- Department of Obstetrics and Gynecology, Peking University First Hospital, Beijing, China
Mixed vaginitis is the result of the simultaneous presence of different pathogenic processes mediated by at least two types of vaginal pathogens. Among the various types of mixed vaginitis presentations, bacterial vaginosis (BV) plus vulvovaginal candidiasis (VVC) presents to be the most prevalent form. Mixed vaginitis affects the health of women of all ages worldwide. However, few studies have focused on clinical manifestations, pathogenesis, diagnostic criteria, or therapy of mixed vaginitis. We recruited 48 symptomatic patients with clinical diagnoses of VVC complicated with BV, they were treated with oral metronidazole combined with local clotrimazole and followed to assess the drug efficacy and vaginal microbiome alterations before and after treatment. The vaginal microbiome in BV+VVC mixed vaginitis patients was altered significantly after the combined drug treatment within a unique form different from a simple overlay mode of BV and VVC, the key bacteria including Gardnerella and Atopobium, Lactobacillus. The combined drug therapy for the mixed vaginitis in this study was effective and enhanced treatment for BV may be more favorable because of more difficulty in dealing with BV according to the treatment outcome. The abundance of Lactobacillus in patients with mixed vaginitis affects the recovery of the vaginal microbiome as well as the prognosis, and the abundance should be actively restored. This is the first study to investigate the composition, diversity, and other characteristics of the vaginal microbiome in patients with BV+VVC mixed vaginitis before and after drug treatment, our results provide clues to improving the cure rate and reducing recurrences.
Introduction
Mixed vaginitis, a syndrome combining symptoms of different pathogenic processes is mediated by at least two types of vaginal pathogens that cause vaginal inflammation. Common vaginitis includes bacterial vaginosis (BV), vaginal trichomoniasis (TV), vulvovaginal candidiasis (VVC), and aerobic vaginitis or desquamative inflammatory vaginitis (AV/DIV) (Paavonen and Brunham, 2018). Although some microorganisms always exhibit asymptomatic colonization, such as Candida, they are actually opportunistic pathogens and able to cause clinical symptoms when the immune system of the host is weakened. Mixed vaginitis presentations may be divided into various types such as BV+VVC, BV+TV, and AV+VVC according to their causative pathogenic processes, they require multiple therapies targeting all pathogens causing symptoms. Few studies on mixed vaginitis have focused on clinical manifestations, pathogenesis, diagnostic criteria and therapy. Mixed vaginitis needs to be distinguished from vaginal coinfections, which present clinical manifestations and signs caused by a single pathogen with asymptomatic concomitant colonization by another pathogen and, therefore, require treatments targeting only the pathogen causing symptoms (Sobel et al., 2013; Benyas and Sobel, 2022).
Attention has been drawn to the increasing prevalence of mixed vaginitis in recent years. According to a literature review summarizing mixed vaginitis advances, the proportion of symptomatic women with mixed vaginitis, most frequently presenting as genital itching, burning pain, and changes in the characteristics of discharge (odor, color, consistency), ranges from 4.44% to 35.06%; moreover, BV+VVC is the most prevalent form (Qi et al., 2021). During the past decade, the overall prevalence of mixed vaginitis in different regions of China varied greatly, fluctuating within a range from 7.33% to 41.87%, with a prevalence for the most common BV+VVC reaching 20.95% to 74.89% (Zhang and Liu, 2020). Regardless of the regional differences in feminine hygiene and the lack of global epidemiological studies, mixed vaginitis seems to have become much more common.
Even after considering mixed vaginitis as a separate vaginal infectious disease, many relevant facts remain unknown, especially the corresponding vaginal microbiome. The vaginal microbiome is characteristic of specific vaginitis types and determines both pathogenic and therapeutic outcomes; for instance, BV is a polymicrobial disorder of the vaginal microbiome that is characterized by the absence of vaginal lactobacilli (Paavonen and Brunham, 2018) and VVC by a Candida dominant vaginal microbiome (Kalia et al., 2020). Whether the vaginal microbiome of BV+VVC mixed vaginitis consists of a simple overlay microbiome or presents unique characteristics is unclear. For this study, we enrolled patients with BV+VVC mixed vaginitis and described their vaginal microbiome before and after treatment with oral metronidazole and local vaginal clotrimazole to provide evidence for improving the cure rate and reducing recurrences (van Schalkwyk and Yudin, 2015; Cooperative Group of Infectious Disease, 2021b; Workowski et al., 2021).
Materials and Methods
Study Design and Study Population
We selected record data from 48 18-to-50-year-old patients clinically diagnosed as having VVC complicated with BV, who were admitted to The First Hospital of Peking University from January 2017 to December 2020. BV was diagnosed using Amsel’s diagnostic criteria and identification of a Nugent score ≥7 from a vaginal Gram stain. Referring to the modified Amsel’s diagnostic criteria, BV was diagnosed when three of the following were present: a thin homogeneous discharge, elevated vaginal pH above 4.5, release of amines on the addition of 10% potassium hydroxide to vaginal fluid, and the presence of “clue” cells. VVC was diagnosed by identification of budding yeasts, hyphae, or pseudo-hyphae in a wet preparation (saline, 10% KOH) of vaginal discharge, or by identification of a culture or Gram staining yielding a positive result for a yeast species (Kalia et al., 2015; Xiao et al., 2016; Workowski et al., 2021). Two experienced microscopists blinded to the patients’ clinical information scored all the vaginal smears independently. The exclusion criteria included patients younger than 18 years or older than 50, Trichomonas vaginalis or AV/DIV, cervicitis or pelvic inflammation caused by Neisseria gonorrhoeae or Chlamydia trachomatis, pregnancy or lactation, menopause, innate or acquired immunodeficiency, and use of corticosteroid or antibiotics during the prior month.
No international medical standards for mixed vaginitis have been developed yet due to the lack of adequate studies. Referring to the latest version of Sexually Transmitted Infections Treatment Guidelines, 2021 and the Expert Consensus on Diagnosis and Treatment of Mixed vaginitis, we adopted oral metronidazole combined with local clotrimazole to treat the BV+VVC mixed vaginitis (Cooperative Group of Infectious Disease, 2021a; Workowski et al., 2021). All patients were treated with metronidazole (400 mg orally twice per day for 7 days) combined with clotrimazole (500 mg intravaginally once per day on days 1, 4, and 7). We obtained informed consents from patients to perform follow-ups 7 ± 3 days after treatment termination; in addition, we also performed secondary follow-ups of patients with incomplete remission of symptoms after their second treatment. The follow-up observation indicators included a general examination and detailed anamnesis, a routine gynecological examination, a routine examination of discharge, vaginal discharge pH determination, Nugent scoring, and microecological detection of vaginal discharge.
Vaginal samples were collected before and after the drug treatment as well as at the time of follow-up. Two vaginal swabs were placed into the vagina at a standard anatomical site (one third of the lateral vaginal wall) and rubbed against the vaginal wall at each visit, one for microecological detection of vaginal secretions and the other for subsequent vaginal microbiota analysis. At every visit, a specimen was taken from each patient for a vaginal fungal culture and drug sensitivity test. To evaluate drug efficacy, we used fungal microscopic examinations for VVC (cured patients had negative results while the uncured ones had positive results) and Amsel’s diagnostic criteria combining Nugent scores for BV (scores between 0 and 3 identified cured patients, scores between 4 and 6 identified those with intermediate BV, scores between 7 and 10 identified uncured patients).
