- 1Foodborne and Waterborne Diseases Research Center, Research Institute for Gastroenterology and Liver Diseases, Shahid Beheshti University of Medical Sciences, Tehran, Iran
- 2Basic and Molecular Epidemiology of Gastrointestinal Disorders Research Center, Research Institute for Gastroenterology and Liver Diseases, Shahid Beheshti University of Medical Sciences, Tehran, Iran
- 3National Institute for Health Research Nottingham Biomedical Research Centre, University of Nottingham, Nottingham, United Kingdom
- 4Nottingham Digestive Diseases Centre, School of Medicine, University of Nottingham, Nottingham, United Kingdom
- 5Gastroenterology and Liver Diseases Research Center, Research Institute for Gastroenterology and Liver Diseases, Shahid Beheshti University of Medical Sciences, Tehran, Iran
Background: Owing to the emergence and spread of multidrug resistance mechanisms in Helicobacter pylori, achieving a successful eradication has become exceedingly difficult. Thus, this study for the first time determines the effect of a combination of vitamin D3 and probiotic on the pathogenesis and treatment of H. pylori.
Methods: We established an in vitro experimental system using AGS human gastric carcinoma cells and explored the synergistic effect of Levilactobacillus brevis IBRC-M10790 and vitamin D3 on H. pylori. Live and pasteurized L. brevis, L. brevis-derived membrane vesicles (MVs), and L. brevis cell-free supernatant (CFS), as well as their combination with vitamin D3 were used during this study. We assessed the anti-inflammatory and anti-oxidative effects of these combinations using RT-qPCR and ELISA, respectively. We further performed an adhesion assay to evaluate the influence of L. brevis and vitamin D3 on the adherence rate of H. pylori to AGS cells.
Results: Our results demonstrated that L. brevis and vitamin D3 possess anti-inflammatory and anti-oxidative effects against H. pylori infection in AGS cells. The combination of vitamin D3 with the probiotic strain (particularly live L. brevis and its CFS) can more efficiently reduce the expression of pro-inflammatory cytokines IL-6, IL-8, IFN-γ, and TNF-α in the AGS cells. Moreover, vitamin D3 and L. brevis exhibited an additive impact preserving the integrity of the epithelial barrier by increasing the expression of the tight junction protein ZO-1. Furthermore, this combination can potentially reduce H. pylori adherence to AGS cells.
Conclusions: This study indicates the advantage of combining vitamin D3 and probiotic to attenuate H. pylori-induced inflammation and oxidative stress. Consequently, probiotic and vitamin D3 co-supplementation can be considered as a novel therapeutic approach to manage and prevent H. pylori infection.
Introduction
Infection with Helicobacter pylori (H. pylori) is the primary causative factor in the development of chronic gastritis, peptic ulcer, and gastric carcinoma (Fakharian et al., 2022). H. pylori might be the most successful of human pathogens, considering the global colonization prevalence of almost 50%. Owing to the profound influence of socioeconomic factors on the prevalence of H. pylori infection, its colonization rates exceeds 80% in parts of Eastern Asia and European countries (O’Connor et al., 2017). This in principle has resulted in the recommendation that all H. pylori-infected patients should receive treatment. Therefore, all international consensus conferences have advocated a cost-effective H. pylori test-and-treat strategy for dyspeptic patients, which is mainly based on a non-invasive H. pylori diagnostic test and subsequent eradication when detected (Beresniak et al., 2020). The standard H. pylori eradication regimen consists of two or three types of antibiotics (mostly clarithromycin, amoxicillin, and metronidazole) and a proton-pump inhibitor (PPI) for two weeks (Suzuki et al., 2020).
The test-and-treat strategy has become challenging owing to the increasing rate of single-drug and multi-drug resistance of H. pylori to previously effective antibiotic-based treatments (Malfertheiner et al., 2022). Consequently, the interest in unconventional therapeutic strategies is growing by the day. In the last decade, probiotic co-supplementation to conventional antibiotic therapies has been demonstrated to attenuate the deleterious effects of antibiotics, modulate the host immune response, and orchestrate an anti-inflammatory response. The regular uptake of probiotics has been suggested to reduce the available bacterial attachment sites, therefore reducing H. pylori adhesion to gastric epithelial cells (Nabavi-Rad et al., 2022b). Consequently, probiotic administration, especially Lactobacillus spp., is currently considered to be an effective concomitant treatment strategy for H. pylori infection (Ji and Yang, 2020). The expanding supplementation of probiotics to vulnerable population require concise and actionable guidelines on how to prevent long-term risks and adverse events, such as disrupting the inherent structure of the gut microbiome and transferring antibiotic resistance genes and toxins (Merenstein et al., 2023). While the administration of live biotherapeutics may give rise to safety concerns, postbiotics which are preparations of inanimate microorganisms and/or their components, can confer selective health benefits, and may be safer and more stable alternatives to probiotics (Hitch et al., 2022; Mosca et al., 2022).
Micronutrient malabsorption or deficiency is a well-known characteristic of H. pylori infection (Nabavi-Rad et al., 2022a). Recent studies have shed light on the inverse correlation between the serum levels of certain micronutrients, particularly vitamin D, and the risk of H. pylori infection and eradication failure (El Shahawy et al., 2018; Han et al., 2019). A number of studies further investigated the beneficial effect of vitamin D supplementation and demonstrated its potential modulatory activity on H. pylori pathogenicity (Yang et al., 2019). Owing to its high stability, cholecalciferol (vitamin D3) is the primary supplementary form of vitamin D that more efficiently increases the serum levels of vitamin D, compared to ergocalciferol (vitamin D2) (Vieth, 2020).
Evidence of synergistic health benefits of vitamin D and probiotic co-supplementation is emerging. A systematic review of randomized controlled trials has shown that co-supplementation of vitamin D with probiotic strains of Lactobacillus, Bifidobacterium, and Streptococcus yielded greater health benefits than its comparators, which were placebo, vitamin D, lower vitamin D dose, and probiotics and lower vitamin D dose (Abboud et al., 2020). Considering the tight interaction of H. pylori with the gastric concentration of vitamin D and the presence of probiotic strains among indigenous gastric microbiota, we have evaluated for the first time, the synergistic effects of vitamin D3 and the probiotic strain Levilactobacillus brevis IBRC-M10790 on the inflammatory and oxidative activity of H. pylori in vitro. To this end, we prepared and utilized live L. brevis, pasteurized L. brevis, L. brevis-derived membrane vesicles (MVs), and L. brevis cell-free supernatant (CFS) in this study.
Materials and methods
Bacterial strains and culture
A H. pylori clinical strain BY-1 was obtained from the H. pylori biobank of Helicobacter Research Laboratory in the Research Institute for Gastroenterology and Liver Diseases, Shahid Beheshti University of Medical Sciences, Tehran, Iran. The isolate was cultured on Brucella agar plates containing 5% sheep blood for 2-3 days under microaerophilic conditions (5% O2, 10% CO2, 85% N2) at 37°C.
L. brevis strain IBRC-M10790 was kindly provided by Takgene Zist Company (Tehran, Iran). The probiotic strain was grown on MRS (De Man-Rogosa-Sharpe) agar (Merck, Darmstadt, Germany) for 48-72 h at 37°C under anaerobic conditions (85% N2, 10% CO2 and 5% H2) created by Anoxomat® Gas Exchange System (Mart Microbiology BV, Holland).
Pasteurization of L. brevis
The MRS broth culture of L. brevis yielded an optical density at a wavelength of 600nm (OD600) of 1.00 ± 0.03, equivalent to 109 colony-forming units (CFU) per ml, following 72 h incubation under anaerobic conditions. The bacterial culture was then heat treated (pasteurized) at 70°C for 30 min and immediately stored at -80°C for at least one hour before use.
Preparation of L. brevis CFS
The MRS broth culture of L. brevis was adjusted after 48 h to an optical density OD600 of 1.00 ± 0.03, corresponding to 109 CFU/ml. Following incubation, bacterial cells were eliminated by centrifugation at 18,928×g for 15 min and the supernatant was harvested. The obtained supernatant was then filtered through 0.22 μm filters to remove any remaining bacterial cells. L. brevis CFS aliquots were stored at -80°C until further use.
Isolation of MVs from L. brevis
The isolation of L. brevis MVs was carried out by culturing a loopful of grown colonies on MRS agar in BHI (brain heart infusion) broth supplemented with 0.5% yeast extract, 0.05% (w/v) L-cysteine (Sigma Aldrich, St. Louis, MO, USA), 5 µg/ml hemin, 1 µg/ml vitamin K1 for 24 h under anaerobic conditions. The turbidity of grown cultures was measured with a spectrophotometer and the bacterial concentration was calculated by optical density conversion factor OD600 of 0.5, corresponding to 1.5×108 CFU/ml. A bacterial suspension of 1000 ml was centrifuged at 10000×g for 20 min at 4°C. The resulting supernatant was filtered through 0.22 μm filters to eliminate any remaining residual bacteria. The MVs were acquired by ultracentrifugation of 36 ml of the samples at 150,000×g for 5 h at 4°C. The obtained pellet was washed in PBS (phosphate-buffered saline, pH=7), ultracentrifuged, resuspended in 300 μl of PBS, and stored at -80°C. Prior to freezing, the protein content of the purified MVs was measured using the BCA (bicinchoninic acid) method (DNAbiotech, Tehran, Iran). The absence of LPS (lipopolysaccharide) in the isolated MVs was confirmed using LAL Chromogenic Endotoxin Quantitation Kit (Thermo Fisher Scientific, MA, USA). The protein pattern of MVs was assessed by SDS-PAGE (sodium dodecyl-sulfate polyacrylamide gel electrophoresis).
MVs characterization
Transmission electron microscopy
The isolated MV samples were applied to a 400-mesh copper grid carbon-coated formvar film and stained with 2% uranyl acetate. The structure of samples was examined in a Philips EM208 TEM system with an accelerating voltage up to 100 Kv and the image magnification of 89 kX.
DLS
The purified MVs were analyzed by DLS (dynamic light scattering) in a particle size analyzer (Nano-ZS, ZEN3600, Malvern Instruments, UK) to determine the size distribution of the MVs (Vargoorani et al., 2020).
