- 1Department of Parasitology, Faculty of Science, Charles University, Prague, Czechia
- 2Transmission Biology, The Pirbright Institute, Surrey, United Kingdom
- 3Departamento de Parasitologia, Instituto de Ciências Biomédicas, Cidade Universitária, São Paulo, Brazil
Parasites of the genus Porcisia, together with the genus Endotrypanum, form a sister clade to the species-rich and medically important genus Leishmania. Both Porcisia species, P. hertigi and P. deanei, are dixenous parasites of Neotropical porcupines. Almost 50 years after their first discovery, knowledge of their life cycle remains poor and their insect vectors are unknown. Because competent vectors of their closest phylogenetic relatives, genera Endotrypanum and Leishmania, are phlebotomine sand flies (Diptera: Psychodidae) and/or biting midges (Diptera: Ceratopogonidae), we examined here the potential of both sand flies and biting midges to transmit Porcisia parasites. The insects (Lutzomyia longipalpis, L. migonei and Culicoides sonorensis) were exposed to parasites through the chicken skin membrane and dissected at various time intervals post bloodmeal. Potentially infected females were also allowed to feed on the ears of anaesthetized BALB/c mice and the presence of parasite DNA was subsequently confirmed in the mice by PCR. Porcisia hertigi did not survive defecation in L. longipalpis or L. migonei, suggesting that these sand fly species are unlikely to serve as natural vectors of this parasite. Similarly, P. hertigi infections were lost in Culicoides midges. In contrast, mature P. deanei infections developed in 51-61% of L. longipalpis females, 7.3% of L. migonei females and 7.7% of Culicoides sonorensis females. In all three vector species, P. deanei colonized predominantly Malpighian tubules and produced metacyclic infective forms. Transmission of P. daenei to BALB/c mice was demonstrated via the prediuresis of L. longipalpis females. This mode of transmission, as well the colonization of Malpighian tubules as the dominant tissue of the vector, is unique among trypanosomatids. In conclusion, we demonstrated the vector competence of L. longipalpis for P. deanei but not for P. hertigi, and further studies are needed to evaluate competence of other Neotropical vectors for these neglected parasites.
Introduction
Porcisia are dixenous parasites of Neotropical porcupines. The genus was established in 2016 for two species, originally described as Leishmania hertigi and L. deanei (Espinosa et al., 2016). These two species are found in different porcupine species, in different geographical regions, and are clearly separated by molecular markers (Espinosa et al., 2016).
Leishmania hertigi was originally found in Coendou rothschildi (according to the taxonomical revision of the genus Coendou (Voss, 2015) the species is now called Coendou quichua) from Central Panama (Herrer, 1971) and later in Coendou mexicanus from Costa Rica (Zeledón et al., 1977). While the parasite was widespread in Panama and showed very high prevalence rates (75 - 100%), it was not so common in Costa Rica, where it was detected in a single individual out of 25 porcupines captured in three different localities (Herrer, 1971; Zeledón et al., 1977). Parasites are harmless to the mammalian host. Although they spread in the skin throughout the body and often enter internal organs, they do not cause any gross skin alteration (Herrer, 1971). It also appears to be host-specific, despite extensive searches, it has not been detected in hosts other than Coendou (Herrer et al., 1966) and laboratory infections of Mesocricetus auratus, Sigmodon hispidus, Jaculus jaculus and Cavia porcellus have been short-lasting and limited to the inoculation site (Herrer, 1971).
Similar parasites of porcupines (Coendou prehensilis, C. nycthemera and Coendou sp.) have been reported from Brazil (Deane et al., 1974; Lainson and Shaw, 1977) and named first Leishmania hertigi deanei (Lainson and Shaw, 1977), later raised to the specific status of L. deanei by Lainson and Shaw (1987). Parasites later described in Brazil (da Silva et al., 2013; Thies et al., 2018) as L. hertigi are probably also L. deanei. Their species identity had been based on 18S rDNA sequences, which are conserved and may not distinguish between these closely related species (Espinosa et al., 2016). Similar to L. hertigi, L. deanei did not produce any visible pathological effects in its natural host and attempts to infect hamsters and guinea-pigs were unsuccessful (Lainson and Shaw, 1977).
Almost 50 years after their first discovery; the vectors of both Porcisia species remain unknown. Since Porcisia are closely related to genera Endotrypanum and Leishmania (Espinosa et al., 2016), they are most likely transmitted by sand flies of the family Psychodidae (proven vectors of most species of the genus Leishmania and likely vectors of the genus Endotrypanum; Franco et al., 1997; Maroli et al., 2013) or biting midges of the family Ceratopogonidae (recently proven to be competent vectors of the Leishmania (Mundinia) subgenus; Dougall et al., 2011; Chanmol et al., 2019; Becvar et al., 2021). Experimental studies from the 1960s-1970s described the poor development of P. hertigi and P. deanei in L. longipalpis (Lainson et al., 1977; Croft and Molyneux, 1979) and L. sanquinaria (Herrer et al., 1966). In Sinop, Brazil, Porcisia DNA was recently detected in Lutzomyia antunesi (Thies et al., 2018). However, whether the parasite is able to complete development in this Lutzomyia species is unclear.
Our study was aimed at detailed assessment of the vector competence of two Neotropical sand fly species, L. longipalpis and L. migonei, known as vectors of Leishmania infantum and Leishmania braziliensis (Guimarães et al., 2016; Dvořák et al., 2018; Alexandre et al., 2020). According to Shimabukuro et al. (2017), they share a distribution range with P. deanei and L. longipalpis also with P. hertigi (Figure 1). In addition, we tested development of both Porcisia species in Culicoides sonorensis, North American biting midge known to experimentally transmit L. (Mundinia) sp. (Chanmol et al., 2019; Becvar et al., 2021).
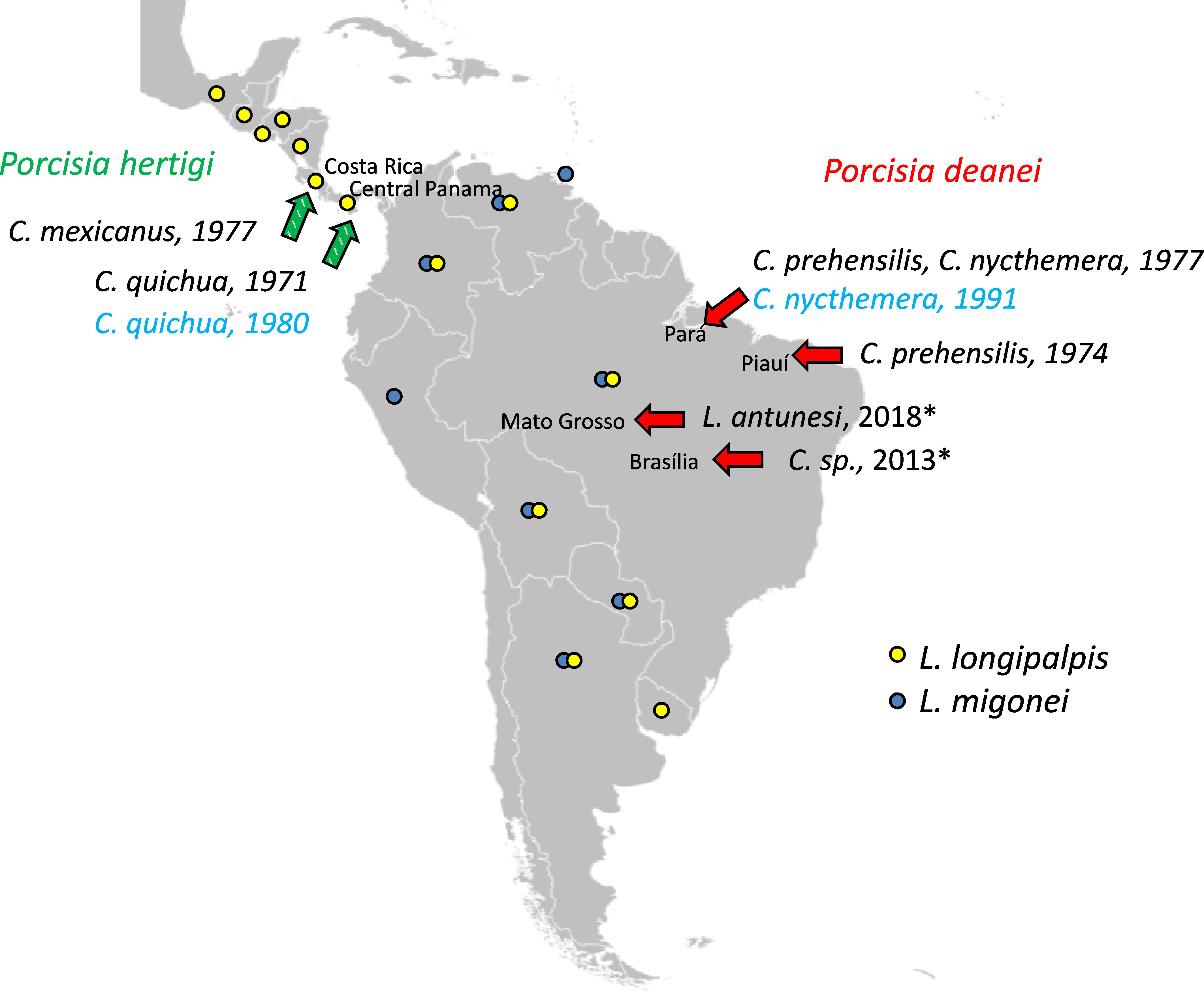
Figure 1 Locations of Porcisia reports. Green arrows indicate P. hertigi records and red arrows P. deanei records with publication/isolation dates and host species, based on (Herrer, 1971; Deane et al., 1974; Zeledón et al., 1977; Lainson and Shaw, 1977; da Silva et al., 2013). Asterisks indicate two Porcisia records identified as P. hertigi, but the species identification method was not sufficient to distinguish the two Porcisia species, and based on geographic region and host species, both are P. deanei (Espinosa et al., 2016). Strains used in this study are indicated in blue. Countries with records of L. longipalpis and L. migonei according to Shimabukuro et al., 2017 are marked with yellow and blue circles, respectively. The blank map source – https://commons.wikimedia.org.
