- 1Southern Regional Station, Indian Council of Agricultural Research (ICAR)-National Dairy Research Institute (NDRI), Bengaluru, Karnataka, India
- 2Institute of Advanced Virology, Bio 360 Life Sciences Park, Trivandrum, Kerala, India
Multidrug-resistant (MDR) bacteria in farm animal products threaten human health by causing zoonotic infections. The complete genome sequences of MDR bacteria from subclinical mastitis-affected cow’s milk have not yet been comprehensively documented in India. In this study, using a bacterial metagenomic sequencing approach, we detected the nearly complete genome sequences of Pseudomonas veronii and Staphylococcus xylosus from subclinical mastitis-affected cow’s milk. Furthermore, we sequenced the nearly complete genome sequences of Escherichia coli, Klebsiella pneumoniae, Staphylococcus hominis, and S. xylosus from subclinical mastitis-affected cow’s milk using a whole-genome metagenomic sequencing method. Our analysis subsequently revealed that the complete genome sequences of the identified bacteria contained MDR genes and genes for multiple virulence factors. These MDR bacteria may pose a public health risk through exposure to milkers, milk handlers, and farm workers or through the handling and consumption of unpasteurized milk.
Introduction
Recently, multidrug-resistant (MDR) bacteria have been detected in cow’s milk in many parts of the world (Tempini et al., 2018; Yang et al., 2021; Hassani et al., 2022; Ashraf et al., 2023; Badawy et al., 2023; Haq et al., 2024), and this poses a threat to public health. Among the MDR bacteria, the ESKAPE pathogens, which include Enterococcus faecium, Staphylococcus aureus, Klebsiella pneumoniae, Acinetobacter baumannii, Pseudomonas aeruginosa, and Enterobacter spp., are emerging as a public health crisis (De Oliveira et al., 2020; Miller and Arias, 2024). The environmental reservoirs and contaminated food of animal or plant origin are considered a source of ESKAPE pathogens spreading to humans (Patil et al., 2021). Continuous use/misuse/overuse of antibiotics in clinical and non-clinical settings, animals, plants, and environments is considered to be the driving force for the emergence of antibiotic-resistant bacteria (Pillay et al., 2022).
In recent years, the complete genome sequencing of bacteria using next-generation sequencing (NGS) has emerged as a powerful tool for monitoring emerging pathogens, particularly those with virulence and antibiotic resistance genes (Boolchandani et al., 2019; Pillay et al., 2022). This approach enables researchers to identify emerging pathogens in various geographical locations and to trace their sources of dissemination (Boolchandani et al., 2019; Pillay et al., 2022). As a result, it assists in the development of diagnostics and preventive and control measures and the formulation of policies for the judicious use of antibiotics (Boolchandani et al., 2019; Pillay et al., 2022). In line with this, recently, the complete genome sequence of S. aureus and non-aureus staphylococci and mammaliicocci (NASM) from clinical mastitis bovine milk in India was identified using the NGS approach, and the MDR genes in them were determined (Sivakumar et al., 2023; Ramesh et al., 2024). Moreover, virulence and MDR genes were identified through complete genome sequencing of S. aureus collected from the milk of cows infected with subclinical mastitis in South Africa (Khasapane et al., 2024), Rwanda (Ndahetuye et al., 2021), and Brazil (Pizauro et al., 2019). Similarly, the complete genome sequences of Escherichia coli, K. pneumoniae, and Bordetella bronchiseptica with MDR genes have been determined in subclinical mastitis-affected cow’s milk in Bangladesh (Anika et al., 2023), Egypt (Tartor et al., 2021), and New Zealand (Munn et al., 2025), respectively. In this context, the complete genome sequencing of bacterial pathogens with MDR and virulent genes in subclinical mastitis-affected cow’s milk is not fully documented in India. In this context, the present study aimed to utilize the NGS approach to determine the complete genome sequences of bacterial pathogens found in subclinical mastitis-affected cow’s milk in India. Additionally, the study planned to identify MDR genes and virulence genes from the complete genome sequences of these bacteria.
Materials and methods
Sample collection and subclinical and clinical mastitis detection using the California mastitis test
The clinical examination of animal, udder, and milk was carried out for the diagnosis of clinical and subclinical mastitis by the veterinary clinician, and milk samples from subclinical mastitis-affected cows were collected from Devanahalli taluk of Bengaluru rural district of Karnataka and Livestock Research Centre, Southern Regional Station of the Indian Council of Agricultural Research (ICAR)-National Dairy Research Institute (NDRI), Bengaluru. Changes in milk (e.g., watery, bloody, curd-like) and udder parenchyma (swelling and redness), with or without systemic symptoms like inappetence and fever, were considered clinical mastitis-affected animals. Animals diagnosed with clinical mastitis by dairy farmers were treated and recorded by veterinarians as per standard practices. In the case of subclinical mastitis, milk and the udder appeared normal, but somatic cell counts (SCCs) increased in milk, which were indirectly detected through the California mastitis test (CMT). The CMT was carried out as per the previous standardized protocols (Dingwell et al., 2003; Rust et al., 2021). Briefly, an equal volume of milk and the CMT reagent was mixed in the CMT paddle, and the mixture was rotated in the horizontal position. The mixture with normal fluidity and no thickening was considered healthy, while the mixture with thickening or gel formation was considered subclinical mastitis-affected.
The bacterial culture; Gram staining; and indole, methyl red, Voges-Proskauer, and citrate tests
The milk samples positive for the CMT were subjected to bacterial isolation in MacConkey agar [M7408; M/s HiMedia Laboratories Pvt. Ltd., Thane (West), Maharashtra, India], Edwards Medium Base [M748; M/s HiMedia Laboratories Pvt. Ltd., Thane (West), Maharashtra, India], and mannitol salt agar (MSA) [MU118; M/s HiMedia Laboratories Pvt. Ltd., Thane (West), Maharashtra, India] as per the previous standard protocol (Sawant et al., 2002; Gebremedhin et al., 2022; Geletu et al., 2022). Then, bacterial colonies grown in MacConkey agar, Edwards Medium Base, and MSA were used for Gram staining characterization. Bacterial Gram staining was performed using the Gram stains kit [K001; M/s HiMedia Laboratories Pvt. Ltd., Thane (West), Maharashtra, India] as per the manufacturer’s protocols. The indole, methyl red, Voges-Proskauer, citrate + and H2S (IMViC) [KB001; M/s HiMedia Laboratories Pvt. Ltd., Thane (West), Maharashtra, India] biochemical tests were performed to differentially identify the Enterobacteriaceae bacteria with standard protocols (https://microbenotes.com/imvic-tests/).
The metagenomic and whole-genome metagenomic sequencing of bacteria and analysis
For metagenomic sequencing, DNA was extracted from the subclinical mastitis-affected cow’s milk. Then, preparation of the bacterial metagenomic DNA library was carried out using the QIASeq FX DNA Library (Cat#180475; QIAGEN, Hilden, Germany) protocol as follows: enzymatic fragmentation, end-repair, 3′ adenylation, adapter ligation, six cycles of indexing-PCR, and purification using CamSelect magnetic beads. Sequencing was carried out using NovaSeq 6000. The whole-genome metagenomic sequencing of bacteria was also performed similar to the bacterial metagenomic protocol, but the DNA used for sequencing was extracted from the bacterial culture. The quality control of the sequences was carried out using FastQC (version 0.11.5). Then, Trimmomatic (Bolger et al., 2014) was used for quality control to filter, identify, and remove potential adapters and low-quality read sequences. The bacteria-specific reads were filtered using the protein-based alignment method DIAMOND (Buchfink et al., 2015) and subsequently de-novo assembled in the metaSPAdes (Bankevich et al., 2012). The bacteria-specific sequences in the de-novo assembled sequences were recognized by Blastx and Blastn in the NCBI RefSeq database for bacteria, and the bacteria-specific contig was aligned using an advanced genome aligner (AGA) (Deforche, 2017), via the Needleman–Wunsch (Smith and Waterman, 1981), Gotoh (1982), and Smith–Waterman algorithms (Smith and Waterman, 1981). Finally, the variant caller GATK/BCFtools (https://github.com/broadinstitute/gatk/releases; https://samtools.github.io/bcftools/howtos/variant-calling.html) was used for optimal alignment and consensus.