Cell culture conditions
The AGS human gastric adenocarcinoma cell line was obtained from the Iranian Biological Resource Center (accession cell no. C10071). The AGS cells were maintained in media containing RPMI-1640 supplemented with 10% FBS (Gibco-Invitrogen, Carlsbad, CA), 1% penicillin–streptomycin and 2 mM l-glutamine under humidified atmosphere with 5% CO2 at 37°C. Sub-culturing of cells was carried out by trypsinization when cells reached 80% confluency.
Cell viability assay
AGS cells were seeded in 96-well plates at a density of 1×105 cells/well and reached 80% confluent monolayers. Thereafter, cells were treated with the H. pylori isolate at a multiplicity of infection of 100 bacteria per cell (MOI 100), live L. brevis (MOI 10, 50, and 100), L. brevis-derived MVs (1, 10, 50, and 100 μg/ml), L. brevis CFS (20% (v/v), pasteurized L. brevis (109 CFU/ml), and vitamin D3 (20, 50, 100, 150 nmol) for 24 h. AGS cells were consequently incubated with MTT solution (Sigma Aldrich, St. Louis, MO, USA) at concentration of 5 mg/L for 4 h and then dissolved in 100 μL of DMSO. The cell viability was measured with an absorbance at 570 nm and a reference wavelength of 630 using a microplate reader (Eon, BioTek Instruments, USA). Vitamin D3 treatments were prepared from cholecalciferol dissolved in cell culture grade DMSO (dimethyl sulfoxide). In addition, DMSO solvent control was included in each experiment.
Infection and treatment of AGS cells
Exponentially growing AGS control and experimental cultures were seeded at a density of 1×106 cells/well and incubated for 48 h at 37°C. Firstly, AGS cells were infected with the H. pylori isolate or treated with vitamin D3, live L. brevis, pasteurized L. brevis, L. brevis-derived MVs, or L. brevis CFS for 24 h. Secondly, based on the cell viability results, AGS cells were treated with H. pylori and simultaneously with one of the followings: vitamin D3, live L. brevis, pasteurized L. brevis, L. brevis-derived MVs, or L. brevis CFS for 24 h. Finally, other wells of AGS cells were simultaneously treated with H. pylori, vitamin D3, and one of the followings: live L. brevis, pasteurized L. brevis, L. brevis-derived MVs, or L. brevis CFS for 24 h.
Adhesion assay
AGS cells were seeded in 96-well plates at a density of 1×105 cells/well and incubated to reach 80% confluent monolayers. Cells were infected with the H. pylori isolate and treated with L. brevis with or without vitamin D3 for 2 h. The AGS cell monolayer was washed three times with PBS (pH=7) to eliminate the unattached bacteria. To evaluate the number of adhered bacteria, AGS cells were treated with 0.1% saponin for 5 min at room temperature and consequently spread on Brucella agar supplemented with 5% sheep blood. The bacterial CFU and percentage of adhesion was calculated after 3 days of incubation.
Oxidative stress assay
Oxidative stress was evaluated in the supernatant of AGS cells using NO (nitric oxide) assay kit (KPG, Tehran, Iran) and MDA (lipid peroxidation) assay kit (KPG, Tehran, Iran). All experiments were performed in triplicate.
Total RNA extraction and RT-qPCR
According to the manufacture’s instruction, total RNA was extracted from treated AGS cells using RNeasy Plus Mini Kit (Qiagen, GmbH, Germany) and the acquired RNA samples were assessed by ultraviolet spectroscopy (NanoDrop spectrophotometer, ND-1000, Thermo Scientific, MA, USA). cDNA was synthesized using a BioFACT™ RT-Kit (BIOFACT CO., Ltd. Daejeon, South Korea). The RT-qPCR was performed by Rotor-Gene® Q (Qiagen, GmbH, Germany) real-time PCR system using BioFACT™ 2X Real-Time PCR Master Mix (BIOFACT CO., Ltd. Daejeon, South Korea). The oligonucleotide sequence of primers used for RT-qPCR are listed in Table 1.
Statistical analysis
The results are expressed as mean ± SD (standard deviation) of three independent experiments. Statistical differences were determined using one-way ANOVA, performed by GraphPad Prism 5 software version 5.04 (GraphPad Software, Inc., San Diego, CA, USA). Statistical differences are indicated by asterisks (*P <0.05, **P <0.01, ***P <0.001, and ****P <0.0001).
Results
Characterization of L. brevis-derived MVs
L. brevis-derived MVs were isolated from the culture supernatant by filtration and ultracentrifugation. The protein profile of L. brevis-derived MVs was observed by SDS-PAGE ranging from 10-100 kDa (Figure 1A). The isolated MVs were morphologically characterized by TEM analysis, revealing nanosized, spherical, membrane-bound vesicles (Figure 1B).
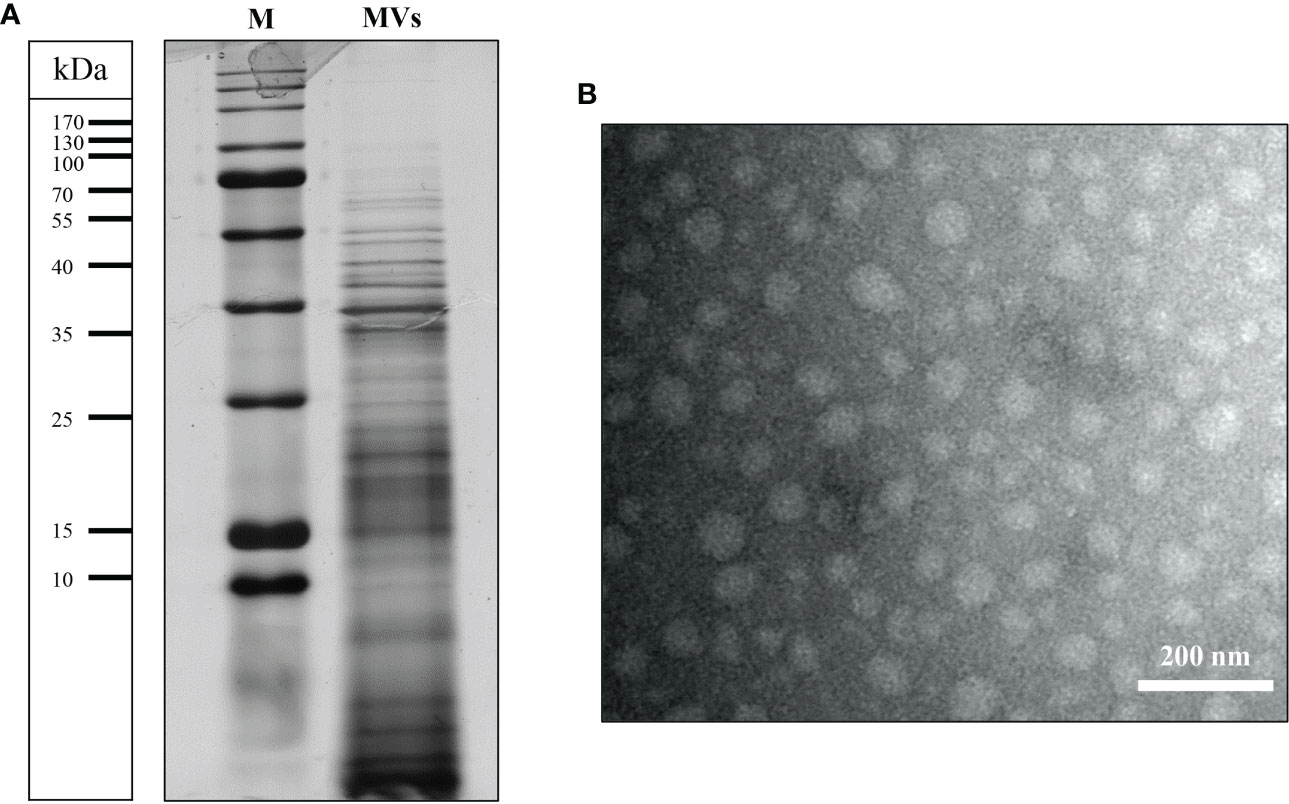
Figure 1 Characterization of the L. brevis strain IBRC-M10790-derived MVs isolated in this study. (A) Protein profiles of MVs separated by 12% SDS-PAGE followed by visualization of proteins using Coomassie blue staining. Lines to the left indicate the molecular masses of the protein standards in kDa. (B) Representative transmission electron micrographs of MVs. Scale bar (lower right) represent: 200 nm. M, molecular weight marker.
Cell viability
To evaluate the toxicity of vitamin D3, H. pylori, L. brevis, and L. brevis derivatives, the MTT assay was carried out measuring the viability of AGS cells treated with H. pylori (MOI 100), vitamin D3 (20, 50, 100, 150 nmol), live L. brevis (MOI 10, 50, and 100), pasteurized L. brevis (109 CFU/ml), L. brevis-derived MVs (1, 10, 50, and 100 μg/ml), and L. brevis CFS (20% (v/v) compared to the untreated control. As demonstrated in Figure S1, the viability of AGS cells slightly reduced following H. pylori infection and increased after probiotic or vitamin D3 treatment; however, there was no significant alteration in the viability of AGS cells in either group compared to the control. Therefore, considering the results from previous studies (Hu et al., 2019; Zhou et al., 2020), L. brevis at MOI 100, L. brevis-derived MVs at concentration of 100 μg/ml, and 150 nmol of vitamin D3 were used as the selected concentrations for further cell culture experiments.