16S rRNA Gene Sequencing
Total genomic DNA was extracted from samples using the SDS method (Lim et al., 2016). 16S rRNA genes (V3-V4) were amplified using specific primers with a barcode. PCR products were mixed at equal ratios. Next, the PCR products mix was purified using a Qiagen Gel Extraction Kit (Qiagen, Germany). Sequencing libraries were generated using a TruSeq® DNA PCR-Free Sample Preparation Kit (Illumina, USA) following manufacturer’s recommendations, and index codes were added. The library was sequenced on an Illumina NovaSeq using 2 x 250 base paired-end technology on an SP flow cell. This process generated an average of 93,000 reads per sample. Paired-end reads were merged using FLASH (VI.2.7, http://ccb.jhu.edu/software/FLASH/) (Magoč and Salzberg, 2011), and the splicing sequences were called raw tags. High quality tags were obtained (Bokulich et al., 2013) in a quality controlled process of QIIME (V1.9.1, http://qiime.org/scripts/splitlibrariesfastq.html) (Caporaso et al., 2010). The tags were compared with the reference database(Silva database, https://www.arb-silva.de/) using UCHIME algorithm (UCHIME Algorithm, http://www.drive5.com/usearch/manual/uchime_algo.html) (Edgar et al., 2011) to detect chimera sequences, and then the chimera sequences were removed (Haas et al., 2011). Then the Effective Tags finally obtained.
UHPLC-MS/MS Analysis
UHPLC-MS/MS analyses were performed using a Vanquish UHPLC system (ThermoFisher, Germany) coupled with an Orbitrap Q ExactiveTM HF mass spectrometer (Thermo Fisher, Germany). Samples were injected onto a Hypesil Goldcolumn (100×2.1 mm, 1.9 μm) using a 17-min linear gradient at a flow rate of 0.2 mL/min. The Orbitrap Q ExactiveTM HF mass spectrometer was operated in positive/negative polarity modes. The raw data files generated by the UHPLC-MS/MS were processed using Compound Discoverer 3.1 (CD3.1, Thermo Fisher) to perform peak alignment, peak picking, and quantitation for each metabolite. Statistical analyses were performed using the statistical software R (R version R-3.4.3), Python (Python 2.7.6 version) and CentOS (CentOS release 6.6). We attempted normal transformations using an area normalization method for non-normally distributed data.
Metabolite Analysis
The samples were placed into EP tubes and resuspended with pre-chilled 80% methanol using a vortex. Next, the samples were incubated on ice for 5 min and centrifuged at 15,000 g (4°C for 20 min). The supernatants were diluted to a final concentration containing 53% methanol by LC-MS grade water. The samples were subsequently transferred to fresh Eppendorf tubes and were then centrifuged at 15,000 g, 4°C for 20 min. Finally, the supernatants were injected into the LC-MS/MS system analysis (Want et al., 2006; Barri and Dragsted, 2013).
Metabolites were annotated using the KEGG (https://www.genome.jp/kegg/pathway.html), HMDB (https://hmdb.ca/metabolites) and LIPIDMaps (http://www.lipidmaps.org/) databases. Principal components analysis (PCA) and partial least squares discriminant analysis (PLS-DA) were performed with metaX (Wen et al., 2017) (a flexible and comprehensive software for processing metabolomics data). Volcano plots were used to filter metabolites of interest based on log2 (FoldChange), a log10 (P-value) of metabolites by ggplot2 in R language. For clustering heat maps, the data were normalized using z-scores of the intensity areas of differential metabolites and were plotted using a Pheatmap package in R language.
Statistical Analysis
SPSS19.0 software was used for statistical analyses. We analyzed changes in vaginal pH and Nugent scores using t tests, the improvement of main symptoms and efficacy were analyzed by χ² and U tests (all conducted using bilateral tests). In addition to the main efficacy indicators, pairwise comparisons were not carried out between groups for other indicators, and P<0.05 indicated significant differences between groups. The description of quantitative indicators is a calculation of the mean, standard deviation, median, minimum, and maximum. Classification indicators and grade indicators are described in terms of the number and percentage of each category.
For community clustering analyses, the clustering of communities was based on community composition and abundance using complete linkage hierarchical clustering with four clusters using R (version 4.0.0) (Zhou et al., 2007). The PCoA and heatmap of main genus abundance figures of vaginal microbial communities were generated using the ade4 and ComplexHeatmap package in R (version 4.0.0). For alpha diversity analyses, the Shannon index at the operational taxonomic units (OTU) level was calculated with QIIME (Version 1.9.1). We used a Wilcoxon rank sum test for comparing Shannon index differences between groups. Marker vaginal bacteria analyses were performed using a differential abundance of bacteria by LDA Effect Size (Segata et al., 2011), and we used a t-test to compare bacteria abundances between groups. Only genera with LDA scores >3 and p<0.05 were regarded as significant. Statistical analyses were performed using R (version 4.0.0).
For marker metabolite analyses, we applied univariate analyses (t-tests) to calculate the statistical significance (P-value) between groups. The metabolites with VIP > 1 and P-values < 0.01 and log2 fold changes ≥1 or ≤ -1 were considered to be differential metabolites. Volcano plots were used to filter metabolites of interest based on log2 (FoldChange) and log10 (P-value) of metabolites by ggplot2 in R (version 4.0.0). Genera–metabolome correlations were obtained by computing pairwise correlation coefficients using Spearman’s correlation coefficients between marker genera and marker metabolites for each group. We focused on genera–metabolite pairs for which the correlation coefficients were greater than or equal to 0.4 (Benjamin–Hochberg adjusted p <0.05).
Results
Grouping Information and Clinical Characteristics of the Study Population
We enrolled 48 patients in this study. We obtained data from all patients before and after treatment and at follow-ups, 39 patients did not undergo recurrences (groups M1–M4 in Table 1) but 9 patients experienced recurrences (group MR in Table 2). Recurrence in our study was defined as that either of BV or VVC relapsed after drug treatment, while merely the recurrence of VVC was observed in our study.
The mean age of the 48 patients was 32.92 ± 6.17 years; 38 patients had had previous VVC episodes (24 with a history of RVVC) and 13 patients had had previous BV episodes, while 9 patients had both. We observed that the symptoms and signs of patients with BV+VVC decreased significantly after treatment, indicating that this treatment plan can effectively relieve symptoms. The abundance of Lactobacillus and dominant bacteria in vaginal samples changed with the remission of symptoms. The vaginal pHs were lower than 4.5 after treatment (and significantly lower than the pHs before treatment).
Analysis and Comparison of Microecology Between Groups Before and After Treatment
We analyzed the scores of clinical symptoms and signs before treatment, during follow-up and recurrence, as well as the results of microscopic microecological evaluations, and we found similar indicators before treatment and Nugent score decreases after treatment. Overall, the BV cure rate was only 47.9% (23/48) while that of VVC reached 56.3% (27/48), this significant difference (p<0.05) indicates that this treatment may not be sufficient against bacterial vaginosis. Further analysis of the BV treatment outcomes showed that Nugent scores increased in 4 individuals, remained unchanged in 9, and decreased in 35 individuals after treatment, suggesting that 72.9% of patients with BV presented improvement after treatment (Supplementary Tables 1–3).
All vaginal fungal cultures were Candida albicans sensitive to clotrimazole. The microecological indicators of the patients in the MR group after relapse did not change significantly compared to those after treatment, therefore, we could not identify the microecological changes that promoted fungal relapses at a microscopic level.
Drug Treatment Affected the Composition of the Vaginal Microbiome
We classified the vaginal microbiome of all patients into clusters based on correlations between the members of their microbiome at the species level. As shown in Figure 1A, we divided each individual vaginal microbiome into four clusters with significant differences in their composition based on the species abundances at the species level. The heat map in Figure 1B shows the distribution of the horizontal abundance of each species in the four main clusters converted to log10. Figure 1C shows the bacterial Shannon diversity index distribution in different clusters. Among the four clusters, the highest diversity index was found in cluster 3 (Figure 1C, Cluster3), which was mainly enriched in Prevotella (Figure 1B, Cluster3). The Shannon diversity index of Cluster 1 was the lowest (Figure 1C, Cluster1), resulting in no enrichment of any single dominant genus (Figure 1B, Cluster1). By contrast, the Shannon diversity index of clusters 2 and 4 were higher than that of cluster 1 (Figure 1C, Cluster2, Cluster4), and both clusters were dominated by Lactobacillus microorganisms. Cluster 2 was mainly enriched in Lactobacillus iners (Figure 1B, Cluster2), while Cluster 4 was enriched in Lactobacillus crispatus (Figure 1B, Cluster4).