Bacterial genome annotation and detection of antibiotic resistance and virulence factor genes
The sequences of the nearly complete genome of bacteria detected by the NGS approach were genome annotated with Bakta v1.8.2 (DB: v5.0 - Light) (Schwengers et al., 2021) and Prokka 1.14.6 (Seemann, 2014) and visualized in Proksee (Grant et al., 2023). Then, the antibiotic resistance genes in these bacteria were determined using the Comprehensive Antibiotic Resistance Database (CARD) and the Resistance Gene Identifier (RGI) 6.0.3 (Alcock et al., 2023), and the results were validated using the NCBI public database. Furthermore, the virulence factor genes in these bacteria were determined using the Virulence Factor Database (VFDB) (Liu et al., 2022).
Results
In this study, we documented both clinical and subclinical cases of mastitis that occurred in Bengaluru, Karnataka, India, from 2017 to 2020 (Table 1). Accordingly, it was revealed that clinical mastitis cases occurred at almost the same rate in the Bengaluru region in all the years of the study periods and different seasons of the year (Table 1). It was also revealed that subclinical mastitis cases were more common than clinical mastitis cases in this region (Table 1). In cases of clinical mastitis, farmers observed distinct abnormalities in the udder (Figure 1A) as well as noticeable changes in the milk. These conditions were typically addressed through appropriate treatments administered by veterinarians. Conversely, subclinical mastitis may not show visible signs in the udder or milk, necessitating diagnosis by a veterinary clinician. As a result, most mastitis cases were subclinical and were often undetected in the rural areas of India. Handling milk from cows with subclinical mastitis can elevate the risk of spreading infectious agents to farm workers, and the sale of unpasteurized milk to households poses a public health threat. Despite this, traditional hand milking persists in rural India, where it is common to give newborns or children locally available, unpackaged boiled cow’s milk instead of pasteurized milk (personal observation). Therefore, this study aimed to identify antibiotic-resistant bacteria using a metagenomic approach in cow’s milk affected by subclinical mastitis, which presents a health hazard in the country.
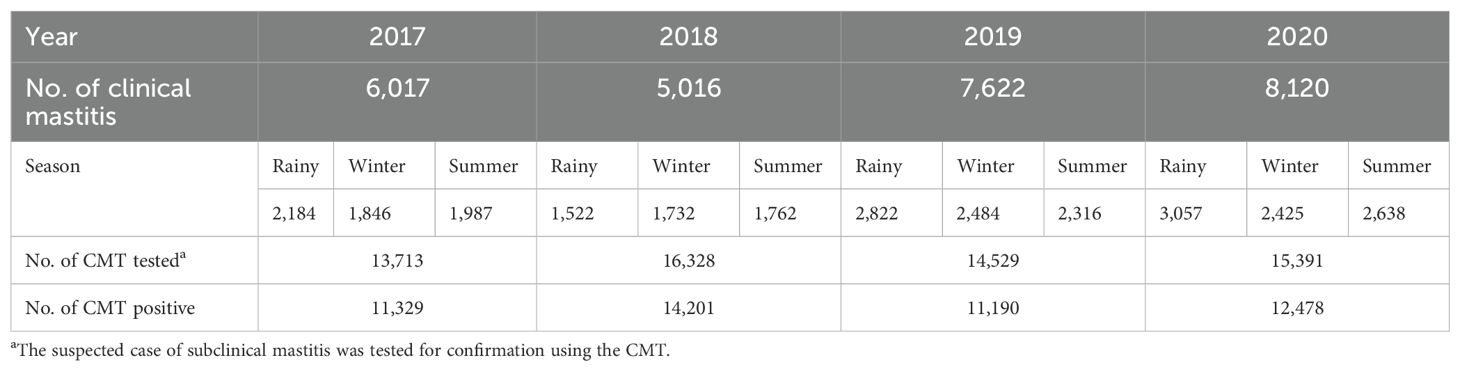
Table 1. Clinical and subclinical mastitis cases recorded from 2017 to 2020 in Bengaluru, Karnataka, India.
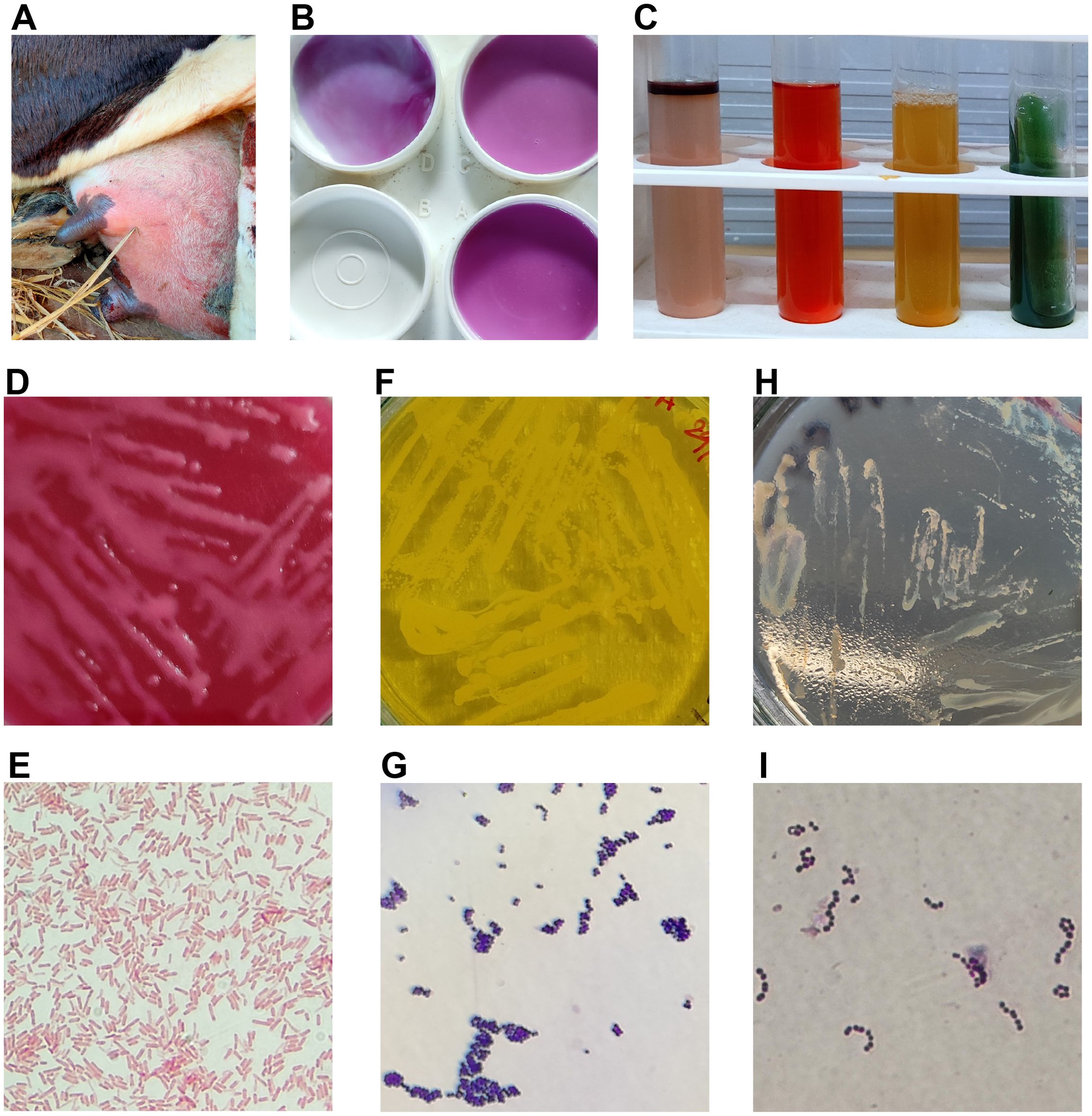
Figure 1. (A) Cow with clinical mastitis-affected udder. (B) Formation of gel in the paddle containing an equal amount of milk and CMT solution, indicating the SCM in the left front quarter of the cow. (C) IMViC tests show positive for the indole and methyl red tests and negative for the remaining tests. It is indicative of Escherichia coli-positive. (D) Pink colonies of E coli growth on MacConkey agar. (E) Pink color rod-shaped E coli in Gram staining. (F) Yellow color Staphylococcus spp. colony growth in MSA. (G) Bunch of grape-like purple color Staphylococcus spp. cocci in Gram staining. (H) Colorless Streptococcus spp. colony growth in Edward’s medium. (I) Purple color chains of Streptococcus spp. cocci in Gram staining.