The impact of vitamin D3 and L. brevis on H. pylori adhesion
H. pylori adhesion to AGS cells was screened following 2 h of exposure to different vitamin D3 and probiotic treatment groups. Live and pasteurized L. brevis, L. brevis-derived MVs, and L. brevis CFS significantly reduced H. pylori adhesion (Figure 2). Vitamin D3 treatment slightly decreased H. pylori adhesion by approximately 5.5% and was considered statistically significant, which might not be substantial in animal models and clinical trials. The combination of vitamin D3 with live L. brevis, pasteurized L. brevis, or L. brevis-derived MVs significantly reduced H. pylori adhesion to AGS cells compared to the same probiotic treatment groups without vitamin D3. The anti-adhesion activity of L. brevis CFS, however, presented no significant alteration in combination with vitamin D3. All complementary groups exhibited significantly higher anti-adhesion activity compared to the vitamin D3-treated group. Overall, the adhesion of H. pylori to AGS cells was significantly interfered by vitamin D3 or probiotic treatment, especially L. brevis CFS. Given the high efficacy of L. brevis CFS, vitamin D3 and L. brevis CFS combination presented no synergistic effect in preventing H. pylori adhesion. However, the combination of vitamin D3 with other L. brevis derivatives demonstrated a more significant reduction (up to 30%) in H. pylori adhesion.
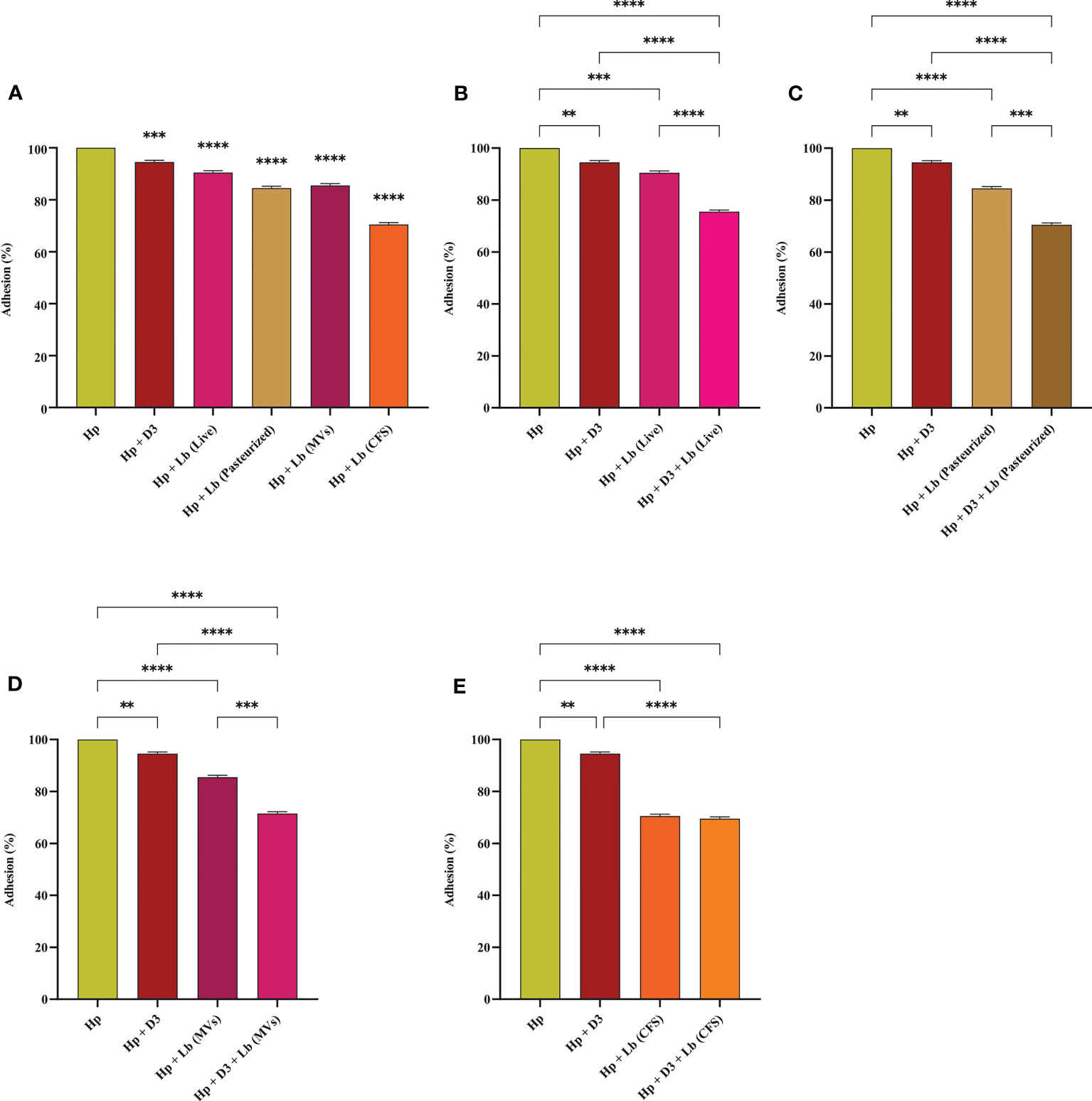
Figure 2 Adherence rate of H. pylori to AGS cells. (A) Adherence rate of H. pylori treated with vitamin D3, live and pasteurized L. brevis, L. brevis-derived MVs, and L. brevis CFS. Adherence rate of H. pylori treated with vitamin D3 and live L. brevis (B), pasteurized L. brevis (C), L. brevis-derived MVs (D), or L. brevis CFS (E). (*P <0.05; **P <0.01; ***P <0.001; ****P <0.0001).
The effect of vitamin D3 and L. brevis on H. pylori-mediated oxidative stress
We measured NO and MDA levels in AGS cells to evaluate the antioxidant capacity of vitamin D3 and L. brevis. As depicted in Figure 3, H. pylori infection significantly increased NO concentration in AGS cells, whereas vitamin D3 and probiotic treatment substantially lowered NO levels, compared to H. pylori-infected cells. Notably, the combination of vitamin D3 with each type of probiotic treatment group (especially live L. brevis) presented a significant reduction in oxidative stress, compared to vitamin D3 or probiotic treatment alone. Likewise, the infection of AGS cells with H. pylori resulted in significantly higher levels of MDA, compared to the untreated control (Figure 3). Vitamin D3 or probiotic treatment, however, substantially reduced MDA levels, compared to the H. pylori-infected group. Compared to vitamin D3 treatment, the combination of vitamin D3 with live L. brevis, pasteurized L. brevis, and L. brevis CFS demonstrated a synergistic effect in lowering MDA concentration. Additionally, live L. brevis and vitamin D3 combination exhibited a synergistic effect, compared to probiotic treatment alone. Hence, vitamin D3 and probiotic treatment together (particularly live L. brevis) might present a more significant impact on reducing H. pylori-induced oxidative stress.
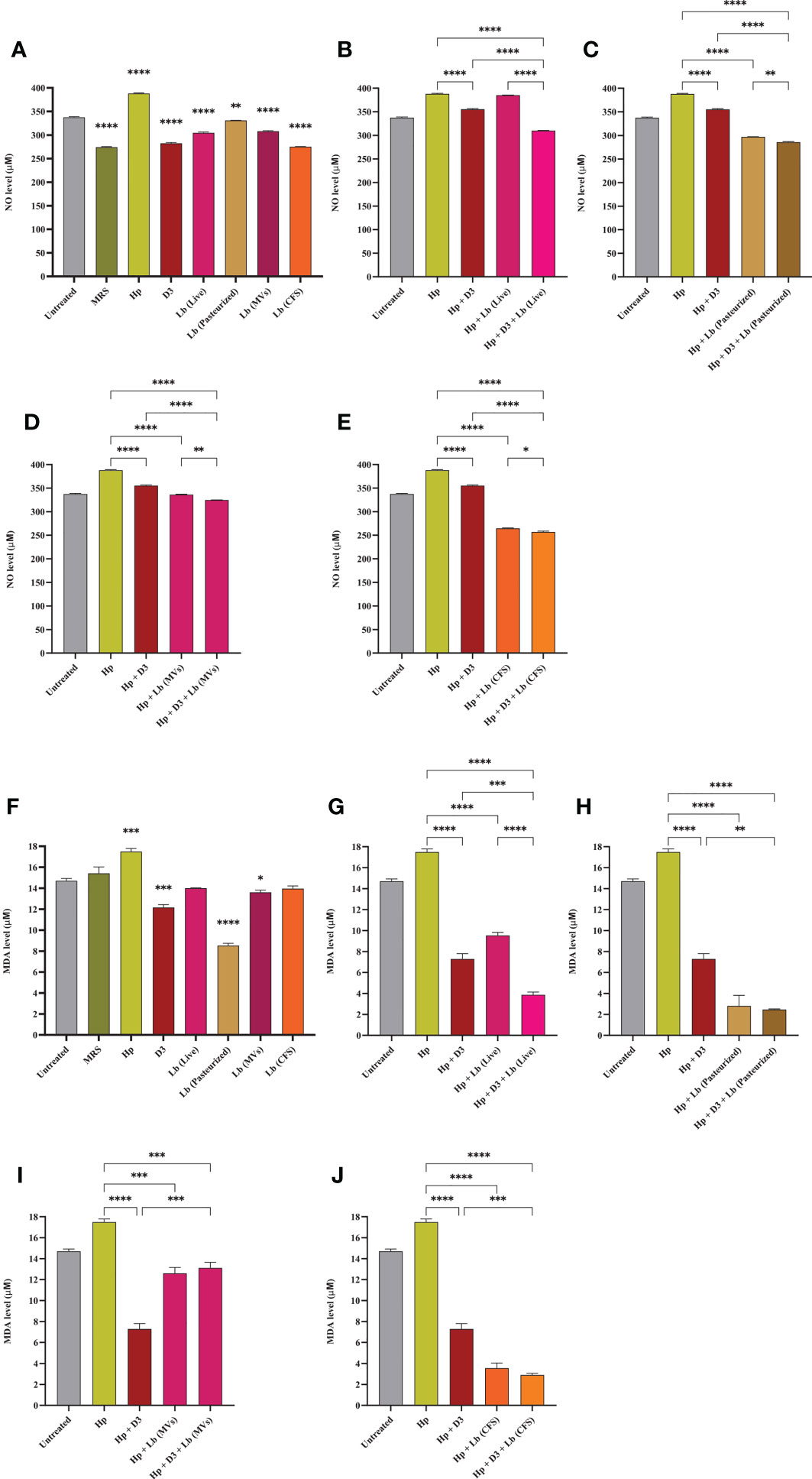
Figure 3 NO and MDA concentration in the culture supernatant of AGS cells. (A) NO concentration in the presence of H. pylori, vitamin D3, live and pasteurized L. brevis, L. brevis-derived MVs, and L. brevis CFS. NO concentration in the culture supernatant of AGS cells during exposure to H. pylori and simultaneous treatment with vitamin D3 and live L. brevis (B), pasteurized L. brevis (C), L. brevis-derived MVs (D), or L. brevis CFS (E). (F) MDA concentration in the presence of H. pylori, vitamin D3, live and pasteurized L. brevis, L. brevis-derived MVs, and L. brevis CFS. MDA concentration in the culture supernatant of AGS cells during exposure to H. pylori and simultaneous treatment with vitamin D3 and live L. brevis (G), pasteurized L. brevis (H), L. brevis-derived MVs (I), or L. brevis CFS (J). (*P <0.05; **P <0.01; ***P <0.001; ****P <0.0001).
