Faecal carriage of ESBL producing and colistin resistant Escherichia coli in avian species over a 2-year period (2017-2019) in Zimbabwe
- 1Department of Medical Microbiology, University of Pretoria, Pretoria, South Africa
- 2National Microbiology Reference Laboratory, Harare, Zimbabwe
- 3Department of Microbiology, Alberta Precision Laboratories, Department Pathology and Laboratory Medicine, Cummings School of Medicine, University of Calgary, Calgary, AB, Canada
- 4Quadram Institute Biosciences, Norwich, United Kingdom
- 5World Health Organization (WHO), Geneva, Switzerland
- 6World Health Organization (WHO), Harare, Zimbabwe
- 7World Health Organization (WHO), Brazzaville, Republic of Congo
- 8Division of Infection and Immunity, The Roslin Institute, The University of Edinburgh, Edinburgh, Scotland, United Kingdom
- 9University of Zimbabwe College of Health Sciences, Health Professions Education, Harare, Zimbabwe
- 10Tshwane Academic Division, National Health Laboratory Service, Pretoria, South Africa
Introduction: Extended spectrum beta-lactamase (ESBL) producing Escherichia coli have become widespread among food producing animals. These strains serve as a reservoir of antibiotic resistance genes (ARGs) and act as a possible source of infection to humans as transmission can occur by direct or indirect contact.
Methods: This study investigated the faecal carriage of ESBL producing and colistin resistant E. coli in poultry over a 2-year period (2017-2019) from Zimbabwe. A total of 21 ESBL positive isolates from poultry cloacal specimens were selected for whole genome sequencing from animal E. coli isolates bio-banked at the National Microbiology Reference laboratory using phenotypic susceptibility testing results from the National Escherichia coli Surveillance Program to provide representation of different geographical regions and year of isolation. Cloacal swabs were collected from 3000 broiler live birds from farm 1 and from farm 2, 40 backyard chickens and 10 ducks were sampled. Antimicrobial susceptibility and ESBL testing were performed as per Clinical Laboratory Standards Institute guidelines. Whole genome sequencing of ESBL producing isolates was used to determine sequence types (STs), ARGs, and phylogroups.
Results: Twenty-one of the included E. coli isolates were confirmed as ESBL producers. Three defined sequence type clonal complexes (CCs) were identified (ST10CC, ST155CC and ST23CC), with ST10CC associated with the most antibiotic resistant profile. The ESBL phenotype was linked to the presence of either cefotaximase-Munich-14 (CTX-M-14) or CTX-M-79. Plasmid mediated quinolone resistant determinants identified were qnrB19 and qnrS1 and one ST10CC isolate from farm 1 broiler chickens harbored a mobile colistin resistance gene (mcr-1). Phylogenetic groups most identified were B1, A and unknown.
Discussions: The avian ESBL producing E. coli belonged to a diverse group of strains. The detection of several ARGs highlights the importance of implementing enhanced control measures to limit the spread in animals, environment, and humans. This is the first report of mcr-1 in Zimbabwe, which further underscores the importance of the One Health approach to control the spread and development of AMR.
Introduction
Antimicrobial resistance (AMR) surveillance on Escherichia coli is a global priority to curb an ever-increasing range of infections caused by bacteria that are no longer susceptible to the common or first –line antibiotics that are used to treat them. The spread of these resistant E. coli strains is a serious threat to public health worldwide. The World Health Organization (WHO) has recognised the need for an improved and coordinated global effort to contain AMR (World Health Organization, 2014). The high levels of AMR have been attributed to the extensive and irrational use of antimicrobials in different sectors and has in turn led to the emergence, evolution and spread of numerous antibiotic resistant bacteria (Caruso et al., 2018). The magnitude of antimicrobial usage is expected to increase considerably over the coming years due to the intensification of farming practises in much of the developing world. The AMR in foodborne pathogens is therefore of concern, especially when antibiotics are widely used on commercial farms and backyard farms (Foster-Nyarko et al., 2021b).
Poultry farming is one of the most widespread animal husbandry industries worldwide. Chickens are the most farmed species, with more than 90 billion tonnes of chicken meat produced per year (Food and Agriculture Organisation, 2020). In Zimbabwe, chickens are mostly reared in the backyard and are regarded as a valuable food source. However, the proximity between backyard poultry and humans may facilitate transmission of pathogens such as E. coli between these two host species. There are several antimicrobials that are used to raise poultry in several countries (Agunos et al., 2012; Landoni and Albarellos, 2015) including in Zimbabwe, with the aim to prevent and treat diseases, but also to enhance growth and productivity (Page and Gautier, 2012). The same type of antimicrobials is critical and of high importance for human medicine (World Health Organization, 2017). The AMR in avian pathogens may lead to economic losses, derived from the expenditure on ineffective antimicrobials, as well as the burden of untreated poultry diseases (Nhung et al., 2017).
The emergence and spread of cephalosporin and carbapenem resistance, primarily mediated by plasmid-encoded beta-lactamases, is therefore of great concern (Dagher et al., 2018). Colistin is considered a last resort for the treatment of infections caused by carbapenemase-producing members of the Enterobacterales (Perreten et al., 2016). The use of polymyxins (colistin and polymyxin B) has been revived in recent years since it emerged as the drug of choice in the face of the emergence of multidrug-resistant (MDR) Gram-negative bacteria (Qiu et al., 2019). Colistin has been widely used in the animal production industry to enhance productivity and control diseases (Van et al., 2020). This led to the emergence of colistin resistance in E. coli. Several studies have reported the worldwide dissemination of the plasmid-mediated mobilised colistin resistance gene (mcr-1) in humans, animals, and the environment, ever since its first detection in November 2015 in China]. To date, nine different plasmid-mediated mcr variants have been described, designated as mcr-1-9, isolated from humans, animals, and the environment (Hernández et al., 2015; Carattoli et al., 2017; Nishino et al., 2017).
