecycline (Saeed et al., 2019). A study from Saudi Arabia reported 43.4% colistin resistance among ICU isolates (Al Mayahi et al., 2019). An article by Paris et al. mentions the presence of 590 colistin-resistant K. pneumoniae isolates from six Middle East countries, including Saudi Arabia (24), Kuwait (5), United Arab Emirates (31), Iran (86), Turkey (438), Lebanon (3), and Israel (3) between 2013 and 2018, none reported from Bahrain (Aris et al., 2020). In a multicenter observational cohort of hospitalized patients with CRKP in US hospitals, Rojas et al. noted a 13% colistin resistance rate (Rojas et al., 2017). Colistin-resistant CRKP was the subject of a sustained outbreak in Brazil, according to a study, in which 83.9% were from the same cluster and 67.6% hadn’t used polymyxin, indicating the likelihood of cross-transmission of colistin-resistant CRKP isolates (Rocha et al., 2022). According to Sharma et al., all K. pneumoniae from NICU and 94.4% from ICU were colistin-resistant CRKP (Sharma et al., 2022). K. pneumoniae clinical isolates in the SENTRY Antimicrobial Surveillance Program in 2014 and 2015 showed 4.4% resistant to colistin (Castanheira et al., 2016). Although colistin is effective in treating infections brought on by CRKP, colistin resistance is known to be induced during colistin treatment and can be brought on by mutations and genetic changes in chromosomal genes (Berglund, 2019).
In Africa-Middle East countries, K. pneumoniae is reported to show in vitro tigecycline susceptibility rates of 96.8% (Renteria et al., 2014). The present study showed 44% intermediate susceptibility to tigecycline whereas a study in Lebanon reported 3% tigecycline-resistant and 16% intermediate findings in K. pneumoniae (Araj and Ibrahim, 2008), and research by Park et al, found that CRKP isolates exhibited a 37.8% tigecycline resistance rate (Park et al., 2020). Another study from Egypt stated 10.9% and 36.1% of CRKP isolates were colistin- and tigecycline-resistant, respectively (Gandor et al., 2022). In South Korea, it was revealed that CRKP had a 14.5% tigecycline resistance rate (Jeong et al., 2016). In the United States, multicenter research found that CRKP isolates had an 18.0% tigecycline resistance rate.
The class B β-lactamase NDM-1 has recently raised significant concerns. Additionally, numerous studies have documented the discovery of enterobacterial isolates that produce NDM-1 in various regions of the world (Poirel et al., 2011a). Our study isolates 95.8% (n=23) also were NDM-1 producers along with other carbapenemases (OXA-23, OXA-48, and OXA-51). None of our isolates carried KPC gene. Whereas a study from Saudi Arabia reported 80.9% of isolates with triple resistance genes KPC/NDM-1/OXA-48 while 19.04% carried double resistance genes (KPC/OXA-48) or (NDM-1/OXA-48) (Khan et al., 2019). A study in Turkey reported 38.9% and 81.05% of CRKP isolates as NDM-1 and OXA-48 producers (Genç et al., 2022). Another study from Tehran found 11.5% of isolates as NDM producers along with other beta-lactamases (Sharahi et al., 2021). According to a study from Egypt, 56.2%, and 41.0% of the CRKP isolates showed the presence of blaNDM, and blaOXA-48 respectively.
Beyond sporadic isolates seen in the USA, Denmark, and India, double carbapenemase producers, particularly OXA and NDM co-producing K. pneumoniae, are on the rise (Balm et al., 2013; Doi et al., 2014). Additionally, K. pneumoniae isolates from Saudi hospitals and numerous other countries in the Arabian Peninsula have both been found to be OXA-48 and NDM positive (Jamal et al., 2016; Al-Agamy et al., 2018).This study also found the co-existence (87.5% (n=21) of blaNDM-1 and blaOXA-48 among CRKP isolates. According to a study from the Arabian Peninsula, UAE had 8.9% of CRE isolates that were NDM-1 and OXA-48 co-producers, and other nations in the region reported 1.9% in the Kingdom of Saudi Arabia, 1.6% in Kuwait, and 5.4% in Oman respectively (Sonnevend et al., 2015) which is comparatively low as compared to the present study. A study from Morocco also reported 34% of K. pneumoniae as NDM-1 and OXA-48 co-producers (Loqman et al., 2021). Even though three OXA types viz. OXA-23, OXA-48, and OXA-51 were found in our cohort, none of the isolates had simultaneous presence of all three OXA types. However, two OXA types were present together (OXA-23 + OXA-48 & OXA-48 + OXA-51), the more common combination was (OXA-48 + OXA-51, 45.8%).