Materials and methods
Sand flies, parasites and mice
Colonies of sand flies L. longipalpis and L. migonei (both originally from Brazil) were maintained in the insectary of the Department of Parasitology, Charles University in Prague, under standardized conditions (26°C, fed on 50% sucrose and photoperiod 14 h light/10 h dark) as described previously (Volf and Volfova, 2011). Culicoides sonorensis biting midges were sent to Charles University from The Pirbright Institute, UK and kept at the same conditions as the sand flies.
Promastigotes of Porcisia deanei (MCOE/BR/91/M13541; TCC 258) isolated from C. nycthemera in Acara region, Brazil, P. hertigi (MCOE/PA/80/C8; TCC 260) isolated from C. quichua in 1980 in central Panama and L. infantum (MHOM/TR/2000/OG-VL) were cultured in M199 medium (Sigma) containing 10% heat-inactivated foetal calf serum (FBS, Gibco) and 2% sterile human urine (provided by J.Sa.) supplemented with 1% BME vitamins (Basal Medium Eagle, Sigma) and 250 µl/mL amikacin (Amikin, Bristol-Myers Squibb).
BALB/c mice originated from AnLab. s.r.o. (Harlan Laboratories, USA, Nederland). Animals were housed in T3 breeding containers (Velaz) equipped with bedding (German Horse Span Pferde) and breeding material (Woodwool), receiving standard ST-1 feed (Velaz) and water ad libitum, with a 12 h light/12 h dark photoperiod, temperature 22–25°C and humidity 40–60%.
Experimental infections of sand flies and biting midges
Promastigotes from log-phase cultures were resuspended in heat-inactivated defibrinated ram blood at a concentration of 1 × 106 promastigotes/ml. Female insects were infected by feeding through a chick-skin membrane and engorged individuals were maintained in the same conditions as the colony for subsequent dissections at different time intervals. Evaluation of infections was difficult in freshly engorged females (day 1 PBM): while the presence of control L. infantum was evident under the light microscope, both Porcisia species were indistinguishable from red blood cells due to their small rounded body and very short or absent flagella. Therefore, the infection rate at this time point was estimated from Giemsa-stained gut smears and infection intensity was scored as moderate. The intensity and localisation of infection in later time intervals were evaluated under the light microscope; the infections were scored as light (<100 parasites per gut), moderate (100-1000 parasites per gut) or heavy (>1000 parasites per gut) (Myskova et al., 2008) Differences in infection intensity were tested by Chi-Square test using SPSS Version 27.
Morphological analysis of parasites
Morphology of parasites from infected guts was evaluated from methanol-fixed and Giemsa-stained gut smears. The stained gut smears were also used to assess the infection rate of Porcisia on day 1 PBM. Promastigotes were examined by light microscopy with an oil immersion objective and photographed using the Olympus DP70 camera. Body length, body width and flagellar length of parasites were measured using Image J software. Promastigotes were scored as procyclic forms (present in the bloodmeal) when flagellum length ≤ body length, metacyclic forms when flagellum length ≥ 2 times body length, leptomonad forms when flagellum length < 2 times body length and body length was < 14 µm and < 8 µm in Leishmania and Porcisia, respectively, and elongated nectomonads when flagellum length < 2 times body length and body length ≥ 14 µm and ≥ 8 µm in Leishmania and Porcisia, respectively, according to (Sadlova et al., 2010). In Leishmania species, a threshold of 14 µm is used to distinguish elongated nectomonads from leptomonads (Sadlova et al., 2010). However, this threshold cannot be used for the smaller promastigotes of the genus Porcisia, so we set a threshold of 8 µm by comparing measurements of the two genera (Supplementary File 1). Haptomonads characterized by a disc-shaped extension of the flagellar tip were recorded but not quantified because these forms, attached to the sand fly gut, are underscored on gut smears.
Examination of urine droplets
Female Lutzomyia longipalpis, 10 - 14 days after infective bloodmeal, were individually forced to feed on microcapillaries containing M199 medium according to (Hertig and Mcconnell, 1963). During feeding, droplets excreted from abdomen were captured on a cover - slip and immediately after feeding, engorged flies were dissected to detect Porcisia infections. Droplets from infected flies were air dried, fixed with methanol and stained with Giemsa. The presence of parasites was examined by light microscopy using an oil immersion objective and their morphology was evaluated as described above.
Transmission experiments and PCR detection of parasites
Experimentally infected insects were maintained for 10 days at 26°C and then allowed to feed on BALB/c mouse anaesthetized with a mixture of ketamin and xylazine (62 mg/kg and 25 mg/kg). The female insects were placed in small plastic tubules covered with fine mesh and the tubules were held on the pinnae of the anaesthetized mouse for one hour. Mice were sacrificed immediately or 4 days after the experiment by cervical dislocation under anesthesia. The pinnae (biting site) were excised and stored at -20 °C for PCR. In a single mouse dissected 4 days post exposure, ears draining lymph nodes were also collected. Because Porcisia spp. are strongly adapted to porcupines and do not survive for long periods of time in other model hosts (Herrer, 1971), infections could not be monitored over the long term. Insects were dissected immediately post bloodmeal and checked for the presence of parasites under a light microscope. DNA extraction from animal tissues was performed using the High Pure PCR Template Preparation Kit (Roche Diagnostics, Indianapolis, IN) according to the manufacturer’s instructions. The total DNA was used as a template for a nested PCR amplification with the outer primers amplifying 332 bp long 18S sequence (forward primer 18SN1F 5´- GGA TAA CAA AGG AGC AGC CTC TA-3´ and reverse primer 18SN1R 5´– CTC CAC ACT TTG GTT CTT GAT TGA-3´) and inner primers amplifying 226 bp long 18S sequence (forward primer 18SN2F 5′-AGA TTA TGG AGC TGT GCG ACA A-3′ and reverse primer 18SN2R 5′-TAG TTC GTC TTG GTG CGG TC-3′). Samples were subsequently analysed using 1% agarose gel. Reaction mixtures and cycling conditions were as follows:
1. Step of PCR: 3 µl of genomic DNA, 0,5 µl forward primer 18SN1F (10 µM), 0,5 µl reverse primer 18SN1R (10 µM), 10 µl of 2x EmeraldAmp® GT PCR Master Mix (Takara Bio), 6 µl of ddH2O.
step 1, 94 °C for 3 min 30 s; step 2, 94 °C for 30 s; step 3, 60 °C for 30 s; step 4, 72 °C for 25 s; step 5, 72 °C for 7 min; followed by cooling at 12 °C. Steps 2–4 were repeated 35 times.
2. Step of PCR: 1 µl of 1. step PCR reaction, 0,5 µl forward primer 18SN2F (10 µM), 0,5 µl reverse primer 18SN2R (10 µM), 10 µl 2x EmeraldAmp® GT PCR Master Mix (Takara Bio), 8 µl ddH2O
Animal experimentation guidelines
The mice were kept and handled in the animal facility of Charles University in Prague in accordance with institutional guidelines and Czech legislation (Act No. 246/1992 and 359/2012 coll. on Protection of Animals against Cruelty in present statutes at large), which is in compliance with all relevant European Union and international guidelines for experimental animals. All experiments were approved by the Committee on the Ethics of Laboratory Experiments of Charles University in Prague and were performed under permission no. MSMT-7831/2020-3 of the Ministry of Education, Youth and Sports. The investigators are certificated for animal experiments by the Ministry of Agriculture of the Czech Republic.
Results
Development of Porcisia in Culicoides sonorensis
Engorged C. sonorensis were dissected on days 1, 3, 6 and 10 PBM. In total, 115 females were analysed. On day 1, the infection rates were 69% (9 positive from 13 gut smears examined) for P. deanei and 45% (9 positive from 20 gut smears) for P. hertigi. Post defecation, P. hertigi infections were lost (we did not detect any positive flies out of 50 females dissected at various time intervals PBM). Porcisia deanei promastigotes survived in three females out of 39 dissected; they were detected twice on day 6 PBM and once on day 10 PBM. All infections were localized exclusively in Malpighian tubules (MT) and parasite numbers were moderate (several hundred per female). The representation of metacyclic forms increased with time post bloodmeal, accounting for 33% in females dissected on day 6 PBM and 71% on day 10 PBM, but the sample of promastigotes measured at this late time interval was limited (Supplementary File 2, 3).
Development of Porcisia in L. migonei
Female L. migonei were dissected on days 1, 4 and 7 PBM [as they would not survive until later control days (Guimarães et al., 2016; Becvar et al., 2021)]. A total of 131 females infected with P. deanei, 118 females infected with P. hertigi and 136 females infected with L. infantum were checked. Control L. infantum showed a typical suprapylarian development, resulting in heavy infections and colonization of the stomodeal valve in 87% of infected females on day 7 PBM. The infection rate of Porcisia species was not significantly different from the control L. infantum on day 1 PBM (P = 0.344, d.f. = 2, Chi-Square = 2.132) but decreased significantly with time after feeding (P = 0.003, d.f. = 2, Chi-Square = 11.770 on day 4 PBM and P < 0.001, d.f. = 2, Chi-Square = 23.500 on day 7 PBM).