The incidence of AMR Enterobacterales in Africa is high. The high incidence especially of ESBL producers has been contributed largely by weak regulations in human and animal health practices, lack of policies and regulation regarding disposal of antimicrobials, despite the limited resources. Most studies on the African continent concentrate on the human clinical and community setting (Storberg, 2014; Sangare et al., 2015), while no data on the molecular epidemiology of E. coli in animals have been reported from Zimbabwe. As such the aim of this study was to provide baseline data on the faecal carriage of ESBL producing and colistin resistant E. coli in avian species from Zimbabwe.
Results
Baseline characteristics of sequenced isolates
A total of 21 ESBL positive cloacal samples were obtained from two major farms from Chitungwiza (2 ducks, 6 backyard chickens) and Harare 13 broiler chickens for analysis. All these ESBL positive carriage isolates displayed MDR phenotypes. The most common MDR phenotype was resistance to ampicillin, cefotaxime, ceftriaxone, ciprofloxacin, nalidixic acid, and tetracycline (AMP-CTX-CRO-CIP-NAL-TET) that was observed in 11 isolates as shown in Table 1. The isolates were all susceptible to carbapenems.
Genomic assembly, quality control and phylogenetic tree
Twenty-one isolates had a combined length of contigs of assembled genomes ranging from ~4.7 Mbp to 9.8 Mbp, with a minimum contig length required to cover 50% of the genome (N50), ranging between 84 kbp to 340 kbp. The SNP matrix output table with sequenced isolates aligned and compared to reference genomes were used to build a phylogenetic tree as shown in Figures 1, 2.
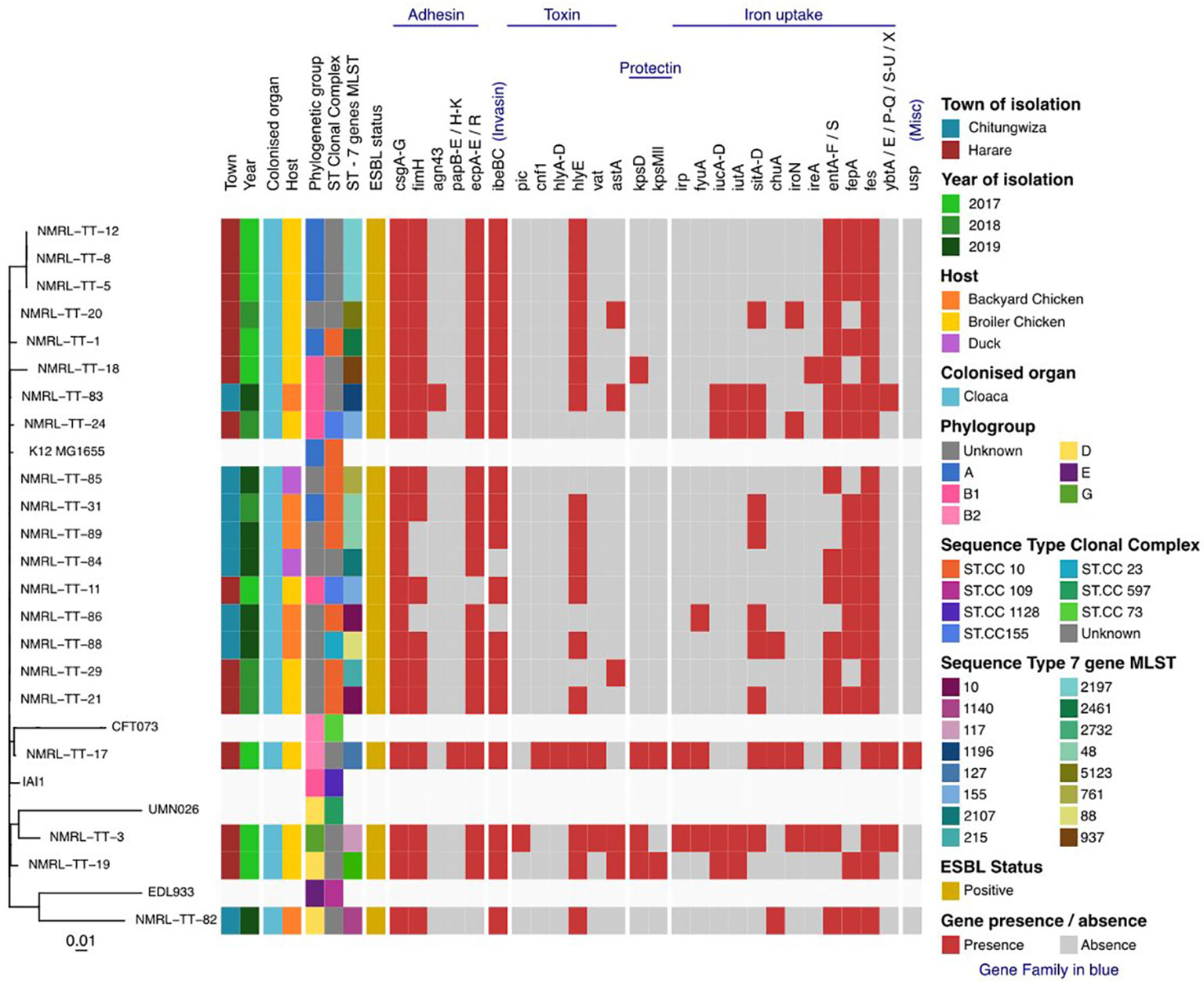
Figure 2 Maximum-likelihood phylogeny of the study isolates reconstructed with RAxML based on non-repetitive, non-recombinant core SNPs.