The present study results indicated that most clinically suspected cases of subclinical mastitis in apparently healthy animals, as identified by veterinarians, tested positive for the CMT (Table 1; Figure 1B). Bacteria were subsequently isolated from the cow’s milk with subclinical mastitis on MacConkey agar, with Gram staining and IMViC tests performed, suggesting the presence of E. coli in the milk (Figures 1C–E). Likewise, bacteria isolated on Edwards Medium Base and MSA, followed by Gram staining, suggested the likely presence of Streptococcus spp. and Staphylococcus spp., respectively (Figures 1F–I). In light of these findings, we employed a bacterial metagenomic method to explore further additional bacteria present in the milk from cows with subclinical mastitis. Through this approach, we discovered nearly complete genomes of Pseudomonas veronii and Staphylococcus xylosus (Supplementary Data Sheet 1). Next, we proceeded with whole-genome sequencing of bacteria isolated from MacConkey agar, Edwards Medium Base, and MSA. The bacterial whole metagenomic sequencing yielded nearly complete genomes for E. coli, K. pneumoniae, Staphylococcus hominis, and S. xylosus (Supplementary Data Sheet 1).
The genome annotation of these sequences revealed numerous open reading frames (ORFs) in E. coli (Figure 2A), K. pneumoniae (Figure 2B), S. hominis (Figure 2C), S. xylosus (Figure 3A), P. veronii (Figure 3B), and S. xylosus (detected by the metagenomic approach) (Figure 3C). The nearly complete genomes were also used to predict antibiotic-resistant genes. Specifically, E. coli exhibited 320 antibiotic resistance genes, categorized as perfect (12), strict (41), and loose (267) matches in the CARD analysis (Table 2; Supplementary Data Sheet 2). Notably, E. coli displayed multiple MDR genes with perfect and strict matches, including those related to carbapenem, penam, cephalosporin, macrolide, cephamycin, tetracycline, aminoglycoside, aminocoumarin, fluoroquinolone, rifamycin, elfamycin, nitroimidazole, phosphonic acid, monobactam, glycylcycline, phenicol, lincosamide, diaminopyrimidine, nucleoside antibiotic, peptide antibiotic, disinfecting agents, and antiseptics (Table 2; Supplementary Data Sheet 2). Additionally, the detected E. coli showed a single nucleotide polymorphism (SNP) in AcrAB-TolC with MarR mutations that confer resistance to ciprofloxacin and tetracycline associated with Y137H and G103S (Table 2; Supplementary Data Sheet 2). Other mutations were identified, including those in E. coli EF-Tu linked to pulvomycin resistance (R234F), mutations in Haemophilus influenzae PBP3 affecting beta-lactam antibiotics (D350N, S357N), and E. coli GlpT mutations conferring resistance to fosfomycin (E448K) (Table 2; Supplementary Data Sheet 2). The plasmid sequence identified in E. coli showed a TEM beta-lactamase and tet(A) tetracycline antibiotic resistance genes (Table 2; Supplementary Data Sheet 2).
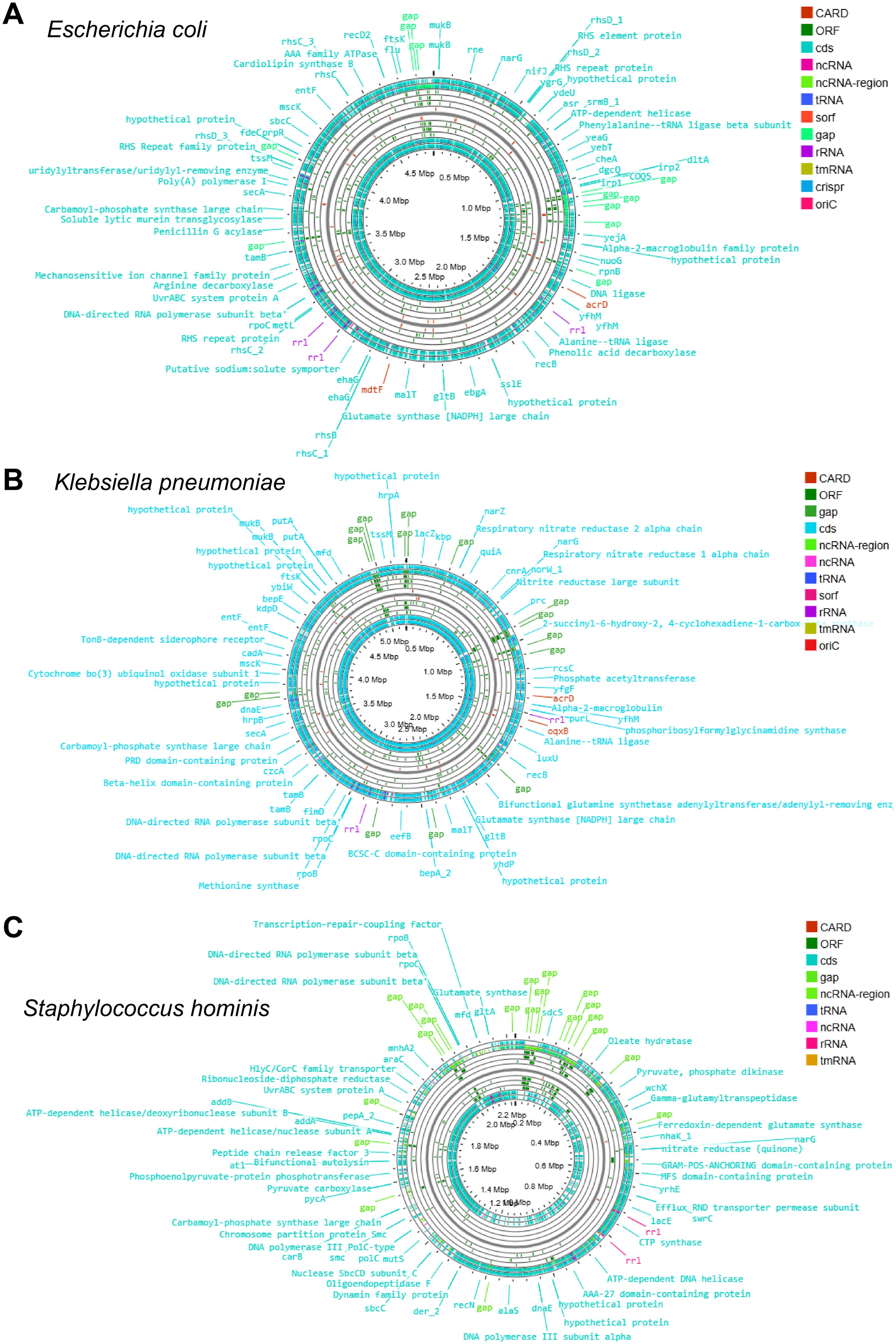
Figure 2. The circular genome map depicting the ORFs, CARDs, rRNAs, ncRNAs, and tRNAs of (A) Escherichia coli, (B) Klebsiella pneumoniae, and (C) Staphylococcus hominis.