Among the study isolates, 45.8% and 41.6% were positive for OXA-51, and OXA-23 respectively which is very high as compared to El-Badawy et al. from Egypt, where they noticed 5.3% and 10.5% of the CRKP were capable of producing OXA-23 and OXA-51 like β-lactamases (El-Badawy et al., 2020). In contrast, OXA-23-like and OXA-51-like enzymes were not found in CRKP isolates by Cetinkol et al. (Cetinkol et al., 2016). There is a dearth of information in the literature about NDM-1 and OXA-51, or OXA-23 co-producers in K. pneumoniae.
Carbapenems and fluoroquinolones are routinely used to treat severe infections caused by MDR K. pneumoniae. The limited treatment options for these strains are caused by the co-existence of plasmid-mediated quinolone resistance (PMQR) determinants and carbapenemases (El-Feky et al., 2017). In the present study, carbapenemases and PMQR co-existed in 100% of our isolates. Whereas a study from Saudi Arabia reported 57% of isolates with co-existence of carbapenemases and qnrS genes (Al-Agamy et al., 2018). However, a study from Egypt reported co-existence of carbapenemases and PMQR genes in 79% of their isolates (El-Feky et al., 2017). A study from China reported that 100% of their carbapenemase-producing K. pneumoniae harbored PMQR genes wherein qnrS was present in 56% of the isolates (Liu et al., 2014). As commonly observed for carbapenemase producers, all the study strains showed multidrug resistance. All NDM and OXA carbapenemases were highly associated with other resistance genes such as qnrS and various mobile elements including class 1 integron, which is comparable with the findings of Liu et al. (Liu et al., 2014) and Huang et al. (Huang et al., 2012).
The transferrable nature of antibiotic resistance is its most worrisome aspect. Multiple mechanisms exist for how plasmids can influence antibiotic resistance (Dong et al., 2018). In this study, we found that majority of the isolates carried five plasmids and every isolate has plasmids ranging in molecular weight from 1 to 18.1 kb. Accordingly, research by Dong et al. found that each isolate included five plasmids, and each measuring 178,177.5, 99.7, 11.9, and 5.6 kb in size (Dong et al., 2018). Another study from the Neonatal intensive care unit of an Indian hospital reported that the 66 kb, 38 kb, and 6 kb plasmid sizes were identified in carbapenem-resistant K. pneumoniae clinical isolates (Orole and Hadi, 2020). The presence of many plasmids in these isolates may serve as a potential source for the spread of highly resistant genes to other bacteria and humans, which poses a risk to the commonly used antibiotics to treat infections.
Detection of class 1, 2, and 3 integrons in CRKP isolates in this study showed the majority carrying class 1 integron. The significant association between the presence of class 1 integrons and the occurrence of MDR among Klebsiella pneumoniae were previously reported (Li et al., 2013; Malek et al., 2015). Additionally, the fact that the majority of the isolates had the entire ISAba125 sequence upstream of blaNDM-1 (Figure 3) suggests that this element may be crucial in the horizontal gene transfer of the blaNDM among members of the Enterobacteriaceae family of bacteria (Poirel et al., 2011b). Moreover, in the current study bleMBL was found at its downstream in all blaNDM-1 producing CRKP isolates. The high rate of association of the bleMBL and blaNDM-1 genes suggests that they might have mobilized together from a common progenitor, which many thought to protect blaNDM (Dortet et al., 2012). These results conform with previous reports that clarified the horizontal transfer of plasmids in CRKP isolates (Dortet et al., 2012).
A few limitations apply to this study. The size of the study isolates included was relatively small and this may not be an accurate representation of all CRKP isolates at SMC, Bahrain. Hence a longer-term, further-extended multi-center study is required. In addition, the analysis of resistance determinants by molecular methods was limited to CRKP isolates and did not include the details of ESBL and other antibiotic resistance mechanisms. However, to the best of the authors’ knowledge, this is the first report thoroughly describing the susceptibility profiles, antibiotic-resistant genes and the genetic environment of CRKP isolates in this region.