Porcisia deanei promastigotes were detected in 50% of females on the first day PBM, when the infection was localized in the endoperitrophic space surrounded by the peritrophic matrix, but infection rates decreased to 21% on day 4 PBM and 7% on day 7 PBM. On day 4 PBM, three females had moderate or low-intensity infections localized in MT and the abdominal midgut (AMG) pre-defecation of bloodmeal remnants and 9 females post-defecation had low-intensity infections localized predominantly in MT (out of 9 females, only once were parasites detected in the AMG and the thoracic midgut (TMG)). On day 7 PBM, P. deanei was detected in four females, in three of which it was localized in MT in low or moderate numbers (less than 100 or 1000 promastigotes, respectively) and once in AMG, TMG and MT (heavy infection, more than 1000 promastigotes). Metacyclic forms were detected on day 4 post bloodmeal, comprising 14% of the sample (Supplementary File 3).
Porcisia hertigi caused a 48% infection rate on the first day PBM, which dropped to 13% on day 4 PBM and to zero on day 7 PBM. On day 4 PBM, only seven females were infected, all prior to defecation: one with moderate intensity infection in MT and AMG and the remainder with low intensity infection (5 females) or moderate intensity infection (1 female) in the AMG. Among the few promastigotes available for morphological analysis, metacyclic forms were present (Supplementary File 3). On day 7 PBM, no infection was detected in 40 females.
Development of Porcisia in L. longipalpis
Female sand flies were dissected on days 1, 4, 7 and 11 post bloodmeal (PBM) and their guts were examined for infection under light microscope. A total of 117 females infected with P. deanei and 171 females with P. hertigi were dissected. In addition, 117 females infected with L. infantum were used as a control group.
On the first day PBM, parasites were localized in the endoperitrophic space. The infection rates of both Porcisia species were comparable to the 95% infection rate of L. infantum, with 69% and 82% females infected with P. deanei and P. hertigi, respectively (P = 0.102, d.f. = 2, Chi-Square = 4.562).
On the fourth day PBM, the infection rate and intensity of P. deanei infection did not differ significantly from those of L. infantum, (P = 0.579, d.f. = 1, Chi-Square = 0.307) while the infection rate of P. hertigi was significantly lower (P < 0.001, d.f. = 1, Chi-Square = 36.177), falling below 10% (Figure 2). Leishmania infantum promastigotes were predominantly localized in the cardia (59%), less frequently remaining restricted to the AMG (10%) or the beginning of the TMG (17%) and in 14% of females, the stomodeal valve (SV) was already colonized. The localization of P. deanei infection was quite different (Figure 3): in all infected females, MT were infected, either as the only tissue (62% of females) or in combination with AMG and TMG (13%), AMG (8%), AMG and hindgut (HG, 4%) or HG (13%). Midgut infections were mostly light or moderate, while most parasites were always packed in MT.
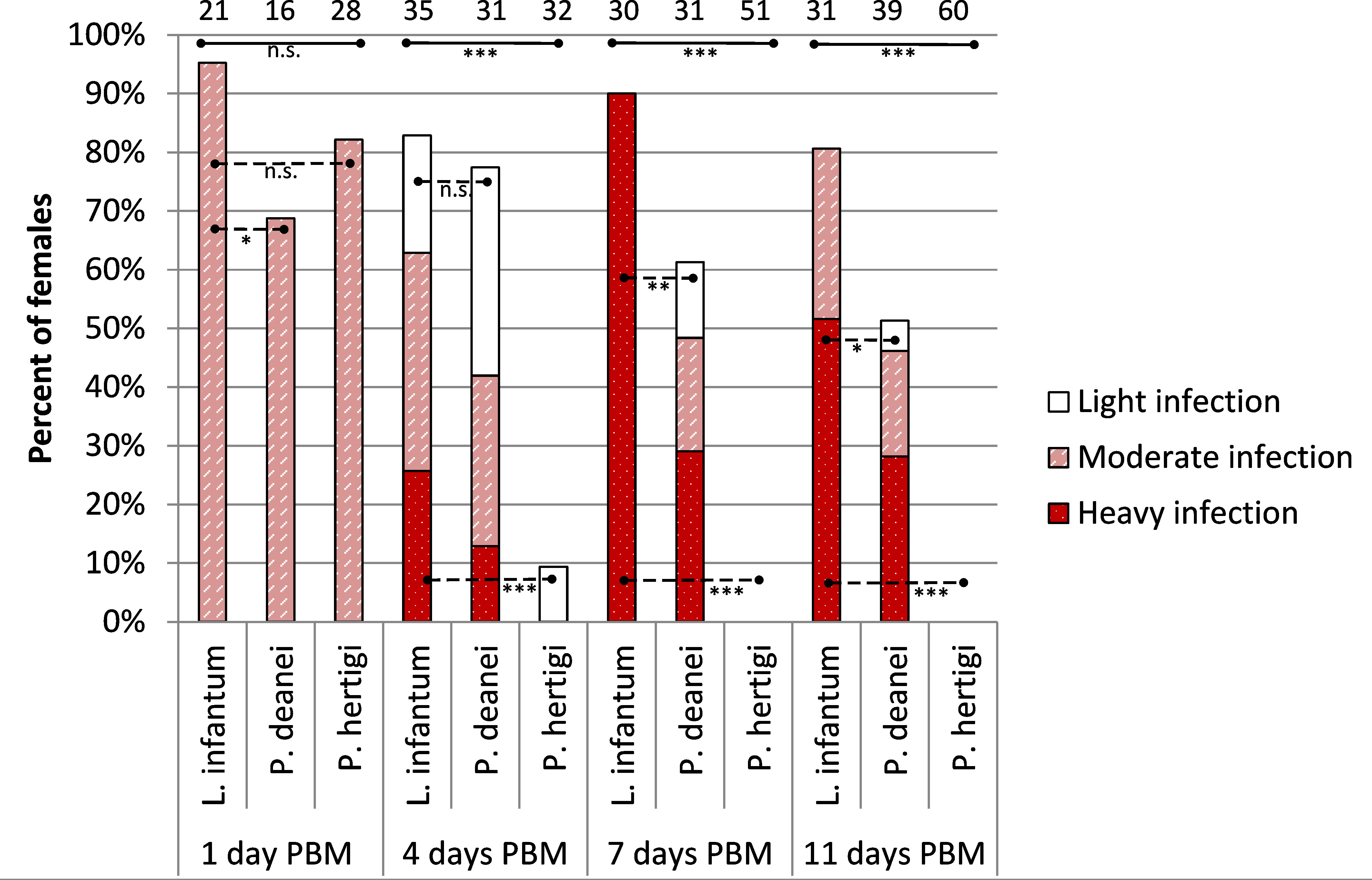
Figure 2 Infection rates and intensity of P. deanei, P. hertigi and L. infantum in Lutzomyia longipalpis. Numbers of dissected females are shown above the bars. Significance of between species differences in infection rates were evaluated using Chi-Square test. n.s, P > 0.05; *,P < 0.05; **P < 0.01; ***P < 0.001. Full lines, difference among all three tested species; dashed lines, difference between two interconnected species.
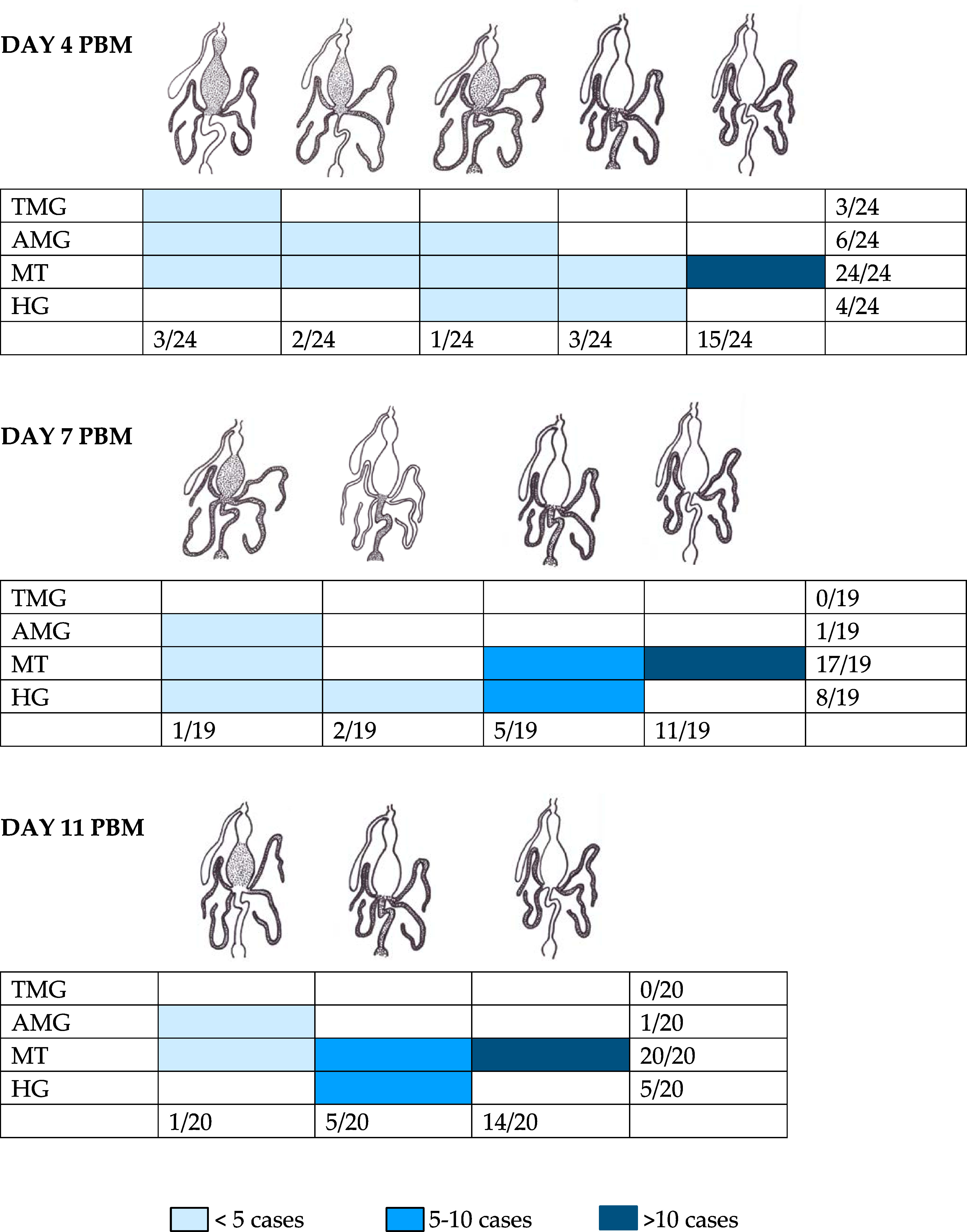
Figure 3 Localization of Porcisia deanei in infected Lutzomyia longipalpis. TMG, thoracic midgut; AMG, abdominal midgut; MT, Malpighian tubules; HG, hindgut.