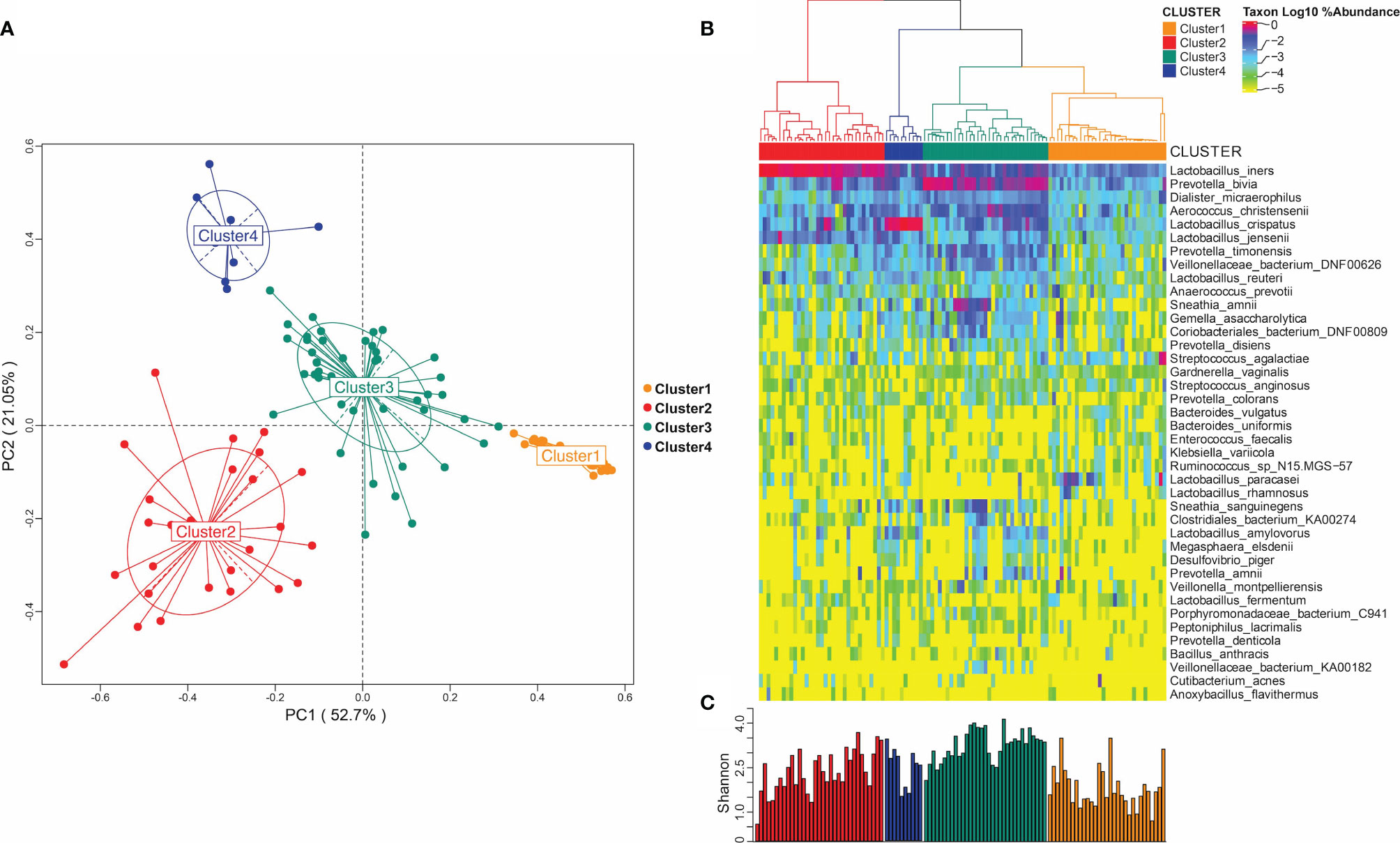
Figure 1 Microbiome of vaginal bacterial community. (A) Microbiome distribution obtained by PCoA clustering of the vaginal microbiota of all subjects; (B) heat maps of vaginal microbiome abundance distribution and the distribution of microbial community obtained by hierarchical clustering. (C) Shannon diversity index distribution in different microbial groups.
Figure 2A shows the vaginal microbiota distribution changes in different groups of patients after drug treatment. M1, M2 and M3 groups at different status were respectively represented as M1.1, M2.1, M3.1 (before treatment) and M1.2, M2.2, M3.2 (after treatment). MR group at different status were represented as MR.1 (before treatment), MR.2 (after treatment) and MR.3 (after recurrence). The microbiomes in patients of the M1 group were dominated by the genus Cluster2 and Cluster3 before treatment, while Cluster3 predominated in other groups of patients before the drug treatment (M2.1, M3.1, and MR.1). However, the vaginal microbiota changed significantly after the drug treatment and showed significant decreases in the proportions of Cluster3 in patient groups M1.2, M2.2, M3.2, and MR.2. In addition, the proportion of Cluster2 increased in patients of the M3 group after treatment (M3.2). By contrast, in the case of the patients of the relapse group (MR.1, MR.2, and MR.3), Cluster3 was predominant before treatment (MR. 1) and its proportion decreased or disappeared after treatment, while the proportions of Cluster2 and Cluster4 (dominated by Lactobacillus) increased significantly. However, after the relapse (MR.3), the proportion of Cluster 3 increased and the proportion of Cluster 2 decreased significantly.
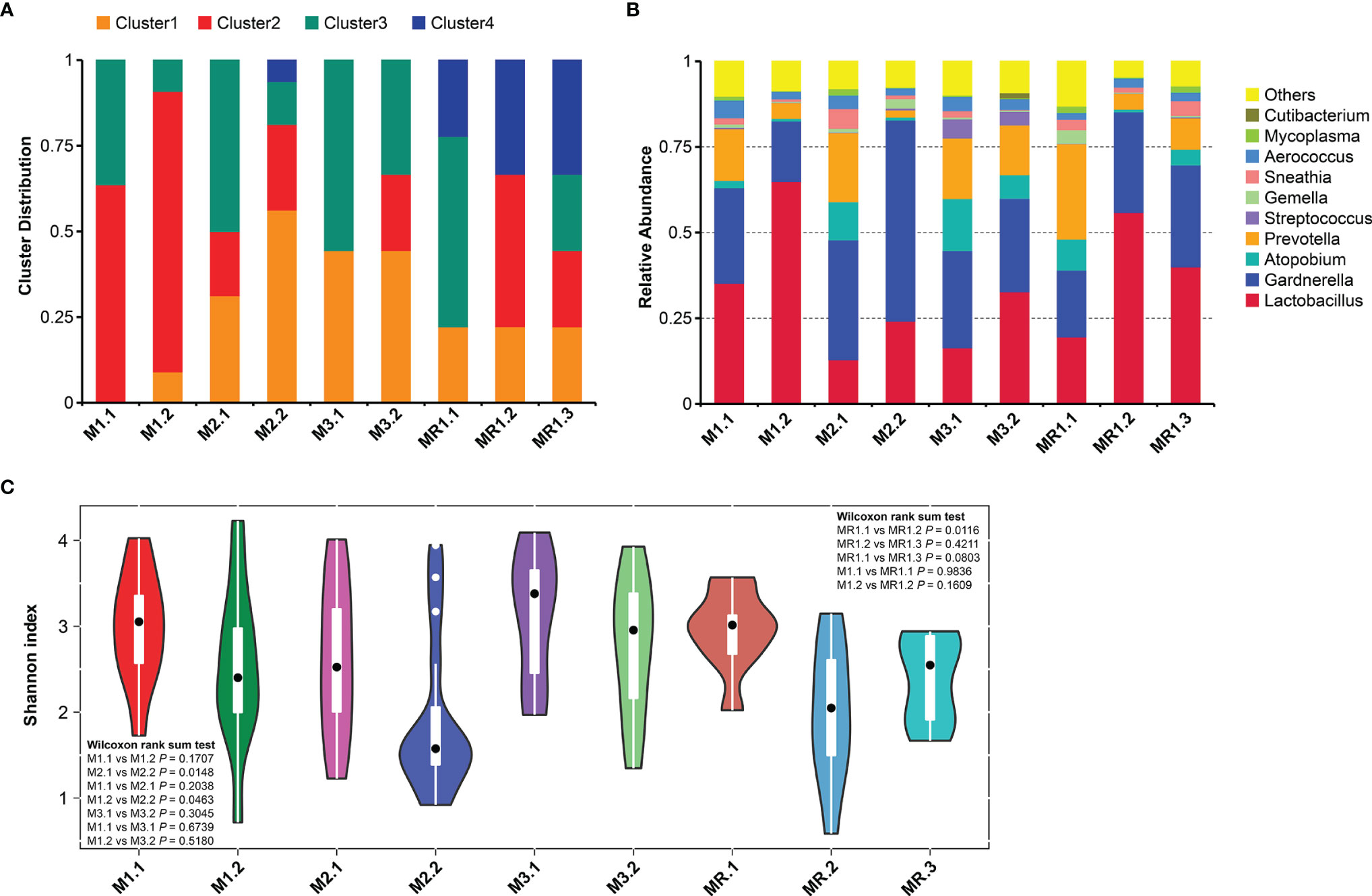
Figure 2 Changes in bacterial vaginal compositions in different patient groups. (A) Microbiota distributions before and after drug treatment. (B) Genus abundances before and after drug treatment. (C) Vaginal microbiome Shannon diversity indexes before and after drug treatment.
