- 1New Iberia Research Center, University of Louisiana at Lafayette, New Iberia, LA, United States
- 2Laboratoire Pathogénèse et Vaccination Lentivirales (PAVAL) Lab., Institut National de Recherche d’Agriculture et Environnement, Université Grenoble Alpes, Saint Martin d’Hères, France
Background: HIV remains a major public health issue in spite of antiretroviral therapy (ART). An innovative vaccine that can induce long-lasting and effective immunity is required to curb the persistently high numbers of new infections worldwide.
Methods: A novel DNA vaccine was generated using a Simian-Human Immunodeficiency Virus (SHIV) backbone with a Zambian T/F clade C envelope and under the control of the caprine arthritis encephalitis virus long terminal repeats (LTRs) for constitutive expression. Due to the deleted integrase, this DNA vaccine “CSH-DIN-T/F Z331” performs only a single replication cycle. To increase immunogenicity, the co-expression of apoptotic genes (BAX, BAK, or caspase 8) incorporated at the end of Pol was tested to promote the release of apoptotic bodies taken up by dendritic cells leading to cross-presentation of antigen. The three vaccines (CSH-DIN-T/F Z331-BAX, CSH-DIN-T/F Z331-BAK, and CSH-DIN-T/F Z331-Cas8) were tested in vitro for expression and in vivo in BALB/cJ mice for immunogenicity.
Results: Transduced HEK293 cells co-cultured with CEMx174 confirmed the single replication cycle of the DNA vaccine and the induction of apoptosis by CSH-DIN-T/F Z331-Cas8 based on Annexin V expression. BALB/cJ mice were immunized with a combined intramuscular + intradermal/electroporation approach. Intracellular cytokine staining (ICS) from splenocytes collected 12 weeks post-prime/6 weeks post-boost demonstrated a clear superiority of caspase 8 expressing construct over the others, with higher proportions of IFN-γ-, IL-2-, and IL-21-producing CD8 T cells specific to Env, Gag, and Nef. The kinetics of immune response after various immunization schedules were also investigated.
Conclusion: This novel single-cycle DNA vaccine with apoptotic genes demonstrated an enhanced immunogenicity primarily for antigen-specific CD8+ T-cell responses.
1 Introduction
According to United Nations Programme on HIV and AIDS (UNAIDS) statistics, by the end of 2023, 39.9 million people were living with HIV globally, with 1.3 million new infections and 9.2 million people still without access to antiretroviral therapy (ART) (https://www.unaids.org/en/resources/fact-sheet). Daily ART has been highly effective in controlling virus replication and bringing virus replication down to undetectable and untransmittable (U=U) levels. However, ART has failed to fully eliminate HIV due to the rapid establishment of persistent reservoirs in lymphoid organs and anatomical sanctuaries such as the brain, testis, and gut-associated lymphoid tissues (Castro-Gonzalez et al., 2018; Whyte-Allman and Bendayan, 2020).
An ideal HIV vaccine would protect people from acquiring infection and control the virus post-infection in people living with HIV. There have been 12 HIV vaccine and passive antibody efficacy clinical trials over 20 years, with only two having had positive outcomes. The 2009 Thai RV144 Prime-Boost, ALVAC-AIDSVAX, B/E trial had a modest 31.2% efficacy (Vaccari et al., 2010), and the 2021 Antibody Mediated Prevention (AMP) studies using the VRCO1 monoclonal antibody reduced the risk of acquisition for patients exposed to HIV strains highly sensitive to VRCO1 neutralization but not to the majority of strains (Corey et al., 2021).
It is essential to develop innovative strategies for vaccines against HIV. In this regard, a vaccination strategy consisting of DNA is safe, simple, stable, and scalable and has minimal storage and transport requirements. However, immunization with HIV DNA vaccines, while effective in rodents, has induced only marginal responses in humans or large animal models. However, these responses are improved when DNA immunization is combined with electroporation to promote cell transfection (Sarwar et al., 2015; Hannaman et al., 2016; Todorova et al., 2017; Elizaga et al., 2018; Tebas et al., 2019; Hooper et al., 2020; Sefidi-Heris et al., 2020; Ahn et al., 2022; Houser et al., 2022; Kraynyak et al., 2022). We developed a SHIV DNA vaccine based on modified lentiviral genomes to better mimic the early stages of natural exposure to HIV and the production of viral proteins.
Previously, a unique SHIV DNA vaccine CAL-SHIV-IN− was developed with i) deleted integrase limiting replication to a single cycle in target cells without integration (Arrode-Bruses et al., 2014; Moussa et al., 2015). This single cycle leads to the production of viral antigens that assemble into virus-like particles (VLPs) and pseudo-infectious particles (Arrode-Bruses et al., 2014; Moussa et al., 2015). ii) The vaccine is under the control of caprine arthritis encephalitis virus (CAEV) long terminal repeats (LTRs), which deliver a constitutive expression of viral proteins. CAEV LTRs are Tat independent and are reviewed in more detail in the review (Bose et al., 2015). In vivo studies with a previous version of this DNA vaccine using combined intramuscular (i.m.) and intradermal (i.d.) injections plus electroporation have demonstrated the enhancement of DNA uptake up to 10- to 100-fold into cells while also causing low-level inflammation (Diehl et al., 2013; Kisakov et al., 2024). This DNA vaccine led to durable central and effector SHIV-specific memory T-cell responses elicited after a single immunization, which endured up to 80 weeks in the absence of a booster (Arrode-Bruses et al., 2014). All vaccinated cynomolgus macaques rapidly controlled viral replication up to 1 year after the challenge. Remarkably, this challenge control correlated with vaccine-specific T-cell precursors with high proliferation capacity (PHPC) in all vaccinated macaques (Arrode-Bruses et al., 2014). The addition of IL-7 or IL-15 in the construct brought marginal improvement in the immune responses (Leroy et al., 2022). This vaccine nevertheless left room for improvement, in particular, if a more virulent model (e.g., rhesus macaques infected with novel SHIV isolates) was to be used. Thus, to further enhance cytotoxic T-cell immunity, we hypothesized that adding an apoptotic gene in the vaccine may trigger rapid apoptosis of transduced cells, leading to the uptake of apoptotic bodies by dendritic cells, thereby increasing antigen presentation as well as cross-presentation of antigen (Sasaki et al., 2002; Scheffer et al., 2003; Kim et al., 2004; Parsania et al., 2010; Mekonnen et al., 2020). Apoptosis is mediated by either the extrinsic cell death pathway (via caspase 8) or the mitochondrial/intrinsic pathway (via BCL2 family member BAK/BAX).
For this study, we developed a novel DNA vaccine using a SHIV backbone with a transmitted founder (T/F) Z331 clade C envelope from a Zambian cohort (GenBank KR820323) (Deymier et al., 2015). T/F viruses were selected through the genetic bottleneck during transmission, as they are CCR5 tropic, exhibit less Env glycosylation, and preferentially bind α4β7 (Parrish et al., 2013; Deymier et al., 2015). The novel DNA vaccine under the control of CAEV LTRs (CSH-DIN-T/F Z331) ensures the constitutive expression of viral proteins during its single-cycle replication with no integration due to the deletion of the integrase gene. Additionally, the apoptotic genes BAX, BAK, and caspase 8 were included with a T2A at the end of Pol. The three vaccines—CSH-DIN-T/F Z331-BAX, CSH-DIN-T/F Z331-BAK, and CSH-DIN-T/F Z331-Cas8—were tested in vitro for expression, and CSH-DIN-T/F Z331-BAX and CSH-DIN-T/F Z331-Cas8 were tested in vivo in BALB/cJ mice for immunogenicity.
2 Materials and methods
2.1 DNA vaccine construction
The CSH-DIN-T/F Z331 DNA vaccine construction details were previously published (Bose, 2017). Briefly, the CSH-DIN-T/F Z331 DNA vaccine was generated using the SHIV backbone under the control of CAEV LTRs and deleted integrase gene (Arrode-Bruses et al., 2014) with the substitution of the tat to nef region containing the env gene of the T/F clade C envelope (Deymier et al., 2014). Similar to the parent CAL-SHIV-IN−, this DNA vaccine can only achieve a single-cycle replication without integration (Figure 1A).