Vitamin D3 and L. brevis promote the integrity of gastric epithelial barrier
We assessed the expression level of ZO-1 in AGS cells upon H. pylori infection and vitamin D3 and/or probiotic treatment. As presented in Figure 4, H. pylori infection of AGS cells was associated with a substantial reduction in the expression of ZO-1. On the contrary, vitamin D3, live L. brevis, and L. brevis-derived MVs significantly elevated the expression level of ZO-1 compared to the untreated control. Pasteurized L. brevis and L. brevis CFS, however, demonstrated no significant alteration in expression of this gene compared to the untreated control. The treatment of H. pylori-infected cells with vitamin D3, live L. brevis, pasteurized L. brevis, and L. brevis-derived MVs substantially promoted the expression of ZO-1 compared to the H. pylori control group. Furthermore, combination of live L. brevis and L. brevis-derived MVs with vitamin D3 exhibited a more significant elevation compared to the same probiotic treatment groups. Taken together, live, and pasteurized L. brevis, L. brevis-derived MVs, and vitamin D3 compensate the reduction of ZO-1 expression upon H. pylori infection. The combination of vitamin D3 with live L. brevis and L. brevis-derived MVs might exert a synergistic effect on the expression of ZO-1 in AGS cells.
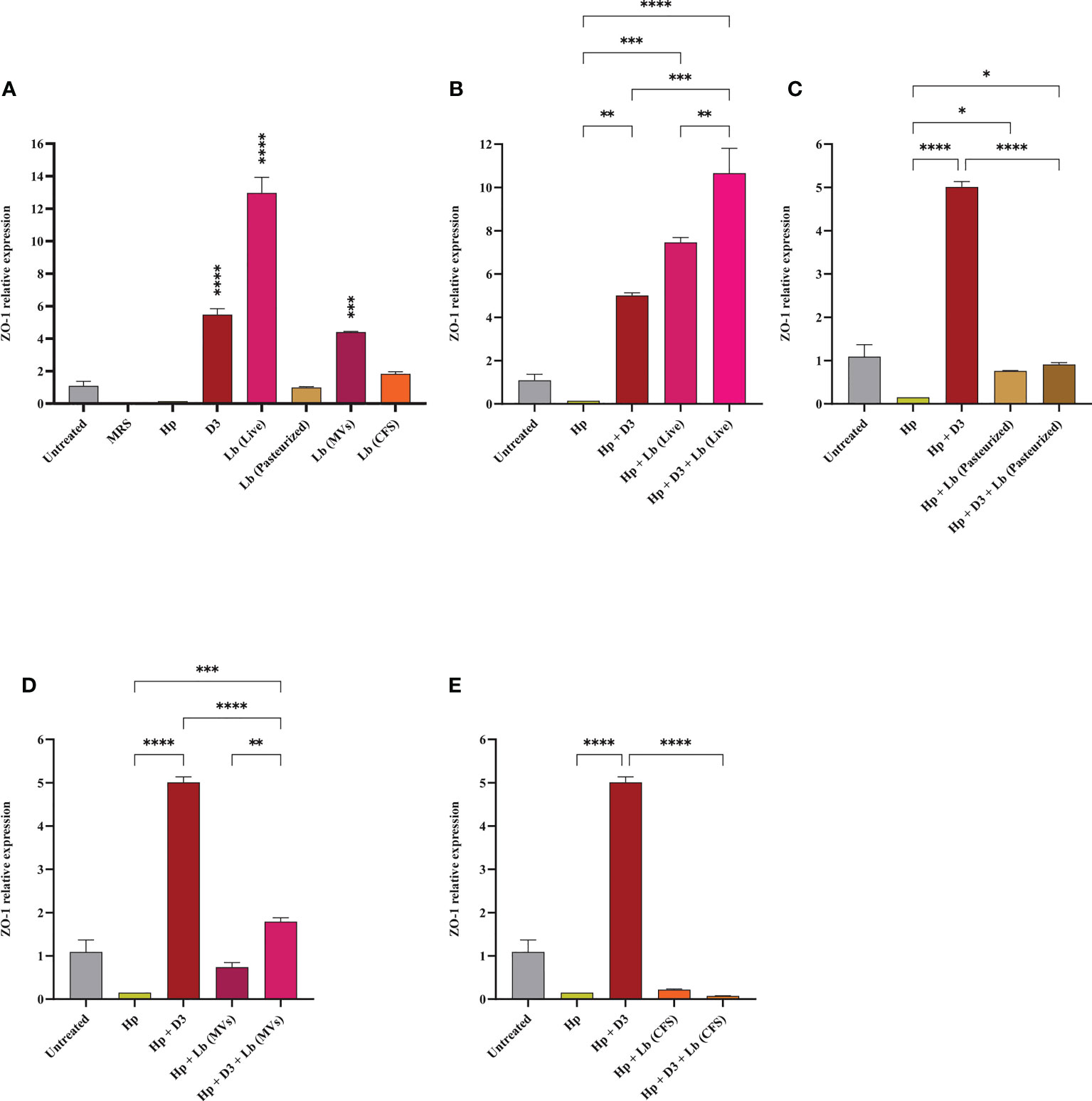
Figure 4 The expression level of ZO-1 in AGS cells. (A) ZO-1 expression level in AGS cells treated with H. pylori, vitamin D3, live and pasteurized L. brevis, L. brevis-derived MVs, and L. brevis CFS. ZO-1 expression level in AGS cells during exposure to H. pylori and simultaneous treatment with vitamin D3 and live L. brevis (B), pasteurized L. brevis (C), L. brevis-derived MVs (D), or L. brevis CFS (E). (*P <0.05; **P <0.01; ***P <0.001; ****P <0.0001).
Vitamin D3 downregulates H. pylori-induced expression of inflammatory genes
In this study, we evaluated the potential capacity of vitamin D3 in modulating the expression of pro-inflammatory cytokines IL-6, IL-8, TNF-α, and IFN-γ in AGS cells during H. pylori infection (Figures 5, 6). The co-culture of AGS cells with the clinical isolate of H. pylori significantly promoted the expression of pro-inflammatory cytokines compared to the untreated control. Treatment of AGS cells with vitamin D3 resulted in a substantial reduction in the expression of TNF-α and IFN-γ compared to the untreated control. Furthermore, treatment of H. pylori-infected cells with 150 nmol of vitamin D3 for 24 h substantially lowered the expression of pro-inflammatory cytokines IL-6, IL-8, TNF-α, and IFN-γ.
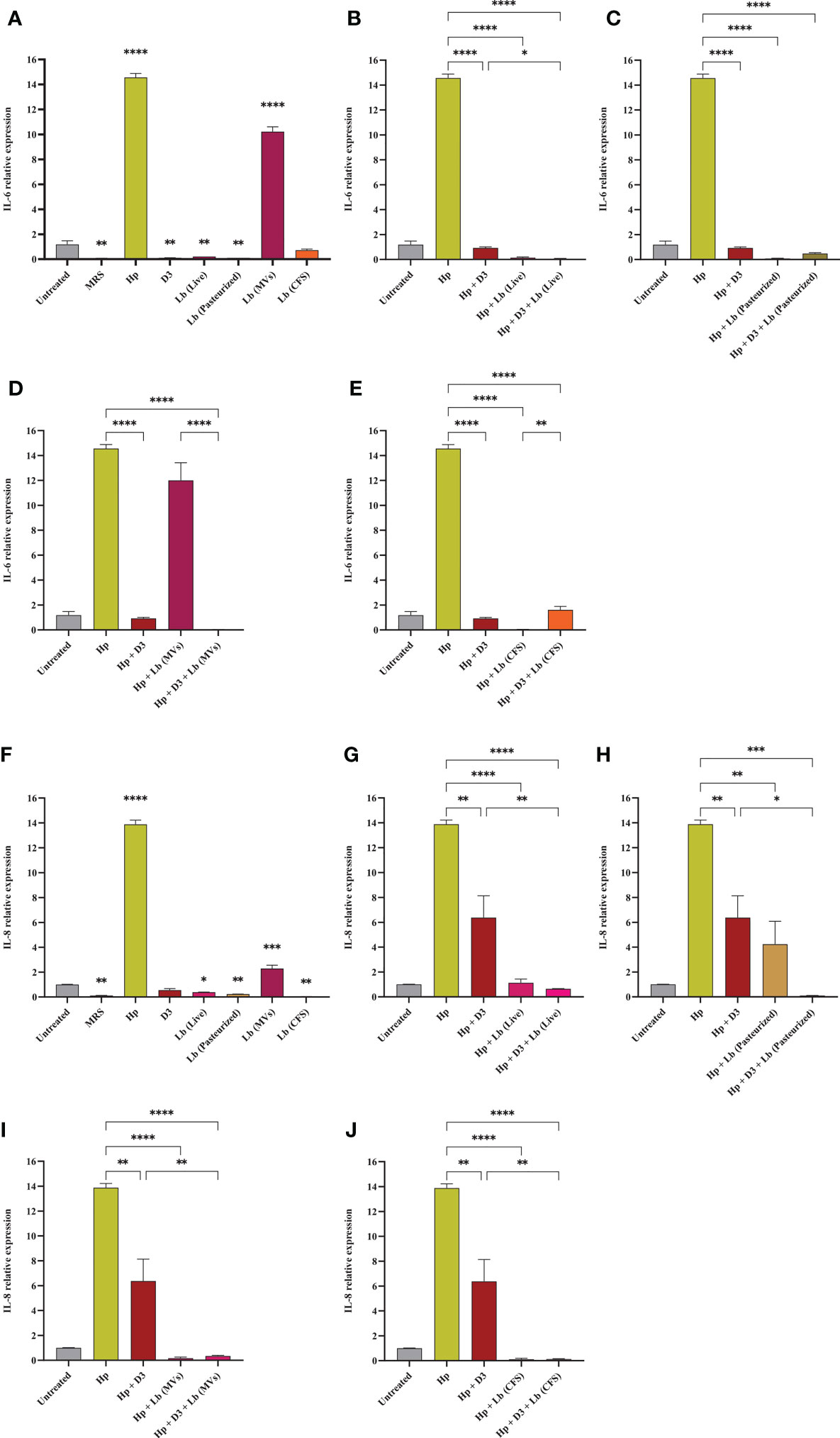
Figure 5 The expression level of IL-6 and IL-8 in AGS cells. (A) IL-6 expression level in AGS cells treated with H. pylori, vitamin D3, live and pasteurized L. brevis, L. brevis-derived MVs, and L. brevis CFS. IL-6 expression level in AGS cells during exposure to H. pylori and simultaneous treatment with vitamin D3 and live L. brevis (B), pasteurized L. brevis (C), L. brevis-derived MVs (D), or L. brevis CFS (E). (F) IL-8 expression level in AGS cells treated with H. pylori, vitamin D3, live and pasteurized L. brevis, L. brevis-derived MVs, and L. brevis CFS. IL-8 expression level in AGS cells during exposure to H. pylori and simultaneous treatment with vitamin D3 and live L. brevis (G), pasteurized L. brevis (H), L. brevis-derived MVs (I), or L. brevis CFS (J). (*P <0.05; **P <0.01; ***P <0.001; ****P <0.0001).