Beta-lactamases, plasmid-mediated quinolone resistance determinants and phylogenetic groups
The 21 avian ESBL E. coli isolates included in this study presented with diverse ESBL genes, where six harboured the cefotaximase-Munich (CTX-M)-14 gene, one harboured the CTX-M-15 gene, two harboured CTX-M-55, two harboured CTX-M-79 and other Temoneira (TEM) and OXA gene variants. No sulfhydryl variable (SHV) variants were detected as shown in Table 1. Illustrates isolates that harboured TEM-1 and OXA-1 genes in addition to CTX-M gene variants. Different plasmid-mediated quinolone resistance determinants were detected in this study, which included the following: qnrS19 (13), qnrB1 (12), qnrD1 (2) and aac (6’)-lb-cr genes respectively. One isolate was positive for the mcr-1 gene. Phylogroups identified were as follows: group unknown (8), group A (5); group B1 (4); group D (2); group B2 (1) and group G (1) respectively.
Multilocus sequence typing
Multilocus sequence typing identified three diverse ST clonal complexes (CCs), which included the following: ST10CC; ST155CC and ST23. Among the ST10CC the STs included ST10, ST48 (2), ST215, ST761, ST2461, while ST155CC included ST155 and ST23CC had ST88. Most of the isolates (12/21, 58%) belonged to one of these defined CCs. The remaining proportion (42%) of isolates in this study represents the undefined clonal complexes referred to as “unknown CCs”. These ST unknown CCs are represented by ST1140, ST1196, ST2197, ST127, ST937, ST5123, ST117, ST2732 and ST2107 (Table 1). The farm location, year of collection, antimicrobial susceptibility profile, plasmid mediated quinolone resistant (PMQR) determinants, ESBL types and phylogenetic groups of the above defined and undefined CCs are shown in Table 1 and Addendum A - Supplementary File 1 - Table 1.1AA. All the ESBL isolates were resistant to multiple antimicrobials with most resistant STs being recorded in ST2197, ST10, ST48 and ST155.
Plasmid replicon types
The most predominant plasmids were Col156, Col440I, ColMG828 and Col pHAD28 that were widely distributed among the ST10 and ST2197 strains. All strains characterised in this study harboured incompatibility group F (IncF) plasmids. The other replicon types detected in this study included IncY, Incl1, Incl2, IncH, IncQ, Inc X, p0111. The plasmid replicons represented as IncFlB were sixteen (n=16) followed by IncFll (n =11) and IncFlA (n = 5).
Single nucleotide polymorphisms distance comparison of ST10 clonal complex from human isolates to those in avian population
A total of nine isolates [three from human isolates (NMRL-TT-54, NMRL-TT-61 and NMRL-TT-93) and six from poultry isolates (NMRL-TT-1, NMRL-TT-21, NMRL-TT-29, NMRL-TT-31, NMRL-TT-85 and NMRL-TT-86] as shown in Supplementary File - Table 4 were included for comparison to assess the genetic relatedness of these ST10CC isolates detected in both animals and humans. The snpdist difference between human and poultry isolates ranged between 728 and 17397 SNPs. A comparison of poultry isolates showed SNP differences ranging between 0 and 30 SNPs while human isolates had SNP differences ranging from 908 to 17397 SNPs.
Discussion
Antimicrobial resistance is one of the biggest threats to food safety and considered a One Health issue with the potential of spreading to other countries, since resistant pathogens do not recognise borders (Mathers et al., 2015). This study reports on the faecal carriage of ESBL producing and colistin resistant E. coli in poultry over a 2-year period (2017-2019) in Zimbabwe. The isolates from this study revealed the prevalence of faecal carriage of ESBL E. coli in poultry to be 11%, which is comparable to similar studies that were done in León, Nicaragua (13%) (Hasan et al., 2016) and Lebanon (20.6%) (Iman et al., 2018). Extensive use of antimicrobials is recognised as the most important factor selecting for AMR in bacteria (Centers for Disease Control and Prevention et al., 2013) according to the European Surveillance of Veterinary Antimicrobial Consumption (ESVAC) report, sales of veterinary antimicrobial agents in 2016 varied from 0.7 to 2726.5 tons in the 30 participating countries in the European Medicine Agency (EMA) and ESVAC (European Centre for Disease Prevention and Control (ECDC) et al., 2017). Notably, polymyxins were the fifth most sold group of antimicrobials in 2015–2016 (European Centre for Disease Prevention and Control (ECDC) et al., 2017).
A technique called in silico MLST was used to determine the circulating clones of importance in the faecal carriage ESBL E. coli from Zimbabwean avian species. The most common STs determined were ST10CC, ST155CC and ST88CC, which are known to be interspecies clones (Flament-Simon et al., 2020). Interspecies clones are defined as E. coli strains/clones capable of being transmitted among humans, wildlife, companion animals, etc. (Roer et al., 2018). The ST10CC results are consistent with findings from other studies where E. coli clones belonging to ST10CC, ST23CC and phylogroup A are increasingly reported in association with ESBL production (Usein et al., 2016; Seni et al., 2021). The ST10CC are known as the largest interspecies clonal complex suggesting that antimicrobial resistant bacteria could be transferred by multiple sources like environment, human or animal contact (Qiu et al., 2019). Given the environment (grass bedding, feeding on kitchen waste and contact with handlers) in which the backyard poultry were exposed to, there is a possibility of transmission of resistant bacteria (Samanta et al., 2018).
Mobile genetic elements (MGEs) play an important role in the transmission of virulence and resistance genes of ESBL producing isolates (Partridge et al., 2018). The different CCs in this study had different ESBL resistance genes ranging from blaTEM, blaOXA, and blaCTX-M variants and virulence genes. The following virulence genes were detected in this study and includes adhesion factors, evasion/invasion genes, toxins, iron acquisition genes and genes associated with serum resistance. These virulence genes are encoded by fimH, csg, agn43, kpsD, kpsMll, ibeBC, fes, fepA, iucA, gspL, and hlyE and can be transmitted through HGT among different Gram-negative bacteria, thus contributing to diseases in humans should they gain access to the appropriate tissue (Hussain et al., 2019).