In the following days PBM (days 7 and 11), the infection rates of L. infantum remained above 80%, infections were mostly heavy and the SV was colonized in all infected females (Figure 2). Porcisia hertigi was not detected in any dissected female. In contrast, promastigotes of Porcisia deanei were observed in 61% and 51% of females on day 7 and 11 PBM, respectively, and nearly 30% were heavily infected. In the majority of females, parasites remained localized only in MT (Figures 3, 4), less frequently and in smaller numbers they were also present in the AMG and/or HG.
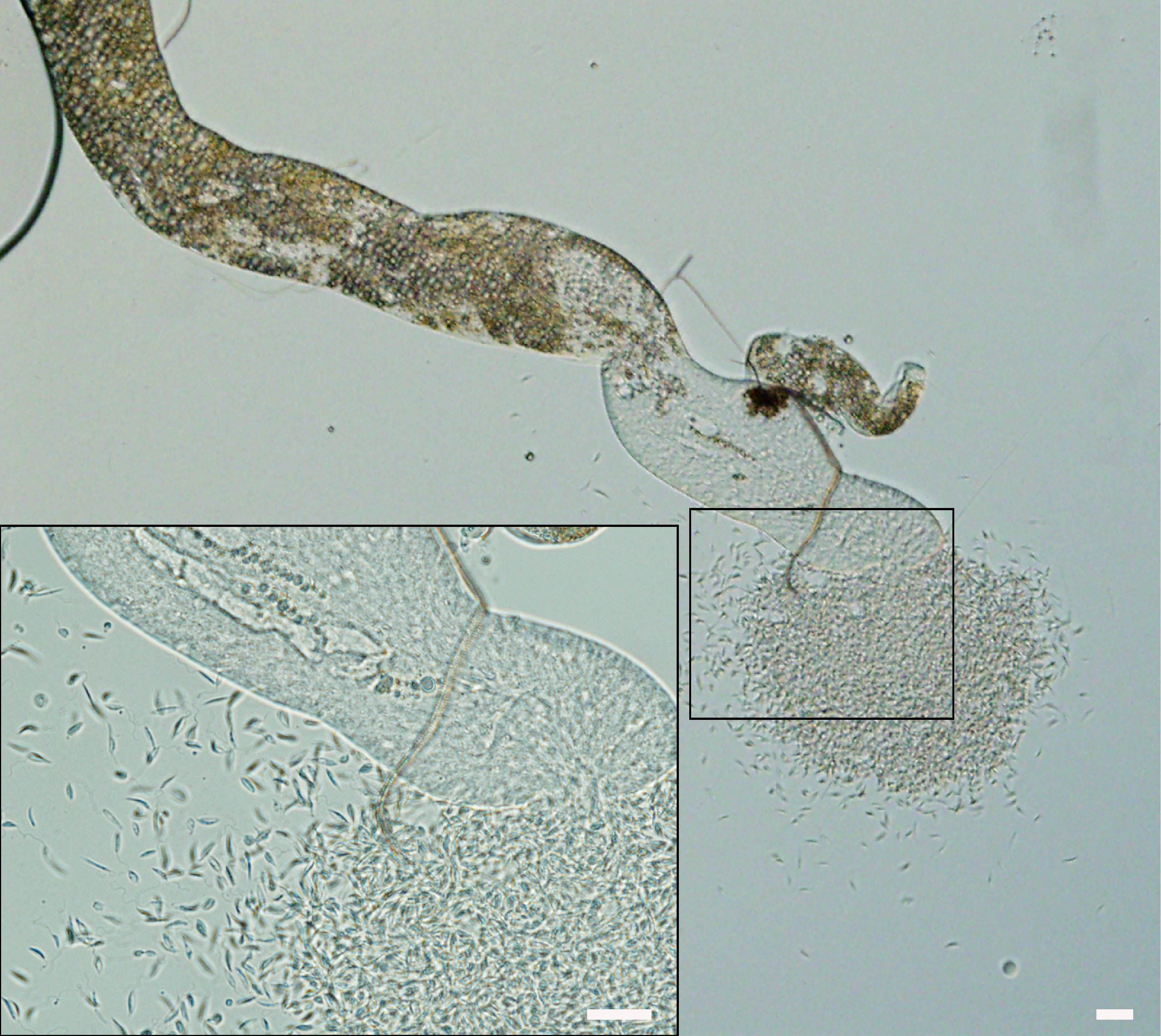
Figure 4 Porcisia deanei colonizing Malpighian tubules of L. longipalpis on day 7 PBM. Scale bars indicate 20 µm.
Morphological forms present in L. longipalpis
According to criteria described in Material and Methods, four morphological forms were distinguished on gut smears of infected L. longipalpis: procyclic promastigotes, elongated nectomonads, leptomonads and metacyclic forms. Representatives of each form are shown in Figure 5 and their measurements are provided in Table 1.
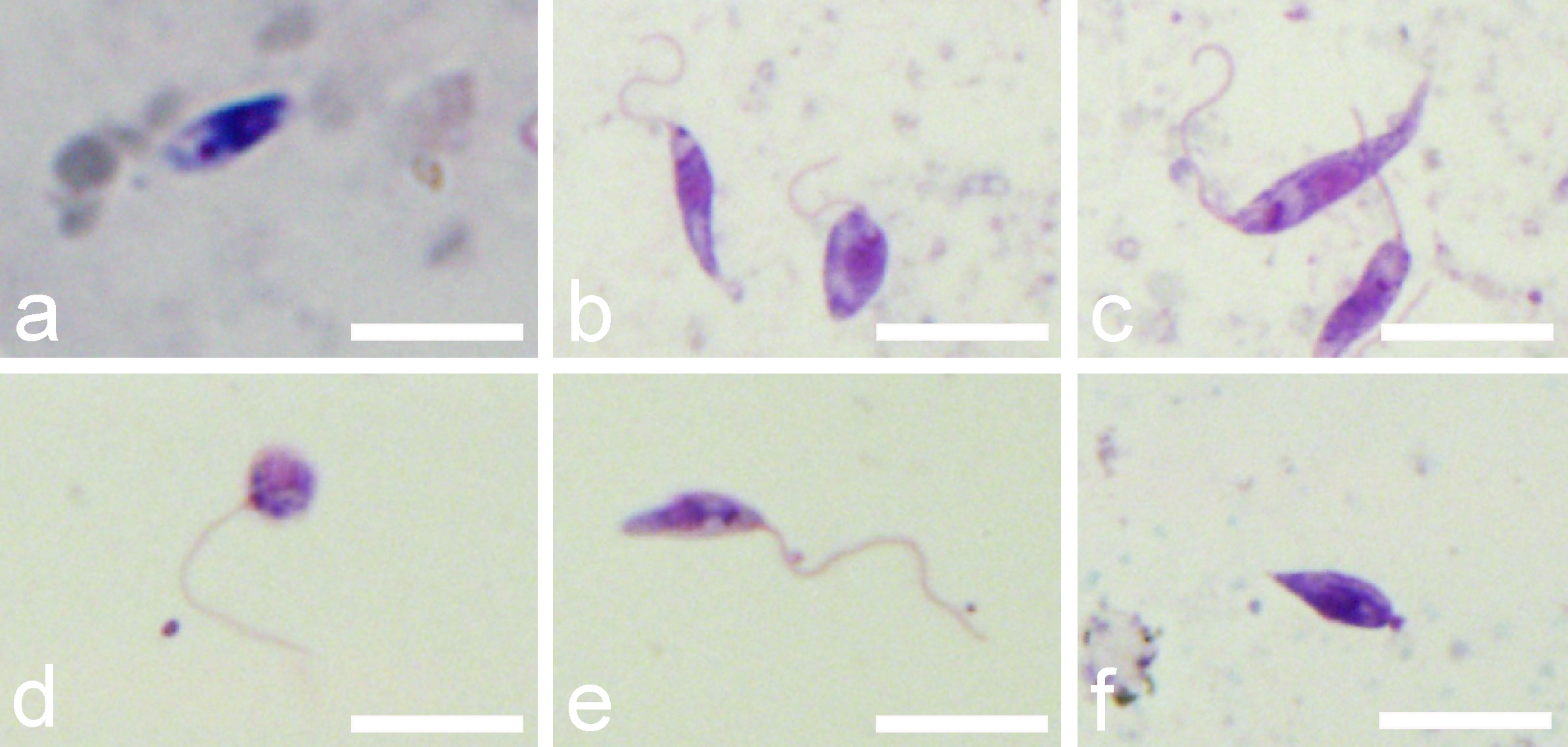
Figure 5 Morphological forms of P. deanei present in gut smears of infected L. longipalpis. (A), procyclic promastigote; (B), leptomonad forms; (C), elongated nectomonad; (D), round metacyclic form; (E), slender metacyclic form; (F), haptomonad. Scale bars indicate 10 µm.