Conclusion
Despite the small sample size, the results are alarming. The resistance rate to most antibiotics is very high in our region, including colistin and tigecycline, and the genetic environment of CRKP is complex with the carriage of multiple resistance markers and multiple plasmids. However, without active surveillance, it is impossible to be convinced that these patterns indicate widespread persistence. Resistance to ceftazidime/avibactam is uncommon and hence can be used as a valuable option for empirical therapy. Molecular data on resistance markers and the genetic environment of CRKP is lacking from this geographical region; this would be the first report addressing the subject matter. Our study emphasizes the significance of surveillance programs and strict infection control strategies backed by potent molecular epidemiological tools in clinical settings to curb the emergence and spread of such isolates.
Data availability statement
The datasets presented in this study can be found in online repositories. The names of the repository/repositories and accession number(s) can be found below: https://www.ncbi.nlm.nih.gov/genbank/, GenBank nucleotide database: ON506904, ON506905, ON506906, ON506907, ON506908, ON506909, ON506910, ON506911, ON506912, ON506913, ON506914, ON506915, ON506916, ON506917, ON506918, ON493161, ON493162, ON493163, ON755345 and ON755346.
Author contributions
MS conceived the project proposal. NS provided clinical CRKP isolates, identified them and performed antibiotic susceptibility on automated systems. NA, MHS and AAM performed further experiments including molecular experimentation. MS, FD, NS, KB, and KT evaluated the data and provided expertise and feedback. NA, RJ and MS wrote the preliminary draft of the manuscript. All authors contributed to the article and approved the submitted version.
Funding
Internal funding for the research grant (G06/AGU-11/19) from the Arabian Gulf University.
Acknowledgments
All authors acknowledge and thank AGU for providing research grant. Dr. Nayeem Ahmad acknowledge and gratefully thank AGU for providing Postdoc Research Fellowship. The authors also acknowledge Genoscreen Lab, France for providing sequence facility support. The authors would like to thank Dr. Amer Almarabheh, PhD, for assistance with statistical analysis. The part of this work was presented as poster presentation at the 70th Annual Conference of the Canadian Society of Microbiologists, University of Guelph, Guelph, Ontario, Canada (June 20- 23, 2022).
Conflict of interest
The authors declare that the research was conducted in the absence of any commercial or financial relationships that could be construed as a potential conflict of interest.
Publisher’s note
All claims expressed in this article are solely those of the authors and do not necessarily represent those of their affiliated organizations, or those of the publisher, the editors and the reviewers. Any product that may be evaluated in this article, or claim that may be made by its manufacturer, is not guaranteed or endorsed by the publisher.
Supplementary material
The Supplementary Material for this article can be found online at: https://www.frontiersin.org/articles/10.3389/fcimb.2022.1033305/full#supplementary-material
References
Ahmad, N., Khalid, S., Ali, S. M., Khan, A. U. (2018). Occurrence of blaNDM variants among enterobacteriaceae from a neonatal intensive care unit in a northern India hospital. Front. Microbiol. 9. doi: 10.3389/fmicb.2018.00407
Al-Agamy, M. H., Aljallal, A., Radwan, H. H., Shibl, A. M. (2018). Characterization of carbapenemases, ESBLs, and plasmid-mediated quinolone determinants in carbapenem-insensitive escherichia coli and klebsiella pneumoniae in Riyadh hospitals. J. Infect. Public Health 11 (1), 64–68. doi: 10.1016/j.jiph.2017.03.010
Alatoom, A., Elsayed, H., Lawlor, K., AbdelWareth, L., El-Lababidi, R., Cardona, L., et al. (2017). Comparison of antimicrobial activity between ceftolozane-tazobactam and ceftazidime-avibactam against multidrug-resistant isolates of escherichia coli, klebsiella pneumoniae, and pseudomonas aeruginosa. Int. J. Infect. Dis. 62, 39–43. doi: 10.1016/j.ijid.2017.06.007
Al Mayahi, Z., Kamel, S., Amer, H., Beatty, M. (2019). Outbreak of colistin-resistant organisms at a tertiary hospital in Riyadh, Saudi arabi. Pan. Afr. Med. J. 34, 162. doi: 10.11604/pamj.2019.34.162.19998
Al-Zahrani, I. A., Alsiri, B. A. (2018). The emergence of carbapenem-resistant klebsiella pneumoniae isolates producing OXA-48 and NDM in the southern (Asir) province, Saudi Arabia. Saudi Med. J. 39 (1), 23–30. doi: 10.15537/smj.2018.1.21094
Araj, G. F., Ibrahim, G. Y. (2008). Tigecycline in vitro activity against commonly encountered multidrug-resistant gram-negative pathogens in a middle Eastern country. Diagn. Microbiol. Infect. Dis. 62 (4), 411–415. doi: 10.1016/j.diagmicrobio.2008.08.011
Aris, P., Robatjazi, S., Nikkhahi, F., Amin Marashi, S. M. (2020). Molecular mechanisms and prevalence of colistin resistance of klebsiella pneumoniae in the middle East region: A review over the last 5 years. J. Glob. Antimicrob. Resist. 22, 625–630. doi: 10.1016/j.jgar.2020.06.009
(2019). Available at: https://www.ecdc.europa.eu/en/publications-data/outbreak-Klebsiella-pneumoniae-Germany (Accessed 14/9/2022).