All the ESBL isolates in this study harboured IncF plasmids, which agrees with other epidemiological studies that revealed an association of blaTEM, blaOXA, and blaCTX-M genes to IncF plasmids (Zhao and Hu, 2013; Sukmawinata et al., 2020). Moreover, genes encoding resistance to aminoglycosides, chloramphenicol, quinolones (qnr), tetracyclines (tet), and trimethoprim have been observed to co-exist with blaTEM, blaOXA, and blaCTX-M variant genes in this study. The co-existence of two or more beta-lactamases in the same strain was observed in ST10 and ST155 isolates as reported in other studies (Salah et al., 2019; Obayiuwana and Ibekwe, 2020). There is little doubt that the overuse of cephalosporins and related compounds in poultry farming has been the driving forces in the persistence and dissemination of ESBL producing bacteria with the use of other antimicrobial compounds also exerting the same selective force (Aslam et al., 2018; Hedman et al., 2020). It is worrisome that commensal E. coli bacteria, i.e., ST10 phylogroup A from food producing animals may contribute to the dissemination of ESBL resistance and to the transference of resistance genes to other human pathogenic bacteria such as Salmonella spp (Ramos et al., 2020).
Epidemiological studies have shown a close genetic relationship between commensal E. coli, APEC and human ExPEC (Foster-Nyarko et al., 2021a). A substantial overlap of phylogenetic groups has been observed between the APEC in this study (A, B1 and unknown) and human ExPEC results (B2 and D) observed from a study by Lee et al., 2016 (Lee et al., 2016). This overlap of phylogenetic groups between humans and chickens is worrying given the high consumption of chicken meat around the world and the virulence properties associated with phylogenetic group B2 strains in chickens (Papouskova et al., 2020).
To the best of our knowledge, this is the first report of the finding of plasmid-mediated colistin resistance (mcr-1) in Zimbabwe. The mcr 1.1 positive E. coli isolate belongs to the ST10 clone. Furthermore, our findings suggest that the diversity among the ST10CC in terms of harboring several AMR determinants, is responsible for the high resistance profiles found in this study. The presence of other AMR determinants on the same plasmid as the one harboring mcr-1 gene may pose a huge public health threat due to the existing risk of spread of resistance genes e.g., mcr-1 among bacterial strains, to other food producing animals, environment, and humans (Aworh et al., 2020).
A limitation of this study was the small sample size of only 21 avian ESBL E. coli isolates with most isolates selected from Harare; however, results from this study contributed to the baseline molecular information for isolates/clones currently and previously linked to resistance in Zimbabwe. Short reads plasmid profile analysis was explored, which does not give a full description of the plasmids as compared to long read sequencing, although this technique provides some indication of the different plasmids within each isolate. Plasmid conjugation experiments could not be performed due to funding and time constraints, therefore future studies should focus on such experiments to get an in-depth understanding of the characteristics of the ESBL -encoding plasmids and the colistin resistance carrying plasmids. As far as we are aware this is the first study to be done in Zimbabwe to provide baseline data on virulence, antimicrobial resistance, and detection of specific lineages of ExPEC circulating in poultry using WGS.
Extended spectrum beta-lactamase producing E. coli and mcr-1 gene detection for colistin resistance is a public health concern, since these genes are harboured on MGEs, i.e., plasmids, they can easily spread in communities and lead to establishment of mcr resistant clones within E. coli. It is well-known that MGEs from E. coli can spread to other Enterobacterales like Klebsiella pneumoniae. Since Zimbabwe is a significant poultry importer in Africa, research on this aspect should be widely conducted in Zimbabwe and constantly improved. It is essential to monitor colistin-resistant E. coli strains for understanding the prevalence of colistin resistance genes in both human and veterinary medicine, including poultry production.
Materials and methods
Selection of bacterial isolates for genomic evaluation
A total of 21 cloacal swabs of ESBL positive avian (broiler chickens, backyard chickens and backyard ducks) E. coli was selected from the two farms included in this study. The selection was based on phenotypic susceptibility testing results from 12 240 animal E coli isolates bio-banked at the National Microbiology Reference Laboratory. The sampling strategy for broiler chickens in farm 1 with 3000 chickens was as follows. The broiler chickens were caged in 20s therefore a total of 150 compartments. One chicken per compartment was randomly selected for cloacal sampling. Therefore 150 samples underwent culturing and antimicrobial resistance testing
This selection provided representation of different geographical region and years of isolation (2017, 2018, 2019). (Addendum A - Supplementary File 1 - Table 1.1AA). The sampling locations/geographical regions included were Chitungwiza (2 ducks, 6 backyard chickens) and Harare (13) due to ease of accessibility and convenience as shown in the Flow diagram (Figure 1) below. No information on previous use of antibiotics was collected.
Study population phenotypic antibiotic resistance profiling
All the selected isolates were sub-cultured on MacConkey or eosin methylene blue (EMB) (Mast Group, Merseyside, UK), incubated at 37°C (Memmert ICH110, Memmert, Germany) for 18 to 24 hours and then stored in 20% glycerol broth at -80°C. Antimicrobial susceptibility testing was determined by the Kirby Bauer disc diffusion method using the Clinical Laboratory Standards Institute (CLSI) guidelines (Clinical and Laboratory Standards Institute, 2018). The antimicrobial drugs tested included ampicillin, trimethoprim-sulfamethoxazole, ciprofloxacin, ceftriaxone, tetracycline, ceftazidime, nalidixic acid, cefepime and ertapenem; results were interpreted as described by the CLSI (Clinical and Laboratory Standards Institute, 2018). The presence of ESBLs was confirmed according to the CLSI criteria for ESBL screening and confirmation (Clinical and Laboratory Standards Institute, 2018). Escherichia coli ATCC 25922 was used as quality control strain. Additional data included on collection of each isolate were the year, location of isolation, and city (Addendum A - Supplementary File 1 - Table 1.1AA).