Elongated nectomonads prevailed in the P. deanei culture used for experimental infection of sand flies (76.5%), leptomonads and metacyclic forms were less represented (17% and 6.5%, respectively). In P. hertigi, nectomonads also prevailed (62%) while in L. infantum, the predominant forms were leptomonads (61%) (Figure 6).
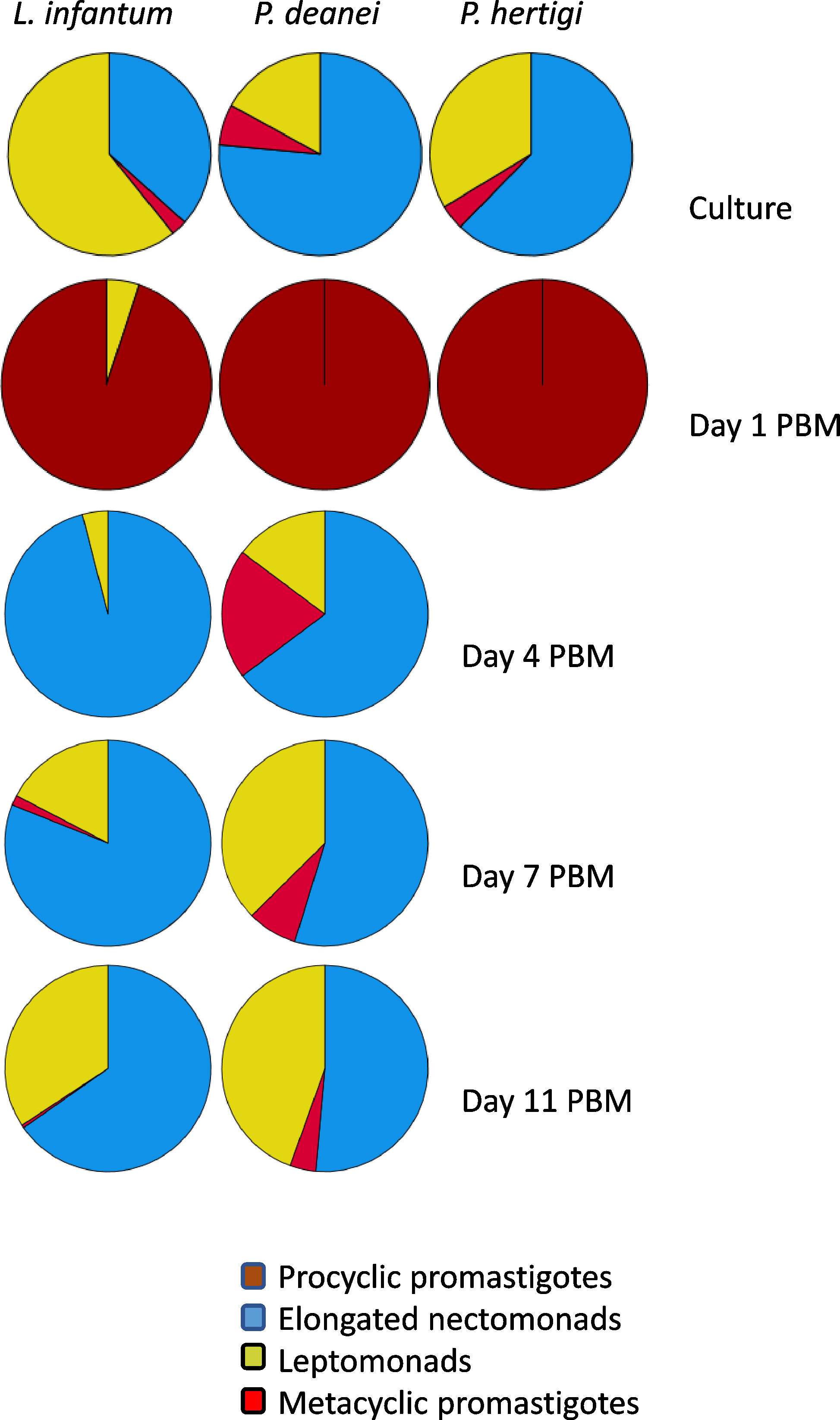
Figure 6 Representation of morphological forms on sand fly guts smears. Haptomonads were not quantified because these attached forms are underscored on gut smears. The numbers of promastigotes can be found in Table 1.
First day PBM, when the infection was restricted to the endoperitrophic space, the population of P. deanei homogenously consisted of small procyclic promastigotes with very short or unapparent flagellum (resembling amastigotes, Figure 5A). These procyclic forms were sometimes seen in dividing rosettes. Porcisia deanei showed the same morphology while in procyclic forms of L. infantum, the flagellum was mostly apparent. In L. infantum, also forms with the flagellum longer than the body length (leptomonads) appeared in 5%.
On day 4 PBM, nectomonads comprised 64%, leptomonads 15% and metacyclics 20% of P. deanei population while in L. infantum; nectomonads highly prevailed over leptomonads (96%). In following days PBM, representation of nectomonads decreased in both species while representation of leptomonads increased. Two forms of metacyclics were seen in P. deanei – round and slender (Figures 5D, E) and in these late infections, metacyclics were more represented in P. deanei compare to L. infantum (Figure 6).
On days 7 and 11 PBM, also several haptomonads were found. These forms were not included to the analysis as these attached forms are principally underestimated on gut smears.
Presence of P. deanei in urine of L. longipalpis
Microcapillary feeding (Hertig and Mcconnell, 1963) was used for testing of parasite presence in the urine droplets discharged with prediuresis during the feeding of female sand flies 10 - 14 days post infective bloodmeal. Engorged flies were dissected immediately after the experiment to confirm Porcisia infections by light microscopy and droplets produced by uninfected females were not further analysed. From five females with P. daenei infection confirmed by microscopy, 3 females discharged parasites in urine droplets. They were present in each droplet produced, in numbers ranging from 40 to 350 parasites per droplet. Among discharged parasites, metacyclic forms represented 20-29 percent (Table 2; Figure 7).
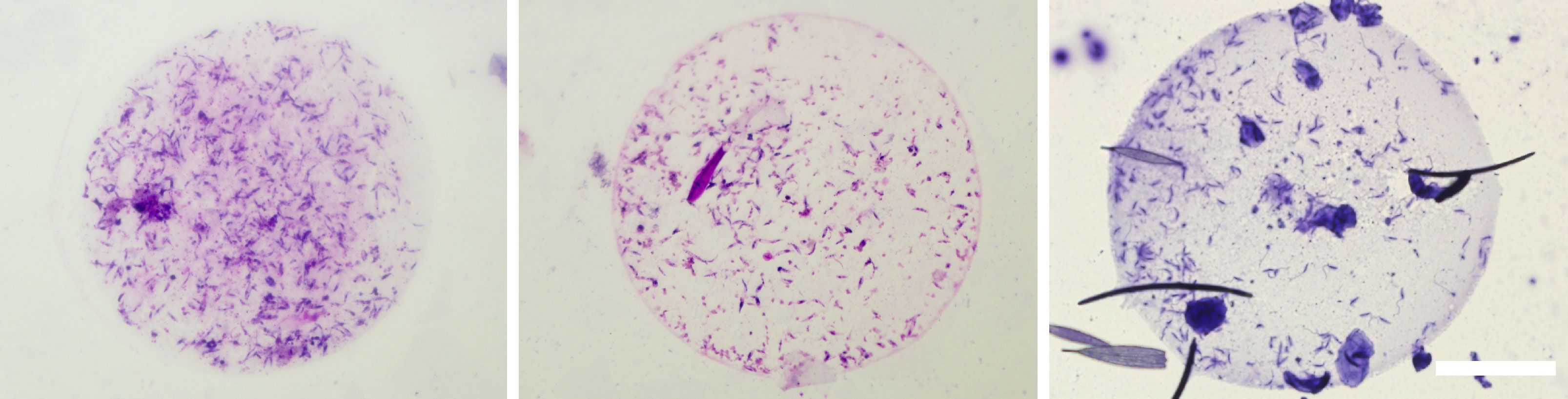
Figure 7 Porcisia deanei promastigotes in urine droplets of Lutzomyia longipalpis. Scale bar indicates 100 µm.
Transmission of P. deanei to BALB/c mice
To prove the transmission potential of L. longipalpis infected with P. deanei, females 10 days post infective blood meal were allowed to feed on the ear pinnae of anaesthetized BALB/c mice. As a control, sand flies infected with L. infantum were used. Numbers of engorged infected females are summarized in Table 3. For detection of parasites in mice tissues, a nested PCR assay with primers flanking a 226 bp 18S sequence was used. The presence of Leishmania DNA was confirmed in 2/3 ears and all 4 ears of mice exposed to L. longipalpis infected with P. deanei and L. infantum, respectively, sacrificed immediately post exposition. In a following experiment, the mouse exposed to sand flies infected with P. deanei was sacrificed later, 4 days post experiment, and also ear draining lymph nodes were sampled. Parasites were detected in both ears and both ear draining lymph nodes (Table 3; Figure 8).