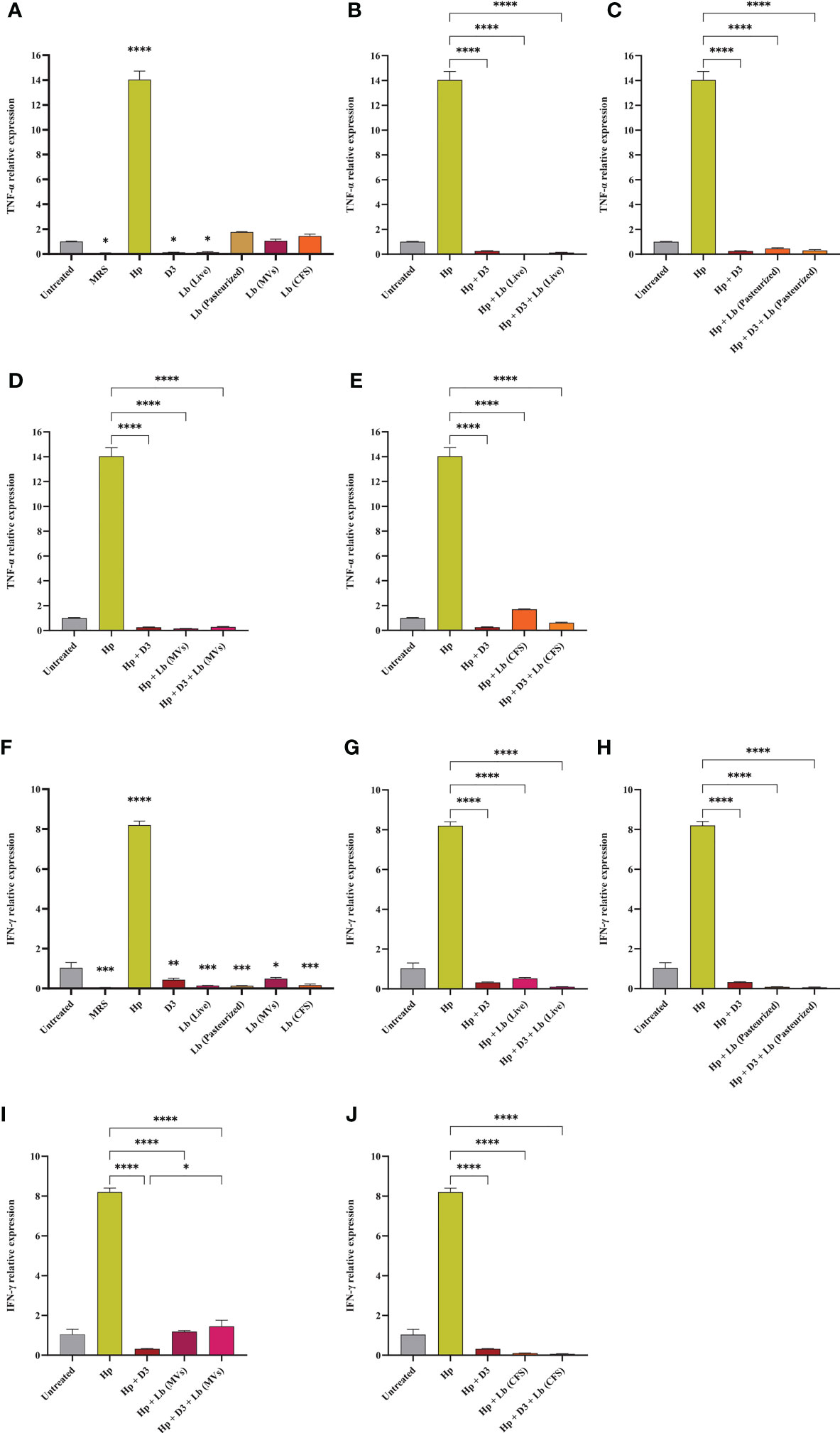
Figure 6 The expression level of TNF-α and IFN-γ in AGS cells. (A) TNF-α expression level in AGS cells treated with H. pylori, vitamin D3, live and pasteurized L. brevis, L. brevis-derived MVs, and L. brevis CFS. TNF-α expression level in AGS cells during exposure to H. pylori and simultaneous treatment with vitamin D3 and live L. brevis (B), pasteurized L. brevis (C), L. brevis-derived MVs (D), or L. brevis CFS (E). (F) IFN-γ expression level in AGS cells treated with H. pylori, vitamin D3, live and pasteurized L. brevis, L. brevis-derived MVs, and L. brevis CFS. IFN-γ expression level in AGS cells during exposure to H. pylori and simultaneous treatment with vitamin D3 and live L. brevis (G), pasteurized L. brevis (H), L. brevis-derived MVs (I), or L. brevis CFS (J). (*P <0.05; **P <0.01; ***P <0.001; ****P <0.0001).
L. brevis suppresses H. pylori-induced expression of inflammatory genes
Here, we investigated the capacity of the local strain L. brevis IBRC-M10790 to reduce the inflammatory activity of H. pylori. In this regard, live L. brevis, pasteurized L. brevis, and L. brevis CFS significantly lowered the expression level of IL-8 and IFN-γ in uninfected AGS cells compared to the untreated control (Figures 5, 6). However, only live L. brevis could substantially reduce the expression of pro-inflammatory cytokine TNF-α (Figure 6). On the contrary, L. brevis-derived MVs presented a significant overexpression of IL-6 and IL-8 in uninfected AGS cells (Figure 5). Live and pasteurized L. brevis profoundly, but not statistically significantly, decreased the IL-6 expression (Figure 5). Yet, the exposure of H. pylori-infected AGS cells to either probiotic treatment groups led to a substantial reduction in the expression of IL-6, IL-8, TNF-α, and IFN-γ.
The synergistic effect of vitamin D3 and L. brevis on H. pylori-induced inflammation
Vitamin D3 combination with L. brevis CFS significantly promoted the anti-inflammatory activity of this probiotic treatment group in reducing the expression level of IL-6, IL8, and IFN-γ. Evaluating the expression level of IFN-γ, vitamin D3 could substantially boost the anti-inflammatory effect of live L. brevis in H. pylori-infected AGS cells (Figure 6G). Similarly, the combination of vitamin D3 and L. brevis-derived MVs exhibited a more significant reduction in the expression of IL-6 in H. pylori-infected AGS cells compared to the same probiotic treatment group (Figure 5D). On the other hand, each probiotic treatment groups significantly promoted the suppressive activity of vitamin D3 against the expression of IL-6 in H. pylori-infected AGS cells (Figure 5). Consequently, the combination of vitamin D3 and L. brevis to some extent presented a synergistic anti-inflammatory activity during H. pylori infection.