Balm, M. N., La, M. V., Krishnan, P., Jureen, R., Lin, R. T., Teo, J. W. (2013). Emergence of klebsiella pneumoniae co-producing NDM-type and OXA-181 carbapenemases. Clin. Microbiol. Infect. 19 (9), E421–E423. doi: 10.1111/1469-0691.12247
Band, V. I., Satola, S. W., Burd, E. M., Farley, M. M., Jacob, J. T., Weiss, D. S., et al. (2018). Carbapenem-resistant klebsiella pneumoniae exhibiting clinically undetected colistin heteroresistance leads to treatment failure in a murine model of infection. mBio 9 (2), e02448–e02417. doi: 10.1128/mBio.02448-17
Berglund, B. (2019). Acquired resistance to colistin via chromosomal and plasmid-mediated mechanisms in klebsiella pneumoniae. Infect. Microbes Dis. 1 (1), 10–19. doi: 10.1097/IM9.0000000000000002
Bush, K. (2015). A resurgence of β-lactamase inhibitor combinations effective against multidrug-resistant gram-negative pathogens. Int. J. Antimicrob. Agents 46 (5), 483–493. doi: 10.1016/j.ijantimicag.2015.08.011
Bush, K., Bradford, P. A. (2020). Epidemiology of beta-Lactamase-Producing pathogens. Clin. Microbiol. Rev. 33 (2), e00047–e00019. doi: 10.1128/CMR.00047-19
Cao, X., Xu, X., Zhang, Z., Shen, H., Chen, J., Zhang, K. (2014). Molecular characterization of clinical multidrug-resistant klebsiella pneumoniae isolates. Ann. Clin. Microbiol. Antimicrob. 13, 16–16. doi: 10.1186/1476-0711-13-16
Carlet, J., Jarlier, V., Harbarth, S., Voss, A., Goossens, H., Pittet, D., et al. (2012). Ready for a world without antibiotics? the pensieres antibiotic resistance call to action. Antimicrob. Resist. Infect. Control 1 (1), 11. doi: 10.1186/2047-2994-1-11
Castanheira, M., Griffin, M. A., Deshpande, L. M., Mendes, R. E., Jones, R. N., Flamm, R. K. (2016). Detection of mcr-1 among escherichia coli clinical isolates collected worldwide as part of the SENTRY antimicrobial surveillance program in 2014 and 2015. Antimicrob. Agents Chemother. 60 (9), 5623–5624. doi: 10.1128/aac.01267-16
Cetinkol, Y., Yildirim, A. A., Telli, M., Calgin, M. K. (2016). The investigation of oxacillinase/metallo-beta-lactamase genes and clonal analysis in carbapenem-resistant klebsiella pneumoniae. Infez Med. 24 (1), 48–53.