Figure 2B shows the variation in vaginal microbial abundance distributions at the genus level among all groups of patients. We found significant vaginal microbial composition imbalances before drug treatment in all subgroups (M1.1, M2.1, M3.1, and MR.1) with low levels of Lactobacillus and high levels of BV-associated microorganisms, such as Atopobium, Prevotella, and Gardnerella. The vaginal microbiome genus distributions changed significantly after drug treatment. The abundance of Lactobacillus increased significantly while that of BV-related Atopobium and Prevotella decreased in all subjects after drug treatment. However, the abundance of Gardnerella decreased only in the M1 group (M1.2) and did not improve significantly in the other groups; in fact the abundance of Gardnerella increased in the M2 group (M2.1, M2.2).
Drug Therapy Changes the Vaginal Microbiome Diversity
We conducted Shannon diversity analyses based on the distribution of at the vaginal species OTU for all patients, Figure 2 shows the Shannon diversity index changes for different patient groups before and after drug treatment. The Shannon diversity results of all patients differed before and after drug treatment, and were mainly manifested by a decreased diversity of vaginal bacteria after drug treatment; in addition, we found a statistically significant difference between the results of the M2 group (Figure 2C, M2.1 vs. M2.2, p=0.0148) and those of the MR group (Figure 2C; MR.1 vs. MR.2; p=0.0116). By contrast, we found differences in the vaginal microbiota diversity in different groups of patients on the basis of their treatment outcomes. As shown in Figure 2C, the diversities of M1 group patients (M1.1 and M1.2) were higher than that of the M2 group patients (M2.1 and M2.2) before and after treatment. Figure 2C shows that the diversity of the M1 group patients (M1.1 and M1.2) was lower than that of the M3 group patients (M3.1 and M3.2) before and after treatment, but the difference was not statistically significant; in addition, the diversities of M3 group patients before and after treatment were similar (M3.1 vs. M3.2, p=0.3045). As shown in Figure 2C, the bacterial diversities of the MR group patients decreased significantly after treatment (MR.1 vs. MR.2, p=0.0116), but increased after relapse, and we found no significant differences when compared to those before treatment (MR.1 vs. MR.3, p=0.0803).
Key Vaginal Microorganism Changes Associated With the Effect of Drug Therapy
We used linear discriminant analysis (LDA) and t-tests to analyze the changes in key vaginal microorganisms on the basis of different treatment outcomes and on the microbial abundance distributions at the genus and species levels for the patients’ groups (Figure 3).
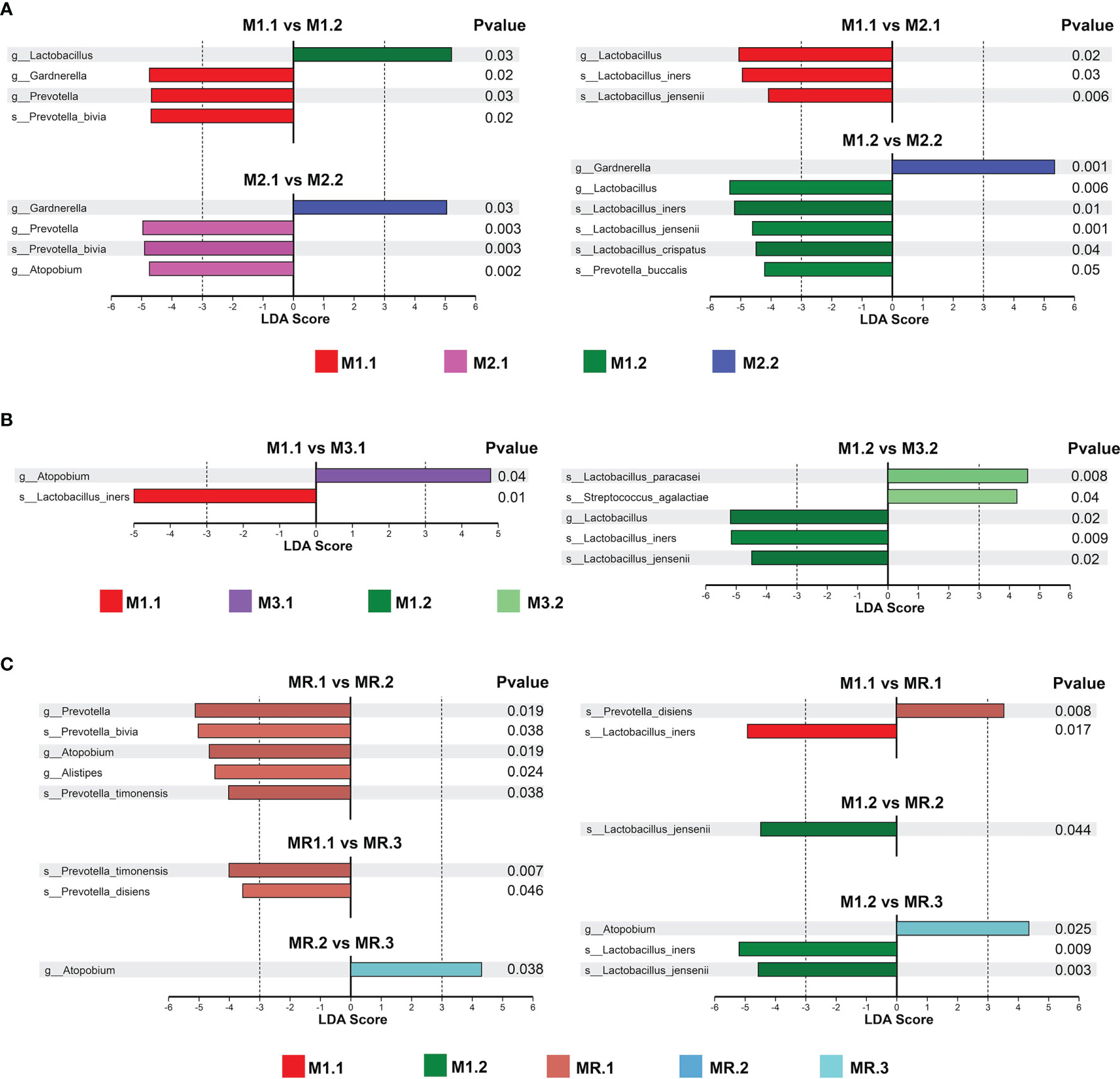
Figure 3 Key vaginal microorganism changes before and after treatment. LDA and T-test identified the most differentially abundant taxons between (A) patients completely cured (M1.1, M1.2) and patients with cured VVC plus uncured BV (M2.1, M2.2), (B) patients completely cured(M1.1, M1.2) and patients with cured BV plus uncured VVC (M3.1, M3.2), (C) patients completely cured (M1.1, M1.2) and patients with recurrence (MR.1, MR.2, MR.3).