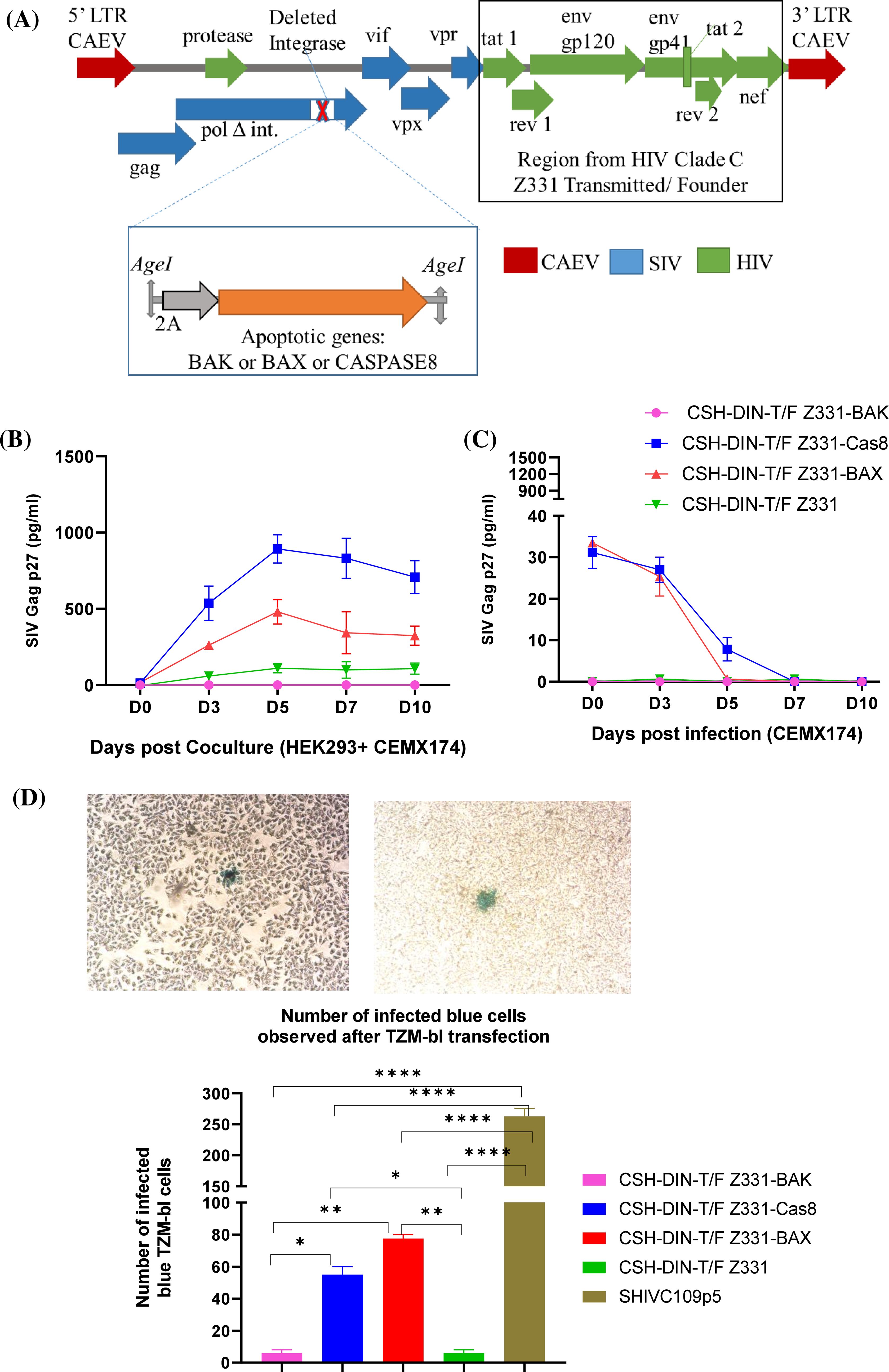
Figure 1. Development and in vitro characterization of lenti DNA vaccines. (A) Schematics of lenti DNA vaccine CSH-DIN-T/F Z331 with the backbone of SHIV, CAEV LTRs, and deleted integrase; the region from tat–nef is derived from T/F Z331 clade C HIV. Using the unique restriction site AgeI, the cassette including apoptotic genes with upstream T2A is inserted to develop CSH-DIN-T/F Z331-BAX, CSH-DIN-T/F Z331-BAK, or CSH-DIN-T/F Z331-Cas8. (B) For all the lenti DNA vaccine prototypes, SIV Gag p27 was determined from the supernatants of the transiently transfected HEK293 co-cultured with CEMx174 cells in two independent experiments (C) and with the supernatants of CEMx174 infected with day 2 HEK293 transiently transfected cells. (D) Infectivity of all the lenti vector DNA vaccines was determined by transiently transfecting the TZM-bl cells and staining after 72 hrs to quantitate the blue infected cells. Representative image of blue infected cell from CSH-DIN-T/F Z331 is in the top panel. The number of infected blue cells from two independent experiments is summarized in the graph. SHIV-C109p5, a replication-competent virus, is a positive control. Results were compared using Tukey’s multiple comparison test and one-way ANOVA. Error bars represent the standard error of mean (s.e.m). *p < 0.05, **p < 0.01, ****p < 0.0001. CAEV, caprine arthritis encephalitis virus; LTRs, long terminal repeats.
The three vaccines CSH-DIN-T/F Z331-BAX, CSH-DIN-T/F Z331-BAK, and CSH-DIN-T/F Z331-Cas8 were constructed by gene synthesis by inserting a T2A coding sequence driving the translation of BAX, BAK, or caspase 8 at the end of the reverse transcriptase ORF using the AgeI restriction site (GenBank references for BAX, BAK, and caspase 8 are XM_015124399.2, XM_015136057.2, and NM_001284129.1, respectively) (Thermo Fisher Scientific, Waltham, MA, USA; GENEART).
The synthesized gene inserts were digested with AgeI and ligated to the vector CSH-DIN-T/F Z331. The ligated products were transformed in One Shot Stbl3 chemically competent Escherichia coli (Invitrogen, Carlsbad, CA, USA; #C737303). The colonies were screened and amplified using midi-preps, and the inserted region was Sanger sequenced (Eurofins Genomics, Louisville, KY, USA). Each plasmid sequence was verified further via whole plasmid sequencing using Next-Generation Sequencing (NSG) generation 3 technology by Eurofins Genomics.
2.2 Cell culture
Cell lines (HEK293, TZM-bl, and CEMx174) were obtained from National Institutes of Health (NIH) (AIDS Reagent Program, NIAID, Bethesda, MD, USA), and cells were cultured according to the recommended culture conditions.
2.2.1 HEK293 transfection, co-culture, and infection of CEMx174
In a 6-well plate, 5 × 105 adherent HEK293 cells were transiently transfected using 9 µg polyethylenimine (PEI) and 3 µg DNA (CSH-DIN-T/F Z331-BAX, CSH-DIN-T/F Z331-BAK, CSH-DIN-T/F Z331-Cas8, or CSH-DIN-T/F Z331) and incubated in tissue culture conditions for 8 hrs. The transfection medium was removed, and a fresh medium was added. Forty-eight hours post-transfection, CEMx174 cells were added to co-culture with the HEK293 cells. For infection, 100 µL of 48-hrs post-transfection supernatant was used to inoculate CEMx174. Days 3, 5, 7, and 10 supernatants were collected from the co-cultures and infections for SIV Gag p27 protein quantification using ELISA (ABL, Inc., Rockville, MD, USA; #SKU 5436). ELISA was conducted according to the manufacturer’s instructions followed by reading the absorbance at 450 nm using a BioTek (Winooski, VT, USA) Synergy HT microplate reader.
2.2.2 TZM-bl transfection/infected and quantifying number of infected cells
The TZM-bl indicator cell line was used to quantitate the viral construct using β-gal as a reporter. In a 6-well plate, 5 × 105 adherent TZM-bl cells were transiently transfected using 9 µg PEI and 3 µg DNA (CSH-DIN-T/F Z331-BAX, CSH-DIN-T/F Z331-BAK, CSH-DIN-T/F Z331-Cas8, or CSH-DIN-T/F Z331). In parallel, an infectious virus SHIV-C109p5 was used to infect the TZM-bl cells as the positive control (Bose et al., 2024). The transfection medium was removed, and fresh medium was added after 8 hrs. Seventy-two hours post-transfection, the cells were washed twice with Phosphate-buffered saline (PBS) and fixed with 4% Paraformaldehyde (PFA). Cells were rinsed once and incubated in PBS containing 5 mM MgCl2. Cells were stained with 1 mg/mL X-Gal in a staining solution containing 5 mM K3Fe(CN)6 and 2 mM K4Fe(CN)6. The cells were incubated for 1 hr at 37°C and then fixed again in 4% PFA for 15 min before washing in PBS and drying. The number of blue cells was counted and reported.
2.2.3 Evaluating transcription and translation of apoptotic genes
Half a million HEK293 cells were transiently transfected using 9 µg PEI and 3 µg DNA (CSH-DIN-T/F Z331-BAX, CSH-DIN-T/F Z331-BAK, CSH-DIN-T/F Z331-Cas8, or CSH-DIN-T/F Z331). Forty-eight hours post-transfection, the cells were collected and washed with PBS, and cell lysates were prepared in either RNAzol for RNA extraction or Radioimmunoprecipitation assay (RIPA) buffer for Western blotting.
RT-PCR using 1 µg RNA was conducted with the following primer pairs: F2: 5′-GACCACCTAGTTAGTCAAGG-3′ (end of Pol) and R1: 5′-CTTCTGGGTACTACCTTAATGTC-3′ (in Tat) using the Superscript III One-step RT-PCR system with platinum Taq DNA polymerase (Invitrogen; #12574-018). The thermocycler cDNA synthesis and pre-denaturation program was 45°C for 30 min; 94°C for 2 min; 40 cycles of denaturation at 94°C for 15 sec, annealing at 58°C for 30 sec, and extension at 68°C for 60 sec; and final extension at 68°C for 5 min.
Cell lysates were prepared in Radioimmunoprecipitation assay (RIPA) buffer, denatured at 95°C for 5 min, separated through polyacrylamide gel electrophoresis (PAGE), and transferred to polyvinylidene difluoride (PVDF) membranes. Afterward, they were washed with Tris-buffered saline (TBS) tween for 5 min and blocked for 1 hr at room temperature in TBS plus 5% milk. Next, 1:1,000 dilutions of the primary rabbit antibodies were used (Abeomics, San Diego, CA, USA; activated caspase 8 #20-1043, BAX #20-1022, and BAK #20-1019) along with mouse antibody to the control GAPDH (1:2,000) protein (Invitrogen; #MA5-15738) and incubated overnight. The next day, the membranes were washed thrice with the Tris-buffered saline tween (TBST) and incubated with 1:5,000 anti-rabbit AP and anti-mouse AP secondary antibody for 1 hr. The membranes were washed again thrice with TBST and developed using Promega (Madison, WI, USA) Western Blue Stabilized Substrate for Alkaline Phosphate #S3841.
2.2.4 Annexin V and live/dead staining
In a 6-well plate, 5 × 105 adherent HEK293 cells were transiently transfected using 9 µg PEI and 3 µg DNA (CSH-DIN-T/F Z331-BAX, CSH-DIN-T/F Z331-Cas8, or CSH-DIN-T/F Z331). The transfection medium was removed, and fresh medium was added after 8 hrs. Seventy-two hours post-transfection, 1 million cells were washed twice, stained with the Alexa Fluor 430 NHS Ester to exclude dead cells, and incubated for 15 min at room temperature. The cells were washed and stained in 25 mM HEPES buffer (100 µL) with Annexin V-FITC (5 µL) (BioLegend, San Diego, CA, USA; #640905), incubated at room temperature for 15 min, and washed twice, and a minimum of 10,000 events were acquired on a BD FACSAria Fusion (BD Biosciences, San Jose, CA, USA) instrument with the FACSDiva software package, and the data were analyzed using FlowJo Version 10.7.1 (Treestar, Woodburn, OR, USA).