Choudhury, S., Medina-Lara, A., Smith, R. (2022). Antimicrobial resistance and the COVID-19 pandemic. Bull. World Health Organ 100 (5), 295–295a. doi: 10.2471/blt.21.287752
Doi, Y., Hazen, T. H., Boitano, M., Tsai, Y. C., Clark, T. A., Korlach, J., et al. (2014). Whole-genome assembly of klebsiella pneumoniae coproducing NDM-1 and OXA-232 carbapenemases using single-molecule, real-time sequencing. Antimicrob. Agents Chemother. 58 (10), 5947–5953. doi: 10.1128/aac.03180-14
Dong, N., Yang, X., Zhang, R., Chan, E. W., Chen, S. (2018). Tracking microevolution events among ST11 carbapenemase-producing hypervirulent klebsiella pneumoniae outbreak strains. Emerg. Microbes Infect. 7 (1), 146. doi: 10.1038/s41426-018-0146-6
Dortet, L., Nordmann, P., Poirel, L. (2012). Association of the emerging carbapenemase NDM-1 with a bleomycin resistance protein in enterobacteriaceae and acinetobacter baumannii. Antimicrob. Agents Chemother. 56 (4), 1693–1697. doi: 10.1128/aac.05583-11
El-Badawy, M. F., El-Far, S. W., Althobaiti, S. S., Abou-Elazm, F. I., Shohayeb, M. M. (2020). The first Egyptian report showing the Co-existence of bla (NDM-25), bla (OXA-23), bla (OXA-181), and bla (GES-1) among carbapenem-resistant k. pneumoniae clinical isolates genotyped by BOX-PCR. Infect. Drug Resist. 13, 1237–1250. doi: 10.2147/idr.s244064
El-Feky, M., Hammad, H., Hadiya, S. (2017). Co-Occurrence of plasmid-mediated quinolone resistance and carbapenemases in klebsiella pneumoniae isolates in assiut, Egypt. Egypt J. Med. Microbiol. 26, 1–7. doi: 10.12816/0046244
Elshamy, A. A., Aboshanab, K. M. (2020). A review on bacterial resistance to carbapenems: epidemiology, detection and treatment options. Future Sci. OA. Stockholm (Sweden): ECDC. 6 (3), FSO438. doi: 10.2144/fsoa-2019-0098
European Centre for Disease Prevention and Control (2019). Outbreak of carbapenemase-producing (NDM-1 and OXA-48) and colistin-resistant klebsiella pneumoniae ST307, north-east Germany 2019. Stockholm, Sweden: ECDC.
Farrell, D. J., Sader, H. S., Flamm, R. K., Jones, R. N. (2014). Ceftolozane/tazobactam activity tested against gram-negative bacterial isolates from hospitalised patients with pneumonia in US and European medical centres, (2012). Int. J. Antimicrob. Agents 43 (6), 533–539. doi: 10.1016/j.ijantimicag.2014.01.032
Flamm, R. K., Farrell, D. J., Sader, H. S., Jones, R. N. (2014). Ceftazidime/avibactam activity tested against gram-negative bacteria isolated from bloodstream, pneumonia, intra-abdominal and urinary tract infections in US medical centres, (2012). J. Antimicrob. Chemother. 69 (6), 1589–1598. doi: 10.1093/jac/dku025
Friedman, N. D., Temkin, E., Carmeli, Y. (2016). The negative impact of antibiotic resistance. Clin. Microbiol. Infect. 22 (5), 416–422. doi: 10.1016/j.cmi.2015.12.002
Gandor, N. H. M., Amr, G. E., Eldin Algammal, S. M. S., Ahmed, A. A. (2022). Characterization of carbapenem-resistant k. pneumoniae isolated from intensive care units of zagazig university hospitals. Antibiotics (Basel) 11 (8), 1108. doi: 10.3390/antibiotics11081108
Genç, S., Kolaylı, F., Özçelik, E. Y. (2022). Molecular characterization of carbapenemase producing klebsiella pneumoniae strains by multiplex PCR and PFGE methods: The first k.pneumoniae isolates co-producing OXA-48/KPC and KPC/NDM in Turkey. J. Infect. Chemother. 28 (2), 192–198. doi: 10.1016/j.jiac.2021.10.009
Huang, S., Dai, W., Sun, S., Zhang, X., Zhang, L. (2012). Prevalence of plasmid-mediated quinolone resistance and aminoglycoside resistance determinants among carbapeneme non-susceptible enterobacter cloacae. PloS One 7 (10), e47636. doi: 10.1371/journal.pone.0047636