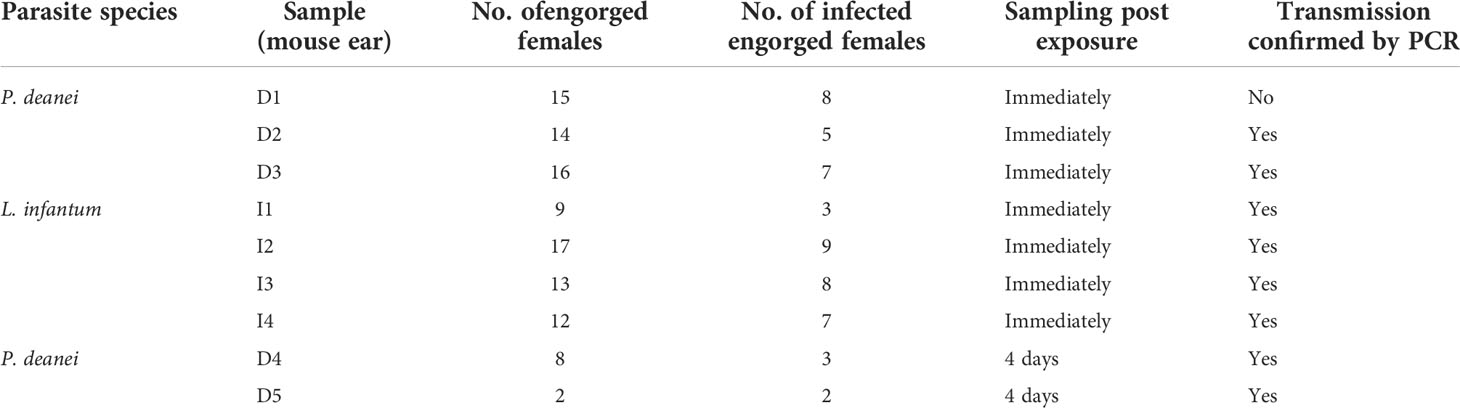
Table 3 Microscopical examination of L. longipalpis females allowed to feed on BALB/c mice for transmission of parasites and result of PCR detection of Leishmania minicircle kDNA in mouse ears.
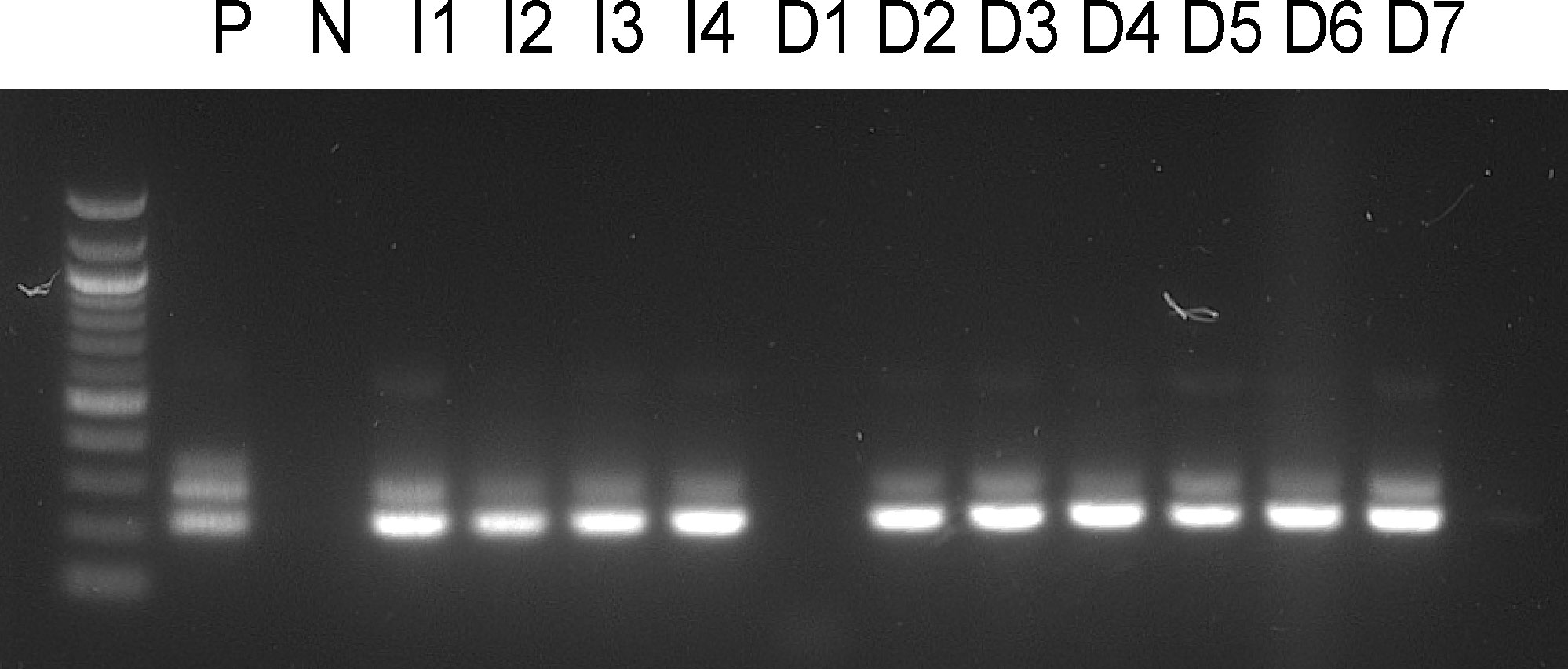
Figure 8 Amplification of a 226 bp Leishmania 18S sequence. P, positive control from cultured parasites; N, negative control; I1-4, mice ears exposed to L. longipalpis infected with L. infantum, mice killed immediately post experiment; D1-3, mice ears exposed to L. longipalpis infected with P. deanei, mice killed immediately post experiment; D4-5, mice ears exposed to L. longipalpis infected with P. deanei and D6-7, their draining lymph nodes, mice killed 4 days post experiment.
Discussion
While the biology of the genus Leishmania with its many medically important species has been extensively studied, knowledge of the life cycle of the genus Porcisia remains poor. These flagellates are apparently harmless to their hosts, the Neotropical porcupines, although they are found in the skin throughout the body and also visceralize (Herrer, 1971). They are highly host specific and well adapted to porcupines and are capable of inducing extremely high infection rates in host populations (Herrer et al., 1966; Lainson and Shaw, 1977). However, the vectors of Porcisia remained a mystery.
In nature, Porcisia DNA was detected by nested PCR in Lutzomyia antunesi in Brazil (Thies et al., 2018). However, this finding does not imply that L. antunesi is a competent vector. Only microscopical observation can document the localization of infection and the time post bloodmeal, and evidence that the parasites survived a non-specific early phase of the infection that may be lost with defecation of blood remnants.
Laboratory experimental infections of various sand fly species with Porcisia have not yet demonstrated a vectorial role for sand flies. Lainson et al. (1977) described the poor development of P. deanei and P. hertigi in L. longipalpis, but their study examined the hindgut development of 14 New World’s Leishmania species and only 2 and 9 female L. longipalpis were infected with P. hertigi and P. deanei, respectively. After an initial period of low multiplication in the midgut, the sparse parasites migrated to the hindgut and MT where they were observed to be free swimming without attachment to any part of the gut wall (Lainson et al., 1977). Similarly, Croft and Molyneux (1979) reported a low infection rate of 10% (5/50) for L. longipalpis. Infections were weak and consisted of a few promastigotes in the posterior and anterior midgut, pylorus and MT (Croft and Molyneux, 1979). Very weak development of P. hertigi has also been reported in the local sand fly species Lutzomyia sanguinaria (Herrer et al., 1966) and Lu. gomezi (Lainson and Shaw, 1977).
In our experiments, P. hertigi failed to form mature infections in all three vector species: L. migonei, L. longipalpis and C sonorensis while P. deanei survived defecation in all three tested vectors. However, while P. deanei only infected a low percentage of L. migonei and C. sonorensis females, it caused heavy infections in L. longipalpis females, localized predominantly in MT with the presence of a high percentage of metacyclic forms.
The morphology of Porcisia parasites has so far been thoroughly described only in cells from culture and vertebrate hosts. Amastigotes of P. deanei have been reported to be considerably large, averaging 6.1 x 3.1 - 3.7 µm with an elongated kinetoplast (Deane et al., 1974; Lainson and Shaw, 1977) whereas amastigotes of L. hertigi are smaller, 3.5 - 4.8 x 1.2 - 2.5 µm (Herrer, 1971) with a round kinetoplast (Herrer, 1971). Promastigotes in culture showed a diversity of short and elongate forms in both species, measuring 6.0 - 18.0 x 1.5 - 2.5 µm with a flagellum of 7 - 24 µm in P. deanei (Lainson and Shaw, 1977) and 7.5 - 20.5 x 1.3 - 4.1 µm with a flagellum of 9.1 - 35.5 µm in P. hertigi (Herrer, 1971). In our study, the promastigotes of P. deanei in culture were larger (5.4 - 17.6 x 1.2 – 3.3 µm) than those of P. hertigi (4.2 - 12.2 x 1.3 - 2.8 µm), and the same was true for procyclic promastigotes in L. longipalpis on the first day PBM with 4.7 - 12.8 x 1.6 - 7.4 µm in P. deanei compared to 3.6 - 9.2 x 1.9 – 4.4 µm in P. hertigi. Procyclic promastigotes developing within the peritrophic matrix were characterized by very short or even invisible flagella in both Porcisia species (average 2.9 µm in P. deanei and 3.0 µm in P. hertigi) and resembled sphaeromastigotes or amastigotes reported as the first Trypanosoma cruzi stages present in the bloodmeal of reduviid bugs (Tyler and Engman, 2001). Post defecation of L. longipalpis females, P. deanei developed late infections with a persistent dominance of elongated nectomonads (8.0 - 19.4 x 1.0 - 5.5 µm) and a lower representation of short leptomonads (2.7 – 7.9 x 1.3 – 8.2 µm). However, it should be noted that division of promastigotes into nectomonads and leptomonads according to their body length is arbitrary (see Supplementary File 1). Importantly, metacyclic forms with an ellipsoid or rounded body shape and flagellum at least twice the body length accounted for a significant proportion of promastigotes in late infections, whereas haptomonads were very rarely found on gut smears and were never observed in vivo. Similarly, Lainson et al. (1977) did not find attached forms of P. deanei on any occasion on any part of the gut wall of L. longipalpis.