2.3 Evaluation of safety, toxicity, and immunogenicity in mice
2.3.1 Ethical statement regarding animal studies
Mice were housed in compliance with the “Guide”, the Animal Welfare Act (AWA), and Animal Welfare Regulations (AWR). All studies were approved by the University of Louisiana at Lafayette IACUC (2019-8802-030). Five mice were housed per cage to allow social interactions, with enrichment such as cellulose, pieces of wood, or cardboard rolls. The housing of mice was under controlled conditions of humidity, temperature, and light (12-hr white light/12-hr red light). Water and dry pellets were provided. Mice were anesthetized with isoflurane vapor (Patterson Veterinary) and monitored until awake. A group of 4–6-week-old BALB/cJ male/female mice were purchased from (Jackson Laboratory, Bay Harbor, ME, USA). They were housed in the New Iberia Research Center (NIRC).
To test for the absence of the integration of the vaccines, BALB/cJ mice were immunized i.m. and i.d. + electroporation with the CSH-DIN-T/F CAS8 DNA vaccine. Two weeks post-immunization, the mice were sacrificed, and DNA was extracted from the skin and muscle areas corresponding to the immunization sites and from the tail, spleen, and blood. PCR was conducted to amplify the end of Pol–beginning of Tat region with the primers F1 (5′-GCACACAAAGGTATAGGAGGAA-3′)—Primer R2 (5′-CTTCTGGGTACTACCTTAATGTC-3′). Mouse GAPDH housekeeping gene used as a sample loading control mus GAPDH Fwd 5′-AGGTCGGTGTGAACGGATTT-3′ mus GAPDH Rev2-5′-TGTAGACCATGTAGTTGAGGTCA-3′.
Skin and muscle tissues corresponding to the immunization sites were also fixed, embedded, and stained with hematoxylin and eosin (H&E) to evaluate for potential toxicity/inflammation 2 weeks post-immunization. Tissues were fixed in 4% PFA and embedded in paraffin (formalin-fixed, paraffin-embedded (FFPE) blocks). H&E slides from FFPE tissue blocks were analyzed, and the images were acquired on an Olympus IX71 microscope using the cellSens software.
The mice were distributed in groups. The mice were immunized with i.m. 50 µg DNA and i.d. 50 µg DNA with electroporation as described by Leroy et al. (Leroy et al., 2022). Different prime and boost approaches were tested with CSH-DIN-T/F Z331-BAX, CSH-DIN-T/F Z331-Cas8, or CSH-DIN-T/F Z331 DNA. At necropsy, the spleen was collected to isolate splenocytes for evaluation of the cell-mediated immune responses, while the blood was collected by intra-cardiac puncture to collect the serum for evaluation of the humoral immune responses.
2.3.2 SHIV-specific intracellular cytokine determination
Mouse splenocytes were isolated and used to evaluate SHIV C-specific T-cell immune responses by multiparametric flow cytometry as previously described (Leroy et al., 2022). Briefly, 1 million cells were stimulated for 6 hrs with 2 µg/mL of each peptide pool (overlapping 15-mer with 11-aa overlaps), spanning the entire SIV Gag, HIV clade C Env [Env N terminal pool (1–435), HIV Env C terminal pool (436–857), or HIV clade C–Nef (AIDS Reagent Repository, NIH-NIAID catalog numbers 6204, 9499, and 13091, respectively)], or with phorbol myristate acetate (PMA)/ionomycin as positive control to assess the phenotype and cytokine production of vaccine-specific T cells. Non-stimulated cells were used to subtract the basal secretion signal. Splenocytes were stimulated for 6 hrs at 37°C. Anti-CD28 and CD49d antibodies (0.5 µg/mL) were added for co-stimulation, and Brefeldin A was added to prevent cytokine excretion (Leroy et al., 2022). To avoid the CD62L cleavage, 35 µg/mL of TAPI-2 (Sigma, Burlington, MA, USA) was added after 1-hr incubation with peptide pools. Splenocytes were then stained with surface and intracellular antibodies as described by Leroy et al. (Leroy et al., 2022). Antibodies were purchased from BD and eBioscience (San Diego, CA, USA) (Table 1). A minimum of 200,000 events were acquired on a BD FACSAria Fusion instrument with the FACSDiva software package, and the data were analyzed using FlowJo Version 10.7.1 (Treestar, USA).
2.3.3 Systemic HIV clade C Env-ELISA-specific IgG binding antibody responses
Plasma binding antibodies to HIV-1 clade C Env protein were assessed by ELISA as described previously (Bose et al., 2024). Briefly, 96 wells (Nunc MaxiSorp™ flat bottom, Thermo Fisher Scientific, Waltham, MA, USA) were coated overnight at 4°C with HIV-1/Clade C gp120 recombinant protein (Acro, Newark, DE, USA; #16055) 2 μg/mL in bicarbonate buffer pH 9.6. It was then washed and blocked with 2% casein in PBS. The mouse serum was 1:4 diluted in casein and incubated for 1 hr at 37°C. Horseradish peroxidase (HRP)-conjugated goat anti-mouse IgG (1:20,000 dilution; Invitrogen; #31430) and the TMB substrate (KPL) were used in sequential steps, followed by reading the absorbance at 450 nm using a BioTek Synergy HT microplate reader.
2.4 Statistics
Statistical analysis was determined using the GraphPad Prism 8 software (Version 8.4.3). Medians were used for the different graphic presentations. Non-parametric Mann–Whitney, parametric one-way ANOVA, two-way ANOVA, and Tukey’s multiple comparison tests were used to determine the significance.
3 Results
3.1 In vitro characterization of CSH-DIN-T/F DNA vaccines with apoptotic genes
3.1.1 HEK293 transfections and CEMx174 infections
All three prototype vaccines were tested in vitro to characterize them. The DNA vaccine constructs CSH-DIN-T/F Z331-BAX, CSH-DIN-T/F Z331-BAK, CSH-DIN-T/F Z331-Cas8, and CSH-DIN-T/F Z331 were transfected into HEK293 and co-cultured with CEMx174. As seen in Figure 1B, the SIV Gag p27 production peaked by day 5 post-transfection, with the highest concentration for CSH-DIN-T/F Z331-Cas8 followed by CSH-DIN-T/F Z331-BAX and the parent CSH-DIN-T/F Z331, while CSH-DIN-T/F Z331-BAK did not produce any measurable SIV Gag p27. Overall, these levels were lower than those obtained with a more active promoter such as the Cytomegalovirus Virus (CMV) one (Liu et al., 2007; Ganguly et al., 2010). Next, a passage of 100-µL day 2-infected HEK293 supernatants to CEMx174 cells showed only decreased input virus but no true reinfection, confirming the single-cycle nature of these DNA vaccines that are unable to integrate (Figure 1C).
3.1.2 TZM-bl transfections and infections
Next, the number of cells transfected by CSH-DIN-T/F Z331-BAX, CSH-DIN-T/F Z331-BAK, CSH-DIN-T/F Z331-Cas8, and CSH-DIN-T/F Z331 were compared by exposure to TZM-bl cells. One hundred microliters of (4.8 × 108 TCID50) the infectious replication-competent virus SHIV-C109p5 (Bose et al., 2024) was run in parallel as a positive control. At 72 hrs post-transfection, a total of 276 infected blue cells were counted for replication-competent virus SHIV-C109p5, while the blue-stained cells for CSH-DIN-T/F Z331-BAX and CSH-DIN-T/F Z331-Cas8 were 80 and 60, respectively. In contrast, CSH-DIN-T/F Z331-BAK and CSH-DIN-T/F Z331-Z331 had only four blue cells each (Figure 1D), reflecting the amount of p27 for the various constructs (Figure 1B).
3.1.3 Detection of apoptotic gene products and function
3.1.3.1 RT-PCR and Western blotting
The RT-PCR products amplified from RNA extracted from 48 hrs post-transfected HEK293 cells corresponded to the expected sizes of the BAK, Cas8, and BAX (875, 1,679, and 1,004 bp, respectively) gene inserts (Figure 2A).