As shown in Figure 3A, Prevotella and Gardnerella were predominant in M1.1 group patients before treatment, while Lactobacillus was predominant after treatment in M1.2 group patients. Prevotella and Atopobium were predominant in M2.1 group patients before treatment, while the Prevotella abundance decreased significantly after treatment in M2.2 group patients, but that of Gardnerella increased. Compared with the abundance in M2.1 group patients, the abundance of Lactobacillus was higher in M1.1 patients, suggesting that the failure to cure the BV may have been associated with the low baseline abundance of Lactobacillus. In addition, the abundance of Lactobacillus, especially those of Lactobacillus iners, Lactobacillus jensenii and Lactobacillus crispatus, was higher in M1.2 group patients than in M2.2 group patients with predominant Gardnerella. Moreover, the high abundance of Gardnerella probably correlates with the failure to cure BV.
As shown in Figure 3B, we found similar microbiome compositions in M3 group patients before and after treatment (M3.2 vs. M3.1), suggesting that the drug treatment failed to significantly change the vaginal microbiome of the patients in this group. The abundance of Lactobacillus, especially that of Lactobacillus iners, was lower in M3 group patients (M3.1 and M3.2) than in M1 group patients (M1.1 and M1.2) before and after treatment, suggesting a possible association between the treatment failure and a low Lactobacillus abundance. In addition, the abundance of Atopobium was high in M3 group patients before treatment (M3.1).
As shown in Figure 3C, in the recurrence group, the abundances of Prevotella and Atopobium were significantly decreased after treatment (MR.2) compared with those before treatment (MR.1); however, after the recurrence (MR.3), the abundance of Atopobium increased to a level similar to that before treatment (MR.1). By contrast, patients in the MR group (MR.1, MR.2 and MR.3) had lower Lactobacillus abundances (especially lower Lactobacillus iners and Lactobacillus jensenii abundances) and a higher Atopobium abundance than patients in the M1 group (M1.1 and M1.2) before and after treatment.
Correlation Between Metabolites and Key Vaginal Microbiota Associated With the Therapy Outcomes
The key bacteria found to be associated with the treatment outcomes included Lactobacillus, Atopobium, Prevotella and Gardnerella. In addition, we identified metabolites that were differentially expressed in vaginal discharge of different patients, including 2-hydroxyvaleric acid, tyramine, acetophenone, styrene, LysoPE, oleoyl ethanolamide, 16−hydroxyhexadecanoic acid, N−acetylmethionine, propionyl−L−carnitine, glycerol 1−hexadecanoate, α -linolenoyl ethanolamide, 4−hydroxybutyric acid (GHB), sedanolide, and thymidine (Supplementary Figures 1–5). We conducted correlation analyses to assess the potential association between key vaginal bacteria (Lactobacillus, Atopobium, Prevotella and Gardnerella) associated with the treatment outcomes and the differential metabolites in vaginal secretions (Figure 4).
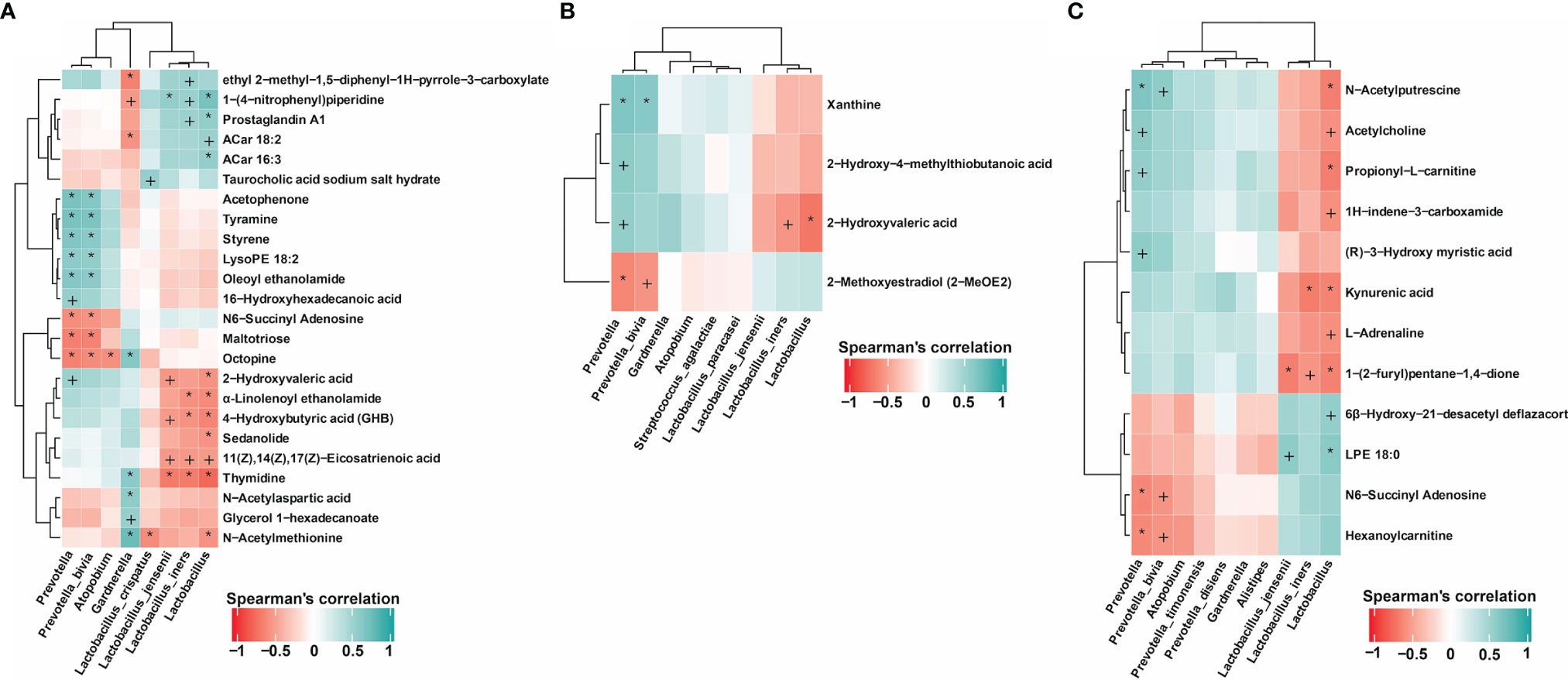
Figure 4 Correlation between metabolites and key vaginal microbiota associated with the drug therapy outcomes. (A) Association of key vaginal microorganisms and metabolites in patients completely cured (M1.1, M1.2) and in patients with cured VVC and untreated BV (M2.1, M2.2). (B) Associations between key vaginal microorganisms and metabolites in completely cured patients (M1.1, M1.2) and in uncured patients (M3.1, M3.2). (C) Association of key vaginal microorganisms and metabolites in completely cured patients (M1.1, M1.2) and in patients with recurrence (MR.1, MR.2, MR.3). P value of Spearman’s correlation: +<0.05, *<0.01.