Jamal, W. Y., Albert, M. J., Rotimi, V. O. (2016). High prevalence of new Delhi metallo-β-Lactamase-1 (NDM-1) producers among carbapenem-resistant enterobacteriaceae in Kuwait. PloS One 11 (3), e0152638. doi: 10.1371/journal.pone.0152638
Jeong, S. H., Kim, H.-S., Kim, J.-S., Shin, D. H., Kim, H. S., Park, M.-J., et al. (2016). Prevalence and molecular characteristics of carbapenemase-producing enterobacteriaceae from five hospitals in Korea. Ann. Lab. Med. 36 (6), 529. doi: 10.3343/alm.2016.36.6.529
Kazmierczak, K. M., Biedenbach, D. J., Hackel, M., Rabine, S., de Jonge, B. L., Bouchillon, S. K., et al. (2016). Global dissemination of blaKPC into bacterial species beyond klebsiella pneumoniae and In vitro susceptibility to ceftazidime-avibactam and aztreonam-avibactam. Antimicrob. Agents Chemother. 60 (8), 4490–4500. doi: 10.1128/aac.00107-16
Khan, F., Chaudhary, B., AU, K. (2022). “Class d type beta-lactamases," in beta-lactam resistance,” in Gram-negative bacteria: Threats and challenges. Eds. Shahid, M., Singh, A., Sami, H. (Springer Nature Singapore) 126–140. doi: 10.1007/978-981-16-9097-6
Khan, A. U., Maryam, L., Zarrilli, R. (2017). Structure, genetics and worldwide spread of new Delhi metallo-β-lactamase (NDM): a threat to public health. BMC Microbiol. 17 (1), 1–12. doi: 10.1186/s12866-017-1012-8
Khan, M. A., Mohamed, A. M., Faiz, A., Ahmad, J. (2019). Enterobacterial infection in Saudi Arabia: First record of klebsiella pneumoniae with triple carbapenemase genes resistance. J. Infect. Dev. Ctries 13 (4), 334–341. doi: 10.3855/jidc.11056
Lagacé-Wiens, P., Walkty, A., Karlowsky, J. A. (2014). Ceftazidime–avibactam: an evidence-based review of its pharmacology and potential use in the treatment of gram-negative bacterial infections. Core Evid. 9, 13. doi: 10.2147/CE.S40698
Li, B., Hu, Y., Wang, Q., Yi, Y., Woo, P. C., Jing, H., et al. (2013). Structural diversity of class 1 integrons and their associated gene cassettes in klebsiella pneumoniae isolates from a hospital in China. PloS One 8 (9), e75805. doi: 10.1371/journal.pone.0075805
Liu, Y., Wan, L. G., Deng, Q., Cao, X. W., Yu, Y., Xu, Q. F. (2014). First description of NDM-1-, KPC-2-, VIM-2- and IMP-4-producing klebsiella pneumoniae strains in a single Chinese teaching hospital. Epidemiol. Infect. 143 (2), 376–384. doi: 10.1017/S0950268814000995
Logan, L. K., Weinstein, R. A. (2017). The epidemiology of carbapenem-resistant enterobacteriaceae: The impact and evolution of a global menace. J. Infect. Dis. 215 (suppl_1), S28–s36. doi: 10.1093/infdis/jiw282
Lombardi, F., Gaia, P., Valaperta, R., Cornetta, M., Tejada, M. R., Di Girolamo, L., et al. (2015). Emergence of carbapenem-resistant klebsiella pneumoniae: Progressive spread and four-year period of observation in a cardiac surgery division. BioMed. Res. Int. 2015, 871947. doi: 10.1155/2015/871947
Loqman, S., Soraa, N., Diene, S. M., Rolain, J. M. (2021). Dissemination of carbapenemases (OXA-48, NDM and VIM) producing enterobacteriaceae isolated from the Mohamed VI university hospital in marrakech, Morocco. Antibiotics (Basel) 10 (5), 492 . doi: 10.3390/antibiotics10050492
Mairi, A., Pantel, A., Sotto, A., Lavigne, J.-P., Touati, A. (2018). OXA-48-like carbapenemases producing enterobacteriaceae in different niches. Eur. J. Clin. Microbiol. Infect. Dis. 37 (4), 587–604. doi: 10.1007/s10096-017-3112-7
Malek, M. M., Amer, F. A., Allam, A. A., El-Sokkary, R. H., Gheith, T., Arafa, M. A. (2015). Occurrence of classes I and II integrons in enterobacteriaceae collected from zagazig university hospitals, Egypt. Front. Microbiol. 6. doi: 10.3389/fmicb.2015.00601
Orole, O. O., Hadi, N. (2020). Characterization and plasmid profile of resistant klebsiella pneumoniae isolates in patients with urinary tract infection in nasarawa state, Nigeria. Int. J. Appl. Sci. Biotechnol. 8 (1), 21–28. doi: 10.3126/ijasbt.v8i1.28252