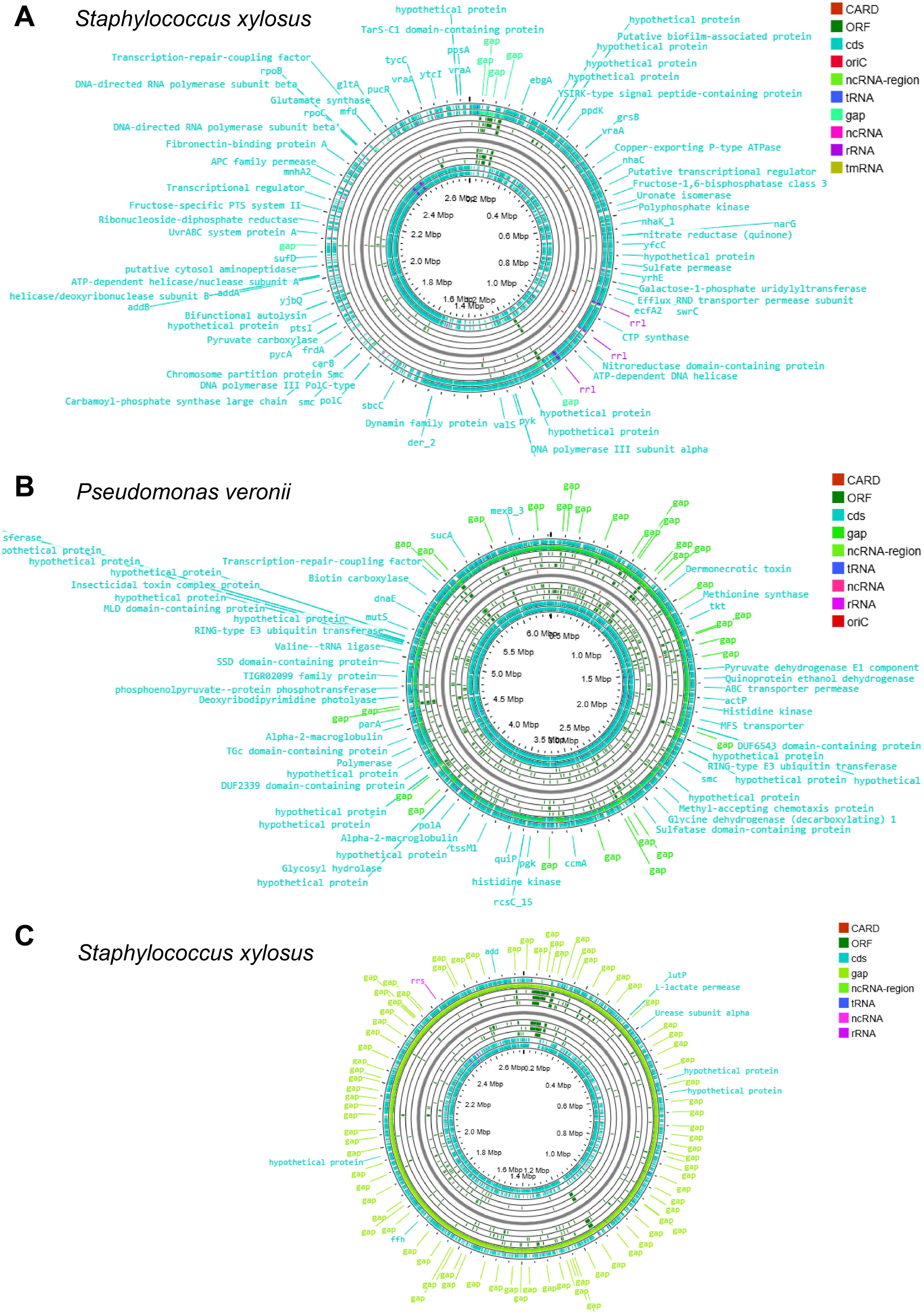
Figure 3. The circular genome map depicting the ORFs, CARDs, rRNAs, ncRNAs, and tRNAs of (A) Staphylococcus xylosus, (B) Pseudomonas veronii, and (C) Staphylococcus xylosus (detected using the metagenomic approach).
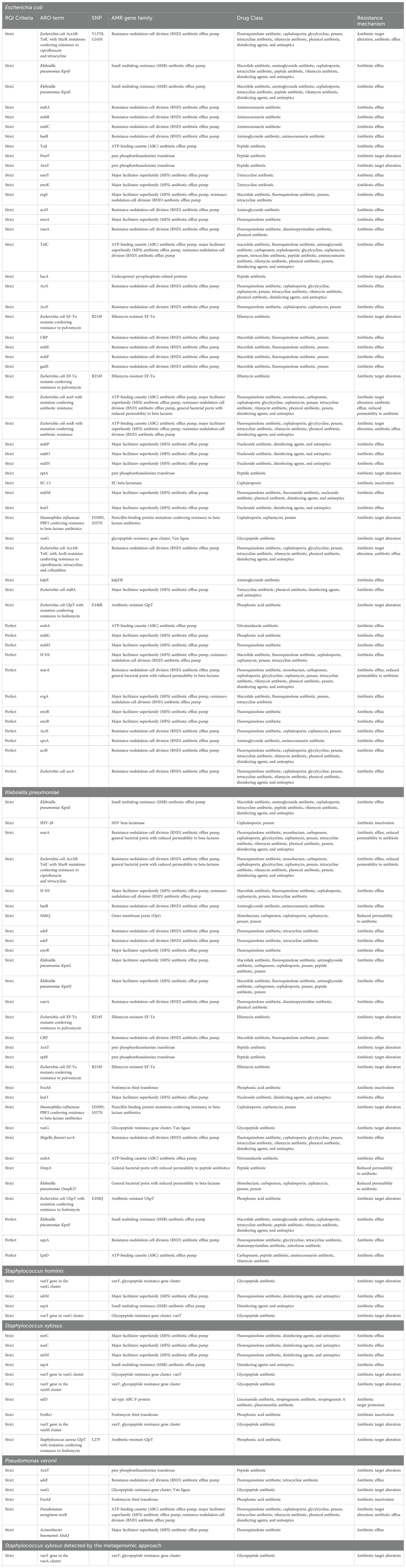
Table 2. A comprehensive depiction of the antibiotic resistance ontology (ARO), including SNPs, AMR gene families, drug classes, and their corresponding resistance mechanisms in the identified bacteria.
Likewise, the K. pneumoniae genome exhibited the following MDR genes with perfect and strict matches: carbapenem, penam, cephalosporin, macrolide, cephamycin, tetracycline, aminoglycoside, aminocoumarin, fluoroquinolone, rifamycin, elfamycin, nitroimidazole, nitrofuran, phosphonic acid, monobactam, glycylcycline, glycopeptide, phenicol, diaminopyrimidine, nucleoside antibiotic, peptide antibiotic, disinfecting agents, and antiseptics (Table 2; Supplementary Data Sheet 2). Similarly, K. pneumoniae also revealed an SNP in EF-Tu mutants that impart resistance to pulvomycin (R234F) and in H. influenzae PBP3 correlating with beta-lactam resistance (D350N, S357N). Additionally, a mutation in E. coli UhpT was noted, granting resistance to fosfomycin (E350Q) (Table 2; Supplementary Data Sheet 2).
The S. hominis genome indicated resistance genes for glycopeptide, fluoroquinolone, disinfecting agents, and antiseptics (Table 2; Supplementary Data Sheet 2). The S. xylosus genome presented resistance genes for glycopeptide, fluoroquinolone, phosphonic acid, lincosamide, streptogramin, pleuromutilin, disinfecting agents, and antiseptics (Table 2; Supplementary Data Sheet 2). It also harbored an SNP in the S. aureus GlpT conferring resistance to fosfomycin (L27F). The P. veronii genome exhibited resistance genes for cephalosporin, penam, tetracycline, rifamycin, fluoroquinolone, glycopeptide, phosphonic acid, glycylcycline, phenicol, disinfecting agents, and antiseptics (Table 2; Supplementary Data Sheet 2). The S. xylosus genome identified through metagenomic analysis contained the glycopeptide antibiotic resistance gene (Table 2; Supplementary Data Sheet 2).
Furthermore, the nearly complete genome sequences were utilized to predict the presence of virulence factor genes. The E. coli found in the subclinical mastitis-affected cow possessed nearly all virulent genes analogous to those of Enteroaggregative Escherichia coli (EAEC) (Figure 4; Supplementary Data Sheet 3). Klebsiella pneumoniae identified in the study exhibited virulent genes similar to K. pneumoniae 342/K. pneumoniae JM45/K. pneumoniae KCTC 2242 (Figure 4; Supplementary Data Sheet 3). Both S. hominis and S. xylosus presented virulent genes equivalent to those of Staphylococcus haemolyticus JCSC1435 and Staphylococcus epidermidis RP62A, respectively (Figure 4; Supplementary Data Sheet 3). Although P. veronii exhibited virulence genes found in other Pseudomonas species, the LPS O-antigen (P. aeruginosa) was not detected (Figure 4; Supplementary Data Sheet 3).