As shown in Figure 4A, in the patients completely cured (M1.1 and M1.2) and in those with cured VVC and untreated BV (M2.1 and M2.2), the association of differential metabolites with the presence of key vaginal microorganisms showed that metabolites including tyramine, acetophenone, styrene, LysoPE, oleoyl ethanolamide, 16− hydroxyhexanoic acid, 2−hydroxyvaleric acid, thymidine, glycerol 1−hexadecanoate, N−acetylaspartic acid, and N−acetylmethionine were positively correlated with the presence of BV-related microorganisms including Atopobium, Prevotella and Gardnerella. In addition, 2−hydroxyvaleric acid, α-linolenoyl ethanolamide, 4−hydroxybutyric acid (GHB), sedanolide, thymidine, and N−acetylmethionine were negatively correlated with the presence of Lactobacillus. Moreover, 2-hydroxyvaleric acid was not only positively correlated with the presence of Prevotella but also negatively correlated with Lactobacillus and Lactobacillus iners. By contrast, we found amine metabolites, benzoones and acids such as tyramine, acetophenone, 16−hydroxyhexadecanoic acid, and 2−hydroxyvaleric acid to be positively correlated with the presence of Prevotella. Thymidine and N−acetylmethionine were also positively correlated with the presence of Gardnerella and negatively correlated with the presence of Lactobacillus, especially of Lactobacillus crispatus. Gardnerella was the predominant microorganism after treatment in the patients with cured VVC and untreated BV.
In completely cured patients (M1.1 and M1.2) and in uncured patients (M3.1, M3.2), the association analysis of differential metabolites with key vaginal microorganisms (Figure 4B) showed that 2−hydroxyvaleric acid was positively correlated with the presence of Prevotella and negatively correlated with the presence of Lactobacillus and Lactobacillus iners, further illustrating the important role of 2−hydroxyvaleric acid in the treatment of BV and VVC. By contrast, in completely cured patients (M1.1 and M1.2) and in patients with recurrences (MR.1, MR.2, and MR.3), our association analysis of differential metabolites and differential species revealed positive correlations between N−acetylputrescine, acetylcholine, and propionyl−L−carnitine and the presence of Prevotella and negative correlations with the presence of Lactobacillus (Figure 4C).
Discussion
For this study, we investigated the composition, diversity and other characteristics of the vaginal microbiome in patients with BV+VVC mixed vaginitis before and after drug treatment. As mentioned, mixed vaginitis and vaginal co-infections are different entities. Based on the literature and our own results, patients presenting BV and VVC simultaneously have their own characteristic symptoms and signs of vaginitis rather than a simple overlay microbiome, Lactobacillus iners and Prevotella predominate in their vaginal microbiome and the abundances of Gardnerella and Atopobium are also higher than those in healthy women. Previous studies have focused on single types of vaginitis or in asymptomatic co-infections with multiple pathogenic micro-organisms. For example, in 2005, Fredricks et al. (Fredricks et al., 2005) found that compared with the vaginal microbiome of healthy women, that of women with BV had a significant increase in its diversity, with low abundance of Lactobacillus crispatus (significantly decreased to almost inexistent) but detection of Lactobacillus iners in most patients. In addition, the abundances of Gardnerella, Atopobium, Megasphaera, Leptotrichia, and BVAB 1-3 were significantly increased and correlated with the presence of BV (p <0.001). McKloud et al. (McKloud et al., 2021) found that the vaginal microbiome of women with recurrent VVC was dominated by bacteria of the genus Lactobacillus with a significant increase in the Candida abundance compared with the abundance in healthy women. The levels of bacterial diversity and the abundances of vaginal anaerobic bacteria such as Gardnerella and Prevotella were similar, but slightly higher in healthy women. The abundances of Lactobacillus crispatus and Lactobacillus jensenii dropped from 44% in healthy women to 30% in women with RVVC, while the abundance of Lactobacillus iners increased from 19% in healthy women to 40% in women with RVVC. The abundances of Lactobacillus iners in the two groups were significantly different.
On the basis of the high abundances of BV-related pathogens in the treatment failure and recurrence groups in this study, we believe that BV-related pathogens play a major pathogenic role in patients with BV+VVC mixed vaginitis and determine their treatment outcome and the occurrence of relapses. We found that Gardnerella abundance increased in the VVC cured but BV uncured group after treatment (M2.2); patients in the MR recurrence group had lower Lactobacillus abundances (especially Lactobacillus iners and Lactobacillus jensenii) and a higher Prevotella and Atopobium abundance than patients in the M1 group before and after treatment. Gardnerella, Prevotella, and Atopobium were the main pathogenic bacteria of BV in our study, and other studies have identified synergistic effects between them, allowing them to form multi-strain biofilms and to further lead to refractory infections and recurrences. Moreover, each bacterium plays a different role during the infections. Prevotella can secrete proteases to degrade host antibodies and transfer ammonia to Gardnerella, leading to excessive secretion of ammonia in the host vagina, reducing mucosal immunity (Xie et al., 2020), and promoting the growth of other anaerobic bacteria. However, in the course of our limited follow-up, we found that the abundance of Prevotella decreased significantly after metronidazole treatment and did not increase again, a finding consistent with the reported high drug sensitivity of Prevotella to metronidazole (Ulger Toprak et al., 2018). Thus, we believe that Prevotella probably did not play a significant role in recurrences. Gardnerella, the main bacteria in the formation of BV biofilms, can adhere to vaginal epithelial cells and acts as a scaffold for the formation of biofilms. Dense biofilms can tolerate high concentrations of H2O2 and lactic acid, and have enhanced resistance to antibiotics and host mucosal immune defenses (Hardy et al., 2017). The presence of this specific type of biofilm can lead to resistance of Gardnerella to metronidazole, causing BV treatment difficulties and recurrences (He et al., 2021). In the BV pathogenesis model (Muzny et al., 2020), Gardnerella and Prevotella, as initially colonizing bacteria, are highly abundant in the vagina of patients with BV, but the formation of a biofilm does not induce a strong inflammatory response in vaginal epithelial cells and instead promotes immune escape. The secondary colonizer Atopobium is more likely to effectively stimulate the host to produce a strong immune response against the BV, leading to the corresponding signs and symptoms. A synergistic effect may exist between the two types of colonizers (Castro et al., 2019; Vestby et al., 2020). In addition, previous studies have found that the sensitivity of different clinical isolates of Atopobium to metronidazole varies greatly and more than half of the isolates display drug resistance to metronidazole (Schuyler et al., 2015; Shaskolskiy et al., 2016; Mendling et al., 2019), which suggests that targeted drugs with high sensitivity to Atopobium are needed to reduce recurrences during the treatment of patients with BV+VVC mixed vaginitis.
Although multi-species biofilms play a role in recurrences, the type and abundance of Lactobacillus also had a considerable influence on infection and recurrences (Ceccarani et al., 2019; Redelinghuys et al., 2020). The results of this study showed that before treatment the completely cured patients (M1.1) had a higher abundance of Lactobacillus iners than the patients with recurrences (MR.1), whereas the significantly higher abundance of Lactobacillus jensenii was established earlier in the completely cured patients after treatment (M1.2) than in the patients with recurrences (MR.2), suggesting that the abundance of Lactobacillus iners and Lactobacillus jensenii correlate with the recurrence of BV+VVC mixed vaginitis after a cure. On the basis of existing findings and our results, we can speculate that the absence of Lactobacillus crispatus and other protective Lactobacillus from the vaginal microenvironment of patients with mixed vaginitis, Lactobacillus iners may tend to act as the protector of the vaginal epithelium. Verwijs et al. (Verwijs et al., 2020) assessed the vaginal microbiota of 68 women with BV who received metronidazole treatment and found results similar to ours: Lactobacillus iners was the most common Lactobacillus species before and after treatment, the abundance of L. jensenii was also significantly increased after treatment, and that of Lactobacillus crispatus was significantly decreased before treatment and only slightly increased after treatment. After analyzing the vaginal microbiota of patients treated with metronidazole gel, Lactobacillus iners was the only dominant strain 30 days after treatment, indicating that Lactobacillus iners was resistant to metronidazole, while Lactobacillus crispatus was probably more susceptible to metronidazole in the BV environment (Petrova et al., 2017). Moreover, due to the ineffectiveness of metronidazole against Lactobacillus iners, the removal of BV-related pathogens with therapy results in a vaginal microbiome with predominant Lactobacillus iners. Therefore, combining with our results, assessing the level of Lactobacillus iners in the vagina of patients with mixed vaginitis before treatment may have a predictive value for the treatment outcome and, promoting the recovery of the Lactobacillus abundance in the vagina may help to enhance the treatment effects beyond antibiotic treatment.