Park, Y., Choi, Q., Kwon, G. C., Koo, S. H. (2020). Molecular epidemiology and mechanisms of tigecycline resistance in carbapenem-resistant klebsiella pneumoniae isolates. J. Clin. Lab. Anal. 34 (12), e23506. doi: 10.1002/jcla.23506
Pfeifer, Y., Schlatterer, K., Engelmann, E., Schiller, R. A., Frangenberg, H. R., Stiewe, D., et al. (2012). Emergence of OXA-48-type carbapenemase-producing enterobacteriaceae in German hospitals. Antimicrob. Agents Chemother. 56 (4), 2125–2128. doi: 10.1128/AAC.05315-11
Pitout, J. D., Nordmann, P., Poirel, L. (2015). Carbapenemase-producing klebsiella pneumoniae, a key pathogen set for global nosocomial dominance. Antimicrob. Agents Chemother. 59 (10), 5873–5884. doi: 10.1128/AAC.01019-15
Pitout, J. D., Peirano, G., Kock, M. M., Strydom, K.-A., Matsumura, Y. (2019). The global ascendency of OXA-48-type carbapenemases. Clin. Microbiol. Rev. 33 (1), e00102–e00119. doi: 10.1128/CMR.00102-19
Poirel, L., Benouda, A., Hays, C., Nordmann, P. (2011a). Emergence of NDM-1-producing klebsiella pneumoniae in Morocco. J. Antimicrob. Chemother. 66 (12), 2781–2783. doi: 10.1093/jac/dkr384
Poirel, L., Dortet, L., Bernabeu, S., Nordmann, P. (2011b). Genetic features of blaNDM-1-positive enterobacteriaceae. Antimicrob. Agents Chemother. 55 (11), 5403–5407. doi: 10.1128/aac.00585-11
Renteria, M., Biedenbach, D., Bouchillon, S., Hoban, D., Raghubir, N., Sajben, P. (2014). In vitro activity of tigecycline and comparators against carbapenem-resistant enterobacteriaceae in Africa–middle East countries: TEST 2007–2012. J. Glob. Antimicrob. Resist. 2 (3), 179–182. doi: 10.1016/j.jgar.2014.03.002
Rocha, V. F. D., Barbosa, M. S., Leal, H. F., Silva, G. E. O., Sales, N. M. M. D., Monteiro, A., et al. (2022). Prolonged outbreak of carbapenem and colistin-resistant klebsiella pneumoniae at a Large tertiary hospital in Brazil. Front. Microbiol. 13. doi: 10.3389/fmicb.2022.831770
Rojas, L. J., Salim, M., Cober, E., Richter, S. S., Perez, F., Salata, R. A., et al. (2017). Colistin resistance in carbapenem-resistant klebsiella pneumoniae: Laboratory detection and impact on mortality. Clin. Infect. Dis. an Off. Publ. Infect. Dis. Soc. America 64 (6), 711–718. doi: 10.1093/cid/ciw805
Sader, H. S., Carvalhaes, C. G., Streit, J. M., Doyle, T. B., Castanheira, M. (2021). Antimicrobial activity of ceftazidime-avibactam, ceftolozane-tazobactam and comparators tested against pseudomonas aeruginosa and klebsiella pneumoniae isolates from united states medical centers in 2016-2018. Microb. Drug Resist. 27 (3), 342–349. doi: 10.1089/mdr.2020.0217
Saeed, N. K., Alkhawaja, S., Azam, N., Alaradi, K., Al-Biltagi, M. (2019). Epidemiology of carbapenem-resistant enterobacteriaceae in a tertiary care center in the kingdom of Bahrain. J. Lab. Physicians 11 (2), 111–117. doi: 10.4103/jlp.jlp_101_18
Sharahi, J. Y., Hashemi, A., Ardebili, A., Davoudabadi, S. (2021). Molecular characteristics of antibiotic-resistant escherichia coli and klebsiella pneumoniae strains isolated from hospitalized patients in Tehran, Iran. Ann. Clin. Microbiol. Antimicrob. 20 (1), 32. doi: 10.1186/s12941-021-00437-8
Sharma, S., Banerjee, T., Kumar, A., Yadav, G., Basu, S. (2022). Extensive outbreak of colistin resistant, carbapenemase (blaOXA-48, blaNDM) producing klebsiella pneumoniae in a large tertiary care hospital, India. Antimicrob. Resist. Infect. Control 11 (1), 1. doi: 10.1186/s13756-021-01048-w
Shibl, A., Al-Agamy, M., Memish, Z., Senok, A., Khader, S. A., Assiri, A. (2013). The emergence of OXA-48- and NDM-1-positive klebsiella pneumoniae in Riyadh, Saudi Arabia. Int. J. Infect. Dis. 17 (12), e1130–e1133. doi: 10.1016/j.ijid.2013.06.016