Discussion
The increasing incidence of antibiotic resistance in H. pylori is a global threat, leading to a significant reduction in sufficient efficacy of H. pylori eradication (>80-90% efficacy level) and an elevation in the risk of clinical complications. All recommended regimens in treatment guidelines as first-line and rescue therapies encounter failure in 10-30% of H. pylori-infected subjects (Tshibangu-Kabamba and Yamaoka, 2021). In the context of antibiotic resistance, alternative and complementary therapeutic strategies are being given considerable attention. Probiotic supplementation has demonstrated several beneficial effects by eliminating pathogenic bacteria, promoting host immunity, and attenuating antibiotic side effects (Nabavi-Rad et al., 2022b). Given the beneficial yet insufficient influence of probiotic monotherapy on H. pylori eradication, probiotics are taken alongside antibiotics (Losurdo et al., 2018). In an effort to promote probiotic efficacy, recent studies exhibited a synergistic effect of combining probiotics with minerals and vitamins, especially vitamin D (Abboud et al., 2020). Jamilian et al. reported the beneficial influence of probiotics (Lactobacillus acidophilus, Bifidobacterium lactis, Bifidobacterium bifidum, and Bifidobacterium longum) and selenium co-supplementation on the general score and insulin, homeostasis model of assessment-insulin resistance (HOMA-IR), quantitative insulin sensitivity check index (QUICKI), fasting plasma glucose (FPG), high-sensitivity C-reactive protein (hs-CRP), total antioxidant capacity (TAC), and glutathione (GSH) serum levels in chronic schizophrenia (Jamilian and Ghaderi, 2021). Additionally, Ghaderi et al. demonstrated the favorable influence of probiotics (L. acidophilus, B. bifidum, Lactobacillus reuteri, and Lactobacillus fermentum) and vitamin D administration on the serum MDA levels, triglycerides levels, and total cholesterol/HDL (high-density lipoprotein)-cholesterol ratio in schizophrenia patients (Ghaderi et al., 2019). Raygan and colleagues presented the improvement of mental health and insulin sensitivity of type 2 diabetic patients following 12 weeks of probiotics (L. acidophilus, L. reuteri, L. fermentum, and B. bifidum) and selenium co-supplementation (Raygan et al., 2019). Furthermore, the combination of probiotics (L. acidophilus, B. bifidum, and Bifidobacterium animalis) with vitamin C exhibited a beneficial impact on the prevention and treatment of the upper respiratory tract infection (Garaiova et al., 2015). Vitamin D and probiotics (L. acidophilus, B. bifidum, L. reuteri, and L. fermentum) co-supplementation was also reported to reduce serum levels of inflammation and oxidative stress biomarkers (hs-CRP and MDA) in gestational diabetes patients (Jamilian et al., 2019). Vitamin D supplementation has been suggested with the capacity to attenuate the serum levels of several inflammatory biomarkers by interacting with the vitamin D receptor (VDR) (Alvarez et al., 2013; Gregório et al., 2021). Vitamin D-VDR interaction was reported to further induce the expression of cathelicidin antimicrobial peptide (CAMP) and inhibit H. pylori infection (Zhou et al., 2020). Furthermore, vitamin D activates the autolysosomal degradation of H. pylori by interacting with the protein disulfide isomerase family A member 3 (PDIA3) receptor (Hu et al., 2019). Given the negative correlation between H. pylori infection and serum levels of vitamin D and the beneficial impact of probiotic supplementation on H. pylori eradication, we evaluated the synergistic effect of probiotic and vitamin D treatment on the inflammatory and oxidative activity of H. pylori. We further evaluated the influence of probiotic and vitamin D treatment on the integrity of AGS cells and the adherence rate of H. pylori to these cells (Figure 7). To this end, we utilized live and pasteurized L. brevis, L. brevis-derived MVs, and L. brevis CFS, as well as vitamin D3 in this work.
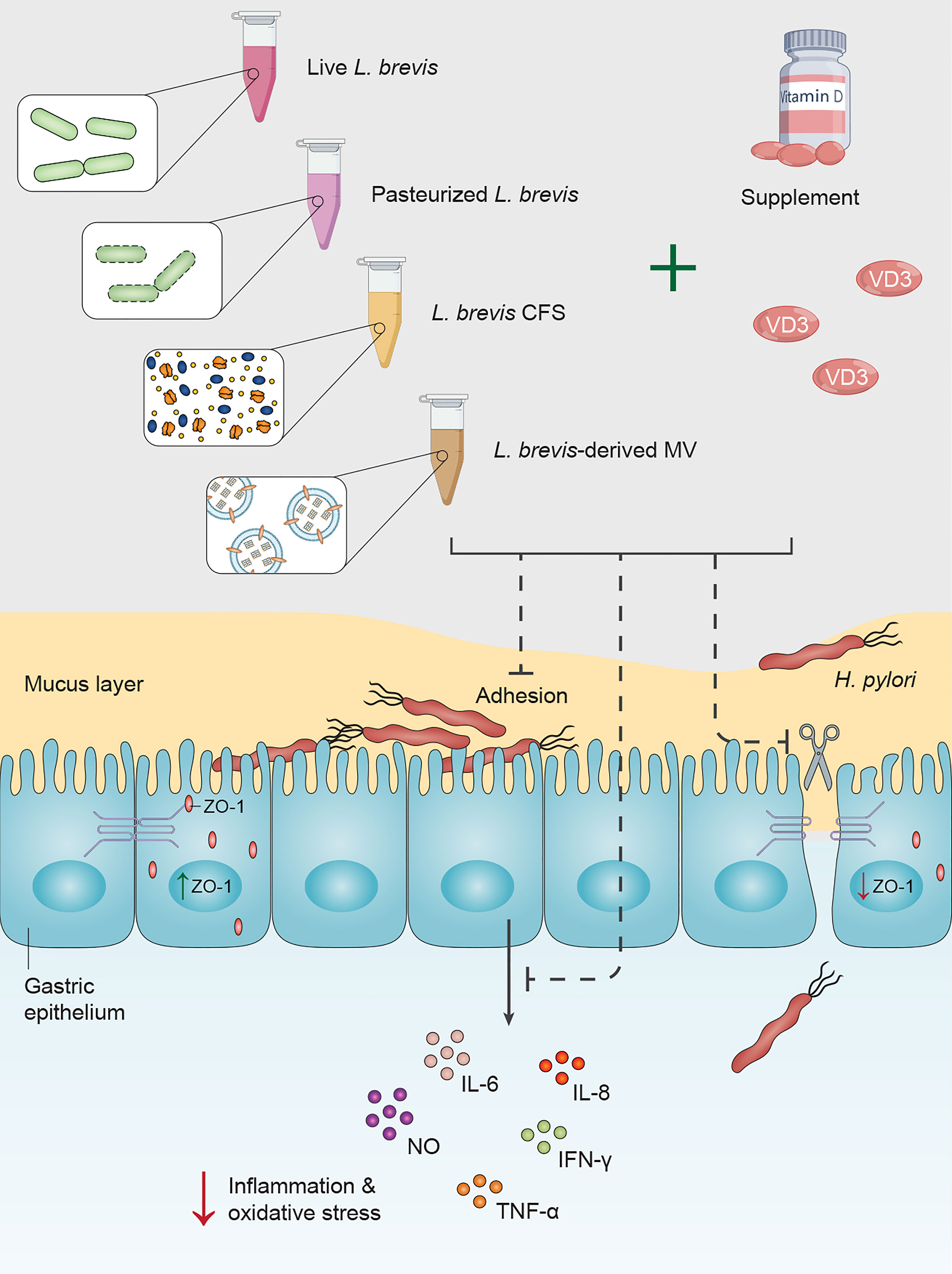
Figure 7 Vitamin D3 and L. brevis co-treatment can prevent H. pylori attachment to the gastric epithelium, attenuate H. pylori-induce inflammation and oxidative stress, and promote the integrity of the gastric epithelial barrier.
Upon contacting the host gastric mucus layer, H. pylori protects itself by swimming freely through the viscous layer of gastric mucus, attaching to gastric epithelial cells, and colonizing the gastric mucosa epithelium (Huang et al., 2016). Given the significance of H. pylori attachment to gastric epithelial cells for its survival, colonization, and pathogenesis, we assessed the effect of L. brevis and vitamin D3 treatment on the adherence rate of H. pylori to AGS cells. Consistent with previous studies (Chen et al., 2019; Song et al., 2019), L. brevis or vitamin D3 treatment substantially reduced H. pylori attachment to AGS cells. Furthermore, vitamin D3 co-treatment with live and pasteurized L. brevis and L. brevis-derived MVs presented a synergistic impact on the adherence rate of H. pylori. One potential mechanistic action of live probiotics is competitive exclusion of H. pylori, which refers to rigorous competition for attachment sites and available nutrients (van Zyl et al., 2020). As for probiotic derivatives and vitamin D3, they possibly can reduce the number of H. pylori bacteria through bactericidal activity and further decrease H. pylori adherence rate by regulating the integrity of the gastric epithelial barrier (Raheem et al., 2021; Nabavi-Rad et al., 2022a).
The gastric epithelial barrier, which is a major obstacle preventing H. pylori penetration to lamina propria, plays a key role in preserving gastric homeostasis. The integrity of this barrier highly relies on the expression and production of tight junction proteins (Moonwiriyakit et al., 2022). The tight junction protein 1 (TJP1)/ZO-1 has a critical activity in maintaining the integrity of the gastric barrier and repairing the mucus layer (Cario et al., 2004; Kuo et al., 2021). H. pylori separates the epithelial tight junction proteins, disrupts the membrane distribution of ZO-1 in gastric epithelial cells, and leads to the accumulation of ZO-1 in small vesicles (Wroblewski and Peek, 2011). Yeung et al. reported a negative correlation between vitamin D deficiency and the production of tight junction proteins in the gut epithelium of mouse models (Yeung et al., 2021). Zhao et al. also demonstrated the protective role of vitamin D3 in reducing mucosal injury and maintaining the structural integrity of colonic epithelial cells in acute colitis mouse models (Zhao et al., 2012). Similarly, our results exhibited the substantial influence of vitamin D3 treatment on the expression level of ZO-1 in AGS cells. Moreover, the combination of vitamin D3 with live L. brevis or L. brevis-derived MVs had a remarkable impact on H. pylori-infected AGS cells, compared to probiotic treatment alone.
Following H. pylori attachment to the gastric epithelium and penetration to the lamina propria, the excessive production of reactive oxygen species (ROS), reactive nitrogen species (RNS), and pro-inflammatory cytokines from the epithelium and immune cells have a particular significance in H. pylori pathogenesis (Jain et al., 2021). H. pylori-induced activation of pro-inflammatory pathways acts mainly through nuclear factor kappa B (NF-κB), which leads to the accumulation of reactive radicals and the development of precancerous lesions (He et al., 2022). Continuous oxidative stress can induce DNA damage, cellular apoptosis, and consequently gastric carcinogenesis (Butcher et al., 2017). Using VDR knockdown mouse models, Wu et al. reported that VDR expression negatively regulates pathogen-induced NF-κB activity and attenuates the inflammation (Wu et al., 2010). Furthermore, different strains of L. brevis bacteria have been reported to present a beneficial impact by modulating the immune response and attenuating the intensity of oxidative stress and inflammation (Lim et al., 2016; Jiang et al., 2018; Brandi et al., 2020). Compared to the beneficial effect of live L. brevis, the combination of L. brevis with vitamin D3 demonstrated a significant reduction in the concentration of oxidative biomarkers. On the other hand, vitamin D3 combination with live L. brevis, pasteurized L. brevis, or L. brevis CFS presented a synergistic effect on H. pylori-infected AGS cells, compared to the vitamin D3 treatment group. Although vitamin D is better known for its capacity in eradicating H. pylori infection, this supplement has potent anti-inflammatory traits by regulating the expression of different pro- and anti-inflammatory biomarkers (Krajewska et al., 2022). In this study, vitamin D3 treatment resulted in a lower expression level of pro-inflammatory cytokines in H. pylori-infected AGS cells with a significant reduction in TNF-α and IFN-γ expression levels. Additionally, vitamin D3 co-treatment with L. brevis to some extent could boost the anti-inflammatory properties of this probiotic bacteria.