This study was limited mainly by the size of the patient cohort, and larger prospective studies are needed to confirm our results. This is our first study to explore the vaginal microbiome of the patients with BV+VVC mixed vaginitis, so we merely measured the relative abundances. Apart from this, all our fungal culture results showed Candida albicans, preventing us from studying more on various vaginal fungus. In addition, the final cure rates of BV and VVC in this study were 47.92% (23/48) and 56.25% (27/48), respectively, revealing a better therapeutic effect for VVC than for BV. The cure rates in our study were low probably owing to our therapy targeting VVC with more potency than BV, future studies should apply longer periods of BV-targeted treatments.
In conclusion, we observed significant vaginal microbiome alterations in patients with BV+VVC mixed vaginitis before and after drug treatment, with the key causative bacteria including Gardnerella, Atopobium, and Lactobacillus. We have found some special bacterial in this study and we will consider proceeding with further quantitative research in the subsequent studies. The therapy of oral metronidazole with local clotrimazole to treat BV+VVC mixed vaginitis was effective in our study. And to enhance treatment for BV may be more favorable for prognosis of patients with BV+VVC mixed vaginitis. The abundance of Lactobacillus in women with mixed vaginitis has a great influence on the recovery of a normal vaginal microbiome and on the prognosis, and it should be actively restored.
Data Availability Statement
The datasets presented in this study can be found in online repositories. The names of the repository/repositories and accession number(s) can be found below: NCBI SRA database, accession PRJNA801439 and EMBL-EBI MetaboLights database, accession MTBLS4222.
Ethics Statement
The studies involving human participants were reviewed and approved by The Ethics Committee of Peking University First Hospital (V2.0/201504.20). The patients/participants provided their written informed consent to participate in this study. Written informed consent was obtained from the individual(s) for the publication of any potentially identifiable images or data included in this article.
Author Contributions
BX and DZ conceived the study design. BX, LM, and DZ recruited volunteers and collected samples. LM and DZ were responsible for performing the laboratory assays. BX, DA, and HQ performed the data analysis. DA wrote the initial manuscript. BX and DZ revised the manuscript. All authors contributed to the article and approved the submitted version.
Funding
This work was supported by the grants of the National Natural Science Foundation of China (No. 81971342 and No. 81200411).
Conflict of Interest
The authors declare that the research was conducted in the absence of any commercial or financial relationships that could be construed as a potential conflict of interest.
Publisher’s Note
All claims expressed in this article are solely those of the authors and do not necessarily represent those of their affiliated organizations, or those of the publisher, the editors and the reviewers. Any product that may be evaluated in this article, or claim that may be made by its manufacturer, is not guaranteed or endorsed by the publisher.
Acknowledgments
We would like to thank all the participants for their support and cooperation. We would also like to thank all the researchers, clinicians, and technicians involved in our study for their participation and technical support.
Supplementary Material
The Supplementary Material for this article can be found online at: https://www.frontiersin.org/articles/10.3389/fcimb.2022.860589/full#supplementary-material
References
Barri, T., Dragsted, L. O. (2013). UPLC-ESI-QTOF/MS and Multivariate Data Analysis for Blood Plasma and Serum Metabolomics: Effect of Experimental Artefacts and Anticoagulant. Anal. Chim. Acta 768, 118–128. doi: 10.1016/j.aca.2013.01.015
Benyas, D., Sobel, J. D. (2022). Mixed Vaginitis Due to Bacterial Vaginosis and Candidiasis. J. Low Genit. Tract. Dis. 26 (1), 68–70. doi: 10.1097/lgt.0000000000000641
Bokulich, N. A., Subramanian, S., Faith, J. J., Gevers, D., Gordon, J. I., Knight, R., et al. (2013). Quality-Filtering Vastly Improves Diversity Estimates From Illumina Amplicon Sequencing. Nat. Methods 10 (1), 57–59. doi: 10.1038/nmeth.2276
Caporaso, J. G., Kuczynski, J., Stombaugh, J., Bittinger, K., Bushman, F. D., Costello, E. K., et al. (2010). QIIME Allows Analysis of High-Throughput Community Sequencing Data. Nat. Methods 7 (5), 335–336. doi: 10.1038/nmeth.f.303
Castro, J., Machado, D., Cerca, N. (2019). Unveiling the Role of Gardnerella Vaginalis in Polymicrobial Bacterial Vaginosis Biofilms: The Impact of Other Vaginal Pathogens Living as Neighbors. Isme. J. 13 (5), 1306–1317. doi: 10.1038/s41396-018-0337-0
Ceccarani, C., Foschi, C., Parolin, C., D'Antuono, A., Gaspari, V., Consolandi, C., et al. (2019). Diversity of Vaginal Microbiome and Metabolome During Genital Infections. Sci. Rep. 9 (1), 14095. doi: 10.1038/s41598-019-50410-x
Cooperative Group of Infectious Disease, C.S.o.O.a.G, Chinese Medical Association (2021a). Consensus on the Diagnosis and Treatment of Mixed Vaginitis, (2021 Edition). Chin. J. Obstet. Gynecol. 56 (01), 15–18. doi: 10.3760/cma.j.cn112141-20200603-00472
Cooperative Group of Infectious Disease, C.S.o.O.a.G, Chinese Medical Association (2021b). Guideline for Diagnosis and Treatment of Bacterial Vaginosis, (2021 Revised Edition). Chin. J. Obstet. Gynecol. 56 (01), 3–6. doi: 10.3760/cma.j.cn112141-20200717-00583
Edgar, R. C., Haas, B. J., Clemente, J. C., Quince, C., Knight, R. (2011). UCHIME Improves Sensitivity and Speed of Chimera Detection. Bioinformatics 27 (16), 2194–2200. doi: 10.1093/bioinformatics/btr381
Fredricks, D. N., Fiedler, T. L., Marrazzo, J. M. (2005). Molecular Identification of Bacteria Associated With Bacterial Vaginosis. N. Engl. J. Med. 353 (18), 1899–1911. doi: 10.1056/NEJMoa043802
Haas, B. J., Gevers, D., Earl, A. M., Feldgarden, M., Ward, D. V., Giannoukos, G., et al. (2011). Chimeric 16s rRNA Sequence Formation and Detection in Sanger and 454-Pyrosequenced PCR Amplicons. Genome Res. 21 (3), 494–504. doi: 10.1101/gr.112730.110
Hardy, L., Cerca, N., Jespers, V., Vaneechoutte, M., Crucitti, T. (2017). Bacterial Biofilms in the Vagina. Res. Microbiol. 168 (9-10), 865–874. doi: 10.1016/j.resmic.2017.02.001
He, Y., Na, R., Niu, X., Xiao, B., Yang, H. (2021). Lactobacillus Rhamnosus and Lactobacillus Casei Affect Various Stages of Gardnerella Species Biofilm Formation. Front. Cell Infect. Microbiol. 11, 568178. doi: 10.3389/fcimb.2021.568178
Kalia, N., Singh, J., Kaur, M. (2020). Microbiota in Vaginal Health and Pathogenesis of Recurrent Vulvovaginal Infections: A Critical Review. Ann. Clin. Microbiol. Antimicrob. 19 (1), 5. doi: 10.1186/s12941-020-0347-4
Kalia, N., Singh, J., Sharma, S., Kamboj, S. S., Kaur, M. (2015). Prevalence of Vulvovaginal Infections and Species Specific Distribution of Vulvovaginal Candidiasis in Married Women of North India. Int. J. Curr. Microbiol. App. Sci. 4 (8), 253–266.