Genomic DNA isolation and whole genome sequencing
Genomic DNA (gDNA) of avian E. coli was extracted using the Wizard® Genomic DNA Purification Kit (Promega, Madison, WI, USA) according to the manufacturer’s instructions (Promega Corporation, 2019) and stored at -20°C. Library preparation was performed using the Nextera XT DNA Library Preparation Kit (Illumina, San Diego, CA, USA) and sequenced on a MiSeq benchtop sequencer (Illumina, San Diego, CA, USA) at Quadram Biosciences Institute, United Kingdom. The data was uploaded to BaseSpace (http://www.basespace.illumina.com) and then converted to FASTQ files.
Genomic sequence analyses
The sequences were analysed on the Cloud Infrastructure for Microbial Bioinformatics (O’Connor and Moon, 2016). Paired-end short read sequences were concatenated, and quality-checked using FastQC v0.11.7. De novo assembly was performed with SPAdes 3.11 (Bankevich et al., 2012), and quality assessed using QUAST 4.5 (Gurevich et al., 2013). Snippy v4.3.2 (Seemann, 2020) was used to generate a core single nucleotide polymorphism (SNP) alignment using default parameters. The complete genome sequence of E. coli strain K12 sub-strain MG1655 was used as reference genome (NCBI accession: NC_000913.3). After the core-genome alignment, a reconstruction of maximum likelihood phylogeny with 1000 bootstrap replicates with RAxML v8.2.4 based on a general time-reversible nucleotide substitution model was performed (Stamatakis, 2014; Kozlov et al., 2019). The phylogenetic tree was rooted using E. fergusonii as an outgroup (NCBI accession: GCA_000026225.1). The phylogenetic tree was visualised in Figtree v1.4.4 (Beghain et al., 2018) and annotated in RStudio v3.5.2 and Adobe illustrator v 23.0.3 (Adobe Inc. San Jose, California). Recombination was detected and masked using Gubbins before the phylogenetic tree reconstruction (Croucher and Didelot, 2015). The pairwise SNP distances were computed between genomes from the core-genome alignment using snp-dists v0.6 (Seemann, 2018). The ST10 clonal complex isolates identified in a study of 48 ESBL urine isolates from humans (Takawira et al., 2021), were compared to similar (ST10) clonal complex isolates from this study. The assembled draft genomes underwent similar procedures as above to produce pairwise SNP distances (Seemann, 2018; Seemann, 2020).
Comparative genomics analysis
The assembled draft genomes were used to define the presence of genes and their alleles. The following databases or typing schemes were used to determine: (i) STs (multi-locus sequence typing; Achtman scheme) (Wirth et al., 2006); (ii) phylogenetic groups (ClermonTyper v1.0.0) (Beghain et al., 2018); (iii) resistance genes (ARIBA database) (Hunt et al., 2017); (iv) virulence factors (virulence factor database, VFDB) (Liu et al., 2019); and (v) plasmids (PlasmidFinder) (Wirth et al., 2006).
Data availability statement
The data presented in this study are deposited in the NCBI BioProject Number PRJNA 799483 with accession numbers listed in the Supplementary Datasheet 1.
Ethics statement
Ethical clearance was obtained from the Faculty of Health Sciences Research Ethics Committee, University of Pretoria (Ethics Reference Number: 782/2018) and the Medical Research Council of Zimbabwe (Approval Number: MRCZ/A/2394).
Author contributions
All authors provided critical input and contributed to the manuscript writing and approved its final version. Conception or design of the work FT, JP, MK, SZ, TM, LM. Methodology FT, JP, MK, JM, SM, LWM, AT. Formal analysis and interpretation FT, JP, MK, RK, GT, AG, GP, DG, TM. Writing – original draft preparation FT, JP, MK. Writing – review and editing of the article FT, JP, MK, ME, AT, TM. Final approval of the version to be published FT, JP, MK, SZ, TM, LWM, GT, AG, RK, GP, JM, SM, LM, DG, AT, ME. All authors contributed to the article and approved the submitted version.
Funding
This project was funded by the National Health Laboratory Service (NHLS) and the University of Pretoria, South Africa, a strategic partnership between National Microbiology Reference Laboratory and Quadram BioSciences Institute. Opinions expressed and conclusions arrived at, are those of the authors and are not necessarily to be attributed to the funders. The funders of the study had no role in the study design, data collection, data analysis, interpretation or writing of the article.
Acknowledgments
The authors would like to thank the Department of Medical Microbiology, University of Pretoria (South Africa), the staff at Central Veterinary Laboratory (Zimbabwe) for their contributions in making the national surveillance a success and contributing to the bio-banking of these isolates for future studies. We would also like to thank the sequencing team at Quadram Institute Biosciences United Kingdom, for the help and assistance offered.
Conflict of interest
The authors declare that the research was conducted in the absence of any commercial or financial relationships that could be construed as a potential conflict of interest.
Publisher’s note
All claims expressed in this article are solely those of the authors and do not necessarily represent those of their affiliated organizations, or those of the publisher, the editors and the reviewers. Any product that may be evaluated in this article, or claim that may be made by its manufacturer, is not guaranteed or endorsed by the publisher.
Supplementary material
The Supplementary Material for this article can be found online at: https://www.frontiersin.org/articles/10.3389/fcimb.2022.1035145/full#supplementary-material
References
Agunos, A., Léger, D., Carson, C. (2012). Review of antimicrobial therapy of selected bacterial diseases in broiler chickens in Canada. Can. Vet. J. 53 (12), 1289–1300.