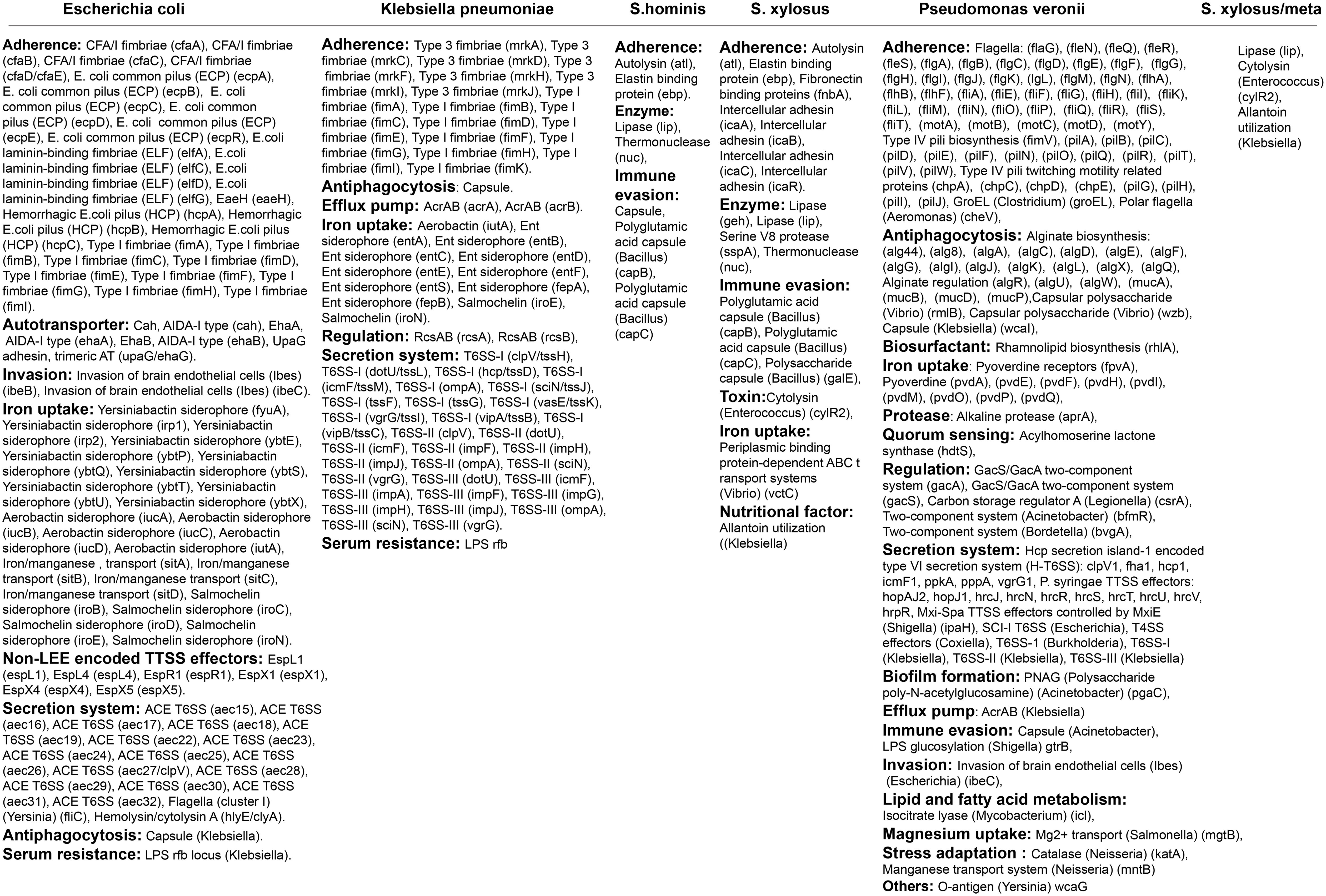
Figure 4. The table represents the virulence genes detected in Escherichia coli, Klebsiella pneumoniae, Staphylococcus hominis, Staphylococcus xylosus, and Pseudomonas veronii (details are provided in Supplementary Data Sheet 3).
Discussion
In the present study, we detected nearly complete genomes of various virulent and antibiotic-resistant bacterial pathogens, including E. coli, K. pneumoniae, P. veronii, S. hominis, and S. xylosus, from subclinical mastitis-affected cow’s milk in India. It is worth noting that among the bacteria identified in the present study, E. coli and K. pneumoniae are members of the ESKAPE pathogens (De Oliveira et al., 2020; Miller and Arias, 2024). Furthermore, E. coli, K. pneumoniae, and S. hominis are pathogenic in humans, but S. xylosus and P. veronii may not cause significant human infections (Kaur et al., 2010; Brand and Rufer, 2021; Chang et al., 2021; Szemraj et al., 2025).
In the case of treatment of the clinical condition caused by the EAEC bacteria, the widely used/recommended antibiotics are azithromycin, ciprofloxacin, and rifaximin (Hebbelstrup Jensen et al., 2018); however, the detected EAEC bacteria have the resistance gene for all these antibiotics. The EAEC bacteria are the emerging pathogens that cause acute and persistent diarrhea in children and adults in developing countries and cause acute diarrhea in travelers who travel to these regions (Nataro and Kaper, 1998; Durrer et al., 2000; Estrada-Garcia and Navarro-Garcia, 2012; Ellis et al., 2020). Recent studies indicate that only a few virulence factors are conserved in E. coli associated with bovine mastitis, and these conserved virulence factors are also found in commensal E. coli (Kempf et al., 2016; Alawneh et al., 2020). In Bangladesh, a neighboring country of India, the complete genome of E. coli found in clinical and subclinical mastitis bovine milk contains genes for multiple virulence factors (Anika et al., 2023; Rahman et al., 2025), and E. coli detected in the present study was also found to have various virulent factor genes. Furthermore, plasmids in E. coli, in particular, contain MDR genes (Alawneh et al., 2020). It is noteworthy that the E. coli plasmid identified in this present study also contains resistance genes for beta-lactam and tetracycline antibiotics. Beta-lactamase producing E. coli poses a global health threat to humans (Bajaj et al., 2016; Mahmud et al., 2020), and the beta-lactamase gene in the E. coli plasmid identified in this present study has the potential to spread vertically and horizontally in E. coli, posing a health threat. Furthermore, the genome of E. coli identified in this study contains 53 resistance genes that confer resistance to various antibiotics. This could potentially lead to health issues in humans if these bacteria spread through milk. Therefore, there is a need for large-scale surveillance to monitor such E. coli strains in India.
Klebsiella pneumoniae presents significant challenges in healthcare settings, especially for neonates, the elderly, and immunocompromised persons (Bengoechea and Sa Pessoa, 2019; Grubwieser et al., 2023; Bai and Guo, 2024). The identification of K. pneumoniae in bovine clinical mastitis across various regions of the world underscores its potential for zoonotic transmission (Munoz et al., 2007; Salauddin et al., 2019; Fu et al., 2022; Zheng et al., 2022; Bai and Guo, 2024). Thus, the World Health Organization (WHO) is giving priority to K. pneumoniae that contains carbapenemase genes in the Global Antimicrobial Resistance and Surveillance System on Emerging Antimicrobial Resistance Reporting (GLASS-EAR) (https://www.who.int/emergencies/disease-outbreak-news/item/2024-DON527), and K. pneumoniae identified in the present study also contains a carbapenem-resistant gene. The treatment of K. pneumoniae is challenging due to its thick capsule; however, it can be treated with cephalosporins, quinolones, and carbapenems (Prince et al., 1997). Klebsiella pneumoniae detected in the subclinical mastitis-affected cow’s milk in this study has capsules and carries antibiotic resistance genes for all these antibiotics. Additionally, the genome of K. pneumoniae identified in this study carries 30 resistance genes that confer resistance to various antibiotics, potentially posing a public health threat in India.