Lim, H. J., Lee, E. H., Yoon, Y., Chua, B., Son, A. (2016). Portable Lysis Apparatus for Rapid Single-Step DNA Extraction of Bacillus Subtilis. J. Appl. Microbiol. 120 (2), 379–387. doi: 10.1111/jam.13011
Magoč, T., Salzberg, S. L. (2011). FLASH: Fast Length Adjustment of Short Reads to Improve Genome Assemblies. Bioinformatics 27 (21), 2957–2963. doi: 10.1093/bioinformatics/btr507
McKloud, E., Delaney, C., Sherry, L., Kean, R., Williams, S., Metcalfe, R., et al. (2021). Recurrent Vulvovaginal Candidiasis: A Dynamic Interkingdom Biofilm Disease of Candida and Lactobacillus. mSystems 6 (4), e0062221. doi: 10.1128/mSystems.00622-21
Mendling, W., Palmeira-de-Oliveira, A., Biber, S., Prasauskas, V. (2019). An Update on the Role of Atopobium Vaginae in Bacterial Vaginosis: What to Consider When Choosing a Treatment? A Mini Review. Arch. Gynecol. Obstet. 300 (1), 1–6. doi: 10.1007/s00404-019-05142-8
Muzny, C. A., Łaniewski, P., Schwebke, J. R., Herbst-Kralovetz, M. M. (2020). Host-Vaginal Microbiota Interactions in the Pathogenesis of Bacterial Vaginosis. Curr. Opin. Infect. Dis. 33 (1), 59–65. doi: 10.1097/qco.0000000000000620
Paavonen, J., Brunham, R. C. (2018). Bacterial Vaginosis and Desquamative Inflammatory Vaginitis. N. Engl. J. Med. 379 (23), 2246–2254. doi: 10.1056/NEJMra1808418
Petrova, M. I., Reid, G., Vaneechoutte, M., Lebeer, S. (2017). Lactobacillus Iners: Friend or Foe? Trends Microbiol. 25 (3), 182–191. doi: 10.1016/j.tim.2016.11.007
Qi, W., Li, H., Wang, C., Li, H., Zhang, B., Dong, M., et al. (2021). Recent Advances in Presentation, Diagnosis and Treatment for Mixed Vaginitis. Front. Cell Infect. Microbiol. 11, 759795. doi: 10.3389/fcimb.2021.759795
Redelinghuys, M. J., Geldenhuys, J., Jung, H., Kock, M. M. (2020). Bacterial Vaginosis: Current Diagnostic Avenues and Future Opportunities. Front. Cell Infect. Microbiol. 10, 354. doi: 10.3389/fcimb.2020.00354
Schuyler, J. A., Mordechai, E., Adelson, M. E., Gygax, S. E., Hilbert, D. W. (2015). Draft Genome Sequence of a Metronidazole-Susceptible Atopobium Vaginae Isolate. Genome Announc. 3 (5), e00991–00915. doi: 10.1128/genomeA.00991-15
Segata, N., Izard, J., Waldron, L., Gevers, D., Miropolsky, L., Garrett, W. S., et al. (2011). Metagenomic Biomarker Discovery and Explanation. Genome Biol. 12 (6), R60. doi: 10.1186/gb-2011-12-6-r60
Shaskolskiy, B., Dementieva, E., Leinsoo, A., Runina, A., Vorobyev, D., Plakhova, X., et al. (2016). Drug Resistance Mechanisms in Bacteria Causing Sexually Transmitted Diseases and Associated With Vaginosis. Front. Microbiol. 7, 747. doi: 10.3389/fmicb.2016.00747
Sobel, J. D., Subramanian, C., Foxman, B., Fairfax, M., Gygax, S. E. (2013). Mixed Vaginitis-More Than Coinfection and With Therapeutic Implications. Curr. Infect. Dis. Rep. 15 (2), 104–108. doi: 10.1007/s11908-013-0325-5
Ulger Toprak, N., Veloo, A. C. M., Urban, E., Wybo, I., Justesen, U. S., Jean-Pierre, H., et al. (2018). A Multicenter Survey of Antimicrobial Susceptibility of Prevotella Species as Determined by Etest Methodology. Anaerobe 52, 9–15. doi: 10.1016/j.anaerobe.2018.05.005
van Schalkwyk, J., Yudin, M. H. (2015). Vulvovaginitis: Screening for and Management of Trichomoniasis, Vulvovaginal Candidiasis, and Bacterial Vaginosis. J. Obstet. Gynaecol. Can. 37 (3), 266–274. doi: 10.1016/s1701-2163(15)30316-9
Verwijs, M. C., Agaba, S. K., Darby, A. C., van de Wijgert, J. (2020). Impact of Oral Metronidazole Treatment on the Vaginal Microbiota and Correlates of Treatment Failure. Am. J. Obstet. Gynecol. 222 (2), 157.e151–157.e113. doi: 10.1016/j.ajog.2019.08.008
Vestby, L. K., Grønseth, T., Simm, R., Nesse, L. L. (2020). Bacterial Biofilm and Its Role in the Pathogenesis of Disease. Antibiot. (Basel) 9 (2), 59. doi: 10.3390/antibiotics9020059
Want, E. J., O'Maille, G., Smith, C. A., Brandon, T. R., Uritboonthai, W., Qin, C., et al. (2006). Solvent-Dependent Metabolite Distribution, Clustering, and Protein Extraction for Serum Profiling With Mass Spectrometry. Anal. Chem. 78 (3), 743–752. doi: 10.1021/ac051312t
Wen, B., Mei, Z., Zeng, C., Liu, S. (2017). Metax: A Flexible and Comprehensive Software for Processing Metabolomics Data. BMC Bioinf. 18 (1), 183. doi: 10.1186/s12859-017-1579-y
Workowski, K. A., Bachmann, L. H., Chan, P. A., Johnston, C. M., Muzny, C. A., Park, I., et al. (2021). Sexually Transmitted Infections Treatment Guidelines 2021. MMWR Recomm. Rep. 70 (4), 1–187. doi: 10.15585/mmwr.rr7004a1
Xiao, B., Niu, X., Han, N., Wang, B., Du, P., Na, R., et al. (2016). Predictive Value of the Composition of the Vaginal Microbiota in Bacterial Vaginosis, a Dynamic Study to Identify Recurrence-Related Flora. Sci. Rep. 6, 26674. doi: 10.1038/srep26674
Xie, Y., Feng, Y., Li, W., Zhan, F., Huang, G., Hu, H., et al. (2020). Revealing the Disturbed Vaginal Micobiota Caused by Cervical Cancer Using High-Throughput Sequencing Technology. Front. Cell Infect. Microbiol. 10, 538336. doi: 10.3389/fcimb.2020.538336
Zhang, Z., Liu, Z. (2020). Mixed Vaginitis and Vaginal Microecology. Chin. J. Pract. Gynecol. Obstet. 36 (02), 185–189. doi: 10.19538/j.fk2020020122
Keywords: mixed vaginitis, vulvovaginal candidiasis (VVC), vaginal microbiome, Gardnerella, Atopobium, Lactobacillus, bacterial vaginosis (BV)
Citation: Xiao B, A D, Qin H, Mi L and Zhang D (2022) Correlation Analysis of Vaginal Microbiome Changes and Bacterial Vaginosis Plus Vulvovaginal Candidiasis Mixed Vaginitis Prognosis. Front. Cell. Infect. Microbiol. 12:860589. doi: 10.3389/fcimb.2022.860589
Received: 23 January 2022; Accepted: 15 February 2022;
Published: 08 March 2022.
Edited by:
Claudio Foschi, University of Bologna, ItalyReviewed by:
Manpreet Kaur, Guru Nanak Dev University, IndiaGregory A. Buck, Virginia Commonwealth University, United States
Copyright © 2022 Xiao, A, Qin, Mi and Zhang. This is an open-access article distributed under the terms of the Creative Commons Attribution License (CC BY). The use, distribution or reproduction in other forums is permitted, provided the original author(s) and the copyright owner(s) are credited and that the original publication in this journal is cited, in accordance with accepted academic practice. No use, distribution or reproduction is permitted which does not comply with these terms.
*Correspondence: Dai Zhang, bWFpbHpoYW5nZGFpQHNpbmEuY29t