Conclusions
This study demonstrates a synergistic effect for vitamin D3 and L. brevis co-treatment in reducing H. pylori inflammatory and oxidative activity as well as adherence ratio in AGS cells. However, limitations to our work include the absence of in vivo experiments and proteomics/metabolomics analysis. The metabolite composition of L. brevis-derived MVs, the bactericidal mechanistic action of L. brevis against H. pylori, and the efficacy of vitamin D3 combination with multi-strain probiotic consortia on H. pylori infection need further elucidation. Furthermore, studying the influence of vitamin D3 and L. brevis on gastric and fecal bacterial metabolites, especially H. pylori metabolites, could bring up important insights about H. pylori treatment. Therefore, in vivo studies and mechanism-oriented clinical trials are required to investigate the influence of probiotics and vitamin D co-supplementation on different aspects of H. pylori infection.
Data availability statement
The original contributions presented in the study are included in the article/Supplementary Material. Further inquiries can be directed to the corresponding author.
Author contributions
AN-R, SJ, and MA performed the H. pylori and probiotic culture, cell culture and molecular assays. AN-R reviewed the literature and wrote the manuscript draft. AY contributed to study design, conceptualization and methodology. AN-R and AY analyzed and interpreted the data. AY, KR, TM, and MZ critically edited the manuscript. All authors contributed to the article and approved the submitted version.
Funding
This research was financially supported by the Foodborne and Waterborne Diseases Research Center, Research Institute for Gastroenterology and Liver Diseases, Shahid Beheshti University of Medical Sciences, Tehran, Iran (Project No. RIGLD 1128, IR.SBMU.RIGLD.REC.1399.046).
Conflict of interest
The authors declare that the research was conducted in the absence of any commercial or financial relationships that could be construed as a potential conflict of interest.
Publisher’s note
All claims expressed in this article are solely those of the authors and do not necessarily represent those of their affiliated organizations, or those of the publisher, the editors and the reviewers. Any product that may be evaluated in this article, or claim that may be made by its manufacturer, is not guaranteed or endorsed by the publisher.
Supplementary material
The Supplementary Material for this article can be found online at: https://www.frontiersin.org/articles/10.3389/fcimb.2023.1171469/full#supplementary-material
References
Abboud, M., Rizk, R., AlAnouti, F., Papandreou, D., Haidar, S., Mahboub, N. (2020). The health effects of vitamin d and probiotic Co-supplementation: a systematic review of randomized controlled trials. Nutrients 13 (1), 111. doi: 10.3390/nu13010111
Alvarez, J. A., Zughaier, S. M., Law, J., Hao, L., Wasse, H., Ziegler, T. R., et al. (2013). Effects of high-dose cholecalciferol on serum markers of inflammation and immunity in patients with early chronic kidney disease. Eur. J. Clin. Nutr. 67 (3), 264–269. doi: 10.1038/ejcn.2012.217
Beresniak, A., Malfertheiner, P., Franceschi, F., Liebaert, F., Salhi, H., Gisbert, J. P. (2020). Helicobacter pylori “Test-and-Treat” strategy with urea breath test: a cost-effective strategy for the management of dyspepsia and the prevention of ulcer and gastric cancer in Spain-results of the hp-breath initiative. Helicobacter 25 (4), e12693. doi: 10.1111/hel.12693
Brandi, J., Cheri, S., Manfredi, M., Di Carlo, C., Vita Vanella, V., Federici, F., et al. (2020). Exploring the wound healing, anti-inflammatory, anti-pathogenic and proteomic effects of lactic acid bacteria on keratinocytes. Sci. Rep. 10 (1), 11572. doi: 10.1038/s41598-020-68483-4
Butcher, L. D., den Hartog, G., Ernst, P. B., Crowe, S. E. (2017). Oxidative stress resulting from helicobacter pylori infection contributes to gastric carcinogenesis. Cell Mol. Gastroenterol. Hepatol. 3 (3), 316–322. doi: 10.1016/j.jcmgh.2017.02.002
Cario, E., Gerken, G., Podolsky, D. K. (2004). Toll-like receptor 2 enhances ZO-1-associated intestinal epithelial barrier integrity via protein kinase c. Gastroenterology 127 (1), 224–238. doi: 10.1053/j.gastro.2004.04.015
Caruso, R., Marafini, I., Sedda, S., Del Vecchio Blanco, G., Giuffrida, P., MacDonald, T. T., et al. (2014). Analysis of the cytokine profile in the duodenal mucosa of refractory coeliac disease patients. Clin. Sci. (Lond) 126 (6), 451–458. doi: 10.1042/CS20130478
Chen, Y.-H., Tsai, W.-H., Wu, H.-Y., Chen, C.-Y., Yeh, W.-L., Chen, Y.-H., et al. (2019). Probiotic lactobacillus spp. act against helicobacter pylori-induced inflammation. J. Clin. Med. 8 (1), 90. doi: 10.3390/jcm8010090
El Shahawy, M. S., Hemida, M. H., El Metwaly, I., Shady, Z. M. (2018). The effect of vitamin d deficiency on eradication rates of helicobacter pylori infection. JGH Open 2 (6), 270–275. doi: 10.1002/jgh3.12081
Fakharian, F., Asgari, B., Nabavi-Rad, A., Sadeghi, A., Soleimani, N., Yadegar, A., et al. (2022). The interplay between helicobacter pylori and the gut microbiota: an emerging driver influencing the immune system homeostasis and gastric carcinogenesis. Front. Cell Infect. Microbiol. 12, 953718. doi: 10.3389/fcimb.2022.953718
Garaiova, I., Muchová, J., Nagyová, Z., Wang, D., Li, J. V., Országhová, Z., et al. (2015). Probiotics and vitamin c for the prevention of respiratory tract infections in children attending preschool: a randomised controlled pilot study. Eur. J. Clin. Nutr. 69 (3), 373–379. doi: 10.1038/ejcn.2014.174
Ghaderi, A., Banafshe, H. R., Mirhosseini, N., Moradi, M., Karimi, M. A., Mehrzad, F., et al. (2019). Clinical and metabolic response to vitamin d plus probiotic in schizophrenia patients. BMC Psychiatry 19 (1), 77. doi: 10.1186/s12888-019-2059-x
Gregório, P. C., Bucharles, S., Cunha, R. S. D., Braga, T., Almeida, A. C., Henneberg, R., et al. (2021). In vitro anti-inflammatory effects of vitamin d supplementation may be blurred in hemodialysis patients. Clinics (Sao Paulo) 76, e1821. doi: 10.6061/clinics/2021/e1821
Han, C., Ni, Z., Yuan, T., Zhang, J., Wang, C., Wang, X., et al. (2019). Influence of serum vitamin d level on helicobacter pylori eradication: a multi-center, observational, prospective and cohort study. J. Dig Dis. 20 (8), 421–426. doi: 10.1111/1751-2980.12793
He, C., Peng, C., Xu, X., Li, N., Ouyang, Y., Zhu, Y., et al. (2022). Probiotics mitigate helicobacter pylori-induced gastric inflammation and premalignant lesions in INS-GAS mice with the modulation of gastrointestinal microbiota. Helicobacter 27 (4), e12898. doi: 10.1111/hel.12898
Hitch, T. C. A., Hall, L. J., Walsh, S. K., Leventhal, G. E., Slack, E., de Wouters, T., et al. (2022). Microbiome-based interventions to modulate gut ecology and the immune system. Mucosal Immunol. 15 (6), 1095–1113. doi: 10.1038/s41385-022-00564-1
Hu, W., Zhang, L., Li, M. X., Shen, J., Liu, X. D., Xiao, Z. G., et al. (2019). Vitamin D3 activates the autolysosomal degradation function against helicobacter pylori through the PDIA3 receptor in gastric epithelial cells. Autophagy 15 (4), 707–725. doi: 10.1080/15548627.2018.1557835
Huang, Y., Wang, Q. L., Cheng, D. D., Xu, W. T., Lu, N. H. (2016). Adhesion and invasion of gastric mucosa epithelial cells by helicobacter pylori. Front. Cell Infect. Microbiol. 6, 159. doi: 10.3389/fcimb.2016.00159
Jain, U., Saxena, K., Chauhan, N. (2021). Helicobacter pylori induced reactive oxygen species: a new and developing platform for detection. Helicobacter 26 (3), e12796. doi: 10.1111/hel.12796
Jamilian, M., Amirani, E., Asemi, Z. (2019). The effects of vitamin d and probiotic co-supplementation on glucose homeostasis, inflammation, oxidative stress and pregnancy outcomes in gestational diabetes: a randomized, double-blind, placebo-controlled trial. Clin. Nutr. 38 (5), 2098–2105. doi: 10.1016/j.clnu.2018.10.028
Jamilian, H., Ghaderi, A. (2021). The effects of probiotic and selenium Co-supplementation on clinical and metabolic scales in chronic schizophrenia: a randomized, double-blind, placebo-controlled trial. Biol. Trace Elem Res. 199 (12), 4430–4438. doi: 10.1007/s12011-020-02572-3
JanssenDuijghuijsen, L. M., Grefte, S., de Boer, V. C. J., Zeper, L., van Dartel, D. A. M., van der Stelt, I., et al. (2017). Mitochondrial ATP depletion disrupts caco-2 monolayer integrity and internalizes claudin 7. Front. Physiol. 8, 794. doi: 10.3389/fphys.2017.00794