Aslam, B., Wang, W., Arshad, M. I., Khurshid, M., Muzammil, S., Rasool, M. H. (2018). Antibiotic resistance: A rundown of a global crisis. Infect. Drug Resist. 11, 1645–1658. doi: 10.2147/IDR.S173867
Aworh, M. K., Kwaga, J., Okolocha, E., Harden, L., Hull, D., Hendriksen, R. S. (2020). Extended-spectrum ß-lactamase-producing Escherichia coli among humans, chickens and poultry environments in Abuja, Nigeria. One Health Outlook. 2, 8. doi: 10.1186/s42522-020-00014-
Bankevich, A., Nurk, S., Antipov, D., Gurevich, A. A., Dvorkin, M., Kulikov, A. S., et al. (2012). SPAdes: A new genome assembly algorithm and its applications to single-cell sequencing. J. Comput. Biol. 19 (5), 455–477. doi: 10.1089/cmb.2012.0021
Beghain, J., Bridier-Nahmias, A., Le Nagard, H., Denamur, E., Clermont, O. (2018). ClermonTyping: An easy-to-use and accurate in silico method for Escherichia genus strain phylotyping. Microb. Genom. 4 (7), e000192. doi: 10.1099/mgen.0.000192
Carattoli, A., Villa, L., Feudi, C., Curcio, L., Orsini, S., Luppi, A., et al. (2017). Novel plasmid-mediated colistin resistance mcr-4 gene in Salmonella and Escherichia coli, Italy 2013, Spain and Belgium, 2015 to 2016. Euro Surveill. 22 (31), 30589. doi: 10.2807/1560-7917.ES.2017.22.31.30589
Caruso, G., Giammanco, A., Cardamone, C., Oliveri, G., Mascarella, C., Capra, G., et al. (2018). Extra-intestinal fluoroquinolone-resistant Escherichia coli strains isolated from meat. BioMed. Res. Int. doi: 10.1155/2018/8714975
Centers for Disease Control and Prevention, US Department of Health, Human Services (2013). Antibiotic resistance threats in the united states (Atlanta: CDC). Available at: http://www.cdc.gov/drugresistance/pdf/ar-threats-2013-508.pdf.
Clinical and Laboratory Standards Institute (2018). Performance standards for antimicrobial susceptibility testing, 28th ed (CLSI: Wayne, PA, USA: CLSI Supplement Document M100).
Croucher, N. J., Didelot, X. (2015). The application of genomics to tracing bacterial pathogen transmission. Cur Opin. ln Microbiol. 23, 62–67. doi: 10.1016/j.mib.2014.11.004
Dagher, C., Salloum, T., Sahar, A., Harout, A., George, F. A., Sima, T. (2018). Molecular characterization of carbapenem resistant Escherichia coli recovered from a tertiary hospital in Lebanon. PloS One 13 (9), 1–13. doi: 10.1371/journal.pone.0203323
European Centre for Disease Prevention and Control (ECDC), European Food Safety Authority (EFSA), European Medicines Agency (EMA) (2017). ECDC/EFSA/EMA second joint report on the integrated analysis of the consumption of antimicrobial agents and occurrence of antimicrobial resistance in bacteria from humans and food-producing animals: Joint interagency antimicrobial consumption and resistance analysis (JIACRA) report. EFSA J. 15 (7), e04872. doi: 10.2903/j.efsa.2017.4872
Flament-Simon, S. C., Nicolas-Chanoine, M. H., García, V., Duprilot, M., Mayer, N., Alonso, M. P., et al. (2020). Clonal structure, virulence factor-encoding genes and antibiotic resistance of Escherichia coli, causing urinary tract infections and other extraintestinal infections in humans in Spain and France during 2016. Antibiotics (Basel). 9 (4), 161. doi: 10.3390/antibiotics9040161
Food and Agriculture Organisation (2020). World food and agriculture - statistical yearbook 2020 (Rome:Food and Agriculture Organisation). Available at: http://www.fao.org/faostat/en. doi: 10.4060/cb1329en
Foster-Nyarko, E., Alikhan, N. F., Ikumapayi, U. N., Sarwar, G., Okoi, C., Tientcheu, P. M., et al. (2021a). Genomic diversity of Escherichia coli from healthy children in rural Gambia. PeerJ 9, e10572. doi: 10.7717/peerj.10572
Foster-Nyarko, E., Alikhan, N. F., Ravi, A., Thomson, N. M., Jarju, S., Kwambana-Adams, B. A., et al. (2021b). Genomic diversity of Escherichia coli isolates from backyard chickens and guinea fowl in the Gambia. Microb. Genom. 7 (1), mgen000484. doi: 10.1099/mgen.0.000484
Gurevich, A., Saveliev, V., Vyahhi, N., Tesler, G. (2013). QUAST: quality assessment tool for genome assemblies. Bioinformatics 29 (8), 1072–1075. doi: 10.1093/bioinformatics/btt086
Hasan, B., Laurell, K., Rakib, M. M., Ahlstedt, E., Hernandez, J., Caceres, M. (2016). Fecal carriage of extended-spectrum beta-lactamases in healthy humans, poultry, and wild birds in león, Nicaragua-a shared pool of blaCTX-m genes and possible interspecies clonal spread of extended-spectrum beta-lactamases-producing. Escherichia coli. Microb. Drug Resist. 22 (8), 682–687. doi: 10.1089/mdr.2015.0323
Hedman, H. D., Vasco, K. A., Zhang, L. A. (2020). Review of antimicrobial resistance in poultry farming within low-resource settings. Anim. (Basel). 10 (8), 1264. doi: 10.3390/ani10081264
Hernández, M., Iglesias, M. R., Rodríguez-Lázaro, D., Gallardo, A., Quijada, N., Miguela-Villoldo, P., et al. (2015). Co-Occurrence of colistin-resistance genes mcr-1 and mcr-3 among multidrug-resistant Escherichia coli isolated from cattle, Spain. Euro Surveill. 22 (31), 30586. doi: 10.2807/1560-7917.ES.2017.22.31.30586
Hunt, M., Mather, A. E., Sánchez-Busó, L., Page, A. J., Parkhill, J., Keane, J. A., et al. (2017). ARIBA: Rapid antimicrobial resistance genotyping directly from sequencing reads. Microb. Genom. 43(10), e000131. doi: 10.1099/mgen.0.000131