Staphylococcus hominis and S. xylosus detected in this study have comparatively lesser numbers of antibiotic resistance genes than E. coli and K. pneumoniae. Recently, complete genome sequencing of the S. xylosus strains K19 and K46, derived from clinical mastitis-affected cow’s milk in India, revealed the presence of the salE and fosBx1 resistance genes, and the S. xylosus strain SMG24 was found to have the vanY resistance gene (Ramesh et al., 2024). In the present study, it is noteworthy that S. xylosus detected in subclinical mastitis-affected cow’s milk contained resistance genes such as norC, sdrM, sepA, and vanT in the vanG cluster; the vanY gene in the vanM cluster; salD; and FosBx1. In addition, antibiotic-resistant S. hominis and S. xylosus have been identified in Brazil from subclinical mastitis milk and milkers’ hands, respectively (Pizauro et al., 2019). Furthermore, the identification of the vancomycin-resistant S. hominis in endophthalmitis in humans also highlights its importance (Won and Kim, 2013). In this line, multiple strains of the complete genome of S. hominis detected from clinical mastitis-affected cow’s milk in India have been found to contain several resistance genes such as sepA, mdeA, norC, sdrM, and vanY in the vanB cluster; msrA; PC1 beta-lactamase (blaZ); and tet(K) (Ramesh et al., 2024). It is important to note that S. xylosus, detected in the subclinical mastitis-affected cow’s milk in the present study, contained resistance genes such as the vanY gene in the vanG cluster, the vanT gene in the vanG cluster, and sdrM and sepA. Next, there are no reports of P. veronii infecting humans (Mullaeva et al., 2022; Zavala-Meneses et al., 2024). The O antigen of lipopolysaccharides (LPS) in Pseudomonas bacteria is crucial for their virulence and pathogenesis (Rocchetta et al., 1999; Lam et al., 2011; Huszczynski et al., 2019; Azimi et al., 2021). However, as this study found that P. veronii lacks the LPS O antigen, it is unlikely to be virulent in humans/animals.
In conclusion, the present study reports the presence of multiple virulent and antibiotic-resistant genes containing bacteria in the subclinical mastitis cases in India, which poses a public health threat. Early detection of subclinical mastitis and subclinical mastitis milk management training are very important for Indian cow farmers. If such training is not provided, these MDR bacteria are likely to spread to humans from hand milking, milk storage and transportation to boiling, and consumption, posing a public health threat. Large-scale surveillance needs to be conducted to determine how MDR bacteria cause subclinical mastitis and how they accumulate MDR genes in subclinical mastitis, and policies for control, prevention, and treatment measures need to be designed accordingly.
Data availability statement
The datasets presented in this study can be found in online repositories. The names of the repository/repositories and accession number(s) can be found in the article/Supplementary Material. BioProject ID PRJNA1188839 and PRJNA1189268, Submitted in NCBI.
Ethics statement
The collection and processing of mastitis milk samples were carried out at the Southern Regional Station of the Indian Council of Agricultural Research (ICAR)-National Dairy Research Institute (NDRI), Bengaluru, Karnataka, India. The study was conducted as per the guidelines and approval of the Institutional Animals Ethics Committee (IAEC), NDRI.
Author contributions
AM: Conceptualization, Funding acquisition, Investigation, Methodology, Project administration, Supervision, Writing – original draft, Writing – review & editing. PADe: Conceptualization, Data curation, Formal analysis, Investigation, Methodology, Resources, Software, Validation, Visualization, Writing – original draft, Writing – review & editing. AK: Data curation, Writing – review & editing. PS: Investigation, Writing – review & editing. KS: Investigation, Writing – review & editing. PD: Investigation, Writing – review & editing. PADi: Investigation, Methodology, Writing – review & editing.
Funding
The author(s) declare that financial support was received for the research and/or publication of this article. Funding of this study was provided by ICAR-National Agricultural Science Fund, SRS, ICAR-(NASF).
Acknowledgments
The authors acknowledge the Southern Regional Station, ICAR-National Dairy Research Institute (NDRI), Adugodi, Bengaluru-560 030, Karnataka, India, and the Institute of Advanced Virology, Bio 360 Life Sciences Park, Thonnakkal, Trivandrum, Kerala-695 317 for providing the research facility to carry out this study.
Conflict of interest
The authors declare that the research was conducted in the absence of any commercial or financial relationships that could be construed as a potential conflict of interest.
Generative AI statement
The author(s) declare that no Generative AI was used in the creation of this manuscript.
Publisher’s note
All claims expressed in this article are solely those of the authors and do not necessarily represent those of their affiliated organizations, or those of the publisher, the editors and the reviewers. Any product that may be evaluated in this article, or claim that may be made by its manufacturer, is not guaranteed or endorsed by the publisher.
Supplementary material
The Supplementary Material for this article can be found online at: https://www.frontiersin.org/articles/10.3389/fcimb.2025.1549523/full#supplementary-material
References
Alawneh, J. I., Vezina, B., Ramay, H. R., Al-Harbi, H., James, A. S., Soust, M., et al. (2020). Survey and sequence characterization of bovine mastitis-associated escherichia coli in dairy herds. Front. Vet. Sci. 7. doi: 10.3389/fvets.2020.582297
Alcock, B. P., Huynh, W., Chalil, R., Smith, K. W., Raphenya, A. R., Wlodarski, M. A., et al. (2023). CARD 2023: expanded curation, support for machine learning, and resistome prediction at the Comprehensive Antibiotic Resistance Database. Nucleic Acids Res. 51, D690–D699. doi: 10.1093/nar/gkac920
Anika, T. T., Noman, Z. A., Islam, M. S., Sultana, N., Ashraf, M. N., Pervin, M., et al. (2023). Draft genome sequence of multidrug-resistant Escherichia coli MAHK_SCM_BAU_30A strain isolated from a subclinical mastitis cow in Bangladesh. Microbiol. Resour. Announc. 12, e0071323. doi: 10.1128/MRA.00713-23
Ashraf, D., Ombarak, R. A., Samir, A., Abdel-Salam, A. B. (2023). Characterization of multidrug-resistant potential pathogens isolated from milk and some dairy products in Egypt. J. Adv. Vet. Anim. Res. 10, 275–283. doi: 10.5455/javar.2023.j679
Azimi, S., Thomas, J., Cleland, S. E., Curtis, J. E., Goldberg, J. B., Diggle, S. P. (2021). O-specific antigen-dependent surface hydrophobicity mediates aggregate assembly type in pseudomonas aeruginosa. mBio 12, e0086021. doi: 10.1128/mBio.00860-21
Badawy, B., Moustafa, S., Shata, R., Sayed-Ahmed, M. Z., Alqahtani, S. S., Ali, M. S., et al. (2023). Prevalence of multidrug-resistant pseudomonas aeruginosa isolated from dairy cattle, milk, environment, and workers’ Hands. Microorganisms 11, 2775. doi: 10.3390/microorganisms11112775
Bai, R., Guo, J. (2024). Interactions and implications of klebsiella pneumoniae with human immune responses and metabolic pathways: A comprehensive review. Infect. Drug Resist. 17, 449–462. doi: 10.2147/IDR.S451013
Bajaj, P., Singh, N. S., Virdi, J. S. (2016). Escherichia coli beta-Lactamases: What Really Matters. Front. Microbiol. 7. doi: 10.3389/fmicb.2016.00417
Bankevich, A., Nurk, S., Antipov, D., Gurevich, A. A., Dvorkin, M., Kulikov, A. S., et al. (2012). SPAdes: a new genome assembly algorithm and its applications to single-cell sequencing. J. Comput. biol.: J. Comput. Mol. Cell Biol. 19, 455–477. doi: 10.1089/cmb.2012.0021
Bengoechea, J. A., Sa Pessoa, J. (2019). Klebsiella pneumoniae infection biology: living to counteract host defences. FEMS Microbiol. Rev. 43, 123–144. doi: 10.1093/femsre/fuy043
Bolger, A. M., Lohse, M., Usadel, B. (2014). Trimmomatic: a flexible trimmer for Illumina sequence data. Bioinformatics 30, 2114–2120. doi: 10.1093/bioinformatics/btu170
Boolchandani, M., D’Souza, A. W., Dantas, G. (2019). Sequencing-based methods and resources to study antimicrobial resistance. Nat. Rev. Genet. 20, 356–370. doi: 10.1038/s41576-019-0108-4
Brand, Y. E., Rufer, B. (2021). Late prosthetic knee joint infection with Staphylococcus xylosus. IDCases 24, e01160. doi: 10.1016/j.idcr.2021.e01160
Buchfink, B., Xie, C., Huson, D. H. (2015). Fast and sensitive protein alignment using DIAMOND. Nat. Methods 12, 59–60. doi: 10.1038/nmeth.3176
Chang, D., Sharma, L., Dela Cruz, C. S., Zhang, D. (2021). Clinical epidemiology, risk factors, and control strategies of klebsiella pneumoniae infection. Front. Microbiol. 12. doi: 10.3389/fmicb.2021.750662
Deforche, K. (2017). An alignment method for nucleic acid sequences against annotated genomes. bioRxiv. doi: 10.1101/200394
De Oliveira, D. M. P., Forde, B. M., Kidd, T. J., Harris, P. N.A., Schembri, M. A., Beatson, S. A., et al. (2020). Antimicrobial resistance in ESKAPE pathogens. Clin. Microbiol. Rev. 33, e00181-19. doi: 10.1128/CMR.00181-19
Dingwell, R. T., Leslie, K. E., Schukken, Y. H., Sargeant, J. M., Timms, L. L. (2003). Evaluation of the California mastitis test to detect an intramammary infection with a major pathogen in early lactation dairy cows. Can. Vet. J. 44, 413–415.