Ji, J., Yang, H. (2020). Using probiotics as supplementation for helicobacter pylori antibiotic therapy. Int. J. Mol. Sci. 21 (3), 1136. doi: 10.3390/ijms21031136
Jiang, X., Gu, S., Liu, D., Zhao, L., Xia, S., He, X., et al. (2018). Lactobacillus brevis 23017 relieves mercury toxicity in the colon by modulation of oxidative stress and inflammation through the interplay of MAPK and NF-κB signaling cascades. Front. Microbiol. 9, 2425. doi: 10.3389/fmicb.2018.02425
Krajewska, M., Witkowska-Sędek, E., Rumińska, M., Stelmaszczyk-Emmel, A., Sobol, M., Majcher, A., et al. (2022). Vitamin d effects on selected anti-inflammatory and pro-inflammatory markers of obesity-related chronic inflammation. Front. Endocrinol. 13. doi: 10.3389/fendo.2022.920340
Kuo, W. T., Zuo, L., Odenwald, M. A., Madha, S., Singh, G., Gurniak, C. B., et al. (2021). The tight junction protein ZO-1 is dispensable for barrier function but critical for effective mucosal repair. Gastroenterology 161 (6), 1924–1939. doi: 10.1053/j.gastro.2021.08.047
Lim, S.-M., Jeong, J.-J., Jang, S.-E., Han, M. J., Kim, D.-H. (2016). A mixture of the probiotic strains bifidobacterium longum CH57 and lactobacillus brevis CH23 ameliorates colitis in mice by inhibiting macrophage activation and restoring the Th17/Treg balance. J. Funct. Foods 27, 295–309. doi: 10.1016/j.jff.2016.09.011
Losurdo, G., Cubisino, R., Barone, M., Principi, M., Leandro, G., Ierardi, E., et al. (2018). Probiotic monotherapy and helicobacter pylori eradication: a systematic review with pooled-data analysis. World J. Gastroenterol. 24 (1), 139–149. doi: 10.3748/wjg.v24.i1.139
Malfertheiner, P., Megraud, F., Rokkas, T., Gisbert, J. P., Liou, J. M., Schulz, C., et al. (2022). Management of helicobacter pylori infection: the maastricht VI/Florence consensus report. Gut. 71, 1724–1762. doi: 10.1136/gutjnl-2022-327745
Merenstein, D., Pot, B., Leyer, G., Ouwehand, A. C., Preidis, G. A., Elkins, C. A., et al. (2023). Emerging issues in probiotic safety: 2023 perspectives. Gut Microbes 15 (1), 2185034. doi: 10.1080/19490976.2023.2185034
Moonwiriyakit, A., Pathomthongtaweechai, N., Steinhagen, P. R., Chantawichitwong, P., Satianrapapong, W., Pongkorpsakol, P. (2022). Tight junctions: from molecules to gastrointestinal diseases. Tissue Barriers 2077620. doi: 10.1080/21688370.2022.2077620
Mosca, A., Abreu Y Abreu, A. T., Gwee, K. A., Ianiro, G., Tack, J., Nguyen, T. V. H., et al. (2022). The clinical evidence for postbiotics as microbial therapeutics. Gut Microbes 14 (1), 2117508. doi: 10.1080/19490976.2022.2117508
Nabavi-Rad, A., Azizi, M., Jamshidizadeh, S., Sadeghi, A., Aghdaei, H. A., Yadegar, A., et al. (2022a). The effects of vitamins and micronutrients on helicobacter pylori pathogenicity, survival, and eradication: a crosstalk between micronutrients and immune system. J. Immunol. Res. 2022, 4713684. doi: 10.1155/2022/4713684
Nabavi-Rad, A., Sadeghi, A., Asadzadeh Aghdaei, H., Yadegar, A., Smith, S. M., Zali, M. R. (2022b). The double-edged sword of probiotic supplementation on gut microbiota structure in helicobacter pylori management. Gut Microbes 14 (1), 2108655. doi: 10.1080/19490976.2022.2108655
O’Connor, A., O’Morain, C. A., Ford, A. C. (2017). Population screening and treatment of helicobacter pylori infection. Nat. Rev. Gastroenterol. Hepatol. 14 (4), 230–240. doi: 10.1038/nrgastro.2016.195
Raheem, A., Liang, L., Zhang, G., Cui, S. (2021). Modulatory effects of probiotics during pathogenic infections with emphasis on immune regulation. Front. Immunol. 12, 616713. doi: 10.3389/fimmu.2021.616713
Raygan, F., Ostadmohammadi, V., Asemi, Z. (2019). The effects of probiotic and selenium co-supplementation on mental health parameters and metabolic profiles in type 2 diabetic patients with coronary heart disease: a randomized, double-blind, placebo-controlled trial. Clin. Nutr. 38 (4), 1594–1598. doi: 10.1016/j.clnu.2018.07.017
Shan, X., Zhang, Y., Chen, H., Dong, L., Wu, B., Xu, T., et al. (2017). Inhibition of epidermal growth factor receptor attenuates LPS-induced inflammation and acute lung injury in rats. Oncotarget 8 (16), 26648–26661. doi: 10.18632/oncotarget.15790
Song, H., Zhou, L., Liu, D., Ge, L., Li, Y. (2019). Probiotic effect on helicobacter pylori attachment and inhibition of inflammation in human gastric epithelial cells. Exp. Ther. Med. 18 (3), 1551–1562. doi: 10.3892/etm.2019.7742
Suzuki, S., Gotoda, T., Kusano, C., Ikehara, H., Ichijima, R., Ohyauchi, M., et al. (2020). Seven-day vonoprazan and low-dose amoxicillin dual therapy as first-line helicobacter pylori treatment: a multicentre randomised trial in Japan. Gut 69 (6), 1019–1026. doi: 10.1136/gutjnl-2019-319954
Tshibangu-Kabamba, E., Yamaoka, Y. (2021). Helicobacter pylori infection and antibiotic resistance — from biology to clinical implications. Nat. Rev. Gastroenterol. Hepatol 18 (9), 613–629. doi: 10.1038/s41575-021-00449-x
van Zyl, W. F., Deane, S. M., Dicks, L. M. T. (2020). Molecular insights into probiotic mechanisms of action employed against intestinal pathogenic bacteria. Gut Microbes 12 (1), 1831339. doi: 10.1080/19490976.2020.1831339
Vargoorani, M. E., Modarressi, M. H., Vaziri, F., Motevaseli, E., Siadat, S. D. (2020). Stimulatory effects of lactobacillus casei derived extracellular vesicles on toll-like receptor 9 gene expression and cytokine profile in human intestinal epithelial cells. J. Diabetes Metab. Disord. 19 (1), 223–231. doi: 10.1007/s40200-020-00495-3
Vieth, R. (2020). Vitamin d supplementation: cholecalciferol, calcifediol, and calcitriol. Eur. J. Clin. Nutr. 74 (11), 1493–1497. doi: 10.1038/s41430-020-0697-1
Wroblewski, L. E., Peek, R. M., Jr. (2011). “Targeted disruption of the epithelial-barrier by helicobacter pylori”. Cell Commun. Signal. 9 (1), 29. doi: 10.1186/1478-811X-9-29
Wu, S., Liao, A. P., Xia, Y., Li, Y. C., Li, J. D., Sartor, R. B., et al. (2010). Vitamin d receptor negatively regulates bacterial-stimulated NF-kappaB activity in intestine. Am. J. Pathol. 177 (2), 686–697. doi: 10.2353/ajpath.2010.090998
Yang, L., He, X., Li, L., Lu, C. (2019). Effect of vitamin d on helicobacter pylori infection and eradication: a meta-analysis. Helicobacter 24 (5), e12655. doi: 10.1111/hel.12655
Yeung, C.-Y., Chiang Chiau, J.-S., Cheng, M.-L., Chan, W.-T., Jiang, C.-B., Chang, S.-W., et al. (2021). Effects of vitamin d-deficient diet on intestinal epithelial integrity and zonulin expression in a C57BL/6 mouse model. Front. Med. 8. doi: 10.3389/fmed.2021.649818
Zhao, H., Zhang, H., Wu, H., Li, H., Liu, L., Guo, J., et al. (2012). Protective role of 1,25(OH)2 vitamin D3 in the mucosal injury and epithelial barrier disruption in DSS-induced acute colitis in mice. BMC Gastroenterol. 12, 57. doi: 10.1186/1471-230X-12-57
Keywords: Helicobacter pylori, Levilactobacillus brevis, vitamin D3, extracellular vesicles, synergistic effect, AGS cells
Citation: Nabavi-Rad A, Jamshidizadeh S, Azizi M, Yadegar A, Robinson K, Monaghan TM and Zali MR (2023) The synergistic effect of Levilactobacillus brevis IBRC-M10790 and vitamin D3 on Helicobacter pylori-induced inflammation. Front. Cell. Infect. Microbiol. 13:1171469. doi: 10.3389/fcimb.2023.1171469
Received: 22 February 2023; Accepted: 24 April 2023;
Published: 05 May 2023.
Edited by:
Amir Peymani, Qazvin University of Medical Sciences, IranReviewed by:
Musaab Al Ameer, Saint Luke’s Hospital, United StatesMohammad Yaghoubi-Avini, Shahid Beheshti University, Iran
Mohammad Hossein Mehraban, University of California, San Francisco, United States
Copyright © 2023 Nabavi-Rad, Jamshidizadeh, Azizi, Yadegar, Robinson, Monaghan and Zali. This is an open-access article distributed under the terms of the Creative Commons Attribution License (CC BY). The use, distribution or reproduction in other forums is permitted, provided the original author(s) and the copyright owner(s) are credited and that the original publication in this journal is cited, in accordance with accepted academic practice. No use, distribution or reproduction is permitted which does not comply with these terms.
*Correspondence: Abbas Yadegar, YS55YWRlZ2FyQHNibXUuYWMuaXI=; YmFiYWtfeTE5ODNAeWFob28uY29t
†ORCID: Ali Nabavi-Rad, orcid.org/0000-0001-7799-9404
Shaghayegh Jamshidizadeh, orcid.org/0000-0001-8368-9226
Mahsa Azizi, orcid.org/0000-0001-7388-1935
Abbas Yadegar, orcid.org/0000-0002-2135-7581
Karen Robinson, orcid.org/0000-0002-6329-9340
Tanya M. Monaghan, orcid.org/0000-0001-7622-3997