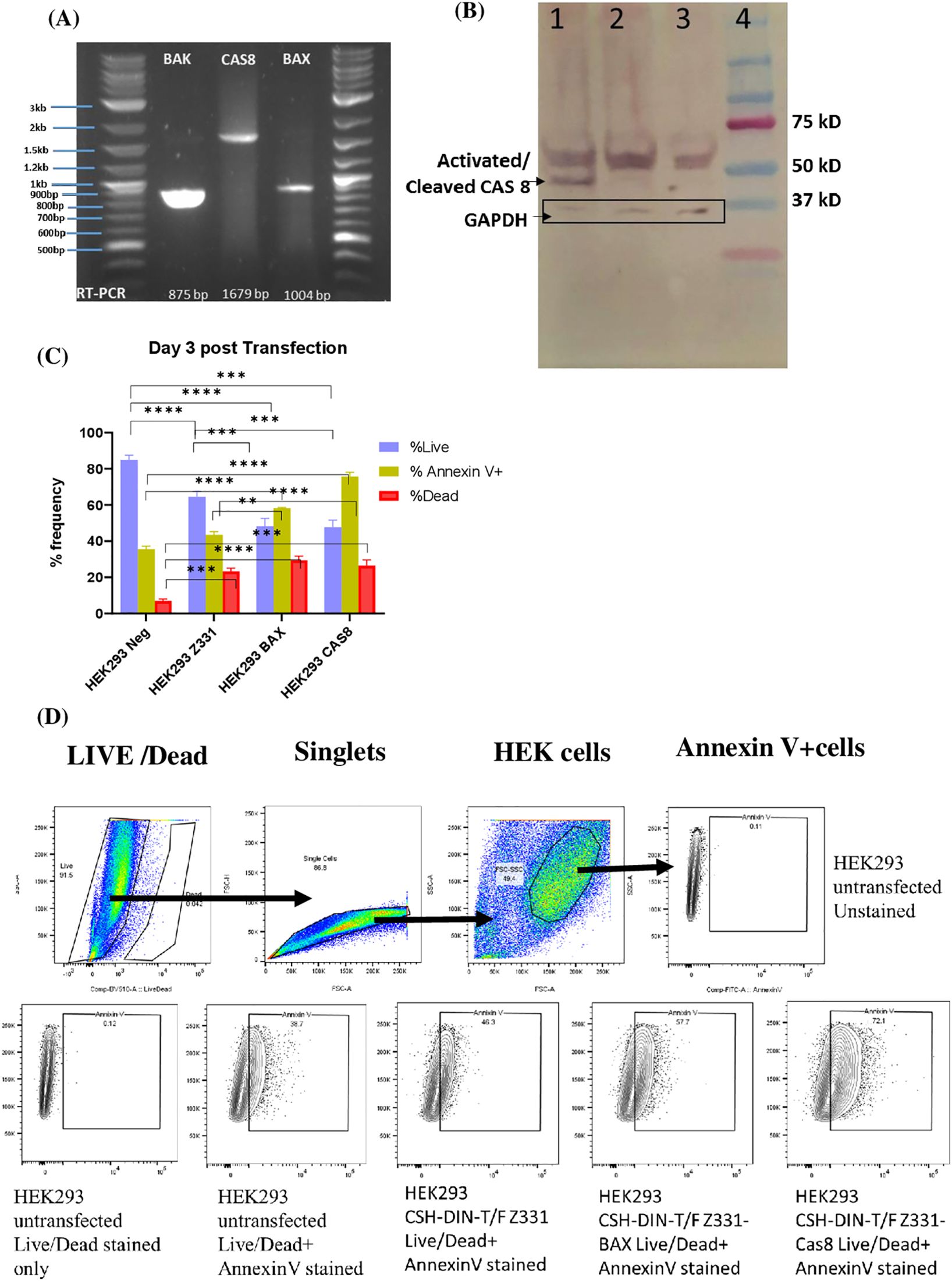
Figure 2. (A) Forty-eight hours post-transfection, HEK293 cells RNA was extracted to perform RT-PCR (1 µg RNA) using primer pairs complementary to the end of pol and the beginning of tat. The expected product sizes of the inserted apoptotic genes BAK, Cas8, and BAX were 875, 1,679, and 1,004 bp, respectively. (B) Western blotting was conducted with lysates from HEK293 cells transfected with the vaccine constructs CSH-DIN-T/F Z331-Cas8, CSH-DIN-T/F Z331, and un-transfected cells. Western blotting with the 48 hrs post-transfection cell lysates. Primary antibody: Anti-CAS8 Rabbit IgG Abeomics #20-1043(1:1,000) + Anti-GAPDH Mouse IgG Invitrogen #MA5-15738 (1:2,000). Secondary antibody: Goat anti-Rabbit AP + Goat anti-mouse AP (1:5,000). Lane 1: HEK293T Cell lysates from 48-hr post-transfected CSH-DIN-T/F Z331-Cas8 DNA. Lane 2: HEK293T Cell lysates from 48-hr post-transfected CSH-DIN-T/F Z331 DNA. Lane 3: Un-transfected HEK293T cell lysates. Lane 4: Precision Plus Protein Dual Color Standards (Bio-Rad, Hercules, CA, USA). (C) Transiently transfected cells with the lenti vector DNA vaccines were stained with the live/dead marker and Annexin V at 72 hrs post-transfection, and data were acquired on FACSAria. The graph summarizes the percentage of live cells, dead cells, and Annexin V-positive cells based on gated HEK cells. Experiments were conducted in triplicates, and the results were compared using Tukey’s multiple comparison test and two-way ANOVA. Error bars represent standard errors of the mean (s.e.m). **p < 0.01, ***p < 0.001, ****p < 0.0001. (D) The cells were gated first to select the live singlet cells and then gated for Annexin V positive. The gating was based on the unstained controls.
Western blotting from HEK29 cell lysates collected 48 hrs post-transfection with CSH-DIN-T/F Z331-Cas8 and the parent CSH-DIN-T/F Z331 was analyzed as described (Figure 2B). While both lysates comprised the full-length 55-kDa caspase 8 protein, only the CSH-DIN-T/F Z331-Cas8 cell lysate exhibited the activated caspase (p43/p41) 46.5-kDa band, demonstrating the activation of the apoptotic pathway. Similar analyses of BAX and BAK did not show any measurable difference, and these were constitutively expressed and did not have a distinct activated form (data not shown).
3.1.3.2 Annexin V apoptosis assay
Annexin V detection was performed on HEK293 transfected with CSH-DIN-T/F Z331-BAX, CSH-DIN-T/F Z331-Cas8, and CSH-DIN-T/F Z331 at 72 hrs post-transfection. Owing to its minimal p27 production in vitro, the CSH-DIN-T/F Z331-BAK vaccine prototype was dropped from further analyses. Percentages of Annexin V-positive apoptotic cells were higher at 72.1% in CSH-DIN-T/F Z331-Cas8 followed by 57.7% in CSH-DIN-T/F Z331-BAX and 46.3% in CSH-DIN-T/F Z331. The percentage of dead cells was 29.3%, 33%, and 25.9% in CSH-DIN-T/F Z331-Cas8, CSH-DIN-T/F Z331-BAX, and CSH-DIN-T/F Z331, respectively (Figures 2C, D), confirming the enhanced induction of apoptosis with the vaccine prototypes with apoptotic genes.
3.2 Vaccine safety and immune responses in BALB/cJ mice
BALB/cJ mice were immunized intramuscularly (50 µg) and intradermally (50 µg) + electroporation with the CSH-DIN-T/F CAS8 DNA vaccine. Two weeks post-immunization, the mice were sacrificed, and DNA was extracted from the immunization sites, with muscles collected from the immunized area along with the tail, spleen, and blood. There was no evidence of DNA vaccine persistence (i.e., integrated DNA) from in the sites of injection: tail, spleen, or blood (Supplementary Figure S1). Further, 2 weeks post-immunization, the vaccine was tested for evidence of inflammation. There was no evidence of leucocyte infiltration at either site of immunization with the CSH-DIN-T/F CAS8 DNA vaccine, suggesting an absence of toxicity (Supplementary Figure S2).
3.2.1 CSH-DIN-T/F Z331-Cas8 vaccine induces enhanced Env and Nef-specific cytotoxic T-cell responses
The first set of BALB/cJ mouse experiments were conducted by immunizing five mice each with CSH-DIN-T/F Z331-BAX, CSH-DIN-T/F Z331-Cas8, and CSH-DIN-T/F Z331. Due to the lack of p27 production by CSH-DIN-T/F Z331-BAK in in vitro studies, this vaccine prototype was not tested in vivo. Vaccines were delivered intramuscularly (50 µg) and intradermally (50 µg) with electroporation at week 0 and boosted at week 6. Mice were euthanized at week 12 post-vaccination (Figure 3A), and serum and splenocytes were collected at necropsy. The HIV clade C Env-specific humoral responses at the end of the experiment were barely above the background for all three vaccine prototypes tested (Figure 3B). Antigen-specific cellular responses were, however, readily detected in restimulated splenocytes. CD4+ and CD8+ T-cell responses were compared between vaccine prototypes for IL-2, IFN-γ, and IL-21 cytokine responses to Gag, Env N terminal (aa 1–435), HIV Env C terminal (aa 436–857), or HIV clade C–Nef peptide pools.
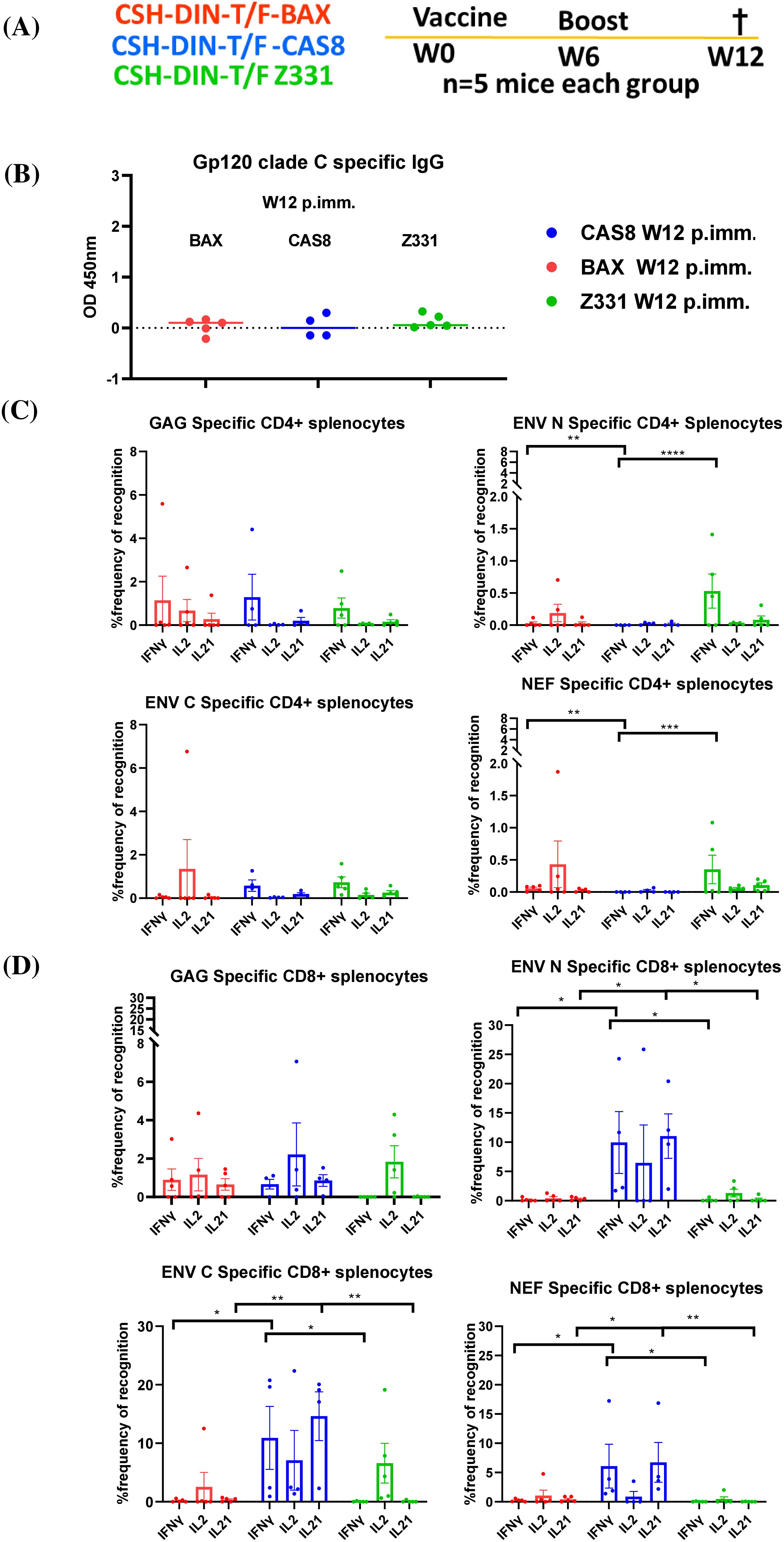
Figure 3. (A) Schematic of the in vivo studies on BALB/cJ. Lenti DNA vaccines were used to immunize mice i.m., and i.d. + electroporation at week 0, boosted at week 6, and mice were euthanized at week 12 post-immunization (W12 p. imm.); the serum and splenocytes were collected at necropsy. (B) gp120 clade C-specific IgG humoral responses of the different mouse groups were determined in the serum samples collected at week 12. (C) SHIV-specific IFN-γ-, IL-2-, and IL-21-producing CD4+ T cells. (D) CD8+ T cells from splenocytes of immunized BALB/cJ mice at week 12 post-immunization. Results were compared using paired t-test. Error bars represent standard error of mean (s.e.m), n = 5. *p < 0.05, **p < 0.01, ***p < 0.001, ****p < 0.0001, NS, not significant (p > 0.05). Statistical differences between total responses of each of the groups are indicated on the graph.