Durrer, P., Zbinden, R., Fleisch, F., Altwegg, M., Ledergerber, B., Karch, H., et al. (2000). Intestinal infection due to enteroaggregative Escherichia coli among human immunodeficiency virus-infected persons. J. Infect. Dis. 182, 1540–1544. doi: 10.1086/315885
Ellis, S. J., Crossman, L. C., McGrath, C. J., Chattaway, M. A., Hölken, J. M., Brett, B., et al. (2020). Identification and characterisation of enteroaggregative Escherichia coli subtypes associated with human disease. Sci. Rep. 10, 7475. doi: 10.1038/s41598-020-64424-3
Estrada-Garcia, T., Navarro-Garcia, F. (2012). Enteroaggregative Escherichia coli pathotype: a genetically heterogeneous emerging foodborne enteropathogen. FEMS Immunol. Med. Microbiol. 66, 281–298. doi: 10.1111/j.1574-695X.2012.01008.x
Fu, S., Wen, C., Wang, Z., Qiu, Y., Zhang, Y., Zuo, J., et al. (2022). Molecular epidemiology and antimicrobial resistance of outbreaks of klebsiella pneumoniae clinical mastitis in Chinese dairy farms. Microbiol. Spectr. 10, e0299722. doi: 10.1128/spectrum.02997-22
Gebremedhin, E. Z., Ararso, A. B., Borana, B. M., Kelbesa, K. A., Tadese, N. D., Marami, L. M., et al. (2022). Isolation and identification of staphylococcus aureus from milk and milk products, associated factors for contamination, and their antibiogram in Holeta, central Ethiopia. Vet. Med. Int. 2022, 6544705. doi: 10.1155/2022/6544705
Geletu, U. S., Usmael, M. A., Ibrahim, A. M. (2022). Isolation, Identification, and Susceptibility Profile of E. coli, Salmonella and S. aureus in Dairy Farm and Their Public Health Implication in Central Ethiopia. Vet. Med. Int. 2022, 1887977. doi: 10.1155/2022/1887977
Gotoh, O. (1982). An improved algorithm for matching biological sequences. J. Mol. Biol. 162, 705–708. doi: 10.1016/0022-2836(82)90398-9
Grant, J. R., Enns, E., Marinier, E., Mandal, A., Herman, E. K., Chen, C. Y., et al. (2023). Proksee: in-depth characterization and visualization of bacterial genomes. Nucleic Acids Res. 51, W484–W492. doi: 10.1093/nar/gkad326
Grubwieser, P., Hilbe, R., Gehrer, C. M., Grander, M., Brigo, N., Hoffmann, A., et al. (2023). Klebsiella pneumoniae manipulates human macrophages to acquire iron. Front. Microbiol. 14. doi: 10.3389/fmicb.2023.1223113
Haq, I. U., Kamal, M., Swelum, A. A., Khan, S., Ríos-Escalante, P. R.L., Usman, T. (2024). Alarming multidrug resistance in Staphylococcus aureus isolated from raw milk of cows with subclinical mastitis: Antibiotic resistance patterns and occurrence of selected resistance genes. PloS One 19, e0301200. doi: 10.1371/journal.pone.0301200
Hassani, S., Moosavy, M. H., Gharajalar, S. N., Khatibi, S. A., Hajibemani, A., Barabadi, Z. (2022). High prevalence of antibiotic resistance in pathogenic foodborne bacteria isolated from bovine milk. Sci. Rep. 12, 3878. doi: 10.1038/s41598-022-07845-6
Hebbelstrup Jensen, B., Adler Sørensen, C., Hebbelstrup Rye Rasmussen, S., Rejkjær Holm, D., Friis-Møller, A., Engberg, J., et al. (2018). Characterization of diarrheagenic enteroaggregative escherichia coli in Danish adults-antibiotic treatment does not reduce duration of diarrhea. Front. Cell Infect. Microbiol. 8. doi: 10.3389/fcimb.2018.00306
https://github.com/broadinstitute/gatk/releases. Available online at: https://github.com/broadinstitute/gatk/releases (Accessed November 8, 2024).
https://microbenotes.com/imvic-tests/. Available online at: https://microbenotes.com/imvic-tests/ (Accessed November 8, 2024).
https://samtools.github.io/bcftools/howtos/variant-calling.html. Available online at: https://samtools.github.io/bcftools/howtos/variant-calling.html (Accessed November 8, 2024).
https://www.who.int/emergencies/disease-outbreak-news/item/2024-DON527. Available online at: https://www.who.int/emergencies/disease-outbreak-news/item/2024-DON527 (Accessed November 8, 2024).
Huszczynski, S. M., Lam, J. S., Khursigara, C. M. (2019). The role of pseudomonas aeruginosa lipopolysaccharide in bacterial pathogenesis and physiology. Pathogens 9, 6. doi: 10.3390/pathogens9010006
Kaur, P., Chakraborti, A., Asea, A. (2010). Enteroaggregative escherichia coli: an emerging enteric food borne pathogen. Interdiscip. Perspect. Infect. Dis. 2010, 254159. doi: 10.1155/2010/254159
Kempf, F., Slugocki, C., Blum, S. E., Leitner, G., Germon, P. (2016). Genomic comparative study of bovine mastitis escherichia coli. PloS One 11, e0147954. doi: 10.1371/journal.pone.0147954
Khasapane, N. G., Nkhebenyane, J., Mnisi, Z., Kwenda, S., Thekisoe, O. (2024). Comprehensive whole genome analysis of Staphylococcus aureus isolates from dairy cows with subclinical mastitis. Front. Microbiol. 15. doi: 10.3389/fmicb.2024.1376620
Lam, J. S., Taylor, V. L., Islam, S. T., Hao, Y., Kocincova, D. (2011). Genetic and functional diversity of pseudomonas aeruginosa lipopolysaccharide. Front. Microbiol. 2. doi: 10.3389/fmicb.2011.00118
Liu, B., Zheng, D., Zhou, S., Chen, L., Yang, J. (2022). VFDB 2022: a general classification scheme for bacterial virulence factors. Nucleic Acids Res. 50, D912–D917. doi: 10.1093/nar/gkab1107
Mahmud, Z. H., Kabir, M. H., Ali, S., Moniruzzaman, M., Imran, K. M., Nafiz, T. N., et al. (2020). Extended-spectrum beta-lactamase-producing escherichia coli in drinking water samples from a forcibly displaced, densely populated community setting in Bangladesh. Front. Public Health 8. doi: 10.3389/fpubh.2020.00228
Miller, W. R., Arias, C. A. (2024). ESKAPE pathogens: antimicrobial resistance, epidemiology, clinical impact and therapeutics. Nat. Rev. Microbiol. 22, 598–616. doi: 10.1038/s41579-024-01054-w
Mullaeva, S. A., Delegan, Y. A., Streletskii, R. A., Sazonova, O. I., Petrikov, K. V., Ivanova, A. A., et al. (2022). Pseudomonas veronii strain 7-41 degrading medium-chain n-alkanes and polycyclic aromatic hydrocarbons. Sci. Rep. 12, 20527. doi: 10.1038/s41598-022-25191-5
Munn, R. R., MacPherson, Y., Karkaba, A., McDougall, S. (2025). First identification of Bordetella bronchiseptica subclinical mastitis in a lactating dairy cow in New Zealand. N Z Vet. J. 1-6, 219–224. doi: 10.1080/00480169.2025.2456244
Munoz, M. A., Welcome, F. L., Schukken, Y. H., Zadoks, R. N. (2007). Molecular epidemiology of two Klebsiella pneumoniae mastitis outbreaks on a dairy farm in New York State. J. Clin. Microbiol. 45, 3964–3971. doi: 10.1128/JCM.00795-07
Nataro, J. P., Kaper, J. B. (1998). Diarrheagenic escherichia coli. Clin. Microbiol. Rev. 11, 142–201. doi: 10.1128/CMR.11.1.142
Ndahetuye, J. B., Leijon, M., Bage, R., Artursson, K., Persson, Y. (2021). Genetic characterization of staphylococcus aureus from subclinical mastitis cases in dairy cows in Rwanda. Front. Vet. Sci. 8. doi: 10.3389/fvets.2021.751229