The predominant localization of promastigotes in MT was surprising because MT are rarely used during the development of both monoxenous and dixenous trypanosomatids in their vectors. MT infections account for only 2% of infections of monoxenous trypanosomatids in Heteropteran and Dipteran hosts, being combined with parasite presence in the adjacent mid and/or hindgut (Lukeš et al., 2018). In the dixenous Leishmania genus, three types of development have been recognized: suprapylarian development, typical of the subgenus Leishmania, is restricted to the mesenteron; peripylarian development, known in the subgenus Viannia and some Sauroleishmania species, also involves multiplication in the hindgut prior to the anterior migration and colonization of the stomodeal valve; and finally, hypopylarian development in other Sauroleishmania species is restricted to the hind gut (Lainson and Shaw, 1987). Occurrence of low numbers of Leishmania promastigotes in MT, reported in natural or experimental infections, has been interpreted as a consequence of peristaltic backwash from the midgut (Lainson and Shaw, 1968) and invasion of MT by Leishmania has been more frequently observed in unnatural vector- parasite pairs or atypical experimental conditions like maltose or fructose diet and reduced temperature (Rangel et al., 1985; Walters et al., 1987; Anez et al., 1989; Nieves and Pimenta, 2000; Ticha et al., 2021). In the dixenous genus Endotrypanum, which is closely relative to Porcisia, development in MT has been observed more frequently than in Leishmania, but in variable proportion in different sand fly species and in a lower percentage of females compared with midgut infections (Shaw, 1981; Franco et al., 1997). The type of development observed in this study - colonization of MT as the dominant body part of the vector, was not described yet.
We sought to answer the important question of whether this curious localization is an artefact resulting from the fact that L. longipalpis is not a natural vector of P. deanei, or whether this sand fly species can serve as a competent vector and the infection can be transmitted to vertebrates. Malpighian tubules are the major osmoregulatory and excretory organs of insects, responsible for the production of isosmotic filtrate from the hemolymph, the primary urine that carries excretory products of metabolism and toxic compounds into the hindgut (Nocelli et al., 2016). Sand flies begin urine production immediately upon feeding on the host to concentrate the proteins of the bloodmeal and restore weight and water balance (Sádlová et al., 1998). During this process, called prediuresis, parasites may be excreted in urine droplets, allowing for contaminative transmission, as demonstrated in a model of Phlebotomus duboscqi infected with L. major (Sádlová and Volf, 1999). Therefore, free-swimming Porcisia promastigotes can theoretically be passed from MT of L. longipalpis to the hindgut and reach the host skin in urine droplets during prediuresis. To prove this theory, we inspected urine droplets of infected L. longipalpis exposed to capillary feeding and observed the droplets packed with hundreds of parasites with 20-29 percent of metacyclics.
This finding encouraged us to perform the following step, transmission to the mammalian hosts. Female L. longipalpis infected with P. deanei were allowed to feed on ears of anaesthetized BALB/c mice and PCR confirmed successful transmission - DNA of P. deanei was detected in the ear tissue not only immediately post feeding but also 4 days post experiment, in samples from both the ear and the ear draining lymph node. These results demonstrated that L. longipalpis is a competent vector of P. deanei, but the involvement of other phlebotomine species in its transmission is not excluded. In C. sonorensis, although surviving in a small percentage of females, P. deanei formed heavy infections containing metacyclic stages, also localized in the MT. Therefore, the search for Neotropical vectors of Porcisia parasites may also involve biting midges.
Contaminative transmission is rather rare in trypanosomatids. Transmission by excretion of metacyclic forms into the reduviid bite wound is typical for Trypanosoma cruzi (Tyler and Engman, 2001). In Leishmania, the predominant route by which promastigotes enter the host is certainly the transmission by bite. Contaminative transmission by ingestion of infected female sand flies presumably takes place in the subgenus Sauroleishmania (Lainson and Shaw, 1987) and may occur in transmission of Leishmania to dogs while in man, rather little importance is attributed to occasional contaminative transmission by crushing the fly on the skin as it bites (reviewed by (Killick-Kendrick, 1979). However, the frequent occurrence of live L. major have been confirmed in the prediuretic liquid of P. duboscqi and transmission of metacyclic forms by mosquito prediuretic fluid has also been considered for mammalian T. congolense (Mutero and Mutinga, 1993) and avian T. theileri (Brotánková et al., 2022). Contaminative transmission is not as conditioned by the number or localization of parasites in the gut of the vector like as is the case for transmission by bite (Sádlová and Volf, 1999). Therefore, delivery of infective forms to the host in urine may be a primitive mode of transmission to which Porcisia adapted by its unique localization in vector´s Malpighian tubules.
Data availability statement
The raw data supporting the conclusions of this article will be made available by the authors, without undue reservation.
Ethics statement
The animal study was reviewed and approved by The Committee on the Ethics of Laboratory Experiments of Charles University in Prague.
Author contributions
Conceptualization, methodology, formal analysis, writing - original draft preparation, JSa; investigation, DB, JSa, TB, and BV; writing—review and editing – PV, JSh, and ME; supervision, JSa, and PV; funding acquisition, PV and JSa. All authors have read and agreed to the published version of the manuscript.
Funding
JSa and PV were funded by ERD Funds, project CePaViP (https://ec.europa.eu/regional_policy/en/funding/erdf/, grant No. CZ.02.1.01/0.0/0.0/16_019/0000759). Shipment of Culicoides was funded by Research Infrastructures for the control of vector-borne diseases (Infravec2, https://infravec2.eu/), which has received funding from the European Union’s Horizon 2020 research and innovation programme under grant agreement No 731060 by JSa. ME is funded by the Defra national Culicoides laboratory.
Acknowledgments
We thank to Lenka Krejcirikova and Kristyna Srstkova for the administrative and technical support.
Conflict of interest
The authors declare that the research was conducted in the absence of any commercial or financial relationships that could be construed as a potential conflict of interest.
Publisher’s note
All claims expressed in this article are solely those of the authors and do not necessarily represent those of their affiliated organizations, or those of the publisher, the editors and the reviewers. Any product that may be evaluated in this article, or claim that may be made by its manufacturer, is not guaranteed or endorsed by the publisher.
Supplementary material
The Supplementary Material for this article can be found online at: https://www.frontiersin.org/articles/10.3389/fcimb.2022.981071/full#supplementary-material
References
Alexandre, J., Sadlova, J., Lestinova, T., Vojtkova, B., Jancarova, M., Podesvova, L., et al. (2020). Experimental infections and co-infections with leishmania braziliensis and Leishmania infantum in two sand fly species, Lutzomyia migonei and Lutzomyia longipalpis. Sci. Rep. 10, 1–8. doi: 10.1038/s41598-020-60600-7
Anez, N., Nieves, E., Cazorla, D. (1989). The validity of the developmental pattern in the sandfly gut for classification of Leishmania. Trans. R. Soc Trop. Med. Hyg. 83, 634–635. doi: 10.1016/0035-9203(89)90378-7
Becvar, T., Vojtkova, B., Siriyasatien, P., Votypka, J., Modry, D., Jahn, P., et al. (2021). Experimental transmission of Leishmania (Mundinia) parasites by biting midges (Diptera: Ceratopogonidae). PloS Pathog. 17, 1–18. doi: 10.1371/journal.ppat.1009654
Brotánková, A., Fialová, M., Čepička, I., Brzoňová, J., Svobodová, M. (2022). Trypanosomes of the Trypanosoma theileri group: Phylogeny and new potential vectors. Microorganisms 10 (2), 294. doi: 10.3390/microorganisms10020294
Chanmol, W., Jariyapan, N., Somboon, P., Bates, M. D., Bates, P. A. (2019). Development of Leishmania orientalis in the sand fly Lutzomyia longipalpis (Diptera: Psychodidae) and the biting midge Culicoides soronensis (Diptera: Ceratopogonidae). Acta Trop. 199, 105157. doi: 10.1016/j.actatropica.2019.105157
Croft, S. L., Molyneux, D. H. (1979). Studies on the ultrastructure, virus-like particles and infectivity of Leishmania hertigi. Ann. Trop. Med. Parasitol. 73, 213–226. doi: 10.1080/00034983.1979.11687251
da Silva, D. A., de Madeira, F., Filho, C. J. L. B., Schubach, E. Y. P., da S, J. H., Figueiredo, F. B. (2013). Leishmania (Leishmania) hertigi in a porcupine (Coendou sp.) found in brasília, federal district, Brazil. Rev. Bras. Parasitol. Vet. 22, 297–299. doi: 10.1590/s1984-29612013005000014
Deane, L. M., da Silva, J. E., de Figueiredo, P. Z. (1974). Leishmaniae in the viscera of porcupines from the state of piaui, Brazil. Rev. Inst. Med. Trop. Sao Paulo 16, 68–69.