CSH-DIN-T/F Z331-BAX and CSH-DIN-T/F Z331-Cas8 induced higher CD4+ T cell Gag-specific IFN-γ responses compared to CSH-DIN-T/F Z331. There were also significantly higher CD4+ T cell IFN-γ immune responses specific to Env (N term) and Nef in cells from CSH-DIN-T/F Z331-BAX and CSH-DIN-T/F Z331 immunized mice compared to CSH-DIN-T/F Z331-Cas8 (Figure 3C).
The CSH-DIN-T/F Z331 parent vector induced IL-2 CD8+ T-cell responses to Gag and Env. CSH-DIN-T/F Z331-BAX induced polyclonal Gag-specific CD8+ responses but relatively few responses to Env or Nef. In contrast, CSH-DIN-T/F Z331-Cas8 induced the highest responses overall to all antigens (Gag, Env, and Nef), responding with IFN-γ, IL-2, and uniquely IL-21 (Figure 3D).
3.2.2 CSH-DIN-T/F Z331-Cas8 induces memory late recall responses
The ability to induce memory responses after early-boost (week 6 boost/necropsy collection at week 18; that is, 12 weeks post-boost) vs. late-boost (week 24/necropsy collection at week 26; that is, 2 weeks post-boost) recall responses were tested comparing CSH-DIN-T/F Z331-Cas8 with CSH-DIN-T/F Z331 (Figure 4A). Unexpectedly, no significant difference was observed in antibody titers to HIV clade C Env between the two vaccines CSH-DIN-T/F Z331-Cas8 and CSH-DIN-T/F Z331, indicating that the addition of the apoptotic genes did not influence the generation of humoral immune responses (Figure 4B). However, there was a marked difference in the kinetics of immune responses with the serum samples collected 12 weeks after the early boost given markedly higher titers than those collected 2 weeks after the late boost, perhaps due to a slower antigen expression or the decay of primed B cells. In these immunization schedules, late CD4+ T-cell responses to an early boost showed predominantly IFN-γ responses against all three antigens, although higher for CSH-DIN-T/F Z331 compared to CSH-DIN-T/F Z331-Cas8 (Figure 4C). Responses to the late boost, however, did not seem to generate higher recall responses with CSH-DIN-T/F Z331, while CSH-DIN-T/F Z331 Cas8 induced polyclonal responses to all three antigens, although perhaps the collections were not conducted at the peak responses. CD8 responses by contrast clearly reflected a far more potent immunization delivered by CSH-DIN-T/F Z331-Cas8 (Figure 4D). Not only did the late IFN-γ and IL-21 responses to an early boost persist for 3 months post-boost, but the late boost also recalled high responses for this construct, while responses to the parent CSH-DIN-T/F Z331 were very low overall.
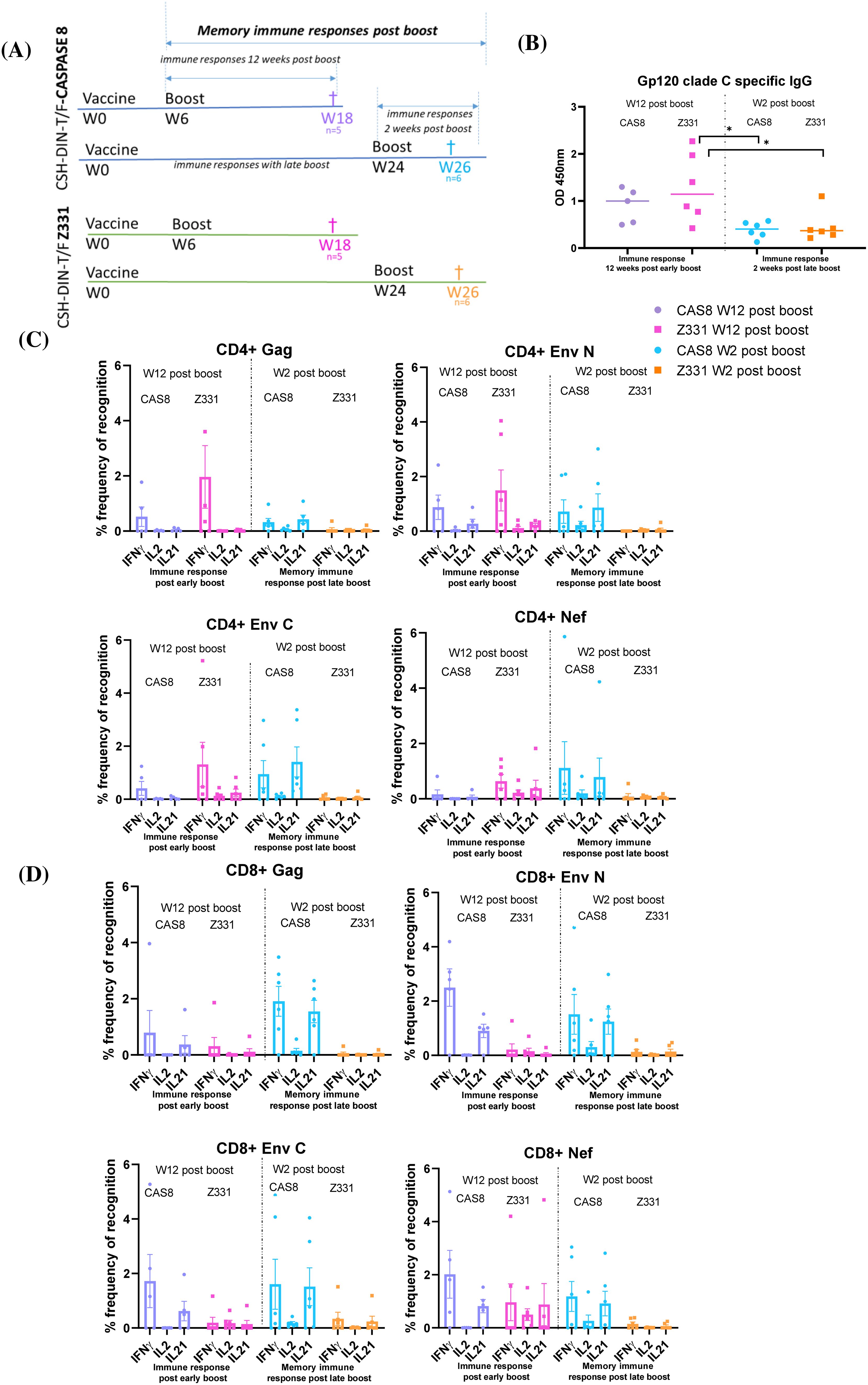
Figure 4. Schematics representing CSH-DIN-T/F Z331-Cas8 and CSH-DIN-T/F Z331 groups of BALB/cJ mice immunized to compare immune responses of early boost at week 6 (euthanized at week 12 post-boost) and late week 24 boost (euthanized week 2 post-boost). (B) gp120 clade C-specific IgG humoral responses of the different mouse groups were determined using the serum samples taken at week 18 post-immunization (week 12 post-boost) or week 26 post-immunization (week 2 post-boost). (C) SHIV-specific IFN-γ-, IL-2-, and IL-21-producing CD4+ T cells. (D) CD8+ T cells from splenocytes of immunized BALB/cJ mice at week 18 post-immunization (week 12 post-boost) or week 26 post-immunization (week 2 post-boost). Results were compared using paired t-test. Error bars represent the standard error of the mean (s.e.m), n = 5. *p < 0.05. Statistical differences between the total responses of each of the groups are indicated on the graph.
3.2.3 CSH-DIN-T/F Z331-Cas8 induces durable and late recall responses
To further examine the longevity of the immune responses induced by CSH-DIN-T/F Z331-Cas8, immune responses observed 3 weeks after primary immunization were compared with memory responses 6 months after an early boost (Figure 5A). No antibody responses were detected 3 weeks after the prime immunization, but the highest HIV clade C Env antibody titers were observed with the CSH-DIN-T/F Z331-Cas8 vaccine 12 weeks post-first boost (Figures 4B, 5B), suggesting the need for time to develop such antibodies after this type of immunization (Figure 5B). Memory humoral responses also showed marked decay since the mice collected at week 33, 6 months after an early boost, had markedly lower titers. Somewhat surprisingly, CD4+ IFN-γ responses to Env and Nef were high 3 weeks after the prime immunization, although with marked individual differences between animals from the same group (Figure 5C). Similarly, the CD8+ Env N or C term-stimulated splenocytes had higher IFN-γ and IL-21 responses at 3 weeks post-prime immunization, compared to memory responses post-boost (Figure 5D). In contrast, both CD4+ and especially CD8+ Gag responses were undetectable after the prime immunization while showing strong responses 6 months after the boost.