Patil, A., Banerji, R., Kanojiya, P., Saroj, S. D. (2021). Foodborne ESKAPE biofilms and antimicrobial resistance: lessons learned from clinical isolates. Pathog. Glob Health 115, 339–356. doi: 10.1080/20477724.2021.1916158
Pillay, S., Calderon-Franco, D., Urhan, A., Abeel, T. (2022). Metagenomic-based surveillance systems for antibiotic resistance in non-clinical settings. Front. Microbiol. 13. doi: 10.3389/fmicb.2022.1066995
Pizauro, L. J. L., de Almeida, C. C., Gohari, I. M., MacInnes, J. I., Zafalon, L. F., Kropinski, A. M., et al. (2019). Complete genome sequences of 11 staphylococcus sp. Strains isolated from buffalo milk and milkers’ Hands. Microbiol. Resour. Announc. 8, e01264-19. doi: 10.1128/MRA.01264-19
Prince, S. E., Dominger, K. A., Cunha, B. A., Klein, N. C. (1997). Klebsiella pneumoniae pneumonia. Heart Lung 26, 413–417. doi: 10.1016/s0147-9563(97)90028-5
Rahman, M. M., Siddique, N., Arafat, K. Y., Homa, S. F., Das, Z. C., Islam, T., et al. (2025). Draft genome sequencing of multidrug-resistant Escherichia coli strains isolated from mastitic cow milk. Microbiol. Resour. Announc. 14, e0120524. doi: 10.1128/mra.01205-24
Ramesh, V., Sivakumar, R., Annamanedi, M., Chandrapriya, S., Isloor, S., Rajendhran, J., et al. (2024). Genome sequencing and comparative genomic analysis of bovine mastitis-associated non-aureus staphylococci and mammaliicocci (NASM) strains from India. Sci. Rep. 14, 29019. doi: 10.1038/s41598-024-80533-9
Rocchetta, H. L., Burrows, L. L., Lam, J. S. (1999). Genetics of O-antigen biosynthesis in Pseudomonas aeruginosa. Microbiol. Mol. Biol. Rev. 63, 523–553. doi: 10.1128/MMBR.63.3.523-553.1999
Rust, J. D., Christian, M. J., Vance, C. J., Bolajoko, M. B., Wong, J. T., Suarez-Martinez, J., et al. (2021). A study of the effectiveness of a detergent-based California mastitis test (CMT), using Ethiopian and Nigerian domestic detergents, for the detection of high somatic cell counts in milk and their reliability compared to the commercial UK CMT. Gates Open Res. 5, 146. doi: 10.12688/gatesopenres.13369.2
Salauddin, M., Akter, M. R., Hossain, M. K., Rahman, M. M. (2019). Isolation of multi-drug resistant Klebsiella sp. from bovine mastitis samples in Rangpur, Bangladesh. J. Adv. Vet. Anim. Res. 6, 362–365. doi: 10.5455/javar.2019.f355
Sawant, A. A., Pillai, S. R., Jayarao, B. M. (2002). Evaluation of five selective media for isolation of catalase-negative gram-positive cocci from bulk tank milk. J. Dairy Sci. 85, 1127–1132. doi: 10.3168/jds.S0022-0302(02)74174-X
Schwengers, O., Jelonek, L., Dieckmann, M. A., Beyvers, S., Blom, J., Goesmann, A. (2021). Bakta: rapid and standardized annotation of bacterial genomes via alignment-free sequence identification. Microb. Genom. 7, 000685. doi: 10.1099/mgen.0.000685
Seemann, T. (2014). Prokka: rapid prokaryotic genome annotation. Bioinformatics 30, 2068–2069. doi: 10.1093/bioinformatics/btu153
Sivakumar, R., Pranav, P. S., Annamanedi, M., Chandrapriya, S., Isloor, S., Rajendhran, J., et al. (2023). Genome sequencing and comparative genomic analysis of bovine mastitis-associated Staphylococcus aureus strains from India. BMC Genomics 24, 44. doi: 10.1186/s12864-022-09090-7
Smith, T. F., Waterman, M. S. (1981). Identification of common molecular subsequences. J. Mol. Biol. 147, 195–197. doi: 10.1016/0022-2836(81)90087-5
Szemraj, M., Glajzner, P., Olszowiec, K., Sienkiewicz, M. (2025). The prevalence of multidrug resistance in Staphylococcus hominis isolated from clinical materials. Sci. Rep. 15, 414. doi: 10.1038/s41598-024-84500-2
Tartor, Y. H., Abd El-Aziz, N. K., Gharieb, R. M.A., El Damaty, H. M., Enany, S., Soliman, E. A., et al. (2021). Whole-Genome Sequencing of Gram-Negative Bacteria Isolated From Bovine Mastitis and Raw Milk: The First Emergence of Colistin mcr-10 and Fosfomycin fosA5 Resistance Genes in Klebsiella pneumoniae in Middle East. Front. Microbiol. 12. doi: 10.3389/fmicb.2021.770813
Tempini, P. N., Aly, S. S., Karle, B. M., Pereira, R. V. (2018). Multidrug residues and antimicrobial resistance patterns in waste milk from dairy farms in Central California. J. Dairy Sci. 101, 8110–8122. doi: 10.3168/jds.2018-14398
Won, J. Y., Kim, M. (2013). Vancomycin-resistant Staphylococcus hominis endophthalmitis following cataract surgery. Clin. Ophthalmol. 7, 1193–1195. doi: 10.2147/OPTH.S46792
Yang, Y., Peng, Y., Jiang, J., Gong, Z., Zhu, H., Wang, K., et al. (2021). Isolation and characterization of multidrug-resistant Klebsiella pneumoniae from raw cow milk in Jiangsu and Shandong provinces, China. Transbound Emerg. Dis. 68, 1033–1039. doi: 10.1111/tbed.13787
Zavala-Meneses, S. G., Firrincieli, A., Chalova, P., Pajer, P., Checcucci, A., Skultety, L., et al. (2024). Proteogenomic characterization of pseudomonas veronii SM-20 growing on phenanthrene as only carbon and energy source. Microorganisms 12, 753. doi: 10.3390/microorganisms12040753
Keywords: ESKAPE pathogens, pathogenic bacteria, multidrug-resistant bacteria, mastitis-affected cow’s milk, E. coli
Citation: Manimaran A, Desingu PA, Kumaresan A, Singh P, Subramanya K, Dodamani P and Dineshbhai PA (2025) The metagenomic and whole-genome metagenomic detection of multidrug-resistant bacteria from subclinical mastitis-affected cow’s milk in India. Front. Cell. Infect. Microbiol. 15:1549523. doi: 10.3389/fcimb.2025.1549523
Received: 31 December 2024; Accepted: 21 March 2025;
Published: 22 April 2025.
Edited by:
Bo Peng, Sun Yat-sen University, ChinaReviewed by:
Daniel Jesuwenu Ajose, North-West University, South AfricaTulsi Ram Gompo, Central Veterinary Laboratory, Nepal
Copyright © 2025 Manimaran, Desingu, Kumaresan, Singh, Subramanya, Dodamani and Dineshbhai. This is an open-access article distributed under the terms of the Creative Commons Attribution License (CC BY). The use, distribution or reproduction in other forums is permitted, provided the original author(s) and the copyright owner(s) are credited and that the original publication in this journal is cited, in accordance with accepted academic practice. No use, distribution or reproduction is permitted which does not comply with these terms.
*Correspondence: Perumal Arumugam Desingu, cGFkZXNpbmd1QGdtYWlsLmNvbQ==; cGFkZXNpbmd1QGlhdi5yZXMuaW4=; Ayyasamy Manimaran, bWFyYW5waGFybWFAZ21haWwuY29t; YS5tYW5pbWFyYW5AaWNhci5nb3YuaW4=
†ORCID: Ayyasamy Manimaran, orcid.org/0000-0001-9063-2711
Perumal Arumugam Desingu, orcid.org/0000-0003-4395-4472