Sonnevend, Á., Ghazawi, A. A., Hashmey, R., Jamal, W., Rotimi, V. O., Shibl, A. M., et al. (2015). Characterization of carbapenem-resistant enterobacteriaceae with high rate of autochthonous transmission in the Arabian peninsula. PloS One 10 (6), e0131372. doi: 10.1371/journal.pone.0131372
Sutherland, C. A., Nicolau, D. P. (2015). Susceptibility profile of Ceftolozane/Tazobactam and other parenteral antimicrobials against escherichia coli, klebsiella pneumoniae, and pseudomonas aeruginosa from US hospitals. Clin. Ther. 37 (7), 1564–1571. doi: 10.1016/j.clinthera.2015.05.501
Sutherland, C. A., Nicolau, D. P. (2017). Potency of parenteral antimicrobials including ceftolozane/tazobactam against nosocomial respiratory tract pathogens: considerations for empiric and directed therapy. J. Thorac. Dis. 9 (1), 214–221. doi: 10.21037/jtd.2017.01.26
Tanne, J. H. (2022). Covid-19: Antimicrobial resistance rose dangerously in US during pandemic, CDC says. BMJ 378, o1755. doi: 10.1136/bmj.o1755
van Duin, D., Doi, Y. (2017). The global epidemiology of carbapenemase-producing enterobacteriaceae. Virulence 8 (4), 460–469. doi: 10.1080/21505594.2016.1222343
Yin, D., Wu, S., Yang, Y., Shi, Q., Dong, D., Zhu, D., et al. (2019). Results from the China antimicrobial surveillance network (CHINET) in 2017 of the In vitro activities of ceftazidime-avibactam and ceftolozane-tazobactam against clinical isolates of enterobacteriaceae and pseudomonas aeruginosa. Antimicrob. Agents Chemother. 63 (4), e02431-18. doi: 10.1128/aac.02431-18
Yong, D., Toleman, M. A., Giske, C. G., Cho, H. S., Sundman, K., Lee, K., et al. (2009). Characterization of a new metallo-β-lactamase gene, bla NDM-1, and a novel erythromycin esterase gene carried on a unique genetic structure in klebsiella pneumoniae sequence type 14 from India. Antimicrob. Agents Chemother. 53 (12), 5046–5054. doi: 10.1128/AAC.00774-09
Zhang, R., Dong, N., Huang, Y., Zhou, H., Xie, M., Chan, E. W., et al. (2018). Evolution of tigecycline- and colistin-resistant CRKP (carbapenem-resistant klebsiella pneumoniae) in vivo and its persistence in the GI tract. Emerg. Microbes Infect. 7 (1), 127. doi: 10.1038/s41426-018-0129-7
Zhu, J., Sun, L., Ding, B., Yang, Y., Xu, X., Liu, W., et al. (2016). Outbreak of NDM-1-producing klebsiella pneumoniae ST76 and ST37 isolates in neonates. Eur. J. Clin. Microbiol. Infect. Dis. 35 (4), 611–618. doi: 10.1007/s10096-016-2578-z
Keywords: carbapenem-resistant klebsiella pneumoniae, antibiotics, integron, plasmids, hospital, polymerase chain reaction
Citation: Shahid M, Ahmad N, Saeed NK, Shadab M, Joji RM, Al-Mahmeed A, Bindayna KM, Tabbara KS and Dar FK (2022) Clinical carbapenem-resistant Klebsiella pneumoniae isolates simultaneously harboring blaNDM-1, blaOXA types and qnrS genes from the Kingdom of Bahrain: Resistance profile and genetic environment. Front. Cell. Infect. Microbiol. 12:1033305. doi: 10.3389/fcimb.2022.1033305
Received: 31 August 2022; Accepted: 26 September 2022;
Published: 11 October 2022.
Edited by:
Piotr Majewski, Medical University of Bialystok, PolandReviewed by:
Taniela Marli Bes, University of São Paulo, BrazilMahmoud M. Tawfick, Faculty of Pharmacy, Al-Azhar, Egypt
Copyright © 2022 Shahid, Ahmad, Saeed, Shadab, Joji, Al-Mahmeed, Bindayna, Tabbara and Dar. This is an open-access article distributed under the terms of the Creative Commons Attribution License (CC BY). The use, distribution or reproduction in other forums is permitted, provided the original author(s) and the copyright owner(s) are credited and that the original publication in this journal is cited, in accordance with accepted academic practice. No use, distribution or reproduction is permitted which does not comply with these terms.
*Correspondence: Mohammad Shahid, bW9oYW1tZWRzQGFndS5lZHUuYmg=