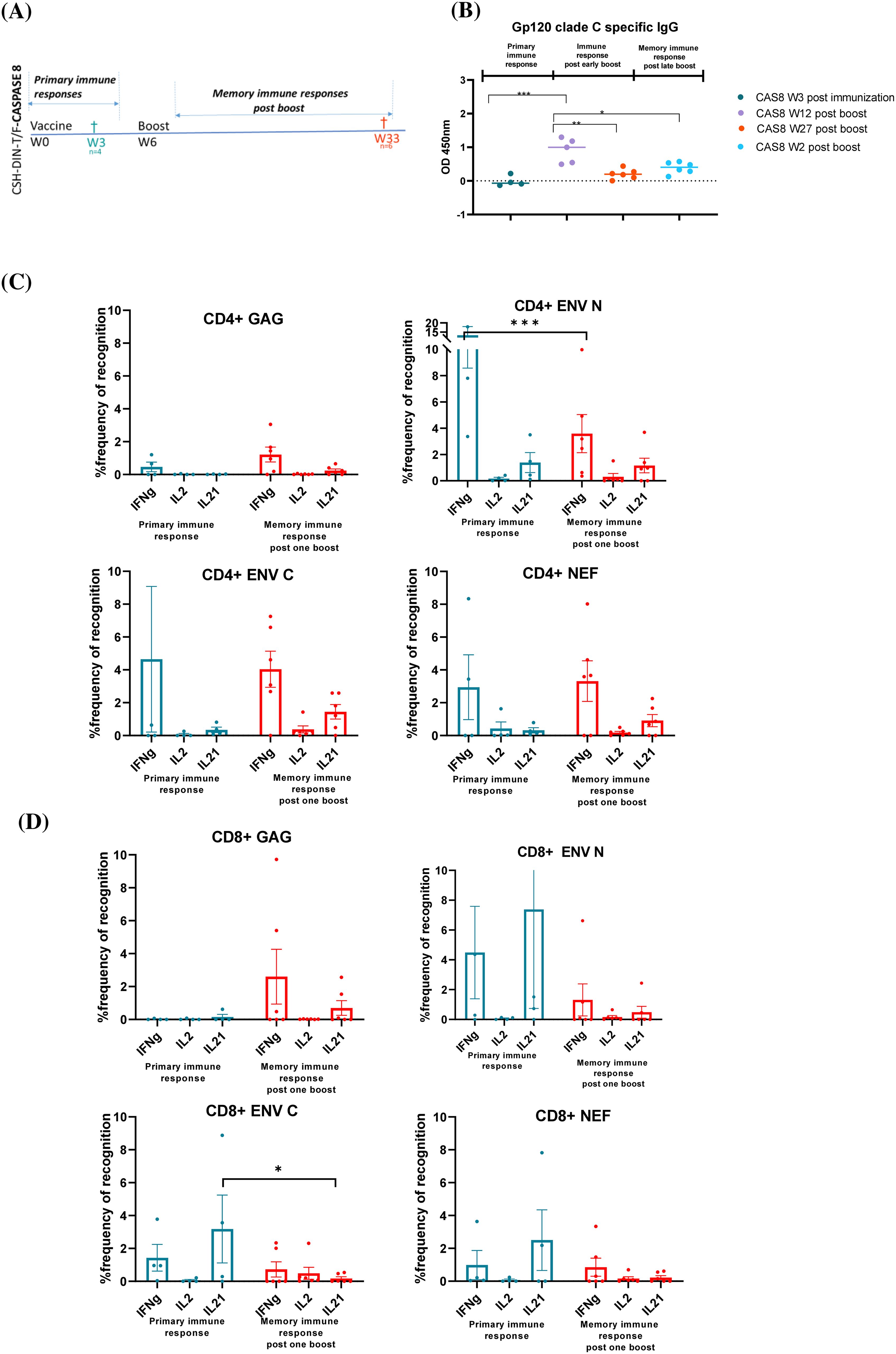
Figure 5. Schematics representing multiple groups of BALB/cJ mouse immunizations to compare the primary immune responses at week 3 post-immunization with memory immune responses at week 33 (week 27 post-boost). (B) gp120 clade C-specific IgG humoral responses of the different groups immunized with CSH-DIN-T/F Z331-Cas8 were compared. (C) SHIV-specific IFN-γ-, IL-2-, and IL-21-producing CD4+ T cells, (D) CD8+ T cells from splenocytes of immunized BALB/cJ mice at week 3 and week 33. Results were compared using the paired t-test. Error bars represent the standard error of mean (s.e.m), n = 5. *p < 0.05, **p < 0.01, ***p < 0.001. Statistical differences between total responses of each of the groups are indicated on the graph.
These data confirm that the addition of an apoptotic gene to the vaccine enhances cytotoxic T cell-specific responses.
4 Discussion
A safe and effective vaccine that prevents the acquisition or controls the replication of HIV remains elusive so far. While strategies to induce broadly neutralizing antibodies are progressing (Haynes et al., 2023; Nkolola and Barouch, 2024), these approaches would likely have minimal benefit past HIV acquisition. Hence, developing parallel strategies designed to enhance T-cell responses remains critical. To that extent, DNA vaccines have shown promise in generating cell-mediated immune responses especially when combined with electroporation to enhance transduction and antigen expression in vivo (Arrode et al., 2007; Arrode-Bruses et al., 2014; Chebloune et al., 2020). Our study was built on the premise that a vaccine expressing most lentiviral antigens may have one of the best abilities to induce broad and protective immune responses to HIV, even if the approach was clearly not able to prevent virus acquisition. The substitution of HIV/SIV LTR for the constitutively active CAEV LTRs has been shown to induce detectable immune responses in mice and monkeys as well as induce a rapid control of SIV infection (Arrode et al., 2007; Arrode-Bruses et al., 2014; Chebloune et al., 2020). This correlated with the presence of antigen-specific highly recallable T cells from precursors named PHPC (Arrode-Bruses et al., 2014). However, the vaccine had ample room for improvement in its induction of antiviral responses. In an initial approach, the inclusion of IL-7 and IL-15 as natural adjuvants was attempted, showing some modest benefits in mice and rhesus macaques (Leroy et al., 2022). In the current approach, the vaccine was updated via the inclusion of a clade C T/F HIV envelope more representative of widely transmitted isolates in the developing world (Deymier et al., 2014). Next, we wanted to combine this approach with the enhanced induction of apoptosis to promote cross-presentation of antigen via MHC Class I (Huang et al., 2007). This approach has not previously been attempted with a lentiviral-based DNA vaccine construct. Three pro-apoptotic genes were selected, two targeting the intrinsic apoptotic pathway (BAX and BAK) and the third one focusing on the extrinsic pathway (caspase 8). The apoptotic cassettes were individually placed after the pol coding sequence to avoid overexpression and premature expression relative to antigen production (Bergmann-Leitner and Leitner, 2004; Kim et al., 2004). Surprisingly, CSH-DIN-T/F Z331-BAK was non-functional, failing to express either antigens or the BAK apoptotic gene (Figure 1) in spite of mRNA presence and fully verified sequence. The reason for such failure remains unclear at present but led us to drop this construct from further evaluation.
The two other vaccine prototypes considered for in vivo studies with BALB/cJ were CSH-DIN-T/F Z331-BAX and CSH-DIN-T/F Z331-Cas8, which appeared to produce more SHIV antigens than the parent CSH-DIN-T/F Z331 devoid of apoptotic gene (Figures 1B, D) in vitro, suggesting gain of fitness, at least in cell culture, especially for CSH-DIN-T/F Z331-Cas8. Moreover, CSH-DIN-T/F Z331-Cas8 was shown to not only express caspase 8 but also generate activated caspase 8 (Figure 2B), leading to the enhanced apoptosis of transduced cells (Figures 2C, D). The superiority of CSH-DIN-T/F Z331-Cas8 was also apparent in vivo, whereby mice immunized with this construct generated markedly higher antigen-specific CD8-mediated responses than mice immunized with CSH-DIN-T/F Z331-BAX or parent CSH-DIN-T/F Z331. Our findings confirm studies by Sasaki et al. in which DNA vaccines can be made more effective by co-delivering antigens of interest with pro-apoptotic genes. In their study, DNA vaccines co-expressing influenza virus hemagglutinin or nucleoprotein genes and mutant caspase genes have markedly increased the T-cell responses (Sasaki et al., 2001). The projected mechanisms targeted were the induction of apoptosis of the initial antigen-producing cells to generate apoptotic bodies that are engulfed by Langerhans cells in the skin and dendritic cells in the draining lymph nodes, leading to higher activation, and cross-presentation of antigen complexed to MHC class I to T cells. One limitation of our study is the lack of demonstration of such apoptosis and cross-presentation in vivo.
However, earlier studies in cancer immunology have adopted the opposite approach, comparing pro-apoptotic and anti-apoptotic genes in DNA vaccines (Kim et al., 2004) based on the assumption that prolonging the life of short-lived dendritic cells (DCs) will enhance antigen presentation, leading to better immune responses (Kim et al., 2003b; Kim et al., 2003a; Huang et al., 2007; Kang et al., 2007; Cheng et al., 2008). However, a subsequent study compared the inclusion of the apoptotic gene Bax with the anti-apoptotic Bcl-XL, co-injected i.d. with DNA expressing the glycoprotein B (gB) of herpes simplex virus (HSV)-1. In this study, mice immunized with pBax and pgB induced higher titers of antibody along with stronger lymphocyte proliferative responses and cytotoxic activity compared to those mice that received pgB alone or with pBcl-xl (Parsania et al., 2010). These data reinforced the idea that cells transfected with plasmid DNA encoding apoptotic genes result in the formation of apoptotic bodies, which are phagocytosed by the dendritic cells resulting in immune responses to the antigen (Kinsey et al., 2004). In addition, having the apoptotic gene incorporated in the same DNA expressing the antigens further ascertains that the pro-apoptotic effect will be exerted on the cells transfected with the antigen-producing construct as opposed to the injection of two separate DNA plasmids (Kinsey et al., 2004).