Hussain, A., Shaik, S., Ranjan, A., Suresh, A., Sarker, N., Semmler, T. (2019). Genomic and functional characterization of poultry Escherichia coli from India revealed diverse extended-spectrum beta-lactamase-producing lineages with shared virulence profiles. Front. Microbiol. 10. doi: 10.3389/fmicb.2019.02766
Iman, D., Chabou, S., Daoud, Z., Rolain, J. M. (2018). Prevalence and emergence of extended-spectrum cephalosporin-, carbapenem- and colistin-resistant gram-negative bacteria of animal origin in the Mediterranean basin. Front. Microbiol. 9. doi: 10.3389/fmicb.2018.02299
Kozlov, A. M., Darriba, D., Flouri, T., Morel, B., Stamatakis, A. (2019). RAxML-NG: a fast, scalable, and user-friendly tool for maximum likelihood phylogenetic inference. Bioinformatics 35 (21), 4453–4455. doi: 10.1093/bioinformatics/btz305
Landoni, M. F., Albarellos, G. (2015). The use of antimicrobial agents in broiler chickens. Vet. J. 205 (1), 21–27. doi: 10.1016/j.tvjl.2015.04.016
Lee, J. H., Subhadra, B., Son, Y. J., Kim, D. H., Park, H. S., Kim, J. M., et al. (2016). Phylogenetic group distributions, virulence factors and antimicrobial resistance properties of uropathogenic Escherichia coli strains isolated from patients with urinary tract infections in south Korea. Lett. Appl. Microbiol. 62 (1), 84–90. doi: 10.1111/lam.12517
Liu, B., Zheng, D., Jin, Q., Chen, L., Yang, J. (2019). VFDB 2019: a comparative pathogenomic platform with an interactive web interface. Nucleic Acids Res. 47 (D1), D687–D692. doi: 10.1093/nar/gky1080
Mathers, A. J., Peirano, G., Pitout, J. D. (2015). The role of epidemic resistance plasmids and international high-risk clones in the spread of multidrug-resistant Enterobacteriaceae. Clin. Microbiol. Rev. 28 (3), 565–591. doi: 10.1128/CMR.00116-14
Nhung, N. T., Chansiripornchai, N., Carrique-Mas, J. J. (2017). Antimicrobial resistance in bacterial poultry pathogens: A review. Front. Vet. Sci. 4. doi: 10.3389/fvets.2017.00126
Nishino, Y., Shimojima, Y., Suzuki, Y., Ida, M., Fukui, R., Kuroda, S. (2017). Detection of the mcr-1 gene in colistin-resistant Escherichia coli from retail meat in Japan. Microbiol. Immunol. 61 (12), 554–557. doi: 10.1111/1348-0421.12549
Obayiuwana, A., Ibekwe, A. M. (2020). Antibiotic resistance genes occurrence in wastewaters from selected pharmaceutical facilities in Nigeria. Water 12 (7), 1897. doi: 10.3390/w12071897
O’Connor, A. H., Moon, T. S. (2016). Development of design rules for reliable antisense RNA behavior in. E. coli. ACS Synthetic Biol. 5 (12), 1441–1454. doi: 10.1021/acssynbio.6b00036
Page, S. W., Gautier, P. (2012). Use of antimicrobial agents in livestock. Rev. Sci. Tech. 31, 145–188. doi: 10.20506/rst.31.1.2106
Papouskova, A., Masarikova, M., Valcek, A., Senk, D., Cejkova, D., Jahodarova, E., et al. (2020). Genomic analysis of Escherichia coli strains isolated from diseased chicken in the Czech republic. BMC Vet. Res. 16, 189. doi: 10.1186/s12917-020-02407-2
Partridge, S. R., Kwong, S. M., Firth, N., Jensen, S. O. (2018). Mobile genetic elements associated with antimicrobial resistance. Clin. Microbiol. Rev. 31 (4), e00088–e00017. doi: 10.1128/CMR.00088-17
Perreten, V., Strauss, C., Collaud, A., Gerber, D. (2016). Colistin resistance gene mcr-1 in avian-pathogenic escherichia coli in south Africa. Antimicrobial Agents Chemother. 60 (7), 4414–4415. doi: 10.1128/AAC.00548-16
Promega Corporation (2019) Technical manual wizard® genomic DNA purification kit wizard® genomic DNA purification kit. technical bulletin, 1–19. Available at: www.promega.com.
Qiu, J., Jiang, Z., Ju, Z., Zhao, X., Yang, J., Guo, H., et al. (2019). Molecular and phenotypic characteristics of Escherichia coli isolates from farmed minks in zhucheng, China. BioMed. Res. Int. 2019. doi: 10.1155/2019/3917841
Ramos, S., Silva, V., Dapkevicius, M. L. E., Caniça, M., Tejedor-Junco, M. T., Igrejas, G., et al. (2020). Escherichia coli as commensal and pathogenic bacteria among food-producing animals: Health implications of extended spectrum beta-lactamase (ESBL) production. Anim. (Basel). 10 (12), 2239. doi: 10.3390/ani10122239
Roer, L., Overballe-Petersen, S., Hansen, F., Schønning, K., Wang, M., Røder, B. L., et al. (2018). Escherichia coli sequence type 410 is causing new international high-risk clones. mSphere 3 (4), e00337–e00318. doi: 10.1128/mSphere.00337-18
Salah, F. D., Soubeiga, S. T., Ouattara, A. K., Sadji, A. Y., Metuor-Dabire, A., Obiri-Yeboah, D., et al. (2019). Distribution of quinolone resistance gene (qnr) in ESBL-producing Escherichia coli and klebsiella spp. in lomé, Togo. Antimicrob. Resist. Infect. Control. 8, 104. doi: 10.1186/s13756-019-0552-0
Samanta, A., Mahanti, A., Chatterjee, S., Joardar, S. N., Bandyopadhyay, S., Sar, T. K., et al. (2018). Pig farm environment as a source of beta-lactamase or AmpC-producing Klebsiella pneumoniae and Escherichia coli. Ann. Microbiol. 68 (11), 781–791. doi: 10.1007/s13213-018-1387-2
Sangare, S. A., Maiga, A. I., Guindo, I., Maiga, A., Camara, N., Savadogo, S., et al. (2015). Prevalence of extended-spectrum beta-lactamase-producing Enterobacteriaceae isolated from blood cultures in Africa. Med. Mal Infect. 45 (9), 374–382. doi: 10.1016/j.medmal.2015.08.003
Seemann, T. (2018) Snp-dists. pairwise SNP distance matrix from a FASTA sequence alignment. Available at: https://github.com/tseemann/snp-dists (Accessed 19 January 2017).