Dougall, A. M., Alexander, B., Holt, D. C., Harris, T., Sultan, A. H., Bates, P. A., et al. (2011). Evidence incriminating midges (Diptera : Ceratopogonidae ) as potential vectors of Leishmania in Australia q. Int. J. Parasitol. 41, 571–579. doi: 10.1016/j.ijpara.2010.12.008
Dvořák, V., Shaw, J. J., Volf, P. (2018). “Parasite biology: The vectors,” in The leishmaniases: Old neglected tropical diseases. Eds. Bruschi, F., Gradoni, L.. Cham, Switzerland:Springer, 31–78. doi: 10.1007/978-3-319-72386-0
Espinosa, O. A., Serrano, M. G., Camargo, E. P., Teixeira, M. M. G., Shaw, J. J. (2016). An appraisal of the taxonomy and nomenclature of trypanosomatids presently classified as Leishmania and Endotrypanum. Parasitology 145, 430–442. doi: 10.1017/S0031182016002092
Franco, A. M. R., Tesh, R. B., Guzman, H., Deane, M. P., Grimaldi, G. J. (1997). Development od Endotrypanum (Kinetoplastida: Trypanosomatidae) in experimentally infected phlebotomine sand flies (Ditera: Psychodidae). J. Med. Entomol. 34, 189–192. doi: 10.1093/jmedent/34.2.189
Guimarães, V. C. F. V., Pruzinova, K., Sadlova, J., Volfova, V., Myskova, J., Filho, S. P. B., et al. (2016). Lutzomyia migonei is a permissive vector competent for Leishmania infantum. Parasites Vectors 9, 1–6. doi: 10.1186/s13071-016-1444-2
Herrer, A. (1971). Leishmania hertigi sp. n., from the tropical porcupine, Coendou rothschildi Thomas. J. Parasitol. 57, 626–629. doi: 10.2307/3277928
Herrer, A., Thatcher, V. E., Johnson, C. M. (1966). Natural infections of Leishmania and trypanosomes demonstrated by skin culture. J. Parasitol. 52, 954–957. doi: 10.2307/3276542
Hertig, M., Mcconnell, P. (1963). Experimental infection of Panamanian phlebotomus sandflies with Leishmania. Exp. Parasitol 14, 92–106. doi: 10.1016/0014-4894(63)90014-6
Killick-Kendrick, R. (1979). “Biology of leishmania in phlebotomine sandflies,” in Biology of kinetoplastida. Eds. Lumsden, W. H., Evans, D. A. (London: Academic Press), 396–460.
Lainson, R., Shaw, J. J. (1968). Leishmaniasis in Brazil: I. observations on enzootic rodent leishmaniasis. incrimination of Lutzomyia flaviscutellata (Mangabeira) as the vector in the lower Amazon basin. Trans. R. Soc. Trop. Med. Hyg. 62, 385–395. doi: 10.1016/0035-9203(68)90090-4
Lainson, R., Shaw, J. J. (1977). Leishmanias of Neotropical porcupines: Leishmania hertigi deanei nov. Subsp. Acta Amaz. 7, 51–57. doi: 10.1590/1809-43921977071051
Lainson, R., Shaw, J. J. (1987). “Evolution, classification and geographical distribution,” in The leishmaniases in biology and medicine: Volume I biology and epidemiology. Eds. Peters, W., Killick-Kendrick, R. (London: Academic Press Inc), 1–120.
Lainson, R., Ward, R. D., Shaw, J. J. (1977). Leishmania in phlebotomid sandflies: VI. importance of hindgut development in distinguishing between parasites of the Leishmania mexicana and L. braziliensis complexes. Proc. R. Soc. Lond. B 199, 309–320. doi: 10.1098/rspb.1977.0141
Lukeš, J., Butenko, A., Hashimi, H., Maslov, D. A., Votýpka, J., Yurchenko, V. (2018). Trypanosomatids are much more than just trypanosomes: Clues from the expanded family tree. Trends Parasitol. 34, 466–480. doi: 10.1016/j.pt.2018.03.002
Maroli, M., Feliciangeli, M. D., Bichaud, L., Charrel, R. N., Gradoni, L. (2013). Phlebotomine sandflies and the spreading of leishmaniases and other diseases of public health concern. Med. Vet. Entomol. 27, 123–147. doi: 10.1111/j.1365-2915.2012.01034.x
Mutero, C. M., Mutinga, M. J. (1993). Defecation by Anopheles arabiensis mosquitoes of host blood infected with live Trypanosoma congolense. Trop. Med. Parasitol. 44, 23–26.
Myskova, J., Votypka, J. A. N., Volf, P. (2008). Leishmania in sand flies: Comparison of quantitative polymerase chain reaction with other techniques to determine the intensity of infection. J. Med. Ento Mol., 45 (1) 133–138. doi: 10.1093/jmedent/45.1.133
Nieves, E., Pimenta, P. F. P. (2000). Development of Leishmania (Viannia) braziliensis and Leishmania (Leishmania) amazonensis in the sand fly Lutzomyia migonei (Diptera: Psychodidae). J. Med. Entomol. 37, 134–140. doi: 10.1603/0022-2585-37.1.134
Nocelli, R., Cintra-Socolowski, P., Roat, T., Silva-Zacarin, E., Malaspina, O. (2016). Comparative physiology of malpighian tubules: form and function. Open Access Insect Physiol. 6, 13–23. doi: 10.2147/oaip.s72060
Rangel, E. F., Deane, L. M., Grimaldi, G., De Souza, N. A., Wermelinger, E. D., Barbosa, A. F. (1985). Flagellates in the malpighian tubules of laboratory-bred Lutzomyia longipalpis fed on a hamster experimentally infected with Leishmania mexicana amazonensis. Mem. Inst. Oswaldo Cruz 80, 371–372. doi: 10.1590/s0074-02761985000300016
Sadlova, J., Price, H. P., Smith, B. A., Votypka, J., Volf, P., Smith, D. F. (2010). The stage-regulated HASPB and SHERP proteins are essential for differentiation of the protozoan parasite Leishmania major in its sand fly vector, Phlebotomus papatasi. Cell. Microbiol. 12, 1765–1779. doi: 10.1111/j.1462-5822.2010.01507.x
Sádlová, J., Reishig, J., Volf, P. (1998). Prediuresis in female Phlebotomus sandflies (Diptera: Psychodidae). Eur. J. Entomol. 95, 643–647.
Sádlová, J., Volf, P. (1999). Occurrence of Leishmania major in sandfly urine. Parasitology 118, 455–460. doi: 10.1017/S0031182099004254
Shaw, J. J. (1981). “The behaviour of endotrypanum schaudinni (Kinetoplastida: Trypanosomatidae) in three species of laboratory-bred Neotropical sandflies (Diptera: Psychodidae) and its influence on the classification of the genus leishmania,” in Parasitological topics. a presentation volume to p. c. c. garnham, f. r. s., on the occasion of his 80th birthday. Ed. Canning, E. U. (Lawrence, KS, USA: Allen Press), 232–241.
Shimabukuro, P. H. F., De Andrade, A. J., Galati, E. A. B. (2017). Checklist of American sand flies (Diptera, psychodidae, phlebotominae): Genera, species, and their distribution. Zookeys 2017, 67–106. doi: 10.3897/zookeys.660.10508
Thies, S. F., de Bronzoni, R. V. M., Michalsky, É.M., dos Santos, E. S., da Silva, D. J. F., Dias, E. S., et al. (2018). Aspects on the ecology of phlebotomine sand flies and natural infection by Leishmania hertigi in the southeastern Amazon basin of Brazil. Acta Trop. 177, 37–43. doi: 10.1016/j.actatropica.2017.09.023
Ticha, L., Kykalova, B., Sadlova, J., Gramiccia, M., Gradoni, L., Volf, P. (2021). Development of various Leishmania (Sauroleishmania) tarentolae strains in three phlebotomus species. Microorganisms 9, 1–13. doi: 10.3390/microorganisms9112256
Tyler, K. M., Engman, D. M. (2001). The life cycle of Trypanosoma cruzi revisited. Int. J. Parasit. 31, 472–481. doi: 10.1016/s0020-7519(01)00153-9
Volf, P., Volfova, V. (2011). Establishment and maintenance of sand fly colonies. J. Vector Ecol. 36, 1–9. doi: 10.1111/j.1948-7134.2011.00106.x
Voss, R. S. (2015). “Superfamily erethizontoidea Bonaparte 1845,” in Mammals of south America, volume 2 rodents. Eds. Patton, J. L., Pardiñas, U. F. J., D’Elía, G. (Chicago: University of Chcago Press), 786–805
Walters, L. L., Modi, G. B., Tesh, R. B., Burrage, T. (1987). Host-parasite relationship of Leishmania mexicana mexicana and Lutzomyia abonnenci (Diptera: Psychodidae). Am. J. Trop. Med. Hyg. 36, 294–314. doi: 10.4269/ajtmh.1987.36.294
Keywords: Porcisia deanei, Porcisia hertigi, Lutzomyia, Culicoides, prediuresis, Malpighian tubules, contaminative transmission
Citation: Sadlova J, Bacikova D, Becvar T, Vojtkova B, England M, Shaw J and Volf P (2022) Porcisia transmission by prediuresis of sand flies. Front. Cell. Infect. Microbiol. 12:981071. doi: 10.3389/fcimb.2022.981071
Received: 29 June 2022; Accepted: 20 July 2022;
Published: 10 August 2022.
Edited by:
Atchara Phumee, Walailak University, ThailandReviewed by:
Narissara Jariyapan, Chulalongkorn University, ThailandDanilo Ciccone Miguel, State University of Campinas, Brazil
Copyright © 2022 Sadlova, Bacikova, Becvar, Vojtkova, England, Shaw and Volf. This is an open-access article distributed under the terms of the Creative Commons Attribution License (CC BY). The use, distribution or reproduction in other forums is permitted, provided the original author(s) and the copyright owner(s) are credited and that the original publication in this journal is cited, in accordance with accepted academic practice. No use, distribution or reproduction is permitted which does not comply with these terms.
*Correspondence: Jovana Sadlova, c2FkbG92YWpAbmF0dXIuY3VuaS5jeg==