The multiple immunization studies showed not only the early generation of CD8 T cell-mediated immune responses as early as 3 weeks post-prime but also polyclonal responses, attesting to the quality of such responses. Moreover, these responses when boosted were shown to be highly durable, with extensive levels of CD8 T cells producing IFN-γ in response to SIV Gag restimulation even 6 months after the last boost and beyond. While other HIV immunization attempts in mice using plasmid DNA with or without molecular adjuvants gave similar magnitudes of cell-mediated responses (Liu et al., 2007; Ganguly et al., 2010; Kulkarni et al., 2013; Zhou et al., 2013; Xu et al., 2020), our approach induces durable responses that are not restricted to select HIV antigens but incorporate all viral proteins except for the integrase. Even if responses to some of these antigens are below detection or not tested here, priming will have occurred with considerable breadth breath of the responses expected upon infection post-challenge.
In conclusion, we submit that we have generated a safe and promising single-cycle lentiviral DNA vaccine capable of inducing potent and durable CD8 T cell antiviral responses important for protection even post-viral acquisition to control viral loads (Turk et al., 2013). Moreover, our DNA vaccine may be combined with other vaccines promoting the induction of humoral immune response for future testing in a humanized mouse or non-human primate model of HIV infection.
Data availability statement
The raw data supporting the conclusions of this article will be made available by the authors, without undue reservation.
Ethics statement
The animal study was approved by IACUC: University of Louisiana at Lafayette. The study was conducted in accordance with the local legislation and institutional requirements.
Author contributions
DB: Conceptualization, Data curation, Formal Analysis, Investigation, Methodology, Software, Validation, Visualization, Writing – original draft, Writing – review & editing. KR: Writing – review & editing, Investigation. LS: Writing – review & editing, Investigation. YC: Writing – review & editing, Conceptualization. FV: Conceptualization, Funding acquisition, Investigation, Methodology, Project administration, Resources, Supervision, Visualization, Writing – original draft, Validation, Writing – review & editing.
Funding
The author(s) declare that financial support was received for the research and/or publication of this article. This work was funded by the New Iberia Research Center, University of Louisiana at Lafayette.
Acknowledgments
The authors would like to thank NIRC animal care/vets/techs and staff for the care of the animals and the NIH AIDS Research and Reference Reagent Program for providing HIV and SIV overlapping peptides.
Conflict of interest
The authors declare that the research was conducted in the absence of any commercial or financial relationships that could be construed as a potential conflict of interest.
Publisher’s note
All claims expressed in this article are solely those of the authors and do not necessarily represent those of their affiliated organizations, or those of the publisher, the editors and the reviewers. Any product that may be evaluated in this article, or claim that may be made by its manufacturer, is not guaranteed or endorsed by the publisher.
Supplementary material
The Supplementary Material for this article can be found online at: https://www.frontiersin.org/articles/10.3389/fcimb.2025.1481427/full#supplementary-material
References
Ahn, J. Y., Lee, J., Suh, Y. S., Song, Y. G., Choi, Y. J., Lee, K. H., et al. (2022). Safety and immunogenicity of two recombinant DNA COVID-19 vaccines containing the coding regions of the spike or spike and nucleocapsid proteins: an interim analysis of two open-label, non-randomised, phase 1 trials in healthy adults. Lancet Microbe 3, e173–ee83. doi: 10.1016/S2666-5247(21)00358-X
Arrode, G., Hegde, R., Mani, A., Jin, Y., Chebloune, Y., Narayan, O. (2007). Phenotypic and functional analysis of immune CD8+ T cell responses induced by a single injection of a HIV DNA vaccine in mice. J. Immunol. 178, 2318–2327. doi: 10.4049/jimmunol.178.4.2318
Arrode-Bruses, G., Moussa, M., Baccard-Longere, M., Villinger, F., Chebloune, Y. (2014). Long-term central and effector SHIV-specific memory T cell responses elicited after a single immunization with a novel lentivector DNA vaccine. PLoS One 9, e110883. doi: 10.1371/journal.pone.0110883
Bergmann-Leitner, E. S., Leitner, W. W. (2004). Danger, death and DNA vaccines. Microbes Infect. 6, 319–327. doi: 10.1016/j.micinf.2003.11.015
Bose, D. (2017). Tat-independent lentivirus genomes for vaccination and host/pathogen interaction studies. (HAL Thesis). Université Grenoble Alpes, Saint-Martin-d’Hères. Available online at: https://theses.hal.science/tel-01938074/file/BOSE_2017_diffusion.pdf (Acessed February 17, 2023).
Bose, D., Deb Adhikary, N., Xiao, P., Rogers, K. A., Ferrell, D. E., Cheng-Mayer, C., et al. (2024). SHIV-C109p5 NHP induces rapid disease progression in elderly macaques with extensive GI viral replication. J. Virol. 98, e0165223. doi: 10.1128/jvi.01652-23
Bose, D., Gagnon, J., Chebloune, Y. (2015). Comparative analysis of tat-dependent and tat-deficient natural lentiviruses. Vet. Sci. 2, 293–348. doi: 10.3390/vetsci2040293
Castro-Gonzalez, S., Colomer-Lluch, M., Serra-Moreno, R. (2018). Barriers for HIV cure: the latent reservoir. AIDS Res. Hum. Retroviruses 34, 739–759. doi: 10.1089/aid.2018.0118
Chebloune, Y., Moussa, M., Arrode-Bruses, G., Ronfort, C., Bose, D., Gagnon, J., et al. (2020). A single lentivector DNA based immunization contains a late heterologous SIVmac251 mucosal challenge infection. Vaccine. 38, 3729–3739. doi: 10.1016/j.vaccine.2020.03.053
Cheng, W. F., Chang, M. C., Sun, W. Z., Lee, C. N., Lin, H. W., Su, Y. N., et al. (2008). Connective tissue growth factor linked to the E7 tumor antigen generates potent antitumor immune responses mediated by an antiapoptotic mechanism. Gene Ther. 15, 1007–1016. doi: 10.1038/gt.2008.25
Corey, L., Gilbert, P. B., Juraska, M., Montefiori, D. C., Morris, L., Karuna, S. T., et al. (2021). Two randomized trials of neutralizing antibodies to prevent HIV-1 acquisition. N Engl. J. Med. 384, 1003–1014. doi: 10.1056/NEJMoa2031738
Deymier, M. J., Claiborne, D. T., Ende, Z., Ratner, H. K., Kilembe, W., Allen, S., et al. (2014). Particle infectivity of HIV-1 full-length genome infectious molecular clones in a subtype C heterosexual transmission pair following high fidelity amplification and unbiased cloning. Virology 468-470, 454–461. doi: 10.1016/j.virol.2014.08.018
Deymier, M. J., Ende, Z., Fenton-May, A. E., Dilernia, D. A., Kilembe, W., Allen, S. A., et al. (2015). Heterosexual transmission of subtype C HIV-1 selects consensus-like variants without increased replicative capacity or interferon-alpha resistance. PLoS Pathog. 11, e1005154. doi: 10.1371/journal.ppat.1005154
Diehl, M. C., Lee, J. C., Daniels, S. E., Tebas, P., Khan, A. S., Giffear, M., et al. (2013). Tolerability of intramuscular and intradermal delivery by CELLECTRA((R)) adaptive constant current electroporation device in healthy volunteers. Hum. Vaccin Immunother. 9 (10), 2246–2252. doi: 10.4161/hv.24702
Elizaga, M. L., Li, S. S., Kochar, N. K., Wilson, G. J., Allen, M. A., Tieu, H. V. N., et al. (2018). Safety and tolerability of HIV-1 multiantigen pDNA vaccine given with IL-12 plasmid DNA via electroporation, boosted with a recombinant vesicular stomatitis virus HIV Gag vaccine in healthy volunteers in a randomized, controlled clinical trial. PLoS One 13, e0202753. doi: 10.1371/journal.pone.0202753
Ganguly, S., Liu, J., Pillai, V. B., Mittler, R. S., Amara, R. R. (2010). Adjuvantive effects of anti-4-1BB agonist Ab and 4-1BBL DNA for a HIV-1 Gag DNA vaccine: different effects on cellular and humoral immunity. Vaccine. 28, 1300–1309. doi: 10.1016/j.vaccine.2009.11.020
Hannaman, D., Dupuy, L. C., Ellefsen, B., Schmaljohn, C. S. (2016). A Phase 1 clinical trial of a DNA vaccine for Venezuelan equine encephalitis delivered by intramuscular or intradermal electroporation. Vaccine. 34, 3607–3612. doi: 10.1016/j.vaccine.2016.04.077
Haynes, B. F., Wiehe, K., Borrow, P., Saunders, K. O., Korber, B., Wagh, K., et al. (2023). Strategies for HIV-1 vaccines that induce broadly neutralizing antibodies. Nat. Rev. Immunol. 23, 142–158. doi: 10.1038/s41577-022-00753-w
Hooper, J., Paolino, K. M., Mills, K., Kwilas, S., Josleyn, M., Cohen, M., et al. (2020). A phase 2a randomized, double-blind, dose-optimizing study to evaluate the immunogenicity and safety of a bivalent DNA vaccine for hemorrhagic fever with renal syndrome delivered by intramuscular electroporation. Vaccines (Basel) 8 (3), 377, 0-20. doi: 10.3390/vaccines8030377