Seemann, T. (2020) Snippy. rapid haploid variant calling and core genome alignment. Available at: https://github.com/tseemann/snippy (Accessed 20 August 2019).
Seni, J., Peirano, G., Mshana, S. E., Pitout, J. D. D., DeVinney, R. (2021). The importance of Escherichia coli clonal complex 10 and ST131 among Tanzanian patients on antimicrobial resistance surveillance programs. Eur. J. Clin. Microbiol. Infect. Dis. doi: 10.1007/s10096-021-04271-w
Stamatakis, A. (2014). RAxML version 8: A tool for phylogenetic analysis and post-analysis of large phylogenies. Bioinformatics 30 (9), 1312–1313. doi: 10.1093/bioinformatics/btu033
Storberg, V. (2014). ESBL-producing Enterobacteriaceae in Africa - a non-systematic literature review of research published 2008-2012. Infect. Ecol. Epidemiol. 13, 4. doi: 10.3402/iee
Sukmawinata, E., Uemura, R., Sato, W., Mitoma, S., Kanda, T., Sueyoshi, M. (2020). IncI1 plasmid associated with blaCTX-M-2 transmission in ESBL-producing Escherichia coli isolated from healthy thoroughbred racehorse, Japan. Antibiotics (Basel). 9 (2), 70. doi: 10.3390/antibiotics9020070
Takawira, F. T., Pitout, J. D., Thilliez, G., Mashe, T., Gutierrez, A. V., Kingsley, R. A., et al. (2021). Molecular epidemiology of extended-spectrum beta-lactamase-producing extra-intestinal pathogenic Escherichia coli strains over a 2-year period (2017-2019) from Zimbabwe. Eur. J. Clin. Microbiol. Infect. Dis. doi: 10.1007/s10096-021-04379-z
Usein, C. R., Papagheorghe, R., Oprea, M., Condei, M., Strãuţ, M. (2016). Molecular characterization of bacteremic Escherichia coli isolates in Romania. Folia Microbiol. (Praha). 61 (3), 221–226. doi: 10.1007/s12223-015-0427-6
Van, T. T. H., Yidana, Z., Smooker, P. M., Coloe, P. J. (2020). Antibiotic use in food animals worldwide, with a focus on Africa: Pluses and minuses. J. Glob Antimicrob. Resist. 20, 170–177. doi: 10.1016/j.jgar.2019.07.031
Wirth, T., Falush, D., Lan, R., Colles, F., Mensa, P., Wieler, L. H., et al. (2006). Sex and virulence in Escherichia coli: an evolutionary perspective. Mol. Microbiol. 60 (5), 1136–1151. doi: 10.1111/j.1365-2958.2006.05172
World Health Organization (2014) Antimicrobial resistance: global report on surveillance (World Health Organization). Available at: https://apps.who.int/iris/handle/10665/1126 (Accessed 3 January 2014).
World Health Organization (2017) Critically important antimicrobials for human medicine: Ranking of medically important antimicrobials for risk management of antimicrobial resistance due to non-human use. fifth revision. Available at: https://apps.who.int/iris/handle/10665/255027 (Accessed 13 April 2017).
Keywords: Escherichia coli, ST155, ST10, ST2197, CTX-M-14, CTX-M-15, ESBL, MCR-1
Citation: Takawira FT, Pitout JDD, Thilliez G, Mashe T, Gutierrez AV, Kingsley RA, Peirano G, Matheu J, Midzi SM, Mwamakamba LW, Gally DL, Tarupiwa A, Mukavhi L, Ehlers MM, Mtapuri-Zinyowera S and Kock MM (2022) Faecal carriage of ESBL producing and colistin resistant Escherichia coli in avian species over a 2-year period (2017-2019) in Zimbabwe. Front. Cell. Infect. Microbiol. 12:1035145. doi: 10.3389/fcimb.2022.1035145
Received: 02 September 2022; Accepted: 31 October 2022;
Published: 23 December 2022.
Edited by:
Joana Castro, National Institute for Agricultural and Veterinary Research (INIAV), PortugalReviewed by:
Eva Pinho, Instituto Nacional Investigaciao Agraria e Veterinaria (INIAV), PortugalBen Pascoe, University of Oxford, United Kingdom
Copyright © 2022 Takawira, Pitout, Thilliez, Mashe, Gutierrez, Kingsley, Peirano, Matheu, Midzi, Mwamakamba, Gally, Tarupiwa, Mukavhi, Ehlers, Mtapuri-Zinyowera and Kock. This is an open-access article distributed under the terms of the Creative Commons Attribution License (CC BY). The use, distribution or reproduction in other forums is permitted, provided the original author(s) and the copyright owner(s) are credited and that the original publication in this journal is cited, in accordance with accepted academic practice. No use, distribution or reproduction is permitted which does not comply with these terms.
*Correspondence: Marleen M. Kock, marleen.kock@up.ac.za