Houser, K. V., Chen, G. L., Carter, C., Crank, M. C., Nguyen, T. A., Burgos Florez, M. C., et al. (2022). Safety and immunogenicity of a ferritin nanoparticle H2 influenza vaccine in healthy adults: a phase 1 trial. Nat. Med. 28, 383–391. doi: 10.1038/s41591-021-01660-8
Huang, B., Mao, C. P., Peng, S., He, L., Hung, C. F., Wu, T. C. (2007). Intradermal administration of DNA vaccines combining a strategy to bypass antigen processing with a strategy to prolong dendritic cell survival enhances DNA vaccine potency. Vaccine. 25, 7824–7831. doi: 10.1016/j.vaccine.2007.08.036
Kang, T. H., Lee, J. H., Noh, K. H., Han, H. D., Shin, B. C., Choi, E. Y., et al. (2007). Enhancing dendritic cell vaccine potency by combining a BAK/BAX siRNA-mediated antiapoptotic strategy to prolong dendritic cell life with an intracellular strategy to target antigen to lysosomal compartments. Int. J. Cancer. 120, 1696–1703. doi: 10.1002/ijc.v120:8
Kim, T. W., Hung, C. F., Boyd, D., Juang, J., He, L., Kim, J. W., et al. (2003a). Enhancing DNA vaccine potency by combining a strategy to prolong dendritic cell life with intracellular targeting strategies. J. Immunol. 171, 2970–2976. doi: 10.4049/jimmunol.171.6.2970
Kim, T. W., Hung, C. F., Ling, M., Juang, J., He, L., Hardwick, J. M., et al. (2003b). Enhancing DNA vaccine potency by coadministration of DNA encoding antiapoptotic proteins. J. Clin. Invest. 112, 109–117. doi: 10.1172/JCI200317293
Kim, T. W., Hung, C. F., Zheng, M., Boyd, D. A., He, L., Pai, S. I., et al. (2004). A DNA vaccine co-expressing antigen and an anti-apoptotic molecule further enhances the antigen-specific CD8+ T-cell immune response. J. BioMed. Sci. 11, 493–499. doi: 10.1007/BF02256098
Kinsey, B. M., Marcelli, M., Song, L., Bhogal, B. S., Ittmann, M., Orson, F. M. (2004). Enhancement of both cellular and humoral responses to genetic immunization by co-administration of an antigen-expressing plasmid and a plasmid encoding the pro-apoptotic protein Bax. J. Gene Med. 6, 445–454. doi: 10.1002/jgm.v6:4
Kisakov, D. N., Belyakov, I. M., Kisakova, L. A., Yakovlev, V. A., Tigeeva, E. V., Karpenko, L. I. (2024). The use of electroporation to deliver DNA-based vaccines. Expert Rev. Vaccines 23, 102–123. doi: 10.1080/14760584.2023.2292772
Kraynyak, K. A., Blackwood, E., Agnes, J., Tebas, P., Giffear, M., Amante, D., et al. (2022). SARS-coV-2 DNA vaccine INO-4800 induces durable immune responses capable of being boosted in a phase 1 open-label trial. J. Infect. Dis. 225, 1923–1932. doi: 10.1093/infdis/jiac016
Kulkarni, V., Rosati, M., Valentin, A., Ganneru, B., Singh, A. K., Yan, J., et al. (2013). HIV-1 p24(gag) derived conserved element DNA vaccine increases the breadth of immune response in mice. PLoS One 8, e60245. doi: 10.1371/journal.pone.0060245
Leroy, L. A., Mac Donald, A., Kandlur, A., Bose, D., Xiao, P., Gagnon, J., et al. (2022). Cytokine adjuvants IL-7 and IL-15 improve humoral responses of a SHIV lentiDNA vaccine in animal models. Vaccines (Basel) 10 (3), 461 0-22. doi: 10.3390/vaccines10030461
Liu, J., Hellerstein, M., McDonnel, M., Amara, R. R., Wyatt, L. S., Moss, B., et al. (2007). Dose-response studies for the elicitation of CD8 T cells by a DNA vaccine, used alone or as the prime for a modified vaccinia Ankara boost. Vaccine. 25, 2951–2958. doi: 10.1016/j.vaccine.2006.05.081
Mekonnen, Z. A., Masavuli, M. G., Yu, W., Gummow, J., Whelan, D. M., Al-Delfi, Z., et al. (2020). Enhanced T cell responses induced by a necrotic dendritic cell vaccine, expressing HCV NS3. Front. Microbiol. 11, 559105. doi: 10.3389/fmicb.2020.559105
Moussa, M., Arrode-Bruses, G., Manoylov, I., Malogolovkin, A., Mompelat, D., Ishimwe, H., et al. (2015). A novel non-integrative single-cycle chimeric HIV lentivector DNA vaccine. Vaccine. 33, 2273–2282. doi: 10.1016/j.vaccine.2015.03.021
Nkolola, J. P., Barouch, D. H. (2024). Prophylactic HIV-1 vaccine trials: past, present, and future. Lancet HIV. 11, e117–ee24. doi: 10.1016/S2352-3018(23)00264-3
Parrish, N. F., Gao, F., Li, H., Giorgi, E. E., Barbian, H. J., Parrish, E. H., et al. (2013). Phenotypic properties of transmitted founder HIV-1. Proc. Natl. Acad. Sci. U S A. 110, 6626–6633. doi: 10.1073/pnas.1304288110
Parsania, M., Bamdad, T., Hassan, Z. M., Kheirandish, M., Pouriayevali, M. H., Sari, R. D., et al. (2010). Evaluation of apoptotic and anti-apoptotic genes on efficacy of DNA vaccine encoding glycoprotein B of Herpes Simplex Virus type 1. Immunol. Lett. 128, 137–142. doi: 10.1016/j.imlet.2009.12.014
Sarwar, U. N., Costner, P., Enama, M. E., Berkowitz, N., Hu, Z., Hendel, C. S., et al. (2015). Safety and immunogenicity of DNA vaccines encoding Ebolavirus and Marburgvirus wild-type glycoproteins in a phase I clinical trial. J. Infect. Dis. 211, 549–557. doi: 10.1093/infdis/jiu511
Sasaki, S., Amara, R. R., Oran, A. E., Smith, J. M., Robinson, H. L. (2001). Apoptosis-mediated enhancement of DNA-raised immune responses by mutant caspases. Nat. Biotechnol. 19, 543–547. doi: 10.1038/89289
Sasaki, S., Xin, K. Q., Okudela, K., Okuda, K., Ishii, N. (2002). Immunomodulation by apoptosis-inducing caspases for an influenza DNA vaccine delivered by gene gun. Gene Ther. 9, 828–831. doi: 10.1038/sj.gt.3301696
Scheffer, S. R., Nave, H., Korangy, F., Schlote, K., Pabst, R., Jaffee, E. M., et al. (2003). Apoptotic, but not necrotic, tumor cell vaccines induce a potent immune response in vivo. Int. J. Cancer 103, 205–211. doi: 10.1002/ijc.v103:2
Sefidi-Heris, Y., Jahangiri, A., Mokhtarzadeh, A., Shahbazi, M. A., Khalili, S., Baradaran, B., et al. (2020). Recent progress in the design of DNA vaccines against tuberculosis. Drug Discovery Today 25 (11), 1971–1987. doi: 10.1016/j.drudis.2020.09.005
Tebas, P., Kraynyak, K. A., Patel, A., Maslow, J. N., Morrow, M. P., Sylvester, A. J., et al. (2019). Intradermal synCon(R) ebola GP DNA vaccine is temperature stable and safely demonstrates cellular and humoral immunogenicity advantages in healthy volunteers. J. Infect. Dis. 220, 400–410. doi: 10.1093/infdis/jiz132
Todorova, B., Adam, L., Culina, S., Boisgard, R., Martinon, F., Cosma, A., et al. (2017). Electroporation as a vaccine delivery system and a natural adjuvant to intradermal administration of plasmid DNA in macaques. Sci. Rep. 7, 4122. doi: 10.1038/s41598-017-04547-2
Turk, G., Ghiglione, Y., Falivene, J., Socias, M. E., Laufer, N., Coloccini, R. S., et al. (2013). Early Gag immunodominance of the HIV-specific T-cell response during acute/early infection is associated with higher CD8+ T-cell antiviral activity and correlates with preservation of the CD4+ T-cell compartment. J. Virol. 87, 7445–7462. doi: 10.1128/JVI.00865-13
Vaccari, M., Poonam, P., Franchini, G. (2010). Phase III HIV vaccine trial in Thailand: a step toward a protective vaccine for HIV. Expert Rev. Vaccines 9, 997–1005. doi: 10.1586/erv.10.104
Whyte-Allman, S. K., Bendayan, R. (2020). HIV-1 sanctuary sites-the role of membrane-associated drug transporters and drug metabolic enzymes. AAPS J. 22, 118. doi: 10.1208/s12248-020-00498-1
Xu, Z., Wise, M. C., Chokkalingam, N., Walker, S., Tello-Ruiz, E., Elliott, S. T. C., et al. (2020). In vivo assembly of nanoparticles achieved through synergy of structure-based protein engineering and synthetic DNA generates enhanced adaptive immunity. Adv. Sci. (Weinh). 7, 1902802. doi: 10.1002/advs.201902802
Keywords: SHIV, DNA vaccine, lentivector, apoptotic gene, immune responses, mouse immunization
Citation: Bose D, Rogers KA, Shirreff LM, Chebloune Y and Villinger FJ (2025) Enhanced HIV immune responses elicited by an apoptotic single-cycle SHIV lentivector DNA vaccine. Front. Cell. Infect. Microbiol. 15:1481427. doi: 10.3389/fcimb.2025.1481427
Received: 15 August 2024; Accepted: 04 March 2025;
Published: 10 April 2025.
Edited by:
Marina Tuyishime, Duke University, United StatesReviewed by:
Jian Chen, Fudan University, ChinaRoberta Bona, National Institute of Health (ISS), Italy
Copyright © 2025 Bose, Rogers, Shirreff, Chebloune and Villinger. This is an open-access article distributed under the terms of the Creative Commons Attribution License (CC BY). The use, distribution or reproduction in other forums is permitted, provided the original author(s) and the copyright owner(s) are credited and that the original publication in this journal is cited, in accordance with accepted academic practice. No use, distribution or reproduction is permitted which does not comply with these terms.
*Correspondence: Francois J. Villinger, ZnJhbmNvaXMudmlsbGluZ2VyQGxvdWlzaWFuYS5lZHU=